- 1Shimoda Marine Research Centre, Tsukuba University, Shimoda, Shizuoka, Japan
- 2Institute of Anthropic Impacts and Sustainability in Marine Environment (IAS), National Research Council of Italy, Palermo, Italy
- 3Institute for Marine Biological Resources and Biotechnology (IRBIM), National Research Council CNR, Mazara del Vallo, TP, Italy
- 4Department of Biology, University of Bari Aldo Moro, Bari, Italy
- 5Center of Marine Sciences (CCMAR), University of Algarve Campus de Gambelas, Faro, Portugal
In the light of future ocean warming scenarios and habitat fragmentation, coastal fishes must adjust their physiological and behavioral traits to face the combined effect of global warming and ecological interactions. Seahorses (Hippocampus spp.) are charismatic fishes with peculiar life cycle traits, which make them vulnerable to several anthropic pressures and natural disturbances. In this study, we investigated the behavior of two sympatric seahorse species, the long-snouted Hippocampus guttulatus (Cuvier, 1829) and the short-snouted Hippocampus hippocampus (Linnaeus, 1758). Specifically, we carried out two manipulative experiments to assess (i) the effect of temperature and habitat availability on both H. hippocampus and H. guttulatus behavior and (ii) the effect of temperature and an increased density of H. guttulatus (by 3×) on the activity level of congeneric species H. hippocampus. Our results showed that +3°C warming did not affect seahorse behavior in both experiments, suggesting greater behavioral tolerance to thermal variation. However, a significant reduction of the active behavior of H. hippocampus was observed when the artificial habitat was introduced in the tank, while H. guttulatus maintained its activity. Furthermore, a significant decrease of the H. hippocampus activity was observed with an increased relative dominance of H. guttulatus. Our results suggest that both increased density of H. guttulatus and habitat availability, but not ocean warming, will affect the behavior of H. hippocampus. Therefore, different interspecific behavioral strategies may occur, thus affecting the distribution of the two species among shallow habitats when they occur in sympatry.
1 Introduction
The study of animal behavior is critically important in understanding the status and welfare of species and populations (Sih et al., 2004; Clements and Hunt, 2015) and can reveal the ecological processes structuring marine populations and communities (Hay, 2009; Schmidt et al., 2010; Nagelkerken and Munday, 2016). Species behavior is a crucial factor in shaping ecological interactions, thus representing a powerful tool to better understand the combined effects of species interactions, their response in a shifting environment, including in a climate change scenario (Harmon and Barton, 2013; Milazzo et al., 2013; Levine et al., 2017; Boulanger et al., 2022).
Warming has been shown to influence the distribution and behavior of many organisms (Abram et al., 2017; Freitas et al., 2021; Spatafora et al., 2021). Higher temperature may induce behavioral changes by altering activity rate (Biro et al., 2010), foraging (Biro et al., 2007; Hu et al., 2021), habitat use and selection (Freitas et al., 2016; Matis et al., 2018; Freitas et al., 2021), and reproduction (Hopkins et al., 2011; Miller et al., 2015; Spatafora et al., 2021). However, temperature-related changes in the organism’s behavior may in turn affect species interactions and ecological processes (Harmon and Barton, 2013; Freitas et al., 2021; Zhang et al., 2022). For example, higher temperature can create novel interspecific interactions (e.g., invasive vs. native species; Galaiduk et al., 2013; Galasso et al., 2015) or alter the balance of pre-existing antagonistic ones (Nogués-Bravo & Rahbek, 2011; Milazzo et al., 2013; Wernberg et al., 2013; Alexander et al., 2016; Bonaviri et al., 2017; Yeruham et al., 2020). This may allow species coexistence through thermal resource partitioning, thus maintaining their functional roles in the ecosystem (Fey et al., 2019; Freitas et al., 2021). Moreover, organism behavior (e.g., habitat use and selection, foraging and anti-predator responses) can also be indirectly affected via temperature-dependent changes in the abundance or quality of resources (Ockendon et al., 2014; Nagelkerken and Munday, 2016; Matis et al., 2018; Nay et al., 2020; Freitas et al., 2021).
Seahorses are extremely vulnerable to natural and anthropogenic impacts (Foster and Vincent, 2004) due to their distinct life history, including sparse distribution, low mobility, small home ranges, low fecundity, lengthy parental care, and mate fidelity. Over the past few years, populations of seahorses showed a sudden and sharp decline mainly due to habitat loss, fragmentation, illegal trade, and incidental capture in fishing gears. Only recently, seahorse species were listed on Appendix II of the Convention on International Trade in Endangered Species of Wild Fauna and Flora (CITES Convention) (Foster and Vincent, 2004) and in the International Union for Conservation of Nature (IUCN) Red List of Threatened Species (Pollom, 2014; IUCN, 2020; Pollom et al., 2021). Given their extreme vulnerability to natural and anthropogenic impacts, climate changes may have a larger impact on seahorses compared with other fish species (Aurélio et al., 2013; Faleiro et al., 2015; Cohen et al., 2017; Pierri et al., 2021; Pollom et al., 2021; Costa et al., 2023). However, studies investigating seahorses’ ability to cope with future ocean warming and other ecological factors (e.g., habitat availability and species interaction) are still scarce and limited to just a few species (Costa et al., 2023).
In the temperate coastal waters of the Europe, few studies have investigated the effects of ocean warming on the behavioral and physiological performance of Hippocampus guttulatus (Cuvier, 1829) (Aurélio et al., 2013; Faleiro et al., 2015; Costa et al., 2023). Although two of the three prementioned studies reported a normal increase in the metabolic rates (within their thermal sensitivity) and ventilation of adult H. guttulatus individuals with increasing temperature (18°C–26°C–30°C), the authors suggested great behavioral and physiological plasticity to warming (Aurélio et al., 2013; Faleiro et al., 2015). Additionally, Costa et al. (2023) found significant increases in the activity and food intake in the same species exposed to +7°C variation. While some information is present for H. guttulatus, no studies have been conducted to investigate the effect of temperature on behavior of the congeneric species H. hippocampus (Linnaeus, 1758). The two species inhabit lagoons, estuaries, and coastal marine waters (Pierri et al., 2022) where they generally share same habitat but with differences in niche partitioning (Curtis and Vincent, 2005). H. hippocampus has been mainly found in bare and sandy habitats, while H. guttulatus prefers more complex habitats such as seagrass beds (Curtis & Vincent, 2005; Caldwell and Vincent, 2012; Curtis et al., 2017; Correia et al., 2020; Correia, 2022). In most of the European study sites where the two species occurred sympatrically, the density of H. guttulatus was greater than that of H. hippocampus (Woodall et al., 2018; but not in Greece, see Correia et al., 2020). Although very low densities of H. guttulatus (0 to 0.51 ind. m−2) and H. hippocampus (0.001–0.035 ind. m−2) have been recorded in the wild, the two species tend to be patchily distributed and are occasionally found at very high density (e.g., 10 ind. H. guttulatus m2, Foster and Vincent, 2004). Low densities are a recent characteristic of European seahorse populations mainly due to habitat loss, fragmentation, illegal trade, and incidental capture in fishing gears (Correia et al., 2018; Pierri et al., 2021). Only recently, European species were listed on Appendix II of CITES (Appendix II) and the IUCN Red List where they were classified as “Data Deficient” at a global level (Pollom, 2014; Pollom et al., 2021) and “Near Threatened” in the Mediterranean (Pollom, 2017). Indeed, IUCN Species Survival Commission (SSC) retain that the status of “Near Threatened’’ is not appropriate, and the two species should probably be included in the upper categories, as most of the known European populations faced declines of up to 90% in the past decade. Given the high vulnerability of these species and the predicted effects of the climate changes, many aspects of European seahorse biology remain unknown (especially for H. hippocampus), including wide-scale distribution data, abundance rates, and behavior. In addition, given seahorse tendency to be patchily distributed and positively correlated with the percentage of holdfasts’ availability (Correia et al., 2015), the preferred habitat may become scarce, thus forcing these species (e.g., seagrasses) to occupy the same niche. Therefore, mechanisms underpinning the behavioral interaction between the two species need to be addressed to better understand the distribution of the two seahorse species in the European coastal ecosystems (Milazzo et al., 2013).
Here, we first examined the behavioral responses of H. guttulatus and H. hippocampus to increased temperature (+3°C, RCP 8.5, IPCC, 2014) in the presence/absence of an artificial habitat. As H. guttulatus generally prefers more shallow waters (with higher temperature variation) with complex habitats (e.g., seagrass beds) respect to the congeneric H. hippocampus, we predicted that the likely more thermal tolerant species H. guttulatus would maintain its activity levels regardless temperature conditions and habitat availability while H. hippocampus would reduce its activity under the same altered conditions. Second, as no information is available for H. hippocampus in relation to temperature variation and the species interaction with H. guttulatus, we explored the behavioral responses (i.e., activity level) of H. hippocampus to increased density (3×) of the congeneric species and elevated temperature conditions. We forecasted that H. hippocampus would reduce its activity under elevated temperatures and in presence of a higher density of the congeneric, thus, explaining their lower abundances when the two species share the same habitat.
Finally, given that sex-specific behavioral responses have been reported for H. guttulatus (Faleiro et al., 2008; Gristina et al., 2022) and other seahorses species (Freret-Meurer and Alves, 2018), sex effect, and its interaction with other experimental factors (temperature, habitat availability, and species) were also tested.
2 Materials and methods
2.1 Specimens rearing and stocking conditions
H. guttulatus (11 males and seven females) and H. hippocampus (24 males and 20 females), were selected in October 2021 from a captive broodstock held at the breeding structures of Ramalhete Research Station of the Centre of Marine Science—CCMAR (Faro, Portugal). Individuals of both species were randomly chosen from the broodstock and assigned to four holding tanks (100 cm × 70 cm × 50 cm) at two temperatures: two 22°C tanks (H. hippocampus, 11 males and 10 females; H. guttulatus, five males and three females) and two 25°C tanks (H. hippocampus, 13 males and 10 females; H. guttulatus, six males and four females). The mean temperature matched average summer temperature of 21.6°C (Newton and Mudge, 2003) experienced by these species where individuals were collected, while the higher temperature represented a mean temperature increase of +3°C forecasted by the end of the 21st century (RCP 8.5, IPCC, 2014). The elevated temperature (25°C) was reached by an increase of ±0.5°C per hour. The individuals of H. hippocampus (mean standard length ± SE: males = 12.26 ± 0.15 cm and female = 11.8 ± 0.18 cm) and H. guttulatus (mean standard length ± SE: males = 12.84 ± 0.17 cm and female = 12.81 ± 0.22 cm) (Supplementary Table S1) were acclimated to the different temperature conditions for two weeks prior to behavioral testing. Seawater temperature was set to 21.4 ± 0.5°C and 25.2 ± 0.5°C, while salinity and dissolved oxygen were 37.6 ± 0.1‰ and 6.9 ± 0.1 mg L−1 for both treatments. Holding tanks were illuminated from above with 2 × 36W fluorescent tubes with a light intensity of 900 ± 40 l× at the water surface and the photoperiod was set on 14h light/10h dark cycles without twilight periods. Artificial structures made of nautical rope (0.8 cm Ø) were provided as holdfasts. Seahorses were fed ad libitum once a day with a mix of live mysid shrimp (Mesopodopsis slabberi and Leptomysis sp.). Aquariums were daily cleaned by syphoning to remove debris and eventual uneaten food. Seawater parameters, such as ammonia, nitrates, and nitrites, were recorded biweekly and kept stable throughout the experiment.
2.2 Experimental setup
Four experimental tanks (60 cm × 50 cm × 40 cm) were kept in the same conditions (temperature, dissolved oxygen, and light intensity) as the holding tanks and used for two behavioral experiments. Seahorse behavior was observed and recorded using a set of GoPro Hero 8 video cameras each assembled in a PVC frame support placed in each aquarium at ≈15 cm above the water surface. A grid (mesh size: 20 cm × 17 cm) was superimposed on the tank bottom to help behavioral measurements. Fish were randomly assigned to different observation tanks. Behavioral observations were made daily in the morning (09.30h–13.30h.). During video recordings, temperature conditions were kept constant.
2.2.1 Experiment 1: Effect of habitat and temperature on H. guttulatus and H. hippocampus behavior
To determine the effects of elevated temperature and habitat availability on the behavior of H. hippocampus and H. guttulatus, a total of 21 and 18 individuals, respectively, at control temperature (22°C: H. hippocampus, five males and five females; H. guttulatus, five males and three females) and elevated temperature condition (25°C: H. hippocampus, seven males and four females; H. guttulatus, six males and four females) were used (see Supplementary Table S1 for details). One individual (same protocol for both species) was gently taken from the holding tank and introduced into one of the two experimental tanks in relation to the previous temperature condition of fish acclimation (22°C or 25°C). Recording began after 5 min of acclimation once the seahorse was introduced into the experimental tank. Behavioral observations consisted of recording seahorse responses before and after the introduction of an artificial habitat (Supplementary Figure S1). For each replicate, a 10-min pre-introduction period was followed by a 10-min post-introduction period during which each fish was exposed to their new habitat: three nautical rope (0.8 cm Ø) placed in the middle of the experimental tank mimicking the Posidonia oceanica (Delile, 1813) seagrass (Correia et al., 2013). The tested artificial structures used for this experiment were the same as those available inside the holding tanks and have been proven to be a suitable habitat for H. hippocampus and H. guttulatus (Faleiro et al., 2008; Correia et al., 2013).
2.2.2 Experiment 2: Effect of temperature and increased density of H. guttulatus (3×) on H. hippocampus behavior
To investigate the effects of elevated temperature and increased density of H. guttulatus on the behavior of H. hippocampus, a total of 24 individuals of the latter species (22°C: seven males and five females; 25°C: six males and six females) were used (see Supplementary Table S2 in Supplementary Material for details).
A protocol similar to experiment 1 was followed to evaluate the behavior of H. hippocampus with increased densities of H. guttulatus (3×) under two temperature conditions. A single individual of both H. hippocampus and H. guttulatus was carefully taken from the holding tanks (at the same temperature conditions: 22°C or 25°C) and introduced into one experimental tank. Recording began after 5 min of acclimation once the seahorse was introduced into the experimental tank. Behavioral observations consisted of recording H. hippocampus responses before (H. hippocampus and H. guttulatus, density 1:1) and after the introduction of two additional individuals of H. guttulatus (H. hippocampus and H. guttulatus, density 1:3) (Supplementary Figure S2). Ten-minute time frame was recorded for each introduction period (pre- and post-introduction) at different combinations of H. hippocampus to H. guttulatus density (1:1 and 1:3). The 3× density of H. guttulatus was selected to mimicking wild condition where the density of H. guttulatus was greater than that of H. hippocampus and to preserve the welfare of these species under captive conditions (Faleiro et al., 2008). The experiment was run at the two selected temperature conditions (22°C or 25°C) and the same artificial holdfasts (three artificial plastic plants) during the two introduction periods. For this experiment, H. guttulatus were randomly selected with a sex ratio of 2:1 (two males, one female). A total of 12 individuals (a total of four trials) of H. guttulatus were tested daily, and after each trial, the fish were placed back in a separate tank and other fish were used. After four trials, the fish were kept for 24h before being tested again.
2.3 Behavioral analyses
The behavior of seahorses in the two experiments was assessed by a standard continuous focal sampling procedure (Martin and Bateson, 1993) using the VLC software (Software – VLC media player vs 3.0.11: VideoLAN; https://www.videolan.org/vlc/index.it.html). After video analyses, the behavior of seahorses was described through a species ethogram (adapted from Faleiro et al., 2008), and the behaviors were assigned in two categories: Activity and Inactivity. In detail, for the first experiment, a total of five behaviors for both H. hippocampus and H. guttulatus were recorded and grouped into two categories: (i) Inactive: resting and swinging and (ii) Active: swimming, crawling, and body movement (see Table 1A for the detailed description of the behavior). For the second experiment, a total of eight behaviors for H. hippocampus were recorded and grouped into two categories: (i) Inactive: resting, swinging, interactions with H. guttulatus, grasping holdfast and (ii) Active: swimming, crawling, and searching and body movement (see Table 1B for the detailed description of the behavior). For each individual and in both experiments, we recorded the time spent (in seconds, expressed as percentage) by seahorses on all behaviors during the 20-min video observations. In both experiments, the sum of active behaviors (defined as “Active”) was compared between the two introduction periods (pre- and post-) and the two temperature conditions (22°C or 25°C).
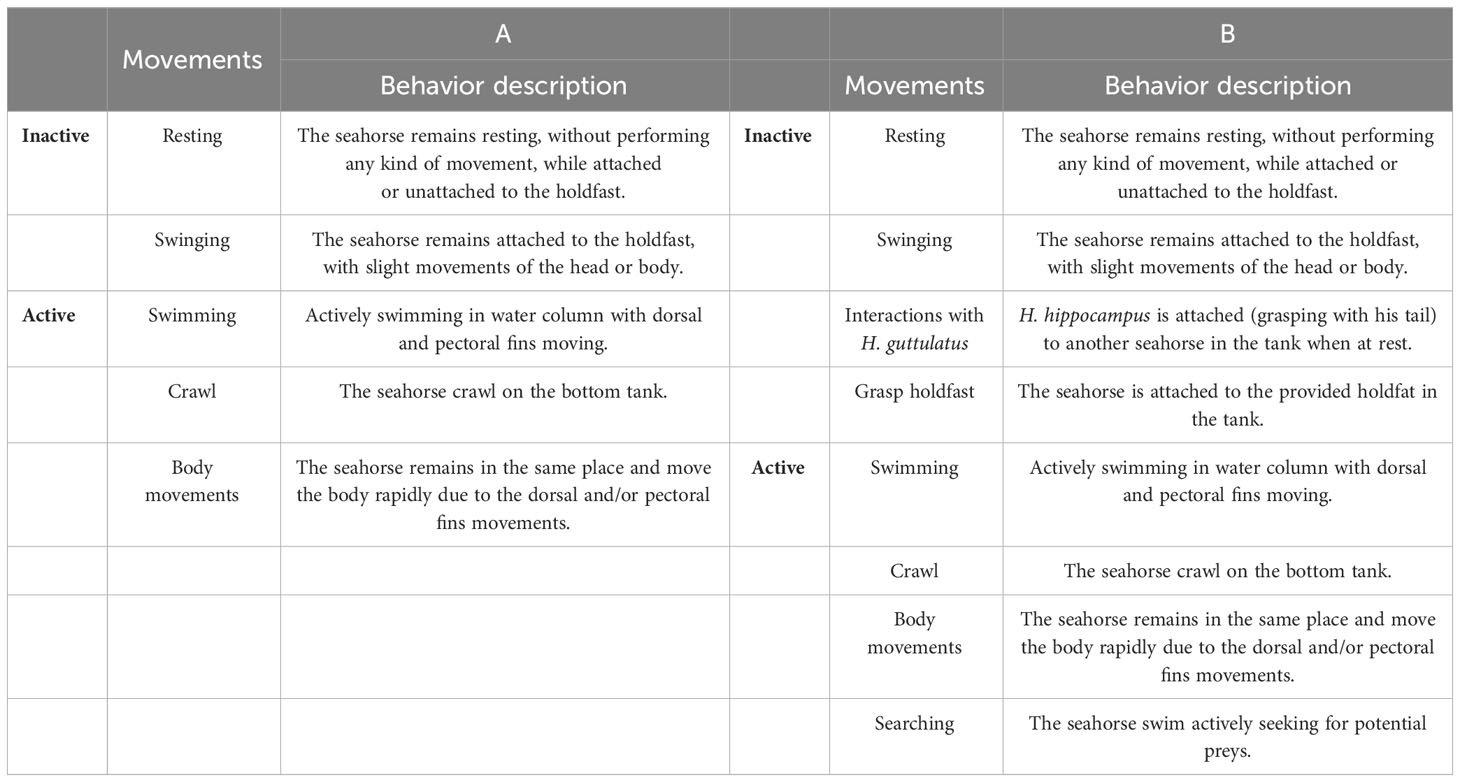
Table 1 Behavioral ethograms reporting the list of the behaviors grouped in the two categories (Active and Inactive) described for H. guttulatus and H. hippocampus (A, first experiment) and for H. hippocampus (B, second experiment).
2.4 Statistical analysis
For both experiments, linear mixed models allowing to account for the correlation between observations coming from the same individual (before and after the introduction of the habitat in the first experiment; before and after the introduction of two individuals of H. guttulatus in the second experiment) were used. In particular, for the first experiment, the differences in the time spent on active behaviors (Active) between the two species (Species) and sex (Sex) at different temperatures (Temperature) and before and after the introduction of the habitat (Habitat) were formulated as follows:
For the second experiment, the differences in the Active behavior of H. hippocampus before and after the introduction of the two H. guttulatus individuals (Density) and between the two sexes (Sex) at the two temperature conditions were modeled as follows:
For both model formulations, the response variable was log +1 transformed to meet the model assumptions of homoscedasticity and normality of the residuals. Specifically, for the first experiment, the log+1-transformed Active of the two species was modeled as an intercept α plus a linear combination of the interaction between Species [fixed factor with two levels: H. hippocampus (HH) and H. guttulatus (HG)], Sex (fixed factor with two levels: male and female), Habitat (fixed factor with two levels: pre- and post- introduction of the habitat) and Temperature (fixed factor with two levels: 22°C and 25°C). While for the second experiment the log+1-transformed Activity of H. hippocampus was modeled as an intercept α plus a linear combination of the interaction between Density (fixed factor with two levels: pre- and post- introduction of two individuals of H. guttulatus), and Temperature (fixed factor with two levels). In both formulations, the response was modeled by adding also a random factor αi (allowing us to account for the correlation of the observations coming from the same individual for the first (H. Hippocampus: n = 21; H. guttulatus: n = 18) and the second experiment (H. hippocampus: n = 24). assumed to be normally distributed with mean 0 and variance σα2 plus the model errors (ϵij) assumed to be normally distributed with mean 0 and variance σ2. The index i refers to a specific individual, while the index j to the observations coming from an individual. The analyses were performed using lme4 library (Bates et al., 2014) in R statistical Software (R Core Team, 2019).
3 Results
3.1 Experiment 1: Effect of habitat and temperature on H. guttulatus and H. hippocampus behavior
Obtained data are presented in Supplementary Table S3. A significant difference in the active behavior between the two species in two introduction periods (Supplementary Table S3; Figure 1A; Habitat x Species: F1,78 = 14.84, p< 0.001) was observed, but neither temperature nor sex or their interaction significantly affected the behavior (Supplementary Table S3). A post hoc test performed on the interaction terms showed that H. hippocampus active behavior (78.4 s ± 26.3 SE) was significantly lower than of H. guttulatus (414.6 s ± 54.5 SE) during the post-introduction period when the artificial habitat was provided (Supplementary Table S3; Figure 1A; t = 3.507, p< 0.01). In addition, H. hippocampus active behavior significantly decreased during the post-introduction period (78.4 s ± 26.3 SE) compared with the pre-introduction period (294.24 s ± 32.7 SE) (Supplementary Table S3; Figure 1A; t = −6.649, p< 0.001). The time spent by H. guttulatus on active behaviors was similar between the two introduction periods (Supplementary Table S3; Figure 1A; t = -0.887; p = 1). Descriptive results of the single behaviors showed that, in the absence of habitat, H. guttulatus spent approximately 81% of the total time performing body movement (36%), crawling (~33%), and swimming (~12%), while H. hippocampus spent 97% of the total time for body movement (~48%) and crawling (~49%). However, when artificial plants were added to the tank, the percentage of the activity of H. hippocampus was reduced to 28% (body movement = ~15%; crawling = ~13%) in favor to the resting behaviors. Finally, the time spent by H. guttulatus (in %) performing active behaviors during the post-introduction period was approximately 70% of the total activity (body movement = 43%, crawling = ~23% and swimming = ~4%) (Figure 1B). In addition, seahorse length did not affect, or change, their behavior (Supplementary Table S3).
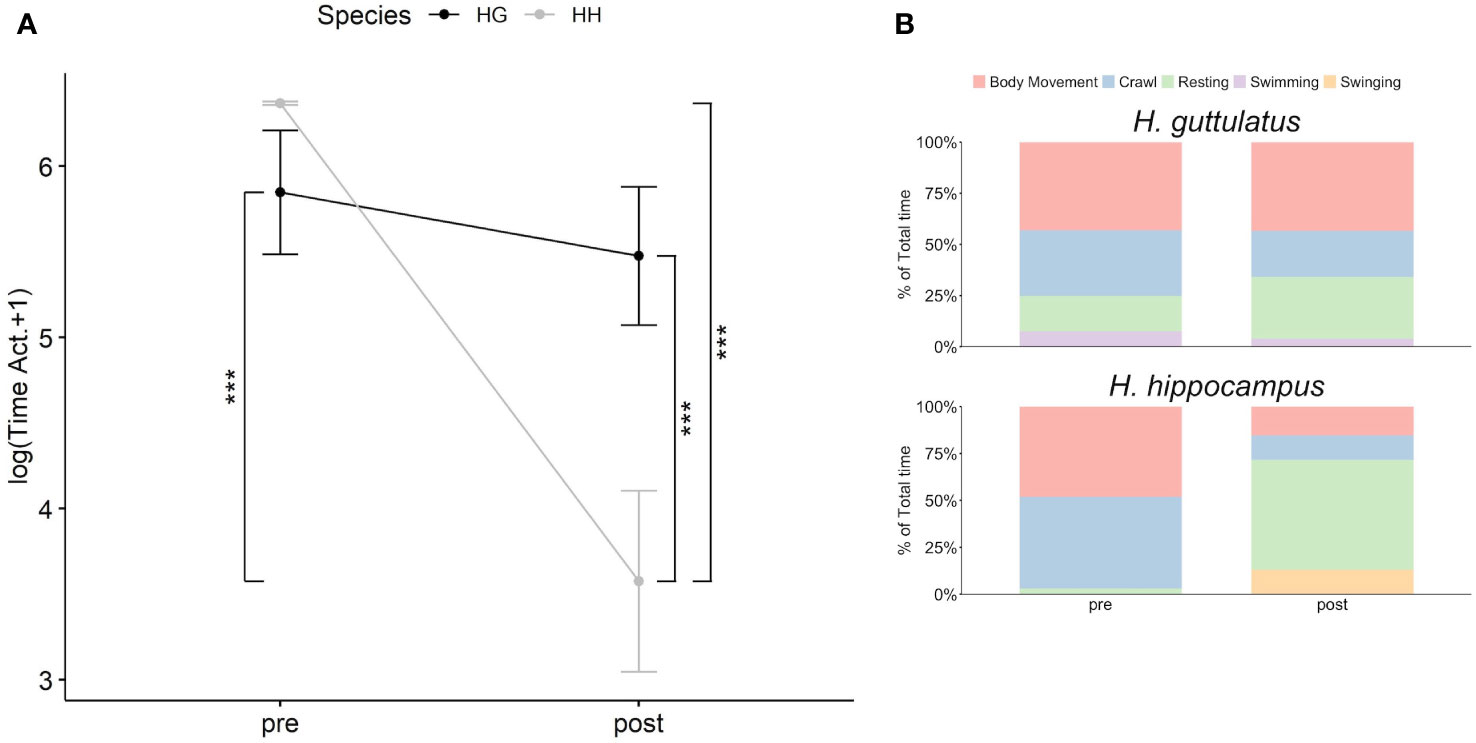
Figure 1 Relationship between the introduction periods (pre- and post-introduction of the habitat) and the sum of the time spent on active behaviors (in log +1) by the two seahorse species: H. hippocampus (gray line; n = 21) and H. guttulatus (black line; n = 18) (A). Solid dots represent the median, top, and bottom vertical whiskers represent quartiles. Asterisk (*) indicates significant differences (p<.05) between the two seahorse species and the two introduction periods for H. hippocampus and H. guttulatus. Stacked bar charts represent the percentage of time spent on the different behaviors (body movement, crawl, resting, swimming, and swinging) by the two seahorse species between the two introduction periods (B).
3.2 Experiment 2: Effect of temperature and increased density of H. guttulatus (3×) on H. hippocampus behavior
The time spent by H. hippocampus on active behavior was significant different (Density: F1,46 = 16.91, p< 0.001) between the two introduction periods (pre- and post-) (Figure 2A; Supplementary Table S4) regardless temperature or sex (Supplementary Table S4). Specifically, a twofold decrease of H. hippocampus active behavior was observed during the postintroduction (164.7 s ± 41.9 SE) compared with the pre-introduction period (362.5 s ± 39.8 SE) (Supplementary Table S4, Figure 2A). Descriptive results of the single behaviors showed that, before the introduction of two individuals of H. guttulatus, the percentage of time spent by H. hippocampus on active behavior was approximately 67% of the total time distributed between crawling (~41%), body movement (~25%), and searching (~1%) (Figure 2B). During the post-introduction period, H. hippocampus spent approximately 30% of the total time for body movement (~18%), crawling (9%), and searching (~3%) (Figure 2B). No swimming activity was recorded in both introduction periods. As previously observed in Experiment 1, seahorse length did not affect, or change, their behavior (Supplementary Table S4).
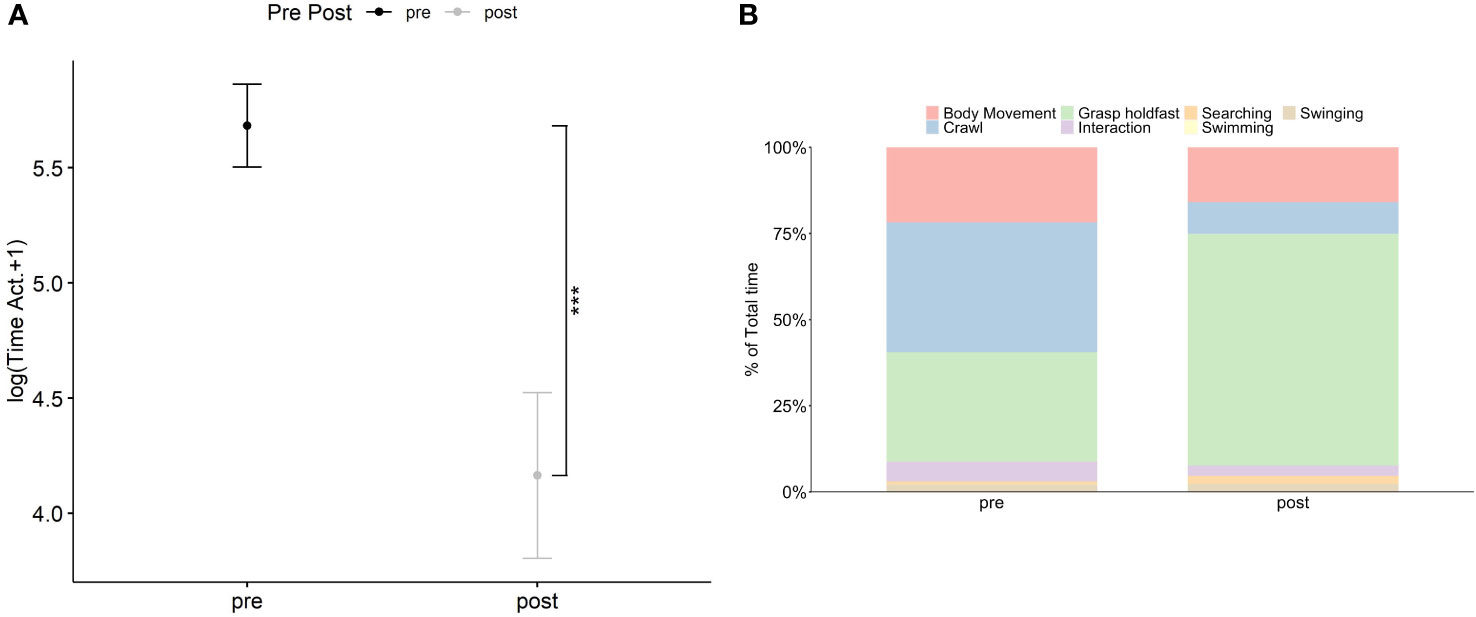
Figure 2 Relationship between the introduction periods (pre- and post-introduction of two ind. of H. guttulatus) and the sum of the time spent on active behaviors (in log +1) by H. hippocampus (n = 24) (A). Solid dots represent the median; top and bottom-vertical whiskers represent quartiles. Asterisk (*) indicates significant differences (p<.05) between the two introduction periods for H. hippocampus Stacked bar chart represent the percentage of time spent on the different behaviors (body movement, crawl, resting, swimming, and swinging) by H. hippocampus between the two introduction periods (B).
4 Discussion
The results of the present study indicated that the two seahorse species reacted differently when a habitat was provided but were not affected by +3°C warming. H. hippocampus significantly reduced its activity in presence of a habitat, while H. guttulatus maintained its activity; additionally, H. hippocampus significantly reduced its activity when exposed to an increased density of the congeneric species regardless temperature.
Higher temperatures can increase fish activity as can raise metabolic rates (Biro et al., 2010; White and Kearney, 2014; Abram et al., 2017) of fish species (Ojanguren and Brañta, 2000; Biro et al., 2010; Laubenstein et al., 2018; Culumber, 2020). However, in our study, behavior of the two seahorse species did not change significantly with an increase in temperature. Wide thermal tolerance of H. guttulatus has been reported in a previous study where the species showed unaltered activity pattern under different temperature conditions (18°C, 26°C, 28°C, and 30°C) (Aurélio et al., 2013; Faleiro et al., 2015). Our findings were in accordance with previous studies conducted on H. guttulatus (Costa et al., 2023) and other seahorses (Qin et al., 2018) where seahorse activity became affected by extreme temperature increase (+7°C and +8°C, respectively) but not by a lower temperature increase (+3°C and +4°C, respectively). Such a result for H. guttulatus was expected as the species inhabits shallow inshore waters (inlets and coastal lagoons) where there are large daily and seasonal temperature fluctuations (see Lourie et al., 2004; Woodall et al., 2018; Pierri et al., 2021); however, the lack of same response for H. hippocampus was somewhat surprising as the species usually lives in deeper and cooler waters (Lourie et al., 2004). Indeed, unaltered activity displayed by the two seahorse species under increased temperature conditions suggests tolerance to warming. Given their poor swimming abilities, seahorse tolerance to +3°C warming may allow them to cope with future increases in water temperature. However, considering limited thermal range used in the present experiment and the captive conditions, further laboratory and field investigations are needed to exclude other negative impacts of long-term warming (Faleiro et al., 2015).
Behavioral differences between the two species were observed in relation to habitat availability. In the absence of habitat, both species had similar activity levels and spent a similar amount of time in body movement, crawling, and swimming. This contrasts with previous evidence of higher activity levels of H. hippocampus compared with H. guttulatus in areas with sparse vegetation (Curtis and Vincent, 2005; Curtis et al., 2007). When artificial habitat was introduced, H. hippocampus was less active than H. guttulatus. This may be related to different aspects of their lifestyle (e.g., locomotor activity or foraging strategy) (James and Heck, 1994; Curtis and Vincent, 2005). The reduction of active behaviors (i.e., lower crawling and higher resting, see Figure 1B) observed in H. hippocampus may reflect the species preference for less vegetated habitat as supported by previous study conducted on the same and other seahorse species (James and Heck, 1994; Curtis and Vincent, 2005). H. guttulatus prefers dense-vegetated habitats (Curtis and Vincent, 2005; Caldwell and Vincent, 2012; Correia, 2022) and reductions in species abundance have been associated with declines in these habitats (Ribeiro et al., 2006; Correia et al., 2015). However, no changes in the behavior of H. guttulatus were observed in presence and absence of the artificial holdfast. Although there were some differences in the experimental design (e.g., the use of different number of holdfast availability), this study findings are in line with previous evidences that failed to detect any effect of habitat availability on H. guttulatus activity (Faleiro et al., 2008). Our results suggest that H. guttulatus have a higher behavioral plasticity compared with congeneric species when a suitable holdfast is provided. This may support the density pattern trend observed in most of the European study sites where H. guttulatus tends to have higher densities than H. hippocampus when co-occuring (Woodall et al., 2018). The capacity of H. guttulatus to remain active in different habitats may allow this species to increase the chance to find prey or reproductive mates thus increasing its competitive ability as reported for other seahorse species (Freret-Meurer and Alves, 2018). Indeed, H. guttulatus can modulate its foraging strategy to different habitats including bare substrates when no potential holdfasts are available (Ape et al., 2019). Evidence of trophic flexibility in this species have been reported in other studies where H. guttulatus was able to switch from a “sit and wait” foraging strategy in vegetated habitat to active feeding while swimming or crawling in uncovered areas (James and Heck, 1994; Felício et al., 2006). On the other hand, a higher activity of H. guttulatus may reduce camouflage and increase predation susceptibility (Foster and Vincent, 2004) with this potentially favoring the less active species. Seahorses used for this study came from a captive broodstock and were maintained under controlled conditions with limited holdfast diversity compared with the wild populations. However, the two seahorse species were kept isolated in different tanks, and the same artificial structures were provided to both species. Therefore, as they were previously adapted to these artificial structures, the difference in the activity level between the two seahorses may be related only to species-specific behavioral responses.
A significant reduction in the active behavior of H. hippocampus was observed when its ecological congeneric species was on patrol at 3× relative dominance. Despite the limited thermal range used in this study, this result was observed independently of the temperature condition thus ulteriorly supporting thermal plasticity of H. hippocampus behavior. The highest density of H. guttulatus along with the reduced activity of H. hippocampus seemed mostly related to the increased time spent, by the latter, grasping artificial substrata. This may be due to the higher risk to lose their holdfasts thus maximizing substrate occupation when more individuals compete for the same habitat in line with the “The competitive exclusion principle” (Gause, 1934). Indeed, the benefit of remaining still enough while grasping the holdfast for camouflage reduce seahorse visibility to both predator and prey (Foster and Vincent, 2004; Webster et al., 2009). Although not tested in this study, the reduced activity may however limit other crucial behavioral activities such as foraging, especially because H. hippocampus is considered an active forager of both planktonic and epibenthic prey (Curtis and Vincent, 2005; Kitsos et al., 2008). Overall, and despite the limited number of information available in literature, our results pointed out that both less-preferred habitat and increased density of the congeneric species H. guttulatus cause changes in the behavior of H. hippocampus. To date, only few information is present in literature regarding similar interspecific interactions among sympatric fish species (e.g., labrids) showing evident behavioral alterations with a reduction of the activity of subordinate species (Milazzo et al., 2013). However, further investigation is needed to determine whether behavioral changes observed in this study affected the intraspecific interactions of the two seahorses.
Broadly, this study emphasized the behavior as a primary mechanism in understanding the effects of warming and other ecological factors (i.e., habitat availability) on H. hippocampus and H. guttulatus. In addition, our results provided preliminary insight into ocean warming and species interaction that may affect patterns of distribution of the two seahorses. The paucity of behavioral and ecological information on seahorses is hampered by their naturally low densities (Foster and Vincent, 2004), low catchability (Curtis et al., 2007) and challenges associated with differentiating species (Lourie and Vincent, 1999; Curtis et al., 2007).
However, given that one of the main threats to seahorse populations on a global scale is represented by degradation of coastal habitats (Olden et al., 2007), this study helps to understand the behavioral traits that drive distribution of these sensitive species and could be therefore useful for future management and conservation projects. Additional factors, which were not explored in this study, could also have some influence on behavioral responses in the wild, including other alternative habitats, predator presence, and abundance (Shelton and Mangel, 2011; Harasti et al., 2014), as well as food availability (Felício et al., 2006; Correia et al., 2015). We believe that future studies should account for these additional factors that could affect the distribution and abundance of seahorse populations.
Data availability statement
The raw data supporting the conclusions of this article will be made available by the authors, without undue reservation.
Ethics statement
The use of the animals was approved by the ethics committee from the Veterinary Medicines Directorate, the Portuguese competent authority for the protection of animals, Ministry of Agriculture, Rural Development and Fisheries, Portugal. The studies were conducted in accordance with the local legislation and institutional requirements. Written informed consent was obtained from the owners for the participation of their animals in this study.
Author contributions
DS and MG: Conceptualization (Lead); data curation (lead); formal analysis (lead); investigation (lead); methodology (lead); writing— original draft (lead). FQ: Data curation (Supporting); formal analysis (support- ing); investigation (supporting); methodology (supporting). CP: Conceptualization (Equal); investigation (equal); methodology (equal). TL: Conceptualization (Equal); investigation (equal); methodology (equal). JP: Conceptualization (Equal); data curation (equal); writing— original draft (lead); investigation (equal); methodology (equal). All authors contributed to the article and approved the submitted version.
Funding
This study received Portuguese national funds from FCT - Foundation for Science and Technology through projects UIDB/04326/2020, UIDP/04326/2020 and LA/P/0101/2020”.
Acknowledgments
The authors would like to thank the Prof. Jason Hall Spencer (https://www.plymouth.ac.uk/staff/jason-hall-spencer) for proofreading the article.
Conflict of interest
The authors declare that the research was conducted in the absence of any commercial or financial relationships that could be construed as a potential conflict of interest.
Publisher’s note
All claims expressed in this article are solely those of the authors and do not necessarily represent those of their affiliated organizations, or those of the publisher, the editors and the reviewers. Any product that may be evaluated in this article, or claim that may be made by its manufacturer, is not guaranteed or endorsed by the publisher.
Supplementary material
The Supplementary Material for this article can be found online at: https://www.frontiersin.org/articles/10.3389/fmars.2023.1138296/full#supplementary-material
References
Abram P. K., Boivin G., Moiroux J., Brodeur J. (2017). Behavioural effects of temperature on ectothermic animals: unifying thermal physiology and behavioural plasticity. Biol. Rev. 92, 1859–1876. doi: 10.1111/brv.12312
Alexander J. M., Diez J. M., Hart S. P., Levine J. M. (2016). When climate reshuffles competitors: A call for experimental macroecology. Trends Ecol. Evol. 31 (11), 831–841. doi: 10.1016/j.tree.2016.08.003
Ape F., Corriero G., Mirto S., Pierri C., Lazic T., Gristina M. (2019). Trophic flexibility and prey selection of the wild long-snouted seahorse Hippocampus guttulatus Cuvier 1829 in three coastal habitats. Estuarine Coast. Shelf Sci. 224, 1–10. doi: 10.1016/j.ecss.2019.04.034
Aurélio M., Faleiro F., Lopes V. M., Pires V., Lopes A. R., Pimentel M. S., et al. (2013). Physiological and behavioral responses of temperate seahorses (Hippocampus guttulatus) to environmental warming. Mar. Biol. 160, 2663–2670. doi: 10.1007/s00227-013-2259-8
Bates D., Mächler M., Bolker B., Walker S. (2014). Fitting Linear Mixed-Effects Models using lme4. J. Stat. Softw. 67, 1–48. doi: 10.48550/arXiv.1406.5823
Biro P. A., Beckmann C., Stamps J. A. (2010). Small within-day increases in temperature affects boldness and alters personality in coral reef fish. Proc. R. Soc. B.: Biol. Sci. 277, 71–77. doi: 10.1098/rspb.2009.1346
Biro P. A., Post J. R., Booth D. J. (2007). Mechanisms for climate-induced mortality of fish populations in whole-lake experiments. PNAS 104, 9715–9719. doi: 10.1073/pnas.0701638104
Bonaviri C., Graham M., Gianguzza P., Shears N. T. (2017). Warmer temperatures reduce the influence of an important keystone predator. J. Anim. Ecol. 86, 490–500. doi: 10.1111/1365-2656.12634
Boulanger E., Benestan L., Guerin P.-E., Dalongeville A., Mouillot D., Manel S. (2022). Climate differently influences the genomic patterns of two sympatric marine fish species. J. Anim. Ecol. 91, 1180–1195. doi: 10.1111/1365-2656.13623
Caldwell R., Vincent A. C. J. (2012). Revisiting two sympatric European seahorse species: Apparent decline in the absence of exploitation. Aquat. Conserv. Mar. Freshw. Ecosyst. 22, 427–435. doi: 10.1002/aqc.2238
Clements J., Hunt H. (2015). Marine animal behaviour in a high CO2 ocean. Mar. Ecol. Prog. Ser. 536, 259–279. doi: 10.3354/meps11426
Cohen F. P. A., Valenti W. C., Planas M., Calado R. (2017). Seahorse aquaculture, biology and conservation: knowledge gaps and research opportunities. Rev. Fish. Sci. Aquac. 25, 100–111. doi: 10.1080/23308249.2016.1237469
Core Team R. (2019). R: A Language and Environment for Statistical Computing (Vienna, Austria: R Foundation for Statistical Computing). Available at: https://www.R-project.org/.
Correia M. (2022). Monitoring of seahorse populations, in the Ria Formosa Lagoon (Portugal), reveals steep fluctuations: potential causes and future mitigations. Proc. Zool. Soc. 75, 190–199. doi: 10.1007/s12595-021-00394-2
Correia M., Caldwell I. R., Koldewey H. J., Andrade J. P., Palma J. (2015). Seahorse (Hippocampinae) population fluctuations in the Ria Formosa Lagoon, south Portugal. J. Fish. Biol. 87, 679–690. doi: 10.1111/jfb.12748
Correia M., Koldewey H. J., Andrade J. P., Esteves E., Palma J. (2018). Identifying key environmental variables of two seahorse species (Hippocampus guttulatus and Hippocampus hippocampus) in the Ria Formosa lagoon. South Portugal. Environm. Biol. Fish. 101, 1357–1368. doi: 10.1007/s10641-018-0782-7
Correia M., Palma J., Koldewey H., Andrade J. P. (2013). Can artificial holdfast units work as a habitat restoration tool for long-snouted seahorse (Hippocampus guttulatus Cuvier). J. Exp. Mar. Biol. Ecol. 448, 258–264. doi: 10.1016/j.jembe.2013.08.001
Correia M., Paulo D., Samara E., Koulouri P., Mentogiannis V., Dounas C. (2020). Field studies of seahorse population density, structure and habitat use in a semi-closed north-eastern Mediterranean marine area (Stratoni, North Aegean Sea). J. Fish. Biol. 97, 314–317. doi: 10.1111/jfb.14371
Costa A. B., Correia M., Silva G., Lopes A. F., Faria A. M. (2023). Performance of the long-snouted seahorse, Hippocampus guttulatus, under warming conditions. Front. Mar. Science. 10. doi: 10.3389/fmars.2023
Culumber Z. W. (2020). Thermal stress increases activity and risk-taking behavior but not anxiety in a livebearing fish. Environm. Biol. Fish. 103, 313–317. doi: 10.1007/s10641-020-00966-9
Curtis J. M. R., Ribeiro J., Erzini K., Vincent A. C. J. (2007). A conservation trade-off? Interspecific differences in seahorse responses to experimental changes in fishing effort. Aquat. Conservation: Mar. Freshw. Ecosyst. 17, 468–484. doi: 10.1002/aqc.798
Curtis J. M. R., Santos S. V., Nadeau J. L., Gunn B., Bigney Wilner K., Balasubramanian H., et al. (2017). Life history and ecology of the elusive European short-snouted seahorse Hippocampus hippocampus. J. Fish. Biol. 91, 1603–1622. doi: 10.1111/jfb.13473
Curtis J. M., Vincent A. C. (2005). Distribution of sympatric seahorse species along a gradient of habitat complexity in a seagrass dominated community. Mar. Ecol. Prog. Ser. 291, 81–91. doi: 10.3354/meps291081
Faleiro F., Baptista M., Santos C., Aurélio M. L., Pimentel M., Pegado M. R., et al. (2015). Seahorses under a changing ocean: the impact of warming and acidification on the behaviour and physiology of a poor-swimming bony-armoured fish. Conserv. Physiol. 3, 1–77. doi: 10.1093/conphys/cov009
Faleiro F., Narciso L., Vicente L. (2008). Seahorse behaviour and aquaculture: How to improve Hippocampus guttulatus husbandry and reproduction? Aquaculture 282, 33–40. doi: 10.1016/j.aquaculture.2008.05.038
Felício A. K. C., Rosa I. L., Souto A., Renato H. A., Freitas R. H. A. (2006). Feeding behavior of the long-snout seahorse Hippocampus reidi Ginsbur. J. Ethol 24, 219–225. doi: 10.1007/s10164-005-0189-8
Fey S. B., Vasseur D. A., Alujević K., Kroeker K. J., Logan M. L., O’Connor M. I., et al. (2019). Opportunities for behavioral rescue under rapid environmental change. Global Change Biol. 25, 3110–3120. doi: 10.1111/gcb.14712
Foster S. J., Vincent A. C. J. (2004). Life history and ecology of seahorses: implications for conservation and management. J. Fish. Biol. 65, 1–61. doi: 10.1111/j.0022-1112.2004.00429.x
Freitas C., Olsen E. M., Knutsen H., Albretsen J., Moland E. (2016). Temperature-associated habitat selection in a cold-water marine fish. J. Anim. Ecol. 85, 628–637. doi: 10.1111/1365-2656.12458
Freitas C., Villegas-Ríos D., Moland E., Olsen E. M. (2021). Sea temperature effects on depth use and habitat selection in a marine fish community. J. Anim. Ecol. 90, 1787–1800. doi: 10.1111/1365-2656.13497
Freret-Meurer N. V., Alves M. A. S. (2018). Personality in the longsnout seahorse, Hippocampus reidi Ginsburg 1933: are males shyer than females? Behav. Processes 157, 106–110. doi: 10.1016/j.beproc.2018.09.006
Galaiduk R., Figueira W., Kingsford M., Curley B. (2013). Factors driving the biogeographic distribution of two temperate Australian damselfishes and ramifications for range shifts. Mar. Ecol. Progr. Ser. 484, 189–202. doi: 10.3354/meps10300
Galasso N. M., Bonaviri C., Trapani F. D., Picciotto M., Gianguzza P., Agnetta D., et al. (2015). Fish-seastar facilitation leads to algal forest restoration on protected rocky reefs. Sci. Rep. 5, 12409. doi: 10.1038/srep12409
Gristina M., Pierri C., Lazic T., Palma J. (2022). “Behavioral traits of captive short-snouted seahorse Hippocampus hippocampus, Linnaeus 1758,” in 2022 IEEE International Workshop on Metrology for the Sea (Learning to Measure Sea Health Parameters (MetroSea), 545–548. doi: 10.1109/MetroSea55331.2022.9950976
Harasti D., Martin-Smith K., Gladstone W. (2014). Ontogenetic and sex-based differences in habitat preferences and site fidelity of White’s seahorse. Hippocampus Whitei. J. Fish. Biol. 85, 1413–1428. doi: 10.1111/jfb.12492
Harmon J. P., Barton B. T. (2013). On their best behavior: how animal behavior can help determine the combined effects of species interactions and climate change. Ann. N. Y. Acad. Sci. 1297, 139–147. doi: 10.1111/nyas.12192
Hay M. E. (2009). Marine chemical ecology: chemical signals and cues structure marine populations, communities, and ecosystems. Ann. Rev. Mar. Sci. 1, 193–212. doi: 10.1146/annurev.marine.010908.163708
Hopkins K., Moss B. R., Gill A. B. (2011). Increased ambient temperature alters the parental care behaviour and reproductive success of the three-spined stickleback (Gasterosteus aculeatus). Environ. Biol. Fish. 90, 121–129. doi: 10.1007/s10641-010-9724-8
Hu N., Yu Z., Huang Y., Liu D. A., Wang. F., Zhang T. (2021). Elevated temperatures increase growth and enhance foraging performances of a marine gastropod. Aquacult. Environ. Interact. 13, 177–188. doi: 10.3354/aei00398
IPCC. (2014). Climate change 2014: synthesis report. Contribution of Working Groups I, II and III to the fifth assessment report of the Intergovernmental Panel on Climate Change. IPCC, Geneva.
IUCN. (2020). 2020 IUCN Red List of Threatened Species (Online). Retrieved from http://www.iucnredlist.org/.
James P. L., Heck K. L. J. (1994). The effects of habitat complexity and light intensity on ambush predation within a simulated seagrass habitat. J. Exp. Mar. Biol. 176, 187–200. doi: 10.1016/0022-0981(94)90184-8
Kitsos M.-S., Tzomos T. H., Anagnostopoulou L., Koukouras A. (2008). Diet composition of the seahorses, Hippocampus guttulatus Cuvier 1829 and Hippocampus hippocampus (L. 1758) (Teleostei, Syngnathidae) in the Aegean Sea. J. Fish. Biol. 72, 1259–1267. doi: 10.1111/j.1095-8649.2007.01789.x
Laubenstein T. D., Rummer J. L., Nicol S., Parsons D. M., Pether S. M. J., Pope S., et al. (2018). Correlated effects of ocean acidification and warming on behavioral and metabolic traits of a large Pelagic fish. Diversity 10, 35. doi: 10.3390/d10020035
Levine J. M., Bascompte J., Adler P. B., Allesina S. (2017). Beyond pairwise mechanisms of species coexistence in complex communities. Nature 546, 56–64. doi: 10.1038/nature22898
Lourie S. A., Foster S. J., Cooper E. W. T., Vincent A. C. J. (2004). A Guide to the Identification of Seahorses (Washington: University of British Columbia and WorldWildlife Fund), 1–114.
Lourie S. A., Vincent A. C. J. (1999). Hall, H. Seahorses: An Identification Guide to the World’s Species and Their Conservation (Project Seahorse: London, UK), 186.
Martin P., Bateson P. (1993). Measuring behaviour—an introductory guide. 2nd edition (Cambridge University Press, Cambridge, UK).
Matis P. A., Donelson J. M., Bush S., Fox R. J., Booth D. J. (2018). Temperature influences habitat preference of coral reef fishes: Will generalists become more specialised in a warming ocean? Global Change Biol. 24, 3158–3169. doi: 10.1111/gcb.14166
Milazzo M., Mirto S., Domenici P., Gristina M. (2013). Climate change exacerbates interspecific interactions in sympatric coastal fishes. J. Anim. Ecol. 82, 468–477. doi: 10.1111/j.1365-2656.2012.02034.x
Miller G. M., Kroon F. J., Metcalfe S., Munday P. L. (2015). Temperature is the evil twin: effects of increased temperature and ocean acidification on reproduction in a reef fish. Ecol. Applicat. 25, 603–620. doi: 10.1890/14-0559.1
Nagelkerken I., Munday P. L. (2016). Animal behaviour shapes the ecological effects of ocean acidification and warming: moving from individual to community-level responses. Global Change Biol. 22, 974–989. doi: 10.1111/gcb.13167
Nay T. J., Johansen J. L., Rummer J. L., Steffensen J. F., Pratchett M. S., Hoey A. S. (2020). Habitat complexity influences selection of thermal environment in a common coral reef fish. Conserv. Physiol. 8, coaa070. doi: 10.1093/conphys/coaa070
Newton A., Mudge S. M. (2003). Temperature and salinity regimes in a shallow, mesotidal lagoon, the Ria Formosa, Portugal. Estuarine. Coast. Shelf Sci. 57, 73–85. doi: 10.1016/S0272-7714(02)00332-3
Nogués-Bravo D., Rahbek C. (2011). Communities under climate change. Science 334, 1070–1071. doi: 10.1126/science.1214833
Ockendon N., Baker D. J., Carr J. A., White E. C., Almond R. E. A., Amano T., et al. (2014). Mechanisms underpinning climatic impacts on natural populations: altered species interactions are more important than direct effects. Global Change Biol. 20, 2221–2229. doi: 10.1111/gcb.12559
Ojanguren A. F., Brañta F. (2000). Thermal dependence of swimming endurance in juvenile brown trout. J. Fish. Biol. 56, 1342–1347. doi: 10.1111/j.1095-8649.2000.tb02147.x
Olden J. D., Hogan Z. S., Vander Zanden M. J. (2007). Small fish, big fish, red fish, blue fish: size biased extinction risk of the world’s freshwater and marine fishes. Global Ecol. Biogeography 16, 694–701. doi: 10.1111/j.1466-8238.2007.00337.x
Pierri C., Cardone F., Corriero G., Lazic T., Quattrocchi F., Alabiso G., et al. (2021). Density decline in a Mediterranean seahorse population: natural fluctuations or new emerging threats? Front. Mar. Sci. 8. doi: 10.3389/fmars.2021.692068
Pierri C., Lazic T., Gristina M., Corriero G., Sinopoli M. (2022). Large-scale distribution of the European Seahorses (Hippocampus Rafinesque 1810): A systematic review. Biology 11, 325. doi: 10.3390/biology11020325
Pollom R. (2014) Hippocampus Hippocampus. The IUCN Red List of Threatened Species. 2014. Available at: https://www.iucnredlist.org/species/10069/54904826 (Accessed 10 September 2021).
Pollom R. (2017)Hippocampus Guttulatus. In: The IUCN Red List of Threatened Species. Available at: https://www.iucnredlist.org/species/41006/67617766 (Accessed 10 September 2021).
Pollom R. A., Ralph G. M., Pollock C. M., Vincent A. C. J. (2021). Global extinction risk for seahorses, pipefishes and their near relatives (Syngnathiformes). Oryx 55, 497–506. doi: 10.1017/S0030605320000782
Qin G., Johnson C., Zhang Y., Zhang H., Yin J., Miller G., et al. (2018). Temperature-induced physiological stress and reproductive characteristics of the migratory seahorse Hippocampus erectus during a thermal stress simulation. Biol. Open 7, bio032888. doi: 10.1242/bio.032888
Ribeiro J., Bentes L., Coelho R., Gonçalves J. M. S., Lino P. G., Monteiro P., et al. (2006). Seasonal, tidal and diurnal changes in fish assemblages in the Ria Formosa lagoon (Portugal). Estuar. Coast. Shelf Sci. 67, 461–474. doi: 10.1016/j.ecss.2005.11.036
Schmidt K. A., Dall S. R. X., Van Gils J. A. (2010). The ecology of information: an overview on the ecological significance of making informed decisions. Oikos 119, 304–316. doi: 10.1111/j.1600-0706.2009.17573.x
Shelton A. O., Mangel M. (2011). Fluctuations of fish populations and the magnifying effects of fishing. PNAS 108, 17. doi: 10.1073/pnas.1100334108
Sih A., Bell A., Johnson J. C. (2004). Behavioral syndromes: an ecological and evolutionary overview. Trends Ecol. Evol. 19, 372–378. doi: 10.1016/j.tree.2004.04.009
Spatafora D., Massamba N’Siala G., Quattrocchi F., Milazzo M., Calosi P. (2021). Plastic adjustments of biparental care behavior across embryonic development under elevated temperature in a marine ectotherm. Ecol. Evol. 11, 11155–11167. doi: 10.1002/ece3.7902
Webster M. M., Ward A. J. W., Hart P. J. B. (2009). Individual boldness affects interspecific interactions in sticklebacks. Behav. Ecol. Sociobiol. 63, 511–520. doi: 10.1007/s00265-008-0685-2
Wernberg T., Smale D. A., Tuya F., Thomsen M. S., Langlois T. J., de Bettignies T., et al. (2013). An extreme climatic event alters marine ecosystem structure in a global biodiversity hotspot. Nat. Climate Change 3 (1), 78–82. doi: 10.1038/nclimate1627
White C. R., Kearney M. R. (2014). Metabolic scaling in animals: methods, empirical results, and theoretical explanations. Compr. Physiol. 4 (1), 231–256. doi: 10.1002/cphy.c110049
Woodall L. C., Otero-Ferrer F., Correia M., Curtis J. M., Garrick-Maidment N., Shaw P. W., et al. (2018). A synthesis of European seahorse taxonomy, population structure, and habitat use as a basis for assessment, monitoring and conservation. Mar. Biol. 165, 1–19. doi: 10.1007/s00227-017-3274-y
Yeruham E., Shpigel M., Abelson A., Rilov G. (2020). Ocean warming and tropical invaders erode the performance of a key herbivore. Ecology 101, e02925. doi: 10.1002/ecy.2925
Keywords: behavioral plasticity, Hippocampus guttulatus, Hippocampus hippocampus, climate change, seahorse, coastal habitat
Citation: Spatafora D, Gristina M, Quattrocchi F, Pierri C, Lazic T and Palma J (2023) Different behavioral strategies of two sympatric seahorses: habitat availability and increased density of Hippocampus guttulatus alter the behavior of Hippocampus hippocampus. Front. Mar. Sci. 10:1138296. doi: 10.3389/fmars.2023.1138296
Received: 05 January 2023; Accepted: 10 August 2023;
Published: 31 August 2023.
Edited by:
Siu Gin Cheung, City University of Hong Kong, Hong Kong SAR, ChinaReviewed by:
Francesca Ape, National Research Council (CNR), ItalyAngus Mitchell, University of Adelaide, Australia
Copyright © 2023 Spatafora, Gristina, Quattrocchi, Pierri, Lazic and Palma. This is an open-access article distributed under the terms of the Creative Commons Attribution License (CC BY). The use, distribution or reproduction in other forums is permitted, provided the original author(s) and the copyright owner(s) are credited and that the original publication in this journal is cited, in accordance with accepted academic practice. No use, distribution or reproduction is permitted which does not comply with these terms.
*Correspondence: Michele Gristina, bWljaGVsZS5ncmlzdGluYUBjbnIuaXQ=
†These authors have contributed equally to this work
‡Present address: Davide Spatafora, Stazione Zoologica Anton Dohrn, Department of Integrative Marine Ecology, Palermo, Italy