- 1Shandong Key Laboratory of Eco-Environmental Science for Yellow River Delta, Binzhou University, Binzhou, China
- 2College of Forestry, Shandong Agricultural University, Taian, China
Introduction: The effects of nitrogen (N) and phosphorus (P) addition on soil microbial diversity have been widely studied, however, the response of bacterial community to N and P imbalance input remains unclear.
Methods: Using a high-throughput Illumina Miseq sequencing platform, N and P imbalance addition experiment was conducted to characterize the rhizosphere and bulk soil bacterial community of Suaeda salsa (S. salsa) in the Yellow River Delta.
Results: The results showed that the rhizosphere soil bacterial community α-diversity was significantly higher than bulk soil. The rhizosphere soil Bacteroidetes and Actinobacteria were higher and lower than bulk soil, respectively. N and P imbalance input had small effects on the composition and α -bacterial diversity of the rhizosphere soil, while significantly increasing the bulk soil bacterial diversity and remarkably changing the community composition. Differences in the response of rhizosphere and bulk soil bacterial community to N and P imbalance input were caused by soil organic matter (SOM) content. The N and P imbalance input increased the relative abundance of bulk soil Eutrophic bacteria and decreased the relative abundance of the predicted oligotrophic bacteria (Acidobacteria,Chorolflexi). Rhizosphere and bulk soil bacterial community α-diversity was significantly correlated with SOM, salt, total carbon (TC) and total N (TN) content, with SOM and salt having the greatest effect on bulk soil bacterial community composition.
Discussion: There may be a threshold N-P input ratio between 15:1 and 45:1. This threshold is the optimal ratio for increasing the diversity of bacterial community.
1 Introduction
Nitrogen (N) input to the ecosystem has increased dramatically from 15.3 Tg yr-1 in 1860 to 175-259 Tg yr-1 in 2011 due to extensive fossil burning, agricultural fertilization, and other human activities (Liu et al., 2019). Meanwhile, compared to the N input, the phosphorus (P) input was lower, increasing from 0.3 Tg yr-1 to 14-16 Tg yr-1 (Penuelas et al., 2013; Liu et al., 2019). Human activity significantly altered the ratio of N and P supply, which may have a series of effects on the ecosystem, such as decreased soil pH, soil acidification, increased soil carbon storage, and altered soil carbon flux (Cusack et al., 2011; Tian and Niu, 2015). These series of subsurface ecological processes are closely related to soil microorganisms. Microorganisms are the most diverse and abundant organisms on Earth and are essential for maintaining plant diversity and ecosystem function (Bardgett and van der Putten, 2014; Bennett et al., 2017).
N addition typically reduces soil bacterial community diversity and changes bacterial community composition, for example, by increasing the relative abundance of eutrophic bacterial taxa and decreasing the abundance of oligotrophic taxa (Ling et al., 2017). Due to direct changes in N availability, the response of soil microbial community to N addition is consistent regardless of soil type and N form (Ramirez et al., 2010). However, it was also found that low-level N addition alleviated the nutrient limitation of soil bacterial community growth and promoted its diversity (Zhang et al., 2017). Relative to N addition, there are fewer reports about the effect of P addition on the bacterial community. Ma et al. (2021) found that P addition directly affected microbial community composition in primary and secondary forests. It has also been found that P addition has less effect on soil microbial community composition (Widdig et al., 2020; Ran et al., 2021). In addition, P forms also have different effects on bacterial community structure. Inorganic P has a stronger effect on bacterial communities compared to organic P (Wang et al., 2022a).
The effect of N and P addition and their interaction on the soil bacterial community has been widely reported. However, the effect of the N-P ratio on the bacterial community is unclear. Previous studies have shown that higher N with lower P input in the short term tends to promote plant growth (Peng et al., 2019). However, the higher N-P ratio may also negatively impact plant growth, particularly the allocation of underground biomass, which may further impact the soil bacterial community (Deegan et al., 2012; Chen et al., 2017; Wang et al., 2019). N addition affects the functional potential of bacteria, such as urea decomposition, N fixation, and respiratory organic matter decomposition processes, while P addition can mitigate or modify the negative effects of N addition on soil microorganisms (Xia et al., 2020). However, this phenomenon is reversed or neutral for the effect of P addition at high-level N addition (Wang et al., 2018b). The combined addition of N and P has a synergistic effect on the ecosystem, where the addition of a single nutrient leads to the limitation of other nutrients, suggesting that in natural ecosystems, the supply of N and P is tight and balanced (Elser et al., 2007). Therefore, it is necessary to study the effect of the N-P addition ratio, rather than a single nutrient, on the bacterial community.
Plants usually influence the nutrient conditions of the rhizosphere microenvironment and thus have a selective effect on rhizosphere microorganisms, thus changing the structure and ecological function of the soil microbial community (Li et al., 2022b). Plant root release secretions provide abundant carbon (C) sources, and N and P addition can promote plant primary productivity, which in turn increases the input of C sources to the subsurface (Liu et al., 2016). The growth of soil microorganisms is usually C-limited (Fierer et al., 2009). Therefore, increased C sources may promote the growth and development of bacterial communities. However, it has also been found that nutrient addition reduces fine root biomass and increases recalcitrant C (Liu et al., 2016; Peng et al., 2017). This results in a potential increase in bacteria with recalcitrant C degradation and a decrease in other species, which can alter the composition of the soil bacterial community. Changes in bacterial community composition lead to changes in functional gene abundance, which in turn affect soil nutrient cycling. Plant-soil-microbe interactions play an important role in nutrient cycling (Freedman et al., 2013). Therefore, it is necessary to combine soil microorganisms, plants and soil to predict the ecosystem processes under nutrient addition (Miki et al., 2010).
The Yellow River Delta (YRD) coastal wetland is the youngest, most complete and most native estuarine wetland ecosystem in the warm temperate zone of the world (Liu et al., 2021), but it is also an ecologically fragile area with a single biological system, poor resilience and threatened biodiversity. In recent years, N deposition in the Yellow River Delta has continued to increase and is at a high level in China (Lu et al., 2021). Increased anthropogenic N input to coastal wetland ecosystems causes a severe imbalance between N and P, which may reduce vegetation diversity and may affect soil biogeochemical cycling in the ecosystem (Penuelas et al., 2013; Peng et al., 2019). However, the effect of N and P imbalance input on the bacterial community remains unclear. Therefore, we designed a field experiment by fertilizing N and P with different N-P addition ratios to the typical dominant halophyte of the YRD wetland, taking rhizosphere and bulk soil, to investigate (1) the rhizosphere effect of S. salsa on soil bacterial community, (2) the effect of N-P addition ratio on bacterial diversity in the rhizosphere and bulk soil, and (3) the relationship between soil environmental factor and bacterial community by 16s rRNA sequencing technique. We predicted that (1) the effect of N and P imbalance input on the bacterial community would be varied due to rhizosphere effects, (2) N and P imbalance input would reduce bacterial diversity and change bacterial community composition, and become more pronounced with increasing N and P ratio. and (3) The nutrient supply would increase the abundance of eutrophic bacteria and decrease the relative abundance of oligotrophic bacteria.
2 Materials and methods
2.1 Site description
The study area was located in the Yellow River Delta Dongying City, Shandong Province (118°44’2”E, 38°1’18”N), which is the temperate semi-humid continental monsoon climate with annual precipitation of 550-640 mm and an average annual temperature of 12.6°C. The dominant plants are Phragmites communis, S. salsa, Aeluropus sinensis, and Tamarix chinensis. The terrain is relatively flat, mostly sandy, and muddy soil, which is easy to compact and has poor ventilation.
2.2 Experiment design
A 1m×1m sample square was set up within a single plant, and a 5m buffer strip was set up between the sample squares for N and P fertilizer addition treatment in May 2021. Five N-P addition ratio treatments CK (0 kg N ha-1, 0 kg P ha-1), N0P (0 kg N ha-1, 10 kg P ha-1), N1P (MN, 50 kg N ha-1, 10 kg P ha-1), N2P (HN, 150 kg N ha-1, 10 kg P ha-1), N3P (HN, 450 kg N ha-1, 10 kg P ha-1), and each treatment consisted of three repetitions. The selection of the N-P addition ratio was referred to a previous study (Liu et al., 2019). Previous studies have found that urea addition has a stronger effect on soil N cycling than ammonia and nitrate N addition (Li et al., 2019a; Wang et al., 2022b). Therefore, urea was used as N fertilizer. KH2PO4 was used as P fertilizer by referring to Li et al. (2019a). Urea and KH2PO4was first dissolved in pure water and then sprayed evenly on the target area, respectively. CK treatment was sprayed with pure water.
2.3 Soil sampling
Sample collection was conducted in July 2021, approximately 3 months after the N and P addition. The rhizosphere soil was carefully collected from soil attached to the root system (0-0.5 cm from the root) of all plants. The bulk soil was collected from the soil at a depth of 15cm on the ground without plant growth. A total of 30 soil samples were collected, and then each soil sample was divided into two parts after removing impurities. One part was naturally dried for the determination of soil physicochemical properties. Another part was stored at -80°C for soil microbial analysis.
2.4 Measurements of soil physical and chemical properties
Soil organic matter (SOM) content was measured by the potassium dichromate volumetric method. Total N (TN) and total carbon (TC) content were measured using an elemental analyzer (Vario EL III, Elementar, Germany). Soil pH and salt were measured by pH meter and conductivity meter, respectively. Total P (TP) content was determined using the molybdenum blue method with an ultraviolet-visible spectrophotometer, and the available P (AP) content was measured by NaHCO3 extraction-molybdenum antimony anti-colorimetry.
2.5 Soil DNA extraction, sequencing and data analysis
Total genomic DNA from samples was extracted using CTAB method by OMEGA DNA kit, and the purity and concentration of DNA were detected by 1% agarose gel electrophoresis, and the samples were diluted to 1 ng/µl with sterile water in a centrifuge tube. The V3-V4 region of the bacterial 16S rRNA gene was amplified with specific primers 341F and 806R. The products were detected by 2% agarose gel electrophoresis, and purified by using AxyPrep DNA Gel Extraction Kit. The MiSeqPE300 platform of Illumina company (Wekemo Tech Group Co., Ltd. Shenzhen China.) was used for high-throughput sequencing. Qiime2 processed the original DNA data, and the obtained effective sequences were clustered by OTU for further analysis.
2.6 Statistical analysis
Two-way ANOVA followed by LSD multiple-comparison test was used to analyze the effects of rhizosphere and N-P addition ratio on soil characteristics, indices of community diversity, and abundance of dominant taxa. The Mantel test was used to determine the effect of soil characteristics on bacterial community diversity, dominant bacterial phyla, and dominant bacterial genera groups. PERMANOVA (per=999) was conducted to test the effect of different N-P addition ratio treatments on bacterial community composition. Pearson analysis was used to correlate soil characteristics with bacterial communities.
All data were processed based on R software (4.1.0), and Two-way ANOVA was performed using the basic command aov. LSD was performed using the agricolae and multcomp package. Mantel test was performed using the linkET package. PERMANOVA and PCoA analyses were performed using the vegan package. The correlation analysis was performed using the corrplot package. Image processing was performed using the ggplot2 package.
3 Results
3.1 Soil bacterial community α-diversity
The results of the α-diversity analysis of the rhizosphere and bulk soil bacterial community in S. salsa under different N-P addition ratio additions were shown in Tables 1, 2, and it was observed that a-diversity indices were significantly different between rhizosphere and bulk soil. N-P addition ratio had a significant effect on Chao1, Observed OTUs and Shannon index (P<0.05), Chao1 and Observed OTUs index were also significantly affected by the interaction of N-P addition ratio and Soil (P<0.05). N and P addition significantly increased the richness of the bulk soil bacterial community, the bacterial evenness was reduced in the high N-P addition ratio treatment.
3.2 PCoA analyses
The PCoA result showed that the first principal components accounted for 78% of the data matrix variance (Figure 1), and the explanations were 56.9% and 21.1% for PCoA1 and PCoA2, respectively. The results show that the rhizosphere and bulk soil were clearly separated, and a significant difference in the distance was found after the PERMANOVA test (Table 3, R2 = 0.364, P=0.001). The rhizosphere bacterial community was more concentrated, while the bulk soil bacterial community was more dispersed, indicating that the N-P addition ratio had less effect on the rhizosphere than bulk soil bacterial community. PERMANOVA test (Table 3, R2 = 0.637 P=0.001) indicates N-P addition ratio has a significant effect on bacterial community composition in bulk soil.
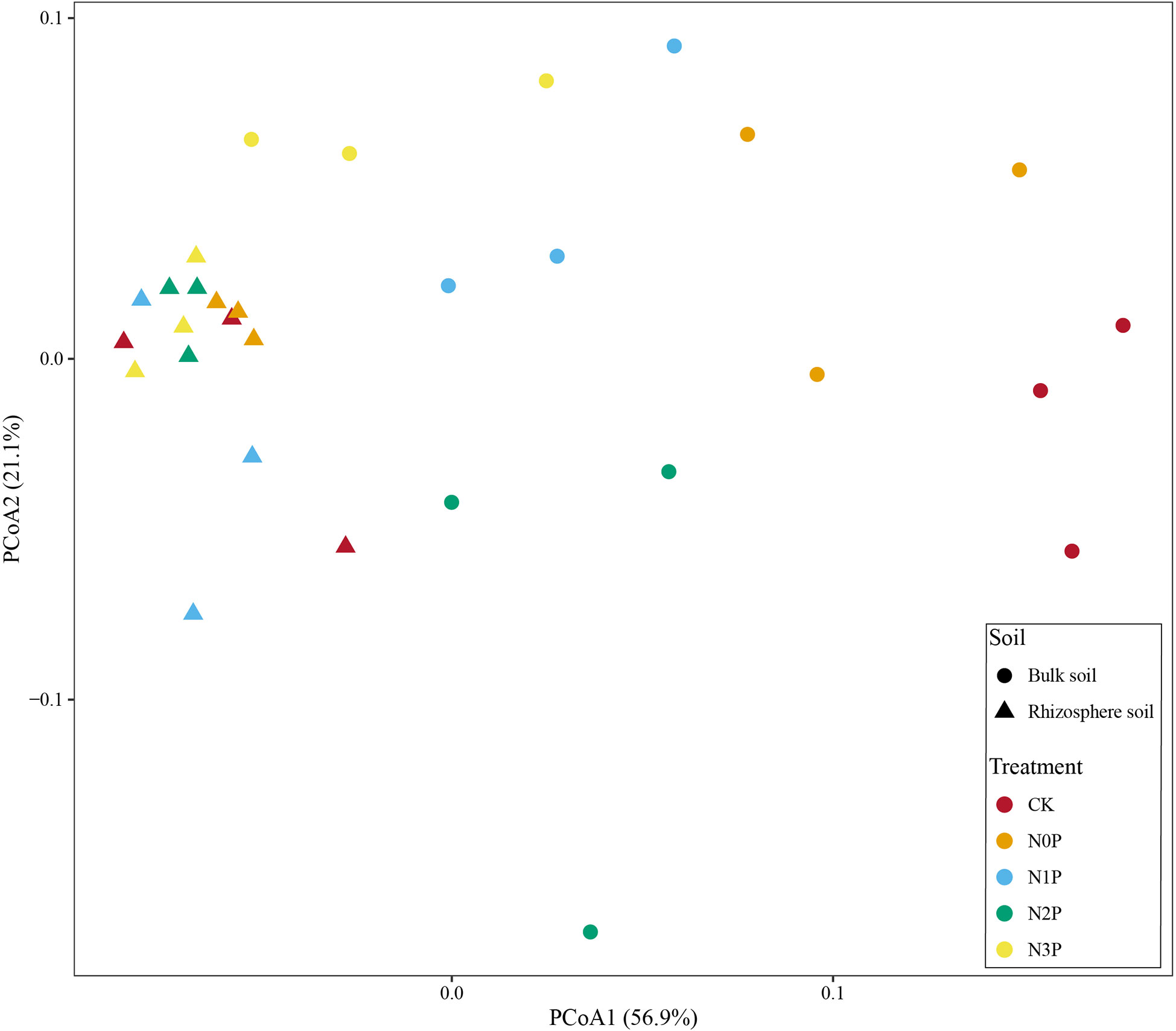
Figure 1 PCoA analysis of the bacterial community structure in the rhizosphere and bulk soil of S. salsa at different N-P addition ratio treatments.
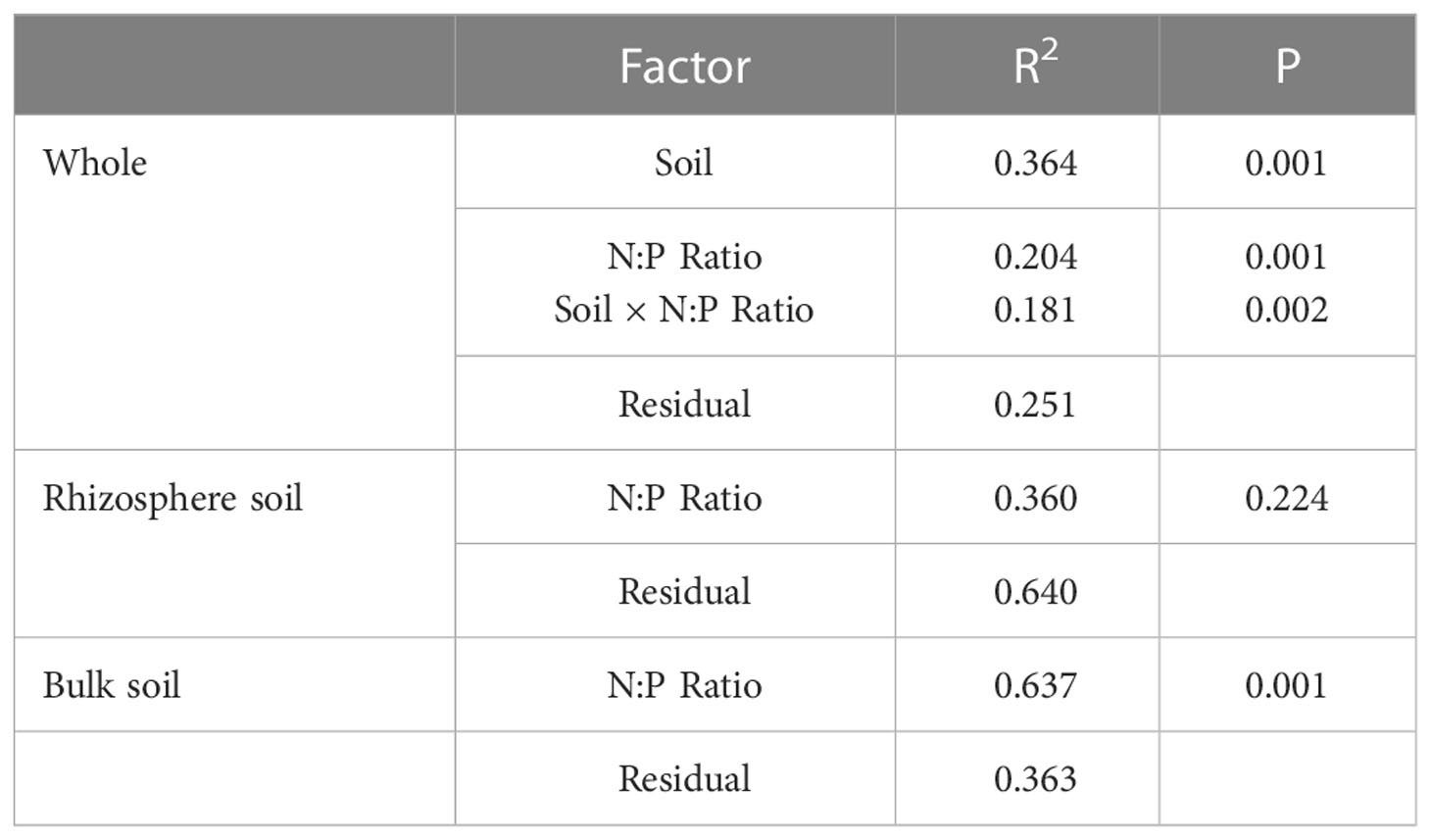
Table 3 PERMANOVA test (per = 999) of bacterial community in rhizosphere and bulk soil of S. salsa in different N-P addition ratio treatments.
3.3 Soil bacterial structure at the phylum level
The Proteobacteria was stable in the rhizosphere and bulk soil with the highest relative abundance (Figure 2), followed by the Bacteroidetes, Actinobacteria, Gemmatimonadetes, Acidobacteria and Chloroflexi phylum. The relative abundance of Bacteroidetes and Verrucomicrobia was significantly higher in the rhizosphere than in bulk soil (P<0.01, P<0.001), while the Actinobacteria, Acidobacteria and Gemmatimonadetes were significantly lower than in bulk soil (P<0.001, P<0.001, P<0.01). Two-way ANOVA (Table 4) showed that the Actinobacteria, Acidobacteria and Gemmatimonadetes relative abundance differed significantly between rhizosphere and bulk soil. In rhizosphere soil, the N-P addition ratio had small effects on the top five bacterial phyla. In contrast, in bulk soil, N and P addition significantly increased the relative abundance of the Gemmatimonadetes and Bacteroidetes (Figure 3). The difference is that the Bacteroidetes increased with increasing N-P addition ratio and the Gemmatimonadetes in contrast. N and P addition reduced the relative abundance of the Acidobacteria. The highest relative abundance of the Acidobacteria was observed under the N0P treatment, while it decreased with the co-addition of N and P.
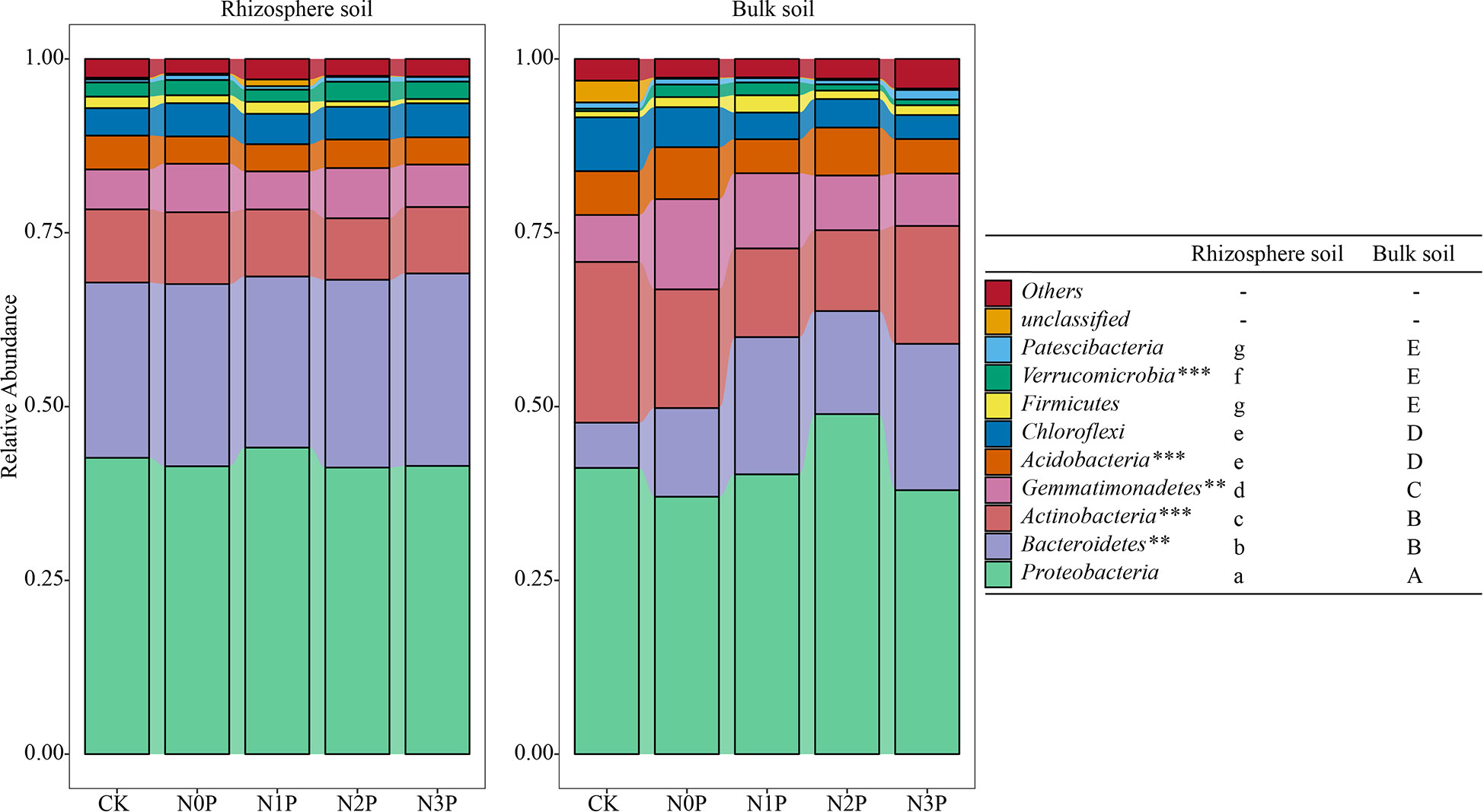
Figure 2 The relative abundance of the top 10 bacterial phyla in the rhizosphere and bulk soil of S. salsa at different N-P addition ratio treatments. Different capital letters indicate that the difference between bulk soil bacterial phyla is significant (P<0.05) and different lowercase letters indicate that the difference between rhizosphere soil bacterial phyla is significant (P<0.05) as well as **, *** indicate that the difference between different soils is significant at 0.01 and 0.001 level, respectively.
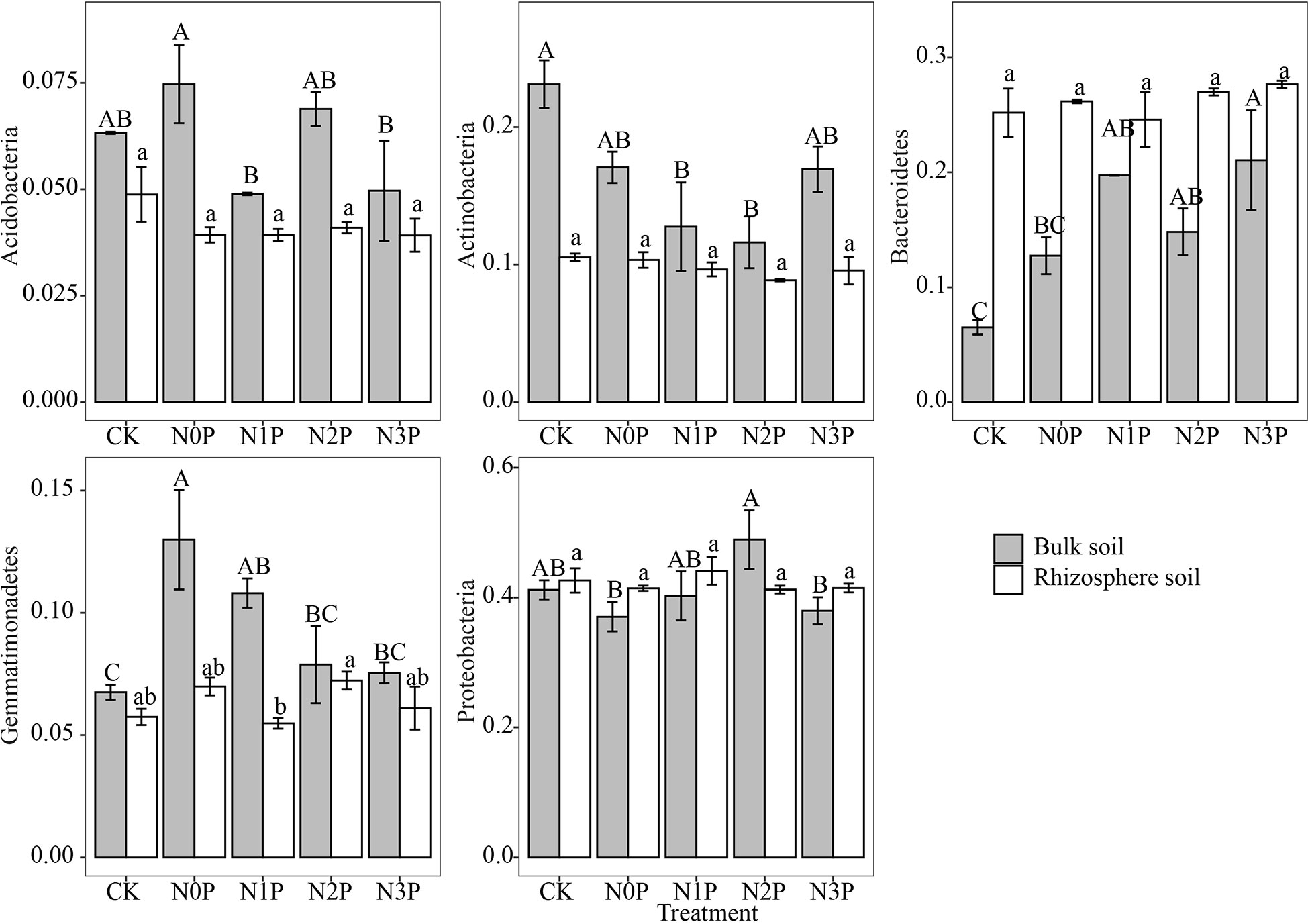
Figure 3 The relative abundance of dominant bacterial phyla in the rhizosphere and bulk soil of S. salsa at different N-P addition ratio treatments. Different capital letters indicate that the difference among N-P addition ratio treatments in bulk soil is significant (P<0.05) and different lowercase letters indicate that the difference among N-P addition ratio treatments in rhizosphere soil is significant (P<0.05).
3.4 Soil bacterial structure at the genus level
The relative abundance of rhizosphere and bulk soil with relative abundance of bacterial genera greater than 1% is shown in Figure 4. The number of abundant genera in the rhizosphere and bulk soil was 6 and 8, respectively, where Marinobacter, Subgroup_10, bacterium_YC_LK_LKJ36 and Woeseia were present in both soils. In rhizosphere soil, the relative abundance of bacterium_YC_LK_LKJ36, Marinobacter and Saccharospirillum were highest in the N0P treatment, while the relative abundance of all three decreased with increasing N-P addition ratio. In contrast, the Salinimicrobium was lowest in the N0P treatment, while N and P addition increased its relative abundance, however, the relative abundance decreased with increasing N-P addition ratio. In bulk soil, N and P addition decreased the relative abundance of MND1 and Marinobacterium and increased the relative abundance of bacterium_YC_LK_LKJ36. The relative abundance of Marinobacter was lower at low N-P addition ratio and higher at the high N-P addition ratio.
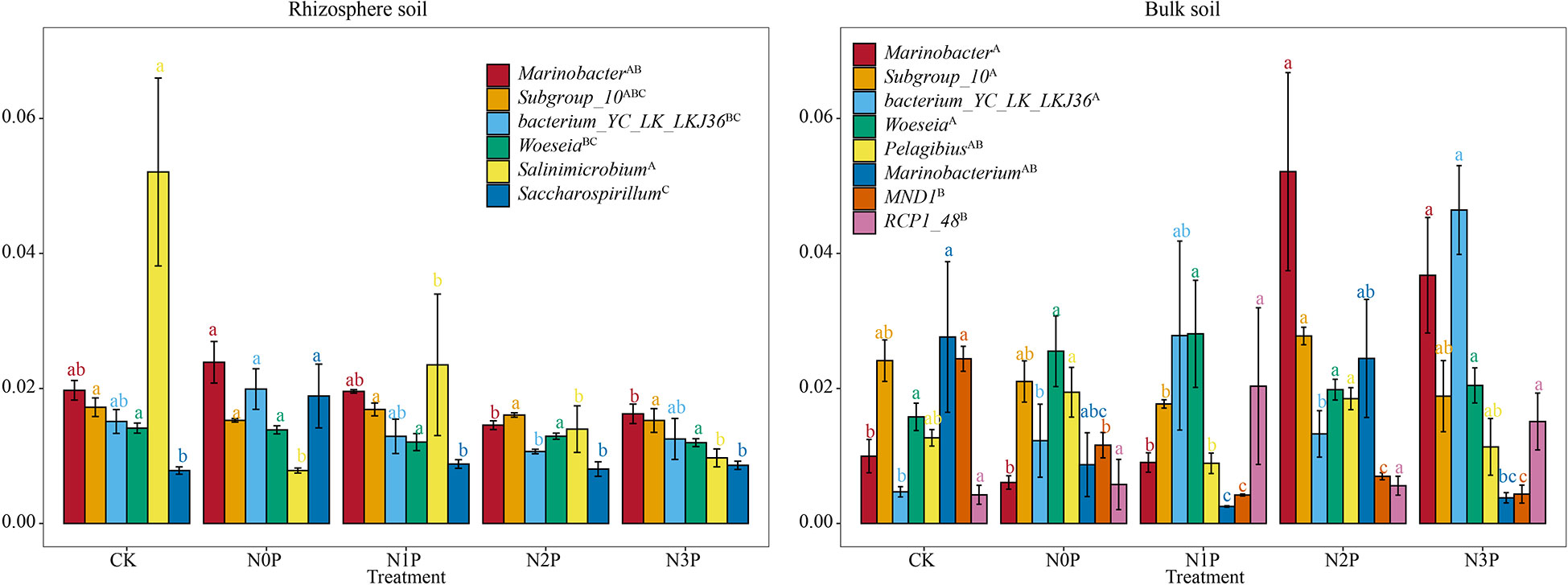
Figure 4 The relative abundance of dominant bacterial genus in the rhizosphere and bulk soil of S. salsa at different N-P addition ratio treatments. Different capital letters indicate that the difference among dominant bacterial genus is significant (P<0.05). Different lowercase letters indicate that the difference among N-P addition ratio treatments is significant (P<0.05).
3.5 Soil physicochemical properties
Soil environmental factors of rhizosphere and bulk soil added with different N-P addition ratios are shown in Figure 5. All environmental factors except C: N were significantly different between the rhizosphere and bulk soil (Figure 5, Table 5, P<0.05). The pH and TP content of rhizosphere soil were lower than those of bulk soil, while the C: N, C: P, N: P and salt, SOM, TC and TN were higher than those of bulk soil. Under CK and N0P treatments, AP showed that rhizosphere soil was larger than bulk soil, while the opposite was found under N and P addition.
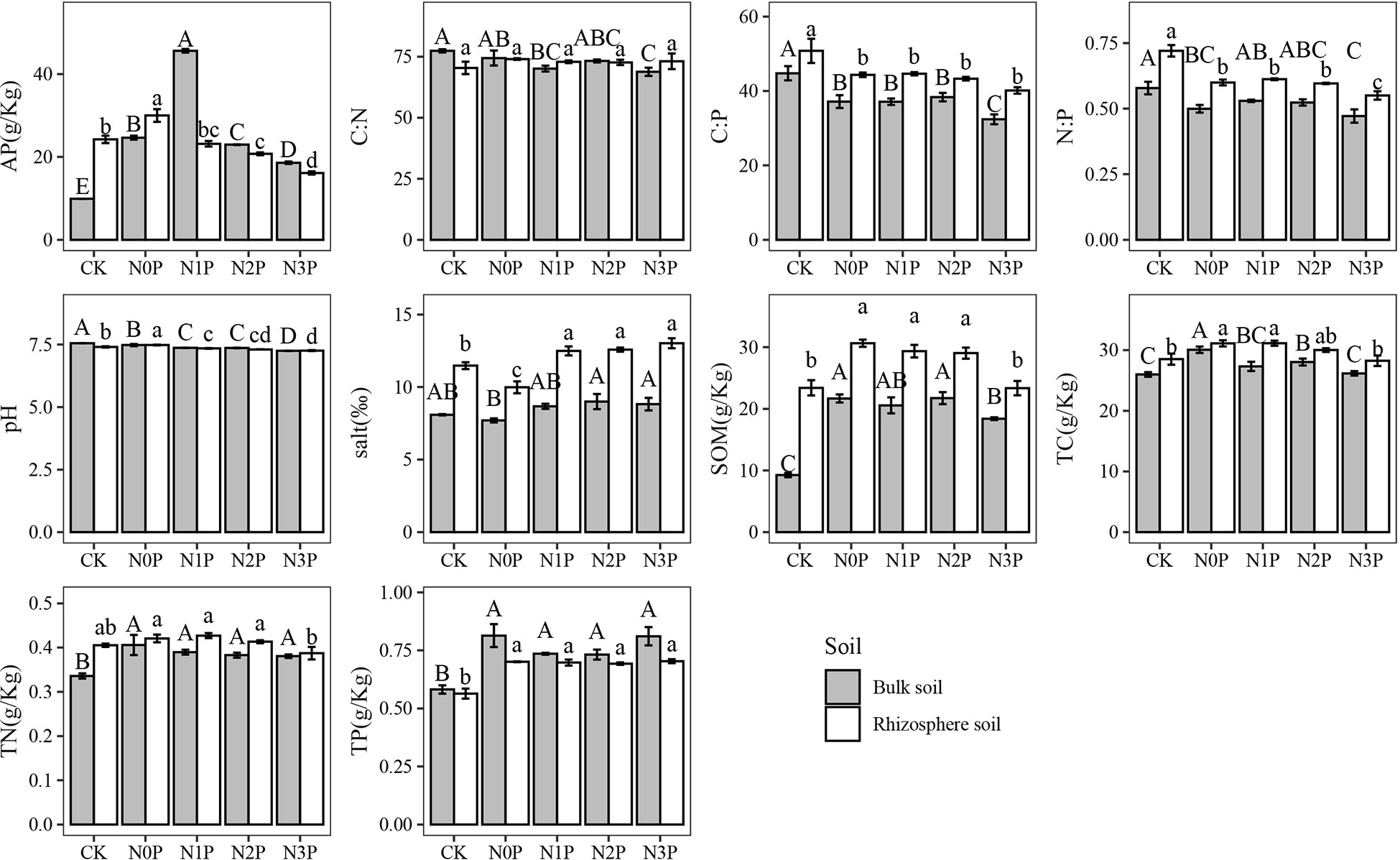
Figure 5 The environmental factors in the rhizosphere and bulk soil of S. salsa at different N-P addition ratio treatments. Different capital letters indicate that the difference among N-P addition ratio treatments in bulk soil is significant (P<0.05) and different lowercase letters indicate that the difference among N-P addition ratio treatments in rhizosphere soil is significant (P<0.05).
In the rhizosphere soil, the AP, pH, SOM, TC, and TN content showed an increase followed by a decrease with increasing N-P addition ratio, with the highest in the N0P treatment and the lowest in the N3P treatment. In bulk soil, pH decreased with increasing N-P addition ratio. N and P addition significantly increased soil AP, SOM, TC, TN, and TP (P<0.05) and decreased soil N:P, C: N, and N:P (P<0.05). SOM content was highest in the N0P and N2P treatment and decreased in the N3P treatment, but all were significantly higher than the CK (P<0.05).
3.6 Soil physicochemical properties and their correlations with bacterial community
The α-diversity index, the top 8 bacterial phyla, and the genera with relative abundance greater than 1% are used as the bacterial community in the Mantel test (Figure 6). In rhizosphere soil, soil environmental factors had no effects on bacterial α-diversity and bacterial community phylum, while TP, C:P, and N:P had significant effects (P<0.05) on bacterial enrichment genus. The bacterial community is strongly influenced by environmental factors in bulk soil, with high contributions from SOM and pH.
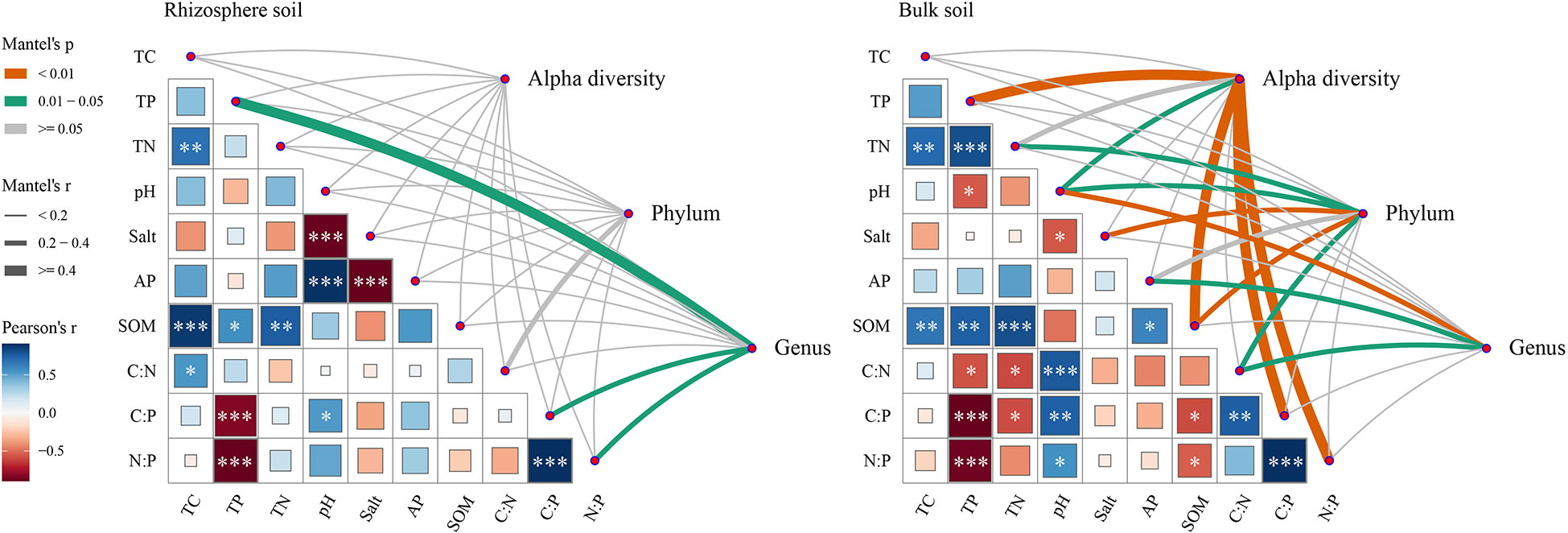
Figure 6 The mantel test between bacterial community and environmental factors in rhizosphere and bulk soil of S. salsa in different N-P addition ratio treatments. Note: Alpha diversity represents the alpha diversity index (Chao1, Shannon, Simpson, Faith_pd and Observer OTUs index) of the bacterial community. Phylum represents the dominant bacterial phyla of the bacterial community. Genus represents the dominant bacterial genus of the bacterial community. *, **, *** indicate that the correlation between the environmental factor is significant at 0.05, 0.01 and 0.001 level, respectively.
The correlation analysis between soil environmental factors and bacterial community (Figure 7) showed that N: P, salt, SOM, TC, and TN content were positively correlated with bacterial α-diversity index (P<0.001), while Chao1 and observed OTUs were negatively correlated with pH (P<0.05). The Bacteroidetes were positively correlated with salt, SOM, TN, and TC, while the Actinobacteria were the opposite of them. The woeseia was most strongly correlated with soil environmental factors.
4 Discussion
4.1 N-P addition ratio and rhizosphere effect on soil environmental factor
Plant root secretion of mucus, shed cells and tissues, and soluble products of ruptured cells all contribute to the TC and SOM of rhizosphere soil (Zhao et al., 2022). In addition, sugars and amino acids secreted by plant roots increase N availability in the soil (Zhao et al., 2022). In this study, the rhizosphere soil contained higher TN, SOM, and TC content compared to the bulk soil, which is evidence that the plant rhizosphere effect is at work. Soil pH was in the range of 7.21 to 7.56, showing weak alkalinity, exhibiting less rhizosphere than bulk soil, while salt content was higher in the rhizosphere than in the bulk soil. S. salsa was a salt aggregating plant, which can gather salt into its body without damage, thus the rhizosphere soil has higher salt content. Previous studies have found that S. salsa is useful in lowering soil pH, and this was again demonstrated in our study.
Both rhizosphere and bulk soil pH gradually decreased with the increase of the N-P addition ratio. Previous research (Huang et al., 2016; Sha et al., 2021) has found that N and P addition leads to soil acidification and pH decrease, which is consistent with the result of this study. As the N-P input ratio increased, the SOM content increased and then decreased. Previous studies have found that low levels of N and P can enhance SOC storage in the nutrient-limited ecosystem (Pascault et al., 2013; Jian et al., 2016). However higher N-P addition ratio may accelerate SOC mineralization. Li et al. (2022a) found that there is a threshold value of the N-P addition ratio between 15:1 and 45:1, which once exceeded, causes the loss of SOC, which is consistent with our study.
At the CK and N0P treatment, the AP content showed higher in the rhizosphere than in bulk soil. This may be due to the root secretion in the rhizosphere soil, which promoted the P activation and availability (Zhao et al., 2022). This may be caused by plant uptake of P. The addition of N reduces the availability of soil P (Yang et al., 2015). Zhu et al. (2016) found that unbalanced input of N and P could potentially alter nutrient availability and enhance P limitation, which is consistent with our study.
4.2 N-P addition ratio and rhizosphere effect on bacterial community α-diversity
We found that the bacterial community α-diversity was significantly higher in rhizosphere soil than in bulk soil, which is consistent with the previous study. This is due to the presence of the plant root system, some organic compounds are deposited into the soil, providing more carbon and N source for the bacterial community, thus promoting bacterial growth and increasing diversity (Ran et al., 2021). In addition, healthy soil environment promote the growth of bacteria. According to the analysis of soil environmental factors, the higher SOM and TN content of rhizosphere soil can provide sufficient nutrients for bacterial growth.
There was no significant effect of N-P ratio addition on the rhizosphere bacterial community diversity. Li et al. (2019b) studied the effect of N addition on the diversity of rhizosphere soil microbial community in different soil types and found that N addition had minimal effects on bacterial community diversity, which is consistent with our study. Previous studies found that the decrease in bacterial diversity due to N addition was mainly caused by the decrease in soil pH (Liu et al., 2020b). In the bulk soil of this study, although pH also decreased significantly as mentioned in previous studies, bacterial community richness increased and bacterial diversity first increased and then decreased with increasing N-P addition ratio. This may be due to the low or medium N-P addition ratio treatment where the pH did not reach the threshold that limits the α-diversity of the bacterial community, while the lower pH under the high N-P addition ratio treatment limited the bacterial growth. The effect of fertilizer supply on microbial diversity was influenced by changes in soil SOC under both original soil conditions and the fertilizer application time, while variations in soil pH had less effect (Wang et al., 2018a). Yu et al. (2016) used structural equation modeling to propose that soil SOC is generally considered as a key factor affecting soil microorganisms. In our study, we found that N and P addition significantly increased soil SOM and decreased at high N-P addition ratio. The Mantel test again proved that SOM was the most important factor affecting the α-diversity of bulk soil bacteria. The rhizosphere soil SOM was high in S. salsa, so bacterial diversity did not change significantly between treatments.
4.3 N-P addition ratio and rhizosphere effect on bacterial community structure
Soil bacterial community composition is related to soil nature and the interaction between plants and microorganisms (Weand et al., 2010). PCoA analysis and PERMANOVA test based on Bray-Curtis distance showed a large difference in bacterial community composition between rhizosphere and bulk soil, which is consistent with previous studies. Liu (Liu et al., 2020a) studied the rhizosphere and bulk soil bacterial community of S. salsa in two habitats of the YRD and suggested that root secretion and apoplast play a key role in shaping the bacterial community composition.
The N-P addition ratio increase due to increased N input had insignificant effects on rhizosphere bacterial community composition, which is consistent with the previous study (Li et al., 2019b). However, the N-P addition ratio significantly altered the bacterial community composition of bulk soil. In general, the lower β-diversity of the rhizosphere bacterial community indicated that it was more stable than bulk soil (Tian et al., 2022). In terms of the ecological building process, the stochastic process contributed more to the rhizosphere than the bulk soil bacterial community, where the difference in SOM content might be the reason for the difference in nutrient addition to the bacterial community construction process (Tian et al., 2022). Both the SOM initial content and the degree of SOM content variation in response to nutrient addition influenced the relative contribution of different ecological construction processes (Feng et al., 2018). Specifically, when soil SOM content was high, nutrient addition homogenized the bacterial community composition. In contrast, when SOM initial content was low or SOM response increased significantly with nutrient addition, nutrient addition changes bacterial community composition (Feng et al., 2018). In this study, bacterial community composition was not significantly altered due to the high SOM content of rhizosphere soil. In addition, microorganisms and plants evolved synergistically and showed high host specificity (Li et al., 2019b), which may also be a key factor contributing to the insignificant effect of the N-P addition ratio on the structure of the inter-rooted microbial community.
4.4 N-P addition ratio and rhizosphere effect on bacterial community phylum and genus
At the phylum level, the highest Proteobacteria relative abundance was found in both soils, which is consistent with previous studies (Liu et al., 2020a), which indicates that Proteobacteria have excellent adaptability to the environment. Bacteroidetes and Actinobacteria are eutrophic taxa. We predict that these two taxa will have relatively high abundance in rhizosphere soil, while oligotrophic taxa represented by Acidobacteria will have high relative abundance in bulk soil (Kanokratana et al., 2011; Fierer et al., 2012; Redding et al., 2016; Ren et al., 2017; Sha et al., 2021). The rhizosphere soil had high soil nutrients, which stimulated the growth of the Bacteroidetes, while limiting the Actinobacteria, which further supports the bacterial nutrient survival strategy. However, in contrast to Bacteroidetes, Actinobacteria had a lower relative abundance in the rhizosphere soil, which may be due to the difference in salt tolerance between them. In the current study, the Actinobacteria and the Bacteroidetes were significantly negatively and positively correlated with salt, respectively, which is consistent with the previous study (Zhao et al., 2020). Bacteroidetes have better salt tolerance and resistance, while Actinobacteria are less salt tolerant or more susceptible to the negative effect of high salt (Zhao et al., 2020). Despite the high nutrient content in rhizosphere soil, high salinity reduces the Actinobacteria competitiveness to compete for nutrients with Bacteroidetes.
With an increasing N-P addition ratio, the bulk soil Gemmatimonadetes and Acidobacteria relative abundance gradually decreased, while Bacteroidetes relative abundance increased, which further reflected the trophic strategy of bacteria. The elevated N-P addition ratio increased the availability of utilizing C sources in the soil, which promoted and inhibited the development and reproduction of the phylum Bacteroidetes and Acidobacteria, respectively. Gemmatimonadetes have a strong denitrification ability with strong denitrification ability (Guo et al., 2017). However, the present study result is contrary to the previous study. The previous study showed that the abundance of Gemmatimonadetes increased with increasing N application in water-N integrated environmental conditions (Guo et al., 2017), therefore, we speculate that Gemmatimonadetes is more sensitive to soil moisture, while in terrestrial, the trend of Gemmatimonadetes decreases with increasing N-P addition ratio due to insufficient moisture.
At the genus level, Marinobacter, Subgroup_10, bacterium_YC_LK_LKJ36, and Woeseia had high abundance in both soils. Marinobacter, Subgroup_10, and Woeseia have salt-tolerant bacteria, indicating that soil salinity has a strong shaping effect on bacterial genera (Li et al., 2017). The Marinobacter, a common denitrifying bacterium in the Yellow River Delta, is capable of denitrification using nitrate or nitrite as electron acceptors (Chi et al., 2021; Zhou et al., 2021). In this study, the relative abundance of Marinobacter was increased in bulk soil, indicating that the imbalanced input of the N-P ratio enhances bacterial denitrification.
5 Conclusion
The N and P imbalance input caused no change in rhizosphere soil bacterial community composition and α-diversity, but altered the composition of bulk soil bacterial community in S. salsa and increased bulk soil bacterial α-diversity, which resulted from N and P addition that increased nutrient availability to soil bacterial community. Higher SOM content in the rhizosphere soil is the reason why the bacterial community is less affected by the N-P imbalance input. There is a threshold between 15:1 and 45:1 for the N-P addition ratio. This threshold is the optimal ratio for increasing the diversity of bulk soil bacterial community.
Data availability statement
The datasets presented in this study can be found in online repositories. The names of the repository/repositories and accession number(s) can be found below: BioProject, PRJNA925463.
Author contributions
ZZ completed data analysis and wrote the manuscript. JS conceived and designed the study, and fund support. TL and PS were in charge of the modification of the manuscript. JM and KD conducted sample collection. All authors contributed to the article and approved the submitted version.
Funding
The funding was supported by the National Natural Science Foundation of China (42171059, 41971119 and 41871089), the Natural Science Foundation of Shandong Province (ZR2019MD024, ZR2020QD004) and the Science and Technology Support Plan for Youth Innovation of Colleges and Universities (2019KJD010).
Conflict of interest
The authors declare that the research was conducted in the absence of any commercial or financial relationships that could be construed as a potential conflict of interest.
Publisher’s note
All claims expressed in this article are solely those of the authors and do not necessarily represent those of their affiliated organizations, or those of the publisher, the editors and the reviewers. Any product that may be evaluated in this article, or claim that may be made by its manufacturer, is not guaranteed or endorsed by the publisher.
References
Bardgett R. D., van der Putten W. H. (2014). Belowground biodiversity and ecosystem functioning. Nature 515, 505–511. doi: 10.1038/nature13855
Bennett J. A., Maherali H., Reinhart K. O., Lekberg Y., Hart M. M., Klironomos J. (2017). Plant-soil feedbacks and mycorrhizal type influence temperate forest population dynamics. Science 355, 181–184. doi: 10.1126/science.aai8212
Chen Q., Wang Y., Zou C. B., Wang Z. L. (2017). Aboveground biomass invariance masks significant belowground productivity changes in response to salinization and nitrogen loading in reed marshes. Wetlands 37, 985–995. doi: 10.1007/s13157-017-0932-2
Chi Z. F., Zhu Y. H., Li H., Wu H. T., Yan B. X. (2021). Unraveling bacterial community structure and function and their links with natural salinity gradient in the yellow river delta. Sci. Total Environ. 773, 145673. doi: 10.1016/j.scitotenv.2021.145673
Cusack D. F., Silver W. L., Torn M. S., Burton S. D., Firestone M. K. (2011). Changes in microbial community characteristics and soil organic matter with nitrogen additions in two tropical forests. Ecology 92, 621–632. doi: 10.1890/10-0459.1
Deegan L. A., Johnson D. S., Warren R. S., Peterson B. J., Fleeger J. W., Fagherazzi S., et al. (2012). Coastal eutrophication as a driver of salt marsh loss. Nature 490, 388–392. doi: 10.1038/nature11533
Elser J. J., Bracken M. E., Cleland E. E., Gruner D. S., Harpole W. S., Hillebrand H., et al. (2007). Global analysis of nitrogen and phosphorus limitation of primary producers in freshwater, marine and terrestrial ecosystems. Ecol. Lett. 10, 1135–1142. doi: 10.1111/j.1461-0248.2007.01113.x
Feng Y. Z., Chen R. R., Stegen J. C., Guo Z. Y., Zhang J. W., Li Z. P., et al. (2018). Two key features influencing community assembly processes at regional scale: Initial state and degree of change in environmental conditions. Mol. Ecol. 27, 5238–5251. doi: 10.1111/mec.14914
Fierer N., Lauber C. L., Ramirez K. S., Zaneveld J., Bradford M. A., Knight R. (2012). Comparative metagenomic, phylogenetic and physiological analyses of soil microbial communities across nitrogen gradients. ISME J. 6, 1007–1017. doi: 10.1038/ismej.2011.159
Fierer N., Strickland M. S., Liptzin D., Bradford M. A., Cleveland C. C. (2009). Global patterns in belowground communities. Ecol. Lett. 12 (11), 1238–1249. doi: 10.1111/j.1461-0248.2009.01360.x
Freedman Z., Eisenlord S. D., Zak D. R., Xue K., He Z. L., Zhou J. Z. (2013). Towards a molecular understanding of n cycling in northern hardwood forests under future rates of n deposition. Soil Biol. Biochem. 66, 130–138. doi: 10.1016/j.soilbio.2013.07.010
Guo W., Qi X., Li P., Li Z., Zhou Y., Xiao Y. (2017). Impact of reclaimed water irrigation and nitrogen fertilization on soil bacterial community structure. Acta Sci. Circumstantiae 37 (01), 280–287. doi: 10.13671/j.hjkxxb.2016.0189
Huang J. S., Hu B., Qi K. B., Chen W. J., Pang X. Y., Bao W. K., et al. (2016). Effects of phosphorus addition on soil microbial biomass and community composition in a subalpine spruce plantation. Eur. J. Soil Biol. 72, 35–41. doi: 10.1016/j.ejsobi.2015.12.007
Jian S., Li J., Chen J., Wang G., Mayes M. A., Dzantor K. E., et al. (2016). Soil extracellular enzyme activities, soil carbon and nitrogen storage under nitrogen fertilization: A meta-analysis. Soil Biol. Biochem. 101, 32–43. doi: 10.1016/j.soilbio.2016.07.003
Kanokratana P., Uengwetwanit T., Rattanachomsri U., Bunterngsook B., Nimchua T., Tangphatsornruang S., et al. (2011). Insights into the phylogeny and metabolic potential of a primary tropical peat swamp forest microbial community by metagenomic analysis. Microb. Ecol. 61, 518–528. doi: 10.1007/s00248-010-9766-7
Li Z., Guo C., Shi J., Lin K., Cao G., Cui C. (2017). Diversity of bacterial community in suaeda roots rhizosphere growth in PAHs-contaminated saline soil estimated by high throughput sequencing method. Microbiol. China 44, 1602–1612. doi: 10.13344/j.microbiol.china.170164
Li J., Han G., Wang G., Liu X., Zhang Q., Chen Y., et al. (2022a). Imbalanced nitrogen–phosphorus input alters soil organic carbon storage and mineralisation in a salt marsh. Catena 208, 105720. doi: 10.1016/j.catena.2021.105720
Li W., Li Y., Lv J., He X., Wang J., Teng D., et al. (2022b). Rhizosphere effect alters the soil microbiome composition and c, n transformation in an arid ecosystem. Appl. Soil Ecol. 170, 104296. doi: 10.1016/j.apsoil.2021.104296
Li L., Liu. B., Gao X., Li X., Li C. (2019a). Nitrogen and phosphorus addition differentially affect plant ecological stoichiometry in desert grassland. Sci. Rep. 9, 18673. doi: 10.1038/s41598-019-55275-8
Li M. S., Wang T. Y., Li L., Gao Q., Gao Y. H., Liu S. X., et al. (2019b). Effects of long-term nitrogen fertilizer application on rhizosphere microorganisms under different soil types. Polish J. Environ. Stud. 28, 1771–1784. doi: 10.15244/pjoes/89583
Ling N., Chen D., Guo H., Wei J., Bai Y., Shen Q., et al. (2017). Differential responses of soil bacterial communities to long-term n and p inputs in a semi-arid steppe. Geoderma 292, 25–33. doi: 10.1016/j.geoderma.2017.01.013
Liu W., Jiang L., Yang S., Wang Z., Tian R., Peng Z., et al. (2020b). Critical transition of soil bacterial diversity and composition triggered by nitrogen enrichment. Ecology 101(8), e03053. doi: 10.1002/ecy.3053
Liu J., Li A., Sun J., Song A., Xia J. (2021). Foliar c, n, and p stoichiometry of dominant shrubs in the chenier wetland of the yellow river delta, China. Acta Ecol. Sin. 41 (10), 3805–3815. doi: 10.5846/stxb202007091789
Liu F., Mo X., Kong W., Song Y. (2020a). Soil bacterial diversity, structure, and function of suaeda salsa in rhizosphere and non-rhizosphere soils in various habitats in the yellow river delta, China. Sci. Total Environ. 740, 140144. doi: 10.1016/j.scitotenv.2020.140144
Liu X., Wang G., Ran Y., Qi D., Han G., Guan B., et al. (2019). Overall supply level, not the relative supply of nitrogen and phosphorus, affects the plant community composition of a supratidal wetland in the yellow river delta. Sci. Total Environ. 695, 133866. doi: 10.1016/j.scitotenv.2019.133866
Liu J., Wu N. N., Wang H., Sun J. F., Peng B., Jiang P., et al. (2016). Nitrogen addition affects chemical compositions of plant tissues, litter and soil organic matter. Ecology 97, 1796–1806. doi: 10.1890/15-1683.1
Lu G., Xie B., Cagle G. A., Wang X., Han G., Wang X., et al. (2021). Effects of simulated nitrogen deposition on soil microbial community diversity in coastal wetland of the yellow river delta. Sci. Total Environ. 757, 143825. doi: 10.1016/j.scitotenv.2020.143825
Ma S., Chen G., Tang W., Xing A., Chen X., Xiao W., et al. (2021). Inconsistent responses of soil microbial community structure and enzyme activity to nitrogen and phosphorus additions in two tropical forests. Plant Soil 460, 453–468. doi: 10.1007/s11104-020-04805-9
Miki T., Ushio M., Fukui S., Kondoh M. (2010). Functional diversity of microbial decomposers facilitates plant coexistence in a plant-microbe-soil feedback model. PNAS 107, 14251–14256. doi: 10.1073/pnas.0914281107
Pascault N., Ranjard L., Kaisermann A., Bachar D., Christen R., Terrat S., et al. (2013). Stimulation of different functional groups of bacteria by various plant residues as a driver of soil priming effect. Ecosystems 16, 810–822. doi: 10.1007/s10021-013-9650-7
Peng Y. F., Guo D. L., Yang Y. H. (2017). Global patterns of root dynamics under nitrogen enrichment. Global Ecol. Biogeogr. 26, 102–114. doi: 10.1111/geb.12508
Peng Y., Peng Z., Zeng X., Houx J. (2019). Effects of nitrogen-phosphorus imbalance on plant biomass production: a global perspective. Plant Soil 436, 245–252. doi: 10.1007/s11104-018-03927-5
Penuelas J., Poulter B., Sardans J., Ciais P., van der Velde M., Bopp L., et al. (2013). Human-induced nitrogen-phosphorus imbalances alter natural and managed ecosystems across the globe. Nat. Commun. 4, 2934. doi: 10.1038/ncomms3934
Ramirez K. S., Craine J. M., Fierer N. (2010). Nitrogen fertilization inhibits soil microbial respiration regardless of the form of nitrogen applied. Soil Biol. Biochemist. 42, 2336–2338. doi: 10.1016/j.soilbio.2010.08.032
Ran J., Liu X., Hui X., Ma Q., Liu J. (2021). Differentiating bacterial community responses to long-term phosphorus fertilization in wheat bulk and rhizosphere soils on the loess plateau. Appl. Soil Ecol. 166, 104090. doi: 10.1016/j.apsoil.2021.104090
Redding M. R., Shorten P. R., Lewis R., Pratt C., Paungfoo-Lonhienne C., Hill J. (2016). Soil n availability, rather than n deposition, controls indirect N2O emissions. Soil Biol. Biochem. 95, 288–298. doi: 10.1016/j.soilbio.2016.01.002
Ren B., Jun-Tao W., Ye D., Ji-Zheng H., Kai F., Li-Mei Z. (2017). Microbial community and functional structure significantly varied among distinct types of paddy soils but responded differently along gradients of soil depth layers. Front. Microbiol. 8. doi: 10.3389/fmicb.2017.00945
Sha M. H., Xu J., Zheng Z. C., Fa K. Y. (2021). Enhanced atmospheric nitrogen deposition triggered little change in soil microbial diversity and structure in a desert ecosystem. Global Ecol. Conserv. 31, e01879. doi: 10.1016/j.gecco.2021.e01879
Tian D. S., Niu S. L. (2015). A global analysis of soil acidification caused by nitrogen addition. Environ. Res. Lett. 10, 24019. doi: 10.1088/1748-9326/10/2/024019
Tian G., Qiu H., Li D., Wang Y., Zhen B., Li H., et al. (2022). Little environmental adaptation and high stability of bacterial communities in rhizosphere rather than bulk soils in rice fields. Appl. Soil Ecol. 169, 104183. doi: 10.1016/j.apsoil.2021.104183
Wang M., Fan F., Yi K., Xu L., Xu Z., Zhang J., et al. (2022a). Effects of different phosphorus forms on characteristics of bacterial community in rich roots. Soil Fertilizer Sci. China 3, 173–181. doi: 10.11838/sfsc.1673-6257.20721
Wang J., Gao Y., Zhang Y., Yang J., Smith M. D., Knapp A. K., et al. (2019). Asymmetry in above- and belowground productivity responses to n addition in a semi-arid temperate steppe. Glob Chang Biol. 25, 2958–2969. doi: 10.1111/gcb.14719
Wang C., Liu D., Bai E. (2018a). Decreasing soil microbial diversity is associated with decreasing microbial biomass under nitrogen addition. Soil Biol. Biochem. 120, 126–133. doi: 10.1016/j.soilbio.2018.02.003
Wang H., Liu S., Zhang X., Mao Q., Li X., You Y., et al. (2018b). Nitrogen addition reduces soil bacterial richness, while phosphorus addition alters community composition in an old-growth n-rich tropical forest in southern China. Soil Biol. Biochem. 127, 22–30. doi: 10.1016/j.soilbio.2018.08.022
Wang Y., Niu G., Wang R., Rousk K., Li A., Hasi M., et al. (2022b). Enhanced foliar 15N enrichment with increasing nitrogen addition rates: Role of plant species and nitrogen compounds. Glob Chang Biol. 00, 1–25. doi: 10.1111/gcb.16555
Weand M. P., Arthur M. A., Lovett G. M., McCulley R. L., Weathers K. C. (2010). Effects of tree species and n additions on forest floor microbial communities and extracellular enzyme activities. Soil Biol. Biochem. 42, 2161–2173. doi: 10.1016/j.foreco.2020.118491
Widdig M., Heintz-Buschart A., Schleuss P.-M., Guhr A., Borer E. T., Seabloom E. W., et al. (2020). Effects of nitrogen and phosphorus addition on microbial community composition and element cycling in a grassland soil. Soil Biol. Biochem. 151, 108041. doi: 10.1016/j.soilbio.2020.108041
Xia Z., Yang J., Sang C., Wang X., Sun L., Jiang P., et al. (2020). Phosphorus reduces negative effects of nitrogen addition on soil microbial communities and functions. Microorganisms 8 (11), 1828. doi: 10.3390/microorganisms8111828
Yang K., Zhu J. J., Gu J. C., Yu L. Z., Wang Z. Q. (2015). Changes in soil phosphorus fractions after 9 years of continuous nitrogen addition in a larix gmelinii plantation. Ann. For. Sci. 72, 435–442. doi: 10.1007/s13595-014-0444-7
Yu H. L., Gao Q., Shao Z. Q., Ying A. N., Sun Y. Y., Liu J. W., et al. (2016). Decreasing nitrogen fertilizer input had little effect on microbial communities in three types of soils. PloS One 11 (3), e0151622. doi: 10.1371/journal.pone.0151622
Zhang J., Ai Z., Liang C., Wang G., Xue S. (2017). Response of soil microbial communities and nitrogen thresholds of bothriochloa ischaemum to short-term nitrogen addition on the loess plateau. Geoderma 308, 112–119. doi: 10.1016/j.geoderma.2017.08.034
Zhao Y., Li T., Shao P., Sun J., Xu W., Zhang Z. (2022). Variation in bacterial community structure in rhizosphere and bulk soils of different halophytes in the yellow river delta. Front. Ecol. Evol. 9. doi: 10.3389/fevo.2021.816918
Zhao J., Xie H., Zhang J. (2020). Microbial diversity and physicochemical properties of rhizosphere microenvironment in saline-alkali soils of the yellow river delta. Acta Sci. Circumstantiae 41 (03), 1449–1455. doi: 10.13227/j.hjkx.201908044
Zhou Y., Chen Y., Zheng Y., Lin S., Xu X., Wang X., et al. (2021). Effect of china’s typical coastal wetlands conversion into aquaculture pond on the soil bacterial diversity and community structure. Acta Sci. Circumstantiae 41 (06), 2402–2413. doi: 10.13671/j.hjkxxb.2020.0447
Keywords: rhizosphere microorganisms, nitrogen and phosphorus imbalance input, bacterial community diversity, halophytes, Yellow River delta
Citation: Zhang Z, Sun J, Li T, Shao P, Ma J and Dong K (2023) Effects of nitrogen and phosphorus imbalance input on rhizosphere and bulk soil bacterial community of Suaeda salsa in the Yellow River Delta. Front. Mar. Sci. 10:1131713. doi: 10.3389/fmars.2023.1131713
Received: 26 December 2022; Accepted: 31 January 2023;
Published: 09 February 2023.
Edited by:
Zhiyong Li, Shanghai Jiao Tong University, ChinaReviewed by:
Jun-Jian Wang, Southern University of Science and Technology, ChinaChunyu Zhao, Dezhou University, China
Copyright © 2023 Zhang, Sun, Li, Shao, Ma and Dong. This is an open-access article distributed under the terms of the Creative Commons Attribution License (CC BY). The use, distribution or reproduction in other forums is permitted, provided the original author(s) and the copyright owner(s) are credited and that the original publication in this journal is cited, in accordance with accepted academic practice. No use, distribution or reproduction is permitted which does not comply with these terms.
*Correspondence: Jingkuan Sun, c3VuamluZ2t1YW5AMTI2LmNvbQ==