- 1Marine Mammal Institute, Oregon State University, Newport, OR, United States
- 2Department of Fisheries, Wildlife, and Conservation Sciences, Oregon State University, Newport, OR, United States
Located in the eastern tropical Pacific, the Galápagos Islands are an oceanic insular ecosystem subject to strong environmental variability driven by local and regional processes. Past research has shown that such conditions can attract and sustain at least 23 cetacean species, out of which 14 are common, including nine Delphinids, one Ziphiid, one Physeterid, and three Balaenopterids. These species occupy both coastal and oceanic habitats, most are present year-round, and a few are migratory. However, research on cetaceans in Galápagos has been sporadic and chronically underfunded and is not currently considered a priority in the research agenda for Galápagos. Based on a review of existing information and an assessment of knowledge gaps, here we identify priorities for ecological research on cetaceans in Galápagos along five topical areas: 1) spatiotemporal occurrence, 2) population assessment, 3) health assessment, 4) social ecology, and 5) trophic ecology. Addressing these knowledge gaps will also help inform actions to preserve cetacean biodiversity and to manage human activities involving or affecting cetaceans in Galápagos. Given the logistical and funding challenges of conducting cetacean research in Galápagos, we recommend optimizing data sampling and accessibility via integrated research protocols and open data repositories. We also recommend capitalizing on local citizen science activities, such as those conducted from cruise ships and whale-watching tours, which can serve as platforms of opportunity for obtaining basic data, thereby contributing to long-term data acquisition. Our proposed priorities should be assessed by Ecuadorian and Galápagos governmental institutions in broad and inclusive consultation with stakeholders and the scientific community prior to development and implementation of a research agenda. Collectively, these efforts will advance our understanding of the ecological role that marine megafauna, such as cetaceans, play in Galápagos and other oceanic islands, including maintaining large-scale connectivity and mitigating climate change.
1 Introduction
A casual glance at oceanic islands might suggest a collection of isolated terrestrial habitats in an otherwise blue desert. A view from underwater, however, reveals that these insular ecosystems are much more than remote oases for terrestrial biota (e.g., Hasegawa et al., 2009; Gove et al., 2016). They provide habitats that attract a wealth of marine life from larval to adult stages and serve as steppingstones for migratory species (e.g., Pinheiro et al., 2017; Fontoura et al., 2022). Due to their long life spans and wide-ranging movements, marine megafauna in particular can play a key role in linking coastal and oceanic insular ecosystems (e.g., Hindell et al., 2020; Klimley et al., 2022). Indeed, ecological connectivity via local, regional, and long-distance movements is increasingly recognized as an essential process in the life history of large fishes, sea turtles, and marine mammals inhabiting oceanic insular ecosystems (e.g., Ketchum et al., 2020; Rooker et al., 2019; Ferreira et al., 2021) that is worth understanding and preserving in and of themselves (Game et al., 2009; Dunn et al., 2019; Klimley et al., 2022), as well as to help preserving the ecological communities these “umbrella” species are part of (Caro and O’Doherty, 1999).
Belonging to Ecuador, the Galápagos Islands are an oceanic insular ecosystem located in the eastern tropical Pacific (ETP) 1,000 km off mainland South America (Figure 1). Despite their equatorial location, the Galápagos are subject to seasonal variability resulting from the annual intensification of the southeast trade winds and equatorial upwelling (Palacios, 2004; Sweet et al., 2007; Forryan et al., 2021), and to strong interannual variability driven by the El Niño-Southern Oscillation (Palacios et al., 2006; Fiedler and Lennert-Cody, 2019), which is intensifying with climate change (Mendelssohn et al., 2005; Dueñas et al., 2021). Variable exposure of the islands to this environmental forcing results in a marked environmental zonation (Harris, 1969; Wellington et al., 2001; Schaeffer et al., 2008), which gives rise to a distinctive biogeographic patterning across the archipelago (Edgar et al., 2004; McKinley et al., 2022). Regarding migratory connectivity, the Galápagos are a well-known stop-over for marine megafauna, including teleosts, elasmobranchs, and chelonians that regularly move among oceanic archipelagos in the ETP (e.g., Todd and Grove, 2010; Cambra et al., 2021; Silver‐Gorges et al., 2020; Klimley et al., 2022).
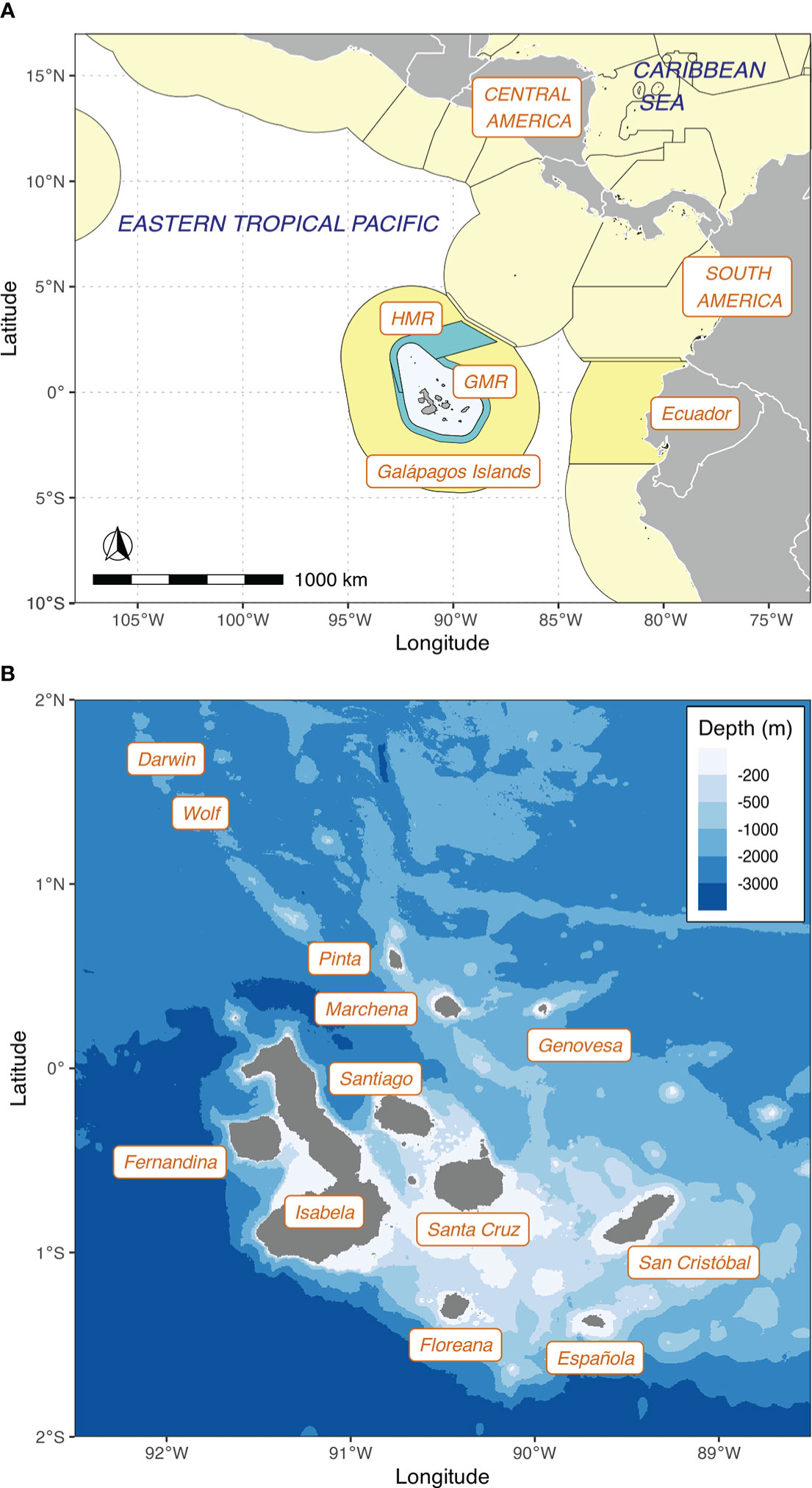
Figure 1 (A) Regional map showing the location of the Galápagos Islands in the eastern tropical Pacific, and the boundaries of the Galápagos Marine Reserve (GMR) and Hermandad Marine Reserve (HMR). The Exclusive Economic Zones (EEZ) of countries bordering the region are shown in yellow. (B) Map of the main islands of the Galápagos Archipelago (labeled) and bathymetry of the surrounding seafloor. The GMR and HMR boundaries are available from Fundación Charles Darwin’s GeoData Portal at https://geodata-fcdgps.opendata.arcgis.com/. EEZ boundaries are available from Flanders Marine Institute (2019), used under Creative Commons license CC BY. Bathymetry data from SRTM15+ (Tozer et al., 2019), available from https://topex.ucsd.edu/WWW_html/srtm15_plus.html.
For the equally highly mobile cetaceans, however, this regional connectivity has not been directly established, except in a few cases (Torres-Florez et al., 2015; Cantor et al., 2016; Hucke-Gaete et al., 2018; Pacheco et al., 2019). Nevertheless, the slow life history, high trophic position, and large-scale movements of cetaceans are such that their ecological roles (Bowen, 1997; Kiszka et al., 2015; Kiszka et al., 2022) could have broader implications within and beyond Galápagos waters. In their role as predators, cetaceans participate in top-down control of the marine communities they inhabit and link oceanic and coastal ecosystems (e.g., Estes et al., 1998). Further, by acting as nutrient recyclers and carbon reservoirs, cetaceans provide ecological services that are key for oceanic productivity and climate change mitigation at global scales (Martin et al., 2021; Pearson et al., 2022).
A collaboratively derived environmental research agenda was recently developed for Galápagos based on a participatory process involving governmental entities, universities, and non-governmental organizations (Izurieta et al., 2018). This process prioritized 50 research questions, including several with a marine or conservation theme, although these questions were selected for their importance for policy makers and practitioners, and the authors acknowledged the value of conducting a parallel exercise for identifying fundamental research questions (Izurieta et al., 2018). This paper is an answer to this call, by identifying research questions relevant to cetaceans in Galápagos. Toward this goal, here we summarize the existing legislative and protective framework of relevance to cetaceans in Galápagos, review the available scientific information on cetacean occurrence in Galápagos, identify knowledge gaps, and provide recommendations for advancing ecological research.
2 Legislative and protective measures
Despite having been settled by humans only relatively recently, the Galápagos Archipelago has a long history of indiscriminate human exploitation, primarily driven by extractive pressures on the rich marine resources, which has resulted in serious conservation issues that continue through today (e.g., Ruttenberg, 2001; Boersma et al., 2005; Awkerman et al., 2006; Jacquet et al., 2008; Sonnenholzner et al., 2009; Schiller et al., 2014; Ruiz et al., 2016; Usseglio et al., 2016; Alava and Paladines, 2017; Cerutti-Pereyra et al., 2020; Bonaccorso et al., 2021). Starting with 18th century whaling, the sperm whale (Physeter macrocephalus) was among the first species to be targeted, and by the mid 19th century the local population had become depleted (Shuster, 1983; Hope and Whitehead, 1991; Whitehead and Hope, 1991; Whitehead et al., 1997). To prevent further whaling, in 1990 the government of Ecuador declared a “whale refuge” in all its jurisdictional waters, that is, the 200-nautical-mile Exclusive Economic Zone (EEZ) (Acuerdo Ministerial No. 196, 1990; Evans, 1991; Merlen, 1992), and subsequent legislation prohibited whale hunting indefinitely in its EEZ (Registro Oficial, 2000; Registro Oficial, 2002).
In 1998, Ecuador created the Galápagos Marine Reserve (GMR; Figure 1A), extending 40 nautical miles seaward from the coastal baseline and covering an area of 138,000-km2 (Ley Orgánica de Régimen Especial Para la Provincia de Galápagos, 1998), making it one of the largest marine protected areas in the world. In 2001, UNESCO designated the GMR as a Natural World Heritage Site, in recognition of the astonishingly rich and diverse marine communities inhabiting it (Heylings et al., 2002). The boundaries of the GMR were revised in 2022, resulting in a slightly larger area of 142,759 km2 (Acuerdo Ministerial No. MAATE-2022-039, 2022).
Despite the protections offered by the GMR, industrial fishing pressures targeting squid, high-trophic level fish, and sharks have continued to increase just beyond the reserve—and sometimes within it due to weak enforcement—and are driving a regional conservation crisis (Edgar et al., 2011; White et al., 2020; Bonaccorso et al., 2021; Global Fishing Watch, 2021). This led the Ecuadorian government to announce in January 2022 that it would create a new marine protected area within its EEZ, named “Hermandad Marine Reserve,” a 60,000-km2 corridor adjacent to the GMR (Decreto Ejecutivo No. 319, 2022; Acuerdo Ministerial No. MAATE-2022-019, 2022; Acuerdo Ministerial No. MAATE-2022-041, 2022) (Figure 1A). The new marine reserve is part of a regional initiative that seeks to connect the insular marine protected areas of Ecuador, Costa Rica, Panama, and Colombia to achieve greater protection of their shared marine resources (Decreto Ejecutivo No. 319, 2022; Acuerdo Ministerial No. MAATE-2022-019, 2022).
Finally, in November 2022, the IUCN Marine Mammal Protected Areas Task Force announced that the Galápagos Archipelago would become one of its Important Marine Mammal Areas in the southeast temperate and tropical Pacific Ocean, recognizing that its waters harbor small and resident populations of endemic pinnipeds, provide habitat for reproductive and feeding activities of vulnerable whale species, and support aggregations of a high diversity of medium and small cetaceans (IUCN-MMPATF, 2022). These criteria provide a basis for further prioritization of conservation measures of relevance to marine mammals in Galápagos (Tetley et al., 2022).
3 A review of cetacean occurrence in Galápagos
Contemporary research on cetaceans in Galápagos waters has been largely sporadic and chronically underfunded. Collection of information on cetacean presence began in the 1970s, primarily as a casual but growing interest by local naturalist guides working aboard cruise ships touring the islands (Day, 1994; Merlen, 1995), an activity that continues through today (e.g., Palacios and Salazar, 2002; Denkinger et al., 2013; Denkinger et al., 2020). Also in the 1970s, a program to monitor dolphin mortality incidental to purse-seine tuna fishing operations in the ETP was established by the U.S.’s National Oceanic and Atmospheric Administration (NOAA) and subsequently implemented by other nations participating in the fishery (Ballance et al., 2021). These programs placed fisheries observers on tuna vessels, who collected cetacean sightings throughout the ETP, including Galápagos waters (Fiedler and Lennert-Cody, 2019). Additionally, between the mid 1980s and mid 2000s, NOAA also conducted dedicated research vessel surveys to estimate dolphin abundance and trends in the ETP, including Galápagos waters (Wade and Gerrodette, 1993; Hamilton et al., 2009).
A long-term study of sperm whales in Galápagos waters began in the mid 1980s, which has provided the basis for much of the contemporary knowledge on the species (e.g., Whitehead, 2003; Eguiguren et al., 2021). Several other research expeditions have been undertaken in Galápagos with the explicit purpose of studying cetaceans since then (Lyrholm et al., 1992; Smith and Whitehead, 1999; Palacios, 1999a; Palacios, 2000; Wise et al., 2009; Félix et al., 2011; Biggs et al., 2017; O’Hern et al., 2017). With the establishment of the GMR, since the early 2000s collaborative efforts between Galápagos-based and international scientists have continued to generate crucial knowledge about marine mammals in the area (Palacios and Salazar, 2002; Palacios et al., 2004; Denkinger et al., 2013; Biggs et al., 2017; O’Hern et al., 2017; Eguiguren et al., 2019; Denkinger et al., 2020; Eguiguren et al., 2021; Páez-Rosas et al., 2021). Finally, cetacean presence in Galápagos has also been gleaned from documentation of live-stranding events, beach-cast specimens, and osteological specimens in museums (Palacios, 1996; Palacios et al., 2004).
Several studies have attempted to characterize cetacean species diversity in Galápagos waters (Day, 1994; Merlen, 1995; Smith and Whitehead, 1999; Palacios and Salazar, 2002; Palacios, 2003; Denkinger et al., 2013). At least 23 species have been documented, although the relative sighting frequency of each species varies between studies because of differences in geographic coverage, sampling effort, and methodological approaches. To objectively assess the spatial and temporal patterns of cetacean occurrence in Galápagos waters, for purposes of this review we used the regional compilation of marine mammal sightings by Palacios (2003) and augmented it with more recent compilations of sightings for humpback whales (Megaptera novaeangliae) by Félix et al. (2011) and sperm whales by Cantor et al. (2017). We defined the geographic extent of our study area as a 4×4-degree box bounded by 88.5-92.5°W and 2°S-2°N (Figure 1B) and limited the marine mammal sightings data to this extent, resulting in a total of 3,227 sightings spanning the period 1973-2014 (Table 1, Figure 2A). As these sightings were collected using different methods, no standardized measure of effort was possible and instead we used the general spatiotemporal pattern in the data as a proxy for coverage (Figures 2A and 3). Out of the 20 cetacean species in our sightings compilation (Table 1), 14 were most common (i.e., species with 10 or more sightings). These included nine Delphinids (Stenella attenuata, Stenella longirostris, Stenella coeruleoalba, Delphinus delphis delphis, Tursiops truncatus, Grampus griseus, Globicephala macrorhynchus, Pseudorca crassidens, and Orcinus orca), one Ziphiid (Ziphius cavirostris), one Physeterid (P. macrocephalus), and three Balaenopterids (Balaenoptera edeni brydei, Balaenoptera musculus, and M. novaeangliae). Among the less commonly seen species, there were three Delphinids (Peponocephala electra, Lagenodelphis hosei, Feresa attenuata), one Balaenopterid (Balaenoptera acutorostrata), one Ziphiid (Mesoplodon peruvianus), and one Kogiid (Kogia sima). The relatively low sighting frequency of the Ziphiids and Kogiid is likely due to their long dive times coupled with their cryptic behavior at the surface, as the stranding record suggests that they may be more common (Palacios et al., 2004; MacLeod and Mitchell, 2006). In fact, one additional Ziphiid species, ginkgo-toothed beaked whale (Mesoplodon ginkgodens), is only known from strandings (Palacios, 1996; Palacios et al., 2004).
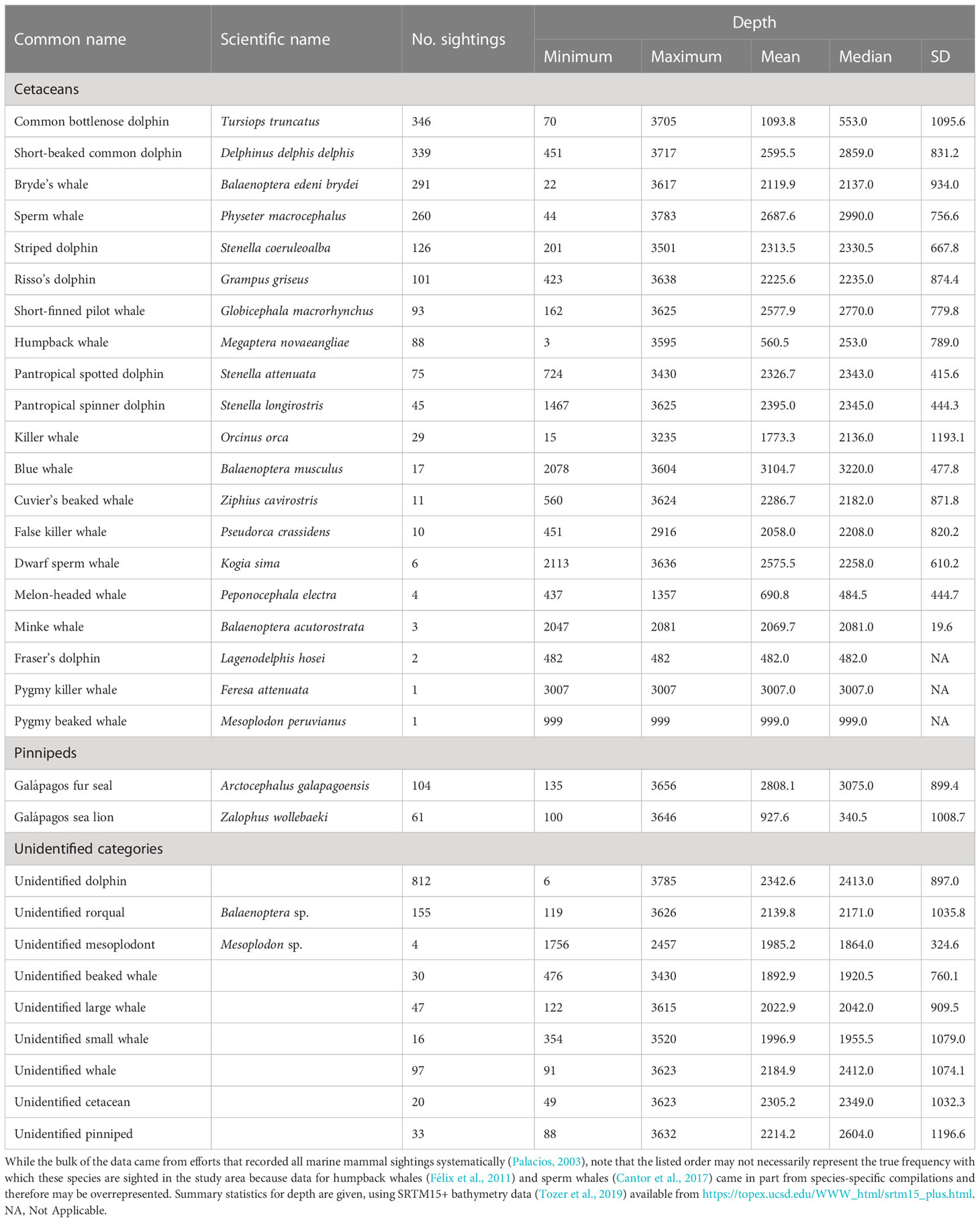
Table 1 Cetacean species reported in Galápagos, listed in decreasing frequency, based on a comprehensive compilation of marine mammal sightings (n = 3,227) in an area defined by a 4×4-degree box bounded by 88.5-92.5°W and 2°S-2°N, spanning the period 1973-2014 (see text for details and Figures 2 and 3 for graphical presentation).
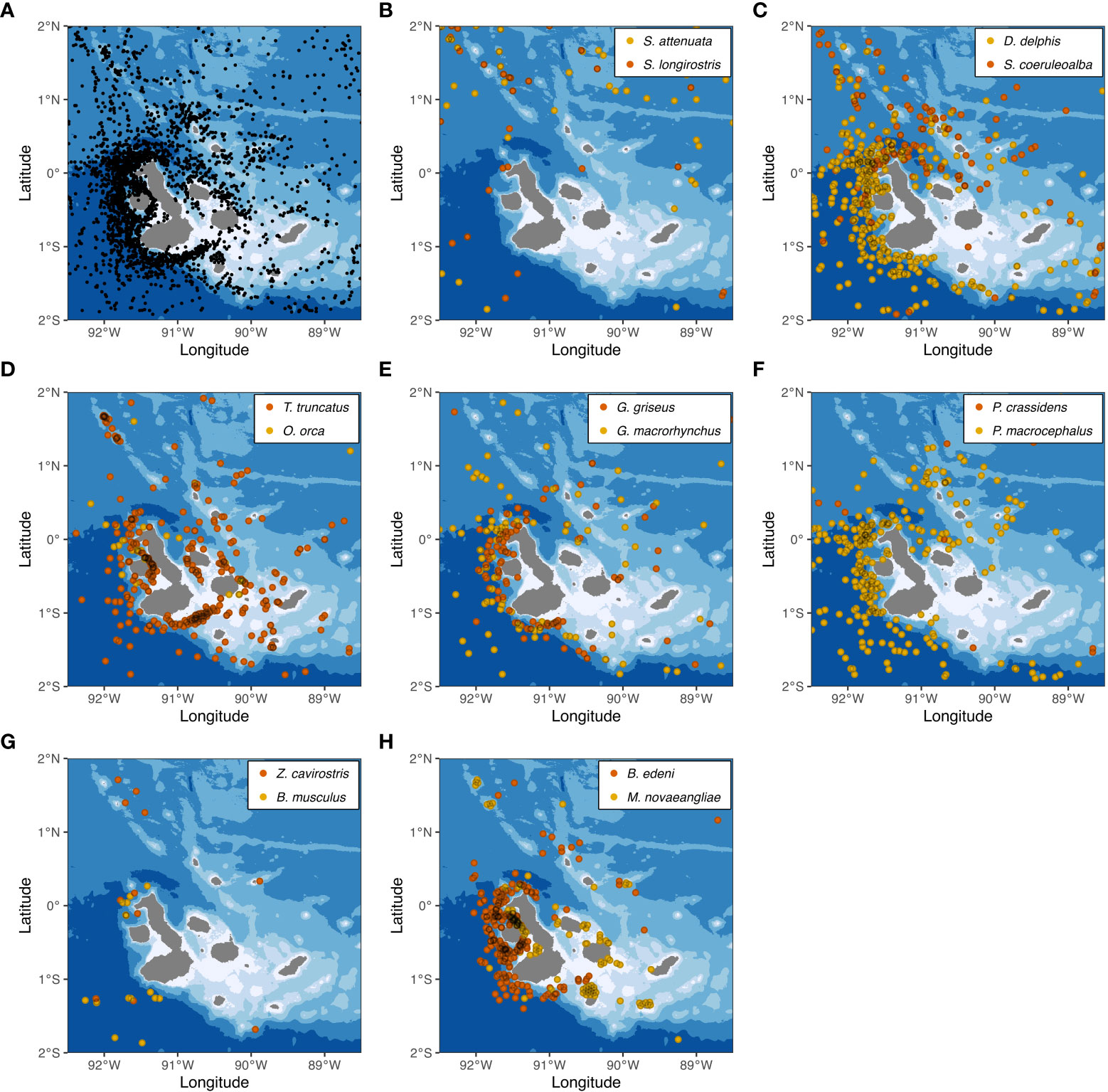
Figure 2 Maps depicting the distribution of 14 cetacean species commonly seen in Galápagos waters. (A) Locations of all marine mammal sightings (identified and unidentified) from a compilation of sightings (n = 3,227), used here as a proxy for search effort. (B) Sighting locations for S. attenuata (n = 75) and S. longirostris (n = 45). (C) Sighting locations for D. delphis delphis (n = 339) and S. coeruleoalba (n = 126). (D) Sighting locations for T. truncatus (n = 346) and O. orca (n = 29). (E) Sighting locations for G. griseus (n = 101) and G. macrorhynchus (n = 93). (F) Sighting locations for P. crassidens (n = 10) and P. macrocephalus (n = 260). (G) Sighting locations for Z. cavirostris (n = 11) and B. musculus (n = 17). (H) Sighting locations for B. edeni brydei (n = 291) and M. novaeangliae (n = 88). Marine mammal sighting data from Palacios (1999b), Palacios and Salazar (2002), Palacios (2003), Félix et al. (2011), and Cantor et al. (2017), used with permission or under Creative Commons licenses CC BY and CC BY-NC. Bathymetry data from SRTM15+ (Tozer et al., 2019), available from https://topex.ucsd.edu/WWW_html/srtm15_plus.html.
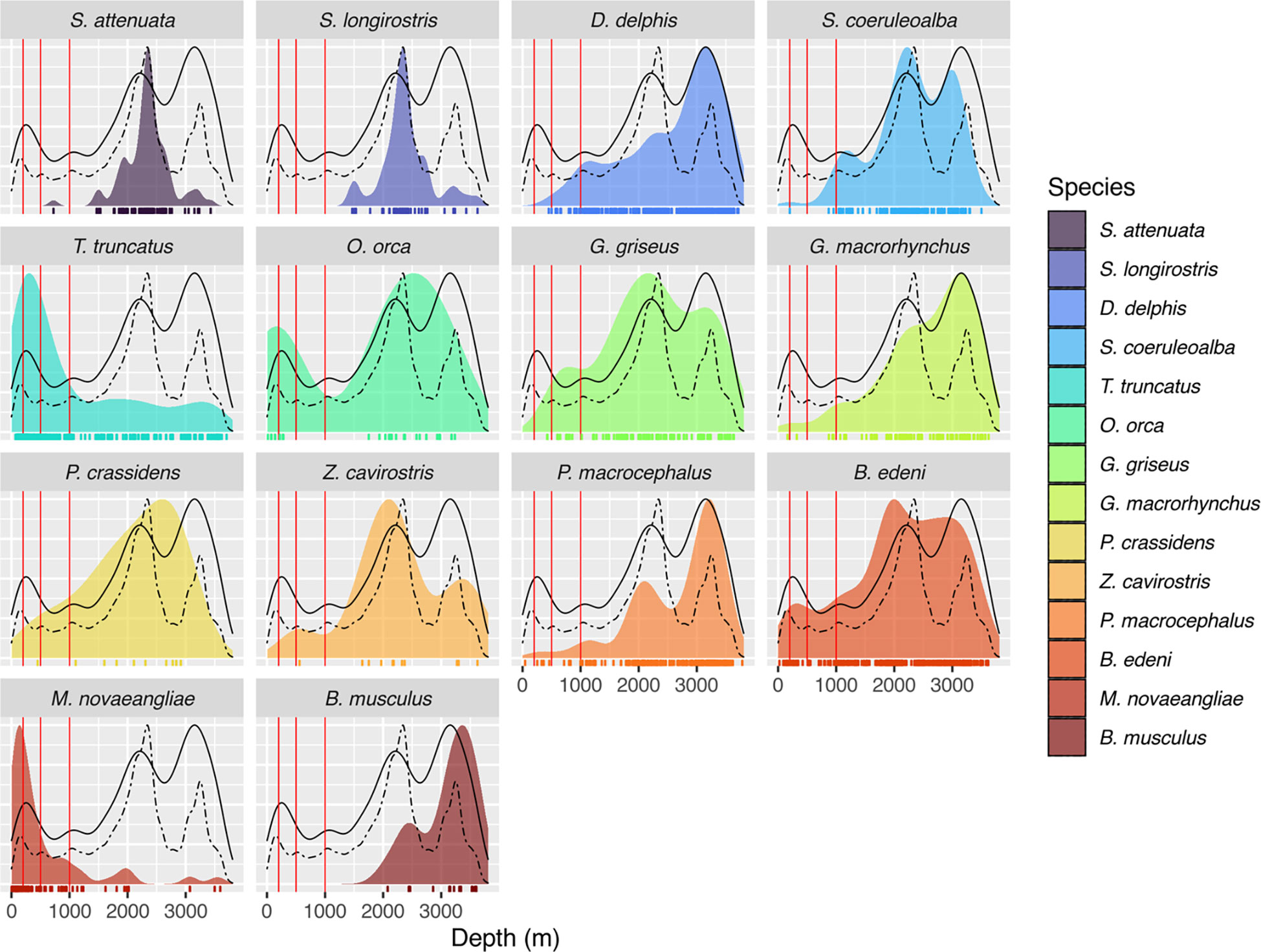
Figure 3 Probability density plots of the distribution of seafloor depth for sighting locations of 14 cetacean species commonly seen in Galápagos waters, as shown on the maps in Figure 2. For reference, black dashed curve is the probability density of seafloor depth for the full study area (see Figure 1B) and black solid curve is the probability density of seafloor depth for all marine mammal sightings, identified and unidentified (see Figure 2A). The three vertical red lines indicate depths of 200, 500, and 1000 m, respectively. Marine mammal sighting data from Palacios (1999b); Palacios and Salazar (2002); Palacios (2003), Félix et al. (2011), and Cantor et al. (2017), used with permission or under Creative Commons licenses CC BY and CC BY-NC. Bathymetry data from SRTM15+ (Tozer et al., 2019), available from https://topex.ucsd.edu/WWW_html/srtm15_plus.html.
The overall spatial distribution of marine mammal sightings in our compilation indicated that the study area was covered reasonably well, although it is apparent that much of the effort has been on the deep waters (> 1500 m) of the western part of the archipelago, especially around Isabela and Fernandina islands (Figures 2A and 3). In contrast, the coastal shallow waters (< 500 m) of the central and eastern part of the archipelago have received comparatively much less effort (Figures 2A and 3). Among the commonly seen species, two had a primarily coastal (< 500 m) distribution (T. truncatus and M. novaeangliae), two occurred in both coastal and oceanic habitats (O. orca and B. edeni brydei), and the remainder were primarily found in deep (> 1000 m) waters (Figures 2 and 3).
In terms of seasonal coverage, more sightings in our compilation were collected in the first part of the year (January-May), when sea state conditions are calm, than in the second part (June-December), when sea conditions are rougher (Figure 4). Nevertheless, most species appear to use waters of the archipelago year-round, with sightings in most or all months of the year (Figure 4). Two of the Balaenopterids (B. musculus and M. novaeangliae) are seasonal migrants, being reported primarily during the second part of the year (Figure 4), coinciding with the low-latitude phase of the migratory cycle for Eastern South Pacific populations (Palacios, 1999b; Félix et al., 2011; Torres-Florez et al., 2015; Hucke-Gaete et al., 2018). Additionally, although the Bryde’s whale (B. edeni brydei) is present year-round, a larger number of sightings during the second part of the year (Figure 4), indicates a preference for the cooler and more productive conditions characteristic of this season, as also reported by Denkinger et al. (2013). Finally, several species had more sightings in the first part of the year (S. attenuata, S. longirostris, S. coeruleoalba, D. delphis delphis, G. griseus, G. macrorhynchus, P. macrocephalus; Figure 4), but this pattern is likely influenced by increased sampling effort when sea conditions are calmer.
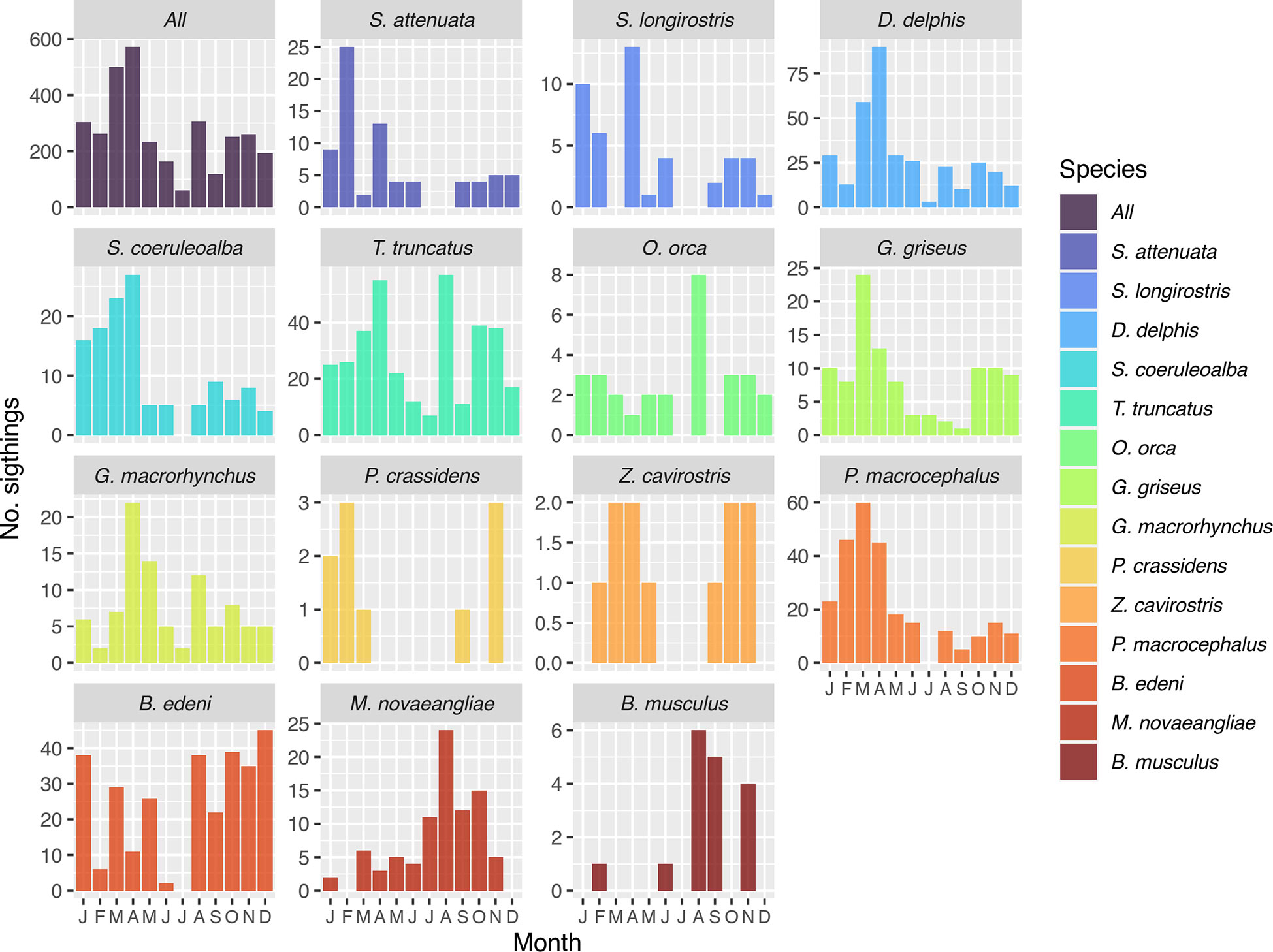
Figure 4 Histogram plots of number of sightings by month for 14 cetacean species commonly seen in Galápagos waters. Marine mammal sighting data from Palacios (1999b); Palacios and Salazar (2002); Palacios (2003), Félix et al. (2011), and Cantor et al. (2017), used with permission or under Creative Commons licenses CC BY and CC BY-NC.
Marked fluctuations in species occurrence and distribution at interannual and long-term scales have also been described. For example, Denkinger et al. (2013) documented the apparent disappearance of Bryde’s whales and short-beaked common dolphins (D. delphis delphis) from the archipelago during the 1997-98 and 2010 El Niño events, when upwelling, productive conditions were suppressed. In the case of the sperm whale, a long-term study spanning the period 1985-2014 revealed large inter-decadal fluctuations in the number of animals visiting Galápagos each year (Cantor et al., 2017; Eguiguren et al., 2021), a result of the movements in and out of the archipelago undertaken by this highly social and widely roaming species (Whitehead et al., 1997; Cantor et al., 2017).
Finally, Palacios (2003) investigated the community ecology of Galápagos cetaceans in relation to oceanographic conditions through non-metric multidimensional scaling, based on nine species with sufficient occurrence data (Figure 5). The ordination provided a two-axis solution (86% and 4.2% of the information in the data, respectively), with axis 1 representing the dominant environmental gradient in the study area, contrasting upwelling conditions close to the islands at the negative end with warm and phytoplankton-poor conditions away from the islands at the positive end, and with sample units sorting out along this gradient (Figure 5A). Species showed distinct functional responses along this gradient, with pantropical spotted (S. attenuata) and spinner dolphin (S. longirostris) occurrence increasing towards the positive end; common bottlenose dolphins (T. truncatus), Risso’s dolphins (G. griseus), and Bryde’s whales increasing towards the negative end; and short-beaked common dolphins, short-finned pilot whales (G. macrorhynchus), sperm whales, and striped dolphins (S. coeruleoalba) peaking somewhere along these two extremes (Figure 5B). Axis 2 only explained a small amount of the information in the data and was unrelated to the environmental variables considered (Palacios, 2003). These results shed insight on how the complex oceanographic conditions around Galápagos support a diverse cetacean community with distinct habitat preferences and distribution patterns (Palacios, 2003).
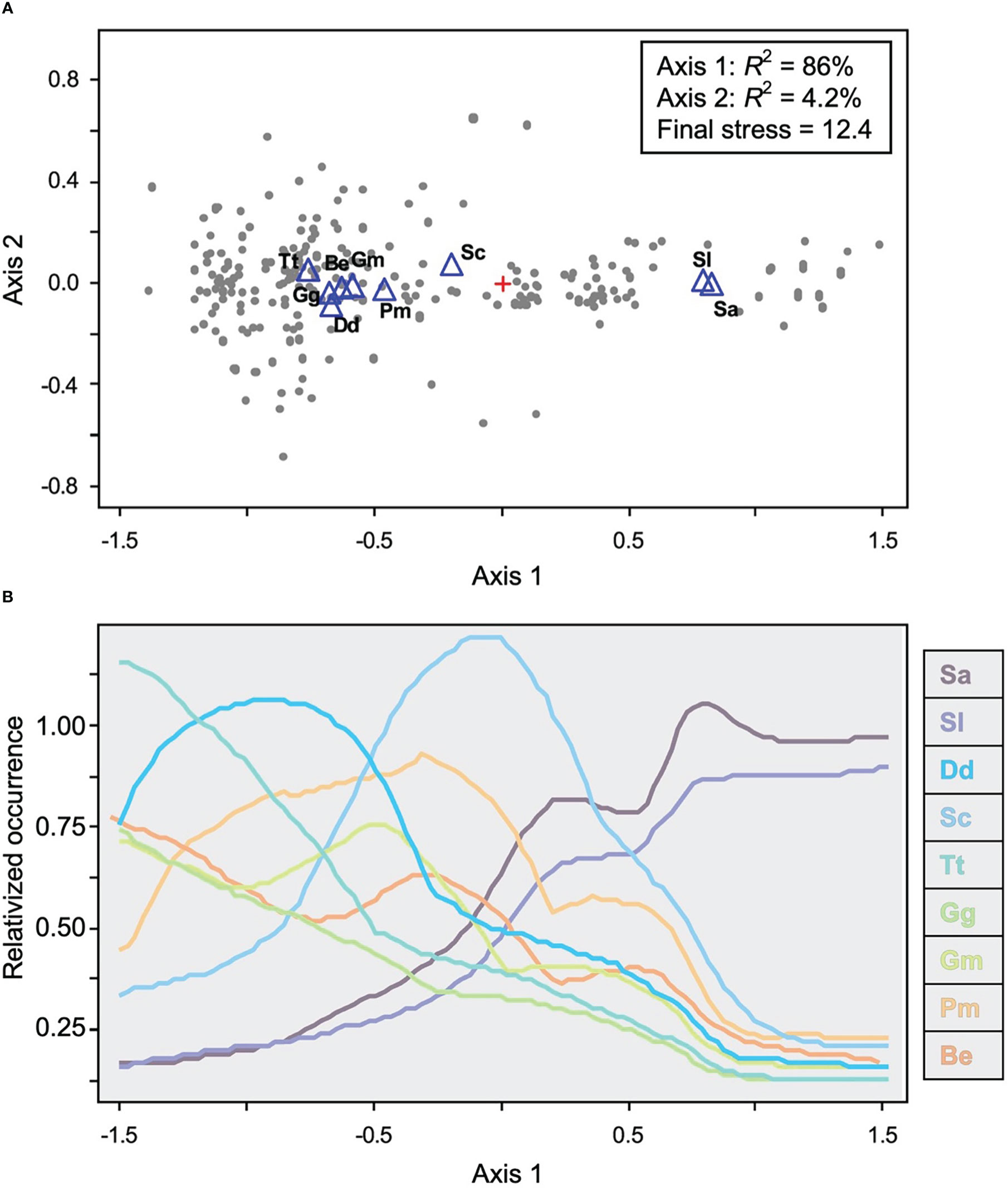
Figure 5 Non-metric multidimensional scaling ordination of the Galápagos cetacean community, based on nine species with acceptable sample size. (A) Biplot of the ordination scores on the two main axes, with gray dots corresponding to 904 sample units (0.25-degree grid cells) where species occurrence was evaluated. Open blue triangles are the average positions of the nine species, calculated by weighted averaging. (B) Cetacean community gradient (coenocline) for the nine species in the ordination, showing species responses (relativized occurrence) along axis 1, the dominant environmental gradient. Curves correspond to an envelope that includes points falling within two standard deviations of a running mean along the axis. Sa, S. attenuata; Sl, S. longirostris; Dd, D delphis delphis; Sc, S. coeruleoalba; Tt, T. truncatus; Gg, G griseus; Gm, G macrorhynchus; Pm, P. macrocephalus; and Be, B edeni brydei. Adapted from Palacios (2003).
4 Research priorities
The above review demonstrates that the biologically productive Galápagos Archipelago is an area that attracts and sustains an outstanding cetacean diversity. However, beyond information on spatial and temporal occurrence, little is known about the biology, ecology, and stock structure of most cetacean species in Galápagos waters or about the connectivity of their movements between the archipelago and other habitats in the ETP. This information is necessary for assessing the status of cetacean populations in Galápagos and for developing appropriate management and conservation measures. In this section, we identify 10 critical knowledge gaps along five topical priority areas for future ecological research on cetaceans in Galápagos based on feasibility and scientific merit: I) spatiotemporal occurrence, II) population assessment, III) health assessment, IV) social ecology, and V) trophic ecology. These knowledge gaps and priorities are summarized in Table 2, with consideration of relevant methodological approaches, species most amenable to these approaches, and the outcomes that may be expected from short- and long-term efforts. Although most of the knowledge gaps require long-term efforts (years to decades), short-term efforts (months to years) will be crucial for generating the necessary baseline information. We emphasize that these priorities are based on our combined experiences and perceptions as international academic scientists conducting cetacean research in Galápagos, as detailed in our Positionality Statement in Section 6. By advancing these priorities, our goal is to catalyze discussion and consultation leading to setting a participative research agenda among relevant institutions, local stakeholders, and the broader scientific community interested in advancing cetacean research, management, and conservation in Galápagos.
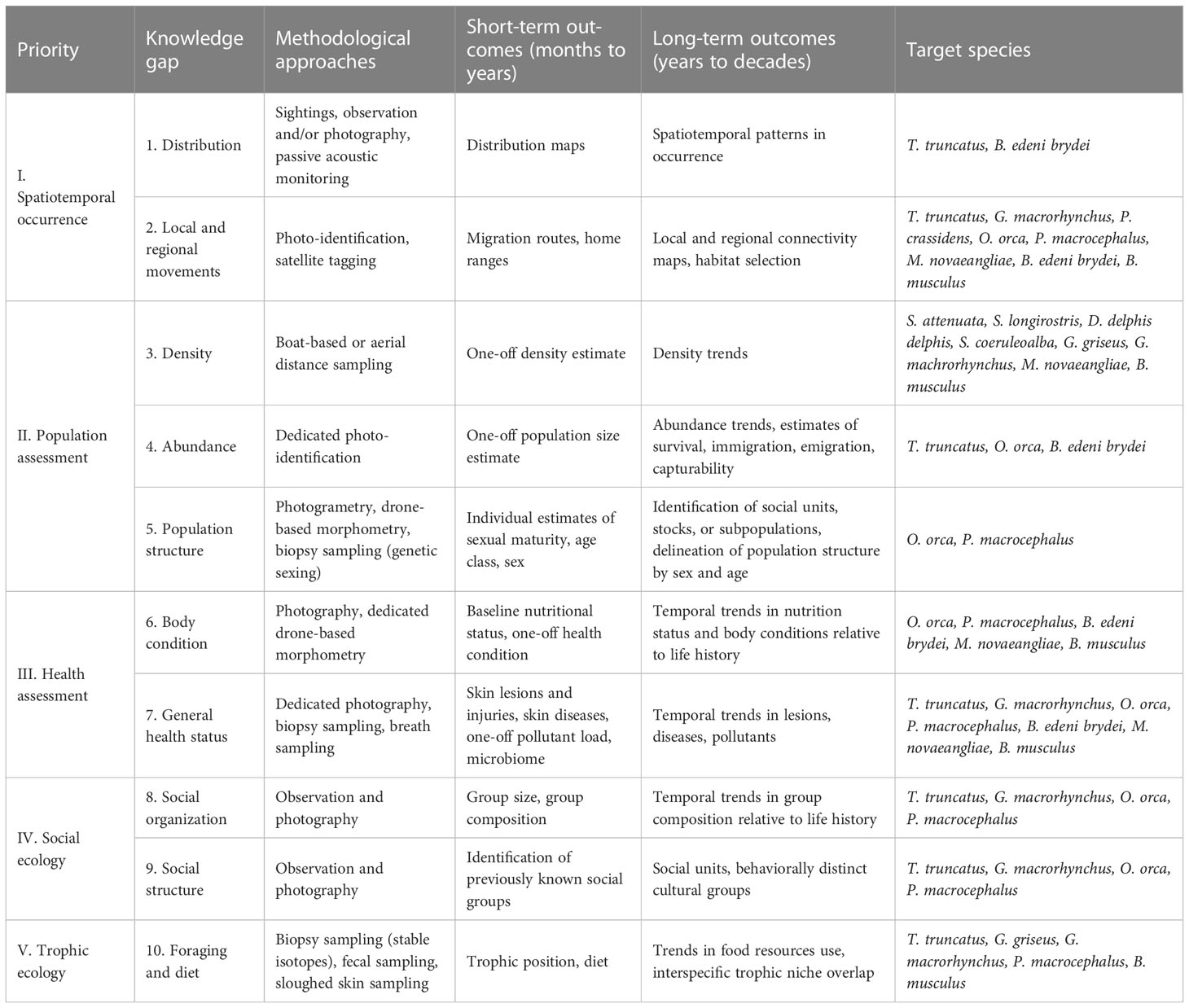
Table 2 Summary of the proposed priorities for ecological research on cetaceans in Galápagos, along with knowledge gaps, suggested methodological approaches, expected outcomes in the short- (months to years) and long-term (years to decades), and species that are best suited for these approaches.
4.1 Priority I: Spatiotemporal occurrence
4.1.1 Knowledge gap 1: Distribution
The shallow (< 500 m) and coastal waters of the central and eastern part of the archipelago have received comparatively less effort than the deep waters (> 1500 m) of the western part (Figure 2A), and therefore our understanding of cetacean occurrence and habitat use in neritic waters is incomplete. For example, common bottlenose dolphins appear to occur primarily in neritic waters throughout the archipelago, but sightings tend to be strongly clustered (Palacios and Salazar, 2002; Palacios, 2003; Figure 2B). Bryde’s whales are common in the highly productive western part of the archipelago (Palacios and Salazar, 2002; Palacios, 2003; Figure 2G), but a temporally variable “hotspot” of occurrence has been also reported off San Cristóbal Island, on the eastern part, that appears to be driven by a localized upwelling (Denkinger et al., 2013; Biggs et al., 2017). These illustrative examples indicate that further work is needed to characterize cetacean distribution and their relationship to fine-scale oceanographic and topographic processes (Houvenaghel, 1978; Schaeffer et al., 2008; Figueroa, 2021; Neave et al., 2021).
4.1.2 Knowledge gap 2: Local and regional movements
Many studies of cetaceans around oceanic islands throughout the world have identified distinct island-associated populations (e.g., Baird et al., 2009a; Baird et al., 2013a; Oremus et al., 2012; Quérouil et al., 2013; Kiszka et al., 2014; Estrade and Dulau, 2020; Panicker et al., 2022; Sambolino et al., 2022). Further work is needed to determine whether there are island-associated cetacean populations in Galápagos, to what islands, their inter-island movements, and their differentiation with pelagic populations. Studies are also needed to characterize the movements of Bryde’s whales within the archipelago and their connection to other areas where the species is seen along the mainland (Castro et al., 2017; Rasmussen and Palacios, in press1) and the offshore ETP (Wade and Gerrodette, 1993; Hamilton et al., 2009).
4.1.3 Methodological approaches
Future studies could combine multiple sampling approaches to advance our understanding of cetacean spatiotemporal occurrence in Galápagos (Table 2). First, for most species, this can be achieved with continued collection of sighting data (time, geographic position, species, group size, and photography for confirmation and archiving). These observational data are straightforward to record by researchers as well as by naturalists and citizen scientists aboard cruise ships (e.g., Palacios and Salazar, 2002; Denkinger et al., 2013; Alves et al., 2018; Denkinger et al., 2020). Second, photo-identification efforts will allow for the study of local and regional movements of individuals within and beyond Galápagos (e.g., Baird et al., 2013a; Baird, 2016; Alves et al., 2018; Alves et al., 2019; Pacheco et al., 2019; Ferreira et al., 2021; Dinis et al., 2021), particularly for species that are easy to identify and often have distinctive markings (T. truncatus, G. macrorhynchus, P. crassidens, O. orca, P. macrocephalus, and M. novaeangliae) (e.g., Cantor et al., 2016; Denkinger et al., 2020). Third, electronic tagging will allow detailed tracking of the movements and migrations of the large whales (B. edeni brydei, B. musculus, M. novaeangliae, P. macrocephalus) as well as the larger-bodied Delphinids (G. griseus, G. macrorhynchus, P. crassidens) and Ziphiids (Z. cavirostris and Mesoplodon spp.) that use Galápagos waters. While more costly, logistically demanding, and requiring welfare considerations, tagging is the most feasible way to reveal the routes of migratory whales (e.g., Silva et al., 2013; Prieto et al., 2014; Hucke-Gaete et al., 2018; Fonseca et al., 2022), which will enable researchers to create connectivity maps across coastal and oceanic habitats in the ETP and beyond (e.g., Davies et al., 2021; Boteler et al., 2022). For the Delphinids and Ziphiids, tagging can provide crucial information on residency, site fidelity, and habitat use (e.g., Baird et al., 2009b; Baird et al., 2013b; Abecassis et al., 2015; Baird, 2016; ). Finally, passive acoustic monitoring with towed or moored hydrophones (Mellinger et al., 2007) would be especially useful for detecting deep-diving and cryptic species (K. sima, Ziphiids), as well as Odontocetes or Balaenopterids with distinctive acoustic signatures, such as clicking sperm whales and singing humpback and blue whales. Relative to visual methods, acoustic data may not always provide accurate species identification or group size estimates, but recent advances on automated detection of broad-band echolocation clicks and tonal sounds (e.g., Gillispie et al., 2009; Frasier et al., 2017), as well as machine learning tools (e.g., Beslin et al., 2018; Bermant et al., 2019; Woodward et al., 2020) offer promising solutions.
Such multiplatform data collection is increasingly being used to generate predictive habitat suitability maps (e.g., Thorne et al., 2012; Bouchet et al., 2015; Tobeña et al., 2016; Tardin et al., 2017; Fiedler et al., 2018; Tardin et al., 2019; Correia et al., 2021; Fernandez et al., 2021), even with short-term efforts (months to years). In the long term (years to decades), the accumulation of such data will increase the coverage, reliability, and scope of these maps and help refine the characterization of species-specific hotspots of occurrence and their fluctuations in response to environmental variation such as El Niño Southern Oscillation and climate change (e.g., Llapapasca et al., 2018; Becker et al., 2019; Becker et al., 2022). This information could be used to identify cetacean areas of significant importance within Galápagos, as has been done for other locally threatened marine taxa (Edgar et al., 2008; Ventura et al., 2019) as well as for the highly migratory elasmobranchs (Hearn et al., 2010; McKinley et al., 2022). Beyond helping researchers improve their ecological understanding of cetacean occurrence patterns, predictive habitat suitability maps are increasingly used as management tools (e.g., Sahri et al., 2021), and they could help to delineate areas of importance within the GMR that have particularly high conservation significance and yet may be subject to ongoing anthropogenic disturbances.
4.2 Priority II: Population assessment
4.2.1 Knowledge gaps 3 and 4: Density and abundance
Unlike pinnipeds, for which population assessments have been conducted on a regular basis throughout Galápagos (e.g., Páez-Rosas et al., 2021), very few studies have reported population assessments for cetaceans in Galápagos (Palacios and Forney, 2008; O’Hern et al., 2017; Whitehead and Shin, 2022), and there is no formal ongoing program for monitoring cetacean populations. Yet, baseline information on demographic parameters, such as density, abundance, survival, and recruitment, is essential for understanding population status and dynamics, and to unravel the relative importance of competition and predation in structuring the local cetacean community relative to the influences of environmental variability. Ultimately, monitoring temporal trends in population parameters provides information that is immediately useful for guiding conservation decisions (e.g., Taylor et al., 2007; Boyd and Punt, 2021).
4.2.2 Knowledge gap 5: Population structure
Studies are needed to assess the relationship of common bottlenose dolphins in Galápagos to the recently recognized subspecies of offshore bottlenose dolphin (T. truncatus nuuanu) or other ecotypes in the ETP (Palacios et al., 2004; Costa et al., 2022) for appropriate management. Other examples include elucidating the relationship of Bryde’s whales and sperm whales seen in Galápagos to those that occur in offshore waters and in the jurisdictions of countries bordering the ETP.
4.2.3 Methodological approaches
To estimate demographic parameters of species whose individuals are likely to be re-identified multiple times, such as local populations (T. truncatus, B. edeni brydei) or those observed in long-lasting groups (O. orca), we suggest designing mark-recapture studies based on photo-identification data (Urian et al., 2015; Wickman et al., 2020; Hammond et al., 2021) (Table 2). In the short term, analyzing photo-identification data with simple mark-recapture models (White and Burnham, 1999) is sufficient to generate one-off estimates of abundance or minimum population size (e.g., Baird et al., 2008; Baird et al., 2009a; Baird et al., 2009b). With an appropriate sampling design for photo-identification effort, long-term data will allow more elaborate mark-recapture modeling to estimate a range of other demographic parameters (e.g., survival, emigration, recruitment; see Pollock et al., 1982; White and Burnham, 1999). Our understanding of population structure and dynamics of cetacean species in Galápagos can be much improved by considering individual covariates, such as sex, age, and/or maturity classes, when estimating parameters with mark-recapture models. This information can be obtained using a variety of field and laboratory approaches: some species are sexually dimorphic (O. orca, P. macrocephalus) and others can be sexed genetically with remote biopsy sampling; hormonal analyses can indicate reproductive condition; epigenetic methods can be used for aging individuals (e.g., Polanowski et al., 2014); and boat-based photogrammetry and drone-based morphometry can generate body length estimates, especially of large Odontocetes (e.g., Kotik et al., 2022).
Although some species are common and/or easy to identify, many are unsuited for photo-identification-based mark-recapture modeling, either because the populations and/or their ranging areas are very large (M. novaeangliae, B. musculus), or because they are typically seen in large, fast-moving groups (D. delphis delphis, S. coeruleoalba, S. attenuata, S. longirostris). For these cases, archipelago-wide efforts would be better suited to distance sampling using line-transect surveys (Buckland et al., 2001; Buckland et al., 2015). This approach can generate one-off density or abundance estimates in the short-term (Palacios and Forney, 2008; O’Hern et al., 2017), as well as trends over time (Table 2). Distance sampling requires dedicated surveys with properly pre-defined transect lines, trained personnel, and careful consideration of assumptions and caveats (Thomas et al., 2007; Faustino et al., 2010), but it remains a cornerstone of cetacean population monitoring. This methodology is typically conducted from large research vessels capable of open-ocean operation (e.g., O’Hern et al., 2017), but has also been adapted to sailboats (e.g., Palacios and Forney, 2008), small boats (Dawson et al., 2008), and aircraft (e.g., Panigada et al., 2011). Line-transect surveys, either boat-based or aerial, can be cost-effective solutions for estimating density and abundance of a wide range of cetacean species in coastal areas (Aragones et al., 1997; Williams and Thomas, 2009; Lambert et al., 2019).
4.3 Priority III: Health assessment
4.3.1 Knowledge gaps 6 and 7: Body condition and general health status
Other than basic documentation of stranding events (Palacios et al., 2004), health assessments for cetaceans in Galápagos are absent. Studies have shown that environmental perturbations such as El Niño as well as exposure to anthropogenic activities near human population centers have impacts on the body condition and general health of teleost fish (Lamb et al., 2018) and pinnipeds (Brock et al., 2013a; Brock et al., 2013b; Paéz-Rosas et al., 2016; Páez-Rosas et al., 2021), suggesting that cetaceans may also be affected by these stressors.
4.3.2 Methodological approaches
Health status in cetaceans can be assessed through monitoring of the nutritional status (Joblon et al., 2014; Christiansen et al., 2020) and the prevalence of cutaneous conditions of individuals and populations (Hart et al., 2012; Soares et al., 2022), which can reveal key insights about population status, the quality of the surrounding environment, and interactions with human activities (e.g., boat traffic, fisheries). A straightforward way to investigate body condition and external lesions and injuries in cetaceans is through visual analyses of photographic and video data. For instance, footage recorded from drones offers a privileged bird’s-eye view from which researchers can extract accurate quantitative morphometric data to evaluate body condition and health status (e.g., Christiansen et al., 2020; Horton et al., 2019; Bierlich et al., 2021) (Table 2). Drones can also be adapted to sample the microbial and viral communities in the breath of small and large cetaceans as a complementary approach to assess their health (e.g., Centelleghe et al., 2020). Drone-based photogrammetry and respiratory microbiota sampling may be more suitable for large whales in Galápagos (B. edeni brydei, B. musculus, M. novaeangliae, P. macrocephalus) (e.g., Dawson et al., 2017), but coupling drone images with laser altimeters may generate suitable high-resolution aerial photogrammetric data for smaller cetaceans as well (see Bierlich et al., 2021).
While drone sampling requires dedicated research efforts and specific safety and sampling protocols (e.g., Raoult et al., 2020), boat-based photography can provide a quick assessment of general body condition (e.g., “emaciated”, “thin”, “good”) based on fat deposition in specific body parts (e.g., Joblon et al., 2014; Soares et al., 2022; Wachtendonk et al., 2022). Such photographic records can be collected even from opportunistic platforms and can generate insights on the cutaneous lesions and diseases of a range of cetacean species. Visual analyses of the gross characteristics of skin conditions from photographs can indicate cases that are potentially related to viral, fungal, or bacterial aetiology (e.g., Van Bressem et al., 2007; Van Bressem et al., 2009). Photographic records can also be used to assess injuries indicative of traumatic events; wounds and scars, for instance, can suggest predation pressure (Best and Photopoulou, 2016; Baird et al., 2022; Capella et al., 2018; Corsi et al., 2022) as well as negative interactions with anthropogenic activities (e.g., Harnish et al., 2019), entanglement in or interactions with fishing gear (e.g., Baird et al., 2014), or collision with boats (e.g., Toms et al., 2020).
Investigating the underlying causes of body and cutaneous conditions in cetaceans, however, requires further laboratory analyses to determine nutritional status and diagnose disease or injury (Table 2). Therefore, we recommend including biological tissue sampling during dedicated surveys whenever feasible. Proper evaluation of cutaneous conditions includes electron microscopy or molecular analyses of skin tissue to confirm a potential pathology (e.g., Groch et al., 2020). Additional analyses of tissue samples should include pollutant loads (e.g., Alava et al., 2009; Remili et al., 2020). On the other hand, although fresh stranded animals can yield tissue samples opportunistically, any dedicated biopsy sampling requires specialized research efforts (i.e., personnel, equipment, storage, and permitting) and are most feasible for the larger-bodied species (i.e., T. truncatus, G. griseus, G. macrorhynchus, O. orca, P. macrocephalus, B. edeni brydei, B. musculus, M. novaeangliae).
4.4 Priority IV: Social ecology
4.4.1 Knowledge gaps 8 and 9: Social organization and social structure
Long-term studies of social ecology in Galápagos marine mammals are among the most world-renowned, including pinnipeds (Wolf et al., 2007), sperm whales (Whitehead, 1985; Whitehead, 2003; Cantor and Whitehead, 2015), and killer whales (Denkinger et al., 2020). While we advocate for the continuation of these efforts, studies on other species or aspects of sociality would advance our understanding of social complexity in cetaceans. Investigating the various social systems present in cetaceans (Mann et al., 2000; Rendell et al., 2019) requires understanding four axes: social organization, social structure, care system, and mating system (Kappeler, 2019). This work requires long-term efforts and can be particularly challenging in the marine realm; however, two of these axes, social structure and social organization, are feasible to measure, even with short-term studies.
The diverse Odontocete fauna of Galápagos represents a variety of unique social systems. Matrilineal species like short-finned pilot whales and killer whales (Denkinger et al., 2020) or species with high levels of fission-fusion social dynamics like common bottlenose dolphins and Risso’s dolphins are found in Galápagos. Studying their social organization, in terms of group size and composition, as well as their social structure, in terms of number and strength of social associations, will make for interesting comparisons with other oceanic islands where these species have been studied (Pinela et al., 2009; Hartman et al., 2015; Alves et al., 2019; Servidio et al., 2019; Dinis et al., 2021). Even the social structure of the well-studied sperm whale would benefit from continued investigation. Despite being widespread in deep waters (> 1000 m) around Galápagos (e.g., Palacios and Salazar, 2002; Palacios, 2003; Figures 2F and 3), sperm whale habitat use has shifted over the past four decades between the western, northern, and southern parts of the archipelago (Cantor et al., 2016; Eguiguren et al., 2019). These fluctuations possibly reflect different space use by socially distinct clans over time, but further studies are needed to elucidate their cultural and environmental drivers.
4.4.2 Methodological approaches
Basic information on social organization, such as group size (number of individuals estimated in the field and double-checked with photography) and composition (age and/or sex classes, at least for sexually dimorphic species: P. macrocephalus, O. orca), can always be recorded during both dedicated surveys and from opportunistic platforms (Table 2). Describing social structure, on the other hand, requires tracking social interactions or associations among individuals identified in groups over time (Whitehead, 2008). The need for recurrent re-sighting makes this effort more suitable for species that are reliably identifiable through standard photo-identification techniques and that are common in Galápagos, such as T. truncatus, P. macrocephalus, O. orca, and G. macrorhynchus (Table 2). As such data accumulate, a major goal will be to describe patterns of social structure, for instance subdivisions of the population into stable or more socially connected social communities (Weiss et al., 2021). For species living in medium to high levels of fission-fusion dynamics (e.g., T. truncatus), subdivision into communities can reflect important aspects of their ecological environment and the level of competition among individuals (e.g., quality and distribution of resources) and reveal key aspects of their social environment (e.g., social preferences and avoidances, spatial overlap, social clustering around behavioral or biological traits; e.g. Machado et al., 2019). For sperm whales, quantifying temporal stability, geographic range, behavioral variation, and acoustic communication will further reveal how social learning and cultural transmission influences the lives of individual whales and erects social barriers across sympatric clans (Rendell and Whitehead, 2003; Cantor and Whitehead, 2015; Cantor et al., 2015; Eguiguren et al., 2019; Eguiguren et al., 2021; Hersh et al., 2022). The delineation of sperm whale populations into culturally driven vocal clans is a key attribute of their population structure to be considered in regional and international conservation efforts to conserve this broadly roaming species (Brakes et al., 2019).
4.5 Priority V: Trophic ecology
4.5.1 Knowledge gap 10: Foraging and diet
Trophic interactions are central for mapping marine food web structures and understanding ecosystem functioning and dynamics, including nutrient cycling and energy flow. Empirical data on predator-resource interactions are the backbone of food web and ecosystem models, which are typically inferred from foraging observations and analysis of stomach contents and feces. For instance, undigested beaks in fecal samples of sperm whales provide a window into the inaccessible guild of demersal and mesopelagic cephalopods (Smith and Whitehead, 2000; Smith and Whitehead, 2001; Whitehead et al., 2001), and are a reliable proxy of foraging success (Whitehead and Rendell, 2004). In contrast to pinnipeds (Páez-Rosas et al., 2012; Urquía and Páez-Rosas, 2019), elasmobranchs (Páez-Rosas et al., 2018; Salinas-de-León et al., 2019), and seabirds (Jiménez-Uzcátegui et al., 2019), studies of diet in cetaceans in Galápagos have been rarely performed (Smith and Whitehead, 2000; Smith and Whitehead, 2001; Palacios et al., 2004). While fresh specimens (e.g., stomach contents) from stranding events are rarely available in Galápagos (Palacios et al., 2004), the establishment of basic protocols for sample collection from stranded cetaceans by designated and properly trained personnel could help fill this gap, while also informing monitoring and conservation strategies (e.g., Peltier et al., 2014).
Among the oceanic Delphinids occurring in Galápagos, ecologically similar species pairs have somewhat distinct distribution patterns, while mixed-species aggregations are relatively rare. For example, short-beaked common dolphins are predominant in the western and southern parts of the archipelago, while striped dolphins occur more often in the northwestern and northern part (Palacios and Salazar, 2002; Palacios, 2003; Figures 2C and 3). Similarly, Risso’s dolphins and short-finned pilot whales are both found in the deep waters surrounding the margins of the archipelago, but Risso’s dolphins appear to be more closely associated with the steep slopes of the western part of the archipelago (Palacios and Salazar, 2002; Palacios, 2003; Figures 2E and 3). These intriguing patterns suggest some level of habitat selection or niche partitioning, possibly mediated by foraging specializations (e.g., Whitehead et al., 2001; Quérouil et al., 2008) that deserve further study through methodologies such as those described below.
4.5.2 Methodological approaches
The investigation of the role of prey and predators in ecosystem dynamics can be largely expanded by biochemical tracer analyses (e.g., Boecklen et al., 2011; Pethybridge et al., 2018). Stable isotopes, fatty acid signatures, and other trace elements can complement our understanding of the role of cetaceans as both prey and predators. For instance, combining carbon and nitrogen stable isotopic analysis of bulk tissue and their constituent amino acids can reveal key insights on cetacean diet at the population level, and trophic position and interactions at the community level, over time (Teixeira et al., 2022). Such biomarkers can be accessed from stranded and preserved animals in scientific collections, but also from tissue samples of living animals (e.g., Teixeira et al., 2022). We suggest increased effort for collecting skin and blubber tissues of medium species (T. truncatus, G. griseus, G. macrorhynchus) and large species (B. musculus), both with remote biopsy systems and non-invasively, such as collecting sloughed skin of Odontocetes that frequently shed epithelial tissue (P. macrocephalus; e.g., Marcoux et al., 2007) (Table 2). Long-term data on biochemical tracers will provide a unique opportunity for assessing trends in resource use over time and space, and for relating these trends to environmental variation (e.g., El Niño Southern Oscillation; Arnés-Urgellés et al., 2021). Beyond contributing to mapping the local Galápagos food webs (e.g., Okey et al., 2004; Alava, 2009), this approach will also allow us to quantify trophic niche overlap between and within species (Enríquez-García et al., 2022), and investigate individual and ontogenetic variation in foraging, and the extent to which such variation is driven by resource partitioning and intra- and interspecific competition (e.g., Teixeira et al., 2022).
5 Discussion
The biologically productive waters surrounding the Galápagos Archipelago form a distinct oceanic insular ecosystem within the greater ETP. Our review confirmed that this ecosystem supports a large and diverse cetacean community, but beyond presence and distribution information, for most species not much more is known about habitat use, population structure, health, and social ecology. In contrast, significantly more efforts have been devoted to the study and monitoring of Galápagos invertebrates (e.g., Edgar et al., 2004; Edgar et al., 2008; Edgar et al., 2011), fishes (e.g., Schiller et al., 2014; Lamb et al., 2018; Salinas-de-León et al., 2019), pinnipeds (e.g., Páez-Rosas et al., 2012; Brock et al., 2013a; Brock et al., 2013b; Paéz-Rosas et al., 2016; Páez-Rosas et al., 2018; Urquía and Páez-Rosas, 2019; Páez-Rosas et al., 2021), and seabirds (e.g., Anchundia et al., 2014; Tompkins et al., 2017; Jiménez-Uzcátegui et al., 2019; Arauco-Shapiro et al., 2020). Admittedly, cetacean research is comparatively more expensive and more logistically demanding. However, at present, the study of cetaceans in Galápagos lags other tropical and subtropical oceanic insular ecosystems around the world, especially the Hawaiian (e.g., Baird et al., 2013b; Baird, 2016; Kratofil et al., 2023) and Macaronesian (e.g., Silva et al., 2014; Hartman et al., 2015; Fais et al., 2016; Tobeña et al., 2016; Alves et al., 2018; Alves et al., 2019; Romagosa et al., 2020; Dinis et al., 2021; Ferreira et al., 2021; Herrera et al., 2021) archipelagoes, where long-term studies have been generating significant new information in recent years. We acknowledge that, being located in the global north, the latter two ecosystems have benefited from established expertise, access to financial resources, and robust legislation mandating marine mammal research and protection that are generally not available in the global south. Further, as cetaceans are not considered extractive resources in Galápagos and additionally receive some level of legal protection throughout Ecuador (whales, at least), they are generally not “on the radar” of government entities in charge of marine resource management.
Given the logistical and funding challenges of conducting cetacean research in Galápagos, we suggest that the development of a collaborative research agenda should include specific actions aimed at establishing and strengthening meaningful partnerships between foreign and local researchers, and include the involvement of governmental and non-governmental entities, as well as stakeholders. Some existing examples of such partnerships include the long-term monitoring of cetacean presence using opportunistic sightings collected by naturalist guides working aboard cruise ships through a program implemented by the Galápagos National Park and the Charles Darwin Foundation (Palacios and Salazar, 2002; Denkinger et al., 2013; Denkinger et al., 2020), or the population assessment surveys for cetaceans conducted through a collaboration between U.S. academics and the Ecuadorian Navy through INOCAR, its institute for oceanographic and Antarctic research (Biggs et al., 2017; O’Hern et al., 2017). Continued partnership with the local tourism industry, in particular, would provide additional opportunities for participation by trained naturalists and citizen scientists in opportunistic but valuable data collection that would enhance and enrich research goals in a relatively inexpensive manner (e.g., Van Cise et al., 2021). An increased presence of researchers and observers in the field would have a side benefit related to the management of human activities in Galápagos by promoting better-guided ecotourism, better regulated vessel traffic, and enhanced surveillance and reporting of illegal fishing activities within the GMR (e.g., Cerutti-Pereyra et al., 2020; Bonaccorso et al., 2021; Global Fishing Watch, 2021).
Other aspects to be considered during development of a collaborative research agenda include capacity building and training of local researchers; optimization the research permit application required by the Galápagos National Park based on the specific needs and requirements cetacean research, including vessel operation and sample collection and export (if appropriate); and fostering the adoption of integrative data sampling and data sharing protocols. On the topic of data sharing, efforts can be optimized if research groups contribute to centralized repositories that provide the technological infrastructure, data access permissions, and terms of use. Such data repositories include those that are taxa-specific but otherwise accept a wide variety of data types (e.g., Halpin et al., 2006), as well as those specializing on photo-identification (e.g., Olson et al., 2020; Berger-Wolf et al., 2017) or tracking data (e.g., Block et al., 2016; Kays et al., 2022). Centralized repositories have the additional advantage of facilitating the curation of citizen science data; for instance, photographs from the public could be perused by specialists to verify species identification (e.g., Jarić et al., 2020), individual photo-identification (e.g., Cheeseman et al., 2021), and assessment of cutaneous and body conditions (e.g., Hart et al., 2012). Ultimately, these collective efforts will foster collaborative research, accelerate discovery, maximize the use of funds, promote the training of local scientists, and create opportunities for the professional development of early-career researchers as well as established scientists and conservation practitioners.
In conclusion, an improved understanding of Galápagos cetaceans will help quantify the ecological role that megafauna plays in connecting oceanic insular ecosystems (Boteler et al., 2022) and further establish the importance of marine reserves for transboundary conservation (e.g., Halpern, 2003; Game et al., 2009; Tetley et al., 2022). Indeed, in the marine realm, cetaceans are firm favorites for raising public awareness about the need for conservation actions (i.e., “flagship species”; Veríssimo et al., 2011) and for expanding these conservation actions to their habitats and ecological communities (“umbrella species”; Caro and O’Doherty, 1999). The information generated from our proposed approaches will also inform the management of the effects of climate change (Dueñas et al., 2021) and of human activities in Galápagos, including the impact of illegal fishing within the GMR, which not only can lead to direct negative interactions (such as megafauna bycatch; Alava et al., 2019; Cerutti-Pereyra et al., 2020) but also contribute to the overexploitation of marine resources (e.g., Usseglio et al., 2016; Bonaccorso et al., 2021). Avoiding such downstream cascading effects on Galápagos’ unique biota, environment, and socio-ecological systems (Denkinger and Vinueza, 2014; Walsh and Mena, 2016) will require tailored conservation plans that consider cetaceans as integral components of the ecosystem.
6 Positionality statement
We are biologists and academics in the fields of marine ecology, population ecology, and behavioral ecology, with a focus on marine mammals and other marine megafauna. We share a keen interest in the study of Galápagos cetaceans. As international scientists, we have a variety of experiences conducting cetacean research in Galápagos, but we are neither Ecuadorian citizens nor Galápagos residents. As such, we are not active participants in these communities, nor do we depend, economically or otherwise, from research conducted in Galápagos. We do share cultural aspects with the Ecuadorian people and have professional ties with its research community.
Born and raised in Colombia, DP is a marine ecologist and oceanographer with Ph.D. studies and post-doctoral appointments from institutions in the U.S., where he has resided for 27 years. He has participated in research expeditions in Galápagos in 1993-94, 2000, and 2005, and has additionally visited Galápagos on multiple occasions to participate in scientific workshops. DP is currently an Associate Professor at Oregon State University and remains actively involved in scientific research and conservation throughout Latin America.
MC Is a behavioral ecologist, born and raised in Brazil, who completed undergraduate and graduate degrees at Brazilian institutions before undertaking Ph.D. studies and post-doctoral appointments in universities in the global north. MC has been participating in research expeditions in Galápagos since 2013, starting with his Ph.D. research on the social ecology of sperm whales, as part of a multi-decadal collaboration between a Canadian university and several Ecuadorian and Galápagos institutions. MC is currently an Assistant Professor at Oregon State University in the U.S. and remains an active collaborator in Latin American research projects, including the long-term sperm whale research program in Galápagos.
Despite our collective experience in Galápagos, we acknowledge that we are still external actors. As such, our understanding of the priorities for research in the area is unintentionally influenced by our worldviews, which are themselves biased by our own social and cultural origins, privileges, academic trajectories, and personal experiences. For these reasons, we emphasize that our proposed priorities should be assessed by Ecuadorian and Galápagos governmental institutions in broad and inclusive consultation with stakeholders and the scientific community prior to development and implementation of a research agenda.
Author contributions
DP conceived the study, which was further developed in collaboration with MC. DP prepared Table 1 and Figures 1–5. MC prepared Table 2. DP and MC wrote the manuscript and contributed to revisions. All authors contributed to the article and approved the submitted version.
Funding
DP was supported by the Endowed Professorship in Whale Habitats at Oregon State University. MC was supported by the Marine Mammal Research Program Fund and the Jungers Faculty Development and Research Fund, the Marine Studies Initiative, and the College of Agricultural Sciences, all at Oregon State University.
Acknowledgments
DP is grateful to Iain Kerr, Kim Marshall-Tilas, and the Ocean Alliance for the opportunity to join the Galápagos 1993-94 Odyssey Expedition. We thank the many researchers who have carried out cetacean research in Galápagos, especially those who were involved in the collection of the published data used here (David Day, Godfrey Merlen, Fernando Félix, Sandie Salazar, Hal Whitehead). For our own past fieldwork, we thank the following Ecuadorian Government agencies: Ministerio de Defensa Nacional (DIGEIM), the Ministerio de Ambiente, Agua y Transición Ecológica (MAATE), and the Dirección del Parque Nacional Galápagos (DPNG) for the permits to operate in Ecuadorian waters and to carry out research within the Galápagos Marine Reserve. We also acknowledge the invaluable logistical support of the Charles Darwin Foundation through its Charles Darwin Research Station. We thank Michaela A. Kratofil, Aldo S. Pacheco, and Guido J. Parra for their constructive feedback on the draft manuscript.
Conflict of interest
The authors declare that the research was conducted in the absence of any commercial or financial relationships that could be construed as a potential conflict of interest.
Publisher’s note
All claims expressed in this article are solely those of the authors and do not necessarily represent those of their affiliated organizations, or those of the publisher, the editors and the reviewers. Any product that may be evaluated in this article, or claim that may be made by its manufacturer, is not guaranteed or endorsed by the publisher.
Footnotes
- ^ Rasmussen, K., and Palacios, D. M. Bryde’s whale (Balaenoptera edeni) aggregation area in the gulf of chiriqui, Panama. Rev. Biol. Trop.
References
Abecassis M., Polovina J., Baird R. W., Copeland A., Drazen J. C., Domokos R., et al. (2015). Characterizing a foraging hotspot for short-finned pilot whales and blainville’s beaked whales located off the west side of hawai’i island by using tagging and oceanographic data. PLoS One 10 (11), e0142628-22. doi: 10.1371/journal.pone.0142628
Acuerdo Ministerial No. 196 (1990). Ministerio de industrias (Corte Constitucional del Ecuador). Registro Oficial No. 458.
Acuerdo Ministerial No. MAATE-2022-019 (2022). Ministerio de ambiente, agua y transición ecológica. Corte Constitucional del Ecuador. Registro Oficial No. 32, 3–11.
Acuerdo Ministerial No. MAATE-2022-039 (2022). Ministerio de ambiente, agua y transición ecológica. Corte Constitucional del Ecuador 59, 2–12. Registro Oficial No.
Acuerdo Ministerial No. MAATE-2022-041 (2022). Ministerio de ambiente, agua y transición ecológica. Corte Constitucional del Ecuador 59, 17–31. Registro Oficial No.
Alava J. J. (2009). Carbon productivity and flux in the marine ecosystems of the Galapagos marine reserve based on cetacean abundances and trophic indices. Rev. Biol. Mar. Oceanogr. 44 (1), 109–122. doi: 10.4067/S0718-19572009000100010
Alava J. J., Ikonomou M. G., Ross P. S., Costa D., Salazar S., Aurioles-Gamboa D., et al. (2009). Polychlorinated biphenyls and polybrominated diphenyl ethers in Galapagos sea lions (Zalophus wollebaeki). Environ. Toxicol. Chem. 28 (11), 2271–2282. doi: 10.1897/08-331.1
Alava J. J., Paladines F. (2017). Illegal fishing on the galápagos high seas. Science 357, 1362.1–131362. doi: 10.1126/science.aap7832
Alava J. J., Tatar B., Barragán M. J., Castro C., Rosero P., Denkinger J., et al. (2019). Mitigating cetacean bycatch in coastal Ecuador: governance challenges for small-scale fisheries. Mar. Pol. 110, 102769. doi: 10.1016/j.marpol.2017.05.025
Alves F., Alessandrini A., Servidio A., Mendonça A. S., Hartman K. L., Prieto R., et al. (2019). Complex biogeographical patterns support an ecological connectivity network of a large marine predator in the north-east Atlantic. Divers. Distrib. 25 (2), 269–284. doi: 10.1111/ddi.12848
Alves F., Ferreira R., Fernandes M., Halicka Z., Dias L., Dinis A. (2018). Analysis of occurrence patterns and biological factors of cetaceans based on long-term and fine-scale data from platforms of opportunity: Madeira island as a case study. Mar. Ecol. 39 (2), e12499-13. doi: 10.1111/maec.12499
Anchundia D., Huyvaert K. P., Anderson D. J. (2014). Chronic lack of breeding by galápagos blue-footed boobies and associated population decline. Avian Conserv. Ecol. 9 (1), art6–ar15. doi: 10.5751/ace-00650-090106
Aragones L. V., Jefferson T. A., Marsh H. (1997). Marine mammal survey techniques applicable in developing countries. Asian Mar. Biol. 14, 15–39.
Arauco-Shapiro G., Schumacher K. I., Boersma D., Bouzat J. L. (2020). The role of demographic history and selection in shaping genetic diversity of the galápagos penguin (Spheniscus mendiculus). PloS One 15, e0226439. doi: 10.1371/journal.pone.0226439
Arnés-Urgellés C., Salinas-de-León P., Rastoin-Laplane E., Vaca-Pita L., Suárez-Moncada J., Páez-Rosas D. (2021). The effects of climatic variability on the feeding ecology of the scalloped hammerhead shark (Sphyrna lewini) in the tropical eastern pacific. Front. Mar. Sci. 8. doi: 10.3389/fmars.2021.625748
Awkerman J. A., Huyvaert K. P., Mangel J., Alfaro-Shigueto J., Anderson D. J. (2006). Incidental and intentional catch threatens galápagos waved albatross. Biol. Cons. 133 (4), 483–489. doi: 10.1016/j.biocon.2006.07.010
Baird R. W. (2016). The lives of hawai’i’s dolphins and whales (Honolulu, Hawai’i: University of Hawai’I Press), 341.
Baird R. W., Gorgone A. M., McSweeney D. J., Ligon A. D., Deakos M. H., Webster D. L., et al. (2009a). Population structure of island-associated dolphins: Evidence from photo-identification of common bottlenose dolphins (Tursiops truncatus) in the main Hawaiian islands. Mar. Mamm. Sci. 25, 251–274. doi: 10.1111/j.1748-7692.2008.00257.x
Baird R. W., Mahaffy S. D., Gorgone A. M., Cullins T., McSweeney D. J., Oleson E. M., et al. (2014). False killer whales and fisheries interactions in Hawaiian waters: Evidence for sex bias and variation among populations and social groups. Mar. Mamm. Sci. 31 (2), 579–590. doi: 10.1111/mms.12177
Baird R. W., Mahaffy S. D., Lerma J. K. (2022). Site fidelity, spatial use, and behavior of dwarf sperm whales in Hawaiian waters: using small-boat surveys, photo-identification, and unmanned aerial systems to study a difficult-to-study species. Mar. Mamm. Sci. 38 (1), 326–348. doi: 10.1111/mms.12861
Baird R. W., McSweeney D. J., Schorr G. S., Mahaffy S. D., Webster D. L., Barlow J., et al. (2009b). “Studies of beaked whales in hawai’i: population size, movements, trophic ecology, social 18rganization and behaviour,” in Proceedings of the ECS workshop “Beaked whale research”. Eds. Dolman S. J., MacLeod C. D., Evans P. G. H. (San Sebastián, Spain: the European Cetacean Society’s 21st Annual Conference), 23–25.
Baird R. W., Oleson E. M., Barlow J., Ligon A. D., Gorgone A. M., Mahaffy S. D. (2013a). Evidence of an island-associated population of false killer whales (Pseudorca crassidens) in the northwestern Hawaiian islands. Pac. Sci. 67, 513–521. doi: 10.2984/67.4.2
Baird R. W., Webster D. L., Aschettino J. M., Schorr G. S., McSweeney D. J. (2013b). Odontocete cetaceans around the main Hawaiian islands: Habitat use and relative abundance from small-boat sighting surveys. Aquat. Mamm. 39 (3), 253–269. doi: 10.1578/am.39.3.2013.253
Baird R. W., Webster D. L., Mahaffy S. D., McSweeney D. J., Schorr G. S., Ligon A. D. (2008). Site fidelity and association patterns in a deep-water dolphin: Rough-toothed dolphins (Steno bredanensis) in the Hawaiian archipelago. Mar. Mamm. Sci. 24, 535–553. doi: 10.1111/j.1748-7692.2008.00201.x
Ballance L. T., Gerrodette T., Lennert-Cody C. E., Pitman R. L., Squires D. (2021). A history of the tuna-dolphin problem: successes, failures, and lessons learned. Front. Mar. Sci. 8. doi: 10.3389/fmars.2021.754755
Becker E. A., Forney K. A., Miller D. L., Barlow J., Rojas-Bracho L., Urbán-R. J., et al. (2022). Dynamic habitat models reflect interannual movement of cetaceans within the California current ecosystem. Front. Mar. Sci. 9. doi: 10.3389/fmars.2022.829523
Becker E. A., Forney K. A., Redfern J. V., Barlow J., Jacox M. G., Roberts J. J., et al. (2019). Predicting cetacean abundance and distribution in a changing climate. Divers. Distrib. 25, 626–643. doi: 10.1111/ddi.12867
Berger-Wolf T. Y., Rubenstein D. I., Stewart C. V., Holmberg J. A., Parham J., Menon S., et al. (2017). Wildbook: Crowdsourcing, computer vision, and data science for conservation. doi: 10.48550/arXiv.1710.08880 (Accessed January 19, 2023).
Bermant P. C., Bronstein M. M., Wood R. J., Gero S., Gruber D. F. (2019). Deep machine learning techniques for the detection and classification of sperm whale bioacoustics. Sci. Rep. 9, 12588. doi: 10.1038/s41598-019-48909-4
Beslin W. A., Whitehead H., Gero S. (2018). Automatic acoustic estimation of sperm whale size distributions achieved through machine recognition of on-axis clicks. J. Acoust. Soc Am. 144, 3485–3495. doi: 10.1121/1.5082291
Best P. B., Photopoulou T. (2016). Identifying the “demon whale-biter”: Patterns of scarring on large whales attributed to a cookie-cutter shark isistius sp. PLoS One 11 (4), e0152643-20. doi: 10.1371/journal.pone.0152643
Biggs D. C., Durkacz S. M., Martin L. M., Narvaez M., de la Garza A., Lombraña Z., et al. (2017). Bryde’s whales (Balaneoptera brydei) in an area of upwelling off isla San cristóbal, galápagos. Neotrop. Biodivers. 3 (1), 189–195. doi: 10.1080/23766808.2017.1368309
Bierlich K., Schick R., Hewitt J., Dale J., Goldbogen J., Friedlaender A., et al. (2021). Bayesian Approach for predicting photogrammetric uncertainty in morphometric measurements derived from drones. Mar. Ecol. Prog. Ser. 673, 193–210. doi: 10.3354/meps13814
Block B. A., Holbrook C. M., Simmons S. E., Holland K. N., Ault J. S., Costa D. P., et al. (2016). Toward a national animal telemetry network for aquatic observations in the united states. Anim. Biotelemetry 4, 6. doi: 10.1186/s40317-015-0092-1
Boersma P. D., Vargas H., Merlen G. (2005). Living laboratory in peril. Science 308, 925. doi: 10.1126/science.1114395
Boecklen W. J., Yarnes C. T., Cook B. A., James A. C. (2011). On the use of stable isotopes in trophic ecology. Annu. Rev. Ecol. Evol. Syst. 42, 411–440. doi: 10.1146/annurev-ecolsys-102209-144726
Bonaccorso E., Ordóñez-Garza N., Pazmiño D. A., Hearn A., Páez-Rosas D., Cruz S., et al. (2021). International fisheries threaten globally endangered sharks in the Eastern tropical pacific ocean: the case of the fu Yuan yu leng 999 reefer vessel seized within the galápagos marine reserve. Sci. Rep. 11, 14959. doi: 10.1038/s41598-021-94126-3
Boteler B., Wagner D., Durussel C., Stokes E., Gaymer C. F., Friedlander A. M., et al. (2022). Borderless conservation: Integrating connectivity into high seas conservation efforts for the Salas y gómez and nazca ridges. Front. Mar. Sci. 9. doi: 10.3389/fmars.2022.915983
Bouchet P. J., Meeuwig J. J., Salgado Kent C. P., Letessier T. B., Jenner C. K. (2015). Topographic determinants of mobile vertebrate predator hotspots: current knowledge and future directions. Biol. Rev. 90, 699–728. doi: 10.1111/brv.12130
Bowen W. D. (1997). Role of marine mammals in aquatic ecosystems. Mar. Ecol. Progr. Ser. 158, 267–274. doi: 10.3354/meps158267
Boyd C., Punt A. E. (2021). Shifting trends: Detecting changes in cetacean population dynamics in shifting habitat. PloS One 16 (5), e0251522. doi: 10.1371/journal.pone.0251522
Brakes P., Dall S. R. X., Aplin L. M., Bearhop S., Carroll E. L., Ciucci P., et al. (2019). Animal cultures matter for conservation. Science 363, 1032–1034. doi: 10.1126/science.aaw3557
Brock P., Hall A., Goodman S. J., Cruz M., Acevedo-Whitehouse K. (2013a). Applying the tools of ecological immunology to conservation: a test case in the Galapagos sea lion. Anim. Conserv. 16, 19–31. doi: 10.1111/j.1469-1795.2012.00567.x
Brock P. M., Hall A. J., Goodman S. J., Cruz M., Acevedo-Whitehouse K. (2013b). Immune activity, body condition and human-associated environmental impacts in a wild marine mammal. PLoS One 8 (6), e67132. doi: 10.1371/journal.pone.0067132.s003
Buckland S. T., Anderson D. R., Burnham K. P., Laake J. L., Borchers D. L., Thomas L. (2001). Introduction to distance sampling (Oxford: Oxford University Press), 432.
Buckland S. T., Rexstad E., Marques T. A., Oedekoven C. S. (2015). Distance sampling: Methods and applications (Heidelberg: Springer).
Cambra M., Lara-Lizardi F., Peñaherrera-Palma C., Hearn A., Ketchum J. T., Zarate P., et al. (2021). A first assessment of the distribution and abundance of large pelagic species at Cocos ridge seamounts (Eastern tropical pacific) using drifting pelagic baited remote cameras. PloS One 16, e0244343. doi: 10.1371/journal.pone.0244343
Cantor M., Shoemaker L. G., Cabral R. B., Flores C. O., Varga M., Whitehead H. (2015). Multilevel animal societies can emerge from cultural transmission. Nat. Commun. 6, 8091. doi: 10.1038/ncomms9091
Cantor M., Whitehead H. (2015). How does social behavior differ among sperm whale clans? Mar. Mamm. Sci. 31, 1275–1290. doi: 10.1111/mms.12218
Cantor M., Eguiguren A., Merlen G., Whitehead H. (2017). Galápagos sperm whales (Physeter macrocephalus): waxing and waning over three decades. Can. J. Zool. 95 (9), 645–652. doi: 10.1139/cjz-2016-0266
Cantor M., Whitehead H., Gero S., Rendell L. (2016). Cultural turnover among galápagos sperm whales. R. Soc Open Sci. 3 (10), 160615. doi: 10.1098/rsos.160615
Capella J. J., Félix F., Florez-González L., Gibbons J., Haase B., Guzman H. M. (2018). Geographic and temporal patterns of non-lethal attacks on humpback whales by killer whales in the eastern south pacific and the Antarctic peninsula. Endanger. Species Res. 37, 207–218. doi: 10.3354/esr00924
Caro T. M., O’Doherty G. (1999). On the use of surrogate species in conservation biology. Conserv. Biol. 13, 805–814. doi: 10.1046/j.1523-1739.1999.98338.x
Castro C. A., Rasmussen K., Pacheco A. S., Cardenas D., Carnero-Huaman R., Echeverria G., et al. (2017). Bryde’s whale Balaenoptera edeni occurrence and movements in coastal 21ater off Ecuador, Peru and Panama. A preliminary report. paper SC/67a/SH/15 presented to the international whaling commission scientific committee annual meeting (Bled, Slovenia: International Whaling Commission), 11pp.
Centelleghe C., Carraro L., Gonzalvo J., Rosso M., Esposti E., Gili C., et al. (2020). The use of unmanned aerial vehicles (UAVs) 21aters21os the blow microbiome of small cetaceans. PLoS One 15 (7), e0235537. doi: 10.1371/journal.pone.0246177
Cerutti-Pereyra F., Moity N., Dureuil M., Ramírez-González J., Reyes H., Budd K., et al. (2020). Artisanal longline fishing the Galapagos islands–effects on vulnerable megafauna in a UNESCO world heritage site. Ocean Coast. Manage. 183, 104995. doi: 10.1016/j.ocecoaman.2019.104995
Cheeseman T., Southerland K., Park J., Olio M., Flynn K., Calambokidis J., et al. (2021). Advanced image recognition: a fully automated, high-accuracy photo-identification matching system for humpback whales. Mamm. Biol. 102, 915–929. doi: 10.1007/s42991-021-00180-9
Christiansen F., Dawson S. M., Durban J. W., Fearnbach H., Miller C. A., Bejder L., et al. (2020). Population comparison of right whale body condition reveals poor state of the north Atlantic right whale. Mar. Ecol. Prog. Ser. 640, 1–16. doi: 10.3354/meps13299
Correia A. M., Sousa-Guedes D., Gil Á., Valente R., Rosso M., Sousa-Pinto I., et al. (2021). Predicting cetacean distributions in the eastern north Atlantic to support marine management. Front. Mar. Sci. 8. doi: 10.3389/fmars.2021.643569
Corsi E., Calambokidis J., Flynn K. R., Steiger G. H. (2022). Killer whale predatory scarring on mysticetes: A comparison of rake marks among blue, humpback, and gray whales in the eastern north pacific. Mar. Mamm. Sci. 38 (1), 223–234. doi: 10.1111/mms.12863
Costa A. P. B., Archer F. I., Rosel P. E., Perrin W. F. (2022). Tursiops truncatus nuuanu, a new subspecies of the common bottlenose dolphin from the eastern tropical pacific. J. Mamm. Evol., 1–17. doi: 10.1007/s10914-022-09641-5
Davies T. E., Carneiro A. P. B., Campos B., Hazin C., Dunn D. C., Gjerde K. M., et al. (2021). Tracking data and the conservation of the high seas: Opportunities and challenges. J. Appl. Ecol. 58 (12), 2703–2710. doi: 10.1111/1365-2664.14032
Dawson S. M., Bowman M. H., Leunissen E., Sirguey P. (2017). Inexpensive aerial photogrammetry for studies of whales and large marine animals. Front. Mar. Sci. 4. doi: 10.3389/fmars.2017.00366
Dawson S., Wade P., Slooten E., Barlow J. (2008). Design and field methods for sighting surveys of cetaceans in coastal and riverine habitats. Mamm. Rev. 38 (1), 19–49. doi: 10.1111/j.1365-2907.2008.00119.x
Decreto Ejecutivo No. 319 (2022) Gobierno de la república del Ecuador. Available at: https://www.comunicacion.gob.ec/decreto-ejecutivo-no-319/.
Denkinger J., Alarcon D., Espinosa B., Fowler L., Manning C., Oña J., et al. (2020). Social structure of killer whales (Orcinus orca) in a variable low-latitude environment, the galápagos archipelago. Mar. Mamm. Sci. 36 (3), 774–785. doi: 10.1111/mms.12672
Denkinger J., Oña J., Alarcón D., Merlen G., Salazar S., Palacios D. M. (2013). “From whaling to whale watching: Cetacean presence and species diversity in the galápagos marine reserve,” in Science and conservation in the galápagos islands: Frameworks and perspectives, social and ecological interactions in the galápagos islands 1. Eds. Walsh S. J., Mena C. F. (New York, NY: Springer Science+Business Media, LCC), 217–235. doi: 10.1007/978-1-4614-5794-7_14
Denkinger J., Vinueza L. (2014). The Galapagos marine reserve: A dynamic social-ecological system (London: Springer).
Dinis A., Molina C., Tobeña M., Sambolino A., Hartman K., Fernandez M., et al. (2021). Large-Scale movements of common bottlenose dolphins in the Atlantic: dolphins with an international courtyard. PeerJ 9, e11069. doi: 10.7717/peerj.11069
Dueñas A., Jiménez-Uzcátegui G., Bosker T. (2021). The effects of climate change on wildlife biodiversity of the 22aters22os islands. Clim. Change. Ecol. 2, 100026. doi: 10.1016/j.ecochg.2021.100026
Dunn D. C., Harrison A.-L., Curtice C., DeLand S., Donnelly B., Fujioka E., et al. (2019). The importance of migratory connectivity for global ocean policy. Proc. R. Soc B 286, 20191472. doi: 10.1098/rspb.2019.1472
Edgar G. J., Banks S., Bensted-Smith R., Calvopiña M., Chiriboga A., Garske L. E., et al. (2008). Conservation of threatened species in the Galapagos marine reserve through identification and protection of marine key biodiversity areas. Aquat. Conserv.: Mar. Freshw. Ecosyst. 18, 955–968. doi: 10.1002/aqc.901
Edgar G. J., Banks S. A., Bessudo S., Cortés J., Guzmán H. M., Henderson S., et al. (2011). Variation in reef fish and invertebrate communities with level of protection from fishing across the Eastern tropical pacific seascape. Global Ecol. Biogeogr. 20, 730–743. doi: 10.1111/j.1466-8238.2010.00642.x
Edgar G. J., Banks S., Fariña J. M., Calvopiña M., Martínez C. (2004). Regional biogeography of shallow reef fish and macro-invertebrate communities in the Galapagos archipelago. J. Biogeogr. 31, 1107–1124. doi: 10.1111/j.1365-2699.2004.01055.x
Eguiguren A., Pirotta E., Boerder K., Cantor M., Merlen G., Whitehead H. (2021). Historical and contemporary habitat use of sperm whales around the galápagos archipelago: Implications for conservation. Aquat. Conserv.: Mar. Freshw. Ecosyst. 31 (6), 1466–1481. doi: 10.1002/aqc.3496
Eguiguren A., Pirotta E., Cantor M., Rendell L., Whitehead H. (2019). Habitat use of culturally distinct galápagos sperm whale Physeter macrocephalus clans. Mar. Ecol. Progr. Ser. 609, 257–270. doi: 10.3354/meps12822
Enríquez-García A. B., Villegas-Zurita F., Tripp-Valdez A., Moreno-Sánchez X. G., Galván-Magaña F., Elorriaga-Verplancken F. R., et al. (2022). Foraging segregation between spotted (Stenella attenuata) and spinner (Stenella longirostris) dolphins in the Mexican south pacific. Mar. Mamm. Sci. 38 (3), 1070–1087. doi: 10.1111/mms.12912
Estrade V., Dulau V. (2020). Abundance and site fidelity of bottlenose dolphins off a remote oceanic island (Reunion island, southwest Indian ocean). Mar. Mamm. Sci. 36 (3), 871–896. doi: 10.1111/mms.12693
Estes J. A., Tinker M. T., Williams T. M., Doak D. F. (1998). Killer whale predation on sea otters linking oceanic and nearshore ecosystems. Science 282, 473–476. doi: 10.1126/science.282.5388.473
Evans D. (1991). Ecuadorian Whale sanctuary is created providing protection for the galápagos islands. Ambio 20 (2), 97.
Fais A., Lewis T. P., Zitterbart D. P., Álvarez O., Tejedor A., Soto N. A. (2016). Abundance and distribution of sperm whales in the canary islands: Can sperm whales in the archipelago sustain the current level of ship-strike mortalities? PloS One 11 (3), e0150660-16. doi: 10.1371/journal.pone.0150660
Faustino C. E. S., Silva M. A., Marques T. A., Thomas L. (2010). Designing a shipboard line transect survey to estimate cetacean abundance off the Azores archipelago. Arquipelago (Life Mar. Sciences) 27, 49–58.
Félix F., Palacios D. M., Salazar S. K., Caballero S., Haase B., Falconí J. (2011). The 2005 galápagos humpback whale expedition: A first attempt to assess and characterize the population in the archipelago. J. Cetacean Res. Manage. Special Issue 3, 291–299.
Fernandez M., Alves F., Ferreira R., Fischer J., Thake P., Nunes N., et al. (2021). Modeling fine-scale cetaceans’ distributions in oceanic islands: Madeira archipelago as a case study. Front. Mar. Sci. 8. doi: 10.3389/fmars.2021.688248
Ferreira R., Dinis A., Badenas A., Sambolino A., Marrero-Pérez J., Crespo A., et al. (2021). Bryde’s whales in the north-East Atlantic: New insights on site fidelity and connectivity between oceanic archipelagos. Aquat. Conserv.: Mar. Freshw. Ecosyst. 31 (10), 2938–2950. doi: 10.1002/aqc.3665
Fiedler P. C., Lennert-Cody C. E. (2019). Seasonal and interannual variations in the distributions of tuna-associated dolphins in the eastern tropical pacific ocean. J. Cetacean Res. Manage. 20, 67–79. doi: 10.47536/jcrm.v20i1.240
Fiedler P. C., Redfern J. V., Forney K. A., Palacios D. M., Sheredy C., Rasmussen K., et al. (2018). Prediction of large whale distributions: a comparison of presence–absence and presence-only modeling techniques. Front. Mar. Sci. 5. doi: 10.3389/fmars.2018.00419
Figueroa D. (2021). Environmental forcing on zooplankton distribution in the coastal waters of the galápagos islands: spatial and seasonal patterns in the copepod community structure. Mar. Ecol. Progr. Ser. 661, 49–69. doi: 10.3354/meps13617
Flanders Marine Institute (2019). Maritime boundaries geodatabase. In: Maritime boundaries and exclusive economic zones (200NM), version 11. Available at: https://www.marineregions.org/ (Accessed 6 December 2022).
Fonseca C. T., Pérez-Jorge S., Prieto R., Oliveira C., Tobeña M., Scheffer A., et al. (2022). Dive behavior and activity patterns of fin whales in a migratory habitat. Front. Mar. Sci. 9. doi: 10.3389/fmars.2022.875731
Fontoura L., D’agata S., Gamoyo M., Barneche D. R., Luiz O. J., Madin E. M., et al. (2022). Protecting connectivity promotes successful biodiversity and fisheries conservation. Science 375, 336–340. doi: 10.1126/science.abg4351
Forryan A., Garabato A. C. N., Vic C., Nurser A. J. G., Hearn A. R. (2021). Galápagos upwelling driven by localized wind–front interactions. Sci. Rep. 11, 1277. doi: 10.1038/s41598-020-80609-2
Frasier K. E., Roch M. A., Soldevilla M. S., Wiggins S. M., Garrison L. P., Hildebrand J. A. (2017). Automated classification of dolphin echolocation click types from the gulf of Mexico. PloS Comput. Biol. 13, e1005823. doi: 10.1371/journal.pcbi.1005823
Game E. T., Grantham H. S., Hobday A. J., Pressey R. L., Lombard A. T., Beckley L. E., et al. (2009). Pelagic protected areas: the missing dimension in ocean conservation. Trends Ecol. Evol. 24, 360–369. doi: 10.1016/j.tree.2009.01.011
Gillespie D., Mellinger D. K., Gordon J., McLaren D., Redmond P., McHugh R., et al. (2009). PAMGUARD: Semiautomated, open source software for real-time acoustic detection and localisation of cetaceans. J. Acoust. Soc Am. 125, 2547. doi: 10.1121/1.4808713
Global Fishing Watch (2021) Analysis of the southeast pacific distant water squid fleet. GFW-2021-FA-SQUID2020. Available at: https://globalfishingwatch.org/research/squid-smarts-jumbo-squid-in-the-pacific-ocean/ (Accessed 5 December 2022).
Gove J. M., McManus M. A., Neuheimer A. B., Polovina J. J., Drazen J. C., Smith C. R., et al. (2016). Near-island biological hotspots in barren ocean basins. Nat. Commun. 7, 10581. doi: 10.1038/ncomms10581
Groch K. R., Díaz-Delgado J., Santos-Neto E. B., Ikeda J. M., Carvalho R. R., Oliveira R. B., et al. (2020). The pathology of cetacean morbillivirus infection and comorbidities in Guiana dolphins during an unusual mortality event (Brazil 2017–2018). Vet. Pathol. 57 (6), 845–857. doi: 10.1177/0300985820954550
Halpern B. S. (2003). The impact of marine reserves: do reserves work and does reserve size matter? Ecol. Appl. 13, 117–137. doi: 10.1890/1051-0761(2003)013[0117:TIOMRD]2.0.CO;2
Halpin P. N., Read A., Best B. D., Hyrenbach K. D., Fujioka E., Coyne M. S., et al. (2006). OBIS-SEAMAP: developing a biogeographic research data commons for the ecological studies of marine mammals, seabirds, and sea turtles. Mar. Ecol. Progr. Ser. 316, 239–246. doi: 10.3354/meps316239
Hamilton T. A., Redfern J. V., Barlow J., Ballance L. T., Gerrodette T., Holt R. S., et al. (2009). Atlas of cetacean sightings from southwest fisheries science center cetacean and ecosystem surveys: U.S. Department of Commerce, National Oceanic and Atmospheric Administration, National Marine Fisheries Service, Southwest Fisheries Science Center. 1986 – 2005. 70.
Hammond P. S., Francis T. B., Heinemann D., Long K. J., Moore J. E., Punt A. E., et al. (2021). Estimating the abundance of marine mammal populations. Front. Mar. Sci. 8. doi: 10.3389/fmars.2021.735770
Harnish A. E., Ault J., Babbitt C., Gulland F. M. D., Johnson P. C., Shaughnessy N. L., et al. (2019). Survival of a common bottlenose dolphin (Tursiops truncatus) calf with a presumptive gunshot wound to the head. Aquat. Mamm. 45 (5), 543–548. doi: 10.1578/AM.45.5.2019.543
Harris M. P. (1969). Breeding seasons of sea-birds in the Galapagos islands. J. Zool. 159, 145–165. doi: 10.1111/j.1469-7998.1969.tb03076.x
Hart L. B., Rotstein D. S., Wells R. S., Allen J., Barleycorn A., Balmer B. C., et al. (2012). Skin lesions on common bottlenose dolphins (Tursiops truncatus) from three sites in the Northwest Atlantic, USA. PLoS ONE 7, e33081. doi: 10.1371/journal.pone.0033081
Hartman K. L., Fernández M., Wittich A., Azevedo J. M. N. (2015). Sex differences in residency patterns of risso’s dolphins (Grampus griseus) in the Azores: Causes and management implications. Mar. Mamm. Sci. 31 (3), 1153–1167. doi: 10.1111/mms.12209
Hasegawa D., Lewis M. R., Gangopadhyay A. (2009). How islands cause phytoplankton to bloom in their wakes. Geophys. Res. Lett. 36 (20), L20605. doi: 10.1029/2009gl039743
Hearn A., Ketchum J., Klimley A. P., Espinoza E., Peñaherrera C. (2010). Hotspots within hotspots? hammerhead shark movements around wolf island, Galapagos marine reserve. Mar. Biol. 157 (9), 1899–1915. doi: 10.1007/s00227-010-1460-2
Herrera I., Carrillo M., Esteban M. C., Haroun R. (2021). Distribution of cetaceans in the canary islands (northeast Atlantic ocean): implications for the natura 2000 network and future conservation measures. Front. Mar. Sci. 8. doi: 10.3389/fmars.2021.669790
Hersh T. A., Gero S., Rendell L., Cantor M., Weilgart L., Amano M., et al. (2022). Evidence from sperm whale clans of symbolic marking in non-human cultures. Proc. Natl. Acad. Sci. U.S.A. 119 (37), e2201692119. doi: 10.1073/pnas.220169211
Heylings P., Bensted-Smith R., Altamirano M. (2002). “Zonificación e historia de la reserva Marina de galápagos,” in Reserva Marina de galápagos, linea base de la bioversidad. Eds. Danulat E., Edgar G. J. (Santa Cruz, Galapagos, Ecuador: Fundación Charles Darwin/Servicio Parque Nacional Galápagos), 10–21.
Hindell M. A., Reisinger R. R., Ropert-Coudert Y., Hückstädt L. A., Trathan P. N., Bornemann H., et al. (2020). Tracking of marine predators to protect southern ocean ecosystems. Nature 580, 87–92. doi: 10.1038/s41586-020-2126-y
Hope P. L., Whitehead H. (1991). Sperm whales off the galápagos islands from 1830–50 and comparisons with modern studies. Rep. Int. Whal. Commn. 41, 273–283.
Horton T. W., Hauser N., Cassel S., Klaus K. F., Fettermann T., Key N. (2019). Doctor drone: Non-invasive measurement of humpback whale vital signs using unoccupied aerial system infrared thermography. Front. Mar. Sci. 6. doi: 10.3389/fmars.2019.00466
Houvenaghel G. T. (1978). “Oceanographic conditions in the galápagos archipelago and their relationships with life on the islands,” in Upwelling ecosystems. Eds. Boje R., Tomczak M. (Berlin: Springer-Verlag), 181–200.
Hucke-Gaete R., Bedrinana-Romano L., Viddi F. A., Ruiz J. E., Torres-Florez J. P., Zerbini A. N. (2018). From Chilean Patagonia to Galapagos, Ecuador: novel insights on blue whale migratory pathways along the Eastern south pacific. PeerJ 6, e4695. doi: 10.7717/peerj.4695
IUCN-MMPATF (2022). Galápagos archipelago IMMA. In: Marine mammal protected areas task force (MMPATF). Available at: https://www.marinemammalhabitat.org/portfolio-item/galapagos-archipelago-imma/ (Accessed 27 November 2022).
Izurieta A., Delgado B., Moity N., Calvopiña M., Cedeño I., Banda-Cruz G., et al. (2018). A collaboratively derived environmental research agenda for galápagos. Pac. Conserv. Biol. 24 (2), 207–207. doi: 10.1071/pc17053_co
Jacquet J., Alava J. J., Pramod G., Henderson S., Zeller D. (2008). In hot soup: sharks captured in ecuador’s waters. Env. Sci. 5 (4), 269–283. doi: 10.1080/15693430802466325
Jarić I., Correia R. A., Brook B. W., Buettel J. C., Courchamp F., Di Minin E., et al. (2020). iEcology: harnessing large online resources to generate ecological insights. Trends Ecol. Evol. 35, 630–639. doi: 10.1016/j.tree.2020.03.003
Jiménez-Uzcátegui G., Vaca L., Cotín J., García C., Costales A., Sevilla C., et al. (2019). Using referential values of δ13C and δ15N to infer the foraging ecology of galápagos seabirds. Mar. Ornithol. 47, 5–10.
Joblon M. J., Pokras M. A., Morse B., Harry C. T., Rose K. S., Sharp S. M., et al. (2014). Body condition scoring system for delphinids based on short-beaked common dolphins (Delphinus delphis). J. Mar. Anim. Ecol. 7 (2), 5–13.
Kappeler P. M. A. (2019). A framework for studying social complexity. Behav. Ecol. Sociobiol. 73, 13. doi: 10.1007/s00265-018-2601-8
Kays R., Davidson S. C., Berger M., Bohrer G., Fiedler W., Flack A., et al. (2022). The movebank system for studying global animal movement and demography. Methods Ecol. Evol. 13 (2), 419–431. doi: 10.1111/2041-210X.13767
Ketchum J. T., Hoyos-Padilla M., Aldana-Moreno A., Ayres K., Galván-Magaña F., Hearn A., et al. (2020). Shark movement patterns in the Mexican pacific: A conservation and management perspective. Adv. Mar. Biol. 85, 1–37. doi: 10.1016/bs.amb.2020.03.002
Kiszka J. J., Heithaus M. R., Wirsing A. J. (2015). Behavioural drivers of the ecological roles and importance of marine mammals. Mar. Ecol. Progr. Ser. 523, 267–281. doi: 10.3354/meps11180
Kiszka J. J., Méndez-Fernandez P., Heithaus M. R., Ridoux V. (2014). The foraging ecology of coastal bottlenose dolphins based on stable isotope mixing models and behavioural sampling. Mar. Biol. 161, 953–961. doi: 10.1007/s00227-014-2395-9
Kiszka J. J., Woodstock M. S., Heithaus M. R. (2022). Functional roles and ecological importance of small cetaceans in aquatic ecosystems. Front. Mar. Sci. 9. doi: 10.3389/fmars.2022.803173
Klimley A. P., Arauz R., Bessudo S., Chávez E. J., Chinacalle N., Espinoza E., et al. (2022). Studies of the movement ecology of sharks justify the existence and expansion of marine protected areas in the Eastern pacific ocean. Environ. Biol. Fish. 105, 2133–2153. doi: 10.1007/s10641-021-01204-6
Kotik C., Durban J. W., Fearnbach H., Barrett-Lennard L. G. (2022). Morphometrics of mammal-eating killer whales from drone photogrammetry, with comparison to sympatric fish-eating killer whales in the eastern north pacific. Mar. Mamm. Sci. 39 (1), 42–58. doi: 10.1111/mms.12965
Kratofil M. A., Harnish A. E., Mahaffy S. D., Henderson E. E., Bradford A. L., Martin S. W., et al. (2023). Biologically important areas II for cetaceans within U.S. and adjacent waters – Hawaiʻi Region. Front. Mar. Sci. 10, 1053581. doi: 10.3389/fmars.2023.1053581
Lambert C., Authier M., Dorémus G., Gilles A., Hammond P., Laran S., et al. (2019). The effect of a multi-target protocol on cetacean detection and abundance estimation in aerial surveys. R. Soc Open Sci. 6, 190296. doi: 10.1098/rsos.190296
Lamb R. W., Smith F., Aued A. W., Salinas-de-León P., Suarez J., Gomez-Chiarri M., et al. (2018). El Niño drives a widespread ulcerative skin disease outbreak in Galapagos marine fishes. Sci. Rep. 8, 16602. doi: 10.1038/s41598-018-34929-z
Llapapasca M. A., Pacheco A. S., Fiedler P., Goya E., Ledesma J., Peña C., et al. (2018). Modeling the potential habitats of dusky, commons and bottlenose dolphins in the Humboldt current system off Peru: The influence of non-El niño vs. El niño 1997-98 conditions and potential prey availability. Prog. Oceanogr. 168, 169–181. doi: 10.1016/j.pocean.2018.09.003
Lyrholm T., Kerr I., Galley L., Payne R. (1992). “Report of the “Expedición siben”, Ecuador 1988/89,” in Final report submitted by the Whale Conservation Institute to the Charles Darwin research station and the galápagos national park service (Puerto Ayora, Islas Galápagos, Ecuador: Whale Conservation Institute), 38.
Machado A. M. S., Cantor M., Costa A. P., Righetti B. P., Bezamat C., Valle-Pereira J., et al. (2019). Homophily around specialized foraging underlies dolphin social preferences. Biol. Lett. 15 (4), 20180909. doi: 10.1098/rsbl.2018.0909
MacLeod C. D., Mitchell G. (2006). Key areas for beaked whales worldwide. J. Cetacean Res. Manage. 7 (3), 309–322.
Mann J., Connor R. C., Tyack P. L., Whitehead H. (Eds.) (2000). Cetacean societies: Field studies of dolphins and whales (Chicago: University of Chicago Press).
Marcoux M., Whitehead H., Rendell L. (2007). Sperm whale feeding variation by location, year, social group and clan: Evidence from stable isotopes. Mar. Ecol. Progr. Ser. 333, 309–314. doi: 10.3354/meps333309
Martin A. H., Pearson H. C., Saba G. K., Olsen E. M. (2021). Integral functions of marine vertebrates in the ocean carbon cycle and climate change mitigation. One Earth 4 (5), 680–693. doi: 10.1016/j.oneear.2021.04.019
McKinley S. J., Saunders B. J., Rastoin-Laplane E., Salinas-de-León P., Harvey E. S. (2022). Functional diversity of reef fish assemblages in the Galapagos archipelago. J. Exp. Mar. Biol. Ecol. 549, 151695. doi: 10.1016/j.jembe.2022.151695
Mellinger D. K., Stafford K. M., Moore S. E., Dziak R. P., Matsumoto H. (2007). An overview of fixed passive acoustic observation methods for cetaceans. Oceanography 20 (4), 36–45. doi: 10.5670/oceanog.2007.03
Mendelssohn R., Bograd S. J., Schwing F. B., Palacios D. M. (2005). Teaching old indices new tricks: A state-space analysis of El niño related climate indices. Geophys. Res. Lett. 32, L07709. doi: 10.1029/2005gl022350
Merlen G. (1995). A field guide to the marine mammals of Galapagos (Guayaquil, Ecuador: Instituto Nacional de Pesca), 130.
Neave E. F., Seim H., Gifford S. M., Torano O., Johnson Z. I., Páez-Rosas D., et al. (2021). Protistan plankton communities in the galápagos archipelago respond to changes in deep water masses resulting from the 2015/16 El niño. Environ. Microbiol. 24, 1746–1759. doi: 10.1111/1462-2920.15863
O’Hern J. E., Sculley M., Jean-Smith K., Biggs D., Slowey N., Ruales D. A., et al. (2017). Marine mammal distribution in Ecuador: surveys aboard a ship of opportunity as a means of monitoring relative abundance. Lat. Am. J. Aquat. Mamm. 12 (1-2), 28–39. doi: 10.5597/lajam00235
Okey T. A., Banks S., Born A. F., Bustamante R. H., Calvopiña M., Edgar G. J., et al. (2004). A trophic model of a galápagos subtidal rocky reef for evaluating fisheries and conservation strategies. Ecol. Modell. 172 (2–4), 383–401. doi: 10.1016/j.ecolmodel.2003.09.019
Olson P. A., Blount D., Cheeseman T., Holmberg J., Minton G. (2020). A cross-reference of flukebook and happywhale platforms (International Whaling Commission Scientific Committee Annual Meeting), 11pp.
Oremus M., Poole M. M., Albertson G. R., Baker C. S. (2012). Pelagic or insular? genetic differentiation of rough-toothed dolphins in the society islands, French Polynesia. J. Exp. Mar. Biol. Ecol. 432–433, 37–46. doi: 10.1016/j.jembe.2012.06.027
Pacheco A. S., Castro C., Carnero-Huaman R., Villagra D., Pinilla S., Denkinger J., et al. (2019). Sightings of an adult male killer whale match humpback whale breeding seasons in both hemispheres in the eastern tropical pacific. Aquat. Mamm. 45 (3), 320–326. doi: 10.1578/am.45.3.2019.320
Páez-Rosas D., Aurioles-Gamboa D., Alava J. J., Palacios D. M. (2012). Stable isotopes indicate differing foraging strategies in two sympatric otariids of the Galapagos islands. J. Exp. Mar. Biol. Ecol. 424, 44–52. doi: 10.1016/j.jembe.2012.05.001
Paéz-Rosas D., Hirschfeld M., Deresienski D., Lewbart G. A. (2016). Health status of galápagos sea lions (Zalophus wollebaeki) on San cristóbal island rookeries determined by hematology, biochemistry, blood gases, and physical examination. J. Wildl. Dis. 52, 100–105. doi: 10.7589/2015-04-084
Páez-Rosas D., Insuasti-Zarate P., Riofrío-Lazo M., Galván-Magaña F. (2018). Feeding behavior and trophic interaction of three shark species in the Galapagos marine reserve. PeerJ 6 (8), e4818–e4821. doi: 10.7717/peerj.4818
Páez-Rosas D., Torres J., Espinoza E., Marchetti A., Seim H., Riofrío-Lazo M. (2021). Declines and recovery in endangered Galapagos pinnipeds during the El niño event. Sci. Rep. 11, 8785. doi: 10.1038/s41598-021-88350-0
Palacios D. M. (1996). On the specimen of the ginkgo-toothed beaked whale, Mesoplodon ginkgodens, from the galápagos islands. Mar. Mamm. Sci. 12 (3), 444–446. doi: 10.1111/j.1748-7692.1996.tb00596.x
Palacios D. M. (1999a). “Marine mammal research in the Galápagos Islands: The 1993-94 Odyssey expedition” Final report submitted by the Whale Conservation Institute to galápagos national park service and Charles Darwin research station(Puerto Ayora, Is. Galápagos, Ecuador), 6.
Palacios D. M. (1999b). Blue whale (Balaenoptera musculus) occurrence off the galápagos islands 1978-1995. J. Cetacean Res. Manage. 1 (1), 41–51.
Palacios D. M. (2000). “GalCet2K: A line-transect survey for cetaceans across an environmental gradient off the galápagos islands, 5–19 April 2000,” in Final report submitted to Ocean Alliance to Galápagos National Park Service, Charles Darwin Research Station, Capitanía de Puerto Ayora, and Dirección General de Intereses Marítimos de la Armada Naciona Puerto ayora, and dirección general de intereses marítimos de la armada nacional(Puerto Ayora, Is. Galápagos, Ecuador: Ocean Alliance), 9.
Palacios D. M. (2003). Oceanographic conditions around the Galápagos Archipelago and their influence on cetacean community structure. (Corvallis (OR): Oregon State University http://ir.library.oregonstate.edu/concern/graduate_thesis_or_dissertations/xp68kk78c
Palacios D. M. (2004). Seasonal patterns of sea-surface temperature and ocean color around the galápagos: regional and local influences. Deep-Sea Res. II: Top. Stud. Oceanogr. 51 (1-3), 43–57. doi: 10.1016/j.dsr2.2003.08.001
Palacios D. M., Bograd S. J., Foley D. G., Schwing F. B. (2006). Oceanographic characteristics of biological hot spots in the north pacific: A remote sensing perspective. Deep-Sea Res. II: Top. Stud. Oceanogr. 53 (3-4), 250–269. doi: 10.1016/j.dsr2.2006.03.004
Palacios D. M., Forney K. A. (2008). Cetacean abundance on the western side of the galápagos islands during April 2000. SC/60/SM7 (Santiago, Chile: International Whaling Commission Scientific Committee), 1–12.
Palacios D. M., Salazar S. (2002). “Cetáceos,” in Reserva Marina de galápagos, línea base de la biodiversidad. Eds. Danulat E., Edgar G. J. (Santa Cruz, Galápagos, Ecuador: Fundación Charles Darwin/Servicio Parque Nacional Galápagos), 291–304.
Palacios D. M., Salazar S. K., Day D. (2004). Cetacean remains and strandings in the galápagos islands 1923-2003. Lat. Am. J. Aquat. Mamm. 3, 127–150. doi: 10.5597/lajam00058
Panicker D., Baumgartner M., Stafford K. (2022). Fine-scale spatial and temporal acoustic occurrence of island-associated odontocetes near a mid-oceanic atoll in the northern Indian ocean. Mar. Ecol. Prog. Ser. 683, 195–208. doi: 10.3354/meps13947
Panigada S., Lauriano G., Burt L., Pierantonio N., Donovan G. (2011). Monitoring winter and summer abundance of cetaceans in the pelagos sanctuary (Northwestern Mediterranean Sea) through aerial surveys. PLoS One 6, e22878. doi: 10.1371/journal.pone.0022878.t003
Pearson H. C., Savoca M. S., Costa D. P., Lomas M. W., Molina R., Pershing A. J., et al. (2022). Whales in the carbon cycle: can recovery remove carbon dioxide? Trends Ecol. Evol. 3074, 1–12. doi: 10.1016/j.tree.2022.10.012
Peltier H., Jepson P. D., Dabin W., Deaville R., Daniel P., Canneyt O. V., et al. (2014). The contribution of stranding data to monitoring and conservation strategies for cetaceans: Developing spatially explicit mortality indicators for common dolphins (Delphinus delphis) in the eastern north-Atlantic. Ecol. Indic. 39, 203–214. doi: 10.1016/j.ecolind.2013.12.019
Pethybridge H. R., Choy C. A., Polovina J. J. (2018). Improving marine ecosystem models with biochemical tracers. Ann. Rev. Mar. Sci. 10, 199–228. doi: 10.1146/annurev-marine-121916-063256
Pinheiro H. T., Bernardi G., Simon T., Joyeux J. C., Macieira R. M., Gasparini J. L., et al. (2017). Island biogeography of marine organisms. Nature 549, 82–85. doi: 10.1038/nature23680
Pinela A. M., Quérouil S., Magalhes S., Silva M. A., Prieto R., Matos J. A., et al. (2009). Population genetics and social organization of the sperm whale (Physeter macrocephalus) in the Azores inferred by microsatellite analyses. Can. J. Zoology 87 (9), 802–813. doi: 10.1139/z09-066
Polanowski A. M., Robbins J., Chandler D., Jarman S. N. (2014). Epigenetic estimation of age in humpback whales. Mol. Ecol. Resour. 14 (5), 976–987. doi: 10.1111/1755-0998.12247
Pollock K. H. (1982). A capture-recapture design robust to unequal probability of capture. J. Wildl. Manage. 46, 752–757. doi: 10.2307/3808568
Prieto R., Silva M. A., Waring G. T., Gonçalves J. (2014). Sei whale movements and behaviour in the north Atlantic inferred from satellite telemetry. Endanger. Species Res. 26 (2), 103–113. doi: 10.3354/esr00630
Quérouil S., Kiszka J., Cordeiro A. R., Cascão I., Freitas L., Dinis A., et al. (2013). Investigating stock structure and trophic relationships among island-associated dolphins in the oceanic waters of the north Atlantic using fatty acid and stable isotope analyses. Mar. Biol. 160 (6), 1325–1337. doi: 10.1007/s00227-013-2184-x
Quérouil S., Silva M. A., Cascão I., Magalhães S., Seabra M. I., Machete M. A., et al. (2008). Why do dolphins form mixed-species associations in the Azores? Ethology 114, 1183–1194. doi: 10.1111/j.1439-0310.2008.01570.x
Raoult V., Colefax A. P., Allan B. M., Cagnazzi D., Castelblanco-Martínez N., Ierodiaconou D., et al. (2020). Operational protocols for the use of drones in marine animal research. Drones 4, 64. doi: 10.3390/drones4040064
Registro Oficial (2000). “Corte constitucional del Ecuador,” in Registro oficial no. 5 del 28 de enero del 2000.
Registro Oficial (2002). “Corte constitucional del Ecuador,” in Registro oficial no. 679 del 8 de octubre de 2002.
Remili A., Gallego P., Pinzone M., Castro C., Jauniaux T., Garigliany M.-M., et al. (2020). Humpback whales (Megaptera novaeangliae) breeding off Mozambique and Ecuador show geographic variation of persistent organic pollutants and isotopic niches. Environ. pollut. 267, 115575. doi: 10.1016/j.envpol.2020.115575
Rendell L. E., Whitehead H. (2003). Vocal clans in sperm whales (Physeter macrocephalus). Proc. R. Soc B: Biol. Sci. 270 (1512), 225–231. doi: 10.1098/rspb.2002.2239
Rendell L., Cantor M., Gero S., Whitehead H., Mann J. (2019). Causes and consequences of female centrality in cetacean societies. Philos. Trans. R. Soc B: Biol. Sci. 374, 20180066. doi: 10.1098/rstb.2018.0066
Rooker J. R., Dance M. A., Wells R. D., Ajemian M. J., Block B. A., Castleton M. R., et al. (2019). Population connectivity of pelagic megafauna in the Cuba-Mexico-United states triangle. Sci. Rep. 9, 1663. doi: 10.1038/s41598-018-38144-8
Romagosa M., Baumgartner M., Cascão I., Lammers M. O., Marques T. A., Santos R. S., et al. (2020). Baleen whale acoustic presence and behaviour at a mid-Atlantic migratory habitat, the Azores archipelago. Sci. Rep. 10 (1), 4766. doi: 10.1038/s41598-020-61849-8
Ruiz D. J., Banks S., Wolff M. (2016). Elucidating fishing effects in a large-predator dominated system: The case of Darwin and wolf islands (Galápagos). J. Sea Res. 107, 1–11. doi: 10.1016/j.seares.2015.11.001
Ruttenberg B. I. (2001). Effects of artisanal fishing on marine communities in the galápagos islands. Conserv. Biol. 15, 1691–1699. doi: 10.1046/j.1523-1739.2001.99556.x
Sahri A., Putra M. I. H., Mustika P. L. K., Kreb D., Murk A. J. (2021). Cetacean habitat modelling to inform conservation management, marine spatial planning, and as a basis for anthropogenic threat mitigation in Indonesia. Ocean Coast. Manage. 205, 105555. doi: 10.1016/j.ocecoaman.2021.105555
Salinas-de-León P., Fierro-Arcos D., Suarez-Moncada J., Proaño A., Guachisaca-Salinas J., Páez-Rosas D. (2019). A matter of taste: Spatial and ontogenetic variations on the trophic ecology of the tiger shark at the Galapagos marine reserve. PLoS One 14 (9), e0222754. doi: 10.1371/journal.pone.0222754
Sambolino A., Alves F., Fernandez M., Krakauer A. B., Ferreira R., Dinis A. (2022). Spatial and temporal characterization of the exposure of island-associated cetacean populations to whale-watching in Madeira island (NE Atlantic). Reg. Stud. Mar. Sci. 49, 102084. doi: 10.1016/j.rsma.2021.102084
Schaeffer B. A., Morrison J. M., Kamykowski D., Feldman G. C., Xie L., Liu Y., et al. (2008). Phytoplankton biomass distribution and identification of productive habitats within the Galapagos marine reserve by MODIS, a surface acquisition system, and in-situ measurements. Remote Sens. Environ. 112 (6), 3044–3054. doi: 10.1016/j.rse.2008.03.005
Schiller L., Alava J. J., Grove J., Reck G., Pauly D. (2014). The demise of darwin’s fishes: evidence of fishing down and illegal shark finning in the galápagos islands. Aquat. Conserv.: Mar. Freshw. Ecosyst. 25 (3), 431–446. doi: 10.1002/aqc.2458
Servidio A., Pérez-Gil E., Pérez-Gil M., Cañadas A., Hammond P. S., Martín V. (2019). Site fidelity and movement patterns of short-finned pilot whales within the canary islands: Evidence for resident and transient populations. Aquat. Conserv.: Mar. Freshw. Ecosyst. 29 (S1), 227–241. doi: 10.1002/aqc.3135
Shuster G. W. (1983). The Galapagos islands: a preliminary study of the effects of sperm whaling on a specific whaling ground. Rep. Int. Whal Commn Special Issue 5, 81–82.
Silva M. A., Prieto R., Cascão I., Seabra M. I., Machete M., Baumgartner M., et al. (2014). Spatial and temporal distribution of cetaceans in the mid-Atlantic waters around the Azores. Mar. Biol. Res. 10 (2), 123–137. doi: 10.1080/17451000.2013.793814
Silva M. A., Prieto R., Jonsen I., Baumgartner M. F., Santos R. S. (2013). North Atlantic blue and fin whales suspend their spring migration to forage in middle latitudes: building up energy reserves for the journey? PloS One 8 (10), e76507. doi: 10.1371/journal.pone.0076507.s004
Silver-Gorges I., Koval J., Rodriguez-Zarate C. J., Paladino F. V., Jordan M. (2020). Large-Scale connectivity, cryptic population structure, and relatedness in Eastern pacific olive ridley sea turtles (Lepidochelys olivacea). Ecol. Evol. 10, 8688–8704. doi: 10.1002/ece3.6564
Smith S. D., Whitehead H. (1999). Distribution of dolphins in galápagos waters. Mar. Mamm. Sci. 15 (2), 550–555. doi: 10.1111/j.1748-7692.1999.tb00819.x
Smith S. C., Whitehead H. (2000). The diet of Galapagos sperm whales Physeter macrocephalus as indicated by fecal sample analysis. Mar. Mamm. Sci. 16, 315–325. doi: 10.1111/j.1748-7692.2000.tb00927.x
Smith S. C., Whitehead H. (2001). Reply to r. Clarke and paliza’s comment: “The food of sperm whales in the southeast pacific”. Mar. Mamm. Sci. 17, 430–431. doi: 10.1111/j.1748-7692.2001.tb01288.x
Soares E. D., Cantor M., Bracarense A. P. F. R. L., Groch K. R., Domit C. (2022). Health conditions of Guiana dolphins facing cumulative anthropogenic impacts. Mamm. Biol., 1–16. doi: 10.1007/s42991-022-00299-3
Sonnenholzner J., Ladah L., Lafferty K. (2009). Cascading effects of fishing on Galapagos rocky reef communities: reanalysis using corrected data. Mar. Ecol. Prog. Ser. 375, 209–218. doi: 10.3354/meps07890
Sweet W. V., Morrison J. M., Kamykowski D., Schaeffer B. A., Banks S., McCulloch A. (2007). Water mass seasonal variability in the galápagos archipelago. Deep-Sea Res. I: Oceanogr. Res. Pap. 54 (12), 2023–2035. doi: 10.1016/j.dsr.2007.09.009
Tardin R. H., Chun Y., Simão S. M., Alves M. (2017). Modeling habitat use by bryde’s whale Balaenoptera edeni off southeastern Brazil. Mar. Ecol. Progr. Ser. 576, 89–103. doi: 10.3354/meps12228
Tardin R. H., Chun Y., Simão S. M., Alves M. A. S. (2019). Habitat use models of spatially auto-correlated data: a case study of the common bottlenose dolphin, Tursiops truncatus truncatus, in southeastern Brazil. Mar. Biol. Res. 15, 305–316. doi: 10.1080/17451000.2019.1644773
Taylor B. L., Martinez M., Gerrodette T., Barlow J., Hrovat Y. N. (2007). Lessons from monitoring trends in abundance of marine mammals. Mar. Mamm. Sci. 23 (1), 157–175. doi: 10.1111/j.1748-7692.2006.00092.x
Tetley M. J., Braulik G. T., Lanfredi C., Minton G., Panigada S., Politi E., et al. (2022). The important marine mammal area network: a tool for systematic spatial planning in response to the marine mammal habitat conservation crisis. Front. Mar. Sci. 9. doi: 10.3389/fmars.2022.841789
Thomas L., Williams R., Sandilands D. (2007). Survey design for complex survey regions. J. Cetacean Res. Manage. 9 (1), 1–13.
Thorne L. H., Johnston D. W., Urban D. L., Tyne J., Bejder L., Baird R. W., et al. (2012). Predictive modeling of spinner dolphin (Stenella longirostris) resting habitat in the main Hawaiian islands. PloS One 7 (8), e43167. doi: 10.1371/journal.pone.0043167.t003
Tobeña M., Prieto R., Machete M., Silva M. A. (2016). Modeling the potential distribution and richness of cetaceans in the Azores from fisheries observer program data. Front. Mar. Sci. 3. doi: 10.3389/fmars.2016.00202
Todd V. L. G., Grove J. S. (2010). First records of golden trevally (Gnathodon speciosus, carangidae), sharp-tail mola (Masturus lanceolatus, molidae) and evidence for white shark (Carcharodon carcharias, lamnidae) in the galápagos islands, Ecuador. Mar. Biodivers. Rec. 3, E104. doi: 10.1017/s1755267210000771
Tompkins E. M., Townsend H. M., Anderson D. J. (2017). Decadal-scale variation in diet forecasts persistently poor breeding under ocean warming in a tropical seabird. PloS One 12 (8), e0182545-24. doi: 10.1371/journal.pone.0182545
Toms C. N., Stone T., Och-Adams T. (2020). Visual-only assessments of skin lesions on free-ranging common bottlenose dolphins (Tursiops truncatus): Reliability and utility of quantitative tools. Mar. Mamm. Sci. 36 (3), 744–773. doi: 10.1111/mms.12670
Torres-Florez J. P., Olson P. A., Bedriñana-Romano L., Rosenbaum H., Ruiz J., LeDuc R., et al. (2015). First documented migratory destination for eastern south pacific blue whales. Mar. Mamm. Sci. 31 (4), 1580–1586. doi: 10.1111/mms.12239
Tozer B., Sandwell D. T., Smith W. H. F., Olson C., Beale J. R., Wessel P. (2019). Global bathymetry and topography at 15 arc sec: SRTM15+. Earth Space Sci. 6, 1847–1864. doi: 10.1029/2019EA000658
Teixeira C. R., Troina G. C., Daura-Jorge F. G., Simões-Lopes P. C., Botta S. (2022). A practical guide on stable isotope analysis for cetacean research. Mar. Mamm. Sci. 38, 1200–1228. doi: 10.1111/mms.12911
Urian K., Gorgone A., Read A., Balmer B., Wells R. S., Berggren P., et al. (2015). Recommendations for photo-identification methods used in capture-recapture models with cetaceans. Mar. Mamm. Sci. 31 (1), 298–321. doi: 10.1111/mms.12141
Urquía D. O., Páez-Rosas D. (2019). Δ13C and δ15N values in pup whiskers as a proxy for the trophic behavior of Galapagos sea lion females. Mamm. Biol. 96 (1), 28–36. doi: 10.1016/j.mambio.2019.03.012
Usseglio P., Friedlander A. M., Koike H., Zimmerhackel J., Schuhbauer A., Eddy T., et al. (2016). So long and thanks for all the fish: overexploitation of the regionally endemic Galapagos grouper mycteroperca olfax (Jenyns 1840). PloS One 11 (10), e0165167. doi: 10.1371/journal.pone.0165167
Van Bressem M.-F., Van Waerebeek K., Reyes J. C., Félix F., Echegaray M., Siciliano S., et al. (2007). A preliminary overview of skin and skeletal diseases and traumata in small cetaceans from south American waters. Lat. Am. J. Aquat. Mamm. 6, 7–42. doi: 10.5597/lajam00108
Van Bressem M.-F., Raga A., Guardo G. D., Jepson P., Duignan P., Siebert U., et al. (2009). Emerging infectious diseases in cetaceans worldwide and the possible role of environmental stressors. Dis. Aquat. Org. 86, 143–157. doi: 10.3354/dao02101
Van Cise A., Baird R., Harnish A., Currie J., Stack S., Cullins T., et al. (2021). Mark-recapture estimates suggest declines in abundance of common bottlenose dolphin stocks in the main Hawaiian islands. Endanger. Species Res. 45, 37–53. doi: 10.3354/esr01117
Ventura F., Matthiopoulos J., Jeglinski J. W. E. (2019). Minimal overlap between areas of high conservation priority for endangered Galapagos pinnipeds and the conservation zone of the Galapagos marine reserve. Aquat. Conserv.: Mar. Freshw. Ecosyst. 29 (1), 115–126. doi: 10.1002/aqc.2943
Veríssimo D., MacMillan D. C., Smith R. J. (2011). Toward a systematic approach for identifying conservation flagships. Conserv. Lett. 4 (1), 1–8. doi: 10.1111/j.1755-263x.2010.00151.x
Wachtendonk R., Calambokidis J., Flynn K. (2022). Blue whale body condition assessed over a 14-year period in the NE pacific: Annual variation and connection to measures of ocean productivity. Front. Mar. Sci. 9. doi: 10.3389/fmars.2022.847032
Wade P. R., Gerrodette T. (1993). Estimates of cetacean abundance and distribution in the eastern tropical pacific. Rep. Int. Whal. Commn. 43, 477–493.
Walsh S. J., Mena C. F. (2016). Interactions of social, terrestrial, and marine sub-systems in the Galapagos islands, Ecuador. Proc. Natl. Acad. Sci. U.S.A. 113 (51), 14536–14543. doi: 10.1073/pnas.1604990113
Weiss M. N., Ellis S., Croft D. P. (2021). Diversity and consequences of social network structure in toothed whales. Front. Mar. Sci. 8, 688842. doi: 10.3389/fmars.2021.688842
Wellington G. M., Strong A. E., Merlen G. (2001). Sea Surface temperature variation in the galápagos archipelago: a comparison between AVHRR nighttime satellite data and in-situ instrumentation (1982–1988). Bull. Mar. Sci. 69, 27–42.
Whitehead H. (1985). Studying sperm whales on the galápagos grounds. Not. Gal. (Gal. Res.) 42, 18–21.
White G. C., Burnham K. P. (1999). Program MARK: survival estimation from populations of marked animals. Bird Study 46 (sup1), S120–S139. doi: 10.1080/00063659909477239
Whitehead H., Christal J., Dufault S. (1997). Past and distant whaling and the rapid decline of sperm whales off the Galapagos islands. Conserv. Biol. 11 (6), 1387–1396. doi: 10.1046/j.1523-1739.1997.96246.x
Whitehead H., Hope P. L. (1991). Sperm whalers off the galápagos islands and in the western north pacific 1830–1850: Ideal free whalers? Ethol. Sociobiol. 12 (2), 147–161. doi: 10.1016/0162-3095(91)90018-L
Whitehead H., MacLeod C. D., Rodhouse P. (2001). Differences in niche breadth among some teuthivorous mesopelagic marine mammals. Mar. Mamm. Sci. 19 (2), 400–406. doi: 10.1111/j.1748-7692.2003.tb01118.x
Whitehead H. (2003). Sperm whales: social evolution in the ocean (Chicago: University of Chicago Press).
Whitehead H. (2008). Analyzing animal societies: quantitative methods for vertebrate social analysis (Chicago: University of Chicago Press).
Whitehead H., Rendell L. (2004). Movements, habitat use and feeding success of cultural clans of south pacific sperm whales. J. Anim. Ecol. 73, 190–196. doi: 10.1111/j.1365-2656.2004.00798.x
Whitehead H., Shin M. (2022). Current global population size, post-whaling trend and historical trajectory of sperm whales. Sci. Rep. 12, 19468. doi: 10.1038/s41598-022-24107-7
White T. D., Ong T., Ferretti F., Block B. A., McCauley D. J., Micheli F., et al. (2020). Tracking the response of industrial fishing fleets to large marine protected areas in the pacific ocean. Cons. Biol. 34, 1571–1578. doi: 10.1111/cobi.13584
Wickman L., Rayment W., Slooten E., Dawson S. M. (2020). Recommendations for estimating mark rate of cetaceans in photo-ID research: A critique of field sampling protocols and variance estimation. Mar. Mamm. Sci. 37 (1), 328–343. doi: 10.1111/mms.12723
Williams R., Thomas L. (2009). Cost-effective abundance estimation of rare animals: Testing performance of small-boat surveys for killer whales in British Columbia. Biol. Conserv. 142 (7), 1542–1547. doi: 10.1016/j.biocon.2008.12.028
Wise J. J. P., Payne R., Wise S. S., LaCerte C., Wise J. J. P., Gianios C., et al. (2009). A global assessment of chromium pollution using sperm whales (Physeter macrocephalus) as an indicator species. Chemosphere 75, 1461–1467. doi: 10.1016/j.chemosphere.2009.02.044
Wolf J. B., Mawdsley D., Trillmich F., James R. (2007). Social structure in a colonial mammal: unravelling hidden structural layers and their foundations by network analysis. Anim. Behav. 74 (5), 1293–1302. doi: 10.1016/j.anbehav.2007.02.024
Keywords: cetaceans, megafauna, Galápagos Islands, eastern tropical Pacific, oceanic insular ecosystems, migratory connectivity, research priorities/questions, participatory research agenda
Citation: Palacios DM and Cantor M (2023) Priorities for ecological research on cetaceans in the Galápagos Islands. Front. Mar. Sci. 10:1084057. doi: 10.3389/fmars.2023.1084057
Received: 29 October 2022; Accepted: 19 January 2023;
Published: 14 February 2023.
Edited by:
Filipe Alves, Center for Marine and Environmental Sciences (MARE), PortugalReviewed by:
Aldo S. Pacheco, National University of San Marcos, PeruGuido J. Parra, Flinders University, Australia
Copyright © 2023 Palacios and Cantor. This is an open-access article distributed under the terms of the Creative Commons Attribution License (CC BY). The use, distribution or reproduction in other forums is permitted, provided the original author(s) and the copyright owner(s) are credited and that the original publication in this journal is cited, in accordance with accepted academic practice. No use, distribution or reproduction is permitted which does not comply with these terms.
*Correspondence: Daniel M. Palacios, daniel.palacios@oregonstate.edu