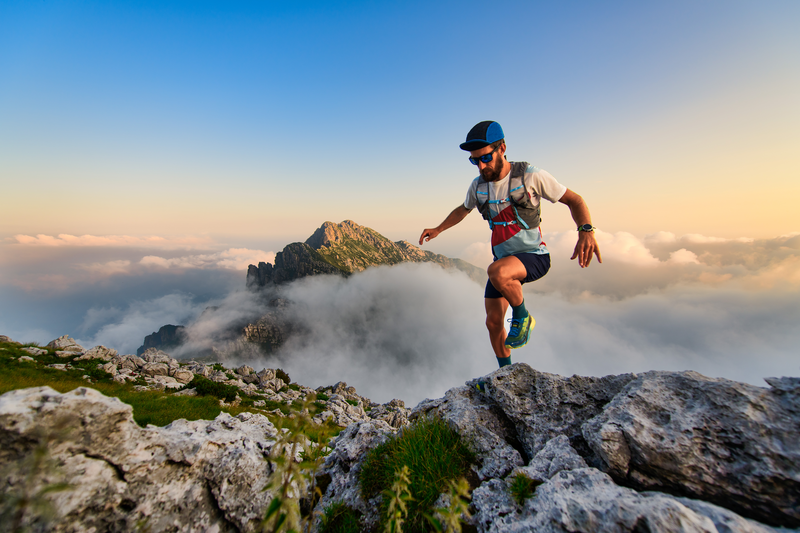
94% of researchers rate our articles as excellent or good
Learn more about the work of our research integrity team to safeguard the quality of each article we publish.
Find out more
ORIGINAL RESEARCH article
Front. Mar. Sci. , 02 March 2023
Sec. Marine Fisheries, Aquaculture and Living Resources
Volume 10 - 2023 | https://doi.org/10.3389/fmars.2023.1131522
This article is part of the Research Topic Offshore Aquacultural Engineering and Its Interaction with the Marine Environment View all 18 articles
A correction has been applied to this article in:
Corrigendum: Study on feeding behavior and biological sound of Sebastes schlegelii
The construction of marine ranches can enrich and conserve the fishery resources and improve the marine ecosystem, which helps realize the sustainable utilization of these resources. Sebastes schlegelii is a major breeding and releasing fish species in the marine ranches of North China. Its behavioral characteristics can be understood better by researching its vocalization, which will provide data support for constructing acoustic taming marine ranches with S. schlegelii as the target fish species. However, there are few studies focusing on its sounds and behaviors. Therefore, based on the passive acoustic monitoring technology, the audios of underwater noises made by S. schlegelii were extracted using an AQH hydrophone. The high-definition internet protocol camera was used to monitor the behavior change of S. schlegelii. Then, by collating and replaying the collected audios and videos, the feeding behavior and biological noises of S. schlegelii were matched to analyze their relationship. Results are as follows: (1) The noise of chewing settling granular baits (Φ5.0 mm) has a main frequency band and sound pressure level of 2000~4500 Hz and 96.53 ± 0.65 dB, respectively; in this feeding process, the main frequency band and sound pressure level of the swimming noise are 25~400 Hz and 95.63 ± 0.38 dB, respectively; the values are 500~700 Hz and 97.34 ± 4.91 dB, respectively, for the noise of flapping the water with the tail. (2) The sound signals emitted by S. schlegelii are mostly presented as single pulses during normal habitation or ingestion of baits on the surface of the water tank. However, S. schlegelii will attack and fight against each other when scrambling for baits, during which these signals are presented as continuous pulses. To sum up, the vocalization of S. schlegelii is closely related to feeding activities, and the sounds produced under different behaviors have specific biological significance.
The classification and identification of economic fish is of great significance to the development and utilization of marine fishery resources. It is a feasible method to identify fish through the characteristics of fish vocal spectrum (Chen et al., 2021). The vocalization of fish usually associates with specific behaviors, so monitoring this vocalization can help identify different behavioral characteristics (Myrberg et al., 1965; Luh and Mok, 1986; Lobel and Kerr, 1999; Bertucci et al., 2010). Fish can make different sounds by various methods, such as the sound of whirlpool or spoondrift produced by swinging fins or tails, the sound of chewing baits, the sound of moving vertebrae, and the sound from the resonance and squeeze of swim bladders, of which the last one is the loudest. There are two types of fish: physostomi and physoclisti. The former refers to the fish whose swim bladders are connected to the esophagus through a pneumatic duct. It is categorized as the lower-grade Osteichthyes, such as carps. The latter refers to the fish without a pneumatic duct. It is categorized as the higher-grade Osteichthyes, such as perches (Fish and Mowbray, 1971; Sprague, 2000a; Sprague et al., 2000b). Vocation is an important part of fish behavior. Different behaviors correspond to different sounds. Fish’s courtship, breeding, feeding, swimming and other behaviors will produce different sounds (Holt and Johnston, 2014; Picciulin et al., 2020; Mackiewicz et al., 2021). In addition, some vocal fish make sounds when a predator is approaching or when it is caught (Ladich, 2022).The characteristics of sound intensity and frequency produced by different behaviors of fish are different (Qu et al., 2021). Thus, much work is needed to adequately characterize vocal species, acoustic features, and behavioral contexts, and to build sound repertoires, which will contribute to researching the soundscape of fish shoals and provide important vocal library data for identifying the distribution of fish populations (Tricas and Boyle, 2014).
The passive acoustic monitoring (PAM) technology is also known as passive sonar. Without a special sound emission system, this technology detects the underwater targets and determines their states and natures by receiving the radiated noise made by themselves, thus locating, tracking, and identifying them. It is a non-intrusive and non-invasive observation method (noiseless input) (Cato et al., 2005) used to evaluate the biodiversity, monitor the habitat environment, and locate the spawning fish (Lindseth and Lobel, 2018). In 2008, Anderson et al. first investigated the vocalization of fish in freshwater areas using this method and found that Oyster Toadfish, Ophidion marginatum, Ameiurus nebulosus, and Ietalurus Punetaus could produce sounds (Anderson et al., 2008). In 2008, Aalbers and Drawbridge first combined the underwater video surveillance with this method, with a finding that the size and gender of Atractoscion nobilis could be distinguished by its vocalization(Aalbers and Drawbridge, 2008).
Many scholars carried out time-domain and frequency analyses on the acoustic spectra of fish and proposed that the acoustic frequency was significantly different in diverse types of fish. The frequency of Anguilli Formes is 350 Hz, and that of Stromateus can reach 2000 Hz (Shen et al., 2014). The main frequency range of Bahaba taipingensis vocalization is 0~1000 Hz The main frequency segment of Sebasticus marmoratus’ vocalization ranges from 68 to 175 Hz. (Zhang et al., 2013).
In marine environments, the energy in the forms of light, heat, or electromagnetic wave will attenuate quickly, but sound signals can be transmitted over long distances, which differs from the situation in air environments on land. For this reason, the PAM technology has become the best method to research fish vocalization by recording and analyzing underwater sound signals. In addition, PAM technology can not only monitor fish, but also be used to locate the spawning grounds of some fish based on the identified sound frequency (Picciulin et al., 2020). However, this technology can only detect the underwater noises in marine environments and the sounds produced by marine species, which merely reflects a part of the sonic characteristics of these species.In some cases, the acoustic spectra of vocal species are unidentified or misidentified (Sprague and Luczkovich, 2001; Mann and Jarvis, 2004; Anderson et al., 2008). Under such circumstances, the soundscape in fish habitats can be monitored by combining passive acoustics and optics to match the collected acoustic spectra with corresponding species and behaviors, thus exploring the relationship between the soundscape and these behaviors. This lays a foundation for researching the fish vocalization and the impact of man-made noise on this soundscape (Tricas and Boyle, 2014).
Korean rockfish (Sebastes schlegelii), belonging to the Sebastes of Scorpaenidae in the Scorpaeniformes (Xu et al., 2018), mainly lives in the northwest Pacific Ocean. It is an important commercial fish species in China, primarily distributed in the Bohai Sea, Yellow Sea, and East China Sea (Feng et al., 2021). In 1974, Hallacher studied the swim bladder muscle of nearly 30 types of fish in the Scorpaeniformes and speculated that the fish of Sebastes with this muscle could produce sounds (Hallacher, 1974). However, the fish of Scorpaenidae are rarely reported in respect of vocalization (Nichols, 2005; Širović and Demer, 2009).
S. schlegelii is a major breeding and releasing fish species in typical marine ranches of North China. There is also vocal behavior in the wild population of S.schlegelii, but there are few studies on using acoustic technology to monitor the wild population of it. This work seeks to analyze the relationship between biological noises and behaviors of S. schlegelii. PAM was used to obtain the acoustic spectrum characteristics of the indoor S.schlegelii, aiming to provide data support for identifying the S.schlegelii population and studying its cluster behavior in the marine ranch demonstration area. At the same time, it provides scientific basis for controlling fish population activities, establishing a modern marine fishery production model of ecological health, environmental friendliness and resource protection, and optimizing and improving the construction of marine ranch with S.schlegelii as the target in the future.
The test fish, S. schlegelii, were purchased from a fish farm in Dalian City, Liaoning Province, China. They were provisionally cultured in a cylinder-shaped canvas water tank (Φ200cm×150cm) for 7 d and fed with settling granular baits (Φ5.0 mm) at 8:00 every day. The water was changed daily by half, before which the residual baits and feces at the bottom of the tank were cleaned using a bottom suction pump. Seven days later, 90 healthy fish with the body length of 22.44 ± 0.90 cm and the weight of 185.13 ± 15.45 g were selected for our experiment and averagely divided into 3 groups. Then, these fish were raised in open cylinder-shaped fiberglass reinforced plastic (FRP) water tanks (Φ100cm×90cm). In each tank, there were 3 air stones (diameter: 43 mm; height: 43 mm; pore size: 8 mm) connected to the aerator (ACO-007; power: 185 W; voltage: 220 V) through plastic hoses. In addition, a water pump (power: 80 W) was connected to each tank through PVC tubes, thus forming a circulating water system. The water temperature was 20.41 ± 0.87 °C; the salinity was 25.85 ± 0.77%.
The high-definition internet protocol camera (Hikvision, China) was used to monitor the behaviors of test fish. The behavioral surveillance videos and underwater noise audios were collected synchronously. Thereinto, videos were collated and saved using the HIK VISION software. By replaying and analyzing these videos and audios, the fish behaviors were matched with biological noises. The monitoring method is that each camera monitors one experimental water tank, and the height and monitoring Angle of each group of cameras are consistent to ensure that the whole water tank picture can be recorded completely. The population dynamics and behavioral characteristics of test fishes were obtained by video playback.
According to the methods proposed by Yin et al. (2017), hydrophones were set at 2 measuring points in each water tank where the water was 70 cm deep in each group of experiments and were connected to a lead weight to make them vertically fixed to two positions at the center of the tank, as shown in Figure 1 (Yin et al., 2017). The two points refer to surface measuring point and bottom measuring point, of which the former is 20 cm away from the surface, located in a water layer for the feeding activities of S. schlegelii, and the latter is 60 cm away from the surface, located in a water layer for the normal habitation of this fish species.
In this experiment, the behaviors and noises were monitored for a total of 15 days, during which audios were recorded at 8:00~8:30 and 16:00~16:30 every day. we collected the circulating water system as well as the feeding and swimming noises of S. schlegelii under the shutdown of this aerator and system. Within the frequency band of 20 Hz~20 kHz, these noises were measured 5 times at each point at an interval of over 10 min, with each time lasting for 120 s. The AQH20k-1062 hydrophone (sensitivity: -193 dB re 1V μPa-1, Aquasound, Japan) was connected to a system-provided professional recorder to collect noise audios, which were saved offline in the file format of Wav. according to the bit rate of 16 bits·s-1 and the sampling frequency of 96 kHz.
Based on the methods suggested by Craven et al. (2009), these audios were replayed and discriminated by iZotope RX 5 Audio Editor to remove the man-made noise (Craven et al., 2009). Then, in combination with the behavioral surveillance videos previously recorded by a high-definition internet protocol camera, the audios were analyzed and processed using the AQ Level Meter1607 to draw frequency spectra. Finally, we carried out the 1/3 octave analysis to study the test fish’s swimming noise, feeding noise, and vocalization.
After measuring the body length and weight of S. schlegelii, the X-ray image was collected. Before shooting, calibrate, preheat and adjust the parameters of the equipment according to the operation manual. According to the method of Guan et al. (2011), use MS-222 to anesthetize Sebastian Hsu. After S. schlegelii enters the deep anesthesia period, place it on the self-made bracket to obtain the high-definition X-ray image of S. schlegelii.
The biological noises produced by S. schlegelii were collected after the aerator and circulating water system were shut down. The noises of chewing settling granular baits and swimming as well as the vocalization data were extracted for spectral characteristic and 1/3 octave analyses, with the frequency bandwidth set at 20~5000 Hz. After the equipment shutdown, the feeding noise made by S. schlegelii has an SPL of about 103.06 dB, which is 13 dB higher than that of the background noise (90.85 dB) in the water tank.
In the whole feeding process (Figures 2, 3), the noise in the water tank shows a primary peak of 80 Hz and 3150 Hz at low and high frequency bands, respectively. The sound detected at the low frequency band of 25~400 Hz is caused by swimming. The sound detected within 1000~2000 Hz is produced by S. schlegelii when it flaps the water with its tail during feeding or stirs the water during ingesting. This fish species can make a “click” sound when chewing baits, which is detected at the frequency band of 2000~4500 Hz.
After feeding, S. schlegelii swims back to the bottom of the water tank. When it swims without vocalization, the noise has an SPL of about 95.29 dB at the low frequency band of 25~400 Hz. It makes a “coo” sound when swimming at the bottom of the tank, and this sound is monitored at the main frequency band of 80~315 Hz, showing an SPL of about 95.23 dB. It can be seen that this SPL is close to that of the swimming noise (95.29 dB) but is approximately 5 dB higher than that of the background noise (90.85 dB). The main biological noises made by S. schlegelii in the water tank are classified in Table 1.
Table 1 Biological noise classification and main acoustic frequency bands of S. schlegelii in the water tank.
When the aerator and circulating water system are turned on, the background noise in the water tank without any test fish shows an SPL of 92.04 dB at the frequency band of 80~100 Hz, an SPL of 95.86 dB at the frequency band of 1000~2500 Hz, and an SPL of 92.09 dB at the frequency band of 2500~4500 Hz. The overall SPL is 7.41 dB higher than that of the feeding noise made by S. schlegelii.
According to the X-ray image and anatomy map of S. schlegelii, its swim bladders are located behind the skull and right below the vertebrae, as shown in Figure 4. The “coo” sound made by it during the experiment presents a sound wave composed of several single pulses and a set of continuous pulses, which, with similar waveforms, have the same frequency band of about 140 Hz (Figure 5A). As shown in Figure 5B, the mean width and average pulse interval of single pulses are 15.83 ± 2.11 ms and 175.63 ± 67.01 ms, respectively; those of continuous pulses are 15.92 ± 2.06 ms and 161.57 ± 22.69 ms, respectively.
Figure 4 (A) X-ray image of S.schlegelii; (B) Schematic diagram of the location of the swim bladder and swimbladder muscle of S.schlegelii.
Figure 5 Vocalization of S. schlegelii [(A) oscillogram and spectrogram of single pulses; (B) oscillogram and spectrogram of continuous pulses].
The behavior change of S. schlegelii was synchronously recorded using a high-definition internet protocol camera during the collection of underwater noises, as shown in Figure 6. We obtained 30 audio clips, in all clips, the swimming noise could be detected. It was found that the feeding and swimming noises produced by S. schlegelii could be detected in 15 clips recorded within 8:00~8:30.
Figure 6 Behavioral surveillance of S. schlegelii in the FRP water tank. [(A) Before bait casting, the test fish moved freely at the bottom of the water tank; (B) During bait casting, the test fish swam to the surface of the water tank to eat baits; (C) After feeding, a single test fish slapped the water with its tail; (D) After feeding, several test fish slapped the water with their tails].
By analyzing the audio data of 120 s collected during the bait casting on the first day and replaying the video data about fish behaviors using a high-definition internet protocol camera at the speed of ×0.5, we obtained 17 clips of chewing baits, and 11 clips of flapping the water with the tail during feeding. The vocalization of S. schlegelii was detected in 31 clips, of which 28 ones presented single pulses and 2 ones presented continuous pulses. The test fish swam back to the bottom of the water tank and moved freely after eating the baits on the water surface, making a single-pulse “coo” sound.
At the time intervals of 88 s~90 s and 105 s~108 s, the test fish scrambled for the baits sunk into the bottom, and larger fish chased and attacked smaller ones (Figure 7). Meanwhile, a “coo” sound with continuous pulses was detected. In combination with the video data, we found that this sound was made by the test fish when they attacked others. In this 15-day experiment, the sound with continuous pulses could be detected in 14 days, except the 9th day, and it emerged when the test fish scrambled for baits at the bottom of the water tank.
Figure 7 Behavior change of S. schlegelii at the bottom of the water tank (▴ for larger fish; • for smaller fish. (A) Larger fish chased smaller fish; (B) Larger fish ingested the baits at the bottom of the tank; (C) Larger fish continued to chase smaller fish; (D) Smaller fish escaped faster, and larger fish stopped chasing and attacking.).
The sounds of chewing baits or flapping the water with the tail were not monitored in all 15 audio clips recorded at 16:00~16:30. We analyzed the audio data of 120 s collected at this time interval on the first day and obtained 15 clips containing the swimming noise and 6 clips containing the single-pulse “coo” sound. In the whole experiment, this sound could be detected at the bottom of the water tank in 13 days, except the 4th, 7th, and 12th days. Thereinto, the sound with continuous pulses was also monitored on the 3rd and 8th days. In combination with the video data, it was found that S. schlegelii may chase and fight against each other in the water tank during daily activities, during which it also made a sound with continuous pulses.
In this experiment, we collected a total of 30 audio clips, of which half recorded the noises during the feeding of S. schlegelii, and the other half recorded the noises during its daily activities. Thereinto, those collected during feeding include: the noises of chewing baits and swimming, the vocalization (with single and continuous pulses), and the noise of flapping the water with the tail; those collected during daily activities include: the swimming noise, and the single-pulse sound.
Within 120 s at the time interval of 8:00~8:30, the noises of chewing baits and swimming occurred 14.06 ± 3.17 and 16.40 ± 2.44 times, respectively; the sounds with single and continuous pulses were detected 20.67 ± 5.04 and 2.53 ± 1.31 times, respectively; the noise of flapping the water with the tail occurred 11.47 ± 2.65 times. Within 120 s at the time interval of 16:00~16:30, the swimming noise was monitored 11.60 ± 2.47 times; the single-pulse sound was detected 4.20 ± 2.66 times, as shown in Figure 8. These observations showed that S. schlegelii was more active between 8:00 and 8:30, during which the swimming noise and its vocalization occurred more frequently than those at the time interval of 16:00~16:30. The noise of flapping the water with the tail was not monitored within 16:00~16:30, because the test fish all moved freely at the bottom of the water tank when no baits were cast.
Under the equipment shutdown, the low-frequency noise (<1 kHz) is mainly caused by ground vibration and water flow (Bart et al., 2001), which may mute the feeding and swimming noises at low frequencies (Takemura, 1988; Fujieda et al., 1993). S. schlegelii eats fast when baits are cast into the water tank, with the following manifestations: it swims faster to ingest baits, producing the sounds caused by the splashing of water and the swinging of tail. This feeding mode indicates that the test fish are vivacious (Phillips, 1989). The feeding noise is primarily influenced by the properties of baits: the peak frequency of noise made by chewing varies with the hardness of baits.Furthermore, the duration of feeding is determined by the amount and particle distribution of baits, which refer to the settling granular baits in this experiment. During bait casting, the test fish swim faster to the surface of the water tank for feeding. The “click” sound produced by S. schlegelii during chewing and swallowing is detected at the main frequency band of 2~4.5 kHz. By contrast, the main frequency band of the feeding noise made by Oncorhynchus mykiss and Scophthalmus maximus is measured to be 4~6 kHz when they swim faster to the surface of the water tank to eat granular baits (Lagardere et al., 2004), which is similar to that of the feeding noise made by S. schlegelii within 6 kHz.
However, Scophthalmus maximus has a special feeding frequency of 7~9 kHz, which is attributed to its exclusive suction feeding mode (Lagardere et al., 2004). This mode is also observed in Cyprinus carpio, with the maximum acoustic energy of 7~9 kHz (Takemura, 1988). However, S. schlegelii does not eat in this mode. The bony fish have the most diverse vocalization mechanisms in all vertebrates (Ladich and Fine, 2006). In 1974, Hallacher studied nearly 30 types of fish in the Scorpaeniformes and speculated that the fish of Sebastes with a swim bladder muscle could produce sounds (Hallacher, 1974). This hypothesis is supported by the observations of Zhang et al. (2013) made during the study on morphological structures of the acoustic system and acoustic features of Sebasticus marmoratus(Zhang et al., 2013). The “glouglou” sound with continuous pulses produced by this fish species is monitored at the main frequency band of 68~175 Hz. Based on the morphological and behavioral analyses, it is deduced that Sebasticus marmoratus makes a sound by contracting its swim bladder muscle.
In this study, S. schlegelii can produce a “coo” sound with continuous pulses, and the primary peak of this sound at the low frequency band is basically consistent with that of Sebasticus marmoratus. The reason may be that both S. schlegelii and Sebasticus marmoratus belong to the Scorpaenidae, with similar swim bladders and vocal muscles. The sounds produced by them are caused by the vibration of swim bladders and retractor muscles. However, the fish in different families and genera have distinct acoustic frequencies, and there are also certain differences in their vocalization mechanisms. For example, the pharyngeal jaw apparatus also involves in sound production in the Cichlidae and Pomacentridae (Rice and Lobel, 2003). The Doradidae, Pimelodidae, Mochokidae, and Ariidae have two kinds of sound-production mechanisms (Ladich and Fine, 2006). They can make a stridulation through pectoral spines and produce a low-frequency sound by vibrating swim bladders (Fine and Ladich, 2003).
The Sciaenidae can also make a sound, with the main frequency band of 300~800 Hz (Chen et al., 2021). The main frequency bands of sounds produced by Nibea albiflora and cultured Larimichthys crocea are 300~800 Hz and 550~800 Hz, respectively (Ren et al., 2016). In addition to the difference in vocalization mechanisms, the vocalization modes also vary with different fish species or with different behaviors of the same species (Akamatsu et al., 2002; Zhang et al., 2013; Lindseth and Lobel, 2018; Chen et al., 2021). For instance, the longsnout seahorse can produce two types of “click” sounds (50~800 Hz) during feeding and courting. Zhang et al. (2013) believed that the sounds with single and continuous pulses made by Sebasticus marmoratus in a territorial invasion test could reflect its different states in the face of invasion (Zhang et al., 2013).
Our study shows that S. schlegelii can produce sounds both with single and continuous pulses, which are speculated to be made under different behaviors. By matching the video and audio data, we obtained that the two kinds of sounds were produced during the normal feeding and the scrambling for baits, which verified the above conjecture. The sound signals emitted by S. schlegelii are mostly presented as single pulses during normal habitation and ingestion but presented as continuous pulses during attacking. Fish can produce sounds when they are interfered or threatened. In the same species, fish usually vocalize during attacking and fighting, but rarely make a sound during defending and escaping (Ladich, 1997). This phenomenon is also verified in this study.
The PAM technology can be used to monitor the underwater soundscape and collect the vocalization of underwater organisms in indoor and outdoor environments, including fish, marine mammals, crustaceans, etc (Kikuchi et al., 2015; Putland et al., 2018; Emmons et al., 2021; Muñoz-Duque et al., 2021). Aalbers and Drawbridge (2008) proposed that this technology could be combined with behavioral surveillance to make up for its deficiency of non-visualization (Aalbers and Drawbridge, 2008). The combination was performed in this study to research the feeding behavior and biological noises of S. schlegelii by matching sounds with behaviors, which solved the problem of non-visualization during acoustic monitoring. However, these sounds and behaviors were only monitored during feeding. Therefore, the behavioral surveillance shall be combined with PAM technology in future studies to explore the acoustic features of S. schlegelii in the face of territorial invasion. Meanwhile, the vocalization mechanisms of this fish species shall be further explored using morphological and behavioral methods, thus providing better data support for the research on the sounds and behaviors of S. schlegelii.
In summary, taking S. schlegelii as an example, analyzing the relationship between its biological noise information and its behavior can provide scientific basis for controlling the action of fish stocks in the future, creating a modern marine fishery production mode of ecological health, environmental friendliness and resource conservation, and optimizing and improving the construction of marine pasture with S. schlegelii as the target fish species.
The original contributions presented in the study are included in the article/supplementary material. Further inquiries can be directed to the corresponding authors.
The animal study was reviewed and approved by Dalian Ocean University. Written informed consent was obtained from the owners for the participation of their animals in this study.
MS and DG conceived and designed the experiment. BX and LY conducted the experiment. XC, XY and PX are involved in data analysis. MS and DG wrote the manuscript. MS and DG contributed equally to this work and share first authorship. All authors contributed to the article and approved the submitted version.
This research was supported by National Natural Science Foundation of China (No. 42030408) and the Science and Technology Innovation Fund of Dalian, China (No. 2021JJ11CG001).
We would like to thank the Fish Behavior Laboratory of Dalian Ocean University and the Center for Marine Ranching Engineering Science Research for providing experimental sites and technical support for this study. Thank all the authors for their valuable time. Without the hard work of all the authors, we could not complete this study.
The authors declare that the research was conducted in the absence of any commercial or financial relationships that could be construed as a potential conflict of interest.
The reviewer YL declared a shared parent affiliation with the authors to the handling editor at the time of review.
All claims expressed in this article are solely those of the authors and do not necessarily represent those of their affiliated organizations, or those of the publisher, the editors and the reviewers. Any product that may be evaluated in this article, or claim that may be made by its manufacturer, is not guaranteed or endorsed by the publisher.
Aalbers S. A., Drawbridge M. A. (2008). White seabass spawning behavior and sound production. Trans. Am. Fish. Soc. 137 (2), 542–550. doi: 10.1577/T04-058.1
Akamatsu T., Okumura T., Novarini N., Yan H. Y. (2002). Empirical refinements applicable to the recording of fish sounds in small tanks. J. Acoustical Soc. America 112 (6), 3073–3082. doi: 10.1121/1.1515799
Anderson K. A., Rountree R. A., Juanes F. (2008). Soniferous fishes in the Hudson river. Trans. Am. Fish. Soc. 137 (2), 616–626. doi: 10.1577/T05-220.1
Bart A. N., Clark J., Young J., Zohar Y. (2001). Underwater ambient noise measurements in aquaculture systems: A survey. Aquacult. Eng. 25 (2), 99–110. doi: 10.1016/S0144-8609(01)00074-7
Bertucci F., Beauchaud M., Attia J., Mathevon N. (2010). Sounds modulate males’ aggressiveness in a cichlid fish. Ethology 116 (12), 1179–1188. doi: 10.1111/j.1439-0310.2010.01841.x
Cato D. H., Noad M. J., McCauley R. D. (2005). Passive acoustics as a key to the study of marine animals (New York: Cambridge University Press).
Chen D. H., Lin J. H., Yi X. J., Jiang P. F. (2021). Research on band energy extraction and classification of three kinds of fishes sound signals. Tech. Acoustics. 40 (2), 254–259. doi: 10.16300/j.cnki.1000-3630.2021.02.017
Craven A., Carton A. G., McPherson C. R., McPherson G. (2009). Determining and quantifying components of an aquaculture soundscape. Aquacult. Eng. 41 (3), 158–165. doi: 10.1016/j.aquaeng.2009.07.003
Emmons C. K., Hanson M. B., Lammers M. O. (2021). Passive acoustic monitoring reveals spatiotemporal segregation of two fish-eating killer whale orcinus orca populations in proposed critical habitat. Endangered Species Res. 44, 253–261. doi: 10.3354/esr01099
Feng A. X., Wang F., Sun C. F., Zhang Y. G., Liu Y. X. (2021). Artificial breeding techniques and breeding and releasing methods of sebastes schlegelii. Sci. Fish. Farming 04, 64–65. doi: 10.14184/j.cnki.issn1004-843x.2021.04.039
Fine M. L., Ladich F. (2003). Sound production, spine locking and related adaptations. Catfishes 1, 249–290. doi: 10.1007/978-94-007-1060-3_10
Fish M. P., Mowbray W. H. (1971). Sounds of western north Atlantic fishes. Copeia 1, 190–190. doi: 10.2307/1441636
Fujieda S., Matsuno Y., Yamanaka Y., Chung Y. J., Kishimoto C. (1993). Feature of the swimming sound for fishes in the netting cages at the culture ground (Memoirs of Faculty of Fisheries Kagoshima University).
Guan S. G., Guan J., Liu H. J., Zheng Y. Y., Liu M. X., Gao X., et al. (2011). Anesthetic effect of MS-222 on juveniles of sebastes schlegeli. Mar. Sci. 35 (05), 100–105.
Hallacher L. E. (1974). The comparative morphology of extrinsic gasbladder musculature in the scorpionfish genus sebastes (Pisces: Scorpaenidae). Proc. California Acad. Sci. 4th Ser. 40, 59–86.
Holt D., Johnston C. (2014). Sound production and associated behaviours in blacktail shiner cyprinella venusta: A comparison between field and lab. Environ. Biol. Fishes 97, 1207–1219. doi: 10.1007/s10641-013-0208-5
Kikuchi M., Akamatsu T., Takase T. (2015). Passive acoustic monitoring of Japanese spiny lobster stridulating sounds. Fish. Sci. 81 (2), 229–234. doi: 10.1007/s12562-014-0835-6
Ladich F. (1997). Agonistic behaviour and significance of sounds in vocalizing fish. Mar. Freshw. Behav. Phy. 29 (1-4), 87–108. doi: 10.1080/10236249709379002
Ladich F. (2022). Shut up or shout loudly: Predation threat and sound production in fishes. Fish. Fish. 23 (1), 227–238. doi: 10.1111/faf.12612
Ladich F., Fine M. L. (2006). Sound-generating mechanisms in fishes: a unique diversity in vertebrates. Commun. Fishes 1, 3–43.
Lagardere J. P., Mallekh R., Mariani A. (2004). Acoustic characteristics of two feeding modes used by brown trout (Salmo trutta), rainbow trout (Oncorhynchus mykiss) and turbot (Scophthalmus maximus). Aquaculture 240 (1-4), 607–616. doi: 10.1016/j.aquaculture.2004.01.033
Lindseth A. V., Lobel P. S. (2018). Underwater soundscape monitoring and fish bioacoustics: A review. Fishes 3 (3), 36. doi: 10.3390/fishes3030036
Lobel P. S., Kerr L. M. (1999). Courtship sounds of the pacific damselfish, abudefduf sordidus (Pomacentridae). Biol. Bull. 197 (2), 242–244. doi: 10.2307/1542627
Luh H. K., Mok H. K. (1986). Sound production in the domino damselfish, dascyllus trimaculatus (Pomacentridae) under laboratory conditions. Japanese J. Ichthyol. 33 (1), 70–74. doi: 10.11369/jji1950.33.70
Mackiewicz A., Putland R., Mensinger A. (2021). Effects of vessel sound on oyster toadfish opsanus tau calling behavior. Mar. Ecol. Prog. Ser. 662, 115–124. doi: 10.3354/meps13634
Mann D. A., Jarvis S. M. (2004). Potential sound production by a deep-sea fish. J. Acoustical Soc. America 115 (5), 2331–2333. doi: 10.1121/1.1694992
Muñoz-Duque S., López-Casas S., Rivera-Gutiérrez H., Jiménez-Segura L. (2021). Bioacoustic characterization of mating calls of a freshwater fish (Prochilodus magdalenae) for passive acoustic monitoring. Biota Colombiana 22 (1), 108–121. doi: 10.21068/c2021.v22n01a07
Myrberg A. A. Jr., Kramer E., Heinecke P. (1965). Sound production by cichlid fishes. Science 149 (3683), 555–558. doi: 10.1126/science.149.3683.555
Nichols B. (2005). Characterizing sound production in nearshore rockfishes (Sebastes spp.) (USF Tampa Graduate Theses and Dissertations).
Phillips M. J. (1989). The feeding sounds of rainbow trout, salmo gairdneri Richardson. J. Fish. Biol. 35 (4), 589–592. doi: 10.1111/j.1095-8649.1989.tb03008.x
Picciulin M., Fiorin R., Facca C., Malavasi S. (2020). Sound features and vocal rhythms as a proxy for locating the spawning ground of sciaena umbra in the wild. Aquat. Conservation: Mar. Freshw. Ecosyst. 30 (7), 1299–1312. doi: 10.1002/aqc.3340
Putland R. L., Mackiewicz A. G., Mensinger A. F. (2018). Localizing individual soniferous fish using passive acoustic monitoring. Ecol. Inf. 48, 60–68. doi: 10.1016/j.ecoinf.2018.08.004
Qu R., Liu H., Liu J. W., Zhang Y. L. (2021). Acoustic signal characteristics of largemouth bass in feeding process and the effects of breeding. Fishery Modernization. 48 (6), 55–63. doi: 10.3969/j.issn.1007-9580.2021.06.008
Ren X. M., Gao D. Z., Yao Y. L., Yang F., Liu J. F., Xie F. J. (2016). Occurrence and characteristic of sound in large yellow croaker ( pseudosciaena crocea ). J. Dalian Ocean Univ. 22 (2), 123–128. doi: 10.16535/j.cnki.dlhyxb.2007.02.009
Rice A. N., Lobel P. S. (2003). The pharyngeal jaw apparatus of the cichlidae and pomacentridae: Function in feeding and sound production. Rev. Fish. Biol. Fish. 13 (4), 433–444. doi: 10.1007/s11160-004-8794-0
Shen W. X., Hu Q. S., Li C. X., Shentu J. K. (2014). Fish voice spectrum analysis and research. BioTechnol.: Indian J. 10 (23).
Širović A., Demer D. A. (2009). Sounds of captive rockfishes. Copeia 2009 (3), 502–509. doi: 10.1643/CP-08-121
Sprague M. W. (2000a). The single sonic muscle twitch model for the sound-production mechanism in the weakfish, cynoscion regalis. J. Acoustical Soc. America 108 (5), 2430–2437. doi: 10.1121/1.1315296
Sprague M. W., Luczkovich J. J. (2001). Do striped cusk-eels ophidion marginatum (Ophidiidae) produce the “chatter” sound attributed to weakfish cynoscion regalis (Sciaenidae)? Copeia 2001 (3), 854–859. doi: 10.1643/0045-8511(2001)001[0854:dsceom]2.0.co;2
Sprague M. W., Luczkovich J. J., Pullinger R. C., Johnson S. E., Jenkins T., DANIEL H. J. III (2000b). Using spectral analysis to identify drumming sounds of some north Carolina fishes in the family sciaenidae. J. Elisha Mitchell Sci. Soc. 116(2), 124–145.
Takemura A. (1988). The attraction effect of natural feeding sound in fish. Bull. Fac. Fish. Nagasaki Univ. 63, 1–4.
Tricas T. C., Boyle K. S. (2014). Acoustic behaviors in Hawaiian coral reef fish communities. Mar. Ecol. Prog. Ser. 511, 1–16. doi: 10.3354/meps10930
Xu G. C., Li J. J., Li X. S., Wang X. Y., Wang H. B. (2018). The offshore cage culture technology of sebastes schlegelii. J. Aquacult. 39 (6), 23–26. doi: 10.3969/j.issn.1004-2091.2018.06.010
Yin L. M., Chen X. Z., Zhang X. G., Li L. Z., Huang H. L. (2017). The morphology of sonic system and sound characteristics of the marbled rockfish sebasticus marmoratus. Mar. Fish. 39 (3), 314–321. doi: 10.13233/j.cnki.mar.fish.2017.03.009
Keywords: Sebastes schlegelii, biological sound, behavior, marine ranch, passive acoustics
Citation: Song M, Gong D, Cui X, Yu X, Xu P, Xing B and Yin L (2023) Study on feeding behavior and biological sound of Sebastes schlegelii. Front. Mar. Sci. 10:1131522. doi: 10.3389/fmars.2023.1131522
Received: 25 December 2022; Accepted: 20 February 2023;
Published: 02 March 2023.
Edited by:
Fukun Gui, Zhejiang Ocean University, ChinaReviewed by:
Yong Tang, Shanghai Ocean University, ChinaCopyright © 2023 Song, Gong, Cui, Yu, Xu, Xing and Yin. This is an open-access article distributed under the terms of the Creative Commons Attribution License (CC BY). The use, distribution or reproduction in other forums is permitted, provided the original author(s) and the copyright owner(s) are credited and that the original publication in this journal is cited, in accordance with accepted academic practice. No use, distribution or reproduction is permitted which does not comply with these terms.
*Correspondence: Binbin Xing, xingbinbin@dlou.edu.cn; Leiming Yin, yinleiming@dlou.edu.cn
†These authors share first authorship
Disclaimer: All claims expressed in this article are solely those of the authors and do not necessarily represent those of their affiliated organizations, or those of the publisher, the editors and the reviewers. Any product that may be evaluated in this article or claim that may be made by its manufacturer is not guaranteed or endorsed by the publisher.
Research integrity at Frontiers
Learn more about the work of our research integrity team to safeguard the quality of each article we publish.