- 1Institute of Marine Environment and Ecology, National Taiwan Ocean University, Keelung, Taiwan
- 2Center of Excellence for the Oceans, National Taiwan Ocean University, Keelung, Taiwan
- 3Graduate Institute of Marine Biology, National Dong Hwa University, Pingtung, Taiwan
- 4Department of Oceanography, National Sun Yat-sen University, Kaohsiung, Taiwan
- 5Department of Applied Science, Republic of China (R.O.C.) Naval Academy, Kaohsiung, Taiwan
Dissolved oxygen (DO) and partial pressure of CO2 (pCO2) were measured at half-hourly intervals from June 29 to September 9, 2019, in a seagrass meadow in the Southeast Asia archipelagos region. The open water mass balance of the O2 approach was used to calculate metabolic rates (i.e., gross primary production (GPP), community respiration (CR), and net community production (NCP). The calculations show that GPP and CR rates in the seagrass meadow of Dongsha Island were approximately 2.5 times higher than the global means (GPP, 507 ± 173 vs. 225 ± 11 mmol O2 m-2 d-1; CR, 497 ± 171 vs. 188 ± 10 mmol O2 m-2 d-1), while NCP was similar to the global mean (8 ± 61 vs. 27 ± 6 mmol O2 m-2 d-1), suggesting that seagrass meadows with high GPP may not necessarily hold high potential for carbon sequestration. The current data set also reveal that NCP tended to increase with GPP only at lower GPP levels, while NCP did not increase with GPP anymore at higher GPP levels. Moreover, the autotrophic/heterotrophic status did not correspond well to the sink/source behavior of CO2, suggesting that organic carbon metabolism could not be the only dominant factor in determining the sink/source status in a typical seagrass meadow underlain by carbonate sediments, which was further supported by the observed decrease in the trend of pCO2 with a relatively stable NCP level over the study period. These results demonstrate that the metabolism and the relationship between NCP and pCO2 in the seagrass meadows of Dongsha Island may deviate greatly from the global mean condition. To obtain a better assessment of the global potential of seagrass meadows as a nature-based solution for carbon sequestration, more regional-specific studies are still needed in the key regions, such as Indonesia and the Pacific archipelagos, that support extensive seagrass meadows but have not been charted.
1 Introduction
Seagrass meadows are highly productive habitats in shallow coastal waters and rank among the most productive ecosystems (Duarte and Chiscano, 1999). Recently, they have received substantial attention as a ‘blue carbon’ sink due to their high rates of primary production and carbon burial in sediments (Duarte et al., 2010; Fourqurean et al., 2012). As such, seagrass conservation and restoration are considered potential climate change mitigation and adaptation strategies (Nellemann et al., 2009; McLeod et al., 2011; Duarte et al., 2013). Yet, the high production and carbon input also render the seagrass meadow as sites of elevated abundance and activity of heterotrophs, which support high community respiration rates (Middelburg et al., 2005). As a result, the status of seagrass meadows as a source or a sink of carbon may largely depend on the metabolic balance of the primary producers (including seagrass itself, epiphytic algae, and microphytobenthos) and the associated heterotrophs (including the pelagic and benthic compartments) in the community.
The community metabolic status in seagrass meadows can be described by net community production (NCP), referring to the difference between gross primary production (GPP) and community respiration (CR). Autotrophic meadows (NCP > 0) fix organic carbon in excess to local demand (GPP > CR) and may, therefore, either store or export organic carbon to adjacent communities, while heterotrophic meadows (NCP< 0) require additional organic carbon and that the community may be sustained by allochthonous organic carbon (Duarte et al., 2010). Determining the metabolic status of seagrass meadows is therefore crucial for a better understanding of their potential in “blue carbon” sink and thus in climate change mitigation (Champenois and Borges, 2021).
Seagrass meadows support a large range of metabolic rates from autotrophy (Barrón et al., 2006) to heterotrophy (Van Dam et al., 2019), which vary significantly among different community types and species along with seasonal variations (Duarte et al., 2010). In fact, earlier efforts at assessing seagrass metabolism existed a large imbalance in the geographic distributions of the data available. In particular, there is a considerable gap in reports of seagrass community metabolism in the Indo-Pacific, Africa, and South America (Duarte et al., 2013). To gain a better sink potential of seagrass meadows on a global scale, observations are required from the key regions where supporting extensive seagrass meadows have not been reported.
Despite being a hot spot for the diversity of seagrass species and habitat types, the seagrass meadows in Southeast Asia archipelagos have been poorly studied (Fortes et al., 2018), and to our knowledge, metabolic rates in this region have not yet been reported. Lee et al. (2017) have reported the community-scale metabolism of seagrass meadows in East Asia (Korea) for the first time as autotrophic state, but their study site was located in temperate area. In the present study, we report a data set of O2, partial pressure of CO2 (pCO2), and pH measurements at half-hourly intervals, which have been recorded continuously from June 29 to September 9, 2019, in a seagrass meadow on the northern shore of Dongsha Island, located in the largest tropical marginal sea in Southeast Asia (i.e., the South China Sea). Community metabolic rates (GPP, CR, and NCP) were estimated on a daily basis from this data set using the open water mass balance of the O2 approach (Odum, 1956; Champenois and Borges, 2021). We also investigated the relationship between metabolism and concurrent concentrations of pCO2 in the water column. Overall, the objectives of this study are (i) to document the metabolism rates and the relationships between them for the first time in the seagrass meadows in the Southeast Asia archipelagos region, (ii) to compare the present result with previously global synthesis, and (iii) to provide new insight into the relationship between metabolism and pCO2 dynamics for tropical seagrass meadows.
2 Materials and methods
2.1 The study site
Dongsha Island (also known as Pratas Island) is a coral island located on the western side of Dongsha Atoll, a ring-shaped coral reef ecosystem in the northern South China Sea (Figure 1). The island is quite small around 2.80 km long and 0.87 km wide with an area of approximately 1.74 km2, and it is made up of coral atolls and reef flats. Vines and bushes cover some of the island, and the rest is surrounded by white coral sand (Dai, 2006). The East Asia Monsoon prevails over Dongsha Island with distinct seasonality: the southwesterly monsoon starts in June and lasts until August in the summer, while the northeasterly monsoon starts in October and predominates over the winter and early spring. The transition periods are April-May for the southwesterly monsoon and September for the northeasterly monsoon (Chai et al., 2009). As a military control zone, there is no permanent inhabitants on the island, and it is visited only by military personnel, researchers and fishermen. Furthermore, there is no freshwater flow into the sea from the island, and most of freshwater on the island is produced by seawater desalination and recycled. Therefore, the inflow from the artifact at Dongsha Island nearly could be negligible.
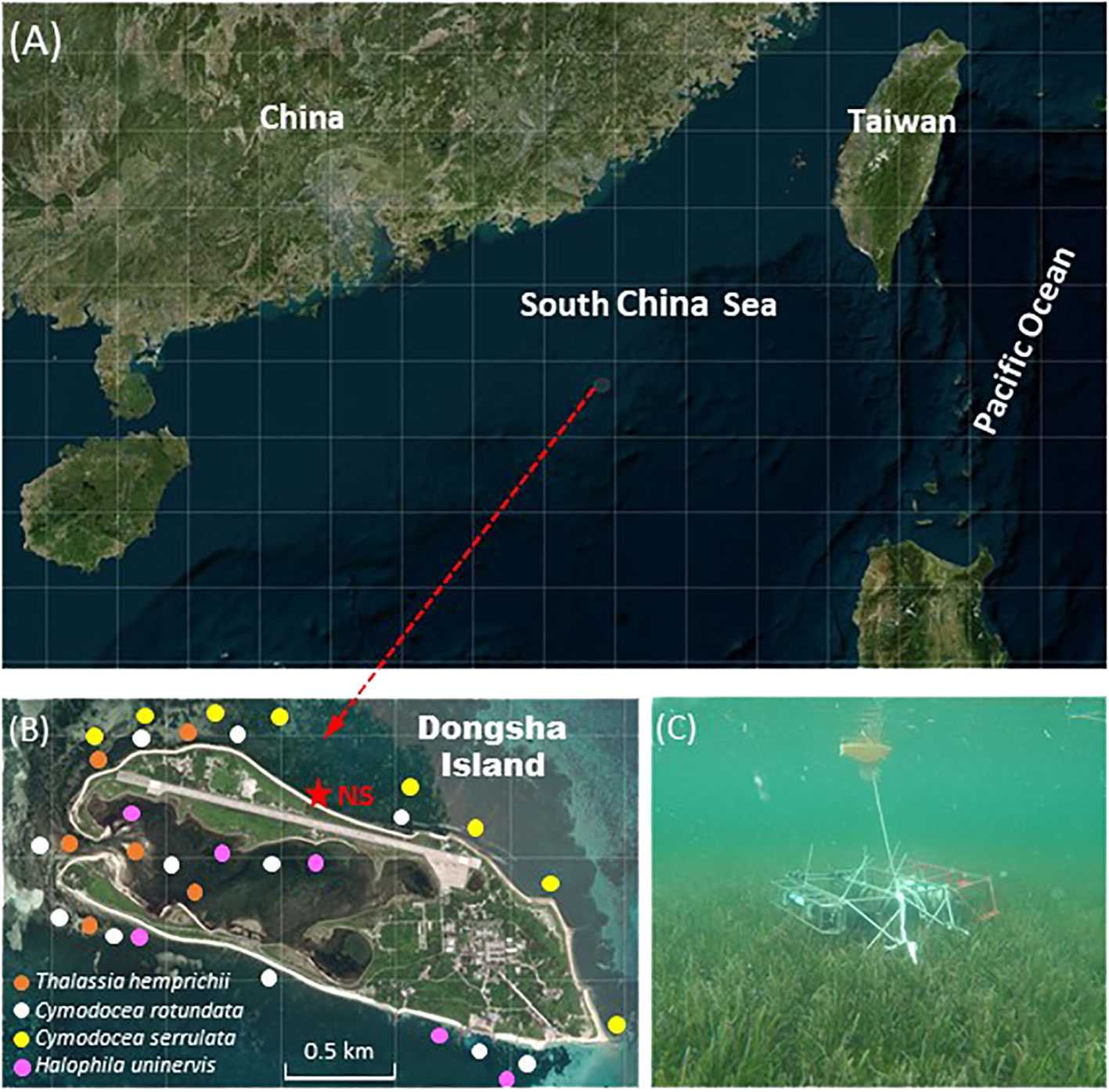
Figure 1 Map showing (A) the location of Dongsha Atoll, (B) the distribution of dominant seagrass (studies by Lin et al., 2005) and the study site on the northern shore of Dongsha Island (NS, asterisk), and (C) a photo of the sensors deployed within the seagrass canopy.
Despite the small area of Dongsha Island, massive seagrass meadows extend from the intertidal to the subtidal zones with a total coverage area of 11.85 km2 around the entire island (Huang et al., 2015). A total of seven seagrass species from six genera and two families have been identified at Dongsha Island, including Thalassia hemprichii, Halophila ovalis, Cymodocea rotundata, C. serrulata, Halodule uninervis, Syringodium isoetifolium, and Thalassodentron ciliatum (Lin et al., 2005), and the coverage of seagrass meadows was generally >75% around the island (Lee et al., 2021). Among them, the most abundant species around Dongsha Island are T. hemprichii, H. uninervis, C. rotundata, and C. serrulata (Huang et al., 2015), and which are also popular species in Indo-Pacific (Short et al., 2007). Besides, T. hemprichii and H. uninervis are widely appeared in Hengchun Peninsula and surrounding islands of Taiwan, but C. rotundata, and C. serrulata are only recorded in Dongsha and Penghu Islands (Lin et al., 2005).This study was conducted in the seagrass meadow on the northern shore (NS, Figure 1) of Dongsha Island, which is a multispecies seagrass meadow with three dominant species of T. hemprichii, C. rotundata, and C. serrulata. The average total seagrass biomass, total seagrass production, seagrass cover, and shoot density were previously reported to be 949.4 ± 62.1 (g DW m−2), 8.47 ± 2.92 (g DW m−2), 81.91 ± 2.13 (%), and 2677 ± 485 (shoots m-2), respectively, based on four seasonal surveys in April, August, October 2010, and February 2011 (Lee et al., 2015). The large area of the seagrass meadow, the high shoot density and coverage of seagrasses indicate that the seagrass on Dongsha Island is in a good heath condition (Lin et al., 2005). The epiphytic algae cover on seagrass leaves was previously reported to be approximately 4%, 5%, 9%, and 5% in April, June, August, and October 2010, respectively (Chang, 2012). The seasonal variations in salinity and chlorophyll a concentration in water column around the Dongsha Island were relatively minor, which were 33.8 ± 0.4, 33.8 ± 0.5, 33.8 ± 0.5, 34.6 ± 0.3 for salinity (Chou et al., 2021), and 0.81 ± 0.37, 0.70 ± 0.20, 0.60 ± 0.25, 0.41 ± 0.13 mg m-3 for Chlorophyll a (Liang, 2012) in spring, summer, autumn, and winter, respectively. Furthermore, only 4 rainy days were recorded during the entire study period with tiny precipitation less than 2 mm d-1, suggesting that salinity could remain within a relatively stable level in the study site during the study period.
2.2 Dissolved oxygen, pCO2, pH, and light intensity measurements
High‐resolution dissolved O2 (DO), pCO2, and pH were measured simultaneously at intervals of 30 min, 1 h, and 30 min using HOBO U26 Dissolved Oxygen Data Logger (Onset, MA, USA) and Submersible Autonomous Moored Instrument sensors (SAMI‐CO2 and SAMI‐pH; Sunburst Sensors, LLC, MT, USA), respectively. To avoid biofouling, SAMI-CO2 and SAMI-pH are designed to isolate the sensor in an enclosed cell that is periodically flushed with sample that has been exposed to a biocide such as tributyl tin or copper, which has been proven to be useful in keeping biofouling to a minimum (Lai et al., 2018). Furthermore, the sensors using in this study were covered by copper mesh to protect from fish bites and other sources of damages (Lai et al., 2018). Before deploying, the DO logger was first calibrated to 100% saturation by placing it in water-saturated air and then to 0% saturation by placing it in sodium sulfite solution. The accuracy and resolution of DO measurement were 6 and 0.6 μM, respectively. SAMI‐CO2 and SAMI‐pH sensors were returned to Sunburst Sensors LLC for re-calibration and refurbishment just before their deployments. The accuracy and resolution of pCO2 and pH measurements were ±3 μatm and<1 μatm and ±0.003 and<0.001 pH units, respectively. The long-term drift of SAMI‐CO2 and SAMI‐pH sensors was<1 μatm and<0.001, respectively, over six months. Light intensity was recorded by a HOBO UA-002-64 Data Logger (Onset, MA, USA) at an interval of 30 min, and the measured lux was converted to photosynthetically active radiation (PAR, μmol/m2/s) by dividing lux by 52.63 (Langhans and Tibbitts, 1997). These sensors were deployed within the seagrass canopy 0.1 m above the bottom (Figure 1C), for a period of 73 days, from 6/29 to 9/9 2019. The water column depth is approximately 1 m at the study site. Furthermore, the wind, wave and tide data during the study period were collected by Central Weather Bureau of Taiwan (Buoy Observation Data Annual Report 2019 and Tide Tables 2019).
2.3 Gross primary production, community respiration, and net community production calculations
The metabolic rates (i.e., gross primary production, GPP; community respiration, CR; net community production, NCP) were computed using the open water mass balance of O2 (Odum, 1956). First, the hourly respiration was calculated as the temporal excursion in DO during the nighttime hours (18:00–06:00) with corrections for air-water gas exchange using the following equation:
where is the rate of change in DO between two consecutive measurements (0.5 h); h is the water depth (m); and air-water O2 flux is the air-water exchange flux of O2, calculated with a bulk transfer approach using the formula of Wanninkhof (1992):
where k600 was the gas transfer velocity estimated using the parametrization of Ho et al. (2006), in which wind data was obtained from the meteorology station on Dongsha Island; Sc was Schmidt numbers calculated from in situ salinity and temperature data (Wanninkhof, 1992); O2(water) and O2(air) were measured and saturated DO concentrations, respectively. Time-series variations in k600 were shown in Figure S1, and an error analysis on the calculations of CR, GPP and NCP resulted from the uncertainty in k600 were given in Figure S2. Likewise, the change in the rate of DO between two consecutive measurements corrected for the exchange of O2 with the atmosphere during daytime (06:00–18:00) corresponds to the hourly rate of NCP. Assuming a constant hourly rate of CR through the diel cycle, the hourly rate of GPP was calculated as NCP-CR (Champenois and Borges, 2012). Daily integrated GPP was computed by multiplying the average hourly rate by 12 (i.e., the daytime hours); daily integrated CR was calculated by multiplying the average hourly rate by 24 (i.e., the daytime + nighttime hours); and daily integrated NCP was computed as the sum of daily integrated GPP and daily integrated CR.
3 Results
3.1 Site characteristics: Wind, wave, and tide
The daily wind, wave and tide variations are showed in Figure 2. Daily mean wind speed ranged from 1.6 to 11 m s-1 with an average speed of 6.0 m s-1 during the study periods. Southwesterly wind direction was dominant before Julian day 232 and wind direction was variated after that day. These results reflect that southwesterly monsoon was prevailed during the summer (July and August) but ceased in the early autumn (September), which is consistent with the general pattern of the East Asia Monsoon. Daily mean wave was within a range of 0.56 – 3.37 m with an average level of 1.9 m, and most of daily wave were southwesterly direction. Tidal range was from 0.04 m of neap tide to 1.42 m of spring tide with an average level of 0.73 m.
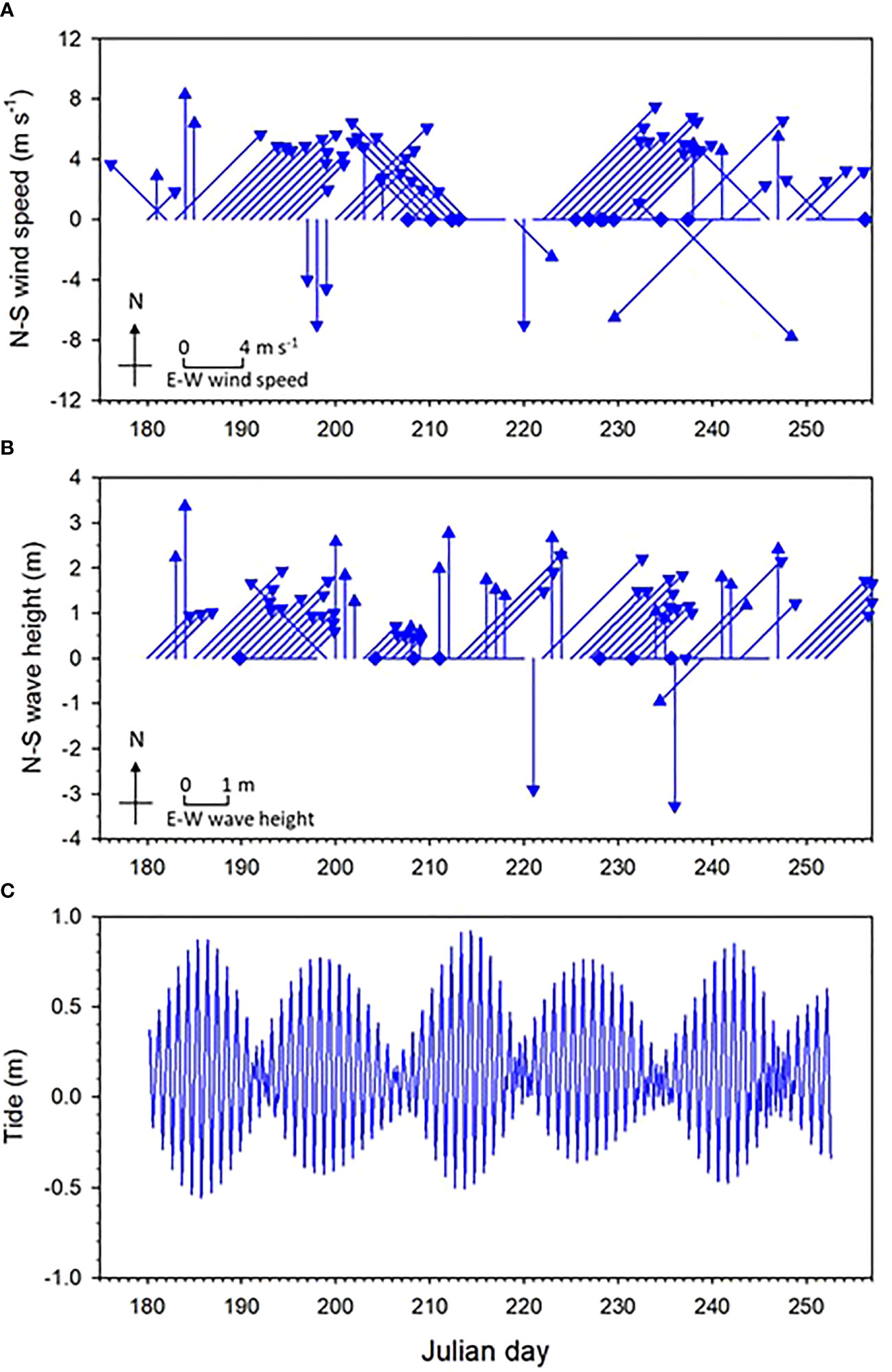
Figure 2 Time-series variations in (A) wind, (B), wave, and (C) tide variation during the study period. Wind and wave are showed in eight directions.
3.2 In-situ monitoring of temperature, light intensity, dissolved oxygen, pCO2, and pH
The diel patterns of temperature, light intensity, DO, pCO2, and pH are shown in Figure 3. Over the study period, water temperature varied between 27.5 and 35.0 °C and averaged 30.4 ± 1.4 °C with a regular diurnal trend (i.e., increasing during daytime and decreasing during nighttime). The lowest temperature period occurred on Julian days 213–214 and 232–233, which corresponded well with the lowest PAR intensity period, suggesting light intensity may exert a strong control over water temperature variation. The variation ranges and the means ( ± SD) of DO, pCO2, and pH were 16–529 μM and 195 ± 102 μM, 39–1565 μatm and 438 ± 252 μatm, and 7.55–8.38 and 7.97 ± 0.16 pH units, respectively. Generally, DO, pCO2, and pH revealed distinct diurnal patterns, with DO and pH increasing but pCO2 decreasing during the day and DO and pH decreasing but pCO2 increasing during the night. As shown in Figure S3, both DO saturation and pCO2 closely followed the diurnal cycle of photosynthesis and respiration, suggesting that organic carbon metabolism could play an important role in regulating the CO2 dynamics in the seagrass meadows of Dongsha Island (Chou et al., 2018). Furthermore, similar to the temperature variation, the smallest amplitude of diel variation in DO corresponded to the lowest PAR intensity period, implying a possible important role of light intensity in regulating metabolic activities (i.e., photosynthesis and respiration) in seagrass meadows.
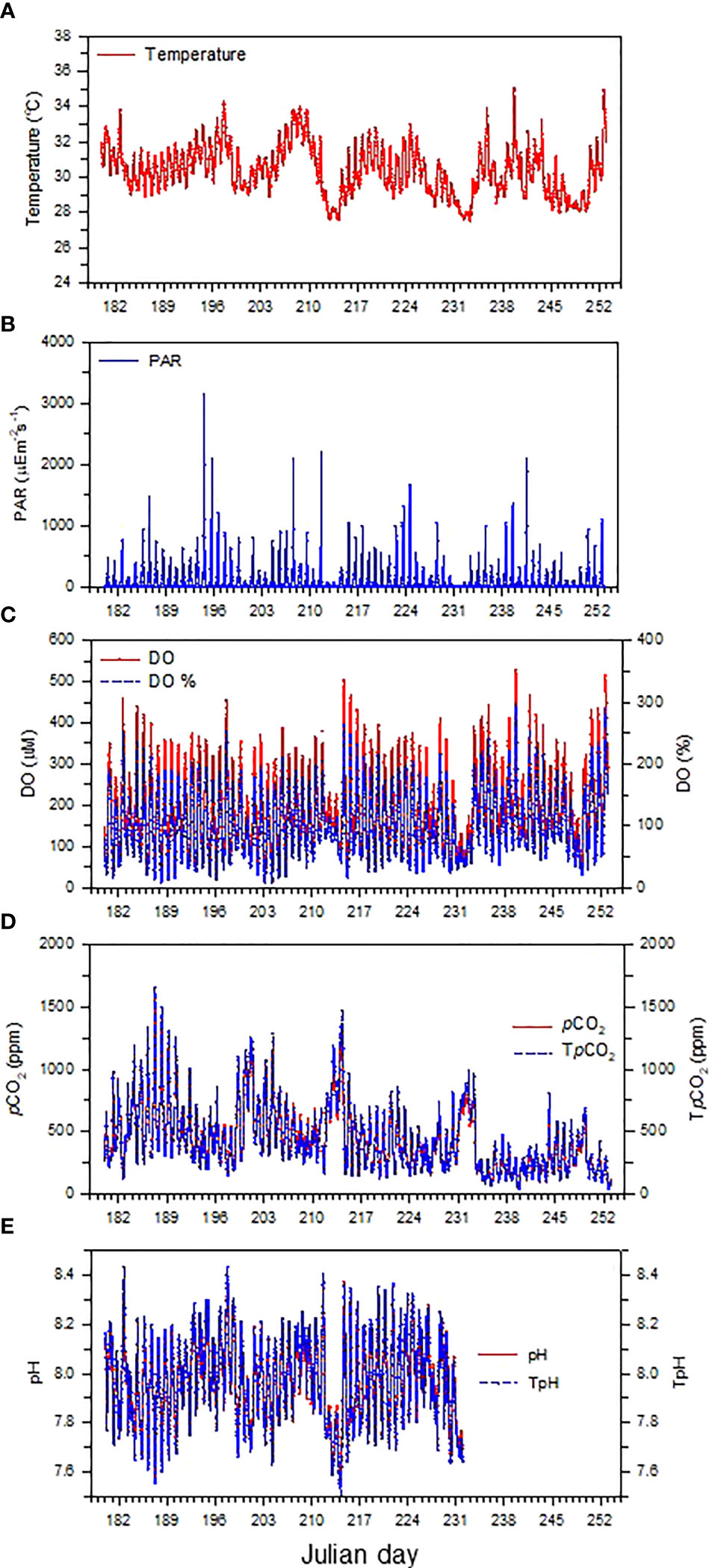
Figure 3 Time-series variations in (A) temperature, (B) PAR, (C) DO and DO saturation level (DO%), (D) pCO2 (red solid line) and temperature normalized pCO2 (TpCO2, blue dashed line), and (E) pH (red solid line) and temperature normalized pH (TpH, blue dashed line). TpCO2 and TpH were normalized to a constant temperature of 30.4 °C, the average temperature during the study period.
Provided that the average atmospheric pCO2 with 100% humidity was 395 μatm during the study period, seawater pCO2 was lower and higher than the atmospheric pCO2 in up to 52% and 48% of the time, respectively, indicating contrasting times when seagrass meadows could either act as a CO2 sink or source for the atmosphere. Likewise, assuming that the pH value of the source water was 8.0, pH was lower and higher than the source water pH in up to 55% and 45% of the time, respectively, during the entire study period, demonstrating divergent times when seagrass meadows would either enhance or mitigate ocean acidification.
3.3 Daily variability of gross primary production, community respiration, and net community production
The daily variability of GPP, CR, and NCP is presented in Figure 4. Over the study period, GPP was characterized by strong day-to-day variability, ranging between 82 and 832 mmol O2 m−2 d−1 with an average of 507 ± 173 mmol O2 m−2 d−1. The lower GPP corresponded well with the lower PAR intensity, suggesting that GPP variations could be closely related to the daily variability of irradiance. CR ranged between 99 and 900 mmol O2 m−2 d−1 with an average of 497 ± 171 mmol O2 m−2 d−1, and its variations closely tracked those of GPP, implying that high GPP could also support high CR. NCP also showed a strong day-to-day variability but did not track those of either GPP or CR. During the study period, NCP ranged between −138 and 197 mmol O2 m−2 d−1 and averaged 8 ± 61 mmol O2 m−2 d−1, indicating the range of ecosystem metabolism from a distinctly autotrophic to a clearly heterotrophic status and might be near metabolic balance, in general.
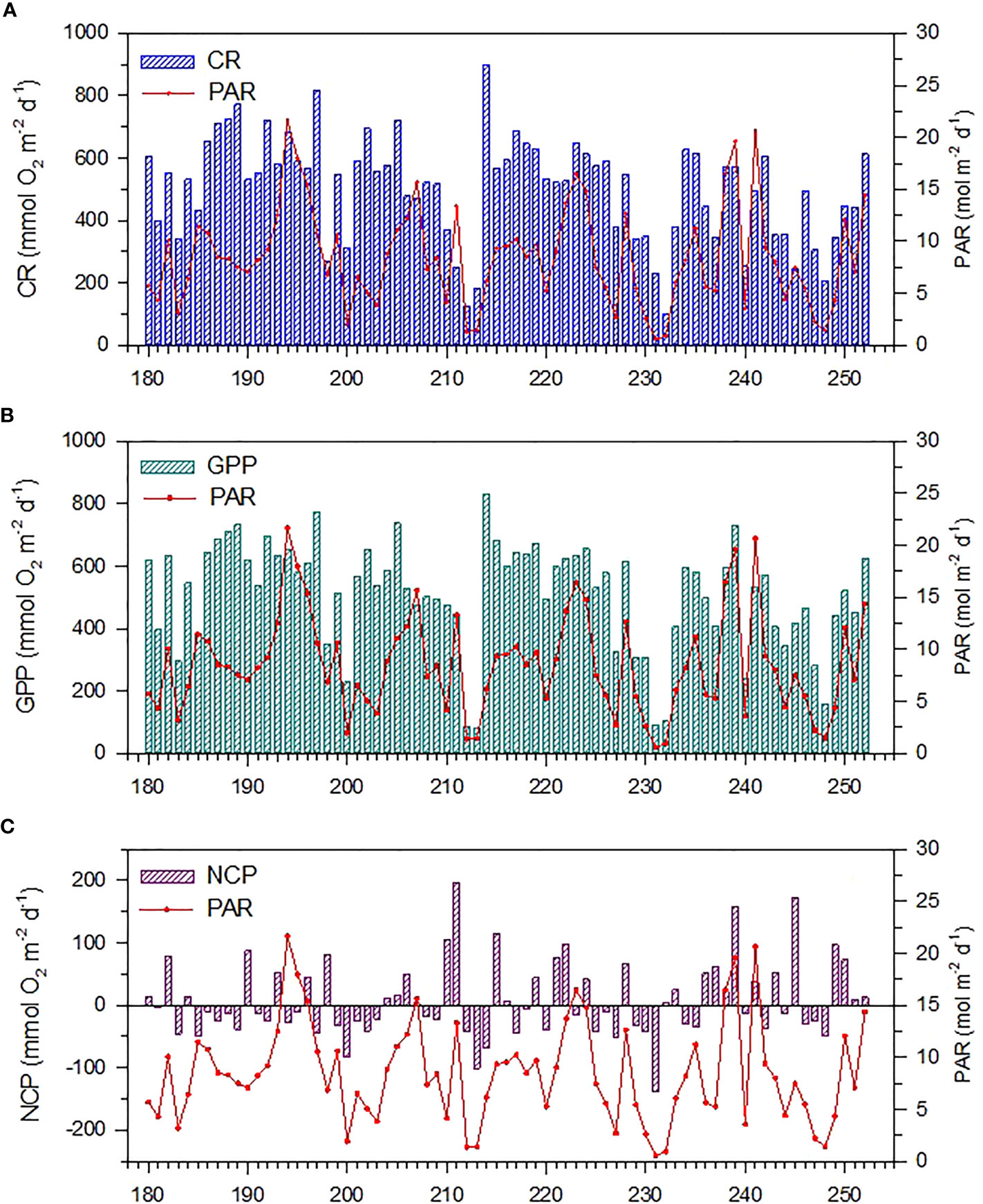
Figure 4 Daily (A) community respiration (CR), (B) gross primary production (GPP), and (C) net community production (NCP) from June 29 to September 9, 2019 (Julian day 180–252) over a seagrass meadow on the northern shore of Dongsha Island. The superimposed red line represents the daily photosynthetically active radiation (PAR).
4 Discussion
4.1 Comparison of seagrass community metabolism between the present study and global synthesis
Based on a data set containing 403 individual estimates derived from a total of 155 different sites, Duarte et al. (2010) estimated the global mean of GPP, CR, and NCP (mean ± SE) in seagrass meadows to be 225 ± 11, 188 ± 10, and 27 ± 6 mmol O2 m−2 d−1, respectively, while these metabolic rates were 507 ± 173, 497 ± 171, and 8 ± 61 mmol O2 m−2 d−1, respectively, in the present study (Table 1). This comparison clearly demonstrates that the averaged GPP and CR in the seagrass meadows on the northern shore of Dongsha Island were nearly 2.5 times higher than the global means, in contrast, the average NCP was similar to the global mean. This finding may not be surprising because the previous syntheses have shown that GPP, CR, and NCP reveal significant differences across different biogeographic locations and that tropical seagrass meadows tended to support higher metabolic rates, with somewhat lower NCP than temperate ones (Duarte et al., 2010). As Dongsha Island is located in the largest tropical marginal sea (the South China Sea) in the northwest Pacific, higher GPP and CR but lower NCP could be expected. Additionally, it is worth noting that the average total biomass of seagrass meadows on Dongsha Island (947-1047 g DW m-2, Huang et al., 2015) is within the high end of the global dataset (716 ± 140 g DW m-2, Fourqurean et al., 2012; Table 1). Furthermore, previous study has found that Thalassia hemprichii has the highest specific GPP to seagrass biomass ratio for meadows dominated by different species, including Cymodocea nodosa, Cymodocea rotundata, Enhalus acoroides, Halodule uninervis, Halodule wrightii, Halophila ovalis, Posidonia oceanica, Ruppia maritime, Syringodium filiforme, Syringodium isoetifolium, Thalassia hemprichii, Thalassia testudinum and Zostera marina (Duarte et al., 2010), and Thalassia hemprichii is one of the dominant species in the seagrass meadows on the northern shore of Dongsha Island (Lee et al., 2015). Therefore, the high biomass and high species-specific GPP to biomass ratio may partially explain the observed high GPP in the present study.
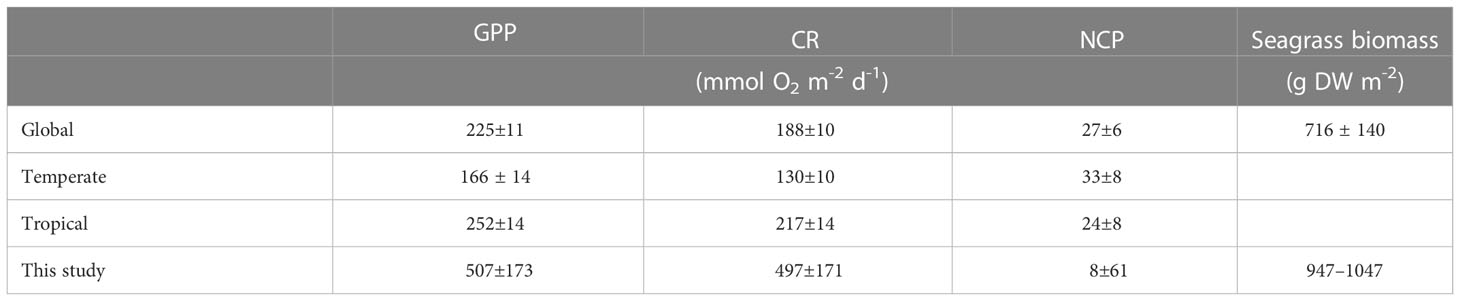
Table 1 Comparison of gross primary production (GPP), community respiration (CR), net community production (NCP), and seagrass biomass in dry weight units of Dongsha Island (this study) with those for global, temperate, and tropical seagrass meadows as studied by Duarte et al. (2010) for GPP,CR and NCP, and Fourqurean et al. (2012) for total biomass.
With the increasing recognition of their importance for carbon sequestration, seagrass conservation and restoration have been widely proposed as blue carbon strategies to mitigate and adapt to climate change (Nellemann et al., 2009; McLeod et al., 2011; Macreadie et al., 2019). Nevertheless, the present study suggests that seagrass meadows with high GPP in the tropical northwest Pacific do not necessarily hold a high potential for carbon sequestration due to the lower NCP. Accordingly, to obtain a better assessment of the potential of seagrass meadows as a nature-based solution for carbon sequestration, more regional-specific metabolic studies are imperatively needed before implementing any seagrass conservation and restoration plans, particularly in the northwestern Pacific archipelagos, where are hot spots of seagrass meadows but with very few record of community metabolism.
4.2 Relationships between community respiration, net community production, and gross primary production
A strong quadratic relationship was observed between CR and GPP (CR = 129 + 0.42xGPP + 0.0006xGPP2, r=0.94; Figure 5A), indicating that high production rates may support high community respiration rates. In seagrass meadows, CR comprises both autotrophic respiration (AR) and heterotrophic respiration (HR). Generally, AR is linked to GPP at the time scale of minutes to hours, whereas HR is linked to GPP at the time scale of hours to days for bacteria and days to weeks for metazoans (Del Giorgio and Williams, 2005; Champenois and Borges, 2021). We observed a strong coupling between daily CR and GPP (Figure 5A), thus suggesting that HR from metazoans is less likely the dominant contributor to the observed strong coupling of GPP and CR in seagrass meadows of Dongsha Island. In contrast, seagrasses could release a substantial amount of DOC (Barrón and Duarte, 2009), which can support bacterial HR, so enhanced HR in response to DOC release associated with high GPP might contribute to the close coupling of GPP and CR. It is then likely that the tight coupling between CR and GPP is mainly due to a close coupling between AR and GPP and/or HR for bacteria and GPP. Furthermore, the quadratic increasing trend between CR and GPP suggest that the rate of increase in CR with GPP would be lower at lower GPP than that at higher GPP. The different CR increasing rates at various GPP levels implies that the individual contribution of AR and HR to CR could respond differently to the variation in GPP. In fact, the separation of the individual contribution of AR and HR to CR remains a challenge to date in the metabolism studies of seagrass meadows (Champenois and Borges, 2021). The current results further suggest that how the AR and HR may respond differently to GPP variations is also an open question that needs to be carefully examined for a better understanding of the relationship between CR and GPP.
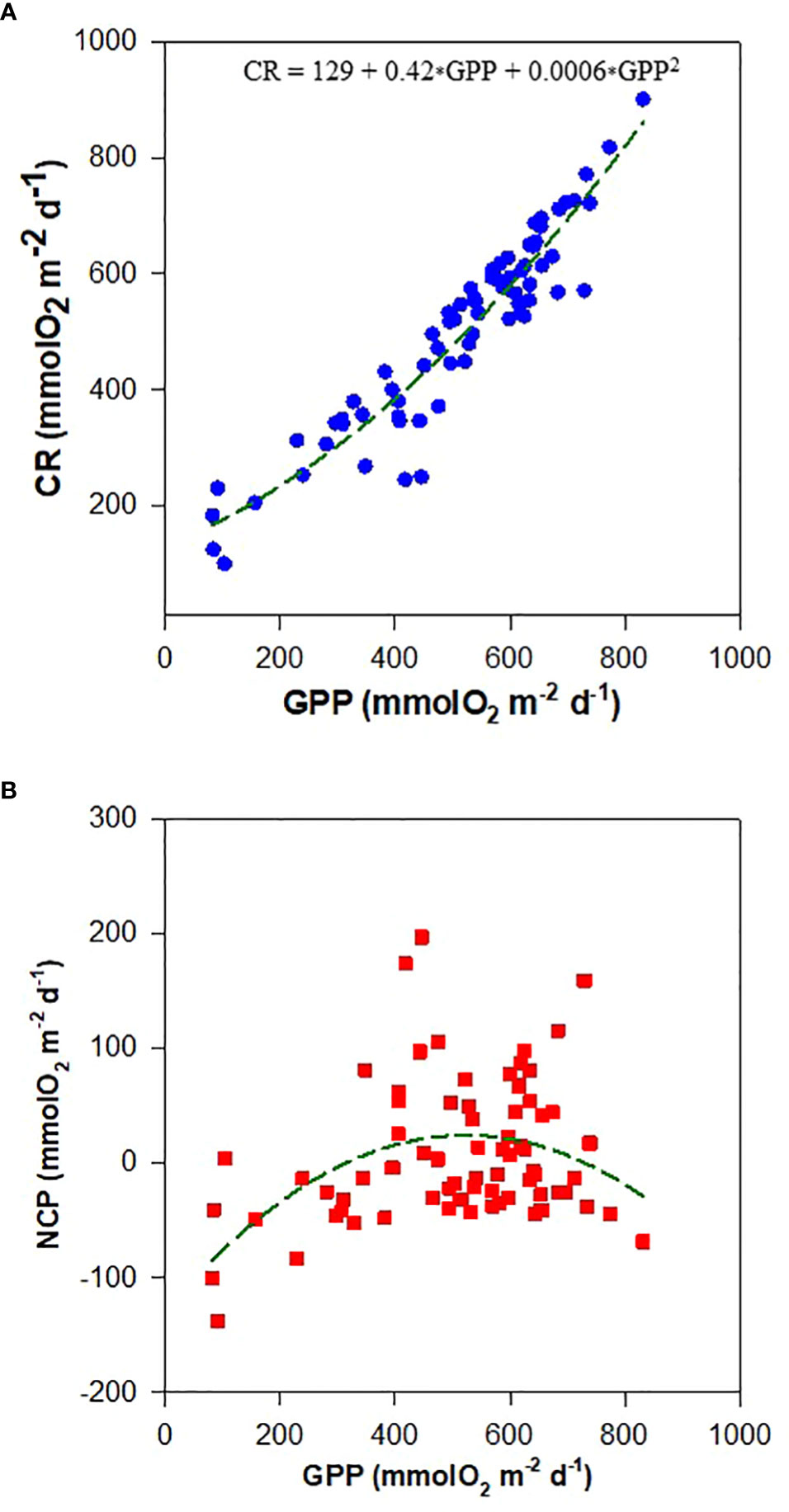
Figure 5 The relationship between (A) community respiration (CR) and gross community production (GPP) and (B) net community production (NCP) and GPP in seagrass meadows of Dongsha Island.
Unlike the strong correlation between CR and GPP, variability was observed in the relationship between NCP and GPP (Figure 5B). Although previous studies have suggested that NCP tends to increase with GPP (Duarte and Agustí, 1998; Champenois and Borges, 2021), the present dataset revealed that the increasing trend of NCP with GPP only held true at lower GPP (GPP<~500 mmol O2 m-2 d-1), and NCP did not increase with GPP anymore at higher GPP (GPP>~500 mmol O2 m-2 d-1; Figure 5B). Interestingly, the lower rate of increase in CR at low GPP but a higher rate of increase in CR at high GPP can reasonably explain the observed divergent relationships between NCP and GPP at different GPP levels.
An average threshold GPP of 186 mmol O2 m-2 d-1 on a global scale was proposed by Duarte et al. (2010), above which the seagrass meadows tended to be autotrophic (NCP>0) and shifted to heterotrophy (NCP<0) at lower GPP values. The average GPP in the current study was higher than 186 mmol O2 m-2 d-1, and NCP was positive and thus qualitatively in agreement with the global assessment. In spite of this agreement, the present dataset revealed a quite different GPP threshold from the global average; the present results show that seagrass meadows in the study area tended to be heterotrophic when GPP< 347 and GPP > 620 mmol O2 m-2 d-1, but they tended to be autotropic when GPP between 347 and 620 mmol O2 m-2 d-1 (Figure 5B). These results demonstrate again that the metabolic relationship in the seagrass meadows of Dongsha Island may deviate greatly from the global mean condition reported in previous synthesis studies, and thus more regional-specific studies are still needed in the key regions where support extensive seagrass meadows but have not yet been charted, such as Indonesia and Pacific archipelagos.
4.3 Relationship between net community production and pCO2
Although NCP and daily average pCO2 showed an expected negative correlation (Champenois and Borges, 2021; Figure 6A), the autotrophic/heterotrophic status did not correspond well to the sink/source behavior of CO2 in the seagrass meadow of Dongsha Island on a daily scale. As shown in Figure 6A, when NCP<0 (heterotrophic), pCO2 could fluctuate from a strong sink status (pCO2< 200 μatm) to a strong source status (pCO2 > 900 μatm), providing air pCO2 = 395 μatm. Similarly, when NCP>0 (autotrophic), pCO2 could also demonstrate either a sink or a source status. These results suggest that the organic carbon metabolism could not be the only dominant factor in determining the sink/source status of seagrass meadows (Macreadie et al., 2017; Saderne et al., 2019). This proposition can be further supported by the observed divergent temporal trends of NCP and pCO2 in the present dataset. As shown in Figure 6B, NCP did not show either a decrease or an increasing trend over the study period, while pCO2 revealed a significant decreasing trend with time.
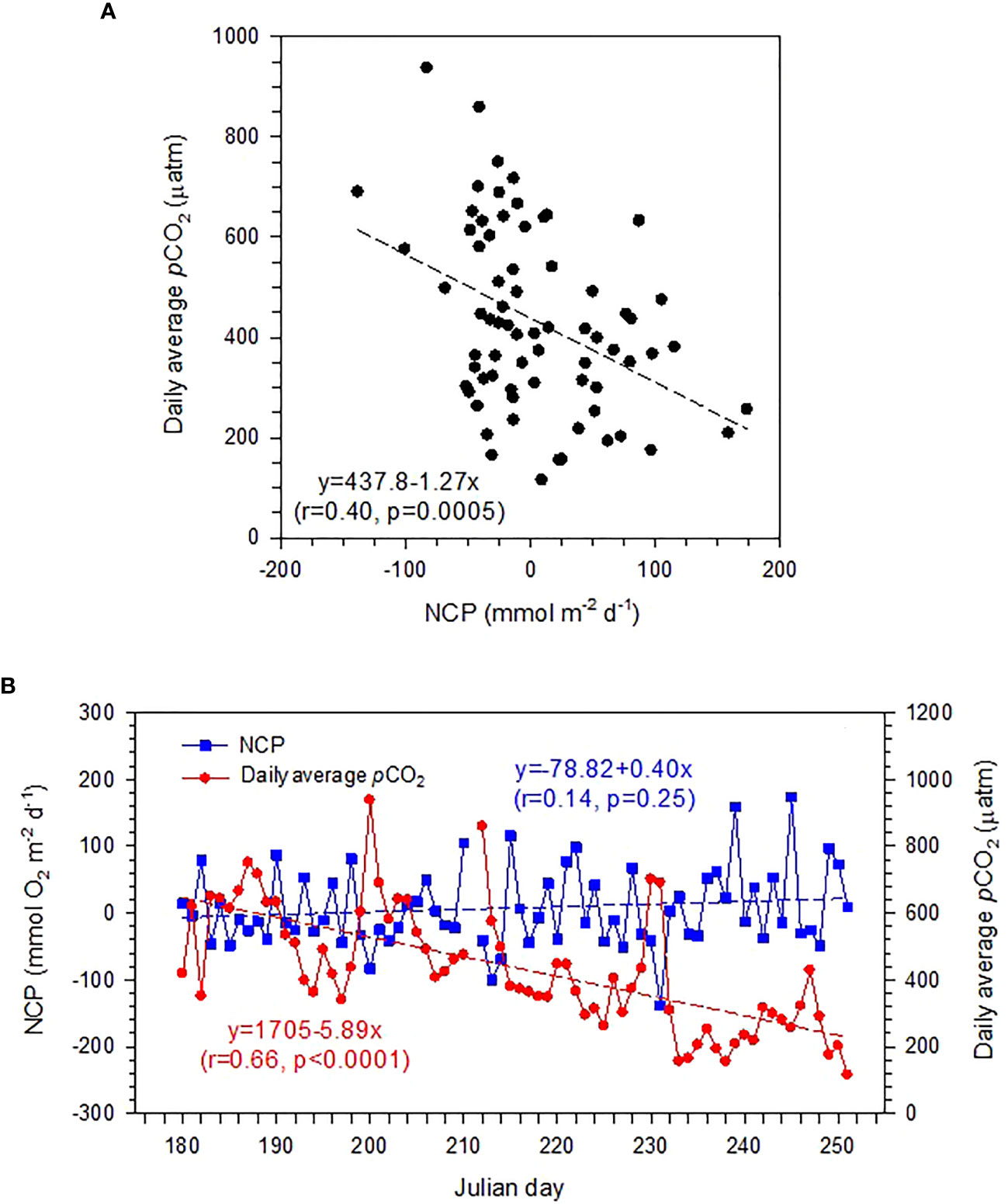
Figure 6 (A) The relationship between daily average pCO2 and net community production (NCP), and (B) daily variations in NCP and average pCO2 during the study period (June 29 to September 9, 2019, Julian day 180–252).
Several recent studies have highlighted that in addition to organic metabolism, inorganic carbon processing (i.e., calcium carbonate precipitation/dissolution; Macreadie et al., 2017; Saderne et al., 2019) and the external sources of land-based pollutants (Banerjee et al., 2018) may also play a pivotal role in regulating the capacity of CO2 sequestration of seagrass meadows. Since Dongsha Island is a military control zone, there is no permanent inhabitants neither no freshwater flow into the sea from the island, the impact of land-based pollution sources could be negligible. In terms of carbonate precipitation/dissolution, Van Dam et al. (2021) recently reported that calcification-induced CO2 emissions may exceed organic carbon sequestration in the seagrass-dominated estuary in central Florida Bay, and they argued that net carbon sequestration of seagrasses might be overestimated if calcification-induced CO2 emissions are not accounted for. On the contrary, our previous studies around Dongsha Island revealed that the enhancement of total alkalinity (TA) coupled with carbonate dissolution may enhance the CO2 sequestration capacity in seagrass meadows (Chou et al., 2018; Chou et al., 2021). We suggest that the lower pCO2 in late August and early September (Julian day 230–250) compared with that in late June and early July (Julian day 180–200) could be associated with the favorable condition for TA accumulation in water column deriving from sedimentary carbonate dissolution and/or anaerobic metabolism. During the monsoon transition period, the weak wind results in less energetic hydrodynamics and longer residence time for the waters around Dongsha Island, making it favorable for the occurrence of sedimentary metabolic carbonate dissolution and/or anaerobic TA generation and the subsequent TA accumulation in the overlying waters (Chou et al., 2018; Chou et al., 2021). The elevated TA may lead to a lower pCO2 level at a relatively stable NCP level. Unfortunately, TA data were unavailable during the monsoon transition period for northeasterly in September, but Chou et al. (2021) indeed found significantly higher TA during the monsoon transition period for southwesterly in April than in summer. This result implies that the different hydrodynamic regimes may lead to divergent sedimentary organic and inorganic metabolic processes, which may exert an important influence on regulating the carbon sequestration capacity of seagrass meadows. Therefore, the focus on organic carbon metabolism alone may not be enough, and hydrodynamics and calcium carbonate cycling remain key uncertainties that will need to be addressed to more accurately estimate the net carbon sequestration potential of seagrass meadows, especially for seagrass meadows with underlying carbonate sediments.
In summary, the present study is the first to report the metabolic rates of seagrass meadows in the Southeast Asia region. The results show that although daily NCP varied largely from autotrophy to heterotrophy, the average NCP over the entire study period was nearly in balance. Furthermore, the calculated GPP and CR in the present study were significantly higher, but NCP was similar to the global means, suggesting that seagrass meadows with higher GPP may not necessarily hold higher blue carbon potential. We also found that organic carbon metabolism was not the only dominant factor in determining the sink/source status in seagrass meadows of Dongsha Island, and hydrodynamics and calcium carbonate cycling may exert an important influence on regulating the carbon sequestration capacity of carbonate seagrass meadows. Since the present work was done mainly during the southwesterly monsoon period, more studies with better temporal coverage, in particular during the northeasterly monsoon and monsoon transition period are still needed to elucidate the linkage between monsoon-driven change in hydrodynamic and biogeochemical processes controlling CO2 variation in seagrass meadow on Dongsha Island. Overall, the current results in the Southeast Asia region deviate greatly from the global mean condition, and thus regional-specific studies are imperatively needed before implementing any seagrass conservation and restoration as a nature-based solution for carbon sequestration.
Data availability statement
The raw data supporting the conclusions of this article will be made available by the authors, without undue reservation.
Author contributions
W-CC conceived the study design, performed the field work, and wrote the manuscript. L-FF, C-CH, Y-YS, W-JH, H-KL, and T-YC contributed to the data collection and interpretation. All authors contributed to the article and approved the submitted version.
Funding
Funding was provided by the Ministry of Science and Technology of Taiwan, under grants no. #110-2611-M-019 -012 – and #111-2119-M-019 -002, awarded to W-CC.
Acknowledgments
We are grateful to the Dongsha Atoll Research Station, Dongsha Atoll National Park, and Coast Guard Administration for assistance in field sampling. We would also like to thank Ying-Hsuan Chen, Hui-Chuan Chu, Kuan-Chieh Wu, and Chang-Chang Yang for their help with the field and laboratory work.
Conflict of interest
The authors declare that the research was conducted in the absence of any commercial or financial relationships that could be construed as a potential conflict of interest.
Publisher’s note
All claims expressed in this article are solely those of the authors and do not necessarily represent those of their affiliated organizations, or those of the publisher, the editors and the reviewers. Any product that may be evaluated in this article, or claim that may be made by its manufacturer, is not guaranteed or endorsed by the publisher.
Supplementary material
The Supplementary Material for this article can be found online at: https://www.frontiersin.org/articles/10.3389/fmars.2023.1076991/full#supplementary-material
References
Banerjee K., Paneerselvam A., Ramachandran P., Ganguly D., Singh G., Ramesh R. (2018). Seagrass and macrophyte mediated CO2 and CH4 dynamics in shallow coastal waters. PloS One 13 (10), e0203922. doi: 10.1371/journal.pone.0203922
Barrón C., Duarte C. M. (2009). Dissolved organic matter release in a Posidonia oceanica meadow. Mar. Ecol. Prog. Ser. 374, 75–84. doi: 10.3354/meps07715
Barrón C., Duarte C. M., Frankignoulle M., Borges A. V. (2006). Organic carbon metabolism and carbonate dynamics in a Mediterranean seagrass (Posidonia oceanica) meadow. Estuar. Coast. 29, 417–426. doi: 10.1007/BF02784990
Chai F., Liu G., Xue H., Shi L., Chao Y., Tseng C. M., et al. (2009). Seasonal and interannual variability of carbon cycle in south China Sea: a three-dimensional physical-biogeochemical modeling study. J. Oceanogr. 65, 703–720. doi: 10.1007/s10872-009-0061-5
Champenois W., Borges A. V. (2012). Seasonal and interannual variations of community metabolism rates of a Posidonia oceanica seagrass meadow. Limnol. Oceanogr. 57, 347–361. doi: 10.4319/lo.2012.57.1.0347
Champenois W., Borges A. V. (2021). Net community metabolism of a Posidonia oceanica meadow. Limnol. Oceanogr. 66, 2126–2140. doi: 10.1002/lno.11724
Chang H.-Y. (2012). Spatial-temporal variation of epiphytic assemblages on seagrass leaves around dongsha island (Taichung, Taiwan: National Chung Hsing University). Master thesis (in Chinese with English abstract).
Chou W.-C., Chu H.-C., Chen Y.-H., Syu R.-W., Hung C.-C., Soong K. (2018). Short-term variability of carbon chemistry in two contrasting seagrass meadows at dongsha island: Implications for pH buffering and CO2 sequestration. Estuar. Coast. Shelf Sci. 210, 36–44. doi: 10.1016/j.ecss.2018.06.006
Chou W.-C., Fan L.-F., Yang C.-C., Chen Y.-H., Hung C.-C., Huang W.-J., et al. (2021). A unique diel pattern in carbonate chemistry in the seagrass meadows of dongsha island: the enhancement of metabolic carbonate dissolution in a semienclosed lagoon. Front. Mar. Sci. 8. doi: 10.3389/fmars.2021.717685
Dai C.-F. (2006). “Dongsha atoll in the South China Sea: Past, present and future,” in Proceeding of 10th International Coral Reef Symposium, Okinawa, Japan. June 28-July 2, 2004. 1587–1592 pp.
Del Giorgio P. A., Williams P. J. L. B. (2005). Respiration in aquatic ecosystems (New York: Oxford Univ. Press), 315. doi: 10.1093/acprof:oso/9780198527084
Duarte C. M., Agustí S. (1998). The CO2 balance of unproductive aquatic ecosystems. Science 281, 234–236. doi: 10.1126/science.281.5374.234
Duarte C. M., Chiscano C. L. (1999). Seagrass biomass and production: a reassessment. Aquat. Bot. 65, 159–174. doi: 10.1016/S0304-3770(99)00038-38
Duarte C. M., Kennedy H. A., Marbá N., Hendriks I. (2013). Assessing the capacity of seagrass meadows for carbon burial: Current limitations and future strategies. Ocean Coast. Manage. 83, 32–38. doi: 10.1016/j.ocecoaman.2011.09.001
Duarte C. M., Marbà N., Gacia E., Fourqurean J. W., Beggins J., Barrón C., et al. (2010). Seagrass community metabolism: Assessing the carbon sink capacity of seagrass meadows. Global Biogeochem. Cycles 24, GB4032. doi: 10.1029/2010GB003793
Fortes M. D., Ooi J. L. S., Tan Y. M., Prathep A., Bujang J. S., Yaakub S. M. (2018). Seagrass in southeast Asia: a review of status and knowledge gaps, and a road map for conservation. Botanica Marina; 61, 269–288. doi: 10.1515/bot-2018-0008
Fourqurean J. W., Duarte C. M., Kennedy H., Marbà N., Holmer M., Mateo M. A., et al. (2012). Seagrass ecosystems as a globally significant carbon stock. Nat. Geosci. 5, 505–509. doi: 10.1038/ngeo1477
Ho D. T., Law C. S., Smith M. J., Schlosser P., Harvey M., Hill P. (2006). Measurements of air-sea gas exchange at high wind speeds in the southern ocean: Implications for global parameterizations. Geophys. Res. Lett. 33, L16611. doi: 10.1029/2006GL026817
Huang Y. H., Lee C. L., Chung C. Y., Hsiao S. C., Lin H. J. (2015). Carbon budgets of multispecies seagrass beds at dongsha island in the south China sea. Mar. Environ. Res. 106, 92–102. doi: 10.1016/j.marenvres.2015.03.004
Lai C.-Z., DeGrandpre M. D., Darlington R. C. (2018). Autonomous optofluidic chemical analyzers for marine applications: insights from the submersible autonomous moored instruments (SAMI) for pH and pCO2. Front. Mar. Sci. 4. doi: 10.3389/fmars.2017.00438
Langhans R. W., Tibbitts T. W. (1997). Plant Growth Chamber Handbook. (Iowa Agriculture and Home Economics Experiment Station Special Report No. 99 (SR-99) and North Central Regional Research Publication No. 340.). Langhans R. W., Tibbitts T. W. Eds. (Ames, IA, USA: Iowa State University).
Lee C.-L., Huang Y.-H., Chung C.-Y., Hsiao S.-C., Lin H.-J. (2015). Herbivory in multi-species, tropical seagrass beds. Mar. Ecol. Prog. Ser. 525, 65–80. doi: 10.3354/meps11220
Lee J. S., Kang D.-J., Hineva E., Slabakova V., Todorova V., Park J., et al. (2017). Estimation of net ecosystem metabolism of seagrass meadows in the coastal waters of the East Sea and black Sea using the noninvasive eddy covariance technique. Ocean Sci. J. 52, 243–256. doi: 10.1007/s12601-017-0032-5
Lee C.-L., Lin W.-J., Liu P.-J., Shao K.-T., Lin H.-J. (2021). Highly productive tropical seagrass beds support diverse consumers and a Large organic carbon pool in the sediments. Diversity 13, 544. doi: 10.3390/d13110544
Liang C.-H. (2012). Temporal-spatial variation of heterotrophic bacterioplankton production and its growth controlling factor in dong-sha atoll (Keelung, Taiwan: National Taiwan Ocean University). Master thesis (in traditional Chinese with English abstract).
Lin H. J., Hsieh L. Y., Liu P. J. (2005). Seagrasses of tongsha island, with descriptions of four new records to Taiwan. Bot. Bull. Acad. 46, 163–168.
Macreadie P. I., Anton A., Raven J. A., Beaumont N., Connolly R. M., Friess D. A., et al. (2019). The future of blue carbon science. Nat. Commun. 10, 3998. doi: 10.1038/s41467-019-11693-w
Macreadie P. I., Serrano O., Maher D. T., Duarte C. M., Beardall J. (2017). Addressing calcium carbonate cycling in blue carbon accounting. Limnol. Oceanogr. Lett. 2, 195–192. doi: 10.1002/lol2.10052
McLeod E., Chmura G. L., Bouillon S., Salm R., Björk M., Duarte C. M., et al. (2011). A blueprint for blue carbon: toward an improved understanding of the role of vegetated coastal habitats in sequestering CO2. Front. Ecol. Environ. 9, 552–560. doi: 10.1890/110004
Middelburg J. J., Duarte C. M., Gattuso. J.-P. (2005). “Respiration in coastal benthic communities,” in Respiration in aquatic ecosystems. Eds. del Giorgio P. A., Williams P.J.l. (Oxford, U. K.: Oxford Univ. Press), 206–224.
Nellemann C., Corcoran E., Duarte C. M., Valdes L., DeYoung C., Fonseca L., et al. (2009). Blue carbon, the role of healthy oceans in binding carbon, a rapid response assessment, united nations environment programme, GRID-arendal. (Birkeland Trykkeri AS: Norway) 80.
Odum H. T. (1956). Primary production in flowing waters. Limnol. Oceanogr. 1, 102–117. doi: 10.4319/lo.1956.1.2.0102
Saderne V., Geraldi N. R., Macreadie P. I., Maher D. T., Middelburg J. J., Serrano O., et al. (2019). Role of carbonate burial in blue carbon budgets. Nat. Commun. 10, 1106. doi: 10.1038/s41467-019-08842-6
Short F., Carruthers T., Dennison W., Waycott M. (2007). Global seagrass distribution and diversity: A bioregional model. J. Exp. Mar. Biol. Ecol. 350, 3–20. doi: 10.1016/j.jembe.2007.06.012
Van Dam B. R., Zeller M. A., Lopes C., Smyth A. R., Böttcher M. E., Osburn C. L. (2007). Calcification-driven CO2 emissions exceed “Blue Carbon” sequestration in a carbonate seagrass meadow. Sci. Adv. 7, eabj1372. doi: 10.1126/sciadv.abj1372
Van Dam B. R., Lopes C., Osburn C. L., Fourqurean J. W. (2019). Net heterotrophy and carbonate dissolution in two subtropical seagrass meadows. Biogeosciences 16, 4411–4428. doi: 10.5194/bg-16-4411-2019
Keywords: seagrass, Dongsha Island, blue carbon, Southeast Asia, dissolved oxygen, carbon dioxide
Citation: Chou W-C, Fan L-F, Hung C-C, Shih Y-Y, Huang W-J, Lui H-K and Chen T-Y (2023) Dynamics of O2 and pCO2 in a Southeast Asia seagrass meadow: Metabolic rates and carbon sink capacity. Front. Mar. Sci. 10:1076991. doi: 10.3389/fmars.2023.1076991
Received: 22 October 2022; Accepted: 05 January 2023;
Published: 26 January 2023.
Edited by:
Tim Jennerjahn, Leibniz Centre for Tropical Marine Research (LG), GermanyReviewed by:
Dirk Jacob Koopmans, University of Virginia, United StatesDipnarayan Ganguly, National Centre for Sustainable Coastal Management, India
Zhijian Jiang, South China Sea Institute of Oceanology (CAS), China
Copyright © 2023 Chou, Fan, Hung, Shih, Huang, Lui and Chen. This is an open-access article distributed under the terms of the Creative Commons Attribution License (CC BY). The use, distribution or reproduction in other forums is permitted, provided the original author(s) and the copyright owner(s) are credited and that the original publication in this journal is cited, in accordance with accepted academic practice. No use, distribution or reproduction is permitted which does not comply with these terms.
*Correspondence: Wen-Chen Chou, d2NjaG91QG1haWwubnRvdS5lZHUudHc=