- 1Key Laboratory of Aquaculture Nutrition and Feed (Ministry of Agriculture and Rural Affairs), Key Laboratory of Mariculture (Ministry of Education), Ocean University of China, Qingdao, Shandong, China
- 2Shandong Longchang Animal Health Product Co., Ltd, Dezhou, Shandong, China
- 3Laboratory for Marine Fisheries Science and Food Production Processes, Qingdao National Laboratory for Marine Science and Technology, Qingdao, Shandong, China
Now the replacement of fish oil (FO) with vegetable oils (VOs) has been broadly applied in aquatic feed, but studies reported that there were negative effects on growth, skin color, flavor and muscle texture. A 10-week feeding trial was conducted to investigate the effects of dietary mixed bile acids (BA) on growth performance, body composition, digestive enzyme activities, skin color and flesh quality of juvenile large yellow croaker (initial weight, 13.10 ± 0.18 g). Four iso-nitrogenous and iso-lipidic experimental diets were formulated and designated as soybean oil (SO), SO supplemented with 300 (BA300), 600 (BA600), and 1200 (BA1200) mg/kg bile acids. Two hundred and forty fish were randomly allocated into 12 floating net cages (1 m × 1 m × 1.5 m) that were located at marine fishing rafts. Each diet was randomly distributed in triplicate (three replicates per treatment, 20 fish per replicate). Fish were hand-fed to apparent satiation twice daily (05:30 and 17:30) for 10 weeks. Results showed that specific growth rate presented quadratic pattern with supplemental bile acids level, peaking at BA600 group. Meanwhile, feed conversion rate of cultured fish was significantly improved in BA600 group (P < 0.05). The redness (a*) of dorsal and lateral line skin and the yellowness (b*) of abdominal skin showed significantly quadratic pattern with the increase of supplemental BA level (P < 0.05), peaking at BA600 group. In terms of dorsal muscle texture, springiness showed a decreasing trend in significantly linear pattern with the increase of supplemental BA level, bottoming at BA600 group. However, cohesiveness and gumminess were significantly linear increased with the increase of supplemental BA level (P < 0.05). No significant differences were observed in lipase, amylase, and trypsin activities (P > 0.05). Total proportion of muscle n-3LCPUFA showed a linearly increasing trend with the increase of supplemental BA level. Muscle TG content was linearly increased with the increase of supplemental BA level (P < 0.05), and significant differences occurred as compared to fish fed diets with SO when supplemental BA level were reached 600 mg/kg and 1200 mg/kg (P < 0.05). The gene expression of acyl-CoA oxidase (aco) was significantly decreased in fish fed diets supplemented with 600 mg/kg bile acids compared with 300 mg/kg other than those fed diets with SO. In conclusion, these results suggested that mixed bile acids supplemented to SO-based diets could improve feed conversion ratio, skin color and flesh quality to some extent.
1 Introduction
In recent years, capture fisheries are gradually declining, which induces a steady increase in aquaculture production for meeting global demands for seafood (Watanabe, 2002; Turchini et al., 2010; Fawole et al., 2021). Thus, the demand for commercial aquatic feeds continue to increase. As an important lipid source in aquatic feeds, fish oil (FO) is abundant in n-3 polyunsaturated fatty acids, such as eicosapentaenoic acid (EPA) and docosahexaenoic acid (DHA) (Yu et al., 2017), which are indispensable for growth and development of farmed marine animals. However, with the rapid expansion of aquaculture and the sharp increase of aquatic feed, the contradiction of FO between supply and demand becomes more and more prominent (Nasopoulou and Zabetakis, 2012). Compared with FO, vegetable oils (VOs) have many advantages including easy availability, relatively stable output and acceptable price (Bell et al., 2005; Tan et al., 2016), therefore they are considered as the best alternative lipid sources in aquafeed (Montero et al., 2015). Some studies reported that partial replacement of FO with VOs had no effects on growth and feed utilization in large yellow croaker (Duan et al., 2014), yellowtail (Aoki et al., 2000), Atlantic salmon (Bell et al., 2003), gilthead seabream (Menoyo et al., 2004; Fountoulaki et al., 2009), turbot (Regost et al., 2003b), cobia (Trushenski et al., 2011). However, high level VOs replacing FO had significantly negative effects on growth performance, body composition, fatty acid profiles, skin color, flavor and muscle texture in turbot (Regost et al., 2003a), gilthead seabream (Fountoulaki et al., 2009), red spotted grouper (Wang et al., 2016), large yellow croaker (Mu et al., 2018). Therefore, it is necessary to alleviate or eliminate the negative effects of FO substituted with VOs by nutrition regulation.
Bile acids are the main component of bile and synthesized in liver from cholesterol (Houten et al., 2006; Hofmann and Hagey, 2008). They have been well recognized to be able to emulsify lipids (Wang and Hartsuck, 1993; Alrefai and Gill, 2007; Romano et al., 2020), activate intestinal lipase (Adhami et al., 2017; Romano et al., 2020; Li et al., 2021b) and combine to long chain fatty acids to form a water-soluble complex (Einarsson et al., 1991) so as to improve lipids efficiency. Moreover, bile acids play vital role in emulsification, digestion and absorption of lipids. Meanwhile, they are considered as signaling molecules to regulate metabolism of triglyceride, cholesterol and maintain energy and glucose homeostasis (Insull, 2006; Nguyen and Bouscarel, 2008; Pols et al., 2011; Swann et al., 2011; Burrin et al., 2013; Di Ciaula et al., 2017; Molinaro et al., 2018). Previous studies in mammals and aquatic animals have shown that bile acids could effectively promote growth, improve lipids metabolism and reduce fat accumulation such as broiler (Lai et al., 2018; Ge et al., 2019), grass carp (Zhou et al., 2018), tilapia (Jiang et al., 2018), large yellow croaker (Du et al., 2017; Ding et al., 2020), largemouth bass (Yu et al., 2019; Yin et al., 2021) and improve body quality such as broiler (Lai et al., 2018), Wagyu heifer (Irie et al., 2011), grass carp (Zeng et al., 2017) and large yellow croaker (Yi et al., 2013). Given the functional properties of bile acids, dietary supplementary bile acids are gaining increasing concern in fish and providing the feasibility for improving the negative effects described above of FO replaced by VOs.
Large yellow croaker (Larimichthys crocea)is an important marine fish species with high economic value which has been widely farmed in China (Duan et al., 2014; Du et al., 2017; Ding et al., 2020; He et al., 2022). Previous studies indicated that VOs replacing FO had negative effects on growth, skin color and flesh quality etc. (Yi et al., 2013; Mu et al., 2018), which hindered the development of aquaculture industry and consumer market of large yellow croaker to some extent. In the previous study of our laboratory, supplemental chenodeoxycholic acid (CDCA) in diet replacing fish oil with soybean oil could significantly improve growth performance (Du et al., 2017). However, on the one hand, the price of CDCA is higher than mixed bile acids and it hasn’t been extensively applied in aquaculture. On the other hand, no study was conducted on flesh quality of large yellow croaker in above-mentioned study. Considering this, the purpose of the study was to comprehensively investigate the effects of the exogenous supplementation of mixed bile acids on growth performance, skin color and flesh quality of large yellow croaker.
2 Materials and methods
2.1 Ethics statement
The present study was conducted in strict accordance with the Management Rule of Laboratory Animals (Chinese Order No.676 of the State Council, revised 1 March 2017) and approved by the Committee on the Ethics of Animal Experiments of Ocean University of China.
2.2 Feed ingredients and diets formulation
Four iso-nitrogenous (42% crude protein) and iso-lipidic (12% crude lipid) experimental diets were formulated, including SO (formulation diet with soybean oil as the lipid source), BA300 (formulation diet with SO supplemented with 300 mg/kg mixed bile acids), BA600 (formulation diet with SO supplemented with 600 mg/kg mixed bile acids), and BA1200 (formulation diet with SO supplemented with 1200 mg/kg mixed bile acids). White fish meal, krill meal, and soybean meal were used as the main protein sources. Soybean oil was used as the main oil source. Ingredients were grinded to fine powder and mixed thoroughly to make pellets using pellet mill (EL-220, Haiyang City Huatong Machinery Co., Ltd., Shandong, China). After drying, diets were sieved into a proper size (diameter: 2 mm, length: 8 mm). Analysis of dry matter (105 C, 24 h), crude protein (Kjeldahl nitrogen × 6.25) and crude lipid (ether extraction by Soxhlet method) in experimental diets were performed according to the standard methods of Association of Official Analytical Chemists (AOAC, 2005). Feed formulation and proximate composition are presented in Table 1. All formulated diets were packed in separate bags and stored at -20° until further use.
2.3 Fish and experimental procedures
Hatchery produced juvenile large yellow croaker were purchased from Xiangshan Harbor Aquatic Seeds Company (Ningbo, China). Before the feeding trail, fish were reared for 2 weeks to acclimate the experimental diet and rearing condition. After acclimation, two hundred and forty fish (initial weight, 13.10 ± 0.18 g) were randomly allocated into 12 floating net cages (1 m × 1 m × 1.5 m) that were located at marine fishing rafts of Ningbo Marine Fishery Science and Technology Innovation Base. Each diet was randomly distributed in triplicate (three replicates per treatment, 20 fish per replicate). Fish were hand-fed to apparent satiation twice daily (05:30 and 17:30) for 10 weeks. During the feeding period, the water salinity, temperature, and dissolved oxygen were maintained at 25-28‰, 22-29°, 6.2-7.5 mg/L, respectively.
2.4 Sample collection
At the end of the experiment, all fish were fasted for 24 h and anesthetized with diluted MS-222 (Sigma-Aldrich, USA) before sampling. All fish were counted and weighed for measuring the survival rate、specific growth rate, respectively. Three fish from each cage were randomly sampled for the analysis of the body composition. The liver and viscera of six fish from each cage were weighed for measuring the hepatosomatic index and viscerosomatic index. Blood samples of three fish from each cage were obtained from the caudal vein using heparinized syringes and centrifuged at 3,000×g at 4°C for 10 min. The plasma was collected and stored at -80°C for subsequent analysis. Dorsal muscle and mid-intestine samples were flash-frozen in liquid nitrogen for determination of muscle quality and enzyme activities, respectively.
2.5 Body composition analysis
Proximate compositions of diets and whole fish were both analyzed by the standard methods of Association of Official Analytical Chemists (AOAC, 2005). Moisture was measured by drying at 105°C until constant weight in the oven. Crude protein (nitrogen × 6.25) was determined by the micro-Kjeldahl method using an Auto Kjeldahl System (FOSS KT260, Switzerland). Crude lipid was measured by solvent extraction using a Soxtec System (Soxtec System HT6; Tecator, Höganäs, Sweden). Ash was analyzed by combustion at 550°C for 4 h.
2.6 Skin color measurement
Skin color measurements were performed with a portable Minolta Chroma Meter CR-400 (Minolta, Osaka, Japan). According to CIE (CIE, 1976), the color parameters were L* for lightness, a* for redness or greenness, and b* for yellowness or blueness, respectively.
2.7 Dorsal muscle texture analysis
Texture analysis (hardness, adhesiveness, springiness, cohesiveness, gumminess, chewiness, resilience) was performed by a texture analyzer (TMS-PRO, FTC, USA). A texture profile analysis (TPA) was conducted using an 8-mm cylinder probe. The test parameters were set as follows: 0.1 N with the initial force, 30 mm/min of compression speed, and 60% of deformation of the original length (Gines et al., 2004).
2.8 Biochemical analysis of plasma and muscle
Plasma total bile acid (TBA), total cholesterol (TC), glucose (GLU), total protein (TP) content and muscle triglyceride (TG) level were measured by colorimetric enzymatic methods using commercial kits (Nanjing Jiancheng Bioengineering Institute, China).
2.9 Digestive enzyme activities assay
The intestine of fish was weighed (0.2 g) and then homogenized in 2 mL ice-cold PBS followed by centrifugation at 3,000×g for 10 min. The supernatant was subject to digestive enzyme activities assay. Lipase, amylase, and trypsin activities were measured by colorimetric enzymatic methods using commercial kits (Nanjing Jiancheng Bioengineering Institute, China). The total protein was measured by using commercial kits (Nanjing Jiancheng Bioengineering Institute, China).
2.10 Real-time quantitative polymerase chain reaction
In the present study, the sequences of primers were designed based on nucleotide sequences from GeneBank using software Primer Premier 5, and the method of total RNA extraction and complementary DNA (cDNA) synthesis were as described in the previous publication (Li et al., 2021a). qPCR measurements were performed in a total volume of 20 μL, containing 1 μL of each primer, 2 μL of cDNA product, 10 μL of SYBR-Premix ExTaqII (Takara, Japan) and 6 μL of RNA-free water and was carried out in a CFX Connect Real-Time System (Bio-Rad). The amplification procedure was employed as follows: 95°C for 2 min, followed by 39 cycles of 95°C for 10 s, 58°C for 10 s, and 72°C for 20 s. Primer of each gene for RT-qPCR was designed according to mRNA sequences of large yellow croaker, amplification efficiencies of all genes ranged from 0.95 to 1.05, and β-actin was chosen as the internal control. The expression level of genes was calculated and normalized using the 2−ΔΔCt method (Livak and Schmittgen, 2001). The primers were referred in previous study and listed in Table 2.
2.11 Calculations and statistical analysis
Where Nt was the number of fish in each cage at the end of this experiment and N0 was the number of fish in each cage at the beginning of this experiment.
Where Wt was the final wet body weight (g), W0 was the initial wet body weight and d was the experimental period in days.
One-way ANOVA was used for data statistics (SPSS 25.0, IBM, United States), and Tukey test was used for significance comparison between groups. Before Tukey’s multiple range test, data were examined for normality and homogeneity of variances. Polynomial contrasts analysis was also performed in the pattern of linear and quadratic. P < 0.05 indicated significant difference. All results were expressed as the mean ± SEM.
3 Results
3.1 Survival, growth performance and body index
No significant differences were observed in survival rate, final weight, specific growth rate, feed intake, hepatosomatic index, viscerasomatic index, and condition factor among different dietary treatments (P > 0.05), but specific growth rate showed quadratic pattern with supplemental mixed bile acids (BA) level, peaking at fish fed diets supplemented with 600 mg/kg (Table 3). Compared with fish fed diets with SO, FCR of those fed diets supplemented with 600 mg/kg BA was significantly improved (P < 0.05).
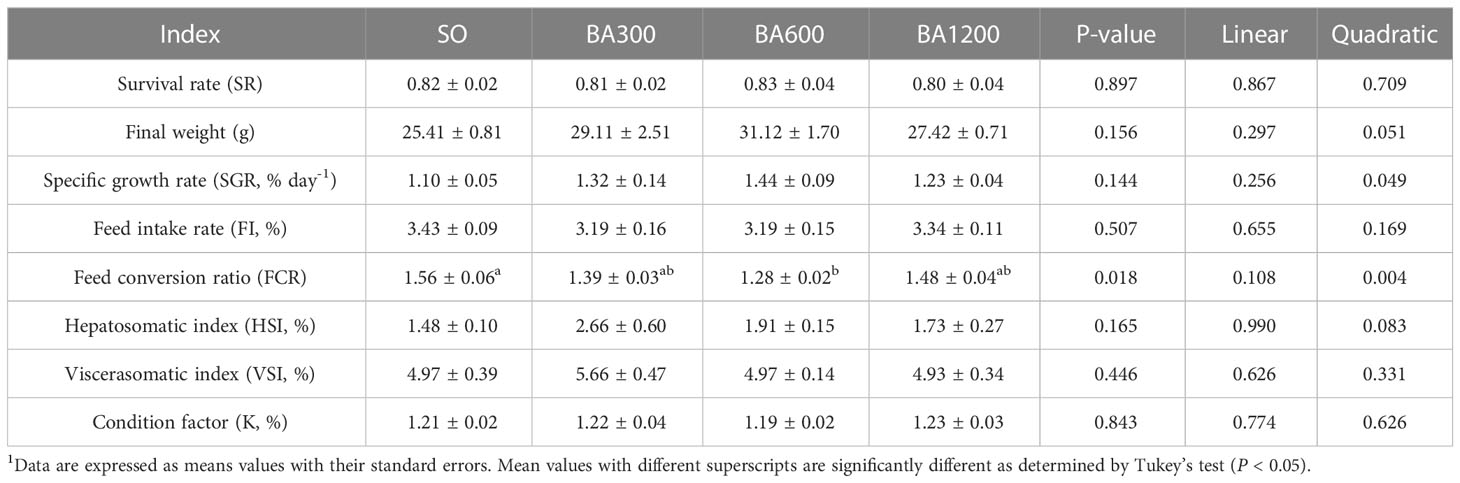
Table 3 Survival, growth performance and body index of juvenile large yellow croaker in different treatments (Means ± SEM, n = 3)1.
3.2 Whole body proximate composition and muscle TG content
Whole body proximate composition and muscle TG content of juvenile large yellow croaker are shown in Table 4. In terms of whole body proximate composition, no significant differences were observed in moisture, crude protein, crude fat, and crude ash among different dietary treatments (P > 0.05), but moisture exerted a decreasing trend in linear pattern with the increase of supplemental BA level. Muscle TG content was linearly increased with the increase of dietary BA level (P < 0.05), and significant differences occurred as compared with fish fed diets with SO when supplemental BA level were reached 600 mg/kg and 1200 mg/kg (P < 0.05), respectively.
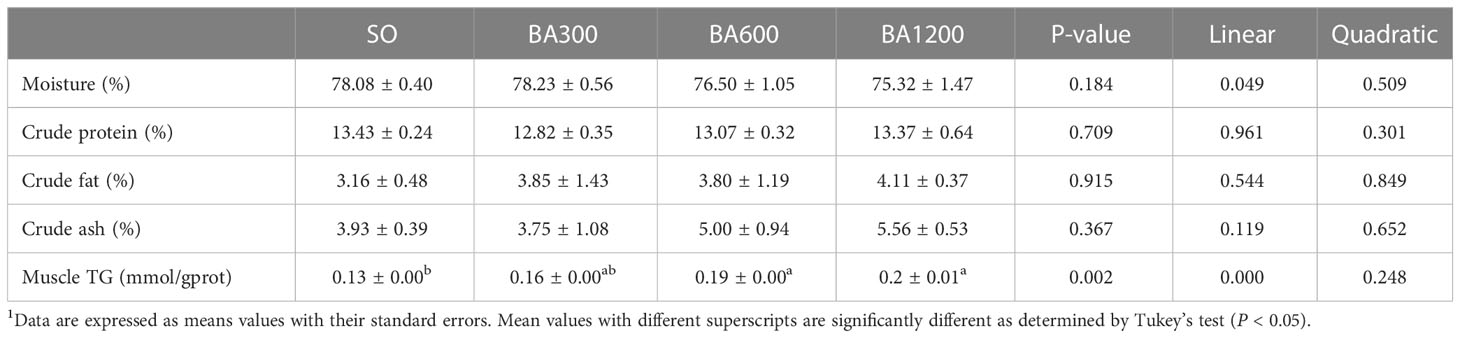
Table 4 Body composition of juvenile large yellow croaker in different treatments (Means ± SEM, n = 3)1.
3.3 Skin color
Skin color of juvenile large yellow croaker is shown in Table 5. No significant differences were observed in the lightness (L*) of dorsal, lateral line, and abdominal skin among different dietary treatments (P > 0.05). The b* of dorsal skin showed no significant difference among different dietary treatments. The redness (a*) of dorsal and lateral line skin and the b* of abdominal skin showed significantly quadratic pattern with the increase of supplemental BA level (P < 0.05), peaking at fish fed diets supplemented with 600 mg/kg. The b* of lateral line skin was significantly linearly decreased with the increase of supplemental BA level (P < 0.05), and significant differences occurred in fish fed diets supplemented with 600 mg/kg and 1200 mg/kg BA compared with those fed diets with SO (P < 0.05). The a* of abdominal skin showed both significantly linear and quadratic decreasing pattern with the increase of supplemental BA level (P < 0.05), and it was significantly higher in fish fed diets supplemented with 1200 mg/kg BA compared with those supplemented with 300 mg/kg or 600 mg/kg (P < 0.05).
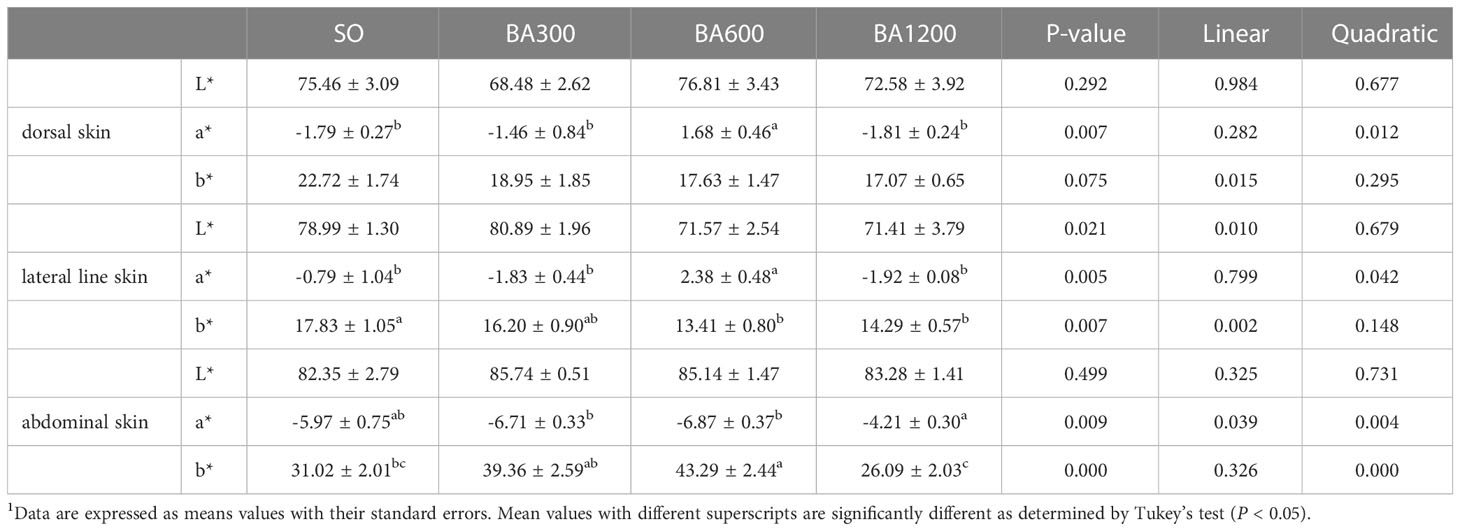
Table 5 Skin color parameters of juvenile large yellow croaker in different treatments (Means ± SEM, n = 3)1.
3.4 Dorsal muscle texture
Muscle texture of juvenile large yellow croaker fed with different diets is shown in Table 6. No significant differences were observed in hardness, adhesiveness, chewiness, and resilience among different dietary treatments (P > 0.05). Springiness showed a significantly decreasing trend in significantly linear and quadratic pattern with the increase of supplemental BA level, bottoming at fish fed diets supplemented with 600 mg/kg. However, cohesiveness and gumminess were significantly linear increased with the increase of supplemental BA level (P < 0.05), and significant differences were found in the fish fed diets supplemented with 1200 mg/kg BA compared with those fed diets with SO and 300 mg/kg BA (P < 0.05).
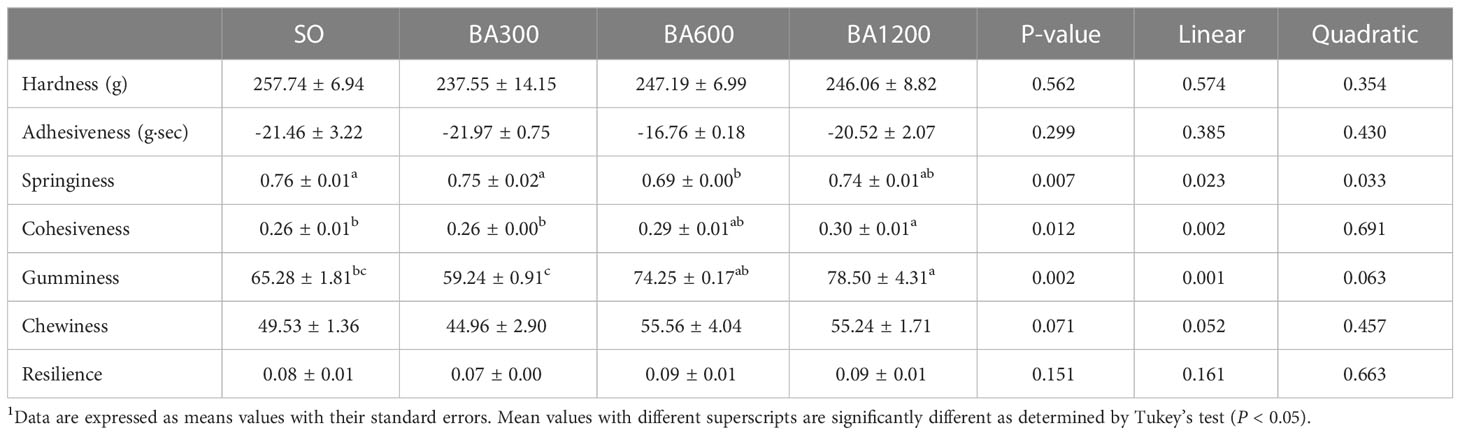
Table 6 Dorsal muscle texture of juvenile large yellow croaker in different treatments (Means ± SEM, n = 3)1.
3.5 Plasma metabolites
Effect of dietary BA on plasma metabolites of juvenile large yellow croaker is shown in Figure 1. The contents of plasma total bile acid (TBA), total cholesterol (TC), glucose (GLU), and total protein (TP) showed no statistical difference among all treatments (Figures 1A–D) (P > 0.05).
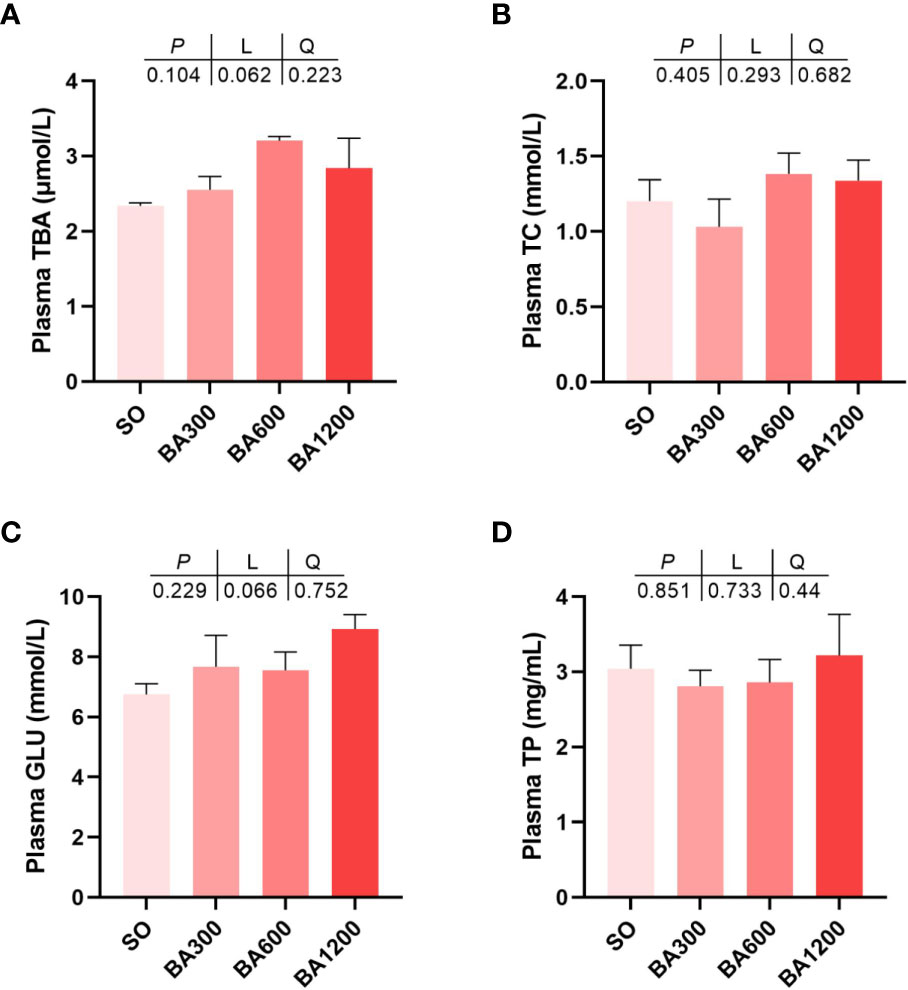
Figure 1 Plasma metabolites of juvenile large yellow croaker in different treatments. (A), Plasma TBA; (B), Plasma TC; (C), Plasma GLU; (D), Plasma TP. TBA, total bile acid; TC, total cholesterol; GLU, glucose; TP, total protein. Values are represented as mean ± SEM (n = 3).
3.6 Digestive enzyme activities
Effects of dietary BA on main digestive enzyme activities in the intestine of juvenile large yellow croaker are shown in Figure 2. No significant differences were observed in lipase, amylase, and trypsin activities (Figures 2A–C) (P > 0.05).
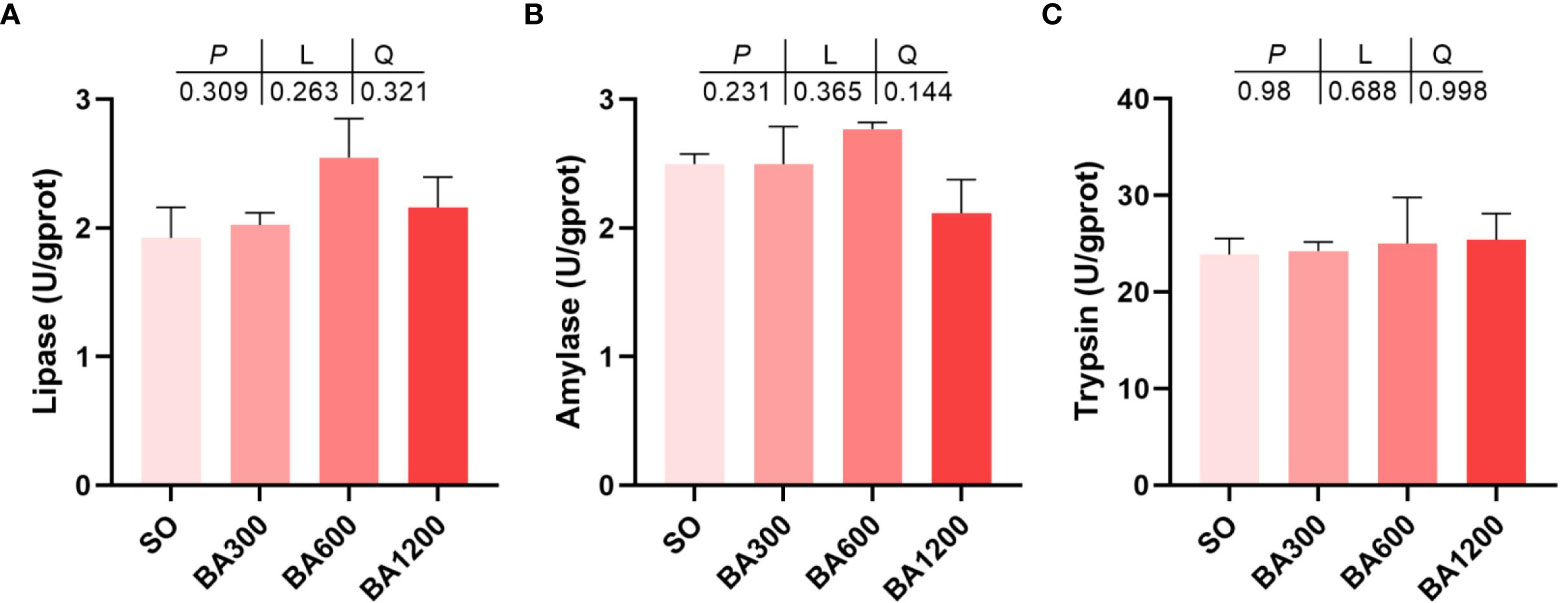
Figure 2 Activities of main digestive enzymes in the intestine of juvenile large yellow croaker in different treatments. (A), Lipase; (B), Amylase; (C), Trypsin. Values are represented as mean ± SEM (n = 3).
3.7 Muscle fatty acids profiles
Fatty acids profile in the muscle of large yellow croaker is measured using a HP6890 gas chromatograph (Table 7). Muscle fatty acids profile showed no statistical difference among all treatments (P > 0.05). However, Total proportion of n-3LCPUFA showed a linearly increasing trend with the increase of supplemental BA level.
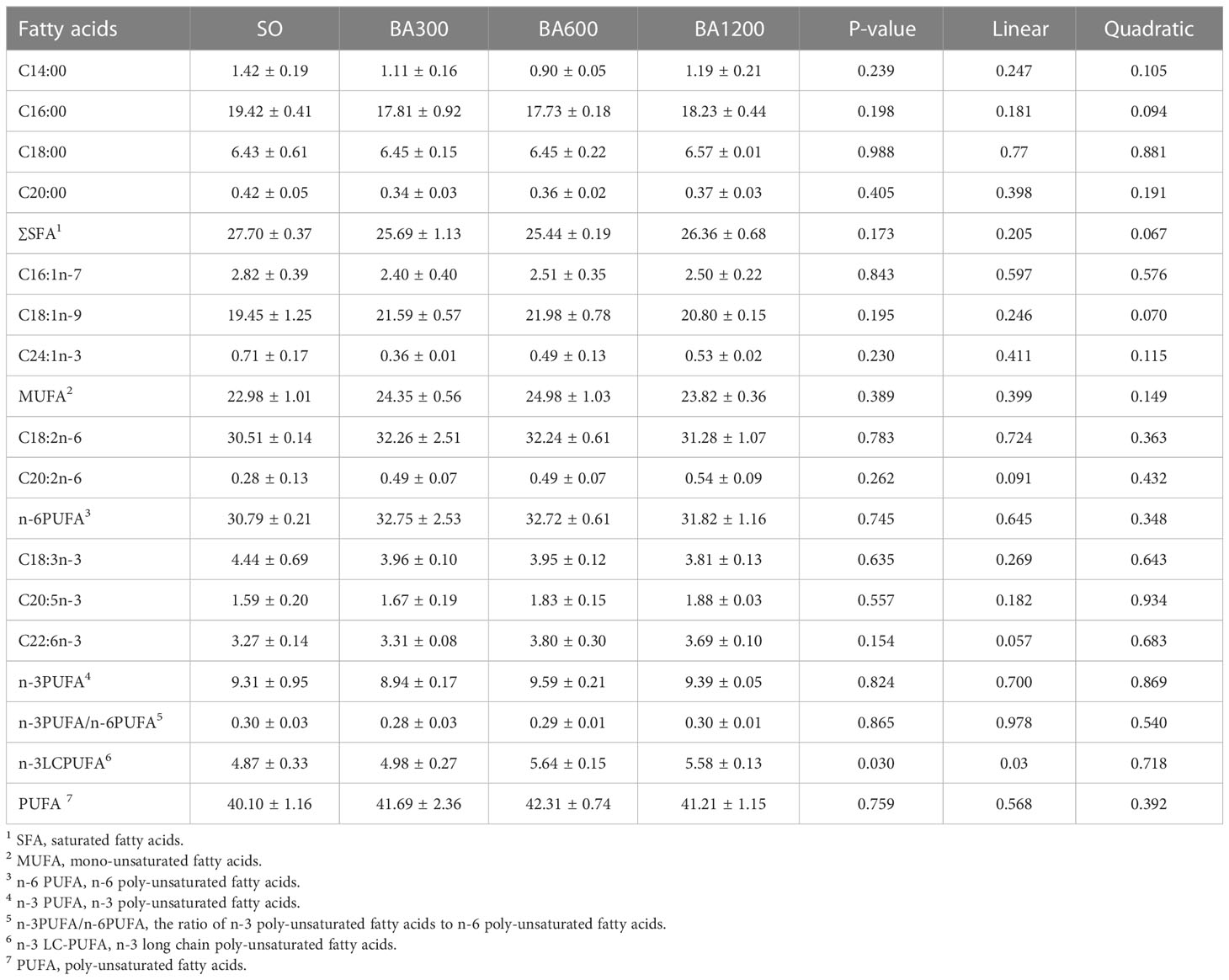
Table 7 Fatty acids profile (% total fatty acids) in muscles of juvenile large yellow croaker in different treatments.
3.8 Real-time quantitative polymerase chain reaction
Gene expression of cd36, fas, scd, and cpt1 showed no statistical difference among all treatments (Figures 3A–D) (P > 0.05). However, gene expression of aco was significantly decreased in fish fed diets supplemented with 600 mg/kg BA compared with 300 mg/kg other than those fed diets with SO (Figure 3E) (P < 0.05).
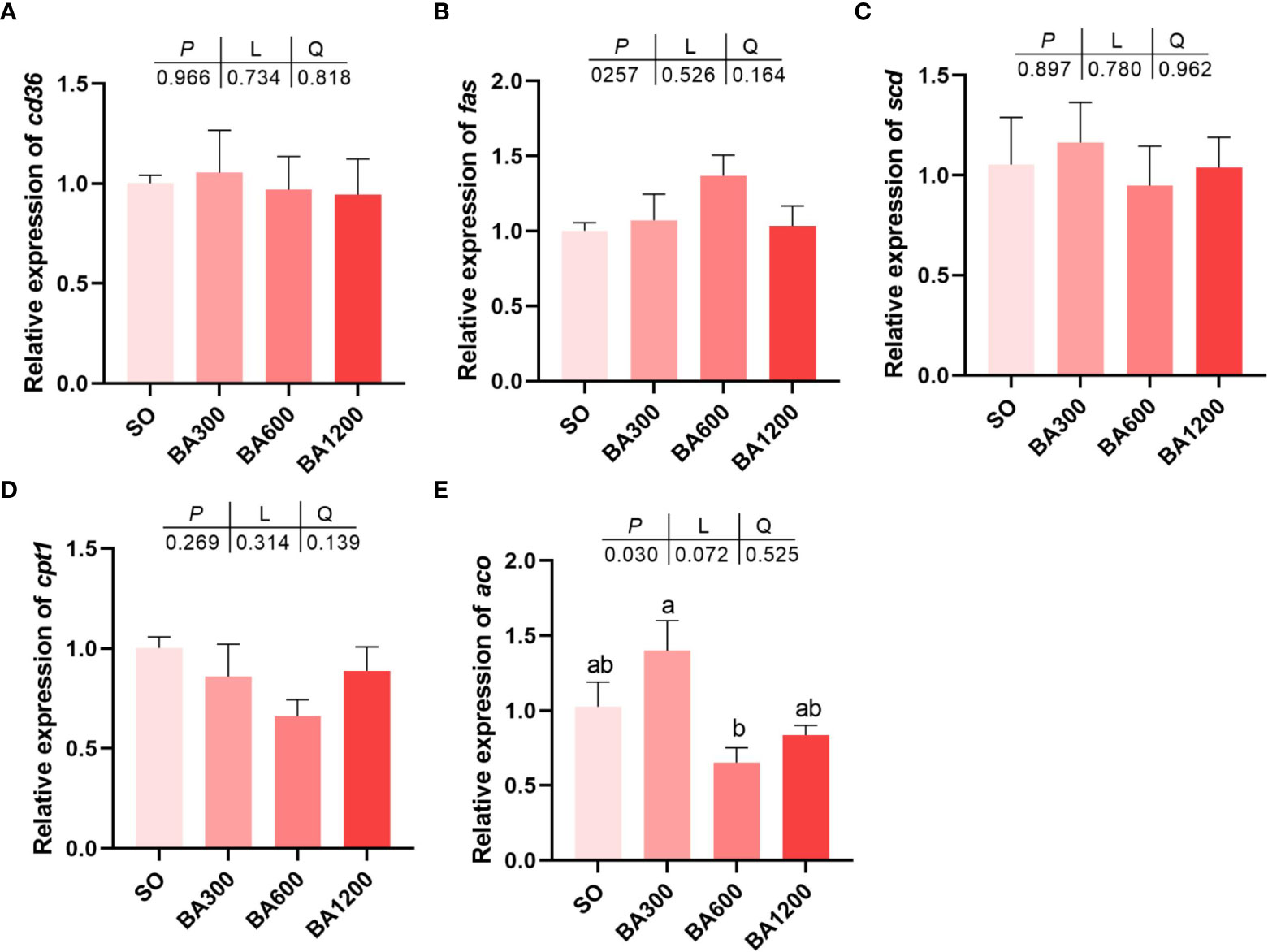
Figure 3 Gene expression of lipid metabolism (A-E) in the muscle of juvenile large yellow croaker in different treatments. Values are represented as mean ± SEM (n = 3). Bars with different lowercase letters represent significant difference (P < 0.05). cd36, cluster of differentiation 36; fas, fatty acid synthase; scd, stearoyl-coenzyme A desaturase; cpt1, carnitine palmitoyl transferase 1; aco, acyl-CoA oxidase.
4 Discussion
In the present study, feed conversion rate (FCR) was significantly improved with the supplementation of mixed bile acids (BA) compared with fish fed diets with soybean oil (SO). The optimum dosage was 600 mg/kg and not in agreement with a previous finding in juvenile large yellow croaker (optimum dosage: 300 mg/kg) (Du et al., 2017; Ding et al., 2020). This discrepancy might be attributed to the differences of feed formations or ingredients of bile acids. It was reported that dietary bile acids could effectively increase feed utilization (Yamamoto et al., 2007; Jiang et al., 2018) and improve the growth performance of fish including largemouth bass (Micropterus salmoides) (Yu et al., 2019), rainbow trout (Oncorhynchus mykiss) (Yamamoto et al., 2007), and large yellow croaker (Larimichthys crocea) (Du et al., 2017). The reason why bile acids are important in improving FCR is that it can effectively improve the emulsification and transportation of lipids (Romanski, 2007).
Lipase activity can be recognized as an indicator of lipid utilization for animals. Previous studies showed dietary bile acids supplementation improved lipase activity as described in European eels (Zhai et al., 2020), rainbow trout (Adhami et al., 2017), large yellow croker (Ding et al., 2020), tilapia (Jiang et al., 2018), tongue sole (Li et al., 2021b), prenant’s schizothoracin (Zeng et al., 2016; Xiang et al., 2019). Generally, bile acids are composed of a convex hydrophobic face and a concave hydrophilic face, this amphipathic structure is facilitated to lipid emulsification and breaks down large lipid molecules into small globules (Jiang et al., 2018), which provides lipase a higher surface area to digest lipids by catalyzing the hydrolysis of ester bonds (Romano et al., 2020). However, in this study, although lipase activity showed an upward trend, the difference was not significant. So we could attribute the improving FCR to the promoting emulsification of exogenous bile acids.
From the perspective of mixed bile acids, the main compositions are HDCA and CDCA. However, these components have seldom been studied in aquatic animals. Liao et al. (2020) reported that dietary bile acid (HDCA=69.9%) supplementation did not affect the growth performance of juvenile tiger puffer fed normal or high-lipid diets. Yin et al. (2021) found that dietary CDCA attenuated the adverse effects induced by high-fat diet on growth performance of largemouth bass, they attributed it to improving intestinal health, which conduced to the absorption and utilization of lipids. Du et al. (2017) reported that growth was significantly increased by dietary CDCA supplementation in large yellow croaker fed a soybean oil diet, they speculated that dietary CDCA might improve the growth performance by reducing inflammatory and improving health. Further studies are needed to explore the underlying mechanisms.
In addition to the improving feed conversion ratio, bile acids could also improve skin color and flesh quality of large yellow croaker in this study. Skin color is adopted as an index for assessing the quality of large yellow croaker, because it can directly affect the purchase of customers (Jiang et al., 2021). In this study, compared with fish fed diets with SO, supplementation of 600 mg/kg BA can significantly improve the redness (a*) of dorsal skin and the a* of lateral line skin. As a kind of fish characterized by yellow skin, the increase of skin color, especially yellowness (b*) of abdominal skin of large yellow croaker would improve commercial value. Moreover, muscle texture is also an important index for assessing the flesh quality of large yellow croaker. In the present study, the springness was decreased in fish fed diets supplemented with BA compared with those fed diets with SO. The reason should be attributed to the increase of triglyceride in muscle, this was in consistent with previous study in largemouth bass (Geng et al., 2018). Meanwhile, the increase of cohesiveness and gumminess could contribute to chewiness, suggesting that the taste of fish muscle was more tender, palatable and not sticky. As to the reason for the improvement of skin color and muscle texture, it was speculated that bile acids promoted the absorption of lipids and lipid-soluble substances such as pigments (Insull, 2006; Islam et al., 2011; Yang et al., 2022). This process was achieved through solubilizing dietary lipids and lipid-soluble substances as micelle by bile acids to facilitate their easily diffuse across the intestinal mucosa (de Aguiar Vallim et al., 2013). Synthesis and catabolism of triglyceride are very pivotal for lipid accumulation in a specific tissue (Li et al., 2013). The present study found that triglyceride (TG) content in muscle was markedly increased when supplemented 600 mg/kg and 1200 mg/kg bile acids. Similarly, previous studies have verified that dietary bile acids play an important role in regulating triglyceride metabolism (Ding et al., 2020). Consequently, we examined the gene expression related to fat metabolism in muscle. ACO is a key enzyme of fatty acid oxidation, the gene expression level of aco in muscle of fish fed diets 600 mg/kg BA was down-regulated in the present study. Therefore, we inferred that supplemented dietary bile acids enhanced TG deposit in muscle by inhibiting fatty acids oxidation, and thereby improve the flesh quality of large yellow croaker. Inclusion of bile acids could activate FXR (Du et al., 2017). FXR plays a central role in the regulation of bile acid synthesis, excretion and transport (Chiang, 2009), and fxr expression has been reported to reduce bile acids synthesis by inhibiting the expression of cyp7α1 in the liver (Zhou et al., 2018). Moreover, the activation of FXR could improve pparα expression and promote the β-oxidation of fatty acids (Xu et al., 2014). Therefore, in this study, the reason for lack of significant change in total bile acids (TBA) levels could be due to the down-regulation of cyp7a1 expression and up-regulation of pparα expression, resulting in a reduction of BA synthesis and increase of fatty acids oxidation.
The fatty acids profile of diets could affect the fatty acids composition of fish muscle, which was well documented for gilthead sea bream (Regost et al., 2003a; Grigorakis, 2007) and large yellow croaker (Li et al., 2018). The substitution of fish oil by vegetable oils affected the fatty acids composition of fish, leading to increase of n-6PUFA and decrease of n-3PUFA in muscle and affecting flesh quality (Mu et al., 2018), In present study, compared with fish fed diets with SO, the level of total n-3LCPUFA showed a linearly increasing trend with the increase of supplemental bile acids level, which was consistent with study in large yellow croaker (Ding et al., 2020). n-3LCPUFA, especially, DHA and EPA play very important role in improving immunity, decreasing the risk of cardiovascular disease (Li et al., 2017). Therefore, promoting the increase of n-3LCPUFA by dietary bile acids inclusion will be beneficial for health in human.
5 Conclusion
In summary, the results of the present study showed that dietary 600 mg/kg mixed bile acids supplementation could improve feed conversion ratio, skin color and muscle texture of juvenile large yellow croaker. These findings would contribute to the long-term sustainable development of large yellow croaker farming. We will intensively study the effects of bile acids on large yellow croaker through more comprehensive work such as adding fish oil group as control, increasing the quantity of sampled fish or selecting other different bile acids etc. in the future.
Data availability statement
The original contributions presented in the study are included in the article/supplementary materials, further inquiries can be directed to the corresponding author.
Ethics statement
The animal study was reviewed and approved by Committee on the Ethics of Animal Experiments of Ocean University of China. Written informed consent was obtained from the owners for the participation of their animals in this study.
Author contributions
JL: Conceptualization, Data curation, Formal analysis, Writing - original draft, Writing - review and editing. ZW: Software, Methodology, Writing - review and editing. XC: Formal analysis, Methodology, Writing - review and editing. JW: Writing - review and editing. YG: Software, Methodology. XW: Software, Methodology. WL: Writing - review and editing. XB: Writing - review and editing. JZ: Writing - review and editing. KM: Supervision. QA: Conceptualization, Funding acquisition, Supervision, Writing - review and editing. All authors contributed to the article and approved the submitted version.
Funding
This research was financially supported by Scientific and Technological Innovation of Blue Granary with grant number (2019YFD0900202), the China Agriculture Research System (CARS47-11) and Leading Talent of Technological Innovation of Ten-Thousands Talents Program (CS31117200001).
Acknowledgments
We thank Jie Xue and Miao Weng for their help in diet preparation and the sample collection.
Conflict of interest
Author JW was employed by Shandong Longchang Animal Health Product Co., Ltd.
The authors declare that the research was conducted in the absence of any commercial or financial relationships that could be construed as a potential conflict of interest.
Publisher’s note
All claims expressed in this article are solely those of the authors and do not necessarily represent those of their affiliated organizations, or those of the publisher, the editors and the reviewers. Any product that may be evaluated in this article, or claim that may be made by its manufacturer, is not guaranteed or endorsed by the publisher.
References
Adhami B., Amirkolaie A. K., Oraji H., Kenari R. E. (2017). Growth performance, nutrient digestibility and lipase activity in juvenile rainbow trout (Oncorhynchus mykiss) fed fat powder in diet containing emulsifiers (cholic acid and tween-80). Aquac. Nutr. 23, 1153–1159. doi: 10.1111/anu.12484
Alrefai W. A., Gill R. K. (2007). Bile acid transporters: Structure, function, regulation and pathophysiological implications. Pharm. Res. 24, 1803–1823. doi: 10.1007/s11095-007-9289-1
AOAC. (2005). Official methods of analysis of the association analytical chemists (Gaithersburg, Maryland, USA: Association of Official Analytical Chemists).
Aoki H., Watanabe T., Sanada Y., Yamauchi K. (2000). Use of alternate protein and lipid sources in practical feeds for yellowtial. Aquac. Sci. 48, 81–90. doi: 10.11233/AQUACULTURESCI1953.48.81
Bell J. G., McGhee F., Dick J. R., Tocher D. R. (2005). Dioxin and dixon-like polychlorinated biphenyls (PCBS) in Scottish farmed salmon (Salmo salar): Effects of replacement of dietary marine fish oil vegetable oils. Aquaculture 243, 305–314. doi: 10.1016/j.aquaculture.2004.10.016
Bell J. G., Tocher D. R., Henderson R. J., Dick J. R., Crampton V. O. (2003). Altered fatty acid compositions in Atlantic salmon (Salmo salar) fed diets containing linseed and rapeseed oils can be partially restored by a subsequent fish oil finishing diet. J. Nutr. 133, 2793–2801. doi: 10.1093/jn/133.9.2793
Burrin D., Stoll B., Moore D. (2013). Digestive physiology of the pig symposium: Intestinal bile acid sensing is linked to key endocrine and metabolic signaling pathways. J. Anim. Sci. 91, 1991–2000. doi: 10.2527/jas.2013-6331
Cai Z., Feng S., Xiang X., Mai K., Ai Q. (2016). Effects of dietary phospholipid on lipase activity, antioxidant capacity and lipid metabolism-related gene expression in large yellow croaker larvae (Larimichthys crocea). Comp. Biochem. Physiol. Part B: Biochem. Mol. Biol. 201, 46–52. doi: 10.1016/j.cbpb.2016.06.007
Chiang J. Y. (2009). Bile acids: Regulation of synthesis. J. Lipid Res. 50, 1955–1966. doi: 10.1194/jlr.R900010-JLR200
CIE. (1976). Official recommendations on uniform colour space, colour difference equations and metric olour terms (Colorimetry, Paris: Color Research & Application). Suppl. No.2 to CIE Publication No.15.
de Aguiar Vallim T. Q., Tarling E. J., Edwards P. A. (2013). Pleiotropic roles of bile acids in metabolism. Cell Metab. 17, 657–669. doi: 10.1016/j.cmet.2013.03.013
Di Ciaula A., Garruti G., Lunardi Baccetto R., Molina-Molina E., Bonfrate L., Wang D. Q., et al. (2017). Bile acid physiology. Ann. Hepatol. 16 Suppl 1, S4–S14. doi: 10.5604/01.3001.0010.5493
Ding T., Xu N., Liu Y., Du J., Xiang X., Xu D., et al. (2020). Effect of dietary bile acid (BA) on the growth performance, body composition, antioxidant responses and expression of lipid metabolism-related genes of juvenile large yellow croaker (Larimichthys crocea) fed high-lipid diets. Aquaculture 518, 734768. doi: 10.1016/j.aquaculture.2019.734768
Du J., Xu H., Li S., Cai Z., Mai K., Ai Q. (2017). Effects of dietary chenodeoxycholic acid on growth performance, body composition and related gene expression in large yellow croaker (Larimichthys crocea) fed diets with high replacement of fish oil with soybean oil. Aquaculture 479, 584–590. doi: 10.1016/j.aquaculture.2017.06.023
Duan Q., Mai K., Shentu J., Ai Q., Zhong H., Jiang Y., et al. (2014). Replacement of dietary fish oil with vegetable oils improves the growth and flesh quality of large yellow croaker (Larmichthys crocea). J. Ocean Univ. China 13, 445–452. doi: 10.1007/s11802-014-2188-2
Einarsson K., Ericsson S., Ewerth S., Reihnér E., Rudling M., Ståhlberg D., et al. (1991). Bile acid sequestrants: Mechanisms of action on bile acid and cholesterol metabolism. Eur. J. Clin. Pharmacol. 40 Suppl 1, S53–S58. doi: 10.1007/BF03216291
Fawole F. J., Labh S. N., Hossain M. S., Overturf K., Small B. C., Welker T. L., et al. (2021). Insect (black soldier fly larvae) oil as a potential substitute for fish or soy oil in the fish meal-based diet of juvenile rainbow trout (Oncorhynchus mykiss). Anim. Nutr. 7, 1360–1370. doi: 10.1016/j.aninu.2021.07.008
Fountoulaki E., Vasilaki A., Hurtado R., Grigorakis K., Karacostas I., Nengas I., et al. (2009). Fish oil substitution by vegetable oils in commercial diets for gilthead sea bream (Sparus aurata l.); effects on growth performance, flesh quality and fillet fatty acid profile recovery of fatty acid profiles by a fish oil finishing diet under fluctuating water temperatures. Aquaculture 289, 317–326. doi: 10.1016/j.aquaculture.2009.01.023
Ge X., Wang A., Ying Z., Zhang L., Su W., Cheng K., et al. (2019). Effects of diets with different energy and bile acids levels on growth performance and lipid metabolism in broilers. Poult Sci. 98, 887–895. doi: 10.3382/ps/pey434
Geng Z., Zhang X., Zheng H., Xu S., Li X., Tang X. (2018). Comparative on muscle quality characteristics of micropterus salmoides between in-pond raceway system and traditional pond sytstem. Science Technol. Food Industry 39, 95–98. doi: 10.13386/j.issn1002-0306.2018.23.017
Gines R., Valdimarsdottir T., Sveinsdottir K., Thorarensen H. (2004). Effects of rearing temperature and strain on sensory characteristics, texture, colour and fat of Arctic charr (Salvelinus alpinus). Food Qual. Preference 15, 177–185. doi: 10.1016/s0950-3293(03)00056-9
Grigorakis K. (2007). Compositional and organoleptic quality of fanned and wild gilthead sea bream (Sparus aurata) and sea bass (Dicentrarchus labrax) and factors affecting it: A review. Aquaculture 272, 55–75. doi: 10.1016/j.aquaculture.2007.04.062
He Y., Tang Y., Xu N., Yao C., Gong Y., Yin Z., et al. (2022). Effects of supplemental phytosterol on growth performance, body composition, serum biochemical indexes and lipid metabolism of juvenile large yellow croaker (Larimichthys crocea) fed with high lipid diet. Aquaculture 551, 737889. doi: 10.1016/j.aquaculture.2022.737889
Hofmann A. F., Hagey L. R. (2008). Bile acids: chemistry, pathochemistry, biology, pathobiology, and therapeutics. Cell Mol. Life Sci. 65, 2461–2483. doi: 10.1007/s00018-008-7568-6
Houten S. M., Watanabe M., Auwerx J. (2006). Endocrine functions of bile acids. EMBO J. 25, 1419–1425. doi: 10.1038/sj.emboj.7601049
Insull W. Jr. (2006). Clinical utility of bile acid sequestrants in the treatment of dyslipidemia: a scientific review. South Med. J. 99, 257–273. doi: 10.1097/01.smj.0000208120.73327.db
Irie M., Kouda M., Matono H. (2011). Effect of ursodeoxycholic acid supplementation on growth, carcass characteristics, and meat quality of wagyu heifers (Japanese black cattle). J. Anim. Sci. 89, 4221–4226. doi: 10.2527/jas.2011-4211
Islam K. B. M. S., Fukiya S., Hagio M., Fujii N., Ishizuka S., Ooka T., et al. (2011). Bile acid is a host factor that regulates the composition of the cecal microbiota in rats. Gastroenterology 141, 1773–1781. doi: 10.1053/j.gastro.2011.07.046
Jiang H., Wang J., Wang Y., Feng F. (2021). Research progress of flesh quality evaluation and nutrition regulation of farmed large yellow croaker. J. Zhejiang University. Agric. Life Sci. 47, 275–283. doi: 10.3785/j.issn.1008-9209.2020.08.071
Jiang M., Wen H., Gou G., Liu T., Lu X., Deng D. (2018). Preliminary study to evaluate the effects of dietary bile acids on growth performance and lipid metabolism of juvenile genetically improved farmed tilapia (Oreochromis niloticus) fed plant ingredient-based diets. Aquac. Nutr. 24, 1175–1183. doi: 10.1111/anu.12656
Lai W., Huang W., Dong B., Cao A., Zhang W., Li J., et al. (2018). Effects of dietary supplemental bile acids on performance, carcass characteristics, serum lipid metabolites and intestinal enzyme activities of broiler chickens. Poultry Sci. 97, 196–202. doi: 10.3382/ps/pex288
Li Q., Cui K., Xu D., Wu M., Mai K., Ai Q. (2021a). Molecular identification of peptidoglycan recognition protein 5 and its functional characterization in innate immunity of large yellow croaker, Larimichthys crocea. Dev. Comp. Immunol. 124, 104130. doi: 10.1016/j.dci.2021.104130
Li X., Jiang G., Qian Y., Xu W., Liu W. (2013). Molecular characterization of lipoprotein lipase from blunt snout bream Megalobrama amblycephala and the regulation of its activity and expression by dietary lipid levels. Aquaculture 416, 23–32. doi: 10.1016/j.aquaculture.2013.08.020
Li J., Li X., Ji R., Xu W., Mai K., Ai Q. (2018). The effects of linseed oil and soybean oil on fatty acid composition and Δ6FAD gene experession in liver and muscle of large yellow croaker (Larimichthys crocea). Acta Hydrobiologica Sin 42, 232–239. doi: 10.7541/2018.029
Li S., Monroig Ó., Wang T., Yuan Y., Carlos Navarro J., Hontoria F., et al. (2017). Functional characterization and differential nutritional regulation of putative Elovl5 and Elovl4 elongases in large yellow croaker (Larimichthys crocea). Sci. Rep. 7, 2303. doi: 10.1038/s41598-017-02646-8
Li Y., Wang S., Hu Y., Cheng J., Cheng X., Cheng P., et al. (2021b). Dietary bile acid supplementation reveals beneficial effects on intestinal healthy status of tongue sole (Cynoglossus semiliaevis). Fish Shellfish Immunol. 116, 52–60. doi: 10.1016/j.fsi.2021.06.020
Liao Z., Sun B., Zhang Q., Jia L., Wei Y., Liang M., et al. (2020). Dietary bile acids regulate the hepatic lipid homeostasis in tiger puffer fed normal or high-lipid diets. Aquaculture 519, 1–10. doi: 10.1016/j.aquaculture.2020.734935
Livak K. J., Schmittgen T. D. (2001). Analysis of relative gene expression data using real-time quantitative PCR and the 2−ΔΔCT method. Methods 25, 402–408. doi: 10.1006/meth.2001.1262
Menoyo D., Izquierdo M. S., Robaina L., Gines R., Lopez-Bote C. J., Bautista J. M. (2004). Adaptation of lipid metabolism, tissue composition and flesh quality in gilthead sea bream (Sparus aurata) to the replacement of dietary fish oil by linseed and soyabean oils. Br. J. Nutr. 92, 41–52. doi: 10.1079/bjn20041165
Molinaro A., Wahlström A., Marschall H. U. (2018). Role of bile acids in metabolic control. Trends Endocrinol. Metab. 29, 31–41. doi: 10.1016/j.tem.2017.11.002
Montero D., Benitez-Dorta V., Caballero M. J., Ponce M., Torrecillas S., Izquierdo M., et al. (2015). Dietary vegetable oils: effects on the expression of immune-related genes in Senegalese sole (Solea senegalensis) intestine. Fish Shellfish Immunol. 44, 100–108. doi: 10.1016/j.fsi.2015.01.020
Mu H., Shen H., Liu J., Xie F., Zhang W., Mai K. (2018). High level of dietary soybean oil depresses the growth and anti-oxidative capacity and induces inflammatory response in large yellow croaker Larimichthys crocea. Fish Shellfish Immunol. 77, 465–473. doi: 10.1016/j.fsi.2018.04.017
Nasopoulou C., Zabetakis I. (2012). Benefits of fish oil replacement by plant originated oils in compounded fish feeds. a review. Lwt-Food Sci. Technol. 47, 217–224. doi: 10.1016/j.lwt.2012.01.018
Nguyen A., Bouscarel B. (2008). Bile acids and signal transduction: Role in glucose homeostasis. Cell. Signal 20, 2180–2197. doi: 10.1016/j.cellsig.2008.06.014
Pols T. W., Noriega L. G., Nomura M., Auwerx J., Schoonjans K. (2011). The bile acid membrane receptor TGR5 as an emerging target in metabolism and inflammation. J. Hepatol. 54, 1263–1272. doi: 10.1016/j.jhep.2010.12.004
Regost C., Arzel J., Cardinal M., Rosenlund G., Kaushik S. J. (2003a). Total replacement of fish oil by soybean or linseed oil with a return to fish oil in turbot (Psetta maxima) 2. flesh quality properties. Aquaculture 220, 737–747. doi: 10.1016/s0044-8486(02)00655-5
Regost C., Arzel J., Robin J., Rosenlund G., Kaushik S. J. (2003b). Total replacement of fish oil by soybean or linseed oil with a return to fish oil in turbot (Psetta maxima) - 1. growth performance, flesh fatty acid profile, and lipid metabolism. Aquaculture 217, 465–482. doi: 10.1016/s0044-8486(02)00259-4
Romano N., Kumar V., Yang G., Kajbaf K., Rubio M. B., Overturf K., et al. (2020). Bile acid metabolism in fish: Disturbances caused by fishmeal alternatives and some mitigating effects from dietary bile inclusions. Rev. Aquac. 12, 1792–1817. doi: 10.1111/raq.12410
Romański K. W. (2007). The role and mechanism of action of bile acids within the digestive system - bile acids in the liver and bile. Adv. Clin. Exp. Med. 16, 793–799. doi: 10.1007/978-3-540-45456-4_43
Swann J. R., Want E. J., Geier F. M., Spagou K., Wilson I. D., Sidaway J. E., et al. (2011). Systemic gut microbial modulation of bile acid metabolism in host tissue compartments. Proc. Natl. Acad. Sci. U.S.A. 108 Suppl 1, 4523–4530. doi: 10.1073/pnas.1006734107
Tan P., Dong X., Mai K., Xu W., Ai Q. (2016). Vegetable oil induced inflammatory response by altering TLR-NF-κB signalling, macrophages infiltration and polarization in adipose tissue of large yellow croaker (Larimichthys crocea). Fish Shellfish Immunol. 59, 398–405. doi: 10.1016/j.fsi.2016.11.009
Trushenski J., Schwarz M., Lewis H., Laporte J., Delbos B., Takeuchi R., et al. (2011). Effect of replacing dietary fish oil with soybean oil on production performance and fillet lipid and fatty acid composition of juvenile cobia Rachycentron canadum. Aquac. Nutr. 17, E437–E447. doi: 10.1111/j.1365-2095.2010.00779.x
Turchini G. M., Torstensen B. E., Ng W.-K. (2010). Fish oil replacement in finfish nutrition. Rev. Aquaculture 1, 10–57. doi: 10.1111/j.1753-5131.2008.01001.x
Wang C. S., Hartsuck J. A. (1993). Bile salt-activated lipase - a multiple functional lipolytic enzyme. Biochim. Et Biophys. Acta 1166, 1–19. doi: 10.1016/0005-2760(93)90277-g
Wang J., Jiang Y., Yang Y., Han T., Yang M., Zheng P., et al. (2016). Effects of dietary of fish oil replacement by soybean oil on growth performance, body composition and body fatty acid composition of red spotted grouper Epinephelus akaara. Oceanologia Limnologia Sin. 47, 640–646. doi: 10.11693/hyhz20160300054
Watanabe T. (2002). Strategies for further development of aquatic feeds. Fisheries Sci 68, 101–104. doi: 10.1046/j.1444-2906.2002.00418.x
Xiang X., Zeng B., Yang L., Zhou X., Chen J., Wang W. (2019). Effects of high-lipid diet supplemented with bile acid on growth performance, muscle nutritional component contents, digestive enzyme activities and serum biochemical indices of juvenile Schizothorax prenanti. Chin. J. Anim. Nutr. 31, 5089–5099. doi: 10.3969/j.issn.1006-267x.2019.11.024
Xu J., Li Y., Chen W., Xu Y., Yin L., Ge X., et al. (2014). Hepatic carboxylesterase 1 is essential for both normal and farnesoid X receptor-controlled lipid homeostasis. Hepatology 59, 1761–1771. doi: 10.1002/hep.26714
Yamamoto T., Suzuki N., Furuita H., Sugita T., Tanaka N., Goto T. (2007). Supplemental effect of bile salts to soybean meal-based diet on growth and feed utilization of rainbow trout Oncorhynchus mykiss. Fisheries Sci. 73, 123–131. doi: 10.1111/j.1444-2906.2007.01310.x
Yan J., Liao K., Wang T., Mai K., Xu W., Ai Q. (2015). Dietary lipid levels influence lipid deposition in the liver of large yellow croaker (Larimichthys crocea) by regulating lipoprotein receptors, fatty acid uptake and triacylglycerol synthesis and catabolism at the transcriptional level. PloS One 10, e0129937. doi: 10.1371/journal.pone.0129937
Yang B., Huang S., Yang N., Cao A., Zhao L., Zhang J., et al. (2022). Porcine bile acids promote the utilization of fat and vitamin a under low-fat diets. Front. Nutr. 9. doi: 10.3389/fnut.2022.1005195
Yi X., Zhang W., Mai K., Shentu J. (2013). Effects of dietary fish oil replaced with rapeseed oil on the growth,fatty acid composition and skin color of large yellow croaker (Larimichthys crocea). J. Fisheries China 37, 751–760. doi: 10.3724/SP.J.1231.2013.38430
Yin P., Xie S., Zhuang Z., He X., Tang X., Tian L., et al. (2021). Dietary supplementation of bile acid attenuate adverse effects of high-fat diet on growth performance, antioxidant ability, lipid accumulation and intestinal health in juvenile largemouth bass (Micropterus salmoides). Aquaculture 531, 735864. doi: 10.1016/j.aquaculture.2020.735864
Yu D., Chang J., Dong G., Liu J. (2017). Replacement of fish oil with soybean oil in diets for juvenile Chinese sucker (Myxocyprinus asiaticus): Effects on liver lipid peroxidation and biochemical composition. Fish Physiol. Biochem. 43, 1413–1420. doi: 10.1007/s10695-017-0381-0
Yu H., Zhang L., Chen P., Liang X., Cao A., Han J., et al. (2019). Dietary bile acids enhance growth, and alleviate hepatic gibrosis induced by a high starch diet via AKT/FOXO1 and cAMP/AMPK/SREBP1 pathway in Micropterus salmoides. Front. Physiol. 10. doi: 10.3389/fphys.2019.01430
Zeng B., Liao Z., Xiang X., Wenxiao H., Cen M., Shancheng H. (2017). Effects of bile acids on growth performance, muscle composition and digestive enzyme activities of Ctenopharyngodon idellus. Prog. Fishery Sci. 38, 99–106. doi: 10.11758/yykxjz.20160215001
Zeng B., Xiang X., Zhou X., Ren S., Chen J., Lu G., et al. (2016). Effect of bile acid level in high lipid diet on the intestinal structure and lipid metabolic enzymes activities of juvenile Schizothorax prenanti. J. Fisheries China 40, 1340–1348. doi: 10.11964/jfc.20160110268
Zhai S., Zhao P., Huang L. (2020). Dietary bile acids supplementation improves the growth performance with regulation of serum biochemical parameters and intestinal microbiota of growth retarded European eels (Anguilla anguilla) cultured in cement tanks. Israeli J. Aquaculture-Bamidgeh 72, 1–12. doi: 10.46989/001c.19138
Keywords: mixed bile acids, growth performance, body composition, digestive enzyme activities, flesh quality, gene expression, Larimichthys crocea
Citation: Li J, Wang Z, Cao X, Wang J, Gong Y, Wang X, Lai W, Bu X, Zheng J, Mai K and Ai Q (2023) Effects of supplemental mixed bile acids on growth performance, body composition, digestive enzyme activities, skin color, and flesh quality of juvenile large yellow croaker (Larimichthys crocea) in soybean oil based diet. Front. Mar. Sci. 10:1149887. doi: 10.3389/fmars.2023.1149887
Received: 23 January 2023; Accepted: 27 February 2023;
Published: 13 March 2023.
Edited by:
Aires Oliva-Teles, University of Porto, PortugalReviewed by:
Jingjing Tian, Pearl River Fisheries Research Institute (CAFS), ChinaShan He, Huazhong Agricultural University, China
Copyright © 2023 Li, Wang, Cao, Wang, Gong, Wang, Lai, Bu, Zheng, Mai and Ai. This is an open-access article distributed under the terms of the Creative Commons Attribution License (CC BY). The use, distribution or reproduction in other forums is permitted, provided the original author(s) and the copyright owner(s) are credited and that the original publication in this journal is cited, in accordance with accepted academic practice. No use, distribution or reproduction is permitted which does not comply with these terms.
*Correspondence: Qinghui Ai, qhai@ouc.edu.cn