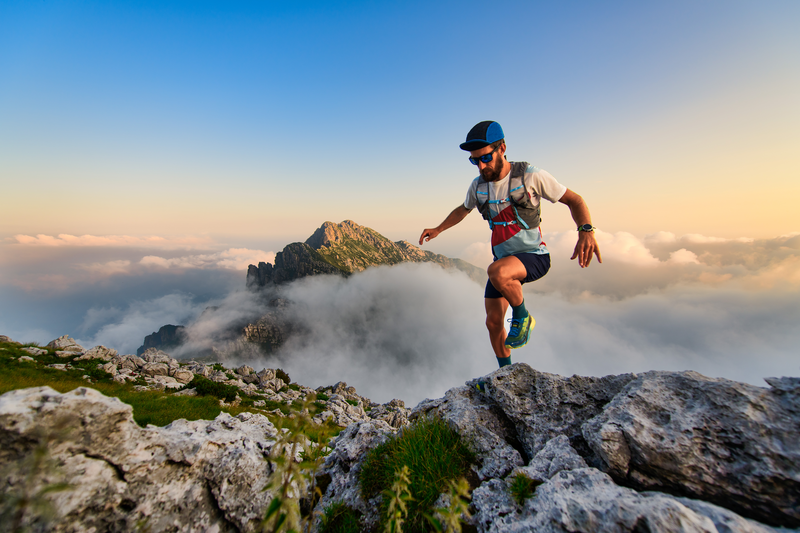
94% of researchers rate our articles as excellent or good
Learn more about the work of our research integrity team to safeguard the quality of each article we publish.
Find out more
ORIGINAL RESEARCH article
Front. Mar. Sci. , 20 February 2023
Sec. Marine Conservation and Sustainability
Volume 10 - 2023 | https://doi.org/10.3389/fmars.2023.1118894
This article is part of the Research Topic Carbon Sinks in Coastal Wetlands: Influences From Multiple Factors View all 5 articles
Coastal reclamation is becoming a common land restoration trend all over the world as a result of the rising demand for land. Though restoring coastal wetlands has significant economic advantages, a slew of adverse ecological effects threatens the carbon functions of coastal wetlands. For the objective of making appropriate land use policymaking, the ecological-economic trade-offs of reclamation initiatives must be carefully considered. In this study, we utilized appropriate models to estimate the ecosystem service values and economic benefits of invasive alien plants and embankment seawall coastal reclamation in China. We centered on three main ecology-related ecosystem services: greenhouse gas regulation, storm-flood-erosion control, and waste treatment/habitat preservation. Coastal reclamation intensity index and financial analysis were utilized to assess the trade-offs between ecological degradation and economic benefit resulting from land reclamation. Findings reveal that a total of 26,322 ha and 10,731 km of coastal wetlands were reclaimed by Spartina alterniflora and seawalls respectively from 2000 to 2020 in China. S. alterniflora reclamation resulted in a significant decline of ESV loss of 5,702,454 Yuan ha−1, while seawalls reclamation yielded some Ecosystem service value (ESV) gain of 4,800, 111 Yuan km-1 from 2000-2020. The combined effects of coastal armoring and invasive S. alterniflora reclamation led to a loss of about 32.2 billion Yuan in ESV for the study duration. Economic gains failed to make up for the ecosystem service value ESV loss, since the cumulative ESV loss significantly exceeded the economic gains across the period studied. This correlation of trade-offs emerged from reclamation development that favored quick economic gains over long-lasting ecological value, posing a potential long-term threat to the ecological integrity and carbon sinks in coastal wetlands. To establish an equilibrium between seawall reclamation and Invasive alien plant species spread in coastal wetlands, stakeholders could use this scientific knowledge as leverage to avert future irreparable losses.
Coastal reclamation is a prospective land reclamation approach for creating new land or enclosing the sea and tidal flats for multiple uses. Two of the most widely used coastal land reclamation measures include invasive alien plant species (IAPS) and seawalls; which are walls or embankments constructed to prevent the sea from infringing on or degrading a piece of land. Reclamation of coastal wetlands on a large scale has now become a major land use concern around the world (Qiu et al., 2021; Wang et al., 2021). Although coastal reclamation has significant economic benefits and promotes regional development, manmade environmental change drivers such as pollution, altered land use, overexploitation, and ecosystem degradation have negative impacts on the structure and function of coastal ecosystems (Bowen et al., 2019; Wang et al., 2021; Yu et al., 2021). IAPS and coastal armoring (CA) structures have been introduced, imported, and spread throughout key coastal habitats around the world as a result of efforts to reclaim and preserve these depleted coastal habitats in recent times (Ma et al., 2014; Li et al., 2021).
According to the Intergovernmental Science-Policy Platform on Biodiversity and Ecosystem Services (IPBES) of the United Nations (UN), IAPS threatens 20% of the earth’s surface, including biodiversity hotspots (Ngo et al., 2019). Concurrently, coastlines have changed dramatically, prompting the armoring of coastlines through the construction of seawalls, which have been vital since ancient times (Ma et al., 2014). As a result, ecosystems and the ecosystem services they provide have been significantly depleted (Dugan et al., 2018; Jaramillo et al., 2021). Global reports of declining coastal biodiversity have been related to anthropogenic activities (MEA, 2005; IPBES, 2019; Newton et al., 2020; Sandoval Gallardo et al., 2021). On a global scale, three most comprehensive studies have reported declines in coastal biodiversity. These studies revealed coastal biodiversity loss as being predominantly caused by IAPS and coastal armoring (MEA, 2005; IPBES, 2019; Newton et al., 2020). Additionally, current worldwide studies have underlined the importance of wetland ecosystems, especially the knowledge of coastal wetlands, in assessing global ecosystem effects (Brown et al., 2021; Zeng et al., 2021). Some comprehensive studies (Schindler et al., 2018; Bartz and Kowarik, 2019; Kumar Rai and Singh, 2020), have reported IAPS to cause a reduction in native plant biodiversity, which have consequential implications for environmental, health, ecosystem, and global warming. The adverse effects of coastal armoring on coastal ecosystems around the world, on the other hand, are swiftly becoming widely recognized (Dugan et al., 2018; Jaramillo et al., 2021).
A global overview of coastal land reclamation reveals that massive land reclamation initiatives have been particularly noticeable in China. Since the 1950s, China’s clamor for coastal reclamation has increased significantly due to its economic growth (Ma et al., 2014). From 1979 to 2014, 11,162 km2 of land has been reclaimed (Meng et al., 2017), while the loss of coastal wetlands in China since 1949 is estimated to be on a scale of 22,000 km2 (Sengupta et al., 2018), with the conversion of coastal wetland vegetation to tidal mudflats and sub-tidal zones attributable to the adoption of geo-engineering construction techniques (Zhu et al., 2016). The rapid spread of IAPS and coastal armored structures may cause irreparable damage to coastal wetland ecosystems, putting a strain on the long-term viability of coastal wetland resources. As a result, thorough scrutiny of IAPS and coastal armoring and their trade-offs are required to adequately grasp how their continued expansion in China’s coastal ecosystems will shape community structure, biodiversity, and habitat in Asia and worldwide.
World regions have in recent times resorted to natural solutions to coastal erosion that reduce sediment loss and promote ecosystem function. This prompted the introduction of S. alterniflora and other IAPS to China. S. alterniflora was brought into China in 1979 as a tide barrier and to speed up the formation of coastal wetlands. Ever since, spontaneous dispersal and replication have caused this invasive plant to spread rapidly across coastal China, with typically detrimental ecological consequences (Meng et al., 2020). S. alterniflora was declared an invasive species in China in 2003 and was listed in the first inventory of alien invasive species based on a collaboration between China’s State Environmental Protection Administration and the Chinese Academy of Sciences (Wang et al., 2006). By 2007, it had expanded to 34,178 ha, with Jiangsu’s coastal areas responsible for about 17,842 ha (52%) (Lu and Zhang, 2013). It now occupies 50,000 ha of China’s land compared to ~260 ha in 1985 (Meng et al., 2020).
Because of its diverse habitats and environmental conditions, China is particularly prone to the spread of invasive species from other countries. S. alterniflora spread has presently impacted Chinese wetlands, affecting productivity, soil hydrology, hydrodynamics, sedimentation, soil structure, and nutrient loading. The usage of Sonneratia apetala as an alternate plant species to replace S. alterniflora is effective on some China islands (Tang et al., 2007). The benefits and drawbacks of these alterations vary according to the area and habitat quality. In certain areas, S. alterniflora, for instance, plays a vital role in coastline conservation, while in others, it has hampered aquaculture activity (Wan et al., 2009; Chen et al., 2018; Gao et al., 2018). Numerous studies have explored its advantages and disadvantages, as well as evolutionary processes and management (Jiang et al., 2009; Chen et al., 2012).
The implications of IAPS as a fast-growing risk to ecosystem delivery of services and local biodiversity loss are widely acknowledged (Kumar Rai and Singh, 2020). By mending putative gaps or displacing native species, IAPS have occupied the phenotypic and operational space within the diverse array of species (Loiola et al., 2018; Dalle Fratte et al., 2019). According to an assessment of the globe’s ecosystem services, 60% are in poor condition due to anthropogenic causes such as loss of habitat and alterations, invasive alien species, and climate change (MEA, 2005). Certain species have been reported as being very invasive globally, especially S. alterniflora, S. anglica, and S. densiflora, and are listed in the IUCN’s International Invasive Species Database (ISSG, 2018).
The largest invasion in China is by S. alterniflora, which was introduced from the Atlantic coasts of the United States, and has quickly spread across China, covering about 50,000 acres of land (Meng et al., 2020). These IAPS are a global problem that threatens ecosystem biodiversity by causing the extinction of native species and interrupting anthropogenic activities due to habitat damage (Chen, 2019). IAPS are known to have harmful consequences on biodiversity. Depending on the region, maturity, and kind of habitat concerned, the invasive tendency of S. alterniflora in China’s coastal wetlands could have varying consequences on biodiversity. S. alterniflora spreads and outcompetes native plants for growth area due to its biotic features and tolerance. The invasion by S. alterniflora altered the main macrobenthos species in the native habitats and affected macrobenthos diversity in seagrass and unvegetated areas (Quan et al., 2016; Su et al., 2020). By altering carbon sequestration and impacting biodiversity, S. alterniflora invasion has the potential to influence the ecological integrity of southern China’s coastal habitats (Su et al., 2020).
Coastal ecosystems regulate gas emissions in two ways. Thus, gas control plays a vital role in minimizing climate change by enabling carbon to be sequestered. Negative gas regulation, on the other hand, might result in higher amounts of CH4 and N2O emissions in wetland environments. The average carbon dioxide (CO2) methane (CH4), and nitrous oxide (N2O), fluxes over China’s coastal wetlands were estimated to be 3.41 x 106 mg m-2 yr-1, 1.92 x 104 mg m-2 yr-1, and 1.44 x 105 µgm-2yr-1, respectively (Hu et al., 2020). A significant link between CO2 fluxes and SOC, which was linked to higher levels of C feedstock and availability of nutrients, could well have resulted in increased C emission by vegetation and microbial activity control. The invasive S. alterniflora s associated with more CH4 and CO2 emissions than native plants, increasing the risk of global warming. Furthermore, in coastal wetlands, CH4 and N2O fluxes have a significant response to global warming, which is mostly mediated by CH4 emissions (Hu et al., 2020). According to China’s national wetland conservation action plan, wetland reclamation has a carbon sequestration capacity of 6.57 Gg C a-1 (Xiaonan et al., 2008). Particulate organic carbon POC sequestration rates were 141 ± 17 g C m-2 y-1 at sites above mean sea level and 381± 10 g C m-2 y-1 at sites below mean sea level. The high rates of PIC accumulation 916 ± 133 g C m-2 y-1 indicate the Yellow River delta’s high concentration of dissolved inorganic carbon (Ye et al., 2015). Compared to native species populated marshes, S. Alterniflora’s introduction in China’s coastal marshes has a remarkably higher CO2 assimilation potential (3.16 Mg C ha-1 yr-1), a 1: 4 ratio increase (Yuan et al., 2015).
Stored CO2 had an approximate average value of 1200 Yuan ton-1 in 2010, whereas released O2 had an approximate average value of 376 Yuan ton-1 (Lin et al., 2019). Currently, the value of gas flux and gas control in the coastal environment ranges from 0.24 x 104 Yuan ha-1 in Mariculture to 2.10 x 104 Yuan ha-1in croplands, attributable to, the transformation of farmlands to developed land resulting in increased weakening of the greenhouse gas control function of coastal ecosystems (Qiu et al., 2021).
Waves can be dissipated by vegetation plants, such that the more noticeably the wave height is attenuated, the broader the breadth of the plants connected (Barbier et al., 2008). Due to its dense plant population and superior wave-damping capabilities, S. alterniflora had a wave-damping capacity that is ten times greater than that of the mangrove (Qin et al., 1998). A perennial grass native to the Atlantic coast of the Americas called S. alterniflora has been introduced to many coastal areas of China primarily to prevent coastal wetland erosion, shoreline protection from storm damage, and enhance coastal reclamation. Exotic S. alterniflora seems to enhance soil erosion resilience (Yang and Guo, 2018). It accomplishes this by diminishing the energy of tidal waves, retaining sediment, and promoting vertical accretion (Zhang et al., 2017; Meng et al., 2020). S. alterniflora has been observed to preserve seawalls, compared to seawalls without S. alterniflora distribution. Additionally, S. alterniflora elevates the ground by forming biomass in its roots and deposits plant and organic debris on the ground’s surface. In Jiangsu coastal areas, salt marshes with S. alterniflora coverage gain more than 1000 ha of new land each year compared to mudflats (Meng et al., 2020). In comparison to S. alterniflora, which was less negatively impacted, increased inundation salinity dramatically reduced the physicochemical properties of native plants. Increased flooding depths ha rmed S. alterniflora’s ability to set seeds, but a significant positive impact on its ability to survive. Increased flooding rates had a negative impact on S. alterniflora’s height, but a positive impact on the production of new stems (Xue et al., 2018). Even though a large portion of China’s population (> 60%) lives in coastal regions, modifying enormous territories through reclamation to accommodate urban growth and industrialization, S. alterniflora wetlands have the potential to conserve these lands (Meng et al., 2020).
In China, invasive plants outcompete native plants in significantly N-limited habitats, and invasiveness increases with N addition, implying that continuing eutrophication of many ecosystems will likely drive exotic plant invasions even further (Wang et al., 2019). Exotic plant invasions in coastal wetland habitats cause significant phosphorus (P) accumulation in the soils, resulting in substantial pollution and eutrophication (Li et al., 2018). The mean Total phosphorous concentration for different S. alterniflora invasion times was 675.37 mg kg-1, and ranges from 160.33–1071 mg kg-1 (Li et al., 2018). S. alterniflora’s ability to accumulate ≥ 70 and ≥15 kg P by ha in soil and standing plant biomass respectively, demonstrates that the ecosystem N:P ratio may drop, with implications for below- and aboveground food cycles in the long term. Furthermore, the depletion of carbon and nutrients reduces the system’s overall reproductive capacity, making future reemergence of dense mangrove ecosystems difficult (Wang et al., 2019). The displacement of native marsh habitat by exotic plant Phragmites australis, leads to the deterioration of the soil’s ability to retain Phosphorus (Wang et al., 2016). Nutrient augmentation of exotic A. philoxeroides’ allelopathic effects on native species suggests that attributable to a unique pathway, eutrophication could significantly boost exotic plant invasion potential and speed up their territorial spread (Xiao et al., 2019).
In China and other parts of Asia, the rapid expansion of coastal armoring and the reclamation of intertidal zones through the deployment of seawalls and other manmade coastal structures has been unparalleled in terms of frequency and intensity (Choi et al., 2018). The recent loss of over two-thirds of tidal flats in the Yellow Sea, a globally unique environment with tremendous ecological significance, is one of the most conspicuous occurrences (Yim et al., 2018). While the most damaging consequences of China’s recent major coastal land reclamation activity on biodiversity have been extensively reported, several recent research has stressed the ecological benefits afforded by such manmade coastal intervention in China (Choi et al., 2018). This could be worrisome because, while exploring the “modern ecology” resulting from coastal alteration is beneficial, drawing general conclusions about the ecological position or sustainability gains from coastal embankment development without sufficient conceptualization trivializes the ecological impacts of mega coastal reclamation projects and may jeopardize biodiversity conservation efforts. The domination by essentially “new” seawalls throughout the China coastline is due to the rising extent and pace of conversion of original coastal wetlands into fishponds and other enterprises. Coastal expansion seawalls erected primarily to create additional land on a huge scale in China have more rapid yields compared to seawalls established primarily as permanent defenses for existing coastlines in other world regions (Asselen et al., 2013). With major additional seawall development currently slated and approved, at least 3,387 km2 of tidal flats are planned to be transformed between 2005 and 2020 in China’s Yellow Sea (Wang et al., 2014). As a result, protecting declining tidal flat ecosystems, restoring degraded habitats, and mitigating irreparable losses will become increasingly important.
Since the 1960s, coastal armoring has caused the loss of an estimated 10,520 km2 of China’s tidal flats and shallow waters (Choi et al., 2018). The span of these seawalls has increased over the last twenty years, surpassing 11,000 km of the entire 18,000 km coastline in 2010 (Guan, 2013). Such enormous coastal reclamation, frequently accompanied by the incorporation of sediment-promoting exotic plants like S. alterniflora, and sand churning has resulted in a significant reduction in biodiversity and related ecological processes, with even worse repercussions anticipated in the not-too-distant future. The accelerated loss of shorebird communities in the East Asian–Australasian flyway, which is presently the migratory route for waterbirds, is due to the deterioration of wetlands, which now encompasses the largest percentage (19%) of vulnerable waterbird populations of the worldwide flyways (Piersma et al., 2016; Studds et al., 2017). After reclamation, wetlands are transformed from pollution sinks to sources, generating contaminants from industrial and agricultural activities and chemicals from aquacultures, like antibiotics, leading to the degradation of onshore and marine habitats (CCICED, 2018). In 2010, it was anticipated that the reclamation of China’s coastal wetlands would result in a loss of $31 billion in ecosystem services each year, accounting for nearly 6% of China’s overall marine products (CCICED, 2018). By the year 2020, 250,000 ha of coastal wetlands will be enclosed for infrastructural projects and other wetlands activities, with the reclamation pace, predicted to accelerate to 60,000 ha/year leading to the loss of more coastal biodiversity (Ma et al., 2014).
Embanked reservoirs and coastal ecosystems emit considerable amounts of greenhouse gases; thus, they ought not be taken for granted (Yuguda et al., 2020). The reclamation of coastal wetlands has been observed to modify the physiochemical characteristics of coastal sediments (Wang et al., 2014) and may impact the balance and accumulation capacity of soil organic carbon pools in coastal wetlands (Bu et al., 2015). Reclamation by sea embankments resulted in significant reductions in total organic carbon and total organic nitrogen, and labile and recalcitrant organic carbon and nitrogen levels were also reduced in invasive S. alterniflora salt marsh (Li et al., 2021). By reducing S. alterniflora remains input into the soil and lowering soil salinity and moisture, embankment construction could significantly reduce soil C and N buildup in invasive plant salt marshes, further reducing soil microbial biomass and limiting available soil C and N in invasive plant salt marshes (Qiao et al., 2018). Coastal embankment has been shown to increase CO2 and N2O emissions in coastal wetlands while curbing CH4 emissions (Li et al., 2021). Essentially, the C and N sinks of native and invasive saltmarshes are weakened by coastal embankment reclamation, effectively reducing C and N sinks in coastal wetlands and spurring CO2 and N2O emissions while providing some CH4 pollution curtailment benefits.
For at least a thousand years now, embankments have been constructed to protect the coastline from erosion and flooding. Almost the whole Chinese coastline has currently been embanked as a precaution (Wang et al., 2021). Embankments exceeding the top-water level are believed to be beneficial for flood mitigation (Zhang et al., 2021), while dike systems must be maintained for inundation and coastal flood management in the major delta (Yin et al., 2010). Although reclamation by coastal embankments has provided protection from moderately severe storm surges and extremely high tidal water levels, they have worsened wave run-ups on the barrier and increased the risk of combined wave overtopping extreme storm surges (Zhang et al., 2021). Accelerating coastline erosion also causes other issues like salinization and harm to seawalls. Because a tropical storm has an elevated base owing to projected sea level rise, the coast will likely be significantly more susceptible to adverse flooding (Yin et al., 2012). Storm surge toppled a seawall, creating floods and having a significant effect on Chongming Island (Chen et al., 2018). Studies show that flooding mostly affects coastal areas and barely reaches inland attributable to the conservative protection provided by embankment systems (Yin et al., 2010). However, due to how slope failure affects the height of seawalls and embankments, it is predicted that 46% of these structures will have been breached by 2100, flooding half of Shanghai (Wang et al., 2012). In addition, it is estimated that without defense upgrades, 0.7–20.0 million people could experience flooding each year and damages would range from US$67 to US$3,308 billion (Fang et al., 2020). Though protection from typhoon damage provided by seawalls is 90% cost-effective compared to paddy fields (Geng et al., 2021), it is more likely that China will be able to establish a longer-lasting “ecological civilization” by preserving and reclaiming coastal wetlands, instead of building an additional 500 km of seawalls (Liu et al., 2019).
The formulation of a systematic framework and characterization of environmental parameters related to reclamation were based on the ecosystem services (ES) framework, which is extensively deployed (MEA, 2005). Current findings have shown that the ES framework can be used to explore coastal reclamation, including Economic and ecological trade-offs (Qiu et al., 2021), habitat depletion (Zhang et al., 2020), resource supply (Yang et al., 2018), treatment of water (Sun et al., 2017) and blue carbon storage (O’Connor et al., 2020). The rationale for using our conceptual approach based on the ES framework is illustrated in Figure 1; (1) Reclamation alters the dynamics and ecosystems along the coastline, from natural to artificial habitats. (2) Landscape alteration has an impact on all four processes; hydrology, the gas cycle, photosynthesis, and respiration, which are different in natural and artificial wetland habitats. (3) As a result of an individual ES, direct and indirect interactions may have an impact. For instance, subterranean water contamination and freshwater eutrophication can be caused by intensive farming and the excessive use of chemical fertilizers and pesticides.
This research explored the impact of reclamation on biodiversity loss, greenhouse gas dynamics, and eutrophication pollutants, with respect to IAPS and coastal armoring. In July, of 2022, the ISI Web of Science database and the China Knowledge Resource Integrated Database (http://www.cnki.net/) were used to conduct a literature search of peer-reviewed papers assessing the effects of invasive alien species and coastal armoring on biodiversity loss, greenhouse gas dynamics, and nutrient cycling. Table 1 shows the search phrases that were used without a limitation on publication year. These keywords were selected research based on measured or inferred invasion and coastal armoring effects at the ecosystem scale. Our search turned up 217, 124, and 32 reports (21,54 and 14 included) on S. alterniflora-biodiversity loss, S. alterniflora-greenhouse gas dynamics, and S. alterniflora-storm/flood/erosion control, respectively. We further identified 113, 106, and 37 reports (18,16, and 15 included) on CA-biodiversity loss, CA-greenhouse gas dynamics, and CA-storm/flood/erosion control as shown in Table 1, from which the ensuing data for evaluation were applied. Figure 2A shows the changes in reclamation areas due to S. alterniflora invasion and seawall expansion, while Figures 2B–D presents the trends in greehnhouse gas fluxes and stocks of CO2, CH4 and N2O from year 2000 – 2020.
Figure 2 (A) coastlines changes due to S. alterniflora and seawall expansion (B) CO2 fluxes due to S. alterniflora and seawall reclamation (C) N2O fluxes due to S. alterniflora and seawall reclamation (D) CH4 fluxes due to S. alterniflora and seawall reclamation3.1 Coastal reclamation intensity index.
Table 1 keyword combinations utilized in the original bibliography for this synthesis. For each key combination, the database was retrieved using the search terms: (China), (coastal wetland OR seagrass meadows OR intertidal flats OR tidal saltmarshes OR mangrove forests OR tidal freshwater), (Spartina alterniflora OR invader* OR invasive* OR invasion* OR exotic species OR alien species OR nonnative species)/(coastal armor OR coastal structures OR coastal embankments OR seawalls OR bulkheads OR dikes OR dams OR revetments) AND (ISI keyword sequences specified below).
The Coastal Reclamation Intensity Index (CRI) was established to assess the extent of reclamation in various areas along China’s coasts over time. The CRI is a basic, approximate quantitative approach that is often used in coastal reclamation investigations as a measure of reclamation area per unit of coastline length over time (Yang et al., 2018; Qiu et al., 2021). The CRI expressed in (ha year-1 km-1) is calculated using the following formula:
where to and t are the study period’s outset and endpoint periods (years), respectively; The entire distances (km) of the study area’s coastline at time to and t, respectively, are lt and lt0, while the entire reclamation area (ha) between to and t is st-to.
In order to produce one gram of organic matter, plants must absorb 1.63 g of carbon dioxide and release 1.19 g of oxygen (He et al., 2012).
where P1 represents the yearly loss of gas regulation function, and C1 represents the cost of fixing carbon dioxide, based on China’s afforestation costs and the global carbon tax requirement (C1 = 750 yuan/t.). C2 represents the cost of generating oxygen, or the cost of producing industrial oxygen to substitute it, which is 400 yuan/t. X is the yield per unit sea area, given as 929. 645mg.C/m2. d, and S is the total S. alterniflora reclamation area in China (He et al., 2012).
To calculate the value of gas control, this study employed the carbon tax approach and the cost of industrial oxygen generation for coastal ecosystems (Qiu et al., 2021).
The net biomass per unit area of varied geographical classifications was used to compute the amount of stored CO2 and emitted O2 for reed marshes (Biomass 25 ton ha−1, CO2 absorbed 40.74 ton ha−1, O2 emission 29.78 ton ha−1, CH4 emission 0.46 ton CO2eq ha−1, N2O emission 0.38 ton CO2eq ha−1, Gas regulation value 5.91×104 Yuan ha−1) (Ma et al., 2019). The approximate annual rate of emitted O2 was 376 Yuan ton-1 (Lin et al., 2019), whereas the approximate annual rate of stored CO2 was 1200 Yuan ton-1 in 2010 (Qiu et al., 2021). CO2 equivalent (CO2-eq ha-1) values for N2O and CH4 were divided by 296 and 23, respectively (IPCC, 2006; Hu et al., 2020). Their costs were calculated by converting them to CO2 equivalents (IPCC, 2014), and the cumulative worth of gas management in the research region was calculated by adding positive and negative values (Qiu et al., 2021).
The effectiveness of natural wetlands and constructed seawalls for averting mortality and safeguarding property from disasters in China’s coastal zones are referred to as storm/flood protection value. The effectiveness of mudflats, saltmarshes and coastal embankments in storm/flood mitigation was investigated in this study, employing the assessment approach developed by (Liu et al., 2019). Given that the average yearly benefits of coastal wetlands and seawalls in preventing storm damage were 892.5 million Yuan ha-1 and 37.2 million Yuan km-1 between 1989 and 2016, a realistic evaluation was conducted using median values of 11.87 million Yuan ha-1 and 4.8 million Yuan km-1 (Liu et al., 2019).
The value of COD treatment is taken into account as the value of N and P regeneration is only evaluated in the context of nutrient cycling activity (He et al., 2012).
Where P2 represents the value of waste treatment, while C represents the price of removing COD, which is 4300 yuan/t according to (Chen et al., 1999). X denotes the COD capability, which ought to be 2 mg/l (He et al., 2012). V stands for reclamation volume (tonnes) of S. alterniflora which is equal to the product of “net primary productivity” NPP and “reclamation area”.
N and P recycling from the water body is valued as follows:
Where P3 is the value of nutrient cycling; CN represents the cost of N removal per unit; CP represents the cost of P removal per unit which is 5000 yuan/t for P as well as for N (Zhang and Sun, 2009). XN and XP represent the capacities of N and P, which is 2 mg/l (He et al., 2012); V represents the volume of reclamation of S. alterniflora defined above.
In order to improve the biomass, (Xie et al., 2015) proposed incorporating the mean annual temperature and rainfall and utilized the Thornthwaite Memoria model to determine the biological community’s net primary productivity (NPP). The equation is given by (Yin et al., 2020) as:
where NPP (t/h m2) is the net primary productivity of the spartina community in the study region; V is the exact yearly evaporation, with V = 1.05pre/[1 + (1.05pre/L)2]1/2; L (mm) is the average annual evapotranspiration, with L = 3000 + 25T + 0.05T3; and T (°C) is the annual average temperature, which is ∼ 14.25°C (Yuguda et al., 2022b).
There are three levels of value that contribute to the overall ecological and economic benefits of the Spartina plants (Qin et al., 1997):
Although S. alterniflora has a range of uses in the natural environment, including advantages for biodiversity, sturdiness, and long-term growth in salt marsh systems, the plantation’s greatest value comes from the money it saves on shoreline protection. The equation for shoreline protection value is as follows (Qin et al., 1997):
Where Ti is the coefficient of typhoon damage, which is equivalent to 0.5 (Qin et al., 1997); Ef is the coefficient of environmental factors, which is equivalent to 0.5 (Qin et al., 1997); Fp is the planting fee coefficient, which is equivalent to 0.1 (Qin et al., 1997); Ms is the plant’s cost savings per hectare, which is equivalent to 10,000 yuan (Qin et al., 1997).
The use of biomineral liquid BML from Spartina culms for food production was approved by the Chinese Ministry of Health in 1990, and the country has since produced several biomineral products (Qin et al., 1997). The extraction value equation is given by (Qin et al., 1997):
Where W represents the annual total biomass of Spartina culm per ha, which is equal to NPP, ∼ 75 tonnes ha−1 as corroborated by (Lu and Zhang, 2013); Cg stands for the coefficient of transition from grass to BML, which is equivalent to 0.1 (Qin et al., 1997); Pn represents the profit from BML, which is equivalent to 30 yuan/kg (Qin et al., 1997).
The grass residue left over after extraction has significant nutritional content and can be utilized. The equation for the residue value is given by (Qin et al., 1997):
Where Ru is the residue utilization ratio, which is equivalent to 0.1 (Qin et al., 1997); Pr1 and Pr2 are the net profit from the mushroom (5 yuan/kg) and fodder (1 yuan/kg) residues respectively (Qin et al., 1997); W and Cg are defined previously in equation (6).
Based on a synthesis of the worldwide estimates for coastal ecosystem services, six landscape groups were found to provide effluent treatment and habitat preservation in the case study (de Groot et al., 2012).
According to (Liu et al., 2019), the cost of building seawalls could reach CNY 6,397,500/km, with the cost of routine maintenance ranging from CNY 15,622/km to CNY 74,216/km. As a result, the average cost of building and maintaining seawalls is CNY 172,969 km/year, which when compared to the projected value of storm protection provided by seawalls of CNY 848,312 km/year yields a benefit/cost ratio of 4.9. These rates were adopted to estimate the ecological-economic benefits of coastal armoring in this study, given the Spatio-temporal variations of storm types and robustness of data utilized by (Liu et al., 2019).
To date, more than 50,000 ha of China’s coastal wetlands have been reclaimed by S. alterniflora since its introduction. On the other hand, approximately 13, 830 km of embankment seawalls have been erected in China for coastal protection since the 20th century. In the past two decades (2000-2020), the average annual coastal reclamation to S. alterniflora is 1,316 ha, and 536.58 km to seawalls, with an average CRI of 12.29 ha yr−1 km−1. With gross reclaimed land areas of 43,061 and 51,970 ha and CRIs of 11.32 and 13.26 ha year−1 km−1 per decade, reclamation area accelerated between 2000 and 2020 Figure 2A. Coastal land reclamation by invasive S. alterniflora and embankment seawalls is pertinent to the four central provinces of China; Jiangsu, Shanghai, Zhejiang, and Fujian, whereas the northernmost and southernmost provinces, account for minimal reclamation Table 1.
Over 579.59 × 104 ha of coastal wetlands make up China’s coastline, which is about 1.8 × 104 km long and accounts for 12.42% of the country’s total natural wetlands (Sun et al., 2015). However, China’s coastal wetlands have drastically shrunk in size as a result of the country’s rapid economic growth and persistent anthropogenic activity, endangering carbon sequestration and other ecological functions (Hu et al., 2020). Consequently, different forms of reclamation have different effects on the sizes and patterns of GHG fluxes across China’s coastal wetlands. In the S. alterniflora marshes, CO2, N2O fluxes, and CH4 fluxes ranged from 256-1202 mg·m-2·h-1, 32.59-101.27 µg·m-2·h-1, and 4.85-14.88 mg·m-2·h-1 respectively, whereas the contribution of seawalls to GHG fluxes ranged from 97.28-456.76 mg·m-2·h-1 for CO2, 5.54-17.22 µg·m-2·h-1 for N2O and 1.65-5.06 mg·m-2·h-1 for CH4. Overall, GHG fluxes were higher in the spartina-dominated wetlands compared to the seawalls reclaimed wetlands and increased with increasing reclamation areas and coastline length, as shown in Figures 2B–D.
Table 2 depicts the areas of S. alterniflora in various years as well as changes in those areas through time. S. alterniflora was found to be continuously spreading throughout the coast of mainland China, increasing from 25,648 ± 201 ha in 2000 to 43,061 ± 405 ha in 2010, and 51,970 ± 115 ha in 2020. Between 2000 and 2020, S. alterniflora increased by 26,322 ha which corresponds to an average total increase of 1316.1 ha yr-1 or a 10.26% annual rate of change. Throughout the entire span of 2000-2020, Jiangsu Province experienced the most expansive spread of S. alterniflora, with an invasive rate of 715 ha yr-1, followed by Zhejiang with a 563 ha yr-1 rate (Mao et al., 2019). In China, seawalls now span between 58 and 61% of the coastline (Luo et al., 2015). As shown in Table 3, a rapid rate of seawall construction occurred between 2000 and 2010, with an increase of 7,902 km at 25.5% per decade compared to other periods. Over the entire period of 2000-2020, seawall construction increased by 10,732 km, corresponding to an average increase rate of 536.6 km yr-1 or 21.26% annual rate of change. Although the cumulative increase in invasive spartina and seawall expansion mostly infringed on mudflat and shallow water, the landscape change revealed a fairly irreversible process of a gradual shift from natural to constructed wetlands, and eventually to impermeable surfaces.
Table 2 Areas and changes in S. alterniflora invasion over the last two decades in China’s coastalines and wetlands.
Table 3 Length and changes in seawalls construction over the last two decades along China’s coastlines.
From the year 2000 to 2020, the estimated distributions of total ESV and economic benefit were estimated. As shown in Figures 3A–C, it can be seen that the ESV of spartina gradually declined while the ESV of seawalls marginally increased. However, the economic benefit of spartina dramatically increased while the economic value of seawalls moderately declined, demonstrating a clear spatial trade-off pattern throughout the investigation. Intriguing trends of greater modification composition were observed for both ESV and economic benefit for seawalls and IAPS, demonstrating that the fragmentation of the ecosystem brought on by both types of reclamation has a growing effect on ESV and economic benefit. Particularly, over the course of two decades, both types of reclamation maintained a rapid change rate with a final total ESV that far exceeded the total economic value Figures 3A, B,. This suggests an apparent trade-off between the economic gains and ecological consequences of the invasion of S. alterniflora, i.e., that the economic boom is linked with long-term loss of ecological value in China’s coastal ecosystems.
Figure 3 (A) the total ecosystem services values of reclamation by S. alterniflora and seawall expansion from 2000 to 2020 (B) the economic benefit of reclamation by S. alterniflora and seawall expansion between 2000 to 2020 (C) the total ecosystem services loss of reclamation by S. alterniflora and seawall expansion between 2000 to 2020.
Throughout the research period, ESV loss and economic gain for the spartina and seawall expansion projects showed opposing trends with no equilibrium point reached between, and ESV loss was greater than economic gain. The study period saw a total net increase from 75.39 billion Yuan in the first decade to 101.34 billion Yuan in the second, indicating that economic growth was unable to make up for the ESV loss caused by the S. alterniflora and seawalls’ reclamation in China. According to the results of Pearson’s correlation analysis shown in Figure 4, there were trade-offs between specific ecosystem services and economic advantage for the study period of 2000–2020, as indicated by a moderately negative correlation between the two variables (P< 0.01). Economic benefits and ESV trade-off characteristics generally showed a steady trend for the study period.
Figure 4 Pearson’s correlation coefficients between ecosystem services: total ESV ecosystem services value; GR, gas regulation; SP, storm protection; WT, waste treatment; HM, habitat maintenance; and EB, economic benefit.
Persistent wetland reclamation has resulted in the steady shrinkage of coastal wetland areas, weakening marine dynamics, habitat degradation, and declines in biodiversity (Cui et al., 2018; Yan et al., 2022). In China, the rate of embankment erection and S. alterniflora invasion spread has increased by more than double as much during the previous few decades with the primary objective of coastal land reclamation (Li et al., 2021). This led to the loss of at least 10,520 km2 of ecosystems in the Yellow Sea, as well as additional ecological effects like global warming, GHG emissions, marine pollution, etc. (Choi et al., 2018). Evidence suggests that continuing conversion of the coastal habitats from natural to engineered has exacerbated the protracted ecological damage, even while reclamation in coastal wetlands has escalated ecologic and environmental losses. The expenses of regulating gas, preventing storms, treating waste, and maintaining the ecosystem were examined in this study with respect to reclamation by spartina and seawalls.
Reclamation by invasive alien plants and embankment seawalls has seriously harmed the coastal ecosystem’s structure and function in sequestering carbon, absorbing waves and storms, reducing water pollution, and enabling breeding habitats for flora and fauna (Feng et al., 2021; Feng et al., 2022). The introduction of embankment seawalls in invasive S. alterniflora-dominated salt marshes significantly altered the GHG regulation capacity of coastal marshes. Sea embankment reclamation has been reported to significantly decreased soil total organic C & N by > 50% (Yang et al., 2016; Li et al., 2021), increasing CO2 emissions by > 30%, and N2O emission by over 40% in coastal saltmarshes and reducing CH4 emissions by > 30% (Li et al., 2021). Due to coastal embankment in a conventional coastal saltmarsh ecosystem, soil nitrogen immobilization and microbial mineralization affected the growth and activity of soil microbes, which were principally related to alterations in the organic and inorganic Nitrogen pools (Feng et al., 2022). Although invasive plant saltmarshes function as filters to remove significant amounts of nitrogen and phosphorus before discharging wastewater into the sea (Qiu et al., 2021), The impounding of salt marshes by embankment walls has been associated with lower nutrient accretion rates (Sturdevant et al., 2002), while salinity and dissolved oxygen levels diminished (Bolduc and Afton, 2004).
According to this study’s trade-off analysis, the ecological consequences of seawalls and invasive spartina reclamation outweigh the economic benefits. Similar trade-off trends were also reported in other coastal ecosystems. For instance, in areas where the shoreline border of the marsh is eroding, the extent of marsh promoting the coastal protection works reduces with time. This is because the possible loss of coastal marshes near seawall structures that border marshes limit the onshore movement of marshes as the sea level rises (Foster et al., 2013). Anthropogenic activities have a significant impact on the growth of tidal flats outside of seawalls as they advance seaward, supporting solely pioneer wetland plants like S. mariqueter (Huang et al., 2020). Jiangsu’s coastal wetlands have high environmental potential, and there are few prospects for further land reclamation, which will provide difficult problems given that the area is a major hub for urban growth and a major producer of food (Cai et al., 2017). The stark contrast between total ESV loss and economic benefits in Hangzhou Bay implies that a trade-off between protracted ecological value and quick economic benefits from reclamation was detrimental (Qiu et al., 2021). Even though erecting hard coastal defenses will always be necessary to ensure a certain degree of protection for the coastal ecosystem, it is also important to take into account the geographic context, economic needs, cultural values, and interactions between sophisticated ecological processes when making decisions about how to reduce flood risk and sustainably develop our beautiful coastlines (Hoggart et al., 2015). Ultimately, preserving and restoring coastal wetlands by introducing invasive species instead of building more seawalls is more likely to guarantee longer-lasting ecological sustainability (Liu et al., 2019).
Coastal reclamation still has the potential to increase economic efficiency by balancing the trade-offs between reclamation by invasive plants and seawalls until the overall costs of ecosystem service loss are adequately offset by economic benefits. This is true even though trade-offs between conservation and restoration of ecosystem services continue to be difficult problems for decision-makers. Nonetheless, the complex problem is that when deciding how to utilize and enhance coastal ecosystems, we fail to value the numerous services they bring (Barbier, 2019).
Though S. alterniflora has a variety of functions in nature, such as benefits for biodiversity, sturdiness, and long-term growth in salt marsh systems, we only considered the plantation’s greatest value which comes from the money it saves on shoreline protection costs, followed by BML extraction value and residue value. This study adopted improved ESV models to assist in attaining robust management of reclamation by invasive plants and coastal armoring rather than relying on the configuration of varying coefficients. Additionally, it made an effort to combine its ESV with ecological components. To maintain the consistency of the annual calculation findings, the prices estimated in this article are derived from the national retail price index and were utilized to adjust the various wetland ESVs in the years 2000 and 2010 into similar values in 2020. Though, the cost of production might vary spatiotemporally, with customers’ excesses, the availability of more preservable or restorable coastal wetland ecosystems may curb the cost of production dramatically. However, because there is a significant awareness gap regarding their advantages, it is still difficult to value many crucial services that have no obvious pricing (Costanza et al., 2017).
Although the long-term ecological cost of reclamation is still grossly overestimated, spatially explicit fate factors in life cycle assessment (LCA) might come in handy, given the well-developed modeling techniques for forecasting the spatial pattern and intensity of anthropogenic activities in the coastal ecosystem (Yuguda et al., 2022a). LCA and life cycle costing (LCC) offer opportunities for the investigation of the environmental and economic consequences associated with invasive plants and seawalls over their entire lifecycle. Therefore, it is strongly recommended to integrate LCA with other approaches such as comprehensive life cycle costing (Yuguda et al., 2020), so that LCA may be a key input in decisions involving coastal ecosystems. While this study centered on the ecology-related ecosystem services of coastal reclamation, it is suggested that future studies take into account other agriculture and bioenergy-related ecosystem services of reclamation, to enable drawing more thorough and reliable conclusions.
Since S. alterniflora was labeled as an invasive plant in the early 2000s and now covers ~50,000 ha of China’s coastal wetlands, seawalls have increasingly been constructed to control its invasion and spread. Previous research primarily documented the adverse effects of S. alterniflora invasion (Meng et al., 2020) and seawall expansion (Feng et al., 2021; Li et al., 2021). However, both positive and adverse aspects are assessed concerning S. alterniflora invasion and expansion of coastal embankments. Construction of seawalls may have detrimental impacts on invasive plant-dominated saltmarshes, including reduced soil organic carbon and nitrogen accumulation, which may weaken sediment nitrification/denitrification, among other effects (Li et al., 2021). Contrarily, when established in front of seawalls and coastal defenses, salt marshes can provide a buffer that greatly reduces waves and storm damage (Liu et al., 2019; Zhu et al., 2020). Open embankments safeguard native wetlands, resulting in limited loss of ecosystem services (Wu et al., 2018), whereas croplands and seawalls can substantially reduce the economic loss caused by strong storms by restoring wetlands outside the seawalls (Geng et al., 2021).
In native and invasive salt marshes, sea embankment reclamation dramatically reduced soil total organic C and total organic N, which in turn decreased plant biomass, soil moisture, and soil salinity (Yang et al., 2016; Li et al., 2021). It considerably enhanced N2O emissions in native plant marshes, and decreased CH4 emissions in invasive plant marshes, while increasing CO2 emissions in both saltmarshes (Li et al., 2021). In China, seawall costs versus estimated storm protection value led to a benefit/cost ratio of ∼ 5.0, implying that coastal embankment is delivering a positive net present value. However, plans to build 500 km of new coastal embankments in the next ten years, suggest that over CNY 30 billion will be invested in new seawalls, placing an undue financial strain on municipal governments (Liu et al., 2019). While findings continue to stress that coastal armored structures altered the Physico-chemical, and biological processes of ecosystems while also promoting habitat loss, it is suggested that stakeholders shift their attention from artificial seawalls to a natural alternative that uses wetland marsh plants for storm mitigation. In this study, the ESV value of invasive S. alterniflora declines while that of seawalls increases, suggesting that coastal wetlands are not guaranteed to substitute seawalls as aforementioned. Furthermore, the ESV loss of alien plant invasion increased as opposed to seawalls in this study, indicating that economic gains are insufficient to offset the ecological costs of reclamation. This supports the notion that seawall-based coastal reclamation could reduce S. alterniflora invasion while putting at risk biodiversity roles related to reducing the effects of climate change, and the long-term viability of coastal wetlands. Consequently, in order to evaluate the ramifications of coastal reclamation management approaches from the perspective of mitigating global warming, decision-makers responsible for ecological sustainability measures and other types of restoration must specify their goals and standards for success. This is particularly paramount given the complexity of today’s critically endangered ecosystems.
This study explored the ecological-economic implications of coastal reclamation by invasive S. alterniflora and coastal armoring in China. A total of 26,322 ha of coastal wetlands were reclaimed by S. alterniflora, and 10,731 km of reclamation seawalls were built between 2000 to 2020 in China. Reclamation by invasive S. alterniflora resulted in a significant decline of ESV loss of 5,702,454 Yuan ha−1, while seawalls reclamation yielded some ESV gain of 4,800, 111 Yuan km-1 from 2000-2020. Overall, reclamation by invasive S. alterniflora and coastal armoring collectively resulted in a total ESV loss of 32.2 billion Yuan between 2000 and 2020. For this study, the total ESV loss greatly outweighed the economic gains from reclamation in China, suggesting that the trade-off correlation was brought on by the surge of quick economic benefits at the detriment of protracted ecological value. Plants to erect more seawalls, coupled with S. alterniflora expansion, will continue to exacerbate the biodiversity ESV losses of the coastal ecosystem while increasing the financial burden on local administrations.
We, therefore, propose that robust wetland conservation strategies are required at both the federal and local governments, towards achieving the objective of “zero net loss” and longer-lasting sustainability of coastal wetlands. Global efforts to counteract global warming and climate change can be boosted by compensating for manmade perturbations and restoring degraded wetlands through sustained Carbon budgets and limiting seawall expansion. This scientific evidence provides stakeholders leverage to strike a balance between seawall reclamation and IAPS spread in coastal wetlands, hence opening up possibilities for future investigations on weighing the trade-offs of the synergistic impacts of coastal armoring and IAPS.
The original contributions presented in the study are included in the article/Supplementary Material. Further inquiries can be directed to the corresponding authors.
TY, JL: Conceptualization, Methodology. DD, ZL, LW: Data curation, Writing- Original draft preparation. JX, CZ, ZN: Visualization, Investigation. DD: Supervision. ZL, CZ: Software, Validation.: TY, DD: Writing- Reviewing and Editing. All authors contributed to the article and approved the submitted version.
This work was supported by the National Natural Science Foundation of China (31800429 and 32071521), Key Research and Development Program of Jiangsu Province-Carbon peak and carbon neutrality technology innovation foundation of Jiangsu Province (BK20220030, BK20170540) and Jiangsu Collaborative Innovation Center of Technology and Material of Water Treatment, China.
The authors declare that the research was conducted in the absence of any commercial or financial relationships that could be construed as a potential conflict of interest.
All claims expressed in this article are solely those of the authors and do not necessarily represent those of their affiliated organizations, or those of the publisher, the editors and the reviewers. Any product that may be evaluated in this article, or claim that may be made by its manufacturer, is not guaranteed or endorsed by the publisher.
The Supplementary Material for this article can be found online at: https://www.frontiersin.org/articles/10.3389/fmars.2023.1118894/full#supplementary-material
Asselen S. V., Verburg P. H., Vermaat J. E., Janse J. H.. (2013). Drivers ofwetland conversion: A global meta-analysis. PloS One 8 (11), e81292. doi: 10.1371/journal.pone.008129
Barbier E. B. (2019). “The value of coastal wetland ecosystem services,” in: Coastal Wetlands: An integrated ecosystem approach. Eds. Perillo G. M. E., Wolanski E., Cahoon D. R., Hopkinson C. S. (Elsevier), 947–964. doi: 10.1016/B978-0-444-63893-9.00027-7
Barbier E. B., Koch E. W., Silliman B. R., Hacker S. D., Wolanski E., Primavera J., et al. (2008). Coastal ecosystem-based management with nonlinear ecological functions and values. Science 319, 321–323. doi: 10.1126/SCIENCE.1150349/SUPPL_FILE/BARBIER.SOM.PDF
Bartz R., Kowarik I. (2019). Assessing the environmental impacts of invasive alien plants: A review of assessment approaches. NeoBiota 43, 69–99. doi: 10.3897/neobiota.43.30122
Bolduc F., Afton A. D. (2004). Hydrologic aspects of marsh ponds during winter on the gulf coast chenier plain, USA: Effects of structural marsh management. Mar. Ecol. Prog. Ser. 266, 35–42. doi: 10.3354/meps266035
Bowen J. L., Baillie C. J., Grabowski J. H., Hughes A. R., Scyphers S. B., Gilbert K. R., et al. (2019). Boston Harbor, Boston, Massachusetts, USA: Transformation from ‘the harbor of shame’ to a vibrant coastal resource. Reg. Stud. Mar. Sci. 25, 100482. doi: 10.1016/j.rsma.2018.100482
Brown C. J., Adame M. F., Buelow C. A., Frassl M. A., Lee S. Y., Mackey B., et al. (2021). Opportunities for improving recognition of coastal wetlands in global ecosystem assessment frameworks. Ecol. Indic 126, 107694. doi: 10.1016/J.ECOLIND.2021.107694
Bu N. S., Qu J. F., Li G., Zhao B., Zhang R. J., Fang C. M. (2015). Reclamation of coastal salt marshes promoted carbon loss from previously-sequestered soil carbon pool. Ecol. Eng. 81, 335–339. doi: 10.1016/J.ECOLENG.2015.04.051
Cai F., van Vliet J., Verburg P. H., Pu L. (2017). Land use change and farmer behavior in reclaimed land in the middle jiangsu coast, China. Ocean Coast. Manag 137, 107–117. doi: 10.1016/j.ocecoaman.2016.12.015
CCICED. (2018). The China council for international cooperation on environment: The second meeting of the sixth phase, innovation for a green new era, Annual Policy Report: Ecosystem Management and Green Development. Beijing, China. Published by the International Institute for Sustainable Development (IISD), Manitoba, Canada for the China Council for International Cooperation on Environment and Development (CCICED).
Chen L. (2019). Invasive plants in coastal wetlands: Patterns and mechanisms (Cham: Springer), 97–128. doi: 10.1007/978-3-030-14861-4_5
Chen X. P., Sun J., Wang Y., Zhang H. Y., Quan He C., Liu X. Y., et al. (2018). Temporal and spatial impact of spartina alterniflora invasion on methanogens community in chongming island, China §. J. Microbiol. 56, 507–515. doi: 10.1007/s12275-018-8062-y
Chen J., Wang L., Li Y., Zhang W., Fu X., Le Y. (2012). Effect of spartina alterniflora invasion and its controlling technologies on soil microbial respiration of a tidal wetland in chongming dongtan, China. Ecol. Eng. 41, 52–59. doi: 10.1016/j.ecoleng.2012.01.024
Chen W., Zhang L., Hong H., Xue X. (1999). A preliminary study on evaluation of coastal sea water environmental capacity. J. Xiamen Univ.(Nat. Sci.)/Xiamen Daxue Xuebao 38, 896–901.
Chen R., Zhang Y., Xu D., Liu M. (2018). Climate change and coastal megacities: Disaster risk assessment and responses in shanghai city. Climate Change, Extreme Events and Disaster Risk Reduction: Towards Sustainable Development Goals, 203–216. Springer, Cham. doi: 10.1007/978-3-319-56469-2_14
Choi C. Y., Jackson M. V., Gallo-Cajiao E., Murray N. J., Clemens R. S., Gan X., et al. (2018). Biodiversity and china’s new great wall. Divers. Distrib 24, 137–143. doi: 10.1111/ddi.12675
Costanza R., de Groot R., Braat L., Kubiszewski I., Fioramonti L., Sutton P., et al. (2017). Twenty years of ecosystem services: how far have we come and how far do we still need to go? Ecosyst. Serv. 28, 1–16. doi: 10.1016/j.ecoser.2017.09.008
Cui L., Li G., Ouyang N., Mu F., Yan F., Zhang Y., et al. (2018). Analyzing coastal wetland degradation and its key restoration technologies in the coastal area of jiangsu, China. Wetlands 38, 525–537. doi: 10.1007/s13157-018-0997-6
Dalle Fratte M., Bolpagni R., Brusa G., Caccianiga M., Pierce S., Zanzottera M., et al. (2019). Alien plant species invade by occupying similar functional spaces to native species. Flora: Morphology Distribution Funct. Ecol. Plants 257, 151419. doi: 10.1016/j.flora.2019.151419
de Groot R., Brander L., van der Ploeg S., Costanza R., Bernard F., Braat L., et al. (2012). Global estimates of the value of ecosystems and their services in monetary units. Ecosyst. Serv. 1, 50–61. doi: 10.1016/J.ECOSER.2012.07.005
Dugan J. E., Emery K. A., Alber M., Alexander C. R., Byers J. E., Gehman A. M., et al. (2018). Generalizing ecological effects of shoreline armoring across soft sediment environments. Estuaries Coasts 41, 180–196. doi: 10.1007/s12237-017-0254-x
Fang J., Lincke D., Brown S., Nicholls R. J., Wolff C., Merkens J. L., et al. (2020). Coastal flood risks in China through the 21st century – an application of DIVA. Sci. Total Environ. 704, 135311. doi: 10.1016/J.SCITOTENV.2019.135311
Feng H., Qiao Y., Xia L., Yang W., Zhao Y., Jeelani N. (2021). Alteration of nutrient substrates and absence of seawater due to coastal embankments affects soil microbial communities in salt marshes of Eastern China. Research Square, PPR, PPR372140, 1–32. doi: 10.21203/rs.3.rs-707097/v1
Feng H., Zhao H., Xia L., Yang W., Zhao Y., Jeelani N., et al. (2022). Nitrogen cycling in plant and soil subsystems is driven by changes in soil salinity following coastal embankment in typical coastal saltmarsh ecosystems of Eastern China. Ecol. Eng. 174, 106467. doi: 10.1016/j.ecoleng.2021.106467
Foster N. M., Hudson M. D., Bray S., Nicholls R. J. (2013). Intertidal mudflat and saltmarsh conservation and sustainable use in the UK: A review. J. Environ. Manage 126, 96–104. doi: 10.1016/J.JENVMAN.2013.04.015
Gao G. F., Li P. F., Shen Z. J., Qin Y. Y., Zhang X. M., Ghoto K., et al. (2018). Exotic spartina alterniflora invasion increases CH4 while reduces CO2 emissions from mangrove wetland soils in southeastern China. Sci. Rep. 8, 1–10. doi: 10.1038/s41598-018-27625-5
Geng R., Liu X., Lv X., Gao Z., Xu N. (2021). Comparing cost-effectiveness of paddy fields and seawalls for coastal protection to reduce economic damage of typhoons in China. Ecosyst. Serv. 47, 101232. doi: 10.1016/J.ECOSER.2020.101232
Guan D. M. A. D.. (2013). Study on the national marine functional zoning: General report on the national marine functional zoning research. (Beijing: Ocean Press).
He Y., Xue X., Kong H. (2012). The Application of Costs and Benefits Analysis in Coastal Land-Reclamation. Adv. Mater. Res. (Switzerland: Trans Tech Publications Ltd.), 518–523, 5232–5237. doi: 10.4028/www.scientific.net/AMR.518-523.5232
Hoggart S., Hawkins S. J., Bohn K., Airoldi L., van Belzen J., Bichot A., et al. (2015). “Ecological approaches to coastal risk mitigation,” in Coastal risk management in a changing climate. (Butterworth-Heinemann), 171–236. doi: 10.1016/B978-0-12-397310-8.00004-X
Hu M., Sardans J., Yang X., Peñuelas J., Tong C. (2020). Patterns and environmental drivers of greenhouse gas fluxes in the coastal wetlands of China: A systematic review and synthesis. Environ. Res. 186, 109576. doi: 10.1016/j.envres.2020.109576
Huang S. L., Chen Y. N., Li Y. (2020). Spatial dynamic patterns of saltmarsh vegetation in southern hangzhou bay: Exotic and native species. Water Sci. Eng. 13, 34–44. doi: 10.1016/J.WSE.2020.03.003
IPBES. (2019). Summary for policymakers of the global assessment report on biodiversity and ecosystem services of the Intergovernmental Science-Policy Platform on Biodiversity and Ecosystem Services. Eds. Díaz S., Settele J., Brondízio E. S., Ngo H. T., Guèze M., Agard J., et al (Bonn, Germany: IPBES Secretariat), 56. doi: 10.5281/zenodo.3553579
IPCC. (2006). “Intergovernmental Panel on Climate Change; guidelines for national greenhouse gas inventories,” in Expert meeting report. (Hayama, Kanagawa, Japan: Institute for Global Environmental Strategies), 1–20.
IPCC. (2014). “IPCC 2014: Impacts, adaptation, and vulnerability. Part b: Regional aspects,” in Contribution of working group II to the fifth assessment report of the intergovernmental panel on climate change (New York, NY, USA: Tech. rep. Cambridge University Press).
Jaramillo E., Dugan J., Hubbard D., Manzano M., Duarte C. (2021). Ranking the ecological effects of coastal armoring on mobile macroinvertebrates across intertidal zones on sandy beaches. Sci. Total Environ. 755, 142573. doi: 10.1016/j.scitotenv.2020.142573
Jiang L. F., Luo Y. Q., Chen J. K., Li B. (2009). Ecophysiological characteristics of invasive spartina alterniflora and native species in salt marshes of Yangtze river estuary, China. Estuar. Coast. Shelf Sci. 81, 74–82. doi: 10.1016/j.ecss.2008.09.018
Kumar Rai P., Singh J. S. (2020). Invasive alien plant species: Their impact on environment, ecosystem services and human health. Ecol. Indic 111, 106020. doi: 10.1016/j.ecolind.2019.106020
Li J., Lai Y., Xie R., Ding X., Wu C. (2018). Sediment phosphorus speciation and retention process affected by invasion time of spartina alterniflora in a subtropical coastal wetland of China. Environ. Sci. pollut. Res. 25, 35365–35375. doi: 10.1007/S11356-018-3447-3/TABLES/2
Li J., Leng Z., Wu Y., Li G., Ren G., Wu G., et al. (2021). The impact of Sea embankment reclamation on greenhouse gas GHG fluxes and stocks in invasive spartina alterniflora and native phragmites australis wetland marshes of East China. Sustainability 13, 12740. doi: 10.3390/SU132212740
Lin W., Xu D., Guo P., Wang D., Li L., Gao J. (2019). Exploring variations of ecosystem service value in hangzhou bay wetland, Eastern China. Ecosyst. Serv. 37, 100944. doi: 10.1016/j.ecoser.2019.100944
Liu X., Wang Y., Costanza R., Kubiszewski I., Xu N., Yuan M., et al. (2019). The value of china’s coastal wetlands and seawalls for storm protection. Ecosyst. Serv. 36, 100905. doi: 10.1016/J.ECOSER.2019.100905
Loiola P. P., de Bello F., Chytrý M., Götzenberger L., Carmona C. P., Pyšek P., et al. (2018). Invaders among locals: Alien species decrease phylogenetic and functional diversity while increasing dissimilarity among native community members. J. Ecol. 106, 2230–2241. doi: 10.1111/1365-2745.12986
Lu J., Zhang Y. (2013). Spatial distribution of an invasive plant spartina alterniflora and its potential as biofuels in China. Ecol. Eng. 52, 175–181. doi: 10.1016/j.ecoleng.2012.12.107
Luo S., Cai F., Liu H., Lei G., Qi H., Su X. (2015). Adaptive measures adopted for risk reduction of coastal erosion in the people’s republic of China. Ocean Coast. Manag. 103, 134–145. doi: 10.1016/j.ocecoaman.2014.08.008
Ma T., Li X., Bai J., Ding S., Zhou F., Cui B. (2019). Four decades’ dynamics of coastal blue carbon storage driven by land use/land cover transformation under natural and anthropogenic processes in the yellow river delta, China. Sci. Total Environ. 655, 741–750. doi: 10.1016/j.scitotenv.2018.11.287
Ma Z., Melville D. S., Liu J., Chen Y., Yang H., Ren W., et al. (2014). Rethinking china’s new great wall. Science 346 (6212), 912–914. doi: 10.1126/science.1257258
Mao D., Liu M., Wang Z., Li L., Man W., Jia M., et al. (2019). Rapid invasion of spartina alterniflora in the coastal zone of mainland China: Spatiotemporal patterns and human prevention. Sensors 19 (10), 2308. doi: 10.3390/S19102308
MEA (2005). “Ecosystems and human well-being : synthesis,” in Assessment of climate change in the southwest united states: A report prepared for the national climate assessment (Washington, DC: Island Press).
Meng W., Feagin R. A., Innocenti R. A., Hu B., He M., Li H. (2020). Invasion and ecological effects of exotic smooth cordgrass spartina alterniflora in China. Ecol. Eng. 143, 105670. doi: 10.1016/J.ECOLENG.2019.105670
Meng W., He M., Hu B., Mo X., Li H., Liu B., et al. (2017). Status of wetlands in China: A review of extent, degradation, issues and recommendations for improvement. Ocean Coast. Manag. 146, 50–59. doi: 10.1016/j.ocecoaman.2017.06.003
Newton A., Icely J., Cristina S., Perillo G. M. E., Turner R. E., Ashan D., et al. (2020). Anthropogenic, direct pressures on coastal wetlands. Front. Ecol. Evol. 8. doi: 10.3389/FEVO.2020.00144/BIBTEX
Ngo H. T., Guèze M., Agard Trinidad J., Arneth A., Balvanera P., Brauman K., et al. (2019). Summary for policymakers of the global assessment report on biodiversity and ecosystem services of the Intergovernmental Science-Policy Platform on Biodiversity and Ecosystem Services-ADVANCE UNEDITED VERSION-Members of the management committee who provided guidance for the production of this assessment Overall review editors. (Bonn, Germany: Secretariat of the Intergovernmental Science-Policy Platform on Biodiversity and Ecosystem Services).
O’Connor J. J., Fest B. J., Sievers M., Swearer S. E. (2020). Impacts of land management practices on blue carbon stocks and greenhouse gas fluxes in coastal ecosystems–a meta-analysis. Glob Chang Biol. 26, 1354–1366. doi: 10.1111/GCB.14946
Piersma T., Lok T., Chen Y., Hassell C. J., Yang H.-Y., Boyle A., et al. (2016). Simultaneous declines in summer survival of three shorebird species signals a flyway at risk. J. Appl. Ecol. 53, 479–490. doi: 10.1111/1365-2664.12582
Qiao Y., Yang W., Zhao Y., Jeelani N., Xu L., Zhao H., et al. (2018). How spartina alterniflora adapts to a new environment created by embankment reclamation through c-N-P stoichiometry in the coastal wetlands of eastern China. Mar. Freshw. Res. 69, 823–832. doi: 10.1071/MF17374
Qin P., Xie M., Jiang Y. (1998). Spartina green food ecological engineering. Ecol. Eng. 11, 147–156. doi: 10.1016/S0925-8574(98)00030-5
Qin P., Xie M., Jiang Y., Chung C.-H. (1997). Estimation of the ecological-economic benefits of two spartina alterniflora plantations in north jiangsu, China. Ecol. Eng. 8, 5–17. doi: 10.1016/S0925-8574(96)00227-3
Qiu L., Zhang M., Zhou B., Cui Y., Yu Z., Liu T., et al. (2021). Economic and ecological trade-offs of coastal reclamation in the hangzhou bay, China. Ecol. Indic 125, 107477. doi: 10.1016/j.ecolind.2021.107477
Quan W., Zhang H., Wu Z., Jin S., Tang F., Dong J. (2016). Does invasion of spartina alterniflora alter microhabitats and benthic communities of salt marshes in Yangtze river estuary? Ecol. Eng. 88, 153–164. doi: 10.1016/J.ECOLENG.2015.12.026
Sandoval Gallardo S., Fossile T., Herbst D. F., Begossi A., Silva L. G., Colonese A. C. (2021). 150 years of anthropogenic impact on coastal and ocean ecosystems in Brazil revealed by historical newspapers. Ocean Coast. Manag 209, 105662. doi: 10.1016/J.OCECOAMAN.2021.105662
Schindler S., Rabitsch W., Essl F. (2018). “Climate change and increase of impacts on human health by alien species,” in Invasive species and human health. (Wallingford UK: CAB International), 151–166. doi: 10.1079/9781786390981.0151
Sengupta D., Chen R., Meadows M. E. (2018). Building beyond land: An overview of coastal land reclamation in 16 global megacities. Appl. Geogr. 90, 229–238. doi: 10.1016/j.apgeog.2017.12.015
Studds C. E., Kendall B. E., Murray N. J., Wilson H. B., Rogers D. I., Clemens R. S., et al. (2017). Rapid population decline in migratory shorebirds relying on yellow Sea tidal mudflats as stopover sites. Nat. Commun. 8 (1), 14895. doi: 10.1038/ncomms14895
Sturdevant A., Craft C., Sacco J. (2002). Ecological functions of an impounded marsh and three natural estuarine marshes along woodbridge river, NY/NJ harbor. Urban Ecosyst. 6, 163–181. doi: 10.1023/A:1026356211862
Su Z., Qiu G., Fan H., Li M., Fang C. (2020). Changes in carbon storage and macrobenthic communities in a mangrove-seagrass ecosystem after the invasion of smooth cordgrass in southern China. Mar. pollut. Bull. 152, 110887. doi: 10.1016/j.marpolbul.2020.110887
Sun X., Li Y., Zhu X., Cao K., Feng L. (2017). Integrative assessment and management implications on ecosystem services loss of coastal wetlands due to reclamation. J. Clean Prod 163, S101–S112. doi: 10.1016/j.jclepro.2015.10.048
Sun Z., Sun W., Tong C., Zeng C., Yu X., Mou X. (2015). China’s coastal wetlands: Conservation history, implementation efforts, existing issues and strategies for future improvement. Environ. Int. 79, 25–41. doi: 10.1016/J.ENVINT.2015.02.017
Tang G., Shen L., Weng W. (2007). Effects of using sonneratia apetala to control the growth of spartina alterniflora loisel. J. South China Agric. Univ. 28, 10–13. doi: 10.7671/j.issn.1001-411X.2007.01.003
Wan S., Qin P., Liu J., Zhou H. (2009). The positive and negative effects of exotic spartina alterniflora in China. Ecol. Eng. 35, 444–452. doi: 10.1016/j.ecoleng.2008.05.020
Wang Q., An S. Q., Ma Z. J., Zhao B., Chen J. K., Li B. (2006). Invasive spartina alterniflora: Biology, ecology and management. Acta Phytotaxonomica Sin. 44, 559–588. doi: 10.1360/aps06044
Wang Y. J., Chen D., Yan R., Yu F. H., van Kleunen M. (2019). Invasive alien clonal plants are competitively superior over co-occurring native clonal plants. Perspect. Plant Ecol. Evol. Syst. 40, 125484. doi: 10.1016/j.ppees.2019.125484
Wang J., Gao W., Xu S., Yu L. (2012). Evaluation of the combined risk of sea level rise, land subsidence, and storm surges on the coastal areas of shanghai, China. Climatic Change 115, 537–558. doi: 10.1007/S10584-012-0468-7
Wang W., Liu H., Li Y., Su J. (2014). Development and management of land reclamation in China. Ocean Coast. Manag 102, 415–425. doi: 10.1016/j.ocecoaman.2014.03.009
Wang W., Sardans J., Wang C., Zeng C., Tong C., Chen G., et al. (2019). The response of stocks of c, n, and p to plant invasion in the coastal wetlands of China. Glob Chang Biol. 25, 733–743. doi: 10.1111/gcb.14491
Wang W. Q., Sardans J., Zeng C. S., Tong C., Wang C., Peñuelas J. (2016). Impact of plant invasion and increasing floods on total soil phosphorus and its fractions in the minjiang river estuarine wetlands, China. Wetlands 36, 21–36. doi: 10.1007/s13157-015-0712-9
Wang Y., Wang Z.-L., Feng X., Guo C., Chen Q. (2014). Long-term effect of agricultural reclamation on soil chemical properties of a coastal saline marsh in bohai rim, northern China. PloS One 9, e93727. doi: 10.1371/JOURNAL.PONE.0093727
Wang X., Yan F., Su F. (2021). Changes in coastline and coastal reclamation in the three most developed areas of China 1980–2018. Ocean Coast. Manag 204, 105542. doi: 10.1016/j.ocecoaman.2021.105542
Wang X., Zhang W., Yin J., Wang J., Ge J., Wu J., et al. (2021). Assessment of coastal erosion vulnerability and socio-economic impact along the Yangtze river delta. Ocean Coast. Manag 215, 105953. doi: 10.1016/J.OCECOAMAN.2021.105953
Wu W., Yang Z., Tian B., Huang Y., Zhou Y., Zhang T. (2018). Impacts of coastal reclamation on wetlands: Loss, resilience, and sustainable management. Estuar. Coast. Shelf Sci. 210, 153–161. doi: 10.1016/J.ECSS.2018.06.013
Xiao T., Yu H., Song Y., Jiang Y. P., Zeng B., Dong M. (2019). Nutrient enhancement of allelopathic effects of exotic invasive on native plant species. PloS One 14, e0206165. doi: 10.1371/journal.pone.0206165
Xiaonan D., Xiaoke W., Lu F., Zhiyun O. (2008). Primary evaluation of carbon sequestration potential of wetlands in China. Acta Ecologica Sin. 28, 463–469. doi: 10.1016/S1872-2032(08)60025-6
Xie G., Zhang C., Zhang L., Chen W., Li S. (2015). Improvement of ecosystem service valorization method based on unit area value equivalent factor. J. Nat. Resour 30, 1243–1254. (In Chinese). doi: 10.11849/zrzyxb.2015.08.001
Xue L., Li X., Zhang Q., Yan Z., Ding W., Huang X., et al. (2018). Elevated salinity and inundation will facilitate the spread of invasive spartina alterniflora in the Yangtze river estuary, China. J. Exp. Mar. Biol. Ecol. 506, 144–154. doi: 10.1016/j.jembe.2018.06.008
Yan J., Du J., Su F., Zhao S., Zhang S., Feng P. (2022). Reclamation and ecological service value evaluation of coastal wetlands using multispectral satellite imagery. Wetlands 42, 1–15. doi: 10.1007/s13157-022-01537-7
Yang R.-M., Guo W.-W. (2018). Exotic spartina alterniflora enhances the soil functions of a coastal ecosystem. Soil Sci. Soc. America J. 82, 901–909. doi: 10.2136/sssaj2017.12.0411
Yang W., Jin Y., Sun T., Yang Z., Cai Y., Yi Y. (2018). Trade-offs among ecosystem services in coastal wetlands under the effects of reclamation activities. Ecol. Indic 92, 354–366. doi: 10.1016/j.ecolind.2017.05.005
Yang W., Li N., Leng X., Qiao Y., Cheng X., An S. (2016). The impact of sea embankment reclamation on soil organic carbon and nitrogen pools in invasive spartina alterniflora and native suaeda salsa salt marshes in eastern China. Ecol. Eng. 97, 582–592. doi: 10.1016/J.ECOLENG.2016.10.064
Ye S., Laws E. A., Yuknis N., Ding X., Yuan H., Zhao G., et al. (2015). Carbon sequestration and soil accretion in coastal wetland communities of the Yellow River Delta and Liaohe Delta, China. Estuaries Coast. 38, 1885–1897. doi: 10.1007/s12237-015-0012-x
Yim J., Kwon B. O., Nam J., Hwang J. H., Choi K., Khim J. S. (2018). Analysis of forty years long changes in coastal land use and land cover of the yellow Sea: The gains or losses in ecosystem services. Environ. pollut. 241, 74–84. doi: 10.1016/J.ENVPOL.2018.05.058
Yin L., Dai E., Zheng D., Wang Y., Ma L., Tong M. (2020). What drives the vegetation dynamics in the hengduan mountain region, southwest China: Climate change or human activity? Ecol. Indic 112, 106013. doi: 10.1016/j.ecolind.2019.106013
Yin J., Xu S., Wang J., Zhong H., Hu Y., Yin Z., et al. (2010). “Vulnerability assessment of combined impacts of sea level rise and coastal flooding for china’s coastal region using remote sensing and GIS,” in 2010 18th International Conference on Geoinformatics, Geoinformatics. (Beijing, China: Institute of Electrical and Electronics Engineers IEEE), 1–4. doi: 10.1109/GEOINFORMATICS.2010.5567562
Yin J., Yin Z., Wang J., Xu S. (2012). National assessment of coastal vulnerability to sea-level rise for the Chinese coast. J. Coast. Conserv. 16, 123–133. doi: 10.1007/S11852-012-0180-9/FIGUREURES/4
Yu S., Cui B., Xie C., Ma X., Man Y., Yan J., et al. (2021). A quantitative approach for offsetting the coastal reclamation impacts on multiple ecosystem services in the yellow river delta. Ecosyst. Serv. 52, 101382. doi: 10.1016/j.ecoser.2021.101382
Yuan J., Ding W., Liu D., Kang H., Freeman C., Xiang J., et al. (2015). Exotic spartina alterniflora invasion alters ecosystem-atmosphere exchange of CH4 and N2O and carbon sequestration in a coastal salt marsh in China. Glob Chang Biol. 21, 1567–1580. doi: 10.1111/gcb.12797
Yuguda T. K., Leng Z., Wu Y., Jia H., Zhang S., Dai Z., et al. (2022a). Consequences of coastal wetlands reclamation and the need for integrating impact assessment of invasive alien plants species and coastal armoring in life cycle assessment (LCA). Int. J. Environ. Res. 16, 1–18. doi: 10.1007/s41742-022-00461-2
Yuguda T. K., Li Y., Luka B. S., Dzarma G. W. (2020). Incorporating reservoir greenhouse gas emissions into carbon footprint of sugar produced from irrigated sugarcane in northeastern Nigeria. Sustainability 12, 10380. doi: 10.3390/SU122410380
Yuguda T. K., Li Y., Xiong W., Zhang W. (2020). Life cycle assessment of options for retrofitting an existing dam to generate hydro-electricity. Int. J. Life Cycle Assess. 25, 57–72. doi: 10.1007/s11367-019-01671-1
Yuguda T. K., Wu Y., Leng Z., Gao G., Li G., Dai Z., et al. (2022b). Impact of spartina alterniflora invasion on evapotranspiration water loss in phragmites australis dominated coastal wetlands of east China. Ecol. Eng. 179, 106605. doi: 10.1016/J.ECOLENG.2022.106605
Zeng Y., Friess D. A., Sarira T. V., Siman K., Koh L. P. (2021). Global potential and limits of mangrove blue carbon for climate change mitigation. Curr. Biol. 31, 1737–1743.e3. doi: 10.1016/j.cub.2021.01.070
Zhang Y., Chen R., Wang Y. (2020). Tendency of land reclamation in coastal areas of shanghai from 1998 to 2015. Land Use Policy 91, 104370. doi: 10.1016/j.landusepol.2019.104370
Zhang M., Dai Z., Bouma T. J., Bricker J., Townend I., Wen J., et al. (2021). Tidal-flat reclamation aggravates potential risk from storm impacts. Coast. Eng. 166, 103868. doi: 10.1016/J.COASTALENG.2021.103868
Zhang D., Hu Y., Liu M., Chang Y., Yan X., Bu R., et al. (2017). Introduction and spread of an exotic plant, spartina alterniflora, along coastal marshes of China. Wetlands 37, 1181–1193. doi: 10.1007/S13157-017-0950-0/FIGUREURES/7
Zhang H., Sun Y. L. (2009). Loss appraisal on the value of marine ecosystem services of sea reclamation for qianwan. Trans. Oceanology Limnology 3, 34–38. doi: 10.13984/j.cnki.cn37-1141.2009.03.015
Zhu Z., Vuik V., Visser P. J., Soens T., van Wesenbeeck B., van de Koppel J., et al. (2020). Historic storms and the hidden value of coastal wetlands for nature-based flood defence. Nat. Sustainability 2020 (3), 853–862. doi: 10.1038/s41893-020-0556-z
Keywords: land reclamation, ecosystem services, coastal reclamation, wetland degradation, invasive plants, coastal armoring, climate change
Citation: Li J, Leng Z, Yuguda TK, Wei L, Xia J, Zhuo C, Nie Z and Du D (2023) Increasing coastal reclamation by Invasive alien plants and coastal armoring threatens the ecological sustainability of coastal wetlands. Front. Mar. Sci. 10:1118894. doi: 10.3389/fmars.2023.1118894
Received: 08 December 2022; Accepted: 06 February 2023;
Published: 20 February 2023.
Edited by:
Sijia Li, Northeast Institute of Geography and Agroecology (CAS), ChinaReviewed by:
Chuanpeng Zhao, Chinese Academy of Sciences (CAS), ChinaCopyright © 2023 Li, Leng, Yuguda, Wei, Xia, Zhuo, Nie and Du. This is an open-access article distributed under the terms of the Creative Commons Attribution License (CC BY). The use, distribution or reproduction in other forums is permitted, provided the original author(s) and the copyright owner(s) are credited and that the original publication in this journal is cited, in accordance with accepted academic practice. No use, distribution or reproduction is permitted which does not comply with these terms.
*Correspondence: Taitiya Kenneth Yuguda, dGFpdGl5YXl1Z3VkYUBvdXRsb29rLmNvbQ==; Daolin Du, ZGRsQHVqcy5lZHUuY24=
Disclaimer: All claims expressed in this article are solely those of the authors and do not necessarily represent those of their affiliated organizations, or those of the publisher, the editors and the reviewers. Any product that may be evaluated in this article or claim that may be made by its manufacturer is not guaranteed or endorsed by the publisher.
Research integrity at Frontiers
Learn more about the work of our research integrity team to safeguard the quality of each article we publish.