- 1Fisheries College, Guangdong Ocean University, Zhanjiang, China
- 2Guangdong Provincial Key Laboratory of Aquatic Animal Disease Control and Healthy Culture, Guangdong Ocean University, Zhanjiang, China
Cold spells, characterized by prolonged periods of low temperature, have become increasingly frequent, intense, and prolonged due to the ongoing effects of climate change, resulting in devastating consequences for marine ecosystems and significant socio-economic impacts. As ectothermic organisms, bivalves are dependent on their environment for regulating body temperature, and thus, cold spells can disrupt their normal functioning, leading to mass mortalities. This review comprehensively summarizes the effects of cold spells on bivalves and proposes mitigation measures to be considered in future bivalve farming and management plans. Scientific evidence has indicated that cold spells can alter bivalve metabolism, leading to an increase in stress protein production and a decrease in the activity of energy metabolism-related enzymes, which can negatively impact the bivalve immune system and increase the risk of disease. To mitigate the effects of cold spells on bivalves, a number of strategies can be employed, including the use of thermal shelters such as floating covers, selective breeding of more cold-tolerant bivalves, and genetic engineering to enhance the expression of heat-shock proteins in bivalves. The impacts of cold spells on bivalves are significant, affecting both their physiological and molecular processes. Through the implementation of thermal shelters, selective breeding, and genetic engineering, the effects of cold spells on bivalves can be reduced, improving their survival and growth. Further research is required to fully understand cold spells’ impacts on bivalves and develop effective mitigation measures.
1 Introduction
As climate change progresses, extreme climatic events such as heat waves, droughts, cyclones, and cold spells are expected to become more frequent and intense (Weilnhammer et al., 2021). Bivalve species, a crucial component of marine environments, are frequently exposed to such fluctuations and are vulnerable to the impacts of these events (He et al., 2023). Extreme low water temperatures, specifically cold spells, can significantly affect the various levels of biological organization in bivalves, leading to declines or cessation of vital activities (Gosling, 2015). Moreover, in aquaculture and natural waters, extreme cold spells can even cause substantial mortality among bivalve populations (Ferreira et al., 2021). Bivalves are of vital importance to marine ecosystems, both ecologically and economically. As a vital food source for many species and a key element in aquaculture, understanding their responses to environmental stressors, such as cold spells, is crucial. Although much has been studied about high-temperature stress responses in bivalves, knowledge about their response to cold stress is limited (Liu et al., 2016).
The effects of cold stress on bivalves have been investigated at different levels of biological organization, and the advances in molecular-genetic, physiological, and biochemical methods have improved our understanding of these effects (Figure 1). This review aims to synthesize the current knowledge on the physiological and molecular mechanisms underlying the effects of cold spells on bivalve species. The focus will be on the changes that occur in various levels of biological organization in response to cold stress and on identifying adaptation and mitigation strategies to cope with these events.
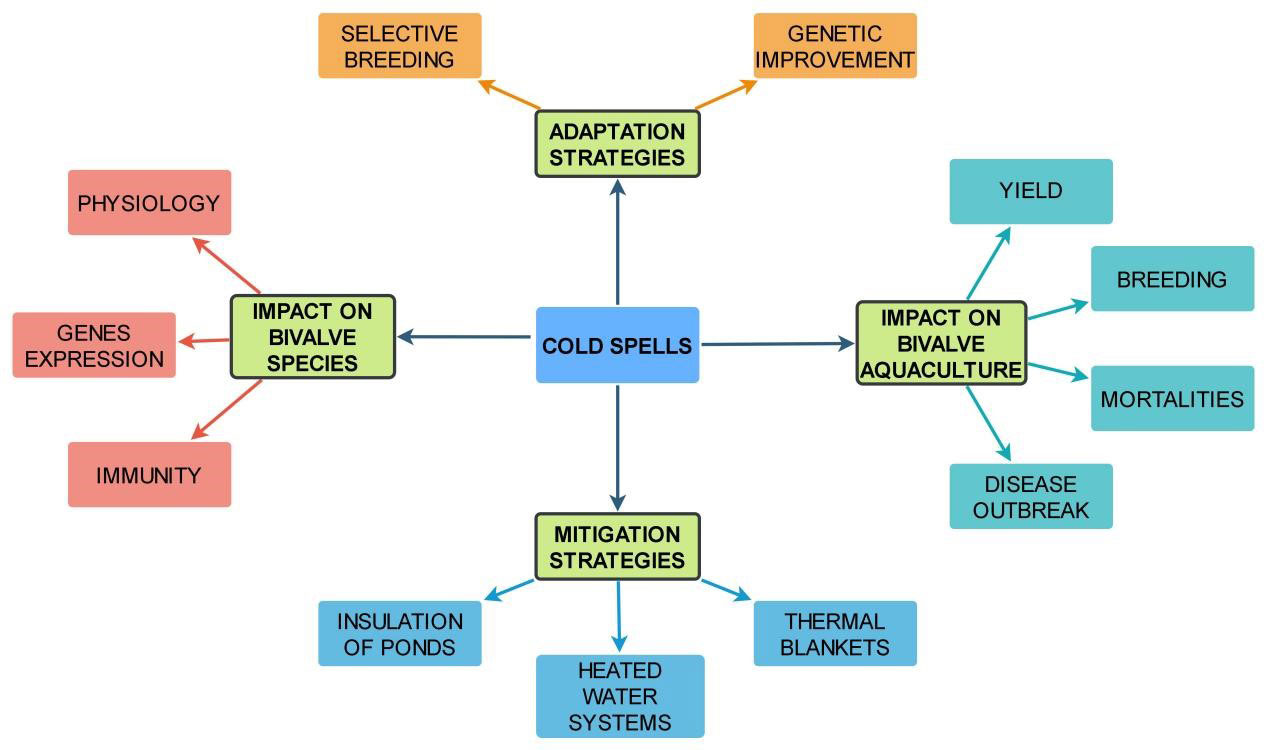
Figure 1 Effects of cold spells on bivalve species physiology, aquaculture yield, and adaptation/mitigation strategies. This figure summarizes the impact of cold spells on bivalve aquaculture, focusing on their effects on the physiology of bivalve species, and aquaculture yield, as well as the various adaptation and mitigation strategies employed to mitigate these impacts.
1.1 Cold spells
Marine cold spells are regional and prolonged instances of anomalously cold ocean water (Schlegel et al., 2021; Figure 2). Although these events have profound ecological and economic impacts, including shifts in species distribution and declines in coastal fisheries (Schlegel et al., 2021), they have received less attention compared to marine heatwaves, which are characterized by elevated ocean temperatures and have been linked to global warming (Hobday et al., 2016). Notably, severe cold spells have resulted in significant fish mortalities, coral bleaching, and macroinvertebrate mortalities in areas such as the North Atlantic Subtropical Gyre (Josey et al., 2018) and the Taiwan Strait (Chang et al., 2013). These events have resulted in economic losses estimated at USD 10 million (Schlegel et al., 2021).
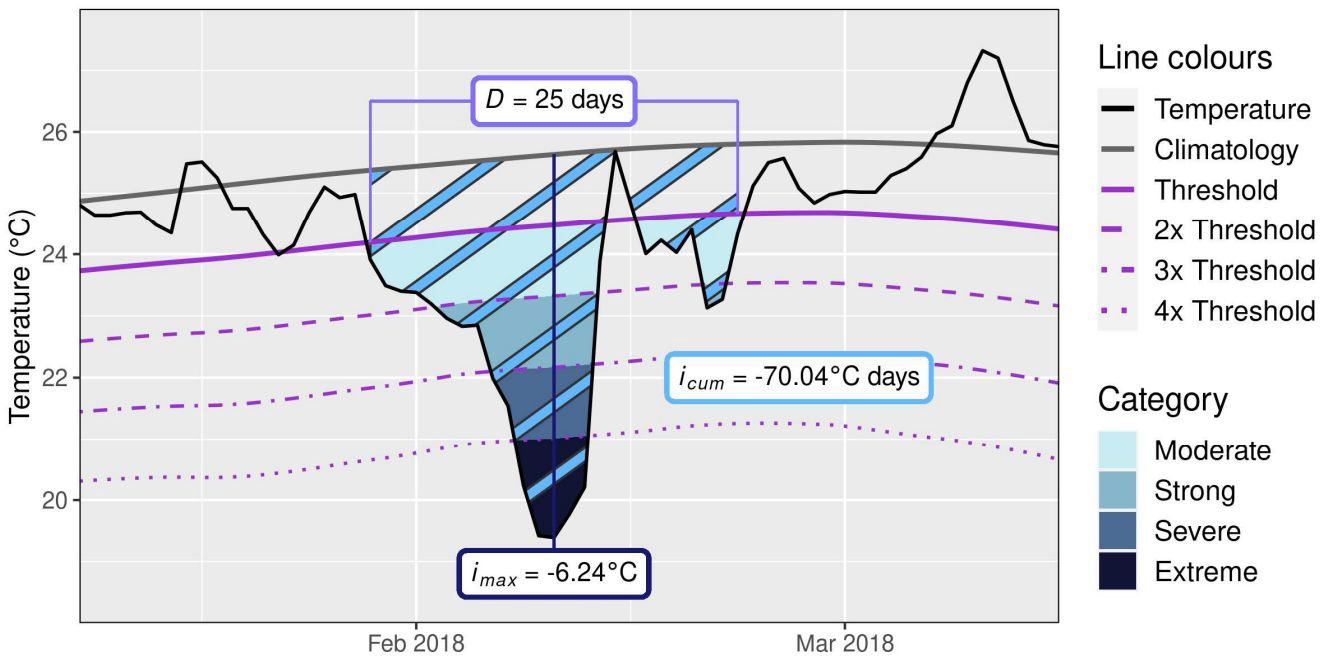
Figure 2 An example of a marine cold spell (MCS). The metrics shown are duration (D; days), maximum intensity (imax; °C), and cumulative intensity (icum; °C days) (Schlegel et al.,2021).
1.1.1 Ecological impacts
The effects of cold spells on bivalves have become an increasingly important area of investigation in the field of ecology. Cold spells have been shown to have a detrimental impact on bivalve development and reproduction, with studies demonstrating reduced growth rates and a decrease in the number of juveniles produced (Cheng et al., 2018; Boroda et al., 2020). This is particularly evident in areas such as China and the North Atlantic coast, where high mortality rates of oysters and mussels have been observed in response to cold spell events (Liu et al., 2016; Baden et al., 2021). The impacts of cold spells on bivalves also have indirect effects on other species within the ecosystem. For example, a cold spell event in China resulted in a reduction in the populations of fish and crabs that rely on bivalves as a food source, potentially leading to a cascading effect throughout the ecosystem (Wakelin et al., 2021). It is clear that the impact of cold spells on bivalves represents a critical issue with far-reaching ecological consequences. Further research is necessary to fully comprehend the effects of cold spells on bivalve populations and the ecosystems in which they reside.
1.1.2 Economic impacts
The impact of cold spells on bivalve populations has far-reaching economic consequences, as bivalves, particularly oysters and mussels, are a critical component of the global aquaculture industry valued at over $10 billion (FAO, 2018). Cold spells can result in a significant decline in the growth and reproduction of bivalves, thereby reducing yields for commercial bivalve fisheries and leading to economic losses for both fishers and the fishing industry. Studies by Möllmann (2019) and Whitfield et al. (2016) have reported that cold spells in the Baltic Sea and Western Cape, South Africa, respectively, caused reductions in the growth of the common Baltic clam (Macoma balthica), Western Cape rock lobster (Jasus lalandii) by up to 50% and 20% respectively; The Pacific oyster (Crassostrea gigas) has been extensively studied in terms of the impacts of marine cold spells, and studies have indicated that prolonged cold spells can cause decreased growth and increased mortality in oysters (Büttger et al., 2011), leading to reduced yields for oyster aquaculture operations and economic losses for farmers and related industries. An extensive literature search was conducted using the terms “cold spell,” “cold wave,” “cold event,” “cold water,” “cold-extreme,” “cold shock,” “cold stress,” and “cold temperature” in Google Scholar, Research Gate, and Semantic Scholar to provide a comprehensive examination of the topic.
2 Impacts of cold spells on bivalve physiology
The impact of cold spells on bivalve physiology, with significant findings indicating alterations in growth, reproduction, decrease in immunity, and metabolism (Carneiro et al., 2020). Studies by Lesser et al. (2010) and Brumbaugh et al. (2010) have shown that cold spells can reduce the growth rate of the blue mussel (Mytilus edulis) and decrease the number of eggs produced by the Atlantic oyster (Crassostrea virginica) by up to 80%, respectively. These impacts can negatively affect the species’ population size and, thus, the ecosystem. The reduction in fertility observed in bivalves during cold spells has been attributed to changes in the production of reproductive hormones, such as estrogen and testosterone (Liu et al., 2016). The disruption of hormone production is linked to the down-regulation of genes involved in their synthesis and alterations in the signaling pathways that regulate reproduction, including the hypothalamic-pituitary-gonadal axis (Yan et al., 2018).
The decrease in immunity leading to higher disease incidence is an important physiological effect of the cold spell on bivalves. Cold exposure can suppress several components of the immune response. This can make bivalves more vulnerable to infections by pathogens, such as bacteria, viruses, and parasites. For example, a study on the clam (Mactra veeriformis) showed that clams underwater temperature stress change at 10°C, 20°C, or 30°C for 24 h. Viable bacterial counts (VBC), total haemocyte count (THC), phagocytic activity, lysozyme activity, Neutral red retention (NRR) times, and superoxide dismutase (SOD) activity were assessed in three different water temperature groups. Clams held at 10°C decreased in THC, lysozyme activity, and NRR (Yu et al., 2009). Another study on Mussel (Mytilus galloprovincialis) kept at 10°C shows lower phagocytic activity than at 20°C and 30°C (Carballal et al., 1997). Therefore, cold spell events can have significant impacts on the health and survival of bivalve populations. In conclusion, cold spells significantly impact the physiology of bivalve species, particularly regarding growth, reproduction, immunity, and hormone production. Further research is necessary to better understand the mechanisms behind these impacts and the potential ecological consequences.
3 Impacts of cold spells on bivalve molecular level
3.1 Gene expression
The alteration of gene expression is a significant effect of cold spells on bivalves. Exposure to low temperatures can activate a multitude of molecular pathways, including those involved in stress response, immunity, and metabolism. For instance, Zhu et al. (2016) reported a substantial increase in the expression of genes related to stress response, such as heat shock proteins (HSPs) and antioxidant enzymes, in Pacific oysters (Crassostrea gigas) after 24 hours of exposure to cold temperatures. This modulation of gene expression may play a role in the oysters’ coping mechanisms against cold stress by offering cellular protection and facilitating repair and recovery.
Similarly, a study by Li et al. (2020) demonstrated a significant increase in the expression of genes related to immunity, including antimicrobial peptides and lectins, in blue mussels (Mytilus edulis) after 48 hours of exposure to cold temperatures. This change in gene expression could help mussels defend against more prevalent pathogens in cold environments. In conclusion, these findings highlight the importance of further investigating the molecular mechanisms underlying the effects of cold spells on bivalves, as they may provide insight into the adaptation strategies of these organisms.
3.2 Biochemical composition
Cold stress in bivalves is characterized by alterations in temperature-sensitive enzymes and increased production of HSPs (Boroda et al., 2020). HSPs are a group of proteins that are activated in response to stress and play a crucial role in safeguarding cells from damage (Kregel, 2002). The expression of HSPs is a widespread response to stress in various organisms, including bivalves (Fabbri et al., 2008). One of the essential physiological responses to cold stress in bivalves is the synthesis of antifreeze proteins (AFPs) (Storey and Storey, 2013). These proteins bind to ice crystals, inhibiting their growth and thereby protecting cells from freezing damage (Storey and Storey, 2013). Bivalves have been shown to produce several types of AFPs, including type I and type II (Dong et al., 2022). The effects of cold stress on bivalves also involve changes in biomolecule levels, such as lipids and carbohydrates (Margesin et al., 2007). For instance, the levels of certain lipid types, such as wax esters and phospholipids, increase in response to cold stress (Copeman and Parrish, 2003; Margesin et al., 2007). This is believed to aid bivalves in maintaining cell membrane integrity and shielding against freezing damage (Storey and Storey, 2013). In the blue mussel (Mytilus edulis), cold stress has been observed to increase the expression of HSP70 and HSP90, which are involved in protein folding and repairing damaged proteins (Ioannou et al., 2009). Similarly, the Pacific oyster (Crassostrea gigas) experiences an increase in the expression of HSP70 and HSP60 during cold stress, which protects cellular proteins from damage (Chen et al., 2018). Besides HSP activation, bivalves utilize other molecular mechanisms to cope with cold stress. For example, the expression of antioxidant enzymes, such as superoxide dismutase (SOD) and catalase, is elevated in response to cold stress to protect cells from oxidative damage (Storey and Storey, 2013; Boroda et al., 2020). Additionally, bivalves increase the expression of cold-shock proteins (CSPs) to adapt to low temperatures. CSPs are a family of proteins that counteract some harmful effects of temperature downshift and thus help the cells to adapt example is Ybox (Karlson et al., 2002; Kohno et al., 2003), which regulates mRNA translation and protect cellular proteins from damage (Dong et al., 2020). The study on the long-term effects of low temperature on mussel species revealed significant changes in the gill membrane composition, which were found to be associated with alterations in the fatty acid profile. Specifically, the analysis showed an increase in the levels of unsaturated fatty acids, including non-methylene-interrupted ones (NMIFA), and a decrease in the concentration of saturated fatty acids in the gills of the mussels (Chao et al., 2020). Moreover, we observed a notable rise in the cholesterol level in mussels exposed to a temperature of 5°C (Chao et al., 2020). These findings suggest that low temperatures can significantly impact the biochemical composition of mussel gills, potentially affecting their physiological functions. Further research is needed to explore the mechanisms underlying these changes and their potential ecological implications.
3.3 Impacts on immune responses
Several investigations have evaluated the effects of marine cold spells on the immune system of bivalves. Findings indicate that such events can impair the immune function of bivalves and increase their vulnerability to diseases. Tan et al. (2020) observed a decrease in the expression of immune genes in the (Chlamys farreri) scallop due to a marine cold spell. In addition to gene expression changes, cold spells can also affect the levels of immune proteins. Chen et al. (2007) reported a reduction in the hemocyte lysate protein levels in (Chlamys farreri) scallops following a cold spell. The reduced immune response of bivalves resulting from marine cold spells may result in increased disease incidence, as demonstrated by Tan et al. (2020) in the (Chlamys farreri) scallop, where a cold spell resulted in a higher occurrence of (Vibrio splendidus) disease. Long-term exposure to low temperatures can have significant impacts on the immune response and gene expression of bivalves, potentially leading to changes in their physiological functions and survival. One study on the blue mussel (Mytilus edulis) found that exposure to cold temperatures caused changes in the expression of immune-related genes, including genes involved in immune recognition, phagocytosis, and oxidative stress response (Boroda et al., 2020). The current review highlights the detrimental effects of marine cold spells on the immune response of bivalves, causing an increase in their susceptibility to diseases. However, further research is necessary to comprehend the underlying mechanisms. Future studies should particularly focus on exploring the effects of cold spells on diverse bivalve species.
4 Mitigation and adaptation strategies to reduce the impacts of cold spells
4.1 Mitigation strategies
Mitigation strategies aim to reduce the negative impacts of cold spells on bivalve populations by preventing or reducing the occurrence of cold spells. Some potential mitigation strategies include mitigating the impacts of cold spells on bivalves, including using thermal blankets, heated water systems, and insulation of ponds and tanks. Thermal blankets, made of materials such as polyethylene or fiberglass, can be placed on the surface of ponds or tanks to reduce heat loss. Heated water systems can also be used to maintain water temperatures at optimal levels for bivalve growth. Insulation of ponds and tanks can also help to reduce heat loss and maintain optimal water temperatures. In addition, habitat restoration can increase the resilience of bivalve populations to cold spells by creating more suitable conditions for bivalves to survive and grow. This can be achieved through the restoration of seagrass beds, oyster reefs, and other habitats that provide protection from cold temperatures. For example, seagrass beds can act as a thermal buffer, reducing the effects of cold spells on bivalve populations (McCay and Rowe, 2003). Finally, temperature monitoring can provide early warning of cold spells, allowing for proactive management of bivalve populations. For example, by monitoring the temperature in oyster aquaculture sites, farmers can take measures to protect their stock from cold spells (Mark et al., 2003; Atindana et al., 2020).
4.2 Adaptation strategies
Adaptation strategies for reducing the impacts of cold spells on bivalves include selecting cold-tolerant species and using genetic improvement programs (Aarset,1982; Paget et al., 2014). Cold-tolerant species, such as the Pacific oyster (Crassostrea gigas) and the European flat oyster (Ostrea edulis), are better able to withstand cold temperatures and are, therefore, less susceptible to cold-related mortality. Genetic improvement programs, such as selective breeding, can also be used to improve the cold tolerance of bivalve populations. Overwintering is when some organisms survive the winter season by either passing through it or waiting it out. During this time, conditions such as cold temperatures, ice, snow, and limited food supplies make survival difficult, notably in insects (Bale and Hayward, 2010), birds (Latta and Faaborg,2009), and plants (Adams et al., 2004), this a strategy that can be used to protect bivalve populations from cold spells. This can be achieved by moving the bivalves to a location where the water temperature is warmer or by providing them with a suitable overwintering habitat. Mitigating the impacts of cold spells on bivalves is a critical issue for the aquaculture industry, as these events can result in significant mortality and reduced growth rates.
5 Conclusion
In conclusion, cold events have a substantial impact on bivalve species, leading to reductions in growth and reproductive capacity, feeding and metabolic rates, and heightened mortality rates. To cope with these adverse conditions, bivalves have evolved mechanisms such as burrowing into the sediment, altering the composition of their bodily fluids, and modulating their physiology and molecular pathways. However, the effects of cold events on bivalve species are variable and contingent upon the severity, duration, and physiological and molecular resilience. While global trends suggest a decline in the frequency of cold events, additional research is required to fully comprehend the effects on bivalve populations and to devise more effective research and management strategies that mitigate these impacts.
Author contributions
We hereby declare the credited author states as follows: FM: Writing – original draft, data curation, investigation, validation. YX: Data curation, Writing – review & editing, Validation. KY: Data curation, Writing – review & editing, Validation. RM: Writing – review & editing. YD: Conceptualization, Resources. LZ: Conceptualization, Writing-original draft preparation, Project administration. Kind regards, LZ on behalf of all other co-authors. All authors contributed to the article and approved the submitted version.
Funding
The current research has been facilitated by funding from the following organizations: Department of Education of Guangdong Province (grant numbers 2020KTSCX050 and 2022ZDZX4012), Guangdong Zhujiang Talents Program (grant number 2021QN02H665), National Science Foundation of China (grant numbers 42076121, M-0163, and 42211530423), Modern Agro-industry Technology Research System (earmarked fund CARS-49), and the Scientific Research Start-up Funds program of Guangdong Ocean University.
Conflict of interest
The authors declare that the research was conducted in the absence of any commercial or financial relationships that could be construed as a potential conflict of interest.
Publisher’s note
All claims expressed in this article are solely those of the authors and do not necessarily represent those of their affiliated organizations, or those of the publisher, the editors and the reviewers. Any product that may be evaluated in this article, or claim that may be made by its manufacturer, is not guaranteed or endorsed by the publisher.
References
Aarset A. V. (1982). Freezing tolerance in intertidal invertebrates (a review). Comp. Biochem. Physiol. Part A: Physiol. 73.4, 571–580. doi: 10.1016/0300-9629(82)90264-X
Adams W. W., Zarter C. R., Ebbert V., Demmig-Adams B. (2004). Photoprotective strategies of overwintering evergreens. Bioscience 54.1, 41–49. doi: 10.1641/0006-3568(2004)054[0041:PSOOE]2.0.CO;2
Atindana S. A., Fagbola O., Ajani E., Alhassan E. H., Ampofo-Yeboah A. (2020). Coping with climate variability and non-climate stressors in the West African oyster (Crassostrea tulipa) fishery in coastal Ghana. Maritime Stud. 19.1, 81–92. doi: 10.1007/s40152-019-00132-7
Baden S., Hernroth B., Lindahl O. (2021). Declining populations of mytilus spp. in north Atlantic coastal waters–a Swedish perspective. J. Shellfish Res. 40 (2), 269–296. doi: 10.2983/035.040.0207
Bale J. S., Hayward S. A. L. (2010). Insect overwintering in a changing climate. J. Exp. Biol. 213.6, 980–994. doi: 10.1242/jeb.037911
Brumbaugh R., Beck M. W., Hancock B., Meadows A., Spalding M., zu Ermgassen P. (2010). Changing a management paradigm and rescuing a globally imperiled habitat. National Wetlands Newsletter 32, 16–20.
Boroda A. V., Kipryushina Y. O., Odintsova N. A. (2020). The effects of cold stress on Mytilus species in the natural environment. Cell Stress Chaperones 25.6, 821–832. doi: 10.1007/s12192-020-01109-w
Büttger H., Nehls G., Witte S. (2011). High mortality of pacific oysters in a cold winter in the north-Frisian wadden Sea. Helgoland Mar. Res. 65.4, 525–532. doi: 10.1007/s10152-011-0272-1
Carballal M. J., López C., Azevedo C., Villalba A. (1997). In vitro Study of phagocytic ability of mytilus galloprovincialis lmk haemocytes. Fish Shellfish Immunol. 7, 403–416. doi: 10.1006/fsim.1997.0094
Carneiro A. P., Soares C. H. L., Manso P. R. J., Pagliosa P. R. (2020). Impact of marine heat waves and cold spell events on the bivalve anomalocardia flexuosa: a seasonal comparison. Mar. Environ. Res. 156, 104898. doi: 10.1016/j.marenvres.2020.104898
Chang Y., Lee M. A., Lee K. T., Shao K. T. (2013). Adaptation of fisheries andmariculture management to extreme oceanic environmental changes and climate variability in Taiwan. Mar. Policy 38, 476–482. doi: 10.1016/j.marpol.2012.08.002
Chao Y. C., Merritt M., Schaefferkoetter D., Evans T. G. (2020). High-throughput quantification of protein structural change reveals potential mechanisms of temperature adaptation in mytilus mussels. BMC Evol. Biol. 20 (1), 28. doi: 10.1186/s12862-020-1593-y
Chen B., Feder M. E., Kang L. (2018). Evolution of heat-shock protein expression underlying adaptive responses to environmental stress. Mol. Ecol. 27.15, 3040–3054. doi: 10.1111/mec.14769
Chen M. Y., Yang H. S., Delaporte M., Zhao S. J., Xing K. (2007). Immune responses of the scallop Chlamys farreri after air exposure to different temperatures. J. Exp. Mar. Biol. Ecol. 345.1, 52–60. doi: 10.1016/j.jembe.2007.01.007
Cheng M. C., Sarà G., Williams G. A. (2018). Combined effects of thermal conditions and food availability on thermal tolerance of the marine bivalve, perna viridis. J. thermal Biol. 78, 270–276. doi: 10.1016/j.jtherbio.2018.10.014
Copeman L. A., Parrish C. C. (2003). Marine lipids in a cold coastal ecosystem: Gilbert bay, Labrador. Mar. Biol. 143 (6), 1213–1227. doi: 10.1007/s00227-003-1156-y
Dong S., Nie H., Li D., Cai Z., Sun X., Huo Z., et al. (2020). Molecular cloning and characterization of y-box gene (Rpybx) from Manila clam and its expression analysis in different strains under low-temperature stress. Anim. Genet. 51.3, 430–438. doi: 10.1111/age.12919
Dong S., Wang C., Nie H., Yin Z., Zhang Y., Jiang K., et al. (2022). Type II ice structuring protein (ISP II) gene and its potential role in low-temperature tolerance in Manila clam, Ruditapes philippinarum. Aquaculture 549, 737723. doi: 10.1016/j.aquaculture.2021.737723
Fabbri E., Valbonesi P., Franzellitti S. (2008). HSP expression in bivalves. Invertebrate survival J. 5.2, 135–161.
FAO (2018). The state of world fisheries and aquaculture 2018 - meeting the sustainable development goals (Rome) Licence: CC BY-NC-SA 3.0 IGO.
Ferreira J. G., Taylor N. G., Cubillo A., Lencart-Silva J., Pastres R., Bergh Ø., et al. (2021). An integrated model for aquaculture production, pathogen interaction, and environmental effects. Aquaculture 536, 736438. doi: 10.1016/j.aquaculture.2021.736438
Gosling E. (2015). Ecology of bivalves. In Marine bivalve molluscs, Gosling E. (Ed.). doi: 10.1002/9781119045212.ch3
He G., Xiong X., Peng Y., Yang C., Xu Y., Liu X., et al. (2023). Transcriptomic responses reveal impaired physiological performance of the pearl oyster following repeated exposure to marine heatwaves. Sci. Total Environ. 854, 158726. doi: 10.1016/j.scitotenv.2022.158726
Hobday A. J., Alexander L. V., Perkins S. E., Smale D. A., Straub S. C., Oliver E. C., et al. (2016). A hierarchical approach to defining marine heatwaves. Prog. Oceanography 141, 227–238. doi: 10.1016/j.pocean.2015.12.014
Ioannou S., Anestis A., Pörtner H. O., Michaelidis B. (2009). Seasonal patterns of metabolism and the heat shock response (HSR) in farmed mussels Mytilus galloprovincialis. J. Exp. Mar. Biol. Ecol. 381.2, 136–144. doi: 10.1016/j.jembe.2009.09.014
Josey S. A., Hirschi J. J. M., Sinha B., Duchez A., Grist J. P., Marsh R. (2018). The recent Atlantic cold anomaly: causes, consequences, and related phenomena. Annu. Rev. Mar. Sci. 10, 475–501. doi: 10.1146/annurev-marine-121916-063102
Karlson D., Nakaminami K., Toyomasu T., Imai R. (2002). A cold-regulated nucleic acid-binding protein of winter wheat shares a domain with bacterial cold shock proteins. J. Biol. Chem. 277, 35248–35256. doi: 10.1074/jbc.M205774200
Kohno K., Izumi H., Uchiumi T., Ashizuka M., Kuwano M. (2003). The pleiotropic functions of the y-box-binding protein, YB-1. BioEssays 25, 691–698. doi: 10.1002/bies.10300
Kregel K. C. (2002). Invited review: heat shock proteins: modifying factors in physiological stress responses and acquired thermotolerance. J. Appl. Physiol. 92.5, 2177–2186. doi: 10.1152/japplphysiol.01267.2001
Latta S. C., Faaborg J. (2009). Benefits of studies of overwintering birds for understanding resident bird ecology and promoting development of conservation capacity. Conserv. Biol. 23.2, 286–293. doi: 10.1111/j.1523-1739.2008.01098.x
Lesser M. P., Bailey M. A., Merselis D. G., Morrison J. R. (2010). Physiological response of the blue mussel Mytilus edulis to differences in food and temperature in the gulf of Maine. Comp. Biochem. Physiol. Part A: Mol. Integr. Physiol. 156.4, 541–551. doi: 10.1016/j.cbpa.2010.04.012
Li D., Nie H., Jahan K., Yan X. (2020). Expression analyses of c-type lectins (CTLs) in Manila clam under cold stress provide insights for its potential function in cold resistance of Ruditapes philippinarum. Comp. Biochem. Physiol. Part C: Toxicol. Pharmacol. 230, 108708. doi: 10.1016/j.cbpc.2020.108708
Liu Y., Wang M., Wang W., Fu H., Lu C. (2016). Chilling damage to mangrove mollusk species by the 2008 cold event in southern China. Ecosphere 7.6, e01312. doi: 10.1002/ecs2.1312
Margesin R., Neuner G., Storey K. B. (2007). Cold-loving microbes, plants, and animals–fundamental and applied aspects. Naturwissenschaften 94.2, 77–99. doi: 10.1007/s00114-006-0162-6
Mark S., Provencher L., Munro J. (2003). Approach for the assessment and monitoring of marine ecosystem health with application to the mya-macoma community. Can. Tech Rep. Fish Aquat Sci./Rapp Tech Can. Sci. Halieut Aquat 2491), 87.
McCay D. P. F., Rowe J. J. (2003). Habitat restoration as mitigation for lost production at multiple trophic levels. Mar. Ecol. Prog. Ser. 264, 233–247. doi: 10.3354/meps264233
Möllmann C. (2019). “Effects of climate change and fisheries on the marine ecosystem of the Baltic Sea,” in Oxford Research encyclopedia of climate science. doi: 10.1093/acrefore/9780190228620.013.682
Paget C. M., Schwartz J. M., Delneri D. (2014). Environmental systems biology of cold-tolerant phenotype in saccharomyces species adapted to grow at different temperatures. Mol. Ecol. 23.21, 5241–5257. doi: 10.1111/mec.12930
Schlegel R. W., Darmaraki S., Benthuysen J. A., Filbee-Dexter K., Oliver E. C. (2021). Marine cold-spells. Prog. Oceanography 198, 102684. doi: 10.1016/j.pocean.2021.102684
Storey K. B., Storey J. M. (2013). Molecular biology of freezing tolerance. Compr. Physiol. 3.3, 1283–1308. doi: 10.1002/cphy.c130007
Tan K., Zhang H., Lim L., Zheng H. (2020). Selection breeding program of nan'ao golden scallop chlamys nobilis with higher nutritional values and less susceptible to stress. Aquaculture 517, 734769. doi: 10.1016/j.aquaculture.2019.734769
Wakelin S., Townhill B., Engelhard G., Holt J., Renshaw R. (2021). Marine heatwaves and cold-spells, and their impact on fisheries in the southern North Sea. doi: 10.5194/egusphere-egu21-7329
Weilnhammer V., Schmid J., Mittermeier I., Schreiber F., Jiang L., Pastuhovic V., et al. (2021). Extreme weather events in Europe and their health consequences–a systematic review. Int. J. Hygiene Environ. Health 233, 113688. doi: 10.1016/j.ijheh.2021.113688
Whitfield A. K., James N. C., Lamberth S. J., Adams J. B., Perissinotto R., Rajkaran A., et al. (2016). The role of pioneers as indicators of biogeographic range expansion caused by global change in southern African coastal waters. Estuarine Coast. Shelf Sci. 172, 138–153. doi: 10.1016/j.ecss.2016.02.008
Yan L., Li Y., Wang Z., Su J., Yu R., Yan X., et al. (2018). Stress response to low temperature: transcriptomic characterization in Crassostrea sikamea× Crassostrea angulata hybrids. Aquaculture Res. 49 (10), 3374–3385. doi: 10.1111/are.13801
Yu J. H., Song J. H., Choi M. C., Park S. W. (2009). Effects of water temperature change on immune function in surf clams, Mactra veneriformis (Bivalvia: mactridae). J. invertebrate Pathol. 102 (1), 30–35. doi: 10.1016/j.jip.2009.06.002
Keywords: cold spells, bivalve, physiology, mitigation, climate change
Citation: Masanja F, Xu Y, Yang K, Mkuye R, Deng Y and Zhao L (2023) Surviving the cold: a review of the effects of cold spells on bivalves and mitigation measures. Front. Mar. Sci. 10:1158649. doi: 10.3389/fmars.2023.1158649
Received: 04 February 2023; Accepted: 10 April 2023;
Published: 21 April 2023.
Edited by:
Vladimir Laptikhovsky, Centre for Environment, Fisheries and Aquaculture Science (CEFAS), United KingdomReviewed by:
Xizhi Huang, Johannes Gutenberg University Mainz, GermanyYuan Wang, Dalian Ocean University, China
Copyright © 2023 Masanja, Xu, Yang, Mkuye, Deng and Zhao. This is an open-access article distributed under the terms of the Creative Commons Attribution License (CC BY). The use, distribution or reproduction in other forums is permitted, provided the original author(s) and the copyright owner(s) are credited and that the original publication in this journal is cited, in accordance with accepted academic practice. No use, distribution or reproduction is permitted which does not comply with these terms.
*Correspondence: Liqiang Zhao, bHpoYW9AZ2RvdS5lZHUuY24=