- 1Australian Research Council (ARC) Centre of Excellence for Coral Reef Studies, James Cook University, Townsville, QLD, Australia
- 2Centre for Tropical Bioinformatics and Molecular Biology, James Cook University, Townsville, QLD, Australia
- 3School of Zoology, The George S. Wise Faculty of Life Sciences, Tel-Aviv University, Tel-Aviv, Israel
- 4The Interuniversity Institute for Marine Sciences, Eilat, Israel
- 5Australian Research Council (ARC) Centre of Excellence for Coral Reef Studies and School of Biological Sciences, The University of Queensland, St. Lucia, QLD, Australia
- 6The Mina & Everard Goodman Faculty of Life Sciences, Bar-Ilan University, Ramat Gan, Israel
- 7Tropical Biosphere Research Center, University of the Ryukyus, Motobu, Okinawa, Japan
- 8Department of Environ & Social Affairs, School of Marine Sci & Techno, Tokai University, Shimizu, Shizuoka, Japan
To compete effectively, living organisms must adjust the allocation of available energy resources for growth, survival, maintenance, and reproduction throughout their life histories. Energy demands and allocations change throughout the life history of an organism, and understanding their energy allocation strategies requires determination of the relative age of individuals. As most scleractinian corals are colonial, the relationship between age and mass/size is complicated by colony fragmentation, partial mortality, and asexual reproduction. To overcome these limitations, solitary mushroom corals, Herpolitha limax from Okinawa, Japan and Fungia fungites from Okinawa and the Great Barrier Reef (GBR), Australia, were used to investigate how energy allocation between these fundamental processes varies as a function of age. Measurements of the relative growth, biochemical profiles, fecundity of individuals of different sizes, and the settlement success of their progeny have revealed physiological trade-offs between growth and reproduction, with increasing body mass ultimately leading to senescence. The importance of energy allocation for reproduction led us to examine the reproductive strategies and sex allocation in the two studied species. In the present study, the smallest individuals of both species studied were found to invest most of their energy in relative growth, showing higher lipid and carbohydrate content than the later stages. In medium-sized corals, this pattern was overturned in favour of reproduction, manifesting in terms of both the highest fecundity and settlement success of the resulting brooded larvae. Finally, a phase of apparent senescence was observed in the largest individuals, characterized by a decrease in most of the parameters measured. In addition, complex reproductive plasticity has been revealed in F. fungites in the GBR, with individual females releasing eggs, embryos, planulae, or a combination of these. These data provide the most direct estimates currently available for physiological, age-related trade-offs during the life history of a coral. The unusual reproductive characteristics of the GBR F. fungites indicate previously unknown layers of complexity in the reproductive biology of corals and have implications for their adaptive potential across a wide geographical scale.
1 Introduction
The major premise in the theory of reproductive cost is that there are trade-offs at the physiological level of resource allocation between growth and reproduction (Obeso, 2002). These trade-offs are central to understanding the life-history traits and adaptation of organisms to their environment (Schaffer, 1983; Calow, 1987). Patterns of energy allocation may change in accordance with the stage of sexual maturity of the individual (Tytler and Calow, 1985) and are often size-dependent processes (Weiner, 2004). Because reproductive investment and growth are often used as indicators of health or stress at the organism level (Maltby, 1999), knowledge of how such allocation varies intrinsically among species or morphological types is crucial for the interpretation of the physiological responses of animals to environmental factors. The reproductive energy allocation strategy of an organism affects virtually all other life-history traits. Because resources are finite, reproduction itself can incur a cost in that it can reduce the survival probability or growth rate of the individual. As such, the rules governing reproductive allocation, (the proportion of resources allocated to reproduction; Cheplick, 2005), are generally assessed from within a cost–benefit framework (Kozłowski, 1992; Stearns, 1992; Obeso, 2002; Bradshaw and McMahon, 2008).
External stressors can affect the allocation of energy towards different functions. For example, in corals, extrinsic factors such as sedimentation, competition by macroalgae (Kojis and Quinn, 1984; Tanner, 1995; Foster et al., 2008) or intra-and inter-specific aggression with other corals (Rinkevich and Loya, 1985; Tanner, 1997) have been shown to reduce both growth and fecundity. Moreover, reduced levels of storage lipids were found following both natural bleaching events (Grottoli et al., 2004; Yamashiro et al., 2005), and short-term experimental sediment stress (Niebuhr, 1999). Furthermore, a wide range of biochemical and physiological parameters, including chlorophyll a, lipid, carbohydrate and protein content, tissue biomass, and photosynthesis, were impaired following experimental bleaching (Rodrigues and Grottoli, 2007). Understanding the biochemical composition of organisms is crucial for understanding energy budgets not only within individuals but also in ecological communities (Ben-David-Zaslow and Benayahu, 1999). To date, little is known about the relationships between body composition and energy allocation to competing metabolic processes at the different life-stages of corals.
In addition to the environmental pressures, in iteroparous organisms (i.e. species that reproduce more than once during their lifetime) such as scleractinian corals, fecundity often increases with age following reproductive maturity and may then decline during later stages (i.e. reproductive senescence) (Bradshaw and McMahon, 2008). Therefore, understanding the age specificity of reproduction is an essential element for understanding the evolution of life-history strategies (Bradshaw and McMahon, 2008). Aging processes are generally assumed to explain declines in polyp fecundity and growth (Rinkevich and Loya, 1986), as well as the increased susceptibility to stress observed in older corals (Brown et al., 2014). In the case of colonial corals, complex life history traits including fission, fusion and partial mortality of colonies can lead to an uncoupling of relationships between age and size (Chadwick-Furman et al., 2000). Furthermore, evidence for senescence in clonal organisms is scant (Silvertown et al., 2001; Ally et al., 2010) and it has been widely argued that clonality essentially enables (colonial) corals to escape the aging process (Fadlallah, 1983; Harrison and Wallace, 1990; Hall and Hughes, 1996).
Declining growth rates in mature colonies have been documented for a wide range of coral species of different growth forms, including those that form branching colonies: Pocillopora bulbosa (Stephenson and Stephenson, 1933), P. cespitosa (Tamura and Hada, 1932), and P. meandrina (Grigg and Maragos, 1974); massive colonies: Goniastrea aspera (Motoda, 1940; Sakai, 1998); attached solitary polyps: Balanophyllia elegans, Paracyathus stearnsii and Balanophyllia europaea (Gerrodette, 1979; Goffredo et al., 2004); free-living colonies: Manicina areolata (Johnson, 1992), and free-living solitary polyps: the mushroom corals Ctenactis echinata (Goffredo, 1995), Diaseris distorta (Yamashiro and Nishihira, 1998), Fungia actiniformis (Tamura and Hada, 1932), F. granulosa (Chadwick-Furman et al., 2000), F. paumotensis (Bablet, 1985) and F. fungites (Stephenson and Stephenson, 1933). In theory, the shifts in energy investment from growth to reproduction that are predicted to occur with increasing body mass (Kozłowski and Wiegert, 1986; Hall and Hughes, 1996) should, at least to some extent, be reflected in the growth trajectories of mature colonies. However, evidence for such trends is scant – in fact many studies have failed to detect reduced growth rates in older colonies (reviewed in Buddemeier and Kinzie, 1976; Hughes and Jackson, 1985), providing circumstantial support for the hypothesis that corals may be immortal at the level of the asexual lineage (Bythell et al., 2018). Finally, given the extreme lifespan of some corals, it is neither practical nor feasible to follow traits such as fecundity or growth over their complete lifespan.
While different growth forms such as colonial and solitary may affect those life-history events, a representative of each can be sometimes found in a single coral genus. One example is the fungiid Cycloseris sp. in which morphologic characters such as growth form (colonial and solitary), as well as number of mouths (polystomatous or monostomatous), based on genetical analyses, no longer define a distinct population (Benzoni et al., 2012). Since most solitary fungiid corals do not divide asexually, the approximate age of an individual can be inferred from its size (Chadwick-Furman et al., 2000). Hence parameters such as the age at which maturity is achieved, age-specific fecundity, growth rates, reproductive lifespan, and senescence characteristics can be determined relatively simply and accurately. Consequently, we chose the mushroom corals H. limax (solitary polystomatous) and F. fungites (solitary monostomatous) as our model organisms to study these life history parameters.
Sexual reproduction is an energetically costly process, and the energy allocated to gamete production by an organism may be subject to energetic constraints (Loya and Sakai, 2008). Moreover, while planulae sourced from a stressed coral previously showed low lipid content (Rivest et al., 2017), the effect of age on the settelment is yet to be explored. Successful sexual reproduction followed by settlement and metamorphosis is crucial for local adaptation and the sustainability of a species (Richmond, 1987; Harii et al., 2002; Rivest et al., 2017).
Another poorly understood area of coral reproductive biology is that of the relative energetic demands of egg (broadcast spawning) or planulae production (brooding), simply as most coral species exhibit only one strategy. In line with this, H. limax is thought to reproduce exclusively by broadcast spawning (Eyal-Shaham et al., 2019). However, the literature suggested that F. fungites might differ in reproductive mode across its range, potentially enabling a comparison of energy investment between spawning and brooding for a single species. F. fungites is common in Okinawa (Loya and Sakai, 2008) where it has a protracted, four-month reproductive season, during which brooded planulae are released daily for 4-6 weeks (Munasik, 1999; Loya et al., 2009; Eyal-Shaham et al., 2020). In contrast to the Okinawa population, the only record to date for reproduction of F. fungites on the GBR implies that there it behaves as a broadcast spawner (Willis et al., 1985; but note that no available data on the reproductive cycle of this species on the GBR). While a recent study (Grinblat et al., 2021) indicated that the Okinawan and GBR F. fungites populations are distinct at the genetic level, these populations permit comparison of the reproductive energy allocation as a function of age in brooding and broadcast spawning individuals of a single species. Since reproductive strategy is likely to affect survival, distribution and life-history traits of the organism, an additional aim of the work described here was to more thoroughly investigate the reproductive biology of F. fungites on the GBR.
Here, we examine hypotheses derived from life-history models of size-specific energy-allocation patterns by characterizing trade-offs between reproduction, growth, and survival of individuals of different sizes. Additionally, we provide records of settlement success and energy available as biochemical components (i.e. lipids, carbohydrates, and proteins) for all coral sizes. We also investigate the possible benefits of the reproductive plasticity of the F. fungites populations on the GBR and in Okinawa.
2 Methods
2.1 Study site and specimen collection, tagging and measurements
The first part of the study took place on several patch reefs near the Sesoko Marine Station, (26°39’N, 127°52’E), Okinawa (Japan). In this area, the F. fungites and H. limax populations are concentrated at a few sites that are inhabited by tens of thousands of fungiid corals (> 12 spp.) (Loya and Sakai, 2008). Specimens of both species, from all sizes (3-17 cm in diameter - F. fungites and 9-54 cm in length - H. limax), were randomly collected from the reef in 2004 and transferred to open-system running seawater at the station. Individuals were tagged with a numeric plastic tag as previously described (Loya and Sakai, 2008). To avoid damage to the small-size corals of F. fungites (<5cm), they were individually kept in marked containers.
2.2 Sex and size-frequency distribution
The relationship between sex and size frequency distribution was assessed for both species. For H. limax, since individual corals in the population changed sex in consecutive years, the calculation of size-frequency distribution was performed as an accumulation of data from 2014-2017. In F. fungites, due to the labile sex ratio of the population, the calculation of size-frequency distribution was based on data collected in 2015, in which observations were conducted continuously for 2.5 months and accurately represent the true sex of an individual.
During the reproduction season, the corals were kept in large containers with constant seawater inflow during the day and were transferred to separate individual containers for observations at night. As eggs and planulae of both H. limax and F. fungites were found to emit green fluorescence when exposed to UV or blue light, a blue flashlight (~450 nm) and a yellow long-pass filter (NightSea) were used to confirm their presence. Sperm were detected by observation of the opacity of the water in the container and verified microscopically (Loya & Sakai, 2008; Loya et al., 2009).
Following each annual set of measurements and observations, the tagged corals were returned to their natural habitat and retrieved the following year, one to two weeks before the expected breeding season.
2.3 Growth rate
Growth rates in both species were determined by means of annual (2004, 2006, 2007, 2010, 2014 and 2015-2017 for H. limax and 2015-2017 for F. fungites) size measurements of individual corals. In order to be able to discuss the energy investment in growth rather than the absolute growth, we present growth relative to the size of the coral (i.e. relative growth per individual coral) as suggested in Pratchett et al. (2015). Using a tape measure, length (L, mm) was measured to the nearest 1 mm and weight (W, gr) was measured to the nearest 1 gr, following careful removal of excess moisture. A correlation test between weight and length or diameter was performed and as correlation was found (P-values< 0.05 were considered statistically significant), size was represented by weight only. As we were unable to retrieve a portion of the corals over the years, new individuals were added every year. Overall, we recorded measurements of 73 F. fungites and 106 H. limax individuals over several years. Individuals were only included in the analysis if the gap between the measurements was 3 years or less to avoid major changes in energy investment patterns.
Growth rate; was calculated according to equation I:
Equation I:
ΔWx (gr) – Weight difference (gr) between the initial Wx and its weight after N years.
Wx – Weight (gr) of individual coral x when first collected and measured
N – Total number of years between consecutive weight measurements
The N ranged between 1-2 years for F. fungites and 1-3 years for H. limax
2.4 Female fecundity of H. limax and F. fungites in Okinawa
Female fecundity measurements of 27 H. limax individuals were carried out during July 2016. Following spawning, the water in each aquarium containing female gametes was homogenized by stirring and 10 replicates of 1 ml sample of water containing eggs were taken for counting, using a Stempel pipette. The counting was done in a Petri dish (against a dark background) with the aid of a blue flashlight (~450 nm) and a yellow long-pass filter (NightSea) for easy detection of the fluorescent eggs and was carried out no later than two hours post spawning to avoid eggs disintegration. Fecundity was determined as the total egg production per individual coral per month and was calculated by multiplying the averaged egg count per individual by the volume of water (in ml) in the corresponding aquarium. Egg count was repeated daily until the end of the July reproduction season and the number of eggs released by each individual was summed for the entire reproductive cycle (seven consecutive days starting from five nights after the full moon of July 2016). For F. fungites the number of planulae released by each individual coral was counted every morning after the reproduction hours (06:30-08:30 AM) for 35 consecutive days (July 2-August 5, 2015, n=250). Although few individuals released small numbers of eggs, planulation was the predominant reproduction mode of this population. After removing the coral from its container, we gradually transferred the water from the container to a square glass-measuring container in which we were able to easily count the fluorescent planulae. We summed the number of planulae released from each individual for the entire period and determined fecundity as the average planulae number released per individual coral per day.
2.5 Male fecundity of F. fungites in Okinawa
Male fecundity measurements for F. fungites (n=22) were conducted in 2010 (July 28-31) and in 2015 (July 2-3, 11, 14). Following sperm release, the water in each container containing male gametes was homogenized by stirring and 5 replicates of 1 ml water samples containing sperm were taken for counting, using a Stempel pipette. Sperm were counted on a haemocytometer under a microscope. Male fecundity was determined as the total sperm production per individual coral per day and was calculated by multiplying the average sample count by the volume of water (in ml) in the relevant container.
2.6 Biochemical profiles
At the beginning of the 2015 reproduction season, a slice (2-3 cm wide) was cut through the middle of the polyp from individuals of H. limax and F. fungites from all sizes, in order to examine the biochemical content of the coral tissues: (i) carbohydrates, (ii) lipids, and (iii) proteins. The fragments were immediately dipped in liquid nitrogen and stored at −80°C until processing.
Coral tissue was brushed off with an airbrush using 0.2-μm filtered seawater on ice and the tissue was immediately afterwards homogenized using a mini-handheld homogenizer (Pellet Pestle Motor, KONTES). The slurry was centrifuged (5000 rpm, 5 min, 4°C) and various aliquots were taken from the host tissue for subsequent biochemical analyses. Aliquots were frozen at −80°C. Coral skeletons were dried, and coral surface area was determined by a single wax dipping (Veal et al., 2010). (i) The total carbohydrate content of coral tissue was assessed using an adapted protocol of the sulfuric acid-phenol method (Masuko et al., 2005; Krueger et al., 2017) using glucose as standard. (ii) Lipid extraction was modified from Harii et al. (2007); aliquots of 1 ml coral slurry were freeze-dried and lipids were extracted 5 times with 5 min rounds of ultrasonication in the presence of 5 ml dichloromethane-methanol (6:4). The lipid extracts were concentrated and passed through a short bed of Na2SO4 to remove water and then weighed. (iii) Total soluble protein content of coral tissue was determined using the Bradford reagent, with bovine serum albumin as protein standard (Bradford, 1976). The biochemical contents for each sample were standardized to the determined coral surface area (cm2).
2.7 Settlement success
To determine whether settlement success of F. fungites planulae is influenced by individual size, we compared settlement success of planulae originating from individuals of different weight. Approximately two months prior to the experiment, 24 clay tiles were placed in a flow-through aquarium with unfiltered sea water in order to biologically condition them for coral settlement. In July 2015, 200 planulae per individual were collected and placed in separate buckets with filtered seawater and a pre-conditioned clay tile. We monitored the number of settled fluorescent planulae on the clay tiles on days 1 to 7, 10, and 13 when the total number of recruits present on each tile was recorded. Since the buckets lacked water circulation, 95% of the water was replaced daily using a mesh to avoid planulae removal.
2.8 Site and sample collection of F. fungites on the GBR
The second part of the study took place in the inner part of the GBR around Orpheus and Pelorus islands (18°36’S 146°29’E) which are a part of the Palm group of islands and are separated from one another by a channel. In October 2017, 80 F. fungites specimens of all sizes (7-26 cm in diameter) were randomly collected from the reefs located on the western sides of Orpheus and Pelorus islands. This area features mostly a fringing reef with partial stony-coral cover down to ~15m depth followed by a sandy bottom. The western side of both islands is relatively protected from the weather. The corals were transferred to aquaria with flow-through filtered seawater (5 μm) located at the Orpheus Island Research Station (OIRS) and were tagged as previously described.
Prior to the November and December 2017 spawning events, the tagged individuals were placed in separate containers isolated from the flow-through sea water. The sexually reproductive individuals were identified by observing the gametes released and their sex was recorded. This took place at 17:00 until midnight on the day of the full moon and was repeated daily until the seventh night after the full moon in both November and December. The observations were made at 20 min intervals. Individuals that released both female and male gametes on different nights were classified as hermaphrodites. Since only corals larger than 9 cm spawned in November 2017, 20 additional individuals larger than 10 cm were collected before the December spawning event in order to increase the sample size of sexually reproductive individuals. Similar to section 1.2.1, a correlation test between weight and diameter was performed and as correlation was found (P-values< 0.05 were considered statistically significant), size was represented by weight only.
2.9 Fertilization and early development of F. fungites on the GBR
Within 20 minutes after observed male and female spawning in December 2017, water samples containing sperm and eggs (~500 ml of each) were mixed in the lab and kept in a temperature-controlled room at ambient seawater temperature. A small sample of the gametes was observed under a microscope at 10 minute intervals and the dominant developmental stage was recorded. The time post-fertilization for each developmental stage was determined when at least 25% of the embryos in the sample had reached this stage.
2.10 Statistical analyses
Spearman’s non-parametric correlation test between coral length/diameter and weight was performed using the cor.test function from the Stats package (Best and Roberts, 1975; Hollander and Wolfe, 1973).
For the analyses of growth rate, female and male fecundity and biochemical profiles in both species, linear model (lm) was applied to fit the best curvilinear regression as described in Mangiafico (2015). Briefly, models with different polynomial degrees were compared using one-way analysis of variance. If a model with an additional polynomial degree did not differ significantly from the reduced model, it was not considered. Best fitting model was selected based on lowest Akaike information criterion (AIC) which measures the relatively quality of the model for the data set (Supplementary Table 1). In addition, for growth rate calculation, AIC was used to determine the best fitting random effects (Supplementary Table 1). Linear mixed-effects (lme) and AIC were used to fit the best curvilinear model for planulae settlement success, with individual coral as a repeated measure (Supplementary Table 2). One-way analysis of variance (ANOVA) was used post hoc to investigate each of the measured parameters and P-values< 0.05 were considered statistically significant. All data were evaluated graphically for normality and homogeneity of variance. When these conditions were not met, a transformation was used (Supplementary Table 1). The relationships between size and sex was evaluated using a non-parametric analysis of variance and Dunn’s tests considering P-values< 0.05 as significant. All analyses were done using R software (R Core Team, 2020).
3 Results
Diameter (longest axis) and weight were strongly correlated in H. limax and F. fungites from Okinawa (Figures S1 A, B; Spearman’s non-parametric correlation test, p-value< 2.2e-16, ρ = 0.94, and p-value< 2.2e-16, ρ = 0.965147, respectively) and this relationship also held for F. fungites on the GBR (Figure S1C; Spearman’s non-parametric correlation test, p-value< 2.2e-16, ρ = 0.94). Hence, weight alone was used as a proxy for size from this point on.
3.1 Relative annual growth rate of H. limax and F. fungites
Relative annual growth rates (per individual coral) were calculated for H. limax and F. fungites from Okinawa. In both species, relative growth rate declined with increasing size (Supplementary Table 1; Figures 1A, B; lm, F 3/102 = 74.8, p< 0.05, R2 = 0.68 and F2/69 = 31.95, p<0.001, R2 = 0.48, for H. limax and F. fungites, respectively).
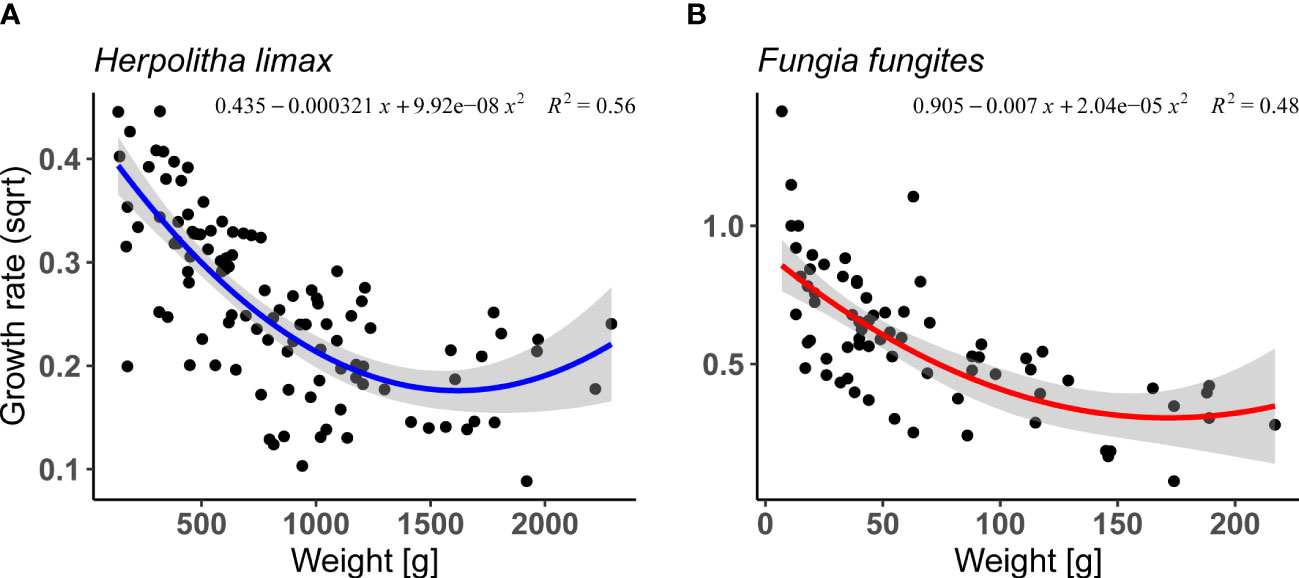
Figure 1 Annual growth rate as a function of weight (g) in (A) Herpolitha limax (2004, 2006, 2007, 2010, 2014 and 2015-2017, n=106) and (B) Fungia fungites (2015-2017,n=73). Shaded area represents 95% confidence intervals.
3.2 Fecundity and settlement success of H. limax and F. fungites
In both H. limax and F. fungites from Okinawa, female fecundity (i.e. total number of eggs or planulae released per individual throughout the season, respectively) differed significantly between sizes. In the case of H. limax, fecundity peaked in the intermediate sized individuals (Supplementary Table 1; Figure 2A; lm, F2/24 = 5.52, p = 0.011, R2 = 0.32). F. fungites showed an increase in intermediate sized individuals however high proportion of variance (Supplementary Table 1; Figure 2B; lm, F3/246 = 6.83, p<.001, R2 = 0.08).
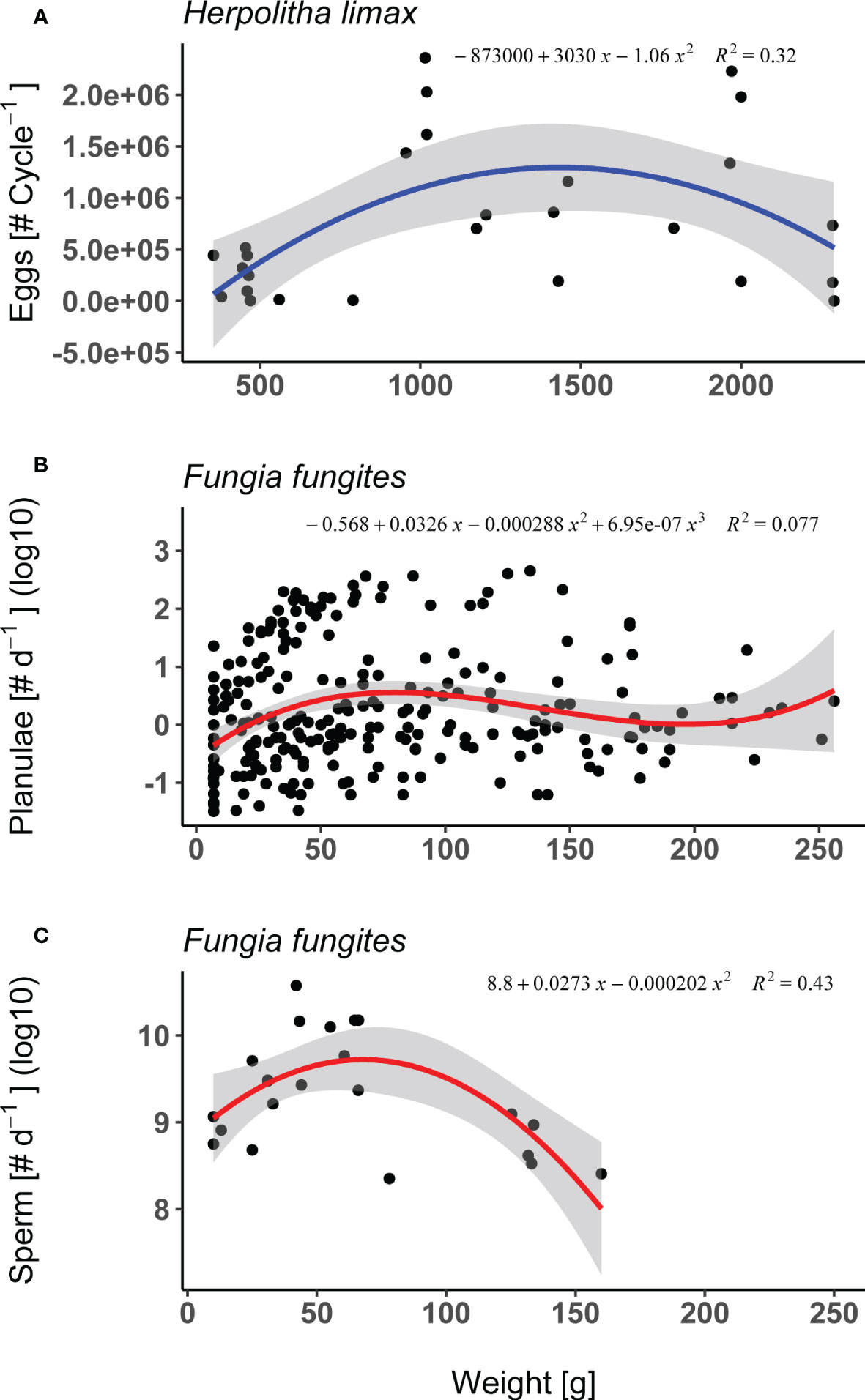
Figure 2 Fecundity measurements: (A) Female fecundity of Herpolitha limax (i.e. total # of eggs released per individual per month, n=27) as a function of weight (g), measured during the July 2016 reproductive cycle. (B) Female fecundity of Fungia fungites (i.e. total # of planulae released per individual per day, log10 scale, n=250) as a function of weight (g), measured during the reproductive season of 2015. (C) Male fecundity of F. fungites (i.e. total # of sperm released per individual per day, n=22) as a function of weight (g), measured during the reproductive seasons of 2010, 2015. Shaded area represents 95% confidence intervals.
F. fungites male fecundity, measured as the total number of sperm released per individual per day, differed significantly between sizes (Supplementary Table 1; Figure 2C; lm, F2/19 = 7.24, p = 0.005, R2 = 0.43), being lower in the smallest and largest individuals compared to the intermediate sized corals.
Settlement success of planulae released by F. fungites of different sizes followed a similar pattern with significantly higher settlement success of planulae released from individuals of intermediate sizes (Figure 3; lme, p<.001, R2 = 0.75).
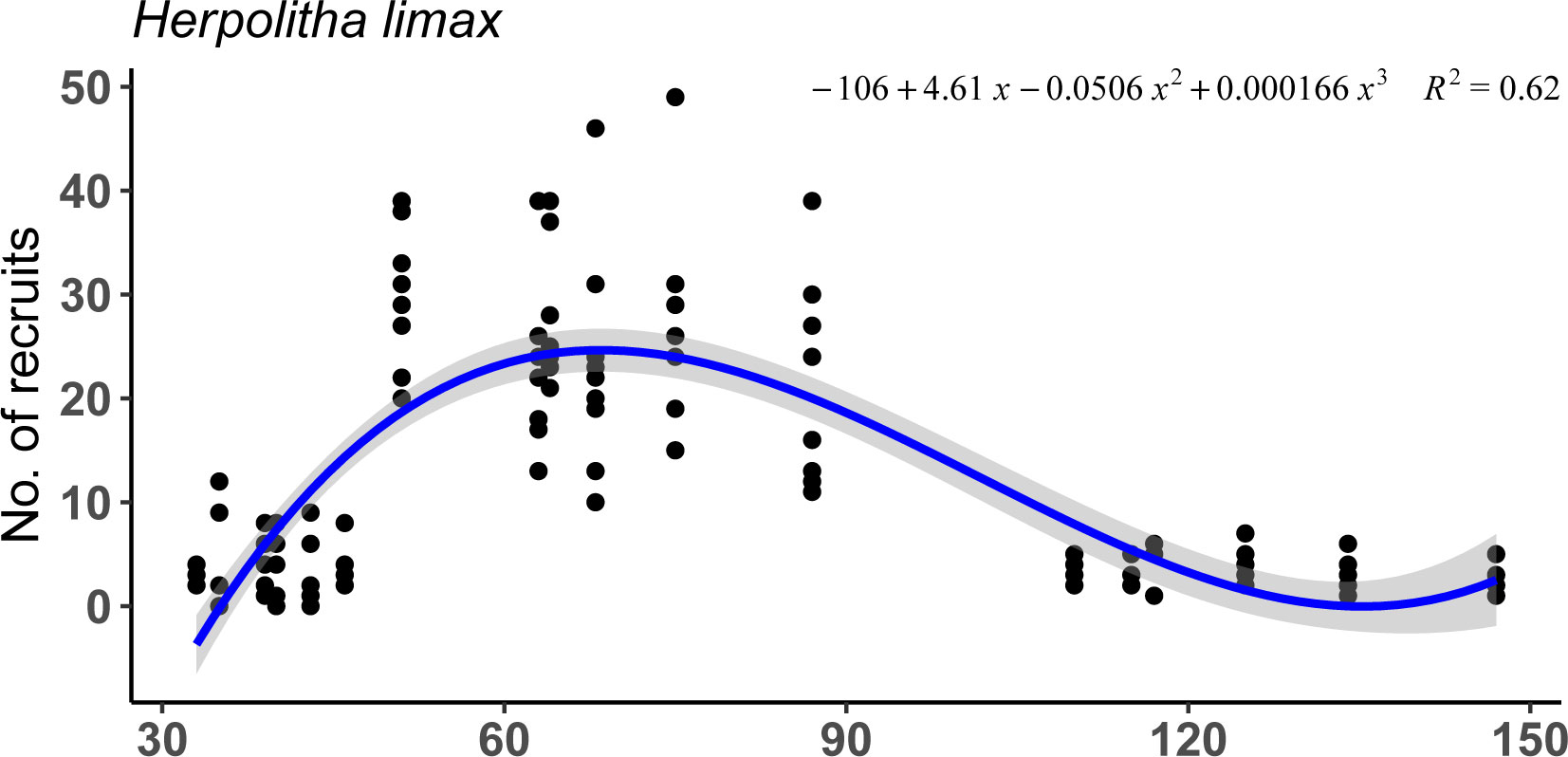
Figure 3 Settlement success and survivorship of planulae shed by 18 Fungia fungites individuals of different sizes on days 1 to 7, 10, and 13 (July 2015).
3.3 Biochemical profiles of H. limax and F. fungites
Biochemical profiles of carbohydrate, lipids and protein were evaluated in both species.
Carbohydrate levels differed significantly as a function of weight in both species where in H. limax, a decrease was observed between the smallest and intermediate individuals, followed by a slight increase at the largest (Supplementary Table 1; Figure 4A; lm, F2/43 = 15.88, p<.001, R2 = 0.42) while in F. fungites, carbohydrate levels showed a significant decrease with size (Supplementary Table 1; Figure 4B; lm, F1/93 = 10.29, p = 0.002, R2 = 0.10).
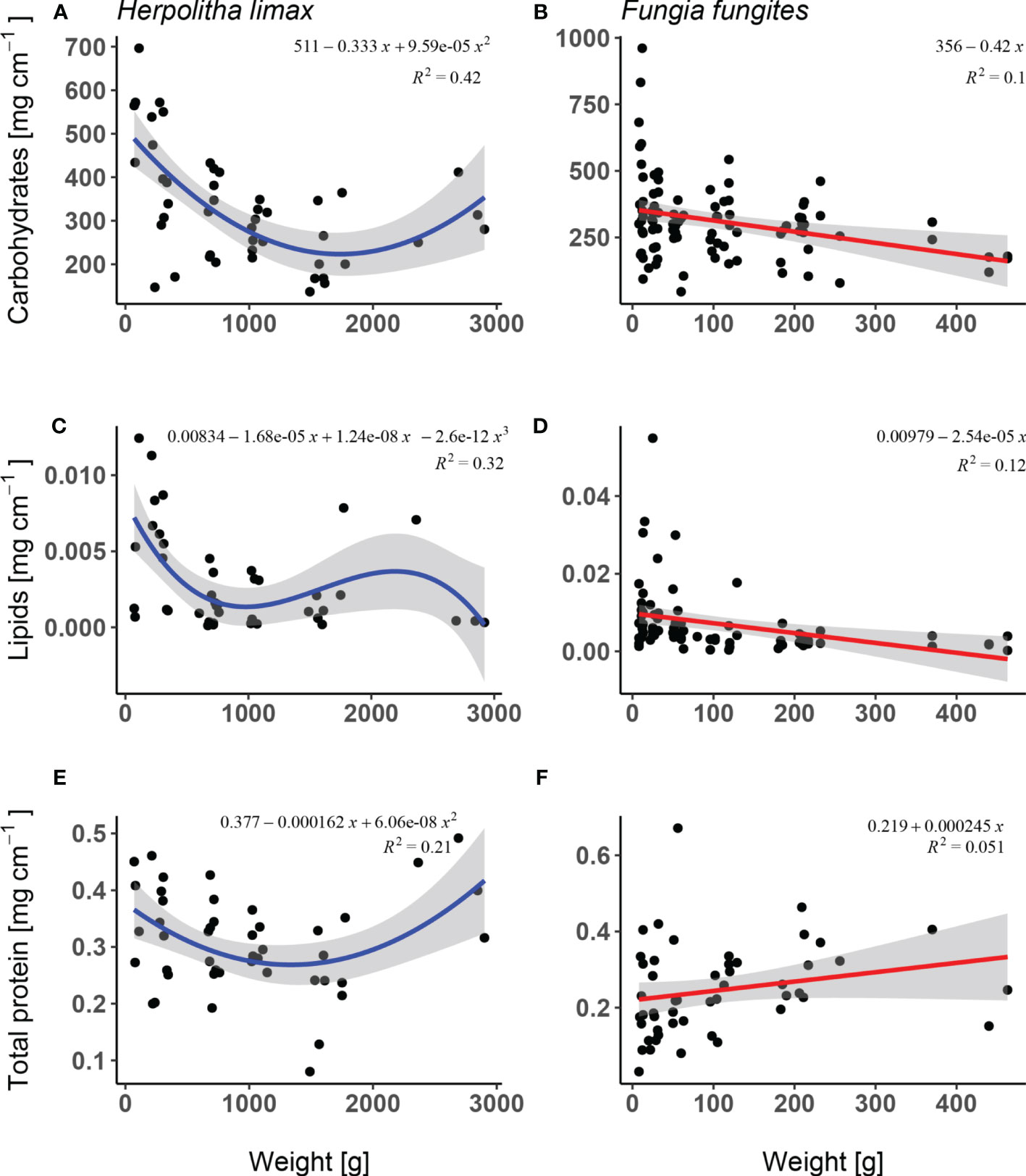
Figure 4 Biochemical content: (i) carbohydrates, (ii) lipids, and (iii) proteins; of: (A, C, E) Herpolitha limax and ( (B, D, F) Fungia fungites as a function of weight (g) sampled at the beginning of July 2015, prior to onset of H. limax reproductive season and in the middle of F. fungites reproductive season.
Lipid concentration in H. limax decreased significantly with increasing weight with a slight increase at intermediately large individuals (Supplementary Table 1; Figure 4C; lm, F3/39 = 6.08, p = 0.002, R2 = 0.32) while F. fungites showed a decreasing lipid concentration with increase in weight (Supplementary Table 1; Figure 4D; lm, F1/77 = 10.42, p = 0.002, R2 = 0.12). In both species the proportion of variance was high.
Protein concentration in H. limax was significantly lower in intermediate sized corals and showed higher values in smallest and largest corals with a moderate proportion of variance (Supplementary Table 1; Figures 4E, F; lm, F2/43 = 5.72, p = 0.006, R2 = 0.21) and showed an increase however statistically non-significant in F. fungites (Supplementary Table 1; Figure 4; lm, F1/48 = 2.59, p = 0.114, R2 = 0.05).
3.4 Relationships between size and sex
The population of H. limax consisted of males, females and a few rare individuals that released male or female gametes in different lunar months within the same reproduction season. A significant difference was found in the sex ratio as a function of weight (Figure 5A; Kruskal-Wallis chi-squared = 21.205, df = 2, p-value = 0). Although sexual dimorphism is not evident in H. limax, a strong bias existed amongst the smallest individuals towards males rather than hermaphrodites and females (Dunn’s Method, Q= 2.697325, P=0.007 and Q= 4.240882, P<0.05, respectively). No significant difference was found in number of females and hermaphrodites in relation to their weight (Dunn’s Method, Q= -0.331621, P=0.74). By contrast, in both Okinawa and the GBR, no significant biases in sex ratios were observed in F. fungites across sizes (Kruskal-Wallis, Okinawa: chi-squared = 3.1984, df = 2, p-value = 0.2, Figure 5B; GBR: chi-squared = 4.8431, df = 2, p-value = 0.09, Figure 5C).
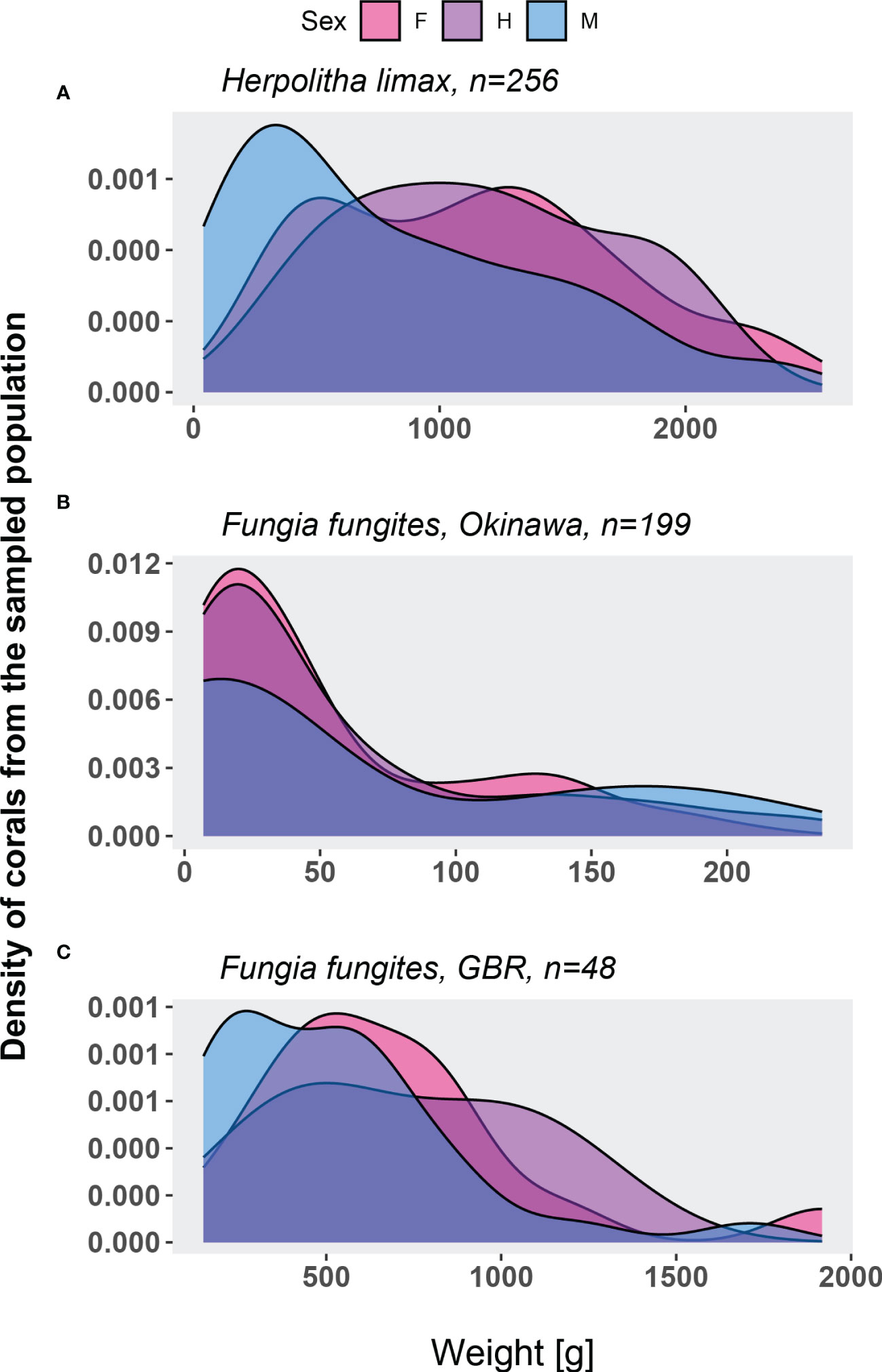
Figure 5 Size-frequency distribution of Herpolitha limax (2014-2017, A) Fungia fungites in Okinawa (2015, B) and Fungia fungites from the GBR (2017, C) individuals for which sex was determined.
3.5 Reproduction of F. fungites on the GBR
Whereas Okinawan F. fungites individuals released planulae daily for a few months, in F. fungites on the GBR spawning occurred 1-5 nights after the full moon in November and on the night of the full moon until day 4 after the full moon in December 2017, with most reproductive individuals spawning in both November and December. A high proportion of individuals spawned between 20:40-22:00, some of the individuals spawned over more than one night and some released either sperm or eggs on different nights. The smallest reproductive individuals were found to be 179 gr (male) and 149 gr (female). Female individuals exhibited a mixed mode of reproduction in which some released unfertilized eggs, some released embryos that were at early stages of development (2 and 4 cell stages), and one individual released both eggs and planulae simultaneously while others released swimming planulae. The predominant reproductive mode differed between months; in November ~85% of the females released eggs, while in December planula release was more common (54%; Figure S2).
Sperm collected and measured under a microscope (n=10) displayed a length of ~ 50 μm and head diameter of 7 μm. Mature eggs were ~150 μm diameter and exhibited green fluorescence when illuminated with blue light. Fertilized eggs developed rapidly, reaching the early planula stage within 9 hours post-fertilization (HPF) (Figure 6).
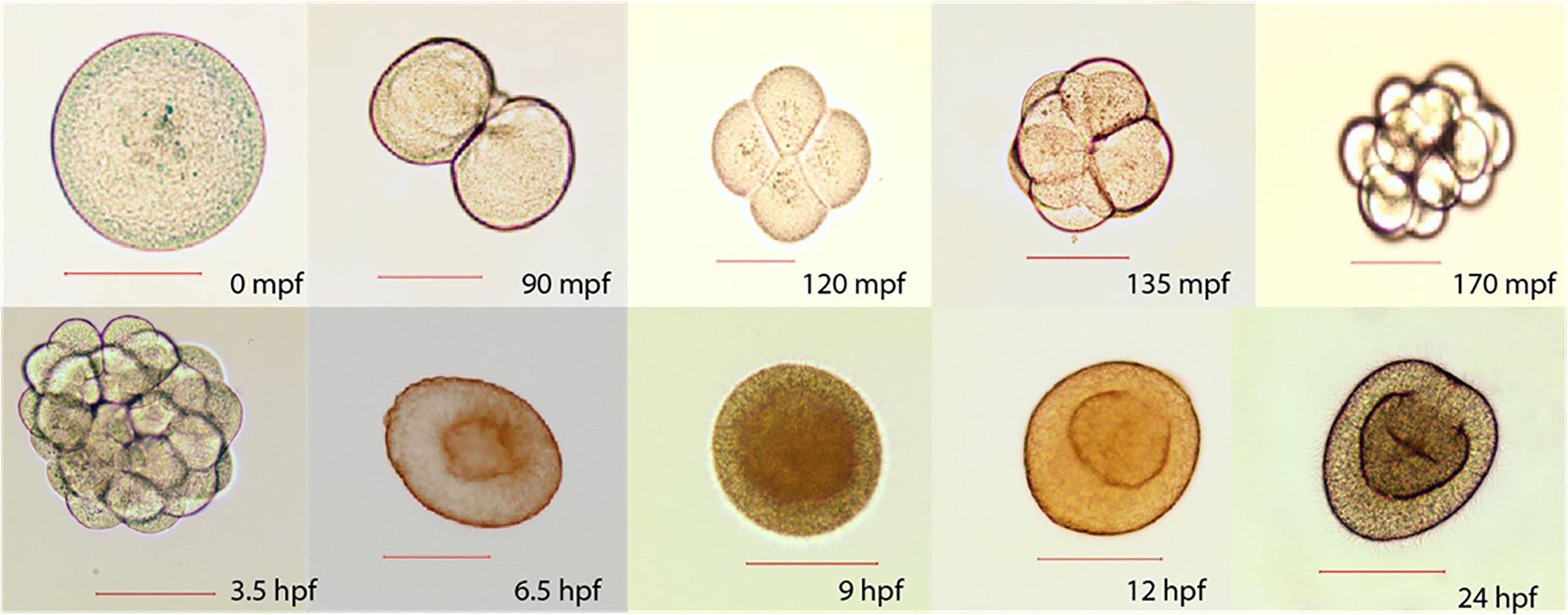
Figure 6 Early development stages sampled at different time points: minutes post-fertilization (mpf) and hours post-fertilization (hpf). 0 mpf: unfertilized egg, 90 mpf: 2 cell stage, 120 mpf: 4 cell stage, 135 mpf: 8 cell stage, 170 mpf: 16 cellstage, 3.5 hpf: 32 cell stage, 6.5 hpf: gastrula, 9 hpf: early planula, 12 hpf: planula mesentery growth, 24 hpf: mature planula. Scale represents 100 µm.
4 Discussion
Life-history traits such as growth, survival, and reproduction, are linked through constraining relationships, implying that adaptation to specific ecological conditions requires compromises (Obeso, 2002), often referred to as trade-offs, between life-history variables (Reznick, 1985). In the case of the solitary corals, F. fungites and H. limax, the size of individuals more reliably reflects their age than is the case of colonial corals (Chadwick-Furman et al., 2000). Consequently, by sampling specimens from a range of sizes, we were able to infer life-history traits from early life to adulthood and study the dynamics of these traits as manifested in these populations.
4.1 The shift in resources allocation from growth to reproduction
In general, we found a large inherent variability in fecundity and growth rates of both species, relative growth rate per individual being highest in the smallest individuals and declining thereafter with increasing size (Figure 1). Consistent with previous work on corals (Johnson, 1992; Goffredo et al., 2004), this relationship indicates that small colonies invest relatively more energy in growth than in other needs. In colonial corals the rapid growth of juveniles may increase the probability of survival while competing with adjacent reef organisms. Because free-living fungiid corals can avoid strong competition by changing their position, for these organisms, early investment in body mass may be driven by the need to reduce the probability of damage by preventing inversion. These high growth rates come at the cost of reduced female reproductive performance; in this case, the smallest individuals display the lowest fecundity (Figure 2). Thus, after heavy early investment in growth at the expense of reproduction, the balance shifts towards reproduction as corals mature.
The ability of both species to undergo bi-directional sex change (Loya and Sakai, 2008) facilitated investigation of the relationships between size, energy investment, and sex of the individuals. According to our findings, H. limax reproduced primarily as males during the early life-history stages (Figure 5). This may be due to the limited energy investment by small corals in reproduction and the higher energetic costs of being a female (Schärer, 2009; Ward, 1995). The increase in the numbers of female H. limax in the intermediate sized corals was associated with a decrease in relative growth, consistent with this shift in resources allocation to female reproduction. Because resource requirements for reproduction differ between the sexes, the expectation is that when resources become limiting during growth, males and females will adjust their allocation to resource-harvesting differently (Tonnabel et al., 2017). While the shift in energy allocation (Figures 2A, B) and biochemical parameters (Figures 4A, C, E) with increasing size was clear in the case of H. limax, F. fungites did not follow the same pattern, either in Okinawa or on the GBR, instead displaying no significant effect of size on the sex ratio (Figures 5 B, C). Presumably this is a consequence of the complex reproductive behaviour of this species.
There are precedents for this: for example, energy allocation towards sexual reproduction varied considerably among hermaphroditic colonies of Stylophora pistillata, in which some colonies with high fecundity becoming male or sterile in subsequent breeding seasons, presumably due to low remaining energy resources (Rinkevich and Loya, 1986). A considerable shift in energy allocation from growth to reproduction has been long suggested to occur at the onset of reproduction at an early life stage in colonial corals (Loya, 1976; Hall and Hughes, 1996; Sakai, 1998). However, some of the results presented here imply that, in the solitary fungiid corals, such shifts in resource allocation towards reproduction at the cost of growth may continue well beyond the juvenile stages.
4.2 Reproductive declines and senescence in corals
Although the topic of coral senescence is controversial, declines in both growth and reproductive output were observed in the largest cohorts of both coral species, potentially as consequences of the physiological deterioration that is typically observed during aging processes. To better understand the physiology underlying growth and reproduction of corals, we investigated the biochemical composition of different sizes of both species. Our results show that levels of lipids decline in larger size individuals in H. limax (Figure 4C), while carbohydrates and protein content show a slight increase in larger corals, showing an opposite pattern to female reproduction (Figures 2A, 4A, E). In F. fungites on the other hand, the decline in both lipid and carbohydrate levels with size increase was significant however weak with no significant change in protein content (Figures 4B, D, F). It appears that energy trade-off between reproduction and other life functions in F. fungites is less defined than in H. limax. One potential explanation is the difference in size between the two species. Large size such as of H. limax, may impose disproportionately high investments of energy in maintenance and repair, reducing the energy available for growth and reproduction. These size/age-specific differences in energy reserves, together with the decline in fecundity and growth at old age, support the hypothesis that senescence occurs in at least some fungiid corals. Rinkevich and Loya (1986) documented decreases in the calcification rate, which began 6-9 months before the death of S. pistillata colonies. These findings, with the results presented here, suggest that a gradual diminution of physiological processes can manifest in old/dying corals, colonial or solitary. How widespread is the relationship observed here between age and energy reserves in corals, awaits quantification of the biochemical composition and physiological status of a representative range of other species.
It should be noted that the lipid contents of both species studied here were significantly higher than values reported in the literature for scleractinian corals (reviewed in Lesser (2013). Although lipid content is thought to vary considerably both within and between coral species (10–40% of dry weight; reviewed in Lesser (2013)), estimates for the two species studied here (average of 80% of dry weight) were exceptionally high in comparison to values reported for other species. Since reproductive material is known to be rich in lipids (Ward, 1995), we assume that these high values are affected, inter alia, by the reproductive state of the corals (the sampling process was performed during the reproduction season). We consider it unlikely, however, that reproductive status is the sole factor underlying this difference (Ward, 1995; Leuzinger et al., 2003), and argue that the solitary nature and structure of fungiid species could be a contributing factor.
It is often assumed that the fecundity of colonial corals increases with age as a result of increased numbers of polyps and/or increased polyp fecundity (Kojis and Quinn, 1984; Szmant, 1986; Harrison and Wallace, 1990; Hall and Hughes, 1996; Sweet et al., 2017), suggesting that only the death of the genet halts this increase. However, exceptions to this (i.e. reproductive senescence) have been documented in both colonial and solitary corals (reviewed in Bythell et al., 2018). Examples of this include remarkable decreases in the rate of reproduction of mature colonies of S. pistillata (Rinkevich and Loya, 1986) and the reduction in fecundity of aged parts of Acropora spp. colonies (Irikawa et al., 2011; Nozawa and Lin, 2014). A decreases in the rate of reproduction with increasing size was also reports in the solitary Lithophyllon repanda, formerly known as Fungia repanda (Loya and Sakai, 2008), suggesting a similar pettern between colonial and solitary corals. Here we have demonstrated a reduction in the energy investment in both the relative growth rate per individual coral and fecundity in the oldest/largest individuals of both H. limax and F. fungites, regardless of their reproductive strategy.
4.3 Energy investment in the next generation
With reproduction known to constitute a major energy consuming trait, the difference in energy allocation towards broadcast spawning or brooding is currently unclear as most species only exhibit one strategy. The brooding reproductive mode is associated with higher recruitment success, enabling replenishment of populations following local disturbance events. Brooded larvae are usually better provisioned and competent to undergo settlement more rapidly than larvae developed from broadcast gametes (Bastidas et al., 2002; Harii and Kayanne, 2003; Nishikawa et al., 2003; Goffredo et al., 2004; Goodbody-Gringley and Putron, 2016) thus avoiding the higher mortality rates to which planktonic stages are often subject. However, the cost of brooding is unknown. With F. fungites in Okinawa known to reproduce as a brooder and on the GBR, as a broadcast spawner, we chose to investigate in depth the reproductive biology of F. fungites from the GBR population in order to compare the energy investment levels of the different strategies. Surprisingly, on the GBR F. fungites individuals exhibited a mixed reproductive mode, female individuals releasing either unfertilized eggs, embryos at an early developmental stage or swimming planulae, sometimes simultaneously from a single (female) individual. In Okinawa, F. fungites released planulae in various developmental stages (Munasik, 1999), a strategy that presumably allows greater variation in dispersal distance (Rinkevich and Loya, 1979). Planulae released by Okinawan F. fungites were noticeably larger than those released by individuals from the GBR (~400 µm and ~150 µm, respectively; Eyal-Shaham et al., 2020, Figure 6), suggesting higher competency for settlement and also higher energetic allocation towards the offspring in the former. It appears that reproductive mode it is not the only factor influencing dispersal and settlement potential. In a previous study, planulae were found to exhibit lower lipid content in response to environmental stressors (Rivest et al., 2017), which is likely to lead to lower buoyancy, affecting the potential distance of dispersal, and planulae ability to settle and successfully metamorphose (Richmond, 1987; Harii et al., 2002; Rivest et al., 2017). In the present study, although lipid content of the larvae was not examined, the increased rate of settlement in planulae of intermediate size corals (Figure 3) suggests high-resource allocation to the female gametes of corals in these sizes, providing the next generation with higher survival chances. A similar settlement experiment on the GBR population could be informative but would require a larger sample size than was used in the present work.
It appears that, during the reproduction season, F. fungites individuals can regulate their investment in reproduction in response to environmental (sperm unavailability) or energetic (energy resources) limitations. The flexibility between external and internal fertilization (i.e. the release of eggs or planula) may also constitute an energy conservation mechanism. However, the energy costs associated with the production of planulae and eggs within the same species are as yet, unknown.
5 Conclusions
In this study, we have described how energy is allocated to different functions over the lifetime of the two fungiid species studied. Our results provide the strongest evidence to date of the well-established life-history trade-offs hypothesis in solitary corals, previously demonstrated only in other colonial species. In addition to changes in growth and reproduction, we document age-specific changes in the biochemical composition and provide further evidence of senescence or aging processes in stony corals. However, given the unique characteristics of fungiid corals, the extent to which conclusions based on fungiids can be generalized to other corals requires follow-up studies.
Data availability statement
The raw data supporting the conclusions of this article will be made available by the authors, without undue reservation.
Author contributions
YL initiated the study. MG, LE-S, DM, and YL designed the experiments. GE and OB-Z assisted in the field and lab work. All authors edited and approved the final version of this manuscript. All authors contributed to the article.
Funding
This research was funded through the Israel Science Foundation (1191/16) and (640/21) to YL and the ARC Centre of Excellence Program (CE140100020) to DJM. LE-S was partially supported by the WDHOF graduate scholarships in marine conservation, the MERCI travel grant, the PADI Foundation and by the Ministry of Science, Technology & Space fellowship.
Acknowledgments
We thank Sesoko Station, Tropical Biosphere Research Center, University of the Ryukyus, Orpheus Island research station and James Cook University for the logistical support. We are indebted to I. Hoskin, T. Shlesinger, Y. Nakano, R. Prasetia, Y. Rosenberg, J. Bergman and T. Eyal for help with the fieldwork in Okinawa and to M. Espinheira, G. Torda, A. Moya, R. Brunner, C. Whalen, I. Cooke, C. Boote and B. Luz for fieldwork assistance on the GBR, Australia. All coral reef samples were collected and treated according to regulations of Okinawa Prefecture, Japan and the GBRMPA permit restrictions.
Conflict of interest
The authors declare that the research was conducted in the absence of any commercial or financial relationships that could be construed as a potential conflict of interest.
Publisher’s note
All claims expressed in this article are solely those of the authors and do not necessarily represent those of their affiliated organizations, or those of the publisher, the editors and the reviewers. Any product that may be evaluated in this article, or claim that may be made by its manufacturer, is not guaranteed or endorsed by the publisher.
Supplementary material
The Supplementary Material for this article can be found online at: https://www.frontiersin.org/articles/10.3389/fmars.2023.1113987/full#supplementary-material
References
Ally D., Ritland K., Otto S. P. (2010). Aging in a long-lived clonal tree. PloS Biol. 8 (8), e1000454. doi: 10.1371/journal.pbio.1000454
Bablet J.-P. (1985). Report on the growth of a scleractinia (Fungia paumotensis). Proc. 5th. Int. Coral. Reef. Congress. 4, 361–366.
Bastidas C., Benzie J. A. H., Fabricius K. E. (2002). Genetic differentiation among populations of the brooding soft coral clavularia koellikeri on the great barrier reef. Coral Reefs 21, 233–241. doi: 10.1007/s00338-002-0236-7
Ben-David-Zaslow R., Benayahu Y. (1999). Temporal variation in lipid, protein and carbohydrate content in the red Sea soft coral heteroxenia fuscescens. J. Mar. Biol. Assoc. United. Kingdom. 79 (6), 1001–1006. doi: 10.1017/S002531549900123X
Benzoni F., Arrigoni R., Stefani F., Reijnen B. T., Montano S., Hoeksema B. W. (2012). Phylogenetic position and taxonomy of cycloseris explanulata and C. wellsi (Scleractinia: fungiidae): lost mushroom corals find their way home. Contributions. to. Zool. 81 (3), 125–146. doi: 10.1163/18759866-08103001
Best D. J., Roberts D. E. (1975). Algorithm AS 89: the upper tail probabilities of spearman's rho. J. R. Stat. Soc. Ser. C. (Applied. Statistics). 24 (3), 377–379. doi: 10.2307/2347111
Bradford M. M. (1976). A rapid and sensitive method for the quantitation of microgram quantities of protein utilizing the principle of protein-dye binding. Analytical. Biochem. 72 (1-2), 248–254. doi: 10.1006/ABIO.1976.9999
Bradshaw C. J. A., McMahon C. R. (2008). “Fecundity,” Encyclopedia of ecology (Second Edition) Ed. Fath B. (Elsevier Inc), 93–101. doi: 10.1016/B978-0-444-63768-0.00645-4
Brown B. E., Dunne R. P., Phongsuwan N., Patchim L., Hawkridge J. M. (2014). The reef coral goniastrea aspera: a ‘winner’becomes a ‘loser’during a severe bleaching event in Thailand. Coral. Reefs. 33 (2), 395–401. doi: 10.1007/s00338-013-1120-3
Buddemeier R. W., Kinzie R. A. (1976). Coral growth. Oceanogr. Mar. Biol. Annu. Rev. 14, 183–225. Available at: https://www.vliz.be/imisdocs/publications/252445.pdf&sa=U&ei=xEprVbXlCIW2oQTnYKoAw&ved=0CCIQFjAD&usg=.
Bythell J. C., Brown B. E., Kirkwood T. B. (2018). Do reef corals age? Biol. Rev. 93 (2), 1192–1202. doi: 10.1111/brv.12391
Chadwick-Furman N. E., Goffredo S., Loya Y. (2000). Growth and population dynamic model of the reef coral fungia granulosa klunzinger 1879 at eilat, northern red Sea. J. Exp. Mar. Biol. Ecol. 249 (2), 199–218. doi: 10.1016/S0022-0981(00)00204-5
Cheplick G. P. (2005). “4 - The allometry of reproductive allocation,” in Physiological ecology, reproductive allocation in plants. Eds. Reekie E. G., Bazzaz F. A. (Academic Press), 97–128. doi: 10.1016/B978-012088386-8/50004-1
Eyal-Shaham L., Eyal G., Ben-Zvi O., Sakai K., Harii S., Sinniger F., et al. (2020). A unique reproductive strategy in the mushroom coral fungia fungites. Coral. Reefs. 39 (6), pp.1793–1804. doi: 10.1007/s00338-020-02004-7
Eyal-Shaham L., Eyal G., Sakai K., Nozawa Y., Harii S., Sinniger F., et al. (2019). Repetitive sex change in the stony coral herpolitha limax across a wide geographic range. Sci. Rep. 9 (1), pp.1–pp12. doi: 10.1038/s41598-018-37619-y
Fadlallah Y. H. (1983). Population dynamics and life history of a solitary coral, balanophyllia elegans, from central California. Oecologia 58 (2), pp.200–pp.207. doi: 10.1007/BF00399217
Foster N. L., Box S. J., Mumby P. J. (2008). Competitive effects of macroalgae on the fecundity of the reef-building coral montastraea annularis. Mar. Ecol. Prog. Ser. 367, 143–152. doi: 10.3354/MEPS07594
Gerrodette T. (1979). Ecological studies of two temperate solitary corals (San Diego: University of California), 112. Available at: https://www.worldcat.org/title/ecological-studies-of-two-temperate-solitary-corals/oclc/9844615.
Goffredo S. (1995). Growth of Ctenactis echinata (Pallas, 1766) and Fungia fungites (Linnaeus, 1758) (Scleractinia, Fungiidae) on a coral reef at Sharm el Sheikh, Red Sea, South Sinai, Egypt Dissertation, University of Bologna.
Goffredo S., Mattioli G., Zaccanti F. (2004). Growth and population dynamics model of the Mediterranean solitary coral balanophyllia europaea (Scleractinia, dendrophylliidae). Coral. Reefs. 23 (3), 433–443. doi: 10.1007/s00338-004-0395-9
Goodbody-Gringley G., Putron S. J. D. (2016). “Brooding corals: planulation patterns, larval behavior, and recruitment dynamics in the face of environmental change,” in The cnidaria, past, present and future (Cham: Springer), 279–289. doi: 10.1007/978-3-319-31305-4_18
Grigg R. W., Maragos J. E. (1974). Recolonization of hermatypic corals on submerged lava flows in Hawaii. Ecology 55 (2), 387–395. doi: 10.2307/1935226
Grinblat M., Cooke I., Shlesinger T., Ben-Zvi O., Loya Y., Miller D. J., et al. (2021). Biogeography, reproductive biology and phylogenetic divergence within the fungiidae (mushroom corals). Mol. Phylogenet. Evol. 164, 107265. doi: 10.1016/j.ympev.2021.107265
Grottoli A. G., Rodrigues L. J., Juarez C. (2004). Lipids and stable carbon isotopes in two species of Hawaiian corals, porites compressa and montipora verrucosa, following a bleaching event. Mar. Biol. 145 (3), 621–631. doi: 10.1007/S00227-004-1337-3
Hall V. R., Hughes T. P. (1996). Reproductive strategies of modular organisms: comparative studies of reef-building corals. Ecology 77 (3), 950–963. doi: 10.2307/2265514
Harii S., Kayanne H. (2003). Larval dispersal, recruitment, and adult distribution of the brooding stony octocoral heliopora coerulea on ishigaki island, southwest Japan. Coral. Reefs. 22 (2), 188–196. doi: 10.1007/s00338-003-0302-9
Harii S., Kayanne H., Takigawa H., Hayashibara T., Yamamoto M. (2002). Larval survivorship, competency periods and settlement of two brooding corals, heliopora coerulea and pocillopora damicornis. Mar. Biol. 141 (1), 39–46. doi: 10.1007/S00227-002-0812-Y
Harii S., Nadaoka K., Yamamoto M., Iwao K. (2007). Temporal changes in settlement, lipid content and lipid composition of larvae of the spawning hermatypic coral acropora tenuis. Mar. Ecol. Prog. Ser. 346, 89–96. doi: 10.3354/MEPS07114
Harrison P. L., Wallace C. C. (1990). Reproduction, dispersal and recruitment of scleractinian corals. Ecosyst. World 25, 133–207.
Hollander M., Wolfe D. A. (1973). Nonparametric statistical methods (New York: John Wiley & Sons). 185–194. (Kendall and Spearman tests). Available at: https://www.rdocumentation.org/packages/stats/versions/3.6.2/topics/cor.test.
Hughes T. P., Jackson J. B. C. (1985). Population dynamics and life histories of foliaceous corals. Ecol. Monogr. 55 (2), 141–166. doi: 10.2307/1942555
Irikawa A., Casareto B. E., Suzuki Y., Agostini S., Hidaka M., van Woesik R. (2011). Growth anomalies on acropora cytherea corals. Mar. pollut. Bull. 62 (8), 1702–1707. doi: 10.1016/j.marpolbul.2011.05.033
Johnson K. G. (1992). Population dynamics of a free-living coral: recruitment, growth and survivorship of manicina areolata (Linnaeus) on the Caribbean coast of Panama. J. Exp. Mar. Biol. Ecol. 164 (2), 171–191. doi: 10.1016/0022-0981(92)90173-8
Kojis B. L., Quinn N. J. (1984). Seasonal and depth variation in fecundity of acropora palifera at two reefs in Papua new Guinea. Coral. Reefs. 3 (3), 165–172. doi: 10.1007/BF00301961
Kozłowski J. (1992). Optimal allocation of resources to growth and reproduction: implications for age and size at maturity. Trends Ecol. Evol. 7 (1), 15–19. doi: 10.1016/0169-5347(92)90192-E
Kozłowski J., Wiegert R. G. (1986). Optimal allocation of energy to growth and reproduction. Theor. Population. Biol. 29 (1), 16–37. doi: 10.1016/0040-5809(86)90003-1
Krueger T., Horwitz N., Bodin J., Giovani M. E., Escrig S., Meibom A., et al. (2017). Common reef-building coral in the northern red Sea resistant to elevated temperature and acidification. R. Soc. Open Sci. 4 (5), 170038. doi: 10.1098/rsos.170038
Lesser M. P. (2013). Using energetic budgets to assess the effects of environmental stress on corals: are we measuring the right things? Coral. Reefs. 32 (1), 25–33. doi: 10.1007/s00338-012-0993-x
Leuzinger S., Anthony K., Willis B. L. (2003). Reproductive energy investment in corals: scaling with module size. Oecologia 136 (4), 524–531. doi: 10.1007/s00442-003-1305-5
Loya Y. (1976). The red Sea coral Stylophora pistillata is an r strategist. Nature 259, 478. doi: 10.1038/259478a0
Loya Y., Sakai K. (2008). Bidirectional sex change in mushroom stony corals. Proc. R. Soc. B.: Biol. Sci. 275 (1649), 2335–2343. doi: 10.1098/rspb.2008.0675
Loya Y., Sakai K., Heyward A. (2009). Reproductive patterns of fungiid corals in Okinawa, japan. galaxea. J. Coral. Reef. Stud. 11 (2), 119–129. doi: 10.3755/galaxea.11.119
Maltby L. (1999). Studying stress: the importance of organism-level responses. Ecol. Appl. 9 (2), 431–440. doi: 10.2307/2641131
Mangiafico S. S. (2015) An R companion for the handbook of biological statistics, version 1.3.9, revised 2023. Available at: https://rcompanion.org/rcompanion/a_02.html.
Masuko T., Minami A., Iwasaki N., Majima T., Nishimura S. I., Lee Y. C. (2005). Carbohydrate analysis by a phenol–sulfuric acid method in microplate format. Analytical. Biochem. 339 (1), 69–72. doi: 10.1016/J.AB.2004.12.001
Motoda S. (1940). The environment and the life of massive reef coral, goniastrea aspera verrill, inhabiting the reef flat in palao. Palao. Trop. Biol. Stn. Stud. 2, 61–104.
Munasik (1999). Sexual reproduction of the solitary coral Fungia fungites in Okinawa. Japan: University of Ryukyus, 37. Available at: https://opac.lib.uryukyu.ac.jp/opc/en/recordID/catalog.bib/RB00395880?hit=3&ca.
Niebuhr D. H. (1999). Environmental stress in hard coral: evaluating lipid as an indicator of sublethal stress on short time scales (The College of William and Mary). doi: 10.25773/v5-sv8z-2g73
Nishikawa A., Katoh M., Sakai K. (2003). Larval settlement rates and gene flow of broadcast-spawning (Acropora tenuis) and planula-brooding (Stylophora pistillata) corals. Mar. Ecol. Prog. Ser. 256, 87–97. doi: 10.3354/meps256087
Nozawa Y., Lin C. H. (2014). Effects of colony size and polyp position on polyp fecundity in the scleractinian coral genus acropora. Coral. Reefs. 33 (4), 1057–1066. doi: 10.1007/S00338-014-1185-7
Obeso J. R. (2002). The costs of reproduction in plants. New Phytol. 155 (3), 321–348. doi: 10.1046/J.1469-8137.2002.00477.X
Pratchett M. S., Anderson K. D., Hoogenboom M. O., Widman E., Baird A. H., Pandolfi J. M., et al. (2015). Spatial, temporal and taxonomic variation in coral growth–implications for the structure and function of coral reef ecosystems. Oceanogr. Mar. Biol.: Annu. Rev. 53, 215–295. doi: 10.1201/B18733-7
R Core Team (2020). “A language and environment for statistical computing,” in R foundation for statistical computing. Vienna, Austria. Available at: http://www.r-project.org.
Reznick D. (1985). Costs of reproduction: an evaluation of the empirical evidence. Oikos 44 (2), 257–267. doi: 10.2307/3544698
Richmond R. H. (1987). Energetics, competency, and long-distance dispersal of planula larvae of the coral pocillopora damicornis. Mar. Biol. 93 (4), 527–533. doi: 10.1007/BF00392790
Rinkevich B., Loya Y. (1979). The reproduction of the red Sea coral Stylophora pistillata. i. gonads and planulae. Mar. Ecol. Prog. Ser. 1, 133–144. doi: 10.3354/meps001133
Rinkevich B., Loya Y. (1985). Intraspecific competition in a reef coral: effects on growth and reproduction. Oecologia 66 (1), 100–105. doi: 10.1007/BF00378559
Rinkevich B., Loya Y. (1986). Senescence and dying signals in a reef building coral. Experientia 42 (3), 320–322. doi: 10.1007/BF01942521
Rivest E. B., Chen C. S., Fan T. Y., Li H. H., Hofmann G. E. (2017). Lipid consumption in coral larvae differs among sites: a consideration of environmental history in a global ocean change scenario. Proc. R. Soc. B.: Biol. Sci. 284 (1853), 20162825. doi: 10.1098/rspb.2016.2825
Rodrigues L. J., Grottoli A. G. (2007). Energy reserves and metabolism as indicators of coral recovery from bleaching. Limnol. Eceanogr. 52 (5), 1874–1882. doi: 10.4319/lo.2007.52.5.1874
Sakai K. (1998). Delayed maturation in the colonial coral Goniastrea aspera (Scleractinia): whole-colony mortality, colony growth and polyp egg production. Population. Ecol. 40 (3), 287–292. doi: 10.1007/BF02763460
Schaffer W. M. (1983). The application of optimal control theory to the general life history problem. Am. Nat. 121 (3), 418–431. doi: 10.1086/284070
Schärer L. (2009). Tests of sex allocation theory in simultaneously hermaphroditic animals. Evolution 63 (6), 1377–1405. doi: 10.1111/j.1558-5646.2009.00669.x
Silvertown J., Franco M., Perez-Ishiwara R. (2001). Evolution of senescence in iteroparous perennial plants. Evol. Ecol. Res. 3 (4), 393–412.
Stearns S. C. (1992). The evolution of life histories Vol. 249 (Oxford: Oxford university press), xii. doi: 10.1046/j.1420-9101.1993.6020304.x
Stephenson T. A., Stephenson A. (1933). Growth and asexual reproduction in corals. British museum. Expedition 1928–29, 167–217.
Sweet M. J., Brown B. E., Dunne R. P., Singleton I., Bulling M. (2017). Evidence for rapid, tide-related shifts in the microbiome of the coral coelastrea aspera. Coral. Reefs. 36 (3), 815–828. doi: 10.1007/s00338-017-1572-y
Szmant A. M. (1986). Reproductive ecology of Caribbean reef corals. Coral. Reefs. 5 (1), 43–53. doi: 10.1007/BF00302170
Tamura T., Hada Y. (1932). Growth rate of reef building corals inhabiting in the south Sea island. Sci. Rep. Tohoku. Imp. Univ.(Ser 4) 7, 433–455.
Tanner J. E. (1995). Competition between scleractinian corals and macroalgae: an experimental investigation of coral growth, survival and reproduction. J. Exp. Mar. Biol. Ecol. 190 (2), 151–168. doi: 10.1016/0022-0981(95)00027-O
Tanner J. E. (1997). Interspecific competition reduces fitness in scleractinian corals. J. Exp. Mar. Biol. Ecol. 214 (1-2), 19–34. doi: 10.1016/S0022-0981(97)00024-5
Tonnabel J., David P., Pannell J. R. (2017). Sex-specific strategies of resource allocation in response to competition for light in a dioecious plant. Oecologia 185, 675–686. doi: 10.1007/s00442-017-3966-5
Tytler P., Calow P. (1985). Fish energetics: new perspectives (Springer Science & Business Media). doi: 10.1007/978-94-011-7918-8
Veal C. J., Carmi M., Fine M., Hoegh-Guldberg O. (2010). Increasing the accuracy of surface area estimation using single wax dipping of coral fragments. Coral. Reefs. 29 (4), 893–897. doi: 10.1007/S00338-010-0647-9
Ward S. (1995). Two patterns of energy allocation for growth, reproduction and lipid storage in the scleractinian coral pocillopora damicornis. Coral. Reefs. 14 (2), 87–90. doi: 10.1007/BF00303428
Weiner J. (2004). Allocation, plasticity and allometry in plants. Perspect. Plant Ecol. Evol. Syst. 6 (4), 207–215. doi: 10.1078/1433-8319-00083
Willis B. L., Babcock R. C., Harrison P. L., Oliver J. K., Wallace C. C. (1985). Patterns in the mass spawning of corals on the great barrier reef from 1981 to 1984. Proc. Of. Fifth. Int. Coral. Reef. Congress. 4, 343–348.
Yamashiro H., Nishihira M. (1998). Experimental study of growth and asexual reproduction in diaseris distorta (Michelin 1843), a free-living fungiid coral. J. Exp. Mar. Biol. Ecol. 225 (2), 253–267. doi: 10.1016/S0022-0981(97)00229-3
Keywords: reproductive allocation, senescence, sex-allocation, biochemical profiles, fecundity, Fungia fungites, Herpolitha limax
Citation: Grinblat M, Eyal-Shaham L, Eyal G, Ben-Zvi O, Harii S, Morita M, Sakai K, Hirose M, Miller DJ and Loya Y (2023) Energy allocation trade-offs as a function of age in fungiid corals. Front. Mar. Sci. 10:1113987. doi: 10.3389/fmars.2023.1113987
Received: 02 December 2022; Accepted: 25 May 2023;
Published: 14 June 2023.
Edited by:
Cliff Ross, University of North Florida, United StatesReviewed by:
Will Ryan, Towson University, United StatesDaniel Pech, El Colegio de la Frontera Sur, Mexico
Copyright © 2023 Grinblat, Eyal-Shaham, Eyal, Ben-Zvi, Harii, Morita, Sakai, Hirose, Miller and Loya. This is an open-access article distributed under the terms of the Creative Commons Attribution License (CC BY). The use, distribution or reproduction in other forums is permitted, provided the original author(s) and the copyright owner(s) are credited and that the original publication in this journal is cited, in accordance with accepted academic practice. No use, distribution or reproduction is permitted which does not comply with these terms.
*Correspondence: Yossi Loya, eW9zaWxveWFAZ21haWwuY29t
†These authors share first authorship