- Escuela de Biología en Acuicultura, Universidad Nacional del Santa, Av. Universitaria S/N, Nuevo Chimbote, Peru
The development of industrial mariculture in Peruvian coasts will continue to grow according to global trends; however, it is characterized by being mostly monospecific, resulting in ecosystemic impacts due to organic matter, which deteriorate the benthic system and encourage eutrophication, negatively affecting biodiversity and fishery resources, which in a long term could turn the activity into an unsustainable practice. Therefore, it is imperative to adopt new production models, focused on the sustainability principle, such as Integrated Multi-Trophic Aquaculture (IMTA), that allow for greater efficiency, competitiveness, and profitability, while guaranteeing environmental balance. In this context, the present study has addressed the problem of Argopecten purpuratus cultivation in Samanco Bay, in which, based on a diagnosis of the bay's conditions, details of the cultivation, and a thorough analysis of the IMTA concept, a strategic model for mariculture is proposed in order to be adopted by private companies, after a validation process. These concepts can be transferred for their adaptation to other scenarios. Furthermore, it is expected that the academic sector, private companies, and the competent authority will be able to intervene synergistically in this process.
1 Introduction
Aquaculture is an activity that has provided food for humanity since the ancient ages (Beveridge and Little, 2002). Its origins date back to 3500 B.C. from ancient China (Vela and Ojeda, 2007). However, as a productive-economic activity, it has experienced great growth in recent years, becoming one of the most important economic activities in the world (Asche et al., 2022). Moreover, a major contribution of aquatic resources from aquaculture is expected in the future (Fiorella et al., 2021) since capture fishing has stabilized at levels that are practically impossible to overcome (Gephart et al., 2023). In addition to this, the growing demand for fish (FAO, 2022a), together with global population growth, which is about to reach the milestone of 10 billion people (FAO, 2020), requires that aquaculture activities be developed efficiently and sustainably in order to achieve global food security.
Globally, aquaculture reached a record of 122.6 million tons in 2020, which represents a value of USD 28.5 billion, and is estimated to grow by 13% in 2030 (FAO, 2022b). In 2022, aquaculture provided 50% of the total fish for direct human consumption in the world, being the Asia-Pacific region the major producer (Bartley, 2022). Regarding the diversity of cultivated species, macroalgae currently represent the largest production (51.3%), followed by mollusks (27.4%), crustaceans (9.1%), and other aquatic animals (0.6%) (Chopin and Tacon, 2021). It is important to highlight that modern aquaculture has developed to an advanced technological level that can be extended to all types of ecosystems (Goswami et al., 2023). But it must be recognized that most of the worldwide aquaculture operations are highly dependent on the environment and ecosystem performances (Pailan and Biswas, 2022). Despite this, traditional aquaculture, which is mainly developed in the form of monocultures Park et al. (2018), is disturbing these environments to such a degree that in many regions the activity has been affected to nearly disappear (Han, 2002; Thomsen et al., 2020). Therefore, the current global trend is developing strategies aimed at reducing the impacts of aquaculture to conserve natural capital, thus making it an environmentally friendly, efficient, profitable, and socially beneficial activity (Buschmann et al., 2001).
In Peru, aquaculture is sustained on monoculture: Argopecten purpuratus (36.4%), Oncorhynchus mykiss (34.2%), and Litopenaeus vannamei (24.2%) (PRODUCE, 2022). Here, the culture of the mollusk A. purpuratus notoriously outstands in the bays of Sechura and Samanco, which in 2018, with a production of 89,872 t, ranked 1 in Latin America and 4 in the world (Kluger et al., 2018). During the 20 century, aquaculture development has been characterized by intensive monospecific cultivation, based on species of high commercial value and high economic benefit. However, this aquaculture approach generates an important ecological footprint due to the organic waste produced in the cultivation and processing stages, threatening the integrity of ecosystems and the future viability of the sector (González Henríquez et al., 2015). As a result, aquaculture is often associated with pollution (Vela and Ojeda, 2007).
For producers, it is necessary to find measures that are compatible with profitability, as well as sustainability. In addition, it is imperative to migrate from traditional monoculture practices to integrated and sustainable models (Chopin, 2013), in order to ensure the continuity of the aquaculture activity. Under this need, the ecosystem approach to aquaculture (EAA) concept has emerged, which is considered as a strategy aimed at integrating the activity into a broader ecosystem that articulates sustainable development, equity, and resilience of interconnected socio-ecological systems (Brugère et al., 2019). In this sense, associating two or more species from different trophic levels is the most optimal way to reduce the environmental impacts of aquaculture. This aquaculture approach has the advantage of not generating competition among the organisms in captivity, and additionally, improving the flow of matter and energy in the culture. Therefore, the worldwide trend is to implement integrated multi-trophic aquaculture (IMTA) (Chopin et al., 2001; Buschmann et al., 2013).
The IMTA represents the integration of four types of aquaculture: a) feed (fish), b) organic extractive (filter-feeding and suspension-feeding invertebrates), c) inorganic extractive (macroalgae), and d) deposit extractive (suspension-feeding and sediment-feeding invertebrates) (Chopin et al., 2001; Chopin, 2010; Chopin et al., 2010). Nevertheless, to integrate them it is necessary to establish the proportion between the species, according to their specific roles and the abiotic conditions of the ecosystem. This approach is addressed to maximize the benefits of different trophic levels, diversifying the activity, ensuring environmental sustainability and economic viability, while also improving the aquaculture image across society (Salinas-Morrondo, 2012).
The application of IMTA has been successful in several regions. In China, IMTA has been developed in coastal inlets integrating the culture of Chlamys farreri, Crassostrea gigas and Laminaria japonica, allowing them to estimate the exploitation load capacity of C. farreri and C. gigas in bays and the harvest potential, demonstrating that it is possible to control phytoplankton abundance by managing the density of extractive species (Buschmann et al., 2008b). In Dalian (China), the potential of the marine sponge Hymeniacidon perleve has been investigated, revealing a removal capacity between 44-61% of total organic carbon (TOC) in IMTA in co-culture with the marine fish Fugu rubripes, and also determining an increase in sponge biomass of 22.8% (Fu et al., 2007). Moreover, in Canada, it has been integrated macroalgae Laminariales and mussel Mytilus edulis into the culture of Atlantic salmon in cages, observing that the algae and mussels grow 50% faster than in control sites, proving that the food and nutrient residues generated in the cages have economic potential (Chopin and Robinson, 2004). In that sense, macroalgae can remove dissolved N between 35-100% (Thomas, 2010).
Under the scope of improving aquaculture in accordance with world trends, it is necessary to introduce the IMTA concept in order to be applied in the A. purpuratus cultures developed in Peru. Therefore, the purpose of this work is to present the problems of marine aquaculture in Peru and to develop the concepts and advantages of the IMTA model. This model is vital for the continuity A. purpuratus culture in Samanco Bay, in addition to providing other economic advantages for the aquaculture producers. The model presented here is also suitable for application to other types of aquaculture cultures in other regions of the world.
2 Aquaculture problems in PERU
2.1 Context of the A. purpuratus culture in Peru
The culture of this filter-feeding species is successful due to the high phytoplankton productivity of the Peruvian coast (Guillén and Rondán, 1968). Cultivation is performed in bays such as Sechura, Nonura, Lobos de Tierra (Piura region), Samanco, Guaynuma, Los Chimus, Salinas, Tortugas, Casma (Ancash region), Independencia, and Paracas (Ica region). In total, the culture of A. purpuratus is developed in around 15,999.67 ha, corresponding to 374 rights in modalities of Micro and Small Enterprise Aquaculture (AMYPE, <150 t, 82%), Medium and Large Enterprise Aquaculture (AMYGE, >150 t, 18%) and Aquaculture of Limited Resources (AREL, <3.5 t, <1%) (PRODUCE, 2021). The main form of culture is performed in suspended lines with longline systems, but corrals under the seabed are also used by small-scale producers.
Suspended cultivation is practiced in long-line systems of 100 m, located at a depth of 6 m. Here, lanterns of 2 m in height and 0.5 m in diameter are suspended in the long line systems every 1 m. As a general rule, 3 lines are installed in 1 ha, which remains immovable. The growth process lasts between 12-14 months, and the final phase is carried out at a density of 250 organisms per lantern, employing approximately 49.2 million organisms in 1000 ha approximately. In the final stage of culture, A. purpuratus is harvested at 106.5 ± 23.6 g each.
The evolution of the A. purpuratus culture shows two stages (Figure 1): a slow growth between 1996-2009, and a fast growth from 2010 onwards. The latest is explained mainly by the formalization of a large number of fishermen’s associations that were previously considered informal and were not recorded in the statistics at that time. Notably, the 2010-2018 period is characterized by great instability due to massive mortalities of cultured organisms in Sechura Bay, associated with oxygen deficiency due to harmful algal blooms (HAB) (Gonzales and Yepez, 2007; IMARPE, 2019), the occurrence of El Nino event, such as the one in 2017 (Salazar Camacho, 2023), and deficiencies in the supply of seed from the natural environment.
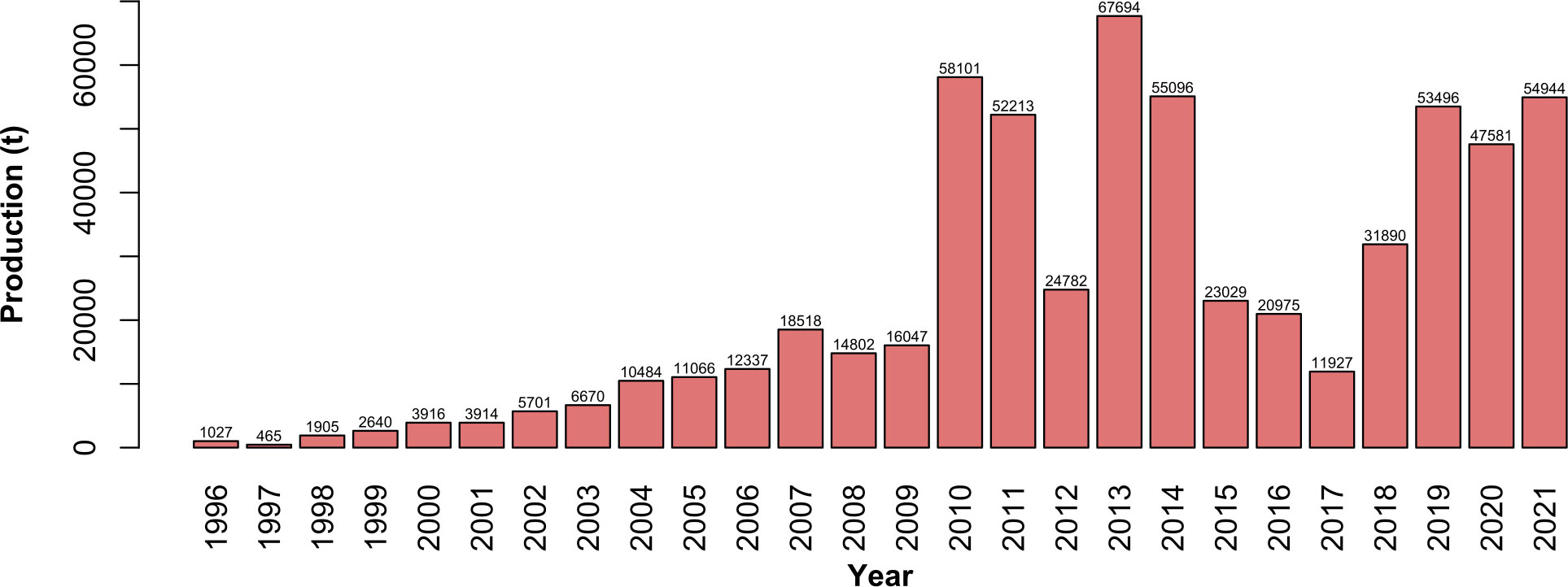
Figure 1 Production of A. purpuratus from aquaculture in Peru, during the period 1996 to 2021 (Cavero and Rodríguez, 2008; PRODUCE, 2015; PRODUCE, 2020; PRODUCE, 2021; PRODUCE, 2022). The production of A. purpuratus had an important growth since 2010, however, due to extraordinary events, such as the El Nino phenomenon, ˜ in 2012 and during the period 2015-17, the production declined significantly. The production remains sustained in the last years despite environmental concerns.
Despite this, the activity generated more than 10,162 new jobs in 2019 (PRODUCE, 2020), and incomes of USD 420.7 million in 2021, from exportation (PRODUCE, 2022), contributing foreign exchange to the country. Although the economic advantages of this activity are significant, it has also caused serious problems derived from organic contamination, with repercussions on water quality, eutrophication, and impacts on benthic biodiversity. Thus, this activity is clearly not aligned with the principles of sustainability and, if not accounted for, could restrict the continuity of the activity in the medium to long term. In addition, it will have repercussions on other economic value chains, small producers, and the recreational landscape of the bays.
2.2 Environmental impacts of A. purpuratus culture in Samanco Bay
The production process generates impacts due to: a) ammonia excretion products from cultured organisms and biofouling, b) biodeposition (feces and pseudofeces) by A. purpuratus and biofouling, and c) disposal of biofouling in situ, due to bad practices, not yet quantified (Loayza Aguilar, 2018). Further contamination is contributed by the disposal of lines, buoys, lanterns, and perl net (broken or unusable), not yet quantified as well.
Biofouling represents the main source of contamination during cultivation. This is composed of algae and invertebrates that develop attached to the culture structures (lanterns, lines, buoys, floating structures). It has been estimated that in the final phase of culture, a lantern can produce between 68.04 to 131.87 kg of biofouling (Loayza and Tresierra, 2014; Loayza Aguilar, 2018). The filter-feeding species Semimytilus algosus and Ciona intestinalis have been identified as the main biofouling components, which account for 58.6 to 73.0 and 22.3 to 50.0% of the biofouling per lantern, respectively (Loayza Aguilar, 2018). Likewise, Uribe et al. (2008) and Uribe and Blanco (2001), reported that in Tongoy Bay (Chile), a lantern can produce between 100 to 120 kg of biofouling every 3 months. These data show the magnitude of impacts by A. purpuratus cultivation.
Besides, feces and pseudofeces are produced by A. purpuratus in conjunction with the biofouling organisms represent an important source of pollution. These pollutants release NH4, NO3, and PO4, which are initially deposited in the water column and then distributed throughout the environment by the effect of marine currents (Buschmann et al., 2001), promoting primary productivity. The release of feces and pseudofeces also promotes the biodeposition of organic matter (OM) in the water column, decreasing transparency. In addition, these particulate residues precipitate to the seabed where they accumulate and modify the granulometric composition of the substrate. This has proven to be the main source of sludge generation at the bottom of the bays. As a consequence, a decrease in dissolved oxygen in the marine environment has been observed, with the release of toxic substances such as H2S and NO2. Naturally, biodiversity and natural banks of interest to small-scale fishing are significantly affected. On the subject, there are no data on the rates of biodeposition and excretion of NH4 from A. purpuratus and biofouling, or about the production of NO3 and PO4 by bacterial action, which we could have a deeper understanding of their impacts.
Regarding the impact of biodeposition, assuming the biodeposition rate recorded in Tongoy Bay (Chile) of 3.9 times the weight of the organisms (Uribe and Blanco, 2001), we can estimate that Samanco Bay would be producing 5,239.8 t in feces and pseudofeces every 12-14 months. This unavoidable quantity, directly related to production volumes, is of great concern, considering that dissipation in the bay is slow according to the currents. Biofouling biodepositions (due to detachment) should be added to this, although due to lack of information, it is not possible to provide accurate estimations.
Among other impacts, we have the disturbance of the benthic ecosystem. Herein, a key aspect to consider is the calcareous valves that are deposited on the seabed, which quantities have not yet been quantified. However, it is known that 67% of the biomass of A. purpuratus is composed by valves (Loayza Aguilar, 2018). The valves cause capping of the substrate, trapping of OM, and increase bacterial activity on the seabed. This event drives an exacerbated production of H2S and NO2, limiting oxygen exchange at the water-sediment interface (anoxic conditions). Studies have been conducted to reduce biofouling production bacteria (Colunche Díaz et al., 2016) and doubling lantern exchange (Loayza Aguilar, 2018), but remain as proposals.
The organic matter released also encourages the eutrophication of the bay. Excessive phytoplankton biomass reduces water transparency and it sediments to the bottom when is not consumed, increasing OM and therefore a microbial activity that demands oxygen in specific areas referred to as “dead zones”. This obviously alters the diversity in the water column and on the seafloor (Buschmann et al., 2008b; Buschmann et al., 2009; Chopin et al., 2012), causing mortality of benthic-demersal and pelagic resources as reported in Sechura Bay in 2019 (IMARPE, 2019). In addition, nutrients promote excess biomass of macroscopic algae, with increased OM decomposition and repercussions on fisheries, and loss of the landscape value of the coasts (Camargo and Alonso, 2007; Buschmann et al., 2008b) This is related to the exuberant production of macroalgae in the bay, such as Caulerpa filiformis and Ulva spp. which, by accumulating on the beaches, affect the habitat and the intertidal and subtidal benthic community, affecting fishery resources such as Donax marincovichi, Emerita analoga, and others of ecological importance, such as Excirolana braziliensis and Lepidopa chilensis, which are food for seabirds.
2.3 Water and sediment quality in Samanco bay
Due to the importance of the aquaculture activity, studies on water and sediment quality are conducted periodically in Samanco Bay. The following tables present the 13-year historical evolution of the conditions concerning water quality and sediment (Tables 1, 2), and those of the A. purpuratus culture concession area (Table 3), compared to the Environmental Quality Standards (EQS) for Water (MINAM, 2017), and the standards of the Canadian Environmental Quality Guidelines (Guidelines CEQ, 1999).
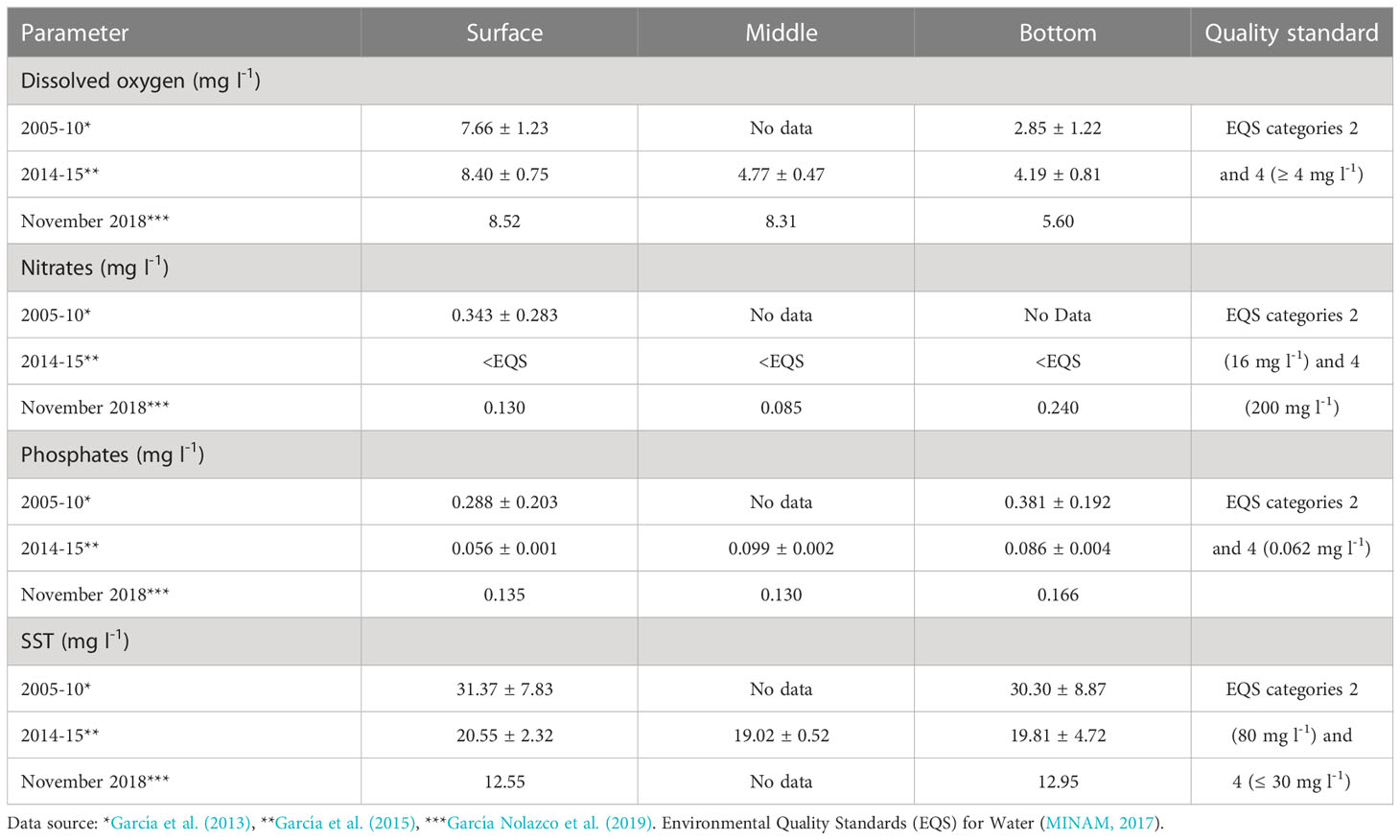
Table 1 Physico-chemical parameters of water in Samanco Bay during the period 2005-10, 2014-15, and November 2018.
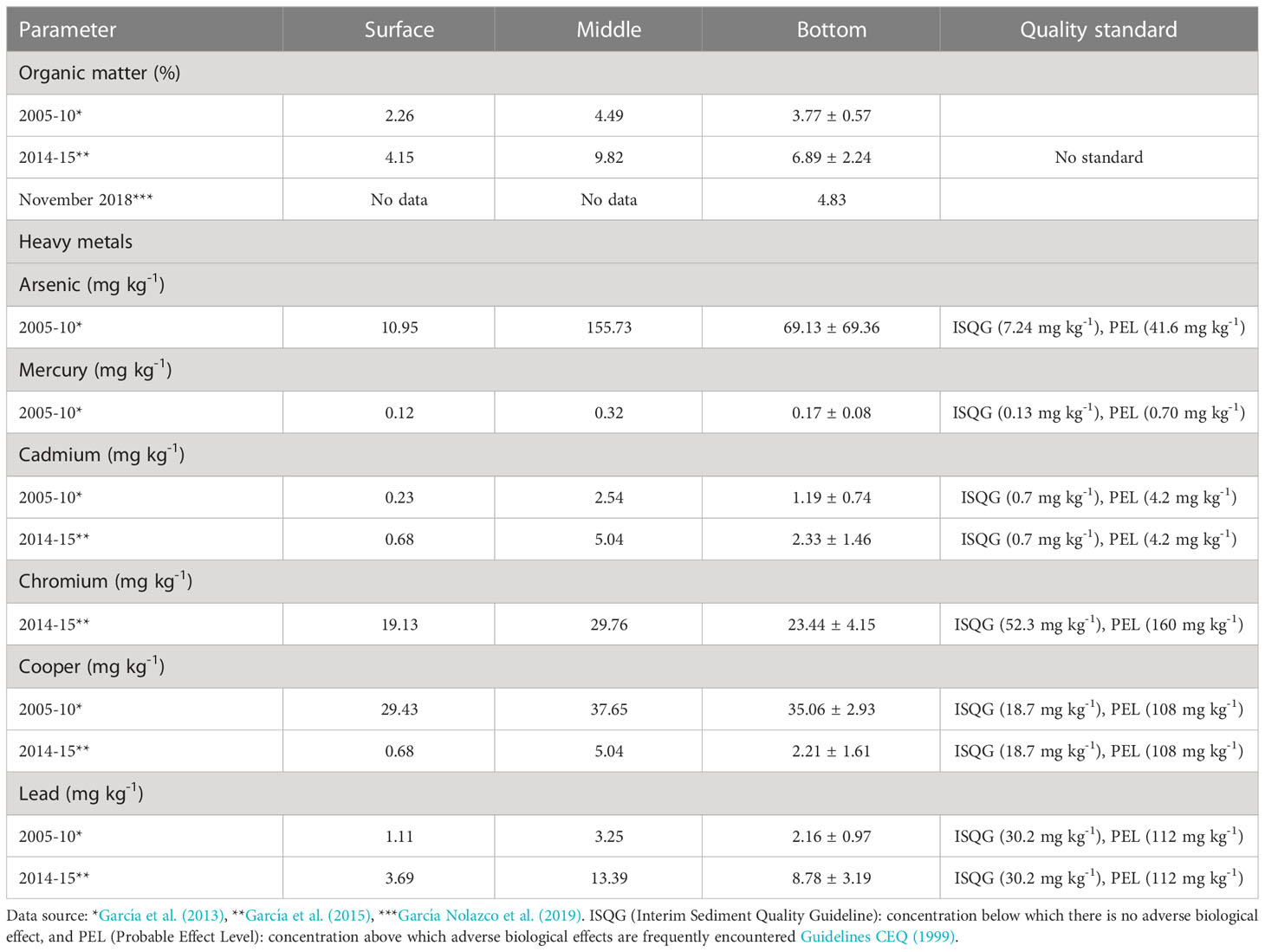
Table 2 Organic and inorganic water pollution in Samanco Bay during the period 2005-10, 2014-15, and November 2018.
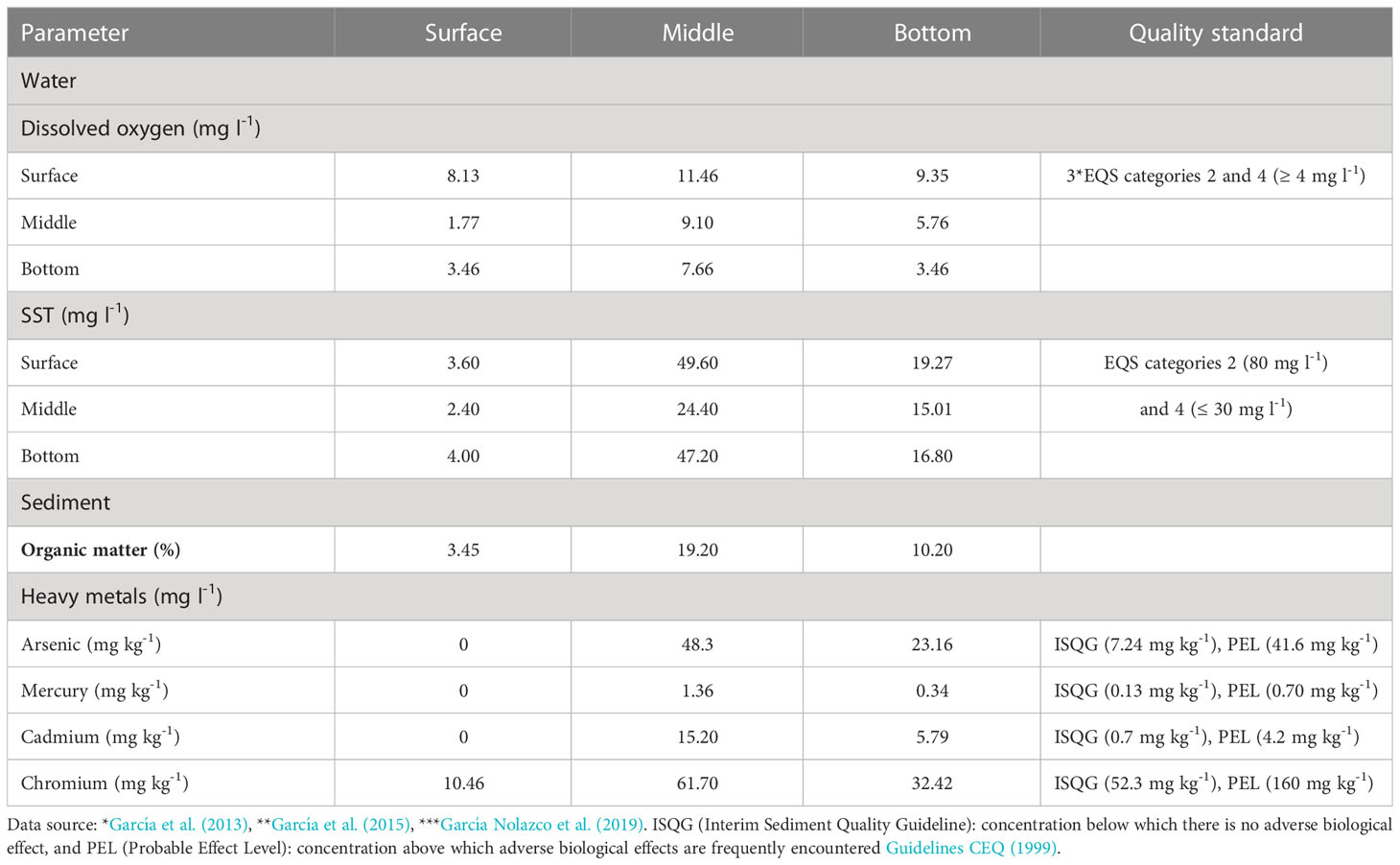
Table 3 Water and sediment quality values in Samanco Bay during the period 2005-10, 2014-15, and November 2018.
Oxygen in the water column tends to increase, exceeding EQS category 2C1 values (Table 1), which can be attributed to a process of eutrophication, linked to an accumulation of nutrients (N and P) due to bacterial mineralization of biodepositions and biofouling in the concession area. Two aspects characterize eutrophication: a) dissolved P is present in excess and dissolved N (NH4, NO2, and NO3) near or below detection levels (Ryther and Dunstan, 1971), and b) levels above 440 µg NT l (0.44 mg l) and 30 µg PT l (0.03 mg l), can generate eutrophication in coastal areas (Swedish, 2000). As shown in Table 1, P is 2.68 to 6.15 times higher than EQS, and is no longer a limiting factor for phytoplankton productivity, hence any excess of N will stimulate eutrophication.
A study evaluated the behavior of oxygen for the period 2013-18, in one of the companies dedicated to the production of A. purpuratus in Samanco Bay, encountering 2.8 ± 0.96 mg l-1 at the bottom (16 m) (Ramos Alfaro and Villegas Ruiz, 2019). This coincides with the data for the periods 2005-10 and 2014-15 (Table 1). As these values are below the EQS category 4E3, benthic community organisms, including those of artisanal fishing, would be affected, with social and economic repercussions for the fishing community. In the cultivation area, OM (Table 3) was between 1.48 and 2.71 times higher than in the rest of the bay (Table 2), confirmed by IMARPE (IMARPE, 2009b) and OEFA (OEFA, 2013), that found values of 7.18% in 2000, 28.17% in 2008, and 20.8% in 2012, with respect to the baseline of 2.14%. In addition, for the period 2013-18, it has been reported that sediment from the cultivation area of one of the companies dedicated to the production of A. purpuratus was characterized by fine-black sludge with a sulfurous odor, and in 75% of the biannual samplings, no live organisms were recorded, but empty shells of S. algosus and A. purpuratus were found (Ramos Alfaro and Villegas Ruiz, 2019). These characteristics are related to the concentration of 279 mg kg-1 of H2S found in 2013 by OEFA (2013), a value 266 times higher than that reported in the baseline (1.05 mg kg-1). These findings demonstrate the impact that the culture exerts, altering not only the chemical and granulometric composition of the sediment but also benthic biodiversity and abundance, including some fishery interest, as A. purpuratus cultures, as well as socio-economic repercussions, in addition to the economic profitability of the company (Figure 2).
The accumulation of biodepositions, biofouling, and phytoplankton biomass on the bottom of Samanco Bay would be increased by the resistance of the anchoring system of the cultivation lines (700 kg weight and 1 m height), from operational and abandoned concessions to the velocity of the bottom currents, retaining OM and calcareous valvae. On the other hand, cultivation operations are carried out in approximately 65% of each concession, which implies the use of roughly 196,800 lanterns, which generate a field of resistance to the current velocity, and therefore an increase in the sedimentation velocity of the seston generated by the cultivation, contributing to the accumulation of OM on the seabed.
There are few studies on the impacts of biodeposition from shellfish culture. In Canada, Richard et al. (2007), observed the accumulation of feces and pseudofeces in the M. edulis and Placopecten magellanicus culture area were twice as high as in the control area, and the abundance of benthic macrofauna was 6 times lower than in the control area. Heavy metals such as Cd, Pb, Cr, As, and Hg in marine sediments are of concern because they bioaccumulate and biomagnify, with high toxicity (Sadiq, 2021), and affect communities (Kennish, 2002), biogeochemical cycles (Sadiq, 2021), and humans who use them in their consumption. In hydrodynamically stable environments, such as Samanco Bay, heavy metals have a strong affinity for finer sediments (Calderón and Valdés, 2012), such as clay, humic acid, OM, Fe, Mn, and CaCO3 oxides, capable of forming complexes with metal ions and being adsorbed (Kennish, 2002; Sadiq, 2021). Deep-sea currents, waves, storms, and activities such as dredging, shipping, and commercial fishing can resuspend heavy metals (Kennish, 2002), and re-enter in food chains.
In Samanco Bay, the sources of heavy metals (Table 2) may be lixiviated from the bedrock area and contributions from the Nepeña River, which irrigates 9,000 ha of agricultural land (ANA, 2009), and its flows discharge in the southeastern part very close to the mouth of the bay (García Nolazco et al., 2019). This river has a small discharge, however, during El Nino events, it has a great influence and may be transporting As, Se, Ba, Cd, Cr, Pb, Hg, and Ag, pollutants that may come from agricultural and livestock farming activities (Metcalf and Eddy, 1995), especially Cd that is an ingredient of phosphate fertilizers (Arnous and Hassan, 2015). The values of Cr, Cu, and Pb in sediments in the bay (Table 2) and within the marine cultivation area are below the Interim Sediment Quality Guideline (ISQG) and Probable Effect Level (PEL); however, As, Cd and Hg are at risk levels, since in the sediment outside the marine concessions they represent, respectively, 9.55, 3.33 and 1.31 times the ISQG, and within the concessions 3.2, 8.27 and 2.62 times, respectively, the ISQG. It is advisable to study the dynamics of these metals in sediments, water, and the bioaccumulation and biomagnification in the biota of Samanco Bay, and in the organisms in culture.
Due to its biological, bathymetric, and marine dynamic qualities, Samanco Bay is suitable for greater development of mariculture. The diagnosis made in previous paragraphs reveals that it urgently requires a new approach. It should focus on two fundamental aspects: a) within the scope of the General Aquaculture Law which invokes two principles: (a1) sustainability, e.g. profitable and competitive aquaculture, but in harmony with the conservation of resources and the ecosystem, which guarantees the satisfaction of the necessities of future generations, and (a2) the ecosystem approach, which considers the environmental, social and institutional dimensions, which encourages equity in the distribution of benefits, respect for the integrity and functionality of ecosystems; and, (b) adopt technologies to satisfy these principles, through the IMTA concept.
3 Integrated multi-trophic aquaculture (IMTA)
3.1 Foundations of the IMTA model
Integrated multi-trophic aquaculture (IMTA) involves the culture of two or more aquatic species from different trophic levels to improve efficiency, reduce waste and provide ecosystem services, such as bioremediation FAO (2022). IMTA is the practice of combining, in appropriate proportions, the culture of fed aquaculture species (e.g. fish and shrimp) with organic extractive aquaculture species (e.g. shellfish and detritivores) and inorganic extractive aquaculture species (e.g. seaweed) (Sasikumar and Viji, 2015). The IMTA’s goal is to create balanced systems for environmental sustainability (biomitigation), economic stability (product diversification and risk reduction), and social acceptability (best management practices). Currently, IMTA is an appropriate method for developing economically viable and socially beneficial coastal aquaculture Hossain et al. (2022).
A fundamental aspect of developing the IMTA model is species selection. Careful consideration must be given to the suitability of species in a particular habitat/farming unit, and they must be economically viable as aquaculture products Sukhdhane et al. (2018). To this end, the following aspects are recommended for the selection of species in the IMTA model: a) the selected species must have complementary roles with the other species in the system, b) they must be species adaptable to the cultivation technologies and environmental conditions of the site, c) they must possess the capacity to provide efficient biomitigation, and d) market demand and an attractive price (Sasikumar and Viji, 2015).
3.2 Perception
Mariculture in the 20 century did not acknowledge that when the development of any economic activity exceeds natural limits, it implies the deterioration of ecosystems, which can be irreversible and hinder the permanence of the activity that originates them (Martínez and Roca, 2015). For instance, in the Bay of Fundy (Canada), the production of 35,000 t of salmon had an exogenous input of 1,225 t of N and 245 t of P, a load that is retained, since the residence time of the water within the bay is about 76 days (Chopin et al., 2007). At fish cage culture locations, N can be 1.8-2.3 times more compared to a control site (Wen et al., 2007), accumulate sediment within 100 m and elevate N and P by 8-25 times up to 1,000 m from the cages (Tolga et al., 2017), promoting eutrophication and affecting the resilient capacity of the ecosystem.
In view of the situation described above, a new approach is required, which contemplates the ecosystemic balance, using species of multiple trophic levels. The approach of culturing organisms as primary producers, filter feeders, detritivores, and carnivores in a defined area, allows the recycling of the waste produced, minimizing environmental deterioration, under a circular economy approach (Buschmann et al., 2013; González Henríquez et al., 2015; Correia et al., 2020). This brings us to the point of addressing the IMTA concept, which guarantees the viability of aquaculture in the long term, generating social acceptability.
3.3 IMTA model proposal for Samanco Bay
The proposed IMTA model (Figure 3) is a design for environmental sustainability, and implies using cultures in proximity, with commercially valuable species of different trophic levels and with complementary ecosystemic functions, which synergistically act in the recycling and biomitigation processes (Chopin et al., 2010; Chopin et al., 2012; González Henríquez et al., 2015). In this sense, the system would be integrated by: a) extractive aquaculture of organic suspension, which represents the production of A. purpuratus, b) extractive aquaculture of inorganic suspension, based on macroalgae, and c) extractive aquaculture of deposit, using holothurians (Figure 4). In this model, the OM (feces, pseudofeces, biofouling organisms) are disposed into the water by the main culture, and the nutrients released, such as N and P, are no longer contaminants as they are biotransformed into valuable resources by the other organisms.
As a result of the implementation of the IMTA model, we would have: biodiversity preserved, aquaculture diversified and profitability improved, as the proposed biotransformer species have current or potential economic value (Table 4). As an additional advantage, the main culture will be more efficient by reducing the impacts of eutrophication and HAB, and therefore more profitable. These advantages are transferred to the ecosystem, since, as less OM reaches the bottom and the accumulated is used by the organisms in the benthic system; therefore, the artisanal fishing activity and the balneability of the summer beaches would be recovered. This translates into social benefits for the activity, thus improving its image in society. This principle, which is practiced on a commercial scale mainly in China, improves the sustainability of the aquaculture systems, clearly reducing environmental risks and increasing profits (Han et al., 2016).
It is worth mentioning that, in the IMTA context, marine macroalgae are an indispensable and mandatory component to solve nutrient accumulation, since they constitute the primary organisms that require solar energy and sequester C, N, and P from the environment (Neori et al., 2004). In this sense, its great bioremediation capacity is highlighted. (Buschmann et al., 2013). The best criterion for choosing algal species for integration into the IMTA is to combine commercial value with high growth rates and N concentration in their tissues (Neori et al., 2004).
On the other hand, holothurians or sea cucumbers as deep-sea extractive organisms, act as nutrient recyclers and bioturbation agents, allowing bottom oxygenation and preventing OM stratification. Hence, their participation is indispensable for the management of marine ecosystems (Han et al., 2016; Tolga et al., 2017), and enhances the detrimental effects of coastal mariculture (Purcell et al., 2012).
An aspect of relevance in terms of economic value is related to the internalization of nutrient removal costs. This is aimed at encouraging the development of technologies to reduce environmental threats (Buschmann et al., 1996; Neori et al., 2007; Buschmann et al., 2008a; Buschmann et al., 2013), and political strategies, such as the idea of developing N bonds, as is currently practiced in the municipality of Lysekil (Sweden), which pays USD 10 kg of N extracted by M. edulis. Furthermore, the creation of incentives for cultivation under the IMTA model can be a policy that promotes the recovery of disturbed environments (Buschmann et al., 2008a) especially in Samanco Bay.
3.4 Conclusion
The industrial cultivation of A. purpuratus, in Samanco Bay, has generated jobs and related activities that stimulate the local economy and contribute to foreign exchange in Peru. However, it is being developed under a classic model (monospecific cultivation), prioritizing economic profits with almost no environmental responsibility. We anticipate that if this situation persists, it could exacerbate the impacts on the ecosystem, but at the same time generate inadequate physical and chemical conditions for the sustainability of the activity itself.
The proposed IMTA model has great potential to be an economically much more profitable activity, but in a balanced ecosystem, for which it is necessary to adopt this new production model with an ecological engineering approach. The integration of species from different trophic levels is the key concept for their adaptation. This model will allow them to diversify their production, improve their economic and social profitability, and guarantee their sustainability.
Finally, we consider that the adaptation of the proposed model will not be an effortless task. The main challenges will be the technological adaptation in addition to government policies that encourage the transition to an IMTA model. It will also be necessary to study the physical and chemical characteristics of the water mass, oceanographic dynamics, physical and chemical description of the bottom, and a better understanding of the socioeconomic conditions for businesses. It is therefore imperative to involve academia, the competent authority, and the business community.
Author contributions
RL-A conceived the idea, conducted the research, obtained the grant, wrote the original draft, edited, revised, and supervised the work. YH-P investigated, wrote, reviewed, edited, and administrated the project. GS-R investigated, wrote, edited, reviewed, and supervised the work. GO-R reviewed, wrote, edited, and produced the images. All authors contributed to the article and approved the submitted version.
Funding
This work was funded by the PNIPA-CU-SFOCA-PP-000045 Subproject “Capacity building for the management of multi-trophic crops in Samanco Bay (Ancash, Peru)”, Contract N° 021-2018-PNIPA-SUBPROYECTOS.
Acknowledgments
We would like to express our gratitude to the Vicerrectorado de Investigación at Universidad Nacional del Santa (VRIN-UNS), who supported us in the publication process.
Conflict of interest
The authors declare that the research was conducted in the absence of any commercial or financial relationships that could be construed as a potential conflict of interest.
Publisher’s note
All claims expressed in this article are solely those of the authors and do not necessarily represent those of their affiliated organizations, or those of the publisher, the editors and the reviewers. Any product that may be evaluated in this article, or claim that may be made by its manufacturer, is not guaranteed or endorsed by the publisher.
References
ANA (Autoridad Nacional del Agua) (2009). Evaluación de los recursos hídricos en las cuencas de los ríos santa, lacramarca y nepeña: Estudio hidrológico de la cuenca del río nepeña (informe final).
Arnous M. O., Hassan M. A. (2015). Heavy metals risk assessment in water and bottom sediments of the eastern part of lake manzala, egypt, based on remote sensing and GIS. Arab J Geosci 8, 7899–7918. doi: 10.1007/s12517-014-1763-6
Asche F., Eggert H., Oglend A., Roheim C. A., Smith M. D. (2022). Aquaculture: Externalities and policy options. Rev. Environ. Economics Policy 16, 282–305. doi: 10.1086/721055
Avila M., Piel M. I., Caceres J. H., Alveal K. (2011). Cultivation of the red alga chondracanthus chamissoi: sexual reproduction and seedling production in culture under controlled conditions Vol. 23 (Springer), 529–536.
Avila-Peltroche J., Padilla-Vallejos J. (2020). The seaweed resources of peru. Botanixa Marin 63, 381–394. doi: 10.1515/bot-2020-0026
Bartley D. M. (2022). World aquaculture 2020 - a brief overview (FAO Fisheries and Aquaculture Circular No. 1233). doi: 10.4060/cb7669en
Beveridge M. C., Little D. C. (2002). “The history of aquaculture in traditional societies,” in Ecological aquaculture. the evolution of the blue revolution 1, 1–29. doi: 10.1002/9780470995051.ch1
Bolton J. J., Robertson-Andersson D. V., Shuuluka D., Kandjengo L. (2009). Growing ulva (chlorophyta) in integrated systems as a commercial crop for abalone feed in south africa: a SWOT analysis. J Appl Phycol, 21, 575–583. doi: 10.1007/s10811-008-9385-6
Brugère C., Aguilar-Manjarrez J., Beveridge M. C. M., Soto D. (2019). The ecosystem approach to aquaculture 10 years on - a critical review and consideration of its future role in blue growth. Reviews in Aquaculture11. 493–514. doi: 10.1111/raq.12242
Buschmann A. H., Cabello F., Young K., Carvajal J., Varela D. A., HenrÃquez L. (2009). Salmon aquaculture and coastal ecosystem health in chile: analysis of regulations, environmental impacts and bioremediation systems. Ocean & Coastal Management 52 (5), 243–249. doi: 10.1016/j.ocecoaman.2009.03.002
Buschmann A. H., Correa J. A., Westermeier R., Hernández-González M., Norambuena R. (2001). Red algal farming in chile: a review. Aquaculture 194 (3-4) , 203–220. doi: 10.1016/S0044-8486(00)00518-4
Buschmann A. H., Gonzalez M. H., Varela D. (2008b). Seaweed future cultivation in chile: perspectives and challenges. International Journal of Environment and Pollution 33 (4), 432-456. doi: 10.1504/IJEP.2008.020571
Buschmann A., Hernández-González M., Aranda C., Chopin T., Neori A., Halling C., et al. (2008a). “Mariculture waste management,” in Encyclopedia of ecology (Elsevier) Ecological Engineering, 5, 2211–2217. doi: 10.1016/B978-008045405-4.00045-8
Buschmann A. H., López D. A., Medina A. (1996). A review of the environmental effects and alternative production strategies of marine aquaculture in chile. Aquacultural Eng. 15, 397–421. doi: 10.1016/S0144-8609(96)01006-0
Buschmann A. H., Stead R. A., Hernández-González M., Pereda S. V., Paredes J. E., Maldonado M. A. (2013). Un análisis crÃtico sobre el uso de macroalgas como base para una acuicultura sustentable. Rev. chil. hist. nat. 86 (3), 251–264. doi: 10.4067/S0716-078X2013000300003
Calderón C., Valdés J. (2012). Contenido de metales en sedimentos y organismos bentónicos de la bahÃa san jorge, antofagasta, chile. Rev. biol. mar. oceanogr, 47 (1), 121–133. doi: 10.4067/S0718-19572012000100011
Camargo J. A., Alonso A. (2007). Contaminación por nitrógeno inorgánico en los ecosistemas acuáticos: problemas medioambientales, criterios de calidad del agua, e implicaciones del cambio climático.16 (2), 8-110.
Cerrato P. C., Rodríguez P. P. (2008). Producción sostenida de moluscos bivalvos en el perú: Acuicultura y repoblamiento, en. Lovatelli A., Farías A., Uriarte e. I. (eds). Estado actual del cultivo y manejo de moluscos bivalvos y su proyección futura: factores que afectan su sustentabilidad en América Latina. Taller Técnico Regional de la FAO. 20-24 de agosto de 2007, Puerto M ontt, Chile. FAO Actas de Pesca y Acuicultura. FAO 12,209-218.
OECD, Chopin T. (2010). Integrated multi-trophic aquaculture. Advancing the aquaculture agenda: Workshop proceedings (Paris: OECD Publishing), doi: 10.1787/9789264088726-15-en
Chopin T. (2013). “Aquaculture, integrated multi-trophic (IMTA),” in Sustainable food production. Eds. Christou P., Savin R., Costa-Pierce B. A., Misztal I., Whitelaw C. B. A. (Springer New York), 184–205. doi: 10.1007/978-1-4614-5797-8\s\do5(1)73
Chopin T., Buschmann A. H., Halling C., Troell M., Kautsky N., Neori A., et al. (2001). Integrating seaweeds into marine aquaculture systems: a key toward sustainability. Journal of Phycology 37, 975–986. doi: 10.1046/j.1529-8817.2001.01137.x
Chopin T., Cooper J. A., Reid G., Cross S., Moore C. (2012). Open-water integrated multi-trophic aquaculture: environmental biomitigation and economic diversification of fed aquaculture by extractive aquaculture. Reviews in Aquaculture 4, 209–220. doi: 10.1111/j.1753-5131.2012.01074.x
Chopin T., Robinson S. M. (2004). Defining the appropriate regulatory and policy framework for the development of integrated multi-trophic aquaculture practices: introduction to the workshop and positioning of the issues. Bull. Aquacul. Assoc. Canada 104–3.
Chopin T., Tacon A. G. (2021). Importance of seaweeds and extractive species in global aquaculture production. Rev. Fisheries Sci. Aquaculture 29, 139–148. doi: 10.1080/23308249.2020.1810626
Chopin T., Troell M., Reid G. K., Knowler D., Robinson S., Neori A., et al. (2010). Integrated multi-trophic aquaculture. part i. responsible practice provides diversified products, biomitigation.
Chopin T., Yarish C., Sharp G. (2007). “Beyond the monospecific approach to animal aquaculture—the light of integrated multi-trophic aquaculture. Methods and Technologies in Fish Biology and Fisheries, vol 6. Springer, Dordrecht, 447–458. doi: 10.1007/978-1-4020-6148-6_25
Colunche Díaz J. L., Gonzales Veintimilla F., Quiñones Paredes P., Terán Iparraguirre R. (2016). Efecto del antifouling en la abundancia de ciona intestinalis y en el crecimiento de argopecten purpuratus. Arnaldoa 23, 631–648. doi: 10.22497/arnaldoa.232.23214
Correia M., Azevedo I. C., Peres H., Magalhaes R., Oliva-Teles A., Almeida C. M. R., et al. (2020). Integrated multi-trophic aquaculture: a laboratory and hands-on experimental activity to promote environmental sustainability awareness and value of aquaculture products . Front. Mar. Sci., 7, 156. doi: 10.3389/fmars.2020.00156
Coste O., Malta E.-j., López J. C., Fernández-Díaz C. (2015). Production of sulfated oligosaccharides from the seaweed ulva sp. using a new ulvan-degrading enzymatic bacterial crude extract. Algal Research, 10, 224–231.
Felix N., Brindo R. A. (2014). Evaluation of raw and fermented seaweed, ulva lactuca as feed ingredient in giant freshwater prawn macrobrachium rosenbergii. Int J Fish Aquat Stud 1 (3), 199–204.
Fiorella K. J., Okronipa H., Baker K., Heilpern S. (2021). Contemporary aquaculture: implications for human nutrition. Curr. Opin. Biotechnol. 70, 83–90. doi: 10.1016/j.copbio.2020.11.014
Fu W., Wu Y., Sun L., Zhang W. (2007). Efficient bioremediation of total organic carbon (toc) in integrated aquaculture system by marine sponge hymeniacidon perleve. Biotechnol. Bioengineering 97, 1387–1397. doi: 10.1002/bit.21352
García F., Amaya Rojas C. M., Gonzales Rossel J. A., Monzón Anticona J. A., Aldave Agüero S. S., Ventura Miranda F. A., et al. (2015). Informe de evaluación ambiental de la bahía de samanco, ejecutado durante los años 2014 y 2015.
García V., Huerto Rengifo M., Cervantes Renjifo C., León J., Córdova N. (2013). Caracterización ambiental y potencial pesquero 2004-2011: Bahía de samanco, chimbote, perú. Inf Inst Mar Perú, (Callao), 40 (1-2), 8–30.
García Nolazco V., de la Matta P., Orozco M. R. (2019). Calidad del ambiente marino y costero en la región à ncash 2018. Bol Inst Mar Perú (Instituto del Mar del Perú), 34 (2), 406-431.
Gephart J., Gephart J. A., Bejarano R. A., Gorospe K., Godwin A., Golden C. D., et al. (2023). Globalization of wild capture and farmed aquatic foods (Authorea Preprints).
Gonzales E., Yepez V. (2007). Estudio de línea base del ámbito marino de la bahía de sechura 14-28 enero 2007 (Callao: Instituto del Mar del Peru).
González Henríquez M. N., Rey-Méndez M., Pérez Y., Bilbao A., Pavón N., Louzara G., et al. (2015). Cultivos multitróficos: complementos a la explotación de otras especies de fuerte presencia en el mercado (Universidad católica de Oriente (UCO).
Goswami M., Trudeau V. L., Lakra W. S. (2023). “Chapter 1 - biotechnology in modern aquaculture: Innovations, advancements, and challenges,” in Frontiers in aquaculture biotechnology. Eds. Lakra W. S., Goswami M., Trudeau V. L. (Academic Press), 1–13. doi: 10.1016/B978-0-323-91240-2.00003-8
Guillén O., Rondán R. I. D. (1968). Producción primaria de las aguas costeras del perú en el año 1964. Bol. Inst. Mar Perú, 1 (7), 349–376.
Han M. (2002). “Chapter twelve human influences on muddy coasts,” in Muddy coasts of the world. Eds. Healy T., Wang Y., Healy J.-A. (Elsevier) 4, 293–317. doi: 10.1016/S1568-2692(02)80086-9
Han Q., Keesing J. K., Liu D. (2016). A review of sea cucumber aquaculture, ranching, and stock enhancement in china. Rev. Fisheries Sci. Aquaculture 24, 326–341. doi: 10.1080/23308249.2016.1193472
Hossain A., Senff P., Glaser M. (2022). Lessons for coastal applications of imta as a way towards sustainable development: A review. Appl. Sci. 12, 11920. doi: 10.3390/app122311920
IMARPE (2009b). Informe final de la línea base en el ambito marino de la bahía de samanco 13 - 24 diciembre 2008.
IMARPE (2019). IMARPE registra mortandad de concha de abanico argopecten purpuratus y varazón de peces en bahía de sechura.
Kennish M. J. (2002). Environmental threats and environmental future of estuaries. Environmental Conservation (Cambridge University Press) 29 (1), 78–107.
Kluger L. C., Taylor M. H., Wolff M., Stotz W., Mendo J. (2018). From an open-access fishery to a regulated aquaculture business: the case of the most important latin american bay scallop (argopecten purpuratus). Rev. Aquaculture 11, 187–203. doi: 10.1111/raq.12234
Loayza R. E., Tresierra Ã. (2014). Variación del “biofouling” en linternas de cultivo de “concha de abanico” argopecten purpuratus en bahía samanco, ancash, perú. Revista Ciencia y Tecnología 2, 19.
Loayza Aguilar R. E. (2018). Duplicación del recambio de linternas y su efecto biológico y economico en la etapa final del cultivo suspendido de argopecten purpuratus, en la bahía de samanco (ancash, perú), Universidad Nacional del Santa.
Martínez J., Roca J. (2015). Economía ecológica y política ambiental. Nueva antropología 21 (68), 131–138.
Metcalf and Eddy (1995). Ingeniería de aguas residuales: Tratamiento, vertido y reutilización. Madrid (Spain), McGraw-Hil 3a ed.
Neori A., Chopin T., Troell M., Buschmann A. H., Kraemer G. P., Halling C., et al. (2004). Integrated aquaculture: rationale, evolution and state of the art emphasizing seaweed biofiltration in modern mariculture. Aquaculture, 231 (1-4), 361–391.
Neori A., Troell M., Chopin T., Yarish C., Critchley A., Buschmann A. H. (2007). “The need for a balanced ecosystem approach to blue revolution aquaculture,” in Environment: science and policy for sustainable development, vol. 49. , 36–43. doi: 10.1016/j.aquaculture.2003.11.015
OEFA (2013). Reporte público del informe n°00120-2013-OEFA/DS-PES. supervisión ambiental especial en concesión acuícola en la bahía de samanco. reporte público del informe del organismo de evaluación y fiscalización ambiental (RPI-OEFA), Lima, Perú.
Pailan G. H., Biswas G. (2022). Dealing the hardship in aquaculture nutrition in a changing climatic condition (Singapore: Springer Nature Singapore), 311–322. doi: 10.1007/978-981-19-5500-6\s\do5(2)0
Pang Q., Sui Z., Kang K. H., Kong F., Zhang X. (2010). Application of SSR and AFLP to the analysis of genetic diversity in gracilariopsis lemaneiformis (rhodophyta). J. Appl. Phycol. 22, 607–612. doi: 10.1007/s10811-009-9500-3
Park M., Shin S. K., Do Y. H., Yarish C., Kim J. K. (2018). Application of open water integrated multi-trophic aquaculture to intensive monoculture: A review of the current status and challenges in korea. Aquaculture 497, 174–183. doi: 10.1016/j.aquaculture.2018.07.051
PRODUCE (2015). Anuario estadÃstico pesquero y acuÃcola 2015. Available at: https://sinia.minam.gob.pe/documentos/anuario-estadistico-pesquero-acuicola-2015
PRODUCE (2020). Anuario estadÃstico pesquero y acuÃcola 2019. Available at: https://ogeiee.produce.gob.pe/index.php/en/shortcode/oee-documentos-publicaciones/publicaciones-anuales/item/949-anuario-estadistico-pesquero-y-acuicola-2019
PRODUCE (2021). Anuario estadÃstico pesquero y acuÃcola 2020. Available at: https://ogeiee.produce.gob.pe/index.php/en/shortcode/oee-documentos-publicaciones/publicaciones-anuales/item/1001-anuario-estadisticoo-pesquero-y-acuicola-2020
PRODUCE (2022). Anuario estadÃstico pesquero y acuÃcola 2021. Available at: https://ogeiee.produce.gob.pe/index.php/en/shortcode/oee-documentos-publicaciones/publicaciones-anuales/item/1080-anuario-estadistico-pesquero-y-acuicola-2021
Purcell S. W., Samyn Y., Conand C. (2012). Commercially important sea cucumbers of the world (FAO) Species Catalogue for Fishery Purposes No. 6.
Ramírez M. E., Tapia L. (1991). Gracilariopsis lemaneiformis (bory) dawson, acleto et foldvik en el norte de chile (rhodophyta, gracilariaceae). Revista Chilena de Historia Natural 64, 323–330.
Ramos Alfaro K. J., Villegas Ruiz R. I. (2019). Evaluación de monitoreos ambientales de una concesión de cultivo suspendido de argopecten purpuratus en la bahía de samanco (Áncash, perú), periodo 2013–2018, y su relación con el marco legal (Universidad Nacional del Santa).
Richard M., Archambault P., Thouzeau G., McKindsey C. W., Desrosiers G. (2007). Influence of suspended scallop cages and mussel lines on pelagic and benthic biogeochemical fluxes in havre-aux-maisons lagoon, Îles-de-la-madeleine (quebec, canada). Can. J. Fisheries Aquat. Sci. 64, 1491–1505. doi: 10.1139/f07-116
Romo H., Ávila M., Candia M., Núñez M., Oyarzo C., Galleguillos F., et al. (2005). Manual de técnicas de cultivo de luche (porphyra sp.). Proyecto Fondef D01I.1148.
Ruzafa A. P., Diego C. M. (1985). Técnicas de recolección y estudio en la clase holothuroidea. 1. generalidades, sistemática, ecología, biología y comportamiento. Anales de biología 3, 13–36.
Ryther J. H., Dunstan W. M. (1971). Nitrogen, phosphorus, and eutrophication in the coastal marine environment. Science, 171 (3975), 1008–1013. doi: 10.1126/science.171.3975.1008
Saavedra S., Henríquez L., Leal P., Galleguillos F., Cook S., Cárcamo F. (2019). “Cultivo de macroalgas: Diversificación de la acuicultura de pequeña escala en chile,” in Convenio de desempeño, subsecretaría de economía y empresas de menor tamaño (Instituto de Fomento Pesquero).
Sadiq M. (2021). Toxic metal chemistry in marine environments (1st ed.). (CRC Press). doi: 10.1201/9781003210214
Salazar Camacho A. I. (2023). Evaluación del riesgo por inundaciones en las viviendas del asentamiento humano tacala, distrito de castilla, provincia de piura-piura. Bach. Universidad Privada Antenor Orrego.
Salinas-Morrondo J. M. (2012). Acuicultura multitrófica integrada. una alternativa sostenible y de futuro para los cultivos marinos en galicia (Xunta de Galicia, Conselleria do Medio Rural e do Mar).
Sasikumar G., Viji C. (2015). Integrated multi-trophic aquaculture systems (imta). Winter School on Technological Advances in Mariculture for Production Enhancement and Sustainability
Sukhdhane K., Kripa V., Divu D., Vase V., Mojjada S., et al. (2018). Integrated multi-trophic aquaculture systems: a solution for sustainability. Aquaculture Asia Magazine 22, 26–29.
Swedish E. (2000). “Environmental quality criteria: coasts and seas,” in Swedish Environmental protection agency report, vol. 5052. , 138.
Thomas S. A. (2010). “Integrated multi-trophic aquaculture: A workshop,” in White paper summarizing a workshop held at peninsula college(Port Angeles, Washington, USA: Peninsula College Port Angeles, Washington).
Thomsen E., Herbeck L. S., Jennerjahn T. C. (2020). The end of resilience: Surpassed nitrogen thresholds in coastal waters led to severe seagrass loss after decades of exposure to aquaculture effluents. Mar. Environ. Res. 160, 104986. doi: 10.1016/j.marenvres.2020.104986
Tolga M. T., Emiroglu D., Gunay D., Ozgul A. (2017). Sea Cucumber (holothuria tubulosa gmelin 1790) culture under marine fish net cages for potential use in integrated multi-trophic aquaculture (IMTA) (India: NISCAIR-CSIR).
Uribe E., Blanco J. (2001). Capacidad de los sistemas acuáticos para el sostenimiento del cultivo de pectÃnidos: el caso de argopecten purpuratus en la bahÃa de tongoy, chile . Los Moluscos Pectínidos de Iberoamérica: Ciencia y Acuicultura A. N. Maeda-Martínez (ed.) 2001, Cap. 12: 000-00.
Uribe E., Moraga J., Zúñga S., Rosales S., Álvarez G., Ávalos P., et al. (2008). Establecimiento de un protocolo de seguimiento ambiental para la determinación de la capacidad de carga para el cultivo del ostión del norte. informe final. INFORME FINAL PROYECTO FIP N° 2006-37
Uribe R., RodrÃguez J. R., Enzian P. C., Paz P. B. (2013). Invertebrados marinos bentónicos del litoral de la región à ncash, perð, Vol. 28. 136–293.
Vela S., Ojeda J. (2007). Acuicultura: La revolucion azul. publicaciones CientÃficas y Tecnológicas del observatorio Espaà ± ol de acuicultura.
Villalobos J. (2012). Utilización de las macroalgas ulva lactuca y gracilaria parvispora en la formulación de dietas balanceadas para el camarón blanco litopenaeus vannamei Interdisciplinary Research Center for Integral Regional Development Sinaloa Unit (CIIDIR Unidad Sinaloa).
Keywords: integrated multi-trophic aquaculture, mariculture, Samanco bay, organic matter, extractive aquaculture, macroalgae
Citation: Loayza-Aguilar RE, Huamancondor-Paz YP, Saldaña-Rojas GB and Olivos-Ramirez GE (2023) Integrated Multi-Trophic Aquaculture (IMTA): Strategic model for sustainable mariculture in Samanco Bay, Peru. Front. Mar. Sci. 10:1151810. doi: 10.3389/fmars.2023.1151810
Received: 26 January 2023; Accepted: 13 March 2023;
Published: 24 March 2023.
Edited by:
Pablo Pita, University of Santiago de Compostela, SpainReviewed by:
Moslem Sharifinia, Iranian Fisheries Science Research Institute, IranXiujuan Shan, Yellow Sea Fisheries Research Institute (CAFS), China
Copyright © 2023 Loayza-Aguilar, Huamancondor-Paz, Saldaña-Rojas and Olivos-Ramirez. This is an open-access article distributed under the terms of the Creative Commons Attribution License (CC BY). The use, distribution or reproduction in other forums is permitted, provided the original author(s) and the copyright owner(s) are credited and that the original publication in this journal is cited, in accordance with accepted academic practice. No use, distribution or reproduction is permitted which does not comply with these terms.
*Correspondence: Rómulo E. Loayza-Aguilar, rloayza@uns.edu.pe