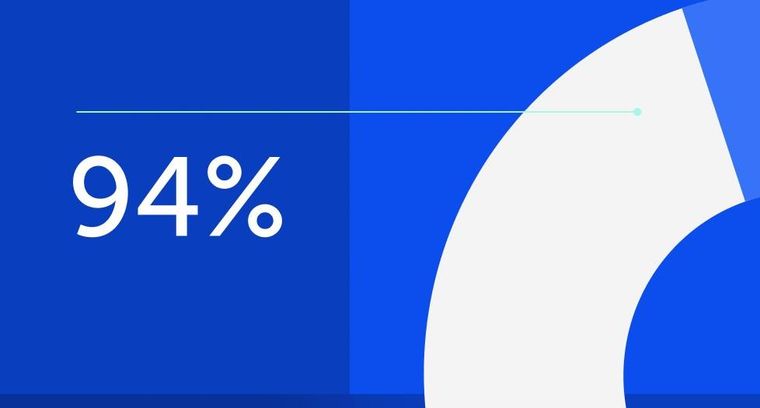
94% of researchers rate our articles as excellent or good
Learn more about the work of our research integrity team to safeguard the quality of each article we publish.
Find out more
ORIGINAL RESEARCH article
Front. Mar. Sci., 28 April 2023
Sec. Marine Ecosystem Ecology
Volume 10 - 2023 | https://doi.org/10.3389/fmars.2023.1146148
This article is part of the Research TopicExtreme Weather Events Induced Coastal Environment Changes under Multiple Anthropogenic ImpactsView all 16 articles
The frequency and duration of marine heat waves (MHWs) have recently increased. There is therefore an urgent need to understand the response of marine organisms to MHWs. However, most estimates of MHW impacts on abundances include the effects of environmental stochasticity other than the MHWs. In addition, although MHWs sometimes persist for year-round or occur repeatedly for shorter periods, the accumulative carryover effects (ACEs)— the effects of sequential events accumulating additively over time— of MHWs on organisms have never been evaluated. Furthermore, the relationship between species niche traits other than thermal niches and susceptibility to MHWs is unknown. We examined the impacts of MHWs in southeastern Hokkaido, northern Japan, in summer from 2010 to 2016 on rocky intertidal communities by distinguishing MHW effects from environmental stochasticity. We asked (1) Did MHWs have ACEs on four major functional groups: macroalgae, sessile invertebrates, herbivorous invertebrates, and carnivorous invertebrates? (2) Does ignoring ACEs lead to biased assessments? (3) How did the effects of the MHWs on functional groups and their subsequent recovery differ? And (4) How does the susceptibility to MHWs differ with species niche (thermal and vertical) traits? We detected ACEs of MHWs and found that if they are ignored, the effects of MHWs can be underestimated. Although MHWs are known to reduce the abundance of macroalgae and increase that of sessile invertebrates in rocky intertidal habitats, our results show that macroalgal abundance increased during and after MHWs, whereas sessile invertebrates showed no change during or after MHWs. The abundance of herbivorous mollusks decreased during and after MHWs. Carnivorous invertebrates declined during MHWs and in the first year after MHWs. During and after MHWs, abundances of species with low thermal niches decreased and those with high thermal niches increased. There were no differences in response to MHWs between vertical niches when accumulative carryover effects were ignored. These results emphasize the importance of considering ACEs when assessing the response of marine organisms to MHWs, and that more studies of these responses are needed for a variety of ecosystems, regions and organisms to predict the responses of marine organisms.
Marine heatwaves (MHWs) are anomalous, sea-surface-temperature warm events and are defined as when an upper determined threshold (the 90th percentile of temperature relative to the long-term climatology) is exceeded for five or more days (Hobday et al., 2016), in which multiples of the 90th percentile difference (2× twice, 3× three times, etc.) from the mean climatology value define each of the category I (Moderate), II (Strong), III (Severe), and IV (Extreme) MHW, respectively (Hobday et al., 2018). MHWs are known to sometimes persist for long periods (year-round) (Hobday et al., 2018) or to recur annually for short periods (e.g., every summer) (Miyama et al., 2021a; Miyama et al., 2021b). From 1925 to 2016, MHW frequency and duration increased by 34% and 17%, respectively, with a 54% increase globally in annual marine heatwave days (Oliver et al., 2018). Also, the number of MHW days is projected to further increase as a result of global warming (Frölicher et al., 2018). Therefore, there is an urgent need to understand the response of marine organisms to MHWs.
In elucidating the response of marine ecosystems to MHWs, it is important to focus on revealing the impacts of MHWs on functional groups and species niche traits for various habitats, organisms, and regions. This is because understanding the differences in responses to MHWs by functional group will lead to a better understanding of the effects of MHWs at the community and ecosystem level. In addition, the relationship between species niche traits such as thermal tolerance and species’ responses to MHWs is important because it will lead to an understanding of the physiological and ecological processes behind differences between species in MHW effects.
The effects of MHWs on marine ecosystems have been reported for various parts of the world such as the Mediterranean, Northeast Pacific, and western Australia (Hobday et al., 2018; Smale et al., 2019). These studies have shown that biological responses to MHWs are diverse (negative, positive, or neutral) across multiple trophic levels (i.e., lower to upper trophic levels) and taxonomic groups (e.g., plankton, algae, invertebrates, fishes, birds, and mammals) (Ruthrof et al., 2018; Sanford et al., 2019; Smale et al., 2019; Suryan et al., 2021). The effects of MHWs vary among species: the abundances of cold-affinity species decreased after one MHW (Smale et al., 2017) and those of warmer-water species increased (Smale et al., 2017; Sanford et al., 2019; Miner et al., 2021) for herbivorous invertebrates (sea urchins and gastropods), barnacles and Rhodophyta. Many studies have reported differences in the response of different functional groups to MHWs (Smale and Wernberg, 2013; Wernberg et al., 2013; Smale et al., 2019; Suryan et al., 2021; Weitzman et al., 2021), and there are also reported cases of indirect spillover of MHW effects to other functional groups (Smale and Wernberg, 2013; Wernberg et al., 2013; Smale et al., 2017). For example, the decline in abundance of herbivorous macroinvertebrates (sea urchins and gastropods) after the 2011 MHW in western Australia is possibly because, in addition to direct thermal stress, invertebrate populations were also likely affected by the indirect effects of loss of habitat and food resources (Smale et al., 2017). Responses to an MHW can vary regionally. For example, in the warm regions along the west coast of Australia, the community structures of algae (Smale and Wernberg, 2013; Wernberg et al., 2013), invertebrates (Wernberg et al., 2013) and fishes (Wernberg et al., 2013), and the abundances of herbivorous macroinvertebrates (Smale et al., 2017) were significantly different after the 2011 MHW, whereas no effects were detected in the cooler regions.
Despite the many studies that have been conducted in recent years, about how MHWs have impacted marine organisms and these studies are lacking from several perspectives. First, most previous studies have evaluated the impact of heat waves using comparisons of abundances from several surveys before and after an MHW (Smale and Wernberg, 2013; Wernberg et al., 2013; Smale et al., 2017; Sanford et al., 2019; Thomsen et al., 2019; Weitzman et al., 2021). Therefore, the estimated impacts of MHWs on abundances include not only the direct effects of MHWs, but also the effects of environmental stochasticity—that is, unpredictable spatiotemporal fluctuation in environmental conditions (Fujiwara and Takada, 2017)— other than the MHWs. Second, accumulative carryover effects—that is, those occurring when the effects of sequential events accumulate over time (Ryo et al., 2019)— of MHWs on organisms are largely unknown. Accumulative carryover effects can occur when the effects of successive events accumulate additively over time, because short intervals between events inhibit the system from recovering to its original state (Ryo et al., 2019). The concept of the accumulative carryover effect is important because it focuses on the cumulative effect of frequent small perturbations that can significantly affect the state of the system, even though the impact of each individual event is small or negligible. An urgent need is to understand the accumulative carryover effects of MHWs on marine ecosystems, because weak MHWs (such as category I and II) occur more frequently than strong ones (Hobday et al., 2018; Miyama et al., 2021a; Miyama et al., 2021b). Third, there has been little investigation of the relationship between species niche traits and susceptibility to MHWs, other than for the thermal niche traits of individual species (Smale et al., 2017; Sanford et al., 2019; Miner et al., 2021; Harvey et al., 2022). Identifying the species niche traits susceptible to MHWs can be useful for understanding and predicting the ecological processes of marine organism responses to MHWs (Smale et al., 2017; Sanford et al., 2019; Miner et al., 2021; Harvey et al., 2022).
The rocky intertidal zone is a habitat where the intensity of desiccation stress varies widely over a narrow vertical range (Noda, 2009). The rocky intertidal benthic assemblages are composed of organisms of various trophic levels, they are classified as algae, sessile animals (most species are suspension feeders), grazers and carnivores (Menge, 1976; Menge et al., 1986; Okuda et al., 2010; Iwasaki et al., 2016). In the rocky intertidal zone, each species inhabits a specific elevation range depending on its desiccation tolerance, with the more desiccation-tolerant species generally being found in the upper intertidal zone. Rocky intertidal communities are some of the best study systems for assessing the response of marine benthic communities to MHWs. First, the component species of rocky intertidal communities have a variety of trophic levels. Second, the abundance of most species can accurately be quantified in a non-destructive way. Therefore, it is relatively easy to continuously obtain highly accurate time-series data for the abundance of community component species before and after MHWs.
Relatively few previous studies have described the effects of MHWs on rocky intertidal communities, with one case in the South Island of New Zealand in 2017–2018 (Thomsen et al., 2019) and another in the northeast Pacific during 2014–2016 (Sanford et al., 2019; Miner et al., 2021; Suryan et al., 2021; Weitzman et al., 2021; Spiecker and Menge, 2022). The impact of MHWs on rocky intertidal organisms was relatively well documented in the latter series of studies, which have revealed that the effects of MHWs vary by functional group. For example, the abundance of macroalgae (Fucus) and that of predatory invertebrates (sea stars) declined precipitously after the onset of the MHW; in contrast, the abundance of sessile invertebrates (mussels) increased (Suryan et al., 2021). In addition, algae cover (Fucus and other fleshy macroalgae) declined during and after the MHW, whereas that of sessile animals (barnacles and mussels) increased (Weitzman et al., 2021). The effects of MHWs may also differ among species depending on their thermal niche, with the geographic distributions of southern species shifting northwards with striking increases in recruitment (Sanford et al., 2019). In these studies, however, the effects of MHWs on abundance are estimated by methods that cannot isolate the effects of MHWs from overall environmental stochasticity, so the estimates are not highly accurate.
Differences in response to an MHW by functional group have also been reported in rocky intertidal communities (Suryan et al., 2021; Weitzman et al., 2021), but so far the differences in response using the abundance of an entire functional group have not been evaluated. Also not yet evaluated are the presence and extent of accumulative carryover effects of MHWs. Furthermore, the relationship between the vertical niches of sessile organisms as a species niche trait and susceptibility to MHWs has never been investigated. In rocky intertidal community, sessile species live in a narrow elevation range of the rock surface, where various environmental stresses change acutely across vertical gradient. Each species lives in a suitable position that is caused by physiological characteristics and outcome of species interaction, which is the vertical niche. Among rocky intertidal sessile organisms, species with higher vertical niches (i.e., species living in the upper intertidal zone) may be less susceptible to the effects of anomalous sea surface warming due to MHWs. First, the upper intertidal zone has a shorter immersion period than the lower intertidal zone. Second, thermal extremes and variation increase from low to high elevations in intertidal habitats (Benedetti-Cecchi et al., 2006; Watt and Scrosati, 2013). Therefore, species with higher vertical niches may have acclimated to higher temperature environments.
In the summer (July–September) of all years from 2010 to 2016, MHWs classified as category I or II occurred in the Oyashio water off southeast Hokkaido, northern Japan (Miyama et al., 2021a; Miyama et al., 2021b). The sea surface temperature in summer of all of these years was continuously higher by more than 1°C than its climatology, 17.5°C, as defined for the period between 1993 and 2009, and the average summer sea surface temperature between 2010 and 2016 was 18.9°C (Figure 1; Miyama et al., 2021a). The MHW events have been attributed to a weakening of the influence from cold Oyashio water resulting from an increase in anticyclonic eddies from the warm Kuroshio Extension (Miyama et al., 2021a; Miyama et al., 2021b). After the end of this series of MHWs, sea surface temperatures declined to normal in 2017 and 2018 (Figure 1; Miyama et al., 2021a; Miyama et al., 2021b). Subsequently, anticyclonic eddies returned in 2019 and 2020, and sea surface temperatures again increased (Miyama et al., 2021b). In this study, we examined the impacts of the MHWs that occurred in southeastern Hokkaido from 2010 to 2016 on community abundance (i.e., the sum of the abundances of all species in the community) of the four main functional groups of rocky intertidal communities and on the abundances of individual species during the MHWs from 2010 to 2016 and for two years after the end of the MHWs (when sea surface temperatures were at normal levels). Although nearshore temperature dynamics vary at smaller spatial scales (Krumhansl et al., 2020; Krumhansl et al., 2021), we estimated the effect size of the MHWs at the regional scale, covering an entire metapopulation of most of rocky intertidal organisms (along shorelines at scales of several to tens of kilometers). This is because population persistence are generally maintained by persistence of metapopulation, a group of local populations connected each other with dispersal, not by persistence of individual local population. Therefore, if the spatial extent of a stressor encompasses an entire metapopulation, its impacts at the metapopulation scale is crucial to understand the ecological consequence of the stressor.
Figure 1 Time series of the July–September mean sea surface temperature (°C) from 1982 to 2018 southeast of Hokkaido, Japan (143–147°E, 40–43°N). The blue line shows the 1993–2009 mean; the red line shows the 2010–2016 mean. (From Miyama et al., 2021a).
We included only two years after the MHWs (2017 and 2018) for evaluation; the marine conditions in the study area were far from normal in the three subsequent years because anticyclonic eddies again raised sea surface temperatures in 2019 and 2020 (Miyama et al., 2021b), and in 2021 there was an outbreak of harmful algae (Misaka and Ando, 2021; Iwataki et al., 2022). We specifically asked the following questions: (1) Did MHWs have accumulative carryover effects at the regional scale on the community abundance of the four functional groups and the abundance of individual species? (2) If accumulative carryover effects of MHW are found, we also examine how ignoring accumulative carryover effects leads to biased assessments. (3) How did the effects of the MHWs on these community abundances at the regional scale differ between the MHWs (2010–2016) and the subsequent recovery in the first two years afterward (2017 and 2018)? (4) How did the impacts of MHWs on the abundance of each species at the regional scale during the MHWs (2010–2016), and in the first (2017) and second (2018) years after the MHWs differ depending on species niches traits (thermal and vertical niches)? We assessed the effects of MHWs at the regional scale because the thermal environment is expected to have large spatial variation within the range encompassing the metapopulation scale of marine benthos (Helmuth et al., 2002; Helmuth et al., 2006).
The study area is located on the coast along the Northwest Pacific Ocean in southeast Hokkaido, Japan (Figure 2). The area is within the cold temperate provinces (Spalding et al., 2007) and is impacted by the cold Oyashio current (Payne et al., 2012; Ishida et al., 2021; Miyama et al., 2021a; Miyama et al., 2021b). In the study area, the mid-tidal zone is dominated by the native barnacle Chthamalus dalli and perennial seaweeds such as the crustose coralline alga Corallina pilulifera, the red algae Gloiopeltis furcata and Chondrus yendoi, and the brown alga Analipus japonicus (Kanamori et al., 2017; Ishida et al., 2021). Invertebrate predators include the whelk Nucella lima and the starfish Leptasterias ochotensis (Noda and Ohira, 2020). The invasive barnacle Balanus glandula invaded this area in 2004, but it did not become a dominant species, with an average cover of less than 5% (Noda and Ohira, 2020). There was a massive harmful algal bloom in this region during September–November 2021 (Misaka and Ando, 2021; Iwataki et al., 2022). The dominant species of the bloom was identified as Karenia selliformis (Gymnodiniales, Dinophyceae) (Iwataki et al., 2022). Misaka and Ando (2021) have reported on the mortality of marine organisms such as salmon, sea urchins, whelks, octopuses, and Sipuncula caused, by the bloom.
Figure 2 Location of five rocky shores chosen for a census of intertidal organisms along the coast in southeast Hokkaido, Japan, along the Northwest Pacific Ocean.
We used hierarchical nested sampling (Noda, 2004) for the layout of each site. Five rocky shores were chosen for the census of intertidal organisms along the coast in southeast Hokkaido, Japan, by the Northwest Pacific Ocean (Figure 2). Within each shore, we established four or five permanent census plots (the total number of plots was 21) on steep rock walls in semi-exposed locations. Each plot was 50 cm wide by 100 cm high, with the vertical midpoint corresponding to mean tidal level. Abundances of all sessile organisms (macroalgae and sessile invertebrates) were determined by placing 200 grid points at 5-cm intervals both vertically and horizontally on the rock surface within each census plot and identifying and recording the species occupying each grid point. The abundances of all mobile organisms (herbivorous and carnivorous invertebrates) were recorded as the number of individuals of each species that appeared within each census plot. The abundances of sessile and mobile organisms in each census plot just after summer (October or November) from 2004 to 2018 were used to calculate the total abundances summed over all plots at all shores for each species in each year.
To evaluate the impact of MHWs on community abundance and the abundance of each species at the regional scale, we calculated the total abundance over all plots for each species in each year. We used the data for the total abundance of all plots because the thermal environment is expected to have large spatial variation within the range encompassing the metapopulation scale of marine benthos (Helmuth et al., 2002; Helmuth et al., 2006). Because the differences in the number of plots at each shore was small (all shores had 4 or 5 plots), we ignored any differences in abundance between shores and used the same method to obtain the total abundance at the regional scale. We believe that this had little effect on the estimation of regional abundance.
When assessing the effect of MHWs on the abundance of individual species, we selected common species that occurred in more than seven records in surveys during the 15 years. The data for rare species that were recorded only a few times in the 15-year period could contain large observational errors, making it difficult to accurately estimate abundance and the traits of species’ niches. In the case of the invasive barnacle Balanus glandula, whose introduction to the study area occurred relatively recently, the population dynamics may not have reached equilibrium (Noda and Ohira, 2020), making it difficult to precisely assess the effects of MHWs on their population dynamics. They were therefore excluded from the analysis. On the basis of the above criteria, 25 rocky intertidal macro-benthic species were included in analysis of the effects of MHWs at the species level: 12 algae, 5 sessile invertebrates, 6 herbivorous mollusks, and 2 carnivorous invertebrates (Tables 1, 2).
To evaluate the effects of MHWs on each functional group and each species, we calculated the effect sizes (Iwasaki et al., 2016; Noda et al., 2017; Iwasaki and Noda, 2018) of the abundance of each functional group and each species from before the MHWs (2004–2009) to during (2010–2016) and after (2017 and 2018) using the following formulas:
where Fduring or after represents sum of the log10(abundance) of each functional group for each year during or after the MHWs. before and SD(Fbefore) are the mean and standard deviation, respectively, for the sum of log10(abundance) of each functional group for each year before the MHWs. Sduring or after represents the sum of log10(abundance) of each species for each year during or after the MHWs. before and SD(Sbefore) are the mean and standard deviation, respectively, for the sum of log10(abundance + 0.5) of each species for each year before the MHWs. The ± 95% CIs are only available for the MHWs, as these events occurred in several consecutive years. Here, if the 95% confidence interval does not cross the zero line, or if the absolute value of the effect size is greater than 1.96 (where there is no confidence interval for the estimated effect size), then this fact indicates that an abundance is significantly different from that before the MHWs.
While many previous studies assessed effects of MHWs by comparing abundances from a single pre-MHW survey and several post-MHW surveys (Smale and Wernberg, 2013; Wernberg et al., 2013; Smale et al., 2017; Sanford et al., 2019; Thomsen et al., 2019; Weitzman et al., 2021), this study assessed MHW effects based on effect sizes calculated using abundance data from multiple pre- and post-MHW surveys. Thus, this study provides a more accurate assessment of the effects of MHW because the results are less influenced by environmental stochasticity other than MHW than most previous studies. Firstly, because the reference state abundance (abundance when not affected by heatwaves) in this study is determined as a multi-year average, the effect of environmental stochasticity other than heatwaves on the change in abundance before and after MHW is less than in previous studies. Secondly, because the effect of the MHW on abundance is assessed as the relative magnitude of the change in abundance before and after a MHW to the magnitude of the temporal variation of abundance in a normal year.
We estimated the thermal niche for all species as a species niche trait. We also estimated the vertical niche for sessile species.
The position of the thermal niche (i.e., the preferred temperature) for each species was estimated from information on sea surface temperatures in the ecoregion in which each species was distributed (Spalding et al., 2007) using the following formula:
where SSTs are mean annual sea surface temperatures. The mean annual sea surface temperatures for each ecoregion corresponding to the northern and southern range limits were obtained from Payne et al. (2012).
An ecoregion is part of a biogeographic schema, that is, a global hierarchical classification system based on marine coastal environments (Spalding et al., 2007). The biogeographic schema is a nested system of 12 realms, 62 provinces and 232 ecoregions (Spalding et al., 2007). Our study area is included within the Oyashio Current ecoregion within the temperate northern Pacific realm. In previous studies, thermal niches were estimated by defining northern (i.e., cold-affinity) species and southern (i.e., warmer-water) species using species occurrence reports (Smale et al., 2017; Sanford et al., 2019). Thus, we obtained occurrence reports for each species from Hayashi (1943; 1947); Toyokokujigyo Corporation (1980); Pasco Corporation (1988); Korn and Kulikova (1995); Yoshida (1998); Higo et al. (1999); Okutani (2000); Selivanova (2002); Kado (2003); Latypov and Kasyanov (2003); Nishi (2003); Okuda et al. (2010); Yamazaki (2011); Fukui and Kashiwao (2012); Cox et al. (2014); Kanamori et al. (2017); Association for the Research of Littoral Organisms in Osaka Bay (2018); Hanyuda et al. (2018); Ito et al. (2018; 2019); Marko and Zaslavskaya (2019); Klochkova et al. (2020; 2021); Noda and Ohira (2020), and Ishida et al. (2021). Here, we focused on the distribution of each species in four ecoregions along the coastal northwest Pacific within the temperate northern Pacific realm: Kamchatka Shelf and Coast, Oyashio Current, Northeastern Honshu, and Central Kuroshio Current. These four ecoregions with their distinct latitudinal gradients in sea surface temperatures (Payne et al., 2012) are suitable as indicators of biogeographic affinity for each species. For example, for species distributed in the Gulf of Alaska (51.0–64.8°N) and the Oyashio Current (39.6–52.8°N), the northern limit would be the Gulf of Alaska, but the mean annual surface seawater temperature in this ecoregion is higher than that in the Oyashio Current (Payne et al., 2012); this is contrary to the “expected” latitudinal gradient of the colder north and the warmer south. In addition, using these four ecoregions for defining ranges reflects the natural distribution of the area. For example, Ulva pertusa, which is distributed in tropical regions, may have been introduced from temperate regions as a non-indigenous species (Hanyuda et al., 2016). The number of reported occurrences of individual species was well described within these four ecoregions, and the occurrence reports in four ecoregions of many species were similar, so we can accurately determine cold-affinity species and warmer-water species.
For each of the 17 sessile species, the mode of zonation was estimated as a measure of their vertical niche position. First, we estimated the vertical profile of the distribution of each species over the entire vertical extent of the intertidal zone. For this, in May 2017 we extended each survey plot in the 2004–2018 survey by 50 cm vertically above and below the original plot, placed 400 grid points at 5-cm intervals both vertically and horizontally on the rock surface within each extended survey plot as in the 2004–2018 survey, and recorded the species of sessile organisms occupying each grid point. The elevation of each grid point was estimated by using the slope of the rock walls measured at 25-cm vertical intervals on each rock. Thus, we obtained data for the occupancy within each 200 cm high by 50 cm wide census plot (400 grid points) in May 2017, data for the occupancy within each 100 cm high by 50 cm wide census plot (200 grid points) obtained from the surveys conducted in autumn from 2004 to 2018, and an estimated elevation for each grid point. From these data, we determined the mean abundance of each sessile species in the each of 20 elevation classes (100–90 cm, 90–80 cm… –80 to –90 cm, and –90 to –100 cm from mean tidal level), corresponding to the rock surface in each census plot divided into 10-cm elevation intervals. The vertical niche of each sessile species was calculated from the average abundance of all elevation classes obtained using the following formula:
where H is the elevation class defined as 1 at –90 to –100 cm from mean tidal level. SS,H represents the frequency of occurrence of species S in elevation class H. Values of vertical niches are larger in species that inhabit higher elevations.
If there are accumulative carryover effects from MHWs on population and community dynamics, then the difference in abundance during MHWs and under normal conditions (i.e., the absolute value of the effect size) should be small immediately after the MHW, but then increase with the elapsed time from the MHW event. Therefore, to detect whether there was a temporal trend in the changes in the community abundance of the four functional groups or the abundance of each species during the MHWs, we conducted linear regression analysis, with the number of years (1–7) since the onset of the MHWs as the explanatory variable and the effect sizes of the abundances of four functional groups and 25 species from 2010 to 2016 as response variables. Here, a significant slope and a non-significant intercept were considered evidence of accumulative carryover effects. This is because a significant slope indicates a linear increase or decrease, and a non-significant intercept (i.e., the intercept is not different from 0) indicates no change from before the MHWs.
To determine whether ignoring accumulative carryover effects would result in a biased assessment of the effects of MHWs on the abundance of each functional group and each species during the MHWs and for two years after they ended (when sea surface temperatures returned to normal), we calculated both the average effect sizes from 2010–2016 and the accumulative carryover effect sizes. For the latter measure, we used the effect size in the last year of the MHWs (2016) for species for which an accumulative carryover effect was detected, and the average effect size from 2010 to 2016 for species for which no accumulative carryover effect was found. We compared the average effect size from 2010 to 2016 and the effect size in the last year of the MHWs (2016) to determine whether it is important to consider the accumulative carryover effects.
We examined how the effects of MHWs on the abundance of each species of sessile organisms (macroalgae and sessile invertebrates) differed following during the onset of the MHWs (2010–2016) and during the first year (2017) and the second year (2018) after the MHWs, depending on species niche traits (thermal and vertical niches). We performed multiple regression analysis in which the effect size of the abundance of sessile species from 2010–2016, 2017, and 2018, or consideration of the accumulative carryover effect, were treated as response variables, and the species niche traits (standardized thermal niches and standardized vertical niches) were explanatory variables. The two functional groups of sessile species were combined because the number of sessile invertebrate species (5 species) was much smaller than that of macroalgae (12 species).
We examined how the effects of MHWs on the abundance of each species of mobile organism (herbivorous mollusks and carnivorous invertebrates) differed following during the onset (2010–2016) and during the first (2017) and second year (2018) after the MHWs, depending on thermal niche. We performed linear regression analysis, in which the effect size of the abundance of mobile species from 2010–2016, 2017, and 2018, or consideration of the accumulative carryover effects were treated as response variables, and the standardized thermal niches were explanatory variables. The two functional groups were combined as mobile species because the number of species of carnivorous invertebrates was low (2 species) compared to herbivorous mollusks (6 species). All linear and multiple regressions were performed after checking the data for normality by using the Shapiro-Wilk test. In addition, we have checked that the residuals are normally distributed using the Q-Q plot. All statistical analyses were executed using R version 4.1.2.
The accumulative carryover effects of the MHWs on community abundance at the regional scale varied among four functional groups of rocky intertidal organisms. There was a positive carryover effect for macroalgae and a negative one for carnivorous invertebrates (Table 3), whereas there were no apparent accumulative carryover effects for sessile invertebrates or herbivorous mollusks. The interpretation of the impact of MHWs on the community abundance of functional groups was the same with (the last year of the MHWs) or without (during the MHWs) consideration of accumulative carryover effects (Figure 3).
Table 3 Accumulative carryover effects on community abundances of four functional groups of rocky intertidal organisms in southeast Hokkaido, Japan.
Figure 3 Effect sizes of MHWs on the community abundance of four functional groups during the MHWs (2010–2016), in the last year of the MHWs (2016; shown only for functional groups where accumulative carryover effects were detected), and in the first (2017) and second (2018) years after the MHWs. The mean ( ± 95% CI) is only presented for effect sizes during the MHWs. An abundance is significantly different from that before the MHWs if the 95% confidence interval does not cross the zero line or if the absolute value of the effect size exceeds 1.96 (where there is no confidence interval for the estimated effect size). The dotted lines represent 0 and ±95% CI.
The accumulative carryover effects of the MHWs on regional population size varied among functional groups. Accumulative carryover effects were detected for 6 out of the 25 species (24%); i.e., these species increased or decreased in abundance with the number of years after the onset of the MHWs (Tables 1, 2). Negative accumulative carryover effects were detected for 2 out of 12 species of macroalgae (16.7%) and one of the two species of carnivorous invertebrates, whereas a positive accumulative carryover effect was detected for one out of six species of herbivorous mollusks (2%). In contrast, no accumulative carryover effects were detected for sessile invertebrate species. For three of the six species for which accumulative carryover effects were detected, the interpretation of MHW effects on individual species abundance differed when accumulative carryover effects were considered (the last year of the MHWs) or not considered (during the MHWs) (Figures 4, 5). Although the abundance of these species was not significantly affected by the MHW during the MHW period, the effect sizes calculated to account for accumulative carryover effects showed significant decreases for two species (Pterosiphonia bipinnata for large algae and Lottia cassis for herbivorous mollusks) and significant increases for one species (Littorina brevicula for herbivorous mollusks) (Figures 4, 5).
Figure 4 Effect sizes of MHWs on the abundances of 17 sessile species during the MHWs (2010–2016), in the last year of the MHWs (2016; shown only for species where accumulative carryover effects were detected), and in the first (2017) and second (2018) years after the MHWs. The mean ( ± 95% CI) is only presented for effect sizes during the MHWs. An abundance is significantly different from that before the MHWs if the 95% confidence interval does not cross the zero line or if the absolute value of the effect size exceeds 1.96 (where there is no confidence interval for the estimated effect size). The dotted lines represent 0 and ±95% CI.
Figure 5 Effect sizes of MHWs on the abundances of eight mobile species during the MHWs (2010–2016), in the last year of the MHWs (2016; shown only for species where accumulative carryover effects were detected), and in the first (2017) and second (2018) years after the MHWs. The mean ( ± 95% CI) is only presented for effect sizes during the MHWs. An abundance is significantly different from that before the MHWs if the 95% confidence interval does not cross the zero line or if the absolute value of the effect size exceeds 1.96 (where there is no confidence interval for the estimated effect size). The dotted lines represent 0 and ±95% CI.
The effect of the MHWs on community abundance at the regional scale varied among the four functional groups of the rocky intertidal community. There were significant positive effects on macroalgae during the MHWs (2010–2016), in the last year of the MHWs (considering accumulative carryover effects), and in the first and second years after the MHWs (Figure 3). The MHWs did not have any significant impact on sessile invertebrates. MHWs had significant negative effects on herbivorous mollusks during the MHWs (2010–2016), and in the first and second years after the MHWs (Figure 3). MHWs had significant negative effects on carnivorous invertebrates during the MHWs, in the last year of the MHWs (considering accumulative carryover effects), and in the first year after the MHWs.
The effect of the MHWs on regional population size varied among functional groups. For macroalgae, MHWs had significant positive effects on 50% of the species during the MHWs (50.0%), whereas there were no significant effects on the majority of species in 2017 and 2018 (both 66.7%) (Figure 4). MHWs had a significant positive effect on Corallina pilulifera and Hildenbrandia spp. during the MHWs (2010–2016), and in the first and second years after the MHWs. MHWs had positive effects on the majority of species of macroalgae during the MHWs (2010–2016), and in the first and the second year after the MHWs. The effects of the MHWs on regional population size in the last year of the MHWs (considering accumulative carryover effects) differed between two species for which there were negative accumulative carryover effects: the population size of Pterosiphonia bipinnata decreased significantly, but that of Cladophora opaca did not.
For sessile invertebrates, most species (80–100%) were unaffected by MHWs during the MHWs (2010–2016), or in the first or second years after the MHWs (Figure 4).
For herbivorous mollusks, MHWs had significant negative effects on 50% or more of the species during the MHWs (50.0%), and in the first and second years after the MHWs (66.7% and 50.0%, respectively) (Figure 5). The MHWs had a significant negative impact on Littorina sitkana and Stenotis uchidai during the MHWs (2010–2016), and in the first and second years after the MHWs. MHWs had negative effects on the majority of species of herbivorous mollusks during the MHWs (2010–2016), and in the first and second years after the MHWs. MHWs had a positive effect on Littorina brevicula in the last year of the MHWs (considering accumulative carryover effects), and in the first and second years after the MHWs, in which positive accumulative carryover effects were detected. MHWs had a significant negative impact in the last year of the MHWs (considering accumulative carryover effects) for both species for which negative accumulative carryover effects were found.
For the two carnivorous invertebrate species, MHWs had significant negative effects on both during the MHWs (2010–2016) and in the first year after the MHWs (Figure 5). The MHWs also had a significant negative impact on Leptasterias ochotensis similispinis during the second year after the MHWs. MHWs had a significant negative impact in the last year of the MHWs (considering accumulative carryover effects) for Nucella lima, for which there were also negative accumulative carryover effects.
The results of multiple regression analysis for sessile organisms showed significant effects only for thermal niches, regardless of the response variables. That is, the MHWs caused changes in composition of sessile assemblages at a regional scale by reducing the abundances of species with low thermal niches (i.e., cold-affinity species) and increasing those of species with high thermal niches (i.e., warmer-water species) (Table 4 and Figure 6).
Table 4 Results of multiple regression analysis testing how the effects of MHWs on the abundance of each sessile species of rocky intertidal organism (macroalgae and sessile invertebrates) for each period differ depending on thermal and vertical niches.
Figure 6 Relationship between the effect size of MHWs on species abundances of sessile and mobile organisms during the MHWs, and in the first two years after the MHWs, and standardized thermal niches. Each plot includes the regression line for each model. (A) Sessile organisms during the MHWs, (B) sessile organisms in 2017, (C) sessile organisms in 2018, (D) mobile organisms during the MHWs, (E) mobile organisms in 2017, and (F) mobile organisms in 2018. The open circles in (A–C) indicate macroalgae and the filled circles indicate sessile invertebrates. The open circles in (D–F) indicate herbivorous mollusks, and the filled circles indicate carnivorous invertebrates.
The results of linear regression analysis for mobile organisms showed significant effects for thermal niches regardless of the response variables. That is, the MHWs caused changes in the composition of mobile assemblages at a regional scale by reducing the abundances of species with low thermal niches (i.e., cold-affinity species) and increasing those of species with high thermal niches (i.e., warmer-water species) (Table 5 and Figure 6).
Table 5 Results of linear regression analysis testing how the effects of MHWs on the abundance of each mobile species of rocky intertidal organism (herbivorous mollusks and carnivorous invertebrates) for each period differ depending on thermal niche.
The results of multiple regression analysis for each species of sessile organisms when considering accumulative carryover effects showed a significant interaction between thermal and vertical niches (Table 6). This indicates that the increase in abundance due to MHWs in warmer-water species is more pronounced for species with higher vertical niches.
Table 6 Results of multiple regression analysis testing how the effects of MHWs on the abundance of each sessile species of rocky intertidal organism (macroalgae and sessile invertebrates) in 2016 (i.e., considering the accumulative carryover effect) differ depending on thermal and vertical niches.
The results of linear regression analysis for each species of mobile animal when considering the accumulative carryover effects showed no effect by thermal niche (Table 7).
Table 7 Results of linear regression analysis testing how the effects of MHWs on the abundance of each mobile species (herbivorous mollusks and carnivorous invertebrates) in 2016 (i.e., considering the accumulative carryover effect) differ depending on thermal niche.
In this study, we evaluated the impacts of the MHWs in southeastern Hokkaido in summer (July–September) from 2010 to 2016 on the abundances and by species niche traits of rocky intertidal communities (macroalgae, sessile invertebrates, herbivorous mollusks and carnivorous invertebrates) by separating the effects of MHWs from those of other environmental stochasticity. We found positive and negative accumulative carryover effects of MHWs (i.e., a continued increase or decrease in abundance with the number of years elapsed since the onset of the MHWs) in the community abundance of macroalgae and carnivorous invertebrates, respectively (Table 3). At the species level, accumulative carryover effects were detected in 6 out of 25 species (Tables 1, 2). The interpretation of the effect of MHWs on the community abundance of functional groups did not change with or without consideration of accumulative carryover effects (Figure 3). However, it is important to consider the accumulative carryover effects. Because, abundance of some species was underestimated when ignored accumulative carryover effects (Figures 4, 5). In addition, we found differences in the response to MHWs in terms of community abundance among four functional groups. Macroalgae increased during and after the MHWs, whereas sessile invertebrates showed no response (Figure 3). Herbivorous mollusks decreased in abundance during and after the MHWs. Carnivorous invertebrates decreased in abundance during the MHWs and in the first year afterward. Finally, we found reduced abundances of species with low thermal niches (i.e., cold-affinity species) and increased abundances of those with high thermal niches (i.e., warmer-water species) (Tables 4, 5, and Figure 6). The increase in abundance of warmer-water species due to MHWs is more pronounced for species with higher vertical niches, but this became evident only when accumulative carryover effects were considered (Table 6).
The frequency of MHWs has increased in recent years (Hobday et al., 2018; Oliver et al., 2018). Therefore, it is important to clarify whether MHWs have an accumulative carryover effect when they occur continuously or persist over multiple years. In this study, accumulative carryover effects caused by consecutive MHWs were detected in both the community abundance of some functional groups and the abundance of some individual species. These results indicate that repeated weak MHWs, such as category I and II, had cumulative effects on marine communities and populations.
The 5 species out of 25 for which negative accumulative carryover effects were found included four species for which significant negative effects were detected in the last year of the MHWs (2016)—the macroalga Pterosiphonia bipinnata, herbivorous mollusks Lottia cassis and Stenotis uchidai, and the predatory invertebrate Nucella lima—as well as one species for which no MHW effects were detected in 2016: the macroalga Cladophora opaca (Figures 4, 5). This suggests that these five species are particularly susceptible when MHWs occur continuously or persist for a period of time. In Cladophora opaca, the mechanism by which negative accumulative carryover effects were observed but without significant negative effects in 2016 is unknown, but perhaps this species had a higher thermal niche (Table 8) than the other four species for which negative accumulative carryover effects were found, and it was not affected in 2016 because it is less sensitive to MHWs.
The interpretation of the impact of MHWs on the community abundance of functional groups was the same with or without consideration of accumulative carryover effects, with a significant increase in macroalgae and a significant decrease in carnivorous invertebrates (Figure 3). In contrast, the interpretation of the impact of MHWs on the abundance of individual species for which accumulative carryover effects were found differed when those effects were considered. There were no significant effects during the MHWs, but for the effect size calculated considering accumulative carryover effects two of the six species (Pterosiphonia bipinnata in macroalgae and Lottia cassis in herbivorous mollusks) showed a significant decrease and one species (Littorina brevicula in herbivorous mollusks) showed a significant increase (Figures 4, 5). These results emphasize that the response of organisms to MHWs should be evaluated even when accumulative carryover effects are considered.
Our results show that sequential MHWs caused an accumulative carryover effect on some marine community abundance and population size. In some species, the effects of the MHWs could be underestimated if the accumulative carryover effects were not considered. However, there are still outstanding questions regarding the accumulative carryover effects of MHWs on marine organisms. First, it is not clear how the tendency to cause accumulative carryover effects depends on species niche traits. For example, species with longer life histories and smaller dispersal ability are more likely to show accumulative carryover effects on population size when sequential MHWs occur. Unfortunately, we were unable to test this hypothesis in this study because there were fewer annual and non-planktonic species (7 and 4 species, respectively) than perennial and planktonic species. Second, it is not clear which functional groups and species niche traits of the organisms affected are particularly susceptible to the accumulative carryover effects of MHWs. In this study, four of the five species for which negative accumulative carryover effects were found (Tables 1, 2) had significant negative effects detected in the last year of the MHWs (2016), whereas one species showed no significant negative effects in 2016 (Figures 4, 5). This suggests that there are functional groups or species niche traits that are particularly susceptible among those that show accumulative carryover effects. However, this hypothesis could not be tested in this study because of the small number of species showing an accumulative carryover effect.
The response of rocky intertidal communities to MHWs has been mainly investigated for relatively strong MHWs (categories I–III) (Hobday et al., 2018) in the northeast Pacific (Sanford et al., 2019; Miner et al., 2021; Suryan et al., 2021; Weitzman et al., 2021; Spiecker and Menge, 2022). Little is known, therefore, about how relatively weak MHWs (categories I and II) affect rocky intertidal communities. We examined the effects of category I and II MHWs (Miyama et al., 2021a; Miyama et al., 2021b) that occur every summer (July–September) at our study site, thus filling this gap in our knowledge. Despite the relatively weak category I or II MHWs that occurred in southeastern Hokkaido, Japan, from 2010 to 2016, there were few signs of community abundance recovery in the major functional groups by the second year after the MHWs. Of the functional groups that increased or decreased in abundance during and after MHWs (macroalgae, herbivorous mollusks and carnivorous invertebrates), both macroalgae and herbivorous mollusks remained higher and lower in abundance, respectively, in the second year after the MHWs (2018) (Figure 3).
There are two possible reasons for the increase in macroalgal community abundance during the MHWs in southeastern Hokkaido. First, the increase in overall abundance of macroalgae may have been caused by an increase in warmer-water species due to the increase in sea surface temperatures caused by MHWs. This is because the species that comprise the macroalgae are relatively dominated by warmer-water species with thermal niches greater than 10 (Tables 8, 9). Four of these species, which include the dominant crustose coralline algae Corallina pilulifera and the red alga Gloiopeltis furcata (Kanamori et al., 2017; Ishida et al., 2021), showed increases in both the mean effect size during the MHWs, and in the effect size in the first and second years after the MHWs (Figure 4). Second, the increase in community abundance of macroalgae may have been caused by a trophic cascade resulting from a decline in herbivorous invertebrate abundance due to MHWs. This is supported by the fact that the abundance of algal communities in rocky intertidal zones is restricted by grazing pressure (Cubit, 1984; Guerry et al., 2009; Poore et al., 2012). The community abundances of herbivorous mollusks and carnivorous invertebrates showed a negative response to MHWs (Figure 3). Compared to macroalgae and sessile invertebrates, many species of herbivorous mollusks and carnivorous invertebrates have lower thermal niches (Tables 8, 9); also, the higher trophic levels are regulated by environmental stress (Menge and Sutherland, 1987; Menge and Olson, 1990), which may have caused a decline in community abundance.
Our results show a significant positive effect of MHWs for the community abundance of macroalgae in all periods (during and after the MHWs), whereas MHWs had no effect on the community abundance of sessile invertebrates in any period (Figure 3). In contrast, previous studies showed a decrease in algae after the 2014–2016 northeast Pacific MHW in the Gulf of Alaska, but an increase in sessile animals (Suryan et al., 2021; Weitzman et al., 2021). Despite being in the same ecosystem (i.e., rocky intertidal zones), there are a variety of factors that might have caused the differences in responses between this study and those in the Northeast Pacific region. There were differences in MHW intensity (categories I and II, Miyama et al., 2021a; Miyama et al., 2021b vs. categories I–III, Hobday et al., 2018), species composition (Fucus and mussels are not dominant species in our study area; Kanamori et al., 2017; Ishida et al., 2021) and in thermal niches of the component species. More reports on the responses of marine organisms to MHWs are needed for various ecosystems, regions, and organisms, including information on the intensity of MHWs, in order to identify factors that result in differences in responses to MHWs in the same ecosystem. Identification of these factors would facilitate our understanding of the areas and functional groups that are susceptible to MHWs and would thus help in the conservation of marine ecosystems.
Identifying the species niche traits susceptible to MHWs will improve the predictability the responses of marine organisms to MHWs. Our results show that the abundances of species with low thermal niches decreased during and after MHWs, whereas those of species with high thermal niches increased (Tables 4, 5, and Figure 6). Previous studies have suggested a decrease in abundance of cold-affinity species (Smale et al., 2017) and an increase in warmer-water species (Smale et al., 2017; Sanford et al., 2019; Miner et al., 2021). These studies suggest that focusing on thermal niches can be useful for understanding and predicting the ecological processes behind the responses of marine organisms to MHWs. When we considered accumulative carryover effects for mobile animals, there were no differences in the response to MHWs by thermal niche (Table 7), possibly because the abundance of Lottia cassis, which has a relatively high thermal niche among mobile animals (Table 9), was drastically reduced by accumulative carryover effects (Figure 5).
Our examination of the effects of thermal and vertical niches on species-specific responses to MHWs did not support our prediction that sessile rocky intertidal species with higher vertical distributions (i.e., higher vertical niches) would likely be less affected by MHWs. One possible reason why our prediction was not supported by our results is that the MHWs might affect regional-scale population sizes of rocky intertidal sessile species through complex mechanisms, including direct and indirect effects of changes in seawater temperature, which to date have been rarely examined in empirical studies. To deepen our understanding of the influence of MHWs on rocky intertidal communities, studies focusing on the underlying mechanisms that cause interspecific differences in MHW responses are eagerly awaited. As a cause of interspecific differences in responses to MHWs, both this and previous studies emphasize the importance of thermal niches. However, the influence and importance of vertical niches are still unknown. Although the present study demonstrates that the increase in abundance of warmer-water species due to MHWs is more pronounced for species with higher vertical niches, a previous study suggested that vertical niches may not be an important factor for explaining interspecific differences in response to MHWs; in the Northeast Pacific, changes in the percent cover of each rocky intertidal taxon during and after marine heat waves were similar in both the middle and lower strata of the intertidal zone (Weitzman et al., 2021).
In this study, we examined the response of rocky intertidal communities to relatively weak (categories I and II) MHWs that occurred southeast of Hokkaido, northern Japan, in summer (July–September) from 2010 to 2016. The specific questions addressed were (1) Did MHWs have an accumulative carryover effect on the community abundance of the four main functional groups or on the abundance of each species? (2) How did the effects of MHWs on the community abundance of the four functional groups during the MHWs (2010–2016) and subsequent recovery in the first (2017) and second (2018) years after the MHWs differ? (3) How did the susceptibility to MHWs differ depending on two species niche traits, i.e., thermal and vertical niches?
We found that (1) the continuous MHWs had an accumulative carryover effect on both functional group abundance and the abundance of individual species. In addition, some species for which accumulative carryover effects were detected were underestimated when those effects were not considered.
(2) The community abundance of macroalgae and herbivorous mollusks increased and decreased, respectively, during the MHWs (2010–2016), and in the first and second years after the MHWs, and the community abundance of carnivorous invertebrates decreased during the MHWs and in the first year after. The increase in community abundance of macroalgae may have occurred because the macroalgal communities are composed of a relatively large number of warmer-water species, and/or by a trophic cascade resulting from a decrease in the community of herbivorous invertebrates. The decline in community abundance of herbivorous mollusks and carnivorous invertebrates may have been caused by the fact that these communities are composed of a relatively large number of species with more cold-affinity.
(3) The abundance of species distributed in colder water (i.e., lower thermal niches) decreased and those distributed in warmer water (i.e., higher thermal niches) increased. There were no detectable differences in response to MHWs between species distributed at higher vertical positions (i.e., higher vertical niches) and those at lower vertical positions (i.e., lower vertical niches) when accumulative carryover effects were not considered. On the other hand, the increase in abundance due to MHWs in warmer-water species is more pronounced for species with higher vertical niches when accumulative carryover effects are considered.
The results of this study agree with those of a previous study conducted on subtidal communities (Smale et al., 2017) in that the susceptibility to MHWs is related to the thermal niche traits of the species. On the other hand, for the response of functional groups to MHWs, our results are not consistent with those of previous study (Weitzman et al., 2021), despite being in the same intertidal ecosystem (i.e., rocky intertidal). Thus the findings from studies to date are insufficient to predict the effects of MHWs on marine ecosystems, and further studies are needed to elucidate the response of marine organisms to MHW events of various intensities and durations for a variety of ecosystems, regions, and organisms.
The datasets presented in this study are included in the Supplementary Materials.
T.N. designed the census. K.I., M.T., Y.Y., and T.N. conducted field work. K.I. and Y.W. collected the species occurrence reports. M.T. designed the survey of May 2017. K.I. carried out the data analysis. K.I. and M.T. prepared Figure 2. K.I. and T.N. wrote the manuscript. M.T., Y.Y., and Y.W. commented on the manuscript. All authors discussed the results and approved the submitted manuscript.
This research was supported by the Japan Society for the Promotion of Science (KAKENHI grants nos. 20570012, 24570012, 5K07208, and 18H02503 to T.N.).
We are grateful to T. Hagino, Dr. T. Okuda, Dr. K. Fukaya, Dr. M. Tsujino, Dr. A. Iwasaki, Dr. Y. Kanamori, T. Abe, and H. Shimizu for field assistance. We appreciate the help of Drs. M. Nakaoka, I. Koizumi, and M. Senzaki, who reviewed this paper. We also thank Dr. S. Ohdachi, R. Fujii, S. Arai, and H. Ogawa for their valuable comments. We thank the many researchers and students who helped with data collection in the field. This study was made possible by the generous support and encouragement of local fishermen and the fishery officers of the Fisheries Cooperative Associations in Hokkaido. We are grateful to the staff and students at Akkeshi Marine Station of Hokkaido University.
The authors declare that the research was conducted in the absence of any commercial or financial relationships that could be construed as a potential conflict of interest.
All claims expressed in this article are solely those of the authors and do not necessarily represent those of their affiliated organizations, or those of the publisher, the editors and the reviewers. Any product that may be evaluated in this article, or claim that may be made by its manufacturer, is not guaranteed or endorsed by the publisher.
The Supplementary Material for this article can be found online at: https://www.frontiersin.org/articles/10.3389/fmars.2023.1146148/full#supplementary-material
Association for the Research of Littoral Organisms in Osaka Bay. (2018). Rocky shore macrobiota of southeastern Osaka bay: results of surveys carried out in the years 2011–2015. Occasional papers Osaka Museum Natural History. 4, 17–40. doi: 10.20643/00001327
Benedetti-Cecchi L., Bertocci I., Vaselli S., Maggi E. (2006). Temporal variance reverses the impact of high mean intensity of stress in climate change experiments. Ecology. 87, 2489–2499. doi: 10.1890/0012-9658(2006)87[2489:TVRTIO]2.0.CO;2
Cox L. N., Zaslavskaya N. I., Marko P. B. (2014). Phylogeography and trans-pacific divergence of the rocky shore gastropod nucella lima. J. Biogeogr. 41, 615–627. doi: 10.1111/jbi.12217
Cubit J. D. (1984). Herbivory and the seasonal abundance of algae on a high intertidal rocky shore. Ecology. 65, 1904–1917. doi: 10.2307/1937788
Frölicher T. L., Fischer E. M., Gruber N. (2018). Marine heatwaves under global warming. Nature. 560, 360–364. doi: 10.1038/s41586-018-0383-9
Fujiwara M., Takada T. (2017). Environmental stochasticity. in ELS - ecology. Ed. Baxter R. (Chichester: John Wiley & Sons, Ltd.). doi: 10.1002/9780470015902.a0021220.pub2
Fukui S., Kashiwao S. (2012). Molluscan fauna of usujiri marine station field science center for northern biosphere, Hokkaido university. 2nd ed. (Hokkaido University). Available at: https://eprints.lib.hokudai.ac.jp/dspace/handle/2115/38939 (Accessed April 21, 2022).
Guerry A. D., Menge B. A., Dunmore R. A. (2009). Effects of consumers and enrichment on abundance and diversity of benthic algae in a rocky intertidal community. J. Exp. Mar. Biol. Ecol. 369, 155–164. doi: 10.1016/j.jembe.2008.11.011
Hanyuda T., Hansen G. I., Kawai H. (2018). Genetic identification of macroalgal species on Japanese tsunami marine debris and genetic comparisons with their wild populations. Mar. Pollut. Bull. 132, 74–81. doi: 10.1016/j.marpolbul.2017.06.053
Hanyuda T., Heesch S., Nelson W., Sutherland J., Arai S., Boo S. M., et al. (2016). Genetic diversity and biogeography of native and introduced populations of ulva pertusa (Ulvales, chlorophyta). Phycol. Res. 64, 102–109. doi: 10.1111/pre.12123
Harvey B. P., Marshall K. E., Harley C. D., Russell B. D. (2022). Predicting responses to marine heatwaves using functional traits. Trends Ecol. Evol. 37, 20–29. doi: 10.1016/j.tree.2021.09.003
Hayashi R. (1943). Contributions to the classification of the Sea-stars of japan. II. forcipulata, with the note on the relationships between the skeletal structure and respiratory organs of the Sea-stars. J. Fac. Sci. Hokkaido Univ. Ser. 6. Zoology. 8, 133–281. Available at: https://eprints.lib.hokudai.ac.jp/dspace/handle/2115/27045 (Accessed May 7, 2022).
Hayashi R. (1947). The fauna of akkeshi bay: XVI. asteroidea. J. Fac. Sci. Hokkaido Univ. Ser. 6. Zoology. 9, 277–280. Available at: https://eprints.lib.hokudai.ac.jp/dspace/handle/2115/27062 (Accessed May 25, 2022).
Helmuth B., Harley C. D. G., Halpin P. M., O’Donnell M., Hofmann G. E., Blanchette C. A. (2002). Climate change and latitudinal patterns of intertidal thermal stress. Science. 298, 1015–1017. doi: 10.1126/science.1076814
Helmuth B., Mieszkowska N., Moore P., Hawkins S. J. (2006). Living on the edge of two changing worlds: forecasting the responses of rocky intertidal ecosystems to climate change. Annu. Rev. Ecol. Evol. Syst. 37, 373–404. doi: 10.1146/annurev.ecolsys.37.091305.110149
Higo S., Callomon P., Goto Y. (1999). Catalogue and bibliography of the marine shell-bearing mollusca of Japan: gastropoda, bivalvia, polyplacophora, scaphopoda (Yao: Elle Scientific Publications).
Hobday A. J., Alexander L. V., Perkins S. E., Smale D. A., Straub S. C., Oliver E. C., et al. (2016). A hierarchical approach to defining marine heatwaves. Prog. Oceanogr. 141, 227–238. doi: 10.1016/j.pocean.2015.12.014
Hobday A., Oliver E. C. J., Gupta A. S., Benthuysen J. A., Burrows M. T., Donat M., et al. (2018). Categorizing and naming marine heatwaves. Oceanography 31, 63–73. doi: 10.5670/oceanog.2018.205
Ishida K., Tachibana M., Hori M., Okuda T., Yamamoto T., Nakaoka M., et al. (2021). Quantifying the dynamics of rocky intertidal sessile communities along the pacific coast of Japan: implications for ecological resilience. Sci. Rep. 11, 16073. doi: 10.1038/s41598-021-95348-1
Itoh T., Miyadai M., Kobayashi A. (2019). Marine algal flora in the intertidal and shallow infralittoral zones of enoshima island, sagami bay, Japan. Natural History Rep. Kanagawa. 40, 5–14. doi: 10.32225/nkpmnh.2019.40_5
Itoh T., Ueda I., Hagiwara K., Kitajima M., Iwasaki T., Muraishi K., et al. (2018). Intertidal animals found on enoshima island – VII. Natural History Rep. Kanagawa. 39, 13–21. doi: 10.32225/nkpmnh.2018.39_13
Iwasaki A., Fukaya K., Noda T. (2016). “Quantitative evaluation of the impact of the great East Japan earthquake and tsunami on the rocky intertidal community,” in Ecological impacts of tsunamis on coastal ecosystems: lessons from the great East Japan earthquake, ecological research monographs. Eds. Urabe J., Nakashizuka T. (Tokyo: Springer), 35–46.
Iwasaki A., Noda T. (2018). A framework for quantifying the relationship between intensity and severity of impact of disturbance across types of events and species. Sci. Rep. 8, 795. doi: 10.1038/s41598-017-19048-5
Iwataki M., Lum W. M., Kuwata K., Takahashi K., Arima D., Kuribayashi T., et al. (2022). Morphological variation and phylogeny of karenia selliformis (Gymnodiniales, dinophyceae) in an intensive cold-water algal bloom in eastern Hokkaido, Japan. Harmful Algae. 114, 102204. doi: 10.1016/j.hal.2022.102204
Kado R. (2003). Invasion of Japanese shores by the NE pacific barnacle balanus glandula and its ecological and biogeographical impact. Mar. Ecol. Prog. Ser. 249, 199–206. doi: 10.3354/meps249199
Kanamori Y., Fukaya K., Noda T. (2017). Seasonal changes in community structure along a vertical gradient: patterns and processes in rocky intertidal sessile assemblages. Popul. Ecol. 59, 301–313. doi: 10.1007/s10144-017-0596-z
Klochkova N. G., Klochkova T. A., Klimova A. V. (2020). Marine benthic algae from Commander islands (Revision 2020). i. chlorophyta and ochrophyta (Bulletin оf Kamchatka State Technical University), 82–107. doi: 10.17217/2079-0333-2020-54-82-107
Klochkova N. G., Klochkova T. A., Klimova A. V. (2021). Marine benthic algae from Commander islands (Revision 2021). II. rhodophyta (Bulletin оf Kamchatka State Technical University), 41–72. doi: 10.17217/2079-0333-2021-55-41-72
Korn O. M., Kulikova V. A. (1995). Seasonal species composition and distribution of barnacle larvae in avacha inlet (Kamchatka). J. Plankton Res. 17, 221–234. doi: 10.1093/plankt/17.2.221
Krumhansl K. A., Dowd M., Wong M. C. (2020). A characterization of the physical environment at eelgrass (Zostera marina) sites along the Atlantic coast of Nova Scotia. Can. Tech. Rep. Fish. Aquat. Sci, 3361. https://publications.gc.ca/site/eng/9.884622/publication.html (Accessed March 16, 2023).
Krumhansl K. A., Dowd M., Wong M. C. (2021). Multiple metrics of temperature, light, and water motion drive gradients in eelgrass productivity and resilience. Front. Mar. Sci. 8. doi: 10.3389/fmars.2021.597707
Latypov Y. Y., Kasyanov V. L. (2003). “Biodiversity of the Commander islands and Bering Sea coast of the kamchatka peninsula by Russian scientists,” in Proceedings of the Arctic biodiversity workshop: new census of marine life initiative. Eds. Iken K., Konar B. (Alaska Fairbanks: Alaska Sea Grant College Program University), 59–66.
Marko P. B., Zaslavskaya N. I. (2019). Geographic origin and timing of colonization of the pacific coast of north America by the rocky shore gastropod littorina sitkana. PeerJ. 7, e7987. doi: 10.7717/peerj.7987
Menge B. A. (1976). Organization of the new England rocky intertidal community: role of predation, competition, and environmental heterogeneity. Ecol. Monogr. 46, 355–393. doi: 10.2307/1942563
Menge B. A., Lubchenco J., Gaines S. D., Ashkenas R. L. (1986). A test of the menge-Sutherland model of community organization in a tropical rocky intertidal food web. Oecologia. 71, 75–89. doi: 10.1007/BF00377324
Menge B. A., Olson A. M. (1990). Role of scale and environmental factors in regulation of community structure. Trends Ecol. Evol. 5, 52–57. doi: 10.1016/0169-5347(90)90048-I
Menge B. A., Sutherland J. P. (1987). Community regulation: variation in disturbance, competition, and predation in relation to environmental stress and recruitment. Am. Nat. 130, 730–757. doi: 10.1086/284741
Miner C. M., Burnaford J. L., Ammann K., Becker B. H., Fradkin S. C., Ostermann-Kelm S., et al. (2021). Latitudinal variation in long-term stability of north American rocky intertidal communities. J. Anim. Ecol. 90, 2077–2093. doi: 10.1111/1365-2656.13504
Misaka T., Ando Y. (2021) Large-Scale outbreak of harmful algae in the pacific coast of Hokkaido, Japan. Available at: https://www.hro.or.jp/list/fisheries/marine/att/ima943.pdf (Accessed November 1, 2022).
Miyama T., Minobe S., Goto H. (2021a). Marine heatwave of sea surface temperature of the oyashio region in summer in 2010–2016. Front. Mar. Sci. 7. doi: 10.3389/fmars.2020.576240
Miyama T., Minobe S., Goto H. (2021b) Frequent marine heat waves off the coast of Hokkaido and tohoku revealed: relationship between marine heat waves and yellowtail catches. Available at: https://www.hokudai.ac.jp/news/pdf/210114_pr4.pdf (Accessed November 1, 2022).
Nishi E. (2003). “Polychaetes (annelids, polychaetes) from the waters around manazuru peninsula, sagami bay, Japan,” in Actinia: bulletin of the manazuru marine laboratory for science education, faculty of education and human sciences, Yokohama national university. 15, 7–13. Available at: https://ynu.repo.nii.ac.jp/?action=pages_view_main&active_action=repository_view_main_item_detail&item_id=1785&item_no=1&page_id=59&block_id=74 (Accessed May 25, 2022).
Noda T. (2004). Spatial hierarchical approach in community ecology: a way beyond high context-dependency and low predictability in local phenomena. Popul. Ecol. 46, 105–117. doi: 10.1007/s10144-004-0184-x
Noda T. (2009). Metacommunity-level coexistence mechanisms in rocky intertidal sessile assemblages based on a new empirical synthesis. Popul. Ecol. 51, 41–55. doi: 10.1007/s10144-008-0117-1
Noda T., Ohira M. (2020). Transition in population dynamics of the intertidal barnacle balanus glandula after invasion: causes and consequences of change in larval supply. J. Mar. Sci. Eng. 8, 915. doi: 10.3390/jmse8110915
Noda T., Sakaguchi M., Iwasaki A., Fukaya K. (2017). Influence of the 2011 tohoku earthquake on population dynamics of a rocky intertidal barnacle: cause and consequence of alteration in larval recruitment. Coast. Mar. Sci. 40, 35–43. Available at: https://repository.dl.itc.u-tokyo.ac.jp/records/48908 (Accessed Febrary 22, 2022)
Okuda T., Noda T., Yamamoto T., Hori M., Nakaoka M. (2010). Contribution of environmental and spatial processes to rocky intertidal metacommunity structure. Acta Oecol. 36, 413–422. doi: 10.1016/j.actao.2010.04.002
Oliver E. C., Donat M. G., Burrows M. T., Moore P. J., Smale D. A., Alexander L. V., et al. (2018). Longer and more frequent marine heatwaves over the past century. Nat. Commun. 9, 1324. doi: 10.1038/s41467-018-03732-9
Pasco Corporation. (1988). Third basic survey for conservation of the natural environment marine area survey report of marine biological environment survey. Available at: https://www.biodic.go.jp/reports2/3rd/kaiiki/3_kaiiki.pdf (Accessed May 13, 2022).
Payne M. C., Brown C. A., Reusser D. A., Lee H. II. (2012). Ecoregional analysis of nearshore sea-surface temperature in the north pacific. PloS One 7, e30105. doi: 10.1371/journal.pone.0030105
Poore A. G. B., Campbell A. H., Coleman R. A., Edgar G. J., Jormalainen V., Reynolds P. L., et al. (2012). Global patterns in the impact of marine herbivores on benthic primary producers. Ecol. Lett. 15, 912–922. doi: 10.1111/j.1461-0248.2012.01804.x
R Core Team. (2021) R: a language and environment for statistical computing (Vienna, Austria: R Foundation for Statistical Computing). Available at: https://www.R-project.org/ (Accessed December 11, 2021).
Ruthrof K. X., Breshears D. D., Fontaine J. B., Froend R. H., Matusick G., Kala J., et al. (2018). Subcontinental heat wave triggers terrestrial and marine, multi-taxa responses. Sci. Rep. 8, 13094. doi: 10.1038/s41598-018-31236-5
Ryo M., Aguilar-Trigueros C. A., Pinek L., Muller L. A. H., Rillig M. C. (2019). Basic principles of temporal dynamics. Trends Ecol. Evol. 34, 723–733. doi: 10.1016/j.tree.2019.03.007
Sanford E., Sones J. L., García-Reyes M., Goddard J. H., Largier J. L. (2019). Widespread shifts in the coastal biota of northern California during the 2014–2016 marine heatwaves. Sci. Rep. 9, 4216. doi: 10.1038/s41598-019-40784-3
Selivanova O. N. (2002). Marine benthic algae of the Russian coasts of the Bering Sea (from ozernoi gulf to dezhnev bay, including karaginskii island). Constancea 83. Available at: https://ucjeps.berkeley.edu/constancea/83/selivanova/Selivanova.html (Accessed April 14, 2022).
Smale D. A., Wernberg T. (2013). Extreme climatic event drives range contraction of a habitat-forming species. Proc. R. Soc. B. Biol. Sci. 280, 1–9. doi: 10.1098/rspb.2012.2829
Smale D. A., Wernberg T., Oliver E. C. J., Thomsen M., Harvey B. P., Straub S. C., et al. (2019). Marine heatwaves threaten global biodiversity and the provision of ecosystem services. Nat. Clim. Change 9, 306–312. doi: 10.1038/s41558-019-0412-1
Smale D. A., Wernberg T., Vanderklift M. A. (2017). Regional-scale variability in the response of benthic macroinvertebrate assemblages to a marine heatwave. Mar. Ecol. Prog. Ser. 568, 17–30. doi: 10.3354/meps12080
Spalding M. D., Fox H. E., Allen G. R., Davidson N., Ferdaña Z. A., Finlayson M., et al. (2007). Marine ecoregions of the world: a bioregionalization of coastal and shelf areas. BioScience. 57, 573–583. doi: 10.1641/B570707
Spiecker B. J., Menge B. A. (2022). El Niño and marine heatwaves: ecological impacts on Oregon rocky intertidal kelp communities at local to regional scales. Ecol. Monogr. 92, e1504. doi: 10.1002/ecm.1504
Suryan R. M., Arimitsu M. L., Coletti H. A., Hopcroft R. R., Lindeberg M. R., Barbeaux S. J., et al. (2021). Ecosystem response persists after a prolonged marine heatwave. Sci. Rep. 11, 6235. doi: 10.1038/s41598-021-83818-5
Thomsen M. S., Mondardini L., Alestra T., Gerrity S., Tait L., South P., et al. (2019). Local extinction of bull kelp (Durvillaea spp.) due to a marine heatwave. Front. Mar. Sci. 6. doi: 10.3389/fmars.2019.00084
Toyokokujigyo Corporation. (1980). Second basic survey for conservation of the natural environment marine area survey report of marine biological survey. Available at: https://www.biodic.go.jp/reports2/2nd/kaiiki_all/2_kaiiki_all.pdf (Accessed May 13, 2022).
Watt C. A., Scrosati R. A. (2013). Bioengineer effects on understory species richness, diversity, and composition change along an environmental stress gradient: experimental and mensurative evidence. Estuar. Coast. Shelf Sci. 123, 10–18. doi: 10.1016/j.ecss.2013.02.006
Weitzman B., Konar B., Iken K., Coletti H., Monson D., Suryan R., et al. (2021). Changes in rocky intertidal community structure during a marine heatwave in the northern gulf of Alaska. Front. Mar. Sci. 8. doi: 10.3389/fmars.2021.556820
Wernberg T., Smale D. A., Tuya F., Thomsen M. S., Langlois T. J., De Bettignies T., et al. (2013). An extreme climatic event alters marine ecosystem structure in a global biodiversity hotspot. Nat. Clim. Change 3, 78–82. doi: 10.1038/nclimate1627
Yamazaki T. (2011). Molluscan fauna of akkeshi marine station: part 1 (Hokkaido University). Available at: https://eprints.lib.hokudai.ac.jp/dspace/handle/2115/48596 (Accessed April 21, 2022).
Keywords: Marine heatwave, Rocky intertidal, community dynamics, accumulative carryover effects, species niche trait
Citation: Ishida K, Tachibana M, Yao Y, Wada Y and Noda T (2023) The impact of marine heatwaves on rocky intertidal communities: evidence of accumulative carryover effects of marine heatwaves. Front. Mar. Sci. 10:1146148. doi: 10.3389/fmars.2023.1146148
Received: 17 January 2023; Accepted: 13 April 2023;
Published: 28 April 2023.
Edited by:
Yongsheng Wu, Fisheries and Oceans Canada (DFO), Ottawa, CanadaReviewed by:
Maria Pia Miglietta, Texas A&M University at Galveston Galveston, United StatesCopyright © 2023 Ishida, Tachibana, Yao, Wada and Noda. This is an open-access article distributed under the terms of the Creative Commons Attribution License (CC BY). The use, distribution or reproduction in other forums is permitted, provided the original author(s) and the copyright owner(s) are credited and that the original publication in this journal is cited, in accordance with accepted academic practice. No use, distribution or reproduction is permitted which does not comply with these terms.
*Correspondence: Ken Ishida, a2VuLmlzaGlkYS5lY29sb2d5QGdtYWlsLmNvbQ==
†Present address: Ken Ishida, Fisheries Resources Institute, Japan Fisheries Research and Education Agency, Yokohama, Japan
Disclaimer: All claims expressed in this article are solely those of the authors and do not necessarily represent those of their affiliated organizations, or those of the publisher, the editors and the reviewers. Any product that may be evaluated in this article or claim that may be made by its manufacturer is not guaranteed or endorsed by the publisher.
Research integrity at Frontiers
Learn more about the work of our research integrity team to safeguard the quality of each article we publish.