- 1School of Zoology, George S. Wise Faculty of Life Sciences, Tel-Aviv University, Tel Aviv, Israel
- 2The Steinhardt Museum of Natural History and Israel National Center for Biodiversity Studies, Tel-Aviv University, Tel Aviv, Israel
Introduction: Both colonial and solitary ascidians (Chordata, Ascidiacea) have been gathering attention due to their impact on coastal environments. The solitary ascidian Styela plicata was first observed along the coast of Israel in 2014. It is considered a cosmopolitan species of unknown origin, and is well-known for its ability to create large aggregations on artificial substrates in the western Mediterranean Sea, North-Pacific Ocean and the Atlantic. The extreme environmental conditions that characterize the Mediterranean coast of Israel, with a salinity of 40 psu and sea-surface temperature reaching 33°C, may however present a barrier to its future spread.
Methods: We examined the seasonal reproduction patterns and population structure of three populations over two years, in addition to spatial surveys and monitoring oocyte release under laboratory conditions.
Results: Histological sections revealed significant differences in reproduction patterns between seasons and sites, with a higher proportion of the larger oocytes size group observed during the winter for all sites. Along the Israeli coast, with the exception of one site, S. plicata is currently restricted to marinas and ports.
Discussion: With an impressive ability to survive the extreme environmental conditions and sustain reproductive populations. Acquiring data on its spread and establishment is essential for modeling S. plicata’s distribution under the current and predicted global climate changes, and for developing tools for its mitigation and control.
1 Introduction
Biological invasions are considered a major threat to biodiversity in marine ecosystems and can cause severe economic losses (Bax et al., 2003; Molnar et al., 2008). The introduction and spread of marine alien species have become common due to the increased shipping associated with global trade and aquaculture (Carlton, 1989; Naylor et al., 2001).
The Mediterranean Sea is considered the world’s most invaded sea by non-indigenous species (NIS) (Coll et al., 2010). The eastern basin is associated with high evaporation and limited input of freshwater, with the water temperature and salinity increasing from west to east (Malanotte-Rizzoli et al., 2014). The eastern basin is characterized by an annual salinity average of surface waters ranging from 37.5 to 39 psu, and highly fluctuating seasonal temperatures ranging from 14°C in the winter to 30°C in the summer (Galil and Zenetos, 2002). These gradients make the sea’s eastern end more oligotrophic than the western one (Coll et al., 2010). Israel’s coastline is located at the easternmost point of the Levantine Sea and is therefore considered to reflect the Mediterranean Sea’s most extreme environmental conditions (Galil and Zenetos, 2002).
One taxon that is gathering increasing attention in the field of marine bioinvasions is that of the ascidians (Shenkar and Swalla, 2011; Zhan et al., 2015). Ascidians, also known as ‘sea squirts’ (Phylum: Chordata, Subphylum: Tunicata, Class: Ascidiacea) are sessile suspension-feeders that are abundant on artificial substrates and are among the most frequently reported introduced sessile taxa (Aldred and Clare, 2014; Bishop et al., 2015; Pestana et al., 2017). The majority of NIS can tolerate a wide range of fluctuating environmental conditions, such as temperature, salinity, and even pollution (Lambert, 2007). Moreover, they can grow quickly, reaching sexual maturity within a couple of weeks, with a high reproductive output (Lambert, 2001; Carver et al., 2003). As coastal environments become more urbanized, such characteristics suggest that these increasingly abundant alien species in marinas could infiltrate into natural habitats and even thrive there, as some species have already done (Castilla et al., 2004; Osman and Whitlatch, 2007; Bulleri and Chapman, 2010; Gewing et al., 2014; Soares et al., 2020)
Styela plicata (Lesueur, 1823) (Ascidiacea; Stolidobranchia; Styelidae) is a solitary ascidian, considered cosmopolitan and of unknown origin. It is mostly found in marinas and ports in warm and temperate waters and can reach a body length of up to 8 cm (Lambert and Lambert, 1998; Pineda et al., 2011). The species is commonly observed at high densities on artificial substrates such as ropes, buoys, and ships’ hulls (Gewing and Shenkar, 2017; Novak et al., 2017) S. plicata is known to tolerate a wide range of temperatures and salinities, and can thrive in polluted waters (10°- 30°, 18 - 40 psu) (Sims, 1984; Naranjo et al., 1996; Thiyagarajan and Qian, 2003; Wyatt et al., 2005; Pineda et al., 2013; Lord et al., 2015). The majority of S. plicata populations are found on artificial substrates, but in some areas, such as Sakushima Island in Japan and in the northern Gulf of Mexico, this species is also found on natural substrates such as rocks and seagrass (Koplovitz and McClintock, 2011; Pineda et al., 2011). S. plicata was first recorded in the Mediterranean Sea in the 19th century (Pérès, 1958b; Maltagliati et al., 2016). S. plicata was reported in Israel by Pérès (1958b), although it was not subsequently detected until 2014. In 2014 local fishermen reported aggregations of S. plicata on artificial substrates and ropes in marinas along Israel’s coast and, following those reports, an extensive survey was conducted in eight marinas to assess the species’ distribution in Israeli marinas. At that time, only two of the eight marinas did not appear to be infested by S. plicata (Novak et al., 2017).
S. plicata presents different reproductive patterns in different areas. In the Western Mediterranean its reproduction seasons were shown to be throughout the year, with greatest intensity in the spring (Pineda et al., 2013); An extended reproduction period was observed in Italy, Mar Piccolo of Taranto, from spring to autumn (Sciscioli et al., 1978; Tursi and Matarrese, 1981). Given the extreme environmental conditions along the Mediterranean coast of Israel, which include a salinity of 40 psu and temperatures reaching 31°C in the summer (Rilov et al., 2022), in view of the current and predicted global warming it is important to understand the adaptive ability of such a potentially invasive species in reproducing and sustaining large populations. Comprehending such abilities will contribute to a better understanding of the effects of climate change on non-indigenous marine species’ distribution and their adaptive potential (Zhan et al., 2015; Zhang et al., 2020).
Here we conducted seasonal surveys of three populations of S. plicata along the Mediterranean coast of Israel as well as sampling individuals for histology and monitoring oocytes release under laboratory conditions. Additionally, spatial surveys were conducted at 30 sites along the Israeli coast in order to determine the current status of S. plicata’s establishment in the region.
2 Materials and methods
2.1 Spatial distribution survey
From April 2021 to September 2022, spatial distribution surveys of Styela plicata were conducted at 30 sites along the Mediterranean coast of Israel by snorkeling, SCUBA diving (4-15 m depth), and visual screening (marinas). The sites were chosen for their proximity to locations that were already populated by S. plicata and for the characteristics of their substrate, artificial or natural. The sites comprised marinas, fishing harbors, marine protected areas, and public and undeclared beaches (Figure 1; Figures S1, S2).
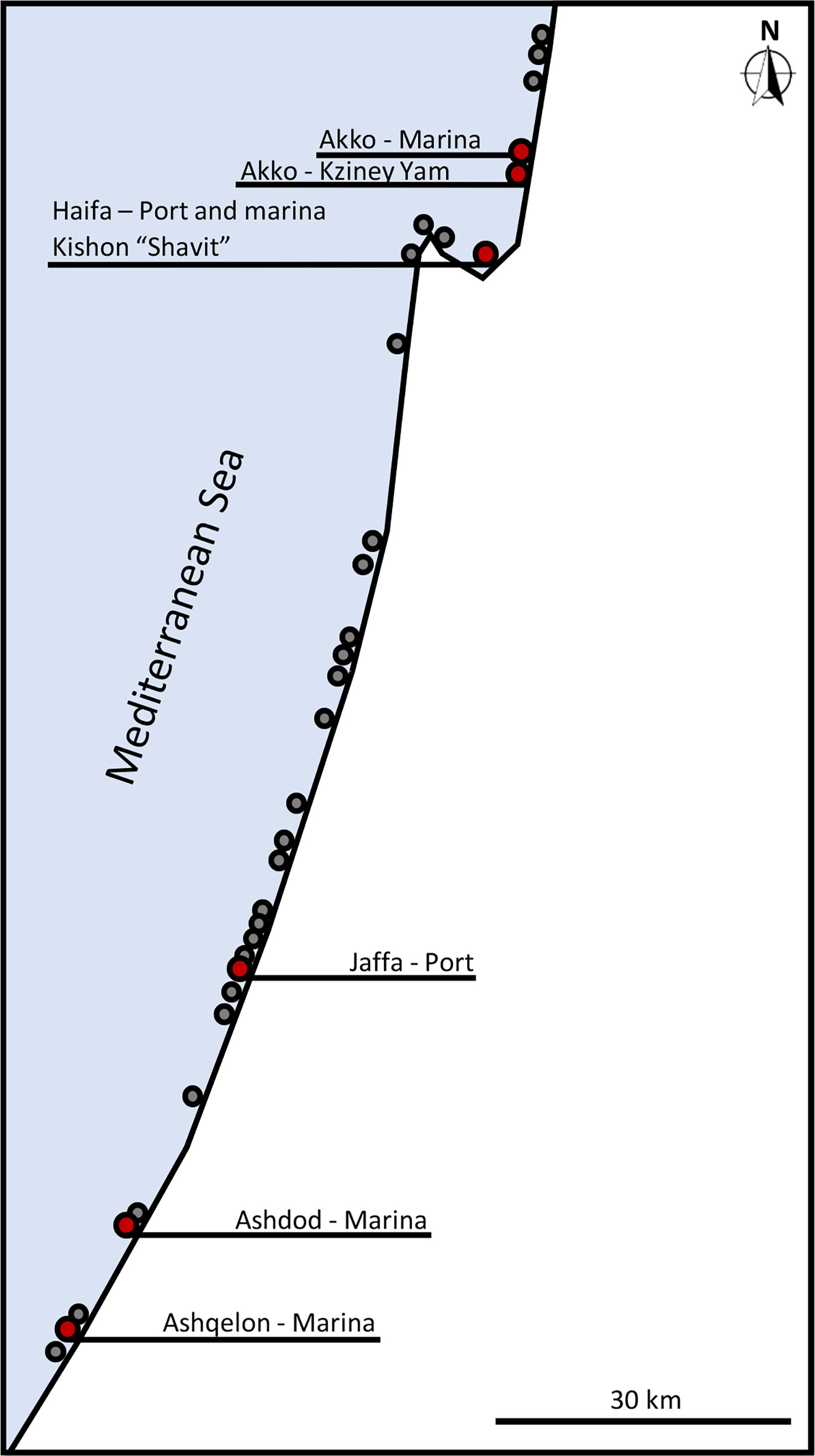
Figure 1 Spatial distribution of S. plicata along the Mediterranean coast of Israel. Red points – presence of S. plicata; gray points – absence of S. plicata.
2.2 Seasonal monitoring of Styela plicata reproduction patterns
2.2.1 Study sites and sampling
To assess the reproduction pattern of the solitary ascidian S. plicata along Israel’s coast, 6-12 individuals were sampled each season for two consecutive years, from three different sites, by pulling up suspended ropes or removing animals from artificial substrates at 0-3.5 meters depth (Tables S1, S2).
a. Akko- “The horse’s beach” (32°55’15”N, 35°04’23”E), is the only population of S. plicata that has succeeded to establish outside marinas and ports in Israel (current study). Samples were collected by snorkeling, from concrete elements, metal fragments, and metal fences (0-2 meter depth). The site is located ~420 meter from Akko marina (32°55’10”N, 35°04’10”E).
b. Haifa- Kishon “Shavit” marina and Israel’s main fishing port (32°48’23”N, 35°01’49”E), is characterized by highly polluted waters and relativly wide fluctuations of salinity and temperature throughout the year, due to the Kishon Stream that flows into the marina (Herut et al., 2023). Samples were collected from suspended ropes or other artificial substrates adjacent to the docks.
c. Jaffa- fishing port and marina (32 03’09” N, 34 44’58” E) is used as an anchorage for mooring yachts and fishing boats. Samples were collected from suspended ropes or other artificial substrates adjacent to the docks.
Upon sampling, individuals were placed in closed containers with seawater and narcotized with menthol crystals to relax the animals’ muscles and prevent stress (Shenkar and Loya, 2009). In the laboratory, samples were washed with artificial seawater, fixed in formaldehyde 4% (formalin) mixed with artificial seawater, and stored at room temperature until further processing. Data loggers (HOBO ® data logger Onset, MA) were placed at each site to measure the temperature every six hours throughout the study period.
2.2.2 Population dynamics
From October 2020 to July 2022, during each sampling period the body length of up to 100 individuals was measured in the field in order to follow population size distribution of the individuals in the Haifa-Kishon “Shavit” and Jaffa ports. At each site, ropes were randomly pulled from the water and up to 20 individuals per rope were measured using calipers.
2.2.3 Gonad index measurements
The gonad index (GI, in percentage) is a popular index used to estimate the size ratio of the gonads relative to the animal’s body size as a proxy for the phase of the reproduction cycle (Pearse and Eernisse, 1982; Ouréns et al., 2012). GI is calculated as the ratio between the gonads’ wet weight and the whole body wet weight without the tunic (Mcclary et al., 2008; Raijman Nagar and Shenkar, 2016). The animal’s tunic was dissected from one of the siphons along the lateral side and separated from the body in order to weigh only the body of each sample. Next, the gonads were carefully separated from the body wall and their weight was recorded. From each specimen two sections from the right body-side gonads were preserved in 70% ethanol solution for histology analysis, following fixation of at least five days.
2.2.4 Histological analysis
To determine the developmental stage of the gonads, histology slides were prepared in order to observe the reproductive system of the sampled animals. After soaking two pieces from the right-side gonads of each individual in 70% ethanol they were dehydrated in 70% ethanol 3 times for an hour each, then placed in butanol for several hours, and embedded in paraffin. For slide preparation, one gonad piece was chosen from each sample and sectioned in the paraffin blocks with a microtome (Shandon MIR by S.C.I.L) to 7 µm thickness and stained with hematoxylin-eosin. Three to five sections were prepared on each microscope slides, with a gap between sections of at least 40 µm to prevent repeat counts of eggs. The histology slides were observed and photographed with a Nikon eclipse Ni light microscope with Nikon DS-Ri2 (Figures 2, 3). Using NIS elements D software synchronized with the light microscope and camera, the diameter of 100 oocytes were measured in each individual through the nucleolus (Bingham, 1997). In addition, the oocytes were classified into four size-classes according to developmental stage (Becerro and Turon, 1992; Wong et al., 2011; Shmuel and Shenkar, 2017): pre-vitellogenic oocytes (<50 µm); vitellogenic oocytes (50-100 µm and 100-150 µm); and mature oocytes (>150). Developmental stages of the testes were classified according to three (subjective) stages: 1. Immature- only spermatogonia; 2. Mature- spermatozoa; and 3. Spawning- empty spaces within the lumen (Figure 3) (Pineda et al., 2013).
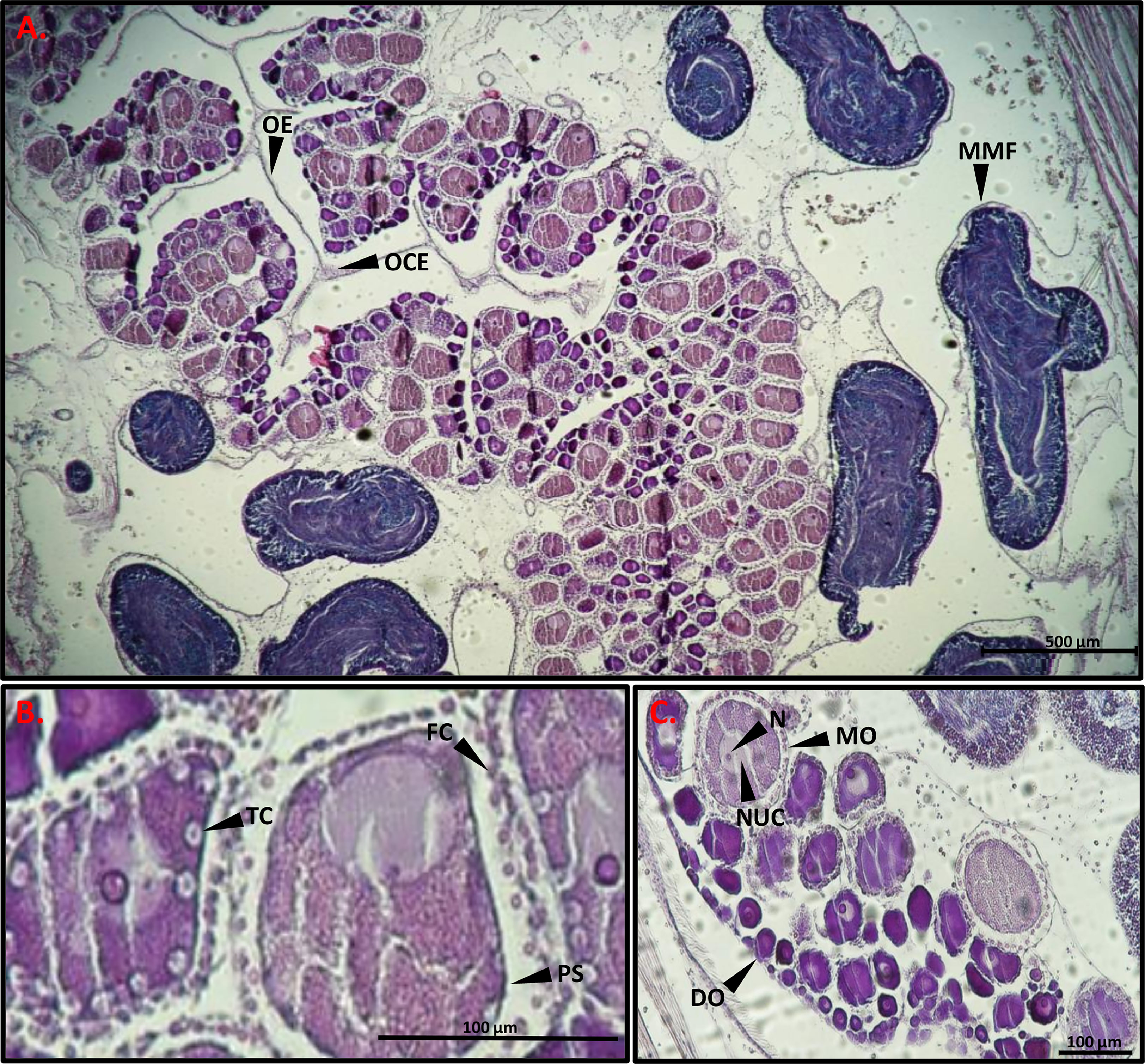
Figure 2 Light micrographs of a gonad histological section stained with hematoxylin-eosin. (A) MMF mature male follicle, OE ovarian epithelium, OCE ovarian ciliated epithelium. (B) TC test cells, FC follicle cells, PS perivitelline space. (C) DO, developing oocyte; MO, mature oocyte; NUC, nucleus; N, nucleolus. Scale bars, 500 µm (A), 100 µm (B, C).
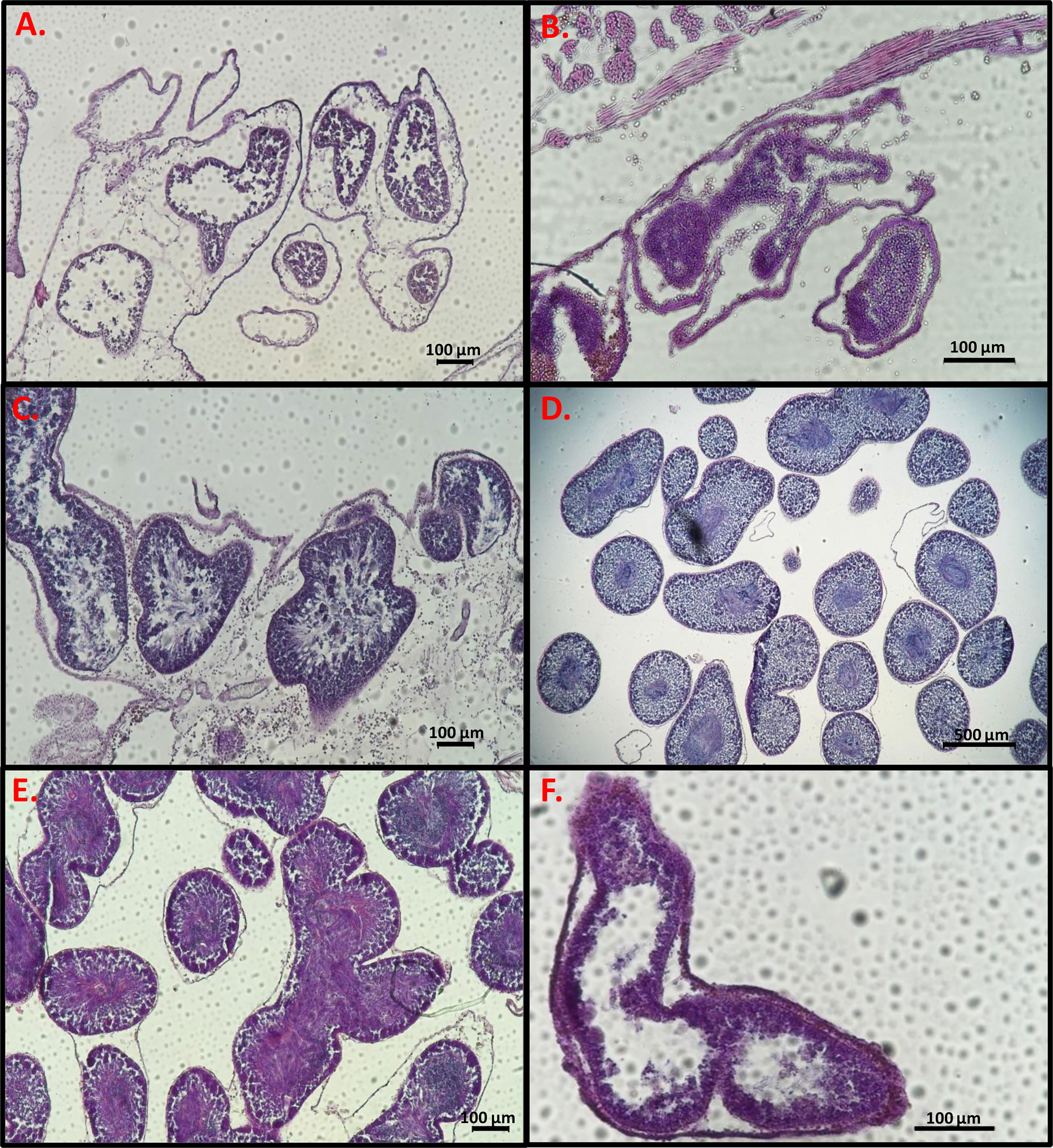
Figure 3 Light micrographs of gonad histological sections. Male follicles stained with hematoxylin-eosin at different stages. (A–C) Immature, (D, E) Mature, (F) Mature and spawning. Scale bars = 100 µm (A–C, E, F), 500 µm (D).
2.2.5 Spontaneous spawning under laboratory conditions
From January 2021 to July 2022, we followed on a seasonal basis the spontaneous spawning of S. plicata in Jaffa port. Each visit 12 individuals were collected and placed in a large jar for transport to the laboratory. In addition, 75 L of seawater were taken from the port and maintained in the aquaria room with an airstone, following filtration with a 50 µm plankton mesh to ensure that the water was free from eggs. The collected individuals were acclimated for 24 hours in four separate aquaria (3 individuals per aquarium, 15 x 28 x 20 cm3, containing 6 L seawater from Jaffa port), and fed with 2 ml of Brightwell Aquatics PhytoChrom. Following acclimation, 2 L of seawater were pumped out of each aquarium on a daily basis and filtered through a 50 µm plankton mesh. Following the filtration, the residue was washed carefully from the mesh and placed into separate Petri dishes for examination using a Nikon SMZ18 Stereo microscope. If spawned eggs were observed, the diameter of a maximum of 100 eggs (from each aquarium) was measured through the egg center to determine whether the eggs were ready for fertilization or had already been fertilized and started cell division (Figures S1A, B, West and Lambert, 1976; Bingham, 1997; Pineda et al., 2013). This routine was repeated for three days (excluding summer and fall 2021). After each pumping, the aquaria were filled with new filtered seawater, and the animals were fed on alternate days with 2 ml of PhytoChrom (Brightwell Aquatics). Air was supplied via an airstone in all aquaria, and the temperature was set using either an electric water heater or a chiller set, to adapt to the ambient temperature. Lamps (LED Submersible lamps, RGB 1350 mm, AQUADECOR) located above the aquarium system were used to establish a day-night cycle (excluding summer and fall 2021, during which the lights were kept on).
2.3 Statistical analysis
Statistical significance was tested using JMP® Pro statistical software (version 15 for Windows). Significant differences in population size structure were tested by Kruskal- Wallis and non-parametric comparison for all pairs of seasons at each site using Stell-Dwass method. Differences in gonad index between the seasons for each site were tested using one-way ANOVA. Square-root transformation was applied to the data obtained from the Jaffa site in order to normalize the results to meet the test assumptions. To compare the GI between sites for each season, square-root transformation was preformed and tested with two-way ANOVA. A nested design ANOVA was used for oocyte diameter analysis. The data from the spontaneous spawning were tested by Kruskal-Wallis and a non-parametric comparison for all pairs of seasons at each site using Stell-Dwass method (from winter 2021 to summer 2022). All tests were performed following Shapiro-Wilk and Anderson-Darling tests for normality, Tukey HSD post-hoc tests were conducted to reveal significant differences between the groups.
3 Results
3.1 Spatial distribution along the coast of Israel
The findings from to the current survey indicate that Styela plicata populations along the Mediterranean coast of Israel still occur only in those marinas in which they had already been recorded in the past (Figure 1, distribution map), as they were not found in any of the other locations investigated (Table S1). The northernmost edge of these populations was the Akko marina, and the southernmost edge was the Ashqelon marina (31°40’54”N, 34°33’20”E). The populations were observed on various artificial substrates, such as suspended ropes, buoys, boats, concrete elements, and fishing traps at depths ranging from 0-3.5 m. The only site at which S. plicata individuals were recorded outside a port/marina was in Akko. However, this population too was found mostly on an artificial substrate – a metal shipwreck and ropes and metal fragments scattered on the adjacent sandy bottom, and with only few anecdotal observations of few (< 5) individuals on the natural rocky substrate.
3.2 Seasonal monitoring of Styela plicata reproduction patterns
3.2.1 Population dynamics
S. plicata individuals were seen to be present throughout the year (excluding winter 2021 Haifa) at both sites on various artificial substrates from October 2020 to July 2022. The Haifa population was dense, with individuals forming numerous aggregations. This population disappeared in winter 2021, probably due to the low salinity conditions of 15 psu (Table S2). The Haifa population recovered in spring 2021, however, and continued to do so during the following year, but did not reach the largest size of the previously recorded individuals. During the study period the Jaffa port was undergoing renovations, resulting in an unstable S. plicata population and an unequal sampling of individuals during each visit. Small individuals (15 mm in diameter) were mostly observed in the spring, while adults (>45 mm), were particularly abundant in the fall and winter (Figure 4). There were no significant seasonal differences in individual size between winter and fall at both sites (p <0.05). Furthermore, the sizes of individuals were found to be smaller during summer and spring compared to those measured during fall, for both locations (Kruskal-Wallis,followed by Stell-Dwass method p <0.05).
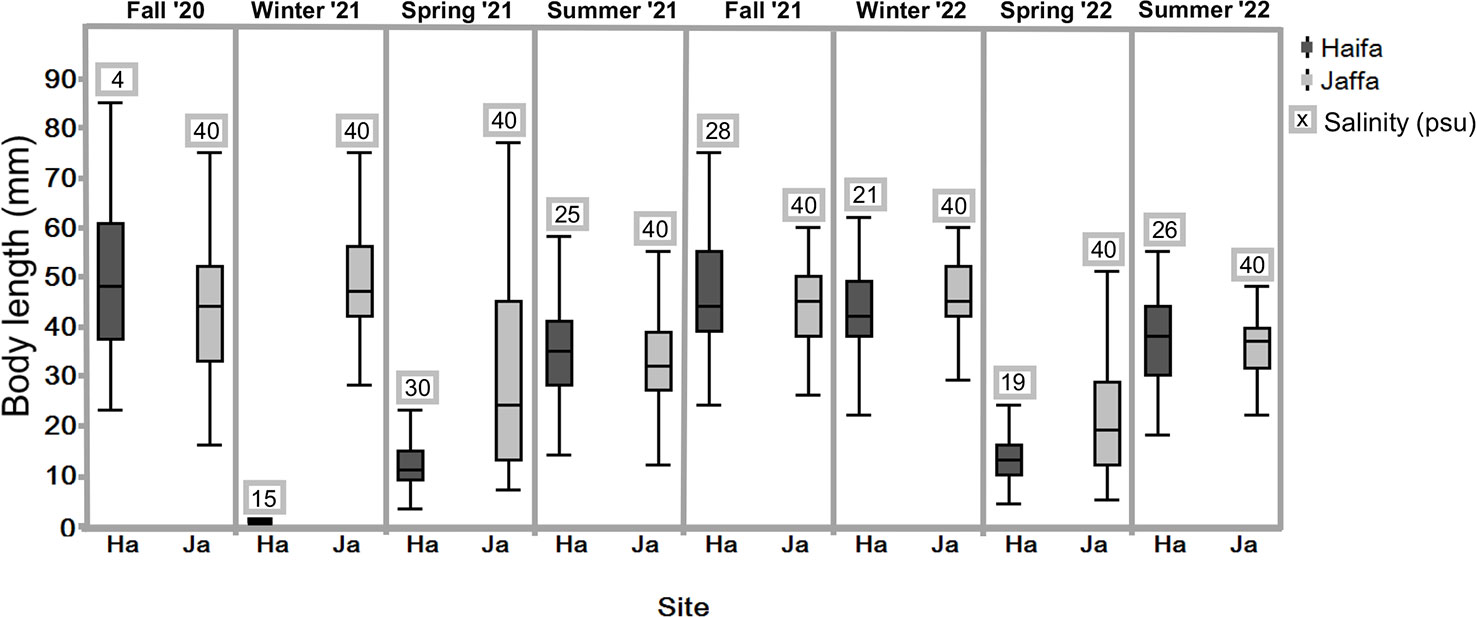
Figure 4 Box-plot showing the size distribution of S. plicata for each season at the Jaffa and Haifa study sites, n=53-100 (excluding winter 2022); Jaffa, n =25 over two years. Dots beyond the whiskers = extreme values; line in box = median of sampled body length; Box = 25th to 75th percentiles: bars = min and max values, excluding outliers. .
3.2.2 Gonad index measurements
The gonad index (GI) box plot represents the gonad weight ratio to the total body weight in percentage for three distinct locations over two years (Figure 5B). GI values during summer 2022 were significantly lower for all sites (one-way ANOVA, p <0.05). In Haifa, significant differences were observed between summer and the other seasons excluding fall 2021 (one-way ANOVA, followed by Tukey-HSD, p <0.05). Similar results were obtained for Jaffa, in which GI values of summer 2021 did not significantly differ from fall 2021 (one-way ANOVA, followed by Tukey-HSD p <0.05). Two-way ANOVA analysis followed by Tukey-HSD revealed significant differences between Jaffa and Akko during winter 2021 and spring 2021 (p <0.05).
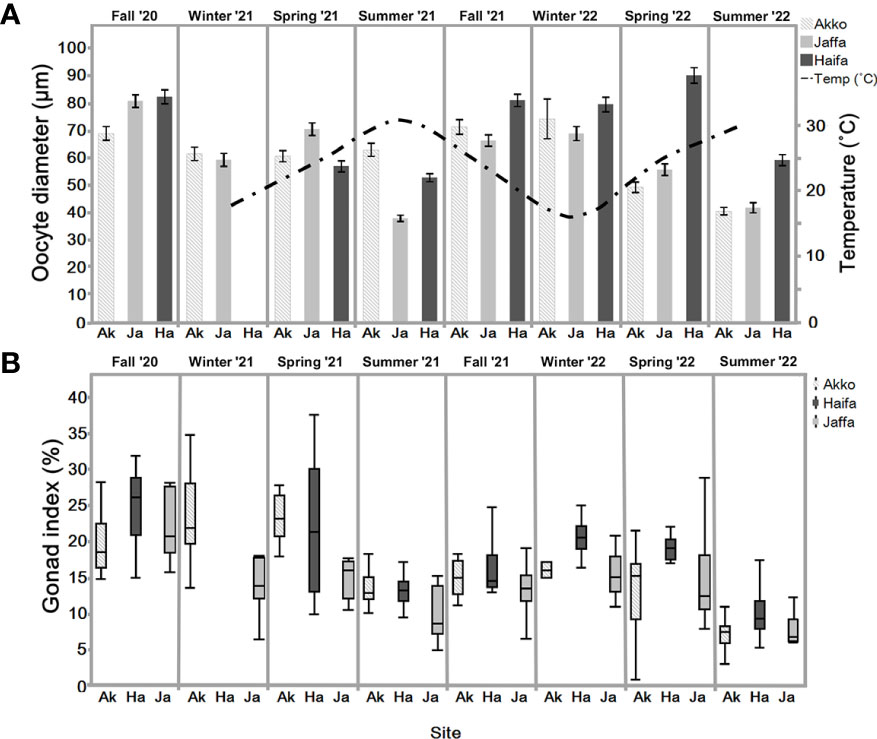
Figure 5 (A) Mean oocyte diameter (with 95% Cis) in sampled individuals N=6-12, n=100 (excluding winter 2022, Akko N=2) of S. plicata over two years, at three sites: Akko, Jaffa, and Haifa. Bar represents standard deviation. Haifa average sea-surface temperature (right Y-axis in ˚C) from 15/02/21-24/07/22. (B) Gonad index (%) of S. plicata from Akko, Jaffa, and Haifa, fall 2020 to summer 2022 (n=6-12 excluding winter 2022, Akko, n =2). Dots beyond the whiskers = extreme values; line in box = median of sampled GI percentage; Box = 25th to 75th percentiles: bars = min and max values excluding outliers.
3.2.3 Gamete development
The oocyte size frequency distribution graph (Figure 6A) reveals that the highest proportion of mature oocytes (>150 µm) appeared in fall of 2020 and winter of 2022. In both summers pre-vitellogenic oocytes (<50 µm) were abundant, with vitellogenic oocytes (50-100 and 100-150 µm) less abundant compared to the other seasons (except spring 2022). All oocyte classes were present year-round. Increasing proportions of vitellogenic and mature oocytes were observed during fall and winter throughout the study period. Their abundance decreased in the summer, when pre-vitellogenic oocytes increased. The sperm follicle frequency analysis (Figure 6B) indicates that all stages were present to various extents all year round. As seen in the pre-vitellogenic oocytes, mature and spawning follicles (stage 3) were abundant during the summer compared to during the other seasons, while immature follicles were mostly abundant during the summer. The measured oocyte results demonstrate a similar trend (Figure 5A), with an increasing oocyte size during the winter and fall, when seawater temperature is low, followed by a decrease in average oocyte diameter in the summer, when temperature increases. Significant differences in oocyte diameter were found in Akko during summer and spring 2022 compared to fall (one-way ANOVA, p <0.05). At the Jaffa and Haifa sites, the lowest mean of oocyte diameter was observed during the summer and increased significantly during the following fall and winter throughout the study (one-way ANOVA, p <0.05). Comparisons between the sampling sites during the same seasons showed that during the summer of 2021, mean oocyte diameter values in individuals sampled in Akko were significantly higher compared to Jaffa, but with no significant difference from Haifa. In addition, in spring 2022 oocyte diameter in individuals from Haifa was significantly higher than those at all other sites (two-way ANOVA followed by Tukey HSD test, p <0.05). These findings indicate that the main spawning events for all sites probably occur during fall and winter, followed by the onset of a new gametogenesis cycle in the summer.
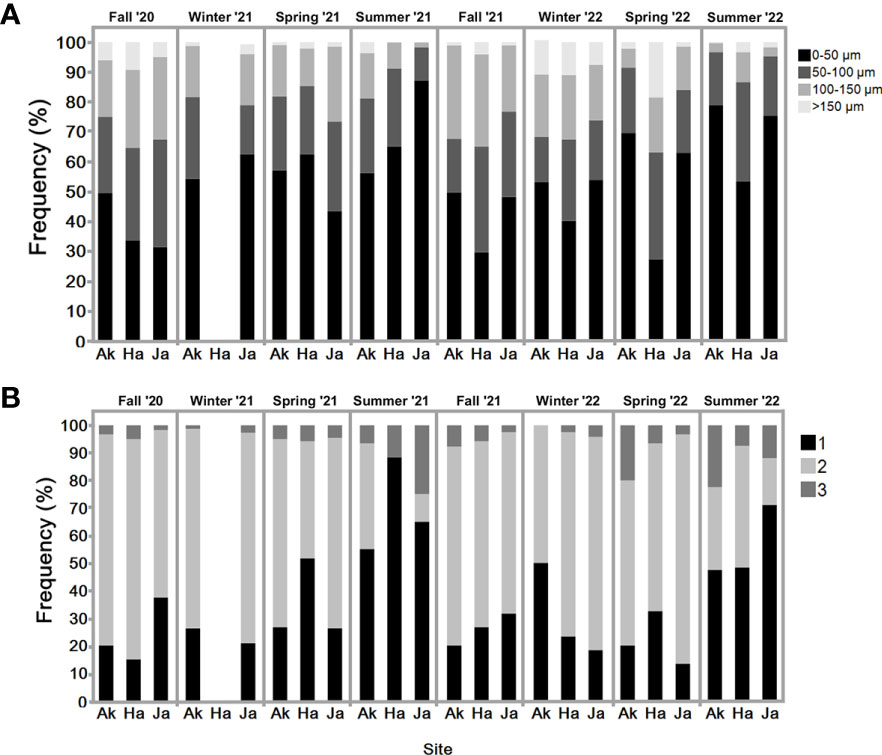
Figure 6 (A) Percentage of each oocyte size-class (pre-vitellogenic <50 μm; 50–100 μm; vitellogenic 100–150 μm; mature >150 μm). in sampled individuals of S. plicata over two years. Three sites: Akko, Jaffa, and Haifa. Sampled individuals N=6-12 (excluding winter 2022, Akko n =2) of S. plicata and eggs from each individual (n=100). (B) Percentage of sperm follicles at different reproductive stage. Sampled individuals N=6-12 (excluding winter 2022, Akko n =2) of S. plicata and sperm follicles from each individual (n=10) over two years. Three sites: Akko, Jaffa, and Haifa. 1-immature male follicles, 2-mature male follicles, 3-mature and spawning.
3.2.4 Spontaneous spawning
The results of spawning under laboratory conditions are presented in Figure S2. Cell division illustrations represent the seasons in which fertilized eggs were observed. Significantly higher amounts of reproductive products (oocytes) were observed in winter 2021 (n=332), compared to the other seasons, while those of summer 2021 (n=112), and spring 2022 (n=115) were similar (Kruskal-Wallis,followed by Stell-Dwass method p <0.05). In fall 2021 and winter 2022 (n=24,0) although very few reproduction products were observed (Figure S1A), four individuals were seen to be spawning only a few weeks after the experiment had ended in winter 2022. Cell divisions had also been observed in summer 2022 (Figure S1B), but they failed to produce motile larvae, while the winter 2021 and spring 2022 reproduction products successfully metamorphosed within two to three days after the spawning event. In spring 2021 only two reproduction products were observed out of the entire set-up.
4 Discussion
The current study documents the presence of thriving populations of the solitary ascidian Styela plicata along the Mediterranean coast of Israel even under extreme seawater temperatures of 31-32°C (in semi-closed sites such as marinas and ports) and 40 psu salinity. Under such extreme environmental conditions S. plicata exhibits a seasonal reproduction cycle, with the main spawning events occurring throughout fall and winter (16-23°C) and a new gametogenesis cycle beginning in the summer. Such adaptive resistance to increasing temperatures signifies this species’ potential to further disperse to additional coastal areas following the predicted rise in sea-surface temperature due to global warming.
4.1 Spatial distribution
Out of the 30 surveyed sites, S. plicata populations were recorded at six sites, all of which were marinas and ports, except for the population in Akko. However, this population too was found only on artificial substrates, such as concrete elements, ropes, and metal fragments scattered on the sandy bottom, and with only few anecdotal observations on the adjacent natural rocky substrate. Similar to other well-known invasive ascidians, no abundance gradient was found, meaning that either the species was present at a site in large numbers – or it was absent (Lambert and Lambert, 1998; Lambert, 2001). In all cases it is still restricted to artificial structures, as also found in the past for the solitary ascidian Herdmania momus. This latter species was first recorded from Israel’s Mediterranean coast in 1958, and later, in the early 2000s, along the eastern basin of the Mediterranean Sea (Pérès, 1958a; Çinar et al., 2006). A meticulous survey of H. momus conducted between 2005–2006 along the Israeli coastline revealed that populations were restricted to artificial substrates (Shenkar and Loya, 2008). However, eight years later, the population had spread to natural habitats, with many individuals spotted in marine protected areas (Gewing et al., 2014). Such a lag in establishment and infiltration into natural habitats was also documented in cases of introduction of the colonial ascidian Didemnum vexillum into the United Kingdom in 2008. Following its initial detection, three to five months later, D. vexillum was already found on 1-10% of the floating docks and ropes in the monitored harbor. Two years after the first record, the species could be found on almost every breakwater next to the harbor, as well as on 50% of the structures within the harbor, despite the numerous efforts to eradicate this species after its initial recognition (Sambrook et al., 2014).
Human-mediated vectors are well known for their contribution to mediating non-native species invasions from one artificial substrate to another (Apte et al., 2000; Goldstien et al., 2010). Much less is known, however, about the relative dynamics of NIS on anthropogenic structures and their invasion of adjacent natural habitats. A study in Canada compared four invasive ascidian species and their dispersal distribution on an artificial substrate and the adjacent natural habitat, as well as a literature review of the species’ distribution. The finding reveals a distinct distribution pattern. While only two of the species were found on natural substrates, their densities were the same on these as those of the populations on artificial substrates. Although all the above-noted species also have records from natural substrates, they have mostly been reported from anthropogenic habitats. These findings suggest that high densities of NIS in anthropogenic habitats do not necessarily contribute to their successful infiltration of the nearby rocky reef (Simkanin et al., 2012). Another study, examining settlement preferences of the solitary ascidian Microcosmus sequamiger on natural and artificial substrates, reported that there was no difference in genetic variability between individuals that grew on artificial versus natural substrates. It has been suggested that the ability of a species to infiltrate into different habitats is driven by its particular characteristics, such as long-lived larvae. (Ordóñez et al., 2013). Interestingly, a study that examined the gamete plasticity in S. plicata revealed that this species produced sperm that were larger, more motile, and viable for a longer period under high-density environments than sperm collected from animals kept under low density. Furthermore, individuals at low densities produced larger eggs, while eggs from low-density individuals had a significantly larger follicle cell area (Crean and Marshall, 2008). These findings emphasize the high adaptive ability of this species, enabling it to form such massive aggregations in newly-introduced sites.
Generally, species richness is lower on artificial substrates compared to on natural ones (Bulleri and Chapman, 2004; Firth et al., 2013). Consequently, natural habitats are suggested to be less prone to invasion (Stachowicz et al., 1999; Tyrrell and Byers, 2007). Predation pressure along the coast of Israel may also be higher in the waters outside the marinas, which also present unfavorable conditions to a larger number of species (Naranjo et al., 1996). In ascidians, adults are more resilient than the post-settlement phase or the juveniles. The early life stages are more exposed both to direct predation and to indirect damage such as trampling by mobile organisms (Oricchio et al., 2016). However, once an individual increases its body size, it can protect itself by means of physical or chemical defenses, such as a tough tunic and natural toxic products in the tunic (Stoecker, 1980). A study comparing two ascidian species’ vulnerability to predation in juveniles and adults, as well as the differences in predator influence at different life stages, revealed that the early life stages, regardless of species, were more vulnerable to predation. Furthermore, the early life stages are exposed to more types of potential predators in comparison to the adults. Sea urchins were the only predators shown to cause significant mortality to adult Ciona robusta, while Ascidia aspersa was found to be less vulnerable to sea urchins. This resistance may be due to A. aspersa’s thicker tunic and ability to develop acidity when abraded (Giachetti et al., 2022), a physiological advantage present in S. plicata, which is primarily protected from predators by its thickened tunic at the adult stage (Koplovitz and McClintock, 2011). We suggest that outside the marinas and protected artificial structures, however, due to the higher predation pressure and competition for settlement space, young juvenile S. plicata exhibit high mortality rates and fail to reach a detectable size.
S. plicata is well-known for its ability to grow and thrive within a variety of salinities, temperatures, and even polluted water conditions (Sims, 1984; Naranjo et al., 1996; Thiyagarajan and Qian, 2003). These abilities can be related to a genetic plastic response to environmental changes, as shown in a study of the solitary ascidian C. savignyi (Huang and Zhan, 2021), which revealed rapid and highly dynamic gene expression shifts, as well as alternative splicing under high and low temperature stress. In addition, S. plicata’s microbial community includes taxa known to be capable of carbon fixation, ammonia oxidation, and heavy metal resistance. This symbiotic microbiome may contribute to its establishment in a variety of environments (Dror et al., 2019). A recent study has demonstrated the ability of S. plicata to survive a 28-day simulated voyage that mimicked changes in temperature, salinity, and food availability (Bereza and Shenkar, 2022), further emphasizing the ability of this species to survive and rapidly expand its distribution following certain global change scenarios that have been shown to have crucial effects on marine ecosystems (Cheung et al., 2009; Walther et al., 2009). Zhang et al. (2020) simulated the future distribution of eight invasive ascidian species, including S. plicata, and demonstrated that this species’ distribution is expected to contract under future climate change. However, their distribution model has several limitations, including the inability to account for unknown factors such as genetic plasticity and evolution. Furthermore, it is possible that the authors of this latter study were unaware of the recently demonstrated high thermal tolerance of S. plicata (Bereza and Shenkar, 2022). Based on the massive development along the Mediterranean coast of Israel is undergoing, including numerous breakwater additions and the deployment of artificial structures (Airoldi et al., 2009; Bulleri and Chapman, 2010; Spanier and Zviely, 2022), we predict that S. plicata populations will proliferate along the Israeli coastline, although, its potential to infiltrate into adjacent natural habitats is yet to be determined.
4.2 Reproduction cycle of Styela plicata along the Mediterranean coast of Israel
Based on four different methods used in the current study, the highest reproductive effort of S. plicata along the Mediterranean coasts of Israel was found to be during fall and winter. The application of all four methods is important in order to achieve an effective understanding of the entire reproduction cycle (Becerro and Turon, 1992; Pineda et al., 2013).Combining measurements of individuals in the field with the Gonad Index (GI) and gamete development is an accepted method for monitoring the reproduction cycle in many species of ascidians (Millar, 1952; Pineda et al., 2013; Raijman Nagar and Shenkar, 2016; Shmuel and Shenkar, 2017). The latter method, which measured the spawning effort in the laboratory, was developed for ascidians in the current study. Other studies on ascidian spawning events have been conducted in the field rather than in laboratories (Dybern, 1965; Yamaguchi, 1975; Svane and Havenhand, 1993). The ‘spawning method’ is commonly used in the field with corals and recently also with mussels (Žuljević et al., 2018; Baird et al., 2021), while in studies of invertebrates such as annelids and sea urchins, observing spawning in the laboratory is widely used (Farke and Berghuis, 1979; Luis et al., 2005). In comparison to field measurements, the spawning in the laboratory approach may be more accessible in regard to some species, and easier for data collection and quantification. Yet, it is important to note that the stress experienced by the individuals transported to the lab may have induced earlier spawning. Following the ability of the spawned gametes to produce motile larvae further contributes to our ability to understand the reproductive stage of individuals in the field. Our results from the spawning experiments show that the high reproduction products observed during winter 2021 support the hypothesis that S. plicata reproduces during the colder months. However, no spawning events were observed during the fall and winter of 2022, probably due to the existence of earlier spawning events. A few studies have shown that, in some cases, this method can provide misleading information due to stress sometimes preventing spawning even when the animal has ripe eggs or sperm (Giese, 1959; Himmelman, 1975; West and Lambert, 1976). The detection of gametes and zygotes during the summer and spring trials was unexpected but corresponds to the observation of mature eggs and sperm follicles all year round. These findings reveal that, strikingly, S. plicata individuals are able to release gametes all year round at varying intensities. In addition, our observation of reproduction products during the summer may indicate that S. plicata adapts to the high temperatures in Israel during the summer, implying the high ability of this species to tolerate global warming (Lambert, 2007; Pineda et al., 2012; Zhang et al., 2020). A study examining several early developmental stages of S. plicata in a population from Hong Kong, under different temperatures and salinities, found no significant difference in embryo survival and metamorphosis at 30°C. Moreover, they demonstrated that increasing the temperature even accelerated development (Thiyagarajan and Qian, 2003).
The results from the population dynamics study in the field revealed that summer and spring differed in individual size from the other seasons, with the smallest individuals measured during spring (mean body length 13.12 and 25.49 cm in Haifa and Jaffa respectively), while the summer saw a growth in young individuals (mean body length 35.41 and 34.09 cm in Haifa and Jaffa, respectively). GI measurements show that the lowest values were found during the summer, supporting the hypothesis that the young individuals measured in the summer are most likely from the same spawning event as those measured in the spring. Furthermore, S. plicata reaches sexual maturity at about 40 mm in body length (Yamaguchi, 1975), and this size (42-48 mm) was common during fall and winter in the current study. These observations may suggest that the majority of the populations that were measured during the spring and summer had not yet reached sexual maturity. However, all oocyte size-classes were observed throughout the seasons, as were all the stages of the sperm follicles. To conclude, S. plicata’s highest reproductive output is thus during the winter and spring, when seawater temperatures range between 16-24°C. Nevertheless, additional spawning events may occur throughout the year, albeit at less intensity, and with a new gametogenesis cycle beginning in the summer.
This finding is consistent with other studies that found that water temperature appears to have a significant influence on ascidians’ growth and reproduction cycles, with some regional variations (Goodbody, 1961; Raijman Nagar and Shenkar, 2016; Gewing et al., 2019). In Spain, for example, no specific reproduction pattern was observed in two populations of S. plicata, suggesting that the species reproduced all year round; while in Japan, S. plicata were found to be ripe in winter with no active spawning during the coldest months (Yamaguchi, 1975; Pineda et al., 2013).
5 Conclusions
Since the ascidian Styela plicata became prominent in Israel in 2014, the species has failed to establish itself on natural substrates. As artificial structures along Israel’s Mediterranean coast become more common, suitable habitats and preferable conditions for S. plicata will increase. The S. plicata population in Akko is the only one in Israel that has succeeded to date to establish outside the marina, with anecdotal occurrences of few individuals on natural substrate. This demonstrates the potential of S. plicata to infiltrate into adjacent habitats, as well as to survive and reproduce under changing conditions, such as currents, sandblasting during transition seasons, and high turbulence. S. plicata has been shown not only to survive the extreme conditions along the coast of Israel, characterized by high salinity (reaching 40 psu) combined with high summer temperatures (up to 33°C) inside the marina, but it can also successfully reproduce there and establish thriving populations. We conclude that in order to prevent the further spread of this potentially invasive species, focused marina maintenance should be considered throughout the summer, prior to S. plicata’s breeding seasons, in order to reduce the abundance of sexually mature adults and population growth prior to the species’ gonad maturation and the re-occurrence of spawning.
Data availability statement
The original contributions presented in the study are included in the article/Supplementary Material. Further inquiries can be directed to the corresponding author.
Author contributions
RP and NS conceived the ideas and designed the study. RP collected the data and preformed the field surveys, histological sections, laboratory experiments and statistical analysis. NS supervised the study and wrote the manuscript together with RP. Both authors contributed to the article and approved the submitted version.
Funding
This research was supported by an ISF-NSFC grant number 3347/20 to NS.
Acknowledgments
We are grateful to the Akko Naval Officers Academy, and to I. Nahum and the management teams of Shavit Kishon marina and Jaffa port for granting access to sample in their facilities. We thank the members of the Shenkar lab for assisting in field sampling, and N. Paz for editorial assistance.
Conflict of interest
The authors declare that the research was conducted in the absence of any commercial or financial relationships that could be construed as a potential conflict of interest.
Publisher’s note
All claims expressed in this article are solely those of the authors and do not necessarily represent those of their affiliated organizations, or those of the publisher, the editors and the reviewers. Any product that may be evaluated in this article, or claim that may be made by its manufacturer, is not guaranteed or endorsed by the publisher.
Supplementary material
The Supplementary Material for this article can be found online at: https://www.frontiersin.org/articles/10.3389/fmars.2023.1159231/full#supplementary-material
References
Airoldi L., Connell S. D., Beck M. W. (2009). “The loss of natural habitats and the addition of artificial substrata,” in Marine hard bottom communities (Heidelberg: Springer, Berlin), 269–280.
Aldred N., Clare A. S. (2014). Mini-review: Impact and dynamics of surface fouling by solitary and compound ascidians. Biofouling. 30, 259–270. doi: 10.1080/08927014.2013.866653
Apte S., Holland B. S., Godwin L. S., Gardner J. P. A. (2000). Jumping ship: a stepping stone event mediating transfer of a non-indigenous species via a potentially unsuitable environment. Biol. Invasions 2, 75–79. doi: 10.1023/A:1010024818644
Baird A. H., Guest J. R., Edwards A. J., Bauman A. G., Bouwmeester J., Mera H., et al. (2021). An indo-pacific coral spawning database. Sci. Data. 8, 35. doi: 10.1038/s41597-020-00793-8
Bax N., Williamson A., Aguero M., Gonzalez E., Geeves W. (2003). Marine invasive alien species: a threat to global biodiversity. Mar. Policy. 27, 313–323. doi: 10.1016/S0308-597X(03)00041-1
Becerro M. A., Turon X. (1992). Reproductive cycles of the ascidians Microcosmus sabatieri and Halocynthia papillosa in the northwestern Mediterranean. Mar. Ecol. 13, 363–373. doi: 10.1111/j.1439-0485.1992.tb00360.x
Bereza D., Shenkar N. (2022). Shipping voyage simulation reveals abiotic barriers to marine bioinvasions. Sci. Total Environ. 837, 155741. doi: 10.1016/j.scitotenv.2022.155741
Bingham B. L. (1997). Light cycles and gametogenesis in three temperate ascidian species. Invertebr. Biol. 116, 61. doi: 10.2307/3226925
Bishop J. D. D., Wood C. A., Yunnie A. L. E., Griffiths C. A. (2015). Unheralded arrivals: non-native sessile invertebrates in marinas on the English coast. Aquat. Invasions. 10, 249–264. doi: 10.3391/ai.2015.10.3.01
Bulleri F., Chapman M. G. (2004). Intertidal assemblages on artificial and natural habitats in marinas on the north-west coast of Italy. Mar. Biol. 145, 381–391. doi: 10.1007/s00227-004-1316-8
Bulleri F., Chapman M. G. (2010). The introduction of coastal infrastructure as a driver of change in marine environments. J. Appl. Ecol. 47, 26–35. doi: 10.1111/j.1365-2664.2009.01751.x
Carlton J. (1989). Man’s role in changing the face of the ocean: Biological invasions and implications for conservation of near-shore environments. Conserv. Biol. 3, 265–273. doi: 10.1111/j.1523-1739.1989.tb00086.x
Carver C. E., Chisholm A., Mallet A. L. (2003). Strategies to mitigate the impact of Ciona intestinalis (L.) biofouling on shellfish production. J. Shellfish Res. 22, 621–631.
Castilla J. C., Guiñez R., Caro A. U., Ortiz V. (2004). Invasion of a rocky intertidal shore by the tunicate Pyura praeputialis in the bay of antofagasta, Chile. Proc. Natl. Acad. Sci. 101, 8517–8524. doi: 10.1073/pnas.0401921101
Cheung W. W. L., Lam V. W., Sarmiento J. L., Kearney K., Watson R., Pauly D. (2009). Projecting global marine biodiversity impacts under climate change scenarios. Fish Fish. 10, 235–251. doi: 10.1111/j.1467-2979.2008.00315.x
Çinar M. E., Bilecenoglu M., Öztürk B., Can A. (2006). New records of alien species on the levantine coast of Turkey. Aquat. invasions. 1, 84–90. doi: 10.3391/ai.2006.1.2.6
Coll M., Piroddi C., Steenbeek J., Kaschner K., Ben Rais Lasram F., Aguzzi J., et al. (2010). The biodiversity of the Mediterranean Sea: Estimates, patterns, and threats. PloS One 5, e11842. doi: 10.1371/journal.pone.0011842
Crean A. J., Marshall D. J. (2008). Gamete plasticity in a broadcast spawning marine invertebrate. Proc. Natl. Acad. Sci. 105, 13508–13513. doi: 10.1007/s00248-018-1276-z
Dror H., Novak L., Evans J. S., et al (2019). Core and dynamic microbial communities of two invasive ascidians: Can host–symbiont dynamics plasticity affect invasion capacity? Microb Ecol. 78, 170–184.
Dybern B. I. (1965). The life cycle of ciona intestinalis (L.) f. typica in relation to the environmental temperature. Oikos 16 (1/2), 109–131. doi: 10.2307/3564870
Farke H., Berghuis E. M. (1979). Spawning, larval development and migration behaviour of Arenicola marina in the laboratory. Netherlands. J. Sea Res. 13, 512–528. doi: 10.1016/0077-7579(79)90022-X
Firth L. B., Thompson R. C., White F. J., Schofield M., Skov M. W., Hoggart S. P. G., et al. (2013). The importance of water-retaining features for biodiversity on artificial intertidal coastal defence structures. Divers. Distrib. 19, 1275–1283. doi: 10.1111/ddi.12079
Galil B. S., Zenetos A. (2002). “A sea change — exotics in the eastern Mediterranean Sea,” in Invasive aquatic species of europe. distribution, impacts and management (Dordrecht: Springer), 325–336.
Gewing M.-T., Goldstein E., Buba Y., Shenkar N. (2019). Temperature resilience facilitates invasion success of the solitary ascidian Herdmania momus. biol. Invasions. 21, 349–361. doi: 10.1007/s10530-018-1827-8
Gewing M.-T., Rothman S. B. S., Raijman Nagar R., Shenkar N. (2014). Early stages of establishment of the non-indigenous ascidian Herdmania momus (Savigny 1816) in shallow and deep water environments on natural substrates in the Mediterranean Sea. BioInvasions Rec. 3, 77–81. doi: 10.3391/bir.2014.3.2.04
Gewing M. T., Shenkar N. (2017). Monitoring the magnitude of marine vessel infestation by non-indigenous ascidians in the Mediterranean. Mar. Pollu. Bull. 121, 52–59. doi: 10.1016/j.marpolbul.2017.05.041
Giachetti C. B., Battini N., Castro K. L., Schwindt E. (2022). The smaller, the most delicious: Differences on vulnerability to predation between juvenile and adult of invasive ascidians. Estuar. Coast. Shelf Sci. 268, 107810. doi: 10.1016/j.ecss.2022.107810
Giese A. C. (1959). Comparative physiology: Annual reproductive cycles of marine invertebrates. Annu. Rev. Physiol. 21, 547–576. doi: 10.1146/annurev.ph.21.030159.002555
Goldstien S. J., Schiel D. R., Gemmell N. J. (2010). Regional connectivity and coastal expansion: differentiating pre-border and post-border vectors for the invasive tunicate Styela clava. mol. Ecol. 19, 874–885. doi: 10.1111/j.1365-294X.2010.04527.x
Goodbody I. (1961). Continuous breeding in three species of tropical ascidians. Proc. Zool. Soc London. 136, 403–409. doi: 10.1111/j.1469-7998.1961.tb05882.x
Herut B., Gertner Y., Segal Y., Sisma-Ventura G., Gordon N., Belkin, et al. (2023). Long-term, (2002–2021) trend in nutrient-related pollution at small stratified inland estuaries, the kishon SE Mediterranean case. Water 15, 484. doi: 10.3390/w15030484
Himmelman J. H. (1975). Phytoplankton as a stimulus for spawning in three marine invertebrates. J. Exp. Mar. Bio. Ecol. 20, 199–214. doi: 10.1016/0022-0981(75)90024-6
Huang X., Zhan A. (2021). Highly dynamic transcriptional reprogramming and shorter isoform shifts under acute stresses during biological invasions. RNA. Biol. 18, 340–353. doi: 10.1080/15476286.2020.1805904
Koplovitz G., McClintock J. B. (2011). An evaluation of chemical and physical defenses against fish predation in a suite of seagrass-associated ascidians. J. Exp. Mar. Bio. Ecol. 407, 48–53. doi: 10.1016/j.jembe.2011.06.038
Lambert G. (2001). “A global overview of ascidian introductions and their possible impact on the endemic fauna,” in The biology of ascidians. Eds. Sawada H., Yokosawa H., Lambert C. C. (Tokyo: Springer). doi: 10.1007/978-4-431-66982-1_40
Lambert G. (2007). Invasive sea squirts : A growing global problem. J. Exp. Mar. Bio. Ecol. 342, 3–4. doi: 10.1016/j.jembe.2006.10.009
Lambert C. C., Lambert G. (1998). Non-indigenous ascidians in southern California harbors and marinas. Mar. Biol. 130, 675–688. doi: 10.1007/s002270050289
Lesueur C. A. (1823). Descriptions of several new species of Ascidia. Proc. Acad. Nat. Sci. Phila. 3, 2–8.
Lord J. P., Calini J. M., Whitlatch R. B. (2015). Influence of seawater temperature and shipping on the spread and establishment of marine fouling species. Mar. Biol. 162, 2481–2492. doi: 10.1007/s00227-015-2737-2
Luis O., Delgado F., Gago J. (2005). Year-round captive spawning performance of the sea urchin Paracentrotus lividus: Relevance for the use of its larvae as live feed. Aquat. Living Resour. 18, 45–54. doi: 10.1051/alr:2005004
Malanotte-Rizzoli P., Artale V., Borzelli-Eusebi G. L., Brenner S., Crise A., Gacic M., et al. (2014). Physical forcing and physical/biochemical variability of the Mediterranean Sea: A review of unresolved issues and directions for future research. Ocean Sci. 10, 281–322. doi: 10.5194/os-10-281-2014
Maltagliati F., Lupi L., Castelli A., Pannacciulli F. G. (2016). The genetic structure of the exotic ascidian Styela plicata (Tunicata) from Italian ports, with a re-appraisal of its worldwide genetic pattern. Mar. Ecol. 37, 492–502. doi: 10.1111/maec.1226
Mcclary D., Phipps C., Golder Associates S. H. (2008). Reproductive behaviour of the clubbed tunicate, styela clava, in northern new Zealand waters. Ministry of Agriculture and Forestry. Biosecurity New Zealand Technical Paper No: 2009/01. Available at: http://www.biosecurity.govt.nz/about-us/our-publications/technical-papers.
Millar R. H. (1952). The annual growth and reproduction cycle in four ascidians. J. Mar. Biol. Assoc. United Kingdom. 31, 41–61. doi: 10.1017/S0025315400003672
Molnar J. L., Gamboa R. L., Revenga C., Spalding M. D. (2008). Assessing the global threat of invasive species to marine biodiversity. Front. Ecol. Environ. 6, 485–492. doi: 10.1890/070064
Naranjo S. A., Carballo J. L., García-Gómez J. C. (1996). Effects of environmental stress on ascidian populations in algeciras bay (southern spain). possible marine bioindicators? Mar. Ecol. Prog. Ser. 144, 119–131. doi: 10.3354/meps144119
Naylor R. L., Williams S. L., Strong D. R. (2001). Aquaculture - a gateway for exotic species. Science. 294, 1655–1656. doi: 10.1126/science.1064875
Novak L., Lopez-Legentil S., Sieradzki E., Shenkar N. (2017). Rapid establishment of the non-indigenous ascidian Styela plicata and its associated bacteria in marinas and fishing harbors along the Mediterranean coast of Israel. Mediterr. Mar. Sci. 18, 324–331. doi: 10.12681/mms.2135
Ordóñez V., Pascual M., Rius M., Turon X. (2013). Mixed but not admixed: A spatial analysis of genetic variation of an invasive ascidian on natural and artificial substrates. Mar. Biol. 160, 1645–1660. doi: 10.1007/s00227-013-2217-5
Oricchio F. T., Flores A. A. V., Dias G. M. (2016). The importance of predation and predator size on the development and structure of a subtropical fouling community. Hydrobiologia. 776, 209–219. doi: 10.1007/s10750-016-2752-4
Osman R. W., Whitlatch R. B. (2007). Variation in the ability of Didemnum sp. to invade established communities. J. Exp. Mar. Bio. Ecol. 342, 40–53. doi: 10.1016/j.jembe.2006.10.013
Ouréns R., Juan F., Luis F. (2012). Definition of a new unbiased gonad index for aquatic invertebrates and fish: its application to the sea urchin Paracentrotus lividus. aquat. Biol. 17, 145–152. doi: 10.3354/ab00476
Pearse J. S., Eernisse D. J. (1982). Photoperiodic regulation of gametogenesis and gonadal growth in the sea star Pisaster ochraceus. Mar. Biol. 67, 121–125. doi: 10.1007/BF00401277
Pérès J. M. (1958a). Ascidies recoltées sur les cotes mediterranées d’Israel. Bull. Res. Counc. Isr. 3-4 (7B), 143–150.
Pérès J. M. (1958b). Ascidies de la baie de Haifa collectées par e. gottlieb. Bull. Res. Counc. Isr. 3-4 (7B), 151–164.
Pestana L. B., Dias G. M., Marques A. C. (2017). A century of introductions by coastal sessile marine invertebrates in Angola, south East Atlantic ocean. Mar. pollut. Bull. 125, 426–432. doi: 10.1016/j.marpolbul.2017.09.041
Pineda M. C., López-Legentil S., Turon X. (2011). The whereabouts of an ancient wanderer: Global phylogeography of the solitary ascidian Styela plicata. PloS One 6, e25495. doi: 10.1371/journal.pone.0025495
Pineda M. C., López-Legentil S., Turon X. (2013). Year-round reproduction in a seasonal sea: Biological cycle of the introduced ascidian Styela plicata in the western Mediterranean. Mar. Biol. 160, 221–230. doi: 10.1007/s00227-012-2082-7
Pineda M. C., Turon X., López-Legentil S. (2012). Stress levels over time in the introduced ascidian Styela plicata: The effects of temperature and salinity variations on hsp70 gene expression. Cell Stress Chaperones. 17, 435–444. doi: 10.1007/s12192-012-0321-y
Raijman Nagar L., Shenkar N. (2016). From tropical to sub-tropical: Prolonged reproductive activity of the invasive ascidian Microcosmus exasperatus in the eastern Mediterranean. Front. Ecol. Evol. 4. doi: 10.3389/fevo.2016.00102
Rilov G., Klein L., Iluz D., Dubinsky Z., Guy-Haim T. (2022). Last snail standing? superior thermal resilience of an alien tropical intertidal gastropod over natives in an ocean-warming hotspot. Biol. Invasions. 24, 3703–3719. doi: 10.1007/s10530-022-02871-x
Sambrook K., Holt R. H. F., Sharp R., Griffith K., Roche R. C., Newstead R. G., et al. (2014). Capacity, capability and cross-border challenges associated with marine eradication programmes in Europe: The attempted eradication of an invasive non-native ascidian, Didemnum vexillum in Wales, united kingdom. Mar. Policy 48, 51–58. doi: 10.1016/j.marpol.2014.03.018
Sciscioli M., Lepaore E., Tursi A. (1978). Relationship between Styela plicata (Les.) (Tunicata) settlement and spawning. Mem. Biol. Mar. oceanogr. 8, 65–75.
Shenkar N., Loya Y. (2008). The solitary ascidian Herdmania momus: Native (Red Sea) versus non-indigenous (Mediterranean) populations. Biol. Invasions 10, 1431–1439. doi: 10.1007/s10530-008-9217-2
Shenkar N., Loya Y. (2009). Non-indigenous ascidians (Chordata: Tunicata) along the Mediterranean coast of Israel. Mar. Biodivers. Rec. 2, e166. doi: 10.1017/S1755267209990753
Shenkar N., Swalla B. J. (2011). Global diversity of ascidiacea. PloS One 6, e20657. doi: 10.1371/journal.pone.0020657
Shmuel Y., Shenkar N. (2017). Reproductive cycle and ecology of the tropical ascidian halocynthia spinosa in the red Sea. Mar. Biol. 164, 1–12. doi: 10.1007/s00227-017-3179-9
Simkanin C., Davidson I. C., Dower J. F., Jamieson G., Therriault T. W. (2012). Anthropogenic structures and the infiltration of natural benthos by invasive ascidians. Mar. Ecol. 33, 499–511. doi: 10.1111/j.1439-0485.2012.00516.x
Sims L. L. (1984). Osmoregulatory capabilities of three macrosympatric stolidobranch ascidians, Styela clava herdman, s. plicata (Lesueur), and S. montereyensis (Dall). J. Exp. Mar. Bio. Ecol. 82, 117–129. doi: 10.1016/0022-0981(84)90098-4
Soares M. O., Salani S., Paiva S. V., Braga M. D. A. (2020). Shipwrecks help invasive coral to expand range in the Atlantic ocean. Mar. pollut. Bull. 158, 111394. doi: 10.1016/j.marpolbul.2020.111394
Spanier E., Zviely D. (2022). Key environmental impacts along the Mediterranean coast of Israel in the last 100 years. J. Mar. Sci. Eng. 11, 2. doi: 10.3390/jmse11010002
Stachowicz J. J., Whitlatch R. B., Osman R. W. (1999). Species diversity and invasion resistance in a marine ecosystem. Science 286, 1577–1579. doi: 10.1126/science.286.5444.157
Stoecker D. (1980). Relationships between chemical defense and ecology in benthic ascidians. Mar. Ecol. Prog. Ser. 3, 257–265. doi: 10.3354/meps003257
Svane I., Havenhand J. N. (1993). Spawning and dispersal in Ciona intestinalis (L.). Mar. Ecol. 14, 53–66. doi: 10.1111/j.1439-0485.1993.tb00364.x
Thiyagarajan V., Qian P. Y. (2003). Effect of temperature, salinity and delayed attachment on development of the solitary ascidian Styela plicata (Lesueur). J. Exp. Mar. Bio. Ecol. 290, 133–146. doi: 10.1016/S0022-0981(03)00071-6
Tursi A., Matarrese A. (1981). Phenomena of settling in Styela plicata (Les.) (Tunicata). Mem. Biol. Mar. Oceanogr. 11, 117–130.
Tyrrell M. C., Byers J. E. (2007). Do artificial substrates favor nonindigenous fouling species over native species? J. Exp. Mar. Bio. Ecol. 342, 54–60. doi: 10.1016/j.jembe.2006.10.014
Walther G. R., Roques A., Hulme P. E., Sykes M. T., Pyšek P., Kühn I., et al. (2009). Alien species in a warmer world: risks and opportunities. Trends Ecol. Evol. 24, 686–693. doi: 10.1016/j.tree.2009.06.008
West A. B., Lambert C. C. (1976). Control of spawning in the tunicate Styela plicata by variations in a natural light regime. J. Exp. Zool. 195, 263–270. doi: 10.1002/jez.1401950211
Wong N. A., McClary D., Sewell M. A. (2011). The reproductive ecology of the invasive ascidian, Styela clava, in Auckland harbour, new Zealand. Mar. Biol. 158, 2775–2785. doi: 10.1007/s00227-011-
Wyatt A. S. J., Hewitt C. L., Walker D. I., Ward T. J. (2005). Marine introductions in the shark bay world heritage property, Western Australia: a preliminary assessment. Divers. Distrib. 11, 33–44. doi: 10.1111/j.1366-9516.2005.00109.x
Yamaguchi M. (1975). Growth and reproductive cycles of the marine fouling ascidians Ciona intestinalis, Styela plicata, Botrylloides violaceus, and Leptoclinum mitsukurii at aburatsubo-moroiso inlet (central Japan). Mar. Biol. 29, 253–259. doi: 10.1007/BF00391851
Zhan A., Briski E., Bock D. G., Ghabooli S., MacIsaac H. J. (2015). Ascidians as models for studying invasion success. Mar. Biol. 162, 2449–2470. doi: 10.1007/s00227-015-2734-5
Zhang Z., Capinha C., Karger D. N., Turon X., MacIsaac H. J., Zhan A. (2020). Impacts of climate change on geographical distributions of invasive ascidians. Mar. Environ. Res. 159, 104993. doi: 10.1016/j.marenvres.2020.104993
Keywords: global warming, tunicates, non-indigenous species, environmental tolerance, local adaptation
Citation: Platin R and Shenkar N (2023) Can stand the heat – ecology of the potentially invasive ascidian Styela plicata along the Mediterranean coast of Israel. Front. Mar. Sci. 10:1159231. doi: 10.3389/fmars.2023.1159231
Received: 05 February 2023; Accepted: 21 March 2023;
Published: 03 April 2023.
Edited by:
Fabio Crocetta, Anton Dohrn Zoological Station Naples, ItalyReviewed by:
Alfonso Angel Ramos-Esplá, University of Alicante, SpainS. López-Legentil, University of North Carolina Wilmington, United States
Copyright © 2023 Platin and Shenkar. This is an open-access article distributed under the terms of the Creative Commons Attribution License (CC BY). The use, distribution or reproduction in other forums is permitted, provided the original author(s) and the copyright owner(s) are credited and that the original publication in this journal is cited, in accordance with accepted academic practice. No use, distribution or reproduction is permitted which does not comply with these terms.
*Correspondence: Noa Shenkar, shenkarn@tauex.tau.ac.il