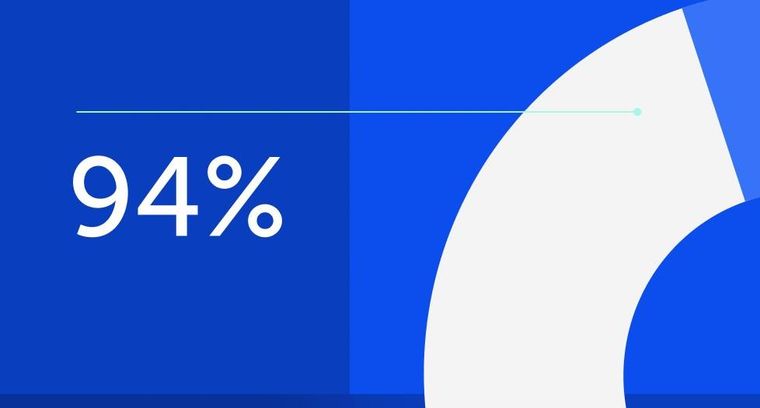
94% of researchers rate our articles as excellent or good
Learn more about the work of our research integrity team to safeguard the quality of each article we publish.
Find out more
ORIGINAL RESEARCH article
Front. Mar. Sci., 31 May 2023
Sec. Marine Evolutionary Biology, Biogeography and Species Diversity
Volume 10 - 2023 | https://doi.org/10.3389/fmars.2023.1125629
This article is part of the Research TopicThe 3Ds of Marine Viruses: Discovery, Diversity, and DynamicsView all 7 articles
Nowadays, zoos and aquariums, along with the constant advancement of sociocultural moral values, are proactively committed to ensuring and safeguarding cetacean health standards. This entails developing new approaches to health assessments by embracing minimally invasive sampling methods and enhanced animal handling and management, among other aspects. Hence, in the present survey, to appraise skin diseases, the implementation of cytology cell samplers as a non-invasive skin sampling device on 18 bottlenose dolphins housed in two facilities in the Canary Islands during the months of April, October, and December 2019 was performed to isolate cetacean poxvirus in tattoo-like lesions through a real-time PCR-based method using the DNA polymerase gene. Samples were repeatedly collected over time from eleven tattoo-like lesions and from apparently healthy skin to serve as a control for all study animals. From a total of 55 skin samples, detection of the poxvirus was attained in 31 (56.36%); specifically, on 20 of 21 samples collected from tattoo-like lesions (95.23%) and on 11 of 34 samples acquired from apparently healthy skin (32.35%). Correspondingly, the current study constitutes the first report of the isolation of cetacean poxvirus in skin samples without macroscopical signs of tattoo lesions in cetaceans. Likewise, ten of the eleven dolphins that showed tattoo lesions housed in Facility 1 were positive for tattoo skin disease, while four dolphins held in Facility 2 were positive for cetacean poxvirus without ever showing clinical evidence of the disease. This raises the question of whether this pathogen can produce latent infections and whether progression of the disease may depend on environmental stimuli, viral load, or the good health/immunological status of individual animals. Accordingly, further scientific research on cetaceans under human care could provide the knowledge, skills, and resources to understand the host–pathogen dynamics of cetacean poxviruses and their effect on cetaceans’ health.
During the past few decades, growing social concern regarding cetacean well-being has been accompanied by a remarkable ethical awareness of their maintenance in public display facilities (Jiang et al., 2008; Brando et al., 2018; Makecha and Highfill, 2018; Miller et al., 2018). This has forced these establishments to undergo reinvention to adapt to the demands of a constant change in social moral values and culture (Fraser and Switzer, 2021; Miranda et al., 2022). By this means, current modern zoo and aquarium objectives are not only based on ensuring animal conservation but also on promoting public education and recreation and taking part in scientific investigation (Draper and Harris, 2012; Gross, 2015; EAAM, 2019; EAZA, 2019; Rose and Riley, 2022). What these four goals have in common is that, in order to attain them, cetaceans under human care must be provided with the best health conditions possible (Daoust et al., 2014; Brando et al., 2018; Samelius, 2018; Wolfensohn et al., 2018).
A common approach to assessing animal health in zoos is to perform qualitative assessments through animal-based resources that relate to physical health and/or the prevalence and incidence of diseases and injuries, among others (Broom, 1991; Lerner, 2008; Clegg et al., 2015; Salas and Manteca, 2016; Whitham et al., 2017). For instance, the body condition score, commonly used in farm animals, is beginning to be applied to bottlenose dolphins under human care with the aim of assessing their nutritional status and overall health (Wells et al., 2004; Clegg et al., 2015; Patterson, 2016; Castrillon and Nash, 2020). Another proposed physical bodily measure of health is the quantification of rake marks as an indirect measure of aggression in cetaceans. This has already been applied to other studies (Pettis et al., 2004; Scott et al., 2005; Clegg et al., 2015). Further, best animal management and handling practices, along with the fulfillment of ethical matters, go hand in hand with animal health assessments (Lauderdale et al., 2021a; Schilling et al., 2022). Thus, the goal of any investigation developed in those facilities might be conducted along with the enhancement of innovative approaches and/or the improvement of sampling techniques to assess their well-being through minimally- or non-invasive methods, usually aiming to avoid long-lasting manipulations of animals (Zemanova, 2020; Schilling et al., 2022). Hence, different methods that minimize impact while sampling marine mammals in managed care have been reported, including the use of exhaled breath condensate to examine respiratory-associated microbial microorganisms (Lima et al., 2012), rubberized scrapers to obtain sloughed skin to further determine skin cortisol concentrations (Bechshoft et al., 2015), or swabs to collect saliva (Rickert et al., 2022), among others.
Accordingly, a previous study (Segura-Göthlin et al., 2021) proved the feasibility of using cytology cell samplers (CCS) as a skin sampling method to detect cetacean poxvirus (CePV) on sloughed skin when compared to skin biopsies on stranded cetaceans. The results showed that slightly increased sensitivity to further molecular isolation of this pathogen was gained through sloughed skin collected with this device in contrast to biopsied samples. Thus, CCS could serve as a novel and reliable skin sampling technique for cetaceans under human care, with the view that it might be a potential alternative to avoid invasive and enduring manipulations as well as minimize the risk of affecting their well-being during the procedure. Although CePV is currently not known to be lethal (van Elk et al., 2000), it is thought to be a potential health indicator due to the relative ability to distinguish their characteristic skin blemishes known as “tattoo-like” lesions, which show gray, black, or yellowish color with an irregular stippled pattern (Powell et al., 2018; Rodrigues et al., 2020; Luciani et al., 2022) and because its clinical manifestation has been implied to be the reflection of long-term environmental pressures, making the animals more susceptible to disease (Van Bressem et al., 2009b; Bossart and Duignan, 2018; Koch et al., 2018). In line with the aforementioned and because of their distinctive macroscopical appearance, a significant extent of what has been reported regarding CePV consists of photographic surveys identifying tattoo-like lesions in free-ranging cetaceans, assuming their emergence is related to CePV infection (Van Bressem et al., 2003; Riggin and Maldini, 2010; Fury and Reif, 2012; Powell et al., 2020). However, despite being highly recognizable, it is important to diagnose CePV in those lesions using diagnostic-based methods to confirm tattoo skin disease (TSD) and avoid subjective assumptions.
Since its first description through transmission electron microscopy (TEM) about several decades ago (Flom and Houk, 1979; Geraci et al., 1979; Van Bressem et al., 1993; Van Bressem and Van Waerebeek, 1996), which is considered, together with PCR techniques, the key diagnostic methods for CePV identification (Blacklaws et al., 2013; Barnett et al., 2015; Luciani et al., 2022), poxvirus-like lesions have been reported worldwide (Van Bressem et al., 2022). Through these assays, despite the limited available sequencing data, it has been possible to ascribe this viral pathogen to an unclassified genus within the Chordopoxvirinae subfamily, which subsequently includes two described groups: CePV-1 in odontocetes and CePV-2 in mysticetes (Sacristán et al., 2018b). Hence, these characteristic skin blemishes have been distinguished in a notable number of cetacean species, from small cetaceans such as porpoises to larger ones like southern right whales (Baker, 1992; Van Bressem et al., 1993; Raga et al., 1999; Bracht et al., 2006; Fiorito et al., 2015; Yang et al., 2015; Cocumelli et al., 2018). Even though this skin disease does not exclusively affect free-ranging cetaceans, only a few studies have reported its emergence in cetaceans under human care, in contrast to what has been described in wild populations (Flom and Houk, 1979; Ridgway, 1984; Cao et al., 2017; Terio et al., 2018). Nevertheless, the accessibility of marine mammals in housed conditions might provide an opportunity to gain a more detailed view of a wide range of features, which in this regard may contribute to a better understanding of CePV host–pathogen interactions and their possible effects on cetaceans’ health. Hence, the aim of the present study was to implement the use of CCS, a non-invasive skin sampling device, as an alternative to skin biopsies by validating their efficacy in detecting CePV in “tattoo-like” lesions in cetaceans in managed facilities. This is intended to corroborate tattoo skin disease (TSD) through evidence-based methods and prove cetaceans in house conditions to serve as an attainable model to improve our knowledge of the health of these marine mammals.
Eighteen bottlenose dolphins (Tursiops truncatus), which were housed in two different outdoor pool enclosures in two public display facilities in the Canary Islands, Spain, participated in the present survey. In addition, eight dolphins were held in Rancho Texas Lanzarote Park (Facility 1, FAC1), forming a pod only composed of males. Loro Parque (Facility 2, FAC2) housed the remaining ten animals, where both female and male dolphins formed the group. Dolphins vary in age, establishing three different categories based on their reproductive history (Robeck et al., 2008; Ijsseldijk et al., 2019). Generally, most of the animals were born under human care, specifically second-generation offspring, and they have been kept in almost two different zoos throughout their lives. Both establishments held bottlenose dolphins in five interconnected closed life support pools with a total volume of salt water of more than 7 million liters and a support system of chlorine and ozone. Aside from the temperature of the water, which was regularly monitored to prevent it from exceeding 25°C, dolphins experienced normal fluctuations in environmental conditions, including the day/night cycle and weather temperature.
In FAC1, two study animals (FAC1-N6 and FAC1-N7) had recently been moved from an indoor facility in Germany precisely one month before starting this study. At first, these animals were maintained separately from the main group to comply with appropriate quarantine procedures. During the study, they were gradually allowed to join the social group and subsequently allowed to mix with the other animals, always being controlled by trained animal care staff. Regarding dolphins held in FAC2, dolphin enclosures were freely connected, leaving the gates open, allowing animals to voluntarily perform social mixes within the female and male groups. Sometimes, staff coordinated those mixes to avoid unpredictable breeding or to manage training and medical sessions.
Skin sample collection was performed at different times throughout the year in 2019. Further, three different visits to FAC1 were conducted in the months of April, October, and December, while one visit was undertaken to FAC2 at the end of October. Unlike animals from this last facility, those housed in FAC1 showed tattoo-like lesions that appeared over different periods of time, which allowed for both macroscopic appraisal and sampling over time. Table 1 provides an overview of general animal information and their individual contributions to the current survey. Skin was sampled from both tattoo-like lesions and apparently healthy skin using CCS, which are sterile plastic swabs with a tip coated with a brush with soft-like texture that rapidly and efficiently dislodge cells in much the same way as described in Segura-Göthlin et al. (2021) (Segura-Göthlin et al., 2021), but with the difference that the present study was accomplished on bottlenose dolphins under professional care in place of stranded cetaceans. Samples were collected by the same person on every visit: the head dolphin trainer or the principal investigator of the present study. Moreover, they were collected individually and on a one-to-one basis in order to prevent cross-contamination. Collecting samples from apparently healthy skin was performed with the aim that they could serve as a control in each sampling procedure.
Sloughed skin was collected by gently brushing the surface of the epidermis of the lesions (Figures 1A, B). With the objective of standardizing the sampling protocol, samples of skin that presumably did not show any lesions macroscopically were intended to be collected at the same location for each individual. Thus, a considerable amount of loose skin was easier to obtain from the dorsal fin of the individuals, in addition to being an area where samples can be collected without the risk of being submerged in water. Consecutively, CCS tips from each sample were introduced into 1.5 ml sterile RNAse- and DNA-se-free microcentrifuge tubes (Thermofisher Scientific, Madrid, Spain), to which 1 ml of RNAlater Stabilization Solution (Thermofisher Scientific, Madrid, Spain) had previously been added, aiming to preserve both RNA and DNA qualities. Plastic stems were cut to the level of the microcentrifuge tubes’ tops, allowing the closure of the vials with the bristled top of the swabs embedded in RNAlater inside of them. Microcentrifuge tubes were stored at room temperature until their subsequent molecular analysis, which was performed within a working week.
Figure 1 Non-invasive skin sampling of tattoo-like lesions in a housed bottlenose dolphin (FAC1-N7) with cytology cell samplers at Facility 1. (A) FAC1-N7 is voluntarily rearing its head on the surface of the water by the edge of the pool, allowing a trainer to collect skin samples. Lower inset: close-up detail of the gentle brushing of two near tattoo-like lesions located adjacent to the blowhole. (B) While FAC1-N7 is maintaining the same position, the trainer can collect sloughed skin from a second tattoo-like lesion that is located over the right eye.
Skin samples from this study were collected in parallel with photo identification of the skin lesions and animals. Firstly, whole body images were taken from each side of the dolphins, aiming to have a correct perspective of the localization as well as the size of the lesions. To achieve this, dolphins were voluntarily beached or positioned on the surface of the water along one side of the edge of the pool. Furthermore, close-up images allowed for an improved evaluation of the appearance of the tattoo-like lesions.
DNA extraction from sloughed skin samples was carried out through the DNeasy™ Blood and Tissue Kit (Qiagen, Inc., Valencia, CA, USA) with some adaptations as thoroughly explained in Segura-Göthlin et al. (2021) (Segura-Göthlin et al., 2021). Subsequently, the molecular detection of CePV-1 was performed using a real-time polymerase chain reaction (q-PCR) method to amplify a conserved region (150 bp) of the DNA polymerase gene by using the degenerate primer sets designed by Sacristán et al. (2018a) (Odontopox-F: 5’-CARGAAATMAAAAAGAARTTTCCATC-3’, and Odontopox-R: 5’-ACGTTCTGTTAARA AYCGTCTTAGTA-3’). Negative (nuclease-free water) and positive controls previously confirmed by our group for both extraction and amplification were included. The PCR products from positive lesions were purified using a commercial kit (Real Clean Spin kit 50 Test-REAL) and then sequenced using Sanger DNA sequencing (Secugen S.L., Madrid, Spain). The amplicon identities were confirmed with BLAST (www.ncbi.nlm.nih.gov/blast/Blast.cgi).
In the present survey, eleven samples from tattoo-like skin lesions that were evolving and/or showed different stages that appeared and remitted during three different periods of 2019 were repeatedly collected, obtaining, therefore, a total of 21 samples from those lesions. Furthermore, ten apparently healthy skin samples from animals belonging to FAC2 were collected with eight periodically collected skin samples from the housed dolphins in FAC1. In this way, 34 skin samples were acquired without macroscopical evidence of being affected. This resulted in the collection of 55 skin samples.
A first sample collection was undertaken in April 2019 at FAC1. In this instance, ten skin samples were collected: two from tattoo-like lesions and eight from apparently healthy skin. FAC1-N6, one of the males that came from a facility in Germany one month before starting the present study, showed multiple and variable tattoo-like skin lesions all over the body on the first day it joined the dolphinarium. However, by the time the study started, only two remaining tattoo-like lesions on the left ear (A1) and the right side of the peduncle (A2) were present. Macroscopically, both lesions were oval-shaped, well delimited with a stippled pattern in the center, and associated with rake marks (Figure 2). The first lesion (Figure 2A) was smaller in size (3.5 × 2.2 cm) and slightly clearer than the second one (4.5 × 4.5 cm), which was gray in color with black borders (Figure 2D). Similarly, in the case of FAC1-N7, another male that had also recently arrived at the dolphinarium, all tattoo-like lesions apparently went into remission. Thus, after just one month since these individuals joined the dolphinarium, almost all lesions disappeared. Accordingly, both tattoo-like lesions from FAC1-N6 were collected using CCS. Additionally, eight other samples of apparently healthy skin collected from the dorsal fins of each of the eight animals that formed the pod were also sampled. By this time, FAC1-N6 and FAC1-N7 were independent of the rest of the social group.
Figure 2 Macroscopic development of two tattoo-like lesions between April and December 2019 in a bottlenose dolphin (FAC1-N6) at Facility 1. (A–C) Progress of lesion A1: a delimited oval tattoo-like lesion with a stippled pattern in the center that appeared to be associated with the left ear of the dolphin. (A) The lesion shows a black border with an evident pattern of dark dots in the center, but with some unaffected clear areas (3.5 × 2.2 cm) in April. (B) In October, a newly formed oval tattoo-like lesion appears associated with the initial one, increasing its size (5.5 × 3.5 cm). The border is still evident, but its black color is not that accentuated. A copious stippled pattern affecting the whole interior area of the lesion is observed. (C) The lesion has slightly increased in size (6 × 4 cm). The area where the new lesion appeared is slightly raised with marked dark dots in contrast to the rest of the blemish. (D–F) Progress of lesion A2: a delimited oval tattoo-like lesion with a stippled pattern in the center, associated with rake marks, and located on the peduncle of the dolphin. (D) The lesion is well-delimited with a thick black border, and an inner pinpoint pattern that is darker in the center than the closer areas of the margins (4.5 × 4.5 cm). (E) In October, the lesion apparently did not evolve significantly. (F) The lesion shows a slight increase in size with a thinner border and a diffuse stippled pattern (5 × 6.5 cm).
A total of 26 skin samples were collected in October 2019. Eight samples were from tattoo-like lesions and 18 from supposedly healthy skin from both FAC1 and FAC2 individuals. Lesions from FAC1-N6 were systematically sampled. The lesion associated with the left ear doubled in size (5.5 × 3.5 cm), apparently coalescing with another new tattoo-like lesion that seemingly appeared and was superimposed over the initial lesion (Figure 2B). On the other hand, a lesion localized on the peduncle apparently remained in the same aspect (Figure 2E). Rake marks remained perceptible in both lesions. Furthermore, a third tattoo-like lesion appeared on the right dorsal flank of the animal and was collected (1.5 × 1 cm). On this second visit, FAC1-N7 showed multiple tattoo-like lesions on the melon and both flanks. They were different in size, and most were associated with rake marks. Two of those lesions were sampled, which were situated on the right side of the melon just beneath the blowhole and over the right eye. The first was two close-up tattoo-like lesions (1.5 × 1 cm and 2 × 1 cm) (Figure 3A), and the second seemed to show up as three tattoo-like lesions that had coalesced between each other (2.5 × 1 cm) (Figure 3C). During the summer and part of autumn, these two bottlenose dolphins were gradually introduced to the main group during staff-controlled sessions, participating in training and medical sessions as well as the show. Other two animals of the pod (FAC1-N2 and FAC1-N8) showed tattoo-like lesions that were particularly small (1 × 0.5 cm and 0.5 × 0.5 cm, respectively) (Figures 3E, G). Generally, they appeared on the melon of the dolphins and were also associated with rake marks. A total of two skin samples were collected from the lesions of both animals. A fourth individual (FAC1-N5) presented an irregular pale blemish that corresponded to the regression stage of a tattoo-like lesion and was situated dorsal to the right pectoral fin. Additionally, apparently healthy skin samples were collected from all the animals that were integrated into the group. It is important to highlight that the increase in interaction marks in every animal in the pod was notable compared to the beginning of the study.
Figure 3 Macroscopical development of four tattoo-like lesions between October and December 2019 in three bottlenose dolphins (FAC1-N7, FAC1-N2, and FAC1-N8) at Facility 1. (A, B) Progression of lesion A1; tattoo-like lesion on the melon of dolphin FAC1-N2. (A) An oval tattoo-like lesion with a marked dark pinpoint pattern on the dorsal part of it, which could correspond to the beginning of the emergence of the margins of the lesion (1 × 0.5 cm). (B) In December, the lesion appeared well-delimited and slightly bigger in size (1.8 × 1.2 cm). (C, D) Progression of lesion A1; two nearby tattoo-like lesions located close to the blowhole and associated with rake marks on dolphin FAC1-N7. (C) Lesions showed slender black margins with a pronounced inner pinpoint pattern and were almost the same size (left lesion: 1.5 × 1 cm; right lesion: 2 × 1 cm). (D) In December, both lesions showed hardly any changes, neither in aspect nor size. (E, F) Progression of lesion A2: three coalesced tattoo-like lesions associated with rake marks and located next to the right eye on dolphin FAC1-N7. (E) In October, three oval tattoo-like lesions that apparently are coalescing between each other can be clearly distinguished, still appreciating the black outer margins of each lesion (2.5 × 1 cm). (F) In December, those lesions had almost completely merged with each other, with the margins that visually separated them (2.5 × 1 cm). (G, H) Progression of lesion A1; tattoo-like lesion on the melon of dolphin FAC1-N8. (G) Small tattoo-like lesion with marked margins that seemingly does not fully limit the lesion. It shows a dark center without a stippled pattern, and it is associated with rake marks (0.5 × 0.5 cm). (H) In December, the lesion appears to have coalesced with another with the same appearance and an increase in size (1.2 × 0.8 cm). Rake marks were no longer perceptible.
In December 2019, the eight skin lesions described previously were consistently collected among the other three new ones, in addition to the other eight samples obtained from skin without macroscopical evidence of being affected, obtaining in this manner a total of 19 skin samples. Concerning tattoo-like lesions from FAC1-N6, both lesions from the ear and peduncle continued to increase. The lesion associated with the left ear reached 6 × 4 cm at the end (Figure 2C), and the one on the peduncle was 5 × 6.5 cm (Figure 2F). In addition, the third tattoo-like lesion that appeared on the dorsal right flank associated with a significant incision apparently maintained the same size but darkened slightly in color. Apparently, lesions collected from the melon of FAC1-N7 remained the same (Figure 3B) in contrast to the others that appeared on the right eye, which, by this time, appeared almost completely coalesced (2.5 × 1 cm) (Figure 3D). It must be noted that we observed the increased presence of rake marks with associated tattoo-like lesions on both flanks (Figure 4A). Lesions from FAC1-N2 and FAC1-N8 also continued to evolve until the day of sampling (Figures 3F, H). The lesion on FAC1-N2 located on the melon was darker in color and slightly enlarged (1.8 × 1.2 cm) with a well-marked dark border (Figure 3F). The associated rake marks were no longer perceptible. A small, new tattoo-like lesion was also observed on the tip of the same individual. The lesion from FAC1-N8 appeared to coalesce with another tattoo-like lesion with the same aspect as the initial one (1.2 × 0.8 cm) (Figure 4A). Furthermore, the amount of interaction marks in the melon and flank areas of this individual was remarkable, which, in turn, presented multiple small, associated pinpoint lesions (Figures 4B, C). By this time, the apparently regressive tattoo-like lesion that FAC1-N5 previously showed was no longer observable. Despite the foregoing, skin samples from this area were collected. The other three remaining individuals in the group also showed tattoo-like lesions, which were systematically sampled. FAC1-N3 and FAC1-N4 presented multiple pinpoint lesions associated with rake marks all over the body (Figure 4D). FAC1-N1 showed small tattoo-like lesions on the melon. Moreover, up to this point, all animals had formed a unique group and shared all the pools.
Figure 4 Rake marks with associated tattoo-like and pinpoint lesions in three bottlenose dolphins at Facility 1. (A) Several short and nearly healed discontinuous interaction marks were followed one by another along the right lateral side of FAC1-N3. Lower inset: zoomed-in image of two coalesced tattoo-like lesions associated with barely noticeable interaction marks. (B) Several apparently superficial rake marks are randomly arranged, tracing different trajectories over the right lateral flank of FAC1-N7. Lower inset: note some small, tattoo-like lesions disposed close to rake marks. (C) Numerous rake marks showing different healing stages on the caudal area of the blowhole of FAC1-N8. A few almost imperceptible, small, pinpoint, and tattoo-like lesions are associated with the wounds. Lower inset: zoomed-in image of three coalesced tattoo-like lesions associated with rake marks. (D) Nearly healed, clear rake marks distributed in different directions on the right flank of FAC1-N8. Lower inset: with this interaction, small tattoo-like lesions are associated.
From the 55 skin samples collected in the present survey, a total of 31 resulted in positive identification of CePV-1 (56.36%). Specifically, this pathogen was molecularly isolated in 20 of the 21 total samples collected from the eleven tattoo lesions in the present study (95.23%) and in eleven of the 34 total apparently healthy skin samples (32.35%). Table 2 specifies the samples, individual animals, and time at which CePV-1 was detected. On the first visit to FAC1, the two tattoo-like lesions that were observable on FAC1-N6 were positive for CePV-1. Subsequently, in October, there were five more tattoo lesions from four different individuals (FAC1-N2, FAC1-N5, FAC1-N7, and FAC1-N8) and the resurging of a new lesion from FAC1-N6, from which CePV-1 was isolated. Thus, considering that detection of CePV-1 was continuedly achieved in the two previous lesions from FAC1-N6, CePV-1 was isolated in a total of eight samples by this time. Those lesions continued to be positive on the third visit. Additionally, at that time, samples from three newly tattoo-like lesions that appeared on three more dolphins from the pod (FAC1-N1, FAC1-N3, and FAC1-N4) were collected and were also positive for CePV-1. In this way, a total of ten samples tested positive for CePV-1 on this last visit. To summarize, 20 samples collected from eleven tattoo-like lesions at different timepoints were positive for CePV-1. On the other hand, CePV-1 was also isolated in eleven of the 34 skin samples collected from which tattoo lesions were not macroscopically identified. Those samples were collected in every visit performed at FAC1 and FAC2. They were sampled from the same body part of each dolphin, specifically the dorsal fin, aiming to standardize the sampling method and avoid areas where lesions frequently appeared. In this manner, despite CePV-1 not being detected on either of the skin samples collected at the first visit, its isolation was achieved in October 2019 from six individuals: two bottlenose dolphins from FAC1 and four from FAC2. Furthermore, on the last visit carried out on FAC1, another five samples obtained from presumed healthy skin were positive for CePV-1. Consequently, it appears that, over time, isolation of CePV-1 from more skin samples is achieved, whether from tattoo-like lesions or apparently healthy skin from individuals with FAC1.
A wide range of cycle threshold values (Ct) are observed. Among samples collected from tattoo-like lesions, the one from which higher viral loads were obtained was the lesion that appeared on the melon of FAC1-N2 on the second visit performed, with a Ct value of 15.35. In contrast, FAC1-N5 presented with lesions with a lower viral load and a Ct value of 35.25 in December. It is important to note that this lesion appeared in an apparently regressive state, showing a slightly paler, almost imperceptible color than the surrounding skin. Focusing on the dolphins whose skin lesions persisted and were sampled more than once, it is notable that they generally showed lower Ct values during the first sampling compared to the following collections, which could indicate that viral loads decreased over time. Hence, while lesions A1 from FAC1-N2 and FAC1-N8 showed Ct values of 15.35 and 18.20 in October, both values slightly increased to 17.94 and 23.13, respectively. Moreover, lesions A1 and A2 collected from FAC1-N6 remained with similar Ct values during the two first collections but were slightly raised in December. Additionally, a third lesion that appeared on the tip of the same animal in October and was repeatedly sampled in December presented a noted decrease in viral loads, changing from showing 16.10 to 30.55 Ct values, respectively. Accordingly, an evident correlation between these molecular results and the gross appearance of the latter lesion can be established, as it macroscopically evolved from a tattoo-like pattern into an irregular pale regressive lesion, which could explain the lower viral load from the last collection. The same association could be made with the regressed lesion of FAC1-N5, which turned out to show Ct values of 30.19 to 35.25 when it was barely distinguishable. Importantly, FAC1-N7 was the only animal that showed an increased viral load in one of its lesions (A2) at the end of the study, which might suggest possible reactivation of the lesion. Notwithstanding the observed slight increases in Ct values in tattoo lesions over time, lesions generally maintained a high viral load.
Regarding samples collected from areas of skin where lesions were macroscopically not distinguishable, CePV-1 was detected in almost one-third of the samples (32.35%). All samples from which the pathogen was detected showed low viral loads, with Ct values ranging from 34.24 to 39.33. However, there was one sample that showed a high viral load with a Ct value of 24.89 and was collected from FAC1-N2. Further interpretation of molecular results and their association with the macroscopic appearance of the lesions is considered in the Discussion section.
A CePV-1 DNA polymerase product of 98 bp from the 31 positive skin samples of the present survey was obtained. Accordingly, the same sequence was acquired from the eleven TSD-positive bottlenose dolphins of both zoological parks, whether they showed clinical evidence of the disease or not.
So far, cetacean skin has been considered an essential matrix for understanding and acquiring an approximate approach to the overall health of these marine mammals (Mouton and Botha, 2012; Aubail et al., 2013; Barlow et al., 2019; Van Cise et al., 2020). Among the range of information that it can provide in relation to health, skin diseases constitute one of the most documented concerns, having their emergence related to environmental stressors such as anthropogenic threats, the exposure to persistent pollutants, and climate change, among others (Wilson et al., 1999; Van Bressem et al., 2009a; Bressem et al., 2015; Koch et al., 2018). However, little has been reported regarding skin diseases in cetaceans living in managed facilities (Thurman et al., 1983; Leamaster and Ostrowski, 1988; Ueda et al., 2013; Duignan et al., 2020). Much of the available information is related to free-ranging cetaceans. In view of the above, together with the fact that skin diseases might be both considerably visible and feasible to study in housed conditions in contrast to wild environments, appraising skin diseases in marine mammals under human care could potentially have great value not only in acquiring a better comprehension of their epidemiology and host-pathogen dynamics but also in assessing the overall health of cetaceans (Clegg et al., 2015).
Skin biopsies and sloughed skin collected with scalpels have been the sampling methods of choice with the aim of molecular diagnosis of specific pathogens in skin lesions in cetaceans, whether in the wild or under human care (Flom and Houk, 1979; Palmer et al., 1991; Esperón et al., 2012). Nevertheless, the employment of those techniques has been questioned because of their invasiveness (Harlin et al., 1999; Bearzi, 2000; Parsons et al., 2003; Kiszka et al., 2010; Noren and Mocklin, 2012; Schilling et al., 2022). This is largely due to ethical advances in the research field, which have advocated the refinement of sampling methods to limit the presumed discomfort that they may induce in the animals and safeguard their well-being, among other approaches. Thus, the present survey is one of the few studies that tries to address the importance of monitoring skin diseases in cetaceans under human care by managing to scientifically corroborate the presence of CePV-1 in tattoo-like lesions without compromising the well-being of individuals through the employment of a non-invasive sampling device.
Through CCS, sufficient desquamating epidermis was obtained by rubbing the surface of the skin several times without compromising it. This is in contrast to other skin sampling procedures used on cetaceans under human care that require deep sampling to maximize material recovery, thereby damaging the skin. As has previously been reported (Raga et al., 1999; Geraci and Lounsbury, 2005; Bracht et al., 2006; Van Bressem et al., 2009a; Powell et al., 2018), most tattoo-like lesions were located in the dorsal areas of the animal, mainly on the melon and lateral flanks. In this manner, whether the animals were voluntarily beached or remained at the exterior of the water at any place of the pool, each skin sample was collected without directly touching the water. This likely improved the adherence of sloughed skin to the bristles. Moreover, collection was neither time-consuming nor laborious. This is due, in part, to the high cellular turnover rate of cetacean skin, which facilitates the collection of sloughed skin (Flom and Houk, 1979; Geraci et al., 1979). Additionally, trainers at both participating facilities completed the skin sampling procedure without the need for dolphins to have undergone previous operant conditioning training (Lauderdale et al., 2021b). Thus, gently rubbing their skin with the bristles of the CCS was rather similar to scratching, which is a positive stimulus commonly used in training sessions at dolphinariums as a supportive reward (Clegg et al., 2019). Likewise, through the employment of CCS, further isolation of CePV-1 in the present survey was attained. Hence, this device could be considered a reference for an innovative sampling method to collect enough epidermal material for the molecular isolation of pathogens without creating discomfort or perturbing animal wellbeing.
Almost all bottlenose dolphins from FAC1, at different times, showed similar tattoo lesions with their characteristic round to oval shape and stippled pattern at the center. Generally, lesions were small and appeared solitary, mostly on the melon or lateral flanks of the animals. Macroscopically, almost all the sampled lesions did not generally undergo any substantial change other than a part of them turning slightly darker with widening borders and barely enlarging as previously described (Sacristán et al., 2018b), and certain ones coalescing between each other. However, there was one individual (FAC1-N5) that showed a tattoo lesion that, at the time of collection, was hardly perceptible and had apparently evolved to a healed stage, according to the denotation given in prior descriptions (Van Bressem et al., 2009b; Sacristán et al., 2018b), to finally disappear on the third visit. With this, it is understood that the macroscopic progression of the lesions of a housed social group continuously sharing the same environment might be divergent and independent of the rest of the pod, observing different stages of the lesions between animals. Thus, while some lesions remained persistent during the same period, others had disappeared. This suggests that what has previously been reported about tattoo lesions can progress in a distinctive manner over time (Geraci et al., 1979; Van Bressem et al., 2008). Furthermore, the might also be applied from an individual standpoint because, besides showing persistent tattoo lesions, animals also presented other poxvirus-like lesions that appeared and rapidly remitted in a short period of time, impeding their collection on time.
Between the months of April and December 2019, molecular isolation of CePV-1 was attained from ten tattoo-like lesions repeatedly collected over time from seven bottlenose dolphins held in FAC1. Thus, molecular corroboration of TSD was achieved in seven of the eight animals. Correspondingly, there was one individual (FAC1-N1), for whom, despite the identification of tattoo lesions at the end of the study, isolation of CePV-1 could not be achieved. However, notwithstanding this negative result, it is highly likely that this dolphin presented with TSD as all the members of the social group contracted the infection over time. This is likely since an inappropriate sampling collection was carried out, resulting in the collection of insufficient sloughed skin. Another possible explanation may be that an incorrect genomic DNA procedure on this skin sample was unintentionally conducted. Either way, excluding the latter, all tattoo lesions sampled in the present study resulted in a positive diagnosis of CePV-1.
One of the most notable findings of the present study was the molecular isolation of CePV-1 from apparently healthy skin. Thus, skin that does not show macroscopic evidence of lesions compatible with poxvirus from the dorsal fin of the 18 bottlenose dolphins in the current study was collected along with the sampling of tattoo-like lesions, aiming to serve as a control for further DNA extraction. Unexpectedly, this resulted in the detection of CePV-1 in eleven apparently healthy skin samples collected from five individuals from FAC1 and four dolphins housed in FAC2. To our knowledge, this is the first report in which the isolation of CePV-1 from apparently healthy skin in cetaceans is achieved. Further, in a prior study carried out by Melero and co-workers (Melero et al., 2014), performed on another marine mammal, specifically a Pacific walrus (Odobenus rosmarus divergens) held under human care, the authors detected poxvirus in the skin and in other organs such as the pre-scapular and tracheobronchial lymph nodes and tonsils, without the animal showing gross lesions. The amplicons obtained had a striking similarity to CePV-1. Inevitably, the molecular detection of CePV-1 in the absence of clinical evidence raises several questions. A possible explanation for these results might be related to the high sensitivity of q-PCRs, which can sometimes highlight increased probabilities of contamination and possible subsequent false-positive data (Opota et al., 2015). Contamination can be due to the circulating cell-free DNA brought from the environment, which raises another question of whether the detection of CePV-1 was due to the presence of viral particles in the water interfering with the sample rather than its isolation in apparently healthy skin. However, in order to detect the virus in this medium, specific protocols for pathogen concentration are normally required, as has been proven in multiple studies focused on the isolation of infectious agents that persist and are transmitted by aquatic means (Albinana-Gimenez et al., 2009; Girones et al., 2010). In addition, even though PCR is a very sensitive detection technique, in zoological conditions, pools are subjected to continuous disinfection treatments, which might significantly reduce the concentration of the virus (Girones et al., 2010; Lanrewaju et al., 2022). However, CePV-1 was also detected in apparently healthy skin samples from bottlenose dolphins from FAC2, a completely different scenario where tattoo-like lesions had not been reported in any of the dolphins. To date, thanks to the medical history carried out both by veterinarians and with the help of trainers, none of the dolphins on this establishment have ever shown clinical signs of the disease, and no molecular diagnostics of CePV have been performed. Accordingly, these results suggest that the latter individuals may have been developing the skin disease in a subclinical manner. Thus, CePV-1 could be present or even proliferating, resulting in the absence of clinical signs of the disease, which may be due to a low infective dose of the viral agent, and/or good animal health and/or the immunological status of the infected individuals, thus impeding disease progression. Correspondingly, Ct values from these apparently healthy skin samples showed remarkably low viral loads, ranging from 35.29 to 39.14, which could be one of the reasons why the skin disease did not eventually develop. With this last hypothesis, it could be deduced that low infective concentrations of the pathogen could have been present in areas of skin where there was no clinical evidence of poxvirus-like lesions in the bottlenose dolphins from FAC1, and that a route of entry through damaged skin may have been necessary for the development of the lesion. In this context, it is observed that Ct values obtained from these supposedly healthy skin samples indicated significant low viral loads, with values ranging from 30.89 to 38.8, which could support the hypothesis above stated. However, caution must be exercised when making these assumptions, as the present study, to our knowledge, is the first reported to date that has attempted to perform molecular analyses to detect poxvirus in apparently healthy skin in cetaceans.
Interestingly, the same sequence was derived from both tattoo lesions and skin samples without clinical evidence of the disease in dolphins from both FAC1 and FAC2 facilities. Besides, this amplicon showed a high homology of 99.10% and a query cover of 100% to the published sequence obtained from bottlenose dolphins in Brazil by Sacristán and co-workers (GenBank accession no. KU726612). A plausible interpretation of the fact that bottlenose dolphins kept under managed care showed a strain of CePV-1 with such remarkable similarities to another obtained from wild populations might reside in the likelihood that this pathogen has persisted through generations among housed cetaceans worldwide since the introduction of original wild-caught individuals around the 90s (Van Waerebeek et al., 2006), with the capability to be latent and cause subclinical infections in individuals without giving rise to the skin disease until reactivation by certain stimuli. Although there is no scientific evidence that corroborates TSD in the originally introduced and captured wild bottlenose dolphins, most of which came from Caribbean populations (Fisher and Reeves, 2007; Brownell and Reeves, 2008), prior reports have isolated CePV-1 sequences with high similarities to the one in question from social pods throughout the North and South Atlantic Seas (Sacristán et al., 2018a; Luciani et al., 2022), strengthening the possibility of the persistence of the virus along this geographical area. Furthermore, apparently this strain does not exclusively infect bottlenose dolphins, as quite homologous sequences have been detected in an Atlantic spotted dolphin and in a striped dolphin stranded along the Canary and Mediterranean coasts (Sacristán et al., 2018a; Segura-Göthlin et al., 2021). In this manner, the fact that it might have been transferred among different free-ranging cetacean species could indicate a high incidence of infection in wild populations. Moreover, supporting the latter hypothesis, a recent study from Rodrigues and co-workers (Rodrigues et al., 2020) achieved the isolation of a CePV-1 sequence from classic tattoo-like lesions from a managed Indo-Pacific bottlenose dolphin (Tursiops aduncus) kept in an oceanarium in Hong Kong, which also showed maximum likelihood with the above-mentioned strain (Bracht et al., 2006). The latter not only supports what is already known about the high distribution of this viral skin disease and the wide range of cetacean species that may be affected in the wild, but also raises the presumption that the same could also be happening in cetaceans held in zoos and aquariums around the world.
Little has so far been reported about the incidence of TSD in cetaceans under human care, which could represent a breakthrough in our understanding of the disease and the health of animal populations in captivity and in the wild. Up until now, some studies have suggested that environmental factors could influence the emergence of tattoo-like lesions in housed individuals. Thus, it has been found that water temperature changes at facilities that are in places where they experience notable fluctuations in the weather contribute to the emergence of tattoo-like lesions. In this way, elevated water temperatures favor the remission of the lesions, while reduced temperatures presumably encourage their presence (Gulland et al., 2018; St Leger et al., 2018). Comparing these observations with our current findings, it is assumed that the present survey was carried out in two facilities located on the Canary Islands, where subtropical temperatures are mild and stable throughout the year within the range of 18–24°C without noticeable interference with the water conditions (Bechtel, 2016). Certainly, bottlenose dolphins from FAC1 were gradually contracting the skin disease between the months of April and December, being that almost all animals were TSD-positive at the end of the year, coinciding with the winter season. However, it is very likely that this may be due to the progressive introduction of two CePV-1-positive bottlenose dolphins (FAC1-N6 and FAC1-N7) to the rest of the group, which could have led to the spread of the disease rather than mere variations in water temperature. Nevertheless, it is important to note the almost complete disappearance of the tattoo lesions in these two individuals during their first weeks of arrival at the enclosure, which could have been linked to environmental differences between the facility these dolphins came from and the one they were introduced to. Furthermore, the introduction of two new members of the pod caused a sustained reestablishment of the social hierarchy and, in turn, a gradual increase in interaction marks, which is normal behavior among this species (Scott et al., 2005; Clegg et al., 2015; Clegg et al., 2019). This survey reinforces what has previously been described in studies of cetaceans under managed care, noting that a great part of the emerging tattoo lesions in the present study were associated with rake marks (Van Bressem et al., 2017; St Leger et al., 2018). This suggests these discontinuities in the skin as a potential route of entry for the pathogen (Van Bressem et al., 2008; Mouton and Botha, 2012; Savini et al., 2017). In this manner, as interaction marks between animals increased, a higher prevalence of lesions was observed, as well as TSD-positive individuals.
Correspondingly, the current survey has served to probe whether CCS is a practical device in that it allows skin samples to be taken promptly and non-invasively without causing harm or affecting the well-being of the animals during the collection, being assertive in isolating CePV-1 from sloughed skin. Moreover, it is adaptable for trainers and caretakers due to the ease of performing the sampling and the unnecessary need to restrain or even capture the animals during clinical/health assessments, avoiding the stress that these procedures entail. Taken together, this CCS is a conceivable and innovative tool to enhance veterinary and husbandry practices to assess the health of captive cetaceans, which is an important target for zoos and aquariums. Furthermore, through this device, it has been possible to confirm with scientific corroboration what has previously been reported in relation to the epidemiology of this skin disease. Thus, tattoo lesions macroscopically evolve and persist on cetacean skin in an independent and indefinite manner and seem to recurrently disappear and appear. This supports hypotheses regarding the persistent nature of CePV infections due to a possible latent phase for a considerable period, where the virus is quiescent until reactivation is triggered by environmental stimuli or health conditions, as it could occur with the increase of intraspecific interactions due to imbalances in the social environment or fluctuations in water temperatures. However, even though this research constitutes one of the few prospective studies held on CePV in cetaceans under human care, it must be noted that additional longitudinal studies of this skin disease should be carried out to gain better scientific knowledge concerning host–pathogen interaction dynamics. Hence, in this article, we illustrate the significance of developing research in cetaceans under human care, which could be of significant value in taking steps towards improving and understanding animal health both in housed conditions and in the wild, and to progress on the conservation of these marine mammals.
The datasets presented in this study can be found in online repositories. The names of the repository/repositories and accession number(s) can be found below: GenBank, OQ199540.
Ethical review and approval was not required for the animal study because Skin sample collection from the housed cetaceans was carried out by the first author of the present manuscript as a qualified researcher and veterinarian, and the trainers authorized by Loro Parque and Lanzarote Rancho Texas Park zoos, within their routine medical program, as well as their use for the present scientific study, in compliance with the requirements established on the articles 3, 4 and 5 of the “Ley 31/2003, de 27 de octubre, de conservación de la fauna silvestre en los parques zoológicos” (BOE-A-2003-19800) and the Council Directive 1999/22/EC of 29 March 1999 relating to the keeping of wild animals in zoos (EUR-Lex -31999L0022). In addition, the use of this skin samples for the isolation of cetacean poxvirus was for the current investigation was expressly authorized by the Directors of Loro Parque and Lanzarote Rancho Texas Park zoos.
Conceptualization, ES, AF, and JA. Methodology and formal analysis, SS-G, ES, AF, MA, IF-J, and AC-R. Writing-original draft preparation, SS-G and ES. Supervision, ES, AF, MA, and JA. Funding acquisition, AF, ES, MA, and JA. All authors listed have made a substantial, direct, and intellectual contribution to the work and approved it for publication.
This research was partially supported by a National Project [ref. PID2021-127687NB-I00]; by the Canary Islands Government, which has founded and provided support to the stranding network; the Agencia Canaria de Investigación, Innovación y Sociedad de la Información [ref. CEI2020-05]; and by the Fundación Loro Parque.
The authors would like to thank the directors of Loro Parque Fundación and Lanzarote Rancho Texas Park for allowing the development of the present study in both zoos. Likewise, gratitude is expressed to the trainers and veterinarians of both establishments for collaborating and supporting the collection of the skin samples. Finally, we would like to make a special mention to Lorenzo von Fersen and Katrin Baumgartner for their initial guidance on the present survey.
The authors declare that the research was conducted in the absence of any commercial or financial relationships that could be construed as a potential conflict of interest.
All claims expressed in this article are solely those of the authors and do not necessarily represent those of their affiliated organizations, or those of the publisher, the editors and the reviewers. Any product that may be evaluated in this article, or claim that may be made by its manufacturer, is not guaranteed or endorsed by the publisher.
Albinana-Gimenez N., Clemente-Casares P., Calgua B., Huguet J. M., Courtois S., Girones R. (2009). Comparison of methods for concentrating human adenoviruses, polyomavirus JC and noroviruses in source waters and drinking water using quantitative PCR. J. Virol. Methods 158 (1–2), 104–109. doi: 10.1016/j.jviromet.2009.02.004
Aubail A., Méndez-Fernandez P., Bustamante P., Churlaud C., Ferreira M., Vingada J. V., et al. (2013). Use of skin and blubber tissues of small cetaceans to assess the trace element content of internal organs. Mar. Pollut. Bull. 76 (2013), 158–169. doi: 10.1016/j.marpolbul.2013.09.008
Barlow D. R., Pepper A. L., Torres L. G. (2019). Skin deep: an assessment of new Zealand blue whale skin condition. Front. Mar. Sci. 6, 757. doi: 10.3389/fmars.2019.00757
Barnett J., Dastjerdi A., Davison N., Deaville R., Everest D., Peake J., et al. (2015). Identification of novel cetacean poxviruses in cetaceans stranded in south West England. PloS One 10 (6), e0124315. doi: 10.1371/journal.pone.0124315
Bearzi G. (2000). First report of a common dolphin (Delphinus delphis) death following penetration of a biopsy dart. J. Cetacean Res. Manag 2 (3), 217–221. doi: 10.1579/0044-7447-38.2.66
Bechshoft T., Wright A. J., Weisser J. J., Teilmann J., Dietz R., Hansen M., et al. (2015). Developing a new research tool for use in free-ranging cetaceans: recovering cortisol from harbour porpoise skin. Conserv. Physiol. 3 (1), 16. doi: 10.1093/conphys/cov016
Bechtel B. (2016). The climate of the canary islands by annual cycle parameters. Int. Arch. Photogramm Remote Sens Spat Inf Sci. XLI-B8, 243–250. doi: 10.5194/isprs-archives-XLI-B8-243-2016
Blacklaws B. A., Gajda A. M., Tippelt S., Jepson P. D., Deaville R. (2013). Molecular characterization of poxviruses associated with tattoo skin lesions in UK cetaceans. PloS One 8 (8), 71734. doi: 10.1371/journal.pone.0071734
Bossart G. D., Duignan P. J. (2018). Emerging viruses in marine mammals. CAB Rev. 13 (52), 1–17. doi: 10.1079/PAVSNNR201813052
Bracht A. J., Brudek R. L., Ewing R. Y., Manire C. A., Burek K. A., Rosa C., et al. (2006). Genetic identification of novel poxviruses of cetaceans and pinnipeds. Arch. Virol. 151 (3), 423–438. doi: 10.1007/s00705-005-0679-6
Brando S., Broom D. M., Acasuso-Rivero C., Clark F. (2018). Optimal marine mammal welfare under human care: current efforts and future directions. Behav. Processes 156, 16–36. doi: 10.1016/j.beproc.2017.09.011
Bressem V., Flach M.-F., Reyes L., Echegaray J. C., Santos M., Félix F., et al. (2015). Epidemiological characteristics of skin disorders in cetaceans from south American waters. Lat Am. J. Aquat. Mamm. 10 (1), 20–32. doi: 10.5597/lajam00190
Broom D. M. (1991). Animal welfare: concepts and measurement. J. Anim. Sci. 69 (10), 4167–4175. doi: 10.2527/1991.69104167x
Brownell R., Reeves R. (2008). Biological background on bottlenose dolphins ( tursiops spp.) in the “Live-capture” trade and specifically on the indo-pacific bottlenose dolphin, t. aduncus (USA: IUCN).
Cao S., Realegeno S., Pant A., Satheshkumar P. S., Yang Z. (2017). Suppression of poxvirus replication by resveratrol. Front. Microbiol. 8. doi: 10.3389/fmicb.2017.02196/full
Castrillon J., Nash S. B. (2020). Evaluating cetacean body condition; a review of traditional approaches and new developments. Ecol. Evol. 10 (12), 6144–6162. doi: 10.1002/ece3.6301
Clegg I. L. K., Borger-Turner J. L., Eskelinen H. C. (2015). C-well: the development of a welfare assessment index for captive bottlenose dolphins (Tursiops truncatus). Anim. Welf. 24 (3), 267–282. doi: 10.7120/09627286.24.3.267
Clegg I. L. K., Rödel H. G., Mercera B., van der Heul S., Schrijvers T., de Laender P., et al. (2019). Dolphins’ willingness to participate (WtP) in positive reinforcement training as a potential welfare indicator, where WtP predicts early changes in health status. Front. Psychol. 10. doi: 10.3389/FPSYG.2019.02112
Cocumelli C., Fichi G., Marsili L., Senese M., Cardeti G., Cersini A., et al. (2018). Cetacean poxvirus in two striped dolphins (Stenella coeruleoalba) stranded on the tyrrhenian coast of Italy: histopathological, ultrastructural, biomolecular, and ecotoxicological findings. Front. Veterinary Sci. 5. doi: 10.3389/fvets.2018.00219
Daoust P.-Y., Ford J., Kreiberg H., Lanthier C., Mehren K., Stewart T., et al. (2014). Recommendations for the care and maintenance of marine mammals (Ottawa: Canadian Council on Animal Care). Available at: http://www.ccac.ca.
Draper C., Harris S. (2012). The assessment of animal welfare in British zoos by government-appointed inspectors. Animals 2, 507–528. doi: 10.3390/ani2040507
Duignan P. J., Stephens N. S., Robb K. (2020). Fresh water skin disease in dolphins: a case definition based on pathology and environmental factors in Australia. Sci. Rep. 10 (1), 17. doi: 10.1038/s41598-020-78858-2
EAZA (2019). Standards for the accommodation and care of animals in zoos and aquaria. Available at: http://www.eaza.net/assets/Uploads/Standards-and-policies/Standards-for-the-Accommodation-and-Care-of-Animals-2014.pdf.
Esperón F., García-Párraga D., Belliére E. N., Sánchez-Vizcaíno J. M. (2012). Molecular diagnosis of lobomycosis-like disease in a bottlenose dolphin in captivity. Med. Mycol 50 (1), 106–109. doi: 10.3109/13693786.2011.594100
Fiorito C., Palacios C., Golemba M., Bratanich A., Argüelles M. B., Fazio A., et al. (2015). Identification, molecular and phylogenetic analysis of poxvirus in skin lesions of southern right whale. Dis. Aquat Organ 116 (2), 157–163. doi: 10.3354/dao02918
Fisher S. J., Reeves R. R. (2007). The global trade in live cetaceans: implications for conservation. J. Int. Wildl. Law Policy 8, 4, 315–340. doi: 10.1080/13880290500343624
Flom J., Houk E. J. (1979). Morphologic evidence of poxvirus in “Tattoo” lesions from captive bottlenosed dolphins. J. Wildl Dis. 15 (4), 593–596. doi: 10.7589/0090-3558-15.4.593
Fraser J., Switzer T. (2021). The social value of zoos. Soc. Value Zoos 1, 300. doi: 10.1017/9781108644679
Fury C. A., Reif J. S. (2012). Incidence of poxvirus-like lesions in two estuarine dolphin populations in Australia: links to flood events. Sci. Total Environ. 416, 536–540. doi: 10.1016/j.scitotenv.2011.11.056
Geraci J. R., Hicks B. D., St Aubin D. J. (1979). Dolphin pox: a skin disease of cetaceans. Can. J. Comp. Med. 43 (4), 399–404.
Geraci J. R., Lounsbury V. J. (2005). Marine mammals ashore: a field guide for strandings. 2nd ed (Baltimore, MD: National Aquarium in Baltimore, Inc), 371. Available at: https://books.google.es/books/about/Marine_Mammals_Ashore.html?id=AIwWScWoP6MC&redir_esc=y.
Girones R., Ferrús M. A., Alonso J. L., Rodriguez-Manzano J., Calgua B., de Abreu Corrêa A., et al. (2010). Molecular detection of pathogens in water–the pros and cons of molecular techniques. Water Res. 44 (15), 4325–4339. doi: 10.1016/j.watres.2010.06.030
Gross M. (2015). Can zoos offer more than entertainment? Curr. Biol. 25, 391–394. doi: 10.1016/j.cub.2015.04.056
Gulland F. M. D., Dierauf L. A., Whitman K. L. (2018). “CRC Handbook of marine mammal medicine,” in Broken sound parkway, 3rd ed. Eds. Gulland F. M. D., Dierauf L. A., Whitman K. L. (New York, NY: CRC Press), 63–75 p. Available at: https://lccn.loc.gov/2017024598.
Harlin A. D., Würsig B., Baker C. S., Markowitz T. M. (1999). Skin swabbing for genetic analysis: application to dusky dolphins (Lagenorhynchus obscurus). Mar. Mamm. Sci. 15 (2), 409–425.
Ijsseldijk L. L., Brownlow A. C., Mazzariol S. (2019). Best practice on cetacean post mortem investigation and tissue sampling (Istanbul: Republic of Turkey), 72.
Jiang Y., Lück M., Parsons E. C. M. (2008). Public awareness, education, and marine mammals in captivity. Tour Rev. Int. 11 (3), 237–249. doi: 10.3727/154427207783948829
Kiszka J., Simon-Bouhet B., Charlier F., Pusineri C., Ridoux V. (2010). Individual and group behavioural reactions of small delphinids to remote biopsy sampling. Anim. Welfare 19, 411–417. doi: 10.1017/S0962728600001895
Koch M. S., De Pasquale Da Silva V., Rodrigues Loureiro Bracarense A. P. F., Domit C. (2018). Environmental aspects and diseases related to immunosuppression in cetaceans: a concise review. (Semina: Ciências Agrárias, Londrina, Brasil: Universidade Estadual de Londrina) 39, 2897–2918.
Lanrewaju A. A., Enitan-Folami A. M., Sabiu S., Swalaha F. M. (2022). A review on disinfection methods for inactivation of waterborne viruses. Front. Microbiol. 13, 3622. doi: 10.3389/fmicb.2022.991856
Lauderdale L. K., Mellen J. D., Walsh M. T., Granger D. A., Miller L. J. (2021a). Towards understanding the welfare of cetaceans in accredited zoos and aquariums. PloS One 16 (8), e0255506. doi: 10.1371/journal.pone.0255506
Lauderdale L. K., Samuelson M. M., Xitco M. J. (2021b). Modern applications of operant conditioning through the training of a beaching behaviour with bottlenose dolphins (Tursiops truncatus). J. Zoo Aquarium Res. 9 (2), 81–87. doi: 10.1371/JOURNAL.PONE.0255506
Leamaster B., Ostrowski A. (1988). Vibriosis in captive dolphins. progress fish-culturist. Prog. Fish-Cult. 50, 4, 251–254. doi: 10.1577/1548-8640(1988)050<0251:VICD>2.3.CO;2
Lerner H. (2008). “The concepts of health, well-being and welfare as applied to animals,” in A philosophical analysis of the concepts with the regard to the differences between animals (Linköpings Universitet, Department of Medical and Health Sciences Linköping 2008. Linköping Studies in Arts and Science), 438.
Lima N., Rogers T., Acevedo-Whitehouse K., Brown M. V. (2012). Temporal stability and species specificity in bacteria associated with the bottlenose dolphins respiratory system. Environ. Microbiol. Rep. 4 (1), 89–96. doi: 10.1111/j.1758-2229.2011.00306.x
Luciani L., Piorkowski G., De Lamballerie X., Van Waerebeek K., Van Bressem M. F. (2022). Detection of cetacean poxvirus in Peruvian common bottlenose dolphins (Tursiops truncatus) using a pan-poxvirus PCR. Viruses 14 (9), 4. doi: 10.3390/v14091850
Makecha R. N., Highfill L. E. (2018). Environmental enrichment, marine mammals, and animal welfare: a brief review. Aquat Mamm. 44 (2), 221–230. doi: 10.1578/AM.44.2.2018.221
Melero M., García-Párraga D., Corpa J. M., Ortega J., Rubio-Guerri C., Crespo J. L., et al. (2014). First molecular detection and characterization of herpesvirus and poxvirus in a pacific walrus (Odobenus rosmarus divergens). BMC Vet. Res. 10 (1), 968. doi: 10.1186/s12917-014-0308-2
Miller D. S., Anthony R., Golab G. (2018). Assessing aquatic mammal welfare while assessing differing values and imperfect tradeoffs. Aquat Mamm. 44 (2), 116–141. doi: 10.1578/AM.44.2.2018.116
Miranda R., Escribano N., Casas M., Pino-del-Carpio A., Villarroya A. (2022). The role of zoos and aquariums in a changing world. Annu. Rev. Anim. Biosci. 11 (1), 1–20. doi: 10.1146/annurev-animal-050622-104306
Mouton M., Botha A. (2012). “Cutaneous lesions in Cetaceans: an indicator of ecosystem Status?,” in New approaches to the study of marine mammals. Eds. Aldemaro R., Keith E. (Rijeka, Croatia: In Tech), 232.
Noren D. P., Mocklin J. A. (2012). Review of cetacean biopsy techniques: factors contributing to successful sample collection and physiological and behavioral impacts. Mar. Mammal Sci. 28, 154–199. doi: 10.1111/j.1748-7692.2011.00469.x
Opota O., Jaton K., Greub G. (2015). Microbial diagnosis of bloodstream infection: towards molecular diagnosis directly from blood. Clin. Microbiol. Infect. 21 (4), 323–331. doi: 10.1016/j.cmi.2015.02.005
Palmer C. J., Schroeder P. J., Fujioka R. S., Douglas J. T. (1991). Staphylococcus aureus infection in newly captured pacific bottlenose dolphins (Tursiops truncatus gilli). J. Zoo Wildl Med. 22 (3), 330–338.
Parsons K. M., Durban J. W., Claridge D. E. (2003). Comparing two alternative methods for sampling small cetaceans for molecular analysis. Mar. Mammal Sci. 19 (1), 224–231. doi: 10.1111/j.1748-7692.2003.tb01104.x
Patterson K. (2016). Validating bottlenose dolphins (Tursiops truncatus) body condition score. Available at: https://scholarship.miami.edu/esploro/outputs/report/Validating-bottlenose-dolphins-Tursiops-truncatus-body-condition-score/991031447786102976.
Pettis H. M., Rolland R. M., Hamilton P. K., Brault S., Knowlton A. R., Kraus S. D. (2004). Visual health assessment of north Atlantic right whales (Eubalaena glacialis) using photographs. Can. J. Zool 82 (1), 8–19. doi: 10.1139/z03-207
Powell S. N., Wallen M. M., Bansal S., Mann J. (2018). Epidemiological investigation of tattoo-like skin lesions among bottlenose dolphins in shark bay, Australia. Sci. Total Environ. 630, 774–780. doi: 10.1016/j.scitotenv.2018.02.202
Powell S. N., Wallen M. M., Miketa M. L., Krzyszczyk E., Foroughirad V., Bansal S., et al. (2020). Sociality and tattoo skin disease among bottlenose dolphins in shark bay, Australia. Behav. Ecol. 31 (2), 459–466. doi: 10.1093/beheco/arz207
Raga J. A., Van Waerebeek K., Van Bressem M.-F. (1999). A review of virus infections of cetaceans and the potential impact of morbilliviruses, poxviruses and papillomaviruses on host population dynamics. Dis. Aquat. Org. 83, 53–65.
Rickert D., Simon R., von Fersen L., Baumgartner K., Bertsch T., Kirschbaum C., et al. (2022). Saliva and blood cortisol measurement in bottlenose dolphins (Tursiops truncatus): methodology, application, and limitations. Anim. an Open Access J. MDPI 12 (1), 21. doi: 10.3390/ANI12010022
Ridgway S. H. (1984). Regression of cetacean tattoo lesions concurrent with conversion of precipitin antibody against a poxvirus comparison of dolphin brains view project dolphin biosonar view project. Article J. Am. Veterinary Med. Assoc. 183, 2.
Riggin J., Maldini D. (2010). Photographic case studies of skin conditions in wild-ranging bottlenose dolphin (Tursiops truncatus) calves. J. Mar. Anim. Their Ecol. 3 (1), 5–9.
Robeck T. R., Atkinson S., Brook F. (2008) “Reproduction,” in CRC Handbook of marine mammal medicine second edition, 2nd ed. Eds. Dierauf L. A., Gulland F. M. D. (Boca Raton, Florida: CRC Press), 193–236 Available at: https://www.researchgate.net/publication/263457537_Reproduction_CRC_Handbook_of_Marine_Mammal_Medicine_Second_Edition.
Rodrigues T. C. S., Subramaniam K., Varsani A., McFadden G., Schaefer A. M., Bossart G. D., et al. (2020). Genome characterization of cetaceanpox virus from a managed indo-pacific bottlenose dolphin (Tursiops aduncus). Virus Res. 278, 197861. doi: 10.1016/j.virusres.2020.197861
Rose P. E., Riley L. M. (2022). Expanding the role of the future zoo: wellbeing should become the fifth aim for modern zoos. Front. Psychol. 13. doi: 10.3389/fpsyg.2022.1018722/full
Sacristán C., Catão-Dias J. L., Ewbank A. C., Ferreira-Machado E., Neves E., Santos-Neto E. B., et al. (2018a). Novel and highly sensitive SYBR® green real-time pcr for poxvirus detection in odontocete cetaceans. J. Virol. Methods 259, 45–49. doi: 10.1016/j.jviromet.2018.06.002
Sacristán C., Esperón F., Marigo J., Ewbank A. C., De Carvalho R. R., Groch K. R., et al. (2018b). Molecular identification and microscopic characterization of poxvirus in a Guiana dolphin and a common bottlenose dolphin, Brazil. Dis. Aquat Organ 130 (3), 177–185. doi: 10.3354/dao03271
Salas M., Manteca X. (2016). Assessing welfare in zoo animals: animal-based indicators. Welfare Educ. Centre 4, 2.
Samelius V. (2018). Zoo animal welfare assessment a review of current research and protocols concerning welfare assessment and feasibility of application in a zoo environment (Uppsala: Swedish Univeristy of Agricultural Sciences). Available at: https://stud.epsilon.slu.se/13776/1/Samelius_V_180830.pdf.
Savini V., Marrollo R., Nigro R., Fusella C., Fazii P. (2017). “Skin and soft tissue infections following marine injuries,” in The microbiology of skin tissue, bone and joint infections. Eds. Kon K., Rai M. (Spirito Santo Hospital, Pescara, Italy: Elsevier Inc), 338. doi: 10.1016/B978-0-12-811079-9.00006-9
Schilling A.-K., Mazzamuto M. V., Romeo C. (2022). A review of non-invasive sampling in wildlife disease and health research: what’s new? Animals 12 (13), 1719. doi: 10.3390/ani12131719
Scott E. M., Mann J., Watson-Capps J. J., Sargeant B. L., Connor R. C. (2005). Aggression in bottlenose dolphins: evidence for sexual coercion, male-male competition, and female tolerance through analysis of tooth-rake marks and behaviour. Behaviour. 142 (1), 21–44. doi: 10.1163/1568539053627712
Segura-Göthlin S., Fernández A., Arbelo M., Felipe-Jiménez I., Colom-Rivero A., Almunia J., et al. (2021). The validation of a non-invasive skin sampling device for detecting cetacean poxvirus. Anim 11 (10), 2814. doi: 10.3390/ANI11102814
St Leger J., Raverty S., Mena A. (2018). “Cetacea,” in Pathology of wildlife and zoo animals. Eds. St Leger J., Raverty S., Mena A. (SeaWorld Parks and Entertainment, San Diego, CA, United States; Animal Health Center, Abbottsford, BC, Canada: Elsevier), 533–568.
Terio K. A., McAloose D., St. Leger J. (2018). Pathology of wildlife and zoo animals. 1st edition (Cambridge: Academic Press), 1136 p.
Thurman G., Downes S., Forthergill M., Goodwin N., Hegarty M. (1983). Diagnosis and successful treatment of subacute erysipelas in a captive dolphin. J. S Afr Vet. Assoc. 54 (3), 193–200. doi: 10.1007/s00203-014-1020-0
Ueda K., Sano A., Yamate J., Nakagawa E. I., Kuwamura M., Izawa T., et al. (2013). Two cases of lacaziosis in bottlenose dolphins (Tursiops truncatus) in Japan. Case Rep. Vet. Med. 2013, 9. doi: 10.1155/2013/318548
Van Bressem M. F., Gaspar R., Aznar F. J. (2003). Epidemiology of tattoo skin disease in bottlenose dolphins tursiops truncatus from the sado estuary, Portugal. Dis. Aquat. Organ 56 (2), 171–179. doi: 10.3354/dao056171
Van Bressem M. F., Raga J. A., Di Guardo G., Jepson P. D., Duignan P. J., Siebert U., et al. (2009a). Emerging infectious diseases in cetaceans worldwide and the possible role of environmental stressors. Dis. Aquat. Organisms 85, 225-237. doi: 10.3354/dao02101
Van Bressem M. F., Raga J. A., Guardo Di, Giovanni, Jepson P. D., Duigman P. J., et al. (2008). Emerging and recurring diseases in cetaceans worldwide and the role of environmental stressors. Dis. Aquat. Organ. 2008.
Van Bressem M. F., Van Waerebeek K. (1996). Epidemiology of poxvirus in small cetaceans from the Eastern south pacific. Mar. Mammal Sci. 12 (3), 371–382. doi: 10.1111/j.1748-7692.1996.tb00590.x
Van Bressem M. F., Van Waerebeek K., Aznar F. J., Raga J. A., Jepson P. D., Duignan P., et al. (2009b). Epidemiological pattern of tattoo skin disease: a potential general health indicator for cetaceans. Dis. Aquat Organ 85 (3), 225–237. doi: 10.3354/dao02080
Van Bressem M., Van Waerebeek K., Duignan P. J. (2017). Sex differences in the epidemiology of tattoo skin disease in captive common bottlenose dolphins (Tursiops truncatus): are males more vulnerable than females? bioRxiv, 101915. doi: 10.1101/101915v1
Van Bressem M. F., Van Waerebeek K., Duignan P. J. (2022). Tattoo skin disease in cetacea: a review, with new cases for the northeast pacific. Animals (Semina: Ciências Agrárias Londrina, Brasil) 14 (12), 24. doi: 10.3390/ani12243581
Van Bressem M. F., Van Waerebeek K., Reyes J. C., Dekegel D., Pastoret P. P. (1993). Evidence of poxvirus in dusky dolphin (Lagenorhynchus obscurus) and burmeister’s porpoise (Phocoena spinipinnis) from coastal Peru. J. Wildl Dis. 29 (1), 109–113. doi: 10.7589/0090-3558-29.1.109
Van Cise A. M., Wade P. R., Goertz C. E. C., Burek-Huntington K., Parsons K. M., Clauss T., et al. (2020). Skin microbiome of beluga whales: spatial, temporal, and health-related dynamics. Anim. Microbiome 2 (1), 18. doi: 10.1098/rsos.192046
van Elk N., Siebert U., Desportes G., Anderson K. (2000). “Skin pathology, possibly caused by pox virus, leading to severe health problems in harbor porpoises from European waters,” in 31st International Association for Aquatic Animal Medicine Congress, (New Orleans LA, USA).
Van Waerebeek K., Sequeira M., Williamson C., Sanino G. P., Gallego P., Carmo P. (2006). Live-captures of common bottlenose dolphins tursiops truncatus and unassessed bycatch in Cuban waters: evidence of sustainability found wanting. Lat Am. J. Aquat. Mamm. 5 (1), 39-48. doi: 10.5597/lajam00090
Wells R., Rhinehart H., Hansen L., Sweeney J., Townsend F., Stone R., et al. (2004). Bottlenose dolphins as marine ecosystem sentinels: developing a health monitoring system. Ecohealth. 1 (3), 246–254. doi: 10.1007/s10393-004-0094-6
Whitham J. C., Society C. Z., Wielebnowski N., Zoo O. (2017). “Animal-based welfare monitoring,” in Using keeper ratings as an assessment tool (Brookfield, Illinois: Chicago Zoological Society).
Wilson B., Arnold H., Bearzi G., Fortuna C. M., Gaspar R., Ingram S., et al. (1999). Epidermal diseases in bottlenose dolphins: impacts of natural and anthropogenic factors. Proc. R Soc. B Biol. Sci. 266 (1423), 1077–1083. doi: 10.1098/RSPB.1999.0746
Wolfensohn S., Shotton J., Bowley H., Davies S., Thompson S., Justice W. S. M. (2018). Assessment of welfare in zoo animals: towards optimum quality of life. Anim 8 (7), 110. doi: 10.3390/ani8070110
Yang W.-C., Chou L. S., Chiou H. Y., Hsu P.-C., Chin S.-C. (2015). “Poxvirus infection in a rough-toothed dolphin (Steno bredanensis) stranded in Taiwan: a pathological and molecular study,” in 38th International Association for Aquatic Animal Medicine Congress, (Disney's The Seas at Epcot Orlando, Florida).
Keywords: bottlenose dolphins, cetacean poxvirus, cytology cell sampler, health, PCR, skin lesions, under human care
Citation: Segura-Göthlin S, Fernández A, Arbelo M, Almunia J, von Fersen L, Baumgartner K, Garcés JG, Llanos AG, Felipe-Jiménez I, Colom-Rivero A and Sierra E (2023) Towards understanding host–pathogen dynamics of cetacean poxvirus: attainable approach through the application of a repetitive non-invasive skin sampling in bottlenose dolphins (Tursiops truncatus) under human care. Front. Mar. Sci. 10:1125629. doi: 10.3389/fmars.2023.1125629
Received: 13 January 2023; Accepted: 08 May 2023;
Published: 31 May 2023.
Edited by:
Lusheng Xin, Chinese Academy of Fishery Sciences, ChinaReviewed by:
Salvatore Siciliano, Fundação Oswaldo Cruz (Fiocruz), BrazilCopyright © 2023 Segura-Göthlin, Fernández, Arbelo, Almunia, von Fersen, Baumgartner, Garcés, Llanos, Felipe-Jiménez, Colom-Rivero and Sierra. This is an open-access article distributed under the terms of the Creative Commons Attribution License (CC BY). The use, distribution or reproduction in other forums is permitted, provided the original author(s) and the copyright owner(s) are credited and that the original publication in this journal is cited, in accordance with accepted academic practice. No use, distribution or reproduction is permitted which does not comply with these terms.
*Correspondence: Antonio Fernández, YW50b25pby5mZXJuYW5kZXpAdWxwZ2MuZXM=
Disclaimer: All claims expressed in this article are solely those of the authors and do not necessarily represent those of their affiliated organizations, or those of the publisher, the editors and the reviewers. Any product that may be evaluated in this article or claim that may be made by its manufacturer is not guaranteed or endorsed by the publisher.
Research integrity at Frontiers
Learn more about the work of our research integrity team to safeguard the quality of each article we publish.