- 1Biosciences, Faculty of Health and Life Sciences, University of Exeter, Exeter, United Kingdom
- 2Greenpeace Research Laboratories, College of Life and Environmental Sciences, University of Exeter, Exeter, United Kingdom
- 3Biosciences, Faculty of Environment, Science and Economy, University of Exeter, Penryn, Cornwall, United Kingdom
- 4Marine Mammal Institute, Department of Fisheries, Wildlife, and Conservation Sciences, Oregon State University, Newport, OR, United States
Deep seabed mining operations, if permitted, could present significant risks to ocean ecosystems. Disturbance on any scale is likely to be long lasting and irreversible. Scant research to date has examined the impact that deep sea minerals extraction would have on cetaceans. The Clarion-Clipperton Zone (CCZ) is of particular interest to mining companies aiming to exploit polymetallic nodules. The CCZ, with an average depth of 5,500 m and an area of approximately 11,650,000 km2, is a habitat for cetaceans including baleen (mysticetes) and toothed whales (odontocetes). Of particular concern is anthropogenic noise. If permitted, commercial-scale mining is expected to operate 24-hours a day, at varying depths. The sounds produced from mining operations, including from remotely operated vehicles on the seafloor, overlap with the frequencies at which cetaceans communicate, which can cause auditory masking and behavior change in marine mammals. Cetaceans are already facing numerous stressors, including climate change, and many species are still recovering from centuries of exploitation. We argue the need for urgent research to assess more fully the potential impact of deep seabed mining on cetaceans.
1 Introduction
Increasing commercial interest in seabed mineral resources has been driven in part by a perceived need for metals to enable the ‘green transition’ (Miller et al., 2021). A legal framework governs human activities in the marine environment, including rights to marine minerals (see Miller et al., 2018 and Thompson et al., 2018). Broadly, coastal states have rights to resources that fall within the exclusive economic zone (EEZ) within 200 nautical miles (370 km) of their coastline. Some commercial shallow seabed mining has already taken place within EEZs, such as offshore diamond mining in Namibia by Diamond Fields International Ltd. Mineral deposits on the deep seabed in the area beyond national jurisdiction (ABNJ) are regulated by the International Seabed Authority (ISA), a United Nations body. In this Perspective we focus on deep seabed mining in the ABNJ, commonly known as the Area.
The mineral deposits of greatest commercial interest are: (i) polymetallic nodules on the abyssal plains; (ii) seafloor massive (polymetallic) sulfides at hydrothermal vents; and (iii) ferromanganese crusts on seamounts. Of the three deposit types, nodules are most likely to be exploited first because of the even greater technical challenges associated with mining vents and seamounts (Figure 1A) (Miller et al., 2018). Deep seabed mining operations have the potential to pose significant risks to ocean ecosystems and not enough is known about the deep seabed mining industry. Although substantial uncertainties and unknowns remain, it is likely that commercial extraction of marine minerals at any scale will have a negative impact on the local ecosystem and potentially further afield. Potential impacts on cetaceans are not well studied to date.
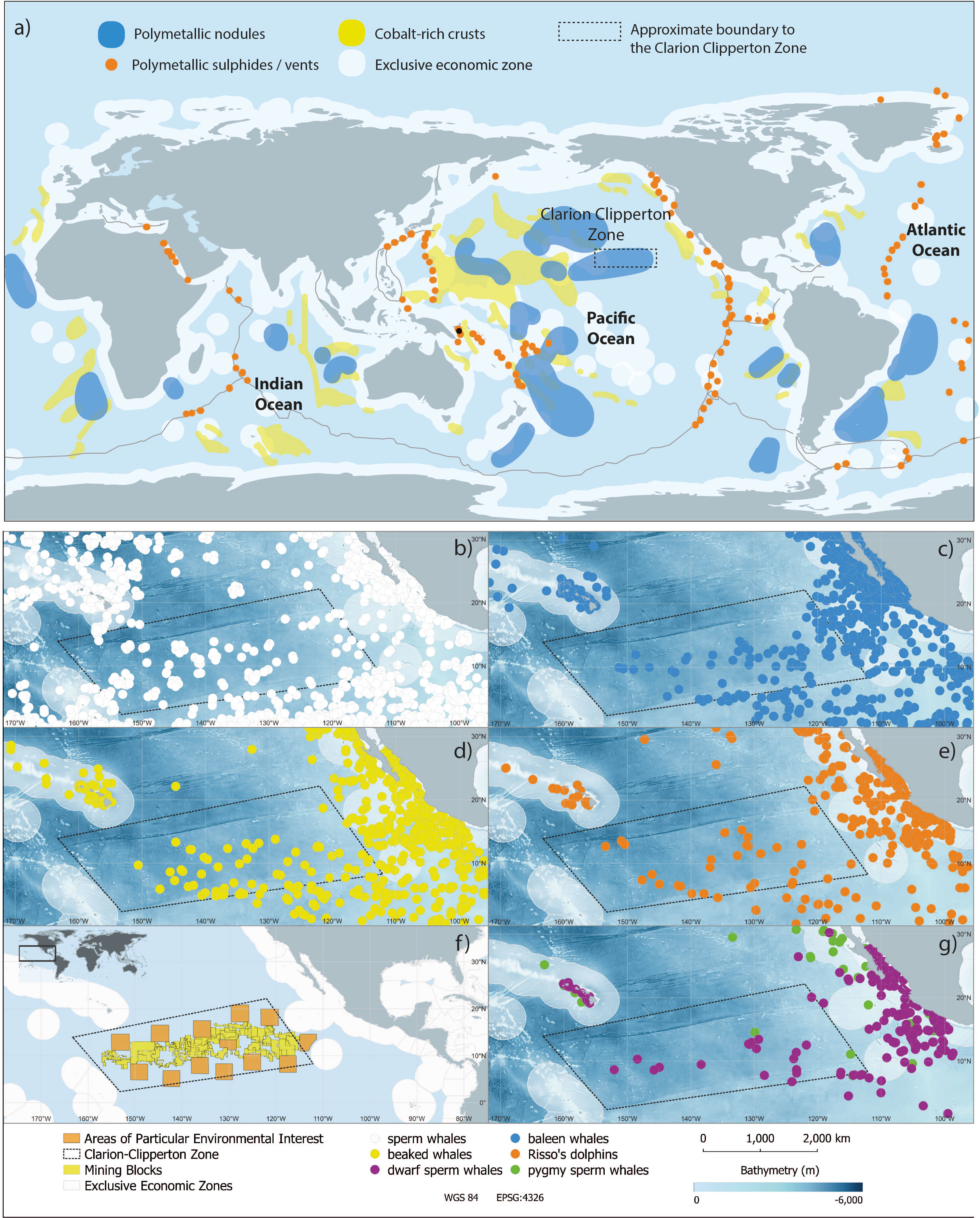
Figure 1 Deep seabed mining areas and with a focus on the spatial overlap of the Clarion Clipperton Zone (CCZ) with data on cetacean occurrence as reported in the OBIS-SEAMAP (https://seamap.env.duke.edu/) repository. (A) A global map of areas of the seabed with known regions of mineral resources. (Source: Miller et al., 2018. Licensed for use under Creative Commons: http://creativecommons.org/licenses/by/4.0/). (B) sperm whale (Physeter macrocephalus) occurrence (in white) (C) occurrence of baleen whales (in blue), (D) beaked whale occurrence (in yellow), (E) Risso’s dolphin (Grampus griseus) occurrence (in orange), (F) the rectangular box marks the extent of cetacean data accessed from OBIS-SEAMAP and approximate location of the boundaries of the CCZ, mining blocks and areas of particular environmental interest and (G) occurrence of Kogia spp. (Kogia sima in dark green, Kogia breviceps in purple). Note that occurrence information include data from multiple sources (see supplementary information for more details). Baleen whale data refer to the following species: blue (Balaenoptera musculus), fin (Balaenoptera physalus), humpback (Megaptera novaeangliae), gray (Eschrichtius robustus), Bryde’s (Balaenoptera edeni) and minke whales (Balaenoptera acutorostrata) and unidentified baleanopterids. Beaked whale data refer to the following species: Cuvier’s (Ziphius cavirostris), Longman’s (Indopacetus pacificus), Blainville’s (Mesoplodon densirostris), pygmy (Mesoplodon peruvianus) and Baird’s (Berardius bairdii) and unidentified beaked whales. All depth data are derived from GEBCO (https://www.gebco.net/).
No commercial-scale deep seabed mining operations have yet occurred. In June 2021, however, Narau informed the ISA of its intention to trigger the so-called 'two-year rule', meaning that seabed mining could be permitted in 2023 with whatever rules are in place at that time. The ISA is (as of February 2023) continuing to work on draft regulations for exploitation of minerals in the Area. In spite of significant financial and logistical setbacks – and concerns voiced by marine scientists and conservation groups – seabed mining companies continue efforts to develop commercial operations (Miller et al., 2018).
The general consensus of marine ecologists that have published on this subject is that commercial extraction of minerals from the deep seabed would cause lasting and irreversible damage to fragile ecosystems (Jones et al., 2017; Niner et al., 2018; Levin et al., 2020). Anticipated impacts from mining include noise and light pollution and dispersal of sediment plumes, in addition to direct effects on species and habitats (Miller et al., 2018). Arguably the greatest impact will be habitat loss because mining removes mineral substrate, though predicting the scale and extent of the damage to fragile marine habitats is extremely difficult. Although technological advances have led to improved ocean mapping and scientific understanding of deep-sea biodiversity, ecosystem dynamics and connectivity, much remains unknown. A ten-year global census concluding in 2010 estimated that only approximately 20% of marine species had been described by science (Costello et al., 2010).
Cetaceans, including baleen (mysticetes) and toothed whales (odontocetes), inhabit areas of the oceans where mineral deposits of commercial interest are located. For example, the Clarion Clipperton Zone (CCZ) in the Eastern North Pacific is a key target for manganese nodule extraction and contains complex bathymetry including abyssal plains and seamounts (Cuvelier et al., 2020; Leitner et al., 2021). The CCZ is within the distribution range for many cetacean populations, some of which are globally threatened according to the International Union for the Conservation of Nature (IUCN) Red List of Threatened Species. Seamounts are areas of high productivity and provide important nutrition and navigation cues for cetaceans (Garrigue et al., 2015). In other areas of the ocean, cetaceans have long been negatively impacted by marine industrial activities – many species have been reported as sensitive to chemical pollution, habitat degradation and anthropogenic noise associated with industries such as oil and gas exploration and with commercial shipping (Kavanagh et al., 2019; Pires et al., 2021; Sahri et al., 2021). To date, assessments of impacts from deep seabed mining on biodiversity have focused on those species associated with the seabed and not mobile marine megafauna, many of which have poorly described distributions and ecology in the open ocean areas where mining is likely to occur.
This Perspective aims to provide a preliminary evaluation of the potential impact of deep seabed mining on cetaceans. In particular, we highlight the need for extensive research to address the lack of empirical data related to cetacean ecology in offshore areas and commercial-scale deep seabed mining. Given such knowledge gaps we hope that this Perspective will provide the basis for further discussion and research – and the application of the Precautionary Principle.
2 Spatial overlap between cetaceans and proposed deep seabed mining
Oceanic cetaceans inhabit deep regions of the ocean from the shelf break to the abyssal plains, either as primary habitat (for example, the beaked whales and oceanic dolphins) or during migrations (for example, humpback whales, Megaptera novaeangliea). Gathering data on species offshore presents logistical challenges resulting in considerable knowledge gaps relating to their distributions and ecology. Nonetheless, cetacean distribution data available from online repositories, such as OBIS-Seamaps (https://seamap.env.duke.edu/), indicate significant spatial overlap between cetacean habitats and potential deep seabed mining sites.
The CCZ is in the eastern Pacific range, covering an area of approximately 11,650,000 km2 at an average depth of 5,500 m (Wedding et al., 2015; Marsh et al., 2018), and provides habitat to an estimated 22–30 species of cetacean, including dolphins, sperm whales, kogiids and ziphiids (Carwardine, 2020). Population densities are largely unknown. Even though systematic surveys of the CCZ area are sparse, data from multiple research cruises reported in the OBIS-SEAMAP repository show multiple cetacean species within the CCZ (Figures 1B–G), including sperm whales (Physeter macrocephalus), multiple baleen and beaked whale species, Risso’s dolphins (Grampus griseus) and dwarf and pygmy sperm whales (Kogia spp.). Although more survey data are needed to determine temporal and spatial use of the CCZ by cetaceans, available data clearly confirm the presence of the aforementioned species in the region.
Seamounts are found in numerous locations around the globe and are also a potential target for deep seabed mining (particularly those whose summits are relatively shallow), as well as being important pelagic biodiversity hotspots. For example, seamounts in the deep waters of the Azores are feeding stations for dolphins (Cascão et al., 2020) and for humpback whales during migration (Ross-Marsh et al., 2022). Data from bottom-mounted hydrophones deployed on Vema seamount, southeast Atlantic, indicated that numerous baleen whale species were present around the seamount (Elwen et al., in press). Similar data collected at Antigonia seamount, South Pacific, indicated the constant presence of odontocetes, with these animals likely moving from deeper to shallower waters to forage at night (Barcock, 2022). Humpbacks tracked across the South Pacific have been recorded spending extended periods of time at seamounts and regrouping in great numbers there, either for feeding or socializing (Derville et al., 2020). Other studies suggest that oceanic seamounts are also important for humpback whale mating, calving and nursing (Derville et al., 2018).
3 Anthropogenic noise from deep seabed mining
One of the major potential impacts on cetaceans from deep seabed mining activity is expected to be from noise produced by commercial operations, and for that reason is the primary focus of this Perspective. Anthropogenic noise in the ocean travels, or propagates, differently depending on the frequency and amplitude of the source. Ocean sound can propagate far from a point source of production, especially in sound fixing and ranging (SOFAR) channels created through interactions between temperature and pressure, which cause additional internal refraction and horizontal propagation (Prideaux & Prideaux, 2016). Ocean noise is characterized into three frequency bands: low (10 to 500 Hz); medium (500 Hz to 25 kHz); and high (>25 kHz) (Hildebrand, 2009). Low-frequency noise arises predominantly from human sources (commercial shipping and seismic exploration) and has potential for long-range propagation – for example, seismic low frequency airgun noise has been detected over a distance of 3,000 km from source using an array of autonomous bottom-mounted hydrophones (Nieukirk et al., 2004). Medium-frequency sounds have the potential to travel tens of kilometers; high-frequency noise is attenuated within a few kilometers (Hildebrand, 2009).
The frequency, amplitude and duration of noise that could be generated by deep seabed mining will depend upon how minerals are extracted and/or how many companies might carry out extraction in a given area. A reasonable expectation is that commercial-scale mining would operate 24-hours a day, at varying depths at the seabed (Miller et al., 2018), and that noise emitted would depend upon the collection process, specific location and bathymetry. Some deposits could be mined using remotely operated vehicles (ROVs), with a resulting slurry of seabed material and seawater pumped to surface support vessels for primary processing, and with deposition of waste sediment back into the water column (Miller et al., 2018). Noise is likely to be concentrated at the seabed and surface, although midwater pumps, slurry flow and ROV equipment would distribute noise throughout the water column (Christiansen et al., 2020). Surface support vessels produce low frequency and broadband sound (over a broad range of frequencies) similar to that produced by commercial shipping (Hildebrand, 2009). At the seabed, ore extraction is likely to produce a broad range of frequencies, some of which will be at the lower frequencies and could travel for at least several hundred kilometers. Pumps on surface vessels and material transfer between surface vessels may be analogous to noise emitted by standard dredging and drilling although sound propagation could be quite different at greater depths (McQueen et al., 2019). According to Kyhn et al. (2014), drillships that operate at circa 500 m depth introduce broadband noise ranging from 184–190 dB re 1 µPa at 1 m. Acoustic telemetry, used to coordinate ROV equipment, uses loud, repetitive, high frequency sound that can be 40–60 dB louder than dolphins at frequencies around 17 kHz, which is within their audible range. Telemetry is beyond the upper audible range for many adult humans (around 15 kHz on average, Markandeya et al., 2018), but is well within the range that dolphins, porpoises and beaked whales use to communicate.
Indeed, cetaceans produce and detect sounds within a number of specific frequency bands, for conspecific communication (for example, to coordinate group behavior during foraging, social interactions and breeding, Bailey et al., 2009) and to navigate with echolocation, and with significant overlap with a variety of anthropogenic sources of noise (Figure 2) (Allen et al., 2013).
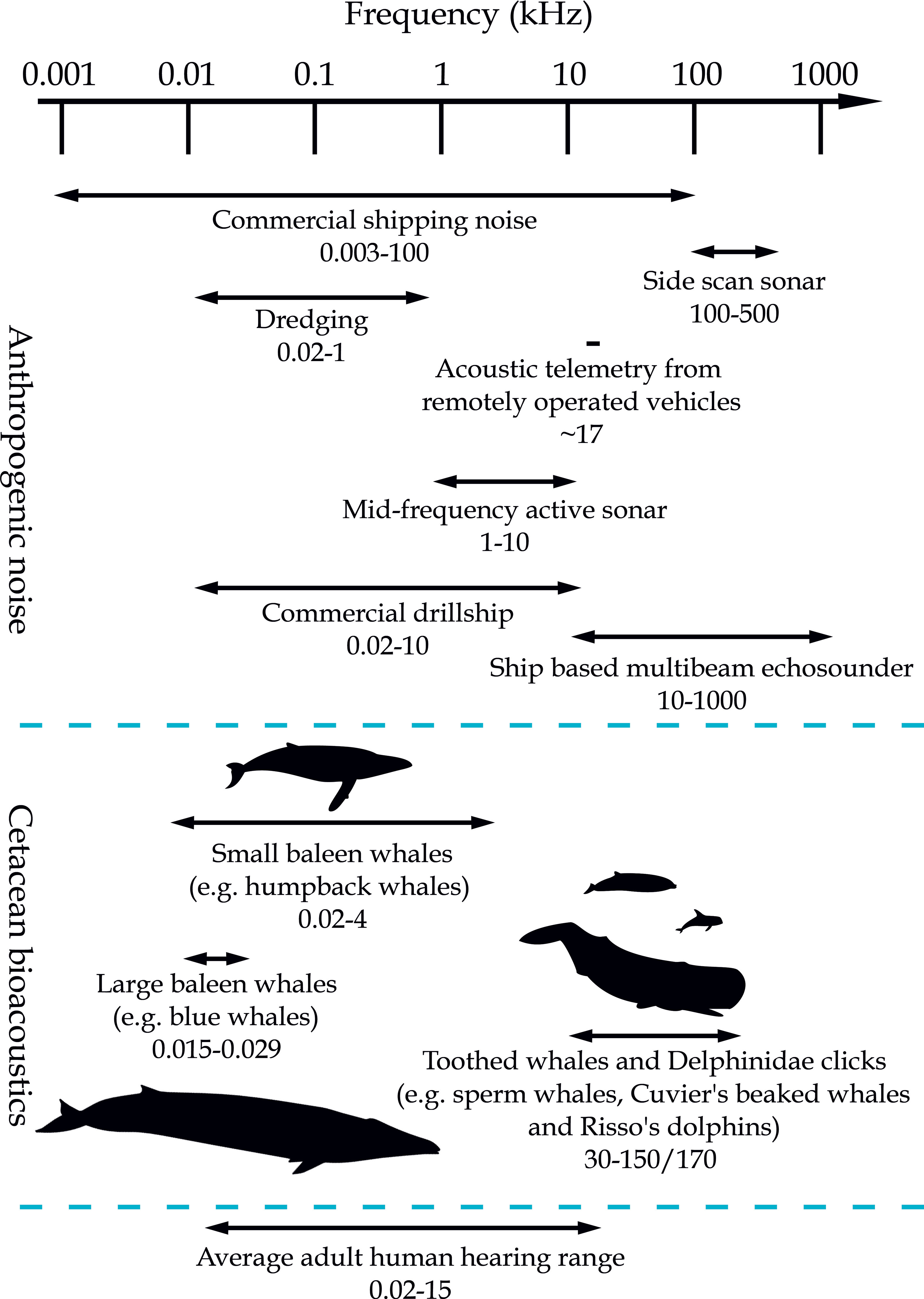
Figure 2 A schematic showing cetacean bioacoustics, anthropogenic noise (as a proxy for noise likely to be generated by deep seabed mining) and acoustic telemetry (which is the noise generated by remotely operated vehicles) (Erbe, 2003; Hildebrand, 2009; Kyhn et al., 2014; Leroy et al., 2018; Markandeya et al., 2018; Teague et al., 2018; Galatius et al., 2019; McQueen et al., 2019). Silhouettes of Risso’s dolphin, humpback and Cuvier’s beaked whales by Chris Huh from Phylopic (https://creativecommons.org/licenses/by-sa/3.0/), blue whale from Freepik (https://www.freepik.com/).
Interference of ocean noise with the sound perception of animals is termed auditory masking and can cause a variety of behavioral changes (Erbe et al., 2016). Masking occurs when anthropogenic noise degrades the perception of biologically important signals, such as those used for communication or navigation (Terhune & Killorn, 2021). The degree of masking is determined by the extent to which the frequency and amplitude of the received noise overlaps with cetacean bioacoustics (Erbe et al., 2016). Depending on the frequency of the noise, individuals close to the source of the sound may be more affected by auditory masking because there is less sound attenuation across short distances than long distances (Erbe et al., 2016). Cetaceans reliant on low frequency sound are more likely to experience masking over long distances because low frequency sound travels the furthest (Hildebrand, 2009; Prideaux and Prideaux, 2016).
Research into the sounds made by whales suggests that increased anthropogenic noise, such as from commercial ships, military sonar or seismic surveys, can mask calls between mating fin whales (Balaenoptera physalus) (Croll et al., 2002). Another study concluded that anthropogenic noise increased the risk of humpback whale mother–calf separation because normal vocalizations between them are very quiet (Videsen et al., 2017). Toothed whales such as sperm whales that rely on higher frequencies of 15 kHz can be negatively affected by sonar (von Benda-Beckmann et al., 2021). Anthropogenic noise, particularly from mid- and low-frequency sonar, seems to elicit either a startle response (foraging beaked whales surface quickly, increasing risk of gas emboli, sometimes followed by stranding) or a cessation in foraging (Frantzis, 1998; Cox et al., 2006; Hooker et al., 2019). Blackwell et al. (2015) found that bowhead whales (Balaena mysticetus) can initially shift calling frequencies to outcompete, but ultimately stop calling in response to increasingly loud airgun noise.
The density, abundance and ecology of most beaked whale species are poorly understood but the spatial overlap between deep seabed mining regions and beaked whale habitat is a concern. Beaked whales such as northern bottlenose whales (Hyperoodon ampullatus) and Cuvier’s beaked whales have been shown to respond to mid-frequency active sonar (1–10 kHz, between 117 – 126 dB re 1 µPa at 1 m) (DeRuiter et al., 2013; Bernaldo de Quirós et al., 2019; Wensveen et al., 2019). Not all cetaceans respond in the same way to a given sound. For example, noise from dredging and shipping prompted avoidance responses in minke whales (Balaenoptera bonaerensis), which are sensitive to low-frequency sound, but did not deter bottlenose dolphins (Tursiops truncatus), perhaps because odontocetes are less sensitive at low frequencies (Anderwald et al., 2013). There is, nonetheless, a clear need for further research into the potential for sound from commercial mining activities to alter the behavior of marine mammals and the implications of this at a population level.
4 Habitat degradation and removal
4.1 Plumes
Settlement of sediment plumes generated by mining vehicles could smother benthic species in the vicinity, while sediment discharged from processing vessels would increase turbidity in the water column and could mobilize contaminants. Sediment plumes are likely to cause both immediate and longer-term impacts, depending on specific location characteristics (Miller et al., 2018), and while their potential impact on cetaceans is currently unknown, future detailed assessments should consider the possible interaction of cetaceans and their prey with plumes.
4.2 Degradation of seamounts and habitat used for foraging
Recovery of deep seabed habitats from any form of mineral exploitation is likely to be extremely slow, or impossible (Niner et al., 2018). Few large-scale, long-term experiments have been conducted, though one notable experiment on nodule mining found that benthic disturbance was still evident more than 20 years later (Gausepohl et al., 2020).
Sessile organisms increase seabed complexity and provide habitat for benthopelagic species. Removal of habitat provided by mineral deposits themselves will cause mortality to organisms that live on the deposits – as well as having an impact on the wider food web (Levin et al., 2016; Vanreusel et al., 2016). Many seamounts have higher species richness and enhanced productivity than coastal or abyssal areas and, as a result, can aggregate pelagic species, providing food sources for cetaceans. For example, humpbacks were observed feeding at the Vema seamount on their migration to the Southern Ocean (Elwen et al., in press). Seamount mining is expected to remove at least the upper 10 cm of benthic material in mineral-rich areas, obliterating physical features that could in turn even influence productivity at the summit of seamounts (Miller et al., 2018; Levin et al., 2020). The damage caused by commercial trawling of seamounts provides perhaps the best proxy we have so far for the impacts that could arise from mineral extraction, including habitat destruction and the resuspension of sediments into the water column (Pusceddu et al., 2014; Clark et al., 2019; Goode et al., 2020). In such cases, increased light attenuation from resuspended sediments can decrease productivity and, in turn, diminish pelagic species biomass (Shi and Wang, 2010; Levin et al., 2016). If mining operations remove sediment in a similar way to prolonged trawling, it is plausible that foraging regions for pelagic species could be irreversibly damaged.
5 Governance of marine minerals
The current fragmented nature of ocean governance in relation to mining activities hinders conservation effort because the seabed is partly under ISA jurisdiction and partly under the jurisdiction of coastal states. The governance of deep seabed mining exploration (and exploitation) in areas beyond national jurisdiction falls under the purview of the ISA, which has responsibility to manage anthropogenic activities in the Area. The ISA has, as of February 2023, issued 31 exploration contracts in the ABNJ for the three main mineral types. In spite of it being an international UN organization, the transparency of the ISA’s work has been called into question (Ardron et al., 2018; Gerber and Grogan, 2020).
More broadly, the lack of coherent ocean protection mechanisms means that marine flora and fauna are vulnerable to increasing anthropogenic threats. The UN negotiations to develop a legally binding instrument for conservation and sustainable use of marine biological diversity in areas beyond national jurisdiction provide an opportunity to consolidate commitments to marine conservation, improve governance of human activities in the high seas and implement dynamic management approaches (Crespo et al., 2020). How such developments may influence the exploration for and exploitation of seabed mineral resources remain to be seen.
6 Conclusion
Oceans globally are facing increasing pressure from anthropogenic activities. Deep seabed mining would add to those stressors. There are concerns that exploiting non-renewable resources from the deep sea runs counter to United Nations (UN) Sustainable Development Goal 14, which aims “to protect the health of the ocean”, and also brings into question Article 140 of the United Nations Convention on the Law of the Sea (UNCLOS), which stipulates that activities within the ABNJ are to be carried out for “the benefit of mankind as a whole” (UNCLOS, 1982). If deep seabed mining companies begin commercial-scale exploitation in 2023, the industry could damage the oceans in ways we do not fully understand before germane regulations are even developed, and perhaps at the expense of species that have been the focus of conservation effort for many years. Far-from-sight impacts on cetaceans could go largely unquantified and unnoticed, along with those on other pelagic predator species that rely on deep ocean areas, including sharks.
The demand for minerals to support green energy and transport is often cited as justification for seabed mining. This link is questionable and highlights the importance of deeper scrutiny into the drivers underlying the pursuit of seabed minerals and the need to address them as far as possible through more sustainable means (Miller et al., 2021). The IUCN Cetacean Specialist Group has classified five cetacean species as ‘critically endangered’, including the North Atlantic Right whale (Eubalaena glacialis) and the newly described Rice’s whale (Balaenoptera ricei), as well as an additional 19 sub-species or populations. Currently 12 species are listed as ‘endangered’, including the sei whale (Balaenoptera borealis) and blue whale (Balaenoptera musculus) (IUCN, 2022). Insufficient data are available to assess the population status of a significant number of species, though the distributions of at least some of these species include areas being explored for commercial seabed mining. In this Perspective we aim to catalyze focused discussions between cetacean and deep ocean specialists to act with urgency to assess the impacts that deep seabed mining could have on cetacean populations, including through collaboration to cement new research partnerships. An integrated research partnership or consortium could be developed through existing networks, perhaps building on the example of the Southern Ocean Research Partnership that aims to maximize conservation-oriented outcomes for Southern Ocean cetaceans. Given the imminent threat that the two-year rule presents to ocean conservation, we suggest there is no time to waste.
Author contributions
KT, JW and KM conceived the perspective. KT, KM, JW wrote the manuscript. SD, CL, DS, PJ critically reviewed the paper. All authors contributed to the article and approved the submitted version.
Funding
The preparation of this manuscript was funded by Umweltstiftung Greenpeace to provide independent scientific advice and analytic services to that non-governmental organization.
Acknowledgments
We would like to thank Dr Mónica de Silva, Dr Rui Prieto, Dr Jaime Sutcliffe and Dr Tim Smyth. We extend thanks to Thomas Webber and Ildiko Kriston for help with designing the two figures. In addition, we thank two reviewers for their helpful comments that improved the manuscript.
Conflict of interest
The authors declare that the research was conducted in the absence of any commercial or financial relationships that could be construed as a potential conflict of interest.
Publisher’s note
All claims expressed in this article are solely those of the authors and do not necessarily represent those of their affiliated organizations, or those of the publisher, the editors and the reviewers. Any product that may be evaluated in this article, or claim that may be made by its manufacturer, is not guaranteed or endorsed by the publisher.
Supplementary material
The Supplementary Material for this article can be found online at: https://www.frontiersin.org/articles/10.3389/fmars.2023.1095930/full#supplementary-material
References
Allen J., Weinrich M., Hoppitt W., Rendell L. (2013). Network-based diffusion analysis reveals cultural transmission of lobtail feeding in humpback whales. Science 340, 485–488. doi: 10.1126/science.1231976
Anderwald P., Brandecker A., Coleman M., Collins C., Denniston H., Haberlin D., et al. (2013). Displacement responses of a mysticete, an odontocete, and a phocid seal to construction-related vessel traffic. Endang. Sp. Res. 21, 231. doi: 10.3354/esr00523
Ardron J. A., Ruhl H. A., Jones D. O. (2018). Incorporating transparency into the governance of deep-seabed mining in the area beyond national jurisdiction. Mar. Pol. 89, 58–66. doi: 10.1016/j.marpol.2017.11.021
Bailey H., Mate B. R., Palacios D. M., Irvine L., Bograd S. J., Costa D. P. (2009). Behavioural estimation of blue whale movements in the northeast pacific from state-space model analysis of satellite tracks. Endang. Species Res. 10, 93–106. doi: 10.3354/esr00239
Barcock C. (2022). The call of the seamount: Temporal and diel variations in odontocete vocalisations at antigonia seamount (Scotland: University of St Andrews).
Bernaldo de Quirós Y., Fernandez A., Barid R. W., Brownell R. L., Anguilar de Soto. N., Allen D., et al. (2019). Advances in research on the impacts of anti-submarine sonar on beaked whales. Proc. R. Soc B: Biol. Sci. 286, 20182533. doi: 10.1098/rspb.2018.2533
Blackwell S. B., Nations C. S., McDonald T. L., Thode A. M., Mathias D., Kim K. H., et al. (2015). Effects of airgun sounds on bowhead whale calling rates: Evidence for two behavioral thresholds. PloS One 10, e0125720. doi: 10.1371/journal.pone.0125720
Cascão I., Lammers M. O., Prieto R., Santos R., de Silva M. (2020). Temporal patterns in acoustic presence and foraging activity of oceanic dolphins at seamounts in the Azores. Sci. Rep. 10, 1–11. doi: 10.1038/s41598-020-60441-4
Christiansen B., Denda A., Christiansen S. (2020). Potential effects of deep seabed mining on pelagic and benthopelagic biota. Mar. Pol. 114, 103442. doi: 10.1016/j.marpol.2019.02.014
Clark M. R., Bowden D. A., Rowden A. A., Stewart R. (2019). Little evidence of benthic community resilience to bottom trawling on seamounts after 15 years. Front. Mar. Sci. 6. doi: 10.3389/fmars.2019.00063
Costello M. J., Coll M., Danovaro R., Halpin P., Ojaveer H., Miloslavich P. (2010). A census of marine biodiversity knowledge, resources, and future challenges. PloS One 5, e12110. doi: 10.1371/journal.pone.0012110
Cox T. M., Ragen T. J., Read A. J., Vos E., Baird R. W., Balcomb K., et al. (2006). Understanding the impacts of anthropogenic sound on beaked whales. J. Cetacean Res. Manage. 7, 177–187.
Crespo G. O., Mossop J., Dunn D., Gjerde K., Hazen E., Reygondeau G., et al. (2020). Beyond static spatial management: Scientific and legal considerations for dynamic management in the high seas. Mar. Pol. 122, 104102. doi: 10.1016/j.marpol.2020.104102
Croll D. A., Clark C., Acebedo A., Tershy B., Flores S., Gedamke J., et al. (2002). Only male fin whales sing loud songs. Nature 417, 809–809. doi: 10.1038/417809a
Cuvelier D., Ribeiro P. A., Ramalho S. P., Kersken D., Martinez Arbizu P., Colaço A. (2020). Are seamounts refuge areas for fauna from polymetallic nodule fields? Biogeosci 17, 2657–2680. doi: 10.5194/bg-17-2657-2020
DeRuiter S. L., Southall B. L., Calambokidis J., Zimmer W. M. X., Sadykova D., Falcone E. A., et al. (2013). First direct measurements of behavioural responses by cuvier’s beaked whales to mid-frequency active sonar. Biol. Lett. 9, 20130223. doi: 10.1098/rsbl.2013.0223
Derville S., Torres L. G., Garrigue C. (2018). Social segregation of humpback whales in contrasted coastal and oceanic breeding habitats. J. Mamm. 99, 41–54. doi: 10.1093/jmammal/gyx185
Derville S., Torres L. G., Zerbini A. N., Oremus M., Garrigue C. (2020). Horizontal and vertical movements of humpback whales inform the use of critical pelagic habitats in the western south pacific. Sci. Rep. 10, 4871. doi: 10.1038/s41598-020-61771-z
Elwen S., Fearey J., Ross-Marsh E., Thompson K. F., Maack T., Webber T., et al. Cetacean diversity of the eastern south Atlantic ocean and vema In press seamount detected during a visual and passive acoustic survey. J. Mar. Biol. Assoc. U.K.
Erbe C. (2003). “Assessment of bioacoustic impact of ships on humpback whales in glacier bay, Alaska,” in Gustavus A. Report to Glacier Bay National Park and Preserve, vol. 38. .
Erbe C., Reichmuth C., Cunningham K., Lucke K., Dooling R. (2016). Communication masking in marine mammals: A review and research strategy. Mar. Poll. Bull. 103, 15–38. doi: 10.1016/j.marpolbul.2015.12.007
Galatius A., Olsen M. T., Steeman M. E., Racicot R. A., Bradshaw C. D., Kyhn L. A., et al. (2019). Raising your voice: Evolution of narrow-band high-frequency signals in toothed whales (Odontoceti). Biol. J. Linn. Soc 126, 213–224. doi: 10.1093/biolinnean/bly194
Garrigue C., Clapham P. J., Geyer Y., Kennedy A. S., Zerbini A. N. (2015). Satellite tracking reveals novel migratory patterns and the importance of seamounts for endangered south pacific humpback whales. R. Soc Open Sci. 2, 150489. doi: 10.1098/rsos.150489
Gausepohl F., Hennke A., Schoening T., Köser K., Greinert J. (2020). Scars in the abyss: Reconstructing sequence, location and temporal change of the 78 plough tracks of the 1989 DISCOL deep-sea disturbance experiment in the Peru basin. Biogeosci 17, 1463–1493. doi: 10.5194/bg-17-1463-2020
Gerber L., Grogan R. (2020). Challenges of operationalising good industry practice and best environmental practice in deep seabed mining regulation. Mar. Pol. 114. doi: 10.1016/j.marpol.2018.09.002
Goode S. L., Rowden A. A., Bowden D. A., Clark M. R. (2020). Resilience of seamount benthic communities to trawling disturbance. Mar. Environ. Res. 161, 105086. doi: 10.1016/j.marenvres.2020.105086
Hildebrand J. (2009). Anthropogenic and natural sources of ambient noise in the ocean. Mar. Ecol. Prog. Seri. 395, 5–20. doi: 10.3354/meps08353
Hooker S. K., de Soto N. A., Baird R. W., Carroll E. L., Claridge D., Feyrer L., et al. (2019). Future directions in research on beaked whales. Front. Mar. Sci. 5. doi: 10.3389/fmars.2018.00514
International Seabed Authority (2021) Exploration for minerals in the area. Available at: https://www.isa.org.jm/index.php/minerals/exploration-areas (Accessed December 13, 2021).
International Union for the Conservation of Nature (2022) Status of the world’s cetaceans. Available at: https://iucn-csg.org/status-of-the-worlds-cetaceans/ (Accessed September 30, 2022).
Jones D. O. B., Kaiser S., Sweetman A. K., Smith C. R., Menot L., Vink A., et al. (2017). Biological responses to disturbance from simulated deep-sea polymetallic nodule mining. PloS One 12, e0171750. doi: 10.1371/journal.pone.0171750
Kavanagh A. S., Nykänen M., Hunt W., Richardson N., Jessopp M. J. (2019). Seismic surveys reduce cetacean sightings across a large marine ecosystem. Sci. Rep. 9, 19164. doi: 10.1038/s41598-019-55500-4
Kyhn L. A., Sveegaard S., Tougaard J. (2014). Underwater noise emissions from a drillship in the Arctic. Mar. Poll. Bull. 86, 424–433. doi: 10.1016/j.marpolbul.2014.06.037
Leitner A. B., Drazen J. C., Smith C. R. (2021). Testing the seamount refuge hypothesis for predators and scavengers in the Western clarion-clipperton zone. Front. Mar. Sci. 8. doi: 10.3389/fmars.2021.636305
Leroy E. C., Royer J.-Y., Bonnel J., Samaran F. (2018). Long-term and seasonal changes of Large whale call frequency in the southern Indian ocean. J. Geophys. Res.: Oceans 123, 8568–8580. doi: 10.1029/2018JC014352
Levin L. A., Amon D. J., Lily H. (2020). Challenges to the sustainability of deep-seabed mining. Nat. Sustain. 3, 784–794. doi: 10.1038/s41893-020-0558-x
Levin L. A., Mengerink K., Gjerde K. M., Rowden A. A., Van Dover C. L., Clark M. R., et al. (2016). Defining “serious harm” to the marine environment in the context of deep-seabed mining. Mar. Pol. 74, 245–259. doi: 10.1016/j.marpol.2016.09.032
Markandeya M. N., Abeyratne U. R., Hukins C. (2018). Characterisation of upper airway obstructions using wide-band snoring sounds. BioMed. Signal Process. Control 46, 201–211. doi: 10.1016/j.bspc.2018.07.013
Marsh L., Huvenne V. A. I., Jones D. O. B. (2018). Geomorphological evidence of large vertebrates interacting with the seafloor at abyssal depths in a region designated for deep-sea mining. R. Soc Open Sci. 5, 180286. doi: 10.1098/rsos.180286
McQueen A., Suedel B., Wilkens J. (2019). Review of the adverse biological effects of dredging-induced underwater sounds. Technical Report (Engineer Research and Development Center (U.S.)) ; no. ERDC/EL TR-19-18. http://dx.doi.org/10.21079/11681/34245.
Miller K. A., Brigden K., Santillo D., Currie D., Johnston P., Thompson K. F. (2021). Challenging the need for deep seabed mining from the perspective of metal demand, biodiversity, ecosystems services, and benefit sharing. Front. Mar. Sci. 8. doi: 10.3389/fmars.2021.706161
Miller K. A., Thompson K. F., Johnston P., Santillo D. (2018). An overview of seabed mining including the current state of development, environmental impacts, and knowledge gaps. Front. Mar. Sci. 4. doi: 10.3389/fmars.2017.00418
Nieukirk S. L., Stafford K. M., Mellinger D. K., Dziak R. P., Fox C. G. (2004). Low-frequency whale and seismic airgun sounds recorded in the mid-Atlantic ocean. J. Acoust. Soc Am. 115, 1832–1843. doi: 10.1121/1.1675816
Niner H. J., Ardron J. A., Escobar E. G., Gianni M., Jaeckel A., Jones D. O. B., et al. (2018). Deep-sea mining with no net loss of biodiversity–an impossible aim. Front. Mar. Sci. 5. doi: 10.3389/fmars.2018.00053
Pires A. L. M. S., de Sá Maciel I., dos Santos Alves M. A., Tardin R. H. (2021). The effects of anthropogenic noise on cetaceans in Brazil: the need to consider recent scientific advances in environmental licensing. J. Coast. Conserv. 25, 45. doi: 10.1007/s11852-021-00832-5
Prideaux G., Prideaux M. (2016). Environmental impact assessment guidelines for offshore petroleum exploration seismic surveys. Impact Assess. Proj. Apprais. 34, 33–43. doi: 10.1080/14615517.2015.1096038
Pusceddu A., Bianchelli S., Martín J., Puig P., Palanques A., Masqué P., et al. (2014). Chronic and intensive bottom trawling impairs deep-sea biodiversity and ecosystem functioning. Proc. Natl. Acad. Sci. U.S.A. 111, 8861–8866. doi: 10.1073/pnas.1405454111
Ross-Marsh E. C., Elwen S. H., Fearey J., Thompson K. F., Maack T., Gridley T. (2022). Detection of humpback whale (Megaptera novaeangliae) non-song vocalizations around the vema seamount, southeast Atlantic ocean. JASA Express Lett. 2, 041201. doi: 10.1121/10.0010072
Sahri A., Putra M. I. H., Mustika P. L. K., Kreb D., Murk A. J. (2021). Cetacean habitat modelling to inform conservation management, marine spatial planning, and as a basis for anthropogenic threat mitigation in Indonesia. Ocean Coast. Manage. 205, 105555. doi: 10.1016/j.ocecoaman.2021.105555
Shi W., Wang M. (2010). Characterization of global ocean turbidity from moderate resolution imaging spectroradiometer ocean color observations. J. Geophys. Res.: Oceans 115, C11. doi: 10.1029/2010JC006160
Teague J., Allen M. J., Scott T. B. (2018). The potential of low-cost ROV for use in deep-sea mineral, ore prospecting and monitoring. Ocean Engineer. 147, 333–339. doi: 10.1016/j.oceaneng.2017.10.046
Terhune J. M., Killorn D. (2021). A method for preliminary assessment of the masking potential of anthropogenic noise to baleen whale calls. Aquat. Mamm. 47, 283–291. doi: 10.1578/AM.47.3.2021.283
Thompson K. F., Miller K. A., Currie D., Johnston P., Santillo D. (2018). Seabed mining and approaches to governance of the deep seabed. Front. Mar. Sci. 5. doi: 10.3389/fmars.2018.00480
United Nations Convention on the Law of the Sea [UNCLOS] (1982). Montego bay, 10 December 1982, in force 6 November 1994, 21 ILM 1261–1354, 1982, article 140. montego bay: United nations convention on the law of the Sea.
Vanreusel A., Hilario A., Ribeiro P. A., Menot L., Arbizo P. M. (2016). Threatened by mining, polymetallic nodules are required to preserve abyssal epifauna. Sci. Rep. 6, 26808. doi: 10.1038/srep26808
Videsen S. K. A., Bejder L., Johnson M., Madsen P. T. (2017). High suckling rates and acoustic crypsis of humpback whale neonates maximise potential for mother–calf energy transfer. Funct. Ecol. 31, 1561–1573. doi: 10.1111/1365-2435.12871
von Benda-Beckmann A. M., Isojunno S., Zandvliet M., Ainslie M. A., Wensveen P. J., Tyack P. L., et al. (2021). Modeling potential masking of echolocating sperm whales exposed to continuous 1–2 kHz naval sonar. J. Acoust. Soc Am. 149, 2908–2925. doi: 10.1121/10.0004769
Wedding L. M., Reiter S. M., Smith C. R., Gjerde K. M., Kittinger J. M., Friedlander A. M., et al. (2015). Managing mining of the deep seabed. Science 349, 144–145. doi: 10.1126/science.aac6647
Keywords: marine minerals, marine mammals, International Seabed Authority, anthropogenic noise, seamounts, anthropogenic impacts, two-year rule, Clarion Clipperton Zone
Citation: Thompson KF, Miller KA, Wacker J, Derville S, Laing C, Santillo D and Johnston P (2023) Urgent assessment needed to evaluate potential impacts on cetaceans from deep seabed mining. Front. Mar. Sci. 10:1095930. doi: 10.3389/fmars.2023.1095930
Received: 11 November 2022; Accepted: 20 January 2023;
Published: 14 February 2023.
Edited by:
Dianne McLean, Australian Institute of Marine Science (AIMS), AustraliaReviewed by:
Michele Thums, Australian Institute of Marine Science (AIMS), AustraliaVictoria Louise Georgia Todd, Ocean Science Consulting Ltd., United Kingdom
Copyright © 2023 Thompson, Miller, Wacker, Derville, Laing, Santillo and Johnston. This is an open-access article distributed under the terms of the Creative Commons Attribution License (CC BY). The use, distribution or reproduction in other forums is permitted, provided the original author(s) and the copyright owner(s) are credited and that the original publication in this journal is cited, in accordance with accepted academic practice. No use, distribution or reproduction is permitted which does not comply with these terms.
*Correspondence: Kirsten F. Thompson, ay5mLnRob21wc29uQGV4ZXRlci5hYy51aw==