- 1WMU-Sasakawa Global Ocean Institute, World Maritime University (WMU), Malmö, Sweden
- 2Centre for Resource Management and Environmental Studies, The University of the West Indies, Bridgetown, Barbados
- 3Department of Biological and Chemical Sciences, The University of the West Indies, Bridgetown, Barbados
Mass accumulations of pelagic sargassum (Sargassum natans and S. fluitans) in the Tropical Atlantic, across the Caribbean and off the coast of West Africa, are causing extensive ecological and socioeconomic harm. The extraordinary volumes of sargassum influxes could also provide a business opportunity if innovative ways are developed to utilise the raw material. In-water harvesting provides the best opportunity to collect substantial amounts of ‘fresh’ sargassum that can be used in a variety of applications. However, sargassum rafts are living and diverse ecosystems with a range of associated fauna including fish that are targeted by fishers. The consequences of in-water harvesting of sargassum on the biodiversity, including associated fishes, remain poorly understood. Characterisation of this biodiversity within nearshore and offshore environments is needed to help guide best harvest practices and assess possible impacts on fishing opportunities. We assessed the free-swimming fauna associated with sargassum rafts at various distances from shore with the use of underwater video recordings. Over a three-month period, a total of 35 underwater surveys were conducted off the eastern and southern coastline of Barbados. Thirteen species (12 fishes and one comb jelly) from 8 families were identified, with the family Carangidae representing the greatest number of species (n=6). Application of the MaxN metric (maximum number of individuals of a species seen during deployment) revealed significant correlations with raft characteristics notably raft volume, raft distance from shore and water depth. The three environmental variables accounted for 9% of the variation (adjusted R2) in the free-swimming community composition with raft volume being the major driver of species richness. This aligns with ecological theory and supports our hypothesis that larger rafts would host greater species richness. The results demonstrate a strong affiliation between pelagic sargassum rafts and species biodiversity and abundance that will need to be considered by managers when seeking a best compromise between protecting beaches from inundation by sargassum and protecting biodiversity and fishing opportunities.
Introduction
Vast quantities of pelagic Sargassum spp. (Sargassum natans and S. fluitans) subsequently referred to simply as ‘sargassum’ continue to inundate the coasts of Caribbean, South American and West African countries (Wang et al., 2019; Chávez et al., 2020; Cox et al., 2021). This proliferation of sargassum in the Tropical Atlantic has been linked to a new source region known as the North Equatorial Recirculation Region (NERR) (Franks et al., 2016; Wang et al., 2019). Influx events are episodic in nature with volumes varying from year to year (Cox et al., 2021). In 2018, the Caribbean recorded the highest volumes of sargassum influxes leading to states of national emergency being declared in some countries. This has continued in 2022 with strandings just as extreme as 2018, if not worse in places (The Sargassum Watch System (SaWS), 2022; Centre for Resource Management and Environmental Studies, 2022).
Sargassum influx events are now considered the new ‘normal’ for the region (Desrochers et al., 2020) and show a trend of increasing severity (The Sargassum Watch System (SaWS), 2022). As the region continues to adapt to this new reality, there has been a surge in research and publications on impacts (Milledge and Harvey, 2016; Ramlogan et al., 2017; van Tussenbroek et al., 2017; United Nations Environment Programme, 2018; Resiere et al., 2019), forecasting (Wang and Hu, 2017; Johnson et al., 2020; Marsh et al., 2021; Marsh et al., 2022), potential uses (Desrochers et al., 2020; Thompson et al., 2020; Amador-Castro et al., 2021; Oxenford et al., 2021; United Nations Environment Programme - Caribbean Environment Programme et al., 2021) and chemical composition (Devault et al., 2019; Davis et al., 2020; Nielsen et al., 2021; Devault et al., 2022; Tonon et al., 2022). Research into the sargassum phenomenon reveals considerable spatial and temporal complexities associated with management due to its transboundary nature and unpredictability (Cox et al., 2021). Management efforts to date have been largely focused on protecting fisheries and tourism sectors as these are severely impacted during influx events and contribute approximately US$370 million (Patil et al., 2016) and US$29.2 billion (Milledge and Harvey, 2016), respectively, to the region’s economy. Considerable attention is also now being given to the valorization of pelagic sargassum to ameliorate the economic damage generated in the region (Desrochers et al., 2020; Oxenford et al., 2021; United Nations Environment Programme - Caribbean Environment Programme, 2021; Robledo et al., 2021).
In-water harvesting provides an opportunity to reduce mass shoreline inundations and provide large volumes of sargassum for valorization (Robledo et al., 2021). Government agencies and the private sector have been working together to carry out clean-ups at considerable cost (running into tens of millions of US dollars per year), to help reduce the impacts of influx events (Milledge and Harvey, 2016; Chávez et al., 2020). These harvesting efforts were initially carried out in response to stranding events and placed significant strain on the economy of Caribbean Small Island Developing States (SIDS) (Cox et al., 2021; Liranzo-Gómez et al., 2021). Furthermore, shoreline harvesting contributed to coastal erosion (Liranzo-Gómez et al., 2021) and negatively impacted sea turtle nests and hatchlings (Maurer et al., 2015; Schiariti and Salmon, 2022). In-water harvesting is currently being developed to reduce the impacts associated with mechanical shoreline harvesting and provide the large quantities of fresh clean (high quality) sargassum required for many applications (Liranzo-Gómez et al., 2021; Webber and Maddix, 2021).
While urgent solutions to sargassum strandings are needed and turning a challenge into an opportunity is commendable, it should also be recognized that pelagic sargassum hosts a diverse assemblage of endemic and associated fauna that rely on the sargassum for food and shelter (Dooley, 1972; Bortone et al., 1977; Butler et al., 1983; Settle, 1993; Moser et al., 1998; Wells and Rooker, 2003; Hoffmayer et al., 2005; Huffard et al., 2014). Whilst studies from the Sargasso Sea highlight the importance of pelagic sargassum to a diverse assemblage of fauna, there have been very few studies (Schell et al., 2016; Monroy-Velázquez et al., 2019; Mendoza-Becerril et al., 2020; Martin et al., 2021) focused on understanding the biodiversity associated with pelagic sargassum from the new source region. Recent evidence (Martin et al., 2021) suggests important differences in biodiversity associated with the three prevalent sargassum morphotypes (S. natans I, S. natans VIII and S. fluitans III). Given the seasonal and interannual shifts in morphotype composition (Schell et al., 2016; García-Sánchez et al., 2020; Machado et al., 2022) and the influence of sub-origins on morphotype composition (Alleyne et al.; unpublished data) addressing the current knowledge gaps on biodiversity can play a pivotal role in guiding best harvest practices.
Fishes have long been known to associate with the rafts of sargassum, attracting fishers, but the sheer scale of the recent influxes has also caused problems for fishers. Fishers across the region have reported damage to fishing gear, predominantly propeller and net entanglements, and damage to boat engines from overheating when navigating through extensive sargassum accumulations (Speede et al., 2018). Sargassum also hinders fishers’ ability to launch their boats leading to loss of fishing days (Franks et al., 2012; Caribbean Regional Fisheries Mechanism and Japanese International Cooperation Agency, 2019). In Barbados, there has also been a reduction in catches of key fisheries species like flyingfish and adult dolphinfish; however, there have been increases in catch of other species such as almaco jacks (Caribbean Regional Fisheries Mechanism and Japanese International Cooperation Agency, 2019). These impacts are especially concerning since most Caribbean countries are categorised as SIDS, meaning that they already experience unique social, economic and environmental challenges (United Nations, no date), and their economic growth is intrinsically linked to the marine environment. It is therefore a priority to understand the association of fishes with sargassum influxes and thus be able to advise managers on the impacts of interventions such as in-water harvesting on fishing opportunities.
The purpose of this study was to characterise the free-swimming fauna associated with pelagic sargassum arriving in Barbados and identify what factors (if any) drive changes in community assemblage. Following Alleyne (2022), free-swimming fauna in this study refer to motile species that swim inside and beneath floating sargassum such as juvenile turtles, adult turtles, vertical migrating fishes and pelagic fishes. Drawing from ecological theory, we hypothesized that larger rafts would host greater species richness (Simberloff, 1976; Lomolino, 2000).
Materials and methods
Sampling method
During three periods of high sargassum abundance (August 2021, March, and May 2022), a total of 35 underwater videos of sargassum rafts were conducted offshore between (613 and 2,368 m off) the eastern and southern coastline of Barbados. Videos were conducted from a small open boat (length 5 m) with an outboard engine, using a free-floating underwater recording device. Each recording device consisted of a U-shape aluminum frame on which a GoPro camera HERO 8 (1920 x 1080 pixels with 30 frames per second) or HERO 9 (1920 x 1080 pixels with 60 frames per second) was mounted. Cameras were set to ‘wide- angle’ view and suspended below the water surface by two one-meter lengths of rope attached to the aluminum frame that was kept afloat with a surface buoy (Figure 1).
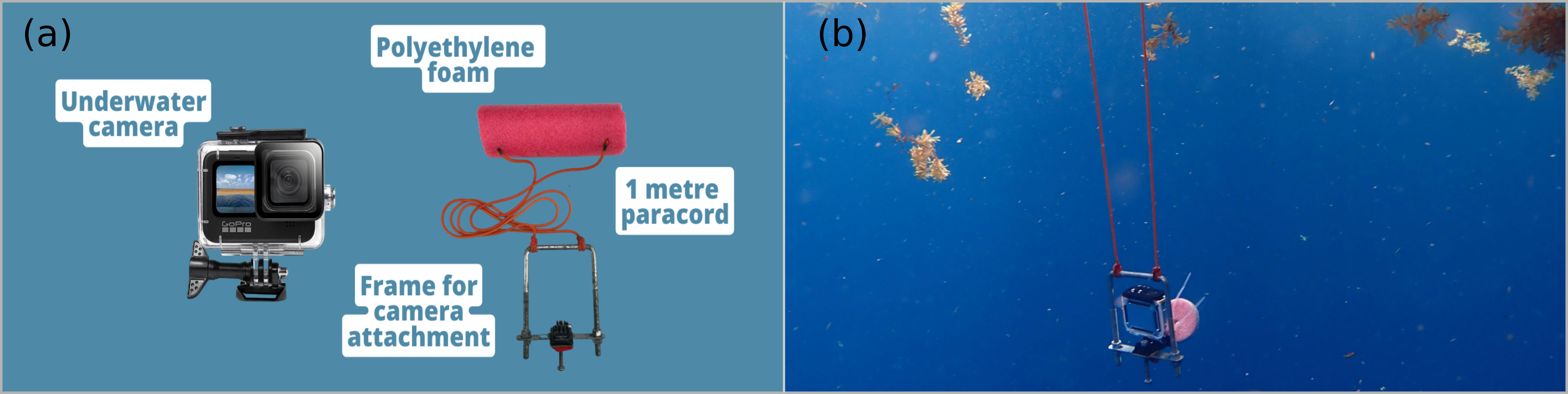
Figure 1 Materials used to construct underwater recording device (A) and a still image of the device deployed underwater (B).
Cameras were deployed under two types of pelagic sargassum aggregation states: mats and windrows (Figure 2). Mats were classified as densely packed aggregations of sargassum with an irregular to round shape and measured 5 to 100s of meters in diameter (distance across) (Marmorino et al., 2011; Goodwin et al., 2022). Windrows were defined as aggregations of sargassum generally arranged in a line and ranged from 0.5 meter to several meters in diameter (Marmorino et al., 2011; Goodwin et al., 2022). The term ‘raft’ is hereinafter used to refer to aggregations of mats and/or windrows.
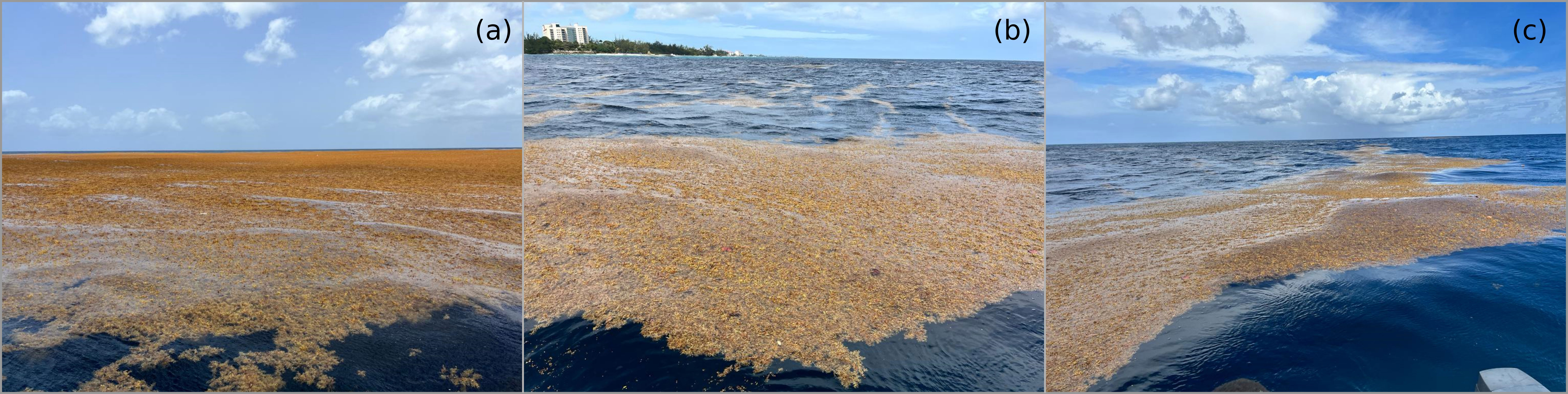
Figure 2 Pelagic sargassum aggregation states sampled off the coast of Barbados. (A) Large mat (>100 m in diameter), (B) small mat (10 m in diameter) and (C) windrows (7 m in diameter).
Each targeted sargassum raft was defined as a sampling station. Small stations (5-25 m length) were sampled with one camera and larger stations (> 40 m length) were sampled with 2 to 3 cameras spanning the extent of the sampling station. Stations ranging between 26-40 m in length were not encountered. Camera deployments on larger stations were done at a minimal distance of 30 m apart and each deployment was treated as an individual sample during video analysis. For each deployment the camera was set to record video footage and submerged approximately 1 m below the water’s surface (angled slightly upwards) to record the free-swimming fauna in the water column directly beneath the sargassum raft. After deployment, cameras were left adrift for a period of 15 minutes and the boat maintained a minimal distance of 10 m from the raft to limit interference.
At each sampling station several dimensions were recorded: the length of the sargassum raft; the diameter of the sargassum raft; the thickness of the sargassum raft; the depth of the water; and the distance from shore. The length and diameter of the station was estimated using the boat length as a reference. The thickness of the sargassum raft was determined (to the nearest 5 cm) with the use of a graduated pole. Water depth was recorded (to the nearest 0.1 m) with a handheld depth sounder (Speedtech SM-5A) and GPS coordinates were recorded via a handheld GPS device (Garmin GPS 72). Subsequent to field sampling, GPS coordinates were uploaded into Google Earth to determine the distance from shore of each sampling station.
Video analysis
To minimise any interference caused by the sampling boat during camera deployment and retrieval, 2.5 minutes of footage were removed from the beginning and end of each video, resulting in 10-minute surveys. Following Priede et al. (1994), the maximum number of individuals sighted in any one field of view (i.e., frame) (hereafter referred to as MaxN) was recorded for each species within each survey. Within each survey free-swimming fauna were identified to species level where possible, using FishBase (Froese and Pauly, 2011) or the FAO species identification guides (Carpenter, 2002) where necessary.
MaxN was chosen as a conservative measure of abundance as it avoids repeated counts of individuals (Priede et al., 1994; Willis and Babcock, 2000; Harvey et al., 2007). For each species, relative abundance was determined by dividing the MaxN of an individual species by the sum of MaxN values across all species.
The total number of species observed (species richness) and the species frequency (as number of surveys in which a species occurred) across surveys were also recorded. The relative frequency of each species was calculated by dividing its frequency by the sum of frequencies across all species.
To assess the dominance and the spatiotemporal consistency of dominant species within the community, we used Sanders Biological Value Index (BVI). BVI assesses dominance within a community by assigning ranks (based on the number of species) and corresponding points to individual species (Loya-Salinas and Escofet, 1990). The number of species to be considered in the overall calculation was identified by assessing patterns of dominance across the 35 surveys. Within each survey the number of species making up 95% of the cumulative relative abundance was identified and the maximum number of species across all surveys that accounted for 95% of the cumulative relative abundance (in our case 6 species) was used to assign ranks and points to species across all surveys (Supplementary Table S1).
Within each survey, ranks from 1 to 6 were assigned to species in order of abundance in a survey with a rank of 1 being the highest and equivalent to a value of 6 points (Supplementary Table S1). If there were more than 6 species in any survey, species after the 6th species received a value of 0 for that sample. With this method if a species ranks first across all surveys (35) it would receive a value of 210, that is, the maximum possible value.
Statistical analysis
The 35 surveys yielded species abundance estimates that differed by more than three orders of magnitude (Supplementary Figure 2). Since, ceteris paribus, species richness estimates will tend to increase non-linearly with individual abundance, we standardized species richness estimates across the surveys using rarefaction curves to facilitate cross-survey comparisons. We used a sample size of eight individuals to generate the rarefied species richness for each survey, since the maximum number of species observed in any given survey was eight. Two surveys had zero individuals observed, and so their species richness estimates were manually set to zero. Six other surveys had fewer than eight individuals (but more than zero); these surveys were manually and conservatively set to one species, since this is the minimum value that can be produced via rarefaction. Rarefied species richness was produced using the rarefy function in “vegan” (Oksanen et al., 2018) in R (R Core Team, 2022). Associations between the rarefied species richness estimates and distance from shore, water depth, and raft volume were assessed using Spearman rank correlations. Raft volume was calculated by multiplying raft length, raft width and raft depth, which provided a single estimate of raft size.
We also performed a redundancy analysis (RDA) to assess the effects of depth, distance from shore, and raft volume on community assemblage composition. Prior to running the RDA, the species data were first turned into species presence/absence matrix and subsequently Hellinger-transformed. These analyses were conducted using the “vegan” package in R (Oksanen et al., 2018). To assess the independent and shared effects of raft volume, distance from shore, and depth on assemblage composition, we conducted a variance partitioning (Borcard et al., 1992) using the varpart command in the “vegan” package.
Finally, we also assessed the extent to which changes in assemblage composition across surveys, i.e., beta diversity, reflected species replacement versus losses in species richness and whether any such potential process was associated with any of the three environmental variables. This implied first decomposing beta diversity into species replacement and species losses variance components using the beta.div.comp function in the “adespatial” package in R (Dray et al., 2022). These two variance components were subsequently and separately used to create a response matrix in a distance-based redundancy analysis where each environmental variable was used as predictor (after removing the effect of the two other variables via linear regression).
Results
A total of 35 underwater surveys were conducted off the eastern and southern coastline of Barbados during August 2021, March 2022 and May 2022. Species assemblages and abundances (0-250 individuals) varied across surveys (Figure 3); some rafts were teeming with life while 6% of rafts were unoccupied. Species richness was low (0-8 species) across surveys, with the majority (74%) of sargassum rafts occupied by 5 or fewer species.
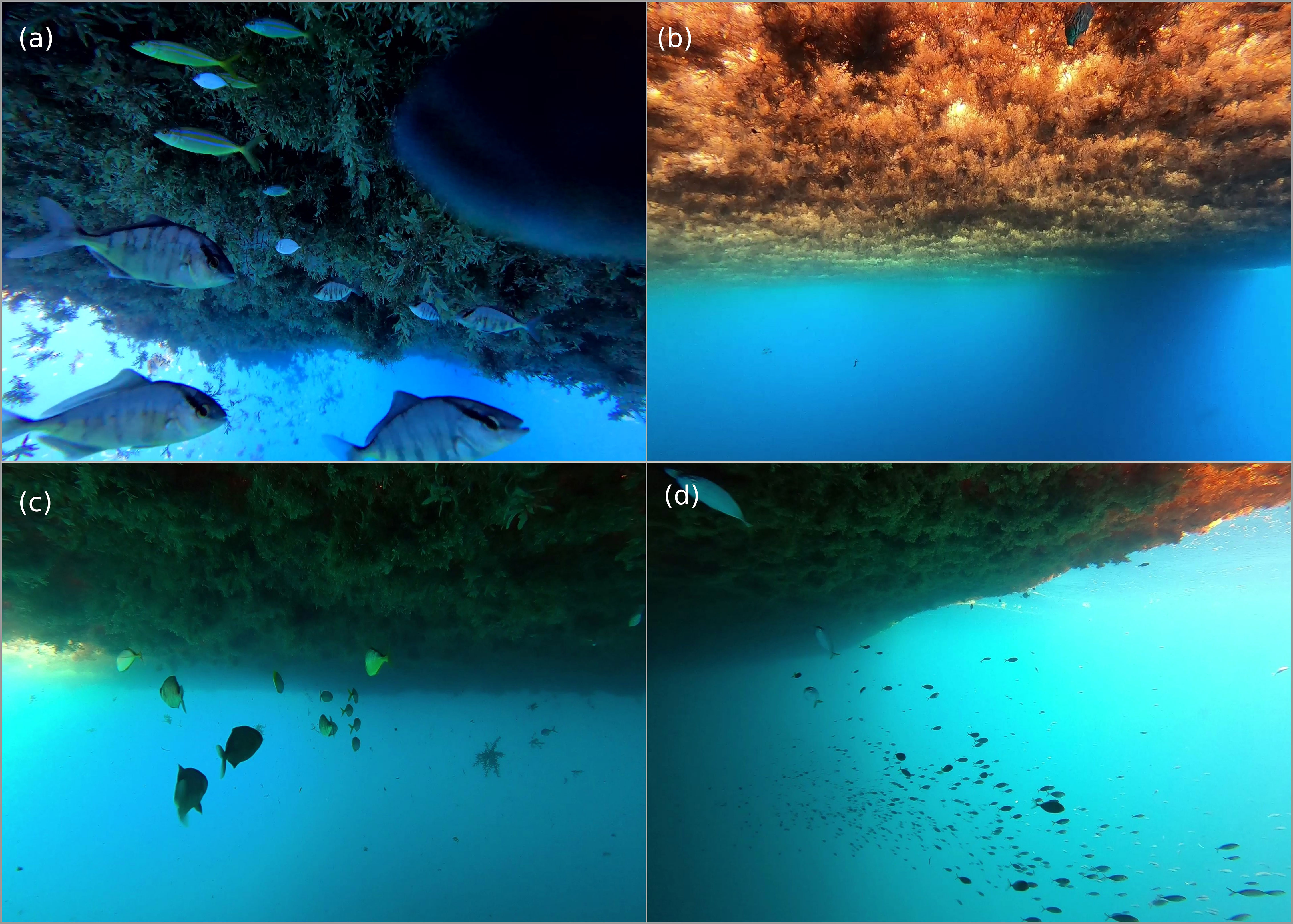
Figure 3 Still images collected from underwater videos of pelagic sargassum rafts. Smaller rafts (A) (5 m in diameter) and (B) (7 m in diameter). Larger rafts (C) (20 m in diameter) and (D) (100 m in diameter).
Relative abundance, relative frequency, and Sanders biological value index for observed species
We identified 1958 individuals representing 13 species (12 fishes and one comb jelly) across 8 families (Table 1 and Supplementary Figure 1). Carangidae represented the majority (96.1%) of species observed with pelagic sargassum and had high frequencies across all surveys.
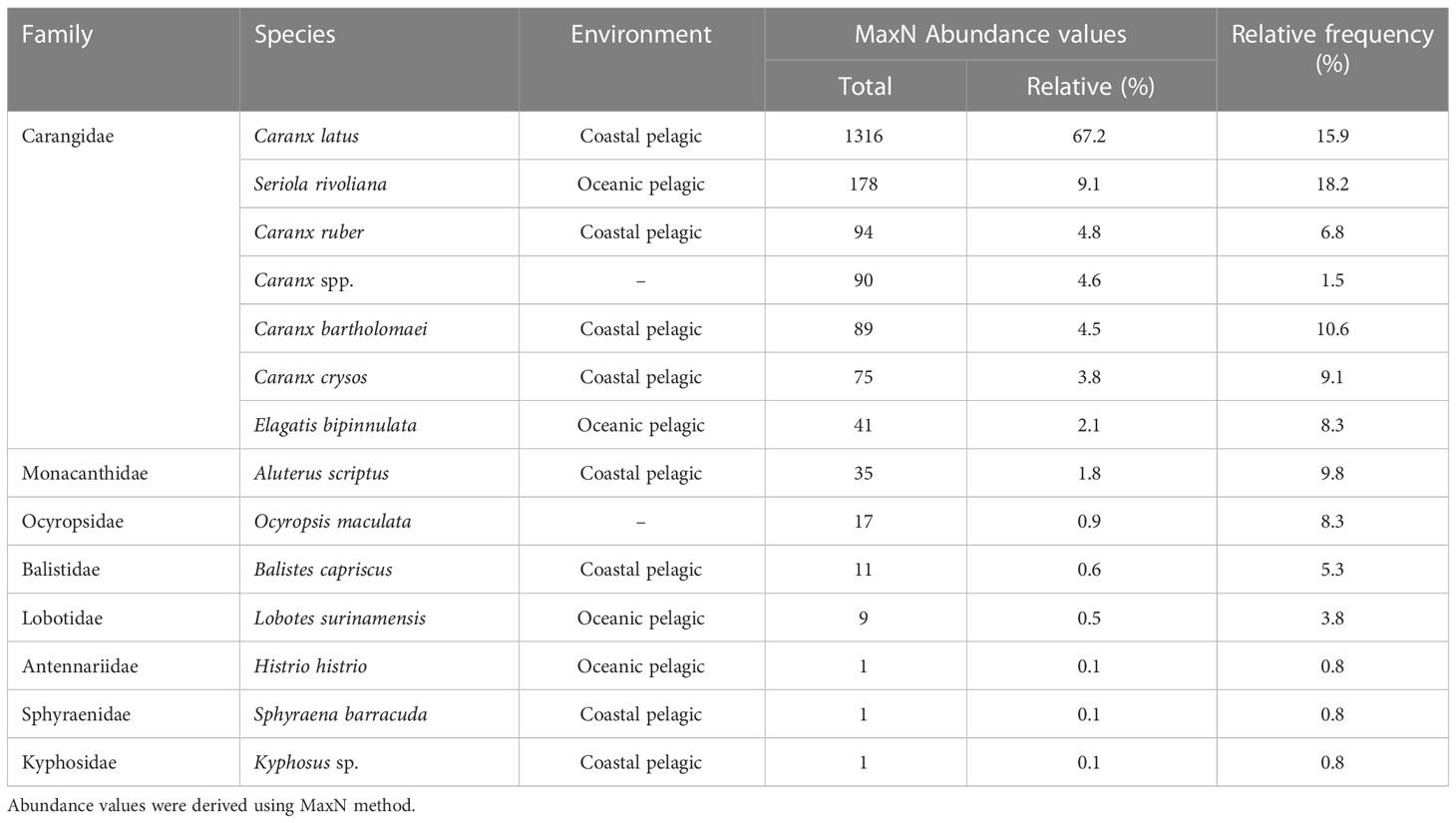
Table 1 Total and relative abundance and frequency of free-swimming fauna observed under pelagic sargassum by family and species.
Across the 35 surveys, Caranx latus (horse-eye jack) had the highest overall abundance but obtained a lower BVI score than Seriola rivoliana (almaco jack), which had a higher frequency across surveys resulting in spatiotemporal dominance (Table 2). Irrespective of total abundance or BVI ranking, Carangidae species dominated pelagic sargassum arriving in Barbados.
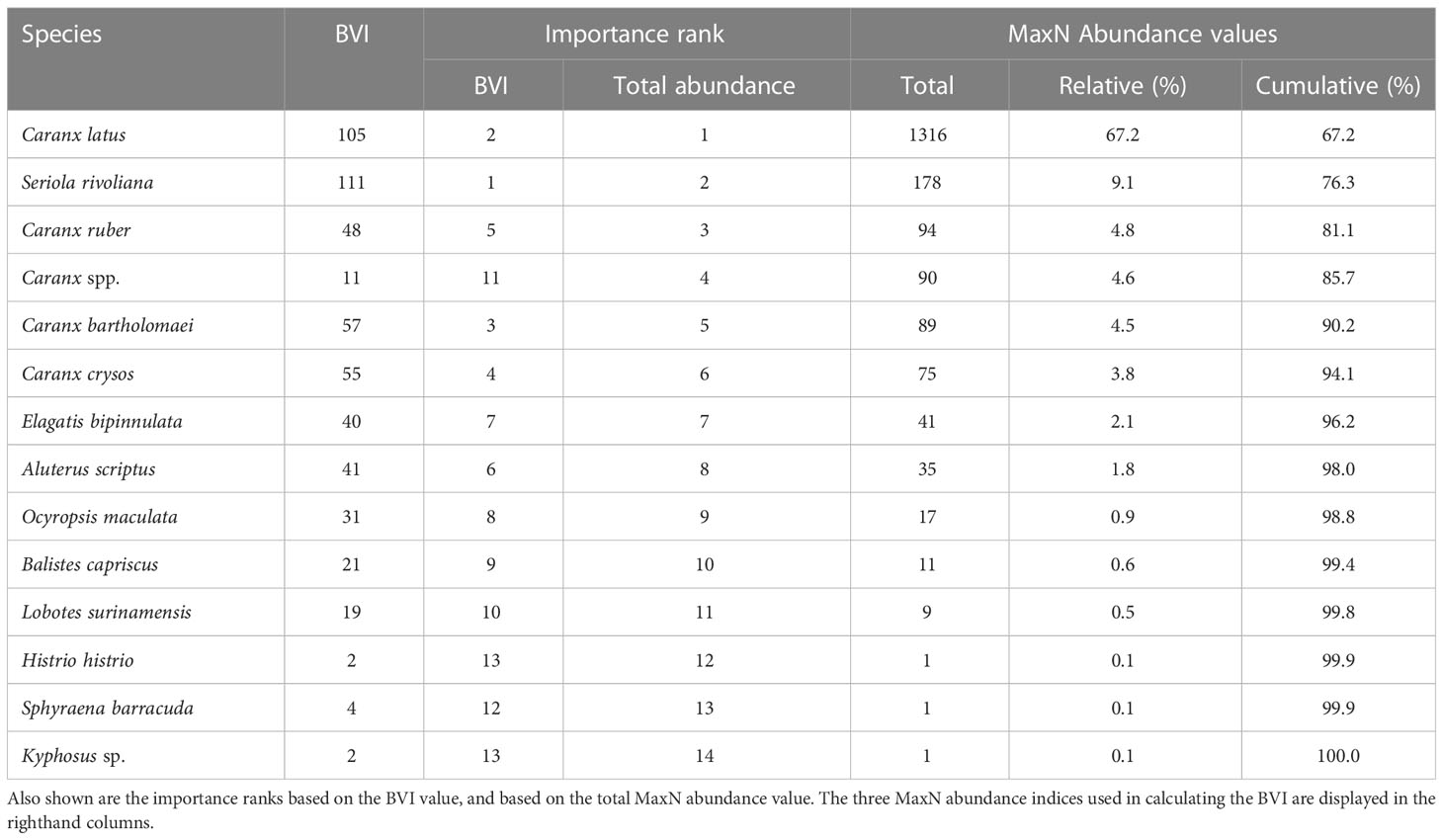
Table 2 Sanders biological value index (BVI) for species observed under pelagic sargassum, based on the total points obtained per species from 35 underwater surveys.
Effect of distance from shore, depth, and raft volume on species assemblage composition
The RDA revealed that 16.9% of the variance across surveys in species assemblage composition could be significantly explained by the environmental data (Pseudo-F= 1.96, p=0.008). The main axis of the RDA accounted for 51% of the explained variance and reflected primarily raft volume effects and secondarily depth effects (Figure 4). The second axis accounted for an additional 29% of the explained variance and reflected primarily the effect of distance from shore and secondarily depth effects (Figure 4). Testing the effect of distance from shore, depth, and raft volume separately indicated that species assemblage composition was significantly related to raft volume and depth (Pseudo-F≥ 2.94, p ≤ 0.006), whereas the relationship with distance from shore was marginally non-significant (Pseudo-F=2.05, p=0.053).
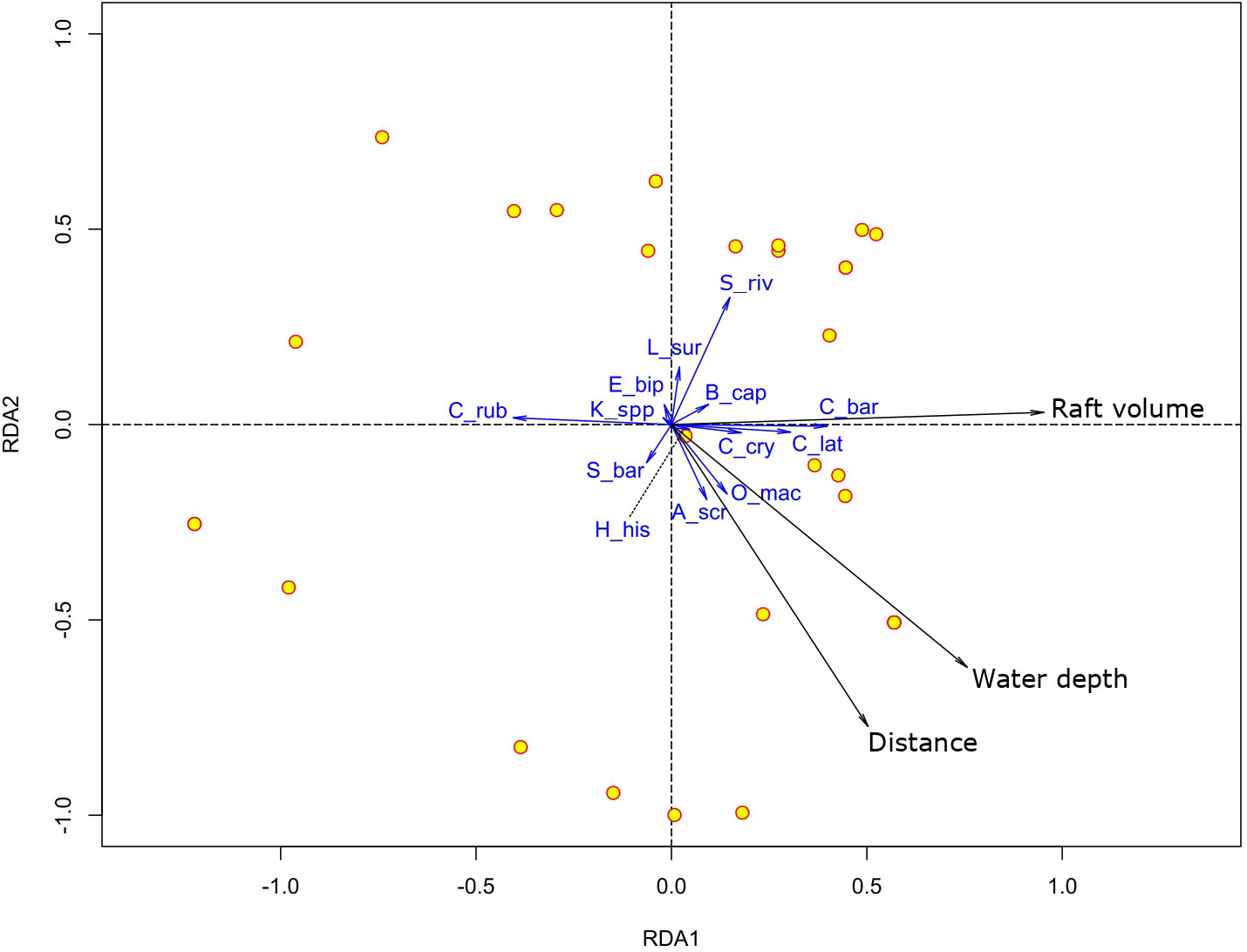
Figure 4 Redundancy analysis (RDA) ordination plot showing the effect of distance, water depth and raft volume on species composition of the free-swimming fauna associated with pelagic sargassum. S. rivoliana (S_riv), B. capriscus (B_cap), C. bartholomaei (C_bar), L. surinamensis (L_sur), C. latus (C_lat), C. crysos (C_cry), C. ruber (C_rub), E. bipinnulata (E_bip), Kyphosus spp. (K_spp), S. barracuda (S_bar), H. histrio (H_his), A. scriptus (A_scr), O. maculata (O_mac).
A variance partitioning to assess the independent and shared contributions of the three environmental variables indicated that they jointly accounted for 8.5% of the adjusted variance (adjusted R2) in species assemblage composition (Figure 5). Of this, 2.1% of the explained variance was shared among the three variables, whereas approximately 1.0% was shared between distance from shore and depth and between depth and raft volume, respectively (Figure 5). Raft volume independently accounted for the biggest fraction of the explained variance (2.5%; Figure 5), which was statistically significant (Pseudo-F=2.56, p=0.007). Depth and distance from shore independently accounted for 1.7% and 1.0% of the explained variance (Figure 5), respectively, but these independent fractions were not statistically significant (Pseudo-F ≤ 1.29, p≥0.228).
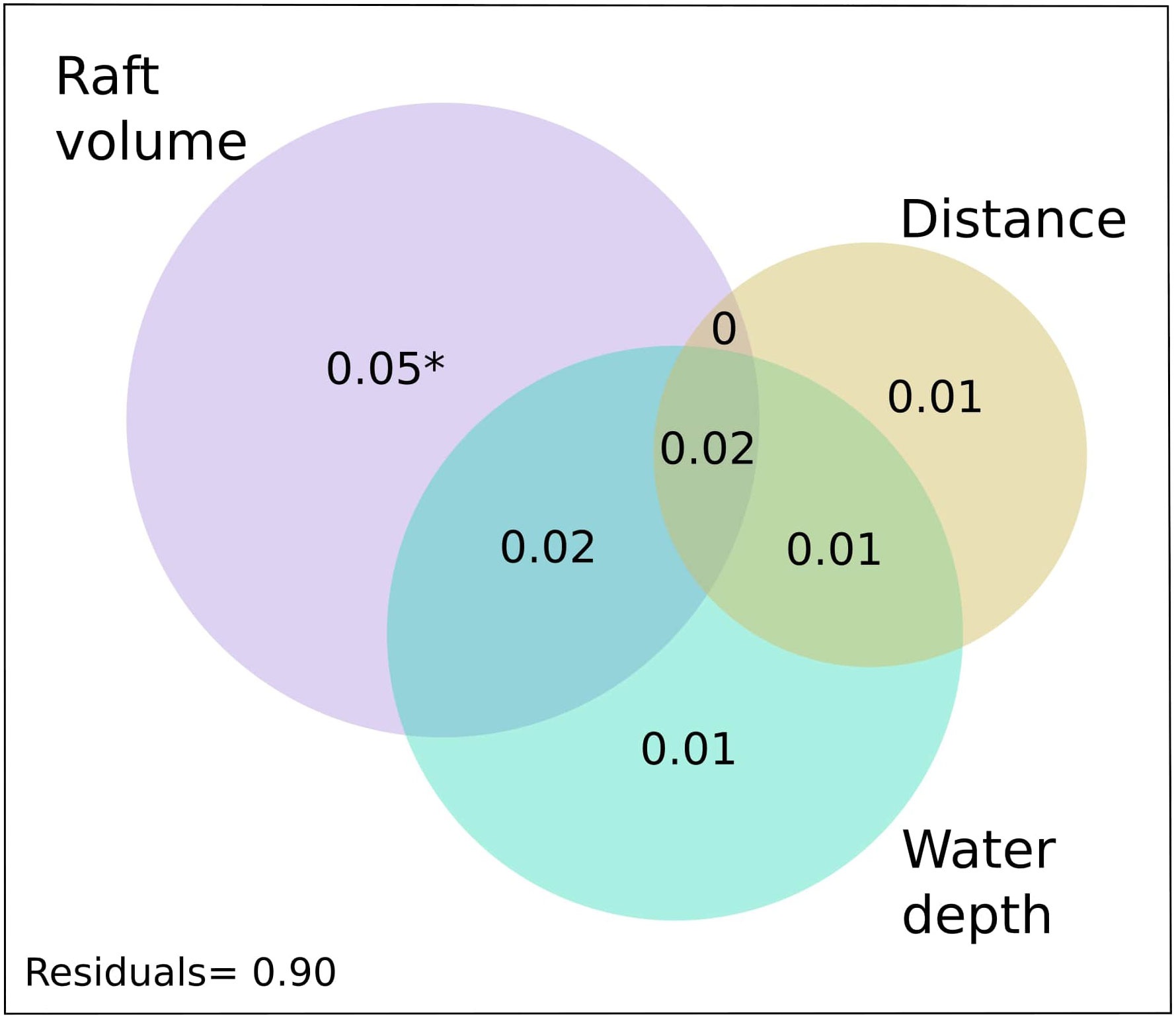
Figure 5 Venn diagram representing the shared and independent effects of each environmental variable on the assemblage of free-swimming fauna associated with pelagic sargassum. The variance jointly explained by distance, water depth and raft volume is represented in the region where all three circles overlap. Note that the sizes of the various shared and non-shared effects are only approximations.
Depth was highly positively correlated with distance from shore (rs=0.76, p<0.001) and raft volume (rs =0.43, p=0.009), but raft volume and distance from shore were not significantly correlated (rs =0.25, p=0.146) (Supplementary Figure 3). Raft volume was positively correlated with species richness (p<0.010) (Supplementary Figure 3), rarefied species richness (p= 0.001), and individual abundance (p<0.001) (Figure 6). In contrast, there was no significant correlation between distance or depth with species richness or rarefied species richness (p>0.050) (Figure 6 and Supplementary Figure 3). Distance and depth were positively correlated with abundance (p<0.05) (Figure 6).
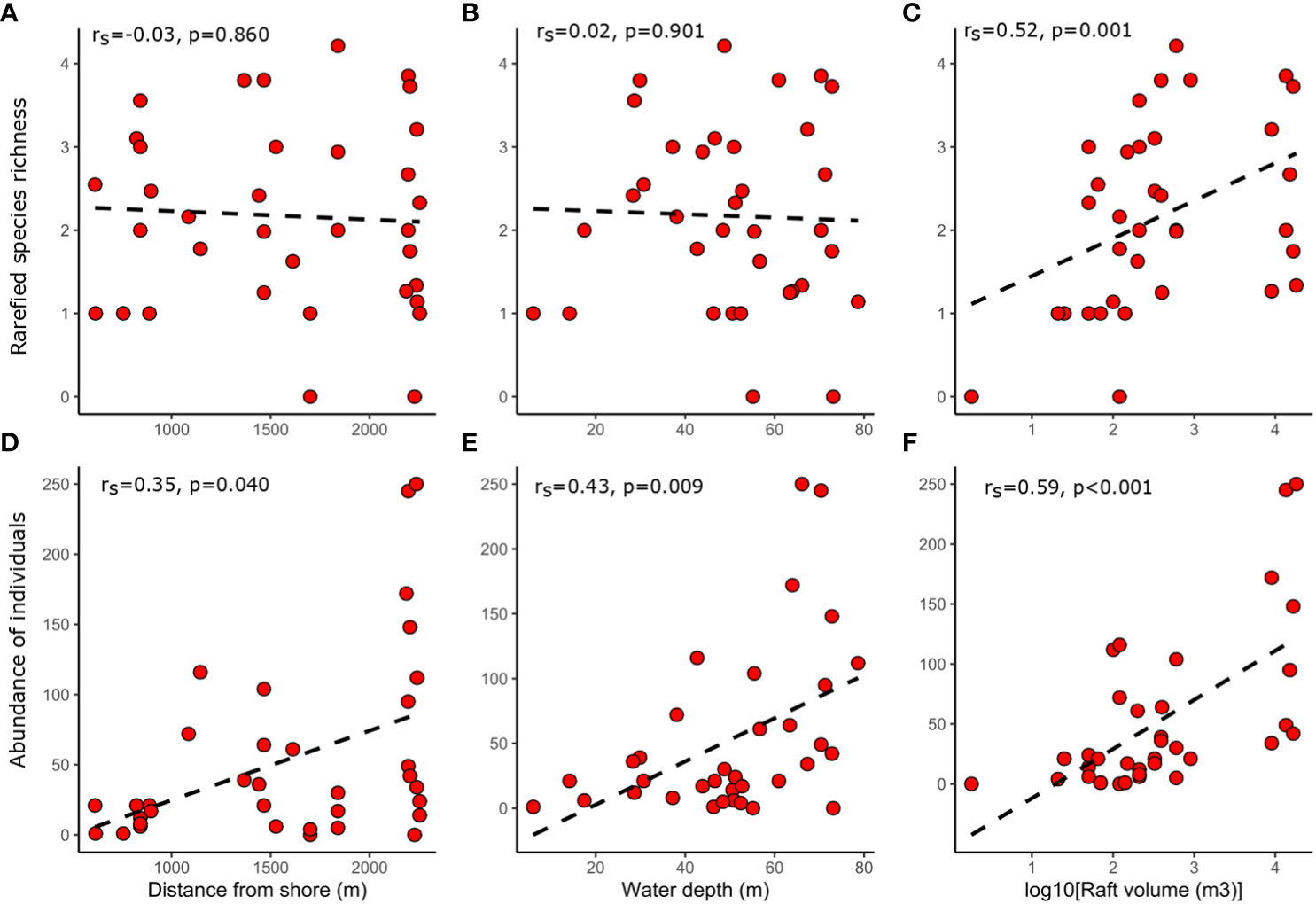
Figure 6 Scatter plots showing relationships between distance vs rarefied species richness (A), water depth vs rarefied species richness (B), raft volume vs rarefied species richness (C), distance vs abundance of individuals (D), water depth vs abundance of individuals (E) and raft volume vs abundance of individuals (F). Spearman rank correlation coefficients and associated p-values are also shown. A best fit line was inserted to help interpret trends.
The analysis of beta-diversity revealed that species replacement and species richness losses were equally important as each accounted for half (50%) of the variation in beta-diversity. The species replacement component was not significantly linked to any of the three environmental variables (Pseudo-F ≤ 1.09, p≥0.125). In contrast, the species richness loss component was significantly linked to raft volume (Pseudo-F=1.97, p=0.011), but not to depth nor distance from shore (Pseudo-F ≤ 0.79, p≥0.809), supporting that species were being lost from the original species assemblage as the rafts got smaller.
Discussion
Pelagic sargassum arriving in Barbados during 2021 and 2022 influx events was associated with 12 fish species (predominantly (67%) coastal pelagic) and one comb jelly (Ocyropsis maculata). This first account of free-swimming fauna associated with pelagic sargassum for the Eastern Caribbean is considerably lower than that reported by earlier studies (36-110 species) conducted across the Gulf of Mexico, the Gulf Stream, and various parts of the North Atlantic (Dooley, 1972; Bortone et al., 1977; Settle, 1993; Wells and Rooker, 2003; Hoffmayer et al., 2005). This was also the case when compared with Moser et al. (1998) (29 species, Gulf Stream) despite the use of video surveys in both studies. Differences in sampling methodologies between the present study and earlier studies (Dooley, 1972; Bortone et al., 1977; Settle, 1993; Wells and Rooker, 2003; Hoffmayer et al., 2005) may have contributed to the differences in species diversity (Alleyne, 2022). However, given the fact that species diversity increases with the amount of time a drifting structure, such as pelagic sargassum, is available (Kingsford, 1992), we postulate that earlier studies with ties to the well-established Sargasso Sea would have greater diversity when compared to the newly established bloom region in the Tropical Atlantic. This observation is supported by anecdotal information from local fishers in the Eastern Caribbean, who report finding increasing numbers and greater diversity of fish species associated with sargassum over time since the first influxes in 2011.
Across surveys, Carangidae was the dominant family observed making up 50% of the total fish species. Similarly, earlier studies (Moser et al., 1998; Hoffmayer et al., 2005) have also reported high abundances of Carangidae associated with pelagic sargassum. Within the Carangidae family, C. latus (horse-eye jack) was the most abundant species with large schools observed under some rafts. In Barbados, Carangidae are important to the coastal pelagic fishery (Maraj et al., 2011; Food and Agriculture Organization (FAO), 2022) making up 98.4% of the total catch in the seine fishery (Maraj et al., 2011). These species help to support the livelihood of fishers during the oceanic pelagic fishery ‘off-season’ and contribute to the island’s food security (Maraj et al., 2011).
The fisheries sector of Barbados, like much of the Eastern Caribbean, is an integral component of the culture, economy and food security (Oxenford and Monnereau, 2018). The mass proliferations and subsequent influx events of pelagic sargassum brought new navigation and harvesting challenges to the fishery sector (Ramlogan et al., 2017; Speede et al., 2018). Prior to the onset of large-scale sargassum strandings in 2011, Hirundichthys affinis (flyingfish) made up approximately 60% of the annual fish landings in Barbados and accounted for the highest value-added benefits of all landed species (Oxenford et al., 2019). By 2019, prolonged sargassum influx events resulted in extremely low catches in the flyingfish industry with a 51.5% decrease in the mean monthly landings (Oxenford et al., 2019). This decrease was followed by a rapid increase in catches of almaco jack (S. rivoliana) not previously known to Eastern Caribbean fishers or consumers (Caribbean Regional Fisheries Mechanism and Japanese International Cooperation Agency, 2019). Within this study, almaco jacks showed the highest spatiotemporal dominance of the 13 identified species. The association of almaco jacks with pelagic sargassum is now widely recognised in Barbados with iceboat and longline fishers actively targeting almaco jacks in periods of low flyingfish abundance. Over the last two years, Barbadian fishers have been using satellite images, provided by the University of South Florida, to target large sargassum rafts in pursuit of almaco jacks (Dr. Shelly-Ann Cox, pers. comm., Barbados).
Within this study, distance from shore, water-depth and/or raft volume jointly accounted for approximately 9% of the variation (adjusted R2) in the free-swimming community associated with pelagic sargassum. The effect of raft volume, distance from shore and water depth on community composition is complicated by the fact that water depth is correlated with both distance from shore and raft volume. That said, the variance partitioning and pairwise correlation tests do support that raft volume, a proxy for raft size, is the major structuring factor (among those measured here) of the free-swimming community associated with pelagic sargassum arriving in Barbados. As raft size decreased, individual abundance and species richness also decreased, as expected by ecological theory (Simberloff, 1976; Lomolino, 2000). The observed positive relationships between sargassum raft size and species richness and abundance is consistent with the earlier studies (Settle, 1993; Moser et al., 1998; Casazza and Ross, 2008; Martin et al., 2021; Goodwin et al., 2022) and explains why Barbadian fishers target larger rafts. Our results also support the findings of Wells and Rooker (2003) and Monroy-Velázquez et al. (2019), who reported reduced abundances within nearshore environments. Moreover, our beta-diversity analyses supported that the losses in species richness with decreasing raft size were due to a gradual loss of resident species (nestedness) rather than to progressively having smaller assemblages of new species (species replacement) (Legendre, 2014).
Interestingly, Monroy-Velázquez et al. (2019) noted that the occurrence of sargassum-brown-tide events in nearshore waters may account for the lower abundance of motile sargassum-associated fauna close to shore. They suggested that the reduction in available oxygen in these brown-tides may force fish to abandon the rafts as they approach the shore. In our study, however, none of the surveys were close enough to shore to experience the sargassum-brown-tide phenomenon. Regardless of cause, reduced abundances of associated biodiversity nearshore have been put forth as an argument for encouraging in-water harvesting of sargassum close to shore, rather than in the open sea. Furthermore, nearshore rafts are more likely to strand, resulting in the loss of any remaining biodiversity (Hinds et al., 2016; López-Contreras et al., 2022).
In order to reduce the environmental and economic impacts associated with shoreline accumulation of sargassum, affected countries need integrated management plans with adaptive strategies geared towards the creation of sustainable sargassum opportunities (Liranzo-Gómez et al., 2021; Robledo et al., 2021). Harvesting at sea will likely be required in the future to obtain the large quantities of sargassum needed for industrial-scale applications, or the high quality clean sargassum needed for many other applications, given the issues associated with separating partially decayed sargassum from fresh sargassum, other macroalgae, seagrasses and sand after stranding (Oxenford et al., 2021). However, a key question remains for providing best practice guidance: at what point (distance from shore) should sargassum be harvested to minimize biodiversity loss? The answer will likely vary on a case-by-case basis as coastal morphology, hydrography and presence of nearshore habitats will likely influence the distance from shore at which biodiversity decreases (Alleyne, 2022).
To date, the destruction of biodiversity associated with pelagic sargassum has been the primary concern raised with in-water harvesting (Dutch Caribbean Nature Alliance, 2019; Robledo et al., 2021). However, given the utilization of pelagic sargassum by Eastern Caribbean fishers, large-scale harvesting of pelagic sargassum will result in the loss of fishing opportunities. Fishers in this region are no longer merely ‘coping’ with sargassum as they did in the initial years (Speede et al., 2018) but rather they are adapting and now depend on the incoming rafts to target alternative species that are now well accepted by the market (e.g., almaco jacks, C. bartholomaei (yellow jacks). In fact, all species (with the exception of Histrio histrio (sargassum frogfish), and Ocyropsis maculata (comb jelly) found to be closely associated with pelagic sargassum in this study are considered commercial species and provide fishing opportunities, and contribute to the local economy and food security. In addition to reducing fishing opportunities, large-scale removal of sargassum could potentially affect commercially important flyingfish populations since they are known to use sargassum as a spawning substrate (Oxenford et al., 2019). A recent study in West Africa by Ofori and Rouleau (2021) using agent-based modelling to demonstrate the effects of sargassum harvesting on fisheries, showed that when all of the incoming sargassum is harvested, the fishery sector is denied the opportunities that sargassum offers to enhance fish growth and abundance. On the other hand, if no sargassum is removed, fishers will likely experience challenging fishing conditions with high volumes of sargassum in nearshore environments, and tourism sectors will also be negatively affected (Ofori and Rouleau, 2021). These findings, supported by our own results suggest that a best comprise should be sought with regard to in-water sargassum harvesting and preserving fishing opportunities. Therefore, as the region explores the use of in-water harvesting to minimize beaching events and increase valorization, efforts should also be made to maintain fishing opportunities. Additionally, if large-scale harvesting is developed, endangered species that use sargassum rafts, such as turtle hatchlings (known to be present as part of the clinging-fauna community in the rafts off Barbados but not captured in our video surveys of the free-swimming community) will need to be considered (Oxenford et al., 2021). Such concerns have already led to pelagic sargassum being given special management attention in the USA through its designation as ‘essential fish habitat’ resulting in regulations restricting the harvesting of sargassum in waters under the jurisdiction of the South Atlantic Fishery Management Council (South Atlantic Fishery Management Council (SAFMC), 2002; National Marine Fisheries Service (NMFS), 2003). Furthermore in 2014 sargassum was designated as ‘critical habitat’ for loggerhead turtles in the US Gulf of Mexico and NW Atlantic (National Oceanic and Atmospheric Administration (NOAA), 2014) resulting in its legal protection.
A significant conundrum highlighted here in the management of sargassum is the conflicting needs of two important commercial sectors. Large scale in-water removal of sargassum has the potential to reduce fishing opportunities but it is also likely to greatly improve tourist experience. It is well established that clean beaches, clear waters and healthy coral reefs provide the principal settings for tourism activity in Barbados (Schuhmann et al., 2017) and play a critical role in tourists’ return visitation decisions (Schuhmann et al., 2019).
In conclusion, this preliminary study provides the first insights for the Eastern Caribbean on the free-swimming fauna associated with incoming pelagic sargassum rafts originating from the NERR, and reveals the management dilemma between the fisheries and tourism sectors. We have also demonstrated the usefulness of the relatively simple and low-cost video technique for recording free-swimming fauna associated with sargassum. Even with the use of a conservative assessment of the associated biodiversity (MaxN) and the crude estimates for raft size, we have revealed a significant positive correlation between raft size and community assemblage that aligns well with ecological theory that biodiversity should increase with patch/habitat size (e.g., Simberloff, 1976; Kohn and Walsh, 1994; Lomolino, 2000; Munguía-Rosas and Montiel, 2014).
However, there are limitations to our study and generalisations across years or countries should not be made on the basis of samples taken over a limited period. Additionally, our camera design would have inherently missed some of the cryptically colored species swimming directly beneath the raft. Within this study, about 90% of the variation in the free-swimming community associated with pelagic sargassum was not explained by distance from shore, water-depth and/or raft volume. This suggests that there are likely to be other important unmeasured variable(s) influencing the community associated with pelagic sargassum. These could include variability in sargassum morphotype composition of the rafts (Martin et al., 2021), age of the sargassum (Stoner and Greening, 1984) source area of sargassum within the NERR which varies with season (Beron-Vera et al., 2022), oxygen levels and changes in hydrography.
To better understand where, when or if in-water harvesting of sargassum should be permitted, future studies should compare the fish community in offshore and nearshore environments over a greater range of distance and over a longer time period than the current study. Ideally sampling should be carried out closer than 500 m from shore for applicability of assessing the impact of harvesting sargassum along the outside of barriers deployed to prevent sargassum reaching the shore. Sampling should also occur in oceanic waters for applicability of assessing the impacts of industrial-scale harvesting or sinking of sargassum. Likewise, sampling should be expanded to cover summer and winter influx events that bring sargassum from different source areas in the NERR (Beron-Vera et al., 2022). Moreover, studies that quantify the utilization of pelagic sargassum by fishers in Barbados and the Eastern Caribbean are needed to better assess the impacts of loss of fishing opportunities.
Data availability statement
The original contributions presented in the study are included in the article/Supplementary Material. Further inquiries can be directed to the corresponding author.
Author contributions
KA, MC and HO conceptualized the work. KA, MC and MS participated in the data collection process and the data was analyzed by KA and HV. All authors contributed to the writing of the article and approved the submitted version.
Funding
This work was supported by The Nippon Foundation of the World Maritime University (WMU) - Sasakawa Global Ocean Institute as well as The Nippon Foundation and WMU-Sasakawa Global Ocean Institute’s ‘Closing the Circle Programme: Marine Debris, Sargassum and Marine Spatial Planning’ in the Eastern Caribbean. Further support was provided by the Caribbean Biodiversity Fund Ecosystem based Adaptation Facility with financing from the International Climate Initiative (IKI) under the SargAdapt “Adapting to a new reality: Managing responses to influxes of sargassum seaweed in the Eastern Caribbean as ecosystem hazards and opportunities” project.
Acknowledgments
The authors acknowledge Chè Alleyne, Gary Marshall and Rudolph Brathwaite for assisting with the data collection process and further acknowledges Shelly-Ann Cox for her valuable insights. We extend our gratitude to Verónica Monroy-Velázquez and Brigitta van Tussenbroek for providing assistance with species identification and guidance on BVI calculations. This work would not have been possible without the generous funding by The Nippon Foundation of the World Maritime University (WMU) - Sasakawa Global Ocean Institute as well as for The Nippon Foundation & WMU-Sasakawa Global Ocean Institute's 'Closing the Circle Programme: Marine Debris, Sargassum and Marine Spatial Planning’ in the Eastern Caribbean. We further acknowledge the Caribbean Biodiversity Fund Ecosystem based Adaptation Facility with financing from the International Climate Initiative (IKI) under the SargAdapt “Adapting to a new reality: Managing responses to influxes of sargassum seaweed in the Eastern Caribbean as ecosystem hazards and opportunities” project for their support with this study.
Conflict of interest
The authors declare that the research was conducted in the absence of any commercial or financial relationships that could be construed as a potential conflict of interest.
Publisher’s note
All claims expressed in this article are solely those of the authors and do not necessarily represent those of their affiliated organizations, or those of the publisher, the editors and the reviewers. Any product that may be evaluated in this article, or claim that may be made by its manufacturer, is not guaranteed or endorsed by the publisher.
Supplementary material
The Supplementary Material for this article can be found online at: https://www.frontiersin.org/articles/10.3389/fmars.2023.1090742/full#supplementary-material
References
Alleyne K. S. (2022). How is pelagic sargassum-associated biodiversity assessed? insights from the literature.“. Gulf Caribbean Res. 33 (1): GCFI 14-GCFI 23. doi: 10.18785/gcr.3301.08
Amador-Castro F., García-Cayuela T., Alper H. S., Rodriguez-Martinez V., Carrillo-Nieves D. (2021). Valorization of pelagic sargassum biomass into sustainable applications: Current trends and challenges. J. Environ. Manage. 283, 112013. doi: 10.1016/j.jenvman.2021.112013
Beron-Vera F. J., Olascoaga M. J., Putman N. F., Triñanes J., Goni G. J., Lumpkin R. (2022). Dynamical geography and transition paths of Sargassum in the Tropical Atlantic. AIP Adv. 12 (10), 105107. doi: 10.1063/5.0117623
Borcard D., Legendre P., Drapeau P. (1992). "Partialling out the spatial?component of ecological variation.". Ecology 73, 1045–1055. doi: 10.2307/1940179
Bortone S. A., Hastings P. A., Collard S. B. (1977). The pelagic - sargassum ichthyofauna of the Eastern Gulf of Mexico. Northeast Gulf Sci. 1 (2). doi: 10.18785/negs.0102.02
Butler J. N., Morris B. F., Cadwallader J., Stoner A. (1983). “Studies of sargassum and the sargassum community,” in Special publication 22 (St. George's, Bermuda: Bermuda Biological Station for Research, Inc.), 307 p.
Caribbean Regional Fisheries Mechanism, and Japanese International Cooperation Agency (2019). “Fact-finding survey regarding the influx and impacts of sargassum seaweed in the Caribbean region,” in Draft report (Belize: CRFM-JICA).
Carpenter K. E. (2002). “FAO species identification guide for fishery purposes and American society of ichthyologists and herpetologists special publication no. 5,” in The living marine resources of the Western central atlantic. volume 3: Bony fishes part 2 (Opistognathidae to molidae), sea turtles and marine mammals (Rome: FAO), 1375–2127 pp.
Casazza T. L., Ross S. W. (2008). Fishes associated with pelagic sargassum and open water lacking sargassum in the Gulf Stream off North Carolina. Fishery Bull. 106 (4), 348–635.
Centre for Resource Management and Environmental Studies (2022). “Sargassum Sub-regional outlook bulletin,” in Volume 2 issue 5 (Bridgetown: Centre for Resource Management and Environmental Studies, The University of the West Indies, Cave Hill Campus).
Chávez V., Uribe-Martínez A., Cuevas E., Rodríguez-Martínez R. E., van Tussenbroek B. I., Francisco V., et al. (2020). Massive influx of pelagic sargassum spp. on the coasts of the Mexican Caribbean 2014–2020: Challenges and opportunities. Water 12 (10), 2908. doi: 10.3390/w12102908
Cox S.-A., Degia A.K., Lopez I. C. (2021). “Sargassum: Brown tide or golden jewel?,” in Communication brief 024. UNEP foresight briefs (United Nations Environment Programme).
Davis D., Simister R., Campbell S., Marston M., Bose S., McQueen-Mason S. J., et al. (2020). Biomass composition of the golden tide pelagic seaweeds Sargassum fluitans and S. natans (Morphotypes I and VIII) to inform valorisation pathways. Sci. Total Environ. 762, 143134. doi: 10.1016/j.scitotenv.2020.143134
Desrochers A., Cox S.-A., Oxenford H. A., van Tussenbroek B. (2020). “Sargassum uses guide: A resource for Caribbean researchers, entrepreneurs and policy makers,” in Report prepared for the climate change adaptation in the Eastern Caribbean fisheries sector (CC4FISH) project of the Food and Agriculture Organization (FAO) and the Global Environment Facility (GEF) (Bridgetown: Barbados: Centre for Resource Management and Environmental Studies (CERMES), University of the West Indies, Cave Hill Campus), 159 pp.
Devault D. A., Massat F., Dupuy L., Lambourdière J., Ménez F., Maridakis C., et al. (2019). Contamination of sargassum by chlordecone: the other challenge of strandings in the West indies (Montpellier: 49e congrès du Groupe Français de recherche sur les Pesticides), 102.
Devault D. A., Massat F., Lambourdière J., Maridakis C., Dupuy L., Péné-Annette A., et al. (2022). Micropollutant content of sargassum drifted ashore: Arsenic and chlordecone threat assessment and management recommendations for the Caribbean (Preprint). doi: 10.21203/rs.3.rs-1210563/v1.
Dooley J. K. (1972). Fishes associated with the pelagic sargassum complex, with a discussion of the sargassum community.“. Contributions Mar. Sci. 16, 1–32.
Dray S., Bauman D., Blanchet G., Borcard D., Clappe S., Guénard G., et al. (2022). “Adespatial: Multivariate multiscale spatial analysis,” in R package version 0.3-19. Available at: https://CRAN.R-project.org/package=adespatial.
Dutch Caribbean Nature Alliance (2019). Prevention and clean-up of sargassum in the Dutch Caribbean (Bonaire: Dutch Caribbean Nature Alliance), 30pp. Available at: http://dx.doi.org/10.25607/OBP-796.
Food and Agriculture Organization (FAO) (2022). Fishery and aquaculture country profiles. Barbados. In: Country profile fact sheets (Rome: Fisheries and Aquaculture Division). Available at: https://www.fao.org/fishery/en/facp/brb?lang=en (Accessed 18th October 2022).
Franks J. S., Johnson D. R., Ko D.-S., Sanchez-Rubio G., Hendon J. R. (2012). Unprecedented influx of pelagic Sargassum along Caribbean island coastlines during summer 2011. 64th Gulf and Caribbean Fisheries Institute, 6–8.
Franks J. S., Johnson D. R., S. Ko D. (2016). Pelagic sargassum in the tropical north Atlantic. Gulf Caribbean Res 27(1), SC6–SC11. doi: 10.18785/gcr.2701.08
Froese R., Pauly D. (2011) FishBase. Available at: http://www.fishbase.org/Summary/speciesSummary.php?ID=12858&genusname=Leptonotus&speciesname=blainvilleanus&AT=Leptonotus+blainvilleanus&lang=English (October 21st 2022).
García-Sánchez M., Graham C., Vera E., Escalante-Mancera E., Álvarez-Filip L., van Tussenbroek B. I. (2020). Temporal changes in the composition and biomass of beached pelagic sargassum species in the Mexican Caribbean. Aquat. Bot. 167, 103275. doi: 10.1016/j.aquabot.2020.103275
Goodwin D. S., Siuda A. N. S., Schell J. M. (2022). In situ observation of holopelagic sargassum distribution and aggregation state across the entire North Atlantic from 2011 to 2020. PeerJ 10, e14079. doi: 10.7717/peerj.14079
Harvey E. S., Cappo M., J. Butler J., Hall N., Kendrick G. A. (2007). Bait attraction affects the performance of remote underwater video stations in assessment of demersal fish community structure. Mar. Ecol. Prog. Ser. 350, 245–254. doi: 10.3354/meps07192
Hinds C., Oxenford H. A., Cumberbatch J., Fardin F., Doyle E., Cashman A. (2016). “Golden tides: Management best practices for influxes of sargassum in the Caribbean with a focus on clean-up,” in Sargassum management brief (Barbados: Centre for Resource Management and Environmental Studies). Available at: https://www.cavehill.uwi.edu/cermes/getdoc/123bf91c-1565-414d-8e21-e59fb6f7ca2d/cermes_sargassum_management_brief_2016_08_24.aspx.
Hoffmayer E. R., Franks J. S., Comyns B. H., Hendon J.R., Waller R. S. (2005). “Larval and juvenile fishes associated with pelagic sargassum in the northcentral Gulf of Mexico,” in 56th Gulf and Caribbean fisheries institute (British Virgin Islands: GCFI), 259–269.
Huffard C. L., von Thun S., Sherman A. D., Sealey K., Smith K. L. (2014). Pelagic Sargassum community change over a 40-year period: Temporal and spatial variability. Mar. Biol. 161, 2735–2751. doi: 10.1007/s00227-014-2539-y
Johnson D. R., Franks J. S., Oxenford H. A., Cox S.-A. L. (2020). Pelagic sargassum prediction and marine connectivity in the Tropical Atlantic. Gulf Caribbean Res. 31, GCFI20–GCFI30. doi: 10.18785/gcr.3101.15
Kingsford M. J. (1992). Drift algae and small fish in coastal waters of northeastern New Zealand. Mar. Ecol. Prog. Ser. 80, 41–55. doi: 10.3354/meps080041
Kohn D. D., Walsh D. M. (1994). Plant species richness-the effect of island size and habitat diversity. J. Ecol. 82 (2), 367–377. doi: 10.2307/2261304
Legendre P. (2014). Interpreting the replacement and richness difference components of beta diversity: Replacement and richness difference components. Global Ecol. Biogeography 23 (11), 1324–1334. doi: 10.1111/geb.12207
Liranzo-Gómez R. E., García-Cortés D., Jáuregui U. (2021). Adaptation and sustainable management of massive influx of sargassum in the Caribbean. Proc. Environ. Science Eng. Manage. 8 (2), 543–535.
Lomolino M. V. (2000). Ecology's most general, yet protean pattern: The species-area relationship. J. Biogeography 27 (1), 17–26. doi: 10.1046/j.1365-2699.2000.00377.x
López-Contreras A. M., Núñez Valenzuela P., Celis García B., Driegen J., Huerta Lwanga E., Domin P., et al. (2022). Sargassum in Mexico: From environmental problem to valuable resource. WUR Rep. 2319. doi: 10.18174/574423
Loya-Salinas D. H., Escofet A. (1990). Contribution to the calculation of the biological value index (Sander). Cienc. Marinas 16 (2), 97–115. doi: 10.7773/cm.v16i2.688
Machado C. B., Maddix G.-M., Francis P., Thomas S.-L., Burton J.-A., Langer S., et al. (2022). Pelagic sargassum events in Jamaica: Provenance, morphotype abundance, and influence of sample processing on biochemical composition of the biomass. Sci. Total Environ. 817 (April), 152761. doi: 10.1016/j.scitotenv.2021.152761
Maraj V., Cox S.-A., Oxenford H. A. (2011). The current status of the small-scale seine fishery in Barbados. Proceedings of the Gulf and Caribbean Fisheries Institute 63, 411–419.
Marmorino G. O., Miller W. D., Smith G. B., Bowles J. H. (2011). Airborne imagery of a disintegrating sargassum drift line. Deep Sea Res. Part I: Oceanographic Res. Papers 58 (3), 316–215. doi: 10.1016/j.dsr.2011.01.001
Marsh R., Addo K. A., Jayson-Quashigah P.-N., Oxenford H. A., Maxam A., Anderson R., et al. (2021). Seasonal predictions of holopelagic sargassum across the Tropical Atlantic accounting for uncertainty in drivers and processes: The SARTRAC ensemble forecast system. Front. Mar. Sci. 8. doi: 10.3389/fmars.2021.722524
Marsh R., Oxenford H. A., Cox S.-A. L., Johnson D. R., Bellamy J. (2022). Forecasting seasonal sargassum events across the Tropical Atlantic: Overview and challenges. Front. Mar. Sci. 9. doi: 10.3389/fmars.2022.914501
Martin L. M., Taylor M., Huston G., Goodwin D. S., Schell J. M., Siuda A. N. S. (2021). Pelagic sargassum morphotypes support different rafting motile epifauna communities. Mar. Biol. 168 (7), 1155. doi: 10.1007/s00227-021-03910-2
Maurer A. S., Neef E. D., Stapleton S. (2015). Sargassum accumulation may spell trouble for nesting sea turtles. Front. Ecol. Environ. 13 (7), 394–965. doi: 10.1890/1540-9295-13.7.394
Mendoza-Becerril M. A., Serviere-Zaragoza E., Mazariegos-Villarreal A., Rivera-Perez C., Calder D. R., Vázquez-Delfín E. F., et al. (2020). Epibiont hydroids on beachcast Sargassum in the Mexican Caribbean. PeerJ 8, e9795. doi: 10.7717/peerj.9795
Milledge J. J., Harvey P. J. (2016). Golden tides: Problem or golden opportunity? the valorisation of sargassum from beach inundations. J. Mar. Sci. Eng. 4 (60). doi: 10.3390/jmse4030060
Monroy-Velázquez L. V., Rodríguez-Martínez R. E., van Tussenbroek B. I., Aguiar T., Solís-Weiss V., Briones-Fourzán P. (2019). Motile macrofauna associated with pelagic sargassum in a Mexican reef lagoon. J. Environ. Manage. 252. doi: 10.1016/j.jenvman.2019.109650
Moser M. L., Auster P. J., Bichy J. B (1998). Effects of mat morphology on large sargassum-associated fishes: Observations from a remotely operated vehicle (ROV) and free-floating video camcorders. Environ. Biol. Fishes 51, 391–398. doi: 10.1023/A:1007493412854
Munguía-Rosas M. A., Montiel S. (2014). Patch size and isolation predict plant species density in a naturally fragmented forest. PloS One 9 (10), e111742. doi: 10.1371/journal.pone.0111742
National Marine Fisheries Service (NMFS) (2003). Fisheries of the Caribbean, Gulf of Mexico and South Atlantic: Pelagic sargassum habitat of the South Atlantic region (Final rule). Federal Register 68:192, 57375. doi: 10.1037/e722922011-001
National Oceanic and Atmospheric Administration (NOAA) (2014). Endangered and threatened species: Critical habitat for the Northwest Atlantic ocean loggerhead sea turtle distinct population segment (DPS) and determination regarding critical habitat for the north pacific ocean loggerhead DPS; final rule. Federal Register 79 (132), 39856–39912.
Nielsen B. V., Milledge J. J., Hertler H., Maneein S., Mahmud Al Farid Md, Bartlett D. (2021). Chemical characterisation of sargassum inundation from the Turks and Caicos: Seasonal and post stranding changes. Phycology 1 (2), 143–625. doi: 10.3390/phycology1020011
Ofori R. O., Rouleau M. D. (2021). Modeling the impacts of floating seaweeds on fisheries sustainability in Ghana. Mar. Policy 127. doi: 10.1016/j.marpol.2021.104427
Oksanen J., Simpson G., Blanchet F., Kindt R., Legendre P., Minchin P., et al. (2018). “Vegan: community ecology package,” in R package version 2, 5–3. Available at: https://CRAN.R-project.org/package=vegan.
Oxenford H. A., Cox S.-A., van Tussenbroek B. I, Desrochers A. (2021). Challenges of turning the sargassum crisis into gold: Current constraints and implications for the Caribbean. Phycology 1 (1), 27–485. doi: 10.3390/phycology1010003
Oxenford H. A., Johnson D., Cox S.-A., Franks J. (2019). Report on the Relationships between Sargassum Events. Oceanic variables and Dolphinfish and Flyingfish Fisheries. Centre for Resource Management and Environmental Studies, University of the West Indies Cave Hill Campus Bridgetown: Barbados. 32.
Oxenford H. A., Monnereau I. (2018). “Chapter 9: Climate change impacts, vulnerabilities and adaptations,” in Western Central Atlantic marine fisheries, vol. 627. (Rome: FAO Fisheries & Aquaculture Technical Paper, no), 185–206.
Patil P. G., Virdin J., Michele Diez S., Roberts J., Singh A. (2016). Toward a blue economy: A promise for sustainable growth in the Caribbean (Washington D.C: The World Bank).
Priede I. G., Bagley P. M., Smith A., Creasey S., Merrett N. R. (1994). Scavenging deep demersal fishes of the porcupine seabight, north-east Atlantic: observations by baited camera, trap, and trawl. J. Mar. Biol. Assoc. United Kingdom 74 (3), 481–4985. doi: 10.1017/S0025315400047615
Ramlogan N. R., McConney P., Oxenford H. A. (2017). “Socio-economic impacts of sargassum influx events on the fishery sector of Barbados,” in 81. CERMES technical report (Bridgetown, Barbados: Centre for Resource Management and Environmental Studies, University of the West Indies, Cave Hill Campus).
R Core Team (2022). R: A language and environment for statistical computing (Vienna, Austria: R Foundation for Statistical Computing). Available at: https://www.R-project.org/.
Resiere D., Mehdaoui H., Nevière R., Mégarbane B. (2019). Sargassum invasion in the Caribbean: The role of medical and scientific cooperation. Rev. Panamericana Salud Pública 43, e52. doi: 10.26633/RPSP.2019.52
Robledo D., Vázquez-Delfín E., Freile-Pelegrín Y., Vásquez-Elizondo R. M., Qui-Minet Z. N., Salazar-Garibay A. (2021). Challenges and opportunities in relation to sargassum events along the Caribbean Sea. Front. Mar. Sci. 8. doi: 10.3389/fmars.2021.699664
Schell J., Siuda A. N. S., Goodwin D. S. (2016). “Shipboard observation of pelagic sargassum spp. reveals proliferation of a rare form and differences in associated mobile fauna community structure,” in 68th Gulf and Caribbean fisheries institute (Panama City, Panama: GCFI), 421–423.
Schiariti J. P., Salmon M. (2022). Impact of sargassum accumulations on loggerhead (Caretta caretta) hatchling recruitment in SE Florida, united states. J. Coast. Res. 00 (0), 115. doi: 10.2112/JCOASTRES-D-21-00134.1
Schuhmann P., Skeete R., Waite R. (2017) The economic importance of coastal and marine to tourism in Barbados. Available at: https://www.onecaribbean.org/wp-content/uploads/Economic-Importance-of-Coastal-and-Marine-Resources-to-Tourism-in-Barbados.pdf.
Schuhmann P., Skeete R., Waite R., Bangwayo-Skeete P., Casey J., Oxenford H. A., et al. (2019). Coastal and marine quality and tourists’ stated intention to return to Barbados. Water 11 (6), 12655. doi: 10.3390/w11061265
Settle L. R. (1993). “Spatial and temporal variability in the distribution and abundance of larval and juvenile fishes associated with pelagic sargassum,” in MSc (USA: University of North Carolina at Wilmington).
Simberloff D. (1976). Experimental zoogeography of islands: Effects of island size. Ecology 57 (4), 629–648. doi: 10.2307/1936179
South Atlantic Fishery Management Council (SAFMC) (2002). Fishery management plan for pelagic sargassum habitat of the South Atlantic region (Charleston, South Carolina, USA: SAFMC).
Speede R. F., Cox A. L., Oxenford H. A. (2018). “Best practices for Caribbean fishers coping with sargassum influx events,” in 71st Gulf and Caribbean fisheries institute, vol. 71. (San Andrés, Colombia: GCFI), 315–316.
Stoner A. W., Greening H. S. (1984). Geographic variation in the macrofaunal associates of pelagic Sargassum and some biogeographic implications. Mar. Ecol. Prog. Ser. 20, 185–192. doi: 10.3354/meps020185
The Sargassum Watch System (SaWS) (2022). Sargassum blooms in the Caribbean Sea. In: Optical oceanography laboratory (University of South Florida). Available at: https://optics.marine.usf.edu/projects/saws.html (Accessed 3rd September 2022).
Thompson T. M., Young B. R., Baroutian S. (2020). Pelagic sargassum for energy and fertiliser production in the Caribbean: A case study on Barbados. Renewable Sustain. Energy Rev. 118, 109564. doi: 10.1016/j.rser.2019.109564
Tonon T., Machado C. B., Webber M., Webber D., Smith J., Pilsbury A., et al. (2022). Biochemical and elemental composition of pelagic sargassum biomass harvested across the Caribbean. Phycology 2 (1), 204–215. doi: 10.3390/phycology2010011
United Nations Environment Programme (2018). Sargassum White Paper – Turning the crisis into an opportunity. Ninth Meeting of the Scientific and Technical Advisory Committee (STAC) to the Protocol Concerning Specially Protected Areas and Wildlife (SPAW) in the Wider Caribbean Region (Kingston, Jamaica).
United Nations Environment Programme- Caribbean Environment Programme (2021). Sargassum White Paper – Turning the crisis into an opportunity. Ninth Meeting of the Scientific and Technical Advisory Committee (STAC) to the Protocol Concerning Specially Protected Areas and Wildlife (SPAW) in the Wider Caribbean Region (Kingston, Jamaica: United Nations Environment Programme - Caribbean Environment Programme).
van Tussenbroek B. I., Hernández-Arana H. A., Rodríguez-Martínez R. E., Espinoza-Avalos J., Canizales-Flores H. M., González-Godoy C. E., et al. (2017). Severe impacts of brown tides caused by sargassum spp. on near-shore Caribbean seagrass communities. Mar. Pollut. Bull. 122 (1–2), 272–281. doi: 10.1016/j.marpolbul.2017.06.057
Wang M., Hu C. (2017). Predicting sargassum blooms in the Caribbean Sea from MODIS observations. Geophysical Res. Lett. 44 (7), 3265–3735. doi: 10.1002/2017GL072932
Wang M., Hu C., Barnes B. B., Mitchum G., Lapointe B., Montoya J. P. (2019). The great Atlantic sargassum belt. Science 365, 83–87. doi: 10.1126/science.aaw7912
Webber M., Maddix G.-M. (2021). “User manual & field guide for bulk harvesting of sargassum,” in CRFM technical & advisory document (Belize: CRFM).
Wells R. J. D., Rooker J. R. (2003). “Distribution and abundance of fishes associated with sargassum mats in the NW Gulf of Mexico,” in 54th Gulf and Caribbean fisheries institute (GCFI).
Keywords: pelagic sargassum, associated biodiversity, motile fauna, Barbados, fishing opportunities
Citation: Alleyne KST, Small M, Corbin M, Vallès H and Oxenford HA (2023) Free-swimming fauna associated with influxes of pelagic sargassum: Implications for management and harvesting. Front. Mar. Sci. 10:1090742. doi: 10.3389/fmars.2023.1090742
Received: 05 November 2022; Accepted: 06 February 2023;
Published: 28 February 2023.
Edited by:
Aleke Stöfen-O’Brien, World Maritime University, SwedenReviewed by:
Lydia Ladah, Center for Scientific Research and Higher Education in Ensenada (CICESE), MexicoLindsay Martin, National Science Foundation (NSF), United States
Copyright © 2023 Alleyne, Small, Corbin, Vallès and Oxenford. This is an open-access article distributed under the terms of the Creative Commons Attribution License (CC BY). The use, distribution or reproduction in other forums is permitted, provided the original author(s) and the copyright owner(s) are credited and that the original publication in this journal is cited, in accordance with accepted academic practice. No use, distribution or reproduction is permitted which does not comply with these terms.
*Correspondence: Kristie S. T. Alleyne, w2005361@wmu.se