- 1Centro Universitario Regional Este (CURE), Universidad de la República, Maldonado, Uruguay
- 2Dirección Nacional de Recursos Acuáticos (DINARA), Ministerio de Ambiente, Montevideo, Uruguay
- 3Dirección Nacional de Biodiversidad y Servicios Ecosistémicos (DINABISE-MA), Ministerio de Ambiente, Montevideo, Uruguay
- 4Ciencia Y Tecnología Marina (CINCYTEMA), Sección Oceanología, Instituto de Ecología y Ciencias Ambientales, Facultad de Ciencias, Universidad de la República, Montevideo, Uruguay
- 5Área Biodiversidad y Conservación, Museo Nacional de Historia Natural, Montevideo, Uruguay
Introduction: The Uruguayan Exclusive Economic Zone (U-EEZ) is still lacking a technically sound and institutionally agreed system for classifying marine benthic ecosystems. This fact, added to the growing anthropic pressure marine ecosystems face in the U-EEZ, increases the vulnerability of these ecosystems and their associated biodiversity. Despite this, there is valuable and abundant disaggregated environmental and biological information for benthic systems.
Methods: In this work, we used ca. 4000 records of all major Phyla of benthic invertebrates to determine discrete benthic regions for the U-EEZ. We looked at the structuring forces of bathymetry, salinity, and water masses. First, we categorized the multivariate structure according to statistical significance to test a priori categories for univariate descriptors. Then, we mapped unique combinations of categories of structuring variables, which largely determine the structure and composition of benthic communities. Operationally, the variables to be analyzed were classified, and subsequently, group (cluster) and SIMPROF analyzes were performed.
Results: The results suggest seven bathymetric categories, two of water masses and two of salinity, significantly structuring the benthic communities. The combination of these categories suggests nine discrete benthic marine regions for the U-EEZ, with heterogeneous structural characteristics. Our results provide an initial model of distinct benthic ecological complexes in U-EEZ.
Discussion: However, caution should be exerted when using this model during decision-making. For example, implementing use restrictions of benthic areas should rely on further groundtruthing, including direct seafloor observations and collections. The latter is relevant since our system is based on the analysis of accumulated historical data that may or may not represent the current condition of habitats of interest.
1 Introduction
Recognizing the current and potential threats affecting the provision of ecosystem services of the world oceans, and failing to comply with the Aichi Target 11 at a global scale (CBD, 2020), the UN Convention on Biological Diversity (CBD) released the first official draft of a new Global Biodiversity Framework to guide actions worldwide towards new goals to ensure more effectiveness in conservation. The framework includes 21 targets for 2030 that calls, among others, for at least 30% of sea areas (focusing on those of particular importance for biodiversity and its contributions to people) to be conserved through effective and equitable management (Nicholson et al., 2021). In this line, the Uruguayan Government has recently announced the intention of reaching the 10% target by the end of 2022. Thus, a favorable international and national context should join scientists and policy makers’ work, helping to protect ecologically representative and well-connected systems of particular importance for marine biodiversity integrated into the regional seascape.
The Uruguayan Government is committed to increasing the protected marine area from 0.7% to 10% by 2022 and to reach the 30% goal by 2030, through a network of well connected and representative marine protected areas (MPAs). The recently created Ministry of the Environment (ME) will lead a comprehensive and participatory process of spatial prioritization, identifying and mapping focal conservation targets for the design and implementation of the network of MPAs based on the best available science, as part of an integral Marine Spatial Planning (MSP) process. In order to achieve support among stakeholders, this process will incorporate scientific and technical advice and explicitly consider other uses of the marine environment.
The Uruguayan Exclusive Economic Zone (U-EEZ) is located in the subtropical region (i.e., between 33 S and 38 S) of the Southwestern Atlantic margin, positioned in a critical transitional region concerning global ocean circulation, and is part of the Patagonian Shelf Large Marine Ecosystem (Bisbal, 1995; Hempel & Sherman, 2003). Therefore, from a global perspective for marine conservation planning, this area is also classified as the Uruguay–Buenos Aires shelf ecoregion (Marine Ecoregions of the World, MEOW No183; Spalding et al., 2007) included in the Warm-Temperate Southwestern Atlantic. Likewise, according to the categorization of the Pelagic Provinces of the World scheme (PPOW), the Uruguayan EEZ is located in the Malvinas Current Province.
The Uruguay-Buenos Aires Shelf received the highest score on conservation concerns and biological importance amongst Latin American Marine Ecoregions (see Table 2.10 in Sullivan and Bustamante, 1999). A wide array of human activities, including deep water fisheries, submarine cables and exploration for hydrocarbons, is performed in the region and in Uruguayan waters (Marin et al., 2021), also occurring at a hotspot of climate warming (Gianelli et al., 2019). Most fishery resources are vulnerable to climate change (Gianelli, under review), and climate velocities are faster in the deep oceans than in surface waters (Brito-Morales et al., 2020). This concerns fisheries that would shift to deeper water in search of new, unexploited stocks or following the habitat tracking of traditional fisheries species (Vilela et al., 2018). Thus, habitat mapping will be a step toward implementing a much-needed MPA network aiming at the conservation of Marine Ecosystems within the U- EEZ.
However, as Stirling & Burgman (2021) pointed out, conservation science can be understood as a “crisis discipline”, and often relies on unavailable, incomplete, outdated, or biased data. These categories can be applied to some of the information needed to classify and map benthic environments within the U-EEZ. MPAs should be based on high quality data on habitat distribution, larval dispersal patterns, adult movement ranges, and oceanography (Botsford et al., 2001; Gaines et al., 2003; Gaines et al., 2010). However, in the absence of this information, developing coarse-grained classification systems based on what is currently known and available can be seen as the first step in a data-poor scenario, particularly concerning seabed assessments. In this vein, here we propose a preliminary bioregionalization of benthic environments within U-EEZ, which should be helpful to support prioritization process, decision making and the design of MPAs a future MPAs network. It will also highlight regions with high priority for scientific research programs to generate knowledge of the seafloor and their benthic communities (Harris and Baker, 2012).
2 Material and methods
2.1 Study area
Located in the Southwestern Atlantic margin’s subtropical region, the U-EEZ is positioned in a critical transitional region regarding the global ocean circulation. It is characterized by the Río de la Plata (RdlP) contribution and the confluence of water masses with contrasting thermohaline features determining the formation of well-marked fronts (Ortega and Martínez, 2007). In the continental shelf, this characteristic is represented by the Subtropical Shelf Front (STSF), a shallow extension of the Brazil-Malvinas Confluence (BMC) that occurs in deep water off the shelf (Matano et al., 2010). Despite its small size compared to neighboring countries, the U-EEZ is considered one of the most productive areas of the southern hemisphere (Burone et al., 2021; de Mello et al., 2014), owing to regional oceanic circulation patterns and interactions with the RdlP freshwater discharge. Offshore, in the Brazil–Malvinas Confluence Zone, the southward-flowing subtropical Brazil Current (which transports: Tropical Water (TW) and South Atlantic Central Water (SACW)) meets the northward-flowing Malvinas/Falkland Current, displacing Subantarctic Water (SW) and Antarctic Intermediate Water (AAIW).
The U-EEZ presents a wide continental shelf (ca. 142,000 km2), that widens towards the south. It has a gentle slope and the shelf break occurs on average water depth of 160 to 200m (Lantzsch et al., 2014; Urien and Ewing, 1974). The inner continental shelf is characterized by the paleo-valley (SW-NE direction) and located 40km from the Uruguayan coast (Lantzsch et al., 2014; Urien et al., 1980) which constitutes a mud depocenter (Lantzsch et al., 2014; Martins et al., 2003). The outer to mid shelf is characterized by a terrace-like shape and a smooth relief. Relict sand and/or shelly bottoms cover the shelf.
Straddling the continental margins of Uruguay and Argentina, the RdlP submarine canyon system is present between 35°S and 38°S (Lonardi and Ewing, 1971), comprising six canyons on the middle and upper slope, including a mega slide canyon (Cabo Polonio Mega Slide; Franco-Fraguas et al., 2014; Hernández-Molina et al., 2016). A well-developed Contourite Depositional System (CDS) showing erosive and depositional features associated with the action of Antarctic water masses and their interface is present in the slope and rise (Hernández-Molina et al., 2016; Hernández-Molina et al., 2009).
The lower slope and abyssal plain are dominated by mud deposits (Burone et al., 2021). This system must contribute to a high benthic species richness and emerging patterns of endemism at basin scales. For example, results from the 1971 cruise of the RV ATLANTIS II in the U-EEZ yielded several of the 112 bivalve species known between 200 m and 5,500 m depth, incorporating almost every known group occurring in other deep-ocean basins globally (Allen and Sanders, 1997; Allen, 2008). Of these, 38 species have only been recorded in the Argentine Basin, suggesting a potentially high degree of endemism, at least for deep-sea bivalves (Scarabino et al., 2015). Similarly, recent work synthesizing information on the known diversity of deep-water Ascidiacea (Scarabino et al., 2018), Pycnogonida (Scarabino et al., 2019), and Bryozoa (Ramalho et al., 2022) supports this general pattern.
2.2 What are we mapping?
Our broad-scale benthic mapping is aimed at delimiting preliminary large Benthic Regions (BRs) comprising a variety of benthic habitats, defined as physically distinct areas of the sea bottom associated with (and shaped by) benthic communities or assemblages (sensu Fauth et al., 1996). These communities consistently occur along the same key environmental or ecological variables. Thus, our BRs can be analogous to “Biotopes”, a commonly used term referring to the combination of both biotic and abiotic elements, and “Seascapes” (i.e., suites of habitats that consistently occur together). We used environmental surrogates and biological data to define large-scale regions within the U-EEZ. These large-scale BRs should represent “areas of relatively homogeneous species composition, clearly distinct from adjacent systems…”, and their “…species composition is likely to be determined by the predominance of a small number of ecosystems and/or a distinct suite of oceanographic or topographic features” (Spalding et al., 2007). Thus, the dominant forcing agents defining the benthic community structure of BRs are depth, salinity, and temperature, interplaying with the straddling geomorphological settings, surface sedimentation, and other processes. In this line, we combined biological data and the spatial distribution of environmental variables to define our BRs.
2.3 Environmental data
The following benthic environmental input data were selected: Bathymetry, Water Mass Structure, and Salinity. Ancillary variables used for interpretation at region scale include Geomorphology, Surface Sediments, Fine bathymetric position index (FBPI) and current velocity. The bathymetry of the study area was obtained from the General Bathymetric Map of the Oceans (GEBCO, 2021), which have a resolution of c.a. 450 m. Mean benthic salinity at mean depth for 2000-2014 period, at 5 x 5 arc min resolution, was obtained from Bio-ORACLE v2. Finally, the McQuaid et al. (In Press) criteria were used to interpret the effect of temperature and to produce a spatially explicit model of the distribution of bottom water masses at the Atlantic Ocean benthic scale.
These Environmental variables were categorized a priori based on already established criteria. Regarding bathymetry, 11 depth range categories were used. For the continental shelf, up to 50 m depth, and following Scarabino (2006), bathymetric categories from 0 m to 10 m and 10 m to 50 m were defined. From 50 m deep, were used those isobaths commonly used as reference in the scientific literature of the area (Rodriguez et al., 2014; Burone et al., 2021) (Figure 1). For salinity, average bottom salinity values were used, and three salinity categories were considered, from 27 to 31.5, from 31.5 to 33.5, and from 33.5 to 36, following Defeo et al. (2009). For dominant water masses, the McQuaid et al. (In Press) model of the distribution of bottom water masses, which considered 8 water masses categories in the study area was used. These water masses categories were interpreted according to Burone et al. (2021), based on Sverdrup et al. (1942), Stramma & England (1999) and Peterson & Stramma (1991), from which surface (0 m-500 m) and intermediate (500 m-1200 m) water masses are reported, corresponding to Tropical Water (TW), South Atlantic Central Water (SACW), and Antarctic Intermediate Water (AAIW) respectively. From 1,200 m to 4,000m, the seafloor is under the influence of Upper Circumpolar Deep Water (UCDW), North Atlantic Deep Water (NADW), and Lower Circumpolar Deep Water (LCDW), in descending order. Finally, Antarctic Bottom Water (AABW) dominates the seabed from 4,000 m deep and up.
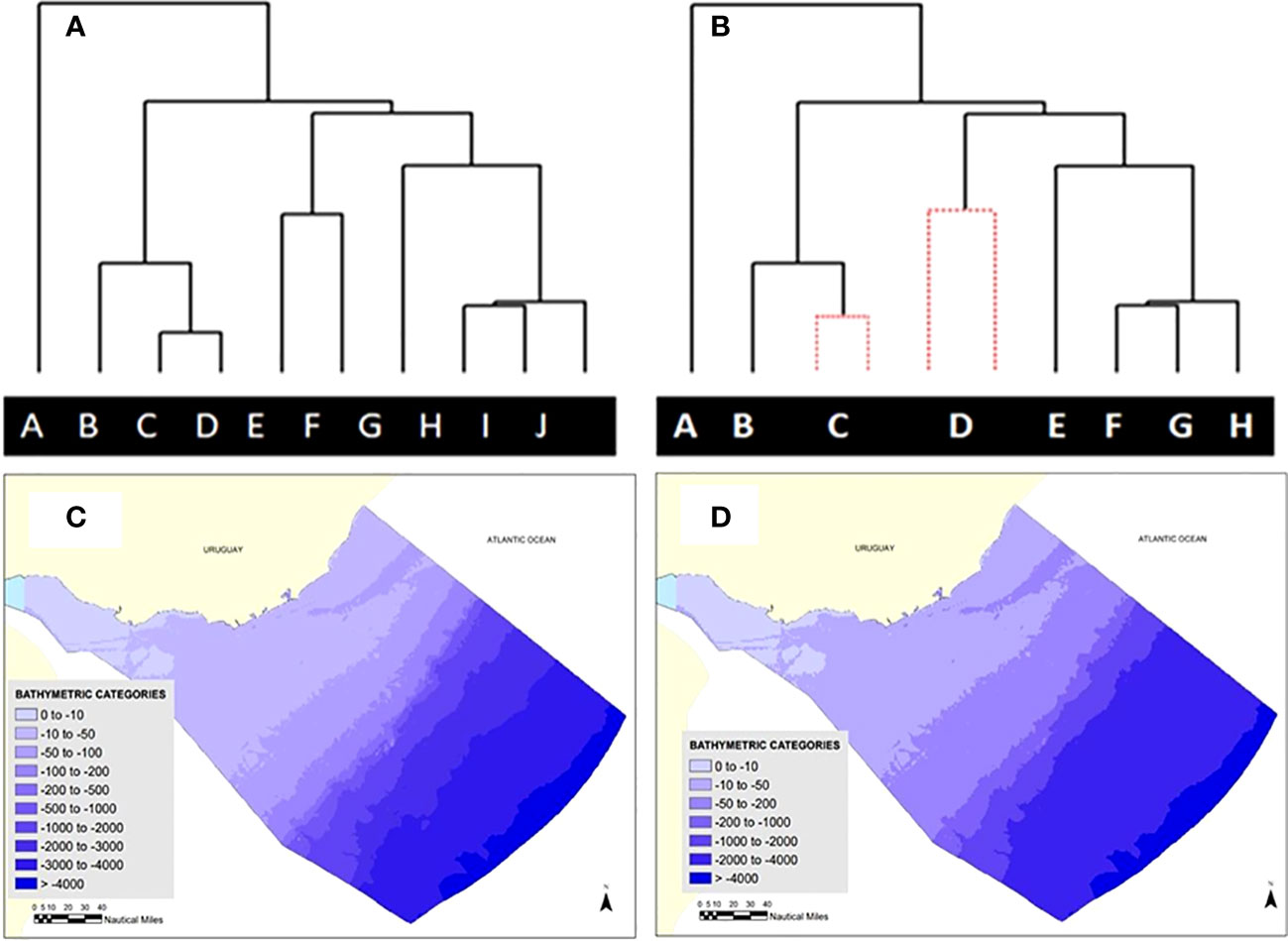
Figure 1 (A) Original cluster (Group Average Link) using the Bray-Curtis Similarity Index, of categories of environmental variables according to the multivariate community structure based on Primary Biodiversity Records (PBRs); (B) SIMPROF routine highlights no remaining structure for some pairs of the original categories (no significant difference in community structure). New categories are constructed merging two or more original categories (C) Maps of the distribution of original depth categories and (D) recategorized according to their effects in community structure. This procedure was applied also for Salinity and Water Masses categories. Each of the uppercase letters in Figure 1 represents a bathymetric category.
Ancillary variables were used a posteriori to aid in the description of BRs. For Geomorphological features, data from Harris et al. (2014) digital seafloor geomorphic features map (GSFM) of the global ocean were used. The GSFM includes 131,192 separate polygons in 29 geomorphic feature categories, used here to assess differences between passive and active continental margins as well as between 8 major ocean regions (the Arctic, Indian, North Atlantic, North Pacific, South Atlantic, South Pacific and the Southern Oceans and the Mediterranean and Black Seas).
Shapefiles for surface sediments for the continental shelf and the upper part of the slope were obtained from the National Environmental Observatory (FREPLATA, 2004). At this stage, we were unable to use the sediment shapefiles for the definition of benthic regions due to the incomplete coverage of the EEZ, the coarse grain of the sediment categories, and the lack of correlations between mapped substrate type and species traits (e.g., hard-substrate vs. soft substrate species) at small spatial scales. Mean particulate organic carbon flux to the seafloor was used as a proxy of primary productivity. For the period 2006-2015 (mmol. C m-2 d-1; 5 x 5 arc min), output from the MEDUSA model (Yool et al., 2013) re-gridded from ORCA0083 to NEMO 5 arc min. Finally, the mean bottom current velocity at mean depth for the period 2000-2014 (m.s-1) at 5 x 5 arc min resolution was obtained from Bio-ORACLE v2.
2.4 Biological data
A database with Primary Biodiversity Data (PBD) for benthic invertebrates of the U-EEZ was collected. Specifically, only georeferenced records of benthic species, emphasizing those taxa/groups that are useful indicators of particular benthic environments were included. A primary database with 4,942 records was obtained. Of these, 44.5% were obtained from biodiversity information networks (OBIS and GBIF), while the remaining 55.5% were obtained from scientific literature and other databases, including fishery-related data. After quality checking, 3,585 records of benthic invertebrates remained. This database includes 2,065 records from the phylum Mollusca, followed in decreasing order by Arthropoda, Annelida, Echinodermata, Cnidaria, Porifera, Chordata, Bryozoa, Nematoda, Hemichordata, and Brachiopoda (Table 1). The database includes 1,126 lower taxa (included in 753 genera) throughout the study area (Figure 2A).
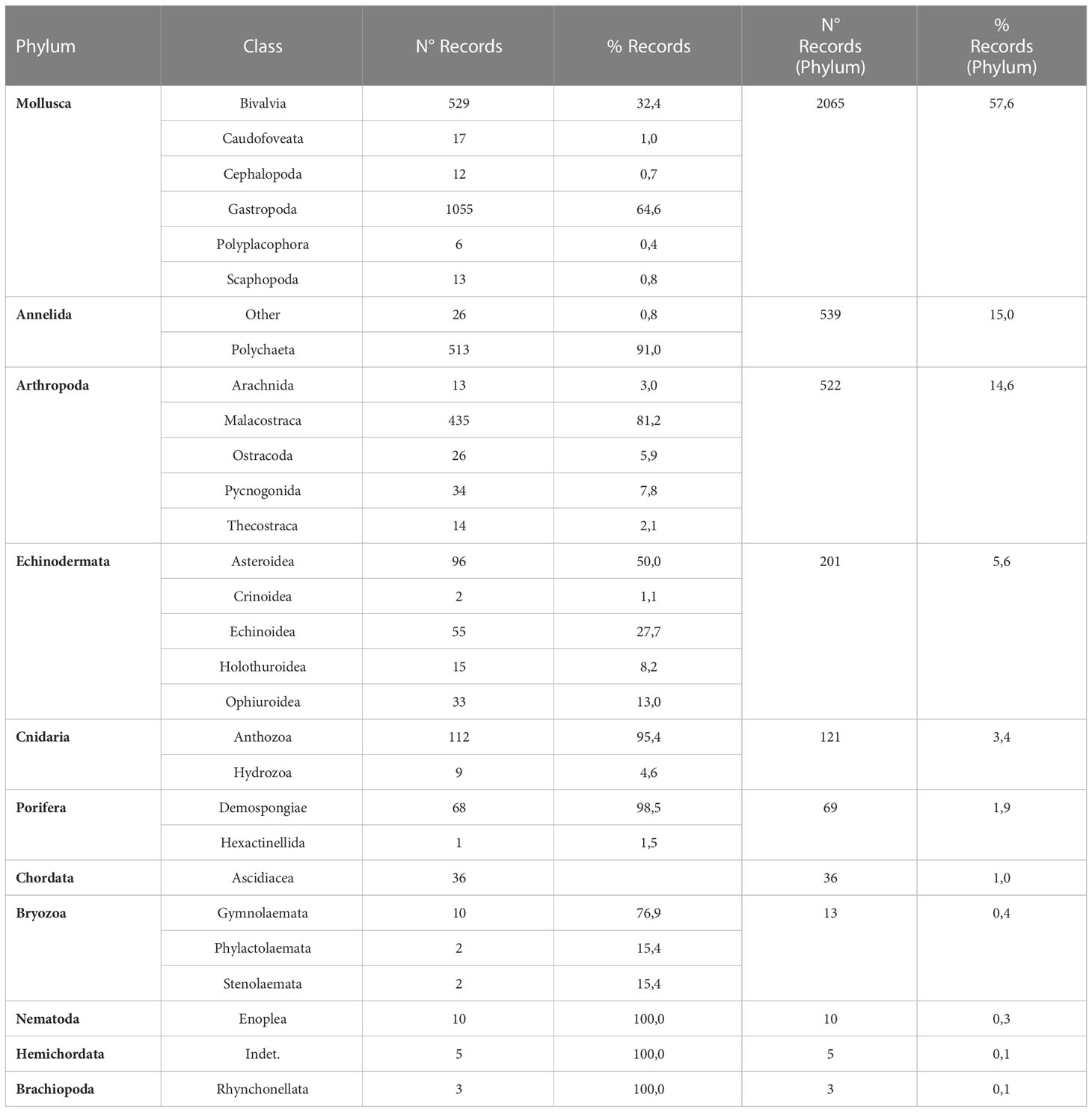
Table 1 Number and percentage of records included in the curated biological database, according to phylum and class.
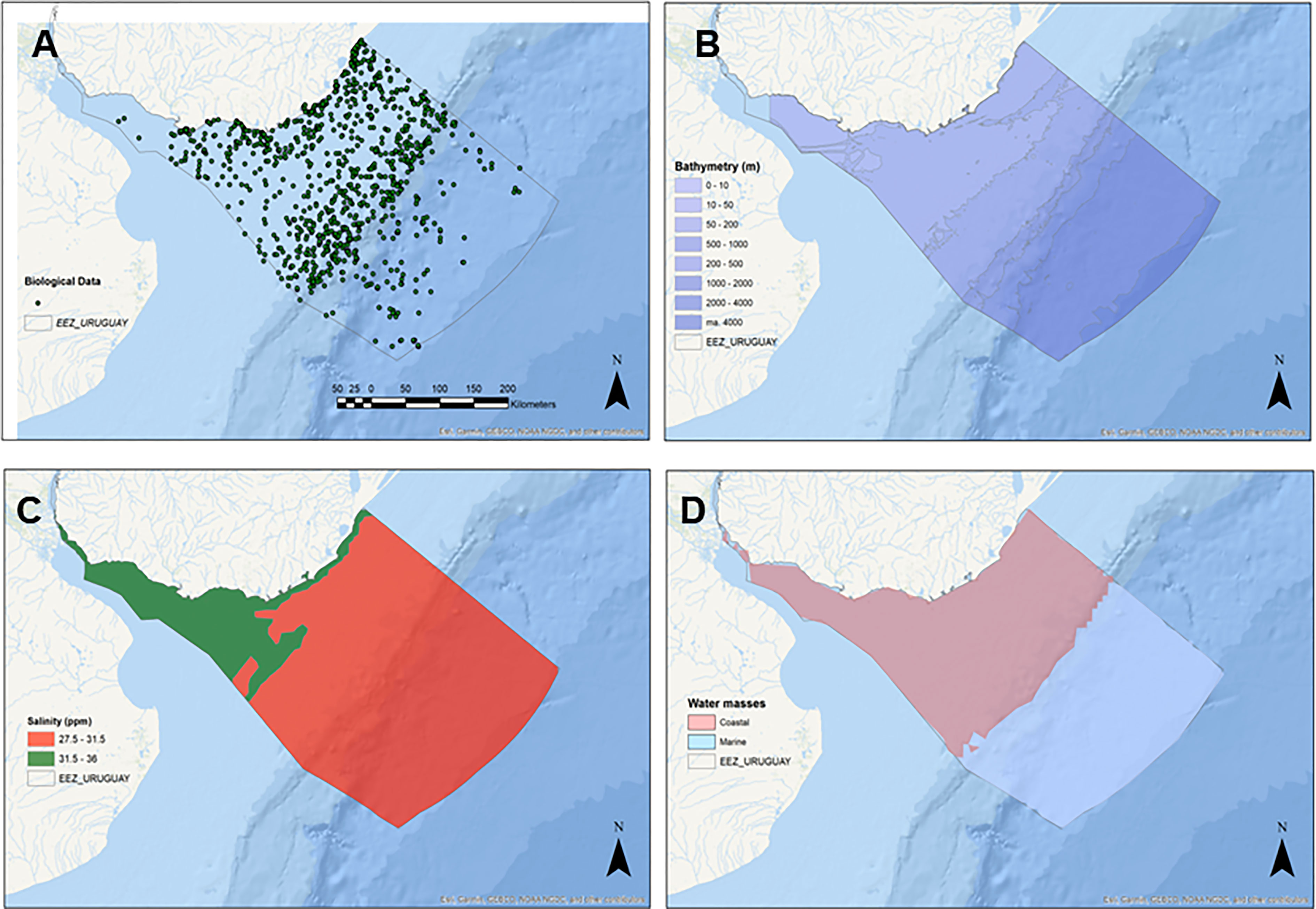
Figure 2 (A) Spatial distributions of Primary Biodiversity Records (PBRs) representing 3,585 records from 1,126 “Taxa” across 11 metazoan Phyla; Significant bathymetric (B), Water masses (C) and salinity (D) regions according to the multivariate structure of the benthic community.
2.5 Benthic regions definition
The structuring variables were analyzed using the PRIMER v7 software (Plymouth Routines in Multivariate Ecological Research) (Clarke et al., 2008) to assess the potential effect of the categories defined a priori on the multivariate structure of the benthic species assemblage. As defined above, each category in the environmental variables was treated as “samples” in a CLUSTER analysis. Operatively, a similarity matrix (from each Taxa by Category matrix) was created, and subsequently, the similarity between “samples” was calculated using the Bray-Curtis index (Clarke et al., 2006). This index is used in ecology to quantify the compositional dissimilarity between two sites. For cluster analysis, agglomerative hierarchical clustering was used. Then, the significance of the clusters was evaluated using the similarity profile (SIMPROF) routine to assess whether there is a natural structure in the subset of data corresponding to each branch of the dendrogram. The (SIMPROF) is a permutation procedure that orders the similarities in the composition of a group of samples and then orders these similarities based on their ranks (Clarke et al., 2008). The structure can be considered an objective basis for exploring those subgroups of each structuring variable. For each of the environmental variables (bathymetry, water mass structure, and salinity), 6 CLUSTER/SIMPROF analyzes were performed using the following datasets: 1) Raw data, species level 2) Presence/absence, species level 3) Raw data, genera level 4) Presence/absence, genera level 5) Raw data, without rare species (only species with more than one record) and 6) Presence/absence without rare species (only species with more than one record). Presence/absence analysis minimize biases in the database due to overrepresentation of certain species (e.g., over-represented species). After SIMPROF, only those groupings with statistical support (that is, at least supported by 60% of all the analysis, see Supplementary Table 1) represented new categories and were robust against known sampling biases. Based on this criterion, the initial categories of bathymetry, salinity, and water masses were redefined (Figure 2).
Once the new categories were obtained, each biological record can be associated with a combination of different bathymetric, salinity and water mass categories. Each unique occurring combination was interpreted as a potential benthic region. Finally, the statistical significance of the different possible combinations was evaluated based on the clusters obtained through the non-parametric analysis of similarity (ANOSIM). The statistical significance of this coefficient was obtained through a randomization process, and a reference value of p < 0.05 was chosen ANOSIM (Somerfield et al., 2021).
2.6 Approaches for defining boundaries and connection with existing ecological classification systems
Several respected and widely utilized global and regional ecological classification systems exist, and the lack of agreement between such systems can be problematic. In developing a new system, we sought to minimize further divergence from existing systems yet still obtain a truly useful national bioregionalization. We did this by (a) defining boundaries utilizing the Nature Conservancy’s system (Lateral limits of the Uruguay Bs Aires Shelf Ecoregion) and McQuaid et al. (In Press) for Abyssal regions within the U-EEZ and Continental Shelf extension. In this vein, Spalding et al. (2007) commented on the heavy influence of data from nearshore and intertidal biotas, recognizing that deep sea biogeographic patterns may hide the patterns represented by MEOW. In this line, the deeper most section of our study area was explicitly correlated with the South Atlantic Benthic regionalization presented by McQuaid et al. (In Press), and several boundaries are based in layers also used there to increase correspondence between both systems. Further, our model can be nested within the MEOW scheme but also fits neatly within the Large Patagonia Marine Ecosystem.
3 Results
A system comprising 9 BRs, obtained by combining the 7 “significant” bathymetric categories with the two water masses and two salinity categories is here proposed (Table 2 and Figure 3). Previously, from the original 11 resultant BRs, 2 were merged with adjacent regions due to the small spatial extent and the very low number of biological records. The grouping obtained shows statistical significance regarding group similarity (ANOSIM; p < 0.05). The BR system, which covers the sea bottom within the Uruguay-Buenos Aires Shelf Ecoregion, limiting westwards with the Argentinean EEZ and extending 370 kilometers (200 nautical miles, nm) offshore. At this scale, this classification is thus exclusively based on the benthos and the spatial distribution of key structuring variables. Coastal areas and the outer region of the RdlP Ecoregion were included in the present classification. However, our system is intended to be used beyond the Exclusive jurisdiction strip in the RdlP (7 nm) and Territorial Sea (12 nm) in the Atlantic Ocean. This is due to both practical issues (e.g., different management regimes) and a stronger emphasis on collating Primary Biodiversity Data from the “offshore” areas. There is, however, a wealth of biological and environmental data for the RdlP, gathered by different institutions in Argentina and Uruguay and bilateral agencies. GIS-ready layers including biological and environmental data, as well as the Benthic Regions model, are provided as Supplementary Data.
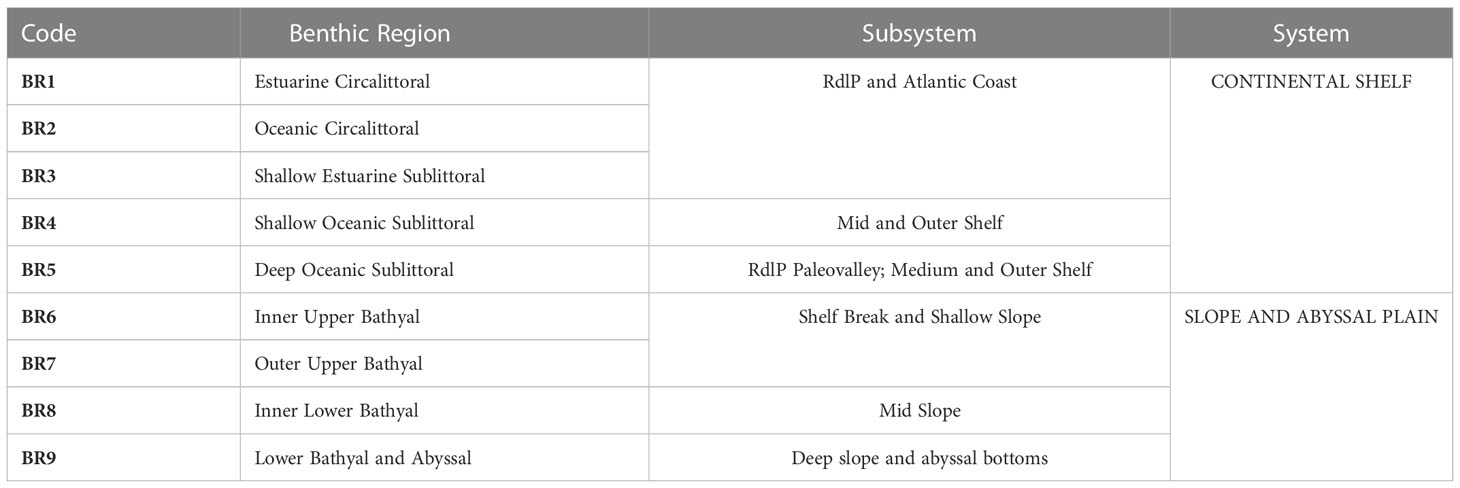
Table 2 Summary of Benthic Regions for the Uruguayan EEZ, according to Systems and Subsystems recognized in the Region.
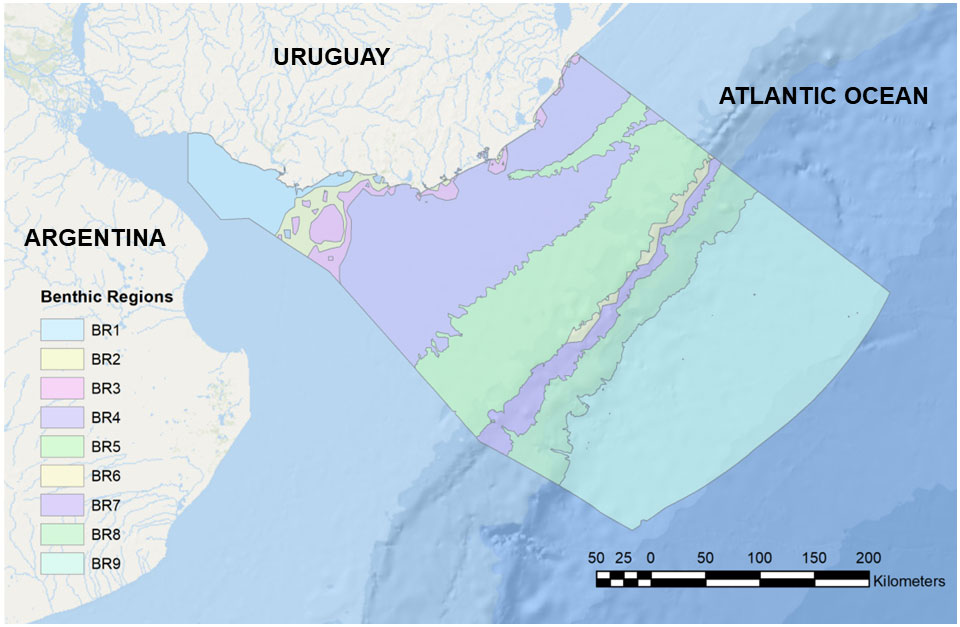
Figure 3 Benthic Regions (BRs) for the Uruguayan EEZ, with outlined boundaries. BRs are numbered and named in Table 1.
Some three bioregions (BR1 to BR3) are predominantly coastal and include areas within the RdlP. These were defined by the interplay between different bathymetric and salinity conditions, and included often intertidal or semi/terrestrial PBD. BR1 and BR2 are limited by the 10 m isobath and dominated by salinities less than 31.5. BR2 occupies part of the RdlP estuary and the Atlantic coast. BR3 (2,367.6 km2) is located between 10 m and 50 m deep, with salinities below 31.5. This BR is distributed in patches along the shallow sublittoral, presenting the largest patch towards the RdlP estuary, extending towards the shelf near the maritime limit with Argentina. The patchy nature of their distribution along the coast is related to local freshwater discharge from coastal lagoons and rivers. As stated above, we will not deal in detail with this set of Coastal and Estuarine BRs.
The remaining portions of the inner, middle and outer Continental Shelf are included chiefly in two BR (BR4 and BR5). The shallowest region, BR4 (Oceanic Shallow Sublittoral) is found between 10m and 50m deep, with a predominance of “Surface” waters (TW and SASW) and salinities above 31.5 ppm (“Marine”). This BR encompasses 32,989.529 km2, equivalent to approximately 27% of the area of the study zone. This BR is dominated by sand and muddy sediments. In the core of the region, the action of the STSF generates high-energy areas which favor the predominance of coarser sediments (Franco-Fraguas et al., 2014). Regarding the geomorphological characteristics of the region, 4 small basins occupy 375.4 km2 and scattered and poorly mapped patches of “hard” bottoms are also present. Unfortunately, due to limitations in our biological database, we could not explicitly correlate these different sedimentological and geomorphological features with benthic community structure.
In deeper waters, the Deep Oceanic Sublittoral (BR5) is located between 50 m and 200 m deep, encompassing the inner shelf zone and the edge of shelf break and slope, with an extension of 27,332.12 km2, (22.3% of the study area). There is a predominance of “Surface” waters (TW, SACW, SASW, and Subtropical Shelf Water (STSW)), although there are also areas under the influence of Subantarctic Waters (SAW), but with salinities greater than 31.5 ppm. This BR is particularly heterogeneous, presenting a great diversity of species and different geomorphological processes. The BR5 is also dominated by soft bottoms, mainly sand, followed by silt. A large part of the continental discharge of the RdlP is deposited along clayey-silt facies known as “mud belts” (Piola et al., 2005; Campos et al., 2008). Two “mud belts” are identified. The largest constitute the RdlP Paleo-valley (Urien and Ewing, 1974), which occupies 7.9% of the BR. Further, 23 “basins” (as defined by Harris et al., 2014) of various sizes have been identified, ranging from 3.9 km2 to 342.5 km2, occupying 7.2% of the BR. Other relevant geomorphological features include the heads of 5 Submarine canyons (Including the Cabo Polonio Mega-Slide canyon), which are dominated by fine sands (Franco-Fraguas et al., 2014). Additionally, nine pockmarks, possibly associated with methane release or cold seeps (eg. Suess, 2020) were recently described for this BR (López-Orrego et al., 2011). Regarding the benthic composition, 370 taxa representing 9 phyla were registered in the database. Some biogeographic patterns in gastropod assemblages are also observed within BR4. Adelomelon beckii, Tonna galea, and Z. dufresnei are distributed in the shallower and eastern portion, whereas species of Subantarctic or Magellanic origin (Odontocymbiola magellanica, Adelomelon ancilla, Fusitriton magellanicus) are distributed in deeper and southern regions (e.g., Carranza et al., 2008). This can be correlated with the interplay between different water masses, in particular, TW, which dominate the East of the shelf and the SACW, flowing north and occupying mainly the southern area of the shelf (Figure 4).
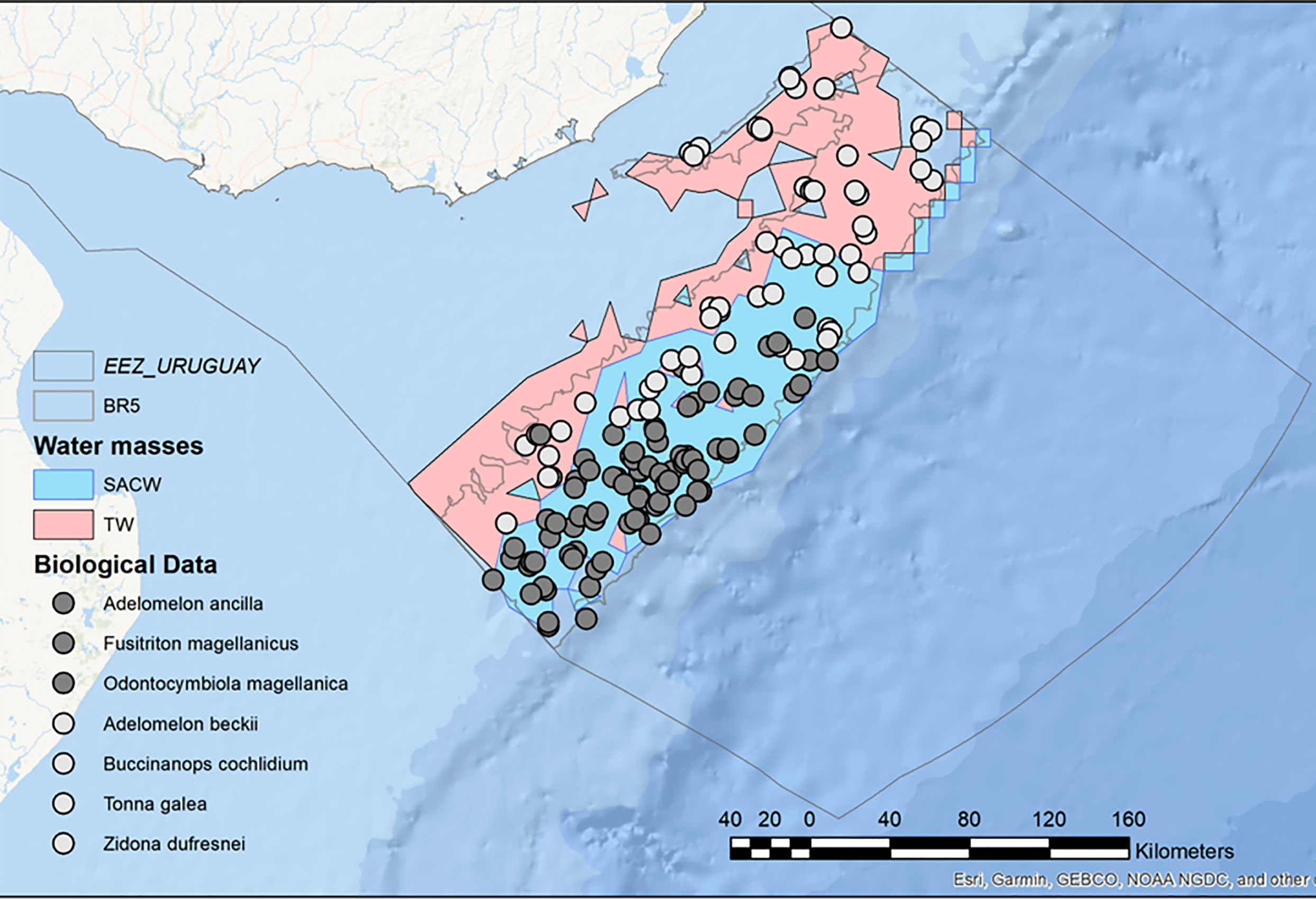
Figure 4 Detail of Benthic Region 5 (BR5, Deep Oceanic Sublittoral) for the Uruguayan EEZ, highlighting the existence of two different benthic assemblages associated with contrasting water masses.
The remaining benthic regions (BR6 to BR9) include the continental margin, from the shelf break and slope to the abyssal plain. BR6 (Inner Upper Bathyal) covers an area of 1,475.70 km2, equivalent to 1.2% of the study area. It is found between 200 m and 1000 m deep, bathed by “Deep” water masses, possibly associated with the STSF and AAIW, and salinities above 31.5 ppm. It occupies the area of the continental shelf break and the beginning of the slope; despite its small size, this is one of the BR with the greatest heterogeneity of biological records per area unit, as well as geomorphological and hydrological processes. The high energy associated with the STSF and AAIW water masses, mainly to the northeast of the U-EEZ, promotes erosion in the shelf break and the head of the canyons to the northeast (Franco-Fraguas et al., 2014). Biological records from this BR included species of economic interest such as Chaceon notialis (red crab) and Zygochlamys patagonica (scallop), and several Vulnerable Marine Ecosystems (VME) indicator species, such as the Scleractinian reef-forming coral Desmophylum pertusum (Carranza et al., 2012), associated with mounds and areas with high current velocities (Hebbeln et al., 2016; Figure 5). Additionally, the presence of 24 pockmarks is worth noting. The sedimentological composition is predominantly sandy, mainly towards the south of the BR (Franco-Fraguas et al., 2014). The shelf break and shallow slope are high-energy zones associated with strong hydrological processes (Franco-Fraguas et al., 2014; Bender et al., 2013). In this context, BR6 experience the highest bottom current speed, probably associated with SACW and TW water masses (Ortega and Martínez, 2007; Franco-Fraguas et al., 2014).
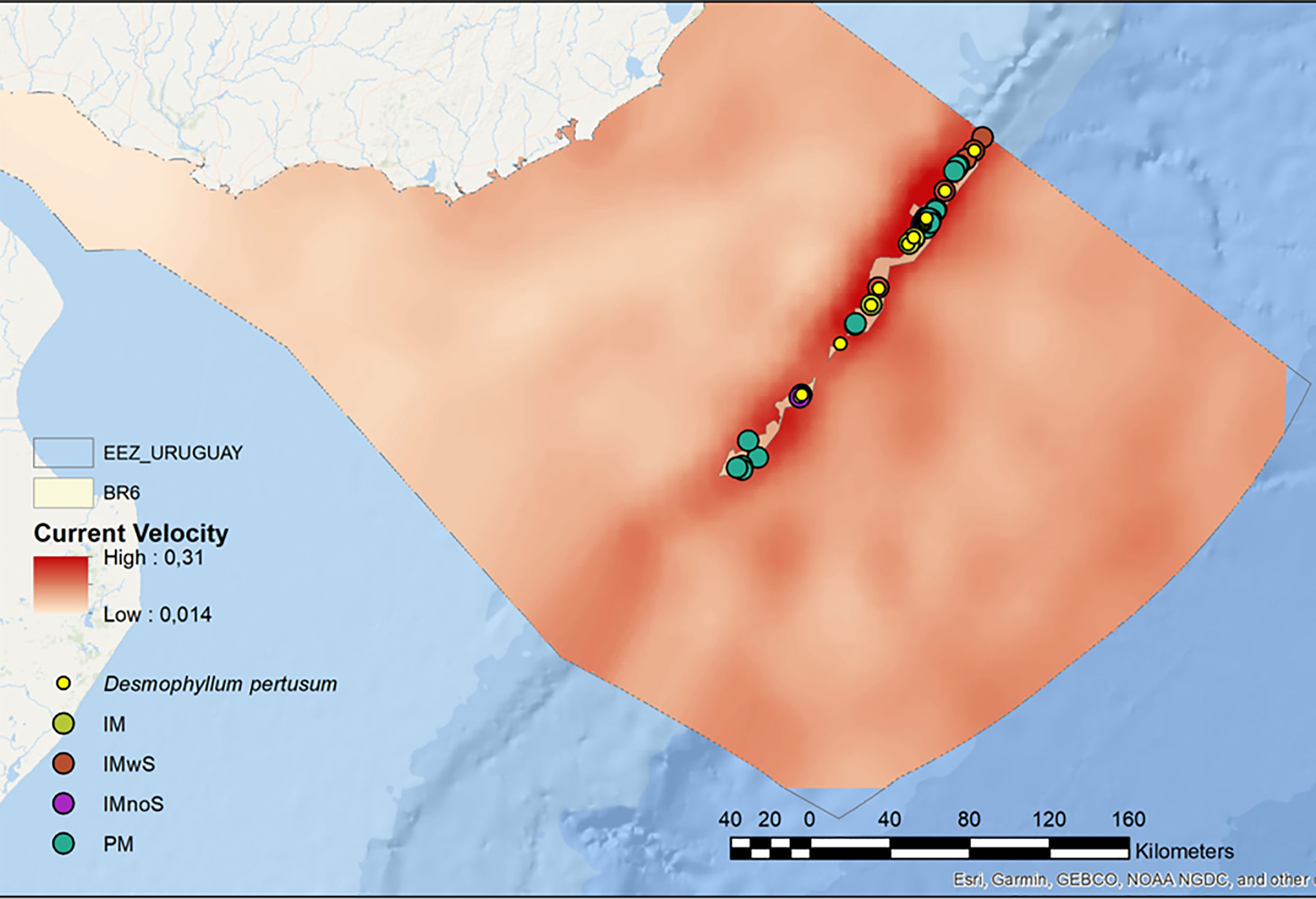
Figure 5 Benthic Region 6 (BR6, Inner Upper Bathyal) for the Uruguayan EEZ, showing the association of cold-water corals with high bottom current velocities. IM = Isolated Mound; IMwS= Isolated mound with scarpment; IMnoS= Isolated mound whithout scarpment and PM= Pockmarks.
The Outer Upper Bathyal region (BR7) is found between 200 m and 1000 m deep, and has an extension of 5,278.04 km2, which corresponds to 4.3% of the study area. There is a predominance of “Deep” water masses (same as in BR6) and salinities above 31.5 ppm. BR7 includes submarine canyons, which occupy 21.5% of the surface area and the steepest area of the shelf break. As in BR6, this BR has areas with high current speeds, where some VME indicator species are found, such as the sea pen Umbellula indet. (Penatulacea), the Scleractinian corals Flabellum spp. and Bathelia candida, as well as a single record of the chemosynthetic polychaete Lamellibrachia victori (Mañé-Garzón and Montero, 1986), which is associated with chemosynthetic environments such as cold seeps or methane releases (Gardiner and Hourdez, 2003; Cowart et al., 2014).
The Inner Lower Bathyal, BR8 covers an area of 11,745.45 km2, which is equivalent to 9.6% of the study area, in depths ranging from 1000 m to 2000 m, under the influence of “Deep” water masses associated with UCDW, NCAW and LCDW and salinities greater than 31.5 ppm. Submarine canyons occupy 26.7% of the BR, and geomorphological layers pointed to the existence of a seamount. A total of 111 taxa were recorded, represented by 7 phyla. Within this BR are several records of VME indicator species associated with reducing and/or chemosynthetic environments and species of economic interest such as the Red Crab Chaceon notialis.
Outer Bathyal and Abyssal areas are represented in BR9. This BR occupies part of the lower slope and abyssal bottom, with an extension of 49,240.6 km2, (40.3% of the study area). The oceanographic system is governed by the BMC, with a predominance of UCDW (2000 m to 2500 m deep), NADW (2500 m to 3500 m), LCDW (3500 m to 4000 m), and AABW (4000 m to 5000 m). From the geomorphological point of view, submarine canyons occupy 22% of the BR, and large extensions of submarine terraces are noteworthy (6.6% of surface area). The region is influenced by a large contour depositional system (CDS), with erosive characteristics from the south and progradation to the north (Franco-Fraguas et al., 2014; Burone et al., 2021). Surprisingly, 326 taxa are reported for this BR, represented by 9 phyla, mainly Mollusca and Arthropoda.
4 Discussion and perspectives
Uruguay has recently decided to advance towards the goal of 30% of marine protected areas by 2030 in a marine spatial planning process named Uruguay Azul 2030. It is of utmost importance that, during the process, the government has access to (and participates in generating) the best information and technical solutions available to prevent the implementation of ill-designed and poorly supported MPAs. Thus, aside from the research community, the most important end-users of our results will be the national agencies National Directorate of Biodiversity and Ecosystem Services (DINABISE, ME) and the National Directorate of Aquatic Resources (DINARA) of the Ministry of Livestock, Agriculture, and Fisheries (MGAP). In addition, ongoing collaborative activities related with the design and implementation of novel MPAs are being undertaken with technical staff from the National Museum of Natural History (MEC -MNHN), the Uruguayan Navy, and University of the Republic.
In this process, the access to Primary Biodiversity Data of certain benthic organisms may be particularly relevant, and several Regional Fisheries Management Organizations (RFMOs) are currently using an agreed list of VME indicator species, and relationships can also be identified between those species and listed habitat types and biotopes. For example, the EUNIS (Moss, 2008) habitat classification scheme comprises over a thousand biotope-based habitats nested over multiple scales. It provides full benthic habitat coverage for the marine environment. Although our study area is not included under RFMOs schemes, the Commission for the Conservation of Antarctic Marine Living Resources (CCAMLR) area or the Southwestern Atlantic has developed some basic guidance on VME indicator species. Thus, we based our VME indicator species and putative habitat types on the recommendations made by EUNIS (ICES, 2016) and CCAMLR.
Our results provide an initial model of distinct ecological complexes in U-EEZ. However, caution should be exerted when using this model during the decision-making process. For example, implementing use restrictions of benthic areas should rely on further ground-truthing, including direct seafloor observations and collections. It is of utmost importance since our system analyzes accumulated historical data that may or may not represent the current condition of habitats of interest. In this line, an interesting feature of our study area is the relative wealth of PBD in deeper areas since data availability tends to be commonly reduced with distance from coastal habitats. However, the northern portion of the shell break and the upper slope has been more thoroughly mapped, and high-resolution bottom topography is only available for this area.
Finally, protecting representative samples of all habitat types, ideally including areas with high density, biomass, significant three-dimensional structures, and chemosynthetic discrete environments, should be the priority target of a National MPA system, also taking into consideration other features of the areas such as the development of human activities (e.g. fisheries) and the links with ecosystem processes and demersal or non-benthic biota (Cordes et al., 2016). This tool and other measures must be urgently developed to avoid or minimize adverse impacts of current and potential impacts of anthropic activities within the U- EEZ.
Data availability statement
The original contributions presented in the study are included in the article/Supplementary Material. Further inquiries can be directed to the corresponding author.
Author contributions
PL is a MSc. Student supervised by AC and LB. AC and PL collected the data, designed and performed the research, analyzed the data, and wrote the paper. SH contributed with GIS analysis and figures. LO contributed with oceanographic data. All authors contributed to the article and approved the submitted version.
Funding
PL acknowledges partial Msc Scholarship from CAP (Comisión Académica de Posgrado), Universidad de la Republica, UdelaR, Uruguay.
Acknowledgments
A. Masello, Y. Marín and F. Scarabino are acknowledged for providing fruitful discussion and insights.
Conflict of interest
The authors declare that the research was conducted in the absence of any commercial or financial relationships that could be construed as a potential conflict of interest.
Publisher’s note
All claims expressed in this article are solely those of the authors and do not necessarily represent those of their affiliated organizations, or those of the publisher, the editors and the reviewers. Any product that may be evaluated in this article, or claim that may be made by its manufacturer, is not guaranteed or endorsed by the publisher.
Supplementary material
The Supplementary Material for this article can be found online at: https://www.frontiersin.org/articles/10.3389/fmars.2023.1130827/full#supplementary-material
References
Allen J. A., Sanders H. L. (1997). The zoogeography, diversity and origin of the deep-sea protobranch bivalves of the Atlantic. Epilogue: Prog. Oceanogr. 38, 95–153. doi: 10.1016/S0079-6611(96)00011-0
Bender V. B., Hanebuth T. J., Chiessi C. M. (2013). Holocene shifts of the subtropical shelf front off southeastern south america controlled by high and low latitude atmospheric forcings. Paleoceanography 28 (3), 481–490.
Bisbal G. A. (1995). The southeast south American shelf large marine ecosystem: Evolution and components. Mar. Policy 19, 21–38. doi: 10.1016/0308-597X(95)92570-W
Botsford L. W., Hastings A., Gaines S. D. (2001). Dependence of sustainability on the configuration of marine reserves and larval dispersal distance. Ecol. Lett. 4, 144–150. doi: 10.1046/j.1461-0248.2001.00208.x
Brito-Morales I., Schoeman D. S., Molinos J. G., Burrows M. T., Klein C. J., Arafeh-Dalmau N., et al. (2020). Climate velocity reveals increasing exposure of deep-ocean biodiversity to future warming. Nat. Climate Change 10, 576–581. doi: 10.1038/s41558-020-0773-5
Burone L., Franco-Fraguas P., Carranza A., Calliari D., de Mahiques M., Gómez M., et al. (2021). Physical drivers and dominant oceanographic processes on the Uruguayan margin (Southwestern atlantic): A review and a conceptual model. J. Mar. Sci. Eng. 9, 304. doi: 10.3390/jmse9030304
Carranza A., Scarabino F., Brazeiro A., Ortega L., Martinez S. (2008). Assemblages of megabenthic gastropods from uruguayan and northern argentinean shelf: Spatial structure and environmental controls. Continental Shelf Res. 28 (6), 788–796.
Campos E. J. D., Mulkherjee S., Piola A. R., Carvalho F. (2008). A note on a mineralogical analysis of the sediments associated with the plata river and patos lagoon outflows. Continental Shelf Res. 28, 1687–1691. doi: 10.1016/j.csr.2008.03.014
Carranza A., Recio A. M., Kitahara M., Scarabino F., Ortega L., López G., et al. (2012). Deep-water coral reefs from the Uruguayan outer shelf and slope. Mar. Biodivers. Records 42, 411–414. doi: 10.1007/s12526-012-0115-6
CBD (2020). Global biodiversity outlook 5 - summary for policy makers (Montréal: Secretariat of the Convention on Biological Diversity).
Clarke K. R., Somerfield P. J., Chapman M. G. (2006). On resemblance measures for ecological studies, including taxonomic dissimilarities and a zero-adjusted bray-Curtis coefficient for denuded assemblages. J. Exp. Mar. Biol. Ecol. 330, 55–60. doi: 10.1016/j.jembe.2005.12.017
Clarke K. R., Somerfield P. J., Gorley R. N. (2008). Testing of null hypotheses in exploratory community analyses: Similarity profiles and biota-environment linkage. J. Exp. Mar. Biol. Ecol. 366, 56–69. doi: 10.1016/j.jembe.2008.07.009
Cordes E. E., Jones D. O., Schlacher T. A., Amon D. J., Bernardino A. F., Brooke S., et al. (2016). Environmental impacts of the deep-water oil and gas industry: A review to guide management strategies. Front. Environ. Sci. 4. doi: 10.3389/fenvs.2016.00058
Cowart D. A., Halanych K. M., Schaeffer S. W., Fisher C. R. (2014). Depth-dependent gene flow in gulf of Mexico cold seep lamellibrachia tubeworms (Annelida, siboglinidae). Hydrobiologia 736, 139–154. doi: 10.1007/s10750-014-1900-y
Defeo O., Horta S., Carranza A., Lercari D., de Álava A., Gómez J., et al. (2009). Hacia un manejo ecosistémico de pesquerías (Áreas Marinas Protegidas en Uruguay: Montevideo, Facultad de Ciencias-DINARA), 122 p.
de Mello C., Burone L., Ortega L., Franco-Fraguas P., Lahuerta N., Mahiques M., et al. (2014). Benthic foraminiferal distributions on the uruguayan continental margin (South-western atlantic) and controlling environmental factors. Continental Shelf Res. 91, 120–133.
Fauth J. E., Bernardo J., Camara M., Resetarits W. J. Jr., Van Buskirk J., McCollum S. A. (1996). Simplifyng the jargon of community ecology: A conceptual approach. Am. Nat. 147, 282–286. doi: 10.1086/285850
Franco-Fraguas P., Burone L., Mahiques M., Ortega L., Urien C., Muñoz A., et al. (2014). Hydrodynamic and geomorphological controls on surface sedimentation at the subtropical shelf Front/Brazil-malvinas confluence transition off Uruguay (Southwestern Atlantic continental margin). Mar. Geol. 349, 24–36. doi: 10.1016/j.margeo.2013.12.010
FREPLATA. (2004). “Análisis Diagnóstico Transfronterizo del Río de la Plata y su Frente Marítimo”. Technical Report. Proyecto Protección Ambiental del Río de la Plata y su Frente MarÍtimo. PNUD/GEF/RLA/99/G3
Gaines S., Gaylord B., Largier J. (2003). Avoiding current oversights in marine reserve design. Ecol. Appl. 13 (1), 32–46. doi: 10.1890/1051-0761(2003)013[0032:ACOIMR]2.0.CO;2
Gaines S. D., Lester S. E., Grorud-Colvert K., Costello C., Pollnac R. (2010). Evolving science of marine reserves: New developments and emerging research frontiers. Proc. Natl. Acad. Sci. U.S.A. 107, 18251–18255. doi: 10.1073/pnas.1002098107
Gardiner S. L., Hourdez S. (2003). On the occurrence of the vestimentiferan tube worm lamellibrachia luymesi van der land and nørrevang 1975 (Annelida: Pogonophora) in hydrocarbon seep communities in the gulf of Mexico. Proc. Biol. Soc. Washington 116, 380–394.
GEBCO Compilation Group (2021). GEBCO 2021 Grid (GEBCO Compilation Group). doi: 10.5285/c6612cbe-50b3-0cff-e053-6c86abc09f8f
Gianelli I., Ortega L., Marín Y., Piola A. R., Defeo O. (2019). Evidence of ocean warming in uruguay’s fisheries landings: The mean temperature of the catch approach. Mar. Ecol. Prog. Ser. 625, 115–125.
Harris P. T., Baker E. K. (2012). “Why map benthic habitats? in Seafloor geomorphology as benthic hábitat.” in GeoHab Atlas of Seafloor Geomorphic Features and Benthic Habitats (Amsterdam, Netherlands; Cambridge, MA: Elsevier), 3–22.
Harris P. T., Macmillan-Lawler M., Rupp J., Baker E. K. (2014). Geomorphology of the oceans. Mar. Geol. 352, 4–24. doi: 10.1016/j.margeo.2014.01.011
Hebbeln D., Van Rooij D., Wienberg C. (2016). Good neighbours shaped by vigorous currents: Cold-water coral mounds and contourites in the north Atlantic. Mar. Geol. 378, 171–185. doi: 10.1016/j.margeo.2016.01.014
Hempel G., Sherman K. (2003). Large Marine ecosystems of the world: Trends in exploitation, protection, and research (Amsterdam: Elsevier).
Hernández-Molina F. J., Soto M., Piola A. R., Tomasini J., Preu B., Thompson P., et al. (2016). A contourite depositional system along the Uruguayan continental margin: Sedimentary, oceanographic and paleoceanographic implications. Mar. Geol. 378, 333–349. doi: 10.1016/j.margeo.2015.10.008
Hernández-Molina F. J., Paterlini M., Violante R., Marshall P., de Isasi M., Somoza L., et al. (2009). Contourite depositional system on the argentine slope: An exceptional record of the influence of antarctic water masses. Geology 37, 507–510.
ICES (2016). Report of the workshop on the vulnerable marine systems database (WKVME) (Peterborough, UK: ICES CM 2015/ACOM), 62.
Lantzsch H., Hanebuth T. J., Chiessi C. M., Schwenk T., Violante R. A. (2014). The high-supply, current-dominated continental margin of southeastern south america during the late quaternary. Quaternary Res. 81 (2), 339–354.
Lonardi A. G., Ewing M. (1971). “Sediment transport and distribution in the Argentine basin. 4. bathymetry of the continental margin. Argentine basin and other related provinces. canyons and sources of sediments,” in Physics and chemistry of the earth (Oxford: Pergamon Press), 79–121.
López-Orrego G., De Mello C., Marín Y. (2011). “Mapeo del fondo marino del talud continental superior y medio de Uruguay,” in Reunión regional de acústica - AUA 2011 (Uruguay: Montevideo). Available at: http://hdl.handle.net/1834/4336.
Mañé-Garzón F., Montero R. (1986). “Sobre una nueva forma de verme tubícola lamellibrachia victori n. sp. (Vestimentifera),” in Proposición de un nuevo phylum: Mesoneurophora (Revista de Biologia de Uruguay), vol. 8 (1), 1–28.
Marín Y. H., Defeo O., Horta S. (2021). So far and so close: Opportunities for marine spatial planning in the southwest atlantic ocean. Ocean Coast. Manage. 211, 105737.
Martins L. R., Martins I. R., Urien C. M. (2003). Aspectos sedimentares da plataforma continental na área de influência do rio de la plata. Gravel 1, 68–80.
Matano R. P., Palma E. D., Piola A. R. (2010). The influence of the brazil and malvinas currents on the southwestern atlantic shelf circulation. Ocean Sci. 6 (4), 983–995.
McQuaid K., Bridges A. E. H., Howell K. L., Gandra T. B. R., Souza V.D., Currie J. C., et al. “Broad-scale benthic habitat classification of the south Atlantic,” in Progress in oceanography. In Press.
Moss D. (2008). EUNIS habitat classification–a guide for users (European Topic Centre on Biological Diversity).
Nicholson E., Watermeyer K. E., Rowland J. A., Sato C. F., Stevenson S. L., Andrade A., et al. (2021). Scientific foundations for an ecosystem goal, milestones and indicators for the post-2020 global biodiversity framework. Nat. Ecol. Evol. 5, 1338–1349. doi: 10.1038/s41559-021-01538-5
Ortega L., Martínez A. (2007). Multiannual and seasonal variability of water masses and fronts over the Uruguayan shelf. J. Coast. Res. 23, 681–629. doi: 10.2112/04-0221.1
Peterson R. G., Stramma L. (1991). Upper-level circulation in the south Atlantic ocean. Prog. Oceanogr. 26, 1–73. doi: 10.1016/0079-6611(91)90006-8
Piola A. R., Matano R. P., Palma E. D., Möller O. O., Campos E. J. D. (2005). The influence of the plata river discharge on the western south atlantic shelf. Geophys. Res. Lett. 32, L01603. doi: 10.1029/2004GL021638
Ramalho L. V., López-Gappa J., Scarabino F. (2022). Checklist of the marine bryozoa from Uruguay (Southwest Atlantic). Rev. del Museo Argentino Cienc. Naturales (Nueva serie) 24, 09–134.
Rodriguez M., Lafanconi A., Scarabino F., Carranza A., Muñiz P. (2014). “Bentos, Uruguay, mar territorial,” in Programa oceanográfico de caracterización del margen continental de la república oriental del Uruguay (Montevideo, Uruguay: ANCAP), 255–288.
Scarabino F. (2006). “Faunística y taxonomía de invertebrados bentónicos marinos y estuarinos de la costa uruguaya,” in Bases para la conservación y el manejo de la costa uruguaya. Eds. Menafra R., Rodríguez-Gallego L., Scarabino F., Conde D. (Montevideo, Uruguay: VIDA SILVESTRE (Sociedad Uruguaya para la Conservación de la Naturaleza), 113–142.
Scarabino F., Maggioni T., Taverna A., Lagger C., Schwindt E., Orensanz L., et al. (2018). Ascidiacea (Chordata, tunicata) from Uruguay (SW atlantic): Checklist and zoogeographic considerations." revista del museo argentino de ciencias naturales Bernardino rivadavia. Hidrobiología 20, 251–270.
Scarabino F., morim Lucena R., Munilla T., Soler-Membrives A., Ortega L., Schwindt E., et al. (2019). Pycnogonida (Arthropoda) from Uruguayan waters (Southwest atlantic): Annotated checklist and biogeographic considerations. Zootaxa 4550 (2), 185–200.
Scarabino F., Zelaya D. G., Orensanz J. M. L., Ortega L., Defeo O., Schwindt E., et al. (2015). Cold, warm, temperate and brackish: Bivalve biodiversity in a complex oceanographic scenario (Uruguay, southwestern Atlantic). Am. Malacological Bull. 33, 1–18. doi: 10.4003/006.033.0219
Somerfield P. J., Robert Clarke K., Gorley R. N. (2021). A generalised analysis of similarities (ANOSIM) statistic for designs with ordered factors. Austral Ecol. 46, 901–910. doi: 10.1111/aec.13043
Spalding M. D., Fox H. E., Allen G. R., Davidson N., Ferdaña Z. A., Finlayson M., et al. (2007). Marine ecoregions of the world: A bioregionalization of coastal and shelf areas. BioScience 57, 573–583. doi: 10.1641/B570707
Stirling A., Burgman M. A. (2021). Strengthening conservation science as a crisis discipline by addressing challenges of precaution, privilege, and individualism: Conservation biology. J. Soc. Conserv. Biol. 35, 1738–1746. doi: 10.1111/cobi.13809
Stramma L., England M. (1999). On the water masses and mean circulation of the south atlantic ocean. . J. Geophysical Research: Oceans 104 (C9), 20863–20883.
Suess E. (2020). “Marine cold seeps: Background and recent advances,” in Hydrocarbons, oils and lipids: Diversity, origin, chemistry and fate, 747–767.
Sullivan K., Bustamante G. (1999). Setting geographic priorities for marine conservation in Latin America and the Caribbean. (Arlington, VA: The Nature Conservancy).
Sverdrup H. U., Johnson M. W., Fleming R. H. (1942). The oceans: Their physics, chemistry, and general biology Vol. 1087 (New York: Prentice-Hall).
Urien C. M., Ewing M. (1974). “Recent sediments and environments of southern Brazil, Uruguay, Buenos Aires and río Negro continental shelf,” in The geology of continental margins. Eds. Burk C., Drake C. H. (New York, NY, USA: Springer), 157–177.
Urien C. M., Martins L. R., Martins I. D. R. (1980). Evolução geológica do quaternário do litoral atlântico uruguaio, plataforma continental e regiões vizinhas. CECO/UFRGS Notas Técnicas 3, 1–43.
Vilela R., Conesa D., Del Rio J. L., López-Quílez A., Portela J., Bellido J. M. (2018). Integrating fishing spatial patterns and strategies to improve high seas fisheries management. Mar. Policy 94, 132–142. doi: 10.1016/j.marpol.2018.04.016
Keywords: marine spatial planning, benthic ecology, primary biodiversity data, southwestern Atlantic Ocean, benthic ecosystems
Citation: Limongi P, Ortega L, Horta S, Burone L and Carranza A (2023) Bioregionalization in a data-poor situation: Mapping of Uruguayan marine benthic regions. Front. Mar. Sci. 10:1130827. doi: 10.3389/fmars.2023.1130827
Received: 23 December 2022; Accepted: 30 January 2023;
Published: 17 February 2023.
Edited by:
Wen-Cheng Wang, National Taiwan Normal University, TaiwanReviewed by:
Judith Gobin, The University of the West Indies St. Augustine, Trinidad and TobagoThadickal V. Joydas, King Fahd University of Petroleum and Minerals, Saudi Arabia
Copyright © 2023 Limongi, Ortega, Horta, Burone and Carranza. This is an open-access article distributed under the terms of the Creative Commons Attribution License (CC BY). The use, distribution or reproduction in other forums is permitted, provided the original author(s) and the copyright owner(s) are credited and that the original publication in this journal is cited, in accordance with accepted academic practice. No use, distribution or reproduction is permitted which does not comply with these terms.
*Correspondence: Alvar Carranza, YWx2YXJkb2NAZmNpZW4uZWR1LnV5