- 1Guangdong Provincial Key Laboratory of Fishery Ecology and Environment, South China Sea Fisheries Research Institute, Chinese Academy of Fishery Sciences, Guangzhou, China
- 2Laboratory of Marine Biodiversity, Third Institute of Oceanography, Ministry of Natural Resources, Xiamen, China
Changes in zooplankton composition, abundance, and some species in response to environmental variation were investigated over four seasons (2020) in Daya Bay. In total, 129 taxa of zooplankton (16 groups of planktonic larvae and 20 indeterminate species) were identified. Zooplankton communities exhibited a significant seasonal shift in abundance and taxonomic composition. The maximum number of zooplankton species was recorded in winter (72 species) and the lowest in spring (42 species). However, the abundance was highest in spring (1,372.01 ± 1,071.14 individuals/m³) and lowest in autumn (50.93 ± 34.05 individuals/m³). Pearson correlation analyses demonstrated that the zooplankton abundance and the variations of indicator species were obviously correlated with environmental parameters (e.g., salinity, temperature, pH, and chlorophyll-a). Based on specificity and occupancy analysis, a total of eight species were selected as indicator species. It is noteworthy that some kollaplankton (such as Dolioletta gegenbauri and Doliolum denticulatum) could potentially cause disaster to the nuclear power plant cooling system because of their relatively large body size and huge blooms in spring. In addition, Centropages tenuiremis blooms in spring and Penilia avirostris blooms in summer could attract assemblages of larval or adult pelagic fish, which would also threaten the cooling system security in Daya Bay. In conclusion, our results suggest that zooplankton communities and some species may be considered as favorable indicators of the marine environment.
Introduction
Coastal waters, which lie at the transition zones between oceans and continents, are the most relevant to human society. They also support rich fishing grounds and provide economic benefits due to their relatively higher productivity (Herrera-Silveira and Morales-Ojeda, 2009; Lange et al., 2010). Coastal areas support relatively greater plankton production and degradation and also play a key role in biogeochemical processes. However, their biodiversity and ecological function are threatened by global changes and anthropogenic pressures which cause the degradation of biotopes and biocenosis (Kemp and Boynton, 2012; Zhang, et al., 2021). Increased anthropogenic activities have accelerated the process of eutrophication, leading to dystrophic crises and/or irreversible deterioration—for example, a decrease in the mean size of dominant copepods from the late 1940s to 2012 was detected in the Central Basin of Long Island Sound, Japan (Rice et al., 2015).
Daya Bay is a semi-enclosed, shallow embayment with depths ranging from 5 to 20 m and an area of 650 km2, which is located in the northern part of the South China Sea (Song et al., 2004). It contains complex habitats, tremendous biological resources, abundant biodiversity, and plentiful marine products, which make it one of the most important treasure houses for sub-tropical aquatic resources. In recent years, rapid social and economic developments have resulted in environmental degradation in Daya Bay. Studies have shown that degradation and habitat modification have significantly increased (Wu et al., 2009; Liu et al., 2018), while eutrophication has reduced the zooplankton species richness by about 50% (Li et al., 2014). Dominant species in the spring have changed because of the rapid increase in water temperature and abundant food supply in Daya Bay (Xiang et al., 2021; Zhao et al., 2022), and Li et al. (2021) reported that the zooplankton community was undergoing great changes, showing tendencies of miniaturization and gelatinization over the past 30 years. Several large-scale zooplankton blooms have occurred in Daya Bay, which were related to changes in water temperature, salinity, and food supply variation (Zeng et al., 2019; An et al., 2021; Zeng et al., 2021; Liu et al., 2022).
Zooplankton are sensitive to chemical, physical, and biological factors in marine systems (Sun et al., 2010; Shi et al., 2015). The composition and abundance of zooplankton are highly dependent on environmental conditions and respond quickly to environmental variations. Consequently, zooplankton are considered as bio-indicators of environmental quality and water mass (Dam, 2013; Carter et al., 2017; Wang et al., 2020; Wang et al., 2022). Zooplankton are not only a key link in energy transfer from primary production to higher trophic levels but also a key factor in controlling the harmful effects of eutrophication and proliferation of microalgae (Rissik et al., 2009)—for example, dinoflagellates and prasinophytes are the most preferred phytoplankton groups by the mesozooplankton in Daya Bay (Li et al., 2018).
Daya Bay Nuclear Power Station and Ling-Ao Nuclear Power Station were built in 1994 and 2002, respectively. They are located in the inner regions of west Daya Bay. The thermal discharge is affected by tidal currents, which depend on the topography of Daya Bay (Yuan et al., 2021). The residual current is relatively small within the bay in summer but is stronger with the stronger current fields in winter, and the self-purification capacity of this area is weak (Wu et al., 2007). In addition, other studies have demonstrated that the thermal discharge has a significant impact on the Daya Bay ecosystem, including levels of nutrients, and zooplankton and phytoplankton (Jiang and Wang, 2020). Therefore, it is vital to monitor the zooplankton composition and variation in Daya Bay.
The aims of this research were to (1) investigate whether there is significant variation in the zooplankton composition in the research area, (2) identify the indicator composition under different environmental conditions, (3) measure the correlation of indicator species with the environmental parameters in the research area, and (4) discuss whether the indicator species caused a potential threat to the safety of the nuclear power plant cooling systems in the research area.
Materials and methods
Field sampling
Twenty-four sampling stations were set up in Daya Bay during winter (January), spring (March), summer (August), and autumn (November) of 2020 (Figure 1). Zooplankton samples were collected at each station during every survey. In total, 96 zooplankton samples were obtained by vertical tow from 1 m above the bottom to the surface at a speed of 0.5 m/s using a plankton net (mesh size, 505 μm; mouth diameter, 50 cm; and net length, 145 cm). A Hydro-Bios flow meter (Hydro-Bios Apparatebau GmbH., Altenholz, Germany) was mounted at the mouth of the net to calculate the filtered water volume. Then, zooplankton samples were immediately preserved with 5% formalin seawater solution on board at each station. Environmental factors, such as temperature, pH, salinity, and dissolved oxygen (DO), were measured in situ using a multi-parameter sensor (YSI Professional Plus, Xylem Analytics, Beijing, China) at each station where the zooplankton samples were collected. In addition, 5,000 ml of seawater samples for nutrient analysis was collected from each station with a Niskin water sampler at a depth of 0.5 m and preserved immediately upon collection by placing them at -20°C in the dark. Simultaneously, approximately 500 ml of sampled seawater was filtered through GF/F filters. These filters were frozen and later extracted with 90% acetone at −20°C in the dark for 24 h to determine the concentration of chlorophyll-a (Chl-a) using a fluorometer (Tuner-10-AU).
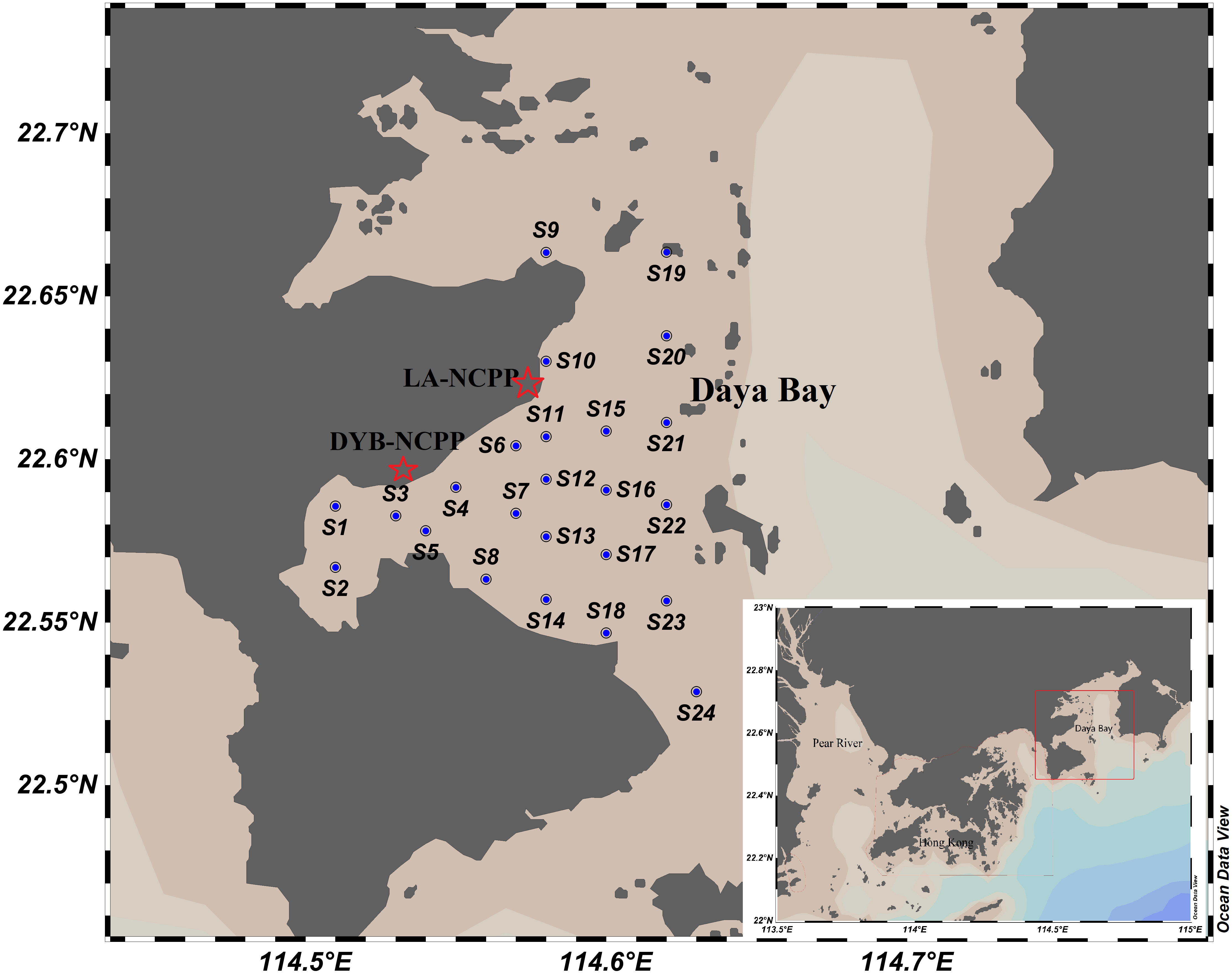
Figure 1 Study area and stations located at western Daya Bay. DYB-NCPP, Daya Bay Nuclear Power Plant; LA-NCPP, Ling’ao Nuclear Power Plant.
Data analysis
In the laboratory, zooplankton samples were split into sub-samples with a Folsom plankton sample splitter until each subsample contained about 200 individuals. All individuals in the subsamples were quantified and identified to species level where possible under a stereomicroscope (Leica MZ95, Leica Microsystems Ltd, Wetzlar, Germany) according to the descriptions of Chen and Zhang (1965), Zheng et al. (1984), Chen (1992); Chihara and Murano (1997), and Lian et al. (2019). Abundance was calculated by the individuals’ numbers to be divided by filtered water volume at each station. Total abundance was equal to the sum of all individuals’ abundance in that station. Total average abundance means the summation of total abundance in each station to be divided by station numbers in each survey. Relative abundance represents the percentage of a certain species’ average abundance in the total average abundance.
One-way analysis of variance with Tukey’s post-hoc test was used to estimate the seasonal variation of environmental characteristics, and the correlation of different parameters was analyzed via Pearson correlation analysis using SPSS software (IBM SPSS statistics for Windows, Armonk, NY, USA). The dominance (Y) of each species was calculated using the formula Y=(ni/N)*fi , where ni represents the mean number of species i, and N represents the total mean number of zooplankton. The specificity and occupancy were calculated using the following formula with reference to Dufrene and Legendre (1997). The figure of specificity–occupancy was finished by ggplot 2 in R language.
Where Nindividuals i,j means the mean abundance of species i in survey j. Nindividualsi means the sum of the mean abundance of species i in different surveys. Occupancy also means occurrence rate. Nsitesi,j means the station numbers of species i occurrence in survey j and Nsitesj means all stations in survey j.
Results
Environmental parameters
The year-round mean surface water temperature was 24.59 ± 3.87°C, and the water temperature followed a clear seasonal pattern. The maximum was recorded in summer (30.48 ± 0.48°C), and the minimum was in winter (20.01 ± 0.93°C). The temperature was close to the year-round average surface water temperature in spring and autumn at 23.73 ± 1.00°C and 24.13 ± 0.85°C, respectively (Figure 2A). The values of salinity and pH peaked in spring but were relatively lower in summer (Figures 2B, C). Additionally, the values of DO ranged from 6.32 ± 0.45 mg/L in summer to 7.76 ± 0.67 mg/L in autumn, with a mean of 7.11 ± 0.70 mg/L (Figure 2D). The values of Chl-a varied consistently with DO—the maximum value was recorded in autumn, and the minimum value was recorded in summer. There were no obvious differences in Chl-a concentration between the spring, summer, and winter surveys (Figure 2E).
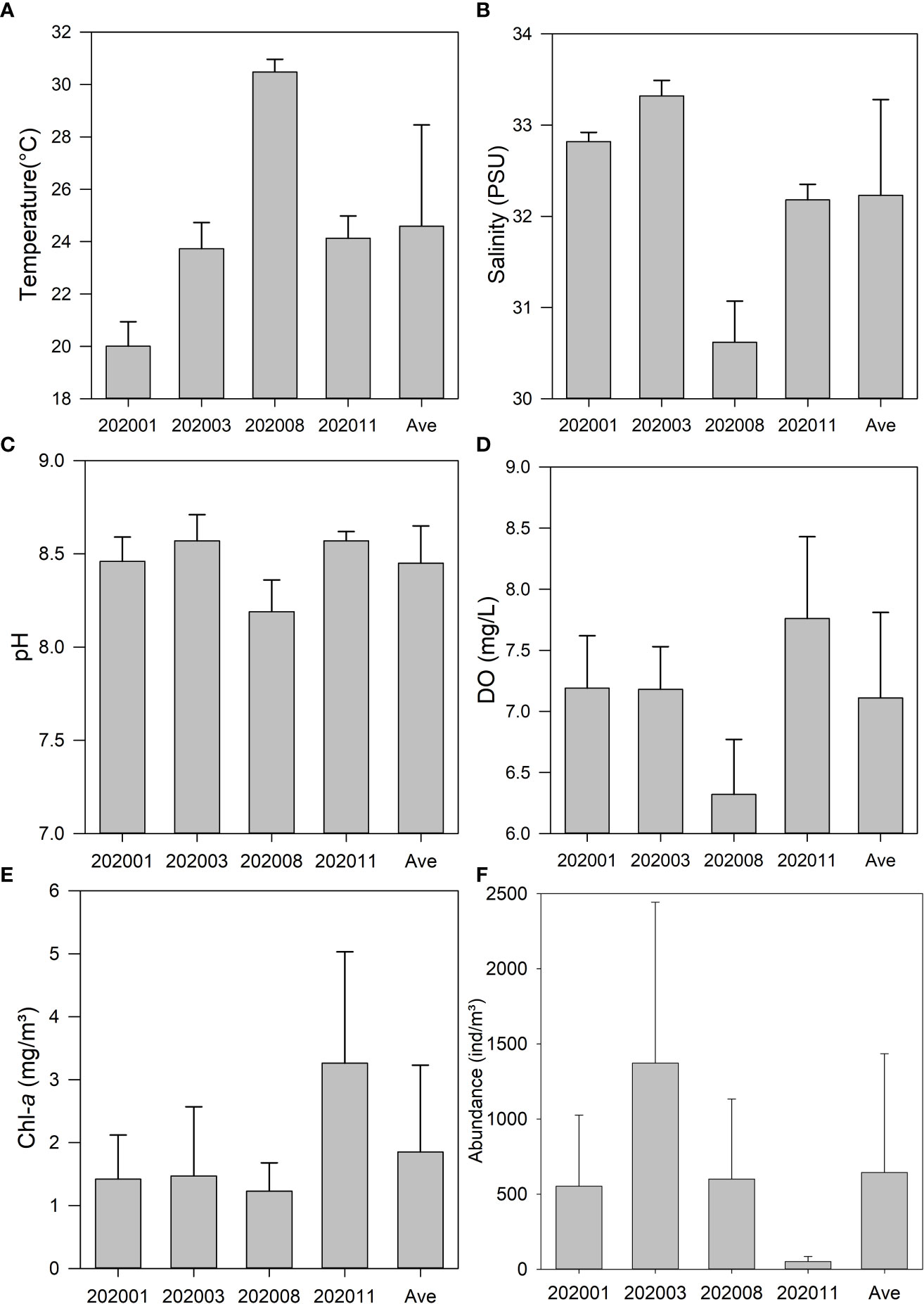
Figure 2 Bar plot of the temperature (A), salinity (B), pH (C), dissolved oxygen (D), chlorophyll-a (E), and total average abundance (F) in the research area.
Zooplankton composition and seasonal variation
A total of 129 zooplankton species (16 groups of planktonic larvae and 20 indeterminate species) were identified in this study. All species were arranged into cladocera, copepoda, kollaplankton, large crustacean, meroplankton, other crustacean, and other plankton groups, based on the systematics and their function in the marine environment. Kollaplankton included tunicates, cnidaria, ctenophores, and chaetognatha. Mysidacea, euphausiacea, and decapoda were classified as large crustaceans. The other crustaceans included amphipoda and ostracoda. Annelida and petropoda were classified as other plankton in this study. The species composition of zooplankton showed obvious seasonal variation (Figure 3). In winter, 72 species were recorded at the species level. This was followed by autumn, summer, and spring, with 54 species, 44 species, and 42 species, respectively. Copepods accounted for the highest number of species, and the percentage ranged from 43.18% to 62.96%.
The total average zooplankton abundance showed a significant seasonal variation. The greatest average abundance was in spring, and the lowest in autumn (Figure 2F). In spring, the average abundance of zooplankton was 1,372.01 individuals/m³, while the zooplankton assemblage was largely dominated by kollaplankton (46.36% of total average abundance), meroplankton (27.04% of total average abundance), and copepods (19.95% of total average abundance). In summer, the average abundance of zooplankton was significantly reduced to 601.10 individuals/m³, with the average abundance of cladocera (58.21% of total average abundance) being the highest, followed by copepods (28.20% of total average abundance) and meroplankton (10.97% of total average abundance). In autumn, zooplankton average abundance was only 50.93 individuals/m³, with copepods and meroplankton dominating, accounting for 57.22% and 21.93% of total average abundance, respectively (Figure 3). However, the zooplankton average abundance increased in winter, reaching 553.06 individuals/m³. The dominant species were cladocera and copepods, with abundance values of 233.14 individuals/m³ (42.15% of total average abundance) and 225.86 individuals/m³ (40.84% of total average abundance), respectively.
The Pearson correlation analysis results showed that the total abundance of zooplankton was significantly positively correlated with surface water salinity and markedly negatively correlated with Chl-a (p< 0.01) (Table 1). In addition, the abundance of cladocera and copepods was significantly negatively correlated with Chl-a (p< 0.01). Similarly, there was a significant negative correlation between the abundance of kollaplankton, large crustaceans, meroplankton, and Chl-a (p< 0.05). However, cladocera abundance showed a significantly positive correlation with surface water temperature and a notable negative correlation with DO. The abundance of cladocera showed a notable negative correlation with pH, while kollaplankton abundance was positively correlated with pH (p< 0.01). The salinity showed a significantly negative correlation with the abundance of cladocera and a notably positive correlation with abundance of kollaplankton and meroplankton (p< 0.01). Both the abundance of other crustaceans and that of other plankton showed no obvious correlation with the environmental parameters in the research area.
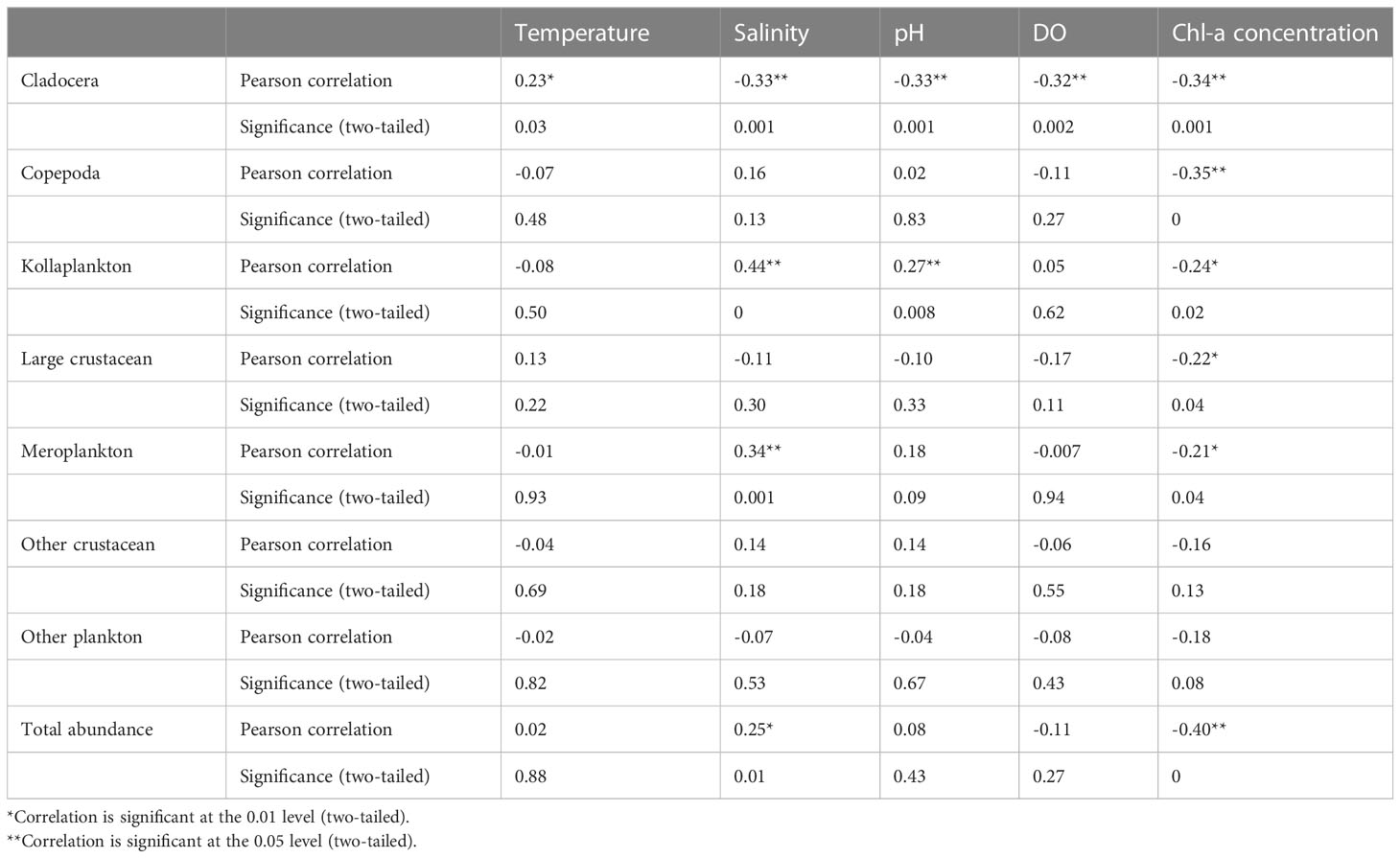
Table 1 Correlation analysis of environmental parameters with different functional groups in the research area.
Dominance was calculated when the individual was identified to the species level. A total of 13 species were considered as dominant species, with dominance values higher than 0.02 (Table 2). In spring, Dolioletta gegenbauri was the most dominant species. Its mean abundance and relative abundance (RA) were 435.10 individuals/m³ and 44.15%, respectively. It was followed by Doliolum denticulatum and Centropages tenuiremis, with mean abundance of 177.25 individuals/m³ and 130.23 individuals/m³, respectively. In summer, there were three new dominant species—Peniliaavirostris, Evadne tergestina, and Acartia clausi—with P. avirostris being the most dominant species in summer. Its abundance was 257.14 individuals/m³, and its RA was 49.21%. Eight species were defined as dominant species in autumn, and the most dominant species was A.clausi, with an average abundance of 8.38 individuals/m³ and RA of 22.88%. In winter, the dominant species were E.tergestina and Temora turbinata. Their abundance and RA were 231.48 individuals/m³ and 46.76%, and 90.84 individuals/m³ and 18.35%, respectively. In addition, the most dominant species showed an obvious seasonal variation in the research area and was abundant with a relatively high occurrence rate only in one or two surveys. In contrast, both the occurrence rate and the relative abundance of T. turbinata showed relatively higher values during the 1-year survey.
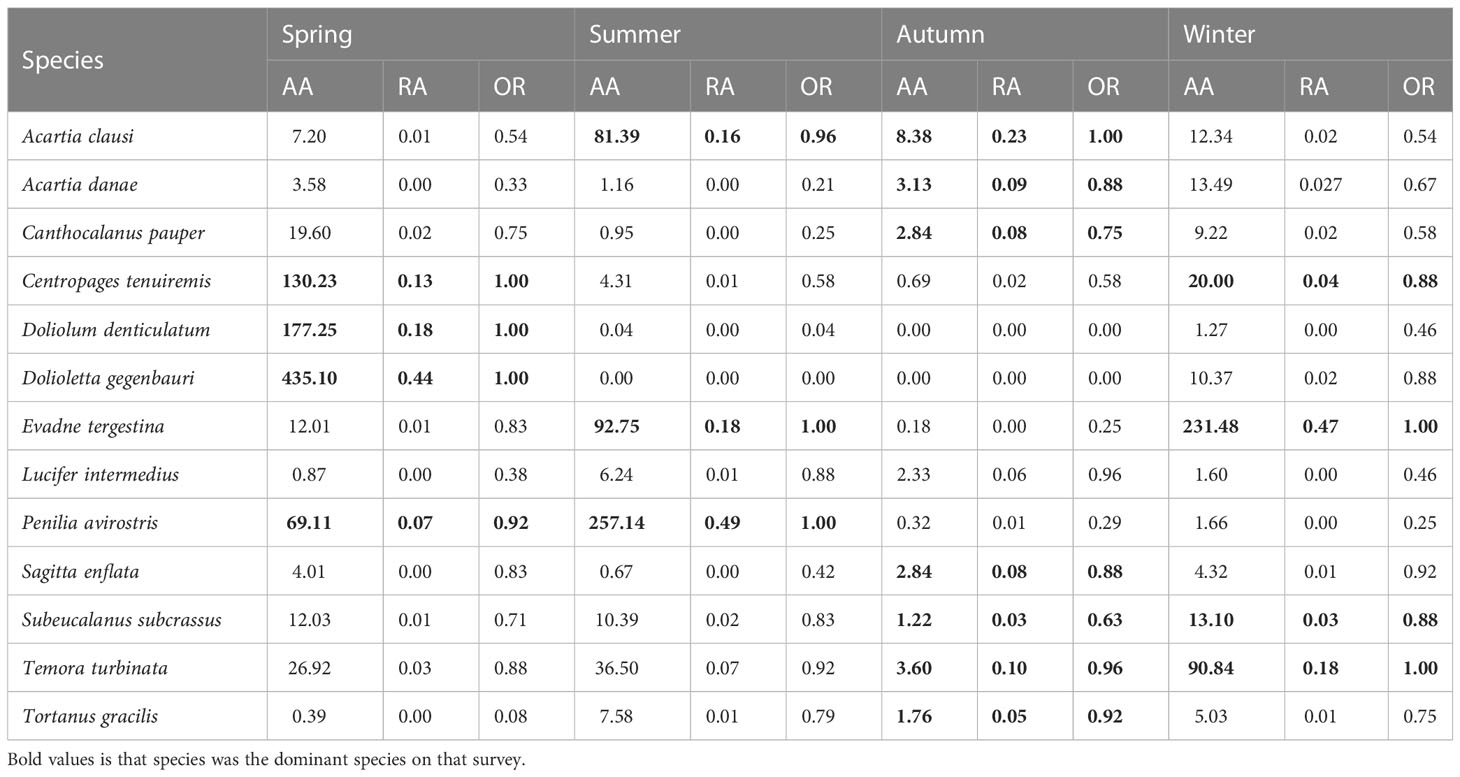
Table 2 The average abundance (AA), relative abundance (RA), and occurrence rate (OR) of dominant species in the research area.
Specificity and occupancy of the zooplankton
The specificity and occupancy were calculated and projected onto a plot (Figure 4). In the plot, the x-axis indicates occupancy, which means how the species was distributed across all stations in that survey. As indicated by the spread across the x-axis, zooplankton displayed a highly varied occupancy in all surveys. Fewer species were present in all stations. The y-axis represents the specificity, which means whether that species was found in other surveys. The highest number of habitat-specialist species (20 species) was found in the winter survey, while there were eight, six, and two specialist species in autumn, spring, and summer, respectively.
Species which were specific to a habitat and common in their habitat at most sites were selected as indicator species of each survey when specificity and occupancy were greater or equal to 0.7 (dotted boxes in Figure 4). The number of indicator species differed significantly between different surveys (Table 3). Dolioletta gegenbauri, D. denticulatum, Diphyes chamissonis, Sagitta bedoti, and C. tenuiremis were selected as indicator species in spring. In summer, the indicator species were A. clausi and P. avirostris. Aglaura hemistoma was the only indicator species in winter. There was no species suitable as an indicator species in autumn.
The Pearson correlation analysis results revealed that zooplankton abundance was negatively associated with Chl-a (p< 0.01) and positively associated with salinity (p< 0.05). Nevertheless, the variations of indicator species were mainly affected by salinity and pH—for example, D. gegenbauri, D. chamissonis, S. bedoti, and C. tenuiremis had a significantly positive correlation with salinity (p< 0.01), whereas A. clausi and P. avirostris showed a significantly negative correlation with salinity (p< 0.01). Simultaneously, other significantly positive correlations included those between D. denticulatum and salinity and between D. chamissonis, S. bedoti, C. tenuiremis, and D. gegenbauri and pH. However, A. clausi and P. avirostris showed negative correlations with pH. In addition, the water temperature was significantly negatively correlated with A. hemistoma but positively correlated with A. clausi and P. avirostris. The DO showed a significantly negative correlation with A. clausi and P. avirostris. The Chl-a concentration was significantly negatively correlated with D. gegenbauri, D. chamissonis, S. bedoti, C. tenuiremis, and P. avirostris.
Discussion
Daya Bay, which is located in a subtropical zone, is a semi-closed bay. Due to the influence of the monsoon, there is plenty of rainfall from May to October and less from November to April (Wu and Wang, 2007). In the dry season (winter), the northeast monsoon prevails, with low temperatures and precipitation. In contrast, in the wet season (summer), the southwest monsoon predominates, with relatively high levels of precipitation and temperature. Spring and autumn are the monsoon transition seasons in the research area (Xu, 1989; Wu et al., 2009; Wu et al., 2010, Wu et al., 2011, Wu et al., 2012; Wu et al., 2016). The highest surface water temperature was observed during summer in this study. Owing to the precipitation and runoff, the surface water was diluted, resulting in relatively low salinity, pH, DO, and Chl-a concentration in summer. The correlation analysis showed that there was a significantly positive correlation between salinity, DO, and pH. Nevertheless, a significantly negative correlation was observed between DO and Chl-a in summer (Supplementary), which was consistent with the results reported for the northern region of the South China Sea during autumn (Long et al., 2006). Shen et al. (2017) reported that a significantly negative correlation was also observed between salinity and Chl-a in Daya Bay. In contrast, we found a notably positive correlation between Chl-a and salinity in autumn and a negative correlation in spring. However, no obvious correlation was detected between Chl-a and salinity in summer or winter in this study. During the dry and monsoon transition seasons, the values of surface water salinity, pH, and DO remained fairly constant without the effects of rainfall dilution in the research area.
Zooplankton species composition and abundance are known to change seasonally in the marine environment (Sammacro and Crenshaw, 1984). Zooplankton diversity and abundance are also greatly affected by environmental parameters (Wang et al., 2018; Aguilera, 2020; Wang et al., 2020; Wang et al., 2022). In this study, there was a significantly negative correlation between the total zooplankton abundance and Chl-a concentration. This finding was in accordance with the result of Jiang and Wang (2020). They found that the seasonal change of zooplankton had its own characteristic pattern, which did not coincide with the phytoplankton but lagged behind by nearly 1 month (Jiang and Wang, 2020). Similarly, in our research, there was a significant seasonal variation in zooplankton composition, and the highest species number was observed in winter. Interestingly, many warm temperature species appeared in winter. This warm temperature species intrusion could be carried by the China Coastal Current—for instance, Salpa fusiformis has been defined as a warm temperature indicator species in California and displays a massive occurrence in the Southern Yellow Sea during summer (Silver, 1975; Liu et al., 2012). Calanus sinicus is the indicator species of the China Coastal Current in the Taiwan Strait and was collected only during winter in the research area (Hwang and Wong, 2005; Wang et al., 2020). In addition, the zooplankton abundance was highest in spring. Dolioletta gegenbauri is a warm-water species which was the most dominant species in spring. It commonly appears in productive subtropical neritic regions worldwide. The abundance of D. gegenbauri is usually at bloom concentrations, which could consume a large fraction of daily primary production (Paffenhöfer and Köster, 2011; Walters et al., 2019).
Temora turbinata is regarded as the predominant species in mesozooplankton communities in various environments around the world. It is able to adapt to various habitats, such as eutrophic lagoons, polluted and eutrophicated waters, outfall areas, and nuclear power plant discharge areas (Hsu et al., 2008; Tseng et al., 2011; Wang et al., 2021). In particular, T. turbinate and Karenia brevis can co-occur in the Gulf of Mexico. Karenia brevis is a toxic dinoflagellate, which often causes blooms (Lester et al., 2008). Copepodites and adults of T. turbinata are often found in upwelled water masses which are usually preferred by D. gegenbauri (Paffenhöfer and Köster, 2011). Li et al. (2018) reported that T. turbinanta ranges in abundance from 1 individual/m³ to 1,185 individuals/m³ in Daya Bay, and the highest abundance was recorded during a winter survey in 2017. Consistent with previous results, T. turbinata was the only dominant species present throughout the year. Temora turbinanta was recorded during our 1-year survey, and the average abundance ranged from 3.60 individuals/m³ (autumn) to 90.84 individuals/m³ (winter) in this study. T. turbinanta abundance changed significantly with season, but it was the dominant species throughout the year. Thus, we suggest that T. turbinanta could be considered as a potential indicator species to monitor its aquatic habitat. Notably, T. turbinanta abundance was obviously lower in the research area than in the northeast coastal area of Taiwan (Tseng et al., 2011; Wang et al., 2021). The seasonal variation characteristics of T. turbinanta abundance were also different. Undoubtedly, these differences could be due to differences in sampling method, local conditions, or the thermal discharge from the nuclear power plant in Daya Bay. Additionally, the other indicator species, P. avirostris, is not only a quality live food supply for marine pelagic fish but also an important component of the zooplankton community of many tropical, subtropical, and temperate waters and occurs seasonally with especially high abundance in summertime (Calbet et al., 2001; Fernández de Puelles et al., 2003; Marazzo and Valentin, 2003; Rose et al., 2004). The body length of female P. avirostris ranges from 0.70 to 1.09 mm (Zhou et al., 2022). Thus, P. avirostris blooms could block the cooling system of a nuclear power plant by attracting huge numbers of larva and adult pelagic fish (such as herring, mackerel, sardine, horse mackerel, etc.). Centropages tenuiremis is an important common copepod in the neritic mesozooplankton assemblage. It is an obviously omnivorous feeding species and can expand its food spectrum under stressful conditions (Xu et al., 2020). Dolioletta gegenbauri and D. denticulatum have been reported to form dense blooms in shallow waters of the continental shelf (Paffenhöfer et al., 1995; Nakamura, 1998; Tew and Lo, 2005). Our results are consistent with previous reports (Lian et al., 2011; Li et al., 2011; Li et al., 2021). These species mentioned above have also become the dominant species in Daya Bay. Additionally, our research revealed that P. avirostris blooms and C. tenuiremis blooms might also threaten the cooling system by acting as the food supply for pelagic fish in spring. It is noteworthy that most of the above-mentioned indicator species in spring belong to kollaplankton. Thus, we suggest that kollaplankton could cause potential disaster to the nuclear power plant cooling system due to their relatively large body size and potential to form huge blooms in spring. Moreover, C. tenuiremis blooms in spring and P. avirostris blooms in summer could also threaten the cooling system security by attracting larva or adult pelagic fish assemblages into Daya Bay. Therefore, these species should be considered as key species in future ecological studies.
Conclusion
In summary, the zooplankton communities showed a significant seasonal variation in terms of species composition, abundance, and dominant species in Daya Bay. Seasonal variations in the dominant species were observably related to environmental variations, such as thermal discharge, pH, salinity, and Chl-a. Thus, zooplankton could be considered as a favorable indicator of the marine environment. In addition, some kollaplankton, cladocera, and copepod species might directly or indirectly affect nuclear power plant cooling systems.
Data availability statement
The original contributions presented in the study are included in the article/Supplementary Material. Further inquiries can be directed to the corresponding authors.
Author contributions
F-XW: conceptualization, data curation, writing—original draft, and writing—review and editing. H-HH: Writing—review and editing and funding acquisition. Y-GW: Writing—review and editing, validation, and visualization. Y-GG: investigation and supervision. Q-XL, S-FZ, Y-YR, H-XL, and MD: investigation and formal analysis. All authors contributed to the article and approved the submitted version.
Funding
We gratefully acknowledge the National Key Research and Development Program of China (2018YFC1407501), Central Public-interest Scientific Institution Basal Research Fund, CAFS (NO.2020TD15), Central Public-interest Scientific Institution Basal Research FundSouth China Sea Fisheries Research Institute, Chinese Academy of Fishery Sciences (2021SD03), Guangdong Basic and Applied Basic Research Foundation (2020A1515010331, 2022A1515110603), Guangzhou Basic and Applied Basic Research Foundation (202201010306) and Fund of Guangdong Provincial Key Laboratory of Fishery Ecology and Environment (FEEL-2022-9).
Acknowledgments
We thank Xian Zhou, Xiao-Qing Qin, Sheng-Qi Du, and Zhi-Hui Zhong for their help in sample collection and Zhen Tan, Gang Hou, and Chuang-Hao Pan for their help in data analysis. In addition, we thank International Science Editing (http://www.internationalscienceediting.com) for editing this manuscript.
Conflict of interest
The authors declare that the research was conducted in the absence of any commercial or financial relationships that could be construed as a potential conflict of interest.
Publisher’s note
All claims expressed in this article are solely those of the authors and do not necessarily represent those of their affiliated organizations, or those of the publisher, the editors and the reviewers. Any product that may be evaluated in this article, or claim that may be made by its manufacturer, is not guaranteed or endorsed by the publisher.
Supplementary material
The Supplementary Material for this article can be found online at: https://www.frontiersin.org/articles/10.3389/fmars.2023.1110160/full#supplementary-material
References
Aguilera V. M. (2020). pH and other upwelling hydrographic drivers in regulating copepod reproduction during the 2015 El nino event: A follow-up study. Estuar. Coast. Shelf S. 234, 106640. doi: 10.1016/j.ecss.2020.106640
An L. N., Wang L., Huang H., Ou D. Y., Li W. W. (2021). Population dynamics of acetes chiensis and its response to environmental factors in western daya bay. J. of. Appl. Oceanography. 40 (3), 403–412.
Calbet A., Garrido S., Saiz E., Alcaraz M., Duarte M. (2001). Annual zooplankton succession in coastal NW Mediterranean waters: The importance of the smaller size fractions. J. Plankton Res. 23 (3), 319–331. doi: 10.1093/plankt/23.3.319
Carter J. L., Schindler D. E., Francis T. B. (2017). Effects of climate change on zooplankton community interactions in an alaskan lake. Climate Change Responses. 4 (3), 1–12. doi: 10.1186/s40665-017-0031-x
Chen Q. C., Zhang S. Z. (1965). The planktonic copepods of the yellow Sea and the East China sea. i. calanoida. Studia Mar. Sin. 7, 20–131.
Chihara M., Murano M. (1997). An illustrated guide to marine plankton in Japan (Tokyo, Japan: Tokai University Press), pp1–1000.
Dam H. G. (2013). Evolutionary adaptation of marine zooplankton to global change. Annu. Rev. Mar. Sci. 5, 349–370. doi: 10.1146/annurev-marine-121211-172229
Dufrene M., Legendre P. (1997). Species assemblages and indicator species: the need for a flexible asymmetrical approach. Ecological Monographs 67 (3), 345–366.
Fernández de Puelles M. L., Pinot J. M., Valencia J. (2003). Seasonal and inter-annual variability of zooplankton community in water off mallorca island (Balearic Sea, Western mediterranean): 1994-1999. Oceanolog. Acta 26, 673–686. doi: 10.1016/j.oceact.2003.07.001
Herrera-Silveira J. A., Morales-Ojeda S. M. (2009). Evaluation of the health status of a coastal ecosystem in southeast Mexico: Assessment of water quality, phytoplankton and submerged aquatic vegetation. Mar. pollut. Bull. 59, 72–86. doi: 10.1016/j.marpolbul.2008.11.017
Hsu P. K., Lo W. T., Shih C. T. (2008). The coupling of copepod assemblages and hydrography in a eutrophic lagoon in Taiwan: Seasonal and spatial variations. Zool. Stud. 47, 172–184.
Hwang J. S., Wong C. K. (2005). The China coastal current as a driving force for transporting Calans sinicus (Copepoda: Calanioida) from its population centers to waters off Taiwan and Hong Kong during the winter northeast monsoon period. J. Plankton Res. 27, 205–210. doi: 10.1093/plankt/fbh162
Jiang R., Wang Y. S. (2020). Modeling the ecosystem response of the semi-closed daya bay to the thermal discharge from two nearby nuclear power plants. Ecotoxicology. 29, 736–750. doi: 10.1007/s10646-020-02229-w
Kemp W. M., Boynton W. R. (2012). Synthesis in estuarine and coastal ecological research: What is it, why is it important, and how do we teach it? Estuar. Coast. 35, 1–22. doi: 10.1007/s12237-011-9464-9
Lange H. J. D., Sala S., Vighi M., Faber J. H. (2010). Ecological vulnerability in risk assessment-a review and perspectives. Sci. Total Environ. 408 (18), 3871. doi: 10.1016/j.scitotenv.2009.11.009
Lester K. M., Heil C. A., Neely M. B., Spence D. N., Murasko S., Hopkins T. L., et al. (2008). Zooplankton and Karenia brevis in the gulf of Mexico. Cont. Shelf Res. 28, 99–111. doi: 10.1016/j.csr.2007.04.009
Lian X. P., Tan Y. H., Huang L. M., Chen Q. C., Li K. Z., Liu Y. H. (2011). Space-time variations and impact factors of macro-meso zooplankton in daya bay (in Chinese). Mar. Environ. Science. 30 (5), 640–645.
Lian G. S., Wang Y. G., Sun R. X., Hwang J. S. (2019). Species diversity of marine planktonic copepods in china’s seas (Beijing, China: China Ocean Press), Pp 868.
Li Y. M., Han L. Y., Chen M. R., Tong M. M. (2018). The mesozooplankton community structure and their herbivory in daya bay. Oceanologia Limnologia Sinica. 49 (4), 839–850. doi: 10.11693/hyhz20180100020
Li K. Z., Ma J., Huang L. M., Tan Y. H., Song X. Y. (2021). Environmental drivers of temporal and spatial fluctuations of mesozooplankton community in daya bay, northern south China Sea. J. Ocean. U. China. 20 (4), 1013–1026. doi: 10.1007/s11802-021-4602-x
Liu J. J., Ni Z. X., Diao Z. H., Hu Y. X., Xu X. R. (2018). Contamination level, chemical fraction and ecological risk of heavy metals in sediments from daya bay, south China Sea. Mar. pollut. Bull. 128, 132–139. doi: 10.1016/j.marpolbul.2018.01.021
Liu Y. Q., Sun S., Zhang G. T. (2012). Seasonal variation in abundance, diel vertical migration and body size of pelagic tunicate Salp fusiformis in the southern yellow Sea. Chin. J. Oceanology Limnology. 30 (1), 92–104. doi: 10.1007/s00343-012-1048-4
Liu Q. X., Zhou L. B., Zhang W. R., Zhang L., Tan Y. H., Han T. T., et al. (2022). Rising temperature contributed to the outbreak of macrozooplankton Creseis acicula by enhancing its feeding and assimilation for algal food nearby the coastal daya bay nuclear power plant. Ecotox. Environ. Safe. 238, 113606. doi: 10.1016/j.ecoenv.2022.113606
Li K. Z., Yin J. Q., Huang L. M., Zhang J. L., Lian S. M., Liu C. W. (2011). Distribution and abundance of thaliaceans in the northwest continental shelf of south China Sea, with response to environmental factors driven by monsoon. Cont. Shelf Res. 31 (9), 979–989. doi: 10.1016/j.csr.2011.03.004
Li K. Z., Yin J. Q., Tan Y. H., Huang L. M., Song X. Y. (2014). Short-term variation in zooplankton community from daya bay with outbreaks of Penilia avirostris. Oceanologia. 56, 583–602. doi: 10.5697/oc.56-3.583
Long A. M., Chen S. Y., Zhou W. H., Xu J. R., Sun C. C., Zhang F. Q., et al. (2006). Distribution of macro-nutrients, dissolved oxygen, pH and chl a and their relationships in northern south China Sea. Mar. Sci. Bulletin. 25 (5), 9–16.
Marazzo A., Valentin J. L. (2003). Penilia avirostris (Crustacea, ctenopoda) in a tropical bay: variations in density and aspects of reproduction. Acta Oecologica. 24, 251–257. doi: 10.1016/S1146-609X(03)00019-5
Nakamura Y. (1998). Blooms of tunicates oikopleura spp. and Dolioletta gegenbauri in the seto inland Sea, Japan, during summer. Hydrobiogia. 385 (1-3), 183–192.
Paffenhöfer G. A., Atkinson L. P., Lee T. N., Verity P. G., Bulluck L. R. (1995). Distribution and abundance of thaliaceans and copepods off the southeastern USA during winter. Cont. Shelf Res. 15 (2-3), 255–280. doi: 10.1016/0278-4343(94)E0004-6
Paffenhöfer G. A., Köster M. (2011). From one to many: On the life cycle of Dolioletta gegenbauri uljanin (Tunicata, thaliacea). J. Plankton Res. 33 (7), 1139–1145. doi: 10.1093/plankt/fbr001
Rice E., Dam H. G., Stewart G. (2015). Impact of climate change on estuarine zooplankton surface water warming in long island sound is associated with changes in copepod size and community structure. Estuar. Coast. 38, 13–23. doi: 10.1007/s12237-014-9770-0
Rissik D., Shon E. H., Newell B., Baird M. E., Suthers I. M. (2009). Plankton dynamics due to rainfall, eutrophication, dilution, grazing and assimilation in an urbanized coastal lagoon. Estuar. Coast. Shelf S. 84, 99–107. doi: 10.1016/j.ecss.2009.06.009
Rose K., Roff J. C., Hopcrof R. R. (2004). Production of Penilia avirostris in Kingston harbour, Jamaica. J. Plankton Res. 26 (3), 1–11. doi: 10.1093/plankt/fbh059
Sammacro P. W., Crenshaw H. (1984). Plankton community dynamics of the central great barrier reef lagoon: analysis of data from ikeda et al. Mar. Biol. 82 (2), 167–180.
Shen C. Y., Chen H. S., Zhao H. (2017). The satellite remotely-sensed analysis of temporal and spatial variation of surface chlorophyll in the daya bay. J. Guangdong Ocean University. 37 (6), 57–64.
Shi Y. Q., Sun S., Zhang G. T., Wang S. W., Li C. L. (2015). Distribution pattern of zooplankton functional groups in the yellow Sea in June: A possible cause for geographical separation of giant jellyfish species. Hydrobiologia. 754 (1), 43–58. doi: 10.1007/s10750-014-2070-7
Silver M. W. (1975). The habitat of Salpa fusiformis in the California current as defined by indicator assemblages. Limnol. Oceanogr. 20 (2), 230–237. doi: 10.4319/lo.1975.20.2.0230
Song X. Y., Huang L. M., Zhang J. L., Huang X. P., Zhang J. B., Yin J. Q., et al. (2004). Variation of phytoplankton biomass and primary production in daya bay during spring and summer. Mar. pollut. Bull. 49 (11-12), 1036–1044. doi: 10.1016/j.marpolbul.2004.07.008
Sun S., Huo Y., Yang B. (2010). Zooplankton functional groups on the continental shelf of the yellow Sea. Deep-Sea Res. PT. II. 57 (11), 1006–1016. doi: 10.1016/j.dsr2.2010.02.002
Tew K. S., Lo W. T. (2005). Distribution of thaliacea in SW Taiwan coastal water in 1997, with special reference to Doliolum denticulatum, Thalia democratica and T. orientalis. Mar. Ecol. Prog. Ser. 292, 181–193. doi: 10.3354/meps292181
Tseng L. C., Kumar R., Chen Q. C., Hwang J. S. (2011). Faunal shift between two copepod congeners (Temora discaudata and T. turbinata) in the vicinity of two nuclear power plants in southern East China Sea: Spatiotemporal patterns of population trajectories over a decade. Hydrobiologia. 666, 301–315. doi: 10.1007/s10750-011-0616-5
Walters T. L., Gibson D. M., Frischer M. E. (2019). Cultivation of the marine pelagic tunicate Dolioletta gegenbauri (Uljanin 1884) for experimental studies. Jove.-J. Vis. Exp. 9, 150.
Wang Y. G., Chen X. Y., Xing B. P., Sun R. X., Fitria N., Xiang P., et al. (2018). Zooplankton composition and distribution in the lembeh strait of north sulawesi, Indonesia. Acta Oceanol. Sin. 37 (12), 35–44. doi: 10.1007/s13131-018-1286-1
Wang Y. G., Tseng L. C., Sun R. X., Chen X. Y., Xiang P., Wang C. G., et al. (2022). Copepods as indicators of different water masses during the northeast monsoon prevailing period in the northeast Taiwan. Biology. 11, 1–16, 1357. doi: 10.3390/biology11091357
Wang Y. G., Tseng L. C., Xing B. P., Sun R. X., Chen X. Y., Wang C. G., et al. (2021). Seasonal population structure of the copepod Temora turbinata (Dana 1849) in the kuroshio current edge, southeastern East China Sea. Appl. Sci. 11, 7545. doi: 10.3390/app11167545
Wang Y. G., Zeng L. C., Sun R. X., Liu Z. Y., Lin M., Hwang J. S. (2020). Effects of the China coastal current on the community structure of planktonic copepods in early spring, with note on eurytemora pacifica sato 1913 in the Western Taiwan strait. Crustaceana. 93 (3-5), 487–506. doi: 10.1163/15685403-00004010
Wu R. H., Cai S. Q., Wang S. A., Zhang W. J. (2007). Three-dimensional numerical simulation of tidal current and residual current at daya bay. J. Trop. Oceanography. 26 (3), 18–23.
Wu M. L., Wang Y. S. (2007). Using chemometrics to evaluate anthropogenic effects in daya bay, china. estuar. Coast. Shelf Sci. 72, 732–742. doi: 10.1016/j.ecss.2006.11.032
Wu M. L., Wang Y. S., Dong J. D., Sun C. C., Wang Y. T., Sun F. L., et al. (2011). Investigation of spatial and temporal trends in water quality in daya bay, south China Sea. Int. J. Environ. Res. Public Health 8, 2352–2365. doi: 10.3390/ijerph8062352
Wu M. L., Wang Y. S., Sun C. C., Sun F. L., Cheng H., Wang Y. T., et al. (2012). Monsoon-driven dynamics of water quality by multivariate statistical methods in daya bay, south China sea. Oceanol. Hydrobiol. Stud. 41, 66–76. doi: 10.2478/s13545-012-0040-0
Wu M. L., Wang Y. S., Sun C. C., Wang H., Dong J. D., Han S. H. (2009). Identification of anthropogenic effects and seasonality on water quality in daya bay, south China Sea. J. Environ. Manage. 90 (10), 3082–3090. doi: 10.1016/j.jenvman.2009.04.017
Wu M. L., Wang Y. S., Sun C. C., Wang H. L., Dong J. D., Yin J. P., et al. (2010). Identification of coastal water quality by statistical analysis methods in daya bay, south China Sea. Mar. pollut. Bull. 60, 852–860. doi: 10.1016/j.marpolbul.2010.01.007
Wu M. L., Wang Y. S., Wang Y. T., Sun F.-L., Sun C. C., Cheng H., et al. (2016). Seasonal and spatial variations of water quality and trophic status in daya bay, south China Sea. Mar. pollut. Bull. 112, 341–348. doi: 10.1016/j.marpolbul.2016.07.042
Xiang C. H., Ke Z. X., Li K. Z., Liu J. X., Zhou L. B., Lian X. P., et al. (2021). Effects of terrestrial inputs and seawater intrusion on zooplankton community structure in daya bay, south China Sea. Mar. pollut. Bull. 167, 112331. doi: 10.1016/j.marpolbul.2021.112331
Xu G. Z. (1989). Environments and resources of daya bay (HeFei, China: Anhui Science Publishing House).
Xu C. J., Hu S. M., Guo Z. L., Li T., Huang H., Chan L. L., et al. (2020). Flexible feeding patterns of copepod Centropages tenuiremis in fluctuating conditions: A possible survival strategy to cope with disturbance. Acta Oceanol. Sin. 39 (2), 59–68. doi: 10.1007/s13131-020-1553-9
Yuan K. R., Wang J. J., Lin J. Y., Han Y., Wang K. M., Xu Y. C., et al. (2021). Analysis of the seasonal variation of the residual currents by HF surface wave radar data in adjacent waters of daya bay. J. Appl. Oceanography. 40 (2), 271–283.
Zeng L., Chen G. B., Wang T., Yang B. Z., Yu J., Liao X. L., et al. (2019). Acoustic detection and analysis of Acetes chinensis in the adjacent waters of the daya bay nuclear power plant. J. fishery Sci. China. 26 (6), 1029–1039.
Zeng L., Chen G. B., Wang T., Zhang S. F., Dai M., Yu J., et al. (2021). Acoustic study on the outbreak of Creseise acicula nearby the daya bay nuclear power plant base during the summer of 2020. Mar. pollut. Bull. 165, 112144. doi: 10.1016/j.marpolbul.2021.112144
Zhang L., Xiong L., Li J., Huang X. (2021). Long-term changes of nutrients and biocenoses indicating the anthropogenic influences on ecosystem in jiaozhou bay and daya bay, China. Mar. pollut. Bull. 168, 112406. doi: 10.1016/j.marpolbul.2021.112406
Zhao J. J., Zhang H. C., Liu J. X., Ke Z. X., Xiang C. H., Zhang L. M., et al. (2022). Role of jellyfish in mesozooplankton community stability in a subtropical bay under the long-term impacts of temperature changes. Sci. Total Environ. 849, 157627. doi: 10.1016/j.scitotenv.2022.157627
Zheng Z., Li S. J., Xu Z. Z. (1984). Marine planktology (In Chinese) (Beijing: China Ocean Press), 1–653.
Keywords: zooplankton, indicator species, Daya Bay, nuclear power, disaster-causing organism
Citation: Wu F-X, Gu Y-G, Liu Q-X, Zhang S-F, Rao Y-Y, Liu H-X, Dai M, Wang Y-G and Huang H-H (2023) Research on the seasonal variation of zooplankton community in Daya Bay, South China Sea. Front. Mar. Sci. 10:1110160. doi: 10.3389/fmars.2023.1110160
Received: 28 November 2022; Accepted: 10 February 2023;
Published: 13 March 2023.
Edited by:
Ángel J. Gutiérrez Fernández, University of La Laguna, SpainReviewed by:
Wentao Li, Ocean University of China, ChinaChao Song, Freshwater Fisheries Research Center (CAFS), China
Copyright © 2023 Wu, Gu, Liu, Zhang, Rao, Liu, Dai, Wang and Huang. This is an open-access article distributed under the terms of the Creative Commons Attribution License (CC BY). The use, distribution or reproduction in other forums is permitted, provided the original author(s) and the copyright owner(s) are credited and that the original publication in this journal is cited, in accordance with accepted academic practice. No use, distribution or reproduction is permitted which does not comply with these terms.
*Correspondence: Yan-Guo Wang, d2FuZ3lnQHRpby5vcmcuY24=; Hong-Hui Huang, aHVhbmdoaEBzY3NmcmkuYWMuY24=
†These authors have contributed equally to this work and share corresponding authorship