- 1Department of Marine Ecological Environment Evaluation, School of Earth Sciences, China University of Geosciences (Wuhan), Wuhan, China
- 2Key Laboratory of Marine Mineral Resources Ministry of Natural Resources, Guangzhou Marine Geological Survey, China Geological Survey, Guangzhou, China
- 3Department of Marine Ecological Environment Evaluation, National Engineering Research Center for Gas Hydrate Exploration and Development, Guangzhou, China
This work aimed to investigate the distribution of benthic foraminiferal assemblages in Houshui and Yangpu Bays, Hainan Island, and assess the relative effects of heavy metals, total organic carbon (TOC), and polycyclic aromatic hydrocarbons (PAHs) on foraminiferal assemblages. A total of 30 sites were sampled and analyzed for benthic foraminiferal assemblages, heavy metals, TOC, and PAHs. The organic matter in the study was derived primarily from algae according to the C/N ratios, and PAHs were generated by petroleum and combustion of petroleum products, as inferred from the diagnostic ratios of PAH isomers. Two-way hierarchical cluster analysis was used to classify the 35 common species into two species groups, and the 30 sites were classified based on their geographical location. CCA was used to investigate the effects of pollution on foraminiferal assemblages. The results showed that the effects of pollution on foraminiferal assemblages were in the order of heavy metals > TOC > PAHs. Furthermore, the species group I, which consisted of agglutinated taxa including Bigenerina nodosaria, Ammobaculites agglutinans, Textularia spp., T. foliacea, Haplophragmoides canariensis, and several tolerant species of hyaline forms, including Ammonia beccarii, Elphidium advenum, E. hispidulum, Quinqueloculina laevigata, and Q. seminula, was found to be positively influenced by heavy metals. In contrast, species group II, primarily composed of porcelaneous taxa such as Massilina laevigata, Spiroloculina communis, and some hyaline taxa including E. crispum and Elphidium spp., was found to be positively influenced by TOC and PAHs. This work suggest that heavy metal pollution is the main factor influencing foraminiferal assemblages in the study area. The observed positive effect of heavy metals on species group I may indicate the presence of metal-tolerant taxa, whereas the positive effect of TOC and PAHs on species group II suggests that these taxa may be more tolerant of organic pollution. The study highlights the potential of specific foraminiferal assemblages as bioindicators for assessing the impact of different types of pollution on marine ecosystems.
1. Introduction
Benthic foraminifera have good potential as indicators of the environment and are widely used to monitor environmental changes and assess the effects of environmental factors on community structure (Murray, 2006). How foraminiferal assemblages change in response to environmental changes and why the change occurs has long been a major objective for ecologists and environmental scientists. Both pollution and natural factors modify benthic foraminiferal assemblages. To what extent a change in foraminiferal assemblages is due to pollution or to natural factors is an unresolved issue because it is impossible to take all environmental factors into account. In contrast, many ecologists prefer to determine the relationship between foraminiferal assemblages and pollution and consider that a positive relationship represents a beneficial effect, while a negative relationship represents a harmful one.
Many kinds of pollutants influence benthic foraminiferal distribution, including heavy metals, organic carbon, toxic organic components, and crude oil (Alve, 1995; Frontalini and Coccioni, 2011). Among pollutants, heavy metals are the most threatening to marine organisms (Teresa Losada Ros et al., 2020) and are thus frequently studied. Overall, the impacts of heavy metals on foraminiferal assemblages include reduced species abundance and diversity, increase in morphological abnormalities, and modified community composition and structure (Alve, 1991; Yanko et al., 1998; Samir, 2000; Debenay et al., 2001; Bergin et al., 2006; Ferraro et al., 2006; Carnahan et al., 2008; Coccioni et al., 2009; Frontalini et al., 2009; Martins et al., 2010; Cosentino et al., 2013; Li et al., 2014; Price et al., 2019). In particular, increasing heavy metal concentrations cause an increase in several species typically tolerant to heavy metals and a decrease in most species that are sensitive. Some tolerant species are distributed worldwide, making it possible to compare the biological effects of pollution around the world. The response of certain species (e.g., Rosalina leei, Nigam et al. (2009); Ammonia species, Le Cadre and Debenay (2006), and Teresa Losada Ros et al. (2020) or an entire assemblage of species (Frontalini et al., 2017) to single heavy metals (Hg, Cu, or Cd) has been confirmed by laboratory culture.
Polycyclic aromatic hydrocarbons (PAHs) are an important kind of anthropogenic organic contaminant. The PAHs are generated mainly from incomplete combustion of fossil fuel and biomass, forest fires, volcano activities, and petroleum seeps (Wang et al., 2007; Liu et al., 2012). The influence of PAHs on benthic foraminifera has been tested in various studies (Armynot du Châtelet et al., 2004; Bergamin et al., 2009; Romano et al., 2009; Vilela et al., 2011; Schintu et al., 2015; Machain-Castillo et al., 2019). A few foraminiferal species, such as Haynesina germanica (Armynot du Châtelet et al., 2004), Cornuspira involvens and Bolivina variabilis (Romano et al., 2009), are considered tolerant to PAHs.
In this study, we investigated the distribution of benthic foraminifera, heavy metals, and PAHs in Houshui and Yangpu Bays, Hainan Island, and established the relationship between benthic foraminiferal assemblages and pollution. The main objectives are to investigate (1) the concentrations, distribution, and sources of heavy metals, TOC, and PAHs; (2) the diversity and species composition of foraminiferal assemblages in two bays and a comparison between them; and (3) the relative effects of heavy metals, TOC, and PAHs on foraminiferal assemblages and individual species.
2. Study area
Hainan Island is located on the northwest side of the South China Sea, to the east side of the Beibu Gulf, and to the south side of the Qiongzhou Strait (Figure 1). The central area covered by mountains and coastal plains and hills surrounding the mountainous area, and rivers and streams originate from the central mountains and flow into the plains (Wang et al., 2006). Three large rivers, Nandu, Changhua, and Wanquan, flow northward, westward, and southeastward into the Qiongzhou Strait, the Beibu Gulf, and the South China Sea. The mean annual runoff of the Nandu River is 296.7×109 m3, and it carries an annual suspended sediment load of 60×104 t/yr. The mean annual runoff of the Changhua River varies greatly, and the annual suspended sediment load is 88–298×104 t/yr. The mean annual runoff of the Wanquan River is 17–83×109 m3, and the annual suspended sediment load is estimated to be 60×104 t/yr. The drainage area of the three rivers accounts for 47% of the entire island.
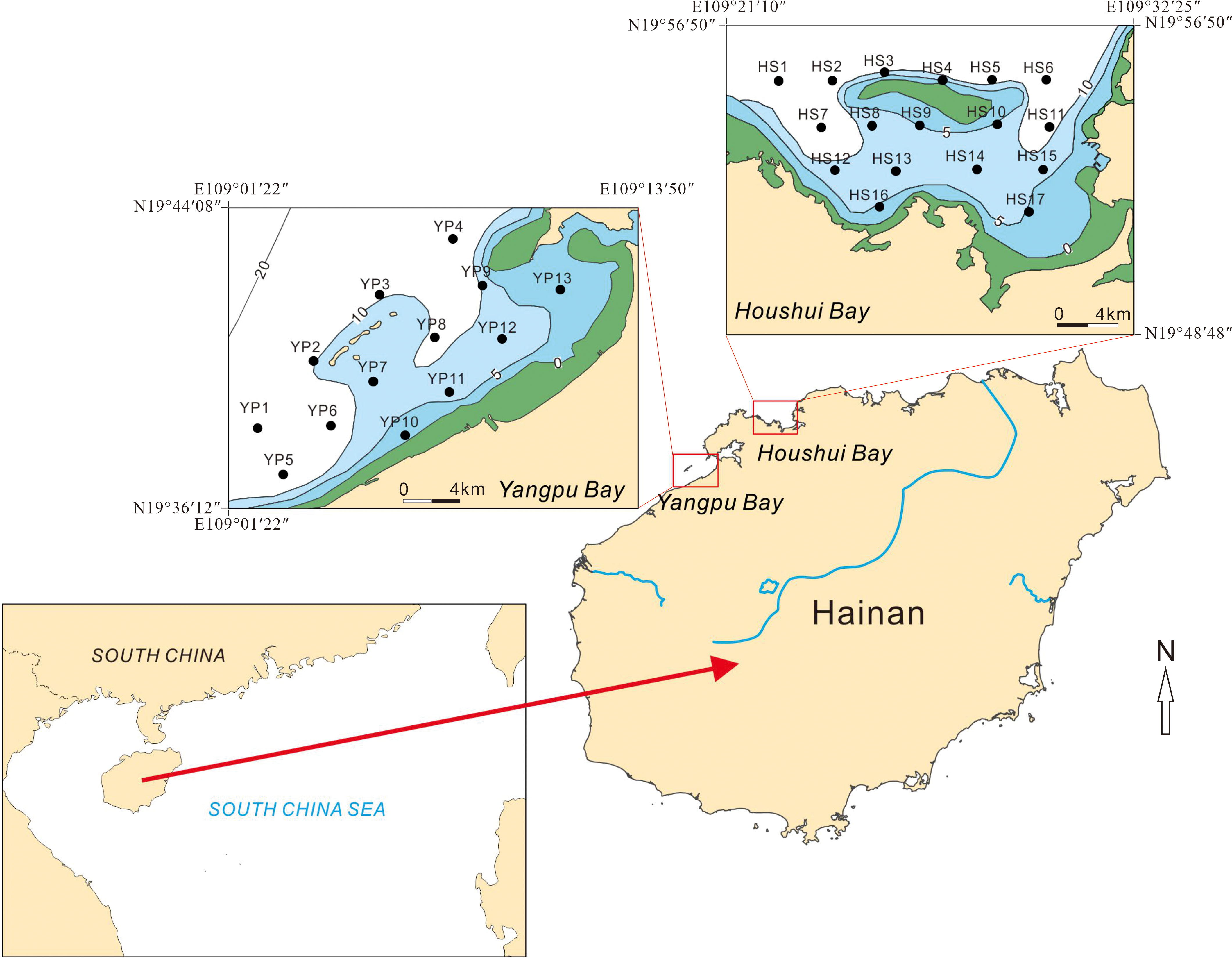
Figure 1 Maps showing Hainan Island, the locations of Yangpu and Houshui Bays, and the distribution of sampling sites in the two bays.
Houshui and Yangpu Bays are situated on the western coasts of Hainan Island. Their geomorphologic conditions, sedimentary properties, and hydrodynamic settings have been summarized by Wang et al. (2006) and The Annals of Chinese Gulfs Compiling Committee (1999) and can be briefly described as follows. Houshui Bay extends from east to west. It is 27 km long and 11.5 km wide and is mainly composed of sandy beaches, rocky coasts, and reef coasts. The bay bottom is flat and mostly covered with silty sand and fine sand. Water depths range from 5 m to 12 m and generally increase from south to north. Diurnal tides exist in the bay, and the annual average tidal range is 1.89 m (maximum of 3.47 m). Yangpu Bay spreads from east to west. It is 26 km long (from east to west) and 16.5 km wide (from south to north). This bay consists of an inner bay and an outer bay, each of which accounts for 35% and 65% of the entire bay. The bathymetry of the bay bottom is complex, and shoals and troughs criss-cross the bottom surface. Sediments are highly heterogeneous, and various types of sediments are found in this bay. Generally, sediments tend to become finer with increasing distance from the coast. Wind-induced waves dominate the bay. The dominant wave directions are ENE and SW, and the maximum wave height is 2.4 m. Diurnal tides occur in the bay, and the annual average tidal range is 1.8 m with a minimum range of 1.47 m and a maximum of 2.18 m.
3. Materials and methods
3.1. Sediment sampling
The current survey was carried out from 19 to 23 April 2019, and 30 surface sediments were collected from Houshui Bay and the outer part of Yangpu Bay (Figure 1). The geographic locations of the sampling sites were recorded by using a global positioning system (GPS). Surface sediments were collected with a grab sampler, the grab was carefully opened in a container, and sediments were laid down in their initial position to avoid sediment disturbance (Debenay et al., 2001). The upper 2 cm layer of undisturbed sediment was sampled for laboratory analyses. Two sets of samples were prepared on the boat: one set was for foraminiferal analysis, and the other was for chemical analyses. For the preparation of samples for foraminiferal analysis, approximately 100 cm3 of sediment was placed into plastic bottles containing 4% neutrally buffered formaldehyde solution (Tonghe Biotechnology, Zhejiang, China) and rose Bengal stain (2 g/l). All samples were kept on ice until transport to the laboratory for further processing.
3.2. Chemical analyses
Samples were dried, ground, and reduced to fine powder for the measurement of heavy metals. To detect the concentrations of Cu, Pb, Zn, Cd, Cr, Mn, Mo, and Co, approximately 0.1 g samples were digested by 2 ml of HNO3 (65%, v/v) and 1 ml of HF (40%, v/v) at 190°C for 48 hours. Then, samples were digested by 1 ml of HNO3 (65%, v/v) and 0.5 ml of HClO4 (70%, v/v) and diluted to 100 ml using Milli-Q water. The concentrations were measured with an inductively coupled plasma mass spectrometer (ICP-MS, Agilent 7900, Agilent Technologies Japan, Ltd., Japan). To detect concentrations of Sb, Hg, and As, samples were digested by aqua regia at a controlled temperature (step 1: 120°C for 2 min; step 2: 150°C for 5 min; step 3: 185°C for 40 min). Then, the concentrations were measured by using an atomic fluorescence spectrophotometer (AFS-930, Beijing Jitian Instruments, Beijing, China). Duplicates, blanks, and standard reference materials (GBW07333 for marine sediment) were used for quality assurance and quality control. The measured concentrations of the reference materials were in satisfactory agreement with the certified values. The detection limits for the heavy metals were as follows: Cu (0.6 mg kg−1), Pb (2 mg kg−1), Zn (1 mg kg−1), Cd (0.09 mg kg−1), Cr (2 mg kg−1), Mn (0.4 mg kg−1), Mo (1 mg kg−1), Co: 0.04 mg kg−1, Sb: 0.01 mg kg−1, Hg: 0.002 mg kg−1, and As: 0.01 mg kg−1. Metal concentrations below the detection limit were replaced with half of the detection limit.
For the measurement of total organic carbon (TOC), samples were freeze-dried, homogenized and acidified with diluted HCl (1%) until all carbonates were removed. TOC and total nitrogen (TN) contents were determined through an Elementar Vario Micro Cube elemental analyzer (Elementar Analysensysteme GmbH, Hanau, Germany).
The 16 USEPA priority PAHs were analyzed, including naphthalene (Nap), acenaphthylene (Acy), acenaphthene (Ac), anthracene (Ant), phenanthrene (Phe), fluorene (Flu), fluoranthene (Fla), pyrene (Pyr), benz[a]anthracene (BaA), chrysene (Chr), benzo(a)-pyrene (BaP), benz[b]fluoranthene (BbF), benz[k]fluoranthene (BkF), dibenz[a,h]anthracene (DahA), indeno[1,2,3-cd]pyrene (InP), and benzo(ghi)-perylene (BgP). Approximately 10 g of sample was weighed and dried over anhydrous sodium sulfate and put into a glass tube with 50 μl of decafluorobiphenyl solution. The glass tube was then placed in a Soxhlet extractor, and 100 ml of acetone/n-hexane mixture (1/1 v/v) was added for extraction. The extract was filtered through a glass fiber membrane and cleaned up by an acetone/n-hexane mixture. After adding 5 ml of n-hexane, the eluent was collected and concentrated to 1 ml and further purified by silica gel column chromatography using anhydrous sodium sulfate and a small amount of dichloromethane. The 16 PAHs were detected using a Shimadzu LC-2010A HPLC system (Shimadzu Co., Kyoto, Japan). PAHs were identified by comparing the retention time of the chromatographic peak between the sample and standard solutions. The contents were calculated from the calibration curve and then converted to ng g−1 in the dry weight of the sample. The detection limits of PAHs were 0.3–0.5 ng g−1. PAH concentrations below the detection limit were set at one-half the detection limit.
3.3. Foraminiferal analysis
Samples were washed through a 63-μm sieve to remove mud and debris and then dried at 40°C in an oven. Foraminifera (rose Bengal-stained) were identified under a Nikon SMZ1000 stereomicroscope. The minimum number of individuals was 150 per sample, while for those samples with fewer than 150 individuals, all individuals were counted. Species classification was based on some previous work in the China Seas (Zheng, 1979; Wang et al., 1988; Lei and Li, 2016) and the taxonomy of the World Modern Foraminifera Database (Hayward et al., 2020).
Diversity indices, e.g., foraminiferal density (individuals per 10 cm3), species richness (number of species), Shannon–Weaver index H(S), and Fisher α index, were calculated using PRIMER v5 software (Plymouth Routines In Multivariate Ecological Research; Clarke and Gorley (2001)).
3.4. Statistical analyses
A two-way (simultaneous R- and Q-modes) hierarchical cluster analysis based on the relative abundance of each species was performed by using PCORD version 5.0 (mJm Software). The Sørensen distance (or similarity) coefficients were used for distance measurements, and clusters were defined according to Ward’s merging criterion. Canonical correspondence analysis (CCA) was performed using CANOCO (v. 5.0) software to determine the variation in species composition explained by environmental variables (Šmilauer and Lepš, 2014). To eliminate the effects of stochastic variations, species abundance was ln(abundance + 1)-transformed prior to the CCA analysis (Morey et al., 2005), and environmental variables (heavy metals, TOC, and PAHs) were standardized to a mean of zero and standard deviation of unity.
4. Results
4.1. Heavy metal concentration and spatial distribution
A total of 11 heavy metals (Cu, Pb, Zn, Cd, Cr, Mn, Mo, Co, Sb, Hg, and As) were measured in 30 sediments from Houshui (17 sites) and Yangpu (13 sites) Bays. Table 1 highlights the ranges and average concentrations of these elements. In Houshui Bay, the average concentrations were Cu, 16.9 mg kg−1; Pb, 28.1 mg kg−1; Zn, 85.3 mg kg−1; Cd, 0.082 mg kg−1; Cr, 63.6 mg kg−1; Mn, 601 mg kg−1; Mo, 32.1 mg kg−1; Co, 13.5 mg kg−1; Sb, 0.32 mg kg−1; Hg, 0.097 mg kg−1; and As, 20 mg kg−1. In Yangpu Bay, the average concentrations were Cu, 7 mg kg−1; Pb, 23.9 mg kg−1; Zn, 59 mg kg−1; Cd, 0.04 mg kg−1; Cr, 39.1 mg kg−1; Mn, 494 mg kg−1; Mo, 22.4 mg kg−1; Co, 10 mg kg−1; Sb, 0.04 mg kg−1; Hg, 0.044 mg kg−1; and As, 15.2 mg kg−1. Obviously, heavy metal concentrations in Houshui Bay were higher than those in Yangpu Bay; in particular, the average concentrations of Cu, Cd, Sb, and Hg in Houshui Bay were more than twice those in Yangpu Bay. Compared with previous investigations of Cu, Pb, Zn, Cd, Cr, Hg, and As in the eastern and western continental shelves of Hainan Island (Hu et al., 2013; Xu et al., 2015), the average concentrations of Cu, Pb, Zn, Cd, Cr, and Hg were at the same levels in Houshui Bay, while they were lower in Yangpu Bay. The As concentration in the two bays was remarkably higher than its levels in the shelves.
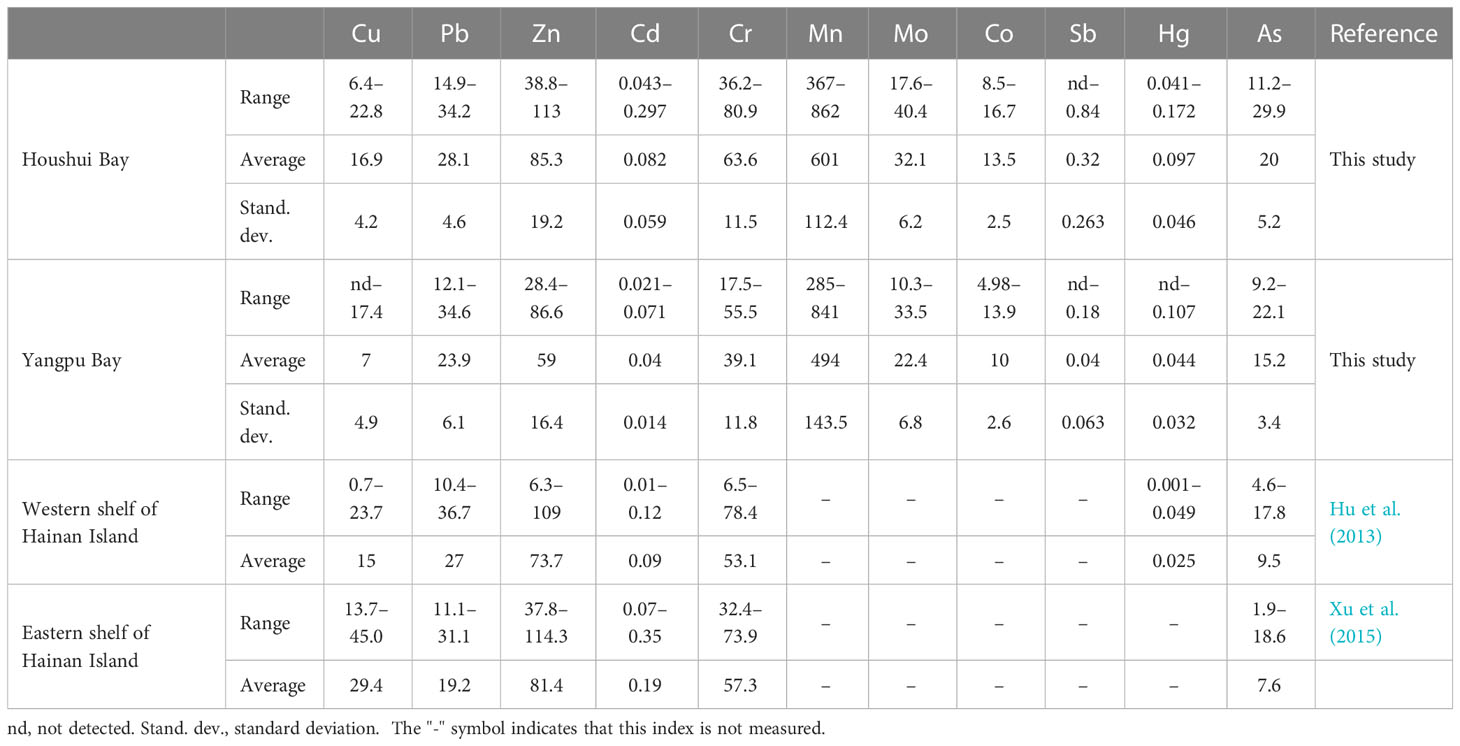
Table 1 Summary of heavy metal concentrations in Yangpu and Houshui Bays and comparisons with river and coastal sediments of Hainan Island, unit: mg kg−1.
The spatial patterns of heavy metals are shown in Figure 2, and all metals exhibited a similar distribution pattern. In Houshui Bay, higher concentrations were observed in the northwest and southeast areas, and relatively lower concentrations were observed in the central area; in Yangpu Bay, heavy metal concentrations gradually increased from northeast to southwest.
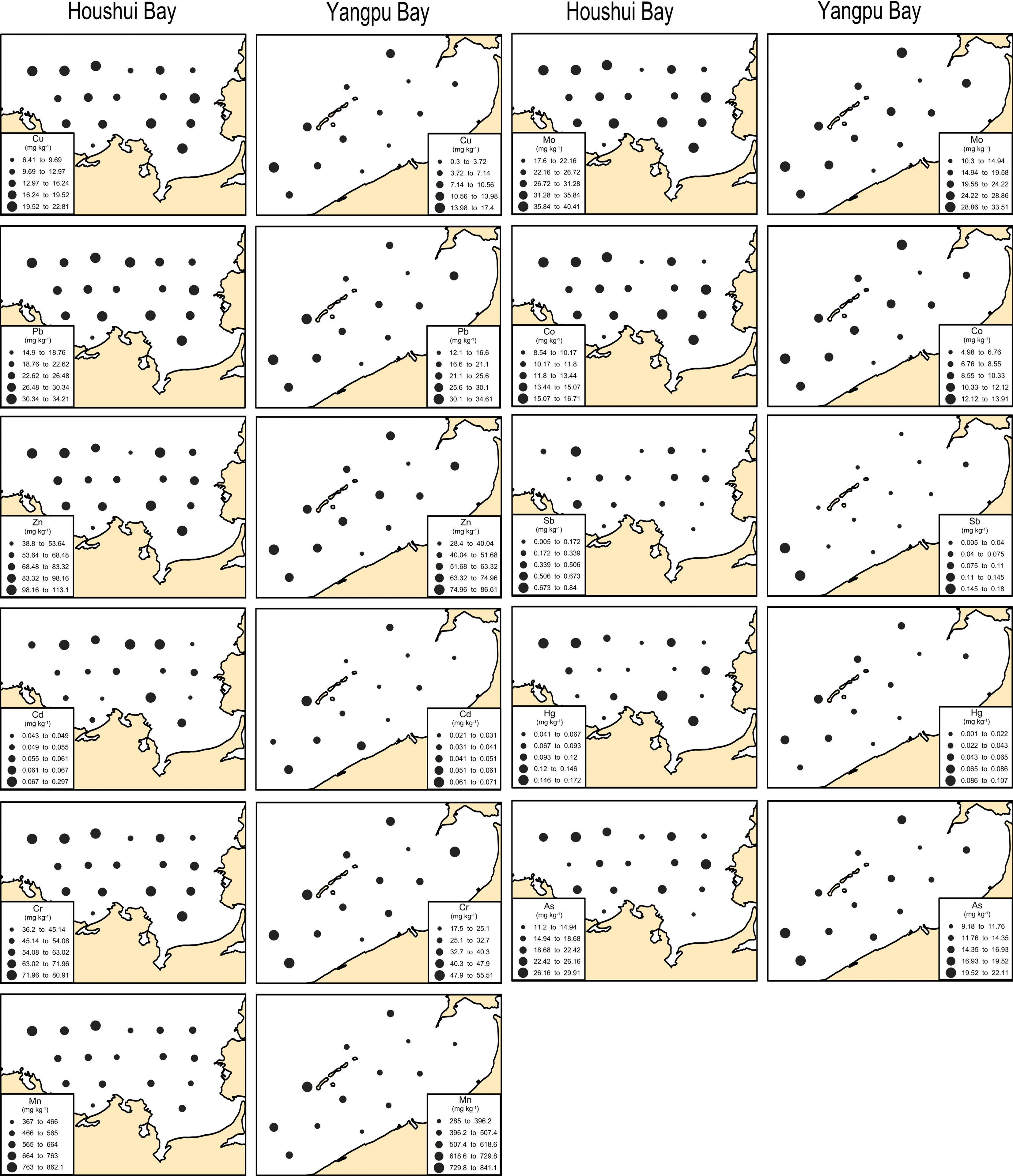
Figure 2 Spatial distributions of heavy metals (Cu, Pb, Zn, Cd, Cr, Mn, Mo, Co, Sb, Hg, and As) in Yangpu and Houshui Bays.
4.2. Concentration of TOC and PAHs and spatial distribution
In total, the 30 sediment samples were measured to determine the content of TOC. The TOC content was higher in Houshui Bay (ranging from 0.06% to 0.73%, mean 0.45) and relatively lower in Yangpu Bay (ranging from 0.05% to 0.55%, mean 0.32). The spatial pattern of the TOC content was similar to that of heavy metals: in Houshui Bay, the TOC content reached its highest level in the northwest and southeast areas, and the lowest content was found in the central area; in Yanpu Bay, the TOC content roughly increased from northeast to southwest (Figure 3).
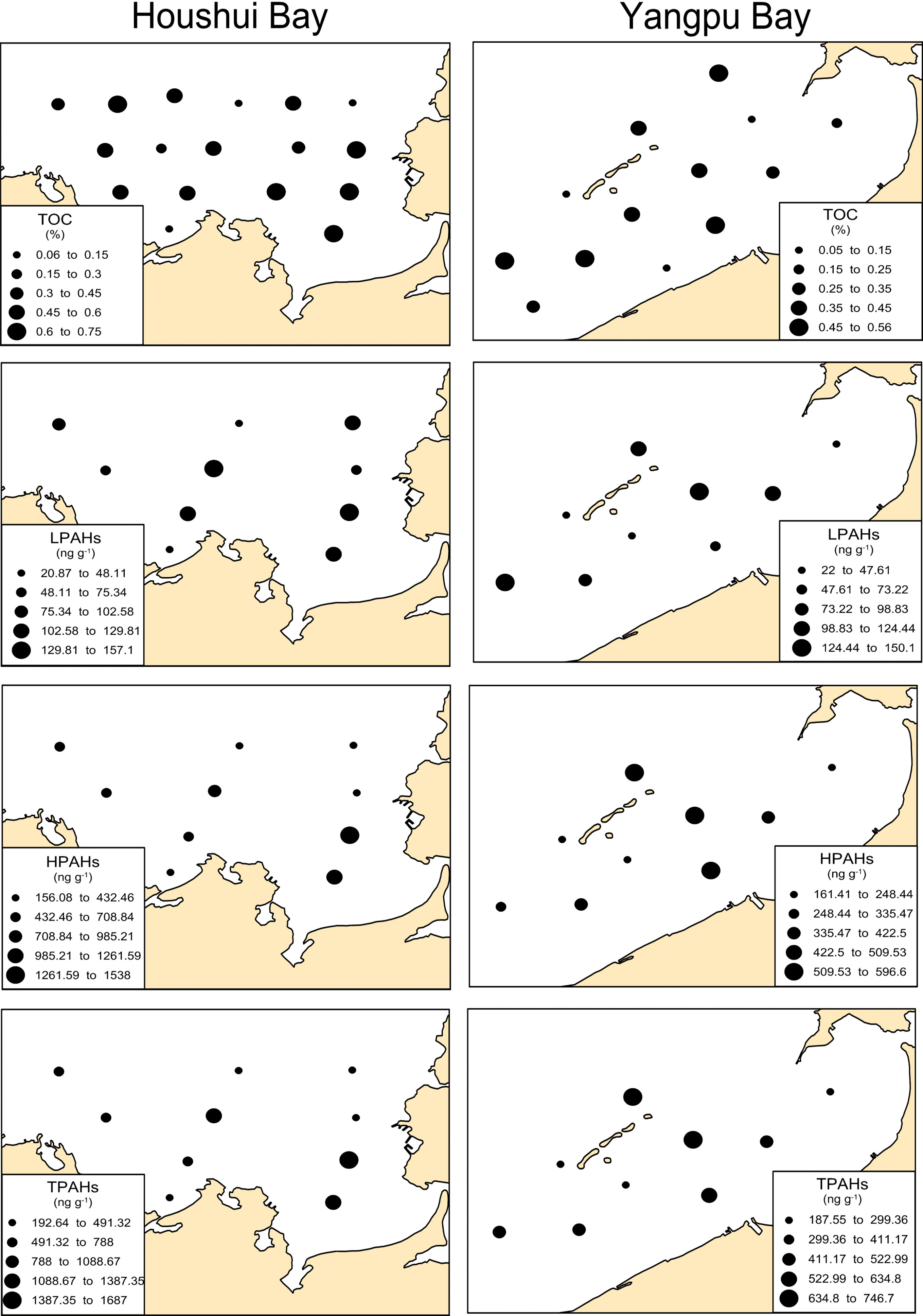
Figure 3 Spatial distributions of total organic carbon (TOC), low-molecular weight PAHs (LPAHs), high-molecular weight PAHs (HPAHs), and total PAHs (TPAHs) in Yangpu and Houshui Bays.
Sixteen PAHs were detected in 19 sediments from the bays of Houshui (10 sites) and Yangpu (9 sites). The concentrations of 18 PAHs (the measured 16 PAHs and two other PAHs) in sediments of the two bays and river and coastal sediments of Hainan Island are summarized in Table 2. A comparison of the average concentrations of TPAHs in different regions of Hainan Island showed a descending order of Houshui Bay, Yangpu Bay, rivers, and offshore areas. In particular, the concentrations of Ace, BaA, BbF, BkF, and BgP were remarkably higher in the two bays than in rivers and offshore areas.
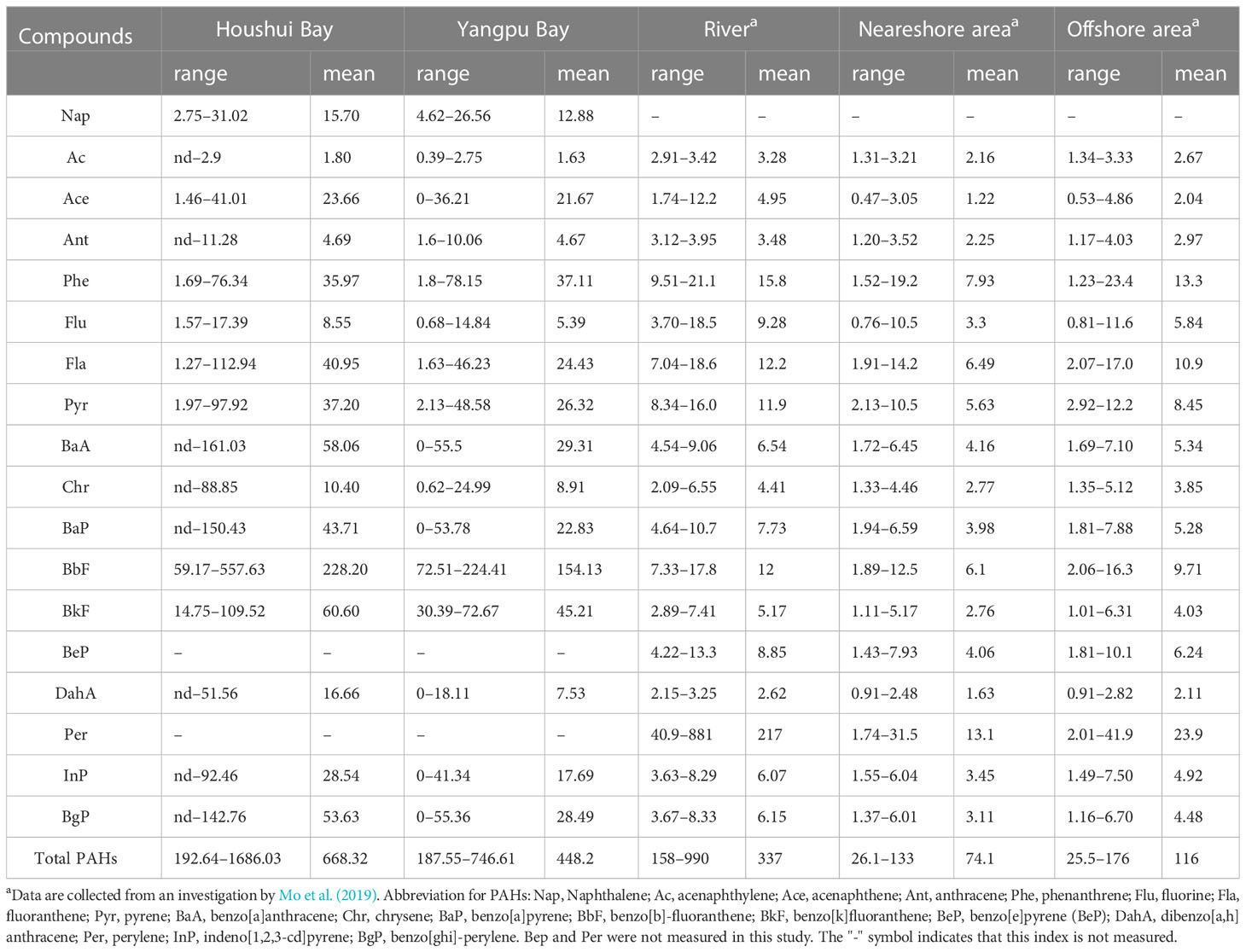
Table 2 Summary of the concentrations of PAHs in Yangpu and Houshui Bays and comparisons with coastal sediments of Hainan Island, unit: ng g−1.
Spatial distributions of low-molecular weight PAHs (LPAHs, 2- to 3-ring PAHs), high-molecular weight PAHs (HPAHs, 4- to 6-ring PAHs), and TPAHs are presented in Figure 3, showing a very similar pattern among them. In Houshui Bay, the concentrations of LPAHs, HPAHs, and TPAHs tended to increase from northwest to southeast; in Yangpu Bay, their concentrations were higher in the central part than in the northeast and southwest areas.
4.3. Benthic foraminiferal community structure and diversity
Quantitative analyses of benthic foraminifera revealed 127 species belonging to 59 genera and 38 families. Among these species, 112 were found in Houshui Bay (17 sites), and 87 were found in Yangpu Bay (13 sites). Thirty-five species exhibited a relative abundance higher than 3% at least three sites and were thus considered commonly occurring species (see Table 3). The foraminiferal communities in both bays were dominated by hyaline-perforate and porcelaneous taxa, which accounted for 47.7% and 50.1% of the average percentages in Yangpu Bay and 48.5% and 37.5% in Houshui Bay, respectively (Figure 4). Table 4 lists the 14 most abundant species (average percentage > 2%) in these two taxa: 7 porcelaneous species (Adelosina colomi, Adelosina longirostra, Massilina laevigata, Quinqueloculina laevigata, Quinqueloculina seminula, Quinqueloculina spp., and Spiroloculina communis) and 7 hyaline species (Ammonia beccarii, Amphistegina exquisite, Amphistegina sp., Elphidium advenum, Elphidium crispum, Elphidium spp., Nonion commune, and Rotalidium annectens). Among these, the dominant species of porcelaneous types were Massilina laevigata, Adelosina laevigata, Quinqueloculina spp., Spiroloculina communis, which occured mainly closer to the coast in southeast of Yangpu Bay and central to outer region of Houshui Bay (Figure 5). The dominant species of hyaline-perforate types were Elphidium crispum, Amphistegina exquisita, Amphistegina sp., Rotalidium annectens, which occured mainly occur in outer of Yangpu Bay. But in Houshui Bay, the dominant hyaline-perforate species were different from those in Yangpu Bay, mainly Elphidium advenum, Rotalidium annectens, and Ammonia beccarii. Elphidium advenum were the most widely distributed and dominant in almost the whole sites, Rotalidium annectens was significantly dominated in the outer bay area, while Ammonia beccarii was also widely distributed but less abundant than the first two in terms of individual stations (Figure 6).
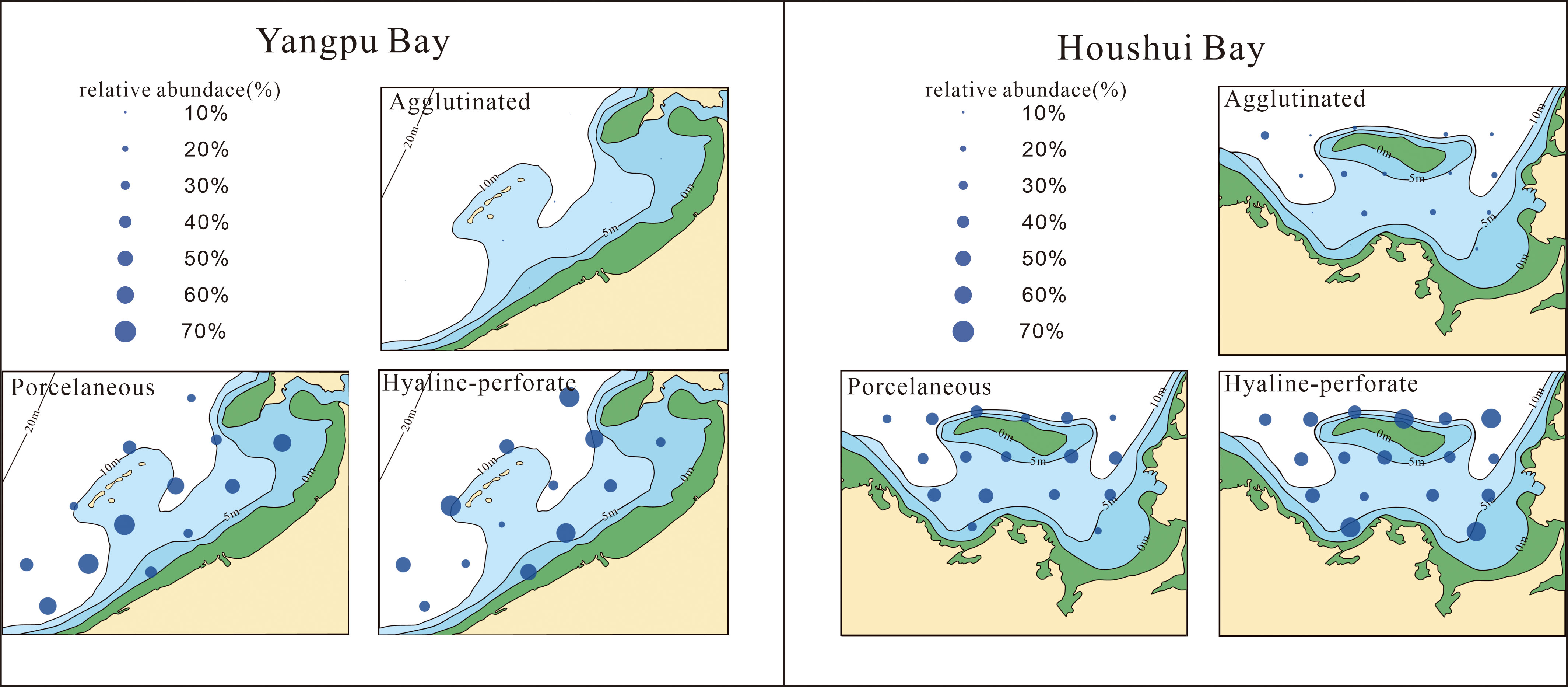
Figure 4 Distribution of agglutinated, porcelaneous and hyaline-perforate taxa in Yangpu and Houshui Bays.
The agglutinated foraminifera were much less diverse and less abundant in the two bays. In Houshui Bay, the agglutinated taxa contribute 2.1% to 28% to the total assemblage at each site, accounting for 14% of the average percentage in relative abundance. In Yangpu Bay, the agglutinated taxa were extremely poor and only 0.1% to 3.5% at each site, accounting for 2.2% of the average percentage in relative abundance. Only 5 species listed in Table 4 were more than 1% of average percentage, including Bigenerina nodosaria, Ammobaculites agglutinans, Textularia spp., Textularia foliacea, Haplophragmoides canariensis, which were significantly dominated in central and outer region of Houshui Bay than in Yangpu Bay (Figure 7).
The average percentages of the dominant species were different in the two bays, and the dominance of the dominant species was stronger in Yangpu Bay than in Houshui Bay (Figures 5–7).
The diversity indices showed that species richness, Shannon–Weaver index (H(S)) and Fisher α index were similar in the two bays (3.2 and 14 in Houshui Bay, 3.1 and 12 in Yangpu Bay, respectively) whereas foraminiferal density was much lower in Yangpu Bay (210 per 10cm2) than in Houshui Bay (48 per 10cm2). The spatial patterns of these indices are shown in Figure 8, and all shared a similar pattern. In Houshui Bay, higher indices were found in the central area, and relatively lower indices occurred in the northwest and southeast areas; in Yangpu Bay, these indices reached their maximum near the coast and decreased with distance away.
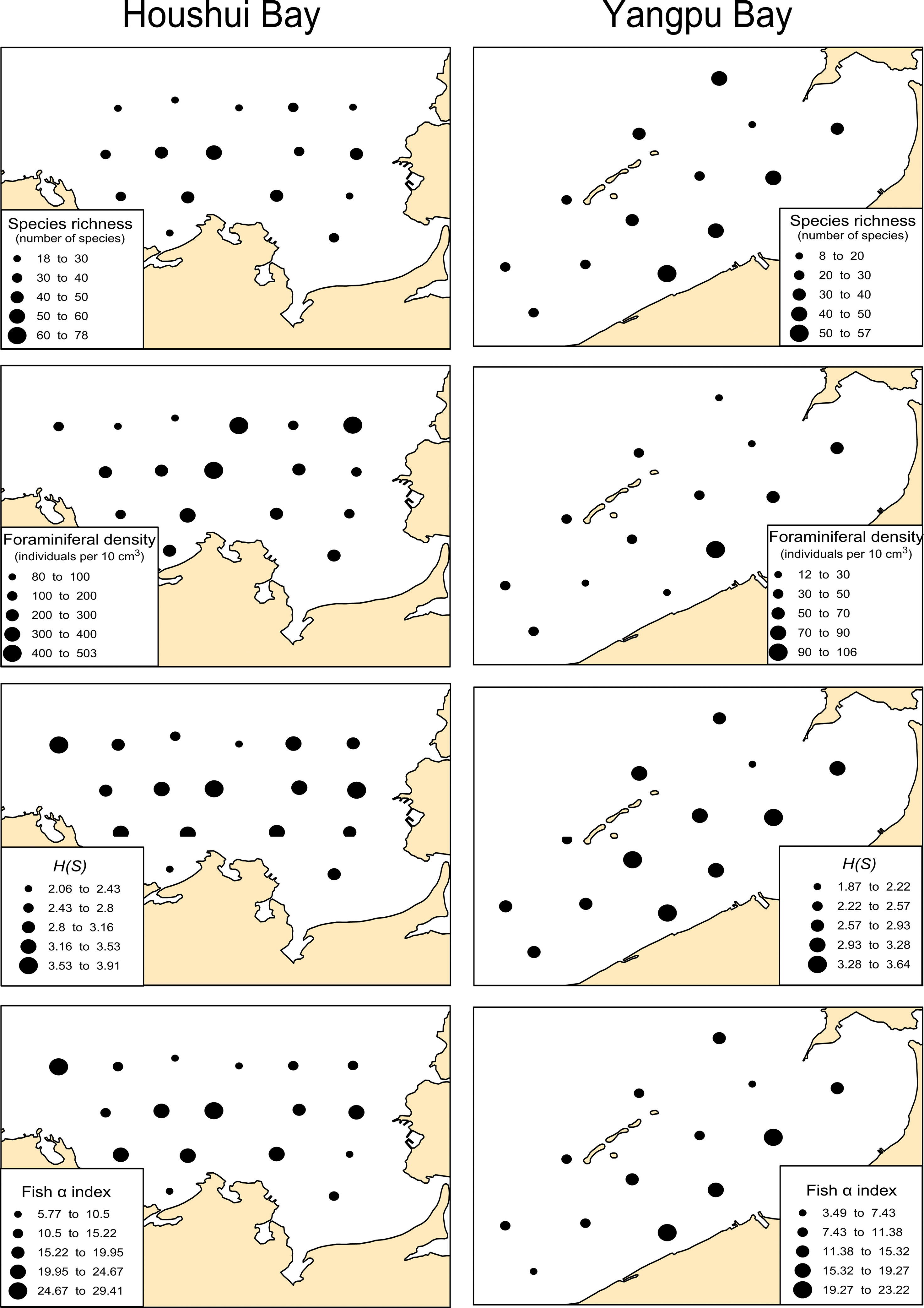
Figure 8 Spatial distributions of diversity indices, species richness, abundance, Shannon–Weaver Index (H(S)) and Fisher α index in Yangpu and Houshui Bays.
4.4. Cluster analysis
All sites and the 35 common species were clustered into two groups by using two-way hierarchical cluster analysis (Figure 9). Sites were roughly classified according to their locations (the bays), which revealed that foraminiferal assemblages within a bay were more similar to each other than to those from different bays. Two species groups (I and II) were divided, each of which was dominated by certain abundant species. For example, R. annectens, Q. laevigata, Q. seminula, A. beccarii, and E. advenum were assigned to group I, and M. laevigata, S. communis, E. crispum, and Elphidium spp. belonged to group II. The agglutinated species Bigenerina nodosaria, Ammobaculites agglutinans, Textularia spp., Textularia foliacea, and Haplophragmoides canariensis were clustered into group I, and most porcelaneous taxa (e.g., Massilina laevigata, Spiroloculina communis) and some hyaline taxa (E. crispum, and Elphidium spp.) were found in group II, while none of agglutinated forms were found in group II. A heat map of the relative abundance and distribution of individual species demonstrated that group I tended to be more abundant in Houshui Bay and that group II was richer in Yangpu Bay (Figure 9B).
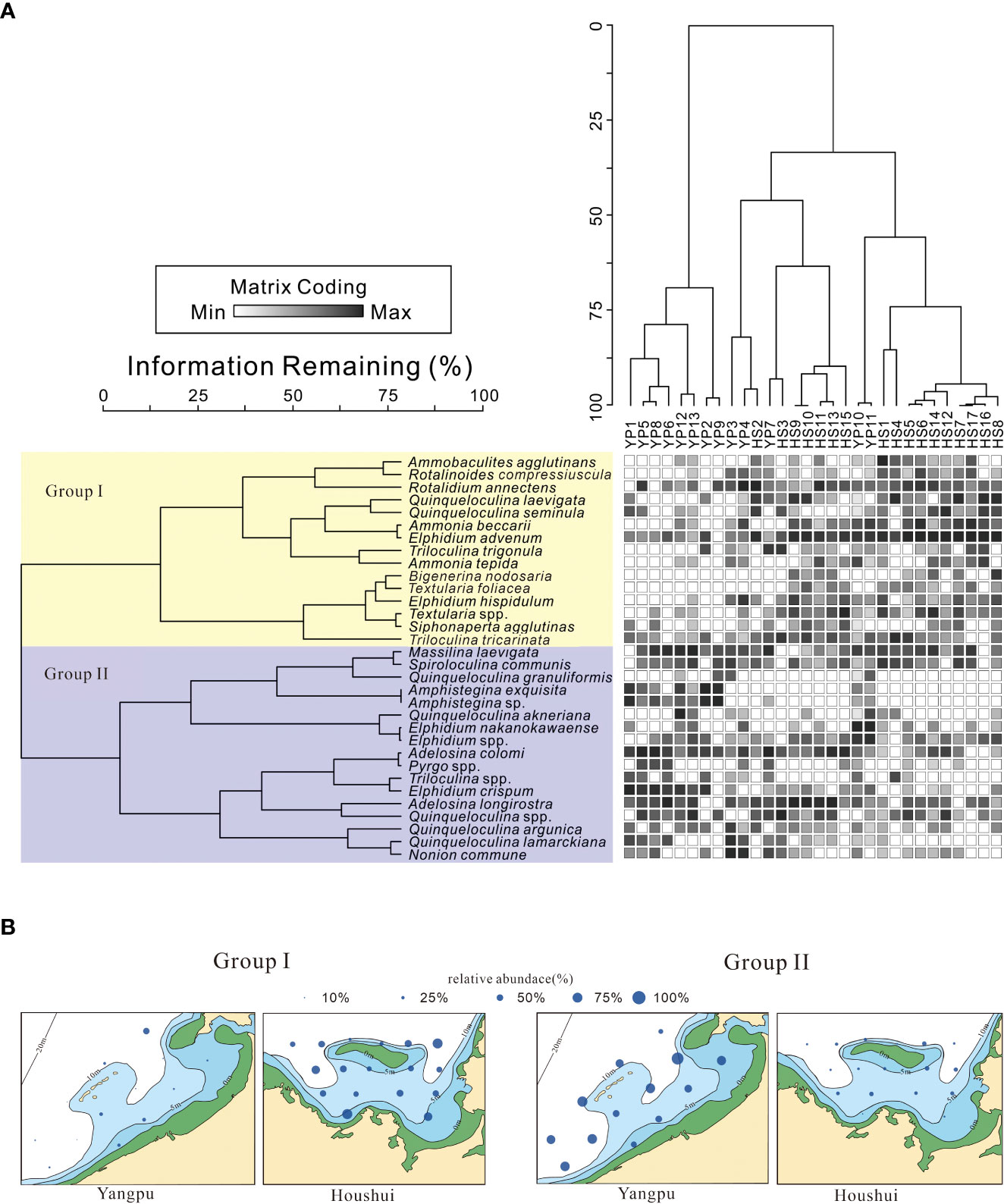
Figure 9 (A) - A two-way hierarchical cluster analysis of foraminiferal assemblages in Yangpu and Houshui Bays. The Q-mode analysis divides sites into two groups, each corresponding to a single bay. Two species groups were classified by R-mode analysis. A heat map of the relative abundance of individual species is also shown in the map; (B) - The relative abundance distribution of Group I and Group II (divided by Q-mode analysis) in Yangpu and Houshui Bays.
4.5. Canonical correspondence analysis
CCA was applied to reveal the relationship of sites/species to heavy metals (Cu, Pb, Zn, Cd, Cr, Mn, Mo, Co, Sb, Hg, As), TOC, and PAHs. Regarding the different generation processes of HPAHs and LPAHs (Liu et al., 2000), we evaluated their effects separately. To eliminate the adverse influence of rare species (Marchant, 2002), only the 35 common species were used for the CCA analysis. Four axes were identified, and the first two together explained 41% of the variation in foraminiferal assemblages (Table 5). The first axis explained a larger fraction (27.7%) of the total variation, whereas the second axis explained a smaller fraction (13.3%). The variation in the assemblages can be explained by the following environmental variables: Cr (13.8%) > Pb (10%) > Mn (9.9%) > Cd (8.4%) > Mo (8.9%) > Cu (4.8%) > Hg (4.3%) > TOC (3.5%) > Co (4.2%) > Zn (4.1%) > As (3.9%) > HPAHs (3.4%) > LPAHs (3.3%) > Sb (2.2%). Overall, heavy metals had the largest effects on foraminiferal assemblages, followed by TOC, while the effects of PAHs were lower.
Figure 10 shows the positioning of sites and species projected on the CCA biplots, where the arrows represent gradient directions of environmental variables. Along the first axis of the site-environment plot (Figure 10A), the sites of Houshui Bay were separated from those of Yangpu Bay. The former appeared on the left side, and the latter appeared on the right side. In the species-environment plot (Figure 10B), the 35 common species were divided into two groups, which was consistent with the cluster of species (Figure 9), and the distribution of the two groups were also similarly in two bays (Figure 10C). Members of group I were closely located at the upper left, while members of group II were scattered on the right half of the plot.
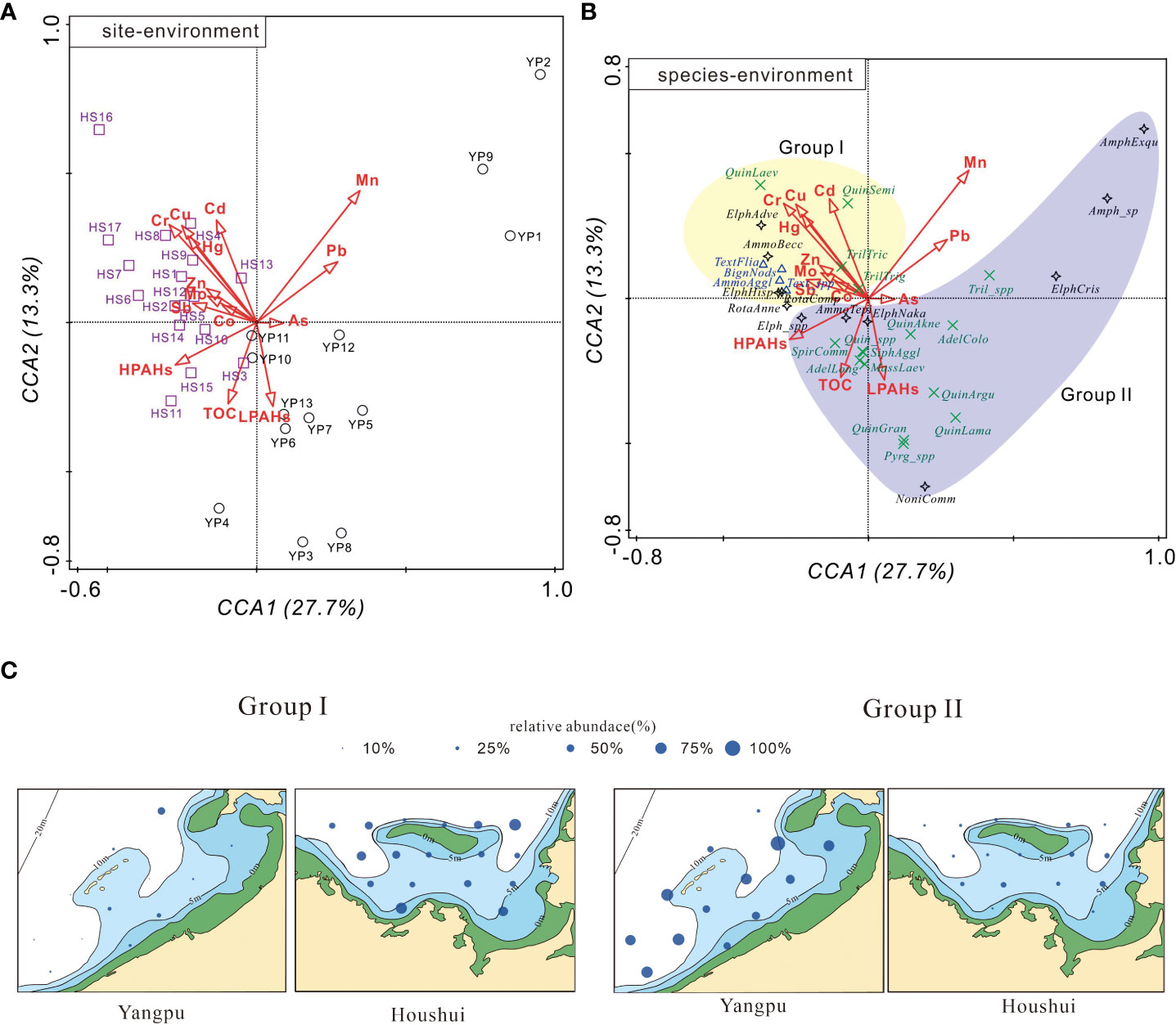
Figure 10 (A) Site-environment and (B) species-environment biplots (the first two axes) of the CCA outputs. The two bays, Yangpu (YPW) and Houshui (HSW), and three wall structure types, agglutinated, porcelaneous, and perforate, are distinguished by different colored symbols, and species belonging to a group are bounded by solid circles. Species names are abbreviated as in Table 2. (C) The relative abundance distribution of Group I and Group II (divided by CCA analysis) in Yangpu and Houshui Bays.
The relationship of site/species to explanatory variables can be inferred from the projection of the sites/species points onto arrows of the environmental variables (Šmilauer and Lepš, 2014). As shown in Figure 10A, most sites from Houshui Bay were positively related to Cu, Zn, Cd, Cr, Mo, Co, Sb, and Hg, and most sites from Yangpu Bay were positively associated with HPAHs, LPAHs, and TOC. In Figure 10B, a positive relationship existed between species group I and heavy metals such as Cu, Zn, Cd, Cr, Mo, Co, Sb, and Hg and between species group II and HPAHs, LPAHs, and TOC. These two groups had an insignificant correlation with Mn, Pb, and As.
5. Discussion
5.1. Distribution of heavy metals, TOC, and PAHs
A previous study of a sediment core from the northern South China Sea (near Hainan Island) indicated that concentrations of heavy metals (e.g., As, Pb, Cu, and Mo) are significantly increased by 2–3 times after 1800 cal yr B.P., and human activities contribute largely to the accumulation of heavy metals (Wan et al., 2015). To date, marine culture, port development, shipping, and tourism have already become pillar industries in Hainan (The Annals of Chinese Gulfs Compiling Committee, 1999). The release of heavy metals into the island’s coastal areas can be attributed to industrial activities, agricultural processes, domestic wastes, and vehicle emissions (Hu et al., 2013). A previous survey revealed that industries such as petrochemical products, paper manufacturing, metal smelting, and oil refining surrounding Yangpu Bay discharge industrial wastes, such as Pb, Cr, and other toxic substances, into the nearshore area (He et al., 2014). Natural processes also play an important role in the enrichment of heavy metals in the coastal areas of Hainan Island (Xu et al., 2015). Rock fragments and mineral debris, which originate from weathering of rocks in the central mountain range of the island, are delivered to river networks and ultimately transported to coastal environments (bays, estuaries, and coastal zones).
The source of sedimentary organic matter can be identified from C/N values. Fresh organic matter produced by microalgae has C/N values that are usually between 4 and 10, whereas organic matter from vascular land plants commonly has C/N ratios of 20 and greater (Meyers, 1994). The C/N values in the two bays ranged from 5.2 to 8.1, indicating that organic matter was derived primarily from algae.
PAHs in marine environments can originate from accidental oil spills, offshore production, discharge from routine tanker operations, and municipal and urban runoff (Dachs et al., 1997; Zakaria et al., 2002). Potential sources of PAHs can be inferred from diagnostic ratios of PAH isomers, for example, the proportions of “kinetic” PAHs (less stable) versus their “thermodynamic” isomers (more stable) (Yunker et al., 2002). Three diagnostic ratios were used in this study, including BaA/(BaA+Chr), InP/(InP+BgP), and Fl/(Fl+Py). These ratios varied between 0.26 and 1, between 0.1 and 0.62, and between 0.1 and 0.5, respectively. According to Viñas et al. (2010), a BaA/(BaA+Chr) ratio < 0.2 indicates petroleum, 0.2–0.35 indicates petroleum and combustion (mixed sources), and > 0.35 indicates combustion; InP/(Inp+BgP) < 0.2 petroleum, 0.2–0.5 indicates petroleum combustion, and > 0.5 indicates combustion of coal, grasses and wood; and Fl/(Fl+Py) < 0.4 indicates petroleum, 0.4–0.5 indicates petroleum combustion, and > 0.5 indicates combustion of coal, grasses and wood. As shown in the scatter diagrams (Figures 11A, B), PAHs were generated primarily by petroleum and combustion of petroleum products. This result is consistent with the previous investigation of PAHs in seawater and sediment from Hainan Island, which was performed by Xiang et al. (2018). Considering that fishing, shipping, and tourism have developed rapidly in recent years in Hainan, we infer that commercial shipping and tourism lead to enhanced concentrations of PAHs in the sediments.
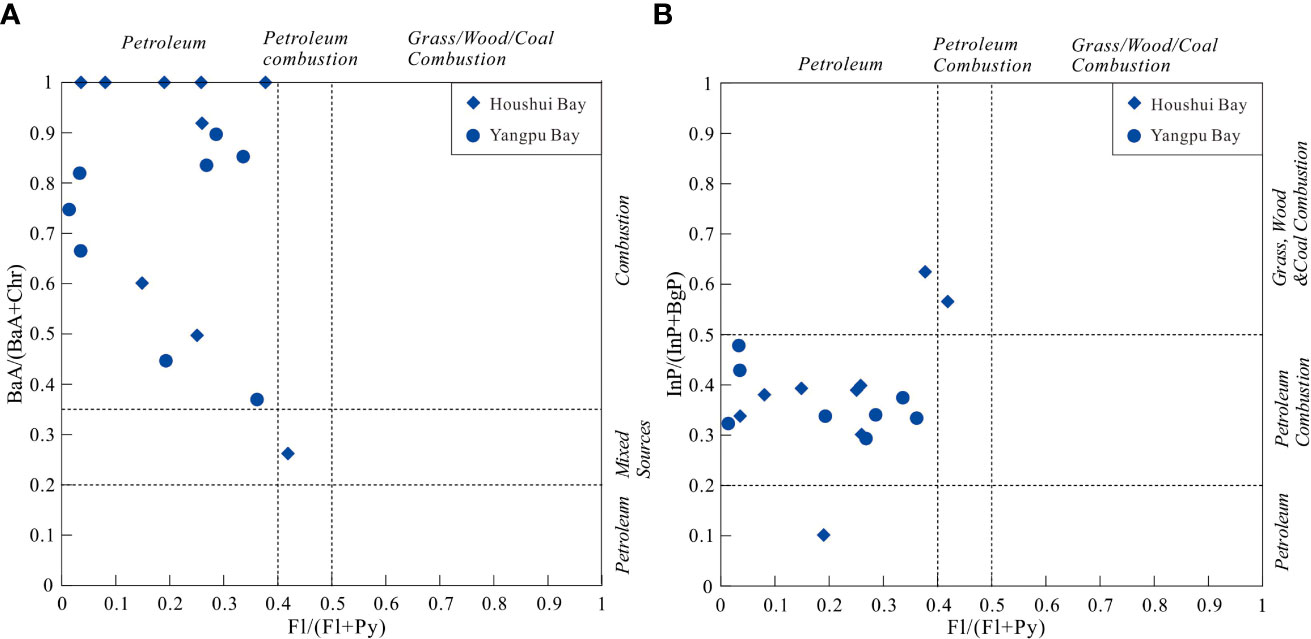
Figure 11 Scatter diagrams for the ratio (A) BaA/(BaA + Chr) and (B) InP/(InP+ BgP) vs. Fl/(Fl + Py).
5.2. Effects of heavy metals, TOC, and PAHs on benthic foraminifera
The present study used CCA to assess the effects of heavy metals, TOC, and PAHs on species distribution and habitats. Sites located in Yangpu and Houshui Bays were separated by the first CCA axis (Figure 10A), showing spatial differences in species composition (also indicated by the heat map of species abundance). Two species groups, divided by cluster analysis, were also identified by the ordination of species on CCA (Figure 10B). With respect to the species-environment relationship, group I was positively related to heavy metals (Cu, Zn, Cd, Cr, Mo, Co, Sb, and Hg), while group II had a positive correlation with HPAHs, LPAHs, and TOC. These relationships provide a means to explain species’ tolerance to different pollutants.
The tolerance and sensitivity of foraminiferal species to heavy metals have been widely discussed. It is known that Ammonia beccarii and A. tepida, the two most frequently observed nearshore species, are tolerant to heavy metals and widely accepted as indicators for heavy metals worldwide (Armynot du Châtelet et al., 2004; Le Cadre and Debenay, 2006; Frontalini and Coccioni, 2008; Debenay et al., 2009; Donnici et al., 2012; Barras et al., 2014; Li et al., 2015). However, Jorissen et al. (2018) considered A. beccarii to be sensitive to pollution. In this study, A. beccarii was strongly and positively related to heavy metals, while the relationship between A. tepida and heavy metals was relatively weak. The genus Elphidium is also abundant and widespread in marginal marine environments (Sen Gupta, 1999). Ammonia−Elphidium assemblages sometimes dominate in polluted environments [e.g., Vilela et al. (2011)]. But the effect of heavy metals on the Elphidium group is less clearly defined and may be positive, null, or negative (Bergin et al., 2006; Romano et al., 2009; Aloulou et al., 2012). Here, only two members of Elphidium, Elphidium advenum and E. hispidulum, had a positive correlation with heavy metals, whereas other members exhibited negative correlations.
Benthic agglutinated Textulariida appeared to respond positively to heavy metals, as shown in the species-environment biplot (Figure 10B). Unfortunately, the interaction between agglutinated foraminifera and heavy metals is rarely reported in the literature. It is known that agglutinated taxa tolerate higher freshwater flux, higher sediment and organic carbon flux, and lower oxygen availability than their calcareous counterparts (Jones, 2013). This study provides a case in which agglutinated foraminifera are somewhat tolerant to heavy metals, and a detailed understanding of their tolerance requires further investigation.
Porcelaneous foraminifera prefer natural, oligotrophic, unpolluted environments and cannot tolerate heavy metals (Elshanawany et al., 2011; Cosentino et al., 2013; Jorissen et al., 2018); this is roughly in accordance with the present study showing that most porcelaneous foraminifera were negatively related to heavy metals (see Figure 10B). The exception is that two abundant species, Quinqueloculina laevigata and Q. seminula, exhibited a positive correlation with heavy metals, but a previous study in a polluted lagoon reflected a negative correlation (Frontalini et al., 2009). Perhaps the two species are flexible to adapt to the surrounding environment and change their autecological requirements to respond to environmental stress (Zettler et al., 2013; Bouchet et al., 2018).
The benefits and adverse effects of organic matter on benthic foraminifera are well documented (Alve, 1991; Armynot du Châtelet et al., 2009; Armynot du Châtelet et al., 2018): organic matter can serve as a food source for benthic foraminifera, but when its content exceeds the optimum value, the influence becomes adverse, mainly due to oxygen depletion under nutrient-enriched conditions. Thus, whether organic matter is beneficial or harmful is highly dependent on its content. In addition to the amount, the kind and quality of organic matter modifies foraminiferal assemblages (Murray, 2006). The calculated C/N ratios suggested that algae supplied organic matter to sediments, while this labile organic matter was bioavailable to benthic foraminifera (Martins et al., 2015). This could explain the positive response of porcelaneous taxa to the increase in TOC content. Furthermore, the TOC content was not high enough to cause pH lowering, which is particularly harmful for porcelaneous taxa (Li et al., 2014).
Much attention has been given to the influence of PAHs on benthic foraminiferal assemblages [e.g., Armynot du Châtelet et al. (2004); Bergamin et al. (2009); Romano et al. (2009); Schintu et al. (2015); Machain-Castillo et al. (2019), and Vilela et al. (2011)]; however, it is still hard to evaluate in a quantitative manner. Machain-Castillo et al. (2019) showed that very high PAH concentrations, e.g., above 5000 ng g−1 (a level far higher than that in the study area), could cause large amounts of damage to foraminiferal assemblages. The problem is how to evaluate their effect at moderate concentrations. In this study, the impact of PAHs (LPAHs and HPAHs) was not as strong as that of heavy metals, probably because the PAH concentration was not sufficiently high. According to the species-environment relationship, porcelaneous taxa appeared to benefit from PAHs, while they were harmful to other species. The reason was yet unclear.
5.3. Limitations
What thickness of the surface sediment layer is required to obtain a reliable dataset of benthic foraminiferal assemblages for biomonitoring studies? In many studies, researchers have chosen thicknesses of 1, 2, 4, and 5 cm [e.g., Frontalini and Coccioni (2008); Bergamin et al. (2009); Cosentino et al. (2013), and Martins et al. (2010)]. Barras et al. (2014) compared foraminiferal assemblages in the 0–1 cm layer and in the 0–4 cm layer and found no significant difference in community parameters and dominant species between the two intervals, although the latter’s density was much higher. However, the FOBIMO group strongly recommends the first centimeter of sediment for biomonitoring purposes because if an interval is too thick, surface-dwelling foraminifera will be diluted by a large number of dead individuals from deeper layers (Schönfeld et al., 2012). In this study, we chose the top 2 cm of sediments for foraminiferal analysis, while a more accurate investigation should be performed in the future.
This work emphasized the effects of pollution on benthic foraminiferal assemblages, including heavy metals, TOC, and PAHs. It should be mentioned that various natural factors, e.g., salinity, temperature, oxygen, nutrients, substrate, and food supply, also influence the distribution of benthic foraminifera (Murray, 2006). The influence of natural factors is not evaluated in this work and should be considered for future work. To measure the association between foraminiferal assemblages and environmental variables, we chose CCA as the primary analysis method. In CCA, environmental variables are used to describe the most important community gradients, but environmental variables are often measured for reasons of convenience rather than the “best” variables from the viewpoint of biology (McCune, 1997). Furthermore, environmental variables can be noisy or irrelevant and are sensitive to CCA (McCune, 1997).
6. Conclusion
This work assessed the relative effects of heavy metals, TOC, and PAHs on benthic foraminifera in two bays of Hainan Island: Houshui and Yangpu. Heavy metals had the largest effects, followed by TOC, while the effects of PAHs were fairly lower. Foraminiferal assemblages in the two bays were significantly different in diversity and species composition (and could be separated from each other) and likely influenced by different concentrations of pollution.
Two groups of sites and two species groups (I and II) were identified by both cluster analysis and CCA. According to CCA, heavy metals, TOC, and PAHs had positive and adverse effects on foraminiferal assemblages (or species groups). Heavy metals had positive effects on species group I, which consisted of agglutinated taxa Bigenerina nodosaria, Ammobaculites agglutinans, Textularia spp., T. foliacea, Haplophragmoides canariensis and several tolerant species (e.g., A. beccarii, E. advenum, E. hispidulum, Q. laevigata and Q. seminula), and negative effects on species group II, which was mainly composed of porcelaneous taxa, indicating that Heavy metals pollution should be more harmful to porcelaneous forms and inhibited the survival of certain species, but provided beneficial conditions for the survival of most agglutinated and some tolerant hyaline species. It appeared that porcelaneous taxa took advantage of the increase in TOC and PAHs, which were harmful to other species.
In addition, the sources of heavy metals, TOC, and PAHs were evaluated. Heavy metals were released from anthropogenic and natural processes as described by a previous investigation. The C/N ratios suggested that organic matter was derived primarily from algae. The diagnostic ratios of PAH isomers provided clues that PAHs were generated by petroleum and combustion of petroleum products.
Data availability statement
The original contributions presented in the study are included in the article/supplementary material. Further inquiries can be directed to the corresponding authors.
Author contributions
MZ and CY designed the study, performed the analysis, and took the lead in writing the manuscript. CY and GC supervised the project. SL and XN performed sample collection and chemical analysis; MZ and SZ identified and analyzed the foraminiferal assemblages. All authors reviewed the manuscript. All authors contributed to the article and approved the submitted version.
Funding
This work was financially supported by the National Natural Science Foundation of China (41776112), the Key Special Project for Introduced Talents Team of Southern Marine Science and Engineering Guangdong Laboratory (Guangzhou) (GML2019ZD0209), and the Regional Marine Geological Survey Projects of China Geological Survey (DD20221712, DD20221719, DD20191002, DD20190627).
Acknowledgments
We express our gratitude to the editors and reviewers for their helpful feedback and suggestions, which greatly improved the quality of this manuscript. In addition, we would like to extend our appreciation to Jiale Chen and Liqi Zhou for their contributions in collecting and handling the samples.
Conflict of interest
The authors declare that the research was conducted in the absence of any commercial or financial relationships that could be construed as a potential conflict of interest.
Publisher’s note
All claims expressed in this article are solely those of the authors and do not necessarily represent those of their affiliated organizations, or those of the publisher, the editors and the reviewers. Any product that may be evaluated in this article, or claim that may be made by its manufacturer, is not guaranteed or endorsed by the publisher.
References
Aloulou F., EllEuch B., Kallel M. (2012). Benthic foraminiferal assemblages as pollution proxies in the northern coast of gabes gulf, Tunisia. Environ. Monit. Assess. 184, 777–795. doi: 10.1007/s10661-011-2001-2
Alve E. (1991). Benthic foraminifera in sediment cores reflecting heavy metal pollution in sorfjord, western Norway. J. Foraminiferal Res. 21, 1–19. doi: 10.2113/gsjfr.21.1.1
Alve E. (1995). Benthic foraminiferal responses to estuarine pollution: A review. J. Foraminiferal Res. 25, 190–203. doi: 10.2113/gsjfr.25.3.190
Armynot du Châtelet É., Bout-Roumazeilles V., Riboulleau A., Trentesaux A. (2009). Sediment (grain size and clay mineralogy) and organic matter quality control on living benthic foraminifera. Rev. micropaléontologie 52, 75–84. doi: 10.1016/j.revmic.2008.10.002
Armynot du Châtelet E., Debenay J. P., Soulard R. (2004). Foraminiferal proxies for pollution monitoring in moderately polluted harbours. Environ. pollut. 127, 27–40. doi: 10.1016/S0269-7491(03)00256-2
Armynot du Châtelet E., Francescangeli F., Bouchet V. M. P., Frontalini F. (2018). Benthic foraminifera in transitional environments in the English channel and the southern north Sea: A proxy for regional-scale environmental and paleo-environmental characterisations. Mar. Environ. Res. 137, 37–48. doi: 10.1016/j.marenvres.2018.02.021
Barras C., Jorissen F. J., Labrune C., Andral B., Boissery P. (2014). Live benthic foraminiferal faunas from the French MediterraneanCoast: Towards a new biotic index of environmental quality. Ecol. Indic. 36, 719–743. doi: 10.1016/j.ecolind.2013.09.028
Bergamin L., Romano E., Finoia M. G., Venti F., Bianchi J., Colasanti A., et al. (2009). Benthic foraminifera from the coastal zone of baia (Naples, italy): Assemblage distribution and modification as tools for environmental characterisation. Mar. pollut. Bull. 59, 234–244. doi: 10.1016/j.marpolbul.2009.09.015
Bergin F., Kucuksezgin F., Uluturhan E., Barut I. F., Meric E., Avsar N., et al. (2006). The response of benthic foraminifera and ostracoda to heavy metal pollution in gulf of izmir (Eastern Aegean Sea). Estuarine Coast. Shelf Sci. 66, 368–386. doi: 10.1016/j.ecss.2005.09.013
Bouchet V. M. P., Telford R. J., Rygg B., Oug E., Alve E. (2018). Can benthic foraminifera serve as proxies for changes in benthic macrofaunal community structure? implications for the definition of reference conditions. Mar. Environ. Res. 137, 24–36. doi: 10.1016/j.marenvres.2018.02.023
Carnahan E. A., Hoare A. M., Hallock P., Lidz B. H., Reich C. D. (2008). Distribution of heavy metals and foraminiferal assemblages in sediments of Biscayne bay, Florida, USA. J. Coast. Res. 24, 159–169. doi: 10.2112/06-0666.1
Coccioni R., Frontalini F., Marsili A., Mana D. (2009). Benthic foraminifera and trace element distribution: A case-study from the heavily polluted lagoon of Venice (Italy). Mar. pollut. Bull. 59, 257–267. doi: 10.1016/j.marpolbul.2009.08.009
Cosentino C., Pepe F., Scopelliti G., Calabrò M., Caruso A. (2013). Benthic foraminiferal response to trace element pollution–the case study of the gulf of milazzo, NE Sicily (Central Mediterranean Sea). Environ. Monit. Assess. 185, 8777–8802. doi: 10.1007/s10661-013-3292-2
Dachs J., Bayona J. M., Raoux C., Albaigés J. (1997). Spatial, vertical distribution and budget of polycyclic aromatic hydrocarbons in the Western Mediterranean seawater. Environental Sci. Technol. 31, 682–688. doi: 10.1021/es960233j
Debenay J. P., Patrona L. D., Goguenheim H. (2009). Colonization of coastal environments by foraminifera: Insight from shrimp ponds in new Caledonia (SW pacific). J. Foraminiferal Res. 39, 249–266. doi: 10.2113/gsjfr.39.4.249
Debenay J.-P., Tsakiridis E., Soulard R., Grossel H. (2001). Factors determining the distribution of foraminiferal assemblages in port joinville harbor (Ile d'Yeu, france): The influence of pollution. Mar. Micropaleontology 43, 75–118. doi: 10.1016/S0377-8398(01)00023-8
Donnici S., Serandrei-Barbero R., Bonardi M., Sperle M. (2012). Benthic foraminifera as proxies of pollution: The case of guanabara bay (Brazil). Mar. pollut. Bull. 64, 2015–2028. doi: 10.1016/j.marpolbul.2012.06.024
Elshanawany R., Ibrahim M. I., Milker Y., Schmiedl G., Badr N., Kholeif S. E. A., et al. (2011). Anthropogenic impact on benthic foraminifera, Abu-qir bay, Alexandria, Egypt. J. Foraminiferal Res. 41, 326–348. doi: 10.2113/gsjfr.41.4.326
Ferraro L., Sprovieri M., Alberico I., Lirer F., Prevedello L., Marsella E. (2006). Benthic foraminifera and heavy metals distribution: A case study from the Naples harbour (Tyrrhenian Sea, southern Italy). Environ. Pollut. 142, 274–287. doi: 10.1016/j.envpol.2005.10.026
Frontalini F., Buosi C., Da Pelo S., Coccioni R., Cherchi A., Bucci C. (2009). Benthic foraminifera as bio-indicators of trace element pollution in the heavily contaminated Santa gilla lagoon (Cagliari, Italy). Mar. Pollut. Bull. 58, 858–877. doi: 10.1016/j.marpolbul.2009.01.015
Frontalini F., Coccioni R. (2008). Benthic foraminifera for heavy metal pollution monitoring: A case study from the central Adriatic Sea coast of Italy. Estuarine Coast. Shelf Sci. 76, 404–417. doi: 10.1016/j.ecss.2007.07.024
Frontalini F., Coccioni R. (2011). Benthic foraminifera as bioindicators of pollution: A review of Italian research over the last three decades. Rev. micropaléontologie 54, 115–127. doi: 10.1016/j.revmic.2011.03.001
Frontalini F., Greco M., Bella L. D., Lejzerowicz F., Reo E., Caruso A., et al. (2017). Assessing the effect of mercury pollution on cultured benthic foraminifera community using morphological and eDNA metabarcoding approaches. Mar. pollut. Bull. 129, 512–524. doi: 10.1016/j.marpolbul.2017.10.022
Hayward B. W., Le Coze F., Vachard D., Gross O. (2020). World foraminifera database. Jones. doi: 10.14284/305
He L., Zhou S., Li X., Lin W. (2014). Coastal marine pollution situation and the impacts on biodiversity in hainan island. J. Qiongzhou Univ. 21, 99–103. doi: 10.13307/j.issn.1008-6722.2014.05.19
Hu B., Cui R., Li J., Wei H., Zhao J., Bai F., et al. (2013). Occurrence and distribution of heavy metals in surface sediments of the changhua river estuary and adjacent shelf (Hainan island). Mar. pollut. Bull. 76, 400–405. doi: 10.1016/j.marpolbul.2013.08.020
Jones R. W. (2013). Foraminifera and their applications (Cambridge: Cambridge University Press). doi: 10.1017/CBO9781139567619
Jorissen F., Nardelli M. P., Almogi-Labin A., Barras C., Bergamin L., Bicchi E., et al. (2018). Developing foram-AMBI for biomonitoring in the Mediterranean: Species assignments to ecological categories. Mar. Micropaleontology 140, 33–45. doi: 10.1016/j.marmicro.2017.12.006
Le Cadre V., Debenay J.-P. (2006). Morphological and cytological responses of Ammonia (foraminifera) to copper contamination: Implication for the use of foraminifera as bioindicators of pollution. Environ. Pollut. 143, 304–317. doi: 10.1016/j.envpol.2005.11.033
Lei Y., Li T. (2016). Atlas of benthic foraminifera from China seas: The bohai Sea and the yellow Sea (Berlin Heidelberg: Springer).
Li T., Li X., Zhong H., Yang C., Sun G., Luo W. (2015). Distribution of trace metals and the benthic foraminiferal assemblage as a characterization of the environment in the north minjiang river estuary (Fujian, China). Mar. pollut. Bull. 90, 227–241. doi: 10.1016/j.marpolbul.2014.10.047
Li T., Xiang R., Li T. (2014). Influence of trace metals in recent benthic foraminifera distribution in the pearl river estuary. Mar. Micropaleontology 108, 13–27. doi: 10.1016/j.marmicro.2014.02.003
Liu M., Baugh P. J., Hutchinson S. M., Yu L., Xu S. (2000). Historical record and sources of polycyclic aromatic hydrocarbons in core sediments from the Yangtze estuary, China. Environ. pollut. 110, 357–365. doi: 10.1016/S0269-7491(99)00292-4
Liu L.-Y., Wang J.-Z., Wei G.-L., Guan Y.-F., Wong C. S., Zeng E. Y. (2012). Sediment records of polycyclic aromatic hydrocarbons (PAHs) in the continental shelf of China: Implications for evolving anthropogenic impacts. Environ. Sci. Technol. 46, 6497–6504. doi: 10.1021/es300474z
Machain-Castillo M. L., Ruiz-Fernández A. C., Gracia A., Sanchez-Cabeza J. A., Rodríguez-Ramírez A., Alexander-Valdés H. M., et al. (2019). Natural and anthropogenic oil impacts on benthic foraminifera in the southern gulf of Mexico. Mar. Environ. Res. 149, 111–125. doi: 10.1016/j.marenvres.2019.06.006
Marchant R. (2002). Do rare species have any place in multivariate analysis for bioassessment? J. North Am. Benthological Soc. 21, 311–313. doi: 10.2307/1468417
Martins V., de Silva E. F., Sequeira C., Rocha F., Duarte A. C. (2010). Evaluation of the ecological effects of heavy metals on the assemblages of benthic foraminifera of the canals of aveiro (Portugal). Estuarine Coast. Shelf Sci. 87, 293–304. doi: 10.1016/j.ecss.2010.01.011
Martins M. V. A., Silva F., Laut L. L. M., Frontalini F., Clemente I. M. M. M., Miranda P., et al. (2015). Response of benthic foraminifera to organic matter quantity and quality and bioavailable concentrations of metals in aveiro lagoon (Portugal). PLoS One 10, e0118077. doi: 10.1371/journal.pone.0118077
McCune B. (1997). Influence of noisy environmental data on canonical correspondence analysis. Ecology 78, 2617–2623. doi: 10.1890/0012-9658(1997)078[2617:IONEDO]2.0.CO;2
Meyers P. A. (1994). Preservation of elemental and isotopic source identification of sedimentary organic matter. Chem. Geology 114, 289–302. doi: 10.1016/0009-2541(94)90059-0
Mo L., Zheng J., Wang T., Shi Y.G., Chen B.J., Liu B., et al (2019). egacy and emerging contaminants in coastal surface sediments around Hainan Island in South China. Chemosphere 215, 133–141. doi: 10.1016/j.chemosphere.2018.10.022
Morey A. E., Mix A. C., Pisias N. G. (2005). Planktonic foraminiferal assemblages preserved in surface sediments correspond to multiple environment variables. Quaternary Sci. Rev. 24, 925–950. doi: 10.1016/j.quascirev.2003.09.011
Murray J. W. (2006). Ecology and applications of benthic foraminifera (New York: Cambridge University Press).
Nigam R., Linshy V. N., Kurtarkar S. R., Saraswat R. (2009). Effects of sudden stress due to heavy metal mercury on benthic foraminifer rosalina leei: laboratory culture experiment. Mar. Pollut. Bull. 59, 362–368. doi: 10.1016/j.marpolbul.2009.08.014
Price E. B., Kabengi N., Goldstein S. T. (2019). Effects of heavy-metal contaminants (Cd, Pb, zn) on benthic foraminiferal assemblages grown from propagules, sapelo island, Georgia (USA). Mar. Micropaleontology 147, 1–11. doi: 10.1016/j.marmicro.2019.01.004
Romano E., Bergamin L., Ausili A., Pierfranceschi G., Maggi C., Sesta G., et al. (2009). The impact of the bagnoli industrial site (Naples, Italy) on sea-bottom environment. chemical and textural features of sediments and the related response of benthic foraminifera. Mar. pollut. Bull. 59, 245–256. doi: 10.1016/j.marpolbul.2009.09.017
Samir A. M. (2000). The response of benthic foraminifera and ostracods to various pollution sources: A study from two lagoons in Egypt. J. Foraminiferal Res. 30, 83–98. doi: 10.2113/0300083
Schintu M., Buosi C., Galgani F., Marrucci A., Marras B., Ibba A., et al. (2015). Interpretation of coastal sediment quality based on trace metal and PAH analysis, benthic foraminifera, and toxicity tests (Sardinia, Western Mediterranean). Mar. pollut. Bull. 94, 72–83. doi: 10.1016/j.marpolbul.2015.03.007
Schönfeld J., Alve E., Geslin E., Jorissen F., Korsun S., Spezzaferri S., et al. (2012). The FOBIMO (FOraminiferal BIo-MOnitoring) initiative–towards a standardized protocol for soft-bottom benthic foraminiferal monitoring studies. Mar. Micropaleontology 94–95, 1–13. doi: 10.1016/j.marmicro.2012.06.001
Šmilauer P., Lepš J. (2014). Multivariate analysis of ecological data using canoco 5 (Cambridge, UK: Cambridge University Press).
Teresa Losada Ros M., Al-Enezi E., Cesarini E., Canonico B., Bucci C., Martins M. V. A., et al. (2020). Assessing the cadmium effects on the benthic foraminifer Ammonia cf. parkinsoniana: An acute toxicity test. Water 12, 1018. doi: 10.3390/w12041018
The Annals of Chinese Gulfs Compiling Committee (1999). Annals of Chinese gulfs (11th fascicule): Gulfs in hainan (Beijing: China Ocean Press), 426.
Vilela C. G., Batista D. S., Neto J. A. B., Ghiselli R. O. Jr. (2011). Benthic foraminifera distribution in a tourist lagoon in Rio de Janeiro, Brazil: A response to anthropogenic impacts. Mar. Pollut. Bull. 62, 2055–2074. doi: 10.1016/j.marpolbul.2011.07.023
Viñas L., Franco M. A., Soriano J. A., González J. J., Pon J., Albaigés J. (2010). Sources and distribution of polycyclic aromatic hydrocarbons in sediments from the Spanish northern continental shelf. assessment of spatial and temporal trends. Environ. pollut. 158, 1551–1560. doi: 10.1016/j.envpol.2009.12.023
Wan S., Toucanne S., Clift P. D., Zhao D., Bayon G., Yu Z., et al. (2015). Human impact overwhelms long-term climate control of weathering and erosion in southwest China. Geology 43, 439–442. doi: 10.1130/G36570.1
Wang B., Chen S., Gong W., Lin W., Xu Y. (2006). Morphology and evolution of coastal bays of hainan island (Beijing: Ocean Press), 255.
Wang J.-Z., Guan Y.-F., Ni H.-G., Luo X.-L., Zeng E. Y. (2007). Polycyclic aromatic hydrocarbons in riverine runoff of the pearl river delta (China): concentrations, fluxes, and fate. Environ. Sci. Technol. 41, 5614–5619. doi: 10.1021/es070964r
Wang P., Zhang J., Zhao Q. (1988). Foraminifera and ostracod in sediments of the East Sea (Beijing, China: China Ocean Press).
Xiang N., Jiang C., Yang T., Li P., Wang H., Xie Y., et al. (2018). Occurrence and distribution of polycyclic aromatic hydrocarbons (PAHs) in seawater, sediments and corals from hainan island, China. Ecotoxicology Environ. Saf. 152, 8–15. doi: 10.1016/j.ecoenv.2018.01.006
Xu F., Tian X., Yin X., Yan H., Yin F., Liu Z. (2015). Trace metals in the surface sediments of the eastern continental shelf of hainan island: Sources and contamination. Mar. pollut. Bull. 99, 276–283. doi: 10.1016/j.marpolbul.2015.07.055
Yanko V., Ahmad M., Kaminsky M. (1998). Morphological deformities of benthic foraminiferal tests in response to pollution by heavy metals: Implications for pollution monitoring. J. Foraminiferal Res. 28, 177–200.
Yunker M. B., Macdonald R. W., Vingarzan R., Mitchell R. H., Goyette D., Sylvestre S. (2002). PAHs in the Fraser river basin: A critical appraisal of PAH ratios as indicators of PAH source and composition. Organic Geochemistry 33, 489–515. doi: 10.1016/S0146-6380(02)00002-5
Zakaria M. P., Takada H., Tsutsumi S., Ohno K., Yamada J., Kouno E., et al. (2002). Distribution of polycyclic aromatic hydrocarbons (PAHs) in rivers and estuaries in Malaysia: A widespread input of petrogenic PAHs. Environental Sci. Technol. 36, 1907–1918. doi: 10.1021/es011278+
Zettler M. L., Proffitt C. E., Darr A., Degraer S., Devriese L., Greathead C., et al. (2013). On the myths of indicator species: Issues and further consideration in the use of static concepts for ecological applications. PLoS One 8, e78219. doi: 10.1371/journal.pone.0078219
Keywords: benthic foraminifera, marine pollution, community structure and diversity, heavy metals, TOC, PAHs, canonical correspondence analysis, cluster analysis
Citation: Zhang M, Yang C, Cai G, Li S, Nie X and Zhou S (2023) Assessing the effects of heavy metals and polycyclic aromatic hydrocarbons on benthic foraminifera: The case of Houshui and Yangpu Bays, Hainan Island, China. Front. Mar. Sci. 10:1123453. doi: 10.3389/fmars.2023.1123453
Received: 14 December 2022; Accepted: 07 February 2023;
Published: 23 February 2023.
Edited by:
Lihua Yang, Sun Yat-sen University, ChinaReviewed by:
Rong Xiang, South China Sea Institute of Oceanology (CAS), ChinaJingfang Lu, Qingdao Institute of Marine Geology (QIMG), China
Copyright © 2023 Zhang, Yang, Cai, Li, Nie and Zhou. This is an open-access article distributed under the terms of the Creative Commons Attribution License (CC BY). The use, distribution or reproduction in other forums is permitted, provided the original author(s) and the copyright owner(s) are credited and that the original publication in this journal is cited, in accordance with accepted academic practice. No use, distribution or reproduction is permitted which does not comply with these terms.
*Correspondence: Muhui Zhang, muhui.zhang@cug.edu.cn; Chupeng Yang, GMGS_yang@foxmail.com