- 1Key Laboratory of Aquatic Science of Chongqing, College of Fisheries, Southwest University, Chongqing, China
- 2Chongqing Fishery Technology Promotion Station, Chongqing Agriculture and Rural Affairs Committee, Chongqing, China
Introduction: Perilla oil is a natural vegetable oil rich in alpha-linolenic acid extracted from Perilla seeds. Fish oil is a commonly used animal fat containing a large amount of n-3 polyunsaturated fatty acids. Soybean oil is a commonly used vegetable oil rich in linoleic acid. This study aimed to determine whether perilla, fish, or soybean oil can be used as a lipid source in the diet of the Chinese giant salamander (Andrias davidianus).
Methods: Three isonitrogenous and isolipidic diets were created by adding 3.0% each of soybean oil (SO), fish oil (FO), and perilla seed oil (PSO). Eight adolescent giant salamanders were fed in rectangular tanks for 98 days with a total of 96 giant salamanders (mean body weight = 75.20g) and were fed an experimental diet until satiation.
Results and Discussion: The viscerosomatic index and crude lipid content of muscle were found to be considerably lower and the muscle crude protein was substantially higher in the PSO group than in the other groups (P<0.05). Linoleic acid levels were highest in SO, eicosapentaenoic acid and docosahexaenoic acid levels were highest in FO, and α-linolenic acid levels were highest in PSO in A. davidianus muscle tissue (P<0.05). The results of study indicate that the PSO diet can significantly increase the total antioxidative capabilities in the liver and intestine, by elevating the activities of total superoxide dismutase, carnitine transferase-1, and acetyl-CoA carboxylase in the liver. Meanwhile, the immunoglobulin M and high-density lipoprotein cholesterol levels were higher in the blood (P<0.05). Furthermore, it significantly reduced hepatic malondialdehyde, plasma endotoxin, D-lactic acid, and total cholesterol levels, and plasma alkaline phosphatase, diamine oxidase, aspartate transaminase, and alanine transaminase activities (P<0.05). Therefore, perilla, fish, and soybean oils can be used as single lipid sources for A. davidianus with respect to growth performance. On the other hand, perilla oil can enhance crude protein content of muscle, increase Immunoglobulin M(IgM) content and Alkaline phosphatase(AKP) enzyme activity, promote lipid metabolism, and maintain the health of the liver and intestine in A. davidianus.
1 Introduction
Lipids are involved in several vital physiological activities, including providing energy for biological activity and essential fatty acids, building tissue, functioning as a carrier of lipid-soluble vitamins, and being a basic nutrient for animal growth (Segatto & Pallottini, 2020). An appropriate lipid source in the diet will help in saving protein, increasing growth performance and feed utilization efficiency, and managing immune and lipid metabolism in farm animals (Trichet, 2010).
Perilla seed oil is a natural oil produced from ripe perilla seeds and is rich in unsaturated fatty acids. Linoleic acid (C18:3n-3) is its most important component, accounting for more than 50% of the total content. PSO currently contains the most α-linolenic acid among all natural vegetable oils (Dhyani et al., 2019; Zhao et al., 2021), An important fatty acid found in freshwater fish. Desaturation and carbon chain extension can be used to transform it into long-chain, highly-unsaturated fatty acids. It is also important to the physiological, metabolic, and cytological processes in fish (Kanazawa et al., 1979). Perilla oil has great potential as a high-quality dietary oil source.
Fish oil is high in n-3 polyunsaturated fatty acids (such as eicosapentaenoic acid, EPA, and docosahexaenoic acid, DHA) (Ghasemi Fard et al., 2018), which promote the growth of aquaculture animals, improve muscle quality, boost immunity, etc(Sargent et al., 1999). It is one of the most important lipid sources in the aquatic feed sector (Peng et al., 2014). Soybean oil contains the highest amount (up to 57%) of linoleic acid (C18:2n-6), as compared to other natural vegetable oils (Ivanov et al., 2010). Many studies have proved that linoleic acid is one of the essential fatty acids of aquatic animals. Soybean oil, as one of the vegetable oils rich in linoleic acid, is widely used in aquatic animal feed. Like the shortfin corvina (Cynoscion parvipinnis), Nile tilapia (Oreochromis niloticus), largemouth bass (Micropterus salmoides) and other aquatic animals (Gonzalez-Felix et al., 2016; Godoy et al., 2019; Chen et al., 2020).
Giant salamander is a carnivorous, cold-water amphibian widely distributed in mountainous areas of China. Based on successful artificial breeding and feeding, our laboratory pioneered its special compound feed. Its tender meat, and high nutritional value, known as “ginseng in water”. It is a national second class protected aquatic wild animal, and also a key development species of agricultural industrialization and characteristic agriculture. (Wen-Bo et al., 2021) Chinese giant salamander has a high economic value, and has a wide range of development and utilization prospects in food, health care, medicine, ornamental and other aspects, so it is quite concerned by the community (Deng et al., 2019; Jiang et al., 2021). Currently, there has been a breakthrough in the availability and breeding of A. davidianus progeny; however, feed supply issues persist, and few studies have been conducted (Li et al., 2020).
As a protected animal, the Chinese giant salamander has achieved effective conservation and sustainable use of resources because of artificial breeding. With the continuous expansion of the scale of farming, the increase in breeding costs and the decline in commodity prices, The need for compound feed development and nutritional research has become urgent. To further optimize the feed formulation,this study aimed to determine perilla seed oil, fish oil and soybean oil the growth, lipid metabolism, oxidation state, and immunity of Andrias davidianus.
2 Material and methods
2.1 Experimental diet
The diets in this trial were prepared with fish and chicken meals as fundamental raw materials, in accordance with the nutritional demands of A. davidianus (Wang et al., 2020). PSO, FO, and SO constitute three isonitrogenous and isolipidic diet groups prepared by adding perilla, fish, or soybean oil as the only lipid source, respectively. A mixed pellet-making device crushed the raw materials using a 40-mesh screen and pelletized them into 4 mm-diameter particles. They were air-dried before being packaged, wrapped in double-layer plastic bags, and stored at -20°C until further use. The details of the experimental feed mix and fundamental nutritional makeup are listed in Table 1. Table 2 shows the fatty acid makeup of the various oil sources and diets.
2.2 Experimental procedure and samples collection
Juvenile A. davidianus were procured from the Luyuan Giant Salamander Farm in Hanzhong, Shaanxi Province, China (Shaanxi species, second generation, and in compliance with relevant breeding regulations), and allowed to rest for 2 days until the formal experiment. They were sterilized using 1% NaCl solution for 5 min and then acclimated with basic feed (Diet FO) in an indoor freshwater tank for 2 weeks. A total of 96 healthy A. davidianus with an initial body weight of 75.20 ± 1.18 g were randomly divided into three experimental groups. Each group was further divided into four replicates, with eight salamanders in each. They were reared in blue plastic square boxes (70×45×17.5 cm) from the Southwest University Aquaculture System of College of Fisheries. The water was thoroughly aerated and maintained at a depth of 5 cm. The water was refilled every day in the morning and evening. During the transient period, A. davidianus were fed only once a day at 17:00 until there were residual bait to determine the satiety level of A. davidianus. During the formal experiment, feeding was done for two days (satiety) and stopped for one day, as they need longer time to digest the food.Every day, residual baits were collected, and their weights were recorded. The A. davidianus were grown and fed the diets in darkness during the 12-week study, with water at 19-20°C, 6.5–7.5 pH, 5.5-6.0 mg/L of dissolved oxygen levels, and less than 0.1 mg/L of total ammonia levels.
As determined by our laboratory, the intestinal emptying time of A. davidianus was 4 days, therefore, A. davidianus were fasted for four days at the end of the route before sampling (Li et al., 2020). Only is presented a sampling at the end of the trial, 8 A. davidianus were randomly selected and anesthetized with MS-222 (75 mg/L; Sigma, St Louis, MO, USA) to collect blood from the abdominal artery using heparinized sterile syringes in each replicate. Blood samples were centrifuged for 10 minutes at 4°C and 1970xg(centrifuge model 5430 R; Eppendorf, Hamburg, Germany), and plasma was extracted and stored at −80°C until further analysis. Another 12 tails after anesthesia are taken to remove viscera.The liver was then excised. The stomach and intestine contents were emptied out and rinsed with phosphate-buffered saline (PBS) (0.064 mol/L, pH 7.4). The tissues were immediately frozen in liquid nitrogen and stored in a refrigerator at −80°C to evaluate tissue enzyme activity. Dorsal samples of the liver and muscle samples were obtained and stored at −20°C until future investigation. All tests were performed in accordance with China’s standard protocol for the care and use of experimental animals. This study was approved by the Animal Ethics Committee of the Southwest University.
2.3 Growth performance and morphologic indices
The growth parameters were calculated as follows:
Weight gain rate (WGR, %)=100×(final body weight, FBW-initial body weight, IBW)/initial body weight
Specific growth rate (SGR, %)=100×(Ln FBW-Ln IBW)/number of feeding days
Feed intake (FI, g/tail)=total feed intake (g)/number of A.davidianus
Feed conversion ratio (FCR)=dry diet intakefeed (g)/wet weight gain (g)
Survival (%)=100×(final number of A.davidianus)/(initial number of A.davidianus).
Viscerosomatic Index (VSI, %)=(weight of viscera/FBW)×100
Hepatosomatic Index (HSI, %)=(weight of liver/FBW)×100
Condition Factor (CF, %)=(weight of A.davidianus/body length3)×100
2.4 Determination of the nutrient composition of diets and muscle
Crude protein, lipid, ash, and moisture contents of diets and muscle were determined according to the methods described by the Association of Official Analytical Chemists, 2005 (AOAC) (Feldsine et al., 2002). Moisture content was determined by drying to constant weight at 105°C. Crude protein content (N×6.25) was determined by the Kjeldahl method using a nitrogen analyzer (KDN-812, Shanghai, China) after acid digestion. Lipid content was determined using petroleum ether (BP, at 30-60°C for 3 h) and a fat analyzer (SZC-D, Shanghai, China). Fatty acid composition of lipid sources, diets, and muscle was determined with the help of Baishilu Biotechnology Co., Ltd, Guangzhou, China.
2.5 Determination of plasma biochemical indexes
The activities of diamine oxidase (DAO), endotoxin (ET), D-lactic acid (D-LA), and immunoglobulin M (IgM) were measured by double antibody sandwich ELISA method(ELISA kits of Shanghai Enzyme-linked Biotechnology). Plasma aspartate transaminase (AST), alanine transaminase (ALT), alkaline phosphatase (ALP), triglyceride (TG), total cholesterol (TC), high-density lipoprotein cholesterol (HDL-C), low-density lipoprotein cholesterol (LDL-C) levels were measured by Multifunctional enzyme marker (Multiskan Sky, China) using a commercial test kit (Nanjing Jiancheng Institute of Biological Engineering) according to the manufacturer’s instructions.
2.6 Determination of antioxidant enzymes in liver, stomach, and intestinal tissues
Total protein(TP) was determined by coomassie blue staining method (Deng et al., 2013). Total antioxidant capacity (T-AOC) and total superoxide dismutase (T-SOD) of liver, stomach and intestine were measured according to the method described by (Wei et al., 2010); malondialdehyde (MDA) levels were measured according to the method of (Loh et al., 2010), all using a commercial kit (Nanjing Jiancheng Bioengineering Institute, Nanjing, China). The unit of TAOC is (U/mgprot), (U) is a unit of total antioxidant capacity (U) per milligram of tissue protein per minute at 37°C, which increases the absorbance (OD) value of the reaction system by 0.01.Carnitine palmitoyltransferase-1 (CPT-1), and acetyl-CoA carboxylase (ACC) activities in liver were detected by enzyme-linked immunosorbent assay (ELISA kits of Shanghai Enzyme-linked Biotechnology).
2.7 Statistical analysis
All data were subjected to a one-way analysis of variance (ANOVA) after Duncan’s multiple range test. P<0.05 was considered statistically significant, and the results were expressed as mean ± standard deviation (SD) of quadruplicate tanks. All statistical analyses were performed using SPSS 22.0 for Windows (v. 13.0; SPSS).
3 Results
3.1 Effects of three kinds of lipid source on growth performance and morphologic index of A. davidianus
The effects of the three kinds of lipid sources on the growth performance and morphologic index of A. davidianus are shown in Table 3. FBW, WGR, SGR, FCR, HSI, CF, and the survival rate were not significantly different among treatments (P>0.05). The VSI of PSO was significantly lower than that of SO and FO (P<0.05).
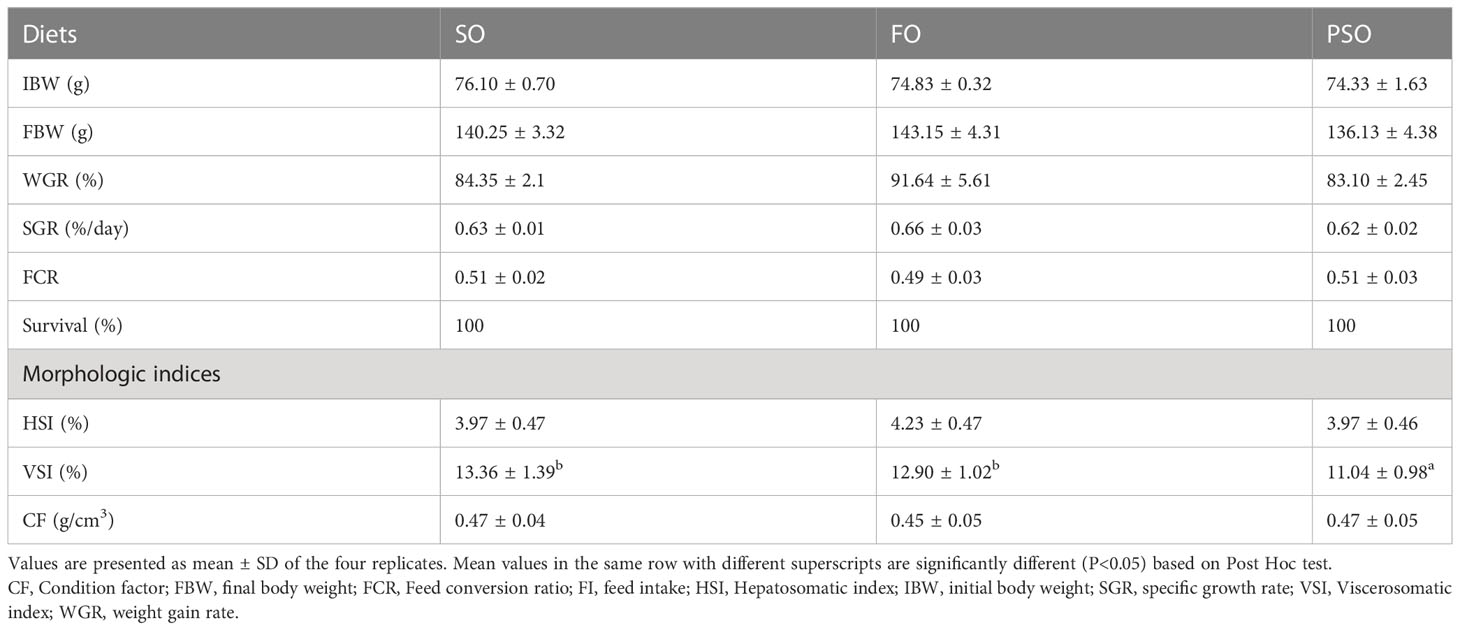
Table 3 Effects of three kinds of lipid source on growth performance and morphologic indices of Andrias davidianus.
3.2 Effects of three kinds of lipid source on composition of nutrients in muscle of A. davidianus
Crude protein content of muscle in PSO was significantly higher than that in SO and FO (P<0.05) (Table 4). Crude lipid content of muscle was significantly lower than that of SO but was not significantly different between PSO and FO (P>0.05). The moisture content in muscles was not significantly different between the groups (P>0.05).
3.3 Effects of three kinds of lipid source on muscle fatty acid of A. davidianus
The effects of the three lipid sources on the muscle fatty acids of A. davidianus are shown in Table 5. The monounsaturated fatty acid(NUFA) content in the PSO group was significantly higher than that in the FO group (P<0.05), but the difference between the SO and FO groups was not significant. The polyunsaturated fatty acid content in SO was higher than that in PSO and FO (P<0.05). The level of C18:2n-6 in SO was significantly higher than that in the other groups (P<0.05), while that of C18:3n-3 in PSO was significantly higher than that in SO and FO (P<0.05), indicating improvement of 235.59% and 318.31%, respectively. EPA and DHA were the highest in FO, EPA was significantly higher in FO than in other groups (P<0.05), and DHA was significantly higher in FO than in PSO (P<0.05). However, the difference between FO and SO was not significant (P>0.05). The n-3 and n-6 polyunsaturated fatty acids (PUFAs) were higher in PSO and SO than in the other two groups, respectively (P<0.05). Regression and correlation analyses of muscle fatty acid (Yi) and the corresponding dietary fatty acid (Xi) from each treatment showed that there was a highly significant linear correlation between muscle fatty acid composition and forage fatty acid composition in the SO and FO groups, with correlation coefficients of 0.920 and 0.962, respectively, and relatively poor in PSO (Table 6).
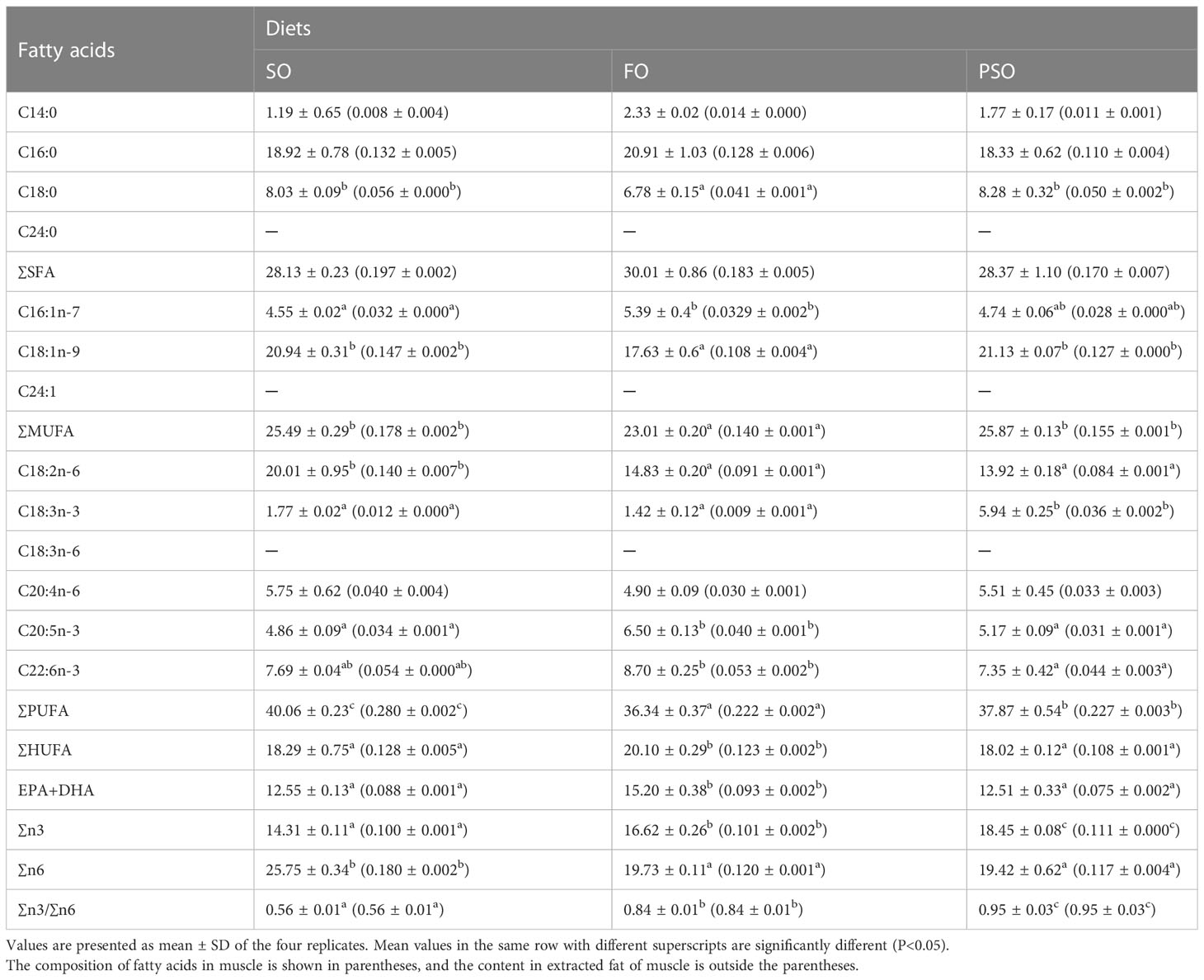
Table 5 Effects of three kinds of lipid source on muscle fatty acid (total fatty acids, %) of A. davidianus.

Table 6 Regression and correlation analysis of essential fatty acids between muscle and experimental diets.
3.4 Effects of three kinds of lipid source on antioxidant ability of A. davidianus
The effects of the three lipid sources on the antioxidant ability of A. davidianus are shown in Figure 1. The activities of liver T-AOC and T-SOD in PSO were significantly higher than those in SO (P<0.05). The MDA content in FO was significantly lower than that in SO (P<0.05), but was not significantly different as compared to PSO (P>0.05). The order of T-SOD activity in gastric tissue is FO>PSO>SO, and with significant differences among treatment groups.T-AOC of gastric tissue in FO group was remarkably higher than that in SO group(P<0.05), but there was no significant difference between FO and PSO (P>0.05).The MDA content was not significantly different among the three treatment groups (P>0.05). T-AOC of intestinal tract was significantly higher in PSO than in SO (P<0.05), but not significantly different between PSO and FO (P>0.05). T-SOD activity in FO group was significantly higher than that in the other treatment groups (P<0.05). There was no significant difference in MDA content among all groups (P>0.05).
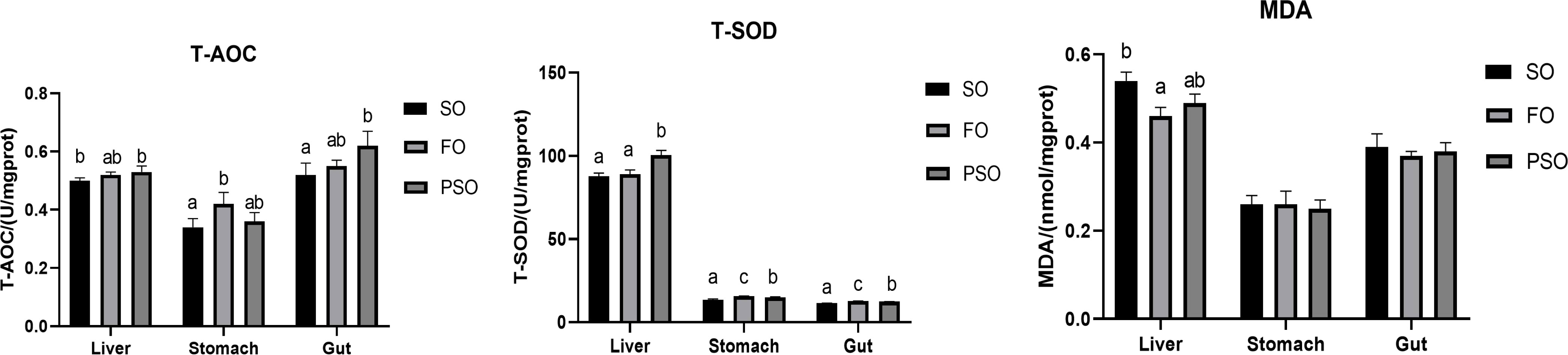
Figure 1 Effects of three kinds of lipid source on antioxidant ability of A. davidianus. Values in each column with different superscripts have significant differences (p< 0.05). T-AOC, Total antioxidant capacity; T-SOD, Total superoxide dismutase; MDA, Malondialdehyde.
3.5 Effects of three kinds of lipid source on immunity and liver function of A. davidianus
As shown in Figure 2, in plasma biochemical indices, IgM content in PSO was significantly different from that in SO and FO (P<0.05), increasing by 51.83% and 21.88%, respectively.The activity of ALP was significantly lower in PSO than that in SO (P<0.05), but was not significantly different between PSO and FO. The activity of ALT was significantly lower in PSO than that in FO (P<0.05), but was not significantly different between PSO and SO. The activity of AST was significantly lower in PSO than that in SO and FO (P<0.05).
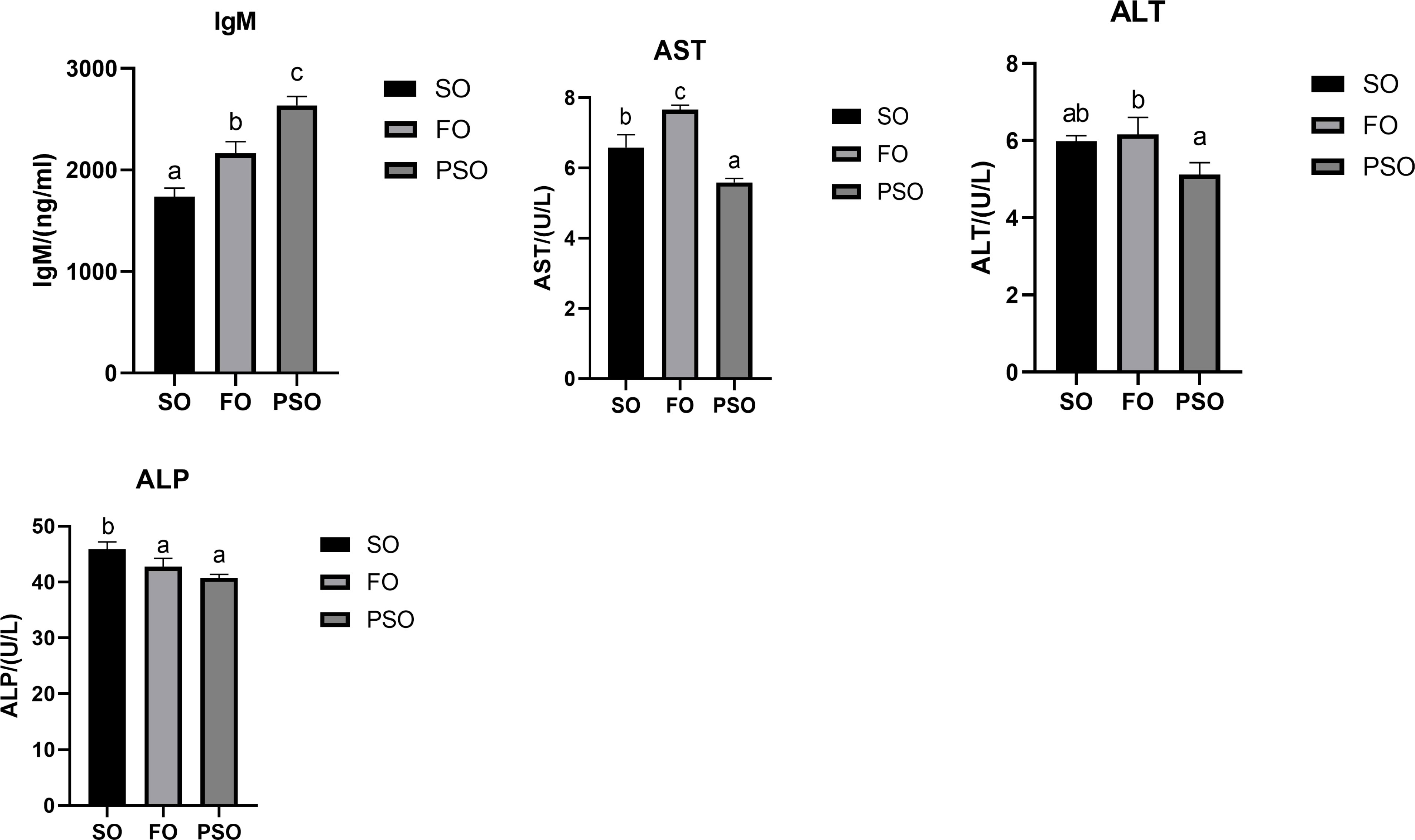
Figure 2 Effects of three kinds of lipid source on plasma immune indices of A.davidianus. Values in each column with different superscripts have significant differences (p< 0.05). IgM, Immunoglobulin M; AST, Aspartate aminotransferase; ALT, Alanine aminotransferase; ALP, Alkaline phosphatase.
3.6 Effects of three kinds of lipid source on intestinal mucosal permeability of A. davidianus
The content of ET and D-LA, and the activity of DAO in PSO were significantly reduced by 22.12% and 14.43%, respectively (P<0.05) (Table 7), and that of D-LA was significantly lower (P<0.05) than SO and FO by 23.27% and 11.27%, respectively (Table 7).
3.7 Effects of three kinds of lipid source on lipid metabolism of A. davidianus
The effects of the three lipid sources on A. davidianus lipid metabolism are shown in Table 8. In plasma biochemical indices,the TC and TG contents in FO were significantly lower than those in SO (P<0.05), but not significantly different between SO and PSO (P>0.05). HDL-C level was significantly higher in PSO than in SO (P<0.05). The LDL-C levels were not significantly different between the treatment groups (P > 0.05). In the liver, the activities of CPT-I and ACC were highest in PSO(P<0.05). In addition, the former’s activity increased by 96.59% and 32.84%, and that of the latter by 59.86% and 24.94%, as compared to SO and FO, respectively (P<0.05).
4 Discussion
The difference between different types of lipid lies in fatty acid composition, fat structure, processing methods, etc. The lipid source selection in aquatic animal feed often depends on the utilization ability of different fatty acids.In the experiment of using different Marine lipid sources on the growth performance of Large Yellow Croaker(Larimichthys crocea) (Yao et al., 2022), replacing fish oil with Schizochytrium sp.oil(SSO) will have an inhibitory effect on appetite and growth of young large yellow croakers.In a study on the growth performance of spotted sea bass (Lateolabrax maculatus) using different dietary fat sources (Jin et al., 2023), compared with FO, the 16:0, 18:0, 18:1n-9, 18:2n-6 and 18:3n-3 series of fatty acids were more present in vegetable oil. The final results showed that substituting FO with vegetable oil did not have any negative effect on the growth performance of spotted sea bass. Similar results were obtained in the study (Liang et al., 2022). When coconut oil (CO), rapeseed oil (RO), linseed oil (LO) and FO were used as lipid sources, there were no significant differences in FBW, SGR, FE, survival rate, HIS and VSI of fish among all groups. This may be because LO is rich in C18:3n-3, which can be used well by freshwater fish.RO is rich in 18:1n-9 and 18:2n-6 essential fatty acids of freshwater fish, and CO is rich in medium chain fatty acids (C10:0, C12:0 and C14:0), which can regulate lipid metabolism. Therefore, replacing FO with CO, RO and LO will not affect the growth performance of Micropterus salmoides.In our study, the protein and lipid contents of the experimental diets were approximately the same, which all met the basic nutritional requirements of juvenile giant salamander. After the end of breeding, no obvious fatty acid deficiency symptoms and no significant difference in growth performance were found in all experimental groups. According to the fatty acids content of the three oils identified in this study, Perilla oil is rich in alpha-linolenic acid, soybean oil is rich in linoleic acid, and fish oil is rich in EPA, DHA and other highly unsaturated fatty acids. Therefore, the authors suggested that the giant salamander had the ability to convert alpha-linolenic acid (ALA) and linoleic acid (LA) into long-chain polyunsaturated fatty acids (LC-PUFA).A vital method for animals to meet their requirements of essential fatty acids is through feeding. Animals digest dietary lipids and break them down into fatty acids, which are then used to resynthesize their own fat. Dietary fatty acid composition can affect fatty acid composition in animal muscles (Ji et al., 2011; Li et al., 2016), which has also been confirmed by the present study. In this experiment, after feeding A. davidianus a diet containing different oils for 14 weeks, the muscle fatty acids showed a significant difference. The α-linolenic acid content of the muscles in PSO was much higher than that in the other two groups. The α-linolenic acid is an essential unsaturated fatty acid for humans, is known as vitamin F, is an important structural and metabolic regulatory substance, and can also be converted into DHA, DPA, EPA, etc. to maintain normal physiological functions of the body (Roesch et al., 2007). The muscles of A. davidianus that are rich in linolenic acid exhibit better quality and meet human nutritional needs well. The highest content of linoleic acid was observed in SO, and that of EPA and DHA in FO because of their possible role in the formation of cell membranes, effect on the activity of some enzymes and expression of some genes, and the organisms’ preference for it to be deposited and stored. While there was a significant linear correlation between muscle fatty acid composition and dietary fatty acids in SO and FO, the correlation in PSO was poor, possibly due to the high content of α-linolenic acid in the diet. A. davidianus was unable to absorb and utilize the linolenic acid completely, resulting in its wastage and requiring further analysis of its optimal amount in the diet. Overall, the difference in fatty acids in A. davidianus muscle was less amongst its diet groups of the three treatments, indicating that it can selectively deposit or utilize fatty acids from diets.
Perilla oil is rich in α-linolenic acid and its blood lipid-lowering effects have been previously described extensively (Winnik et al., 2011; Tsukamoto & Sugawara, 2018). It reduces 3-hydroxy-3-methylglutaryl-coenzyme A reductase (HMG COA) activity, enhances acyl-coenzyme A cholesterol acyltransferase (ACAT) activity, inhibits endogenous cholesterol synthesis, and lowers blood plasma cholesterol levels by reducing triglycerides and apolipoprotein B of very low-density lipoprotein biosynthesis to reduce serum triglyceride levels. Simultaneously, the breakdown of the low-density lipoprotein cholesterol (LDL-C) and high-density lipoprotein cholesterol (HDL-C) synthesis mechanisms exhibited an efficient inhibitory effect, thereby improving the body’s lipid metabolism. EPA and DHA can also reduce the synthesis of endogenous cholesterol and inhibit triglycerides, lowering the levels of arterial stiffness factor (cholesterol, triglycerides, and low-density lipoprotein). Lecithin cholesterol transfer improves lipoprotein enzyme activity, inhibition of liver endothelial lipase activity, and resistance to hardening of arterial factor (HDL) to improve lipid metabolism. In this study, the lipid-lowering effects of perilla and fish oils were also elucidated, and the lipid contents in PSO and FO were lower than those in SO. The key rate-limiting enzymes of fatty acid degradation (CPT-1) and synthesis (ACC) in the liver increased as SO>FO> PSO. However, the rate of increase of CPT-1 was significantly higher than that of ACC, suggesting that the liver lipid deposition in FO and PSO was lower than that in SO, and that perilla oil and fish oil can reduce liver fat deposition and improve liver health, which is also reflected in the classic assessment indicators of liver health (plasma AST and ALT activities). In addition, the muscle fat measurement results showed that the fish and perilla oils also reduced the amount of fat deposited in muscle tissue.
Lipids, particularly PUFAs, contain multiple double bonds and are easily oxidized by free radicals to produce several harmful substances (Tocher et al., 2002). Studies have shown that superoxide dismutase(SOD) and catalase (CAT) are two important free radical scavenging enzymes in the cellular antioxidant system. The body can reduce the damage caused by peroxidation by scavenging the oxygen free radicals produced by SOD, CAT and other enzymes (Mourente et al., 2007). The addition of different types of fatty acids to the diet has different effects on the antioxidant system of the body. Perilla oil had the highest content of PUFAs amongst the three oils used in this study, followed by soybean oil, with the lowest content in fish oil. However, according to the antioxidant indices obtained from the tissues of the three groups in this study, the antioxidant capacity of PSO was the highest, followed by FO, and that of SO was relatively poor. This may be due to the freshness of the oil used in this experiment and the addition of sufficient antioxidants to the feed, ensuring that the level of oxidation in the feed was low before it was ingested by A. davidianus.
Additionally, the antioxidant activity of lipids is closely related to the position of the double bond. A previous study has shown that n-3 PUFA can react with oxidative free radicals in the organism and protect it from oxidative damage (Collison et al., 2005). Chen also reached a similar conclusion in the study of black carp(Mylopharyngodon piceus)(Yanting et al., 2022). The experimental results show that the diet with rapeseed oil as fat source is rich in n-3 LC-PUFA, which can inhibit the production of H2O2 in cells through the nuclear factor-related factor 2 (Nrf2) pathway, so as to improve the antioxidant capacity of black carp.In this experiment, the percentage of n-3 PUFA in perilla oil was the highest due to the abundance of α-linolenic acid, fish oil was also rich in EPA, DHA, and other n-3 PUFA, and the linoleic acid in soybean oil was n-6 PUFA, which is a possible reason for the difference in antioxidant performance among the three treatment groups of A. davidianus.The immunity of cultured animals is affected by the type, dosage, and ratio of dietary fatty acids. In fish, appropriate levels of PUFAs, especially n-3 PUFA, can significantly improve immunity (Wang et al., 2006). The study of Ferreira (Ferreira et al., 2015) showed that linseed oil(LO), which is rich in n-3 PUFA, had immunomodulatory effects on juvenile Nile tilapia when added to the low-fat diet. In addition, some studies have shown that EPA and DHA in diets can also significantly affect specific and non-specific immunity of fish (Puangkaew et al., 2004) and the expression of immune-related genes (Zuo et al., 2012). IgM is an immunoglobulin present in body fluids, which plays an important role in humoral immune response (Awad et al., 2015). In this experiment, the level of IgM in PSO group was the highest, followed by FO group, and the lowest in SO group, which was the same as the content trend of n-3PUFA in the experimental feed group. Therefore, we suggest that n-3PUFA can enhance the immune function of A. davidianus, and the ability of A. davidianus to convert α-linolenic acid into EPA and DHA may be one of the reasons for the highest IgM levels in the PSO group, but the mechanism of action needs to be further investigated.A previous study found that n-3 PUFA can reduce intestinal inflammation (Calder, 2008), promote repair of the intestinal mucosa, and improve intestinal barrier function. Plasma levels of endotoxin, diamine oxidase, and D-lactic acid are three indicators of gut barrier function, and we speculate their changes to also be related to n-3 PUFA levels in the experimental diets of this study.
5 Conclusion
Perilla, fish, and soybean oils can all be used as a single dietary lipid source for A. davidianus when growth performance is used as a reference. Perilla oil can enhance muscle quality and antioxidant capacity, boost immunity, promote lipid metabolism, and maintain liver and intestinal health as fat source of A. davidianus compound feed. Therefore, we strongly recommend the addition of perilla oil to their diet.
Data availability statement
The original contributions presented in the study are included in the article/supplementary material. Further inquiries can be directed to the corresponding author.
Ethics statement
The animal study was reviewed and approved by Southwest University’s Animal Ethics Committee.
Author contributions
The authors confirm contribution to the paper as follows: LL: study conception and design. Y-HG: performed the experiment, analyzed the data, writing—original draft, writing—review and editing. J-KM: performed the experiment, analyzed the data, writing—original draft, writing. H-ZX, W-LL, C-JL and HLu: performed the experiment and writing—review and editing. HLi: funding acquisition. All authors reviewed the results and approved the final version of the manuscript. All authors contributed to the article and approved the submitted version.
Funding
The funding for this paper is supported by the Chongqing Eco-Fishery Industry innovation Technology System Project (2016-2022) and Chongqing Science and Technology Bureau “Social Rural Science and Technology Innovation Project -Chinese Giant Salamander Artificial Compound Feed Manufacturing and Breeding Demonstration” (cstc2016shmszx80084).
Acknowledgments
The authors are grateful for the financial support, the demonstration and promotion of compound feed and breeding technology of giant salamander from Chongqing Aquatic Technology Extension Station; The authors also would like to thank Editage (www.editage.com) for English language editing.
Conflict of interest
The authors declare that the research was conducted in the absence of any commercial or financial relationships that could be construed as a potential conflict of interest.
Publisher’s note
All claims expressed in this article are solely those of the authors and do not necessarily represent those of their affiliated organizations, or those of the publisher, the editors and the reviewers. Any product that may be evaluated in this article, or claim that may be made by its manufacturer, is not guaranteed or endorsed by the publisher.
References
Awad E., Cerezuela R., Esteban M. A. (2015). Effects of fenugreek (Trigonella foenum graecum) on gilthead seabream (Sparus aurata l.) immune status and growth performance. Fish Shellfish Immunol. 45 (2), 454–464. doi: 10.1016/j.fsi.2015.04.035
Calder P. C. (2008). Polyunsaturated fatty acids, inflammatory processes and inflammatory bowel diseases. Mol. Nutr. Food Res. 52 (8), 885–897. doi: 10.1002/mnfr.200700289
Chen Y. F., Sun Z. Z., Liang Z. M., Xie Y. D., Su J. L., Luo Q. L., et al. (2020). Effects of dietary fish oil replacement by soybean oil and l-carnitine supplementation on growth performance, fatty acid composition, lipid metabolism and liver health of juvenile largemouth bass, micropterus salmoides. Aquaculture 516 (734596). doi: 10.1016/j.aquaculture.2019.734596
Collison L. W., Collison R. E., Murphy E. J., Jolly C. A. (2005). Dietary n-3 polyunsaturated fatty acids increase T-lymphocyte phospholipid mass and acyl-CoA binding protein expression. Lipids 40 (1), 81–87. doi: 10.1007/s11745-005-1362-8
Coutinho F., Peres H., Guerreiro I., Pousao-Ferreira P., Oliva-Teles A. (2012). Dietary protein requirement of sharpsnout sea bream (Diplodus puntazzo, cetti 1777) juveniles. Aquaculture 356, 391–397. doi: 10.1016/j.aquaculture.2012.04.037
Deng J., Tang Y. Y., Zhang Q., Wang C., Liao M., Ji P., et al. (2019). A bioinspired medical adhesive derived from skin secretion of andrias davidianus for wound healing. Adv Funct. Mater 29 (31), 13. doi: 10.1002/adfm.201809110
Deng Y., Wang W., Yu P. F., Xi Z. J., Xu L. J., Li X. L., et al. (2013). Comparison of taurine, GABA, glu, and asp as scavengers of malondialdehyde in vitro and in vivo. Nanoscale Res. Lett. 8(9), 190. doi: 10.1186/1556-276x-8-190
Dhyani A., Chopra R., Garg M. (2019). A review on nutritional value, functional properties and pharmacological application of perilla (Perilla frutescens l.). Biomed. Pharmacol. J. 12 (2), 649–660. doi: 10.13005/bpj/1685
Feldsine P., Abeyta C., Andrews W. H. (2002). AOAC International methods committee guidelines for validation of qualitative and quantitative food microbiological official methods of analysis. J. AOAC Int. 85(5). doi: 10.1016/0924-2244(95)90022-5
Ferreira M. W., da Costa D. V., Leal C. A. G., Figueiredo H. C. P., Rosa P. V. (2015). Dietary oil sources on the innate immunity and resistance of Nile tilapia, oreochromis niloticus, to streptococcus agalactiae challenge. J. World Aquacult Soc. 46 (3), 252–262. doi: 10.1111/jwas.12192
Ghasemi Fard S., Wang F., Sinclair A. J., Elliott G., Turchini G. M. (2018). How does high DHA fish oil affect health? a systematic review of evidence. Crit. Rev. Food Sci. Nutr. 59 (11), 1684–1727. doi: 10.1080/10408398.2018.1425978
Godoy A. C., Santos O. O., Oxford J. H., Melo I. W. D., Rodrigues R. B., Neu D., et al. (2019). Soybean oil for Nile tilapia (Oreochromis niloticus) in finishing diets: economic, zootechnical and nutritional meat improvements. Aquaculture 512 (10), 734324. doi: 10.1016/j.aquaculture.2019.734324
Gonzalez-Felix M. L., Maldonado-Othon C. A., Perez-Velazquez M. (2016). Effect of dietary lipid level and replacement of fish oil by soybean oil in compound feeds for the shortfin corvina (Cynoscion parvipinnis). Aquaculture 454, 217–228. doi: 10.1016/j.aquaculture.2015.12.021
Ivanov D. S., Lević J. D., Sredanović S. A. (2010). Fatty acid composition of various soybean products. Food Feed Res. 37 (2), 65–70-65-70.
Ji H., Li J., Liu P. (2011). Regulation of growth performance and lipid metabolism by dietary n-3 highly unsaturated fatty acids in juvenile grass carp, ctenopharyngodon idellus. Comp. Biochem. Physiol. Part B: Biochem. Mol. Biol. 159 (1), 49–56. doi: 10.1016/j.cbpb.2011.01.009
Jiang N., Fan Y. D., Zhou Y., Meng Y., Liu W. Z., Li Y. Q., et al. (2021). The immune system and the antiviral responses in Chinese giant salamander, andrias davidianus. Front. Immunol. 12 (12). doi: 10.3389/fimmu.2021.718627
Jin G. X., Zhang L., Mai K. S., Chen X. R., Xu S. D., Ai Q. H. (2023). Effects of different dietary lipid sources on growth performance, hepatic lipid deposition and transcriptome response in spotted sea bass (Lateolabrax maculatus). Aquaculture 566 (10), 739143. doi: 10.1016/j.aquaculture.2022.739143
Kanazawa A., Teshima S. I., Ono K. (1979). Relationship between essential fatty-acid requirements of aquatic animals and the capacity for bioconversion of linolenic acid to highly unsaturated fatty-acids. Comp. Biochem. Physiol. B-Biochem Mol. Biol. 63 (3), 295–298. doi: 10.1016/0305-0491(79)90251-7
Li Z. F., Chen X. C., Chen Y. J., Li W. L., Feng Q. F., Zhang H. D., et al. (2020). Effects of dietary mulberry leaf extract on the growth, gastrointestinal, hepatic functions of Chinese giant salamander (Andrias davidianus). Aquacult Res. 51 (6), 2613–2623. doi: 10.1111/are.14639
Li F. J., Lin X., Lin S. M., Chen W. Y., Guan Y. (2016). Effects of dietary fish oil substitution with linseed oil on growth, muscle fatty acid and metabolism of tilapia (Oreochromis niloticus). Aquacult Nutr. 22 (3), 499–508. doi: 10.1111/anu.12270
Liang C., Zhao X. Y., Jiao L. F., Shen Y. D., Luo J. X., Zhu T. T., et al. (2022). Effects of different lipid sources on growth performance, fatty acids composition in tissue and expression of genes related to lipid metabolism in largemouth bass (Micropterus salmoides). Aquacult Rep. 23 (8). doi: 10.1016/j.aqrep.2022.101013
Loh K. P., Qi J., Tan B. K. H., Liu X. H., Wei B. G., Zhu Y. Z. (2010). Leonurine protects middle cerebral artery occluded rats through antioxidant effect and regulation of mitochondrial function. Stroke 41 (11), 2661–2668. doi: 10.1161/strokeaha.110.589895
Mourente G., Bell J. G., Tocher D. R. (2007). Does dietary tocopherol level affect fatty acid metabolism in fish? Fish Physiol. Biochem. 33 (3), 269–280. doi: 10.1007/s10695-007-9139-4
Peng M., Xu W., Mai K. S., Zhou H. H., Zhang Y. J., Liufu Z. G., et al. (2014). Growth performance, lipid deposition and hepatic lipid metabolism related gene expression in juvenile turbot (Scophthalmus maximus l.) fed diets with various fish oil substitution levels by soybean oil. Aquaculture 433, 442–449. doi: 10.1016/j.aquaculture.2014.07.005
Puangkaew J., Kiron V., Somamoto T., Okamoto N., Satoh S., Takeuchi T., et al. (2004). Nonspecific immune response of rainbow trout (Oncorhynchus mykiss walbaum) in relation to different status of vitamin e and highly unsaturated fatty acids. Fish Shellfish Immunol. 16 (1), 25–39. doi: 10.1016/s1050-4648(03)00028-7
Roesch L. F., Fulthorpe R. R., Riva A., Casella G., Hadwin A. K. M., Kent A. D., et al. (2007). Pyrosequencing enumerates and contrasts soil microbial diversity. Isme J. 1 (4), 283–290. doi: 10.1038/ismej.2007.53
Sargent J., Bell G., McEvoy L., Tocher D., Estevez A. (1999). Recent developments in the essential fatty acid nutrition of fish. Aquaculture 177 (1-4), 191–199. doi: 10.1016/s0044-8486(99)00083-6
Segatto M., Pallottini V. (2020). Facts about fats: new insights into the role of lipids in metabolism, disease and therapy. Int. J. Mol. Sci. 21 (18), 5. doi: 10.3390/ijms21186651
Tocher D. R., Mourente G., van der Eecken A., Evjemo J. O., Diaz E., Bell J. G., et al. (2002). Effects of dietary vitamin e on antioxidant defence mechanisms of juvenile turbot (Scophthalmus maximus l.), halibut (Hippoglossus hippoglossus l.) and sea bream (Sparus aurata l.). Aquacult Nutr. 8 (3), 195–207. doi: 10.1046/j.1365-2095.2002.00205.x
Trichet V. V. (2010). Nutrition and immunity: an update. Aquacult Res. 41 (3), 356–372. doi: 10.1111/j.1365-2109.2009.02374.x
Tsukamoto I., Sugawara S. (2018). Low levels of linoleic acid and α-linolenic acid and high levels of arachidonic acid in plasma phospholipids are associated with hypertension. Biomed. Rep. 8 (1), 69–76. doi: 10.3892/br.2018.1056
Wang S., Li Z., Li H., Li X., Lei D., Zhu C., et al. (2020). Dietary protein requirement of juvenile giant salamander (Andrias davidianus). J. Fish China 44 (01), 99–110. doi: 10.11964/jfc.20181111533
Wang Z. L., Mai K. S., Liufu Z. G., Ma H. M., Xu W., Ai Q. H., et al. (2006). Effect of high dietary intakes of vitamin e and n-3 HUFA on immune responses and resistance to edwardsiella tarda challenge in Japanese flounder (Paralichthys olivaceus, temminck and schlegel). Aquacult Res. 37 (7), 681–692. doi: 10.1111/j.1365-2109.2006.01481.x
Wei M., Wu Y., Chen D., Gu Y. (2010). Changes of free radicals and digestive enzymes in saliva in cases with deficiency in spleen-yin syndrome. J. Biomed. Res. 24 (3), 250–255. doi: 10.1016/s1674-8301(10)60035-8
Wen-Bo W., Pin L., Ling-Ling D., Ping W., Yue N., Rui-Min X., et al. (2021). A survey of Chinese giant salamander (Andrias davidianus). Acta Hydrobiol Sin. 45 (02), 464–472. doi: 10.7541/2021.2020.184
Winnik S., Lohmann C., Richter E. K., Schäfer N., Song W.-L., Leiber F., et al. (2011). Dietary α-linolenic acid diminishes experimental atherogenesis and restricts T cell-driven inflammation. Eur. Heart J. 32 (20), 2573–2584. doi: 10.1093/eurheartj/ehq501
Yanting C., Xiaowei J., Pengcheng Q., Chen Z., Chenglong W., Jinyun Y. (2022). Effects of dietary different lipid sources on growth performance, serum biochemical parameters and muscle quality of black carp (Mylopharyngodon piceus). Chin. J. Anim. Nutr. 34 (07), 4657–4673. doi: 10.3969/j.issn.1006-267x.2022.07.053
Yao C. W., Huang W. X., Liu Y. T., Miao Y. Q., Yin Z. Y., Mai K. S., et al. (2022). Effects of different marine-based lipid sources on growth performance, activities of digestive enzyme, antioxidant responses, and lipid metabolism of Large yellow croaker (Larimichthys crocea) larvae. Aquacult Nutr. 2022, 12. doi: 10.1155/2022/6314978
Zhao B. B., Fu S. J., Li H., Chen Z. C. (2021). Chemical characterization of Chinese perilla seed oil. J. Oleo Sci. 70 (11), 1575–1583. doi: 10.5650/jos.ess21076
Zuo R. T., Ai Q. H., Mai K. S., Xu W., Wang J., Xu H. G., et al. (2012). Effects of dietary n-3 highly unsaturated fatty acids on growth, nonspecific immunity, expression of some immune related genes and disease resistance of large yellow croaker (Larmichthys crocea) following natural infestation of parasites (Cryptocaryon irritans). Fish Shellfish Immunol. 32 (2), 249–258. doi: 10.1016/j.fsi.2011.11.005
Keywords: Andrias davidianus, antioxidant, fatty acid composition, growth performance, immunity, perilla oil
Citation: Guo Y-H, Ma J-K, Xu H-z, Li W-l, Liu C-J, Lu H, Li H, Zhai X-l, Xue Y and Luo L (2023) Effects of different dietary lipid sources (perilla, fish, and soybean oils) on growth, lipid metabolism, antioxidant, and immune status in Chinese giant salamander (Andrias davidianus). Front. Mar. Sci. 10:1139651. doi: 10.3389/fmars.2023.1139651
Received: 07 January 2023; Accepted: 03 May 2023;
Published: 18 May 2023.
Edited by:
Leila Ktari, Institut National des Sciences et Technologies de la Mer, TunisiaReviewed by:
Amalia Pérez-Jiménez, University of Granada, SpainImtiaz Ahmed, University of Kashmir, India
Seyed Pezhman Hosseini Shekarabi, Islamic Azad University, Iran
Copyright © 2023 Guo, Ma, Xu, Li, Liu, Lu, Li, Zhai, Xue and Luo. This is an open-access article distributed under the terms of the Creative Commons Attribution License (CC BY). The use, distribution or reproduction in other forums is permitted, provided the original author(s) and the copyright owner(s) are credited and that the original publication in this journal is cited, in accordance with accepted academic practice. No use, distribution or reproduction is permitted which does not comply with these terms.
*Correspondence: Li Luo, bHVvbGkxOTcyQDE2My5jb20=