- 1Programa de Pós-Graduação em Biologia Marinha e Ecossistemas Costeiros, Instituto de Biologia, Universidade Federal Fluminense, Rio de Janeiro, Brazil
- 2Laboratório de Produtos Naturais de Algas Marinhas (ALGAMAR), Departamento de Biologia Marinha, Instituto de Biologia, Universidade Federal Fluminense, Rio de Janeiro, Brazil
- 3Laboratório de Ecologia e Biogeografia, Departamento de Ecologia e Recursos Marinhos, Instituto de Biociências, Centro de Ciências Biológicas e da Saúde, Universidade Federal do Estado do Rio de Janeiro, Rio de Janeiro, Brazil
- 4Programa de Geoquímica, Central Analítica de Facilidades Geoquímica, Instituto de Química, Universidade Federal Fluminense, Rio de Janeiro, Brazil
- 5Laboratório de Fisiologia e Cultivo de Algas Marinhas (LFCA), Departamento de Biologia Marinha, Instituto de Biologia, Universidade Federal Fluminense, Rio de Janeiro, Brazil
- 6Programa de Pós-Graduação em Biodiversidade Neotropical, Instituto de Biociências, Universidade Federal do Estado do Rio de Janeiro, Rio de Janeiro, Brazil
The seaweed Dictyotaceae family has a great wealth of diterpenes with important biological activities. Information on these diterpenes qualitative and quantitative aspects in conjunction with physiological data and abiotic parameters are essential to obtain an effective algal culture system that is a sustainable resource. In this study, the diterpene profile of Dictyota menstrualis and Canistrocarpus cervicornis were analyzed as a function of in vitro maintenance time and the influence of culture medium. The algae were collected in the state of Rio de Janeiro and subjected to an acclimation period in the laboratory. Then, the species were acclimated in aquaria containing seawater from the collection sites. Then biomass algal was divided into (i) control experiment (CC): Growth in sterilized seawater; (ii) enriched experiment (EE): Growth in sterilized seawater enriched with Provasoli/2. Triplicate experiments were maintained in vitro (2.4% m/v), and the medium was changed every 7 days. Diterpenes were monitored using dichloromethane extracts from dried algae: (a) collected in the field, (b) after the acclimation period, and (c) from experimental units taken every 7 days for a total of 21 days. Among the main results, we found response variables such as specific growth rate (SGR); moreover, positive data were obtained for the alga C. cervicornis in CC, and this was evident showing after 7 days of cultivation in the experimental control unit (CU). For D. menstrualis, the SGRs for the treatments were negative at the monitored time, which was due to biomass losses, frond breakage, and rapid decomposition of some individuals that did not withstand the stress conditions during their collection. The analysis of the chemical profile by GC-MS made it possible to monitor 12 diterpenes and one sterol in the alga D. menstrualis and 7 diterpenes in C. cervicorni. The correlations found by Generalized Linear Models (GLMs) and redundancy analysis (RDA) statistical analyzes between the diterpene profile with the monitored variables (Nitrate, ammonia and pH), showed correlations for 10 diterpenes of the species D. menstrualis and 1 of the C. cervicornis, our analyses are important for the discussion of metabolic plasticity of the Dictyotaceae family
1 Introduction
Marine natural products are the subject of much study and scientific interest due to their diversity and chemical complexity, as well as their potential biological activities. Brown algae (Phaeophyta) of the Dictyotaceae family are characterized by the fact that they produce a variety of new metabolites, especially terpenoid compounds, that are useful because of their bioactive capabilities. There is particular interest in several species of this family, being notable Dictyota menstrualis and Canistrocarpus cerviconis. Extracts of these algae, which are typically found in shallow waters and intertidal communities in subtropical and tropical areas (Sadeghi et al., 2019), exhibit a remarkably wide range of properties (Chen et al., 2018; Bogaert et al., 2020), effects of the following types: antiviral (Pereira et al., 2004; Abrantes et al., 2010; Vallim et al., 2010; Cavalcanti et al., 2011; de Souza Barros et al., 2016; Cirne-Santos et al., 2019; Cirne-Santos et al., 2020a; Cirne-Santos et al., 2020b; Ribeiro et al., 2021), cytotoxic (Jongaramruong and Kongkam, 2007; Caamal-Fuentes et al., 2014), antibacterial (Viano et al., 2009; Ibraheem et al., 2017; de Figueiredo et al., 2019; Rubiano-Buitrago et al., 2019), antitumoral (Gomes et al., 2015), anti-snake venom effect (Domingos et al., 2011), anti-inflammatory (Albuquerque et al., 2013; do Nascimento Ávila et al., 2019), anticoagulant (Albuquerque et al., 2004; Camara et al., 2011; de Andrade Moura et al., 2011), antioxidant (Rodrigues et al., 2020), and antileishmanial (Dos Santos et al., 2011). Thus, D. menstrualis and C. cervicornis were selected as the target of the present study because they are species with widely demonstrated different bioactivities and pharmacological potential. Moreover, they are species that produce group I and III diterpenes according to hypothetical biogenetic pathway for ‘‘Dictyota’’ diterpenes (Teixeira and Kelecom, 1988), thus covering the study of different types of diterpene skeletons.
A major obstacle in bioprocess engineering for the production of new compounds from marine macroalgae is the development of in vitro culture systems suitable for cultivation in bioreactors (Rorrer et al., 1997; Rorrer and Cheney, 2004). In addition, the potential of these algae is always limited to obtaining biomass for isolating substances in sufficient quantities, which generally prevents the continuation of studies of bioactivities in the laboratory, such as their use on a larger scale. For this reason, it is a major challenge for these algal species to find sustainable options that avoid the continuous removal of populations from their native stocks.
The growing demand and sustainable supply and availability of high-quality seaweed seedlings for metabolite obtaining still face many barriers. One solution for producing high quality seaweed seedlings is tissue culture or micropropagation (Obando et al., 2022b). However, there is a path to follow before embarking on this type of cultivation. For example, in species where culture techniques are poorly understood, such as species in the Dictyotacea family (Bogaert et al., 2016; Martins et al., 2016; Obando et al., 2022a), experiments to maintain individuals in the laboratory in addition to simultaneously monitoring the metabolite of interest can provide important results and conclusions in the “in vitro culture of macroalgae” line of research. In order to try to predict the behavior of an alga under certain conditions and thus make it a sustainable source of innovative pharmaceutical compounds/ingredients, it is necessary to know its behavior and evolution under optimal laboratory conditions. In this article, the growth responses and, in particular, the production of diterpenes of D. menstrualis and C. cervicornis are described in laboratory experiments with the aim of evaluating the aim of evaluating the influence of nutrient enrichment in culture systems on the diterpene profile. In addition, with the aid of multivariate statistical tools, it was possible to make inferences about how the variations of nutrients dissolved in the laboratory system are correlated with the diterpene profile of the seaweed. It is important to remark that the production of diterpenes of the dolastane and secodolastane type from the alga C. cervicornis under laboratory conditions is reported for the first time.
2 Materials and methods
2.1 Sample collection
D. menstrualis specimens were collected in Enseada do Forno, Armação dos Búzios (22°44′00′′ S, 41°57′25′′ W), and C. cervicornis in the southeastern region between Ensenada de Araçatiba and Praia Vermelha, Ilha Grande - Angra dos Reis (23°11,600 íS, 44°38,630 íW) (Rio de Janeiro State, Brazil) in shallow water at a depth of 2.0 to 5.0 m by diving. The visible epiphytic organisms were removed on site, and the collected algal biomass was immediately filled in plastic trays with seawater, stored in a cool box and taken to the laboratory of the Universidade Federal Fluminense for processing. Voucher specimens were deposited in the herbarium of the Universidade Federal do Estado do Rio de Janeiro - (Herb´ario Prof. Jorge Pedro Pereira Carauta) under the numbers HUNI 6810 and HUNI 6811.
2.2 Maintenance conditions
Macroalgae were acclimated for one week in a culture room under controlled conditions (temperature of 20 ± 1°C, adequate and constant ventilation, light intensity of 80 μE.m-2. s-1 provided for bottom-up culture from below by 40-W fluorescent lamps (Sylvania daylight tubes) on a 12:12 h light-dark cycle. The average temperature in the experiments was 21 ± 1°C and salinity was 33.0 psu.
The culture medium consisted of seawater taken from the same macroalgae collection site (salinity of 32 psu and pH of 8.2), sterilized by autoclaving at 15 lbs pressure (121°C) and filtered through a 0.45 µm pore size membrane (Millipore HA). Species preservation was performed on a laboratory scale using 500 mL Erlenmeyer flasks as growth vessels. For both experiments, 400 mL seawater and 10% distilled water were used as the basic medium. Subsequently, individuals were divided into: (i) control experiment (CC), medium sterilized seawater; (ii) enriched experiment (EE), medium sterilized seawater enriched with Provasoli/2 (Provasoli, 1968). Triplicates (C1, C2, C3 and E1, E2, E3) of the experiments were kept under controlled laboratory conditions (described above). An experimental control unit (CU) for each treatment was kept under the same conditions to evaluate the response variables such as biomass and specific growth rate (SGR), without the influence of the removal of individuals. The culture was maintained by weekly medium changes (Pellizzari et al., 2008). Biomass, pH, nutrients dissolved in the culture, and chemical analysis of the diterpene profile were monitored each week for 21 days, and cultures were left under controlled conditions to evaluate their development over time up to 56 days.
2.3 Biomass and pH
pH values were monitored weekly using a previously calibrated digital pH-meter to check for diurnal pH fluctuations. Fresh biomass was recorded at weekly intervals before medium renewal. Macroalgae were dried with absorbent paper and wet weights (g/L) were determined on a digital balance, with four consecutive weights of the algal group recorded for each replicate. The average (n = 4) biomass values were used to calculate the specific growth rate (SGR) (Luhan and Sollesta, 2010; Yong et al., 2013), which was estimated using the formula SGR = (ln Mt – ln Mo)/T x 100%, where R = relative growth rate, Mo = initial dry mass, Mt = dry mass measured on day t, and T = number of days.
2.4 Analysis of dissolved organic nutrients and water-soluble proteins under laboratory conditions
At weekly media renewal intervals, 250 mL of water was collected from the samples (n = 3) of the system for dissolved nutrient analysis. Water samples were filtered through cellulose membrane filters (Millipore R HAWP 0.45 μm pore) and stored at -20°C until spectrophotometric determinations of ammonia/ammonium (NH4+) following Aminot and Chaussepied (1983) methodology, nitrate (NO3-), and nitrite (NO2-) following Strickland and Parsons, 1972 modify by Aminot & Chaussepied, 1983 and phosphate (PO43-) following Grasshoff et al., 1983. Each sample was quantified in triplicate. The precision of these analyses and the detection limit were estimated using triplicate samples. Concentrations of N-NH4+, N-NO2-, N-NO3- and P-PO4-3 were expressed in μM. Water-soluble proteins were determined according to the method proposed by Lowry (1951), adopting the protein extraction procedures developed by Barbarino & Lourenço (2005).
2.5 Chemical analysis of the diterpene profile
The diterpene mixture was analyzed by thin-layer chromatography (TLC) using mobile phase hexane-acetone (7:3); subsequently, the mixtures were analyzed by gas chromatography-mass spectrometry (GC-MS), using an Agilent 7890A/5973C equipped with a model HP5- MS column (30 m length, 0.25 mm diameter and 0.25 μm path length) and a mass-selective detector performed in electron impact mode (70 eV) (Obando et al., 2022a). The chromatograms and mass spectra obtained in the experiments were analyzed using the open-source software Openchrom (Wenig and Odermatt, 2010). The composition of diterpenes in the fractions was confirmed by 1H NMR. Chemical shifts (ppm) in units relative to tetramethylsilane (TMS), intensity, and integration of the peaks in the NMR spectra were registered. All NMR spectra were analyzed using MestreNova 6.0.2 and baseline corrected. The chemical components were identified by comparing the mass spectral data with those of standards in the laboratory and previous studies (Cavalcanti et al., 2006; Ortiz-Ramírez et al., 2008; Obando et al., 2022a).
2.6 Statistical analysis
All data were tested for normality and homoscedasticity. Comparisons of variables that did not meet variance or distributional assumptions were performed with Kruskal-Wallis nonparametric tests. Where applicable, results are reported as mean ± standard error of the mean (SEM). Differences were considered statistically significant at the 0.05 level (p < 0.05) using GraphPad Prism 8 software (Graphpad Software, Inc, San Diego, CA, USA).
To test for the possible effects of dissolved organic nutrients on each diterpenoid abundance of each algae, we run Generalized Linear Models (GLMs) for each dependent variable with all set of variables selected after eliminated collinearity between them. Before building the models, we tested the normality of our response variables (each diterpenoid of each alga) with the Shapiro-Wilk test. We standardized all predictors variables using z-scores and tested the collinearity among the predictors variables (Zuur et al., 2010). The collinearity among the predictor’s variables was tested by Pearson’s correlation coefficient, considering a correlation coefficient threshold of 0.70 as a cut-off value for the exclusion of predictors in the models (Dormann et al., 2013). The GLMs were fitted with gaussian or gamma distributions applying the log, inverse, and identity link functions. The choice of link function was settled by the lowest Akaike’s information criterion, corrected for small sample size (AICc). An additional procedure was performed for the elimination of multicollinear variables, using the variance inflation factors (VIFs). The environmental variables that presented high VIF values were eliminated one by one until all had VIF less than 4 (Zuur et al., 2010). After establishing the minimum set of environmental variables, the best set of models was selected based on AICc and included the models presenting ΔAICc ≤ 2 (Burnham and Anderson, 2002). The best model was selected according to the following hierarchical criteria: 1 – The residuals of the model must be normally distributed (Hartig, 2019). Residual diagnostic qqplots were used to examine the assumption of the regression; 2 – Contribution of predictors variables. At least, the inclusion of a variable with a significant (p > 0.05) contribution; 3 - Model goodness-of-fit, considering explained deviance.
To investigate the influence of dissolved organic nutrients on diterpenoid profile of each alga, we performed a redundancy analysis (RDA). To test the RDA significance, we realized a subsequent analysis of variance (ANOVA) of the canonical axes, using the diterpenoid profile of each alga as a response variable and dissolved organic nutrients composition as explanatory variable. Adjusted R2 was used to determine the fit of each model. Prior to RDA, all explanatory variables were tested for normality (Shapiro-Wilk Test) and collinearity (Pearson’s Correlation), considering |0.7| as a remove threshold for the correlation coefficient between each pair of variables (Zuur et al., 2010). Nitrate and phosphate concentration were highly correlated (0.92), thus nitrate concentration was excluded from all subsequent analysis. Furthermore, all explanatory variables were standardized, and diterpenes abundances were Hellinger-transformed to account for the presence of rare species and zero values within the dataset.
All analyses were performed with the statistical platform R (R Core Team, 2021) using the packages ‘AICcmodavg’ (Mazerolle, 2020), ‘car’ (Fox and Weisberg, 2019), ‘DHARMa’ (Hartig, 2019), “labdsv” (Roberts, 2019), ‘MuMIn’ (Barton, 2009), ‘MASS’ (Venables & Ripley, 2002) and “vegan” (Oksanen et al., 2013) packages of R software (R Core Team, 2019).
3 Results
3.1 Physiological parameters
In the laboratory system, D. menstrualis and C. cervicornis were acclimated for 7 days, plus 21 days determined in the experiments, and as additional data, the species were kept for up to 56 days. The choice of cultivation conditions for species was based on temperature and salinity data recorded in the field, as well as previous studies in which brown algal species were maintained in the laboratory, such as D. menstrualis itself and the type species of the genus, Dictyota dichotoma. Much of the work consulted opted to maintain specimens in Provasoli culture media (Soe Pa Kyaw et al., 2009; Bogaert et al., 2016), at a temperature of 20°C, constant aeration, and a light intensity of 80 µE.m-2.s-1 (Bogaert et al., 2016; Martins et al., 2016; Obando et al., 2022b)
Photographic images were taken of some individuals within the 21 days of cultivation to monitor conditions and morphological changes (Figure 1). In the field, individuals of D. menstrualis showed very thin thalli with abnormal tips and margins and had a slimy texture, which was also reflected in the morphology of individuals in the experimental units, because the individuals monitored in vitro continued to present such characteristics, a result of their health condition in the natural environment and accentuated in the process of adaptation to the system (Figures 1F, G). In C. cervicornis, on the other hand, the observed individuals had healthier characteristics, such as greater stiffness of fronds, preserved and homogeneous color, and low production of mucilage (Figures 1H, O).
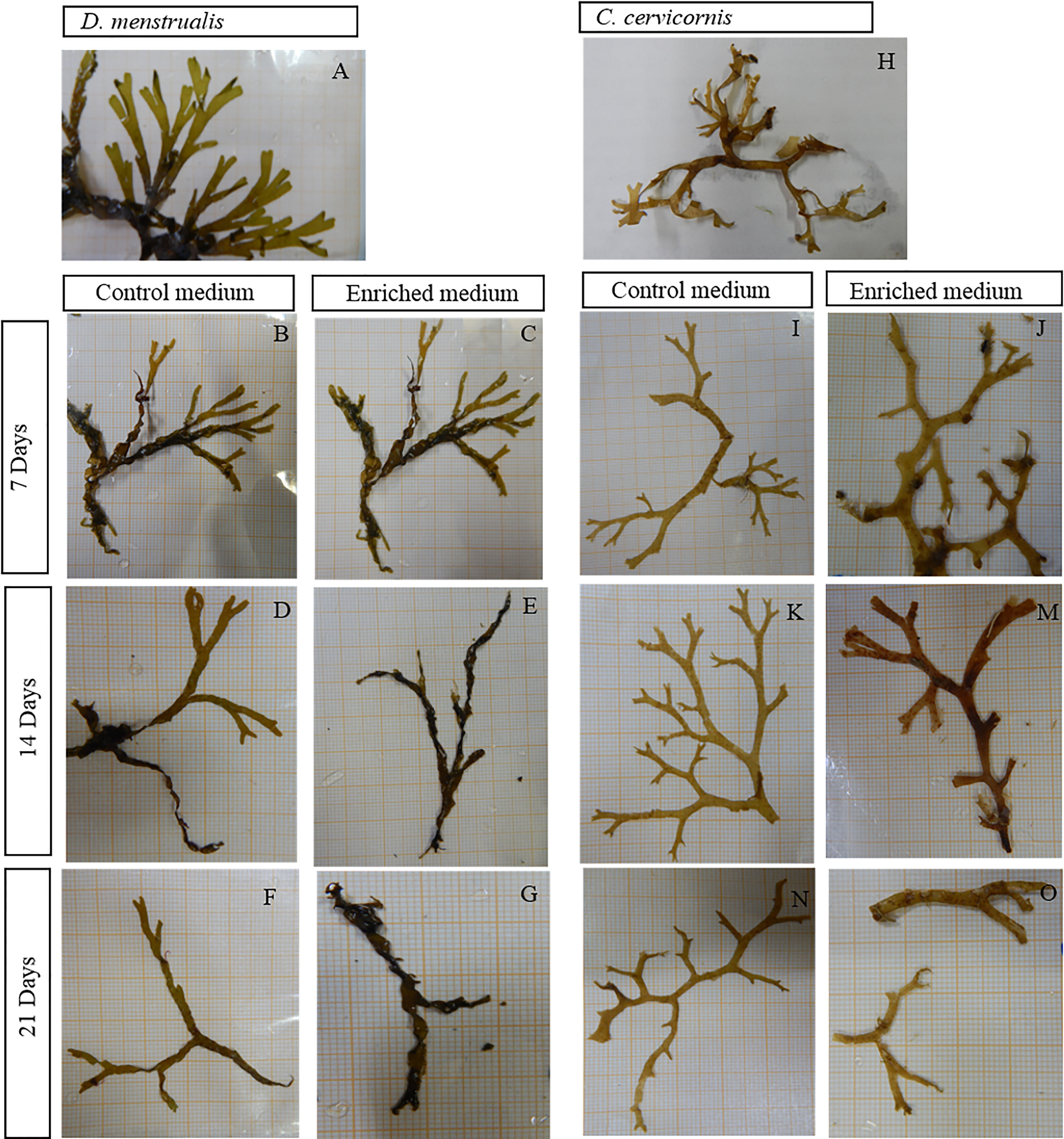
Figure 1 Representative photographs of the algae D. menstrualis (A–G) and C. cervicornis (H–O) taken every 7 days under controlled laboratory conditions.
During the maintenance period of the two species, some response variables were recorded, such as organic extract yield (%), biomass in g wet weight (g/L), SGR and pH (Figure 2). For the species D. menstrualis, statistically significant differences in the yield of dichloromethane extracts were found over the maintenance period, but it was not possible to determine differences between treatments. In particular, differences were found between the field extract and the last time (Figure 2A). The loss of dichloromethane extract yield in the experiments could be related to the adaptation and conditioning process in laboratory, where the production of various substances, both from algae and the associated microbiota, can be reduced. It is known that macroalgae-associated bacteria may play a role in their morphogenesis, reproduction, and chemical defense against other micro- and macrofauna (de Mesquita et al., 2019). Several points associated with this loss have been discussed in previous studies (Obando et al., 2022a).
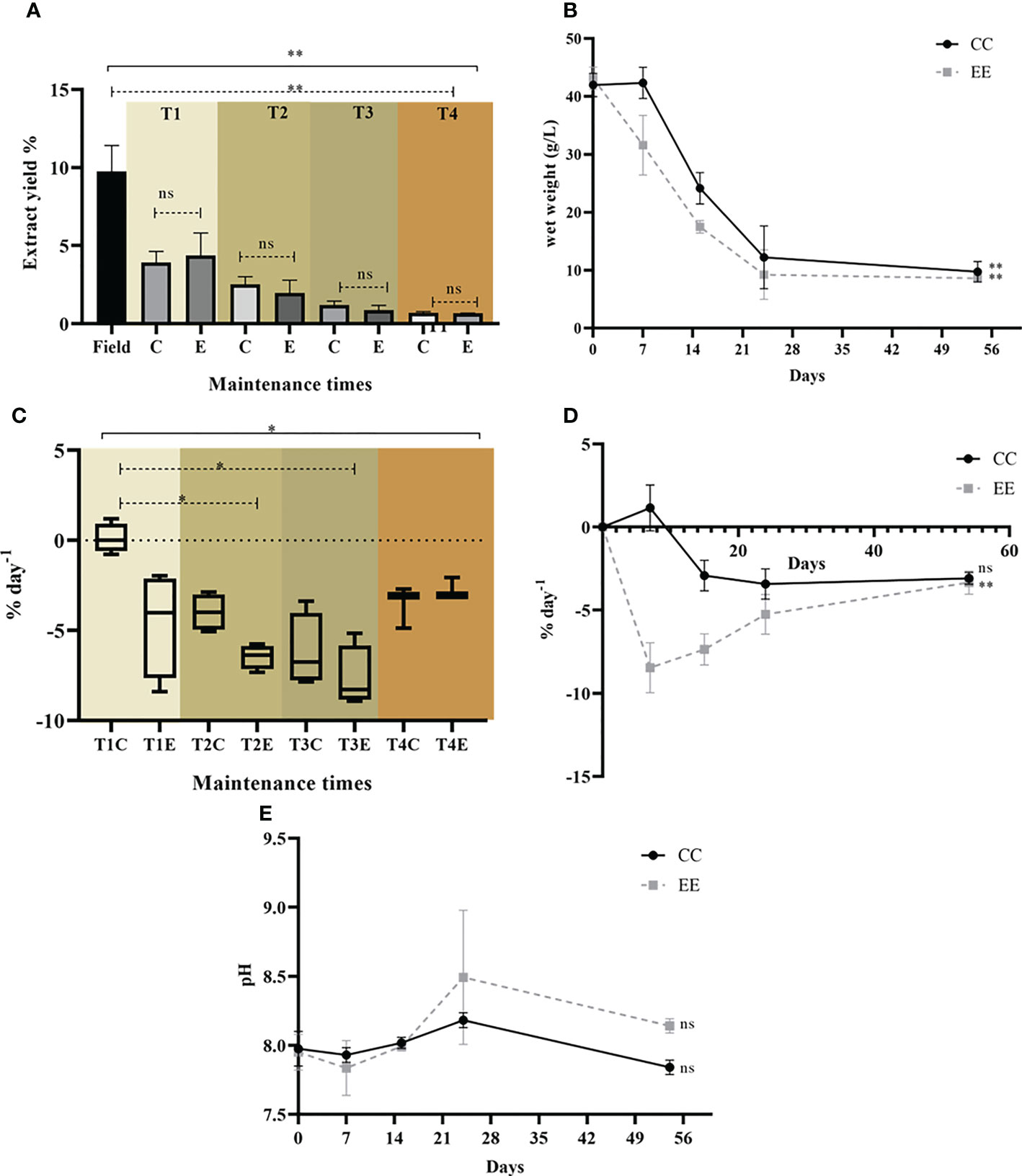
Figure 2 Dictyota menstrualis maintenance response variables under controlled laboratory conditions for both treatments CC and EE in the defined times. (A) Comparison yield of dichloromethane extracts; (B) Biomass in wet weight (g/L) in the 4 defined time periods; (C) SGR (% day -1) at defined time points; (D) SGR (% day -1) in the control unit (CU); (E) pH monitoring at defined time points. Solid line: Kruskal-Wallis test and dashed line: Mann Whitney test. *p < 0.05; **p < 0.005, ns: not significant. All bars represent mean standard deviation (+/- SD, n=4).
For the CC and EE treatments, biomass values show a decrease in biomass (g) over time and the data are statistically significant (Figure 2B). All SGR values for the treatments at the specified time points were negative (Figures 2C, D), reflecting biomass losses due to frond breakage and rapid decomposition of some individuals that did not withstand the stress conditions in the water. Only the experimental control unit of the CC treatment consistently showed positive SGR after 7 days of culture.
In the species C. cervicornis, statistically significant differences in the yield of dichloromethane extracts by maintenance time were observed, but it was not possible to determine differences between treatments (Figure 3A). In the CC treatment, C. cervicornis biomass remained stable until time 3 (21 days of the experiment), and biomass increased in the experimental unit until day 56 (Figure 3B). A decrease in algal biomass in g is observed for the EE treatment (Figure 3B). Most SGRs for the treatments at the defined time points were zero or negative, which can be attributed to biomass losses due to frond breakage (Cunha et al., 1999). However, positive SGR was observed in some triplicates, e.g.: 1.39% day -1 in treatment C1-T1 and 0.24% day -1 in E1-T1. Also, the highest TBI of 3.19% day-1 was observed in treatment E3-T1. However, the data for these experimental units were not stable during the experiment (Figure 3C).
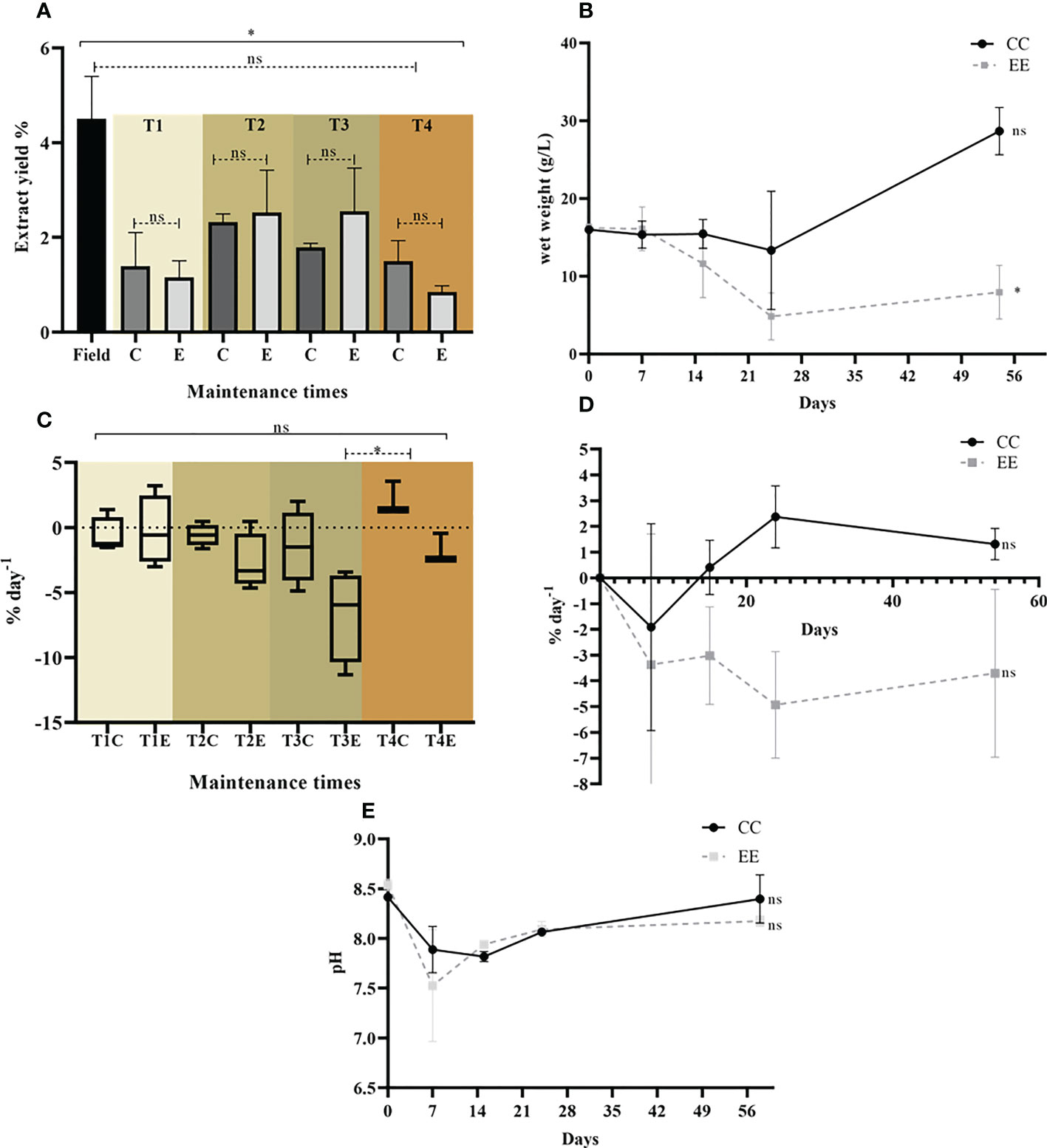
Figure 3 Canistrocarpus cervicornis maintenance response variables under controlled laboratory conditions for both treatments CC and EE in the defined times. (A) Comparison yield of dichloromethane extracts; (B) Biomass in wet weight (g/L) in the 4 defined time periods; (C) SGR (% day-1) at defined time points; (D) SGR (% day -1) in the control unit (CU); (E) pH monitoring at defined time points. Solid line: Kruskal-Wallis test and dashed line: Mann Whitney test. *p < 0.05; **p < 0.005, ns: not significant. All bars represent mean standard deviation (+/- SD, n=4).
The experimental control unit of treatment CC showed positive SGR throughout the experiment from the second week, e.g., 0.40 ± 1.05% day -1 in CC -T2 and 2.37 ± 1.21% day -1 in CC -T3, and at the last time point (56 days) an increase at 1.31 ± 0.60% day -1 could be detected without statistically significant differences (p: value 0.2916) (Figure 3D). It is important to highlight that no individuals were removed in the control experimental units during the experiment. The low handling of the algae in conjunction with the conditions and the culture medium may be factors that contribute to an increase in SGR and promote the growth of algae in the system. The monitored pH data did not show significant differences over time in the system for the two treatments (Figure 3E).
From these results, it is clear that the nutrient medium used as a nutrient source in EE has no significant differences in growth and biomass production compared to CC seawater. From this study, it can be concluded that the growth and production of biomass are not directly proportional to the amount of nitrogen in the medium, as no positive growth rates or increase in biomass were observed in the experimental units obtained in EE (g) (Figures 2D, 3D). The descriptive statistical analyzes performed for dissolved nutrients, ammonium (19,613x+0,0172), nitrate (19,613x+0,0172), nitrite (19,613x+0,0172) and phosphate (47,781x + 0,0481) ions under controlled laboratory conditions are shown in Figure 4.
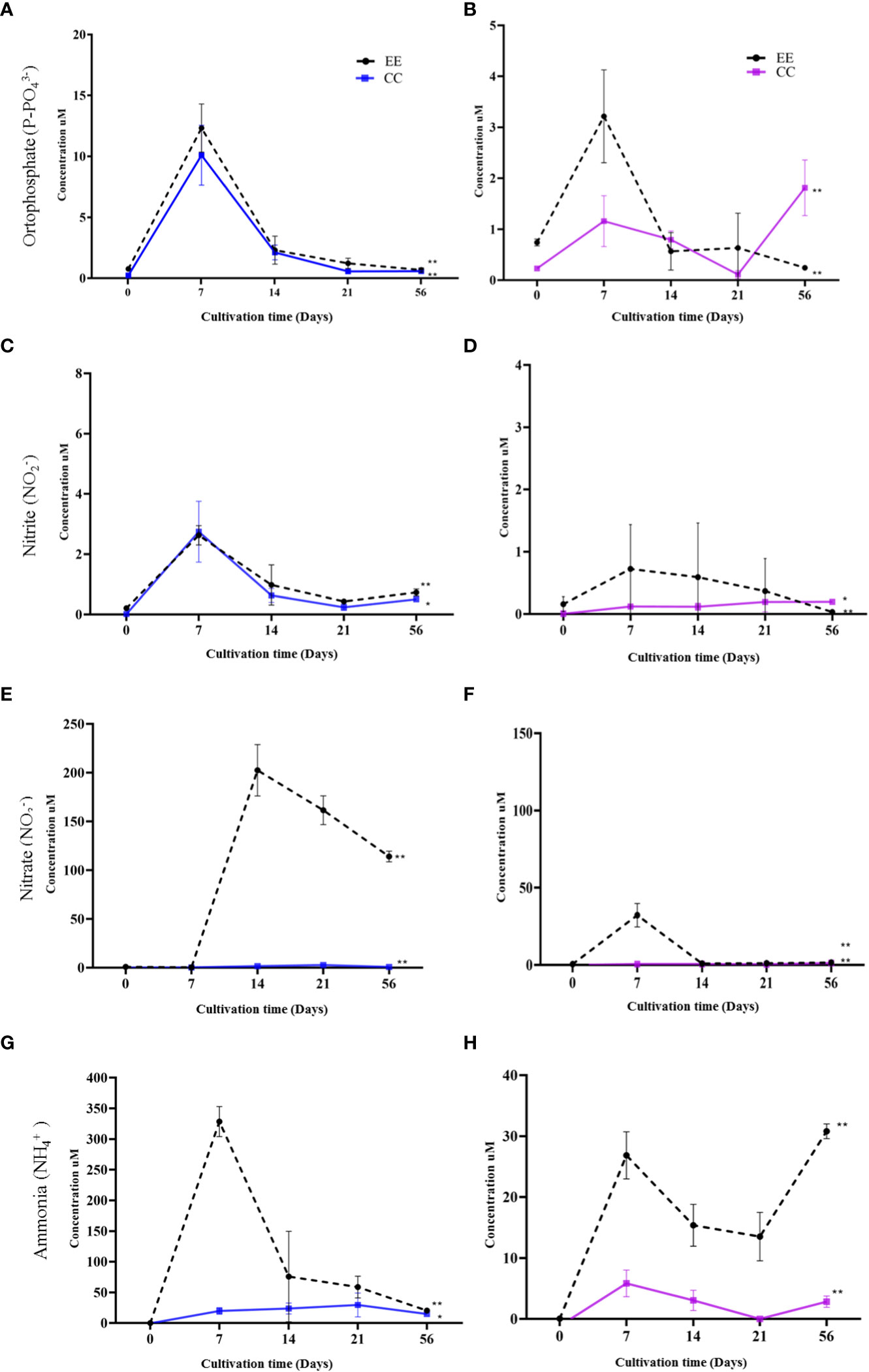
Figure 4 Monitoring and analysis of dissolved nutrients for water samples obtained from the culture system Dictyota menstrualis (A) phosphate, (C) nitrate, (E) nitrite, (G) ammonia. And system Canistrocarpus cervicornis (B) phosphate, (D) nitrate, (F) nitrite, (H) ammonia. Kruskal Wallis test *p<0.05; **p <0.005, ns: no significance. The bars represent the mean standard deviation (+/- SD, n=4).
For the D. menstrualis orthophosphate, it is noteworthy that compared to time 0 (field), there was an increase in concentration for all time points evaluated in culture, with the highest concentrations (μM) at 7 days being 10.09 ± 0.04 and 12.33± 1.97 for CC and EE, respectively (Figure 4A). For nitrite, maximum concentrations after 7 days were observed for both EE and CC, namely 2.65 ± 0.32 and 2.74 ± 0.09, respectively (Figure 4B). For the nitrate, it was found that the treatment with Provasoli/2 (EE) medium showed high concentration values from the 14th day of the experiment, with a maximum value of 202.5 ± 26.32 after 7 days of cultivation. For the CC treatment, concentrations remained stable, with a maximum of 2.76 ± 0.24 after 7 days of cultivation (Figure 4C). For ammonia in the EE treatment, a maximum value of 345.8 ± 29.54 and for CC of 29.44 ± 19.48 after 7 days was recorded (Figure 4D).
In C. cervicornis, the concentrations of dissolved nutrients did not show higher values than 35 μmol. L-1 over time. The concentrations in the experiments of CC showed stability and reached their maximum only at the end of the experiment with orthophosphate 1.53μM ± 0.31, nitrite 0.21 μM ± 0.005, nitrate 1.25 μM ± 0.009, except for ammonia that the maximum was 5.83 μM ± 2.18 at 7 days. In the EE treatment, some nutrient peaks were observed with maximum values of 31.94 μM ± 9.21 for nitrate and 26.86 μM ± 3.86 for ammonia at 7 days of culture (Figures 4B, H).
Regarding, the water-soluble proteins in the culture system for D. menstrualis, maximum concentrations (% PTN tallus) were 13. 83 ± 1.18 at 14 days and 13. 93 ± 1.91 at 21 days for EE and CC, respectively. For C. cervicornis, maximum values at 21 days were 16.03 ± 0.09 for EE treatment and 15.35 ± 0.29 for CC (Figure 5). In general, brown algae contain lower protein concentration (5 to 20% of DW) than red and green algae (5 to 30% of DW) (Rohani-Ghadikolaei et al., 2012). Notably, protein content tends to be low (5-10% of dry weight) in macroalgae from tropical and subtropical coastal environments, such as Brazilian ecosystems, where this profile is more pronounced due to the oligotrophic characteristic of these ecosystems (Banerjee et al., 2009). Our protein data are consistent with these conditions.
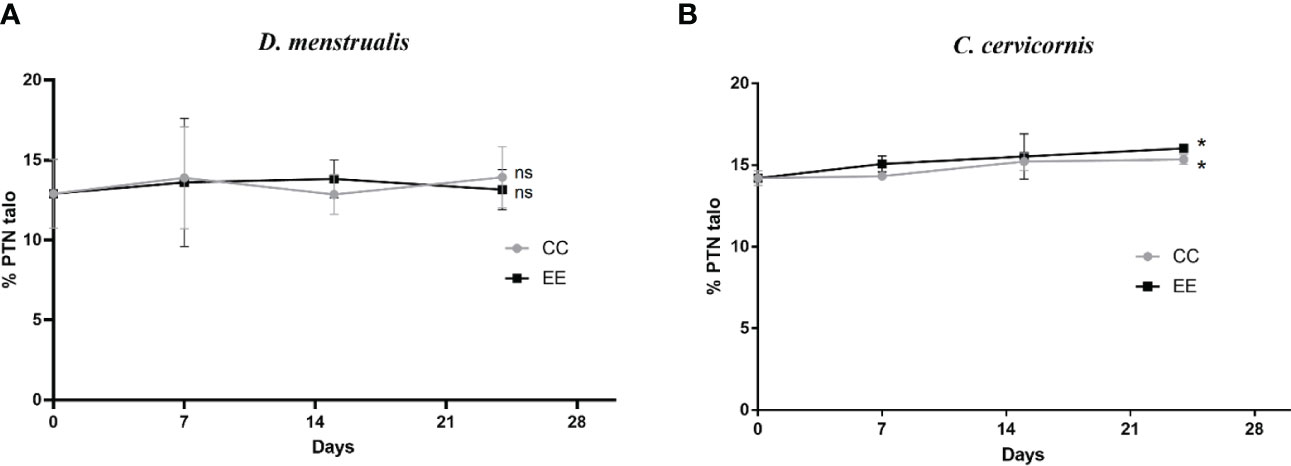
Figure 5 Monitoring and analysis of Water-soluble proteins. (A) Dictyota menstrualis, (B) Canistrocarpus cervicornis. Kruskal Wallis test *p<0.05; **p <0.005, ns: no significance. The bars represent the mean standard deviation (+/- SD, n=4).
3.2 Chemical profile of diterpenes
Chemical analysis of D. menstrualis extract by TLC revealed the presence of bands with terpenoid characteristics in the treatments and experimental units. retention factor (Rf) of each chemical class: sterols (0.21 to 0.28), cycloxeniane (0.34 to 0.36), dichotomane (0.42 to 0.48), dichotomane acetate (0, 51 to 0.54) and prenylated guaiane (0.75 to 0.81) (Supplementary material; Figure S1-A). The alga C. cervicornis, showed the presence of diterpenes. This was recognized by a chromatographic pattern of pink color, characteristic of diterpenes with skeleton of the dolastane and secodolastane type Among them, the pink bands correspond to the most oxidized diterpenes of the types dolastane (Rf - 0.35) and secodolastane (Rf - 0.24), characteristic of these algae (Supplementary material; Figure S1-B). The GC-MS analyses, enabled us to monitor 12 diterpenes and 1 sterol (fucosterol) for D. menstrualis, and 7 diterpenes for C. cervicornis, of which 17 diterpenes have been previously eluded and are shown in Figure 6.
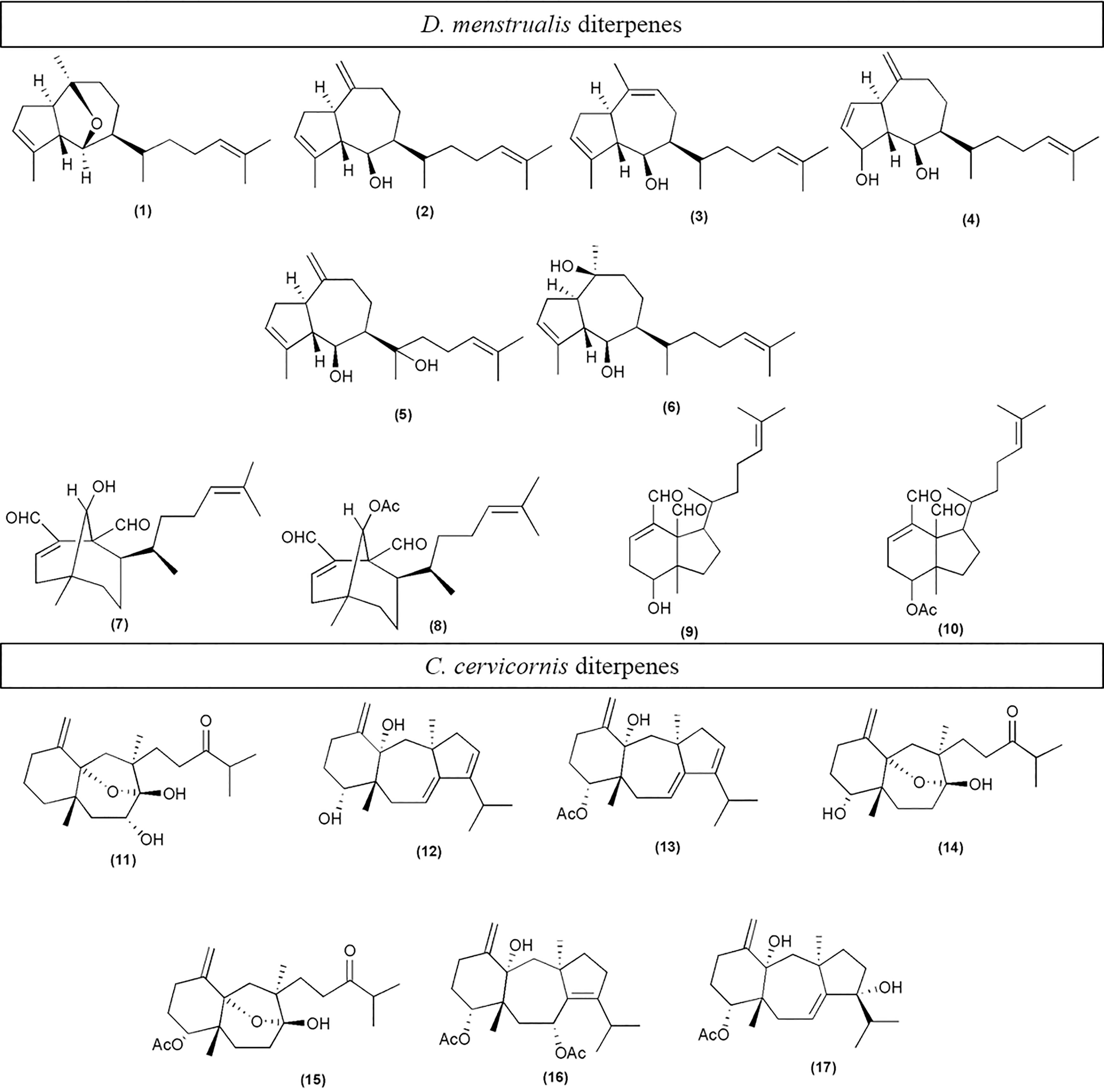
Figure 6 Diterpenes identified as chemical components of Dictyota menstrualis: Dictyoxide (1) (Amico et al., 1979); Pachydictyol A (2) (Hirschfeld et al., 1973); Isopachydictyol A (3) (Durán et al., 1997; Dictyotadiol (4) (Vazquez et al., 1988); Dictyol E (5) (Hay et al., 1987); Dictyol C (6) (Vazquez et al., 1988); 6-hydroxy-dichotomane-3-14- 1,17-dial (7)(Teixeira and Kelecom, 1988; Cavalcanti et al., 2006); 6-acetoxy dichotomane-3-14-diene-1,17 (8) (Teixeira and Kelecom, 1988; Cavalcanti et al., 2006); 5-hydroxy-1,6-cycloxenia-2,13-diene-16,17-dial (9) (Cavalcanti et al., 2006); 5-acetoxy-1,6-cycloxenia-2,13-diene-16,17 (10) (Cavalcanti et al., 2006; Ortiz-Ramírez et al., 2008. Diterpenes identified as chemical components of Canistrocarpus cervicornis: 2-deoxiisolinearol (11); 4,14-diidroxy-dolastane-1(15),7,9-triene (12); 4,14-diidroxy-dolastane-1(15),7,9-triene (13); Isolinearol (14); Acetoxy isolinearol (15); 4,7 diacetoxy-14-hidroxydolastane-1, 8-diene (16); 4-acetoxy-9,14-diidroxy-1,9-dolastane-1,9-diene (17) (De Oliveira et al., 2008). Spectroscopic data of CGMS (Supplementary material; Tables S1,S2 and Figures S2, S3).
Relative abundances of diterpene profiles obtained from GC-MS were plotted to observe changes and differences both over time and between treatments (Figures 7–9). In D. menstrualis, six prenylated guaiane-type diterpenes (1) (2) (3) (4) (5) (6) (Figure 7) and four xeniane-type diterpenes (7) (8) (9) (10) were observed, two unidentified xeniane-like diterpenes and fucosterol were also observed (Figure 8). It is important to emphasize that throughout the 21 days of the experiment, the most important diterpene was the prenylated guaiane, pachydictyol A (1), with no significant differences between treatments, followed by cycloxeniane-acetoxy (10) and dichotomane hydroxyl (7), results observed in previous studies, these diterpenes were also the majority under laboratory conditions during the first 30 days of maintenance (Obando et al., 2022a).
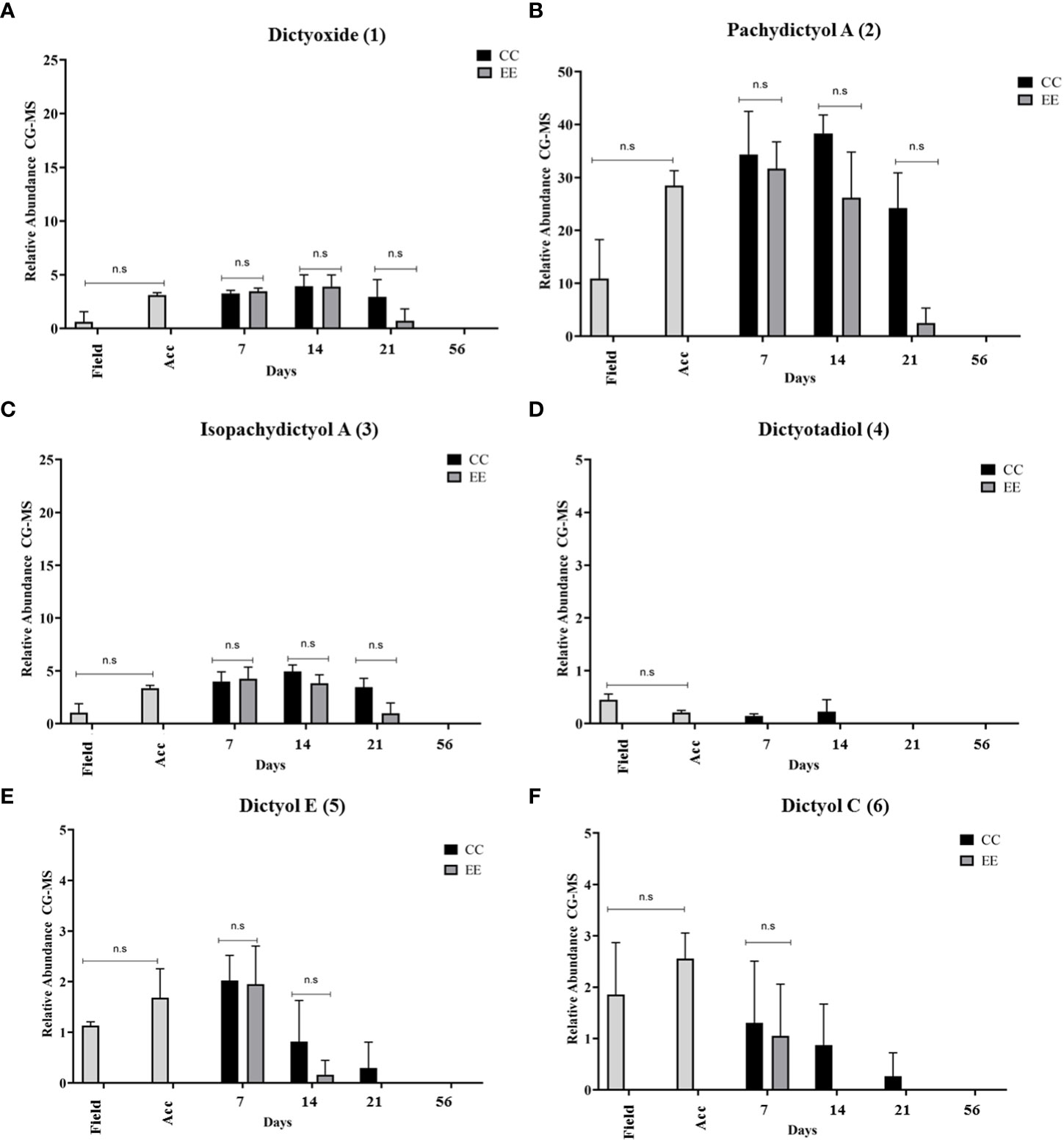
Figure 7 Analysis of GC-MS chromatograms of the chemical profile of Dictyota menstrualis. Comparison of the relative abundance of major diterpenes of the prenylated guaiane type across time and between treatments. (A) Dictyoxide, (B) Pachydictyol A , (C) Isopachydictyol A, (D) Dictyotadiol , (E) Dictyol E, (F) Dictyol C. Kruskal Wallis test *p<0.05; **p <0.005, ns: no significance. The bars represent the mean standard deviation (+/- SD, n=3). Acc (acclimation period).
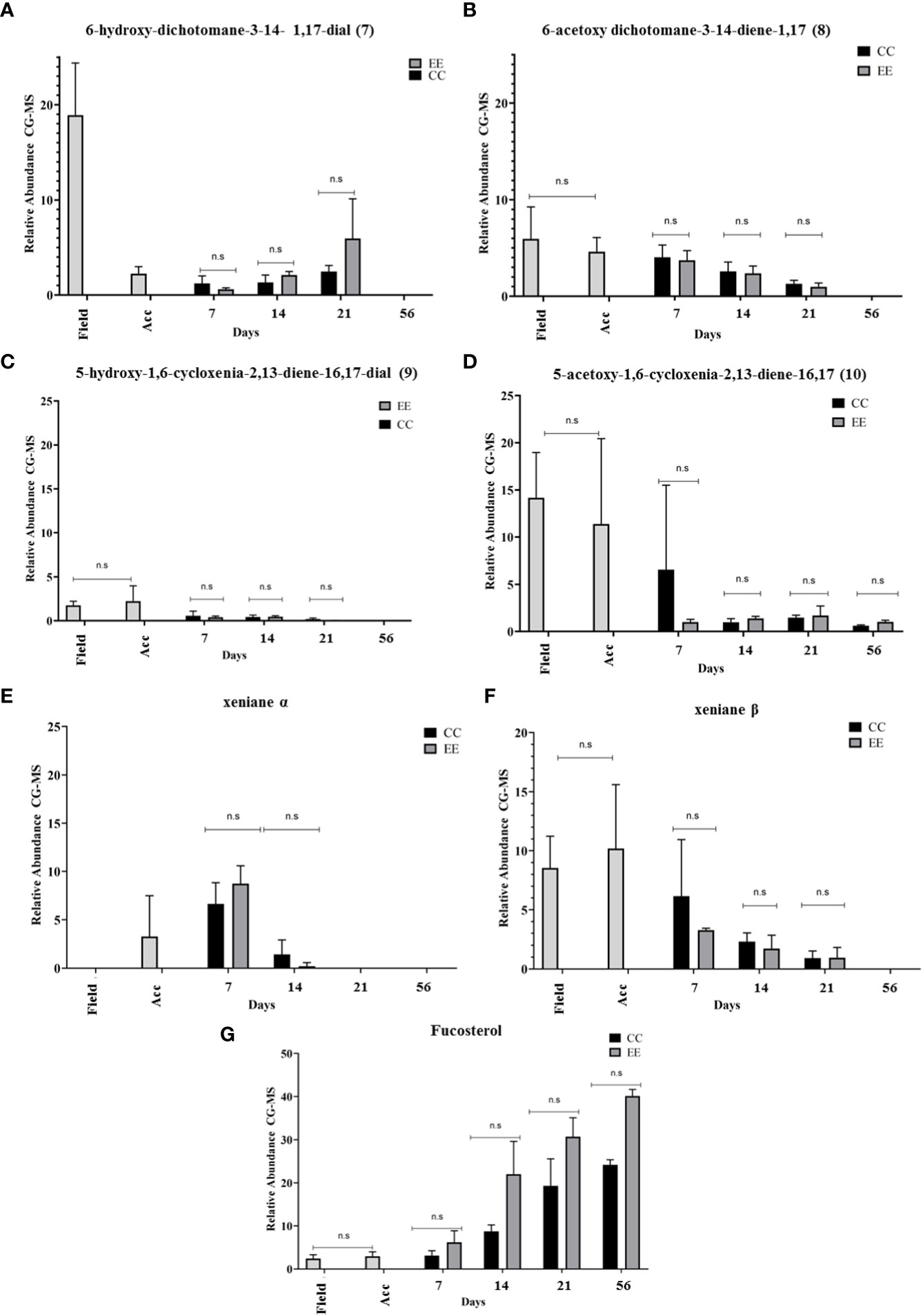
Figure 8 Analysis of GC-MS chromatograms of the chemical profile of Dictyota menstrualis. Comparison of the relative abundance of major xeniane-type diterpenes and fucosterol across time and between treatments. (A) 6-hydroxy-dichotomane-3-14- 1,17-dial, (B) 6-acetoxy dichotomane-3-14-diene-1,17, (C) 5-hydroxy-1,6-cycloxenia-2,13-diene-16,17-dial, (D) 5-acetoxy-1,6-cycloxenia-2,13-diene-16,17, (E) xeniane α, (F) xeniane β, (G) Fucosterol. Kruskal Wallis test *p<0.05; **p <0.005, ns: no significance. The bars represent the mean standard deviation (+/- SD, n = 3). Acc (acclimation period).
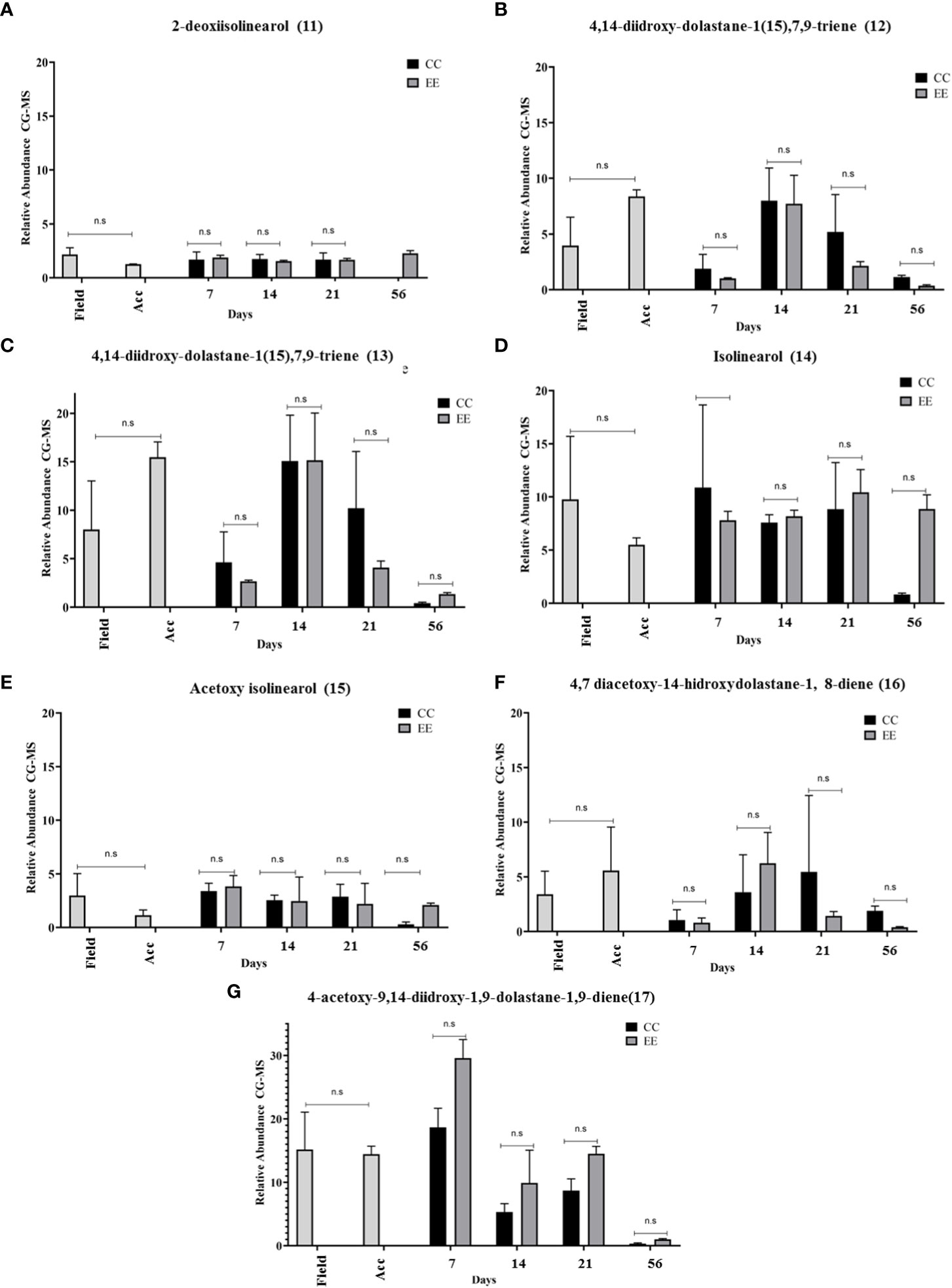
Figure 9 Analysis of GC-MS chromatograms of the chemical profile of Canistrocarpus cervicornis. Comparison of the relative abundance of major diterpenes dolastane e secodolastane type across time and between treatments. (A) 2-deoxiisolinearol, (B) 4,14-diidroxy-dolastane-1(15),7,9-triene, (C) 4,14-diidroxy-dolastane-1(15),7,9-triene, (D) Isolinearol, (E) Acetoxy isolinearol, (F) 4,7 diacetoxy-14-hidroxydolastane-1, 8-diene, (G) 4-acetoxy-9,14-diidroxy-1,9-dolastane-1,9-diene. Kruskal Wallis test *p<0.05; **p <0.005, ns: no significance. The bars represent the mean standard deviation (+/- SD, n = 3). Acc (acclimation period).
In the individual analysis of diterpenes in the maintenance time, it can be noted that at 56 days not all diterpenes were identified, while an increase in the amount of fucosterol was observed both in the treatment CC (24.19 ± 1.162) and EE (40.12 ± 1.52), indicating a choice of metabolic pathway to obtain primary metabolites during stress events (Obando et al., 2022a) and highlighting that the enriched medium favors this production over the control, showing that excess nutrients can cause greater stress. The production of diterpenes is higher than that of sterols at the observed time points, confirming previous studies (Fleury et al., 1994; Ortiz-Ramírez et al., 2008; Obando et al., 2022a)
No significant differences in the relative abundances of the 12 diterpenes were observed in the first 21 days set aside for the experiments, so a larger number of replicates is recommended to obtain meaningful data. There were also no significant differences between the field samples and the samples obtained after 7 days of acclimation, but there is a tendency for the diterpenes dictyoxide (1), pachydictyol A (2) and its isomer isopachydictyol (3), dictyol E (5), and dictyol C (6) to increase in their relative abundance after acclimation (Figure 7).
Notably, for diterpene (1), the highest abundance was observed after 14 days of cultivation in CC (3.927 ± 1.006) and EE (3.90 ± 1.075) (Figure 7A). For the diterpene (2), the highest relative abundance was detected after 14 days at CC (38.307 ± 3.485) and after 7 days at EE (31.683 ± 5.034) (Figure 7B). For the diterpene (3), the highest identified abundance was detected after 14 days of cultivation at CC (4.947 ± 0.606) and EE (4.253 ± 1.101) (Figure 7C). The diterpene (4) was registered only in CC and its greater amount in the field (0.453 ± 0.105) (Figure 7D), possibly because this diterpene is produced under specific physicochemical conditions that can be provided only by the natural environment, and that the excess of nutrients does not favor their production in the laboratory. The diterpene (5) showed its highest relative abundances (2.024 ± 0.494 and 1.950 ± 0.754) after 7 days at CC and EE, respectively (Figure 7E); in turn, the diterpene (6) recorded its highest relative abundance after the acclimation period (2.558 ± 0.287) (Figure 7F).
The results found for the xeniane-type diterpenes, are highlighted (Figure 8) as follows: The diterpene (7), according to previous studies (Ortiz-Ramírez et al., 2008), has its highest abundance in samples obtained in the field (18.99 ± 5.48), and under controlled conditions, after 21 days it is possible to register the highest abundance for the treatment EE (5.950 ± 4.176) (Figure 8A). In turn, its acetylated version (8) has the highest abundances for CC (4.050 ± 1.262) and EE (3.727 ± 1.009) in field samples (5.940 ± 3.321) and in the laboratory after 7 days (Figure 8B). The diterpene (9) has the lowest relative abundances among the xeniane-type diterpenes, with the highest production after acclimation (2,234 ± 1,742) (Figure 8C), while its acetylated version (10) has the highest yields in the field (14,173 ± 4.791) and in the acclimation period (11.372 ± 9.057), maintaining its production up to 56 days in CC (6.549 ± 8.952-0.607 ± 0.092) and in the EE treatment (1.689 ± 1.011- 0.999 ± 0.296) and identified as the only diterpene produced by the algae up to 56 days (Figure 8D).
It is important to emphasize that as of 21 days, few individuals were still participating in the experiments, and the few that remained were already weakened due to the constant sampling of the system for chemical analysis. The health of the algae and the handling of the material are reflected in the results of this last period. However, it is worth noting that the production of this acetylated versions can be maintained in the system. Under the conditions of the culture system, the alga can direct its metabolism to produce more stable molecules with acetyl groups, preventing the transformation or loss of these diterpenes and leading to an improvement in specificity with respect to their function, as previously reported (Obando et al., 2022a).
We also detected the production of a diterpene (xeniane α) with a xeniane-like skeleton with a retention time close to diterpene (10) and with a similar mass fragmentation pattern. This structure was detected only under controlled laboratory conditions up to 14 days, with higher production at 7 days of cultivation in CC (6,648 ± 2,187) and EE (8,736 ± 1,850). Another diterpene (xeniane β), previously identified but not elucidated by Obando et al., 2022a, was identified in this field study to day 21 of maintenance and recorded the highest abundance after acclimation (10,187 ± 5,426). Isolation and purification processes of these diterpenes will be necessary to proceed with structural elucidation studies.
In the alga C. cervicornis, 7 diterpenes such as dolastane (12)(13)(16)(17) and secodolastane (11)(14)(15) (Figure 9) were observed. The major diterpene in the field was diterpene 4-acetoxy-9,14-dihydroxy-1,9-dolastane-1,9-diene (17), followed by diterpene isolinearol (14) and diterpene 4-acetoxy-14-hydroxy-dolastane-1(15),7,9-triene (13). Individual analysis of diterpenes in the maintenance time identified all diterpenes produced up to 56 days. This finding, also corroborates the data obtained from the specific growth analyzed above.
No significant differences in the relative abundances of the 7 diterpenes were observed in the 21 days set aside for the experiments. However, after including the 56-day data, diterpenes (12), (13), and (17) showed significant differences with respect to time. The data obtained from the field samples and those obtained after 7 days of acclimation did not show significant differences, but diterpenes (12), (13), and (16) show a tendency to increase their relative abundances with acclimation time (Figure 9). In particular, for diterpene (11), the highest abundance was observed in the field (2.167 ± 0.618) and after 56 days in the EE treatment (2.276 ± 0.251) (Figure 9A); for diterpene (12), the highest abundances were observed in the acclimation period (8.387 ± 0.591) and at 14 days of cultivation for EE (7.720 ± 2.543) (Figure 9B), for the diterpene (13) the highest abundances were detected in the acclimation period (15.4.70 ± 1.578), at 14 days in CC (15.078 ± 4.737) and in EE (15.155 ± 4.875) (Figure 9C). The relative abundance of diterpenes (14) was highest at 7 days in CC (10,878 ± 7,779) and at 21 days in EE (10,424 ± 2,143) (Figure 9D).
The diterpene (15) had the lowest abundances of all diterpenes, with the highest abundances after 7 days CC (3.389 ± 0.739) and EE (3.821 ± 1.020) (Figure 9E). The diterpene (16) showed the highest abundances in the acclimation phase (5.574 ± 3.971) and after 14 days in EE treatment (6.242 ± 2.815) (Figure 9F). Finally, the diterpene (17), which showed the highest abundances during the cultivation period, recorded its highest production after 7 days for CC (18.662 ± 3.003) and for EE (29.573 ± 2.928). The latter diterpenes were identified as major constituents in field samples of C. cervicornis (De Oliveira et al., 2008).
The presence of diterpenes from the brown alga D. menstrualis was confirmed by the 1H NMR spectra. Four of the major diterpenes identified in previous studies (Obando et al., 2022a) were used to evaluate the chemical profile: Pachydictyol A (1), 5-acetoxy-1,6-cycloxenia-2,13-diene-16,17-dial (2), 9-hydroxy-dichotoma-2,13-diene-16,17-dial (3), and 9-acetoxy-dichotoma-2,13-diene-16,17-dia (4). During the experiments, it is observed that the typical signals of singletts are retained in the range between δ 9.40-9.70 ppm, indicating aldehydes, methyl doubles at δ 0.46-0.48, and methyl singletts between 2.0 and 1.0 ppm (Supplementary material; Figure S4). In the 1H NMR spectra of the alga C. cervicornis, the main diterpenes already identified in other studies (De Oliveira et al., 2008) were detected: 4,7-diacetoxy-14-hydroxydolastane-1(15), 8-diene (5), followed by dolastane (6) and secodolastane-isolinearol (7). The intensity of the characteristic signals of these diterpenes decreased significantly after 21 days compared to the spectra obtained from the field samples (Supplementary material; Figure S5).
Although the univariate statistical analyzes comparing the enriched treatment with the control treatment did not show significant differences in relation to the abundance of diterpenes, and taking into account that other variables could intrinsically influence the metabolite profile, multivariate statistical analyzes were performed using the models GLMs and RDA, to understand whether the variability of dissolved nutrients in the experiments correlates with the abundance of some of the compounds.
The set of predictors selected for the algae diterpenoids concentrations models showed a moderate to a high explanation power, ranging from 0.32 (diterpene 2) to 0.68 (diterpene 6) for D. menstrualis and moderate power to the unique model selected for the (diterpene 17) of C. cervicornis (Table 1). From 13 diterpene of D. menstrualis, only 3 hadn’t usefull models, two (diterpene 5 and α) excluded becaude they did not attained criterias 1 and 2, and the diterpene 1 to not attained the criteria 1. Almost all diterpene model for C. cervicornis (diterpene 11, 12, 13, 14, 15 and 16) hadn’t usefull models, because they did not attained the criteria 2, with the diterpene 13 not attaining the criteria 1 too. Most of D. menstrualis diterpenes presented usefull models, with most of then incluiding a nitrogen organic compound and pH as significant predictors (Table 1). In opposite direction, a unique model, with a lower prediction power (0.29) and including a phosphor compound, explained the C. cervicornis diterpene concentration (Table 1). Only the diterpene 3 of D. menstrualis did not include a nitrogen compound as a significant predictor, which was substituted by pH. Most of the D. menstrualis models included a nitrogen compound and pH as significant contributions (diterpenes 2, 7, 9, β and Fuc), four of then showing a higher contribution for pH (diterpenes 2, 9, β and Fuc) (Table 1).
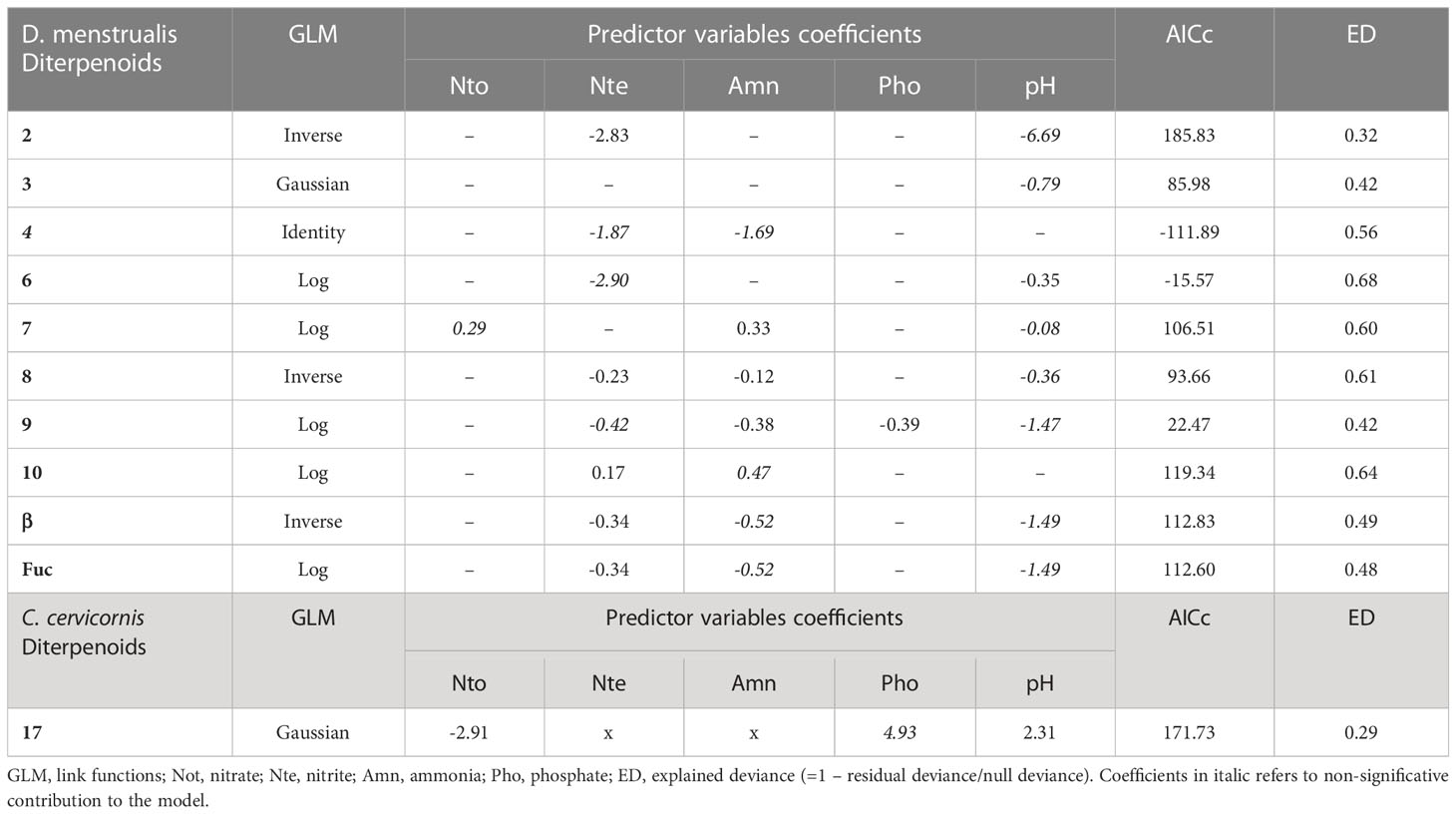
Table 1 Statistical parameters of the best GLM showing the effects of organic nutrients compounds on algae diterpenoids concentrations.
The significant redundancy analysis (RDA) for D. menstrualis explained 30.7% (adjusted R2) and 42.7% (proportion explained) of the total variation within the diterpene data, with the first axis accounting for 31.9% of the proportion explained variation (74.6% of total explained power). Along the rda1 axis, pH (-0.95) and nitrite (-0.48) presented the higher significant contributions, and three diterpene had scores above 0.20 and were considered strongly associated with this axis, the diterpene 7 (-0.56), α (0.35) and 2 (0.33). The Field samples (-0.51, -0.57, -0.25), experiment samples after 8 days (0.40, 0.36, 0.35) and 24 days (-0.23, -1.05, -0.54) presented a strong association between their diterpenes profile composition and the rda1 axis. The redundancy analysis (RDA) for C. cervicornis wasn´t statistically significant (Figure 10).
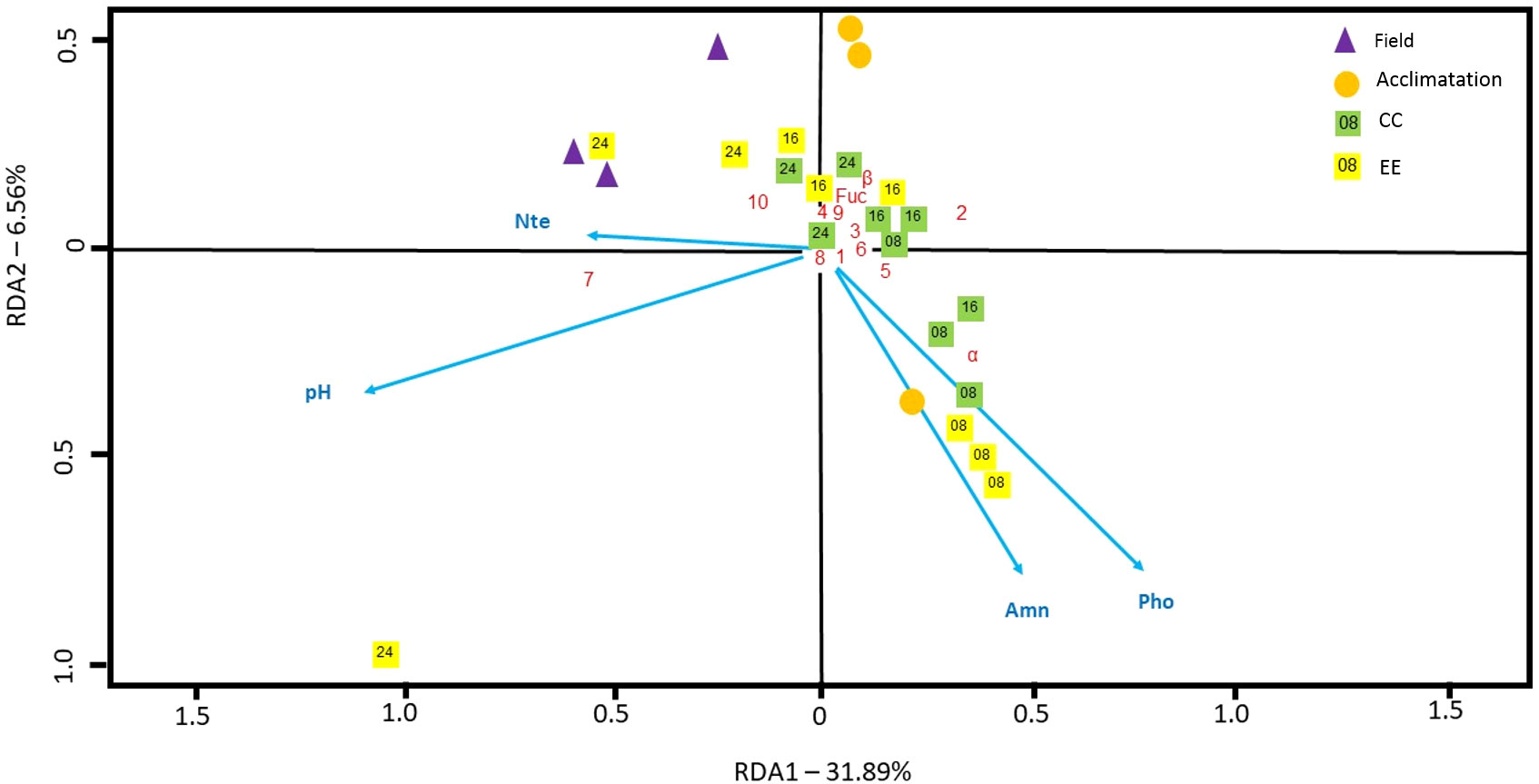
Figure 10 RDA analyses to investigate the relationship between dissolved inorganic nutrients and diterpenoid profile of the brown alga D. menstrualis. Nutrients compounds: Amn, ammonia; Nte, nitrite; Pho, phosphate. Numbers inside the squares means the treatment cultivation time.
4 Discussion
EE experiments show lower rates of relative growth and loss of biomass over time for both D. menstrualis and C. cervicornis. This addition of nutrients can be discussed from previous studies where experiments performed with Dictyota spp. showed percent cover was not significantly affected by nutrient addition like in thallus height results (Ramseyer et al., 2021), such nutrient addition had a significant negative effect on the mean percent change in Dictyota biomass. Although some previous studies have demonstrated a positive effect of nutrient enrichment on the growth of Dictyota (Sotka and Hay, 2009; Martins et al., 2016). Under natural conditions Dictyota spp. may have evolved to take advantage of periodic pulses of nutrients for survival in low-nutrient environments such as coral reefs (Ramseyer et al., 2021). Events called “luxury uptake” can cause growth inhibition at higher nutrient levels. This luxury uptake is nutrient uptake, and is not associated with biomass production, which allows algae to rapidly exploit nutrient pulses and store them for future growth (Reef et al., 2012). The ability to store nutrients during periodic pulses can be advantageous in an environment where nutrient availability is inconsistent (Den Haan et al., 2016).
The results of nutrient analyses from CC and EE showed high initial and low final concentrations of nitrates, nitrites and ammonium with a significant influence of the time period. The largest difference between the average initial concentrations was observed in the first 14 days. It can be seen that the NO3- concentration is high at the beginning and that this level decreases over time due to the intracellular reduction of NO3- to NO2- within the algal cells. It is important to note that the cells initially take up large concentrations of nitrate and yet high amounts are reduced intracellularly, leading to saturation of NO2- within the cells, which they then eliminate and expel into the extracellular medium, allowing these high NO2- peaks to be detected in the culture medium. In general, these higher nutrient concentrations are indicative of cell death and subsequent cell lysis, which removes nutrients and metabolites from the culture medium. These inhibitory effects of higher nitrogen concentrations are due to their toxic influence on all vital processes, leading to the death of the alga. For example, the excess ions in the cells can lead to an osmotic imbalance when the algae are nutrient-rich; water is absorbed and the cells can burst, leading to thallus collapse (Lobban and Harrison, 1994). This deterioration of health due to apparent thallus breakdown was observed more frequently in the enriched treatments in this study, especially for D. menstrualis.
In the experiments treated with EE, the maximum levels of dissolved nutrients were measured in D. menstrualis and C. cervicornis between the 7th and 14th days of culture, but it should be emphasized that these levels were quite high in D. menstrualis in comparison with C. cervicornis. In parallel, negative growth taxa are also recorded on these days, thus indicating apparent cell death and loss of individuals, or that it is reflected in the nitrogen concentration in the culture medium. Our study showed no significant differences in the increase in specific growth rate with Provasoli/2 treatment, which had an initial nitrogen availability per week, NO2- (0.1 μM), NO3- (0.98 μmol.L-1) and NH4+ (0.001 μmol.L-1). These concentrations were not optimal to obtain significant differences in contrast to the control experiment.
In the other hand, our multivariate analysis results showed that the presence of Nitrate and Ammonia in the culture medium over time can influence the production of the diterpenes isopachydictyol (2) and Dictyol C (6), plus 8 compounds including fucosterol, with less power of explanation for the species D. menstrualis. In addition, pH has a high contribution to the content of isopachydictyol, 5-hydroxy-1,6-cycloxenia-2,13-diene-16,17-dial and fucosterol, signaling pH as an important environmental variable in the in vitro system for variation in the profile of diterpenoids. Opposed, the macroalgae C. cervicornis, only 4-acetoxy-9,14-dihydroxy-1,9-dolastane-1,9-diene presented a useful model, with phosphorus being the contributing variable.
It is known that the metabolic profile of macroalgae adapts and evolves to adjust to a dynamic environment, since in the natural environment they are exposed to various stresses and their metabolome must then constantly adapt to new conditions, this phenomenon is given the name of metabolic plasticity (Gaubert et al., 2019). Genetic and environmental factors interact to drive the plasticity of chemicals in marine macroalgae, whose mechanisms have yet to be elucidated (Sudatti et al., 2021). Reports on metabolic variability of species of the Dictyotaceae family began to be hypothesized and studied from biogenic proposals for the differentiation of diterpene skeletons within the group (Teixeira and Kelecom, 1988). Our results corroborate previous reports that pointed to the plasticity of algae of the Dictyotace family (Ortiz-Ramírez et al., 2008; Gaubert et al., 2019), and therefore, it is the first proof under laboratory conditions of the degree of metabolic plasticity of these species. We emphasize that the species C. cervirconis, by not showing associations between the diterpene profile and the variables studied (dissolved inorganic nutrients and pH), may suggest that the variation of diterpenoids are related to other biotic/abiotic factors and/or the genetics of the seaweed.
Furthermore, it is observed that in the last days of the experiments, there was an increase in sterols to the detriment of diterpenes. After studying the metabolic pathway of steroids and terpenes, it was found that the common precursor farnesyl diphosphate is used in both, so that under biotic/abiotic stress the choice of production of these compounds depends on the metabolic needs at a particular time in the life cycle of the alga, especially under conditions of cell regeneration (Obando et al., 2022a). Some studies have speculated that the increase in fucosterol content may be the algae’s response to maintaining cell membrane integrity during periods of lower temperatures and restricted photosynthetic periods (Terasaki et al., 2017). It is important to highlight that fucosterol is the main phytohormone present in macroalgae of the Phaeophyceae class, accounting for up to 97% of the total content of compounds in this class (Men et al., 2022). In addition, it has shown therapeutic potential, being able to act as an anti-inflammatory, antioxidant, anti-diabetic, anti-Alzheimer’s, anticancer and hepatoprotective agent (Bakar et al., 2019; Meinita et al., 2021; Wang et al., 2022). Based on our results, we can then propose that laboratory conditions tested in enriched mediums favored the production of high levels of fucosterol between days 21 and 51, demonstrating, however, that stress conditions are optimal for choosing this biosynthetic route (Obando et al., 2022a), and that in vitro conditions have the biotechnological potential for obtaining this important metabolite.
5 Conclusion
The profile diterpenes from two species of the Dictyotaceae family, which have been widely studied for their recognized bioactivity, was examined under controlled laboratory conditions, using two treatments with respect to the basic culture medium. The results for the dolastane- and secodolastane-type diterpenes from the species C. cervicornis are the first report of laboratory-produced secondary metabolites for this species. Temporal variations in the relative abundances in the profile of the diterpenes of the algae were detected, but with no statistically significant difference between the two treatments, indicating that the enriched culture medium (Provasoli/2) has no effect on the production of diterpenes. The base medium with sterilized seawater is sufficient to maintain the production of diterpenes and increase the biomass of the species, especially for C. cervicornis, which showed specific positive SGRs in the different periods monitored. Biomass data and dissolved nutrient (nitrate and phosphate) monitoring contributed to our understanding of health and some aspects of algal physiology in a laboratory microcosm. The correlations found by the statistical analyzes between the diterpene profile with the monitored variables (dissolved nutrients and pH), showed strong correlations for the diterpenes of the species D. menstrualis, which was only observed for a diterpene of the species C. cervicornis, opening a window field of discussion on the metabolic platicity of the Dictyotaceae family. Analysis of the data will allow us to improve experiments for sustainable biomass recovery of these species and consequently for diterpene and fucosterol recovery.
Data availability statement
The original contributions presented in the study are included in the article/Supplementary Material. Further inquiries can be directed to the corresponding author.
Author contributions
JO: Conceptualization, Methodology, Writing - Original draft preparation, Chemical analysis (1H> NMR and CG-MS) and Physiological data analysis, and Critical review. TDS: Data analysis 1H NMR and Critical review. RF: Statistical analysis and Critical review. EB: Conceptualization, Methodology and Critical review. DC: Supervision, Funding acquisition, Critical review. VLT: Funding acquisition and Critical review. RV: Material Collection and Critical review. MB: data acquisition CG-MS, Critical review. NN: data acquisition CG-MS. All authors contributed to the article and approved the submitted version.
Funding
This work was supported by the Conselho Nacional do Desenvolvimento Científico e Tecnológico (CNPq) (Grants No. 443930/2014-7 and Productivity Fellowship No. 304070/2014-9 to (VLT) and the Fundação de Amparo à Pesquisa Carlos Chagas Filho do Estado do Rio de Janeiro (FAPERJ), Grants No E-26/110.205/2013, No. E-26/111.220/2014, No. E-26/211.069/2019, No. E-26/211.651/2021 and E-26/205.005/2022 (DC).
Acknowledgments
We wold like to thank the Coordenação de Aperfeiçoamento de Pessoal de Nível Superior (CAPES) for PhD (JO) and MSt (TCS) fellowship and Fundação de Amparo à Pesquisa Carlos Chagas Filho do Estado do Rio de Janeiro (FAPERJ) for Training and Technical Qualification fellowship TCT-5-(JO). The comments and suggestions from Mauricio Lorenzo Cerda Lema during in statistical analysis were greatly appreciated.
Conflict of interest
The authors declare that the research was conducted in the absence of any commercial or financial relationships that could be construed as a potential conflict of interest.
Publisher’s note
All claims expressed in this article are solely those of the authors and do not necessarily represent those of their affiliated organizations, or those of the publisher, the editors and the reviewers. Any product that may be evaluated in this article, or claim that may be made by its manufacturer, is not guaranteed or endorsed by the publisher.
Supplementary material
The Supplementary Material for this article can be found online at: https://www.frontiersin.org/articles/10.3389/fmars.2023.1150719/full#supplementary-material
References
Abrantes J. L., Barbosa J., Cavalcanti D. N., Pereira R. C., Fontes C. L. F., Teixeira V. L., et al. (2010). The effects of the diterpenes isolated from the Brazilian brown algae Dictyota pfaffii and Dictyota menstrualis against the herpes simplex type-1 replicative cycle. Planta Med. 76, 339–344. doi: 10.1055/s-0029-1186144
Albuquerque I. R. L., Cordeiro S. L., Gomes D. L., Dreyfuss J. L., Filgueira L. G. A., Leite E. L., et al. (2013). Evaluation of anti-nociceptive and anti-inflammatory activities of a heterofucan from Dictyota menstrualis. mar. Drugs. 11, 2722–2740. doi: 10.3390/md11082722
Albuquerque I. R. L., Queiroz K. C. S., Alves L. G., Santos E. A. D., Leite E. L., Rocha H. A. O. (2004). Anticoagulant heterofucans from Dictyota menstrualis have anticoagulant activity. Braz. J. Med. Biol. 37, 167–171. doi: 10.1590/S0100-879X2004000200002
Amico V., Oriente G., Piattelli M., Tringali C. (1979). Dictyoxide, a new diterpene from the brown alga Dilophus ligulatus. Phytochemistry. 18, 1895–1897. doi: 10.1016/0031-9422(79)83087-3
Aminot A., Chaussepied M. (1983). Manuel Des analyses chimiques en milieu marin. (Paris: Editions Jouve, CNEXO).
Bakar K., Mohamad H., Tan H. S., Latip J. (2019). Sterols compositions, antibacterial, and antifouling properties from two Malaysian seaweeds: Dictyota dichotoma and Sargassum granuliferum. J. Appl. Pharm. Sci. 9 (10), 047–053. doi: 10.7324/JAPS.2019.91006
Banerjee K., Ghosh R., Homechaudhuri S., Mitra A. (2009). Biochemical composition of marine macroalgae from gangetic delta at the apex of bay of Bengal. Afr. J. @ Basic Appl. Sci. 1, 96–104.
Barbarino E., Lourenço S. O. (2005). An evaluation of methods for extraction and quantification of protein from marine macro-and microalgae. J. Appl. Phy. 17 (5), 447–460. doi: 10.1007/s10811-005-1641-4
Barton K. (2009). “Mu-MIn: Multi-model inference,” in the R package version 0.12.2/r18. Available at: http://R-Forge.R-project.org/projects/mumin/.
Bogaert K., Beeckman T., De Clerck O. (2016). Abiotic regulation of growth and fertility in the sporophyte of Dictyota dichotoma (Hudson) J.V. lamouroux (Dictyotales, phaeophyceae). J. Appl. Phycol. 28, 2915–2924. doi: 10.1007/s10811-016-0801-z
Bogaert K. A., Delva S., De Clerck O. (2020). Concise review of the genus dictyota JV lamouroux. J. Appl. Phycol. 32, 1521–1543. doi: 10.1104/pp.18.01041
Burnham K. P., Anderson D. R. (2002). Model selection and multi-model inference – a practical information-theoretic approach, 2nd ed. (New York: Springer).
Caamal-Fuentes E., Moo-Puc R., Freile-Pelegrín Y., Robledo D. (2014). Cytotoxic and antiproliferative constituents from Dictyota ciliolata, Padina sanctae-crucis and Turbinaria tricostata. pharm. Biol. 52, 1244–1248. doi: 10.3109/13880209.2014.886273
Camara R. B. G., Costa L. S., Fidelis G. P., Nobre L. T. D. B., Dantas-Santos N., Cordeiro S. L., et al. (2011). Heterofucans from the brown seaweed Canistrocarpus cervicornis with anticoagulant and antioxidant activities. Mar. Drugs 9, 124–138. doi: 10.3390/md9010124
Cavalcanti D. N., de Oliveira M. A. R., de-Paula J. C., Barbosa L. S., Fogel T., Pinto M. A., et al. (2011). Variability of a diterpene with potential anti-HIV activity isolated from the Brazilian brown alga Dictyota menstrualis. J. Appl. Phycol. 23 (5), 873–876. doi: 10.1007/s10811-010-9601-z
Cavalcanti D. N., Rezende C. M., Pinto A. C., Teixeira V. L. (2006). Diterpenoid constituents from the brown alga Dictyota menstrualis (Dictyotaceae, phaeophyta). Nat. Prod. Commun. 1, 609–611. doi: 10.1177/1934578X0600100802
Chen J., Li H., Zhao Z., Xia X., Li B., Zhang J., et al. (2018). Diterpenes from the marine algae of the genus Dictyota. mar. Drugs. 16, 159. doi: 10.3390/md16050159
Cirne-Santos C. C., Barros C. D. S., Gomes M. W. L., Gomes R., Cavalcanti D. N., Obando J. M., et al. (2019). In vitro antiviral activity against zika virus from a natural product of the Brazilian brown seaweed Dictyota menstrualis. Nat. Prod. Commun. 14 (7):1–7. doi: 10.1177/1934578X19859128
Cirne-Santos C. C., de Souza Barros C., de Oliveira M. C., Rabelo V. W. H., Azebedoet R. C., Teixeira V., et al. (2020a). In vitro studies on the inhibition of replication of zika and chikungunya viruses by dolastane isolated from seaweed Canistrocarpus cervicornis. sci. Rep. 10 (1), 1–10. doi: 10.1038/s41598-020-65357-7
Cirne-Santos C. C., de Souza Barros C., Esteves P. O., Gomes M. W. L., Gomes R., Cavalcanti D. N., et al. (2020b). Antiviral activity against chikungunya virus of diterpenes from the seaweed Dictyota menstrualis. braz. J. Pharmacog. 30 (5), 709–714. doi: 10.1007/s43450-020-00083-9
Cunha S. R., Pazeto F., Crestani D., Lima G. B., Nascimento J., Santanna F. L., et al. (1999). Potencial de crescimento de macroalgas cultiváveis presentes na enseada de armação do itapocoroy (Penha, SC): avaliação preliminar. BJAST. 3, 17–25. doi: 10.14210/bjast.v3n1.p17-25
de Andrade Moura L., Bianco E. M., Pereira R. C., Teixeira V. L., Fuly A. L. (2011). Anticoagulation and antiplatelet effects of a dolastane diterpene isolated from the marine brown alga Canistrocarpus cervicornis. J. Thromb. Thrombolysis. 31 (2), 235–240. doi: 10.1007/s11239-010-0545-6
de Figueiredo C. S., Menezes Silva S. M. P., Abreu L. S., da Silva E. F., da Silva M. S., Cavalcanti de Miranda G. E., et al. (2019). Dolastane diterpenes from Canistrocarpus cervicornis and their effects in modulation of drug resistance in Staphylococcus aureus. nat. Prod. Res. 33, 3231–3239. doi: 10.1080/14786419.2018.1470512
de Mesquita M. M. F., Crapez M. A., Teixeira V. L., Cavalcanti D. N. (2019). Potential interactions bacteria-brown algae. J. Appl. Phycol. 31, 867–883. doi: 10.1007/s10811-018-1573-4
Den Haan J., Huisman J., Brocke H. J., Goehlich H., Latijnhouwers K. R., Van Heeringen S. L., et al. (2016). Nitrogen and phosphorus uptake rates of different species from a coral reef community after a nutrient pulse. Sci. Rep. 6, 1–13. doi: 10.1038/srep28821
De Oliveira A. S., Cavalcanti D. N., Bianco Έ.M., De Paula J. C., Pereira R. C., Yoneshigue-Valentin Y., et al. (2008). Chemical composition of diterpenes from the brown alga Canistrocarpus cervicornis (Dictyotaceae, phaeophyceae). Nat. Prod. Commun. 3, 1469–1472. doi: 10.1177/1934578X0800300913
de Souza Barros C., Cirne-Santos C. C., Garrido V., Barcelos I., Stephens P. R. S., Giongo V., et al. (2016). Anti-HIV-1 activity of compounds derived from marine alga Canistrocarpus cervicornis. J. Appl. Phycol. 28, 2523–2527. doi: 10.1007/s10811-015-0776-1
Domingos T. F. S., Vallim M. A., Carvalho C., Sanchez E. F., Teixeira V. L., Fuly A. L. (2011). Anti-snake venom effect of secodolastane diterpenes isolated from Brazilian marine brown alga Canistrocarpus cervicornis against lachesis muta venom. Braz. J. Pharmacog. 21, 234–238. doi: 10.1590/S0102-695X2011005000048
do Nascimento Ávila F., da Silva Souza L. G., de Macedo Carneiro P. B., Santos F. A., Sasahara G. L., Marinho Filho J. D. B., et al. (2019). Anti-inflammatory diterpenoids from the Brazilian alga Dictyota menstrualis. Algal. Res. 44, 101695. doi: 10.1016/j.algal.2019.101695
Dormann C. F., Elith J., Bacher S., Buchmann C., Carl G., Carré G., et al. (2013). Collinearity: A review of methods to deal with it and a simula-tion study evaluating their performance. Ecography; 36 (1), 27–46. doi: 10.1111/j.1600-0587.2012.07348.x
Dos Santos A. O., Britta E. A., Bianco E. M., Ueda-Nakamura T., Filho B. P. D., Pereira R. C., et al. (2011). 4-acetoxydolastane diterpene from the Brazilian brown alga Canistrocarpus cervicornis as antileishmanial agent. Mar. Drugs 9 (11), 2369–2383. doi: 10.3390/md9112369
Durán R., Zubía E., Ortega M. J., Salvá J. (1997). New diterpenoids from the alga Dictyota dichotoma. Tetrahedron. 53, 8675–8688. doi: 10.1016/S0040-4020(97)00537-1
Fleury B. G., Kelecom A., Pereira R. C., Teixeira V. L. (1994). Polyphenols, terpenes and sterols in Brazilian dictyotales and fucales (Phaeophyta). Bot. Mar. 37, 457–462. doi: 10.1515/botm.1994.37.5.457
Fox J., Weisberg S. (2019). An r companion to applied regression. 3rd ed. (Thousand Oaks CA: Sage). Available at: https://socialsciences.mcmaster.ca/jfox/Books/Companion/.
Gaubert J., Payri C. E., Vieira C., Solanki H., Thomas O. P. (2019). High metabolic variation for seaweeds in response to environmental changes: a case study of the brown algae Lobophora in coral reefs. Sci. Rep. 9 (1), 1–12. doi: 10.1038/s41598-018-38177-z
Gomes D. L., Telles C. B. S., Costa M. S. S. P., Almeida-Lima J., Costa L. S., Keesen T. S. L., et al. (2015). Methanolic extracts from brown seaweeds Dictyota cilliolata and Dictyota menstrualis induce apoptosis in human cervical adenocarcinoma HeLa cells. Molecules. 20, 6573–6591. doi: 10.3390/molecules20046573
Grasshoff K., Kremling K., Ehrhardt M. (Eds.) (1983). Methods of seawater analysis. 2nd ed (Weinheim: Verlag Chemie), 401.
Hartig F. (2019) DHARMa: Residual diagnostics for hierarchical (Multi-level /Mixed) regression models (R package version 0.2.6). Available at: https://cran.r-project.org/package=DHARM.
Hay M. E., Duffy J. E., Pfister C. A., Fenical W. (1987). Chemical defense against different marine Herbivores : Are amphipods insect equivalents. Ecology. 68, 1567–1580. doi: 10.2307/1939849
Hirschfeld D. R., Fenical W., Lin G. H., Wing R. M., Radlick P., Sims J. J. (1973). Marine natural products. VIII. pachydictyol a, an exceptional diterpene alcohol from the brown alga, Pachydictyon coriaceum. J. Am. Chem. Soc 95 (12), 4049–4050. doi: 10.1021/ja00793a037
Ibraheem I., Abdel-Raouf N., Mohamed H. M., Fassihy R., Hamed S. (2017). Impact of the microbial suppression by using the brown alga Dictyota dichotoma extract. Egypt. J. Bot. 57, 205–214. doi: 10.21608/ejbo.2017.912.1072
Jongaramruong J., Kongkam N. (2007). Novel diterpenes with cytotoxic, anti-malarial and anti-tuberculosis activities from a brown alga dictyota sp. J. Asian. Nat. Prod. Res. 9 (8), 743–751. doi: 10.1080/10286020701189203
Kyaw S. P. P., Wai M. K., Nyunt T., Aye M. M., Soe-Htun U. (2009). Effects of light intensity on the formation and growth of the secondary branches of Dictyota adnata zanardini grown in different salinity and temperature regimes. Jour. Myan. Acad. Arts Sc. 7, 321–332.
Lobban C. S., Harrison P. J. (1994). Seaweed ecology and physiology (New York, NY: Cambridge University Press).
Luhan M. R. J., Sollesta H. (2010). Growing the reproductive cells (carpospores) of the seaweed, Kappaphycus striatum, in the laboratory until outplanting in the field and maturation to tetrasporophyte. J. Appl. Phycol. 22, 579–585. doi: 10.1007/s10811-009-9497-7
Martins A. P., Yokoya N. S., Colepicolo P. (2016). Biochemical modulation by carbon and nitrogen addition in cultures of Dictyota menstrualis (Dictyotales, phaeophyceae) to generate oil-based bioproducts. Mar. Biotechnol. 18, 314–326. doi: 10.1007/s10126-016-9693-9
Mazerolle M. J. (2020). “AICcmodavg: Model selection and multimodel inference based on (Q) AIC(c),” in R package version 2.3-1. Available at: https://cran.rproject.org/package=AICcmodavg.
Meinita M. D. N., Harwanto D., Tirtawijaya G., Negara B. F. S. P., Sohn J. H., Kim J. S., et al. (2021). Fucosterol of marine macroalgae: Bioactivity, safety and toxicity on organism. Mar. Drugs 19 (10), 545. doi: 10.3390/md19100545
Men X., Han X., Lee S. J., Oh G., Jin H., Oh H. J., et al. (2022). In-depth understanding of Ecklonia stolonifera okamura: A review of its bioactivities and bioactive compounds. Mar. Drugs 20 (10), 607. doi: 10.3390/md20100607
Obando J. M. C., dos Santos T. C., Bernardes M., Nascimento N., Villaça R. C., Teixeira V. L., et al. (2022a). Chemical variation and analysis of diterpenes from seaweed Dictyota menstrualis under controlled conditions. Algal. Res. 62, 102637. doi: 10.1016/j.algal.2022.102637
Obando J. M. C., dos Santos T. C., Martins R. C. C., Teixeira V. L., Barbarino E., Cavalcanti D. N. (2022b). Current and promising applications of seaweed culture in laboratory conditions. Aquaculture. 560, 738596. doi: 10.1016/j.aquaculture.2022.738596
Oksanen J. F., Blanchet G., Kindt R., Legendre P., Minchin P. R., O'Hara R. B., et al. (2013) Vegan: community ecology package. Available at: http://CRAN.R-project.org/package=vegan.
Ortiz-Ramírez F. A., Cavalcanti D. N., Villaça R. C., de Paula J. C., Yoneshigue-Valentin Y., Teixeira V. L. (2008). Chemical variation in the diterpenes from the Brazilian brown alga Dictyota menstrualis (Dictyotaceae, phaeophyceae). Nat. Prod. Commun. 3, 1879–1884. doi: 10.1177/1934578X0800301117
Pellizzari F., Oliveira E. C., Yokoya N. S. (2008). Life-history, thallus ontogeny, and the effects of temperature, irradiance and salinity on growth of the edible green seaweed gayralia spp. (Chlorophyta) South. Brazil. J. Appl. Phycol. 20, 75–82. doi: 10.1007/s10811-007-9183-6
Pereira H. S., Leão-Ferreira L. R., Moussatché N., Teixeira V. L., Cavalcanti D. N., Costa L. J., et al. (2004). Antiviral activity of diterpenes isolated from the Brazilian marine alga Dictyota menstrualis against human immunodeficiency virus type 1 (HIV-1). Antiviral. Res. 64, 69–76. doi: 10.1016/j.antiviral.2004.06.006
Provasoli L. (1968). Media and Prospects for the Cultivation of Marine Algae. (Hakone: Proceedings of the US-Japan Conference), 63–75.
Ramseyer T. N., Tronholm A., Turner T., Brandt M. E., Smith T. B. (2021). Elevated nutrients and herbivory negatively affect dictyota growth dynamics. Mar. Ecol. Prog. Ser. 671, 81–95. doi: 10.3354/meps13788
R Core Team (2019). R: A language and environment for statistical computing (Vienna, Austria: R Foundation for Statistical Computing). Available at: https://www.R-project.org/.
R Core Team (2021). R: A language and environment for statistical computing (Vienna: R Foundation for Statistical Computing). Available at: https://www.R-project.org.
Reef R., Pandolfi J. M., Lovelock C. E. (2012). The effect of nutrient enrichment on the growth, nucleic acid concentrations, and elemental stoichiometry of coral reef macroalgae. Ecol Evol. 2, 1985–1995. doi: 10.1002/ece3.330
Ribeiro M. M. J., Sodero A. C. R., Díaz N. C., Teixeira V. L., Rodrigues C. R., Souza A. M. T. D. (2021). Diterpenes isolated from Canistrocarpus cervicornis with virucidal activity against HIV-1: an in silico evaluation. Nat. Prod. Res. 36 (22), 1–5. doi: 10.1080/14786419.2021.2016745
Roberts D. W. (2019) Labdsv: Ordination and multivariate analysis for ecology. Available at: https://CRAN.R-project.org/package=labdsv.
Rodrigues S., Harb T. B., Falcão E. P. D. S., Pereira S. M. B., Chow F. (2020). Antioxidant activity of dictyotales from tropical reefs of Brazil. Appl. Biochem. Biotechnol. 192, 665–679. doi: 10.1007/s12010-020-03344-4
Rohani-Ghadikolaei K., Abdulalian E., Ng W. K. (2012). Evaluation of the proximate, fatty acid and mineral composition of representative green, brown and red seaweeds from the Persian gulf of Iran as potential food and feed resources. J. Food. Sci. Technol. 49, 774–780. doi: 10.1007/s13197-010-0220-0
Rorrer G. L., Cheney D. P. (2004). Bioprocess engineering of cell and tissue cultures for marine seaweeds. Aquac. Eng. 32, 11–41. doi: 10.1016/j.aquaeng.2004.03.007
Rorrer G. L., Yoo H. D., Huang Y. M., Hayden C., Gerwick W. H. (1997). Production of hydroxy fatty acids by cell suspension cultures of the marine brown alga Laminaria saccharina. Phytochemistry. 46, 871–877. doi: 10.1016/S0031-9422(97)81273-3
Rubiano-Buitrago P., Duque F., Puyana M., Ramos F. A., Castellanos L. (2019). Bacterial biofilm inhibitor diterpenes from dictyota pinnatifida collected from the Colombian Caribbean. Phytochem. Lett. 30, 74–80. doi: 10.1016/j.phytol.2019.01.021
Sadeghi M., Fakheri B. A., Sohrabipour J., Sohrabipour J., Emamjomeh A., Samsampour D. (2019). Notes on the genus dictyota (Dictyotaceae, phaeophyceae) in the Persian gulf, Iran. Iran. J. Bot. 25, 61–71. doi: 10.22092/ijb.2019.124520.1227
Sotka E. E., Hay M. E. (2009). Effects of herbivores, nutrient enrichment, and their interactions on macroalgal proliferation and coral growth. Coral. Reefs. 28, 555–568. doi: 10.1007/s00338-009-0529-1
Strickland J. D. H., Parsons T. R. (1972). A practical handbook of seawater analysis, 2nd ed. Vol. 167. (Canada: Bull. Fish. Res. Bd Can), 1–310.
Sudatti D. B., Oliveira A. S., Da Gama B. A. P., Fujii M. T., Rodrigues S. V., Pereira R. C. (2021). Variability in seaweed chemical defense and growth under common garden conditions. Front. Mar. Sci 8, 720711. doi: 10.3389/fmars.2021.72071
Teixeira V. L., Kelecom A. (1988). A chemotaxonomic study of diterpenes from marine brown algae of the genus dictyota. Sci. Total Environ. 75, 271–283. doi: 10.1016/0048-9697(88)90040-X
Terasaki M., Kawagoe C., Ito A., Kumon H., Narayan B., Hosokawa M., et al. (2017). Spatial and seasonal variations in the biofunctional lipid substances (fucoxanthin and fucosterol) of the laboratory-grown edible Japanese seaweed (Sargassum horneri turner) cultured in the open sea Saudi. J. Biol. Sci. 24, 1475–1482. doi: 10.1016/j.sjbs.2016.01.009
Vallim M. A., Barbosa J. E., Cavalcanti D. N., De-Paula J. C., da Silva V. A., Teixeira V. L., et al. (2010). In vitro antiviral activity of diterpenes isolated from the Brazilian brown alga Canistrocarpus cervicornis. J. Med. Plant Res. 4, 2379–2382. doi: 10.5897/JMPR10.564
Vazquez J. T., Chang M., Nakanishi K., Manta E., Perez C., Martin J. D. (1988). Structure of hydroazulenoid diterpenes from a marine alga and their absolute configuration based on circular dichroism. J. Org. Chem. 53, 4797–4800. doi: 10.1021/jo00255a025
Venables W. N., Ripley B. D. (2002). Modern applied statistics with SF. 4th ed (New York, NY: Springer).
Viano Y., Bonhomme D., Camps M., Briand J. F., Ortalo-Magné A., Blache Y., et al. (2009). Diterpenoids from the Mediterranean brown alga dictyota sp. evaluated as antifouling substances against a marine bacterial biofilm. J. Nat. Prod. 72, 1299–1304. doi: 10.1021/np900102f
Wang P., Zhang J., Zhan N., Yang S., Yu M., Liu H. (2022). The pharmacokinetic characteristics and excretion studies of fucosterol from Sargasssum fusiforme in rats. Biomed. Chromatogr. 36 (4), e5309. doi: 10.1002/bmc.5309
Wenig P., Odermatt J. (2010). OpenChrom: a cross-platform open source software for the mass spectrometric analysis of chromatographic data. BMC Bioinform. 11 (1), 1–9. doi: 10.1186/1471-2105-11-405
Yong Y. S., Yong W. T. L., Anton A. (2013). Analysis of formulae for determination of seaweed growth rate. J. Appl. Phycol. 25, 1831–1834. doi: 10.1007/s10811-013-0022-7
Keywords: macroalgae, ochrophyta, valued-added products, physiological seaweed, laboratory conditions culture
Citation: Obando JMC, dos Santos TC, Fortes RdR, Bernardes M, Nascimento N, Villaça RC, Teixeira VL, Barbarino E and Cavalcanti DN (2023) Influence of laboratory conditions on two species Dictyotaceae family (Phaeophyceae): Diterpenoid profile and physiological response. Front. Mar. Sci. 10:1150719. doi: 10.3389/fmars.2023.1150719
Received: 24 January 2023; Accepted: 23 February 2023;
Published: 14 March 2023.
Edited by:
Rafael R. Robaina, University of Las Palmas de Gran Canaria, SpainReviewed by:
Leonel Pereira, University of Coimbra, PortugalBalu Alagar Venmathi Maran, University Malaysia Sabah, Malaysia
Copyright © 2023 Obando, dos Santos, Fortes, Bernardes, Nascimento, Villaça, Teixeira, Barbarino and Cavalcanti. This is an open-access article distributed under the terms of the Creative Commons Attribution License (CC BY). The use, distribution or reproduction in other forums is permitted, provided the original author(s) and the copyright owner(s) are credited and that the original publication in this journal is cited, in accordance with accepted academic practice. No use, distribution or reproduction is permitted which does not comply with these terms.
*Correspondence: Johana Marcela Concha Obando, mc1226@gmail.com