- Centre for Marine and Coastal Studies (CEMACS), Universiti Sains Malaysia, Gelugor, Penang, Malaysia
Introduction: This study reports the abundance and distribution of microplastics in tropical estuarine mangrove areas in Penang, Malaysia.
Methods: Samples were collected by using a neuston net and Ponar grab at four study sites (Seberang Perai, Kuala Muda, Penaga and Balik Pulau).
Results and Discussion: A total of 8775 pieces were collected from the four sites, with abundance in coastal surface water, coastal bottom sediment, and estuarine sediment ranging between 201 ± 21.214 - 1407 ± 124.265 pcs/L, 255 ± 22.368 - 350 ± 25.892 pcs/kg, and 430 ± 7.234 - 4000 ± 29.174 pcs/kg respectively. Seberang Perai was found with the highest microplastics abundance in all compartments while fragment is the dominant morphology throughout all study sites. Stereomicroscopic analysis showed that microplastics are of numerous colours and irregular in shape while Field Emission Scanning Electron Microscope (FESEM) observed severe surficial weathering. Polyethylene is the most common identified polymer type via Fourier Transform Infrared Spectroscopy (FTIR) analysis and inorganic chemical compounds such as dimethylmercury were detected which pose a threat to organisms and the environment. The overall outcome suggests that human activities play a significant role in microplastic input into the estuarine environment and that the dominant polymer type in respective study site are closely related to the nature of human activities. This baseline study presents fundamental knowledge on microplastic pollution in the estuarine environment of Penang and encourages similar research to be conducted in the future for pollution control and management.
1 Introduction
In today’s world, plastic can be found everywhere in the ocean because of improper waste management on land that brings them into nearby water bodies (Hammer et al., 2012). Plastic pollution is borderless as debris can be washed on and offshore to and from different countries, travelling throughout different water bodies, and even carried to remote locations such as the poles by water and wind action (Klien et al., 2018). Plastics are widely used because they are cheap, light-weight, and highly durable, thereby creating huge convenience in everyday life (Zhou et al., 2022). High consumption of non-reusable single-use plastics (SUPs) is the major contributor to plastic pollution because of their minimal lifespan, with 40% of plastic production coming from them (Chen et al., 2021). Plastic pollution has become more severe since the COVID-19 pandemic with high consumption of single-use PPE and masks globally. Face masks have been estimated to contribute at least 40000 kg of plastics into the ocean monthly (10 million pieces of 4 g face mask) (Ricciardi et al., 2021). Marine organisms ingesting plastic debris are often reported to suffer from physical entanglement, drowning, and blockage of air and food pathways, ultimately leading to death (GESAMP, 2015).
Exposure of larger plastic to sunlight, mechanical, biological, and chemical degrading mechanisms increases the formation of microplastics (MPs) (GESAMP, 2015). MPs are small, fragmented polymers with sizes up to 1 µm-5 mm (GESAMP, 2015). There are two types of MPs based on their origin: primary and secondary MPs. Primary MPs are commercially produced standard-shaped plastics while secondary MPs are irregular pieces formed from the degradation of large plastic debris into small fragments (Zhang, 2017). Meanwhile, there are five morphological classifications of MPs as suggested by GESAMP (2019): foam, film, fragment, fibre, and pellet. The presence of MPs in the aquatic environment is expected to rise concurrently with the input of plastic waste because a single piece of large plastic may produce up to a thousand pieces of MPs (Gimiliani et al., 2020). High abundance of MPs recovered in water bodies near to land implies that terrestrial input is significant to the environment (Luo et al., 2018). Compared to larger plastics which can be collected through clean-up activities or deploying vessels, MPs are nearly impossible to recover once they enter into the aquatic environment because of their small size (Klien et al., 2018). The density of MPs influences their occupancy in different compartments of a water body, in turn affecting their distribution vertically and horizontally (Zhang, 2017). Low density MPs tend to float on surface waters or stay suspended in water columns while high density MPs often settle to the bottom of the ocean floor (Kumar et al., 2021). When MPs float on surface water, they can travel a longer distance following surface currents while particles that settle in the bottom sediment stay relatively static unless particle buoyancy changes which then allows them to resuspend into water columns or float on water surfaces (GESAMP, 2015). The discovery of MPs in aquatic organisms across different trophic levels is a huge concern to human health as they are not biodegradable and may accumulate in the body if contaminated sea-based food and beverages are accidentally consumed (Ricciardi et al., 2021). Nevertheless, research on the effects of MPs towards human health remains unclear, unlike the understanding towards aquatic animals and plants (Campanale et al., 2020).
Estuaries sit between the land and ocean, making them highly vulnerable to pollution, including from MPs (Govender et al., 2020). Estuaries can accumulate MPs from direct terrestrial input, riverine flow, and back washing of coastal currents that carries them into the estuaries (Ricciardi et al., 2021). Riverine flow is considered as one of the most important MP sources carried from land (Li et al., 2021). In fact, the hydrodynamics of estuaries encourage accumulation of larger and heavier MPs which makes them a potential sink for MPs (Defontaine et al., 2020). For example, the presence of a strong cohesive force in sediment due to finer sediment grains and muddy conditions of estuaries may increase MPs accumulation in estuaries (Furukawa & Wolanski, 1996; Lamichhance et al., 2021). Moreover, mangrove-dominated estuaries with numerous protruding roots that hold sediment and reduce coastal erosion would help build up MPs abundance as their roots can help filter out MPs from river input before entering nearby coastal waters (Govender et al., 2020). Mangrove-dominated estuaries are important nurseries and habitats for numerous terrestrial and estuarine organisms (Bujang & Zakaria, 2020). Due to their high productivity and uniqueness of fresh-saltwater mixture, they also support a wide variety of fish species and serve as fishing grounds for fishery communities (Omar & Misman, 2020). Thus, MPs invasion into estuarine environment can be harmful to both animals and humans as accidental ingestion may affect development and health (Talbot et al., 2022).
To the best of our knowledge, our study is the first work to assess MP pollution in tropical mangrove estuaries of Penang. This study is significant to the environment as quantifying MP abundance in the mangrove estuaries would present an insight on the pollution status that will reflect the influence of nearby anthropogenic activities towards the severity of MP pollution. Our study sites were reported to have a relatively high diversity of mangrove tree species, compared to the well-conserved Matang Mangrove Forest and mangrove park in Klang, even though they are located around highly urbanized areas and are unprotected by law (Stiepani et al., 2021). Our study is driven by the emerging reports of MPs in different compartments of estuarine environment in Malaysia. All study sites have been reported to provide ecological benefits and services to respective communities and organisms. The estuarine environment in Penang harbours significant mangrove tree species and marine birds that require conservation and protection (Foong et al., 2016). There is also high reliance of local communities on the estuarine environment as fishery activities are important to provide a source of income and supply seafood to nearby states. Therefore, four study sites located around Penang with ecological and community importance were selected: Seberang Perai, Teluk Air Tawar-Kuala Muda (TAT-KM), and Balik Pulau. Specifically, TAT-KM was recently considered as a new Ramsar Site for conservation purposes (Aziz, 2022). A lack of supporting data and understanding on the pollution status of these study sites may hamper conservation and preservation effort in the near future. With our study, more data will be available to suggest conservation and preservation efforts to protect the ecosystem and maintain its environmental quality. This study is important for future pollution studies on MPs to contribute to data comparison.
The vulnerability of estuarine mangroves towards pollution is also one of the concerns of our study. These areas are facing threats from conversion for human settlement, industrial, aquaculture, and agriculture activities, coastal reclamation, and unsustainable tourism that would release pollutants into the water (Foong et al., 2016). These estuaries are experiencing busy shipping traffic and fishing boat activities, particularly in Seberang Perai which is near to the Perai Free Industrial Zone (FIZ). On top of that, the mangroves are located near highly populated settlements and industrial sites along Sungai Perai (Yii et al., 2020). Meanwhile, in mangroves estuaries of Kuala Muda, gears used in the fishing industry may release synthetic polymers such as fragments and fibres if submerged in the water for a long time. These activities may contribute to the increase of MPs abundance in the water and threaten public health as fish caught from this area are supplied to local markets.
Our study aims to provide a basic understanding on the abundance of MPs present in mangrove estuaries around Penang and assess the extent of its pollution. We are interested to see whether the influence of anthropogenic activities coincides with the severity of MP pollution of the study sites. Our study also aims to provide meaningful data that can be used for pollution management and control. Furthermore, we aim to narrow the knowledge gap on MP pollution in Malaysia that will encourage mitigation efforts in the near future.
The scope of study for MPs involved sample collection from coastal surface waters, coastal bottom sediment, and estuarine sediment from four estuarine mangrove in Penang. All samples are used to assess and compare the abundance between each study site, followed by physical characterization and chemical analysis of representative MP samples. Samples were classified based on five morphologies as suggested by GESAMP (2019).
Therefore, the objectives of this study are:
a. To determine the abundance of microplastics in coastal surface water, coastal bottom sediment, and estuarine sediment of tropical estuarine mangroves in Penang.
b. To assess the physical changes and chemical composition of microplastics collected from coastal surface water, coastal bottom sediment, and estuarine sediment of tropical estuarine mangroves in Penang.
2 Materials and methods
2.1 Study sites
Figure 1 shows the location of Penang, situated at the north of Peninsular Malaysia and along the Straits of Malacca, and the sampling station at each study site.
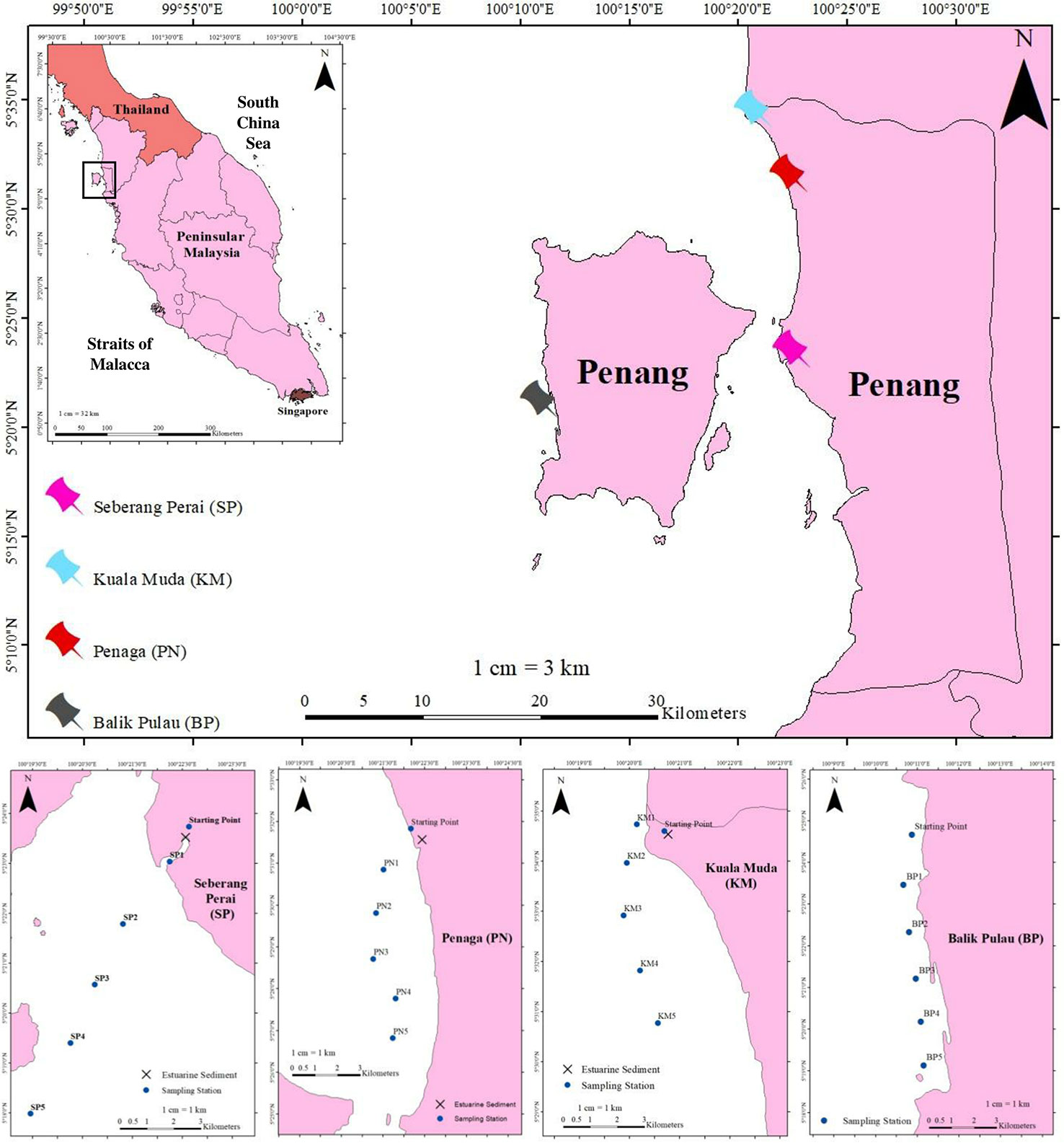
Figure 1 Location of Penang at Peninsular Malaysia, and study sites and sampling stations at the estuarine environment of Penang Seberang Perai, Penaga, Kuala Muda, and Balik Pulau (clockwise).
2.1.1 Seberang Perai
Sungai Perai is approximately 60.5 km long, the longest river in Penang, with mangrove swamps at the river mouth (Think City, 2021). Weeds are distributed along the river with mangrove trees dominating the estuary (Johan et al., 2012). The river flows through Perai Free Industrial Zone, a dense residential area and with local scale fishing activities (Johan et al., 2012). Perai River is also an important international shipping route as the 56 hectares of Perai Bulk Cargo Terminal was built near Perai FIZ (PPC, 2018).
2.1.2 Teluk Air Tawar-Kuala Muda (TAT-KM)
The Teluk Air Tawar-Kuala Muda (TAT-KM) coastal region is located at Seberang Perai Utara, the northern region of mainland Penang, covering about 200 hectares of mangrove and mudflats (Foong et al., 2016). It was recognized by BirdLife International in 2007 as an Important Bird & Biodiversity Area (IBA). TAT-KM IBA coast is about 8 km long, covering the mouth of Sungai Muda north to Butterworth in the south (MNS, 2015; Foong et al., 2016). The Kuala Muda estuary receives riverine input from Sungai Muda, one of the largest rivers (180 km) in the northern Peninsular Malaysia, and forms a 30 km boarder with Penang (Sim et al., 2019). This area is dominated by dense fishing activities at Kuala Muda (Foong et al., 2016). The Kuala Muda coastline is a bird sanctuary area and haven for thousands of birds each season as their stopover site (MPSP, 2020).
2.1.3 Balik Pulau
The mangrove patch of Balik Pulau is located along the west coast of Penang Island, where urbanization is slightly less intense compared to the east coast of the island. The mangrove-dominated estuarine environment is biologically and ecologically important because they are breeding grounds for fishes that provide a source of living to local fishing communities (SAM, 2019). However, the mangrove patch is threatened by the Penang South Reclamation Project (PSRP) which wants to build highways to enhance accessibility around Penang Island (Chee et al., 2020).
2.2 Sample collection
Samples were collected during high tide for boat accessibility. All samples were collected in 2022 on different days for each location. Sampling was carried out at Seberang Perai on 3rd January, Kuala Muda and Penaga on 16th February, and Balik Pulau on 17th March. All glass containers were washed, rinsed with filtered distilled water, and stored in a clean ice chest before sampling. The following was carried out with modifications of a literature review method described by Masura et al. (2015) and Li et al. (2021).
2.2.1 Coastal surface waters
Samples were collected using a neuston net with mesh size 330 µm. The net was towed along the coast of each study site covering an approximate 10 ± 2 km (estimated distance of 2 ± 0.5km between each sampling points) with boat speed controlled at 3-4 knots. The average time spent at each sampling site was about 3 hrs. The net was ensured to float on the water surface when towing. At each sampling point, the net was lifted and flushed with site water before collection to ensure samples enter the collector at the bottom of the net. Collected samples were kept in an ice chest at ambient temperature until analysis. For contamination control, blank samples were prepared by filling double-filtered distilled water in a clean sampling glass bottle before net towing started.
2.2.2 Coastal bottom sediment
Together with water samples, sediment samples were collected alongside the coastal area of each study site using a Ponar grab sampler. During sampling, the coordinate and depth of each station was taken using a handheld GPS and depth sounder respectively. Precaution steps were taken to reduce any contamination of the samples. During sample retrieval, only the inner part of the sediment sample was collected using a metal shovel. Collected samples were placed in glass containers and kept in an ice chest at ambient temperature until analysis.
2.2.3 Estuarine sediment
A random selected transect of 200 m was selected at each study site to collect 10 sediment samples 20 m apart from each other. Surficial sediment (top 5 cm layer) was only considered and scoped approximately 200-300 g sediments using a metal shovel. The transect was selected in-situ depending on boat accessibility and GPS coordinates was recorded. Samples was kept in tightly sealed glass bottles and an ice chest at ambient temperature until analysis.
2.3 Laboratory analysis
All blank samples were treated similarly to field samples. The following was carried out with modification of a literature review method describes by Lusher et al. (2020).
2.3.1 Water
Potassium hydroxide (KOH) was added to the samples for organic matter digestion and the solution was left for 24 hours at room temperature. Once organic matter had completely sunk to the bottom, the clear solution formed on the top layer was pipetted into a beaker and filtered using 0.45µm GC/F Whatman filter paper. MPs present on the filter paper were kept in clean and dry petri dishes for further analysis.
2.3.2 Sediment
Samples were dried in an oven at 60°C for 36-72 hours. For organic matter digestion, 200 g of dried sample was added to 300 mL of Fenton’s solution (30% Hydrogen peroxide, H2O2: Iron (II) sulphate, FeSO4 at ratio 1:2) and left for 24 hours. The clear solution formed in the mixture was pipetted into a clean beaker and filtered using 0.45µm GC/F Whatman filter paper. The remaining mixture was transferred into a funnel, stirred thoroughly after adding zinc chloride (ZnCl2) (density:1.8g/cm3), and left overnight to maximise MP extraction. The clear solution formed above the sediment layer was collected and filtered with 0.45µm GC/F Whatman filter paper. All filter papers were kept in clean, dry petri dishes for further analysis. For contamination control, blank wet filter paper was prepared by wetting dry filter paper with double-filtered distilled water, placed in a rinsed clean petri dish, and left aside when samples processed were exposed to a laboratory environment.
2.4 Instrumental analysis
The following was carried out with a modification of a literature review method describes by Li et al. (2021).
MPs collected on filter papers were observed under an Olympus SZ61 stereomicroscope to calculate their abundance and photographs of representative samples were simultaneously taken. 30 representative MP samples were selected for identification and chemical composition analysis. FEI Quanta 650 FEG Field Emission Scanning Electron Microscopy-Energy Dispersive X-Ray (FESEM-EDX) located at the Center for Global Archaeological Research, Universiti Sains Malaysia was used to study the surface morphology and texture of the MPs respectively while Bruker LUMOS II Fourier Transform Infrared Spectrometry (FTIR) was used to detect chemical compounds present in the MPs. Microplastic samples were rinsed with distilled water, air-dried, and coated with gold prior to FESEM-EDX analysis. The FTIR operates at a spectra range between 400-400cm-1 with a scanning rate of 64x per analysis.
2.5 Data analysis
MP abundance was calculated using Microsoft Excel and statistically analysed using IBM SPSS version 27. All tabular and graphical data were created using Microsoft Excel while study site maps were created using ArcGIS 10.7. MP abundance is expressed in pieces of MPs/L in waters and pieces of MPs/kg in sediments.
All data on MP abundance were statistically analysed based on respective study sites and sampling compartments for significance differences. One-way ANOVA was used when assumptions for normality and homoscedasticity met (Shapiro-Wilk and Welch’s test respectively) and significant ANOVA test (p<0.05) was tested using Games-Howell post-hoc test to compare pollution status of the study sites.
3 Results
3.1 Microplastics abundance
Microplastics (MPs) were found in all sampling sites and compartments of the estuarine environment. This section reports the MPs abundance recovered at three different compartments (coastal surface waters, coastal bottom sediment, and estuarine sediment) from each study site.
3.1.1 Coastal surface waters
Figure 2 presents the total MPs abundance at coastal surface waters in respective study sites. The total abundance decreases from Seberang Perai (1407 ± 124.265 pcs/L) > Penaga (358 ± 50.991 pcs/L) > Balik Pulau (273 ± 24.943 pcs/L) > Kuala Muda (201 ± 21.214 pcs/L). Comparing the morphological composition at each site (Figure 2), Seberang Perai is dominated by fragments at 677 pcs/L (48%) while Penaga, Kuala Muda, and Balik Pulau are dominated by foam at 272 pcs/L (76%), 120 pcs/L (60%), and 131 pcs/L (48%) respectively.
Meanwhile, the MPs abundance in coastal surface waters was analysed using one-way ANOVA, showing no significant difference between each sampling site with F(3, 16) = 3.697, p=0.054 (> 0.05) (Table 1).

Table 1 One-way ANOVA output on assessing MP abundance at coastal surface waters of four different estuarine mangroves in Penang.
3.1.2 Coastal bottom sediment
Coastal bottom sediment samples were collected alongside coastal surface waters. Figure 3 presents the average MP abundance in coastal bottom sediments of all sampling sites. The average MP abundance decreases from Seberang Perai (350 ± 25.892 pcs/kg) > Kuala Muda (290 ± 24.505 pcs/kg) > Penaga (270 ± 18.070 pcs/kg) > Balik Pulau (255 ± 22.368 pcs/kg). The morphological composition of MPs at Seberang Perai and Penaga is dominated by fragments at 217 pcs/kg (62%) and 142 pcs/kg (53%) while Kuala Muda and Balik Pulau are dominated by fibre at 220 pcs/kg (76%) and 200 pcs/kg (78%) respectively (Figure 3).
The MP abundance in coastal bottom sediment was analysed using one-way ANOVA, showing no significant difference between each sampling site, F(3, 56) = 2.030, p= 0.120 (> 0.05) (Table 2).

Table 2 One-way ANOVA output assessing the MP abundance at coastal bottom sediment of different estuarine mangroves in Penang.
3.1.3 Estuarine sediment
Figure 4 presents the total MP abundance in estuarine sediment of three sampling sites: Seberang Perai, Penaga, and Kuala Muda. The total MP abundance in estuarine sediments decrease from Seberang Perai (4000 ± 29.174 pcs/kg) > Penaga (940 ± 15.773 pcs/kg) > Kuala Muda (430 ± 7.234 pcs/kg). Figure 4 shows the MP morphological composition at three sampling sites. Fragments dominate both Seberang Perai and Penaga at 2765 pcs/kg (69%) and 465 pcs/kg (50%) respectively while fibre dominates Kuala Muda at 280 pcs/kg (65%).
Unlike coastal surface waters and bottom sediment, MP abundance of estuarine sediment between each study sites shows statistical significance. Table 3 shows the analysis of one-way ANOVA F(2, 27) = 97.067, p < 0.001 (<0.05). The Games-Howell post-hoc test shows that Seberang Perai and Penaga, Seberang Perai, and Kuala Muda is significantly different at p<0.001 while Penaga and Kuala Muda is significant at p=0.030 (Table 4).
3.2 Instrumental analysis
3.2.1 Stereomicroscope photographs
Figure 5 shows the representative microscopic image of MPs collected from the study sites.
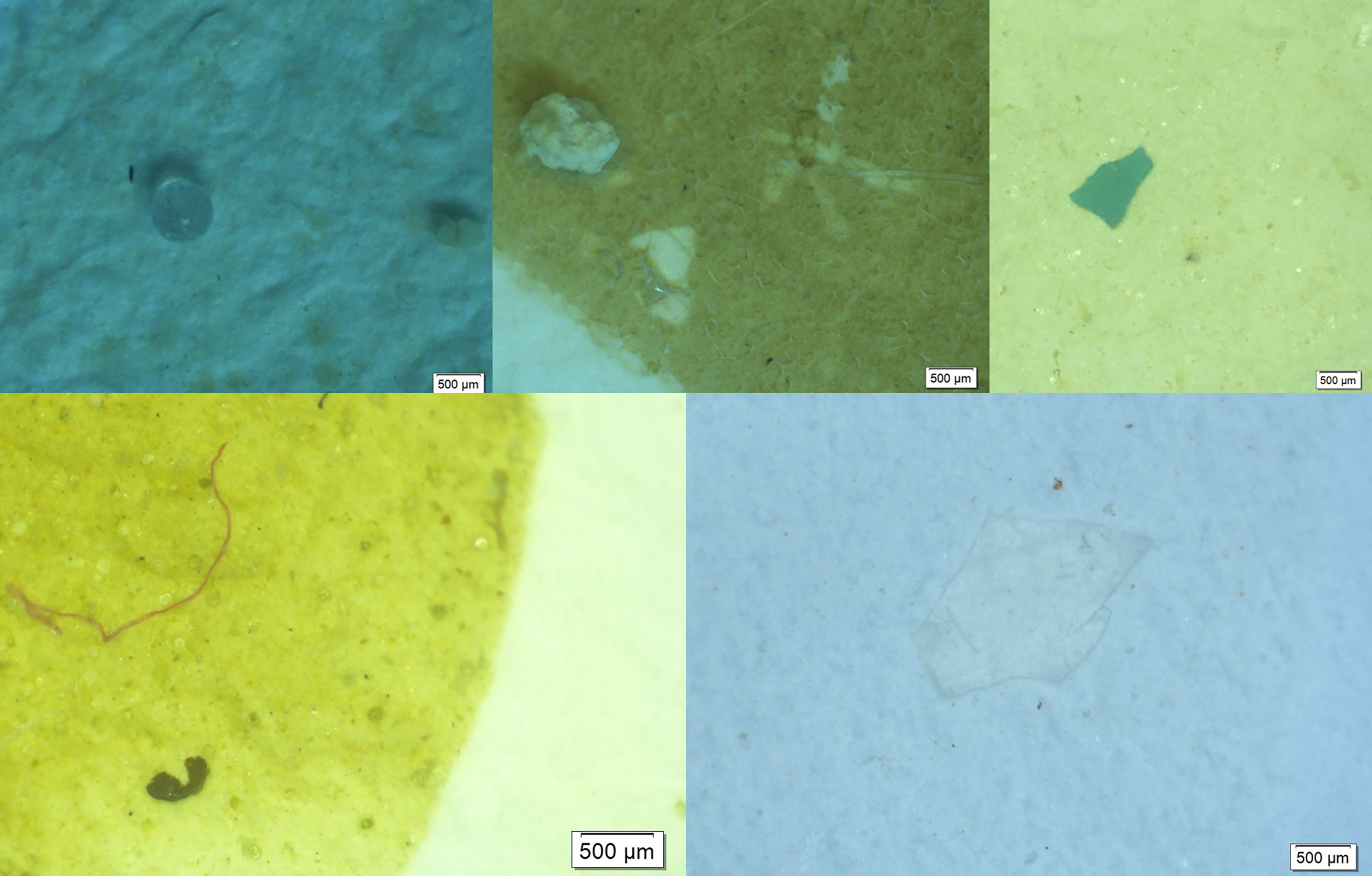
Figure 5 Image of recovered colourless pellet, white foam, green fragment, red and black fibre, and transparent film of this study (clockwise).
3.2.2 Field emission scanned electron microscope-energy dispersive X-ray (FESEM-EDX)
Figures and spectrums below show the surface characteristics and state of the MPs and inorganic elements present in each sample respectively. The morphology captured includes pellet (Figure 6), fibre (Figure 7), film (Figure 8), foam (Figure 9), and fragment (Figure 10). All samples showed obvious signs of wear and tear while appearing extremely rough and uneven on the surface. There were prominent cracks, flakes, and peeling of the outer layer. There was often obvious degradation on the surface of MPs. Most of the MP samples were irregular in shapes except for the pellet which had a more distinctive round shape. All samples analysed under the EDX showed strong signals of carbon (72.54-91.42%) and oxygen (7.38-17.47%), confirming that they are polymers.
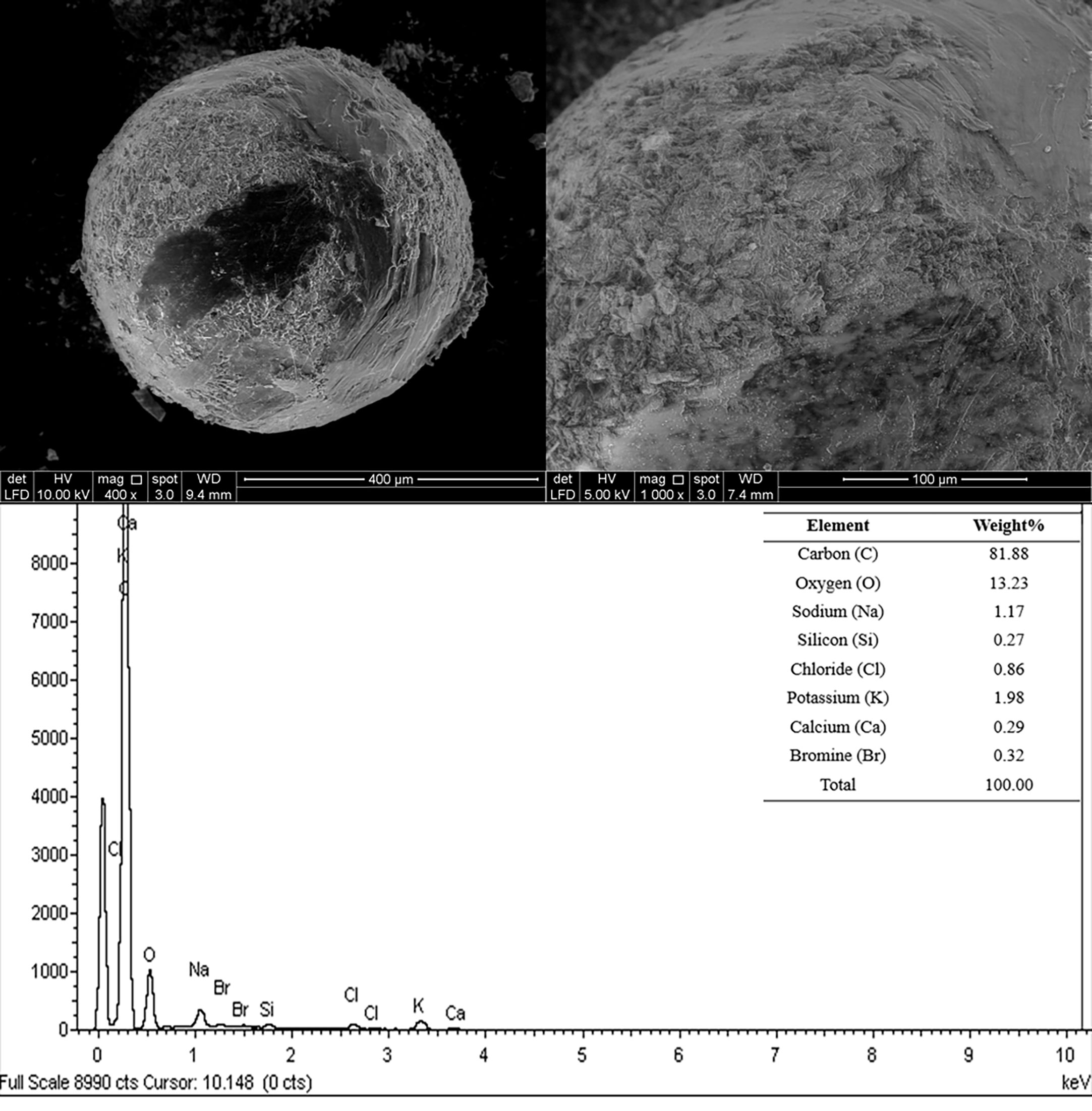
Figure 6 FESEM images of aged pellet and the corresponding EDX spectra recovered from the estuarine environment of Penang.
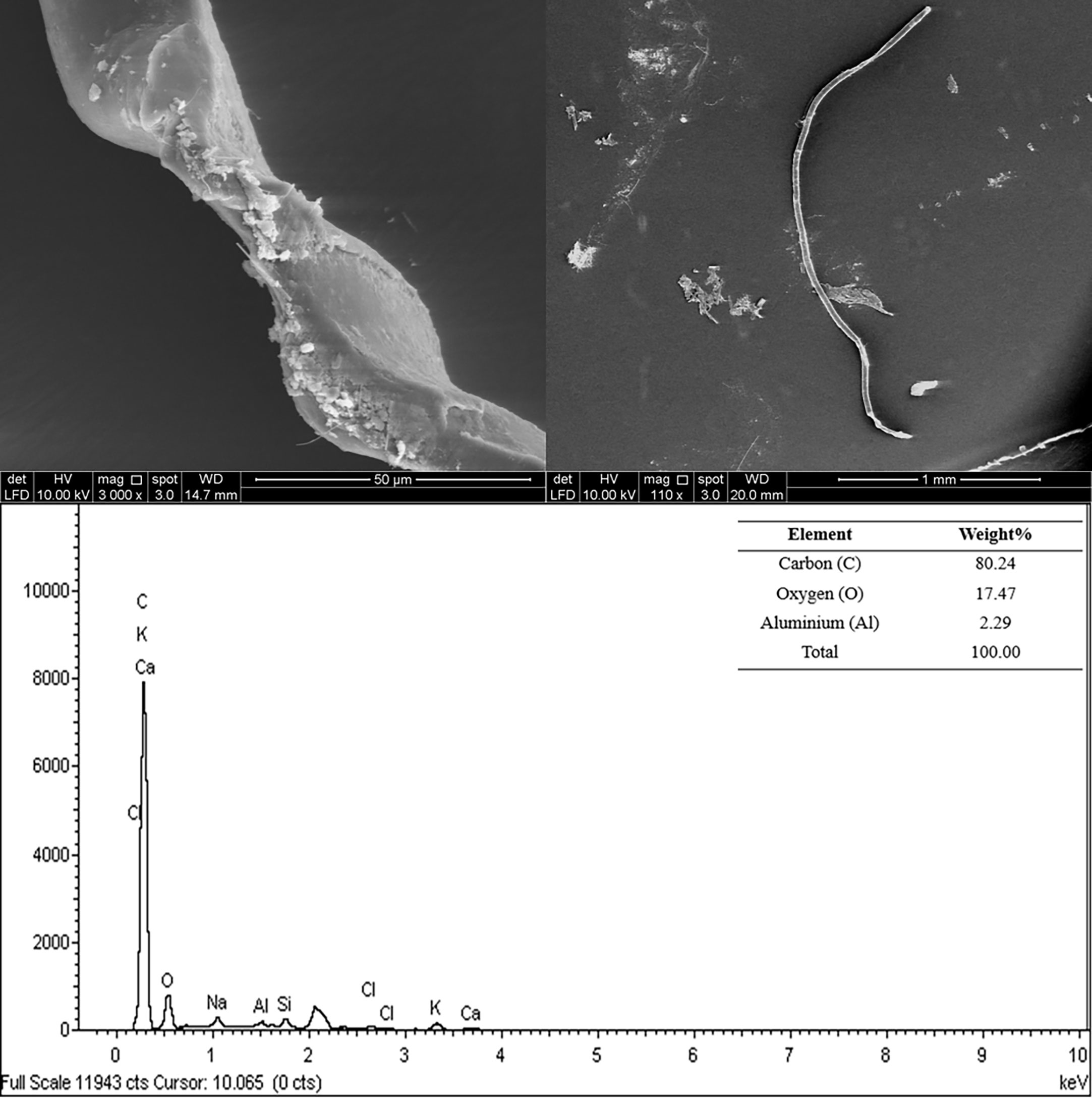
Figure 7 FESEM images of aged fibre and the corresponding EDX spectra of aged fibre recovered from the estuarine environment of Penang.
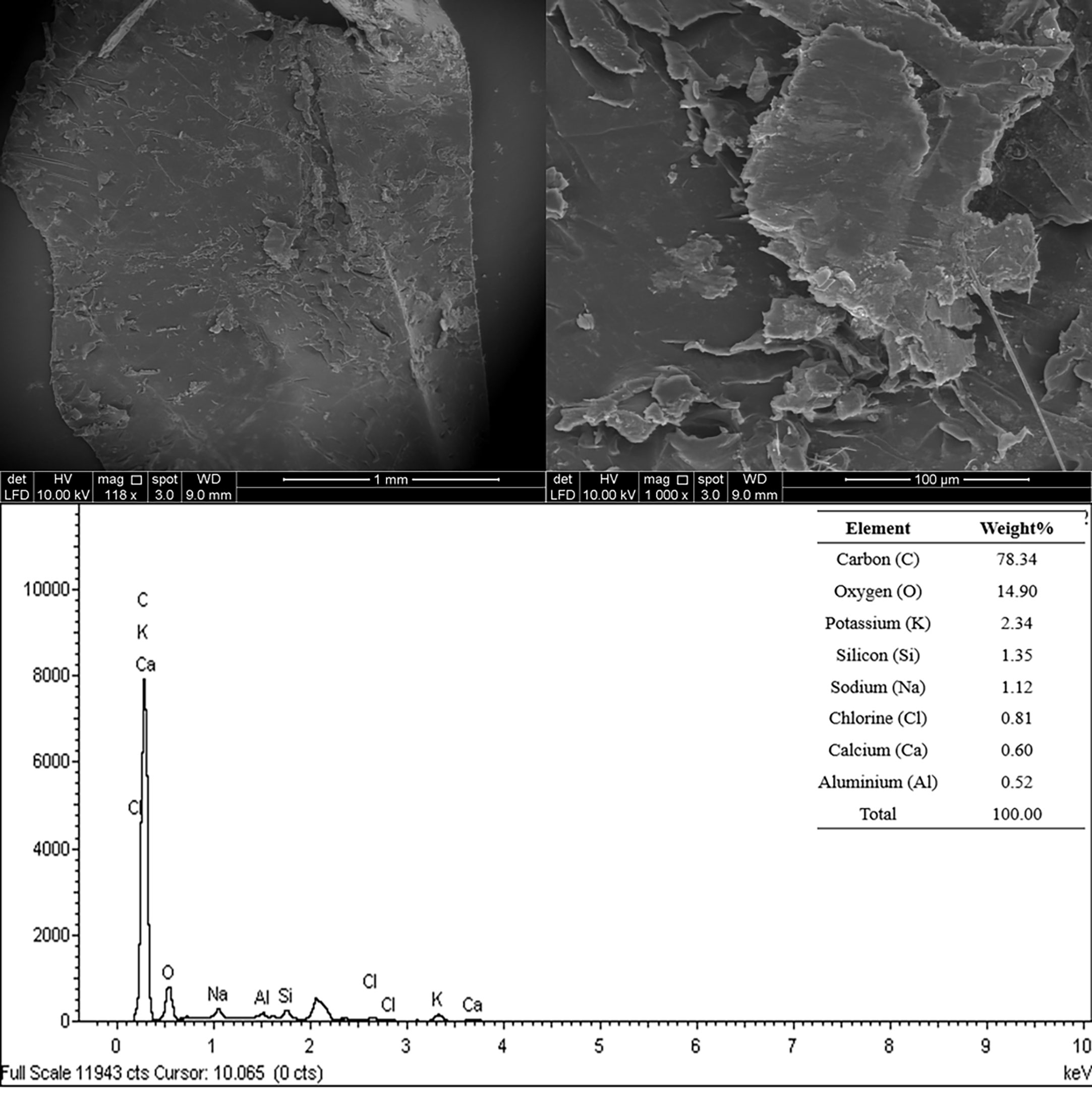
Figure 8 FESEM images of aged film and the corresponding EDX spectra of aged film recovered from the estuarine environment of Penang.
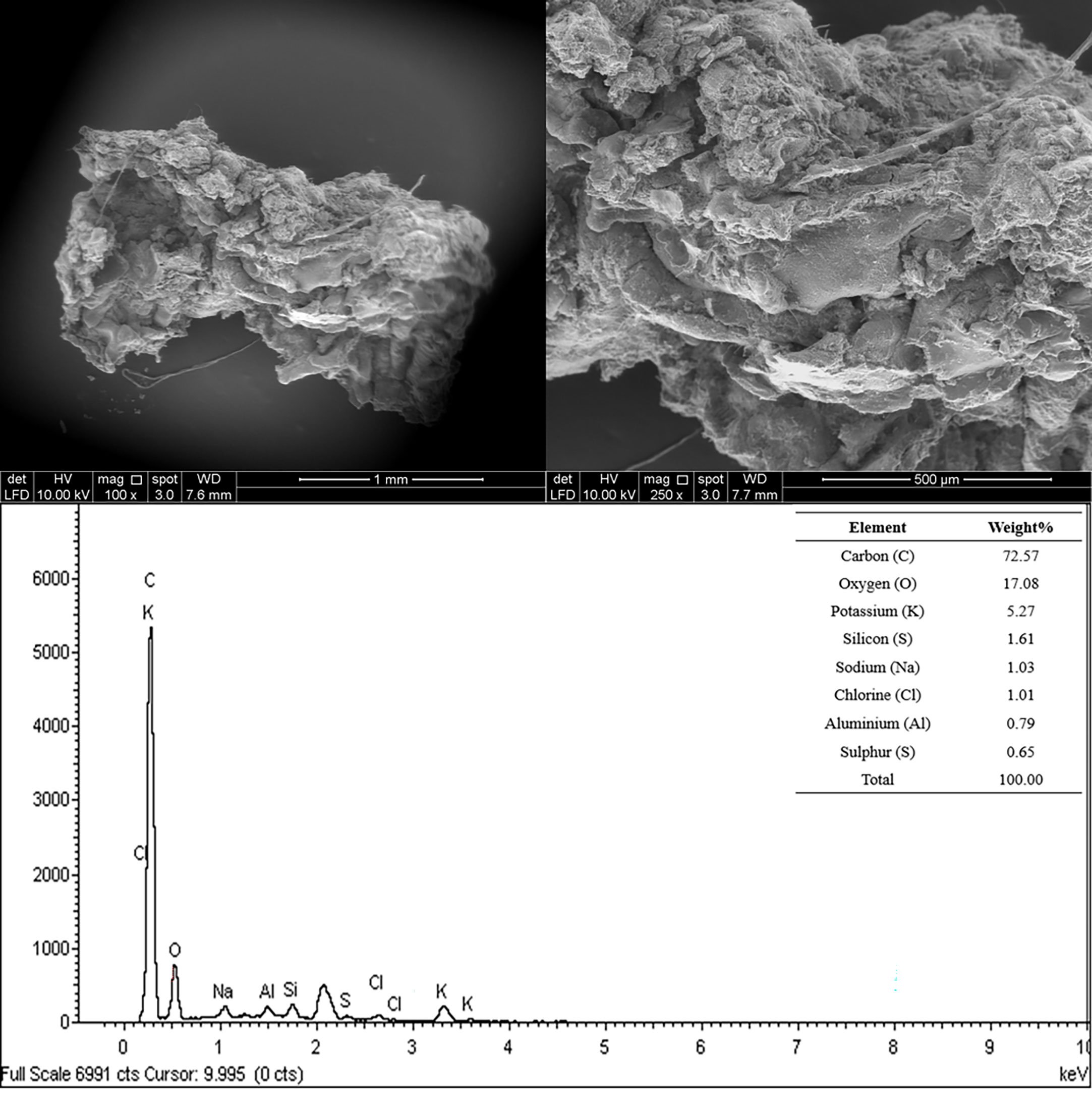
Figure 9 FESEM images of aged foam and the corresponding EDX spectra of aged foam recovered from the estuarine environment of Penang.
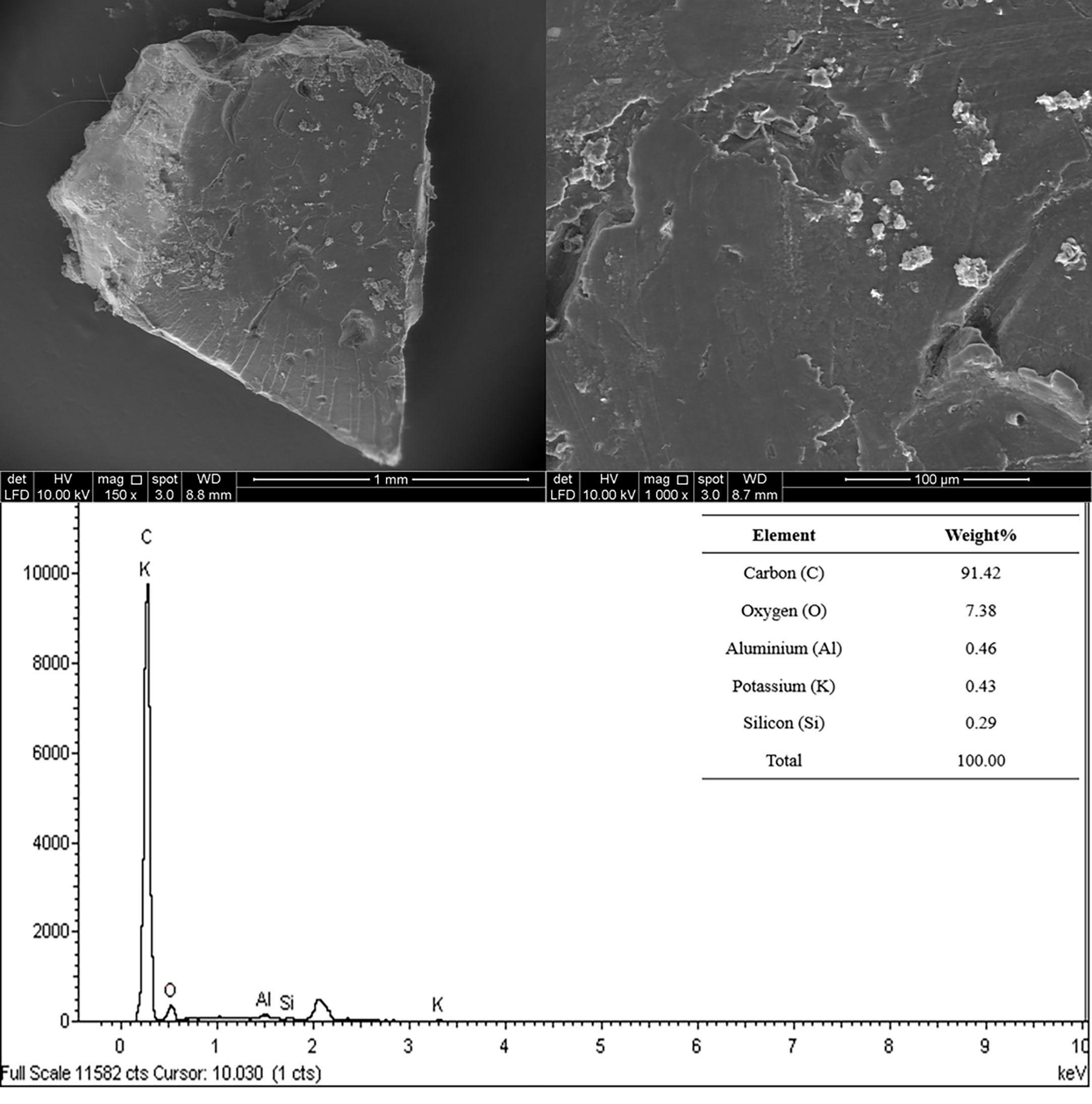
Figure 10 FESEM images of aged fragment and the corresponding EDX spectra of aged fragment recovered from the estuarine environment of Penang.
3.2.3 Fourier Transformed Infrared Spectroscopy (FTIR)
Fourier Transmission Infrared Spectroscopy (FTIR) was used to detect organic compounds present in the environment. For the analysis, 30 representative MP samples ranging between 500 µm-5 mm were selected from various study sites and sampling compartments. Additionally, we acknowledge the challenge in this work to quantitatively analyse the chemical composition in MPs as these FTIR results are based on a limited number of samples (n=30). Inorganic compounds detected included adamantane (C10H16), dimethylmercury [(CH3)2Hg], docosane (C22H46), docosanol-1 (C22H46O), glutaric anhydride (C5H6O3), indene (C9H8), mesityl oxide (MSO), and Mowiol-04. Polymer types detected included polyethylene (PE) (30%), polystyrene (PS) (19%), polyacetal (19%), polypropylene (PP) (9%), polybutadiene (6%), polychloroprene (6%), polyformaldehyde (4%), polyvinyl chloride (PVC) (2%), polyamide-6 (2%), and polyethylene oxide (PEO) (2%). Table 5 includes details and the FTIR spectra of detected compounds.
4 Discussion
4.1 Abundance and distribution
The pollution status of a specific environment can reflect the intensity of on-going anthropogenic activities at its proximity (Zhao et al., 2014; Tibbettes et al., 2018; Defontaine et al., 2020). Estuaries are recognized as pollutant sinks where they can filter riverine input upon receiving before releasing it into the ocean (López et al., 2021). Our study shows a distinct characteristic on the influence of surrounding urbanization activities and land use towards the compartment of the estuaries. Tibbettes et al. (2018) added that high occurrence of specific MPs in a particular area mostly originate from a local point source.
The coastal surface waters, coastal bottom sediment, and estuarine sediment of Seberang Perai have the highest MP abundance. This may due to the rapid urbanization and industrialization at Seberang Perai with Perai Free Industrial Zone located near to the estuary (Lenaker et al., 2019; Defontaine et al., 2020; Schuyler et al., 2021). The proximity of the developed industrial zone, coupled with dense human settlements and a port specialized in bulk cargo, may contribute MPs into nearby waters and sediments of the mangrove area (Ogunola & Palanisami, 2016; AlfaroNúñez et al., 2021; Li et al., 2021; Manoharam et al., 2021). Furthermore, the domination of secondary fragments suggests that they originate from larger plastics from households, industrial usage, or accidental release from landfills (Tibbettes et al., 2018).
Kuala Muda has the lowest MP abundance in coastal surface waters and estuarine sediment while Balik Pulau has the lowest abundance in coastal bottom sediment. Both areas are less urbanized and industrialized compared to Seberang Perai. Fibre is the most abundant morphology in coastal bottom and estuarine sediments of Kuala Muda and Balik Pulau as both sites are dominated by local-scale fishery activities. Fibre can be released from the breakdown of fishing gears, ropes, and nets (Lusher et al., 2017; Osorio et al., 2021; Rebelein et al., 2021). Phaksopa et al. (2021) reported a high abundance of fibre recovered from fishing ground in Thailand, supporting that the degradation of fishing gear can significantly contribute to fibre enrichments in aquatic environments. It is ecologically important that a low abundance of MPs were reported in both sites as they supply substantial amounts of seafood to neighbouring states (Zairudin et al., 2021). This would ensure the food safety for human consumption because seafood is one of the crucial sources of protein in Malaysian households (Karbalaei et al., 2019).
Overall, the coastal surface waters of estuaries are dominated by foams. Ongoing fishery activities that often uses Polystyrene (PS) boxes to pack and transport their catch and buoys to keep fishing nets and ropes afloat in water columns may contribute to the enrichment of foams on surface waters (Lee et al., 2015). Polystyrene (PS) can easily float on water surfaces as they are positively buoyant (Yin et al., 2019). When exposed to sunlight and temperature for a long time on water surface, they can easily become fragmented and produce micro-sized foams (Turner, 2020). Foams are chemically dangerous as they may release hazardous monomers such as styrene or its monomers that may exert a carcinogenic effect on humans (Farrelly & Shaw, 2017).
Meanwhile, fibre is the dominating morphology in coastal bottom sediment. This enrichment suggests that fibre may come from terrestrial sewage runoff, as well as from fishery activities as mentioned above as fabrics can release a high amount of fibre during washing (Pequeno et al., 2021). Their dominance can also be attributed to the properties of fibre that affects its distribution in aquatic environment (Defontaine et al., 2020). Fine fibre has a large surface area to volume ratio which can promote more microbial activities on the particle. Biofouling and aggregation formation due to entanglement of fibrous particle increases its density and will ultimately cause it to sink to the bottom of the ocean (Zhang, 2017; Pequeno et al., 2021). Gimiliani et al. (2020) and Kumar et al. (2021) added that fibre can be easily dragged down by sediments when they collide and become trapped between the grains in the sediment floor. Fibres are commonly discovered and widely distributed in bottom sediment floors (Zhang, 2017; Defontaine et al., 2020; Gimiliani et al., 2020).
The accumulation of fragments in estuarine sediments can be attributed to the less energetic condition of estuaries as they are semi-enclosed (Chapman & Wang, 2001). Li et al. (2020) recovered large plastic objects from food packaging in estuarine sediment from the Yangtze Estuary and explained the vulnerability of estuarine sediments to accumulate plastic waste easily in the environment. It has also been reported that aerial mangrove roots are effective in trapping as they indirectly filter large plastic objects coming from the river (Martin et al., 2019). The trapped plastic waste in mangrove forests will have greater and longer exposure to degradation conditions which further promote fragmentation, thus resulting in a higher abundance of fragments in the estuarine sediment (Defontaine et al., 2020). Furthermore, Govender et al. (2020) added that the formation of fragments can be accelerated by high microbial activities in the estuarine sediments.
There is no statistical significance of MP abundance in coastal surface waters and coastal bottom sediments for any study site. However, there is a significant difference between the Ms abundance of estuarine sediments between the study sites. Estuarine sediment of Seberang Perai is the most polluted while Kuala Muda is the least polluted. This strengthens our hypothesis that urbanization and development in Seberang Perai contributes to the MP abundance in estuarine sediment and the extent of urbanization could possibly influence the MP abundance of the estuarine mangrove in Penang.
The overall pattern of this study demonstrates that the estuarine environment of Penang is most dominated by fragments and least dominated by pellets. With continuous input from plastic waste, more fragments can form and accumulate in the ocean as they are further exposed to degrading factors (sunlight, hydrodynamics, and biological activities) in open waters (Li et al., 2020). Fragments can occupy different compartments of water column and sediments according to their properties (Zhang, 2017). For fragments that are less dense than seawater, they float on surfaces or stay suspended in water columns. Meanwhile, fragments with greater density will sink to the bottom of the ocean floor and accumulate (Tang et al., 2022). The occasional presence of pellets throughout all study sites suggests that terrestrial waste released from treatment plants may not have been effectively treated (Li et al., 2021).
4.2 Field emission scanning electron microscope-energy dispersive X-ray (FESEM-EDX)
The irregular surface and edges observed on most MP samples indicates that they are secondary MPs which come from the breakdown of larger plastic debris (Cooper & Corcoran, 2010). the pellet, on the other hand, looked round and angular, suggesting it is a primary MP which is purposely manufactured for personal care products (Eriksen et al., 2013). Our observation on the FESEM results suggested that the MPs may have experienced both mechanical and oxidative degradation. Mechanical degradation is often characterised with gouges, grooves, and fractures formed due to physical collision between mechanical forces such as wave action or abrasion with natural particles and are observed in the samples (Wang et al., 2017). Meanwhile, chemical degradation associated with degraded surface textures such as flakes, granules, and solution pits due to the exposure of sunlight or air was also observed on the samples (Wu et al., 2018). UV weakens polymer bonds and allows the introduction of oxygen into polymer structures, causing brittleness (Liu et al., 2019). Cooper and Corcoran (2010) added that surface textures that result from mechanical oxidation may serve as a favourable site to initiate chemical degradation. Chemical degradation occurs with the presence of additives while some polymers with antioxidants may lower the rate of degradation (Klien et al., 2018). In this study, the high supply of UV radiation that is available together with warmer temperatures and higher salinity may enhance formation of MPs in the estuarine environment (Wang et al., 2017). It has been reported that higher water salinity can accelerate the degradation process of MPs (Corcoran, 2022). Consistent UV radiation was also reported to experience rapid photodegradation of polymers (Chamas et al., 2020). Samples collected are comparable to the tropical conditions experienced in a study conducted by Zbyszewski et al. (2014) where their samples were collected at the gyres of the Pacific and Atlantic. When MPs are washed up on shorelines and beaches, this further encourages degradation as the conditions are more extreme compared to water columns with more particle collision for example dragging and saltation (Cooper and Corcoran, 2010; Corcoran, 2022).
The elemental analysis of all samples shows that they are dominated by carbon and oxygen, indicating that they are plastics. Both inorganic compounds are the basic unit of fossil fuels, which plastic are made out of (Pandey et al., 2022). It is common to observe high composition of carbon and oxygen (Girão, 2020) and the presence of inorganic compounds such as Al, Ca, bromine (Br), and sulphur (S) (EFSA CONTAM Panel, 2016; Campanale et al., 2020) in the EDX spectrum of MPs. They may originate from plastic manufacturing where they are commonly used as additives (Chamas et al., 2020) or adsorbed onto the surface of MPs (Liu et al., 2019). It has been reported that weathered MPs with a rough surface layer can act as a vector to allow pollutants to attach more easily on the surface and enhance toxicity (Brennecke et al., 2016).
4.3 Fourier Transform Infrared Spectroscopy (FTIR)
Non-biodegradable secondary MPs originating from larger plastics are increasing their persistency in the environment and their sorption capabilities (Tang et al., 2022). Their large surface area to volume ratio and increased surface porosity due to weathering may enhance polymer affinity for the binding of organic and inorganic pollutants (Barboza et al., 2018). The problem with polymers and associated pollutants is their insolubility in water and ability to bioaccumulate (Fu et al., 2021). The emergence of MPs as a pollutant vector is worrisome as MPs can be mistakenly and easily ingested by organisms which can bioaccumulate in the food web and threaten human food security (De-la-Torre, 2020). Passive feeders such as benthic organisms are at high risk as MPs particles sink to the bottom after biofouling (Tang et al., 2022). Even though certain polymers and associated monomers are degradable in water, it should not be overlooked as they may also exhibit toxicity in the environment (Alfonso- López et al., 2021). Hence, it is important to study the chemical compounds found in MPs to better understand the toxicity MPs exhibit towards the environment. Here we report the detected chemical compounds and associated toxicity towards the aquatic environment.
Polymer detection in this study showed common polymer types that are commercially produced and highly consumed, including PE, polypropylene, polystyrene, and polyvinyl chloride (Wang et al., 2021; Yuan et al., 2022). They are commonly found in the environment because of their frequent disposal (short service life) and light weight, meaning they can easily be carried in the environment (Pinheiro et al., 2021). All of the above polymers were categorized by Brown (2022) as hazardous agents produced from plastic processing. The adsorption of pollutants by polymers is dependent on their structural characteristics where some pollutants may directly penetrate into the matrix of PE and polyacetal while others would just attach onto polymer surfaces (Rochman et al., 2014).
Polyacetal, which shares the name of polyoxymethylene and polyformaldehyde, rarely degrades in the environment and may cause monomers such as formaldehyde and ethylene oxide to be released into the environment (Yuan et al., 2022). Although formaldehyde is known to be highly dangerous towards organisms if exposed, it can rapidly breakdown in water, as can ethylene oxide, indicating they will not bioaccumulate in the environment (Laly et al., 2018; ATSDR, 2022). Polychloroprene is another type of synthetic rubber used as a protective layer to delay the corrosion of maritime structures (Tatiana et al., 2017). Hurst (2014) reported that its monomer, chloroprene, is a non-accumulative compound in the environment.
Polybutadiene are synthetic rubbers commonly used in the production of tires (Valentini and Lopez-Manchado, 2020). The polymer was also listed by Brown (2022) as a hazardous agent from plastic processing. Although Magni et al. (2022) found that they do not hold a great threat towards freshwater organisms and the environment, the authors suggested more studies to be conducted due to its gradual emergence in the aquatic environment.
Polyethylene oxide (PEO) has a wide range of applications in various industries, from medical and personal care products to production of resins and plastics, which explains its omnipresence in aquatic environment (Nascimento et al., 2021). PEO has been observed to alter enzymatic activities and negatively influence metabolism, inducing oxidative stress in tadpoles.
Polyamide-6, commonly known as Nylon-6, is used in mechanical and textile industries. They are widespread in the marine environment because of their relative neutral buoyancy in water which causes them to suspend in water columns and makes them highly available to marine organisms. Uptake of this compound was reported to induce cellular and metabolism changes on suspension feeders (Rodrigues et al., 2022).
Docosane (C22H46) is an aliphatic hydrocarbon compound that can be found in petroleum (Akinola et al., 2019). They have a tolerance towards high temperatures and are commonly used in manufacturing of products for extreme conditions such as solar and electrical devices (Fredi et al., 2019). Kaur et al. (2018) reported the presence of docosane in the freshwater environment and added that this compound can remain in water for years. In Nigeria, Docosane was also found in shrimps recovered from petroleum-polluted water, posing a substantial threat to organisms and the environment through reduced reproduction and shift of ecosystem population and balance (Akinola et al., 2019).
Indene together with coumarone forms the Coumarone Indene Resin (CIR), commonly used in coating and rubber industries (Trumbo, 2000; Tian et al., 2018). CIR was widely used before the emergence of petroleum-based resins in the market which eventually replaced them (Trumbo, 2000; Zohuriaan-Mehr & Omidian, 2000). Its insolubility in water may be harmful to aquatic organisms and the environment (Gooch, 2007). CIR was found to release substantial monomers into water that violates recommended environmental safety levels and induces acute toxicity in Daphnia magna (Woods et al., 2007).
Mowiol-04 is the chemical name of polyvinyl alcohol and is used in the medical field (Soltani et al., 2007; Perfetti et al., 2012). In Alfonso-López et al. (2021), polyvinyl alcohol polymers were found to exhibit toxicity towards sea urchin larvae. The usage of Mowiol-04 will be sustainable towards the environment in the future considering its solubility and biodegradability in water (Jambaladinni & Bhat, 2021). Biodegradable polyvinyl acetate also has good electrical insulating properties as they are used in electrical appliances and medical industries (Wang et al., 2021; Tang et al., 2022).
Adamantane (C10H16)-based compounds are very insoluble in water due to their diamondiod structure that enhances its resistance, which may increase its persistency in aquatic settings (Lamoureux & Artavia, 2010). In particular, compounds of adamantane-based carboxylic acids recovered from oil sand process-affected water (OSPW) were reported to exhibit cellular toxicity in organisms (Rundle et al., 2021).
Mesityl Oxide (MSO) is a sweet-scented colourless organic compound primarily used as solvents in the production of engineered polymers such as rubber and resins (Herrador et al., 2021). Although MSO has been listed as a hazardous agent towards humans by Brown (2022); EDQM (2017) reported that the chemical does not pose a threat to the environment. Further studies will be required to learn about and confirm its toxicity.
1-Docosanol (C22H460) is an alcohol-based organic compound manufactured for plastics and rubbers (NCBI, 2022a). They are reported to have inhibitive properties towards viral infection and are found occurring in stems of a coastal plant (Sheela & Uthayakumari, 2013). Unlike docosane, 1-Docosanol as its alcohol derivatives can degrade in the environment via aerobic or anaerobic conditions, and thus do not accumulate in the environment (OPPT, 2020).
Glutaric anhydride (C5H6O3) is used as intermediate materials for polymer synthesis (NCBI, 2022c). It was found to be acutely toxic upon ingestion in rats and dermal exposure of rabbits (NCBI, 2022b). No information is available on its accumulation availability (Thermo Fisher Scientific, 2021), which may imply low possibility of chemical persistency and accumulation in aquatic setting as they also are soluble in water (Chemoxy, 2021).
Dimethylmercury [(CH3)2Hg] is formed from the methylation of inorganic Hg (Lian et al., 2021). The presence of (CH3)2Hg on MPs is worrying as it is very toxic to organisms and the environment where it causes persistent neurological effects and can remain in the environment for a very long time (Barboza et al., 2018). An exemplary case of Hg pollution is Minamata Bay disease in Japan, with side effects seen across generations (Minamata Disease Municipal Museum, 2007).
Small-sized MPs with a large surface area to volume ratio may accelerate chemical reactions, in turn enhancing their surficial properties optimal for pollutant adsorption (Zhao et al., 2022). As pollutant-coated MPs travel from one point to another in water, accidental release of the pollutants may be dangerous to the surrounding environment (Liu et al., 2022). MPs are also considered a direct source of chemicals because they are added during plastic manufacturing to enhance market and functional value (Verla et al., 2019). When digested internally by organisms, MPs were found to hamper normal development and growth, inhibit photosynthesis, alter normal behaviour, and impede reproduction (Zhang et al., 2019). Therefore, understanding the presence of chemicals added into MPs is crucial to understanding their possible toxicity towards the marine environment and improve our knowledge on chemical presence in plastic.
5 Conclusions and recommendations
MP pollution is a serious threat to the environment as they are continuously being discovered in every compartment of the aquatic environment. Its abundance is expected to rise steadily in the near future as the world struggles to cope with the overwhelming usage of plastic products and improving waste management system. It is crucial to understand pollution status in the estuarine environment; these are the transition points for most pollutants before entering into larger water bodies and ecologically provide food and refuge to diverse biodiversity. In this study, the abundance and distribution of MPs in the estuarine mangroves area of Penang is reported for the first time. MP abundance and dominant morphology in each study site is highly influenced by the type and intensity of human activities around the estuarine environment. In general, the results indicate that highly industrialized and urbanized sites such as Seberang Perai have higher MP abundance while less urbanized sites such as Kuala Muda and Balik Pulau have lower abundance. Meanwhile, dominating MP morphology in different compartments can be attributed to polymer properties, such as light-weighted foam on coastal surface waters, and large surface area to small ratio fibre that promotes biofouling and sinking and accumulating in coastal bottom sediment and estuarine sediment. Meanwhile, fragments as the overall dominating morphology suggest MPs mostly originate from the breakdown of large plastic debris, indicating that mangrove aerial roots likely play an important role in accumulating sediments like MPs. The FESEM-EDX results confirm that MPs experienced substantial mechanical and oxidative degradation. The exposures of MP towards tidal influence and yearlong sunlight radiation in Malaysia’s tropical weather may enhance the degradation of large plastic pieces into irregular small-sized MPs. Our FTIR results confirmed polyethylene (PE) as the most common polymer type and detected inorganic chemical compounds that are recognized by NCBI as hazardous agents to human. They are problematic when entering the environment because their hydrophobicity makes them insoluble in water, and thus they may bioaccumulate in organisms and environment.
As our work is a baseline study, we recommend further scientific research during different monsoon seasons to observe temporal influences towards MP occurrence of the estuarine environment of Penang. We also suggest conducting hydrological studies to better understand possible transport pathways of MPs as they have been reported to behave distinctively within the estuaries owing to different water salinities. We also recommend launching long-term clean-up programs to protect the biodiversity of the estuarine area.
Data availability statement
The original contributions presented in the study are included in the article/supplementary material. Further inquiries can be directed to the corresponding author.
Author contributions
Substantial contributions to the conception or design of the work: TE and NM. Drafting the article and revising it critically for important intellectual content: TE and NM. All authors contributed to the article and approved the submitted version.
Funding
This work was supported by Universiti Sains Malaysia, Short-Term Grant with Project No: 304/PPANTAI/6315669.
Conflict of interest
The authors declare that the research was conducted in the absence of any commercial or financial relationships that could be construed as a potential conflict of interest.
Publisher’s note
All claims expressed in this article are solely those of the authors and do not necessarily represent those of their affiliated organizations, or those of the publisher, the editors and the reviewers. Any product that may be evaluated in this article, or claim that may be made by its manufacturer, is not guaranteed or endorsed by the publisher.
References
Akinola J. O., Olawusi-Peters O. O., Akpambang V. O. E. (2019). Ecological hazards of total petroleum hydrocarbon in brackish water white shrimp Nematopalaemon withtatus (AURIVILLUS 1898). Egyptian J. Aquat. Res. 45 (3), 205–210. doi: 10.1016/j.ejar.2019.07.004
AlfaroNúñez A., Astorga D., CáceresFarías L., Bastidas L., Villegas C. S., Macay K. C., et al. (2021). Microplastic pollution in seawater and marine organisms across the tropical Eastern pacific and galápagos. Sci. Rep. 11 (1), 6424. doi: 10.1038/s41598-021-85939-3
Alfonso-López O., López-Ibáñez S., Beiras R. (2021). Assessment of toxicity and biodegradability of poly(vinyl alcohol)-based materials in marine water. Polymers 13 (21), 3742. doi: 10.3390/polym13213742
ATSDR (2022). Toxicology profile for ethylene oxide. august 2022 (Atlanta, Georgia, USA: Agency for Toxic Substances and Disease Registry (ATSDR), 243 pp.
Aziz I. H. (2022) Syor warta teluk air tawar-Kuala muda tapak ramsar. berita harian. Available at: https://www.bharian.com.my/berita/nasional/2022/01/909036/syor-warta-teluk-air-tawar-kuala-muda-tapak-ramsar.
Barboza L. G. A., Vieira L. R., Branco V., Carvalho C., Guilhermino L. (2018). Microplastics increase mercury bioconcentration in gills and bioaccumulation in the liver, and cause oxidative stress and damage in Dicentrarchus labrax juveniles. Sci. Rep. 8, 15655. doi: 10.1038/s41598-018-34125-z
Brennecke D., Duarte B., Paiva F., Caçador I., Canning-Clode J. (2016). Microplastics as vector for heavy metal contamination from the marine environment. Estuarine Coast. Shelf Sci. 178, 189–195. doi: 10.1016/j.ecss.2015.12.003
Brown J. A. (2022) Plastic composites manufacturing. haz-map. Available at: https://haz-map.com/Processes/222.
Bujang J. S., Zakaria M. H. (2020). “The flora of mangrove mudflats,” in Status of mangroves in Malaysia. Eds. Omar H., Husin T. M., Parlan I. (Selangor, Malaysia: Forest Research Institute Malaysia), (pp 43–(pp50).
Campanale C., Massarelli C., Savino I., Locaputo V., Uricchio V. F. (2020). A detailed review study on potential effects of microplastics and additives of concern on human health. International Journal of Environmental Research and Public Health 17, 4, 1212. doi: 10.3390/ijerph17041212
Chamas A., Moon H., Zheng J., Qiu Y., Tabassum T., Jang J. H., et al. (2020). Degradation rates of plastics in the environment. ACS Sustain. Chem. Eng. 8, 3494–3511. doi: 10.1021/acssuschemeng.9b06635
Chapman P., Wang F. (2001). Assessing sediment contamination in estuaries. Environ. Toxicol. Chem. 20 (1), 3–22. doi: 10.1002/etc.5620200102
Chee S. Y., Chai Y. J., Danielle C., Yusri Y., Barry G. J. (2020). Anthropogenic marine debris accumulation in mangroves on penang island, Malaysia. J. Sustainability Sci. Manage. 15 (6), 36–60. doi: 10.46754/jssm.2020.08.004
Chemoxy (2021) Glutaric anhydride. Available at: https://www.chemoxy.com/products-and-applications/products/intermediates/glutaric-anhydride/.
Chen H. L., Nath T. K., Chong S., Foo V., Gibbons C., Lechner A. M. (2021). The plastic waste problem in Malaysia: management, recycling and disposal of local and global plastic waste. SN Appl. Sci. 3, 437. doi: 10.1007/s42452-021-04234-y
Cooper D. A., Corcoran P. L. (2010). Effects of mechanical and chemical processes on the degradation of plastic beach debris on the island of Kauai, Hawaii. Mar. pollut. Bull. 60 (5), 650–654. doi: 10.1016/j.marpolbul.2009.12.026
Corcoran P. L. (2022). “Degradation of microplastics in the environment,” in Handbook of microplastics in the environment. Eds. Rocha-Santos T., Costa M., Mouneyrac C. (Cham: Springer), pp 531–pp 542. doi: 10.1007/978-3-030-39041-9_10
Defontaine S., Sous D., Tesan J., Monperrus M., Lenoble V., Lanceleur L. (2020). Microplastics in a salt-wedge estuary: Vertical structure and tidal dynamics. Mar. pollut. Bull. 160, 111688. doi: 10.1016/j.marpolbul.2020.111688ff.ffhal-02945061f
De-la-Torre G. E. (2020). Microplastics: an emerging threat to food security and human health. J. Food Sci. Technol. 57 (5), 1601–1608. doi: 10.1007/s13197-019-04138-1
EDQM (2017). Mesityl oxide safety data sheet (Strasbourg, France: European Directorate for the Quality of Medicines & Healthcare).
EFSA CONTAM Panel (2016). Statement on the presence of microplastics and nanoplastics in food, with particular focus on seafood. EFSA J. 14 (6), 4501, 30. doi: 10.2903/j.efsa.2016.4501
Eriksen M., Mason S., Wilson S., Box C., Zellers A., Edwards W., et al. (2013). Microplastic pollution in the surface waters of the laurentian great lakes. Mar. pollut. Bull. 771–2, 177–182. doi: 10.1016/j.marpolbul.2013.10.007
Farrelly T. A., Shaw I. C. (2017). “Polystrene as hazardous household waste,” in Household hazardous waste management. Ed. Mmereki D. (Vienna, Austria: IntechOpen), pp 45–pp 60. doi: 10.5772/65865
Foong S. Y., Yeap C. A., Butt C. (2016). A pilot rapid assessment of selected ecosystem services provided by the teluk air tawar-Kuala muda coast IBA in pulau pinang (Kuala Lumpur: Malaysian Nature Society).
Fredi G., Dirè S., Callone E., Ceccato R., Mondadori F., Alessandro P. (2019). Docosane-organosilica microcapsules for structural composites with thermal energy Storage/Release capability. Materials 12 (8), 1286. doi: 10.3390/ma12081286
Fu L., Li J., Wang G., Luan Y., Dai W. (2021). Adsorption behavior of organic pollutants on microplastics. Ecotoxicology Environ. Saf. 217, 112207. doi: 10.1016/j.ecoenv.2021.112207
Furukawa K., Wolanski E. (1996). Sedimentation in mangrove forests. Mangroves Salt Marshes 1 (1), 3–10. doi: 10.1023/A:1025973426404
GESAMP (2015). Sources, fate and effects of microplastics in the marine environment: a global assessment. Ed. Kershaw P. J. (London, UK: IMO/FAO/UNESCO-IOC/UNIDO/WMO/IAEA/UN/UNEP/UNDP Joint Group of Experts on the Scientific Aspects of Marine Environmental Protection), 96.
GESAMP (2019). Guidelines or the monitoring and assessment of plastic litter and microplastics in the ocean. Eds. Kershaw P. J., Turra A., Galgani F. (United Nations Office Nairobi (UNON), Africa: IMO/FAO/UNESCO-IOC/UNIDO/WMO/IAEA/UN/UNEP/UNDP/ISA Joint Group of Experts on the Scientific Aspects of Marine Environmental Protection), 130.
Gimiliani G. T., Fornari M., Redígolo M. M., Bustillos J. O. W. V., de Souza Abessa D. M., Pires M. A. F. (2020). Simple and cost-effective method for microplastic quantification in estuarine sediment: A case study of the santos and sao Vicente estuarine system. Case Stud. Chem. Environ. Eng. 2, 100020. doi: 10.1016/j.cscee.2020.100020
Girão A. V. (2020). “SEM/EDS and optical microscopy analysis of microplastics,” in Handbook of microplastics in the environment. Eds. Rocha-Santos T., Costa M., Mouneyrac C. (Switzerland: Springer), 1–22. doi: 10.1007/978-3-030-10618-8
Gooch J. W. (2007). “Coumarone-indene resin (Cumar),” in Encyclopedic dictionary of polymers. Ed. Gooch J. W. (New York, NY: Springer), 235. doi: 10.1007/978-0-387-30160-0_2941
Govender J., Naidoo T., Rajkaran A., Cebekhulu S., Bhugeloo A., Sershen (2020). Towards characterising microplastic abundance, typology and retention in mangrove-dominated estuaries. Water 12, 10, 2802. doi: 10.3390/w12102802
Hammer J., Kraak M. H. S., Parsons J. R. (2012). Plastics in the marine environment: the dark side of a modern gift. Rev. Environ. Contamination Toxicol. 220, 1–44. doi: 10.1007/978-1-4614-3414-6_1
Herrador J. M., Tišler Z., Kocík J., Fratczak J., Hradecká I., Velvarská R., et al. (2021). Mesityl oxide reduction by using acid-modified phonolite supported NiW, NiMo, and CoMo catalysts. Catalysts 11 (9), 1101. doi: 10.3390/catal11091101
Hurst H. E. (2014). “Choloroprene,” in Encyclopedia of toxicology, 3rd ed. Ed. Wexler P. (London, UK: Academic Press), pp 907–pp 910. doi: 10.1016/B978-0-12-386454-3.01116-7
Jambaladinni S., Bhat J. S. (2021). Enrichment a study of structural, optical and dielectric properties of mowiol 4-88 (Pva) filled zno nanocomposites. Walailak J. 18 (14), 21445. doi: 10.48048/wjst.2021.21445
Johan I., Wan Maznah W. O., Mashhor M., Abu Hena M. K., Amin S. M. N. (2012). Spatial distribution of copepods along the salinity gradient of perai river estuary, penang, Malaysia. Pakistan J. Biol. Sci. 15 (13), 647–652. doi: 10.3923/pjbs.2012.647.652
Karbalaei S., Golieskardi A., Hamzah H. B., Abdulwahid S., Hanachi P., Karami A. (2019). Abundance and characteristics of microplastics in commercial marine fish from Malaysia. Mar. pollut. Bull. 148, 5–15. doi: 10.1016/j.marpolbul.2019.07.072
Kaur R., Akuley-Amenyenu A., Deng Q., Dennis S. O. (2018). Incidence of personal care products in surface water of middle Tennessee urban and rural Sub-watersheds. J. Water Resource Prot. 10, 507–521. doi: 10.4236/jwarp.2018.105027
Klien S., Dimzon I. K., Knepper T. P. (2018). “Analysis, occurrence, and degradation of microplastics in the aqueous environment,” in Freshwater microplastics, vol. 58 . Eds. Wagner M., Lambert S. (Cham, Switzerland: The Handbook of Environmental Chemistry), pp 51–pp 67. doi: 10.1007/978-3-319-61615-5_3
Kumar R., Sharma P., Verma A., Jha P. K., Singh P., Gupta P. K., et al. (2021). Effect of physical characteristics and hydrodynamic conditions on transport and deposition of microplastics in riverine ecosystem. Water 13 (19), 2710. doi: 10.3390/w13192710
Lagerström M., Ytreberga E., Wiklund A. E., Granhaga L. (2020). Antifouling paints leach copper in excess – study of metal release rates and efficacy along a salinity gradient. Water Res. 186, 116383. doi: 10.1016/j.watres.2020.116383
Laly S. J., Priya E. R., Panda S. K., Zynudheen A. A. (2018). Formaldehyde in seafood: A review. Fishery Technol. 55, 87–93. Retrieved from: https://krishi.icar.gov.in/jspui/bitstream/123456789/20617/1/Formaldehyde%20in%20Seafood%20A%20review.pdf
Lamichhance G., Acharya A., Marahatha R., Modi B., Oaudel R., Adhikari A., et al. (2021). Microplastics in environment: global concern, challenges, and controlling measures. Int. J. Environ. Sci. Technol. 20(4), 4673–4694. doi: 10.1007/s13762-022-04261-1
Lamoureux G., Artavia G. (2010). Use of the adamantane structure in medicinal chemistry. Curr. Medicinal Chem. 17, 2967–2978. doi: 10.2174/092986710792065027
Lee J., Hong S., Jang Y. C., Lee M. J., Kang D., Shim W. J. (2015). Finding solutions for the styrofoam buoy debris problem through participatory workshops. Mar. Policy 51, 182–189. doi: 10.1016/j.marpol.2014.08.008
Lenaker P. L., Baldwin A. K., Corsi S. R., Mason S. A., Reneau P. C., Scott J. W. (2019). Vertical distribution of microplastics in the water column and surficial sediment from the Milwaukee river basin to lake Michigan. Environ. Sci. Technol. 53, 12227–12237. doi: 10.1021/acs.est.9b03850
Li Y., Lu Z., Zheng H., Wang. J., Chen C. (2020). Microplastics in surface water and sediments of chongming island in the Yangtze estuary, China. Environ. Sci. Europe 32 (1), 15. doi: 10.1186/s12302-020-0297-7
Li Y., Zhang Y., Chen G., Xu K., Gong H., Huang K., et al. (2021). Microplastics in surface waters and sediments from guangdong coastal areas, south China. Sustainability 13 (5), 2691. doi: 10.3390/su13052691
Lian P., Mou Z., Copper C. J., Johnston R. C., Brooks S. C., Gu B., et al. (2021). Mechanistic investigation of dimethylmercury formation mediated by a sulfide mineral surface. J. Phys. Chem. A 125 (24), 5397–5405. doi: 10.1021/acs.jpca.1c04014
Liu S., Jian M., Zhou L., Li W. (2019). Distribution and characteristics of microplastics in the sediments of poyang lake, China. Water Sci. Technol. 79 (10), 1868–1877. doi: 10.2166/wst.2019.185
Liu Y., Wu L., Shi G., Cao S., Li Y. (2022). Characteristics and sources of microplastic pollution in the water and sediment of the jinjiang river basin, fujian province, China. China Geology 5 (3), 429–438. doi: 10.31035/cg2022051
López A. G., Najjar R. G., Friedrichs M. A. M., Hickner M. A., Wardrop D. H. (2021). Estuaries as filters for riverine microplastics: Stimulation in a Large, coastal-plain estuary. Front. Mar. Sci. 8. doi: 10.3389/fmars.2021.715924
Luo W., Su L., Craig N. J., Du F., Wu C., Shi H. (2018). Comparison of microplastic pollution in different water bodies from urban creeks to coastal waters. Environ. pollut. 246, 174–182. doi: 10.1016/j.envpol.2018.11.081
Lusher A., Hollman P., Mendoza-Hill J. (2017). Microplastics in fisheries and aquaculture: Status of knowledge on their occurrence and implications for aquatic organisms and food safety (Rome, Italy: FAO Fisheries and Aquaculture Technical Paper No. 615). Available at: https://www.fao.org/3/I7677E/I7677E.pdf.
Lusher A. L., Munno K., Hermabessiere L., Carr S. (2020). Isolation and extraction of microplastics from environmental samples: An evaluation of practical approaches and recommendations for further harmonization. Appl. Spectrosc. 74 (9), 1049–1065. doi: 10.1177/0003702820938993
Magni S., Tediosi E., Maggioni D., Sbarberi R., Noé F., Rossetti F., et al. (2022). Ecological impact of end-of-Life-Tire (ELT)-derived rubbers: Acute and chronic effects at organism and population levels. Toxics 10 (5), 201. doi: 10.3390/toxics10050201
Manoharam G., Ismail M. T., Abir I. A., Ali M. K. M. (2021). Efficient solid waste management prai industrial area through GIS using Dijkstra and travelling salesman problem algorithm. Pertanika J. Sci. Technol. 29 (3), 1297–1418. doi: 10.47836/pjst.29.3.02
Martin C., Almawithheer H., Duarte C. M. (2019). Mangrove forests as traps for marine litter. Environ. pollut. 247, 499–508. doi: 10.1016/j.envpol.2019.01.067
Masura J., Baker J., Foster G., Arthuer C. (2015). Laboratory methods for the analysis of microplastics in the marine environment: Recommendations for quantifying synthetic particles in waters and sediments (Silver Spring, MD, USA: NOAA Technical Memorandum NOS-OR&R-48). Available at: https://marinedebris.noaa.gov/sites/default/files/publications-files/noaa_microplastics_methods_manual.pdf.
Minamata Disease Municipal Museum (2007) Minamata disease its history and lessons [Brochure]. Available at: https://minamata195651.jp/pdf/kyoukun_en/kyoukun_eng_all.pdf.
MNS (2015). A concept paper on the proposed establishment of a “Wetland NatureReserve” and development of nature-based initiative in the teluk air tawar-Kuala muda coast IBA (Kuala Lumpur and Penang: MNS Conservation and MNS Penang Branch).
MPSP (2020) Kuala Muda district local plan 2035 (Replacement) – executive summary. Available at: http://epublisiti.townplan.gov.my/turun/rt_kualamuda/ringkasanbi.pdf.
Nascimento I. F., Guimaraes A. T. B., Ribeiro F., de Lima Rodrigues A. S., Estrela F. N., da Luz T. M., et al. (2021). Polyethylene glycol acute and sub-lethal toxicity in neotropical Physalaemus cuvieri tadpoles (Anura, leptodactylidae). Environ. pollut. 283, 117054. doi: 10.1016/j.envpol.2021.117054
National Geographic Society (2022) Tropics. Available at: https://education.nationalgeographic.org/resource/tropics.
NCBI (2022a). PubChem annotation record for 1-docosanol (Bethesda, Maryland, USA: Hazardous Substances Data Bank (HSDB). Available at: https://pubchem.ncbi.nlm.nih.gov/source/hsdb/5739.
NCBI (2022b) PubChem patent summary for CN-114181384-A. Available at: https://pubchem.ncbi.nlm.nih.gov/patent/CN-114181384-A.
NCBI (2022c) PubChem compound summary for CID 7940, glutaric anhydride. Available at: https://pubchem.ncbi.nlm.nih.gov/compound/Glutaric-anhydride.
Ogunola O. S., Palanisami T. (2016). Microplastics in the marine environment: Current status, assessment methodologies, impacts and solutions. J. pollut. Effects Control 4 (2), 161. doi: 10.4172/2375-4397.1000161
Omar H., Misman M. A. (2020). “Extent and distribution of mangroves in Malaysia,” in Status of mangroves in Malaysia. Eds. Omar H., Husin T. M., Parlan I. (Selangor, Malaysia: Forest Research Institute Malaysia, Ministry of Energy and Natural Resources), pp 1–pp42.
OPPT (2020). Supporting information for low-priority substance 1-docosanol (CASRN 661-19-8) final designation (Washington, D.C., USA: U. S. Environmental Protection Agency). U. S. Environmental Protection Agency.
Osorio E. D., Tanchuling M. A. N., Diola M. B. L. D. (2021). Microplastics occurrence in surface waters and sediments in five river mouths of Manila bay. Front. Environ. Sci. 9. doi: 10.3389/fenvs.2021.719274
Pandey D., Banerjee T., Badola N., Chauhan J. S. (2022). Evidences of microplastic in air and Street dust: A case study of varanasi city, India. Environ. Sci. pollut. Res. 29, 82006–82013. doi: 10.1007/s11356-022-21514-1
Pequeno J., Antunes J., Dhimmer V., Bessa F., Sobral P. (2021). Microplastics in marine and estuarine species from the coast of Portugal. Front. Environ. Sci. 9. doi: 10.3389/fenvs.2021.579127
Perfetti G., Alphazan T., Wildeboer W. J., Meesters G. M. H. (2012). Thermo-physical characterization of pharmacoat® 603, pharmacoat® 615 and mowiol® 4-98. J. Thermal Anal. Calorimetry 109, 203–215. doi: 10.1007/s10973-011-1664-9
Phaksopa J., Sukhsangchan R., Keawsang R., Tanapivattanakul K., Thamrongnawasawat T., Worachananant S., et al. (2021). Presence and characterization of microplastics in coastal fish around the Eastern coast of Thailand. Sustainability 13 (23), 13110. doi: 10.3390/su132313110
Pinheiro L. M., Agostini V. O., Lima A. R. A., Ward R. D., Pinho G. L. L. (2021). The fate of plastic litter within estuarine compartments: An overview of current knowledge for the transboundary issue to guide future assessments. Environ. pollut. 279, 116908. doi: 10.1016/j.envpol.2021.116908
PPC (2018) Prai bulk cargo terminal. Available at: https://www.penangport.gov.my/en/facilities-services/prai-bulk-cargo-terminal.
Rebelein A., Int-Venn I., Kamman U., Scharsack J. P. (2021). Microplastic fibres — underestimated threat to aquatic organisms? Sci. Total Environ. 777, 146045. doi: 10.1016/j.scitotenv.2021.146045
Ricciardi M., Pironti C., Motta O., Miele Y., Proto A., Montano L. (2021). Microplastics in the aquatic environment: Occurrence, persistence, analysis, and human exposure. Water 13 (7), 973. doi: 10.3390/w13070973
Rochman C. M., Hentschel B. T., The S. J. (2014). Long-term sorption of metals is similar among plastic types: Implications for plastic debris in aquatic environments. PloS One 9 (1), e85433. doi: 10.1371/journal.pone.0085433
Rodrigues F. G., Vieira H. C., Campos D., Pires S. F. S., Rodrigues A. C. M., Silva A. L. P., et al. (2022). Co-Exposure with an invasive seaweed exudate increases toxicity of polyamide microplastics in the marine mussel. Mytilus galloprovincialis. Toxics 10 (2), 43. doi: 10.3390/toxics10020043
Rundle K. I., Sharaf M. S., Stevens S., Kamunde C., van den Huevel M. R. (2021). Adamantane carboxylic acids demonstrate mitochondrial toxicity consistent with oil sands-derived naphthenic acids. Environ. Adv. 5, 100092. doi: 10.1016/j.envadv.2021.100092
SAM (2019) Impact of coastal reclamation in Malaysia. Available at: https://foe-malaysia.org/wp-content/uploads/2020/12/190226_Impacts_of_Coastal_Reclamation_in_Malaysia-compressed.pdf.
Schuyler Q., Wilcox C., Lawson T. J., Ranatunga R. R. M. K. P., Hu C., Global Plastics Project Partners, et al. (2021). Human population density is a poor predictor of debris in the environment. Front. Environ. Sci. 9. doi: 10.3389/fenvs.2021.583454
Sheela D., Uthayakumari F. (2013). GC-MS analysis of bioactive constituents from coastal sand dune taxon – Sesuvium portulacastrum (L.). Bioscience Discovery 4 (1), 47–53. Retrieved from: http://biosciencediscovery.com/Volume%204%20No1%20Jan%2013/D%20Sheela%2047-53.pdf
Sim L. M., Chan N. W., Tan M. L., Daud N., Ismail W. R., Phang W. L. (2019). Socio-economic and livelihood assessment of inland fishermen in muda river basin. Eur. Proc. Soc. Behav. Sci. Int. Conf. Humanities 2019 89, 586–594. doi: 10.15405/epsbs.2020.10.02.53
Soltani S., Asempour H., Jamshidi H. (2007). Investigation of reaction conditions for preparation of medium molecular weight poly(vinyl alcohol) as emulsifier. Iranian Polymer J. 16 (7), 439–447. Retrieved from https://www.sid.ir/FileServer/JE/81320070701.pdf
Stiepani J., Gillis L. G., Chee S. Y., Pfeiffer M., Nordhaud I. (2021). Impacts of urbanization on mangrove forests and brachyuran crabs in penang, Malaysia. Regional Environ. Change 31, 69. doi: 10.1007/s10113-021-01800-3
Talbot R., Granek E., Chang H., Wood R., Nramder S. (2022). Spatial and temporal cariations of microplastic concentrations in portland’s freshwater ecosystems. Sci. Total Environ. 833, 155143. doi: 10.1016/j.scitotenv.2022.155143
Tan E., Jaafar N. F., Tan S. H. A., Zanuri N. B. M. (2021). A review of plastic and microplastic pollution towards the Malaysian marine environment. IOP Conf. Series: Earth and Environmental Science 1013, 1, 012012. doi: 10.1088/1755-1315/1013/1/012012
Tang C.-C., Chen Y., Zhang Y., Chen H., Brimblecombe P., Lee C.-L. (2022). Cracking and photo-oxidation of polyoxymethylene degraded in terrestrial and simulated marine environments. Front. Mar. Sci. 9. doi: 10.3389/fmars.2022.843295
Tatiana T. T., Pierre-Yves L. G., Maurin R., Créac'Hcadec R. (2017). Polychloroprene behaviour in a marine environment: Role of silica fillers. polymer. Degradation Stability 139, 28–37. doi: 10.1016/j.polymdegradstab.2017.03.011
Thermo Fisher Scientific (2021). Glutaric anhydride (NJ: Thermo Fisher Scientific: Fair Lawn). Available at: https://www.fishersci.com/store/msds?partNumber=AC119970050&countryCode=US&language=en.
Think City (2021) Sungai perai programme. Available at: https://thinkcity.com.my/work/sungai-perai-programme/.
Tian K., Zhu T., Lan P., We Z., Hu W., Xie F., et al. (2018). Massive preparation of coumarone-indene resin-based hyper-crosslinked polymers for gas adsorption. Chin. J. Polymer Sci. 36, 1168–1174. doi: 10.1007/s10118-018-2127-6
Tibbettes J., Krause S., Lynch I., Smith G. H. S. (2018). Abundance, distribution, and drivers of microplastics contamination in urban river environments. Water 10 (11), 1597. doi: 10.3390/w10111597
Trumbo D. (2000). Functionalization of coumarone-indene resins i. acetoacetylation. Polymer Bull. 44, 151–158. doi: 10.1007/s002890050586
Turner A. (2020). Foamed polystyrene in the marine environment: Sources, additives, transport, behavior, and impacts. Environ. Sci. Technol. 54, 17, 10411–10420. doi: 10.1021/acs.est.0c03221
Valentini L., Lopez-Manchado M. A. (2020). “Classification of rubbers and components for harsh environmental systems,” in High-performance elastomeric materials reinforced by nanocarbons: Multifunctional properties and industrial applications. Eds. Valentini L., Lopez-Manchado M. A. (Amsterdam: Elsevier), pp 1–pp14. doi: 10.1016/B978-0-12-816198-2.00001-3
Verla A. W., Enyoh C. E., Verla E. N., Nwarnorh K. O. (2019). Microplastic–toxic chemical interaction: a review study on quantified levels, mechanism and implication. SN Appl. Sci. 1 (1400). doi: 10.1007/s42452-019-1352-0
Wang G. X., Huang D., Ji J. H., Volker C., Wurm F. R. (2021). Seawater-degradable polymers-fighting the marine plastic pollution. Advanced Sci. 8 (1), 2001121. doi: 10.1002/advs.202001121
Wang Z., Wagner J., Ghosal S., Bedi G., Wall S. (2017). SEM/EDS and optical microscopy analyses of microplastics in ocean trawl and fish guts. Sci. total Environ. 603-604, 616–626. doi: 10.1016/j.scitotenv.2017.06.047
Woods R. W., Letinski D. J., Febbo E. J., Szamba C. L., Connelly M. J., Parkerton T. F. (2007). Assessing the aquatic hazard of commercial hydrocarbon resins. Ecotoxicology Environ. Saf. 66 (2), 159–168. doi: 10.1016/j.ecoenv.2005.11.004
Wu C., Zhang K., Xiong X. (2018). “Microplastic pollution in inland waters focusing on Asia,” in Freshwater microplastics, the handbook of environmental chemistry, vol. 58 . Eds. Wagner M., Lambert S. (Cham: Springer), pp 85–pp 99. doi: 10.1007/978-3-319-61615-5_5
Yii M. W., Hashim A., Wan Mahmood Z. U. (2020). Assessment of elements in surface sediment of perai river, penang using neutron activation analysis technique. Jurnal Sains Nuklear Malaysia 32 (2), 31–48. Retrieved from: https://jsnm2.nuclearmalaysia.gov.my/index.php/jsnm/article/view/171
Yin L., Jiang C., Wen X., Du C., Zhong W., Feng Z., et al. (2019). Microplastic pollution in surface water of urban lakes in changsha, China. Int. J. Environ. Res. Public Health 16 (9), 1650. doi: 10.3390/ijerph16091650
Yuan Z., Nag R., Cummins E. (2022). Ranking of potential hazards from microplastics polymers in the marine environment. J. Hazardous Materials 429, 128399. doi: 10.1016/j.jhazmat.2022.128399
Zairudin N. R. F., Withsan A. S., Arab Y., Witchayangkoon B., Saengklieng K. (2021). Layout plan analysis on urban pathways, nodes and edges in Kuala muda fishing village. Int. Arch. Photogrammetry Remote Sens. Spatial Inf. Sci. XLVI-4/W5-2021, 565–571. doi: 10.5194/isprs-archives-XLVI-4-W5-2021-565-2021
Zbyszewski M., Corcoran P. L., Hockin A. (2014). Comparison of the distribution and degradation of plastic debris along shorelines of the great lakes, north America. J. Great Lakes Res. 40 (2), 288–299. doi: 10.1016/j.jglr.2014.02.012
Zhang H. (2017). Transport of microplastics in coastal seas. Estuarine Coast. Shelf Sci. 199, 74–86. doi: 10.1016/j.ecss.2017.09.032
Zhang S., Wang J., Liu X., Qu F., Wang X., Wang X., et al. (2019). Microplastics in the environment: A review of analytical methods, distribution, and biological effects. Trends Analytical Chem. 111, 62–72. doi: 10.1016/j.trac.2018.12.002
Zhao M., Huang L., Arulmani S. R. B., Yan J., Wu L., Wu T., et al. (2022). Adsorption of different pollutants by using microplastic with different influencing factors and mechanisms in wastewater: A review. Nanomaterials 12, 2256. doi: 10.3390/nano12132256
Zhao S., Zhu L., Wang T., Li D. (2014). Suspended microplastics in the surface water of the yante estuary system, China: First observations on occurrence, distribution. Mar. pollut. Bull. 86 (1-2), 562–568. doi: 10.1016/j.marpolbul.2014.06.032
Zhou X., Wang J., Ren J. (2022). Analysis of microplastics in takeaway food containers in China using FPA-FTIR whole filter analysis. Molecules 27, 2646. doi: 10.3390/molecules27092646
Keywords: microplastics (MPs), estuarine mangrove, field emission scanning electron microscope (FESEM), Fourier Transform Infrared Spectroscopy (FTIR), Malaysia
Citation: Tan E and Mohd Zanuri NB (2023) Abundance and distribution of microplastics in tropical estuarine mangrove areas around Penang, Malaysia. Front. Mar. Sci. 10:1148804. doi: 10.3389/fmars.2023.1148804
Received: 20 January 2023; Accepted: 20 March 2023;
Published: 04 April 2023.
Edited by:
Chawalit Charoenpong, Chulalongkorn University, ThailandReviewed by:
Mohamed Mohsen, Jimei University, ChinaAnish Kumar Warrier, Manipal Academy of Higher Education, India
Copyright © 2023 Tan and Mohd Zanuri. This is an open-access article distributed under the terms of the Creative Commons Attribution License (CC BY). The use, distribution or reproduction in other forums is permitted, provided the original author(s) and the copyright owner(s) are credited and that the original publication in this journal is cited, in accordance with accepted academic practice. No use, distribution or reproduction is permitted which does not comply with these terms.
*Correspondence: Norlaila Binti Mohd Zanuri, lailazanuri@usm.my