- 1Laboratório de Genética Aplicada, Instituto de Estudos Costeiros, Universidade Federal do Pará, Bragança, Brazil
- 2Secretaria de Aquicultura e Pesca, Ministério da Agricultura, Pecuária e Abastecimento, Brasília, Brazil
- 3RARE Brasil Organização, Belém, Brazil
- 4Instituto de Estudos Costeiros, Universidade Federal do Pará, Bragança, Brazil
- 5Laboratório de Química do Pescado, Instituto de Estudos Costeiros, Universidade Federal do Pará, Bragança, Brazil
- 6Laboratório de Qualidade de Água, Instituto de Estudos Costeiros, Universidade Federal do Pará, Bragança, Brazil
- 7Núcleo de Ecologia Aquática e Pesca da Amazônia, Universidade Federal do Pará, Belém, Brazil
In recent years, the populations of many shark species have been depleted drastically around the world. In the present study, we analyzed the shark bycatch in the monthly landing data of the acoupa weakfish (Cynoscion acoupa) gillnet fisheries of the state of Pará, on the northern coast of Brazil, between January 1995 and December 2007. Based on 4,659 landings, we estimated that a total of 1,972.50 tons of shark were taken as bycatch during the study period. The acoupa weakfish fisheries operate on the Amazon Shelf, an important fishing ground, and we analyzed the shark landings in relation to the Amazon River Discharge anomaly (ARD) and the climatic variability in the Atlantic Ocean. We applied cross-correlation, cross-wavelet, wavelet coherence, and redundancy analysis techniques to the analysis of the data time series. The shark bycatch landings peaked between 1998 and 2000, a period associated with an increase in fishing effort by the acoupa weakfish fisheries, in particular during the dry season of the Amazon basin. The cross-correlation analysis indicated that shark landings were associated with Sea Surface Temperatures (SSTs), the characteristics of the wind, and the Atlantic Multidecadal Oscillation (AMO), while the fishing effort of the acoupa weakfish fisheries was associated with the meridional wind component, the AMO, and the ARD. The cross-wavelet and coherence wavelet analyses indicated that environmental variability was linked systematically with shark landings and acoupa weakfish fishing effort. We observed a phase change in this signal between 1998 and 2000, due to a strong and persistent La Niña event. Despite the resistance from the fishing industry, development and deployment of devices designed to reduce bycatch should be incentivized in order to reduce the unintentional capture of endangered species such as sharks. The findings of the present study highlight the importance of a continuous and accurate fishery database, and the need for continuous fishery statistics to ensure adequate management practices. Adequate public fishery management policies must be implemented urgently to guarantee the survival of shark species, with the effective participation of all the actors involved in the process, including managers, researchers, and fishers.
1 Introduction
Since 2005, the global elasmobranch harvest has been sustained at approximately 760 thousand tons per year (Dent and Clark, 2015), while catches of sharks have ranged from 63 to 273 million individuals (Worm et al., 2013). These data are especially preoccupying due to the biological characteristics of most elasmobranchs, including their slow growth rate, late sexual maturity, low fecundity, and the small effective size of their populations, which make them highly vulnerable to fishing pressure (Dulvy et al., 2021). Shark populations have been declining worldwide since the 1970s, and conservation initiatives are poorly supported, due primarily to the high market values of shark byproducts, in particular, fins (Brown and Roff, 2019; Pacoureau et al., 2021).
In northern Brazil, the states of Amapá, Pará, and Maranhão are considered to be a global hotspot for elasmobranch conservation due to their high irreplaceability score (Dulvy et al., 2014), although the incidental catch rates of this region are the country’s highest (Oliver et al., 2015). The region’s elasmobranchs are especially vulnerable to the region’s artisanal and industrial fisheries that target high-value teleosts (Marceniuk et al., 2019), including serra spanish mackerel, Scomberomorus brasiliensis, the smooth weakfish (Cynoscion leiarchus) and acoupa weakfish (Cynoscion acoupa) (Lessa et al., 1999a), the laulao catfish, Brachyplatystoma vaillantii (Lessa et al., 1999a), and the gillbacker sea catfish Sciades parkeri (Pinheiro and Frédou, 2004), as well as industrial shrimp trawling operations (Feitosa et al., 2018; Guimarães-Costa et al., 2020). All these fisheries are extremely important sectors of the local economy, and support many of the region’s populations.
Based on an analysis of onboard observations, zoological collections, and the available literature, Marceniuk et al. (2019) identified 69 elasmobranch species from the northern coast of Brazil, representing 20 families and nine orders of the class Chondrichthyes. Eleven of these species are endemic to the Amazon-Orinoco plume. This elasmobranch diversity reinforces the importance of the Amazonian reef system as a habitat for sharks and rays (Moura et al., 2016). At least 32 of these elasmobranchs, including both rare and endemic species, are captured as bycatch by the local industrial trawler fisheries (Marceniuk et al., 2019). These species inhabit a variety of environments, ranging from shallow coastal waters to oceanic habitats. These authors also noted that 65–90% of the total species recorded in the study are exploited as bycatch.
A variety of different types of fishery are found in the Brazilian Amazon region, operating at varying scales, including industrial fleets, although most of the operations are artisanal (Freire et al., 2021). While the industrial fisheries generally target a single fish species, artisanal fisheries tend to be less specialized, exploiting an ample diversity of fish species (Bentes et al., 2012). In Pará state, acoupa weakfish are targeted primarily by small and medium-sized boats operating in estuarine, coastal, and ocean zones. This type of vessel accounts for approximately 80% of the national production of this weakfish (Almeida et al., 2011; Mourão et al., 2018). The use of gillnets by these fisheries means that they catch many other species indiscriminately, including many sharks (Mourão et al., 2018).
In Brazil, there have been few studies of shark populations, and the vast majority of the available data refer to the country’s northeastern (Lessa et al., 1999b; Castro and Rosa, 2005; Freitas et al., 2009; Oliveira et al., 2011; Santander-Neto et al., 2011) and eastern/southern coasts (Andrade et al., 2008; Mendonça et al., 2009). On the northern coast of Brazil, Lessa et al. (2016) found evidence that the local stocks of Isogomphodon oxyrhynchus were in decline, but no other studies have evaluated the status of the shark populations of the coastal Amazon region. Shark populations are notoriously difficult to study, given the complexity of obtaining data, the inaccessibility of most populations, and their interrelationships with other species (Huveneers et al., 2018; Ju et al., 2020). In this case, indirect, alternative study methods are widely used, including simulations and modeling based on the bycatch data from commercial or traditional fisheries (Duffy et al., 2019; Xu et al., 2022). Despite certain limitations, this approach can provide reliable indicators for predictive analyses (Carvalho et al., 2018).
Recent studies indicate that spatial and temporal variation in Sea Surface Temperature (SST) influence the life cycle of the acoupa weakfish, whose juveniles and subadults develop in the coastal estuaries of Pará and Maranhão states before being transported on the North Brazilian current to the region of the Amazon plume off the coast of Amapá state, where the adult stocks are targeted by the local fisheries (Soares et al., 2014; Freire, 2019). Up to now, however, there have been no studies of the dynamics of shark fishing, whether intentional or incidental, and related environmental variables in the region of the Amazon coast.
Historically, Brazilian fishery statistics have been marked by the lack of any robust or continuous dataset. In fact, the systematic collection of fishery statistics depends on the investment of public resources, which may vary considerably over time, preventing the continuous acquisition of reliable data (Salas et al., 2011), and compromising the quality of the data available for most fisheries (Isaac et al., 2006). Given the ongoing decline in shark catches off the Brazilian coast reported by fishers (Barbosa-Filho et al., 2017; Leduc et al., 2021) and the total lack of studies that have investigated the interaction between these catches and environmental factors (Pinaya et al., 2016), this present study investigated shark bycatch landings on the Amazon coast in the context of climatic and oceanographic variables.
The primary objective of this study is to understand the level of influence of environmental factors on shark catches in the study area. Obviously, the effects of fishing effort should also be considered in relation to the spatial and temporal abundance of the species, although the lack of appropriate bio-ecological data prevents the formulation of effective models for the systematic reduction of uncertainties.
Given this, the study analyzed 12 years of data on sharks landed as bycatch by the acoupa weakfish gillnet fisheries operating on the Amazon Shelf fishing grounds. These data were analyzed in the context of the Amazon River Discharge anomaly (ARD), and the variability in marine climatic variables related to the Sea Surface Temperature (SST), zonal (u) and meridional (v) wind components, the Atlantic Ocean Inter-hemispheric Sea Surface Temperature Gradient (GITA), and the Multivariate ENSO Index (MEI) of the variability of the El Niño/Southern Oscillation (ENSO). Even though the shark species were not identified (the official statistics do not provide this information, although some species were recognized by fishers), the investigation of groups (such as sharks) that are threatened by specific pressures can provide important insights for the development of effective conservation strategies.
2 Materials and methods
2.1 Study area
The study area is located on the Amazon Shelf (1°08’S–3.83’N, 50°53’–46°14’W; Figure 1). This area has an extensive coastal plain (up to 70 km wide) that extends onto the adjacent continental shelf, which is almost 200 km wide, and slopes gradually down to a depth of 80 m (Souza Filho, 2005). The climate is humid tropical, which influences the hydrological cycle of the Amazon River, determining a flood season from December to May and a dry season from July to November, which typically coincide with the intensity of local rainfall. The salinity of the waters of this region is also influenced by the plume of freshwater from the Amazon River. The mean Sea Surface Temperature (SST) varies from 27°C in the dry season to 24°C in the rainy season. Southeasterly trade winds predominate, and are more intense at the onset of the dry season (Fisch et al., 1998).
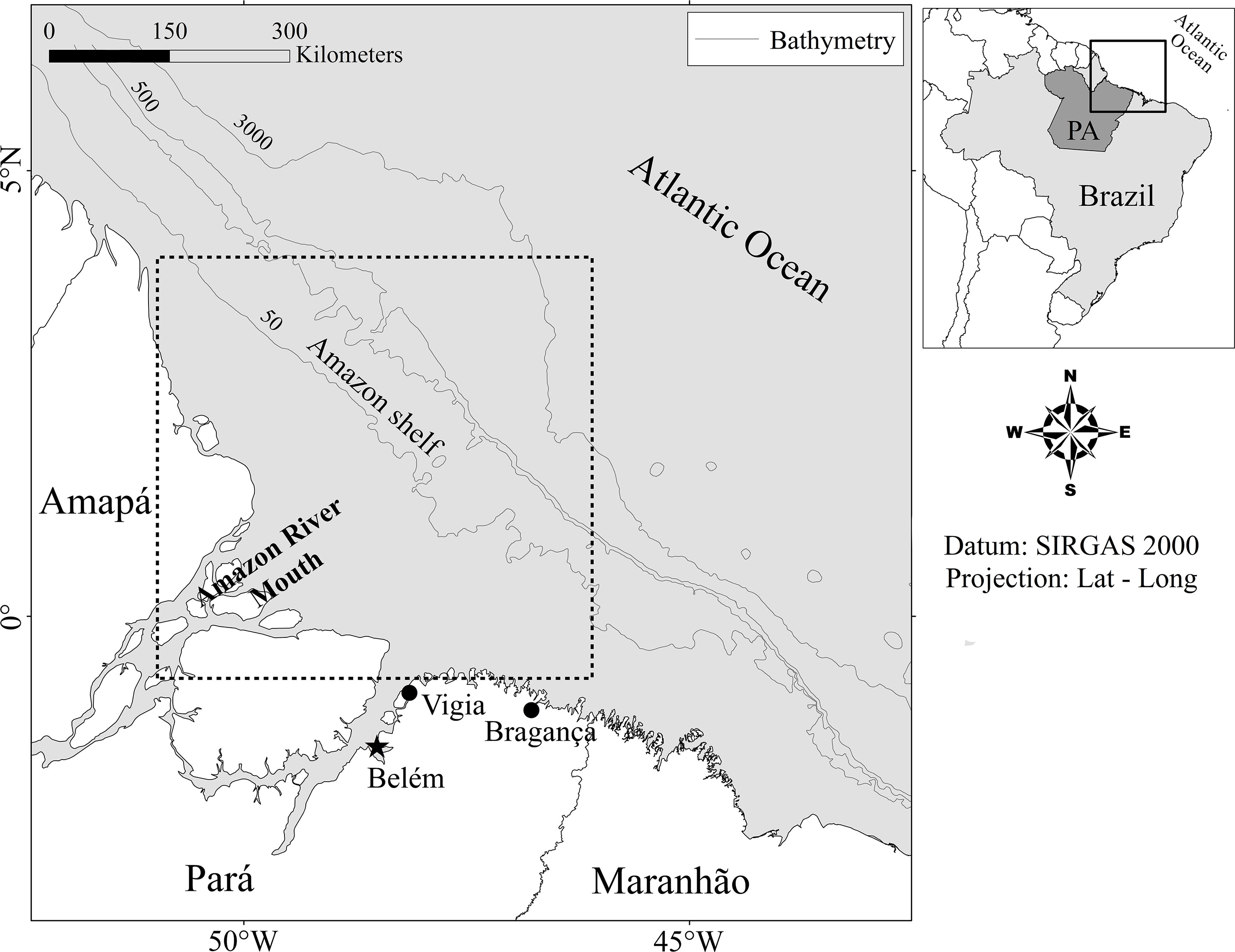
Figure 1 Study area on the Amazon Shelf and the principal fishing ports in the northern Brazilian state of Pará at which the acoupa weakfish (Cynoscion acoupa) gillnet fisheries landed their shark bycatch. The major ports of Vigia, Bragança, and Belém are also identified.
2.2 Data analysis
Daily data (grouped by month) on the shark bycatch landed by acoupa weakfish fisheries (in tons, t) and their fishing effort (days spent fishing by all vessels at sea) were compiled from the Fishing Statistics Project of the Centro Nacional de Pesquisa e Conservação da Biodiversidade Marinha do Norte - CEPNOR/Instituto Chico Mendes de Conservação da Biodiversidade - ICMBio.
This database was compiled by data collectors who gathered information on catches landed at the principal fishing ports on the Amazon coast. The data were tabulated in an electronic spreadsheet, in which each line represents a catch landed, its composition and the fishing effort employed. This database permitted the selection of catches obtained with the gear used to target acoupa weakfish, as well as extracting the specific data on the shark catch. Fishing trips targeting acoupa weakfish could also be identified due to the relative contribution of C. acoupa to the total catch. As the bycatch was identified only by the vernacular name of the fish type, the catches of tubarão (shark) and cação (common name for small sharks used by fishers) were considered for analysis here. The data time series covered the period between January 1995 and December 2007. Fishing effort was quantified as effective fishing days (days at sea), which were directed at the capture of acoupa weakfish, and do not represent any effort to target sharks specifically. Given this, the Catch Per Unit of Effort (CPUE) was not considered as an effective parameter, given that this effort was directed at C. acoupa, rather than sharks. As shark was a relatively large component of the total catch, the data do provide reliable indirect evidence of the pressure on elasmobranch stocks, and this is the first study of its kind for the region.
The oscillations in the shark bycatch were analyzed in relation to the variation in climatological events and other environmental factors that affect the study region, including the discharge of the Amazon River, the Sea Surface Temperature (SST), zonal (u) and meridional (v) wind components, and other climate indices. Monthly data on the flow of the Amazon River (ARD, in m³.s-1) were obtained from the Agência Nacional de Águas e Saneamento Básico (https://www.ana.gov.br), with the SST, u and v were obtained from NCEP Reanalysis data (https://www.esrl.noaa.gov/psd/data/timeseries/). To produce a series with a constant mean and variance, and no trends, it was necessary to transform the anomalies into normalized parameters (Wilks, 2006). That is, the normalized ARD, SST, u and v anomalies were estimated for the study period (January 1995 through December 2007), which made the non-dimensional data mutually comparable, following the approach used by Pinaya et al. (2016) for similar areas in the Amazon region. The climatological parameters were derived from the data available for the period between January 1976 and December 2005. Given the known links between climatic events such as the El Niño-Southern Oscillation (ENSO) and the SST of the Atlantic Ocean, the Multivariate ENSO index (MEI) and Atlantic Ocean Inter-hemispheric Sea Surface Temperature Gradient (GITA) were used to represent these processes. The MEI time series was obtained from https://www.esrl.noaa.gov/psd/data/climateindices/list/, while the GITA values were calculated from the difference between the SSTs of the North and South Atlantic oceans (Souza et al., 2000).
To avoid possible distortions of the results and weak conclusions, all the biological and environmental variables were tested for collinearity using robust statistical methods, following Belsley et al. (2005). Evidence of high levels of correlation (based on Pearson’s correlation analysis – see Figure 2) between variables can be used to exclude some parameters from the analysis, to avoid compromising the interpretation of the data. Overall, catches were correlated most with the wind components (u and v), followed by the AMO index, the SST, and the ARD (Table 1), while fishing effort was correlated primarily with v, ARD, and SST. As expected, shark catches were correlated with fishing effort, i.e., the number of fishing days (R² = 0.74). Based on the collinearity analysis, the environmental variables selected for further analysis here were: the Amazon River Discharge Anomaly (ARD), SST, u, v, and the MEI, GITA, and AMO climate indices.
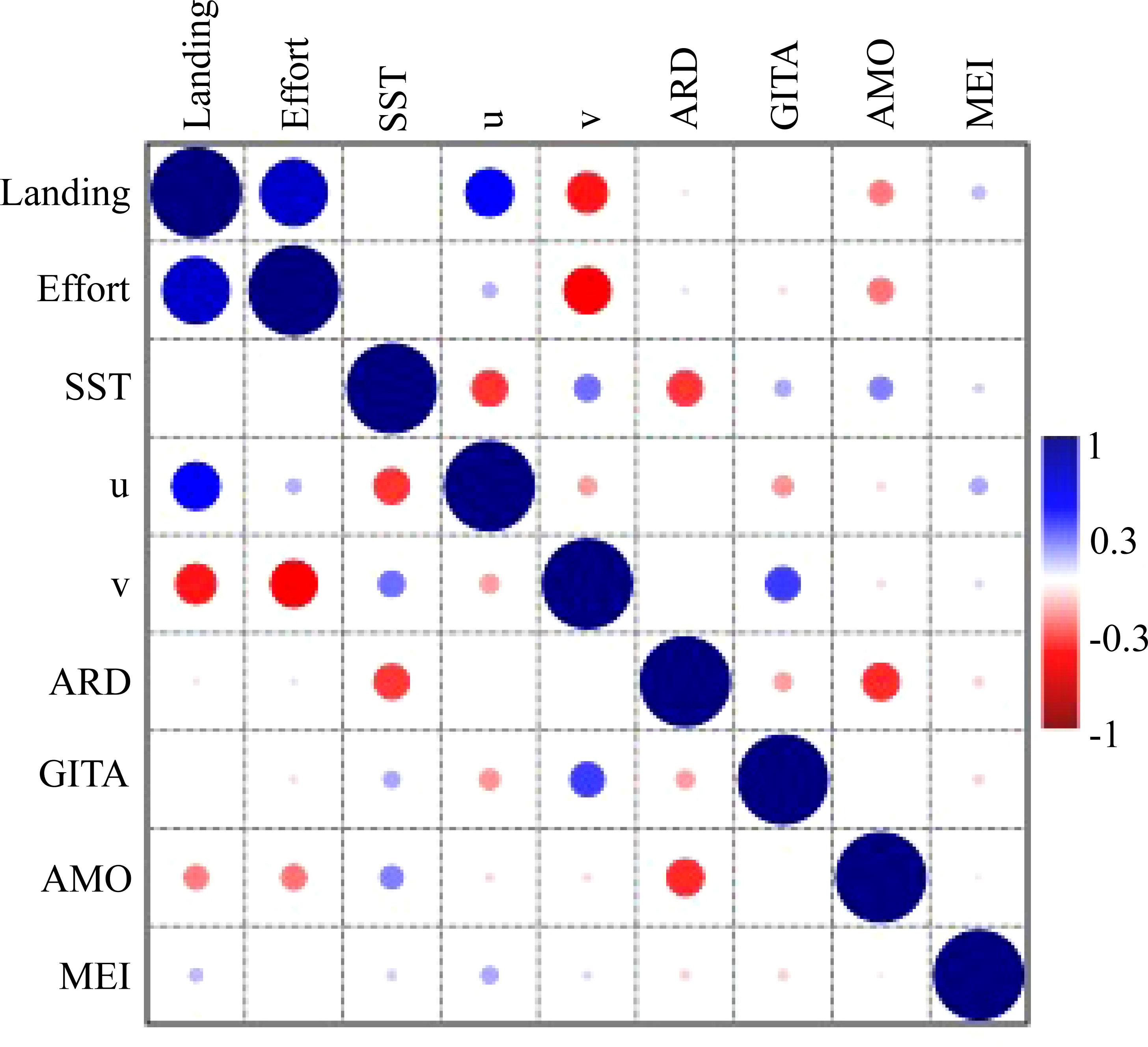
Figure 2 Dispersal plots and correlations between the environmental variables. Sea Surface Temperature (SST), wind components u and v, Amazon River Discharge (ARD), Atlantic Ocean Inter-hemispheric Sea Surface Temperature Gradient (GITA), Atlantic multidecadal oscillation (AMO), and the Multivariate ENSO Index (MEI).
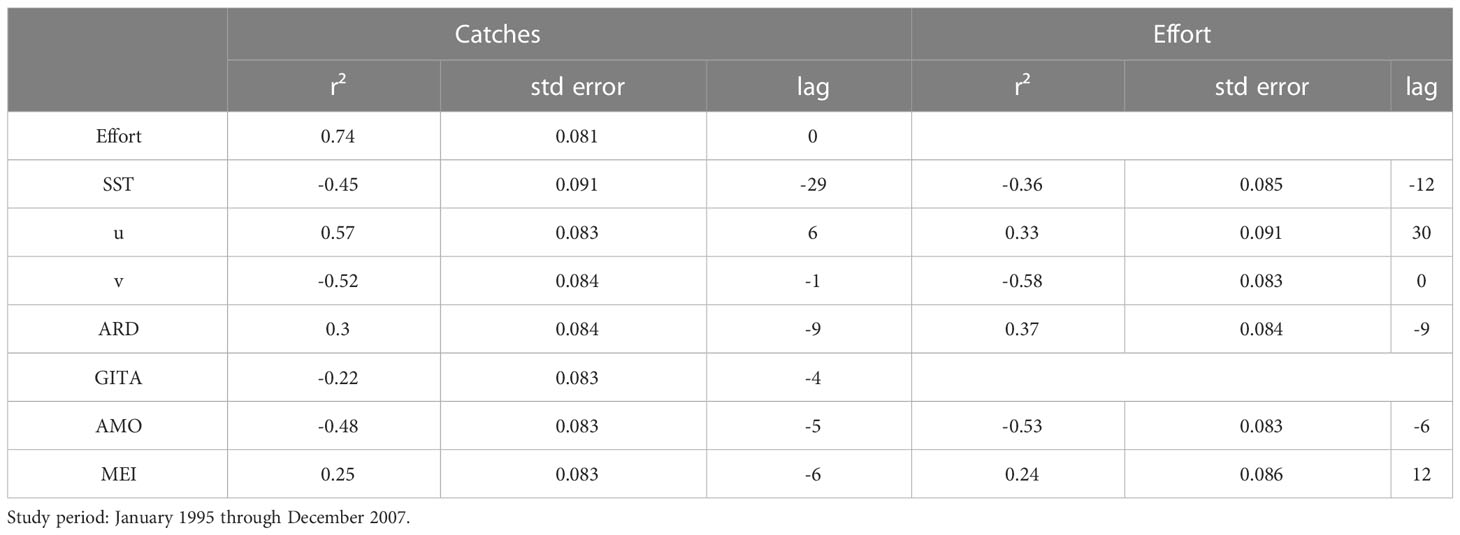
Table 1 Results of the cross correlation (r², standard error, and lag) between catches, fishing effort, and the environmental variables: Sea Surface Temperature anomalies (SST), zonal and meridional wind components (u, v), Amazon River Discharge (ARD), the Atlantic Multidecadal Oscillation (AMO), Atlantic Ocean Inter-hemispheric Sea Surface Temperature Gradient Index (GITA), and Multivariate ENSO Index (MEI).
Trends in the catches were analyzed using scatterplots (heat maps – darker blue representing increasing density). Cross-wavelet, coherence wavelet, and Redundancy Analysis (RDA) techniques were used to determine the relationship between catches and the variation in environmental parameters. The cross-wavelet analysis compares the wavelet spectra of two data series, and has been widely used in fishery research to relate fishing and environmental variables (Ménard et al., 2007; Polanco et al., 2011; Pinaya et al., 2018). This permits the detection of similarities between the local fluctuation of two time series and the estimation of the phase between these fluctuations. The cone of influence on the scalogram plot indicates the region not influenced by edge effects. In this analysis, the wavelet cross-power spectrum emphasizes the commonness of the two series, while the wavelet coherence emphasizes the correlation between these series, i.e., fluctuations in coherence. The interpretation of the crossed and coherence wavelet vectors is based on the angle between the two study variables (see Torrence and Compo, 1998; Grinsted et al., 2004): (a) an angle of 0° indicates that the variables are in phase; (b) 45° indicates a difference of one eighth; (c) 90° indicates a difference of one quarter; (d) 135° indicates a difference of three eighths, and e) 180° indicates that the variables are in completely opposite phases.
Wavelet, cross-wavelet, and coherence wavelet approaches are robust tools for the analysis of time series, and are widely used in geophysical, environmental, and fishery studies (Lan et al., 2012; Pinaya et al., 2018; Shuai et al., 2018). Wavelets are suitable for decomposing other functions in a manner comparable to the sine and cosine functions that form the basis for the Fourier transformation (Polikar, 2001).
While wavelets are considered to be versatile tools for harmonic analysis, cross-wavelets diagnose the gaps to determine the periods and locations at which the high-energy signals coincide (Grinsted et al., 2004). Cross-wavelet analysis reveals common areas of high power and determine how coherent the cross-wavelet transformation is in time-frequency space. The coherence ondelet output resembles a traditional correlation coefficient, and it is useful to consider it exactly as a correlation coefficient located in time-frequency space. The significance of ondelet can be estimated by the Monte Carlo method. The coherence spectra were estimated for the same time series used in the cross-wavelet spectrum analysis. The covariance has a value of between zero (0) and one (1), which provides a measure of the covariance between two time series as a function of both frequency and time, regardless of their phase difference. This analysis evaluates the local correlation of the significant oscillations observed in the initial analysis. To ensure the reliability of this analysis, a Ranging standardization was applied, given that Milligan and Cooper (1988) concluded that this approach may be more effective than other methods (e.g., z-scores). In addition to limiting the magnitude of the attributes to between zero (0) and one (1), the Ranging standardization adjusts the variability of the attributes to a common scale: Xranged = [X − Xmin]/[Xmax − Xmin], where Xranged is the new Ranged value, X is the actual value of the attribute, and Xmin and Xmax are its minimum and maximum values, respectively (Sneath and Sokal, 1973).
The Redundancy Analysis (RDA), which is widely used in ecological studies to analyze time series of data was also applied here. When applied to fishery studies, this method verifies the variability between environmental factors and the capacity of the fishers (Vallejos et al., 2013). In this approach, the response variables are projected onto a set of axes, in which the first axis explains the largest part of the variability found in the dataset, the second axis explains the second largest portion, and so on. A separate matrix was compiled for the dependent variable (catches), with each line representing a catch. This matrix was compared with a second (treatment) matrix in which the independent variables (the environmental data) were ranked according to the procedure proposed by Legendre and Anderson (1999) and Makarenkov and Legendre (2002). Monte Carlo tests (with 9999 permutations) were used for the forward inclusion of the treatment variables. The variables were then included manually in the analysis, successively, based on the significance (p<0.05) of each test. Given this, the spatial dispersal of the points (samples) reflects the best correlations with the abiotic variables tested. The wavelet analysis was run in MATLAB 8.1® and the RDA in CANOCO 5.0®. The spreadsheet containing the full set of data on catches and effort, and the environmental variables is available in Supplementary Material 1.
3 Results
A total of 4,659 landings of catches by the acoupa weakfish gillnet fisheries were recorded in the present study, with a total shark bycatch of 1,972.50 t. Shark bycatch landings peaked between 1998 and 2000, when acoupa weakfish fishing effort also reached its maximum level. The scatterplot (Figure 3) shows a tendency for a reduction in shark bycatch by the acoupa weakfish fishery over time. The largest shark catches were observed in December and were associated with the flood pulse of the Amazon River (Figure 4). Catches were smallest in September, in the dry period of the Amazon River. A persistent, strong La Niña, from 1998 to 2000, was also associated with increased shark bycatch (Figure 4).
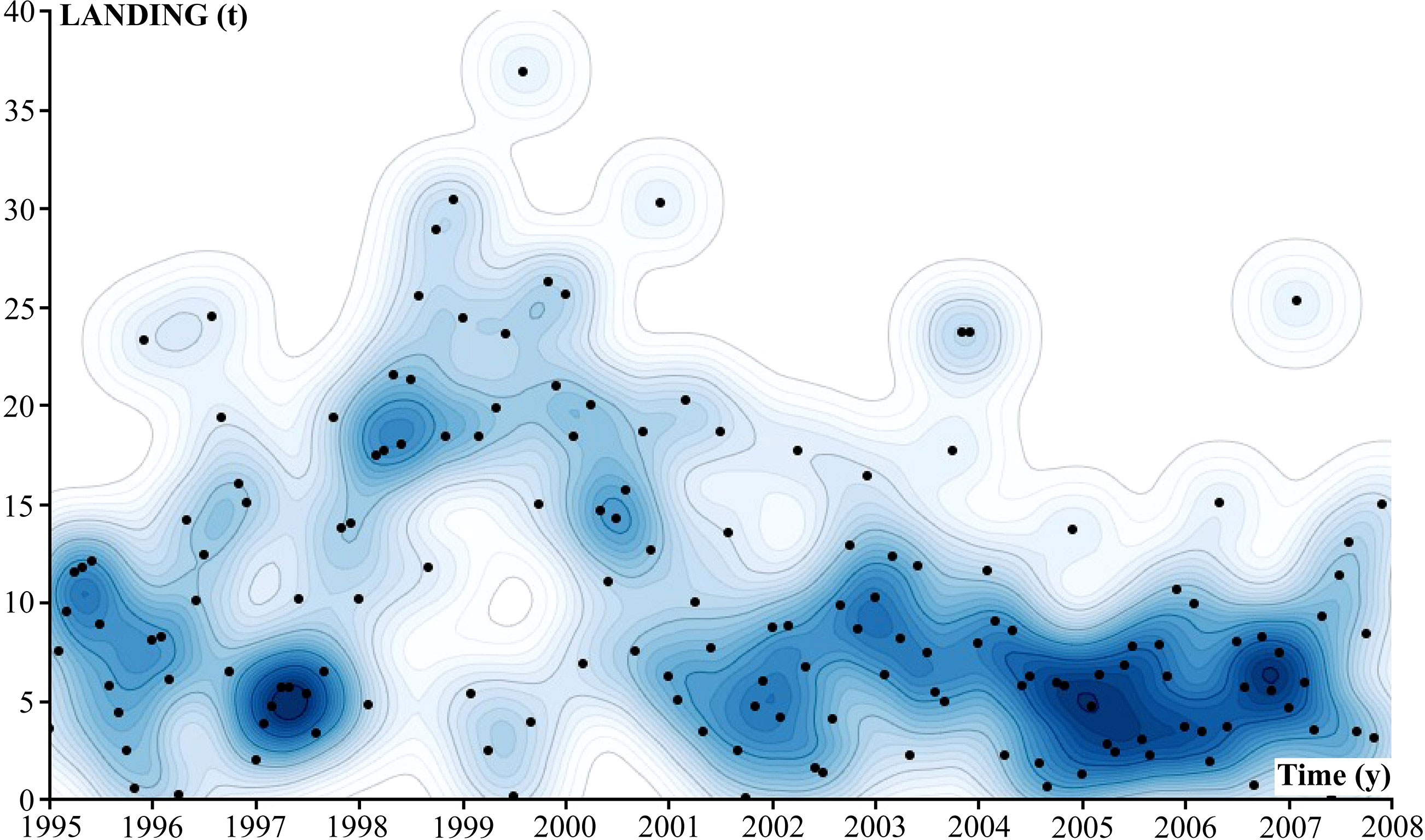
Figure 3 Scatterplot of the shark bycatch landed by the acoupa weakfish (Cynoscion acoupa) gillnet fisheries in Pará state between January 1995 and December 2007. The blue color gradient represents the density of shark catches (darker blue = higher density).
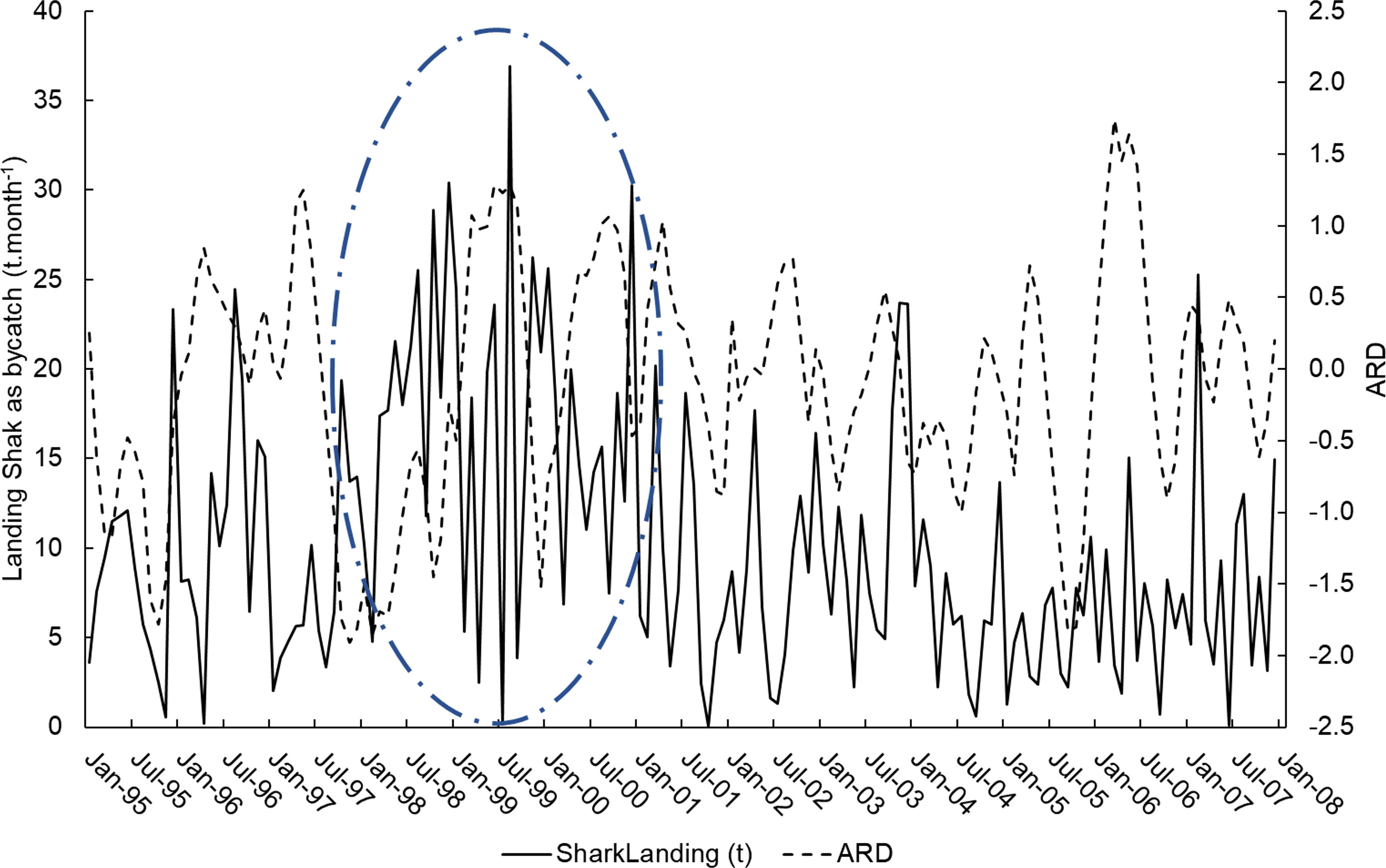
Figure 4 Monthly shark bycatch landed by the acoupa weakfish (Cynoscion acoupa) gillnet fisheries between January 1995 and December 2007 (solid line) and the Amazon River Discharge (ARD) for the same period (dashed line). The dot-dash line represents the persistent, strong La Niña recorded between 1998 and 2000.
The cross-wavelet analysis between the shark bycatch and SST indicated three energy peaks (Figures 5A, B). The first peak was annual, in the opposite phase (180°), up to 2001, after which there was a shift to in phase (0°). This energy band also presented a phase delayed by 45°, i.e., catches that responded to shifts in the SST with a delay of approximately 1.5 months (one eighth of the peak energy). The second peak was quasi-triennial, and was in phase until 2000, subsequently losing strength toward the end of the study (Figure 5A). By contrast, the third peak was quasi-triennial and inversely related (180°). The coherence wavelet analysis also indicated a high level of correlation (0.8–1.0) for the annual energy band and a more moderate correlation (0.6–0.7) for the biannual and quasi-triennial energy bands (Figure 5B). Two principal energy bands in the period/time field can be seen in the cross wavelet and coherence wavelet plots between the shark bycatch and meridional wind component, v (Figures 5C, D). The first band was the annual band of energy, which lagged with at 135°, i.e., the catches responded approximately after 4 months after the variation in the wind (Figure 5C). The second peak was quasi-triennial and in the opposite phase (180°). The coherence wavelet analysis presented a high correlation (0.8–1.0) for the annual and quasi-annual energy bands, especially after 2001 (Figure 5D).
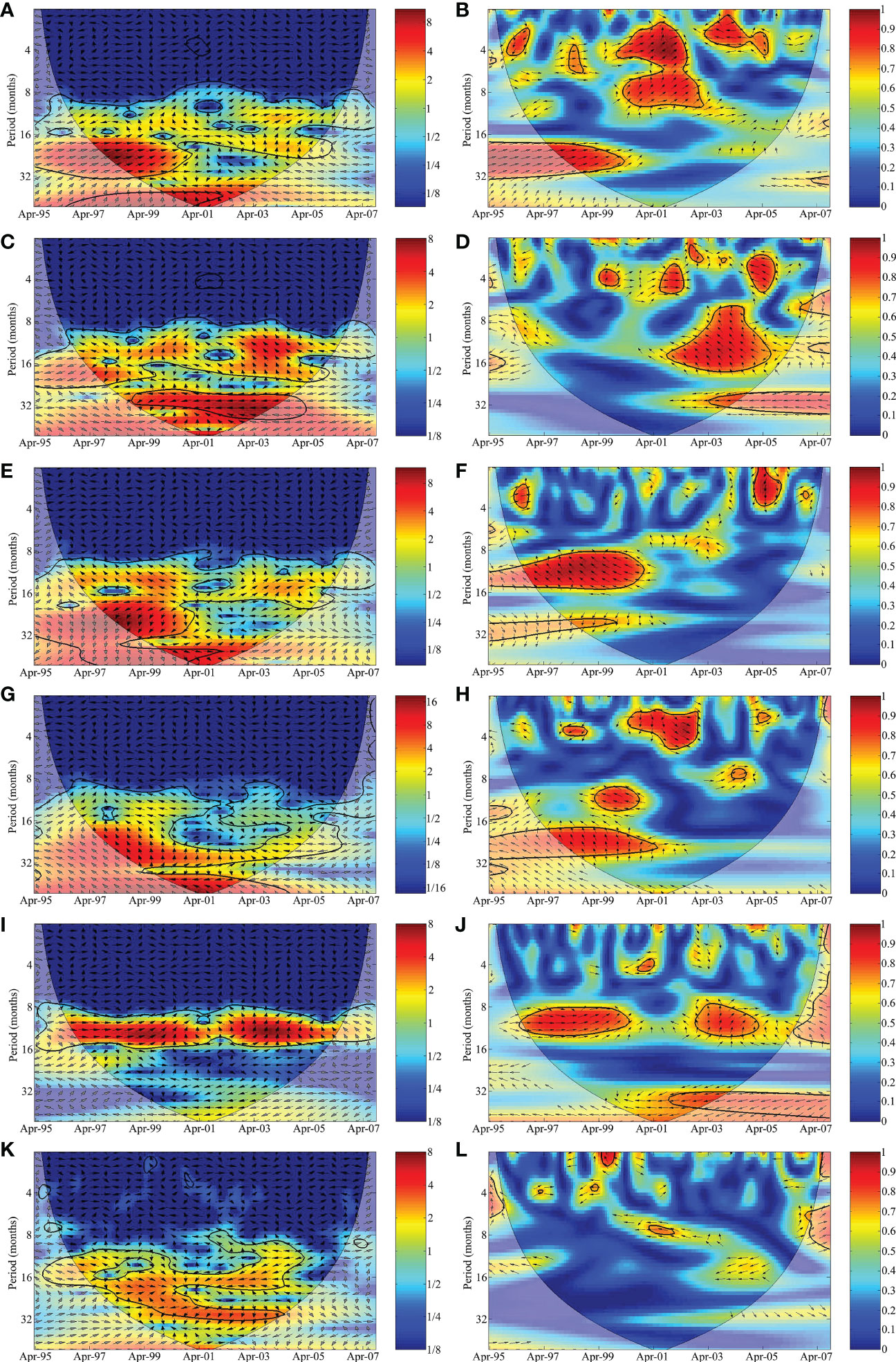
Figure 5 Cross-wavelet and coherence wavelet analysis between the shark bycatch landed by the acoupa weakfish (Cynoscion acoupa) gillnet fisheries in Pará state and the anomalies of (A, B) sea surface temperature (SST), (C, D) the meridional wind component (v), (E, F) the discharge of the Amazon River (ARD), (G, H) the Atlantic multidecadal oscillation (AMO), (I, J) Atlantic Ocean Inter-hemispheric Sea Surface Temperature Gradient Index (GITA), and (K, L) the Multivariate El Niño Index (MEI). The 5% significance level for red noise is shown as a thick contour (the cone of influence). The relative phases are shown as vectors.
The cross-wavelet analysis of the mutual variability between the shark bycatch and the ARD indicated two energy peaks. The first was annual, from 1997 to 2001, lagging with a phase at 135°, i.e., responding to the shift in ARD with a delay of approximately 3 months (three-eights of the peak energy period), while the second peak lagged at 225°, i.e., a delay of approximately 9 months, or three-eighths of the peak energy (Figure 5E). The coherence wavelet also found a high level of correlation (0.9–1.0) for the annual energy band and a more moderate 0.6–0.7 for the biannual energy band. The wavelet of coherence had the same phases as those observed in the cross-wavelet (Figure 5F).
The cross-wavelet analysis of the mutual variability between the shark bycatch and the AMO indicated only one energy peak, which was phase-lagged at 135°, i.e., with a delay of approximately 9 months (three-eighths of the peak energy period) in relation to the shifts in the AMO (Figure 5G). Once again, the coherence wavelet presented the same phase as that observed in the cross-wavelet, with a correlation of 0.7–0.9 for the biannual energy peak (Figure 5H). The cross-wavelet analysis between the shark bycatch and the GITA events indicated an annual peak of energy in phase (0°) up to 1999, after which the phase shifted to 45°, i.e., a delay of approximately 1.5 months (one eighth of the energy peak) in the response to the variability in the GITA (Figures 5I, J). The coherence wavelet presented the same phases as those observed in the cross-wavelet. The cross-wavelet analysis between the shark bycatch and the MEI revealed two energy peaks. The first was annual and the second was a quasi-triennial, both lagging with opposite phases (Figure 5K). The coherence wavelet had a medium correlation (0.5–0.7) with the annual energy band and presented the same phases observed in the cross-wavelet analysis (Figure 5L).
The cross-wavelet analysis between the fishing effort of the acoupa weakfish fleet and the SST indicated a single energy peak (Figures 6A, B), which was biannual and in phase (0°) up to 1998, after which it shifted to the opposite phase, i.e., 180° (Figure 6A). The coherence wavelet presented correlations of 0.7–0.9 for the quasi-seasonal energy band, 0.6–0.8 for the annual energy band, and 0.5–0.7 for the biannual energy band (Figure 6B). The cross-wavelet analysis of the mutual variability between the acoupa weakfish fishing effort and the v wind component indicated one peak of energy, a quasi-triennial peak in the opposite phase at 180° (Figure 6C). The coherence wavelet showed high correlation (0.7–0.9) with the quasi-triennial energy band, and the phase was the same as that recorded in the cross-wavelet analysis (Figure 6D). Two principal energy bands can be seen in the period/time field in both the cross and coherence wavelets between the acoupa weakfish fishing effort and the ARD, reflecting a common pattern in these variables (Figures 6E, F). The first energy band was biannual and showed a defined phase, a lag angle of 225° up to 2000, i.e., the fishing effort responded with a delay of three-eights of the period or 9 months of the variability in the ARD, after which it shifted to in phase. The second band was quasi-triennial with a predominance of the opposite phase (Figure 6E). The coherence wavelet had a correlation of 0.5–0.7 with the biannual energy peak (Figure 6F).
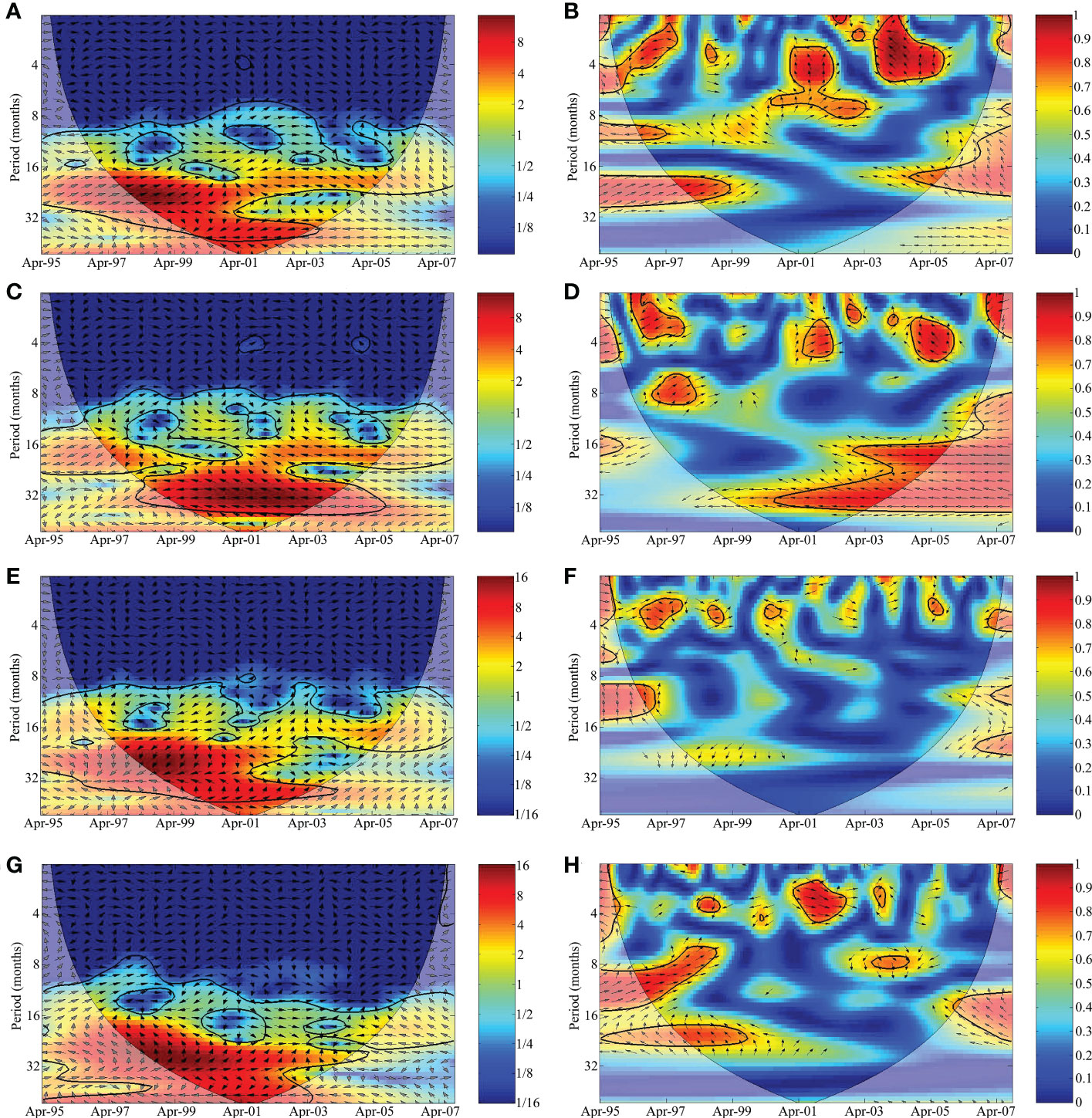
Figure 6 Cross-wavelet and coherence wavelet analysis between the shark bycatch landed by the acoupa weakfish (Cynoscion acoupa) gillnet fisheries in Pará state and the anomalies of (A, B) sea surface temperature (SST), (C, D) the meridional wind component (v), (E, F) the discharge of the Amazon River (ARD), and (G, H) the Atlantic multidecadal oscillation (AMO). The contours outline the variance units, and the 5% significance level for red noise is shown as a thick contour (the cone of influence). The relative phases are shown as vectors.
Considering the cross-wavelet between the acoupa weakfish fishing effort and the AMO index, the principal energy band was biannual, with a lag of 90°, that is, a delay of one quarter in the period (9 months) of variability of the AMO (Figure 6G). This was the same configuration observed in the cross and coherence wavelet analyses of the bycatch and AMO. The coherence wavelet presented a high correlation (0.7–0.9) in the biannual energy band (Figure 6H). The findings of the cross-wavelet and coherence wavelet analyses are summarized in Figure 7.
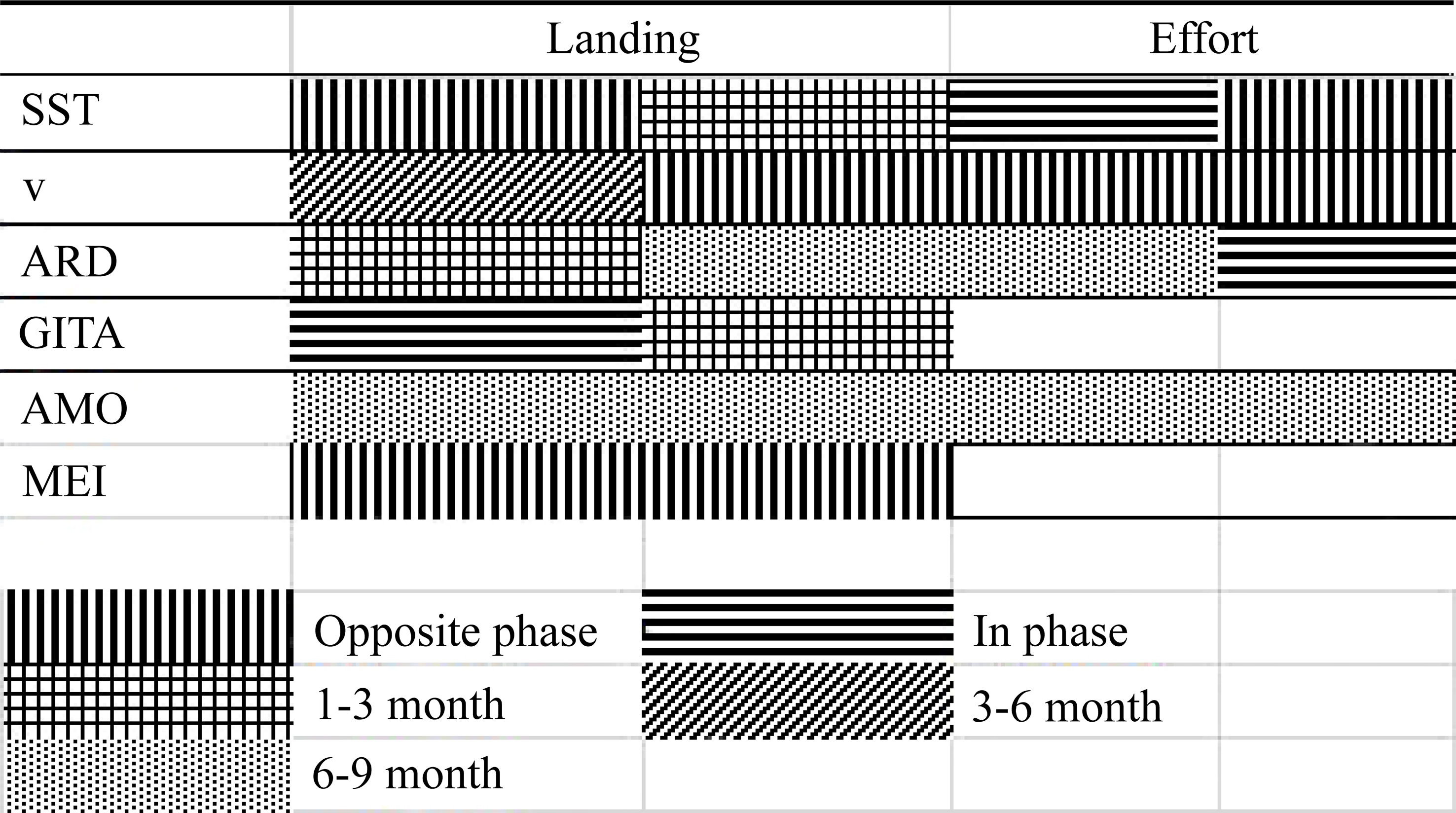
Figure 7 Summary of the results of the cross-wavelet and coherence wavelet analyses. Environmental variables: Sea Surface Temperature (SST), the meridional wind component (v), the Amazon River Discharge (ARD), Atlantic Ocean Inter-hemispheric Sea Surface Temperature Gradient Index (GITA), Atlantic multidecadal oscillation (AMO), and Multivariate ENSO Index (MEI).
The RDA showed that 63.15% (the adjusted explained variation is 61.3%) of the variability in the data on shark bycatch and the fishing effort of the acoupa weakfish fisheries was explained by the variables analyzed on the first and second canonical axes (Figure 8; Table 2). None of the hydrological patterns analyzed, such as the discharge of the Amazon River, had any direct relationship with the variation in the shark catches, either the lowest or the highest volumes landed (Figure 8). However, the lowest shark catches were correlated with the GITA along the first axis (Figure 8). The largest catches, shown in the fourth (bottom right) quadrant of the plot (Figure 8), were correlated more strongly with the MEI (Figure 8). Overall, then, none of the variables examined in this analysis had any direct effect on the largest or smallest shark catches, although correlations were found with specific environmental, depending on the season (Figure 8).
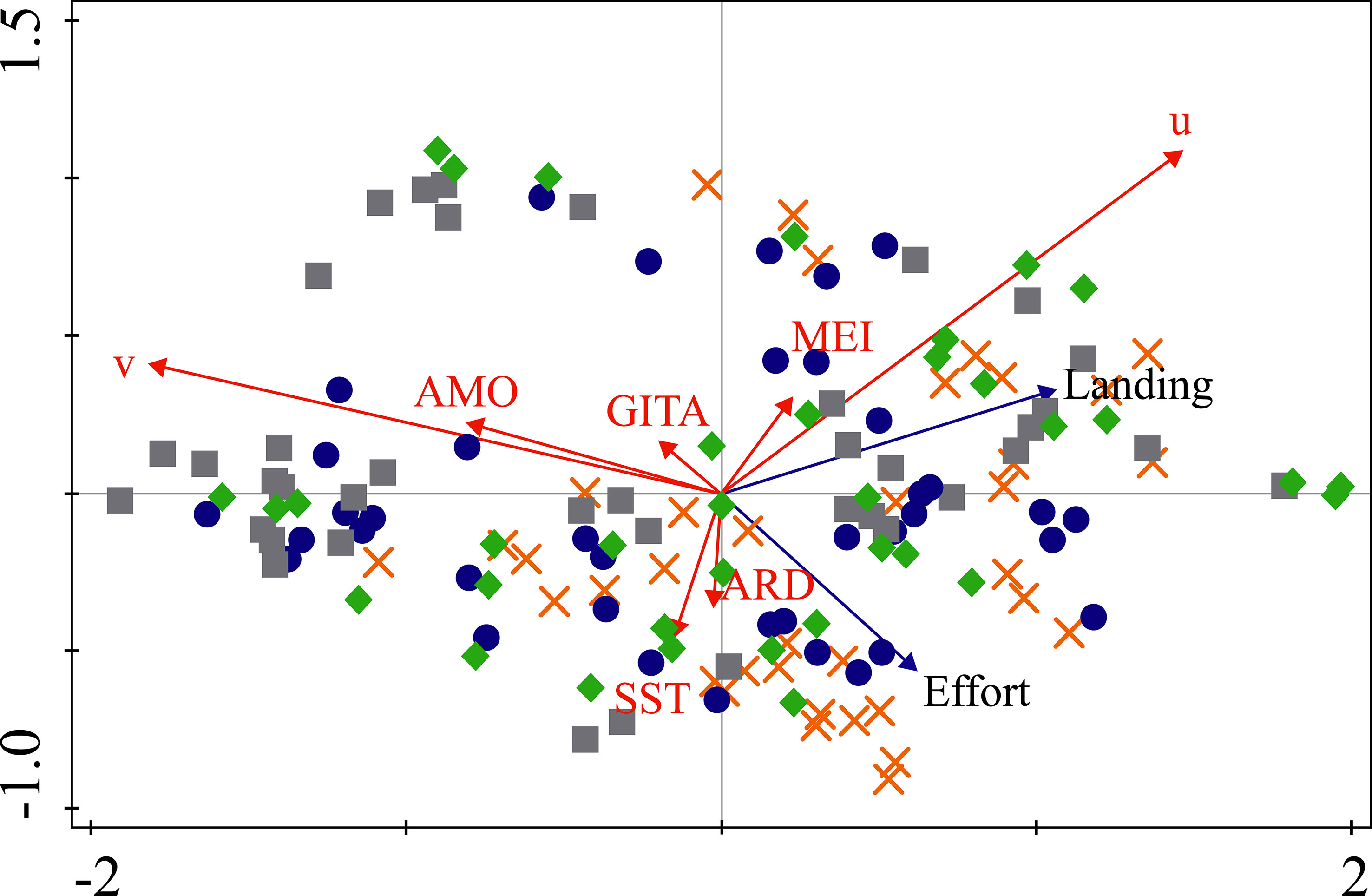
Figure 8 Results of the Redundancy Analysis (RDA) of the shark bycatch landed by the acoupa weakfish (Cynoscion acoupa) gillnet fisheries and the environmental variables. The dark blue arrows indicate the shark bycatch, while the red arrows represent the environmental variables considered in the present study. Categorical variables: hydrological cycle of the Amazon River: wet, flood, ebb, and dry. Environmental variables: Amazon River Discharge (ARD); zonal and meridional wind components (u, v), Sea Surface Temperature (SST); Atlantic Ocean Inter-hemispheric Sea Surface Temperature Gradient Index (GITA), and Multivariate El Niño Index (MEI). Symbols: orange x = wet; dark blue circle = flood; gray square = ebb, and green diamond = dry.
4 Discussion
Defined as the incidental or unintentional capture of non-target species, bycatch is a major obstacle to the sustainability of fisheries, as shown by innumerable previous studies. Bycatch is especially deleterious for sensitive fish that have long life cycles and late sexual maturity, such as elasmobranchs (Pardo et al., 2016), that tend to be captured at the juvenile stage (Kelleher, 2005; Oliver et al., 2015). Sharks are a common component of the bycatch of any different types of fishery around the world (Barker and Schluessel, 2005; Mandelman et al., 2008), including those of the northern coast of Brazil, especially those that operate most intensively (Isaac and Ferrari, 2017). As most elasmobranchs are taken as bycatch, rather than targeted directly, increasing effort on other target species will result in an increase in the level of shark bycatch (Clarke et al., 2014).
In Brazil, a major problem for the analysis of shark catches is the identification of the species harvested (Freire et al., 2021). The catch data analyzed in the present study represent the only information of this kind available for the northern coast of Brazil, and as there was no species-level classification, either in the on-board logs or the landings, virtually nothing is known of the taxonomic diversity of this bycatch. In the few official fishery statistics that are available, the only identification of the catch is the vernacular names tubarão or cação (Freire et al., 2021). This lack of information obscures the true diversity of the fish fauna exploited by these fisheries. Marceniuk et al. (2019), for example, registered 69 elasmobranch species in the region of the Amazon coast, of which eleven are endemic to this region, and 65–90% are taken as bycatch. In an analysis of sharks sold in the fish market in Bragança, an important fishing port in Pará state, Martins et al. (2021) identified 11 taxa of the orders Orectolobiformes and Carcharhiniformes, of which, eight are classified as endangered. Clearly, then, the generic classification of catches by their vernacular names does not reflect the true diversity of the impacted fauna, and limit the more effective analysis of the dynamics of existing stocks and their response to fishing pressure, whether targeted specifically or indirectly, as bycatch (Davidson et al., 2016).
Despite this limitation of the study, it was possible to confirm that both environmental and economic factors influence the variation in the acoupa weakfish fishery operations and, in turn, the harvesting of bycatch. The acoupa weakfish fisheries that operate off the northern coast of Brazil mostly use gillnets, and sharks are a common component of the bycatch obtained with this gear (Lessa et al., 1999a; Mourão et al., 2018). This bycatch accompanies the dynamics of the effort invested in the pursuit of the target species. The productivity of C. acoupa peaks during periods of high salinity (Mourão et al., 2018), when these fish approach coastal areas, a pattern that is likely duplicated by the sharks during the same period. This period is associated with the dry phase of the Amazon River, when the estuary retracts at the mouth and the Amazon plume is weak.
The analysis of the time series revealed that the peak in productivity recorded in 1998 was associated primarily with both environmental volatility and government subsidies that were implemented to provide incentives for an increase in acoupa weakfish fishing effort and the improvement of catch volumes. The financial assistance provided by the Brazilian government is regulated by federal law number 9,445 of March 14th, 1997. The introduction of measures such as fuel subsidies for fishing vessels without adequate fishery management planning can result in excess production capacity, overfishing, and, ultimately, a decline in the sustainability of fisheries (Schuhbauer et al., 2017; Sumaila et al., 2019). In the subsequent years, while catches decreased, fishing effort continued to grow, which indicates that the sustainability of the fishing system was beginning to decline (Sparre and Venema, 1997). One consequence is that shark bycatch has also declined since 2000, as observed in the analysis of the data from 2007.
Amazon fisheries are intimately related with climatic and hydrological variables. The fish fauna that inhabits coastal zones and estuaries, such as the acoupa weakfish, is the most affected by environmental variability (Freire, 2019), especially in rainfall patterns. A number of characteristics of aquatic species are affected by rainfall. For example, fluctuations in the spatial distribution of species and their interactions, life cycles, and demographic parameters, can impact local fisheries and the communities that depend on the resources they provide, with profound implications for economic income and food security (Castello et al., 2015; Isaac and Ferrari, 2017; Lima et al., 2017).
Climate variables presented a clear relationship with acoupa weakfish catches and, consequently, shark bycatch patterns. Precipitation patterns in the Amazon basin respond in an interannual manner to ENSO weather effects and the SST gradient in the tropical Atlantic (Marengo et al., 2013; Pinaya et al., 2016). La Niña events provoke an increase in precipitation in the Amazon basin which, in turn, affects the volume of the Amazon plume (Tyaquiçã et al., 2017). The increase in both fishing effort and the amount of shark bycatch may thus be associated with the atypical climate period between 1998 and 2000, when a persistent La Niña dominated the climate scenario (Figure 4).
During the 1998–2001 La Niña, a phase shift was observed in the relationship between fisheries and environmental variables, as demonstrated by the cross-wavelet analysis. La Niña is responsible for heavy rainfall in northern Brazil, which increases the volume of the Amazon plume and the strength of the trade winds, which also interferes with the dynamics of the local fisheries. Recent studies have revealed a “new” type of ENSO pattern in the central Pacific, known as the Modoki ENSO, which has become more frequent since the late 20th century (Ashok and Yamagata, 2009; Kao and Yu, 2009; Yu et al., 2010; Li et al., 2011; Mcphaden et al., 2011). In this context, it would appear to be necessary to address the Modoki ENSO to obtain a better understanding of the relationship between Amazon shelf fisheries and environmental variability. The Modoki ENSO is considered to be a new driver of climate change (Xie et al., 2014) and is closely linked to global warming (Yeh et al., 2009).
The principal pattern was found between the shark bycatch acoupa weakfish fishery and the ARD, which are inversely related with a 1–3 month lag in relation to shifts in the hydrological cycle of the Amazon River, with a correlation of more than 0.6. The shark bycatch and GITA events are in phase, that is, the variation in the GITA is reflected immediately in fishery productivity. Similarly, shark bycatch, fishing effort, and the ARD have an inverse, but delayed relationship of 6–9 months with shifts in the hydrological cycle of the Amazon River and changes in fishing effort, with a correlation of more than 0.5.
Climate change, and other anthropogenic impacts have drastic effects on both freshwater and marine organisms, including the degradation and loss of habitats, and a decline in productivity (Hollowed et al., 2013). The harvesting of shark, whether targeted specifically or taken as bycatch, should be studied in much greater detail to ensure the conservation of these extremely vulnerable fish (Davidson et al., 2016). As most shark species are K life-history strategists, these findings are of great importance and extreme concern, especially considering that many shark taxa are also impacted by pollution, habitat loss, and other anthropogenic pressures that affect the natural dynamic of the environments they inhabit (Jackson et al., 2001; Halpern et al., 2008; Dodds et al., 2013; Halpern et al., 2015; Dulvy et al., 2021).
Sustainability usually only becomes a concern when fish stocks reach critical levels, and the implementation of sustainable practises often requires profound changes in the production systems that are not easily accepted by the fishery sector. The development of new bycatch reduction methods and their deployment by fisheries, including the Amazonian acoupa weakfish fleet, can greatly reduce the impact of these operations on threatened fauna, including sharks, turtles, dolphins, and whales (Jordan et al., 2013; Wakefield et al., 2017). However, any reduction in fishing effort tends to be met with disapproval from entrepreneurs and investors, who will often lobby policy-makers in favor of their commercial interests (Daw et al., 2012; Watson et al., 2013).
The adequate inspection of fishing vessels is determined by the size and diversity of a fleet, which may include both industrial vessels able to spend long periods at sea, and much smaller artisanal operations, which nevertheless make a significant contribution to total catches (Bentes et al., 2012). These difficulties are exacerbated by both clandestine operations and the practise of finning (removal of the valuable fins, with the body being discarded in the sea), which can greatly reduce the potential for the collection of accurate catch data (Stevens et al., 2000; Clarke et al., 2006; Clarke et al., 2013).
Fishery statistics have not been collected systematically in Brazil since 2007, which means that little reliable information is available on the country’s shark stocks. This highlights the urgent need for investment in programs that guarantee the of reliable and adequate data on fishery catches (Barreto et al., 2017). Although the data discussed in the present study are gross estimates of the shark bycatch of an important fishery system (and their relationship with environmental variables and fishing effort), the results highlight the urgent need for fishery management programs, which should be prioritized in regions such as northern Brazil, where Pará has the second most productive state fishery sector. The collection of more accurate and detailed data, in particular, on the taxonomic diversity of the sharks captured, will be fundamental to the establishment of reliable predictions on future fishing scenarios that represent local scenarios reliably, and provide effective guidance for the conservation and management of the stocks of the more threatened species.
Data availability statement
The original contributions presented in the study are included in the article/Supplementary Material. Further inquiries can be directed to the corresponding author.
Ethics statement
The data were from monitored fishing landings, so the animal study was reviewed and approved by Comissão de Ética no Uso de Animais.
Author contributions
IL: Conceptualization, data collection and curation, visualization, and writing – original draft. WP: Methods, formal analysis, software, data collection, validation, and writing – review and editing. MN: Data curation. WL: Data curation. ES: Data curation and software development. ZN: Project administration, validation, and visualization. BB: Conceptualization, methods, formal analyses, funding acquisition, investigation, supervision, validation, resources, and writing – review and editing. All authors contributed to the article and approved the submitted version.
Funding
The Article Processing Charges were granted by the Programa de Apoio à Publicação Qualificada (PAPQ) of the Universidade Federal do Pará (UFPA).
Acknowledgments
We are grateful to the Centro Nacional de Pesquisa e Conservação da Biodiversidade Marinha do Norte (CEPNOR) and the Instituto Chico Mendes de Conservação da Biodiversidade (ICMBio) for releasing the fishery data analyzed in the present study. The environmental data were obtained from the Agência Nacional de Águas e Saneamento Básico (ANA).
Conflict of interest
The authors declare that the research was conducted in the absence of any commercial or financial relationships that could be construed as a potential conflict of interest.
Publisher’s note
All claims expressed in this article are solely those of the authors and do not necessarily represent those of their affiliated organizations, or those of the publisher, the editors and the reviewers. Any product that may be evaluated in this article, or claim that may be made by its manufacturer, is not guaranteed or endorsed by the publisher.
Supplementary material
The Supplementary Material for this article can be found online at: https://www.frontiersin.org/articles/10.3389/fmars.2023.1101020/full#supplementary-material
References
Almeida Z. D. S., Isaac V. J., Paz A. C., Morais G. C., Porto H. L. R. (2011). Avaliação do potencial de produção pesqueira do sistema da pescada-amarela (Cynoscion acoupa) capturada pela frota comercial do araçagi, raposa, maranhão. Bol. Lab. Hidrobiol. 24, 35–42.
Andrade A. C., Silva-Junior L. C., Vianna M. (2008). Reproductive biology and population variables of the Brazilian sharpnose shark Rhizoprionodon lalandii (Müller & henle 1839) captured in coastal waters of south-eastern Brazil. J. Fish Biol. 72, 473–484. doi: 10.1111/j.1095-8649.2007.01706.x
Ashok K., Yamagata T. (2009). Climate change: The El niño with a difference. Nature 461, 481–484. doi: 10.1038/461481a
Barbosa-Filho M. L. V., Costa-Neto E. M., Siciliano S. (2017). Knowledge and practices of expert fishermen of south bahia, Brazil, regarding the international shark fin market. Hum. Ecol. 45, 67–75. doi: 10.1007/s10745-016-9873-2
Barker M. J., Schluessel V. (2005). Managing global shark fisheries: Suggestions for prioritizing management strategies. Aquat. Conserv.: Mar. Freshw. Ecosyst. 15, 325–347. doi: 10.1002/aqc.660
Barreto R. R., Bornatowski H., Motta F. D. S., Santander-Neto J., Vianna G. M. S., Lessa R. (2017). Rethinking use and trade of pelagic sharks from Brazil. Mar. Policy 85, 114–122. doi: 10.1016/j.marpol.2017.08.016
Belsley D. A., Kuh E., Welsch R. E. (2005). Regression diagnostics: Identifying influential data and sources of collinearity (London: John Wiley & Sons).
Bentes B., Isaac V. J., Espírito Santo R. V., Fredou T., Almeida M. C., Mourão K. R. M., et al. (2012). Multidisciplinary approach to identification of fishery production systems on the northern coast of Brazil. Biota Neotrop. 12, 81–92. doi: 10.1590/S1676-06032012000100006
Brown C. J., Roff G. (2019). Life-history traits inform population trends when assessing the conservation status of a declining tiger shark population. Biol. Conserv. 239, 108230. doi: 10.1016/j.biocon.2019.108230
Carvalho F., Lee H. H., Piner K. R., Kapur M., Clarke S. C. (2018). Can the status of pelagic shark populations be determined using simple fishery indicators? Biol. Conserv. 228, 195–204. doi: 10.1016/j.biocon.2018.09.034
Castello L., Isaac V. J., Thapa R. (2015). Flood pulse effects on multispecies fishery yields in the lower Amazon. R. Soc Open Sci. 2, 150299. doi: 10.1098/rsos.150299
Castro A. L., Rosa R. S. (2005). Use of natural marks on population estimates of the nurse shark, Ginglymostoma cirratum, at atol das rocas biological reserve, Brazil. Environ. Biol. Fishes 72, 213–221. doi: 10.1007/s10641-004-1479-7
Clarke S. C., Harley S. J., Hoyle S. D., Rice J. S. (2013). Population trends in pacific oceanic sharks and the utility of regulations on shark finning. Conserv. Biolo. 27, 197–209. doi: 10.1111/j.1523-1739.2012.01943.x
Clarke S. C., Magnussen J. E., Abercrombie D. L., Mcallister M. K., Shivji M. S. (2006). Identification of shark species composition and proportion in the Hong Kong shark fin market based on molecular genetics and trade records. Conserv. Biolo. 20, 201–211. doi: 10.1111/j.1523-1739.2005.00247.x
Clarke S., Sato M., Small C., Sullivan B., Inoue Y., Ochi D. (2014). Bycatch in longline fisheries for tuna and tuna-like species: A global review of status and mitigation measures. FAO Fisheries and Aquaculture Technical Paper No. 588 (Rome: FAO).
Davidson L. N., Krawchuk M. A., Dulvy N. K. (2016). Why have global shark and ray landings declined: improved management or overfishing? Fish Fish. 17, 438–458. doi: 10.1111/faf.12119
Daw T. M., Cinner J. E., McClanahan T. R., Brown K., Stead S. M., Graham N. A., et al. (2012). To fish or not to fish: Factors at multiple scales affecting artisanal fishers' readiness to exit a declining fishery. PloS One 7, e31460. doi: 10.1371/journal.pone.0031460
Dodds W. K., Perkin J. S., Gerken J. E. (2013). Human impact on freshwater ecosystem services: a global perspective. Environ. Sci. Technol. 47, 9061–9068. doi: 10.1021/es4021052
Duffy L. M., Lennert-Cody C. E., Olson R. J., Minte-Vera C. V., Griffiths S. P. (2019). Assessing vulnerability of bycatch species in the tuna purse-seine fisheries of the eastern pacific ocean. Fish. Res. 219, 105316. doi: 10.1016/j.fishres.2019.105316
Dulvy N. K., Fowler S. L., Musick J. A., Cavanagh R. D., Kyne P. M., Harrison L. R., et al. (2014). Extinction risk and conservation of the world’s sharks and rays. Elife 3, e00590. doi: 10.7554/eLife
Dulvy N. K., Pacoureau N., Rigby C. L., Pollom R. A., Jabado R. W., Ebert D. A., et al. (2021). Overfishing drives over one-third of all sharks and rays toward a global extinction crisis. Curr. Biol. 31, 4773–4787. doi: 10.1016/j.cub.2021.08.062
Feitosa L. M., Martins A. P. B., Giarrizzo T., Macedo W., Monteiro I. L., Gemaque R. (2018). DNA-Based identification reveals illegal trade of threatened shark species in a global elasmobranch conservation hotspot. Sci. Rep. 8, 1–11. doi: 10.1038/s41598-018-21683-5
Fisch G., Marengo J. A., Nobre C. A. (1998). Uma revisão geral sobre o clima da amazônia. Acta Amazon. 28, 101–126. doi: 10.1590/1809-43921998282126
Freire J. N. (2019). Aspectos da pesca e análise da abundância relativa da cynoscion acoupa, lacépède 1801 e suas relações com a temperatura da superfície do mar na plataforma continental norte do brasil (Belém (PA: Universidade Federal Rural da Amazônia).
Freire K. M. F., Almeida Z. D. S. D., Amador J. R. E. T., Aragão J. A., Araújo A. R. D. R., Ávila-da-Silva A. O., et al. (2021). Reconstruction of marine commercial landings for the Brazilian industrial and artisanal fisheries from 1950 to 2015. Front. Mar. Sci. 8. doi: 10.3389/fmars.2021.659110
Freitas R. H. A. D., Rosa R. S., Wetherbee B. M., Gruber S. H. (2009). Population size and survivorship for juvenile lemon sharks (Negaprion brevirostris) on their nursery grounds at a marine protected area in Brazil. Neotrop. Ichthyol. 7, 205–212. doi: 10.1590/S1679-62252009000200011
Grinsted A., Moore J. C., Jevrejeva S. (2004). Application of cross wavelet transform and wavelet coherence to geophysical time series. Nonlinear Process. Geophys. 11. doi: 10.3389/10.5194/npg-11-561-2004
Guimarães-Costa A., Machado F. S., Reis-Filho J. A., Andrade M., Araújo R. G., Corrêa E. M. R. (2020). DNA Barcoding for the assessment of the taxonomy and conservation status of the fish bycatch of the northern Brazilian shrimp trawl fishery. Front. Mar. Sci. 7. doi: 10.3389/fmars.2020.566021
Halpern B. S., Frazier M., Potapenko J., Casey K. S., Koenig K., Longo C., et al. (2015). Spatial and temporal changes in cumulative human impacts on the world/'s ocean. Nat. Commun. 6, 1–7. doi: 10.1038/ncomms8615
Halpern B. S., Walbridge S., Selkoe K. A., Kappel C. V., Micheli F., D'agrosa C., et al. (2008). A global map of human impact on marine ecosystems. Science 319, 948–952. doi: 10.1126/science.1149345
Hollowed A. B., Barange M., Beamish R. J., Brander K., Cochrane K., Drinkwater K., et al. (2013). Projected impacts of climate change on marine fish and fisheries. ICES J. Mar. Sci. 70, 1023–1037. doi: 10.1093/icesjms/fst081
Huveneers C., Apps K., Becerril-García E. E., Bruce B., Butcher P. A., Carlisle A. B., et al. (2018). Future research directions on the “elusive“ white shark. Front. Mar. Sci. 5. doi: 10.3389/fmars.2018.00455
Isaac V. J., Ferrari S. F. (2017). Assessment and management of the north Brazil shelf large marine ecosystem. Environ. Dev. 22, 97–110. doi: 10.1016/j.envdev.2016.11.004
Isaac V. J., Martins A. S., Haimovici M., Andriguetto-Filho J. M. (2006). A pesca marinha e estuarina do brasil no início do século XXI: recursos, tecnologias, aspectos socioeconômicos e institucionais (Belém: Editora Universitária UFPA).
Jackson J. B., Kirby M. X., Berger W. H., Bjorndal K. A., Botsford L. W., Bourque B. J., et al. (2001). Historical overfishing and the recent collapse of coastal ecosystems. Science 293, 629–637. doi: 10.1126/science.1059199
Jordan L. K., Mandelman J. W., McComb D. M., Fordham S. V., Carlson J. K., Werner T. B. (2013). Linking sensory biology and fisheries bycatch reduction in elasmobranch fishes: A review with new directions for research. Conserv. Physiol. 1:1–12. doi: 10.1093/conphys/cot002
Ju P., Chen M., Tian Y., Zhao Y., Yang S., Xiao J. (2020). Stock status estimating of 5 shark species in the waters around Taiwan using a length-based Bayesian biomass estimation (LBB) method. Front. Mar. Sci. 7. doi: 10.3389/fmars.2020.00632
Kao H. Y., Yu J. Y. (2009). Contrasting eastern-pacific and central-pacific types of ENSO. J. Clim. 22, 615–632. doi: 10.1175/2008JCLI2309.1
Kelleher K. (2005). Discards in the world's marine fisheries: An update. FAO Fisheries and Aquaculture Technical paper No. 470. (Rome: FAO).
Lan K. W., Lee M. A., Nishida T., Lu H. J., Weng J. S., Chang Y. (2012). Environmental effects on yellowfin tuna catch by the Taiwan longline fishery in the Arabian Sea. Int. J. Remote Sens. 33, 7491–7506. doi: 10.1080/01431161.2012.685971
Leduc A. O., De Carvalho F. H., Hussey N. E., Reis-Filho J. A., Longo G. O., Lopes P. F. (2021). Local ecological knowledge to assist conservation status assessments in data poor contexts: A case study with the threatened sharks of the Brazilian northeast. Biodivers. Conserv. 30, 819–845. doi: 10.1007/s10531-021-02119-5
Lessa R., Batista V. S., Santana F. M. (2016). Close to extinction? The collapse of the endemic daggernose shark (Isogomphodon oxyrhynchus) off Brazil. Glob. Ecol. Conserv. 7, 70–81. doi: 10.1016/j.gecco.2016.04.003
Lessa R., Santana F. M., Rincón G., Gadig O. B. F., El-Deir A. C. A. (1999a). Biodiversidade de elasmobrânquios do brasil. relatório para o programa nacional da diversidade biológica (PRONABIO) – necton - elasmobrânquios (Recife: Ministério do Meio Ambiente, dos Recursos Hídricos e da Amazônia Legal (MMA).
Lessa R., Santana F., Menni R., Almeida Z. (1999b). Population structure and reproductive biology of the smalltail shark (Carcharhinus porosus) off maranhão (Brazil). Mar. Freshw. Res. 50, 383–388. doi: 10.1071/MF98127
Li W., Zhang P., Ye J., LI L., Baker P. A. (2011). Impact of two different types of El niño events on the amazon climate and ecosystem productivity. J. Plant Ecol. 4, 91–99. doi: 10.1093/jpe/rtq039
Lima M. A. L., Kaplan D. A., Doria C. R. C. (2017). Hydrological controls of fisheries production in a major Amazonian tributary. Ecohydrology 10, e1899. doi: 10.1002/eco.1899
Makarenkov V., Legendre P. (2002). Nonlinear redundancy analysis and canonical correspondence analysis based on polynomial regression. Ecology 83, 1146–1161. doi: 10.1890/0012-9658(2002)083[1146:NRAACC]2.0.CO;2
Mandelman J. W., Cooper P. W., Werner T. B., Lagueux K. M. (2008). Shark bycatch and depredation in the US Atlantic pelagic longline fishery. Rev. Fish Biol. Fish. 18, 427. doi: 10.1007/s11160-008-9084-z
Marceniuk A. P., Barthem R. B., Wosiacki W. B., Klautau A. G. C. M., Junior T. V., Rotundo M. M., et al. (2019). Sharks and batoids (Subclass elasmobranchii) caught in the industrial fisheries off the Brazilian north coast. Rev. Nord. Biol. 27, 120–142. doi: 10.22478/ufpb.2236-1480.2019v27n1.47112
Marengo J. A., Alves L. M., Soares W. R., Rodriguez D. A., Camargo H., Riveros M. P., et al. (2013). Two contrasting severe seasonal extremes in tropical south America in 2012: flood in Amazonia and drought in northeast Brazil. J. Clim. 26, 9137–9154. doi: 10.1175/JCLI-D-12-00642.1
Martins T., Santana P., Lutz Í., Silva R., Guimarães-Costa A., Vallinoto M., et al. (2021). Intensive commercialization of endangered sharks and rays (Elasmobranchii) along the coastal Amazon as revealed by DNA barcode. Front. Mar. Sci. 8. doi: 10.3389/fmars.2021.769908
Mcphaden M. J., Lee T., Mcclurg D. (2011). El Niño and its relationship to changing background conditions in the tropical pacific ocean. Geophys. Res. Lett. 38, L15709. doi: 10.1029/2011GL048275
Ménard F., Marsac F., Bellier E., Cazelles B. (2007). Climatic oscillations and tuna catch rates in the Indian ocean: A wavelet approach to time series analysis. Fish. Oceanogr. 16, 95–104. doi: 10.1111/j.1365-2419.2006.00415.x
Mendonça F. F., Oliveira C., Gadig O. B. F., Foresti F. (2009). Populations analysis of the Brazilian sharpnose shark Rhizoprionodon lalandii (Chondrichthyes: Carcharhinidae) on the são paulo coast, southern Brazil: Inferences from mt DNA sequences. Neotrop. Ichthyol. 7, 213–216. doi: 10.1590/S1679-62252009000200012
Milligan G. W., Cooper M. C. (1988). A study of standardization of variables in cluster analysis. J. Classif. 5, 181–204. doi: 10.1007/BF01897163
Moura R. L., Amado-Filho G. M., Moraes F. C., Brasileiro P. S., Salomon P. S., Mahiques M. M., et al. (2016). An extensive reef system at the Amazon river mouth. Sci. Adv. 2, e1501252. doi: 10.1126/sciadv.1501252
Mourão K. R. M., Frédou F. L., Espírito-Santo R. V., Almeida M. C., Silva B. B., Frédou T., et al. (2018). Sistema de produção pesqueira pescada amarela-Cynoscion acoupa lacèpede, (1802): Um estudo de caso no litoral nordeste do pará-brasil. Bol. Inst. Pesca 35, 497–511.
Oliveira P. G., de Oliveira D. S., Pinheiro P. B., Hazin F. H., Carvalho F. C., Veras D. P., et al. (2011). Population structure and growth of young lemon shark, Negaprion brevirostris (Poey 1868), at the atol das rocas biological reserve, Brazil. J. Integr. Coast. Zone Manage. 11, 389–395. doi: 10.5894/rgci236
Oliver S., Braccini M., Newman S. J., Harvey E. S. (2015). Global patterns in the bycatch of sharks and rays. Mar. Policy 54, 86–97. doi: 10.1016/j.marpol.2014.12.017
Pacoureau N., Rigby C. L., Kyne P. M., Sherley R. B., Winker H., Carlson J. K., et al. (2021). Half a century of global decline in oceanic sharks and rays. Nature 589, 567–571. doi: 10.1038/s41586-020-03173-9
Pardo S. A., Kindsvater H. K., Cuevas-Zimbrón E., Sosa-Nishizaki O., Pérez-Jiménez J. C., Dulvy N. K. (2016). Growth, productivity and relative extinction risk of a data-sparse devil ray. Sci. Rep. 6, 33745. doi: 10.1038/srep33745
Pinaya W. H. D., Lobon-Cervia F. J., Pita P., Buss De Souza R., Freire J., Isaac V. J. (2016). Multispecies fisheries in the lower Amazon river and its relationship with the regional and global climate variability. PloS One 11, e0157050. doi: 10.1371/journal.pone.0157050
Pinaya W. H. D., Pita P., Souza R. B., Lobon-Cérvia F. J., Freire J., Isaac V. J. (2018). The catfish fishing in the Amazon floodplain lakes. Oceanogr. Fish. Open Access J. 7, 555720. doi: 10.19080/OFOAJ.2018.07.555720
Pinheiro L. A., Frédou F. L. (2004). Caracterização geral da pesca industrial desembarcada no estado do pará. Rev. Científica da UFPA 4, 1–16.
Polanco J., Ganzedo U., Sáenz J., Caballero-Alfonso A. M., Castro-Hernández J. J. (2011). Wavelet analysis of correlation among canary islands octopus captures per unit effort, sea-surface temperatures and the north Atlantic oscillation. Fish. Res. 107, 177–183. doi: 10.1016/j.fishres.2010.10.019
Polikar R. (2001) The wavelet tutorial. Available at: https://users.rowan.edu/~polikar/WTtutorial.html (Accessed January 23, 2023).
Salas S., Chuenpagdee R., Charles A. T., Seijo J. C. (2011). Coastal fisheries of Latin America and the Caribbean. FAO Fisheries and Aquaculture Technical paper No. 544. (Rome: FAO).
Santander-Neto J., Shinozaki-Mendes R. A., Silveira L. M., Jucá-Queiroz B., Furtado-Neto M. A., Faria V. V. (2011). Population structure of nurse sharks, Ginglymostoma cirratum (Orectolobiformes), caught off ceará state, Brazil, south-western equatorial Atlantic. J. Mar. Biol. Assoc. 91, 1193–1196. doi: 10.1017/S0025315410001293
Schuhbauer A., Chuenpagdee R., Cheung W. W., Greer K., Sumaila U. R. (2017). How subsidies affect the economic viability of small-scale fisheries. Mar. Policy 82, 114–121. doi: 10.1016/j.marpol.2017.05.013
Shuai F., Lek S., Baehr C., Park Y. S., Li Y., Li X. (2018). Silver carp larva abundance in response to river flow rate revealed by cross-wavelet modelling. Ecol. Modell. 383, 98–105. doi: 10.1016/j.ecolmodel.2018.05.020
Sneath P. H. A., Sokal R. R. (1973). Numerical taxonomy. the principles and practice of numerical classification (San Francisco: W.H. Freeman and Company).
Soares H. C., Gherardi D. F. M., Pezzi L. P., Kayano M. T., Paes E. T. (2014). Patterns of interannual climate variability in large marine ecosystems. J. Mar. Syst. 134, 57–68. doi: 10.1016/j.jmarsys.2014.03.004
Souza E. B., Kayano M. T., Tota J., Pezzi. L., Fisch G., Nobre C. (2000). On the influences of the El niño, la niña and Atlantic dipole pattern on the Amazonian rainfall during 1960-1998. Acta Amazon. 30, 305–318. doi: 10.1590/1809-43922000302318
Souza Filho P. W. M. (2005). Costa De manguezais de macromaré da amazônia: Cenários morfológicos, mapeamento e quantificação a partir de dados de sensores remotos. Rev. Bras. Geofís. 23, 427–435. doi: 10.1590/S0102-261X2005000400006
Sparre P., Venema S. C. (1997). Introdução à avaliação de mananciais de peixes tropicais. parte 1: Manual. FAO Fisheries and Aquaculture Technical paper No. 306. (Rome: FAO).
Stevens J. D., Bonfil R., Dulvy N. K., Walker P. A. (2000). The effects of fishing on sharks, rays, and chimaeras (chondrichthyans), and the implications for marine ecosystems. ICES J. Mar. Sci. 57, 476–494. doi: 10.1006/jmsc.2000.0724
Sumaila U. R., Ebrahim N., Schuhbauer A., Skerritt D., Li Y., Kim H. S., et al. (2019). Updated estimates and analysis of global fisheries subsidies. Mar. Policy 109, 103695. doi: 10.1016/j.marpol.2019.103695
Torrence C., Compo G. P. (1998). A practical guide to wavelet analysis. Bull. Am. Meteorol. Soc 79, 61–78. doi: 10.1175/1520-0477(1998)079<0061:APGTWA>2.0.CO;2
Tyaquiçã P., Veleda D., Lefèvre N., Araujo M., Noriega C., Caniaux G., et al. (2017). Amazon Plume salinity response to ocean teleconnections. Front. Mar. Sci. 4. doi: 10.3389/fmars.2017.00250
Vallejos R. O., Fabré N. N., Batista V. S., Acosta J. (2013). The application of a general time series model to floodplain fisheries in the amazon. Environ. Model. Software 48, 202–212. doi: 10.1016/j.envsoft.2013.07.004
Wakefield C. B., Santana-Garcon J., Dorman S. R., Blight S., Denham A., Wakeford J., et al. (2017). Performance of bycatch reduction devices varies for chondrichthyan, reptile, and cetacean mitigation in demersal fish trawls: Assimilating subsurface interactions and unaccounted mortality. ICES J. Mar. Sci. 74, 343–358. doi: 10.1093/icesjms/fsw143
Watson R. A., Cheung W. W., Anticamara J. A., Sumaila R. U., Zeller D., Pauly D. (2013). Global marine yield halved as fishing intensity redoubles. Fish Fish. 14, 493–503. doi: 10.1111/j.1467-2979.2012.00483.x
Wilks D. S. (2006). Statistical methods in the atmospheric sciences. 2nd Edition (USA: Elsevier), 649.
Worm B., Davis B., Kettemer L., Ward-Paige C. A., Chapman D., Heithaus M. R., et al. (2013). Global catches, exploitation rates, and rebuilding options for sharks. Mar. Policy 40, 194–204. doi: 10.1016/j.marpol.2012.12.034
Xie F., Li J., Tian W., Li Y., Feng J. (2014). Indo-pacific warm pool area expansion, modoki activity, and tropical cold-point tropopause temperature variations. Sci. Rep. 4, 4552. doi: 10.1038/srep04552
Xu Y., Dai X., Huang Z., Sun M., Chen Z., Zhang K. (2022). Stock assessment of four dominant shark bycatch species in bottom trawl fisheries in the northern south China Sea. Sustainability 14, 3722. doi: 10.3390/su14073722
Yeh S.-W., Kug J.-S., Dewitte B., Kwon M.-H., Kirtman B. P., Jin F. F. (2009). El Niño in a changing climate. Nature 461, 511–514. doi: 10.1038/nature08316
Keywords: Amazon, fishery management, gillnet, incidental catch, inshore fishing, ODS-14, sustainability
Citation: Lutz Í, Pinaya WHD, Nascimento M, Lima W, Silva E, Nunes Z and Bentes B (2023) Shark bycatch of the acoupa weakfish, Cynoscion acoupa (Lacèpede, 1801), fisheries of the Amazon Shelf. Front. Mar. Sci. 10:1101020. doi: 10.3389/fmars.2023.1101020
Received: 17 November 2022; Accepted: 14 March 2023;
Published: 03 April 2023.
Edited by:
Francesco Colloca, Anton Dohrn Zoological Station Naples, ItalyReviewed by:
Stefano Moro, Sapienza University of Rome, ItalyPablo Del Monte-Luna, Centro Interdisciplinario de Ciencias Marinas (IPN), Mexico
Copyright © 2023 Lutz, Pinaya, Nascimento, Lima, Silva, Nunes and Bentes. This is an open-access article distributed under the terms of the Creative Commons Attribution License (CC BY). The use, distribution or reproduction in other forums is permitted, provided the original author(s) and the copyright owner(s) are credited and that the original publication in this journal is cited, in accordance with accepted academic practice. No use, distribution or reproduction is permitted which does not comply with these terms.
*Correspondence: Ítalo Lutz, aXRhbG9mcmVpdGFzOTFAaG90bWFpbC5jb20=