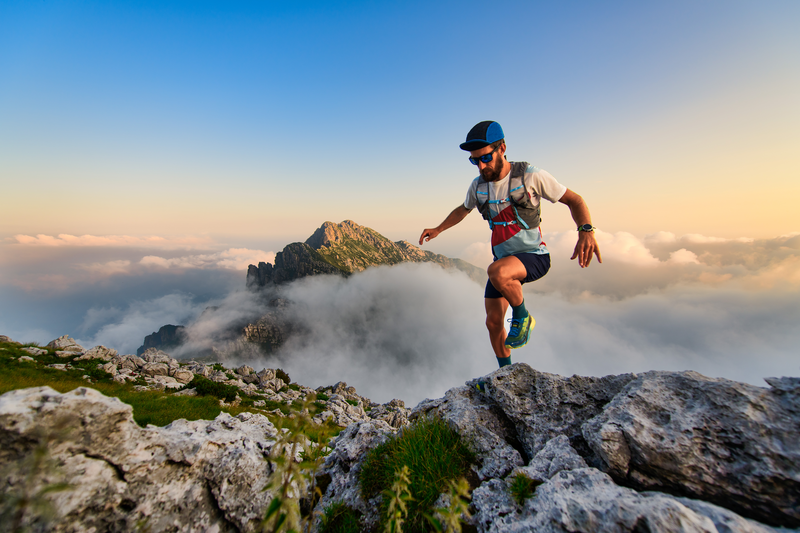
94% of researchers rate our articles as excellent or good
Learn more about the work of our research integrity team to safeguard the quality of each article we publish.
Find out more
ORIGINAL RESEARCH article
Front. Mar. Sci. , 24 February 2023
Sec. Deep-Sea Environments and Ecology
Volume 10 - 2023 | https://doi.org/10.3389/fmars.2023.1098927
This article is part of the Research Topic Submarine Canyons: Human Connections to the Deep Sea View all 12 articles
Introduction and methods: Marine litter density, distribution and potential sources, and the impact on canyon seafloor habitats were investigated in the Motril, Carchuna and Calahonda canyons, located along the northern margin of the Alboran Sea. During the ALSSOMAR-S2S oceanographic survey carried out in 2019, canyon floor imagery was collected by a Remotely Operated Vehicle along 5 km in the Motril Canyon, 10 km in the Carchuna Canyon, and 3 km in Calahonda Canyon, together with 41 surficial sediment samples. Additionally, coastal uses, maritime traffic and fishing activity data were analyzed. A 50 m resolution multibeam bathymetry served as base map.
Results: In the Motril and Calahonda canyons, the density of marine litter was low and the material was dispersed, very degraded and partially buried. In contrast, the Carchuna Canyon contained a greater amount and variety of litter. The Carchuna Canyon thalweg exhibited a density of marine litter up to 8.66 items·100 m-1, and litter hotspots with a density of up to 42 items·m2 are found along the upper reaches of the canyon thalweg.
Discussion: Low litter abundances found in the studied canyons most likely reflect low population densities and the absence of direct connections with streams in the nearby coasts. The high shelf incision of the Carchuna Canyon and its proximity to the coastline favor littoral sediment remobilization and capture as well as the formation of gravity flows that transport the marine litter along the thalweg toward the distal termination of the channel. Litter hotspots are favored by the canyon morphology and the occurrence of rocky outcrops. Most debris is of coastal origin and related to beach occupation and agricultural practices in the adjacent coastal plain. A third origin was represented by fishing gear in the study area. Fishing activity may be producing an impact through physical damage to the skeletons of the colonial scleractinians located in the walls of the Carchuna Canyon. In contrast, the Motril and Calahonda canyons can be considered passive systems that have mainly acted as depositional sinks in the recent past, as evidenced by buried marine litter.
The occurrence and accumulation of litter in the marine realm is an environmental problem with potential consequences for marine and coastal ecosystems (Richmond, 1993; Adams, 2005). Of particular importance is the long-lasting presence of plastic litter in the oceans, which exhibits high concentrations and has accumulated in diverse seafloor features, such as submarine canyons, channels and sedimentary drift deposits (Goldberg, 1997; Thompson et al., 2004; Kane and Fildani, 2021). Considering the global trends in plastic production, the increased fluxes and areal coverage of plastics can impact the seafloor and alter its nature, therefore affecting its habitats and associated benthic communities (Goldberg, 1997; Thushari and Senevirathna, 2020).
Studies on litter composition provide information about its origin. Plastics usually come from coastal and land sources, whereas other types of marine debris —such as those coming from fishing gears— may indicate human activity in the marine realm (Pham et al., 2014a; Vieira et al., 2015; Alves et al., 2021; Morales-Caselles et al., 2021). On continental margins, litter density, distribution, and composition are variable at different scales (Sánchez et al., 2013). Specifically, the spatial distribution of litter is influenced by a multiplicity of factors, including waves and oceanographic currents, seafloor geomorphology, anthropogenic activities, shipping routes, and river inputs (Galgani et al., 2000; Ramirez-Llodra et al., 2013; Pham et al., 2014b; Angiolillo et al., 2015; Angiolillo, 2019; Gerigny et al., 2019; Dominguez-Carrió et al., 2020; Canals et al., 2021; Bergmann et al., 2022; Hernandez et al., 2022). Interactions of litter artefacts with the seafloor biota are very complex and may have an impact on benthic and demersal organisms, since litter can provide habitat or coverage for diverse marine species (e.g., Goldberg, 1997; Sánchez et al., 2013; Mecho et al., 2020).
Channeled seafloor features such as submarine canyons may display significantly higher concentrations of marine debris (Galgani et al., 2000; Pham et al., 2014b), and tend to show patchy but pervasive distributions (Pierdomenico et al., 2019). Canyon heads, incised in the shelf at a short distance from the coast, can act as sinks of diverse types of land-derived litter (Wei et al., 2012; Ramirez-Llodra et al., 2013; Mecho et al., 2020); for this reason, coastal urbanization, population density, and fluvial inputs would be critical factors in determining litter concentrations in shelf-incised canyons (Mordecai et al., 2011; Neves et al., 2015; Tubau et al., 2015). In shelf-incised submarine canyons located at longer distances from the coast, litter is mainly marine-sourced (Mordecai et al., 2011). Yet submarine canyons can locally receive human discards from ships if they are located along shipping routes (Wei et al., 2012; van den Beld et al., 2017).
Along submarine canyons, litter can be entrained by diverse deep-water flows, such as downslope near-bottom currents (Tubau et al., 2015; Pierdomenico et al., 2020) or turbidity currents with strong erosional activity (Zhong and Peng, 2021). In addition, geomorphological canyon complexity may favor litter accumulations along the pathway of the submarine canyons (Tubau et al., 2015).
Because of the high densities of marine litter usually found in submarine canyons, some benthic habitats and species found in these seafloor features are particularly vulnerable, and interactions between marine debris and sessile organisms are varied, including physical contact and entanglements (Mordecai et al., 2011; Oliveira et al., 2015). Major disturbances to Vulnerable Marine Ecosystems (VMEs) of submarine canyons such as cold-water coral banks or gorgonian aggregations appear to be caused by different types of lost fishing gear (Fabri et al., 2014).
The Alboran Sea is a narrow and elongated basin located in the westernmost part of the Mediterranean Sea (Figures 1A, B) that receives moderate amounts of marine plastic litter (García-Rivera et al., 2018; Liubartseva et al., 2018). The occurrence of marine litter in the northern margin of the Alboran Sea is high by the coast at 0–50 m water depths and in open waters at 500–800 m water depths (García-Rivera et al., 2018). It is hypothesized that the abrupt character of the northern margin, with a narrow shelf and the sculpting of specific sectors by submarine canyons and other valley systems (Vázquez et al., 2015), is conducive to a relatively efficient seaward dispersal of marine litter. The Motril, Carchuna, and Calahonda shelf-incised submarine canyons lie in the central sector of the northern Alboran Sea margin (Figures 1B, C). In this study, marine litter has been observed along the canyon floor and the flanks of the three submarine canyons.
Figure 1 (A) Geographic location of the study area in the southern Iberian Peninsula. (B) Overview topo-bathymetric map of the Alboran Sea indicating the location of the study area on the northern margin. (C) Bathymetric map (“Ministerio de Pesca, Agricultura y Alimentación”, Spanish government) of the study area showing the three studied submarine canyons and the location of the ROV underwater dives (Dives 01 to 11) executed along the canyon thalwegs and flanks. Inland aerial photography shows the streams and towns. (D, E) Zoomed-in bathymetric maps of the study area showing the location of sediment cores collected in the canyons during the oceanographic survey ALSSOMAR-S2S 2019. IPW, infralittoral prograding wedges. Bathymetric contours in meters.
This study aims to analyze the litter distribution, density and origin in the Motril, Carchuna, and Calahonda shelf-incised submarine canyons. The specific objectives of this study are: (1) to characterize and quantify litter type and density in Motril, Carchuna and Calahonda canyons and, in view of other worldwide submarine canyons, to understand the major factors behind litter accumulation; (2) to understand the factors that control the distribution of marine litter in the studied submarine canyons; (3) to discern the litter origin (land- versus marine-sourced); and (4) to analyze the potential impact of litter on the benthic habitats and communities in the three studied submarine canyons.
Sediment supply to the coastlines of the northern Alboran Sea is mainly provided via relatively short, mountainous rivers (Liquete et al., 2005) and ephemeral creeks, active during autumn and winter (Stanley et al., 1975; Fabres et al., 2002; Palanques et al., 2005). Inshore of the submarine canyons, there is no significant river or creek-delta system at present (Liquete et al., 2005). Only two small dry streams of a torrential character in the rainy season debouch in the proximity of the study area: Villanueva Ravine inland from the Motril Canyon, and Gualchos Ravine east of the study area (Figure 1C). These ravines are short (<20 km) and feature high slopes (>3.6°), and they occur in small basins (<120 km2) (Bárcenas and Macías, 2009). The Guadalfeo River is a major regional fluvial system located west of the study area (Figure 1C), with an average water discharge of 23.4 m3·s-1 and an average sediment load of 83 kg·s-1 (Liquete et al., 2005; Bárcenas et al., 2015).
The coastal geomorphology of the study area is characterized by beaches and coastal plains that are unusual on this stretch of coast, where the mountains and the sea are in contact (Godoy et al., 2020). The coastal sedimentary record in the study area contains alluvial fans (Lario et al., 1999; Fernández-Salas et al., 2009), along with sandy deposits, such as spit bars and infralittoral prograding wedges (IPW) that constitute major progradational phases separated by erosional gaps, generated by coastal drift during the Holocene (Lario et al., 1999; Fernández-Salas et al., 2009; Bárcenas et al., 2011).
The northern Alboran Sea shelf is narrow (2-15 km) and the shelf break is located at an average water depth of 115 m (Ercilla et al., 1994; Vázquez, 2001). The study area comprises the narrowest shelf (2 km) of the entire northern margin, with a gradient <1° (Figures 1B, C) (Ercilla et al., 1994; Fernández-Salas et al., 2009). The western shelf of the study area is characterized by the south-eastward thinning of the Guadalfeo River deltaic wedge (Figure 1C) (Bárcenas and Macías, 2009; Lobo et al., 2015). Infralittoral prograding wedges (defined according to Hernández-Molina et al., 2000) have been identified in the eastern shelf off the coastal segment fed by ephemeral streams between Carchuna and Calahonda towns (Figure 1D) (Ortega-Sánchez et al., 2014).
The shelf-to-slope transition around the study area is marked by diverse erosive features. The Motril Canyon is located 3 km south-east of the Guadalfeo River mouth at 70 m water depth and its main valley exhibits a sinuous morphology on the slope (Figures 1C-E). The straight Carchuna Canyon (Figures 1C-E) crosses the shelf and is located 200 m off Cape Sacratif at 25 m water depth. Both canyons debouch into large lobes fed by distributary channels (Pérez-Belzuz and Alonso, 2000; Pérez-Belzuz et al., 2000). The Calahonda Canyon (Figures 1C, D) is a shelf-incised canyon located 2.5 km south of Calahonda town with a sinuous valley along the slope.
The Carchuna Canyon head (25-250 m water depths) influences the coastal swell and storm-related processes as it increases nearshore wave heights, particularly of westerly waves, favoring long-term coastal erosion due to energy concentration (Ortega-Sánchez et al., 2014). The shelf around the Carchuna Canyon head is affected by relatively high current velocity values (above 0.1 m·s-1) in the upper layer, under easterlies dominance, which causes high values of shear stress. Under westerlies dominance, a northeastward flow of <0.1 m·s-1 occurs in the upper layer of the water column (Bárcenas et al., 2011). A bottom flow distinct from the wind-driven surface flow has been detected in the Carchuna Canyon; in the bottom layer, the currents tend to flow downcanyon, particularly during easterlies dominance, where bottom flow velocities are between 20-30 cm·s-1 (Serrano et al., 2020).
The cliffs and marine bottoms along the rocky coastlines between Calahonda and Castell de Ferro towns constitute the Special Area of Conservation (SAC) “Acantilados y Fondos Marinos de Calahonda-Castell de Ferro” (Cliffs and Marine Bottoms of Calahonda-Castell de Ferro) (ES6140014) since 2015 (Mateo-Ramírez et al., 2021). The SAC covers an area of ca. 9 km2 between the coastline and ca. 65 m water depth, and contains some littoral habitats, e.g., sandbanks (Habitat Code [HC] 1110 from the EU Habitat Directive), Posidonia beds (HC 1120), large shallow inlets and bays (HC 1160), reefs (HC 1170), and submerged or partially submerged sea caves (HC 8330) (Mateo-Ramírez et al., 2021). Knowledge of the habitats and associated communities of bathyal areas where the three studied canyons are located is very scarce, except for general studies on fish, decapod and mollusc assemblages of the northern Alboran Sea (Abelló et al., 2002; García-Ruiz et al., 2015; Ciércoles et al., 2018; Ciércoles et al., 2022).
The beaches located along the coast of the northern Alboran Sea exhibit high plastic item percentages (ca. 88% on the coast of Málaga and ca. 80% on the coast of Almería) (Ministerio para la Transición Ecológica y el Reto Demográfico (MITECO-DGCM), 2021). Inland the study area, most litter is composed of plastics in Carchuna beach (Figure 1D): 62.4% of >50 cm size items and 80.9% of <50 cm size items. In the vicinity of the study area, Motril beach (Figure 1D) exhibits a microplastic concentration of ca. 32 particles·kg-1 (Godoy et al., 2020). In addition, the massive use of agricultural activities (greenhouses and farming) along the coast in the study area can generate large amounts of waste that are not always managed correctly, and can undoubtedly lead to greater pollution of beaches (Junta de Andalucía. Consejería de Medio Ambiente, 2019). The Port of Motril accounts for 5.3% of artisanal vessels of the total number of vessels (318) along the northern coast of the Alboran Sea (Baro et al., 2021). In the Port of Motril, the most relevant fishing techniques are bottom trawl (67.1%), artisanal activity (21.7%), purse seine (10%) and surface longline activity (1.2%) (Baro et al., 2021).
Recent datasets, submarine imagery and sediment samples were collected during the ALSSOMAR-S2S multidisciplinary expedition carried out from 29 August to 19 September 2019 on board the RV “Sarmiento de Gamboa”. The bathymetric, coastal uses, and fishing activity data were acquired from different Spanish governmental sources.
Acquisition of the optical submarine imagery was carried out with the Remotely Operating Vehicle (ROV) Luso (EMEPC—Portuguese Task Group for the Extension of the Continental Shelf) during the ALSSOMAR-S2S oceanographic cruise (2019). The ROV Luso was equipped with an Argus HD-SDI 1/3” standard definition video camera. The high-resolution photos and video footage obtained amount to a total of 70 hours of video recording. The ROV Luso was equipped with two parallel laser beams at a fixed width of 62 cm that provided a reference scale to measure targets during subsequent video analysis. Besides, the ROV was equipped with an ultra-short baseline positioning system (USBL) to ensure detailed records of the ROV tracks where the device moved at an average speed of 0.3 knots.
Eleven submarine video dives explored almost 18 km track length of the seafloor in the Motril, Carchuna, and Calahonda canyons (Figure 1C; Table 1). Two dives covering a total length of ~5 km were conducted in the upper reaches of the Motril Canyon along the thalweg and the western flank at a water depth range of 106-388 m (Table 1). Seven dives covering a total length of ~10 km were conducted in the Carchuna Canyon in a 107-740 m water depth range (Table 1). Finally, two dives covering a total length of ~3 km were conducted in the upper reaches of the Calahonda Canyon along its eastern flank and thalweg in the 127-324 m water depth range (Table 1).
Table 1 Summary of ROV dives used in this study including their water depth range, length, the amount of litter detected, and litter density recorded in each dive.
The submarine imagery was used to characterize and quantify marine litter type and density, and to analyze the potential impact of litter on the benthic habitats in the three submarine canyons. The length of the ROV dives was calculated and the total number of items for each dive was converted and quantified as linear litter density, in items·100 m-1. Subsequently, the identified marine litter was georeferenced in ArcGIS™. Marine litter classification was based on the protocol of the Guidance on Monitoring of Marine Litter in European Seas put forth by the Technical Subgroup on Marine Litter (MSFD) (European Commission of the European Union, 2013). A standardized litter classification considers five main categories of material (Table 2): plastic, metal, rubber, glass/ceramics, and textiles/natural fibers; and four additional categories in the Mediterranean Sea: wood, paper/cardboard, other, unspecific.
Table 2 Litter categories of the Guidance on Monitoring of Marine Litter in Mediterranean Sea (European Commission of the European Union, 2013).
Seafloor habitats displaying the greatest concentrations of anthropogenic activity were characterized by combining the substrate type and the dominant habitat-structuring species identified in ROV underwater images. Annotations of the type of substrate were made every time a substrate change was detected along the dive transects and in some sedimentary areas, substrate type annotations from the underwater images were calibrated with data coming from grain size analyses from the sediment cores from the push corer and box corer. Six categories of substrates were identified and compared with the surficial sediment samples and grain-size analysis whenever possible (rock, detritic sediment with bioclasts, sand, muddy sand, sandy mud, and mud). The habitat-structuring taxa (mainly megabenthic taxa) and other associated taxa observed in the video dives were ranked using a system to quantify them (0 = absence of species; 1 = 1 individual of that taxa; 2 = 2-5 individuals; 3 = 5-25 individuals; 4 = 25-100 individuals; 5 >100 individuals). Those taxa showing the highest average rank along sections of the same dive were interpreted as dominant, but only the dominant habitat-structuring taxa were selected to determine the biological component of the seafloor habitat type. Habitat characterization and nomenclature was carried out whenever it was possible by combining depth, substrate type and dominant taxa information. In some cases, facies (interpreted as subtypes or varieties) of the same habitat were detected in some of the underwater images. Habitat classification and nomenclature followed the Reference List of Marine Habitats located in Spain (Templado et al., 2013), which follows a similar hierarchical habitat classification scheme to the EUNIS pan-European habitat identification system from the European Environment Agency. Whenever possible, some habitat types were also linked to the habitat classification of the Barcelona Convention and/or the EU Habitats Directive (Council Directive 92/43/EEC).
A total of 25 sediment cores up to 30 cm long were collected during the ALSSOMAR-S2S oceanographic cruise (2019) with a push corer mounted on the ROV and handled by a plier arm (Figures 1D, E). Additionally, 16 sediment cores up to 50 cm long were collected by deploying a box corer from the vessel (Figures 1D, E). Grain size analysis was executed in the uppermost centimeter of 41 sediment cores (Supplementary Table 1) using 10-15 g of dried sediment (60°C) pre-treated with 20% H2O2 to remove organic matter, and sodium hexametaphosphate as a dispersing agent. Samples were wet sieved to separate the coarse fraction (gravel) using a 2 mm mesh size sieve. Particle sizes <2 mm (sand, silt and clay) were determined using a laser diffraction analyzer (Mastersizer 3000, Malvern®). Textural classification of the sediments was based on Folk (1954) ternary diagrams.
A 50 m resolution multibeam bathymetric grid (“Ministerio de Pesca, Agricultura y Alimentación”, Spanish government) was imported in a geographic information system using ArcGIS™ (Figures 1C-E) and QPS Fledermaus™ software to serve as base maps where the rest of the data could be integrated. The multibeam bathymetry acquisition took place in 2002 during cruise Alborán-03 aboard the R/V Vizconde de Eza in the framework of the project entitled Fishing Charts of the Mediterranean (CAPERMA), carried out by the Spanish Institute of Oceanography (IEO) and the General Secretary of Maritime Fisheries (SGPM). These surveys were carried out using a Simrad EM 300 multibeam echosounder in conjunction with differential GPS and the inertial aided system Seapath 200. The slope parameter was calculated from the bathymetric grid to describe the flanks, lateral deposits and axial channels of the studied submarine canyons. The terminology defined by Paull et al. (2013) was used to describe the major geomorphic features of the studied submarine canyons (Figure 2).
Figure 2 Geomorphologic map of the Motril, Carchuna and Calahonda submarine canyons showing the segment distribution within each canyon and the texture of surficial sediment samples. Bathymetric contours in meters. See Supplementary Table 1 in which location and depth of the sediment cores sampled for grain size analysis are included.
Land use data regarding the coastal plain adjacent to the study area (“Consejería de Agricultura, Ganadería, Pesca y Desarrollo Sostenible, Junta de Andalucía”, regional government) were mapped in ArcGIS™. These datasets extend from the Guadalfeo River delta to the east of Calahonda town and cover an extension of 8706 ha. Eleven land uses were considered for classification purposes: beaches, forest, farming, scrubs and pastures, wet areas, areas with low vegetation coverage, greenhouses, roads, industrial infrastructures, mining extraction, and urban. These data were analyzed by calculating the area of each individual use and the minimal distance from each use to the coastline in order to help us to explain the origin of marine litter.
A maritime traffic density map of the number of routes·km-2 in the study area for the year 2018 was downloaded from the MarineTraffic© website (www.marinetraffic.com). This website provides information about the current geographic positions of ships, ship characteristics, and weather conditions, among other data. This map was imported and georeferenced in the ArcGIS™ project to describe the position of the ships and the major maritime routes in the study area.
Vessel Monitoring System (VMS) data of the main fishing fleets operating in the study area were assessed for the period 2018-2019 (“Ministerio de Pesca, Agricultura y Alimentación”, Spanish government). The VMS database covers the geographic position, date, time (approximately every two hours), and instantaneous velocity of each boat. The VMS data included separate data organized by fishing gear of each fleet type, including vessels with licenses for a single gear. Fishing fleets in the study area include artisanal fishing boats, bottom trawling, purse seine, and longline fishing boats. For this study, the fishing activity could only be analyzed for bottom trawling, purse seine, and longline fishing boats, as VMS data were not available for the remaining fishing fleets. The three studied fishing fleets are responsible for the largest landings in the Port of Motril, which is the main one in the study area.
This VMS database was imported and georeferenced in the ArcGIS™ project to describe the fishing activity along the studied canyons. The fishing activity in the study area was quantified in five sectors, from west to east: Western Sector, the Motril Canyon, Carchuna Canyon, Calahonda Canyon, and Eastern Sector. Depth ranges within each sector included the shelf (0-100 m water depths), shelf edge and uppermost slope (100-200 m water depths), upper slope (200-500 m water depths), and middle slope (below 500 m water depth). The fishing effort was estimated as the average number of fishing days per km2 in 2018 and 2019.
The shelf in the study area is up to 3 km wide, narrowing due to the occurrence of shelf-incised canyon heads (Figures 1, 2). Shelf surface sediments vary from coarse silts close to fine sands on the shelf edge (Figure 2).
Three geomorphological segments were identified in the Motril Canyon. The upper segment is defined by a 4.3 km wide amphitheater-like canyon head incised in the outer shelf and upper slope. The canyon head is located 2 km off the coastline and connects to a 950 m long and 140 m wide short and straight shelf channel (Figures 1C, 2). The canyon valley is bounded by gentle flanks (ca. 3°), and it widens and increases in sinuosity downward to 400 m water depth. Surface sediment grain size decreases down the canyon thalweg, from very fine sands to medium silts (Figure 2). The middle segment is characterized by an abrupt change in the direction of the valley from N-S to NW-SE down to 500 m water depth. The canyon valley shows a sinuous path with a gentle south-western flank (ca. 2°), whereas the north-eastern flank is steeper (ca. 8°) (Figure 2). The lower segment is characterized by a meandering channel limited by steep walls with continuous adjacent deposits over the slope.
Two geomorphological segments were identified in the Carchuna Canyon. In the upper segment, the canyon is deeply incised in the shelf, as its head is located just 200 m off the coastline. It is characterized by a 100 m wide narrow axial channel with large steep flanks (Figures 1C, 2). The straight canyon increases its width up to 430 m water depth and it is bounded by a narrow and steep eastern flank and large terraces in the western flank. The axial channel in the upper segment is characterized by fine sands in the canyon head that change downslope to coarse silts, while the flanks are characterized by fine sands (Figure 2). The landward tip of the lower segment is marked by an abrupt change of orientation of the canyon from NE-SW to WNW-ESE. This lower segment has a straight and wide valley (up to 550 m) bounded by steep walls (ca. 20°) and continuous lateral slope deposits. Grain sizes in the lower segment range from very fine sands in the axial channel to medium silts in lateral deposits (Figure 2).
Two geomorphological segments were identified in the Calahonda Canyon. In the upper segment, the canyon incises the shelf edge, where the canyon head is located 2.5 km off the coastline (Figures 1C, 2). The canyon head is 150 m wide at the shelf edge and has steep flanks. In the lower segment, the canyon exhibits a meandering pattern and is bounded by smooth flanks. The axial channel is characterized by fine sands in the canyon head that change downslope to medium silts, while the eastern flank is characterized by fine sands (Figure 2).
A total of 454 litter items were observed in the 11 ROV video dives (Figure 3A; Table 1). Plastics predominate (almost 75% of occurrences), and they include bottles, bags, fishing nets, sheets, seedbeds and other objects with sizes from centimeters to meters. Other minor components of marine litter in the studied canyons include metal items, building material, and rubber items (Figures 3A, 4). Overall, the marine litter is partially buried, covered by a thin layer of mud and in a degraded state.
Figure 3 Litter composition in the three studied submarine canyons. Percentages of litter items are divided into five litter categories and six plastic subcategories as defined by the Guidance on Monitoring of the Marine Litter in the Mediterranean Sea (European Commission of the European Union, 2013): (A) studied submarine canyons; (B) upper segment of Motril Canyon; (C) upper segment of Calahonda Canyon; (D) Carchuna Canyon; and (E) Carchuna Canyon accumulations. n: total number of litter items counted.
Figure 4 Spatial distribution and composition of marine litter along the canyon thalwegs and flanks of the three studied submarine canyons. The size of the pie charts is proportional to the abundance of litter (expressed as number of items·100 m-1). The maximum litter density (8.66 items·100 m-1) was found in the thalweg of the Carchuna Canyon. Bathymetric contours in meters.
The lowest average abundances of litter were found in the Motril and Calahonda canyons (Figures 3B, 4; Table 1). In the Motril Canyon, the litter density along the thalweg was 0.12 items·100 m-1. A higher litter density (0.52 items·100 m-1) was observed along the western flank of the canyon (Figure 4). Marine litter in the upper segment of the Motril Canyon included plastics (60%), metals (6.67%), and unspecified items (33.33%) (Figures 3B, 4). The litter appeared dispersed, degraded and frequently broken, both in the thalweg (Figure 5A) and the western flank.
Figure 5 Examples of litter items observed during ROV dives in the Motril, Calahonda, and Carchuna canyons, and litter accumulations found in Dive 04 along the thalweg of the Carchuna Canyon. Dive number, water depth and relative location within the canyons (T, canyon thalweg; F, canyon flank) are indicated. (A) Plastic bag (arrow) on the thalweg of the Motril Canyon. (B) Two concrete blocks used in longline fishing as weights, colonized by serpulid polychaetes and N. cochlear aggregations, together with two P. bogaraveo individuals as well as a soda can and small plastic fragments on the thalweg of the Calahonda Canyon. (C) A filled plastic bag (used as weight in longline fishing) on rocks colonized by unidentified colonial scleractinians, serpulid polychaetes and Ophiotrix sp., together with individuals of the black-spot seabream Pagellus bogaraveo (one of the target species of longline fishing). (D) Two bricks colonized by serpulids on the eastern flank of the Carchuna Canyon, with some fishes (Serranus cabrilla and Capros aper). (E) Sunbed over the seafloor on the thalweg of the Carchuna Canyon. (F) Accumulation characterized by a large number of bottles. (G) Accumulation composed of bottles, bags and fishing nets blocked behind a seafloor rocky outcrop. (H) Accumulation with bricks, a broken sheet, various bags and bottles, fishing nets, a metal tube and diverse plastic items, some of them colonized by N. cochlear, but also providing shelter to the greater forkbeard Phycis blennoides and Munida sp. B, Burrows made by decapods; C, Caryophyllia sp.; Ca, Capros aper; Mu, Munida sp.; Nc, Neopycnodonte cochlear; Op, Ophiothrix sp.; P, Pagellus bogaraveo; S, Scleractinians; Sc, Serranus cabrilla; Se, Serpulid polychaetes.
In the Calahonda Canyon, litter density was higher along the thalweg (1.13 items·100 m-1) than in the eastern flank (0.19 items·100 m-1) (Figure 4; Table 1). Marine litter in the Calahonda Canyon was composed of plastics (almost 70%), metals, building material, and unspecified items in the upper segment of the canyon (Figures 3C, 4). Marine litter generally occurred as 2-5 item accumulations (Figure 5B) or dispersed along the thalweg and eastern canyon flank. Marine litter on the seafloor was found degraded and partially buried.
The highest litter abundance was detected in the Carchuna Canyon (Figures 3D, 4; Table 1). Litter densities along the flanks of the upper segment varied between 0.35 and 2.3 items·100 m-1. The highest litter density was detected along the thalweg in the upper canyon with 8.66 items·100 m-1. In contrast, the lowest densities of marine litter occurred in the lower canyon up to 0.35 items·100 m-1 in the thalweg and the lateral deposits (Figure 4). In the Carchuna Canyon, marine litter was made up of plastics (almost 76%), metals, building material, rubber, and unspecified items (Figures 3D, 4). Plastic items mainly included bottles, fishing nets, bags, sheets, and seedbeds (Figures 3D, 4). Marine litter on the flanks was composed of plastics such as fishing nets (Figure 5C), and dispersed and degraded building items (Figure 5D). In the lower canyon, marine litter was largely composed of very degraded and buried plastic items.
In the Carchuna Canyon, marine litter mainly occurred as large accumulations or litter hotspots of up to 6 m2 (Accumulations 17, 18, and 20) in extent or containing up to 42 items·m-2 (Accumulation 19) (Table 3) that alternate with isolated items (Figure 5E). Three different types of accumulations were observed along the canyon thalweg: (1) marine litter accumulated in smooth canyon floor depressions (Figure 5F); (2) marine litter accumulated by rocky floor blocking (Figure 5G); (3) marine litter accumulated by tangling of fishing nets (Figure 5H). These accumulations were composed of plastics (70%), metals, building material, and unspecified items (Figure 3E) and they were detected along the canyon thalweg between 339-493 m water depths (Figure 6). Overall, the marine litter accumulations appeared degraded and partially buried.
Table 3 Characterization of litter accumulations found in the Carchuna Canyon in terms of extent, item density and number of items.
Figure 6 Zoomed-in bathymetric map of the study area (“Ministerio de Pesca, Agricultura y Alimentación”, Spanish government) showing the location of the litter accumulations found in the Carchuna Canyon. Bathymetric contours in meters.
Ten straight, regular, and continuous bottom trawling marks were detected on the seafloor of the studied canyons: six in the Motril Canyon (370-180 m water depths), two in the Carchuna Canyon (450-400 m water depths), and two in the Calahonda Canyon (290 m water depth) (Supplementary Table 2). These marks were more frequent in the thalweg and the western flank of the Motril Canyon, but they were also detected in the thalwegs of Calahonda and Carchuna canyons (Figures 7A, B). Some bottom trawling marks exhibited a well-defined groove having straight marks with an extensive and lateral continuous mound (Figure 7A). In some places, irregular broken fragments of organisms were observed over the muddy seafloor of the grooves (Figure 7A). Other mark types were smoother, comprising various parallel lines with straight margins, which may correspond to marks of the weights attached to the bottom part of the opening of bottom trawling nets (Figure 7B).
Figure 7 Examples of seafloor trawling marks observed during ROV dives along the flank of the Motril Canyon (A) and the thalweg of the Calahonda Canyon (B), and benthic and demersal fauna detected in Motril (C), Calahonda (D) and Carchuna (E-G) canyons. (A) Seafloor groove made by the otter board of a bottom trawling gear with broken fragments (red arrow) and a lateral mound (white arrow) on the western flank of the Motril Canyon. (B) Seafloor groove made by the otter board of a bottom trawling gear on the thalweg of the Calahonda Canyon. The bottom trawling marks seen on video footage collected by the ROV in the three submarine canyons is shown in Figure 10D. (C) The soft octocoral Alcyonum palmatum. (D) Aggregation of the cerianthiid Cerianthus sp. (E) Muddy bottoms with decapod burrows and the sea-pen Funiculina quadrangularis, with a detail of its polyps. (F) The colonial scleractinian Dendrophyllia cornigera providing shelter to the ophiurid Ophiothrix sp. (G) Aggregation of unidentified colonial scleractinians, together with solitary Caryophyllia sp. and individuals of the echinoid Cidaris cidaris and the ophiuroid Ophiothrix sp. Ap, Alcyonum palmatum; B, Burrows made by decapods; Ca, Caryophyllia sp.; Cc, Cidaris cidaris; Ce, Cerianthus sp.; Dc, Dendrophyllia cornigera; Fq, Funiculina quadrangularis; Op, Ophiothrix sp.; Pl, Plesionika sp.; S, unidentified colonial scleractinians.
A total of 13 habitat types have been detected in the 11 ROV video dives, however, 6 of those habitats may represent facies of the detected habitats but occur at differents depths (e.g., Circalittoral and bathyal detritic bottom vs. Bathyal detritic bottom with bivalve and cold-water coral remains) and sediment types (e.g., Bathyal muddy sand and sandy mud bottom with burrowing megafauna vs. Bathyal sandy silt bottom with burrowing megafauna). The detected habitats ranged from highly complex ones with a high biogenic component (e.g., Bathyal rocky bottom with scleractinians) to habitats with low complexity and low biogenic component (e.g., Bathyal sandy mud bottom with burrowing megafauna) (Figures 7, 8). Regarding the main habitats detected, the most common ones in the explored canyons (based on their length along the analysed video transects) were: (1) bathyal muddy sand and sandy mud bottom with burrowing megafauna (including the different facies) (Total Length = 8420 m, %Length of all habitats = 50.4%); (2) circalittoral and bathyal sandy silt bottoms with alcyonaceans (mainly dominated by Alcyonum palmatum) (2964 m, 17.7%) (Figure 7C); (3) bathyal sandy silt bottoms with ceriantharians (1739 m, 10.4%) (Figure 7D); (4) bathyal sandy mud and muddy sand with ceriantharians and Funiculina quadrangularis (mainly dominated by Cerianthus spp.) (1279 m, 7.7%) (Figure 7E); and (5) circalittoral and bathyal detritic bottom (1154 m, 6.9%). Data on the length of the different habitats and their facies as well as the percentage of the habitat length within each dive and submarine canyon are given in Figure 8, together with annotations of the main dominant taxa of each habitat.
Figure 8 Types and length (in ROV underwater image transects) of habitats detected along the Motril (A), Calahonda (C), and Carchuna (E) canyons, with annotations of the dominant taxa and mean water depth (blue dotted line) of each habitat. Litter density and types of litter and other anthropic activity indicators along the Motril (B), Calahonda (D), and Carchuna (F) canyons is also shown. Stars indicate the presence of seafloor marks from bottom trawling in a particular habitat.
The habitats that displayed the highest average densities of anthropogenic activity indicators when combining all density values obtained in the same habitat at different canyons and sectors of the canyon (i.e., the 18 km of survey track) were (Figure 8): (1) bathyal detritic bottoms (occurring at the head of the three canyons, ca. 100-200 m water depths) (average 2.14 items·100 m-1), which mainly contained plastics and fishing nets; (2) bathyal sandy mud and muddy sand bottoms with burrowing megafauna (generally in the thalwegs of the studied canyons, ca. 200-500 m water depths) (2.13 items·100 m-1) affected by plastics, bottom trawling marks and fishing nets, among others; and (3) bathyal rocky bottoms with scleractinians (western flank of the Carchuna Canyon head, 193-222 m water depths) (2.1 items·100 m-1) that mainly contained bags (Figures 5C, 7F, G, 8). In contrast, the habitats that displayed the lowest average densities of anthropogenic activity indicators were (Figure 8): (1) bathyal muddy sand and sandy mud bottoms with alcyonaceans (at the flanks of the three canyons, 100-250 m water depths) (average 0.44 items·100 m-1), mostly containing plastics, bottom trawling marks, cables, and fishing nets; (2) bathyal sandy mud and muddy sand bottoms with large echinoderms as well as bathyal sandy mud and muddy sand bottoms with ceriantharians and Funiculina quadrangularis (deep part of the Carchuna thalweg, 630-730 m water depths) (0.34 items·100 m-1) mainly containing plastics; and (3) bathyal sandy mud bottoms with ceriantharians (Motril and Calahonda canyon thalwegs, 273-377 m water depths) (0.15 items·100 m-1) with plastics and bottom trawling marks (Figures 5, 7, 8).
Prevailing land uses along the coasts adjacent to the study area (approximate area of 8706 ha) are agricultural (ca. 48%): farming (ca. 34%) and greenhouses (ca. 13%); scrubs and pastures (ca. 25%); and urban (ca. 8%) (Figure 9A). Motril Town is located 2 km north of the coastline, with a population density of 567.68 people·km-2 (“Instituto de Estadística y Cartografía de Andalucía, Junta de Andalucía”, regional government) and at least 83% of the population residing in the town. Motril Town is surrounded by pastures, industrial infrastructures and greenhouses and crops that extend up to the coastline.
Figure 9 Depiction of coastal uses and maritime traffic in the study area. (A) Mapping of main land uses in the adjacencies of the three studied submarine canyons (“Consejería de Agricultura, Ganadería, Pesca y Desarrollo Sostenible, Junta de Andalucía”, regional government). The area and the minimum distance to the coastline of each land use are shown in the legend. (B) Density (number of routes·0.08 km-2·y-1) of maritime traffic in 2018 (www.marinetraffic.com) with the drawing axial channel of the three studied submarine canyons. Bathymetric contours in meters.
The Motril Canyon head is located 2 km offshore the Port of Motril. The Carchuna Canyon head is located off a small bay limited laterally by beaches, greenhouses and Torrenueva and Carchuna towns. The Calahonda Canyon head is located 2.5 km off the beach of Calahonda Town. The coastline close to the studied canyons is mainly composed of gravel beaches interrupted by the Port of Motril (200,000 m2) and its surrounding industrial area, some greenhouses (mostly concentrated between Calahonda and Carchuna towns) and urban land uses (Torrenueva, Carchuna and Calahonda towns). The population density in Torrenueva Town is 432 people·km-2 while Carchuna and Calahonda towns have lower densities (72 and 53 people·km-2, respectively) (“Instituto de Estadística y Cartografía de Andalucía, Junta de Andalucía”, regional government). Urban land uses on the coastline are typical of a touristic area, with summerhouses and various commercial uses, absorbing a duplication in population during summer that causes a triplication of urban litter (Ayuntamiento de Motril. Medio Ambiente Urbano, 2021).
The Port of Motril is an important commercial and fishing infrastructure offering freight and passenger traffic between Spain and northern Africa. The port has four maritime connections crossing the Alboran Sea (Figure 9B): (1) a south-southeast route that crosses the Carchuna Canyon towards Melilla and Nador cities; (2) a south-west route that crosses the Motril Canyon head towards the Port of Tanger Med; (3) a south-southwest route that crosses the upper segment of the Motril Canyon towards the Port of Alhucemas; and (4) an east route that crosses the Carchuna Canyon head towards the Mediterranean Sea. Additional straight and short routes with north-south and west-east orientations along the slope can be identified in the study area.
The fishing fleet of Motril Port comprised ca. 53 fishing boats in 2019 (from the 640 fishing boats operating in GSA1- Northern Alboran Sea fishing area). The main fishing fleets were artisanal fishing boats (ca. 50%) using set gillnets, trammel nets and traps, followed by bottom otter trawling (ca. 26%), purse seine (ca. 22%), and set longline (ca. 2%) fishing boats.
The spatial distribution of purse seine fishing, bottom trawling, and longline fishing displayed contrasting patterns (Figure 10). Purse seine fishing was mainly detected on the shelf outside the canyons (Figures 10A, B). Bottom trawling was higher in the shelf areas affected by the submarine canyons and in the upper slope of the Motril and Calahonda canyons (Figures 10C, D), where bottom trawling marks were identified (Figure 10D; Supplementary Table 2). Longline fishing showed a very low number of records due to a low number of boats and their highly seasonal trend (mainly in summer and autumn), but the highest activity was detected on the shelf close to the Motril Canyon head (Figures 10E, F).
Figure 10 Data from Vessel Monitoring System during 2018-2019 (“Ministerio de Pesca, Agricultura y Alimentación”, Spanish government). Spatial distribution of purse seine fishing fleets (A), bottom trawling (C) and longline fishing (E). Fishing effort (in days·km-2) for purse seine fishing (B), bottom trawling (D) and longline fishing (F). The bottom trawling marks seen on video footage collected by the ROV in the three submarine canyons is shown (D). See Supplementary Table 2 to location and depth of the bottom trawling marks and Figures 7A, B for examples of seafloor trawling marks along the flank of Motril Canyon and the thalweg of Calahonda Canyon. OCW, Western sector of the study area; M, Motril Canyon; CC, Carchuna Canyon; CH, Calahonda Canyon; OCE, Eastern sector of the study area; E1: Shelf; E2: Outer shelf-uppermost slope; E3: Upper slope; E4: Middle slope. Bathymetric contours in meters.
Gillnet fishing boats operated at 0-100 m water depths. Cadufo fishing boats generally operated at ca. 50 m depth. Crab pot fishing boats operated in upper and middle slope areas are different from those used by bottom trawling boats. Some of these areas could be located within the submarine canyons, where large numbers of individuals of the main target species —the deep-water pandalid shrimp Plesionika edwardsii— were detected in the underwater images.
In the study area of the Northern Alboran Sea, average values of marine litter density in the Motril, Calahonda and Carchuna canyons (0.32, 0.66, and 1.8 items·100 m-1, respectively) are lower than most of the values found in Mediterranean canyons (Table 4), where noteworthy amounts of marine litter have been reported, particularly as large accumulations or litter hotspots along the thalwegs (e.g., Pham et al., 2014b; Tubau et al., 2015; Pierdomenico et al., 2020). The values of marine litter found in Italian, Calabrian and Sardinian canyons are much higher than values found in Spanish and French canyons. Litter density values in the studied canyons are in the range of the values found in the Portuguese canyons (0.083-3.31 items·100 m-1; Mordecai et al., 2011; Oliveira et al., 2015), French Atlantic canyons (0.32-0.86 items·100 m-1; van den Beld et al., 2017), and French Mediterranean canyons (0.5-1.3 items·100 m-1; Fabri et al., 2014) (Table 4). Within the study area, the upper segment of the Carchuna Canyon displayed a relatively high litter density (8.6 items·100 m-1), which is similar to the values registered in the Cap de Creus Canyon off the Catalan coast (up to 8.6 items·100 m-1; Tubau et al., 2015) (Table 4).
Table 4 Values of density of marine litter reported in linear measurements in different submarine canyons of the Atlantic Ocean and the Mediterranean Sea.
The study area has a population density of up to 567 inhabitants·km-2, and over 80% of the population around the study area is located 2 km inshore from the coastline. Such population density is low in comparison with other areas featuring submarine canyons close to the coastline which exhibit higher densities of marine litter. For example, the heads of the Blanes, La Fonera and Cap de Creus canyons are located on the Catalan margin with a coastal population density of up to 1091 inhabitants·km-2, and exhibit marine litter densities of up to 18 items·100 m-1 (Tubau et al., 2015). As an extreme case, the Messina Strait and Gioia canyons have the highest densities of marine litter in the Mediterranean Sea (up to 56.3 items·100 m-1; Pierdomenico et al., 2020; Table 4), and exhibit a coastal population density of up to 800 inhabitants·km-2. As high densities of marine litter generally display a strong correlation with the proximity of canyon heads to densely populated coasts (Hernandez et al., 2022; Taviani et al., 2023), we interpret that in the study area the relatively low amounts of litter found in the studied canyons are primarily determined by low coastal population densities.
Another factor that needs to be considered in explaining marine litter densities is the connectivity of submarine canyons to fluvial streams. The studied canyons are not directly connected to fluvial systems, and the nearby creeks exhibit very reduced dimensions and seasonal patterns (Liquete et al., 2005; Liquete et al., 2009). In contrast, very high litter densities have been registered in shelf-incised submarine canyons with heads connected to fluvial systems or with subaerial streams, for example, up to 130 items·100 m-1 in the Messina Strait canyons (Pierdomenico et al., 2019). Therefore, the relatively low litter densities detected in the studied canyons are interpreted as the result of a combination of the low coastal population densities and the lack of connection of canyon heads with major streams (Figures 1C, 2). These regional conditions broadly resemble those of canyons located off the Catalan coast (Table 4), which incise narrow shelves and generally have heads near the coast, where population densities are relatively low and seasonally variable (Tubau et al., 2015).
The proximity of the Carchuna Canyon head to the coastline (<200 m) implies that the litter density in this canyon is considerably higher than in the Motril and Calahonda canyons, located >2 km from the coastline. This observation agrees with reports from other Mediterranean canyons, where the highest marine litter densities occur in shelf-incised submarine canyons with canyons dissecting the entire shelf (e.g., Calabria coast; Pierdomenico et al., 2020), or in shelf-incised canyons located in very narrow shelves (e.g., Sardinia western coast; Cau et al., 2017). In contrast, the lowest values of marine litter density occur in canyons incised in wide shelves and distant from the coastline (e.g., Gulf of Lion; Fabri et al., 2014). Therefore, we also suggest that differences in marine litter densities observed among the three studied submarine canyons should mainly reflect the distance between canyon heads and the coastline.
The transport and/or accumulation of litter along submarine canyons is considered to be determined by two major factors, downcanyon gravity flows (Dominguez-Carrió et al., 2020; Angiolillo et al., 2021; Zhong and Peng, 2021), and canyon geomorphological settings (Gerigny et al., 2019; Mecho et al., 2020; Pierdomenico et al., 2020).
The identification of litter hotspots along the upper segment of the Carchuna Canyon, the identification of marine litter along the thalweg in the lowest segment, even at the termination of the canyon (740 m water depth), and the occurrence of very fine sands in the lower segment canyon floor, are regarded as compelling evidences of the occurrence of deep transport by gravity flows along the Carchuna Canyon thalweg. Such sediment transport pulses in this canyon may be driven by downslope bottom flows, described during easterlies dominance (Serrano et al., 2020). Therefore, we consider that the Carchuna Canyon is an active system —it acts preferentially as a conduit, favouring the transport of marine litter from the deeply incised shelf (25 m water depth) to the termination of the channel in the transition towards a distal lobular deposit (740 m water depth) (Figure 1). In contrast to the Carchuna Canyon, evidences of gravity flow activity in the Motril and Calahonda canyons are lacking. Instead, the low densities of mainly buried litter by muddy sediments in those canyons can be interpreted as evidence that the canyons have behaved as sinks of marine litter along their thalweg and flanks, possibly because of their relatively high distance from the coastline.
The geomorphological setting of the Carchuna Canyon seems also to have played a role in litter transport and/or accumulation in two ways, inducing the generation of (1) the above-mentioned gravity flows, and (2) litter accumulations along its course. On the one hand, the fact that the canyon head is located very close to the coast has been previously related to energy concentration in the canyon head due to coastal waves and storm focusing (Ortega-Sánchez et al., 2014). Those processes have been observed in other canyon heads close to coastlines, where they lead to increased shear stress that frequently mobilizes coarse-grained sediments (Smith et al., 2018). A similar situation is plausible in the Carchuna Canyon head, where energy concentration in the canyon head could erode proximal sandy infralittoral prograding wedges (IPW; Figure 1D) defined along the coastline, providing a coarse-grained sediment source for the development of gravity flows.
On the other hand, litter hotspots are identified in the upper segment of the Carchuna Canyon favoured by geomorphological features such as smooth seafloor depressions and rocky outcrops blocking the thalweg (Figures 5F-H, 6; Table 3). We consider that those evidences indicate the generation of litter sinks in the Carchuna Canyon, as evidenced elsewhere (Galgani et al., 1996; Galgani et al., 2000; Watters et al., 2010; Miyake et al., 2011; Schlining et al., 2013). In canyon depressions, litter accumulations result from changes in the morphodynamic conditions of bottom currents (Carvajal et al., 2017; Zhong and Peng, 2021), whereas rocky bottoms may act as obstacles to litter items carried by gravity flows (Galgani et al., 2000; Watters et al., 2010; Schlining et al., 2013).
Marine litter in the studied submarine canyons is overwhelmingly dominated by plastics, accounting for 75% of the total litter (Figures 3, 4). This value is similar to the plastic percentages registered in most Mediterranean canyons (Table 4), and suggests a dominant coastal origin (at least 50%) in the studied canyons. Of the different coastal uses in the study area, the influence of coastal recreational uses linked to beaches and coastal urbanization would appear to be outstanding. In fact, most of the coastal fringe at both sides of the Carchuna Canyon head is delineated by several kilometers long beaches, such as Motril and Carchuna beaches (Figure 9A). Although these beaches are periodically cleaned, because they are deemed suitable for tourism, abundant litter can be found on them (Godoy et al., 2020; Martín-Lara et al., 2021; MITECO-DGCM, 2021). Therefore, deficiencies in waste management in the beaches of the study area can increase the total amount of marine litter that can be eventually trapped by the canyons. The fact that 35% of marine litter in the studied canyons is made up of plastic bottles and bags constituted compelling evidence for coastal recreational origin. Several metal items, including beverage and food cans, can also be unequivocally attributed to beach occupation.
Almost 8% of marine litter in the study area consists of plastic sheets connected with remote pieces of greenhouses infrastructures, and seedbeds derived from agricultural practices. Both plastic types can be linked to the greenhouses and farming exploitations in the adjacent coastal plain, the main land use in the coastal domain surrounding the beaches (ca. 48%). Specifically, greenhouses are widespread in the backshore of Carchuna Beach, which is closer to the Carchuna Canyon head, whereas greenhouses in the backshore of Motril Beach are more scattered and they alternate with the farming land uses that are located backshore between the Guadalfeo River and Torrenueva town (Figure 9A). This interpretation is supported by several additional evidences that attest to the occurrence of litter derived from agricultural practices on the northern coast of the Alboran Sea. For example, microplastics found by Godoy et al. (2020) on the beaches of the coast of Granada are low-density polyethylene, high-density polyethylene, polypropylene, and high-strength polystyrene, all common plastic types used in agriculture. In addition, whales consuming greenhouse marine litter items have been found off Almeria and Granada coasts, which are severely occupied by the greenhouse industry (de Stephanis et al., 2013).
Finally, we consider that sporadic inputs of litter from creeks mainly draining farming areas (Figure 9A) would provide marine litter from both urban and agriculture uses due to the torrential behaviour of the ravines close to the Carchuna Canyon head (Liquete et al., 2005). The coast of the Carchuna Canyon head is moreover affected by long-term erosion associated with extreme events (Ortega-Sánchez et al., 2014) and by relatively high current velocity values, causing high values of shear stress (Bárcenas et al., 2011); both processes would favour the transport of marine litter to the Carchuna Canyon head. In contrast, fluvial influence should be minor in the Motril Canyon head, as this submarine feature is relatively far (i.e., several kilometers) from the Guadalfeo River mouth; in any case, the lateral redistribution of Guadalfeo River sediment plumes under westerlies dominance (Bárcenas et al., 2011) could eventually lead to moderate amounts of marine litter from urban uses, considering the occurrence of several urban areas in the vicinity of the Port of Motril.
Submarine canyons can also locally receive human discards from ships if they are located along shipping routes (Stefatos et al., 1999; Wei et al., 2012; van den Beld et al., 2017; Morales-Caselles et al., 2021). In the study area, marine litter such as sunbeds and pipes found in the upper segment of the Carchuna Canyon can be attributed to shipping traffic because of the large size of these items and the fact that the canyon segment is crossed by a south-southeast shipping route (Figure 9B).
The high number of unspecified items found in the Motril Canyon makes it difficult to discern the origin of marine litter. However, the marine litter found in the upper segment of the Motril Canyon coincides with a high concentration of maritime traffic owing to the proximity of the Port of Motril and the junction of both south-west and south-southwest routes above the canyon (Figure 9B). Considering the distance between the Motril Canyon and the coastline (ca. 2 km), we infer that the marine litter in this canyon is mostly marine sourced.
Some evidence suggests the influence of fishing activities in the accumulation of marine litter in the studied canyons, considering that other types of marine debris, such as fishing gear, indicate an origin linked to marine extractive activities (Pham et al., 2014a; Vieira et al., 2015; Hernandez et al., 2022). Indeed, nearly 16% of marine litter is related to fishing activities in the study area, i.e., fishing nets and gear used by the artisanal fishing fleet. In addition, rubber items related to the floating defence of fishing boats and building items that are used as support for longline fishing can be found.
Fishing activity apparently produces a differential effect on the studied canyons, due to their distinctive geomorphological and sedimentological characteristics. The upper and middle segments of the Motril Canyon and the entire Calahonda Canyon contain smooth flanks and muddy bottoms that favour fishing activities; this is reflected by abundant evidence of high fishing efforts by bottom trawling, purse seine and longline fishing in those canyons (e.g., numerous marks by bottom trawling in the upper segment of the Motril Canyon; Figures 7A, B, 10D). However, in the Motril and Calahonda canyons, no marine litter derived from fishing activities (e.g., fishing nets) was observed. This likely indicates an efficient sediment burial and subsequent alteration in muddy environments. In contrast, fishing effort, especially bottom trawling, is lower in the Carchuna Canyon (Figure 10D) owing to the abrupt seafloor morphology, featuring very steep flanks and the local occurrence of rocky outcrops. These seafloor features might favour purse seine and longline fishing practices in the upper segment of the Carchuna Canyon. This is reflected by abundant evidence of high fishing efforts of purse seine and longline fishing (Figures 10B, F). In addition, it is evidenced by the high number of fishing nets, with several building and rubber items related to the fishing activities, found along both flanks and thalweg of the upper segment of the Carchuna Canyon (Figures 4, 11). Indeed, the tangling of fishing nets along the canyon thalweg favours the formation of litter hotspots (Figure 5H).
Figure 11 Chart showing the ranges of fishing effort (days·km-2) for each of the Carchuna Canyon sections (see Figure 10). Litter density (expressed as number of items·100 m-1), and litter composition (as pie charts) are also shown. T, canyon thalweg; F, canyon flank; D, lateral deposit.
The interaction of anthropogenic activities with marine habitats, and especially with certain species, may entail both positive and negative effects (Katsanevakis et al., 2007; Gregory, 2009; Browne et al., 2015; Deudero and Alomar, 2015). Such effects were identified in the study area. Among the positive ones, some litter items provide a hard substrate and refuge for specific sessile (e.g., the bivalve Neopycnodonte cochlear, hydrozoans) and mobile species (e.g., the decapod Munida sp., the greater forkbeard Phycis blennoides) on the sedimentary bottoms of the studied canyons (Figure 5H). The role of some types of litter as artificial hard substrates for sessile species is widely known —particularly floating objects that may act as vectors of dispersion for some species, including indigenous invasive species (Thiel and Gutow, 2005). Among the species settling and growing on these artificial substrates (e.g., N. cochlear), some are generally considered ‘opportunistic’ and may represent pioneering species in the colonization of new natural substrates in circalittoral and bathyal bottoms (Sotomayor-García et al., 2019). The provision of refuge made by litter for mobile species has scarcely been investigated, although an increase in the total abundance and the number of species in soft bottoms with litter has been linked to litter that created refuge or reproduction sites for mobile species (Katsanevakis et al., 2007). In the submarine canyons studied here, some large pieces of litter served as refuge for squat lobsters Munida spp. or the greater forkbeard Phycis blennoides (Figure 5H). These species seem to benefit from the crevices and complexity provided by litter items, because they play a role similar to that of isolated rocks or coral rubble in sedimentary habitats (Uiblein et al., 2003; Poore et al., 2011; Rueda et al., 2021). The use of litter as a refuge by squat lobsters could be related to the fact that they commonly live in burrows or are associated with large sessile invertebrates, which would influence their resistance to physical pressures and their protection against predation and/or acquisition of food (Trenkel et al., 2007; Poore et al., 2011). In the case of the greater forkbeard, large litter affords cavities for these active station holders with an effective foraging strategy for capturing their main prey, such as epibenthic and benthopelagic crustaceans (Du Buit, 1978; Mauchline and Gordon, 1984; Uiblein et al., 2003).
Negative effects of anthropogenic activities on the seabed are widely known, and may include physical abrasion, damage, entanglement, and/or burial of sessile and mobile species, chemical transfer (including microplastics) to organisms and the food chain, as well as declines of important commercial stocks, among others (Gregory, 2009; Ramirez-Llodra et al., 2011; Browne et al., 2015; Clark et al., 2016; Trestrail et al., 2020). Fishing activity in submarine canyons was described as the main source of impacts on their seafloor and associated biota, whether habitat-forming species or those with important economic value (Puig et al., 2015; Cau et al., 2017; Fernandez-Arcaya et al., 2017; Oberle et al., 2018; Giusti et al., 2019; Paradis et al., 2021). Some of the indirect impacts from bottom trawling are generally caused by the resuspension of shelf (and nearby) fine sediments during the trawling operations and their deposition in the seabed of the underlying canyons, which may cause siltation of some sessile habitat-forming species (Puig et al., 2012; Martín et al., 2014). Indeed, in the analyzed underwater images, some of these benthic species seemed to be silted up in different parts of the canyon. In the present study, the largest average densities of anthropogenic activity indicators were detected in some of the shallowest habitats, including detritic bottoms and scleractinian aggregations (Figure 5C), considered vulnerable habitats with high ecological importance (Bellan-Santini et al., 2007; Rueda et al., 2021). Detritic bottoms host a biocoenosis of great diversity and abundance (Bellan-Santini et al., 2007; Rueda et al., 2021). The biocoenosis is favoured by the mixture of different substrate types, which enhances the substrate heterogeneity and the diversification of organisms and food sources. Despite their unquestionable species richness, detritic bottoms do not benefit from any kind of formal protection other than being included in marine protected areas designated for other components. Perspectives for specific protection are scant because these bottoms are a major target for trawling and because they can be very extensive (Figures 7A, B). Meanwhile, scleractinian aggregations in hard substrates correspond to Habitat 1170 “Reefs” from the EU Habitat Directive (92/43/EEC), and this study detected them in the western wall of the Carchuna Canyon head, together with a high number of cloth bags and lines generally used in local longline fisheries (Figure 5C). Interaction of longline fishing with benthic fauna can be very localized to specific areas but still can cause a severe impact on slow-growing species that provide a high three-dimensional complexity (e.g., aggregations of colonial scleractinians) (Fabri et al., 2014; Tubau et al., 2015; Ragnarsson et al., 2017). Longline fishing might alter the seabed to a lesser extent than bottom trawling, in view of its much narrower bottom mark (Pham et al., 2014a; Fabri et al., 2022). A negative impact may stem from the cloth bags filled with sediments or stones used as weights for the longline fisheries, and which, together with the lines, can easily break the skeletons of the scleractinians, inducing the decline and final loss of the habitat they conform (Fosså et al., 2002; Clark et al., 2016). In this sense, urgent protection measures are needed to preserve this vulnerable habitat structured by colonial scleractinians in the studied submarine canyons. Finally, it is important to mention that this study did not provide information on the potential ingestion of microplastics by the detected taxa. It is known that microplastic ingestion in some organisms (e.g., invertebrates) reduces somatic and reproductive growth (Trestrail et al., 2020). Considering this, further studies should provide information on those taxa that may contain high rates of microplastics in their bodies as well as on the potential negative effects on them.
The detailed analysis of the origin and driving mechanisms of marine litter density and distribution and of the interactions between anthropogenic impacts and marine habitats and species, in this case in the Motril, Carchuna, and Calahonda canyons (northern margin of the Alboran Sea), stands as a contribution to cataloguing marine litter in submarine canyons worldwide, and to understanding the role of submarine canyons as conduits or sinks for litter, with its subsequent impact on benthic habitats. The main conclusions of this study are:
1. The Motril, Calahonda and Carchuna canyons exhibit low litter densities in comparison with other submarine canyons located in the Mediterranean Sea, due to the combination of relatively low coastal population densities and the lack of connection of canyon heads to major streams. The proximity of the Carchuna Canyon head (<200 m) to the coastline implies that the litter density in this canyon is considerably higher than in the Motril and Calahonda canyons, at longer distances from the coast.
2. The Motril and Calahonda canyons can be considered passive systems that have acted as mainly depositional sinks in the recent past, as evidenced by the occurrence of buried marine litter and mostly muddy sediments on the canyon floors. In contrast, the high shelf incision of the Carchuna Canyon and its proximity to the coastline favour the capture of littoral sediment transport, and the formation of gravity flows that transport the marine litter downslope, evidenced by marine litter along the thalweg (up to the termination of the canyon; 740 m water depth) and the occurrence of very fine sand in the lower segment; litter hotspots are favoured by the canyon morphology and the occurrence of rocky outcrops.
3. The main sources of marine litter in the study area (at least 35%) can be traced to coastal recreational uses, such as beaches and coastal urbanization as well as the pressure from tourism. Another coastal origin of the marine litter in the study area could be linked to agricultural practices (at least 8%) that is the main land use in the study area and characterized by greenhouse exploitation in the adjacent coastal plain. A third origin is related to fishing techniques and litter discards from ships. The Motril and Calahonda canyons have smooth flanks and muddy bottoms that favour fishing activities, as opposed to the Carchuna Canyon, having an abrupt seafloor morphology.
4. In the studied canyons, some large pieces of litter are used as refuges by some species against predation and/or for food acquisition. In contrast, fishing activity involving the use of cloth bags, gears, and lines may be producing an impact through entanglement and physical damage to erect benthic fauna, including some aggregations of colonial scleractinians on the walls of the Carchuna Canyon.
The original contributions presented in the study are included in the article/Supplementary Material. Further inquiries can be directed to the corresponding author.
JC-E wrote the manuscript draft and elaborated most of the figures. FL and ÁP-B provided the conceptual framework of the study and rewrote extensively the manuscript. JR wrote sections of the manuscript. PB performed the grain size analysis. OS-G, AL-Q, IM, JP-A, and JC-H contributed to figure making. JS, JP, IM, JP-A and YM performed the statistical analysis. All authors contributed to manuscript revision, reading, and approved the submitted version.
This research was funded by the projects Alboran Shelf-Slope cOupling processes and deep sediMent trAnsfeR: Source To Sink approaches and implications for biodiversity‒ALSSOMAR S2S (CTM2017-88237-P) (Ministerio de Economía y Competitividad, Spanish goverment), and Sediment gravity flows and ANthropogenic Impacts in a MEDiterranean deltaic-and-canyon environment: Causal relationships and consequences‒SANIMED (PID2021-125489OB-I00) (Ministerio de Ciencia e Innovación, Spanish goverment). This study is also part of Cerrillo-Escoriza’s PhD project, which is supported by Grant PRE2018-084812 funded by MCIN/AEI/10.13039/501100011033 and FSE Invierte en tu futuro.
The authors wish to thank the captain and crew of R/V Sarmiento de Gamboa for their dedication and constant support for the execution of activities onboard, and to the participants of the ALSSOMAR-S2S expedition for their help during data acquisition. Multibeam bathymetry and fishing activity data were provided by the “Ministerio de Pesca, Agricultura y Alimentación”, Spanish government. Land use data were provided by the “Consejería de Agricultura, Ganadería, Pesca y Desarrollo Sostenible, Junta de Andalucía”, regional government. JR acknowledges partial support from the 18-ESMARES2-CIRCA project of the Instituto Español de Oceanografía (IEO-CSIC), under the framework of the tasks commissioned to the IEO by the Ministerio de Transición Ecológica y Reto Demográfico (MITERD) of the Spanish government for the application of the Marine Strategy Framework Directive (MSFD) in Spanish waters. IM acknowledges to Fundação para a Ciência e a Tecnologia for Research Assistant contract DL57/2016/CP1361/CT0009 and project UID/0350/2020 CIMA. Very constructive and detailed reviews of an initial manuscript version were provided by two reviewers and by Guest Associate Editor Veerle Huvenne. We are grateful to Jean Sanders for correcting the English text.
The authors declare that the research was conducted in the absence of any commercial or financial relationships that could be construed as a potential conflict of interest.
All claims expressed in this article are solely those of the authors and do not necessarily represent those of their affiliated organizations, or those of the publisher, the editors and the reviewers. Any product that may be evaluated in this article, or claim that may be made by its manufacturer, is not guaranteed or endorsed by the publisher.
The Supplementary Material for this article can be found online at: https://www.frontiersin.org/articles/10.3389/fmars.2023.1098927/full#supplementary-material
Abelló P., Carbonell A., Torres P. (2002). Biogeography of epibenthic crustaceans on the shelf and upper slope off the Iberian peninsula Mediterranean coasts: Implications for the establishment of natural management areas. Sci. Mar. 66, 183–198. doi: 10.3989/scimar.2002.66s2183
Adams S. M. (2005). Assessing cause and effect of multiple stressors on marine systems. Mar. Pollut. Bull. 51, 649–657. doi: 10.1016/j.marpolbul.2004.11.040
Alves T. M., Kokinou E., Ekström M., Nikolaidis A., Georgiou G. C., Miliou A. (2021). Scientific, societal and pedagogical approaches to tackle the impact of climate change on marine pollution. Sci. Rep. 11, 1–15. doi: 10.1038/s41598-021-82421-y
Angiolillo M. (2019). Chapter 14 - debris in deep water, world Seas.Volume III: Ecological issues and environmental impacts. 2nd ed. (Cambridge: Academic Press), 251–268. doi: 10.1016/B978-0-12-805052-1.00015-2
Angiolillo M., di Lorenzo B., Farcomeni A., Bo M., Bavestrello G., Santangelo G., et al. (2015). Distribution and assessment of marine debris in the deep tyrrhenian Sea (NW Mediterranean Sea, Italy). Mar. Pollut. Bull. 92, 149–159. doi: 10.1016/j.marpolbul.2014.12.044
Angiolillo M., Gérigny O., Valente T., Fabri M. C., Tambute E., Rouanet E., et al. (2021). Distribution of seafloor litter and its interaction with benthic organisms in deep waters of the ligurian Sea (Northwestern Mediterranean). Sci. Total Environ. 788, 147745. doi: 10.1016/j.scitotenv.2021.147745
Ayuntamiento de Motril. Medio Ambiente Urbano (2021). Anexo 2: Residuos urbanos y asimilables a urbanos. Motril Agenda 21, 45–73.
Bárcenas P., Lobo F. J., Macías J., Fernández-Salas L. M., Díaz del Río V. (2011). Spatial variability of surficial sediments on the northern shelf of the alboran Sea: the effects of hydrodynamic forcing and supply of sediment by rivers. J. Iber. Geol. 37, 195–214. doi: 10.5209/rev_JIGE.2011.v37.n2.8
Bárcenas P., Lobo F. J., Macías J., Fernández-Salas L. M., López-González N., Díaz del Río V. (2015). Submarine deltaic geometries linked to steep, mountainous drainage basins in the northern shelf of the alboran Sea: Filling the gaps in the spectrum of deltaic deposition. Geomorphology 232, 125–144. doi: 10.1016/j.geomorph.2014.11.028
Bárcenas P., Macías J. (2009). Estudio morfométrico comparativo entre las ondulaciones de los prodeltas de los ríos de andalucía oriental. Rev. Soc Geol. Esp. 22, 43–56.
Baro J., García-Jiménez T., Serna-Quintero J. M. (2021). “Description of artisanal fisheries in northern alboran Sea,” in Alboran Sea - ecosystems and marine resources, vol. 15 . Eds. Báez J. C., Vázquez J. T., Camiñas J. A., Malouli Idrissi M. (Cham: Springer), 524–542.
Bellan-Santini D., Bellan G., Bitar G., Harmelin J. G., Pergent G. (2007). Handbook for interpreting types of marine habitat for the selection of sites to be included in the national inventories of natural sites of conservation interest Vol. 168 (Tunisia: UNEP-MAP RAC/SPA).
Bergmann M., Collard F., Fabres J., Gabrielsen G. W., Provencher J. F., Rochman C. M., et al. (2022). Plastic pollution in the Arctic. Nat. Rev. Earth Environ. 3, 323–337. doi: 10.1038/s43017-022-00279-8
Browne M. A., Underwood A. J., Chapman M. G., Williams R., Thompson R. C., Van Franeker J. A. (2015). Linking effects of anthropogenic debris to ecological impacts. Proc. R. Soc B. 282, 20142929. doi: 10.1098/rspb.2014.2929
Canals M., Pham C. K., Bergmann M., Gutow L., Hanke G., Van Sebille E., et al. (2021). The quest for seafloor macrolitter: A critical review of background knowledge, current methods and future prospects. Environ. Res. Lett. 16, 023001. doi: 10.1088/1748-9326/abc6d4
Carvajal C., Paull C. K., Caress D. W., Fildani A., Lundsten E., Anderson K., et al. (2017). Unraveling the channel-lobe transition zone with high-resolution AUV bathymetry: Navy fan, offshore Baja California, Mexico. J. Sediment. Res. 87, 1049–1059. doi: 10.2110/jsr.2017.58
Cau A., Alvito A., Moccia D., Canese S., Pusceddu A., Rita C., et al. (2017). Submarine canyons along the upper sardinian slope (Central Western Mediterranean) as repositories for derelict fishing gears. Mar. Pollut. Bull. 123, 357–364. doi: 10.1016/j.marpolbul.2017.09.010
Ciércoles C., García-Ruíz C., Abelló P., Hidalgo M., Torres P., González M., et al. (2022). Decapod crustacean assemblages on trawlable grounds in the northern alboran Sea and gulf of Vera. Sci. Mar. 86, e039. doi: 10.3989/scimar.05265.039
Ciércoles C., García-Ruiz C., González-Aguilar M., Ortiz de Urbina Gutiérrez J., López-González N., Urra-Recuero J., et al. (2018). Molluscs collected with otter trawl in the northern alboran Sea: main assemblages, spatial distribution and environmental linkage. Mediterr. Mar. Sci. 19, 209–222. doi: 10.12681/mms.2124
Clark M. R., Althaus F., Schlacher T. A., Williams A., Bowden D. A., Rowden A. A. (2016). The impacts of deep-sea fisheries on benthic communities: A review. Ices J. Mar. Sci. 73, 51–69. doi: 10.1093/icesjms/fsv123
de Stephanis R., Giménez J., Carpinelli E., Gutierrez-Exposito C., Canadas A. (2013). As main meal for sperm whales: Plastics debris. Mar. Pollut. Bull. 69, 206–214. doi: 10.1016/j.marpolbul.2013.01.033
Deudero S., Alomar C. (2015). Mediterranean Marine biodiversity under threat: Reviewing influence of marine litter on species. Mar. Pollut. Bull. 98, 58–68. doi: 10.1016/j.marpolbul.2015.07.012
Dominguez-Carrió C., Sanchez-Vidal A., Estournel C., Corbera G., Riera J. L., Orejas C., et al. (2020). Seafloor litter sorting in different domains of cap de creus continental shelf and submarine canyon (NW Mediterranean Sea). Mar. Pollut. Bull. 161, 111744. doi: 10.1016/j.marpolbul.2020.111744
Du Buit M. (1978). Alimentation de quelques poissons téléostéens de profondeur dans la zone du seuil de wyville Thomson. Oceanol. Acta 1, 129–134.
Ercilla G., Alonso B., Baraza J. (1994). Post-calabrian sequence stratigraphy of the northwestern alboran Sea (southwestern Mediterranean). Mar. Geol. 120, 249–265. doi: 10.1016/0025-3227(94)90061-2
European Commission of the European Union (2013). MSDF guidance on monitoring marine litter (Luxembourg: Publications Office of the European Union).
Fabres J., Calafat A., Sanchez-Vidal A., Canals M., Heussner S. (2002). Composition and spatio-temporal variability of particle fluxes in the Western alboran gyre, Mediterranean Sea. J. Mar. Syst. 33–34, 431–456. doi: 10.1016/S0924-7963(02)00070-2
Fabri M. C., Dugornay O., de la Bernardie X., Guerin C., Sanchez P., Arnaubec A., et al. (2022). 3D-representations for studying deep-sea coral habitats in the lacaze-duthiers canyon, from geological settings to individual specimens. Deep. Res. Part I. Oceanogr. Res. Pap. 187, 103831. doi: 10.1016/j.dsr.2022.103831
Fabri M. C., Pedel L., Beuck L., Galgani F., Hebbeln D., Freiwald A. (2014). Megafauna of vulnerable marine ecosystems in French mediterranean submarine canyons: Spatial distribution and anthropogenic impacts. Deep-sea Res. Pt. II. 104, 184–207. doi: 10.1016/j.dsr2.2013.06.016
Fernandez-Arcaya U., Ramirez-Llodra E., Aguzzi J., Allcock A. L., Davies J. S., Dissanayake A., et al. (2017). Ecological role of submarine canyons and need for canyon conservation: A review. Front. Mar. Sci. 4. doi: 10.3389/fmars.2017.00005
Fernández-Salas L. M., Dabrio C. J., Goy J. L., Díaz del Río V., Zazo C., Lobo F. J., et al. (2009). Land-sea correlation between late Holocene coastal and infralittoral deposits in the SE Iberian peninsula (Western Mediterranean). Geomorphology 104, 4–11. doi: 10.1016/j.geomorph.2008.05.013
Folk R. L. (1954). The distinction between grain size and mineral composition in sedimentary rock nomenclature. J. Geol. 62, 344–359. doi: 10.1086/626171
Fosså J. H., Mortensen P. B., Furevik D. M. (2002). The deep-water coral lophelia pertusa in Norwegian waters: Distribution and fishery impacts. Hydrobiologia 471, 1–12. doi: 10.1023/A:1016504430684
Galgani F., Leaute J. P., Moguedet P., Souplet A., Verin Y., Carpentier A., et al. (2000). Litter on the Sea floor along European coasts. Mar. Pollut. Bull. 40, 516–527. doi: 10.1016/S0025-326X(99)00234-9
Galgani F., Souplet A., Cadiou Y. (1996). Accumulation of debris on the deep sea floor off the French Mediterranean coast. Mar. Ecol. Prog. Ser. 142, 225–234. doi: 10.3354/meps142225
García-Rivera S., Lizaso J. L. S., Millán J. M. B. (2018). Spatial and temporal trends of marine litter in the Spanish Mediterranean seafloor. Mar. Pollut. Bull. 137, 252–261. doi: 10.1016/j.marpolbul.2018.09.051
García-Ruiz C., Lloris D., Rueda J. L., García-Martínez M. C., Gil de Sola L. (2015). Spatial distribution of ichthyofauna in the northern alboran Sea (western Mediterranean). J. Nat. Hist. 49, 1191–1224. doi: 10.1080/00222933.2014.1001457
Gerigny O., Brun M., Fabri M. C., Tomasino C., Le Moigne M., Jadaud A., et al. (2019). Seafloor litter from the continental shelf and canyons in French Mediterranean water: Distribution, typologies and trends. Mar. Pollut. Bull. 146, 653–666. doi: 10.1016/j.marpolbul.2019.07.030
Giusti M., Canese S., Fourt M., Bo M., Innocenti C., Goujard A., et al. (2019). Coral forests and derelict fishing gears in submarine canyon systems of the ligurian Sea. Prog. Oceanogr. 178, 102186. doi: 10.1016/j.pocean.2019.102186
Godoy V., Prata J. C., Blázquez G., Almendros A. I., Duarte A. C., Rocha-Santos T., et al. (2020). Effects of distance to the sea and geomorphological characteristics on the quantity and distribution of microplastics in beach sediments of Granada (Spain). Sci. Total Environ. 746, 142023. doi: 10.1016/j.scitotenv.2020.142023
Goldberg E. D. (1997). Plasticizing the seafloor: An overview. Environ. Technol. 18, 195–201. doi: 10.1080/09593331808616527
Gregory M. R. (2009). Environmental implications of plastic debris in marine settings- entanglement, ingestion, smothering, hangers-on, hitch-hiking and alien invasions. Phil. Trans. R. Soc B. 364, 2013–2025. doi: 10.1098/rstb.2008.0265
Hernandez I., Davies J. S., Huvenne V. A. I., Dissanayake A. (2022). Marine litter in submarine canyons: A systematic review and critical synthesis. Front. Mar. Sci. 9. doi: 10.3389/fmars.2022.965612
Hernández-Molina F. J., Fernández-Salas L. M., Lobo F., Somoza L., Díaz-del-Río V., Alveirinho Dias J. M. (2000). The infralittoral prograding wedge: A new large-scale progradational sedimentary body in shallow marine environments. Geo-Mar. Lett. 20, 109–117. doi: 10.1007/s003670000040
Junta de Andalucía, Consejería de Medio Ambiente A. Y. P. (2019). Plan director territorial de gestión de residuos no peligrosos de andalucía 2010–2019. Junta de Andalucía, BOJA 168, 5–6.
Kane I. A., Fildani A. (2021). Anthropogenic pollution in deep-marine sedimentary systems–a geological perspective on the plastic problem. Geology 49, 607–608. doi: 10.1130/focus052021.1
Katsanevakis S., Verriopoulos G., Nicolaidou A., Thessalou-Legaki M. (2007). Effect of marine litter on the benthic megafauna of coastal soft bottoms: A manipulative field experiment. Mar. Pollut. Bull. 54, 771–778. doi: 10.1016/j.marpolbul.2006.12.016
Lario J., Zazo C., Goy J. L. (1999). Fases de progradación y evolución morfosedimentaria de la flecha litoral de calahonda (Granada) durante el holoceno. Estud. Geol. 55, 247–250. doi: 10.3989/egeol.99555-6164
Liquete C., Arnau P., Canals M., Colas S. (2005). Mediterranean River systems of Andalusia, southern Spain, and associated deltas: A source to sink approach. Mar. Geol. 222-223, 471–495. doi: 10.1016/j.margeo.2005.06.033
Liquete C., Canals M., Ludwig W., Arnau P. (2009). Sediment discharge of the rivers of Catalonia, NE Spain, and the influence of human impacts. J. Hydrol. 366, 76–88. doi: 10.1016/j.jhydrol.2008.12.013
Liubartseva S., Coppini G., Lecci R., Clementi E. (2018). Tracking plastics in the Mediterranean: 2D Lagrangian model. Mar. Pollut. Bull. 129, 151–162. doi: 10.1016/j.marpolbul.2018.02.019
Lobo F. J., Goff J. A., Mendes I., Bárcenas P., Fernández-Salas L. M., Martín-Rosales W., et al. (2015). Spatial variability of prodeltaic undulations on the guadalfeo river prodelta: Support to the genetic interpretation as hyperpycnal flow deposits. Mar. Geophys. Res. 36, 309–333. doi: 10.1007/s11001-014-9233-9
Martín J., Puig P., Palanques A., Ribó M. (2014). Trawling-induced daily sediment resuspension in the flank of a Mediterranean submarine canyon. Deep. Res. Part II. Top. Stud. Oceanogr. 104, 174–183. doi: 10.1016/j.dsr2.2013.05.036
Martín-Lara M. A., Godoy V., Quesada L., Lozano E. J., Calero M. (2021). Environmental status of marine plastic pollution in Spain. Mar. Pollut. Bull. 170, 112677. doi: 10.1016/j.marpolbul.2021.112677
Mateo-Ramírez Á., Marina P., Moreno D., Valero Alcántara, Aguilar R., Báez J. C., et al. (2021). “Marine protected areas and key biodiversity areas of the alboran Sea and adjacent areas,” in Alboran Sea – ecosystems and marine resources, vol. 25 . Eds. Báez J. C., Vázquez J. T., Camiñas J. A., Malouli Idrissi M. (Cham: Springer), 819–923.
Mauchline J., Gordon J. D. (1984). Feeding and bathymetric distribution of the gadoid and morid fish of the rockall trough. J. Mar. Biol. Assoc. Uk. 64, 657–665. doi: 10.1017/S0025315400030320
Mecho A., Francescangeli M., Ercilla G., Fanelli E., Estrada F., Valencia J., et al. (2020). Deep-sea litter in the gulf of cadiz (Northeastern Atlantic, Spain). Mar. Pollut. Bull. 153, 110969. doi: 10.1016/j.marpolbul.2020.110969
Ministerio para la Transición Ecológica y el Reto Demográfico (MITECO-DGCM) (2021). Programa de seguimiento de basuras marinas en playas. Informe de resultados. Dirección general de la costa y el mar, 290 pp.
Miyake H., Shibata H., Furushima Y. (2011). “Deep-sea litter study using deep-sea observation tools,” in Interdisciplinary studies on environmental chemistry — marine environmental modeling and analysis. Eds. Omori K., Guo X., Yoshie N., Fujii N., Handoh I. C., Isobe A., Tanabe S. (Tokyo: TERRAPUB), 261–269.
Morales-Caselles C., Viejo J., Martí E., González-Fernández D., Pragnell-Raasch H., González-Gordillo J. I., et al. (2021). An inshore–offshore sorting system revealed from global classification of ocean litter. Nat. Sustain. 4, 484–493. doi: 10.1038/s41893-021-00720-8
Mordecai G., Tyler P. A., Masson D. G., Huvenne V. A. I. (2011). Litter in submarine canyons off the west coast of Portugal. Deep-sea Res. Pt. II. 58, 2489–2496. doi: 10.1016/j.dsr2.2011.08.009
Neves D., Sobral P., Pereira T. (2015). Marine litter in bottom trawls off the Portuguese coast. Mar. Pollut. Bull. 99, 301–304. doi: 10.1016/j.marpolbul.2015.07.044
Oberle F. K. J., Puig P., Martín J. (2018). “Fishing activities,” in Submarine geomorphology. Eds. Micallef A., Krastel S., Savini A. (Cham: Springer), 503–534.
Oliveira F., Monteiro P., Bentes L., Henriques N. S., Aguilar R., Gonçalves J. M. S. (2015). Marine litter in the upper são Vicente submarine canyon (SW portugal): Abundance, distribution, composition and fauna interactions. Mar. Pollut. Bull. 97, 401–407. doi: 10.1016/j.marpolbul.2015.05.060
Ortega-Sánchez M., Lobo F. J., López-Ruiz A., Losada M. A., Fernández-Salas L. M. (2014). The influence of shelf-indenting canyons and infralittoral prograding wedges on coastal morphology: The carchuna system in southern Spain. Mar. Geol. 347, 107–122. doi: 10.1016/j.margeo.2013.11.006
Palanques A., El Khatab M., Puig P., Masqué P., Sánchez-Cabeza J. A., Isla E. (2005). Downward particle fluxes in the guadiaro submarine canyon depositional system (north-western alboran Sea), a river flood dominated system. Mar. Geol. 220, 23–40. doi: 10.1016/j.margeo.2005.07.004
Paradis S., Lo Iacono C., Masqué P., Puig P., Palanques A., Russo T. (2021). Evidence of large increases in sedimentation rates due to fish trawling in submarine canyons of the gulf of Palermo (SW Mediterranean). Mar. Pollut. Bull. 172, 112861. doi: 10.1016/j.marpolbul.2021.112861
Paull C. K., Caress D. W., Lundsten E., Gwiazda R., Anderson K., McGann M., et al. (2013). Anatomy of the la Jolla submarine canyon system; offshore southern California. Mar. Geol. 335, 16–34. doi: 10.1016/j.margeo.2012.10.003
Pérez-Belzuz F., Alonso B. (2000). Evolución sedimentaria reciente de dos sistemas turbidíticos del área de motril (NE alborán). parte II: sistema turbidítico de sacratif. Geotemas 1, 207–211.
Pérez-Belzuz F., Alonso B., Ercilla G. (2000). Modelos de sistemas turbidíticos en el Área de motril (NE alborán). Geotemas 1, 213–216.
Pham C. K., Diogo H., Menezes G., Porteiro F., Braga-Henriques A., Vandeperre F., et al. (2014a). Deep-water longline fishing has reduced impact on vulnerable marine ecosystems. Sci. Rep. 4, 4837. doi: 10.1038/srep04837
Pham C. K., Ramirez-Llodra E., Alt C. H. S., Amaro T., Bergmann M., Canals M., et al. (2014b). Marine litter distribution and density in European seas, from the shelves to deep basins. PloS One 9, e95839. doi: 10.1371/journal.pone.0095839
Pierdomenico M., Casalbore D., Chiocci F. L. (2019). Massive benthic litter funnelled to deep sea by flash-flood generated hyperpycnal flows. Sci. Rep. 9(5330). doi: 10.1038/s41598-019-41816-8
Pierdomenico M., Casalbore D., Chiocci F. L. (2020). The key role of canyons in funnelling litter to the deep sea: A study of the gioia canyon (Southern tyrrhenian Sea). Anthropocene 30, 100237. doi: 10.1016/j.ancene.2020.100237
Poore G. C. B., Ahyong S. T., Taylor J. (2011). The biology of squat lobsters. CSIRO 363 p. doi: 10.1071/9780643104341
Puig P., Canals M., Company J. B., Martín J., Amblas D., Lastras G., et al. (2012). Ploughing the deep sea floor. Nature 489, 286–289. doi: 10.1038/nature11410
Puig P., Martín J., Masqué P., Palanques A. (2015). Increasing sediment accumulation rates in la fonera (Palamós) submarine canyon axis and their relationship with bottom trawling activities. Geophys. Res. Lett. 42, 8106–8113. doi: 10.1002/2015GL065052
Ragnarsson S.Á., Burgos J. M., Kutti T., van den Beld I., Egilsdóttir H., Arnaud-Haond S., et al. (2017). “The impact of anthropogenic activity on cold-water corals,” in Marine animal forests: the ecology of benthic biodiversity hotspots. Eds. Rossi S., Bramanti L., Gori A., Orejas C. (Cham: Springer), 989–1023.
Ramirez-Llodra E., De Mol B., Company J. B., Coll M., Sardà F. (2013). Effects of natural and anthropogenic processes in the distribution of marine litter in the deep Mediterranean Sea. Prog. Oceanogr. 118, 273–287. doi: 10.1016/j.pocean.2013.07.027
Ramirez-Llodra E., Tyler P. A., Baker M. C., Bergstad O. A., Clark M. R., Escobar E., et al. (2011). Man and the last great wilderness: Human impact on the deep sea. PloS One 6, e22588. doi: 10.1371/journal.pone.0022588
Richmond R. H. (1993). Coral reefs: Present problems and future concerns resulting from anthropogenic disturbance. Integr. Comp. Biol. 33, 524–536. doi: 10.1093/icb/33.6.524
Rueda J. L., Gofas S., Aguilar R., de la Torriente A., García Raso J. E., Lo Iacono C., et al. (2021). “Benthic fauna of littoral and deep-Sea habitats of the alboran Sea: A hotspot of biodiversity BT,” in Alboran Sea – ecosystems and marine resources, vol. 9 . Eds. Báez J. C., Vázquez J. T., Camiñas J. A., Malouli Idrissi M. (Cham: Springer), 285–358.
Sánchez P., Masó M., Sáez R., de Juan S., Muntadas A., Demestre M. (2013). Baseline study of the distribution of marine debris on soft-bottom habitats associated with trawling grounds in the northern Mediterranean. Sci. Mar. 77, 247–255. doi: 10.3989/scimar03702.10A
Schlining K., von Thun S., Kuhnz L., Schlining B., Lundsten L., Jacobsen Stout N., et al. (2013). Debris in the deep: Using a 22-year video annotation database to survey marine litter in Monterey canyon, central California, USA. Deep-sea Res. Pt. I. 79, 96–105. doi: 10.1016/j.dsr.2013.05.006
Serrano M. A., Díez-Minguito M., Valle-Levinson A., Ortega-Sanchez M. (2020). Circulation in a short, microtidal submarine canyon in the alborán Sea. J. Coast. Res. 95, 1531–1535. doi: 10.2112/SI95-295.1
Smith M. E., Werner S. H., Buscombe D., Finnegan N. J., Sumner E. J., Mueller E. R. (2018). Seeking the shore: Evidence for active submarine canyon head incision due to coarse sediment supply and focusing of wave energy. Geophys. Res. Lett. 45, 12,403–12,413. doi: 10.1029/2018GL080396
Sotomayor-García A., Rueda J. L., Sánchez-Guillamón O., Urra J., Vázquez J. T., Palomino D., et al. (2019). First macro-colonizers and survivors around tagoro submarine volcano, canary islands, Spain. Geosciences 9, 52. doi: 10.3390/geosciences9010052
Stanley D. J., Kelling G., Vera J. A., Sheng H. (1975). Sands in the alboran Sea: A model of input in a deep marine basin. Sm. C. Earth Sc. 15, 1–51. doi: 10.5479/si.00810274.15.1
Stefatos A., Charalampakis M., Papatheodorou G., Ferentinos G. (1999). Marine debris on the seafloor of the Mediterranean Sea: Examples from two enclosed gulfs in western Greece. Mar. Pollut. Bull. 38, 389–393. doi: 10.1016/S0025-326X(98)00141-6
Taviani M., Foglini F., Castellan G., Montagna P., McCulloch M. T., Trotter J. A. (2023). First assessment of anthropogenic impacts in submarine canyon systems off southwestern Australia. Sci. Total Environ. 857, 159243. doi: 10.1016/j.scitotenv.2022.159243
Templado J., Ballesteros E., Galparsoro I., Borja Á., Serrano A., Martín L., et al. (2013). Guía interpretativa. Inventario Español de Hábitats Marinos. Ministerio de Agricultura, Alimentación y Medio Ambiente, Spain, 230 pp.
Thiel M., Gutow L. (2005). The ecology of rafting in the marine environment. II. the rafting organisms and community. Oceanogr. Mar. Biol. 43, 279–418. doi: 10.1201/9781420037449.ch7
Thompson R. C., Olsen Y., Mitchell R. P., Davis A., Rowland S. J., John A. W. G., et al. (2004). Lost at Sea: Where is all the plastic? Science 304, 838. doi: 10.1126/science.1094559
Thushari G. G. N., Senevirathna J. D. M. (2020). Plastic pollution in the marine environment. Heliyon 6, e04709. doi: 10.1016/j.heliyon.2020.e04709
Trenkel V. M., Le Loc’h F., Rochet M. J. (2007). Small-scale spatial and temporal interactions among benthic crustaceans and one fish species in the bay of Biscay. Mar. Biol. 151, 2207–2215. doi: 10.1007/s00227-007-0655-7
Trestrail C., Nugegoda D., Shimeta J. (2020). Invertebrate responses to microplastic ingestion: Reviewing the role of the antioxidant system. Sci. Total Environ. 734, 138559. doi: 10.1016/j.scitotenv.2020.138559
Tubau X., Canals M., Lastras G., Rayo X., Rivera J., Amblas D. (2015). Marine litter on the floor of deep submarine canyons of the northwestern Mediterranean Sea: The role of hydrodynamic processes. Prog. Oceanogr. 134, 379–403. doi: 10.1016/j.pocean.2015.03.013
Uiblein F., Lorance P., Latrouite D. (2003). Behaviour and habitat utilisation of seven demersal fish species on the bay of Biscay continental slope, NE Atlantic. Mar. Ecol. Prog. Ser. 257, 223–232. doi: 10.3354/meps257223
van den Beld I. M. J., Guillaumont B., Menot L., Bayle C., Arnaud-Haond S., Bourillet J. F. (2017). Marine litter in submarine canyons of the bay of Biscay. Deep-sea Res. Pt. II. 145, 142–152. doi: 10.1016/j.dsr2.2016.04.013
Vázquez J. T. (2001). Estructura del margen continental del mar de alborán. PhD Thesis (Madrid: Universidad Complutense de Madrid).
Vázquez J. T., Ercilla G., Alonso B., Juan C., Rueda J. L., Palomino D., et al. (2015). “Submarine canyons and related features in the alboran Sea: continental margins and major isolated reliefs,” in Submarine canyon dynamics, vol. 47 . Ed. Briand F. (Italy: CIESM Workshop Monographs), 183–196.
Vieira R. P., Raposo I. P., Sobral P., Gonçalves J. M. S., Bell K. L. C., Cunha M. R. (2015). Lost fishing gear and litter at gorringe bank (NE Atlantic). J. Sea Res. 100, 91–98. doi: 10.1016/j.seares.2014.10.005
Watters D. L., Yoklavich M. M., Love M. S., Schroeder D. M. (2010). Assessing marine debris in deep seafloor habitats off California. Mar. Pollut. Bull. 60, 131–138. doi: 10.1016/j.marpolbul.2009.08.019
Wei C. L., Rowe G. T., Nunnally C. C., Wicksten M. K. (2012). Anthropogenic “Litter” and macrophyte detritus in the deep northern gulf of Mexico. Mar. Pollut. Bull. 64, 966–973. doi: 10.1016/j.marpolbul.2012.02.015
Keywords: submarine canyons, marine litter, seafloor imagery, litter hotspots, marine litter-habitats interaction, fishing gear, fishing effort, Alboran Sea
Citation: Cerrillo-Escoriza J, Lobo FJ, Puga-Bernabéu Á, Rueda JL, Bárcenas P, Sánchez-Guillamón O, Serna Quintero JM, Pérez Gil JL, Murillo Y, Caballero-Herrera JA, López-Quirós A, Mendes I and Pérez-Asensio JN (2023) Origin and driving mechanisms of marine litter in the shelf-incised Motril, Carchuna, and Calahonda canyons (northern Alboran Sea). Front. Mar. Sci. 10:1098927. doi: 10.3389/fmars.2023.1098927
Received: 15 November 2022; Accepted: 07 February 2023;
Published: 24 February 2023.
Edited by:
Veerle Ann Ida Huvenne, University of Southampton, United KingdomReviewed by:
Marie-Claire Fabri, Ifremer Centre de Méditerranée, FranceCopyright © 2023 Cerrillo-Escoriza, Lobo, Puga-Bernabéu, Rueda, Bárcenas, Sánchez-Guillamón, Serna Quintero, Pérez Gil, Murillo, Caballero-Herrera, López-Quirós, Mendes and Pérez-Asensio. This is an open-access article distributed under the terms of the Creative Commons Attribution License (CC BY). The use, distribution or reproduction in other forums is permitted, provided the original author(s) and the copyright owner(s) are credited and that the original publication in this journal is cited, in accordance with accepted academic practice. No use, distribution or reproduction is permitted which does not comply with these terms.
*Correspondence: Javier Cerrillo-Escoriza, amF2aWVyLmNlcnJpbGxvQGNzaWMuZXM=
Disclaimer: All claims expressed in this article are solely those of the authors and do not necessarily represent those of their affiliated organizations, or those of the publisher, the editors and the reviewers. Any product that may be evaluated in this article or claim that may be made by its manufacturer is not guaranteed or endorsed by the publisher.
Research integrity at Frontiers
Learn more about the work of our research integrity team to safeguard the quality of each article we publish.