- 1Department of Earth, Ocean and Atmospheric Science, Florida State University, Tallahassee, FL, United States
- 2College of Veterinary Medicine, University of Florida, Gainesville, FL, United States
- 3Center for Marine Science, University of North Carolina Wilmington, Wilmington, NC, United States
Introduction: Microplastics (i.e., plastic debris smaller than 5mm) found in coastal areas can impact the marine habitat used by endangered species since they may alter sand properties including temperature and permeability. Such alterations may pose a significant threat to marine turtle populations as nest productivity, sexual development, and hatchling fitness are dependent on conditions within the nest, which incubate in the sand. Given that there is a record of microplastic presence at marine turtle nesting sites, this study was conducted to explore the potential influence of microplastics on the thermal profile of sediment typical of marine turtle nesting habitat.
Methods: The experiment was conducted at the Florida State University Coastal and Marine Laboratory where the temperatures of containers of sand mixed with 5-30% v/v of either black or white microplastics were recorded from July to September 2018.
Results: The addition of microplastics in the sand resulted in an increase in temperature – 0.017°C for each 1% v/v increase in microplastic. However, the color of the microplastic did not have a significant effect on sand temperature. Overall, the container with 30% v/v black particles had the highest mean temperature increase of 0.58°C (± 0.34°C) over the control.
Discussion: The results obtained from this study indicate that extreme concentrations of microplastics could be an issue for marine turtles as any changes in sand temperature may affect the sex ratio of hatchlings and/or alter nest productivity.
1 Introduction
A substantial amount of debris has accumulated in the marine environment over the past decades as a result of anthropogenic activities with 80% of the debris stemming from land-based sources (Barnes, 2002; Gregory, 2009; Lebreton et al., 2012; Lavers and Bond, 2017). The most common debris type found in coastal and marine environments is plastic (Derraik, 2002; Carson et al., 2011; Wessel et al., 2016; Garrison and Fuentes, 2019; Thushari and Senevirathna, 2020). Plastics are being produced at approximately 381 million metric tons per year to meet human demands and roughly 10% of that is making its way into the ocean (Mendenhall, 2018; Royer et al., 2018). Being extremely durable, plastics take decades or longer to break down (Law et al., 2010), resulting in accumulation in terrestrial and marine environments (Andrady, 2011). Plastics can be made in a wide range of sizes, shapes, and colors, with sizes smaller than 5mm referred to as microplastics and anything larger referred to as macroplastics (Arthur et al., 2009; Wessel et al., 2016; Lahens et al., 2018; Frias and Nash, 2019; Chen et al., 2021). Microplastics are typically manufactured for consumer products or created by the breakdown of larger plastic pieces into smaller ones (Andrady, 2011; Hidalgo-Ruz et al., 2012; Ceccarini et al., 2018).
Macro- and microplastics can impact marine life in multiple ways including entanglement, ingestion, and as a barrier (Derraik, 2002; Rios et al., 2007; Law et al., 2010; Andrades et al., 2018; Wilcox et al., 2018; Cartraud et al., 2019). The entanglement of marine species in macroplastics can lead to drowning, strangulation, loss of blood flow, reduced fitness, and ability to find food, and higher probability of predation (Gregory and Andrady, 2003; Gall and Thompson, 2015). Ingesting large pieces of plastic can cause intestinal blockage, internal puncture, loss of appetite, endocrine disruption, failure to reproduce, and toxicity to vital organs (Gregory, 2009; Gall and Thompson, 2015). Plastics can contain hydrophobic organic contaminants that dissociate after they have been ingested, leading to biological issues such as abnormal cell growth which may be transferred to offspring (Kanu and Anyanwu, 2005; Cole et al., 2013; Shim and Thomposon, 2015). The ingestion of microplastics by lower trophic level species can result in the bioaccumulation of discharged toxicants through predator-prey interaction (Auta et al., 2017; Sun et al., 2017; Williams, 2017; Rios-Fuster et al., 2022). In addition, macroplastics can impact beach-dwelling or -nesting species by causing habitat destruction, entanglement, or obstruction of emergence, nesting, or movement (Nelms et al., 2016; Aguilera et al., 2018; Gündoğdu et al., 2019). This makes plastics extremely threatening to marine animals because they are found throughout all the oceans and in coastal areas (Fendall and Sewell, 2009; Graham and Thompson, 2009; Imhof et al., 2013; Shim and Thomposon, 2015; Wessel et al., 2016; Beckwith and Fuentes, 2018).
Plastic can also alter the sediment of coastal areas used by beach-nesting species, such as marine turtles and seabirds (Carson et al., 2011; Nelms et al., 2016; Lavers et al., 2021). Recent studies have found macro- and microplastics at seabird and marine turtle nesting grounds in the northern Gulf of Mexico (Beckwith and Fuentes, 2018; Garrison and Fuentes, 2019), Samandağ coast of Turkey (Gündoğdu et al., 2019), the coastline of Cyprus (Duncan et al., 2018), northern New Zealand (Buxton et al., 2013), northwest Denmark (Hartwig et al., 2007), the east coast of Australia (Verlis et al., 2018), in Galapagos (Jones et al., 2022), Sir Bu Na’ir Island, United Arab Emirates (Yaghmour and Al Marashda, 2020) and in China (Zhang et al., 2021; Zhang et al., 2022), among others. The largest abundance of plastic in beach sediment has been reported along dunes – the preferred habitat for several beach-nesting species (Meager et al., 2012; Šilc et al., 2018; Beckwith and Fuentes, 2018; Ceccarini et al., 2018). It has been speculated that the presence of microplastics in the sediment of coastal areas can cause changes to the thermal profile and permeability of that sediment (Carson et al., 2011; Beckwith and Fuentes, 2018; Duncan et al., 2018; Lavers et al., 2021). Since microplastics have a higher specific heat than the sediment, increased microplastic concentrations in coastal sediments may alter the temperature of the sediment (Wen, 2007; Andrady, 2011; Lavers et al., 2021). This could be an issue to several species (Carson et al., 2011), particularly for marine turtles since the environment in which their eggs incubate can influence hatchling development, sex ratio, and morphology (Yntema and Mrosovsky, 1982; Davenport, 1997). Successful incubation of marine turtle eggs only occurs within a thermal range of ~24 to 35°C (Mrosovsky and Yntema, 1980; Matsuzawa et al., 2002; Howard et al., 2014). Additionally, marine turtles have temperature-dependent sex determination with warmer temperatures producing females and colder temperatures producing males (Georges et al., 1994).
Given the growing presence of microplastic in coastal areas and the potential impacts that it might have on beach-dwelling and -nesting species, it is crucial to understand how the presence of microplastic affects the thermal profile of the sediment. To address this, we conducted preliminary experiments to examine the effects that microplastic abundance and color may have on the thermal profile of beach sediment.
2 Materials and methods
The effect of microplastics on beach sediment was explored within an experimental setting at the Florida State University Coastal and Marine Laboratory in St. Teresa, Florida in the northern Gulf of Mexico from July 16th to July 26th and August 20th to September 3rd, 2018, which aligns with the marine turtle nesting season in the northern hemisphere (Fuentes et al., 2016; Silver-Gorges et al., 2021; Ware et al., 2021). The experimental design consisted of seven 55-gallon white polyethylene drums (Uline Model No. S-10757NAT) with the tops removed and filled with 40 gallons of silicate beach sand comparable to that from a typical nesting beach in the region (Figures 1, 2). To avoid the accumulation of rainfall, three holes were made down three sides of the drums approximately 20cm, 40cm, and 60cm below the sand surface to provide drainage. Each hole was covered with a piece of small mesh screen (diameter = 0.011mm; mesh count = 18 x 16) and glued on using silicon.
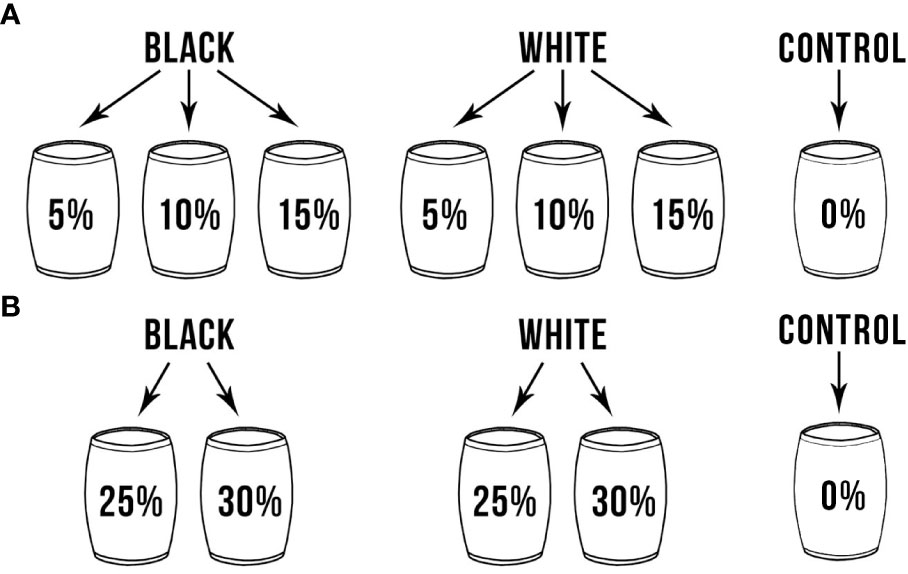
Figure 1 Treatment containers with different colors and concentrations of microplastics: (A) First experiment, July 16th to July 26th, 2018, (B) Second experiment, August 20th to September 3rd, 2018.
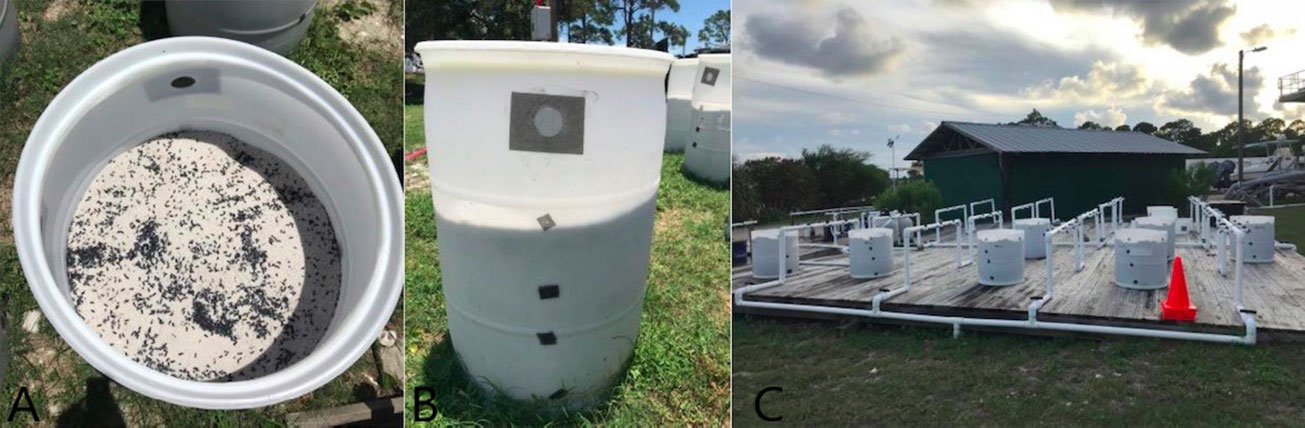
Figure 2 Containers with microplastic in the sand at the experiment site: (A) top view of container, (B). container with screen over holes and, (C). sampling design.
One drum was used as a control with no microplastic added. The remaining six containers had either black or white polyethylene microplastics, created by shredding polyethylene plates using a micro-cut shredder, mixed into the sand in different quantities using a concrete mixer. Polyethylene was used as this type of microplastic is commonly found in the marine environment (Ng and Obbard, 2006; Fries et al., 2013; Wessel et al., 2016; Tiwari et al., 2019). The amount of sand added to the mixer decreased as microplastics increased to keep the total volume of the sand/microplastics mixture at 40 gallons. During the first experiment (July 16th to July 26th, 2018), the microplastic concentrations were 5%, 10%, and 15% of microplastic by volume (Figure 1A), and 25% and 30% by volume in the second experiment (August 20th to September 3rd, 2018, Figure 1B), respectively. Microplastic concentrations are often reported in the literature as pieces per square meter (pc m-2) or per cubic meter (pc m-3). Supplementary Table 1 provides a conversion between microplastic concentrations used in this experiment for reference.
Two iButton temperature data loggers (DS1922L-F5, accuracy ± 0.5°C) per drum were each placed at 40cm below the sand surface and 30cm from the drum wall to record sediment temperature. This depth is typical for loggerhead marine turtle egg chambers (Montero et al., 2018). Note that the drums were stored on a wooden deck and not directly on the ground. Two loggers per drum were used to provide redundancy in case one of the loggers failed during deployment. The temperature was recorded every 30 minutes during each deployment. As mean differences between the paired iButtons were small (0.16°C ± 0.14°C), the temperature readings from both loggers were averaged at each sampling interval to generate one dataset per treatment. The black 30% treatment during the second experiment was an exception as one of the iButtons in the treatment failed, so only the temperature data from the functioning logger was used for analysis. Local atmospheric data (i.e., daily mean air temperature, daily mean solar radiation, and daily accumulated rainfall) during each deployment were collected by the Florida State University Coastal and Marine Laboratory’s weather station (data available from https://franklin.weatherstem.com/data). A linear regression (Equation 1) was conducted in R (version 4.2.2) to determine whether these environmental variables, as well as color and concentration of microplastic were significantly related to daily mean sediment temperature through both hypothesis testing and a stepwise AIC comparison (Burnham and Anderson, 2002). All numerical explanatory variables were scaled to a mean of 0 and a standard deviation of 1 due to the large discrepancies in scale between such variables.
3 Results
Between the first and second experiments, there was no significant difference in air temperature (Experiment 1: 27.52°C ± 0.11°C, Experiment 2: 27.27°C ± 0.10°C, p = 0.087) nor solar radiation (Experiment 1: 204.72 Wm-2 ± 13.68 Wm-2, Experiment 2: 202.10 Wm-2 ± 11.58 Wm-2, p = 0.884). There was a significant difference in daily rainfall (Experiment 1: 0.12 cm ± 0.01 cm, Experiment 2: 0.54 cm ± 0.07 cm, p<< 0.001).
Treatments with higher concentrations of microplastic tended to have larger temperature differences relative to the control treatment (Figures 3, 4, see Supplemental Material for analysis specifics). The largest average temperature difference within the black microplastic treatments was found in the containers with 30% of microplastic (0.58°C ± 0.34°C) followed by the 25% (0.45°C ± 0.24°C). Similarly, within the white microplastic treatments, the largest average temperature difference was in the container with 30% (0.43°C ± 0.37°C) followed by the 25% (0.25°C ± 0.19°C). The black microplastic treatments tended to have greater temperature differences than the white treatments; however, model evaluation was ambiguous as to its significance. Hypothesis testing suggested the inclusion of color as a significant variable (p<< 0.001), but its removal resulted in an improved AIC score (ΔAIC = -1.22). A similar pattern was observed for the interaction between microplastic color and concentration: hypothesis testing suggested inclusion (p<< 0.001) while AIC comparisons suggested exclusion (ΔAIC = -1.78).
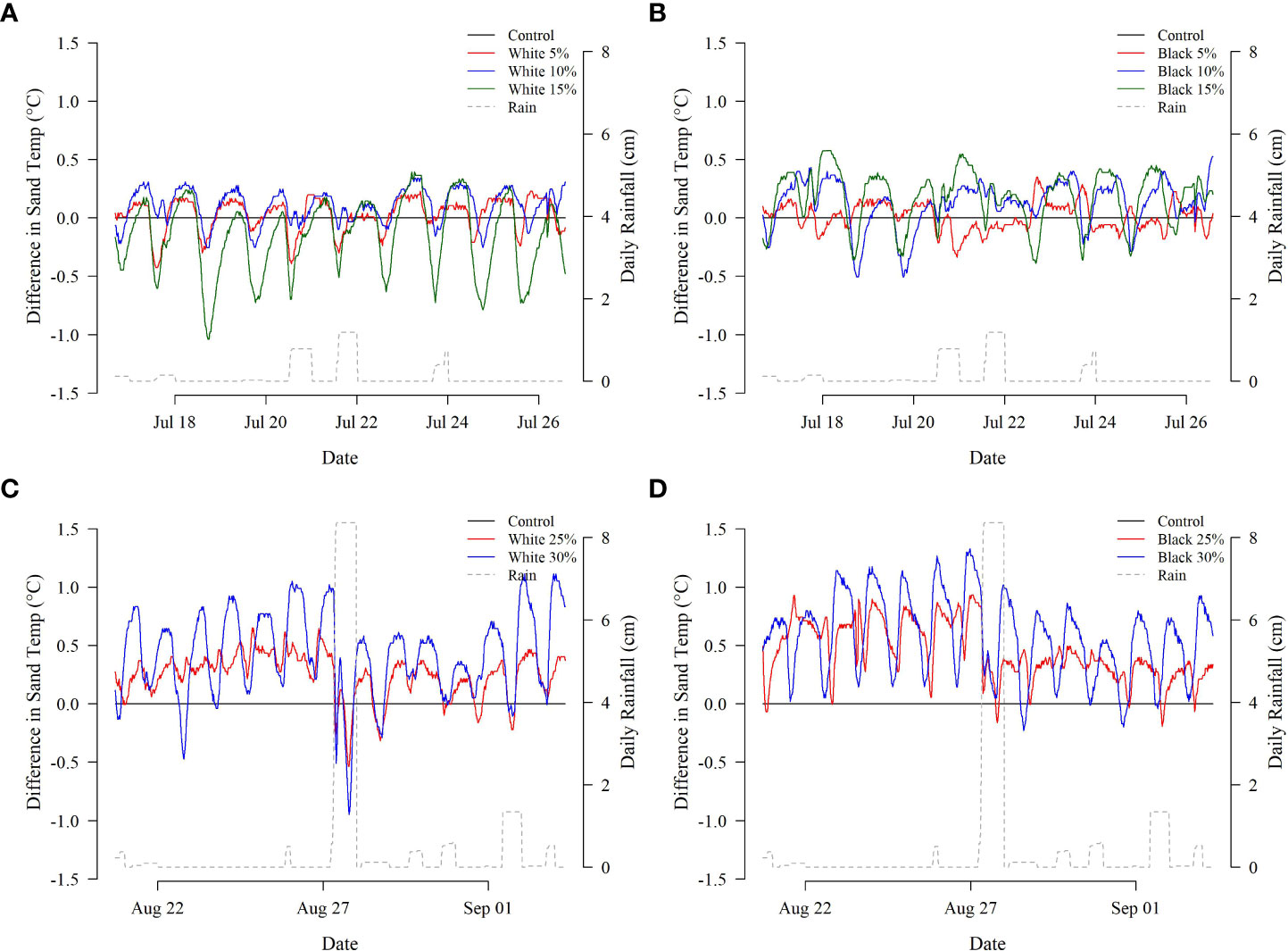
Figure 3 Hourly temperature differences (°C) between the control and treatment [(A) white microplastics, Deployment 1; (B) black microplastics, Deployment 1; (C) white microplastics, Deployment 2; (D) black microplastics, Deployment 2]. Daily rainfall measurements are provided on the secondary y-axis for reference.
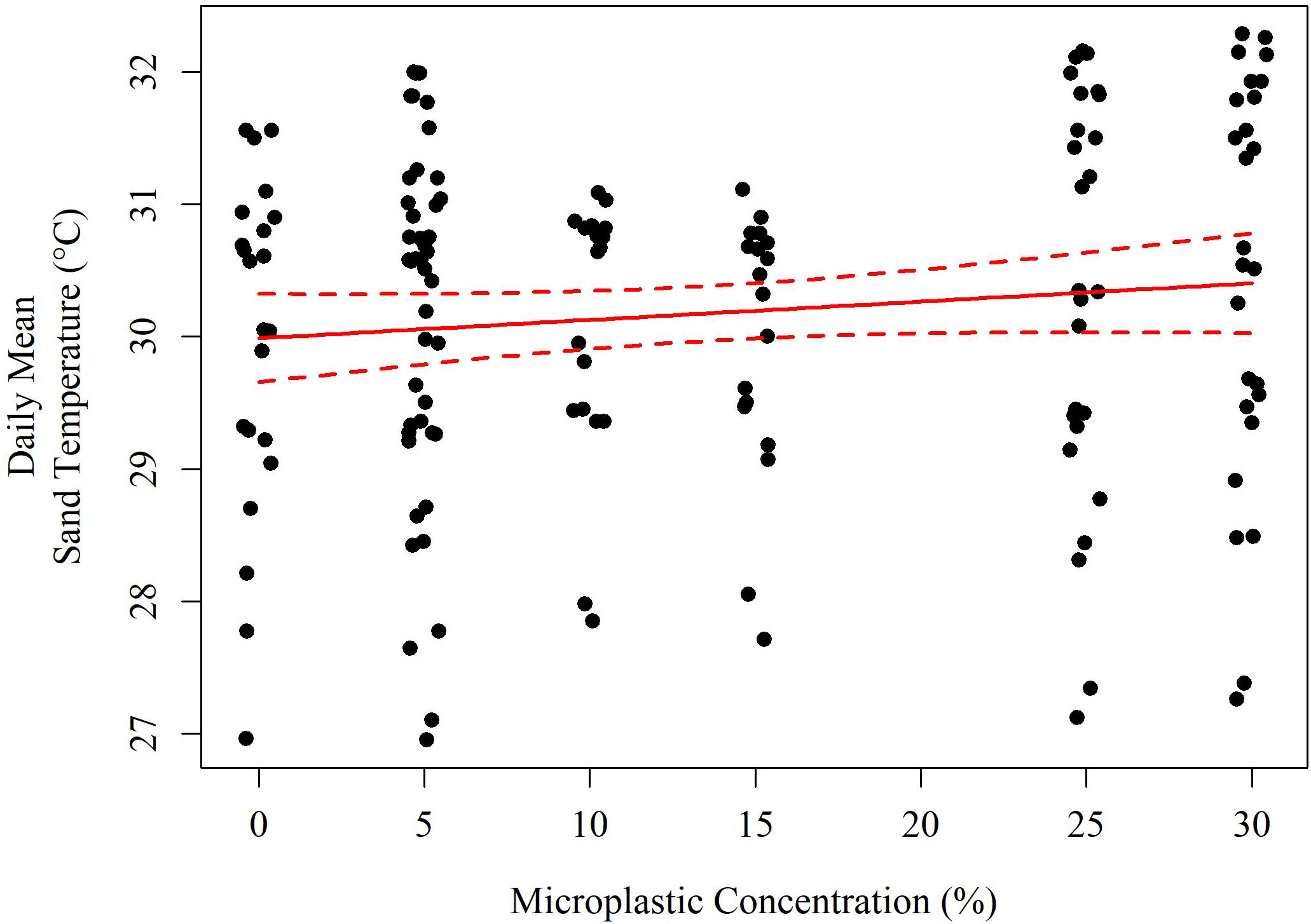
Figure 4 A model of the daily mean sediment temperature (°C) based on the concentration of microplastics (solid red line). The red dashed lines indicate the 95% confidence interval of the model.
The best performing model by AIC (Equation 2) included air temperature (°C), solar radiation (Wm-2), their interaction, daily rainfall (cm), and microplastic concentration (% v/v). Each of these variables was highly significant in hypothesis testing (p<< 0.001) with the exception of rainfall (p = 0.058) but its removal reduced AIC performance (ΔAIC = +1.21), as did removing the other variables in the reduced model (ΔAIC > +10 each). The reduced model is described below including a scale conversion for each term. Both the reduced and the full model explained 70.8% of the variation in daily mean sediment temperature. As the concentration of microplastics increased by 1% v/v, there was an increase in temperature by 0.017°C (Figure 4).
4 Discussion
The concentration of microplastic in the sediment had a greater influence on the sediment’s temperature than the color of the microplastic. Sediment with higher microplastic concentrations had greater increases in temperature relative to the control, with the black 30% v/v treatment having the highest mean difference in temperature at 0.58°C – an increase which could significantly alter sea turtle hatchling sex ratios, physiological performance, and embryonic mortality (Fuentes et al., 2011; Santidrián Tomillo et al., 2015; Patrício et al., 2019; Patrício et al., 2021). The difference in the thermal profile of the sediment between the control and each treatment may be due to the specific heat (i.e., the amount of heat required to change the temperature of a unit mass by one degree) of polyethylene. Polyethylene has a higher specific heat than that of silicate sand, causing heat in the sediment to be gained or lost more slowly (Wen, 2007; Andrady, 2011). This thermal inertia would prevent high-microplastic trials from losing heat at night to the atmosphere or ground as rapidly as low-concentration or control trials, resulting in a relative net increase in mean temperature.
In contrast, the color of the microplastic had an ambiguous effect on sand temperature. As the microplastics in this experiment were well mixed within the sediment, there were only minor changes in overall color – and, therefore, potential absorption of solar visible and infrared radiation – at the sediment surface, limiting the potential for additional surface-generated heat (Shaw and Day, 1994; Andrady, 2011). Microplastics are commonly concentrated in the top few centimeters of the sediment (Carson et al., 2011; Yu et al., 2016; Duncan et al., 2018). Changes in sediment surface temperature may thus be greater under such distributions compared to a uniform distribution as used in this study, which could affect several species including crustaceans, mollusks, polychaetes, and shorebirds (Dexter, 1992; Booth and Astill, 2001; Carson et al., 2011; Verlis et al., 2018). With respect to sea turtle clutches and other subterranean fauna, if the microplastics were concentrated on the surface, additional heat generated as a result of the darker color could have the potential to conduct into deeper sediment layers throughout the day, although significant temperature changes due to the presence of surface macroplastics were not recorded at depth lower than 30cm in a study conducted by Lavers et al. (2021).
Only one other study (Carson et al., 2011) explored how microplastic affects sediment temperature, finding that the presence of microplastics may cause the sediments to warm more slowly and reach lower maximum temperatures. This is likely a result from increased permeability and reduced bulk density as plastic fragments increased the mean grain size of sediment samples (Carson et al., 2011; de Souza Machado et al., 2018). A potential reason for the difference between Carson et al. (2011) lab-based study and this study is the time period over which the temperature was recorded and the construction of the experimental treatments. The experiments performed by Carson et al. (2011) utilized 5x20cm artificial cores with microplastics concentrated in the top 5cm and subjected to heat lamps for four hours. This study was conducted outside over consecutive days using large volumes of silicate sand well-mixed with microplastics throughout, so that any differences in temperature during each part of the day and under different weather conditions could be recorded. The large volume of each trial used in this experiment increased the volumetric heat capacity and thermal inertia of each replicate which could support greater mean temperatures at increasing microplastic concentrations. Diurnal patterns in thermal sources and sinks paired with large-volume observations support this study as a closer model for in situ effects of microplastic concentration on temperature profiles.
However, caution is advised considering the concentrations of microplastics necessary to induce the temperature changes observed in this study (Burns and Boxall, 2018; Bucci et al., 2020; Weis and Palmquist, 2021). The maximum concentration of microplastics here (30% v/v) equates to over 9,788,000 pc m-3/53,000 pc m-2. No studies have reported concentrations this high. Extreme values currently reported in the literature include 42,560 pc m-3/851 pc m-2 in Taiwan (Kunz et al., 2016), 131,939 pc m-3/2,639 pc m-2 in Cyprus (Duncan et al., 2018), 782,000 pc m-3/3,128 pc m-2 at Qilianyu, China (Zhang et al., 2022), and 1,750,500 pc m-3/17,505 pc m-2 in Guangdong, China (Fok et al., 2017). Microplastics at nesting sites have only recently been explored and could be higher in locations that haven’t been studied yet. As human populations increase, so will the demand for plastic and potentially the abundance of plastics in the marine environment (Browne et al., 2011; Cózar et al., 2014). With a possible increase of microplastics at marine turtle nesting grounds, the temperature change due to the addition of microplastics at extreme concentrations could be problematic for the marine turtle reproductive cycle.
A successful loggerhead marine turtle egg incubation temperature ranges between ~24 to 35°C with warmer temperatures producing more females and cooler temperatures producing more males (Mrosovsky and Yntema, 1980; Matsuzawa et al., 2002; Howard et al., 2014). Above 29°C, mainly females are produced while incubation below this temperature produces mainly males (Yntema and Mrosovsky, 1982). Linear modeling suggested that as the amount of microplastics increased by 1% v/v, sand temperature increased by 0.017°C, meaning that populations currently approaching critical thermal thresholds (e.g., pivotal temperature, upper physiological thermal limit) could be particularly sensitive to changes in microplastic concentration. An increase in temperature by 0.58°C as observed in the black 30% v/v treatment could significantly affect the recovery of this endangered species, particularly in combination with climate change and increases in atmospheric temperatures (Fuentes et al., 2011; Santidrián Tomillo et al., 2015; Patrício et al., 2019; Patrício et al., 2021).
Further, the methods presented here did not take into consideration the change in temperature caused by incubating turtle eggs themselves. During the incubation period, the temperature of the clutch increases due to metabolic activity (Kaska et al., 1998; Zbinden et al., 2006). This change can cause a temperature increase in the nest that is higher than the normal beach sediment (Kaska et al., 1998; García-Grajales et al., 2019). The increase in temperature due to the concentration of microplastics and clutch temperature could potentially cause the nest to reach a temperature higher than observed during this experiment.
Additional studies should be conducted to understand both the mechanisms (e.g., heat capacity, conductivity, diffusivity, density, and infrared absorption of the resulting mixture) and consequences of microplastic-linked changes in sediment characteristics (Burns and Boxall, 2018; Bucci et al., 2020; Weis and Palmquist, 2021). Such studies should record the sediment temperature over a longer period than what was conducted here (25 days), such as a full nesting season. To record the temperature of the sand, this study used iButtons which have an accuracy of ±0.5°C and could have contributed to slightly different temperature readings than that of the environment. Future studies should use a more sensitive temperature logger to obtain a more precise result as this study indicates that sand temperature changes may be minor at more realistic concentrations of microplastic contamination.
Despite these limitations, this study provides baseline information on the effects of microplastics on the thermal profile of sand and the implications it could have to a marine turtle nesting site. Additional research should be conducted to examine how different types of polymers and sediment can influence the thermal profile of sand, as well as how the potential exposure to toxicants could affect the nesting environment. This experiment forms the basis for future studies and brings awareness to the effects that microplastics have on coastal environments and endangered species.
Data availability statement
The datasets generated and analyzed for this study can be found in the FuentesLab github repository https://github.com/FuentesLab/Effects-of-Microplastic-on-Thermal-Profile-of-Sand.
Author contributions
MF, VB contributed to the conception and design of the study, obtained the resources to conduct the study, and wrote the first draft of the article. VB collected the data. VB and MW performed the analysis. All authors contributed to the article and approved the submitted version.
Funding
This work was supported by the Garnet and Gold Scholar Society at Florida State University.
Acknowledgments
We wish to thank all the individuals that helped make this project happen including William Beckwith, Travis Mohrman, and Katrina Bayliss. Additionally, we would like to thank the staff at the Florida State University Coastal and Marine Laboratory, and the Florida State University Marine Turtle Research, Ecology, and Conservation Group.
Conflict of interest
The authors declare that the research was conducted in the absence of any commercial or financial relationships that could be construed as a potential conflict of interest.
Publisher’s note
All claims expressed in this article are solely those of the authors and do not necessarily represent those of their affiliated organizations, or those of the publisher, the editors and the reviewers. Any product that may be evaluated in this article, or claim that may be made by its manufacturer, is not guaranteed or endorsed by the publisher.
Supplementary material
The Supplementary Material for this article can be found online at: https://www.frontiersin.org/articles/10.3389/fmars.2023.1146556/full#supplementary-material
References
Aguilera M., Medina-Suárez M., Pinós J., Liria-Loza A., Benejam L. (2018). Marine debris as a barrier: assessing the impacts on sea turtle hatchlings on their way to the ocean. Mar. Pollut. Bull. 137, 481–487. doi: 10.1016/j.marpolbul.2018.10.054
Andrades R., Santos R. G., Joyeux J. C., Chelazzi D., Cincinelli A., Giarrizzo T. (2018). Marine debris in trindade island, a remote island of the south Atlantic. Mar. Pollut. Bull. 137, 180–184. doi: 10.1016/j.marpolbul.2018.10.003
Andrady A. L. (2011). Microplastics in the marine environment. Mar. Pollut. Bull. 62, 1596–1605. doi: 10.1016/j.marpolbul.2011.05.030
Arthur C., Baker J., Bamford H. (2009). Proceedings of the international research workshop on the occurrence, effects and fate of microplastic marine debris (Tacoma WA, United States of America: NOAA Technical Memorandum NOS-OR&R-30), 530. doi: 10.1186/1744-9081-10-32
Auta H. S., Emenike C. U., Fauziah S. H. (2017). Distribution and importance of microplastics in the marine environment: a review of the sources, fate, effects, and potential solutions. Environ. Int. 102, 165–176. doi: 10.1016/j.envint.2017.02.013
Barnes D. K. A. (2002). Invasions by marine life on plastic debris. Nature 416, 808–809. doi: 10.1038/416808a
Beckwith V. K., Fuentes M. M. P. B. (2018). Microplastic at nesting grounds used by the northern gulf of Mexico loggerhead recovery unit. Mar. Pollut. Bull. 131A, 32–37. doi: 10.1016/j.marpolbul.2018.04.001
Booth D. T., Astill K. (2001). Temperature variation within and between nests of the green sea turtle, chelonia mydas (Chelonia: cheloniidae) on heron island, great barrier reef. Aust. J. Zool. 49, 71–84. doi: 10.1071/ZO00059
Browne M. A., Crump P., Niven S. J., Teuten E., Tonkin A., Galloway T. S., et al. (2011). Accumulation of microplastic on shorelines worldwide: sources and sinks. Environ. Sci. Technol. 45, 9175–9179. doi: 10.1021/es201811s
Bucci K., Tulio M., Rochman C. M. (2020). What is known and unknown about the effects of plastic pollution: a meta-analysis and systematic review. Ecol. Appl. 30, e02044. doi: 10.1002/eap.2044
Burnham K. P., Anderson D. R. (2002). Model selection and multimodel inference: a practical information-theoretic approach. 2nd ed (New York: NY: Springer-Verlag). doi: 10.1007/b97636
Burns E. E., Boxall A. B. A. (2018). Microplastics in the aquatic environment: evidence for or against adverse impacts and major knowledge gaps. Environ. Toxicol. Chem. 37, 2776–2796. doi: 10.1002/etc.4268
Buxton R. T., Currey C. A., Lyver P. O. B., Jones C. J. (2013). Incidence of plastic fragments among burrow-nesting seabird colonies on offshore islands in northern new Zealand. Mar. Pollut. Bull. 74, 420–424. doi: 10.1016/j.marpolbul.2013.07.011
Carson H. S., Colbert S. L., Kaylor M. J., McDermid K. J. (2011). Small plastic debris changes water movement and heat transfer through beach sediments. Mar. Pollut. Bull. 62, 1708–1713. doi: 10.1016/j.marpolbul.2011.05.032
Cartraud A. E., le Corre M., Turquet J., Tourmetz J. (2019). Plastic ingestion in seabirds of the western Indian ocean. Mar. Pollut. Bull. 140, 308–314. doi: 10.1016/j.marpolbul.2019.01.065
Ceccarini A., Corti A., Erba F., Modugno F., la Nasa J., Bianchi S., et al. (2018). The hidden microplastics: new insights and figures from the thorough separation and characterization of microplastics and of their degradation byproducts in coastal sediments. Environ. Sci. Technol. 52, 5634–5643. doi: 10.1021/acs.est.8b01487
Chen Y., Awasthi A. K., Wei F., Tan Q., Li J. (2021). Single-use plastics: production, usage, disposal, and adverse impacts. Sci. Total Environ. 752, 141772. doi: 10.1016/J.SCITOTENV.2020.141772
Cole M., Lindeque P., Fileman E., Halsband C., Goodhead R., Moger J., et al. (2013). Microplastic ingestion by zooplankton. Environ. Sci. Technol. 47, 6646–6655. doi: 10.1021/es400663f
Cózar A., Echevarría F., González-Gordillo J. I., Irigoien X., Úbeda B., Hernandez-Leon S., et al. (2014). Plastic debris in the open ocean. Proc. Natl. Acad. Sci. 111, 10239–10244. doi: 10.1073/pnas.1314705111
Davenport J. (1997). Temperature and the life-history strategies of sea turtles. J. Therm. Biol. 22, 479–488. doi: 10.1016/S0306-4565(97)00066-1
Derraik J. G. B. (2002). The pollution of the marine environment by plastic debris: a review. Mar. Pollut. Bull. 44, 842–852. doi: 10.1016/S0025-326X(02)00220-5
de Souza Machado A. A., Lau C. W., Till J., Kloas W., Lehmann A., Becker R., et al. (2018). Impacts of microplastics on the soil biophysical environment. Environ. Sci. Technol. 52, 9656–9665. doi: 10.1021/ACS.EST.8B02212/ASSET/IMAGES/LARGE/ES-2018-02212S_0007.JPEG
Dexter D. M. (1992). Sandy beach community structure: the role of exposure and latitude. J. Biogeogr. 19, 59–66. doi: 10.2307/2845620
Duncan E. M., Arrowsmith J. A., Bain C. E., Broderick A. C., Lee J. H., Metcalfe K., et al. (2018). The true depth of the Mediterranean plastic problem: extreme microplastic pollution on marine turtle nesting beaches in Cyprus. Mar. Pollut. Bull. 136, 334–340. doi: 10.1016/J.MARPOLBUL.2018.09.019
Fendall L. S., Sewell M. A. (2009). Contributing to marine pollution by washing your face: microplastics in facial cleansers. Mar. Pollut. Bull. 58, 1225–1228. doi: 10.1016/j.marpolbul.2009.04.025
Fok L., Cheung P. K., Tang G., Li W. C. (2017). Size distribution of stranded small plastic debris on the coast of guangdong, south China. Environ. Pollut. 220, 407–412. doi: 10.1016/j.envpol.2016.09.079
Frias J. P. G. L., Nash R. (2019). Microplastics: finding a consensus on the definition. Mar. Pollut. Bull. 138, 145–147. doi: 10.1016/j.marpolbul.2018.11.022
Fries E., Dekiff J. H., Willmeyer J., Nuelle M. T., Ebert M., Remy D. (2013). Identification of polymer types and additives in marine microplastic particles using pyrolysis-GC/MS and scanning electron microscopy. Environ. Sci.: Process. Impacts 15, 1949–1956. doi: 10.1039/c3em00214d
Fuentes M. M. P. B., Gredzens C., Bateman B. L., Boettcher R., Ceriani S. A., Godfrey M. H., et al. (2016). Conservation hotspots for marine turtle nesting in the united states based on coastal development. Ecol. Appl. 26, 2706–2717. doi: 10.1002/eap.1386
Fuentes M. M. P. B., Limpus C. J., Hamann M. (2011). Vulnerability of sea turtle nesting grounds to climate change. Glob. Chang. Biol. 17, 140–153. doi: 10.1111/j.1365-2486.2010.02192.x
Gall S. C., Thompson R. C. (2015). The impact of debris on marine life. Mar. Pollut. Bull. 92, 170–179. doi: 10.1016/j.marpolbul.2014.12.041
García-Grajales J., Meraz Hernando J. F., Arcos García J. L., Ramírez Fuentes E. (2019). Incubation temperatures, sex ratio and hatching success of leatherback turtles (Dermochelys coriacea) in two protected hatcheries on the central Mexican coast of the Eastern tropical pacific ocean. Anim. Biodivers. Conserv. 42.1, 143–152. doi: 10.32800/abc.2019.42.0143
Garrison S. R., Fuentes M. M. P. B. (2019). Marine debris at nesting grounds used by the northern gulf of Mexico loggerhead recovery unit. Mar. Pollut. Bull. 139, 59–64. doi: 10.1016/j.marpolbul.2018.12.019
Georges A., Limpus C. J., Stoutjesdijk R. (1994). Hatchling sex in the marine turtle caretta caretta is determined by proportion of development at a temperature, not daily duration of exposure. J. Exp. Zool. 270, 432–444. doi: 10.1002/jez.1402700504
Graham E. R., Thompson J. T. (2009). Deposit- and suspension-feeding sea cucumbers (Echinodermata) ingest plastic fragments. J. Exp. Mar. Biol. Ecol. 368, 22–29. doi: 10.1016/j.jembe.2008.09.007
Gregory M. R. (2009). Environmental implications of plastic debris in marine settings - entanglement, ingestion, smothering, hangers-on, hitch-hiking and alien invasions. Philos. Trans. R. Soc. B: Biol. Sci. 364, 2013–2025. doi: 10.1098/rstb.2008.0265
Gregory M. R., Andrady A. L. (2003). “Plastics in the marine environment,” in Plastics and the environment. Ed. Andrady A. L., 379–401. doi: 10.1002/0471721557.ch10
Gündoğdu S., Nur İ., Erbaş C. (2019). Potential interaction between plastic litter and green turtle chelonia mydas during nesting in an extremely polluted beach. Mar. Pollut. Bull. 140, 138–145. doi: 10.1016/j.marpolbul.2019.01.032
Hartwig E., Clemens T., Heckroth M. (2007). Plastic debris as nesting material in a kittiwake-(Rissa tridactyla)-colony at the jammerbugt, Northwest Denmark. Mar. Pollut. Bull. 54, 595–597. doi: 10.1016/j.marpolbul.2007.01.027
Hidalgo-Ruz V., Gutow L., Thompson R. C., Thiel M. (2012). Microplastics in the marine environment: a review of the methods used for identification and quantification. Environ. Sci. Technol. 46, 3060–3075. doi: 10.1021/es2031505
Howard R., Bell I. P., Pike D. A. (2014). Thermal tolerances of sea turtle embryos: current understanding and future directions. Endanger. Species Res. 26, 75–86. doi: 10.3354/esr00636
Imhof H. K., Ivleva N. P., Schmid J., Niessner R., Laforsch C. (2013). Contamination of beach sediments of a subalpine lake with microplastic particles. Curr. Biol. 23, 867–868. doi: 10.1016/j.cub.2013.09.001
Jones J. S., Guézou A., Medor S., Nickson C., Savage G., Alarcón-Ruales D., et al. (2022). Microplastic distribution and composition on two galápagos island beaches, Ecuador: verifying the use of citizen science derived data in long-term monitoring. Environ. Pollut. 311, 120011. doi: 10.1016/J.ENVPOL.2022.120011
Kanu I., Anyanwu E. (2005). Impact of hydrophobic pollutants’ behavior on occupational and environmental health. Sci. World 5, 211–220. doi: 10.1100/tsw.2005.28
Kaska Y., Downie R., Tippett R., Furness R. W. (1998). Natural temperature regimes for loggerhead and green turtle nests in the eastern Mediterranean. Can. J. Zool. 76, 723–729. doi: 10.1139/z97-245
Kunz A., Walther B. A., Löwemark L., Lee Y. C. (2016). Distribution and quantity of microplastic on sandy beaches along the northern coast of Taiwan. Mar. Pollut. Bull. 111, 126–135. doi: 10.1016/j.marpolbul.2016.07.022
Lahens L., Strady E., Kieu-Le T. C., Dris R., Boukerma K., Rinnert E., et al. (2018). Macroplastic and microplastic contamination assessment of a tropical river (Saigon river, Vietnam) transversed by a developing megacity. Environ. Pollut. 236, 661–671. doi: 10.1016/j.envpol.2018.02.005
Lavers J. L., Bond A. L. (2017). Exceptional and rapid accumulation of anthropogenic debris on one of the world’s most remote and pristine islands. Proc. Natl. Acad. Sci. 114, 201619818. doi: 10.1073/pnas.1619818114
Lavers J. L., Rivers-Auty J., Bond A. L. (2021). Plastic debris increases circadian temperature extremes in beach sediments. J. Hazard. Mater. 416, 126140. doi: 10.1016/J.JHAZMAT.2021.126140
Law K. L., Moret-Ferguson S., Maximenko N. A., Proskurowski G., Peacock E. E., Hafner J., et al. (2010). Plastic accumulation in the north Atlantic subtropical gyre. Science (1979) 329, 1185–1188. doi: 10.1126/science.1191224
Lebreton L. C. M., Greer S. D., Borrero J. C. (2012). Numerical modelling of floating debris in the world’s oceans. Mar. Pollut. Bull. 64, 653–661. doi: 10.1016/j.marpolbul.2011.10.027
Matsuzawa Y., Sato K., Sakamoto W., Bjorndal K. A. (2002). Seasonal fluctuations in sand temperature: effects on the incubation period and mortality of loggerhead sea turtle (Caretta caretta) pre-emergent hatchlings in minabe, Japan. Mar. Biol. 140, 639–646. doi: 10.1007/s00227-001-0724-2
Meager J. J., Schlacher T. A., Nielsen T. (2012). Humans alter habitat selection of birds on ocean-exposed sandy beaches. Divers. Distrib. 18, 294–306. doi: 10.1111/j.1472-4642.2011.00873.x
Mendenhall E. (2018). Oceans of plastic: a research agenda to propel policy development. Mar. Policy 96, 291–298. doi: 10.1016/j.marpol.2018.05.005
Montero N., Ceriani S. A., Graham K., Fuentes M. M. P. B. (2018). Influences of the local climate on loggerhead hatchling production in north Florida: implications from climate change. Front. Mar. Sci. 5. doi: 10.3389/fmars.2018.00262
Mrosovsky N., Yntema C. L. (1980). Temperature dependence of sexual differentiation in sea turtles: implications for conservation practices. Biol. Conserv. 18, 271–280. doi: 10.1016/0006-3207(80)90003-8
Nelms S. E., Duncan E. M., Broderick A. C., Galloway T. S., Godfrey M. H., Hamann M., et al. (2016). Plastic and marine turtles: a review and call for research. ICES J. Mar. Sci. 73, 165–181. doi: 10.1093/icesjms/fsv165
Ng K. L., Obbard J. P. (2006). Prevalence of microplastics in singapore’s coastal marine environment. Mar. Pollut. Bull. 52, 761–767. doi: 10.1016/j.marpolbul.2005.11.017
Patrício A. R., Hawkes L. A., Monsinjon J., Godley B. J., Fuentes M. M. P. B. (2021). Climate change and marine turtles: recent advances and future directions. Endanger. Species Res. 44, 363–395. doi: 10.3354/esr01110
Patrício A. R., Varela M. R., Barbosa C., Broderick A. C., Catry P., Hawkes L. A., et al. (2019). Climate change resilience of a globally important sea turtle nesting population. Glob. Chang. Biol. 25, 522–535. doi: 10.1111/gcb.14520
Rios L. M., Moore C., Jones P. R. (2007). Persistent organic pollutants carried by synthetic polymers in the ocean environment. Mar. Pollut. Bull. 54, 1230–1237. doi: 10.1016/j.marpolbul.2007.03.022
Rios-Fuster B., Alomar C., Paniagua González G., Garcinuño Martínez R. M., Soliz Rojas D. L., Fernández Hernando P., et al. (2022). Assessing microplastic ingestion and occurrence of bisphenols and phthalates in bivalves, fish and holothurians from a Mediterranean marine protected area. Environ. Res. 214, 114034. doi: 10.1016/J.ENVRES.2022.114034
Royer S. J., Ferrón S., Wilson S. T., Karl D. M. (2018). Production of methane and ethylene from plastic in the environment. PloS One 13, e0200574. doi: 10.1371/journal.pone.0200574
Santidrián Tomillo P., Genovart M., Paladino F. V., Spotila J. R., Oro D. (2015). Climate change overruns resilience conferred by temperature-dependent sex determination in sea turtles and threatens their survival. Glob. Chang. Biol. 21, 2980–2988. doi: 10.1111/gcb.12918
Shaw D. G., Day R. H. (1994). Colour- and form-dependent loss of plastic micro-debris from the north pacific ocean. Mar. Pollut. Bull. 28, 39–43. doi: 10.1016/0025-326X(94)90184-8
Shim W. J., Thomposon R. C. (2015). Microplastics in the ocean. Arch. Environ. Contam. Toxicol. 69, 265–268. doi: 10.1007/s00244-015-0216-x
Šilc U., Küzmič F., Caković D., Stešević D. (2018). Beach litter along various sand dune habitats in the southern Adriatic (E Mediterranean). Mar. Pollut. Bull. 128, 353–360. doi: 10.1016/j.marpolbul.2018.01.045
Silver-Gorges I., Ceriani S. A., Ware M., Lamb M., Lamont M. M., Becker J., et al. (2021). Using systems thinking to inform management of imperiled species: a case study with sea turtles. Biol. Conserv. 260, 109201. doi: 10.1016/j.biocon.2021109201
Sun X., Li Q., Zhu M., Liang J., Zheng S., Zhao Y. (2017). Ingestion of microplastics by natural zooplankton groups in the northern south China Sea. Mar. Pollut. Bull. 115, 217–224. doi: 10.1016/j.marpolbul.2016.12.004
Thushari G. G. N., Senevirathna J. D. M. (2020). Plastic pollution in the marine environment. Heliyon 6, e04709. doi: 10.1016/J.HELIYON.2020.E04709
Tiwari M., Rathod T. D., Ajmal P. Y., Bhangare R. C., Sahu S. K. (2019). Distribution and characterization of microplastics in beach sand from three different Indian coastal environments. Mar. Pollut. Bull. 140, 262–273. doi: 10.1016/j.marpolbul.2019.01.055
Verlis K. M., Campbell M. L., Wilson S. P. (2018). Seabirds and plastics don’t mix: examining the differences in marine plastic ingestion in wedge-tailed shearwater chicks at near-shore and offshore locations. Mar. Pollut. Bull. 135, 852–861. doi: 10.1016/j.marpolbul.2018.08.016
Ware M., Ceriani S. A., Long J. W., Fuentes M. M. P. B. (2021). Exposure of loggerhead sea turtle nests to waves in the Florida panhandle. Remote Sens. (Basel) 13, 2654. doi: 10.3390/rs13142654
Weis J. S., Palmquist K. H. (2021). Reality check: experimental studies on microplastics lack realism. Appl. Sci. 11, 8529. doi: 10.3390/app11188529
Wen J. (2007). “Heat capacities of polymers,” in Physical properties of polymers handbook (New York: NY: Springer New York), 145–154. doi: 10.1007/978-0-387-69002-5_9
Wessel C. C., Lockridge G. R., Battiste D., Cebrian J. (2016). Abundance and characteristics of microplastics in beach sediments: insights into microplastic accumulation in northern gulf of Mexico estuaries. Mar. Pollut. Bull. 109, 178–183. doi: 10.1016/j.marpolbul.2016.06.002
Wilcox C., Puckridge M., Schuyler Q. A., Townsend K. A., Hardesty B. D. (2018). A quantitative analysis linking sea turtle mortality and plastic debris ingestion. Sci. Rep. 8, 1–11. doi: 10.1038/s41598-018-30038-z
Williams S. T. (2017). Trophic transfer of microplastics in the marine food web. Master’s Degree (Non-Thesis) in environmental toxicology. Texas Southern University.
Yaghmour F., Al Marashda A. S. (2020). Frequency and composition of anthropogenic debris in the nests of sooty gulls Larus (Adelarus) hemprichii Bruch 1853 from Sir Bu na'ir Island, United Arab Emirates. Mar. Pollut. Bull. 150, 110715. doi: 10.1016/j.envpol.2016.04.080
Yntema C. L., Mrosovsky N. (1982). Critical periods and pivotal temperatures for sexual differentiation in loggerhead sea turtles. Can. J. Zool. 60, 1012–1016. doi: 10.1139/z82-141
Yu X., Peng J., Wang J., Wang K., Bao S. (2016). Occurrence of microplastics in the beach sand of the Chinese inner sea: the Bohai Sea. Environ. Pollut. 214, 722–730.
Zbinden J. A., Margaritoulis D., Arlettaz R. (2006). Metabolic heating in Mediterranean loggerhead sea turtle clutches. J. Exp. Mar. Biol. Ecol. 334, 151–157. doi: 10.1016/j.jembe.2006.01.021
Zhang T., Lin L., Li D., Wang J., Liu Y., Li R., et al. (2022). Microplastic pollution at qilianyu, the largest green sea turtle nesting grounds in the northern south China Sea. PeerJ 10, e13536. doi: 10.7717/peerj.13536
Keywords: sea turtle, pollution, plastic, temperature, marine debris, nesting ground
Citation: Fuentes MMPB, Beckwidth V and Ware M (2023) The effects of microplastic on the thermal profile of sand: implications for marine turtle nesting grounds. Front. Mar. Sci. 10:1146556. doi: 10.3389/fmars.2023.1146556
Received: 17 January 2023; Accepted: 19 May 2023;
Published: 29 May 2023.
Edited by:
Andrea D. Phillott, Flame University, IndiaReviewed by:
Giuseppe Suaria, National Research Council, ItalyFadi Yaghmour, Environment and Protected Areas Authority, United Arab Emirates
Copyright © 2023 Fuentes, Beckwidth and Ware. This is an open-access article distributed under the terms of the Creative Commons Attribution License (CC BY). The use, distribution or reproduction in other forums is permitted, provided the original author(s) and the copyright owner(s) are credited and that the original publication in this journal is cited, in accordance with accepted academic practice. No use, distribution or reproduction is permitted which does not comply with these terms.
*Correspondence: Mariana M. P. B. Fuentes, bWZ1ZW50ZXNAZnN1LmVkdQ==