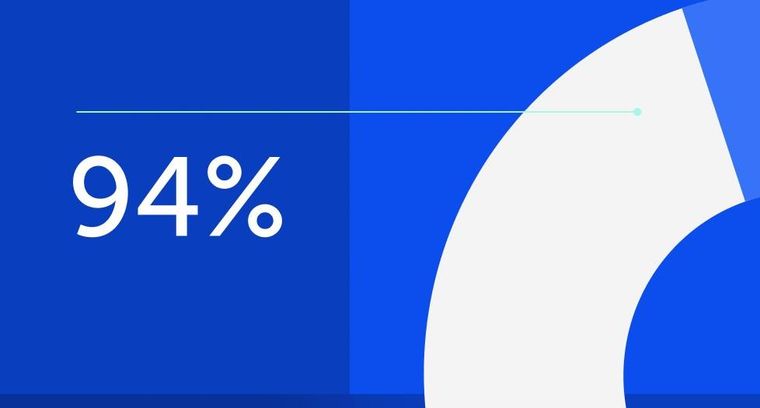
94% of researchers rate our articles as excellent or good
Learn more about the work of our research integrity team to safeguard the quality of each article we publish.
Find out more
ORIGINAL RESEARCH article
Front. Mar. Sci., 17 January 2023
Sec. Aquatic Microbiology
Volume 10 - 2023 | https://doi.org/10.3389/fmars.2023.1105929
This article is part of the Research TopicMicrobial Diversity and Resources in Tidal FlatsView all 17 articles
Five novel halophilic archaeal strains, named BND22T, ZY10T, ZY41T, ZY58T, and ZY43T, were isolated from the coastal saline sediment of the intertidal zone located in Qingdao and the natural sea salt produced from Huanghua marine solar saltern, PRChina. These five strains demonstrated the typical morphology and growth characteristics of haloarchaea. The comparison of 16S rRNA gene revealed that strain BDN22T was associated with Salinirubellus salinus ZS-35-S2T (95.2% similarity), strain ZY10T was related to Halosegnis rubeus F17-44T (95.1% similarity), both strains ZY41T and ZY58T were closely related to Haloglomus irregulare F16-60T (98.1% and 98.2% similarities, respectively), and strain ZY43T was close to Natronomonas salina YPL13T (98.0% similarity). In addition to the 16S rRNA gene, the rpoB′ gene is undoubtedly another important molecular marker for the identification of halophilic archaea, and concatenated-conserved-protein phylogeny was widely used in archaeal classification in recent years. Phylogenetic and phylogenomic analyses based on rpoB′ genes and 122 concatenated archaeal protein genes showed that these haloarchaea construct three different clades and gathered together with the current members of Haloarculaceae. Strains BND22T and ZY10T formed two distinct clades separated from Salinirubellus salinus ZS-35-S2T and Halosegnis members, strains ZY41T and ZY58T clustered with Haloglomus irregulare F16-60T, and strain ZY43T gathered with the current members of Natronomonas. The ANI, DDH, and AAI values of these five strains against phylogenetic neighbours were no more than 91%, 45%, and 92%, respectively, far below the cut-off values for species delineation, supporting their placements in new taxa. Based on the phenotypic, chemotaxonomic, phylogenetic, and phylogenomic properties, these five strains represent five novel taxa of the family Haloarculaceae, Halorarius litoreus gen. nov., sp. nov. (type strain BND22T = CGMCC 1.18780T = JCM 34966T), Halorarius halobius sp. nov. (type strain ZY10T = CGMCC 1.17475T = JCM 34319T), Haloglomus halophilum sp. nov. (type strain ZY41T = CGMCC 1.17030T = JCM 34161T), Haloglomus salinum sp. nov. (type strain ZY58T = CGMCC 1.17216T = JCM 34163T), and Natronomonas marina sp. nov. (type strain ZY43T = CGMCC 1.17202T = JCM 34162T). This is the first report of description of a novel haloarchaeon isolated from a marine intertidal zone.
Halophilic archaea of class Halobacteria, belonging to phylum Euryarchaeota of domain Archaea, flourish in different hypersaline habitats, including salt lakes, marine solar salterns, salted brown algae, and coarse sea salt (Cui and Dyall-Smith, 2021; Han and Cui, 2020; Wang et al., 2022). Currently, there are 73 genera and 318 species with validly published names assigned in the class Halobacteria (List of Prokaryotic names with Standing in Nomenclature, https://lpsn.dsmz.de/class/halobacteria). None of these Halobacteria members was isolated from the marine intertidal zone. In the previous surveys on the halophilic archaeal diversity of coastal beaches located in Shandong and coarse sea salt produced from the coastal solar saltern of Hebei, five novel halophilic archaeal strains were isolated and purified. Strains BND22T and ZY10T were related to each other, then to Salinirubellus salinus ZS-35-S2T and Halosegnis members, strains ZY41T and ZY58T were close to Haloglomus irregulare F16-60T, and strain ZY43T was found to be a member of genus Natronomonas, according to 16S rRNA gene comparison.
The genera Haloglomus, Halosegnis, Natronomonas, and Salinirubellus are four phylogenetically-related groups within the order Halobacteriales. The genus Haloglomus was established by Durán-Viseras in 2020, and only contained one species Haloglomus irregulare F16-60T which was cultivated from the brine of Isla Cristina saltern, Huelva, Spain (Durán-Viseras et al., 2020a). And based on the strains Halosegnis longus F12-1T and Halosegnis rubeus F17-44T, the genus Halosegnis was described in 2021 (Durán-Viseras et al., 2021). As one of the oldest and most famous halophilic archaeal genera, Natronomonas was established in 1997 and at present comprises six species: Natronomonas pharaonis (Kamekura et al., 1997), Natronomonas moolapensis (Burns et al., 2010), Natronomonas gomsonensis (Kim et al., 2013), Natronomonas salsuginis (Durán-Viseras et al., 2020b), Natronomonas halophila and Natronomonas salina (Yin et al., 2020). The genus Salinirubellus related to the above three genera was proposed based on a strain isolated from the sediment sample of a marine solar saltern in Zhejiang, PRChina (Hou et al., 2018). Most of these halophilic archaea cannot hydrolyze starch, gelatin, casein, and Tween 80, but can utilize simple sugars for aerobic growth. The polar lipid profiles of them were diverse, the members of Halosegnis, Haloglomus, and Natronomonas contained phosphatidylglycerol sulphate (PGS) but that of Salinirubellus did not, and Halosegnis members contained S-TGD-1 (sulfated galactosyl mannosyl glucosyl diether) in addition to S-DGD-1 (sulfated mannosyl glucosyl diether), the common glycolipid of these four genera. In this study, two novel species of a new genus, and three novel species of the genera Haloglomus and Natronomonas within the family Haloarculaceae are proposed to accommodate strains BND22T, ZY10T, ZY41T, ZY58T, and ZY43T, respectively.
Strain BND22T was cultivated from the coastal saline soil sample of the intertidal zone located in Qingdao, PRChina (36°12′36″ N, 120°22′10″ E; elevation, 0 m) in 2019. The pH, salt concentration, and organic matter content of the saline soil were 7.9, 5.3 g/kg, and 1.3 g/kg, respectively. Strains ZY10T, ZY41T, ZY58T, and ZY43T were isolated from the natural sea salt produced from Huanghua marine solar saltern in Hebei, PRChina, in 2018. These samples were diluted with liquid NHM, spread on NHM agar plates, and incubated aerobically at 37°C for 30 days (Sun et al., 2022). Single colonies with a shade of red were selected and streaked on fresh NHM agar slants, and the pure colonies were formed after three-time repeated. For further use, the purified strains were preserved in liquid NHM with 15% (w/v) glycerol at -20°C. Halobacterium salinarum CGMCC 1.2367, Haloglomus irregulare JCM 33318T, Halosegnis longus JCM 33319T, Natronomonas gomsonensis KCTC 4088T, Natronomonas halophila C90T, Natronomonas salina YPL13T, and Salinirubellus salinus ZS-35-S2T were used as reference strains and routinely grown aerobically in NHM liquid medium.
The genomic DNA of strains BND22T, ZY10T, ZY41T, ZY58T, and ZY43T was prepared using a DNA extraction kit for bacteria (Jiangsu Cowin Biotech Co., Ltd.). The PCR amplification of the 16S rRNA genes and the rpoB′ (RNA polymerase subunit B′) genes were carried out by two pairs of primers, 20F (5’-ATTCCGGTTGATCCTGCCGG-3’) and 1452R (5’-AGGAGGTGATCCAGCCGCAG-3’), and HrpoB2 1420F and HrpoA 153R, respectively (Cui et al., 2009; Minegishi et al., 2010). The sequencing of purified PCR amplicons was performed by SinoGenoMax (Beijing). The 16S rRNA and rpoB′ genes of related species of the Haloarculaceae were downloaded from the NCBI database. Phylogenetic trees were constructed with MEGA 6 (Tamura et al., 2013) based on three different algorithms, ML (maximum-likelihood) (Felsenstein, 1981), MP (maximum-parsimony) (Fitch, 1971) and NJ (neighbor-joining) (Saitou and Nei, 1987). The calculation of pairwise sequence similarities of 16S rRNA and rpoB′ gene between strains BND22T, ZY10T, ZY41T, ZY58T, ZY43T, and related species was performed by using the online pairwise sequence alignment tool of EzBioCloud (Yoon et al., 2017).
The PacBio Sequel platform was used to sequence the complete genomes of these five strains. CheckM was exploited for the evaluation of the quality of assembled genome sequences (Parks et al., 2015). The 122 conserved archaeal protein marker genes were extracted from the genomes of the isolated five strains and related halophilic archaea for phylogenomic analyses according to the protocol of GTDB (Parks et al., 2018). The sequences of these core orthologous genes were aligned using GTDB-Tk and trimmed by trimAL version 1.4.1 (Capella-Gutiérrez et al., 2009; Chaumeil et al., 2019). The ML phylogenomic tree was constructed based on a standard model by IQ-TREE (Nguyen et al., 2015), and Natronobacterium gregoryi SP2T was selected as the outgroup.
The annotation of these genomes was performed by means of RAST Annotation Sever (Aziz et al., 2008). The metabolic pathways were figured out based on the KEGG database (Kanehisa et al., 2004). To explore the industrial applications of these halophilic archaea, the biotechnological potential of functional genes was identified following the methodology described previously (Sun et al., 2022). The online OrthoVenn was used to compare orthologous clusters (OCs) of related species and generate the Venn diagrams (Xu et al., 2019).
The whole genomic indexes, ANI (average nucleotide identity), DDH (DNA–DNA hybridization), and AAI (average amino acid identity) among strains BND22T, ZY10T, ZY41T, ZY58T, ZY43T, and the related members were estimated by using JSpeciesWS online ANI calculator (https://jspecies.ribohost.com/jspeciesws/) (Richter et al., 2016), Genome-to-Genome Distance Calculator 3.0 (formula 2) (http://ggdc.dsmz.de/ggdc.php) (Meier-Kolthoff et al., 2013), and AAI calculator (http://enve-omics.ce.gatech.edu/aai/) (Luo et al., 2014), respectively.
For microscopical examination, these five isolates were grown in liquid NHM for 7–10 days. The cells of these haloarchaea were observed under a phase-contrast microscope (Eclipse Ci-L, Nikon). Gram staining was performed according to an improved technique developed by Dussault (Dussault, 1955). The pigmentation of the colonies was examined on NHM agar plates after aerobic incubation for 10–15 days at 37°C. The ranges of NaCl and MgCl2 concentrations, temperature, and pH for growth were determined following the methodology described previously (Sun et al., 2022). The remaining phenotypic features of the five isolates and related members were based on the minimal criteria for the description of halophilic archaeal new taxa (Oren et al., 1997). Each test was carried out in triplicates.
Polar lipids of strains BND22T, ZY10T, ZY41T, ZY58T, and ZY43T were extracted with chloroform and methanol mixture, then precipitated by ten volumes of cold acetone after concentration. The polar lipid profiles of isolated halophilic archaea were analyzed by one-dimensional and two-dimensional thin-layer chromatography (TLC) (Cui et al., 2010). The phosphate stain reagent was prepared to detect phospholipids (Vaskovsky and Kostetsk, 1968). Glycolipids and phospholipids were shown with sulfuric acid-ethanol (1:2, v/v) followed by heating at 150 °C for 3 min. The polar lipids of these halophilic archaea were characterized by MALDI-TOF/MS (Xin et al., 2022).
Strains BND22T, ZY10T, ZY41T, ZY58T, and ZY43T all had a single copy of the 16S rRNA gene (1,467 bp, 1,467 bp, 1,472 bp, 1,472 bp, and 1,466 bp, respectively). These sequences are identical to the same genes in their genomes. Based on the 16S rRNA gene similarity, the closest relatives of strains BND22T, ZY10T, ZY41T, ZY58T, and ZY43T were Salinirubellus salinus ZS-35-S2T (95.2%), Halosegnis rubeus F17-44T (95.1%), Haloglomus irregulare F16-60T (98.1%), Haloglomus irregulare F16-60T (98.2%), Natronomonas salina YPL13T (98.0%), respectively (Table S1). Except for 99.6% gene similarity between strains ZY41T and ZY58T, those among strains BND22T, ZY10T, and ZY43T were 92.9–96.8%, less than 98.65%, the suggested threshold for separating different archaeal or bacterial species (Kim et al., 2014). The 16S rRNA gene phylogeny indicated that strains BND22T and ZY10T formed two distinct clades separated from Salinirubellus salinus ZS-35-S2T and Halosegnis members, strains ZY41T and ZY58T clustered with Haloglomus irregulare F16-60T, and strain ZY43T gathered with the current members of Natronomonas (Figure 1A).
Figure 1 ML phylogenetic tree reconstructions based on 16S rRNA gene sequence (A), rpoB′ gene sequence (B) and 122 conserved archaeal protein marker genes (C), showing the relationship among strains BND22T, ZY10T, ZY41T, ZY58T, ZY43T and related species within Haloarculaceae. The numbers at the nodes represent bootstrap values of 1,000 replicates and support ≥ 70% bootstrap. Filled circles highlight branches that were supported by the neighbour-joining, maximum-likelihood and maximum-parsimony algorithms. Empty circles highlight branches that were supported by the maximum-likelihood and maximum-parsimony algorithms or the maximum-likelihood and neighbour-joining algorithms. The bar represents expected substitutions per nucleotide position.
The full length of the rpoB′ genes from strains BND22T, ZY10T, ZY41T, ZY58T, and ZY43T were 1,827 bp. The gene similarities among these five strains were 84.9–95.2%, and they shared 81.1–91.7% sequence similarities with related members of the family Haloarculaceae (Table S2). Strains BND22T, ZY10T, ZY41T, ZY58T, and ZY43T shared maximum sequence similarities with Sala cibi CBA1133T (88.3%), Halosegnis longus F12-1T (89.4%), Haloglomus irregulare F16-60T (91.4%), Haloglomus irregulare F16-60T (91.6%), Natronomonas salina YPL13T (91.7%), respectively. The rpoB’ gene phylogeny revealed that strains BND22T and ZY10T clustered together and separated from the genus Halosegnis, strains ZY41T and ZY58T formed a monophyletic branch closely clustered with Haloglomus irregulare F16-60T, strain ZY43T was a close relative of Natronomonas salina YPL13T within Natronomonas (Figure 1B).
The complete genomes of strains BND22T, ZY10T, ZY41T, ZY58T, and ZY43T were de novo assembled in a total of 2, 5, 3, 1, and 2 contigs, respectively. The complete genome size of new strains ranged from 4.0 to 4.4 Mb, within those of the current Haloarculaceae members (2.6–5.1 Mb). These five strains had one ribosomal RNA operon in their genomes and had different numbers (47, 47, 45, 43, and 48, respectively) of tRNA genes. The DNA G+C content of strains BND22T, ZY10T, ZY41T, ZY58T, and ZY43T are 66.6%, 66.8%, 67.9%, 68.5%, and 66.9%, respectively (Table S3). These values are higher than those of Halosegnis members (64.7–66.0 mol%) and Haloglomus (68.0 mol%), within the range of the genus Natronomonas (63.2–67.4 mol%), and less than that of Salinirubellus salinus ZS-35-S2T (69.0 mol%). Other genomic features were detailed in Table S3.
The ML phylogenomic tree based on 122 concatenated archaeal protein genes indicated that strain BND22T gathered with ZY10T and distinctly separated from Halosegnis and Sala, strains ZY41T and ZY58T formed a tight clade with Haloglomus irregulare F16-60T, and strain ZY43T tightly clustered with the current Natronomonas members (Figure 1C).
The ANI, AAI, and DDH values among these five strains and Haloarculaceae members were 70–91%, 56–92%, and 18–45% (Tables S4-S6), significantly below the cut-off values, 95–96% (ANI), 95% (AAI), and 70% (DDH), for species delineation (Goris et al., 2007; Richter and Rosselló-Móra, 2009; Luo et al., 2014). This suggested that strains BND22T, ZY10T, ZY41T, ZY58T, and ZY43T represent five different species. The AAI values were proved to be useful for genera demarcation and a cut-off value (≤ 76%) was proposed to differentiate genera within the family Natrialbaceae (de la Haba et al., 2021); but the value was inappropriate to be used in grouping the current Haloarculaceae members. If the cut-off value was used to separate the genera within the family Haloarculaceae, the current six Natronomonas species should represent six different genera (Table S6). A cut-off value of < 69% is appropriate to distinguish genera within Haloarculaceae according to the AAI values and evolutionary relationships among the current members of this family. Thus, strains BND22T and ZY10T represent two novel species of a new genus, strains ZY41T and ZY58T two novel different species of Haloglomus, and strain ZY43T a new species of Natronomonas based on the AAI cut-off (< 69%).
It must be noted that the ANI, AAI, and DDH values between Halosegnis longus F12-1T and Sala cibi CBA1133T described recently are 99%, 94%, and 98%, much more than the threshold values of the species boundary. This close relatedness was supported by the 16S rRNA, rpoB’, and 122 conserved archaeal protein marker genes -based phylogenies between Halosegnis longus F12-1T and Sala cibi CBA1133T (Figure 1). According to these results Sala cibi (Song et al., 2022) should be proposed as a later heterotypic synonym of Halosegnis longus (Durán-Viseras et al., 2021).
The subsystem category distribution of novel isolates was annotated by the RAST server (Figure S1). The amino acids and derivatives, and carbohydrates and protein metabolism were the richest RAST subsystems of these five isolates, indicating their possibility to metabolize diverse proteins and carbohydrates. Based on the KEGG database, strains BND22T and ZY58T, ZY41T and ZY43T contained the genes involved in both glycolysis and pyruvate oxidation pathways, respectively, corresponding to their capability to utilize glucose and pyruvate for growth. In contrast, the genes related to fructose utilization, casein, starch, or Tween 80 hydrolysis were not found in all these strains, which was confirmed by the results of phenotypic tests. Additionally, none of the four key enzymes involved in complete denitrification, NarG (nitrate reductase), NirK (copper-containing nitrite reductase), Nor (nitric oxide reductase), and Nos (nitrous-oxide reductase), was found in the genomes of these five strains, this confirmed by their inability to produce nitrogen growing with nitrate anaerobically.
Total of 3,788 orthologous clusters, 566 core clusters, 3,077 accessory clusters, and 145 unique clusters were found in the genomes of strains BND22T, ZY10T, Salinirubellus salinus ZS-35-S2T, Halosegnis longus F12-1T, and Halosegnis rubeus F17-44T. The isolates BND22T and ZY10T contained 62 and 61 unique clusters, while the related members only contained 9, 9, and 4 unique clusters, respectively (Figure S2A). Strains ZY41T, ZY58T, and Haloglomus irregulare F16-60T had 3,850 OCs, including 2,427 core OCs, 1,320 accessory OCs and 103 unique clusters, and contained 22, 13, and 68 unique clusters, respectively (Figure S2B). Strain ZY43T, Natronomonas moolapensis 8.8.11T, Natronomonas pharaonis DSM 2160T, Natronomonas salina YPL13T and Natronomonas salsuginis F20-122T had 3,472 clusters, including 1,427 core OCs, 1,851 accessory OCs and 194 unique clusters. These strains contained 63, 24, 16, 70, and 21 unique clusters, respectively (Figure S2C). The patterns of shared and unique OCs differentiated these novel strains from their relatives.
Many previous studies reported that haloarchaea could produce valuable products of biotechnological aspects, such as haloenzymes, bacteriorhodopsin, biodegradable polyhydroxyalkanoates (PHAs), and carotenoids (Han et al., 2010; Amoozegar et al., 2017; Ashwini et al., 2017; Serrano et al., 2022). Strains ZY10T, ZY41T, ZY58T, and ZY43T possessed bacteriorhodopsin protein genes, indicating their capacity for light-mediated ATP synthesis. Strain BND22T contained the gene cluster encoding for PHA synthesis regulatory protein (PhaR), PHA granule binding protein (PhaP), and type IIIA PHA synthase (PhaE and PhaC subunits), and strain ZY41T contained the gene cluster encoding for acetoacetyl CoA reductase (PhaB), PhaC, PhaE and PhaR (Figure S3), indicating the probable synthesis of PHAs by the new isolates. Additionally, the genes encoded the enzymes involved in the production of β-carotene, phytoene synthase (CrtB), phytoene desaturase (CrtI), and lycopene cyclase (CrtY), were detected in strains ZY41T and ZY58T (Serrano et al., 2022). Moreover, one β-carotene monooxygenase (Brp) was detected in the vicinity of the crtY gene, suggesting the possibility of strain ZY41T to produce retinal from β-carotene (Figure S4). These results indicate that these novel halophilic archaeal strains have much potential in biotechnological applications.
Cells of strains BND22T, ZY10T, ZY41T, ZY58T, and ZY43T were motile and Gram-stain-negative. Except for strains BND22T and ZY58T were pleomorphic, while other strains were coccoid (Figure S5). The colonies of all strains were red and their cells lysed in distilled water. The growth of strains BND22T, ZY10T, ZY41T, ZY58T, and ZY43T occurred in NHM with NaCl concentrations of 1.7–4.8 M, 3.1–5.1 M, 2.1–4.8 M, 2.6–4.3 M, and 2.1–4.8 M, respectively; the five strains grew best at 3.1–4.8 M NaCl. The optimum magnesium concentration varied between 0.05 and 0.7 M, even if magnesium was not necessary for their growth. The growth temperature ranges for these five strains were 20–55 °C, 30–50 °C, 25–60 °C, 25–55 °C, and 30–60 °C, respectively, with optima between 35 and 40°C. These five strains are mainly neutrophilic with pH optima at 6.5–7.5. These strains could not grow with arginine or DMSO anaerobically. Reduction of nitrate to nitrite was found in strains BND22T, ZY10T, ZY41T, and ZY43T but not in strain ZY58T. Indole and H2S were not produced by these strains, and none of the casein, gelatin, starch, or Tween 80 was found to be hydrolyzed. Of the 19 antibiotics tested, only bacitracin (0.04 IU per disc), nitrofurantoin (300 µg), novobiocin (30 µg per disc), nystatin (100 µg), rifampin (5 µg), and trimethoprim (5) could inhibit growth. The remained antibiotics, ampicillin (10 µg), chloramphenicol (30 µg), ciprofloxacin (5 µg), erythromycin (15 µg), gentamicin (10 µg), kanamycin (30 µg), nalidixic acid (30 µg), neomycin (30 µg), norfloxacin (10 µg), penicillin G (10 IU), streptomycin (10 µg), tetracycline (30 µg), or vancomycin (30 µg), were not found to be effective. The distinct phenotypic characteristics differentiating isolates BND22T and ZY10T from the related species of the family Haloarculaceae (Salinirubellus salinus ZS-35-S2T, Halosegnis longus JCM 33317T, Halosegnis rubeus JCM 33319T, and Haloglomus irregulare JCM 33318T) were optimum NaCl, utilization of specific carbon sources, and presence of PGS. Strains ZY41T and ZY58T could be differentiated from Haloglomus irregulare JCM 33318T based on cell motility, gelatin hydrolysis and presence of PGS. The difference between strain ZY43T and the current members of the Natronomonas included H2S formation, cell morphology, catalase, and oxidase activity (Tables 1, 2) (Kamekura et al., 1997; Burns et al., 2010; Kim et al., 2013; Durán-Viseras et al., 2020a; Durán-Viseras et al., 2020b; Yin et al., 2020; Durán-Viseras et al., 2021).
Table 1 Differential characteristics of strains BND22T, ZY10T, ZY41T, ZY58T and their closely related species within the family Haloarculaceae.
The common phospholipids, phosphatidylglycerol (PG) and phosphatidylglycerol phosphate methyl ester (PGP-Me), were detected in strains BND22T, ZY10T, ZY41T, and ZY58T. It was remarkable that the absence of phosphatidylglycerol sulfate (PGS) in these four strains compared with the Halosegnis and Haloglomus members. The phospholipid profile of strain ZY43T was PG, PGP-Me, and PGS, similar to that of the Natronomonas members (Figure S6). Diverse glycolipids were detected in these five strains, three glycolipids (GL1, GL-PL1, and GL-PL2) in strain BND22T, two glycolipids (GL1, GL2) in strain ZY10T, one glycolipid (GL1) in strains ZY41T and ZY58T, and two different glycolipids (GL01, GL02) in strain ZY43T. The TLC mobilities of GL1, GL2 (GL-PL1), and GL02 were similar to those of sulfated mannosyl glucosyl diether (S-DGD-1), mannosyl glucosyl diether (DGD-1), and sulfated galactosyl mannosyl glucosyl diether (S-TGD-1), respectively, based on one-dimensional TLC (Figure S6).
The MALDI-TOF/MS patterns of the polar lipids of strains BND22T, ZY10T, ZY41T, ZY58T, and ZY43T were obtained based on the mode of negative ion (Figure S7). The signals at m/z 731.6, 805.7, 875.7, 885.6, 899.6, 955.7, and 969.6 represented PA, PG, PG (C20C25), PGS, PGP-Me, PGS (C20C25), and PGP-Me (C20C25), respectively. Except for strains ZY41T and ZY58T, the ion of m/z 991.7 appearing in others represented DGD-1. Strains BND22T, ZY10T, ZY41T, and ZY58T showed S-DGD-1 signals at m/z 1055.7, 1071.7, and 1125.7. The ion at m/z 1217.7 detected in strain ZY43T corresponded to S-TGD-1. In addition, several glycosyl-cardiolipins, DGD-PA, S-DGD-PA, and S-DGD-PA (+Na) were detected and judged by the signals of m/z 1690.4, 1770.3, and 1792.3, respectively (Lobasso et al., 2015). These results were mostly in accordance with those detected by TLC.
The polyphasic and genome-based classification indicated that strains BND22T (= CGMCC 1.18780T = JCM 34966T) and ZY10T (= CGMCC 1.17475T = JCM 34319T), represent two different new species of a novel genus within Haloarculaceae, Halorarius litoreus gen. nov., sp. nov. Halorarius halobius sp. nov.; strains ZY41T (= CGMCC 1.17030T = JCM 34161T) and ZY58T (= CGMCC 1.17216T = JCM 34163T) represent two different new species of Haloglomus, Haloglomus halophilum sp. nov. and Haloglomus salinum sp. nov.; and strain ZY43T (= CGMCC 1.17202T = JCM 34162T) represents a novel species of Natronomonas, Natronomonas marina sp. nov.
We propose Sala cibi Song et al., 2022 as a later heterotypic synonym of Halosegnis longus (Durán-Viseras et al., 2021). (type strain F12-1T = CECT 9685T = JCM 33317T; another reference strain of this species, CBA1133 = KACC 22148 = JCM 34265).
Halorarius (Hal.o.ra’ri.us. Gr. masc. n. hals halos, salt; L. masc. adj. orarius, belonging to the seashore; N.L. masc. n. Halorarius salt microorganism isolated from the seashore).
Cells are Gram-stain-negative, motile, pleomorphic and coccoid under optimal growth conditions. Cells are found to be lysed in distilled water. Colonies on NHM agar plate are red, moist and round. Growth was found at 20–55°C, 1.7–5.1 M NaCl, 0–1.0 M MgCl2 and pH 6.5–8.5. The activity of catalase was positive while that of oxidase was negative. Some sugars can be utilized for growth and with the production of acids. The cultures contain PA, PG, PGP-Me, S-DGD-1, and DGD-1. The type species is Halorarius litoreus. Recommended three-letter abbreviation: Hor.
Halorarius litoreus (li.to’re.us. L. masc. adj. litoreus, of or belonging to the seashore).
The cells of type strain are pleomorphic (1.0–1.2×1.0–3.0 μm), motile, and Gram-negative. Colonies are red and circular, about 5 mm in diameter following incubation for 14 days at 37°C. The type strain could grow in NHM with NaCl concentrations of 1.7–4.8 M and grew best at 3.4 M NaCl. The optimum magnesium concentration was 0.03 M, even if magnesium was not necessary for growth. The growth temperature range for the type strain was 20–55 °C, with optimum at 35°C. The type strain is neutrophilic with pH optima at 7.5. The type strain can grow anaerobically with nitrate but not with arginine or DMSO. Reduction of nitrate to nitrite was found but gas was not produced. Diverse substrates, D-glucose, sucrose, D-mannitol, D-sorbitol, acetate, succinate, L-aspartate, L-glutamate, L-lysine and L-ornithine, can be utilized for growth, while D-fructose, D-galactose, lactose, maltose, D-mannose, D-ribose, L-sorbose, D-xylose, glycerol, citrate, fumarate, DL-lactate, L-malate, pyruvate, L-arginine, glycine or L-alanine do not support growth. The catalase test is positive while the oxidase is negative. Indole and H2S formation are not detected. None of casein, gelatin, starch or Tween 80 was found to be hydrolyzed. The type strain contains PA, PG, PGP-Me, DGD-1 (DGD-PA), and S-DGD-1 (S-DGD-PA).
The type strain, BND22T (= CGMCC 1.18780T = JCM 34966T), was isolated from the coastal saline soil sample of the intertidal zone located in Qingdao, PRChina. The DNA G+C content of the type strain is 66.6% (genome). The GenBank/EMBL/DDBJ accession numbers for the 16S rRNA gene and genome of strain BND22T are MW338920 and CP101454–CP101455, respectively.
Halorarius halobius (ha.lo’bi.us. Gr. masc. n. hals, halos, salt; Gr. masc. n. bios, life; N.L. masc. adj. halobius, growing in salt).
The cells of type strain are coccoid (0.5–2.0 μm), motile, and Gram-negative. Colonies are red and circular, about 0.5 mm in diameter following incubation for 14 days at 37°C. The type strain could grow in NHM with NaCl concentrations of 3.1–5.1 M and grew best at 4.8 M NaCl. The optimum magnesium concentration was 0.5 M, even if magnesium was not necessary for growth. The growth temperature range for the type strain was 30–50 °C, with optimum at 37°C. The type strain is neutrophilic with pH optima at 7.5. The type strain can grow anaerobically with nitrate but not with arginine or DMSO. Reduction of nitrate to nitrite was found but gas was not produced. Diverse substrates, D-mannose, sucrose, glycerol, D-mannitol, D-sorbitol, fumarate, DL-lactate, L-malate, pyruvate, succinate, glycine, L-aspartate, L-glutamate and L-lysine, can be utilized for growth, while D-fructose, D-galactose, D-glucose, lactose, maltose, D-ribose, L-sorbose, D-xylose, acetate, citrate, L-arginine, L-alanine or L-ornithine do not support growth. Positive for catalase and oxidase activity. Indole and H2S formation are not detected. None of casein, gelatin, starch or Tween 80 was found to be hydrolyzed. The type strain contains PG, PGP-Me, DGD-1 and S-DGD-1.
The type strain, ZY10T (= CGMCC 1.17475T = JCM 34319T), was isolated from the natural sea salt produced from Huanghua marine solar saltern in Hebei, PRChina. The DNA G+C content of the type strain is 66.8% (genome). The GenBank/EMBL/DDBJ accession numbers for the 16S rRNA gene and whole genome of strain ZY10T are MN589739 and CP101161–CP101165, respectively.
Haloglomus halophilum (ha.lo’phi.lum. Gr. masc. n. hals, halos salt; Gr. masc. adj. philos loving; N.L. neut. adj. halophilum salt-loving, concerning the requirement for salt).
The cells of type strain are coccoid (0.5–2.0 μm), motile, and Gram-negative. Colonies are red and circular, about 0.5 mm in diameter following incubation for 14 days at 37°C. The type strain could grow in NHM with NaCl concentrations of 2.1–4.8 M and grew best at 3.1 M NaCl. The optimum magnesium concentration was 0.7 M, even if magnesium was not necessary for growth. The growth temperature range for the type strain was 25–60 °C, with optimum at 40°C. The type strain is neutrophilic with pH optima at 7.5. The type strain cannot grow anaerobically with arginine, DMSO, or nitrate. Reduction of nitrate to nitrite was found but gas was not produced. Diverse substrates, glycerol, D-mannitol, D-sorbitol, acetate, DL-lactate, pyruvate, succinate, L-ornithine and L-lysine, can be utilized for growth, while D-fructose, D-galactose, D-glucose, lactose, maltose, D-mannose, D-ribose, L-sorbose, sucrose, D-xylose, citrate, fumarate, L-alanine, L-arginine, L-aspartate, L-glutamate, glycine, or L-malate do not support growth. Catalase positive and oxidase negative. Indole and H2S are not produced. None of casein, gelatin, starch or Tween 80 was found to be hydrolyzed. The type strain contains PG, PGP-Me, and S-DGD-1.
The type strain, ZY41T (= CGMCC 1.17030T = JCM 34161T), was isolated from the natural sea salt produced from Huanghua marine solar saltern in Hebei, PRChina. The DNA G+C content of the type strain is 67.9% (genome). The GenBank/EMBL/DDBJ accession numbers for the 16S rRNA gene and whole genome of strain ZY41T are MK721000 and CP101166–CP101168, respectively.
Haloglomus salinum (sa.li’num. N.L. neut. adj. salinum belonging to salt works)
The cells of type strain are pleomorphic (0.8–1.0×1.0–2.0 μm), motile, and Gram-negative. Colonies are red and circular, about 0.5 mm in diameter following incubation for 14 days at 37°C. The type strain could grow in NHM with NaCl concentrations of 2.6–4.3 M and grew best at 3.1 M NaCl. The optimum magnesium concentration was 0.03 M, even if magnesium was not necessary for growth. The growth temperature range for the type strain was 25–55 °C, with optimum at 37°C. The type strain is neutrophilic with pH optima at 7.0. The type strain cannot grow anaerobically with arginine, DMSO, or nitrate. Reduction of nitrate to nitrite was not found and gas was not produced. Diverse substrates, D-galactose, D-glucose, D-mannose, sucrose, acetate, pyruvate, DL-lactate, can be utilized for growth, while D-fructose, lactose, maltose, D-ribose, L-sorbose, D-xylose, glycerol, D-mannitol, D-sorbitol, citrate, fumarate, L-malate, succinate, L-aspartate, L-alanine, L-arginine, L-glutamate, glycine, L-lysine, or L-ornithine do not support growth. Catalase positive and oxidase negative. Indole and H2S production are not detected. None of casein, gelatin, starch or Tween 80 was found to be hydrolyzed. The type strain contains PG, PGP-Me, and S-DGD-1.
The type strain, ZY58T (= CGMCC 1.17216T = JCM 34163T), was isolated from the natural sea salt produced from Huanghua marine solar saltern in Hebei, PRChina. The DNA G+C content of the type strain is 68.5% (genome). The GenBank/EMBL/DDBJ accession numbers for the 16S rRNA gene and whole genome of strain ZY58T are MK920153 and CP101153, respectively.
Natronomonas marina (ma.ri’na. L. fem. adj. marina of the marine).
The cells of type strain are coccoid (0.5–2.0 μm), motile, and Gram-negative. Colonies are red and circular, about 0.5 mm in diameter following incubation for 14 days at 37°C. The type strain could grow in NHM with NaCl concentrations of 2.1–4.8 M and grew best at 3.1 M NaCl. The optimum magnesium concentration was 0.05 M, even if magnesium was not necessary for growth. The growth temperature range for the type strain was 30–60 °C, with optimum at 40°C. The type strain is neutrophilic with pH optima at 6.5. The type strain cannot grow anaerobically with arginine, DMSO, or nitrate. Reduction of nitrate to nitrite was found but gas was not produced. Diverse substrates, glycerol, D-sorbitol, acetate, DL-lactate, pyruvate, succinate, L-aspartate, L-alanine, L-lysine, and L-ornithine can be utilized for growth, while D-fructose, D-galactose, D-glucose, lactose, maltose, D-mannose, D-ribose, L-sorbose, sucrose, D-xylose, D-mannitol, acetate, citrate, fumarate, L-arginine, L-glutamate glycine or L-malate do not support growth. The catalase test is positive while the oxidase is negative. Indole and H2S production are not detected. None of casein, gelatin, starch or Tween 80 was found to be hydrolyzed. The type strain contains PG, PGP-Me, PGS, DGD-1, and S-TGD-1.
The type strain, ZY43T (= CGMCC 1.17202T = JCM 34162T), was isolated from the natural sea salt produced from Huanghua marine solar saltern in Hebei, PRChina. The DNA G+C content of the type strain is 66.9% (genome). The GenBank/EMBL/DDBJ accession numbers for the 16S rRNA gene and whole genome of strain ZY43T are MK920152 and CP101154–CP101155, respectively.
The sequence data of the study are deposited in the NCBI database with accession numbers MW338920, MN589739, MK721000, MK920153, MK920152, CP101454-CP101455, CP101161-CP101165, CP101166-CP101168, CP101153, and CP101154-CP101155.
Y-PS, B-BW, Z-PW, X-WZ performed the experiments and Y-PS drafted the manuscript. JH and H-LC designed and supervised the study. JH and H-LC revised the manuscript. All authors contributed to the article and approved the submitted version.
This work was financially supported by Science & Technology Fundamental Resources Investigation Program (Grant No. 2019FY100700; No. 2021FY100900), and the National Natural Science Foundation of China (No. 32070003).
We are grateful to Prof. Aharon Oren (The Hebrew University of Jerusalem) for his generous and meaningful comments.
The authors declare that the research was conducted in the absence of any commercial or financial relationships that could be construed as a potential conflict of interest.
All claims expressed in this article are solely those of the authors and do not necessarily represent those of their affiliated organizations, or those of the publisher, the editors and the reviewers. Any product that may be evaluated in this article, or claim that may be made by its manufacturer, is not guaranteed or endorsed by the publisher.
The Supplementary Material for this article can be found online at: https://www.frontiersin.org/articles/10.3389/fmars.2023.1105929/full#supplementary-material
Amoozegar M. A., Siroosi M., Atashgahi S., Smidt H., Ventosa A. (2017). Systematics of haloarchaea and biotechnological potential of their hydrolytic enzymes. Microbiology 163, 623–645. doi: 10.1099/mic.0.000463
Ashwini R., Vijayanand S., Hemapriya J. (2017). Photonic potential of haloarchaeal pigment bacteriorhodopsin for future electronics: A review. Curr. Microbiol. 74, 996–1002. doi: 10.1007/s00284-017-1271-5
Aziz R. K., Bartels D., Best A. A., DeJongh M., Disz T., Edwards R. A., et al. (2008). The RAST server: rapid annotations using subsystems technology. BMC Genomics 9, 75. doi: 10.1186/1471-2164-9-75
Burns D. G., Janssen P. H., Itoh T., Minegishi H., Usami R., Kamekura M., et al. (2010). Natronomonas moolapensis sp. nov., non-alkaliphilic isolates recovered from a solar saltern crystallizer pond, and emended description of the genus Natronomonas. Int. J. Syst. Evol. Microbiol. 60, 1173–1176. doi: 10.1099/ijs.0.010132-0
Capella-Gutiérrez S., Silla-Martínez J. M., Gabaldón T. (2009). trimAl: a tool for automated alignment trimming in large-scale phylogenetic analyses. Bioinformatics 25, 1972–1973. doi: 10.1093/bioinformatics/btp348
Chaumeil P. A., Mussig A. J., Hugenholtz P., Parks D. H. (2019). GTDB-tk: a toolkit to classify genomes with the genome taxonomy database. Bioinformatics 36, 1925–1927. doi: 10.1093/bioinformatics/btz848
Cui H. L., Dyall-Smith M. L. (2021). Cultivation of halophilic archaea (class Halobacteria ) from thalassohaline and athalassohaline environments. Mar. Life Sci. Technol. 3, 243–251. doi: 10.1007/s42995-020-00087-3
Cui H. L., Gao X., Yang X., Xu X. W. (2010). Halorussus rarus gen. nov., sp. nov., a new member of the family Halobacteriaceae isolated from a marine solar saltern. Extremophiles 14, 493–499. doi: 10.1007/s00792-010-0329-0
Cui H. L., Zhou P. J., Oren A., Liu S. J. (2009). Intraspecific polymorphism of 16S rRNA genes in two halophilic archaeal genera, Haloarcula and Halomicrobium. Extremophiles 13, 31–37. doi: 10.1007/s00792-008-0194-2
de la Haba R. R., Minegishi H., Kamekura M., Shimane Y., Ventosa A. (2021). Phylogenomics of haloarchaea: The controversy of the genera Natrinema-Haloterrigena. Front. Microbiol. 12. doi: 10.3389/fmicb.2021.740909
Durán-Viseras A., Andrei A.Ş., Vera-Gargallo B., Ghai R., Sánchez-Porro C., Ventosa A. (2021). Culturomics-based genomics sheds light on the ecology of the new haloarchaeal genus Halosegnis. Environ. Microbiology 23, 3418–3434. doi: 10.1111/1462-2920.15082
Durán-Viseras A., Sánchez-Porro C., Ventosa A. (2020a). Haloglomus irregulare gen. nov., sp. nov., a new halophilic archaeon isolated from a marine saltern. Microorganisms 8, 206. doi: 10.3390/microorganisms8020206
Durán-Viseras A., Sánchez-Porro C., Ventosa A. (2020b). Natronomonas salsuginis sp. nov., a new inhabitant of a marine solar saltern. Microorganisms 8, 605. doi: 10.3390/microorganisms8040605
Dussault H. P. (1955). An improved technique for staining red halophilic bacteria. J. Bacteriol. 70, 484–485. doi: 10.1128/jb.70.4.484-485.1955
Felsenstein J. (1981). Evolutionary trees from DNA sequences: A maximum likelihood approach. J. Mol. Evol. 17, 368–376. doi: 10.1007/BF01734359
Fitch W. M. (1971). Toward defining the course of evolution: Minimum change for a specific tree topology. Syst. Biol. 20, 406–416. doi: 10.2307/2412116
Goris J., Konstantinidis K. T., Klappenbach J. A., Coenye T., Vandamme P., Tiedje J. M. (2007). DNA-DNA Hybridization values and their relationship to whole-genome sequence similarities. Int. J. Syst. Evol. Microbiol. 57, 81–91. doi: 10.1099/ijs.0.64483-0
Han D., Cui H. L. (2020). Halostella pelagica sp. nov. and Halostella litorea sp. nov., isolated from salted brown alga Laminaria. Int. J. Syst. Evol. Microbiol. 70, 1969–1976. doi: 10.1099/ijsem.0.004003
Han J., Hou J., Liu H., Cai S., Feng B., Zhou J., et al. (2010). Wide distribution among halophilic archaea of a novel polyhydroxyalkanoate synthase subtype with homology to bacterial type III synthases. Appl. Environ. Microbiol. 76, 7811–7819. doi: 10.1128/AEM.01117-10
Hou J., Zhao. Y. J., Zhu L., Cui H. L. (2018). Salinirubellus salinus gen. nov., sp. nov., isolated from a marine solar saltern. Int. J. Syst. Evol. Microbiol. 68, 1874–1878. doi: 10.1099/ijsem.0.002757
Kamekura M., Dyall-Smith M. L., Upasani V., Ventosa A., Kates M. (1997). Diversity of alkaliphilic halobacteria: Proposals for transfer of Natronobacterium vacuolatum, Natronobacterium magadii, and Natronobacterium pharaonis to Halorubrum, Natrialba, and Natronomonas gen. nov., respectively, as Halorubrum vacuolatum comb. nov., Natrialba magadii comb. nov., and Natronomonas pharaonis comb. nov., respectively. Int. J. Syst. Bacteriol. 47, 853–857. doi: 10.1099/00207713-47-3-853
Kanehisa M., Goto S., Kawashima S., Okuno Y., Hattori M. (2004). The KEGG resource for deciphering the genome. Nucleic Acids Res. 32, D277–D280. doi: 10.1093/nar/gkh063
Kim T. Y., Kim S. J., Park S. J., Kim J. G., Cha I. T., Jung M. Y., et al. (2013). Natronomonas gomsonensis sp. nov., isolated from a solar saltern. Antonie van Leeuwenhoek 104, 627–635. doi: 10.1007/s10482-013-9970-9
Kim M., Oh H. S., Park S. C., Chun J. (2014). Towards a taxonomic coherence between average nucleotide identity and 16S rRNA gene sequence similarity for species demarcation of prokaryotes. Int. J. Syst. Evol. Microbiol. 64, 346–351. doi: 10.1099/ijs.0.059774-0
Lobasso S., Pérez-Davó A., Vitale R., Sánchez M. M., Corcelli A. (2015). Deciphering archaeal glycolipids of an extremely halophilic archaeon of the genus Halobellus by MALDI-TOF/MS. Chem. Phys. Lipids 186, 1–8. doi: 10.1016/j.chemphyslip.2014.11.002
Luo C., Rodriguez-R L. M., Konstantinidis K. T. (2014). MyTaxa: An advanced taxonomic classifier for genomic and metagenomic sequences. Nucleic Acids Res. 42, e73. doi: 10.1093/nar/gku169
Meier-Kolthoff J. P., Auch A. F., Klenk H. P., Göker M. (2013). Genome sequence-based species delimitation with confidence intervals and improved distance functions. BMC Bioinf. 14, 60. doi: 10.1186/1471-2105-14-60
Minegishi H., Kamekura M., Itoh T., Echigo A., Usami R., Hashimoto T. (2010). Further refinement of the phylogeny of the Halobacteriaceae based on the full-length RNA polymerase subunit b′ (rpoB′) gene. Int. J. Syst. Evol. Microbiol. 60, 2398–2408. doi: 10.1099/ijs.0.017160-0
Nguyen L. T., Schmidt H. A., von Haeseler A., Minh B. Q. (2015). IQ-TREE: A fast and effective stochastic algorithm for estimating maximum-likelihood phylogenies. Mol. Biol. Evol. 32, 268–274. doi: 10.1093/molbev/msu300
Oren A., Ventosa A., Grant W. D. (1997). Proposed minimal standards for description of new taxa in the order Halobacteriales. Int. J. Syst. Evol. Microbiol. 47, 233–238. doi: 10.1099/00207713-47-1-233
Parks D. H., Chuvochina M., Waite D. W., Rinke C., Skarshewski A., Chaumeil P. A., et al. (2018). A standardized bacterial taxonomy based on genome phylogeny substantially revises the tree of life. Nat. Biotechnology 36, 996–1004. doi: 10.1038/nbt.4229
Parks D. H., Imelfort M., Skennerton C. T., Hugenholtz P., Tyson G. W. (2015). CheckM: assessing the quality of microbial genomes recovered from isolates, single cells, and metagenomes. Genome Res. 25, 1043–1055. doi: 10.1101/gr.186072.114
Richter M., Rosselló-Móra R. (2009). Shifting the genomic gold standard for the prokaryotic species definition. Proc. Natl. Acad. Sci. U.S.A. 106, 19126–19131. doi: 10.1073/pnas.0906412106
Richter M., Rosselló-Móra R., Glöckner F. O., Peplies J. (2016). JSpeciesWS: a web server for prokaryotic species circumscription based on pairwise genome comparison. Bioinformatics 32, 929–931. doi: 10.1093/bioinformatics/btv681
Saitou N., Nei M. (1987). The neighbor-joining method: A new method for reconstructing phylogenetic trees. Mol. Biol. Evol. 4, 406–425. doi: 10.1093/oxfordjournals.molbev.a040454
Serrano S., Mendo S., Caetano T. (2022). Haloarchaea have a high genomic diversity for the biosynthesis of carotenoids of biotechnological interest. Res. Microbiology 173, 103919. doi: 10.1016/j.resmic.2021.103919
Song H. S., Kim J., Kim Y. B., Lee S. H., Whon T. W., Roh S. W. (2022). Sala cibi gen. nov., sp. nov., an extremely halophilic archaeon isolated from solar salt. J. Microbiol. 60, 899–904. doi: 10.1007/s12275-022-2137-5
Sun Y. P., Wang B. B., Zheng X. W., Wu Z. P., Hou J., Cui H. L. (2022). Description of Halosolutus amylolyticus gen. nov., sp. nov., Halosolutus halophilus sp. nov. and Halosolutus gelatinilyticus sp. nov., and genome-based taxonomy of genera Natribaculum and Halovarius. Int. J. Syst. Evol. Microbiol. 72, 5598. doi: 10.1099/ijsem.0.005598
Tamura K., Stecher G., Peterson D., Filipski A., Kumar S. (2013). MEGA6: molecular evolutionary genetics analysis version 6.0. Mol. Biol. Evol. 30, 2725–2729. doi: 10.1093/molbev/mst197
Vaskovsky V. E., Kostetsk E. Y. (1968). Modified spray for the detection of phospholipids on thin-layer chromatograms. J. Lipid Res. 9, 396. doi: 10.1016/S0022-2275(20)43111-6
Wang B. B., Sun Y. P., Wu Z. P., Zheng X. W., Hou J., Cui H. L. (2022). Halorientalis salina sp. nov., Halorientalis marina sp. nov., Halorientalis litorea sp. nov.: Three extremely halophilic archaea isolated from a salt lake and coarse sea salt. Extremophiles 26, 26. doi: 10.1007/s00792-022-01275-y
Xin Y. J., Bao C. X., Li S. Y., Hu X. Y., Zhu L., Wei W., et al. (2022). Genome-based taxonomy of genera Halomicrobium and Halosiccatus, and description of Halomicrobium salinisoli sp. nov. Syst. Appl. Microbiol. 45, 126308. doi: 10.1016/j.syapm.2022.126308
Xu L., Dong Z., Fang L., Luo Y., Wei Z., Guo H., et al. (2019). OrthoVenn2: A web server for whole-genome comparison and annotation of orthologous clusters across multiple species. Nucleic Acids Res. 47, W52–W58. doi: 10.1093/nar/gkz333
Yin X. M., Yang X. Y., Hou J., Zhu L., Cui H. L. (2020). Natronomonas halophila sp. nov. and Natronomonas salina sp. nov., two novel halophilic archaea. Int. J. Syst. Evol. Microbiol. 70, 5686–5692. doi: 10.1099/ijsem.0.004463
Keywords: Halorarius, Haloglomus, Hatronomonas, halophilic archaea, tidal flat, marine solar salt
Citation: Sun Y-P, Wang B-B, Wu Z-P, Zheng X-W, Hou J and Cui H-L (2023) Halorarius litoreus gen. nov., sp. nov., Halorarius halobius sp. nov., Haloglomus halophilum sp. nov., Haloglomus salinum sp. nov., and Natronomonas marina sp. nov., extremely halophilic archaea isolated from tidal flat and marine solar salt. Front. Mar. Sci. 10:1105929. doi: 10.3389/fmars.2023.1105929
Received: 23 November 2022; Accepted: 02 January 2023;
Published: 17 January 2023.
Edited by:
Xue-Wei Xu, Ministry of Natural Resources, ChinaReviewed by:
Satya P. Singh, Saurashtra University, IndiaCopyright © 2023 Sun, Wang, Wu, Zheng, Hou and Cui. This is an open-access article distributed under the terms of the Creative Commons Attribution License (CC BY). The use, distribution or reproduction in other forums is permitted, provided the original author(s) and the copyright owner(s) are credited and that the original publication in this journal is cited, in accordance with accepted academic practice. No use, distribution or reproduction is permitted which does not comply with these terms.
*Correspondence: Heng-Lin Cui, Y3VpaGVuZ2xpbkB1anMuZWR1LmNu
†These authors have contributed equally to this work
Disclaimer: All claims expressed in this article are solely those of the authors and do not necessarily represent those of their affiliated organizations, or those of the publisher, the editors and the reviewers. Any product that may be evaluated in this article or claim that may be made by its manufacturer is not guaranteed or endorsed by the publisher.
Research integrity at Frontiers
Learn more about the work of our research integrity team to safeguard the quality of each article we publish.