- 1AAssociação BIOPOLIS, Centro de Investigação em Biodiversidade e Recursos Genéticos, Laboratório Associado (CIBIO-InBIO), University of Porto, Vairão, Portugal
- 2Seahorse, Pipefish and Seadragon Specialist Group, International Union for Conservation of Nature (IUCN) Species Survival Commission, Gland, Switzerland
- 3Department of Biology, Faculty of Sciences, University of Porto, Porto, Portugal
- 4Project Seahorse, Institute for the Oceans and Fisheries, The University of British Columbia, Vancouver, BC, Canada
At the latitudinal interval that encompasses Europe, due to the gradual seawater warming imposed by climate change, there is now continuing evidence for a widespread pattern of poleward range shifts, often translated by expansions in the northern leading edges of distribution and contraction episodes at the southern boundaries. As the distribution of biodiversity is far from static, it seems imperative that we can predict changes in species’ geographic ranges to potentially circumvent severe biodiversity losses in the near future. Here, we focus on syngnathids, flagship species and umbrella taxa for coastal marine ecosystems, and attempt to identify areas of environmental suitability in Europe over the next century, according to distinct greenhouse gas concentration trajectories. Our results seamlessly match the general and region-specific impacts of climate change projections for European seas. Especially within the Atlantic Coast, species are expected to continue poleward range shifts, with the hotspot of syngnathid diversity remaining around the Celtic-Biscay Shelf, albeit with slightly distinct species composition from that of present day. The most dramatic changes are expected within semi-enclosed areas such as the Baltic, the Mediterranean and the Black Sea, where present-day endemic species diversity is likely to plunge, especially under a ‘business-as-usual’ scenario. When looking at the potential protective role of ‘no-take’ marine protected areas (MPAs) on European syngnathids, a clear latitudinal asymmetry emerged. From the Atlantic diversity hotspot downwards, and especially in the Mediterranean and Black Sea, the current ‘no-take’ MPA layout, if it remains static, will not be especially effective in either safeguarding syngnathid diversity or delaying the expected gradual species disappearance. Europe needs to be prepared to implement, at a continental scale, coordinated conservation measures aimed at preventing (or delaying, at the very least) the loss of its marine endemic species. As umbrella species, conservation efforts aimed at syngnathids will surely have a positive impact on coastal ecosystems.
1 Introduction
Climate change is impacting all studied ecosystems, terrestrial and aquatic, with animals and plants alike forced to experience environments swiftly diverging from the physiological tolerance limits of the species they harbour (Parmesan, 2006; Doney et al., 2012; Pecl et al., 2017). As environmental change progresses faster than species can adapt, a wave of range shifts and extinction episodes ensues (Loarie et al., 2009; Burrows et al., 2011). Especially noticeable at the latitudinal interval that encompasses Europe, range shifts are being continuously documented in a broad variety of taxa, from algae to mammals (Lenoir and Svenning, 2015). At these latitudes, there is now recurrent evidence for a widespread pattern of poleward range shifts, often translated by expansions in the leading edges of distribution and extinction episodes at the trailing edges (e.g., Parmesan et al., 1999; Hickling et al., 2006).
Given that - now probably more than ever - the distribution of biodiversity is far from static, it seems imperative that we can predict changes in species’ geographic ranges to minimize severe biodiversity losses in the near future (Moritz and Agudo, 2013). It is important to be mindful of two caveats when projecting species’ geographic ranges: (i) forecasts tend to predict severe declines, a pattern that is often inconsistent with palaeoecological records that show resilience to past climatic warming (e.g., Warren et al., 2013), and ii) forecasts largely ignore how adaptation to new hypothetically suitable environments can be impacted by the eco-evolutionary feedbacks mediated by selective forces which are difficult to integrate in our models (e.g., sexual selection; García-Roa et al., 2020). Even so, there is still considerable value in predicting scenarios for species distribution in the future. High on the list is the opportunity to assess and adapt ongoing conservation strategies - for instance, by simultaneously evaluating the efficacy of existing protected areas (where species are) and deciding if new areas are required (where species will be).
Detailed information on current and future species distribution is vital in Europe, as elsewhere, to prepare robust conservation action for a range of possible climatic scenarios. To uphold the health of its seas, European Union (EU) initially committed to protect 10% of their coastal and marine areas by 2020 (Aichi Target 11, Convention on Biological Diversity), while recognizing that a larger area was required (especially in the Macaronesia region and the Mediterranean Sea). Now, the EU Biodiversity Strategy has set the goal of protecting 30% of its sea by 2030 (European Commission, Directorate-General for Environment, 2021). However, even considering this notable increase in coverage, there is concern that climate change may weaken the protective value of marine protected areas (MPAs), especially given the static boundaries of most (Cashion et al., 2020). Moreover, there is growing concern that conservation objectives for certain hotspots of climate change (e.g., semi-enclosed marine regions, especially in the eastern Mediterranean Sea) are unrealistic given the current trend of populations decline (Hermoso et al., 2022).
Known to act as umbrella taxa for coastal marine ecosystems (Shokri et al., 2009), seahorses and their close relatives recently evolved into powerful ambassadors for marine conservation (Vincent et al., 2011). Syngnathids (i.e., seahorses, pipefish, pipehorses and seadragons) belong to a large family of teleost fish (Syngnathidae), with 57 genera and approximately 300 species (Hamilton et al., 2017), that primarily inhabit shallow marine, brackish and freshwater habitats. Their extraordinary life histories (Ahnesjö and Craig, 2011), and particularly their truly unique mode of reproduction (male pregnancy), have long made syngnathids model organisms to address a diverse array of questions related to sexual selection (Berglund and Rosenqvist, 2003; Monteiro et al., 2017) and the evolution of reproductive complexity (Roth et al., 2020; Parker et al., 2023).
Even though syngnathids are iconic flagship species, they face numerous threats, including tremendous fishing pressure (Lawson et al., 2017) and notable habitat degradation and loss (e.g., Tiralongo and Baldacconi, 2014), not least from climate change (Pollom et al., 2021). Such global problems are certainly bearing on Europe’s 19 syngnathid species: 15 pipefish (Cosmocampus retropinnis, Entelurus aequoreus, Minyichthys sentus, Nerophis lumbriciformis, Nerophis maculatus, Nerophis ophidion, Syngnathus abaster, Syngnathus acus, Syngnathus phlegon, Syngnathus rostellatus, Syngnathus schmidti, Syngnathus taenionotus, Syngnathus tenuirostris, Syngnathus typhle and Syngnathus variegatus) and 4 seahorse species (Hippocampus algiricus, Hippocampus erectus, Hippocampus guttulatus and Hippocampus hippocampus). From these, the International Union for Conservation of Nature (IUCN) lists 53% of the European syngnathids as Data Deficient, with current population status is unknown for 79% of the species (IUCN, 2022). These numbers showcase the knowledge gap on the health of European coastal ecosystems which severely restricts our ability to detect, reverse or avoid hypothetical perturbations, namely those imposed by climate change.
Evidence shows that European syngnathids are responding to the gradual warming of seawater imposed by climate change. The worm pipefish, Nerophis lumbriciformis, can now be found mid Norway (Monteiro et al., 2017), several kilometres north of the geographical limit described by Dawson (1986). The West African seahorse, Hippocampus algiricus, is also expanding its distribution northwards, and can now be found in the Canary Islands (Otero-Ferrer et al., 2015). Having recently crossed the Atlantic, the lined seahorse, Hippocampus erectus, has currently reached the Azores (Woodall et al., 2009). The black-striped pipefish, Syngnathus abaster, is gradually moving north, either through the Eastern Atlantic Coast or by colonising freshwater ecosystems in the east of the Mediterranean (Monteiro and Vieira, 2017). Although expansions have been more frequently reported, contractions in European syngnathid distributions are also occurring. For instance, current warming scenarios are raising concern for at least one population of the worm pipefish in the coldest part of the Mediterranean (Iglesias et al., 2021).
Here, using species distribution modelling, we attempt to identify areas of environmental suitability for European syngnathids over the next century, according to four scenarios representing distinct Representative Concentration Pathways (RCPs - greenhouse gas concentration trajectories adopted by the Intergovernmental Panel on Climate Change). Attempting precise estimates of the area of future syngnathid distributions would require detailed information that we lack, on habitat suitability, species interactions, limits for phenotypic plasticity or potential for adaptation. Instead, our primary aim was to uncover indications of possible contractions, expansions, and distributional shifts. To this extent, we based our work on the invaluable aggregated expert knowledge amassed by IUCN which provides curated expert range maps (ERMs; hand-drawn polygons that illustrate the geographic limits of species) and biological information on syngnathids (IUCN, 2022). Having projected future distributions, we then consider the possible conservation value of current European IUCN category I and II MPAs (Strict Nature Reserves, Wilderness Areas, and National Parks; all no-take areas) for syngnathids, now and in the future.
2 Materials and methods
2.1 Ocean data
In this study, to forecast the impact of climate change on European syngnathid distributions, we examined changes in SST (sea surface temperature), SSS (sea surface salinity) and SSV (sea surface current velocity) over the next century. From the available environmental data layers of Bio-Oracle (Tyberghein et al., 2012; Assis et al., 2018), we selected eight variables that were simultaneously available for the “present” (2000-2014) and projected for future climate scenarios (2040-2050 and 2090-2100) according to four distinct representative concentration pathways (RCP: 2.6, 4.5, 6.0 and 8.5) (Moss et al., 2010). Specifically, we selected SST (mean, minimum, maximum, range), SSS (minimum, maximum), and SSV (minimum, maximum). These variables, single or in combination, are well known to impact syngnathid reproduction (e.g., Monteiro et al., 2005; Silva et al., 2007; Monteiro et al., 2017), development (e.g., Hilomen-Garcia et al., 2003; Martinez-Cardenas et al., 2014), feeding and swimming (e.g., Qin et al., 2014), or even immunity (Birrer et al., 2012), and should be incorporated when attempting to understand current or future syngnathid distributions. Data consisted of rasters with a spatial resolution of 5 arcmin (i.e., approximately 0.08° or 9.2 km at the Equator).
2.2 Species range maps
Instead of trying to, once again, delimit the range of each European syngnathid, we opted to follow the information contained on the curated expert range maps provided by IUCN (IUCN, 2022). ERMs for syngnathids were downloaded as ESRI shapefiles. Where there was more than one record, we merged them into a single entry (e.g., Hippocampus erectus has two entries, the first depicting its distribution in the Atlantic American coast and the second considering solely the Azores). The exceptions were those species represented by records portraying distributions in different ecosystems. Since Bio-Oracle data are limited to the oceans, we did not consider freshwater and estuarine syngnathid distributions in our analysis. Thus, we discarded records exclusively depicting rivers, lakes, or estuaries. For example, Syngnathus abaster has three entries, each displaying a distinct type of distribution (marine, freshwater, or estuarine); we retained only marine. Simultaneously, also from the IUCN Red List of Threatened Species website (www.iucnredlist.org), we recorded information on species upper and lower depth limits. In two instances where depth distribution data was missing from the IUCN database (Syngnathus phlegon and Syngnathus variegatus), we used information from Fishbase (Froese and Pauly, 2021) and Akimova (2009). Given our current knowledge on syngnathid distributions, three ERMs were slightly modified: 1) Syngnathus acus range was trimmed to eliminate southern Africa distribution (where the local species is recognised as Syngnathus temminckii); 2) Nerophis lumbriciformis range was trimmed to eliminate Canary Islands (while the species was once present up to Western Sahara, the southern limit of distribution is currently continental Portugal); and 3) Nerophis maculatus range was trimmed to remove Azores and the continental Portuguese coast (the species presence has previously been questioned; see ICN, 1993).
2.3 Modelling procedures
For each syngnathid species, we refined the IUCN ERM, which exaggerate depth range, to create trimmed IUCN ERMs (tERMs). We constricted depth range by using ocean bathymetric information extracted from ETOPO1 (Amante and Eakins, 2009) with the R package “marmap” (Pante and Simon-Bouhet, 2013). We then profiled species’ environments by defining a climate envelope constrained by the minimum and maximum values of all selected environmental variables within the tERM. Based on IUCN ERMs, we also calculated the maximum distance from the coast using a global dataset of distances from the nearest coastline (OBPG and Stumpf, 2022). This value was used when forecasting potential distributions (i.e., all projected distributions are below the maximum distance to the coast reported in IUCN ERMs).
To forecast distributions into the next century, we had to assume that syngnathids will persist primarily within present-day ranges of temperature, salinity, and water currents (i.e., similar climate niche or envelope). Using R (R Development Core Team, 2022) and the package “raster” (Hijmans and van Etten, 2012), we used i) the depth-corrected distribution, ii) maximum distance to the coast, and iii) present-day environmental variable ranges (i.e., between the maximum and minimum values recorded for each variable in present-day) to crop each of the selected environmental variables projected for each of the four RCP scenarios in the two time intervals (eight combinations). For each combination, the resulting eight environmental variable rasters were stacked, and a consensus area (i.e., the projected range) was extracted embracing only locations where all environmental conditions simultaneously fell between present-day limits (homoclime areas). As a way of validating the performance of our method, we also projected suitable areas in the current conditions (2000-2014), analysing how well the obtained distribution reflected that currently reported by IUCN, after correcting to tERM.
Given the low dispersal potential of most syngnathids (Mendes et al., 2020), we opted to use a partial-dispersal scenario (Bateman et al., 2013) by discarding seemingly suitable areas if they were either very distant or separated by known geographical barriers from current distributions. For instance, Hippocampus erectus is currently found on the Atlantic coast of America but not in the Pacific coast (despite the existence of similar temperature, salinity, and sea current areas right across the Panama Isthmus), so we omitted (i.e., masked) the Pacific for this specific seahorse. Nevertheless, as some syngnathid species have proven able in the past, we allowed for possible ‘migrations’ between the Mediterranean and Atlantic, or the Atlantic and the Baltic. We also indicated hypothetical future presence in Iceland even though today’s currents would hardly allow for the colonisation of the Icelandic Coast (as we are especially interested in describing macro-geographical patterns, the inclusion/exclusion of the Icelandic Coast will not greatly impact our results).
We calculated the extension of the present distributions (IUCN ERMs and tERMs), as well as those of the hypothetically suitable distribution areas (in km2). It is important to stress that our analysis, much like IUCN ERMs, does not take habitat into account (e.g., some species require the existence of seagrass meadows, which are only sparsely available within the distributional area) or species interactions (e.g., some species depend on the occurrence of specific prey or absence of certain predators). Even though range maps aim at defining edges of distribution, they have limited ability to detect gaps within the projected areas (Marsh et al., 2022). Thus, our distribution maps are designed to highlight areas where, if appropriate abiotic conditions are available within its borders, the species can potentially be found with greater probability (i.e., they are not a guarantee of presence). From this perspective, our area estimates, although likely more precise than those suggested by IUCN ERMs (which do not account for species depth distribution and distance to the coast), should still be interpreted with care, as they will necessarily overestimate the distribution.
Once range maps were available for the marine distribution of European syngnathid species (present and future scenarios), we were able to produce spatial descriptors of the European species diversity. With these data, we calculated alpha (i.e., species richness) and beta diversity (Sorenson dissimilarity index, ranging from 0, most similar, to 1, most dissimilar). The beta diversity was calculated per latitude comparing current predictions to the different time frames of the projections and RCPs. European MPAs shapefiles (containing individual polygons for each MPA, with information on its geographical coordinates, area and IUCN protection category) were obtained from UNEP-WCMC-IUCN (2022). After choosing IUCN category Ia, Ib and II MPAs (‘no-take MPAs’), we calculated the number of syngnathid species per selected MPA.
Statistical analysis were conducted in R (R Development Core Team, 2022). Wilcoxon signed rank tests were conducted to evaluate differences in i) the area of IUCN ERMs and tERMS and ii) the % of species present in selected MPAs in the present versus future projections for all RCPs. After assessing data linearity, checking for normality of residuals (Kolmogorov-Smirnov test), homoscedasticity (Breusch-Pagan test) and autocorrelation (Durbin Watson Test), linear regressions were used to examine the relationship between i) tERMS and that our projections for the present areas, ii) Common and merged areas (of tERMS and our projections for the present), iii) species range minimum SST and area contraction for the four projected 2090-2100 RCP scenarios.
3 Results
By simultaneously considering species depth distribution and maximum distance to the coast, tERMs significantly contracted (-83.28 ± 13.70%; average ± standard deviation), when compared with the IUCN ERMS, due to the exclusion of large theoretically unsuitable areas (Wilcoxon signed rank test: V=190, P<0.001). With these newly redefined ranges (Supplementary Figures S1-S19), it was possible to observe with increased clarity that, as for the majority of syngnathids (Hamilton et al., 2017), European seahorses and pipefishes predominantly exist in shallow waters (N=19 species; mean minimum depth -0.79 ± 2.51 m; mean maximum depth: -52.52 ± 44.86 m; range: -170 to 0 m) and, consequently, essentially have coastal distributions (Figure 1).
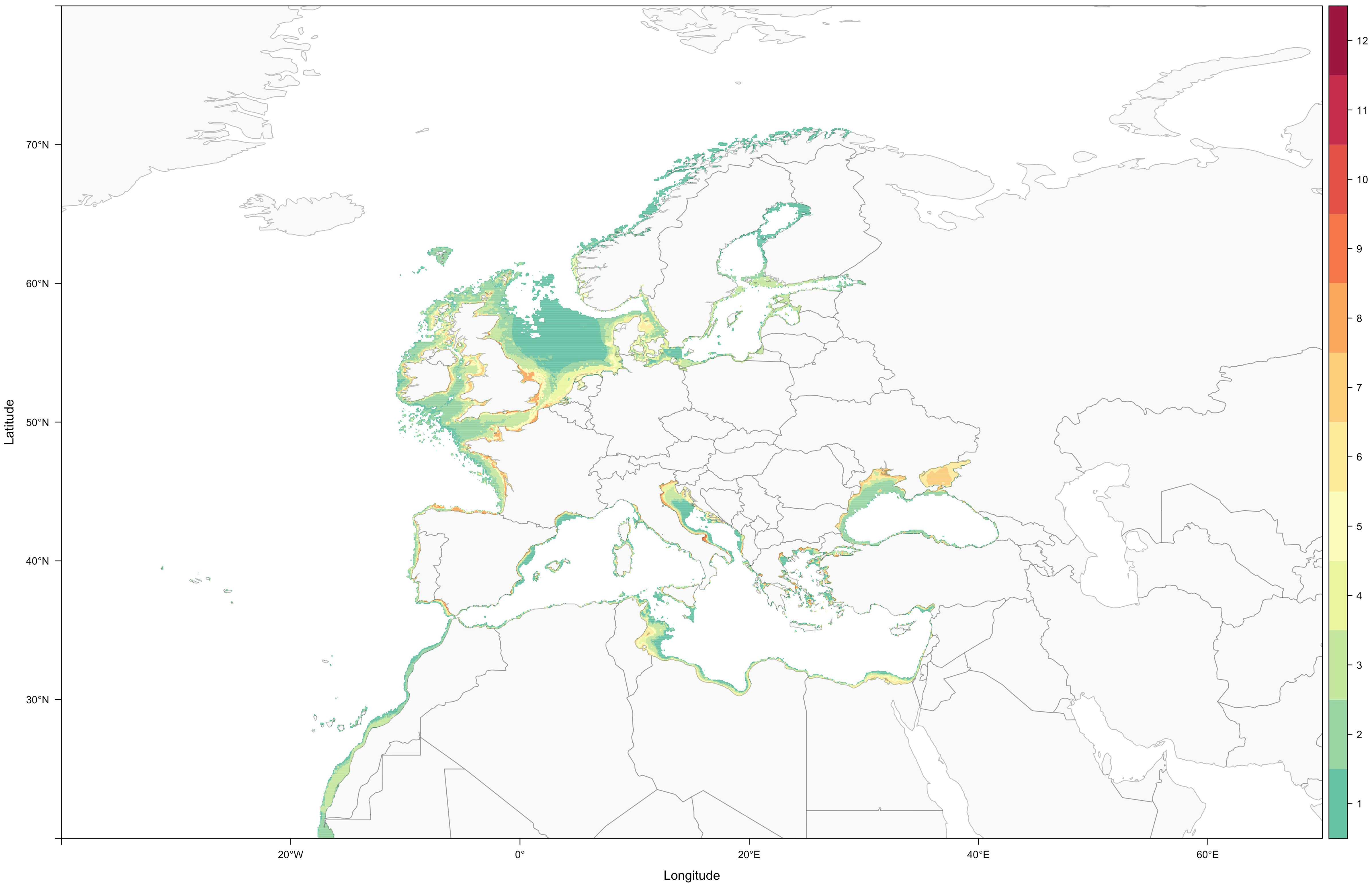
Figure 1 Present-day syngnathid diversity along the European coast, resulting from the sum of all nineteen syngnathid ranges (individual ranges derive from IUCN ERMs that were individually corrected for each species’ depth distribution and maximum distance to the coast; colour scale highlights the number of sympatric species).
Two analyses revealed that our climate envelope approach (i.e., projecting theoretically suitable areas based on sea surface temperature, salinity, and current velocity) closely mapped onto tERMs, thus validating the former. First, a linear regression revealed that our distributional area projections explained nearly 98% of the variation in tERMs (Y = 6742 + 1.179 X, R2 = 0.978, F(1,17) = 766, P < 0.001), with our projections never producing smaller areas than those in tERMS (median = 15% larger). Second, another linear regression showed that the overlapping area of our projections and tERMs also explained approximately 98% of the merged area between both range maps (Y = -3002 + 0.823 X, R2 = 0.978, F(1,17) = 788.4, P < 0.001).
In projecting hypothetically suitable areas across Europe, we found that a progressive range contraction is to be expected for most European syngnathids under all four selected RCP scenarios (2.6, 4.5, 6.0 and 8.5) in each of the two future periods (2040-2050 and 2090-2100) (Table 1). Across all 19 species, nearly a century from now, the forecasted ranges will have shrunk to 70.99 ± 29.27% of their current area in the mildest RCP 2.6. Mean contraction is expected to be even more severe (reduced to 53.78 ± 43.13% of the current area) in the most hostile RCP 8.5 scenario.
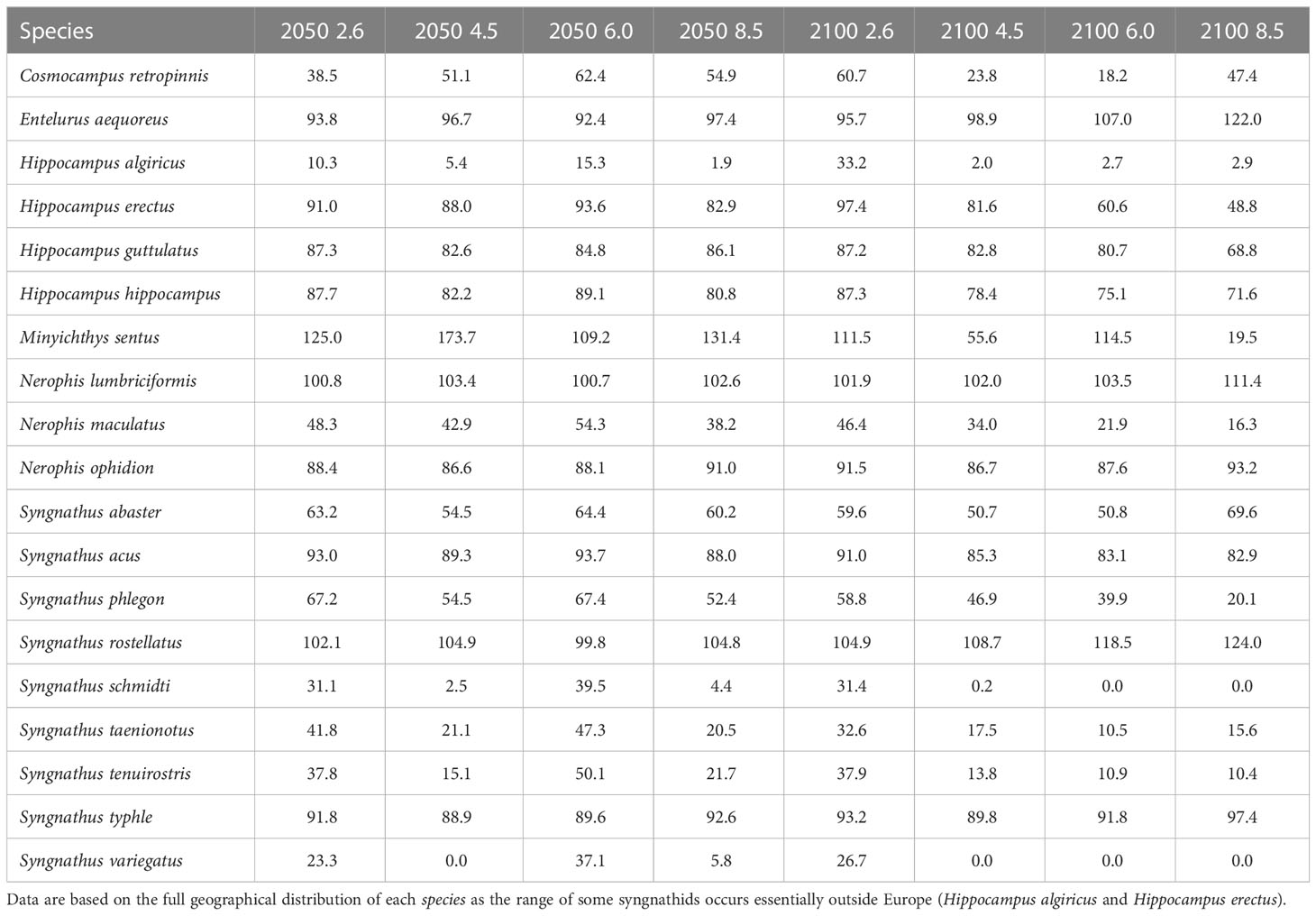
Table 1 Change in potentially suitable areas for European syngnathids (as % of retained present range), in two time periods (2040-2050, 2090-2100) and according to the four selected RCPs (2.6, 4.5, 6.0, 8.5).
As climate change progresses, more northerly species will likely have an increased opportunity to expand their ranges while relatively more southerly species will tend to experience range contractions (Table 1). The most southerly species Hippocampus algiricus, Syngnathus schmidti and Syngnathus variegatus are expected to withstand the most drastic range reductions, especially under the ‘business-as-usual’ scenario (Table 1).
We found noticeable exceptions to the general pattern of range contraction, for five species that are all located at higher latitudes, where water temperature is cooler. Nerophis ophidion and Syngnathus typhle might experience no real range change; they will apparently expand north but may simultaneously lose areas in the enclosed Mediterranean and Black Sea (where northward migration is not possible). Meanwhile, the northerly species found only in the Atlantic - Entelurus aequoreus, Nerophis lumbriciformis and Syngnathus rostellatus – should experience range increases as they expand north into newly suitable areas without losing area in the south. Present day ranges and hypothetical future distributions (i.e., forecasted suitable areas for all combinations of the two periods and four RCP scenarios), for all nineteen European syngnathid species, can be viewed in Supplementary Figures S20-S190.
In general, linear regressions show that the present-day species experience with water temperatures (here, we used minimum SST) appropriately explain future range area reductions in the 2090-2100 interval (RCP 8.5: Y = 16.99 -0.09 X, R2 = 0.72, F(1,17) = 44.06, P < 0.001; RCP 6.0: Y = 16.43 -0.07 X, R2 = 0.49, F(1,17) = 16.12. P < 0.001; RCP 4.5: Y = 17.65 -0.10 X. R2 = 0.67, F(1,17) = 34.37, P < 0.001; RCP 2.6: Y = 19.26 -0.10 X, R2 = 0.42, F(1,17) = 12.20. P < 0.005).
Assuming no invasions by non-indigenous syngnathids, loss of species diversity will be especially apparent in the Mediterranean and Black Sea (Figure 2). Climate change-induced alteration of biotic conditions (e.g., warmer waters) linked to the lack of cooler areas that could allow substantial range shifts, might leave only a handful of refugia for the remaining Mediterranean syngnathids (Supplementary Figures S191-S198). Curiously, one of those refugia (Southern France), among the coolest sections of the Mediterranean, is the same currently occupied by what seems to be the last Mediterranean population of the worm pipefish, Nerophis lumbriciformis.
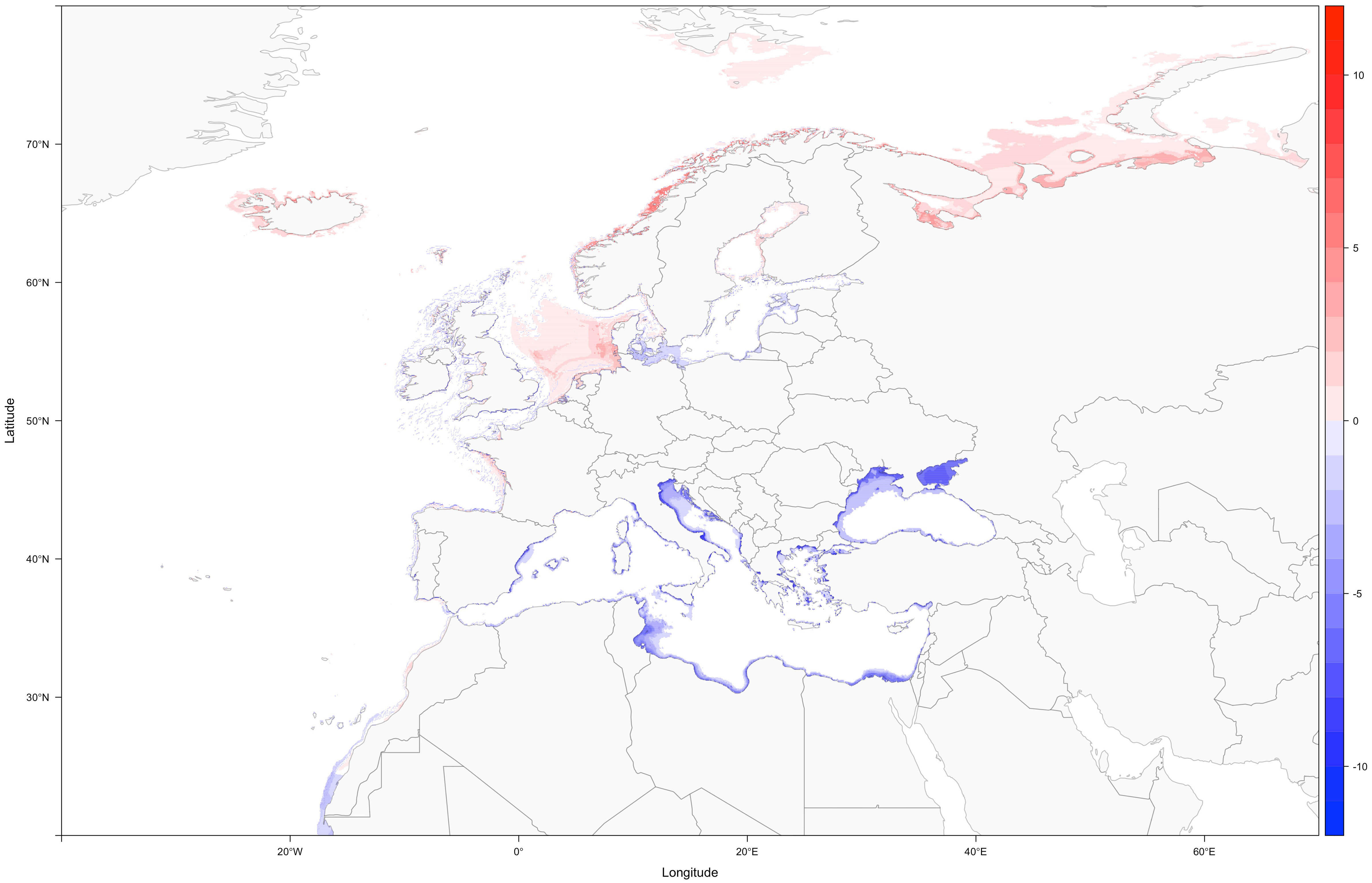
Figure 2 Difference in species richness from the present to the 2090-2100 time period, under RCP 8.5 (blue shows areas with loss of species, and red shows areas of increased diversity).
The forecasted progressive poleward expansions, together with the projected range contractions in the south can be summarised by looking at the latitudinal variation in both alpha diversity (species richness) and beta diversity (Sorensen dissimilarity index). While species richness will remain relatively constant between approximately 50 and 60°N (green bands in Figure 3), progressive alteration of abiotic conditions, according to emission scenarios, will affect species composition at both European latitudinal extremes (Figure 3). Due to range contractions (and possible extinctions) and poleward range shifts, we can predict an increase in the number of species at higher latitudes that contrasts with a decrease in species richness in the South. This generates areas that are predicted to have highly dissimilar composition in relation to the present as a result of increasing species richness in the north and decreasing richness in the south (orange to red bands, Figure 3).
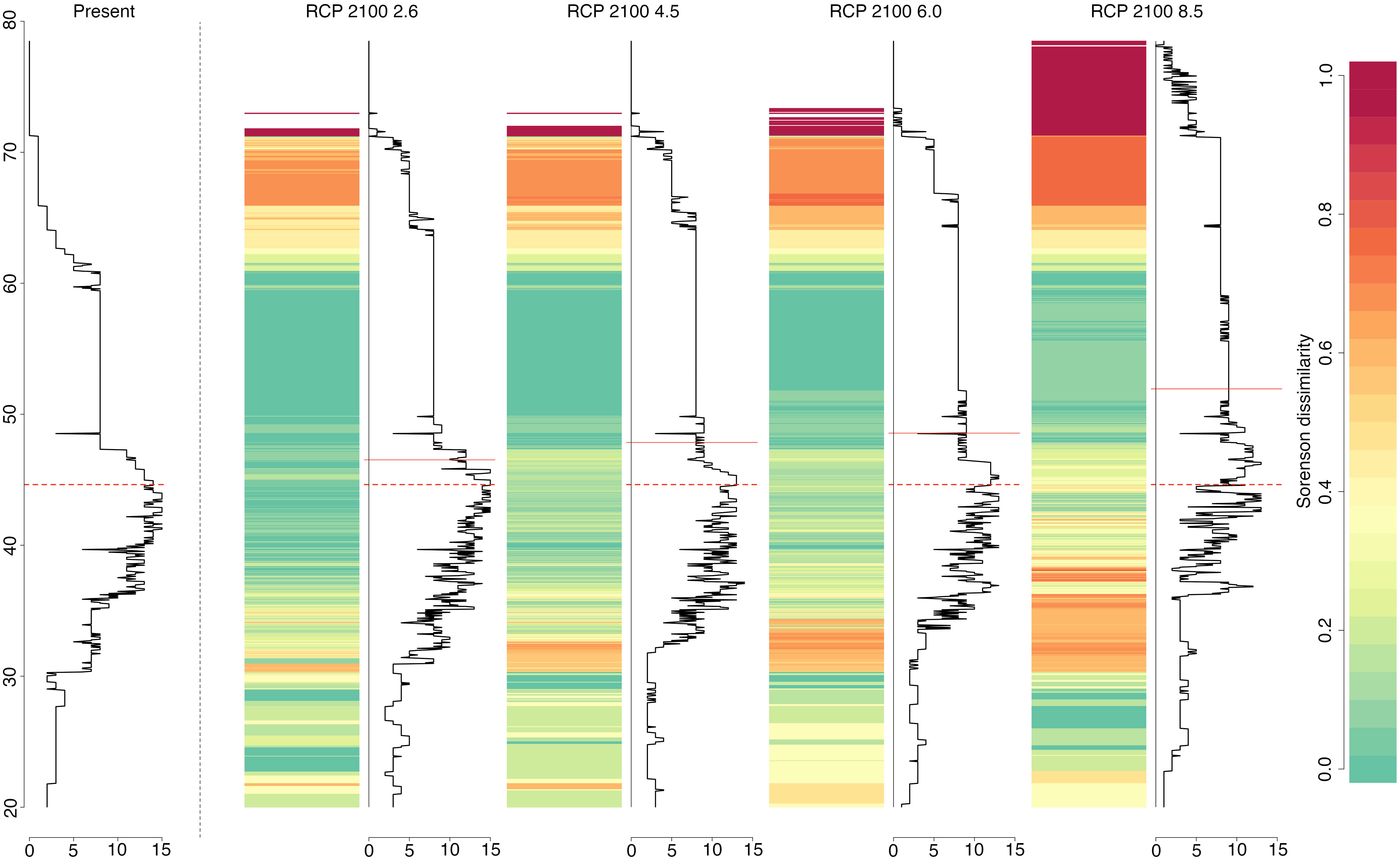
Figure 3 Latitudinal variation in alpha (species richness; solid black line graphs) and beta diversity (Sorensen dissimilarity index; coloured scale), for the present and the 2090-2100 time period, according to the four selected RCP scenarios. Dashed red lines indicate present latitudinal means weighted by species richness and solid red lines considers the same but for each RCP scenario.
For no-take MPAs in Europe between -40° to 70° longitude and 20° to 80° latitude, we found a strong latitudinal asymmetry, with most concentrated in northern Europe (Figure 4, top panels and bottom left panel). With rare exceptions, these MPAs are generally small (N= 849, median = 0.83 Km2, range: 0.002- 86,971.92 Km2; Figure 4, centre panel). In the north, the number and spatial distribution of MPAs covers most syngnathid species richness (and will probably continue to do so in the future, even under the most undesirable RCP scenario). In the south, however, the very few MPAs do not currently cover syngnathid diversity (Figure 4, top panels). Areas with high diversity (i.e., more cooccurring species) are not currently captured by any MPA and probably will continue that way in the future unless new MPAs are designated.
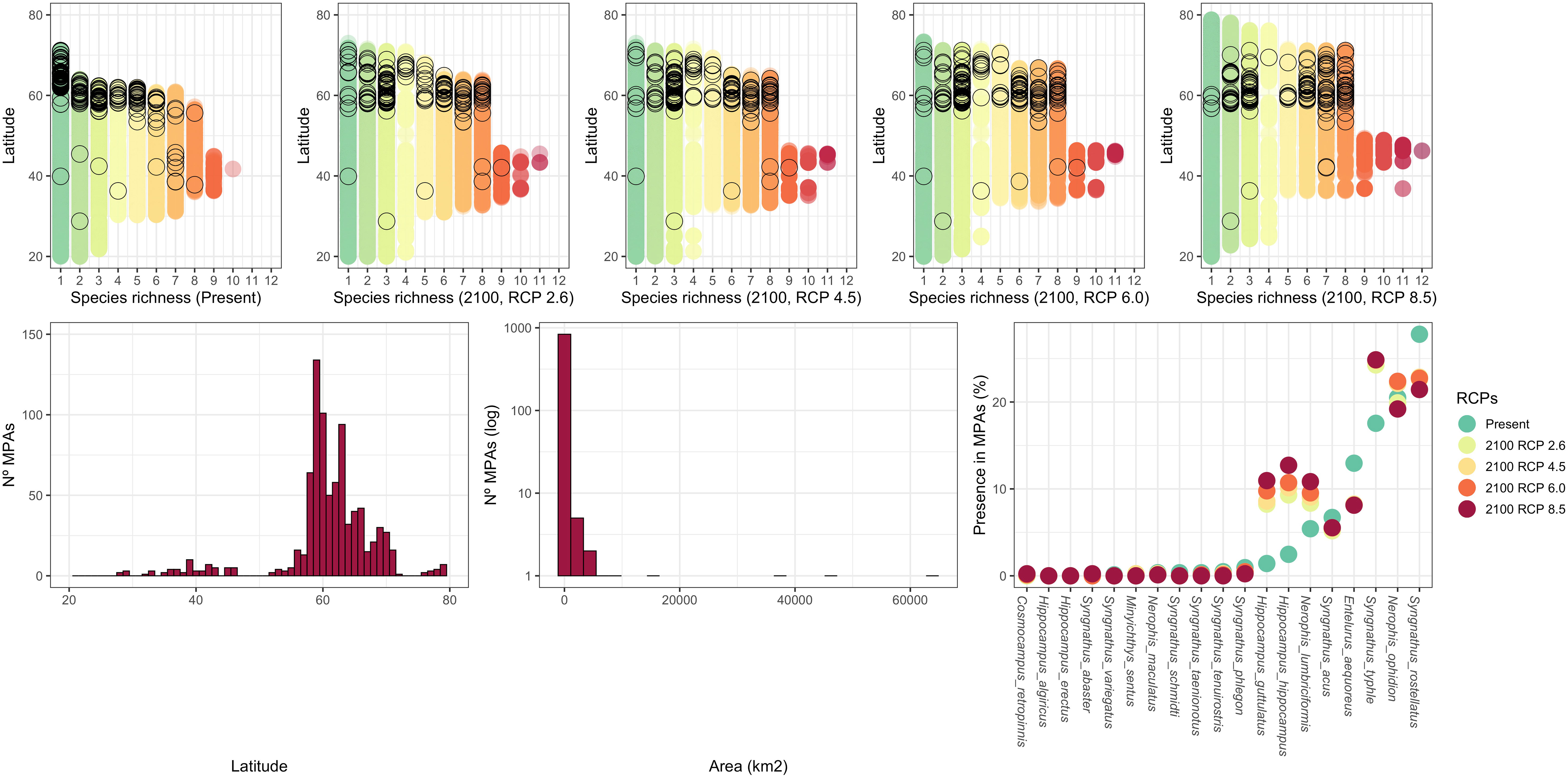
Figure 4 Latitudinal distribution of ‘no-take’ MPAs (black open circles), highlighting the number of sympatric syngnathid species, at the present and in all four considered RCP scenarios for the 2090-2100 time period (top row graphs). Latitudinal distribution of all ‘no-take’ MPAs (bottom left), their area distribution (histogram, log Y axis, bottom centre), and the percentage of ‘no-take’ MPAs where each of the nineteen European syngnathids is currently found or could potentially be found in the 2090-2100 tine-period, according to the four selected RCP scenarios (bottom right).
The skewed latitudinal distribution of MPAs, together with their generally small individual area, means that most European syngnathid species, especially those with a more southerly distribution, will really not benefit from its protection. For instance, eleven species are currently present in fewer than 1% of the ‘no-take’ MPAs (three of which are absent from all; Figure 4, right panel), and only four species are found in more than 10% of ‘no-take’ MPAs (maximum = 27.79%). The relatively small coverage of syngnathid ranges by existing ‘no-take’ MPAs will not significantly change in the future, irrespectively of the RCP scenario (Wilcoxon signed rank test on the % presence in MPAs in the present versus future RCP scenarios; 2050 RCP2.6: V = 50, P = 0.59; 2050 RCP4.5: V = 61, P = 0.98; 2050 RCP6.0: V = 45, P = 0.41; 2050 RCP8.5: V = 73, P = 0.89; 2100 RCP2.6: V = 52, P = 0.67; 2100 RCP4.5: V = 68, P = 1; 2100 RCP6.0: V = 73, P = 0.89; 2100 RCP8.5: V = 66.5, P = 0.65).
4 Discussion
This first dual assessment of current and forecasted distribution, under different climate change scenarios, for the full marine diversity of European syngnathids, reveals results that closely match general and region-specific impacts of climate change projections in Europe (Philippart et al., 2011). Following their climate niche, syngnathids will move north where they can, especially on the Atlantic coast, with some pipefishes, and perhaps even seahorses, extending their ranges beyond Norway, into the Barents Sea. However, in semi-enclosed areas, without real room for manoeuvre, syngnathid populations will most likely decline. Worryingly, the present-day distribution of ‘no-take’ MPAs throughout Europe will provide little support, especially for southern syngnathid species.
Our work has significance for regional understanding and assessment. While the Atlantic hotspot of syngnathid diversity is expected to remain around the Celtic-Biscay Shelf, its exact composition will probably slightly vary from that of present day due to the poleward movement of cold-water species and the progressive substitution by subtropical and Mediterranean species. Semi-enclosed systems such as the Baltic (especially in its southern section, together with the adjacent Kattegat), are expected to experience a considerable diversity loss. Specifically in the Baltic, in addition to the forecasted progressive drop in salinity and increased water temperatures, eutrophication (Skogen et al., 2014) could pose additional challenges to syngnathids, whose reproductive patterns are known to be impacted by turbidity (Sundin et al., 2010). But probably the most dramatic changes are expected within the Mediterranean and the Black Sea, where present-day endemic species diversity is likely to plunge. For instance, the diversity hotspot that is currently observed in the Adriatic Sea, is estimated to stop gathering suitable conditions for most of its syngnathids by 2100, under the ‘business-as-usual’ scenario. Together, the Black Sea and Sea of Azov are expected to experience a similar fate to that of the Mediterranean (i.e., significant loss of species).
Our work can also be significant at a continental level, highlighting the need for concerted action in Europe. The worldwide active legislation for syngnathids was recently reviewed, revealing that most European countries do not have syngnathid-specific regulations (Stanton et al., 2021). Even if currently poorly safeguarded by legislation, European syngnathids still experience some degree of protection, for instance by the action of MPAs whose number is expected to increase in the near future. Nevertheless, even though the EU Biodiversity Strategy has set the goal of significantly increasing the protection of its seas, there is concern that climate change may weaken the protective value of MPAs. As some European syngnathids are shifting their ranges (e.g., Monteiro et al., 2017), and will predictably continue to do so, adaptive management approaches will be increasingly essential (Wilson et al., 2020). Here, when focusing on ‘no-take’ MPAs, areas with stricter controls on fisheries and where conservation of biodiversity should be more extensive (Costello and Ballantine, 2015), the first emerging pattern retained is that there is a clear latitudinal asymmetry in Europe. Although the higher density of ‘no-take’ MPAs in the north (especially above 60° N) will help support connectivity, the panorama becomes quite different towards the south of Europe. From the Atlantic diversity hotspot downwards, and especially in the Mediterranean and Black Sea, ‘no-take’ MPAs are rare and far apart. As a result, syngnathids from southern Europe, those experiencing higher risk of population collapse, are currently much less protected by these MPAs than those from higher latitudes, and the situation will not change in the future, at least according to our distributional projections.
Our analysis on MPAs serves the specific purpose of highlighting the fact that, especially under the dynamic conditions imposed by climate change, the management for biodiversity conservation must be proactive. Especially in southern Europe, the current ‘no-take’ MPA layout, if it remains static, will not be especially effective in either safeguarding syngnathid diversity or delaying the expected gradual species disappearance. As MPAs are warming at roughly the same rate as unprotected areas (Bruno et al., 2018), one could naively conceive that there would be little value in repositioning MPAs or creating new protected areas. However, the establishment of effective corridors that could assist range shifts or the purposeful conservation of climatic refugia could support species persistence. Responses to paleoclimatic change show how refugia can be key landscapes worth protecting (Ashcroft, 2010). Thus, forecasting species distributions under distinct climate scenarios can be a valuable tool for conservation (of syngnathids and other taxa) by informing which MPAs will hold most diversity under climate change scenarios, where MPAs can act as climate refugia, and how MPAs can jointly serve as potential corridors that assist range shifts.
Especially if considered as a group, European syngnathids satisfy the most relevant criteria proposed for selection as umbrella species (Branton and Richardson, 2011). Firstly, they are reasonably common and well distributed around Europe (i.e., most European countries with access to the sea have one or more syngnathid species as part of their national fauna listing), thus covering wide areas that, if adequately preserved, would translate into effective conservation of cooccurring species. Secondly, syngnathids have considerable spatial range overlap with other species of conservation concern. Seagrass meadows, as an example, are hotspots of diversity that are critical for syngnathids and vulnerable early life stages of many marine species. Severely threatened in several European regions (Turschwell et al., 2021), and classified as Vulnerable by the EU, seagrass meadows and all their inhabitants would gain from effective syngnathid protection. Thirdly, we can now see a tight correspondence between the theoretical future scenarios for European coastal ecosystems and those of pipefishes and seahorses. Thus, the implementation of adequate conservation strategies for syngnathids, aimed at mitigating the effects of climate change, would certainly find echo on numerous coastal habitats (Pollom et al., 2021).
Besides the potential to act as umbrella species, syngnathids (especially seahorses) have also the ability to serve as flagship species. These fish are taxonomically distinctive, charismatic, and have successfully proven to be powerful ambassadors for marine conservation and promoters of ocean literacy. Since syngnathids are so easily recognized, and seahorses are historically embedded into European mythology (e.g., as offspring of Poseidon’s steeds or protectors of dead sailors’ souls transitioning into the underworld), their use as flagship species seems like a unique opportunity to better engage with the general European public, thinning potential conflicts with several socioeconomic activities and easing the implementation of conservation measures directed to coastal ecosystems.
5 Conclusion
Predicting the effects of climate change on ecosystems is now, probably more than ever, a fundamental challenge and an unavoidable requirement (Moritz and Agudo, 2013). Even considering that our selection of modelling technique (climate envelope) has limitations (see Hijmans and Graham, 2006), the resulting overall pattern of syngnathid potential distributions throughout the next century is very plausible, unsurprisingly reflecting, at both general and regional levels, the expected climate change impacts on European marine ecosystems (Philippart et al., 2011). At this point in time, a steep decline in European marine endemic species richness, especially in southern Europe, is no longer a question of “if” but rather of “how soon”. Knowing that changes began many decades ago, the results of our species distribution modelling on European syngnathids suggests that the swiftness of the foreseeable diversity decline is inversely linked to our ability to curb emissions. As such, Europe needs to be prepared to implement, at a continental scale, coordinated conservation measures aimed at preventing (or delaying, at the very least) the loss of its marine endemic species. Acting as umbrella species, conservation efforts aimed at syngnathids will surely have a positive impact on coastal ecosystems. Moreover, given syngnathid potential to act as flagship species, these charismatic fish could help decisively enrol the general public into marine ecosystem preservation, leading a much-needed movement aimed at opposing climate change impacts.
Data availability statement
The original contributions presented in the study are included in the article/Supplementary Material. Further inquiries can be directed to the corresponding author.
Author contributions
NM and AV contributed to the conception and design of the study. NM, SP, and SM contributed to data gathering. NM and PT performed the statistical analysis. NM and AV wrote the first draft of the manuscript. All authors contributed to the article and approved the submitted version.
Funding
NM and PT were funded by FCT through DL57/2016/CP1440/CT0015 and DL57/2016/CP1440/CT0008, respectively.
Acknowledgments
We thank members of the IUCN SSC Seahorse, Pipefish and Seadragon Specialist Group for support and helpful suggestions. We also thank the constructive criticism of two reviewers.
Conflict of interest
The authors declare that the research was conducted in the absence of any commercial or financial relationships that could be construed as a potential conflict of interest.
Publisher’s note
All claims expressed in this article are solely those of the authors and do not necessarily represent those of their affiliated organizations, or those of the publisher, the editors and the reviewers. Any product that may be evaluated in this article, or claim that may be made by its manufacturer, is not guaranteed or endorsed by the publisher.
Supplementary material
The Supplementary Material for this article can be found online at: https://www.frontiersin.org/articles/10.3389/fmars.2023.1138657/full#supplementary-material
References
Ahnesjö I., Craig J. F. (2011). The biology of syngnathidae: pipefishes, seadragons and seahorses. J. Fish Biol. 78 (6), 1597–1602. doi: 10.1111/j.1095-8649.2011.03008.x
Amante C., Eakins B. W. (2009). Etopo1 1 arc-minute global relief model: Procedures, data sources and analysis (NOAA technical memorandum).
Ashcroft M. B. (2010). Identifying refugia from climate change. J. Biogeogr. 37 (8), 1407–1413. doi: 10.1111/j.1365-2699.2010.02300.x
Assis J., Tyberghein L., Bosch S., Verbruggen H., Serrão E. A., De Clerck O. (2018). Bio-ORACLE v2.0: Extending marine data layers for bioclimatic modelling. Global Ecol. Biogeogr. 27 (3), 277–284. doi: 10.1111/geb.12693
Bateman B. L., Murphy H. T., Reside A. E., Mokany K., VanDerWal J. (2013). Appropriateness of full-, partial- and no-dispersal scenarios in climate change impact modelling. Diversity Distributions 19 (10), 1224–1234. doi: 10.1111/ddi.12107
Berglund A., Rosenqvist G. (2003). Sex role reversal in pipefish. Adv. Stud. Behav. 32, 131–167. doi: 10.1016/S0065-3454(03)01003-9
Birrer S. C., Reusch T. B. H., Roth O. (2012). Salinity change impairs pipefish immune defence. Fish Shellfish Immunol. 33 (6), 1238–1248. doi: 10.1016/j.fsi.2012.08.028
Branton M., Richardson J. S. (2011). Assessing the value of the umbrella-species concept for conservation planning with meta-analysis. Conserv. Biol. 25 (1), 9–20. doi: 10.1111/j.1523-1739.2010.01606.x
Bruno J. F., Bates A. E., Cacciapaglia C., Pike E. P., Amstrup S. C., van Hooidonk R., et al. (2018). Climate change threatens the world’s marine protected areas. Nat. Climate Change 8 (6), 499–503. doi: 10.1038/s41558-018-0149-2
Burrows M. T., Schoeman D. S., Buckley L. B., Moore P., Poloczanska E. S., Brander K. M., et al. (2011). The pace of shifting climate in marine and terrestrial ecosystems. Science 334 (6056), 652–655. doi: 10.1126/science.1210288
Cashion T., Nguyen T., Ten Brink T., Mook A., Palacios-Abrantes J., Roberts S. M. (2020). Shifting seas, shifting boundaries: Dynamic marine protected area designs for a changing climate. PloS One 15 (11), e0241771. doi: 10.1371/journal.pone.0241771
Costello M. J., Ballantine B. (2015). Biodiversity conservation should focus on no-take marine reserves: 94% of marine protected areas allow fishing. Trends Ecol. Evol. 30 (9), 507–509. doi: 10.1016/j.tree.2015.06.011
Dawson C. E. (1986). “Syngnathidae,” in Fishes of the north-eastern Atlantic and the Mediterranean, vol. 2 . Eds. Whitehead, Hureau J. C., Nielson J., Tortonese E. (Paris: United Nations Educational, Scientific and Cultural Organization), 628–639. M. L. B. P J P.
Doney S. C., Ruckelshaus M., Duffy J. E., Barry J. P., Chan F., English C. A., et al. (2012). Climate change impacts on marine ecosystems. Ann. Rev. Mar. Sci. 4, 11–37. doi: 10.1146/annurev-marine-041911-111611
European Commission, Directorate-General for Environment. (2021). EU Biodiversity strategy for 2030. bringing nature back into our lives Vol. 380 (Brussels : Publications Office of the European Union) Available at: https://data.europa.eu/doi/10.2779/677548.
Froese R., Pauly D. (2021) FishBase. Available at: www.fishbase.org.
García-Roa R., Garcia-Gonzalez F., Noble D. W. A., Carazo P. (2020). Temperature as a modulator of sexual selection. Biol. Rev. 95 (6), 1607–1629. doi: 10.1111/brv.12632
Hamilton H., Saarman N., Short G., Sellas A. B., Moore B., Hoang T., et al. (2017). Molecular phylogeny and patterns of diversification in syngnathid fishes. Mol. Phylogenet Evol. 107, 388–403. doi: 10.1016/j.ympev.2016.10.003
Hermoso V., Carvalho S. B., Giakoumi S., Goldsborough D., Katsanevakis S., Leontiou S., et al. (2022). The EU biodiversity strategy for 2030: Opportunities and challenges on the path towards biodiversity recovery. Environ. Sci. Policy 127, 263–271. doi: 10.1016/j.envsci.2021.10.028
Hickling R., Roy D. B., Hill J. K., Fox R. J., Thomas C. D. (2006). The distributions of a wide range of taxonomic groups are expanding polewards. Global Change Biol. 12 (3), 450–455. doi: 10.1111/j.1365-2486.2006.01116.x
Hijmans R. J., Graham C. H. (2006). The ability of climate envelope models to predict the effect of climate change on species distributions. Global Change Biol. 12 (12), 2272–2281. doi: 10.1111/j.1365-2486.2006.01256.x
Hijmans R. J., van Etten J. (2012). Raster: Geographic analysis and modeling with raster data. (R package version 2.0-12)
Hilomen-Garcia G. V., Delos Reyes R., Garcia C. M. H. (2003). Tolerance of seahorse hippocampus kuda (Bleeker) juveniles to various salinities. J. Appl. Ichthyol. 19 (2), 94–98. doi: 10.1046/j.1439-0426.2003.00357.x
ICN (1993). Livro vermelho dos vertebrados de Portugal Vol. III (Lisboa, Portugal: Peixes Marinhos e Estuarinos). Lisboa, ICN.
Iglesias S. P., Bariche M., Beau F., Berenger L., Beucher R., Chabrolle A., et al. (2021). French Ichthyological records for 2019. Cybium: Rev. Internationale d’Ichtyologie 45 (3), 169–188. doi: 10.26028/cybium/2021-453-001
IUCN (2022). The IUCN red list of threatened species, Version 2021-2. Available at: https://www.iucnredlist.org. Accessed on 04/08/2022.
Lawson J. M., Foster S. J., Vincent A. C. J. (2017). Low bycatch rates add up to big numbers for a genus of small fishes. Fisheries 42 (1), 19–33. doi: 10.1080/03632415.2017.1259944
Lenoir J., Svenning J. C. (2015). Climate-related range shifts – a global multidimensional synthesis and new research directions. Ecography 38 (1), 15–28. doi: 10.1111/ecog.00967
Loarie S. R., Duffy P. B., Hamilton H., Asner G. P., Field C. B., Ackerly D. D. (2009). The velocity of climate change. Nature 462 (7276), 1052–1055. doi: 10.1038/nature08649
Marsh C. J., Sica Y. V., Burgin C. J., Dorman W. A., Anderson R. C., Del Toro Mijares I., et al. (2022). Expert range maps of global mammal distributions harmonised to three taxonomic authorities. J. Biogeogr. 49 (5), 979–992. doi: 10.1111/jbi.14330
Martinez-Cardenas L., Valdez-Hernández E. F., González-Díaz A. A., Soria-Barreto M., Castañeda-Chavez M. R., Lango-Reynoso F., et al. (2014). Effect of salinity on growth and survival in juvenile opossum pipefish, microphis brachyurus, in culture conditions. J. World Aquaculture Soc. 45 (5), 577–585. doi: 10.1111/jwas.12142
Mendes T., Gomes C., Monteiro N., Antunes A. (2020). Strong sexual selection does not induce population differentiation in a fish species with high dispersal potential: The curious case of the worm pipefish nerophis lumbriciformis (Teleostei: Syngnathidae). J. Heredity 111 (7), 585–592. doi: 10.1093/jhered/esaa052
Monteiro N., Almada V., Vieira M. (2005). Temporal patterns of breeding and recruitment in nerophis lumbriciformis (Pisces; syngnathidae) related to seawater temperatures. J. Fish Biol. 67 (5), 1475–1480. doi: 10.1111/j.0022-1112.2005.00818.x
Monteiro N., Cunha M., Ferreira L., Vieira N., Antunes A., Lyons D., et al. (2017). Parabolic variation in sexual selection intensity across the range of a cold-water pipefish: implications for susceptibility to climate change. Global Change Biol. 23 (9), 3600–3609. doi: 10.1111/gcb.13630
Monteiro N., Vieira M. (2017). Rendez-vous at the Baltic? the ongoing dispersion of the black-striped pipefish, syngnathus abaster. Oceanogr. Ocean fish Open Access J. 3 (2), 555608. doi: 10.19080/OFOAJ.2017.03.555608
Moritz C., Agudo R. (2013). The future of species under climate change: resilience or decline? Science 341 (6145), 504–508. doi: 10.1126/science.1237190
Moss R. H., Edmonds J. A., Hibbard K. A., Manning M. R., Rose S. K., van Vuuren D. P., et al. (2010). The next generation of scenarios for climate change research and assessment. Nature 463 (7282), 747–756. doi: 10.1038/nature08823
OBPG, Stumpf R. P. (2022) Distance to nearest coastline: 0.01-degree grid. 2022 Available at: http://pacioos.org/metadata/dist2coast_1deg.html.
Otero-Ferrer F., Herrera R., López A., Socorro J., Molina L., Bouza C. (2015). First records of hippocampus algiricus in the canary islands (north-east Atlantic ocean) with an observation of hybridization with hippocampus hippocampus. J. Fish Biol. 87 (4), 1080–1089. doi: 10.1111/jfb.12760
Pante E., Simon-Bouhet B. (2013). Marmap: A package for importing, plotting and analyzing bathymetric and topographic data in r. PloS One 8 (9), e73051. doi: 10.1371/journal.pone.0073051
Parker J., Dubin A., Schneider R., Wagner K. S., Jentoft S., Bõhne A., et al. (2022). Immunological tolerance in the evolution of male pregnancy. Mol. Ecol. 32, 819–840. doi: 10.1111/mec.16333
Parmesan C. (2006). Ecological and evolutionary responses to recent climate change. Annu. Rev. Of Ecol. Evol. And Systematics 37, 637–669. doi: 10.1146/annurev.ecolsys.37.091305.110100
Parmesan C., Ryrholm N., Stefanescu C., Hill J. K., Thomas C. D., Descimon H., et al. (1999). Poleward shifts in geographical ranges of butterfly species associated with regional warming. Nature 399 (6736), 579–583. doi: 10.1038/21181
Pecl G. T., Araujo M. B., Bell J. D., Blanchard J., Bonebrake T. C., Chen I. C., et al. (2017). Biodiversity redistribution under climate change: Impacts on ecosystems and human well-being. Science 355 (6332):eaai9214. doi: 10.1126/science.aai9214
Philippart C. J. M., Anadón R., Danovaro R., Dippner J. W., Drinkwater K. F., Hawkins S. J., et al. (2011). Impacts of climate change on European marine ecosystems: Observations, expectations and indicators. J. Exp. Mar. Biol. Ecol. 400 (1), 52–69. doi: 10.1016/j.jembe.2011.02.023
Pollom R. A., Ralph G. M., Pollock C. M., Vincent A. C. J. (2021). Global extinction risk for seahorses, pipefishes and their near relatives (Syngnathiformes). Oryx 55 (4), 497–506. doi: 10.1017/S0030605320000782
Qin G., Zhang Y., Huang L., Lin Q. (2014). Effects of water current on swimming performance, ventilation frequency, and feeding behavior of young seahorses (Hippocampus erectus). J. Exp. Mar. Biol. Ecol. 461, 337–343. doi: 10.1016/j.jembe.2014.09.001
R Development Core Team (2022). R: A language and environment for statistical computing (Vienna, Austria: R Foundation for Statistical Computing).
Roth O., Solbakken M. H., Torresen O. K., Bayer T., Matschiner M., Baalsrud H. T., et al. (2020). Evolution of male pregnancy associated with remodeling of canonical vertebrate immunity in seahorses and pipefishes. Proc. Natl. Acad. Sci. U.S.A. 117 (17), 9431–9439. doi: 10.1073/pnas.1916251117
Shokri M. R., Gladstone W., Jelbart J. (2009). The effectiveness of seahorses and pipefish (Pisces: Syngnathidae) as a flagship group to evaluate the conservation value of estuarine seagrass beds. Aquat. Conservation: Mar. Freshw. Ecosyst. 19 (5), 588–595. doi: 10.1002/aqc.1009
Silva K., Vieira M. N., Almada V. C., Monteiro N. M. (2007). The effect of temperature on mate preferences and female–female interactions in syngnathus abaster. Anim. Behav. 74 (5), 1525–1533. doi: 10.1016/j.anbehav.2007.03.008
Skogen M. D., Eilola K., Hansen J. L. S., Meier H. E. M., Molchanov M. S., Ryabchenko V. A. (2014). Eutrophication status of the north Sea, skagerrak, kattegat and the Baltic Sea in present and future climates: A model study. J. Mar. Syst. 132, 174–184. doi: 10.1016/j.jmarsys.2014.02.004
Stanton L. M., Foster S., Vincent A. C. J. (2021). Identifying national conservation status, legislation and priorities for syngnathid fishes globally. Fish. Cent. Res. Rep. 29(2):43. doi: 10.14288/1.0401950
Sundin J., Berglund A., Rosenqvist G. (2010). Turbidity hampers mate choice in a pipefish. Ethology 116 (8), 713–721. doi: 10.14288/1.0401950
Tiralongo F., Baldacconi R. (2014). A conspicuous population of the long-snouted seahorse, hippocampus guttulatus (Actinopterygii: Syngnathiformes: Syngnathidae), in a highly polluted Mediterranean coastal lagoon. Acta Ichthyologica Piscatoria 44 (2):99–104. doi: 10.3750/AIP2014.44.2.02
Turschwell M. P., Connolly R. M., Dunic J. C., Sievers M., Buelow C. A., Pearson R. M., et al. (2021). Anthropogenic pressures and life history predict trajectories of seagrass meadow extent at a global scale. Proc. Natl. Acad. Sci. 118 (45), e2110802118. doi: 10.1073/pnas.2110802118
Tyberghein L., Verbruggen H., Pauly K., Troupin C., Mineur F., De Clerck O. (2012). Bio-ORACLE: a global environmental dataset for marine species distribution modelling. Global Ecol. Biogeogr. 21 (2), 272–281. doi: 10.1111/j.1466-8238.2011.00656.x
UNEP-WCMC-IUCN (2022). Protected planet: The world database on protected areas (WDPA) and world database on other effective area-based conservation measures (WD-OECM) (Cambridge, UK: UNEP-WCMC and IUCN).
Vincent A. C. J., Foster S. J., Koldewey H. J. (2011). Conservation and management of seahorses and other syngnathidae. J. Fish Biol. 78 (6), 1681–1724. doi: 10.1111/j.1095-8649.2011.03003.x
Warren R., VanDerWal J., Price J., Welbergen J. A., Atkinson I., Ramirez-Villegas J., et al. (2013). Quantifying the benefit of early climate change mitigation in avoiding biodiversity loss. Nat. Climate Change 3 (7), 678–682. doi: 10.1038/nclimate1887
Wilson K.L., Tittensor D.P., , Worm B., Lotze H.K., et al. (2020). Incorporating climate change adaptation into marine protected area planning. Glob. Change Biol. 26, 3251–3267. https://doi.org/10.1111/gcb.15094
Keywords: Syngnathidae, climate change, distribution, conservation, marine protected area (MPA)
Citation: Monteiro N, Pinheiro S, Magalhães S, Tarroso P and Vincent A (2023) Predicting the impacts of climate change on the distribution of European syngnathids over the next century. Front. Mar. Sci. 10:1138657. doi: 10.3389/fmars.2023.1138657
Received: 05 January 2023; Accepted: 01 March 2023;
Published: 14 March 2023.
Edited by:
Geng Qin, South China Sea Institute of Oceanology (CAS), ChinaReviewed by:
Zhixin Zhang, South China Sea Institute of Oceanology (CAS), ChinaFrancesco Tiralongo, University of Catania, Italy
Copyright © 2023 Monteiro, Pinheiro, Magalhães, Tarroso and Vincent. This is an open-access article distributed under the terms of the Creative Commons Attribution License (CC BY). The use, distribution or reproduction in other forums is permitted, provided the original author(s) and the copyright owner(s) are credited and that the original publication in this journal is cited, in accordance with accepted academic practice. No use, distribution or reproduction is permitted which does not comply with these terms.
*Correspondence: Nuno Monteiro, nuno.monteiro@cibio.up.pt
†These authors have contributed equally to this work