- 1College of the Environment and Life Science, University of Rhode Island, Kingston, RI, United States
- 2Institute for Socio-Ecological Research, Inc., Lajas, Puerto Rico
- 3Department of Marine Sciences, University of Puerto Rico at Mayagüez, Mayagüez, Puerto Rico
As coral reefs face increasing threats from a variety of stressors, coral restoration has become an important tool to aid coral populations. A novel strategy for restoring boulder corals is microfragmentation, which may enhance coral growth by at least five times, depending on species and conditions. However, mortality rates are still significant during the early weeks after transplanting microfragments to impacted areas. We examined the effects of predation after transplanting fragments by caging Orbicella faveolata microfragments and testing if field survival rates would increase after an acclimation period. We tracked the health and growth of ten genotypes across different acclimation periods from a control group of no acclimation (0 months) to full acclimation (4 months). After four months, we presented a mix of acclimated and unacclimated corals to reef predators. Coral survivorship was highest in acclimation cages (near 100%) compared to the field (p < 0.001), with significant growth differences across genotypes (p < 0.001). Microfragments also grew more in acclimation cages (p < 0.001), with rates slowing down in the first two months after being planted into the substrate. Microfragments that had been acclimated for longer than one month also showed comparatively higher survival rates, further supporting the importance of acclimation during restoration. These results suggest caging fragments boost coral survival during initial stages of restoration by > 50% and increase the persistence of transplanted fragments. Results also highlight the importance of identifying and prioritizing genotypes with high survival and growth rates. Beyond coral restoration, results demonstrate the possible negative ecological effects of corallivores, particularly parrotfishes, on recent transplants of fragments.
Introduction
In recent years, coral reefs have faced extreme reductions in live coral cover due to diseases, overfishing, pollution, ocean acidification, and global warming (Weil et al., 2009; Weil and Rogers, 2011; Bruno et al., 2019). One of the most affected corals is the mountainous coral Orbicella faveolata, a foundational species on Caribbean reefs (Bruckner and Bruckner, 2006; Edmunds, 2015). In Puerto Rico, the loss of high percent live tissue cover of foundational coral species has contributed to the overall decline and community shift from coral dominated to algal dominated habitats at multiple coral reefs (Weil et al., 2009; García-Sais et al., 2017). Since the intense bleaching event and disease outbreak in 2005-2006, some reefs in Puerto Rico have shifted away from an Orbicella annularis complex dominated ecosystem to one dominated by algae, sponges and soft corals (Weil et al., 2009; Williams et al., 2015; García-Sais et al., 2017; Williams and Garcia-Sais, 2020).
In response to the rapid loss of coral cover, coral restoration efforts have become increasingly widespread (Levy et al., 2010; van Oppen et al., 2017). An especially popular technique has been coral gardening, where corals are fragmented and maintained in situ before being replanted on reefs (Levy et al., 2010; Rinkevich, 2015; Lirman and Schopmeyer, 2016). While most restoration efforts have focused on fast-growing branching corals (Shaish et al., 2010), strategies have also been developed for boulder corals. In particular, Forsman et al. (2015) and Page et al. (2018) pioneered microfragmentation, a modification on coral gardening techniques, where boulder corals are cut into 1cm by 1cm pieces that are then glued to a substrate and monitored before replanting on reefs (Forsman et al., 2015; Page et al., 2018). Microfragments have been shown to grow significantly faster (up to 5X) than larger fragments, making the strategy an effective way to produce coral tissue (Forsman et al., 2015; Page et al., 2018).
Despite the increasing adoption of coral restoration, a key finding is that survivorship of fragments is low, especially after five years (Miller and Hay, 1998). It appears that fish corallivory can affect coral fragment survival (Morikawa and Palumbi, 2019; Koval et al., 2020), yet the effects of fish predators on coral fragments have not been quantified. Other corallivores such as fireworms have also been shown to slow the growth of Acropora cervicornis transplants (Miller et al., 2014). And while it has been found that parrotfish predation can have a variety of negative effects on coral colonies (Burkepile, 2012), the factors determining what corals are more or less attractive to corallivores remain unclear (Rotjan and Dimond, 2010). Other restoration strategies have occasionally used protective cages around newly fragmented corals, but the overall effectiveness of these cages as a predator exclusion method has not been specifically studied (Page et al., 2018). While there is a history of predator exclusion experiments on coral reefs, many have focused on fish (Doherty and Sale, 1985) or sponges (Leong and Pawlik, 2010; Pawlik et al., 2013) rather than coral, particularly in a coral restoration context. Understanding the impacts of predation and protective caging on coral microfragments is key to determining the most effective strategies for coral restoration through outplanting.
Genotype variation has been found to be an important factor in coral restoration success, and could also be a factor in predator deterrence (Lirman et al., 2014). A survey of over 1,700 nursery-grown fragments of A. cervicornis found different growth rates between genotypes (Lirman et al., 2014). Differences between genotypes in growth rates and calcification rates of A. cervicornis were also exhibited across multiple attachment strategies (Kuffner et al., 2017; Goergen and Gilliam, 2018). Reciprocal transplant experiments involving A. cervicornis suggest a combination of genotype and location determined colony growth and survival (Drury et al., 2017). Studies that have found no effect from genotype on total linear extension (TLE), have generally been done on small numbers of genotypes (3 or fewer) (Shaish et al., 2008; Ladd et al., 2016). When more genotypes were examined, TLE and calcification rates showed a genotype effect (Kuffner et al., 2017). So while it is clear that different genotypes show significantly different rates of growth and survival, the vast majority of the research done on corals grown for restoration purposes has been done on fast-growing branching species such as A. cervicornis. Little information is available on differences between genotypes of boulder corals such as O. faveolata, although some differences have been observed, but not investigated (Page et al., 2018). Testing difference in survivorship, growth, and predator resistance between genotypes in massive corals like O. faveolata is key for ongoing and future restoration efforts.
Caging corals has been used to examine dynamics such as predation or competition with algae. The effects of caging corals for a portion of the outplanting process has not been specifically investigated. Protecting O. faveolata microfragments in cages near their eventual permanent outplant location seem to improve survival rates, implying that corals need time to acclimate to their new home (Page et al., 2018). In addition, parrotfish behavior may change over time as corallivores adjust to a new presence in their environment. Comparing survival and growth rates of corals caged for multiple different acclimation time periods is key to identify at what stage coral microfragments become more suited to their transplant environment.
A large source of mortality in recently transplanted coral microfragments in the field is fish herbivory (Page et al., 2018). Historically, herbivory has been viewed as a benefit to coral reef ecosystems. Herbivorous fish, such as parrotfish, eat fast-growing macroalgae, which often outcompete slower growing scleractinian corals (Williams and Polunin, 2001; Burkepile and Hay, 2006; Mumby et al., 2006). And there is consensus (among 82 experts on coral bleaching) that protecting parrotfish would protect coral reefs (Rosinkski and Walsh, 2016). However, a more recent meta-analysis has shown that parrotfish may not benefit corals as much as previously thought, as areas with healthy parrotfish levels do not exhibit high coral cover (Bruno et al., 2019). Local presence of herbivorous fish does not seem to have as large an impact on reef health as global stressors such as climate change (Bruno et al., 2019). In addition, parrotfish can significantly contribute to bioerosion (Roff et al., 2015). Certain species of fish, such as the stoplight parrotfish (Sparisoma viride) routinely prey on corals (Scoffin et al., 1980; Harborne and Mumby, 2018). Fish corallivory has even been observed to increase as coral cover declines (Burkepile, 2012). A 2011 survey of various Caribbean reefs found that higher coral density showed a higher density of bite scars (Roff et al., 2015), and initially grazed coral colonies are more likely to be re-grazed (Rotjan and Dimond, 2010) and these more heavily predated colonies show higher mortality rates (Rotjan and Lewis, 2008). Given the key role of fish herbivores on reefs, it is crucial to understand the effect of coral predators in recently transplanted corals in reefs. A variety of complex trophic interactions may be taking place, impacted by substantial unhealthy conditions such as low coral cover, increased algae cover, overfished carnivorous top predators, or in some areas, direct fishing of corallivores such as parrotfish.
To study the effects of genotype and caging in the presence of corallivorous predators, we studied populations of the boulder coral species O. faveolata, using experimental cages, transplant experiments and different acclimation periods. Here we tested: 1) whether the initial mortality in transplanted corals is due to predation, and if increase in acclimation time in cages enhances microfragment survival and growth; 2) whether differences in survival and growth occur across coral genotypes. Results from this study demonstrate the likely utility of acclimation cages, highlight the negative effects of parrotfish on coral growth and survivorship during coral restoration, and provide ways to improve microfragmentation-based restoration strategies.
Methods
Coral collection
To understand the effects of predation on coral growth and survivorship, we collected fragments from tagged Orbicella faveolata from a midshelf reef (~6m) in La Parguera, Puerto Rico (Turrumote reef, 17° 56.1′N, 67° 01.1′W, Figure 1) on June 27 and July 25, 2019 (Collecting permit #: O-VS-PVS15-SJ-01041-16042019). Coral colonies targeted for the collection are part of an ongoing coral monitoring program at the Department of Marine Sciences at the University of Puerto Rico, Mayagüez (DMS-UPRM). These tagged colonies have demonstrated resistance to bleaching events and the biological diseases that affected reefs between 2003 and 2017 (Weil et al., 2009; García-Sais et al., 2017). They have also demonstrated variable responses to other stressors, and are physically separated (5 m) apart to be considered distinct genotypes, as O. faveolata has been shown to exhibit extremely low clonality throughout the Caribbean (although no genetic analysis were performed at this time) (Porto-Hannes et al., 2015). Working in teams, divers used a hammer and chisel to remove skirt pieces from the edge of the large colonies, to minimize damage. Fragments were placed in labeled 1-gallon ziplock bags, which were held in a mesh dive bag until divers were back on the boat. Once pieces from ten colonies were collected, the bags were put in 5-gallon buckets full of fresh seawater. Upon return to the laboratory, colonies were removed from bags and placed in a running sea-water raceway in the on-land coral culture area of the DMS-UPRM. Metal tags stamped with the associated genotype codes (AZ, BY, etc.) were placed near their matching fragments. Raceways were covered with shade cloth to protect newly fragmented corals from heat and light stress. Collected colonies remained in the raceway for at least one week before they were micro-fragmented, with earlier collected colonies fragmented for Acclimation Groups 1 and 2, and later collected colonies fragmented for Acclimation Groups 3, 4, and 5 (acclimation groups further described in Acclimation Treatments section). Colonies were closely monitored, and those that did not transition well were not used for fragmentation.
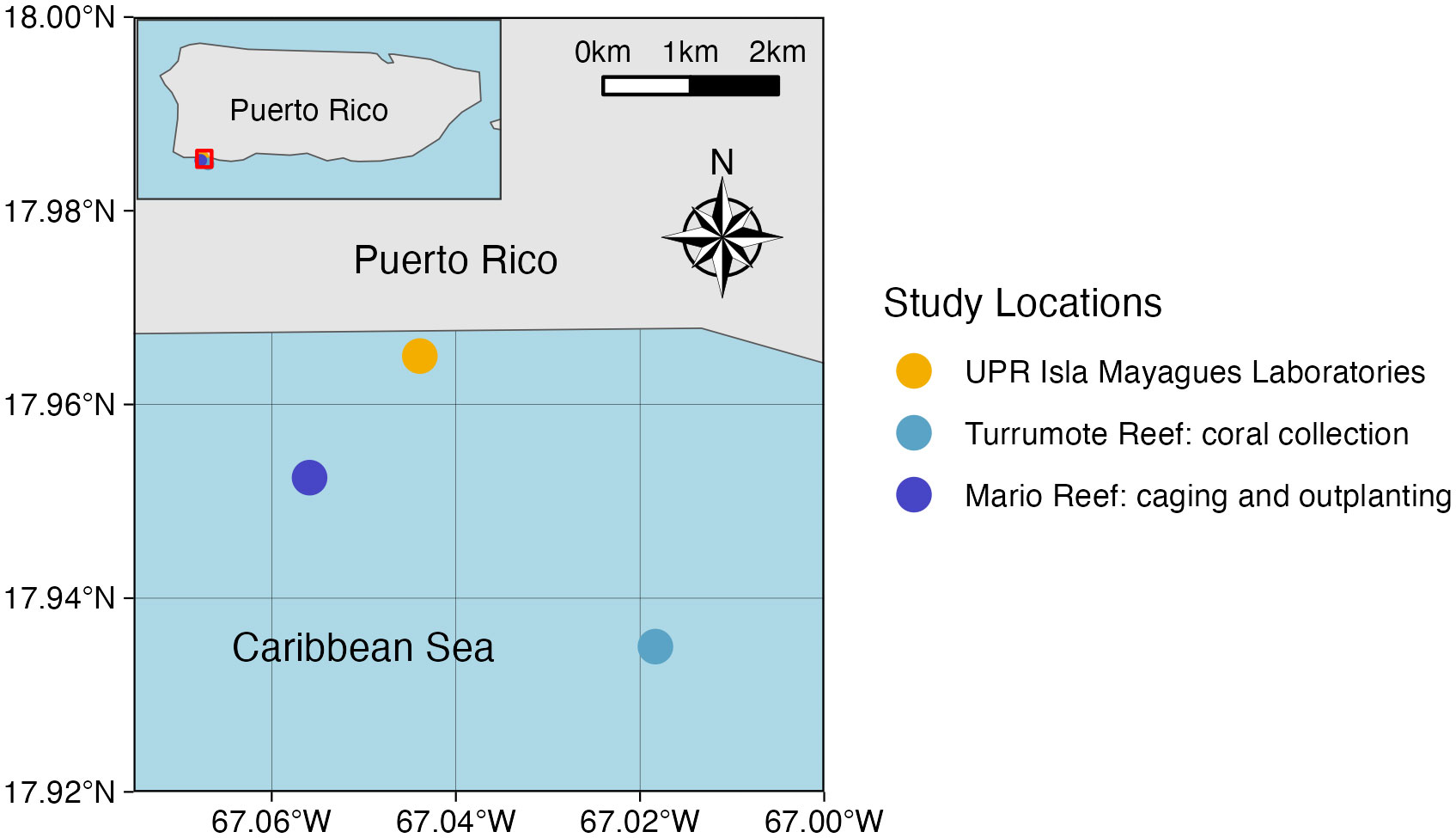
Figure 1 Map of field location showing the locations of the UPR Isla Magueyes research laboratories, the coral collection location, and the acclimaiton and outplanting location.
Microfragmenting
Corals were microfragmented using a Gryphon AquaSaw XL, Model C-40 CR Custom. The saw was fitted with a 42” Gryphon Diamond Band Saw Blade and replaced as needed. Less than one inch of saltwater from the raceway holding the coral fragments was put in the base of the saw. The colony to be fragmented was placed in a bin filled with saltwater. Larger colonies were cut into strips, and then into 1cm by 1cm squares. Excess skeleton off the bottom of the fragments was removed if necessary. Gorilla super glue gel was used to affix microfragments to ceramic plugs made by ReefCreators. Before fragmenting, each plug was labeled with the microfragment’s genotype, acclimation treatment, and individual ID number. Glued microfragments were placed on egg crates in a bin of saltwater. To remove excess mucus off the glued microfragments, a turkey baster or pipet was used. Once finished, fragments were transported back to their original raceway. To standardize the amount of time microfragments spent in the on-shore raceway, fragmenting was staggered. Microfragment groups were generated one month before being moved to the acclimation cage (or in the case of Group 5, one month before planting). This allowed us to assess the total time (from fragmentation to planting) needed to produce successful corals.
Acclimation treatments
To generate different acclimation treatments, coral microfragments were sorted into five groups, with ten microfragments from each collection colony (hereafter referred to as “genotype”) in each group. The acclimation cage was deployed at Mario reef (17°57’08.8”N, 67°03’21.2”W), at around 3 meters of depth. Mario reef was chosen due to the ability to deploy the cage securely, as well as ease of boat access. The cage was 1m wide, 0.5m tall, and 0.5m deep. There were two levels of eggcrate within the cage. The cage was attached to six pieces of rebar that had been hammered into the substrate, both to keep it stationary and to keep it roughly 0.5m above the benthic environment (Figure 2). Coral microfragments were acclimated for the following periods of time to test the effect on outplant survivorship: Group 1 = three months; Group 2 = two months; Group 3 = one month; Group 4 = two weeks and Group 5 = 0 days (control). Each group had 100 fragments (10 replicates X 10 genotypes). Group 1 was put in the acclimation cage on August 8, 2019. All ten microfragments from the AZ genotype died within two weeks, leaving only nine genotypes for this acclimation point. Microfragments in group 2 were put in the acclimation cage on August 22, 2019. Acclimation Group 2 included ten microfragments from each genotype except AZ. Group 3 microfragments were put in the acclimation cage on November 4, 2019, and this group included ten microfragments from all ten genotypes. Group 4 also included ten microfragments from all ten genotypes and were put in the acclimation cage on November 18, 2019. The acclimation cage was checked at least every other week starting August 8, 2019. Coral fragments were cleared of sediment and researchers took photos of the microfragments for later health and growth analysis. Acclimation Group 5 contained ten microfragments from all ten genotypes and remained in the on-shore raceway until outplanting.
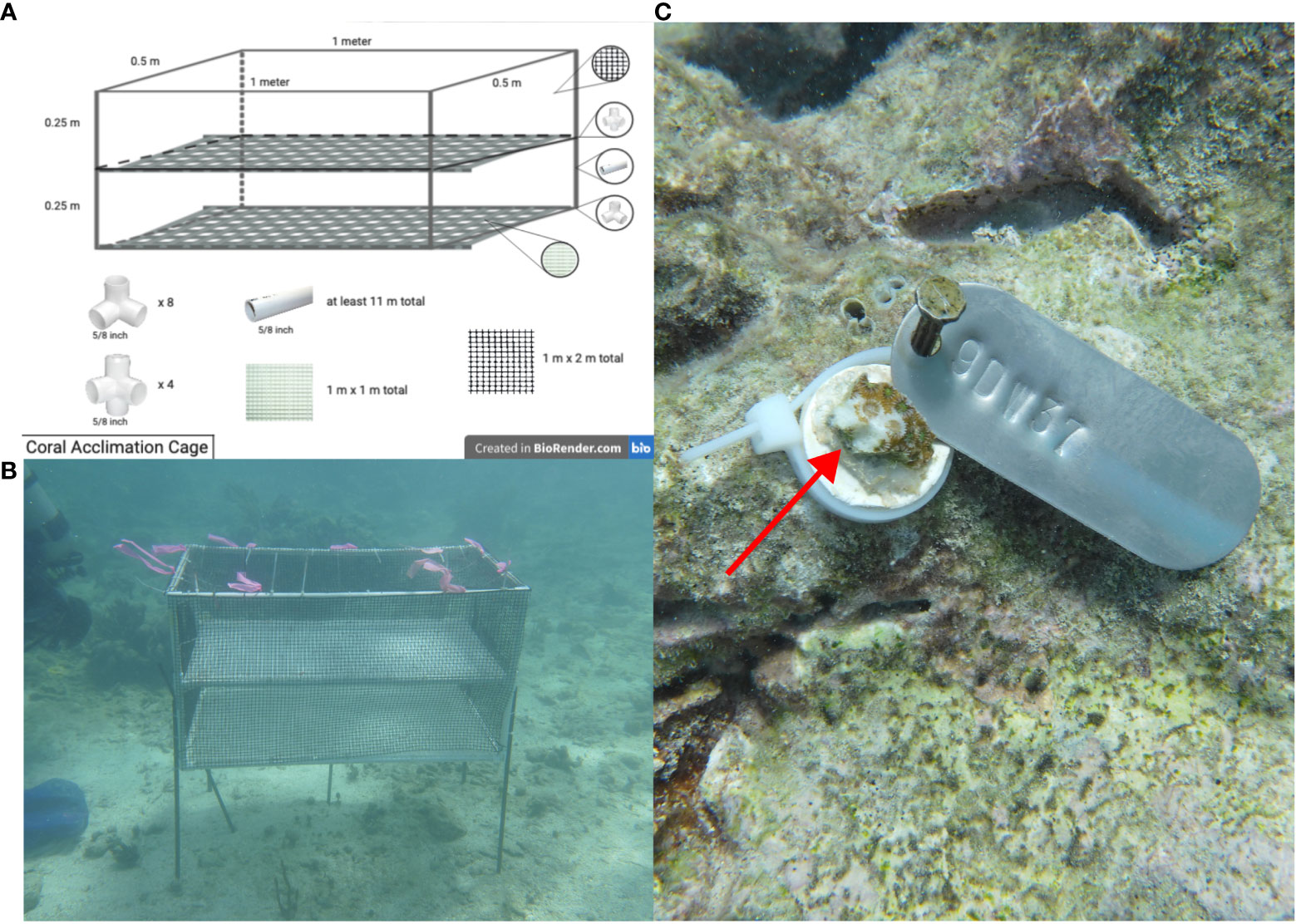
Figure 2 (A). Schematic showing dimensions and materials of coral acclimation cage, (B). photo showing acclimation cage deployed at the study location, secured up out of substrate by six pieces of rebar, and (C). a coral fragment attached to the reef, showing the metal tag as well as evidence of parrotfish predation.
Outplanting
Microfragments were planted at Mario reef, in close proximity and at a similar depth to the acclimation cage. We labeled metal tags with coral fragments’ individual ID numbers using metal stamping rods and a mallet. Tags were then grouped by genotype and further subdivided into groups of 5 IDs with one fragment from each acclimation treatment (ex: one outplant clump would include a BY genotype fragment from each acclimation group). Large pieces of dense coral skeleton from the target outplant site were collected and brought back to shore. Onshore, masonry nails were used to attach groups of tags to the skeleton rubble, with tags spaced at least 5” apart. Nails were hammered at least an inch into the coral skeleton, but not all the way. Labeled pieces were brought onto the boat, along with tools for outplanting (clippers, zip ties, hammers, super glue, and large plastic bins). Upon arrival at the site, the plastic bins were filled with seawater and the water was changed frequently. Two members of the SCUBA team collected coral plugs from the acclimation cage and brought them to the boat. Researchers on the boat clipped the stems off the coral plugs so that they would lie as flat as possible when attached. Using the metal tags and the labels on the bottom of the plugs, the coral fragments were attached to the nail with their matching tag using a zip tie. Any excess zip tie was clipped off. Microfragments were kept submerged in bins as much as possible. Once the microfragments were attached, they were passed back to the SCUBA team, who then placed them around the reef in horizontal, stable substrate. A photo was taken of each fragment and group.
Photo monitoring
To measure growth and quantify mortality across treatments, we took initial photos of fragments in the field upon outplanting, on December 11 and 12, 2019. The next round of photos were not taken until January 30, 2020, due to a stop of diving activities after local earthquakes. Subsequent photos were taken on February 7 and March 3, 2020. Photos of individual or small groups of coral fragments were taken so that the labeled tag was clearly visible, and included a ruler for reference when possible. When a ruler was not present, the size of the tags was used as a calibration reference.
Data analysis
To process all images for growth, we used the ImageJ software. Coral size was measured as a two-dimensional planar area, as fragments remained flat even after growth. Measurements were calibrated using the egg crate, a ruler, or the labeled metal tags, which had been measured previously and were on the same plane as the coral fragments. Microfragment size was recorded in square centimeters. Some coral fragments were missing by the end of the experiment, and these were not included in the final analysis of field survivorship. Survival percentages were calculated by location (on-shore raceway, acclimation cage, and outplanted), and then by genotype. All data, including individual photo processing and survivorship percentages, were uploaded into R for further analysis.
Survivorship data between locations, genotypes, and acclimation treatments were assessed visually, and means were compared using either an unpaired two-samples Wilcoxon test (cage vs. outplanted microfragments) or a Kruskal-Wallis Test (acclimation treatment and genotype comparisons). A non-parametric test was used due to the fact that the data did not show a normal distribution. Data was still transformed to standardize between groups. Survivorship data was further evaluated using the Kaplan-Meier estimate (Goel et al., 2010, R package survminer, https://CRAN.R-project.org/package=survminer). This allowed taking into account coral fragments for which the final date of observation indicated mortality, and coral fragments for which or final date of observation indicated the end of field surveys. Growth rate was calculated by dividing microfragment size by the number of days since planting, and then standardizing to centimeters per month for ease of comparison. Growth rates were then log transformed for further statistical analysis. Growth rates were compared using a Kruskal-Wallis Test (acclimation treatment and genotype comparisons). Survivorship and growth rates were compared between locations, genotypes, acclimation treatments, and predation presence in R (https://cran.r-project.org).
To score predation, visible bite marks were included, as well as samples where the coral was missing off the plug, and samples where the coral and plug were missing but the nail and metal tag remained. This decision was made based on previous literature (Page et al., 2018) and field observations, indicating that fish grazers sometimes prey on coral fragments off the reef structure.
Post-hoc analysis on differences in survivorship and growth was performed using the R-package agricolae (de Mendiburu and Yaseen, 2020). Survival data (measured in days alive) was log transformed to achieve normality. Tukey’s HSD was used to test for pairwise differences between groups. Cox models were used to evaluate variable effects (R package survminer). Two-way ANOVAs were used to rank the different models and choose the one with the best fit to our data
Results
Coral microfragments in the first three acclimation groups show higher survival rates than microfragments in the final two groups, but there was no statistically significant difference in field survivorship between the five acclimation treatments (p = 0.52, Figure 3). Survivorship in the cage was significantly higher than survivorship in the field (96.18% compared to 31.13%, p < 0.001, Figure 4). Kaplan-Meier estimate also showed significant differences between genotypes in the field (p < 0.01, Figure 5). Genotypes AZ and EV die more often than the other genotypes in the field, with AZ performing especially poorly in all locations (pairwise comparisons in supplemental material). All genotypes (except AZ) had similar rates of survival in the acclimation cage. Genotype HS exhibited a significantly higher survivorship probability in the field after outplanting, while genotypes BY, CX, and DW performed well in both the acclimation cage and the field. Nineteen microfragments showed distinct parrotfish bite scars. In total, 105 out of 250 fragments were either missing or bitten, and counted as affected by predation (see Methods). Coral fragments with no evidence of predation survived more than coral fragments by predation (Figure 6).
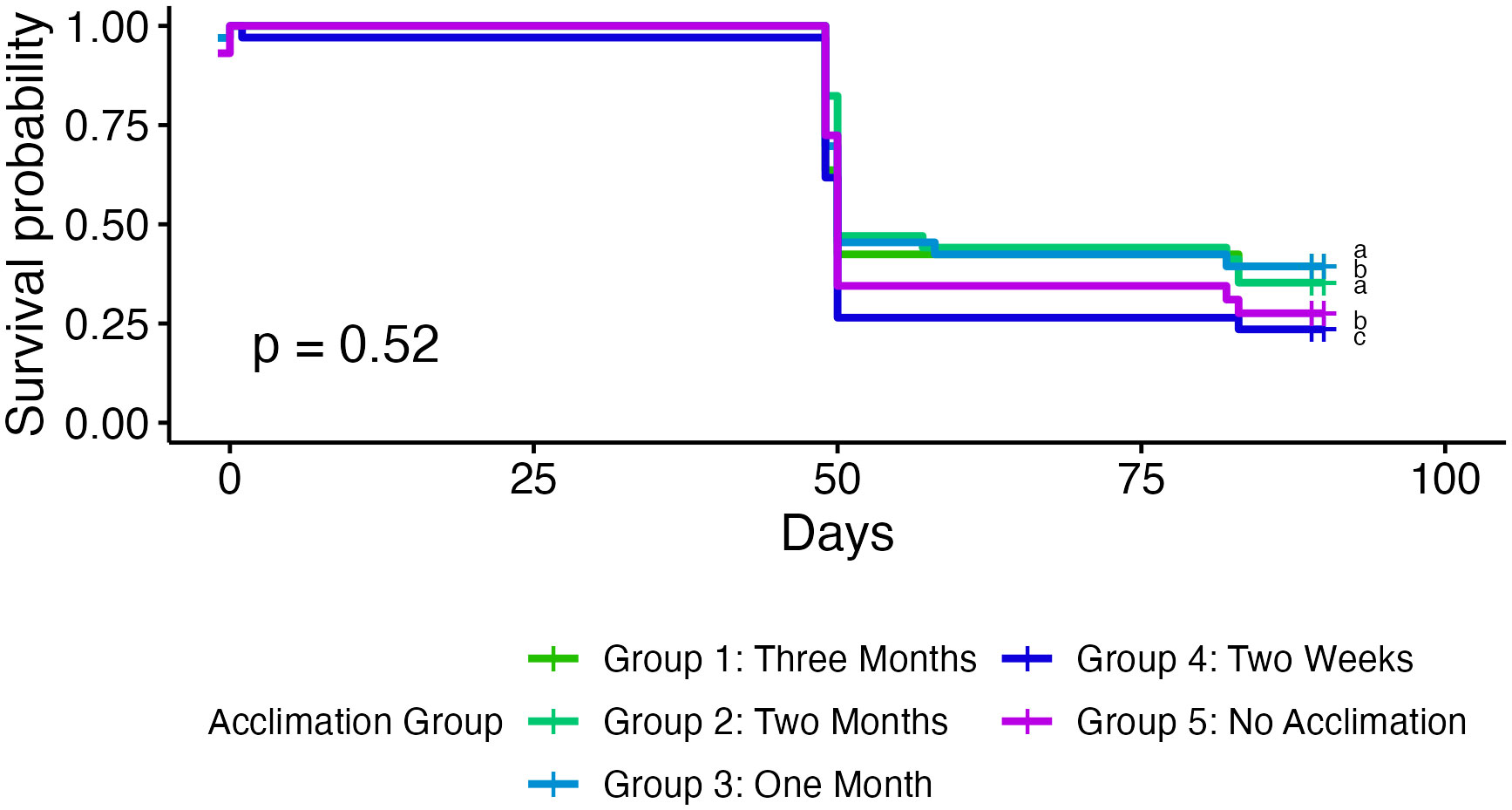
Figure 3 Kaplan-Meier survival curves of coral microfragments in the field grouped by acclimation group. Corals in the first three groups show slightly higher survival rates, but there are no significant differences across acclimation groups.
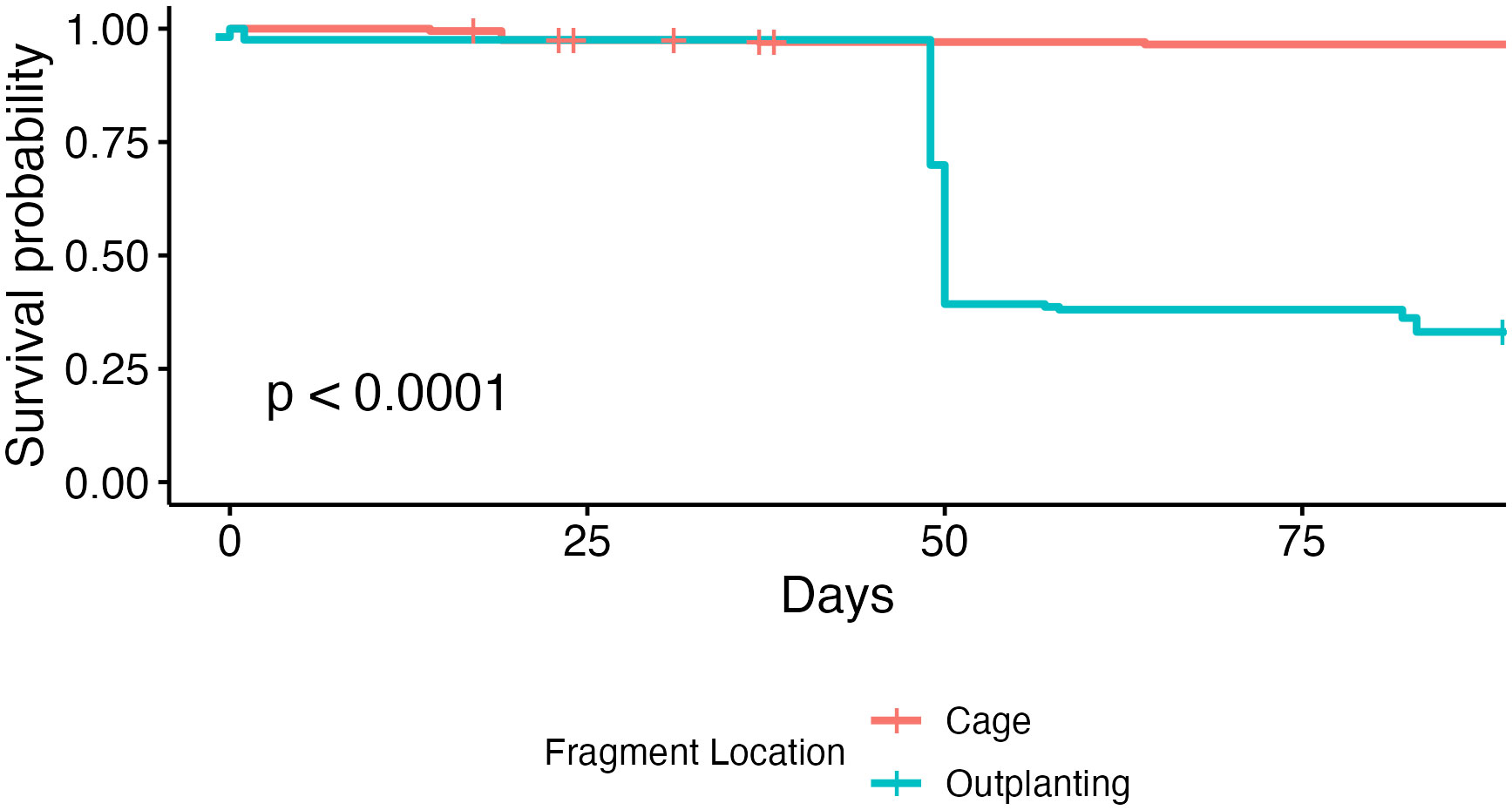
Figure 4 Kaplan-Meier survival curves of coral microfragments over time in both the predator exclusion cage and the field. Corals showed a significantly higher survival rate in the acclimation cage than on the reef.
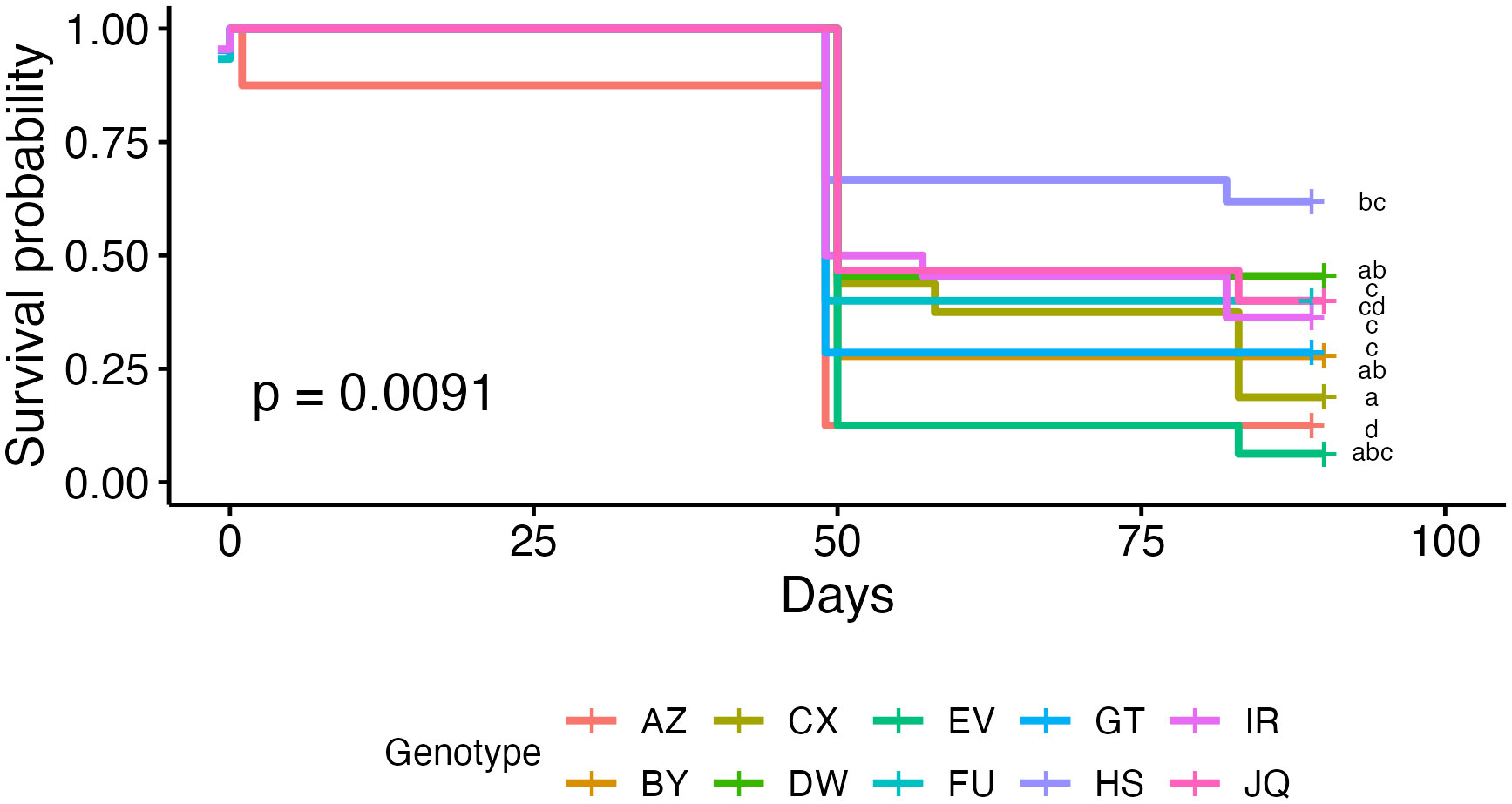
Figure 5 Kaplan-Meier survival curves of coral microfragments in the field grouped by genotype. Curves are grouped by significance using Tukey’s HSD (see pairwise comparisons in supplemental material, Table S1).
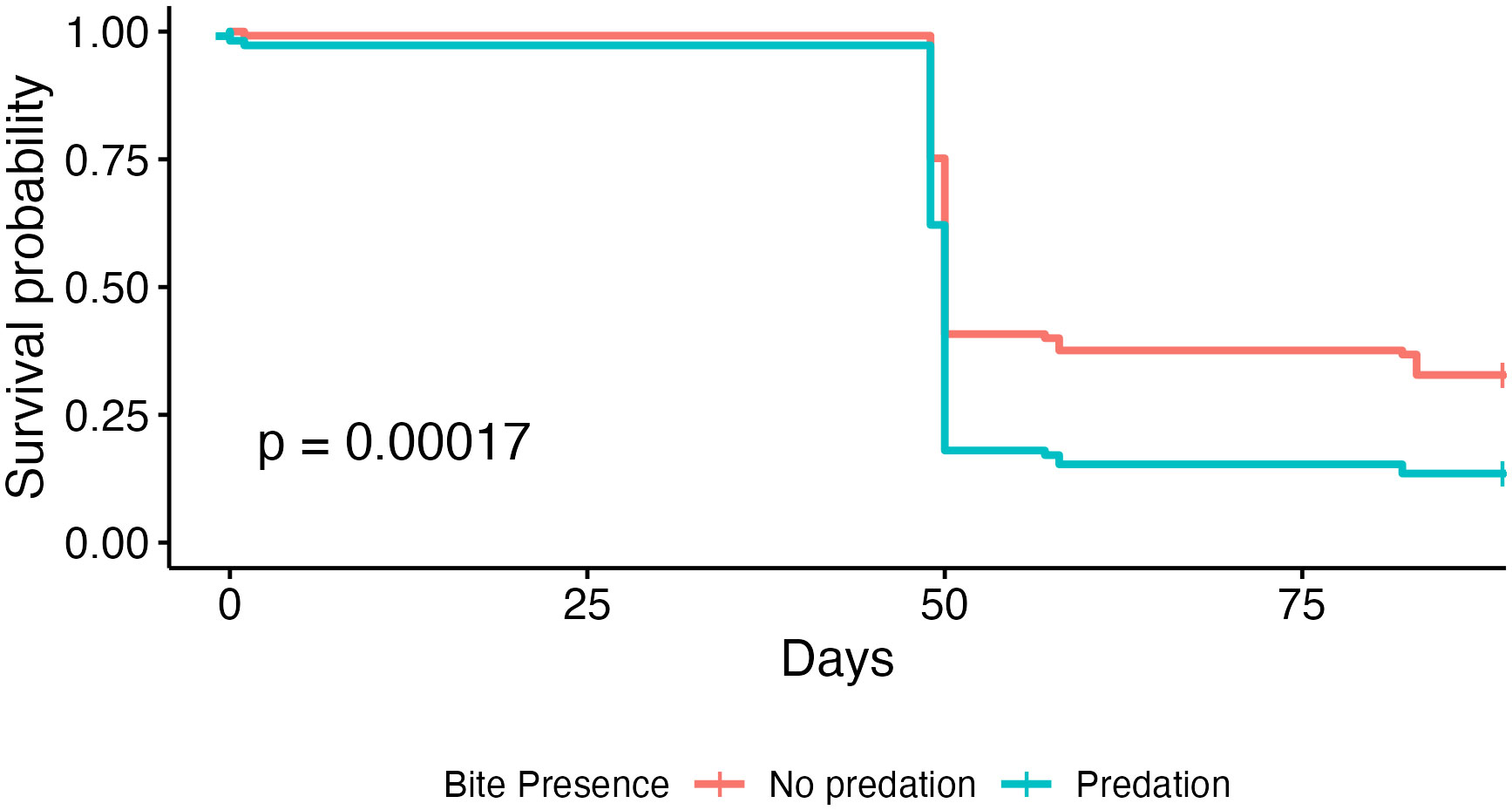
Figure 6 Kaplan-Meier survival curves of coral microfragments in the field grouped by evidence of predation.
There was also a significant difference in growth rates between the five acclimation treatments (p < 0.01). Additionally, coral microfragments also showed significantly higher growth rates in the acclimation cage than in the field (p < 0.001, Figure 7). The average growth rate in the cage was 10.7 mm2/month, while the average growth rate after outplanting was 1.8 mm2/month. In addition, different genotypes grew significantly faster than others (p < 0.001, Figure 8).
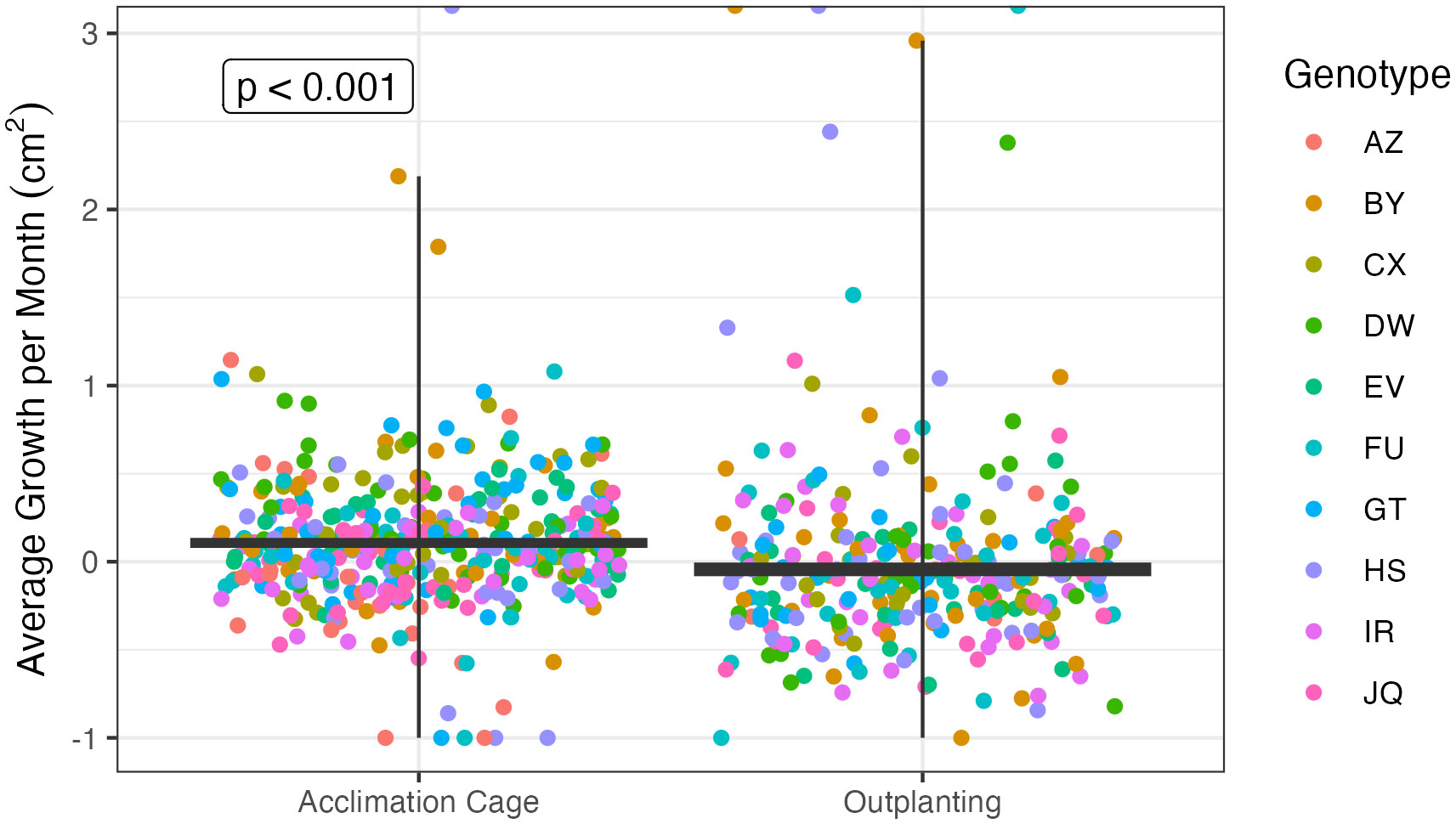
Figure 7 Average growth rates in square centimeters per month between all coral microfragments in the acclimation cages and on the reef after outplanting. Each point represents one microfragment. Corals showed a significantly higher growth rate in the acclimation cage than on the reef.
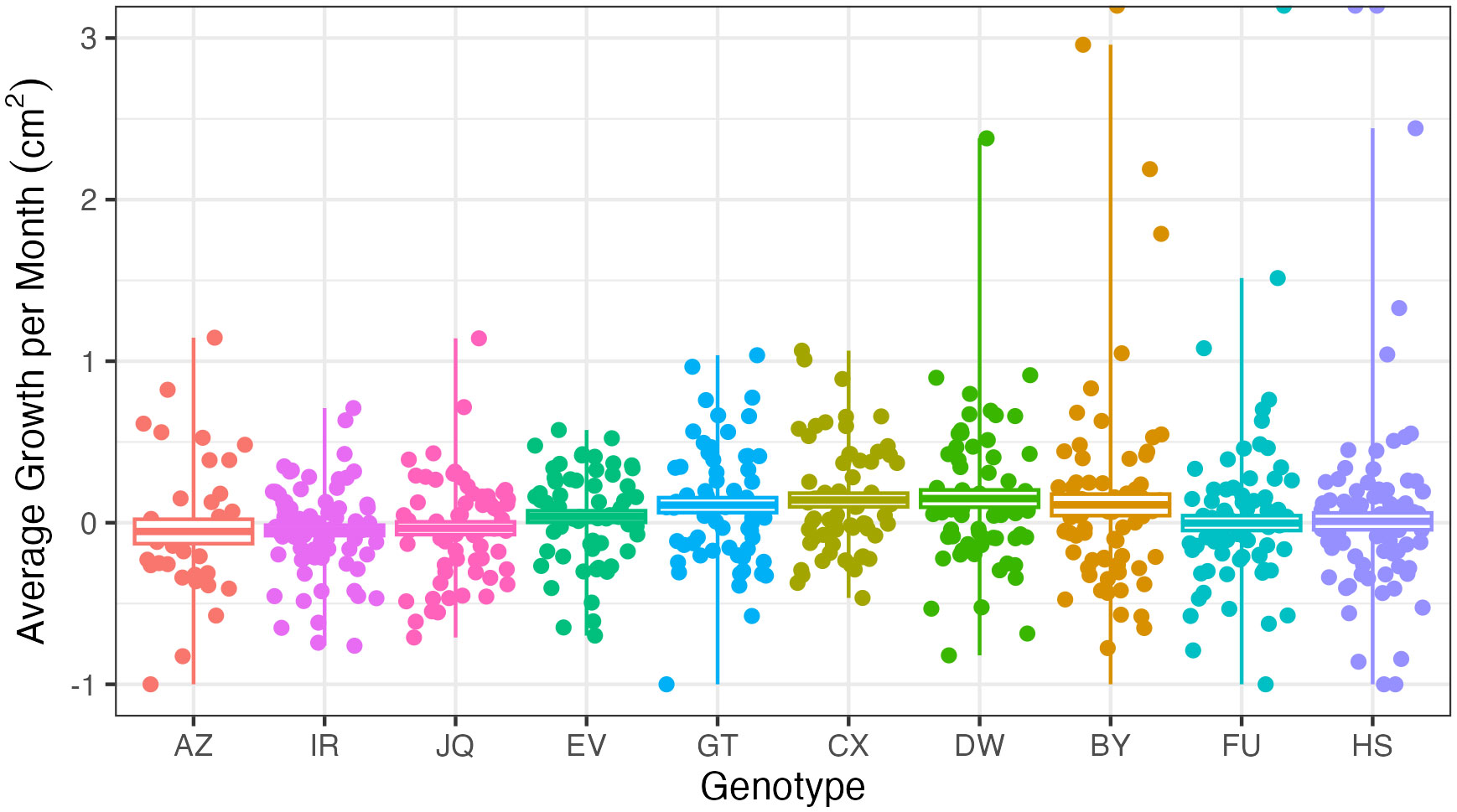
Figure 8 Growth in centimeters per month across genotypes: each point represents one microfragment. Note the large variation within genotypes. Negative growth rates indicate loss of live coral tissue, or coral mortality.
When modeling fragment survivorship over the entire experiment, genotype and fragment location were shown to have significant effects on survival rates. However, adding acclimation treatment as a parameter did not significantly strengthen the model (p = 0.8312). Modeling survivorship of fragments only in the field (i.e. fragment location is not a factor), genotype had a significant effect on survival rates, while including acclimation did not strengthen the model (p = 0.102).
Discussion
Microfragments that were acclimated for longer than one month showed slightly higher survival rates than those that had been acclimated for a shorter length of time or not at all. Our data suggest that initial field mortality is largely due to predation by corallivorous fish. Additionally, microfragments experienced almost zero mortality in the protective cage, which has important implications for future restoration strategies.
Previous studies show that aquacultured corals in protective cages exhibited an 85% survival rate, with most genotypes showing 100% survival in the cages by the end of the acclimation periods (Dela Cruz et al, 2015). The higher survival rate in the acclimation cage is likely due to the exclusion of predators, particularly parrotfish. Schools of juvenile parrotfish were observed to investigate the acclimation cage on a regular basis, and even swim inside while the cage was open to retrieve coral microfragments. While they prefer calcareous algae, juvenile parrotfish do graze on coral (Overholtzer and Motta, 1999; Feitosa and Ferreira, 2015). Adult parrotfish were also frequently observed in the area. Bite marks were observed on coral fragments within 24 hours of being outplanted on the reef. Stoplight parrotfish (Sparisoma viride) was also observed in the area, which is a major coral predator on Caribbean reefs (Scoffin et al., 1980; Harborne and Mumby, 2018). Higher rates of coral predation have been observed on shallow reefs, such as those where our restoration efforts took place. Previous restoration experiments have also observed that predation from reef fish caused the highest mortality rate among transplanted corals (Acropora hyacinthus, Acropora gemmifera, Pocillopora damicornis, and Porites cylindrica) (Morikawa and Palumbi, 2019). Restoration work with Orbicella faveolata in the Florida Keys also observed high predation rates directly after outplanting, although mortality rates were not recorded (Page et al., 2018). Research on O. annularis found that most mortality was from larger bite scars, and that any healing usually occurred in the first month (Rempel et al., 2020). Others found that multiple bite scars are more likely to lead to mortality (Welsh et al., 2014). As coral cover declines, corallivory may increase (Burkepile, 2012), which will greatly impact newly restored corals, as most restoration projects target locations that have already experienced a severe loss in coral cover. Initially grazed colonies are also more likely to be re-grazed (Rotjan and Dimond, 2010), which in the case of small microfragments, would quickly lead to total fragment mortality. We observed 19 distinct bite scars from parrotfish out of 163 corals at the end of three months of observation. As previously mentioned, it seems likely that a portion of “missing” corals were due to parrotfish predation as well, as large parrotfish could dislodge fragments from the rubble pieces. Our low survival rates in the field support the hypothesis that increased presence of herbivores prevent degraded reefs from recovering, as they contribute to high mortality rates of transplanted and recruited corals (Bruno et al., 2019).
Surprisingly, our results showed no significant difference in field survival rates between acclimation treatments (Figure 3). Fragments that had been acclimated in the cage for a longer period of time showed slightly higher survival rates, but not enough to draw significant conclusions. While not statistically significant, these results did exhibit a trend similar to our original hypothesis: that coral microfragments that spent time in a predator exclusion cage would show higher survival rates after outplanting. All microfragments that were protected in the cage for at least one month showed higher survival than microfragments protected in the cage for less than a month. Since the effects of protection from predation was strong, it is possible this caused any differences between acclimations to be too small to be observed. There was, however, a significant difference in growth rate between acclimation treatments (p < 0.01). Growth rates can change significantly during the first year of outplanting (Mahmoud et al., 2019), so time is most likely a confounding factor here. Corals that were fragmented earlier likely saw more changes to their growth rates, not because of acclimation effects, but rather because growth rates change the further away the coral is from fragmenting, regardless of location. If we monitored fragments for a longer period, we may see different results in both differences in growth rates and survival. While most predation on outplanted microfragments and associated mortality has been observed in the first three months (Page et al., 2018), ongoing mortality after this point could show more of a difference between acclimation treatments. Since our study showed a trend rather than significant results, other factors appear to play a bigger role in coral survivorship.
We observed different survival rates between genotypes in the field (Figure 5). Genotype HS performed especially well, while genotype AZ died in both the field and the acclimation cage. In reciprocal transplant experiments performed on Porites lobata, genotype was the most important factor to individual colony’s stress tolerance (Barshis et al., 2010). With the exception of genotype AZ, all genotypes showed near universal survival in the acclimation cage. Other researchers have observed high survivorship across genotypes in Acropora cervicornis nurseries (Goergen and Gilliam, 2018). We also observed significantly different growth rates between genotypes (p < 0.001). This has been well documented in A. cervicornis restoration efforts. In a survey of over 1,700 A. cervicornis fragments in various nurseries around the Caribbean, researchers observed significantly different growth rates between genotypes (Lirman et al., 2014). These differences are still observed when controlling for different locations (Drury et al., 2017). Calcification rates have been found to differ between A. cervicornis genotypes in a nursery environment as well (Kuffner et al., 2017). However, we also observed a large variation in growth rates within genotypes (Figure 8). This fits with some studies that have not observed significant differences between genotypes, largely due to small sample sizes (Shaish et al., 2008; Ladd et al., 2016). So while some genotypes survive at significantly higher rates than others, larger sample sizes are needed to draw conclusions about differences in growth rates. Microfragments also grew significantly faster in the acclimation cage than in the field (Figure 7). In protected environments, microfragments of O. faveolata have been found to produce 6.5 times more tissue than larger fragments (Page et al., 2018). While some studies have found that caged corals grew less than uncaged corals, this was primarily due to an increase in algae presence (Lirman, 2001). As our cages were regularly monitored and cleaned, this was not an issue. Our acclimation cage produced fast growing O. faveolata fragments with high survival rates.
Orbicella faveolata has declined throughout the Caribbean in recent decades. Indeed, the loss of massive coral cover in the Florida Keys has been almost all O. faveolata (Bruno et al., 2019). Further study is required to more comprehensively weight these options. Other studies have found that mixed species plots show higher survival rates after small disturbance events, with single species plots performing better after large scale disturbances (Dizon and Yap, 2006). It would be important to explore the effectiveness of mixing O. faveolata with other species, particularly to examine if this would mitigate the initial stress of outplanting. Previous research has found that low species richness can actually hinder reef recovery (Clements and Hay, 2019). In future restoration efforts focused on O. faveolata, we recommend that if corallivores are present, microfragments are caged for at least one month with periodic cleaning and monitoring to avoid algal overgrowth. While post-outplant survivorship still needs to be improved, caging fragments in a nursery will allow for an improvement in the source material (Dela Cruz et al., 2015). We also recommend identifying successful genotypes before engaging in large scale restoration efforts. Researchers can prioritize survivorship and growth, but should take into account other proxies such as microclimate, origin, and heat tolerance (Morikawa and Palumbi, 2019). Researchers should also be aware of possible impacts of genetic diversity, as there may be a tradeoff between focusing on more successful genotypes and maintaining genetic diversity in the local population.
Future research will delve further into the differences between genotypes by analyzing the corals’ microbiomes. Microbiome research will also allow us to more finely tease apart any differences between the different acclimation treatments. Microbiome analysis will also be useful when examining the impacts of predation. Parrotfish bites can cause a change in the microbial community, and predated corals show higher microbial diversity (Ezzat et al., 2020). Since physiological differences are a response to corallivory rather than a cause (Rotjan and Dimond, 2010), further investigation is needed to describe the relationship between newly restored corals and parrotfish predation. It is also possible that restored corals are producing secondary compounds to deter predators, which could change depending on coral genotype and acclimation treatment (Hay and Fenical, 1988). Changes in metabolomics could also be influenced by changes to the microbiome as a result of stress (Williams et al., 2021).
Coral acclimation cages do not require expensive materials, or complicated training to operate. Additionally, as microfragments survived at such high rates while still within the cage, acclimation cages may be helpful for restoration operations with limited space on shore. While they do require some additional dives, the dives are brief and not labor intensive. Moving forward, practitioners should consider caging corals during the restoration process, and additionally consider prioritizing high performing genotypes. More effective coral restoration strategies will help degraded reefs stand a better chance of recovery from ongoing stress from climate change.
Data availability statement
The datasets presented in this study can be found in online repositories. The names of the repository/repositories and accession number(s) can be found below: https://github.com/ceraker/Orbicella-faveolata-mortalityandgrowth.
Author contributions
CR, CP, MO-S, SW, EW conceived and designed the research. CR, CP, MO-S, EW performed the experiments. CR analyzed the data. CR drafted the manuscript. All authors contributed to the article and approved the submitted version.
Funding
This work was supported by NSF-OIA 2032919, USDA-NIFA 1017848 grants and NOAA NA22OAR4050072.
Acknowledgments
We would like to thank everyone at UPRM for the use of their facilities and their assistance with lab space, raceway setups, boat time, and many other necessities. Especial thank you to Francisco Gonalález-Casañas, Alejandro González-Montes, and Fernando Melendez-Vazquez for their assistance with coral planting. Thank you to Elizabeth Saraf for all her work processing photos. No conflict of interest to declare.
Conflict of interest
The authors declare that the research was conducted in the absence of any commercial or financial relationships that could be construed as a potential conflict of interest.
Publisher’s note
All claims expressed in this article are solely those of the authors and do not necessarily represent those of their affiliated organizations, or those of the publisher, the editors and the reviewers. Any product that may be evaluated in this article, or claim that may be made by its manufacturer, is not guaranteed or endorsed by the publisher.
Supplementary material
The Supplementary Material for this article can be found online at: https://www.frontiersin.org/articles/10.3389/fmars.2023.1122369/full#supplementary-material
References
Barshis D. J., Stillman J. H., Gates R. D., Toonen R. J., Smith L. W., Birkeland C. (2010). Protein expression and genetic structure of the coral porites lobata in an environmentally extreme Samoan back reef: does host genotype limit phenotypic plasticity? Mol. Ecol. 19, 1705–1720. doi: 10.1111/j.1365-294X.2010.04574.x
Bruckner A. W., Bruckner R. J. (2006)The recent decline of montastraea annularis (complex) coral populations in western curaçao: a cause for concern? (Accessed July 2, 2020).
Bruno J. F., Côté I. M., Toth L. T. (2019). Climate change, coral loss, and the curious case of the parrotfish paradigm: why don’t marine protected areas improve reef resilience? Ann. Rev. Mar. Sci. 11, 307–334. doi: 10.1146/annurev-marine-010318-095300
Burkepile D. E. (2012). Context-dependent corallivory by parrotfishes in a Caribbean reef ecosystem. Coral Reefs 31, 111–120. doi: 10.1007/S00338-011-0824-5
Burkepile D. E., Hay M. E. (2006). Herbivore vs. nutrient control of marine primary producers: context-dependent effects. Ecology 87, 3128–3139. doi: 10.1890/0012-9658(2006)87[3128:hvncom]2.0.co;2
Clements C. S., Hay M. E. (2019). Overlooked coral predators suppress foundation species as reefs degrade. Ecol. Appl. 28, 1673–1682. doi: 10.1002/eap.1765.Overlooked
Dela Cruz D. W., Rinkevich B., Gomez E. D., Yap H. T. (2015). Assessing an abridged nursery phase for slow growing corals used in coral restoration restoration of coral on reefs using enhanced larval supply view project coral farming view project assessing an abridged nursery phase for slow growing corals used in co. Ecol. Eng. 84, 408–415. doi: 10.1016/j.ecoleng.2015.09.042
Dizon R. M., Yap H. T. (2006). Effects of multiple perturbations on the survivorship of fragments of three coral species. Mar. Pollut. Bull. 52, 928–934. doi: 10.1016/j.marpolbul.2005.12.009
Doherty P. J., Sale P. F. (1985). Predation on juvenile coral reef fishes: an exclusion experiment. Coral Reefs 4, 225–234. doi: 10.1007/BF00298081
Drury C., Manzello D., Lirman D. (2017). Genotype and local environment dynamically influence growth, disturbance response and survivorship in the threatened coral, acropora cervicornis. PloS One 12. doi: 10.1371/journal.pone.0174000
Edmunds P. J. (2015). A quarter-century demographic analysis of the Caribbean coral, Orbicella annularis , and projections of population size over the next century. Limnol. Oceanogr. 60, 840–855. doi: 10.1002/lno.10075
Ezzat L., Lamy T., Maher R. L., Munsterman K. S., Landfield K. M., Schmeltzer E. R., et al. (2020). Parrotfish predation drives distinct microbial communities in reef-building corals. Anim. Microbiome 2, 1–15. doi: 10.1186/s42523-020-0024-0
Feitosa J. L. L., Ferreira B. P. (2015). Distribution and feeding patterns of juvenile parrotfish on algal-dominated coral reefs. Mar. Ecol. 36, 462–474. doi: 10.1111/MAEC.12154
Forsman Z. H., Page C. A., Toonen R. J., Vaughan D. (2015). Growing coral larger and faster: micro-colony-fusion as a strategy for accelerating coral cover. PeerJ 3, e1313. doi: 10.7717/peerj.1313
García-Sais J. R., Williams S. M., Amirrezvani A. (2017). Mortality, recovery, and community shifts of scleractinian corals in Puerto Rico one decade after the 2005 regional bleaching event. PeerJ 2017. doi: 10.7717/peerj.3611
Goel M. K., Khanna P., Kishore J. (2010). Understanding survival analysis: Kaplan-Meier estimate. Int. J. Ayurveda Res. 1, 274–278. doi: 10.4103/0974-7788.76794
Goergen E. A., Gilliam D. S. (2018). Outplanting technique, host genotype, and site affect the initial success of outplanted acropora cervicornis. PeerJ 2018. doi: 10.7717/peerj.4433
Harborne A. R., Mumby P. J. (2018). FAQs about Caribbean parrotfish management and their role in reef resilience. Biol. Parrotfishes, 383–406. doi: 10.1201/9781315118079-16
Hay M. E., Fenical W. (1988). Marine plant-herbivore interactions: the ecology of chemical defense. Annu. Rev. Ecol. Syst. Vol. 19, 111–145. doi: 10.1146/annurev.es.19.110188.000551
Koval G., Rivas N., D’Alessandro M., Hesley D., Santos R., Lirman D. (2020). Fish predation hinders the success of coral restoration efforts using fragmented massive corals. PeerJ 8. doi: 10.7717/peerj.9978
Kuffner I. B., Bartels E., Stathakopoulos A., Enochs I. C., Kolodziej G., Toth L. T., et al. (2017). Plasticity in skeletal characteristics of nursery-raised staghorn coral, acropora cervicornis. Coral Reefs 36, 679–684. doi: 10.1007/s00338-017-1560-2
Ladd M. C., Shantz A. A., Nedimyer K., Burkepile D. E. (2016). Density dependence drives habitat production and survivorship of acropora cervicornis used for restoration on a caribbean coral reef. Front. Mar. Sci. 3. doi: 10.3389/fmars.2016.00261
Leong W., Pawlik J. R. (2010). Evidence of a resource trade-off between growth and chemical defenses among Caribbean coral reef sponges. Mar. Ecol. Prog. Ser. 406, 71–78. doi: 10.3354/meps08541
Levy G., Shaish L., Haim A., Rinkevich B. (2010). Mid-water rope nursery-testing design and performance of a novel reef restoration instrument. Ecol. Eng. 36, 560–569. doi: 10.1016/j.ecoleng.2009.12.003
Lirman D. (2001). Competition between macroalgae and corals: effects of herbivore exclusion and increased algal biomass on coral survivorship and growth rescue a reef citizen science program view project south Florida MARES project view project. Coral Reefs 19, 392–399. doi: 10.1007/s003380000125
Lirman D., Schopmeyer S. (2016). Ecological solutions to reef degradation: optimizing coral reef restoration in the Caribbean and Western Atlantic. PeerJ 10. doi: 10.7717/PEERJ.2597/FIG-4
Lirman D., Schopmeyer S., Galvan V., Drury C., Baker A. C., Baums I. B. (2014). Growth dynamics of the threatened Caribbean staghorn coral acropora cervicornis: influence of host genotype, symbiont identity, colony size, and environmental setting. PloS One 9, e107253. doi: 10.1371/journal.pone.0107253
Mahmoud M. A. M., Dar M. A., Hussein H. N. M., El-Metwally M. E. A., Maaty M. M., Omar M. Y., et al. (2019)Survivorship and growth rates for some transplanted coral reef building species and their potential for coral reef rehabilitation in the red Sea (Accessed March 10, 2020).
Miller M. W., Hay M. E. (1998). Effects of fish predation and seaweed competition on the survival and growth of corals. Oecologia 113, 231–238. doi: 10.1007/s004420050373
Miller M. W., Marmet C., Cameron C. M., Williams D. E. (2014). Prevalence, consequences, and mitigation of fireworm predation on endangered staghorn coral. Mar. Ecol. Prog. Ser. 516, 187–194. doi: 10.3354/meps10996
Morikawa M. K., Palumbi S. R. (2019). Using naturally occurring climate resilient corals to construct bleaching-resistant nurseries. PNAS 116, 10586–10591. doi: 10.1073/pnas.1721415116
Mumby P. J., Dahlgren C. P., Harborne A. R., Kappel C. V., Micheli F., Brumbaugh D. R., et al. (2006). Fishing, trophic cascades, and the process of grazing on coral reefs. Science 311, 98–101. doi: 10.1126/SCIENCE.1121129
Overholtzer K. L., Motta P. J. (1999). Comparative resource use by juvenile parrotfishes in the Florida keys. Mar. Ecol. Prog. Ser. 177, 177–187. doi: 10.3354/meps177177
Page C. A., Muller E. M., Vaughan D. E. (2018). Microfragmenting for the successful restoration of slow growing massive corals. Ecol. Eng. 123, 86–94. doi: 10.1016/J.ECOLENG.2018.08.017
Pawlik J. R., Loh T.-L., Mcmurray S. E., Finelli C. M. (2013). Sponge communities on Caribbean coral reefs are structured by factors that are top-down, not bottom-up. PloS One 8, 62573. doi: 10.1371/journal.pone.0062573
Porto-Hannes I., Zubillaga A. L., Shearer T. L., Bastidas C., Salazar C., Coffroth M. A., et al. (2015). Population structure of the corals orbicella faveolata and acropora palmata in the mesoamerican barrier reef system with comparisons over Caribbean basin-wide spatial scale. Mar. Biol. 162, 81–98. doi: 10.1007/s00227-014-2560-1
Rempel H. S., Bodwin K. N., Ruttenberg B. I. (2020). Impacts of parrotfish predation on a major reef-building coral: quantifying healing rates and thresholds of coral recovery. Coral Reefs 39, 1441–1452. doi: 10.1007/s00338-020-01977-9
Rinkevich B. (2015). Climate change and active reef restoration–ways of constructing the “Reefs of tomorrow”. J. Mar. Sci. Eng. 3, 111–127. doi: 10.3390/jmse3010111
Roff G., Zhao J. X., Mumby P. J. (2015). Decadal-scale rates of reef erosion following El niño-related mass coral mortality. Glob. Change Biol. 21, 4415–4424. doi: 10.1111/GCB.13006
Rotjan R. D., Dimond J. L. (2010). Discriminating causes from consequences of persistent parrotfish corallivory. J. Exp. Mar. Bio. Ecol. 390, 188–195. doi: 10.1016/j.jembe.2010.04.036
Rotjan R. D., Lewis S. M. (2008). Predators selectively graze reproductive structures in a clonal marine organism. Mar. Biol. doi: 10.1007/s00227-008-1108-7
Scoffin T., Stearn C., Boucher D., Frydl P., Hawkins C., Hunter I., et al. (1980). Calcium carbonate budget of a fringing reef on the west coast of Barbados: part II. Erosion, sediments and internal structure. Bull. Mar. Sci. 30, 475–508.
Shaish L., Levy G., Gomez E., Rinkevich B. (2008). Fixed and suspended coral nurseries in the Philippines: establishing the first step in the “gardening concept” of reef restoration. J. Exp. Mar. Bio. Ecol. 358, 86–97. doi: 10.1016/j.jembe.2008.01.024
Shaish L., Levy G., Katzir G., Rinkevich B. (2010). Employing a highly fragmented, weedy coral species in reef restoration. Ecol. Eng. 36, 1424–1432. doi: 10.1016/j.ecoleng.2010.06.022
van Oppen M. J. H., Gates R. D., Blackall L. L., Cantin N., Chakravarti L. J., Chan W. Y., et al. (2017). Shifting paradigms in restoration of the world’s coral reefs. Glob. Change Biol. 23, 3437–3448. doi: 10.1111/GCB.13647
Weil E., Croquer A., Urreiztieta I. (2009). Temporal variability and impact of coral diseases and bleaching in la parguera, Puerto Rico from 2003–2007. Caribb. J. Sci. 45, 221–246. doi: 10.18475/CJOS.V45I2.A10
Weil E., Rogers C. S. (2011). “Coral reef diseases in the Atlantic-Caribbean,” in Coral reefs: an ecosystem in transition. eds. Dubinsky Z., Stambler N. (Dordrecht: Springer), 465–491. doi: 10.1007/978-94-007-0114-4_27
Welsh J. Q., Bonaldo R. M., Bellwood D. R. (2014). Clustered parrotfish feeding scars trigger partial coral mortality of massive porites colonies on the inshore great barrier reef natural history of fish of the bonito and pantanal-Brazil view project computed tomography view project. Coral Reefs 34, 81–86. doi: 10.1007/s00338-014-1224-4
Williams A., Chiles E. N., Conetta D., Pathmanathan J. S., Cleves P. A., Putnam H. M., et al. (2021). Metabolomic shifts associated with heat stress in coral holobionts. Sci. Adv. 7, eabd4210. doi: 10.1126/sciadv.abd4210
Williams S. M., Mumby P. J., Chollett I., Cortés J. (2015). Importance of differentiating orbicella reefs from gorgonian plains for ecological assessments of Caribbean reefs. Mar. Ecol. Prog. Ser. 530, 93–101. doi: 10.3354/meps11333
Williams I. D., Polunin N. V. C. (2001). Large-Scale associations between macroalgal cover and grazer biomass on mid-depth reefs in the Caribbean. Coral Reefs 19, 358–366. doi: 10.1007/s003380000121
Keywords: coral restoration, corallivory, predator exclusion, Caribbean, reef conservation
Citation: Raker C, Olmeda-Saldaña M, Williams SM, Weil E and Prada C (2023) Use of predator exclusion cages to enhance Orbicella faveolata micro-fragment survivorship and growth during restoration. Front. Mar. Sci. 10:1122369. doi: 10.3389/fmars.2023.1122369
Received: 12 December 2022; Accepted: 31 May 2023;
Published: 15 June 2023.
Edited by:
Carla Zilberberg, Federal University of Rio de Janeiro, BrazilReviewed by:
Marilyn Brandt, University of the Virgin Islands, US Virgin IslandsStephanie Schopmeyer, Florida Fish and Wildlife Research Institute, United States
Thomás Banha, University of São Paulo, Brazil
Copyright © 2023 Raker, Olmeda-Saldaña, Williams, Weil and Prada. This is an open-access article distributed under the terms of the Creative Commons Attribution License (CC BY). The use, distribution or reproduction in other forums is permitted, provided the original author(s) and the copyright owner(s) are credited and that the original publication in this journal is cited, in accordance with accepted academic practice. No use, distribution or reproduction is permitted which does not comply with these terms.
*Correspondence: Catherine Raker, Y2VyYWtlckB1cmkuZWR1