- 1Center for Marine Science, University of North Carolina Wilmington, Wilmington, NC, United States
- 2Department of Biological Sciences, Auburn University, Auburn, AL, United States
- 3Department of Biology, Central Michigan University, Mount Pleasant, MI, United States
The Southern Ocean’s continental shelf communities harbor high benthic biodiversity. However, most census methods have relied on trawling or dredging rather than direct observation. Benthic photographic and videographic transect surveys serve a key role in characterizing marine communities’ abundance and diversity, and they also provide information on the spatial arrangement of species within a community. To investigate diversity and abundance in Southern Ocean benthic communities, we employed photographic transects during cruises aboard the RVIB Nathanial B. Palmer (November 2012) and the ASRV Laurence M. Gould (February 2013). One kilometer long photographic transects were conducted at 8 sites along 6,000 km of Western Antarctica from the tip of the Antarctic Peninsula to the Ross Sea from which epifaunal echinoderms, tunicates, arthropods, cnidarians, poriferans, and annelids were identified and counted allowing estimations of biodiversity. Our results do not support a latitudinal trend in diversity, but rather a decrease in abundance of macrofaunal individuals at higher latitude sites. All communities sampled on the Western Antarctic shelf were primarily dominated by ophiuroids, pycnogonids, holothuroids, and demosponges. However, the most abundant taxon across all sites was Ophionotus victoriae, followed by the symbiotic partners Iophon sp. (demosponge) and Ophioplinthus spp. (ophiuroid). Data also confirm that the Southern Ocean is composed of discretely unique benthic communities. These results provide critical understanding of the current community structure and diversity serving as a baseline as the Antarctic continental shelf changes due to rising ocean temperatures, climate change, and collapse of large ice sheets.
1 Introduction
Waters surrounding the Western Antarctic region house great faunal abundance, richness, and diversity. Although over 8,000 invertebrate species have been described from the Southern Ocean, an estimated 17,000-20,000 invertebrate species may exist there (Gutt et al., 2004) with the highest diversity reported in the Western Antarctic (Gutt and Starmans, 1998; Grange and Smith, 2013; Pineda-Metz et al., 2019). A similar situation exists on Arctic continental shelves, where species diversity is under described in large part due to inaccessibility caused by ice (Piepenburg et al., 2011). Although the Antarctic marine continental shelf biome is sometimes considered one ecoregion, there is considerable variation in the physical environment which can affect organismal distribution and abundance (Convey and Peck, 2019), ultimately impacting community structure. Through photo/video transects, bottom trawls, and box cores, previous research on the Antarctic shelf has revealed great diversity (Gutt et al., 2013) and abundance of epifaunal invertebrates (e.g. Dayton et al., 1974; Grebmeier and Barry, 1991; Gerdes et al., 1992; Gutt and Starmans, 1998; Sumida et al., 2008; Grange and Smith, 2013; Post et al., 2017; Pineda-Metz et al., 2019; Cummings et al., 2021; Zwerschke et al., 2022). These studies describe high numbers of ophiuroids, holothuroids, sponges, cnidarians, and annelids. Similar to destructive techniques, non-destructive imaging technologies (or SCUBA at shallow depths) can accurately characterize spatial relationships and communities of epifaunal species (Pineda-Metz and Gerdes, 2018; Cummings et al., 2021). Previous work in continental shelf regions of the Southern Ocean has revealed more sea cucumbers, urchins, and anthozoans near the Western Antarctic Peninsula and higher numbers of brittle stars and sponges in the Ross Sea (Barry et al., 2003; Sumida et al., 2008). Unlike the Arctic Ocean, where a latitudinal diversity hypothesis is supported, the Southern Ocean has thus far shown little support for a latitudinal decrease in diversity (Gray, 2001; Clarke et al., 2007; Aronson et al., 2009; Griffiths, 2010).
Around the continent, seawater temperature, salinity, and pH remain relatively constant. Antarctic waters are some of the most thermally stable environments on the planet varying on average roughly 1 °C (Clarke, 1988; Arntz et al., 1994; Pineda-Metz et al., 2020). As a result, many organisms in the region are stenothermic, being only able to live in a narrow temperature range (Clarke, 1988; Arntz et al., 1994; Aronson et al., 2009). Close to the edge of the continental shelf however, there are sources of environmental variation (Hellmer et al., 2012; Isla and Gerdes, 2019), such as regions where relatively warm water from the circumpolar deep water (CDW) currents are pushed onto the shelf (e.g., in the Bellingshausen Sea; see Ruan et al., 2021) resulting in different environmental zones (e.g., increased temperature, decreased oxygen) that might harbor distinct benthic communities. Variations in physical ocean characteristics offer insight to faunal diversity, distribution, and abundance in the Western Antarctic (Halanych and Mahon, 2018). Along with the direct influence of CDW influencing physical water parameters, CDW intrusion is currently understood to be an important driver in accelerated melting rates of sea ice (Jacobs and Comiso, 1997; Stern et al., 2013). In the spring time, CDW intrusion effects coupled with higher temperatures cause an annual melting of sea ice (De Jong et al., 2015). Upon sea ice melt in the spring and summer months, iron is released into the water column leading to an annual influx which is an important trigger for phytoplankton blooms that increases primary production, likely influencing the local benthic community (Jacobs and Comiso, 1997; De Jong et al., 2015). Due to the complex oceanography of the region however, mechanisms that cause variation in CDW intrusions annually and inter-annually are poorly understood (Morrison et al., 2020). In the Ross Sea, strong winds in the spring time can create a large area devoid of sea ice, also known as a polynya, which promotes phytoplankton growth (Smith and Gordon, 1997). Predictions of ocean warming driven by climate change threaten the thermal stability of this region, which may lead to detrimental effects on abundance and fitness of regional benthic fauna (Clarke et al., 2007). The warming and freshening of Southern Ocean waters may stress organisms both thermally and nutritionally. Warmer waters can directly affect organismal homeostasis, but these warmer waters also have the potential to alter the quantity and quality of detrital nutrients reaching the seafloor (Wu et al., 2021; Fernández-González et al., 2022). These potential effects may stress organisms and drastically impact the local diversity and abundance of organisms (Pineda-Metz et al., 2020). In addition, phytoplankton blooms stimulated by upwelling, ice-melt, or iron influx from warm water intrusions have been described in Western Antarctica and result in decreased oxygen levels as the phytoplankton decompose (Deppeler and Davidson, 2017; Tripathy and Jena, 2019). In many cases, these environmental factors are influenced by global climate change (Convey and Peck, 2019), further necessitating study and characterization of benthic communities as they experience such variation.
In this study, our goal was to describe epifaunal communities across 6,000km of the Western Antarctic continental shelf, beginning near the tip of the peninsula continuing west, and correlate potential environmental drivers to benthic macrofauna dominance through the use of benthic photographic transects (Figure 1). We expected to see a higher diversity and abundance of epifauna in sites with harder substrates (pebbles and cobbles) and phytodetritus because they provide a stable point of attachment and sustenance, respectively (Post et al., 2017; Gutt et al., 2019). Additionally, we hypothesized a greater relative abundance of brittle stars and sea cucumbers on softer sediments due to their feeding and burrowing behaviors. Understanding environmental influences on communities gives the scientific community valuable insight into how the Western Antarctic (WA) benthos, and the Antarctic ecosystem as a whole, could react to global climate change. Moreover, studies such as this help fill research gaps in global marine communities so latitudinal trends can be better understood.
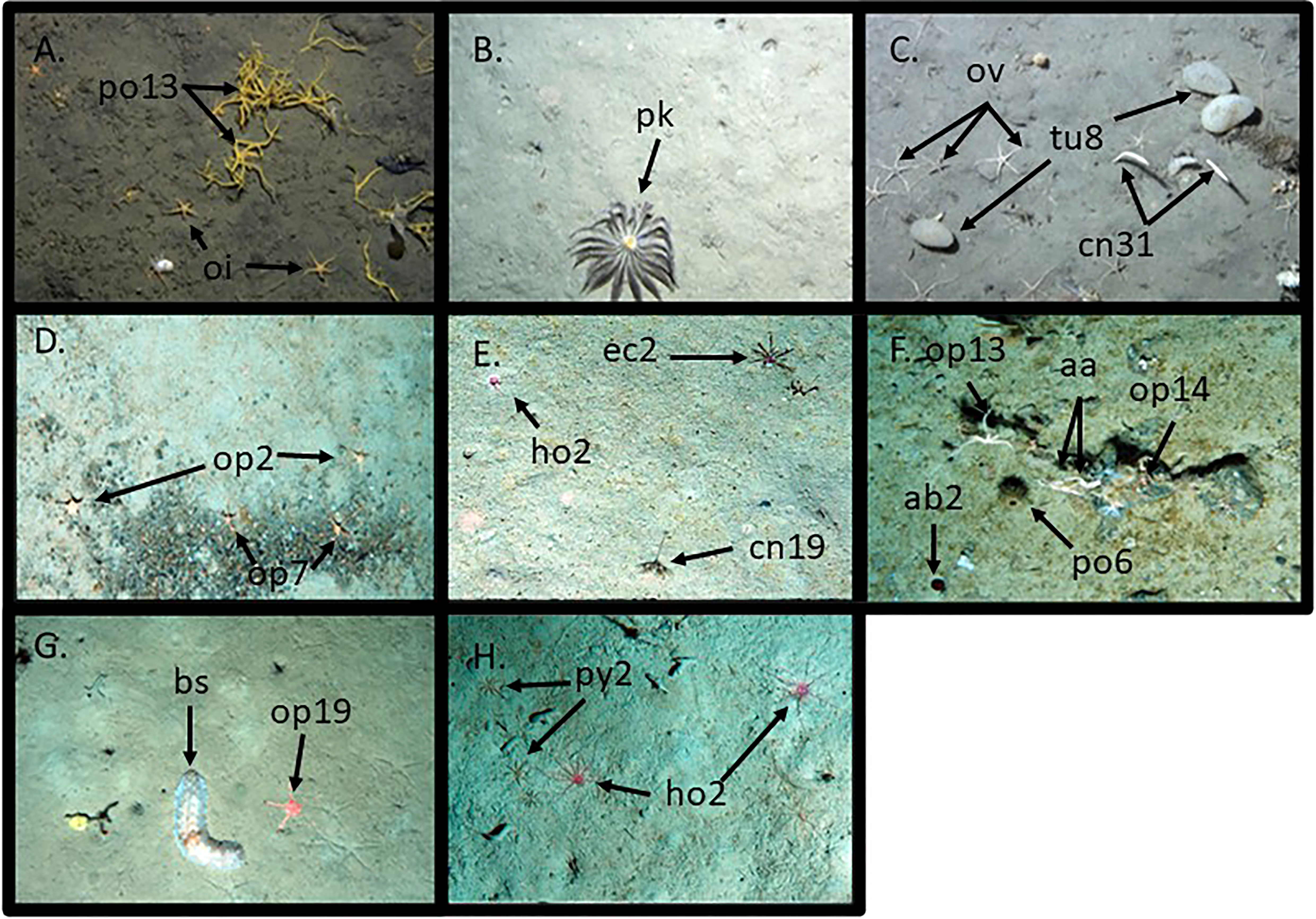
Figure 1 Examples of organismal diversity from each of the sites: (A) Montravel Rock, (B) Recovitza Island, (C) Seymour Island, (D) Bellingshausen, (E) Wright’s Gulf, (F) Eastern Amundsen, (G) Near Ross Sea, (H) Mid Ross Sea. Macrofaunal identifications are: aa = Astrotoma agassizii, ab2 = Abatus sp. 2, bs = Bathyplotes sp. 1, cn19 = cnidarian 19, likely Umbellula sp., cn31 = cnidarian 31, likely Primnoella sp., echinoid 2 = echinoid 2, ho2 = holothurian 2, oi = Ophioplinthus spp. and Iophon sp. symbiosis, op2 =ophiuroid 2, op13 = ophiuroid 13, op14 = ophiuroid 14, ov = Ophionotus victoriae, pk = Promachocrinus kerguluensis, po6 = porifera 6, po13 = porifera 13, py2 = pychnogonid 2, and tu8 = tunicate 8.
2 Materials and methods
During cruise NBP 12-10 aboard the RVIB Nathanial B. Palmer (NBP) and cruise LMG 13-12 on the ASRV Laurence M. Gould (LMG) along Western Antarctica between 2012-2013, photographic transects were conducted via a downward facing camera setup known as the ‘Yoyo’ camera (Smith et al., 2012; Grange and Smith, 2013). The Yoyo camera consists of a steel frame supporting a digital camera (10.2 megapixel, 20mm, Nikon D-80 Camera) and two parallel red lasers 10cm apart (Figure 2A). The unit is fitted with a transmissometer and audible contact that triggers a switch and takes the photo of the seafloor from ~2.5m above (Grange and Smith, 2013) imaging an approximately 3m2 area. The camera unit is repeatedly raised and lowered, hence the yo-yo name, to take multiple images across a transect as the ship moves at approximately 1 knot. Photos were color corrected and white balanced, as well as cropped to the central 135cm x 135cm section, which included the two red laser dots (a scale-bar representing 10 cm on the benthos), to reduce edge effects. Flat and relatively drop stone-free sites were selected based on overall topography to facilitate subsequent organismal collections by Blake Trawl (speed = 1 knot with variable tow durations dependent on tension) to support taxonomic identifications. As a result, sites tended to lack relief and had soft sand and/or pebble bottoms. In this study, photographic data were collected for 1 km long transects between 301-731m depth at 8 sites from the tip of the Western Peninsula to the Ross Sea (Figure 1B). Site position and distance from the mainland were collected via Geographic Positioning System (GPS) to include latitude and longitude as potential factors for analysis.
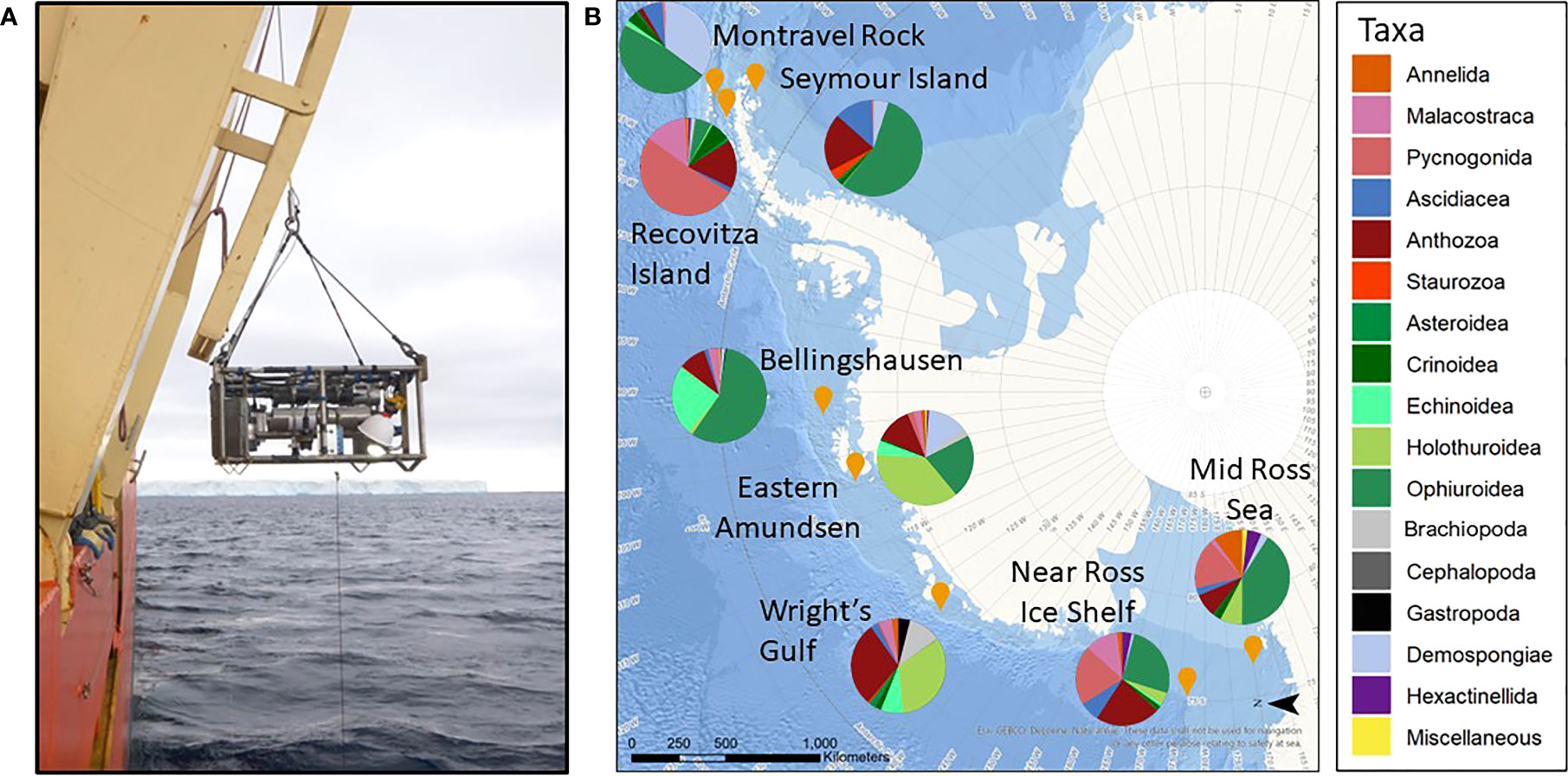
Figure 2 (A) Photograph of the Yoyo camera set up entering the water (Photo by Alex Brett). The weight and contact plate hang down ~2.5m so the images are all taken from the same height above the seafloor. (B) Map of sampling sites with community composition shown with pie charts.
For each transect, benthic environmental data was collected via a Sea-Bird CTD system, providing water column information from the sea surface to the seafloor once per site (Data available at https://www.marine-geo.org: NBP 12-10 doi 10.7284/901177 and LMG 13-12 doi 10.7284/901981). We included maximum fluorescence from the CTD cast in the euphotic zone as a proxy for primary productivity. Sea ice cover and lack of fine-scale satellite coverage across the transect prevented obtaining long-term productivity estimates via satellite imagery. Maximum sea ice coverage for each site was gathered from the National Snow and Ice Data Center (NSIDC, 2023). For each cropped and color-corrected photo, the substrate was classified as sandy, sandy with pebbles (stones < 5cm), or sandy with pebbles and cobble (stones 5-50cm) and examined as a parameter to determine community differences between substrate types. If pebbles and cobbles covered more than 5cm2, then the photo was no longer classified as sandy, instead it was classified as 'sand and pebbles' or 'sand, pebbles, and cobbles', respectively. In addition to the substrate characterization, we also scored whether phytodetritus on the seafloor was present (i.e., visibly covered more than 5 cm2: Figures 1E, F). Highly collinear variables (|r| ≥ 0.80: distance from mainland) were removed from analysis, and trends in abiotic data collected were elucidated via principal component analysis (PCA).
During the 1 km transect at each site, 101-323 photos were taken roughly every 5-10 m. Subsequently, photos were filtered to remove images with substantial turbidity, overlap between photos or poor photo quality. Of the remaining images, 50 photos were randomly selected and analyzed per site to represent the 1 km-long transects following the protocol of Grange and Smith (2013) to allow compatibility. Images were analyzed to identify organismal morphotypes and all morphotypes were assigned to the lowest taxonomic classification possible. For purposes of our analyses, species were also binned into higher taxonomic categories including Annelida, Ascidiacea, Malacostraca, Pycnogonida, Anthozoa, Staurozoa, Asteroidea, Crinoidea, Echinoidea, Holothuroidea, Ophiuroidea, Brachiopoda, Cephalopoda, Gastropoda, Demospongiae, and Hexactinellida. Benthic epifaunal invertebrates were assigned to the “Miscellaneous” group if identification was not possible (e.g., due to viewing angle or obstruction). Colonial fauna were included as individuals if substrate was visible between the zooids. Statistical analyses were run both with and without the miscellaneous group to account for all organisms distinguishable on the bottom, but our primary outcomes were not significantly impacted by the presence of the miscellaneous category (Supplementary Data). Species richness (Margalef, 1958), Pielou’s evenness (Pielou, 1966), and diversity indices (Shannon, 1948; Simpson, 1949) were calculated and compared. Infauna, meiofauna, tube-dwelling annelids (such as chaetopterids) and other taxa (e.g., Bryozoa and Hydrozoa) were not included in the study due to the difficult nature of identifying species/morphotypes based on surface evidence and photo resolution.
Rare taxa that were found at only one or two sites were removed from community analysis. Abundance data for remaining taxa in each photo were 4th-root transformed to reduce the impact of species dominance by a few select species. Then, faunal assemblages were visualized with a distance-based redundancy analysis (dbRDA) based on calculations of a zero-adjusted Bray-Curtis similarity matrix in PRIMER version 7 (Bray and Curtis, 1957; Clarke et al., 2014). We performed a cluster analysis to estimate relatedness of communities and to group sites together based on community compositions and utilized a similarity profile (SIMPROF) to find statistically significant groupings. Then we completed a similarity percentage (SIMPER) analysis to identify taxa responsible for the similarities and dissimilarities between sites and regions. Environmental variables were visualized with a principal component analysis (PCA) then superimposed on the dbRDA to illustrate abiotic influences on community composition. To elucidate abiotic-biotic relationships, we conducted permutational analysis of variance (PERMANOVA) and subsequent PERMDISP to characterize dispersion. We used distance-based linear models with DistLM protocol to determine the environmental factors primarily responsible for community differences based on Akaike information criterion (AIC).
3 Results
A total of 400 photos from 8 transects were included in this study, resulting in a total count of 6,947 organisms belonging to 204 morphotypes (or “taxa”) in the 16 taxonomic groups examined (Tables S1, S2). Benthic communities in the Peninsula region had higher organismal density than other regions (Table 1). Images across all 8 sites varied from having just 1 organism present to 89 individuals of multiple species and tended to have a sandy bottom (Figure 1B) as opposed to a sandy bottom containing pebbles (Figure 1D).
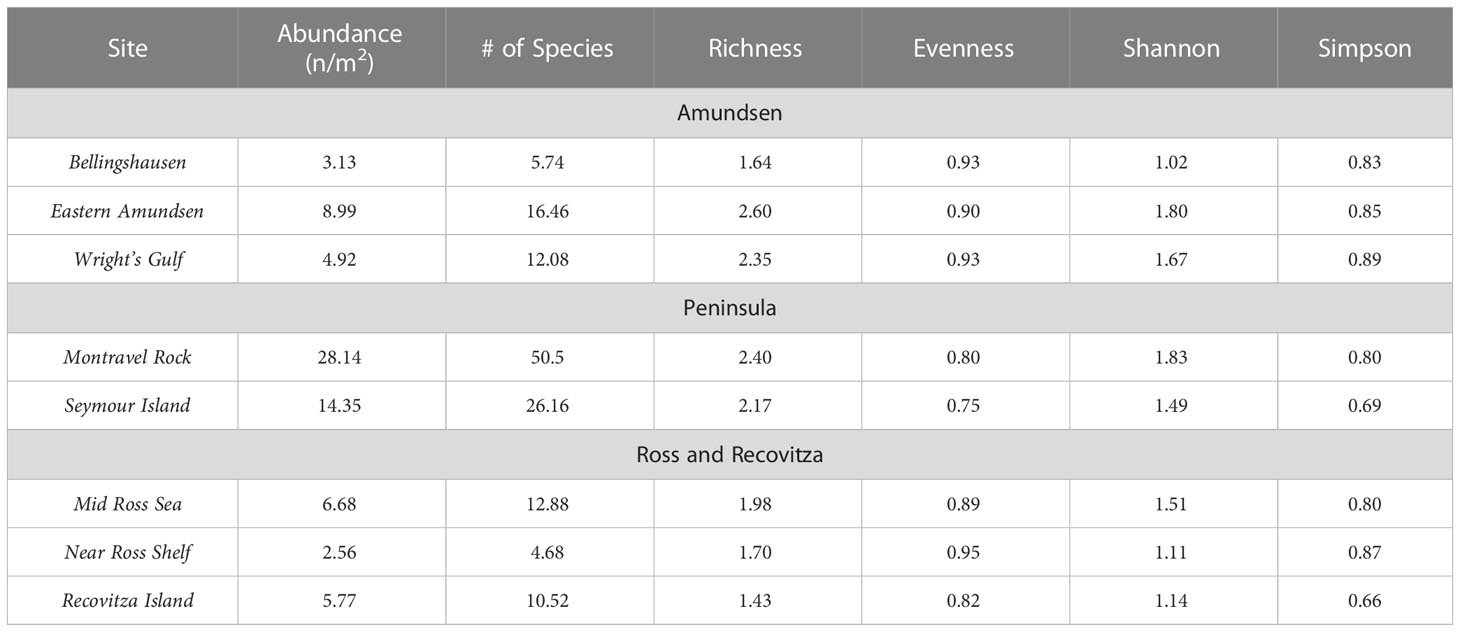
Table 1 Mean values for abundance, number of species, species richness, Pielou’s Evenness, Shannon-Wiener Diversity Index, and Simpson Index for all sites.
3.1 Community assemblage characteristics
Dominant organisms in each site varied between ophiuroids, holothuroids, demosponges, and pycnogonids (Figure 2B and Table 2). Anthozoans, ophiuroids, and ascidians were ubiquitous at all sites, but ophiuroids dominated the Montravel Rock, Seymour Island, and the Bellingshausen Sea sites. Based on the cluster and SIMPROF analysis of benthic communities, we grouped the sites into three regions: the Peninsula region, the Amundsen region, and the Ross Sea region (Figure S1). There were 28 photos (16 Amundsen, 11 Ross Sea, and 1 Peninsula) that did not cluster within the same regions as the rest of the photos from their sites providing evidence of within group dissimilarity (Figure S2). There were 16 photos from the Amundsen in a deeply branching cluster with 15 photos from the Ross Sea indicating the lack of similarity within this particular group indicating patchiness along the transect. These Amundsen region photos were characterized by reduced numbers of cnidarians, ophiuroids, and echinoids compared to other photos of the region. The 11 Ross Sea photos that clustered within the Amundsen Region had less annelids, holothuroids, and pycnogonids than the rest of the photos in the Ross Sea and Recovitza Island region. Generally, sites cluster by relatives location according to epifaunal community assemblages (Figures 3A, S2): the Amundsen region includes the Eastern Amundsen, Bellingshausen, and Wright’s Gulf sites, the Ross region includes the Mid Ross, Near Ross Shelf, and Recovitza Island sites, and the Peninsula region includes the Seymour Island and Montravel Rock sites (Figure 3A). Pycnogonid sp. 1, pycnogonid sp. 2, Ophionotus victoriae, Sterechinus sp. 1, Ophioplinthus spp., Iophon sp., and the yellow branching sponge (Figures 1A, C) were the primary species that helped differentiate the three regions we define here (Figures S3A, B). Based on the cluster and SIMPROF analysis (Figure S2), Recovitza Island communities were more similar to the Ross Region sites and have been included with them in the remainder of the analysis although we note some degree of within group dissimilarity (Figure S1). We saw a higher prevalence of echinoids (e.g., Sterechinus spp.) and holothuroids (e.g., Rhipidothuria racowitzai and sediment dwelling holothuroid 2) in the Amundsen Region than the other two regions. The Iophon sp. (demosponge), and Ophioplinthus spp. (ophiuroid) symbiont pair dominated the Peninsula Region and was significantly less abundant in all other regions (Table 2). The brittle star Ophionotus victoriae is abundant throughout the Southern Ocean, but it typically dominated softer sediments of the Peninsula region and Ross Sea region. The ophiuroid morphotype of Ophiuroid 4, characterized by small spiny pink-red arms with a darker central disc) was common in the Ross Sea region and is possibly Amphiura sp. We also saw an increase in Hexactinellida (glass sponges) in the Ross region compared to other regions (Figure 2B).
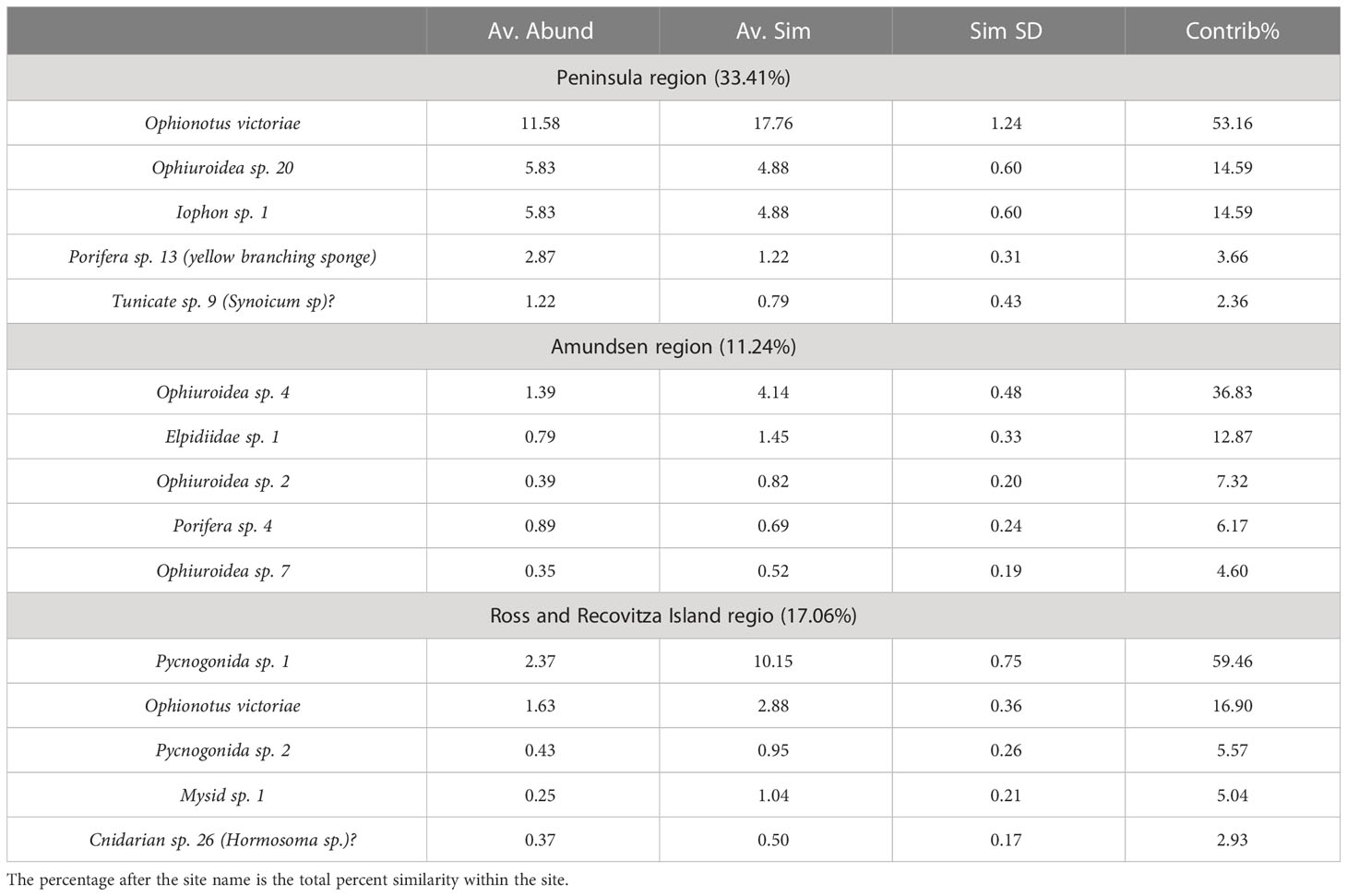
Table 2 Dominant 5 macrofaunal invertebrates based on SIMPER analysis for each region with average abundance (Av. Abund), average similarity within site (Av. Sim) including standard deviation (Sim SD), and percent contribution to the community (Contrib%).
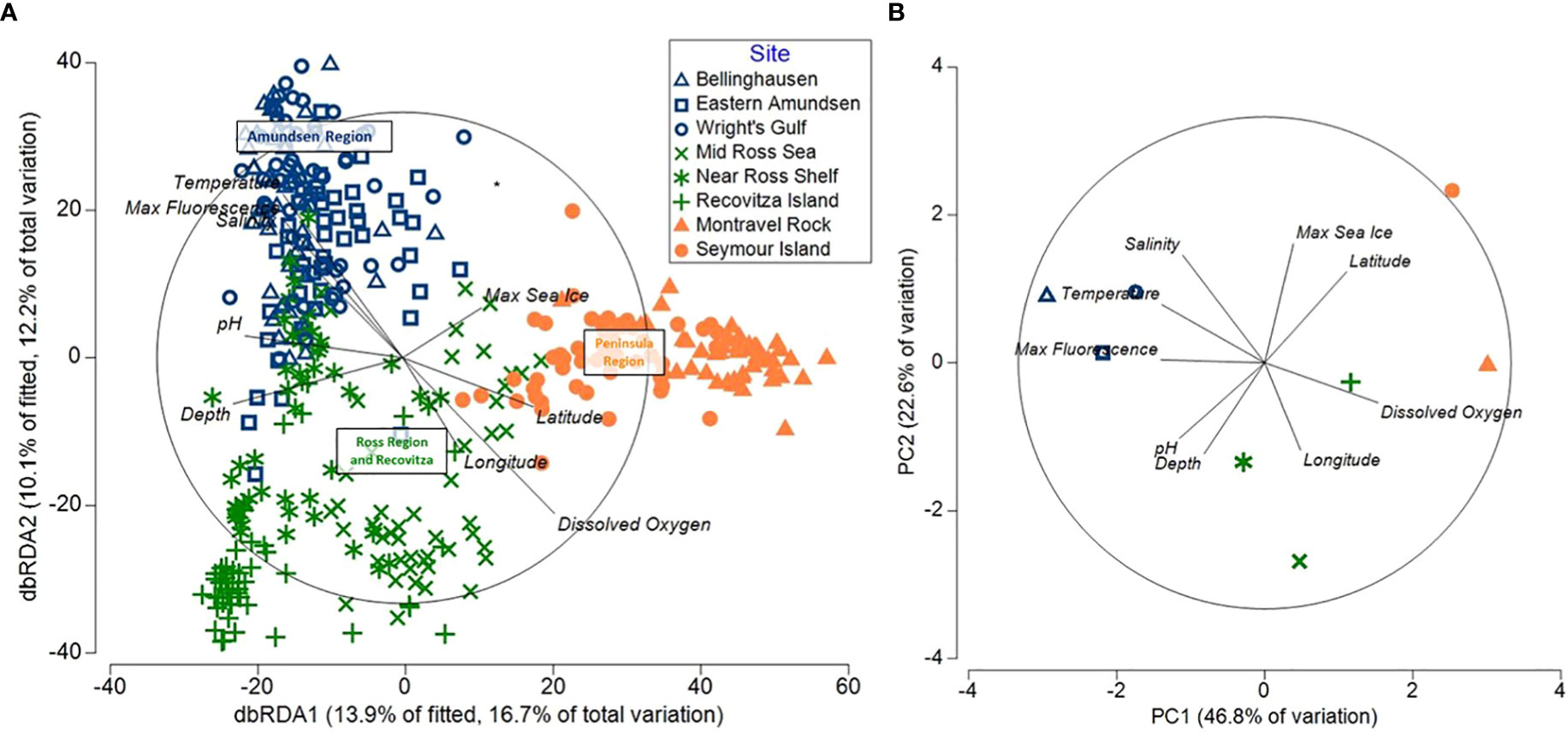
Figure 3 (A) Distance-based redundancy analysis (dbRDA) plot based on the calculations of a zero-adjusted Bray-Curtis similarity matrix showing community composition relationships where proximity estimates community similarity with Principal Component Analysis (PCA) aligned and overlain on top to show environmental trends. Asterisk indicates the one image with a drop stone found in Seymour Island. (B) PCA of the environmental variables.
Our three regions had significant heterogenous dispersions with potentially significant differences between communities (Table 3). Our study regions illustrate distinct community compositions (Figure 2B) and a latitudinal trend in abundance (again with exception of Recovitza Island; Figures 4B, S4). Northernmost sites portrayed higher relative abundances of demosponges and ascidians on average (12.5% and 19%, respectively) than southernmost sites (4% and 3%, respectively) which contained higher percentages echinoids and holothuroids (9% and 18%, respectively). Macrofaunal abundances in the Ross region and Recovitza Island were significantly lower than that of the northernmost Peninsula region sites. Based on a SIMPER analysis between sites (Table 2), epifaunal communities in the Ross Sea and near Recovitza Island significantly differed from other communities near the peninsula as they were dominated by pycnogonids (~65%: pycnogonid 1 and pycnogonid 2 - possibly Nymphon spp.), malacostracan crustaceans (~5%: Mysid sp. 1), and anthozoan cnidarians (3%: possibly Hormosoma sp.). These taxa and those listed in Table 2 for each region are the crucial taxa that may be used to discriminate between the Ross (and Recovitza) region, the Peninsula region, and the Amundsen region (Table S3).
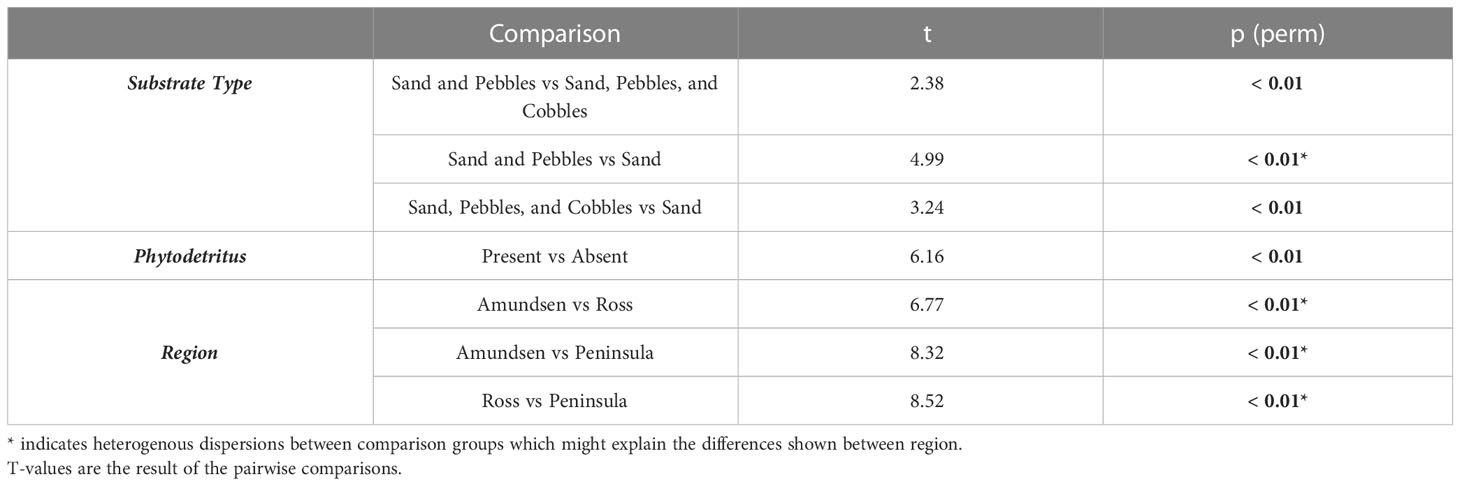
Table 3 PERMANOVA results (no. permutations: 9,999) to compare benthic communities revealing significant differences between substrate type and phytodetritus denoted by bold p values.
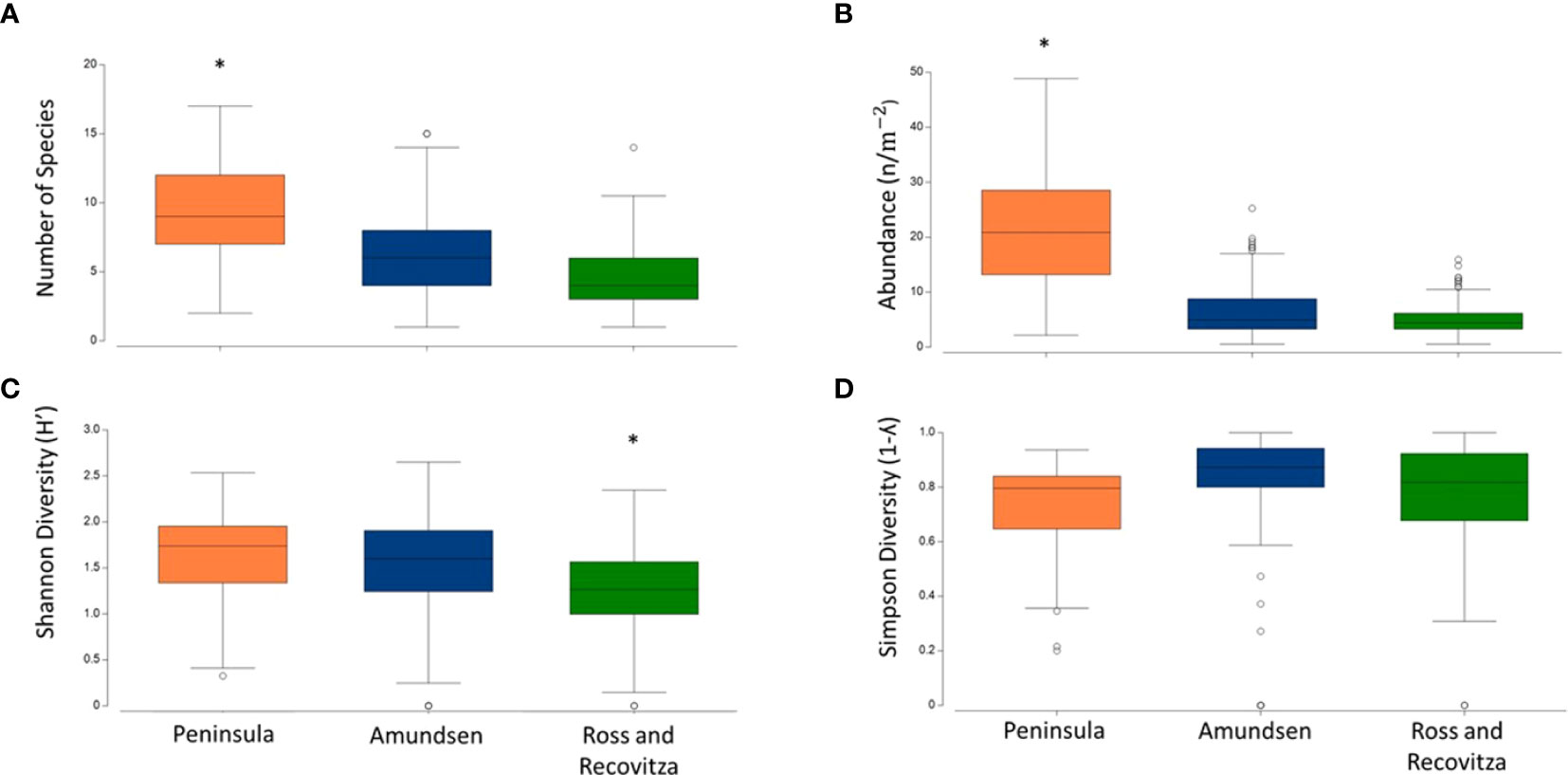
Figure 4 Total number of species (A), Abundance (B), Shannon Diversity Index (C), and Simpson Diversity Index (D) for each region. * indicates significant difference from at least 1 other region based with homogeneous dispersions (Table S4).
The Peninsula region portrayed lower evenness and Simpson diversity values than the Amundsen region as a result of dominance by the yellow rope sponge, brittle stars (Ophioplinthus spp.), and their symbiotic sponge (Iophon sp. [Table 2: Figure 1A]). The Amundsen Region typically had higher Simpson diversity values than the other regions, but there was no difference in this diversity index between the regions. Although the Peninsula region was dominated by a few species (Table 2: Ophioplinthus spp., Iophon sp., and Ophionotus victoriae), it contained the highest species richness and highest Shannon Diversity values, closely followed by the Amundsen region (Figures 4A, C). No significant latitudinal trends can be described here due to the inclusion of the Recovitza Island Site with the Ross region, but there is a discernable decreasing trend when looking at the sites individually (Figure S4). However, species richness, abundance, and Shannon diversity tend to decrease progressing southward (Peninsula region > Amundsen region > Ross Sea region: Figures 4B–D).
3.2 Environmental characteristics
Based on abiotic data (Table 4), sites generally group together based on location along our transect, similar to the assemblages mentioned above. The PCA analysis indicates the strongest driver of differences in PC1 which accounted for 46.8% of the variation (Table S5) was DO content of bottom waters which was negatively correlated with temperature and maximum fluorescence, our proxy for chlorophyll (Table S6). Percent sea ice cover, water depth, latitude, and salinity were the most influential factors for PC2 which accounted for 22.6% of variation in the environmental characteristics (Figure 3B). Phytodetritus presence and pebble substrate were associated with higher chlorophyll, temperatures, and lower oxygen levels near the benthos in the Amundsen region.
Amundsen region sediments included pebbles and cobble stones whereas other regions were primarily comprised of finer sandy sediments, and Amundsen region benthos was also characterized by the presence of algal detritus. One drop stone (>50cm) was encountered at the Seymour Island Site (denoted with an asterisk in Figure 2A) and was classified as containing sand, pebble, and cobble. Salinity and temperature were positively correlated and displayed an inverse relationship with oxygen. For the localities we sampled, Amundsen region sites were also the warmest (average = 1.13 °CC), but there was no difference in temperature between Peninsula region and Ross Sea region sites. Peninsula region sites had the highest DO of all sites (average = 6.95 ml l-1), while Amundsen region sites had the lowest (average = 4.26 ml l-1).
3.3 Community assemblage relationship to environmental characteristics
Macrofaunal communities were significantly different based on the substrate type and presence of phytodetritus (Table 3 and Figures S4A, B). Sites with phytodetritus, cobbles, and pebbles in the Amundsen region were characterized by a higher abundance of echinoids and holothuroids while the sandier sediments without phytodetritus of the Peninsula region contained more ophiuroids and sponges. Sea spiders were the most abundant group on the finer sediments of the Recovitza Island site. PCA vectors illustrate a significant correlation (Spearman Rank: rho = 0.443, p = 0.001; overlain in Figure 3A) between environmental parameters and epifaunal communities. The Amundsen region and Peninsula region are primarily differentiated based on PC1. Therefore, maximum fluorescence, DO, and temperature emerge as important factors driving community distinctness along the Western Antarctic coast (Table S6). In contrast, the Ross region and Recovitza Island were differentiated from the Peninsula and Amundsen regions based on PC2 whose core factors were pH, water depth, and percent sea ice cover. Distance based linear models show the importance DO, depth, temperature, and sea ice cover in predicting community compositions on the western Antarctic shelf at these sites (Tables S7).
4 Discussion
A trend toward decreasing abundances of macrofauna approaching the South Pole was observed (Figure S5), however, a significant latitudinal trend, due to the Recovitza Island outlier, was lacking. Further, Antarctic communities in close proximity are distinct in nature (Montravel Rock vs Recovitza Island). Faunal abundances from the Western Antarctic shelf presented here (2.44 – 28.14 individuals/m2) are similar to previous studies in the Western Antarctic fjords, and other Antarctic shelf sites (2.6 – 46 individuals/m2), but higher than Western Antarctic shelf community estimates (0.38 – 0.5 individuals/m2; (Barry et al., 2003; Sumida et al., 2008; Grange and Smith, 2013). Cummings et al., (2021) reports evidence of comparable megafaunal abundances on the Ross Sea continental shelf through video transects (0.448 - 3.0 individuals/m2), however, they did not find pycnogonids whereas we found them abundant in this region. All 8 sites had ophiuroids (e.g.Ophionotous victoriae and Ophioplinthus spp.) located at the base of anthozoan cnidarians which supports hypotheses of their shade-seeking behavior (Hendler et al., 1995; Johnsen and Kier, 1999). Similar ophiuroid-anemone associations have been described in the Arctic near faunal islands (Syvitski et al., 1989). Although many O. victoriae were observed in the open, this shade-seeking behavior could bias diversity and abundance estimates as not all of those present in the area may be recorded. Throughout these Western Antarctic sites, crinoids (mainly Promachocrinus kerguelensis), and the filter-feeding ophiuroid Astrotoma agassizii were typically seen atop cobble, sponges, cnidarians, and tunicates (Figure 5B) to collect food from water column (Meyer, 1983), but not always (Figure 1 at Recovitza Island).
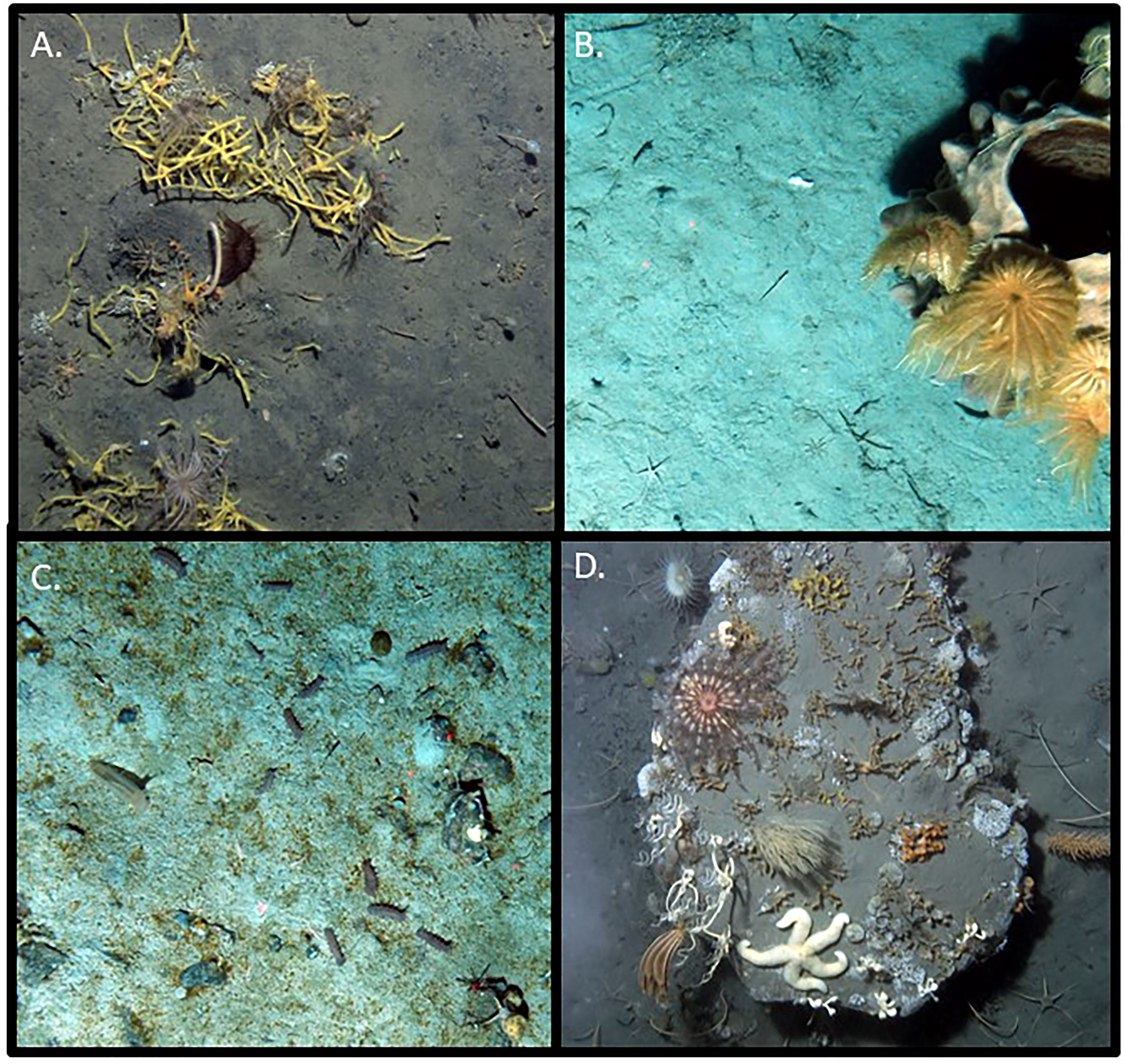
Figure 5 Example of sampling sites (A) Montravel Rock with yellow rope sponge, (B) Mid Ross Sea site, (C) Eastern Amundsen with abundant holothuroids, and (D) the drop stone found at the Seymour Island site.
4.1 Epifaunal communities along the Western Antarctic coast
Echinoderms are one of the most dominant fauna of the Antarctic continental shelf (Dayton et al., 1974; Clarke and Johnston, 2003; Chiantore et al., 2006; Moles et al., 2015). However, cnidarians, sponges, and pycnogonids were abundant enough to influence assemblages along the Western Antarctic Peninsula sites sampled for this investigation. Similar to data presented here from the Peninsula region (Figure 5A), a recent study in the Antarctic peninsula has shown high abundances of ophiuroids, ascidians, and demosponges (Gutt et al., 2019). Within the Bransfield Straight, Gutt et al. (2019) found dominance of varying phyla (e.g. Echinodermata, Hydrozoa, and Bryozoa) which further shows the patchy nature of this region on a relatively small spatial scale (100km). Previous studies on the continental shelf in the Ross Sea denoted high abundances of suspension feeding bryozoans, anthozoans, crinoids, demosponges, and ascidians (Cummings et al., 2021), which is similar to our results except that we also recorded an abundance of pycnogonids which were abundant in the Ross region and dominated Recovitza Island. Further, comparing our Amundsen sites to previous studies is difficult due to lack of data, but the abundance of holothuroids and echinoids at these sites is notable (Figure 5C).
Although we did not see a latitudinal trend in overall diversity across the Western Antarctic benthic communities, we did see a shift in these communities from dominance by a single taxon to a more diverse representation. Previously, higher Shannon diversity was observed on the open continental shelf compared with fjord sites (Grange and Smith, 2013). Communities at similar depths showed higher diversity and evenness on the shelf, but higher species number and abundance in the fjords. Given the proximity of these fjord and shelf sites and the lack of environmental data included, drawing comparisons between these studies is difficult. However, based on our results, predicted changes with climate warming on salinity and temperature in fjords will drastically impact the communities present there (Grange and Smith, 2013).
4.2 Distinct environments driving community composition
Our results indicate Antarctic benthic community variability resulting from abiotic differences on a local scale. Between the Amundsen region and other two regions, differences in maximum fluorescence (>2.3 mg/ml3), temperature (> 1.9 ˚C) and DO (> 1.8 ml/l), compared to salinity (0 PSU at minimum) lead us to emphasize the maximum fluorescence, temperature and DO impacts. The relationships between temperature, salinity, depth, pressure, and DO in marine environments make disentangling singular effects of one factor on a community difficult. Amundsen region sites with higher maximum fluorescence, lower DO, and higher temperature than other regions might have had more echinoids and holothuroids than other sites due to less competition in these zones from organisms that require more oxygen as a result of a higher metabolic rate, such as ophiuroids and crinoids (Woods et al., 2009; Hughes et al., 2011). These environmental characteristics are influenced by decomposition of algal particulates on the seafloor (Iken et al., 2010; Menezes et al., 2017) likely resulting in the community differences observed. These three factors in the Amundsen Sea are typically heavily impacted by algal blooms which was likely occurring during the data collection for this project (Summer 2013). Further, the abundance of holothuroids was higher in the Amundsen region than the Ross or Peninsula region where the maximum fluorescence was highest, potentially indicating a shallower calcium carbonate saturation horizon in this region (Griffiths, 2010). Phytodetritus, higher chlorophyll concentrations in the water column, and a shallower saturation horizon in the Amundsen Sea and Wright’s Gulf would help explain the greater abundance of filter-feeding holothuroids over those organisms more reliant on calcium carbonate exoskeletons, such as ophiuroids or crinoids (Griffiths, 2010). Another important factor in distinguishing communities presented here is the maximum sea ice coverage as it facilitated the differentiation between Peninsula region and Ross Sea regions. Within the Peninsula region, sea ice coverage was increasingly variable as the Montravel Rock site had less than 60% of the sea ice cover than the Seymour Island site, however, these communities present with high abundances of ophiuroids and other filter-feeders (e.g. demosponges, crinoids, and ascidians) as previously described (Sumida et al., 2008; Gutt et al., 2019). Finally, the sea ice coverage for the summer in the Southern Ocean has reached a new historic low (Charctic, 2023) which will likely impact benthic communities.
Because the evidence for algae (maximum fluorescence and phytodetritus absence) was lacking, Recovitza Island’s significantly different community from the Peninsula region sites could be a result of the slight increase in temperature (0.45-0.8 °C) or decreased oxygen (0.6-0.7 ml l-1). Although this site lies within the Bransfield Strait, it is located at the convergence of the transitional waters from the Bellingshausen Sea and water currents from the Weddell Sea (Smith et al., 2012; Deppeler and Davidson, 2017; Tripathy and Jena, 2019). Complexity of the Bransfield water currents leads to environmental fluctuations likely resulting in the community differences presented in our study (e.g., dominance of pycnogonids at Recovitza Island and ophiuroids at Montravel Rock). This distinction in communities on either side of the Bransfield Strait is supported by the clear variation between communities on the northern (mixed assemblage) compared to the southern (more suspension feeders) side of the Bransfield Strait (Gutt et al., 2013). These hydrographic features aid in forming ecoregions in the Southern Ocean which result in these trends in community composition and diversity as shown in the present study (Deppeler and Davidson, 2017). Given impacts of these environmental fluctuations and water currents on the benthic community fluctuations at the local scale, Western Antarctica should be a priority for future climate change studies, especially the Amundsen Region which is the least studied region of all the Southern Ocean (Griffiths et al., 2011).
Although most sites presented in this study were sandy or muddy sediments by design, those that had pebble and cobble substrates in the Amundsen and Peninsula regions, portrayed comparable abundances and Shannon diversity values (Table 1) to those found in other sections of the shelf (Grange and Smith, 2013), the Weddell Sea (e.g. Gutt and Starmans, 1998; Pineda-Metz et al., 2019), and Eastern Antarctica (Post et al., 2017). However, the diversity of benthic communities in the southeastern Weddell Sea were higher than the western Weddell Sea and most of the regions presented in this study (Gutt and Starmans, 1998). Further, in the southeastern Weddell Sea, a greater abundance of holothuroids, bryozoans, and ascidians were found on substrates with less pebbles and a greater abundance of sponges, anthozoans, and other taxa found on finer sandy substrates (Gutt and Starmans, 1998), similar to our Amundsen region and Peninsula region sites, respectively. An increase in arthropods (pycnogonids and others) at the finer sediment sites (Ross Sea and Recovitza region) was found in the Weddell Sea (Gutt and Starmans, 1998) and the Sabrina Coast in Eastern Antarctica (Post et al., 2017). In the Amundsen region and along the Sabrina Coast, on pebble and cobble substrates, there was an increase in brachiopods. Additionally, drop stones and iceberg scour which affect substrate were not accounted for in this study but there was notable abundance and diversity of macrofauna on our one encountered drop stone at Seymour Island (Figure 5D). Drop stones can act as faunal islands in Antarctic and Arctic waters and facilitate dispersal of benthic fauna by serving as steppingstones (Boucot and Carney, 1981; Dale et al., 1989), and in the Arctic, they have been shown to house communities dominated by sponges, ascidians, bryozoans, and crinoids which were found on our one drop stone (Syvitski et al., 1989).
High levels of endemism (Hempel, 1985; Griffiths, 2010; Gutt et al., 2013; Miranda et al., 2021) in the western shelf suggest research is needed to understand how diversity, community composition, and faunal richness will change with climate. Given the higher richness, abundance, and diversity of Peninsula region communities compared to the rest of Western Antarctica, further studies should be employed to better understand changes along the continental shelf and assess regions where benthic community diversity may be of interest (e.g., highly stable or highly variable regions). Diversity trends observed here are similar to those seen in meiofaunal reports from Western Antarctica (Brannock et al., 2018) which show lower richness and diversity in the center of the Bransfield Straight and higher in the Amundsen region. Curiously though, the Eastern Peninsula sites (near our Seymour Island) presented the lowest richness, abundance, and diversity for meiofaunal communities (Brannock et al., 2018) which we believe supports our assertion for localized habitats formed by water currents as seen in the Bransfield Straight. Further microbial diversity was shown to be higher in further west sites (e.g., Bellingshausen Sea and Amundsen Sea) compared to Antarctic Peninsula sites (Learman et al., 2016), however, this might be an artifact of grouping all peninsula sites together.
With predicted decreased sea ice, increased temperature, decreased DO, and algal blooms, polar regions with a dearth of data such as the Amundsen region provide target areas for future research. Studies such as this provide necessary information on benthic communities to aid in characterizing the Southern Ocean’s diverse ecoregions in this remote and isolated habitat without the need for potentially harmful trawling (Konecki and Targett, 1989). The Southern Ocean is a large and diverse habitat with varying benthic communities along the continental shelf. In order to identify economically and ecologically important ecoregions, we must continue to gather more data on biodiversity and spatial relationships in this area.
Data availability statement
The datasets presented in this study can be found in online repositories. The names of the repository/repositories and accession number(s) can be found in the article/Supplementary Material.
Author contributions
KH and AM collected the data. CJG, AM, and KH designed the study. Photo processing and organismal identifications were conducted by CG, KD, CK, and NN. CG, KD, and KH wrote the manuscript. All authors contributed to the article and approved the submitted version.
Funding
Funding for this project was provided by the National Science Foundation’s Antarctic Program (ANT-1043745 to KH) and the Office of Polar Programs (OPP-1916661 to KH and OPP 1916665 to AM).
Acknowledgments
We thank the National Science Foundation for funds to collect and analyze these data. We also thank the ships’ crews, scientific support staff, and scientific crew of the ASRV Laurence M. Gould (LMG 13-12) and RVIB Nathaniel B. Palmer (NBP 12-10). We acknowledge and thank David Branson for initial photo editing steps. Further, we thank the reviewers for their constructive feedback on our manuscript. We especially thank the electronic technicians on the NBP 12-10 and LMG 13-12 cruises who assisted with the deployment of the Yoyo camera.
Conflict of interest
The authors declare that the research was conducted in the absence of any commercial or financial relationships that could be construed as a potential conflict of interest.
Publisher’s note
All claims expressed in this article are solely those of the authors and do not necessarily represent those of their affiliated organizations, or those of the publisher, the editors and the reviewers. Any product that may be evaluated in this article, or claim that may be made by its manufacturer, is not guaranteed or endorsed by the publisher.
Supplementary material
The Supplementary Material for this article can be found online at: https://www.frontiersin.org/articles/10.3389/fmars.2023.1094283/full#supplementary-material
References
Aronson R. B., Moody R. M., Ivany L. C., Blake D. B., Werner J. E., Glass A. (2009). Climate change and trophic response of the Antarctic bottom fauna. PloS One 4, e4385. doi: 10.1371/journal.pone.0004385
Barry J. P., Grebmeier J. M., Smith J., Dunbar R. B. (2003). Oceanographic versus seafloor-habitat control of benthic megafaunal communities in the SW Ross Sea, Antarctica. Biogeochem Ross Sea 78, 327–353. doi: 10.1029/078ARS21
Boucot A. J., Carney R. S. (1981). “Principles of benthic marine paleoecology,” (New York, USA: Academic Press).
Brannock P. M., Learman D. R., Mahon A. R., Santos S. R., Halanych K. M. (2018). Meiobenthic community composition and biodiversity along a 5500 km transect of Western Antarctica: a metabarcoding analysis. Mar. Ecol. Prog. Ser. 603, 47–60. doi: 10.3354/meps12717
Bray J. R., Curtis J. T. (1957). An ordination of the upland forest communities of southern Wisconsin. Ecol. Monogr. 27, 326–349. doi: 10.2307/1942268
Chiantore M., Guidetti M., Cavallero M., De Domenico F., Albertelli G., Cattaneo-Vietti R. (2006). Sea Urchins, sea stars and brittle stars from Terra Nova bay (Ross Sea, Antarctica). Polar Biol. 29, 467–475. doi: 10.1007/s00300-005-0077-2
Clarke A. (1988). Seasonality in the Antarctic marine environment. Comp. Biochem. Physiol. Part B: Comp. Biochem. 90, 461–473.
Clarke K. R., Gorley R., Somerfield P., Warwick R. (2014). Change in marine communities: an approach to statistical analysis and interpretation (Plymouth Marine Laboratory, Plymouth, UK: Primer-E Ltd).
Clarke A., Johnston N. M. (2003). “Antarctic Marine benthic diversity,” in Oceanography and marine biology, an annual review, volume 41 (Boca Raton, Florida, United States: CRC Press), 55–57.
Clarke A., Murphy E. J., Meredith M. P., King J. C., Peck L. S., Barnes D. K., et al. (2007). Climate change and the marine ecosystem of the western Antarctic peninsula. Philos. Trans. R. Soc. B: Biol. Sci. 362, 149–166. doi: 10.1098/rstb.2006.1958
Convey P., Peck L. S. (2019). Antarctic Environmental change and biological responses. Sci. Adv. 5, eaaz0888. doi: 10.1126/sciadv.aaz0888
Cummings V. J., Bowden D. A., Pinkerton M. H., Halliday N. J., Hewitt J. E. (2021). Ross Sea Benthic ecosystems: Macro-and mega-faunal community patterns from a multi-environment survey. Front. Mar. Sci. 8, 629787. doi: 10.3389/fmars.2021.629787
Dale J. E., Aitken A. E., Gilbert R., Risk M. J. (1989). Macrofauna of Canadian arctic fjords. Mar. Geology 85 (2-4), 331–358.
Dayton P. K., Robilliard G. A., Paine R. T., Dayton L. B. (1974). Biological accommodation in the benthic community at McMurdo sound, Antarctica. Ecol. Monogr. 44, 105–128. doi: 10.2307/1942321
De Jong J., Stammerjohn S., Ackley S., Tison J.-L., Mattielli N., Schoemann V. (2015). Sources and fluxes of dissolved iron in the Bellingshausen Sea (West antarctica): The importance of sea ice, icebergs and the continental margin. Mar. Chem. 177, 518–535. doi: 10.1016/j.marchem.2015.08.004
Deppeler S. L., Davidson A. T. (2017). Southern ocean phytoplankton in a changing climate. Front. Mar. Sci. 4, 40. doi: 10.3389/fmars.2017.00040
Fernández-González C., Tarran G. A., Schuback N., Woodward E. M. S., Arístegui J., Marañón E. (2022). Phytoplankton responses to changing temperature and nutrient availability are consistent across the tropical and subtropical Atlantic. Commun. Biol. 5, 1035. doi: 10.1038/s42003-022-03971-z
Gerdes D., Klages M., Arntz W., Herman R., Galéron J., Hain S. (1992). Quantitative investigations on macrobenthos communities of the southeastern Weddell Sea shelf based on multibox corer samples. Polar Biol. 12, 291–301. doi: 10.1007/BF00238272
Grange L. J., Smith C. R. (2013). Megafaunal communities in rapidly warming fjords along the West Antarctic peninsula: hotspots of abundance and beta diversity. PloS One 8, e77917. doi: 10.1371/journal.pone.0077917
Gray J. S. (2001). Antarctic Marine benthic biodiversity in a world-wide latitudinal context. Polar Biol. 24, 633–641. doi: 10.1007/s003000100244
Grebmeier J. M., Barry J. P. (1991). The influence of oceanographic processes on pelagic-benthic coupling in polar regions: a benthic perspective. J. Mar. Syst. 2, 495–518. doi: 10.1016/0924-7963(91)90049-Z
Griffiths H. J. (2010). Antarctic Marine biodiversity–what do we know about the distribution of life in the southern ocean? PloS One 5, e11683. doi: 10.1371/journal.pone.0011683
Griffiths H. J., Arango C. P., Munilla T., Mcinnes S. J. (2011). Biodiversity and biogeography of southern ocean pycnogonids. Ecography 34, 616–627. doi: 10.1111/j.1600-0587.2010.06612.x
Gutt J., Arndt J., Kraan C., Dorschel B., Schröder M., Bracher A., et al. (2019). Benthic communities and their drivers: A spatial analysis off the Antarctic peninsula. Limnol Oceanogr 64, 2341–2357. doi: 10.1002/lno.11187
Gutt J., Griffiths H. J., Jones C. D. (2013). Circumpolar overview and spatial heterogeneity of Antarctic macrobenthic communities. Mar. Biodivers. 43, 481–487. doi: 10.1007/s12526-013-0152-9
Gutt J., Sirenko B. I., Smirnov I. S., Arntz W. E. (2004). How many macrozoobenthic species might inhabit the Antarctic shelf? Antarctic Sci. 16, 11–16. doi: 10.1017/S0954102004001750
Gutt J., Starmans A. (1998). Structure and biodiversity of megabenthos in the Weddell and lazarev seas (Antarctica): ecological role of physical parameters and biological interactions. Polar Biol. 20, 229–247. doi: 10.1007/s003000050300
Halanych K. M., Mahon A. R. (2018). Challenging dogma concerning biogeographic patterns of Antarctica and the southern ocean. Annu. Rev. Ecol Evolution Systematics 49, 355–378. doi: 10.1146/annurev-ecolsys-121415-032139
Hellmer H. H., Kauker F., Timmermann R., Determann J., Rae J. (2012). Twenty-first-century warming of a large Antarctic ice-shelf cavity by a redirected coastal current. Nature 485 (7397), 225–228.
Hempel G. (1985). arine biology of polar regions and effects of stress on marine organisms Gray J. S., Christiansen M. E. eds. Wiley, Chichester, 3–33.
Hendler G., Miller J. E., Pawson D. L., Kier P. M. (1995). Sea Stars, sea urchins, and allies: echinoderms of Florida and the Caribbean (Washington D.C: Smithsonian Institution).
Hughes S. J. M., Ruhl H. A., Hawkins L. E., Hauton C., Boorman B., Billett D. S. (2011). Deep-sea echinoderm oxygen consumption rates and an interclass comparison of metabolic rates in asteroidea, crinoidea, echinoidea, holothuroidea and ophiuroidea. J. Exp. Biol. 214, 2512–2521. doi: 10.1242/jeb.055954
Iken K., Konar B., Benedetti-Cecchi L., Cruz-Motta J. J., Knowlton A., Pohle G., et al. (2010). Large-Scale spatial distribution patterns of echinoderms in nearshore rocky habitats. PloS One 5, e13845. doi: 10.1371/journal.pone.0013845
Isla E., Gerdes D. (2019). Ongoing ocean warming threatens the rich and diverse macrobenthic communities of the Antarctic continental shelf. Prog. Oceanography 178, 102180.
Jacobs S. S., Comiso J. C. (1997). Climate variability in the amundsen and Bellingshausen seas. J. Climate 10, 697–709. doi: 10.1175/1520-0442(1997)010<0697:CVITAA>2.0.CO;2
Johnsen S., Kier W. M. (1999). Shade-seeking behaviour under polarized light by the brittlestar Ophioderma brevispinum (Echinodermata: Ophiuroidea). J. Mar. Biol. Assoc. United Kingdom 79, 761–763. doi: 10.1017/S0025315498000940
Konecki J. T., Targett T. E. (1989). Eggs and larvae of Nototheniops larseni from the spongocoel of a hexactinellid sponge near Hugo island, Antarctic peninsula. Polar Biol. 10, 197–198. doi: 10.1007/BF00238495
Learman D. R., Henson M. W., Thrash J. C., Temperton B., Brannock P. M., Santos S. R., et al. (2016). Biogeochemical and microbial variation across 5500 km of Antarctic surface sediment implicates organic matter as a driver of benthic community structure. Front. Microbiol. 7, 284. doi: 10.3389/fmicb.2016.00284
Menezes V. V., Macdonald A. M., Schatzman C. (2017). Accelerated freshening of Antarctic bottom water over the last decade in the southern Indian ocean. Sci. Adv. 3, e1601426. doi: 10.1126/sciadv.1601426
Miranda T. P., Fernandez M. O., Genzano G. N., Cantero Á.L.P., Collins A. G., Marques A. C. (2021). Biodiversity and biogeography of hydroids across marine ecoregions and provinces of southern south America and Antarctica. Polar Biol. 44, 1669–1689. doi: 10.1007/s00300-021-02909-1
Moles J., Figuerola B., Campanya-Llovet N., Monleon-Getino T., Taboada S., Avila C. (2015). Distribution patterns in Antarctic and subantarctic echinoderms. Polar Biol. 38, 799–813. doi: 10.1007/s00300-014-1640-5
Morrison A., Hogg A. M., England M. H., Spence P. (2020). Warm circumpolar deep water transport toward Antarctica driven by local dense water export in canyons. Sci. Adv. 6, eaav2516. doi: 10.1126/sciadv.aav2516
Pielou E. C. (1966). The measurement of diversity in different types of biological collections. J. Theor. Biol. 13, 131–144. doi: 10.1016/0022-5193(66)90013-0
Piepenburg D., Archambault P., Ambrose W. G., Blanchard A. L., Bluhm B. A., Carroll M. L., et al. (2011). Towards a pan-Arctic inventory of the species diversity of the macro-and megabenthic fauna of the Arctic shelf seas. Mar. Biodivers. 41, 51–70. doi: 10.1007/s12526-010-0059-7
Pineda-Metz S. E., Gerdes D. (2018). Seabed images versus corer sampling: a comparison of two quantitative approaches for the analysis of marine benthic communities in the southern Weddell Sea (Southern ocean). Polar Biol. 41, 515–526. doi: 10.1007/s00300-017-2211-3
Pineda-Metz S. E., Gerdes D., Richter C. (2020). Benthic fauna declined on a whitening Antarctic continental shelf. Nat. Commun. 11, 1–7. doi: 10.1038/s41467-020-16093-z
Pineda-Metz S. E., Isla E., Gerdes D. (2019). Benthic communities of the filchner region (Weddell Sea, Antarctica). Mar. Ecol. Prog. Ser. 628, 37–54. doi: 10.3354/meps13093
Post A. L., Lavoie C., Domack E. W., Leventer A., Shevenell A., Fraser A. D. (2017). Environmental drivers of benthic communities and habitat heterogeneity on an East Antarctic shelf. Antarctic Sci. 29, 17–32. doi: 10.1017/S0954102016000468
Ruan X., Speer K. G., Thompson A. F., Schulze Chretien L. M., Shoosmith D. R. (2021). Ice-shelf meltwater overturning in the Bellingshausen Sea. J. Geophys Research: Oceans 126, e2020JC016957. doi: 10.1029/2020JC016957
Shannon C. E. (1948). A mathematical theory of communication. Bell system Tech. J. 27, 379–423. doi: 10.1002/j.1538-7305.1948.tb01338.x
Smith C. R., Demaster D. J., Thomas C., Sršen P., Grange L., Evrard V., et al. (2012). Pelagic-benthic coupling, food banks, and climate change on the West Antarctic peninsula shelf. Oceanography 25, 188–201. doi: 10.5670/oceanog.2012.94
Smith W. O. Jr., Gordon L. I. (1997). Hyperproductivity of the Ross Sea (Antarctica) polynya during austral spring. Geophys Res. Lett. 24, 233–236. doi: 10.1029/96GL03926
Stern A., Dinniman M., Zagorodnov V., Tyler S., Holland D. (2013). Intrusion of warm surface water beneath the McMurdo ice shelf, Antarctica. J. Geophys Research: Oceans 118, 7036–7048. doi: 10.1002/2013JC008842
Sumida P. Y., Bernardino A. F., Stedall V. P., Glover A. G., Smith C. R. (2008). Temporal changes in benthic megafaunal abundance and composition across the West Antarctic peninsula shelf: results from video surveys. Deep Sea Res. Part II: Topical Stud. Oceanogr 55, 2465–2477.
Syvitski J. P., Farrow G. E., Atkinson R., Moore P., Andrews J. (1989). Baffin Island fjord macrobenthos: bottom communities and environmental significance. Arctic 42, 232–247. doi: 10.14430/arctic1662
Tripathy S., Jena B. (2019). Iron-stimulated phytoplankton blooms in the southern ocean: a brief review. Remote Sens. Earth Syst. Sci. 2, 64–77. doi: 10.1007/s41976-019-00012-y
Woods H. A., Moran A. L., Arango C. P., Mullen L., Shields C. (2009). Oxygen hypothesis of polar gigantism not supported by performance of Antarctic pycnogonids in hypoxia. Proc. R. Soc. B: Biol. Sci. 276, 1069–1075. doi: 10.1098/rspb.2008.1489
Wu X., Niklas K. J., Sun S. (2021). Climate change affects detritus decomposition rates by modifying arthropod performance and species interactions. Curr. Opin. Insect Sci. 47, 62–66. doi: 10.1016/j.cois.2021.05.002
Keywords: Antarctica, benthic biodiversity, photographic surveys, invertebrates, community structure
Citation: Grimes CJ, Donnelly K, Ka C, Noor N, Mahon AR and Halanych KM (2023) Community structure along the Western Antarctic continental shelf and a latitudinal change in epibenthic faunal abundance assessed by photographic surveys. Front. Mar. Sci. 10:1094283. doi: 10.3389/fmars.2023.1094283
Received: 09 November 2022; Accepted: 24 March 2023;
Published: 14 April 2023.
Edited by:
Paris Vasileios Stefanoudis, University of Oxford, United KingdomReviewed by:
Alec Aitken, University of Saskatchewan, CanadaSantiago E. A. Pineda Metz, Alfred Wegener Institute Helmholtz Centre for Polar and Marine Research (AWI), Germany
Copyright © 2023 Grimes, Donnelly, Ka, Noor, Mahon and Halanych. This is an open-access article distributed under the terms of the Creative Commons Attribution License (CC BY). The use, distribution or reproduction in other forums is permitted, provided the original author(s) and the copyright owner(s) are credited and that the original publication in this journal is cited, in accordance with accepted academic practice. No use, distribution or reproduction is permitted which does not comply with these terms.
*Correspondence: Candace J. Grimes, Q0dyaW1lczEzMjdAZ21haWwuY29t; Kenneth M. Halanych, SGFsYW55Y2hLQHVuY3cuZWR1