- 1Key Laboratory of Sustainable Development of Marine Fisheries, Ministry of Agriculture; Yellow Sea Fisheries Research Institute, Chinese Academy of Fishery Sciences, Qingdao, China
- 2Key Laboratory of Fish Conservation and Utilization in the Upper Research of the Yangtze River Sichuan Province, Neijiang Normal University, Neijiang, China
- 3Technology Research and Development Center, Dalian Tianzheng Industry Co., Ltd., Dalian, China
Estrogen exposure during early-life stages has been found to delay ovarian development in female fish, even after a long-term depuration period. However, the mechanisms underlying the disordered ovarian development remain unclear. In this study, the larvae of tiger puffer Takifugu rubripes were exposed to 0 (control) and 10 μg/L 17β-estradiol (E2) from 20 to 90 days post-hatch (dph) and maintained in clear seawater until 180 dph. Genetic females collected at 90 and 180 dph were identified by analyzing a sex-associated SNP. Then, the ovarian structure, gonadosomatic index (GSI), the maximum follicle area and the mRNA levels of genes involving in cell cycle (ckd2, ckd4, cdk6, ccna2, ccnd2, cdkn1b and cdkn2c), meiosis initiation (sycp3, rec8, spo11, and dmc1), follicle formatiaon (bmp2, hnrnpk, hmp15, gdf9, nobox and figla) and apoptosis (bax and bcl-2) were analyzed between control and E2-exposed females. The results show that, no structure difference in ovaries was observed between control and E2-treated females at 90 dph, but the primary growth follicles in E2-treated females were observed to be fewer in number than control at 180 dph. Both ovarian weight and GSI of E2-treated females were significant lower than the control at 90 and 180 dph, while there was no significant different in the maximum follicle area between control and E2-treated females at neither 90 or 180 dph. Additionally, the E2 exposure suppressed the expression of sycp3, rec8, spo11, dmc1, bmp2, hnrnpk and bcl-2 at 90 dph, but the mRNA levels of those genes in E2-treated females showed no significant different with the control at 180 dph. The reduced mRNA levels of sycp3, rec8, spo11 and dmc1 might result in disrupted meiosis, and suppression the expression of bmp2 and hnrnpk affected follicle formation. Then, abnormal meiosis initiation and follicle formation might further promote apoptosis as indicated by the decrease in mRNA levels of bcl-2, which ultimately contributed to less number of follicles and low GSI value in E2-treated females.
Introduction
Tiger puffer Takifugu rubripes is widely grown in East Asia and is regarded as one of the most valuable teraodontiformes fish due to its superb flavor and abundance of nutrients (Kikuchi et al., 2011). Because tiger puffer testes are considered a delicacy, males have a higher commercial value than females (Yoshikawa et al., 2020). Thereby, artificial breeding strategy based on all-male populations is considered as a possible solution to improve economic benefits of tiger puffer industry. The first step in producing all-male tiger puffer is to convert genetic males into physiological females by immersion using high concentration of 17β-estradiol (E2) during gonadal differentiation, thus obtaining pseudo-female individuals (Piferrer, 2001). However, because it is unable to discriminate the sex of fish throughout the hormone induction phase, the genetic female cannot escape being exposed to exogenous estrogen, resulting in the suppression of its ovaries in the later development process (Hu et al., 2017).
Oogenesis in teleost fish is divided in several successive steps: oogonial proliferation (OP), primary growth (PG), secondary growth (SG), maturation (MA) and ovulation (OV) (Luckenbach et al., 2008; García-López et al., 2011a). It is a common phenomenon that oogenesis in fish is disrupted by exogenous estrogens, but the majority studies focused on the adverse effects of exogenous estrogens on the differentiated ovaries, in which oogenesis entered in SG or MA stages (Colli-Dula et al., 2014; Zhang et al., 2014a; Wang et al., 2017). Hypothalamic-pituitary- ovarian-liver (HPGL) axis plays a major role in the regulation of SG and MA. Numerous studies indicated that exogenous estrogens blocked the expression of genes on HPGL axis, which was one of the prime causes of abnormal ovarian development, such as arrested follicle growth and decreased number of follicles (Qin et al., 2014; Lei et al., 2016; Wang et al., 2017). Different with previous studies, the E2 exposure on the tiger puffer occurred on differentiating ovaries, when oogenesis was only at the OP and PG phases (Hu et al., 2017). However, some studies suggested that HPGL axis might not participate in the two processes (Lubzens et al., 2010; Zhang et al., 2015; Tang et al., 2016).
At OP and PG stages, oogonia proliferate mitoticly and subsequently meiosis initiation transforms oogonia into primary oocytes that are surrounded by mitotic somatic cells in the follicles (Nakamura et al., 2010; Tanaka, 2014). Studies have shown that estrogen exposure affected those processes. For example, treatment with BPA (bisphenol A) resulted in mitotic arrest of ovarian granulosa cells in mice (Xu et al., 2002). The expression of genes involving in meiosis initiation was disrupted in black porgy Acanthopagrus schlegelii exposed to E2 and yellow catfish exposed to DES (Lau et al., 2013; Wang et al., 2017). In rare minnow, BPA exposure suppressed the expression of bmp15 and gdf9, which are known as the most important genes required for follicle formation (Zhang et al., 2014b). Additionally, studies in yellow catfish Pelteobagrus fulvidraco exposed to DES and zebrafish exposed to EE2 also suggested that exogenous estrogen exposures leaded to increased apoptotic follicles, and the expression of genes involving in apoptosis pathways were altered (Luzio et al., 2016; Wang et al., 2017). Based on these findings, we proposed a hypothesis that E2 exposure throughout the OP and PG phases would influence the cellular processes of oogonia proliferation, meiosis initiation, follicle formation, or apoptosis, eventually leading to permanent ovarian development retardation in the tiger puffer.
The ovarian differentiation of tiger puffer occurred at 40 days past hatching (dph), and oocytes were observed at 82 dph (Hu et al., 2015). To test the above hypothesis, tiger puffer were exposed to 10 g/L E2 from 20 to 90 dph for 2 hours each day according to the method in the previous study (Hu et al., 2017), and then maintained in clear seawater till 180 dph. The changes of ovarian structure and morphometric parameters were examined. Subsequently, the expression of genes, which have been proved to involve in cell cycles (cdk2, cdk4, cdk6, ccna2, ccnd2, ccne2, cdkn1b, cdkn2c) (Reddy, 1994; Craig et al., 2013), meiosis initiation (sycp3, rec8, spo11, dmc1) (Romanienko and Camerini-Otero, 2000; Iwai et al., 2006; Lau et al., 2013; Cahoon and Hawley, 2016), follicle formatiaon (bmp2, hnrnpk, bmp15, gdf9, figla, nobox) (Soyal et al., 2000; Otsuka et al., 2011; Wang et al., 2011; Bouilly et al., 2014; Chakraborty and Roy, 2015), and follicle apoptosis (bax, bcl-2) (Luzio et al., 2016), were investigated. The aim of this study is to extend our understanding on the molecular mechanisms underlying adverse effects of estrogen immersion during gonadal differentiation on ovarian development of fish.
Materials and methods
Larvae and juveniles rearing
This study was performed at a commercial hatchery of Dalian Tianzheng Industry Co., Ltd in Tangshan City, China. Eggs of tiger puffer were obtained by artificial fertilization and the eggs hatched 8 to 10 days after fertilization. Newly hatched larvae were kept in a larvae rearing tank. The larvae were supplied with live rotifers from 4 to 20 dph, and Artemia nauplius from 20 to 40 dph. From 40 dph, metamorphosed juveniles were fed with commercial pellets (Marine Yu Bao, Hayashikane Sangyo Co., Ltd., Japan) (Rashid et al., 2007). Water temperature was maintained at 18-21°C during the rearing period. At 20 dph, about 4000 larvae were randomly assigned to two 5-m3 aquaria containing ~2000 larvae each.
17β-estradiol immersion
The experiment consisted of one control group and one treatment group. From 20 to 90 dph, juveniles in the treatment aquaria were exposed to 10 μg/L E2 for 2h once every day. Stock solutions were prepared by dissolving 0.5g E2 in 1000 mL absolute ethanol to give a concentration of 0.5 mg/mL E2, and stored in the dark at -4°C. Prior to treatment, the water volume in the aquarium of each treatment group was reduced to 0.5 m3 and 10 mL of the E2 stock solutions was added to each aquarium to give final concentration of 10 μg/L E2. The water volume in the control aquarium was also reduced to 0.5 m3 and treated with 10 mL absolute ethanol only. After 2-h treatment, the water volume in each aquarium was increased to 5 m3 and flowing water was restored.
Sample collection
After E2 immersion, a total of 30 juveniles were collected from each group at 90 and 180 dph, respectively. All fish procedures were conducted according to the guidelines established by the Institutional Animal Care and Use Committee at Yellow Sea Fisheries Research Institute, Chinese Academy of Fishery Science. Fish were first anesthetized with 100 mg/L tricaine methanesulfonate (MS-222, Sigma, St. Louis, Mo). Then, the body weight and gonad weight of each sampled juvenile were recorded to calculate GSI [GSI = (gonad weight/body weight) × 100]. One of the two gonads from each juvenile were fixed in Davidson’s for at least 24h, washed in 50% ethanol, and then stored in 70% ethanol at 4°C until histological analysis. The other gonad were stored at -80°C for RNA extraction. In addition, muscle tissue from each fish was also sampled and stored at -20°C for DNA extraction.
Genetic sex identification
Among the samples, genetic females were selected according to the method in our previous study (Hu et al., 2017). Total DNA from muscle was extracted using a Marine Animals DNA kit (TLANamp, Beijing, China) following the manufacturer’s instructions. DNA quality and quantity were assessed by 1% agarose gel electrophoresis and by UV spectrophotometry (1.8<OD260/280 <2.0), respectively. The sense (F) 5′- TAGACACGATGCACACAAACCAC -3′ and antisense (R) 5′- CGCAAAATGAG GCTCTC TATGGAG -3′ primers for the SNP marker using to identify genetic sex of tiger puffer were designed with Primer Premier 5.0 software (Prenier Biosoft, Palo Alto, CA, USA). The reaction conditions of PCR were 5min at 95°C, followed by 35 cycles of 1min at 94°C, 40 s at 58°C and 50 s at 72°C, with a final extension at 72°C for 5min. The products (625 bp) of PCR were then sequenced by Sangon Biotech Co., Ltd. (Shanghai, China). In the resulted sequence traces, the genetic female (XX) juveniles were homozygous (C/C) in the SNP position (334 bp). 14 genetic females and 15 genetic females were selected from the control group and the treatment group, respectively.
Gonadal histological analysis
Then the ovarian phenotypes of those females were identified for histological analysis. Ovaries were dehydrated in a series of alcohol, clarified in dimethylbenzene, and then embedded in paraffin. Cross-sections were cut at 5-7 μm with a microtome (Leica RM2235, Nussloch, Germany), stained with hematoxylin and eosin, and observed and photographed using a light microscope (Olympus DP72, Tokyo, Japan). The maximum follicle area was measured by the microscope.
Gene expression analysis
Total RNA from ovaries of females was extracted using MiniBEST Universal RNA Extraction kit (Takara, Dalian, China). RNA quality and quantity were assessed by 1% agarose gel electrophoresis (28S:18S>1) and UV spectrophotometry (1.8<OD260/280<2.0), respectively. To avoid contamination of genomic DNA, total RNA was treated with DNase I (Qiagen) for 30min at 37°C. The first-stranded cDNA was synthesized using PrimeScript™ RT reagent kit (Takara, Dalian, China) with 1 μg total RNA according to the manufacturer’s instruction. The cDNA was stored at -20°C.
Relative expression of the candidate genes (Table 1) were determined by quantitative real-time PCR (qRT-PCR) in the ovaries of females from control and treatment groups. The qRT-PCR was performed on an ABI StepOnePluse Sequence Detection System (Applied Biosystems, USA) in accordance with the manufacturer’s instructions. SYBR Premix Ex Taq™ kit (Takara Bio, China) was used for amplification, and the reaction mixture contained 10 μL of SYBR® Premix Ex Taq™, 0.8 μL of each primer (10 μM), 0.4 μL of ROX Dye (50×), 2 μL of cDNA sample (25 ng/μL), and 6 μL of sterile distilled water. Initial denaturation was conducted at 95°C for 10 s, followed by 40 cycles at 95°C for 5 s and at 60°C for 30 s. A dissociation protocol was carried out after thermocycling to determine the target specificity. The stability of six commonly used reference genes 18s, actb, ef1a, ctsd, gapdh, and rpl17 was evaluated using Bestkeeper (version 3.5) and Normfinder (v 0.953) (Andersen et al., 2004; Pfaffl et al., 2004). Evaluation revealed that rpl17 is the most stable reference gene in this study (data not shown). Hence, we selected rpl17 as the internal control, and the relative abundance of the target mRNA was normalized to rpl17 by using the 2-ΔΔCT method. All samples were amplified in triplicates.
Statistical analysis
All data were expressed as mean ± standard error of the mean (SEM). Date were tested for normality of distribution (Shapiroe-Wilk test) and homogeneity of variance (Levene’s test) prior to analysis. Independent-Sample T test was conducted using SPSS 16.0 software (SPSS Inc., USA). Differences were considered significant when P<0.05.
Results
Effects of E2 treatment on histological structure
At 90 dph, no structure difference in ovaries was observed between control and E2-treated groups (Figures 1A, C). Germ cell nests undergoing meiosis at zygotene stage and oocytes at chromatin-nucleolus stage were observed in the ovaries, besides large number of oogonia. A number of follicles at primary growth stage were also observed. At 180 dph, the ovaries in control females were well developed and full of primary growth follicles, with few oogonia and oocytes at chromatin-nucleolus stage (Figure 1B). Similar findings were observed in the ovaries of E2-treated females, with the exception that the number of initial growth follicles in E2-treated females was lower than in controls. (Figure 1D).
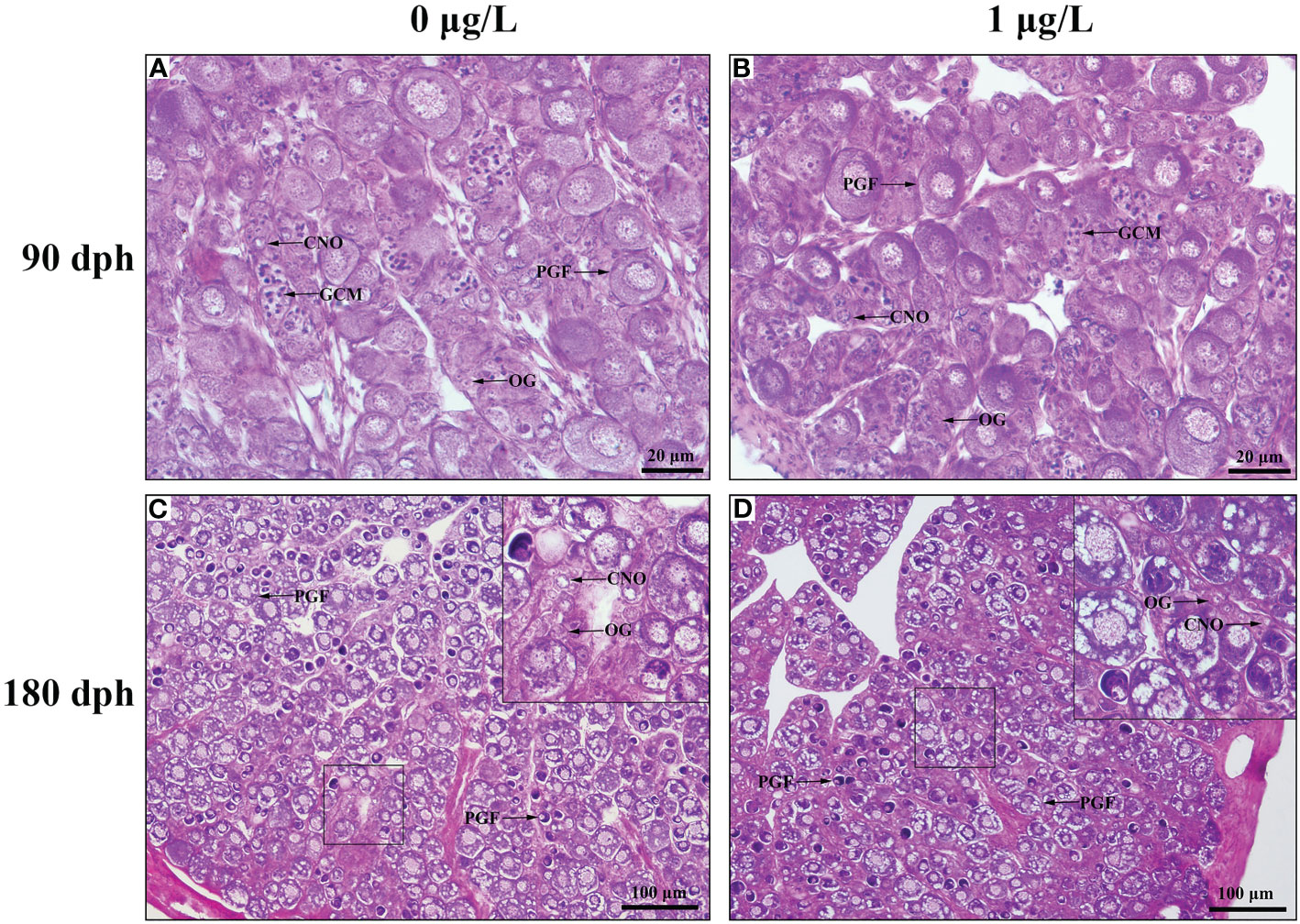
Figure 1 Photomicrographs of ovary in control and E2-exposed female tiger puffer at 90 and 180 dph. (A) Ovary in control female at 90 dph; (B) Ovary in E2-exposed female at 90 dph; (C) Ovary in control female at 180 dph; (D) Ovary in E2-exposed female at 180 dph. CNO, chromatin-necleous oocyte; GCM, germ cells undergoing meiosis at zygotene stage; OG, oogonium; PGF, primary growth follicle.
Effects of E2 treatment on morphological index
After the E2 immersion, there was no significant change in body weight between the control and E2-treated females (Figure 2A). At 90 dph, the ovary weight (F = 0.804, P = 0.01) of E2-treated females were significantly trailing, when compared with the control (Figure 2B). At 180 dph, the ovary weight in both control and E2-treated females were dramatically improved, but the ovary weight (F = 3.156, P = 0.034) of E2-exposed females were still lower than the controls. Similar results were observed in GSI (Figure 2C). The maximum follicle area in both control and E2-treated females was gradually increased after the exposure, and there was no significant difference in maximum follicle area between control and E2-treated groups at neither 90 or 180 dph (Figure 2D).
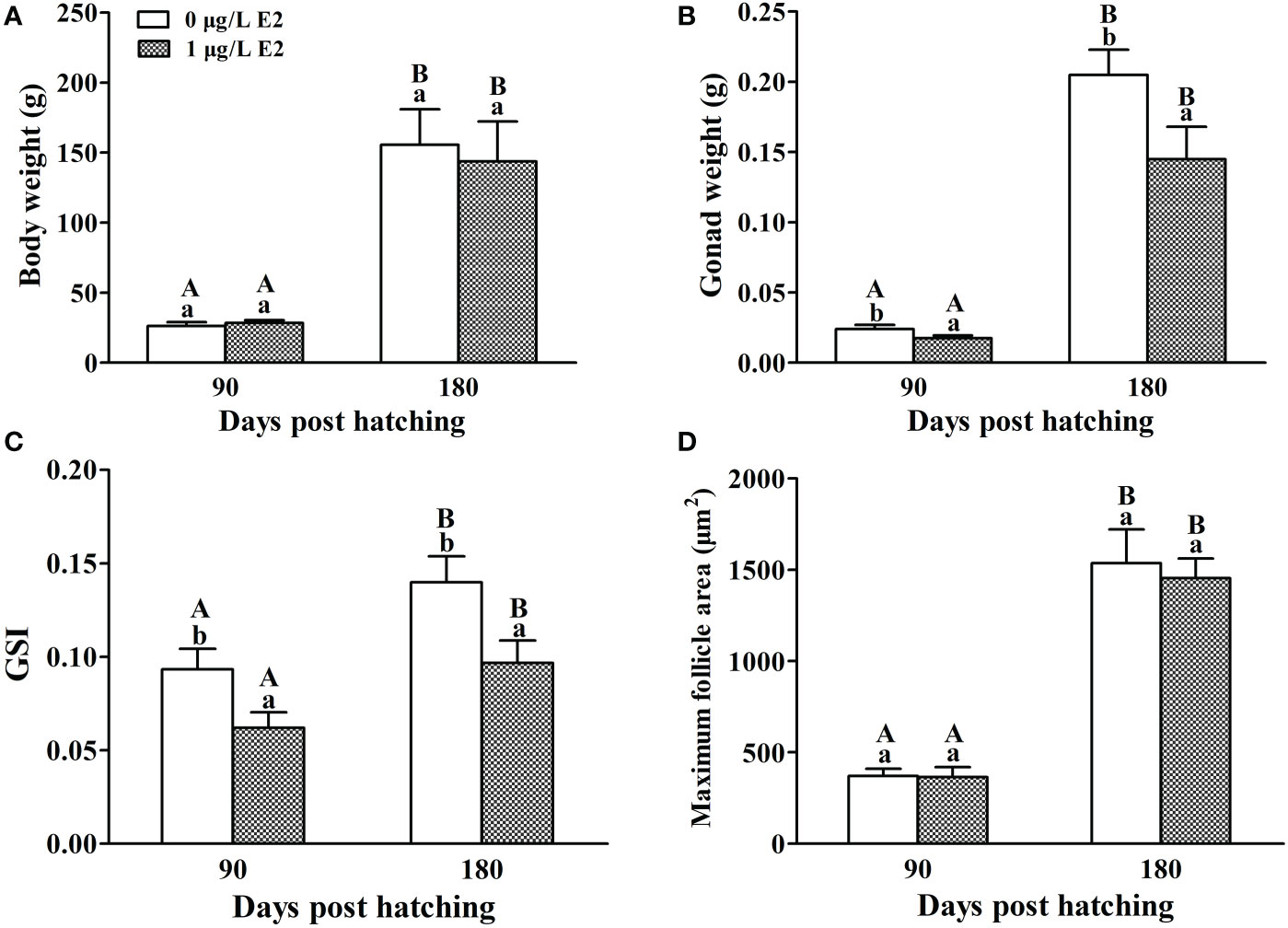
Figure 2 Effects of E2 on body weight (A), ovary weight (B), GSI (C) and maximum follicle area (D) in female tiger puffer after the exposure. Values represent mean ± SEM (n = 6). Uppercase letters indicate significant differences over time within the same group (P < 0.05). Lowercase letters indicate significant differences between groups within the same time (P < 0.05).
Effects of E2 treatment on the expressions of genes involving in cell cycle
Compared with the values expression at 90 dph, the mRNA levels of cdk2, cdk4, ccna2, ccnd2, ccne2, cdkn1b and cdkn2c were observed to increase at 180 dph in the ovaries of control females (Figure 3). Compared with the controls at 90 dph, E2 treatment significantly down-regulated the mRNA levels of cdk2 (F = 1.436, P = 0.000) and ccna2 (F = 0.578, P = 0.004), significantly up-regulated the mRNA levels of ccnd2 (F = 1.846, P = 0.012), and did not significantly alter the mRNA levels of cdk4, cdk6, ccne2, cdkn1b and cdkn2c. At 180 dph, the mRNA levels of ccna2 (F = 1.995, P = 0.002) in the ovaries of E2-treated females were still significantly lower than the control values (Figure 3D). While there was no significant difference in the mRNA levels of cdk4, cdk6, ccne2, cdkn1b and cdkn2c between control and E2-treated females.
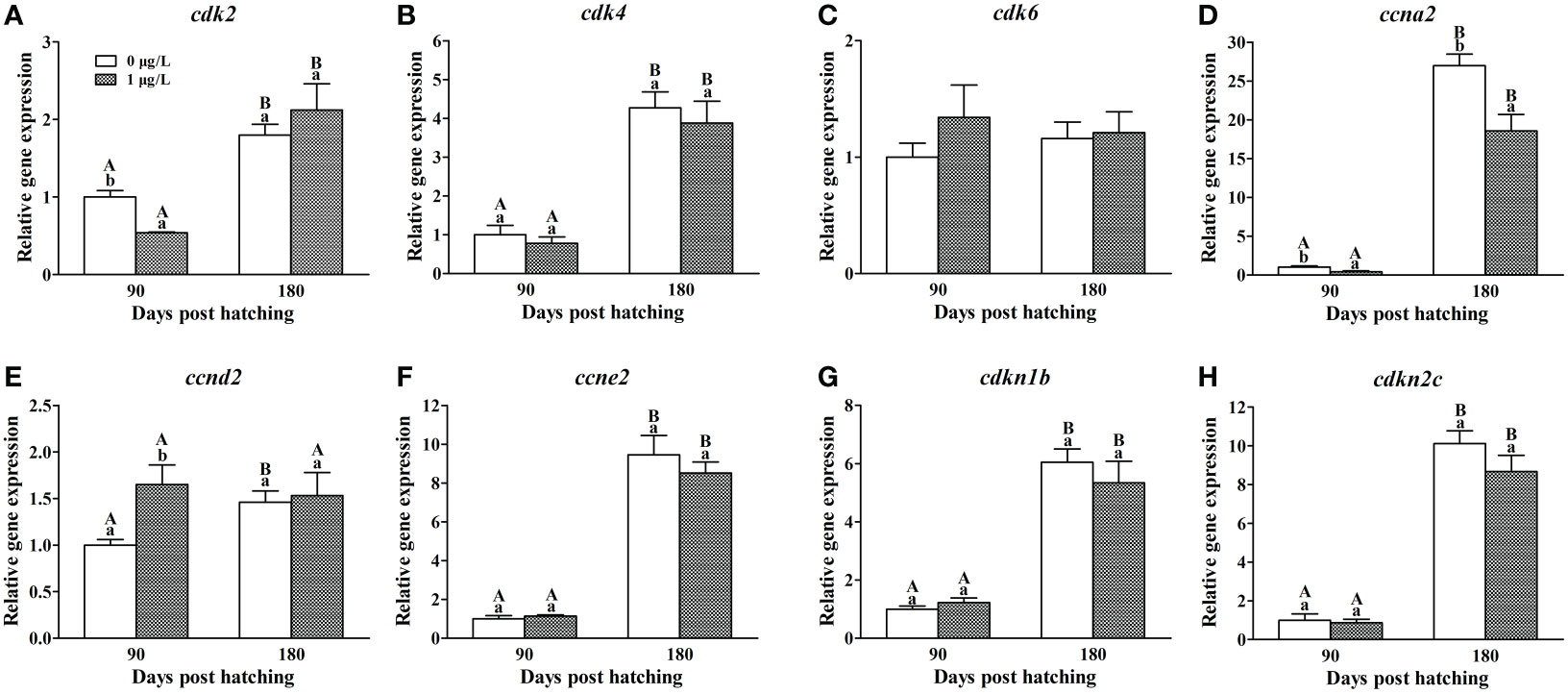
Figure 3 Effects of E2 on the mRNA levels of cdk2 (A), cdk4 (B), cdk6 (C), ccna2 (D), ccnd2 (E), ccne2 (F), cdkn1b (G) and cdkn2c (H) involving in cell cycle. Values represent mean ± SEM (n = 4). Uppercase letters indicate significant differences over time within the same group (P < 0.05). Lowercase letters indicate significant differences between groups within the same time (P < 0.05).
Effects of E2 treatment on the expressions of genes involving in meiosis initiation
In the ovaries of control females, decreased mRNA levels of sycp3 (F = 1.198, P = 0.008), rec8 (F = 0.846, P = 0.013) and dmc1 (F = 1.228, P = 0.006) and unaltered mRNA levels of spo11 were observed at 180 dph compared with the values at 90 dph (Figure 4). E2 treatment resulted in a significant decrease in the mRNA levels of the four genes at 90 dph. While no significant difference was observed in the mRNA levels of all the four genes between E2-treated and control females at 180 dph.
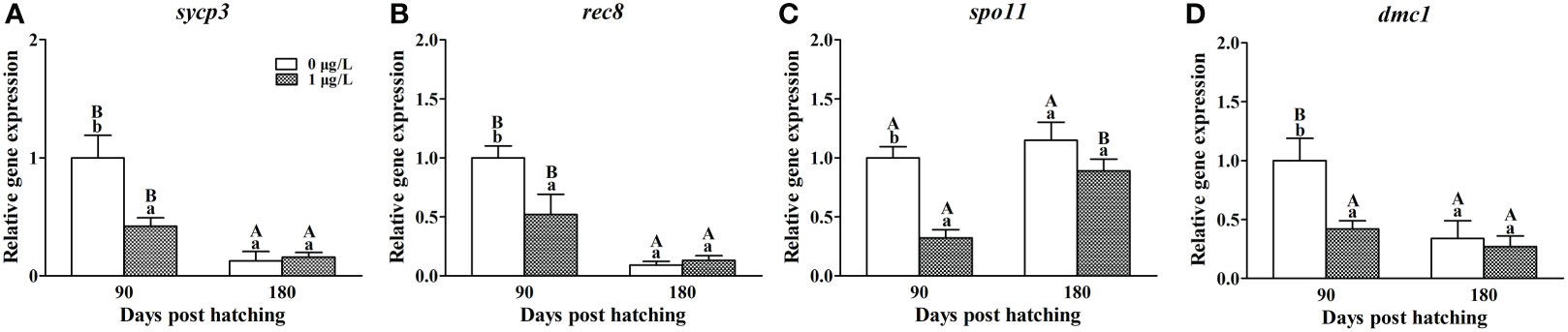
Figure 4 Effects of E2 on the mRNA levels of cdk2 (A), cdk4 (B), cdk6 (C), ccna2 (D), ccnd2 (E), ccne2 (F), cdkn1b (G) and cdkn2c (H) involving in cell cycle. Values represent mean ± SEM (n = 4). Uppercase letters indicate significant differences over time within the same group (P < 0.05). Lowercase letters indicate significant differences between groups within the same time (P < 0.05).
Effects of E2 treatment on the expressions of genes involving in apoptosis
As for apoptosis genes, E2 treatment did not affect the mRNA levels of bax (Figure 5A), but significantly decreased the mRNA levels of bcl-2 (F = 2.463, P = 0.018) at 90 dph, when compared with the controls (Figure 5B). Correspondingly, the ratio of bax/bcl-2 (F = 0.837, P = 0.005) was significantly higher in the ovaries of E2-exposed females than the control values at 90 dph (Figure 5C). At 180 dph, no significant difference was observed in the expression of bax, bcl-2 and bax/bcl-2 ratio between E2-treated and control females.
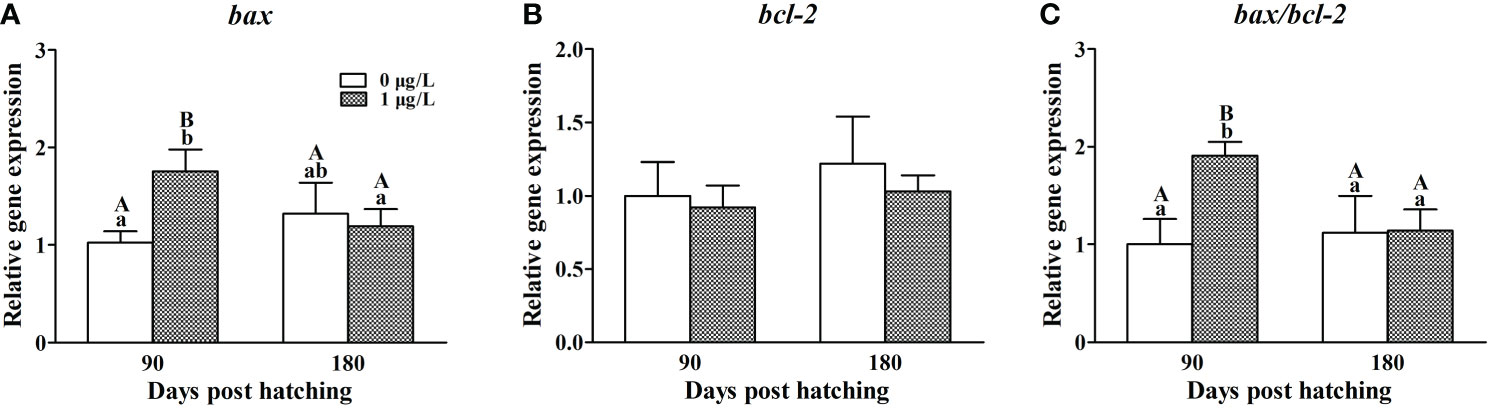
Figure 5 Effects of E2 on the mRNA levels of bax (A), bcl-2 (B) and bax/bcl-2 (C) involving in apoptosis. Values represent mean ± SEM (n = 4). Uppercase letters indicate significant differences over time within the same group (P < 0.05). Lowercase letters indicate significant differences between groups within the same time (P < 0.05).
Effects of E2 treatment on the expressions of genes involving in follicular formation
In the ovaries of control females, the mRNA levels of bmp4 and hnrnpk were found to significantly decrease at 180 dph compared with the values at 90 dph, while contrary expression profile were observed in bmp15, gdf9, figlα and nobox (Figure 6). Compared with the controls at 90 dph, E2 treatment significantly suppressed the mRNA levels of bmp2 (F = 1.826, P = 0.003; Figure 6A) and hnrnpk (F = 1.436, P = 0.006; Figure 6B), significantly elevated the mRNA levels of bmp15 (F = 1.180, P = 0.005; Figure 6C), gdf9 (F = 0.385, P = 0.006; Figure 6D) and figlα (F = 0.243, P = 0.002; Figure 6E), but not alter the mRNA levels of nobox (Figure 6F). At 180 dph, the mRNA levels of all the six genes showed no significant difference between control and E2-treated females.
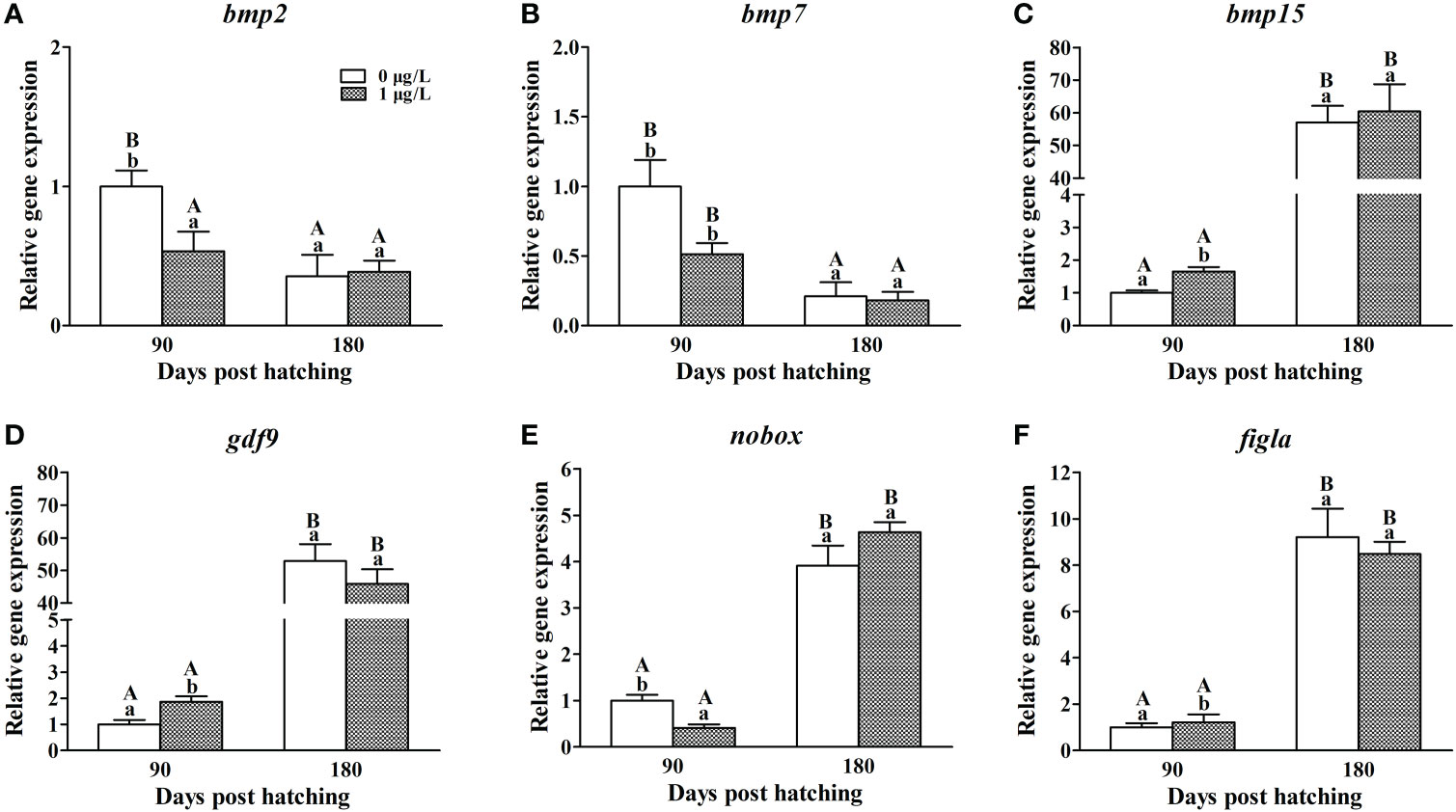
Figure 6 Effects of E2 on the mRNA levels of bmp2 (A), bmp7 (B), bmp15 (C), gdf9 (D), nobox (E) and figla (F) involving in follicle formation. Values represent mean ± SEM (n = 4). Uppercase letters indicate significant differences over time within the same group (P < 0.05). Lowercase letters indicate significant differences between groups within the same time (P < 0.05).
Discussion
As the most important female hormone, estrogens plays a key role to trigger and maintain ovarian differentiation during early life stage of teleost. It was reported that suppression of E2 production during gonad differentiation might result in testicular differentiation of female tiger puffer (Rashid et al., 2007). In this study, on the contrary, female tiger puffer were exposed to high concentration of E2 during gonad differentiation. The results showed that the ovary weight and GSI of E2-exposed female were significantly lower than the values of normal females after the exposure. Similar results were observed in medaka Oryzias latipes exposed to diethylstilbestrol (DES), in which decreased germ cells were also observed in DES-exposed females (Paul-Prasanth et al., 2011). In the present study, although it was difficult to directly count the number of follicles, it could be estimated by measuring the maximum follicle area. There was no significant difference in maximum follicle area between control and E2-treated females at 90 and 180 dph. We hypothesized that E2 exposure during gonad differentiation could limit the number of follicles since the ovary weight of E2-treated animals was considerably lower than the control. This was supported by the result that primary growth follicles in E2-treated females were fewer in number than control at 180 dph.
In order to understand how the follicle number in the ovary of female tiger puffer was affected by the E2 exposure, we firstly detected the mRNA levels of genes involving in germ cell proliferation and meiosis. The results showed that E2 exposure significantly inhibited the expression of cdk2, ccna2, sycp3, rec8, spo11 and dmc1 at 90 dph, and the mRNA levels of ccna2 was still significant low in the ovaries of E2-treated females even after a 90-day depuration in clean seawater. Cdk2 and ccna2 are primarily considered to be involved in G2-M transition in cell cycle. However, gene knockouts in mice showed that the two genes were dispensable for cell cycle progression (Adhikari et al., 2012; Zhang et al., 2017; Chotiner et al., 2019). Cdk2-deficient oocytes resume meiosis and arrest normally at metaphase II (Adhikari et al., 2012). Ccna2-deficient oocytes was also able to progress through the G2-M transition and meiosis I unhindered (Zhang et al., 2017). Thereby, suppression on the expression of cdk2 and ccna2 might not affect cell proliferationn the ovaries of female tiger puffer. Sycp3, rec8, spo11 and dmc1 are key genes participating in the regulation of meiosis initiation. In mice, the loss of any of the four genes caused oocytes and spermatocytes to be stopped at meiosis I with faulty synaptonemal complex formation, resulting in germ cell death and even infertility. (Pittman et al., 1998; Kouznetsova et al., 2005; Xu et al., 2005; Ishiguro, 2019). Similar results were also observed in male medaka in dmc1 mutation (Chen et al., 2016). Based on above researches, the decreased mRNA levels of sycp3, rec8, spo11 and dmc1 in this study implied that the E2 exposure may affect the process of meiosis by suppressing the expression of those genes on meiotic pathway and further lead in decreased oocytes in female tiger puffer.
When the oocytes develop into chromatin nucleolus stage, the oocyte cysts break apart, and somatic cells surround the oocytes forming primordial follicles (Grier et al., 2007; Uribe et al., 2016). Exposure to DES or BPA inhibited cyst breakdown and led to abnormal follicles in female mouse (Iguchi et al., 1990; Susiarjo et al., 2007). A recent study in BPA-exposed mice found that the emergence of aberrant follicles was related with lower levels of bmp15 and gdf9 expression (Wang et al., 2018), which are known as the several of most important genes for follicle formation and development in both mammals and fish (Juengel et al., 2002; García-López et al., 2011b; Otsuka et al., 2011; Liu et al., 2012). In the present study, in contrast, the E2 exposure increased the mRNA levels of bmp15 and gdf9, but decreased the mRNA levels of bmp2 and hnrnpk. Bmp2 promotes the processes of follicle formation by acting on oocyte development and somatic cell differentiation. Its knockdown inhibited primordial follicle formation and promoted apoptosis in hamster Mesocricetus auratus (Chakraborty and Roy, 2015). In mice, disruption of hnrnpk caused a block in cyst breakdown and follicle assembly, subsequently resulting in ovarian cells undergoing abnormal apoptosis (Wang et al., 2011). Meanwhile, the inhibited expression of bmp2 and hnrnpk suggested that the E2 treatment disrupted follicle formation and even caused apoptosis, which was one reason that the follicle number reduced in female tiger puffer.
Now that abnormal meiosis and follicle formation enhanced apoptosis, we further confirmed the effects of E2 exposure on genes on bax/cytochrome c/caspase pathway that is known as the most popular cascade involved in apoptosis. Among those genes, bax is a cell death gene stimulating apoptosis (Perez et al., 1999), and bcl-2 is a cell survival gene blocking apoptosis (Ratts et al., 1995). The bax/bcl-2 ratio is usually used as an important indicator of cell fate (Sánchez and Smitz, 2012; Luzio et al., 2016; Yin et al., 2017). Low bax expression or high bcl-2 expression shifted the balance of bax and bcl-2 toward long survival of cells and apoptosis was observed to be inhibited (Sribnick et al., 2004; Sharma and Mehra, 2008). While elevated mRNA levels of bax and ratio of bax/bcl-2 corresponded well with the atretic follicles in yellow catfish exposed to DES (Wang et al., 2017). In the present study, E2 treatment did not affect bax expression but suppressed the mRNA levels of bcl-2, shifting the balance of bax/bcl-2 toward apoptosis. This result echoed the above inferences that disordered meiosis and follicle formation promoted apoptosis.
In conclusion, our study revealed that E2 exposure from 20 to 90 dph affected female tiger puffer ovarian development by interfering with meiosis initiation, follicle formation, and increasing apoptosis, resulting in fewer follicles and a lower GSI value in E2-treated females. The findings extended to our understanding of the molecular processes underpinning the toxicity of exogenous estrogens on primary ovarian growth in fish.
Data availability statement
The original contributions presented in the study are included in the article/supplementary material. Further inquiries can be directed to the corresponding author.
Ethics statement
The animal study was reviewed and approved by the Institutional Animal Care and Use Committee at Yellow Sea Fisheries Research Institute, Chinese Academy of Fishery Science.
Author contributions
PH: Project administration, writing – original draft, Writing –review and editing. ShuL: Review and editing, Funding acquisition. XL and ZZ: Review and editing. HL and SheL: estrogen immersion experiment. All authors joined the analysis and interpretation of data. All authors contributed to the article and approved the submitted version.
Funding
This work was supported by China Agriculture Research System of MOF and MARA (CARS-47).
Conflict of interest
Authors HL and SL are employed by Dalian Tianzheng Industry Co., Ltd.
The remaining authors declare that the research was conducted in the absence of any commercial or financial relationships that could be construed as a potential conflict of interest.
Publisher’s note
All claims expressed in this article are solely those of the authors and do not necessarily represent those of their affiliated organizations, or those of the publisher, the editors and the reviewers. Any product that may be evaluated in this article, or claim that may be made by its manufacturer, is not guaranteed or endorsed by the publisher.
References
Adhikari D., Zheng W., Shen Y., Gorre N., Ning Y., Halet G., et al. (2012). Cdk1, but not Cdk2, is the sole cdk that is essential and sufficient to drive resumption of meiosis in mouse oocytes. Hum. Mol. Genet. 21, 2476–2484. doi: 10.1093/hmg/dds061
Andersen C. L., Jensen J. L., Ørntoft T. F. (2004). Normalization of real-time quantitative reverse transcription-PCR data: A model-based variance estimation approach to identify genes suited for normalization, applied to bladder and colon cancer data sets. Cancer Res. 64 (15), 5245–5250. doi: 10.1158/0008-5472
Bouilly J., Veitia R. A., Binart N. (2014). NOBOX is a key FOXL2 partner involved in ovarian folliculogenesis. J. Mol. Cell. Biol. 6 (2), 175–177. doi: 10.1093/jmcb/mju006
Cahoon C. K., Hawley R. S. (2016). Meiosis: Cohesins are not just for sisters any more. Curr. Biol. 26 (13), R523–R525. doi: 10.1016/j.cub.2016.05.011
Chakraborty P., Roy S. K. (2015). Bone morphogenetic protein 2 promotes primordial follicle formation in the ovary. Sci. Rep. 5, 12644. doi: 10.1038/srep12664
Chen J., Cui X., Jia S., Luo D., Cao M., Zhang Y. (2016). Disruption of dmc1 produces abnormal sperm in medaka (Oryzias latipes). Sci. Rep. 2 (6), 30912. doi: 10.1038/srep30912
Chotiner J. Y., Wolgemuth D. J., Wang P. J. (2019). Functions of cyclins and CDKs in mammalian gametogenesis. Biol. Reprod. 101 (3), 591–601. doi: 10.1093/biolre/ioz070
Colli-Dula R., Martyniuk C. J., Kroll K. J., Prucha M. S., Kozuch M., Barber D. S., et al. (2014). Dietary exposure of 17-alpha ethinylestradiol modulates physiological endpoints and gene signaling pathways in female largemouth bass (Micropterus salmoides). Aquat. Toxicol. 156, 148–160. doi: 10.1016/j.aquatox.2014.08.008
Craig Z. R., Hannon P. R., Wang W., Ziv-Gal A., Flaws J. A. (2013). Di-n-Butyl phthalate disrupts the expression of genes involved in cell cycles and apoptotic pathways in mouse ovarian antral follicles. Biol. Reprod. 88 (1), 23–21. doi: 10.1095/biolreprod.112.105122
García-López Á., Sànchez-Amaya M. I., Prat F. (2011a). Targeted gene expression profiling in European sea bass (Dicentrarchus labrax, l.) follicles from primary growth to late vitellogenesis. Com. Bio. Phy. A. 160, 374–380. doi: 10.1016/j.cbpa.2011.07.006
García-López Á., Sànchez-Amaya M. I., Halm S., Astola A., Prat F. (2011b). Bone morphogenetic protein 15 and growth differentiation factor 9 expression in the ovary of European sea bass (Dicentrarchus labrax): Cellular localization, developmental profiles, and response to unilateral ovariectomy. Gen. Comp. Endocrinol. 174, 326–334. doi: 10.1016/j.fertnstert.2009.03.014
Grier H. J., Uribe M. C., Parenti L. R. (2007). The germinal epithelium, folliculogenesis, and postovulatory follicles in ovaries of rainbow trout, Oncorhynchus mykiss (Walbaum 1792) (Teleostei, protacanthopterygii, salmoniformes). J. Morphol 268, 293–310. doi: 10.1002/jmor.10518
Hu P., Liu B., Meng Z., Liu X., Jia Y., Yang Z., et al. (2017). Recovery of gonadal development in tiger puffer Takifugu rubripes after exposure to 17β-estradiol during early life stages. Chin. J. Ocean. Limn. 35 (3), 613–623. doi: 10.1007/s00343-017-6016-6
Hu P., Liu X., Liu B., Wen H., Yang Z., Lei J. (2015). Histological observation on the gonadal differentiation of tiger puffer (Takifugu rubripes). Period. Ocean. U. China. 45 (10), 25–30. doi: 10.16441/j.cnki.hdxb.20140237
Iguchi T., Fukazawa Y., Uesugi Y., Takasugi N. (1990). Polyovular follicles in mouse ovaries exposed neonatally to diethylstilbestrol in vivo and in vitro. Polyovular follicles in mouse ovaries exposed neonatally to diethylstilbestrol in vivo and in vitro. Biol. Reprod. 43 (3), 478–484. doi: 10.1095/biolreprod43.3.478
Ishiguro K. (2019). The cohesin complex in mammalian meiosis. Genes Cells 24, 6–30. doi: 10.1111/gtc.12652
Iwai T., Yoshi A., Yokota T., Sakai C., Hori H., Kanamori A., et al. (2006). Structural components of the synaptonemal complex, SYCP1 and SYCP3, in the medaka fish Oryzias latipes. Exp. Cell. Res. 312, 2528–2537. doi: 10.1016/j.cub.2016.05.011
Juengel J. L., Hudson N. L., Heath D. A., Smith P., Reader K. L., Lawrence S. B., et al. (2002). Growth differentiation factor9 and bone morphogenetic protein 15 are essential for ovarian follicular development in sheep. Biol. Reprod. 67 (6), 1777–1789. doi: 10.1095/biolreprod.102.007146
Kikuchi K., Furuta T., Iwata N. A., Onuki K., Noguchi T., Sugita H. (2011). Effect of dietary fatty acid composition on the growth of the tiger puffer Takifugu rubripes. Fish. Sci. 77, 829–837. doi: 10.1007/s12562-011-0393-0
Kouznetsova A., Novak I., Jessberger R., Hoüoüg C. (2005). SYCP2 and SYCP3 are required for cohesin core integrity at diplotene but not for centromere cohesion at the first meiotic division. J. Cell Sci. 118 (10), 2271–2278. doi: 10.1242/jcs.02362
Lau E., Lee M., Chang C. (2013). Conversed sex-specific timing of meiotic initiation during sex differentiation in the protandrous black porgy Acanthopagrus schlegelii. Biol. Reprod. 88 (6), 150. doi: 10.1095/biolreprod.112.105536
Lei B., Peng W., Li W., Yu Y., Xu J., Wang Y. (2016). Diethylstilbestrol at environmental levels affects the development of early life stage and target gene expression in Japanese medaka (Oryzias latipes). Ecotoxicology. 25, 563–573. doi: 10.1007/s10646-016-1615-0
Liu Z., Chen A., Yang Z., Wei H., Leng X. (2012). Molecular characterization of growth differentiation factor 9 and its spatio-temporal expression pattern in gibel carp (Carassius auratus gibelio). Mol. Biol. Rep. 39, 3863–3870. doi: 10.1007/s11033-011-1165-8
Lubzens E., Young G., Bobe J., Cerdà J. (2010). Oogenesis in teleosts: How fish eggs are formed. Gen. Comp. Endocrinol. 165, 367–389. doi: 10.1016/j.ygcen.2009.05.022
Luckenbach J., Iliev D. B., Goetz F. W., Swanson P. (2008). Identification of differentially expressed ovarian genes during primary and early secondary oocyte growth in coho salmon, Oncorhynchus kisutch. Reprod. Biol. Endocrinol. 6, 2. doi: 10.1186/1477-7827-6-2
Luzio A., Matos M., Santos D., Fontaínhas-Fernandes A. A., Monteiro S. M., Coimbra A. M. (2016). Disruption of apoptosis pathways involved in zebrafish gonad differentiation by 17α-ethinylestradiol and fadrozole exposures. Aquat. Toxicol. 177, 269–284. doi: 10.1016/j.aquatox.2016.05.029
Nakamura S., Kobayashi K., Nishimura T., Higashijima S., Tanka M. (2010). Identification of germline stem cells in the ovary of the teleost medaka. Science. 328, 1561. doi: 10.1126/science.1185473
Otsuka F., McTavish K., Shimasaki S. (2011). Integral role of GDF-9 and BMP-15 in ovarian function. Mol. Reprod. Dev. 78 (1), 9–21. doi: 10.1002/mrd.21265
Paul-Prasanth B., Shibata Y., Horiguchi R., Nagahama Y. (2011). Exposure to diethylstilbestrol during embryonic and larval stages of medaka fish (Oryzias latipes) leads to sex reversal in genetic male and reduced gonad weight in genetic females. Endocrinology. 152 (2), 707–717. doi: 10.1210/en.2010-0812
Perez G. I., Robles R., Knudson C. M., Flaws J. A., Korsmeyer S. J., Tilly J. L. (1999). Prolongation of ovarian lifespan into advanced chronological age by bax deficiency. Nat. Genet. 21 (2), 200–203. doi: 10.1038/5985
Pfaffl M. W., Tichopad A., Prgomet C., Neuvians T. P. (2004). Determination of stable housekeeping genes, differentially regulated target genes and sample integrity: BestKeeper–excel-based tool using pair-wise correlations. Biotechnol. Lett. 26, 509–515. doi: 10.1023/b:bile.0000019559.84305.47
Piferrer F. (2001). Endocrine sex control strategies for the feminization of teleost fish. Aquaculture. 197, 229–281. doi: 10.1016/S0044-8486(01)00589-0
Pittman D. L., Cobb J., Schimenti K. J., Wilson L. A., Cooper D. M., Brignull E., et al. (1998). Meiotic prophase arrest with failure of chromosome synapsis in mice deficient for Dmc1, a germline-specific RecA homolog. Mol. Cell. 1, 697–705. doi: 10.1016/s1097-2765(00)80069-6
Qin F., Wang H., Liu S., Zheng Y., Li M., Zhang Y., et al. (2014). Gene expression profiling of key genes in hypothalamus-pituitary-gonad axis of rare minnow Gobiocypris rarus in response to EE2. Gene. 552 (1), 8–17. doi: 10.1016/j.gene.2014.09.006
Rashid H., Kitano. H., Hoon Lee K., Nii S., Shigematsu T., Kadomura K., et al. (2007). Fugu (Takifugu rubripes) sexual differentiation: CYP19 regulation and aromatase inhibitor induced testicular development. Sex Dev. 1, 311–322. doi: 10.1159/000108935
Ratts V. S., Flaws J. A., Kolp R., Sorenson C. M., Tilly J. L. (1995). Ablation of bcl-2 gene expression decreases the number of oocytes and primordial follicles established in the post-natal female mouse gonad. Endocrinology. 136 (8), 3665–3668. doi: 10.1210/endo.136.8.7628407
Reddy G. P. V. (1994). Cell cycle: regulatory events in G1→S transition of mammalian cells. J. Cell. Biochem. 54 (4), 379–386. doi: 10.1002/jcb.240540404
Romanienko P. J., Camerini-Otero R. D. (2000). The mouse Spo11 gene is required for meiotic chromosome synapsis. Mol. Cell. 6, 975–987. doi: 10.1016/S1097-2765(00)00097-6
Sánchez F., Smitz J. (2012). Molecular control of oogenesis. BBA-Mol. Basis Dis. 1822 (12), 1896–1912. doi: 10.1016/j.bbadis.2012.05.013
Sharma K., Mehra R. D. (2008). Long-term administration of estrogen or tamoxifen to ovariectomized rats affords neuroprotection to hippocampal neurons by modulating the expression of bcl-2 and bax. Brain Res. 1204, 1–15. doi: 10.1016/j.brainres.2008.01.080
Soyal S. M., Amleh A., Dean J. (2000). FIGalpha, a germ cell-specific transcription factor required for ovarian follicle formation. Development. 127 (21), 4645–4654. doi: 10.1007/s004290000125
Sribnick E. A., Ray S. K., Nowak M. W., Li L., Banik N. L. (2004). 17beta-estradiol attenuates glutamate-induced apoptosis and preserve electrophysiologic function in primary cortical neurons. J. Neurosci. Res. 76, 688–696. doi: 10.1002/jnr.20124
Susiarjo M., Hassold T. J., Freeman E., Hunt P. A. (2007). Bisphenol a exposure in utero disrupts early oogenesis in the mouse. PLos Genet. 3 (1), e5. doi: 10.1371/journal.pgen.0030005
Tanaka M. (2014). Vertebrate female germline-the acquisition of femaleness. Wiley interdiscip. Rev. Dev. Bio. 3 (3), 231–238. doi: 10.1002/wdev.131
Tang H., Liu Y., Li J., Yin Y., Li G., Chen Y., et al. (2016). Gene knockout of nuclear progesterone receptor provides insights into the regulation of ovulation by LH signaling in zebrafish. Sci. Rep. 6, 28545. doi: 10.1038/srep28545
Uribe M. C., Grier H. J., García-Alarcón A., Parenti L. R. (2016). Oogenesis: from oogonia to ovulation in the flagfish, jordanella floridae goode and bean 1879 (Teleostei: Cyprinodontidae). J. Morphol. 277, 1339–1354. doi: 10.1002/jmor.20580
Wang N., Zhang P., Guo X., Zhou Z., Sha J. (2011). Hnrnpk, a protein differentially expressed in immature rat ovarian development, is required for normal primordial follicle assembly and development. Endocrinology. 3, 1024–1035. doi: 10.1210/en.2010-0797
Wang X., Jiang S., Wang L., Sun Y., Xu F., He H., et al. (2018). Interfering effects of bisphenol a on in vitro growth of preantral follicles and maturation of oocytes. Clin. Chim. Acta 485, 119–125. doi: 10.1016/j.cca.2018.06.041
Wang Y., Li Y., Chen Q., Liu Z. (2017). Diethylstilbestrol impaired oogenesis of yellow catfish juveniles through disrupting hypothalamic–pituitary–gonadal axis and germ cell development. J. Appl. Toxicol. 38, 308–317. doi: 10.1002/jat.3529
Xu H., Beasley M. D., Warren W. D., van der Horst G. T. J., Mckay M. J. (2005). Absence of mouse REC8 cohesin promotes synapsis of sister chromatids in meiosis. Dev. Cell. 8, 949–961. doi: 10.1016/j.devcel.2005.03.018
Xu J., Osuga Y., Yano T., Morita Y., Tang X., Fujiwara T., et al. (2002). Bisphenol a induces apoptosis and G2-to-M arrest of ovarian granulosa cells. Biochem. Biophys. Res. Commun. 292 (2), 456–462. doi: 10.1006/bbrc.2002.6644
Yin P., Li Y., Chen Q., Liu Z. (2017). Diethylstilbestrol, flutamide and their combination impaired the spermatogenesis of male adult zebrafish through disrupting HPG axis, meiosis and apoptosis. Aquat. Toxicol. 185, 129–137. doi: 10.1016/j.aquatox.2017.02.013
Yoshikawa S., Hisashi C., Masaomi H., Kazushi K., Toshiyuki Y., Kiyoshi K., et al. (2020). Precocious maturation in male tiger puferfsh Takifugu rubripes: Genetics and endocrinology. Fisheries Sci. 86 (2), 39–351. doi: 10.1007/s12562-019-01390-4
Zhang Q., Yuen W., Adhikari D., Flegg J., FitzHarris G., Conti M., et al. (2017). Cyclin A2 modulates kinetochore-microtubule attachment in meiosis II. J. Cell Bio. 216 (10), 3133–3143. doi: 10.1083/jcb.201607111
Zhang Y., Gao J., Xu P., Yuan C., Qin F., Liu S., et al. (2014a). Low-dose bisphenol a disrupts gonad development and steroidogenic genes expression in adult female rare minnow Gobiocypris rarus. Chemosphere. 112, 435–442. doi: 10.1016/j.chemosphere.2014.04.089
Zhang Y., Yuan C., Qin F., Hu G., Wang Z. (2014b). Molecular characterization of gdf9 and bmp15 genes in rare minnow Gobiocypris rarus and their expression upon bisphenol a exposure in adult females. Gene. 546 (2), 214–221. doi: 10.1016/j.gene.2014.06.013
Keywords: Takifugu rubripes, 17β-estradiol, early-life exposure, delayed ovarian development, oogenesis
Citation: Hu P, Liu S, Liu X, Liu H, Liu S and Zhuang Z (2023) The adverse effects of 17β-estradiol immersion during gonadal differentiation on ovarian development of female Takifugu rubripes. Front. Mar. Sci. 10:1131041. doi: 10.3389/fmars.2023.1131041
Received: 03 January 2023; Accepted: 07 February 2023;
Published: 20 February 2023.
Edited by:
Hongyu Ma, Shantou University, ChinaReviewed by:
Khor Waiho, University of Malaysia Terengganu, MalaysiaYao Zheng, Freshwater Fisheries Research Center (CAFS), China
Copyright © 2023 Hu, Liu, Liu, Liu, Liu and Zhuang. This is an open-access article distributed under the terms of the Creative Commons Attribution License (CC BY). The use, distribution or reproduction in other forums is permitted, provided the original author(s) and the copyright owner(s) are credited and that the original publication in this journal is cited, in accordance with accepted academic practice. No use, distribution or reproduction is permitted which does not comply with these terms.
*Correspondence: Shufang Liu, bGl1c2ZAeXNmcmkuYWMuY24=