- 1Massachusetts Division of Marine Fisheries, New Bedford, MA, United States
- 2Responsible Offshore Science Alliance, Onset, MA, United States
The massive biomass of Eastern Georges Bank haddock (Melanogrammus aeglefinus) is difficult to harvest without capturing less robust, but still valuable groundfish stocks like Atlantic cod (Gadus morhua) and some flatfish species. Specialized haddock trawls that raise the mouth of the nets off-bottom have reduced bycatch but the very poor status of Atlantic cod prioritizes even greater reduction to prevent exceeding regulatory fishing quotas. Raising the entire fishing gear off-bottom may further reduce bycatch while eliminating benthic impacts, expanding access to grounds previously off-limits to bottom-tending trawls. We evaluated an off-bottom trawl (OBT) to harvest Eastern Georges Bank haddock while reducing catches of overexploited stocks. The OBT net has very large meshes at the front end, made with innovative “helix” twine that produces lateral hydraulic forces while towing, resulting in self-spreading of the meshes. We established optimal gear configurations to achieve the target OBT net shape and distance to the seafloor by using an assortment of mensuration sensors/loggers and cameras. The OBT caught similar amounts of haddock and reduced some bycatch more than a standard bottom “Ruhle trawl”, but also caught fish of the same lengths despite the OBT using a smaller mesh-sized codend. The OBT also demonstrated similar requirements in vessel RPMs as the Ruhle trawl, despite having a larger swept area.
1 Introduction
The New England groundfish fleet has been struggling, in part due to inadequate revenues, with numbers of participating vessels declining over the last decades (Murphy et al., 2014). Haddock (Melanogrammus aeglefinus), an abundant and healthy stock on Georges Bank, could provide additional fishing opportunities but continues to be underexploited, with only 1% of the allowable catch taken in recent years (Finley et al., 2019).
Haddock occupy a narrower range of habitats and less area compared to Atlantic cod (Gadus morhua) (Collette and Klein-MacPhee, 2002), so limits to access to haddock fishing grounds can severely inhibit haddock harvest. At the start of this project in 2016, Closed Areas I and II on Georges Bank had excluded groundfish fishing from roughly 25% of the total area of the bank, or about 10,900 km2 for more than twenty years (Link et al., 2005). Temporal restrictions were also in place: from 2008 to 2010, the USA portion of the Eastern Georges Bank management area was closed from May 1-July 31 to vessels using trawl gear. It is from the trawl gear fleet, exclusively demersal otter trawls, that nearly all US haddock landings are derived (Finley et al., 2019).
Area closures, temporal restrictions, and other management measures are in place, partially to protect and to improve the health of groundfish stocks through effort reduction. Atlantic cod stocks, in particular, appear to be in historically poor condition, with Georges Bank stocks considered to be at very low biomass (NEFSC, 2017) and likely exploited at rates above management targets (NEFSC, 2012). Catch limits for Georges Bank Atlantic cod (or “cod” for short) are consequently quite low at 1,250 MT for 2021 and 2022 (NOAA, 2021). Therefore, fisheries that catch cod, even incidentally, must be conducted cautiously to avoid expensive leasing of additional quota and quota exhaustion.
Access to Georges Bank haddock fishing grounds is permitted under special access programs using several demersal otter trawl designs developed in the region to reduce bycatch of cod when targeting haddock (NOAA, 2004). A relatively commonly used design, the Ruhle trawl (a.k.a. rope trawl or Eliminator trawl) is constructed of very large meshes (320 cm) at the front end, gradually reducing to 16.5 cm or smaller. In experimental trials, this design successfully increased the bycatch ratio of haddock to cod from 3:1 to 20:1 (Beutel et al., 2008). Vessels fishing with bottom-tending trawls equipped with horizontal separator panels are also permitted in the special access program (Nichols et al., 2001; He et al., 2005).
A meta-analysis of multiple studies of separator trawls across the North Atlantic found separation of haddock and cod to be linked to the height of the panel off-bottom, with higher panels increasing the degree of separation of these species (Fryer et al., 2017). Other work investigating raised footropes/fishing lines (with groundgear bottom contact) provides corroborating haddock and cod results (Krag et al., 2010; BIM, 2017). Previously, we developed a haddock trawl design known as the Five-Point Trawl that combined the virtues of cod separation via raising the footrope of the net, with contact with the seafloor minimized to five chains hanging from the fishing line (Chosid et al., 2010). This design elevated the net approximately 1.5 m off the bottom. In field trials, the net fished as well as a net with a horizontal separator panel.
The current status of Georges Bank Atlantic cod is so poor that these various trawl designs, proven to effectively reduce cod bycatch and implemented in special access programs, are not sufficient to prevent exceeding quotas (Eayrs et al., 2020). However, the partial effectiveness of separator trawls and the Five-Point Trawl at reducing cod bycatch suggests that raising the mouth of the trawl net even further off-bottom might decrease cod catch even more. Reductions in groundgear bottom contact, between the doors and the net, have also demonstrated significant reductions in cod catches of all lengths (Sistiaga et al., 2015). Thus, testing of a net to target haddock that fishes completely off, but close to the seafloor and reduces unwanted catch appeared to be a logical work progression.
Modern trawling with bottom trawls began in the early 1900s (Gabriel et al., 2005). Pelagic trawls (or midwater trawls, or off-bottom trawls), in which bottom contact from the trawl doors is eliminated during towing and other contact by the groundgear of the net is reduced or eliminated, were first used in 1948 coinciding with, and relying upon, acoustic sensors to indicate their positions (von Brandt, 1972). These trawls also generally feature much larger fishing circles than demersal trawls and require specially designed pelagic doors to spread the net. They typically represent a substantial increase in complexity in design, construction, and operation compared to demersal trawls. Perhaps due to this complexity, commercial pelagic fishing for groundfish species is rare. It occurs in the Baltic Sea for Atlantic cod (Madsen, 2007), and in the Bering Sea for Alaskan pollock (Gadus chalcogrammus) (Erickson et al., 1996). Semi-pelagic trawling has also been researched in Norway in recent years demonstrating similar haddock catches with different amounts of groundgear seafloor contact (Sistiaga et al., 2016).
An additional potential benefit of the development of pelagic trawls, beyond improved cod avoidance, is reducing seafloor habitat impact. Vessels using pelagic trawl gear could therefore have broader access to fishing areas on Georges Bank than those using bottom tending gears, which are restricted from certain areas due to benthic habitat impact concerns (NOAA Fisheries, 2020), and have greater opportunities to find the sporadic, but highly concentrated haddock.
We developed an off-bottom trawl (OBT) in consultation with our fishing partner and a local net manufacturer and inspired by the “Gloria Trawl” design (Hampiðjan Group, Iceland), intended to be sized and designed for adoption by vessels in our region usually associated with bottom trawls. The OBT incorporated part of an existing trawl net and included innovative helix twine to enhance spreading of the net mouth, kites to reduce drag otherwise caused by headline floats, and trawl doors that could be fished on or off bottom. Generally, the OBT was designed to fish with all components close to, but not contacting the seafloor to harvest Georges Bank haddock along with redfish (Sebastes fasciatus) and pollock (Pollachius virens), while simultaneously avoiding overexploited fish stocks, mainly Atlantic cod, yellowtail flounder (Limanda ferruginea), and windowpane flounder (Scophthalmus aquosus). The combined biomass of the Georges Bank haddock, Gulf of Maine haddock, pollock, and redfish stocks currently constitute more than 90% of the overall groundfish biomass observed in the Northeast Fishery Science Center spring surveys and only small fractions of their respective annual catch limits are landed (NEFSC, 2017).
Our objectives were to evaluate and fish the OBT without seafloor contact and with an optimal net shape and position while both maintaining commercial target catches and further reducing bycatch as compared to a standard groundfish Ruhle trawl net, a net already proven to have low bycatch.
2 Materials and methods
We conducted two at-sea field efforts to evaluate the OBT net: trip 1 was dedicated towards establishing operational parameters (i.e., gear tuning); trip 2 consisted of a structured experiment comparing the OBT net vs a standard, bottom-tending Ruhle trawl. The gear tuning trip preceded comparisons of catch in order for the fishing and scientific crews to understand and control the geometry and performance of the experimental net, gain familiarity with setting, hauling, and fishing the gear, and to identify problems that could have otherwise been avoided by on-shore adjustments. Gear tuning was not constrained by fish availability and therefore, the tuning trip was conducted closer to port, reducing steam time at the start and end. Catch comparisons required working in areas further from shore in deeper waters where haddock are more available. All fieldwork was conducted on-board the F/V Illusion, a 25.3 m (83 ft), 745.7 kW (1000 hp) groundfish Western-rig commercial trawler with a stern ramp and two net reels.
Meshes in the codends for the Ruhle trawl and OBT net were measured wet during the first comparative research trip using an Omega Gauge following recommended procedures including measuring both cross dimensions for square meshes (Fonteyne, 2005).
Environmental data (wave height, wind speed, and weather conditions) were observed and recorded on all tows. For each tow, set time (when trawl winches were locked in place) and retrieval time (when winches began hauling) and respective locations, depths at start, initial warp wire set, and tow speed were recorded. For trip 2, we also recorded the depths at end of tows and RPMs of the vessel soon after start of tows. Position and speed data were provided by the vessel’s Northstar 6100 GPS. Depths during towing were acquired from a Simrad ES70 split-beam sounder (38 and 200 kHz).
2.1 Nets and gear
The OBT net was built with mesh openings greater than 3.5 m in the front-end and made with 8 mm color-coded “helix” twine, an innovative twine that incorporates a thinner twine twisted along the surface of a thicker twine to produce lateral hydraulic forces, resulting in self-spreading of the meshes (Gloria: Self Spreading, 2015; Kebede et al., 2020) (Figure 1). The direction of the spreading force is altered by changing the rotation of the surface twine (clockwise or counterclockwise). Each bar of each mesh was cut to length and swaged with loops at each end. The loops were joined by lashing four of them together to form each diamond mesh.
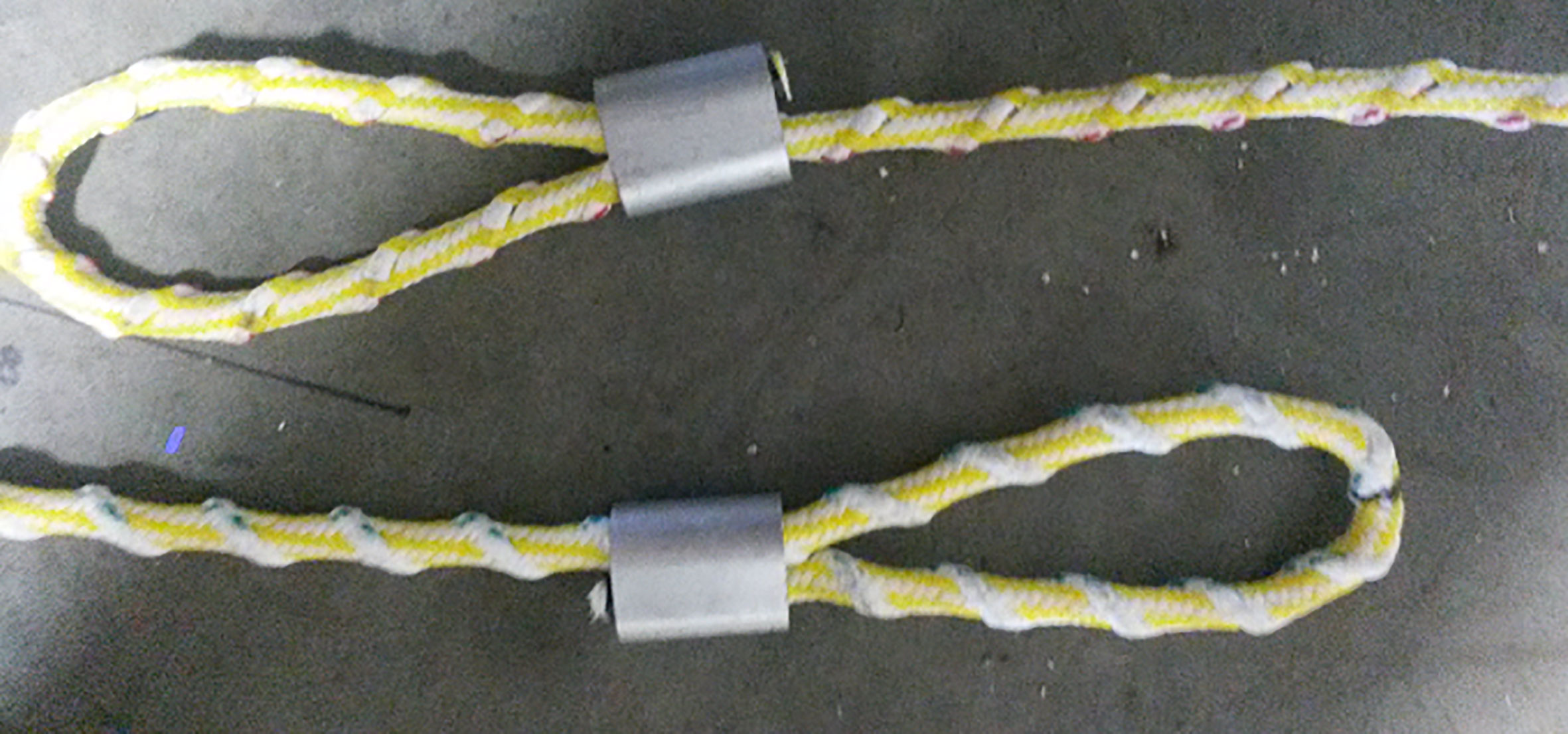
Figure 1 Helix twine sections for the OBT with markings delineating the direction of coils, clockwise (red markings; top) and counterclockwise (green markings; bottom).
The OBT (Figure 2) was modified by Reidar’s Manufacturing (New Bedford, MA) from a net owned by Captain Phillips, owner of the F/V Illusion. The modified net was a four-seam midwater box trawl with a 40.1 m headline and footrope and 28.4 m port and starboard side lines (see Supplementary Material, Table 1). The front end was constructed of meshes made from the helix twine that decrease in mesh size towards the aft. The largest helix twine bar lengths were 3.8 m (150 inch) at the wingends, graduating to 1.6 m (64 inch) aft. The back-end of the trawl (the original net sections) was constructed of nylon twine with five panels that reduce in mesh sizes and twine diameters towards the aft. The fishing circle was 52 meshes around at 2.2 m (85 inch) sized meshes (4th sized meshes to the aft). No floats were used on the headline of the OBT. Rather, a canvas kite, 105 cm wide and 85 cm high, was attached along a false headline to supplement lift. Adjustments to the angle of attack of the kite were made by adding or shortening chain (11 mm/link) near the wingends of the net – fewer links pull the kite more parallel to the angle of the net’s first top panel meshes. The kite was tested at different numbers of links during the tuning trip but was finally settled at setting the chain to three links on each wingend for tow 11 and remained this way for the remainder of that trip and for the comparative research trip. The footrope consisted of 12.7 mm (½ inch) chain. Steel bridles were two legs (12.7 mm (½ inch) on top and 19.1 mm (¾ inch) on bottom), 36.6 m (120 ft) long leading to four legs (12.7 mm (½ inch) on the top three legs and 15.9 mm (5/8 inch) on the bottom), also 36.6 m (120 ft) long.
The control net used was a standard Ruhle trawl design (Beutel et al., 2008) with a PE 15.2 cm (6.0 inch) diamond-shaped mesh codend, double twine, 50 meshes deep and 60 meshes on the round (see Supplementary Material, Table 1). We used 36.6 m (120 ft) ground cables and 73.2 m (240 ft) legs to connect the trawl doors and the wingends. The trawl has a 42.8 m (140.5 ft) headline with fifteen, 20.3 cm (8 inch) floats and 51.4 m (168.6 ft) long groundgear with 7.6 cm (3 inch) cookies. Three kite panels (39.7 cm x 33.0 cm) were located along the center of the headline to help provide lift to the headline, consistent with this net’s prior usage.
The OBT net was equipped with a 13.0 cm (5.1 inch) square mesh-sized codend and composed of braided 5.5 mm PE double twine, 150 bars deep, and 80 meshes on the round which was compared against the Ruhle trawl’s standard 15.2 cm (6.0 inch) diamond mesh codend. The 13.0 cm mesh size was selected to emulate prior research and to match the Canadian fishery across the Hague Line1 (Finley et al., 2019); this mesh size was also easily obtainable from netting manufacturers. Chaffing gear was used on each codend.
Nets were spread using 3.0 m2 Gull Wing doors (Net Systems, AK, USA), configured to fish either on the bottom when towing the Ruhle Trawl or off-bottom when towing the OBT net. The final door configuration was achieved on trip 1, tow 11, after generally demonstrating the doors’ desired heights and positions while towing (see Supplementary Material, Table 2 and Figure 1).
2.2 Net mensuration sensors and data loggers
Gear rigging and geometry were monitored and recorded using multiple net sensors to optimize the positions and shape of the gear (Table 1). Distance between the trawl doors (door spread) of all nets was measured on all trips by a Simrad Fisheries ITI (just Simrad for short; Seattle, Washington) acoustic net mensuration system with a hull-mounted hydrophone. Additionally, on trip 2, we had access to a Simrad headline sensor to obtain the OBT’s headline height off the seafloor, and headline to footrope distance (vertical opening). We were initially unable to automatically log Simrad data due to the age of the system and, as a result, logging on the first trip was by hand; for trip 2, we viewed and logged the data using PuTTY, an open-source terminal emulator, serial console, and network file transfer application (developed by Tatham, 1999; updated version used from 8/2019).
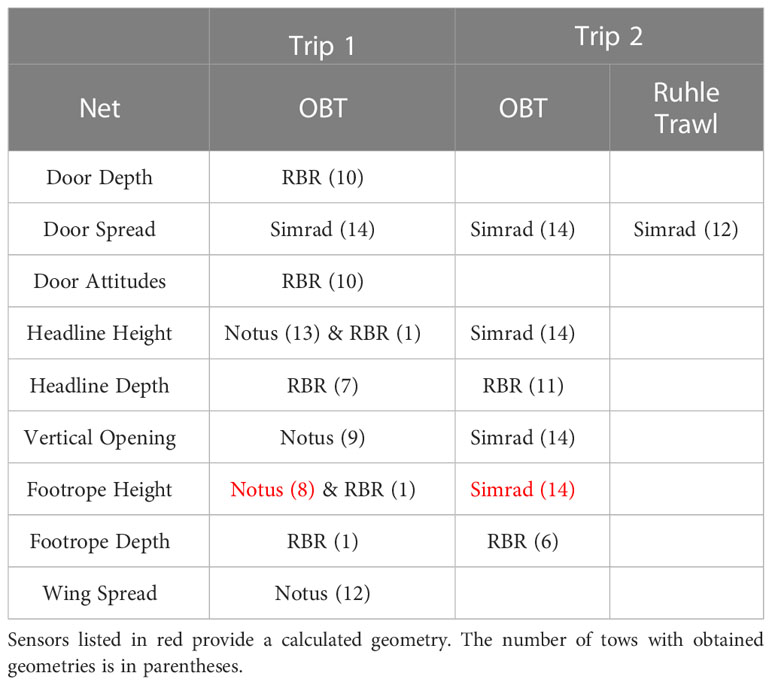
Table 1 Net mensuration sensors used during each trip and for each net towed and their associated measured geometries.
A Notus net mensuration system (just Notus for short; Notus Electronics Ltd., St. John’s, Newfoundland) with a portable hydrophone was used on trip 1 in addition to the Simrad system (Table 1). Notus sensors were attached to the net’s wingends, the mid-points of the headline and the footrope, and recorded the wing spread, the headline height from the seafloor, and the vertical opening. Output from this system was logged to a laptop automatically by the proprietary Notus Trawlmaster software. Footrope height for each tow was calculated from Notus data when available as the difference of the mean distances of the headline heights and vertical openings.
Outputs from Simrad and Notus sensors were live-streamed during each tow using each company’s proprietary software and used by the captain to adjust the vessel’s speed and wire out to achieve target net geometry and height off-bottom, door positions and spreads, and a consistent depth to wire out scope as the depth changed. The captain attempted to maintain the OBT net’s footrope within one-two meters from the seafloor while towing.
The OBT was an unfamiliar gear type, especially when used in conjunction with Gull Wing doors which have more complex ranges of movements than bottom-tending doors. Therefore, we collected attitude data as well as depths to understand door positions in the water column and their relation to the OBT, and the OBT’s position relative to the seafloor. RBR “Concerto” loggers (RBR Ltd., Canada) were mounted on both doors to measure depth and accelerations in three dimensions (x, y, and z) on trip 1 (Table 1). Accelerations were used to derive pitch and roll (see “Data Entry, Processing, and Analyses” below). Door loggers were mounted so that both y-direction accelerations were facing each other, towards the center of net. That is, doors tilting inward (roll) to the center would register with the same sign (positive) for both loggers; tilting from parallel to the vessel direction (pitch) would register as opposite signs. On some tows, a RBR “Duet” logger was mounted on the headline to measure depth and, for one tow, a Concerto logger with an altitude sensor was mounted on the footrope to provide depth and height from the bottom; the altitude was corrected using attitude (tilt) measurements. RBR loggers were set to collect data at one-second intervals.
2.3 Gear tuning, trip 1
Riggings, structures, and positions of each gear type in the water column and presence of fish around the gear on the tuning trip was observed using three cameras: a GoPro Hero 3+ Black in a deep-water Sartek housing, and two low-light cameras: Deep Sea Power and Light (DSP&L) Wide-I SeaCam and a DSP&L Low-Light SeaCam. The low-light cameras were used without illumination to avoid creating an artificial light stimulus. The GoPro camera required additional lighting at depth so two EBL-1200D Sartek LED lights with uniform lumen intensity and a 60° light beam angle were used to provide illumination. Video from GoPro cameras was recorded on SD cards. Video from the low-light cameras was recorded onto a custom, underwater recording system sealed in a deep-water titanium housing (Integrity Systems, Massachusetts, USA). Collected video was viewed immediately afterward to inform gear adjustments on subsequent tows.
The codend remained open during trip 1 and no catch was retained.
2.4 Comparative research, trip 2
Comparative paired tows were conducted on this trip using the Ruhle trawl and the OBT net, fishing for 24-hour periods. Two-hour duration tows were planned but were increased when catches were light or shortened due to large catches or gear problems while towing. Subsequent paired tow durations were matched to the extent possible. When paired, nets were fished in an R-E-E-R (Ruhle trawl = R) and experimental net (OBT = E)) alternating pattern for approximately five days of comparative trials. The second tow of each pair was along roughly the same path although not directly on top of the prior tow’s path. Directions of paired tows were at the discretion of the captain accounting for the lost time to return to the starting side of the previous tow and the effects of changing water currents on the pair.
The captain targeted haddock during each comparative research trip but did not avoid other species in order to show the difference in target catches and non-target catches between compared nets. All fauna in catches were identified to the lowest taxonomic level possible and sorted. Most species were weighed to the nearest 0.1 kg using a Marel M1100 motion-compensated bench scale, or estimated quantitatively by weighing a subset of filled baskets. For rare catches of some larger species, such as sharks, visual weight estimates were made, as needed. Midline lengths of regulated groundfish species were measured to the nearest centimeter, with sub-sampling of at least 100 fish lengths occurring as needed and available. Species of special importance, such as protected species, were weighed, measured, or estimated quickly before returning to sea when possible. Discarding did not occur while towing or during net retrieval or deployment so that discarded catch would not end up in the active tow’s codend and be resampled.
2.5 Data entry, processing, and analyses
A Microsoft Access database was constructed for data entry (trip, tow, and species catch weights and length frequency information), QA/QC, data management, visualization, and analyses.
Catch weights were adjusted by tow lengths (kg/hr) to equalize effort. Sub-samples were scaled to the entire catch weight for analyses. Catch weights of porbeagle sharks (Lamna nasus) were visually estimated or derived from their total lengths (when these data were collected) using calculations provided by the Northeast Fisheries Science Center (https://www.nefsc.noaa.gov/nefsc/Narragansett/sharks/calc.html, accessed 06/2020).
Videos were collected in standard file formats and edited and reviewed using various software including Adobe CC Premiere, FFmpeg (FFmpeg team), VideoRepair Tool (Grau GmbH Hardware & Software Solutions), MediaInfo (developed by Martinez, 2002), HandBrake (HandBrake Team), and VLC player (VideoLAN).
Simrad net mensuration text files were reshaped using R (R Core Team, 2018) so that each line had the prior time stamp appended to it. Files were recompiled by each individual sensor’s output and then imported into the Access database. Height of the footrope off the seafloor was calculated from sensor data during tows and in post-processing by subtracting the headline to footrope height from the headline to seafloor height. When both distances reported the same value, it was assumed that the footrope was close to or contacting the seafloor.
Notus data, logged using Trawlmaster, was exported to an Excel file and then imported into the Access database. Values of headline heights and vertical openings that exceeded 80 m, the maximum depth encountered, were excluded from the data set as unrealistic. Mean footrope heights off-bottom for tows were obtained by subtracting the mean vertical openings from the mean headline heights.
RBR Ruskin proprietary software was used to access RBR data and to operate loggers. Roll and pitch were derived from x, y, and z accelerations using the following equations provided by RBR:
The denominator is limited by values >0.025 (before the sign is applied) due to the sensitivity limitations of the loggers’ accelerometers (RBR, pers. comm.). No gravity vector exists in the x and y planes (the yaw component accelerations) when the logger is turned along the vertical axis. Changes in this axis could not be stabilized by the internal gyroscopes and therefore produced large variations in yaw. For this reason, yaw was not available. Tilt is also calculated to determine the resulting angle based on the roll and pitch together. Sensor tilt greater or less than 0 was used to correct the footrope to seafloor distance measured by the headline sensor for any deviation for the vertical.
Data visualization and analyses were conducted with R and Excel. Box and whisker plots (McGill et al., 1978) were drawn using the 25th and 75th quantiles as lower and upper limits (interquartile range, IQR) of the box, and a bar within the box representing the median of the dataset. Whiskers are drawn to end at observed values at most 1.5 times the length of the IQR. Points plotted beyond the whiskers are greater than 1.5 times the IQR and may be considered outliers (Sokal and Rohlf, 2000). Outliers removed for readability are noted in figure captions. Box widths are proportional to the square roots of the sample sizes within each grouping when indicated in plots.
Catch rates (kg/hr) of regulated, dominant, or selected species were paired in trip 2 using equal catch plots. All pairs of tows where a species was present in at least one of the tows are included. For species that did not show a distinct pattern between the gear types in the equal catch plots, data were checked for normality using Q-Q plots (used as reference and not reported here) and a Shapiro-Wilk Test (p<0.05). The non-parametric Wilcoxon Signed-Rank Test was used to test for significance of difference (p<0.05) between the catch rates in the two gears if the tests for normality failed. For normal data, equal variance was first investigated (p<0.05) and then a paired t-test was applied to check for significance between catches within gear types (p<0.05). Two-sided tests were applied to haddock as the OBT net and Ruhle trawl were hypothesized to catch equally for this species. One-sided tests were applied to species where catch reductions were anticipated in the OBT net as compared to the Ruhle trawl.
Length frequencies are presented for species whose mid-line lengths were measured using box and whisker plots. Length-based differences by species were explored for trip 2 paired data, using generalized linear mixed models (GLMMs) to fit low-order (third or less) polynomials to the proportions of catch-at-length in the experimental net compared to the total catch at length. Our GLMM methodology followed those described by Brooks et al. (2022) using the “selfisher” R package. Lengths of sufficient strength (i.e., where the total count at length was >5) were used as a fixed effect, and haul was a random effect. Where subsampling occurred, we used a “qratio” (intercept term) equal to the inverse of the proportion of the subsample. Data were bootstrapped at 1000 iterations, as recommended by Brooks et al. (2022). A binomial link function was used to fit the most complex model, followed by decreasing complexity. Model terms were assessed for differences using Akaike’s Information Criterion (AIC), again consistent with recommended practices.
3 Results
The gear tuning and comparative research trips were completed from 8/31-9/2/2017 and 8/9-8/15/2019 respectively. Forty-seven tows were completed overall for both trips (Table 2). Tow 18 on trip 2 using the OBT was aborted early due to battery failure in the Simrad headline sensor, instrumental to keeping the net stable and at the desired height, and therefore considered not valid. Thirteen comparative tows of the OBT and the Ruhle trawl were paired successfully by gear type.
Fieldwork was conducted in two general locations (Figure 3). Trip 1 was conducted at a location relatively close to port in southern New England waters with a depth workable for the OBT (mean: 41.5 m) (Table 2). Greater depths were sought for comparative research trips in Georges Bank to obtain preferred door spreads by setting out additional warp wire (limited in more shallow depths) and for the nets to fish as expected; mean depths in trip 2 were 83.1 m for the OBT and 83.5 m for the Ruhle trawl. Depth was not significantly different between nets in trip 2.
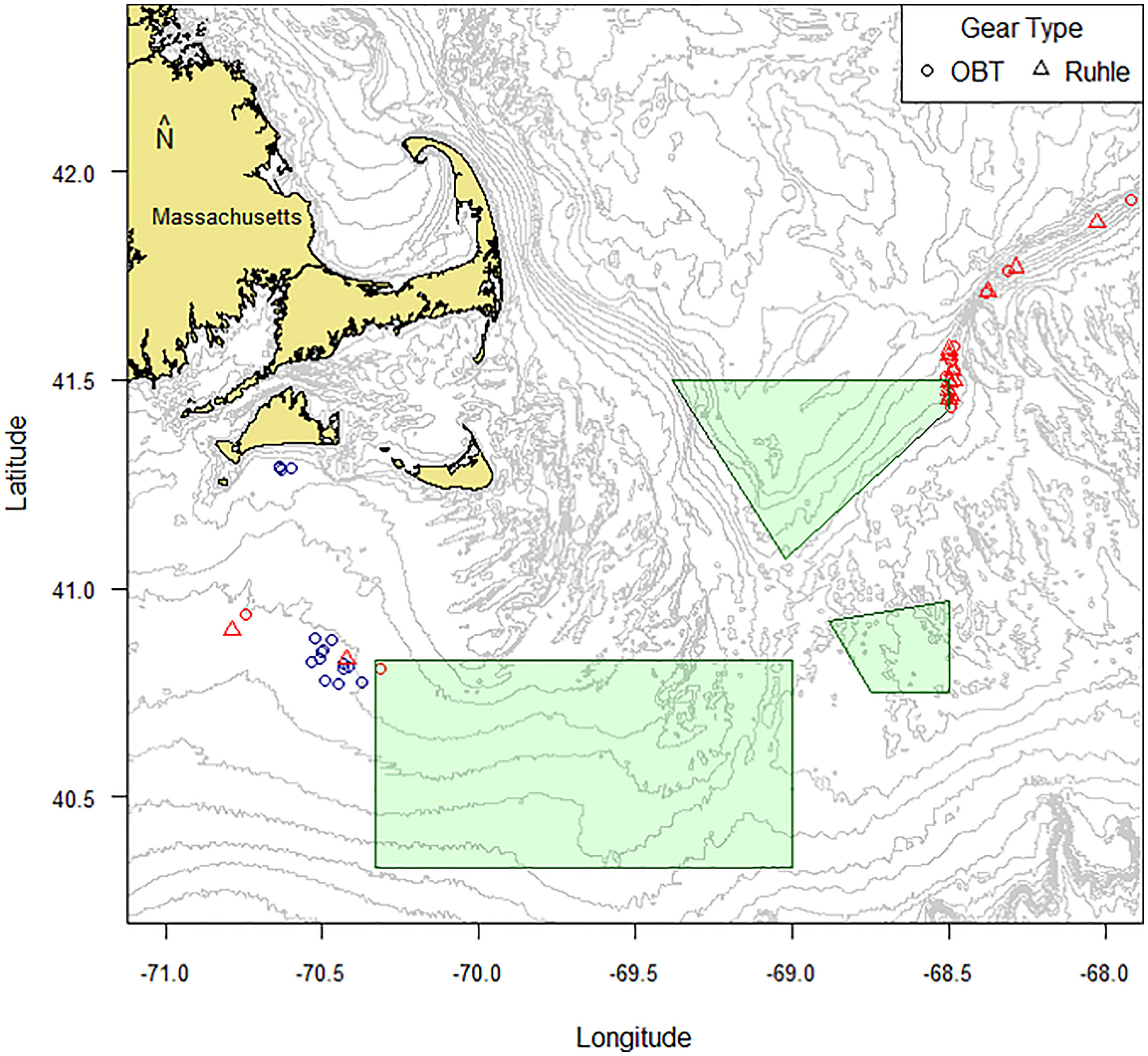
Figure 3 Start point locations of tows during trip 1 (blue marks) and trip 2 (red marks). The key shows symbols for gear types used. Sections shaded in green are regulated closed areas.
Tuning trip 1 generally required shorter tow durations than comparative research trip 2 and occurred at shallower depths and therefore, required less wire out (Table 2). All nets started tows with a mean warp wire to depth ratio of approx. 3:1.
Speed was not significantly different for the OBT between trips 1 and 2 (Table 2). Trip 2 revealed a significantly higher speed while using the OBT over the Ruhle trawl but RPMs were not significantly different, suggesting lower drag while using the OBT. Helix twine increases drag along with spreading forces (Kebede et al., 2020), so lower drag using the OBT is probably a result of reduction in seafloor contact and the significantly smaller amount of wire out. RPMs were not recorded during trip 1.
Square mesh dimensions (inside, knot-to-knot) for the OBT codend were measured for a mean of 121.7 ± 1.2 mm S.E. and 118 ± 1.4 mm S.E., smaller than the nominal 129.5 mm (5.1 inch) mesh size. The Ruhle trawl used diamond-shaped meshes in the codend of 150.5 ± 0.4 mm S.E., which was slightly smaller than the expected 152.4 mm (6 inch) mesh size. Meshes at the front end of both nets could not be measured using conventional gauges due to their large openings.
3.1 Gear modifications
The tuning trip was intended to work out appropriate rigging for the OBT net. Despite this tuning, determining that gear remained off, but still near the seafloor was challenging during the comparative research. Consequently, based on results from the tuning trip and early comparative research tows and Captain Phillips’ previous experience with pelagic trawls, five links of drop chains (22.0 kg total) were added forward of the wingends at the lower bridles for OBT tows 11-30 to reduce instability during comparative research. The added drop chains were occasionally noted to have shine post-tows, presumably from seafloor scouring. Net instability continued to be a concern, despite the presence of drop chains, and minor adjustment of net rigging, tow speed, wire out, and other variables continued throughout much of the field work.
3.2 Gear performance
Gear tuning of the OBT during trip 1 was partially achieved using underwater video. Video were collected from 10 tows from trip 1 which imaged the OBT kite near the headline, the large meshes just behind the center chain sweep, and the chain sweep in relation to the seafloor. From the video, a final position of the kite was set at three links (33 mm; see Supplementary Material, Table 2). Through video review, consistent seafloor visibility indicated an effective operational door configuration; inconsistent seafloor visibility through a tow would indicate that the doors were likely too high in the water column or not in a stable position. The video also helped confirm the stable shape of the helix twine meshes while towing and that the twine was properly spreading. An acceptable gear configuration was achieved on tow 11 of this trip.
The optimal door configuration was set at bail box bracket 5, bail position “A”, and at lower pad position 4 (see Supplementary Material, Table 2 and Figure 1). Connections to the top and lower door pads were set at positions “E” and “C” respectively on trip 1 and at “C” and “D” on trip 2 to account for the difference in depths during trips but still achieve optimal door spreads.
We obtained door spreads using Simrad sensors from 14 tows for trip 1 and 26 tows for trip 2 (Figure 4). Trip 1 door spreads were similar (box and whisker plots’ boxes overlap) for most tows, especially after tow 8. The trip’s median door spread was about 64 m while using the OBT. Door settings were changed after the larger door spreads on tow 1 of this trip and appeared effective at reducing spread (see Supplementary Material, Table 2). It is unknown why tow 16 had a much larger than average door spread. The OBT door spreads were generally smaller in trip 1 than in trip 2, likely due to setting less wire out because of the much shallower depth of trip 1 (Table 2). Trip 2 median door spreads for the Ruhle trawl were generally larger than for the OBT (91.3 m v. 82.5 m) but largely consistent within gear type. Persistent attention was required to maintain a steady door spread while using the OBT by making small adjustments to the RPMs, especially prior to the addition of drop chains. This level of attention was not required while using the Ruhle trawl, perhaps due to bottom contact by the doors.
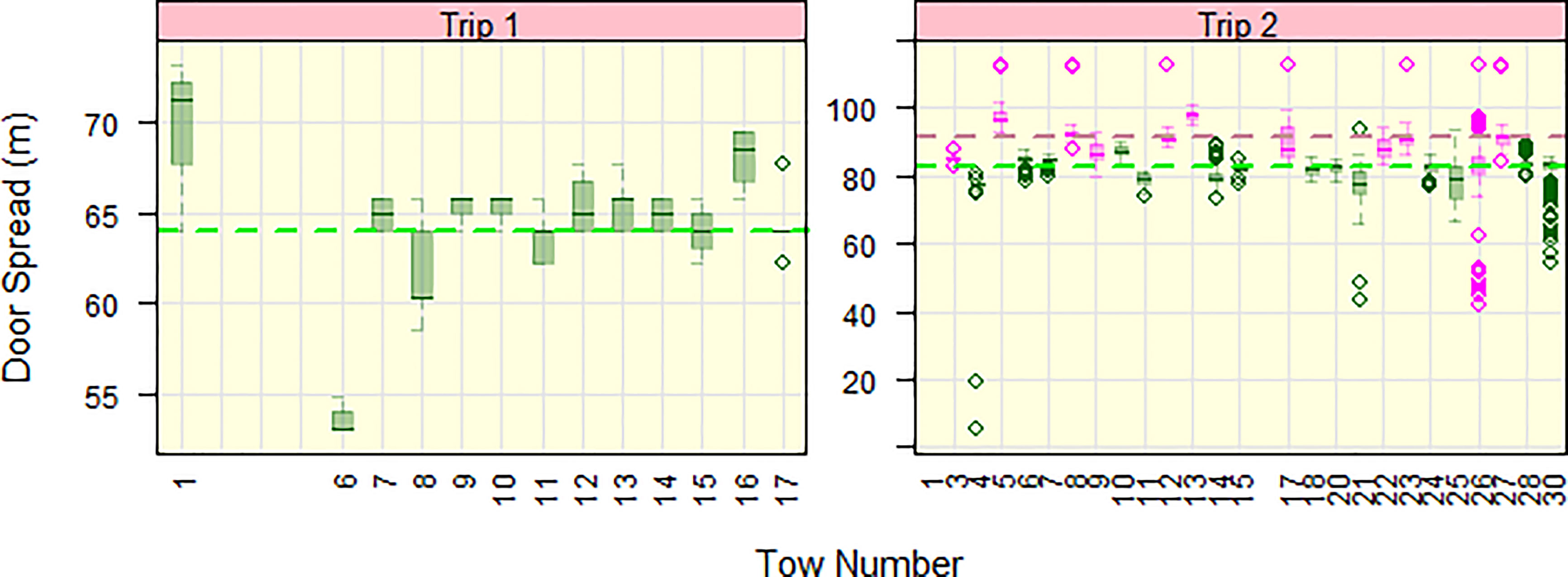
Figure 4 Door spreads (y-axis) by tow (x-axis) and trip (panels) from Simrad sensors. Boxes are colored by net type (green=OBT net, pink=Ruhle trawl). Dashed lines are the panel medians and colors of lines match the associated net type. Box widths represent the sample sizes within tows. Trip 1, tow 5 only had a single data point (23.8 m) and cannot be represented in box and whisker plots.
Roll and pitch of both trawl doors were measured on trip 1 during 10 OBT net tows using RBR loggers (Figure 5). On most tows, pitch and roll for both doors were not significantly different from zero. The port door pitch and roll during tow 10 and the starboard door pitch during tow 16 appeared to be different from the rest, although the medians were still close to zero. We are unaware of what caused deviations in these tows, but as noted above, tow 16 also showed abnormally large door spreads (Figure 4).
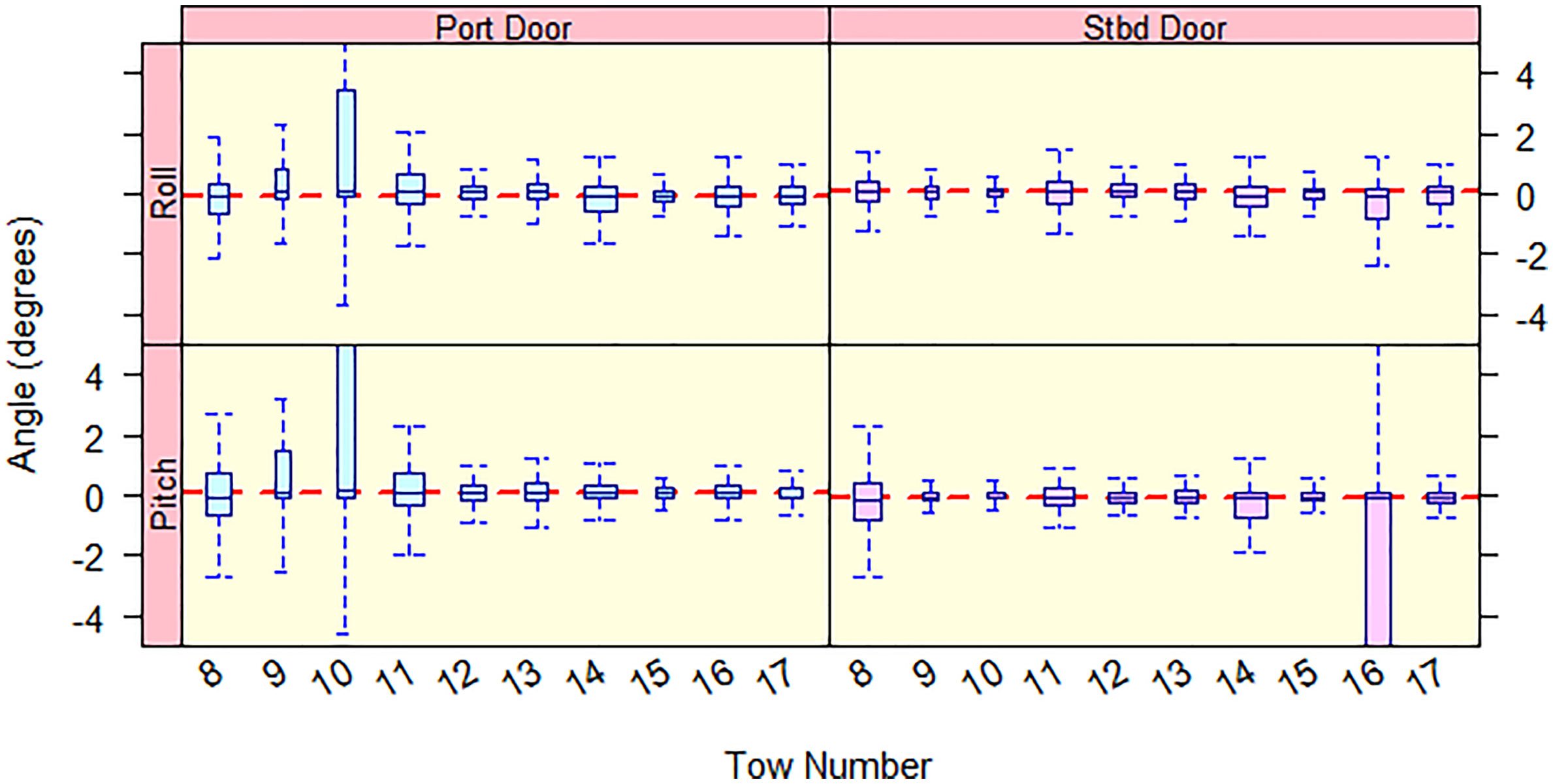
Figure 5 Pitch (bottom panels, forward or backward tilt) and roll (top panels, inward or outward tilt) angles (y-axis) of port door (left, light blue) and starboard (stbd) door (right, pink) for OBT tows (x-axis) during trip 1 from RBR loggers. The y-axis is restricted to -5° to 5° and the outliers are removed for presentation purposes. Dashed red lines are the panel medians. Box widths represent the sample sizes within tows.
The depths of the doors were collected by the RBR loggers during 10 OBT tows on trip 1 (Figure 6). Median door depths ranged from 34.7-41.5 m for the port door and 34.2-42.6 m for the starboard door and were generally similar for both doors as shown by the overlapping boxes of box and whisker plots. The maximum median depth difference occurred on tow 12 at 4.9 m. For this tow, the difference in door depths was as great as 7 m and persisted for more than 30 minutes (within tow details not provided). Overall, whether the starboard or port door was deeper was inconsistent between tows and within tows. It is unclear why this is the case. Changes in depths of one door were usually accompanied by a similar change from the other door and their depths were not usually significantly different by tow.
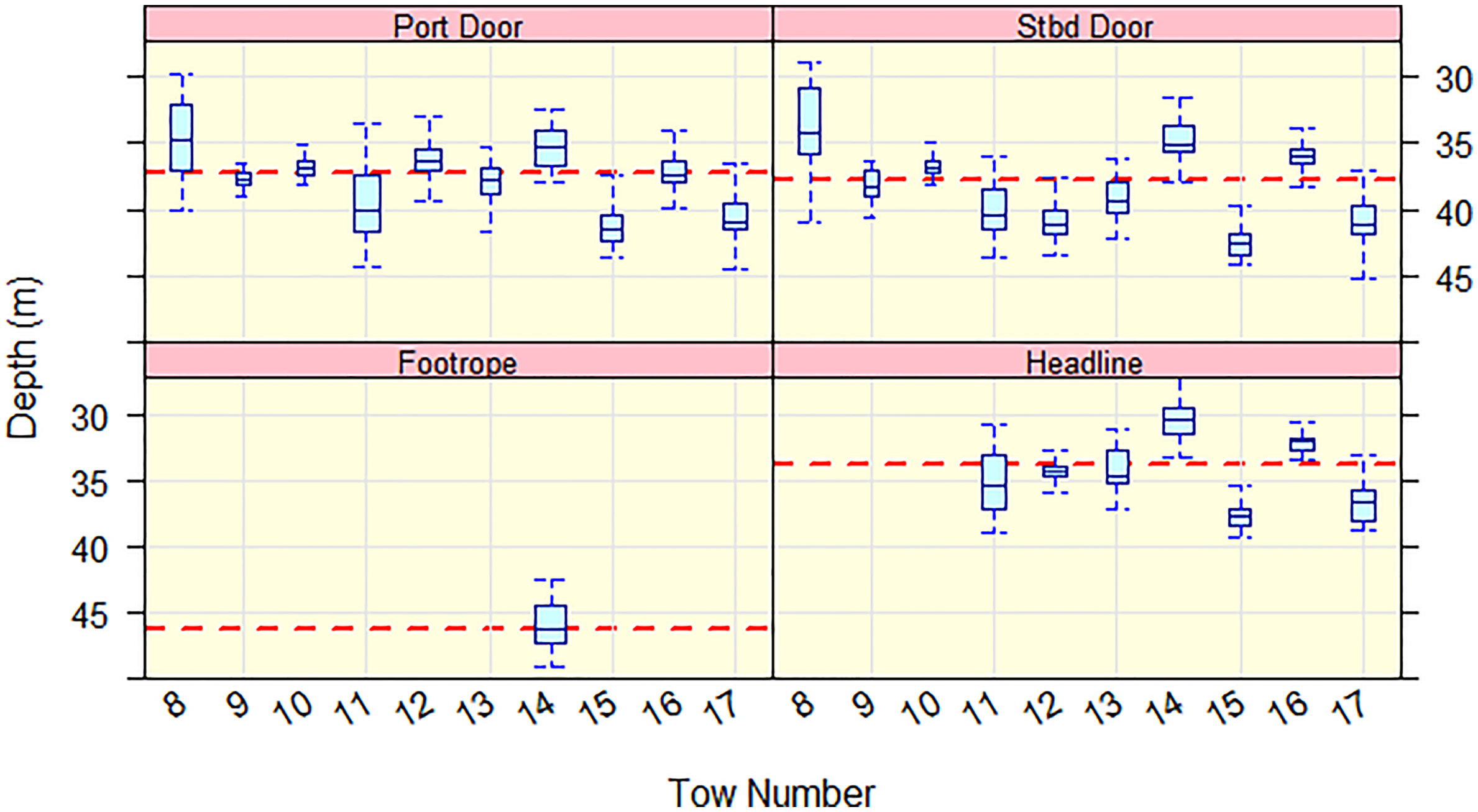
Figure 6 Door, footrope, and headline depths (y-axis) from trip 1 using RBR loggers for OBT tows (x-axis). Panels show individual sensor values with outliers removed for scaling. Dashed red lines are the panel medians. Box widths represent the sample sizes within tows.
Comparisons of door depths to headline and footrope depths for the OBT were possible on trip 2 due to simultaneous deployment of the RBR loggers in three or more locations. The headline was measured to be about 6 m shallower than the doors (Figure 6). On tow 14, an approximate 15 m vertical opening of the OBT was recorded through simultaneous headline and footrope depths. By comparing the relative depths of the doors, headline, and footrope recorded, the net was found to fish with the doors positioned about midway between the headline and footrope. The headline and footrope were observed to vary in depth together (detailed tow data not presented), maintaining a mostly constant opening, which suggests the net was off the seafloor. That is, if the net was contacting the seafloor, the footrope would fluctuate with the topography, while the headline would be more consistent, resulting in a varying opening. Median height of the footrope from the seafloor during tow 14, corrected for tilt, was 5.1 m (data from altitude sensor not presented). Maximum adjustment due to the tilt of the logger was small (0.22 m).
OBT wing spread, headline height, and vertical opening were collected on 14 tows during trip 1 using Notus sensors (Figure 7). Median headline heights varied tow-to-tow, generally from 17-32 m. Tows 4 and 5 were unusual: tow 4 was only eight minutes long and was composed of four data points; tow 5 had a much lower median headline height of 6.7 m for unknown reasons. The headline height became somewhat more stable and consistent within and between tows after tow 11 (the tow where we attained the preferred OBT gear arrangement) when the median vertical opening varied between 14-18 m. This opening is consistent with heights derived from the RBR loggers (Figure 6). The vertical openings are more stable than the headline heights for tows 12-17, with the exception of tow 15, which had a somewhat smaller vertical opening (the box of the box and whisker plot does not overlap with all of these other boxes). The preferred mean footrope heights (<2 m), derived from headline heights and vertical openings, were achieved during tows 12, 13, and 17; five other tows revealed greater mean footrope heights. Wing spread measurements generally varied around 18 m for all tows except tow 6, which was slightly less. Ranges from Notus sensors to the hydrophone from 14 tows were as expected and gave further information on the net geometry regarding the distances from the wingends to the headline through subtraction (data not shown). Means and standard errors of ranges to the headline sensor and wing sensors were 212.0 ± 0.5 m and 199.8 ± 0.4 m, respectively.
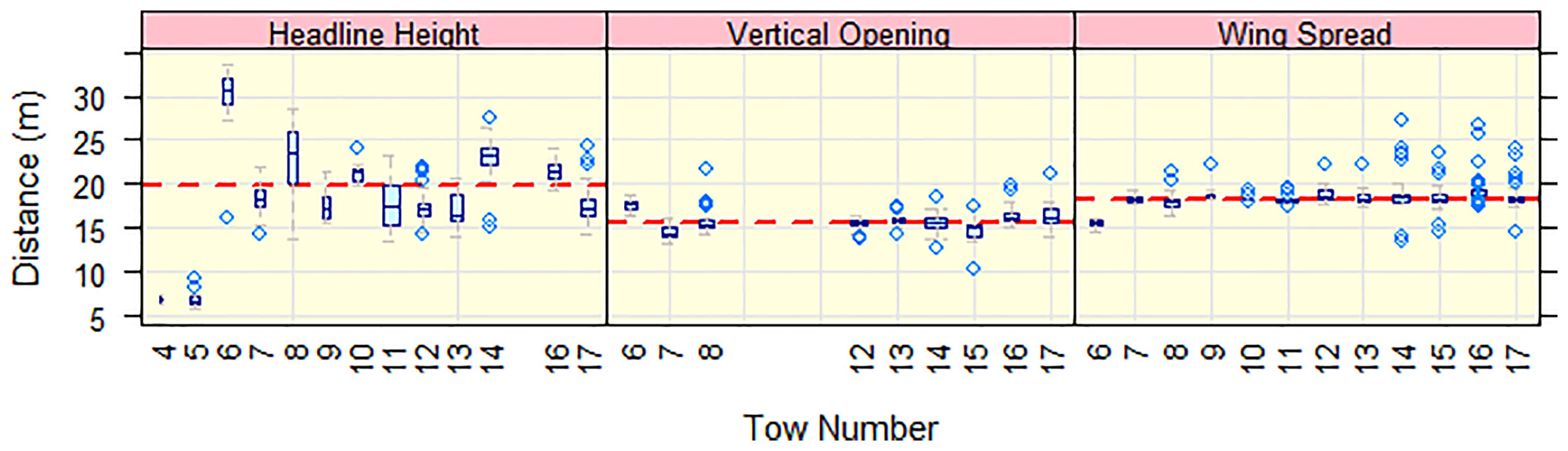
Figure 7 OBT net headline height, vertical opening, and wing spread (y-axis) for tows numbers (x-axis) on trip 1 from Notus data. Dashed red lines are the panel medians. Box widths represent the sample sizes within tows.
Headline heights collected from the vessel’s Simrad ITI system on trip 2 for tows using the OBT were similar (overlapping boxes of box and whisker plots), with medians of about 12 m, except for tow 4 (Figure 8). Headline heights for OBT measured using this system were lower than heights measured during trip 1 from Notus sensors (Figure 7).
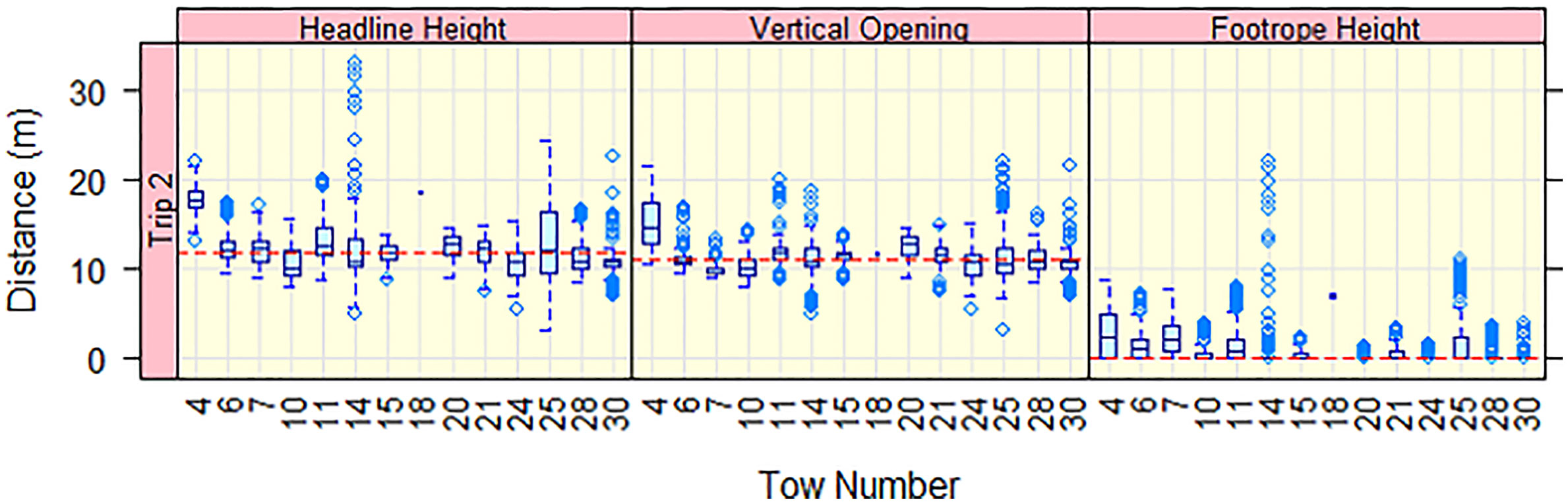
Figure 8 OBT net headline height, vertical opening, and footrope height (y-axis) for tow numbers (x-axis) on trip 2 from Simrad data. Dashed red lines are the panel medians. Box widths represent the sample sizes within tows.
The vertical opening for OBT on trip 2 stabilized to about 12 m after tow 4 (Figure 8). Footrope heights were often <1 m to the bottom for the OBT, particularly later in trip 2, suggesting that the footrope was often on or near the seafloor. The vertical opening measured in trip 2 was also smaller, and the footrope was likely closer to the seafloor than in trip 1 (Figure 7).
On trip 2, headline or footrope depths were collected during 15 tows from RBR loggers for the OBT with total median depths of 80.0 and 90.6 m respectively (data not presented). Depths for both the headline and footrope were only collected on tows 11 and 14 and showed a very consistent net opening of about 12-13 m over the course of the tows, and a headline depth around 80 m.
3.3 Catch results
Catches from the OBT and Ruhle trawl comparisons during trip 2 included 22 species and taxa with aggregate weights of greater than 3 kg (Table 3). Total weight of all species caught was 9,152 kg. Haddock, the target species, was the primary catch species by far, comprising 68.1% of the total weight captured. The next largest catches by weight were short-fin squid, (7.5%), porbeagle sharks (6.6%), winter skate (5.7%), monkfish (3.4%), spiny dogfish (3.0%), and barndoor skate (2.3%). Only 1.5 kg of Atlantic cod were caught using the Ruhle trawl and none in the OBT net. Total flatfish catches in both nets comprised 80.7 kg, mostly of grey sole. Aside from haddock, for the other species that this project was originally intending to target, pollock were barely captured (0.8 kg) and redfish were not caught.
We compared mean catch rates for selected species in 13 valid tow-pairs on trip 2 by net type (Figure 9). Haddock catch rates were highly variable in both the OBT and the Ruhle trawl, ranging up to 259 kg/hr. However, there were no tow-pairs where haddock were caught in one net but not in the other, demonstrating consistency in haddock catches within pairs. For other species, as illustrated by the greater number of points below the equal catch lines, most were clearly caught at lower rates with the OBT. The highest catch rate of any non-target species, excluding porbeagle sharks, was shortfin squid at 21.6 kg/hr in the OBT and 74.0 kg/hr in the Ruhle trawl. Some commercial species were caught at very low rates in both nets such as cod, pollock, and yellowtail flounder.
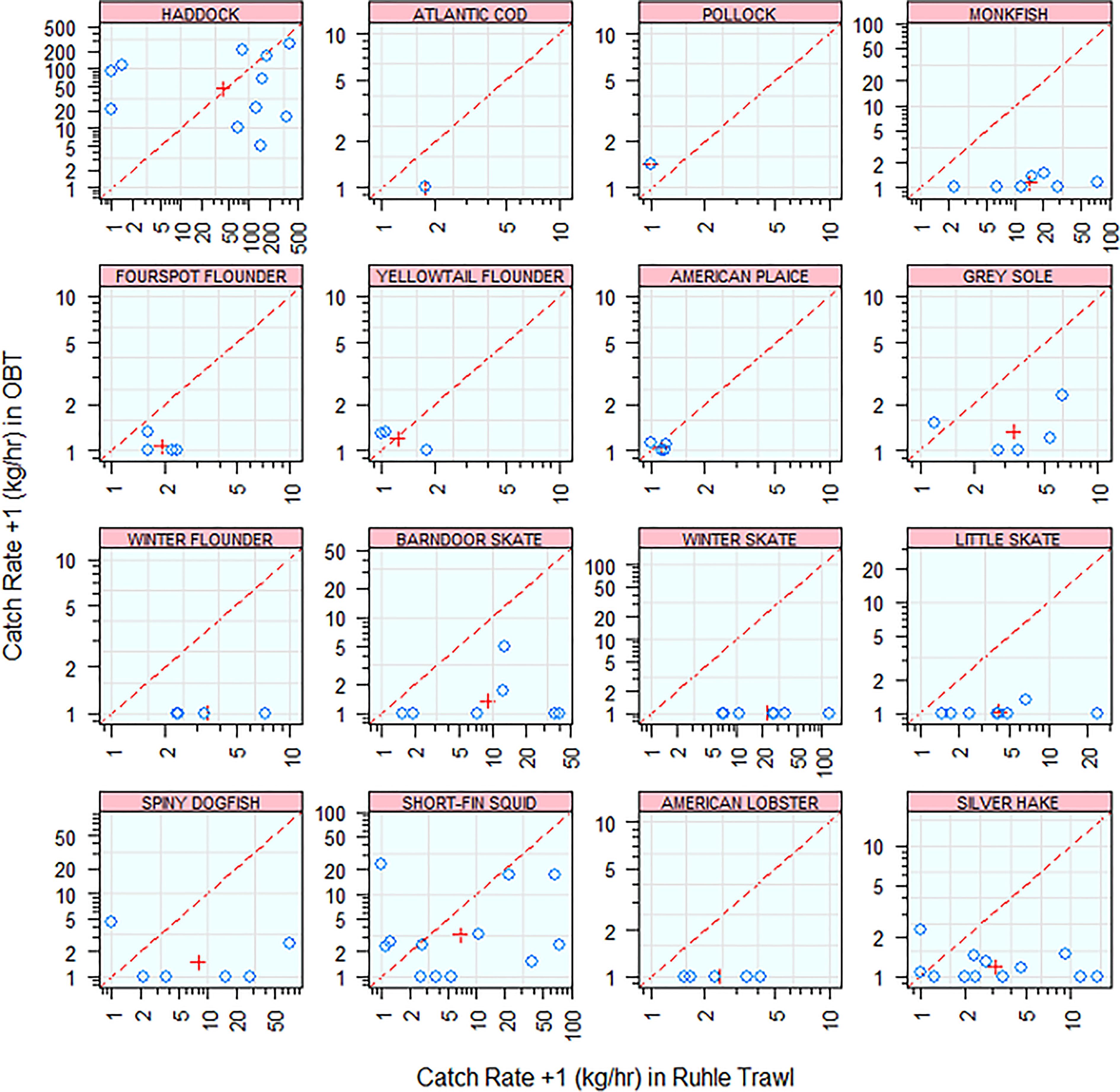
Figure 9 Catch rates (kg/hr) for selected species (panel) by tow-pairs during trip 2. The y-axis shows the OBT catch rates and the x-axis shows the corresponding Ruhle trawl paired catch rates. The “+” symbol shows the means for each species.
Mean catch rates of haddock were not significantly different in the OBT than the Ruhle trawl during trip 2 (Table 4). Mean catch rates of monkfish, grey sole, barndoor skate, and little skate were significantly lower in the OBT. American plaice catch rates were not significantly different between nets but catch rates were very low. Catch rates of other important commercial species that had catches in the Ruhle trawl but zero catch in OBT included winter flounder, Atlantic cod, American lobster, and winter skate and therefore, no tests needed to be completed to demonstrate differences in catch by this gear type (Table 3). Pollock were not caught in the Ruhle trawl and were in low amounts in the OBT and yellowtail flounder catches were also very low so tests for significance were not conducted on these commercially important species.
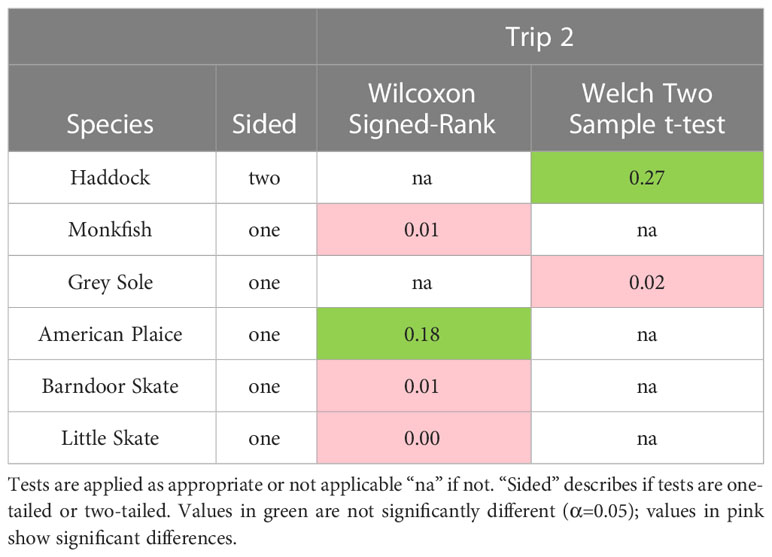
Table 4 Test statistics for significance comparing species’ paired catches between the OBT net and the Ruhle trawl.
Length frequency distributions show no significant differences between gear types for all commercial species measured in adequate numbers in trip 2 tow-pairs (Figure 10). In both nets, most haddock caught were 0-5 cm larger than the minimum legal sizes (MLS). Only haddock were caught in sufficient quantities during trip 2 to examine length-dependent differences using a GLMM (Figure 11). A 2nd order polynomial model was selected as the best fit, in-line with the recommended model comparison selection procedures by Brooks et al. (2022), and no significant difference in proportion at any length between the two nets was found. Most other species were generally caught over the MLSs as illustrated by medians to the right of the MLSs (Figure 10). Sub-legal yellowtail flounder were caught only in the Ruhle trawl and legal ones were caught in both; American plaice above and below the MLS were caught in both nets. Only one pollock was caught; it was below MLS and in the OBT. Also, only one Atlantic cod, above MLS, was caught in the Ruhle trawl.
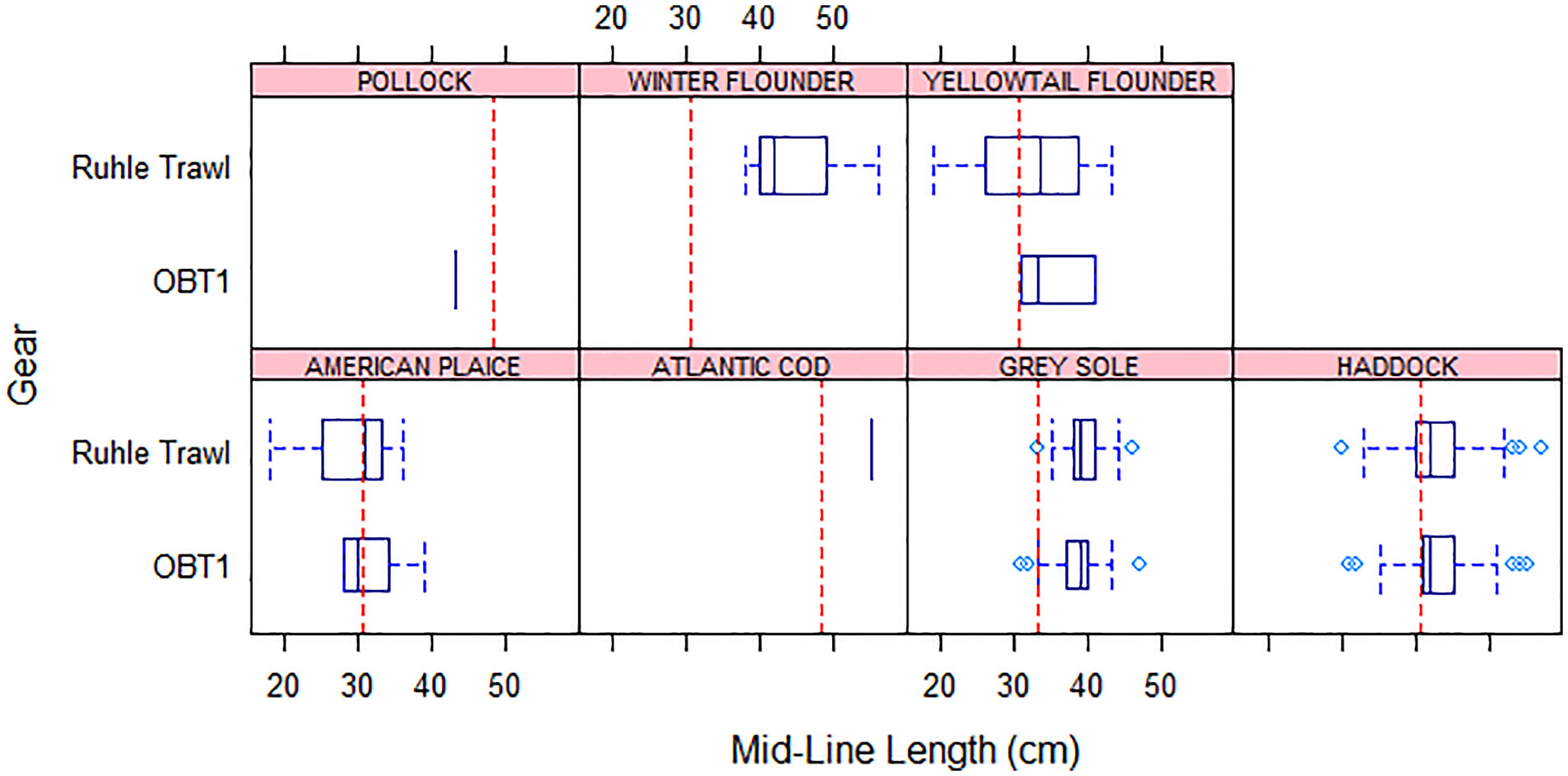
Figure 10 Mid-line lengths (x-axis) of species (panel) by gear type (y-axis) for trip 2. The red line is the minimum legal size for each species. Box widths represent the sample sizes within gear type.
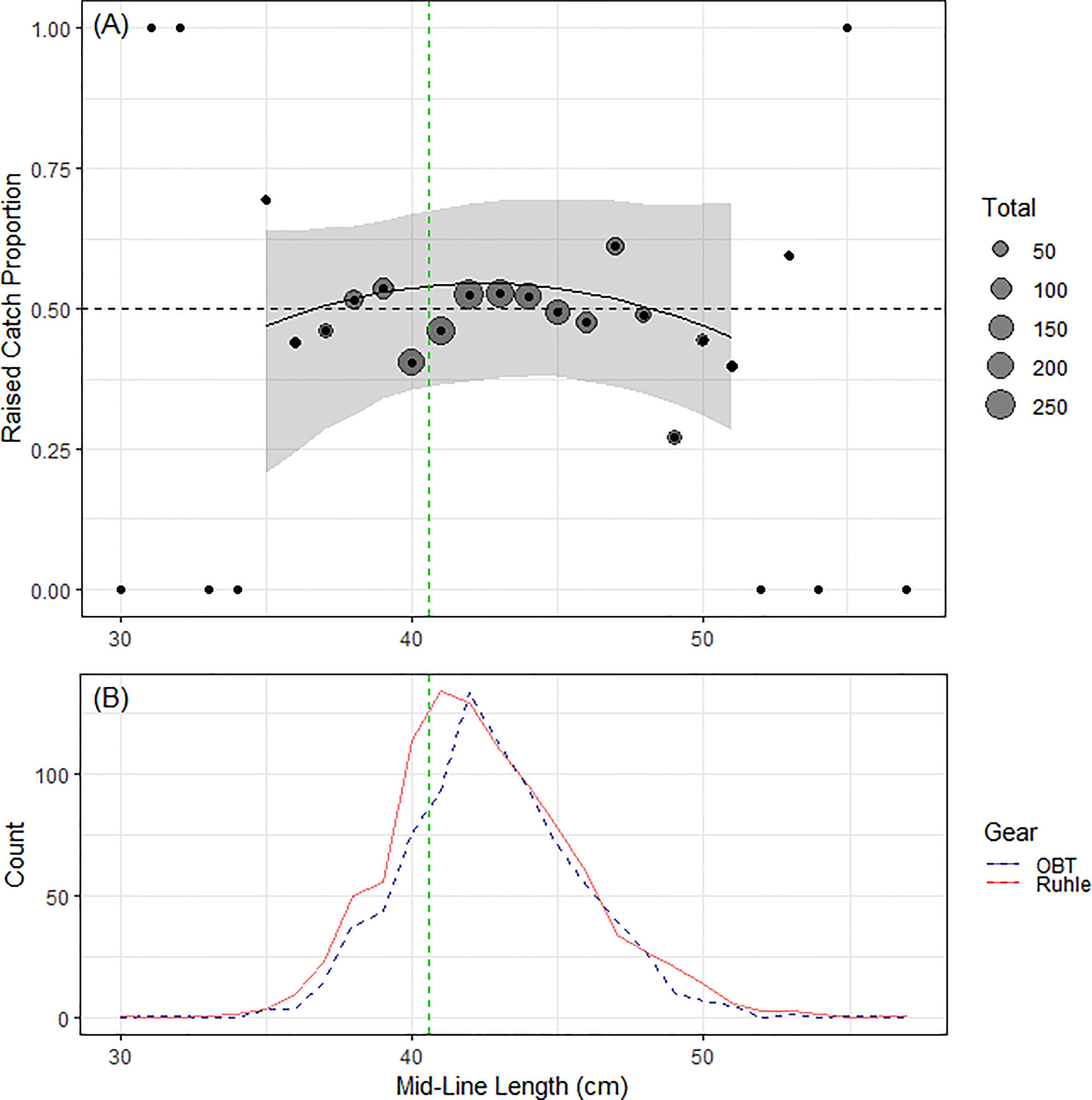
Figure 11 Best fit GLMM model (black line), confidence region (gray area) and catch proportions-at-length (OBT catch/Total catch; open circles) raised by the inverse of the proportion of the subsamples (A) and length frequency distributions (B) for haddock mid-line lengths (x-axes) in the OBT and Ruhle trawls during trip 2. Points outside the gray region in the panel A were not used in the GLMM. Diameters of raised catch proportions are scaled by observation numbers. Dotted green lines are the MLS of haddock.
4 Discussion
This work tested an off-bottom trawl (OBT) net designed to mainly harvest Georges Bank haddock, while simultaneously avoiding overexploited fish stocks, including Atlantic cod, yellowtail flounder, and windowpane flounder. We completed both the gear tuning and comparative research of the OBT net versus a standard Ruhle trawl.
Fishing the OBT net required far more frequent attention and adjustment to speed and wire out than demersal trawling to maintain the targeted door spread and especially net height off-bottom, even with the benefit of a dedicated tuning trip. We relied upon multiple sensors that provided real-time and subsequent information on net location and geometry and a captain experienced with midwater trawling. The frequency of adjustments was lessened with the addition of drop chains near the wingends. For these tows, sensor data, underwater video footage, and post-drop chain shine revealed evidence of a footrope that was close to or touching bottom. Impacts to bottom habitat, therefore, were not entirely eliminated for the OBT due to the use of drop chains but were likely substantially reduced compared to typical demersal haddock trawls like the Ruhle trawl. The outdated Simrad ITI net mensuration system, video footage, and Notus net mensuration data showed that maintaining the OBT’s footrope at the desired height of one–two meters, even without drop chains, was possible. For instance, on trip 2, prior to the addition of drop chains, we observed comparatively stable OBT headline and footrope heights during some tows (Figure 8). Further exploration of net performance and adoption by fishing vessels may benefit from additional technology, such as the use of a third wire cable winch and updated electronics to more easily keep the net in good configuration and off-bottom. These additions may also allow elimination of the drop chains and all seafloor contact.
Setting the main engine to the same RPMs resulted in a faster towing speed for the OBT than the Ruhle trawl. This result suggests that the two nets had the same drag, despite the OBT’s larger mouth opening than standard groundfish trawl nets with similar headline lengths, the presence of helix twine, and the box-shaped construction. The combination of using kites instead of headline floats and reduced contact from doors and ground cables likely counteracted the increased drag from the OBT’s larger size and helix twine (Table 2). Presumably, the OBT filtering a greater volume of water for the same fuel cost, contributed to its catch efficiency. These findings are largely consistent with other research comparing fuel consumptions between bottom, semi-pelagic, and pelagic trawls (Grimaldo et al., 2015) in which bottom trawls demonstrated the highest fuel consumption, using a constant speed (whereas our speeds were different between gear types), despite the differences in rigging and smaller fishing area of the bottom trawl (similar to our research). Due to the large size of the OBT net and despite the presence of helix twine meshes, sufficient depth is still required to attain optimal door spread and net shape. The smaller OBT vertical openings in trip 2 than in trip 1 (Figures 7, 8) coincide with the larger trip 2 door spreads (Figure 4), which are expected due to the flexible shape of the net.
Since the OBT was towed at a faster mean speed than the Ruhle trawl, the total swept areas (or volumes) of the OBT tows would be greater over the same length of time (Table 2). We expected differences in swept areas simply due to the differences in trawl sizes, as the OBT was intentionally designed to be bigger than the Ruhle trawl. For this reason, and because it is more common modifier method, we adjusted catch weights by effort in terms of tow duration (catch/hour).
The OBT successfully maintained haddock catches while reducing several non-target species as compared to the Ruhle trawl (Tables 3, 4 and Figure 9). The reductions of bottom oriented species such as monkfish, grey sole, barndoor skates, and little skates and the elimination of any catch of winter skate imply that the net stayed off-bottom for long periods. Skates are often considered a time-consuming, low value species within this fishery and can reduce the quality of more valuable species by causing abrasion damage in the codend. The Ruhle trawl has been proven to exclude Atlantic cod catch (Beutel et al., 2008) and catches of this species were very low during our trials. The OBT net caught less cod than the Ruhle trawl during testing, although insufficient data was collected to demonstrate a statistical difference. We were unable to capture enough pollock or redfish to make a judgment on the OBT’s effectiveness with catching these other bountiful species.
No significant difference in fish lengths were identified for any species (Figures 10, 11) which was unexpected due to the use of two different sized codends (15.2 cm (6 inch) nominal in the Ruhle trawl vs. 13.0 cm (5.1 inch) nominal in the OBT net). These nets also used two different shaped codend meshes (diamond in the Ruhle trawl and square in the OBT net) which may account for the similar lengths for round fish, such as haddock, which have difficulty passing through less-than-fully-opened diamond-shaped meshes but not for flatfish which are more likely to pass through the diamond-shaped meshes (Graham, 2010). We surmise that either fewer smaller fish entered the OBT net or escape of smaller fish occurred earlier in the OBT net, through the extremely large meshes and before reaching the codend. Fish escape earlier in the fishing process would reduce the physical interactions and physiological fatigue which would likely reduce stress and mortality (Ryer, 2004; Suuronen, 2005; Cook et al., 2019; Pol and Eayrs, 2021).
In addition to the testing of the OBT net described here, we also performed testing of a second OBT net using a similar “Gloria Trawl” design (not presented in this paper) (Hampiðjan Group, Iceland). This net was even larger with approximately double the mouth opening and also utilized helix twine to help spread meshes and to create a large vertical opening, rather than rely on headline floats. Our tuning trips and comparative research trips were performed for both OBT nets and both used the same codend. Drop chains were also added to this other OBT net during research to improve stability and control of the net. The larger OBT net also significantly maintained catches and length frequencies of haddock as compared to the Ruhle trawl and showed significant decreases in catches of some bottom species (monkfish and barndoor skates) as well as a significant decrease in winter skate again implying less bottom contact of the experimental gear. We believe that the larger OBT net requires a sufficiently large depth for proper operation and was not as usable in the locations where our work was conducted; its usage would be potentially more suitable in deeper water targeting redfish on and near Georges Bank. The limited depths that we worked in made it susceptible to interaction with and damage from large pelagic species. Higher RPMs and thus greater fuel consumption were required to operate this larger OBT over the smaller OBT and Ruhle trawl.
5 Conclusions
The OBT net revealed a number of positive characteristics. The large, expansive fishing circle due to the helix twine allowed us to catch haddock in quantities similar to established commercial trawl nets while reducing non-target catches, which is consistent with other research (Sistiaga et al., 2016). Additionally, the lack of the OBT’s apparent contact of the doors and groundgear are, logically, a reduction to the habitat impacts as compared to impacts by typical bottom-tending, groundfish trawl nets. We expected to fish the OBT without bottom contact, to potentially open fishing areas closed to gears with bottom contact. The additions of drop chains near the wingends likely meant that the OBT net touched bottom in some manner. Since the trawl doors and groundgear were still off-bottom, the OBT net likely reduced but did not eliminate seafloor contact, as indicated by the reduction in bottom dwelling species compared to the Ruhle trawl. This outcome may be sufficient to open new areas for access, but perhaps not in all habitats. Additionally, the fuel usage required to fish the OBT net was similar to the Ruhle trawl, based on comparisons of RPMs between the gear types, despite the larger effective swept fishing area of the OBT due to its greater mouth openings and tow speeds.
This project also demonstrated that an outdated net mensuration system on the OBT was adequate to evaluate the net’s positions and shape and could be used to catch haddock with low bycatch. Updated net sensors with live feedback would provide a better representation of the OBT’s positions and shape while towing which can then be used to adjust vessel speed, RPMs, wire out, and other conditions to improve gear parameters; this enhanced knowledge and fine control may lead to the elimination of drop-chains and all bottom contact as the net can be kept more confidently and consistently at the desired heights off-bottom while still maintaining target catches with low bycatch.
Interestingly, despite the OBT net using a smaller codend mesh size than the Ruhle trawl, the OBT net did not lead to higher catches (and discards) of small fish or even increases in less desirable, smaller, but marketable haddock. OBT nets meet the regulation requirements for fishing in areas that allow standard groundfish nets if fished with standard codends. The use of smaller mesh codends in a commercial fishery would require fishing exemptions or changes to regulations.
Our results demonstrate the benefits of fishing with a helix twine OBT, even over an established trawl net designed to reduce Atlantic cod catches, despite using a small codend mesh size. We are optimistic that further exploration with this and other OBT gears in various areas of Georges Bank and the Gulf of Maine, targeting species other than haddock, will yield more positive results.
Data availability statement
The original contributions presented in the study are included in the article/Supplementary Material. Further inquiries can be directed to the corresponding author.
Ethics statement
Ethical review and approval was not required for the animal study because Research was conducted in coordination with normal fishing operations. Federal permits were acquired confirming that all species will be handled in a responsible manner.
Author contributions
DC and MP participated in multiple aspects of this project including the concept and proposal writeup, sampling design and training, permit acquisitions, field testing, data analyses, and report and manuscript writing. DC also was involved in the project coordination, gear purchases, and data entry. All authors contributed to the article and approved the submitted version.
Funding
This study was funded by the 2016/17 NOAA Saltonstall-Kennedy Funding Award NA16NMF4270233. MP and DC received separate salary support under Award NA15NMF4070137 from NOAA Fisheries Service, in cooperation with the Interjurisdictional Fisheries Act. The statements, findings, conclusions, and recommendations are those of the authors and do not necessarily reflect the views of NOAA Fisheries.
Acknowledgments
We would like to thank the crew of the F/V Illusion for field operations. Steve Eayrs and Adam Baukus (GMRI) were originally PIs to this project and contributed greatly to the experimental design and operations of the project. Aaron Whitman, also from GMRI, greatly assisted in the fieldwork, lent research equipment, and data collection. Dr. Pingguo He and Chris Rillahan (SMAST) contributed to the development of the OBT designs and the project’s experimental design. Additional acknowledgements go to Mike Hillers (Simrad Fisheries) for participation in planning of field trials, lending equipment, and assistance in logging ITI data, Reidar’s Manufacturing for gear design consultation, Brad Schondelmeier (MA DMF) for observer data queries, Mollie Brooks (National Institute of Aquatic Resources) for providing and consulting on the R “selfisher” package, and Bob Conrad and Dan Farnham (captain and owner of the F/V Gabby G) for loaning us a Simrad headline ITI sensor and Gullwing doors.
Conflict of interest
The authors declare that the research was conducted in the absence of any commercial or financial relationships that could be construed as a potential conflict of interest.
Publisher’s note
All claims expressed in this article are solely those of the authors and do not necessarily represent those of their affiliated organizations, or those of the publisher, the editors and the reviewers. Any product that may be evaluated in this article, or claim that may be made by its manufacturer, is not guaranteed or endorsed by the publisher.
Supplementary material
The Supplementary Material for this article can be found online at: https://www.frontiersin.org/articles/10.3389/fmars.2023.1118645/full#supplementary-material
Supplementary Table 1 | Characteristics of the OBT and Ruhle trawl.
Supplementary Table 2 | OBT gear configurations during all trips and tows. See figure in “Supplementary Material” for explanations on Gull Wing door settings. Kite links refer to the number of links used to pull the top of the kite inward or let it out.
Supplementary Figure 1 | 3m2 Gull Wing door diagram reference (Net Systems, AK, USA).
Footnotes
- ^ The mesh size in use in the Canadian fishery on Eastern Georges Bank has since decreased to 12.5 cm (4.9 inch).
References
Beutel D., Skrobe L., Castro K., Ruhle P. Sr., Ruhle P. Jr., O’Grady J., et al. (2008). Bycatch reduction in the northeast USA directed haddock bottom trawl fishery. Fish. Res. 94, 190–198. doi: 10.1016/j.fishres.2008.08.008
Bord Iascaigh Mhara (BIM) (2017). “Raising the fishing line to reduce cod catches in demersal trawls targeting fish species,” in Fisheries Conservation Report, 9.
Brooks M. E., Melli V., Savina E. A. C. M., Santos J., Millar R. B., O’Neill F. G., et al. (2022). Introducing selfisher: Open source software for statistical analyses of fishing gear selectivity. Canadian Journal of Fisheries and Aquatic Sciences, 79 (8), 1189–1197. doi: 10.1139/cjfas-2021-0099
Chosid D. M., Pol M. V., Szymanski M. (2010). HadFleet2: Continued testing of the five-point trawl net (1213 Purchase St., Floor 3, New Bedford, MA 02740. USA: Massachusetts Division of Marine Fisheries).
Collette B. B., Klein-MacPhee G. (2002). Bigelow And schroeder’s fishes of the gulf of Maine. 3rd. Ed. Caldwell N. J. (USA: Blackburn Press), 577.
Cook K. V., Reid A. J., Patterson D. A., Robinson K. A., Chapman J. M., Hinch S. G., et al. (2019). A synthesis to understand responses to capture stressors among fish discarded from commercial fisheries and options for mitigating their severity. Fish. Fish. 20 (1), 25–43. doi: 10.1111/faf.12322
Eayrs S., Pol M., Knight J., Ford J. (2020). Development and testing of an ultra-low-opening trawl to improve utilization of quota of Atlantic cod (Gadus morhua) in the groundfish fishery of new England. Fish. Bull. 118 (2), 171–183. doi: 10.7755/FB.118.2.6
Erickson D. L., Perez-Comas J. A., Pikitch E. K., Wallace J. R. (1996). Effects of catch size and codend type on the escapement of walleye pollock (Theragra chalcogramma) from pelagic trawls. Fish. Res. 28 (2), 179–196. doi: 10.1016/0165-7836(96)00497-3
Finley M., Brooks E. N., McCurdy Q., Barrett M. A., Wang Y. (2019) Assessment of haddock on Eastern georges bank for 2019. Available at: http://www.bio.gc.ca/info/intercol/trac-cert/documents/ref/CSAS_Res_Doc_2000_082.pdf.
Fonteyne R. (2005). “Protocol for the use of an objective mesh gauge for scientific purposes,” in ICES coop. res. rep. no. 279, 14 pp. doi: 10.17895/ices.pub.5483
Fryer R. J., Summerbell K., O’Neill F. G. (2017). A meta-analysis of vertical stratification in demersal trawl gears. Can. J. Fish. Aquat. Sci. 74, 1243–1250. doi: 10.1139/cjfas-2016-0391
Gabriel O., Lange K., Dahm E., Wendt T. (2005). Von Brandt’s fish catching methods of the world (9600 Garsington Road, Oxford OX4 2DQ, UK; Blackwell Publishing Ltd), 536.
Gloria: Self Spreading (2015). Hampiðjan group (Skarfagardar 4104 Reykjavík, Iceland), 12. Available at: http://www.hampidjan.is/Media/gloria12pageenlores.pdf.
Graham N. (2010). “Technical measures to reduce bycatch and discards in trawl fisheries,” in Behavior of marine fishes: Capture processes and conservation challenges. Ed. He P. (Ames, Iowa: Wiley-Blackwell).
Grimaldo E., Pedersen R., Sistiaga M. (2015). Energy consumption of three different trawl configurations used in the barents Sea demersal trawl fishery. Fish. Res. 165, 71–73. doi: 10.1016/j.fishres.2014.12.021
He P., Bouchard C., Hamilton R., Smith T., Maxwell S., Bouchard C. (2005). Design and test of a rope separator trawl for haddock and pollack for the Western gulf of Maine (Durham, New Hampshire: University of New Hampshire).
Kebede G. E., Winger P. D., DeLouche H., Legge G., Cheng Z., Kelly D., et al. (2020). Flume tank evaluation of the hydrodynamic lift and drag of helix ropes compared to conventional ropes used in midwater trawls. Ocean Eng. 195, 106674. doi: 10.1016/j.oceaneng.2019.106674
Krag L. A., Holst R., Madsen N., Hansen K., Frandsen R. P. (2010). Selective haddock (Melanogrammus aeglefinus) trawling: Avoiding cod (Gadus morhua) bycatch. Fish. Res. 101 (1–2), 20–26. doi: 10.1016/j.fishres.2009.09.001
Link J. S., Almeida F., Valentine P., Auster P., Reid R., Vitaliano J. (2005). The effects of area closures on georges bank. Am. Fish. Soc. Symp. 41, 345–368.
Madsen N. (2007). Selectivity of fishing gears used in the Baltic Sea cod fishery. Rev. Fish. Biol. Fish. 17 (4), 517–544. doi: 10.1007/s11160-007-9053-y
Martinez J. (2022). MediaInfo. Available at: https://mediaarea.net/en/MediaInfo.
McGill R., Tukey J. W., Larsen W. A. (1978). “Variations of box plots,” in The American statistician, 32(1):12–16. doi: 10.1080/00031305.1978.10479236
Murphy T., Kitts A., Records D., Demarest C., Caless D., Walden J., et al. (2014) 2012 final report on the performance of the northeast multispecies (Groundfish) fishery (May 2012 - April 2013). Available at: https://repository.library.noaa.gov/view/noaa/4594.
National Oceanic and Atmospheric Administration (2004) Magnuson-Stevens fishery conservation and management act provisions; fisheries of the northeastern united states; northeast multispecies fishery; amendment 13. 50 CFR part 648. docket no. 040112010–4114–02, RIN 0648–AN17. Available at: https://www.govinfo.gov/content/pkg/FR-2004-04-27/pdf/04-8884.pdf.
National Oceanic and Atmospheric Administration (2021) Magnuson-Stevens fishery conservation and management act provisions; fisheries of the northeastern united states; northeast multispecies fishery; framework adjustment 61. 50 CFR part 648, docket no. 210723–0150, RIN 0648–BK24. Available at: https://www.govinfo.gov/content/pkg/FR-2021-07-28/pdf/2021-16070.pdf.
National Oceanic and Atmospheric Administration (NOAA) Fisheries (2020) Greater Atlantic region regulations. Available at: https://www.fisheries.noaa.gov/content/greater-atlantic-region-regulations.
Northeast Fisheries Science Center (NEFSC) (2012). Assessment or data updates of 13 northeast groundfish stocks through 2010, Vol. 789.
Northeast Fisheries Science Center (NEFSC) (2017). Operational assessment of 19 northeast groundfish stocks, updated through 2016. 259.
Nichols P., Revill A., Medley P. (2001). “Final report: Evaluation of the state of knowledge concerning selectivity of fishing gear,” (Lymington, UK: MacAlister Elliott and Partners Ltd. for European Commission Directorate-General XIV), 101.
Pol M., Eayrs S. (2021). Behaviour in a bottom trawl: moving forward to limit catches of Atlantic cod. ICES J. Mar. Sci. 28, 2317–2324. doi: 10.1093/icesjms/fsab106
R Core Team (2018). “R: A language and environment for statistical computing,” (Vienna, Austria: R Foundation for Statistical Computing). Available at: https://www.R-project.org.
Ryer C. F. (2004). Laboratory evidence for behavioural impairment of fish escaping trawls: a review. ICES J. Mar. Sci. 61, 1157–1164. doi: 10.1016/j.icesjms.2004.06.004
Sistiaga M., Herrmann B., Grimaldo E., Larsen R. B., Tatone I. (2015). Effect of lifting the sweeps on bottom trawling catch efficiency: A study based on the northeast arctic cod (Gadus morhua) trawl fishery. Fish. Res. 167, 164–173. doi: 10.1016/j.fishres.2015.01.015
Sistiaga M., Herrmann B., Grimaldo E., Larsen R. B., Tatone I. (2016). The effect of sweep bottom contact on the catch efficiency of haddock (Melanogrammus aeglefinus). Fish. Res. 179, 302–307. doi: 10.1016/j.fishres.2016.03.016
Suuronen P. (2005). Mortality of fish escaping trawl gears (Rome: FAO). Available at: http://www.fao.org/tempref/docrep/fao/008/y6981e/y6981e01.pdf.
Tatham S. (1999). PuTTY. Available at: https://www.chiark.greenend.org.uk/~sgtatham/putty/.
Keywords: haddock (Melanogrammus aeglefinus), trawl, mid-water, Georges Bank, Atlantic cod (Gadus morhua), yellowtail flounder (Limanda ferruginea), off-bottom, helix-twine
Citation: Chosid DM and Pol M (2023) Testing of a helix twine off-bottom trawl on Georges Bank. Front. Mar. Sci. 10:1118645. doi: 10.3389/fmars.2023.1118645
Received: 07 December 2022; Accepted: 23 February 2023;
Published: 16 March 2023.
Edited by:
Alessandro Lucchetti, National Research Council (CNR), ItalyReviewed by:
Emilio Notti, National Research Council (CNR), ItalyHao Tang, Shanghai Ocean University, China
Copyright © 2023 Chosid and Pol. This is an open-access article distributed under the terms of the Creative Commons Attribution License (CC BY). The use, distribution or reproduction in other forums is permitted, provided the original author(s) and the copyright owner(s) are credited and that the original publication in this journal is cited, in accordance with accepted academic practice. No use, distribution or reproduction is permitted which does not comply with these terms.
*Correspondence: David M. Chosid, ZGF2aWQuY2hvc2lkQG1hc3MuZ292