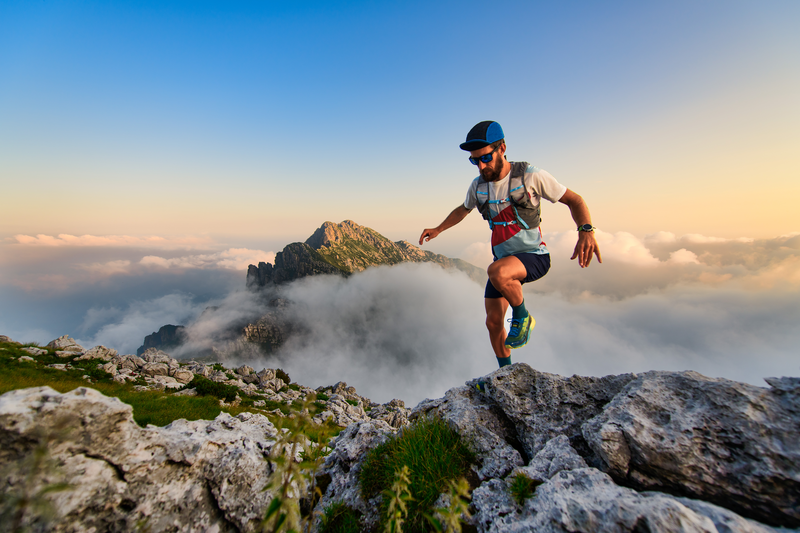
95% of researchers rate our articles as excellent or good
Learn more about the work of our research integrity team to safeguard the quality of each article we publish.
Find out more
ORIGINAL RESEARCH article
Front. Mar. Sci. , 04 April 2023
Sec. Marine Fisheries, Aquaculture and Living Resources
Volume 10 - 2023 | https://doi.org/10.3389/fmars.2023.1151332
This article is part of the Research Topic The World of Seaweeds View all 8 articles
Several studies reported that gametophytes and tetrasporophytes of Gigartinaceae produce different carrageenan types, as observed in Sarcopeltis species although they have isomorphic haploid and diploid phases. Cystocarpic and non-fertile plants of Sarcopeltis (ex Gigartina) skottsbergii produced kappa-carrageenans, while tetrasporophytes produced lambda-carrageenans, and yields were higher in cystocarpic and sterile specimens than in tetrasporophytes. However, comparison on the other primary metabolites among different life history phases still needed to be investigated. Then, we tested the hypothesis if the variation on primary metabolites (pigments, proteins and carbohydrates) are related to the life history phases of Sarcopeltis antarctica and Sarcopeltis skottsbergii, and/or are influenced by abiotic factors. Primary metabolites of S. antarctica varied according to the life-history phases: non-fertile specimens presented higher phycobiliprotein concentrations, tetrasporophytes presented higher chlorophyll a concentrations, and cystocarpic specimens presented higher concentrations of proteins, polysaccharides and floridean starch. However, primary metabolites of S. skottsbergii varied in response to some abiotic factors (nutrients, temperature and salinity), since principal component analysis evidenced two groups; one was related by the higher nitrate and phosphate concentrations, and the other was related to the higher temperatures and lower salinities. Concentrations of floridean starch, low molecular weight carbohydrates, and polysaccharides are higher in S. antarctica than in S. skottsbergii, which could be related to the protective role of these compounds against salinity variation and low temperatures faced in the Antarctic extreme environments.
Benthic marine algae (or seaweeds) are sources of a wide spectrum of compounds of commercial interest, and have been used for direct human consumption, phycocolloid production (agar, carrageenan and alginate), industrial applications (pharmaceuticals, cosmetic and nutraceuticals), and in agriculture as fertilizers and biostimulants (Stirk et al., 2020; Bebianno et al., 2021). Seaweed species adapted to extreme polar ecosystems synthetize bioactive compounds as a result of a long and complex evolutionary and ecological process, which may have several biotechnological and pharmaceutical applications (Oliveira et al., 2020).
The geographic isolation of Antarctica from other continents mainly by the Antarctic Circumpolar Current and extreme climate conditions may explain the low diversity and high degree of endemism observed in Antarctic marine macroalgae (Oliveira et al., 2020). Antarctic marine macroalgae have distinct biochemical and physiological strategies to survive in extreme environments characterized by low temperatures and wide seasonal variations in light with long dark periods during the winter and prolonged light periods with high irradiation during the spring and summer (Weykam et al., 1997). In red algae, these conditions have led to the development of different survival strategies based on the combined activity of several metabolites as for examples: a) phycobilins (phycoerythrin, phycocyanin and allophycocyanin) that act as accessory pigments, increasing the light spectrum absorption for photosynthesis; b) proteins for enzymatic activity, as well as structural and protective functions; c) polysaccharides, such as agar and carrageenan, which can play structural and protective roles in the cell wall against wave action and herbivory (Weykam et al., 1997); and d) reserve carbohydrates (e.g. floridean starch) and low molecular weight carbohydrates (e.g. heterosides, polyols) which act as osmoregulators under salinity variations and desiccation (Wiencke et al., 2007).
The Chilean sub-Antarctic ecoregion of Magallanes (or Magellan) hosts a coastal, benthic community that is highly distinct from other temperate rocky shores on the South American Continent in terms of species composition, richness and structure. Such distinctions could be the result of several factors, such as the geomorphology generated by the glacial erosion during the advance and retreat of ice in the Quaternary (Silva & Calvete, 2002); oceanographic gradients combining unique current flows, salinity, and thermal (Dayton, 1985; Silva & Calvete, 2002), photoperiod and irradiance regimes; presence of glaciers with predominant west-to-east gradients of winds and rainfall (Aravena & Luckman, 2009) and freshwater coastal discharge; variable substrate types with abrupt change in geomorphology of the sub-Antarctic system of fjords and channels (Valdenegro & Silva, 2003), resulting in unique physical and biogeochemical seawater conditions (e.g., nutrient cycles, carbonate system dynamics [Torres et al., 2014]) that generate a distinct algal structure. The environmental heterogeneity governing sub-Antarctic and Antarctic coastal ecosystems has shaped the evolutionary history and physiological adaptations of the local macroalgal flora.
Sarcopeltis Hommersand (Gigartinaceae, Rhodophyta) is a new genus proposed by Hughey et al. (2020), comprising two species: S. skottsbergii (Setchell and N.L. Gardner) Hommersand, Hughey, Leister and P.W. Gabrielson from the southern South America, and a new species S. antarctica Hommersand, Hughey, Leister and P.W. Gabrielson from Antarctic Peninsula. Both Sarcopeltis species have large and peltate thalli (Hughey et al., 2020), and are previously named as Gigartina skottsbergii Setchell and Gardner with geographic distribution in austral region, including Argentina, Chile, Antarctic Islands and Antarctic Peninsula (Ramírez and Santelices, 1991; Wiencke and Clayton, 2002; Oliveira et al., 2020). However, taxonomic studies on Gigartina skottsbergii based on reproductive features (Hommersand et al., 1993), and DNA sequences (Hommersand et al., 1994) suggested that G. skottsbergii may be a new genus. Moreover, divergence on ribulose-bisphosphate carboxylase (rbcL) gene sequences between specimens from Chile and from Antarctic Peninsula indicated that they could be distinct species (Hommersand et al., 1999; Hommersand et al., 2009). This hypothesis was supported by other molecular studies, which reported the genetic divergences between G. skottsbergii from sub-antarctic and Antarctic populations (Billard et al., 2015; Guillemin et al., 2018). Finally, based on phylogenetic analysis of rbcL and the mitochondrial cytochrome c oxidase subunit 1 (cox 1) gene sequences of the lectotype of G. skottsbergii from southern South America and Antarctica, Hughey et al. (2020) described the new species Sarcopeltis antarctica, which is morphologically similar to S. skottsbergii, but with genetic divergences of 2.0 - 2.2% and 7.7% -7.9% for rbcL and cox 1, respectively.
Sarcopeltis antarctica occurs in the Antarctic Peninsula, the South Shetland Islands and the South Orkney Islands (Billard et al., 2015; Pellizzari et al., 2020), with a wide vertical distribution, from the eulittoral and sublittoral zones, up to approximately 30 m depth (Wiencke and Clayton, 2002), which corresponds to the lower distribution limited by the compensation irradiance level (Klöser et al., 1996; Deregibus et al., 2016; Gómez et al., 1997). The life history of S. antarctica is triphasic, with isomorphic diploid tetrasporophytes and haploid gametophytes, and its life strategy is considered as season responder (Wiencke, 1990). Seasonal growth and reproduction as well as culture experiments under daylength variations on S. antarctica were reported by Wiencke (1990), and physiological responses as temperature requirements for spore germination and growth, and susceptibility of tetraspores and carpospores to UV radiation were also investigated (Bischoff-Bäsmann and Wiencke, 1996, Roleda et al., 2008). Due to its large thallus, S. antarctica is commonly associated to invertebrates, and abundance and species composition of gastropod assemblages was reported by Amsler et al. (2022).
Sarcopeltis skottsbergii is a commercially important red alga used as raw material for carrageenan production in Chile (Mansilla et al., 2012; Buschmann et al., 2017), and the economic feasibility of commercial cultivation of S. skottsbergii was evaluated based on dynamic bioeconomic model proposed by Zuniga-Jara et al. (2022) to reduce extractive pressure on natural habitats. Besides carrageenan production, S. skottsbergii revealed to be a source of R-phycoerythrin and antioxidant activity, with potential for application as a bioactive ingredient for the food industry (Castro-Varela et al., 2022). S. skottsbergii occurs frequently in the sublittoral zone, forming beds which are important for the marine biodiversity conservation by providing shelter and breeding places for many species of invertebrates and fishes (Rosenfeld et al., 2015; Rosenfeld et al., 2017). Similar to S. antarctica, the life history of S. skottsbergii is also triphasic with isomorphic diploid tetrasporophytes and haploid gametophytes (Avila et al., 1999). Marambio et al. (2017) reported that photosynthetic rates and pigment contents of S. skottsbergii varied seasonally and according to reproductive stages, and these responses suggested that this species has adopted a strategy allowing it to evolve through selection pressure to survive in the austral polar region.
Several studies reported that gametophytes and tetrasporophytes of Gigartinaceae produce different carrageenan types (e.g., Chen et al., 1973; McCandless et al., 1973; Waaland, 1975; Matulewicz et al., 1989; Usov, 2011). Piriz and Cerezo (1991) reported that cystocarpic and sterile plants of Sarcopeltis skottsbergii (cited as Gigartina skottsbergii) produced kappa-carrageenans, while tetrasporophytes produced lambda-carrageenans, and yields were higher in cystocarpic and sterile specimens than in tetrasporophytes. Other example is Chondrus crispus Stackhouse, which has gametophytes composed primarily of kappa- and iota-carrageenan whereas tetrasporophytes have majority of lambda-carrageenan in the cell walls (Chopin et al., 1987; Tasende et al., 2012). Besides differences on carrageenan types, Westermeier et al. (2021) reported differences between gametophytes and tetrasporophytes of S. skottsbergii in relation to carrageenan yield (15% higher in gametophytes than tetrasporophytes), and viscosity (higher in tetrasporophytes than gametophytes). However, comparison on other primary metabolites, as pigments, proteins and carbohydrates among different life history phases of Gigartinaceae species still needed to be investigated.
The purpose of the present study is to evaluate the variations on primary metabolites (pigments, proteins and carbohydrates) in different life history phases of Sarcopeltis antarctica and S. skottsbergii from different collecting sites. Based on the previous studies on carrageenan in Gigartinaceae species, we tested the hypothesis if other primary metabolites vary according to the life history phases, and/or in response to abiotic factors.
Tetrasporophytic, cystocarpic and non-fertile specimens of Sarcopeltis antarctica from South Shetland Islands, and S. skottsbergii from the southern Chile were collected during low tidal periods. Three specimens (n = 3) of each site and life-history stage were collected in seven sites in the Antarctic Peninsula (from 62°58’44.4” S 60°33’48.6” W to 62°43’54.9” S 61°12’17.1” W), and four sites in the southern Chile (from 43°12’19.12” S 73°31’21.96” W to 43°12’19.12” S 73°31’21.96” W) (Figures 1A–C). Specimens of each life history stage were separated under microscope and stereomicroscope, and samples were stored at -20°C until biochemical analyses. Voucher specimens were deposited in the Herbarium of the Institute of Botany (SP), São Paulo, Brazil (Supplementary Material, Table S1).
Figure 1 (A–C). Sampling sites of Sarcopeltis skottsbergii in the southern Chile (A, B), and S. antarctica in the South Shetland Islands, Antarctica Peninsula (C).
Samples of approximately 1g of fresh weight (FW) were dried in an oven at 105°C until reaching constant dry weight (DW). Percentage of water content (WC) was determined using the equation WC= (DW.FW-1) x 100. Results of pigments, proteins, total soluble sugars (ethanolic and aqueous fractions) and reducing sugars were expressed by DW and calculated using the equation DW = (FW x100).WC-1.
For pigment analysis, fresh algal biomass (FW) was powdered in liquid nitrogen (75 mg FW, n=3), and 1 ml phosphate buffer 50 mM (pH 5.5) was added. Solutions were maintained at 4°C in the dark for 24h. Extracts were centrifuged for 20 min (14,000 ×g at 4°C), and the supernatant containing the phycobiliproteins was withdrawn and kept in the dark until spectrophotometric analysis (Shimadzu - 1800 UV, λ = 498.5, 615 and 651 nm). To extract chlorophyll a, 20 mg of the powdered material were suspended in N,N-Dimethylformamide (DMF) (99.8%). Solutions were maintained at 4°C in the dark for 24h. The supernatant containing chlorophyll a was analyzed in a spectrophotometer at wavelengths 630, 647 and 664 nm. Concentrations of phycobiliproteins (phycoerythrin, phycocyanin and allophycocyanin) and chlorophyll a were determined using equations described by Kursar et al. (1983) and Inskeep and Bloom (1985), respectively.
For total soluble protein (TSP) analysis, the algal biomass (75 mg FW for each replicate, n=3) was powdered in liquid nitrogen, and extractions were carried out at 4°C using 0.2 M phosphate buffer (pH 8) containing 5 mM EDTA and 1 mM DTT. Buffer was added in the proportion of 10 mL g-1 fresh biomass, and solutions were maintained at 4°C in the dark for 24h. The homogenates were centrifuged at 12,000×g for 15 min and 4°C. TSP contents were determined by spectrophotometry at 595 nm after addition of Coomassie Blue solution (Bio-Rad) using BSA as standard, according to the method of Bradford (1976).
The extraction of soluble carbohydrates (n=3) was performed according to Carvalho et al. (1998) with modifications. The ethanolic supernatant and aqueous filtrate were stored separately, concentrated, and then resuspended in deionized water. Quantification of total soluble sugars of ethanolic and aqueous extracts was carried out using the colorimetric method (490 nm) of phenol-sulfuric acid, according to Dubois et al. (1956), and the reducing sugars were quantified using the Somogyi-Nelson colorimetric method (520nm) (Somogyi, 1945). Both analyses used spectrophotometry and glucose as standard. The remaining residues from the extraction of the total soluble sugars were lyophilized and subjected to the quantification of floridean starch by the enzymatic method (Amaral et al., 2007), and the glucose content was determined on an ELISA microplate reader at 490 nm.
Data of environmental factors were obtained from the Bio-Oracle database platform, and Supplementary Material, Table S2 shows the averages from 1997 to 2015 at the seawater surface in Antarctic Peninsula and the southern Chile (Tyberghein et al., 2012; Assis et al., 2018).
The dry weight, photosynthetic pigments, proteins and sugar contents were statistically analyzed by two-way ANOVA, followed by the Student-Newman-Keuls post-hoc test (p<0.05). The Kruskal-Wallis non-parametric test was applied for variables without normal distribution (total soluble proteins, phycocyanin and allophycocyanin). Both tests were performed with Statistica 10.0 software. Principal component analyses (PCA) were performed with all metabolites studied and abiotic data using PCOrd 6.0 software. Data were transformed to log(x+1) to reduce the variability of different metabolites and abiotic factors, and this transformation evidenced the effects of predictor variables on the clusters produced by PCA.
Non-fertile specimens of Sarcopeltis antarctica from Snow Island and Plaza Point had the highest phycoerythrin concentrations, while tetrasporophytes from Dee and Jorge Islands and cystocarpic specimens from Greenwich and Dee Islands presented lower phycoerythrin contents (Figure 2A, Supplementary Material Table S3). In general, non-fertile specimens showed also higher phycocyanin (Figure 2B) and allophycocyanin concentrations (Figure 2C) than tetrasporophytes and cystocarpic specimens, except for tetrasporophytes from Snow and Dee Islands. The highest concentration of chlorophyll a was found in tetrasporophytes and non-fertile samples from Snow and Dee Islands, respectively (Figure 2D).
Figure 2 (A–D). Concentrations of Phycoerythrin (A), Phycocyanin (B), Allophycocyanin (C), and Chlorophyll a (D) in Sarcopeltis antarctica from the South Shetland Islands (DC, Deception Island; SN, Snow Island; GW, Greenwich Island; HM, Halfmoon Island; DE, Dee Island; JG, Jorge Island; HQ, Hennequin Point, King George Island and PP, Plaza Point, King George Island). Mean ± SD (n = 3). Data with distinct letters are significantly different among the sites, following Student-Newman-Keuls post-hoc test (p<0.05).
Higher protein concentrations were found in cystocarpic and non-fertile specimens from Snow Island than other collecting sites (Figure 3A, Supplementary Material Table S3). Low molecular weight carbohydrates (LMCWs) were found in higher concentrations in tetrasporophytes from Halfmoon Island when compared to non-fertile and cystocarpic thallus from the same site (Figure 3B). The tetrasporophytes collected at Snow Island showed higher concentrations of LMWCs than non-fertile thallus from the same site. A similar situation was found in samples from Greenwich Island. Polysaccharide concentrations varied according to the collection sites and life cycle phases (Figure 3C). The cystocarpic thallus from Snow and Jorge Islands presented the highest concentrations of polysaccharides among the S. antarctica samples. In addition, tetrasporophytes showed lower concentrations of polysaccharides than other life history phases from the same sites. The non-fertile thallus of S. antarctica had lower concentration of floridean starch than fertile thallus (Figure 3D).
Figure 3 (A–D). Concentrations of total soluble proteins (A), low molecular weight carbohydrates (LMWC) (B), polysaccharides (C) and floridean starch (D) in Sarcopeltis antarctica from the South Shetland Islands (DC, Deception Island; SN, Snow Island; GW, Greenwich Island; HM, Halfmoon Island; DE, Dee Island; JG, Jorge Island; HQ, Hennequin Point, King George Island and PP, Plaza Point, King George Island). Mean ± SD (n = 3). Data with distinct letters are significantly different among the sites, following Student-Newman-Keuls post-hoc test (p<0.05).
Tetrasporophytes from Tortuoso Channel, followed by cystocarpic thallus from the same site and non-fertile thallus from San Isidro showed higher phycoerythrin concentrations compared to the other phycobiliproteins (Figures 4A–C, Supplementary Material Table S4), while tetrasporophytes and cystocarpic thallus from Cailin Island presented the lowest concentrations. In general, samples from Cailin Island had the lowest phycobiliprotein concentrations (Figures 4A–C), except for chlorophyll a concentration (Figure 4D). On the other hand, S. skottsbergii tetrasporophytes showed lower concentrations of total soluble proteins than the other phases (Figure 5A, Supplementary Material Table S4). Highest concentrations of LMWC were observed in non-fertile and cystocarpic thallus from Port Famine and Cailin Island, respectively (Figure 5B). Concentrations of polysaccharides varied according to life history phases: cystocarpic samples from Cailin Island and San Isidro presented the highest concentrations while tetrasporophytes presented lower concentrations (Figure 5C). Concentrations of floridean starch were higher in all samples from Tortuoso Channel, but also in non-fertile samples from San Isidro and Port Famine as well as in cystocarpic samples from Cailin Island (Figure 5D).
Figure 4 (A–D). Concentration of Phycoerythrin (A), Phycocyanin (B), Allophycocyanin (C) and Chlorophyll a (D) in Sarcopeltis skottsbergii from the southern Chile (SI, San Isidro; PF, Port Famine; TC, Tortuoso Channel and CA, Cailin Island). Mean ± SD (n = 3). Data with distinct letters are significantly different among the sites, following Student-Newman-Keuls post-hoc test (p<0.05).
Figure 5 (A–D). Concentrations of total soluble proteins (A), low molecular weight carbohydrates (LMWC) (B), polysaccharides (C) and floridean starch (D) in Sarcopeltis skottsbergii from the southern Chile (SI, San Isidro; PF, Port Famine; TC, Tortuoso Channel and CA, Cailin Island). Mean ± SD (n = 3). Data with distinct letters are significantly different among the sites, following Student-Newman-Keuls post-hoc test (p<0.05).
Three principal components analyses (PCA) were performed to evaluate the correlation of variables measured (metabolite concentrations) and abiotic factors of collecting sites (Supplementary Material Table S2). For the PCA with Sarcopeltis antarctica data (Figure 6), the first two principal components accounted for 73.602% of total variance (44.132% and 29.470% for axis 1 and 2, respectively). The axis 1 was related to total soluble proteins and polysaccharides, while axis 2 was related to floridean starch, low molecular weight carbohydrates and phycobiliproteins (phycoerythrin, PE; phycocyanin, PC; allophycocyanin, APC), resulting in three groups according to their life history phase: cystocarpic specimens were grouped on the negative side of axes 1 and 2 due to the higher concentrations of LMWC and floridean starch than non-fertile specimens, which were grouped on the positive side of axis 2; tetrasporophytes were grouped in the positive side o axis 1 due to the higher contents of phycobiliproteins. Therefore, primary metabolites of S. antarctica varied according to the life-history phases (Figure 6).
Figure 6 Scatter diagram of plots of the first two axis of the principal components analysis (of abiotic data and metabolites of Sarcopeltis antarctica from the South Shetland Islands (DC, Deception Island; SN, Snow Island; GW, Greenwich Island; HM, Halfmoon Island; DE, Dee Island; JG, Jorge Island; HQ, Hennequin Point, King George Island and PP, Plaza Point, King George Island). Metabolites: FST, Floridean starch; PE, phycoerythrin; PC, phycocyanin; APC, allophycocyanin; CLa, chlorophyll a; TSP, total soluble protein; LMWC, low molecular weight carbohydrate; POLY, Polysaccharides. Abiotic data: TEM, Temperature; SAL, Salinity; NIT, Nitrate; PHO, Phosphate; DOX, Dissolved oxygen; PAR, Photosynthetic active radiation; pH, pH.
For the PCA with Sarcopeltis skottsbergii data (Figure 7), the first two principal components accounted for 79.234% of total variance (54.193% and 25.041% for axis 1 and 2, respectively, Supplementary Material Table S5). The axis 1 was related to nitrate and phosphate concentrations and floridean starch in the opposite side; the axis 2 was also related to temperature and total soluble proteins in the opposite side. This PCA evidenced two groups based on collecting sites: specimens from San Isidro and Port Famine were grouped on the negative side of axis 1 due to the higher nitrate and phosphate concentrations when compared to Cailin Island and Tortuoso Channel, which were grouped on the positive side of axis 1 (Figure 7). In addition, Cailin Island differed from the other Chilean collecting sites by higher temperatures and lower salinities, dissolved oxygen and pH (Supplementary Material Table S5). Therefore, primary metabolites of S. skottsbergii varied in response to some abiotic factors (nutrients, temperature and salinity levels).
Figure 7 Scatter diagram of plots of the first two axis of the principal components analysis (PCA 2) of abiotic data and metabolites of Sarcopeltis skottsbergii from the southern Chile (SI, San Isidro; PF, Port Famine; TC, Tortuoso Channel and CA, Cailin Island). Metabolites: FST, Floridean starch; PE, phycoerythrin; PC, phycocyanin; APC, allophycocyanin; CLa, chlorophyll a; TSP, total soluble protein; LMWC, low molecular weight carbohydrate; POLY, Polysaccharides. Abiotic data: TEM, Temperature; SAL, Salinity; NIT, Nitrate; PHO, Phosphate; DOX, Dissolved oxygen; PAR, Photosynthetic active radiation; pH, pH.
For the PCA with both S. antarctica and S. skottsbergii data (Figure 8), the first two principal components accounted for 84.271% of total variance (71.199% and 13.072% for axis 1 and 2, respectively, Supplementary Material Table S5). The axis 1 was influenced by temperature, and nitrate concentrations and floridean starch in the opposite side, while axis 2 was influenced by nitrate concentrations and floridean starch in the opposite side. This PCA evidenced two groups corresponding to each species: S. antarctica samples were grouped on the positive side of axis 1 related to the abiotic factors: lower temperature and higher nitrate concentrations of Antarctic collecting sites than Chilean ones, and higher concentrations of floridean starch than S. skottsbergii samples, which were grouped in the opposite site.
Figure 8 Scatter diagram of plots of the first two axis of the principal components analysis (PCA 3) of abiotic data and metabolites of Sarcopeltis antarctica and S. skottsbergii. Collecting sites: South Shetland Islands (DC, Deception Island; SN, Snow Island; GW, Greenwich Island; HM, Halfmoon Island; DE, Dee Island; JG, Jorge Island; HQ, Hennequin Point, King George Island and PP, Plaza Point, King George Island), and the southern Chile (SI, San Isidro; PF, Port Famine; TC, Tortuoso Channel and CI, Cailin Island), respectively. Metabolites: FST, Floridean starch; PE, phycoerythrin; PC, phycocyanin; APC, allophycocyanin; CLa, chlorophyll a; TSP, total soluble protein; LMWC, low molecular weight carbohydrate; POLY, Polysaccharides. Abiotic data: TEM, Temperature; SAL, Salinity; NIT, Nitrate; PHO, Phosphate; DOX, Dissolved oxygen; PAR, Photosynthetic active radiation; pH, pH.
Primary metabolites of Sarcopeltis antarctica varied according to the life-history phases: non-fertile specimens presented higher phycobiliprotein concentrations, tetrasporophytes presented higher chlorophyll a concentrations, and cystocarpic specimens presented higher concentrations of proteins, polysaccharides and floridean starch. These findings are unprecedented since no comparative study has looked into the primary metabolites of different life history phases in S. antarctica. Comparing our data to those reported by Peters et al. (2005), S. antarctica (cited as Gigartina skottsbergii) collected during early November to late December from Anvers Island presented protein and carbohydrates concentrations similar to those found in cystocarpic and non-fertile samples collected in December from Snow Island. Higher chlorophyll a concentrations of S. antarctica tetrasporophytes can confer higher photosynthetic performance for this diploid phase, corroborating results that carpospores of S. antarctica (cited as G. skottsbergii) presented higher photosynthetic parameters than tetraspores (Roleda et al., 2008). On the other hand, cystocarpic specimens of S. antarctica showed lower pigment concentrations (phycobiliproteins and chlorophyll a) and higher floridean starch concentrations, and this metabolite regulation could be considered as a life strategy. Similarly, Weykam et al. (1997) and Lüder et al. (2001) observed that carbohydrate concentrations (floridean starch) of the red alga Iridaea cordata (Turner) Bory de Saint-Vincent (Gigartinales) were inversely proportional to the chlorophyll a content, and carbohydrates were synthetized during periods of light to be used as energy source during extended periods of darkness. S. antarctica and I. cordata are considered season responders, and both species grow during the favorable light conditions (spring and summer), showing an opportunistic life strategy (Wiencke et al., 2007).
Unlike S. antarctica, the primary metabolites of S. skottsbergii varied in response to abiotic environmental conditions of collecting sites of the southern Chile. Samples from San Isidro and Port Famine were grouped due to the higher nitrate and phosphate concentrations when compared to samples from Cailin Island and Tortuoso Channel. In addition, samples collected in Cailin Island showed the lowest concentrations of phycobiliproteins and proteins than the other Chilean collecting sites. Besides, this collecting site had higher temperatures and lower salinities, dissolved oxygen and pH than the other sites.
Differences between life history phases were observed in S. skottsbergii collected in Tortuoso Channel, and tetrasporophytes showed higher concentrations of phycoerythrin, phycocyanin and allophycocyanin than cystocarpic and non-fertile samples. Similar results were reported by Marambio et al. (2017), who found higher concentrations of phycocyanin and allophycoccyanin in tetrasporophytes than gametophytes and carposporophytes in S. skottsbergii collected during the winter in Puerto Hambre. However, these differences were not observed in our samples collected at the same site but in the late spring due to the seasonal variations of environmental factors as photoperiod and irradiance (Marambio et al., 2014; Marambio et al., 2017).
Sarcopeltis antarctica and S. skottsbergii are separated by pigment contents, LMWCs and floridean starch. Therefore, some types of LMWCs may be involved in antifreeze processes in S. antarctica, as described for Antarctic diatoms (Gwak et al., 2010) and other organisms, such as fish, fungi and bacteria (Kim et al., 2017). The LMWCs are also involved in osmoregulation processes in red algae (Karsten et al., 1996; Karsten et al., 2005). Floridean starch concentration, LMWC, and, generally, polysaccharides are higher in S. antarctica, which could be related to the Antarctic environmental conditions (higher salinity, higher concentrations of nitrate and phosphate, higher dissolved oxygen and lower temperatures), when compared to S. skottsbergii from the southern Chile. Similarly, Fournet et al. (1999) reported high concentrations of floridean starch in Soliera chordalis (C. Agardh) J. Agardh cultured in sea water enriched with nitrate and phosphate. These results showed that carbohydrate metabolism is an important pathway to survival strategies of Sarcopeltis species in extreme environments.
In conclusion, our hypothesis based on the previous studies on carrageenan in Gigartinaceae species was confirmed since primary metabolites of Sarcopeltis antarctica varied according to the life-history phases: non-fertile specimens presented higher phycobiliprotein concentrations, tetrasporophytes presented higher concentrations of chlorophyll a, and cystocarpic specimens presented higher concentrations of proteins, polysaccharides and floridean starch. Primary metabolites of S. skottsbergii varied in response to some abiotic factors (nutrient, temperature and salinity), with relation to higher nitrate and phosphate concentrations, and higher temperatures and lower salinities. Concentrations of floridean starch, low molecular weight carbohydrates, and polysaccharides are higher in S. antarctica than in S. skottsbergii, which could be related to the protective role of these compounds against salinity variation and low temperatures faced in the Antarctic extreme environments.
The original contributions presented in the study are included in the article/Supplementary Material. Further inquiries can be directed to the corresponding author.
CP: Conceptualization, investigation, methodology, formal analysis, writing – original draft & review. MC: Investigation, methodology, supervision, resources, writing – review. AM: investigation, methodology, resources, review. MA: investigation, methodology, resources, review. PC: Conceptualization, funding acquisition, resources, review. NY: validation, supervision, funding acquisition, writing – review & editing. All authors contributed to the article and approved the submitted version.
This work was supported by the Conselho Nacional de Desenvolvimento Científico e Tecnológico (CNPq/PROANTAR n° 407588/2013-2). CP and NY thank grants from Fundação de Amparo à Pesquisa do Estado de São Paulo (FAPESP n° 2016/03062-5) and CNPq (n° 310672/2016-3), respectively.
We thank the PROANTAR (Brazilian Antarctic Program) for supporting the research project and the Brazilian Navy for the assistance to the field work. The authors thank Mr. Francisco Bahamonde (University of Magallanes, Chile) for helping to design the maps of Antarctica and Chile. The final version of the manuscript was greatly improved based on the comments and suggestions of two reviewers. The present study is part of the PhD thesis presented by the first author to the Graduate Program in Plant Biodiversity and Environment of Institute of Environmental Research, São Paulo, Brazil.
The authors declare that the research was conducted in the absence of any commercial or financial relationships that could be construed as a potential conflict of interest.
All claims expressed in this article are solely those of the authors and do not necessarily represent those of their affiliated organizations, or those of the publisher, the editors and the reviewers. Any product that may be evaluated in this article, or claim that may be made by its manufacturer, is not guaranteed or endorsed by the publisher.
The Supplementary Material for this article can be found online at: https://www.frontiersin.org/articles/10.3389/fmars.2023.1151332/full#supplementary-material
Amaral L. I. V., Costa P. M. F., Aidar M. P. M., Gaspar M., Buckeridge M. S. (2007). Novo método enzimático rápido e sensível de extração e dosagem de amido em materiais vegetais. Hoehnea 34, 425–431. doi: 10.1590/S2236-89062007000400001
Amsler C. D., Miller L. R., Edwards R. A., Amsler M. O., Engl W., Mcclintock J. B., et al. (2022). Gastropod assemblages associated with Himantothallus grandifolius, Sarcopeltis antarctica and other subtidal macroalgae. Antarctic Sci. 34, 246–255. doi: 10.1017/S0954102022000153
Aravena J., Luckman B. (2009). Spatio-temporal rainfall patterns in southern South America. Int. J. Climatol. 29, 2106–2120. doi: 10.1002/joc.1761
Assis J., Tyberghein L., Bosch S., Verbruggen H., Serrão E. A., Clerck O. (2018). Bio-ORACLE v2.0: Extending marine data layers for bioclimatic modelling. Global Ecol. Biogeogr. 27, 277–284. doi: 10.1111/geb.12693
Avila M., Candia A., Nunez M., Romo H. (1999). Reproductive biology of Gigartina skottsbergii (Gigartinaceae, Rhodophyta) from Chile. Hydrobiologia 399, 149–157. doi: 10.1023/A:1017067808892
Bebianno M. J., Calumpong H., Chiba S., Evans K., Garcia-Soto C., Kamara O. K., et al. (2021). “Chapter 1 - overall summary,” in The second world ocean assessment, vol. I . Ed. United Nations (New York: United Nations Publication), 3–36.
Billard E., Reyes J., Mansilla A., Faugeron S., Guillemin M. L. (2015). Deep genetic divergence between austral populations of the red alga Gigartina skottsbergii reveals a cryptic species endemic to the Antarctic continent. Polar Biol. 38, 2021–2034. doi: 10.1007/s00300-015-1762-4
Bischoff-Bäsmann B., Wiencke C. (1996). Temperature requirements for growth and survival of Antarctic Rhodophyta. J. Phycol. 32, 525–535. doi: 10.1111/j.0022-3646.1996.00525.x
Bradford M. M. (1976). A rapid and sensitive method for the quantitation of microgram quantities of protein utilizing the principle of protein-dye binding. Anal. Bioch. 72, 248–254. doi: 10.1016/0003-2697(76)90527-3
Buschmann A. H., Camus C., Infante J., Neori A., Israel A., Hernández-González M. C., et al. (2017). Seaweed production: Overview of the global state of exploitation, farming and emerging research activity. Eur. J. Phycol. 52, 391–406. doi: 10.1080/09670262.2017.1365175
Carvalho M. A. M., Pinto M. M., Figueiredo-Ribeiro R. C. L. (1998). Inulin production by Vernonia herbacea as influenced by mineral fertilization and time of harvest. Rev. Bras. Bot. 21, 275–280. doi: 10.1590/S0100-84041998000300006
Castro-Varela P., Celis-Pla P. S. M., Figueroa F. L., Rubilar M. (2022). Highly efficient water-based extraction of biliprotein r-phycoerythrin from marine the red macroalga Sarcopeltis skottsbergii by ultrasound and high-pressure homogenization methods. Front. Mar. Sci. doi: 10.3389/fmars.2022.877177
Chen L. C. M., Chen. J., Mclachlan A. C., Neish F. R. S., Shacklock P. F. (1973). The ratio of kappa- to lambda-carrageenan in nuclear phases of the rhodophycean algae, Chondrus crispus and Gigartina stellata. J. Mar. Biol. Assoc. UK 53, 11–16. doi: 10.1017/S0025315400056599
Chopin T., Bodeaubellion C., Floch J. Y., Guittet E., Lallemand J. Y. (1987). Seasonal study of carrageenan structures from female gametophytes of Chondrus crispus Stackhouse (Rhodophyta). Hydrobiologia 151, 535–539. doi: 10.1007/BF00046179
Dayton P. (1985). Ecology of kelp communities. An Rev. Ecol. Syst. 16, 215–245. doi: 10.1146/annurev.es.16.110185.001243
Deregibus D., Quartino M. L., Campana G. L., Momo F. R., Wiencke C., Zacher K. (2016). Photosynthetic light requirements and vertical distribution of macroalgae in newly ice-free areas in Potter Cove, South Shetland Islands, Antarctica. Polar Biol. 39, 153–166. doi: 10.1007/s00300-015-1679-y
Dubois M., Gilles K. A., Hamilton J. K., Rebers P. A., Smith F. (1956). Colorimetric method for determination of sugars and related substances. Anal. Chem. 28, 350–356. doi: 10.1021/ac60111a017
Fournet I., Zinoun M., Deslandes E., Diouris M., Floc’h J. Y. (1999). Floridean starch and carrageenan contents as responses of the red alga Solieria chordalis to culture conditions. Eur. J. Phycol. 34, 8. doi: 10.1080/09670269910001736182
Gómez I., Weykam G., Klöser H., Wiencke C. (1997). Photosynthetic light requirements, daily carbon balance and zonation of sublittoral macroalgae from King George Island (Antarctica). Mar. Ecol. Prog. Ser. 148, 281–293. doi: 10.3354/meps148281
Guillemin M. L., Dubrasquet H., Reyes J., Valero M. (2018). Comparative phylogeography of six red algae along the Antarctic peninsula: Extreme genetic depletion linked to historical bottlenecks and recent expansion. Polar Biol. 41, 827–837. doi: 10.1007/s00300-017-2244-7
Gwak I. G., Jung W. S., Kim H. J., Kang S. H., Jin E. (2010). Antifreeze protein in Antarctic marine diatom, Chaetoceros neogracile. Mar. Biotech. 12, 630–639. doi: 10.1007/s10126-009-9250-x
Hommersand M. H., Guiry M. D., Fredericq S., Leister G. L. (1993). "New perspectives in the taxonomy of the Gigartinaceae (Gigartinales, Rhodophyta)", in Fourteenth International Seaweed Symposium, eds. Chapman A. R. O., Brown M. T., Lahaye M. (Belgium: Kluwer Academic Publishers), 105–120. doi: 10.1007/978-94-011-1998-6_13
Hommersand M. H., Fredericq S., Freshwater D. W. (1994). Phylogenetic systematics and biogeography of the Gigartinaceae (Gigartinales, Rhodophyta) based on sequence analysis of rbcL. Bot. Mar. 37, 193–203. doi: 10.1515/botm.1994.37.3.193
Hommersand M. H., Fredericq S., Freshwater D. W., Hughey J. (1999). Recent developments in the systematics of the gigartinaceae (Gigartinales, rhodophyta) based on rbcL sequence analysis and morphological evidence. Phycol. Res. 4, 139–151. doi: 10.1046/j.1440-1835.1999.00168.x
Hommersand M. H., Moe R. L., Amsler C. D., Fredericq S. (2009). Notes on the systematics and biogeographical relationships of Antarctic and sub-Antarctic Rhodophyta with descriptions of four new genera and five new species. Bot. Mar. 52, 509–534. doi: 10.1515/bot.2009.081
Hughey J. R., Leister G. L., Gabrielson P. W., Hommersand M. H. (2020). Sarcopeltis gen. nov. (Gigartinaceae, Rhodophyta), with S. skottsbergii comb. nov. from southern south America and S. antarctica sp. nov. from the Antarctic peninsula. Phytotaxa, 468, 75–88. doi: 10.11646/phytotaxa.468.1.4
Inskeep W. P., Bloom P. R. (1985). Extinction coefficients of chlorophyll a and b in N,N dimethylformamide and 80% acetone. Plant Physiol. 77, 483–458. doi: 10.1104/pp.77.2.483
Karsten U., Barrow K. D., Nixdorf O., King R. J. (1996). The compatibility of unusual organic osmolytes from mangrove red algae with enzyme activity. Aust. J. Plant Physiol. 23, 577–582. doi: 10.1071/PP9960577
Karsten U., Michalik D., Michalik M., West J. A. (2005). A new unusual low molecular weight carbohydrate in the red algal genus Hypoglossum (Delesseriaceae, Ceramiales) and its possible function as an osmolyte. Planta 222, 319–326. doi: 10.1007/S00425-005-1527-3
Kim H. J., Lee J. H., Hur Y. B., Lee C. W., Park S. H., Koo B. W. (2017). Marine antifreeze proteins: Structure, function, and application to cryopreservation as a potential cryoprotectant. Mar. Drugs 15, 27. doi: 10.3390/md15020027
Klöser H., Quartino M. L., Wiencke C. (1996). Distribution of macroalgae and macroalgal communities in gradients of physical conditions in Potter Cove, King George Island Antarctica. Hydrobiologia 333, 1–17. doi: 10.1007/BF00020959
Kursar T. A., van der Meer J., Alberte R. S. (1983). Light-harvesting system of red alga Gracilaria tikvahiae. biochemical analyses of pigment mutations. Plant Physiol. 73, 353–360. doi: 10.1104/pp.73.2.353
Lüder U. H., Knoetzel J., Wiencke C. (2001). Two forms of phycobilisomes in the Antarctic red macroalgae Palmaria decipiens (Palmariales, Florideophyceae). Physiol. Plantarum 112, 572–581. doi: 10.1034/j.1399-3054.2001.1120416.x
Mansilla A., Ávila M., Yokoya N. S. (2012). Current knowledge on biotechnological interesting seaweeds from the Magellan region, Chile. Braz. J. Pharmacog. 22, 760–767. doi: 10.1590/S0102-695X2012005000074
Marambio J., Mansilla A., Ávila M., Rosenfeld A., Ojeda J. (2014). The effects of different light intensities on the culture of Gigartina skottsbergii (Rhodophyta, Gigartinales) tetrasporophytes and gametophytes in the Magellan region, Chile. J. Appl. Phycol. 26, 1963–1969. doi: 10.1007/s10811-014-0316-4
Marambio J., Mendez F., Ocaranza P., Rodriguez J. P., Rosenfeld S., Ojeda J., et al. (2017). Seasonal variations of the photosynthetic activity and pigment concentrations in different reproductive phases of Gigartina skottsbergii (Rhodophyta, Gigartinales) in the Magellan region, sub-Antarctic Chile. J. Appl. Phycol. 29, 721–729. doi: 10.1007/s10811-016-0913-5
Matulewicz M. C., Ciancia M., Noseda M. D., Cerezo A. S. (1989). The carrageenan system from tetrasporic and cystocarpic phases of Gigartina skottsbergii. Phytochemistry 28, 2937–2941. doi: 10.1016/0031-9422(89)80257-2
McCandless E. L., Craigie J. S., Walter J. A. (1973). Carrageenans in the gametophytic and sporophytic stages of Chondrus crispus. Planta 112, 201–212. doi: 10.1007/BF00385324
Oliveira M. C., Pellizzari F., Medeiros A. S., Yokoya N. S. (2020). “Diversity of Antarctic seaweeds,” in Antarctic Seaweeds - diversity, adaptation and ecosystem services. Eds. Gómez I., Huovinen P. (Cham.: Springer Nature), 23–42.
Pellizzari F., Rosa L. H., Yokoya N. S. (2020). “Biogeography of Antarctic seaweeds facing climate changes,” in Antarctic Seaweeds - diversity, adaptation and ecosystem services. Eds. Gómez I., Huovinen P. (Cham: Springer Nature), 83–102.
Peters K. J., Amsler C. D., Amsler M. O., Mcclintock J. B., Dunbar R. B., Bake B. J. (2005). A comparative analysis of the nutritional and elemental composition of macroalgae from the western Antarctic peninsula. Phycologia 44, 453–463. doi: 10.2216/0031-8884(2005)44[453:ACAOTN]2.0.CO;2
Piriz M., Cerezo A. (1991). Seasonal variation of carrageenans in tetrasporic, cystocarpic and “sterile” phases of Gigartina skottsbergii (Rhodophyta, Gigartinales). Hydrobiologia 226, 65–69. doi: 10.1007/BF00006807
Ramírez M. E., Santelices B. (1991). Catálogo de las algas marinas bentónicas de la costa temperada del Pacífico de Sudamérica. Monografías Biol. 5, 1–437.
Roleda M. Y., Zacher K., Wulff A., Hanelt D., Wiencke C. (2008). Susceptibility of spores of different ploidy levels from Antarctic Gigartina skottsbergii (Gigartinales, Rhodophyta) to ultraviolet radiation. Phycologia 47, 361–370. doi: 10.2216/PH07-84.1
Rosenfeld S., Aldea C., Mansilla A., Marambio J., Ojeda J. (2015). Richness, systematics and distribution of mollusks associated with the macroalga Gigartina skottsbergii in the strait of Magellan, Chile: A biogeographic affinity study. Zookeys 519, 49–100. doi: 10.3897/zookeys.519.9676
Rosenfeld S., Aldea C., Ojeda J., Marambio J., Hüne M., Troncoso J. S., et al. (2017). Molluscan assemblages associated with Gigartina beds in the Strait of Magellan and the South Shetland Islands (Antarctica): A comparison of composition and abundance. Polar Res. 36, 1297915. doi: 10.1080/17518369.2017.1297915
Silva N., Calvete C. (2002). Características oceanográficas físicas y químicas de canales australes chilenos entre el Golfo de Penas y el Estrecho de Magallanes. CIMAR dos fiordos. (Physical and chemical oceanographic characteristics of southern Chilean channels between the Gulf of Penas and the Strait of Magellan. CIMAR two fjords). Ciencia y Tecnol. Del. Mar. 25, 23–88.
Somogyi M. (1945). A new reagent for the determination of sugars. J. Biol. Chem. 160, 61–63. doi: 10.1016/S0021-9258(18)43097-9
Stirk W. A., Rengasamy K. R. R., Kulkarni M. G., van Staden J. (2020). “Plant biostimulants from seaweed: an overview,” in The chemical biology of plant biostimulants. Eds. Geelan D., Xu L. (John Wiley and Sons Ltd), 33–55.
Tasende M. G., Cid M., Fraga M. I. (2012). Spatial and temporal variations of Chondrus crispus (Gigartinaceae, Rhodophyta) carrageenan content in natural populations from Galicia (NW Spain). J. Appl. Phycol. 24, 941–951. doi: 10.1007/s10811-011-9715-y
Torres R., Silva N., Reid B., Frangopulos M. (2014). Silicic acid enrichment of subantarctic surface water from continental inputs along the Patagonian archipelago interior sea (41°56 s). Prog. Oceanography 129, 50–61. doi: 10.1016/j.pocean.2014.09.008
Tyberghein L., Verbruggen H., Pauly K., Troupin C., Mineur F., Clerck O. (2012). Bio-ORACLE: A global environmental dataset for marine species distribution modelling. Global Ecol. Biogeog. 21, 272–281. doi: 10.1111/j.1466-8238.2011.00656.x
Usov A. I. (2011). Polysaccharides of the red algae. Adv. Carb. Chem. Bioch. 65, 115–217. doi: 10.1016/B978-0-12-385520-6.00004-2
Valdenegro A., Silva N. (2003). Caracterización oceanográfica física y química de la zona de canales y fiordos australes de Chile entre el Estrecho de Magellan y Cabo de Hornos. CIMAR tres fiordos. (Oceanographic physical and chemical characterization of the area of channels and fjords of Chile between the strait of Magellan and Cape Horn. CIMAR three fjords). Ciencia y Tecnol. Marina 26, 19–60.
Waaland J. R. (1975). Differences in carrageenan in gametophytes and tetrasporophytes of red algae. Phytochem 14, 1359–1362. doi: 10.1016/S0031-9422(00)98626-6
Westermeier R., González C., Murúa P., Morales J., David J., Patiño D. J., et al. (2021). Seasonal variation of carrageenan yield, gel strength and viscosity in Sarcopeltis (ex Gigartina) skottsbergii from southern Chile. Phycol. Res. 70, 42–49. doi: 10.1111/pre.12467
Weykam G., Thomas D. N., Wiencke C. (1997). Growth and photosynthesis of the Antarctic red algae Palmaria decipiens (Palmariales) and Iridaea cordata (Gigartinales) during and following extended periods of darkness. Phycologia 36, 395–405. doi: 10.2216/i0031-8884-36-5-395.1
Wiencke C. (1990). Seasonality of red and green macroalgae from Antarctica – a long-term culture study under fluctuating Antarctic daylengths. Polar Biol. 10, 601–607. doi: 10.1007/BF00239371
Wiencke C., Clayton M. N., Gómez I., Iken K., Luder U. H., Amsler C. D., et al. (2007). Life strategy, ecophysiology and ecology of seaweeds in polar waters. Rev. Environ. Sci. Biotech. 6, 95–126. doi: 10.1007/s11157-006-9106-z
Keywords: abiotic factors, carbohydrates, floridean starch, life history phases, pigments, proteins
Citation: Pasqualetti CB, Carvalho MAM, Mansilla A, Avila M, Colepicolo P and Yokoya NS (2023) Variations on primary metabolites of the carrageenan-producing red algae Sarcopeltis skottsbergii from Chile and Sarcopeltis antarctica from Antarctic Peninsula. Front. Mar. Sci. 10:1151332. doi: 10.3389/fmars.2023.1151332
Received: 26 January 2023; Accepted: 22 March 2023;
Published: 04 April 2023.
Edited by:
Rafael R. Robaina, University of Las Palmas de Gran Canaria, SpainReviewed by:
Carlos Angulo Preckler, King Abdullah University of Science and Technology, Saudi ArabiaCopyright © 2023 Pasqualetti, Carvalho, Mansilla, Avila, Colepicolo and Yokoya. This is an open-access article distributed under the terms of the Creative Commons Attribution License (CC BY). The use, distribution or reproduction in other forums is permitted, provided the original author(s) and the copyright owner(s) are credited and that the original publication in this journal is cited, in accordance with accepted academic practice. No use, distribution or reproduction is permitted which does not comply with these terms.
*Correspondence: Nair S. Yokoya, bnlva295YUBob3RtYWlsLmNvbQ==
Disclaimer: All claims expressed in this article are solely those of the authors and do not necessarily represent those of their affiliated organizations, or those of the publisher, the editors and the reviewers. Any product that may be evaluated in this article or claim that may be made by its manufacturer is not guaranteed or endorsed by the publisher.
Research integrity at Frontiers
Learn more about the work of our research integrity team to safeguard the quality of each article we publish.