- 1Universidad Católica del Norte, Departamento de Biología Marina, Coquimbo, Chile
- 2Centro de Estudios Avanzados en Zonas Áridas, CEAZA, Coquimbo, Chile
- 3Núcleo Milenio de Ecología y Manejo Sustentable (ESMOI), Facultad de Ciencias del Mar, Departamento de Biología Marina, Universidad Católica del Norte, Coquimbo, Chile
- 4Centro de Investigación y Estudios Avanzados del Maule, Vicerrectoría de Investigación y Posgrado, Universidad Católica del Maule, Campus San Miguel, Talca, Chile
- 5J’EAI-CHARISMA, UMNG-Cajicá, Cajicá, Colombia
- 6ANID-Millennium Science Initiative Program Núcleo Milenio UPWELL, La Serena, Chile
- 7Laboratorio de Sedimentología y Paleoambientes, Instituto de Cs Naturales Alexander von Humboldt, Facultad de Ciencias del Mar y de Recursos Biológicos, Universidad de Antofagasta, Antofagasta, Chile
- 8CECI, Université de Toulouse, CERFACS/CNRS, Toulouse, France
The oxygen minimum zone (OMZ) is an essential feature along the Pacific margin, extensively studied for its influence on benthic fauna, acquiring relevance in coastal zones due to its impact on aquaculture species, marine-managed areas, and marine-protected areas. The most evident temporal variability beyond seasonal timescales observed for the OMZ was related to inter-annual variability associated with El Niño, when warmer and more oxygenated waters arrive at shallower zones and the OMZ becomes deeper. However, the impact of oxygen-deficient water that appears sporadically over the shelf is not yet understood. This study provides an integrated view of the oceanographic and climatic conditions behind bottom oxygen conditions along the Chilean continental margin, considering the temporal variability in the South East (SE) Pacific during the last ~2000 years. We organized the information on redox-sensitive metals and δ15N from sedimentary cores obtained from shelf zones from 23°S to 36°S at water depths lower than 100 m. The results demonstrate the variability in the upper part of the OMZ over the shelf, which seems to respond to climatic oscillations. The element distribution indicated in particular a more intense OMZ was developed over the shelf before 1400 AD, followed by more oxygenated bottom conditions afterward, except between 1925 and 1970 off 36°S when the OMZ was intensified, a condition that was not observed at the northern sites. This period mostly coincided with a low Pacific Decadal Oscillation amplitude and reduced El Niño Southern Oscillation (ENSO) activity. In the last 50 years, the proxy data suggests less intense suboxic environment along the Chilean margin. This weakening of the upper boundary of the OMZ in the last decades is interpreted as resulting from the accumulative effect of ventilation processes at different time scales.
Introduction
An essential feature of the eastern margins is the development of zones with low oxygen concentrations or oxygen minimum zones (OMZ), resulting from a high rate of oxygen consumption and limited ventilation. The eastern Pacific (EP) hosts one of the most extended OMZ of the planet that is embedded into the so-called Eastern Boundary Upwelling Systems (EBUS) in which nutrients are brought to the surface by the process of upwelling, making them among the most productive regions of the planet (Pauly and Christensen, 1995; Kämpf and Chapman, 2016). The high biological production associated with a complex nutrient cycle sustained by upwelling induces a significant oxygen consumption mainly through remineralizing organic matter, which, along the weak thermocline ventilation (Luyten et al., 1983) maintains a strong OMZ off Peru and the northern areas of Chile (Helly and Levin, 2004; Paulmier et al., 2006). There dissolved oxygen concentration can be as low as 0.5 mL L-1 (< 22 µM) with a maximum meridional extension of ~3000 km. Off central Peru (15°S), the upper boundary (25–50 m) is shallower and diminishes gradually in thickness and intensity toward the southern areas. Around 37°S, which corresponds to the southern tip of the Southeastern Pacific (SEP) OMZ, it is described as a thin band with a deeper upper boundary (> 100 m) (Schneider et al., 2006; Fuenzalida et al., 2009) (Figure 1).
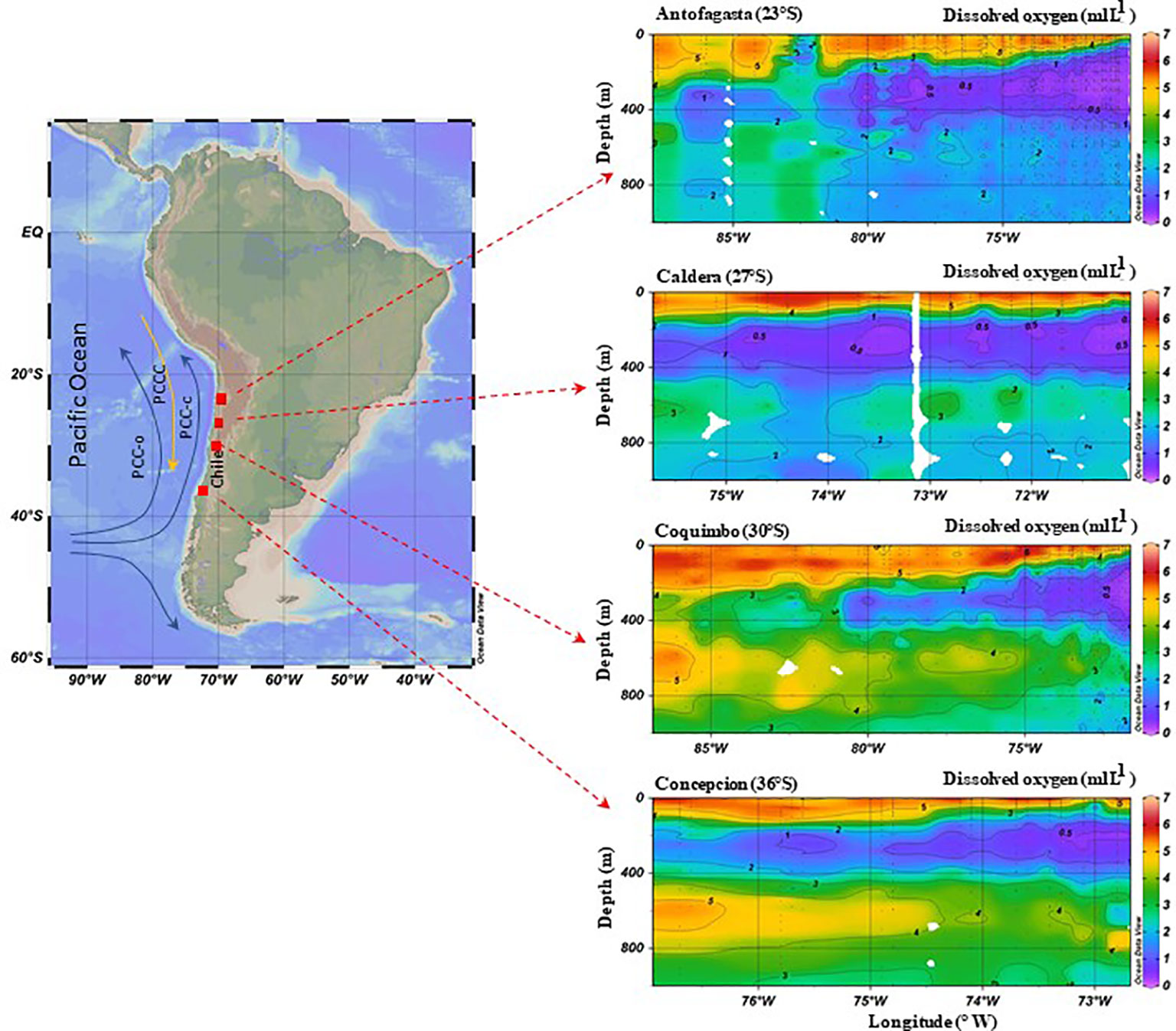
Figure 1 An overview map of South America (left) showing the areas of the cores recovered from the Chilean margin off Antofagasta (23°S; Mejillones Bay), Caldera (27°S; Inglesa Bay), Coquimbo (30°S; Guanaqueros and Tongoy Bay) and Concepcion (36°S; Concepcion shelf). For cores’ information, see Table 1. The main oceanic surface currents are indicated with arrows: the Peru-Chile Current (PCC) coast(c), oceanic(o) branches, and the Peru-Chile Counter Current (PCCC). Dissolved oxygen (DO) concentrations in a transect in front of the study areas show the position of the oxygen minimum zone (OMZ) corresponding to concentrations lower than 0.5 ml L-1. Data were obtained from Auger et al. (2020a); Auger et al. (2020b).
Along the Chilean margin, the OMZ is composed of equatorial subsurface waters (ESSW), which are rich in nutrients and deficient in O2 concentrations, transported southward by the Peru-Chile Undercurrent (PCUC) until ~48°S (Silva and Neshyba, 1979; Pizarro-Koch et al., 2019). It is located above the more oxygenated Antarctic intermediate water (AAIW) formed in the southern regions and moves northward, producing a distinct oxygen front around ~30°S. Nevertheless, the OMZ has limited ventilation because the South Pacific intermediate water (ESPIW) stabilizes the upper part of the water column (Schneider et al., 2003). It has been observed that this zone is highly impacted by remotely forced Kelvin waves originating from the equatorial region and propagating along the coast (Pizarro et al., 2002; Dewitte et al., 2012), which modulates the upwelling and OMZ extension, thereby changing the biogeochemical cycles in the water column and shelf sediments (Gutiérrez et al., 2008; Graco et al., 2017). Thus, the leading causes of their vertical and temporal variability over the contemporary period are tightly linked to the oceanic equatorial teleconnection (Sprintall et al., 2020).
On the other hand, sedimentary records along the SEP suggest that OMZ variability is related to the main atmospheric forcings at secular, decadal, and inter-annual scales (Salvatteci et al., 2014; Srain et al., 2015; Castillo et al., 2017; Ortega et al., 2019; Muñoz et al., 2020; Valdés et al., 2021). The Pacific Decadal Oscillation (PDO) and the Southern Annular Mode (SAM) have established regional cold/warm periods that are indirectly related to changes in bottom oxygenation due to the interaction of upwelling with primary productivity and ventilation. These periods are associated with changes in the position and strength of the westerlies, affecting the winter precipitation and intensity of the upwelling (Quintana and Aceituno, 2012; Ancapichún and Garcés-Vargas, 2015).
These climatic patterns can emulate ENSO-like conditions that affect the primary oceanographic features of the region (upwelling, OMZ, temperature, and productivity). They can exacerbate or weaken the effects of inter-annual variability, which depends on whether the decadal and inter-annual variability is in/out-of-phase (cold/warm). This has been described for the western North Pacific, where the PDO plays a relevant role in the intensity of ENSO (Yang and Oh, 2020). In particular, the cold (positive) phase of PDO (La Niña-like) tends to reduce ENSO variability by favoring a more northward Intertropical Convergence Zone (ITCZ) position and stronger cold tongue in the eastern equatorial Pacific (Hu and Fedorov, 2018). For instance, the northward position of the Intertropical Convergence Zone (ITCZ) was shown to allowed favorable conditions for upwelling and productivity in the early Holocene (Koutavas et al., 2006; Kaiser et al., 2008; Lamy et al., 2010), which in turn can favor a stronger OMZ along the SEP (Salvatteci et al., 2016). Afterward, the mid-Holocene warm period was interrupted by a weakening of ENSO around 6–7 ka when freshwater melting counteracted the insolation regime (Braconnot et al., 2012), driving cold periods associated with active upwelling and increased productivity for the central-Chilean margin (Carré et al., 2012; Carré et al., 2014; Muñoz et al., 2020). In addition, low ENSO activity at 4–5 ka for the Central Pacific, as evidenced by SST proxy from corals and fossil shells, was consistent with precessional forcing (Cobb et al., 2013; Carré et al., 2014). Such a reduced ENSO activity was also consistent with the small increases of diatoms and opal observed in sedimentary records of the north and central Chilean margin that suggested the maintenance of upwelling conditions over this period (Muñoz et al., 2012; Muñoz et al., 2020). However, during that period, the Peruvian margin did not evidence a stronger OMZ, which was explained by an intensification of equatorial subsurface countercurrents (Equatorial undercurrent, South Intermediate current, and Southern subsurface countercurrents) that introduced more oxygenated water and intensifying vertical mixing (Salvatteci et al., 2016; Mollier-Vogel et al., 2019). Recently, an expansion of the OMZ off central Peru was established after 1820 AD (Gutiérrez et al., 2009), while off northern Chile over the same period, the OMZ strengthened and reached shallower depths (Vargas et al., 2007; Valdés et al., 2021). On the other hand, for the central Chilean margin, the OMZ intensity was maintained through the mid-Holocene but experienced a strong reduction over the last 2000 years (Muñoz et al., 2020). The bottom oxygenation suggested by Muñoz et al. (2020) could have been associated with changes in equatorial variability favoring the atmospheric teleconnection off Central Chile. In particular there is a transition zone around 30°S for the ENSO atmospheric teleconnection: to the South of 30°S, upwelling favorable winds tend to reduce during El Niño (Dewitte et al., 2023).
The variability in the OMZ intensity during the late Holocene has been explained by changes in the expansion/contraction of the South Pacific Anticyclone (SPA) and the strength of the Walker Circulation for the Tropical SE Pacific, which determines the intensity of wind and upwelling (Salvatteci et al., 2016; Castillo et al., 2017). However, it has had different effects on the OMZ along the SE Pacific margin, owing to other ventilation mechanisms or local processes (Pitcher et al., 2021). Thus, there is currently still much uncertainty about the mechanisms at play to explain the variability of the SEP OMZ over the last millennia, which calls for revisiting existing proxy data in an integrative way. In this study, we take advantage of sedimentary geochemical records collected at four main upwelling zones in the northern and central Chilean continental shelf, where the OMZ intercepts the bottom: off Antofagasta (23°S), Caldera (27°S), Coquimbo (30°S), and Concepcion (36°S) (Figure 1 and Table 1), to establish the temporal evolution of the OMZ in the SE Pacific over the last 2000 years and discuss the possible processes involved.
Regional settings
The Chilean continental margin has distinct topographical, climatic, and oceanographic characteristics, modulating the primary productivity and chemical composition of the water column. This zone has been characterized as having several sites of high primary production (0.5−9.3 g C m-2 d-1; González et al., 1998; Daneri et al., 2000; Thomas et al., 2001) off Iquique (21°S), Antofagasta (23°S), Coquimbo (30°S) and Concepción (36°S), produced by the influx of waters enriched in nutrients forced by local winds. Differences can be established latitudinally. Upwelling is semi-permanent in the North of Chile (18–30°S) and seasonal in the south (Montecino and Lange, 2009). This productivity occurs close to the coast above the narrow continental shelf in the north, which allows the development of relevant fisheries (Escribano et al., 2004 and references therein). Toward the south, the continental shelf is wider (~36–37°S). The upwelling sustains high primary productivity rates (9.9–19.9 g C m-2 d-1) during the spring-summer period (Bernal et al., 1989; Fossing et al., 1995; Dellarosa, 1998; Daneri et al., 2000).
To the north (at 21°S) and off Peru, the OMZ is permanently present and can extend into the euphotic zone. In the case of northern Chile and southern Peru, it does not interface significantly with the benthic environment because of a narrow continental shelf (Helly and Levin, 2004). Off Central Peru (~11-14°S) and northern Chile (21-23°S), the OMZ extends over the shelf. It has been reported that during the 1997–98 warm ENSO event (El Niño), the appearance of the sediments was less reduced, in some cases, up to a depth of 10 cm, with positive redox potential values at the surface (Muñoz et al., 2004). The most evident response of the benthic fauna was a switch in species composition, deeper penetration of the fauna into the sediments, and increased body size of the organisms (Gutiérrez et al., 2006; Sellanes et al., 2007; Gutiérrez et al., 2008). Following this El Niño, the dissolved oxygen decreased gradually to reach normal low concentrations (< 0.5 ml L-1), dropping well below 0.25 ml L-1 due to repeatedly La Niña events during 1999, 2000, and 2001, reaching considerably lower oxygen concentrations than values reported in previous years (Gonzalez et al., 2007). The oxygen rise at the bottom directly affects biogeochemical cycles that can be observed several months after the event (Ulloa et al., 2001; Escribano et al., 2004).
Methods
Sedimentary records
We reanalyzed previously published information from six sedimentary records at four sites along the Chilean margin: Mejillones del Sur Bay (23°S), Inglesa Bay (27°S), Coquimbo Bay (30°S; Guanaqueros and Tongoy Bay), and Concepcion Bay (36°S) (Muñoz et al., 2012; Guiñez et al., 2014; Srain et al., 2015; Castillo et al., 2017; Muñoz et al., 2020; Valdés et al., 2021). The cores have been dated using short-lived radioisotopes (210Pb, 241Am) by gamma and alpha counting combined with AMS radiocarbon measurements. In the case of Concepción Bay, the geochronology obtained with lineal regression (Muñoz et al., 2012) was updated using the Clam 2.2 software. The background information for each sedimentary record is presented in Table 1.
Cores description
The sedimentological characteristics of the selected cores are summarized below for a better approach to proxy interpretation.
The core located at the northern site (23°S, Mejillones del Sur Bay) was composed of silts (11–15%) and clays (> 70%), presenting an increase in clays in recent times (~90%). The total organic (TOC) content was high, between ~4% in the base of the core, increasing to the surface, reaching 5.5–7%. The sediments were described as olive green, with low stratifications, only visible with X-Rays, and were recognized three sedimentary facies based on color and thickness. Therefore, low bioturbation was observed. The age lower limits for the units were 1880 and 1600 AD, respectively (Guiñez et al., 2014; Valdés et al., 2021).
At 27°S off Inglesa Bay, the sediments also exhibited laminations of alternating light and dark color bands, the latter corresponding to hemipelagic organic material. Four units were recognized based on these laminations. The age lower limits were 1826, 1311, and 1009 AD, with slumps between the last three units. The TOC content was between 4% and 7%, with decreasing values toward the present (Castillo et al., 2017).
The cores retrieved from the southern sites did not show lamination. Off ~30°S (Guanaqueros and Tongoy Bays) were composed of very fine sand and silt, with evidence of weak laminations and low bioturbation. They also contain debris from fish vertebrates and shells, commonly observed in sediments from the northern Chilean shelf areas (Milessi et al., 2005). The sediment colors varied between bays. Guanaqueros exhibited accentuated olive-green colors, whereas Tongoy displayed colors in the range of very dark grayish-brown to dark olive green. In this core, a slight increase in the mean grain size occurred in the last 1000 years owing to a major continental input, which also explains the colors found. In agreement with this, the TOC (%) was lower (1−1.5%) than that in Guanaqueros (< 5%) (Muñoz et al., 2020).
The cores from the shelf of Concepcion (36°S) were composed of dark olive green hemipelagic mud at the surface, followed by distinctive olive green layers alternating with grey clayey mud layers. Differences in colors were related to their composition; olive-green corresponded to low bulk density and higher TOC content, between 1% and 2%, while the gray layers were low in TOC and showed higher bulk densities. This composition is similar to that of the Coquimbo shelf, but the core of Concepcion has a thin gray layer at ~79 cm, corresponding to hemipelagic sediments. These sediments exhibited moderate bioturbation and were not typically laminated (Srain et al., 2015; Muñoz et al., 2020).
The rationale for the selection of proxies δ15N
Water column denitrification has been indirectly inferred from δ15N in sedimentary organic materials (Altabet, 2005; Robinson et al., 2014). Under low subsurface oxygen in the water column (< 2 µmol O2 L-1), the partial NO3 uptake and denitrification process resulted in increased values of δ15N-nitrate, imprinting higher δ15N in sinking organic particles. This signal has been used as a proxy for changes in oxic conditions at the bottoms (Lam et al., 2009; Farmer et al., 2021). Global δ15N variation has been associated with glacial cooling. It is described as a less intense suboxia in denitrification zones due to oxygen supply to the thermocline zone or reduced oxygen consumption due to reduced export production (Robinson et al., 2007 and references therein). This process marks periods of reduced denitrification associated with lower δ15N values during glacial periods and higher values during interglacial periods. This variability has been observed along the SE Pacific in shallower and deeper zones, establishing denitrification zones and their regional variations in surface sediments (De Pol-Holz et al., 2006; Robinson et al., 2007; De Pol-Holz et al., 2009; Mollier-Vogel et al., 2012). Here, we used this tool as a proxy for denitrification intensity, which describes the subsurface variability of oxygen content over the continental shelf.
Redox-sensitive metals (Mo, U)
A few redox-sensitive metals have been used to reconstruct the redox conditions of depositional environments. Under oxygen-deficient subsurface water conditions (reducing conditions), metals show a higher enrichment compared with normoxic conditions (Tribovillard et al., 2006; Bennett and Canfield, 2020; Sánchez et al., 2022). However, the availability of reactive organic material in the column may also control the accumulation and enrichment of redox-sensitive metals on the seafloor (Nameroff et al., 2002; McManus et al., 2006; Algeo and Li, 2020). In the SE Pacific, redox-sensitive metals, such as Mo, Re, and U, have demonstrated moderate to strong enrichment behavior, especially in coastal areas associated with the OMZ (Böning et al., 2004; Böning et al., 2005; Böning et al., 2009; Muñoz et al., 2012; Salvatteci et al., 2014; Valdés et al., 2014; Castillo et al., 2019; Valdés et al., 2021; Muñoz et al., 2022). Here, we used redox-sensitive elements (U and Mo) to interpret the oxidative changes on the bottoms related to oxygen availability, using TOC and stable isotope distribution for complementary interpretations.
Results
δ15N and TOC distribution
The latitudinal distribution of δ15N does not describe a clear pattern (Figure 2). The values vary between ~1.2 and ~13‰, being higher in northern areas (23–27°S). The range of variability of the isotope was narrow for Mejillones and Coquimbo cores, while the cores at 27°S and 36°S showed more variability. In the case of Concepcion, the period of lowest values is concomitant with that of the lowest values for TOC (i.e., ~400 AD), corresponding to sandy sediment below the gray layer. Off-Mejillones (23°S), the maximum values were at the oldest periods (~1400 AD), and in Inglesa Bay, the maxima were around 900−1100 AD. Meanwhile, at the southern sites (30°S−36°S), δ15N values peak during the recent period (~9‰−10‰).
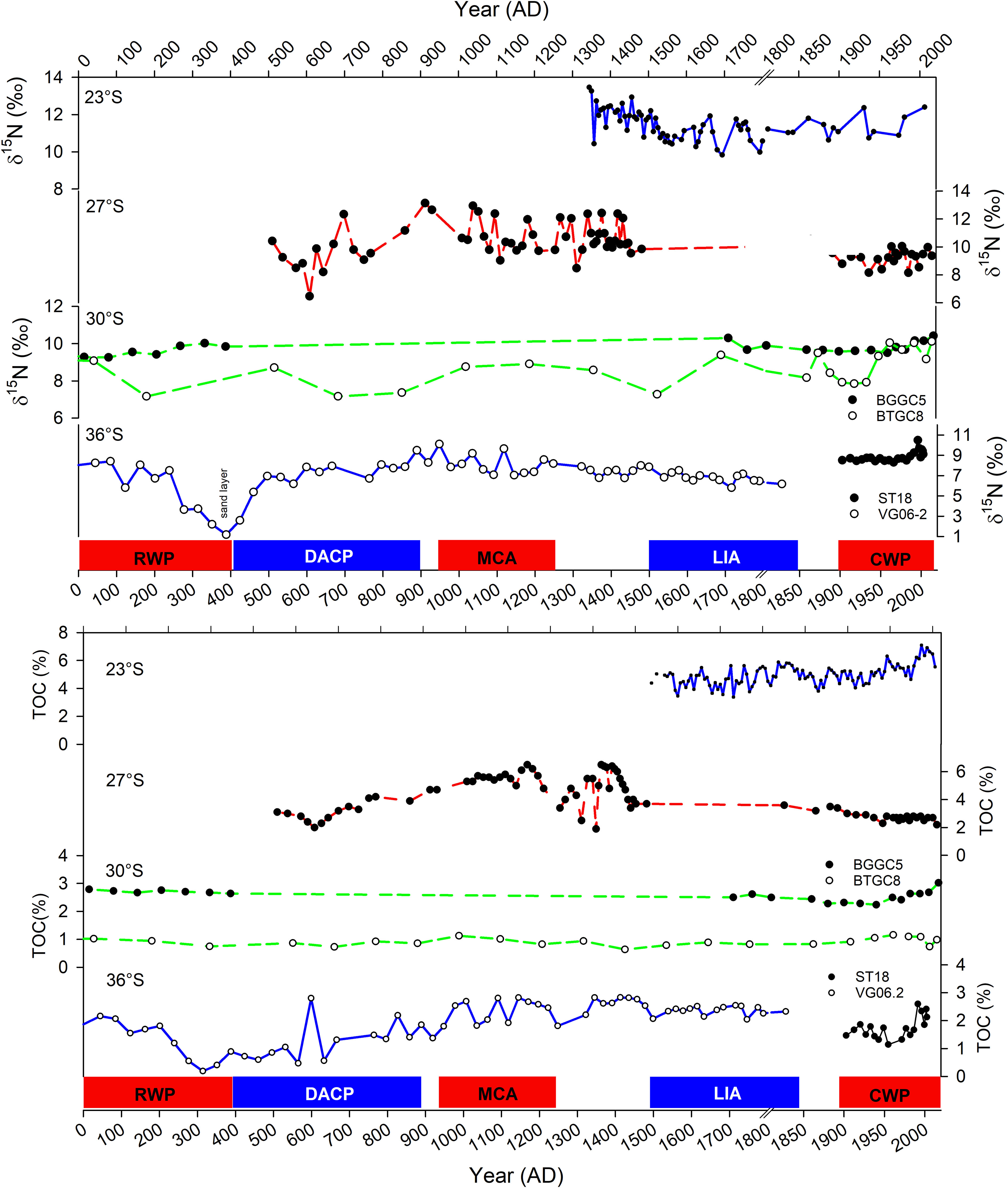
Figure 2 Comparison of δ15N and total organic carbon (TOC%) records along the Chilean margin (23–36°S). The records correspond to the cores indicated in Table 1; Core MEJ2009 (23°S), core BIAC072014 (27°S), core BGGC5 (black dots, 30°S), core BTGC8 (unfilled dots, 30°S), core ST18 (black dots, 36°S) and core VG06-2 (unfilled dots, 36°S). Cold (blue) and warm (red) periods are indicated on the x-axis: RWP (0-400 AD), DACP (400-800 AD), MCA (9501250 AD), LIA (1500-1850 AD) and CWP (> 1900 AD).
Similarly, TOC content did not exhibit a clear latitudinal pattern. The maximum TOC values were in Mejillones (21°S) with high variability, showing an increasing trend in recent times; from 1950 to 2000, the TOC reached 6.5% (~0.5%/decade). Off Coquimbo (30°S), the TOC values were lower and similar amongst the different cores, ~1% and 3% in Tongoy and Guanaqueros Bay, respectively. Other sites showed maximum values between 1000 and 1400 AD, around 6% and 3% off Inglesa Bay and Concepcion, respectively. After this age, the TOC content tended to diminish (~3% and ~1%, respectively) by ~1950−1960. After this date, most sites evidence an increase, except for Inglesa Bay (27°S), which indicates a gradual decrease in the recent period from ~1500 AD.
For a better approach, δ15N related to the denitrification process was standardized to establish its variability at each site (Figure 3). Standardization consisted of estimating the variability around the mean value at each core according to (value-average)/standard deviation. This approach resulted in positive and negative values that helped visualize the intensity of the processes at each site, independently of the latitudinal differences in the proxy concentrations. Figure 3 indicates that, between 0 and 500 AD in Concepcion (36°S), the standardized δ15N evolved from low positive to higher negative values, corresponding to less intense denitrification. In contrast, Coquimbo (30°S) showed high negative values that grew to small positive values, which means that the denitrification was increasing, being slightly more intense at the end of the period. There is no information to compare to northern sites.
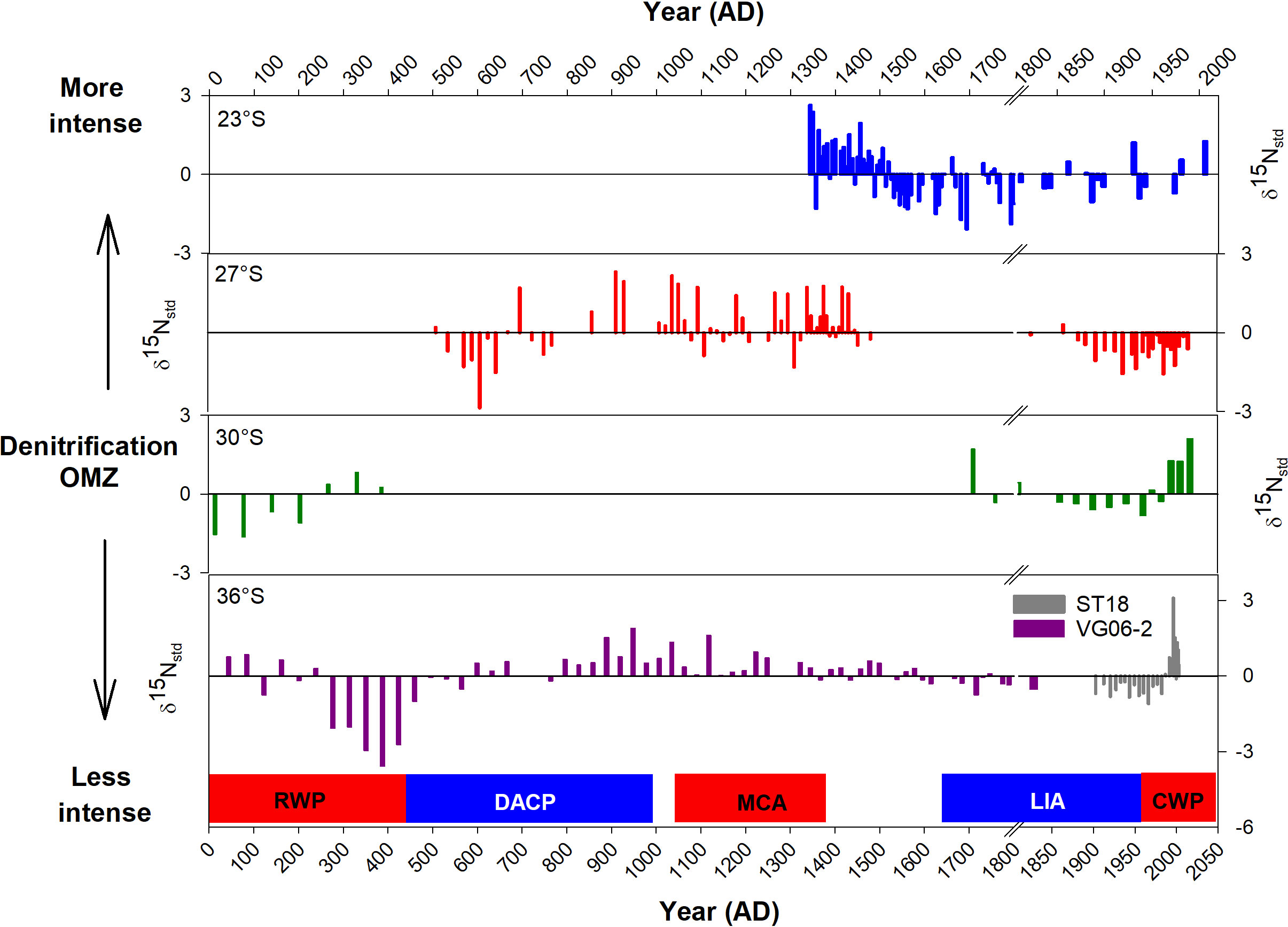
Figure 3 Comparison of standardized isotopic records (δ15Nstd) along the Chilean margin. The core information is indicated in Table 1. (23°S: core Mej2009; 27°S: coreBIAC072014; 30°S: BGGC5 core; 36°S: ST18 and VG06-2 cores).
Between 500 and 1100 AD, Inglesa Bay (27°S) and Concepcion (36°S) displayed a similar trend. The δ15N evolved from strongly negative or “neutral” to positive values; both sites indicated increasing denitrification, peaking at ~900−1000 AD.
Between 1100 and 1500 AD, denitrification was more intense in Mejillones (23°S, only data from ~1300 AD) and Inglesa Bay (27°S), diminishing to the southern sites (36°S) during the same period (there is a gap in information from Coquimbo). The cores of Concepcion showed low variability, low positive values around the mean value. On the other hand, the intensity in denitrification decreased toward 1500 AD in Mejillones, while in Inglesa Bay, this is less clear due to a gap of information in this period, only few data indicated small negative values occurred around 1500 AD, suggesting less intensity in this process.
Between 1500 AD and ~1900 AD, Mejillones showed the lowest intensity in denitrification (higher negative values). Similar to Coquimbo, mostly negative values indicated a diminishing denitrification process; however, only a few pieces of data were available for this period. For Concepcion, the values were also negative but close to the mean intensity. No information is available at Inglesa Bay for this period.
After 1900 AD, Mejillones (23°S) showed alternating positive and negative values with a magnitude similar to the other sites, indicating high variability in the intensity of denitrification. In Inglesa Bay (27°S), they were consistently negative or less intense denitrification. On the other hand, in Coquimbo (30°S), both cores showed an increasing trend to higher positive values indicating an intensified denitrification. Concepcion (36°S) evolved from negative values until ~1970 to very high positive values at the current time.
TOC and metal relationship
We further explored the relationship between Mo and TOC (Figure 4) since Mo indicates reduced conditions closely related to organic matter remineralization in zones with high productivity. Also, we analyzed the relationship between U and Mo (Figure 5) in order to characterize the flux of organic particles. There is a clear linear relationship between U and Mo, independent of the temporal scale of each core (see Table 1). This relationship was more robust in the northern zones (23–27°S; r2 = 0.78–0.81; p < 0.0001) and decreased towards the southern sites, where the relationship between U and Mo weakens but still remains significant (36°S; r2 = 0.24−0.33). TOC was higher in Mejillones (23°S) with maximum values reaching 6–7%, and the relationship with Mo showed a negative correlation (r2 = 0.45). To the south, the TOC values decreased to 3% at Concepción with a positive relationship with Mo (Figure 4), but with a weaker value of correlation (36°S; r2 = 0.13−0.26), probably caused by an increase in detrital inputs that spoilt the relationship between sedimented organic matter and metal precipitation.
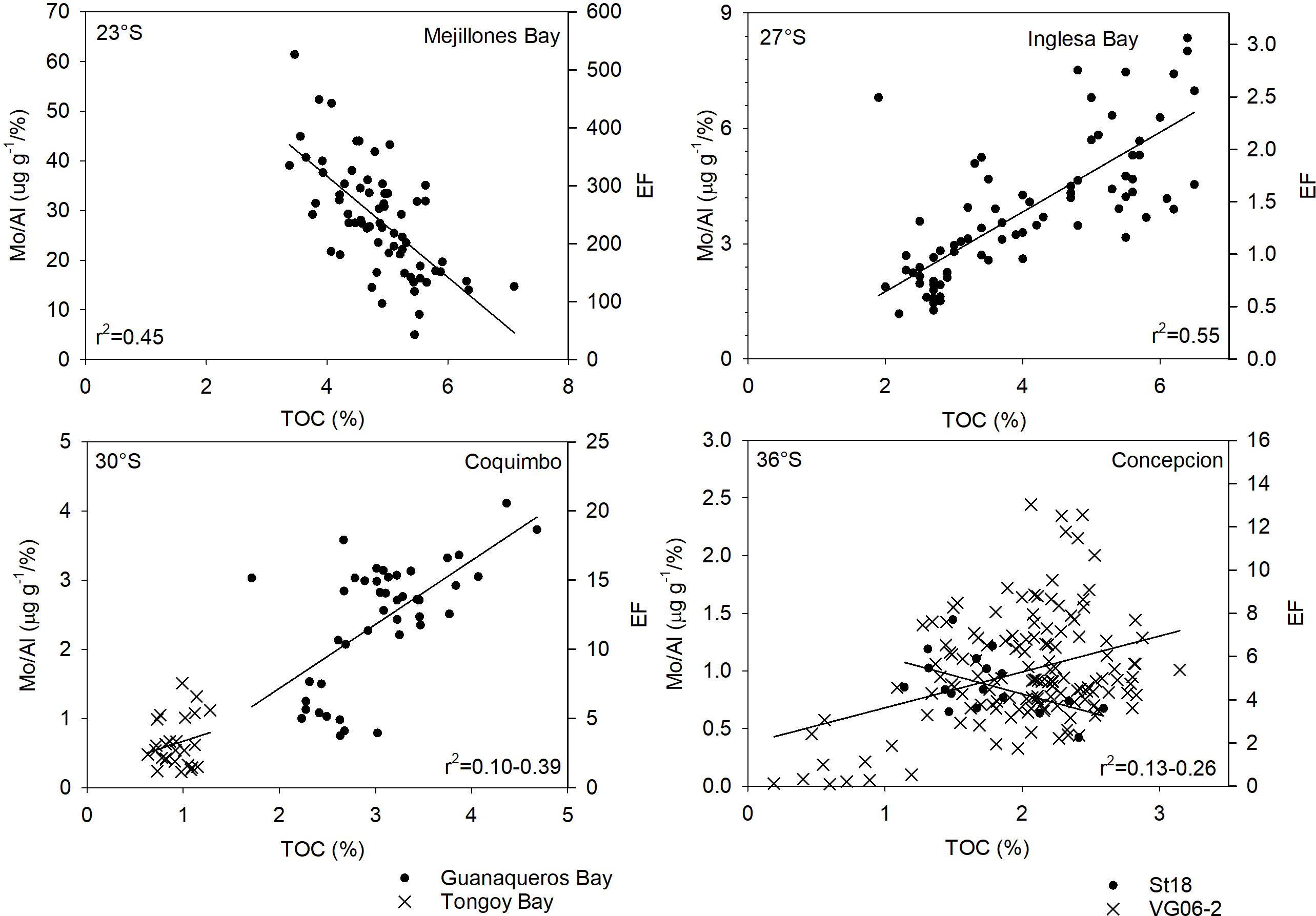
Figure 4 The TOC(%), enrichment factor (EF), and Mo/Al relationship are shown for each core. It considers the complete records of each core (see details in Table 1). r2 = 0.45 at 23°S; r2 = 0.55 at 27°S; r2 = 0.39 at 30°S; r2 = 0.13−0.26 at 36°S, p < 0.0001. The EF values were from Valdés et al. (2021); Muñoz et al. (2012), and Castillo et al. (2017); Castillo et al. (2019).
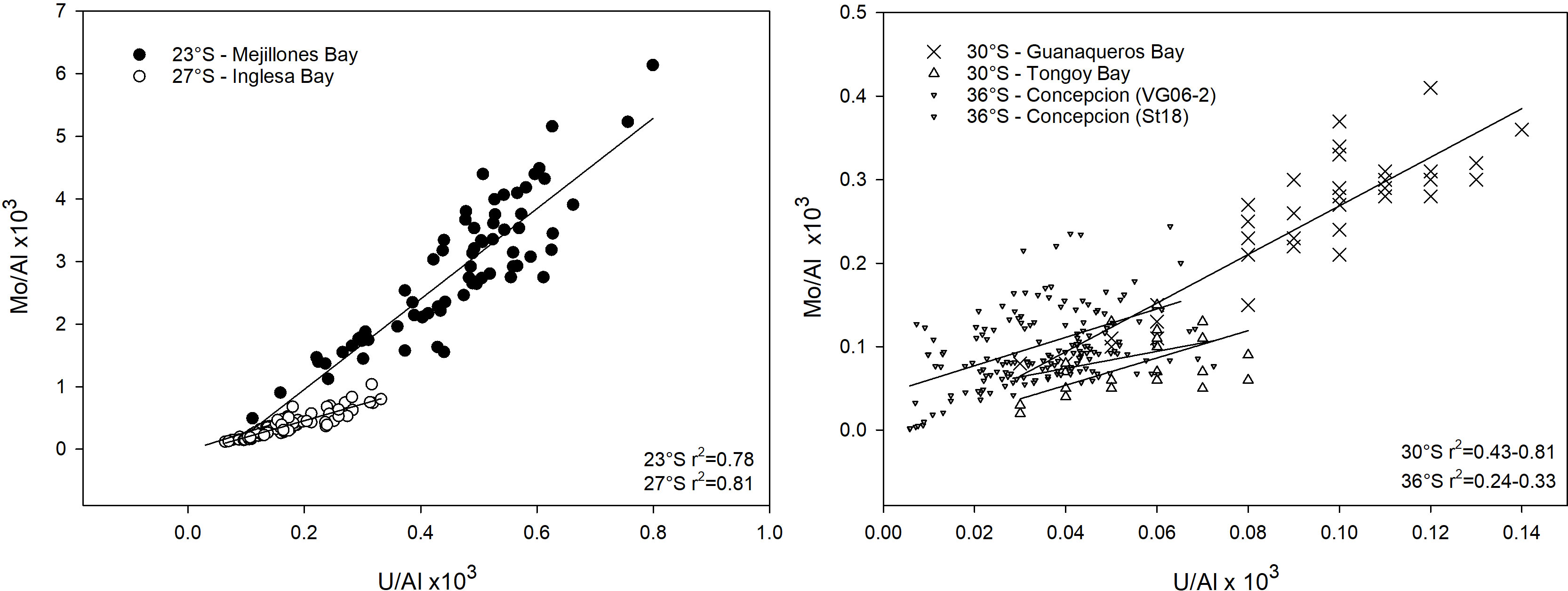
Figure 5 U/Al and Mo/Al relationships, considering the complete record of each core. Cores details are shown in Table 1. r2 = 0.78 at 23°S; r2 = 0.81 at 27°S; r2 = 0.43−0.81 at 30°S; r2 = 0.24−0.33 at 36°S, p < 0.0001.
These EFs were reported as the amount of metal over the crustal concentrations determined in each location (Muñoz et al., 2012; Castillo et al., 2017; Castillo et al., 2019; Valdés et al., 2021) (Table 2). The use of enrichment factors considering local detrital supply has been criticized because several processes in sediment transport may reduce detrital sedimentation. It has been proposed that Me/Al is a better approach (Bennett and Canfield, 2020). However, after reanalyzing the data, the Mo/Al ratio (in µg g-1/%) showed similar behavior to using the EFs, reaching higher values in Mejillones (20–70) and decreasing in southern areas (< 3). Considering the standard EF calculation, the enrichments were high in Mejillones (23°S), one order of magnitude higher (~50−300) than at the southern sites, which was accompanied by a decrease in TOC content. South of 23°S, the EF values peaked to ~25 in Coquimbo and were as low as 3 in Inglesa Bay. This enrichment is probably underestimated because it was calculated using a very high detrital value measured in aeolian dust, enriched in metals by the presence of phosphorites in the zone (Table 2 and Figure 4).
This study used metal ratios to estimate the variability at each site, showing changes that resulted from the redox condition variability at the bottom over time along the margin.
Metal distribution
Similar to δ15N, the metal concentrations were standardized. This information is not complete for all sites (Figure 6), but a similar palpable trend of lower concentrations (below the mean value) of U and Mo was observed from ~1400 AD to the present, suggesting less suboxic conditions, except for Concepcion (36°S) at the southern tip of our study area where the OMZ was more intense between 1900 and 1970 AD, which was not observed in the northern areas. Between 1400 and 1600 AD, significant anoxic conditions in the north sites (23–27°S) were not observed at 36°S, indicating a less intense OMZ. The few data points off Coquimbo also reveals a less severe suboxic environment. Between 800 and 1400 AD, these suboxic conditions were somewhat variable. In the case of 27°S, less intense suboxic conditions covered the period spanning between around 1300 to 600 AD. Both elements suggest less anoxic conditions. Before 800 AD, only the Concepcion site benefits from the complete data. They indicate less intense suboxic conditions between 100 and 600 AD, peaking between 300 and 500 AD. A few data available in Coquimbo 30°S) suggest that this condition was the opposite in this period because U continued to show moderate intensification although mostly negative values of Mo, implying that the sulfidic conditions necessary for Mo precipitation were weak.
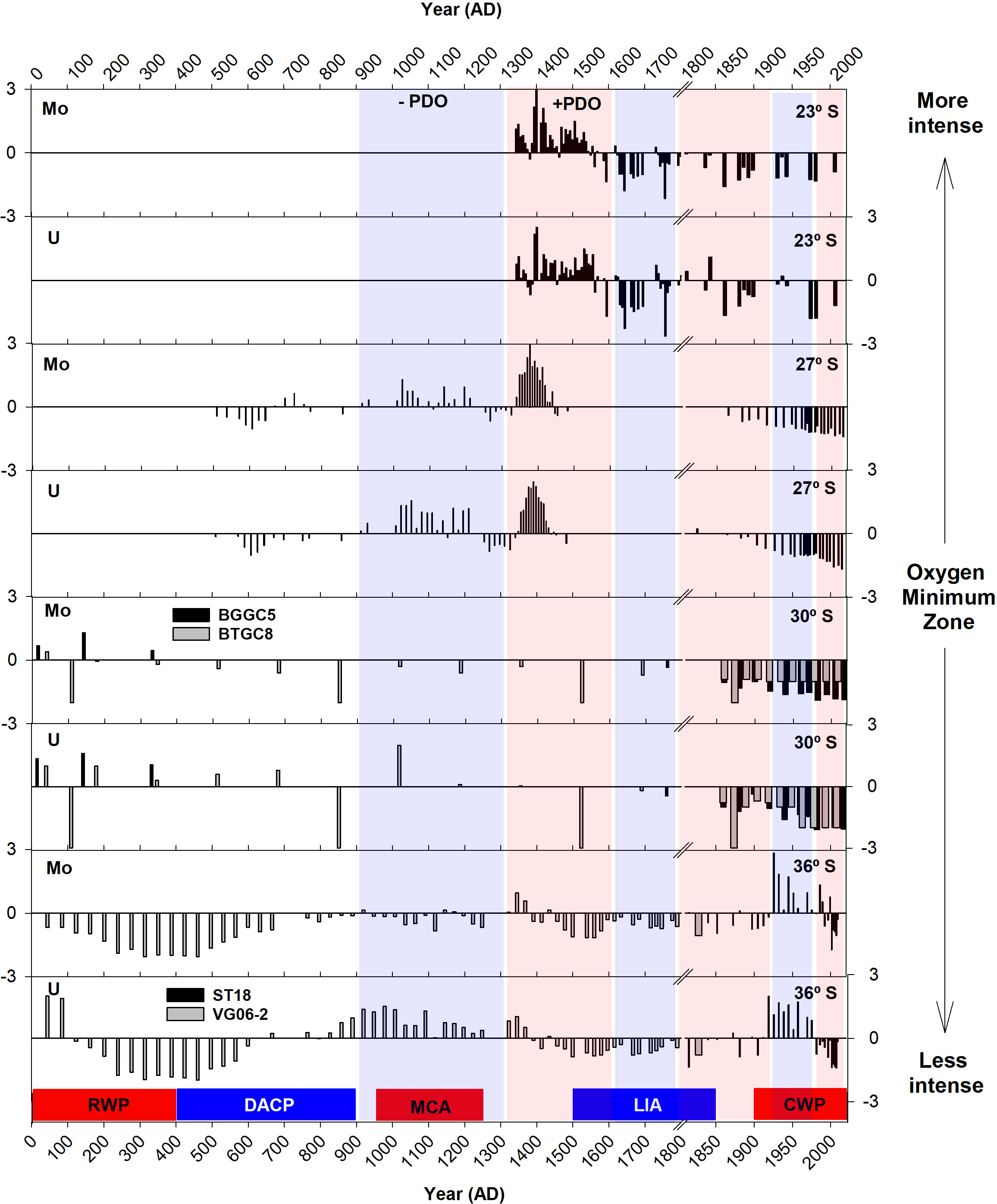
Figure 6 Standardized data records of Mo and U in Mejillones Bay (23°S), Inglesa Bay (27°S), Coquimbo Bay (30°S; Guanaqueros and Tongoy bays), and Concepcion Bay (36°S). Cold (blue) and warm (red) periods are indicated on the x-axis: RWP (0-400 AD), DACP (400-800 AD), MCA (9501250 AD), LIA (1500-1850 AD) and CWP (> 1900 AD). Periods of low (purple) and high (pink) amplitude PDO are indicated by shading. The periods are selected based on the MacDonald and Case (2005)’s reconstructed index (see their Figure 2).
Discussion
δ15N and TOC distribution
Differences in δ15N were established between the northern and southern sites of the Chilean margin, showing a slight latitudinal decrease to the south (Figure 2). The variability in temporal scale at the northern sites (23°S-27°S) were ~3-4‰, in a similar range to that observed in Peru, with a maximum reaching 8–9‰ and a minimum of ~5‰ (Gutiérrez et al., 2009). At the southern sites, the δ15N was lower, between 10‰ and−5‰, except in the sand layer in the core off Concepcion (<3‰), establishing a latitudinal decreasing trend of δ15N toward higher latitudes. This trend could be interpreted as a dilution effect by the increasing input of organic matter from rivers, characterized by low δ15N (2–3‰)(Meyers, 1997; Calvert et al., 2001), which would be absent in the north (23–27°S). However, the organic matter in sediments along the SE Pacific has been described as predominantly marine (De Pol-Holz et al., 2009; Mollier-Vogel et al., 2012). The continental effect would be relevant only in the period corresponding to the sand layer of Concepcion´s core. A better explanation of this light latitudinal gradient may lie from differences in nitrate concentrations and the uptake and assimilation of this nutrient by phytoplankton along the margin (Robinson et al., 2007; De Pol-Holz et al., 2009; Sánchez et al., 2022). The denitrification process domain at shallower depths and any variability could be caused by differences in the utilization of nitrate, such that, north of ~30°S, this nitrate consumption is complete compared with the southern sites, where it could be partial (De Pol-Holz et al., 2009). This signal is translated to the sediments, where the shelf sediments represent the composition of the sinking organic matter favored by the high sedimentation rates (0.24 – 0.15 cm/yr; Muñoz et al., 2004). Therefore, diagenetic alterations would not be relevant for δ15N, this being a robust proxy in sedimentary records to reconstruct denitrification intensity (Robinson et al., 2007; De Pol-Holz et al., 2009). On the other hand, latitudinal increases from Peru to northern Chile were attributed to differences in upwelling and progressive δ15N enrichment associated with the southward flux by the PCUC, which explained the increased values from Peru to Mejillones Bay (23°S) (Vargas et al., 2007; Sifeddine et al., 2008; Gutiérrez et al., 2009). In contrast, the southern sites of Chile showed a decreasing trend that could be attributed to a change in the upwelling pattern, being seasonal off 36°S and almost permanent north of Coquimbo. Therefore, the influence of the denitrification zone would be reduced in the southern sites (Vargas et al., 2007; Sánchez et al., 2012).
Similarly, the TOC content (Figure 2) slightly decreased at the southern sites. The highest values (4–6%) were found in Mejillones, dropping to 3–4% in Inglesa Bay and Coquimbo and off Concepcion, with values ranging between 2% and 3%, except in the sand layer (< 1%). This observation suggests differences in the continental runoff over the shelves, particularly along the coast of Concepcion. The rivers off Concepcion are the primary particle sources that reduce the TOC in the bulk content. However, this contradicts previous analysis of δ13C, which indicates that the organic carbon source is controlled by marine productivity (Hebbeln et al., 2000; De Pol-Holz et al., 2009). However, it has increased the influence of freshwater species on the diatom compositions preserved in sediments in recent times (Sánchez et al., 2012).
The sedimentation rates (SR) estimated in the sediment cores of this study were in the range of values reported previously. Off Concepcion, it is higher (0.27 cm/yr) than the north of 30°S (0.04 and 0.11 cm/yr, in Mejillones and Coquimbo, respectively). However, the organic carbon accumulation rate shows an opposite trend. Off the Mejillones Bay, the value is ~105 gC/cm2yr, and in the southern sites, it is ~38.9 – 24.50 gC/m2yr. Therefore, the differences in organic carbon (OC) accumulation at our sites could result from differences in the detrital runoff. However, it could also respond to enhanced organic preservation in the north because the OMZ is thicker and less affected by seasonal variability (Valdés et al., 2004; Valdés et al., 2009). The buried OC results from organic matter that escapes oxidation referred to as burial efficiency (Middelburg et al., 1993), which depends on oxygen availability and sedimentation. Here, differences in SR should not be enough to explain differences in OC accumulation. Therefore, the differences in its accumulation could be attributed to oxygen availability and primary productivity fluxes at the bottom. Other factors relevant to C preservation include surface area reactivity, grain size composition, and organic carbon reactivity (Burdige, 2007; Katsev and Crowe, 2015), which should be considered but have not been evaluated. The OC content variability over time followed similar patterns at all sites. Considering a larger timescale (> 2000 cal BP) for Coquimbo and Concepcion (Muñoz et al., 2012; Muñoz, et al. 2020), the TOC content showed a slowly decreasing trend after 1400 AD toward the beginning of the Common Era. However, after the 1970s (Figure 2), it rapidly increased, being markedly high in Mejillones and Concepcion, corresponding to the north and south ends of our study area. This change is coincident with the climate shift of the 1970s (Jacques–Coper and Garreaud, 2014), described as a warming shift on the east coast of South America concomitant with a weakening of the subtropical South Pacific High (SPSH). It would be condusive of a decreased organic flux by diminished primary productivity. Notwithstanding, an intensification of coastal upwelling was described for Mejillones (Vargas et al., 2007) and the Peruvian continental margin (Gutiérrez et al., 2011; Salvatteci et al., 2014), explaining the enhanced TOC trend, although others identified this trend earlier, around 1878 (Flores-Aqueveque et al., 2015). These studies identified enhanced wind stress in particular years, suggesting an interplay between inter-annual and decadal variability, which also had an incidence in the manifestation of the 1970s shift (Jacques–Coper and Garreaud, 2014) and would also explain the increased TOC off Concepcion. Even with increased runoff with low metabolizable organic material contributing to enhancing the organic content of these sediments, the δ13C still indicates a marine phytoplankton source (Sánchez et al., 2012). These authors state that the increased river inputs would be a nutrient source that overcompensates for the diminished nutrient inputs from the impoverished upwelling.
Denitrification
The range of the mass accumulation rates was not highly dissimilar. Despite the contribution of rivers in the south that support fresh organic C (highly reactive matter) to shallow depths, phytoplankton sedimentation dominates. Therefore, they maintain the isotopic composition of the settled organic material. The δ15N in the sediments is related to denitrification, linked to the available oxygen content over the bottom. The standardized δ15N data show better the variability of this process over the shelf despite the narrow range of the isotopic values (Figures 2, 3). In general, less intense denitrification, peaking at 300 and 600 AD off Concepción (36°S) and Inglesa Bay, respectively, occurred predominantly in a cold period (Dark Age Cold Period, DACP). However, lower values were also observed in the previous warm period (Roman Warm Period, RWP). Thus less intense denitrification was set before 800 AD; afterward, all sites exhibited enhancement of denitrification (more positive values), peaking during ~1100-1400 AD. The maximum values corresponded predominantly to a warm climate in the medieval climate anomaly (MCA) and the current warm period, while minimum values were during the cold period between 1600 and 1750 AD when the little ice age (LIA) arose. During this cold period, denitrification was less intense, except off Coquimbo (30°S), which has remained positive but more intense in the current time. Previous periods (< 1400 AD) at 23°S were insufficient for comparison. Thus, attenuation of the denitrification process during warmer periods (after 1400 AD) and a less intense OMZ was observed, implying a higher variability of oxygen conditions in the bottom waters. In the last 50 years, the data indicated a more intense denitrification, suggesting a more reduced environment, similar to most sites along the Chilean margin (except at 27°S). These conditions agree with the increase in C accumulation in recent sediments and more reduced conditions on the shelf caused by the intensification of the OMZ, promoting also intensified denitrification. Thus, changes in denitrification could be related to the variability in the upper limit of the OMZ.
Trace metals distribution
U and Mo enrichments (authigenic precipitations) in reduced sediments have been widely used to interpret past redox conditions. U accumulation rate is highly correlated with the organic flux to the ocean bottom, where the precipitation of this element within the sediments could be microbially mediated (Zheng et al., 2002). Still, a substantial fraction can also be lost to the water column during sediment perturbations that affect the redox conditions and release U in the porewater. The U cycling would be similar to Fe cycling, having a similar redox reduction boundary (Zheng et al., 2002). Instead, Mo precipitation requires a sulfidic environment with concentrations >11 µM (Erickson and Helz, 2000). However, in sediments below suboxic waters, Mo-sulfide and Fe-sulfide coprecipitate at very low sulfide concentrations ~ 0.1 µM (Zheng et al., 2000), producing authigenic metal precipitation. Otherwise, Mo could also form intermediate complexes with dissolved organic matter in the bottom waters and sediments in suboxic conditions, contributing to sequestering Mo during early diagenesis (Wagner et al., 2017). This process could be relevant in places where the high primary productivity promotes higher organic flux to the bottoms, as described for the HCS; thus, several pathways can enhance Mo concentrations over the lithogenic background within the sediments. Instead of Mo, for U authigenic enrichment, an oxygen penetration of < 1 cm is necessary to maintain suboxic conditions within the sediments and U in a precipitated form (Morford and Emerson, 1999). Therefore both elements help determine the intensity of the redox changes, establishing suboxic and anoxic conditions.
In the Chilean margin, higher EFs were estimated for Mo over U concentrations, which showed a positive and significative correlation (Figures 4, 5), except at Concepcion, probably due to enhanced input of detrital material (r2 = 0.24-0.33). This situation indicates that the sulfide concentrations were sufficient for Mo precipitation, which are between < 10 and 30 µM off Concepcion (36°S; Muñoz et al., 2012b), and reports for Coquimbo (30°S) indicate similar values of ~10 µM and ~80 µM (Muñoz, unpublished data). There are no sulfide records off Mejillones (23°S) and Inglesa Bay (27°S); however, in both sites, the correlation between metal enrichment and sulfate reduction intensity is poor, and the intensity of this process does not control the metal enrichment by itself (Valdés et al., 2014; Castillo et al., 2019). Thioploca mats on the bottoms probably reduce sulfide availability, diminishing metal-sulfide formation (Valdés et al., 2014). Instead, the metal transport during the Fe reduction/oxidation cycle has been proposed as a relevant mechanism for Mo enrichment (Valdés et al., 2014). Off-Perú, models of Fe shuttle for Mo enrichments would apply for the Chilean margin, even in shallower zones, where Fe has a crucial control over metal accumulation below a nitrogen-rich OMZ (Scholz et al., 2017; Scholz, 2018). The relationship between Mo and U enrichment is presented in Figure 7. It is based on the scheme proposed by Algeo and Tribovillard (2009) that establishes the range for metal enrichment in sulfidic, anoxic, and suboxic conditions. The U/Mo relation exceeds three times the seawater values in Mejillones, indicating a crucial particulate shuttle for metal enrichment here, but not in the other sites, which mostly fall in the suboxic condition area ruled by oxygen content in the bottoms and organic fluxes.
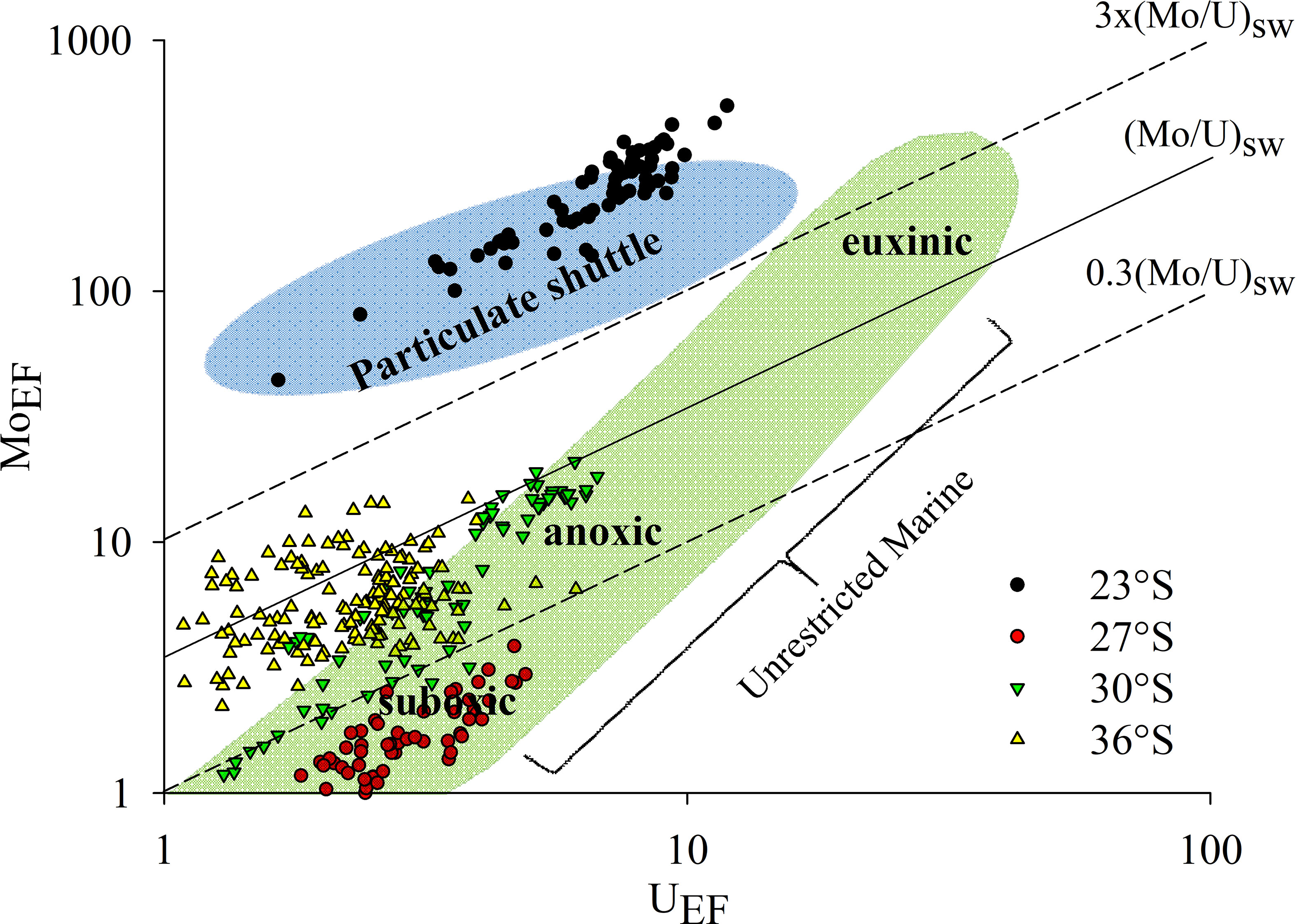
Figure 7 Model adapted from Tribovillard et al. (2012) to interpret the relationship of MoEF vs. UEF of the sedimentary records of the study area (both axes on logarithmic scale): Mejillones del Sur Bay (23°S, black circle), Inglesa Bay (27°S, red circle), Coquimbo (30°S, green triangle), and Concepcion (36°S, yellow triangle). Segmented line identifies Mo/U molar ratios equal to the seawater value (1xSW) and fractions thereof (0.3xSW, 3xSW). The light blue and green sections represent main redox conditions, and the accumulation mechanisms for Mo and U.
The organic fluxes measured in sediments are highly dependent on primary productivity and constitute an essential carrier phase for metal accumulation in surface sediments in the Chilean margin and Peru in deeper zones (McManus et al., 2006; Scholz, 2018). This relationship is negative in Mejillones and positive in the southern sites (Figure 4), suggesting enhanced organic carbon preservation and reduced detrital siliciclastic input in Mejillones. In restricted basins, authigenic enrichment can limit Mo accumulation because the dissolved phase is determined by circulation. This situation diminishes Mo precipitation, producing a lower Mo/Al value related to higher TOC content within the sediments (Tribovillard et al., 2012), explaining the negative correlation. However, this would not apply to upwelling zones; instead, the negative relationship between TOC and Mo in Mejillones (23°S) could result from increased oxygenation. The presence of Fe oxides promotes Mo accumulation during the recycling of oxides, as discussed earlier, and reduces the organic content by remineralization (Figure 4). Besides, it is essential to note that the concentrations of Mo and EFs are very high in Mejillones compared with the bays south of 23°S. Therefore, although the exact mechanism of this metal enrichment has not been established yet, the Fe shuttle is a plausible explanation (Valdés et al., 2014; Castillo et al., 2019).
The temporal evolution of U and Mo (Figure 6) suggests that in Mejillones Bay (23°S), an increasing trend of oxygenation was sustained from ~1600 AD to 1900 AD. While at the southern sites, the trend has been maintained since 1400 AD. No records were obtained at 23°S before 1300 AD. Therefore, while the southern sites (30−36°S) showed cycles of more or less extended periods of suboxic conditions of ~600 years, in the northern areas (23−27°S), this periodicity would be reduced to ~400 years. On the other hand, a reducing condition is observed in all cores but diminishes over time.
Comparing the standardized data of metals and δ15N records, they were relatively in phase until ~1900 AD, suggesting more or less intensity in the OMZ and denitrification. Following this period, an increase in oxygenation does not imply a decrease in denitrification. For example, after 1970, in most sites (except for Inglesa Bay), the less intense OMZ coincided with an intensified denitrification, which appears contradictory. In that case, other variables as the mixing of water mass properties can be considered relevant to adjust the frequency and seasonality of the OMZ upper limit, consistent with the increased occurrence of strong El Niño events after the climate shift of the 70s (Capotondi and Sardeshmukh, 2017).
Temporal variability of OMZ influence above shallows zones
Period 0-800 AD
Before 800 AD, a substantial impact of oxygenation on the southern tip of the study area was observed; metal enrichment was low in Concepcion, peaking around 400 AD, corresponding to the end stage of the RWP, followed by the DACP. To the northern sites, the metal enrichment showed consistently higher oxygenation off Inglesa Bay and Coquimbo (30°). Thus, the less intense OMZ in this period was accompanied by less severe denitrification around 400 AD, except at Coquimbo (30°S), where a moderate intensification of the OMZ and denitrification was observed. The southern position of the westerlies before 400 AD established dryer conditions on northern Patagonia (41-44°S), followed by a northward migration around 600 AD, which determined an intensification of the westerlies, an enhanced runoff by the precipitation, and a northward migration of cooler waters (Mohtadi et al., 2008). It would have a significant impact around 36°S, diminishing toward the northern sites (<30°), establishing the temporal and latitudinal differences at the centennial scale (Figures 3, 6).
Period 800 – 1200 AD
Around 1000–1200 AD, a more intense OMZ was observed, corresponding to the MCA peak that fell during a mostly weaker negative PDO amplitude developed during an extended period (993–1300; MacDonald and Case, 2005). The effects of these environmental conditions in South America have been described based on marine and continental records (Lüning et al., 2019). Marine proxies suggest an intensification of upwelling off Peru and northern Chile concomitant with an intensification of denitrification during this warm period (Salvatteci et al., 2016; Castillo et al., 2017), but others suggest a higher frequency of El Niño episodes in agreement with warmer climatic conditions (Moy et al., 2009, Conroy et al., 2008). A global analysis discussed by Graham et al. (2011) stated that during MCA, the zonal gradient of sea surface temperatures increased in the Tropical Pacific, with cooler conditions in the eastern Pacific and warmer conditions in the western Pacific. This would be in agreement with the intensified upwelling on the eastern side. This situation led to increased metal enrichment in the northern Chilean shelf, as observed at 27°S, related to a rise in organic sedimentation and agreement with denitrification intensification (Figure 3). Conversely, at the southern locations (30 – 36°S), less Mo enrichment was observed. However, U was still higher, indicating less sulfidic conditions but suboxic and less intense denitrification (Figures 3, 6). Therefore, during the MCA period, differences can be established between sites located north and south of 30°S.
Period 1200 – 1600 AD
On decadal scale, cold/warm periods commonly relate to conditions such as La Niña/El Niño-like, intense/reduced upwelling, and more/less reduced conditions would agree with more/less denitrification intensity. However, this cannot be generalized throughout the Chilean margin. During part of the MCA-LIA transitional period and early LIA (1500−1600), denitrification was more intense in northern sites, especially off Mejillones, and marked differences between northern and southern sites were observed (Figure 3). In this period, a mean positive PDO was set up, which implies positive anomalies of SST along the coast and more stratification intensifying the denitrification. In northern sites (23-27°S), the enhanced reduced conditions at the bottoms, resulting in an intensified OMZ, agreed with intensified denitrification (Figures 3, 6). However, south of 30°S, the Mo and U enrichments became opposite to the northern sites (around 1400–1600 AD), suggesting less reduced conditions at the bottoms, less intense OMZ but only minor changes in the denitrification that was still positive (Figures 3, 6).
Period 1600 – 1800 AD
During this cold period, weakened reduced environmental conditions and less intense denitrification were maintained along the Chilean margin but were stronger in the northern sites (Mejillones and Inglesa Bay, 23 – 27°S) (Figure 6). A weakened OMZ and denitrification would be related to the ventilation of subsurface waters, which has been explained by a greater influence of the ESPIW (Eastern South Pacific Intermediate Water) during the northward position of the SPSH. Thermocline ventilation has been proposed as a main mechanism to control the OMZ dynamics at low frequency, highlighting the role of the ESPIW (De Pol-Holz et al., 2006). It has been described for Mejillones ~1870 AD (Gutiérrez et al., 2009) and Inglesa Bay in the last century (Castillo et al., 2017). Thus, the northward migration of westerlies would reduce the latitudinal differences in the sediment redox conditions along the Chilean margin, ventilating the subsurface waters and diminishing the intensity of the OMZ.
Period 1800 AD –recent time
In general, enhanced suboxic conditions have evolved to a less suboxic environment along the Chilean margin in the last century. However, discrepancies with the denitrification process occur after the 1900s. Off Concepcion (36°S), a more intense OMZ deduced from higher Mo and U enrichment was set with less severe denitrification before 1970, which would be explained by enhanced ventilation. The mixing and ventilation of subsurface waters could reduce the denitrification process but enhance the metal accumulation through Mn/Fe oxide transport in the water-sediment interphase (Algeo and Li, 2020; Bennett and Canfield, 2020). However, the Mn-Fe oxide shuttle that increases the metal accumulation does not seem to operate at 36°S (Figure 7). This process has been described in the Arabian Sea OMZ by Kessarkar et al. (2018), explaining that this is valid for other OMZs like the Peruvian margin where the oxides shuttle process occurs under suboxic, not sulfidic conditions (Scholz et al., 2017); but at Concepcion the Mo enrichment still does not reach high enough values to consider this process. Enhanced upwelling was reported in a short period between 1935 and 1970 (Srain et al., 2015), reinforcing the suboxic conditions through intensified vertical advection over the shelf during the negative PDO phase. Others reported for Concepcion a decreasing trend of diatoms in sedimentary records from 1920, accompanied by an increased contribution of warm-temperate and freshwater groups and an increased SST of ~0.7°C (Sánchez et al., 2012). This situation could reduce the denitrification due to a decreased primary productivity, or the δ15N records is showing the continental influence over the shelf at Concepcion (36°S), because it does not match with intensified reduced conditions at bottoms before 1960–70.
Afterward, enhanced denitrification corresponded with a weakened OMZ along the Chilean margin deduced for the lower metal accumulation. It would result from increased stratification but more frequent oxygenation events produced by El Niño. During these events, change the oxide-reduction limits within sediments (Gutiérrez et al., 2000) and reduce the porewaters’ U and Mo content. Additionally, reduced primary productivity would reduce the metal transport to the bottoms and oxygen consumption. In the northern areas, this condition has been variable in recent times; but it showed oxygenation too (Figure 6). This trend was concomitant with intensified denitrification (Figure 3). However, it was not observed in Inglesa Bay, probably due to local differences in the upwelling intensity and the OMZ position (Figure 1). From 1950 to the present, the increased denitrification observed off Mejillones (23°S) and Concepcion (36°S) (Figure 3) was not consistent with the increase in SST (~1°C) established after this date (Vargas et al., 2007; Sánchez et al., 2012). Notwithstanding, a decreased temperature was deduced for Coquimbo (30°S) (Ortega et al., 2019), which would agree with the intensified denitrification due to the OMZ influence over the narrow shelf. Additionally, the upwelling seems to have been intensified, which could be due to local air-sea interaction (e.g. enhanced marine boundary layer mixing and momentum entrainment due to the warmer SST), which promotes phytoplankton development and later oxygen consumption.
Recent evidence suggests that positive ENSO and PDO phases predominated over the last 50 years (Perez et al., 2021), and after the 1970s, an intensified frequency of inter-annual variability (El Niño events) (MacDonald and Case, 2005; Wam et al., 2019). Strong El Niño events induced bottom oxygenations detected from southern Peru to southern Chile at 36°S (Sellanes et al., 2007; Gutiérrez et al., 2008; Graco et al., 2017), supporting oxygen variability in the upper limit of the OMZ that affected the metal records in the last 50 years. Our records indicate that all sites, except for Inglesa Bay (27°S), responded similarly after the 1970s, with intensified denitrification. Although Inglesa Bay showed a slight return to less negative values of denitrification, suggesting a slight intensification of the upwelling. However, they corresponded with a weaker OMZ, which would agree with increased events of ventilation caused by inter-annual variability, producing a cumulative effect of oxygenation over the bottoms.
Latitudinal differences along the Chilean margin can be produced by differences in the hydrographic conditions that allow the ventilation of the OMZ; in the southern regions, the meridional position of the subtropical front and the intensity of the SPSH, which modulates the winds alongshore (Pitcher et al., 2021 and references therein) can modulate the OMZ. Additionally, during the negative phase of the PDO (la Niña-like), the intensity of the inter-annual variability in the EP is reduced; therefore, suboxic conditions would be less affected at the southern tip of our study area, where weakened El Niño causes fewer effects through oceanic teleconnection (at 36°S). Conversely, during the positive phase of the PDO and current global warming, the interannual variability would be intensified, and these latitudinal differences could be reduced.
In the past, the higher/lower denitrification and metal accumulations seem to respond to, probably, by more/less intense primary productivity. It maintains low oxygen concentrations and sulfidic conditions, which have been changing gradually in agreement with the climatic shifts that control the ventilation of subsurface waters. Thus, the current interpretations would be related to a higher stratification and intensified OMZ, in disagreement with higher metal accumulation due to increased oxygenation events.
Conclusions
The suboxic environment settled above the shelf bottoms along the Chilean margin has experienced increased oxygenation over the last 2000 years in response to decadal and inter-annual variability in the circulation in the Pacific. The lowest variability before 1400 AD was highlighted. In this period, denitrification intensified along with the intensification of suboxic conditions. The reconstructed PDO index from 993 (AD) (MacDonald and Case, 2005) indicates that negative anomalies (cold periods) were more common for extended periods before 1300 AD when reduced denitrification and intense OMZ were established. Afterward, positive anomalies with enhanced variability dominated (MacDonald and Case, 2005), which allowed the maintenance of an enhanced denitrification intensity but contributed to diminishing the reduced conditions and OMZ intensity. It showed a decreasing trend toward current times deduced from metal accumulation. The magnitude of the suboxic condition related to more/less OMZ intensity in the upper boundary is affected by remote-forcing oceanic waves that work on different timescales. The effect of this variability is coupled with the inter-annual and decadal variability, whose intensity and periodicity have increased. These processes promote changes in the redox conditions above the bottom of the shelf, leaving less reduced environments. Besides their value for validation purposes, our result challenges projections of current-generation climate models that still indicate a low consensus in the fate of the SEP OMZs in the 21st century (Cabré et al., 2015; Kwiatkowski et al., 2020).
Data availability statement
The original contributions presented in the study are included in the article/Supplementary Material. Further inquiries can be directed to the corresponding author.
Author contributions
PM prepared the manuscript with contributions from all co-authors. All authors drafted the database used in this manuscript. All data used in this manuscript is the result of different projects belonging to the authors of this manuscript. The analysis of this information was discussed with the contribution of all authors of this paper. All authors contributed to the article and approved the submitted version.
Funding
The authors are grateful for the financial support provided by the Anillo Project ACT210071, Fondecyt 1200390 granted to (JV), Núcleo Milenio UPWELL (NCN19_153), and the CLAP program (Concurso de Fortalecimiento al Desarrollo Científico de Centros Regionales 2020-R20F0008-CEAZA). We also extend our gratitude to COPAS Sur-Austral ANID AFB170006 and COPAS COASTAL FB210021, who contributed to the completion of this study.
Conflict of interest
The authors declare that the research was conducted in the absence of any commercial or financial relationships that could be construed as a potential conflict of interest.
Publisher’s note
All claims expressed in this article are solely those of the authors and do not necessarily represent those of their affiliated organizations, or those of the publisher, the editors and the reviewers. Any product that may be evaluated in this article, or claim that may be made by its manufacturer, is not guaranteed or endorsed by the publisher.
Supplementary material
The Supplementary Material for this article can be found online at: https://www.frontiersin.org/articles/10.3389/fmars.2023.1134164/full#supplementary-material
References
Algeo T., Li C. (2020). Redox classification and calibration of redox thresholds in sedimentary systems. Geochim. Cosmochim. Ac. 287, 8–26. doi: 10.1016/j.gca.2020.01.055
Algeo T. J., Tribovillard N. (2009). Environmental analysis of paleoceanographic systems based on molybdenum-uranium covariation. Chem. Geology 268, 211–225. doi: 10.1016/j.chemgeo.2009.09.001
Altabet M. A. (2005). “Isotopic tracers of the marine nitrogen cycle: present and past,” in Marine organic matter: biomarkers, isotopes and DNA. the handbook of environmental chemistry, vol. 2N . Ed. Volkman J. K. (Berlin, Heidelberg: Springer). doi: 10.1007/698_2_008
Ancapichún S., Garcés-Vargas J. (2015). Variability of the southeast pacific subtropical anticyclone and its impact on sea surface temperature off north-central Chile cienc. Mar 41 (1), 1–20. doi: 10.7773/cm.v41i1.2338
Auger P.-A., Bento J. P., Hormazabal S., Morales C. E., Bustamante A. (2020a). Temperature, salinity, density and dissolved oxygen profiles from the world ocean database and argo floats in the eastern south pacific over the 1928-2018 period. SEANOE. doi: 10.17882/74336
Auger P.-A., Bento J. P., Hormazabal S., Morales C. E., Bustamante A. (2020b). Mesoscale variability in the boundaries of the oxygen minimum zone in the eastern south pacific: influence of intrathermocline eddies. J. Geophys. Res. Oceans. doi: 10.1029/2019JC015272
Bennett W., Canfield D. (2020). Redox-sensitive trace metals as paleoredox proxies: a review and analysis of data from modern sediments. Earth Sci. Rev. 204, 103175. doi: 10.1016/j.earscirev.2020.103175
Bernal P., Ahumada R., González H., Pantoja S., Troncoso A. (1989). Carbon flux in a pelagic trophic model for concepción bay, Chile. Biología Pesquera 18, 5−14.
Böning P., Brumsack H.-J., Böttcher M. E., Schnetger B., Kriete C., Kallmeyer J., et al. (2004). Geochemistry of Peruvian near-surface sediments. Geochim. Cosmochim. Ac. 68, 4429–4451. doi: 10.1016/j.gca.2004.04.027
Böning P., Brumsack H.-J., Schnetger B., Grunwald M. (2009). Trace element signatures of Chilean upwelling sediments at 36°S. Mar. Geol. 259, 112–121. doi: 10.1016/j.margeo.2009.01.004
Böning P., Cuypers S., Grunwald M., Schnetger B., Brumsack H.-J. (2005). Geochemical characteristics of Chilean upwelling sediments at 36°S. Mar. Geol. 220, 1–21. doi: 10.1016/j.margeo.2005.07.005
Braconnot P., Luan Y., Brewer S., Zheng W. (2012). Impact of earth's orbit and freshwater fluxes on Holocene climate mean seasonal cycle and ENSO characteristics. Clim. Dyn. 38, 1081–1092. doi: 10.1007/s00382-011-1029-x
Burdige D. (2007). Preservation of organic matter in marine sediments: controls, mechanisms, and an imbalance in sediment organic carbon budgets? Chem. Rev. 107, 467−485.
Cabré A., Marinov I., Bernardello R., Bianchi D. (2015). Oxygen minimum zones in the tropical pacific across CMIP5 models: mean state differences and climate change trends. Biogeosciences 12 (18), 5429–5454. doi: 10.5194/bg-12-5429-2015
Calvert S. E., Pedersen T. F., Karlin R. E. (2001). Geochemical and isotopic evidence for postglacial palaeoceanographic changes in saanich inlet, British Columbia. Mar. Geol. 174, 287–305. doi: 10.1016/S0025-3227(00)00156-0
Capotondi A., Sardeshmukh P. D. (2017). Is El niño really changing? Geophysical Res. Lett. 44 (16), 8548–8556. doi: 10.1002/2017GL074515
Carré M., Azzoug M., Bentaleb I., Chase B. M., Fontugne M., Jackson D., et al. (2012). Mid-Holocene mean climate in the south eastern pacific and its influence on south America. Quat. Int. 253, 55–66. doi: 10.1016/j.quaint.2011.02.004
Carré M., Sachs J. P., Purca S., Schauer A. J., Braconnot P., Falcón R. A., et al. (2014). Holocene History of ENSO variance and asymmetry in the eastern tropical pacific. Science 345, 1045–1048. doi: 10.1126/science.1255768
Castillo A., Valdés J., Sifedine A., Reyss J.-L., Bouloubassi I., Ortlieb L. (2017). Changes in biological productivity and ocean-climatic fluctuations during the last ~1.5 kyr in the Humboldt ecosystem off northern Chile (27°S): a multiproxy approach. Palaeogeogr. Palaeocl. 3, 798–815.b. doi: 10.1016/j.palaeo.2017.07.038
Castillo A., Valdés J., Sifedine A., Vega S.-E., Díaz-Ochoa J., Marambio Y. (2019). Evaluation of redox-sensitive metals in marine surface sediments influenced by the oxygen minimum zone of the Humboldt current system, northern Chile. Int. J. Sediment Res. 179, 2–14. doi: 10.1016/j.ijsrc.2018.08.005
Cobb K. M., Westphal N., Sayani H. R., Watson J. T., Di Lorenzo E., Cheng H., et al. (2013). Highly variable El niño-southern oscillation throughout the Holocene. Science 339 (6115), 67–70. doi: 10.1126/science.1228246
Conroy J., Overpeck J., Cole J. E., Shanahan T. M., Steinitz-Kannan M. (2008). Holocene Changes in eastern tropical pacific climate inferred from a galápagos lake sediment records. Quat. Sci. Rev. 27, 1166–1180.
Crusius J., Thomson J. (2000). Comparative behavior of authigenic re, U, and Mo during reoxidation and subsequent long-term burial in marine sediments. Geochim. Cosmochim Ac. 64, 2233−2242. doi: 10.1016/S0016-7037(99)00433-0
Daneri G., Dellarossa V., Quiñones R., Jacob B., Montero P., Ulloa O. (2000). Primary production and community respiration in the Humboldt current system off Chile and associated oceanic areas, mar. Ecol. Prog. Ser. 197, 41–49. doi: 10.3354/meps197041
Dellarosa V. (1998). Producción primaria anual en sistemas de alta producción biológica (Doctoral dissertation, tesis escuela de graduados (Concepción, Chile: Universidad de Concepción), 149.
De Pol-Holz R., Robinson R. S., Hebbeln D., Sigman D. M., Ulloa O. (20092009). Controls on sedimentary nitrogen isotopes along the Chile margin. Deep-Sea Res. II 56, 1042–1054. doi: 10.1016/j.dsr2.2008.09.014
De Pol-Holz R., Ulloa O., Dezileau L., Kiser J., Lamy F., Hebbeln D. (2006). Melting of the Patagonian ice sheet and deglacial perturbations of the nitrogen cycle in the eastern south pacific. Geophys. Res. Lett. 33, L04704. doi: 10.1029/2005GL024477
Dewitte B., Concha E., Saavedra D., Pizarro O., Martinez-Villalobos C., Gushchina D., et al. (2023). The ENSO-induced south pacific meridional mode. Front. Clim. 4, 143–156. doi: 10.3389/fclim.2022.1080978
Dewitte B., Vazquez-Cuervo J., Goubanova K., Illig S., Takahashi K., Cambon G., et al. (2012). Change in El niño flavours over 1958-2008: implications for the long-term trend of the upwelling off Peru. Deep Sea Res. II. doi: 10.1016/j.dsr2.2012.04.011
Erickson B. E., Helz G. R. (2000). Molybdenum(VI) speciation in sulfidic waters: stability and lability of thiomolybdates. Geochim. Cosmochim. Acta 64 (7), 1149–1158. doi: 10.1016/S0016-7037(99)00423-8
Escribano R., Daneri G., Farías L., Gallardo V. A., González H., Gutiérrez D., et al. (2004). Biological and chemical consequences of the 1997–1998 El ni˜no in the Chilean coastal upwelling system: a synthesis. Deep Sea Res. Part II Top. Stud. Oceanogr. 51, 2389–2411. doi: 10.1016/j.dsr2.2004.08.011
Farmer J. R., Hertsberg J. E., Cardinal D., Fletz S., Hendry K., S. L., et al. (2021). Assessment of c, n, and Si isotopes as tracers of past ocean nutrient and carbon cycling. Global Biochem. Cy. 35. doi: 10.1029/2020GB006775
Flores-Aqueveque V., Alfaro S., Vargas G., Rutllant J. A., Caquineau S. (2015). Aeolian particles in marine cores as a tool for quantitative high-resolution reconstruction of upwelling favorable winds along coastal atacama desert, northern Chile. Progr. Oceanogr. 244–255. doi: 10.1016/j.pocean.2015.02.003
Fossing H., Gallardo V. A., Jørgensen B. B., Hüttel M., Nielsen L. P., Schulz H., et al. (1995). Concentration and transport of nitrate by the matforming sulphur bacterium thioploca. Nature 374, 713–715. doi: 10.1038/374713a0
Fuenzalida R., Schneider W., Garcés-Vargas J., Bravo L., Lange C. (2009). Vertical and horizontal extensión of the oxygen mínimum zone in the eastern south pacific ocean. Deep-Sea Res. Pt.II 56, 992−1003. doi: 10.1016/j.dsr2.2008.11.001
González H., Daneri G., Figueroa D., Iriarte J. L., Pizarro G., Quiñones R., et al. (1998). Primary production and its fate in the pelagic food web and deep-sea, and ocean-atmosphere CO2 exchange in the northern Humboldt current (23°S): possible effects of the 1997–1998 El niño in Chile. Rev. Chil. Hist. Nat. 71, 429–458.
Gonzalez H., Menschel E., Aparicio C., Barría C. (2007). Spatial and temporal variability of microplankton and detritus, and their export to the shelf sediments in the upwelling area off concepción, Chile (36°S), during 2002–2005. Prog. Oceanogr. 75, 435−451. doi: 10.1016/j.pocean.2007.08.025
Graco M., Purca S., Dewitte B., Castro C. G., Moron O., Ledesma J., et al. (2017). The OMZ and nutrients features as a signature of interannual and low frequency variability off the Peruvian upwelling system. Biogeosciences 14, 4601–4617. doi: 10.5194/bg-14-4601-2017
Graham N. E., Ammann C. M., Fleitmann D., Cobb K. M., Luterbacher J. (2011). Support for global climate reorganization during the “Medieval climate anomaly”. Clim. Dyn. 37, 1217–1245.
Guiñez M., Valdés J., Sifedine A., Boussafir M., Dávila P. (2014). Anchovy population and ocean-climatic fluctuations in the Humboldt current system during the last 700 years and their implications. Palaeogeogr. Palaeocl. 415, 210−224. doi: 10.1016/j.palaeo.2014.08.026
Gutiérrez D., Gallardo V. A., Mayor S., Neira C., Vásquez C., Sellanes J., et al. (2000). Effects of dissolved oxygen and fresh organic matter on the bioturbation potential of macrofauna in sublittoral bottoms off central Chile, duringthe 1997–98 El niño. Mar. Ecol. Prog. Ser. 202, 81–99. doi: 10.3354/meps202081
Gutiérrez D., Bouloubassi I., Sifeddine A., Purca S., Goubanova K., Graco M., et al. (2011). Coastal cooling and increased productivity in the main upwelling zone off Peru since the mid-twentieth century. Geophys. Res. Lett. 38, L07603. doi: 10.1029/2010GL046324
Gutiérrez D., Enríquez E., Purca S., Quipuzcoa L., Marquina R., Flores G., et al. (2008). Oxygenation episodes on the continental shelf of central Peru: remote forcing and benthic ecosystem response, prog. Oceanography 79, 177–189.
Gutiérrez D., Sifeddine A., Field D. B., Ortlieb L., Vargas G., Chavez F., et al. (2009). Rapid reorganization in ocean biogeochemistry off Peru towards the end of the little ice age. Biogeosciences 6, 835–848. doi: 10.5194/bg-6-835-2009
Gutiérrez D., Sifeddine A., Reyss J. L., Vargas G., Velazco F., Salvatteci R., et al. (2006). Anoxic sediments off central Peru record interannual to multidecadal changes of climate and upwelling ecosystem during the last two centuries. Adv. Geosci. 6, 119–125. doi: 10.5194/adgeo-6-119-2006
Hebbeln D., Marchant M., Freudenthal T., Wefer G. (2000). Surface distribution along the Chilean continental slope related to upwelling and productivity. Mar. Geol. 164, 119–137. doi: 10.1016/S0025-3227(99)00129-2
Helly J., Levin L. (2004). Global distribution of naturally occurring marine hypoxia on continental margin. Deep-Sea Res. Pt. I 51, 1159–1168. doi: 10.1016/j.dsr.2004.03.009
Hu S., Fedorov A. V. (2018). Cross-equatorial winds control El niño diversity and change. Nat Clim Change 8 (9), 798–802. doi: 10.1038/s41558-018-0248-0
Jacques–Coper M., Garreaud R. (2014). Characterization of the 1970s climate shift in south America. Int. J. Climatol. doi: 10.1002/joc4120
Kaiser J., Schefuß E., Lamy F., Mohtadi M., Hebbeln D. (2008). Glacial to Holocene changes in sea surface temperature and coastal vegetation in north central Chile: high versus low latitude forcing, quat. Sci. Rev. 27, 2064–2075. doi: 10.1016/j.quascirev.2008.08.025
Kämpf J., Chapman P. (2016). “Upwelling systems of the world,” in A scientific journey to the most productive marine ecosystems (Springer). doi: 10.1007/978-3-319-42524-5
Katsev S., Crowe S. (2015). Organic carbon burial efficiencies in sediments: the power law of mineralization revisited. Geology 43, 607−610. doi: 10.1130/G36626.1
Kessarkar P. M., Naqvi S. W. A., Thamban M., Fernandes L. L., Siebert C., Rao V. P., et al. (2018). Variations in denitrification and ventilation within the Arabian Sea oxygen minimum zone during the Holocene. Geochem. Geophys. Geosystems 19, 2179–2193. doi: 10.1029/2017GC007286
Koutavas A., de Menocal P. B., Olive G. C., Lynch-Stieglitz J. (2006). Mid-Holocene El niño–southern oscillation (ENSO) attenuation revealed by individual foraminifera in eastern tropical pacific sediments. Geology 34, 993–996. doi: 10.1130/G22810A.1
Kwiatkowski L., Torres O., Bopp L., Aumont O., Chamberlain M., Christian J. R., et al. (2020). Twenty-first century ocean warming, acidification, deoxygenation, and upper-ocean nutrient and primary production decline from CMIP6 model projections. Biogeosciences 17, 3439–3470. doi: 10.5194/bg-17-3439-2020
Lam P., Lavik G., Jensen M. M., van de Vossenberg J., Schmid M., Woebken D., et al. (2009). Revising the nitrogen cycle in the Peruvian oxygen minimum zone. Proc. Natl. Acad. Sci. USA 106, 4752–4757. doi: 10.1073/pnas.0812444106
Lamy F., Kilian R., Arz H. W., Francois J.-P., Kaiser J., Prange M., et al. (2010). Holocene Changes in the position and intensity of the southern westerly wind belt. Nat. Geosci. 3, 695–699. doi: 10.1038/ngeo959
Lüning S., Gałka M., Bamont F. P., García F., Vahrenholt F. (2019). The medieval climate anomaly in south America. Quat. Int. 508, 70–87. doi: 10.1016/j.quaint.2018.10.041
Luyten J. R., Pedlosky J., Stommel H. (1983). The ventilated thermocline. J. Phys. Oceanogr. 13, 292–309. doi: 10.1175/1520-0485(1983)013<0292:TVT>2.0.CO;2
MacDonald G., Case R. (2005). Variations in the pacific decadal oscillation over the past millennium. Geophys. Res. Lett. 32, L08703. doi: 10.1029/2005GL022478
McManus J., Berelson W. M., Severmann S., Poulson R. L., Hammond D. E., Klinkhammer G. P., et al. (2006). Molybdenum and uranium geochemistry in continental margin sediments: paleoproxy potential. Geochim. Geochim. Cosmochim. Ac. 70, 4643–4662. doi: 10.1016/j.gca.2006.06.1564
Meyers P. A. (1997). Organic geochemical proxies of paleoceanographic, paleolimnologic, and paleoclimatic processes. Org. Geochem. 27, 213–250. doi: 10.1016/S0146-6380(97)00049-1
Middelburg J., Vlug T., Jaco F., van der Nat W. A. (1993). Organic matter mineralization in marine systems. Glob. Planet. Change 8, 47–58. doi: 10.1016/0921-8181(93)90062-S
Milessi A. C., Sellanes J., Gallardo V. A., Lange C. B. (2005). Osseous skeletal material and fish scales in marine sediments under the oxygen minimum zone off northern and central Chile. Estuar. Coast. Shelf Sci. 64 (2–3), 185–190. doi: 10.1016/j.ecss.2005.02.013
Mohtadi M., Rossel P., Lange C. B., Pantoja S., Böning P., Repeta D., et al. (2008). Deglatial pattern of circulation and marine productivity in the upwelling region off central-south Chile. Earth Planet. Sci. Lett. 272, 221–230. doi: 10.1016/j.epsl.2008.04.043
Mollier-Vogel E., Martinez P., Blanz T., Robinson R., Desprat S., Etourneau J., et al. (2019). Mid-Holocene deepening of the southeast pacific oxycline, glob. Planet. Change 172, 365–373. doi: 10.1016/j.gloplacha.2018.10.020
Mollier-Vogel E., Ryabenko E., Martinez P., Wallace D., Altabet M., Schneider R. (2012). Nitrogen isotope gradients off Peru and Ecuador related to upwelling, productivity, nutrient uptake and oxygen deficiency. Deep-Sea Re. Pt.I 70, 14–25. doi: 10.1016/j.dsr.2012.06.003
Montecino V., Lange C. (2009). The Humboldt current system: ecosystem omponents and processes, fisheries, and sediment studies. Prog. Oceanogr. 83, 65–75. doi: 10.1016/j.pocean.2009.07.041
Montero-Serrano J., Martínez-Santana M., Tribovillard N., Riboulleau A., Garbán G. (2009). Geochemical behavior of molybdenum and its isotopes in the sedimentary environment – a bibliographic review. Rev. Biol. Mar. Oceanogr. 44 (2), 263–275.
Morford J., Emerson S. (1999). The geochemistry of redox sensitive trace metals in sediments, geochim. Cosmochim. Ac. 63, 1735–1750. doi: 10.1016/S0016-7037(99)00126-X
Moy C., Moreno P., Dunbar R., Kaplan M., Francois J.-P., Villalba R., et al. (2009). “Past climate variability in south America and surrounding regions,” in Climate change in southern south America during the last two millennia. chapter 15. Ed. Vimeux F. (Springer), 353–393.
Muñoz P., Dezileau L., Lange C., Cárdenas L., Sellanes J., Salamanca M., et al. (2012). Evaluation of sediment trace metal records as paleoproductivity and paleoxygenation proxies in the upwelling center off concepción, Chile (36° s). Prog. Oceanogr. 92–95, 66–80. doi: 10.1016/j.pocean.2011.07.010
Muñoz P., Lange C. B., Gutiérrez D., Hebbeln D., Salamanca M. A., Dezileau L, et al. (2004). Recent sedimentation and mass accumulation rates based on 210Pb along the Peru-Chile continental margin. Deep Sea Res. Pt. II, 2523–2541.
Muñoz P., Rebolledo L., Dezileau L., Maldonado A., Mayr C., C´ardenas P., et al. (2020). Reconstructing past variations in environmental conditions and paleoproductivity over the last ~8000 years off north-central Chile (30°S). Biogeosciences 17, 5763–5785. doi: 10.5194/bg-17-5763-2020
Muñoz P., Hevia-Hormazabal V., Araya K., Maldonado A., Salamanca A. (2022). Metal enrichment evolution in marine sediments influenced by oxygen-deficient waters in a mineral loading zone, atacama, Chile (27°S). Mar. Environ. Res. 177, 105619. doi: 10.1016/j.marenvres.2022.105619
Muratli J. M., Chase Z., Mix A. C., McManus J. (2010). Increased glacial-age ventilation of the Chilean margin by antarctic intermediate water. Nat. Geosci. 3, 2326. doi: 10.1038/NGEO715
Nameroff T., Balistrieri L., Murray W. (2002). Suboxic trace metals geochemistry in the eastern tropical north pacific. Geochim. Cosmochim. Ac. 66, 1139–1158. doi: 10.1016/S0016-7037(01)00843-2
Ortega C., Vargas G., Rojas M., Rutllant J. A., Mu˜noz P., Lange C. B., et al. (2019). Extreme ENSO-driven torrential rainfalls at the southern edge of the atacama desert during the late Holocene and their projection into the 21th century. Glob. Planet. Change 175, 226–237. doi: 10.1016/j.gloplacha.2019.02.011
Paulmier A., Ruiz-Pino D., Garçon V., Farías L. (2006). Maintaining of the East south pacific oxygen minimum zone (OMZ) off Chile. Geophys. Res. Lett. 33 (6), L20601. doi: 10.1029/2006GL026801
Pauly D., Christensen V. (1995). Primary production required to sustain global fisheries. Nature 374, 255–257. doi: 10.1038/374255a0
Perez L., Barreiro M., Etchevers I., Crisci C., García-Rodríguez F. (2021). Centennial hydroclimatic and anthropogenic processes of south East south America modulate interannual and decadal river discharge. Sci. Total Environ. 781, 146733. doi: 10.1016/j.scitotenv.2021.146733
Pitcher G. C., Aguirre-Velarde A., Breitburg D., Cardich J., Carstensen J., Conley D. J, et al. (2021). System controls of coastal and open ocean oxygen depletion. Progr. Oceanogr. doi: 10.1016/j.pocean.2021.102613
Pizarro O., Shaffer G., Dewitte B., Ramos M. (2002). Dynamics of seasonal and interannual variability of the Peru-Chile undercurrent. Geophys. Res. Lett. 29, 28–31. doi: 10.1029/2002GL014790
Pizarro-Koch M., Pizarro O., Dewitte B., Montes I., Ramos M., Paulmier A., et al. (2019). Seasonal variability of the southern tip of the oxygen minimum zone in the Eastern south pacific (30°-38°S): a modeling study. J. Geophys. Res.-Oceans. doi: 10.1029/2019JC015201
Quintana J. M., Aceituno P. (2012). Changes in the rainfall regime along the extratropical west coast of south America (Chile): 30–43 s. Atmosfera 25, 1–22.
Ramos M., Dewitte B., Pizarro O., Garric G. (2008). Vertical propagation of extratropical rossby waves during the 1997–1998 El niño off the west coast of south America in a mediumresolution OGCM simulation. J. Geophys. Res. 113, C08041. doi: 10.1029/2007JC004681
Ramos M., Pizarro O., Bravo L., Dewitte B. (2006). Seasonal variability of the permanent thermocline off northern Chile. Geophys. Res. Lett. 33, L09608. doi: 10.1029/2006GL025882
Robinson R., Etourneau J., Martinez P., Schneider R. (2014). Expansion of pelagic denitrification during early pleistocene cooling. Earth Planet. Sci. Lett. 389, 52–61. doi: 10.1016/j.epsl.2013.12.022
Robinson R. S., Mix A., Martinez P. (2007). Southern ocean control on the extent of denitrification in the southeast pacific over the last 70 ka quaternary. Sci. Rev. 26, 201–212. doi: 10.1016/j.quascirev.2006.08.005
Salvatteci R., Gutiérrez D., Field D., Sifeddine A., Ortlieb L., Bouloubassi I., et al. (2014). The response of the Peruvian upwelling ecosystem to centennial-scale global change during the last two millennia. Clim. Past 10, 1–17. doi: 10.5194/cp-10-715-2014
Salvatteci R., Gutiérrez D., Sifedine A., Ortlieb L., Druffel E., Boussafir M., et al. (2016). Centennial to millennial-scale changes in oxygenation and productivity in the Eastern tropical south pacific during the last 25000 years. Quat. Sci. Rev. 131, 102–117. doi: 10.1016/j.quascirev.2015.10.044
Sánchez G., Lange C., González H., Vargas G., Muñoz P., Cisternas C., et al. (2012). Siliceous microorganisms in the upwelling center off concepción, Chile (36°S): preservation in surface sediments and downcore fluctuations during the past ~150 years. Prog. Oceanogr. 92–95, 50–65. doi: 10.1016/j.pocean.2011.07.014
Sánchez A., Shumilin E., Rodríguez-Figueroa G. (2022). Trace elements V, Ni, Mo and U: a geochemical tool to quantify dissolved oxygen concentration in the oxygen minimum zone of the north-eastern pacific. J. Mar. Syst. 230, 103732. doi: 10.1016/j.jmarsys.2022.103732
Schneider W., Fuenzalida R., Garcés-Vargas J., Bravo L., Lange C. (2006). Vertical and horizontal extensión of the oxygen mínimum zone in the eastern south pacific ocean. Gayana 70, 79–82. doi: 10.4067/S0717-65382006000300016
Schneider W., Fuenzalida R., Rodríguez-Rubio E., Garcés-Vargas J. (2003). Characteristics and formation of Eastern south pacific intermediate water. Geophys. Res. Lett. 30, 158. doi: 10.1029/2003GL017086
Scholz F. (2018). Identifying oxygen minimum zone-type biogeochemical cycling in earth history using inorganic geochemical proxies. Earth. Sci. Rev. 184, 29–45. doi: 10.1016/j.earscirev.2018.08.002
Scholz F., Siebert C., Dale A. W., Frank M. (2017). Intense molybdenum accumulation in sediments underneath a nitrogenous water column and implications for the reconstruction of paleo-redox conditions based on molybdenum isotopes. Geochim. Cosmochim. Ac. 213, 400–417. doi: 10.1016/j.gca.2017.06.048
Sellanes J., Quiroga E., Neira C., Gutiérrez D. (2007). Changes of macrobenthos composition under different ENSO cycle conditions on the continental shelf off central Chile. Continent. Shelf Res. 27, 1002–1016. doi: 10.1016/j.csr.2007.01.001
Sifeddine A., Gutiérrez D., Ortlieb L., Boucher H., Velazco F., Field D., et al. (2008). Laminated sediments from the central Peruvian continental slope: a 500 year record of upwelling system productivity, terrestrial runoff and redox conditions. Prog. Oceanogr. 79, 190–197. doi: 10.1016/j.pocean.2008.10.024
Silva N., Neshyba S. (1979). On the southern most extension of the Peru–Chile undercurrent. Deep-Sea Res. 26A, 1387–1393.
Sprintall J., Cravatte S., Dewitte B., Du Y., Sen Gupta A. (2020). Oceanic teleconnections, chapter 15 in "El nino in a changing climate" AGU book. 528, ISBN: ISBN: 978-1-119-54816-4 Available at: https://agupubs.onlinelibrary.wiley.com/doi/abs/10.1002/9781119548164.ch15.
Srain B., Pantoja S., Sepúlveda J., Lange C. B., Muñoz P., Summons R. E., et al. (2015). Interdecadal changes in intensity of the oxygen minimum zone off concepción, Chile ( _36_ s), over the last century. Biogeosciences 12, 6045–6058. doi: 10.5194/bg-12-6045-2015
Thomas A. C., Blanco J. L., Carr M. E., Strub P. T., Osses J. (2001). Satellite-measured chlorophyll and temperature variability off northern Chile during the 1996–1998 la niña and El niño. J. Geophys. Res. 106, 899–915. doi: 10.1029/1999JC000052
Tribovillard N., Algeo T. J., Baudin F., Riboulleau A. (2012). Analysis of marine environmental conditions based onmolybdenum–uranium covariation–applications to mesozoic paleoceanography. Chem. Geol. 324-325, 46−58. doi: 10.1016/j.chemgeo.2011.09.009
Tribovillard N., Algeo T., Lyons T., Riboulleau A. (2006). Trace metals as paleoredox and paleoproductivity proxies: an update. Chem. Geol. 232, 12–32. doi: 10.1016/j.chemgeo.2006.02.012
Ulloa O., Escribano R., Hormazabal S., Quiñones R. A., Gonzalez R., Ramos M. (2001). Evolution and biological effects of the 1997–98 E1 niño in the upwelling ecosystem off northern Chile, geophys. Res. Lett. 28, 1591–1594. doi: 10.1029/2000GL011548
Valdés J., Sifeddine A., Boussafir M., Ortlieb L. (2014). Redox conditions in a coastal zone of the Humboldt system (Mejillones,23° s). influence on the preservation of redox-sensitive metals. J. Geochem. Explor. 140, 1–10. doi: 10.1016/j.gexplo.2014.01.002
Valdés J., Sifeddine A., Lallier-Verges E., Ortlieb L. (2004). Petrographic and geochemical study of organic matter in surficial sediments from an upwelling system (Mejillones del sur bay, northern Chile). Org. Geochem. 35 (5), 881–894. doi: 10.1016/j.orggeochem.2004.02.009
Valdés J., Sifedine A., Guiñez M., Castillo A. (2021). Oxygen minimum zone variability during the last 700 years in a coastal upwelling area of the Humboldt system (Mejillones, 23° s, chile). a new approach from geochemical signature. Prog. Oceanogr. 193, 102520. doi: 10.1016/j.pocean.2021.102520
Valdés J., Sifedine A., Ortlieb L., Pierre C. (2009). Interplay between sedimentary organic matter and dissolved oxygen availability in a coastal zone of the Humboldt current system; mejillones bay, northern Chile. Mar. Geol. 265, 157–166. doi: 10.1016/j.margeo.2009.07.004
Vargas G., Pantoja S., Rutllant J., Lange C., Ortlieb L. (2007). Enhancement of coastal upwelling and interdecadal ENSO-like variability in the Peru-Chile current since late 19th century. geophys. Res. Lett. 34, L13607. doi: 10.1029/2006GL028812
Wagner M., Chappaz A., Lyons T. W. (2017). Molybdenum speciation and burial pathway in weakly sulfidic environments: insights from XAFS. Geochim. Cosmochim. Acta 206, 18–29. doi: 10.1016/j.gca.2017.02.018
Wam B., Luo X., Yang Y.-M., Sun W., Cane M., Cai W., et al. (2019). Historical change of El niño properties sheds light on future changes of extreme El niño. PNAS 116 (5), 22512–22517. doi: 10.1073/pnas.191113011
Wedepohl K. (1971). “Environmental influences on the chemical composition of shales and clays,” in Physics and chemistry of the earth. Eds. Ahrens L., Press F., Runcorn S., Urey H.(Oxford: Pergamon), 8, 307–333. doi: 10.1016/0079-1946(71)90020-6
Yang S., Oh J.-H. (2020). Effects of modes of climate variability on wave power during boreal summer in the western north pacific. Sci. Rep. 10 (5187), 1–10. doi: 10.1038/s41598-020-62138-0
Zheng Y., Anderson R., Van Geen A., Fleisher M. Q. (2002). Remobilization of authigenic uranium in marine sediments by bioturbation. Geochim. Cosmichim. Acta 66, 1759–1772.
Keywords: oxygen minimum zone, denitrification, sensitive redox metals, enso, late holocene, eastern boundary upwelling system
Citation: Muñoz P, Castillo A, Valdés J and Dewitte B (2023) Oxidative conditions along the continental shelf of the Southeast Pacific during the last two millennia: a multiproxy interpretation of the oxygen minimum zone variability from sedimentary records. Front. Mar. Sci. 10:1134164. doi: 10.3389/fmars.2023.1134164
Received: 04 January 2023; Accepted: 08 June 2023;
Published: 18 July 2023.
Edited by:
Gireesh Kumar T R, Council of Scientific and Industrial Research (CSIR), IndiaReviewed by:
Ejin George, National Centre for Polar and Ocean Research (NCPOR), IndiaAlberto Sánchez-González, National Polytechnic Institute (IPN), Mexico
Copyright © 2023 Muñoz, Castillo, Valdés and Dewitte. This is an open-access article distributed under the terms of the Creative Commons Attribution License (CC BY). The use, distribution or reproduction in other forums is permitted, provided the original author(s) and the copyright owner(s) are credited and that the original publication in this journal is cited, in accordance with accepted academic practice. No use, distribution or reproduction is permitted which does not comply with these terms.
*Correspondence: Praxedes Muñoz, cHJheGVkZXNAdWNuLmNs