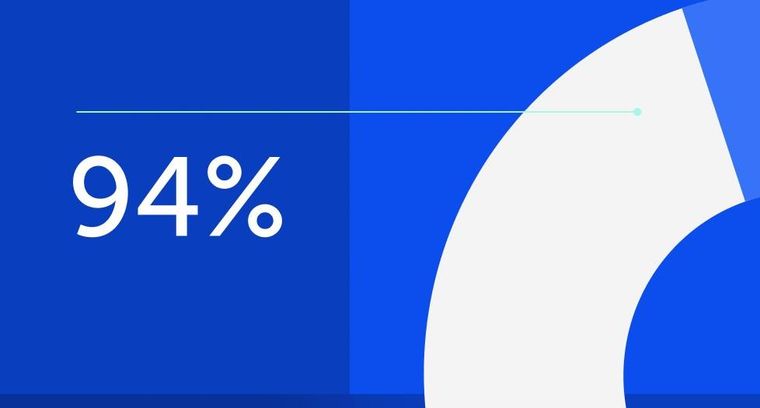
94% of researchers rate our articles as excellent or good
Learn more about the work of our research integrity team to safeguard the quality of each article we publish.
Find out more
ORIGINAL RESEARCH article
Front. Mar. Sci., 28 April 2023
Sec. Marine Fisheries, Aquaculture and Living Resources
Volume 10 - 2023 | https://doi.org/10.3389/fmars.2023.1084386
This article is part of the Research TopicIntegration of Sustainability, Preservation of Biodiversity and Conservation Goals in AquacultureView all 8 articles
Macrobenthic bioturbation affects the environment, and variances in habitat, such as decreased dissolved oxygen concentration and increased ammonia concentration, affects the macrobenthic community. The relationship between macrobenthos and habitat factors may be a mutually causal relationship. The bottom-sowing culture for Manila clam Ruditapes philippinarum in many coastal countries plays an important role in coastal fisheries, and the relationship between Manila clam and other macrobenthos affects the ecological stability of the bottom-sowing culture zone. It is necessary to explore the relationship between them to manage the waters used for the bottom-sowing culture for Manila clam. In this study , from June to October 2021, the field investigation on macrobenthos including Manila clam and their habitat factors, such as particle size, nutrient content, redox potential, and organic matter content, in 21 experimental communities enclosed by bolting-silk net in Shuangdao Bay, Weihai, Shandong, China was conducted, during which macrobenthos functional groups were determined by feed sources and motor behavior. The results showed that Manila clam biomass was 7.215±0.984 g/m2 (calculated by dry soft tissue weight), and it was positively correlated with the biomass of macrobebthos functional groups B1, G1 and P1; water content in sediment; sulfide content in interstitial water; the Shanon-Wiener diversity index; Pielou’s evenness index and the W statistic of the ABC (Abundance-Biomass Comparison) curve (p< 0.05). Moreover, it was negatively correlated with sediment particulate size and HCl-NO3 content in sediment (p< 0.05). The action of Manila clam to habitats was the dominant role of the interaction between clam and habitat. Thus, the bottom-sowing culture for Manila clam does not decrease the macrobenthic community stability, and the invasion of other macrobenthos into the bottom-sowing culture zones for Manila clam may be accidental or inevitable. The results of our study suggest that the management of the bottom-sowing culture for Manila clam should be conducted from ecosystem level; i.e., fishing and aquaculture in the same waters are regarded as two components of an ecosystem; manage them together, rather than treat them separately.
The distribution of macrobenthos is determined by sediment factors, feed abundance, and the tolerance to ecological stresses (Liu et al., 2012; Peng et al., 2019). Nevertheless, when the contents of nutrients, dissolved oxygen (DO), and sulfide change greatly, the community structure varies (Wu et al., 2019; Xu et al., 2021). Macrobenthos remake habitat factors, e.g., the bioturbation of Manila clam Ruditapes philippinarum, affect habitat. These clams filter organic particulate matters and produce biodeposition including feces or false feces (Mesnage et al., 2007), which accelerates the deposition of organic matters and increases the organic matter content in sediment (Liu et al., 2014). This drives the biogeochemical cycles of nitrogen and phosphorus (Zhang et al., 2020), such as the nutrient diffusion flux across the sediment–water interface (Karlson et al., 2005; Wei et al., 2023a), and affects the primary production of microalgae. The mass aggregation of Manila clam reduces DO content (Wu et al., 2020) and redox potential (Eh) in sediments, promotes sulfate reduction reaction, and leads to high sulfide content in sediment (Howard and Evans, 1993; Hatcher et al., 1994; Wang et al., 2006). Moreover, filter feeding by shellfish influences bulk density and the particulate size of sediment (Qin et al., 2010), aggravates sediment resuspension, and affects water content in sediment (Ge and Zhang, 2013). On the other hand, habitat factors of sediment, such as particle size, sulfide content, nutrient content, and organic matter content, affect the distribution, burrowing behavior, and growth of clam (Chen et al., 2010; Li et al., 2017). Thus, the relationship between macrobenthos and habitat factors in natural waters may be a mutually causal relationship.
Manila clam, a filter-feeding species of shellfish, lives in the interior of sediment and it is globally distributed in temperate and subtropical waters. It is also widely cultured; e.g., the annual production of cultured Manila clam in China, which is the largest aquaculture nation for many years, is approximately 3 million tons (FAO, 2021). Asterias rollestoni (Li et al., 2019; Zhao, 2021) and Bullacta exarata (Ysebaert et al., 2009; Liu et al., 2010) invade the bottom-sowing culture zone for Manila clam in Jiaozhou Bay, Shandong Province, China and subsequently reduce the clam yield. This biological invasion is essentially the change of the functional group of macrobenthos and the stability of the macrobenthic community. Is this biological invasion accidental or inevitable? A. rollestoni feed on clam, and B. exarate feed on organic debris. Organic matter and nutrients can be high in areas used for the bottom-sowing culture for Manila clam. Thus, it is considered that the large-scale bottom-sowing culture for Manila clam induces the outbreak of the A. rollestoni or B. exarate. Nevertheless, these claims are not very convincing. Large-scale culture of filter-feeding shellfish leads to either elevated organic debris content or decreased content (Zhang, 2008). The outbreak of organisms could at least have two reasons. One is the increase in the number of bait organisms of the organism, and the other is whether these organisms are preyed upon by other organisms. All these facts imply that the relationship between Manila clam and the outbreak of other macrobenthos is not so simple. To improve the management of the area used for clam culture, their relationship needs to be clarified.
To answer these questions, a field investigation was conducted in the intertidal zone of Shuangdao Bay, Weihai, Shandong, China. Pearson correlation analysis was used to determine the correlation between Manila clam and each factor of habitat or macrobenthos community, such as water content in sediment. The structural equation model (SEM) was used to analyze the direct or indirect effects of Manila clam on macrobenthic community. The results are helpful to further understand the community structure interaction between Manila clam and other macrobenthos, and provide theoretical support for the management of the bottom-sowing culture for Manila clam.
The field investigation was conducted in Shuangdao Bay, Weihai, Shandong Province, China. The bay, a closed lagoon, is surrounded by hills on three sides and the north of the lagoon is connected to the Yellow Sea. It covers an area of approximately 18 km2. The bay mouth has a width of approximately 1.8 km and the longitudinal length of the bay is approximately 6 km. The tide mode belongs to an irregular semi-diurnal tide. The highest tide position reaches 2.89 m and the lowest one is −0.76 m. The bottom sediment is mostly fine sand followed by medium sand. In the early 1980s, the bay had been used for aquaculture of Fenneropenaeus chinensis, Apostichopus japonicus, and Haliotis discus hannai. Moreover, the bay was once used as a Manila clam protection zone.
Twenty-one experimental communities were randomly set in the low-tide region of the intertidal zone of the lagoon (Figure 1) and the mudflat has silt sediments. The mudflat is exposed to air at ebb tide. Moreover, Manila clam with an abundance of 134.71 ind./m2 is naturally distributed in the area. To avoid tourists and fishermen from digging clams, reduce the disturbance from fishing boats and the escape of macrobenthos from these experimental communities; the experimental communities were closed from June to October 2021 by bolting-silk net with a mesh size of 74 μm. Each experimental community covered an area of 1 m × 1 m, and the burial depth of bolting-silk net was 0.2 m and the height of the net from sediment was 0.8 m. During the bottom-sowing culture for Manila clam, human beings no longer interfere with the activity range of Manila clam after the shellfish seeds are sowed, i.e., Manila clam may freely escape or gather in habitat patches. Thus, Manila clam was not artificially sowed in these experiment communities.
In October 2021, macrobenthos and sediment with a depth of 10 cm were collected and the Eh of sediments was measured in situ (State Quality Supervision Bureau, 2007). The sediments of each experimental community were sampled according to the five-point sampling method. Each point was sampled five times in parallel, and the samples of each experimental community were mixed in equal parts and measured. The macrobenthos were preserved in ethanol with a concentration of 75%.
According to Li and Wang (2016), macrobenthos were identified by morphology. These bivalves, crustaceans, gastropods, and polychaetes were dried at 80°C to constant weight to measure their dry weight (with a definition of 0.001 g). The weight of Pagurus ochotensis was dry weight without shell, and the weight of Gastropod was dry weight with shell.
Based on the website of the Biology Traits Information Catalogue (https://www.marlin.ac.uk/biotic/), feeding and exercise modes of macrobenthos were determined (He et al., 2020; Rahman et al., 2022), by which the classification criteria of the functional group of macrobenthos were constructed (Table 1).
The median particulate size (D50) of sediment was analyzed by a laser particulate size analyzer. The sediment bulk density (Dr) was determined by the ring knife soil sampler. The sediment was dried at 105°C to constant weight and the water content (φ) in sediment was calculated by the weight variance. Porosity (Pr) was calculated according to the empirical formula of bulk density and water content (Guo, 1983; Lv et al., 2016; Wei et al., 2023b). The organic matter content in sediment (Org) was determined by cauterization at 450°C for 4.5 h.
The occurrences of nitrogen or phosphorus in sediment, such as exchangeable nitrate nitrogen (Ex-NO3), exchangeable phosphorus (Ex-P) and exchangeable ammonia nitrogen (Ex-NH4), phosphorus that can be soaked out by hydrochloric acid (HCl-P), nitrogen that can be soaked out by hydrochloric acid (HCl-NO3), total phosphorus (TP), and total nitrogen (TN), were determined by the leaching method (Otsuki, 1981; Kan, 2016; Ge et al., 2017). The sulfide content in interstitial water (Sul) was determined by the p-amino dimethylaniline colorimetric method.
The indexes of macrobenthic community, such as the Shanon–Wiener diversity index (H’), Pielou’s evenness index (J’), the W statistic of the ABC (Abundance–Biomass Comparison) curve, and sediment factors, were calculated as follows:
Where, S, P, AAj, BBj, Wwet, W60 and W450 was the number of species, the proportion of species i, the total number of species, the abundance accumulation percentage corresponding to serial number j, biomass accumulation percentage corresponding to serial number j, the sediment wet weight (g), the dry weight of sediment dried at 60°C to constant weight (g), and the ash weight of sediment (g), respectively.
The data were mean ± standard error. The one-sample Kolmogorov–Smirnov normal test was performed on the W statistic of the ABC curve. The R4.2.1 statistical program was used for statistical analysis, during which the Pearson correlation analysis was used to analyze only the relationship between the Manila clam biomass and each habitat factor, such as macrobenthos functional group biomass, and sediment factors, respectively. The significance level was set at 0.05.
According to Ma et al. (2017), Amos24 software was used to construct SEM to explore the influence of Manila clam. Moreover, SEM reduces the dimension of many variables into one principal component through factor analysis. The root mean square error of approximation (RMSEA)<0.05 and the goodness-of-fit index (GFI) >0.9 indicate that the model fits well (Diamantopoulos and Siguaw, 2000). R2 represents the degree of interpretation of the model on the structure and stability of macrobenthic community, and the degree of influence of different paths is compared by the path coefficient (Pc) (Bizzi et al., 2013). The degree of fit of one model is promoted by deleting variables and adding variables (Wu, 2013).
The sediment factors are summarized in Table 2. Water content in sediment ranged from 17% (4th experimental community) to 20.3% (16th community). Maximum porosity was 67.12% (1st community) and minimum was 58.61% (17th community). The median particulate size ranged from 33.60 μm (11th community) to 100.96 μm (4th community). Maximum organic matter content in sediment was 2.254% (5th community) and minimum was 1.058% (4th community). The sulfide content in interstitial water ranged from 0.01 mg/L (20th community) to 0.11 mg/L (5th community). Maximum Eh was −14.2 mv (4th community) and minimum was −181.4 mv (1st community). The exchangeable phosphorus content ranged from 0.77 mg/kg (5th community) to 15.66 mg/kg (7th community). Maximum exchangeable nitrate nitrogen content was 25.46 mg/kg (6th community) and minimum was 2.82 mg/kg (7th community). The exchangeable ammonia nitrogen content ranged from 2.05 mg/kg (8th community) to 86.2 mg/kg (11th community). The maximum phosphorus content that can be soaked out by hydrochloric acid was 87.47 mg/kg (14th community) and the minimum was 5.1 mg/kg (4th community). The nitrogen content that can be soaked out by hydrochloric acid ranged from 148.82 mg/kg (11th community) to 828.71 mg/kg (3rd community). Maximum total phosphorus was 147.55 mg/kg (7th experimental community) and minimum was 80.25 mg/kg (17th community). The total nitrogen ranged from 2,186.44 mg/kg (2nd community) to 2,372.71 mg/kg (4th community).
Table 2 Sediment factors (φ, Pr, D50, Org, Sul, Eh, Ex-P, Ex-NO3, Ex-NH4, HCl-P, HCl-NO3, TP, and TN represent water content in sediment, porosity, particulate size, organic content in sediment, sulfide concentration in interstitial water, redox potential of sediment, exchangeable phosphorus content in sediments, exchangeable nitrate nitrogen content in sediments, exchangeable ammonia nitrogen content in sediments, phosphorus content that can be soaked out by hydrochloric acid in sediments, nitrogen content that can be soaked out by hydrochloric acid in sediments, total phosphorus content in sediment, and total nitrogen in sediment, respectively).
A total of 34 species of 5 orders and 29 genera of macrobenthos were collected (Table 3), in which the functional group M2 was mainly Trachypenaeus curvirostris. The frequency and number of M2 were small, e.g., the number of M2 was six individuals; hence, the role of M2 was excluded from further analysis. The biomass of the macrobenthos functional group G2 ranked the first, followed by the biomass of functional groups C and G3 (Table 4). The value of H’ ranged from 0.66 to 1.27, J’ ranged from 0.23 to 0.42, and W ranged from 0.017 to 0.072 (Table 5).
The biomass of Manila clam was positively correlated with the biomass of functional groups B1, G1, and P1 (p< 0.05); φ and Sul (p< 0.05); and H’, J’, and W (p< 0.05) (Table 6). Moreover, it was negatively correlated with D50 and HCl-NO3 (p< 0.05).
Table 6 Correlation of Manila clam and other functional groups, habitat factors, and community structure (M represents the biomass of Manila clam; φ, Pr, D50, Org, Sul, Eh, Ex-P, Ex-NO3, Ex-NH4, HCl-P, HCl-NO3, TP, and TN represent water content in sediment, porosity, particulate size, organic matter content in sediment, sulfide concentration in interstitial water, redox potential of sediment, exchangeable phosphorus content in sediment, exchangeable nitrate content in sediment, exchangeable ammonia content in sediment, hydrochloric acid leachable phosphorus content in sediment, hydrochloric acid leachable nitrogen in sediment, total phosphorus content in sediment, and total nitrogen in sediment, respectively; B1, B2, G1, G2, G3, G4, P1, P2, P3, M1, and C represent the biomass of functional groups B1, B2, G1, G2, G3, G4, P1, P2, P3, M1, and C, respectively; H’, J’, and W represent the Shanon–Wiener diversity index, Pielou’s evenness index, and the statistics of the ABC curve, respectively).
The SEM of Manila clam-H’ (GFI = 0.999, AIC = 26.036, RMSEA = 0.000) explained 58% (R2 = 0.58) of the influence of Manila clam on .M (Manila clam) directly affected H’ (Pc = 0.25); M influenced H’ (Pc = 0.37) by affecting the functional group B1 (Pc = 0.52); M acted on H’ (Pc = 0.41) by acting on φ (Pc = 0.32); M influenced φ (Pc = −0.48) by acting on D50 (Pc = −0.43); φ acted directly on H’ (Pc = 0.41) or on H’ (Pc = 0.37) by acting on the functional group B1 (Pc = −0.21) (Figure 2).
Figure 2 The SEM (Structural Equation Model) for Manila clam- H' (M 、B1、φ、D50 and H' represent the biomass of Manila clam, the biomass of functional group B1, water content, particle size and Shannon-Wiener diversity index of macrobenthic community, respectively. The black arrow indicates a positive effect, and the red one indicates a negative effect).
The SEM of Manila clam-J’ (GFI = 0.975, AIC = 25.253, RMSEA = 0.000) explained 63% (R2 = 0.63) of the influence of Manila clam on J’. M directly acted on J’ (Pc = 0.79); M acted on J’ (Pc = −0.50) by affecting the functional group G2 (Pc = 0.56); M affected φ (Pc = −0.48) by influencing D50 (Pc = −0.43), or M acted on J’ (Pc = −0.50) by acting directly on φ (Pc = 0.32) and then on G2 (Pc = −0.46) (Figure 3).
Figure 3 The SEM (Structural Equation Model) for Manila clam- J' (M 、G2、φ、D50 and J' represent the biomass of Manila clam, the biomass of functional group G2, water content, particle size and Pielou’s evenness index of macrobenthic community, respectively. The black arrow indicates a positive effect, and the red arrow indicates a negative effect).
The SEM of Manila clam-W (GFI = 0.956, AIC = 26.565, RMSEA = 0.000) explained 33% (R2 = 0.33) of the influence of Manila clam on W. M directly acted on W (Pc = 0.58); M acted on W (Pc = −0.36) by influencing the functional group G2 (Pc = 0.56); M acted on φ (Pc = −0.48) by affecting D50 (Pc = −0.43), or M affected W (Pc = −0.36) by directly influencing φ (Pc = 0.32) and then G2 (Pc = −0.46) (Figure 4).
Figure 4 The SEM (Structural Equation Model) for Manila clam-W (M 、G2、φ、D50 and W represent the biomass of Manila clam, the biomass of functional group G2, water content, particle size and the statistic of ABC curve, respectively. The black arrow indicates a positive effect, and the red one indicates a negative effect).
In the present work, the Manila clam biomass was positively correlated with the water content in sediment, which was consistent with the field investigation conducted in the bottom-sowing culture zone for Manila clam by Ge and Zhang (2013). The sediments with higher water content means that the sediments are softer, which is conducive for Manila clam living in sediments. Moreover, the bioturbation form of Manila clam is diffusive disturbance, which accelerates the diffusion of sediment particles and interstitial water during their activities (Mermillod-Blondin et al., 2005), leading to the vertical mix of sediments. During alternately sucking in and discharging water, the Manila clam leads to the sediment resuspension and increases water diffusion, resulting in an increase in water content in sediment.
As the Manila clam biomass increases, the oxygen consumption caused by clams increases as does the mass of oxygen-consuming biodeposition (Zhou et al., 2003). All these factors further affect sediment hypoxia or anoxia, which promote denitrification, leading to regional nitrogen loss, especially a decrease in nitrate content (Kaspar et al., 1985). Moreover, HCl-NO3 is the nitrogen that can be released from sediments, and the probability of physiological toxicity to macrobenthos increases with increased HCl-NO3, which means that Manila clam will not choose to gather in the area with a high HCl-NO3 content. The experimental community in the present work was closed by bolting-silk net, and the bury depth of bolting-silk net in sediment was much deeper than the diving sand depth of Manila clam (Wei et al., 2023b). The Manila clam in the closed experimental communities could not escape from them. Thus, the reason for the negative correlation between the Manila clam biomass and the HCl-NO3 content in sediment in the investigation was likely mainly caused by Manila clam.
The sediment median particulate size in the natural distribution area of Manila clam was in the range of 16.54–109.14 μm (Zhao et al., 2021), while the sediment median particulate size in the studied area was in the range of 33.60–100.96 μm; i.e., the sediment median particulate size in the present work was beneficial to the survival of Manila clam. In the present work, the Manila clam biomass was negatively correlated with sediment median particulate size, which meant that the correlation was caused by Manila clam as the closure of the experimental communities resulted in difficulty of escape for Manila clam. If the Manila clam biomass is less than the carrying capacity of the region, the quantity of biodeposition produced by clams are positively correlated with the Manila clam biomass (Liu et al., 2014). The carrying capacity of Manila clam can be 2,000 ind./m2 (Li et al., 2015) or 603 ind./m2 (clam with a dry weight of soft tissue of 0.9 g) (Liu et al., 2015). The maximum density of Manila clam in the present work was 246 ind./m2. In addition, according to the owners of the study area, the clams are naturally distributed within this area, and they continuously grow and produce young clams. All these factors meant that the abundance of Manila clam might not be too high to be beyond the carrying capacity of the area for Manila clam. Manila clam produces massive biodeposition composed of feces or false feces, which mainly includes microalgae and organic detritus (Haven and Morales-Alamo, 1966; Kautsky and Evans, 1987), and their accumulation in sediment causes the decrease in median particulate size.
Because of the ecological toxicity of volatile sulfides (AVS) (Liu et al., 2004), Manila clam would not choose areas with a high AVS content to live in, and clams in the experimental community could not escape. With the increase in the Manila clam biomass, biodeposition and the oxygen consumption rate of sediment increase, which accelerates the reduction of sulfide (Otero et al., 2006) and produces AVS subsequently. Thus, the Manila clam biomass was positively correlated with sulfide concentration in interstitial water. In summary, the present work suggested that the role of effects of Manila clam on abiotic habitats is more than the effect of habitats on clams, which reflects that Manila clam chooses abiotic habitats.
With the increase in the Manila clam biomass, the extent of sediment subjected to biodisturbance increases, and the sediment is subsequently locally eroded (Han et al., 2001), which means that sediments are prone to resuspension. Thus, the microhabitat diversity in sediment increases, which provides a basis for the increase in species diversity (Parry et al., 1999). Nutrients caused by the excretion of Manila clam and the regeneration from biodeposition of clams provide better conditions for the proliferation of microalgae such as benthic diatoms (Deng, 2011), which promotes the increase in herbivores and carnivores. Nevertheless, some herbivores are preyed upon by carnivores. As the biodeposition that is rich in organic matter increases, the scavengers increase. Thus, the Manila clam biomass was positively correlated with the biomass of bivalve shellfish of filter feeding and burrowing (B1), carnivorous-crawling gastropods (G3), and burrowing scavenger polychaetes (P1), as well as the Shanon–Wiener diversity index, Pielou’s evenness index, and the W statistic of the ABC curve. Since there is a competitive relationship between Manila clam and the functional group B1, with carnivorous G1 potentially feeding on Manila clam and the functional group P1 potentially feeding on Manila clam biodeposits, Manila clam in natural waters do not actively choose to congregate in areas where these functional groups are concentrated, implying that this positive correlation is caused by variation in the biomass of Manila clam.
Based on these analyses, the framework of the SEM is reasonable; i.e., although Manila clam are naturally distributed and there is not likely to be an escape of Manila clam from the experiment community, the role of Manila clam affecting habitat is dominant during the interaction between the clams and environmental factors.
Because of the biodeposition of Manila clam, the increase in their biomass could lead to the decrease in median particle size of sediment; the disturbance of Manila clam leads to sediment resuspension and an increase in the water diffusion in sediment, resulting in an increase in water content in sediment (Kautsky and Evans, 1987). The water content increases as D50 decreases (Aberle et al., 2004; Flemming and Delafontaine, 2000; Qiao et al., 2020), and an increase in the Manila clam biomass reduces D50 and thus promotes water content in sediment. The increased water content in sediment may lead to an increase in biodiversity (Lv, 2017). The macrobenthos functional group B1 is composed of bivalves, which feed on organic matter such as biodeposition produced by Manila clam (Yang and Zhou, 1998). Thus, the biomass of the functional group B1 increases as the Manila clam biomass increases. Nevertheless, this biodeposition may be diluted by increased water content, which implies that the feed supply for the functional group B1 decreases and the biomass of B1 subsequently decreases with increased water content in sediment.
The larger Pielou’s evenness index implies more uniformity in the spatial distribution of species. The distribution of macrobenthos is influenced by food and predators; they gather for food and disperse to escape from predators (Liu, 2006). Owing to the discharge and water sucking, nutrients, biodeposition, and benthic algae tend to be uniformly distributed in the horizontal direction in sediment. Because of the burrowing behavior of Manila clam, the nutrients, biodeposition, and benthic algae tend to be uniformly distributed in the vertical direction of sediment (Gong and Zhang, 2022). Manila clam provide conditions for the proliferation of microalgae such as benthic diatoms due to excretion and biodeposition (Newell, 1965). The functional group G2 feeds on benthic algae and its biomass increases with increased Manila clam biomass, but the increase in biomass of G2 increases the competition with other phytophagous macrobenthos. Moreover, the group G2 serves as a food source for carnivores, which can trap carnivores and subsequently cause uneven distribution of organisms. Although the increase in the Manila clam biomass promotes the biomass of G2, Manila clam improve Pielou’s evenness index of macrobenthic communities.
The W statistic of the ABC curve > 0 indicates that the community is not disturbed (Warwick, 1986; Arbi et al., 2017). The ABC curves of the macrobenthic communities in all experimental communities in the present work had a statistical W value of 0.05; i.e., the macrobenthic communities were in a stable state, while the competition for food between the functional group G2 and Manila clam, resulting in the increase in the functional group G2, weakens the stability of the macrobenthic community.
Moreover, B. exarata belongs to the functional group G2; thus, it is not a coincidence that there were lots of B. exarata outbreak in the bottom-sowing culture zone for Manila clam in Jiaozhou Bay, and the competition between B. exarata Manila clam reduces the Manila clam production. Although the functional group G1 and the Manila clam biomass were correlated, the SEM showed that the relationship between Manila clam and G1 was not the main relationship, which implied that the outbreak of A. rollestoni in the bottom-sowing culture zone for Manila clam in Jiaozhou Bay was not directly caused by the bottom-sowing culture for Manila clam. In Jiaozhou Bay, A. rollestoni can only be eaten by some carnivorous macrobenthos, such as Paralichthys olivaceus and Cynoglossus robustus, which implies that the biomass of A. rollestoni might increase with decreased biomass of these carnivorous macrobenthos. Nevertheless, the exhaustion of high-quality fishery resources has led to an increase in the biomass of A. rollestoni, and Manila clam serve as their food; thus, they invaded Manila clam bottom-sowing culture areas, which means that the prevention and control of A. rollestoni is a systematic question, i.e., fishing and aquaculture in the same waters are regarded as two components of an ecosystem, during which there are interactions between them. Manage them together, rather than treat them separately.
The interaction between macrobenthos and habitat factors may be a mutual cause-and-effect relationship. Based on the habitat selectivity of Manila clam, the results of the correlation analysis in the present work are considered to be more reflective for the habitat modification effect of Manila clam, which implies that the SEM framework is reasonable. Thus, Manila clam influence the macrobenthic community directly or indirectly through influencing environment factors. Moreover, the B. exarata outbreak was likely caused by the bottom-sowing culture for Manila clam, while the invasion of A. rollestoni was not a direct consequence of the bottom-sowing culture for Manila clam, and the prevention and control of A. rollestoni outbreak based on system-level management are needed.
The original contributions presented in the study are included in the article/supplementary material. Further inquiries can be directed to the corresponding author.
WH: Field sampling, Macrobenthos determination, Determination of environmental factors, Writing and data processing. XW: Field sampling, Macrobenthos determination, Determination of environmental factors, Writing and data processing. WB: Field sampling, Macrobenthos determination. YZ: Field sampling. QT: Macrobenthos determination. ZL: Field sampling. BR: Field sampling. CG: Conceptualization, Methodology, Funning and Writing. All authors contributed to the article and approved the submitted version.
This study was supported by National Natural Science Foundation of China (31972796) and National Key Research and Development Program of China (2018YFD0900704).
The authors declare that the research was conducted in the absence of any commercial or financial relationships that could be construed as a potential conflict of interest.
All claims expressed in this article are solely those of the authors and do not necessarily represent those of their affiliated organizations, or those of the publisher, the editors and the reviewers. Any product that may be evaluated in this article, or claim that may be made by its manufacturer, is not guaranteed or endorsed by the publisher.
Aberle J., Nikora V., Walters R. (2004). Effects of bed material properties on cohesive sediment erosion. Mar. Geology 207 (1), 83–93.
Arbi I., Zhang J., Liu S., Wu Y., Huang X. (2017). Benthic habitat health assessment using macrofauna communities of a sub-tropical semi-enclosed bay under excess nutrients. Mar. Pollut. Bull. 119 (2), 39–49.
Bizzi S., Surridge B. W. J., Lerner D. N. (2013). Structural equation modelling: a novel statistical framework for exploring the spatial distribution of benthic macroinvertebrates in riverine ecosystems. River Res. Appl. 29 (6), 743–759.
Chen A. H., Yao G. X., Zhang Z. W., Wu Y. P., Wu J. P. (2010). Effects of temperature, salinity and sediment on the growth and survival of Solen grandis dunker juvenil. J. Trop. Oceanography 29 (5), 94–97.
Deng K. (2011). Benthic nutrient fluxes at typical areas in Chinese coast, with emphasis on bioturbation (Qingdao: Doctoral dissertation of Ocean University of China).
Diamantopoulos A., Siguaw J. A. (2000). Introducing LISREL a guide for the uninitiated (London: Sage Pubn Inc).
Flemming B. W., Delafontaine M. T. (2000). Mass physical properties of muddy intertidal sediments: some applications, misapplications and non-applications. Continental Shelf Res. 20 (10-11), 1179–1197.
Ge C. Z., Chai Y. C., Wang H. Q., Kan M. M. (2017). Ocean acidification: one potential driver of phosphorus eutrophication. Mar. Pollut. Bull. 115 (1-2), 149–153.
Ge C. Z., Zhang F. (2013). Environmental implication and accumulation flux of organic matters in sediments of mudflat used for bottom sowing culture for Manila clam Ruditapes philippinarum. J. Basic Sci. Eng. 21 (6), 1037–1045.
Gong Z. J., Zhang Q. T. (2022). Influence of macrobenthic bioturbation on the physic-chemical characteristics of sediment: a review. Trans. Oceanology Limnology 44 (2), 166–172.
Han J., Zhang Z. N., Yu Z. S. (2001). Effects of Manila clam (Ruditapes philippinarum) on the benthic-pelagic particle flux in xuejiadao intertidal zone Vol. 5 (Qingdao, China: Journal of Ocean University of Qingdao), 723–729.
Hatcher A., Grant J., Schofield B. (1994). Effects of suspended mussel culture (Mytilus spp.) on sedimentation, benthic respiration and sediment nutrient dynamics in a coastal bay. Mar. Ecol. Prog. Ser. 115, 219–219.
Haven D. S., Morales-Alamo R. (1966). Aspects of biodeposition by oysters and other invertebrate filter feeders. Limnology Oceanography 11 (4), 487–449.
He L. Z., Shou L., Liao Y. B., Tang Y. B., Chen Q. Z., Chen J. F., et al. (2020). The relationship of macrobenthic functional group composition and hypoxia in the changjiang river estuary and its adjacent areas. Aquat. Ecosystem Health Manage. 23 (3), 313–322.
Howard D. E., Evans R. D. (1993). Acid-volatile sulfide (AVS) in a seasonally anoxic mesotrophic lake: seasonal and spatial changes in sediment AVS. Environ. Toxicol. Chem. 12 (6), 1051–1057.
Kan M. M. (2016). Accumulation characteristics of n and p in soils under different vegetations in coastal wetland (Jinan: Master’s thesis of Shandong University).
Karlson K., Hulth S., Ringdahl K., Rosenberg R. (2005). Experimental recolonisation of Baltic Sea reduced sediments: survival of benthic macrofauna and effects on nutrient cycling. Mar. Ecol. Prog. Ser. 294, 35–49.
Kaspar H. F., Gillespie P. A., Boyer I. C., Mackenzie A. L. (1985). Effects of mussel aquaculture on the nitrogen-cycle and benthic communities in kenepuru sounds, Marlborough sounds, new-Zealand. Mar. Biol. 85 (2), 127–136.
Kautsky N., Evans S. (1987). Role of biodeposition by mytilus edulis in the circulation of matter and nutrients in a Baltic coastal ecosystem. Mar. Ecol. Prog. Ser. 38 (3), 201–212.
Li L., Bai Y. C., Huang S. L., Chen Y. S., Zhao Y. C., Xu C. L., et al. (2017). Substrate preference and sand burrowing ability assessment of Meretrix meretrix. Mar. Fisheries 39 (5), 548–553.
Li Y. R., Liang J., Guo Y. J., Xing K. Z. (2015). Culture density of Manila clam Ruditapes philippinarum in the epineritic zone of Tianjin. Mar. Sci. Bull. 4, 470–474.
Li Y. C., Liang J. L., Wu Z. J., Chen S. Q. (2019). Outbreak and prevention of Acanthaster planci. Ocean Dev. Manage. 36 (8), 9–12.
Li X. Z., Wang H. F. (2016). The map of macrobenthos identification in jiaozhou bay (Beijing: Science Press).
Liu X. Q. (2006). Food composition and food webs of zoobenthos in Yangtze lakes (Wuhan: Doctoral dissertation of Institute of Hydrobiology, Chinese Academy of Sciences).
Liu Z. G., Qu X. D., Zhang Y., Ma S. Q., Zhao R., Cao G. P. (2012). Effects of main contaminations on the spatial distribution of macroinvertebrates in the Hun river. J. Environ. Eng. Technol. 2 (2), 116–123.
Liu B., Tao Y., Dang X. X. (2010). Effects of shoaly culturing on the marine ecosystems. Innovation 4 (1), 58–60.
Liu X. H., Wang Z. L., Zhang M. L., Wang B., Sun P. X. (2015). Carrying capacity of Manila clam Ruditapes philippinarum in jiaozhou bay estimated by an ecosystem model. Fisheries Sci. 34 (2), 733–740.
Liu J. C., Yan C. L., Hu J. (2004). A review on the studies of acid-volatile sulfide in aquatic sediments. Acta Ecologica Sin. 4, 812–818.
Liu P., Zhou Y., Wang F., Zhang X. M., Liu B. J., Yang H. S. (2014). In situ Determination of biodeposition by suspension-feeding bivalve in shallow waters (intertidal zone). Oceanologia Limnologia Sin. 45 (2), 253–258.
Lv W. W. (2017). The study on the influence of reclamation and salinity decrease on intertidal macrobenthos in Yangtze river estuary (Shanghai: Doctoral dissertation of East China Normal University).
Lv H. B., Wang S. Q., Liu G. S., Zhang X. M. (2016). Effects of bioturbation on vertical distribution of sediment particles by two macrobenthos species. J. Hydroecol 37 (1), 78–86.
Ma K., Zhang H. P., Qu X. D., Huang X. R., Zhang M., Liu X. B. (2017). Effects of land use patterns on biological indices of macroinvertebrates via multiple pathways in the Luan River watershed. China Environ. Sci. 37 (7), 2674–2683.
Mermillod-Blondin F., Franois-Carcaillet F., Rosenberg R. (2005). Biodiversity of benthic invertebrates and organic matter processing in shallow marine sediments: an experimental study. J. Exp. Mar. Biol. Ecol. 315 (2), 187–209.
Mesnage V., Ogier S., Bally G., Disnar J. R., Lottier N., Dedieu K., et al. (2007). Nutrient dynamics at the sediment–water interface in a Mediterranean lagoon (Thau, france): influence of biodeposition by shellfish farming activities. Mar. Environ. Res. 63 (3), 257–277.
Newell R. C. (1965). “The role of detritus in the nutrition of two marine deposit feeders, the prosobranch hydrobia Ulvae and the bivalve Macoma balthica,” in Proceedings of the zoological society of London (London: Oxford Univ Press), vol. 144, 24–45.
Otero X. L., De-Anta R. M. C., Macias F. (2006). Sulphur partitioning in sediments and biodeposits below mussel rafts in the Rı́a de arousa (Galicia, NW Spain). Mar. Environ. Res. 61 (3), 305–325.
Otsuki A. (1981). Use of ultraviolet spectrophotometric determination of nitrate for the determination of total nitrogen in environmental water samples using alkaline persulfate digestion Vol. 30 (Tokyo, Japan: Bunseki Kagaku), 688–689.
Parry D., Kendall M., Rowden A., Widdicombe S. (1999). Species body size distribution patterns of marine benthic macrofauna assemblages from contrasting sediment types. J. Mar. Biol. Assoc. U. K. 79 (5), 793–801.
Peng S. Y., Lai Z. N., Mai Y. Z. (2019). Distribution of quantity and diversity of macrobenthos in the pearl river estuary. Mar. Fisheries 41 (3), 266–277.
Qiao Y., He Q., Wang X. Y., Meng Y. (2020). Study on surface sediment water-contents and its influence factors in the Yangtze estuary. J. Sediment Res. 45 (1), 29–36.
Qin X. B., Sun H. W., Wu J. Z., Wang R. N., Sun T. H. (2010). Bioturbation of macrobenthos on estuarine sediment. Chin. J. Appl. Ecol. 21 (2), 458–463.
Rahman M. K., Hossain M. B., Majumdar P. R., Mustafa M. G., Noman M. A., Albeshr M. F., et al. (2022). Macrobenthic assemblages, distribution and functional guilds from a freshwater-dominated tropical estuary. Diversity 14 (6), 473.
State Quality Supervision Bureau (2007). Specifications for oceanographic survey (Beijing: Standards Press of China).
Wang J. J., Li X. M., Qu K. M., Sun Y., Ma S. S. (2006). The distributions and variations of sulfide and redox potential in the sediments of rushan bay. Mar. Fisheries Res. 6, 64–70.
Warwick R. M. (1986). A new method for detecting pollution effects on marine macrobenthic communities. Mar. Biol. 92 (4), 557–562.
Wei X. T., Liu Z. J., Ge C. Z., Huang G. Q., Song J. D., Hou W. H., et al. (2023a). Effect of manila clam on nutrients exchange fluxes at sediment-water interface. Fishery Modernization 50 (1), 43–49.
Wei X. T., Liu Z. J., Huang G. Q., Song J. D., Liu X. J., Zheng F. Y., et al. (2023b). Effect of Manila clam ruditapes philippinarum on burial depth of eelgrass zostera marina seeds and sediment porosity. Regional Stud. Mar. Sci. 58, 102799.
Wu M. L. (2013). Structural equation modeling-tips for practical application (Chongqing: Chongqing University Press).
Wu W., Liu J., Bouwman A. F., Wang J., Yin X., Zang J., et al. (2020). Exploring oxygen dynamics and depletion in an intensive bivalve production area in the coastal sea off rushan bay, China. Mar. Ecol. Prog. Ser. 649, 53–65.
Wu C., Xu J., Yin S. L., Tan C. Z., Chen K., Gao J., et al. (2019). The littoral zone macroinvertebrate distribution in relation to environment variables in the lower Yangtze river channel from nanjing to the estuary. Chin. J. Appl. Environ. Biol. 25 (3), 553–560.
Xu F., Wang J. Q., Cai W. Q., Wei J. M., Han X. M. (2021). Macrozoobenthos functional feeding groups and its relationship with environmental factors in the tianjin coastal area. Oceanologia Limnologia Sin. 52 (1), 186–195.
Yang H. S., Zhou Y. (1998). Progress in studies on effect of filter-feeding bivalve on environment of mariculture area. Mar. Sci. 2, 42–44.
Ysebaert T., Hart M., Herman P. M. J. (2009). Impacts of bottom and suspended cultures of mussels. Mytilus surrounding sedimentary Environ. macrobenthic biodiversity. Helgoland Mar. Res. 63, 59–74.
Zhang J. H. (2008). Effect of filter feeding shellfish mariculture on the ecosystem and the evaluation of ecology carrying capacity (Qingdao: Doctoral dissertation of Institute of Oceanography, Chinese Academy of Sciences).
Zhang S., Fang X., Zhang J. B., Yin F., Zhang H., Kitazawa D. (2020). The effect of bioturbation activity of the ark clam Scapharca subcrenata on the fluxes of nutrient exchange at the sediment-water interface. J. Ocean Univ. China 19 (1), 232–240.
Zhao Q. (2021). Hazards and prevention strategies of Asierias rolleston. J. Aquaculture 42 (5), 62–64.
Zhao Y. T., You L. P., Ma Y. Q., Song X. K., Su B., He J. L., et al. (2021). Preliminary analysis of the relationship between sediment grain size and macrobenthos community in laizhou bay. Mar. Sci. Bull. 40 (1), 84–91.
Keywords: aquaculture management, benthic habitat, clam culture, community stability, macrobenthos
Citation: Hou W, Wei X, Bai W, Zheng Y, Tan Q, Liu Z, Rong B and Ge C (2023) Effects of Manila clam Ruditapes philippinarum culture on the macrobenthic community. Front. Mar. Sci. 10:1084386. doi: 10.3389/fmars.2023.1084386
Received: 30 October 2022; Accepted: 10 April 2023;
Published: 28 April 2023.
Edited by:
Michael Frank Tlusty, University of Massachusetts Boston, United StatesReviewed by:
Heidi K. Alleway, The Nature Conservancy, United StatesCopyright © 2023 Hou, Wei, Bai, Zheng, Tan, Liu, Rong and Ge. This is an open-access article distributed under the terms of the Creative Commons Attribution License (CC BY). The use, distribution or reproduction in other forums is permitted, provided the original author(s) and the copyright owner(s) are credited and that the original publication in this journal is cited, in accordance with accepted academic practice. No use, distribution or reproduction is permitted which does not comply with these terms.
*Correspondence: Changzi Ge, Z2VjekBlbWFpbC5zZHUuZWR1LmNu
†These authors have contributed equally to this work
Disclaimer: All claims expressed in this article are solely those of the authors and do not necessarily represent those of their affiliated organizations, or those of the publisher, the editors and the reviewers. Any product that may be evaluated in this article or claim that may be made by its manufacturer is not guaranteed or endorsed by the publisher.
Research integrity at Frontiers
Learn more about the work of our research integrity team to safeguard the quality of each article we publish.