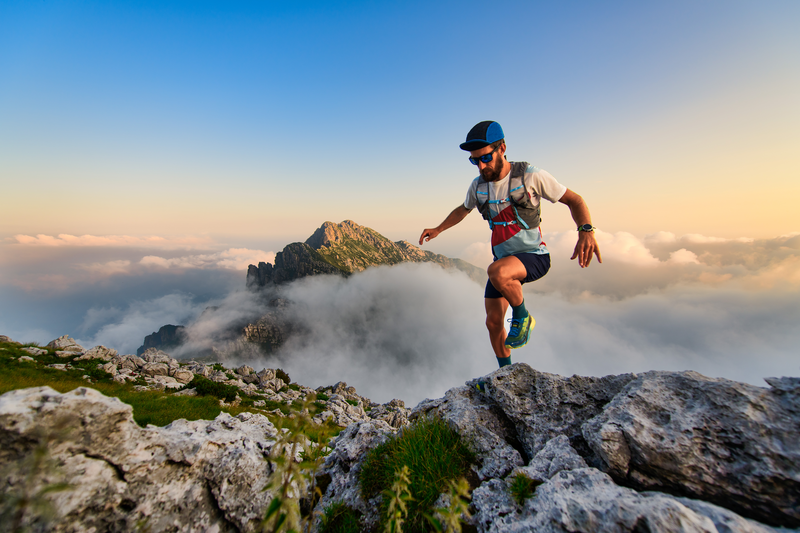
95% of researchers rate our articles as excellent or good
Learn more about the work of our research integrity team to safeguard the quality of each article we publish.
Find out more
ORIGINAL RESEARCH article
Front. Mar. Sci. , 02 May 2023
Sec. Deep-Sea Environments and Ecology
Volume 10 - 2023 | https://doi.org/10.3389/fmars.2023.1111249
This article is part of the Research Topic 16th Deep-Sea Biology Symposium View all 27 articles
Whale carcasses create habitats in the deep sea which are colonized by organisms related to other chemosynthetic environments suggesting that whale falls may act as intermediate refugia for the dispersal and evolution of deep-sea organisms. Such evidence comes mainly from macrofaunal organisms whereas for the smaller meiofauna, data on whalebone assemblages is lacking. In this study, we investigated nematode colonization of whalebones experimentally deployed at 1500 and 3300 m depth and bones from a natural whale carcass found at 4204 m, in the Southwest Atlantic Ocean, off Brazil. By comparing whalebone assemblages to other deep-sea environments, we tested the hypothesis that whale fall nematode assemblages are more similar to those from other cognate chemosynthetic-based habitats, rather than those from sediments surrounding the carcass or other deep-sea habitats. Our results showed that whalebone nematode assemblages resemble those of hydrothermal vents and cold seeps and suggest that organic falls may act as intermediate refugia for meiofauna from chemosynthetic environments. It also showed that oceanographic conditions and the age of the carcasses on the ocean floor could influence nematode assemblage composition and richness. Such findings highlight the importance of organic falls for understanding the connectivity and phylogeny of benthic organisms, including representatives of the meiofauna.
Large whale carcasses create habitats in the deep sea which harbor high species richness and abundance of organisms that live on and inside bones and in surrounding sediments (Smith and Baco, 2003; Smith et al., 2015; Alfaro-Lucas et al., 2017). These are dynamic habitats that pass through different ecological successional stages harboring different faunal assemblages during the decaying carcass process (Smith and Baco, 2003). Particularly during the ‘sulfophilic stage’ (Smith and Baco, 2003), when the production of chemically-reduced compounds supports chemosynthesis (e.g., H2S, CH4), whale falls hold similarities in faunal composition with other deep-sea chemosynthesis-based ecosystems, such as hydrothermal vents and cold seeps (reviewed in Smith et al., 2015). Such discovery of chemolithoautotrophic communities as well as some vent/seep species at whale falls suggested a potential ecological role as intermediate refugia for the dispersal and evolution of sulfide-dependent species in the deep sea (Smith et al., 1989; Smith and Baco, 2003, Fujiwara et al., 2010). Under this view, whale falls facilitate dispersal between remote, isolated vent or seep habitats, expanding species geographic ranges and promoting connectivity among deep-sea chemosynthesis-based communities (Smith and Baco, 2003; Smith et al., 2015; Pereira et al., 2020). As a result, faunal overlaps are observed worldwide across whale falls, vents and seeps communities (Smith et al., 2014; Hilário et al., 2015; Sumida et al., 2016; Smith et al., 2017; Shimabukuro et al., 2019; Pereira et al., 2020).
The distribution patterns described for taxa colonizing whale falls are hitherto based mainly on data from macrofaunal taxa such as polychaetes, bivalves, and gastropods (Smith et al., 2002; Hilário et al., 2015; Smith et al., 2015; Sumida et al., 2016; Souza et al., 2021, Shimabukuro et al., 2022). Although smaller taxa such as nematodes also contribute significantly to the standing stock of these communities, data regarding their species composition and assemblage structure is still lacking (Debenham et al., 2004; Pavlyuk et al., 2009; Alfaro-Lucas et al., 2017). The connectivity between populations of macrofaunal taxa inhabiting whale falls and other chemosynthetic environments is mediated by the dispersal of pelagic larval stages (Siegel et al., 2003). Differently from most macrofaunal taxa, free-living marine nematodes lack pelagic larvae (Ptatscheck and Traunspurger, 2020) and exhibit low swimming ability limiting the active dispersal of these organisms. Thus, large-scale dispersal of nematodes is hypothesized to occur mainly passively through ocean currents, which can resuspend sediments and transport juveniles and adults (Palmer, 1988; Boeckner et al., 2009; Van Gaever et al., 2009). Indeed, the distribution patterns of some nematode species support the idea of long-distance dispersal (Ptatscheck and Traunspurger, 2020) and experimental studies have shown nematode active settlement into selected sites, including sulfidic substrates (Ullberg and Ólafsson, 2003; Lins et al., 2013).
In this study, we investigated the colonization by nematodes of whalebones which were experimentally deployed at 1500 and 3300 m depth and bones from a natural whale carcass found at 4204 m, in the Southwest Atlantic Ocean, off Brazil (Sumida et al., 2016; Souza et al., 2021, Shimabukuro et al., 2022). We have further compared whalebone nematode assemblages with those from adjacent sediments and other deep-sea environments, including hydrothermal vents and cold seeps. Specifically, we tested the hypothesis that whale-fall nematode assemblages are more similar to those from other cognate chemosynthetic-based ecosystems, rather than those from adjacent sediments, considering the assumption that whale falls may act as intermediate refugia for the dispersal of nematodes inhabiting chemosynthetic environments. Additionally, if the hydrodynamic conditions are important factors in dispersal and colonization, we expect higher similarity within assemblages from similar depths (i.e., bathyal vs. abyssal).
Experimental whalebones were deployed along the Southwest Atlantic margin between 21° and 28°S at two different depths, 1500 and 3300 meters (bathyal and abyssal, respectively) - Project BioSuOr (Figure 1) (Table S1) using autonomous structures (landers). The landers consisted of triangular metallic structures containing on each face three open boxes covered with 500µm mesh with an open PVC lid, in a prism shape. The vertebrae were removed and frozen at -20°C until the deployment. Each vertebra was cut in two, and one half of the vertebra was placed inside a mesh box and the other half on the inner lid totaling six whalebone samples for each lander. Since the bone processing for endofauna analysis requires a long time, only one quarter of one vertebra from each lander was considered for this study (please see item 2.2. sample processing). The other two boxes on each side of the lander were filled with wood and synthetic substratum in a similar fashion (half in the box, half on the lid) but were also not considered in this study (Saeedi et al., 2019; Souza et al., 2021). Acoustic releasers and glass floats were installed for retrieval (more details about landers and experimental setup can be found in Saeedi et al., 2019 and Souza et al., 2021).
Figure 1 Study area where landers were deployed at ~1500 and ~3300 m depth, off SE Brazilian continental margin, in front of São Paulo, Rio de Janeiro and Espírito Santo states during the Project BioSuOr. ES = Espírito Santo; RJ = Rio de Janeiro; SP = São Paulo. RJ-1500 lander was lost. The natural whale-fall samples were collected during the Iatá-Piúna Project with the HOV Shinkai 6500 at 4204 m depth.
The deployment cruise was conducted between May 28 and June 6, 2013, aboard the R/V Alpha-Crucis. The lander SP-1500 was recovered after 16 months, on October 9, 2014, aboard the R/V Alpha Delphini, while the remaining landers were sampled after 23 months (between May 18 and 28, 2015) aboard the R/V Almirante Maximiano. The lander RJ-1500 was not recovered due to a battery release failure. After the recovery, the vertebrae were divided in quarters and preserved in 4% formaldehyde. We also analyzed vertebrae from a natural whale fall found at São Paulo Ridge at 4204 meters depth (abyssal), during the Iatá-Piúna Expedition in the SW Atlantic, using the HOV Shinkai 6500. The latter sample was preserved in 99.5% ethanol (Sumida et al., 2016).
From each site, one quarter of one of the three vertebrae which had been deployed within the lander was processed for meiofauna analysis. Each bone sample (i.e. one quarter of one vertebra) was divided into smaller pieces and washed through a 45 µm sieve. From the retained material, meiofauna was extracted by flotation in a colloidal silica solution (Ludox TM50; density: 1.18 g cm-3), and stained with Rose of Bengal. After the flotation procedure, there was still a great volume of bone remains making the sorting process unfeasible, so that subsampling was necessary. As such, each sample was homogenized in 400 ml of water and then a 1 ml aliquot was sub-sampled for counting and identification of nematode individuals. The process was repeated as many times as necessary until 200 nematode individuals were sampled for identification at the genus level. Total numbers of individuals per sample were estimated from the aliquots considering the total volume of the homogenized sample. The effectiveness of the subsampling method was tested by counting an entire sample and comparing it to the density obtained by subsampling. Results of successive subsampling of the 1 ml aliquots were also compared to check the variation between subsamples. Values from six successive subsamples showed very little variation (mean value: 45 ind.10ml-1; standard deviation: 6 individuals). The same subsampling process was repeated for all experimental whalebone samples analyzed in this study. After subsampling, nematodes were picked out, evaporated to anhydrous glycerol, and mounted on permanent slides for identification to genus level. Data on nematodes densities were standardized to 100 cm3 in order to compare bones from the different stations.
Nematode assemblages from whalebones were compared with those from sediments adjacent to the lander at SP-1500 at the Santos Basin slope (25°S and 45°W), and for this purpose we used data collected by the - Santos Project – Santos Basin Environmental Characterization (Brazil), coordinated by PETROBRAS/CENPES (sites D9 and D10, Gallucci et al. in press) (Table S2). These sediment samples were obtained through box corer and meiofauna was sub-sampled with a 5 cm diameter and 10 cm height corer, and fixed with 10% buffered formalin. Samples were washed through a 45 µm sieve and from each sample, 200 individuals were identified to genus level.
A similarity matrix was built from multivariate data on genera abundances to test whether the assemblages are more similar within the same depth. We have built a distance matrix on Hellinger-transformed nematode abundances and ran a hierarchical cluster analysis using the Ward algorithm to compare the similarity between nematode assemblages from deployed bones, from different sites, and the natural whale fall. Hellinger is an appropriate transformation for abundance species data because it is an asymmetric distance measure that properly deals with the “double-zeros” in the comparison of pairs of sites and reduces the influence of most abundant taxa (Borcard et al., 2011). The Ward algorithm favors clusters internal coherence while maximizing the dissimilarities between them being an appropriate choice for finding coherent groups (Kreft and Jetz, 2010; Borcard et al., 2011). We computed a heatmap of nematode genera to visualize their contribution to each whalebone assemblage. Distance matrices were computed using the function vegdist (Legendre and Gallagher, 2001), and with cluster analysis using the Ward algorithm, using the function hclust and the argument methods= “ward.D2” (Murtagh and Legendre, 2014). All analyses were performed using vegan package in R version 4.1.3 (Oksanen et al., 2019; R Core Team, 2020). For each sample, data were extrapolated for its bone volume (Table S1).
Similarly, we also compared the average relative abundances of deployed whalebones and natural whale-fall nematode genera to those of adjacent sediments (Santos slope), and other deep-sea environments such as the continental shelf, continental slope, abyss, nodules, corals, canyons, cold seeps, hydrothermal vents, seamounts, and trenches. We used data from adjacent sediments at Santos slope (Gallucci et al. in press) and data reported in Vanreusel et al. (2010). For the Santos slope, we considered data of all genera which showed relative abundance higher than 2%. For all other deep-sea environments, the genera which were dominantly responsible for the similarities within habitats and the dissimilarity between macrohabitats and the slope habitat based on a SIMPER analysis, (see Table 3 of Vanreusel et al., 2010), were considered. Distance matrices were built using Hellinger distance transformation as described previously. Finally, similarity percentage analysis (SIMPER) was performed to identify which genera were most responsible for differences between non-chemosynthetic and chemosynthetic habitats observed in the cluster analysis, using the software PRIMER (version 6) (Clarke and Gorley, 2006).
Nematode densities varied between 8.8 ind/100 cm3 (SP 3300) and 1093.8 ind/100 cm3 (ES 1500) in the deployed whale bones, while it was more than twice in the natural carcass when compared to ES 1500 sample (2832 ind/100 cm3) (Table 1). A total of 11 nematode genera were found in all whalebone samples, with four genera in each 1500 m depth lander, and five genera in each ~3300 m depth landers, accounting for six and seven genera at each depth respectively (Table 1). Halomonyhystera was the dominant genus at almost all experimentally sampled stations, representing from 41% to 93.8% of the total abundance per site, except for ES-3300, where Anticoma was the predominant genus (~75%). At the natural carcass, Anticoma and Theristus were the dominant genera (~39% and 56%) respectively (Table 1).
Table 1 Densities of nematode genera (ind./100 cm3), total nematode density per vertebra sample and genus richness (number of genera per vertebra sample) found at landers and the natural whale fall.
Nematode assemblages clustered according to depth (Figure 2A), except for the bones deployed at SP-3300 that showed an intermediate position between the two clusters. This separation was mainly due to the high dominance of the genus Halomonhystera at 1500 m depth (90% of total nematode abundance) and the exclusive occurrence of the genera Chromadorita, Microlaimus, and Prismatolaimus at 3300 m depth (Figure 2B). ES-1500 and SP-1500 were the most similar assemblages. Surprisingly, SP-3300, was more similar to the 1500 stations, and was also dominated by Halomonhystera (as landers at 1500 m depth), but shared the exclusive genera found in landers at 3300 m (Figure 2B). Assemblages from the natural carcass (4204 m depth) did not group with any of the experimentally deployed bones (Figure 2A), and were dominated by the genus Theristus, which was exclusive to the natural carcass (Figure 2B).
Figure 2 (A). Hierarchical cluster analysis using Ward algorithm on the Hellinger distance of nematode genera abundances found in landers and the natural whale fall. (B). Heat map of the ordered relative abundance of nematode genera coupled with the cluster analysis. Light colors indicate lower values and dark colors indicate higher values.
When compared to the other deep-sea environments, whalebone assemblages of this study (deployed bones + natural whale fall) clustered with assemblages from hydrothermal vents and cold seeps (Figure 3). Infaunal assemblages from the slope at Santos Basin (Santos slope) were grouped with other non-chemosynthetic-based habitats (Figure 3). Halomonhystera, Thalassomonhystera and Anticoma were the most responsible for the dissimilarity between non-chemosynthetic and chemosynthetic habitats (whalebones, hydrothermal vents and cold seeps) (Table 2).
Figure 3 Hierarchical cluster analysis using Ward algorithm on the Hellinger distance of relative nematode genera abundances found in this study (lander and whale fall pooled data, “Whalebones”) and sediments samples adjacent from the study area (“Santos Slope”), with other deep-sea environments. Data of different deep-sea environments were obtained from Vanreusel et al. (2010).
Table 2 SIMPER analysis of the main nematode genera responsible for the dissimilarity between non-chemosynthetic and chemosynthetic habitats, according to Euclidean distance.
Few studies have assessed the meiofauna associated with deep-sea whale falls (Debenham et al., 2004; Pavlyuk et al., 2009), and even fewer have studied the assemblages colonizing inner bone matrices (Alfaro-Lucas et al., 2017). Our study supports previous findings and shows that free-living nematodes colonize the inner bone matrix showing high densities (up to 1093.8 ind/100cm3, compared to 285 ± 135 ind/100cm3 in adjacent sediments, data not shown). In addition, this study shed light for the first time on whalebone nematode assemblage’s composition and genus richness. In that respect, our results highlight that whale falls shelter less genus richness in comparison with sedimentary deep-sea habitats resembling those assemblages of cognate, chemosynthetic-based environments with dense populations dominated by few species (Vanreusel et al., 2010). Nonetheless, by harboring a distinct nematode community composition compared to surrounding sediments, whale falls increase the turnover of species in deep-sea environments, contributing to the β-diversity of the deep ocean as shown for other taxa (Smith et al., 2015).
In our study, nematode assemblages from whalebones were highly dissimilar between depths (bathyal landers at 1500 vs. abyssal landers at 3300 m depth). Bathymetric variation in nematode assemblages of soft sediments is usually related to sediment characteristics, as well as its physico-chemical properties, physical disturbance, and most importantly, to food availability (Soltwedel, 2000; Rex et al., 2006; Zeppilli et al., 2012; Rosli et al., 2018). At Santos Basin, nematode densities decrease with increasing depth (Gallucci et al. in press), and from the upper to lower slope there is a gradual change in assemblage multivariate structure, although genus composition remains similar within this range of bathymetric change (Yaginuma et al., in prep.). Such bathymetric variations could partly explain the dissimilarity in nematode assemblages from bones deployed at different depths, except from the fact that nematode genera encountered on sediment samples (Table S2; Yaginuma et al., in prep.) were absent from bone samples and vice-versa (Halomonhystera, the dominant genus in the whalebones, was absent in sediment samples). Such results strongly suggest that the colonization of bones takes place by genera that disperse from other habitats, potentially through the water column, rather than the infaunal assemblages from surrounding sediments. If this is true, the observed differences may arise due to the role of the different oceanic currents influencing both depths.
Previous studies suggested that hydrodynamic conditions may influence macrofaunal composition in wood- and whale falls in the deep SW Atlantic Ocean (Saeedi et al., 2019; Shimabukuro and Sumida, 2019; Shimabukuro et al., 2019; Souza et al., 2021; Shimabukuro et al., 2022). The North Atlantic Deep Water (NADW), which is transported southwards by the Deep Western Boundary Current (DWBC), was the main water source at 1500 m depth (Silveira et al., 2020). The abyssal RJ-3300 and ES-3300 landers were most likely in a mixing zone between the NADW and the Antarctic Bottom Water (AABW) associated with a much slower northward transport (Reid, 1989; Mémery et al., 2000; Silveira et al., 2020). The more intense currents influencing the shallow landers may have led to the higher similarity observed at assemblages at 1500 m depth. Such relationships between assemblages and currents give support to the importance of passive dispersal and colonization of new habitats for nematodes (Boeckner et al., 2009). Other depth-related factors, such as the food availability, could influence the different colonization of nematodes on whale bones. However, it is plausible that the establishment of contrasting assemblages between depths, found in this study, may have the influence of passive dispersion through the currents and colonization of specific habitats, such as whale falls.
Assuming the evidence that currents drive nematode colonization on whalebones we should expect a larger similarity between RJ-3300/ES-3300 and SP-3300 than shallower bathyal sites, since the latter site is under DWBC control. However, this study shows that SP-3300 nematode assemblage is more related to bathyal sites than to RJ-3300/ES-3300. This might be related to the presence of the bone-eating worm Osedax at SP-3300 and the bathyal sites (Shimabukuro and Sumida, 2019). Osedax is an ecosystem engineer of bone assemblages controlling whale fall longevity and increasing microhabitat availability within bones (Alfaro-Lucas et al., 2017). Although bones from all sites were colonized by Osedax, it occurred in lower abundances at RJ-3300 and ES-3300, with only a few eroded areas, contrasting with slightly higher density, big animals, and crumbled areas found in shallow bathyal sites and at SP-3300 (Shimabukuro and Sumida, 2019). Nematodes colonize bones either with or without Osedax, but its presence increases the density of fauna living within the bone matrix (Alfaro-Lucas et al., 2017), and our results suggest that they might facilitate the colonization of Halomonhystera which could explain the dominance of this genus in SP-3300, SP-1500 and ES-1500. Moreover, the assemblages present in bones from the natural carcass found at 4204 meters depth were also under the influence of the Antarctic Bottom Water (AABW) and even though they shared some genera with assemblages from experimentally deployed bones, particularly those at 3300 meters depth (Figure 2B), they were grouped separately. This might be partly due to the different colonization stages of experimentally deployed bones (16-23 months) and the natural carcass (estimated at 60-120 months, Sumida et al., 2016) which can influence the composition of whale fall communities (Smith and Baco, 2003; Braby et al., 2007; Lundsten et al., 2010). Furthermore, vertebrae of the natural carcass were smaller, caudal vertebrae belonging to a juvenile minke whale (Balaenoptera bonaerensis) potentially influencing the nematode community composition (Higgs et al., 2011).
The comparison of nematode assemblages inhabiting whale bones with those present in other deep-sea environments highlights whale-fall assemblage’s similarities with those from chemosynthetic-based habitats, such as hydrothermal vents and cold seeps. The similar reducing conditions (low oxygen and the presence of reduced compounds) between chemosynthetic-based habitats, including whale falls during the sulfophilic stage, could lead to convergent community composition (Smith and Baco, 2003). The predominant genus in whalebones was Halomonhystera, which is known to be tolerant to low oxygen and high sulfide concentrations (Van Gaever et al., 2009) and often dominate nematode assemblages at hydrothermal vents and cold seeps (Vanreusel et al., 2010; Zeppilli et al., 2015). This genus was also found in sunken wood and other carcasses where high organic matter degradation occurs (Cuvelier et al., 2014; Zeppilli et al., 2015; Soltwedel et al., 2017). Halomonhystera is usually in association with bacterial mats, typical of these ecosystems (Van Gaever et al., 2006) and, apparently, has important adaptations to these reducing environments such as the ovoviviparity, which is used as a reproductive strategy for securing the survival and development of the brood in anoxic and sulphidic deep-sea habitats (Van Gaever et al., 2006; Grzelak and Kotwicki, 2016). The similarity between whalebone, hydrothermal vent, and cold seep assemblages suggests the same selective forces drive colonization of these environments and whale falls could act as intermediate refugia for dispersal and evolution of deep-sea fauna relying on chemosynthetic production (Smith et al., 1989; Smith and Baco, 2003). Even though marine nematodes lack pelagic larvae, passive dispersion is an important strategy for long-distance dispersal in the deep sea (Palmer, 1988; Boeckner et al., 2009; Lins et al., 2013). Indeed, our results suggest that short-scale active dispersal from adjacent sediments is not important in whalebone nematode colonization.
The present study showed that nematodes colonize whalebones in relatively high density and genera composition corroborated our hypothesis that whale-fall nematode assemblages are more similar to those from other cognate chemosynthetic-based habitats, rather than those from adjacent sediments. Considering only the whalebone assemblages, we observed higher similarity within assemblages from similar depths (i.e., bathyal vs. abyssal), as well as within experimentally deployed bones compared to the natural carcass encountered in the abyssal plain. These results suggest that oceanographic conditions and the age of the carcasses on the ocean floor might influence nematode assemblage composition and richness. Our findings highlight the potential importance of organic falls for meiofauna from chemosynthesis-based ecosystems.
The original contributions presented in the study are included in the article/Supplementary Material. Further inquiries can be directed to the corresponding author.
AA: conceptualization, investigation, methodology, and writing – original draft; MS: designed the study, supervision, writing – review and editing; DC: methodology and sample processing and writing – review and editing; JA-L: data analysis and writing– review and editing; PS: conceptualization, designed the study, funding acquisition, methodology, project administration, resources, supervision, and writing – review and editing; FG: conceptualization, methodology, resources, supervision, and writing – review and editing. All authors contributed to the article and approved the submitted version.
This study was funded by BIOTA-FAPESP grant number 2011/50185-1 to PS. AA was supported by FAPESP scholarships 2019/ 18526-5 and 2022/00411-0. MS was supported by CAPES/Proex PhD scholarship. DC received an undergraduate scholarship from Santander Universidades/PRG-USP.
We would like to thank the master’s and crews of the R/V Alpha-Crucis and R/V Alpha Delphini (IOUSP) during the deployment and the recovery of SP-1500 lander and to the Brazilian Navy and the Master and crew of PRV Almirante Maximiano for the help during the recovery of the other landers. We would like to deeply thank Francisco L.V. Neto and staff of LIO/IOUSP for their assistance in setting up landers and during the deployment cruise. We are deeply grateful to Dr. Hiroshi Kitazato, who was the leader of the Iatá-Piúna expedition where samples of a natural whale fall were collected. We are also indebted to all representatives of Japanese Consulate and Embassy in Brazil, the Brazilian Ministry of Science and Technology and Innovation, the Brazilian Ministry of Foreign Affairs and the Brazilian Navy (during a time when these ministries were supporting Brazilian science and researchers) for the assistance to establish the Brazil-Japan Marine Science Agreement. We are also grateful to Gustavo Fonseca for valuable suggestions in the study conceptualization and Fabio Cop and Danilo Vieira for statistical advice. Finally, we thank Simone Brito for her valuable assistance with nematode identification and Luciana Yaginuma for all support along the project, and to the meiofauna team from the Santos Project – Santos Basin Environmental Characterization (Brazil), coordinated by PETROBRAS/CENPES, for providing nematode assemblage data from the study site (Santos Basin). We also thank the reviewers for their valuable comments that greatly improved the manuscript.
The authors declare that the research was conducted in the absence of any commercial or financial relationships that could be construed as a potential conflict of interest.
All claims expressed in this article are solely those of the authors and do not necessarily represent those of their affiliated organizations, or those of the publisher, the editors and the reviewers. Any product that may be evaluated in this article, or claim that may be made by its manufacturer, is not guaranteed or endorsed by the publisher.
The Supplementary Material for this article can be found online at: https://www.frontiersin.org/articles/10.3389/fmars.2023.1111249/full#supplementary-material
Supplementary Table 1 | Information about the location, depth, bone volume and nematode density for deployed whale bones and the natural carcass, in the South-west Atlantic Ocean.
Supplementary Table 2 | Relative abundance (average abundance ± SD) higher than 2% of nematode assemblages from sediments adjacent to the lander at SP-1500, at the Santos Basin, in the South-west Atlantic Ocean, 25°S and 45°W (Santos slope). Nematode genera not analyzed in this study, with relative abundance less than < 2%, are represented as “others”.
Alfaro-Lucas J. M., Shimabukuro M., Ferreira G. D., Kitazato H., Fujiwara Y., Sumida P. Y. G. (2017). Bone-eating osedax worms (Annelida: siboglinidae) regulate biodiversity of deep-sea whale-fall communities. Deep Sea Res. Part II: Topical Stud. Oceanogr. 146, 4–12. doi: 10.1016/j.dsr2.2017.04.011
Boeckner M. J., Sharma J., Proctor H. C. (2009). Revisiting the meiofauna paradox: dispersal and colonization of nematodes and other meiofaunal organisms in low-and high- energy environments. Hydrobiologia 624, 91–106. doi: 10.1007/s10750-008-9669-5
Borcard D., Gillet F., Legendre P. (2011). Numerical ecology with R (New York: Springer), 688. doi: 10.1007/978-1-4419-7976-6
Braby C. E., Rouse G. W., Johnson S. B., Jones W. J., Vrijenhoek R. C. (2007). Bathymetric and temporal variation among Osedax boneworms and associated megafauna on whale-falls in Monterey bay, California. Deep Sea Res. I 54, 1773–1791. doi: 10.1016/j.dsr.2007.05.014
Cuvelier D., Beesau J., Ivanenko V. N., Zeppilli D., Sarradin P. M., Sarrazin J. (2014). First insights into macro-and meiofaunal colonisation patterns on paired wood/slate substrata at Atlantic deep-sea hydrothermal vents. Deep Sea Res. Part I: Oceanographic Res. Papers 87, 70–81. doi: 10.1016/j.dsr.2014.02.008
Debenham N. J., Lambshead P. J. D., Ferrero T. J., Smith C. R. (2004). The impact of whale falls on nematode abundance in the deep sea. Deep Sea Res. Part I: Oceanographic Res. Papers 51, 701–706. doi: 10.1016/j.dsr.2004.02.004
Fujiwara Y., Kawato M., Noda C., Kinoshita G., Yamanaka T., Fujita Y., et al. (2010). Extracellular and mixotrophic symbiosis in the whale-fall mussel adipicola pacifica: a trend in evolution from extra-to intracellular symbiosis. PloS One 5 (7), e11808. doi: 10.1371/journal.pone.0011808
Gallucci F., Fonseca A., Vieira D. C., Yaginuma L. E., Gheller P. F., Brito S., et al (2023). Predicting large-scale spatial patterns of marine meiofauna: implications for environmental monitoring. Ocean Coast. Res. In press
Grzelak K., Kotwicki L. (2016). Halomonhystera disjuncta–a young-carrying nematode first observed for the Baltic Sea in deep basins within chemical munitions disposal sites. Deep Sea Res. Part II: Topical Stud. Oceanogr. 128, 131–135. doi: 10.1016/j.dsr2.2014.12.007
Higgs N. D., Little C. T. S., Glover A. G. (2011). Bones as biofuel: a review of whale bone composition with implications for deep-sea biology and palaeoanthropology. Proc. R. Soc. B: Biol. Sci. 278, 9–17. doi: 10.1098/rspb.2010.1267
Hilário A., Cunha M. R., Génio L., Marçal A. R., Ravara A., Rodrigues C. F., et al. (2015). First clues on the ecology of whale falls in the deep Atlantic ocean: results from an experiment using cow carcasses. Mar. Ecol. 36(Suppl 1), 82–90. doi: 10.1111/maec.12246
Kreft H., Jetz W. (2010). A framework for delineating biogeographical regions based on species distributions. J. Biogeogr. 37 (11), 2029–2053. doi: 10.1111/j.1365-2699.2010.02375.x
Legendre P., Gallagher E. D. (2001). Ecologically meaningful transformations for ordination of species data. Oecologia 129, 271–280. doi: 10.1007/s004420100716
Lins L., Vanreusel A., Van Campenhout J., Ingels J. (2013). Selective settlement of deep-sea canyon nematodes after resuspension–an experimental approach. J. Exp. Mar. Biol. Ecol. 441, 110–116. doi: 10.1016/j.jembe.2013.01.021
Lundsten L., Schlining K. L., Frasier K., Johnson S. B., Kuhnz L. A., Harvey J. B. J., et al. (2010). Time-series analysis of six whale-fall communities in Monterey canyon, California, USA. Deep-Sea Res. I 57, 1573–1584. doi: 10.1016/j.dsr.2010.09.003
Mémery L., Arhan M., Álvarez-Salgado X. A., Messias M. J., Mercier H., Castro C. G., et al. (2000). The water masses along the western boundary of the south and equatorial Atlantic. Prog. Oceanogr. 47, 69–98. doi: 10.1016/S0079-6611(00)00032-X
Murtagh F., Legendre P. (2014). Ward’s hierarchical agglomerative clustering method: which algorithms implement ward’s criterion? J. classification 31, 274–295. doi: 10.1007/s00357-014-9161-z
Oksanen J., Blanchet G., Friendly M., Kindt R., Legendre P., McGlinn D., et al. (2019). Vegan: community ecology package (R package version 2), 5–6. Available at: https://CRAN.R-project.org/package=vegan.
Palmer M. A. (1988). Dispersal of marine meiofauna: a review and conceptual model explaining passive transport and active emergence with implications for recruitment. Mar. Ecol. Prog. series. Oldendorf 48, 81–91. doi: 10.3354/meps048081
Pavlyuk O. N., Trebukhova Y. A., Tarasov V. G. (2009). The impact of implanted whale carcass on nematode communities in shallow water area of Peter the great bay (East Sea). Ocean Sci. J. 44 (3), 181–188. doi: 10.1007/s12601-009-0016-1
Pereira O. S., Shimabukuro M., Bernardino A. F., Sumida P. Y. (2020). Molecular affinity of southwest Atlantic alvinocaris muricola with Atlantic equatorial belt populations. Deep Sea Res. Part I: Oceanographic Res. Papers 163, 103343. doi: 10.1016/j.dsr.2020.103343
Ptatscheck C., Traunspurger W. (2020). The ability to get everywhere: dispersal modes of free-living, aquatic nematodes. Hydrobiologia 847, 3519–3547. doi: 10.1007/s10750-020-04373-0
R Core Team (2020). R: a language and environment for statistical computing (Vienna, Austria: R Foundation for Statistical Computing). Available at: https://www.r-project.org/.
Reid J. L. (1989). On the total geostrophic circulation of the south Atlantic ocean: flow patterns, tracers, and transports. Prog. Oceanogr. 23, 149–244. doi: 10.1016/0079-6611(89)90001-3
Rex M. A., Eter R. J., Morris J. S., Crouse J., McClain C. R., Johnson N. A., et al. (2006). Global bathymetric patterns of standing stock and body size in the deep-sea benthos. Mar. Ecol. Prog. Ser. 317, 1–8. doi: 10.3354/meps317001
Rosli N., Leduc D., Rowden A. A., Probert P. K. (2018). Review of recent trends in ecological studies of deep-sea meiofauna, with focus on patterns and processes at small to regional spatial scales. Mar. Biodiversity 48, 13–34. doi: 10.1007/s12526-017-0801-5
Saeedi H., Bernardino A. F., Shimabukuro M., Falchetto G., Sumida P. Y. G. (2019). Macrofaunal community structure and biodiversity patterns based on a wood-fall experiment in the deep south-west Atlantic. Deep-Sea Res. I 145, 73–82. doi: 10.1016/j.dsr.2019.01.008
Shimabukuro M., Carrerette O., Alfaro-Lucas J. M., Rizzo A. E., Halanych K. M., Sumida P. Y. G. (2019). Diversity, distribution and phylogeny of hesionidae (Annelida) colonizing whale falls: new species of Sirsoe and connections between ocean basins. Front. Mar. Sci. 6. doi: 10.3389/fmars.2019.00478
Shimabukuro M., Couto D. M., Bernardino A. F., Souza B. H., Carrerette O., Pellizari V. H., et al. (2022). Whale bone communities in the deep southwest Atlantic ocean. Deep Sea Res. Part I: Oceanographic Res. Papers 190, 103916. doi: 10.1016/j.dsr.2022.103916
Shimabukuro M., Sumida P. Y. (2019). Diversity of bone-eating osedax worms on the deep Atlantic whale falls bathymetric variation and inter-basin distributions. Mar. Biodiversity 49 (6), 2587–2599. doi: 10.1007/s12526-019-00988-2
Siegel D. A., Kinlan B. P., Gaylord S. D. (2003). Lagrangian Descriptions of marine larval dispersion. Mar. Ecol. Prog. Ser. 260, 83–96. doi: 10.3354/meps260083
Silveira I. C. A., Napolitano D. C., Farias I. U. (2020). Water masses and oceanic circulation of the Brazilian continental margin and adjacent abyssal plain. Braz. Deep-Sea Biodiversity, 7–36. doi: 10.1007/978-3-030-53222-2_2
Smith C. R., Amon D. J., Higgs N. D., Glover A. G., Young E. L. (2017). Data are inadequate to test whale falls as chemosynthetic stepping-stones using network analysis: faunal overlaps do support a stepping-stone role. Proc. R. Soc. B: Biol. Sci. 284 (1863), 20171281. doi: 10.1098/rspb.2017.1281
Smith C. R., Baco A. R. (2003). Ecology of whale falls at the deep-sea floor. Oceanogr. Mar. Biol. 41, 311–354.
Smith C. R., Baco A. R., Glover A. G. (2002). Faunal succession on replicate deep- sea whale falls: time scales and vent-seep affinities. Cahiers Biologie Mar. 43, 293–298.
Smith C. R., Bernardino A. F., Baco A., Hannides A. K., Altamira I. (2014). The seven-year enrichment: macrofaunal succession in deep-sea sediments around a 30-ton whale fall in the northeast Pacific.Mar. Ecol. Prog. Ser. 515, 133–149. doi: 10.3354/meps10955
Smith C. R., Glover A. G., Treude T., Higgs N. D., Amon D. J. (2015). Whale-fall ecosystems: recent insights into ecology, paleoecology, and evolution. Annu. Rev. Mar. Sci. 7, 571–596. doi: 10.1146/annurev-marine-010213-135144
Smith C. R., Kukert H., Wheatcroft R. A., Jumars P. A., Deming J. W. (1989). Vent fauna on whale remains. Nature 341, 27. doi: 10.1038/341027a0
Soltwedel T. (2000). Metazoan meiobenthos along continental margins: a review. Prog. Oceanogr. 46, 59–84. doi: 10.1016/S0079-6611(00)00030-6
Soltwedel T., Guilini K., Sauter E., Schewe I., Hasemann C. (2017). Local effects of large food-falls on nematode diversity at an arctic deep-sea site: results from an in situ experiment at the deep-sea observatory HAUSGARTEN. J. Exp. Mar. Biol. Ecol. 502, 129–141. doi: 10.1016/j.jembe.2017.03.002
Souza B. H. M., Passos F. D., Shimabukuro M., Sumida P. Y. G. (2021). An integrative approach distinguishes three new species of abyssochrysoidea (Mollusca: caenograstropoda) associated with organic falls of the deep south-west Atlantic. Zool. J. Linn. Society 191, 748–771. doi: 10.1093/zoolinnean/zlaa059
Sumida P. Y., Alfaro-Lucas J. M., Shimabukuro M., Kitazato H., Perez J. A., Soares- Gomes A., et al. (2016). Deep-sea whale fall fauna from the Atlantic resembles that of the pacific ocean. Sci. Rep. 6, 22139. doi: 10.1038/srep22139
Ullberg J., Ólafsson E. (2003). Free-living marine nematodes actively choose habitat when descending from the water column. Mar. Ecol. Prog. Ser. 260, 141–149. doi: 10.3354/meps260141
Van Gaever S., Moodley L., De Beer D., Vanreusel A. (2006). Meiobenthos at the Arctic håkon mosby mud volcano, with a parental-caring nematode thriving in sulphide-rich sediments. Mar. Ecol. Prog. Ser. 321, 143–155. doi: 10.3354/meps321143
Van Gaever S., Olu K., Derycke S., Vanreusel A. (2009). Metazoan meiofaunal communities at cold seeps along the Norwegian margin: influence of habitat heterogeneity and evidence for connection with shallow-water habitats. Deep Sea Res. Part I: Oceanographic Res. Papers 56 (5), 772–785. doi: 10.1016/j.dsr.2008.12.015
Vanreusel A., Fonseca G., Danovaro R., Da Silva M. C., Esteves A. M., Ferrero T., et al. (2010). The contribution of deep-sea macrohabitat heterogeneity to global nematode diversity. Mar. Ecol. 31 (1), 1–15. doi: 10.1111/j.1439-0485.2009.00352.x
Zeppilli D., Canals M., Danovaro R. (2012). Pockmarks enhance deep-sea benthic biodiversity: a case study in the western Mediterranean Sea. Diversity Distributions 18, 832–846. doi: 10.1111/j.1472-4642.2011.00859.x
Keywords: meiofauna, whalebone, dispersion, connectivity, SW Atlantic, organic falls
Citation: Avila AKF, Shimabukuro M, Couto DM, Alfaro-Lucas JM, Sumida PYG and Gallucci F (2023) Whale falls as chemosynthetic refugia: a perspective from free-living deep-sea nematodes. Front. Mar. Sci. 10:1111249. doi: 10.3389/fmars.2023.1111249
Received: 29 November 2022; Accepted: 12 April 2023;
Published: 02 May 2023.
Edited by:
Alexandra Anh-Thu Weber, Swiss Federal Institute of Aquatic Science and Technology, SwitzerlandReviewed by:
Sergi Taboada, Autonomous University of Madrid, SpainCopyright © 2023 Avila, Shimabukuro, Couto, Alfaro-Lucas, Sumida and Gallucci. This is an open-access article distributed under the terms of the Creative Commons Attribution License (CC BY). The use, distribution or reproduction in other forums is permitted, provided the original author(s) and the copyright owner(s) are credited and that the original publication in this journal is cited, in accordance with accepted academic practice. No use, distribution or reproduction is permitted which does not comply with these terms.
*Correspondence: Fabiane Gallucci, ZmdhbGx1Y2NpQHVuaWZlc3AuYnI=
Disclaimer: All claims expressed in this article are solely those of the authors and do not necessarily represent those of their affiliated organizations, or those of the publisher, the editors and the reviewers. Any product that may be evaluated in this article or claim that may be made by its manufacturer is not guaranteed or endorsed by the publisher.
Research integrity at Frontiers
Learn more about the work of our research integrity team to safeguard the quality of each article we publish.