- 1Marine Wildlife Veterinary Care and Research Center, Office of Spill Prevention and Response, California Department of Fish and Wildlife, Santa Cruz, CA, United States
- 2One Health Institute, School of Veterinary Medicine, University of California, Davis, Davis, CA, United States
- 3Department of Pathology, Microbiology, and Immunology, School of Veterinary Medicine, University of California, Davis, Davis, CA, United States
From February 2020 to March 2022, four southern sea otters (Enhydra lutris nereis) stranded in California with severe protozoal steatitis and systemic toxoplasmosis. Three of the infected otters stranded within 26 km of each other, and all four animals died during periods of increased rainfall-driven surface water runoff. High parasite burdens were observed in all tissues except the central nervous system, and toxoplasmosis with severe protozoal steatitis was the primary cause of death for all cases. This lesion pattern differs substantially from all prior reports of toxoplasmosis in sea otters. All cases were T. gondii-positive via serology, immunohistochemistry, and PCR. Multilocus sequence typing at 13 loci revealed that all were infected with the same strain of T. gondii, previously characterized as an atypical and rare genotype in North America (TgCgCa1, or COUG). The COUG genotype was first isolated from mountain lions in British Columbia, Canada during investigation of a waterborne outbreak of toxoplasmosis in humans. This genotype has not been previously reported from sea otters, nor any aquatic species. All prior T. gondii strains obtained from >140 southern sea otters represent Type II or Type X strains, or variants of these genotypes. Archival necropsy data (>1,000 animals over 24 years) were negative for prior cases of severe T. gondii-associated steatitis prior to the cases described herein, and no sublethal COUG T. gondii infections have been previously indentified in sea otters. According to prior studies, the T. gondii COUG genotype is highly virulent in mice and is unusual among T. gondii isolates in eliciting a Type I interferon response in murine and human cells in vitro; this unusual immunomodulatory response could explain the apparent high virulence of this atypical T. gondii strain. Our findings reveal a novel and concerning lesion pattern for sea otters with toxoplasmosis. Due to high zoonotic potential and the risk of infection via shared marine food resources, these findings may also indicate potential health threats for other animals and humans.
Introduction
Toxoplasma gondii is a ubiquitous apicomplexan protozoal parasite of significant importance to human and animal health. Wild and domestic felids are the only definitive hosts, and asymptomatic cats can shed millions of environmentally resistant oocysts in their feces (Dubey and Frenkel, 1972; Fritz et al., 2012). Under optimal conditions, sporulated oocysts can remain viable for months (Dumètre and Dardé, 2003), and are infectious to all warm-blooded vertebrates (Tenter et al., 2000). Toxoplasma gondii infection can occur via consumption of food or water contaminated with sporulated oocysts, ingestion of tissue cysts in intermediate hosts, and transplacental transmission (Tenter et al., 2000).
In intermediate hosts, T. gondii tachyzoites spread systemically, often causing subclinical infection in healthy animals and humans. However, fatal neurological disease can occur, particularly in immunosuppressed individuals. Miscarriage, abortion, and congenital toxoplasmosis are also possible. For individuals surviving initial infection, T. gondii tachyzoites respond to the host immune response by converting to bradyzoite-filled tissue cysts in the central nervous system, muscles, and other tissues. If immunity wanes, quiescent tissue cysts can re-activate, again causing systemic disease (Dellacasa-Lindberg et al., 2007; DaSilva et al., 2010; Miller et al., 2018). These diverse transmission strategies and broad host range, coupled with substantial risk to human and animal health, make T. gondii one of the world’s most globally important pathogens.
Despite being restricted to terrestrial definitive hosts, T. gondii can cause morbidity and mortality in diverse marine mammal intermediate hosts, including phocids, otariids, cetaceans, sirenians, and sea otters (Miller et al., 2018). Southern sea otters (Enhydra lutris nereis) are especially impacted, with T. gondii infecting 62% of sea otters and serving as the primary or contributing cause of death for 8% of otters examined from 1998 through 2012 (Miller et al., 2020). Contributing factors include: 1) Proximity of sea otter habitat to coastal cities, where land-based runoff driven by rainfall can carry freshwater contaminated by oocyst-laden feline feces to nearshore waters, and 2) High consumption of marine bivalves and snails capable of concentrating oocysts from contaminated water (Miller et al., 2008b; Johnson et al., 2009; Krusor et al., 2015).
Although southern sea otters commonly have chronic sublethal T. gondii infections, fatal toxoplasmosis also occurs (Shapiro et al., 2016; Miller et al., 2020). Sea otters that have died due to toxoplasmosis usually have no pathognomonic gross lesions other than non-specific lymphadenosplenomegaly; histopathology is required to confirm infection and disease (Miller et al., 2018). Protozoal tissue cysts and occasional tachyzoites are most common in the central nervous system (CNS), accompanied by non-suppurative meningoencephalitis (Miller et al., 2018). For most T. gondii-infected sea otters, few parasites are observed microscopically outside of the CNS and occasionally the heart, suggestive of chronic infection (Miller et al., 2018). Death is usually attributed to chronic-active or recrudescent, T. gondii-mediated meningoencephalitis and/or myocarditis (Miller et al., 2020).
The four cases described herein exhibited a strikingly different lesion pattern, characterized by grossly apparent, diffuse, severe steatitis affecting all subcutaneous and internal adipose stores. Histopathology revealed severe inflammation and numerous tissue cysts and tachyzoites in most tissues, apart from the central nervous system, where parasite numbers and inflammation were comparatively mild. Multilocus sequence typing revealed that all otters with severe protozoal steatitis were infected with the same atypical T. gondii strain, sharing 100% identity across all examined loci. This previously described genotype (COUG or TgCgCa1), was isolated from two mountain lions (Puma concolor) during investigation of a large community outbreak of waterborne toxoplasmosis in humans in Canada (Aramini et al., 1999; Dubey et al., 2008; Dubey et al., 2020).
This is the first report of T. gondii-associated fatal steatitis and systemic toxoplasmosis in sea otters, and the first report of the COUG T. gondii genotype infecting any aquatic animal. The cases described here highlight detection of a previously unreported and virulent strain of T. gondii in threatened southern sea otters and underscores the need for detailed studies of T. gondii transmission at the land-sea interface.
Materials and methods
Postmortem examination
Stranded southern sea otters were transported to the California Department of Fish and Wildlife’s Marine Wildlife Veterinary Care and Research Center in Santa Cruz, CA, where detailed gross necropsies were performed on fresh and frozen-thawed individuals as previously described (Miller et al., 2020). Sampling methods varied depending on postmortem condition, with formalin fixation of all major tissues for some animals, while others had more limited sampling due to autolysis or freeze-thaw artifact.
Histopathology
Formalin-fixed tissues were embedded, sectioned, and stained with hematoxylin and eosin (H&E) at the Veterinary Medical Teaching Hospital at the University of California, Davis as previously described (Miller et al., 2020). Prepared slides were examined by two veterinary pathologists (MM and DS).
Protozoal immunohistochemistry
Representative paraffin blocks from each case were submitted to the California Animal Health and Food Safety Laboratory at the University of California, Davis for immunohistochemistry using rabbit-derived polyclonal antisera to T. gondii and the related apicomplexan parasite Sarcocystis neurona using established methods (Miller et al., 2001b). Both parasites are confirmed sea otter pathogens that can appear similar on histopathology, and have similar life cycles, except that the definitive host for S. neurona is introduced Virginia opossums (Didelphis virginiana) (Miller et al., 2010; Miller et al., 2018). Known-infected tissues were included as positive controls for each parasite, and completed slides were examined by two veterinary pathologists (MM and DS).
Protozoal serology
Where available, postmortem pericardial fluid was assessed for the presence and concentration of T. gondii and S. neurona IgG antibodies using an indirect fluorescent antibody test (IFAT) as previously described (Miller et al., 2002b), using FITC-conjugated goat anti-ferret IgG antibodies (Bethyl Laboratories Incorporated, Montgomery, Texas). Endpoint titers were determined as the highest dilution that exhibited distinct outline fluorescence of tachyzoites (T. gondii) or merozoites (S. neurona). Titers ≥ 1:320 were used to establish seropositivity (Miller et al., 2002b).
In vitro parasite cultivation
At necropsy, fresh brain tissue was collected aseptically only from Case 2 to be processed for parasite isolation in cell culture as described (Miller et al., 2001a). Briefly, 5 g of brain tissue was placed in antibiotic/antifungal solution overnight and then homogenized. After homogenization, 1 ml of tissue was incubated in 10 ml trypsin-EDTA (0.25%) at 37°C for 1 hr, added to a feeder layer of MA-104 (monkey kidney) cells, and incubated at 37°C and 5% CO2 for 2 hr to optimize isolation of T. gondii. An additional 1 ml of homogenized tissue was also added directly to a feeder layer of MA-104 (monkey kidney) cells without trypsinization and incubated at 37°C and 5% CO2 for 2 hr to optimize for isolation of S. neurona that are more susceptible to trypsin digestion. After 2 hr, both feeder layers were rinsed with RPMI media supplemented with fetal bovine serum. All cultures were examined thrice weekly for parasite growth and media exchange.
Molecular detection and characterization of protozoal parasites
Nucleic acids were extracted from frozen tissue for all four cases, plus parasite-positive culture supernatant from Case 2, using a DNeasy Blood and Tissue Kit (Qiagen, Valencia, CA, USA). Extracted DNA from tissues for each sea otter was initially screened for the presence of T. gondii using a nested PCR assay targeting the B1 locus (Grigg and Boothroyd, 2001). Tissue-derived DNA as well as DNA extracted from culture supernatant from Case 2 were further genotyped using a polymerase chain reaction (PCR)-based multilocus sequence typing approach targeting 12 additional polymorphic loci: SAG1, 5’SAG2, 3’SAG2, altSAG2, SAG3, BTUB, GRA6, C22-8, C29-2, L358, PKI, and Apico (Su et al., 2010).
Amplification at the B1 locus was performed as a nested PCR assay using external and internal primers and cycling conditions as described (Grigg and Boothroyd, 2001; Shapiro et al., 2016; Shapiro et al., 2019). The remaining 12 loci were amplified in a multiplex, nested PCR assay using external and internal primers and cycling conditions as previously described (Su et al., 2010). Two loci, C29-2 and Apico, failed to amplify using the multiplex assay and were repeated as simplex, nested PCR assays using the same primers and cycling conditions.
To evaluate for the possibility of co-infection with S. neurona, DNA extracted from tissues for Case 4 were additionally screened at the ITS1 locus using previously published primers and cycling conditions (Rejmanek et al., 2010). To identify parasites grown in culture, DNA extracted from trypsinized and untreated culture supernatant was also amplified at the ITS1 locus. To assess for possible co-isolation of S. neurona, amplification of the cox1 locus using Sarcocystis spp.-specific primers was performed for both trypsinized and untreated cultures (Gondim et al., 2019).
Products of all PCR assays were sequenced at the UC Davis DNA Sequencing Facility. Sequences were trimmed and aligned to known reference sequences for Type I, Type II, Type III, Type X, and COUG strains at each locus using Geneious software (Biomatters, Auckland, New Zealand). Sequences were further compared to whole genome sequences for reference T. gondii strains available in GenBank using BLAST (NCBI: https://blast.ncbi.nlm.nih.gov/Blast.cgi). Sea otter-derived sequences were deposited in GenBank for all 13 loci characterized in this study and were assigned the following accession numbers: OQ249665 (3’SAG2), OQ249666 (5’SAG2), OQ249667 (altSAG2), OQ249668 (Apico), OQ249669 (B1), OQ249670 (BTUB), OQ249671 (C22-8), OQ249672 (C29-2), OQ249673 (GRA6), OQ249674 (L358), OQ249675 (PKI), OQ249676 (SAG1), OQ249677 (SAG3).
Results
Postmortem examination
From February 2020 through March 2022, four southern sea otters of mixed age and sex (Table 1) presented for necropsy with severe, diffuse, grossly apparent steatitis. Three sea otters stranded within a 26.5 km-span of coastal San Luis Obispo County (two of these animals stranded within 1 km of each other) and one sea otter stranded in Santa Cruz County on the central California coast. The stranding locations for each otter are also shown in a coastal map (Supplementary Figure 1). All four cases died during or after periods of high precipitation in February 2020 (n=1), February 2022 (n=2), and March 2022 (n=1). Only Case 1 had perimortem clinical history (Table 1); all others were found dead. Three otters were sexually mature females, while the other was an immature male. Three animals were classified as good to excellent nutritional condition at necropsy, while one was considered thin, although nutritional condition was difficult to assess in these cases. Three animals were minimally decomposed (one of which had been frozen before necropsy) and one was moderately decomposed and had been frozen before necropsy.
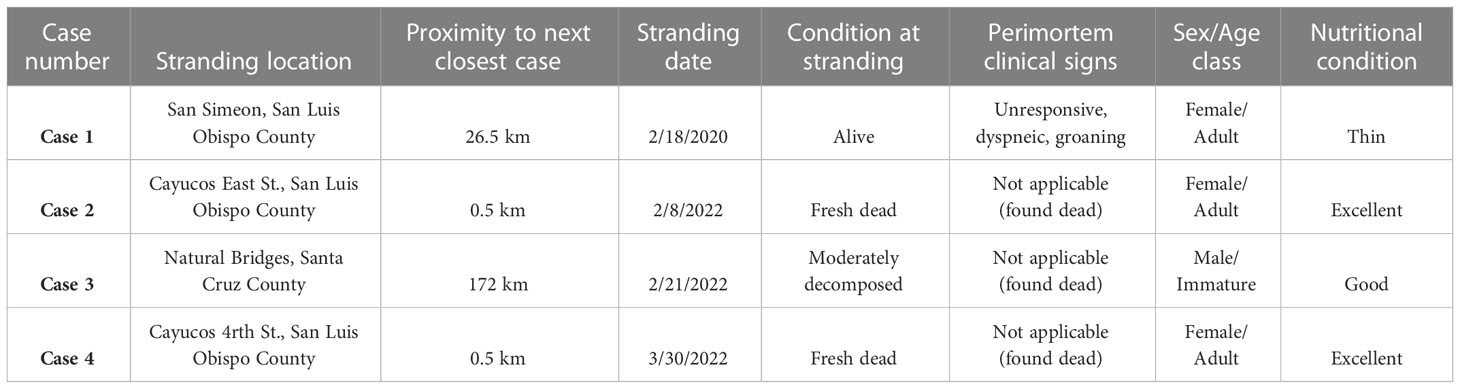
Table 1 Demographical and geographical data for southern sea otters (Enhydra lutris nereis) with fatal Toxoplasma gondii-associated steatitis and toxoplasmosis.
Gross necropsy findings are summarized in Table 2. Putative T. gondii-associated gross pathology included severe, diffuse steatitis affecting subcutaneous, visceral, omental, mesenteric, retroperitoneal, and coronary adipose. Affected adipose was diffusely yellow-tan, finely granular to multinodular, and firm to gritty on palpation (Figures 1A, B, 2A–D and Table 2).
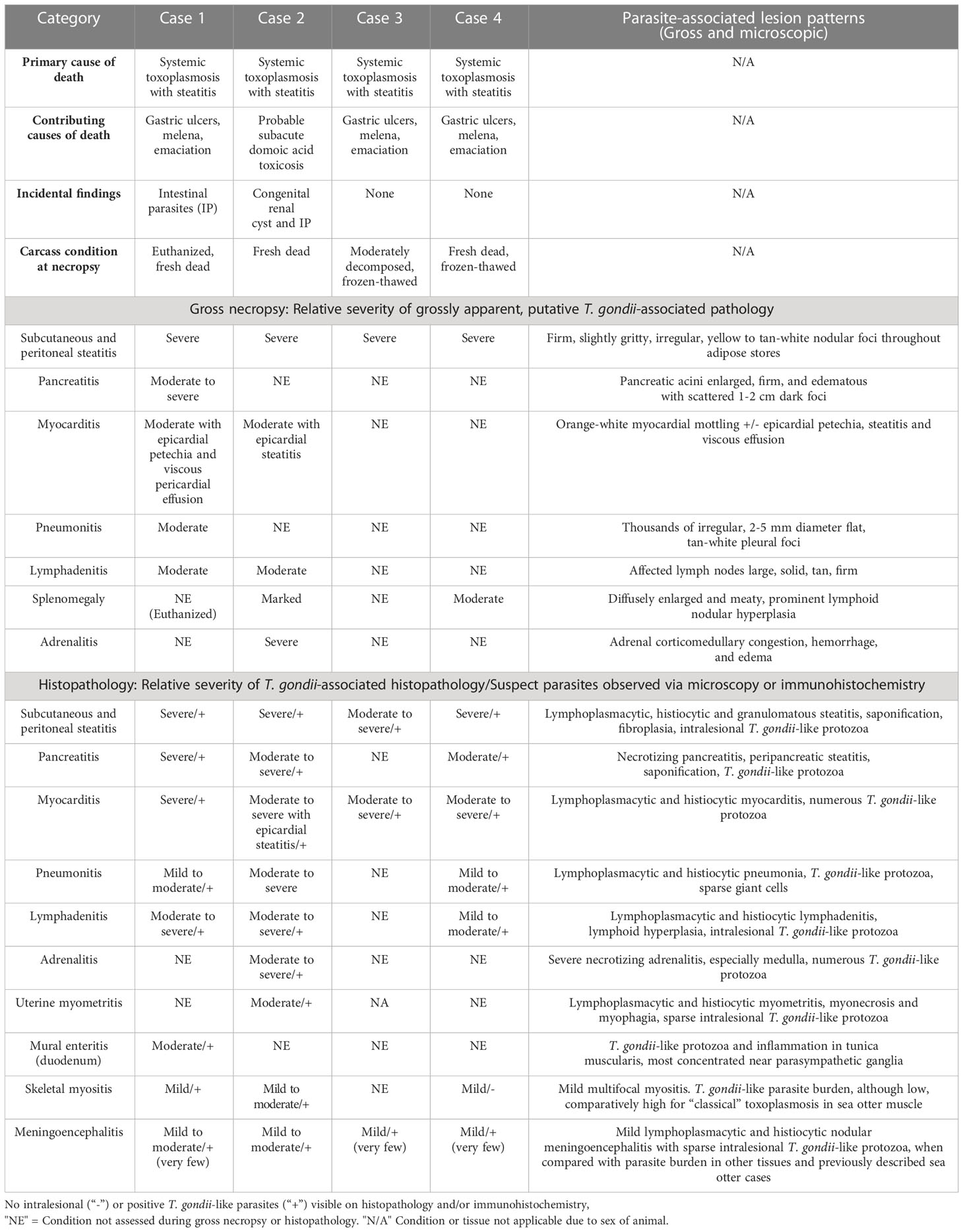
Table 2 Toxoplasma gondii-associated gross and microscopic pathology for southern sea otters (Enhydra lutris nereis) with fatal T. gondii-associated steatitis and toxoplasmosis.
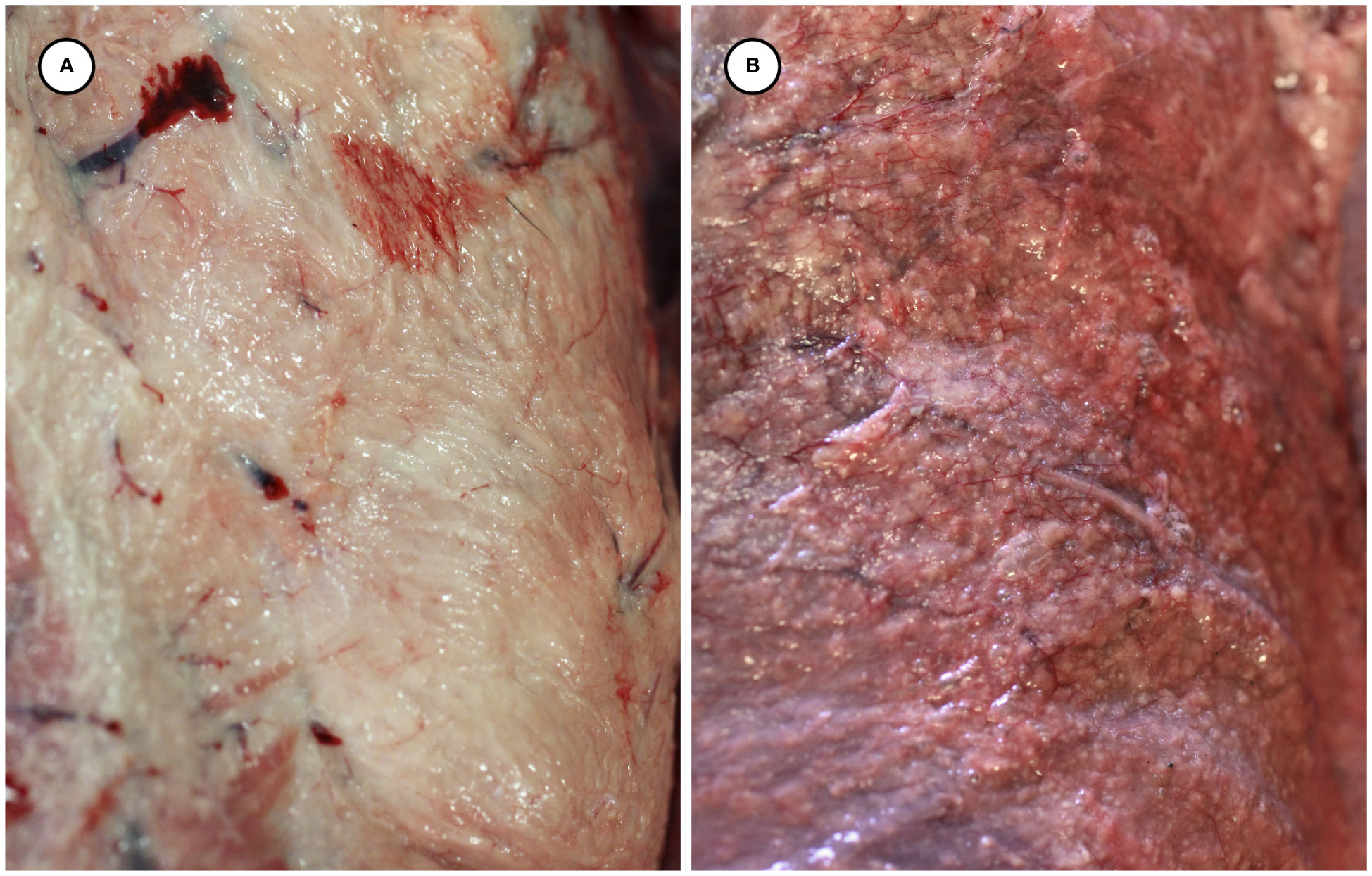
Figure 1 Gross appearance of normal subcutaneous adipose from an unaffected sea otter (A), compared with congested and multinodular-appearing adipose from a sea otter with fatal protozoal steatitis and toxoplasmosis (B).
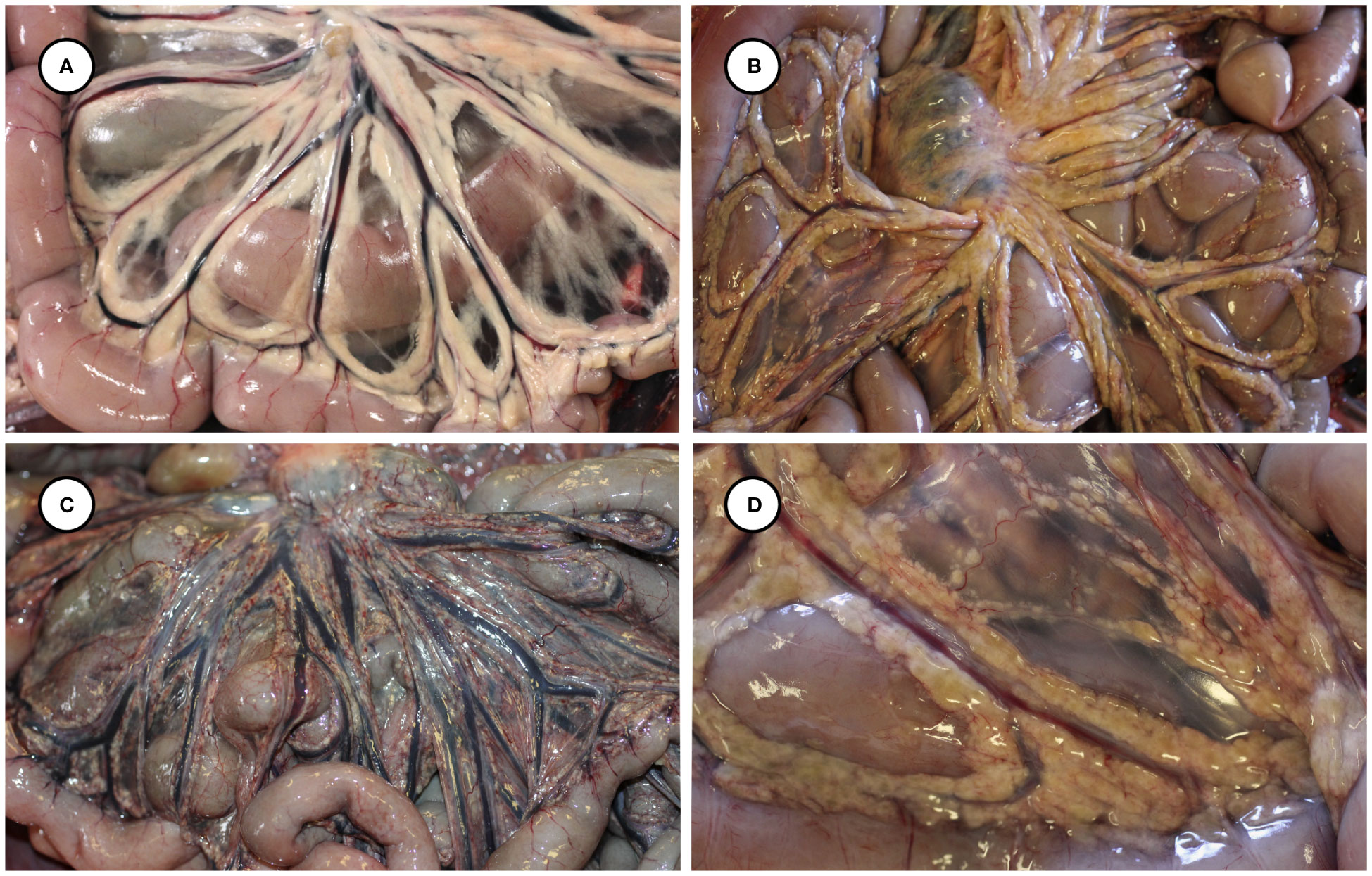
Figure 2 Gross appearance of normal mesenteric adipose from an unaffected sea otter (A), compared with yellow-discolored, variably congested, multinodular-appearing mesenteric adipose at low (B, C) and higher magnification (D) in sea otters with fatal protozoal steatitis and toxoplasmosis.
Several other tissues exhibited grossly apparent T. gondii-associated pathology in addition to systemic adipose (Table 2). Inflamed pancreatic acini were markedly enlarged and firm with scattered intralesional 1-2 cm dark red-black foci (Figures 3A–C). Cardiac involvement was characterized by diffuse orange-white myocardial mottling, epicardial steatitis (Figures 4A, B) and petechia, and viscous pericardial effusion. Lesion overlap between cardiomyopathy associated with domoic acid toxicosis, sarcocystosis, and toxoplasmosis usually precludes discernment between these conditions grossly; histopathology is required (Miller et al., 2020; Miller et al., 2021). However, the severe, grossly apparent epicardial steatitis described herein has not previously been reported from sea otters with fatal toxoplasmosis and may be pathognomonic for this condition. Parasitic pneumonia was characterized grossly by myriad irregular, 2-5 mm diameter, flat, tan-white pleural foci (Figures 5A, B) that were indistinct on cut surface. All lymph nodes were diffusely enlarged, light tan, and firm, with prominent cortical lymphoid hyperplasia on cut surface. Affected spleens were enlarged with prominent lymphoid nodular hyperplasia. In Case 2 the adrenal cortices were congested and hemorrhagic, and copious cloudy, opaque fluid was visible on cut surface especially around the medulla (Figure 6A).
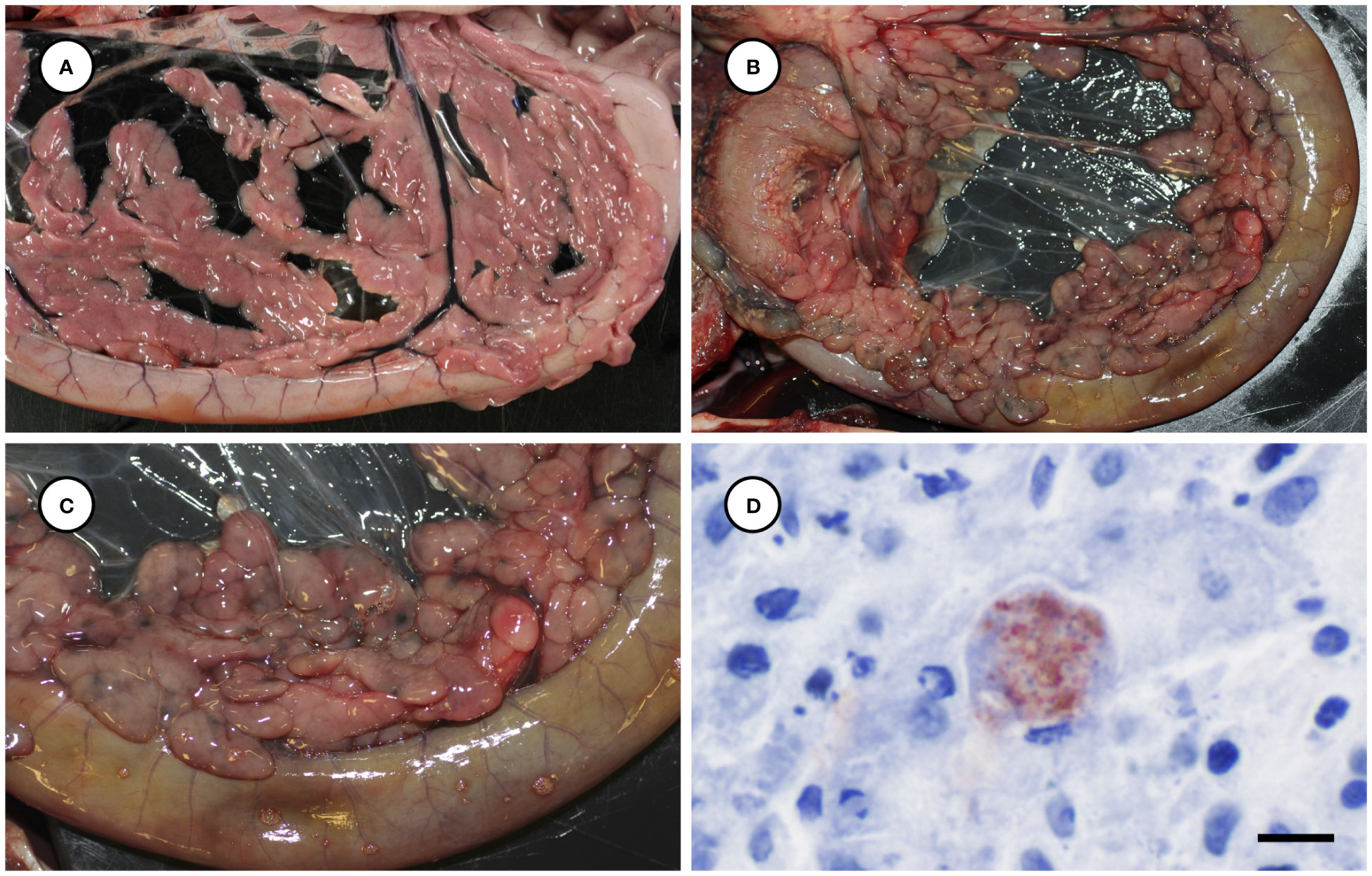
Figure 3 Normal pancreas from an unaffected sea otter (A), compared with low (B) and higher magnification (C) views of swollen, edematous, red-black discolored pancreas from a sea otter with protozoal pancreatitis. Immunohistochemical stain of inflamed pancreas demonstrating strong positive immunostaining of intralesional T. gondii parasites (D: Bar = 10 µm).
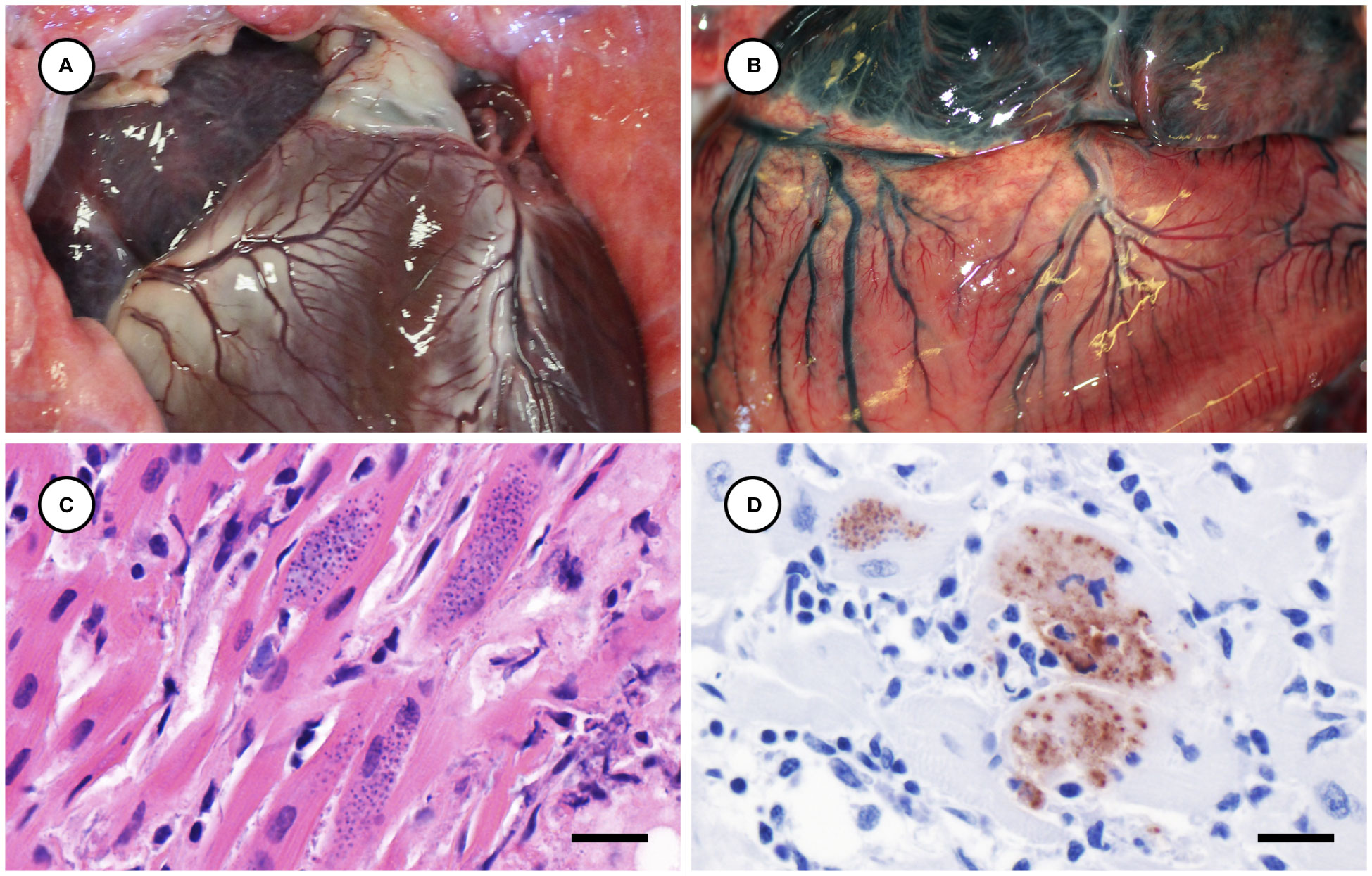
Figure 4 Normal heart from an unaffected sea otter (A), compared with orange-discolored myocardium and pale yellow, multinodular-appearing epicardial adipose from a sea otter with protozoal myocarditis and epicardial steatitis (B). Microscopic view of severely inflamed myocardium (C) with numerous intralesional Toxoplasma gondii-like tissue cysts (H&E stain, bar = 10 µm). Immunohistochemical stain of inflamed myocardium (D) demonstrating strong positive immunostaining of intralesional protozoa for T. gondii (Bar = 10 µm).
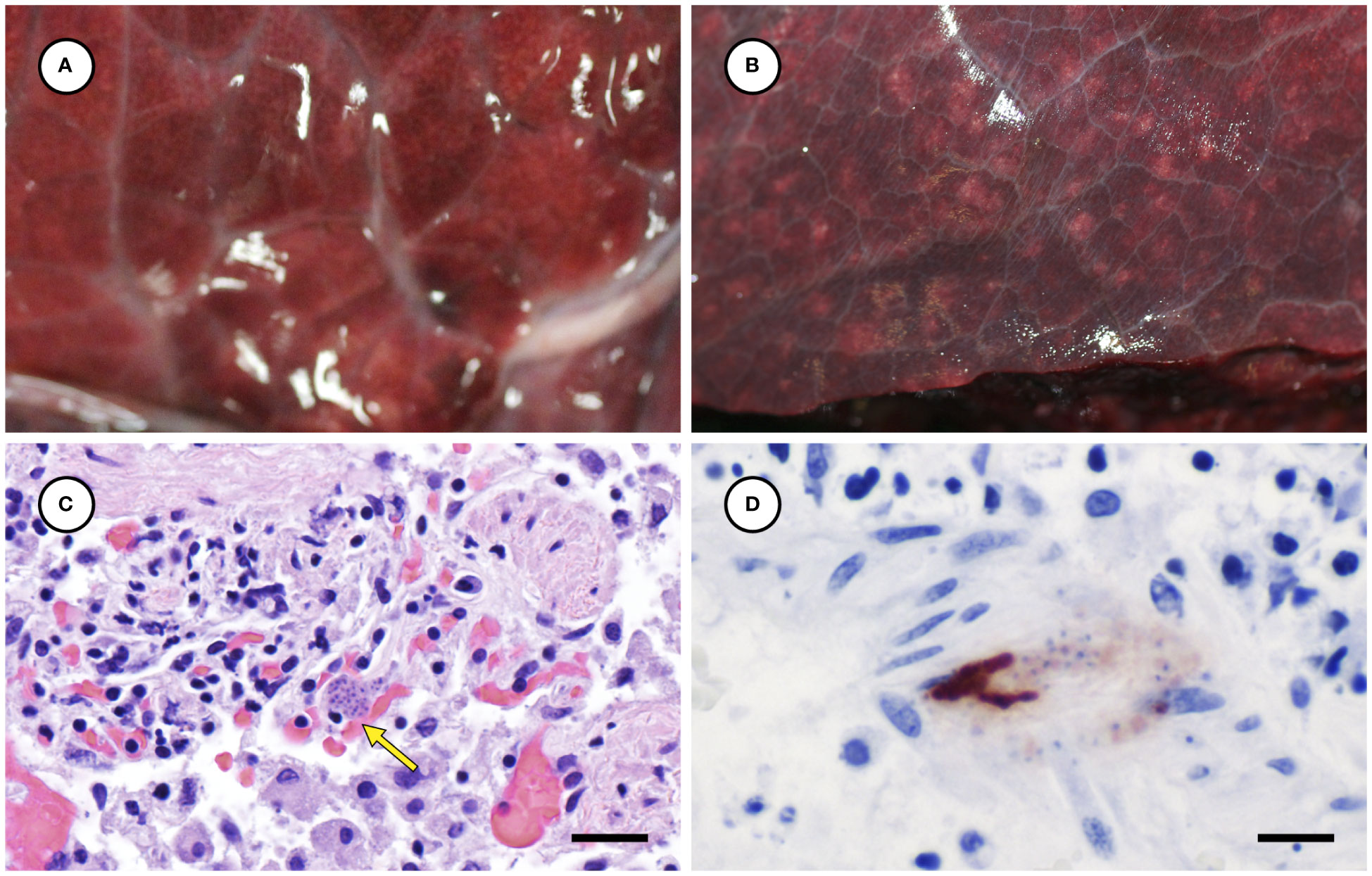
Figure 5 Appearance of normal lung from an unaffected sea otter (A), compared to lung from a sea otter with protozoal pneumonia (B) containing multiple pale irregular pleural foci. Microscopic view of inflamed lung containing intralesional Toxoplasma gondii-like tissue cyst (C: arrow) (H&E stain, bar = 20 µm). Immunohistochemical stain of lung demonstrating strong positive immunostaining of intralesional protozoa for T. gondii (D) (Bar = 10 µm).
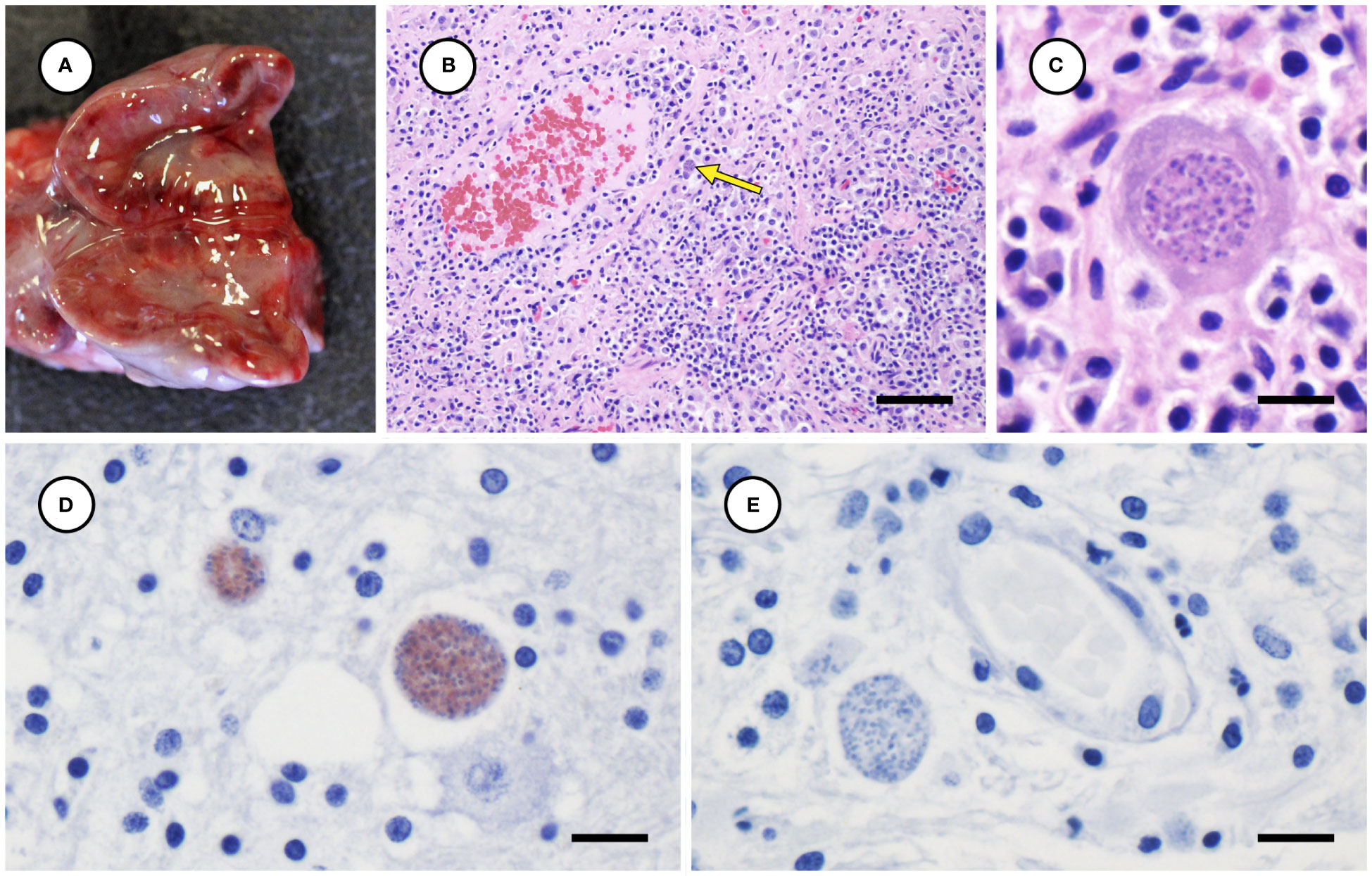
Figure 6 Congested, hemorrhagic and edematous adrenal gland from a sea otter with fatal toxoplasmosis and steatitis (A). Low (B) and high (C) magnification microscopic views of markedly inflamed adrenal medulla from the same sea otter demonstrating intralesional Toxoplasma gondii-like protozoa (indicated by arrow for B) (H&E stain, bar = 40 and 10 µm, respectively). Immunohistochemical stains of mildly inflamed brain tissue from sea otters with fatal toxoplasmosis and steatitis, demonstrating relatively mild inflammation and strong positive immunostaining of intralesional tissue cysts for T. gondii (D), and negative immunostaining of brain parasites for Sarcocystis neurona (E) (D, E: bar = 10 and 10 µm, respectively).
Histopathology
Toxoplasma gondii-associated histopathology findings are summarized in Table 2. Tissues examined microscopically for each case and those positive for T. gondii and S. neurona are summarized in Supplementary Table 1. Lymphoplasmacytic and variably granulomatous inflammation with numerous intralesional protozoa were observed in many tissues, including subcutaneous and peritoneal adipose, pancreas, brain, heart, lung, liver, lymph nodes, adrenal gland, uterine and intestinal smooth muscle, and skeletal muscle (Table 2). Numerous, approximately 4 x 2 µm tachyzoites and small (e.g., 20-50 µm diameter), thin-walled tissue cysts containing short (4 x 2 µm), stout bradyzoites with eosinophilic cytoplasm were common in areas of severe adipose inflammation (Figures 7A–C). Steatitis was accompanied by multifocal necrosis, edema, hemorrhage, and saponification, with scattered intralesional multinucleated giant cells. Protozoa were especially numerous along the periphery of lipid vacuoles within the cytoplasm of infected adipocytes (Figure 7C). Although adipocytes were often somewhat atrophic on histopathology, severe inflammation resulted in an apparent increase in adipose amount at necropsy, complicating accurate gross assessment of nutritional condition.
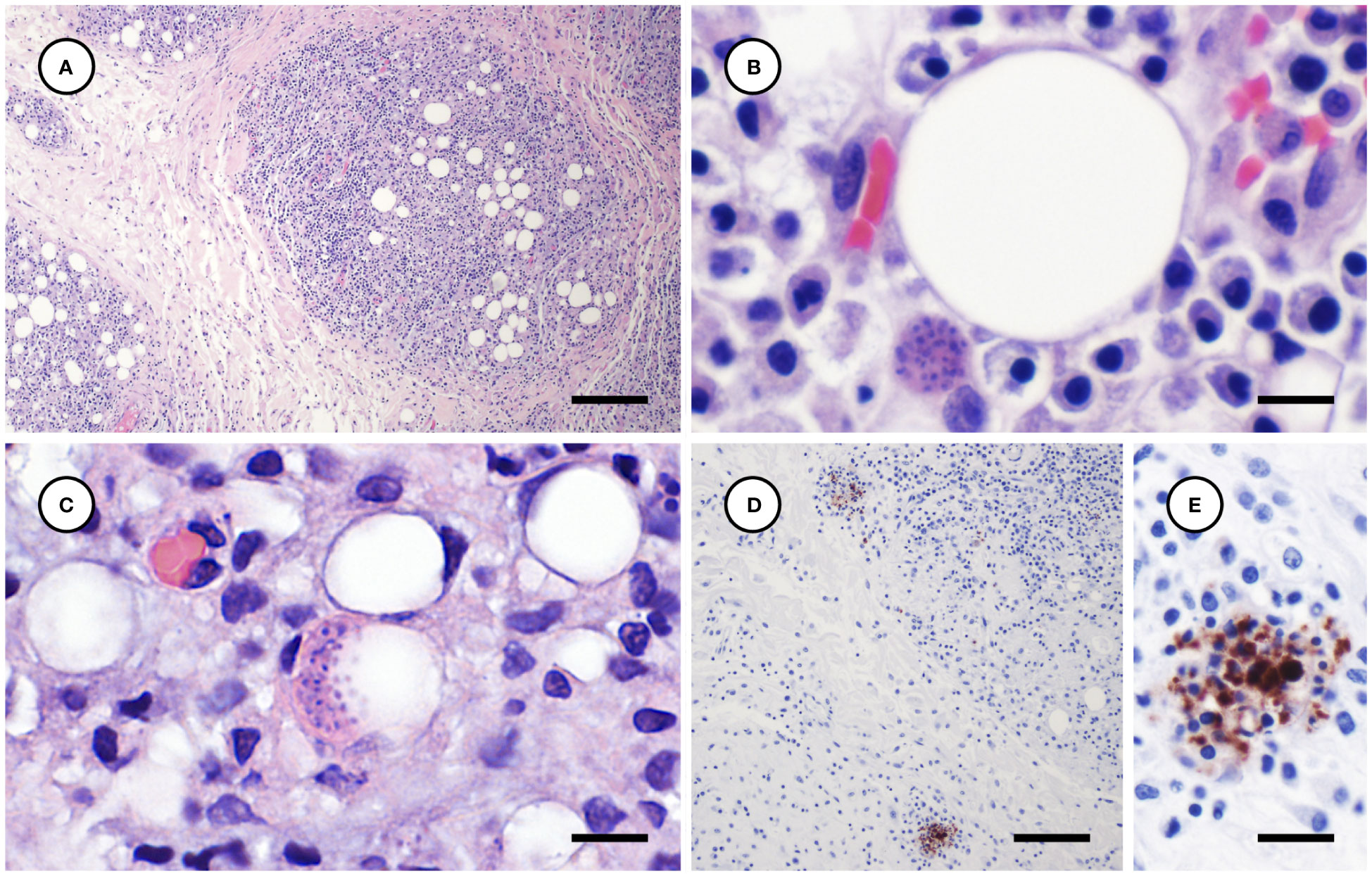
Figure 7 Histological and immunohistochemical appearance of subcutaneous and peritoneal steatitis from sea otters with fatal protozoal steatitis and toxoplasmosis. (A–C): Progressively higher magnification views of severely inflamed adipose tissue (A); a Toxoplasma gondii-like protozoal tissue cyst is located adjacent to a large lipid vacuole (B) and numerous parasites are clustered along the periphery of a cytoplasmic lipid vacuole (C) (H&E stain, bar = 200, 10, and 10 µm, respectively). Immunohistochemical stains of inflamed peritoneal adipose, demonstrating strong positive T. gondii immunostaining of intralesional protozoa at lower (D) and higher (E) magnification (D, E: bar = 100 and 20 µm, respectively).
In addition to widespread steatitis, several other tissues exhibited severe T. gondii-associated lesions (Table 2). Necrotizing pancreatitis and peripancreatic steatitis were associated with numerous intralesional T. gondii-like tachyzoites and tissue cysts. Severe myocarditis was also associated with high numbers of intralesional T. gondii-like protozoa (Figure 4C). White pulmonary pleural foci corresponded microscopically with regions of lymphoplasmacytic, histiocytic, and necrotizing pneumonia with numerous intralesional tachyzoites and tissue cysts (Figure 5C). Protozoal adrenalitis was characterized by marked inflammation and necrosis with intralesional tachyzoites and tissue cysts (Figures 6B, C), especially in the adrenal medulla, corresponding with the location of cloudy fluid observed grossly. Inflamed lymph nodes exhibited marked paracortical lymphoid hyperplasia with numerous intralesional protozoa. Grossly apparent splenomegaly was characterized microscopically by lymphoid hyperplasia and lympholysis, and marked red pulp plasmacytosis, histiocytosis, and extramedullary hematopoiesis, although parasites were sparse.
The left uterine horn of Case 2 was mildly enlarged grossly (Figure 8A), and mild endometrial hemorrhage, hemosiderosis, and partial atrophy of mural arterioles were observed microscopically, suggestive of recent parturition or abortion. The myometrium of the left uterine horn was severely inflamed, with numerous intralesional T. gondii-like tachyzoites and tissue cysts (Figures 8B, C); the endo- and mesometrium exhibited milder inflammation and fewer parasites. The right uterine horn was minimally inflamed, and few parasites were observed microscopically. Moderate inflammation and T. gondii-like tachyzoites and tissue cysts were also observed in the duodenal tunica muscularis in Case 1, with inflammation and parasites most concentrated near parasympathetic ganglia.
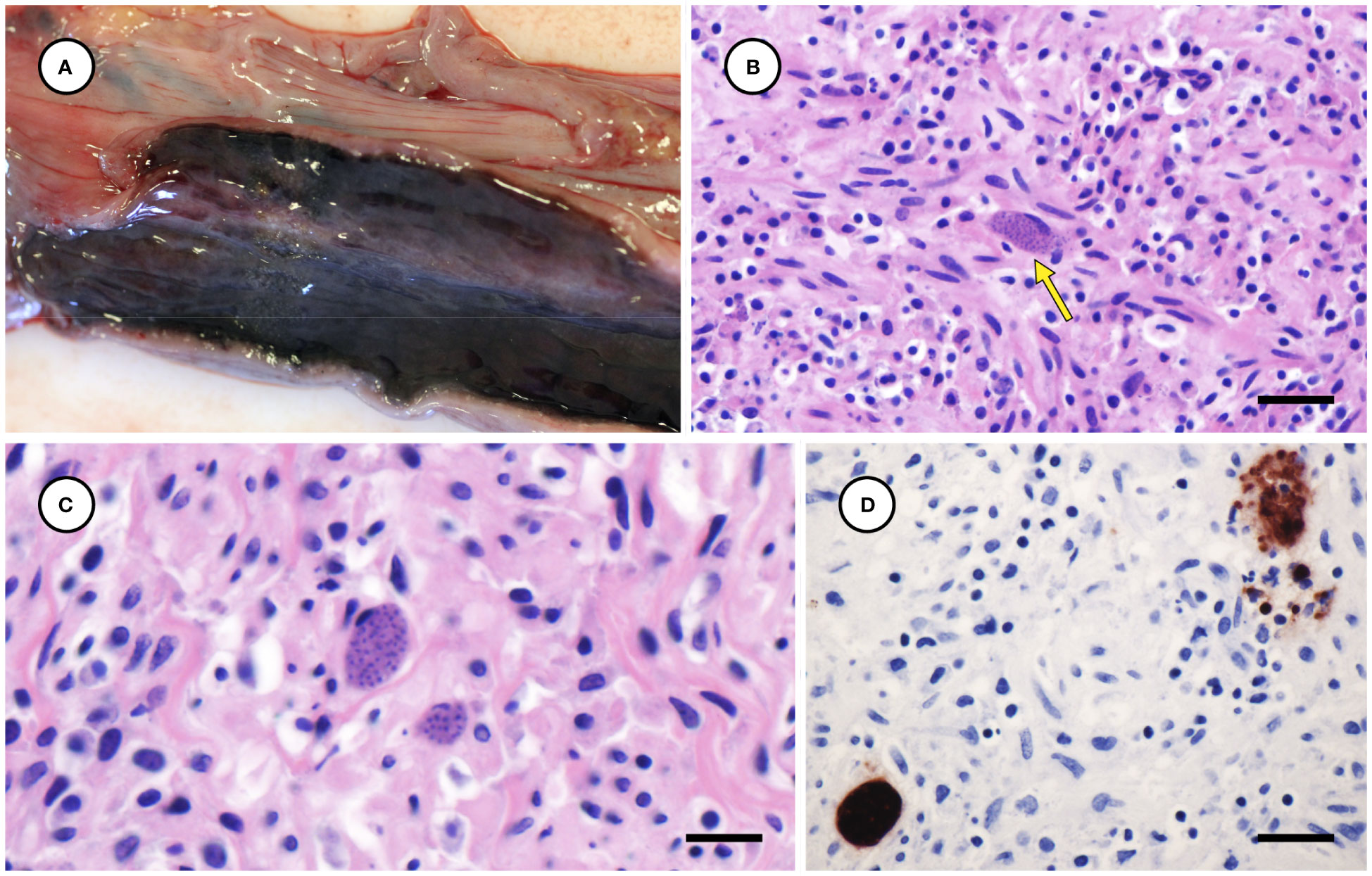
Figure 8 Mildly enlarged and congested left uterine horn from a sea otter with fatal toxoplasmosis and steatitis (A). Low (B) and high (C) magnification microscopic views of severely inflamed uterine myometrium from the same otter with intralesional Toxoplasma gondii-like tissue cysts (indicated by arrow for B) (B, C: H&E stain, bar = 20 and 10 µm, respectively). Immunohistochemical stain of uterine myometrium from the same sea otter demonstrating strong positive immunostaining of intralesional protozoa for T. gondii (D) (Bar = 20 µm).
In contrast with widespread inflammation and high parasite burdens observed in other tissues, brains of affected otters exhibited surprisingly mild meningoencephalitis with sparse intralesional tachyzoites and tissue cysts, unlike typical patterns reported from sea otters with fatal toxoplasmosis (Miller et al., 2018; Miller et al., 2020). Throughout all examined tissues, tissue cysts and tachyzoites were most common on histopathology in the cytoplasm of adipocytes, pancreatic acinar cells, and smooth, skeletal, and cardiac myofibers. Protozoa were also observed within the cytoplasm of fibroblasts, macrophages, monocytes, lymphocytes, hepatocytes, adrenal cortical and chromaffin cells, and cerebral neurons and glia.
Protozoal immunohistochemistry and serology
Results of T. gondii-associated immunohistochemistry are summarized in Table 2, and composite results of protozoal histology, immunohistochemistry, and serology for T. gondii and S. neurona are summarized in Supplementary Table 1. Formalin-fixed tissues from all four cases contained tachyzoites and tissue cysts that were strongly immunopositive for T. gondii. Inflamed subcutaneous and peritoneal adipose (Figures 7D, E), pancreas (Figure 3D), heart (Figure 4D), lymph nodes, and brain (Figure 6D) were T. gondii-immunopositive for all four otters (Table 2). Lung (Figure 5D) and skeletal muscle were T. gondii immunopositive for two of three cases where this tissue was available for microscopic examination. The inflamed adrenal gland and uterine myometrium (Figure 8D) from Case 2 were also strongly T. gondii-immunopositive.
Immunohistochemistry for S. neurona was performed on the same tissues as for T. gondii, and protozoa in all tissues from Cases 1, 2, and 3 were diffusely immunonegative (Figure 6E). Case 4 exhibited sparse S. neurona-positive staining in myocardium, and potential small foci of positive staining in subcutaneous adipose and lung. Pericardial fluid was available for Cases 1, 2, and 4; all had high positive T. gondii IgG (≥1:10,240), and low positive S. neurona titers (≤1:2,560) (Supplementary Table 1).
In vitro parasite cultivation
Parasites morphologically resembling T. gondii and S. neurona were observed in trypsinized and untreated cell cultures from Case 2 within two weeks of inoculating cells with brain tissue. Amplification and sequencing of DNA extracted from supernatant at the ITS1 locus yielded 100% identity to T. gondii in both trypsinized and untreated cultures. Amplification and sequencing of the cox1 locus yielded 100% identity to S. neurona in supernatant from the untreated culture, while DNA amplification at the cox1 locus was not observed for supernatant from the trypsinized culture. These results confirm isolation of T. gondii in the trypsinized and untreated cell cultures, and additional co-isolation of S. neurona in the untreated culture.
Molecular detection and characterization of protozoal parasites
Results of molecular detection and characterization of protozoal parasites are summarized in Table 3. Genotyping across 12 to 13 loci was successfully performed using DNA from subcutaneous adipose from all four sea otters, plus culture supernatant from inoculated brain tissue of Case 2. Initial local sequence alignment revealed that the T. gondii strains from all animals shared 100% sequence identity with each other across all examined loci (Supplementary Figure 2), plus several polymorphisms when compared to known reference sequences for Type I, Type II, Type III, and Type X strains. Subsequent analysis compared the identity of the sea otter T. gondii sequences to whole genome sequences from publicly available T. gondii reference genotypes on GenBank. This analysis revealed 100% identity across the 13 examined loci of T. gondii sequences from all four otters to the COUG strain (TgCgCa1) (Supplementary Figure 2).
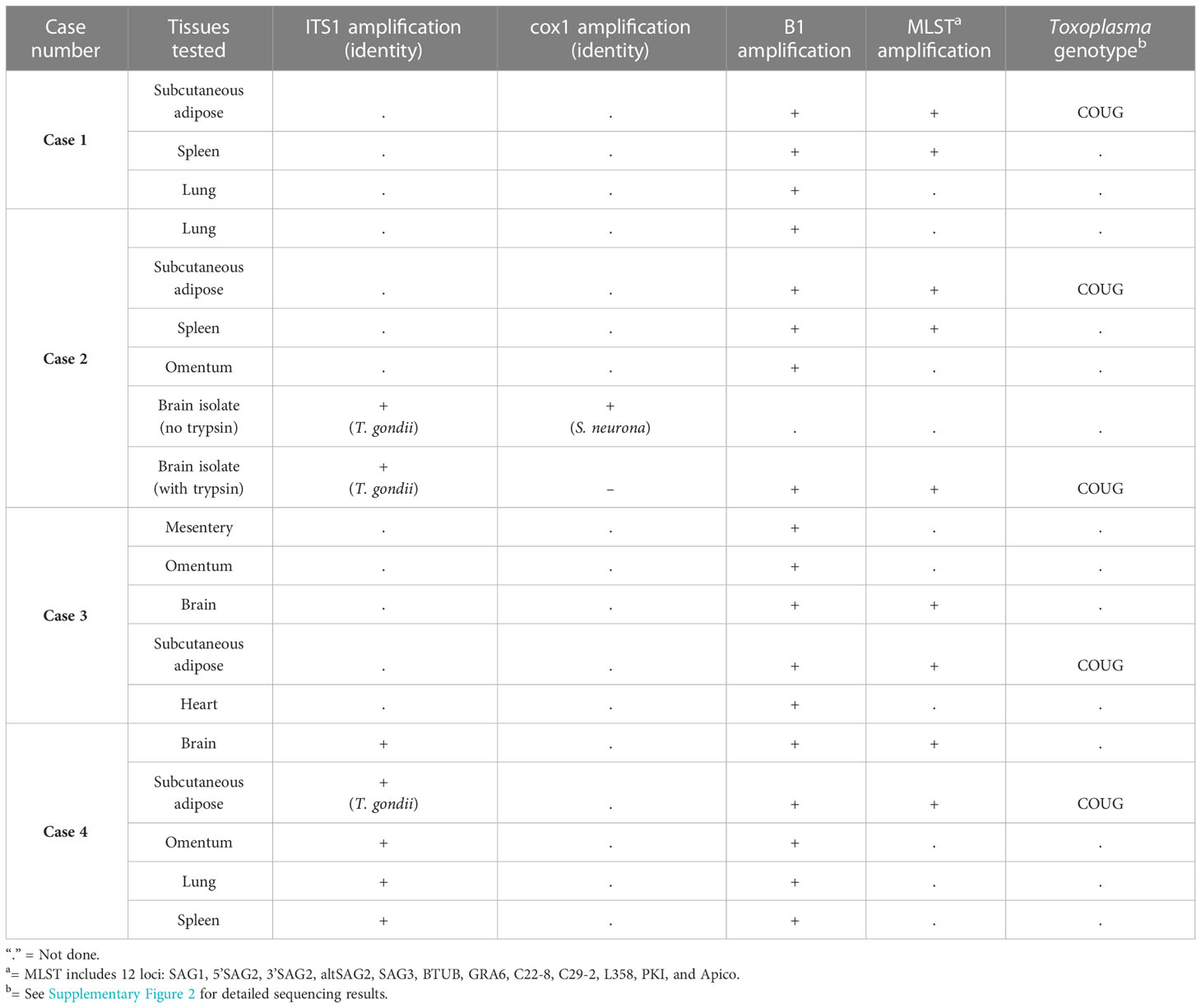
Table 3 Findings from molecular characterization using PCR and MLST for southern sea otters (Enhydra lutris nereis) with fatal Toxoplasma gondii-associated steatitis and toxoplasmosis.
Final diagnoses
Based on cumulative findings from gross necropsy, histopathology, immunohistochemistry, and molecular parasite confirmation, the primary cause of death was systemic toxoplasmosis with severe steatitis for all four cases (Table 2). Given their relatively good nutritional condition, high systemic parasite load, and relative paucity of parasites and parasite-associated inflammation in the CNS, affected animals appear to have died in the acute or subacute stages of T. gondii infection (Miller et al., 2018).
Contributing causes of death and incidental findings are summarized in Table 2. Methods used for lesion assessment and ranking were as previously described (Miller et al., 2020; Miller et al., 2021). Findings suggestive of subacute to chronic domoic acid toxicosis (Miller et al., 2021) in Case 2 were characterized by moderate, diffuse brain congestion; segmental cytoplasmic vacuolation and angularity of hippocampal pyramidal neurons; CA2 sector neuronal depletion; and a mildly shrunken hippocampal profile. Temporally concordant cardiac lesions presumed to be associated with domoic acid toxicosis included patchy myofiber loss, stromal collapse, mural fibrosis, and hyalinization of the tunica media of coronary arterioles. Other contributing findings (gastric ulcers, melena, and emaciation) are common in sick or stressed sea otters (Miller et al., 2020).
Cystic degeneration of the caudal pole of the right kidney was considered an incidental finding for Case 2. The cystic structure was filled with pale yellow urine and sediment and was not patent to the ureter. Histopathology revealed diffuse parenchymal atrophy and mild lymphoplasmacytic inflammation. The inner cyst wall was lined by a single layer of flattened epithelium overlying a dense fibrovascular stroma. No protozoal parasites were observed within the cyst wall, nor in any histological sections of kidney.
Discussion
This study describes an unusual and severe presentation of toxoplasmosis and steatitis in sea otters infected with a rare T. gondii strain not previously reported in any aquatic animal. Observed lesion and case presentation patterns differed substantially from prior descriptions of toxoplasmosis in sea otters, where cases of acute to subacute, severe, disseminated toxoplasmosis were reported mainly from fetuses and neonates (Miller et al., 2008a; Shapiro et al., 2016). In contrast with the cases described herein, sea otters with fatal toxoplasmosis usually have no gross lesions other than non-specific lymphadenosplenomegaly, and histopathology is required to confirm infection (Miller et al., 2018). Protozoa are typically numerous in the CNS, accompanied by non-suppurative meningoencephalitis, but are relatively rare in other tissues on conventional histopathology (Miller et al., 2018). The most common extra-CNS lesion reported from T. gondii-infected sea otters is protozoal myocarditis, and death is usually attributed to chronic, active meningoencephalitis or myocarditis (Miller et al., 2020). Although microscopic foci of T. gondii-associated steatitis, myositis, pancreatitis, and adrenalitis are occasionally observed, this finding is consistently mild in comparison to the brain and cardiac pathology.
The four cases described herein exhibit a strikingly different lesion pattern, characterized by grossly apparent, diffuse, severe steatitis of all subcutaneous and internal adipose stores.
Affected adipose was yellow-mottled and firm with a nodular appearance. Some cases also exhibited grossly visible pancreatic, pulmonary, adrenal, and cardiac pathology. Histopathology revealed severe inflammation with numerous intralesional protozoa in most tissues, apart from the CNS, where parasite numbers and inflammation were comparatively mild. Severe toxoplasmosis and protozoal steatitis was identified as the primary cause of death in all cases and inflamed tissues were strongly immunopositive, confirming the association of systemic inflammation with T. gondii infection.
These unusual cases also differed from prior reports of fatal toxoplasmosis in sea otters in other ways: In previous studies (Kreuder et al., 2003: Miller et al., 2020), sea otter deaths due to toxoplasmosis were relatively sporadic, with little variation by season or month. In contrast, all otters with fatal toxoplasmosis with steatitis stranded in February and March, which corresponds with the peak rainy season in California when land-sea runoff following rainfall events is highest. Wet season protozoal disease outbreaks have also been reported for S. neurona (Miller et al., 2010; Shapiro et al., 2012), and seasonal land-sea transport of infectious oocysts or sporocysts is considered the most likely route of sea otter infection by both parasites (Miller et al., 2002a; Miller et al., 2008b; Miller et al., 2010).
Affected otters were strongly seropositive for T. gondii IgG (≥1:10,240) and multilocus sequence typing at 13 loci revealed that all animals with this unusual lesion pattern were infected with the same atypical COUG strain of T. gondii (TgCgCa1). Despite decades of studies on the molecular epidemiology of T. gondii in coastal California, including genetic characterization of >140 T. gondii isolates obtained from southern sea otters (Miller et al., 2004; Sundar et al., 2008; VanWormer et al., 2014; Shapiro et al., 2015; Shapiro et al., 2016; Shapiro et al., 2019), this is the first report of the COUG strain in sea otters, or any aquatic species. To ensure that prior protozoal steatitis cases had not been missed, archival necropsy data (>1,000 sea otters from 1997 through 2022) from the California Department of Fish and Wildlife was reviewed for additional cases of diffuse, severe T. gondii-associated steatitis. None were found other than the current four cases, the earliest of which occurred in 2020.
Importantly, the COUG T. gondii strain appears to be capable of causing rapid mortality in ostensibly healthy, prime-aged adult sea otters. Three of the four cases were adult females, while one was an immature male. Possible spatial clustering of cases was also noted within a 26.5 km coastal region in San Luis Obispo County. However, given our small sample size, effects of sex, age, and stranding location cannot be statistically evaluated.
The COUG strain (ToxoDB PCR-RFLP genotype #66) is considered a rare, atypical North American strain circulating in wildlife. Phylogenetically, this strain clusters within the same major clade (D) as the archetypal Type II and atypical Type X strain, but within a distinctly characterized haplotype (11) that it shares with another atypical strain derived from a jaguar (GUY-2004-JAG1) (Lorenzi et al., 2016). The COUG strain was originally isolated via mouse bioassay from fecal samples from two mountain lions collected following epidemiological studies of a large waterborne toxoplasmosis outbreak in humans in British Columbia, Canada that occurred in 1995 (Dubey et al., 2008). One sample was found in the environment, while the second was collected per rectum from a mountain lion that was euthanized in the vicinity of the drinking water reservoir implicated in the outbreak; no information was reported regarding T. gondii-associated pathology in the euthanized mountain lion (Dubey et al., 2008). Fecal shedding of oocysts by mountain lions or other wild felids was postulated to have served as the source of the toxoplasmosis outbreak (Bowie et al., 1997; Aramini et al., 1999). However, because no information was published on genotype(s) of T. gondii in infected humans from the outbreak, there is insufficient evidence linking the COUG strain in sympatric felids to the human waterborne toxoplasmosis event. To our knowledge, the only other animal reported to be infected with a COUG genotype is a feral pig (Sus scrofa) from the Sierra Nevada Mountains in eastern California (Dubey et al., 2020).
Although this unique parasite strain could have been present before 2020, no prior molecular studies of T. gondii infection in sea otters, domestic cats, mountain lions, bobcats, canids, and other animals along the California coast identified any animals infected with the COUG T. gondii strain (Miller et al., 2008b; VanWormer et al., 2013; VanWormer et al., 2014; Shapiro et al., 2019). This knowledge, plus detection of a novel T. gondii-associated lesion pattern with high virulence that coincides temporally with detection of COUG T. gondii infection in stranded sea otters suggests that introduction of this highly pathogenic strain into coastal California wildlife occurred recently.
Geographical movement of T. gondii strains may occur via infected migratory birds, migration of terrestrial wildlife, or anthropogenic translocation of wild animals, livestock, and pets, including domestic cats (Galal et al., 2022). Though isolated previously from wild felids, domestic cats have been shown to contribute substantially more oocysts in coastal California watersheds when compared to bobcats and mountain lions (VanWormer et al., 2013), and cats could be exposed to this wildlife-adapted strain by feeding on wild prey.
It is also unclear at present whether multiple point sources of the COUG T. gondii strain may be spreading into the marine environment along the California coast. Multiple sources of exposure could explain the two geographically separate regions where the four cases described in this study were found. Another possibility is that the immature male was initially infected in the same region as the females but stranded elsewhere due to migration post-infection. Previous studies of tagged sea otters revealed that males may travel hundreds of km (Tinker et al., 2017), with immature males frequently dispersing beyond study areas for extended periods (J. Tomoleoni, pers. comm), suggesting that the immature male could have stranded in a different county than the original location of T. gondii infection. Interestingly, the three adult females stranded near Morro Bay, a confirmed, high-risk area for T. gondii Type X infection and mortality in sea otters (Shapiro et al., 2019). Numerous factors appear to contribute to these high-risk zones, including local geography, precipitation, climate, felid density, and other factors (VanWormer et al., 2013; VanWormer et al., 2014; Burgess et al., 2018; Miller et al., 2020). Given the apparent high virulence and unknown source of this atypical strain, as well as the potential health risks for threatened sea otters, other animals and humans, additional research is needed to clarify the host and geographical distribution of the COUG strain in North America.
This is the first report of severe steatitis associated with toxoplasmosis in sea otters, but similar lesions were reported in Hawaiian monk seals (Neomonachus schauinslandi) with fatal toxoplasmosis (Barbieri et al., 2016). Although the monk seal steatitis lesions appear similar grossly and microscopically, the T. gondii genotype(s) associated with monk seal infection were not reported. Protozoal steatitis was also reported in a red kangaroo and aborted goats with fatal toxoplasmosis (Chen and Alley, 1987; Dubey and Hartley, 1992).
Although T. gondii has been traditionally classified into three archetypal genotypes (Type I, II, and III), more advanced genotyping approaches and global characterization of parasite strains have contributed to much broader recognition of non-archetypal or “atypical” genotypes. Associations between infection by specific T. gondii genotypes and more severe disease have been reported in animals and people (Grigg et al., 2001; Khan et al., 2009; Melo et al., 2013). The relationship between strain genotype and virulence is well established in mouse models, with Type I isolates and most South American strains being highly virulent, whereas Type II and III strains are less virulent (Khan et al., 2009; Melo et al., 2013).
The atypical Type X strains (ToxoDB#5) have been classified within haplotype 12 and are predominantly found in North American wildlife (Dubey et al., 2011; Jiang et al., 2018). Southern sea otters are most frequently infected by Type X or X variant strains, which are more pathogenic in sea otters than Type II strains (Miller et al., 2004; Shapiro et al., 2019). In a study of 135 necropsied southern sea otters, 79% of T. gondii isolates were Type X or Type X variants, while the remaining 21% were Type II or Type II/X recombinants. Additionally, all enrolled otters with T. gondii identified as the primary cause of death were infected with Type X or Type X variants (Shapiro et al., 2019). In contrast, a study of mixed marine mammal species in the Pacific Northwest, including five northern sea otters (E. l. kenyoni) revealed no significant associations between T. gondii genotype and disease outcome (Gibson et al., 2011).
Although no assessment of associated pathology or disease outcome was included in descriptions of the COUG T. gondii strain from mountain lions and a wild pig (Dubey et al., 2008; Dubey et al., 2020), the COUG genotype has been shown to be highly virulent in mice and significantly more virulent than Type II strains (Khan et al., 2009). However, it was unclear if this enhanced virulence was due to a high parasite burden or a strong, parasite-associated inflammatory response. Substantial genetic differences between COUG and Type II T. gondii strains have also been reported (Minot et al., 2012).
Another study revealed that the COUG strain is unusual among T. gondii isolates in eliciting a Type I interferon response in both murine macrophages and human fibroblasts (Melo et al., 2013). Type I interferons (IFN), including IFN-α and -β, are immunomodulatory cytokines that serve as potent coordinators of antimicrobial responses. In addition to their well-recognized role in viral immunity, IFNs can also play important roles during parasitic infection by controlling parasite growth through activation of intracellular killing mechanisms or enhancing disease severity. According to Melo et al. (2013), recent reports that Type I IFNs suppress Type II IFN-triggered human anti-mycobacterial responses (Teles et al., 2013) could suggest that the Type I IFN response induced by COUG and other select atypical T. gondii strains could be associated with enhanced pathogenicity. In addition, Type I IFNs can affect host cellular lipid metabolism by inhibiting de novo cholesterol and fatty-acid synthesis and upregulating the uptake of exogenous lipids (Wu et al., 2016). Although Type I IFNs can induce metabolic changes that are critical for immune function (Wu et al., 2016), these changes could also provide selective advantages for highly lipophilic intracellular protozoa.
The striking tropism of the COUG strain of T. gondii for sea otter adipose tissue is unusual and noteworthy. Not only were parasites numerous throughout systemic adipose stores in association with severe inflammation, but on histopathology numerous parasites were concentrated along the periphery of lipid droplets within the cytoplasm of infected adipocytes (Figure 7C). Although T. gondii is known to scavenge host lipids to facilitate parasite replication and survival (Nolan et al., 2017), and host-parasite competition for lipid stores likely contributes to disease pathogenesis (Coppens, 2006), the degree of parasitism within adipose tissue in these cases was extreme when compared with hundreds of previous histologically and molecularly confirmed cases of toxoplasmosis in sea otters.
In addition to the grossly apparent steatitis and pancreatitis reported herein, another fascinating and potentially strain-associated difference for COUG T. gondii-infected sea otters is significant protozoal infection of smooth muscle cells within the uterine myometrium (Figures 8B–D) and duodenal tunica muscularis (Table 2). Despite screening sea otter tissues for 24 years, one author (MM) has never observed this previously. Although T. gondii can infect virtually any nucleated cell type in warm-blooded animals and humans, infection of skeletal and cardiac muscle is most commonly reported, and skeletal muscle appears to be a preferred tissue for persistent T. gondii infection (Swierzy et al., 2014). The parasite load and inflammation in the uterine myometrium was particularly severe and could have been associated with fetal infection and abortion, although this was impossible to confirm at gross necropsy. Interestingly, uterine and gastric mural infection was also reported in Hawaiian Monk seals with fatal toxoplasmosis and protozoal steatitis (Barbieri et al., 2016), although the infecting genotype in the described Monk Seal cases have not been reported to date. Toxoplasma gondii-associated smooth muscle necrosis has also been reported in highly susceptible Australian marsupials (Canfield et al., 1990), and HIV-positive humans with protozoal pneumonia and gastritis (Nash et al., 1994; Alpert et al., 1996).
Although co-infection with S. neurona was identified via parasite isolation, immunohistochemistry, or PCR for two cases (Supplementary Table 1), findings from extensive histopathology and immunohistochemistry confirm T. gondii as the primary cause of death in all four cases. All otters for which pericardial fluid was available for serology had high positive titers for T. gondii and low positive titers for S. neurona. Sarcocystis neurona was isolated in culture from the brain of Case 2; however, no S. neurona organisms were seen associated with inflammation in the brain histologically or immunohistochemically. While Case 4 exhibited sparse S. neurona immunopositive staining in heart, adipose and lung, DNA extracted from the brain, subcutaneous fat, omentum, lung, and spleen for this sea otter only yielded amplification at the ITS1 locus for T. gondii. As the parasite load of T. gondii was far greater and was closely associated with pathology in multiple tissues from all examined otters, S. neurona appeared to be incidental or of minimal clinical significance. However, it cannot be ruled out that S. neurona co-infection may have contributed to the severity of observed lesions.
This study had several limitations including a small sample size, varying postmortem duration, and freeze-thaw conditions for examined animals, which can decrease tissue quality and resolution. Additionally, steatitis altered the gross appearance of adipose stores and likely impacted standardized assessment of nutritional condition.
Our findings reveal a novel and concerning lesion pattern for southern sea otters with systemic toxoplasmosis that appears to be associated with an atypical T. gondii strain described in an aquatic animal for the first time. The unique combination of host, pathogen, and environmental factors contributing to this newly recognized manifestation of systemic toxoplasmosis in sea otters is unknown. Future directions should include expanded research on this seemingly highly pathogenic T. gondii strain, such as genomic studies to identify unique virulence factors, or transcriptomic investigations into host lipid metabolism and tropism. Additional studies are needed to evaluate the presence of this strain in a larger sample of coastal terrestrial animals, sea otters, and other aquatic wildlife, including screening animals with subclinical T. gondii infections. Investigating habitat or climate change factors that may have facilitated the spread of this rare atypical strain to coastal California will shed light on how anthropogenic activities can alter T. gondii ecology and epidemiology. The potential for immunosuppression following exposure to occult viruses, toxins, anthropogenic chemicals, and other causes also warrants investigation. Because T. gondii oocysts can be concentrated in invertebrates and transmitted to susceptible hosts via ingestion (Arkush et al., 2003), there is potential for this virulent pathogen to pose a public health risk for people consuming seafood, or ingesting water contaminated with this parasite. Sea otters have served as important sentinels for recognition of the COUG strain circulating in the coastal California terrestrial and marine environment, highlighting the need for ongoing research into spread and possible emergence of diverse T. gondii strains from coastal terrestrial vertebrates to marine ecosystems.
Data availability statement
The data presented in the study are deposited in GenBank, accession numbers: OQ249665 (3’SAG2), OQ249666 (5’SAG2), OQ249667 (altSAG2), OQ249668 (Apico), OQ249669 (B1), OQ249670 (BTUB), OQ249671 (C22-8), OQ249672 (C29-2), OQ249673 (GRA6), OQ249674 (L358), OQ249675 (PKI), OQ249676 (SAG1), OQ249677 (SAG3).
Ethics statement
In accordance with Section 109(h) of U.S. Marine Mammal Protection Act (MMPA), U.S. Fish and Wildlife Service (Service) regulations implementing the MMPA at 50 CFR 18.22(a), and in accordance with Service regulations implementing the US Endangered Species Act at 50 CFR 17.21(c)(3), samples used to complete this work were collected by an official or employees of the California Department of Fish and Wildlife (CDFW) in the course of their duties as an official or employee of CDFW.
Author contributions
MM: Completed gross necropsies for some enrolled otters; read out all case slides and compiled summary reports; envisioned, coordinated, and oversaw project completion; took gross photos and all photomicrographs; led completion of all summary image plates; substantial contribution to manuscript design, preparation, revision, editing and literature review; designed and contributed Tables 1, 2; Supplementary Figure 2, and Figures 1 through 8. CN: Leadership role in manuscript writing, editing, and polishing of case discussion and literature review; helped coordinate and oversee project completion; assisted with gross necropsy for one enrolled otter and transported samples to UCD for diagnostic workup; provided substantial input regarding project design and completion, and manuscript design, preparation, revision, editing and literature review; final formatting and polishing of manuscript to meet publication guidelines. DS: Extracted DNA and performed PCR for all T. gondii genotyping assays for enrolled otters; established and maintained in vitro cultures for one otter and verified culture ID via PCR; assisted with sequence trimming and alignment; assisted with design and construction of Table 2; reviewed all histopathology slides and immunohistochemistry; designed and contributed Table 3 and Supplementary Figure 2; assistance with literature review, and manuscript writing and editing, especially sections of the discussion related to genotyping and the COUG T. gondii strain. FB: Assisted with gross necropsies, data, and sample collections; coordinated specimen subsampling and shipments, and immunohistochemistry shipments for diagnostic workup; conducted database queries of existing southern sea otter steatitis data; data organization; assisted with manuscript edits and submission. KG: Assisted with gross necropsies, data, and sample collection; coordinated archiving of samples and data to assist completion of summary reports; completed histopathology trimming of 2/4 enrolled otters; assisted with manuscript edits and table completion. AR: Assisted with histopathology trimming of 2/4 enrolled otters; assisted with manuscript edits and completed reformatting and optimization of all image montages for the manuscript. CY: Provided critical field recognition of cases enrolled in the study; assisted with gross necropsies, data, and sample collection; assisted with manuscript edits. Completed gross necropsy on 1 case. MH: Provided critical field recognition of cases enrolled in the study; assisted with gross necropsy for 1 case, data, and sample collection; assisted with manuscript edits. Created Supplementary Figure 1. AP: Completed all serological testing for T. gondii and S. neurona for three cases and provided expertise to facilitate parasite isolation efforts; assisted with manuscript edits. KS: Supervised and funded parasite tissue isolation and protozoal parasite molecular detection and characterization at UC Davis; analyzed sequence data and interpreted molecular results; substantial assistance with literature review and suggestion of applicable manuscripts on genotyping details; substantial assistance with manuscript writing and editing, especially sections of the discussion related to genotyping and the COUG T. gondii strain. All authors contributed to the article and approved the submitted version.
Funding
We thank the taxpayers of California for making this work possible through voluntary contributions to the California Sea Otter Fund (Sea Otter Tax Checkoff). Tax checkoff funding to support this work was made available through the California Department of Fish and Wildlife-Office of Spill Prevention and Response. Funding support for Devinn Sinnott was provided by Morris Animal Foundation Fellowship Award D22ZO-409.
Acknowledgments
This project would not have been possible without assistance from many contributors, including CAHFS staff, especially Mary Williams and Karen Sverlow for preparation of immunohistochemical stains. We also thank the VMTH histology laboratory for processing, cutting, and staining of formalin-fixed tissues. Thank you to the volunteers and staff at California Department of Fish and Wildlife, the U.S. Geological Survey, the Monterey Bay Aquarium, The Marine Mammal Center, and other stranding partners for their efforts to recover sick live and dead sea otters along the central California coast.
Conflict of interest
The authors declare that the research was conducted in the absence of any commercial or financial relationships that could be construed as a potential conflict of interest.
Publisher’s note
All claims expressed in this article are solely those of the authors and do not necessarily represent those of their affiliated organizations, or those of the publisher, the editors and the reviewers. Any product that may be evaluated in this article, or claim that may be made by its manufacturer, is not guaranteed or endorsed by the publisher.
Supplementary material
The Supplementary Material for this article can be found online at: https://www.frontiersin.org/articles/10.3389/fmars.2023.1116899/full#supplementary-material
Supplementary Table 1 | Findings from histopathology, immunohistochemistry, and serology for southern sea otters (Enhydra lutris nereis) with fatal Toxoplasma gondii-associated steatitis and toxoplasmosis.
Supplementary Figure 1 | Map of the central California coast, showing the stranding locations of four southern sea otters (Enhydra lutris nereis) with fatal Toxoplasma gondii-associated steatitis and toxoplasmosis.
Supplementary Figure 2 | Heat maps comparing the sequence identity of Toxoplasma gondii DNA amplified from the four sea otters with systemic toxoplasmosis and reference genotypes including the COUG strain, Type X (bobcat) and the archetypal Types I (RH), II (ME49), and III (CTG). Darker shades of orange indicate higher percent identities between the T. gondii strain infecting sea otters and reference strains across the 13 examined loci.
References
Alpert L., Miller M., Alpert E., Satin R., Lamoureux E., Trudel L. (1996). Gastric toxoplasmosis in acquired immunodeficiency syndrome: Antemortem diagnosis with histopathologic characterization. Gastroenterology 110, 258–264. doi: 10.1053/gast.1996.v110.pm8536865
Aramini J. J., Stephen C., Dubey J. P., Engelstoft C., Schwantje H., Ribble C. S. (1999). Potential contamination of drinking water with Toxoplasma gondii oocysts. Epidemiol. Infect. 122, 305–315. doi: 10.1017/S0950268899002113
Arkush K. D., Miller M. A., Leutenegger C. M., Gardner I. A., Packham A. E., Heckeroth A. R., et al. (2003). Molecular and bioassay-based detection of Toxoplasma gondii oocyst uptake by mussels (Mytilus galloprovincialis). Int. J. Parasitol. 33, 1087–1097. doi: 10.1016/S0020-7519(03)00181-4
Barbieri M. M., Kashinsky L., Rotstein D. S., Colegrove K. M., Haman K. H., Magargal S. L., et al. (2016). Protozoal-related mortalities in endangered Hawaiian monk seals Neomonachus schauinslandi. Dis. Aquat. Org. 121, 85–95. doi: 10.3354/dao03047
Bowie W. R., King A. S., Werker D. H., Isaac-Renton J. L., Bell A., Eng S. B., et al. (1997). Outbreak of toxoplasmosis associated with municipal drinking water. The Lancet. 350, 173–177. doi: 10.1016/s0140-6736(96)11105-3
Burgess T., Tinker M. T., Miller M. A., Bodkin J., Murray M., Saarinen J., et al. (2018). Defining the risk landscape in the context of pathogen pollution: Toxoplasma gondii in sea otters along the Pacific Rim. R. Soc. Open Sci. 5, 171178. doi: 10.1098/rsos.171178
Canfield P. J., Hartley W. J., Dubey J. P. (1990). Lesions of toxoplasmosis in Australian marsupials. J. Comp. Pathol. 103, 159–167. doi: 10.1016/S0021-9975(08)80172-7
Chen W., Alley M. R. (1987). Lesions of toxoplasmosis in the perirenal adipose tissue of aborted kids. N. Z. Vet. J. 35, 176. doi: 10.1080/00480169.1987.35434
Coppens I. (2006). Contribution of host lipids to Toxoplasma pathogenesis. Cell. Microbiol. 8, 1–9. doi: 10.1111/j.1462-5822.2005.00647.x
DaSilva R. C., DaSilva A. V., Langoni H. (2010). Recrudescence of Toxoplasma gondii infection in chronically infected rats (Rattus norvegicus). Exp. Parasitol. 125, 409–412. doi: 10.1016/j.exppara.2010.04.003
Dellacasa-Lindberg I., Hitziger N., Barragan A. (2007). Localized recrudescence of Toxoplasma infections in the Central nervous system of immunocompromised mice assessed by in vivo bioluminescence imaging. Microbes Infect. 9, 1291–1298. doi: 10.1016/j.micinf.2007.06.003
Dubey J. P., Cerqueira-Cézar C. K., Murata F. H. A., Verma S. K., Kwok O. C. H., Pedersen K., et al. (2020). Genotyping of viable Toxoplasma gondii from the first national survey of feral swine revealed evidence for sylvatic transmission cycle, and presence of highly virulent parasite genotypes. Parasitology 147, 295–302. doi: 10.1017/S0031182019001586
Dubey J. P., Frenkel J. K. (1972). Cyst-induced toxoplasmosis in cats. J. Protozool. 19, 155–177. doi: 10.1111/j.1550-7408.1972.tb03431.x
Dubey J. P., Hartley W. J. (1992). Steatitis in a red kangaroo (Macropus rufus) associated with a coccidia-like protozoon. J. Vet. Diagn. Invest. 4, 93–96. doi: 10.1177/104063879200400123
Dubey J. P., Quirk T., Pitt J. A., Sundar N., Velmurugan G. V., Kwok O. C., et al. (2008). Isolation and genetic characterization of Toxoplasma gondii from raccoons (Procyon lotor), cats (Felis domesticus), striped skunk (Mephitis mephitis), black bear (Ursus americanus), and cougar (Puma concolor) from Canada. J. Parasitol. 94, 42–45. doi: 10.1645/ge-1349.1
Dubey J. P., Velmurugan G. V., Rajendran C., Yabsley M. J., Thomas N. J., Beckmen K. B., et al. (2011). Genetic characterisation of Toxoplasma gondii in wildlife from North America revealed widespread and high prevalence of the fourth clonal type. Int. J. Parasitol. 41, 1139–1147. doi: 10.1016/j.ijpara.2011.06.005
Dumètre A., Dardé M. L. (2003). How to detect Toxoplasma gondii oocysts in environmental samples? FEMS Microbiol. Rev. 27, 651–661. doi: 10.1016/S0168-6445(03)00071-8
Fritz H., Barr B., Packham A., Melli A., Conrad P. A. (2012). Methods to produce and safely work with large numbers of Toxoplasma gondii oocysts and bradyzoite cysts. J. Microbiol. Methods. 88, 47–52. doi: 10.1016/j.mimet.2011.10.010
Galal L., Ariey F., Gouilh M. A., Dardé M.-L., Hamidović A., Letourneur F., et al. (2022). A unique Toxoplasma gondii haplotype accompanied the global expansion of cats. Nat. Commun. 13, 5778. doi: 10.1038/s41467-022-33556-7
Gibson A. K., Raverty S., Lambourn D. M., Huggins J., Magargal S. L., Grigg M. E. (2011). Polyparasitism is associated with increased disease severity in Toxoplasma gondii-infected marine sentinel species. PLoS Negl. Trop. Dis. 5, e1142. doi: 10.1371/journal.pntd.0001142
Gondim L. F. P., Soares R. M., Tavares A. S., Borges-Silva W., de Jesus D. F., Llano H. A. B., et al. (2019). Sarcocystis falcatula-like derived from opossum in Northeastern Brazil: In vitro propagation in avian cells, molecular characterization and bioassay in birds. Int. J. Parasitol. Parasites Wildl. 10, 132–137. doi: 10.1016/j.ijppaw.2019.08.008
Grigg M. E., Boothroyd J. C. (2001). Rapid identification of virulent Type I strains of the protozoan pathogen Toxoplasma gondii by PCR-restriction fragment length polymorphism analysis at the B1 gene. J. Clin. Microbiol. 39, 398–400. doi: 10.1128/JCM.39.1.398-400.2001
Grigg M. E., Ganatra J., Boothroyd J. C., Margolis T. P. (2001). Unusual abundance of atypical strains associated with human ocular toxoplasmosis. J. Infect. Dis. 184, 633–639. doi: 10.1086/322800
Jiang T., Shwab E. K., Martin R. M., Gerhold R. W., Rosenthal B. M., Dubey J. P., et al. (2018). A partition of Toxoplasma gondii genotypes across spatial gradients and among host species, and decreased parasite diversity towards areas of human settlement in North America. Int. J. Parasitol. 48, 611–619. doi: 10.1016/j.ijpara.2018.01.008
Johnson C. K., Tinker M. T., Estes J. A., Conrad P. A., Staedler M., Miller M. A., et al. (2009). Prey choice and habitat use drive sea otter pathogen exposure in a resource-limited coastal system. Proc. Natl. Acad. Sci. USA. 106, 2242–2247. doi: 10.1073/pnas.0806449106
Khan A., Taylor S., Ajioka J. W., Rosenthal B. M., Sibley L. D. (2009). Selection at a single locus leads to widespread expansion of Toxoplasma gondii lineages that are virulent in mice. PLoS Genet. 5, e1000404. doi: 10.1371/journal.pgen.1000404
Kreuder C., Miller M. A., Jessup D. A., Lowenstine L. J., Harris M. D., Ames J. A., et al. (2003). Patterns of mortality in southern sea otters (Enhydra lutris nereis) from 1998-2001. J. Wildl. Dis. 39, 495–509. doi: 10.7589/0090-3558-39.3.495
Krusor C., Smith W. A., Tinker M. T., Silver M., Conrad P. A., Shapiro K. (2015). Concentration and retention of Toxoplasma gondii oocysts by marine snails demonstrate a novel mechanism for transmission of terrestrial zoonotic pathogens in coastal ecosystems. Environ. Microbiol. 17, 4527–4537. doi: 10.1111/1462-2920.12927
Lorenzi H., Khan A., Behnke M. S., Namasivayam S., Swapna L. S., Hadjithomas M., et al. (2016). Local admixture of amplified and diversified secreted pathogenesis determinants shapes mosaic Toxoplasma gondii genomes. Nat. Commun. 7, 10147. doi: 10.1038/ncomms10147
Melo M. B., Nguyen Q. P., Cordeiro C., Hassan M. A., Yang N., McKell R., et al. (2013). Transcriptional analysis of murine macrophages infected with different Toxoplasma strains identifies novel regulation of host signaling pathways. PLoS Pathog. 9, e1003779. doi: 10.1371/journal.ppat.1003779
Miller M. A., Conrad P. A., Harris M., Hatfield B., Langlois G., Jessup D. A., et al. (2010). A protozoal-associated epizootic impacting marine wildlife: Mass-mortality of southern sea otters (Enhydra lutris nereis) due to Sarcocystis neurona infection. Vet. Parasitol. 172, 183–194. doi: 10.1016/j.vetpar.2010.05.019
Miller M., Conrad P., James E. R., Packham A., Toy-Choutka S., Murray M. J., et al. (2008a). Transplacental toxoplasmosis in a wild southern sea otter (Enhydra lutris nereis). Vet. Parasitol. 153, 12–18. doi: 10.1016/j.vetpar.2008.01.015
Miller M. A., Crosbie P. R., Sverlow K., Hanni K., Barr B. C., Kock N., et al. (2001b). Isolation and characterization of Sarcocystis from brain tissue of a free-living southern sea otter (Enhydra lutris nereis) with fatal meningoencephalitis. Parasitol. Res. 87, 252–257. doi: 10.1007/s004360000340
Miller M. A., Gardner I. A., Kreuder C., Paradies D. M., Worcester K. R., Jessup D. A., et al. (2002a). Coastal freshwater runoff is a risk factor for Toxoplasma gondii infection of southern sea otters (Enhydra lutris nereis). Int. J. Parasitol. 32, 997–1006. doi: 10.1016/s0020-7519(02)00069-3
Miller M. A., Gardner I. A., Packham A. E., Mazet J. K., Hanni K. D., Jessup D. A., et al. (2002b). Evaluation of an indirect fluorescent antibody test (IFAT) for demonstration of antibodies to Toxoplasma gondii in the sea otter (Enhydra lutris). J. Parasitol. 88, 594–599. doi: 10.2307/3285456
Miller M. A., Grigg M. E., Kreuder C., James E. R., Melli A. C., Crosbie P. R., et al. (2004). An unusual genotype of Toxoplasma gondii is common in California sea otters (Enhydra lutris nereis) and is a cause of mortality. Int. J. Parasitol. 34, 275–284. doi: 10.1016/j.ijpara.2003.12.008
Miller M. A., Miller W. A., Conrad P. A., James E. R., Melli A. C., Leutenegger C. M., et al. (2008b). Type X Toxoplasma gondii in a wild mussel and terrestrial carnivores from coastal California: new linkages between terrestrial mammals, runoff, and toxoplasmosis of sea otters. Int. J. Parasitol. 38, 1319–1328. doi: 10.1016/j.ijpara.2008.02.005
Miller M. A., Moriarty M. E., Duignan P. J., Zabka T. S., Dodd E., Batac F. I., et al. (2021). Clinical signs and pathology associated with domoic acid toxicosis in southern sea otters (Enhydra lutris nereis). Front. Mar. Sci. 8, 585501. doi: 10.3389/fmars.2021.585501
Miller M. A., Moriarty M. E., Henkel L., Tinker M. T., Burgess T. L., Batac F. I., et al. (2020). Predators, disease, and environmental change in the nearshore ecosystem: Mortality in southern sea otters (Enhydra lutris nereis) from 1998–2012. Front. Mar. Sci. 7, 582. doi: 10.3389/fmars.2020.00582
Miller M. A., Raverty S., Shapiro K. (2018). “Protozoan parasites of marine mammals,” in CRC Handbook of marine mammal medicine: Health, disease, and rehabilitation, 3rd Edn. Eds. Dierauf L., Gulland F. M., Whitman K. I. (Boca Raton, FL: CRC Press), 425–470.
Miller M. A., Sverlow K., Crosbie P. R., Barr B. C., Lowenstine L. J., Gulland F. M., et al. (2001a). Isolation and characterization of two parasitic protozoa from a Pacific harbor seal (Phoca vitulina richardsi) with meningoencephalomyelitis. J. Parasitol. 87, 816–822. doi: 10.1645/0022-3395(2001)087[0816:IACOTP]2.0.CO;2
Minot S., Melo M. B., Li F., Lu D., Niedelman W., Levine S. S., et al. (2012). Admixture and recombination among Toxoplasma gondii lineages explain global genome diversity. Proc. Natl. Acad. Sci. USA. 109, 13458–13463. doi: 10.1073/pnas.1117047109
Nash G., Kerschmann R. L., Herndier B., Dubey J. P. (1994). The pathological manifestations of pulmonary toxoplasmosis in the acquired immunodeficiency syndrome. Hum. Pathol. 25, 652–658. doi: 10.1016/0046-8177(94)90297-6
Nolan S. J., Romano J. D., Coppens I. (2017). Host lipid droplets: An important source of lipids salvaged by the intracellular parasite Toxoplasma gondii. PLoS Pathog. 13, e1006362. doi: 10.1371/journal.ppat.1006362
Rejmanek D., Miller M. A., Grigg M. E., Crosbie P. R., Conrad P. A. (2010). Molecular characterization of Sarcocystis neurona strains from opossums (Didelphis virginiana) and intermediate hosts from Central California. Vet. Parasitol. 170, 20–29. doi: 10.1016/j.vetpar.2009.12.045
Shapiro K., Miller M. A., Mazet J. K. (2012). Temporal association between land-based runoff events and California sea otter (Enhydra lutris nereis) protozoal mortalities. J. Wildl. Dis. 48, 394–404. doi: 10.7589/0090-3558-48.2.394
Shapiro K., VanWormer E., Aguilar B., Conrad P. A. (2015). Surveillance for Toxoplasma gondii in California mussels (Mytilus californianus) reveals transmission of atypical genotypes from land to sea. Environ. Microbiol. 17, 4177–4188. doi: 10.1111/1462-2920.12685
Shapiro K., Miller M. A., Packham A. E., Aguilar B., Conrad P. A., Vanwormer E., et al. (2016). Dual congenital transmission of Toxoplasma gondii and Sarcocystis neurona in a late-term aborted pup from a chronically infected southern sea otter (Enhydra lutris nereis). Parasitology 143, 276–288. doi: 10.1017/s0031182015001377
Shapiro K., VanWormer E., Packham A., Dodd E., Conrad P. A., Miller M. (2019). Type X strains of Toxoplasma gondii are virulent for southern sea otters (Enhydra lutris nereis) and present in felids from nearby watersheds. Proc. R. Soc. B. 286, 20191334. doi: 10.1098/rspb.2019.1334
Su C., Shwab E. K., Zhou P., Zhu X. Q., Dubey J. P. (2010). Moving towards an integrated approach to molecular detection and identification of Toxoplasma gondii. Parasitology 137, 1–11. doi: 10.1017/S0031182009991065
Sundar N., Cole R. A., Thomas N. J., Majumdar D., Dubey J. P., Su C. (2008). Genetic diversity among sea otter isolates of Toxoplasma gondii. Vet. Parasitol. 151, 125–132. doi: 10.1016/j.vetpar.2007.11.012
Swierzy I. J., Muhammad M., Kroll J., Abelmann A., Tenter A. M., Lüder C. G. K. (2014). Toxoplasma gondii within skeletal muscle cells: A critical interplay for food-borne parasite transmission. Int. J. Parasitol. 44, 91–98. doi: 10.1016/j.ijpara.2013.10.001
Teles R. M., Graeber T. G., Krutzik S. R., Montoya D., Schenk M., Lee D. J., et al. (2013). Type I interferon suppresses Type II interferon–triggered human anti-mycobacterial responses. Science 339, 1448–1453. doi: 10.1126/science.1233665
Tenter A. M., Heckeroth A. R., Weiss L. M. (2000). Toxoplasma gondii: from animals to humans. Int. J. Parasitol. 30, 1217–1258. doi: 10.1016/S0020-7519(00)00124-7
Tinker M. T., Tomoleoni J., LaRoche N., Bowen L., Miles A. K., Murray M., et al. (2017). Southern sea otter range expansion and habitat use in the Santa Barbara Channel, California: U.S. Geological Survey Open-File Report 2017–1001 (OCS Study BOEM 2017-002), 1–76. doi: 10.3133/ofr20171001
VanWormer E., Conrad P. A., Miller M. A., Melli A. C., Carpenter T. E., Mazet J. A. (2013). Toxoplasma gondii, source to sea: Higher contribution of domestic felids to terrestrial parasite loading despite lower infection prevalence. EcoHealth 10, 277–289. doi: 10.1007/s10393-013-0859-x
VanWormer E., Miller M. A., Conrad P. A., Grigg M. E., Rejmanek D., Carpenter T. E., et al. (2014). Using molecular epidemiology to track Toxoplasma gondii from terrestrial carnivores to marine hosts: Implications for public health and conservation. "PLoS Negl. Trop. Dis. 8, e2852. doi: 10.1371/journal.pntd.0002852
Keywords: Enhydra lutris nereis, genotype, pathology, steatitis, southern sea otter, Toxoplasma gondii, toxoplasmosis, virulence
Citation: Miller MA, Newberry CA, Sinnott DM, Batac FI, Greenwald K, Reed A, Young C, Harris MD, Packham AE and Shapiro K (2023) Newly detected, virulent Toxoplasma gondii COUG strain causing fatal steatitis and toxoplasmosis in southern sea otters (Enhydra lutris nereis). Front. Mar. Sci. 10:1116899. doi: 10.3389/fmars.2023.1116899
Received: 05 December 2022; Accepted: 01 February 2023;
Published: 22 March 2023.
Edited by:
Stephanie Norman, Marine-Med: Marine Research, Epidemiology and Veterinary Medicine, United StatesReviewed by:
Marie-Laure Dardé, University of Limoges, FranceIoannis A Giantsis, University of Western Macedonia, Greece
Copyright © 2023 Miller, Newberry, Sinnott, Batac, Greenwald, Reed, Young, Harris, Packham and Shapiro. This is an open-access article distributed under the terms of the Creative Commons Attribution License (CC BY). The use, distribution or reproduction in other forums is permitted, provided the original author(s) and the copyright owner(s) are credited and that the original publication in this journal is cited, in accordance with accepted academic practice. No use, distribution or reproduction is permitted which does not comply with these terms.
*Correspondence: Melissa Ann Miller, bWVsaXNzYS5taWxsZXJAd2lsZGxpZmUuY2EuZ292
†These authors have contributed equally to this work and share first authorship