- College of Life Sciences, Qufu Normal University, Qufu, China
Linoleic acid (LA), a potentially algae-inhibiting chemical released by macroalgae, has been shown to hinder the growth of numerous bloom-forming species. The allelopathic effects of LA (varying from 100 μg/L to 900 μg/L) on harmful microalgae K. mikimotoi were examined using population growth dynamics and physiological levels of K. mikimotoi. LA (>500 μg/L) strongly inhibited algal growth with most cells halted at the S and G2 phases and an evident drop in photosynthetic pigments (chlorophyll a (chl a), chlorophyll c (chl c) and carotenoids). Furthermore, chlorophyll fluorescence parameters such as Fv/Fm, PI, ETo/RC showed a declining trend whereas ABS/RC, DIo/RC, TRo/RC showed an increasing trend with increasing LA exposure concentrations. The level of intracellular reactive oxygen species (ROS) was considerably higher, indicating that LA promoted oxidative stress in K. mikimotoi. Excessive ROS promoted apoptosis in K. mikimotoi, which was noted by increased activity of caspase-3, caspase-9, and flow cytometry (FCM) data. Furthermore, N-acetylcysteine (NAC) and N-Acetyl-Asp-Glu-Val-Asp-CHO (Ac-DEVD-CHO) lowered the apoptotic rates of the LA-treated algal cells, indicating that the aforementioned inhibitors delayed K. mikimotoi apoptosis under LA treatment. To summarize, cell cycle arrest of K. mikimotoi is less sensitive to ROS, but the overproduction of ROS generated by LA activated caspase-3 and caspase-9, which further promoted the apoptosis of K. mikimotoi. This research showed that LA might have great potential and application prospects in controlling the outbreak of harmful algae.
Highlights
● This study revealed responses of Karenia mikimotoi to linoleic acid (LA) stress.
● LA induced the actual change of photosynthetic pigments.
● Excessive ROS was not the reason to result in cycle arrest of K. mikimotoi.
● Excessive ROS stimulated activities of caspase-3, 9, which caused cell apoptosis.
1. Introduction
Harmful algal blooms (HABs) arisen from eutrophication and have engaged worldwide concern because of its negative effects on financial losses and ecological destruction (Heisler et al., 2008; Glibert, 2017; Zhang et al., 2019). Various red tide algae are able to secret toxic substances, which not only aggravate the mortality of aquatic organisms and imbalance the marine ecosystem, but also threaten the human health through the food chain (Lei and Lu, 2011; Paerl et al., 2016). K. mikimotoi is a dominant dinoflagellate that forms HABs and hemolytic toxins, ichthyotoxins secreted by algal cells have great toxicity effects on some marine organisms (Mooney et al., 2010; Brand et al., 2012; Kim et al., 2020). Besides, the mode of contact dependence may be another reason that K. mikimotoi kills marine organisms, which further illustrates its potential hazards (Li et al., 2019). Considering its hazardousness and frequent outbreaks, more and more researches focus on the prevention and treatment of K. mikimotoi.
It is a great challenge to find an effective and eco-friendly method to prevent or kill K. mikimotoi. Current treatment methods are mostly based on physical and chemical progress (Lu et al., 2015). Modified clay (MC), UV light and the addition of copper-based products have been used for the treatment of K. mikimotoi, which present greatly inhibitory effects on the growth and normal cell activities (Guan and Li, 2017). However, it is unavoidable that above additives cause secondary environmental problems. For example, the removal efficiency of K. mikimotoi reached to 64% within 3 h under the MC treatment, but the left algal cells could grow well, which increased the risk of next bloom (Liu et al., 2018). Moreover, secondary environmental pollution would be caused during the progress of adding the copper compounds because of its toxicity to aquatic organisms (Dethloff et al., 1999). Based on this, allelochemicals secreted by several plants received more attention and some secondary products have been isolated and identified from terrestrial and aquatic plants, which effectively suppress the growth of red tide algae (Nan et al., 2008; Sun et al., 2017; Chen et al., 2021).
Up to now, some fatty acids secreted by macroalgae were regarded as allelochemicals, which provide the possibility to perform in-situ treatment of HABs. Several fatty acids, including Hexadeca-4,7,10,13-tetraenoic acid (HDTA), octadeca-6,9,12,15-tetraenoic acid (ODTA), α-linolenic acid (ALA) and LA, had been extracted from Ulva fasciata and presented remarkable inhibition to K. mikimotoi, Alexandrium tamarense and H. akashiwo (Alamsjah et al., 2008; Oh et al., 2010; Hirao et al., 2012). These allelochemicals inhibit algae mainly via directly inhibiting photosystem II (PSII) components, interrupting the dark respiration and influencing ROS-mediated allelopathic mechanisms (Qian et al., 2009; Yang et al., 2011; D'Abrosca et al., 2013). Excessive ROS induced by environmental stress results in oxidative stress and disrupts the dynamic balance between ROS and the anti-oxidant system, which decreases the activity of superoxide dismutase (SOD), peroxidase (POD) and triggers algal cells death by the action of caspase-3 and -9 when overproduced ROS is unable to clean up in time (Fernández-Herrera et al., 2021; Sun et al., 2021).
LA is a potential substance to inhibit red tide algae and some researchers have preliminarily explained the mechanism of suppressing bloom-forming algae (Ni et al., 2018). However, it is not clear to fully describe the mechanism of K. mikimotoi under LA stresses. Thus, this study illustrated the effects of LA on K. mikimotoi from several aspects: (1) effects on the growth and cell cycle of K. mikimotoi; (2) the responses of photosynthetic system, including the photosynthetic pigment contents (chlorophyll a (chl a), chlorophyll c (chl c) and carotenoids), chlorophyll fluorescence parameters (optimal/maximal quantum yield of PSII (Fv/Fm), performance index on absorption basis (PI)), energy used for electron transfer (ETo/RC), energy absorbed by Antenna chlorophyII (ABS/RC), energy used for heat dissipation (DIo/RC) and energy used for QA (TRo/RC); (3) the relationships among ROS, cell cycle and cell apoptosis of K. mikimotoi.
2. Material and method
2.1. Algal culture and reagents
K. mikimotoi was provided by the Ocean University of China and algal cells were cultured in axenic f/2 medium at 23 ± 3°C under a 12:12 h light: dark cycle with light intensity set to 75 μmol photons m−2 s−1. All related experiments would be carried out using exponential-phase algae with initial cell density diluted to 1 × 105 cells mL−1.
LA (purity > 99%) and acetone (purity ≥ 99.9%) were purchased from Sigma Corporation of America and the LA was dissolved in acetone as a stock solution. NAC and Ac-DEVD-CHO were purchased from Beyotime Institute of Biotechnology (Shanghai, China).
2.2. Population dynamics and cell cycle analysis
LA dissolved in acetone was added to algal cultures at final concentrations of 100, 300, 500, 700, and 900 μg/L. Algal cultures with sterile seawater and acetone treatment were regarded as blank control and solvent control. The cell density of K. mikimotoi at 0, 12, 24, 48, 72 and 96h postexposure was counted with a hemocytometer under optical microscope. The population growth dynamics were monitored according to the method of Guillard (Guillard, 1975).
Cell cycle analysis kit (Beyotime Institute of Biotechnology, Shanghai, China) was used for measuring the cell cycle of the treated and untreated algal cells in a flow cytometer. In brief, the control and LA-treated cells (500 μg/L and 900 μg/L) at 48h postexposure were collected using centrifugation (1200 × g, 4°C) for 15 min and precipitated cells were suspended by 1 mL precooled phosphate-buffered saline (PBS). 0.5 mL propidium iodide (PI) was added to suspend the cells and incubated at 37 °C for 30 min and the fluorescence intensity was detected by the FC 500 MPL flow cytometer (Novocyte2040R, ACEA, USA) under an excitation wavelength of 488 nm (Pokrzywinski et al., 2017).
2.3. Determination of photosynthetic pigments and parameters
35 mL algal cultures at 6, 12, 24 and 48h postexposure from all treatments were centrifuged at 4°C for 15 min at 5000 × g to collect the algal cells and 95% ethanol was used for the extraction of pigments at 4 °C overnight in darkness (Xiao et al., 2010). The absorbance values of supernatant obtained by centrifugation were measured at 470 nm, 646 nm and 663 nm and the photosynthetic pigments were calculated using the following equations:
Chlorophyll fluorescence parameters, including Fv/Fm, PI, ETo/RC ABS/RC, DIo/RC and TRo/RC of algal cells were measured by plant efficiency analyzer (Handy PEA Hansatech Instrument. Ltd, England). In brief, samples after dark treatment for 20 min were measured with the initial fluorescence value F0 at 0.01 μmol photons m−2 s−1 and then Fm, Fv/Fm, PI, ABS/RC, TRo/RC, ETo/RC and DIo/RC at 4000 μmol photons m−2 s−1 for 0.8 s.
2.5. Reactive oxygen species analysis
Detection of intracellular ROS was based on the degree of non-fluorescent DCFH-DA (2′,7′-dichlorodihydrofluorescein diacetate acetyl ester) using a ROS assay kit (Beyotime Institute of Biotechnology, Shanghai, China). In brief, 35 mL LA treated and untreated algal cells at 6, 24 and 48h postexposure were centrifuged at 4°C for 15 min at 1000 × g to collect algal cells. Subsequently, 1 mL DCFH-DA was added to algal cells with incubation treatment at 37 °C in darkness for 30 min and the above cells were washed and suspended. Besides, 200 μL NAC (5 mmol L−1) was added to 500 μg/L, 900 μg/L LA treated groups when they were at 24h postexposure and follow-up steps were carried as above steps. The fluorescence intensity was detected by a flow cytometer (Novocyte 2040R, ACEA, USA) under an excitation wavelength of 488 nm and emission wavelength of 525 nm (Han et al., 2018).
2.6. Caspase-3, 9 activities and cell apoptosis analysis
Caspase-3 and caspase-9 activity detection kit (Beyotime Institute of Biotechnology of Shanghai, China) were used for the detection of caspase-3 and caspase-9 activity of K. mikimotoi with or without LA treatment at 48h postexposure. The fluorescence intensity was measured using Synergy H1 microporous reader (BioTek Instruments, Inc, America) with an excitation wavelength of 485 nm and an emission wavelength of 535 nm (Han et al., 2018).
The apoptosis of K. mikimotoi was detected using an Annexin V-FITC apoptosis detection kit (Beyotime Institute of Biotechnology of Shanghai, China). Early apoptotic cells were stained by Annexin V-FITC and late apoptotic cells were stained by PI, which illustrated the apoptosis of algal cells at different times. Briefly, LA-treated and untreated algal cells at 48h postexposure were collected using centrifugation at 4°C for 15 min at 1200 × g and suspended with 1ml PBS. Annexin V-FITC and PI were added to stain algal cells and cells were incubated at 25°C for 20 min. FITC and PI fluorescence were monitored by the FL-1 channel and FL-2 channel in the FC 500 MPL flow cytometer (Novocyte2040R, ACEA, USA), respectively (Wang and Liu, 2022).
2.7. Determinations of the effects of ROS on cell cycle and apoptosis
NAC is a common antioxidant and Ac-DEVD-CHO is an effective caspase-3 inhibitor. To clear the effects of ROS on cell cycle and apoptosis, 200 μL NAC (5 mmol L−1) and 40 μL Ac-DEVD-CHO (20 mmol L−1) were added and then treated with 900 μg/L LA for 48 h to perform algal cells cycle and apoptosis. Related detecting methods were performed according to the methods above.
2.8. Statistical analysis
Samples in all experiments were performed in triplicate and the data were shown as means ± standard deviations (SD). Results presented in the flow cytometer were analyzed in novocyte. The differences between the control and LA-treated groups were analyzed by one-way ANOVA based on Duncan’s multiple-range test in SPSS 20.0. Significant differences between the control and LA-treated groups are indicated by asterisks: *, p< 0.05; **, p< 0.01; ***, p< 0.001.
3. Results
3.1. Effect of LA on the growth of K. mikimotoi
Figure 1A showed the ability of LA to inhibit the growth of K. mikimotoi. No significant difference in algal growth was observed between the acetone group and the control group (p > 0.05), while LA treatments groups presented different variations. Compared to the control group, high LA concentration (>500 μg/L) significantly inhibited the growth of K. mikimotoi with the inhibitory rate of the LA treatment group (900 μg/L) reached 57.7%, while low-dose LA (100 μg/L) benefited for algal growth (p < 0.05). The effects of LA became more obvious with time prolonged stress and a dose-dependent effect of LA on K. mikimotoi was presented.
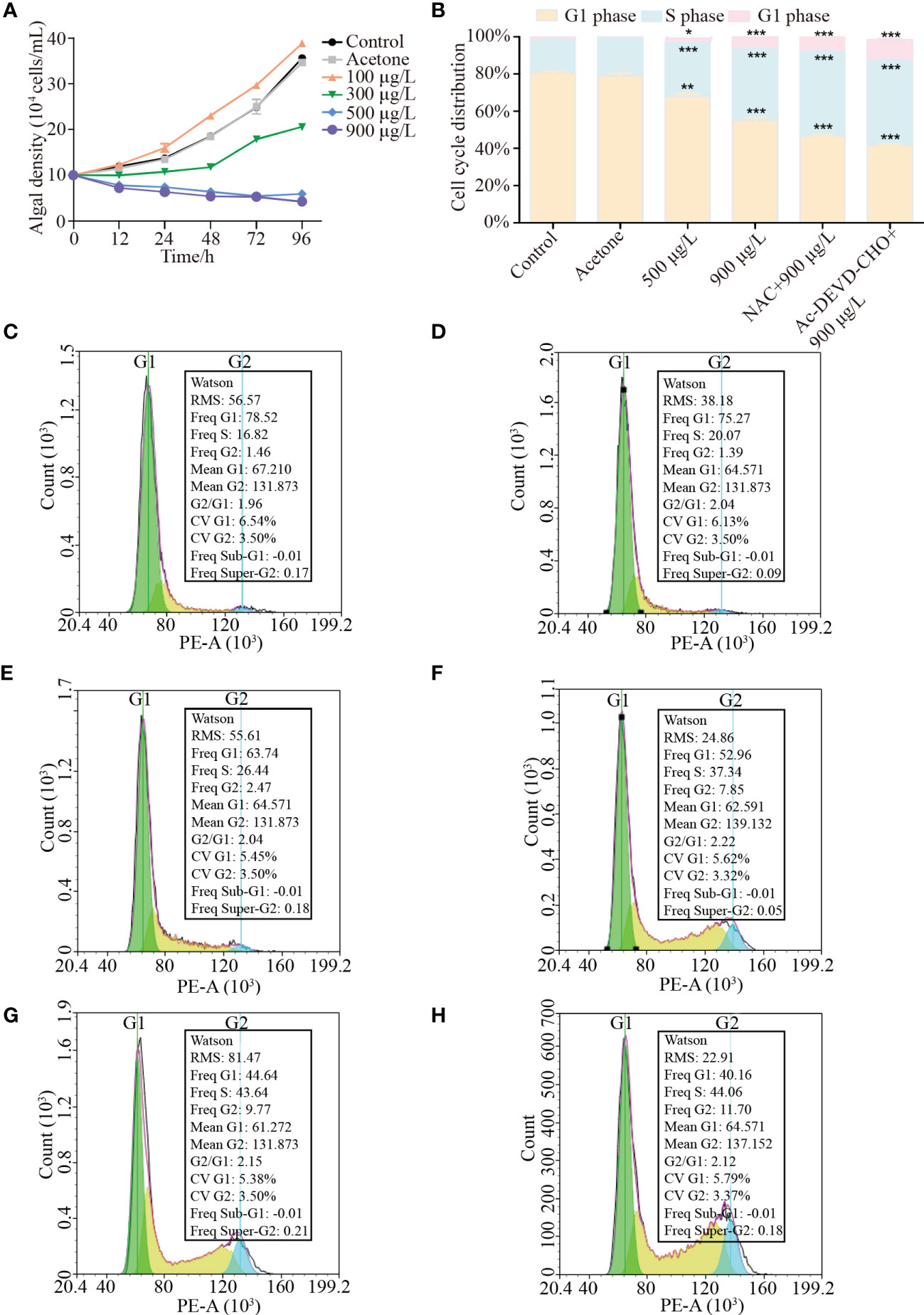
Figure 1 Effects of different treatments with or without LA treatment on the growth and cell cycle arrest of K. mikimotoi. (A) was growth of K. mikimotoi under different treatments (control, acetone, 100 μg/L, 300 μg/L, 500 μg/L and 900 μg/L). (B) showed cell cycle arrest of K. mikimotoi under different treatment. (C–H) showed flow cytometry analysis of K. mikimotoi in different treatment groups (C to H represented groups of control, acetone, 500 μg/L LA treatment, 900 μg/L LA treatment, 900 μg/L LA and NAC treatment and 900 μg/L LA and Ac-DEVD-CHO treatment). * represent p< 0.05, *** represent p< 0.001.
Flow cytometry analysis reflected the effects of different LA levels (500 μg/L, 900 μg/L) on cell cycle of K. mikimotoi. As shown in Figure 1B, cell cycle distribution was significantly changed when exposed to high LA concentrations (500 μg/L, 900 μg/L) (p< 0.001) and more algal cells were arrested at S phase (26.44%, 37.34%) and G2 phase (2.47%, 7.85%), which was higher than cells at S phase (16.82%) and G2 phase (1.46%) of control groups. Moreover, the addition of NAC and Ac-DEVD-CHO could not relieve the occurrence of cell arrest and cell cycle distribution of S phase and G2 phase reached 43.64% and 9.77% respectively in LA combined with NAC treatment, while the ratio reached 44.06% and 11.07% in LA combined with Ac-DEVD-CHO treatment (Figures 1C–H).
3.2. Effects of LA on the photosynthetic pigments and parameters of K. mikimotoi
The responses of photosynthetic pigments (chl a, chl c and carotenoids) and parameters (DIo/RC, ABS/RC, ETo/RC, Fv/Fm, PI and TRo/RC) were measured to illustrate the effects of LA on K. mikimotoi photosynthesis. The results indicated that chl a, chl c and carotenoids of algal cells increased in low-dose LA treatment (100 μg/L-300 μg/L), while a decrease in high-dose LA treatments(500 μg/L-900 μg/L). Moreover, the effects of different LA showed an ongoing trend, which meant that contents change became more pronounced with time went by (Figures 2A–C).
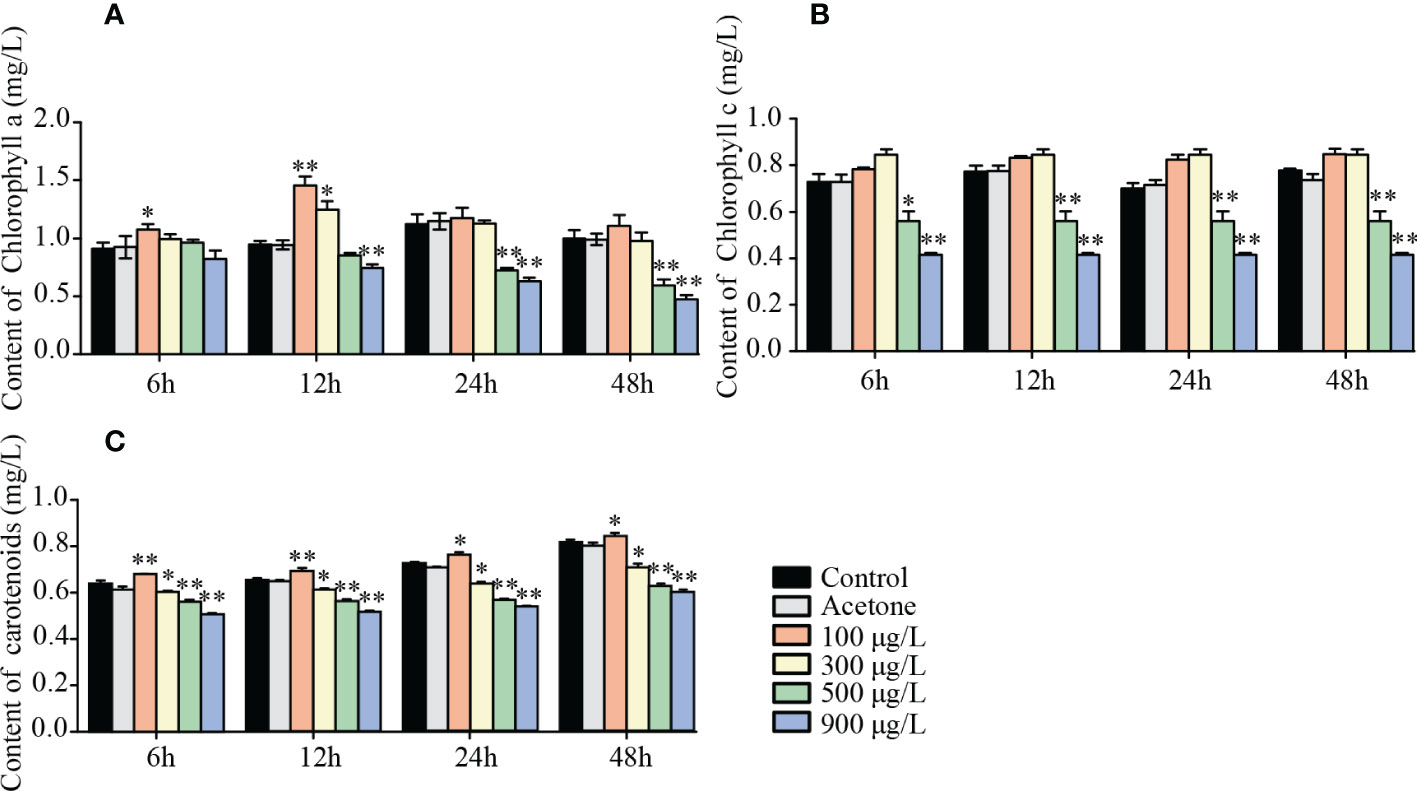
Figure 2 Effects of LA on photosynthetic pigments of K. mikimotoi. (A) was changes of chlorophyll a (chl a) of algal cells in different treatment groups under various exposure time. (B) was changes of chlorophyll c (chl c) of algal cells in different treatment groups under various exposure time. (C) was changes of carotenoids of algal cells in different treatment groups under various exposure time. * represent p< 0.05, ** represent p< 0.01.
A dose-dependent trend was presented in the measurement of photosynthetic parameters. DIo/RC and ABS/RC in the control group increased from 5.1 (DIo/RC) and 14 (ABS/RC) to 5.2- 9.1 and 14.1- 25.0 in different LA treated groups (p< 0.001) (Figures 3A, B). On the contrary, The ETo/RC, Fv/Fm, and PI decreased from 2.8 (ETo/RC), 0.65 (Fv/Fm) and 0.58 (PI) in the control group to 2.4-1.1, 0.58-0.22 and 0.44-0.14 in different LA treated groups (p< 0.001) (Figures 3C–E). Moreover, TRo/RC presented the trend of rising and then falling with increasing LA treatment levels (Figure 3F).
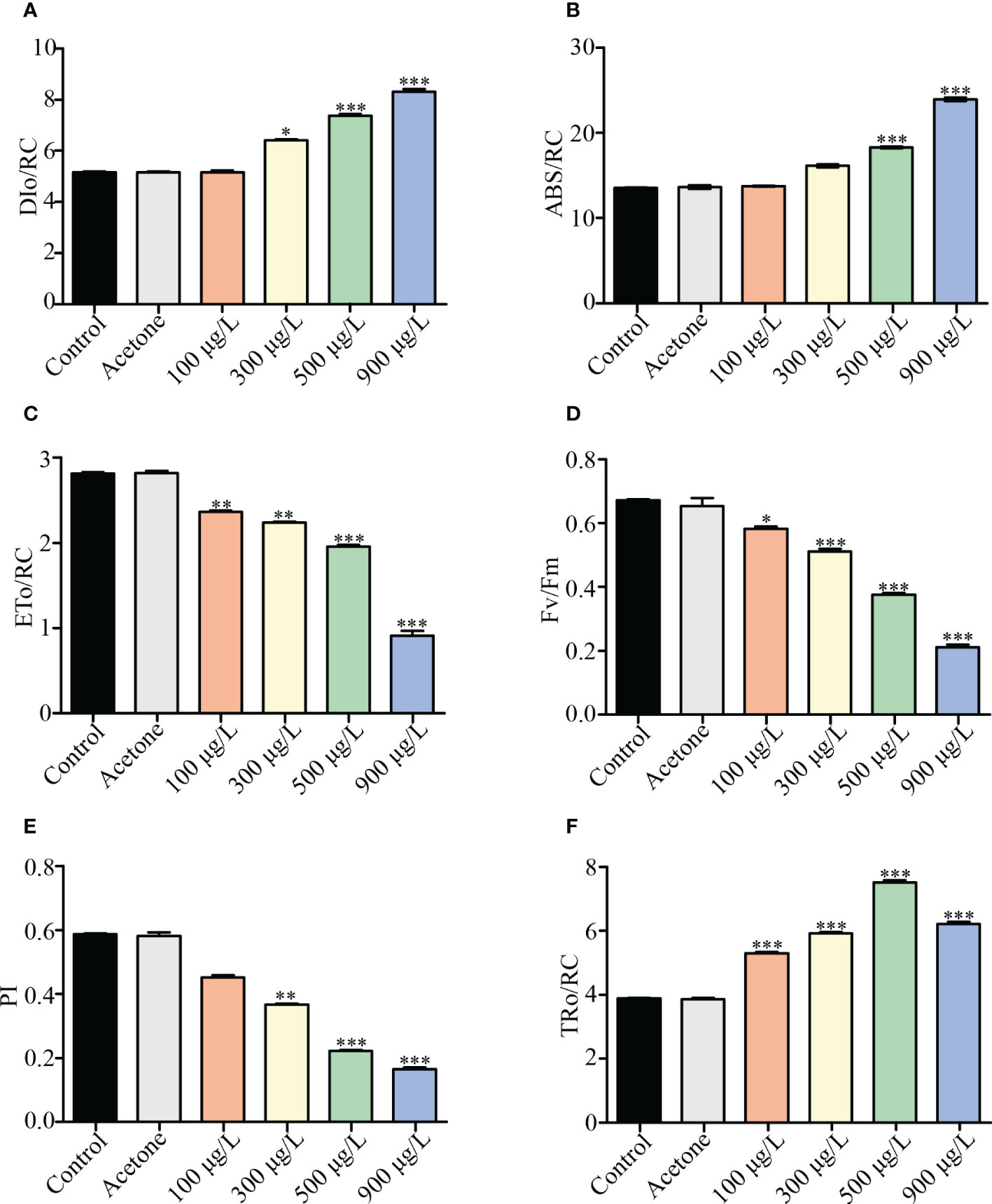
Figure 3 Effects of LA on photosynthetic parameters of K. mikimotoi. (A–F) showed changes of DIo/RC, ABS/RC, ETo/RC, Fv/Fm, PI and TRo/RC in different treatment groups. * represent p< 0.05, ** represent p< 0.01, *** represent p< 0.001.
3.3. Responses of ROS species to LA stress
Figure 4A showed that intracellular ROS significantly increased when algal cells were exposed to high LA-level treatments (>300 μg/L) and the contents of ROS continuously rose over time (p< 0.01). Intracellular ROS reached a higher level after 48h exposure and the highest ROS level was obtained in K. mikimotoi under 900 μg/L LA treatment after 48 h exposure with the DCF fluorescence is about 2.27 fold of the control. And there was a significant decrease in ROS levels for high LA treated groups (500 μg/L, 900 μg/L) when the same amount of NAC was added, while the NAC failed to remove excessive ROS produced in 900 μg/L LA treated group (Figure 4B).
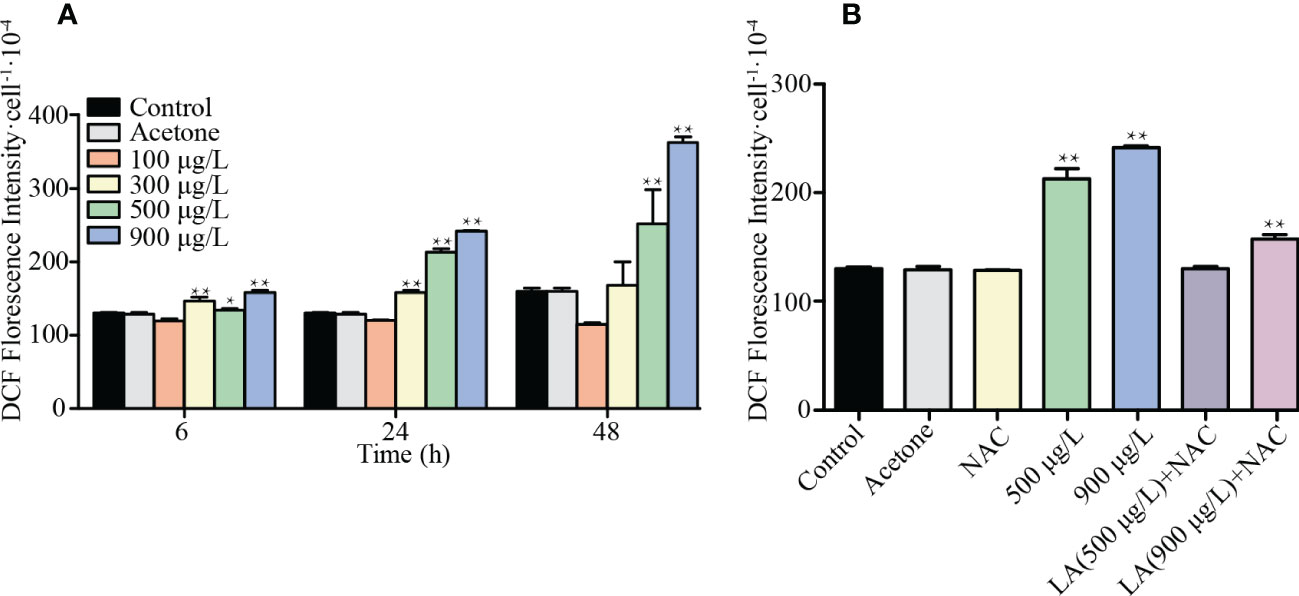
Figure 4 Changes of intracellular ROS contents in different treated group. (A) showed responses of the ROS levels of K. mikimotoi in different treatments for 6h, 24h and 48h. (B) showed ROS levels of K. mikimotoi when were treated by 500 μg/L, 900 μg/L LA and above concentrations with NAC. * represent p< 0.05, ** represent p< 0.01.
3.4. Connection of ROS, caspase and apoptosis of K. mikimotoi
Figures 5A, B showed that the activity of caspase-3 in 500 μg/L and 900 μg/L LA treated groups was roughly 2.81 and 5.27 times higher than that of the control, respectively (p< 0.001). Moreover, the activity of caspase-9 in 900 μg/L LA treated groups was approximately 1.99 times higher than that of the control group (p < 0.001). To illustrate the relationship between ROS and caspase, NAC was added to 900μg/L LA treated group and the activities of caspase-3 and caspase-9 decreased from 5.27 to 0.92 and 2.02 to 0.845, respectively. Figure 5C showed that the apoptosis rate significantly increased after exposure of 900μg/L LA and the addition of NAC and Ac-DEVD-CHO remarkably alleviated cell apoptosis (p< 0.001).
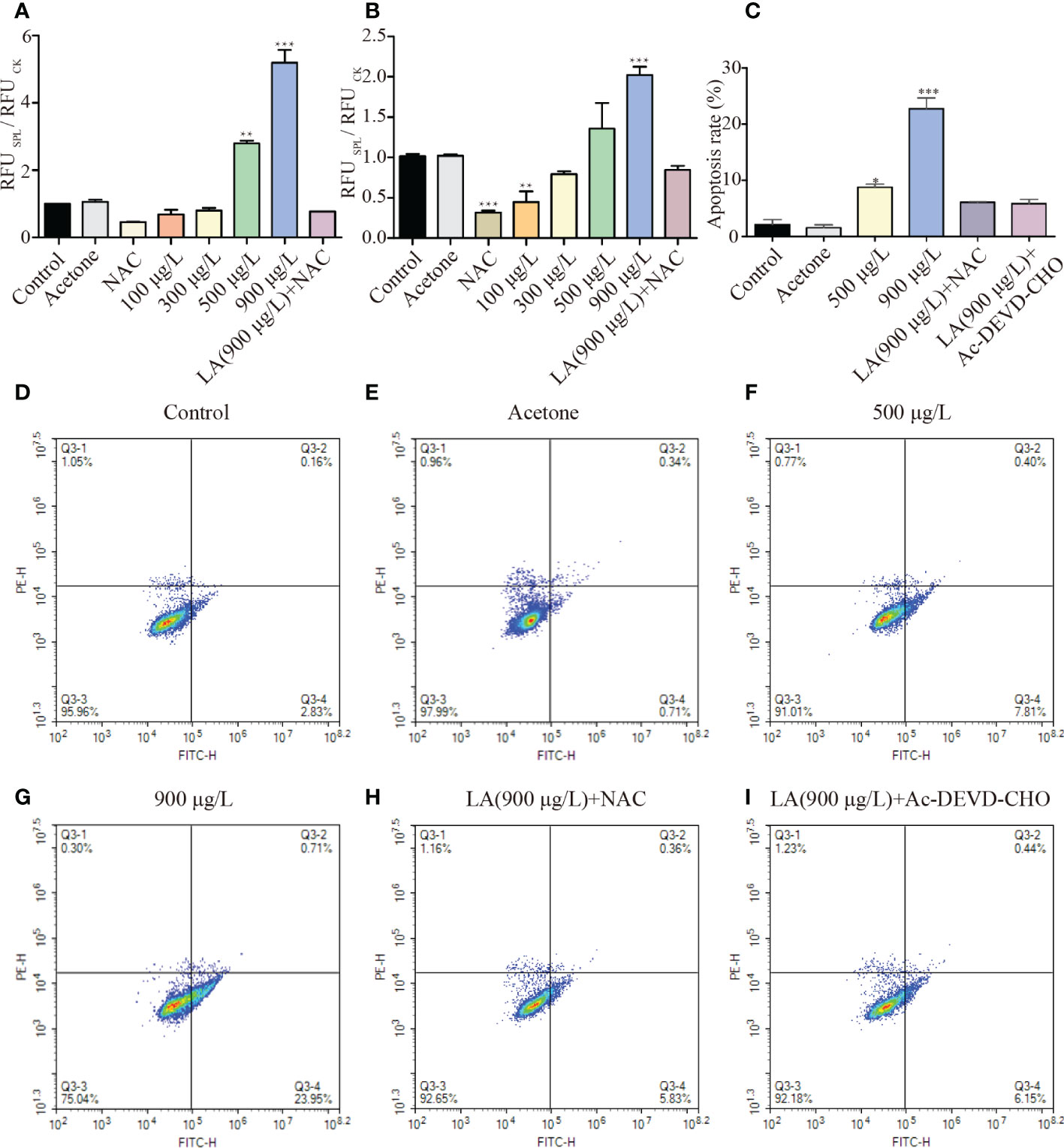
Figure 5 Effects of LA on cell apoptosis of K. mikimotoi. (A, B) showed changes of activities of caspase-3 and caspase-9 when algal cells were under different treatments, respectively. (C) showed apoptosis rate of K. mikimotoi that were treated by 500 μg/L, 900 μg/L LA, 900 μg/L LA +NAC and 900 μg/L LA + Ac-DEVD-CHO. (D–I) represented flow cytometry analysis on different treatments. The Q3-1 quadrant represented the cell debris, Q3-2 quadrant represented the late apoptotic or necrotic cells, Q3-3 quadrant represented the surviving cells, and Q3-4 quadrant represented the early apoptotic cells. * represent p< 0.05, ** represent p< 0.01, *** represent p< 0.001.
AnnexinV-FITC/PI double staining in flow cytometry analysis was adapted to observe phosphatidylserine ectropion and cells strained red, which were marks of early and late apoptotic cells. Most algal cells without LA stress maintained cell activity and algal cells showed varying levels of apoptosis after LA exposure for 48 h, with the proportion of cells in early apoptosis (Annexin V-positive/PI-negative) was greater than late apoptosis cells (Annexin V-positive/PI-positive) (Figures 5D–G). Ratios of early apoptosis cells in algal cells under 900μg/L LA treatment significantly increased, with the apoptosis rate reaching from 2.83% to 23.95% in comparison with the control group and the degree of cell apoptosis was consistent with the concentration of LA stress (p< 0.001). However, the addition of NAC and Ac-DEVD-CHO in advance significantly decreased the ratios of cell apoptosis and cell apoptosis ratio decreased from 23.95% to 5.83% and 6.15%, respectively (Figures 5H, I).
4. Discussion
In recent years, HABs have received widespread attention as it drives great marine environmental crises and K. mikimotoi has been the main casual species of those HABs (Gentien et al., 2007; Griffith and Gobler, 2020). Biological approaches have potential for controlling HABs and some macroalgae have been confirmed to inhibit the growth of many red tide algae using allelochemicals, including LA, ALA and so on (Hirao et al., 2012; Wang and Liu, 2022). In this study, LA was selected as a stress factor to illustrate its effects on K. mikimotoi based on some physiological indicators, which explained the mechanism of algal mortality and revealed the action of ROS to cell cycle and cell apoptosis.
Changes in growth are most likely to be observed once the algae are under various stress factors exposure. In this study, it was discovered that K. mikimotoi showed variable responses to different LA stress, with an increased population density under low LA exposure levels (100 μg/L) and inhibitory effects appeared under high LA exposure levels (>500 μg/L). Flow cytometry analysis indicated that more algal cells were arrested at the S phase and G2 phase with increasing LA exposure concentrations. Moreover, the addition of NAC and Ac-DEVD-CHO failed to relieve the occurrence of cell arrest, which meant that LA directly influenced the growth of K. mikimotoi by S phase and G2 phase cell cycle arrest instead of being mediated by ROS and caspase. Algal cell growth conditions and cell cycle arrest are direct consequences of environmental stress on algal cells. The stability of the cell cycle is the basis for cell proliferation. Some biotic and abiotic factors have been proven to block the cell cycle, which influences the growth of algal cells (Harshkova et al., 2021). Li et al. examined the effects of P deficiency on the cell division cycle and confirmed that cells were arrested in the G1 phase, while the accumulation of proteins and photosynthetically fixed carbon were benefical for the growth of algal cells (Li et al., 2016). Moreover, Pokrzywinski et al. illustrated the effects of IRI-160AA, a secretion of Shewanella sp., on Prorocentrum minimum, Karlodinium veneficum and Gyrodinium instriatum, which indicated that the algicide was benefit for the increase of cells in S phase instead of G1 phase (Pokrzywinski et al., 2017).
Photosynthesis, an important physiological process in sustaining life of algae, is usually affected when algae are under diverse stress factors (Konarzewska et al., 2020). Some algicides simultaneously inhibit algal photosynthesis, which reduces algae biomass to some extent (Zhang et al., 2021). Changes in contents of photosynthetic pigments and some chlorophyll fluorescence parameters reflected the growth of algal cells in an indirect way (Pérez-Pérez et al., 2012; Wang et al., 2017; Lee et al., 2020). A previous study demonstrated that LA inhibited the photosynthetic efficiency of C. pyrenoidosa and the Fv/Fm parameter decreased significantly compared to that of controls (Qian et al., 2018). In this study, high LA concentrations significantly inhibited photosynthetic pigment content, and related chlorophyll fluorescence parameters presented regular variation with increased LA exposure concentration, which was been reported in other related researches (Yang et al., 2013; Ma et al., 2017).
ROS has evolved as a signaling molecule to drive cellular responses to changes in the external environmental (Mullineaux et al., 2018). However, excessive ROS induced by abiotic and biotic stress threatens the redox homeostasis and oxidative stress in algae, which is response for damage to algal cells (Feng et al., 2017; Rezayian et al., 2019; Tziveleka et al., 2021). Results showed that the ROS level in the LA-treated algal cells significantly increased, indicating the occurrence of oxidative stress. The contents of ROS accumulated over time and the addition of NAC alleviated a degree of oxidative stress, which meant the production of excessive ROS was one of the triggers of algae death. Phosphatidylserine (PTS) externalization is one of the indicators of cell apoptosis (Haest, 2003; Bidle and Bender, 2008). Flow cytometry analysis based on AnnexinV-FITC/PI double staining observed more early apoptosis cells and the addition of antioxidants (NAC) and caspase-3 inhibitor (Ac-DEVD-CHO) could slow down the apoptosis of K. mikimotoi under the exposure of LA. Caspase-9 is a cell apoptosis-initiating enzyme and Caspase-3 is a cell apoptosis-executing enzyme (Brentnall et al., 2013). Results also showed that activities of caspase-3, 9 significantly increased in algae cells treated by LA, while its activity could be decreased by adding NAC and Ac-DEVD-CHO, which verified that caspase-3, 9 play key roles in inducing cell apoptosis (Thornberry and Lazebnik, 1998; Bidle and Bender, 2008; Lawrence, 2012).
This study revealed the relationship between ROS, caspase activity to cell cycle and cell apoptosis using ROS scavenger NAC and caspases-3 inhibitor Ac-DEVD-CHO. Excessive ROS induced by LA could not relieve the occurrence of cell arrest, which meant ROS was not a direct cause of cell arrest. The addition of NAC could decrease the activities of Caspase-3, 9 and the cell apoptosis rate decreased after adding NAC and Ac-DEVD-CHO. This phenomenon proved that LA induced the elevation of ROS levels and excessive ROS stimulated the activity of caspase-3 and caspase-9, which resulted in apoptosis of K. mikimotoi.
This research systematically investigated the inhibition mechanism of LA to K. mikimotoi, and improved the understanding about how to control K. mikimotoi using LA. At present, studies of in-situ treatment of HABs using allelochemicals have been carried out (Techer et al., 2016). The investigation of the action mode of LA against K. mikimotoi in this research might provide more possibility to carry out related researches to a certain extent.
5. Conclusion
This study focused on the effects of LA on K. mikimotoi, determined that overproduction of ROS was not responsible for cell cycle arrest and revealed the relationships among ROS, caspase-3, caspase-9 and cell apoptosis. High LA concentrations greatly influenced K. mikimotoi, including a decline in photosynthetic pigments and damage to the photosynthetic system, blocked cell cycle at the S phase and G2 phase, and followed induction of cell apoptosis. It was found that LA stimulated the production of ROS, and excessive ROS increased the activities of caspase-3 and caspase-9, which induced the apoptosis of the algal cells.
Data availability statement
The original contributions presented in the study are included in the article/supplementary material. Further inquiries can be directed to the corresponding author.
Author contributions
PG conceived this project. RW and CW carried out the experiments and analyzed the dates. XL collected the dates. JC, CL, YS, and ND reviewed and edited this writing. All authors contributed to the article and approved the submitted version.
Funding
This work was funded by National Natural Science Foundation of China (31971503, 31901188), Shandong Provincial Natural Science Foundation (ZR2020QC048).
Conflict of interest
The authors declare that the research was conducted in the absence of any commercial or financial relationships that could be construed as a potential conflict of interest.
Publisher’s note
All claims expressed in this article are solely those of the authors and do not necessarily represent those of their affiliated organizations, or those of the publisher, the editors and the reviewers. Any product that may be evaluated in this article, or claim that may be made by its manufacturer, is not guaranteed or endorsed by the publisher.
References
Alamsjah M. A., Hirao S., Ishibashi F., Oda T., Fujita Y. (2008). Algicidal activity of polyunsaturated fatty acids derived from ulva fasciata and u. pertusa (Ulvaceae, chlorophyta) on phytoplankton. J. Appl. Phycol. 20, 713–720. doi: 10.1007/s10811-007-9285-1
Bidle K. D., Bender S. J. (2008). Iron starvation and culture age activate metacaspases and programmed cell death in the marine diatom thalassiosira pseudonana. Eukaryotic Cell. 7, 223–236. doi: 10.1128/EC.00296-07
Brand L. E., Campbell L., Bresnan E. (2012). Karenia: The biology and ecology of a toxic genus. Harmful Algae. 14, 156–178. doi: 10.1016/j.hal.2011.10.020
Brentnall M., Rodriguez-Menocal L., De-Guevara R. L., Cepero E., Boise L. H. (2013). Caspase-9, caspase-3 and caspase-7 have distinct roles during intrinsic apoptosis. BMC Cell Biol. 14, 32. doi: 10.1186/1471-2121-14-32
Chen Q., Sun D., Fang T., Zhu B., Liu W. J., He X. Y., et al. (2021). In vitro allelopathic effects of compounds from cerbera manghas l. @ on three dinophyta species responsible for harmful common red tides. Sci. Total Environ. 754, 142253. doi: 10.1016/j.scitotenv.2020.142253
D'Abrosca B., Scognamiglio M., Fiumano V., Esposito A., Choi Y. H., Verpoorte R., et al. (2013). Plant bioassay to assess the effects of allelochemicals on the metabolome of the target species aegilops geniculata by an NMR-based approach. Phytochemistry 93, 27–40. doi: 10.1016/j.phytochem.2013.03.016
Dethloff G. M., Schlenk D., Khan S., Bailey H. C. (1999). The effects of copper on blood and biochemical parameters of rainbow trout (Oncorhynchus mykiss). Arch. Environ. Contam Toxicol. 36, 415–423. doi: 10.1007/pl00006614
Feng M., Yin H., Peng H., Liu Z. H., Lu G. N., Dang Z. (2017). Hexavalent chromium induced oxidative stress and apoptosis in pycnoporus sanguineus. Environ. Pollut. 228, 128–139. doi: 10.1016/j.envpol.2017.05.012
Fernández-Herrera L. J., Band-Schmidt C. J., Zenteno-Savín T., Leyva-Valencia I., Hernández-Guerrero C. J., Muñoz-Ochoa M. (2021). Cell death and metabolic stress in gymnodinium catenatum induced by allelopathy. Toxins 13, 506. doi: 10.3390/toxins13070506
Gentien P., Lunven M., Lazure P., Youenou A., Crassous M. P. (2007). Motility and autotoxicity in karenia mikimotoi (Dinophyceae). Philos. Trans. R Soc. Lond B Biol. Sci. 362, 1937–1946. doi: 10.1098/rstb.2007.2079
Glibert P. M. (2017). Eutrophication, harmful algae and biodiversity - challenging paradigms in a world of complex nutrient changes. Mar. Pollut. Bull. 124, 591–606. doi: 10.1016/j.marpolbul.2017.04.027
Griffith A. W., Gobler C. J. (2020). Harmful algal blooms: A climate change co-stressor in marine and freshwater ecosystems. Harmful Algae. 91, 101590. doi: 10.1016/j.hal.2019.03.008
Guan W. C., Li P. (2017). Dependency of UVR-induced photoinhibition on atomic ratio of n to p in the dinoflagellate karenia mikimotoi. Mar. Biol. 164, 1–9. doi: 10.1007/s00227-016-3065-x
Guillard R. R. L. (1975). Culture of phytoplankton for feeding marine invertebrates (US: Springer), 29–60.
Haest C. W. M. (2003). Distribution and movement of membrane lipids (Berlin Heidelberg: Springer), 1–25.
Han M. A. X., Wang R. J., Ding N., Liu X. X., Zheng N. N., Fu B. Y., et al. (2018). Reactive oxygen species-mediated caspase-3 pathway involved in cell apoptosis of karenia mikimotoi induced by linoleic acid. Algal Res. 36, 48–56. doi: 10.1016/j.algal.2018.10.013
Harshkova D., Liakh I., Bialevich V., Ondrejmišková K., Aksmann A., Bišová K. (2021). Diclofenac alters the cell cycle progression of the green alga chlamydomonas reinhardtii. Cells 10, 1936. doi: 10.3390/cells10081936
Heisler J., Glibert P. M., Burkholder J. M., Anderson D. M., Cochlan W., Dennison W. C., et al. (2008). Eutrophication and harmful algal blooms: A scientific consensus. Harmful Algae. 8, 3–13. doi: 10.1016/j.hal.2008.08.006
Hirao S., Tara K., Kuwano K., Tanaka J., Ishibashi F. (2012). Algicidal activity of glycerolipids from brown alga ishige sinicola toward red tide microalgae. Bull. Chem. Soc Jpn. 76, 372–374. doi: 10.1271/bbb.110645
Kim D., Li W. C., Matsuyama Y., Matsuo A., Yagi M., Cho K., et al. (2020). Strain-dependent lethal effects on abalone and haemolytic activities of the dinoflagellate karenia mikimotoi. Aquaculture 520, 734953. doi: 10.1016/j.aquaculture.2020.734953
Konarzewska Z., Śliwińska-Wilczewska S., Felpeto A. B., Vasconcelos V., Latała A. (2020). Assessment of the allelochemical activity and biochemical profile of different phenotypes of picocyanobacteria from the genus synechococcus. Mar. Drugs 18, 179. doi: 10.3390/md18040179
Lawrence J. E. (2012). Heat-stress-induced programmed cell death in heterosigma akashiwo (Raphidophyceae). Harmful Algae. 16, 108–116. doi: 10.1016/j.hal.2012.02.003
Lee J., Kim M., Jeong S. E., Park H. Y., Jeon C. O., Park W. (2020). Amentoflavone, a novel cyanobacterial killing agent from selaginella tamariscina. J. Hazard. Mater. 384, 121312. doi: 10.1016/j.jhazmat.2019.121312
Lei Q. Y., Lu S. H. (2011). Molecular ecological responses of the dinoflagellate karenia mikimotoi to phosphate stress. Harmful Algae. 12, 39–45. doi: 10.1016/j.hal.2011.08.010
Li M. Z., Shi X. G., Guo C. T., Lin S. J. (2016). Phosphorus deficiency inhibits cell division but not growth in the dinoflagellate amphidinium carterae. Front. Microbiol. 7. doi: 10.3389/fmicb.2016.00826
Liu S. Y., Yu Z. M., Song X. X., Cao X. H. (2018). Physiological and photosynthetic responses of karenia mikimotoi to the modified clay mitigation method. Mar. Pollut. Bull. 133, 491–499. doi: 10.1016/j.marpolbul.2018.05.044
Li X. D., Yan T., Yu R. C., Zhou M. J. (2019). A review of karenia mikimotoi: Bloom events, physiology, toxicity and toxic mechanism. Harmful Algae. 90. doi: 10.3389/fmicb.2016.00826
Lu G. Y., Song X. X., Yu Z. M., Cao X. H., Yuan Y. Q. (2015). Environmental effects of modified clay flocculation on alexandrium tamarense and paralytic shellfish poisoning toxins (PSTs). Chemosphere 127, 188–194. doi: 10.1016/j.chemosphere.2015.01.039
Ma Z. L., Wu M. J., Lin L. D., Thring R. W., Yu H. G., Zhang X., et al. (2017). Allelopathic interactions between the macroalga hizikia fusiformis (Harvey) and the harmful blooms-forming dinoflagellate karenia mikimotoi. Harmful Algae. 65, 19–26. doi: 10.1016/j.hal.2017.04.003
Mooney B. D., Hallegraeff G. M., Place A. R. (2010). Ichthyotoxicity of four species of gymnodinioid dinoflagellates (Kareniaceae, dinophyta) and purified karlotoxins to larval sheepshead minnow. Harmful Algae. 9, 557–562. doi: 10.1016/j.hal.2010.04.005
Mullineaux P. M., Exposito-Rodriguez M., Laissue P. P., Smirnoff N. (2018). ROS-dependent signalling pathways in plants and algae exposed to high light: Comparisons with other eukaryotes. Free Radic. Biol. Med. 122, 52–64. doi: 10.1016/j.freeradbiomed.2018.01.033
Nan C. R., Zhang H. Z., Lin S. Z., Zhao G. Q., Liu X. Y. (2008). Allelopathic effects of ulva lactuca on selected species of harmful bloom-forming microalgae in laboratory cultures. Aquat. Bot. 89, 9–15. doi: 10.1016/j.hal.2010.04.005
Ni L. X., Rong S. Y., Gu G. X., Hu L. L., Wang P. F., Li D. Y., et al. (2018). Inhibitory effect and mechanism of linoleic acid sustained-release microspheres on microcystis aeruginosa at different growth phases. Chemosphere 212, 654–661. doi: 10.1016/j.chemosphere.2018.08.045
Oh M. Y., Sang B. L., Jin D. H., Hong Y. K., Jin H. J. (2010). Isolation of algicidal compounds from the red alga corallina pilulifera against red tide microalgae. J. Appl. Phycol. 22, 453–458. doi: 10.1016/j.chemosphere.2018.08.045
Paerl H. W., Scott J. T., McCarthy M. J., Newell S. E., Gardner W. S., Havens K. E., et al. (2016). It takes two to tango: When and where dual nutrient (N & p) reductions are needed to protect lakes and downstream ecosystems. Environ. Sci. Technol. 50, 10805–10813. doi: 10.1021/acs.est.6b02575
Pérez-Pérez M. E., Couso I., Crespo J. L. (2012). Carotenoid deficiency triggers autophagy in the model green alga chlamydomonas reinhardtii. Autophagy 8, 376–388. doi: 10.4161/auto.18864
Pokrzywinski K. L., Tilney C. L., Warner M. E., Coyne K. J. (2017). Cell cycle arrest and biochemical changes accompanying cell death in harmful dinoflagellates following exposure to bacterial algicide IRI-160AA. Sci. Rep. 7, 45102. doi: 10.1038/srep45102
Qian H. F., Xu J. H., Lu T., Zhang Q., Qian Q., Yang Z. P., et al. (2018). Responses of unicellular alga chlorella pyrenoidosa to allelochemical linoleic acid. Sci. Total Environ. 625, 1415–1422. doi: 10.1016/j.scitotenv.2018.01.053
Qian H. F., Xu X. Y., Wei C., Jiang H., Jin Y. X., Liu W. P., et al. (2009). Allelochemical stress causes oxidative damage and inhibition of photosynthesis in chlorella vulgaris. Chemosphere 75, 368–375. doi: 10.1016/j.chemosphere.2008.12.040
Rezayian M., Niknam V., Ebrahimzadeh H. (2019). Oxidative damage and antioxidative system in algae. Toxicol. Rep. 6, 1309–1313. doi: 10.1016/j.toxrep.2019.10.001
Sun S. Q., Hu S. S., Zhang B., Sun X., Xu N. J. (2021). Allelopathic effects and potential allelochemical of sargassum fusiforme on red tide microalgae heterosigma akashiwo. Mar. pollut. Bull. 170, 112673. doi: 10.1016/j.marpolbul.2021.112673
Sun Y. Y., Meng K., Su Z. X., Guo G. L., Pu Y. F., Wang C. H. (2017). Isolation and purification of antialgal compounds from the red alga gracilaria lemaneiformis for activity against common harmful red tide microalgae. Environ. Sci. Pollut. Res. Int. 24, 4964–4972. doi: 10.1007/s11356-016-8256-y
Techer D., Fontaine P., Personne A., Viot S., Thomas M. (2016). Allelopathic potential and ecotoxicity evaluation of gallic and nonanoic acids to prevent cyanobacterial growth in lentic systems: A preliminary mesocosm study. Sci. Total Environ. 547, 157–165. doi: 10.1016/j.scitotenv.2015.12.164
Thornberry N. A., Lazebnik Y. (1998). Caspases: Enemies within. Science 281, 1312. doi: 10.1126/science.281.5381.1312
Tziveleka L. A., Tammam M. A., Tzakou O., Roussis V., Ioannou E. (2021). Metabolites with antioxidant activity from marine macroalgae. Antioxidants (Basel). 10, 1431. doi: 10.3390/antiox10091431
Wang R. J., Liu Q. (2022). Responses of bloom-forming heterosigma akashiwo to allelochemical linoleic acid: Growth inhibition, oxidative stress and apoptosis. Front. Mar. Sci. 8. doi: 10.3389/fmars.2021.793567
Wang L. C., Zi J. M., Xu R. B., Hilt S., Hou X. L., Chang X. X. (2017). Allelopathic effects of microcystis aeruginosa on green algae and a diatom: Evidence from exudates addition and co-culturing. Harmful Algae. 61, 56–62. doi: 10.1016/j.hal.2016.11.010
Xiao Y., Liu Y. D., Wang G. H., Hao Z. J., An Y. J. (2010). Simulated microgravity alters growth and microcystin production in microcystis aeruginosa (cyanophyta). Toxicon 56, 1–7. doi: 10.1016/j.toxicon.2010.01.026
Yang C. Y., Liu S. J., Zhou S. W., Wu H. F., Yu J. B., Xia C. H. (2011). Allelochemical ethyl 2-methyl acetoacetate (EMA) induces oxidative damage and antioxidant responses in phaeodactylum tricornutum. Pestic Biochem. Physiol. 99, 93–103. doi: 10.1016/j.pestbp.2011.02.014
Yang C. Y., Zhou J., Liu S. J., Fan P., Wang W. H., Xia C. H. (2013). Allelochemical induces growth and photosynthesis inhibition, oxidative damage in marine diatom phaeodactylum tricornutum. J. Exp. Mar. Biol. Ecol. 444, 16–23. doi: 10.1016/j.jembe.2013.03.005
Zhang X. K., Li B. L., Xu H., Wells M., Tefsen B., Qin B. Q. (2019). Effect of micronutrients on algae in different regions of taihu, a large, spatially diverse, hypereutrophic lake. Water Res. 151, 500–514. doi: 10.1016/j.watres.2018.12.023
Keywords: harmful algal blooms, K. mikimotoi, linoleic acid, reactive oxygen species, cell apoptosis, cell cycle arrest
Citation: Wang R, Wang C, Liu X, Chen J, Liu C, Song Y, Ding N and Gao P (2023) Responses of Karenia mikimotoi to allelochemical linoleic acid: Growth inhibition, photosynthetic damage, oxidative stress and cell apoptosis. Front. Mar. Sci. 10:1105956. doi: 10.3389/fmars.2023.1105956
Received: 23 November 2022; Accepted: 03 January 2023;
Published: 18 January 2023.
Edited by:
You-Shao Wang, South China Sea Institute of Oceanology (CAS), ChinaReviewed by:
Sun Cuici, South China Sea Institute of Oceanology (CAS), ChinaShafi Muhammad, Lasbela University of Agriculture, Water and Marine Sciences, Pakistan
Copyright © 2023 Wang, Wang, Liu, Chen, Liu, Song, Ding and Gao. This is an open-access article distributed under the terms of the Creative Commons Attribution License (CC BY). The use, distribution or reproduction in other forums is permitted, provided the original author(s) and the copyright owner(s) are credited and that the original publication in this journal is cited, in accordance with accepted academic practice. No use, distribution or reproduction is permitted which does not comply with these terms.
*Correspondence: Peike Gao, Z3BreWxsLTAwMUAxNjMuY29t
†These authors have contributed equally to this work