- 1Graduate School of Agricultural and Life Sciences, University of Tokyo, Tokyo, Japan
- 2Fisheries Technology Institute, Japan Fisheries Research and Education Agency, Hatsukaichi, Japan
- 3Bachok Marine Research Station, Institute of Ocean and Earth Sciences, University of Malaya, Bachok, Malaysia
In Asia, four harmful raphidophyte species, Chattonella malayana, C. marina, C. subsalsa, and C. tenuiplastida, coexist in the tropical waters but only C. marina was detected in temperate waters. This occurrence pattern pointed to a potentially distinct ecophysiological niche occupancy and possible species dispersion. The growth physiology of these species isolated from tropical Southeast Asia was investigated using unialgal cultures in ten temperatures (13.0–35.5°C) and five salinities (15–35) to better understand the factors driving their distribution. The highest maximum specific growth rates were observed in C. subsalsa (0.65 ± 0.01 d-1), followed by C. malayana (0.47 ± 0.03 d-1), C. marina (0.45 ± 0.02 d-1), and C. tenuiplastida (0.39 ± 0.01 d-1). Their optimal temperatures were 28.0, 30.5, 25.5, and 30.5°C, respectively, of which C. marina preferred colder water. C. subsalsa exhibited a wider growth temperature range (20.5–35.5°C), followed by C. marina (20.5–30.5°C), C. tenuiplastida (23.0–33.0°C), and C. malayana (25.5–33.0°C). Optimal salinities were similar between C. subsalsa and C. malayana (30), and between C. marina and C. tenuiplastida (25), but C. subsalsa and C. marina exhibited a similar growth salinity range of 15–35, while C. malayana and C. tenuiplastida was 20–35. High values of Fv/Fm were observed in C. subsalsa and C. marina (> 0.5) in all tested conditions, but Fv/Fm of C. malayana and C. tenuiplastida were significantly lower at 20.5°C. All four species achieved a maximum cell density of > 104 cells mL-1 in their optimal conditions. Optimal temperatures in C. subsalsa and C. marina were identical to previous reports. The high adaptability of C. subsalsa in various temperatures and salinities suggests its high competitiveness and bloom potential. The high adaptability of C. marina in colder waters compared to other species likely contributes to its wide distribution in the temperate Asian waters. The narrow temperature window of C. malayana and C. tenuiplastida suggests their endemicity and limited distribution in the tropical waters. This study provides evidence about the occurrences and bloom potential of Chattonella spp. in Asia, but the endemicity versus dispersion issue remains unresolved.
1 Introduction
Global warming has been perceived as a factor in the geographical expansion, intensification, and earlier timing of harmful algal blooms (HABs), especially along the coasts of tropical, subtropical, and temperate countries (Hallegraeff, 2010; Fu et al., 2012; Anderson et al., 2021; Sakamoto et al., 2021). In Southeast Asia, harmful algae are commonly found and records of HABs have been increasing (Maclean, 1984; Edvardsen and Imai, 2006; Lim et al., 2012; Azanza et al., 2017; Furuya et al., 2018; Mohammad-Noor et al., 2018; Yñiguez et al., 2021). Some of these HABs species were newly discovered in the region, and likely were introduced by anthropogenic transport or due to the adaptive strategies in the changing environment. The harmful dinoflagellate Cochlodinium Schütt (= Margalefidinium Gómez, Richlen et Anderson) is a case in point, blooms of the species have been discovered in Indonesia, Malaysia, and Philippines (Iwataki et al., 2007; Anton et al., 2008; Azanza et al., 2008; Iwataki et al., 2008; Iwataki et al., 2015); other example species are harmful raphidophytes Chattonella Biecheler and Heterosigma akashiwo (Hada) Hada ex Hara et Chihara that have been found in Indonesia, Malaysia, and Thailand (Lirdwitayaprasit et al., 1996; Gin et al., 2006; Ayu-Lana-Nafisyah et al., 2018). It is difficult to trace the origin of these HABs species due to a lack of reliable knowledge of their previous distributions, but studies have shown that they can adapt to various environments, e.g., the raphidophyte H. akashiwo was first detected in the USA (Hulburt, 1965), but later has been detected in Japan (Hada, 1967; Hada, 1968; Hara and Chihara, 1987), UK (Leadbeater, 1969), Norway (Throndsen, 1969), Russia and Arctic waters (Konalova, 1995; Ratkova and Wassmann, 2005; Engesmo et al., 2016). The successive adaptation of HAB species could be attributed to favorable environmental conditions such as temperature, salinity, and cyst formation ability (Marshall and Hallegraeff, 1999; Smayda, 2002; Mehnert et al., 2010; Thomas et al., 2012; Boyd et al., 2013).
The raphidophyte Chattonella is one of the noxious microalgae that has caused mass mortalities of coastal marine organisms, particularly farmed fish (Imai and Yamaguchi, 2012; Viana et al., 2019; Sakamoto et al., 2021; Lum et al., 2022). Recently, the wide distribution of Chattonella and associated fisheries damages have been clarified in the ten countries of Southeast Asia (Edvardsen and Imai, 2006; Lum et al., 2019; Lum et al., 2021; Lum et al., 2022). Moreover, recent phylogeographic studies have revealed the presence of four Chattonella species in Southeast Asia, including C. subsalsa Biecheler, C. marina (as the C. marina complex including C. antiqua (Hada) Ono, C. marina, and C. ovata Hara et Chihara), and two newly described species, C. malayana W.M. Lum, H.C. Lim, S.T. Teng, K. Takahashi, Leaw, P.T. Lim et Iwataki, and C. tenuiplastida W.M. Lum, H.C. Lim, K. Takahashi, S.T. Teng, Benico et Iwataki (Bowers et al., 2006; Ayu-Lana-Nafisyah et al., 2018; Lum et al., 2019; Lum et al., 2021; Lum et al., 2022). As physiological responses can vary among species, strains, and populations, the coexistence and interaction between these four Chattonella species arouse scientific interest in their ecophysiological traits because each may have a distinct environmental adaptability and different dispersion background (Marshall and Hallegraeff, 1999; Band-Schmidt et al., 2012; Viana et al., 2019). Furthermore, while the bloom of C. malayana had caused wild fish kills in Malaysia in 2016, and the distribution of C. subsalsa coincided with the locations where fish kills have been reported, the environmental parameters promoting their blooms have not been identified (Lum et al., 2021; Lum et al., 2022).
In situ population dynamics of HABs species are difficult to be understood without long-term observation data in specific habitats or regions, as such, data from laboratory-based growth experiments are essential to understand the effects of environmental parameters on their occurrence patterns and blooms, including Chattonella (Fu et al., 2012; Wells et al., 2015). Due to difficulties in the in situ growth rate assessment, the growth characteristics of Chattonella have been commonly elucidated by laboratory experiments using single or multiple unialgal culture strains under the effects of temperature, salinity, irradiance, and nutrient concentrations, i.e., C. subsalsa from Brazil and USA (Zhang et al., 2006; Viana et al., 2019), and C. marina complex (hereinafter referred to as C. marina) from Australia (Marshall and Hallegraeff, 1999), Japan (e.g., Nakamura and Watanabe, 1983), Korea (Lim et al., 2020), and Mexico (Band-Schmidt et al., 2012). Among these environmental parameters, temperature and salinity are two major factors in promoting/demoting the growth of Chattonella (Nakamura and Watanabe, 1983; Yamaguchi et al., 1991; Noh et al., 2006a; Noh et al., 2006b; Yamatogi et al., 2006; Zhang et al., 2006; Salvitti, 2010). Chattonella marina from different localities in Japan grew in a similar temperature range of 15–30°C (optimum 25°C), but with a slightly different salinity range of 15–35 (Yamaguchi et al., 1991; Khan et al., 1995). On a wider scale, a similar response against temperatures was observed in C. marina from Australia, Japan, and Korea, but they had different optimal salinities (Yamaguchi et al., 1991; Kahn et al., 1998; Marshall and Hallegraeff, 1999; Noh et al., 2006a; Noh et al., 2006b). Chattonella subsalsa from the USA had a wider temperature range of 10–30°C (optimum 30°C) and salinity range of 5–30 (optimum 25) for growth while the same species from Brazil had an optimal salinity of 30 (Zhang et al., 2006; Viana et al., 2019).
In Southeast Asia, there was limited studies investigating the growth characteristics of Chattonella, further, past studies demonstrated inconsistent conclusions (Lee, 2014; Ayu-Lana-Nafisyah et al., 2018). Lee (2014) reported C. marina from Sarawak, Malaysia (strain CtSb02, reported as C. subsalsa without molecular characterization, see Lum et al., 2022) had an optimal growth at salinity 25, which was similar to those in the temperate waters. However, Ayu-Lana-Nafisyah et al. (2018) revealed that C. marina from a mangrove area in Indonesia had a lower optimal salinity of 15. Whether the unique salinity preference of C. marina in Indonesia was a strain-specific, species-specific, or ecotypic adaptation in tropical Asian waters remains unclear. The growth characteristics of C. marina and other Chattonella species in tropical waters, including C. subsalsa and the two new Chattonella species recently described from Southeast Asia (C. tenuiplastida and C. malayana), need to be investigated and clarified to further understand the effects of the abiotic factors on their occurrence patterns and bloom dynamics. This study compared the growth responses of single unialgal cultures of the four Chattonella species in various temperatures and salinities to achieve the following objectives: (1) to provide baseline growth information of Chattonella spp. in tropical waters, (2) to recognize the ecophysiological niches (growth temperature/salinity ranges) of these Chattonella species, and (3) to determine their optimal temperature and/or salinity that may contribute to the formation of HABs in this region. The ecophysiological comparison in this study may reveal the bloom potentials of Chattonella spp. in each respective temperature and salinity and help to specify the factors promoting their blooms in the natural environment.
2 Materials and methods
2.1 Algal cultures
All Chattonella cultures were previously established by Lum et al. (2021; 2022). from Southeast Asia. One representative strain of C. malayana, C. marina, C. subsalsa, and C. tenuiplastida was selected (Table 1). They were grown in IMK medium (Wako, Tokyo, Japan), made up of 0.22 µm filter-sterilized, nutrient-depleted aged oceanic seawater pre-adjusted to a salinity of 30. The cultures were maintained at 23.0°C, a light intensity of 70–100 µmol photons m–2 s–1 of cool, white fluorescent light under a 12:12 h light: dark cycle regime. Note that cultures used in the growth experiments were not axenic, but culture vessels and media were sterilized by autoclaving at 120°C for 15 min, and inoculation was operated aseptically in a laminar flow cabinet.

Table 1 Culture strains of Chattonella species used in this study (Lum et al., 2021; Lum et al., 2022).
2.2 Temperature experiment
The temperature experiment was performed in ten temperature treatments, i.e., 13.0, 15.5, 18.0, 20.5, 23.0, 25.5, 28.0, 30.5, 33.0, and 35.5°C (Yamatogi et al., 2006; Sakamoto et al., 2009). Cells of each strain were inoculated into 50 mL culture flasks with filtered caps (Sumitomo Bakelite, Tokyo, Japan) containing IMK medium prepared from aged offshore seawater pre-adjusted to a salinity of 30 by distilled water. The experiment was conducted at a light intensity of 220 µmol photons m-2 s-1 under a 12:12 h light: dark photocycle. Before the experiment, cells were pre-acclimatized to the targeted temperatures at a rate of ± 2.5°C every two days, starting from 23.0°C. After the acclimatization, an inoculum was transferred to a 50 mL fresh medium to make up for 100 ± 50 cells mL-1 initial cell densities for each flask. The experiment was performed in triplicate for each strain at each temperature treatment for a growth cycle of 20 days.
2.3 Salinity experiment
The salinity experiment was performed in five salinity treatments i.e., 15, 20, 25, 30, and 35. Lower salinity IMK media were prepared by pre-dilution with sterile distilled water. The experiment was conducted at 28.0°C under the same light conditions as described above. Prior to the experiment, cells were pre-acclimatized to the targeted salinities by decreasing/increasing the salinity of five every two days, starting from salinity 30. After the acclimatization, an inoculum was transferred to a 50 mL fresh medium to make up for 100 ± 50 cells mL-1 initial cell densities for each flask. The experiment was performed in triplicate for each strain at each salinity treatment for a growth cycle of 20 days.
2.4 Cross-factorial temperature and salinity experiment
To further understand the combined effects of temperature and salinity on the two recently described Chattonella tenuiplastida and C. malayana (Lum et al., 2022), a cross-factorial experiment was conducted with 12 treatments (in triplicate), by crossing temperatures of 25.5, 28.0, 30.5 and 33.0°C with salinities of 25, 30 and 35 in 50 mL culture flasks with non-filtered caps (Sumitomo Bakelite, Tokyo, Japan). Cells in each treatment were pre-acclimatized as described above and subsequently transferred to 50 mL fresh medium to make up for 100 ± 50 cells mL-1 initial cell densities for each flask. The experiment was carried out for at least 20 days and monitored until day 40.
2.5 Cell count and growth rate
Two milliliters subsamples were collected from each culture flask every two days until day 20 or day 40, and cells were fixed with HEPES-buffered glutaraldehyde before counting (Katano et al., 2009). Fixed samples were manually counted by a Sedgwick-rafter chamber until ≥ 100 cells. Specific growth rate (µ, d-1) was calculated from the following equation, where N is the number of cells and t is time (Hall et al., 2014).
The maximum specific growth rate (µmax) was calculated from the maximum slope over a five-point window (h = 5), in other words, the highest growth rate in any eight days (Hall et al., 2014) using the package growthrates (Petzoldt, 2022) in R (R Core Team, 2022). Growth rates of Chattonella were classified into four levels; (1) negative growth where cells died after the experiment had begun and were omitted from graphs, (2) no growth indicating the survival and mortality of Chattonella were almost equal, and no observable µ, (3) growth where µ > 0.1 d-1, and (4) optimal growth with the highest µ (µmax). Treatments where Chattonella spp. achieved high cell densities (104 cells mL-1) were noted.
2.6 Fv/Fm measurement
To determine the effects of temperature and salinity on the Chattonella cell conditions, the maximum quantum yield of photosystem II (Fv/Fm) was determined by measuring chlorophyll fluorescence from selected conditions, i.e., temperatures 20.5, 25.5, and 30.5°C, and salinities 25, 30, and 35 (Yuasa et al., 2020a; Yuasa et al., 2020b). Subsamples (2 mL) were collected from each culture flask in 4-day intervals, cells were incubated in the dark for 10–15 min, and Fv/Fm values were measured subsequently by a Water-PAM fluorometer (Walz, Effeltrich, Germany). The value of Fv/Fm was calculated by the following equation:
where Fm and Fo are the maximum and minimum fluorescence, respectively. Measurement was conducted in triplicate.
2.7 Statistical analyses
For the single-factor experiments of the effects of temperature and salinity, the µ and Fv/Fm were analyzed by one-way variance analysis (ANOVA), followed by Tukey’s post hoc comparison test to determine the statistical significance at p< 0.01. For the cross-factorial experiment of temperature and salinity, the µ was analyzed by two-way ANOVA. Tukey’s post hoc comparison test was performed at a statistical significance of p< 0.01 when the result of ANOVA was significant. Statistical analyses were conducted in R ver. 4 (R Core Team, 2022).
3 Results
3.1 Effects of temperature
None of the four Chattonella species grew at 13.0°C (Figure 1A). However, the effects of temperature on the growth varied among species when temperature > 13.0°C (Figures 1A, 2A and Tables 2, S1, S2). In general, growth was suppressed at 15.5 and 18.0°C, C. subsalsa and C. marina showed no growth with almost constant cell densities, while C. tenuiplastida and C. malayana exhibited negative growth (Figure 1A).
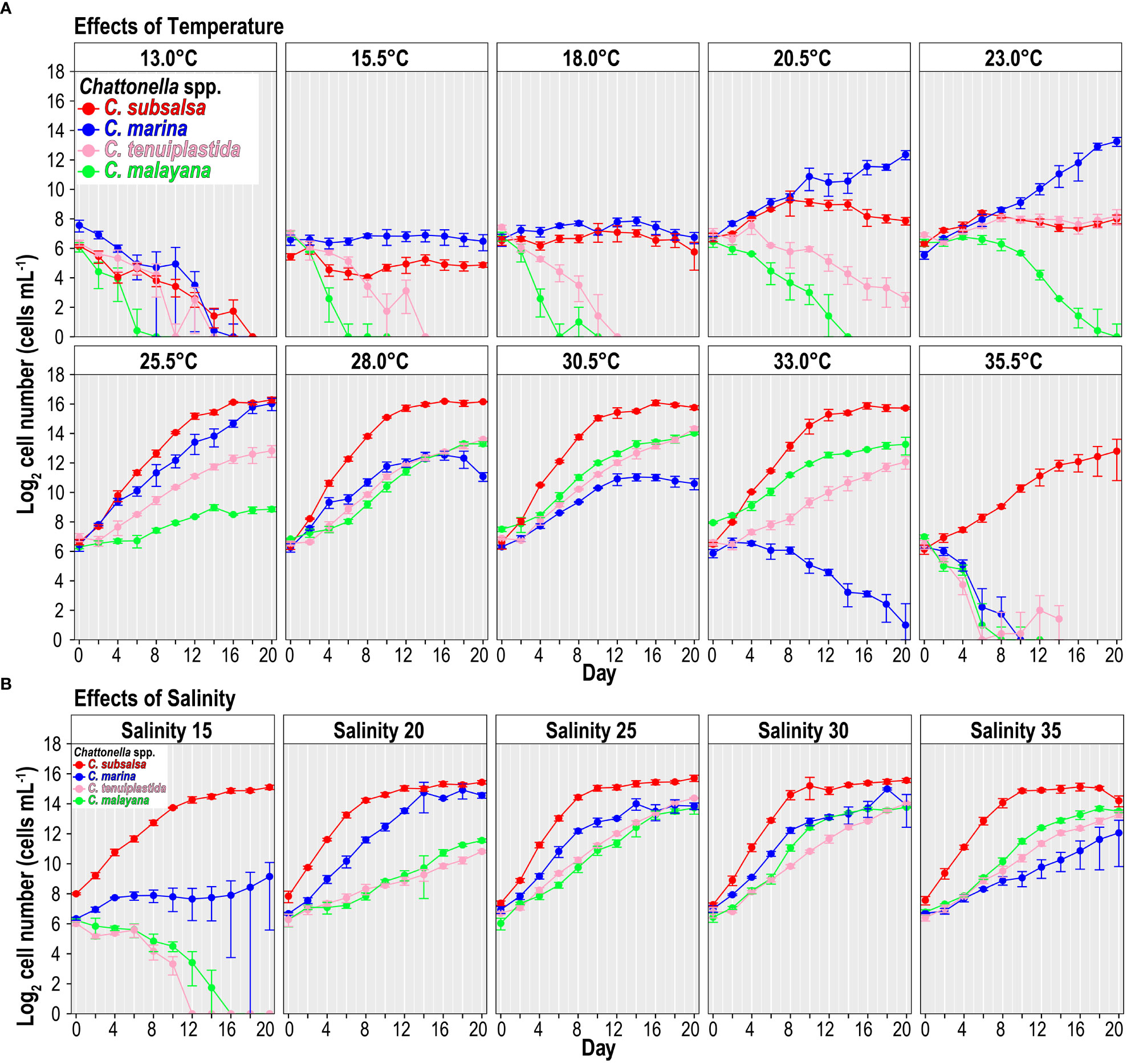
Figure 1 Growth of Chattonella subsalsa, C. marina, C. tenuiplastida, and C. malayana at different temperature (A) and salinity (B) treatments. Error bars represent standard deviations (n = 3).
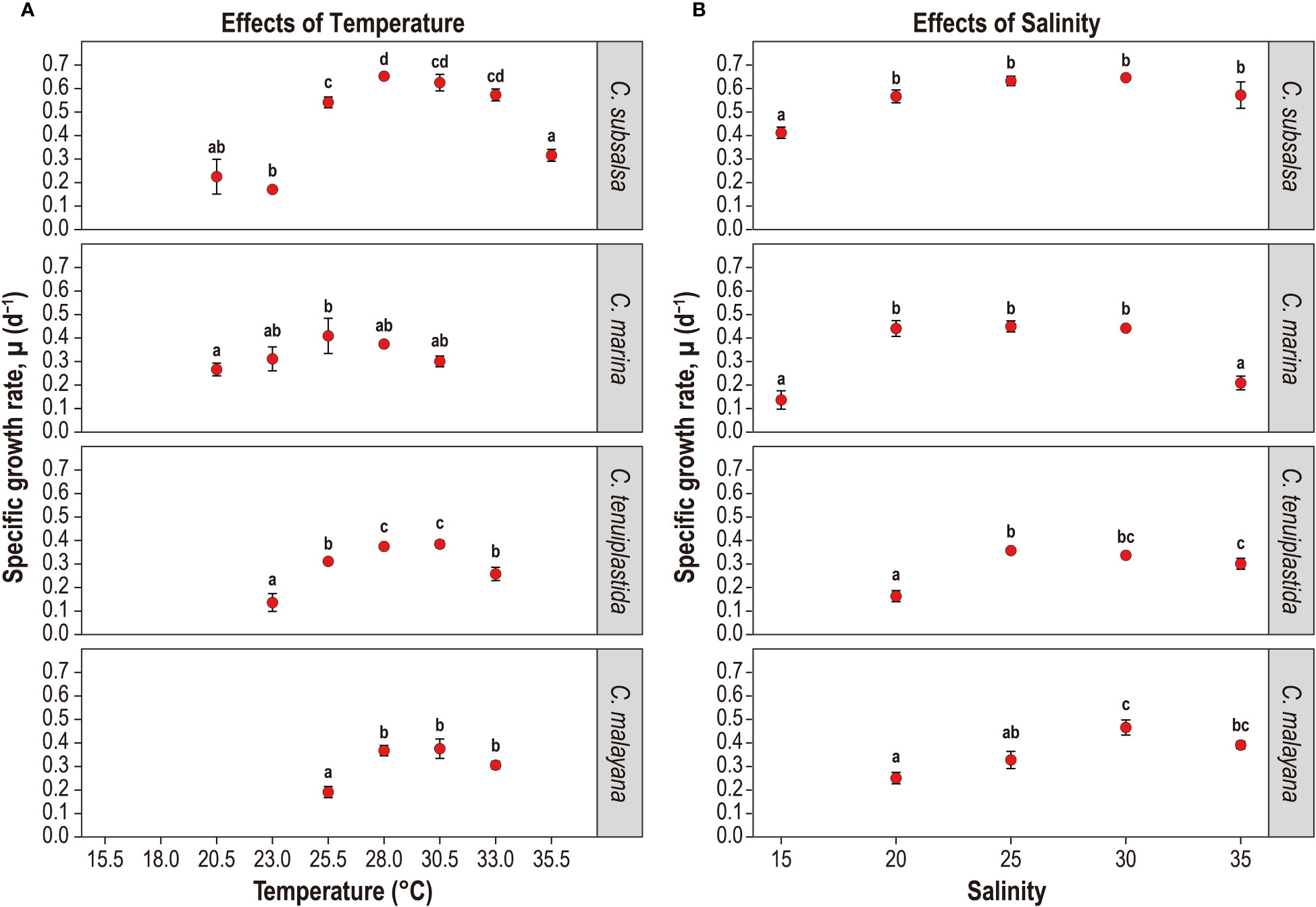
Figure 2 Specific growth rate, µ (d-1) of Chattonella subsalsa, C. marina, C. tenuiplastida, and C. malayana at different temperature (A) and salinity (B) treatments. Note that treatments with negative or no growth were not shown, i.e., C. subsalsa (≤ 18.0°C), C. marina (≤ 18.0°C and ≥ 33.0°C), C. tenuiplastida (≤ 20.5°C and 35.5°C), C. malayana (≤ 23.0°C and 35.5°C), and C. tenuiplastida and C. malayana at salinity 15. Alphabets indicate ANOVA significant level of between-treatment comparisons (p< 0.01, Tukey’s test). Error bars represent standard deviations (n = 3).
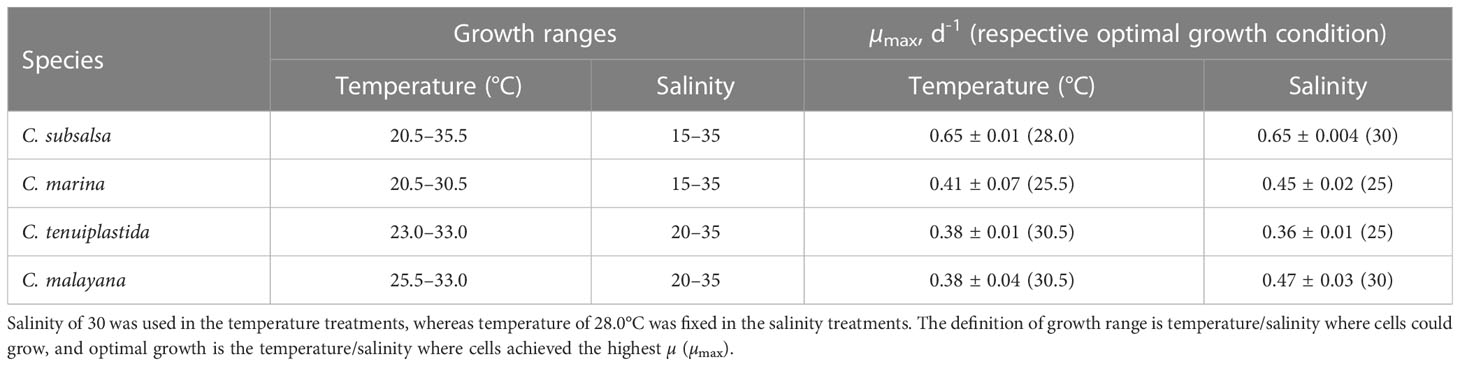
Table 2 Growth ranges and maximum specific growth rates (µmax) on Chattonella spp. in the single-factor experiments.
Growth temperature ranges were different among the Chattonella species. Chattonella subsalsa showed a wide temperature range of 20.5–35.5°C, while C. malayana demonstrated a remarkably narrow range (25.5–33.0°C). Chattonella marina and C. tenuiplastida exhibited moderate temperature ranges of 23.0–30.5°C and 23.0–33.0°C, respectively (Figures 1A, 2A). When comparing the µmax and their respective optimal temperature (Tables 2, S1), C. subsalsa had the highest µmax among all four Chattonella species (0.65 ± 0.01 d-1 at 28.0°C), followed by C. marina (0.41 ± 0.07 d-1 at 25.5°C), and the µmax were similar for C. tenuiplastida (0.38 ± 0.01 d-1 at 30.5°C) and C. malayana (0.38 ± 0.04 d-1 at 30.5°C).
Cells of C. subsalsa entered the exponential phase in day 2–8, which demonstrated the shortest lag phase among all Chattonella species (Figure 1A). Comparable to C. subsalsa, C. marina entered the exponential phase in day 4–10. In contrast, C. tenuiplastida and C. malayana displayed a much longer lag phase and entered the exponential phase after day 8 (Figure 1A).
The culture of C. subsalsa showed the highest maximum cell densities, with the maximum cell yield of 7.9 × 104 cells mL-1 observed at 25.5°C. High cell densities of > 6 × 104 cells mL-1 were recorded throughout the temperature treatments (25.5–33.0°C) (Figure 1A). The highest maximum cell density of C. marina was recorded at 25.5°C (> 6 × 104 cells mL-1), however, cell densities were relatively high at 20.5–30.5°C (> 103 cells mL-1). High cell densities of C. tenuiplastida were recorded at 28.0–30.5°C (> 104 cells mL-1), with the maximum cell density of 2.1 × 104 cells mL-1 observed at 30.5°C. The culture of C. malayana exhibited the lowest maximum cell yield among the species examined, with the highest maximum cell density of 1.7 × 104 cells mL-1 observed at 30.5°C. At 28.0 and 33.0°C, cell densities remained high (104 cells mL-1) on day 20 but observation of whether the cells continued to grow was not available at the time.
The maximum quantum yield of photosystem II (Fv/Fm), an indicator of photosynthetic potential, has been commonly used to determine whether cells are under stress (Vonshak et al., 1994; Qiu et al., 2013; Katayama et al., 2017; Yuasa et al., 2020b). In this experiment, the Fv/Fm values of four Chattonella species were monitored in 20.5, 25.5, and 30.5°C treatments in 4-day intervals. Chattonella subsalsa and C. marina exhibited relatively high Fv/Fm (≥ 0.5) at all temperatures during the experiment period, indicating their photosynthetic potential was retained over the period (Figure 3A and Tables S5, S6). Fv/Fm of C. subsalsa were significantly higher at 25.5°C than that of 20.5°C throughout the experiment period (p< 0.01) (Figure 3A and Table S6). Fv/Fm values of C. marina were significantly different in all temperature treatments starting from day-8 (p< 0.01), the highest Fv/Fm was observed at 30.5°C (Figure 3A and Table S6). The differences of Fv/Fm among treatments were obvious in C. tenuiplastida and C. malayana. Both species showed negative Fv/Fm values at 20.5°C, implying their growth and photosynthetic potential were negatively affected by lower temperatures (Figure 3A and Table S6). At higher temperature treatments, Fv/Fm of C. malayana were significantly different (p< 0.01) between 25.5 and 30.5°C treatments after day 12, but those of C. tenuiplastida were not significantly different between 25.5 and 30.5°C (p ≥ 0.01; Table S6), suggesting that C. malayana might be less tolerant to temperatures lower than 25.5°C.
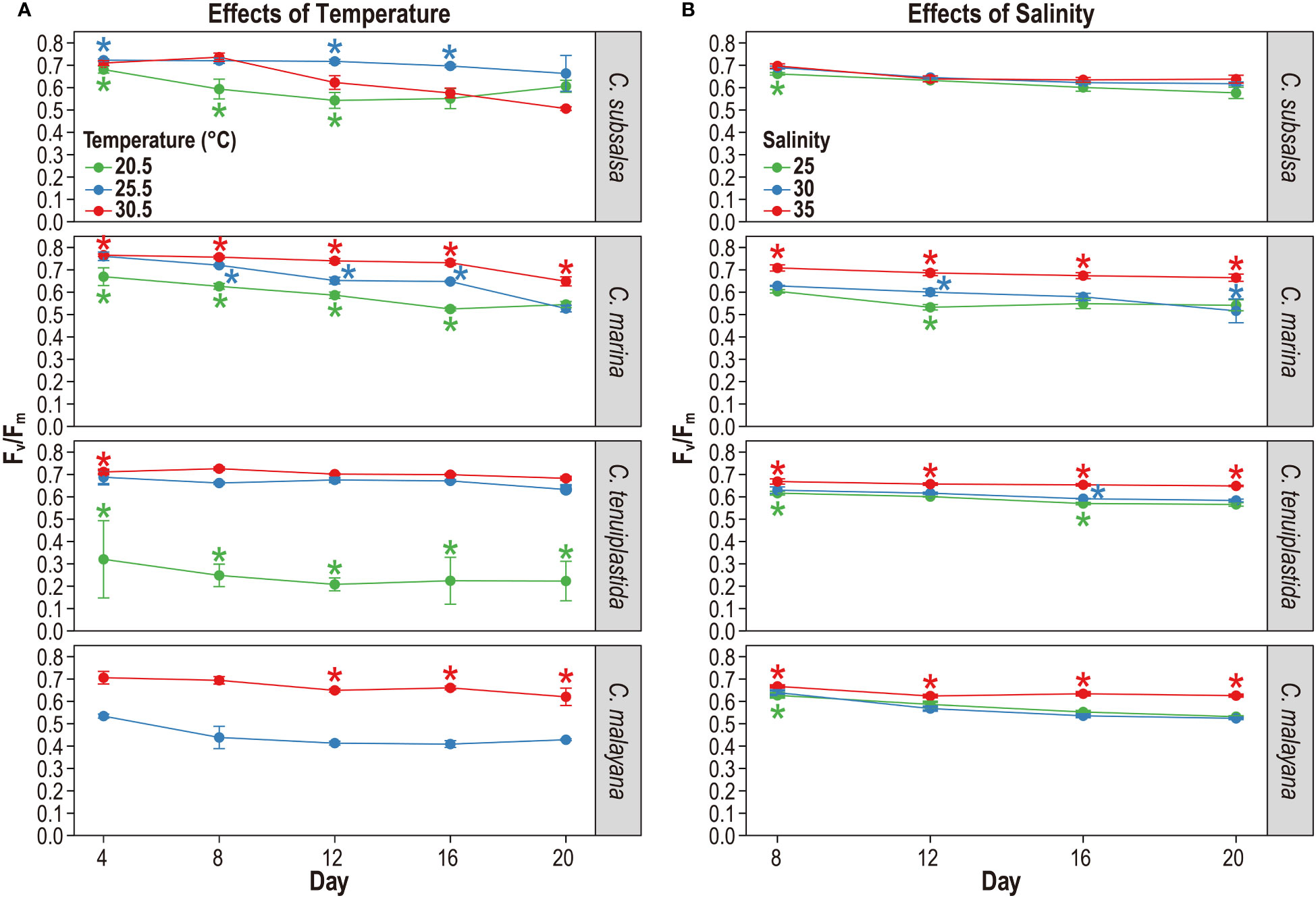
Figure 3 The maximum quantum yield of photosystem II (Fv/Fm) of Chattonella subsalsa, C. marina, C. tenuiplastida, and C. malayana at temperature treatments of 20.5, 25.5, and 30.5°C (A), and salinity treatments of 25, 30, and 35 (B). Asterisks indicate ANOVA significance differences (p< 0.01) between different temperature or salinity treatments on the sampling days. Note that Fv/Fm of C. malayana at 20.5°C (< 0.1) was excluded, and no data for day 4 of the salinity experiment due to equipment failure. Error bars represent standard deviations (n = 3).
3.2 Effects of salinity
Overall, all Chattonella spp. showed similar growth responses to the salinity treatments (Figures 1B, 2B and Tables 2, S3, S4). Growth of C. subsalsa and C. marina was observed in the salinity range of 15–35, while C. tenuiplastida and C. malayana were in the salinity range of 20–35 (Table S3). At salinity 15, the growth of C. tenuiplastida and C. malayana were suppressed and cells died after day 12–14 (Figure 1B). Growth of C. subsalsa was significantly lower at salinity 15 than those at 20–35 (p< 0.01) (Figure 2B and Table S4).
Among all species, the highest µmax was observed in C. subsalsa (0.65 ± 0.004 d-1 at salinity 30, Tables 2, S3), followed by C. malayana (0.47 ± 0.03 d-1 at salinity 30), C. marina (0.45 ± 0.02 d-1 at salinity 25), and C. tenuiplastida (0.36 ± 0.01 d-1 at salinity 25), respectively (Figures 1B, 2B).
For the growth phase, the exponential phase of C. subsalsa started on day 4 across all salinity treatments, which was the shortest among all species (Figure 1B). Chattonella marina entered the exponential phase on day 6 at salinities 20–30, but with a longer lag phase (10 days) at salinities 15 and 35 (Figure 1B). Chattonella malayana also started to grow exponentially on day 6, similar to C. marina, at salinity 30 (Figure 1B). The culture of C. tenuiplastida exhibited the longest lag phase, as its exponential phase only started after day 8 (Figure 1B).
The maximum cell densities of C. subsalsa exceeded 3.5 × 104 cells mL-1 at all salinity treatments, which was much higher than other species (Figure 1B). The maximum cell densities of C. marina exceeding 104 cells mL-1 were recorded at salinities 20–30 but were lower (< 5 × 103 cells mL-1) at salinities 15 and 35. Maximum cell densities of both C. tenuiplastida and C. malayana were comparable to C. subsalsa and C. marina (> 104 cells mL-1) at salinities 25–35.
The maximum quantum yield of photosystem II (Fv/Fm) was observed for salinities 25, 30, and 35 in 4-day intervals, and the results showed that the effects of salinity on the photosynthetic potential of the four Chattonella species were minor (Figure 3B and Tables S7, S8). High Fv/Fm (> 0.6) was observed in C. subsalsa in all salinity treatments, and they were not significantly different (p ≥ 0.01; Figure 3B and Table S8). At salinity 35, C. marina, C. tenuiplastida, and C. malayana had lower µ but higher Fv/Fm as compared to those at salinities 30 and 25, suggesting that the three species could tolerate high salinity of 35 (Figures 2B, 3B and Table S8).
3.3 Cross-factorial effects of temperature and salinity
The combined effects of temperature and salinity produced similar results from the single-factor experiments, and no significant differences were observed among treatments, including the interaction effects of temperature and salinity (Figure 4 and Tables 3, 4). The only statistically significant result was detected in temperature (p = 0.0064, two-way ANOVA) in C. tenuiplastida, but almost all results showed no statistical differences (p ≥ 0.01), except for 25.5-30.5°C (p = 0.0055, Tukey’s test) (Tables 4, S9). Cells of C. tenuiplastida did not grow at 33.0°C across the salinities 25, 30, and 35, except for one replicate, which grew at salinity 35 (µ = 0.12 d-1). Chattonella malayana grew in all tested conditions but µ was extremely low (0.03 ± 0.02 d-1) at 33.0°C and salinity 25. µmax of C. malayana (0.40 ± 0.10 d-1) was higher than that of C. tenuiplastida (0.39 ± 0.05 d-1), comparable to previous experiments (Table 3).
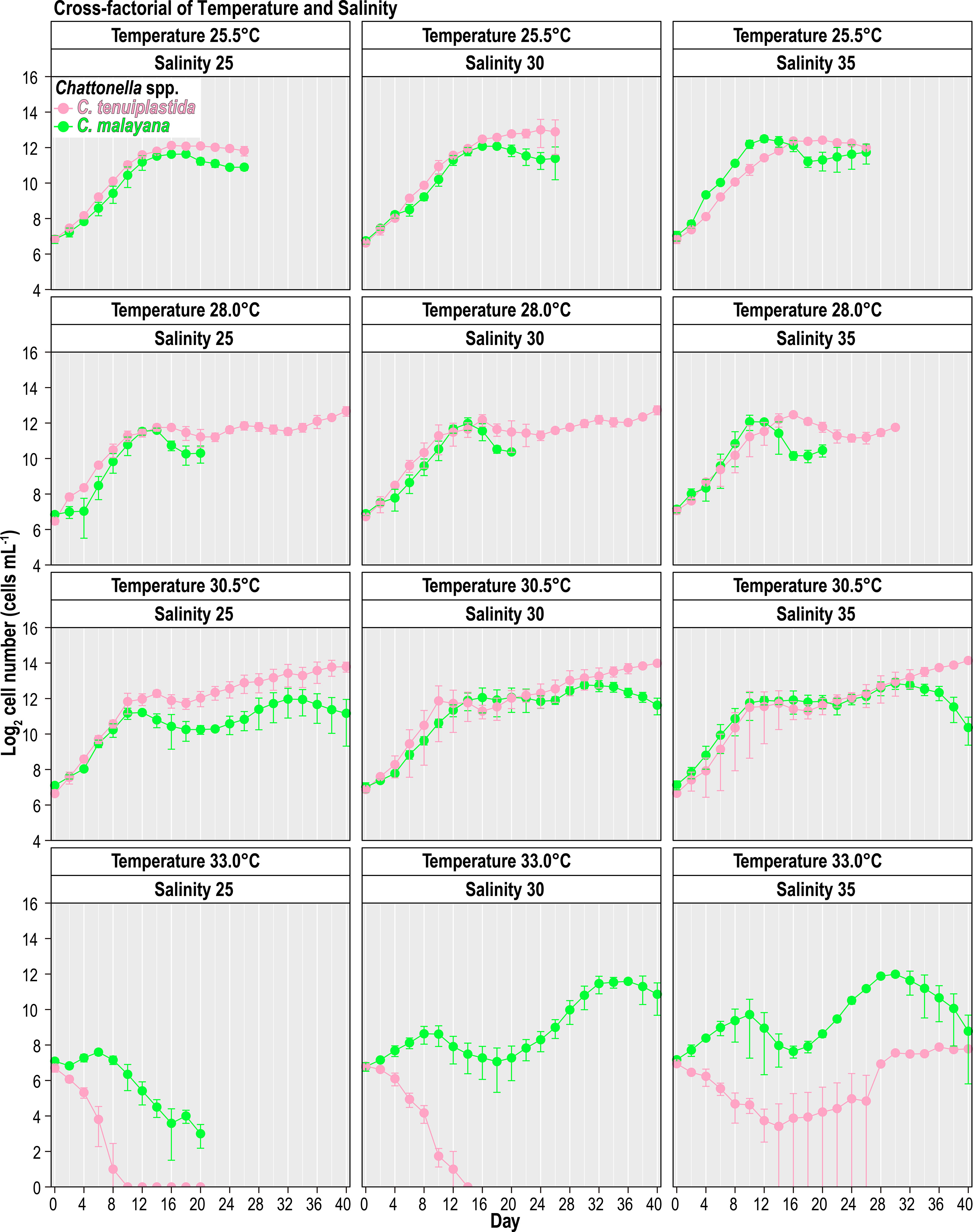
Figure 4 Growth of Chattonella tenuiplastida and C. malayana in the cross-factorial experiment of various temperatures and salinities. Error bars represent standard deviations (n = 3).
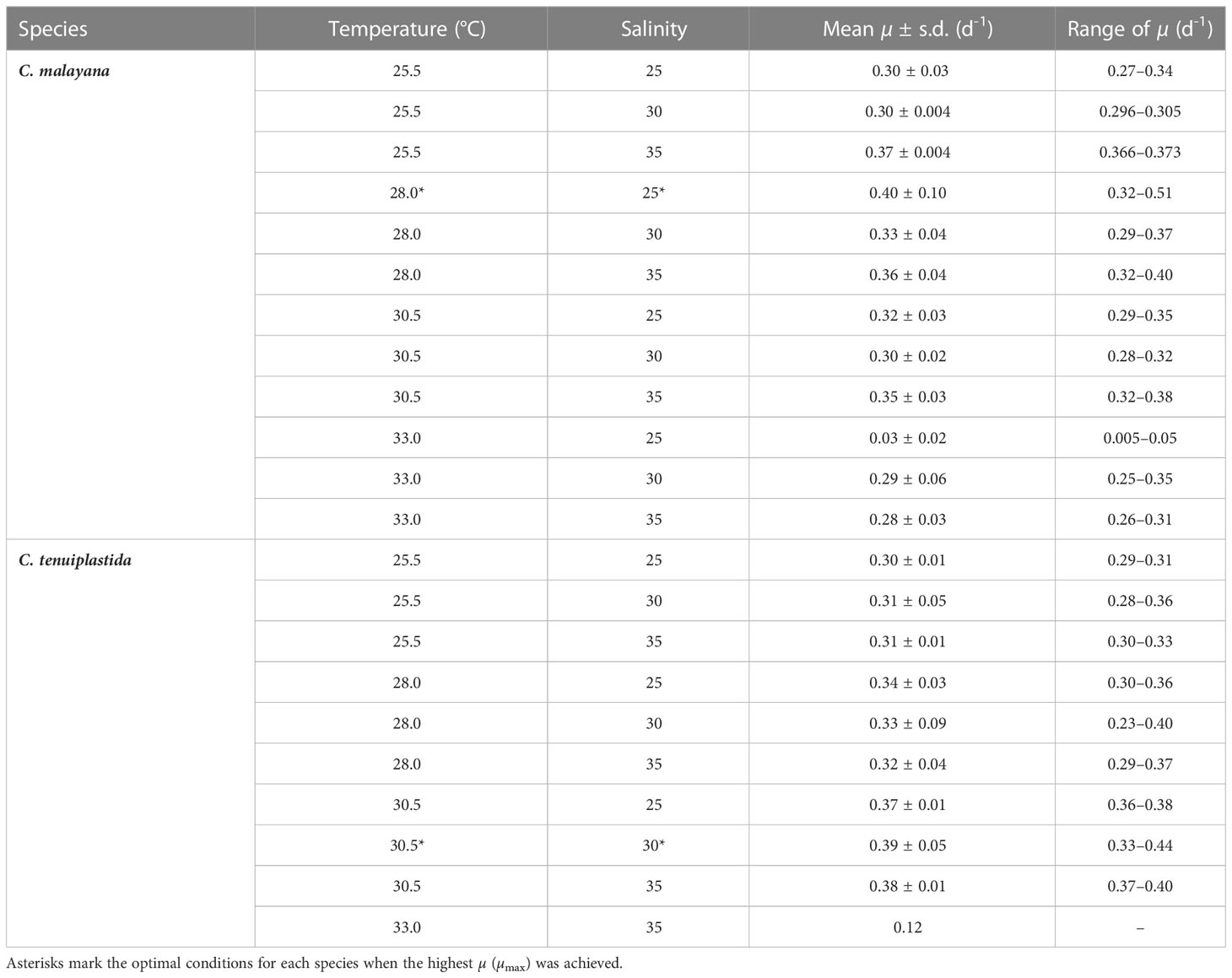
Table 3 Specific growth rate (µ) in the cross-factorial experiments on Chattonella malayana and C. tenuiplastida, n = 3.
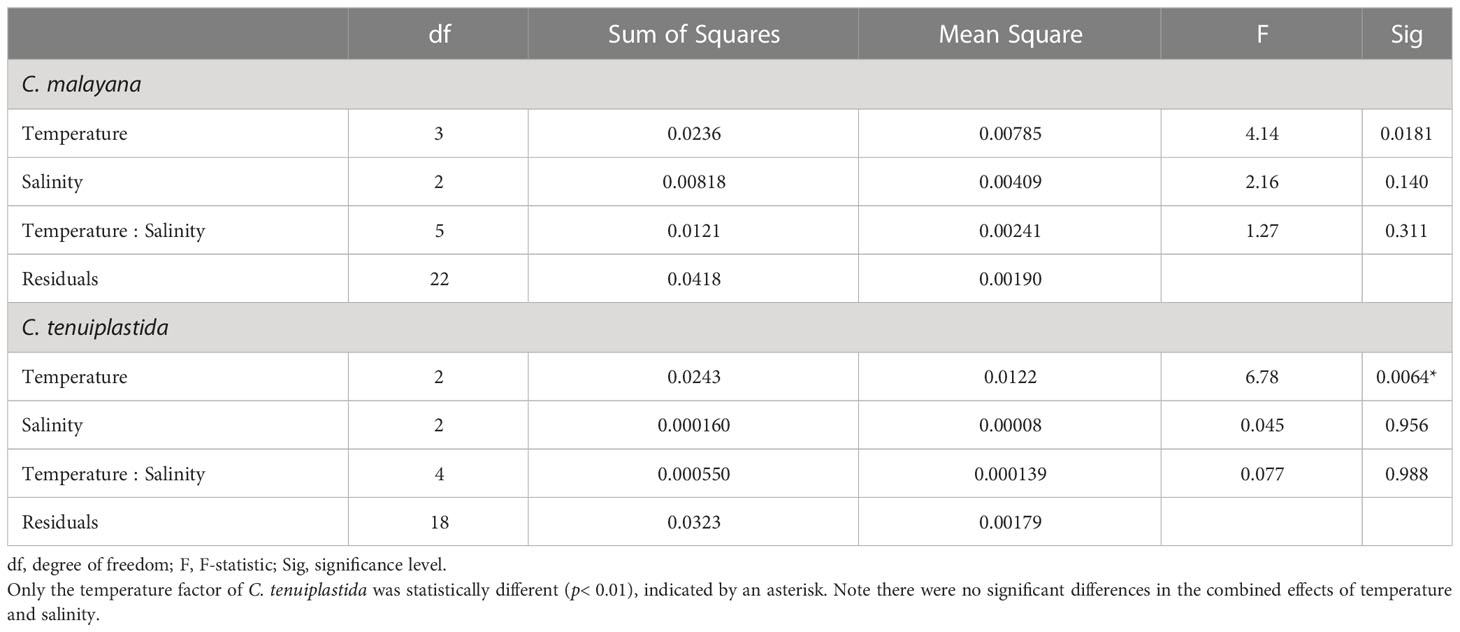
Table 4 Two-way ANOVA for cross-factorial experiments on Chattonella malayana and C. tenuiplastida, n = 3.
The optimal growth of C. malayana was observed at 28.0°C and salinity 25, with a remarkable µ of 0.51 d-1 observed in a replicate (Figure 5A and Table 3). The maximum cell density of 8.3 × 103 cells mL-1 was observed at 30.5°C and salinity 30, which was lower than those observed in the single-factor experiments (Figure 4). In contrast, the optimal growth of C. tenuiplastida was at 30.5°C and salinity 30, with its highest µmax of 0.39 ± 0.05 d-1 (Figure 5B and Table 3). In the cross-factorial experiment with temperatures set at 28.0 and 30.5°C, a fluctuating pattern of growth was observed where cell densities declined slightly around day 20 and gradually increased to higher cell densities, resulting in two peaks in the growth curves (Figure 4). This type of growth curve was not observed at 25.5°C, as cells entered the exponential phase from day 4 and then stationary/death phases before day 20. High cell densities (> 104 cells mL-1) were recorded at their optimal temperature of 30.5°C across all salinities 25–35.
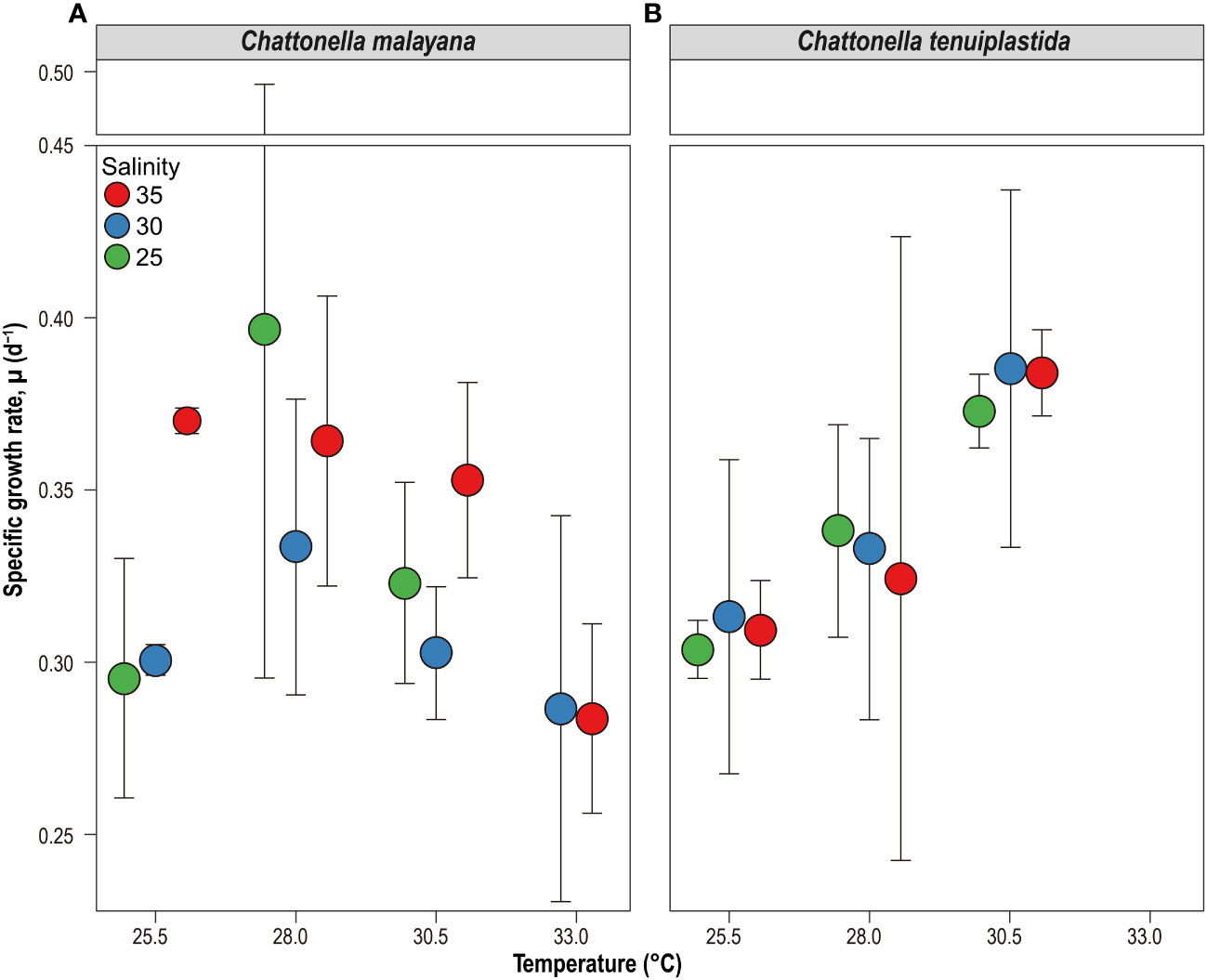
Figure 5 Specific growth rate, µ (d-1) of Chattonella malayana (A) and C. tenuiplastida (B) and in the cross-factorial experiments of temperatures (x-axis) and salinity (colored circles). Note that C. tenuiplastida showed no growth at 33.0°C at salinities of 25 and 30, and only one replicate grew at salinity 35 (µ = 0.12 d-1), which is not included in the plot. Error bars represent standard deviations (n = 3).
4 Discussion
4.1 Chattonella in the tropical and temperate waters
Studies of temperature effects on the growth of C. subsalsa and C. marina focus mainly on temperate waters (Australia, Japan, Korea, and the USA), only a few reports had investigated the growth characteristics of Chattonella in tropical waters (Lee, 2014; Ayu-Lana-Nafisyah et al., 2018; Kok et al., 2019) (Table 5). In this study, we demonstrated that all four species, C. subsalsa, C. malayana, C. marina, and C. tenuiplastida from tropical Asian waters exhibited comparable growth (µmax) and maximum quantum yield of photosystem II (Fv/Fm) to those of temperate C. subsalsa and C. marina (Salvitti, 2010; Qiu et al., 2013; Yuasa et al., 2020b) (Table 5). This study revealed that C. subsalsa achieved its µmax at 28.0°C and salinity 30 (single-factor), C. malayana at 28.0°C and salinity 30 (single-factor), C. marina at 28.0°C and salinity 25 (single-factor), and C. tenuiplastida at 30.5°C and salinity 30 (cross-factor).
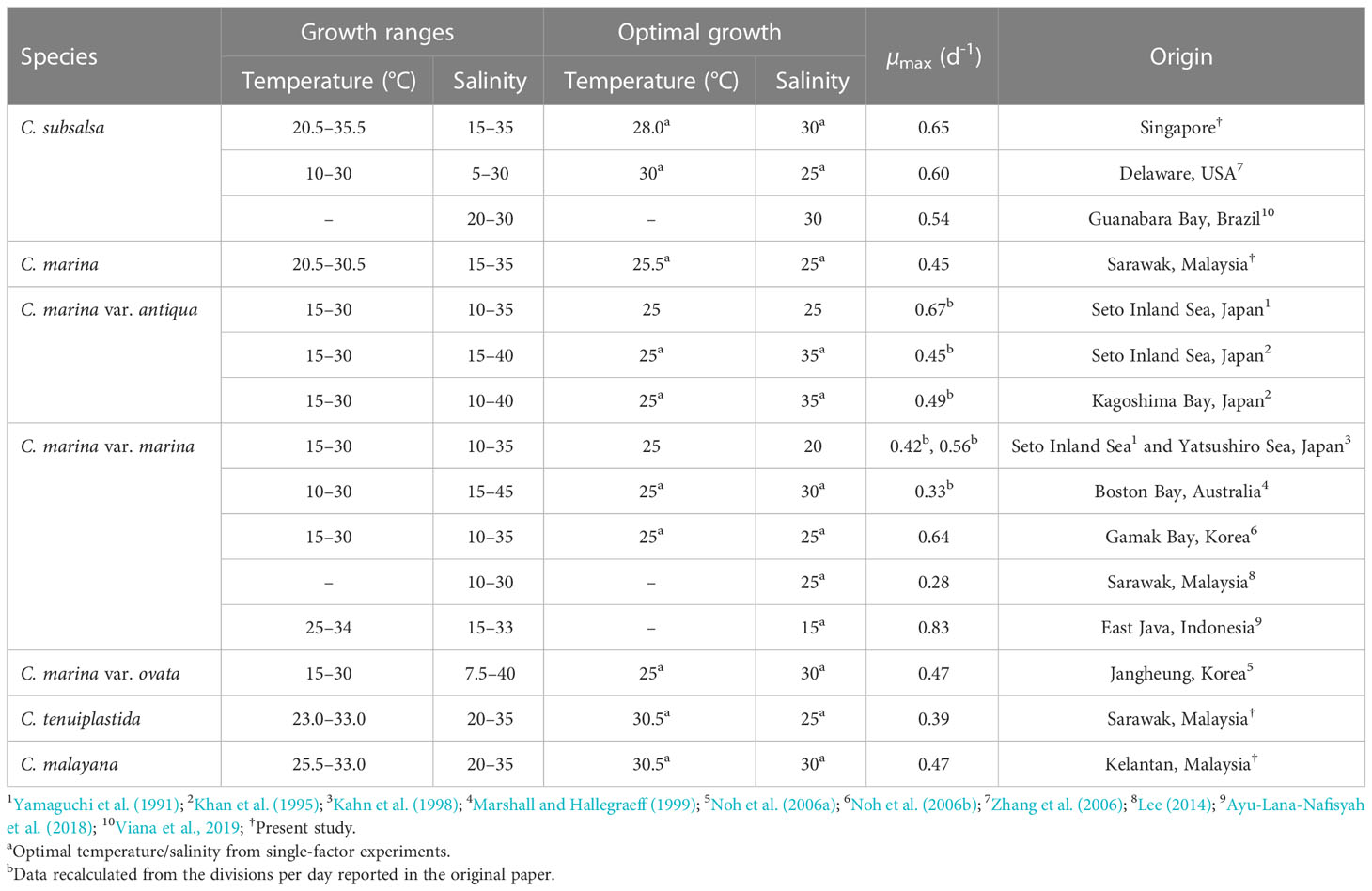
Table 5 Growth ranges, optimum growth conditions, and maximum specific growth rates (µmax) of Chattonella spp.
When comparing the tolerance of two distinct geographical populations of C. subsalsa, the tropical Singapore strain from this study showed a narrower temperature range (20.5–35.5°C), in contrast to the temperate USA strain which showed the range of 10–30°C (Zhang et al., 2006; this study; Table 5). As for C. marina, past studies have revealed a lower temperature tolerance limit (10–30°C) of the temperate strains, while the tropical C. marina strain demonstrated an upper temperature tolerance limit in the range of 20.5–34°C (Yamaguchi et al., 1991; Khan et al., 1995; Ayu-Lana-Nafisyah et al., 2018; this study). In both cases, tropical C. subsalsa and C. marina have higher growth temperature ranges than the temperate ones, suggesting that they have well adapted to the local environment and intraspecific variability by latitudinal differences is prominent.
Previous studies have reported higher optimal temperatures in C. subsalsa (28–30°C) as compared to C. marina, which was 25°C (Yamaguchi et al., 1991; Khan et al., 1995; Kahn et al., 1998; Marshall and Hallegraeff, 1999; Noh et al., 2006a; Noh et al., 2006b; Zhang et al., 2006; Wang et al., 2011; this study). This is in agreement with the present study. A study by Band-Schmidt et al. (2012) also showed that the Mexican C. marina strain grew better in colder water than C. subsalsa strain. The adaptability to a broader temperature range of C. marina explained its wide latitudinal distribution across the tropical Southeast Asia and temperate East Asia.
On the other hand, the salinity ranges for growth were inconclusive, as intraspecific variation occurred in Chattonella species from similar climates (Table 5). For example, the C. subsalsa strain from the USA had a broad salinity range of 5–30 but the C. subsalsa strain from Brazil was unable to grow at a salinity below 20 (Zhang et al., 2006; Viana et al., 2019). As for C. marina, the temperate Korean strains grew in a salinity of 7.5 (Noh et al., 2006a; Noh et al., 2006b), which was the lowest salinity ever reported for C. marina, whereas the Japanese and Australian strains grew in higher salinity ranges (Yamaguchi et al., 1991; Khan et al., 1995; Kahn et al., 1998; Marshall and Hallegraeff, 1999) (Table 5). The tropical C. marina strains also showed slightly different salinity ranges, i.e., C. marina from Malaysia grew at salinity ranges of 10–30 or 15–35 (both were optimal at 25), but those from Indonesia had a salinity range of 15–33, with an optimum salinity of 15 (Lee, 2014; Ayu-Lana-Nafisyah et al., 2018; this study). The specific growth rates of the tropical and temperate C. marina varied at salinity 35, i.e., the tropical strains had lower growth rates (0.2–0.4 d-1) as compared to the Japanese strains (0.4–0.7 d-1) (Nakamura and Watanabe, 1983; Khan et al., 1995; Lee, 2014; Ayu-Lana-Nafisyah et al., 2018; this study). In short, the salinity ranges are not species-specific and could not be explained by the latitudinal differences.
As for C. tenuiplastida and C. malayana, where their ecophysiology was firstly examined in the present study, relatively narrower growth ranges of temperatures and salinities were observed. The strains exhibited longer lag phases, slightly lower growth rates, and affected Fv/Fm at low temperature (20.5°C) when compared to the tropical C. subsalsa and C. marina in this study. This suggested that the two species have lower adaptability to colder water and thus restricted their distributions in warm waters. However, their µmax were comparable to those of C. subsalsa from Brazil, Malaysia, and Mexico, and C. marina from Australia, Japan, and Korea, suggesting competitive growth potentials in the two species (Table 5).
Combined effects of temperature and salinity on the optimal growth have been observed in Chattonella (Smayda, 1969; Tomas, 1978; Yamaguchi et al., 1991; Yamatogi et al., 2006). In our single-factor experiments, results showed that C. tenuiplastida and C. malayana had relatively slower growth (longer lag phase) and relatively lower µ than C. subsalsa and C. marina, but since Chattonella usually could grow very dense (Marshall and Hallegraeff, 1999), the interaction effect of temperature and salinity on these two species had been determined to better understand their growth characteristics. Although our cross-factorial experiments did not show significant differences among all tested conditions (Table 4), the optimal temperature and salinity differed from that observed in the single-factor experiments, i.e., the optimum growth in C. malayana had changed from 30.5°C (when salinity was 30) and salinity 30 (when the temperature was 28.0°C) to the combined factors of 28.0°C and salinity 25 (Table 3). The optimal temperature of C. tenuiplastida remained the same at 30.5°C but its optimal salinity changed from 25 to 30 in the cross-factorial experiments (Table 3). The changes in the optimal temperature and salinity suggested that other factors not considered in this study might have affected the physiological responses, e.g., the use of non-filtered caps that limited the gaseous exchange. The accumulation of high carbon dioxide (CO2) concentration resulting in acidic conditions has produced different growth responses in C. marina var. marina and C. marina var. ovata (Lim et al., 2020). Increased growth of H. akashiwo has also been observed in higher concentrations of CO2 (Fu et al., 2008).
The µmax of these two species, however, was similar to those of the single-factor experiments, further supported their optimal growths and adaptability. In the cross-factorial experiments, there was no significant difference in the growth response of C. tenuiplastida and C. malayana in the treatments of salinities 25, 30, and 35, unlike those demonstrated in the single-factor salinity experiments (Figures 2B, 4 and Tables 4, S4). Unfortunately, no constructive conclusion can be drawn from the cross-factorial experiments, except the evidence that the two species exhibited narrow windows of temperature and salinity tolerances. The results from the cross-factorial experiment somewhat agree with those in the single-factor experiments, which suggested the limited distribution of C. tenuiplastida and C. malayana in the tropical Asian waters.
4.2 Indigenous or alien Chattonella spp.?
It is difficult to ascertain the origin and the geographical introduction of these Chattonella, but the ability to adapt to a new environment has been suggested as an important driver for the widespread distribution of some phytoplankton especially the bloom-forming raphidophytes and dinoflagellates (Richlen et al., 2010; Zerebecki and Sorte, 2011; Sala-Pérez et al., 2021). They can adapt to warmer temperatures, less saline water, lower nutrient availability, and/or high CO2 concentration (Cubillos et al., 2007; Hallegraeff, 2010; Thomas et al., 2012; Sala-Pérez et al., 2021). As a case in point, the harmful raphidophyte H. akashiwo is widely distributed globally, likely owing to its adaptability to a wide growth temperature and salinity range, where it has high growth in warmer temperatures (Zhang et al., 2006; Fu et al., 2008). Another example is Margalefidinium polykrikoides (Margalef) Gómez, Richlen et Anderson, which is a euryhaline and eurythermal bloom-forming dinophyte that could grow in a wide range of temperatures and salinities (Richlen et al., 2010; Kudela and Gobler, 2012). Its widespread global distribution has been well-known, and many newly detected locations have been reported since its first discovery (Richlen et al., 2010; Kudela and Gobler, 2012; Thoha et al., 2019). Similarly, the adaptation of Gymnodinium aureolum (Hulburt) Hansen in the Black Sea since its first report in the USA has also been pointed to its euryhaline traits (Sala-Pérez et al., 2021).
The first reports of Chattonella in Asia and Southeast Asia were in 1969 and 1983, respectively, which were 30 years later than the first discovery of C. subsalsa in France (1933) and 10 years after the discovery of C. marina in India (1949), but the understanding on the origin and dispersion of these Chattonella was limited (Biecheler, 1936; Subrahmanyan, 1954; Khoo, 1985; Okaichi, 2003). Among the four Chattonella species tested in our experiments, we anticipated that C. subsalsa and C. marina could have been transported in or out of tropical Asian waters and adapted to the new environments as they exhibited high adaptability to broad temperature ranges. Cyst formation in C. subsalsa and C. marina has also been observed, and these cysts could survive for months in the dark and be transported by ballast water, further suggesting the chance of them being transported (Imai and Itoh, 1988; Imai, 1989; Portune et al., 2009; Jeong et al., 2013; Satta et al., 2017).
In contrast to C. subsalsa and C. marina, C. tenuiplastida and C. malayana exhibit narrower temperature and salinity ranges for growth (Lum et al., 2022; this study). In addition, their growths were unparalleled to those observed in C. subsalsa, suggesting that these two species may have a weaker ability to spread to other regions. The ability of cyst formation in C. tenuiplastida and C. malayana has yet to be clarified.
4.3 Potential harmful algal bloom formation
Cell densities of Chattonella species ranging from 35 × 103 to 28 × 107 cells L-1 have been reported to be associated with fish kill events (Okaichi, 2003; Barraza-Guardado et al., 2004; Cortés-Altamirano et al., 2006; Martínez-López et al., 2006; Jugnu and Kripa, 2009; Satta et al., 2017). In this study, the cell density of all four Chattonella species exceeded 107 cells L-1 in their respective temperatures and salinities, suggesting that they can form high cell biomass when conditions are favorable. The growth rates of the Chattonella spp. in this study are comparable to those of C. marina estimated from an in situ mesocosm experiment (0.1–0.36 d-1) and during a HAB incident in Yatsushiro Sea, Japan (~1 division d-1, Watanabe et al., 1995; Nakashima et al., 2019).
One of the major factors affecting Chattonella blooms in their natural habitat is the water temperature (Imai and Yamaguchi, 2012; Satta et al., 2017). Blooms of C. subsalsa occurred in tropical Guanabara Bay, Brazil at 21–31°C, and in Santa Giusta Lagoon, Italy at 20–30°C (Satta et al., 2017; Branco et al., 2019; Viana et al., 2019). Chattonella subsalsa in our study demonstrated high growth rates in a wide range of temperatures (25.5–33.0°C), which overlaps with the known bloom temperature of this species. Chattonella tenuiplastida and C. malayana also showed better growth in warmer temperatures, which were ≥ 25.5°C and ≥ 28.0°C, respectively. These three species could possibly achieve high growth and form HABs in Southeast Asia as the coastal water ranges from 27.2–32.5°C fit their preferred temperature (Lau et al., 2017; Hii et al., 2021). Blooms of C. marina have been observed in slightly lower temperatures, such as in southwest India (25–27°C, Sanilkumar et al., 2012), and Mexico (17–22.7°C, Cortés-Altamirano et al., 2006; García-Mendoza et al., 2018). Our tropical C. marina maintained these growth characteristics, where it preferred colder water and may have a lower chance to form HABs in Southeast Asia. However, its ichthyotoxicity should not be underestimated as it had caused fish kills in low cell densities (Nishikawa et al., 2014).
Another factor, salinity, has seldom been related to the blooms of Chattonella, except when there is a nutrient influx from the river discharge (Onitsuka et al., 2011; Aoki et al., 2012). A wide range of salinities has been reported during their blooms, i.e., blooms of C. subsalsa at 38.5 (Mexico, Martínez-López et al., 2006), and 50 (Salton Sea, Tiffany et al., 2001), and blooms of C. marina at 35.3–36.9 (Australia, Marshall, 2002), 30–32.8 (Japan, Nakamura et al., 1988), 11.6–33.2 (Korea, Jeong et al., 2013), 33–38.9 (Mediterranean Sea, Ismael and Halim, 2001), and 34.5–34.7 (Mexico, Cortés-Altamirano et al., 2006). In Southeast Asia, heavy rainfalls and low salinity conditions in the coastal areas are common (Leong et al., 2015; Lau et al., 2017; Razali et al., 2022). In such cases, salinity adaptability is important for the survival of these HABs species and the chance to bloom (Nakamura, 1985; Onitsuka et al., 2011; Aoki et al., 2012; Kok et al., 2019; Hii et al., 2021). In the present study, C. subsalsa and C. marina could grow in lower salinity and the growth ranges were larger than C. tenuiplastida and C. malayana, suggesting the former two had higher potential to proliferate and form blooms in the tropical Southeast Asian region.
Based on the historical records of Chattonella blooms and fisheries damages in Southeast Asia, C. subsalsa has been suggested as the responsible species in the region because of its occurrence in the fish-kill locations (Lum et al., 2021). Its higher growth and maximum cell densities in a wider range of temperatures and salinities as in this study, compared to the other three species, have further supported this claim. Aside from C. subsalsa, C. malayana had been reported to cause a harmful algal bloom resulting in massive wild fish mortality in Malaysia (Lum et al., 2022). It is interesting to note that, however, the cell density of C. malayana examined in this study was relatively low as compared to the other three species. This implied that there are other bloom-promoting factors that may affect the species but have yet to be unveiled. García-Mendoza et al. (2018) suggested that changes in the phytoplankton community must also be considered in understanding the bloom dynamics of Chattonella. In future studies, other factors such as irradiance, nutrient availability, CO2 concentration, strain variability, and their interaction effects should be clarified (Yamaguchi et al., 1991; Marshall and Hallegraeff, 1999; Yamaguchi et al., 2008; Salvitti, 2010; Band-Schmidt et al., 2012; Wells et al., 2015; Kok et al., 2019; Viana et al., 2019; Lim et al., 2020; Yuasa et al., 2020a). To truly explain the ichthyotoxicity of these Chattonella species, the linkage between bloom potential and the production of reactive oxygen species (ROS) or other allelopathic chemicals should also be explored (Liu et al., 2007; Imai and Yamaguchi, 2012; Qiu et al., 2013; Yuasa et al., 2020a; Yuasa et al., 2020b; Ahumada-Fierro et al., 2021; Shikata et al., 2021; Cho et al., 2022).
Data availability statement
The original contributions presented in the study are included in the article/Supplementary Material. Further inquiries can be directed to the corresponding authors.
Author contributions
WL conducted the experiments, and wrote and finalized the manuscript. SS and KY assisted in data collection. TKo, TKa, and KyT assisted in data analyses. KK, CL and PL provided and maintained cultures. KtT and MI conceptualized, reviewed and finalized the manuscript, and funding acquisition. All authors contributed to the article and approved the submitted version.
Funding
This work was partially supported by JSPS Kakenhi 19H03027 and 19KK0160 to MI.
Acknowledgments
We express our deepest gratitude to the staffs at Japan Fisheries Research and Education Agency for sharing the equipment necessary for the experiments. This international collaborative study was conducted under the Core-to-Core Program (B. Asia-Africa Science Platforms) of JSPS and IOC/WESTPAC-HAB project.
Conflict of interest
The authors declare that the research was conducted in the absence of any commercial or financial relationships that could be construed as a potential conflict of interest.
The handling editor ZH declared a past collaboration with the author MI.
Publisher’s note
All claims expressed in this article are solely those of the authors and do not necessarily represent those of their affiliated organizations, or those of the publisher, the editors and the reviewers. Any product that may be evaluated in this article, or claim that may be made by its manufacturer, is not guaranteed or endorsed by the publisher.
Supplementary material
The Supplementary Material for this article can be found online at: https://www.frontiersin.org/articles/10.3389/fmars.2023.1127871/full#supplementary-material
References
Ahumada-Fierro N. V., García-Mendoza E., Sandoval-Gil J. M., Band-Schmidt C. J. (2021). Photosynthesis and photoprotection characteristics related to ROS production in three Chattonella (Raphidophyceae) species. J. Phycol. 57, 941–954. doi: 10.1111/jpy.13138
Anderson D. M., Fensin E., Gobler C. J., Hoeglund A. E., Hubbard K. A., Kulis D. M., et al. (2021). Marine harmful algal blooms (HABs) in the United States: history, current status and future trends. Harmful Algae 102, 101975. doi: 10.1016/j.hal.2021.101975
Anton A., Teoh P. L., Mohd-Shaleh S. R., Mohammad-Noor N. (2008). First occurrence of Cochlodinium blooms in Sabah, Malaysia. Harmful Algae 7, 331–336. doi: 10.1016/j.hal.2007.12.013
Aoki K., Onitsuka G., Shimizu M., Kuroda H., Matsuyama Y., Kimoto K., et al. (2012). Factors controlling the spatio-temporal distribution of the 2009 Chattonella antiqua bloom in the Yatsushiro Sea, Japan. Estuar. Coast. Shelf. Sci. 114, 148–155. doi: 10.1016/j.ecss.2012.08.028
Ayu-Lana-Nafisyah, Endang-Dewi-Masithah, Matsuoka K., Mirni-Lamid, Mochammad-Amin-Alamsjah, O-hara S., et al. (2018). Cryptic occurrence of Chattonella marina var. marina in mangrove sediments in Probolinggo, East Java province, Indonesia. Fish. Sci. 84, 877–887. doi: 10.1007/s12562-018-1219-0
Azanza R., Benico G., Iwataki M., Fukuyo Y. (2017). Harmful marine dinoflagellates in the Philippines (Diliman: The Marine Science Institute, University of the Philippines), 96.
Azanza R. V., David. L. T., Borja R. T., Baula I. U., Fukuyo Y. (2008). An extensive Cochlodinium bloom along the western coast of Palawan, Philippines. Harmful Algae 7, 324–330. doi: 10.1016/j.hal.2007.12.011
Band-Schmidt C. J., Martínez-López A., Bustillos-Guzmán J. J., Carreón-Palau L., Morquecho L., Olguín-Monroy N. O., et al. (2012). Morphology, biochemistry, and growth of raphidophyte strains from the Gulf of California. Hydrobiologia 693, 81–97. doi: 10.1007/s10750-012-1088-y
Barraza-Guardado R., Cortés-Altamirano R., Sierra-Beltrán A. (2004). Marine die-offs from Chattonella marina and Ch. cf. ovata in Kun Kaak Bay, Sonora in the Gulf of California. Harmful Algae News 25, 7–8.
Biecheler B. (1936). Sur une chloromonadine nouvelle d’eau saumatre Chattonella subsalsa n. gen., n. sp. Arch. Zool. Expérimentale. 19énérale. 78, 79–83.
Bowers H. A., Tomas C. R., Tengs T., Kempton J. W., Lewitus A. J., Baruch B. W., et al. (2006). Raphidophyceae [Chadefaud ex Silva] systematics and rapid identification: sequence analyses and real-time PCR assays. J. Phycol. 42, 1333–1348. doi: 10.1111/j.1529-8817.2006.00285.x
Boyd P. W., Rynearson T. A., Armstrong E. A., Fu F., Hayashi K., Hu Z., et al. (2013). Marine phytoplankton temperature versus growth responses from polar to tropical waters – outcome of a scientific community-wide study. PloS One 8, e63091. doi: 10.1371/journal.pone.0063091
Branco S., Almeida L. L., Alves-de-Souza C., Oliveira M. M. M., Proença L. A. O., Menezes M. (2019). Morphological and genetic characterization of bloom-forming raphidophyceae from Brazilian coast. Phycol. Res. 67, 279–290. doi: 10.1111/pre.12377
Cho K., Ueno M., Liang Y., Kim D., Oda T. (2022). Generation of reactive oxygen species (ROS) by harmful algal bloom (HAB)-forming phytoplankton and their potential impact on surrounding living organisms. Antioxidants 11, 206. doi: 10.3390/antiox11020206
Cortés-Altamirano R., Alonso-Rodriguez R., Sierra-Beltrán A. (2006). Fish mortality associated with Chattonella marina and C. cf. ovata (Raphidophyceae) blooms in Sinaloa (Mexico). Harmful Algae News 31, 7–8.
Cubillos J. C., Wright S. W., Nash G., De Salas M. F., Griffiths B., Tilbrook B., et al. (2007). Calcification morphotypes of the coccolithophorid Emiliania huxleyi in the Southern Ocean: changes in 2001 to 2006 compared to historical data. Mar. Ecol. Prog. Ser. 348, 47–54. doi: 10.3354/meps07058
Edvardsen B., Imai I. (2006). “The ecology of harmful flagellates within Prymnesiophyceae and Raphidophyceae,” in Ecology of harmful algae. Eds. Granéli E., Turner J. T. (Berlin: Springer-Verlag), 67–79.
Engesmo A., Eikrem W., Seoane S., Smith K., Edvardsen B., Hofgaard A., et al. (2016). New insights into the morphology and phylogeny of Heterosigma akashiwo (Raphidophyceae), with the description of Heterosigma minor sp. nov. Phycologia 55, 279–294. doi: 10.2216/15-115.1
Fu F. X., Tatters A. O., Hutchins D. A. (2012). Global change and the future of harmful algal blooms in the ocean. Mar. Ecol. Prog. Ser. 470, 207–233. doi: 10.3354/meps10047
Fu F. X., Zhang Y., Warner M. E., Feng Y., Sun J., Hutchins D. A. (2008). A comparison of future increased CO2 and temperature effects on sympatric Heterosigma akashiwo and Prorocentrum minimum. Harmful Algae 7, 76–90. doi: 10.1016/j.hal.2007.05.006
Furuya K., Iwataki M., Lim P. T., Lu S., Leaw C.-P., Azanza R. V., et al. (2018). “Overview of harmful algal blooms in Asia,” in Global ecology and oceanography of harmful algal blooms. Eds. Glibert P. M., Berdalet E., Burford M. A., Pitcher G. C., Zhou M. (Berlin: Springer-Verlag), 289–308.
García-Mendoza E., Cáceres-Martínez J., Rivas D., Fimbres-Martinez M., Sánchez-Bravo Y., Vásquez-Yeomans R., et al. (2018). Mass mortality of cultivated northern bluefin tuna Thunnus thynnus orientalis associated with Chattonella species in Baja California, Mexico. Front. Mar. Sci. 5. doi: 10.3389/fmars.2018.00454
Gin K. Y. H., Holmes M. J., Zhang S., Lin X. (2006). ““Phytoplankton structure in the tropical port waters of Singapore,” in The environment in Asia pacific harbours. Ed. Wolanski E. (Netherlands: Springer), 347–375.
Hada Y. (1967). Protozoan plankton of the Inland Sea, Setonaikai. I. the mastigophora. Bull. Suzugamine. Women’s. Coll. Natural Sci. 13, 1–26.
Hada Y. (1968). Protozoan plankton of the Inland Sea, Setonaikai. II. the mastigophora and sarcodina. Bull. Suzugamine. Women’s. Coll. Natural Sci. 14, 1–28.
Hall B. G., Acar H., Nandipati A., Barlow M. (2014). Growth rates made easy. Mol. Biol. Evol. 31, 232–238. doi: 10.1093/molbev/mst187
Hallegraeff G. M. (2010). Ocean climate change, phytoplankton community responses, and harmful algal blooms: a formidable predictive challenge. J. Phycol. 46, 220–235. doi: 10.1111/j.1529-8817.2010.00815.x
Hara Y., Chihara M. (1987). Morphology, ultrastructure and taxonomy of the raphidophycean alga Heterosigma akashiwo. Bot. Mag. Tokyo. 100, 151–163. doi: 10.1007/BF02488320
Hii K. S., Mohd-Din M., Luo Z., Tan S. N., Lim Z. F., Lee L. K., et al. (2021). Diverse harmful microalgal community assemblages in the Johor Strait and the environmental effects on its community dynamics. Harmful Algae 107, 102077. doi: 10.1016/j.hal.2021.102077
Hulburt E. M. (1965). Flagellates from brackish waters in the vicinity of Woods Hole, Massachusetts. J. Phycol. 1, 87–94. doi: 10.1111/j.1529-8817.1965.tb04563.x
Imai I. (1989). Cyst formation of the noxious red tide flagellate Chattonella marina (Raphidophyceae) in culture. Mar. Biol. 103, 235–239. doi: 10.1007/BF00543353
Imai I., Itoh K. (1988). Cysts of Chattonella antiqua and C. marina (Raphidophyceae) in sediments of the Inland Sea of Japan. Bull. Plankton. Soc Japan. 35, 35–44.
Imai I., Yamaguchi M. (2012). Life cycle, physiology, ecology and red tide occurrences of the fish-killing raphidophyte Chattonella. Harmful Algae 14, 46–70. doi: 10.1016/j.hal.2011.10.014
Ismael A., Halim Y. (2001). “Occurrence and succession of potentially harmful phytoplankton species in the Eastern harbour of Alexandria, Egypt,” in Harmful algal blooms 2000: proceedings of the ninth international conference on harmful algal blooms. Eds. Hallegraeff G. M., Blackburn S. I., Bolch C. J. S., Lewis R. J. (Hobart: Intergovernmental Oceanographic Commission of UNESCO), 141–143.
Iwataki M., Kawami H., Matsuoka K. (2007). Cochlodinium fulvescens sp. nov. (Gymnodiniales, dinophyceae), a new chain-forming unarmored dinoflagellate from Asian coasts. Phycol. Res. 55, 231–239. doi: 10.1111/j.1440-1835.2007.00466.x
Iwataki M., Kawami H., Mizushima K., Mikulski C. M., Doucette G. J., Relox J. R. Jr, et al. (2008). Phylogenetic relationship in the harmful dinoflagellate Cochlodinium polykrikoides (Gymnodiniales, dinophyceae) inferred from LSU rDNA sequences. Harmful Algae 7, 271–277. doi: 10.1016/j.hal.2007.12.003
Iwataki M., Takayama H., Takahashi K., Matsuoka K. (2015). “Taxonomy and distribution of the unarmored dinoflagellates Cochlodinium polykrikoides and C. fulvescens,” in Marine protists: diversity and dynamics. Eds. Ohtsuka S., Suzaki T., Horiguchi T., Suzuki N., Not F. (Tokyo: Springer), 551–565.
Jeong H. J., Yoo Y., Lim A. S., Kim T. W., Lee K., Kang C. K. (2013). Raphidophyte red tides in Korean waters. Harmful Algae 30, S41–S52. doi: 10.1016/j.hal.2013.10.005
Jugnu R., Kripa V. (2009). Effect of Chattonella marina [(Subrahmanyan) Hara et Chihara 1982] bloom on the coastal fishery resources along Kerala coast, India. Indian J. Mar. Sci. 38, 77–88.
Kahn S., Arakawa O., Onoue Y. (1998). Physiological investigations of a neurotoxin-producing phytoflagellate, Chattonella marina (Raphidophyceae). Aquac. Res. 29, 9–17. doi: 10.1046/j.1365-2109.1998.00928.x
Katano T., Yoshida M., Lee J., Han M.-S., Hayami Y. (2009). Fixation of Chattonella antiqua and C. marina (Raphidophyceae) using Hepes-buffered paraformaldehyde and glutaraldehyde for flow cytometry and light microscopy. Phycologia 48, 473–479. doi: 10.2216/08-102.1
Katayama T., Makabe R., Sampei M., Hattori H., Sasaki H., Taguchi S. (2017). Photoprotection and recovery of photosystem II in the Southern Ocean phytoplankton. Polar. Sci. 12, 5–11. doi: 10.1016/j.polar.2016.12.003
Khan S., Arakawa O., Onoue Y. (1995). Effects of physiological factors on morphology and motility of Chattonella antiqua (Raphidophyceae). Bot. Mar. 38, 347–354. doi: 10.1515/botm.1995.38.1-6.347
Khoo E. W. (1985). Occurrences of “red tide” along Johore Straits, Malaysia, resulted in heavy mortality of shrimp. World Maricult. Soc News. 16, 4.
Kok J. W. K., Yeo D. C. J., Leong S. C. Y. (2019). Growth, pigment, and chromophoric dissolved organic matter responses of tropical Chattonella subsalsa (Raphidophyceae) to nitrogen enrichment. Phycol. Res. 67, 134–144. doi: 10.1111/pre.12360
Konalova G. V. (1995). “The dominant and potentially dangerous species of phytoflagellates in the coastal waters of east Kamchatka,” in Harmful marine algal blooms: proceedings of the sixth international conference on toxic marine phytoplankton. Eds. Lassus P., Arzul G., Denn E.E.-L., Gentien P., Marcaillou-Le Baut C. (Nantes, France: Lavoisier Publishing), 169–174.
Kudela R. M., Gobler C. J. (2012). Harmful dinoflagellate blooms caused by Cochlodinium sp.: global expansion and ecological strategies facilitating bloom formation. Harmful Algae 14, 71–86. doi: 10.1016/j.hal.2011.10.015
Lau W. L. S., Law I. K., Liow G. R., Hii K. S., Usup G., Lim P. T., et al. (2017). Life-history stages of natural bloom populations and the bloom dynamics of a tropical Asian ribotype of Alexandrium minutum. Harmful Algae 70, 52–63. doi: 10.1016/j.hal.2017.10.006
Leadbeater B. S. C. (1969). A fine structural study of Olisthodiscus luteus carter. Br. Phycol. J. 4, 3–17. doi: 10.1080/00071616900650021
Lee S. W. (2014). Effect of salinity and light intensity on the growth of Chattonella (Raphidophyceae). [Bachelor’s thesis] (Kota Samarahan (Sarawak: University Malaysia Sarawak).
Leong S. C. Y., Lim L. P., Chew S. M., Kok J. W. K., Teo S. L. M. (2015). Three new records of dinoflagellates in Singapore’s coastal waters, with observations on environmental conditions associated with microalgal growth in the Johor Straits. Raffles. Bull. Zool. 31, 24–36.
Lim M. H., Lee C. H., Min J., Lee H. G., Kim K. Y. (2020). Effect of elevated pCO2 on thermal performance of Chattonella marina and Chattonella ovata (Raphidophyceae). Algae 35, 375–388. doi: 10.4490/ALGAE.2020.35.12.8
Lim P. T., Usup G., Leaw C. P. (2012). Harmful algal blooms in Malaysian waters. Sains. Malaysiana. 41, 1509–1515.
Lirdwitayaprasit T., Ochi T., Montani S. (1996). “Changes in cell chemical composition during the growth cycle of Chattonella sp. and Heterosigma sp. found in the shrimp ponds at Chantaburi, Thailand,” in Harmful and toxic algal blooms. Eds. Yasumoto T., Oshima Y., Fukuyo Y. (Sendai: Intergovernmental Oceanographic Commission of UNESCO), 507–510.
Liu W., Au D. W. T., Anderson D. M., Lam P. K. S., Wu R. S. S. (2007). Effects of nutrients, salinity, pH and light:dark cycle on the production of reactive oxygen species in the alga Chattonella marina. J. Exp. Mar. Bio. Ecol. 346, 76–86. doi: 10.1016/j.jembe.2007.03.007
Lum W. M., Benico G., Azanza R., Furio E., Lim P. T., Lim H. C., et al. (2019). Morphology and molecular phylogeny of the harmful raphidophyte Chattonella subsalsa isolated from Bolinao, Philippines. Philipp. J. Nat. Sci. 24, 50–56.
Lum W. M., Benico G., Doan-Nhu H., Furio E., Leaw C. P., Leong S. C. Y., et al. (2021). The harmful raphidophyte Chattonella (Raphidophyceae) in Western Pacific: its red tides and associated fisheries damage over the past 50 years (1969–2019). Harmful Algae 107, 102070. doi: 10.1016/j.hal.2021.102070
Lum W. M., Lim H. C., Lau W. L. S., Law I. K., Teng S. T., Benico G., et al. (2022). Description of two new species Chattonella tenuiplastida sp. nov. and Chattonella malayana sp. nov. (Raphidophyceae) from South China Sea, with a report of wild fish mortality. Harmful Algae 118, 102322. doi: 10.1016/j.hal.2022.102322
Maclean J. L. (1984). “Indo-pacific toxic red tide occurrences (1972–1984,” in Toxic red tides and shellfish toxicity in southeast Asia: proceedings of a consultative meeting held in Singapore. Eds. White A. W., Anraku M., Hooi K.-K. (Singapore: Southeast Asian Fisheries Development Center and the International Development Research Centre), 92–104. Available at: http://hdl.handle.net/20.500.12066/4873.
Marshall J. (2002). Comparative ecophysiology, chemotaxonomy and ichthyotoxicity of Chattonella marina (Raphidophyceae) from Australia and Japan (Hobart (TAS: University of Tasmania).
Marshall J., Hallegraeff G. M. (1999). Comparative ecophysiology of the harmful alga Chattonella marina (Raphidophyceae) from south Australian and Japanese waters. J. Plankton. Res. 21, 1809–1822. doi: 10.1093/plankt/21.10.1809
Martínez-López A., Band-Schmidt C. J., Escobedo-Urías D., Ulloa-Pérez A. E. (2006). Bloom of Chattonella subsalsa in an impacted coastal lagoon in the Gulf of California. Harmful Algae News 31, 1–12.
Mehnert G., Leunert F., Cirés S., Jöhnk K. D., Rücker J., Nixdorf B., et al. (2010). Competitiveness of invasive and native cyanobacteria from temperate freshwaters under various light and temperature conditions. J. Plankton. Res. 32, 1009–1021. doi: 10.1093/plankt/fbq033
Mohammad-Noor N., Moestrup Ø., Leaw C. P., Lim P. T., Chin G. J. W. L., Usup G. (2018). Harmful algae of Malaysia (Kuala Lumpur: IIUM Press), 80.
Nakamura Y. (1985). Kinetics of nitrogen- or phosphorus-limited growth and effects of growth conditions on nutrient uptake in Chattonella antiqua. J. Oceanogr. Soc Japan. 41, 381–387. doi: 10.1007/BF02109032
Nakamura Y., Takashima J., Watanabe M. (1988). Chemical environment for red tides due to Chattonella antiqua in the Seto Inland Sea, Japan – part 1. growth bioassay of the seawater and dependence of growth rate on nutrient concentrations. J. Oceanogr. Soc Japan. 44, 113–124. doi: 10.1007/BF02302618
Nakamura Y., Watanabe M. M. (1983). Growth characteristics of Chattonella antiqua. J. Oceanogr. Soc Japan. 39, 151–155. doi: 10.1007/BF02070258
Nakashima H., Murata K., Yano K., Nishi H., Yoshimura N., Kuroki Y., et al. (2019). A Chattonella bloom in the Yatsushiro Sea, Japan in summer 2016: environmental characteristics during the bloom and mortality of cultured yellowtail Seriola quinqueradiata. Nippon Suisan Gakkaishi 85, 162–172. doi: 10.2331/suisan.18–00006
Nishikawa T., Hori Y., Nagai S., Miyahara K., Nakamura Y., Harada K., et al. (2014). Long-term (36-year) observations on the dynamics of the fish-killing raphidophyte Chattonella in Harima-Nada, eastern Seto Inland Sea, Japan. J. Oceanogr. 70, 153–164. doi: 10.1007/s10872-014-0219-7
Noh I.-H., Yoon Y.-H., Kim D.-I. (2006a). “Effect of water temperature, salinity and light on the growth of the poisonous acicular algae Chattonella ovata isolated from Jangheung coastal water in South Sea, Korea,” in Proceedings of the 2006 fall conference of the Korean society of marine and environmental energy (Busan, Korea: Korean Society of Marine and Environmental Energy), 184–190.
Noh I.-H., Yoon Y.-H., Kim D.-I., Oh S.-J. (2006b). Effects of water temperature, salinity and irradiance on the growth of the harmful algae Chattonella marina (Subrahmanyan) Hara et Chihara (Raphidophyceae) isolated from Gamak Bay, Korea. Korean. J. Fish. Aquat. Sci. 39, 487–494. doi: 10.5657/kfas.2006.39.6.487
Onitsuka G., Aoki K., Shimizu M., Matsuyama Y., Kimoto K., Matsuo H., et al. (2011). Short-term dynamics of a Chattonella antiqua bloom in the Yatsushiro Sea, Japan, in summer 2010: characteristics of its appearance in the southern area. Bull. Japan. Soc Fish. Oceanogr. 75, 143–153.
Petzoldt T. (2022) Growthrate [Estimate growth rates from experimental data]. Available at: https://github.com/tpetzoldt/growthrates (Accessed September 4, 2022).
Portune K. J., Coyne K. J., Hutchins D. A., Handy S. M., Cary S. C. (2009). Quantitative real-time PCR for detecting germination of Heterosigma akashiwo and Chattonella subsalsa cysts from Delaware’s Inland Bays, USA. Aquat. Microb. Ecol. 55, 229–239. doi: 10.3354/ame01292
Qiu X., Shimasaki Y., Tsuyama M., Yamada T., Kuwahara R., Kawaguchi M., et al. (2013). Growth-phase dependent variation in photosynthetic activity and cellular protein expression profile in the harmful raphidophyte Chattonella antiqua. Biosci. Biotechnol. Biochem. 77, 46–52. doi: 10.1271/bbb.120543
Ratkova T. N., Wassmann P. (2005). Sea Ice algae in the white and barents seas: composition and origin. Polar. Res. 24, 95–110. doi: 10.3402/polar.v24i1.6256
Razali R. M., Mustapa N. I., Md. Noordin W. N., Rahim M. A., Hii K. S., Lim P. T., et al. (2022). Report of a fish kill due to a dinoflagellate bloom in Perak and Penang, Malaysia. Asian Fish. Sci. 35, 257–268. doi: 10.33997/j.afs.2022.35.3.004
R Core Team. (2022) R: a language and environment for statistical computing (R foundation for statistical computing). Available at: https://www.R-project.org.
Richlen M. L., Morton S. L., Jamali E. A., Rajan A., Anderson D. M. (2010). The catastrophic 2008–2009 red tide in the Arabian Gulf region, with observations on the identification and phylogeny of the fish-killing dinoflagellate Cochlodinium polykrikoides. Harmful Algae 9, 163–172. doi: 10.1016/j.hal.2009.08.013
Sakamoto S., Lim W. A., Lu D., Dai X., Orlova T., Iwataki M. (2021). Harmful algal blooms and associated fisheries damage in East Asia: current status and trends in China, Japan, Korea and Russia. Harmful Algae 102, 101787. doi: 10.1016/j.hal.2020.101787
Sakamoto S., Yamaguchi M., Yamatogi T., Kim D. I., Honjo T. (2009). Growth physiology of Cochlodinium polykrikoides. Bull. Plankton. Soc Japan. 56, 32–36.
Sala-Pérez M., Lockyer A. E., Anesio A., Leroy S. A. G. (2021). Effect of temperature and salinity on the growth and cell size of the first cultures of Gymnodinium aureolum from the Black Sea. Bot. Mar. 64, 201–210. doi: 10.1515/bot-2020-0076
Salvitti L. (2010). The effects of temperature, CO2, and nitrogen source on the growth and physiology of the raphidophytes Heterosigma akashiwo and Chattonella subsalsa (Newark (DE: University of Delaware).
Sanilkumar M., Thomas A. M., Vijayalakshmi K. C., Mohamed Hatha A. A., Saramma A. V. (2012). Chattonella marina bloom in the coastal sea off Mahe, southwest India. Curr. Sci. 103, 624–626.
Satta C. T., Padedda B. M., Sechi N., Pulina S., Loria A., Lugliè A. (2017). Multiannual Chattonella subsalsa Biecheler (Raphidophyceae) blooms in a Mediterranean lagoon (Santa giusta lagoon, Sardinia island, Italy). Harmful Algae 67, 61–73. doi: 10.1016/j.hal.2017.06.002
Shikata T., Yuasa K., Kitatsuji S., Sakamoto S., Akita K., Fujinami Y., et al. (2021). Superoxide production by the red tide-producing Chattonella marina complex (Raphidophyceae) correlates with toxicity to aquacultured fishes. Antioxidants 10, 1635. doi: 10.3390/antiox10101635
Smayda T. J. (1969). Experimental observations on the influence of temperature, light, and salinity on cell division of the marine diatom, Detonula confervacea (Cleve) gran. J. Phycol. 5, 150–157. doi: 10.1111/j.1529-8817.1969.tb02596.x
Smayda T. J. (2002). Adaptive ecology, growth strategies and the global bloom expansion of dinoflagellates. J. Oceanogr. 58, 281–294. doi: 10.1023/A:1015861725470
Subrahmanyan R. (1954). On the life-history and ecology of Hornellia marina gen, et sp. nov., (Chloromonadineae), causing green discoloration of the sea and mortality among marine organisms off the Malabar coast. Indian J. Fish. 1, 182–203.
Thoha H., Muawanah, Bayu Intan M. D., Rachman A., Sianturi O. R., Sidabutar T., et al. (2019). Resting cyst distribution and molecular identification of the harmful dinoflagellate Margalefidinium polykrikoides (Gymnodiniales, Dinophyceae) in Lampung Bay, Sumatra, Indonesia. Front. Microbiol. 10. doi: 10.3389/fmicb.2019.00306
Thomas M. K., Kremer C. T., Klausmeier C. A., Litchman E. (2012). A global pattern of thermal adaptation in marine phytoplankton. Science 338, 1085–1088. doi: 10.1126/science.1224836
Tiffany M. A., Barlow S. B., Matey V. E., Hurlbert S. H. (2001). Chattonella marina (Raphidophyceae), a potentially toxic alga in the Salton Sea, California. Hydrobiologia 466, 187–194. doi: 10.1023/A:1014503920898
Tomas C. R. (1978). Olisthodiscus luteus (Chrysophyceae) i. effects of salinity and temperature on growth, motility and survival. J. Phycol. 14, 309–313. doi: 10.1111/j.1529-8817.1978.tb00303.x
Viana T. V., Fistarol G. O., Amario M., Menezes R. B., Carneiro B. L. R., Chaves D. M., et al. (2019). Massive blooms of Chattonella subsalsa Biecheler (Raphidophyceae) in a hypereutrophic, tropical estuary-Guanabara Bay, Brazil. Front. Mar. Sci. 6. doi: 10.3389/fmars.2019.00085
Vonshak A., Torzillo G., Tomaseli L. (1994). Use of chlorophyll fluorescence to estimate the effect of photoinhibition in outdoor cultures of Spirulina platensis. J. Appl. Phycol. 6, 31–34. doi: 10.1007/BF02185901
Wang Z., Yuan M., Liang Y., Lu S. (2011). Effects of temperature and organic and inorganic nutrients on the growth of Chattonella marina (Raphidophyceae) from the Daya Bay, South China Sea. Acta Oceanol. Sin. 30, 124–131. doi: 10.1007/s13131-011-0127-2
Watanabe M., Kohata K., Kimura T., Takamatsu T., Yamaguchi S., Ioriya T. (1995). Generation of a Chattonella antiqua bloom by imposing a shallow nutricline in a mesocosm. Limnol. Oceanogr. 40, 1447–1460. doi: 10.4319/lo.1995.40.8.1447
Wells M. L., Trainer V. L., Smayda T. J., Karlson B. S. O., Trick C. G., Kudela R. M., et al. (2015). Harmful algal blooms and climate change: learning from the past and present to forecast the future. Harmful Algae 49, 68–93. doi: 10.1016/j.hal.2015.07.009
Yamaguchi M., Imai I., Honjo T. (1991). Effects of temperature, salinity and irradiance on the growth rates of the noxious red tide flagellates Chattonella antiqua and C. marina (Raphidophyceae). Nippon Suisan Gakkaishi 57, 1277–1284. doi: 10.2331/suisan.57.1277
Yamaguchi H., Sakamoto S., Yamaguchi M. (2008). Nutrition and growth kinetics in nitrogen- and phosphorus-limited cultures of the novel red tide flagellate Chattonella ovata (Raphidophyceae). Harmful Algae 7, 26–32. doi: 10.1016/j.hal.2007.05.011
Yamatogi T., Sakaguchi M., Iwataki M., Matsuoka K. (2006). Effects of temperature and salinity on the growth of four harmful red tide flagellates occurring in Isahaya Bay in Ariake Sound, Japan. Nippon Suisan Gakkaishi 72, 160–168. doi: 10.2331/suisan.72.160
Yñiguez A. T., Lim P. T., Leaw C. P., Jipanin S. J., Iwataki M., Benico G., et al. (2021). Over 30 years of HABs in the Philippines and Malaysia: what have we learned? Harmful Algae 102, 101776. doi: 10.1016/j.hal.2020.101776
Yuasa K., Shikata T., Ichikawa T., Tamura Y., Nishiyama Y. (2020b). Nutrient deficiency stimulates the production of superoxide in the noxious red-tide-forming raphidophyte Chattonella antiqua. Harmful Algae 99, 101938. doi: 10.1016/j.hal.2020.101938
Yuasa K., Shikata T., Kitatsuji S., Yamasaki Y., Nishiyama Y. (2020a). Extracellular secretion of superoxide is regulated by photosynthetic electron transport in the noxious red-tide-forming raphidophyte Chattonella antiqua. J. Photochem. Photobiol. B.: Biol. 205, 111839. doi: 10.1016/j.jphotobiol.2020.111839
Zerebecki R. A., Sorte C. J. B. (2011). Temperature tolerance and stress proteins as mechanisms of invasive species success. PloS One 6, e14806. doi: 10.1371/journal.pone.0014806
Zhang Y., Fu F. X., Whereat E., Coyne K. J., Hutchins D. A. (2006). Bottom-up controls on a mixed-species HAB assemblage: a comparison of sympatric Chattonella subsalsa and Heterosigma akashiwo (Raphidophyceae) isolates from the Delaware Inland bays, USA. Harmful Algae 5, 310–320. doi: 10.1016/j.hal.2005.09.001
Keywords: bloom potential, endemicity, harmful algae, adaptive ecology, algal growth, Fv/Fm, specific growth rate, Southeast Asia (SEA)
Citation: Lum WM, Sakamoto S, Yuasa K, Takahashi K, Kuwata K, Kodama T, Katayama T, Leaw CP, Lim PT, Takahashi K and Iwataki M (2023) Comparative effects of temperature and salinity on growth of four harmful Chattonella spp. (Raphidophyceae) from tropical Asian waters. Front. Mar. Sci. 10:1127871. doi: 10.3389/fmars.2023.1127871
Received: 20 December 2022; Accepted: 09 May 2023;
Published: 26 May 2023.
Edited by:
Zhangxi Hu, Guangdong Ocean University, ChinaReviewed by:
Ning Xu, Jinan University, ChinaAllan Douglas Cembella, Alfred Wegener Institute Helmholtz Centre for Polar and Marine Research (AWI), Germany
Copyright © 2023 Lum, Sakamoto, Yuasa, Takahashi, Kuwata, Kodama, Katayama, Leaw, Lim, Takahashi and Iwataki. This is an open-access article distributed under the terms of the Creative Commons Attribution License (CC BY). The use, distribution or reproduction in other forums is permitted, provided the original author(s) and the copyright owner(s) are credited and that the original publication in this journal is cited, in accordance with accepted academic practice. No use, distribution or reproduction is permitted which does not comply with these terms.
*Correspondence: Wai Mun Lum, lumwaimun@gmail.com; Mitsunori Iwataki, iwataki@g.ecc.u-tokyo.ac.jp
†Present address: Wai Mun Lum, Fisheries Technology Institute, Japan Fisheries Research and Education Agency, Fukuura, Kanagawa, Japan