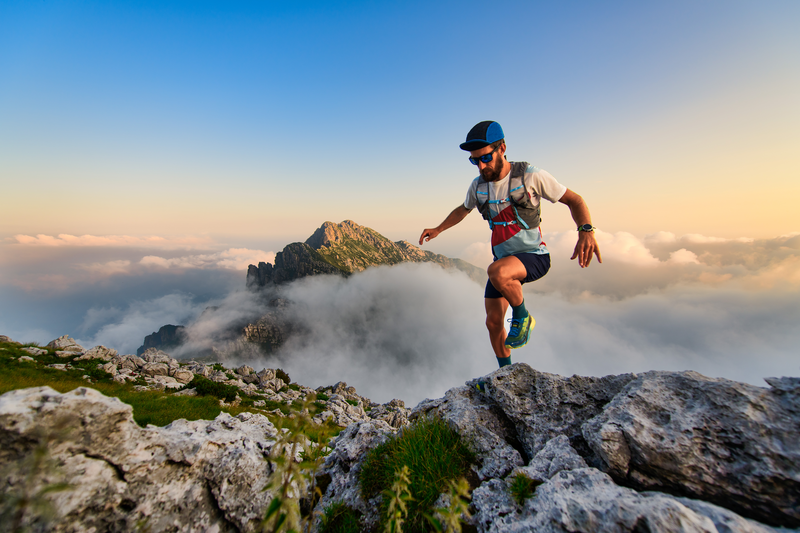
94% of researchers rate our articles as excellent or good
Learn more about the work of our research integrity team to safeguard the quality of each article we publish.
Find out more
ORIGINAL RESEARCH article
Front. Mar. Sci. , 06 February 2023
Sec. Marine Conservation and Sustainability
Volume 10 - 2023 | https://doi.org/10.3389/fmars.2023.1082049
The coastal waters of South Africa are habitat to a diverse composition of sharks that are vulnerable to exploitation, many of which are endemic and/or classified by the International Union for Conservation of Nature (IUCN) Red List as Threatened or Data Deficient. Accordingly, this region has been identified as a global research and conservation priority for elasmobranchs. The De Hoop Marine Protected Area (MPA), in the Western Cape Province of South Africa, provides 288 km2 of no-take protection within its boundaries. However, the region experiences heavy commercial fishing, with two vessels actively operating as dedicated shark longliners (as of 2022). When crossing MPA boundaries, sharks are susceptible to capture by these vessels. Utilizing passive acoustic telemetry, the present study evaluated the movements of a threatened juvenile shark species, the smooth hammerhead (Sphyrna zygaena), both inside and adjacent to the De Hoop MPA, and along the greater coastline. Movement data from 20 tagged sharks were used to explore the effects of spatial, environmental, and management variables on their residency and movement patterns. Results indicate a high reliance of sharks on unprotected waters immediately adjacent to the MPA’s eastern boundary, an area of high biological productivity due to its proximity to the mouth of an estuary. Although some tagged sharks did move regionally along the South African coastline, individuals spent 95% of their days detected just outside the eastern boundary of the MPA, rendering them vulnerable to commercial shark longlining occurring there. These findings have conservation implications for smooth hammerhead sharks in South Africa and present an opportunity to revisit management practices that may optimize spatial protection for an important life stage of this threatened species.
Fisheries and global shark product trade are drivers of population declines for many shark species (Gallagher et al., 2012; Worm et al., 2013; Dulvy et al., 2014). Conservation of shark can be challenging, in large part due to their wide-ranging movements, sparking debate over the suitability of marine protected areas (MPAs) for their protection (Agardy et al., 2011; Acuña-Marrero et al., 2018; Daly et al., 2018). Despite this, 29% of the world’s protected waters have been designated explicitly to achieve shark conservation goals (MacKeracher et al., 2018). While MPAs have been shown to benefit shark conservation (Goetze and Fullwood, 2013; Bond et al., 2017; Speed et al., 2018), the extent of protection afforded depends on biological, ecological, and environmental factors along with MPA configuration and region (MacKeracher et al., 2018; van Zinnicq Bergmann et al., 2022). Since MPAs are often designed without region-specific space-use information, sharks may not be maximally protected in these areas (Dulvy et al., 2017). Thus, conservation outcomes of MPAs for sharks may be improved by utilizing biological and ecological data to identify and protect key areas used by sharks (Davidson and Dulvy, 2017; Daly et al., 2018).
The smooth hammerhead (Sphyrna zygaena) is listed as Vulnerable on the International Union for the Conservation of Nature (IUCN) Red List (Rigby et al., 2019), and appears in Appendix II of both the Convention on International Trade in Endangered Species (CITES) and the Convention on Migratory Species of Wild Animals (CMS), making its conservation an international priority. However, the conservation success of these international treaties is highly dependent upon domestic-level implementation, which is lacking for smooth hammerheads in many regions (Rigby et al., 2019). Targeted or bycatch exploitation of smooth hammerheads remains one of the main risks to this species (Cortés et al., 2010; Rigby et al., 2019), with inaccurate catch data and heavy fishing pressure in data-poor regions obscuring the actual effect of this threat (Rigby et al., 2019).
Smooth hammerheads are known to display high site fidelity to specific areas along the South African coastline (Kuguru et al., 2019). Juvenile (45-120 cm precaudal length, PCL) and year one neonates (35-45 cm PCL) are found seasonally in the summer (December to February) months (Spalding et al., 2008). Mark-recapture studies have shown these life stages likely return to certain areas within their annual activity spaces, while sexually mature individuals (> 160 cm PCL) do not exhibit this characteristic (Diemer et al., 2011; Dicken et al., 2018). This pattern amplifies the need for conservation action if this species is exposed to heavy fishing pressure prior to reaching sexual maturity. While this species is protected in South Africa, it shares a common habitat preference as species targeted by commercial shark fisheries, including smoothhound (Mustelus spp.), soupfin (Galeorhinus galeus), and bronze whaler (Carcharhinus brachyurus) sharks (Albano et al., 2021), making this species vulnerable to bycatch. Accordingly, understanding the residency and movement patterns of juvenile smooth hammerheads in relation to areas exposed to and protected from commercial shark fisheries will help inform their conservation management.
In the present study, we examined the residency patterns of juvenile smooth hammerheads in relation to a no-take MPA along the coast of South Africa. Utilizing passive acoustic telemetry, 20 sharks were tagged and tracked inside and adjacent to the MPA, and along the greater coastline to explore the effects of spatial, environmental, and management variables on their residency and movement patterns. Using these data, we investigated the following questions: (1) Is residency of smooth hammerhead sharks higher inside versus outside the MPA, (2) Are there any locations of increased shark residency and are these locations protected within an MPA, and (3) What is the spatial extent of smooth hammerhead movement outside the MPA? Ultimately, this study sought to better understand the efficacy of an MPA for the protection of juvenile smooth hammerheads in the area and to emphasize the importance of evaluating MPAs for species conservation.
This study was conducted within and around the De Hoop Marine Protected Area: a 288 km2 no-take marine reserve in the Western Cape Province, South Africa (Figure 1). The De Hoop MPA is bordered by the fishing villages of Struisbaai and Arniston (to the west), and Witsand and Stilbaai (to the east), rendering its surrounding waters easily accessed by fishing vessels. The MPA contains several habitat types such as rocky reefs, sandy bottom, and corrugated rock, which are suitable for a variety of shark species (Albano et al., 2021). The unprotected waters adjacent to the MPA contain similar habitat types, which may prompt movement between the MPA and outside areas by mobile shark species.
Figure 1 The De Hoop Marine Protected Area (MPA) boundaries and geographical placement within South Africa (A) and the African continent (B), with acoustic receivers used to monitor the movements of juvenile S. zygaena within and adjacent to the MPA depicted by black circles. Tagging locations are depicted in white diamonds (A). Receiver stations detecting tagged smooth hammerheads in the greater South African region are depicted by white circles (C).
To track the local movement of smooth hammerheads relative to the De Hoop MPA, seven VR2W 69 kHz acoustic receivers (Innovasea, Halifax, Canada) were deployed inside (n = 7), outside (n = 6), and on the eastern boundary (n =1) of the De Hoop MPA (Figure 1A). Additionally, acoustic receiver coverage was complemented by 12 existing 69 kHz V2AR receivers (Innovasea) which formed part of South Africa’s Acoustic Tracking Array Platform – a national array of acoustic receivers spanning approximately 2,200 km (ATAP; Murray et al., 2022), located both inside (n = 5) and outside (n = 7) the border of the De Hoop MPA (Figure 1B). Taken together, we categorized these receivers inside and immediately adjacent to the MPA as “local stations”. To explore possible regional movements of smooth hammerheads, our study benefited from the ATAP. We categorized these acoustic receivers as “regional stations”.
Receivers were deployed using ATAP’s standardized railway anchor moorings (Cowley et al., 2017), and were recovered by divers for maintenance and data download four times throughout the study period (January 2019 - May 2020). The nine receivers deployed specifically for this study were tested to determine their effective detection range following the methods of Kessel et al. (2014) and Rider et al. (2021). Specifically, two V16 continuous pinging sentinel tags (Innovasea) were deployed at distance intervals of 350 m and 650 m from receivers. Tags emitted 69 kHz acoustic signals every 10 seconds and were recovered after 6 hours. Detection probability was calculated by dividing the total number of detections at each distance interval by the total possible detections that station could have received in an hour. Probability of detection was plotted against distance from receiver and a logistic curve was fitted to estimate the range in which detection probability was 50%. Since only two distance intervals were able to be tested due to poor field conditions, the 50% detectability range had to be extrapolated.
Juvenile smooth hammerheads were captured using a hook and handline fishing system consisting of 60 kg test monofilament line attached to 50 cm of 100 kg test wire trace and a 10/0 circle hook. Hooks were baited with either sardine (Sardinops sagax) or Atlantic mackerel (Scomber scombrus). Captured individuals were held in the water for workup and fitted with an external V13 69 kHz acoustic transmitter (Innovasea) via a tethered medium Domeier plastic umbrella dart imbedded in the dorsal musculature following Rogers et al. (2013). Acoustic tags were programmed with a nominal delay of 60 to 120 seconds. In addition to acoustic tagging, shark sex and standardized length measurements (pre-caudal length, PCL; fork length, FL; total length, TL) were recorded for all individuals. All sharks also received a plastic, non-electronic identification (dart) tag as part of South Africa’s nationwide cooperative mark-recapture tagging program – the Oceanographic Research Institute Cooperative Fish Tagging Project (ORI-CFTP, Dunlop et al., 2013).
To facilitate cooperative acoustic telemetry efforts, data were shared with both ATAP and the Ocean Tracking Network (OTN). Detection extracts furnished by the OTN Data Centre were analyzed for this study to be compatible with the GLATOS (Holbrook et al., 2018) package in RStudio. Data extracts were filtered for false detections (i.e. detections occurring from overlapping signals of multiple acoustic transmitters or excessive ambient noise) which were removed when the time between detections for an individual was greater than 60 minutes (McDougall et al., 2013; Kessel et al., 2014).
To account for variation in tagging dates and receiver deployment time, a residency index (RI) was calculated for each individual at each receiver as the number of days an individual shark was detected at a receiver divided by the total number of days it could have been detected at that station (Rider et al., 2021) according to the following formula:
Days detected (DD) is the number of days an individual was detected at a given receiver station. Detection period (DP) refers to the number of days between first and last detection of each individual. Days missing (DM) is the number of days during a detection period that a given receiver was not in the water. The denominator of this formula controlled for variability in receiver deployment time throughout the study period and represents the number of days that each individual could possibility have been detected at a given receiver station. Although acoustic receivers were deployed using a standardized and tested mooring system, large swells and storms during the autumn/winter season caused some receivers to become detached from their moorings and float to shore where they were retrieved. Data from these receivers was analyzed to determine detachment date within a conservative margin of error by validating detection data from detached receivers with neighboring receivers that remained attached. Data were cut off a week before the determined detachment window to ensure reliability of the final dataset.
We compared RIs by management type (inside vs. outside of an MPA), season (spring/summer vs. autumn/winter), and diel period (day vs. night) using a penalized quasi-likelihood generalized linear mixed model (GLMM) with a Poisson distribution for the response variable. Spring/summer was defined as October through March, and autumn/winter was defined as April through September. Day and night were defined using the StreamMetabolism (Sefick, 2016) package in R which calculates sunrise and sunset times in a specified UTC time zone (UTC+2 for this study) for a specified range of dates. To accommodate the Poisson distribution’s requirement for count data in the response variable, DD was used as the response variable in the models with the natural log of (DP – DM) as an offset. Management type, season, and diel period were set as fixed effects in the GLMMs, and individual tag identification was set as the random effect to account for individual variation in residency. Models followed the following structure using the MASS (Venables & Ripley, 2002) package in R:
Post-hoc analysis (pairwise comparison of least squares means) was used to compare differences between each category within the explanatory variables (management type, season, diel period). Best-fit models were chosen using a drop one method and comparing residuals vs. fitted plots for each potential model.
Since sharks were not tagged uniformly throughout the study area, a Pearson R correlation coefficient was calculated to test for association between individual shark RI and the distance (in m) to the closest receiver at the time of tagging. This correlation was plotted and fitted with a linear model. Figure 1 shows tagging locations in relation to receiver stations.
A network analysis was constructed to help answer questions about habitat and protected area utilization following Jacoby et al. (2012). To facilitate this analysis, acoustic receivers were grouped into spatial zones to simplify the network and to account for uneven spatial receiver coverage. 12 zones were drawn according to management type, assumed habitat type, receiver presence, and regional distribution along the coastline (Figure 2). Zones that included acoustic receivers in the local De Hoop MPA area were categorized as “local zones”, whereas zones containing receivers along the greater South African coastline were labeled as “regional zones”. Zones represented the nodes in the network and subsequent detections between nodes were treated as edges (Jacoby et al., 2012). A directed network was constructed for both local and regional nodes by season (spring/summer and autumn/winter) as defined by Diemer et al. (2011). Edge metric “weight” was calculated by summing the number of times that an individual used an edge pathway. The node metric “degree” (e.g., the measure of direct links, or edges, attached to a node) was calculated as an indicator of node importance to the network by its level of connectedness (Jacoby et al., 2012). To provide a measure of node importance that accounts for edge use, node degree was also weighed by edge weights as another indicator of node strength. To quantify number of times that the MPA’s eastern and western boundaries were crossed, edge weights were summed for the pathway that crosses these areas. Edges that crossed through these nodes were summed to quantify boundary-use.
Figure 2 Network analysis nodes represented as zones on (A) local and (B) regional levels. Receiver stations included in each zone are displayed along with boundaries of the De Hoop MPA.
Range testing resulted in a 50% detection probability range of about 200 m. Since only two distances from the test receiver station could be recorded, actual 50% detection probability range could be even narrower than the extrapolated 200 m.
Twenty juvenile smooth hammerheads, ranging in size from 100 to 132 cm TL (13 female, 7 male), were tagged between 11 January 2019 and 14 April 2019 (Table 1). All individuals were detected at least once between January 2019 and May 2020 (Table 1) across a total of 52 different receivers, in both the local and regional arrays, along ~900 km of the South African coastline. Of those 52 receiver stations, sharks were detected on 7 stations inside the De Hoop MPA, one on the De Hoop MPA Eastern boundary, 7 inside another South African MPA (Tsitsikamma MPA, Eastern Cape) approximately 300 km east, and 37 outside of the De Hoop MPA. Tagged sharks were detected on 19 local stations and 33 regional stations (Figure 1). In total, 22,136 detections were collected from all receivers throughout the study period, with individual detections ranging from 89 detections to 7,365 detections. Figure 3 displays individual shark movement over time.
Figure 3 This abacus plot displays detections of each tagged smooth hammerhead over time for the study period (January 2019 - May 2020). Each dot represents a detection on an individual receiver station, which are color-coded by longitude to indicate movement along the greater South African coastline. The center of the De Hoop MPA is located at -34.475454, 20.647058.
Average residency index (RI) varied by management type. Residency was highest outside of any MPA for all receivers (local and regional) (mean RI = 0.053 ± 0.003 SE) and was also highest outside the MPA for just receiver stations local to the De Hoop area (mean RI = 0.087 ± 0.077 SE) (Figure 4). Out of all receiver stations on which individuals were detected (local and regional combined), average residency was greatest (mean RI = 0.204 ± 0.039 SE) on the station which lies outside the east boundary of the De Hoop MPA, east of the Breede River mouth (UM001) (Figure 1A). Smooth hammerheads were also most resident at this station when just the local receiver stations were considered (mean RI = 0.204 ± 0.039 SE). Although tagged sharks were detected on regional receiver stations, average RI for this species was greatest at local De Hoop stations (mean RI = 0.060 ± 0.002 SE) (Figure 1B).
Figure 4 Mean residency index (± SE) by receiver management type for (A) local and regional receivers combined, (B) only local De Hoop MPA receivers, and (C) only regional receivers. In (A, C), residency indces for management type “Inside Reserve” include receivers inside the De Hoop MPA and the Tstisikamma MPA (Eastern Cape), an MPA in the regional array where smooth hammerheads were also detected.
The predictor variables of season and diel period were not found to significantly affect residency in any model combination. The best-fit GLMM model included management type as a fixed effect for predicting residency. This model revealed lower residency at management type “inside reserve” (t = -9.89, p< 0.001) and higher residency at management type “outside reserve” (t = 3.86, p< 0.001) compared to receivers at management type “east boundary” (Table 2). A Pearson correlation revealed a negative correlation between distance from tagging location and RI (R = -0.23, p< 0.001). Post-hoc analysis showed a significant decrease in residency at receiver stations inside the MPA compared to outside the MPA (t = -1.74, p< 0.0001).
Table 2 Parameter estimates and results from penalized quasi-likelihood GLMM models predicting juvenile smooth hammerhead residency relative to management type for all species combined and each species individually.
Network analysis of all tagged smooth hammerheads on local nodes revealed Local Zone 3 (LZ3, Figure 2), which includes the easternmost receiver stations inside the De Hoop MPA, as the most connected node in the local network, with the strongest edge connections (highest weighted degree and strength) on an annual basis (both spring/summer and autumn/winter seasons). By contrast, Local Zone1 (LZ1, Figure 2), which includes the westernmost receiver stations in the local array, was the least connected node in the local network (lowest weighted degree and strength), with the weakest edges connections on an annual basis (both spring/summer and autumn/winter season). Within the regional network, which included all receiver stations that smooth hammerheads were detected on along the South African coastline, Regional Zone 6 (RZ6) and Regional Zone 7 (RZ7, Figure 2) had the highest annual weighted node strength and weighted degree, respectively, making them the strongest nodes in the regional network. Regional Zone 3 (RZ3, Figure 2) represented the weakest node in the regional network. In the overall network, including both local and regional zones, Local Zone 3 (LZ3) was the strongest node and had the highest weighted degree.
The most heavily used pathway within the local network was from Local Zone 4 (LZ4) to Local Zone 5 (LZ5, Figure 5), the easternmost local nodes outside the De Hoop MPA, which occurred 57 times all within the spring/summer season. Edge weights within the regional network were weaker than those of the local network, indicating lighter movement traffic between the farther-reaching areas of the coastline where smooth hammerheads were detected (Figure 5). Overall, tagged smooth hammerheads crossed the De Hoop MPA eastern boundary a total of 13 times throughout the study duration. Greatest extent of movement from tagging location was 724 km by a male with the total length of 127 cm (individual tag ID “A69-1602-22673”). This individual was originally tagged at the mouth of the Breede River, immediately adjacent to receiver at the easternmost boundary of the MPA (UM001) and was detected 724 km northeast at Kei Mouth (KM001) (Figure 1B).
Figure 5 Link map of network analysis conducted on the local and regional level. This directional network has “to” nodes represented by blue circles and “from” nodes represented by red circles. Circles are sized by weighted degree, with larger circles indicating higher degree values and more connectivity with the directed network. A node may be represented more than once in the network if tagged sharks moved both “to” that node and “from” that node at some point throughout the study period. Edges are weighted by the sum of number of pathway uses of each connection between nodes and are referred to here as “visits”. Thicker edged lines indicate increased pathway use. This link map combines the spring/summer and autumn/winter season to visualize the movement network over the entirety of the study period.
As area-focused protection goals continue to promote rapid MPA establishment worldwide, there remains uncertainty in the utility of this tool for protecting the most imperilled shark species (Davidson and Dulvy, 2017; MacKeracher et al., 2018; Derrick et al., 2020);. Although the effectiveness of MPAs for protecting highly mobile shark species has been debated (Mora et al., 2006; Dwyer et al., 2020), there is evidence that this tool can be beneficial for extinction prevention if implemented strategically (Goetze and Fullwood, 2013; Davidson and Dulvy, 2017; Dwyer et al., 2020). The goal of this study was to evaluate the movement and residency patterns of threatened juvenile smooth hammerheads in relation to the De Hoop MPA, South Africa.
While baited remote underwater video surveys in the study area have demonstrated that the De Hoop MPA can benefit the local shark community (Albano et al., 2021), here we found that tagged juvenile smooth hammerheads spent extensive periods of time immediately adjacent to the eastern boundary of the De Hoop MPA, where they may be vulnerable to fishing. In fact, residency was highest in unprotected waters to the east of the MPA (at receiver UM001), an area of high biological productivity due to its proximity to the mouth of an estuary at the Breede River (Figure 2). It’s likely that sharks could be using this site for foraging from high abundance of potential prey found there. These results are of conservation concern as two commercial shark longliners operating near the eastern boundary of the De Hoop MPA have been observed (C.F. Direct Observation.; Global Fishing Watch, 2022), where smooth hammerheads have also been observed to be captured (C.F. Direct Observation). This fishery is likely benefitting from the spillover effect of increased abundances of fish inside the MPA moving into adjacent unprotected waters. It has been well-documented in the literature that, over time, fish abundances increase after spatial closure of a previously fished area (Colléter et al., 2014; Di Lorenzo et al, 2020). Spillover can occur in mobile species that may be reliant on the continuity of habitat types that straddle MPA boundaries, ultimately driving movement between protected and unprotected waters (Barrett et al., 2007; Forcada et al., 2008; Pinillos and Riera, 2022). Previous work (Albano et al., 2021) in this study site identified shared habitat preferences between smooth hammerhead sharks and commercially targeted smooth hound sharks (Mustelus spp.). If this overlapping habitat preference is driving spillover effects outside the De Hoop MPA, this is of conservation concern for this protected species as its likelihood of interaction with the commercial shark longline fishery may be increased.
Network analysis revealed high connectivity between zones in the local array and variation in node metrics for each zone. Zones on the eastern side of the local array were found to be stronger and more connected than those in the middle or on the western side of the local array. Weighted edges revealed the heavy pathway-use between zones around the eastern boundary (LZ3 and LZ4) and outside the eastern boundary (LZ5), with the boundary itself being crossed a total of 13 times (Figure 5). LZ3 is centralized as the strongest in the network, with the most heavily used incoming edge pathways. Given this zone’s proximity to the eastern boundary of the MPA, this finding is significant as sharks could be exposed to the heavy fishing pressure in this area as they cross in and out of the MPA on its eastern boundary. Taken together, these findings point to an opportunity for extending the eastern boundary of the MPA to this area of high residency and connectivity, which would enable protection for an important life stage of this threatened species.
On a regional scale, tagged sharks displayed relatively low residency outside of the local De Hoop Array. These results suggest that although tagged smooth hammerheads are indeed moving along the coastline, those tagged within the local De Hoop area showed high residency and site fidelity to this area. Network analysis of shark movement on a regional scale supports this conclusion. Edge weights indicate that pathway usage between nodes decreased as sharks moved into the regional network, suggesting that broad scale movements may be more migratory in nature as opposed to movement within a core area. This is corroborated by results of mark-recapture movement studies of this species by the ORI-CFTP, which indicate that smooth hammerheads undergo seasonal migrations along the South African coastline, possibly in response to changing sea surface temperature (Diemer et al., 2011). This pattern was also supported by seasonal local and regional network analysis. Sharks were only detected in local zones and one regional node in the spring/summer network, whereas the autumn/winter season saw more northeast movement into the regional network along the assumed seasonal migration route. Additionally, a shift in node metrics between seasons suggests that regional nodes are becoming stronger and more connected during the autumn/winter season than the spring/summer season. This seasonal change in regional connectivity and movement is vitally important to understand if smooth hammerheads are to be sufficiently protected along the South African coastline. The elevated space-use at local receiver stations in waters inside and adjacent to the De Hoop MPA during the spring/summer season emphasizes the importance of this area for smooth hammerheads at a vulnerable time during their life history. If populations of this globally threatened species are to be conserved, critical areas that juveniles rely on must be identified and adequately managed.
Acoustic telemetry allows for the monitoring of many individuals simultaneously while remaining cost-effective. However, this method comes with several limitations, the most prominent being detection range of acoustic receivers (Adams et al., 2012; Brownscombe et al., 2020). Animal detectability is limited to the detection range of receivers, which is a function of technical specifications and characteristics of the study area (Heupel and Simpfendorfer, 2008; Simpfendorfer et al., 2008; Kessel et al., 2014). Additionally, it should be noted that, due to challenging field conditions, receiver coverage was not evenly spatially distributed throughout the De Hoop MPA study area. This should be considered when interpreting residency results. 316 -
Another important consideration of this study when interpreting the results was the significant negative correlation found between residency and distance to the closest receiver at tagging. This means that tagging location had a large impact on residency patterns, whereby sharks exhibited higher residency closer to their tagging location. This is expected as juvenile species are known to be less mobile compared to older conspecifics (Gallagher and Klimley, 2018), especially if the tagging area may be serving as a nursery. Here, tagging was concentrated at or near the eastern boundary of the De Hoop MPA (Figure 1A) since shark longline fishing was observed at or near the eastern boundary of the MPA (C.F. Direct Observation; Global Fishing Watch, 2022). Therefore, caution should be made when extrapolating these results beyond the local De Hoop area. It is possible that if more smooth hammerheads were tagged at or near the western or southern boundary of the MPA, higher residency would have been found at or adjacent to these locations. Nevertheless, we found that juvenile smooth hammerheads tagged at or near the eastern boundary of the De Hoop MPA were spending significantly more time outside the eastern boundary, leaving them vulnerable to fishing pressure here. It is also interesting to note that residency indices for tagged sharks in this study are comparable to other hammerhead species (Guttridge et al., 2017; Rider et al., 2021) in studies that investigated coastal movement patterns.
Future research should build upon this study to gain a comprehensive understanding of the De Hoop MPA’s importance for shark species occurring in the region. As noted above, this study focused tagging effort near the eastern boundary of the De Hoop MPA due to observations of demersal shark longline fishing occurring in this area. Future research could focus on distributing tagging effort more evenly throughout the MPA. This study was not initially designed to answer questions about the seasonality of shark movement and residency outside of the local area. However, to better understand the drivers of these seasonal movement patterns, future studies would benefit from tracking smooth hammerheads over a multiyear period, with environmental loggers deployed on acoustic receivers to capture information like temperature and salinity measurements to explore potential environmental drivers. Such information may provide insights for management, such as whether seasonal closures could provide protective benefit for smooth hammerheads in the waters adjacent to the De Hoop MPA.
The vast majority of MPAs never undergo scientific evaluation to validate their utility for biodiversity preservation (Jameson et al., 2002). As home to many threatened and endemic species, as well as serving as one of the largest aggregations of southern right whales (Eubalaena australis) in the world, the De Hoop MPA has a vested interest in meeting the objectives stated in its management plan to conserve its natural resources (CapeNature, 2016).
Previous research has highlighted the importance of the South African coastline for juvenile smooth hammerheads, citing high site fidelity in areas along the coast such as Mossel Bay and the Wild Coast (Diemer et al., 2011; Kuguru et al., 2019) (Figure 1B). This study reveals that, similarly to other localities along the coastline, tagged smooth hammerheads show a degree of fidelity to the De Hoop MPA and its adjacent waters. Taken together, our findings demonstrate a clear pattern of high utilization and movement of juvenile smooth hammerheads in/near the unprotected waters outside the MPA’s eastern boundary and in the Breede River estuarine area. This area is also home to various life stages of multiple threatened teleost and elasmobranch species, of economic and ecological importance, including dusky kob (Argyrosomus japonicus), spotted grunter (Pomadasys commersonnii), white steenbras (Lithognathus lithognathus), leervis (Lichia amia), diamond ray (Gymnura natalensis), duckbill ray (Aetomylaeus bovinus) and eagle ray (Myliobatis aquila) (Cowley et al., 2008; Dunlop et al., 2015; Murray et al., 2018). The results of this study reveal site fidelity of juvenile smooth hammerheads near the Breede River mouth estuarine area and many of these other local species may benefit from increased spatial protection in this region as well. While previous research (Bennett & Attwood, 1991; Albano et al., 2021) supports the utility of the MPA for increased fish and shark abundance, this study suggests increased residency of juvenile smooth hammerheads on habitat immediately adjacent to the MPA’s eastern boundary. The spatial overlap of commercial shark longline fishing effort (Global Fishing Watch, 2022) and smooth hammerheads to this area renders this species highly vulnerable. Our results suggest that increased spatial protections in this area could provide conservation benefit to this threatened species.
The raw data supporting the conclusions of this article will be made available by the authors, without undue reservation.
The animal study was reviewed and approved by The Institutional Animal Care and Use Committee (IACUC), University of Miami.
PA: methodology, analysis, investigation, data management, writing, project administration. CF: conceptualization, methodology, investigation, resources, review and editing, supervision, project administration, funding acquisition. MF: investigation, resources, review and editing. LW: investigation, review and editing, project administration. TM: methodology, resources, data management, review and editing. OS: investigation, resources. NH: conceptualization, validation, methodology, investigation, resources, review and editing, supervision, project administration, funding acquisition. All authors contributed to the article and approved the submitted version.
Funding for this work was provided by the Shark Conservation Fund and the Rock the Ocean Foundation.
Support for this work was provided by the Western Cape Nature Conservation Board and the Department of Environmental Affairs Oceans and Coast Branch. We specially thank the following people for logistical and field work support/guidance: P. de Villiers, S.K. Tembe, L. Swart, P. Cowley, J.D. Filmalter, M. Rider, S. Cain, and A. Sedgwick. An additional thank you to the staff and students at the University of Miami’s Shark Research and Conservation Program, Apex Shark Expeditions, and South African Institute for Aquatic Biodiversity. Data were collected under permits from the South African Department of Environmental Affairs (RES2018/13) and CapeNature (CN32-31-5459).
The authors declare that the research was conducted in the absence of any commercial or financial relationships that could be construed as a potential conflict of interest.
All claims expressed in this article are solely those of the authors and do not necessarily represent those of their affiliated organizations, or those of the publisher, the editors and the reviewers. Any product that may be evaluated in this article, or claim that may be made by its manufacturer, is not guaranteed or endorsed by the publisher.
Acuña-Marrero D., Smith A. N., Salinas-de-León P., Harvey E. S., Pawley M. D., Anderson M. J. (2018). Spatial patterns of distribution and relative abundance of coastal shark species in the Galapagos marine reserve. Mar. Ecol. Prog. Ser. 593, 73–95. doi: 10.3354/meps12505
Adams N. S., Beeman J. W., Eiler J. H. (2012). Telemetry techniques: A user guide for fisheries research (Bethesda, Maryland: American Fisheries Society).
Agardy T., Di Sciara G. N., Christie P. (2011). Mind the gap: Addressing the shortcomings of marine protected areas through large scale marine spatial planning. Mar. Policy 35 (2), 226–232. doi: 10.1016/j.marpol.2010.10.006
Albano P. S., Fallows C., Fallows M., Schuitema O., Bernard A. T., Sedgwick O., et al. (2021). Successful parks for sharks: No-take marine reserve provides conservation benefits to endemic and threatened sharks off south Africa. Biol. Conserv. 261, 109302. doi: 10.1016/j.biocon.2021.109302
Barrett N. S., Edgar G. J., Buxton C. D., Haddon M. (2007). Changes in fish assemblages following 10 years of protection in Tasmanian marine protected areas. J. Exp. Mar. Biol. Ecol. 345 (2), 141–157. doi: 10.1016/j.jembe.2007.02.007
Bennett B. A., Attwood C. G. (1991). Evidence for recovery of a surf-zone fish assemblage following the establishment of a marine reserve on the southern coast of south Africa. Mar. Ecol. Prog. series. Oldendorf 75 (2), pp.173–pp.181. doi: 10.3354/meps075173
Bond M. E., Valentin-Albanese J., Babcock E. A., Abercrombie D., Lamb N. F., Miranda A., et al. (2017). Abundance and size structure of a reef shark population within a marine reserve has remained stable for more than a decade. Mar. Ecol. Prog. Ser. 576, 1–10. doi: 10.3354/meps12241
Brownscombe J. W., Griffin L. P., Chapman J. M., Morley D., Acosta A., Crossin G. T., et al. (2020). A practical method to account for variation in detection range in acoustic telemetry arrays to accurately quantify the spatial ecology of aquatic animals. Methods Ecol. Evol. 11 (1), 82–94. doi: 10.1111/2041-210X.13322
CapeNature. (2016). De hoop nature reserve complex, protected area management plan 2017-2022. protected area management plan 2017-2022.
Colléter M., Gascuel D., Albouy C., Francour P., de Morais L. T., Valls A., et al. (2014). Fishing inside or outside? A case studies analysis of potential spillover effect from marine protected areas, using food web models. J. Mar. Syst. 139, 383–395. doi: 10.1016/j.jmarsys.2014.07.023
Cortés E., Arocha F., Beerkircher L., Carvalho F., Domingo A., Heupel M., et al. (2010). Ecological risk assessment of pelagic sharks caught in Atlantic pelagic longline fisheries. Aquat. Living Resour. 23 (1), 25–34. doi: 10.1051/alr/2009044
Cowley P. D., Bennett R. H., Childs A. R., Murray T. S. (2017). Reflection on the first five years of south africa’s acoustic tracking array platform (ATAP): Status, challenges and opportunities. Afr. J. Mar. Sci. 39 (4), 363–372. doi: 10.2989/1814232X.2017.1399927
Cowley P. D., Kerwath S. E., Childs A. R., Thorstad E. B., Økland F., Næsje T. F. (2008). Estuarine habitat use by juvenile dusky kob argyrosomus japonicus (Sciaenidae), with implications for management. Afr. J. Mar. Sci. 30 (2), 247–253. doi: 10.2989/AJMS.2008.30.2.5.555
Daly R., Smale M. J., Singh S., Anders D., Shivji M., K. Daly C. A., et al. (2018). Refuges and risks: Evaluating the benefits of an expanded MPA network for mobile apex predators. Diversity Distributions 24 (9), 1217–1230. doi: 10.1111/ddi.12758
Davidson L. N., Dulvy N. K. (2017). Global marine protected areas to prevent extinctions. Nat. Ecol. Evol. 1 (2), pp.1–pp.6. doi: 10.1038/s41559-016-0040
Derrick D. H., Cheok J., Dulvy N. K. (2020). Spatially congruent sites of importance for global shark and ray biodiversity. PloS One 15 (7), e0235559. doi: 10.1371/journal.pone.0235559
Dicken M. L., Winker H., Smale M. J., Cliff G. (2018). Sharks caught in the KwaZulu-Natal bather protection programme, South Africa. 14. The smooth hammerhead shark Sphyrna zygaena (Linnaeus). African Journal of Marine Science 40(2), 157–174.
Diemer K. M., Mann B. Q., Hussey N. E. (2011). Distribution and movement of scalloped hammerhead sphryna lewini and smooth hammerhead sphyrna zygaena sharks along the east coast of southern Africa. Afr. J. Mar. Sci. 33 (2), pp.229–pp.238. doi: 10.2989/1814232X.2011.600291
Di Lorenzo M., Guidetti P., Di Franco A., Calò A., Claudet J. (2020). Assessing spillover from marine protected areas and its drivers: A meta-analytical approach. Fish Fisheries 21 (5), 906–915. doi: 10.1111/faf.12469
Dulvy N. K., Fowler S. L., Musick J. A., Cavanagh R. D., Kyne P. M., Harrison L. R., et al. (2014). Extinction risk and conservation of the world’s sharks and rays. elife 3, e00590. doi: 10.7554/eLife.00590
Dulvy N. K., Simpfendorfer C. A., Davidson L. N., Fordham S. V., Bräutigam A., Sant G., et al. (2017). Challenges and priorities in shark and ray conservation. Curr. Biol. 27 (11), R565–R572. doi: 10.1016/j.cub.2017.04.038
Dunlop S. W., Mann B. Q., Cowley P. D., Murray T. S., Maggs J. Q. (2015). Movement patterns of lichia amia (Teleostei: Carangidae): Results from a long-term cooperative tagging project in south Africa. Afr. Zoology 50 (3), 249–257. doi: 10.1080/15627020.2015.1058724
Dunlop S. W., Mann B. Q., van der Elst R. P. (2013). A review of the oceanographic research institute's cooperative fish tagging project: 27 years down the line. Afr. J. Mar. Sci. 35 (2), 209–221. doi: 10.2989/1814232X.2013.769909
Dwyer R. G., Krueck N. C., Udyawer V., Heupel M. R., Chapman D., Pratt H. L. Jr., et al. (2020). Individual and population benefits of marine reserves for reef sharks. Curr. Biol. 30 (3), 480–489. doi: 10.1016/j.cub.2019.12.005
Forcada A., Bayle-Sempere J. T., Valle C., Sánchez-Jerez P. (2008). Habitat continuity effects on gradients of fish biomass across marine protected area boundaries. Mar. Environ. Res. 66 (5), 536–547. doi: 10.1016/j.marenvres.2008.08.003
Gallagher A. J., Klimley A. P. (2018). The biology and conservation status of the large hammerhead shark complex: the great, scalloped, and smooth hammerheads. Rev. Fish Biol. Fisheries 28 (4), 777–794. doi: 10.1007/s11160-018-9530-5
Gallagher A. J., Kyne P. M., Hammerschlag N. (2012). Ecological risk assessment and its application to elasmobranch conservation and management. J. Fish Biol. 80 (5), 1727–1748. doi: 10.1111/j.1095-8649.2012.03235.x
Global Fishing Watch (2022). Available at: www.globalfishingwatch.org.
Goetze J. S., Fullwood L. A. F. (2013). Fiji’s largest marine reserve benefits reef sharks. Coral Reefs 32 (1), 121–125. doi: 10.1007/s00338-012-0970-4
Guttridge T. L., Van Zinnicq Bergmann M. P., Bolte C., Howey L. A., Finger J. S., Kessel S. T., et al. (2017). Philopatry and regional connectivity of the great hammerhead shark, sphyrna mokarran in the US and Bahamas. Front. Mar. Sci. 4, 3. doi: 10.3389/fmars.2017.00003
Heupel M. R., Simpfendorfer C. A. (2008). Movement and distribution of young bull sharks carcharhinus leucas in a variable estuarine environment. Aquat. Biol. 1 (3), 277–289. doi: 10.3354/ab00030
Holbrook C., Hayden T., Binder T., Pye J., Nunes A. (2018). Glatos: A package for the great lakes acoustic telemetry observation system. R Package version 0.2., 7.9000.
Jacoby D. M., Brooks E. J., Croft D. P., Sims D. W. (2012). Developing a deeper understanding of animal movements and spatial dynamics through novel application of network analyses. Methods Ecol. Evol. 3 (3), 574–583. doi: 10.1111/j.2041-210X.2012.00187.x
Jameson S. C., Tupper M. H., Ridley J. M. (2002). The three screen doors: can marine “protected” areas be effective? Marine pollution bulletin, 44(11), 1177–1183.
Kessel S. T., Cooke S. J., Heupel M. R., Hussey N. E., Simpfendorfer C. A., Vagle S., et al. (2014). A review of detection range testing in aquatic passive acoustic telemetry studies. Rev. Fish Biol. Fisheries 24 (1), 199–218. doi: 10.1007/s11160-013-9328-4
Kuguru G., Gennari E., Wintner S., Dicken M. L., Klein J. D., Rhode C., et al. (2019). Spatio-temporal genetic variation of juvenile smooth hammerhead sharks in south Africa. Mar. Biol. Res. 15 (10), 568–579. doi: 10.1080/17451000.2019.1695058
MacKeracher T., Diedrich A., Simpfendorfer C. A. (2018). Sharks, rays and marine protected areas: A critical evaluation of current perspectives. Fish and Fisheries 20(2), 255–267. doi: 10.1111/faf.12337
McDougall C. A., Blanchfield P. J., Peake S. J., Anderson W. G. (2013). Movement patterns and size-class influence entrainment susceptibility of lake sturgeon in a small hydroelectric reservoir. Trans. Am. Fisheries Soc. 142 (6), 1508–1521. doi: 10.1080/00028487.2013.815659
Mora C., Andréfouët S., Costello M. J., Kranenburg C., Rollo A., Veron J., et al. (2006). Coral reefs and the global network of marine protected areas. Science 312 (5781), 1750–1751. doi: 10.1126/science.1125295
Murray T. S., Cowley P. D., Bennett R. H., Childs A. R. (2018). Fish on the move: connectivity of an estuary-dependent fishery species evaluated using a large-scale acoustic telemetry array. Can. J. Fisheries Aquat. Sci. 75 (11), 2038–2052. doi: 10.1139/cjfas-2017-0361
Murray T. S., Elston C., Parkinson M. C., Filmalter J. D., Cowley P. D. (2022). A decade of south africa’s acoustic tracking array platform: An example of a successful ocean stewardship programme. Front. Mar. Sci., 740.
Pinillos F., Riera R. (2022). The influence of boundary habitat continuity on spillover from a Mediterranean marine protected area. thalassas. Int. J. Mar. Sci. 38 (1), 687–696. doi: 10.1007/s41208-022-00396-7
Rider M. J., Kirsebom O. S., Gallagher A. J., Staaterman E., Ault J. S., Sasso C. R., et al. (2021). Space use patterns of sharks in relation to boat activity in an urbanized coastal waterway. Mar. Environ. Res. 172, 105489. doi: 10.1016/j.marenvres.2021.105489
Rigby C. L., Barreto R., Carlson J., Fernando D., Fordham S., Herman K., et al. (2019) Smooth hammerhead (Sphyrna zygaena). [WWW document]. IUCN red list threat. species 2019 e.T39388A2921825. Available at: https://www.iucnredlist.org/species/39388/2921825 (Accessed 5.3.21).
Rogers P. J., Huveneers C., Goldsworthy S. D., Mitchell J. G., Seuront L. (2013). Broad-scale movements and pelagic habitat of the dusky shark c archarhinus obscurus off s outhern a ustralia determined using pop-up satellite archival tags. Fisheries Oceanography 22 (2), 102–112. doi: 10.1111/fog.12009
Sefick S. Jr. (2016). Stream metabolism-a package for calculating single station metabolism from diurnal oxygen curves. R Package version 1 (2), p.2.
Simpfendorfer C. A., Heupel M. R., Collins A. B. (2008). Variation in the performance of acoustic receivers and its implication for positioning algorithms in a riverine setting. Can. J. Fisheries Aquat. Sci. 65 (3), 482–492. doi: 10.1139/f07-180
Spalding M. D., Fish L., Wood L. J. (2008). Toward representative protection of the world's coasts and oceans–progress, gaps, and opportunities. Conserv. Lett. 1 (5), 217–226. doi: 10.1111/j.1755-263X.2008.00030.x
Speed C. W., Cappo M., Meekan M. G. (2018). Evidence for rapid recovery of shark populations within a coral reef marine protected area. Biol. Conserv. 220, 308–319. doi: 10.1016/j.biocon.2018.01.010
van Zinnicq Bergmann M. P., Guttridge T. L., Smukall M. J., Adams V. M., Bond M. E., Burke P. J., et al. (2022). Using movement models and systematic conservation planning to inform marine protected area design for a multi-species predator community. Biol. Conserv. 266, 109469. doi: 10.1016/j.biocon.2022.109469
Keywords: elasmobranchs, marine protected areas, conservation management, spatial protection, South Africa, acoustic telemetry, ecology
Citation: Albano PS, Fallows C, Fallows M, Williams LH, Murray T, Sedgwick O and Hammerschlag N (2023) Acoustic tracking of a threatened juvenile shark species, the smooth hammerhead (Sphyrna zygaena), reveals vulnerability to exploitation at the boundary of a marine reserve. Front. Mar. Sci. 10:1082049. doi: 10.3389/fmars.2023.1082049
Received: 27 October 2022; Accepted: 09 January 2023;
Published: 06 February 2023.
Edited by:
Trevor Willis, Anton Dohrn Zoological Station Naples, ItalyReviewed by:
Emilio Sperone, University of Calabria, ItalyCopyright © 2023 Albano, Fallows, Fallows, Williams, Murray, Sedgwick and Hammerschlag. This is an open-access article distributed under the terms of the Creative Commons Attribution License (CC BY). The use, distribution or reproduction in other forums is permitted, provided the original author(s) and the copyright owner(s) are credited and that the original publication in this journal is cited, in accordance with accepted academic practice. No use, distribution or reproduction is permitted which does not comply with these terms.
*Correspondence: Patricia S. Albano, cGF0cmljaWFhbGJhbm83QGdtYWlsLmNvbQ==
Disclaimer: All claims expressed in this article are solely those of the authors and do not necessarily represent those of their affiliated organizations, or those of the publisher, the editors and the reviewers. Any product that may be evaluated in this article or claim that may be made by its manufacturer is not guaranteed or endorsed by the publisher.
Research integrity at Frontiers
Learn more about the work of our research integrity team to safeguard the quality of each article we publish.