- 1Groupement d'Intérêt Scientifique (GIS) Posidonie, Observatoire des Sciences de l'Univers (OSU) Pythéas, Marseille, France
- 2Center for Advenced Studies of Blanes, Spanish National Research Council (CSIC) Blanes, Girona, Spain
- 3Aix Marseille University, Université de Toulon, Centre National de la Recherche Scientifique (CNRS), Institut de Recherche pour le Développement (IRD), Mediterranean Institute of Oceanography (MIO) Marseille, France
- 4Seascape Ecology Laboratory, Department of Earth, Environment and Life Sciences (DiSTAV), University of Genoa, Genova, Italy
- 5Department of Integrative Marine Ecology (EMI), Stazione Zoologica Anton Dohrn–National Institute of Marine Biology, Ecology and Biotechnology, Genoa Marine Centre (GMC), Genova, Italy
- 6Institut Méditerranéen de Biodiversité et d'Ecologie Marine et Continentale (IMBE), Centre National de la Recherche Scientifique (CNRS), Institut de Recherche pour le Développement (IRD), Aix-Marseille University, Avignon University, Station Marine d’Endoume, Marseille, France
- 7Natural Marine Park of the Gulf of Lion, Argelès-sur-mer, France
- 8French Office for Biodiversity, Vincennes, France
- 9Université de Corse Pasquale Paoli, Centre National de la Recherche Scientifique (CNRS), Unité Mixte de Recherche (UMR) 6134 Science pour l'Environnement (SPE), Corte, France
Introduction: Coastal detrital bottoms (CDB) are one of the most extensive habitats of the continental shelf worldwide, in the upper levels of the circalittoral zone. Hosting a diverse community structured by sediment grain size, trophic interactions and calcified organisms, CDB exhibit important ecological functions. In the Mediterranean Sea, CDB are constituted by recent elements partly provided by adjacent infralittoral and circalittoral ecosystems. Since the 2010s, the offshore extension of many Marine Protected Areas (MPAs) has resulted in the incorporation of vast areas of CDB, raising the issue of their management. The Marine Strategy Framework Directive (MSFD) has embraced the concept of an ecosystem-based approach involving taking into account the functioning of marine habitats and their related ecosystem services. The purpose of this paper is to propose an ecosystem-based quality index (EBQI) tested on CDB from the north-western Mediterranean Sea, focusing mainly on epibenthic assemblages.
Methods: The first step has been to define a conceptual model of the CDB functioning, including the main trophic compartments and their relative weighting, then to identify appropriate assessment methods and potential descriptors. Twenty-nine sites were sampled along the coast of Provence and French Riviera (Southern France). Study sites were chosen with a view to encompassing a wide range of hydrological conditions and human pressures.
Results: Very well-preserved sites were found in Provence in areas without trawling and terrigenous inputs, while impacted and low-ES sites were located in the vicinity of urbanized areas. The cover of rhodoliths characterizes the seascape and might be an indicator of the good ES of CDB and reduced human pressure. However, the absence of rhodoliths may also be induced by natural phenomena.
Discussion: The EBQI designed for CDB proved representative and useful for a functional assessment based on epibenthic assemblages. However, some descriptors have shown their limitations and should be further explored. We highlight here the priority of establishing an index corresponding to a societal demand (e.g., European Directives, Barcelona convention) as a basis for a broad and large-scale assessment, for practical reasons. We stress the need to better apprehend the role of the macro-infauna and to extend this index over a wider geographical scale.
1 Introduction
Coastal detrital bottoms (CDB), sometimes also referred to as coastal (bio-)detritic bottoms in the literature, are sea bottoms composed of mineral and biogenic particles, characterized by a distinct structural complexity hosting sedimentary infauna together with epifauna and epiflora settled or not on coarser granules (Rees, 1999). They are considered as a distinctive biotope in the circalittoral zone of the continental shelf worldwide (Pérès, 1982; Konar et al., 2006; Amado-Filho and Pereira-Filho, 2012; La Rivière et al., 2021).
In the Mediterranean Sea, CDB are one of the most widespread ecosystems between 30 and 100 m depth, corresponding to the upper part of the circalittoral zone (Pérès and Picard, 1964; Pérès, 1967), and house a high alpha species diversity (Stamouli et al., 2022). Besides a mineral component (typically coarse sand with a variable proportion of finer particles), these bottoms contain significant quantities of recent organogenic and bioclastic sediments (remains from hard bottoms, shell debris, bryozoans or calcified macro-algae), either autogenous or imported from adjacent ecosystems (e.g., coralligenous reefs, photophilic rocky reefs, seagrass meadows). The structure and composition of CDB can therefore differ depending on adjacent ecosystems, light availability and hydrodynamic conditions (Pérès and Picard, 1964; Joher et al., 2015; Agnesi et al., 2020). The pelitic proportion (sediment grain< 63 µm) of the sediment is usually very low, but muddy CDB can be found (Bellan-Santini et al., 1994). Located within the same depth range as CDB, Muddy Detrital Bottoms (MDB) are characterized by a high sedimentation rate influenced by the terrigenous input from coastal rivers. MDB can be constituted by a compact mud including bioclastic shells, muddy sand, or sandy mud (Carpine, 1964; Pérès and Picard, 1964; Picard, 1965; Bellan-Santini et al., 1994; Michez et al., 2014; La Rivière et al., 2021). Deep Detrital Bottoms (DDB), generally between 80 and 250 m depth, correspond to fossilized detrital sediments from terrigenous (fluviatile) and bioclastic inputs corresponding to earlier environmental conditions and a lower sea level (Pérès and Picard, 1964; Bellan-Santini et al., 1994), covering the deepest part of the continental shelf. The sediment is a mix of pebbles, sand and mud but with a mud proportion significantly higher than in the CDB. Coarser sediment is made of calcareous remains from thanatocenoses of the Pleistocene ice ages (Laborel et al., 1961; Pérès and Picard, 1964; Bourcier and Zibrowius, 1973; Michez et al., 2011; Michez et al., 2014). Distinguishing the boundary between CDB and muddy or deep detrital ecosystems is sometime challenging.
Pérès and Picard (1964) based their description of CDB on the infauna, which contain the most characteristic species; however, the same authors and others (Costa, 1960; Joher et al., 2015; La Rivière et al., 2021) identified a number of CDB facies on the basis of epibenthic species, especially macroalgae, either erect or encrusting. The former include perennial and seasonal species, such as Cystoseira spp. C.Agardh, Ericaria spp. Stackhouse, Gongolaria spp. Boehmer, Osmundaria volubilis (L.) R.E.Norris, etc.; the latter are represented by the Corallinales Lithophyllum racemus (Lamarck) Foslie, Lithothamnion corallioides (P.Crouan & H.Crouan) P.Crouan & H.Crouan, L. crispatum Hauck, L. minervae Basso, L. valens Foslie, Neogoniolithon hauckii (Rothpletz) R.A.Townsend & Huisman, Spongites fruticulosa Kützing, the Peyssonneliales Peyssonnelia crispata Boudouresque & Denizot, P. heteromorpha (Zanardini) Athanasiadis, P. rosa-marina Boudouresque & Denizot, P. rubra (Greville) J.Agardh, P. squamaria (S.G.Gmelin) Decaisne ex J.Agardh, and the Sporolithales Sporolithon mediterraneum Heydrich, among others (Huvé, 1954; Huvé, 1956; Carpine, 1958; Jacquotte, 1962; Boudouresque and Denizot, 1973; Ballesteros, 1994). Free-living calcified macroalgae forming nodules of varied morphology, referred to as ‘rhodoliths’ (or more specifically ‘maerl’ in the case of branched growth forms), gave rise to a great interest among benthic ecologists (Sciberras et al., 2009; Basso et al., 2017; Deidun et al., 2022). Their conservation value is recognized under EU rules and international conventions (Barbera et al., 2003; Salomidi et al., 2012). Marion (1883); Meinesz et al. (1983); Holon and Harmelin (2014) and La Rivière et al. (2021) described a CDB facies with large calcified bryozoans, such as Pentapora fascialis (Pallas, 1766), Smittina cervicornis (Pallas, 1766), Turbicellepora avicularis (Hincks, 1860), Hornera spp. Lamouroux, 1821, etc., but the highest diversity of bryozoans on CDB is found among small species (Harmelin, 2017). By analogy with calcareous rhodophytes, free bryozoan nodules are called bryoliths (James et al., 2006; Lombardi et al., 2014). This bryozoan facies is classified as a priority habitat according to the Barcelona Convention (Bianchi, 2009a). Recently, coralliths formed by the scleractinian coral Cladocora caespitosa (L., 1767), also considered of conservation interest (Morri et al., 2000; Bianchi, 2009b), have been described from coarse sandy bottoms in the Balearic Islands (Kersting et al., 2017a; Kersting et al., 2017b). Several octocoral species, such as Eunicella singularis (Esper, 1791), E. verrucosa (Pallas, 1766), Leptogorgia sarmentosa (Esper, 1791), Alcyonium palmatum Pallas, 1766, Pennatula rubra (Ellis, 1764), Pteroeides griseum (Bohadsch, 1761) and others, may also form epibenthic facies on detrital seabeds (Morri et al., 1991). Fauna and flora associated with all these epibenthic facies are sometimes very similar to coralligenous assemblages (Ballesteros, 2006; Pergent et al., 2015). The EUNIS typology defines as coralligenous platforms bioconstructed horizontal formations developing within sedimentary beds subject to currents, at down to at least 100 metres depth in clear waters (Davies et al., 2004; Bajjouk et al., 2015). These formations are not usually built on rock substrata but result from the active development of constructional organisms (e.g., calcified algae, hard-skeleton invertebrates) from scattered elements on loose beds, such as shells, stones, and gravel (Pérès and Picard, 1964).
Important ecological functions and ecosystem services, up to now poorly assessed, are provided by CDB (Ballesteros, 1994; Barbera et al., 2003): (i) primary production (Basso, 2012; Basso et al., 2012); (ii) key habitat and trophic resources for invertebrates and fish; (iii) breeding, spawning and nursery grounds for fish and crustaceans including species of fishery interest, sometimes close to the deep limit of the Posidonia oceanica (L.) Delile meadow (e.g., Mullidae, Sparidae, Scorpaenidae, Palinurus elephas (Fabricius, 1787)) (Harmelin and Harmelin-Vivien, 1976; Verlaque, 1990; Kamenos et al., 2004; Soykan et al., 2010); (iv) fishing ground for trawlers and artisanal fishers (Stamouli et al., 2022); (v) long term carbon sink (Basso, 2012; Basso et al., 2012; Savini et al., 2012; Burrows et al., 2014; Watanabe et al., 2020); (vi) recycling of necromass (e.g., rhizomes and dead Posidonia oceanica leaves and drift macroalgae) (Boudouresque et al., 2016); (vii) exportation and settlement of larvae towards or from adjacent ecosystems (source-sink hypothesis; Levin and Dayton, 2009). In addition, CDB host numerous protected species and other species of conservation interest (Astruch et al., 2012; Joher et al., 2015; Astruch et al., 2019): e.g., the fan shell Pinna nobilis L. 1758 (annexe II Berne convention and UICN Red list: critically endangered), the red coralline alga Lithothamnion corallioides (Annexe 5 of the HD: species of interest with regulation of its exploitation), and several Fucales (Annexe 2 of the Barcelona convention), among others. Management goals for maerl beds are different in the Mediterranean compared to the rest of the world, where extraction activities are carried out (Foster et al., 1997; Steller et al., 2003; Foster et al., 2013; Amado-Filho et al., 2017; Harvey et al., 2017).
Nowadays, like most coastal biocenoses, CDB are facing the impact of human activities and climate change. They are threatened by numerous pressures (Dutertre et al., 2015; Demestre et al., 2017) such as trawling (Bordehore et al., 2000; Bordehore et al., 2003; Fragkopoulou et al., 2021), artisanal or recreational fishing (Rendina et al., 2020), dredging (De Grave and Whitaker, 1999; Bermejo et al., 2018; Bernard et al., 2019), terrigenous input, dumping of sediment and waste (Aliani et al., 1994; Cocito et al., 1994), fish farming (Sanz-Lázaro et al., 2011; Aguado-Giménez and Ruiz-Fernández, 2012), consequences of coastal development, sea water warming, the spread of non-indigenous species (Klein and Verlaque, 2009; Katsanevakis et al., 2014; Martin and Hall-Spencer, 2017; Hall-Spencer and Harvey, 2019) and potentially acidification (Noisette et al., 2013).
Until recently, studies on CDB in the Mediterranean Sea were mainly focused on the description of infauna (e.g., Pérès and Picard, 1964; Picard, 1965; Somaschini et al., 1998), rhodolith beds and associated communities (e.g., Ledoyer, 1966; Ballesteros, 1994; Basso et al., 2017), and taxonomy (e.g., Boudouresque and Denizot, 1973; Souto et al., 2010), without any overview at ecosystem scale (e.g., Gautier and Picard, 1957; Jacquotte, 1962; Boudouresque and Denizot, 1973; Augier and Boudouresque, 1975; Laborel et al., 1976; Augier and Boudouresque, 1978; Harmelin, 1978; Bourcier, 1982; Bourcier, 1985; Bourcier, 1988; Fornos et al., 1988; Joher et al., 2012; Joher et al., 2015).
Despite the high ecological and heritage value of CDB and the many threats facing it, this ecosystem was not considered as a habitat of European interest (Bensettiti et al., 2004) by the Habitats Directive of the European Union (Natura 2000, 92/43/CEE) while some facies of CDB are described in EUNIS typology (Gayet et al., 2018) and are included in the Barcelona convention. Recently, the Habitats Directive has taken a step forward, considering rhodolith beds as a reef habitat (1170) if they host communities characteristic of hard substrates (De Bettignies et al., 2021). In France, the extension of the overall surface areas of Marine Protected Area (MPA) (national parks, Natura 2000 sites, etc.) (Petit, 2019), since the 2010s, has incorporated large expanses of seabed occupied by CDB. In accordance with these new management priorities, it has become a challenge to better take into account this widespread ecosystem.
Even with the improving accuracy of technologies (e.g., side-scan sonar, multibeam, remotely operated vehicles, autonomous underwater vehicles, etc.) (Bianchi et al., 2004; Astruch et al., 2012; Valette-Sansevin et al., 2019) and prediction models (Martin et al., 2014; Vassallo et al., 2018), the mapping of CDB is still challenging. The assessment of coastal water body masses, marine habitats or ecosystems involves numerous indices with different purposes (Bianchi et al., 2022). None of these indices alone offers an adequate basis for solving all the issues: specific descriptors may assess the ecological status linked with water quality (WFD; e.g., Borja et al., 2000; Borja et al., 2003; Ballesteros et al., 2007; Gobert et al., 2009; Lopez y Royo et al., 2010; Blanfuné et al., 2017; Piazzi et al., 2021), ecosystem functioning (descriptor 1 of the MSFD; e.g., Personnic et al., 2014; Montefalcone et al., 2015a; Giakoumi et al., 2015), seafloor integrity (descriptor 6 of the MSFD; e.g., Montefalcone et al., 2007; Gatti et al., 2015; Enrichetti et al., 2019; Piazzi et al., 2019), the conservation status of natural habitats (HD; e.g., Maciejewski et al., 2016; Sartoretto et al., 2017), natural and anthropogenic stressors (Holon et al., 2015; La Rivière et al., 2017; Ruitton et al., 2020).
Obviously, the future of coastal zone management policies will not be based on a single integrative index (Bond, 2001), but an appropriate combination of the most relevant ones (Pikaver et al., 2004; Paoli et al., 2016; Borja et al., 2021; Oprandi et al., 2021). Indicators are currently used to underpin guidelines for managers and stakeholders. In this context, the ecosystem-based approach should be deployed (Laffoley et al., 2004; Boudouresque et al., 2020a), with the aim of achieving a better understanding of ecosystem functioning as a basis for more suitable management strategies. Ecosystem-Based Quality Indices (EBQIs) have been already defined for a number of coastal marine ecosystems, namely Posidonia oceanica seagrass meadows (Personnic et al., 2014), coralligenous reefs (Ruitton et al., 2014), undersea caves (Rastorgueff et al., 2015) and rocky reefs (Thibaut et al., 2017). The aim of the present work is to develop and apply a new EBQI expressly designed for CDB (Astruch et al., 2022a). Although CDB is traditionally identified mostly on the basis of the study of the infaunal component, which requires sampling with grabs or dredges operated from large vessels, the approach presented here will focus on the epibenthic component, which can be studied with a lighter and less costly approach, i.e., photographic, video and/or visual techniques - to be privileged in a conservation context (Bianchi et al., 2022). The effectiveness of this novel index was tested along the coasts of Provence and French Riviera (France, Mediterranean Sea) (Figure 1).
2 Material and methods
2.1 Conceptual model of coastal detrital bottom functioning
A workshop involving 15 experts in benthic ecology (mainly the authors of the present work) was held the 2nd of April 2019 (i) to propose and validate a conceptual model of the CDB functioning, (ii) to define the weight of each functional compartment (=box) corresponding to its importance in the ecosystem, and (iii) to develop a sampling protocol with suitable operational descriptors to assess the different compartments. A DELPHI approach was implemented (Rowe and Wright, 1999). The conceptual model thus defined schematizes the functioning of CDB (Figure 2). The compartments of the conceptual model are described in the following pages.
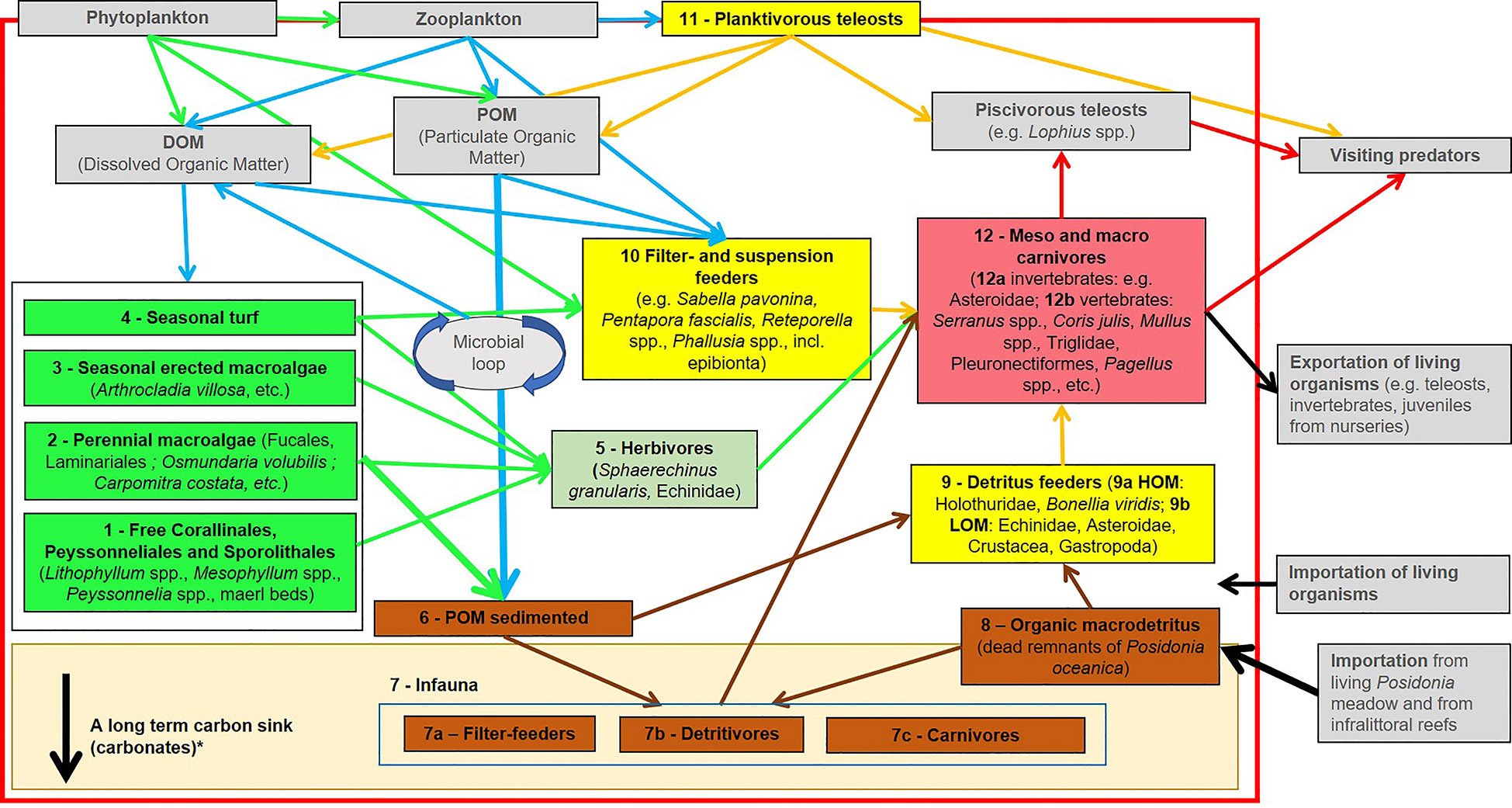
Figure 2 Conceptual functional model of the Coastal Detrital Bottom Ecosystem. Arrows correspond to a flux of organic matter between boxes. Light grey boxes are not assessed directly. *See corresponding paragraph for more details about Carbon sink.
2.2 Sampling strategy
Twenty-nine sampling sites were investigated (Table 1, Figure 1). Sampling took place between June and July 2020 and 2021 during the maximum development of the macroalgal communities (Piazzi et al., 2002). Site selection took into account different levels of pressures linked with human activities, from pristine-like to urbanized sites. The study area presents a gradient in hydrological conditions, involving the Rhône River to the west, the oligotrophic waters of eastern Provence and the run-offs in the urbanized French Riviera.
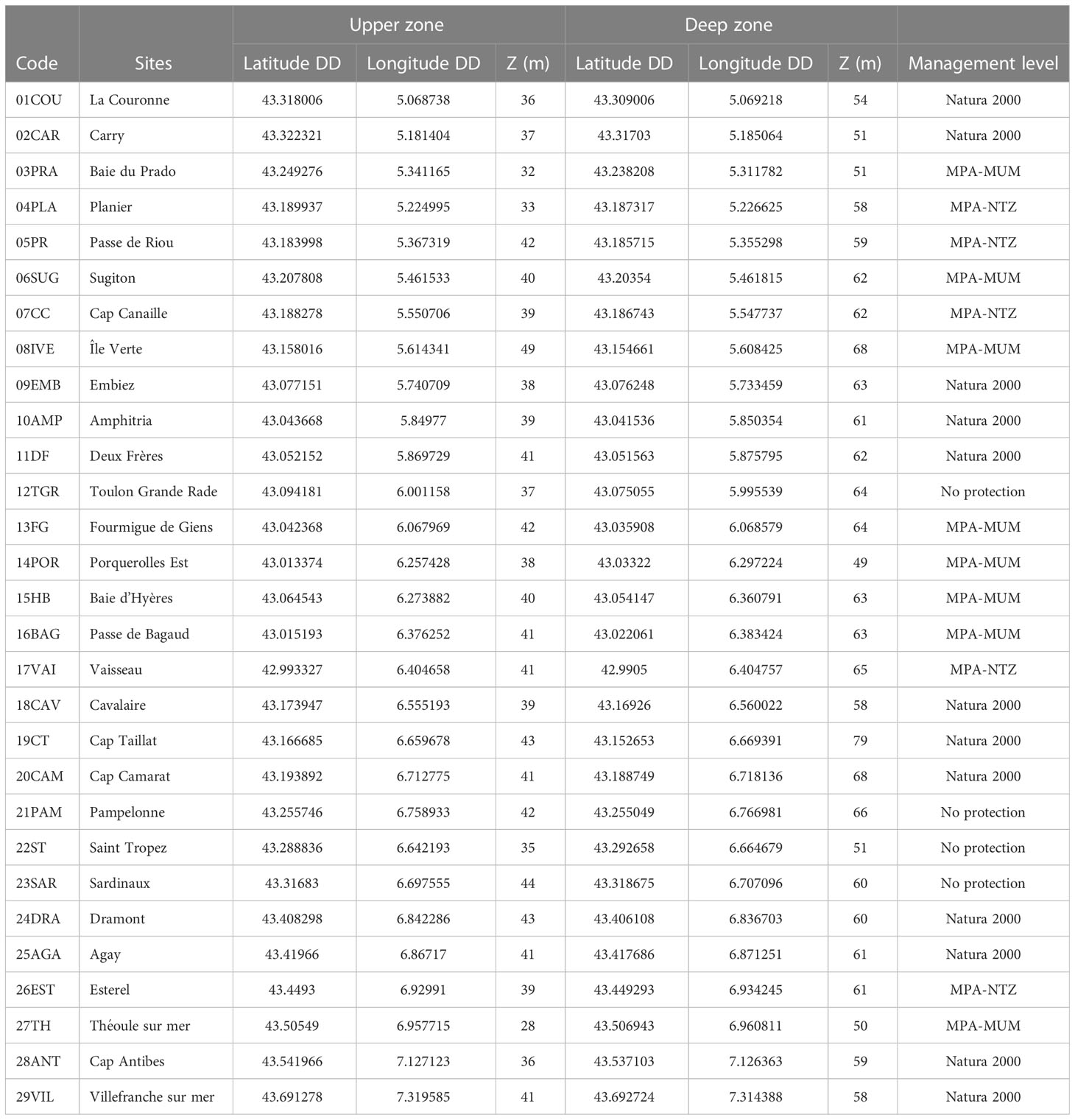
Table 1 Sampling sites position (DD: Decimal degrees), depth at upper and deep zones (m) and management level (MPA, Marine Protected Area; NTZ, No-take Zone; MUM, Multi-use Management).
Two depth ranges were sampled at each site: the upper zone (between 28 and 43 m, according to the lower limit of the P. oceanica meadow), and the deep zone (between 48 and 79 m depth). Upper sites were selected far enough away from P. oceanica meadows to avoid the transitional zone and ensure a correct assessment of CDB compartments.
Sampling was carried out by SCUBA diving, photoquadrats and seascape video. In the upper zone, observations were carried out by scuba divers for inventory of in situ recognizable taxa and assessment of their abundance. Each dive corresponds to the observation of 2 divers during ~12 minutes. Assuming the movements of the divers at an average speed of ~10 m·min-1 (including stops) observing the bottom on a 2 m wide strip, each dive corresponds to an investigated surface area of ~500 m². Three cores of sediment were sampled per site to assess organic matter and sediment grain size. In both upper and deep zone, an original device, the ‘Biocube’ (Figure 3), was deployed to sample: (i) photoquadrats with an 80 cm × 80 cm sized frame using a compact camera with a 15 s time lapse. On-board video monitoring using a GoPro® Hero 3 was used to control the position of the quadrat during sampling. Each quadrat was randomly positioned according to the drift of the operating boat. (ii) Seascape videos were taken during the photoquadrat sampling. The aim of the diversity of sampling methods is to provide complementary observations at the scale of each sampling site for a standardized assessment.
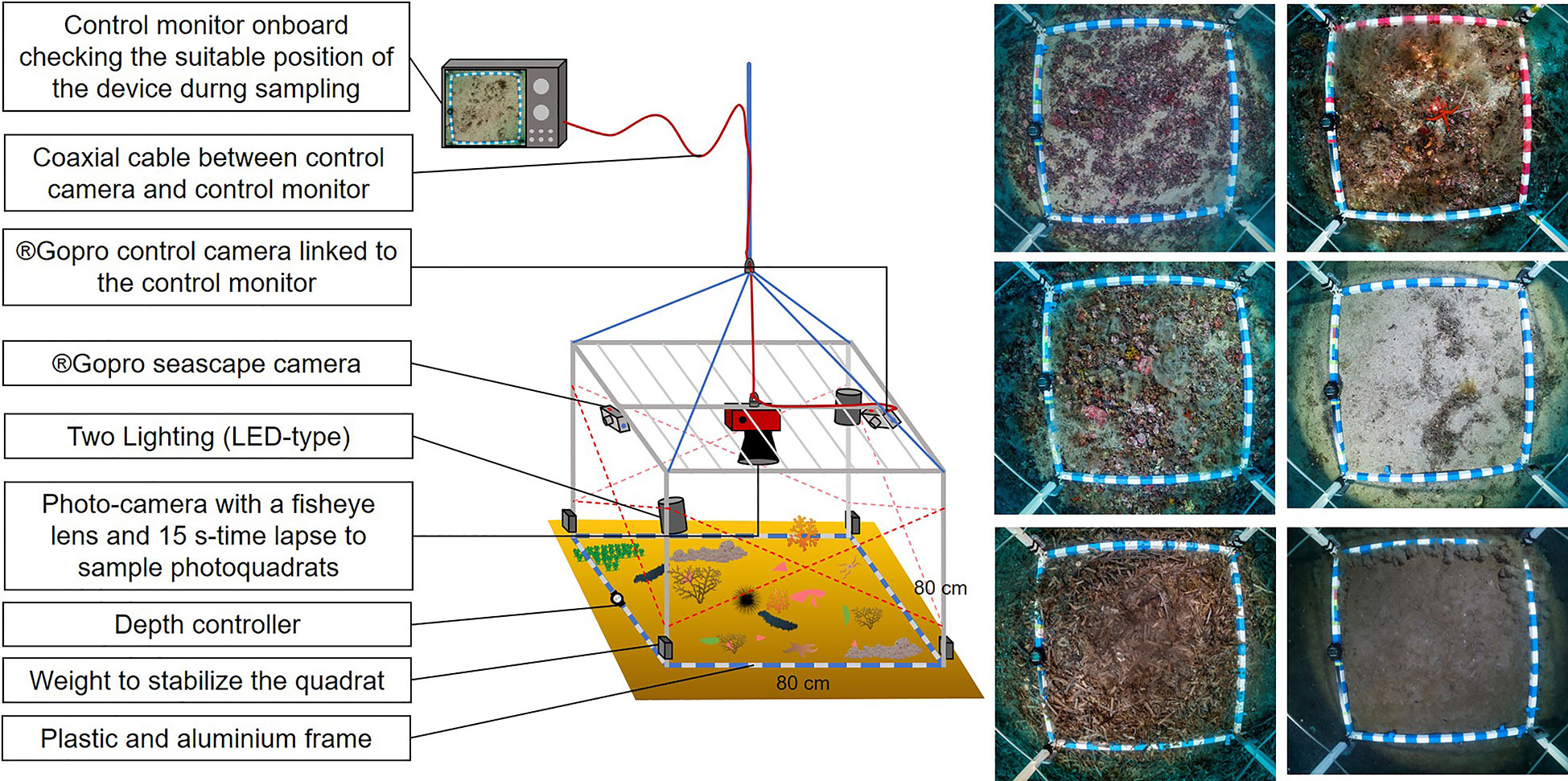
Figure 3 Diagram of the Biocube device. The pictures on the right show different types of CDB sampled on photoquadrats.
2.3 Compartments assessed
2.3.1 Box 1: Rhodoliths: Free living corallinales, peyssonneliales, and sporolithales
This compartment is involved in the structuring of the ecosystem by increasing sediment grain size and providing a support for other compartments (see boxes 2, 3, 10 in Figure 2). Among Corallinales, several species can be found such as Lithothamnion corallioides. Peyssonneliales can be calcareous or not, several species occur in CDB, such as Peyssonnelia rosa-marina (Boudouresque and Denizot, 1973) or P. squamaria. The Sporolithale Sporolithon mediterraneum is a frequent rhodolith species of CDB. For some species, in situ identification is challenging or impossible and collection is needed. The cover naturally decreases according to depth and the corresponding reduction of light availability. In oligotrophic and clear waters, rhodolith beds can be found down to 80 m depth (Ballesteros, 1994). Aguilar et al. (2009) described rhodolith beds at 140-150 m depth around the Balearic Islands. Rhodoliths play an important role in the deep benthic fixing and sequestration of organic and inorganic carbon (Basso, 2012; Basso et al., 2016). The cover (mean percentage) of living rhodoliths appears to be the easiest descriptor to assess the structure of CDB, which conditions its functioning in both the upper and the deep zones (Bosellini and Ginsburg, 1971; Basso et al., 2016).
2.3.2 Box 2: Perennial non-calcified macroalgae
The abundance of perennial non-calcified macroalgae can be linked to good ecological conditions and the absence of significant pressures (mechanical erosion, terrigenous inputs, diminution of light). Fucales (Sargassum spp., Ericaria spp., Gongolaria spp., Cystoseira spp.), Laminariales (Laminaria rodriguezii Bornet), Osmundaria volubilis and Carpomitra costata (Stackhouse) Batters are among the most representative taxa (Gautier and Picard, 1957; Joher et al., 2012; Thibaut et al., 2016; Aouissi et al., 2018; Bermejo et al., 2018; Bruno de Sousa et al., 2019; Jódar-Pérez et al., 2020; Reynes et al., 2021). These long-lived species provide a stand for seasonal macroalgae (boxes 3 and 4) and sessile fauna (box 10), a habitat (boxes 9 and 12) and a trophic resource (box 5). They contribute significantly to the primary production of the ecosystem (Ballesteros, 1990). Codium bursa (Olivi) C.Agardh, C. vermilara (Olivi) Delle Chiaje and Sphaerococcus coronopifolius Stackhouse, perennial species observed during the present work, were not considered. The abundance of these species known as tolerant can be linked to a stressor (Vidondo and Duarte, 1995; Ruitton et al., 2017). In addition, C. fragile (Suringar) Hariot is a non-indigenous species (Provan et al., 2005). The mean percentage cover in the upper zone is a suitable descriptor to assess this compartment.
2.3.3 Box 3: Seasonal erect macroalgae
Macroalgal species from this compartment, characterized by fast growth rate and seasonal cycle, thrive mainly from spring to a maximum development during summer. Seasonal macroalgae also contribute significantly to primary production (Ballesteros, 1989). The most frequent and abundant taxa are the Phaeophyceae Arthrocladia villosa (Hudson) Duby and Sporochnus pedunculatus (Hudson) C.Agardh, widespread in the circalittoral both in Coralligenous reefs and CDB. Some species usually observed in the infralittoral can be found such as Acetabularia acetabulum (L.) P.C.Silva or Padina pavonica (Linnaeus) Thivy. Umbraulva dangeardii M.J.Wynne & G.Furnari is an Ulvophyceae that can be observed often associated with rhodolith beds. Chrysymenia ventricosa (J.V.Lamouroux) J.Agardh and Sebdenia spp. (J.Agardh) Berthold are among the main characteristic Florideophyceae. Identification at species level is impossible based on in situ observation or video and photograph analysis. The mean cover (percentage) of seasonal macroalgae appears to be a suitable descriptor. Non-indigenous and invasive species with a seasonal dynamic can thrive in the CDB: Caulerpa cylindracea Sonder (Klein and Verlaque, 2008), C. taxifolia (M.Vahl) C.Agardh (Meinesz et al., 2001; Montefalcone et al., 2015b), mucilage (several species including NIS: Acinetospora crinita (Carmichael) Sauvageau, Chrysonephos lewisii (W.R.Taylor) W.R.Taylor, Nematochrysopsis marina (J.Feldmann) C.Billard, Zosterocarpus oedogonium (Meneghini) Bornet) (Giuliani et al., 2005; Schiaparelli et al., 2007; Bianchi et al., 2019a). The latter taxa have been considered as stressors and are therefore not taken into account in the assessment of Compartment 3.
2.3.4 Box 4: Seasonal turf
Seasonal turf is defined as an algal community with a low height (a few centimetres at most). Worldwide, turf is more and more often observed replacing more structuring communities, influenced by stressors (Littler and Littler, 1980; Connell et al., 2014). Seasonal turfs include numerous species but very few can be identified in situ like the Ulvophyceae Pseudochlorodesmis furcellata (Zanardini) Børgesen and Valonia macrophysa Kützing. Womersleyella setacea (Hollenberg) R.E.Norris and Acrothamnion preissii (Sonder) E.M.Wollaston, two alien species (Boudouresque and Verlaque, 2002), can be present and are considered as stressors. These two species are often invasive in coralligenous reefs, Posidonia oceanica meadows, and infralittoral photophilic reefs (Piazzi and Cinelli, 2000; Piazzi et al., 2007; Bianchi et al., 2019b), and were found in CDB at Port-Cros National Park (Provence, France; Astruch et al., 2019). The distinction by visual assessment between seasonal turf and seasonal erect macroalgae is difficult. Considering that the two compartments progress with the same dynamics, it is relevant to consider their cumulative cover as the descriptor of their status in both upper and deep zones. The ‘Compartments 3 and 4’ are therefore named hereafter ‘Compartment 3-4’.
2.3.5 Box 5: Herbivores
The high diversity of the macroalgal communities on CDB (boxes 1, 2, 3, 4) influences the abundance and the diversity of epibenthic herbivores. In the present work, the following taxa were considered as herbivores: echinids (Sphaerechinus granularis (Lamarck, 1816), Stylocidaris affinis (Philippi, 1845); occasionnaly Centrostephanus longispinus (Philippi, 1845), Echinus melo Lamarck, 1816), and the gastropods Cerithium sp. Bruguière, 1789 and Aplysia spp. L., 1767. While S. granularis is strictly a herbivore (Weinberg, 1996; Wirtz and Debelius, 2003), S. affinis, C. longispinus and E. melo are omnivores. The latter two are not characteristic of CDB, even if they can be found in the vicinity of coralligenous beds. According to food availability and habitat (Wilkie et al., 1996), they are able to shift into herbivorous-dominant behaviour (De Ridder and Lawrence, 1982; Francour, 1989; Francour, 1991; Bergbauer and Humberg, 2000). Sphaerechinus granularis and S. affinis are known to be observed gathering on detrital bottoms (Harmelin and Duval, 1983). Vertebrate herbivores are mainly represented seasonally by the fish Sarpa salpa (L., 1758) during its breeding period (late summer) (Verlaque, 1990). Considering the short duration of the phenomenon, this species is not taken into account in this compartment. The alpha taxonomic richness (number of taxa per site) is a relevant descriptor to assess the herbivores compartment.
2.3.6 Box 6: Particulate organic matter (POM) of the sediment
Particulate organic matter (POM) of the sediment observed in abundance can be linked to natural eutrophic conditions or to a stressor (Sciberras et al., 2009). Based on our sampling strategy, the assessment of this compartment is feasible by considering the cover of muddy sediment on the bottom observed on the photoquadrats. This descriptor is already applied to assess the POM sedimented on coralligenous reefs (Ruitton et al., 2017). However, its assessment on CDB proved difficult. We therefore propose the percentage of pelitic fraction (<63 µm) as a proxy of the POM of the sediment. Organic matter accumulation is determined by environmental dynamics (currents, fine particles transfer to deep areas) (Orekhova and Ovsyany, 2020). A considerable percentage of pelitic fraction can also correspond to Muddy Detrital Bottoms (MDB).
2.3.7 Box 7: Infauna
Infauna constitutes a key compartment, influenced by sediment grain size and organic matter content (Pérès and Picard, 1964; Word, 1978; Cocito et al., 1990). Part of the Infauna can also be part of the Epifauna. We distinguished here three trophic groups within the infauna: (Box 7a) Filter and suspension-feeders: Represented by Phoronida, bivalves (ie. Laevicardium oblongum (Gmelin, 1791) or Acanthocardia deshayesii (Payraudeau, 1826)), Porifera (e.g., Suberites domuncula (Olivi, 1792)), Ophiuroidae and annelids such as Serpulidae; (Box 7b) Detritivores: Regroup surface and sub-surface deposit feeders such as annelids such as Petta pusilla Malmgren, 1866 (or Capitellidae, particularly in case of organic enrichment) and other different groups of worms (Echiura, Sipuncula, Nematoda), and molluscs such as Turritellinella tricarinata (Brocchi, 1814), Moerella donacina (L., 1758), Abra nitida (O. F. Müller, 1776) or A. prismatica (Montagu, 1808) (Zenetos, 1996); (Box 7c) Carnivores> (including scavengers): annelids such as Nephtyidae, Eunicidae or Polynoidae, molluscs gastropods such as Naticidae or Conidae, decapods and starfishes.
CDB, particularly in the upper circalittoral zone, are characterized by a relatively coarse grain size, decreasing with depth. It directly influences the trophic network in the sediment. Consequently, coarse sediment hosts a lower content in organic matter and a lower abundance of the infauna (Blanchard et al., 2009). According to Bellan et al. (1980), high organic matter content can be a stressor for infauna, by preventing the recycling of organic matter due to polluted water inputs. The organic matter content is often used as a descriptor of the trophic status of infauna (Cocito et al., 1990; Dell’Anno et al., 2002). However, the natural abundance of organic matter can be linked to the vicinity of seagrass meadows, (i) providing dead leaves that partly remain in the sediment, or (ii) with dead matte a few cm under the sediment surface (Boudouresque et al., 2019). In the present work, we assess the whole infauna compartment on the basis of the organic matter content in the sediment (percentage).
2.3.8 Box 8: Organic microdetritus
Posidonia oceanica seagrass meadows produce and export a huge number of dead leaves to adjacent ecosystems, including CDB (Boudouresque et al., 2016). Infralittoral photophilous reefs and coralligenous reefs also export macroalgae, which accumulate or move over circalittoral soft bottoms. Organic macrodetritus composition and biomass vary according to source ecosystems: dead leaves of P. oceanica, macroalgae or terrestrial plant remains. Before reaching greater depth, off the continental shelf, organic macrodetritus are used as a trophic resource by the detritivores of the CDB.
2.3.9 Box 9: Detritivores (epibenthic)
Detritivores play an important role, degrading litter and organic debris on the sediment. This compartment is composed of Echinodermata (Holothuroidea, Echinoidea and Asteroidea), Crustacea and Gastropoda. The abundance of some taxa can be favoured by eutrophic (i.e., HOM: High Organic Matter) or oligotrophic (LOM: Low Organic Matter) conditions. Two categories are distinguished: (9a) HOM Detritivores: Including Holothuria spp. L., 1767, Bonellia viridis Rolando, 1822 and Paguridea; (9b) LOM Detritivores: Represented by Spatangus purpureus O.F. Müller, 1776, Chaetaster longipes (Bruzelius, 1805), Echinaster sepositus (Retzius, 1783), Gastropoda and Crustacea (Mysidacea, Malacostraca). While a high abundance of HOM detritivores can be linked with a CDB that is potentially degraded (eutrophication), the abundance of LOM detritivores, more sensitive to eutrophication, indicates a good status of this compartment. Mean density (individuals per m² observed in the photoquadrats at both depths) is a relevant descriptor to assess detritivores 9a and 9b.
2.3.10 Box 10: Filter and suspension feeders (epibenthic)
Benthic filter and suspension feeders on CDB belong to various taxonomic groups: Annelida, Ascidiacea, Bryozoa, Cnidaria, Porifera) (Harmelin, 1978; Souto et al., 2010). On coralligenous reefs, the abundance of Cliona viridis (Schmidt, 1862) is considered as a consequence of stressors (bioerosion in a eutrophic configuration) (Cerrano et al., 2001). However, the presence of C. viridis with a relatively low density on CDB, as observed in our case study, cannot be considered as an indicator of stress. Mean density of individuals or colonies per m² (observed in the photoquadrats at both depths) seems to be the most suitable descriptor for filter and suspension feeder assessment, unlike taxonomic richness, due to the difficulty of identification in situ or from videos or photographs.
2.3.11 Box 11: Planktivorous teleosts
This compartment includes planktivorous pelagic or demersal fish, with life traits significantly linked with the substrate. Strictly pelagic planktivorous teleosts with no interaction with the bottom (e.g., Clupeidae, Engraulidae) were not considered. The main species observed were Spicara maena (L., 1758), S. smaris (L., 1758), Boops boops (L., 1758), Chromis chromis (L., 1758) and Anthias anthias (L., 1758). The latter two indicate the vicinity of hard substrates. CDB play an important role for these species: a spawning ground for Spicara spp. (Harmelin and Harmelin-Vivien, 1976) and an essential habitat for Boops boops juveniles. Planktivorous species are also essential in the trophic network providing a food supply for high level predators (Bănaru et al., 2013; Cresson et al., 2020). The alpha specific richness (number of species per site, considering all methods) was measured to assess the planktivorous teleosts compartment.
2.3.12 Box 12: Carnivores
This box encompasses all carnivorous species, predators of crustaceans, annelids, fishes and other benthic invertebrates. This box is divided in two: Invertebrate carnivores (12a) and Vertebrate carnivores (12b). (12a) Invertebrate carnivores: This includes mainly motile species: crustaceans, echinids (Asteroidea, Ophiuroidea), Gastropoda, Nudibranchia, Cnidaria, etc. The alpha taxonomic richness of invertebrate carnivores (per site considering all methods) is a suitable descriptor for this compartment. (12b) Vertebrate carnivores: This includes teleost fish species (e.g., Pleuronectidae, Trachinidae, Sparidae, Gobiidae, Blenniidae, Mullidae, Labridae, Triglidae) and chondrichthyans (e.g., Scyliorhinus spp. Blainville, 1816, Rajidae). Carnivorous fish find an important trophic resource at the surface or inside the sediment of CDB. Some use CDB as a nursery habitat (e.g., Serranus hepatus (L., 1758), Blennius ocellaris L., 1758) or a breeding habitat (e.g., Symphodus cinereus (Bonnaterre, 1788), Solea spp. Quensel, 1806). The assessment of abundance or diversity of fish assemblages normally applied in other marine habitats, such as reefs or seagrass meadows (Harmelin-Vivien et al., 1985), proved of little applicability in CDB. The most suitable descriptor to assess the compartment 12b is the alpha taxonomic richness (number of species observed per site using all methods).
2.4 Compartments not assessed
In the frame of an ecosystem-based approach, it is necessary to considerer all the main functional compartments even if some are not assessed.
2.4.1 Phyto- and zooplankton
Together with POM, phytoplankton and zooplankton are the basis of planktivore diet (Khoury, 1987; Cresson et al., 2014; Chen et al., 2022). This compartment can vary very quickly, and its proper assessment would require too much time and resources relative to its weight in the ecosystem functioning (Table 1). In the framework of an index assessment, it is more suitable to assess it indirectly through its consumers, as is done for other ecosystems (see Personnic et al., 2014; Thibaut et al., 2017).
2.4.2 Pelagic dissolved and particulate organic matter (DOM and POM)
Dissolved organic matter (DOM) and particulate organic matter (POM), provided by phytoplankton exudation, grazing by zooplankton and terrestrial inputs, play a key-role in the marine microbial trophic network (Mostajir et al., 2012) and in some coastal food webs (Darnaude, 2005; Bănaru et al., 2007). As far as the CDB ecosystem is concerned, this compartment is considered as indirectly assessed by the filter feeders and suspension feeder compartment (Box 10) and therefore was not assessed directly.
2.4.3 Microbial loop
The microbial loop is driven by the DOM and the POM (Velimirov et al., 1984; Buffoni et al., 1990). DOM is an important substrate for bacterial production. The microbial loop is defined as a community including heterotrophic micro-organisms, namely Archaea, bacteria, flagellated eukaryotes and ciliates (Azam et al., 1983; Boudouresque, 2015). This compartment is not directly assessed in the present work but it is strictly linked with POM and DOM, plankton communities, filter and suspension feeders (Box 10), planktivores (Box 11) and carnivores (Box 12).
2.4.4 Piscivores
Piscivorous teleosts strictly linked with CDB are very few. High level predators that can be found are mostly casual visitors from adjacent ecosystems (see below). Lophius spp. L., 1758 and Scorpaena scrofa L., 1758, two nocturnal and benthic predators, more frequently occupy CDB. At the scale of a given sampling site, the occurrence of piscivores is very low.
2.4.5 Transiting live organisms
Many organisms transit through the CDB mainly from the infralittoral and circalittoral ecosystems to the bathyal and abyssal bottoms. Consequently, numerous species from coralligenous reefs, infralittoral reefs and Posidonia oceanica meadows can be found in CDB. The assessment of this transiting fauna was indirect through the main compartments of CDB assessed (Boxes 1, 2, 3-4, 5, 9, 10, 12).
Numerous high-level predators can visit CDB occasionally (see the compartment ‘Visiting predators’ in Figure 2), such as Dentex dentex (L., 1758), Pagrus pagrus (L., 1758), Zeus faber L., 1758, and feed on planktivorous or mesocarnivorous fish species. Because of a very low and occasional occurrence, this compartment was not taken into account.
2.4.6 Carbon sink
Rhodolith beds act as major carbon sinks through their high storage capacity of both organic (Corg) and inorganic carbon (Cinorg). The effectiveness of this role is strictly associated with the vitality of calcareous organisms living with these ecosystems (Martin et al., 2007; Basso, 2012; Burrows et al., 2014; Watanabe et al., 2020). While the Cinorg sequestration results mainly from the significant calcium carbonate contents of the rhodolith skeleton (Van der Heijden and Kamenos, 2015), the capture and the trapping of Corg within rhodolith beds come from autochthonous and allochthonous sources (Mao et al., 2020). The Corg production is mainly completed through the photosynthesis of rhodolith organisms (B1) and the occurrence of facies of calcareous invertebrates (e.g., Bryozoa, Echinidae, Gastropoda, Annelida, Bivalvia). Though the Corg fixation is well known for rhodolith beds (Watanabe et al., 2020), its long-term sequestration in the sediments is still to be specified. For P. oceanica meadows, the fixation and Corg sequestration capacity proved to be closely related with water depth and its state of conservation. Thus, healthy and shallow meadows are considered as carbon sinks whereas deeper and altered ones may act as carbon sources (Monnier, 2020; Monnier et al., 2021; Pergent-Martini et al., 2021; Monnier et al., 2022).
2.5 Descriptors selection
Fifteen descriptors were selected to assess the 12 boxes of CDB (Table 2). Boxes 3 and 4 were considered together as seasonal macroalgae, so that 11 boxes were actually considered. The weight of each box (W), first defined during the workshop, was successively adjusted according to the results of the test in the field. The compartments selected as providing the main contribution to the functioning of the ecosystem are: Box 1-rhodoliths (W=6), Box 5-infauna (W=5), box 10-filter and suspension feeders, Box 2-perennial and Box 3-4-seasonal macroalgae (W=4).
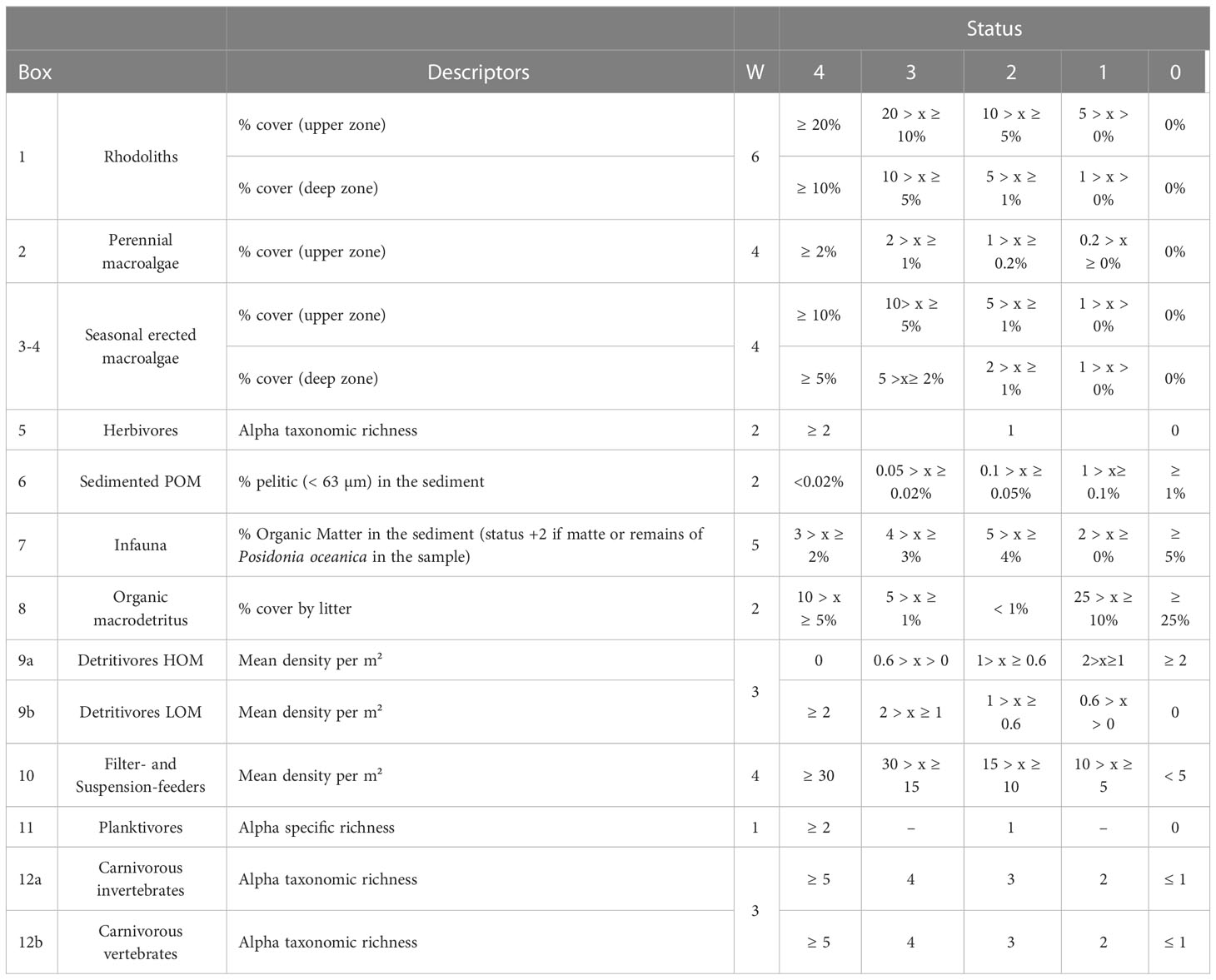
Table 2 Boxes, descriptors, weight (W) and status for the EBQI assessment (POM, Particulate Organic Matter; HOM, High Organic Matter; LOM, Low Organic Matter).
Descriptors for each compartment were selected among a wide range of available descriptors tested during the sampling. The criteria for descriptor selection were (i) its applicability, (ii) a wide enough range of values across the sampled sites, (iii) the absence of redundancy with the descriptors of other compartments in order to reduce the total number of descriptors.
The method to assess each descriptor is presented in Table 3. The monitoring of one sampling site can be carried out in less than one day with a small boat and 3 operators. It involves (i) one dive with two operators in the upper zone with 12 minutes of investigations (sampling of 3 sediment cores, photographs and videos), covering a mean surface of about 500 m², and (ii) the deployment of the Biocube at the two depths (upper and deep zones) to sample 30 photoquadrats per depth and seascape videos. (iii) Analysis of the cores involves measurement of grain size and percentage of organic matter.
2.6 EBQI calculation
The EBQI is then calculated using the following formula:
Wi is the weight of the box, Si the status of the box and Smax maximum status possible (4/4). A notation between 0 and 10 is obtained. An ecological status is defined according to the EBQI notation (Personnic et al., 2014; Table 4). The ecological status limits are similar to those defined for Posidonia oceanica seagrass meadow (Personnic et al., 2014), coralligenous reefs (Ruitton et al., 2014) and rocky reefs (Thibaut et al., 2017).
A confidence index (CI) was used to assess the quality of the data (suitable protocol, recent data, expert judgment) (see Personnic et al., 2014 for CI calculation) (Table 5). The CI allows assessment of the quality of the sampling according to the recommended methods. If a sampling does not combine all the suitable methods, it will still be possible to calculate an EBQI with a low CI (e.g., data available with other protocols, expert judgement).
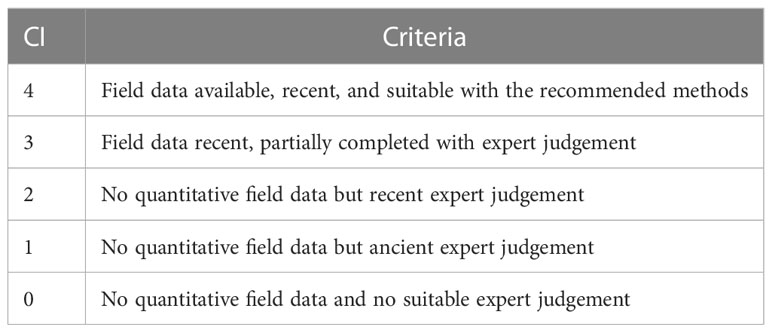
Table 5 Confidence index (CI) related with data quality or expert judgement (Criteria from Personnic et al., 2014).
2.7 Statistical analysis
Correlation between descriptors notation has been tested by calculating Spearman rank-order correlation and assessing its significance with p-value. Principal Component Analysis (PCA) was used without standardization to show the relation between boxes and EBQI sites notations.
The robustness of the EBQI for CDB has been tested with regard to the ecological status per box and the weight per box. A robustness analysis involving 1000 simulations with 25, 50, 75 or 100% of probability of perturbation of ±1 or ±2 (of each ecological status per box and each weighting per box) has been tested. The analysis recalculates the EBQI notation considering the corresponding perturbation ( ± 1 or ±2) and the probability of perturbation (25, 50, 75 or 100%).
Box status has been plotted on radar charts and the uniformity of box status has been assessed through circularity of polygons representing those charts. Circularity is the ratio between the polygon area and the area of a circle having a circumference equal to the polygon perimeter (Oprandi et al., 2021). Perimeter and area have been calculated using ArcGIS Pro 3.0®.
The influence of the management level was tested on EBQI notations and circularity value using PERMANOVA analysis, using Euclidian distance and 999 permutations (Anderson, 2001). Following Thibaut et al. (2017), four management levels were defined: (i) sites without protection (hereafter named ‘No protection’), (ii) Natura 2000 sites (EU Habitats Directives) with no effective protection (hereafter named ‘Natura 2000), (iii) protected sites, including MPA with Multi-Use Management (hereafter named MPA-MUM) and (iv) highly protected, including MPA with No-Take Zone (hereafter named ‘MPA-NTZ’).
P-value threshold was set at 5% for all tests. Analyses were performed with R software (R core team, 2022).
3 Results
3.1 EBQI notation
EBQI notation ranged from 2.05 (27TH) to 8.06 (21PAM) (Table 6; Supplementary Figure 1). Eight sites out of 29 exhibited a Good or Very Good notations while 10 showed a Poor or Bad status. The Confidence CI was lower in Box 7 (infauna) for all sites because of the method used (organic matter assessment instead of macrofauna analysis). The CI was Very Good for all sites, ranging from 88 to 93%.
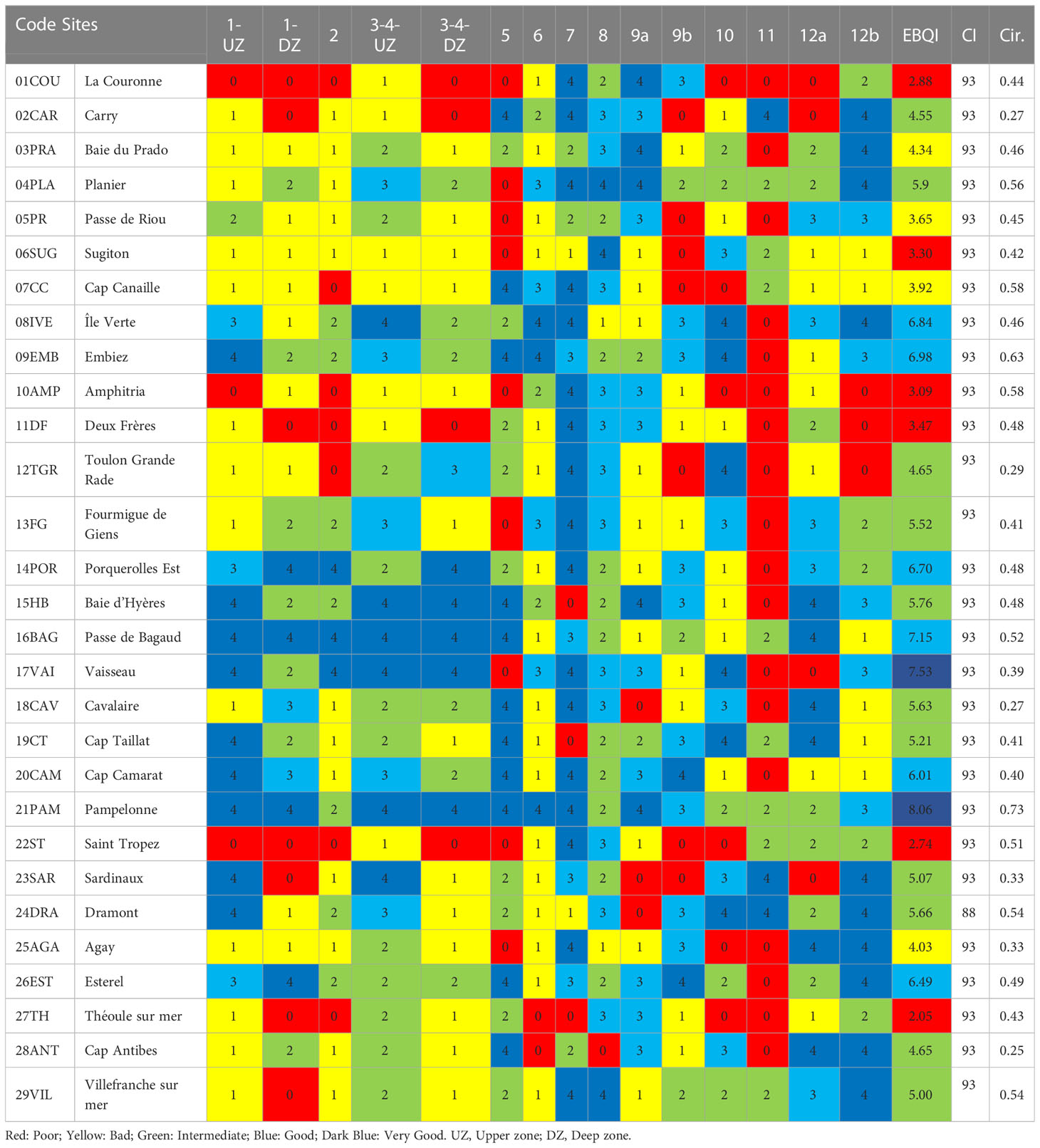
Table 6 EBQI notation, status per box, Confidence Index (CI in %) and Circularity value (Cir.) for the 29 sampling sites (see Figure 1 for location).
EBQI notation and box status attributed to each site are mainly explained by local specificities and the vicinity or not of human activities (i.e., urbanized areas). PCA showed a gradient along Axis 2 (16.8% of the total variance) from Poor to Very Good sites. Good and Very Good EBQI notations were mainly explained by the high status of Boxes 1, 2 and 3-4 (primary producers). Boxes 6 (sedimented POM), 7 (infauna), 8 (organic macrodetritus) and 12 (carnivores) were less discriminating (Figure 4).
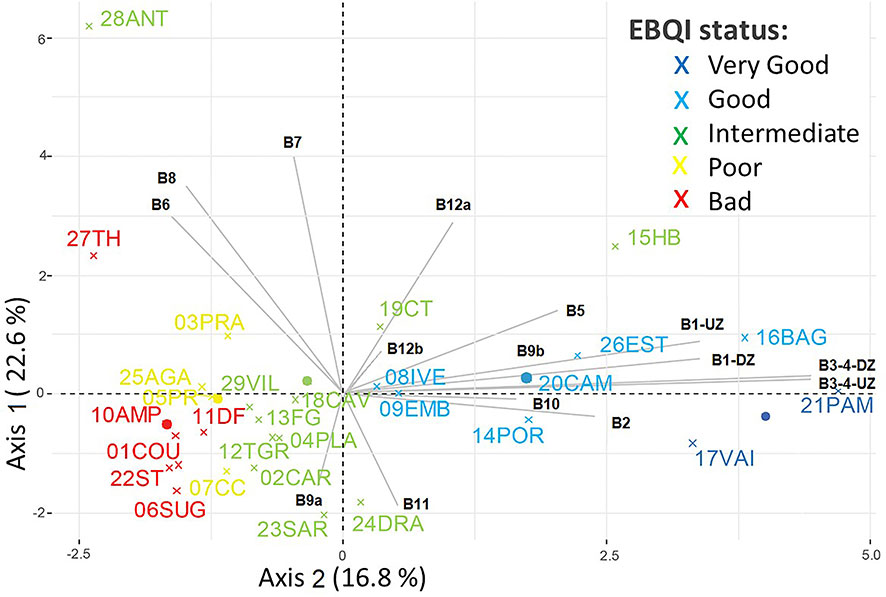
Figure 4 Principal Component Analysis. Sampling sites are coloured according to their EBQI notation. Boxes descriptors are projected in light grey. Red: Poor; Yellow: Bad; Green: Intermediate; Blue: Good; Dark Blue: Very Good.
Radar plot projection helps in assessing the heterogeneity of ecological status per box for each sampling site (Supplementary Figure 2). For example, 21PAM (Pampelonne), with the best notation (EBQI = 8.06), is defined by an ecological status ranging from 2 to 4 while 09EMB (Embiez), also with a good notation (EBQI = 6.98), is characterized by an ecological status between 0 and 4.
A Spearman rank correlation has been tested between the fifteen descriptors used for EBQI calculations (Figure 5). Positive correlations moderately to highly significant are observed between descriptors related to primary producers (Box 1 - rhodoliths, Box 2 - perennial macroalgae, and Box 3-4: seasonal macroalgae and turf). These same descriptors also show significant correlations with the mean density of LOM detritivores (Box 9b). The diversity of herbivores (Box 5) shows a significant negative correlation with the cover of organic macrodetritus (Box 8). The percentage of organic matter (Box 7) shows low but significant correlations (R²< 0.34) with sedimented POM (Box 6). The percentage of pelitic fraction of the sediment (Box 6) shows a significant negative correlation with the cover of perennial macroalgae (Box 2), rhodoliths (Box 1-UZ) and seasonal macroalgae and turf (Box 3-4). HOM detritivores density (Box 9a) shows no correlations with any other descriptor.
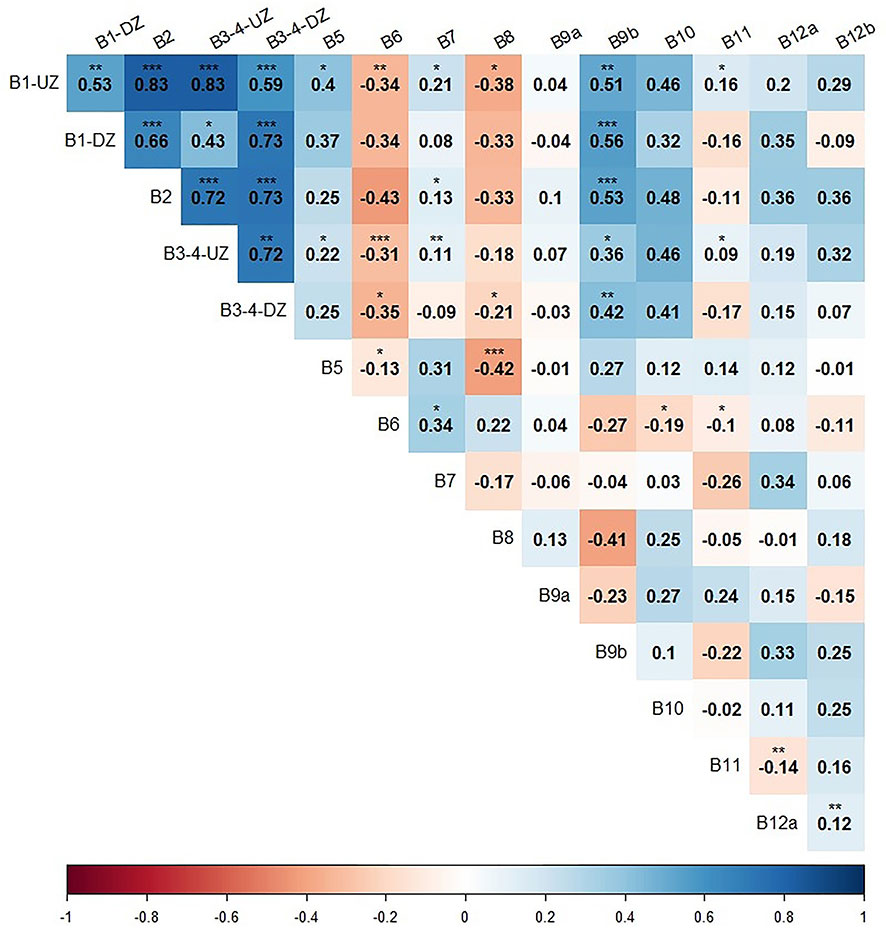
Figure 5 Spearman rank correlation between the fifteen descriptors related to ecosystem boxes of the coastal detrital bottoms. The number in bold is the correlation coefficient R. *: p< 0.05; **: p< 0.01; ***: p< 0.001. Blue, positive correlation; Red, negative correlation.
3.2 Circularity analysis
The circularity of radar charts reflects the uniformity of box status. Values range from 0.25 (28ANT) to 0.73 (21PAM) and are independent of the EBQI notation (Spearman correlation: R=0.242 and p=0.206). According to the EBQI notation and circularity value, 4 different situations are identified (Figure 6): (i) CDB sites with moderate-good notation (EBQI≥5) and high homogeneity of box status (circularity≥0.5) such as 24DRA, 04PLA, 09EMB, 16BAG and 21PAM; (ii) CDB sites with moderate-good notation but low homogeneity of box status such as 13FG, 18CAV, 15HB, 20CAM, 26EST, 24PQR and 17 VAI; (iii) CDB sites with poor notation (EBQI<5) and high homogeneity of box status status like 22ST, 10AMP, 07 CC and 29VIL; (iv) CDB sites with poor overall notation and low homogeneity of quality status such as 27TH, 01COU, 06SUG, 11DF, 05PR, 25AGA, 03PRA, 02CAR, 12TGR, 28ANT, 23SAR and 19CT.
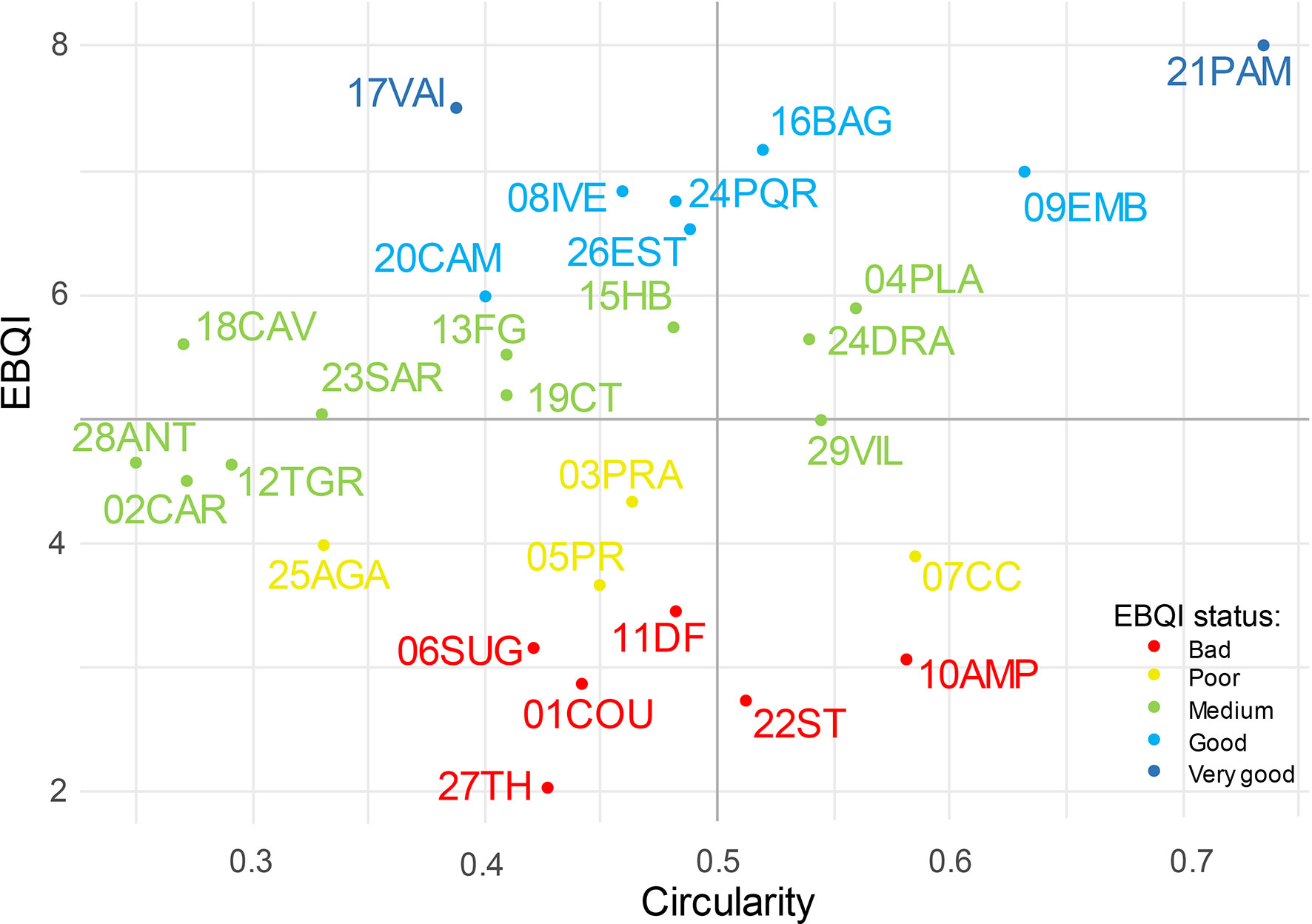
Figure 6 Scores of EBQI and Circularity for the 29 sites. Red: Poor; Yellow: Bad; Green: Intermediate; Blue: Good; Dark Blue: Very Good.
3.3 Influence of the management level
The management level at the 29 sampling sites is heterogeneous. Four sites have no protection, 12 sites are included in Natura 2000 sites, 5 sites are within MPA-MUMs, and 8 are within MPA-NTZs. Analysis of EBQI mean notation and Circularity mean value per management level shows no significant differences between management levels (Permanova: respectively p=0.866 and 0.737; Figure 7).
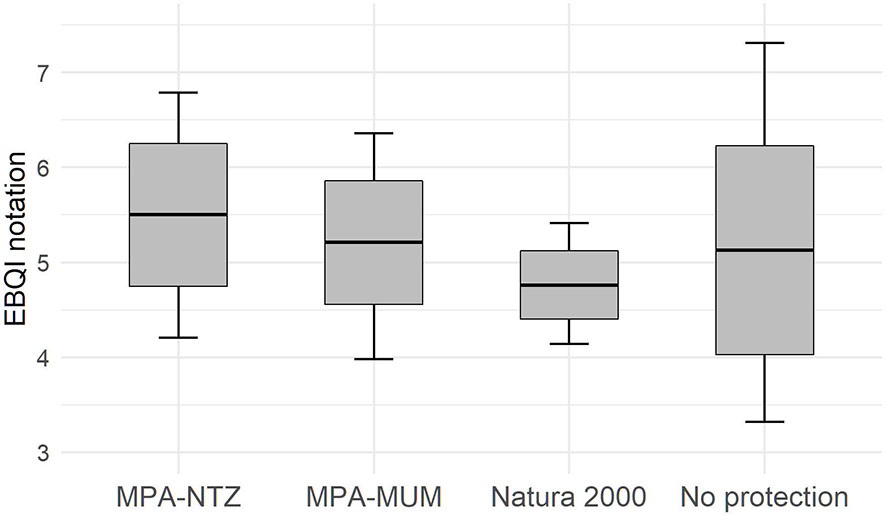
Figure 7 Box plots showing EBQI notation (left) and circularity value (right) according management level. The black crossbar corresponds to the mean EBQI notation, the grey rectangle corresponds to the standard error, the vertical bars correspond to the 95% confidence interval.
3.4 Robustness analysis
3.4.1 Robustness regarding perturbation of the ecological status
The higher the perturbation and the probability of perturbation, the less similarity is observed in the iterations compared to the initial EBQI calculation (Figure 8; Supplementary Table 1). The percentage of similarity decreases with the probability of perturbation or the number of perturbations ( ± 1 or ±2). With perturbation of the ecological status per box, the mean similarity (all sites included) moves from 81.8% (25%) to 61.7% (100%) for a ±1 perturbation and from 68.8% (25%) to 38.8% (100%) for a ±2 perturbation. A high perturbation of the ecological status implies a lower stability of the EBQI.
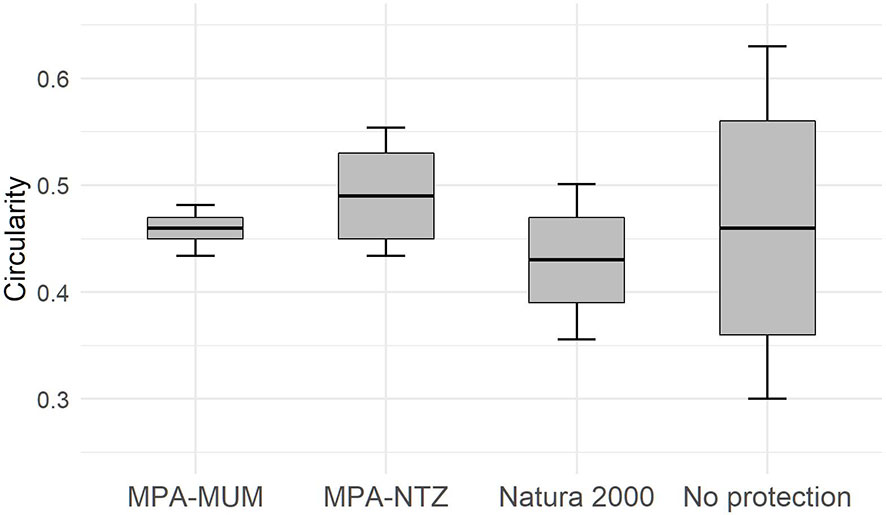
Figure 8 Robustness of the EBQI with regard to the Ecological status per box. Sampling sites (x-axis) are sorted in ascending order according to the EBQI notation from left to right. In order to test the effect of the status per box on the EBQI (robustness), status values have been randomly perturbed (above, ± 1; below, ± 2) 25% (left) and 100% (right) of probability; 1000 iterations were performed. The change of the EBQI notation (Bad through Very Good) of a site, for a given iteration, is shown by the colour of the new class in which it falls). Red: Poor; Yellow: Bad; Green: Intermediate; Blue: Good; Dark Blue: Very Good.
3.4.2 Robustness regarding perturbation of the weighting
When perturbing the weighting per box, the mean similarity is higher, moving from 91.5% (25%) to 84.5% (100%) for a ±1 perturbation and from 86.5% (25%) to 73.3% (100%) for a ±2 perturbation (Figure 9; Supplementary Table 1). The perturbation of the weighting does not affect the stability of the index as much as the perturbation of the ecological status per box.
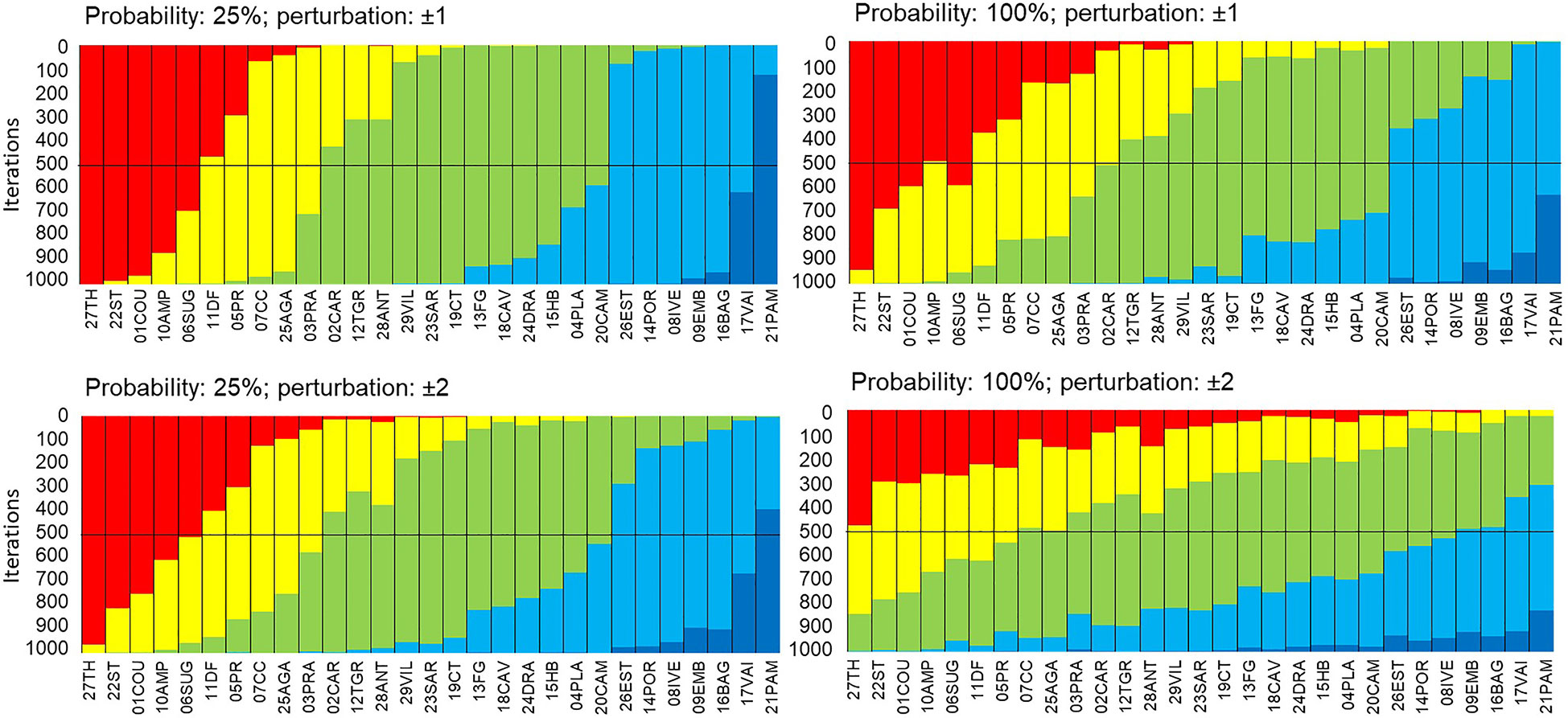
Figure 9 Robustness of the EBQI with regard to the weighting per box. Sampling sites (x-axis) are sorted in ascending order according to the EBQI notation from left to right. In order to test the effect of the weighting per box on the EBQI (robustness), status values have been randomly perturbed (above, ± 1; below, ± 2) 25% (left) and 100% (right) of probability; 1000 iterations were performed. The change of the EBQI notation (Bad through Very Good) of a site, for a given iteration, is shown by the colour of the new class within which it falls. Red: Poor; Yellow: Bad; Green: Intermediate; Blue: Good; Dark Blue: Very Good.
The lower values of similarity for high levels of perturbation ( ± 2) of both the ecological status per box and the weighting confirm the fact that weighting the boxes is consistent and useful, as already shown by Personnic et al. (2014) for Posidonia oceanica seagrass meadows. It is worth noting that the sites with the lowest similarity are often the sites where the initial EBQI notation is close to a threshold between two statuses (e.g., 7.53 is a Very Good notation but very close to the Good status<7.50).
4 Discussion
4.1 An original Ecosystem-Based Quality Index for Coastal Detrital Bottoms
This paper proposes an original Ecosystem-Based Quality Index to assess the health status of the Coastal Detrital Bottoms in the North-Western Mediterranean context. The goal is to provide (i) a useful tool, embracing the concept of the ecosystem-based approach, for coastal managers (MPAs, local authorities), and (ii) arguments for a better consideration of this key habitat according to the MSFD and Barcelona Convention. To determine a relevant biological indicator, the main issue is finding the balance between the most suitable descriptors versus the feasibility of methods of implementation according to available human and material resources. Unlike ecosystems of priority interest such as Posidonia oceanica meadows (Montefalcone, 2009; Lopez y Royo et al., 2010; Personnic et al., 2014; Oprandi et al., 2021), no indices or descriptors are available for the functional or ecosystem-based analysis of CDB.
The EBQI is a suitable tool to broadly assess the functioning of an ecosystem (Boudouresque et al., 2020a; Boudouresque et al., 2020b). It aims at providing a first integrated insight with a general notation and status per compartment, useful at the scale of a sampling site or in the frame of a monitoring network at larger scale. The CI for CDB, between 88 to 93%, is far higher than the mean CI assessed for sites used to defined other EBQI indices: 53% for Posidonia oceanica seagrass meadows (Personnic et al., 2014), 62% for undersea caves (Rastorgueff et al., 2015), and 74% for rocky reefs (Thibaut et al., 2017).
As expected, our analysis highlights the influence of both natural features and anthropogenic activities on the ecological status of CDB. Sites in areas without trawling or dredging activities, far from urbanized zones, show the best notation. The Provence and French Riviera coasts provide a wide range of anthropogenic pressure and local natural specificities. This heterogeneity was the key for defining an EBQI for CDB and testing the reliability of the descriptors of the functional compartments. A further analysis will have the benefit of new and detailed data to identify the causes of such a status: natural feature (hydrological context, geology, adjacent habitats) or pressures linked with human activities (trawling, terrigenous input, coastal development, etc.). As for all point measurement methods to assess the ecological quality, the EBQI requires a high number of replicates per site to give a better insight into the structure of the ecosystem assessed. The aim of this approach is to offer a broader view of the ecosystem functioning of a given ecosystem.
According to our results, the protocol proposed is accurate, using accessible methods involving SCUBA diving, photographs, photoquadrats and videos. The robustness analysis showed an effect of a perturbation of the ecological status per box or weighting. Nevertheless, the percentage of similarity remained high for a relatively moderate perturbation. The probability of 25% was tested to simulate a theoretical application with one in four chances of being wrong, which is consistent and closer to the reality of field application. Keeping in mind that the EBQI aims at a broad assessment of ecosystem quality and functioning, the results confirmed the robustness of the approach.
4.2 Criticism and improvement perspectives
It would have been a much-improved method if the sediment macrofauna had been properly assessed instead of just measuring the organic matter (Box 7). The quality of the assessment would have been improved, at ecosystem scale, but this would have been very time-consuming during the sampling process. The AMBI index (the AZTI’s Marine Biotic Index: Borja et al., 2000; Borja et al., 2003), and other indexes that have been developed, such as e.g., BENTIX (Simboura and Zenetos, 2002), the BQI (Benthic Quality Index; Rosenberg et al., 2004), the GPBI (General Purpose Biological Index; Labrune et al., 2021) and MEDOCC (specifically for Mediterranean assemblages; Pinedo et al., 2015), allow the assessment of the ecological quality of soft bottoms based on sediment macrofauna (see also Labrune et al., 2006). They consider the abundance in the sediment of species sensitive to stressors, tolerant and opportunistic. They can provide reliable information about infauna status to complete and improve the ecosystem-based assessment, but can be ineffective for detection of an impact of physical pressures (e.g., AMBI: see Labrune et al., 2006, but also Borja et al., 2015). The macrofauna of the sediment is of importance in terms of ecological functioning and trophic interactions with epibenthic and demersal communities (Austen et al., 2002), and obviously an assessment considering only the organic matter content is far from being entirely satisfactory (Cocito et al., 1990). It could also be argued that while the proper identification of CDB must be based on the infauna (Pérès and Picard, 1964), the index presented here focuses mostly on the epibenthos. However, characterization and assessment are two distinct steps of environmental diagnostics (Bianchi et al., 2012): assessment criteria can be applied only after the habitat in question has been characterized. We do believe that the health status of the CDB epibenthic facies is an acceptable proxy for the quality of the whole ecosystem; that is why we have decided to assign the highest weighting to Box 1 (Rhodoliths), which may require discussion. All the direct anthropogenic physical pressures mentioned in the previous pages obviously threaten the epibenthos first, rather than the infauna, which is known to be more resilient. Furthermore, as far as we are aware, the ongoing seawater warming in the Western Mediterranean region (Cerrano et al., 2000; Garrabou et al., 2009) is affecting epibenthic species more than the infauna. But it may be true that the degradation of the CDB epibenthic facies may not necessarily imply a bad status for the infauna, and further research is needed. An accurate assessment of the entire ecosystem should imply more than just a selection of functional compartments, as evoked by de Jonge et al. (2019).
Rhodolith beds in CDB can be considered both soft and hard substrata, according to the associated benthic communities (Jacquotte, 1962; Hall-Spencer, 1998; Basso et al., 2017). Rhodolith beds are also described as a step towards the edification of coralligenous banks, from a soft bottom towards a hard bottom, under specific environmental conditions (Basso et al., 2007). The pioneer zoologist André Fortuné Marion named as ‘coralligenous gravels’ the rhodolith beds of the Gulf of Marseille (Marion, 1883). The ‘Coralligène de plateau’ described by Pérès and Picard (1951, 1964); Laborel (1961) and Bellan-Santini et al. (1994) is a coralligenous bank developing from the growth and coalescence of rhodoliths and calcified invertebrates. This highlights the key-role of calcareous macroalgae in inducing autogenic ecological succession (Laborel, 1961; Laubier, 1966; Odum, 1971; Begon et al., 1986; Basso et al., 2007). In some circumstances, the epibenthic associations of the CDB, particularly rhodolith beds, can be considered as being at the threshold between a circalittoral sandy bottom and an actual upcoming coralligenous bank. A CDB facies with small concretionary boulders has also been described by Meinesz et al. (1983). This does not mean that CDB are necessarily hard substrates. The slow evolution towards a coralligenous bank cannot be generalized to all CDB, even in presence of rhodolith beds.
Bryozoan facies, as described by Bianchi (2009a) and Harmelin (2017), have not been found at the 29 sampling sites considered in the present work. The patchiness of epibenthic associations and facies and the rarity of such a feature might explain our result. Yet facies of Pentapora fascialis and other branched bryozoans on CDB are known for example around Port-Cros Island (Laborel et al., 1976; Holon and Harmelin, 2014; Harmelin, 2017) but were not found during our investigations. Such rarity highlights the conservation issue and the importance of protecting and monitoring CDB. Bryozoans are known to contribute significantly to the bioclastic part of soft bottoms worldwide (Halfar et al., 2006).
The assessment of widespread and patchy habitat such as CDB requires a seascape approach. It is known that the seascape can directly influence the trophic network and the ecosystem functioning (Boström et al., 2011; Abadie et al., 2018; Santos et al., 2022). In the light of that, a seascape approach should be a potential suitable descriptor to assess the quality of an ecosystem (e.g., marine forests, habitat structure, etc.; Cheminée et al., 2014; Thiriet et al., 2014). We have proposed a combination of methods to provide as broad and as representative an assessment as possible, involving direct observation via SCUBA diving, photoquadrats and seascape videos, taking into account functional ecosystem compartments structuring the seascape. The compartments assessed exhibited structuring communities (filter and suspension feeders, rhodoliths) which contribute to the seascape. For example, Gobert et al. (2014) proposed the LIMA index, which is based on a topographical and a biological description and could be applied on all types of habitat from 0 to 40 m depth. Such an approach could be of interest for CDB hosting structuring facies and associations; in the case of CDB without such features, a relatively unstructured seascape cannot be interpreted as a low-functioning ecosystem, considering the macrofauna of the sediment, a compartment of importance but with little or barely perceptible consequences for the seascape structure (bioturbation, calcified organisms providing bioclastic elements, etc.).
Our analysis showed strong and expected correlations between some of the descriptors. Defining an indicator implies the selection of suitable descriptors but that are not too numerous for an operational implementation. Our purpose was to assess the main compartments of the ecosystem, as defined in Figure 2, using as few descriptors as possible. The correlation observed between the final set of descriptors could lead us to reduce the number of descriptors and then simplify the assessment and avoid redundancy; at the same time, a combination of correlated descriptors assessing different ecological functions improves the robustness. As reported by Personnic et al. (2014), the removal of correlated boxes might result in a loss of accuracy of the method.
An ecosystem-based approach can be useful for Ecosystem Services assessment, providing quantitative data for the different ecosystem compartments (Seitz et al., 2014; Burgos et al., 2017). Such an approach has already been carried out for several ecosystems, including seagrass meadows and considering different bundles of ecosystem services according to different quality status (Kermagoret et al., 2019; Scémama et al., 2020).
4.3 A tool to support assessment of anthropogenic pressures
The next step should be the design of an anthropogenic pressure assessment tool to estimate the links between ecological status and cumulated anthropogenic pressures (Holon et al., 2015; Guarnieri et al., 2016; Quemmerais-Amice et al., 2020). Assessing anthropogenic pressures is challenging. Moreover, the assessment of human activities can be considered as a good proxy. In the present work, we did not identify visible evidence of trawling or dredging, the main physical pressures affecting CDB. Such evidence can be difficult to find, even in long-time trawled areas (Ordines et al., 2017). The main evidences of stress observed was the occurrence of macrowastes (e.g., glass, plastic, fishing gears) and invasive alien species (e.g., Caulerpa cylindracea) or the transition to Muddy detrital bottoms. A complementary approach is needed to better characterize human pressures. Our study sites are not located in areas with heavy impact of trawling activity, compared to e.g., the Gulf of Lion, the Catalan Coast or Balearic Islands (Farriols et al., 2017; Jac and Vaz, 2020). New data in different configurations with regard to anthropogenic pressures need to be sampled and assessed.
4.4 The need for an effective protection
The increase in the surface area of Marine Protected Areas driven by the European Union since the 2010s has led to the extension of MPAs over the continental shelf, including coastal detrital bottoms. The new borders of these MPAs do not automatically mean an effective protection and management, and it takes time for coastal managers to integrate these new areas in dashboards and management plans (Vassallo et al., 2020). As a result, the ecological status of CDB sites shows no correlation with the management level in contrast with the findings of Thibaut et al. (2017), who evidenced differences in sublittoral rocky reefs according to the level of management. In addition, we observed no statistical difference between Natura 2000 and unprotected sites, pointing some weaknesses already discussed by Gianni et al. (2022). These results highlight the need to enhance the conservation of widespread ecosystems of the continental shelf such as the CDB, requiring the implementation of effective marine protected areas (Sala et al., 2021).
The conservation or the restoration of CDB implies the reduction or at least the regulation of anthropogenic pressures to allow the natural resilience of communities identified as impacted by such activities. In that case, the role of effective MPAs is limited to the regulation or prohibition of certain human activities: e.g., trawling, dredging, artisanal fishing, mooring. The regulation of other pressures (e.g., terrigenous inputs, the spread of NIS, eutrophication, sedimentation) calls for larger scale policies.
4.5 EBQI implementation for CDB
As an indicator embracing the concept of the ecosystem-based approach, the aim of the EBQI defined for CDB is to provide relevant and useful data for both European Directives (MSFD, WFD, HD) and local management purposes. Compared to other indicators dedicated to soft bottoms (e.g., Borja et al., 2000; Labrune et al., 2006; Labrune et al., 2021), using the EBQI does not require a strong expertise in taxonomy even if more parameters are involved for its calculation. Fifteen descriptors are needed to assess the EBQI for CDB, 13 for Posidonia oceanica seagrass meadows (Personnic et al., 2014) and 10 for infralittoral rocky reefs (Thibaut et al., 2017). Data sampling can be carried out in less than one day per site, involving three operators, which is reasonable if planning a long-term monitoring network at regional (e.g., French Mediterranean coast) or local scale (e.g., MPA; Astruch et al., 2022b). The frame proposed by the EBQI can provide a standardized method for assessing the conservation status according to the Habitats Directive (e.g., assessment of the structure and the function of a given habitat), where diagnosis is usually based on expert judgement and broad data. Repeated applications over time would be welcome to assess the suitability of this index for describing change following management actions (Mancini et al., 2020; Oprandi et al., 2022).
The EBQI on CDB should be applied and tested in contexts with other biogeographical specificities and pressures: oligotrophic, warmer and impacted by non-indigenous species, where very little knowledge is available (e.g., Southern and Eastern Mediterranean Sea). The same approach could also be applied on subtidal rhodolith beds in other areas worldwide of high concern with regard to related human activities (e.g., Brittany). The strength of the EBQIs implemented from now in the Mediterranean Sea is the possibility to measure an ecological status even without all the descriptors assessed with the appropriate method, using the Confidence Index (CI) (see Bevilacqua et al., 2020; Güreşen et al., 2020). This point could allow a preliminary evaluation of CDB in areas with limited human and material resources.
5 Conclusion
An original Ecosystem-Based Quality Index (EBQI) for the Coastal Detrital Bottoms (CDB) has been defined, inspired by already existing EBQIs for Posidonia oceanica seagrass meadows, coralligenous reefs, underwater marine caves, and infralittoral rocky reefs, all based mainly on the assessment of their epibenthic assemblages. Relatively easy to implement and robust, the CDB EBQI provides a fit-for-purpose tool to assess the quality of this widespread ecosystem in accordance with public policies at national and European levels. In the context of the increase in the surface areas of Marine Protected Areas, management goals should include CDB in upcoming plans to secure the conservation of a habitat of importance at Mediterranean scale. Our approach is mainly focused on epibenthic assemblages and improvement is needed in order to better take into account the assessment of the macrofauna of the sediment. We suggest applying and testing this approach in other areas within the Mediterranean Sea and beyond.
Data availability statement
The raw data supporting the conclusions of this article will be made available by the authors, without undue reservation.
Author contributions
All authors (excepting AO, NM, BM, and BD) participated in a workshop in Marseille in April 2019. PA, BB, TS, and AO carried out the field investigations and the data sampling in 2020 and 2021. All authors contributed to the content for their associated sections. TS and AO produced the statistical analysis. PA compiled content from all authors and prepared the final version. All authors contributed to the article and approved the submitted version.
Funding
The present work was funded by the French Biodiversity Office in the frame of the ACDSea project (Assessment of Coastal Detrital ecosystem conservation Status: an ecosystem-based approach).
Acknowledgments
This work was carried out in the frame of the ACDSea project (Assessment of Coastal Detrital ecosystem conservation Status: an ecosystem-based approach). We kindly thank the French Biodiversity Office (OFB) for financial support, the experts involved in the working out of the index, Michèle Perret-Boudouresque for documentation assistance, and Michael Paul for proof-reading of the text. This work is dedicated to the memory of our late colleague Boris Daniel, involved in the ACDSea project since the first steps.
Conflict of interest
The authors declare that the research was conducted in the absence of any commercial or financial relationships that could be construed as a potential conflict of interest.
Publisher’s note
All claims expressed in this article are solely those of the authors and do not necessarily represent those of their affiliated organizations, or those of the publisher, the editors and the reviewers. Any product that may be evaluated in this article, or claim that may be made by its manufacturer, is not guaranteed or endorsed by the publisher.
Supplementary material
The Supplementary Material for this article can be found online at: https://www.frontiersin.org/articles/10.3389/fmars.2023.1130540/full#supplementary-material
Glossary
Life Science Identifiers
Taxa are sorted in order of appearance.
Posidonia oceanica (L.) Delile urn:lsid:marinespecies.org:taxname:145794
Cystoseira C. Agardh urn:lsid:marinespecies.org:taxname:144126
Ericaria Stackhouse urn:lsid:marinespecies.org:taxname:1535414
Gongolaria Boehmer urn:lsid:marinespecies.org:taxname:369694
Osmundaria volubilis R.E.Norris urn:lsid:marinespecies.org:taxname:144841
Lithophyllum racemus (Lamarck) Foslie urn:lsid:marinespecies.org:taxname:145160
Lithothamnion corallioides (P.Crouan & H.Crouan) P.Crouan & H.Crouan urn:lsi:marinespecies.org:taxname:145165
Lithothamnion crispatum Hauck urn:lsid:marinespecies.org:taxname:145166
Lithothamnion minervae Basso urn:lsid:marinespecies.org:taxname:145174
Lithothamnion valens Foslie urn:lsid:marinespecies.org:taxname:145180
Neogoniolithon hauckii (Rothpletz) R.A.Townsend & Huisman urn:lsid:marinespecies.org:taxname:169942
Spongites fruticulosa Kützing urn:lsid:marinespecies.org:taxname:494816
Peyssonnelia crispata Boudouresque & Denizot urn:lsid:marinespecies.org:taxname:145277
Peyssonnelia heteromorpha (Zanardini) Athanasiadis urn:lsid:marinespecies.org:taxname:1311383
Peyssonnelia rosa-marina Boudouresque & Denizot urn:lsid:marinespecies.org:taxname:145285
Peyssonnelia rubra (Greville) J.Agardh urn:lsid:marinespecies.org:taxname:145287
Peyssonnelia squamaria (S.G.Gmelin) Decaisne ex J.Agardh urn:lsid:marinespecies.org:taxname:145288
Sporolithon mediterraneum Heydrich urn:lsid:marinespecies.org:taxname:496041
Pentapora fascialis (Pallas, 1766) urn:lsid:marinespecies.org:taxname:111082
Smittina cervicornis (Pallas, 1766) urn:lsid:marinespecies.org:taxname:111551
Turbicellepora avicularis (Hincks, 1860) urn:lsid:marinespecies.org:taxname:111285
Hornera Lamouroux, 1821 urn:lsid:marinespecies.org:taxname:111041
Cladocora caespitosa (Linnaeus, 1767) urn:lsid:marinespecies.org:taxname:135146
Eunicella singularis (Esper, 1791) urn:lsid:marinespecies.org:taxname:125365
Eunicella verrucosa (Pallas, 1766) urn:lsid:marinespecies.org:taxname:125366
Leptogorgia sarmentosa (Esper, 1791) urn:lsid:marinespcies.org:taxname:125369
Alcyonium palmatum Pallas, 1766 urn:lsid:mariespecies.org:taxname:125334
Pennatula rubra (Ellis, 1764) urn:lsid:marinespecies.org:taxname:128519
Pteroeides griseum (Bohadsch, 1761) urn:lsid:marinespecies.org:taxname:181504
Palinurus elephas (Fabricius, 1787) urn:lsid:marinespecies.org:taxname:107703
Pinna nobilis L. 1758 urn:lsid:marinespecies.org:taxname:140780
Laminaria rodriguezii Bornet urn:lsid:marinespecies.org:axname:145729
Carpomitra costata (Stackhouse) Batters urn:lsid:marinespecies.org:taxname:145910
Codium bursa (Olivi) C.Agardh urn:lsid:marinespecies.org:taxname:145079
Codium vermilara (Olivi) Delle Chiaje urn:lsid:marinespecies.org:taxname:145093
Sphaerococcus coronopifolius Stackhouse urn:lsid:marinespecies.org:taxname:145908
Codium fragile (Suringar) Hariot urn:lsid:marinespecies.org:taxname:145086
Arthrocladia villosa (Hudson) Duby urn:lsid:marinespecies.org:taxname:145306
Sporochnus pedunculatus (Hudson) C.Agardh urn:lsid:marinespecies.org:taxname:145915
Acetabularia acetabulum (L.) P.C.Silva urn:lsid:marinespecies.org:taxname:494795
Padina pavonica (Linnaeus) Thivy urn:lsid:marinespecies.org:taxname:145385
Umbraulva dangeardii M.J.Wynne & G.Furnari urn:lsid:marinespecies.org:taxname:840293
Chrysymenia ventricosa (J.V.Lamouroux) J.Agardh urn:lsid:marinespecies.org:taxname:145845
Sebdenia (J.Agardh) Berthold urn:lsid:marinespecies.org:taxname:144177
Caulerpa cylindracea Sonder urn:lsid:marinespecies.org:taxname:660621
Caulerpa taxifolia (M.Vahl) C.Agardh urn:lsid:marinespecies.org:taxname:144476
Acinetospora crinita (Carmichael) Sauvageau urn:lsid:marinespecies.org:taxname:145398
Chrysonephos lewisii (W.R.Taylor) W.R.Taylor urn:lsid:marinespecies.org:taxname:375956
Nematochrysopsis marina (J.Feldmann) C.Billard urn:lsid:marinespecies.org:taxname:375863
Zosterocarpus oedogonium (Meneghini) Bornet urn:lsid:marinespecies.org:taxname:145476
Pseudochlorodesmis furcellata (Zanardini) Børgesen urn:lsid:marinespecies.org:taxname:144485
Valonia macrophysa Kützing urn:lsid:marinespecies.org:taxname:145883
Womersleyella setacea (Hollenberg) R.E.Norris urn:lsid:marinespecies.orgtaxname:146371
Acrothamnion preissii (Sonder) E.M.Wollaston urn:lsid:marinespecies.org:taxname:144488
Sphaerechinus granularis (Lamarck, 1816) urn:lsid:marinespecies.org:taxname:124427
Stylocidaris affinis (Philippi, 1845) urn:lsid:marinespecies.org:taxname:124268
Centrostephanus longispinus (Philippi, 1845) urn:lsid:marinespecies.org:taxname:124331
Echinus melo Lamarck, 1816 urn:lsid:marinespecies.org:taxname:124294
Cerithium Bruguière, 1789 urn:lsid:marinespecies.org:taxname:137760
Aplysia L., 1767 urn:lsid:marinespecies.org:taxname:137654
Sarpa salpa (L., 1758) urn:lsid:marinespecies.org:taxname:127064
Laevicardium oblongum (Gmelin, 1791) urn:lsid:marinespecies.org:taxname:139006
Acanthocardia deshayesii (Payraudeau, 1826) urn:lsid:marinespecies.org:taxname:138991
Suberites domuncula (Olivi, 1792) urn:lsid:marinespecies.org:taxname:134282
Petta pusilla Malmgren, 1866 urn:lsid:marinespecies.org:taxname:130597
Turritellinella tricarinata (Brocchi, 1814) urn:lsid:marinespecies.org:taxname:1381415
Moerella donacina (L., 1758) urn:lsid:marinespecies.org:taxname:147021
Abra nitida (O. F. Müller, 1776) urn:lsid:marinespecies.org:taxname:141435
Abra prismatica (Montagu, 1808) urn:lsid:marinespecies.org:taxname:141436
Holothuria L., 1767 urn:lsid:marinespecies.org:taxname:123456
Bonellia viridis Rolando, 1822 urn:lsid:marinespecies.org:taxname:110363
Spatangus purpureus O.F. Müller, 1776 urn:lsid:marinespecies.org:taxname:124418
Chaetaster longipes (Bruzelius, 1805) urn:lsid:marinespecies.org:taxname:124004
Echinaster sepositus (Retzius, 1783) urn:lsid:marinespecies.org:taxname:125161
Cliona viridis (Schmidt, 1862) urn:lsid:marinespecies.org:taxname:134146
Spicara maena (L., 1758) urn:lsid:marinespecies.org:taxname:126828
Spicara smaris (L., 1758) urn:lsid:marinespecies.org:taxname:126830
Boops boops (L., 1758) urn:lsid:marinespecies.org:taxname:127047
Chromis chromis (L., 1758) urn:lsid:marinespecies.org:taxname:127000
Anthias anthias (L., 1758) urn:lsid:marinespecies.org:taxname:127031
Scyliorhinus Blainville, 1816 urn:lsid:marinespecies.org:taxname:105729
Serranus hepatus (L., 1758) urn:lsid:marinespecies.org:taxname:127042
Blennius ocellaris L., 1758 urn:lsid:marinespecies.org:taxname:126761
Symphodus cinereus (Bonnaterre, 1788) urn:lsid:marinespecies.org:taxname:273567
Solea Quensel, 1806 urn:lsid:marinespecies.org:taxname:126132
Lophius L., 1758 urn:lsid:marinespecies.org:taxname:125802
Scorpaena scrofa L., 1758 urn:lsid:marinespecies.org:taxname:127248
Dentex dentex (L., 1758) urn:lsid:marinespecies.org:taxname:273962
Pagrus pagrus (L., 1758) urn:lsid:marinespecies.org:taxname:127063
Zeus faber L., 1758 urn:lsid:marinespecies.org:taxname:127427
References
Abadie A., Pace M., Gobert S., Borg J. A. (2018). Seascape ecology in Posidonia oceanica seagrass meadows: Linking structure and ecological processes for management. Ecol. Indic. 87, 1–13. doi: 10.1016/j.ecolind.2017.12.029
Agnesi S., Annunziatellis A., Inghilesi R., Mo G., Orasi A. (2020). The contribution of wind-wave energy at sea bottom to the modelling of rhodolith beds distribution in an offshore continental shelf. Mediterr. Mar. Sci. 21 (2), 433–441. doi: 10.12681/mms.22462
Aguado-Giménez F., Ruiz-Fernández J. M. (2012). Influence of an experimental fish farm on the spatio-temporal dynamic of a Mediterranean maerl algae community. Mar. Environ. Res. 74, 47–55. doi: 10.1016/j.marenvres.2011.12.003
Aguilar R., Pastor X., Torriente A., Garcia S. (2009). “Deep-sea coralligenous beds observed with ROV on four seamounts in the western Mediterranean,” in UNEP–MAP–RAC/SPA proceedings of the 1st Mediterranean symposium on the conservation of the coralligenous and other calcareous bio-concretions. Eds. Pergent-Martini C., Brichet M. (Tabarka, Tunisia: RAC/SPA), 148–150.
Aliani S., Bianchi C. N., De Biasi A. M. (1994). Benthos changes in a dumping site. Biol. Marina Mediterr. 1 (1), 265–269.
Amado-Filho G. M., Bahia R. G., Pereira-Filho G. H., Longo L. L. (2017). “South Atlantic rhodolith beds: latitudinal distribution, species composition, structure and ecosystem functions, threats and conservation status,” in Rhodolith/Maërl beds: A global perspective (Cham.: Springer), 299–317. doi: 10.1007/978-3-319-29315-8_12
Amado-Filho G. M., Pereira-Filho G. H. (2012). Rhodolith beds in Brazil: a new potential habitat for marine bioprospection. Rev. Bras. Farmacognosia 22, 782–788. doi: 10.1590/S0102-695X2012005000066
Anderson M. J. (2001). A new method for non-parametric multivariate analysis of variance. Austral Ecol. 26, 32–46.
Aouissi M., Sellam L. N., Boudouresque C. F., Blanfuné A., Derbal F., Frihi H., et al. (2018). Insights into the species diversity of the genus Sargassum (Phaeophyceae) in the Mediterranean Sea, with a focus on a previously unnoticed taxon from Algeria. Mediterr. Mar. Sci. 19 (1), 48–57. doi: 10.12681/mms.14079
Astruch P., Boudouresque C. F., Bonhomme D., Goujard A., Antonioli P. A., Bonhomme P., et al. (2012). Mapping and state of conservation of benthic marine habitats and assemblages of port-cros national park (Provence, France, northwestern Mediterranean Sea). Sci. Rep. Port-Cros Natl. Park 26, 45–90.
Astruch P., Goujard A., Rouanet E., Boudouresque C. F., Verlaque M., Berthier L., et al. (2019). “Assessment of the conservation status of coastal detrital sandy bottoms in the Mediterranean Sea: An ecosystem-based approach in the framework of the ACDSea project,” in Proceedings of the 3rd Mediterranean Symposium on the conservation of coralligenous and other calcareous bio-concretions, Antalya, Turquie, 15-16 january 2019 Eds. Langar H., Ouerghi A. (Tunis: RAC/SPA Publ.) 23–29.
Astruch P., Orts A., Schohn T., Belloni B., Ballesteros E., Bianchi C. N., et al. (2022a). “Towards an ecosystem-based index to assess the ecological status of Mediterranean coastal detrital bottoms,” in Proceedings of the 4th Mediterranean symposium on the conservation of coralligenous & other calcareous bio-concretions. Eds. Bouafif C., Ouerghi A. (Tunis: SPA/RAC), 137–138.
Astruch P., Schohn T., Belloni B., Cassetti O., Cabral M., Ruitton S., et al. (2022b). “Involving managers in the ecosystem-based assessment of marine habitats: A case study in French Catalonia,” in Proceedings of the 7th Mediterranean symposium on marine vegetation Mediterranean symposia on marine vegetation. Eds. Bouafif C., Ouerghi A. (Tunis: SPA/RAC).
Augier H., Boudouresque C. F. (1975). Dix ans de recherches dans la zone marine du parc national de port- cros-troisième partie. Annales la SSNATV 27, 133–170.
Augier H., Boudouresque C. F. (1978). Végétation marine de l’île de port-cros (Parc national) XVI: Contribution à l’étude de l’épiflore du détritique côtier. Travaux Scientifiques du Parc Natl. Port-Cros 4, 101–125.
Austen M. C., Lambshead P. J. D., Hutchings P. A., Boucher G., Snelgrove P. V. R., Heip C., et al. (2002). Biodiversity links above and below the marine sediment–water interface that may influence community stability. Biodiversity Conserv. 11 (1), 113–136. doi: 10.1023/A:1014098917535
Azam F., Fenchel T., Field J. G., Gray J. S., Meyer-Reil L. A., Thingstad F. (1983). The ecological role of water-column microbes in the sea. Mar. Ecol. Prog. Ser. 10, 257–263. doi: 10.3354/meps010257
Bajjouk T., Guillaumont B., Michez N., Thouin B., Croguennec C., Populus J., et al. (2015). Classification EUNIS, système d’information européen sur la nature: Traduction française des habitats benthiques des régions atlantique et méditerranée. Habitats subtidaux complexes d’habitats 2, 237.
Ballesteros E. (1989). Production of seaweeds in northwestern Mediterranean marine communities: its relation with environmental factors. Scientia Marina 53 (2-3), 357–364.
Ballesteros E. (1990). Structure and dynamics of the community of Cystoseira zosteroides (Turner) c. agardh (Fucales, phaeophyceae) in the northwestern Mediterranean. Scientia Marina 54 (3), 217–229.
Ballesteros E. (1994). The deep-water Peyssonnelia beds from the Balearic islands (Western Mediterranean). Mar. Ecol. 15 (3-4), 233–253. doi: 10.1111/j.1439-0485.1994.tb00055.x
Ballesteros E. (2006). Mediterranean Coralligenous assemblages: A synthesis of present knowledge. Oceanogr. Mar. Biol. 44, 123–195. doi: 10.1201/9781420006391.ch4
Ballesteros E., Torras X., Pinedo S., García M., Mangialajo L., De Torres M. (2007). A new methodology based on littoral community cartography dominated by macroalgae for the implementation of the European water framework directive. Mar. Pollut. Bull. 55 (1-6), 172–180. doi: 10.1016/j.marpolbul.2006.08.038
Bănaru D., Harmelin-Vivien M., Gomoiu M. T., Onciu T. M. (2007). Influence of the Danube river inputs on c and n stable isotope ratios of the Romanian coastal waters and sediment (Black Sea). Mar. Pollut. Bull. 54, 1385–1384. doi: 10.1016/j.marpolbul.2007.05.022
Bănaru D., Mellon-Duval C., Roos D., Bigot J. L., Souplet A., Jadaud A., et al. (2013). Trophic interactions in the gulf of lions ecosystem (northwestern Mediterranean) and fishing impacts. ICES J. Mar. Syst. 111-112, 45–68. doi: 10.1016/j.jmarsys.2012.09.010
Barbera C., Bordehore C., Borg J. A., Glémarec M., Grall J., Hall-Spencer J. M., et al. (2003). Conservation and management of northeast Atlantic and Mediterranean maerl beds. Aquat. Conservation: Mar. Freshw. Ecosyst. 13 (S1), S65–S76. doi: 10.1002/aqc.569
Basso D. (2012). Carbonate production by calcareous red algae and global change. Geodiversitas 34 (1), 13–33. doi: 10.5252/g2012n1a2
Basso D., Babbini L., Kaleb S., Bracchi V., Falace A. (2016). Monitoring deep Mediterranean rhodolith beds. Aquat. Conservation: Mar. Freshw. Ecosystem 26, 549–561. doi: 10.1002/aqc.2586
Basso D., Babbini L., Ramos-Esplá A. A., Salomidi M. (2017). “Mediterranean Rhodolith beds,” in Rhodolith/Maerl beds: a global perspective (Cham.: Springer), 281–298. doi: 10.1007/978-3-319-29315-8_11
Basso D., Nalin R., Massari F. (2007). Genesis and composition of the pleistocene coralligène de plateau of the cutro terrace (Calabria, southern Italy). Neues Jahrbuch für Geol. und Paläontologie–Abhandlungen 244 (2), 73–182. doi: 10.1127/0077-7749/2007/0244-0173
Basso D., Quaranta F., Vannucci G., Piazza M. (2012). Quantification of the coralline carbonate from a serravallian rhodolith bed of the tertiary piedmont basin (Stazzano, alessandria, NW Italy). Geodiversitas 34 (1), 137–149. doi: 10.5252/g2012n1a8
Begon M., Harper J. L., Townsend C. R. (1986). Ecology: Individuals, populations and communities (Oxford: Blackwell Scientific Publications), 876.
Bellan G., Bellan-Santini D., Picard J. (1980). Mise en évidence de modèles éco-biologique dans des zones soumises à perturbations par matières organiques. Oceanol. Acta 3 (3), 383–390.
Bellan-Santini D., Lacaze J. C., Poizat C. (1994). Les Biocénoses marines et littorales de méditerranée. synthèse, menaces et perspectives. Collection Patrimoines naturels 19, 246. Secrétariat de la faune et de la flore/MNHN, Paris.
Bensettiti F., Bioret F., Roland J., Lacoste J. P. (2004). Cahiers d’habitats natura 2000. connaissance et gestion des habitats et des espèces d’intérêt communautaire. tome 2 - habitats côtiers (Paris: La Documentation française), 399. MEDD/MAAPAR/MNHN.
Bergbauer M., Humberg B. (2000). “La vie sous-marine en méditerranée,” in Guide vigot (p: Vigot), 318.
Bermejo R., Chefaoui R. M., Engelen A. H., Buonomo R., Neiva J., Ferreira-Costa J., et al. (2018). Marine forests of the Mediterranean-Atlantic Cystoseira tamariscifolia complex show a southern Iberian genetic hotspot and no reproductive isolation in parapatry. Sci. Rep. 8, 10427. doi: 10.1038/s41598-018-28811-1
Bernard G., Romero-Ramirez A., Tauran A., Pantalos M., Deflandre B., Grall J., et al. (2019). Declining maerl vitality and habitat complexity across a dredging gradient: Insights from in situ sediment profile imagery (SPI). Sci. Rep. 9 (1), 1–12. doi: 10.1038/s41598-019-52586-8
Bevilacqua S., Katsanevakis S., Micheli F., Sala E., Rilov G., Sarà G., et al. (2020). The status of coastal benthic ecosystems in the Mediterranean Sea: evidence from ecological indicators. Front. Mar. Sci. 7. doi: 10.3389/fmars.2020.00475
Bianchi C. N. (2009a). Gli habitat prioritari del protocollo SPA/BIO (Convenzione di barcellona) presenti in italia. schede descrittive per l’identificazione. IV. 2. 2. 10. facies with large bryozoans. Biol. Marina Mediterr. 16 (Suppl. 1), 209–212.
Bianchi C. N. (2009b). Gli habitat prioritari del protocollo SPA/BIO (Convenzione di barcellona) presenti in italia. schede descrittive per l’identificazione. III. 6. 1.14. facies with Cladocora caespitosa. Biol. Marina Mediterr. 16 (Suppl. 1), 163–166.
Bianchi C. N., Ardizzone D., Belluscio A., Colantoni P., Diviacco G., Morri C., et al. (2004). Benthic cartography. Biol. Marina Mediterr. 11 (suppl. 1), 347–370.
Bianchi C. N., Azzola A., Bertolino M., Betti F., Bo M., Cattaneo-Vietti R., et al. (2019a). Consequences of the marine climate and ecosystem shift of the 1980-90s on the ligurian Sea biodiversity (NW Mediterranean). Eur. Zool. J. 86 (1), 458–487. doi: 10.1080/24750263.2019.1687765
Bianchi C. N., Azzola A., Cocito S., Morri C., Oprandi A., Peirano A., et al. (2022). Biodiversity monitoring in Mediterranean marine protected areas: scientific and methodological challenges. Diversity 14, 43. doi: 10.3390/d14010043
Bianchi C. N., Azzola A., Parravicini V., Peirano A., Morri C., Montefalcone M. (2019b). Abrupt change in a subtidal rocky reef community coincided with a rapid acceleration of sea water warming. Diversity 11, 215. doi: 10.3390/d11110215
Bianchi C. N., Parravicini V., Montefalcone M., Rovere A., Morri C. (2012). The challenge of managing marine biodiversity: a practical toolkit for a cartographic, territorial approach. Diversity 4, 419–452. doi: 10.3390/d4040419
Blanchard J. L., Jennings S., Law R., Castle M. D., McCloghrie P., Rochet M. J., et al. (2009). How does abundance scale with body size in coupled size-structured food webs? J. Anim. Ecol. 78 (1), 270–280. doi: 10.1111/j.1365-2656.2008.01466.x
Blanfuné A., Thibaut T., Boudouresque C. F., Mačić V., Markovic L., Palomba L., et al. (2017). The CARLIT method for the assessment of the ecological quality of European Mediterranean waters: Relevance, robustness and possible improvements. Ecol. Indic. 72, 249–259. doi: 10.1016/j.ecolind.2016.07.049
Bond W. (2001). Keystone species–hunting the snark? Science 292, 63–64. doi: 10.1126/science.1060793
Bordehore C., Borg J. A., Lanfranco E., Espla A. A., Rizzo M., Schembri P. J. (2000). “Trawling as a major threat to Mediterranean maerl beds,” in First Mediterranean Symposium on Marine Vegetation, Ajaccio. (Tunis: RAC/SPA publ.) 105–109.
Bordehore C., Ramos-Esplá A. A., Riosmena-Rodríguez R. (2003). Comparative study of two maerl beds with different otter trawling history, southeast Iberian peninsula. Aquat. Conservation: Mar. Freshw. Ecosyst. 13 (S1), S43–S54. doi: 10.1002/aqc.567
Borja A., Franco J., Pérez V. (2000). A marine biotic index to establish the ecological quality of soft-bottom benthos within European estuarine and coastal environments. Mar. Pollut. Bull. 40, 1100–1114. doi: 10.1016/S0025-326X(00)00061-8
Borja Á., Marín S. L., Muxika I., Pino L., Rodríguez J. G. (2015). Is there a possibility of ranking benthic quality assessment indices to select the most responsive to different human pressures? Mar. Pollut. Bull. 97 (1-2), 85–94. doi: 10.1016/j.marpolbul.2015.06.030
Borja A., Menchaca I., Garmendia J. M., Franco J., Larreta J., Sagarminaga Y., et al. (2021). Big insights from a small country: The added value of integrated assessment in the marine environmental status evaluation of Malta. Front. Mar. Sci. 8, 375. doi: 10.3389/fmars.2021.638232
Borja A., Muxika I., Franco J. (2003). The application of a marine biotic index to different impact sources affecting soft-bottom benthic communities along European coasts. Mar. Pollut. Bull. 46, 835–845. doi: 10.1016/S0025-326X(03)00090-0
Bosellini A., Ginsburg R. N. (1971). Form and internal structure of recent algal nodules (rhodolites) from Bermuda. J. Geol. 79 (6), 669–682. doi: 10.1086/627697
Boström C., Pittman S. J., Simenstad C., Kneib R. T. (2011). Seascape ecology of coastal biogenic habitats: advances, gaps, and challenges. Mar. Ecol. Prog. Ser. 427, 191–217. doi: 10.3354/meps09051
Boudouresque C. F. (2015). “Taxonomy and phylogeny of unicellular eukaryotes,” in Environmental microbiology: Fundamentals and applications (Dordrecht: Springer), 191–257. doi: 10.1007/978-94-017-9118-2_7
Boudouresque C. F., Astruch P., Bănaru D., Blanfuné A., Belloni B., Changeux T., et al. (2020b). Ecosystem-based quality indices: Valuable tools for environment management. Vie Milieu – Life Environ. 70 (3-4), 2–15.
Boudouresque C. F., Astruch P., Bănaru D., Blanfuné A., Carlotti F., Faget D., et al. (2020a). “Global change and the management of Mediterranean coastal habitats: A plea for a socio-ecosystem-based approach,” in Evolution of marine coastal ecosystems under the pressure of global changes (Cham: Springer International Publishing), 297–320. doi: 10.1007/978-3-030-43484-7_20
Boudouresque C. F., Astruch P., Goujard A., Rouanet E., Bonhomme D., Bonhomme P. (2019). “The withdrawal of the lower limit of the Posidonia oceanica seagrass meadow in the bay of hyères (NW mediterranean): A combination of natural and human-induced recent and ancient phenomena?,” in Proceedings of the 6th Mediterranean symposium on marine vegetation, Antalya, Turkey, 14-15 January 2019. 35–40.
Boudouresque C. F., Denizot M. (1973). Recherches sur le genre Peyssonnelia (Rhodophycées) i. peyssonnelia rosa-marina sp. nov. et Peyssonnelia bornetii sp. nov. Giornale Botanico Italiano 107, 17–27. doi: 10.1080/11263507309426312
Boudouresque C. F., Pergent G., Pergent-Martini C., Ruitton S., Thibaut T., Verlaque M. (2016). The necromass of the Posidonia oceanica seagrass meadow: Fate, role, ecosystem services and vulnerability. Hydrobiologia 781 (1), 25–42. doi: 10.1007/s10750-015-2333-y
Boudouresque C. F., Verlaque M. (2002). Biological pollution in the Mediterranean Sea: invasive versus introduced macrophytes. Mar. Pollut. Bull. 44 (1), 32–38. doi: 10.1016/S0025-326X(01)00150-3
Bourcier M. (1982). Nouvelles localisations et délimitation fine de quelques faciès de la biocénose des fonds détritiques côtiers du PN port-cros. Travaux Scientifiques du Parc Natl. Port-Cros 8, 19–24.
Bourcier M. (1985). Localisation et délimitation de quelques faciès de la biocénose des fonds détritiques côtiers dans la partie nord du PN de port-cros. Parc Natl. Port-Cros Ed. Sci. Rep. Port-Cros Natl. Park 11, 181–183.
Bourcier M. (1988). Macrobenthos de substrat meuble circalittoral autour’ de l’île de port-cros. Parc Natl. Port-Cros Ed. Sci. Rep. Port-Cros Natl. Park 14, 41–64.
Bourcier M., Zibrowius H. (1973). Red muds dumped in the la cassidaigne (Marseille region), observations with the sp 350 dipping saucer (June 1971), and results of dredgings. Bull. Signalétique C.N.R.S. 740-1973 34 11-12), 15190.
Bruno de Sousa C., Cox C. J., Brito L., Pavão M. M., Pereira H., Ferreira A., et al. (2019). Improved phylogeny of brown algae Cystoseira (Fucales) from the Atlantic-Mediterranean region based on mitochondrial sequences. PloS One 14, e0210143. doi: 10.1371/journal.pone.0210143
Buffoni G., Bianchi C. N., Delfanti R., Di Cola G., Morri C., Niccolai I., et al. (1990). Modello di reti trofiche in biocenosi bentiche. applicazione a biocenosi del golfo di gaeta. Atti dell’Associazione Italiana di Oceanol. e Limnol. 8, 227–251.
Burgos E., Montefalcone M., Ferrari M., Paoli C., Vassallo P., Morri C., et al. (2017). Ecosystem functions and economic wealth: Trajectories of change in seagrass meadows. J. Cleaner Production 168, 1108–1119. doi: 10.1016/j.jclepro.2017.09.046
Burrows M. T., Kamenos N. A., Hughes D. J., Stahl H., Howe J. A., Tett P. (2014). “Assessment of carbon budgets and potential blue carbon stores in scotland’s coastal and marine environment,” in Scottish Natural heritage commissioned report no. 761 (Scottish Natural Heritage).
Carpine C. (1958). Recherches sur les fonds à Peyssonnelia polymorpha (Zan.) schmitz de la région de marseille. Bull. l’Institut Océanographique Monaco 1125, 1–50.
Carpine C. (1964). Contribution à l’étude bionomique de la méditerranée occidentale (côtes du var et des alpes maritimes, côtes occidentales de corse). fasc. 3: La côte de l’Estérel, de la pointe des lions à la pointe de l’Aiguille (Région A2). Bull. l’Institut Océanographique Monaco 63 (1312 A et B), 1–52.
Cerrano C., Bavestrello G., Bianchi C. N., Calcinai B., Cattaneo-Vietti R., Morri C., et al. (2001). “The role of sponge bioerosion in Mediterranean coralligenous accretion,” in Mediterranean Ecosystems (Milano: Springer), 235–240.
Cerrano C., Bavestrello G., Bianchi C. N., Cattaneo-Vietti R., Bava S., Morganti C., et al. (2000). A catastrophic mass-mortality episode of gorgonians and other organisms in the ligurian Sea (North-western Mediterranean), summer 1999. Ecol. Lett. 3, 284293. doi: 10.1046/j.1461-0248.2000.00152.x
Cheminée A., Feunteun E., Clerici S., Cousin B., Francour P. (2014). “Management of infralittoral habitats: Towards a seascape scale approach,” in Underwater seascapes (Cham: Springer), 161–183. doi: 10.1007/978-3-319-03440-9
Chen C. T., Carlotti F., Harmelin-Vivien M., Lebreton B., Guillou G., Vassallo L., et al. (2022). Diet and trophic interactions of Mediterranean planktivorous fishes. Mar. Biol. 169 (9), 1–18. doi: 10.1007/s00227-022-04103-1
Cocito S., Fanucci S., Niccolai I., Morri C., Bianchi C. N. (1990). Relationships between trophic organization of benthic communities and organic matter content in tyrrhenian Sea sediments. Hydrobiologia 207 (1), 53–60. doi: 10.1007/BF00041440
Cocito S., Sgorbini S., Bianchi C. N., Morgigni M. (1994). R.O.V. monitoring in a dumping site. Biol. Marina Mediterr. 1 (1), 275–276.
Connell S. D., Foster M. S., Airoldi L. (2014). What are algal turfs? towards a better description of turfs. Mar. Ecol. Prog. Ser. 495, 299–307. doi: 10.3354/meps10513
Costa S. (1960). Recherches sur les fonds à Halarachnion spatulatum de la baie de marseille. Vie Milieu 11, 1–68.
Cresson P., Chouvelon T., Bustamante P., Bănaru D., Baudrier J., Le Loc’h F., et al. (2020). Primary production and depth drive different trophic structure and functioning in French marine ecosystems. Prog. Oceanogr. 186 (102343), 1–14. doi: 10.1016/j.pocean.2020.102343
Cresson P., Ruitton S., Harmelin-Vivien M. (2014). Artificial reefs do increase secondary biomass production: Mechanisms evidenced by stable isotopes. Mar. Ecol. Prog. Ser. 509, 15–26. doi: 10.3354/meps10866
Darnaude A. M. (2005). Fish ecology and terrestrial carbon use in coastal areas: Implications for marine fish production. J. Anim. Ecol. 74, 864–876. doi: 10.1111/j.1365-2656.2005.00978.x
Davies C. E., Moss D., Hill M. O. (2004). EUNIS habitat classification revised 2004 (European environment agency-European topic centre on nature protection and biodiversity), 127–143.
De Bettignies T., La Rivière M., Delavenne J., Dupré S., Gaudillat V., Janson A.-L., et al. (2021). Interprétation française des habitats d’Intérêt communautaire marins (Paris: PatriNat (OFB-CNRS-MNHN), 58.
De Grave S., Whitaker A. (1999). Benthic community re-adjustment following dredging of a muddy-maerl matrix. Mar. Pollut. Bull. 38 (2), 102–108. doi: 10.1016/S0025-326X(98)00103-9
Deidun A., Marrone A., Gauci A., Galdies J., Lorenti M., Mangano M. C., et al. (2022). Structure and biodiversity of a Maltese maerl bed: New insight into the associated assemblage 24 years after the first investigation. Regional Stud. Mar. Sci. 52, 102262. doi: 10.1016/j.rsma.2022.102262
de Jonge V. N., Schückel U., Baird D. (2019). Subsets of food webs cannot be used as a substitute to assess the functioning of entire ecosystems. Mar. Ecol. Prog. Ser. 613, 49–66. doi: 10.3354/meps12863
Dell’Anno A., Mei M. L., Pusceddu A., Danovaro R. (2002). Assessing the trophic state and eutrophication of coastal marine systems: A new approach based on the biochemical composition of sediment organic matter. Mar. Pollut. Bull. 44 (7), 611–622. doi: 10.1016/S0025-326X(01)00302-2
Demestre M., Muntadas A., Sanchez P., Garcia-de-Vinuesa A., Mas J., Franco I., et al. (2017). “Bio and anthropogenic disturbance of maërl communities settled on subaqueous dunes on the mar menor continental shelf (Western Mediterranean),” in Atlas of bedforms in the Western Mediterranean (Cham: Springer International Publishing), 215–219. doi: 10.1007/978-3-319-33940-5_33
De Ridder C., Lawrence J. M. (1982). “Food and feeding mechanisms: Echinoidea,” in Echinoderm nutrition: 57-1 15. Eds. Jangoux M., Lawrence J. M. (Rotterdam: Balkema).
Dutertre M., Grall J., Ehrhold A., Hamon D. (2015). Environmental factors affecting maerl bed structure in Brittany (France). Eur. J. Phycol. 50 (4), 371–383. doi: 10.1080/09670262.2015.1063698
Enrichetti F., Bo M., Morri C., Montefalcone M., Toma M., Bavestrello G., et al. (2019). Assessing the environmental status of temperate mesophotic reefs: a new, integrated methodological approach. Ecol. Indic. 102, 218–229. doi: 10.1016/j.ecolind.2019.02.028
Farriols M. T., Ordines F., Somerfield P. J., Pasqual C., Hidalgo M., Guijarro B., et al. (2017). Bottom trawl impacts on Mediterranean demersal fish diversity: not so obvious or are we too late? Continental Shelf Res. 137, 84–102. doi: 10.1016/j.csr.2016.11.011
Fornos J. J., Ballesteros E., Massutti C., Rodriguez-Perea A. (1988). Red algae sediments in the Balearic shelf. Rapports Procès Verbaux Des. Réunions Commission Internationale pour l’Exploration Scientifique la Mer Méditerranée 31 (2), 86–86.
Foster M. S., Amado Filho G. M., Kamenos N. A., Riosmena-Rodríguez R., Steller D. L. (2013). Rhodoliths and rhodolith beds. Res. discoveries: revolution Sci. through SCUBA. 39, 143–155.
Foster M. S. R., Riosmena-Rodriguez R., Steller D. L., Woelkerling W. J. (1997). Living rhodolith beds in the gulf of California and their implications. pliocene carbonates and related facies flanking the gulf of California, Baja California, Mexico. Geol. Soc. America special paper 318), 127–139.
Fragkopoulou E., Serrão E. A., Horta P. A., Koerich G., Assis J. (2021). Bottom trawling threatens future climate refugia of rhodoliths globally. Front. Mar. Sci. 7. doi: 10.3389/fmars.2020.594537
Francour P. (1989). L’oursin Centrostephanus longispinus en méditerranée occidentale: résultats d’une enquête sur sa répartition et son écologie. Vie Marine H.S. n°10 Actes du VI séminaire Int. sur les Echinodermes 1988), 138–147.
Francour P. (1991). “Statut de centrostephanus longispinus en méditerranée,” in Colloque internation. espèces marines à protéger en méditerranée. Eds. Boudouresque C. F., Avon M., Gravez V. (Marseille: GIS Posidonie publ.), 187–202.
Garrabou J., Coma R., Bensoussan N., Bally M., Chevaldonné P., Cigliano M., et al. (2009). Mass mortality in northwestern Mediterranean rocky benthic communities: effects of the 2003 heat wave. Global Change Biol. 15 (5), 1090–1103. doi: 10.1111/j.1365-2486.2008.01823.x
Gatti G., Bianchi C. N., Morri C., Montefalcone M., Sartoretto S. (2015). Coralligenous reefs state along anthropized coasts: Application and validation of the COARSE index, based on a rapid visual assessment (RVA) approach. Ecol. Indic. 52, 567–576. doi: 10.1016/j.ecolind.2014.12.026
Gautier Y., Picard J. (1957). Bionomie du banc de magaud (Est des iles d’Hyères) (Marseille: Recueil des Travaux de la Station Marine d’Endoume), 28–42.
Gayet G., Baptist F., Maciejewski L., Poncet R., Bensettiti F. (2018). Guide de détermination des habitats terrestres et marins de la typologie EUNIS - version 1.0 (AFB, collection Guides et protocoles), 230.
Giakoumi S., Halpern B. S., Michel L. N., Gobert S., Sini M., Boudouresque C. F., et al. (2015). Towards a framework for assessment and management of cumulative human impacts on marine food webs. Conserv. Biol. 29 (4), 1228–1234. doi: 10.1111/cobi.12468
Gianni F., Manea E., Cataletto B., Pugnetti A., Bergami C., Bongiorni L., et al. (2022). Are we overlooking natura 2000 sites? lessons learned from a transnational project in the Adriatic Sea. Front. Mar. Sci. 9. doi: 10.3389/fmars.2022.1070373
Giuliani S., Lamberti C. V., Sonni C., Pellegrini D. (2005). Mucilage impact on gorgonians in the tyrrhenian Sea. Sci. Total Environ. 353 (1-3), 340–349. doi: 10.1016/j.scitotenv.2005.09.023
Gobert S., Chéry A., Volpon A., Pelaprat C., Lejeune P. (2014). “The seascape as an indicator of environmental interest and quality of the Mediterranean benthos: The in situ development of a description index: the LIMA,” in Underwater seascapes (Cham: Springer International Publishing), 277–291. doi: 10.1007/978-3-319-03440-9_18
Gobert S., Sartoretto S., Rico-Raimondino V., Andral B., Chery A., Lejeune P., et al. (2009). Assessment of the ecological status of Mediterranean French coastal waters as required by the water framework directive using the posidonia oceanica rapid easy index: PREI. Mar. Pollut. Bull. 58 (11), 1727–1733. doi: 10.1016/j.marpolbul.2009.06.012
Guarnieri G., Bevilacqua S., De Leo F., Farella G., Maffia A., Terlizzi A., et al. (2016). The challenge of planning conservation strategies in threatened seascapes: Understanding the role of fine scale assessments of community response to cumulative human pressures. PloS One 11 (2), e0149253. doi: 10.1371/journal.pone.0149253
Güreşen A., Güreşen S. O., Aktan Y. (2020). Combined synthetic and biotic indices of Posidonia oceanica to qualify the status of coastal ecosystems in the north Aegean. Ecol. Indic. 113, 106149. doi: 10.1016/j.ecolind.2020.106149
Halfar J., Strasser M., Riegl B., Godinez-Orta L. (2006). “Oceanography, sedimentology and acoustic mapping of a bryomol carbonate factory in the northern gulf of California, Mexico,” in Cool-water carbonates: Depositional systems and palaeoenvironmental controls, vol. 255 . Eds. Pedley H. M., Carannante G. (London: Geological Society), 197–215.
Hall-Spencer J. M. (1998). Conservation issues relating to maerl beds as habitats for molluscs. J. Conchol. special Publ. 2, 271–286.
Hall-Spencer J. M., Harvey B. P. (2019). Ocean acidification impacts on coastal ecosystem services due to habitat degradation. Emerging Topics Life Sci. 3 (2), 197–206. doi: 10.1042/ETLS20180117
Harmelin J. G. (1978). Bryozoaires des iles d’Hyères; II: inventaire des fonds détritiques. Parc Natl. Port-Cros Ed. Travaux Scientifiques du Parc Natl. Port-Cros 4, 127–148.
Harmelin J. G. (2017). Pentapora fascialis, a bryozoan under stress: Condition on coastal hard bottoms at port-cros island (Port-cros national park, France, Mediterranean) and other sites. Sci. Rep. Port-Cros Natl. Park 31, 125–133.
Harmelin J. G., Duval C. (1983). Localisation et dissémination des jeunes de l’oursin Sphaerechinus granularis (Lamarck) en méditerranée. Rapports Procès-verbaux Des. Réunions Commission Internationale pour l’Exploration Scientifique la Mer Mediterranée Monaco 28, 267–269.
Harmelin J. G., Harmelin-Vivien M. L. (1976). Observations in situ des aires de ponte de Spicara smaris (Pisces, perciformes, centracanthidae) dans les eaux de port-cros. Travaux Scientifiques du Parc Natl. Port-Cros 2, 115–120.
Harmelin-Vivien M. L., Harmelin J. G., Chauvet C., Duval C., Galzin R., Lejeune P., et al. (1985). Evaluation visuelle des peuplements et populations de poisons, méthodes et problèmes. Rev. d’Ecologie 40, 467–539.
Harvey A. S., Harvey R. M., Merton E. (2017). The distribution, significance and vulnerability of Australian rhodolith beds: A review. Mar. Freshw. Res. 68 (3), 411–428. doi: 10.1071/MF15434
Holon F., Harmelin J. G. (2014). Bryozoan reef, an unknown Mediterranean habitat? Int. Bryozool. Assoc. Bull. 10, 3.
Holon F., Mouquet N., Boissery P., Bouchoucha M., Delaruelle G., Tribot A. S., et al. (2015). Fine-scale cartography of human impacts along French Mediterranean coasts: a relevant map for the management of marine ecosystems. PloS One 10 (8), e0135473. doi: 10.1371/journal.pone.0135473
Huvé H. (1954). Contribution à l’étude des fonds à Peyssonnelia polymorpha (Zan.) schmitz de la région de marseille. Recueil Des. Travaux la Station Mar. d’Endoume 12, 119–136.
Huvé H. (1956). Contribution à l’étude des fonds à Lithothamnium solutum foslie de la région de marseille. recueil des. Travaux la Sation Mar. d’Endoume Fr 18 (1), 105–133.
Jac C., Vaz S. (2020). Abrasion superficielle des fonds par les arts trainants – méditerranée (surface swept area ratio). surface abrasion of seabeds by bottom contacting fishing gears - Mediterranean Sea (surface swept area ratio). IFREMER (Marseille), 230. doi: 10.12770/8bed2328-a0fa-4386-8a3e-d6d146cafe54
Jacquotte R. (1962). Etude des fonds de maërl de méditerranée. Recueil Des. Travaux la Station Mar. d’Endoume Fr. 28 (41), 141–235.
James D. W., Foster M. S., O’Sullivan J. (2006). Bryoliths (Bryozoa) in the gulf of California1. Pacific Sci. 60 (1), 117–124. doi: 10.1353/psc.2005.0057
Jódar-Pérez A. B., Terradas-Fernández M., López-Moya F., Asensio-Berbegal L., López-Llorca L. V. (2020). Multidisciplinary analysis of Cystoseira sensu lato (SE Spain) suggest a complex colonization of the Mediterranean. J. Mar. Sci. Eng. 8 (12), 961. doi: 10.3390/jmse8120961
Joher S., Ballesteros E., Cebrian E., Sánchez N., Rodríguez-Prieto C. (2012). Deep-water macroalgal-dominated coastal detritic assemblages on the continental shelf off mallorca and menorca (Balearic islands, Western Mediterranean). Botanica Marina 55 (5), 485–497. doi: 10.1515/bot-2012-0113
Joher S., Ballesteros E., Rodríguez-Prieto C. (2015). Contribution to the study of deep coastal detritic bottoms: the algal communities of the continental shelf off the Balearic islands, Western Mediterranean. Mediterr. Mar. Sci. 16 (3), 573–590. doi: 10.12681/mms.1249
Kamenos N. A., Moore P. G., Hall-Spencer J. M. (2004). Nursery-area function of maerl grounds for juvenile queen scallops Aequipecten opercularis and other invertebrates. Mar. Ecol. Prog. Ser. 274, 183–189. doi: 10.3354/meps274183
Katsanevakis S., Wallentinus I., Zenetos A., Leppäkoski E., Çinar M. E., Oztürk B., et al. (2014). Impacts of invasive alien marine species on ecosystem services and biodiversity: a pan-European review. Aquat. Invasions 9 (4), 391–423. doi: 10.3391/ai.2014.9.4.01
Kermagoret C., Claudet J., Derolez V., Nugues M. M., Ouisse V., Quillien N., et al. (2019). How does eutrophication impact bundles of ecosystem services in multiple coastal habitats using state-and-transition models. Ocean Coast. Manage. 174, 144–153. doi: 10.1016/j.ocecoaman.2019.03.028
Kersting D. K., Cebrian E., Verdura J., Ballesteros E. (2017a). Rolling corals in the Mediterranean Sea. Coral Reefs 36 (1), 245–245. doi: 10.1007/s00338-016-1498-9
Kersting D. K., Cebrian E., Verdura J., Ballesteros E. (2017b). A new Cladocora caespitosa population with unique ecological traits. Mediterr. Mar. Sci. 18 (1), 38–42. doi: 10.12681/mms.1955
Khoury C. (1987). Ichtyofaune des herbiers de posidonies du parc national de port-cros: composition, éthologie alimentaire et rôle dans le réseau trophique (Doctoral dissertation, Aix-Marseille 2), 230.
Klein J., Verlaque M. (2008). The Caulerpa racemosa invasion: A critical review. Mar. Pollut. Bull. 56 (2), 205–225. doi: 10.1016/j.marpolbul.2007.09.043
Klein J. C., Verlaque M. (2009). Macroalgal assemblages of disturbed coastal detritic bottoms subject to invasive species. Estuarine Coast. Shelf Sci. 82 (3), 461–468. doi: 10.1016/j.ecss.2009.02.003
Konar B., Riosmena-Rodriguez R., Iken K. (2006). Rhodolith bed: a newly discovered habitat in the north pacific ocean. Botanica Marina 49, 355–359. doi: 10.1515/BOT.2006.044
Laborel J. (1961). Le concrétionnement algal “coralligène” et son importance geomorphologique en méditerranée. – recueil des travaux de la station marine. Endoume 23, 37–60.
Laborel J., Pérès J., Picard M., Vacelet J. (1961). Etude directe des fonds des parages de marseille de 30 à 300 m avec la soucoupe plongeante Cousteau. Bull. l’Institut Océanographique Monaco 58, 1–5.
Laborel J., Tailliez P., Vacelet J. (1976). Premières observations dans les eaux du parc national de port-cros à l’aide du sous-marin “Griffon” de la marine nationale. Travaux Scientifiques du Parc Natl. Port-Cros 2, 121–130.
Labrune C., Amouroux J. M., Sarda R., Dutrieux E., Thorin S., Rosenberg R., et al. (2006). Characterization of the ecological quality of the coastal gulf of lions (NW mediterranean). a comparative approach based on three biotic indices. Mar. Pollut. Bull. 52 (1), 34–47. doi: 10.1016/j.marpolbul.2005.08.005
Labrune C., Gauthier O., Conde A., Grall J., Blomqvist M., Bernard G., et al. (2021). A general-purpose biotic index to measure changes in benthic habitat quality across several pressure gradients. J. Mar. Sci. Eng. 9 (6), 654. doi: 10.3390/jmse9060654
Laffoley D., Maltby E., Vincent M. A., Mee L., Dunn E., Gilliland P., et al. (2004). The ecosystem approach, coherent actions for marine and coastal environments. a report to the UK government (Peterborough, UK: English Nature), 65.
La Rivière M., Aish A., Auby I., Ar Gall E., Dauvin J. C., de Bettignies T., et al. (2017). Evaluation de la sensibilité des habitats élémentaires (DHFF) d’Atlantique, de manche et de mer du nord aux pressions physiques (Paris: Rapport SPN 2017-4. MNHN), 93.
La Rivière M., Michez N., Delavenne J., Andres S., Fréjefond C., Janson A. L., et al. (2021). Fiches descriptives des biocénoses benthiques de méditerranée (Paris: UMS PatriNat (OFB-CNRS-MNHN), 660.
Laubier L. (1966). Le coralligène des albères Vol. 43/2 (Paris: Monographie biocénotique. – Annales Institut Océanographique), 137–316.
Ledoyer M. (1966). Ecologie de la faune vagile des biotopes méditerranéens accessibles en scaphandre autonome. III. données analytiques sur les biotopes de substrat meuble. Recueil Des. Travaux la Station Mar. d’Endoume 41 (57), 165–186.
Levin L. A., Dayton P. K. (2009). Ecological theory and continental margins: Where shallow meets deep. Trends Ecol. Evol. 24 (11), 606–617. doi: 10.1016/j.tree.2009.04.012
Littler M. M., Littler D. S. (1980). The evolution of thallus form and survival strategies in benthic marine macroalgae: field and laboratory tests of a functional form model. Am. Nat. 116 (1), 25–44. doi: 10.1086/283610
Lombardi C., Taylor P. D., Cocito S. (2014). “Bryozoan constructions in a changing Mediterranean Sea,” in The Mediterranean Sea: Its history and present challenges. Eds. Goffredo S., Dubinsky Z. (Dordrecht: Springer), 373–384. doi: 10.1007/978-94-007-6704-1_21
Lopez y Royo C. L., Casazza G., Pergent-Martini C., Pergent G. (2010). A biotic index using the seagrass Posidonia oceanica (BiPo), to evaluate ecological status of coastal waters. Ecol. Indic. 10 (2), 380–389. doi: 10.1016/j.ecolind.2009.07.005
Maciejewski L., Lepareur F., Viry D., Bensettiti F., Puissauve R., Touroult J. (2016). État de conservation des habitats: propositions de définitions et de concepts pour l’évaluation à l’échelle d’un site natura 2000. Rev. d’Écologie Terre Vie 71 (1), 3–20. doi: 10.3406/revec.2016.1862
Mancini I., Rigo I., Oprandi A., Montefalcone M., Morri C., Peirano A., et al. (2020). What biotic indices tell us about ecosystem change: lessons from the seagrass Posidonia oceanica. indices application on historical data. Vie Milieu - Life Environ. 70 (3-4), 55–61.
Mao J., Burdett H. L., McGill R. A., Newton J., Gulliver P., Kamenos N. A. (2020). Carbon burial over the last four millennia is regulated by both climatic and land use change. Global Change Biol. 26 (4), 2496–2504. doi: 10.1111/gcb.15021
Marion A. F. (1883). Esquisse d’une topographie zoologique du golfe de marseille. Cayer cie. 1 (1), 1–103.
Martin S., Clavier J., Chauvaud L., Thouzeau G. (2007). Community metabolism in temperate maerl beds. I. Carbon and carbonate fluxes. Mar. Ecol. Prog. Ser. 335, 19–29. doi: 10.3354/meps335019
Martin C. S., Giannoulaki M., De Leo F., Scardi M., Salomidi M., Knittweis L., et al. (2014). Coralligenous and maërl habitats: predictive modelling to identify their spatial distributions across the Mediterranean Sea. Sci. Rep. 4 (1), 1–9.
Martin S., Hall-Spencer J. M. (2017). “Effects of ocean warming and acidification on rhodolith/maërl beds,” in Rhodolith/maërl beds: A global perspective (Cham: Springer International Publishing), 55–85. doi: 10.1007/978-3-3319-29315-8
Meinesz A., Belsher T., Thibaut T., Antolic B., Mustapha K. B., Boudouresque C. F., et al. (2001). The introduced green alga Caulerpa taxifolia continues to spread in the Mediterranean. Biol. Invasions 3 (2), 201–210. doi: 10.1023/A:1014549500678
Meinesz A., Boudouresque C. F., Falconetti C., Astier J. M., Bay D., Blanc J. J., et al. (1983). Normalisation des symboles pour la représentation et la cartographie des biocénoses benthiques littorales de méditerranée. Annales l’Institut Océanographique 59 (2), 155–172.
Michez N., Dirberg G., Bellan-Santini D., Verlaque M., Bellan G., Pergent G., et al. (2011). Typologie des biocénoses benthiques de méditerranée, liste de référence française et correspondances (Paris: Rapport SPN–2011 - 13, MNHN), 50.
Michez N., Fourt M., Aish A., Bellan G., Bellan-Santini D., Chevaldonné P., et al. (2014). Typologie des biocénoses benthiques de méditerranée version 2 (Paris: Rapport SPN–2014 - 33, MNHN), 26.
Monnier B. (2020). Quantification et dynamique spatio-temporelle des puits de carbone associés aux herbiers à posidonia oceanica (Corte: Manuscrit de thèse, Université de Corse Pascal Paoli FRES 3041 – UMR CNRS SPE 6134 Faculté des sciences et techniques) 838, 239.
Monnier B., Pergent G., Mateo M.Á., Carbonell R., Clabaut P., Pergent-Martini C. (2021). Sizing the carbon sink associated with Posidonia oceanica seagrass meadows using very high-resolution seismic reflection imaging. Mar. Environ. Res. 170, 105415. doi: 10.1016/j.marenvres.2021.105415
Monnier B., Pergent G., Mateo M.Á., Clabaut P., Pergent-Martini C. (2022). Quantification of blue carbon stocks associated with Posidonia oceanica seagrass meadows in Corsica (NW Mediterranean). Sci. Total Environ. 838 (1), 155864. doi: 10.1016/j.scitotenv.2022.155864
Montefalcone M. (2009). Ecosystem health assessment using the Mediterranean seagrass Posidonia oceanica: A review. Ecol. Indic. 9 (4), 595–604. doi: 10.1016/j.ecolind.2008.09.013
Montefalcone M., Albertelli G., Morri C., Bianchi C. N. (2007). Urban seagrass: status of Posidonia oceanica off Genoa city waterfront (Italy). Mar. Pollut. Bull. 54, 206–213. doi: 10.1016/j.marpolbul.2006.10.005
Montefalcone M., Morri C., Parravicini V., Bianchi C. N. (2015b). A tale of two invaders: divergent spreading kinetics of the alien green algae Caulerpa taxifolia and Caulerpa cylindracea. Biol. Invasions 17 (9), 2717–2728. doi: 10.1007/s10530-015-0908-1
Montefalcone M., Vassallo P., Gatti G., Parravicini V., Paoli C., Morri C., et al. (2015a). The exergy of a phase shift: Ecosystem functioning loss in seagrass meadows of the Mediterranean. Sea. Estuarine Coast. Shelf Sci. 156, 186–194. doi: 10.1016/j.ecss.2014.12.001
Morri C., Bavestrello G., Bianchi C. N. (1991). Faunal and ecological notes on some benthic cnidarian species from the Tuscan archipelago and eastern ligurian Sea (western Mediterranean). Bollettino dei Musei e degli Istituti Biologici dell’Università di Genova 54-55 (1988-1989), 27–47.
Morri C., Peirano A., Bianchi C. N., Rodolfo-Metalpa R. (2000). Cladocora caespitosa: A colonial zooxanthellate Mediterranean coral showing constructional ability. Reef Encounter 27, 22–25.
Mostajir B., Amblard C., Buffan-Dubau E., de Wit R., Lensi R., Sime-Ngando T. (2012). Les Réseaux trophiques microbiens des milieux aquatiques et terrestres. Ed. Bertrand J. C., et al (Pau: Presses Universitaires de Pau et des Pays de l’Adour), 28.
Noisette F., Egilsdottir H., Davoult D., Martin S. (2013). Physiological responses of three temperate coralline algae from contrasting habitats to near-future ocean acidification. J. Exp. Mar. Biol. Ecol. 448, 179–187. doi: 10.1016/j.jembe.2013.07.006
Oprandi A., Bianchi C. N., Karayali O., Morri C., Rigo I., Montefalcone M. (2021). RESQUE: A novel comprehensive approach to compare the performance of different indices in evaluating seagrass health. Ecol. Indic. 131, 108118. doi: 10.1016/j.ecolind.2021.108118
Oprandi A., Mancini I., Bianchi C. N., Morri C., Azzola A., Montefalcone M. (2022). “Indices from the past: relevance in the status assessment of Posidonia oceanica meadows,” in Proceedings of the 7th Mediterranean symposium on marine vegetation. Eds. Bouafif C., Ouerghi A. (Tunis: SPA/RAC, Tunis), 72–77.
Ordines F., Ramón M., Rivera J., Rodríguez-Prieto C., Farriols M. T., Guijarro B., et al. (2017). Why long term trawled red algae beds off Balearic islands (western Mediterranean) still persist? Regional Stud. Mar. Sci. 15, 39–49. doi: 10.1016/j.rsma.2017.07.005
Orekhova N. A., Ovsyany E. I. (2020). Organic carbon and particle-size distribution in the littoral bottom sediments of the laspi bay (the black Sea). Phys. Oceanogr. 27 (3), 266–277. doi: 10.22449/1573-160X-2020-3-266-277
Paoli C., Morten A., Bianchi. C. N., Morri C., Fabiano M., Vassallo P. (2016). Capturing ecological complexity: OCI, a novel combination of ecological indices as applied to benthic marine habitats. Ecol. Indic. 66, 86–102. doi: 10.1016/j.ecolind.2016.01.029
Pérès J. M. (1982). “Zonations and organismic assemblages,” in Marine ecology, vol. V, ocean management, part 1. Ed. Kinne O. (Chichester: Wiley), 9–642.
Pérès J. M., Picard J. (1951). Note sur les fonds coralligènes de la région de marseille. – Arch. Zool. Expérimentale Générale 88, 24–38.
Pérès J. M., Picard J. (1964). Nouveau manuel de bionomie benthique de la mer méditerranée. Recueil Des. Travaux la Station Mar. d Endoume 31 (47), 5–137.
Pergent G., Agnesi S., Antonioli P. A., Babbini L., Belbacha S., Ben Mustapha K., et al. (2015). Standard methods for inventorying and monitoring coralligenous and rhodoliths assemblages (Tunis: RAC/SPA, Tunis), 20.
Pergent-Martini C., Pergent G., Monnier B., Boudouresque C. F., Morri C., Valette-Sansevin A. (2021). Contribution of Posidonia oceanica meadows in the context of climate change mitigation in the Mediterranean Sea. Mar. Environ. Res. 165, 105236. doi: 10.1016/j.marenvres.2020.105236
Personnic S., Boudouresque C. F., Astruch P., Ballesteros E., Blouet S., Bellan-Santini D., et al. (2014). An ecosystem-based approach to assess the status of a Mediterranean ecosystem, the Posidonia oceanica seagrass meadow. PloS One 9 (6), e98994. doi: 10.1371/journal.pone.0098994
Petit A. (2019). Les Aires marines protégées « à la française » un enjeu de politique internationale. Bull. l’Institut Pierre Renouvin 1), 161–171. doi: 10.3917/bipr1.049.0161
Piazzi L., Balata D., Cinelli F. (2007). Invasions of alien macroalgae in Mediterranean coralligenous assemblages. Cryptogamie-Algologie 28 (3), 289–302.
Piazzi L., Cinelli F. (2000). Effet’ de l’expansion des rhodophyceae introduites Acrothamnion preissii et Womersleyella setacea sur les communautés algales des rhizomes de Posidonia oceanica de méditerranée occidentale. Cryptogamie Algol. 21 (3), 291–300. doi: 10.1016/S0181-1568(00)00116-1
Piazzi L., Gennaro P., Cecchi E., Bianchi C. N., Cinti M. F., Gatti G., et al. (2021). Ecological status of coralligenous assemblages: ten years of application of the ESCA index from local to wide scale validation. Ecol. Indic. 121, 107077. doi: 10.1016/j.ecolind.2020.107077
Piazzi L., Gennaro P., Montefalcone M., Bianchi C. N., Cecchi E., Morri C., et al. (2019). STAR: An integrated and standardized procedure to evaluate the ecological status of coralligenous reefs. Aquat. Conservation: Mar. Freshw. Ecosyst. 29, 189–201. doi: 10.1002/aqc.2983
Piazzi L., Pardi G., Balata D., Cecchi E., Cinelli F. (2002). Seasonal dynamics of a subtidal north-western Mediterranean macroalgal community in relation to depth and substrate inclination. Botanica Marina 45, 243–252. doi: 10.1515/BOT.2002.023
Picard J. (1965). Recherches qualitatives sur les biocénoses marines des substrats meubles dragables de la region marseillaise. Recueil Des. Travaux la Station Mar. d’Endoume 52 (36), 1–160 (10).
Pikaver A. H., Gilbert C., Breton F. (2004). An indicator set to measure the progress in the implementation of integrated coastal zone management in Europe. Ocean Coast. Manage. 47, 449–462. doi: 10.1016/j.ocecoaman.2004.06.001
Pinedo S., Jordana E., Ballesteros E. (2015). A critical analysis on the response of macroinvertebrate communities along disturbance gradients: description of MEDOCC (MEDiterranean OCCidental) index. Mar. Ecol. 36, 141–154. doi: 10.1111/maec.12126
Provan J. I. M., Murphy S., Maggs C. A. (2005). Tracking the invasive history of the green alga codium fragile ssp. tomentosoides. Mol. Ecol. 14 (1), 189–194. doi: 10.1111/j.1365-294X.2004.02384.x
Quemmerais-Amice F., Barrere J., La Rivière M., Contin G., Bailly D. (2020). A methodology and tool for mapping the risk of cumulative effects on benthic habitats. Front. Mar. Sci. 7. doi: 10.3389/fmars.2020.569205
Rastorgueff P. A., Bellan-Santini D., Bianchi C. N., Bussotti S., Chevaldonné P., Guidetti P., et al. (2015). An ecosystem-based approach to evaluate the ecological quality of Mediterranean undersea caves. Ecol. Indic. 54, 137–152. doi: 10.1016/j.ecolind.2015.02.014
R core team (2022). R: A language and environment for statistical computing (Vienna, Austria: R Foundation for Statistical Computing). Available at: https://www.R-project.org/.
Rees I. (1999). Lag veneer: a missing piece in the benthic biotopes jigsaw? Newslett. PMNHS 2, 24–26.
Rendina F., Ferrigno F., Appolloni L., Donnarumma L., Sandulli R., Fulvio G. (2020). Anthropic pressure due to lost fishing gears and marine litter on different rhodolith beds off the campania coast (Tyrrhenian Sea, Italy). Ecol. Questions 31 (4), 1–17. doi: 10.12775/EQ.2020.027
Reynes L., Thibaut T., Mauger S., Blanfuné A., Holon F., Cruaud C., et al. (2021). Genomic signatures of clonality in the deep water kelp Laminaria rodriguezii. Mol. Ecol. 30 (8), 1806–1822. doi: 10.1111/mec.15860
Rosenberg R., Blomqvist M., Nilsson H. C., Cederwall H., Dimming A. (2004). Marine quality assessment by use of benthic species-abundance distributions: A proposed new protocol within the European union water framework directive. Mar. Pollut. Bull. 49 (9-10), 728–739. doi: 10.1016/j.marpolbul.2004.05.013
Rowe G., Wright G. (1999). The Delphi technique as a forecasting tool: Issues and analysis. Int. J. Forecasting 15 (4), 353–375. doi: 10.1016/S0169-2070(99)00018-7
Ruitton S., Astruch P., Blanfuné A., Cabral M., Thibaut T., Boudouresque C. F. (2020). Bridging risk assessment of human pressure and ecosystem status. Vie Milieu/Life Environ. 70 (3-4), 37–53.
Ruitton S., Boudouresque C. F., Thibaut T., Rastorgueff P. A., Personnic S., Boissery P., et al. (2017). Guide méthodologique pour l’évaluation écosystémique des habitats marins (Frances: MIO publ.), 161. doi: 10.13140/RG.2.2.23411.02085
Ruitton S., Personnic S., Ballesteros E., Bellan-Santini D., Boudouresque C. F., Chevaldonné P., et al. (2014). “An ecosystem-based approach to assess the status of the Mediterranean coralligenous habitat,” in Proceedings of the 2nd Mediterranean symposium on the conservation of coralligenous and other calcareous bio-concretions. Eds. Bouafif C., Langar H., Ouerghi A. (Tunis: RAC/SPA publ.), 153–158.
Sala E., Mayorga J., Bradley D., Cabral R. B., Atwood T. B., Auber A., et al. (2021). Protecting the global ocean for biodiversity, food and climate. Nature 592, 1–6. doi: 10.1038/s41586-021-03371-z
Salomidi M., Katsanevakis S., Borja A., Braeckman U., Damalas D., Galparsoro I., et al. (2012). Assessment of goods and services, vulnerability, and conservation status of European seabed biotopes: A stepping stone towards ecosystem-based marine spatial management. Mediterr. Mar. Sci. 13 (1), 49–88. doi: 10.12681/mms.23
Santos R. O., James W. R., Nelson J. A., Rehage J. S., Serafy J., Pittman S. J., et al. (2022). Influence of seascape spatial pattern on the trophic niche of an omnivorous fish. Ecosphere 13 (2), e3944. doi: 10.1002/ecs2.3944
Sanz-Lázaro C., Belando M. D., Marín-Guirao L., Navarrete-Mier F., Marín A. (2011). Relationship between sedimentation rates and benthic impact on maerl beds derived from fish farming in the Mediterranean. Mar. Environ. Res. 71 (1), 22–30. doi: 10.1016/j.marenvres.2010.09.005
Sartoretto S., Schohn T., Bianchi C. N., Morri C., Garrabou J., Ballesteros E., et al. (2017). An integrated method to evaluate and monitor the conservation state of coralligenous habitats: the INDEX-COR approach. Mar. Pollut. Bull. 120, 222–231. doi: 10.1016/j.marpolbul.2017.05.020
Savini A., Basso D., Bracchi V. A., Corselli C., Pennetta M. (2012). Maerl-bed mapping and carbonate quantification on submerged terraces offshore the cilento peninsula (Tyrrhenian Sea, Italy). Geodiversitas 34 (1), 77–98. doi: 10.5252/g2012n1a5
Scémama P., Kermagoret C., Accornero-Picon A., Alban F., Astruch P., Boemare C., et al. (2020). A strategic approach to assess the bundle of ecosystem services provided by Posidonia oceanica meadows in the bay of marseille. Vie Milieu/Life Environ. 70 (3-4), 197–207.
Schiaparelli S., Castellano M., Povero P., Sartoni G., Cattaneo-Vietti R. (2007). A benthic mucilage event in north-Western Mediterranean Sea and its possible relationships with the summer 2003 European heatwave: Short term effects on littoral rocky assemblages. Mar. Ecol. 28 (3), 341–353. doi: 10.1111/j.1439-0485.2007.00155.x
Sciberras M., Rizzo M., Mifsud J. R., Camilleri K., Borg J. A., Lanfranco E., et al. (2009). Habitat structure and biological characteristics of maerl bed off the northeastern coast of the Maltese islands (central Mediterranean). Mar. Biodiversity 39, 251–264. doi: 10.1007/s12526-009-0017-4
Seitz R. D., Wennhage H., Bergström U., Lipcius R. N., Ysebaert T. (2014). Ecological value of coastal habitats for commercially and ecologically important species. ICES J. Mar. Sci. 71 (3), 648–665. doi: 10.1093/icesjms/fst152
Simboura N., Zenetos A. (2002). Benthic indicators to use in ecological quality classification of Mediterranean soft bottom marine ecosystems, including a new biotic index. Mediterr. Mar. Sci. 3 (2), 77–111. doi: 10.12681/mms.249
Somaschini A., Martini N., Gravina M. F., Belluscio A., Corsi F., Ardizzone G. D. (1998). Characterization and cartography of some Mediterranean soft-bottom benthic communities (Ligurian Sea, Italy). Scientia Marina 62 (1-2), 27–36.
Souto J., Reverter-Gil O., Fernandez-Pulpeiro E. (2010). Bryozoa from detritic bottoms in the menorca channel (Balearic islands, western Mediterranean), with notes on the genus Cribellopora. Zootaxa 2536, 1, 36–52. doi: 10.11646/zootaxa.2536.1.2
Soykan O., İlkyaz A. T., Metin G., Kinacigil H. T. (2010). Growth and reproduction of blotched picarel (Spicara maena Linnaeus 1758) in the central Aegean Sea, Turkey. Turkish J. Zool. 34 (4), 453–459. doi: 10.3906/zoo-0903-29
Stamouli C., Zenetos A., Kallianiotis A., Voultsiadou E. (2022). Megabenthic invertebrate diversity in Mediterranean trawlable soft bottoms: A synthesis of current knowledge. Mediterr. Mar. Sci. 23 (3), 447–459. doi: 10.12681/mms.29165
Steller D. L., Riosmena-Rodríguez R., Foster M. S., Roberts C. A. (2003). Rhodolith bed diversity in the gulf of California: the importance of rhodolith structure and consequences of disturbance. Aquat. Conservation: Mar. Freshw. Ecosyst. 13 (S1), S5–S20. doi: 10.1002/aqc.564
Thibaut T., Blanfuné A., Boudouresque C. F., Personnic S., Ruitton S., Ballesteros E., et al. (2017). An ecosystem-based approach to assess the status of Mediterranean algae-dominates shallow rocky reefs. Mar. Pollut. Bull. 117, 311–329. doi: 10.1016/j.marpolbul.2017.01.029
Thibaut T., Blanfuné A., Verlaque M., Boudouresque C. F., Ruitton S. (2016). The Sargassum conundrum: Very rare, threatened or locally extinct in the NW Mediterranean and still lacking protection. Hydrobiologia 781 (1), 3–23. doi: 10.1007/s10750-015-2580-y
Thiriet P., Cheminée A., Mangialajo L., Francour P. (2014). “How 3D complexity of macrophyte-formed habitats affect the processes structuring fish assemblages within coastal temperate seascapes?,” in Underwater seascapes (Cham: Springer), 185–199. doi: 10.1007/978-3-319-03440-9_12
Valette-Sansevin A., Pergent G., Buron K., Pergent-Martini C., Damier E. (2019). Continuous mapping of benthic habitats along the coast of Corsica: A tool for the inventory and monitoring of blue carbon ecosystems. Mediterr. Mar. Sci. 20 (3), 585–593. doi: 10.12681/mms.19772
Van der Heijden L. H., Kamenos N. A. (2015). Reviews and syntheses: calculating the global contribution of coralline algae to total carbon burial. Biogeosciences 12 (21), 6429–6441. doi: 10.5194/bg-12-6429-2015
Vassallo P., Bianchi C. N., Paoli C., Holon F., Navone A., Bavestrello G., et al. (2018). A predictive approach to benthic marine habitat mapping: Efficacy and management implications. Mar. Pollut. Bull. 131, 218–232. doi: 10.1016/j.marpolbul.2018.04.016
Vassallo P., Paoli C., Aliani S., Cocito S., Morri C., Bianchi C. N. (2020). Benthic diversity patterns and predictors: a study case with inferences for conservation. Mar. Pollut. Bull. 150, 110748. doi: 10.1016/j.marpolbul.2019.110748
Velimirov B., Herndl G., Kavka G. (1984). “Biomass distribution and physiological capabilities of bacteria in the water column above a sea grass system,” in 2. colloque international de bacteriologie marine, Brest (Brest, France: Ifremer), 1–5.
Verlaque M. (1990). Relations entre Sarpa salpa (Linnaeus 1758) (Téléostéen, sparidae), les autres poissons brouteurs et le phytobenthos algal méditerranéen. Oceanol. Acta 13 (3), 373–388.
Vidondo B., Duarte C. M. (1995). Seasonal growth of Codium bursa, a slow-growing Mediterranean macroalga: In situ experimental evidence of nutrient limitation. Mar. Ecol. Prog. Ser. 123, 185–191. doi: 10.3354/meps123185
Watanabe K., Yoshida G., Hori M., Umezawa Y., Moki H., Kuwae T. (2020). Macroalgal metabolism and lateral carbon flows can create significant carbon sinks. Biogeosciences 17 (9), 2425–2440. doi: 10.5194/bg-17-2425-2020
Wilkie I. C., Carnevali M. C., Andrietti F. (1996). Mechanical properties of the peristomial membrane of the cidaroid sea-urchin Stylocidaris affinis. J. Zool. 238 (3), 557–569. doi: 10.1111/j.1469-7998.1996.tb05413.x
Wirtz P., Debelius H. (2003). Mediterranean And Atlantic invertebrate guide from Spain to Turkey, from Norway to equator (Hackenheim, Allemagne: ConchBooks), 305.
Word J. Q. (1978). “The infaunal trophic index,” in Coastal water research project annual report (El Segundo, CA: southern California coastal water research project), 19–39.
Keywords: coastal detrital bottoms, ecosystem-based approach (EBA), quality assessment, marine habitat, rhodolith beds, epibenthic assemblages
Citation: Astruch P, Orts A, Schohn T, Belloni B, Ballesteros E, Bănaru D, Bianchi CN, Boudouresque C-F, Changeux T, Chevaldonné P, Harmelin J-G, Michez N, Monnier B, Morri C, Thibaut T, Verlaque M and Daniel B (2023) Ecosystem-based assessment of a widespread Mediterranean marine habitat: The Coastal Detrital Bottoms, with a special focus on epibenthic assemblages. Front. Mar. Sci. 10:1130540. doi: 10.3389/fmars.2023.1130540
Received: 23 December 2022; Accepted: 06 March 2023;
Published: 27 March 2023.
Edited by:
Chiara Piroddi, Joint Research Centre, ItalyReviewed by:
Lucia Fanini, University of Salento, ItalyRoberto Carlucci, University of Bari Aldo Moro, Italy
Copyright © 2023 Astruch, Orts, Schohn, Belloni, Ballesteros, Bănaru, Bianchi, Boudouresque, Changeux, Chevaldonné, Harmelin, Michez, Monnier, Morri, Thibaut, Verlaque and Daniel. This is an open-access article distributed under the terms of the Creative Commons Attribution License (CC BY). The use, distribution or reproduction in other forums is permitted, provided the original author(s) and the copyright owner(s) are credited and that the original publication in this journal is cited, in accordance with accepted academic practice. No use, distribution or reproduction is permitted which does not comply with these terms.
*Correspondence: Patrick Astruch, cGF0cmljay5hc3RydWNoQHVuaXYtYW11LmZy
†Deceased