- 1Cooperative Institute for Marine and Atmospheric Research, University of Hawaii, Honolulu, HI, United States
- 2Pacific Islands Fisheries Science Center, National Marine Fisheries Service, Honolulu, HI, United States
- 3Center for Fisheries Research and Development, The University of Southern Mississippi, Ocean Springs, MS, United States
- 4Hawaii Institute of Marine Biology, University of Hawaii, Honolulu, HI, United States
- 5Department of Oceanography, University of Hawaii, Honolulu, HI, United States
Reproductive characteristics of a fish stock provide important tools for assessing population health. Change in length-at-maturity (L50) is a potential indicator of exploited fish populations but when criteria for determining maturity classifications are inconsistent, it is difficult to accurately assess change over time and space. Etelis coruscans is a commercially important eteline snapper found throughout the Indo-Pacific, but its region-specific reproductive information in the main Hawaiian Islands (MHI) is sparse. The present study describes length-specific (fork length: FL) female reproductive characteristics of this deep water snapper in the context of a data-limited fishery. We explored the use of six maturity classification criteria based on a functionally mature (containing vitellogenic oocytes and capable of spawning within the season of collection) or physiologically mature (gonadotropin-dependent maturation initiation) designation combined with seasonality and inclusion of additional reproductive phases. Of these classifications, the largest and therefore most conservative estimate was for functional maturity during the spawning period, September-December (L50F = 65.8 cm). Progressively smaller L50 estimates occurred as we incorporated additional reproductive phases and seasonality, the smallest being for physiological maturity during the entire year (L50PY = 50.0 cm). Both functional and physiological maturity criteria are valid for estimating L50 but can yield drastically different parameter estimates based on the definition of mature or immature reproductive phases. Fish that are relatively slow growing, late to mature, and whose fisheries encompass a wide size range, such as E. coruscans, may be more subject to unreliability in the development of their reproductive indicators (length-at-maturity and spawning period) when inconsistent maturity classification criteria are executed over time. We discuss the reproductive characteristics of E. coruscans in the MHI, the use of alternative maturity classification criteria in L50 estimation, the impact on resultant parameters estimates, and the life history implications for the future fishery.
1 Introduction
Reproductive timing is a complex process occurring on several temporal scales, but the timing of the onset of maturation is particularly important to population dynamics and species-specific life history information (Trippel, 1995; Lowerre-Barbieri et al., 2011a; Lowerre-Barbieri et al., 2011b). Age and size at first maturity, sometimes referred to as “age- or length-at-maturity” or “age- or length-at-50% maturity”, are important life history traits that affect biological processes and individual fitness (Stearns, 1992; Perez and Munch, 2010). These life history traits are also valuable biological reference points often used in fisheries management as harvest controls to conserve a portion of mature females (Trippel and Harvey, 1991; Trippel, 1995; Lowerre-Barbieri et al., 2017). These biological reference points become increasingly important for data-limited fisheries where Spawning Stock Biomass (SSB) is used as a measure of reproductive potential to assess the sustainability of the adult population (Trippel, 1999; Marshall and Browman, 2007; Lowerre-Barbieri et al., 2022). In SSB, maturity is the only reproductive measure used; therefore, consistent and transparent classifications of maturity have more impact on resulting outputs in these circumstances (Lambert, 2008; Agulló Núñez et al., 2015; Lowerre-Barbieri et al., 2022).
Length is a readily accessible piece of biological information, and one of the most important aspects of fish reproductive biology is the length at which 50% of females in a population become sexually mature (median length–at–maturity, L50). Beverton and Holt (1957) first described the use of a logistic curve to estimate the timing of female maturity when 50% of individuals begin to produce gametes in proportion to their body weight in order to describe the point at which sexual maturation begins. This metric then took on several forms for length, age and weight, including L50, A50 or W50. The use of L50 is now commonly accepted in the field of fisheries through wide use in stock assessment models or for setting size limits as a harvest control (Trippel, 1995; Prince et al., 2022). Accurate estimates of L50 allow stock assessment scientists to evaluate the spawning stock for potential effects of fishing mortality on a population (Polovina, 1987; De Roos et al., 2006; Rochet and Marty, 2009). However, there are currently no standardized criteria for assessing the classification of mature vs. immature individuals used to estimate parameter values for L50 (Trippel and Harvey, 1991; Lowerre-Barbieri et al., 2011a; Lowerre-Barbieri et al., 2011b). Further complicating maturity interpretations, “first maturity” appears to be used synonymously with “50% maturity” in some of the literature (Grimes, 1987; Hunter and Macewicz, 2003). To accurately assess reproductive success and fishery impacts on a stock, the application of consistent and transparent maturity data classifications are crucial components for providing accurate maturity parameter estimates that are comparable over time.
In fisheries biology, length-at-maturity is typically estimated using the presence of females with vitellogenic oocytes that are capable of spawning within the given year, functional maturity (West, 1990; Brown-Peterson et al., 2011). This criterion for L50 estimation is widely used in fisheries research because of its ability to easily identify mature, spawning individuals within the spawning period (Hunter and Macewicz, 1985; DeMartini, 2016). However, when spawning capable females are difficult to obtain because of restrictions to sampling effort, fishery dynamics and cost constraints, maturity may still be estimated using alternative approaches. One such approach is based on physiological maturity — the gonadotropin-dependent phase of ovarian development first indicated by cortical alveolar (CA) oocytes and found in the early developing subphase (Wallace and Selman, 1981; Wright, 2007; Brown-Peterson et al., 2011). This appearance of CA oocytes was used previously to define secondary growth (Murua and Saborido-Rey, 2003; Luckenbach et al., 2008; Lowerre-Barbieri et al., 2011b) and is often used as a sexual maturity metric (Saborido-Rey and Junquera, 1998; Piñón et al., 2009; Lesyna and Barnes, 2016). The early developing subphase can be indicative of either first time spawners entering the reproductive cycle from the immature phase or repeat spawners that are already mature fish entering the reproductive period from the regenerating phase (Brown-Peterson et al., 2011). Additionally, the incorporation of regenerating females during the spawning period is a common practice that is generally used to increase sample size and may be considered unofficially as a “hybrid” approach when these data are included in a length-at-maturity estimate (Lowerre-Barbieri et al., 2022; Pacicco et al., 2023). To date, only two studies have compared the effect of a maturity classification criteria on the L50 parameter using macroscopic versus microscopic comparisons (McBride et al., 2013; Agulló Núñez et al., 2015; Salas-Singh et al., 2022). Only Pacicco et al. (2023) has delved into the implications of using different microscopic (histological) maturity classifications and its impact on resulting parameters. In general, the impact of differences between functional and physiological L50 maturity estimates on stock assessments is poorly understood among species.
The deep-water longtail snapper Etelis coruscans (Valenciennes, 1862) — known as “Onaga” in Hawaii — occurs across the tropical and subtropical Indo-Pacific (Anderson, 1981; Randall, 2007), is data-limited and commercially important. Onaga is a component of the Hawaii ‘Deep 7’ fishery, which consists of a complex of six eteline snappers and one grouper that is managed according to the Western Pacific Regional Fishery Management Council’s (WPRFMC) Hawaii Fishery Ecosystem Plan for the Hawaiian Archipelago (WPRFMC 2009). The main Hawaiian Islands (MHI) bottomfish fishery is fished year-round but it is common for commercial fishers to switch their effort from bottomfish to more lucrative migratory pelagic species (e.g., tuna) or Uku (Aprion virescens), a coastal pelagic eteline snapper that forms spawning aggregations, during the spring and summer months (Langseth et al., 2018; Nadon et al., 2020; O’Malley et al., 2021). Although limited quantitative biological information is available for the Hawaiian bottomfish fishery due in part to the difficulty in obtaining specimens, there is a lucrative market for these species.
Of the Hawaii Deep 7, E. coruscans is the second most common species by landings but the most valuable in terms of price per pound (Langseth et al., 2018). Various ecological and biological characteristics have been described for this species, including habitat (Misa et al., 2013; Moore et al., 2016; Oyafuso et al., 2017), diet (Haight et al., 1993b), genetics (Andrews et al., 2014; Loeun et al., 2014), movement behavior (Okuyama et al., 2019), and larval development (Leis and Lee, 1994). This species occupies the deepest water column depth range among the Hawaiian eteline snappers, ranging from 100–400 m (Misa et al., 2013). Similar to other eteline species, E. coruscans is known as long-lived (> 50 years), slow-growing, and late to mature in New Caledonia, Okinawa, and Hawaii (Williams et al., 2013; Uehara et al., 2018; Andrews et al., 2020; Uehara et al., 2020). Reproductive characteristics for E. coruscans in the MHI were first described by Everson et al. (1989) and included estimates of length at first maturity, median length-at-maturity (66.3 cm), and a spawning period of June to November, although the number of females studied was quite small (n=95). In Okinawa, E. coruscans displayed a similar length-at-maturity and a slightly extended spawning period of May to November (Uehara et al., 2018) compared to the Everson et al. (1989) findings for the MHI; however, the maturity classifications used for L50 estimation were not well defined. New data are needed to clearly define maturity criteria that is used to estimate L50 for E. coruscans from the MHI to provide contemporary information for stock assessments and direct species-specific and inter-regional comparisons over time. The purpose of our study is to: (1) provide the best available region-specific information on the reproductive characteristics of E. coruscans in the MHI; (2) identify and quantify the impact of different maturity classifications on L50 estimates; and (3) elucidate the biological reasons each maturity classification leads to differing L50 estimates with recommendations on moving forward.
2 Materials and methods
2.1 Sample collection
Female E. coruscans were opportunistically sampled from various locations among the MHI, including the islands of Oahu, Maui, Molokai, Kauai, and Niihau (Figure 1). Fishery-dependent samples were initially collected during October to April in 2007 and 2008 and sporadically during all months except June in 2012–2017. In an effort to produce comprehensive and updated species-specific reproductive information on females, additional samples were collected from April 2019 to December 2020 in collaboration with fishers and their partners on Maui. Fishery-independent samples were also collected from other projects in 2019 and 2020, including a research cruise in the MHI aboard the NOAA Ship Oscar Elton Sette in June 2019, and the annual bottomfish fishery-independent survey (BFISH) in August to December 2019 and 2020. Both fishery-dependent and -independent collection methods used hook-and-line with electric and hydraulic handline gurdies to fish at depths between 100 and 400 m.
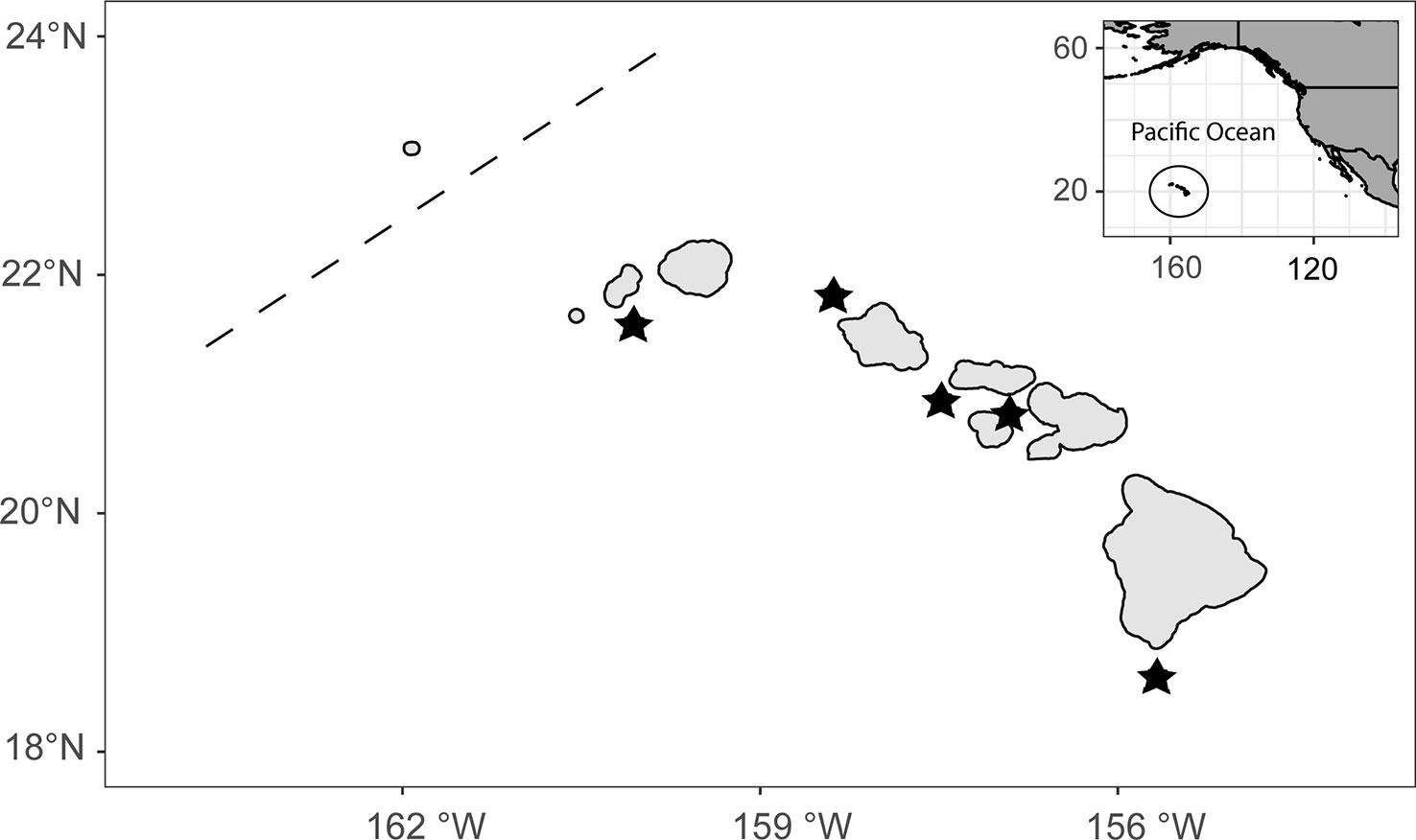
Figure 1 Map of the study region, the main Hawaiian Islands fishing zone (MHI). The inset indicates the position of the MHI in the Pacific Ocean. Poleward of the dashed line is the southernmost end of the Papahānaumokuākea Marine National Monument (PMNM) which separates the MHI from formerly fished bottomfishing areas that no longer are fished in the Mau and Ho’omalu zones of the PMNM. Stars indicate the general fishing locations where samples were obtained.
Once caught, fish were placed on ice for 4–24 h prior to being processed. A general location, date, vessel, species, and species-specific identification number was assigned to each fish. Biological data were recorded for each fish, including fork length (FL, 0.1 cm), weight (W, 0.01 kg), and gonad weight (GW, 0.01 g). Macroscopic sex identification and a gross macroscopic assessment of maturity (immature, mature) was recorded. A 1.0 cm3 section, taken from the mid-section of the right gonad, was preserved in 10% neutral buffered formalin for histological verification of sex and reproductive phase at a later date. During the 2019–2020 collection period, gonads were periodically shipped to the laboratory overnight on ice, thereby increasing the time on ice to 48 hours in some cases. However, all specimens were considered to be in good condition upon arrival at the lab, with negligible tissue degradation, and 1.0 cm3 sections were immediately preserved for subsequent histological processing.
2.2 Reproductive biology
Formalin-fixed tissue was dehydrated in a series of graded alcohols, cleared in xylene, and embedded in paraffin following standard histological protocols. Embedded tissue was sectioned transversely at 6 µm, mounted on slides, and stained with hematoxylin and eosin. Histology slides were viewed with a compound microscope between magnifications 10–40× for verification of sex and determination of reproductive phase and maturity following Brown-Peterson et al. (2011). Female fish were considered functionally mature when ovaries contained vitellogenic oocytes (primary, secondary or tertiary vitellogenesis; Vtg1, Vtg2, Vtg3) or physiologically mature when CA oocytes were present (Brown-Peterson et al., 2011; Lowerre-Barbieri et al., 2011a). Due to the scarcity of vitellogenic oocytes present in our samples and the large proportion of female E. coruscans in a reproductively inactive state across a wide range of sizes, reliance on secondary characteristics and CA oocytes to determine sexual maturity was necessary. However, since the regenerating reproductive phase lacks CA oocytes, secondary characteristics to distinguish between immature and mature but regenerating individuals included the presence of greater spacing among oocytes, developed interstitial tissue, the presence of blood vessels, the presence of atretic vitellogenic oocytes, the thickness of the ovarian wall, and muscle bundles (Brown-Peterson et al., 2011; Pacicco et al., 2023). These same secondary characteristics were used to determine if fish in the early developing sub-phase were virgins (first time to enter the reproductive cycle) or repeat spawners (mature fish reentering the reproductive cycle). Reproductive phase was determined by a combination of the most advanced oocyte stage present and histological indicators of secondary characteristics to describe the reproductive state of an individual fish as their spawning condition changes throughout a spawning cycle (as discussed by Lowerre-Barbieri et al., 2022), and was used for the length–at–maturity analyses.
Spawning seasonality was described using temporal trends in GSI values by plotting mean GSI across months; immature fish were not included in GSI calculations. The mean GSI of females in the developing phase was used as the GSI threshold to identify reproductively active fish for the season, as suggested for red snapper (Lutjanus campechanus; Brown-Peterson et al., 2019) and hardhead catfish (Ariopsis felis; Pensinger et al., 2021). Salas-Singh et al. (2022) similarily assigned a GSI threshold for the bullseye puffer (Sphoeroides annulatus), but considered spawning capable phase fish with Vtg2 or Vtg3 to be reproductivey active rather than developing phase fish with Vtg1. Spawning seasonality was histologically verified by the presence of Vtg3 oocytes or oocytes undergoing maturation (OM).
2.3 Determination of maturity ogives
Six different combinations of female reproductive phases and spawning period were assessed in accordance with our definitions of functional and physiological maturity to estimate length-specific sexual maturity using a logistic curve. All fish in the early developing phase, including repeat spawners, were considered to be immature for functional maturity analyses. For both the hybrid and the full-year maturity estimates, fish in all reproductive phases were used; fish in the regenerating phase were not used for the seasonal maturity estimates. When a spawning period was used to select individuals for the estimation of a length-at-maturity, the beginning of the spawning period was defined as the first presence of spawning capable females (September). The end of the spawning period was based on the last month (December) during which spawning capable females were identified. Table 1 describes each data combination used to derive L50 and L95 (length at 95% maturity) estimates. To address the potential impact of considering early developing repeat spawners as immature on functional maturity, we also calculated maturity ogives for each data combination (seasonal, hybrid and full year) of functional maturity with only early developing virgin females considered to be immature, and repeat spawners as mature.
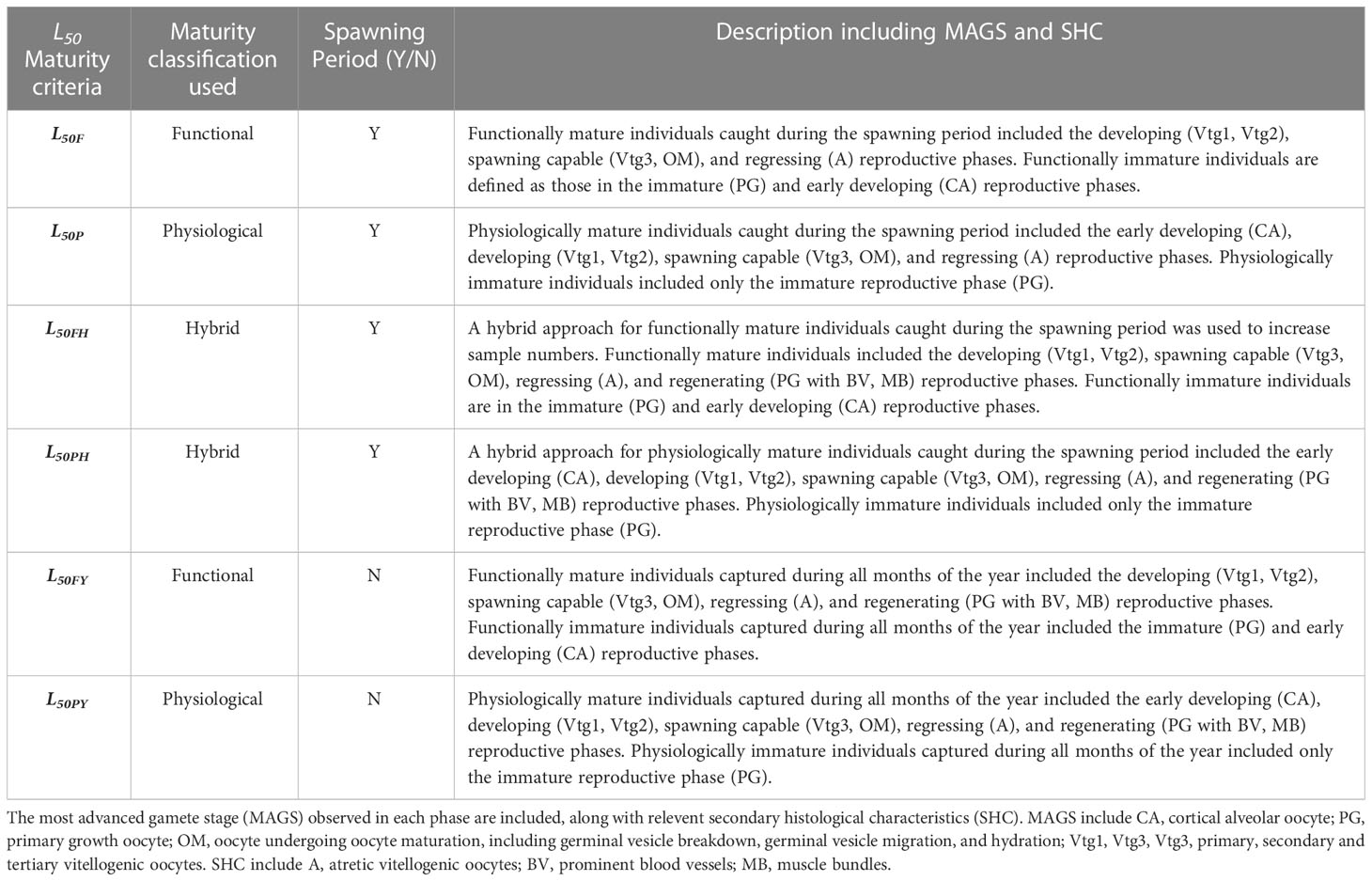
Table 1 Six different combinations of female reproductive phases and spawning periods were assessed in accordance with definitions of functional and physiological maturity to estimate length-specific sexual maturity using a logistic curve.
2.4 Data analysis
To address objective (1), we estimate the spawning period and the L50 of females from the MHI in an effort to provide biological traits of value to stock assessors and fisheries managers. Due to the difficulty in obtaining spawning capable females from the fishery, we address objective (2) by providing alternative estimates of L50 ogives that are based on classifications that differ from the traditional criteria of constructing a length-at-maturity ogive during the spawning period (functional maturity). These alternative classifications for estimation of L50 include additional reproductive phases, both functional and physiological maturity classifications, and inclusion of fish that were captured year-round or only during the spawning period. We address objective (3) by showing how much L50 values differ for the species and if any maturity classification criteria are prone to over- or underestimating the resulting parameter.
Mean GSI of female reproductive phases was plotted by collection month to provide more information on seasonal changes in reproductive status. The gonadosomatic index (GSI) was calculated using females in all histological phases except immature as:
Estimates of L50 and L95 were evaluated by plotting the proportional frequencies of mature and immature individuals by 5-cm length class across all samples. The selection of samples as immature and mature was based on either functional or physiological maturity criteria in a generalized linear model (GLM) with a binomial distribution and a logit-link function using R ver. 4.0.2 (R Core Team, 2020). A two-parameter logistic curve was fit to the proportional frequencies:
where p is the estimated proportion of mature individuals at a given length (L), a describes the width of the interval, and L50 is previously defined. Specifically, a and L50 were estimated by minimizing the negative log-likelihood of the ogive using a binomial density probabilistic function (Brouwer and Griffifths, 2005; Aguirre-Villaseñor et al., 2022) using the glm function from the stats package in R ver. 4.0.2 (R Core Team, 2020):
where ln is the natural logarithm; pi is the proportion of individuals in size class Li; ni is the number of samples in each size class of fish i; mi = the number of mature fish in size class i.
Boostrapping using random sampling with replacement for 2,000 iterations was used to replace the data pairs in our equation, L and the corresponding maturity parameter in each data source for maturity criteria. In doing this we allow for all simulated samples to have an equal probability of being selected. Each boostrap was fit with a negative log-liklihood function using the boot package from R ver. 4.0.2 (R Core Team, 2020) and then simulation of the observed data was conducted for the total number of individuals in each data source for maturity criteria. The uncertainty around the parameters and the confidence intervals were estimated based on nonparametric CI (α =0.05) by using the bias-bias corrected percentile method (Efron, 1979; Haddon, 2001). We implemented this process for each of the six data sources using different maturity classification criteria. The L95 was derived from the output of the model. The descriptive statistics for each parameter θi describe the efficiency of the boostrapping and include the standard error (SE), the mean value (), the coefficient of variation (CV), the bias (B), and the percent bias (%B) (Jacobson et al., 1994).
Due to each of the six generalized linear models using different data sources to explore the effects of different maturity classification criteria on the resulting parameters, the use of AIC was not appropriate to test for model fit. To test for the goodness of fit for each data set with our logistic model we used McFadden’s pseudo R2 to measure the proportion of variance explained which gives a value between 0 and 1 with a higher value indicating the likelihood of the model fitting the data well (McFadden, 1974). A McFadden’s pseudo R2 less than 0.2 is a poor fit (McFadden, 1979). The L0 is the value of the likelihood function for the model with no predictors, LM the likelihood of the model being estimated.
3 Results
3.1 Length summary
A total of 382 female E. coruscans were collected from October 2007 to December 2020 from various fishing locations across the MHI. The length distribution ranged from 21.2 cm FL to a maximum length of 97.0 cm FL with a mean of 59.0 cm FL and median of 61.7 cm FL. The size range of physiologically immature females was 21.2–66.6 cm FL and for physiologically mature females was 32.2–97.0 cm FL (Figure 2); thus, the length at first maturity (Lf) was 32.2 cm FL. The size range for functionally immature females was 21.1–76.0 cm FL and for functionally mature females 45.5–97.0 cm FL (Supplementary Figure 1). For functional maturity, virgin females in the early developing sub-phase ranged from 46.0–76.0 cm FL, while those that were histologically identified with secondary characteristics to be repeat spawners ranged from 50.2–89.9 cm FL.
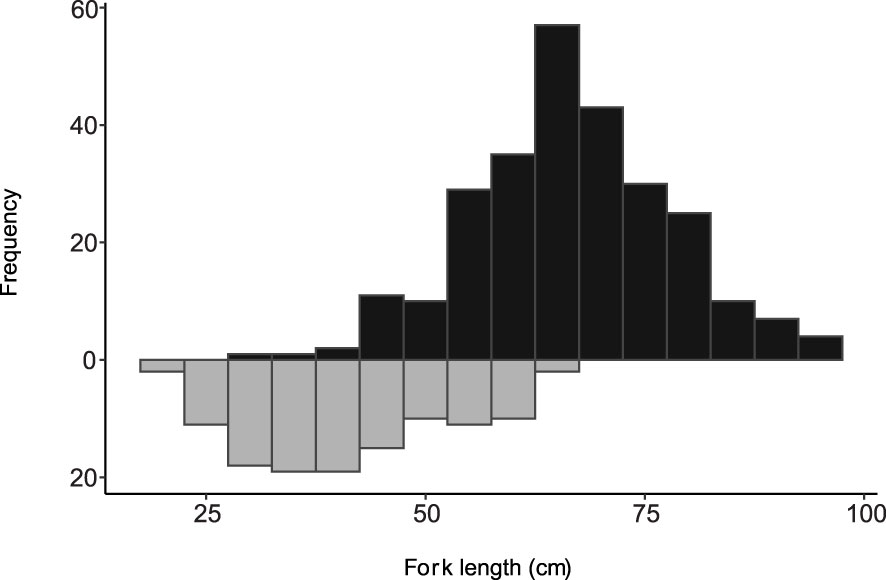
Figure 2 Length frequency distribution of sampled female Etelis coruscans collected during October 2007 to December 2020, from the main Hawaiian Islands, in 5-cm size bins. The black bars above the x-axis indicate the range in sizes of physiologically mature individuals and the grey bars below the x-axis indicate range in sizes of immature individuals.
3.2 Spawning periodicity
Fish in all reproductive phases were histologically analyzed (total n = 382), and this species was found to have asynchronous oocyte development (Figure 3) which is typical of a batch spawner. No evidence of POF (post-ovulatory follicle complex) was seen in any spawning capable fish collected, although this may be due to the small sample size of E. coruscans in this phase (n = 33). The oocyte size range for cells >100 µm was 102.18–642.40 µm, with oocytes undergoing oocyte maturation (OM) being the largest cells observed (Supplemental Table 1).
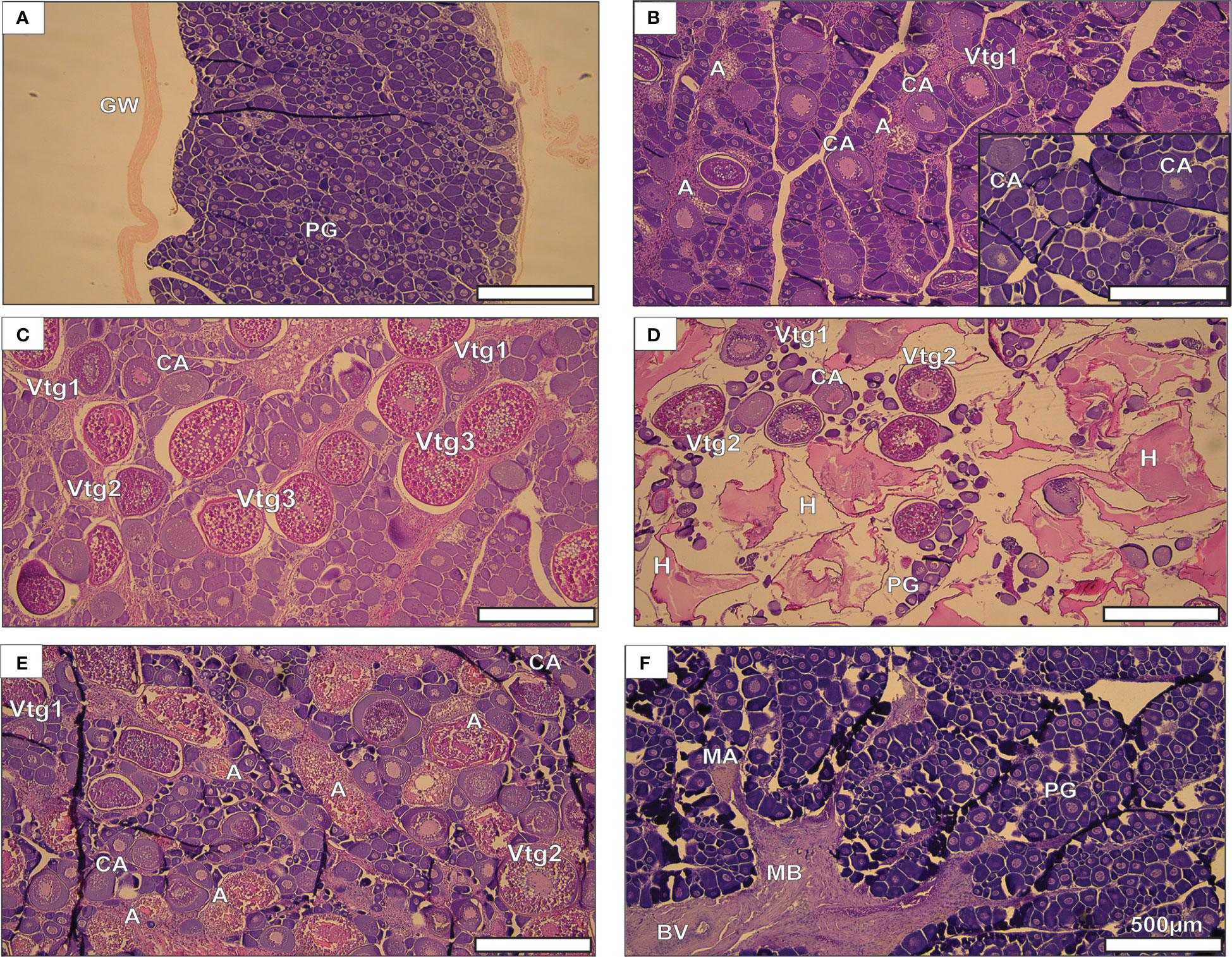
Figure 3 Etelis coruscans gonadal histology depicting reproductive phases. (A) immature, (B) developing, (C) spawning capable, (D) actively spawning sub-phase, (E) regressing, and (F) regenerating. The insert in B) is the early developing subphase and it is to scale with the other images. The scale bar of 500 µm applies to all images. Abbreviations for histological characteristics include: A, atresia; MA, macrophage aggregate; BV, blood vessel; CA, cortical aveoloar; GW, gonad wall; MB, muscle bundle; PG, primary growth; Vtg 1, primary vitellogenic; Vtg2, secondary vitellogenic; Vtg3, tertiary vitellogenic; H, hydrated oocyte.
The change in mean GSI at each reproductive phase shows a steady increase from the early developing sub-phase through the actively spawning sub-phase, with a decrease in the regressing and regenerating phases typical of reproductively inactive females (Table 2). The confidence intervals of immature and regenerating (mature) females do not overlap indicating a detectable difference between these two reproductively inactive phases. The mean GSI of females in the developing phase (0.962) (fish with vitellogenic oocytes corresponding to a functional maturity designation) was used as the threshold for reproductively active fish when using GSI as one method to determine the spawning period for the MHI. Six females in the developing phase and three females in the spawning capable phase fell below this GSI threshold; all the spawning capable fish were small (45.5-60.4 cm FL) and likely first-time spawners, while all the developing fish had very few Vtg1 oocytes and had likely just entered the developing phase. The monthly mean GSI values indicate two peaks, a small one in spring (March–April) and a larger one in the fall (September–December) (Figure 4). However, the smaller peak did not reach the GSI threshold for reproductively active fish (line in Figure 4), although three individuals in the developing phase did have GSI values greater than the cut-off value in March and April. Mean GSI of the first peak likely represents initial oocyte development (CA, Vtg1, Vtg2) and corresponds to mean GSI values for the early developing sub-phase and developing phases (Figure 4; Table 2). A pronounced drop in monthly mean GSI is indicated during the summer months of May–July; however, sample sizes during these months were low and thus summer GSI values should be interpreted with caution. Based on GSI values alone, the spawning period of E. coruscans in the MHI appears to be September–December.
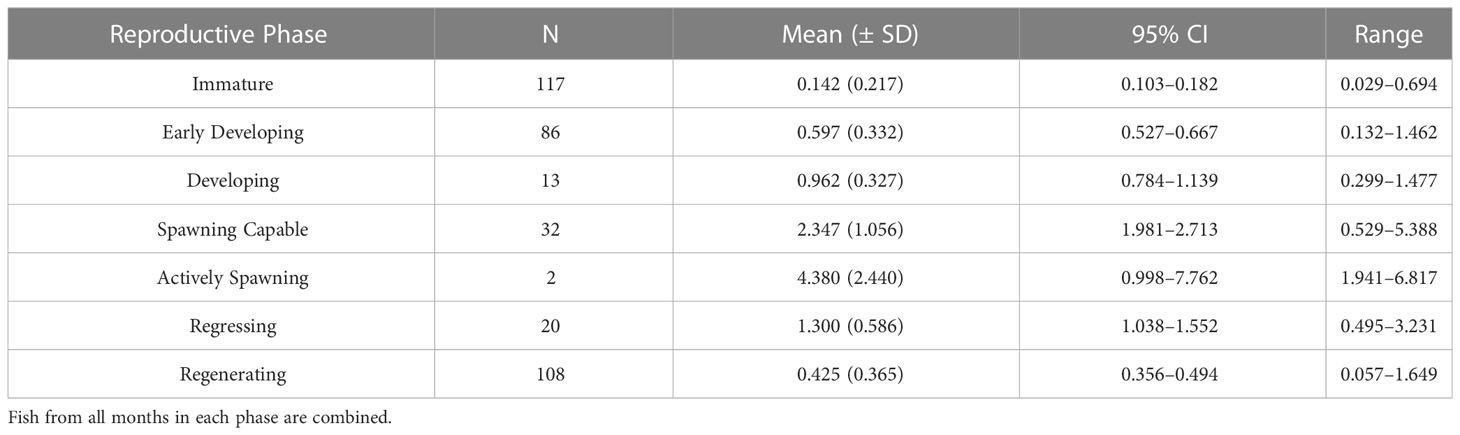
Table 2 The mean (± SD) Gonadosomatic Index (GSI) for each reproductive phase of female Etelis coruscans from the main Hawaiian Islands.
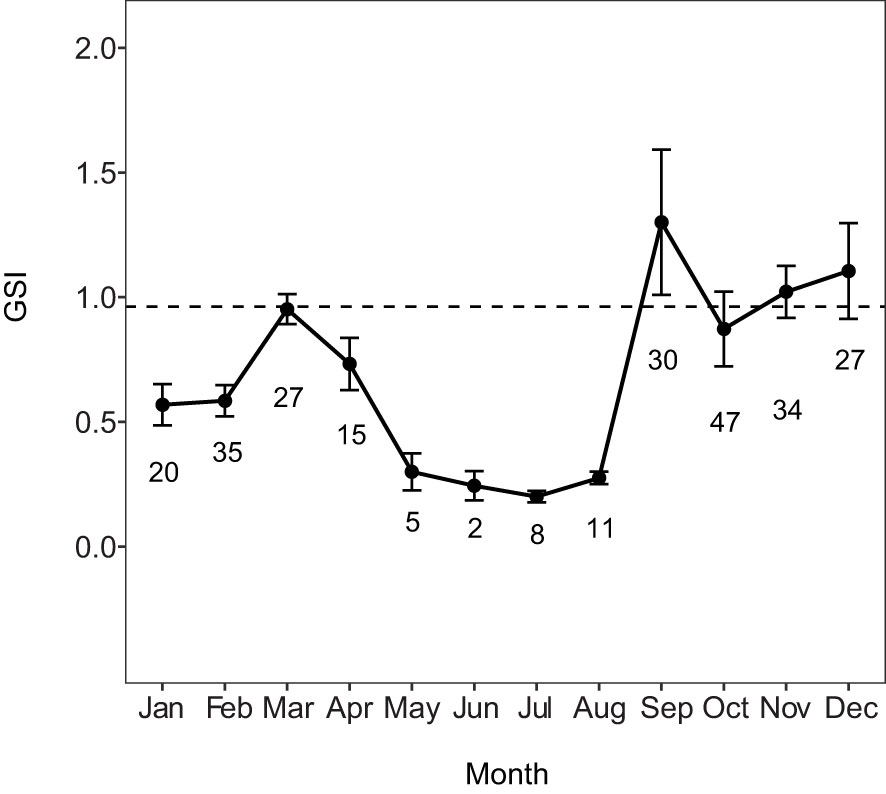
Figure 4 Spawning periodicity of main Hawaiian Islands female Etelis coruscans. Monthly mean ( ± SE) gonadosomatic index (GSI). Plot is restricted to physiologically mature individuals (n = 261). Numbers of fish are indicated adjacent to each monthly mean. Black dashed line indicates the GSI value (0.962) for reproductively active fish.
Histological analysis confirmed fluctuations in GSI and allowed for increased accuracy in determination of the spawning period. Females in the spawning capable phase (Figure 3C) were only seen from September through December (Table 3), corresponding to the peak in GSI values (Figure 4), while the only actively spawning females (Figure 3D, n = 2) were captured in September and October 2020. A relatively high percentage of early developing females (Figure 3B, inset) were found during March and April (Table 3), as well as the presence of some developing females (Figure 3B), indicating ovarian recrudescence begins in the early spring. Our updated characterization of the spawning period based on the occurrence of spawning–capable females and a seasonal increase in mean GSI indicates that the spawning period is September–December for the MHI. The specimen numbers during the summer months (May to July, Table 3) were quite low due to the difficulty in obtaining fish during this time; larger sample sizes may have resulted in the identification of spawning capable fish during these months. Immature fish (Figure 3A, n = 118) were present during all months except December and January and represented the reproductive phase with the most individuals collected. January to April had the highest proportions of early developing individuals, but this phase also occurred in small proportions during the spawning period later in the year (August to December). Overall, few fish with vitellogenic oocytes were encountered at any size or in any month (n = 69, Table 3), a likely explanation for the generally low GSI values (Figure 4). Regressing females (Figure 3E) were observed September to December with the highest percentages in November and December (Table 3), indicating the end of the spawning period. Regenerating females (Figure 3F) were the second most numerous reproductive phase (n = 108) and were present during all months, although lowest percentages were seen from August through October, corresponding to the beginning of the reproductive season (Table 3).
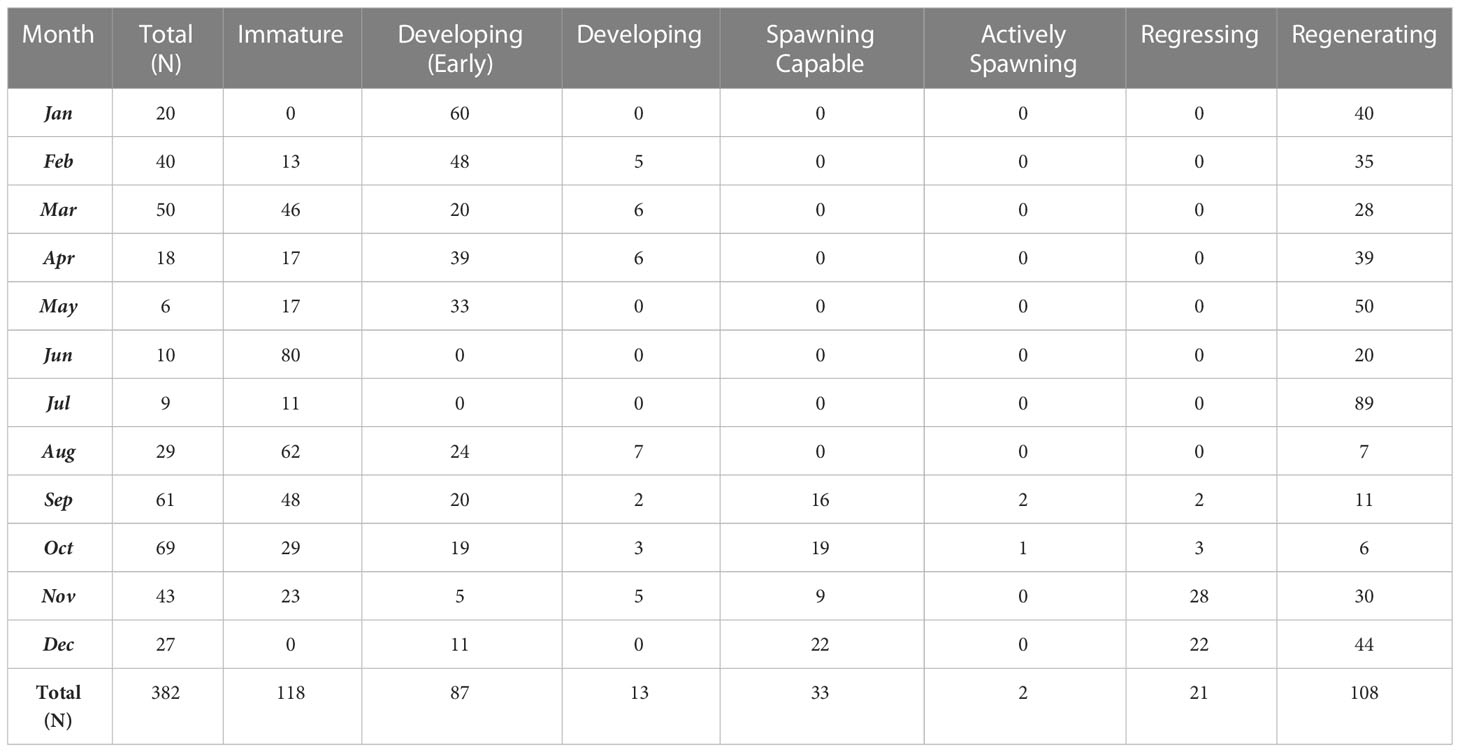
Table 3 Monthly percentages of female Etelis coruscans in each reproductive phase captured from the main Hawaiian Islands during October 2007 to December 2020.
3.3 Size at maturity and statistical measure of fit
The smallest physiologically mature fish (Lf) was a regenerating female 32.2 cm FL and the smallest functionally mature fish was a spawning capable female 45.5 cm FL. A total 149 specimens were used to estimate the physiologically and functionally mature L50 and L95 during the spawning period (September to December). The L50F and L95F estimates for functional maturity during the spawning period (61 mature, 88 immature, Table 4) were 65.79 cm (95% CI, 62.99–68.50 cm; Figure 5A) and 83.98 cm FL (95% CI, 77.22–90.74 cm), respectively. The physiological maturity determinations during the spawning period included 91 mature fish and 58 immature fish (Table 4). The L50P and L95P estimates for physiological maturity in the spawning period were 57.18 cm (95% CI, 54.88–59.45 cm; Figure 5B) and 67.98 cm FL (95% CI, 64.65–71.31cm), respectively. A total of 198 fish were used to determine functional and physiological maturity for the hybrid criteria during the spawning period. Functional maturity included 110 mature fish and 88 immature fish (Table 4), with L50FH and L95FH estimates of 57.9 cm (95% CI, 54.19–61.61 cm; Figure 5C) and 88.64 cm FL (95% CI, 79.48–97.80 cm), respectively. Physiological maturity using the hybrid criteria during the spawning period included 140 mature fish and 58 immature fish (Table 4), with resulting L50PH estimate of 51.61 cm (95% CI, 48.62–54.60 cm; Figure 5D) and L95PH estimate of 68.93 cm FL (95% CI, 64.97–72.89 cm). Functional maturity across all months included 382 specimens (178 mature and 204 immature individuals, Table 4), with a L50FY estimate of 61.97 cm (95% CI, 58.30–65.64 cm; Figure 5E) and a L95FY estimate of 108.88 cm FL (95% CI, 96.06–121.70 cm). Physiological maturity across all months included 265 mature and 117 immature individuals (Table 4); the L50PY estimate was 49.97 cm (95% CI, 48.03–51.91 cm; Figure 5F) and the L95PY estimate was 65.51 cm FL (95% CI, 62.54–68.48 cm). Overall, the smallest L50 estimate was found using physiological maturity for the entire year, while the largest estimate was for functional maturity during the spawning period (Figure 6).
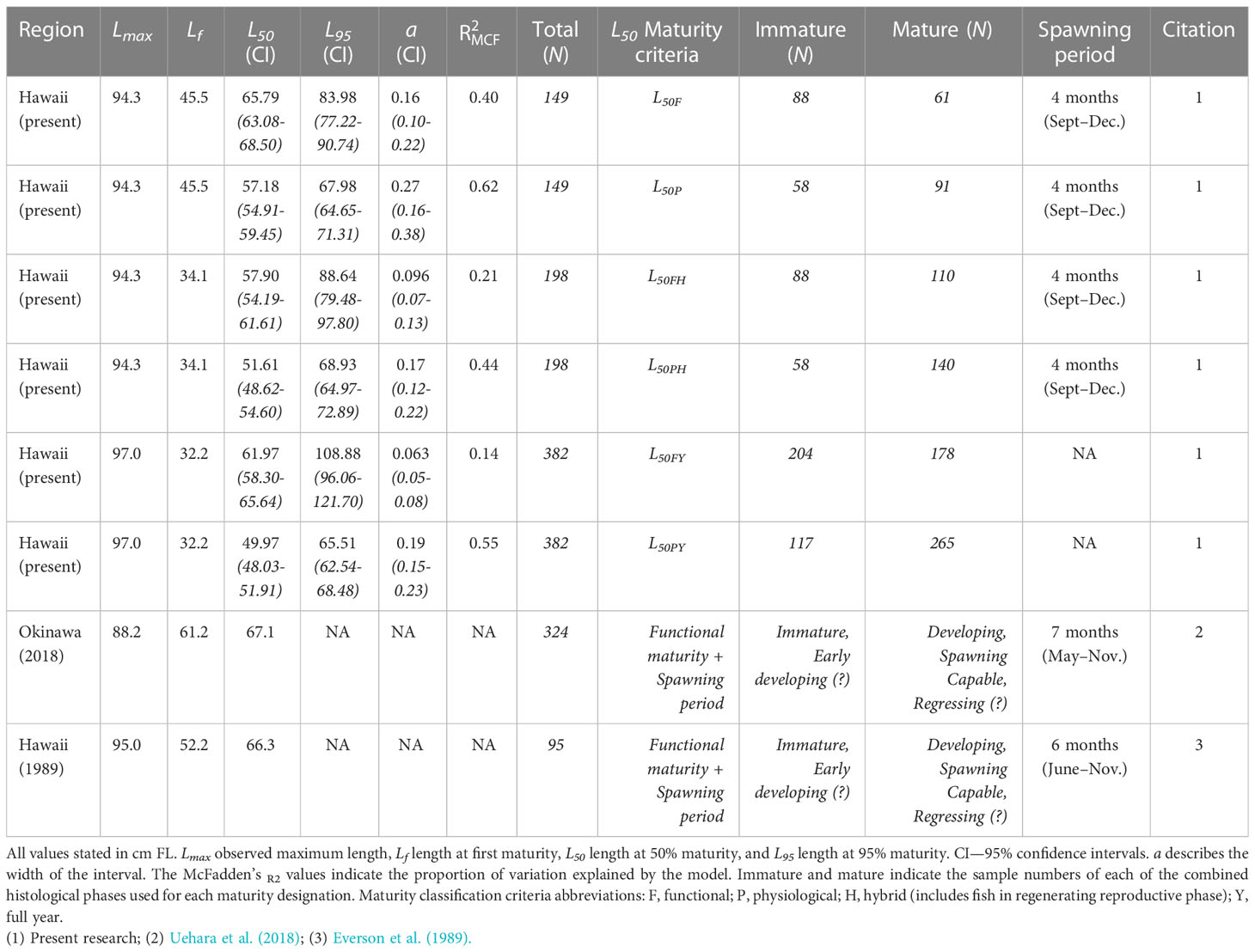
Table 4 Summary of reproductive characteristics for female Etelis coruscans, including previous studies.
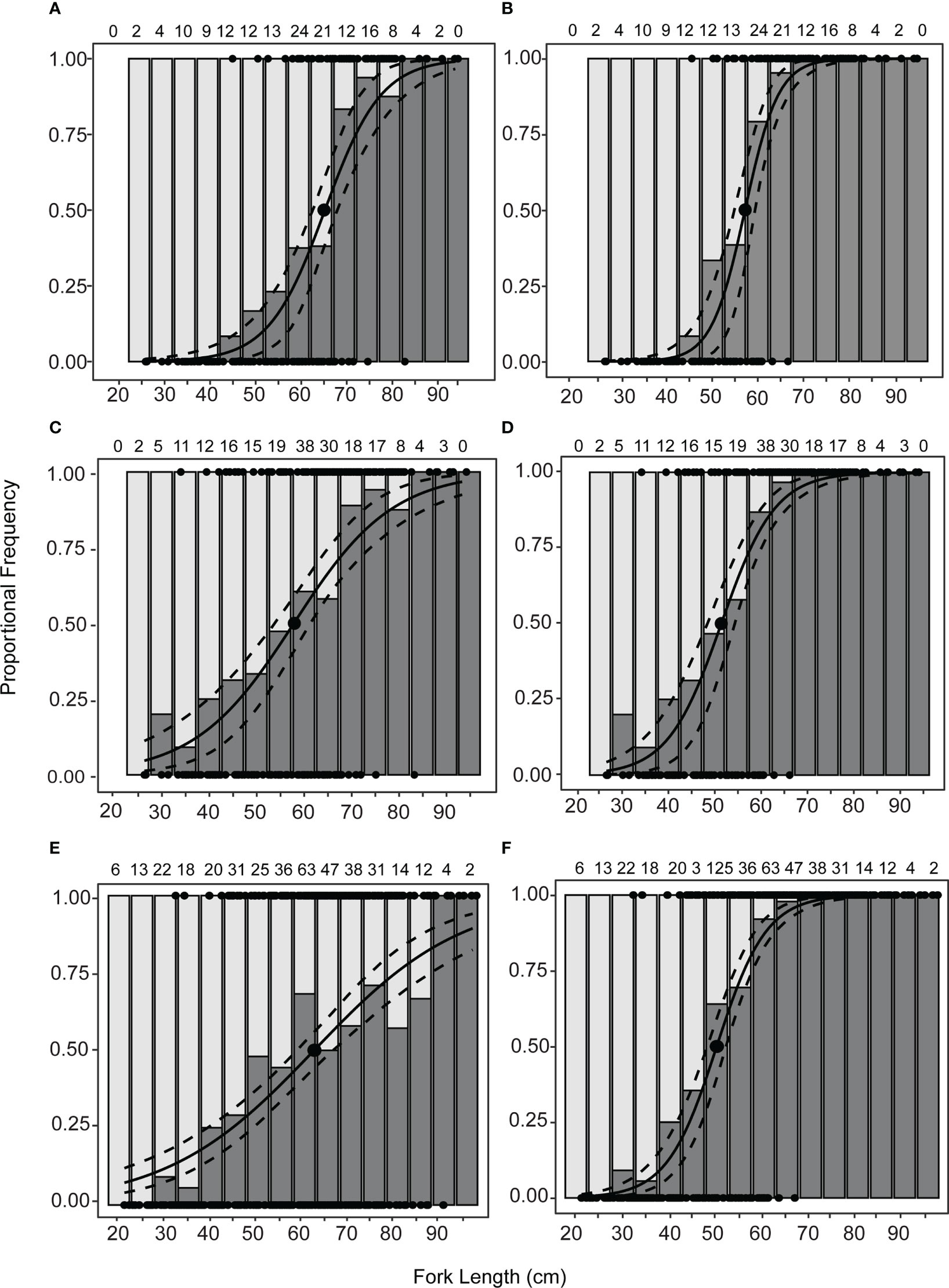
Figure 5 Fitted logistic regression for the proportion of mature female Etelis coruscans and proportional frequency of immature (light grey) and mature (dark grey) by 5-cm length bins. Dashed lines indicate ± 95% confidence intervals. Dark circles indicate L50. Sample size for each length bin is indicated on top of each graph. Maturity classification is based on the histological criteria, functional vs. physiological maturity determination, and inclusion or exclusion of the September–December spawning period. Hybrid estimates include fish in the regenerating reproductive phase. The McFadden’s R2 values indicate the proportion of variation explained by the model. (A) Functional maturity during spawning period; L50F = 65.8 cm FL, R2 = 0.40. (B) Physiological maturity during spawning period; L50P = 57.2 cm FL, R2 = 0.62. (C) Functional hybrid during spawning period; L50FH = 58.0 cm FL, R2 = 0.21. (D) Physiological hybrid during spawning period; L50PH = 51.6 cm FL, R2 = 0.44. (E) Functional maturity during all months; L50FY = 62.1 cm FL, R2 = 0.14. (F) Physiological maturity during all months; L50PY = 50.0 cm FL, R2 = 0.55.
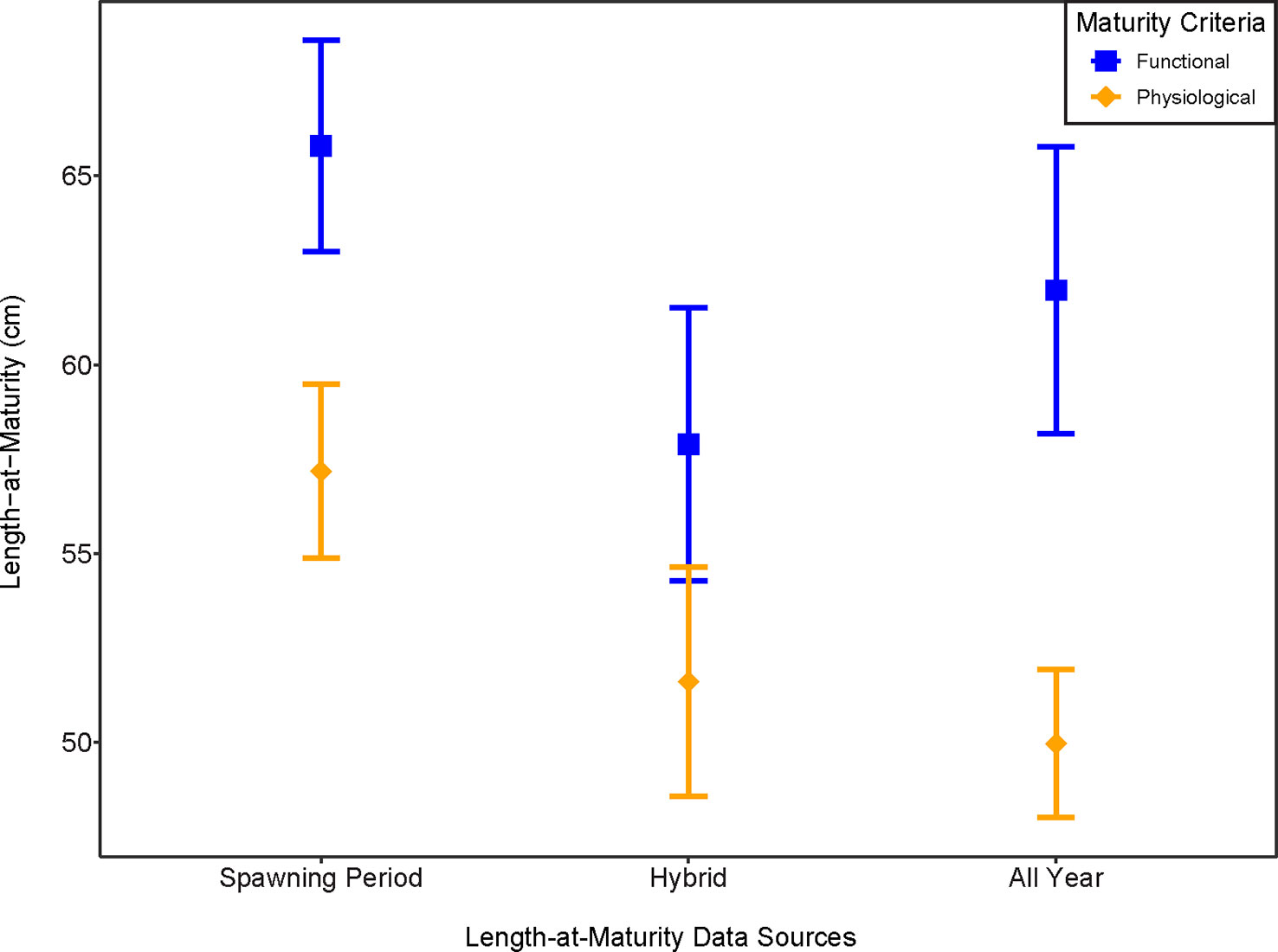
Figure 6 A comparison of length-at-maturity L50 parameters for each of the six maturity classification criteria analyzed. Spawning period was defined by the presence of spawning capable females (September to December). Hybrid was defined as the Spawning period but with the addition of regenerating females. All Year is defined as including all individuals from all months of the year caught. The error bars indicate 95% confidence intervals around the median length at maturity.
Comparison of median L50 by maturity classification criteria shows differences not only among the time-scale used for maturity estimates, but also for physiological vs functional maturity (Figure 6). There was nearly a 9 cm difference between functional maturity L50 (65.8 cm) and physiological maturity L50 (57.2 cm), and the CIs did not overlap (Figure 6). This represents a difference close to 9% of overall maximum fish length. The hybrid maturity criteria showed a slight overlap in CIs by a small margin, 0.41 cm. While small, this overlap indicates that functional and physiological maturity executed in a hybrid method have the most similarity compared to the other two data sources (spawning period or all months of the year collected) (Figure 6). Functional maturity and physiological maturity for all months of the year collected did not have overlapping CIs. Overall, there were progressively smaller values in L50 as our classification system included females that were either early in their gonadal development (early developing phase) and possibly in recruit spawners, or females that were reproductively inactive caught outside of the spawning period as seen in Figure 6. Descriptive statistics from our boostrapping procedure indicated the L50F was most efficiently estimated while the L95 parameters consistently indicated a larger percent bias, suggesting a lack of efficiency in estimating this parameter (Table 5).
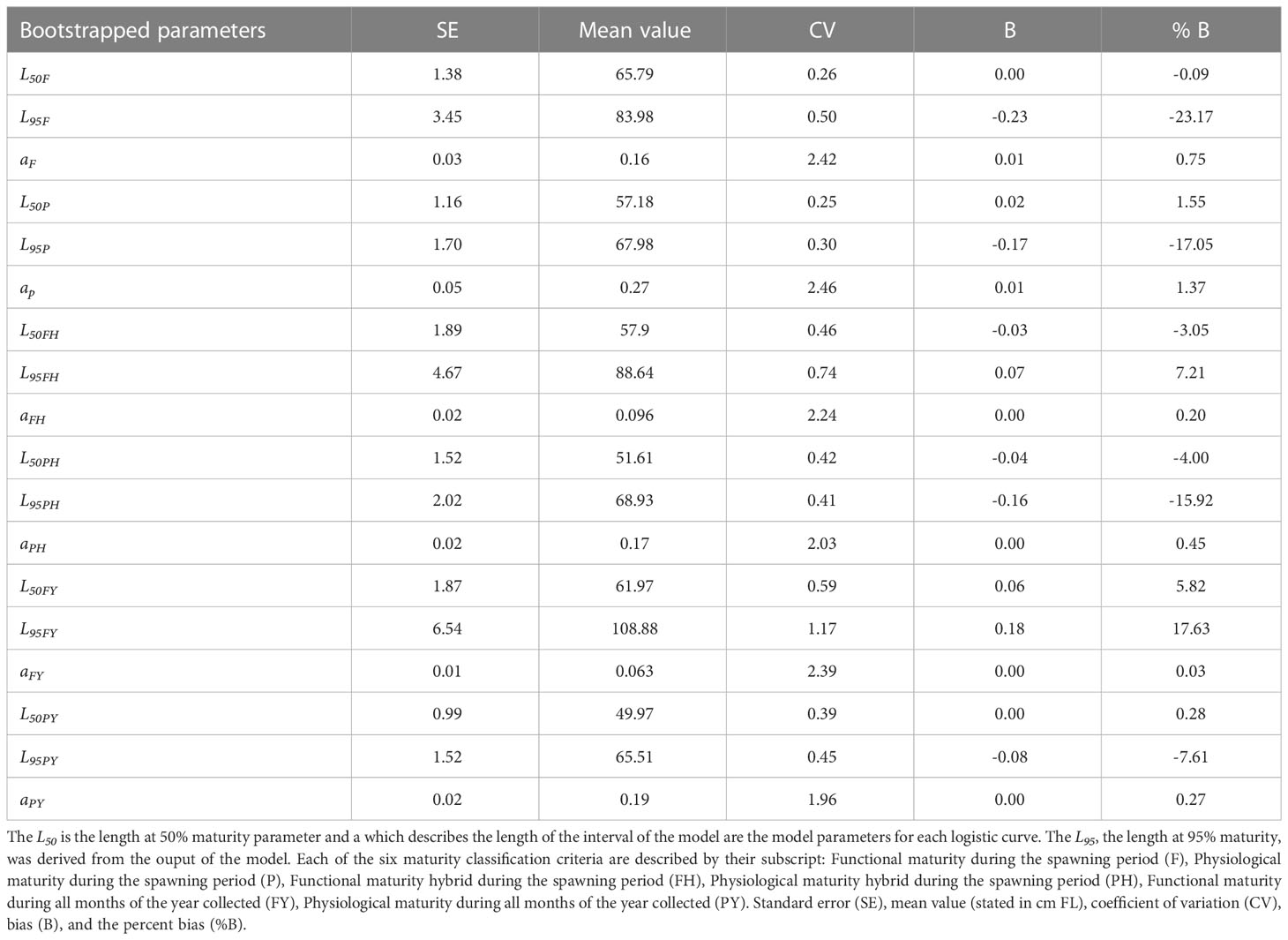
Table 5 Summary of descriptive statistics for boostrapping results of all length at 50% maturity parameters estimated.
We showed a large decrease in size from the L50F when including females in the regenerating phase during the spawning period as mature (L50FH, a 7.9 cm decrease in size). The addition of regenerating females during the spawning period resulted in a decrease of the fit of the model to the data resulting in a poor fit (decrease in R2 from 0.40 to 0.21; Table 4). Similarly, the L50 for physiologically mature females during the spawning period (L50P) also decreased by 5.6 cm when females in the regenerating phase were included (L50PH). In this case the addition of regenerating females to the physiological criteria resulted in a decrease of fit but the overall fit of both the physiological hybrid and all months of the year data remained high. Therefore the proportion of variation was still well explained by the model used despite the different data sets (R2 = 0.62 and 0.44, respectively; Table 4). Physiological maturity assessed from all months of the year fit the data well (R2 = 0.55). All physiological models had relatively high goodness of fit to the data (Table 4). Two of the three functional maturity classification criteria had poor fits to the data, the hybrid classification criteria and functional maturity assessed with samples from all months of the year (R2 of 0.21 and 0.14, respectively; Table 4), therefore the model used did not explain an adequate amount of variation. The most traditional maturity classification criteria using functional maturity during a spawning period had a good fit to our data, L50F R2 = 0.401; although this value is lower than all of the physiological maturity models tested, the boostrapping most efficiently estimated this parameter compared to the other 5 data sources with the least amount of percent bias (Table 5).
Additionally, to investigate what impact including repeat spawners as immature has on functional maturity estimates, we included post-hoc analyses for the functional maturity ogives to differentiate between repeat spawners and virgins who have the same reproductive phase (early developing as denoted by CA oocytes). Functional maturity during the spawning period had 14 fish in the early developing phase that we reclassified by their secondary characteristics to be repeat spawners and therefore mature. The length range of these individuals was 50.2 – 75.2 cm FL and the change in L50F was a decrease of 4.3 cm, to 61.54 cm FL (95% CI, 59.03 – 64.05 cm; Supplementary Figure 2A). The L95F decreased 6.7 cm, to 77.34 cm FL (95% CI, 71.72 – 82.96 cm; Supplementary Figure 2A). For the functional maturity hybrid criteria, the same 14 fish in the early developing phase were reclassified as mature. The change in L50FH was a decrease of 3.3 cm, to 54.69 cm FL (95% CI, 51.40– 57.98 cm; Supplementary Figure 2B). The L95F decreased 4.8 cm FL, to 79.21 cm FL (95% CI, 72.62 – 85.80 cm; Supplementary Figure 2B). The functional maturity during all months of the year had 46 fish that were reclassified as mature repeat spawners, and their size range was 50.2- 89.9 cm FL. The change in L50FY was a decrease of 7.2 cm FL, to 54.92 cm FL (95% CI, 52.61 – 57.23 cm; Supplementary Figure 2C). The L95FY had the largest decrease of 29.1 cm FL, to 79.88 cm FL (95% CI, 75.01 – 84.75 cm; Supplementary Figure 2C). The CI for both the L50F and the L50FH do not overlap and the CI for L50FY has a slight overlap with the L50FH by 0.75 cm. The post hoc testing for repeat spawners as mature yielded an increase in fit for each of the functional maturity classification criteria relative to when repeat spawners were considered immature, L50F R2 = 0.461, L50FH R2 = 0.289, and L50FY, R2 = 0.3419.
4 Discussion
Our results provide the most reliable reproductive characteristics for E. coruscans in the MHI to date with the best available information for determining estimates of maturity. We examined four times the number of fish in this region as compared to Everson et al. (1989) and found the spawning period to be shorter and extended further into winter (September–December) than previously reported. We provide region-specific estimates of the maximum size caught in Hawaii to date, length at first maturity (Lf), L50, and L95. Furthermore, our estimates of L50 using six different maturity classification criteria were smaller than previously reported in either the MHI or Okinawa, Japan (Everson et al., 1989; Uehara et al., 2018; Table 4). Both previous studies on E. coruscans based their estimates of length-at-maturity on fish that were caught during the spawning period and that were observed to be functionally mature (individuals with ovaries containing vitellogenic oocytes) a criteria that we also employed. Fisheries scientists commonly use spawning capable individuals caught during the spawning period to estimate L50 (West, 1990; Brown-Peterson et al., 2011). However, for data-limited species, like E. coruscans where few specimens have been collected containing vitellogenic oocytes during the spawning period, there is a need to investigate alternative approaches for estimating length-at-maturity. To appropriately assess temporal and spatial variability in reproductive characteristics of E. coruscans, a combination of recent information with clear maturity classification criteria provided data inputs.
4.1 Spawning period and size at maturity
We obtained spawning capable and actively spawning females from September through December. Our estimate of a shorter spawning period (4 months) differs from the 6-month period reported previously (Everson et al., 1989; Uehara et al., 2018). This difference may be an artifact of specimen collection difficulties during May–August from the commercial fishery, despite a relatively large overall sample size. The Deep 7 bottomfish fishery encounters competition for more lucrative pelagic species (e.g., tuna and uku) during the summer months, and as a result, samples are difficult to obtain (Chan, 2020; Nadon et al., 2020). We suspect gonadal recrudescence, and thus the start of the reproductive season, likely occurs during the summer based on the presence of early developing and developing females captured in May and August. Furthermore, using a GSI threshold to define the spawning season based on mean GSI of fish in the developing phase (vitellogenic oocytes) was supported by histological observations; similarly defined thresholds have been previously used to determine spawning seasons in other species (Brown-Peterson et al., 2019; Pensinger et al., 2021; Salas-Singh et al., 2022). Spawning periods are known to vary for many reasons including exogenous cues such as temperature and photoperiod, which can switch in primary importance depending on latitude (Pankhurst and Porter, 2003). A spawning period can also vary spatially via species movement patterns both by latitude and with water column depth (Hilborn and Walters, 1992; Lowerre-Barbieri et al., 2011b). The timing of spawning periods may also vary due to population dynamics, whereby fishing pressure reduces the age and size of a population, consequently reducing the average lifetime spawning duration of a fish stock (Wright and Trippel, 2009). However, our shortened spawning period is likely a result of low sample numbers and insufficient sampling of larger individuals (> 65 cm FL) in the summer months, which we interpret as a result of fishery dynamics changing seasonally rather than a change in the structure of the reproductive population. Therefore, we are reporting a four-month spawning period for the MHI that overlaps with the previously reported May or June through November season (Everson et al., 1989; Uehara et al., 2018).
We found an L50F for functionally mature females during the spawning period of 65.8 cm, a value slightly smaller than that found previously in the MHI (L50 = 67.1 cm, Everson et al., 1989) and in Okinawa, Japan (L50 = 66.3 cm; Uehara et al., 2018) (Table 4). However, previous estimates of L50F are within the confidence intervals of our estimates. Even with our sampling challenges and data limitations, we were still able to calculate a parameter using comparable classification criteria to previously published works. It is likely that our L50F was influenced by the relatively small number of females sampled with vitellogenic oocytes during the spawning period. The small difference in size of L50 compared to fish in other regions may not be biologically relevant, but the degree to which a reduction in several centimeters of L50 impacts the estimate of the SSB and other stock assessment outputs requires future work. We found a size at first maturity smaller than previously reported for MHI (32.2 cm FL for physiological maturity, 45.4 cm FL for functional maturity; Table 4). Deep-water snappers such as E. coruscans are particularly sensitive to fisheries-induced evolution (Koslow et al., 2000; Newman et al., 2016), and therefore continued future research estimating L50F for E. coruscans in the MHI is necessary to appropriately interpret this apparent change in size-at-maturity over time.
Another important aspect of E. coruscans in the MHI bottomfish fishery is that it is possible to catch a wide range in sizes and therefore maturity phases at size in a single fishing event. Immature fish are essential for a length-at-maturity ogive, and not having enough immature fish is often problematic in exploited fisheries since a trend in proportion-mature cannot be fit by a logistic curve without immatures (Taylor et al., 2018; Prince et al., 2021). However, our data show the opposite scenario, an abundance of immature fish. The commercial fishery for MHI onaga uses hook and line fishing with electronic reels. It appears this is a relatively nonselective gear based on the wide length distribution of fish and the wide size range of immature individuals. Onaga samples obtained from similar gear as our study (Everson et al., 1989) also found wide length ranges in their datasets (approximately 50.0 – 90.0 cm in the MHI). Okinawa, Japan uses vertical longlines and trotlines to catch their bottomfish and also obtained a similar wide length range for onaga (20.6 – 88.2 in Okinawa; Uehara et al, 2018). Despite the use of different gear across regions to catch the same species, similar length ranges and similar L50F estimates are found. Thus, reports of a large size-at-maturity as well as the upper size-limits of the species is consistent across regions, which suggests there is no gear bias.
Recent research indicates that the type of sigmoidal curve selected for a maturity ogive can influence the variability in the L50 parameter up to 7 cm in size depending on the model that is used based on goodness of fit (Aguirre-Villaseñor et al., 2022). Although we did not compare different model types for a best fit approach, we acknowledge the importance other sources of variability or bias have in estimating a L50 parameter. Testing for a well fit model is another important component for reporting the most accurate L50 (Aguirre-Villaseñor et al., 2022; Lowerre-Barbieri et al., 2022; Salas-Singh et al., 2022), and we have examined our model for each classification criteria thoroughly using a boostrapping routine and McFadden’s pseudo R2 to test for the proportion of variance explained by the model. We maintain that, regardless of the model used, it is most important that consistency and clear determination in the biological classification criteria are used for maturity determination. The clarity in this criteria is likely to be most important for fisheries with wide size ranges simply because the inflection point in the curve is subject to a wider range in sizes when first time spawners or repeat spawners in the early developing phase are classified as immature or mature. Only with transparent and consistent maturity classification criteria can the test of a well fit model be meaningful.
4.2 Effects of alternative data sets
Fishery-dependent sampling constraints and the subsequent lack of samples during the summer from the MHI bottomfish fishery led to an exploration of alternative data sets based on differing maturity classification criteria for estimating an L50 of E. coruscans. Of the six L50 parameters estimated, the largest value was derived using functionally mature females caught during the spawning period. This value was a relatively good fit to the data and its confidence intervals did not overlap with any of the alternative criteria L50 parameters. We show that the maturity classification for estimation of length-at-maturity has a significant impact on the resulting parameter. Although physiological maturity was the best fit model in each of our three model comparisons, it consistently estimated a smaller L50 value when compared to functional maturity. In terms of conserving the spawning population, a smaller L50 value is more likely to allow fish that have yet to spawn for the first time (juveniles) to be subject to fishing pressure. Alternative data sets including fish in the regenerating phase or including all fish captured year-round may be less ideal for current management use because those data sources produce inherently smaller values than the traditional functional maturity parameter (L50F). As fisheries dynamics change, the potential of future scenarios where sampling does not yield large numbers of females in an advanced spawning condition may increase. Therefore, spawning females have the potential to become more elusive. Currently, spawning capable E. coruscans in Okinawa, Japan represent less than 2% of the fish landed (Uehara et al., 2019; Uehara et al., 2020). The authors indicate this is a direct reflection of the stock’s reproductive potential in dire condition (Uehara et al., 2019; Uehara et al., 2020). We do not have reason to believe that this is the current case for the MHI due to reduced fishing effort during the early reproductive season. We do, however, foresee the applicability of reporting multiple values of L50 (a functional and a physiological L50) for species like E. coruscans that have a wide size distribution in the fishery, are considered to be slow growing, and have a large size or old age at 50% maturity. Providing L50 estimates using multiple maturity classification criteria will provide additional information to managers to address current issues and are also valuable as fishery dynamics are likely to change in the future due to fishing pressure or climate change (Planque et al., 2010; Audzijonyte et al., 2016).
One of our key findings is that using physiological or functional maturity could either over– or under–estimate length-at-maturity due primarily to how maturity of individuals were classified, which can therefore bias subsequent analyses. The use of physiologically mature individuals captured during the spawning period yielded an L50P that was 8.8 cm smaller than the functional (L50F) maturity estimate for E. coruscans. However, it is unknown if females with CA oocytes will be able to develop and spawn during the spawning period (Lowerre-Barbieri et al., 2022). Therefore, these fish may not contribute to the spawning stock (Lowerre-Barbieri et al., 2022). Assessing females who are functionally mature during the spawning period produced the largest L50 value, and represents fish that will actually spawn during the current season. Our hybrid criteria resulted in lower L50 estimates for both physiological and functional maturity since they increased sample size by including regenerating females captured during the spawning period. In data-limited situations where sample numbers of reproductively active fish during the spawning period are low and L50 cannot be calculated using the functional maturity criteria (L50F), the hybrid approach may be considered when used with caution and clearly stated. When estimates of length-at-maturity use regenerating females caught just prior to or at the start of the spawning period, these estimates are often smaller than estimates based on data collected throughout the year (Hunter and Macewicz, 2003; Grande et al., 2012). The decrease in the physiological maturity L50 is also likely due in part to inclusion of fish in the early developing phase, which includes smaller recruits or first time spawners as mature; these fish are near the inflection point in size of the maturity ogive and thus bias the L50 parameter toward a smaller size. Additionally, a caveat against including regenerating females in the estimation is the potential difficulty in determining immature from mature inactive (regenerating) fish, particularly for fish close in size to the L50. When faced with the issue of low sample size, this is an important disadvantage to acknowledge when supplementing with regenerating females to increase overall sample size.
When used with the appropriate assumptions, physiological maturity might be applicable to data-poor species for which a spawning period has not yet been described (Reed and Taylor, 2021). In instances when a small number of reproductively active females are captured, the use of physiologically mature females for estimating maturity ogives might provide important baseline information that would not exist otherwise. For instance, 22% of our sample was determined to be in the early developing subphase, with the majority of these individuals found in the months prior to the defined spawning period. It is recognized that the early developing subphase can include recruit (virgin)–spawners as well as larger and older repeat–spawners (Brown-Peterson et al., 2011). Many female E. coruscans found in the months prior to spawning (January–April) are of size classes greater than the L50F, indicating that they are likely repeat spawners preparing to spawn in the coming year. Differentiating between repeat–spawners and first time spawners that often mature later in protracted spawning seasons can be challenging, but misclassification can affect the value of the L50 estimate. We showed that inclusion of repeat spawning females in the early developing phase as mature results in differences in the functional L50 parameter, and reduces the difference in L50 estimates between physiological and functional maturity. However, regardless of how repeat spawners were classified, functional maturity continued to have a larger L50 compared to physiological maturity, and the functional maturity ogive was a good fit to the data only during the spawning season (L50F). We showed that functional maturity must be assessed within a spawning period for this reason to accurately estimate L50 of spawning individuals. Alternatively, physiological maturity may be assessed with or without the use of a spawning period, which is ideal for data-limited or data-poor scenarios. However, it is important to acknowledge that using the physiological maturity classification will likely result in L50 values smaller than using a functional maturity classification which carries an increased risk of overestimation of the SSB if used in a stock assessment context (Agulló Núñez et al., 2015). This might have ramifications to the larger knowledge base of fisheries reproductive biology because it could directly affect how we interpret the length-at-maturity, particularly for slow growing and late-to-mature fishes for which population models are more sensitive to length-at-maturity estimates. Depending on the species, its life history characteristics, and temperature of the water in its environment, the extent to which functional versus physiological maturity classification-derived parameters are different for a species is an important consideration when determining maturity classifications to use in an L50 estimate.
4.3 Implications for the fishery from a life history perspective
The life history of E. coruscans in MHI is characterized by old age, moderately slow growth (Andrews et al., 2020), a large size-at-maturity for females (Everson et al., 1989; present study), and the largest maximum size to date for this species. Eteline snapper of the family Lutjanidae are known for large maximum ages and body sizes (Grimes, 1987). Etelis coruscans is unique in that females mature at a disproportionately large body size (67.8%) when comparing L50F (65.79 cm FL) to the largest fish captured (97 cm, this study). Shallow water species of snappers of Lutjanidae mature at smaller body sizes than deep-water snappers, 43% on average compared to 49% (Grimes, 1987). Furthermore, other deep-water eteline snapper species in the Deep 7 mature at a smaller percentage of maximum body size than onaga, including E. carbunculus (41.4%), Pristipomoides sieboldii (55.3%), and Pristipomoides filamentosus (55.7%) (DeMartini, 2016; Leurs et al., 2017; Nichols, 2019). Onaga in the MHI inhabit the deepest depth range in this complex, up to 400 m. Even when compared to other deep-dwelling eteline snappers at oceanic islands, E. coruscans continues to stand out as maturing at an above-expected proportion of their maximum size (Grimes, 1987; Newman et al., 2016). Our newly estimated L50F supports a large length-at-maturity and likely indicates an A50F in the range of 9–11 years based on empirical estimates of length-at-age (Andrews et al., 2020). The A50 of female E. coruscans in Okinawa, Japan, is also old (11.3 years) (Uehara et al., 2020) which further supports an old age-at-maturity for this species in the MHI. Larger size and older age at maturity is importantly influenced by lower water temperatures, slower growth, and greater longevity at deeper depths (Cailliet et al., 2001).
An older age and larger size at maturity are life history characteristics that can increase vulnerability of a species and its population to overfishing. Analysis of the population biology of E. coruscans in New Caledonia suggests vulnerability to overfishing since fish younger than the age at maturity are captured (Williams et al., 2013). The wide size range of E. coruscans caught in the MHI bottomfish fishery also suggests a parallel capacity for vulnerability to overfishing based on the high percentage of immatures capable of being caught in the fishery. Onaga in the MHI is regularly assessed as a part of the Deep 7 bottomfish fishery complex and to date the fishery is considered not overfished and not experiencing overfishing (Langseth et al., 2018). However, the fishery circumstances are similar in Hawaii to those in New Caledonia with a small allowable size (~30 cm FL), and consequently low age (2–3 years), at capture and retention for the fishery (Andrews et al., 2020), and thus immature fish are legally harvested.
Transparency regarding how maturity was determined (physiological or functional) is necessary for understanding potential changes in stock status. Typically, the presence of vitellogenic oocytes has been used for stock assessments in tropical tunas (Schaefer and Fuller, 2019), even though CA can develop through to vitellogenesis and maturation in the same year in warm water fishes (Wright, 2007). For this reason, the use of CA oocytes to identify maturity is recommended by some because it is closely associated with the timing of the physiological trigger in fish with annual reproductive cycles (Lowerre-Barbieri et al., 2011b). The appearance of CA oocytes was used to define the secondary growth stage of oocytes, and thus sexual maturity, in other studies of warm-temperate species (Murua and Saborido-Rey, 2003; Moncrief et al., 2018; Leontiou et al., 2021a; Leontiou et al., 2021b). Furthermore, the accuracy of a length- or age-at-maturity estimate can also be impacted by the spatial distribution of sampling and the time period over which samples are collected (Hunter and Macewicz, 2003; Murua and Saborido-Rey, 2003; Lowerre-Barbieri et al., 2011a; Lowerre-Barbieri et al., 2011b). The life history characteristics reported here for E. coruscans and the wide difference in a functional versus physiological length-at-maturity leads us to believe that continuing to use a functional maturity classification criteria for L50 estimation in a stock assessment context gives the most reliable value parameter for the spawning population of this species. Regardless of the maturity classification used in an estimation of length-at-maturity, equally as important is clear reporting of reproductive phases and oocyte stages classified as mature for correct interpretation of parameter changes over time.
5 Conclusions
New data suggest that E. coruscans in the MHI have a four-month spawning season, supported by using a GSI threshold based on functional maturity and histological analysis. The wide difference in value and confidence intervals in a functional versus physiological length-at-maturity leads us to believe that continuing to use a functional maturity method for L50 estimation in a stock assessment context gives the most reliable value of the parameter for the spawning population of this species. We provide evidence that the L50 parameter estimate is highly influenced by the maturity classification criteria used and may be most variable for species that have a wide size range caught in the fishery, slower growth, and a large length– or age–at–maturity, similar to E. coruscans. This is particularly important for temporal and spatial interpretations in changes of a L50 parameter. We believe that our exploration of length-at-maturity estimations using alternative datasets for E. coruscans provides the best available data for this species and important guidance for future stock assessment scientists and managers. Regardless of the maturity classification used in an estimation of length-at-maturity, equally as important is clear reporting of reproductive phases and oocyte stages classified as mature for correct interpretation of parameter changes over time. We recommend that future reproduction studies provide transparency in the specifics of how immature vs. mature fish are classified, and over what time periods the data for the estimation of maturity parameters are used, so that accurate comparisons across space and time can be made.
Data availability statement
The datasets presented in this study can be found in online repositories. The names of the repository/repositories and accession number(s) can be found below: NOAA PIFSC Life history program estimates repository. Available: https://inport.nmfs.noaa.gov/inport/item/59002.
Ethics statement
The animal study was reviewed and approved by the University of Hawai’i Animal Care & Use Committee (IACUC # 13-1696-7).
Author contributions
ER: Conceptualization, Methodology, Software, Validation, Formal Analysis, Investigation, Resources, Data Curation, Writing- Original Draft, Writing- Review and Editing, Visualization, and Project Administration. NBP: Conceptualization, Methodology, Writing- Original Draft, Writing- Review and Editing, and Supervision. ED: Conceptualization, Methodology, Investigation, Writing- Review and Editing, and Funding Acquisition. AA: Conceptualization, Writing- Review and Editing, and Funding Acquisition. All authors contributed to the article and approved the submitted version.
Funding
Additional funding for the sample collection was provided by NOAA Improve a Stock Assessment funds and the NOAA US Territorial Biosampling program, project # 6111135 funded the open access publication fees.
Acknowledgments
We sincerely thank all fishers involved with sampling and those that facilitated collection efforts, particularly L. Nakagawa and the restaurant Mama’s Fish House in Paia, Maui, the PIFSC bottomfish fishery-independent survey (BFISH) and Pacific Islands Fishery Group (PIFG) as well as the crew of the NOAA Ship Oscar Elton Sette for providing samples. Additionally, we thank R. Humphreys for fostering this initial study, R. Nichols, J. O’Malley, E. Schemmel, M. Hutchinson, and A. Uyehara for logistical support, the John A. Burns School of Medicine at the University of Hawaii for histology preparations. We thank J. O’Malley and the reviewers for constructive comments that improved the manuscript.
Conflict of interest
The authors declare that they have no known competing financial interests or personal relationships that could have appeared to influence the work reported in this paper.
Publisher’s note
All claims expressed in this article are solely those of the authors and do not necessarily represent those of their affiliated organizations, or those of the publisher, the editors and the reviewers. Any product that may be evaluated in this article, or claim that may be made by its manufacturer, is not guaranteed or endorsed by the publisher.
Supplementary material
The Supplementary Material for this article can be found online at: https://www.frontiersin.org/articles/10.3389/fmars.2023.1102388/full#supplementary-material
References
Aguirre-Villaseñor H., Morales-Bojórquez E., Espino-Barr E. (2022). Implementation of sigmoidal models with different functional forms to estimate length at 50% maturity: A case study of the pacific red snapper Lutjanus peru. Fish. Res. 248, 106204. doi: 10.1016/j.fishres.2021.106204
Agulló Núñez L., Hallfredsson E. H., Falk-Petersen I.-B. (2015). Different maturity scales affect estimations of fecundity, TEP and spawning stock size of Greenland halibut, Reinhardtius hippoglossoides (Walbaum 1792). Mar. Bio. Res. 11 (8), 824–833. doi: 10.1080/17451000.2015.1031797
Andrews A. H., Brodziak J., Demartini E. E., Cruz E. (2020). Long-lived life history for onaga Etelis coruscans in the Hawaiian islands. Mar. Freshw. Res. 71, 1367–1372. doi: 10.1071/MF20243
Andrews K. R., Moriwake V. N., Wilcox C., Grau E. G., Kelley C., Pyle R. L., et al. (2014). Phylogeographic analyses of submesophotic snappers Etelis coruscans and Etelis “marshi” (family Lutjanidae) reveal concordant genetic structure across the Hawaiian archipelago. PloS One 9 (4), e91665. doi: 10.1371/journal.pone.0091665
Anderson W. D. Jr. (1981). A new species of the Indo-west Pacific Etelis (Pisces: Lutjanidae), with comments on other species of the genus. Copeia 1981 (4), 820–825.
Audzijonyte A., Fulton E., Haddon M., Helidoniotis F., Hobday A. J., Kuparinen A., et al. (2016). Trends and management implications of human-influenced life-history changes in marine ectotherms. Fish. Fish. 17, 1005–1028. doi: 10.1111/faf.12156
Beverton R. J., Holt S. J. (1957). On the dynamics of exploited fish populations. Fishery Investigations II 19. (London: UK Ministry of Agriculture. Fisheries, and Food).
Brouwer S. L., Griffifths M. H. (2005). Reproductive biology of carpenter seabream (Argyrozona argyrozona) (Pisces: Sparidae) in a marine protected area. Fish. Bull. 103 (2), 258–269. Available at: http://hdl.handle.net/1834/26221
Brown-Peterson N. J., Peterson C. R., Fitzhugh G. R. (2019). Multidecadal meta-analysis of reproductive parameters of female red snapper (Lutjanus campechanus) in the northern Gulf of Mexico. Fish. Bull. 117, 37–49. doi: 10.7755/FB.117.1.5
Brown-Peterson N. J., Wyanski D. M., Saborido-Rey F., Macewicz B. J., Lowerre-Barbieri S. K. (2011). A standardized terminology for describing reproductive development in fishes. Mar. Coast. Fish. 3, 52–70. doi: 10.1080/19425120.2011.555724
Cailliet G. M., Andrews A. H., Burton E. J., Watters D. L., Kline D. E., Ferry-Graham L. A. (2001). Age determination and validation studies of marine fishes: do deep dwellers live longer? Exp. Gerontol. 36, 739–764. doi: 10.1016/S0531-5565(00)00239-4
Chan H. L. (2020). Economic impacts of Papahānaumokuākea Marine National Monument expansion on the Hawaii longline fishery. Mar. Policy 115, 1–13. doi: 10.1016/j.marpol.2020.103869
DeMartini E. E. (2016). Body size at sexual maturity in the eteline snappers Etelis carbunculus and Pristipomoides sieboldii: Subregional comparisons between the main and north-western Hawaiian islands. Mar. Fresh. Res. 68, 1178–1186. doi: 10.1071/MF16174
De Roos A. M., Boukal D. S., Persson L. (2006). Evolutionary regime shifts in age and size at maturation of exploited fish stocks. Proc. Royal. Soc. B. 273, 1873–1880. doi: 10.1098/rspb.2006.3518
Efron B. (1979). Bootstrap methods: Another look at the jacknife. Ann. Stat 7, 1–26. doi: 10.1214/aos/1176344552
Everson A. R., Williams H. A., Ito B. M. (1989). Maturation and reproduction in 2 Hawaiian eteline snappers, uku, Aprion virescens, and onaga, Etelis coruscans. Fish. Bull. 87, 877–888.
Grande M., Murua H., Zudaire I., Korta M. (2012). Oocyte development and fecundity type of the skipjack, Katsuwonus pelamis, in the Western Indian ocean. J. Sea Res. 73, 117–125. doi: 10.1016/j.seares.2012.06.008
Grimes C. B. (1987). “Reproductive biology of the Lutjanidae: A review,” in Tropical snappers and groupers: Biology and fisheries management. Eds. Polovina J. J., Ralston S. (Boulder, CO: Westview Press), 239–294.
Haddon M. (2001). Modeling and quantitative methods in fisheries. 2nd ed. (Boca Raton: Chapman and Hall).
Haight W. R., Parrish J. D., Hayes T. A. (1993b). Feeding ecology of deepwater lutjanid snappers at penguin bank, Hawaii. Trans. Am. Fish. Soc 122, 328–347. doi: 10.1577/1548-8659(1993)122<0328:FEODLS>2.3.CO;2
Hilborn R., Walters C. J. (1992). “Observing fish populations,” in Quantitative fisheries stock assessment (Boston, MA: Springer). doi: 10.1007/978-1-4615-3598-0_5
Hunter J. R., Macewicz B. J. (1985). “Measurement of spawning frequency in multiple spawning fishes,” in An egg production methods for estimating spawning biomass ofpelagic fish: application to the northern anchovy, Engraulis mordax, vol. 36 . Ed. Lasker R. (La Jolla California: NOAA Tech. Rep. NMFS), 79–97.
Hunter J. R., Macewicz B. J. (2003). “Improving the accuracy and precision of reproductive information used in fisheries,” in Reports of the workshop “Modern approaches to assess maturity and fecundity of warm- and cold-water fish and squids". Eds. Kjesbu O. S., Hunter J. R., Witthames P. R. (Bergen, Norway: Fisken Og Havet), 57–68.
Jacobson L. D., Lo N. C. H., Barnes J. T. (1994). A biomass-based assessment model for northern anchovy, Engraulis mordax. Fish. Bull. 92, 711–724.
Koslow J. A., Boehlert G. W., Gordon J. D. M., Haedrich R. L., Lorance P., Parin N. (2000). Continental slope and deep-sea fisheries: Implications for a fragile ecosystem. ICES J. Mar. Sci. 57, 548–557. doi: 10.1006/jmsc.2000.0722
Lambert Y. (2008). Why should we closely monitor fecundity in marine fish populations? J. Northwest Atl. Fish. Sci. 41, 93–106. doi: 10.2960/J.v41.m628
Langseth B., Syslo J., Yau A., Kapur M., Brodziak J. (2018). Stock assessment for the main Hawaiian islands deep 7 bottomfish complex in 2018, with catch projections through 2022 (Honolulu, Hawaii: NOAA Tech. Memo. NMFS-PIFSC-69), 217 p.
Leis J. M., Lee K. (1994). Larval development in the lutjanid subfamily etelinae (Pisces): The genera Aphareus, Aprion, Etelis and Pristipomoides. Bull. Mar. Sci. 55, 46–125.
Leontiou A. J., Wu W., Brown-Peterson N. J. (2021a). Immature and mature female red snapper habitat use in the north-central Gulf of Mexico. Reg. Stud. Mar. Sci. 44, 1–8. doi: 10.1016/j.rsma.2021.101715
Leontiou A. J., Wu W., Brown-Peterson N. J. (2021b). The role of maturity in the habitat selection of female red snapper. Mar. Coast. Fish. 13, 332–344. doi: 10.1002/mcf2.10160
Lesyna K. M., Barnes C. L. (2016). Assessment of length-and age-at-maturity for California halibut (Paralichthys californicus), including a histologically-based description of the reproductive cycle. California Fish. Game 102, 79–99.
Leurs M. A., DeMartini E. E., Humphreys R. L. (2017). Seasonality, sex ratio, spawning frequency and sexual maturity of the opakapaka Pristipomoides filamentosus (Perciformes: Lutjanidae) from the main Hawaiian islands: Fundamental input to size-at-retention regulations. Mar. Fresh. Res. 69, 325–335. doi: 10.1071/MF17195
Loeun K. L., Goldstien S., Gleeson D., Nicol S. J., Bradshaw C. J. A. (2014). Limited genetic structure among broad-scale regions for two commercially harvested, tropical deep-water snappers in new Caledonia. Fish. Sci. 80, 13–19. doi: 10.1007/s12562-013-0673-y
Lowerre-Barbieri S. K., Brown-Peterson N. J., Murua H., Tomkiewicz J., Wyanski D. M., Saborido-Rey F. (2011a). Emerging issues and methodological advances in fisheries reproductive biology. Mar. Coast. Fish. 3, 32–51. doi: 10.1080/19425120.2011.555725
Lowerre-Barbieri S. K., Decelles G., Pepin P., Catalán I. A., Muhling B., Erisman B., et al. (2017). Reproductive resilience: a paradigm shift in understanding spawner-recruit systems in exploited marine fish. Fish. Fish. 18, 285–312. doi: 10.1111/faf.12180
Lowerre-Barbieri S. K., Friess C., Brown-Peterson N. J., Moncrief-Cox H., Barnett B. (2022). Best practices for standardized reproductive data and methodology to estimate reproductive parameters for red snapper in the Gulf of Mexico. SEDAR74-DW-36 (North Charleston, SC: SEDAR), 43. Available at: http://sedarweb.org/docs/wpapers/S74_DW_36_Repro_best%20practices%20report%20final_30_June_2022.v2.pdf.
Lowerre-Barbieri S. K., Ganias K., Saborido-Rey F., Murua H., Hunter J. R. (2011b). Reproductive timing in marine fishes: Variability, temporal scales, and methods. Mar. Coast. Fish. 3, 71–91. doi: 10.1080/19425120.2011.556932
Luckenbach J. A., Iliev D. B., Goetz F. W., Swanson P. (2008). Identification of differentially expressed ovarian genes during primary and early secondary oocyte growth in coho salmon, Oncorhynchus kisutch. Reprod. Biol. Edocrinol. 6, 1–15. doi: 10.1186/1477-7827-6-2
Marshall C. T., Browman H. I. (2007). Introduction to theme section on disentangling the causes of maturation trends in exploited fish populations. Mar. Ecol. Prog. 335, 249–251. doi: 10.3354/meps335249
McBride R. S., Vidal T. S., Cadrin S. X. (2013). Changes in size and age at maturity of the northern stock of tilefish (Lopholatilus chamaeleonticeps) after a period of overfishing. Fish. Bull. 111 (2), 161–174. doi: 10.7755/FB.111.2.4
McFadden D. (1974). “Conditional logit analysis of qualitative choice behavior,” in Front. Econometrics. Ed. Zarembka P. (New York: Academic Press), 105–142.
McFadden D. (1979). “Quantitative methods for analyzing travel behaviour of individuals: Some recent developments,” in Behavioural travel modelling. Eds. Hensher D., Stopher P. (London: Croom), 279–318.
Misa W. F. X. E., Drazen J. C., Kelley C. D., Moriwake V. N. (2013). Establishing species-habitat associations for 4 eteline snappers with the use of a baited stereo-video camera system. Fish. Bull. 111, 293–308. doi: 10.7755/FB.111.4.1
Moncrief T., Brown-Peterson N. J., Peterson M. S. (2018). Age, growth, and reproduction of vermilion snapper in the north-central Gulf of Mexico. Trans. Am. Fish. Soc 147, 996–1010. doi: 10.1002/tafs.10100
Moore C., Drazen J. C., Radford B. T., Kelley C., Newman S. J. (2016). Improving essential fish habitat designation to support sustainable ecosystem-based fisheries management. Mar. Pol. 69, 32–41. doi: 10.1016/j.marpol.2016.03.021
Murua H., Saborido-Rey F. (2003). Female reproductive strategies of marine fish species of the north Atlantic. J. Northwest Atl. Fish. Sci. 33, 23–31. doi: 10.2960/J.v33.a2
Nadon M. O., Sculley M., Carvalho F. (2020). Stock assessment of uku (Aprion virescens) in Hawaii (U.S. Dept. of Commerce, NOAA Technical Memorandum NOAA-TM-NMFS- PIFSC-100), 120. doi: 10.25923/57nb-8138
Newman S. J., Williams A. J., Wakefield C. B., Nicol S. J., Taylor B. M., O'Malley J. M. (2016). Review of the life history characteristics, ecology and fisheries for deep-water tropical demersal fish in the Indo-Pacific region. Rev. Fish. Biol. Fish. 26, 537–562. doi: 10.1007/s11160-016-9442-1
Nichols R. S. (2019). Sex-specific growth and longevity of 'ehu', Etelis carbunculus (Family Lutjanidae), within the Hawaiian archipelago (University of Hawaii: MS Thesis Marine Biology), 128.
Okuyama J., Shishidou H., Hayashibara T. (2019). Post-release horizontal and vertical behavior and philopatry of deepwater longtail red snapper Etelis coruscans around a bank. J. Fish. Sci. 85, 361–368. doi: 10.1007/s12562-019-01287-2
O’Malley J., Wakefield C. B., Kinney M. J., Newman S. J. (2021). Markedly similar growth and longevity of green jobfish Aprion virescens over an expansive geographic range between the Hawaiian archipelago and the eastern Indian ocean. Mar. Coast. Fish. 13, 253–262. doi: 10.1002/mcf2.10155
Oyafuso Z. S., Drazen J. C., Moore C. H., Franklin E. C. (2017). Habitat-based species distribution modelling of the Hawaiian deepwater snapper-grouper complex. Fish. Res. 195, 19–27. doi: 10.1016/j.fishres.2017.06.011
Pacicco A. E., Brown-Peterson N. J., Murie D. J., Allman R. J., Snodgrass D., Franks J. S. (2023). Reproductive biology of yellowfin tuna (Thunnus albacares) in the northern central U.S. Gulf of Mexico. Fish. Res. 261, 106620. doi: 10.1015/j,fishres.2023.106620
Pankhurst N. W., Porter M. J. R. (2003). Cold and dark or warm and light: Variations on the theme of environmental control of reproduction. Fish. Physiol. Biochem. 28, 385–389. doi: 10.1023/B:FISH.0000030602.51939.50
Pensinger L. G., Brown-Peterson N. J., Green C. C., Midway S. R. (2021). Reproductive biology of hardhead catfish Ariopsis felis: Evidence for overwintering oocytes. J. Fish. Bio. 99, 308–320. doi: 10.1111/jfb.14717
Perez K. O., Munch S. B. (2010). Extreme selection on size in the early lives of fish. Evol 64, 2450–2457. doi: 10.1111/j.1558-5646.2010.00994
Piñón A., Amezcua F., Duncan N. (2009). Reproductive cycle of female yellow snapper Lutjanus argentiventris (Pisces, Actinopterygii, Lutjanidae) in the SW Gulf of California: Gonadic stages, spawning seasonality and length at sexual maturity. J. Appl. Ichthyol. 25, 18–25. doi: 10.1111/j.1439-0426.2008.01178.x
Planque B., Fromentin J. M., Cury P., Drinkwater K. F., Jennings S., Perry R. I., et al. (2010). How does fishing alter marine populations and ecosystems sensitivity to climate? J. Mar. Syst. 79, 403–417. doi: 10.1016/j.jmarsys.2008.12.018
Polovina J. J. (1987). “Assessment and management of deepwater bottom fishes in Hawaii and the Marianas,” in Tropical snappers and groupers: Bio. fish. manag. Eds. Polovina J. J., Ralston S. (Boulder, Colorado: Westview Press), 505–532.
Prince J., Hardford W. J., Taylor B. M., Lindfeild S. J. (2022). Standard histological techniques systematically undestimate the size fish start to spawn. Fish. Fish. 00, 1–10. doi: 10.1111/faf.12702
Randall J. E. (2007). Snappers (Lutjanidae), in: Reef and Shore Fishes from the Hawaian Islands. Sea Grant Coll. Program Univ. Hawai'i Honolulu, 245–254.
R Core Team (2020). R: A language and environment for statistical computing. version 4.0.2 (Vienna: R Foundation for Statistical Computing).
Reed E. M., Taylor B. (2021). Life history of two data-poor but commercially valuable tropical reef fishes, Parupeneus barberinus and Mulloidichthys flavolineatus, from the saipan fishery, northern Mariana islands. Mar. Fresh. Res 72, 383–397. doi: 10.1071/MF20049
Rochet M. J., Marty L. (2009). “Effects of fishing on the population,” in Fish reproductive biology. Eds. Jakobsen T., Fogarty M. J., Megrey B. A., Mokseness E. (Chichester, UK: Wiley-Blackwell Scientific Publications), 188–228. doi: 10.1002/9781444312133
Saborido-Rey F., Junquera S. (1998). Histological assessment of variations in sexual maturity of cod (Gadus morhua l.) at the Flemish cap (north-west Atlantic). ICES J. Mar. Sci. 55, 515–521. doi: 10.1006/jmsc.1997.0344
Salas-Singh C., Morales-Bojórquez E., Aguirre-Villaseñor H. (2022). Reproductive biology of the bullseye puffer Sphoeroides annulatus: Gonadosomatic index and its suitability for estimating length at maturity. J. Fish. Bio. 101 (5), 1119–1133. doi: 10.1111/jfb.15174
Schaefer K. M., Fuller D. W. (2019). Spatiotemporal variability in the reproductive dynamics of skipjack tuna (Katsuwonus pelamis) in the eastern pacific ocean. Fish. Res. 209, 1–13. doi: 10.1016/j.fishres.2018.09.002
Taylor B. M., Oyafuso Z. S., Pardee C. B., Ochavillo D., Newman S. J. (2018). Comparative demography of commercially-harvested snappers and an emperor from American Samoa. PeerJ 6:e5069. doi: 10.7717/peerj.5069
Trippel E. A. (1995). Age at maturity as a stress indicator in fisheries. BioScience 45, 759–771. doi: 10.2307/1312628
Trippel E. A. (1999). Estimation of stock reproductive potential: History and challenges for Canadian Atlantic gadoid stock assessments. J. Northwest. Atl. Fish. Sci. 25, 61–81. doi: 10.2960/J.v25.a6
Trippel E. A., Harvey H. H. (1991). Comparison of methods used to estimate age and length of fishes at sexual maturity using populations of white sucker (Catostomus commersoni). Can. J. Fish. Aquat. Sci. 48, 1446–1459. doi: 10.1139/f91-172
Uehara M., Ebisawa A., Ohta I. (2018). Reproductive traits of deep-sea snappers (Lutjanidae): Implication for Okinawan bottomfish fisheries management. Reg. Stud. Mar. Sci. 17, 112–126. doi: 10.1016/j.rsma.2017.12.002
Uehara M., Ebisawa A., Ohta I (2020). Comparative age-specific demography of four commercially important deep-water snappers: Implication for fishery management of a long-lived lutjanid. J. Fish. Bio. 97, 121–136. doi: 10.1111/jfb.14332
Uehara M., Ebisawa A., Ohta I., Aomuma Y. (2019). Effectiveness of deepwater marine protected areas: Implication for Okinawan demersal fisheries management. Fish. Res. 215, 123–130. doi: 10.1016/j.fishres.2019.03.018
Wallace R. A., Selman K. (1981). Cellular and dynamic aspects of oocyte growth in teleosts. Am. Zool. 21, 325–343. doi: 10.1093/icb/21.2.325
West G. (1990). Methods of assessing ovarian development in fishes: A review. Aust. J. Mar. Freshw. Res. 41, 199–222. doi: 10.1071/MF9900199
Williams A. J., Loeun K., Nicol S. J., Chavance P., Ducrocq M., Harley S. J., et al. (2013). Population biology and vulnerability to fishing of deep-water eteline snappers. J. Appl. Ichthyol. 29, 395–403. doi: 10.1111/jai.12123
WPRFMC (2009). “Fishery ecosystem plan for the Hawaii archipelago,” in Western Pacific regional fisheries management council (Honolulu, Hawaii: Western Pacific Regional Fishery Management Council).
Wright P. J. (2007). Understanding the maturation process for field investigations of fisheries-induced evolution. Mar. Eco. Prog. Ser. 335, 279–283. doi: 10.3354/meps335279
Keywords: Lutjanidae, deep-sea snappers, Indo-Pacific, functional maturity ogive, physiological maturity ogive
Citation: Reed EM, Brown-Peterson NJ, DeMartini EE and Andrews AH (2023) Effects of data sources and biological criteria on length-at-maturity estimates and spawning periodicity of the commercially important Hawaiian snapper, Etelis coruscans. Front. Mar. Sci. 10:1102388. doi: 10.3389/fmars.2023.1102388
Received: 18 November 2022; Accepted: 08 March 2023;
Published: 24 March 2023.
Edited by:
Oscar Sosa-Nishizaki, Center for Scientific Research and Higher Education in Ensenada (CICESE), MexicoReviewed by:
Hugo Aguirre-Villaseñor, Instituto Nacional de Pesca y Acuacultura, MexicoEnrique Morales-Bojórquez, Centro de Investigación Biológica del Noroeste (CIBNOR), Mexico
Agustin Hernandez-Herrera, National Polytechnic Institute (IPN), Mexico
Copyright © 2023 Reed, Brown-Peterson, DeMartini and Andrews. This is an open-access article distributed under the terms of the Creative Commons Attribution License (CC BY). The use, distribution or reproduction in other forums is permitted, provided the original author(s) and the copyright owner(s) are credited and that the original publication in this journal is cited, in accordance with accepted academic practice. No use, distribution or reproduction is permitted which does not comply with these terms.
*Correspondence: Erin M. Reed, ZXJpbi5yZWVkQG5vYWEuZ292