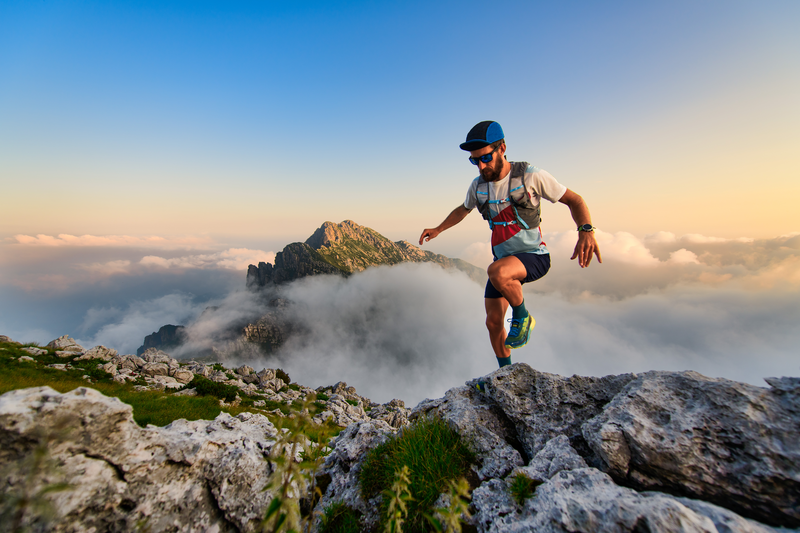
94% of researchers rate our articles as excellent or good
Learn more about the work of our research integrity team to safeguard the quality of each article we publish.
Find out more
ORIGINAL RESEARCH article
Front. Mar. Sci. , 20 February 2023
Sec. Coastal Ocean Processes
Volume 10 - 2023 | https://doi.org/10.3389/fmars.2023.1114337
This article is part of the Research Topic Processes and Management of Altered Estuaries and Deltas in the Anthropocene View all 11 articles
Benthic foraminifera (BF) are utilized in palaeo-environmental reconstruction based on our understanding of how living individuals respond to environmental variations. However, there is still a lack of empirical insight into how non-environmental factors, such as taphonomic processes, influence the preservations of fossil BF in strata. In this study, we compare the spatial distribution and composition of alive and dead BF fauna in surface sediments to elucidate how well fossil foraminiferal fauna mirror quasi-contemporary alive BF groups indicative of different water masses off a mega-river (Changjiang) estuary, which is characterized by intense and complex river-sea interactions. On-site measurements of bottom water salinity, temperature, and dissolved oxygen were conducted in the summer to determine water mass properties. A same-site comparison of alive (Rose Bengal stained) and dead foraminiferal fauna in surface sediment samples over 73 stations was then carried out. Q-mode Hierarchical clustering analysis was used to differentiate foraminiferal assemblages based on the relative abundance of common species. Three distinct regions with different water-mass properties were identified. The distribution pattern of dead foraminiferal fauna is mainly inherited from alive fauna, while the density and diversity of the dead fauna were found to be higher than those of the alive one. Both alive and dead fauna were clustered into four assemblages. A few common alive species (small-agglutinated and thin-calcareous) were rarely found in dead fauna, and a few common dead species (preferring low temperature and indicating allochthonous sources) were rarely present in alive fauna. The alive foraminiferal abundance and diversity were mainly determined by food resources and environmental properties of salinity and temperature. Alive foraminiferal assemblages were separated by different water masses determined by river-sea interactions off the Changjiang Estuary. The “time-averaging” effect was found to be responsible for the higher density and diversity of the dead fauna. Disintegration of agglutinated tests, dissolution of calcareous tests and selective transportation were observed to contribute to the different species compositions between the alive and dead fauna. Nevertheless, indicative species-environment relations in alive and taphocoenose fauna were found to be almost homologous among most common species. This suggests that distinct benthic foraminiferal assemblages can be used to effectively differentiate between different water masses in the study coastal seas.
Benthic foraminifera (BF) are single-celled organisms that are widely distributed in marginal seas and are often used as a reliable proxy to indicate palaeoceanographic changes due to their high sensitivity to environmental conditions (Schönfeld and Zahn, 2000; Evans et al., 2002; Abrantes et al., 2005; Murray, 2006; Dai et al., 2018; Zhao et al., 2018; Ren et al., 2019; Jiang et al., 2021). This time-tested methodology is also highly dependent on baseline data regarding the response and sensitivity of alive BF to various biological, chemical and physical conditions (Wang et al., 1988; Murray, 2006). To understand how taphocoenose BF (including alive and dead BF) are buried and fossilized, hence, a comparative study between alive and dead benthic foraminiferal fauna in surface sediments is needed to explore the effects of taphonomic processes on the composition and preservation of BF, and whether the fossil BF accurately mirror the quasi-contemporary living faunal groups. Such research has been a focus of interest for paleontologists worldwide for the past few decades (Jorissen and Wittling, 1999; Horton and Murray, 2006; Bouchet et al., 2012; Wang et al., 2016; Stefanoudis et al., 2017; Ye et al., 2021).
Previous studies have demonstrated that water-mass properties, such as bottom-water salinity (BWS), temperature (BWT), dissolved oxygen (DO), food availability, and sediment composition, are essential in determining the living benthic foraminiferal composition (Gupta, 1999; Murray, 2006; Lei et al., 2017; Jernas et al., 2018). Furthermore, taphonomic processes, such as the destruction, dissolution, and transportation of tests have been indicated to alter the living and dead faunas (Gooday and Hughes, 2002; Murray, 2006; Berkeley et al., 2007; Glover et al., 2010; Duros et al., 2012). Thus, further research into these taphonomic processes is essential to accurately interpret BF data and associated paleoenvironmental variations.
Comparative studies between living and dead BF in dynamic estuarine and shelf environments are remarkably rare (Mendes et al., 2013; Goineau et al., 2015). The mega-Changjiang Estuary and broad shelf of the eastern China seas are characterized by complex interactions of different water masses with distinct physical and chemical properties (Su, 1998; Naimie et al., 2001; Yang et al., 2018; Liu et al., 2021), hence, serving as an ideal platform to conduct such comparative study. In the East China Sea (ECS), the quantitative relationship between contemporary benthic foraminiferal distributions in surface sediments and the associated controlling environmental factors have been well discussed, significantly improving our understanding of common BF inhabiting the estuary and shelf (Wang et al., 1988; Zheng, 1988; Zhao et al., 2009; Lei and Li, 2016; Xu et al., 2017; Wang et al., 2018; Guo et al., 2019; Zhang et al., 2020). However, the differences between in-situ living and dead BF have so far been little studied. Thus, large data gap exists in the impacts of taphonomic processes on changing the compositions from living to dead foraminiferal fauna.
This study focuses on the Changjiang Estuary and its adjacent coastal sea, which are characterized by notable environmental gradients in terms of oceanographic, biological, and sediment compositions. Except for on-site observations of water-mass properties, analyses of living and dead benthic foraminiferal fauna in surface sediments were conducted in detail to assess their structures (abundance, diversity and species compositions). Multivariate statistical methods were employed to cluster stations into different assemblage types based on species compositions. Comparisons between water-mass and maps of faunal structures were designed to quantify the role of water-mass properties in determining faunal structures. The differences between living and dead faunal structures were used to assess effects of taphonomic processes. Furthermore, relationships among the common species and multiple environmental parameters were established by a linear method of redundance analysis in living and taphocoenose fauna to validate reliability of the marine environmental index.
The study coastal sea is located off the Changjiang Estuary, including the northwestern part of the ECS and the southwestern part of the Yellow Sea (Figure 1A). It is characterized by intense interactions of the Changjiang Diluted Water (CDW), coastal currents and the intrusions of the Kuroshio Current (KC) and its branches, which are strongly affected by seasonal East Asian Monsoon activities. In summer, the southeasterly monsoon wind stress enhances the eastern and northeastern expansion of the CDW, separating the southerly YSCC and the northerly ZFCC (Su, 1998; Naimie et al., 2001; Guan and Fang, 2006). In addition, the warm and salty branched-flows driven by the KC move northwards throughout the year, intruding into the ECS inner shelf at the bottom along the 50 m isobath (Yang et al., 2018). Water masses from diverse sources possess distinct physical and chemical properties, creating a high environmental heterogeneity in the study area.
Figure 1 Schematic diagram of oceanic circulation patterns in summer in the eastern China seas (after Lee et al., 2002; Su and Yuan, 2005; Guan and Fang, 2006; Yang et al., 2012), and (B) distribution of 73 sampling stations off the Changjiang Estuary. Acronyms in (A) and their full expressions are KC, Kuroshio Current; NKBC, nearshore Kuroshio branch current; TSWC, Tsushima warm current; TWC, Taiwan warm current; YSCC, Yellow Sea costal current; ZFCC, Zhejiang-Fujian costal current; KCC, Korean coastal current; and CDW, Changjiang diluted water; those in (B) are NB, the North Branch; SB, the South Branch; NC, the North Channel; and SC, the South Channel of the Changjiang Estuary.
The complex hydrodynamical conditions and sediment sources result in the patchy distribution of fine-grained mud, coarse-grained sand, and mixed deposits off the Changjiang Estuary. Generally, muddy and sandy depositions prevail respectively in the inner and outer belts off the Changjiang Estuary, while the narrow patch of sand and mud mixture lies in between (Qiao et al., 2017). The sedimentation rates deduced from 210Pb data generally decrease both eastward and southward from the Changjiang subaqueous delta where a maximum sedimentation rate of >4.0 cm/yr occurs (Gao et al., 2015; Jia et al., 2018).
A cruise survey was conducted in and off the Changjiang Estuary (Figure 1B) from 6 to 22 July in 2014 on board R/V “Runjiang-1”. Water depth, BWS and BWT were recorded using a CTD (conductivity, temperature and depth; Model: SBE-25, USA). DO in bottom water samples was measured using the Winkler titration method (Dickson, 1994). Seventy-three sediment samples were collected from the water depths of 7.5 m to 67.0 m (Figure 1B using a stainless-steel sediment grab sampler, and the top layers (0-2 cm thick) were taken for grain-size measurements and living and dead benthic foraminiferal analyses.
Grain-size analysis were conducted on all 73 surface sediment samples. All samples were pretreated with 30% hydrogen peroxide to remove organic matter and then with 10% diluted hydrochloric acid to remove carbonates, followed by repeated washing with de-ionized water and then dispersed in an ultrasonic vibrator for several minutes. The particle-size distribution was measured with a laser-diffraction Beckman Coulter LS230, ranging from 0.375 to 2,000 μm. The calculation of the particle sizes relies on the theory of Folk and Ward (1957).
All 73 surface sediment samples for foraminiferal analysis were soaked and thoroughly mixed with a methanol and Rose Bengal solution (1.0 g/L) for the purpose of tinting living individuals to distinguish them from dead ones immediately after collection on board. These samples were dried and weighed, and then were wet-sieved through a 63 μm-sieve in the lab. The coarse fraction (>63 μm) was dried and split, and the split sample was examined completely for foraminiferal compositions. Benthic species were identified and quantified under a stereomicroscope with continuous zooming up to a maximum amplification of 40×. Living specimens were identified by the presence of Rose Bengal-stained protoplasm in their tests, including those that were entirely stained or those with the last one or two chambers been unstained (De Stigter et al., 1998).Taxonomic identification mainly followed the protocol outlined by Zheng (1988); Wang et al. (1988), and Lei and Li (2016).
Faunal abundance (N, individuals ind./g dry weight of sediment) and foraminiferal species richness (H′, Shannon-Wiener diversity index based on calculation of equation (1), Shannon, 1984) were calculated for living and dead foraminiferal communities on raw data. Benthic species with relative abundances of >5% in at least three samples were regarded as common species in the study coastal sea (Wang et al., 1988).
Additionally, we quantitatively interpreted the living and dead foraminiferal ecology by utilizing multivariate statistical methods based on ordination and classification methods such as Q-mode Hierarchical clustering analysis (HCA) and redundancy analysis (RDA). HCA was used to classify the distribution of foraminiferal groups and subgroups into homogeneous assemblage zones. RDA was applied to quantify the relationships between faunal structures and environmental variables.
The distribution patterns of BWS and BWT featured the elongated bands roughly parallel to the coast (Figures 2A, B). The BWS increased seaward from 13.4 to 34.6, while the BWT decreased eastward from 25.2 °C to18.6 °C. The northern coastal water body off the North Branch (NB) and Jiangsu coast had much higher DO than the coastal water body off the Hangzhou Bay and Zhejiang coast. The latter enclosed a subzone with DO values lower than 4.0 mg/L (Figure 2C). Sandy sediments (>63 μm) occupied the northeastern region known as the Yangtze Shoal and the rest area was covered by silty clay or silt (Figure 2D).
Figure 2 Distribution patterns of bottom-water oceanographic indexes including (A) salinity (‰), (B) temperature (°C), and (C) DO (mg/L), and (D) mean grain size (μm) of surface sediments. Detailed data are included in Supporting Information Table S1.
The living abundance varied from 0 to 1,845 ind./g (Supporting Information Table S2-Living BF2), with an average of 76 ind./g. The peak abundance (>100 ind./g) occurred at the inner belt off the South Channel (SC) and the Hangzhou Bay (blue to red in Figure 3A). The intermediate abundance (20-100 ind./g) occurred at south of the peak abundance zone, the inner belt off Zhejiang coast (dark purple to blue in Figure 3A). A narrow belt with an abundance between 10 and 20 ind./g extended north-south at the outer band off the Hangzhou Bay and Zhejiang coast (dark purple in Figure 3A). More than a half of all stations had living abundance of less than 10 ind./g, typically located off the NB and Jiangsu coast (light purple in Figure 3A).
The dead abundance ranged between 0 ind./g and 11,586 ind./g, with a mean of 844 ind./g (Supporting Information Table S2-Dead BF2). The peak abundance (>1000 ind./g) occurred at stations off Zhejiang coast (blue to red in Figure 3B). The intermediate abundance (100-1000 ind./g) occurred in the inner and outer regions off the SC and the Hangzhou Bay (dark purple to green in Figure 3B). The less abundance (<100 ind./g) occurred at coastal zone off the Jiangsu coast and the NB, together with a narrow N-S belt between the inner peak and the outer intermediate abundance belts off the Hangzhou Bay (light purple in Figure 3B).
Shannon-Wiener diversity index (H′) for living fauna ranged from 0.5 to 2.9 (Supporting Information Table S2-Living BF2). The southernmost region had the lowest faunal diversity. The inner bands off the Changjiang Estuary and the Hangzhou Bay had a lower diversity (H′<1.5), while their outer counterparts had a relatively higher diversity (H′>1.5) (Figure 3C).
The H′ values of dead fauna had a relatively higher and wider range (0-3.4) (Supporting Information Table S2-Dead BF2) than the living one. Additionally, there were some differences in their spatial patterns (Figures 3C, D). The inner region off the Changjiang Estuary and the region off Zhejiang coast exhibited a relatively lower diversity (H′<2.0, blue in Figure 3D). The remanent area was characterized by two higher H′ (>2.5) bands, which were interspersed with a lower H′ belt (2.0-2.5) (Figure 3D).
Living species were identified among 15,158 counts of stained individuals (Supporting Information Table S2-Living BF1). Twenty-three species with an abundance over 5% from at least 3 stations were identified, accounting for 87% of living fauna (Figure 4A). The dominant species include Verneuilinulla advena (V. advena), Bolivina striatula (B. striatula) and Nonionella jacksonensis (N. jacksonensis), which were found in over 50 stations.
The resultant dendrograms of Q-mode HCA based on the 23 common species demonstrate four clusters: FI-a, FI-b, FII and FIII (Figure 4A). Twenty-three stations are organized into Cluster FI-a in terms of the dominance of N. jacksonensis (37.2% on average), Cribrononion subincertum (C. subincertum, 12.1%) and Verneuilinulla advena (V. advena, 6.6%), and they generally congregate at the inner band off Jiangsu coast and the Changjiang Estuary (Figure 5A). Cluster FI-b includes 10 stations off the SC and the Hangzhou Bay (Figure 5A), with Florilus atlanticus (F. atlanticus, 17.5%), Polskiammina asiatica (P. asiatica, 15.5%), Ammonia pauciloculata (A. pauciloculata, 7.2%), and N. jacksonensis (5.2%) being the dominate species (Figure 4A). Cluster FII consists of 16 stations dominated by Florilus decorus (F. decorus, 24.7%), A. convexidorsa (13.1%), B. striatula (12.0%), V. advena (11.0%), Ammonia tepida (A. tepida, 10.0%), and N. jacksonensis (9.9%) (Figure 4A), which are mainly located at the outer region off Jiangsu coast and the Changjiang Estuary (Figure 5A). Cluster FIII includes 17 stations, with Ammonia compressiuscula (A. compressiuscula, 27.5%), F. atlanticus (7.2%), Cancris auriculus (C. auriculus, 6.6%), B. marginata (4.7%) and B. robusta (3.4%) being the dominate species (Figure 4A), and they are mainly located at the outer region off the SC and the Hangzhou Bay (Figure 5A).
The species were identified among 16,075 counts of dead individuals (Supporting Information Table S2, Dead BF1). Twenty-eight species with an abundance over 5% from at least 3 stations were identified, accounting for 80% of dead fauna. (Figure 4B). The dominant species that frequently appeared at over 50 stations are Bolivina robusta (B. robusta), Bulimina marginata (B. marginata), A. tepida, A. compressiuscula, Elphidium advenum (E. advenum) and F. atlanticus.
The resultant dendrograms of Q-mode HCA based on 28 common species distinguish four clusters: FI′-a, FI′-b, FII′ and FIII′ (Figure 4B). Thirty-seven stations are regarded as Cluster FI′-a in terms of the dominance of Epistominella naraensis (E. naraensis, 10.9%), B. robusta (7.7%), B. marginata (7.6%), and F. decorus (5.8%) (Figure 4B), and they are majorly distributed in the northern half of study coastal sea (Figure 5B). Thirteen stations of Cluster FI′-b, with F. atlanticus (14.6%), N. jacksonensis (11.8%), and A. tepida (8.2%) being the dominate species (Figure 4B), and they are majorly distributed in the inner belts off the SC, the Hangzhou Bay and Zhejiang coast (Figure 5B). Seven stations are classified as Cluster FII′ based on high abundance of Ammonia ketienziensis (A. ketienziensis, 19.4%), B. robusta (11.3%), E. advenum (7.8%), A. compressiuscula (7.0%) and Q. seminulangulata (5.9%) (Figure 4B), which are scattered in the outer belt off Jiangsu coast and the NB (Figure 5B). Another 16 stations are grouped into Cluster FIII′ showing high abundance of A. compressiuscula (23.4%), Quinqueloculina lamarckiana (Q. lamarckiana, 12.5%), B. marginata (7.1%), A. tepida (6.4%), and B. robusta (6.4%) (Figure 4B), and they are mainly present off the southern Hangzhou Bay and the outer belt off Zhejiang coast (Figure 5B).
The dominant hydrodynamic processes in relatively shallow eastern China seas are strongly affected by massive freshwater outflows, tides, monsoon-related waves and currents, and the KC and its branches (Su, 1998; Naimie et al., 2001). Interactions among the above-mentioned factors result in different circulation regimes and water masses that are generally diagnosed by their varied physical properties, especially temperature and salinity (Park and Chu, 2006; Chen, 2009).
In summer, the inner belt of the study coastal sea is generally occupied by the water mass WI of low BWS (15.0-30.0) and high BWT (21.0–25.0°C) (Figures 2A, B, 6), resulting from a mixture of the Changjiang diluted water and alongshore currents (Chen, 2009). For example, the water mass to the south of the Changjiang Estuary is significantly impacted by the southward fresher CDW and the northward ZFCC (Figure 6; Lee et al., 2002; Su and Yuan, 2005; Liu et al., 2021). The NE offshore area (the outer region off Jiangsu coast and the Changjiang Estuary) is prevailed by the water mass WII with high BWS (30.0-32.5) and low BWT (19.0-21.0°C) (Figures 2A, B, 6), receiving interactive effects of the northeastward CDW, the southward YSCC and the northward TWC (Figure 6; Lee et al., 2002; Su and Yuan, 2005; Liu et al., 2021). The SE offshore area (the outer region off the Hangzhou Bay and Zhejiang coast) is occupied by the water mass WIII with high BWS (32.5-34.6), low BWT (ca. 19.0 °C) and relative lower DO (<4.0 mg/L) (Figures 2A–C, 6), resulting from strong intrusion of salty Kuroshio branch currents (Yang et al., 2018). Meanwhile the relative lower DO values in the WIII waters (Figure 2C) could also be driven by water column stratification and organic matter decomposition near the bottom in summer (Li et al., 2002; Zhu et al., 2017; Wang et al., 2021; Zhang et al., 2021).
Living individuals are short-lived and highly sensitive to ecological conditions during their growing stage. Marine ecological system is complex and multivariate due to dynamic interactions between environmental factors (salinity, temperature, DO, substrate and food supply) and living organisms (Wang et al., 1988; Murray, 2006).
The considerably high abundance (>100 ind./g) was observed at the inner region off the SC and the Hangzhou Bay (Figure 3A). This region has a low salinity and high temperature (Figures 2A, B), whereas abundant nutrient inputs from the densely-populated river plume and coastal upwelling cause frequent algal bloom (Tseng et al., 2014; Hu and Wang, 2016; Wang et al., 2019). In the marine ecosystems, phytoplankton serves as the main food source for BF (Murray, 2006). Being as the most important and leading environment parameters, food quality and quantity can shape the foraminiferal abundance (Gustafsson and Nordberg, 1999; Contreras-Rosales et al., 2010; Mojtahid et al., 2016; Wang et al., 2016).
Figure 3 Distribution of living and dead benthic foraminiferal abundance (A, B), and distribution of Shannon-Wiener diversity (H′) of living and dead benthic foraminiferal fauna (C, D). Detailed data are included in Supporting Information Table S2.
The occurrence of lower abundance (0-20 ind./g) at the inner region off Jiangsu coast and the NB (Figure 3A) was mainly ascribed to lower salinity (Figure 2A) (Lei et al., 2017). Moreover, the inner region is characterized by high suspended sediment concentrations, where low transparency of the water mass limits the growth of primary producers consequently lowering the abundance (Li et al., 2020). To the east of 123°E, the lower abundance (Figure 3A) was constrained by nutrient deficits from the river plumes (Wang et al., 2019).
The occurrence of high diversity (H′>1.5) in the outer region of the studied coastal sea was generally coincident with high BWS (30.0-34.6), low BWT (18.6-21.0 °C), and lower DO (2.3-5.0 mg/L) (Figures 2, 3C). Previous studies indicated that the diversity is positively correlated to salinity (Lei et al., 2017) and the normal salinity (33.0) in the ECS provokes foraminiferal diversity (Wang et al., 1988). In addition, a temperature ranging between 18.0 and 24.0°C could promote the growth of various foraminiferal species, but higher temperature may in turn reduce the species richness (Lei et al., 2017). Moreover, the lower DO in the outer region may also play a side-role in promoting higher foraminiferal diversity (Levin et al., 2009).
Four clusters of living common species in the study coastal sea were determined by Q-mode HCA as FI-a, FI-b, FII and FIII (Figure 4A), and their spatial partitions were closely linked to the distribution of three water masses in this area (Figures 5A, 6).
Figure 4 Relative abundance (%) of common species of living (A) and dead (B) benthic foraminiferal fauna. Species shown in black are common for both living and dead fauna, and those in green or orange are common only for dead or living fauna. The dendrograms of Q-mode HCA are plotted in the left to show four clusters of common species: FI-a (FI′-a), FI-b (FI′-b), FII (FII′) and FIII (FIII′) for living and dead fauna in different station groups (see Figure 1B for their locations). Detailed data are included in Supporting Information Table S2-Living BF3 and Dead BF3.
Figure 5 Partition maps of living (A) and dead (B) benthic foraminiferal assemblages. Blue squares, cyan stars, purple deltas, and green asterisks denote Clusters FI-a, FI-b, FII, and FIII in (A), and FI′-a, FI′-b, FII′, and FIII′ in (B). See Figure 4 for the information of different clusters and their associated stations.
Figure 6 General current circulations (Su and Yuan, 2005; Guan and Fang, 2006; Yang et al., 2018) and the distribution of water masses in coastal sea off the Changjiang Estuary in summer. WI: low BWS and high BWT and DO water mass, WII: high BWS and DO and low BWT water mass, and WIII: high BWS and low BWT and DO water mass.
Cluster FI-a (dominated by N. jacksonensis, C. subincertum, and V. advena) and Cluster FI-b (dominated by F. atlanticus, P. asiatica, A. pauciloculata and N. jacksonensis) (Figure 4A) enriched with low salinity species were prevalent in the inner belt of the study coastal sea, highly coincident with the distribution of the water mass WI (Figures 5A, 6). Previous studies also indicated that these species are specifically keen to the estuarine water mass with lower salinity and higher temperature and DO (Wang et al., 1988; Lei and Li, 2016). Moreover, the F. atlanticus and A. pauciloculata were recognized as species being particularly influenced by the ZFCC (Wang et al., 1988). Thus, the Clusters FI-a and FI-b represent assemblages that are unique to the inner estuarine and coastal sea, which are strongly influenced by the CDW and the ZFCC.
Cluster FII consists of species with a large range of salinity tolerance, from A. convexidorsa, a low salinity species to B. striatula, a high salinity species (Figure 4A), prevailed in the outer region off Jiangsu coast and the Changjiang Estuary. The region was coincident with the distribution of the water mass WII (Figures 5A, 6), where high salinity gradient is produced by the mixture of the YSCC (higher salinity and lower temperature) and the CDW (lower salinity and higher temperature) (Figure 2A, B) (Chen, 2009).
Cluster FIII consisting of species A. compressiuscula, C. auriculus, B. marginata, and B. robusta (Figure 4A), was prevalent in the outer region off the Hangzhou Bay and Zhejiang coast (Figure 5A), where the intrusion of the TWC and the NKBC produces the water mass WIII featuring higher salinity, lower temperature and DO (Figures 2A–C, 6). The species A. compressiuscula and C. auriculus have been recognized to thrive well in high salinity waters, while the species A. compressiuscula, B. marginata and B. robusta could adapt well to a lower DO condition (Wang et al., 1988; Gooday and Jorissed, 2012). Thus, spatial partitions of living assemblages are clearly determined by the distribution of water masses with their distinct oceanographic and ecological properties.
Taphonomic processes change the abundance, diversity and species composition in surface sediments during transition from living to dead faunas in response to sediment accumulation, destruction and transportation (Murray, 2006; Glover et al., 2010).
In general, the abundance and diversity of dead BF are rather higher than those of the living ones. For instance, the dead abundance reached >1000 ind./g in the inner region off the SC and the Hangzhou Bay, but the living abundance was only ca. 100 ind./g (Figures 3A, B). Meanwhile, distribution patterns of the two faunal abundance and diversity are relatively comparable (Figure 3), denoting their close affiliation. This affiliation has been reported to be common in the Bohai Sea, the northern Yellow Sea and the South China Sea (Li et al., 2014, Li et al., 2021).
The 2-cm thick surface sediment analyzed in this study represents a depositional period from 0.5 to 4.0 years according to the 210Pb derived sedimentation rates of 0.5-4.0 cm/yr in the study area (Gao et al., 2015; Jia et al., 2018). In contrast, living fauna has much shorter life spans than the depositional period. Thus, it indicates that dead fauna is a burial accumulation of multiple living faunas over a period of time. Such result offers an interpretation for the dissimilarities between living and dead faunas in the surface sediment samples, known as “time-averaging” effect (Murray, 2006; Glover et al., 2010; Kidwell and Tomasovych, 2013). The sedimentation rates in the inner region off Zhejiang coast are much lower (0.5-1.0 cm/yr) than the others (Gao et al., 2015; Jia et al., 2018), hence, a longer time-averaging effect results in a higher dead BF abundance (Figure 3B). In contrast, the lower diversity (a 2.0-2.5 H′ belt) of dead fauna in the central study area (Figure 3D) was likely due to therein higher sedimentation rates (ca. 2.0-3.0 cm/yr) (Gao et al., 2015; Jia et al., 2018).
In addition to abundance and diversity, the difference of species compositions is quite evident between living and dead fauna in the same sample. Such difference is also obvious in the statistics analytic result of common species over the entire study area. Totally, 23 common species in living fauna are less than 28 in dead fauna (Figure 4). The 7 common living species, including Textularia earlandi (T.earlandi), Bolivina aff. acerosa (B.aff.acerosa), Hopkinsina pacifica (H. pacifica), A. pauciloculata, C. auriculus, Nonionella opima (N. opima) and Protelphidium tuberculatum (P.tuberculatum) were rare or not present in dead fauna (Figure 4A). On the contrary, the 12 common species in dead fauna were rare or not present in living fauna, including Textularia foliacea (T. foliacea), Quinqueloculina akneriana rotunda (Q. akneriana rotunda), Q. lamarckiana, Spiroloculina indica (S. indica), Virgulinella fragilis (V. fragilis), Ammonia confertitesta (A. confertitesta), E. naraensis, Heterolepa subpraecincta (H. subpraecincta), Cribrononion porisuturalis (C. porituralis), Astrononion tasmanensis (A. tasmanensis), E. advenum and Elphidium magellanicum (E. magellanicum) (Figure 4B).
The above-mentioned 7 common species are of small (fine-sand size) agglutinated and thin-shell calcitic tests. Agglutinated tests with small amount of organic cement and loosely cemented walls are susceptible to bacterial or chemical decay of organic matter, to be scarcely fossilized in discernable forms (Murray and Alve, 1999; Kuhnt et al., 2000; Murray, 2006; Lei and Li, 2016; Dessandier et al., 2018). Shells of the calcitic species such as H. pacifica and C. auriculus are very thin and susceptible to destruction and dissolution, leading to a poor preservation (Gooday and Hughes, 2002; Murray, 2006; Duros et al., 2012).
There are a few reasons for why the 12 common species in dead fauna were rare or not present in living fauna. First of all, some species can grow in other seasons besides summer when surface sediments were sampled for this study. For example, T. foliacea and Q. lamarckiana in dead fauna (Figure 4B) are marked by large tests, indicating a low-temperature (even below 4.0 °C) condition, and BF with calcareous tests such as S. indica and E. naraensis prefer a water temperature below 17.5 °C (Lei and Li, 2016). These species probably grow in winter and spring before being buried after death. In addition, external sources of dead fauna are expected to be transported by strong currents although living individuals are less likely to be carried away because their reticulopodial network tends to anchor them firmly in the sediments (Goldstein, 1999; Murray, 2006). For instance, E. advenum and E. magellanicum were reportedly to be a typical coastal species along Jiangsu coast and A. confertitesta a typical supratidal species (Wang et al., 1988). Thus, their common presence in the dead fauna (Figure 4B) demonstrates their allochthonous source via sediment transport.
RDA analysis was employed to explore potential correlations between indicative foraminiferal species and key environmental factors (BWS, BWT, DO and sediment size) for both living (Figure 7A) and taphocoenose (living + dead) fauna (Figure 7B). As discussed above, living fauna can rapidly respond to environmental conditions, but it needs further investigation to reveal whether species-environmental relationships are consistent between living and taphocoenose fauna or valid for palaeo-environmental reconstruction. In RDA ordination diagrams, longer vectors with smaller angles indicate greater correlation between the BF species and environmental parameters.
Figure 7 RDA ordination diagrams of living benthic foraminiferal fauna (A) and taphocoenose benthic foraminiferal fauna (B) with key environmental variables (BWS, BWT, DO and sediment size) measured in the summer of 2014.
There are quite a few common species that show their persistent relationship with certain environmental parameters before and after burial. The relative abundance of A. compressiuscula and H. nipponica are positively correlated with water depth and BWS in both living and taphocoenose fauna (Figure 7). A. compressiuscula is one of the typical species in the middle shelf of the ECS, and H. nipponica tends to live in the middle and outer shelf (Wang et al., 1988; Zheng, 1988; Lei and Li, 2016). C. subincertum, S. bohaiensis and N. jacksonensis build an inverse relationship with salinity, and P. asiatica ties up with temperature (Figure 7). The two species, B. robusta and B. marginata, known as the low oxygen foraminiferal species (Gooday and Jorissed, 2012), show a negative correlation to DO (Figure 7). Abiotic factors such as grain size also impact foraminiferal assemblages (Magno et al., 2012). For instance, A. convexidorsa, A. ketienziensis, V. advena, and F. decorus prefer sandy setting, while F. atlanticus likes clayey seabed (Figure 7). Our results of the RDA explain the same ecological preferences of the same species in the two faunas. This indicates that the environmental signal in living fauna is preserved in the taphocoenose fauna.
There are also some species to show varied relationships with environmental parameters before and after burial (shown in green in Figure 7). Q. seminulangulata (stenohaline) in living fauna is positively associated with salinity (Figure 7A), but this was not revealed in taphocoenose fauna probably due to reworking process after death (Figure 7B) (Wang et al., 1988; Zheng, 1988; Lei and Li, 2016). A. tepida, especially abundant in dead fauna (Figure 4B), shows a little association with other environmental factors except grain size (Figure 7), probably ascribed to its higher capacity of bio-ecological adaptation throughout the year (Debenay et al., 2000), and it prefers living in a sandy setting (Figure 7A), but may be displaced into a muddy setting after death (Figure 7B). The taphocoenose fauna is built up by combined ecological and taphonomic forces over a period of time, hence taphocoenose species provide a time averaged record integrating different seasonal conditions (Glover et al., 2010).
Collectively, our results have demonstrated that living benthic foraminifera (BF) responds sensitively to water-mass properties but dead benthic foraminiferal fauna can be partly reformed by taphonomic forces over a period of time. Food resources (primary productivity) play a key role in affecting living benthic foraminiferal fauna abundance, and the distribution laws of living diversity and salinity are generally consistent. Furthermore, distinct water masses (WI, WII and WIII) with different properties (BWS, BWT and DO) shaped by river-sea interactions explain well the distribution of four living assemblages (FI-a and FI-b, FII, FIII).
The effects of taphonomic processes on dead faunal structures are revealed through comparison between living and dead faunal indices. Because of the “time-averaging” effect, the dead fauna has higher abundance and diversity than the living fauna, in that the former contains a few species that are not present in the sampling time or location. In addition, the dead fauna may lack certain living species due to their poor preservation ability, such as agglutinated-test species and thin-shell calcitic species.
The taphocoenose fauna is the result of both ecological and taphonomic forces within a given temporal framework. Correlations between the most indicative species and environment variables in both living and taphocoenose fauna remain homologous. Thus, taphocoenose BF in core sediments can be effectively used to reconstruct the past marine environments, although care should be taken with a few species that are prone to heavy taphonomic influences.
The original contributions presented in the study are included in the article/Supplementary Material. Further inquiries can be directed to the corresponding author.
FJ: Conceptualization, Methodology, Validation, Formal analysis, Investigation, Writing-Original Draft, Writing-Review & Editing, Visualization. DF: Conceptualization, Resources, Writing-Review & Editing, Supervision, Project administration, Funding acquisition. QZ: Investigation, Writing-Review & Editing. YW: Investigation, Writing-Review & Editing. FR: Investigation, Writing-Review & Editing. AL: Investigation. YL: Writing-Review & Editing. All authors contributed to the article and approved the submitted version.
This research is jointly funded by the Innovation Program of Shanghai Municipal Education Commission (2021-01-07-00-07- E00093), the National Natural Science Foundation of China (NSFC-41976070, 42206052, 41971007), and the Fundamental Research Funds for the Central Universities (No. ZD-21-202101).
The authors declare that the research was conducted in the absence of any commercial or financial relationships that could be construed as a potential conflict of interest.
All claims expressed in this article are solely those of the authors and do not necessarily represent those of their affiliated organizations, or those of the publisher, the editors and the reviewers. Any product that may be evaluated in this article, or claim that may be made by its manufacturer, is not guaranteed or endorsed by the publisher.
The Supplementary Material for this article can be found online at: https://www.frontiersin.org/articles/10.3389/fmars.2023.1114337/full#supplementary-material
Supplementary Table 1 | The Longitude and Latitude of stations, bottom-water oceanographic conditions (water depth, temperature, salinity and dissolve oxygen) and sediment size compositions.
Supplementary Table 2 | Dataset of living and dead benthic foraminifera: sheet of Living BF1 is “Living BF species counted of all stations”, sheet of Living BF2 is “Abundance and Shannon-Wiener diversity index (H′) of living BF”, sheet of Living BF3 is “Relative abundance (%) of common species of living BF”, sheet of Dead BF1 is “Dead BF species counted of all stations”, sheet of Dead BF2 is “Abundance and Shannon-Wiener diversity index (H′) of dead BF”, sheet of Dead BF3 is “Relative abundance (%) of common species of dead BF”.
Abrantes F., Lebreiro S., Rodrigues T., Gil I., Bartels-Jónsdóttir H., Oliveira P., et al. (2005). Shallow-marine sediment cores record climate variability and earthquake activity off Lisbon (Portugal) for the last 2000 years. Quat. Sci. Rev. 24, 2477–2494. doi: 10.1016/j.quascirev.2004.04.009
Berkeley A., Perry C.T., Smithers S.G., Horton B.P., Taylor K.G. (2007). A review of the ecological and taphonomic controls on foraminiferal assemblage development in intertidal environments. Earth-Sci. Rev. 83, 205–230. doi: 10.1016/j.earscirev.2007.04.003
Bouchet V., Alve E., Rygg B., Telford R. (2012). Benthic foraminifera provide a promising tool for ecological quality assessment of marine waters. Ecol. Indic. 23, 66–75. doi: 10.1016/j.ecolind.2012.03.011
Chen C. (2009). Chemical and physical fronts in the bohai, yellow and East China seas. J. Mar. Syst. 78, 394–410. doi: 10.1016/j.jmarsys.2008.11.016
Contreras-Rosales L. A., Koho K. A., Duijnstee I., Strigter H., Epping E. (2010). Living deep-sea benthic foraminifera from the cap de creus canyon (western mediterranean): Faunal–geochemical interactions. Deep. Sea. Res. 1 Oceanogr. Res. Pap. 57, 22–42. doi: 10.1016/j.dsr.2012.01.010
Dai B., Liu Y., Sun Q., Ma F., Chen J., Chen Z. (2018). Foraminiferal evidence for Holocene environmental transitions in the yaojiang valley, south hangzhou bay, eastern China, and its significance for neolithic occupations. Mar. Geol. 404, 15–23. doi: 10.1016/j.margeo.2018.07.001
Debenay J. P., Guillou J. J., Redois F., Geslin E. (2000). ““Distribution trends of foraminiferal assemblages in paralic environments: A base for using foraminifera as bioindicators,”,” in Environmental micropaleontology, vol. 39-67 . Ed. Martin R. E. (New York: Springer US Publication).
Dessandier P., Bonnin J., Kim J., Racine C. (2018). Comparison of living and dead benthic foraminifera on the Portuguese margin: Understanding the taphonomical processes. Mar. Micropaleontol. 140, 1–16. doi: 10.1016/j.marmicro.2018.01.001
De Stigter H. C., Jorissen F. J., van der Zwaan G. J. (1998). Bathymetric distribution and microhabitat partitioning of live (Rose Bengal stained) benthic foraminifera along a shelf to deep sea transect in the southern Adriatic Sea. J. Foraminiferal. Res. 28, 40–65. doi: 10.1016/S0016-6995(98)80113-7
Dickson A. G. (1994). Determination of dissolved oxygen in sea water by winkler titration (Massachusetts: WOCE Hydrographic Program, Operations and Methods Manual. Woods Hole).
Duros P., Fontanier C., De Stigter H. C., Cesbron F., Metzger E., Jorissen F. J. (2012). Live and dead benthic foraminiferal faunas from whittard canyon (NE atlantic): focus on taphonomic processes and paleo-environmental applications. Mar. Micropaleontol. 94, 25–44. doi: 10.1016/j.marmicro.2012.05.004
Evans J., Austin W. E. N., Brew D. S., Wilkinson I. P., Kennedy H. A. (2002). Holocene Shelf sea evolution offshore northeast England. Mar. Geol. 191, 147–164. doi: 10.1016/S0025-3227(02)00529-7
Folk R. L., Ward W. C. (1957). Brazos river bar: A study in the significance of grain size parameters. J. Sediment. Petrol. 31, 514–529. doi: 10.1306/74D70646-2B21-11D7-8648000102C1865D
Gao S., Wang D., Yang Y., Zhou L., Zhao Y., Gao W., et al. (2015). Holocene Sedimentary systems on a broad continental shelf with abundant river input: process-product relationships. Geol. Soc Spec. Publ. 429, 223–259. doi: 10.1144/SP429.4
Glover A. G., Gooday A. J., Bailey D. M., Billett D. S. M., Chevaldonne P., Colaco A., et al. (2010). Temporal change in deep-sea benthic ecosystems: a review of the evidence from recent time-series studies. Adv. Mar. Biol. 58, 1–95. doi: 10.1016/S0065-2881(10)58001-5
Goineau A., Fontanier C., Mojtahid M., Fanget A.-S., Bassetti M.-A., Berné S., et al. (2015). Live-dead comparison of benthic foraminiferal faunas from the rhône prodelta (Gulf of lions, NW mediterranean): Development of a proxy for paleoenvironmental reconstructions. Mar. Micropaleontol. 119, 17–33. doi: 10.1016/j.marmicro.2015.07.002
Goldstein S. T. (1999). ““Foraminifera: a biological overview,”,” in Benthic foraminiferal microhabitats below the sediment-water interface. Ed. Sen Gupta B. K. (Dordrecht: Kluwer Academic Publishers), 37–56.
Gooday A. J., Hughes J. A. (2002). Foraminifera associated with phytodetritus deposits at a bathyal site in the northern rockall trough (NE atlantic): seasonal contrasts and a comparison of stained and dead assemblages. Mar. Micropaleontol. 46, 83–110. doi: 10.1016/S0377-8398(02)00050-6
Gooday A., Jorissed F. (2012). Benthic foraminiferal biogeography: Controls on global distribution patterns in deep-water settings. Ann. Rev. Mar. Sci. 4, 237–262. doi: 10.1146/annurev-marine-120709-142737
Guan B. X., Fang G. H. (2006). Winter counter-wind currents off the southeastern China coast: A review. J. Oceanogr. 62, 1–24. doi: 10.1007/s10872-006-0028-8
Guo X., Xu B., Burnett W. C., Yu Z., Yang S., Huang X., et al. (2019). A potential proxy for seasonal hypoxia: LA-ICP-MS Mn/Ca ratios in benthic foraminifera from the Yangtze river estuary. Geochim. Cosmochim. Acta 245, 290–303. doi: 10.1016/j.gca.2018.11.007
Gustafsson M., Nordberg K. (1999). Benthic foraminifera and their response to hydrography, periodic hypoxic conditions and primary production in the kolö fjordfjord on the Swedish west coast. J. Sea. Res. 41, 163–178. doi: 10.1016/S1385-1101(99)00002-7
Horton B. P., Murray J. W. (2006). Patterns in cumulative increase in live and dead species from foraminiferal time series of cowpen marsh, Tees estuary, UK: implications for sea-level studies. Mar. Micropaleontol. 58, 287–315. doi: 10.1016/j.marmicro.2005.10.006
Hu J., Wang X. (2016). Progress on upwelling studies in the China seas. Rev. Geophysis. 54, 653–673. doi: 10.1002/2015RG000505
Jernas P., Klitgaard-Kristensen D., Husum K., Koc N., Tverberg V., Loubere P., et al. (2018). Annual changes in Arctic fjord environment and modern benthic foraminiferal fauna: Evidence from kongsfjorden, Svalbard. Glob. Planet. Change 163, 119–140. doi: 10.1016/j.gloplacha.2017.11.013
Jia J., Gao J., Cai T., Liu Y., Yang Y., Wang Y., et al. (2018). Sediment accumulation and retention of the changjiang (Yangtze river) subaqueous delta and its distal muds over the last century. Mar. Geol. 401, 2–16. doi: 10.1016/j.margeo.2018.04.005
Jiang F., Wang Y., Zhao X., Liu Y., Chen J., Sun Q., et al. (2021). Reconstruction of the Holocene sedimentary–ecological complex in the incised valley of the Yangtze delta, China. Palaeogeogr. Palaeoclimatol. Palaeoecol. 571, 11038. doi: 10.1016/j.palaeo.2021.110387
Jorissen F. J., Wittling I. (1999). Ecological evidence from live-dead comparisons of benthic foraminiferal faunas off cape blanc (Northwest Africa). Palaeogeogr. Palaeoclimatol. Palaeoecol. 149, 151–170. doi: 10.1016/S0031-0182(98)00198-9
Kidwell S. M., Tomasovych A. (2013). Implications of time-averaged death assemblages for ecology and conservation biology. Annu. Rev. Ecol. Syst. 44, 539–563. doi: 10.1146/annurev-ecolsys-110512-135838
Kuhnt W., Collins E., Scott D. B. (2000). “Deep water agglutinated foraminiferal assemblages across the gulf stream: distribution patterns and taphonomy,” in Proceedings of the fifth international workshop on agglutinated foraminifera (Grzybowski Foundation Special Publication), 261–298.
Lee H. J., Jung K. T., So J. K., Chung J. Y. (2002). A three-dimensional mixed finite-difference galerkin function model for the oceanic circulation in the yellow Sea and the East China Sea in the presence of M2 tide. Continental. Shelf. Res. 22, 67–91. doi: 10.1016/S0278-4343(01)00068-1
Lei Y., Li T. (2016). Atlas of benthic foraminifera from China seas Vol. 345 (Springer Berlin Heidelberg), p.
Lei Y., Li T., Jian Z., Nigam R. (2017). Taxonomy and distribution of benthic foraminifera in an intertidal zone of the yellow Sea, PR China: Correlations with sediment temperature and salinity. Mar. Micropaleontol. 133, 1–20. doi: 10.1016/j.marmicro.2017.04.005
Levin L., Ekau W., Gooday A., Jorssen F., Middelburg J., Naqvi S., et al. (2009). Effects of natural and human-induced hypoxia on coastal benthos. Biogeosciences 6, 2063–2098. doi: 10.5194/bg-6-2063-2009
Li T., Cai G., Zhang M., Li S., Nie X. (2021). The response of benthic foraminifera to heavy metals and grain sizes: A case study from hainan island, China. Mar. pollut. Bull. 167, 112328. doi: 10.1016/j.marpolbul.2021.112328
Li W., Ge J., Ding P., Ma J., Glibert P., Liu D. (2020). Effects of dual fronts on the spatial pattern of chlorophyll-a concentrations in and off the changjiang river estuary. Estuaries. Coast. 44, 1408–1418. doi: 10.1007/s12237-020-00893-z
Li Z., Liu D., Long H. (2014). Living and dead benthic foraminifera assemblages in the bohai and northern yellow seas: Seasonal distributions and paleoenvironmental implications. Quat. Int. 349, 113–126. doi: 10.1016/j.quaint.2014.05.019
Liu Z., Gan J., Wu H., Hu J., Cai Z., Deng Y. (2021). Advances on coastal and estuarine circulations around the changjiang estuary in the recent decades, (2000-2020). Front. Mar. Sci. 8. doi: 10.3389/fmars.2021.615929
Li D., Zhang J., Huang D., Wu Y., Liang J. (2002). Oxygen depletion off the changjiang (Yangtze river) estuary. Sci. China Ser. D. Earth Sci. 45, 1137–1146. doi: 10.1360/02yd9110
Magno M. C., Bergamin L., Finoia M. G., Pierfranceschi G., Venti F., Romano E. (2012). Correlation between textural characteristics of marine sediments and benthic foraminifera in highly anthropogenically-altered coastal areas. Mar. Geol. 315, 143–161. doi: 10.1016/j.margeo.2012.04.002
Mendes I., Dias J. A., Schöffel J., Ferreira Ó., Rosa F., Lobo F. J. (2013). Living, dead and fossil benthic foraminifera on a river dominated shelf (northern gulf of cadiz) and their use for paleoenvironmental reconstruction. Cont. Shelf. Res. 68, 91–111. doi: 10.1016/j.csr.2013.08.013
Mojtahid M., Geslin E., Coynel A., Gorse L., Vella C., Davranche A., et al. (2016). Spatial distribution of living (Rose Bengal stained) benthic foraminifera in the Loire estuary (western France). J. Sea. Res. 118, 1–16. doi: 10.1016/j.seares.2016.02.003
Murray J. W. (2006). Ecology and applications of benthic foraminifera Vol. 426 (Cambridge: Cambridge University Press), p.
Murray J. W., Alve E. (1999). Natural dissolution of modern shallow water benthic foraminifera: taphonomic effects on the paleoecological record. Palaeogeogr. Palaeoclimatol. Palaeoecol. 146, 195–209. doi: 10.1016/S0031-0182(98)00132-1
Naimie B., Blain C., Lynch D. (2001). Seasonal mean circulation in the yellow Sea-a model-generated climatology. Cont. Shelf. Res. 21, 667–695. doi: 10.1016/S0278-4343(00)00102-3
Park S., Chu P. C. (2006). Thermal and haline fronts in the Yellow/East China seas: Surface and subsurface seasonality comparison. J. Oceanogr. 62, 617–638. doi: 10.1007/S10872-006-0081-3
Qiao S., Shi X., Wang G., Zhou L., Hu B., Hu L., et al. (2017). Sediment accumulation and budget in the bohai Sea, yellow Sea and East China Sea. Mar. Geol. 390, 270–281. doi: 10.1016/j.margeo.2017.06.004
Ren F., Fan D., Wu Y., Zhao Q. (2019). The evolution of hypoxia off the changjiang estuary in the last 3000 years: Evidence from benthic foraminifera and elemental geochemistry. Mar. Geol. 417, 106039. doi: 10.1016/j.margeo.2019.106039
Schlitzer R. (2009) Ocean data view. Available at: http://odv.awi.de.
Schönfeld J., Zahn R. (2000). Late glacial to Holocene history of the Mediterranean outflow. evidence from benthic foraminiferal assemblages and stable isotopes at the Portuguese margin. Palaeogeogr. Palaeoclimatol. Palaeoecol. 59, 85–111. doi: 10.1016/S0031-0182(00)00035-3
Shannon C. E. (1984). A mathematical theory of communications. Bell. Syst. Tech. J. 27, 379–423. doi: 10.1002/j.1538-7305.1948.tb01338.x
Stefanoudis P., Bett B., Gooday A. (2017). Relationship between ‘live’ and dead benthic foraminiferal assemblages in the abyssal NE Atlantic. Deep-Sea. Res. 1 121, 190–201. doi: 10.1016/j.dsr.2017.01.014
Su J. (1998). ““Circulation dynamics of the China seas north of 18°N,”,” in The sea. Eds. Robinson A. R., Brink K. H. (New York, NY: Wiley), 483–505.
Su J. L., Yuan Y. L. (2005). Hydrology of Chinese coastal waters Vol. 367 (Beijing: China Ocean Press), p. (In Chinese).
Tseng Y. F., Lin J., Dai M., Kao S. J. (2014). Joint effect of freshwater plume and coastal upwelling on phytoplankton growth off the changjiang river. Biogeosciences 11, 409–423. doi: 10.5194/bg-11-409-2014
Wang S. L., Fan D., Huang W. J., Wu Y., Su J. (2021). Hypoxic effects on the radiocarbon in DIC of the ECS subsurface water. J. Geophys. Res. Oceans. 126, e2020JC016979. doi: 10.1029/2020JC016979
Wang F., Gao M., Liu J., Pei S., Li C., Mei X., et al. (2016). Distribution and environmental significance of live and dead benthic foraminiferal assemblages in surface sediments of laizhou bay, bohai Sea. Mar. Micropaleontol. 123, 1–14. doi: 10.1016/j.marmicro.2015.12.006
Wang Y., Wu H., Gao L., Shen F., Liang X. (2019). Spatial distribution and physical controls of the spring algal blooming off the changjiang river estuary. Estuaries. Coast. 42, 1066–1083. doi: 10.1007/s12237-019-00545-x
Wang F., Yu Z., Xu B., Liu J., Guo X., Nan H. (2018). Nepartak typhoon influenced bottom sediments from the Yangtze river estuary and adjacent East China Sea-foraminiferal evidence. Geochem. Geophys. Geosyst. 19, 1046–1063. doi: 10.1002/2017GC007413
Wang P. X., Zhang J. J., Zhao Q. H., Min Q. B., Bian Y. H., Zheng L. F., et al. (1988). Foraminifera and ostracoda in bottom sediments of the East China Sea Vol. 438 (Beijing: China Ocean Press), p. (In Chinese with English abstract).
Xu Z., Liu S., Xiang R., Song G. (2017). Live benthic foraminifera in the yellow Sea and the East China Sea: vertical distribution, nitrate storage, and potential denitrification. Mar. Ecol. Prog. Ser. 571, 65–81. doi: 10.3354/meps12135
Yang D. Z., Yin B. S., Chai F., Feng X., Xue H., Gao G., et al. (2018). The onshore intrusion of kuroshio subsurface water from February to July. Prog. Oceanogr. 167, 97–115. doi: 10.1016/j.pocean.2018.08.004
Yang D. Z., Yin B. S., Liu Z. L., Bai T., Qi J. F., Chen H. Y. (2012). Numerical study on the pattern and origins of kuroshio branches in the bottom water of southern East China Sea in summer. J. Geophys. Res. Oceans. 117 (C2), C02014. doi: 10.1029/2011JC007528
Ye F., Huang X., Shi Z., Chen B. (2021). The spatial distribution of benthic foraminifera in the pearl river estuary, south China and its environmental significance. Mar. pollut. Bull. 173, 113055. doi: 10.1016/j.marpolbul.2021.113055
Zhang J., Hu G., Ma G., Hui J., Zhang M., Zhang Y., et al. (2020). Distribution of benthic foraminifera in the surficial sediments of changjiang distal delta and its environmental implications. Mar. Geol. Quat. Geol. 40, 127–138. doi: 10.16562/j.cnki.0256-1492.2019101401. (In Chinese with English abstract).
Zhang W., Moriarty J., Wu H., Feng Y. (2021). Response of bottom hypoxia off the changjiang river estuary to multiple factors: A numerical study. Ocean. Model. 159, 101751. doi: 10.1016/j.ocemod.2021.101751
Zhao Q., Jian Z., Zhang Z., Cheng X., Wang K., Zhen H. (2009). Holocene Benthic foraminifera and ostracoda from the shelf mud area of the east China Sea and their paleoenvironmental implication. Acta Micropalaeontol. Sin. 26, 117–128. (In Chinese with English abstract). doi: 10.1360/972009-495
Zhao B., Yan X., Wang Z., Shi Y., Chen Z., Xie J., et al. (2018). Sedimentary evolution of the Yangtze river mouth (East China Sea) over the past 19,000 years, with emphasis on the Holocene variations in coastal currents. Palaeogeogr. Palaeoclimatol. Palaeoecol. 490, 421–449. doi: 10.1016/j.palaeo.2017.11.023
Zheng S. Y. (1988). The agglutinated and porcelaneous foraminifera of the East China Sea Vol. 337 (Beijing: Science Press), p. (In Chinese).
Zhu Z., Wu H., Liu S., Wu Y., Huang D., Zhang J., et al. (2017). Hypoxia off the changjiang (Yangtze river) estuary and in the adjacent East China Sea: Quantitative approaches to estimating the tidal impact and nutrient regeneration. Mar. pollut. Bull. 125, 103–114. doi: 10.1016/j.marpolbul.2017.07.029
Keywords: benthic foraminifera, coastal seas, surface sediment, shelf circulations, postmortem processes, water mass
Citation: Jiang F, Fan D, Zhao Q, Wu Y, Ren F, Liu Y and Li A (2023) Comparison of alive and dead benthic foraminiferal fauna off the Changjiang Estuary: Understanding water-mass properties and taphonomic processes. Front. Mar. Sci. 10:1114337. doi: 10.3389/fmars.2023.1114337
Received: 02 December 2022; Accepted: 27 January 2023;
Published: 20 February 2023.
Edited by:
Yifei Zhao, Nanjing Normal University, ChinaReviewed by:
Qiang Yao, Louisiana State University Agricultural Center, United StatesCopyright © 2023 Jiang, Fan, Zhao, Wu, Ren, Liu and Li. This is an open-access article distributed under the terms of the Creative Commons Attribution License (CC BY). The use, distribution or reproduction in other forums is permitted, provided the original author(s) and the copyright owner(s) are credited and that the original publication in this journal is cited, in accordance with accepted academic practice. No use, distribution or reproduction is permitted which does not comply with these terms.
*Correspondence: Daidu Fan, ZGRmYW5AdG9uZ2ppLmVkdS5jbg==
Disclaimer: All claims expressed in this article are solely those of the authors and do not necessarily represent those of their affiliated organizations, or those of the publisher, the editors and the reviewers. Any product that may be evaluated in this article or claim that may be made by its manufacturer is not guaranteed or endorsed by the publisher.
Research integrity at Frontiers
Learn more about the work of our research integrity team to safeguard the quality of each article we publish.