- 1Jiangsu Key Laboratory of Marine Bioresources and Environment, Jiangsu Ocean University, Lianyungang, China
- 2Co-Innovation Center of Jiangsu Marine Bio-industry Technology, Jiangsu Institute of Marine Resources Development, Lianyungang, China
- 3Jiangsu Institute of Marine Resources Development, Jiangsu Ocean University, Lianyungang, China
Polychlorinated biphenyls (PCBs) are environmentally persistent and highly toxic organochlorine compounds that may cause toxic effects on aquatic animals. In this study we assess the toxic effect of PCBs on a bivalve used in aquaculture, the clam Cyclina sinensis. To this end, individuals of C. sinensis were exposed for 72 h at two PCB concentrations (1 ng/L and 10 ng/L) and control (absence of PCBs). At the end of the exposure, the hemolymph, hepatopancreas, and gills samples of C. sinensis were harvested for analysis of the enzyme activity and histology. The results showed that acute PCBs exposure decreased the survival rate of C. sinensis compared to the control. Acute PCBs exposure up-regulated the enzymatic activity of superoxide dismutase (SOD), catalase (CAT) and glutathione peroxidase (GSH-Px) and the content of malondialdehyde (MDA) in the hemolymph of C. sinensis, while down-regulated the non-specific enzymatic activity of alkaline phosphatase (AKP). For the hepatopancreas, 1 ng/L PCBs exposure up-regulated the enzymatic activity of SOD while down-regulated the enzymatic activity of CAT of C. sinensis. In the gill, the enzymatic activity of CAT decreased significantly and the MDA content increased of C. sinensis after 10 ng/L PCBs exposure. Moreover, histological observations showed that acute exposure to PCBs caused loss of gill filaments and lateral cilia and shortening of their length, in the studied organism. The present study will provide valuable reference data for marine shellfish aquaculture and toxicology research.
1 Introduction
Polychlorinated biphenyls (PCBs) are synthetic organochlorine compounds consisting of 209 congeners that have been used in hundreds of industrial and commercial applications due to their non-flammability, chemical stability, high boiling point and electrical insulation properties in the early years (Rudel et al., 2008; Weis et al., 2011). However, research showed that PCBs are environmentally persistent, highly toxic and bioaccumulate (EUA, 2000; Cao et al., 2008). Thus, concerns over the toxicity and persistence of PCBs in the environment led to the usage of PCBs was banned in the 1970s (Dodoo et al., 2013). Nevertheless, PCBs continue to enter nearby waterways in most developing countries through the uncontrolled spillage, stream transport, surface runoff and atmospheric deposition and that accumulated through the food pyramid (Beate and Ralf, 2004; Lin et al., 2020; Xiao et al., 2021). Recent surveys have found that the concentration of PCBs ranges from 15.1 to 57.9 ng/g (mean: 34.5 ng/g) in the sediment of the Minjiang River in southern China (Zhang et al., 2003).
The most obvious signs of environmental harm caused by PCBs are in the aquatic ecosystems (Environment Canada, 2008). Once PCBs are released into the aquatic environment, they can be bioaccumulate along the food chain and pose potential hazards to other organisms and human consumers (Ashley et al., 2000; Fontenot et al., 2000; Pruell et al., 2000; Dodoo et al., 2013). As the second largest phylum of invertebrates, molluscs are major aquaculture species worldwide and its aquaculture accounts for approximately 27% of the total world aquaculture production (Guo, 2009). With the improvement of people’s understanding of the safety of aquatic products, more and more research focus on the detection of the accumulation level of PCBs in wild and farmed mollusk (Madureira et al., 2014; Sun et al., 2015; Zaynab et al., 2021). Take the oysters Crassostrea tulipa as an example, the content of PCBs in oysters ranged from 2.95-11.41 mg/kg wet weight (Dodoo et al., 2013). Similarly, Milun et al. (2016) reported that the concentrations of PCBs ranged from 1.53 to 21.1(ng g-1 dry weight) in the soft tissue of bivalves. However, the studies regarding the toxic effects of PCB in bivalves is poorly understood.
The clam Cyclina sinensis are widely distributed in the coastal beaches and estuaries of China, Japan and North Korea, and have been widely cultivated and proliferated in China (Ge et al., 2021; Dong et al., 2021; Ge et al., 2022). In recent years, extensive C. sinensis culture have been carried out in coastal areas, but diseases are frequent, including germplasm decline, environmental pollution and pathogenic stimulation have resulted in high mortality in this species and resulted in huge economic losses (Liang et al., 2000; Ni et al., 2021). As a shellfish with typical filter-feeding behavior, PCBs may accumulate in the gills and digestive glands of C. sinensis through their presence in the aquatic environment and in food. Studies have shown that when the concentration of organic contaminants in the environment is above the toxic tolerance limit, it will have serious negative effects on farm animals (Xiao et al., 2021). Several studies have reported that the bivalves in this type of environment suffer from increased mortality, elevated oxidative stress, immune dysfunction, and disturbed energy metabolism (Stewart et al., 2020; Klimova et al., 2021; Balbi et al., 2021).
Antioxidants related to glutathione (GSH) metabolism, and antioxidant enzymes such as superoxide dismutase (SOD) and catalase (CAT), are current topics in biomarker in bivalves during environmental pollutants or factors stress (Liu and Wang, 2016; Danielli et al., 2017; Liang et al., 2022). Some hydrolytic enzymes like alkaline phosphatase (AKP) and ACP (acid phosphatase) also play a role in bivalve non-specific immunity because they may accelerate phagocytosis by modifying pathogen surface molecule (Cheng, 1978; Tang et al., 2010; Adzigbli et al., 2020; Sun et al., 2022). It has been reported that the bivalve gill is the tissue that first comes into contact with foreign particles, which is highly susceptible to damage from environmental pollutants (Cui et al., 2019; Teng et al., 2021; De Campos et al., 2021). The hemolymph and Hepatopancreas also played key role in innate immunity or detoxification for bivalve (Kasturi et al., 2006; Bouallegui, 2019; Zhang et al., 2019). Therefore, the gill, hemolymph and hepatopancreas of mollusks are three target organs that are very valuable to study in acute stress experiments.
To study the toxic effects of PCBs on the bivalves, the clams (Cyclina sinensis) were exposed at three different concentrations of PCBs (control, 1 ng/LPCB, 10 ng/L PCBs) for 72 h in this study. At the end of the exposure, the hemolymph, hepatopancreas, and gills samples of C. sinensis were harvested for analysis of the enzyme activity and histology. These results will explore the PCBs toxicity to C. sinensis, and this study may provide valuable reference data for marine shellfish aquaculture and toxicology research.
2 Materials and methods
2.1 Animal and culture conditions
The C. sinensis (shell lengths: 3.5-4.1 cm; body weight: 17.5-18.7 g) were obtained from seaside aquaculture base of Jiangsu Ocean University, Ganyu District, Lianyungang City, Jiangsu Province, China. The C. sinensis were transported alive to Shellfish Laboratory of Jiangsu Ocean University, Lianyungang, China, and maintained in cement tanks (length × width × depth=100 cm × 100 cm × 80 cm) filled with natural seawater (salinity 25 ± 1 ppt). During acclimatization, the clams were fed with microalgae Chaeroeeros moelleri twice in the 8:30am and 18:00pm. The microalgae Chaeroeeros moelleri was purchased from Wudi Zaocheng Biotechnology company (Shandong, China). The water quality was maintained at: ammonia-N<0.5 mg L−1; nitrite<0.10 mg L−1; DO>5 mg L−1 and pH 7.0–9.0. After acclimated for seven days, the individual C. sinensis of similar size (body weight: 18 ± 0.2g) were selected for the following acute PCBs exposure experiments.
2.2 PCBs exposure and sampling
Polychlorinated biphenyls (P115160, 100μg/mL) were purchased from Bilan Marine Bio-Technology company (Jiangsu, China). Prior to the experiment, the PCBs solution was diluted using the ethanol, the solvent control with 0.001% ethanol was used as the negative control. The exposure experiment is divided into three groups (control, 1 ng/L PCBs treatment and 10 ng/L PCBs treatment) based on a review of the literature (Dong et al., 2017; Zha et al., 2019a; Zha et al., 2019b; Xiao et al., 2021). 63 clams were used in this experiment and each group had three replicates. Throughout the experiment, the seawater with PCBs was changed every 24 h to ensure that the concentration of PCBs in each group remained invariable during the experiment. Moreover, the number of deaths of clams was recorded for subsequent calculation of the survival rate. The survival rate was calculated using the following formulas:
Where X is the number of final clams, X0 is the number of initial clams.
In order to study the effect of acute PCB stress on C. sinensis, we chose the time point of 72 h exposure as the duration of the stress experiment based on previous studies in zebrafish (Licata et al., 2019a; Licata et al., 2019b). After PCBs exposure for 72 h, fifteen clams were sampled randomly from each group. Approximately 500 μL hemolymph was collected from the adductor muscle of each clams using a sterilized syringe and placed in a 1.5 mL centrifuge tube for the measurement of enzyme activities. The hepatopancreas and gills from each sampled clam were divided into two sub-samples: one was fixed in 4% Paraformaldehyde for histological sectioning, and the remaining tissue was frozen in liquid nitrogen and stored at -40°C for following biochemical analysis.
2.3 Determination of biochemical parameters after acute PCBs exposure
Hemolymph samples were firstly thawed and homogenized using an IKA homogenizer (T10B, IKA Co., Germany). The resultant homogenates were then centrifuged at 12000 rpm for 10 min at 4°C, and the supernatant were placed in sterile centrifuge tubes and stored in 4°C refrigerator for subsequent measurement. Around 0.1 g of hepatopancreas and gills was weighted and added to ice-cold physiological saline solution in a proportion of 1:9 (w/v), and then were homogenized using an IKA homogenizer in a 2 mL centrifuge tube. The homogenate was then centrifuged at 10,000 rpm for 10 min at 4°C, and the supernatant phase was taken and stored at 4°C for later analysis. The same volume of supernatant phase from each sample at the same replicate tank were pooled and mixed prior to later analysis. Thus, three pooled samples for each treatment were detected in this study.
The activities of superoxide dismutase (SOD, A001-3), catalase (CAT, A007-1), glutathione peroxidase (GSH-Px, A005-1), acid phosphatase (ACP, A060-2), alkaline phosphatase (AKP, A059-2) and malondialdehyde (MDA, A003-1) content in the gill, hemolymph and hepatopancreas were analyzed with a spectrophotometer and corresponding detection kits (Nanjing Jiancheng Biological Product, China) according to the manufacturer’s guidelines. The total protein contents of the tissue samples were determined using a Coomassie Brilliant Blue Total Protein Assay Kit (Nanjing Jiancheng Bioengineering Research Institute, Nanjing, China). The enzyme activities in the gill and hepatopancreas were expressed as enzyme unit perprotein (U/mg), while the enzyme activities in the hemolymph were expressed as enzyme unit permilliliter (U/mL).
2.4 Histologic observation of gills and hepatopancreas after acute PCBs exposure
The gills and hepatopancreas were fixed in 4% Paraformaldehyde for 24 h and then dehydrated in ascending concentrations of ethanol solutions, cleared in xylene and embedded in paraffin wax. Embedded tissues were cut into 6-μm-thick sections using a rotary microtome (Leica RM2125RTS, Leica Microsystems, Bannockburn, IL, U.S.A.). Sections of gills and hepatopancreas were stained with hematoxylin-eosin for observation under a light microscope (Nikon, Japan) equipped with an automated Leica digital camera system (Nikon, Japan and image manager software (Nikon, Japan). The gill filament and lateral cilia length of C. sinensis were measured with Image J 1.8.0 software. 5 individuals and 15 sections in each group were randomly selected for histological observation. The histological structure of the gills and hepatopancreas of C. sinensis according to the previous study (Cui et al., 2005; Teng et al., 2021; Liang, 2022). Specifically, the gill filaments are arranged parallel, wider on the outside, thinner on the center and inside, and the outer 1/3 to 1/2 of the gill filaments are covered with a single layer of columnar epithelium, and the lateral cilia are longer. Interfilament connections are covered with cuboidal or flattened epithelium, with abundant connective tissue under the epithelium. The hepatopancreas of clam is composed of multiple blind-end tubules with walls consisting of four kinds of cells: the embryonic cell (E cell), blister-like cell (B cell), resorptive cell (R cell) and fibrillar cell (F cell).
2.5 Data analysis
Data were presented as the mean ± standard error (SE). Homogeneity of the variance of relevant data was tested with Levene’s test. When necessary, an arcsine-square root or logarithmic transformation was performed prior to analysis. One-way ANOVA and Duncan’s multiple range determine significant differences between groups. All statistical analysis was performed using SPSS 19.0 statistical software (IBM, USA). The level of significance was set to P< 0.05. The graphs are drawn by software Graphpad Prism 9 (Graphpad Software Inc., USA).
3 Results
3.1 Impacts of acute PCBs exposure on C. sinensis survival rate
As shown in Figure 1, the survival rate of C. sinensis after acute PCBs exposure was decreased. The results showed that the lowest survival rate of 81% for the 10 ng/L PCBs group and the highest survival rate of 95% for the control group. There was no significant difference in the survival rate of C. sinensis among the three treatment groups.
3.2 Impacts of acute PCBs exposure on the non-specific immunity and antioxidant capability
To investigate the effects of acute PCBs exposure on antioxidant capability and non-specific immunity of C. sinensis, the activities of SOD, CAT, GSH-Px and MDA content in hemolymph, hepatopancreas and gills were detected. The results further showed that the SOD activity in the hemolymph of C. sinensis increased gradually with the increasing of PCBs concentration (Figure 2A). Compared to the control group, the CAT, MDA, GSH-Px in the hemolymph increased significantly in 1 ng/L PCBs group (Figures 2B–D). As shown in Figure 3, the hepatopancreatic SOD activity of C. sinensis showed a trend of first increasing and then decreasing after acute PCBs exposure. Compared to the control group, the hepatopancreatic CAT, MDA and GSH-Px decreased in a dose-dependent manner by PCBs groups (Figures 3B–D). In the gills, the SOD, MDA and GSH-Px a trend of first decreasing and then increasing followed by an increase with PCBs concentration, while there was no significant difference among the three groups (Figures 4A, C, D). However, the CAT activity in the gills decreased significantly with the ascending of PCBs concentration (Figure 4B, P<0.05).
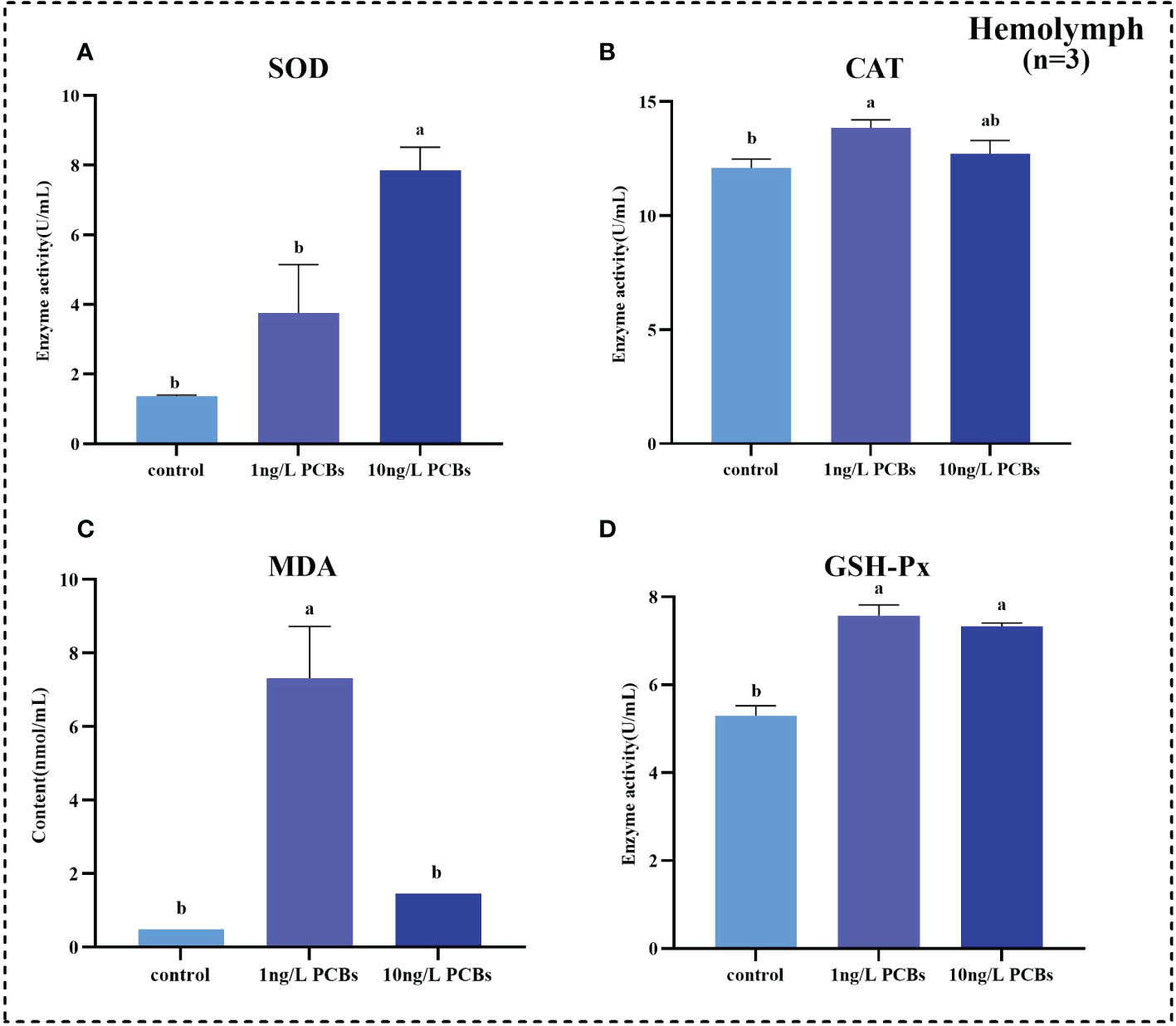
Figure 2 Effect of PCBs on the antioxidant capacity in the hemolymph of C. sinensis. The columns with different letter indicate significant difference (P< 0.05). SOD: superoxide dismutase; CAT: catalase; MDA: malonaldehyde; GSH-Px: glutathione peroxidase.
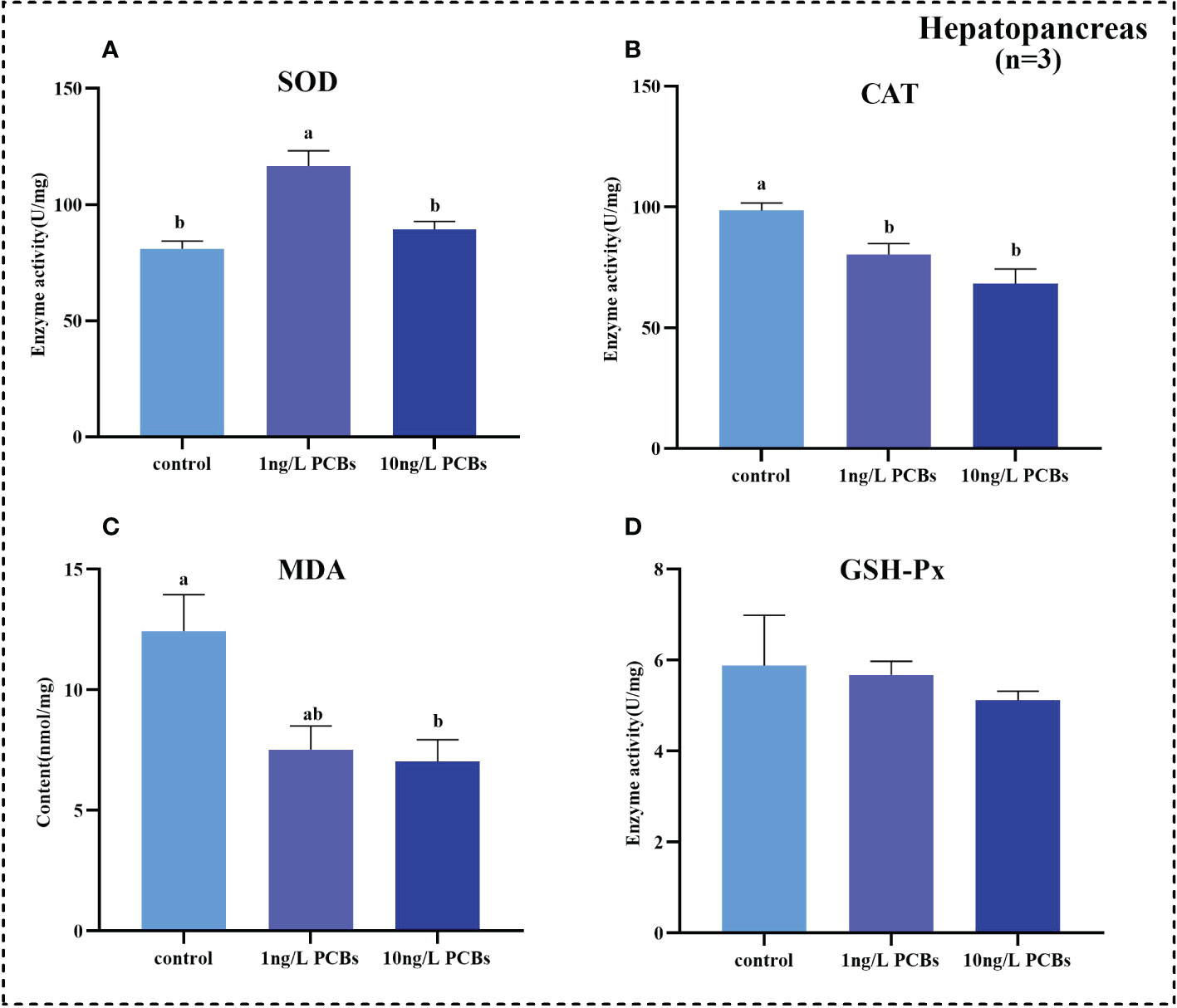
Figure 3 Effect of PCBs on the antioxidant capacity in the hepatopancreas of C. sinensis. The columns with different letter indicate significant difference (P< 0.05). SOD: superoxide dismutase; CAT: catalase; MDA: malonaldehyde; GSH-Px: glutathione peroxidase.
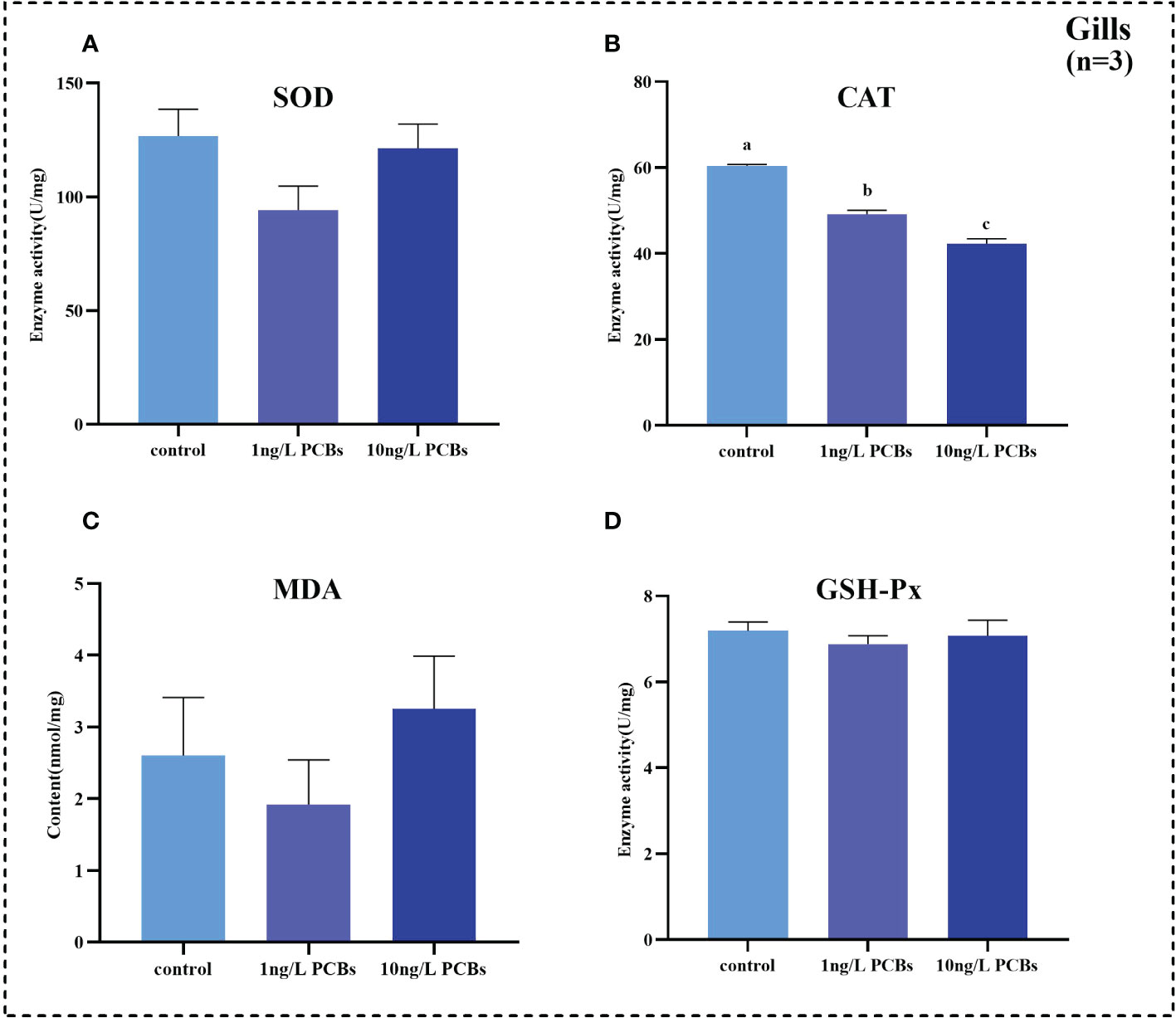
Figure 4 Effect of PCBs on the antioxidant capacity in the gills of C. sinensis. The columns with different letter indicate significant difference (P< 0.05). SOD: superoxide dismutase; CAT: catalase; MDA: malonaldehyde; GSH-Px: glutathione peroxidase.
The enzyme activities of ACP and AKP in hemolymph and hepatopancreas after acute PCBs exposure have also been examined. In the hemolymph, no observable changes were evident in the ACP activity among the three groups (Figure 5A). However, the enzyme activity of AKP in the PCBs treatments significantly lower than that of the control group (Figure 5B). As shown in Figures 5C, D, after 72 h PCBs exposure, the activities of ACP and AKP in the hepatopancreas decreased gradually in comparison to the control, while there was no significant difference among the different groups.
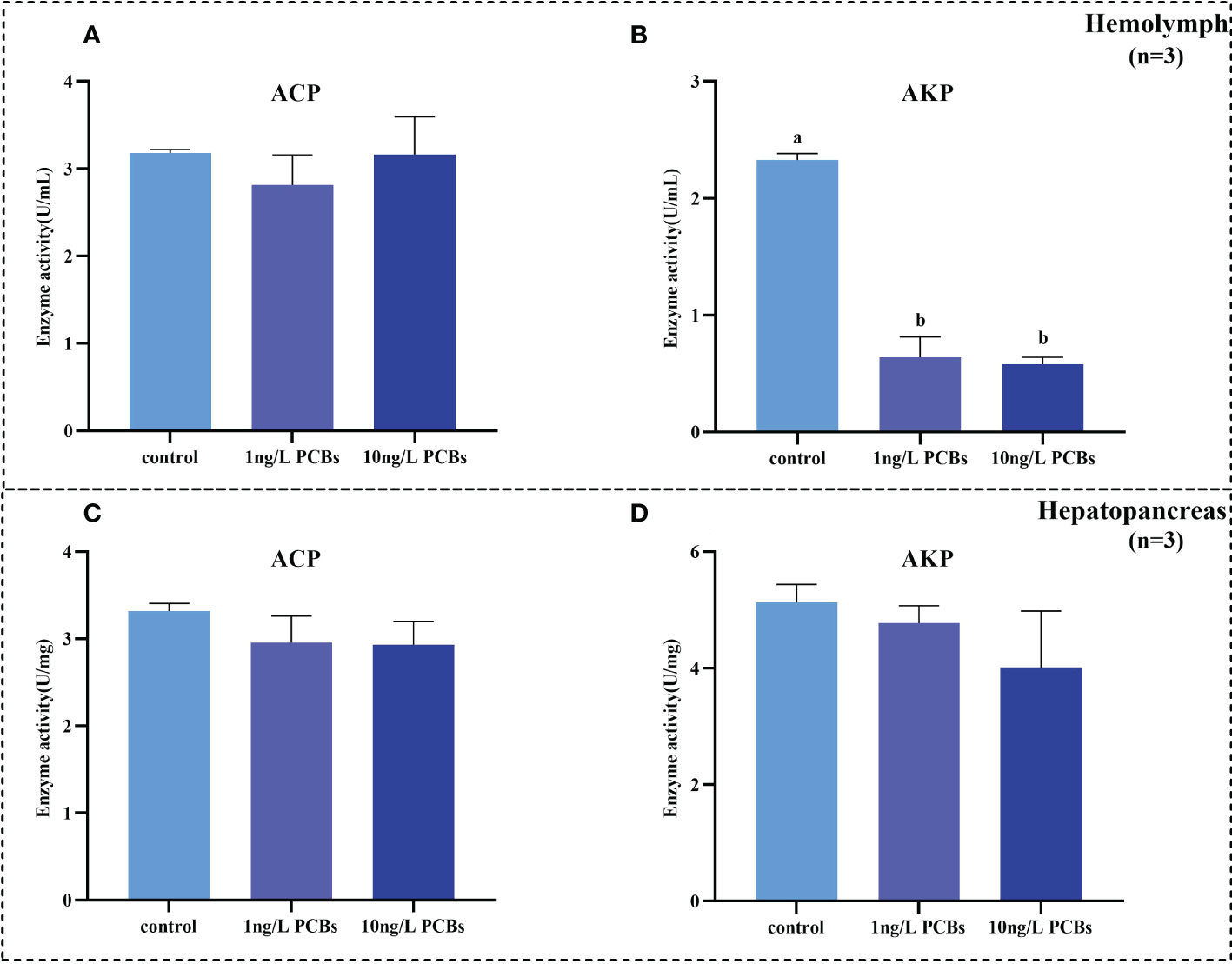
Figure 5 Effect of PCBs on the non-specific immunity indices in the hemolymph and hepatopancreas of C. sinensis. The columns with different letter indicate significant difference (P< 0.05). AKP: alkaline phosphatase; ACP: acid phosphatase.
3.3 Histological effect of acute PCBs exposure on gills and hepatopancreas
Compared with the control group, with the increase of PCBs concentration, the gill plate gap gradually widened and the tissue structure was incomplete and appeared to be detached and defective of C. sinensis (Figure 6). After 72 h PCBs exposure, most of the gill filaments and lateral cilia in the clam gills in the 10 ng/L PCBs group were shed, and the connective tissue inside the columnar cells on the gills basically disappeared (Figure 6C). Meanwhile, the length of gill filament and lateral cilia of C. sinensis in the 10 ng/L PCBs group were significantly shorter than those in the control group (Table 1).
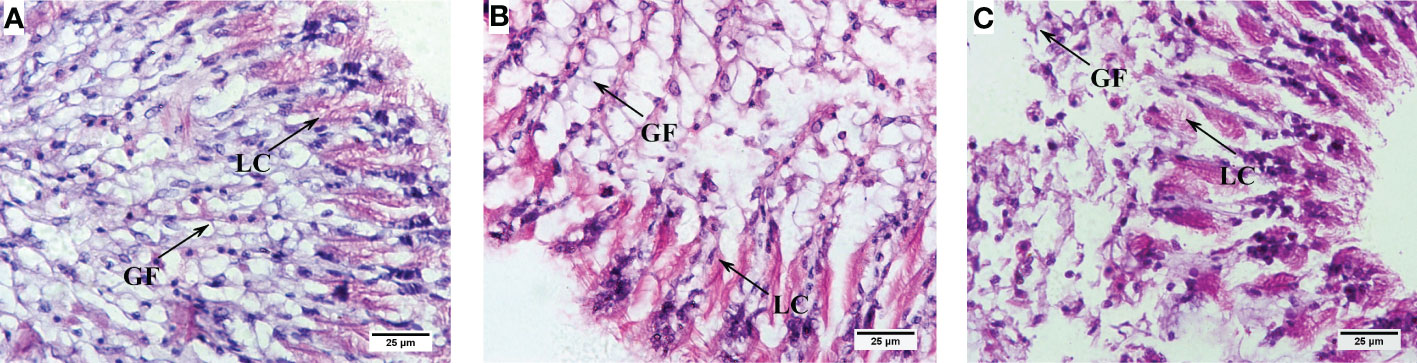
Figure 6 Histological changes in the gills of (C) sinensis under different concentrations of PCBs. Notes: (A) control; (B) 1ng/L PCBs group; (C) 10 ng/L PCBs group; GF: gill filament; LC: lateral cilia.
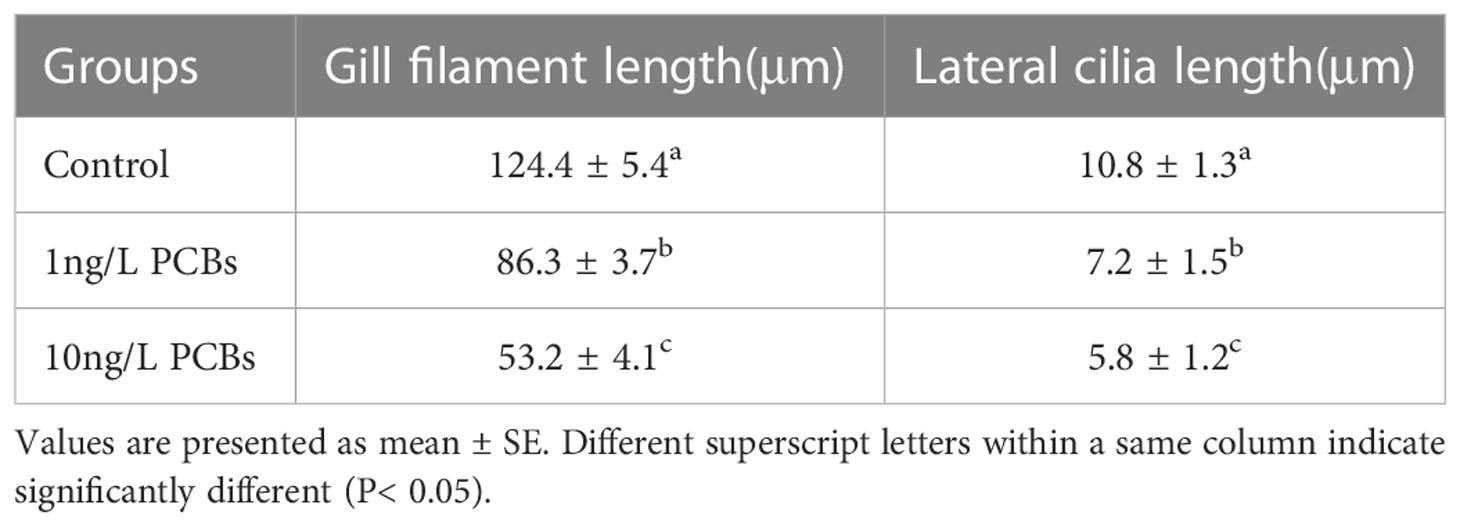
Table 1 The Gill filament and Lateral cilia length of C. sinensis after exposure to different concentration of PCBs for 72 h. (N=5).
The histological results showed that the number of blister-like cells in the hepatopancreatic ducts tended to increase with increasing PCB concentration, but there was no significant difference between the groups (Figure 7). Furthermore, the hepatopancreatic tubular lumen of C. sinensis gradually shrinks with the increase of PCBs concentration (Figure 7).
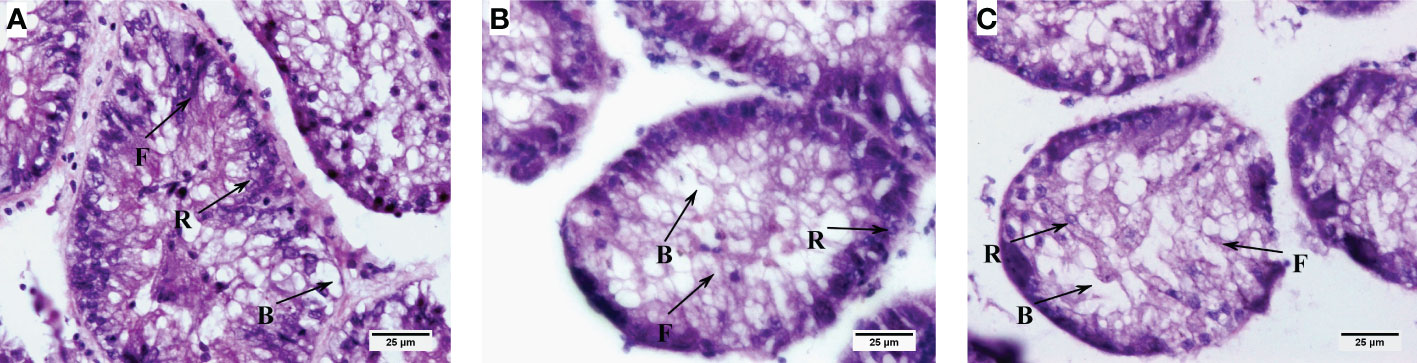
Figure 7 Histological changes in the hepatopancreas of (C) sinensis under different concentrations of PCBs. Notes: (A) control; (B) 1ng/L PCBs group; (C) 10 ng/L PCBs group. B: blister-like cell; F: fibrillar cell; R: resorptive cell.
4 Discussion
In this study, the relationship among the survival rate, non-specific immunity antioxidant capability, histology and concentrations of PCBs were investigated using clams, Cyclina sinensis, as the experimental animal. The results are envisaged to be important towards increasing knowledge of physiological response in the C. sinensis, and also shading some lights on the possible ecotoxicological effects of PCBs contamination in effluent seawaters. PCBs are frequently detected in aquatic environments, which particularly toxic to fishes and invertebrates and are fatal to these animals in even small concentrations (McGraw-Hill, 1987; Encyclopedia, 2003). Previous studies showed that the toxicity of PCBs is thought to be solely mediated through binding to aryl hydrocarbon receptor, AhR (Safe et al., 1985; Yuan et al., 2014). Thus, the adverse effects of PCBs in mammal were reported such as hepatotoxicity, endocrine effects, immunotoxicity, body weight loss, teratogenicity and carcinogenicity (Yuan et al., 2014). Recently, several studies reported that PCBs treatment significantly decreased the survival rate of aquatic animals (He et al., 2010; Adams et al., 2016; Xiao et al., 2021). In present study, the survival rate of C. sinensis also decreased after exposure to PCBs for 72 h, further indicating that PCBs have toxic effects on aquatic organisms even in the low concentration (Berg et al., 2011; Gard et al., 2021).
Identifying how environmental pollutants reduce the antioxidant capacity and innate immunity of farmed animals has become an important topic in the aquaculture research (Xiao et al., 2021). Several studies have reported that the bivalves in organic pollutants of environment suffer from elevated oxidative stress, immune dysfunction, and disturbed energy metabolism (Stewart et al., 2020; Klimova et al., 2021; Balbi et al., 2021). Therefore, in order to protect themselves, the activities of enzymes related to the antioxidant system in the organism are altered in response to oxidative stress (Karakoc et al., 1997). As the important intracellular primary antioxidant enzymes, SOD neutralizes O2− and converts it into the less reactive H2O2, while CAT catalyses the conversion of H2O2 to water and molecular oxygen during the process of ROS elimination (Valko et al., 2006; Newsholme et al., 2016). GSH-Px catalyse the removal of hydroperoxides using the tripeptide glutathione as reducing substrate (Duan et al., 2015). MDA is formed as a byproduct caused by oxidative stress, which is generally used as a biomarker for oxidative damage (Lopes et al., 2001; Aldini et al., 2010). In this study, PCBs exposure up-regulated the enzymatic activities of SOD, CAT and GSH-Px and the content of MDA in the hemolymph of C. sinensis, indicating that hemolymph is very sensitive to PCBs exposure and that it mobilizes a variety of antioxidant enzymes to cope with the oxidative damage caused by PCB to maintain biological homeostasis. Similarly, He and Chen (2010) reported that PCBs treatment significantly increased the enzymatic activities of SOD, CAT and GSH-Px and the content of MDA in the mudskipper Boleophthalmus pectinirostris compared to the control group. Feng et al. (2019) reported that the PCBs treatment significantly increased the enzymatic activities of SOD, CAT and the content of MDA in the hemolymph of Chinese mitten crab Eriocheir sinensis. Moreover, the enzymatic activity of CAT decreased significantly and the MDA content increased in the gills of C. sinensis after 10 ng/L PCBs exposure, indicating that PCBs treatment caused the body to produce excess ROS, which in turn damaged the gills and reduced enzyme activity (Cheng et al., 2020; Cheng et al., 2021). For the hepatopancreas, 1 ng/L PCBs exposure up-regulated the enzymatic activity of SOD while down-regulated the enzymatic activity of CAT of C. sinensis. It was speculated that the hepatopancreas is under low oxidative stress and it can eliminate damage caused by PCBs by regulating the balance of the oxidative system in the hepatopancreas (Vieira et al., 2012; Zheng et al., 2016). The low content of MDA in the hepatopancreas also further indicated that the antioxidant enzymes alleviate oxidative stress, and thereby were likely to have limited the accumulation of MDA (Han et al., 2014).
The innate immune response of marine bivalve molluscs is the main immune system against the stressful conditions (Pourmozaffar et al., 2019). In this study, non-specific immune-related enzymes ACP and AKP were studied to assessment the non-specific immune response to PCBs stress of C. sinensis. ACP and AKP, important lysosomal marker enzymes, played an important role in non-specific immune responses and were often used as indicators to evaluate the immune status of organisms (Rahman and Siddiqui, 2004; Ma et al., 2013; Liang et al., 2014). In bivalve’s species, the activities of ACP and AKP is often measured to evaluate their immune response to adverse environmental and biological factors, such as heat, pH, temperature, heavy metals and virus, etc (Tang et al., 2010; Hu et al., 2015; Yang et al., 2019; Adzigbli et al., 2020; Xu et al., 2021; Yang et al., 2021). Moreover, a previous study in crustacean have shown that the enzyme activities of ACP were decreased in the hemolymph after PCBs treatment (Feng et al., 2019). In agreement with previous results, this study also found that the enzymatic activities of AKP and ACP in the hemolymph and hepatopancreas decline after acute PCBs exposure, indicating that PCB damages the non-specific immune system of C. sinensis through inhibited the enzymatic activities of AKP and ACP.
Gill is the respiratory organ as well as the feeding organ of shellfish. Shellfish can filter food, transport food particles, conduct gas, ion exchange and other activities through gill structures, such as cilia (Li, 2008; De Campos et al., 2021). Biotransformation of organic xenobiotics, excretion of harmful trace metals and food digestion and storage are the main hepatopancreas functions (Hinton et al., 2001; Zhang et al., 2019). Besides the measurement/evaluation of chemical and physical parameters, evidence arising from histo-cytopathological examinations has been increasingly recognised as valuable tool to evaluate the impact of pollutants on aquatic animals (Au, 2004; Carballeira et al., 2011; Sikdokur et al., 2020). Thus, PCBs exposure caused histological changes in the gills and hepatopancreas of clams was also investigated in this study. Based on the histological analysis generated in this study, it was shown that PCBs expose caused the gill plate gap widened and tissue structure appeared to be detached and defective of C. sinensis. These results indicate that PCBs impacts physiological metabolism also by causing damage to structure of gills. Differently to what reported in crustaceans, the present study showed that histological structure of the hepatopancreas in the PCBs treatments of C. sinensis did not show significant changes compared to the control group. The possible reasons for the contradictory results on the PCBs may related to the period of exposure experiment (Feng et al., 2019; Xiao et al., 2021).
5 Conclusion
This study investigated the effect of acute PCBs exposure on the survival, non-specific immunity, antioxidant capability and histology of the C. sinensis. The results showed that acute PCBs exposure down-regulated the survival rate, the CAT activity and MDA content in the hepatopancreas, while up-regulated the CAT, SOD, GSH-Px activities and MDA content in the hemolymph of C. sinensis. Moreover, PCBs exposure caused the gill plate gap widened and tissue structure appeared to be detached and defective of C. sinensis, and down-regulated the CAT activity in the gills (Figure 8). These data will provide valuable reference data for further understanding the effects of PCBs on marine shellfish toxicology. In the future, tailored studies on the toxicological mechanisms of PCBs regulating the physiological metabolism of shellfish are therefore warranted.
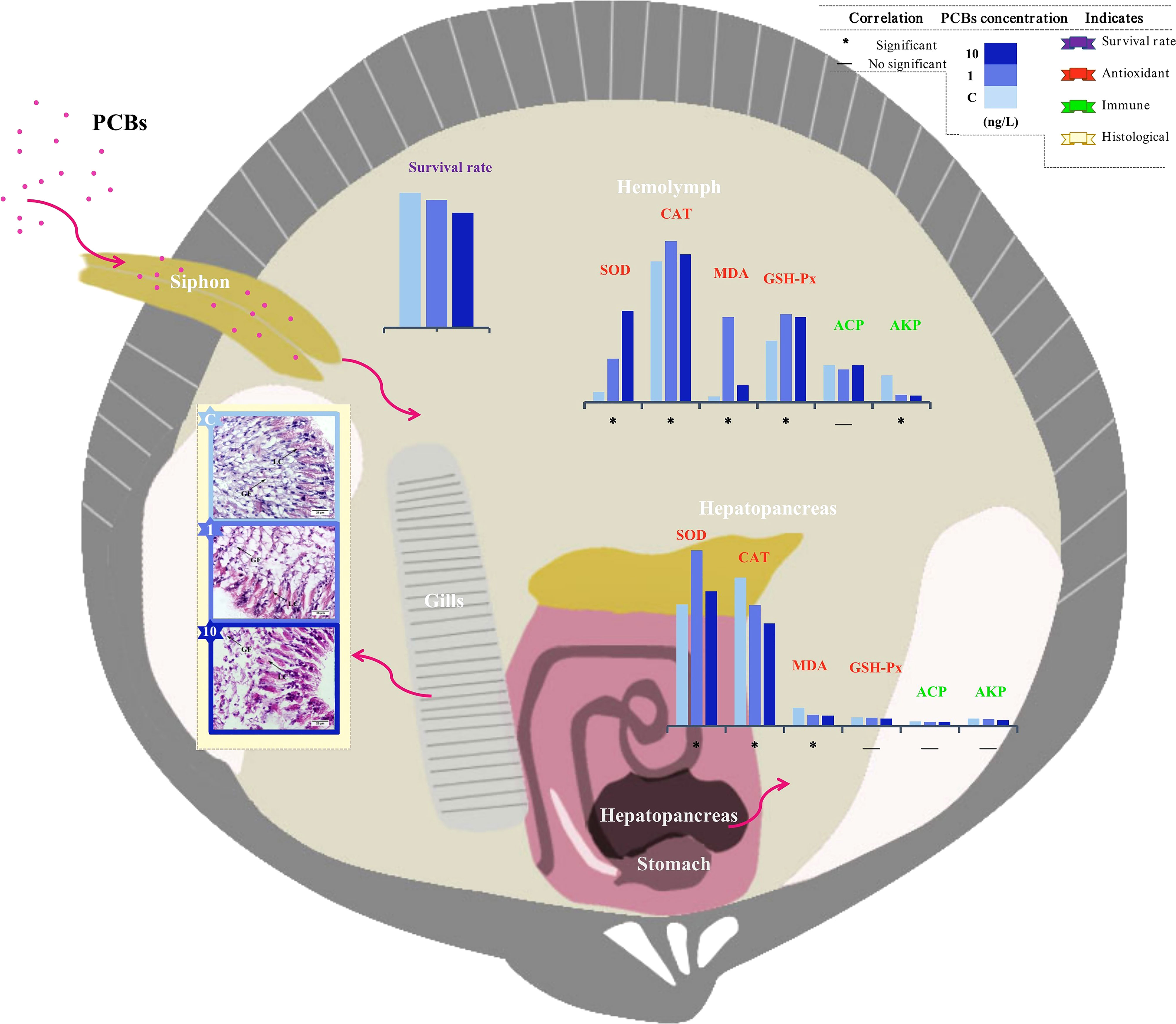
Figure 8 Schematic of the effects of acute PCBs exposure on the histological changes of the gills, and antioxidant capacity and non-specific immunity response of hemolymph as well as hepatopancreas of C. sinensis. SOD: superoxide dismutase; CAT: catalase; MDA: malonaldehyde; GSH-Px: glutathione peroxidase. AKP: alkaline phosphatase; ACP: acid phosphatase.
Data availability statement
The original contributions presented in the study are included in the article/supplementary material. Further inquiries can be directed to the corresponding author.
Author contributions
ML: Experimental design, writing - original draft, data curation. SF: Formal analysis, data curation. ZR: Data curation, validation. HQ: Data curation, visualization. SY: Experimental design, formal analysis. ZD: Writing-editing, funding acquisition. All authors contributed to the article and approved the submitted version.
Funding
This study was funded by the”JBGS”Project of Seed Industry Revitalization in Jiangsu Province(JBGS[2021]034), an Open-end Funds (SH20201205) of Jiangsu Key Laboratory of Marine Bioresources and Environment, two practice innovation training program projects (No. 202111641127Y and No. SY202257X) for the Jiangsu College students. Infrastructure costs were partially supported by the Project of Jiangsu Fisheries Science and Technology (SZ-LYG202029) and Modern Agro-industry Technology Research System (CARS-49).
Conflict of interest
The authors declare that the research was conducted in the absence of any commercial or financial relationships that could be construed as a potential conflict of interest.
Publisher’s note
All claims expressed in this article are solely those of the authors and do not necessarily represent those of their affiliated organizations, or those of the publisher, the editors and the reviewers. Any product that may be evaluated in this article, or claim that may be made by its manufacturer, is not guaranteed or endorsed by the publisher.
Supplementary material
The Supplementary Material for this article can be found online at: https://www.frontiersin.org/articles/10.3389/fmars.2023.1076870/full#supplementary-material
References
Adams M., Baker J., Kjellerup B. (2016). Toxicological effects of polychlorinated biphenyls (PCBs) on freshwater turtles in the united states. Chemosphere. 154, 148–154. doi: 10.1016/j.chemosphere.2016.03.102
Adzigbli L., Wang Z., Li J., Deng Y. (2020). Survival, retention rate and immunity of the black shell colored stocks of pearl oyster Pinctada fucata martensii after grafting operation. Fish. Shellfish Immun. 98, 691–698. doi: 10.1016/j.fsi.2019.11.003
Aldini G., Dalle-Donne I., Facino R., Milzani A., Carini M. (2010). Intervention strategies to inhibit protein carbonylation by lipoxidation-derived reactive carbonyls. Med. Res. Rev. 27 (6), 817–868. doi: 10.1002/med.20073
Ashley J., Secor D., Slokovitz E. (2000). Linking habitat use of Hudson river striped bass to the accumulation of polychlorinated biphenyls. Environ. Sci. Technol. 34, 1023–1029. doi: 10.1021/es990833t
Au D. (2004). The application of histo-cytopathological biomarkers in marine pollution monitoring: a review. Mar. pollut. Bull. 48, 817–834. doi: 10.1016/j.marpolbul.2004.02.032
Balbi T., Auguste M., Ciacci C., Canesi L. (2021). Immunological responses of marine bivalves to contaminant exposure: contribution of the omics approach. Front. Immun. 12. doi: 10.3389/FIMMU.2021.618726
Beate U., Ralf S. (2004). Developmental toxicity of polychlorinated biphenyls (PCBs): a systematic review of experimental data. Arch. Toxicol. 78, 483–487. doi: 10.1007/s00204-004-0583-y
Berg K., Puntervolland P., Klungsøyr J., Goksøyr A. (2011). Brain proteome alterations of atlantic cod (Gadus morhua) exposed to PCB 153. Aquat. Toxicol. 105 (3-4), 206–217. doi: 10.1016/j.aquatox.2011.06.010
Bouallegui Y. (2019). Immunity in mussels: An overview of molecular components and mechanisms with a focus on the functional defenses. Fish Shellfish Immun. 89, 158–169. doi: 10.1016/j.fsi.2019.03.057
Cao X., Chen H., Shen S., Song Y., Lian X. (2008). The nature and environmental impact of PCBs. Sciencepa. On. 5), 375–381.
Carballeira C., Espinosa J., Carballeira A. (2011). Linking δ15n and histopathological effects in molluscs exposed in situ to effluents from land-based marine fish farms. Mar. pollut. Bull. 62 (12), 2633–2641. doi: 10.1016/j.marpolbul.2011.09.034
Cheng T. (1978). The role of lysosmal hydrolases in moluscan cellular response to immunologic challenge (US: Springer). doi: 10.1007/978-1-4757-1278-0_4
Cheng C., Ma H., Deng Y., Feng J., Jie Y., Guo Z. (2021). Oxidative stress, cell cycle arrest, DNA damage and apoptosis in the mud crab (Scylla paramamosain) induced by cadmium exposure. Chemosphere. 263, 128277. doi: 10.1016/j.chemosphere.2020.128277
Cheng C., Su Y., Ma H., Deng Y., Feng J., Chen X., et al. (2020). Effect of nitrite exposure on oxidative stress, DNA damage and apoptosis in mud crab (Scylla paramamosain). Chemosphere. 239, 124668. doi: 10.1016/j.chemosphere.2019.124668
Cui L., Kong J., Zhou X. (2005). Observation on the gill of Mactra chinensis with optical and scanning electron microscopes. Prog. Fish. Sci. 4), 60–63. doi: 10.3969/j.issn.1000-7075.2005.04.010
Cui Q., Qiu L., Yang X. (2019). Transcriptome profiling of the low-salinity stress responses in the gills of the juvenile Pseudopleuronectes yokohamae. Comp. Biochem. Phys. D. 32, 100612. doi: 10.1016/j.cbd.2019.100612
Danielli N., Trevisan R., Mello D., Fischer K., Deconto V., Acosta D., et al. (2017). Upregulating Nrf2-dependent antioxidant defenses in pacific oysters Crassostrea gigas: Investigating the Nrf2/Keap1 pathway in bivalves. Comp. Biochem. Physiol. C. 195, 16–26. doi: 10.1016/j.cbpc.2017.02.004
De Campos B. G., Fontes M. K., Gusso-Choueri P. K., Marinsek G. P., Nobre C. R., Moreno B. B., et al. (2021). A preliminary study on multi-level biomarkers response of the tropical oyster Crassostrea brasiliana to exposure to the antifouling biocide DCOIT. Mar. pollut. Bull. 174, 113241. doi: 10.1016/j.marpolbul.2021.113241
Dodoo D., Essumang D., Jonathan J. (2013). Accumulation profile and seasonal variations of polychlorinated biphenyls (PCBs) in bivalves Crassostrea tulipa (oysters) and Anadara senilis (mussels) at three different aquatic habitats in two seasons in Ghana. Ecotoxicol. Environ. Saf. 88, 26–34. doi: 10.1016/j.ecoenv.2012.10.013
Dong Z., Duan H., Zheng H., Ge H., Wei M., Liu M., et al. (2021). Research progress in genentic resources assessment, culture technique and exploration utilization of Cyclina sinensis. J. Fish. China. 45 (12), 2083–2098. doi: 10.11964/jfc.20201212545
Dong Y., Zhang X., Tian H., Li X., Wang W., Ru S. (2017). Effects of polychlorinated biphenyls on metamorphosis of a marine fish Japanese flounder (Paralichthys olivaceus) in relation to thyroid disruption. Mar. pollut. Bull. 119, 325–331. doi: 10.1016/j.marpolbul.2017.04.033
Duan Y., Zhang J., Dong H., Wang Y., Liu Q., Li H. (2015). Oxidative stress response of the black tiger shrimp Penaeus monodon to Vibrio parahaemolyticus challenge. Fish. Shellfish Immun. 46 (2), 354–365. doi: 10.1016/j.fsi.2015.06.032
EUA (2000). Department of health and human services. agency for toxic substances and disease registry. toxicological profile for polychlorinated biphenyls (Update). ATSDR toxicological profile.
Encyclopedia, B (2003). The New Encyclopedia Britannica (Chicago: Encyclopedia Britannica Inc) 9, 577.
Feng D., Wang X., Li E., Bu X., Qiao F., Qin J., et al. (2019). Dietary aroclor 1254-induced toxicity on antioxidant capacity, immunity and energy metabolism in Chinese mitten crab Eriocheir sinensis: Amelioration by vitamin a. Front. Physiol. 10. doi: 10.3389/fphys.2019.00722
Fontenot L., Noblet G., Atkins J. (2000). Bioaccumulation of polychlorinated biphenyls in ranid frogs and northern water snakes from a hazardous waste site and a contaminated watershed. Chemosphere. 40, 803–809. doi: 10.1016/S0045-6535(99)00329-X
Gard N., Edwards M., Kulacki K., Ginn T. (2021). Limitations on development of polychlorinated biphenyl tissue concentration thresholds for survival, growth, and reproduction in fish. Environ. Toxicol. Chem. 40 (8), 2085–2097. doi: 10.1002/ETC.5071
Ge H., Liu J., Ni Q., Wang F., Dong Z. (2022). Effects of acute ammonia exposure and post-exposure recovery on nonspecific immunity in clam cyclina sinensis. Isr. J. Aquacult-Bamid. 74, 1–10. doi: 10.46989/001c.32647
Ge H., Shi J., Liu J., Liang X., Dong Z. (2021). Combined analysis of mRNA-miRNA reveals the regulatory roles of miRNAs in the metabolism of clam Cyclina sinensis hepatopancreas during acute ammonia nitrogen stress. Aquacult. Res. 53 (4), 1492–1506. doi: 10.1111/ARE.15683
Guo X. (2009). Use and exchange of genetic resources in molluscan aquaculture: Genetic resources in molluscan aquaculture. Rev. Aquacult. 1 (3-4), 251–259. doi: 10.1111/j.1753-5131.2009.01014.x
Han X., Gao B., Wang H., Liu P., Chen P., Li H. (2014). Effects of low salinity stress on microstructure of gill and hepatopancreas and family survival rate of Portunus trituberculatus. Pro. Fish. Sci. 35 (1), 104–110. doi: 10.3969/j.issn.1000-7075.2014.01.015
He S., Chen B. (2010). Effects of aroclor 1248 on oxidative stress and toxicity in Boleophthalmus pectinirostris. Acta Hydrobio. Sini. 34 (2), 442–447. doi: 10.3724/SP.J.1035.2010.00442
Hinton D., Segner H., Braunbeck T. (2001). “Toxic responses of the liver,” in Toxicity in marine and freshwater teleosts, vol. 1 . Eds. Schlenk D., Bensen W. H. (London: Taylor & Francis), 224–268.
Hu M., Li L., Sui Y., Li L., Wang Y., Lu W., et al. (2015). Effect of pH and temperature on antioxidant responses of the thick shell mussel Mytilus coruscus. Fish. Shellfish Immun. 46 (2), 573–583. doi: 10.1016/j.fsi.2015.07.025
Karakoc F., Hewer A., Philips D., Gaines A., Yuregir G. (1997). Biomarkers of marine pollution observed in species of mullet living in two eastern Mediterranean harbours. Biomarkers 2 (5), 303–309. doi: 10.1080/135475097231535
Kasturi R., Lutz A., Peter C. (2006). Ammonia toxicity and its effect on the growth of the south African abalone Haliotis midae Linnaeus. Aquaculture. 261 (2), 678–687. doi: 10.1016/j.aquaculture.2006.06.020
Klimova Y., Chuiko G., Pesnya D., Ivanova E. (2021). Biomarkers of oxidative stress in freshwater bivalve mollusks (Review). Inland Water Biol. 13 (4), 674–683. doi: 10.1134/S1995082920060073
Li J. (2008). Studies on the shell characters, feeding, and digestive systems and nuclear DNA of saxidomus purpuratus (Sowerby) (Shaodong Qingdao: Ocean University of China). Master Thesis.
Liang X. (2022). Effects of ammonia nitrogen stress on detoxification metabolism and functional verification of related genes in cyclina sinensis (Jiangsu Liangyungang: Jiangsu Ocean University). Master Thesis. doi: 10.44354/d.cnki.gjsuy.2022.000260
Liang J., Liu Y., Zhu F., Li Y., Liang S., Guo Y. (2022). Impact of ocean acidification on the physiology of digestive gland of razor clams Sinonovacula constricta. Front. Mar. Sci. 9. doi: 10.3389/FMARS.2022.1010350
Liang S., Luo X., You W., Luo L., Ke C. (2014). The role of hybridization in improving the immune response and thermal tolerance of abalone. Fish. Shellfish Immun. 39 (1), 69–77. doi: 10.1016/j.fsi.2014.04.014
Liang Y., Yang B., Wang L., Zhang X., Wu Z., Jiang Y., et al. (2000). Disease triggering mechanism and protecting policy of the mariculture shellfish in coast of yellow sea. Mar. Environ. Res. 1), 5–10. doi: 10.3969/j.issn.1007-6336.2000.01.002
Licata P., Piccione G., Fazio F., Lauriano E., Calò M. (2019a). Protective effects of genistein on cytochrome p-450 and vitellogenin expression in liver of zebrafish after PCB-126 exposure. Sci. Total Environ. 674, 71–76. doi: 10.1016/j.scitotenv.2019.03.467
Licata P., Tardugno R., Pergolizzi S., Capillo G., Aragona M., Colombo A., et al. (2019b). In vivo effects of PCB-126 and genistein on vitellogenin expression in zebrafish. Nat. Prod. Res. 33 (17), 2507–2514. doi: 10.1080/14786419.2018.1455048
Lin S., Zhao B., Ying Z., Fan S., Hu Z., Xue F., et al. (2020). Residual characteristics and potential health risk assessment of polychlorinated biphenyls (PCBs) in seafood and surface sediments from xiangshan bay, China, (2011-2016). Food Chem. 327, 126994. doi: 10.1016/j.foodchem.2020.126994
Liu X., Wang W. (2016). Antioxidant and detoxification responses of oysters Crassostrea hongkongensis in a multimetal-contaminated estuary. Environ. Toxicol. Chem. 35 (11), 2798–2805. doi: 10.1002/etc.3455
Lopes P., Pinheiro T., Santos M., Da L., Collares-Pereira M., Viegas-Crespo A. (2001). Response of antioxidant enzymes in freshwater fish populations (Leuciscus alburnoides complex) to inorganic pollutants exposure. Sci. Total Environ. 280, 153–163. doi: 10.1016/S0048-9697(01)00822-1
Madureira T., Santos C., Velhote S., Cruzeiro C., Rocha E., Rocha M. (2014). Contamination levels of polychlorinated biphenyls in wild versus cultivated samples of female and male mussels (Mytilus sp.) from the Northwest coast of Iberian peninsula-new application for QuEChERS (Quick, easy, cheap, effective, rugged, and safe) methodology. Environ. Sci. pollut. Res. Int. 21 (2), 1528–1540. doi: 10.1007/s11356-013-2017-y
Ma Y., Liu Z., Yang Z., Li M., Liu J., Song J. (2013). Effects of dietary live yeast Hanseniaspora opuntiae C21 on the immune and disease resistance against Vibrio splendidus infection in juvenile sea cucumber Apostichopus japonicus. Fish. Shellfish Immun. 34 (1), 66–73. doi: 10.1016/j.fsi.2012.10.005
Milun V., Lušić J., Despalatović M. (2016). Polychlorinated biphenyls, organochlorine pesticides and trace metals in cultured and harvested bivalves from the eastern Adriatic coast (Croatia). Chemosphere. 153, 18–27. doi: 10.1016/j.chemosphere.2016.03.039
Newsholme P., Cruzat V., Keane K., Carlessi R., Bittencourt P. (2016). Molecular mechanisms of ROS production and oxidative stress in diabetes. Biochem. J. 473 (24), 4527–4550. doi: 10.1042/BCJ20160503C
Ni Q., Li W., Liang X., Liu J., Ge H., Dong Z. (2021). Gill transcriptome analysis reveals the molecular response to the acute low-salinity stress in Cyclina sinensis. Aquacult. Rep. 19, 100564. doi: 10.1016/J.AQREP.2020.100564
Pourmozaffar S., Tamadoni J., Rameshi H., Sadeghi A., Bagheri T., Behzadi S., et al. (2019). The role of salinity in physiological responses of bivalves. Rev. Aquacult. 12, 1548–1566. doi: 10.1111/raq.12397
Pruell J., Taplin B., Mcgovern D. (2000). Organic contaminant distributions in sediments, polychaetes (Nereis virens) and American lobster (Homarus americanus) from a laboratory food chain experiment. Mar. Environ. Res. 49, 19–36. doi: 10.1016/S0141-1136(99)00046-X
Rahman M., Siddiqui M. (2004). Biochemical effects of vepacide (from azadirachta indica) on wistar rats during subchronic exposure. Ecotoxicol. Environ. Saf. 59 (3), 332–339. doi: 10.1016/j.ecoenv.2003.07.013
Rudel R., Seryak L., Brody J. (2008). PCB-Containing wood floor finish is a likely source of elevated PCBs in resident’s blood, household air and dust: a case study of exposure. Environ. Health 7, 2. doi: 10.1186/1476-069x-7-2
Safe S., Bandiera S., Sawyer T., Robertson L., Safe L., Parkinson A., et al. (1985). PCBs: structure-function relationships and mechanism of action. Environ. Health Perspect. 60, 47–56. doi: 10.2307/3429944
Sikdokur E., Belivermis M., Sezer N., Pekmez M., Bulan O., Kilic O. (2020). Effects of microplastics and mercury on manila clam Ruditapes philippinarum: feeding rate, immunomodulation, histopathology and oxidative stress. Environ. pollut. 7), 262. doi: 10.1016/j.envpol.2020.114247
Stewart B., Jenkins S., Boig C., Sinfield C., Kennington K., Brand A. (2020). Metal pollution as a potential threat to shell strength and survival in marine bivalves. Sci. Total Environ. 755 (1), 143019. doi: 10.1016/j.scitotenv.2020.143019
Sun Y., Guo K., Yu X., Li Y., Yao W., Wu Z. (2022). Molecular and biochemical effects on metabolism and immunity of Hyriopsis cumingii fed with four different microalgae. Front. Mar. Sci. 9. doi: 10.3389/FMARS.2022.970781
Sun X., Hu H., Zhong Z., Jin Y., Zhang X., Guo Y. (2015). Ultrasound-assisted extraction and solid-phase extraction as a cleanup procedure for organochlorinated pesticides and polychlorinated biphenyls determination in aquatic samples by gas chromatography with electron capture detection. J. Sep. Sci. 38 (4), 626–633. doi: 10.1002/jssc.201400880
Tang B., Liu B., Wang X., Yue X., Xiang J. (2010). Physiological and immune responses of zhikong scallop Chlamys farreri to the acute viral necrobiotic virus infection. Fish. Shellfish Immun. 29 (1), 42–48. doi: 10.1016/j.fsi.2010.02.019
Teng J., Zhao J., Zhu X., Shan E., Zhang C., Zhang W., et al. (2021). Toxic effects of exposure to microplastics with environmentally relevant shapes and concentrations: Accumulation, energy metabolism and tissue damage in oyster crassostrea gigas. Environ. pollut. 269, 116169. doi: 10.1016/j.envpol.2020.116169
Valko M., Rhodes C., Moncol J., Izakovic M., Mazur M. (2006). Free radicals, metals and antioxidants in oxidative stress-induced cancer. Chem.-Biol. Interact. 160 (1), 1–40. doi: 10.1016/j.cbi.2005.12.009
Vieira M., Torronteras R., Córdoba F., Canalejo A. (2012). Acute toxicity of manganese in goldfish Carassius auratus is associated with oxidative stress and organ specific antioxidant responses. Ecotoxicol. Environ. Saf. 78, 212–217. doi: 10.1016/j.ecoenv.2011.11.015
Xiao C., Zhang Y., Zhu F. (2021). Immunotoxicity of polychlorinated biphenyls (PCBs) to the marine crustacean species, Scylla paramamosain. Environ. pollut. 291, 118229. doi: 10.1016/j.envpol.2021.118229
Xu Y., Zhang Y. H., Liang J., He G. X., Liu X. L., Zheng Z., et al. (2021). Impacts of marine heatwaves on pearl oysters are alleviated following repeated exposure. Mar. pollut. Bull. 173, 112932. doi: 10.1016/j.marpolbul.2021.112932
Yang C., Du X., Hao R., Wang Q., Deng Y., Sun R. (2019). Effect of vitamin D3 on immunity and antioxidant capacity of pearl oyster Pinctada fucata martensii after transplantation: Insights from LC-MS-based metabolomics analysis. Fish. Shellfish Immun. 94, 271–279. doi: 10.1016/j.fsi.2019.09.017
Yang X., Zhang Y., Liang J., He G., Liu X., Zheng Z. (2021). Impacts of marine heatwaves on pearl oysters are alleviated following repeated exposure. Mar. pollut. Bull. 173, 112932. doi: 10.1016/J.MARPOLBUL.2021.112932
Yuan J., Pu Y., Yin L. (2014). Prediction of binding affinities of PCDDs, PCDFs and PCBs using docking-based comparative molecular similarity indices analysis. Environ. Toxicol. Pharmacol. 38 (1), 1–7. doi: 10.1016/j.etap.2014.04.019
Zaynab M., Fatima M., Sharif Y., Sughra K., Sajid M., Khan K. (2021). Health and environmental effects of silent killers organochlorine pesticides and polychlorinated bipheny. J. King Saud Univ. Sci. 33 (6), 101511. doi: 10.1016/J.JKSUS.2021.101511
Zhang Z., Hong H., Zhou J., Huang J., Yu G. (2003). Fate and assessment of persistent organic pollutants in water and sediment from minjiang river estuary, southeast China. Chemosphere. 52 (9), 1423–1430. doi: 10.1016/S0045-6535(03)00478-8
Zhang T., Yan Z., Zheng X., Fan J., Wang S., Wei Y., et al. (2019). Transcriptome analysis of response mechanism to ammonia stress in Asian clam (Corbicula fluminea). Aquat. Toxicol. 214, 105235. doi: 10.1016/j.aquatox.2019.105235
Zha S., Rong J., Guan X., Tang Y., Han Y., Liu G. (2019a). Immunotoxicity of four nanoparticles to a marine bivalve species, Tegillarca granosa. J. Hazard Mater. 377, 237–248. doi: 10.1016/j.jhazmat.2019.05.071
Zha S., Shi W., Su W., Guan X., Liu G. (2019b). Exposure to 2,3,7,8-tetrachlorodibenzo-paradioxin (TCDD) hampers the host defense capability of a bivalve species, Tegillarca granosa. Fish Shellfish Immun. 86, 368–373. doi: 10.1016/j.fsi.2018.11.058
Keywords: bivalve, ecotoxicology, aquaculture, non-specific immunity, chemical stress
Citation: Liu M, Fan S, Rong Z, Qiu H, Yan S, Ni H and Dong Z (2023) Exposure to polychlorinated biphenyls (PCBs) affects the histology and antioxidant capability of the clam Cyclina sinensis. Front. Mar. Sci. 10:1076870. doi: 10.3389/fmars.2023.1076870
Received: 22 October 2022; Accepted: 16 January 2023;
Published: 27 January 2023.
Edited by:
Thanos Dailianis, Hellenic Centre for Marine Research, GreeceReviewed by:
Yao Zheng, Freshwater Fisheries Research Center, Chinese Academy of Fishery Sciences, ChinaChuangye Yang, Guangdong Ocean University, China
Youji Wang, Shanghai Ocean University, China
Copyright © 2023 Liu, Fan, Rong, Qiu, Yan, Ni and Dong. This is an open-access article distributed under the terms of the Creative Commons Attribution License (CC BY). The use, distribution or reproduction in other forums is permitted, provided the original author(s) and the copyright owner(s) are credited and that the original publication in this journal is cited, in accordance with accepted academic practice. No use, distribution or reproduction is permitted which does not comply with these terms.
*Correspondence: Zhiguo Dong, ZHpnNzcxMkAxNjMuY29t
†These authors have contributed equally to this work