- 1Science for Nature and People Partnership at the National Center for Ecological Analysis and Synthesis, Santa Barbara, CA, United States
- 2Department of Environmental Science and Policy, University of California, Davis, Davis, CA, United States
- 3The Nature Conservancy, Graduate School of Oceanography, University of Rhode Island, Narragansett, RI, United States
- 4SECORE International, Miami, FL, United States
- 5Environmental Studies, University of California, Santa Barbara, Santa Barbara, CA, United States
- 6Ecology, Evolution, and Marine Biology, University of California, Santa Barbara, Santa Barbara, CA, United States
- 7University of Miami, Miami, FL, United States
- 8The Nature Conservancy, Hawai’i & Palmyra Program, Honolulu, HI, United States
- 9Department of Biology, The Pennsylvania State University, University Park, PA, United States
- 10Department of Biological Sciences, University of Rhode Island, Kingston, RI, United States
- 11School for the Environment, University of Massachusetts Boston, Boston, MA, United States
- 12The Nature Conservancy, Global Aquaculture Program, Arlington, VA, United States
- 13Elkhorn Slough National Estuarine Research Reserve, Watsonville, CA, United States
- 14Ecology and Evolutionary Biology, University of California, Santa Cruz, Santa Cruz, CA, United States
Temperate oyster and tropical coral reefs are analogous systems that create habitat for economically, ecologically, and culturally important species, and they provide countless ecosystem services to human coastal communities. Globally, reefs are imperiled by multiple anthropogenic stressors, particularly climate impacts. Using aquaculture to support conservation goals - known as conservation aquaculture - is a relatively new approach for many reef building species, but it shows great promise for promoting species recovery and bolstering resilience to stressors. Concerns about aquaculture-associated risks, both known and potential, have often restricted the implementation of this tool to an emergency intervention following dramatic declines on reefs, when species or systems were unlikely to recover. Here, we combine expertise from coral and oyster reef ecosystems to consider the role of aquaculture as a conservation intervention for reefs, and provide recommendations for its timely development and targeted implementation. We highlight the importance of evaluating reef systems - alongside local stakeholders and Indigenous communities - to determine where and when the benefits of using aquaculture are most likely to outweigh the risks. We spotlight the importance of proactive monitoring to detect reef population declines, and the value of early aquaculture interventions to increase efficacy. Novel aquaculture approaches and technologies specifically designed for reef builders are considered, including techniques for building complex, multi-generational and multi-species reefs. We address the need for scaling up aquaculture-assisted reef recovery, particularly of corals, using high volume methods like those that have been successfully employed for oysters. We also recommend the immediate assessment and development of techniques to increase climate resilience of reef builders and we identify the challenges and trade-offs of these approaches. We highlight the use of proof-of-concept projects to test these promising methods, and we advise tracking of all interventions over time to determine their long-term efficacy. Finally, we outline opportunities to leverage novel partnerships among conservation, industry, and community interests that utilize aquaculture to facilitate the conservation of reefs. Developing conservation aquaculture approaches now is critical to position managers, scientists, and restoration practitioners to implement this intervention in timely and effective ways to support resilient reef and human communities worldwide.
1 Introduction
Temperate and tropical reefs create critical habitat and provide irreplaceable ecosystem functions and services to coastal communities (Table 1A; Grabowski et al., 2012; Wolfe et al., 2020; Bruce et al., 2021). Yet reef builders are at risk globally, due to threats such as overfishing, habitat loss, sedimentation, pollution, and climate change (Bellwood et al., 2004). Oyster reefs have declined by over 85% globally (Beck et al., 2011), with oyster reef ecosystems in Australia and the United States designated as critically endangered (IUCN, 2022). Coral reefs show evidence of significant reductions in coral cover and frequent shifts in community structure worldwide (Gardner et al., 2005; Bruno and Selig, 2007; De’ath et al., 2009), with 30 hard coral species and the Meso-American, Continental Pacific, and Caribbean coral systems currently listed as endangered or critically endangered (IUCN, 2022). Reef building species are particularly vulnerable to climate effects, as evidenced by recent mass mortality of coral reefs following bleaching events (Ainsworth et al., 2016; Hughes et al., 2017) and hypoxia (Altieri et al., 2017; Johnson et al., 2021) and the synergistic effects of increased temperatures on oyster disease outbreaks (Altizer et al., 2013; Burge et al., 2014).
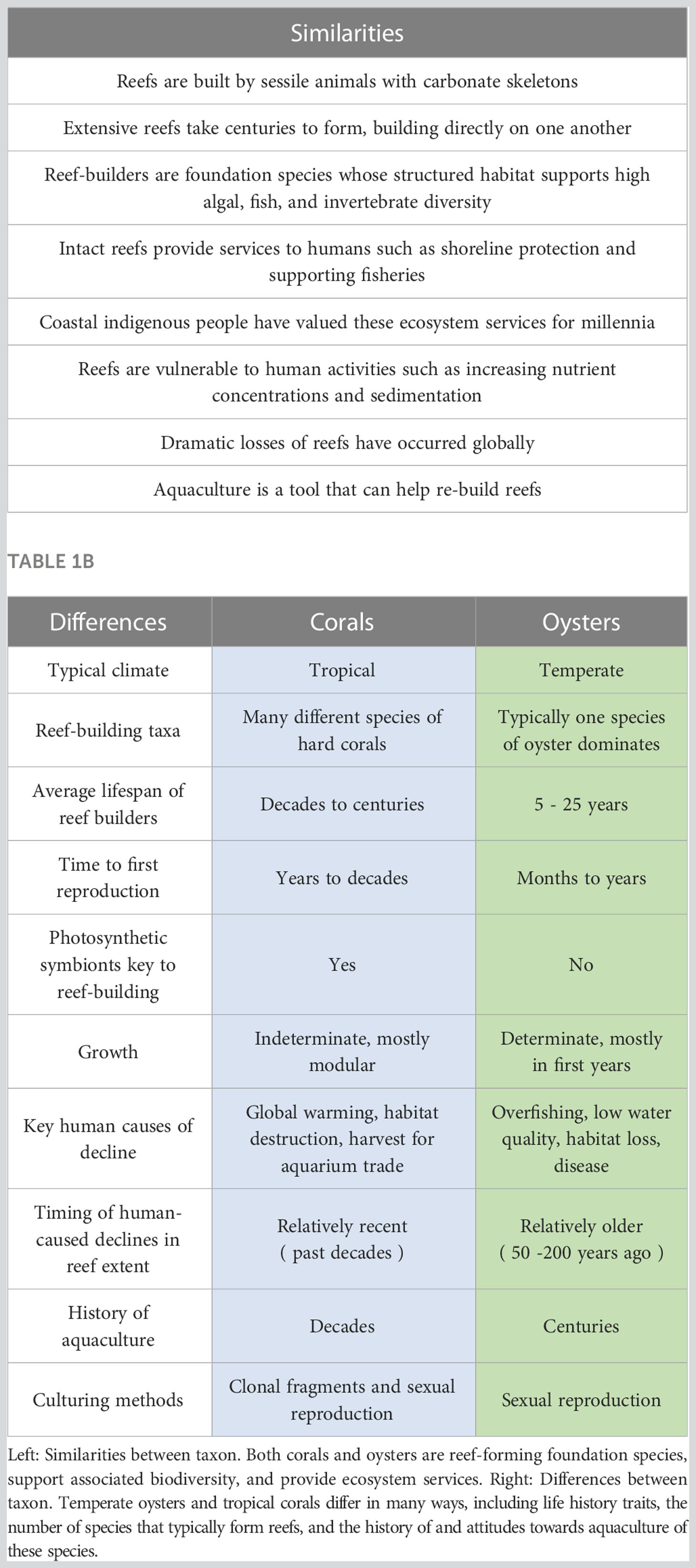
Table 1A Similarities and differences between oysters and corals affect aquaculture approaches, methods, and success.
Aquaculture for supporting conservation goals – often referred to as conservation aquaculture (Box 1; Rinkevich, 2019; Fitzsimons et al., 2020; Gillies et al., 2020; Vanderklift et al., 2020) –is a promising tool for reef builders. Conservation aquaculture may be particularly effective for species recovery and preservation (Tlusty, 2002; Young et al., 2012; Pollock et al., 2017) and holds the potential to confer reef builders with greater resilience to environmental stressors, including climate effects (Tan et al., 2020; Caruso et al., 2021). However, concerns over aquaculture-associated risks - both known and potential - have often delayed implementation past the point when aquaculture can be most effectively used to maintain system integrity and ensure continued provision of substantial services. Though similar as foundational reef builders, corals and oysters differ in their biology, ecology, and market values in ways that have driven different approaches to their conservation and restoration (Tables 1A and B). Here, we identify cross-cutting generalities that apply to the conservation of reefs broadly and highlight the approaches used in one ecosystem that can inform the other.
Box 1. Definitions of aquaculture. We define aquaculture for reef builders to broadly include techniques used to culture both oysters and corals. Conservation aquaculture must support wild populations, and may or may not include commercial aquaculture.
Aquaculture: the manipulation of reproduction and/or the assisted growth or survival of transplanted individuals to include: 1) raising individuals in a hatchery, 2) collection, transportation, and/or care of larvae (e.g., Doropoulos et al. 2019a), and 3) any movement of post-settlement individuals that involves housing them in a nursery, pen, or in/on any artificial enclosure/structure (in situ or in a lab) for a duration adequate to increase growth, development or survival, or to condition individuals for tolerances to environmental conditions.
Conservation aquaculture: aquaculture techniques that directly support conservation of and/or recovery goals for wild populations of native species. This can include commercial production if individuals are simultaneously cultured for sale and restoration, using protocols that support species recovery/conservation goals (Anders, 1998; Froehlich et al., 2017; Ridlon et al. 2021a; The Nature Conservancy, 2021).
Commercial aquaculture: the farming of aquatic organisms including molluscs, which implies some sort of intervention in the rearing process to enhance production, such as regular stocking, feeding and protection from predators, and individual or corporate ownership of the stock being cultivated (Food and Agriculture Organization, 2010).
Perceptions and use of aquaculture for conservation have differed between oyster and coral systems and have shaped the approaches and methods developed in each (Table 1B). Aquaculture has been embraced and used to support oyster reef recovery in various systems worldwide over the past century (Kennedy et al., 2011; Bersoza Hernández et al., 2018). This is in part because people eat oysters, and overfishing has historically been a main driver of the decline of populations, necessitating commercial aquaculture production. The long history of oyster fisheries (Kennedy et al., 2011; Carranza and Zu Ermgassen, 2020) and the relative ease of using aquaculture techniques to build reefs dominated by a single species (Bersoza Hernández et al., 2018) has led to the extensive use of commercial techniques in oyster restoration. As a result of this commercial history, culture of oysters for restoration often does not incorporate conservation protocols, like retaining wild genetic variation or other characteristics that would preserve and help ensure the long-term health of wild populations (Dégremont et al., 2015; McAfee and Connell, 2020). Thus, while effective oyster aquaculture methods are well-established and tested, more thorough assessments of the associated risks are still needed so that conservation protocols can be developed and broadly adopted. It is also important to note that the elimination or restriction of oyster harvesting and improvements in water quality have addressed two of the major sources of oyster decline and contributed to the success of aquaculture-assisted restoration of populations globally (Pogoda et al., 2019).
In contrast, coral reef restoration is generally more recent and smaller in scale (e.g., Smith et al., 2021, although see efforts in Indonesia, Razak et al., 2022). Coral managers and scientists alike have long expressed hesitation towards active human interventions, particularly because of the complexities involved in adequately addressing the varied and often global scale of existing stressors to coral reefs (Morrison et al., 2019). Thus, while the basic techniques of culturing corals have long been in place (Omori, 2019; Smith et al., 2021), cultured corals have historically been deployed only in small areas, often referred to as “coral gardening” (Hancock et al., 2017; Boström-Einarsson et al., 2020). Early aquaculture efforts were also almost exclusively focused on single-species recovery efforts (Johnson et al., 2011; Young et al., 2012; National Marine Fisheries Service, 2015). As a result, aquaculture techniques for corals are relatively expensive, and their efficacy - particularly in successfully outplanting cultured corals to reefs - remains largely untested for most species. These factors have further contributed to hesitancy towards the use of this tool for coral recovery (Precht et al., 2005; Rinkevich, 2014; Omori, 2019), and not until very recently has the scope of aquaculture use for coral reefs begun to expand to include a wider range of species and a more community-level approach (Shaver et al., 2022). However, increasingly severe and frequent climate change effects - such as wide-scale coral bleaching and mortality events around the globe - have combined with existing stressors to necessitate a more proactive and intervention-centered approach to coral reef conservation, even on reefs where restoration actions were not previously considered (Burke et al., 2011; Hardisty et al., 2019). Some aquaculture techniques proposed as human-assisted evolution of corals (Prober et al., 2015; van Oppen et al., 2015) have shown promise in increasing the resilience of corals to climate effects (Anthony et al., 2020; Howells et al., 2021; Shaver et al., 2022). Indeed, for some coral species with limited environmental tolerances, aquaculture may serve as a critical tool for their preservation in aquariums and land-based facilities (Schopmeyer et al., 2012; Zoccola et al., 2020). Thus, while the culture of corals has contributed to reef restoration and been used to prevent loss of certain species, little is known about its efficacy when used with a range of coral species and at larger spatial scales, especially with respect to how aquaculture methods may be used to contribute to reef resilience (DeFilippo et al., 2022; Shaver et al., 2022).
In the past, the conservation community has broadly been cautious about using aquaculture for conservation, until populations are at the point of collapse exacerbated by a disaster (e.g., overharvest, climate, and disease), at which point it is used as an emergency or reactive effort (Brumbaugh et al., 2000; Bersoza Hernández et al., 2018; Williams et al., 2020). Recently, ecologists have increasingly called for an expansion of the use of aquaculture techniques to support species recovery and conservation goals for both oysters (Bersoza Hernández et al., 2018; Gillies et al., 2020) and corals (van Oppen et al., 2017; Caruso et al., 2021; Kleypas et al., 2021). However, there are clear mismatches between the promising applications of this intervention and the capacity for it to be implemented with reef building species (Kleypas et al., 2021), and it will take time to develop effective aquaculture approaches, especially as their use expands to new species or regions. Here, we assembled experts from temperate and tropical reef ecosystems to consider the role of aquaculture as a conservation intervention for imperiled reefs around the world. We address the urgent need to evaluate and target the use of aquaculture on reefs where it is most needed, and to develop aquaculture techniques specific to reef builders that can enhance populations and restore habitat at reef scales. In each section of the paper, we have highlighted the most critical or promising next steps to implementation of these tools with both corals and oysters, and identified the successful approaches or lessons learned with one taxon that might inform restoration for the other, while recognizing that further evaluations of these are urgently needed.
2 Evaluating the appropriateness of aquaculture as a conservation tool
Before aquaculture is implemented as a conservation intervention, steps should be taken to evaluate whether it is the most appropriate tool to reach the specific goals for a given species, reef, and/or system, and whether it can provide unique or added benefits to local human communities (Box 2). This approach ensures that aquaculture is used as a targeted intervention, where and when it is most likely to provide added benefit over other restoration and conservation tools. Of course, the efficacy of aquaculture interventions and their relative contribution to reaching conservation goals should also be evaluated periodically as projects develop in an iterative process (Figure 1). Our subsequent recommendations about the implementation of reef conservation aquaculture (Box 3) assume that these initial evaluations have been made, and that aquaculture has been identified as an appropriate tool to use for a particular goal on a particular reef.
Box 2. Evaluating the appropriateness of aquaculture as a conservation tool. Prior to implementing any aquaculture-assisted project, these three steps should be used to evaluate whether aquaculture is an appropriate tool to use relative to other options. The evaluation should include setting conservation goals with Indigenous communities and/or local stakeholders to enhance benefits to humans.
• Address the stressors that caused or contributed to the decline of reef-builders. Aquaculture should be integrated into a broader ecosystem management approach that addresses the root causes of reef declines.
• Evaluate the risks and benefits of using aquaculture relative to other tools, based on the best available data and expertise for a particular reef population and system. Use aquaculture only when benefits outweigh risks, and other restoration techniques alone are not effective in stabilizing populations.
• Engage Indigenous communities and local stakeholders to set conservation goals and enhance the benefits of aquaculture for people. Indigenous communities should be engaged in the planning and long-term stewardship of the restored reef. Other local stakeholders may also benefit from and contribute to aquacultureassisted projects, and should be consulted early.
Box 3. Recommendations for the implementation of reef conservation aquaculture. Seven recommendations for actions that reef scientists and managers can take now and in an iterative process to assess the need for and timing of conservation aquaculture interventions on reefs, and to develop practical methods of implementation with reef building species.
• Proactively monitor for extreme events and population declines to time the use of aquaculture interventions.
• Engage local partners to set and reach goals in an interative process of planning, implementing and assessing the success of the project.
• Build complex reef systems with novel aquaculture methods and technologies designed for reef-builders, including generating aggregations, using modular structures, and leveraging positive species interactions.
• Conduct small-scale proof-of-concept projects across a diversity of reef systems to determine efficacy with multiple species and methods, and to ensure that efforts can be scaled up as needed before populations and reefs are not recoverable.
• Test methods to increase climate resilience by pursuing aquaculture techniques that have shown success in lab settings or over limited spatial scales, modifying these as needed for practical applications on reefs.
• Match the scale of effort to conservation goals using available data and modeling. Strategically plan small projects for maximum impact, and scale up where possible.
• Implement aquaculture early to stabilize populations, preserve wild genetic diversity, and maintain system integrity.
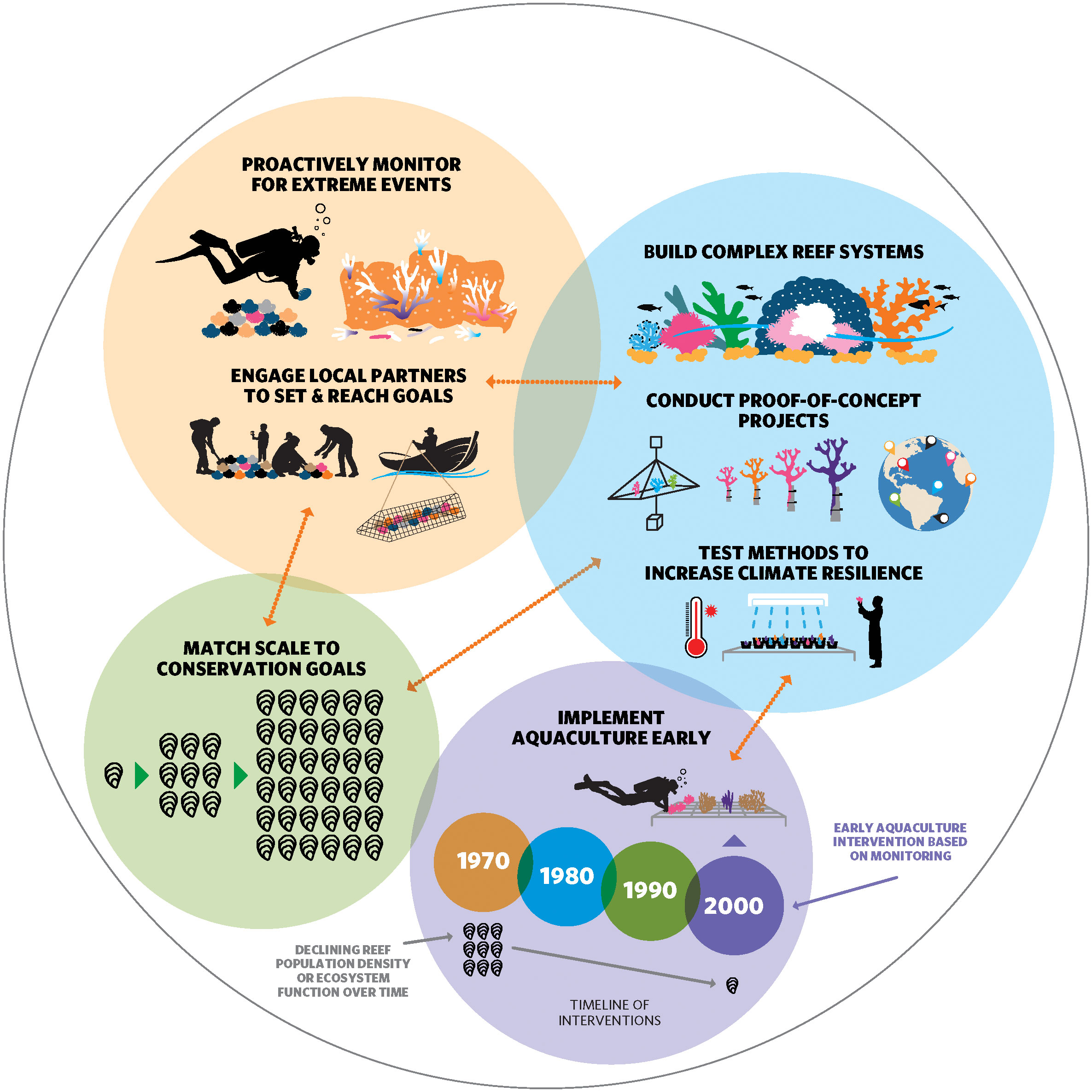
Figure 1 Iterative processes of development and implementation of reef conservation aquaculture. Ongoing and iterative activities (overlapping circles, orange arrows) must be started immediately to assess the need for and timing of aquaculture interventions, and to develop practical methods with sufficient lead time for implementation to be effective. Peach circle: Proactive, long term monitoring for extreme events and reef population declines (top: scuba diver monitoring an oyster bed, bleached and broken coral) and engagement of local partners, including Indigenous communities, to set and reach conservation goals (bottom: community oyster beds, fisher) are critical foundations for aquaculture interventions. Blue circle: Research and development are needed to establish effective methods and technologies for building complex reef systems (top: restored coral reef) and testing promising methods to increase climate resilience on reefs (bottom: coral fragments are exposed to high temperatures to test differences in thermal tolerances). Proof-of-concept projects should be implemented on smaller scales across many reef systems (middle: different coral species are grown, outplanted on reefs in various locales). Green circle: The scale of aquaculture efforts should then be matched to conservation goals for reefs (oyster icons increase in number left to right to depict scaling up), using the data, goals, and methods that have been developed. Purple circle: The early implementation of aquaculture relative to population or ecosystem decline may therefore take decades to prepare for, making it urgent to begin these processes now (dated circles and oyster icons indicate that declines were detected in 1970s, but aquaculture only implemented at large scale 30 years later).
First, aquaculture is most likely to be an effective intervention where the stressors that caused or contributed to the decline of reefs have been or are simultaneously being addressed (van Oppen et al., 2015; van Oppen et al., 2017, though aquaculture can also contribute to ameliorating these stressors, e.g. oysters and diseases: Ben-Horin et al., 2018). As with all restoration, aquaculture-assisted restoration of reef builders cannot replace the preservation of existing reefs and the maintenance or recovery of viable habitat conditions. Thus, the use of aquaculture should be integrated into a broader ecosystem management approach that addresses the root causes of reef declines, and never in isolation from or in lieu of measures that address these factors (Mann and Powell, 2007; Froehlich et al., 2017; Morrison et al., 2019; Anthony et al., 2020). However, it is increasingly clear that historical and current practices alone are not adequately conserving existing reefs. Most restoration efforts seek to recreate historical ecosystem structure and function, and yet active, adaptive management of reefs for resistance and resilience under future climate conditions is now essential (Boström-Einarsson et al., 2020; Schmidt-Roach et al., 2020; Shaver et al., 2022). Aquaculture can be a powerful tool to use for adaptive management, both because it can generate a rapid increase in population size (Fitzsimons et al., 2020) and because it provides new mechanisms to confer resistance and resilience to current and future stressors, including those related to climate change (oysters: Parker et al., 2011; corals: Howells et al., 2021). For example, techniques that aim to facilitate reef builders’ adaptation to future conditions include translocation (Prober et al., 2015), conditioning of individuals to new environmental conditions, (Pereira et al., 2020) and selective breeding (Caruso et al., 2021).
Second, despite its promise, aquaculture carries known and potential risks to both the species being cultured and the broader biological community. Hatchery rearing can result in selective breeding and unintended selection, including the potential loss of genetic diversity and local adaptations. Aquaculture may also increase the spread of diseases and invasive species (Baums, 2008; Lafferty et al., 2015; Tillotson et al., 2019), negatively affecting species that are not being cultured. Thus, assessing the relative benefits and risks of aquaculture based on the best available information for a particular species and system is an essential first step in evaluating its use. Aquaculture should then be used as a targeted intervention where the benefits are most likely to outweigh the risks, and where other restoration techniques alone are not effective, particularly where demographic data indicate that populations are not likely to stabilize without this intervention (van Oppen et al., 2017). For example, aquaculture techniques should be considered for species or populations of reef builders that are endangered or have severely declined (e.g., oysters: Wasson et al., 2020 corals: A. palmata and cervicornis: National Marine Fisheries Service, 2015), have poor natural recruitment and good post-recruitment survival (Hughes and Tanner, 2000; van Woesik et al., 2014), and are sufficiently isolated to prevent the sourcing and mixing of larvae from nearby populations. We recommend using a framework such as the one presented in Ridlon et al. (2021a) to assess the trade-offs of using aquaculture as a conservation intervention on a particular reef or for a particular population.
Finally, using aquaculture for reef conservation can enhance benefits to human communities, where their own goals align with species recovery or restoration goals (Spalding et al., 2016; Section 8). Thus, aquaculture should not be implemented without first working collaboratively with Indigenous communities and local stakeholders to identify shared goals and opportunities. Reefs provide ecosystem services that humans are motivated to preserve and steward, including traditional foods, cultural connections, livelihoods connected to fishing and tourism, coastal protection, and water quality improvement (Cinner et al., 2009; Costanza et al., 2014). Indigenous communities in particular have been maintaining and protecting oyster and coral reefs for millennia (e.g., corals: Cinner et al., 2006; oysters: Thompson et al., 2020). Ecological restoration goals for reefs are typically set using pre-colonial baselines, which were often the result of local and Indigenous resource management, including aquaculture (Millin, 2020; The Nature Conservancy, 2021). Indigenous stewardship often simultaneously meets conservation and harvest goals, for example by maintaining or restoring oysters and their associated species for partial future harvest (Reeder-Myers et al., 2022; Sax, 2022), or by maintaining the biodiversity of a coral reef that sustains fisheries (Cinner and Aswani, 2007). Indigenous communities should always be consulted about aquaculture-assisted projects, particularly those on traditional lands, prior to their planning and implementation (McLeod et al., 2018). Whenever possible, full co-management of reef systems should center on Indigenous communities’ conservation, economic, and cultural goals. In addition to Indigenous community partners, other local stakeholders should be consulted and ideally engaged directly in project planning to maximize benefits to both reefs and human communities. Factors such as which partners are willing and able to contribute, and whether the cost-effectiveness of aquaculture techniques will be improved by or hinder a partnership, for example, should be assessed.
3 Timely implementation and the role of monitoring
To increase the success of aquaculture-assisted projects, and to maintain both population stability and ecosystem integrity, aquaculture techniques should be applied as a proactive intervention before a reef species or entire reef ecosystem is designated as endangered, or a population has collapsed. Doing so increases the likelihood that aquaculture interventions are effective. For example, aquaculture is likely to be more successful when implemented while enough live individuals remain to support a culturing program with a level of genetic diversity that is representative of the wild population (e.g., Baums, 2008). Similarly, aquaculture interventions are more likely to be successful while sufficient underlying substrate is still in place and receptive for settlement (e.g., coral reef is structurally intact and not covered in algae, oyster reef is not buried under sediment). Early interventions also increase the likelihood that populations of reef builders remain stable over time (Lynch et al., 2014) and maintain both the functions of these foundation species and the integrity of the reef ecosystems they support (van Oppen et al., 2017; Hardisty et al., 2019). Figure 2 illustrates the importance of timing in initiating aquaculture interventions in the hypothetical recovery trajectories for coral populations under three scenarios. Early aquaculture implementation may stabilize the population, preserving high wild genetic diversity and integrity in ecosystem structure, function, and services, which would in turn facilitate relatively rapid population growth and recovery to initial population size (Figure 2, green arrow and line). Later interventions, for example after a decade of severe population decline, may still prevent populations from further collapse and allow for modest recovery based on the genetic resources available and status of the ecosystem at the time of implementation (Figure 2, orange arrow and line). When used as an emergency effort in critically declined populations, or once species are endangered, aquaculture interventions may maintain a small fraction of the population and prevent species or population extinction (Figure 2, red arrow and line). Late-stage emergency efforts have also been used or proposed to create genetic repositories to preserve species that are rare or extinct in the wild, as potential future sources for breeding populations once conditions have stabilized (Schopmeyer et al., 2012; Zoccola et al., 2020). However, implementing aquaculture with severely declined populations (Figure 2, red arrow and line) will likely fail to provide broader benefits to the population or reef because much of the original genetic diversity and ecosystem functioning and services will already have been lost.
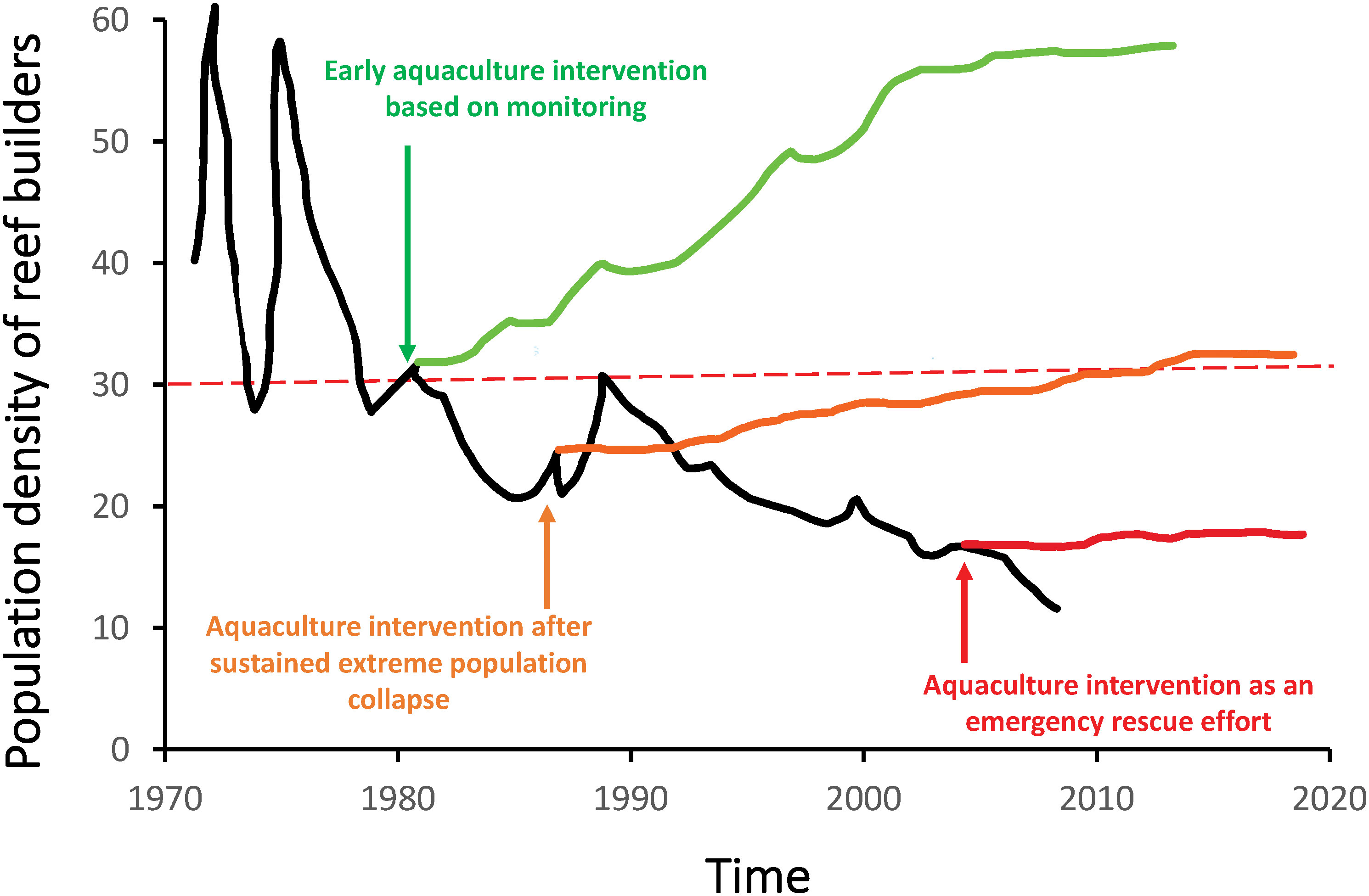
Figure 2 Early aquaculture interventions can increase efficacy by stabilizing larger populations of reef builders and maintaining functions and services that they provide. Early aquaculture interventions (green arrow, at a threshold of <30% coral cover, red dotted line) maintain higher genetic diversity, and integrity in ecosystem structure, function and services, making recovery to former population densities and conditions more likely (green line). Later interventions (orange arrow) may prevent populations from further collapse and allow for more modest recovery (orange line) based on the remaining genetic resources and ecosystem status at the time of implementation. Late-stage emergency efforts may prevent species or population extinction (red arrow), but much genetic diversity, system functioning and services are likely to be lost from remnant populations (solid red line). Population density data (black line) is percent live coral cover in Caribbean coral reefs from 1973 to 2010 (Serge, 2013), but could be any measure of population density (e.g. oysters per m2), reef integrity, or relevant ecosystem function or service.
Determining the point in time at which populations are not likely to recover on their own - and when the benefits of using aquaculture likely outweigh the risks - can be difficult, especially early in a trajectory of population decline. Regular, comprehensive monitoring for early warning signs that reef building populations may be in severe decline is especially important to increase the likelihood of success with aquaculture-assisted projects. This is due both to the need for timely implementation of aquaculture as described above, and to the extra lead time that may be involved in implementing an aquaculture-assisted project, for example in culturing organisms. In general, when monitoring data indicate persistent and severe population declines, consistent recruitment failure over many years, and/or extreme events that are known to trigger mass mortality (e.g., disease outbreak, heat wave), these indicators can be used to identify populations that are at risk of collapse and to trigger the serious consideration of aquaculture interventions (Oliver et al., 2017; O’Neil et al., 2021). Aquaculture can be implemented as a proactive intervention, for example, when a minimum population threshold is reached (e.g., loss of overall live coral cover to below 20% remaining, Figure 3A) or when multiple stressors co-occur with prolonged population declines (e.g. predation, cyclones, and coral bleaching coinciding with years of steady decline in live coral cover, Figure 3B). However, this strategy depends upon appropriate aquaculture methods, materials, and expertise being ready for use. We recommend developing and making available the appropriate broodstock and larvae needed for culturing a broader range of coral and oyster species, focusing efforts first on populations unlikely to recover without intervention based on historical data, or those especially vulnerable to mass mortality and population collapse, such as rare species or those with narrow environmental tolerances.
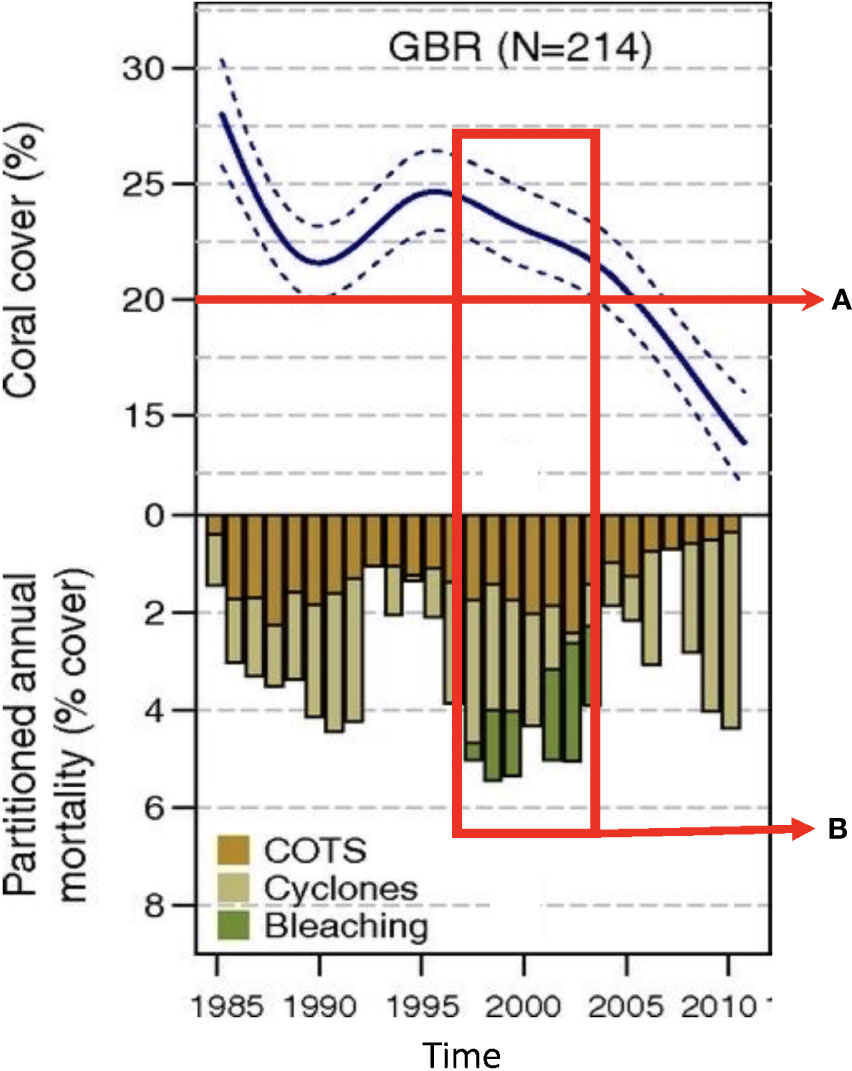
Figure 3 Monitoring for severe population declines and extreme events can inform the timely implementation of aquaculture. Proactive, ongoing monitoring of reef populations and for multiple stressors provides the data with which to assess the need for and timing of aquaculture interventions. Top Panel: temporal trends in coral cover for the Great Barrier Reef over the period 1985–2012 (N, number of reefs). (A) Aquaculture can be implemented proactively when a minimum population threshold is reached, here a loss of overall live coral cover to below 20% remaining (red line), circa 2008. Lower panel: annual coral mortality due to Crown of Thorns Seastar predation (COTS, orange), cyclones (light green), and coral bleaching (dark green). (B) Aquaculture interventions can be triggered by prolonged or multiple extreme events co-occurring with continued or severe decline of populations, here repeated bleaching events co-occurring with COTS, cyclones, and declining coral cover (red box), circa 2000. Figure modified from De’ath et al. PNAS 2012;109:44:17995-17999.
Although the metrics and thresholds used to time aquaculture interventions should be species- and system-specific, some successful models for this type of monitoring already exist. Long-term demographic monitoring can provide population and community-level data on a broad range of reef organisms, providing baselines from which to detect changes and the need for conservation interventions (Glynn and Ault, 2000; De’ath et al., 2009; Darling et al., 2010). These data can be used to predict trajectories for reef populations and their capacity for recovery from environmental stressors and population declines, which can further guide decisions about the nature and timing of interventions, including aquaculture (Gross et al., 2015; Guest et al., 2018; Elahi et al., 2022). More intensive demographic and genotypic monitoring may be required to assess the point at which rare species or severely declined populations are more likely to decline than recover, and to implement aquaculture in time to prevent the loss of rare individuals and already diminished genetic resources (Fisheries, NOAA Coral Reef Watch Homepage and Near Real-Time Products Portal, 2019; Williams et al., 2020). In some cases, this type of intensive monitoring has not only triggered species-specific interventions, but also signaled the need for a broader, multi-species reef recovery effort.
Many programs have engaged recreational users of reefs and new technologies to increase the scale of monitoring and the efficacy of early detection of reef degradation. Recreational scuba divers can be trained to collect long-term demographic data on coral reefs (Hodgson and Liebler, 2002; Done et al., 2017), and these and other recreational users can recognize and report the early signs of extreme events like bleaching, creating a real-time warning system (e.g., Coral Bleaching Early Warning Network of Mote Marine Laboratory and the Florida Key National Marine Sanctuary, coral disease ‘strike teams’ organized by the Virgin Islands Coral Disease Advisory Committee). Volunteers engaged in programs like these can often detect changes in reef builders earlier than scientists or managers, and community scientists may be increasingly relied upon as apps and online databases become more accessible and user-friendly. Additionally, scientists are employing new technologies like remote sensing to provide increasingly accurate warnings of extreme events such as coral bleaching conditions in real time and at a global scale (e.g., Coral Reef Watch satellite monitoring by NOAA, available at: https://coralreefwatch.noaa.gov/).
4 Building complex reefs
Reef builders such as oysters and corals are ecosystem engineers that form unique habitats from interconnected aggregations of individuals comprised of many generations, sizes, and species. These aggregations create extensive, complex structures that support many other species and provide shoreline protection from storms to human coastal communities (Graham and Nash, 2013; La Peyre et al., 2014). Building vertically on a multi-generational framework is also critical for reef sustainability amidst sedimentation or rising seas. One key to the success of aquaculture-assisted restoration of oysters and corals is adopting approaches that can generate such aggregate, sustainable reef structures. This requires a different approach to aquaculture methods, which have primarily been used to generate single individuals intended for human consumption (Stickney, 2009). Most aquaculture techniques are based upon fish or invertebrate species that do not form connected units, but even for reef-forming species, commercial aquaculture has focused on producing single oysters for the plate (Coon et al., 1986) or small individual corals for the aquarium trade (Barton et al., 2017). To date, much of the conservation aquaculture of reef building organisms has adopted these approaches, generating single individuals on cultch, small numbers of oyster spat on shells in the hatchery (Ozbay et al., 2017), or individual pieces of coral grown separately (Omori, 2019). There is an urgent need to develop and test innovative aquaculture approaches that successfully create biogenic, complex reefs.
One approach to generating complex reefs is to provide physical structures that mimic natural reefs and provide a framework for reef builders to grow upon. Using aquaculture techniques to seed those substrates can accelerate the formation of reefs (corals: Omori, 2019; Boström-Einarsson et al., 2020; oysters: Goelz et al., 2020). Modular substrate units are particularly well suited for use with aquaculture techniques, because they allow for settlement and growth of corals and oysters in tanks at early life stages, and for outplanting of those organisms at various life stages to better mimic the natural formation of reefs over time. Modular approaches that allow sequential addition of new units with different cohorts, life stages, or species is particularly critical for corals, to more rapidly mimic complex, mature reef structure than would occur through the slow process of natural succession (Duarte et al., 2009). For example, coral fragments can be attached to metal spider-shaped structures (Figures 4E, F), which can be clustered together to mimic natural reef structure and can be added over time (Combillet et al., 2022). When these units are set directly atop coral rubble, they can stabilize and augment remaining natural reef structure (Williams et al., 2019). Another approach involves individual interlocking modular units with high rugosity meant to simulate dead coral (Figure 4D), which may provide an especially effective approach for restoring multiple life stages of corals onto an artificial reef over time. By comparison, oyster reef structure is often created with durable materials such as shell, concrete, or even plastic, but modular units made of biodegradable material can also support the initial formation of an oyster reef until the oysters themselves begin to provide the substrate for new recruits to build upon (Figures 4A–C; Nitsch et al., 2021). We recommend that coral and oyster reef restoration practitioners expand innovative use of modular physical structures to generate multi-generational and multi-species reefs that are larger and more complex than previous conservation aquaculture efforts for either taxon.
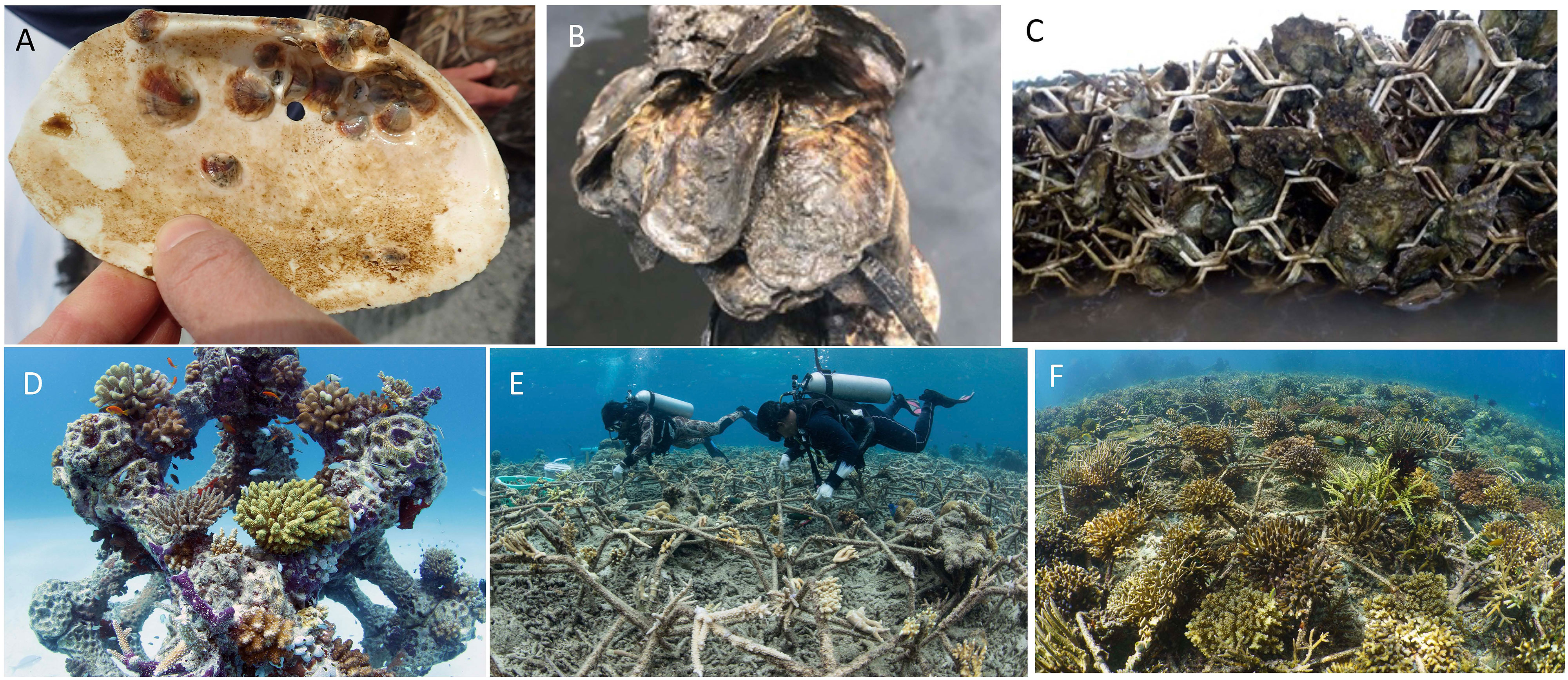
Figure 4 Innovative methods for building complex reefs. (A) Hatchery-raised oyster aggregation on clam shell. Photo credit: (C) Zabin; (B) Cluster resulting from aggregation like the one at right one year after deployment. Photo credit: (K) Wasson; (C) Biodegradable framework seeded with cultured oysters jumpstarts reef formation. Photo credit: Nitsch et al., 2021; (D) Interlocking modular units mimic dead coral, and can facilitate the addition of individuals at multiple life stages. Photo credit: (A) Goad, Reef Design Lab. (E) Coated metal “reef star” structures with coral fragments attached mimic and stabilize coral rubble, Pictured: Mars, Incorporated divers, with permission. Photo credit: Mars, Incorporated (F) Hard corals grow over the “reef star” structures to form a reef, Photo credit: Mars, Incorporated.
Generating aggregations of corals or oysters using aquaculture, including clusters of single or multiple species, provides another approach to create complex reef structure. For oysters, the practice of setting spat-on-shell in a hatchery has become a standard method of producing small oyster clusters for restoration, either on oyster or clam shells that are later deployed to mimic natural bed structure (Figure 4A; Parker et al., 2020; Wasson et al., 2020), but this is rarely scaled up to larger aggregations. Later life stages (i.e., juveniles and adults) of both oysters and corals can also be deployed as clusters. For example, staghorn and elkhorn corals have been placed in dense aggregations to form thickets mimicking natural structural complexity (Johnson et al., 2011). Aquaculture techniques can also facilitate the formation of multiple-species coral aggregations directly onto reefs. Methods that involve collection and transport of larvae from multi-species spawning events, for example, can enhance aggregate settlement of early life stages of coral (Heyward et al., 2002; see Section 6). There is still much to be learned about generating large aggregations in the hatchery, and we recommend further exploration of techniques that can jumpstart the formation of extensive, three-dimensional reefs using groups of reef builders.
Aquaculture techniques can also be used to incorporate positive intraspecific and interspecific interactions, which have been shown to benefit coral and oyster restoration (Shaver and Silliman, 2017; Ladd et al., 2018; Reeves et al., 2020), into three-dimensional self-sustaining reefs. Hatchery techniques can be modified to explicitly incorporate positive interactions at early stages of culturing reef builders, which have continued benefits when organisms are outplanted. Aquaculture techniques can also generate aggregations of conspecific settlers of the same cohort or of different life stages to potentially enhance reef restoration success. For instance, fusion of small hatchery-grown corals appears to enhance rapid growth and thus survival (Raymundo and Maypa, 2004; Forsman et al., 2015). Likewise, clusters of oysters resist predation better than singles (Poirier and Quijón, 2022). Aquatic restoration success can also be enhanced by harnessing mutualisms among different species (Halpern et al., 2007). One obvious example for reef-forming corals is to incorporate the culture and introduction of symbiotic zooxanthellae into the culture of corals. Culturing symbionts along with corals can increase early survival in the hatchery, support later reef building capacity (Suzuki et al., 2013), and provide a potential mechanism for increasing climate resilience (van Oppen et al., 2015; Chakravarti et al., 2017; Buerger et al., 2020). Given their diversity, however, the response of different symbiont species to hatchery rearing, transplantation, and other aquaculture techniques is likely widely variable (Randall et al., 2020), and more research is needed to hone techniques that harness these coral-symbiont interactions in reef aquaculture. Incorporating positive species interactions in the construction of reefs is a relatively new concept for active interventions, but it is likely important for enhanced success. We recommend further investment in mechanisms incorporating facilitation within and among reef builders and their associated species, specifically to generate rich, complex reef structure.
5 Matching scales of impact to conservation goals
To maximize the impact of aquaculture interventions, the methods and scale should match the broader conservation goals for the population, species, and/or ecosystem (Mann and Powell, 2007; Morrison et al., 2019; Boström-Einarsson et al., 2020). This should include considerations of costs and how they relate to project scale, since aquaculture can be more costly than traditional restoration interventions due to the additional resources required, for example, to settle and rear animals (e.g., oysters: Bayraktarov et al., 2019; corals: Fitzsimons et al., 2020). While it is ideal to determine the scale that is needed to match the conservation goal by using data from previous successful aquaculture-assisted projects or models (e.g., oysters: Melbourne-Thomas et al., 2011; Kimbro et al., 2019; corals: Walsworth et al., 2019; McClenachan et al., 2020), these data are often lacking. However, there is evidence for the values of both smaller- and larger-scale aquaculture-assisted projects in relation to different conservation goals, from which we can recommend methods for determining the scale of aquaculture-assisted projects with oysters and corals.
It is broadly accepted that the effective conservation of reefs requires some regional or seascape-scale efforts on the order of kilometers to tens of kilometers (Melbourne-Thomas et al., 2011; Blomberg et al., 2018). Larger projects are needed to restore and increase ecosystem service benefits on a larger scale, to for example support finfish fisheries (Gilby et al., 2018). Larger projects may also be critical to maintain or restore ecosystem processes and functions by optimizing community recovery (Ladd et al., 2019), and increase reef-wide resilience to stressors such as climate change effects (Gibbs, 2021). Conservation aquaculture techniques must also be used at larger scales to increase the overall efficiency of aquaculture as an intervention for reef builders (Bruce et al., 2021). However, to date, evidence for the population- and ecosystem-level effects of reef aquaculture is lacking. Aquaculture techniques have either not been implemented on large enough spatial scales, or they have not been adequately monitored to measure significant effects on reef populations, communities, or systems. This is particularly true for coral reefs, and it contributes to reef scientists and managers skepticism that aquaculture can be successfully implemented at relevant spatial scales (Bellwood et al., 2019). The implementation and monitoring of more large-scale reef aquaculture projects is thus critical to determine whether these techniques can be used to reach broader conservation goals for both corals and oysters.
While the development of methods for scaling up aquaculture-assisted restoration is needed, it is important to recognize the human and ecological benefits of implementing aquaculture on smaller scales. Smaller-scale aquaculture projects may be prioritized because they produce social outcomes and ecosystem services for human populations (Abrina and Bennett, 2021) and because they are commensurate with local logistical capacity, funding, and priorities. For example, aquaculture-assisted restoration of oyster populations is sometimes driven not only by ecological imperatives, but also based on cultural values related to restoring oysters in a particular estuary (Ridlon et al., 2021a, 2 Ridlon et al, 2021b). As discussed, aquaculture can be instrumental in preserving dwindling populations of threatened or endangered species (e.g., oysters: Carne et al., 2006) or in preventing local extinction of a population (e.g., O. edulis Christianen et al., 2018; O. lurida: Wasson et al., 2020). Although smaller-scale projects tend to have more localized effects, their cumulative effects can also be leveraged for greater spatial and temporal impact. Networks of small, connected populations of reef builders, for example, can help maintain larger populations, increasing the overall resilience of reefs in an area as has been documented with Crassostrea virginica reefs (Schulte et al., 2009) and “spawning hot spots” of corals (Zayasu and Suzuki, 2019). These networks can also increase the scale of the ecosystem services they provided (McClenachan et al., 2020). Thus, for smaller scale efforts, we recommend selecting reefs that have a high potential for spill-over of natural recruitment wherever possible (Hock et al., 2017; Walsworth et al., 2019; Kleypas et al., 2021). Empirical data and modeling to locate these networks where they would maximize benefits are lacking for most species, and we recommend developing evidence-based resources for practitioners to use in site selection (Lipcius et al., 2015).
6 Methods for increasing scale
Attitudes and approaches to aquaculture interventions can differ widely between coral and oyster systems, and new methods to address issues of scale are needed for both taxa. In general, much less is known about the efficacy of coral aquaculture techniques, not only with respect to implementing them at reef scales, but with respect to successfully culturing the wide range of hard coral species that are needed to maintain reef biodiversity (Montoya-Maya et al., 2016). In contrast, oyster restoration projects have long employed aquaculture techniques to increase the scale of both production and implementation, but due to a lack of long-term monitoring for reef-scale effects, questions remain about the conservation impacts of those efforts.
A wide range of hard corals serve as foundation species on tropical reefs, and aquaculture techniques for producing and simultaneously outplanting multiple species of hard corals onto a reef are critical for increasing the efficacy of this intervention at reef scales (Montoya-Maya et al., 2016). Yet only recently has the scope of aquaculture use for coral reefs begun to expand to include a wider range of species and a more community-level approach (Shaver et al., 2022). Thus, most available aquaculture approaches were designed to meet species recovery goals with a limited number of coral species, and associated techniques can be expensive and time-consuming (e.g., Becker and Mueller, 2001; Johnson et al., 2011). This has limited the practical scale and applicability of many coral culture methods to restoring the incredibly diverse mix of corals and associated species that make up coral reefs (Bayraktarov et al., 2019). We recommend testing existing aquaculture techniques with a broader range of coral species that represent different forms and functions (DeFilippo et al., 2022), adjusting these approaches, and developing new techniques as needed.
In addition, the current lack of evidence for economically feasible aquaculture approaches that can be implemented at spatial scales that increase or maintain coral reef area is a key challenge for coral restoration efforts (Bayraktarov et al., 2019; Schmidt-Roach et al., 2020). We recommend techniques that employ high volume/high mortality methodologies for raising and outplanting corals analogous to those used for oyster aquaculture be pursued to reduce expense and labor and increase the scales at which corals are cultured (Figure 5). For example, raising large cultures of coral larvae in situ with mobile units is similar in cost and efficiency and is equally efficacious as methods using land-based facilities (Figure 5C; de la Cruz and Harrison, 2017; Sellares-Blasco et al., 2021; Miller et al., 2022). New artificial substrate designs can also increase coral settlement and reduce the time and labor of deploying corals to reefs (Figure 5C), avoiding labor intensive attachment methods which account for 30% of total coral restoration costs (Chamberland et al., 2017; Sellares-Blasco et al., 2021). Projects using each of these approaches should explicitly track the numbers of adult, breeding colonies produced over time to both determine the long-term efficacy of these aquaculture techniques, and to better inform the cost/benefit trade-offs inherent in these methods (Goergen et al., 2020; Shaver et al., 2022).
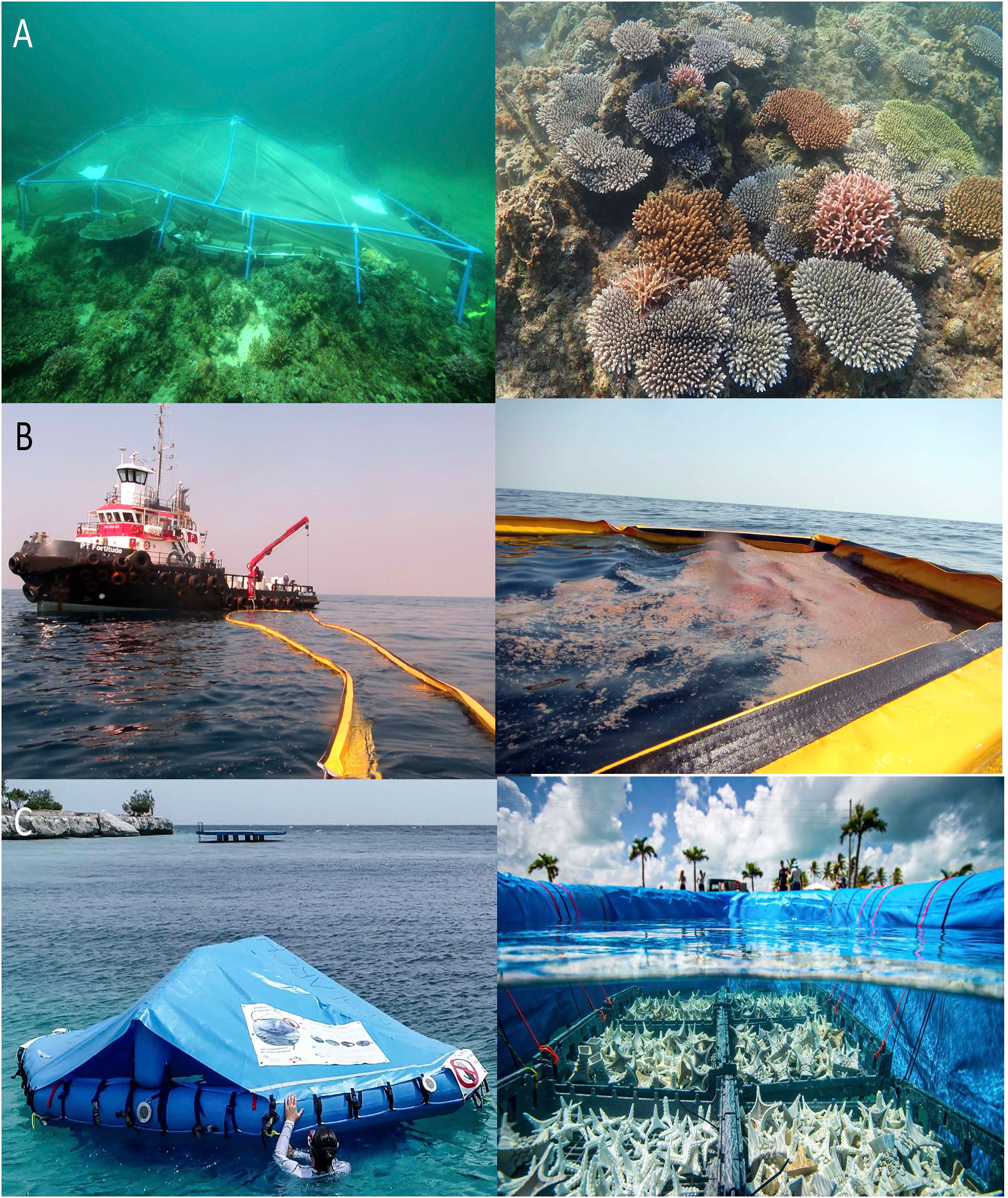
Figure 5 Aquaculture techniques that increase coral recruitment and eliminate nursery time. Row (A) Mesh enclosures effectively retain larvae and enhance recruitment on a small scale, resulting in healthy adult colonies, shown here three years after settlement. Photo credit: Peter Harrison. Row (B) A coral-spawn slick is captured in an oil boom and transfered into large tanks on board a tugboat for transport of the larvae to a degraded area of the reef as part of an industrial-scale effort. Photo credit: Copyright CSIRO. Row (C) Coral Rearing In-situ Basins (CRIB) can increase settlement efficiency without the need for land-based infrastructure, shown deployed in the water and with conditioned substrates in crates within the CRIB. Pictured: Nina LeTrocquer. Photo credit: Zach Ransom, Paul Selvaggio, SECORE.
Aquaculture techniques that facilitate restoration for multiple coral species at once and over larger spatial scales are especially promising. We recommend the further development of techniques that simultaneously increase coral recruitment and settler survivorship, which will both increase the possible project footprint and address the need for seeding multi-species assemblages. For example, where wild propagule supply is limited, reef tenting of settling larvae (Heyward et al., 2002) enhances recruitment to natural substrates, resulting in breeding coral colonies within a few years (Figure 5A; Harrison, 2017; Harrison et al., 2021), although to date efforts have been limited in footprint. Collection and relocation of wild coral larval slicks is another innovative technique for scaling up this method and seeding multiple species to reefs. This can be done at industrial scales by collecting considerable quantities of coral spawn and culturing larvae in pools directly on reefs (Harrison et al., 2021) or in large tanks on vessels (Figure 5B; Doropoulos et al., 2019a; Doropoulos et al, 2019b). Competent larvae can then be seeded on large areas of degraded reef, with minimal impact to wild populations. Where large larval slicks do not occur (e.g., most of the Caribbean), coral spawn can be collected from restored breeding corals using spawn collection cones (Harrison et al., 2021). Subsequent culturing within facilities may facilitate larger scale production of larvae for restoration in the future. Despite demonstrated success in producing breeding Acropora corals with these methods (Baria et al., 2012; Chamberland et al., 2016; Harrison, 2017; Harrison et al., 2021) there remains a dearth of long-term data that demonstrates the overall efficacy of these techniques in contributing to self-sustaining populations for most coral species. We recommend immediate investments to determine the rate of recruitment, post-recruitment survival, and demographic effects on local populations resulting from large-scale, multiple species techniques including larval slick relocation, as these data are critical in evaluating the return on these efforts.
In contrast to coral restoration efforts, high volume/high mortality aquaculture techniques (e.g., spreading spat-on-shell from hatcheries) have been used over large spatial scales in the successful recovery of various oyster and mussel species worldwide (Blomberg et al., 2018; Fitzsimons et al., 2019; Maryland Oyster Restoration Interagency Workgroup, 2020). Long-term evaluations of aquaculture-assisted oyster restoration highlight the factors contributing to their success, including adequately addressing the root causes of population decline (Mann and Powell, 2007). However, even for well-established methods, regional scale impacts, including on adjacent and connected wild oyster reef populations, are poorly understood (La Peyre et al., 2022).
The long history of commercial production of oysters also highlights some unique opportunities and challenges for increasing the scale of their production to meet conservation goals. For example, producing broodstock simultaneously for use in commercial markets and the restoration of wild populations would leverage industry infrastructure and resources at larger scales than are within reach of most conservation organizations. However, many oyster culturing techniques are based on commercial practices that do not align with conservation goals, such as hatchery selection for certain traits which can result in unintended trade-offs including reduced survival or thermal tolerance (McAfee et al., 2017). We recommend that conservation protocols that minimize hatchery selection and genetic concerns be used whenever possible for both commercial and restorative oyster production (e.g., conservation protocols for O. lurida: Blake and Bradbury, 2012). Using commercial oyster aquaculture areas for the shared production of oysters for commercial and restoration purposes could also increase the scale of oyster production for conservation goals. However, many commercial oyster operations are currently under severe limitations for space, and permitting of new grow-out areas can be restrictive. We recommend that areas that address shared conservation and commercial goals, and/or support ecosystem restoration goals (e.g., space for seagrass and oyster culture, Groner et al., 2018), be identified and supported by resource agencies and spatial planning authorities.
7 Climate resilience
Resilience to stressors and extreme events, including those related to the effects of climate change, must be integrated into the current and future restoration of reefs (Shaver et al., 2022; Zabin et al., 2022). Aquaculture can be used to maintain larger (Figure 2) and thus more genetically diverse wild populations, and genetic variation may preserve critical capacity for reef builders to adapt to changing conditions (DeFilippo et al., 2022; Shaver et al., 2022). We recommend prioritizing the development and use of aquaculture techniques where they best support the preservation of wild genetic diversity of reef builders. To maximize their benefits, aquaculture interventions should follow conservation protocols that facilitate the maintenance of genetic diversity, such as those developed for both corals and oysters (Baums, 2008; Blake and Bradbury, 2012; Baums et al., 2019). There are also a range of aquaculture techniques that show promise in conferring resilience by facilitating relatively rapid increases in either more individuals of stress tolerant species (via translocation) or an increase in the phenotypes of more tolerant reef builders (via selection while culturing). The risks and benefits associated with these techniques are unknown for many species, and the efficacy of these methods on reef scales relative to on-going natural selection in the wild is largely untested (see DeFilippo et al., 2022). However, these methods may provide useful mechanisms for the adaptive management of reefs under current and future climate scenarios when used as targeted interventions. We recommend the further development and testing of these techniques where assessments indicate that they are likely to accelerate the natural adaptation of reef building species to changing environmental conditions (e.g., increase the chances of survival and reproduction; Scanes et al., 2020) or more quickly increase the overall resilience of especially imperiled reef communities.
Whether and which aquaculture techniques should be used will differ between oyster and coral reefs based on biological and natural history differences (Table 1), including vulnerability to the effects of climate change. For example, increasing disease tolerance has been more critical for oyster conservation to date than increasing tolerance to rising temperatures or acidification (Dégremont et al., 2015). However, the link between increasing ocean temperature and disease outbreak in a wide range of species (Burge et al., 2014) suggests that climate effects may have synergistic as well as direct effects for oysters, and they deserve attention. In addition, oysters have relatively shorter generation times and wider environmental tolerances than corals, which increases the likelihood that natural selection can keep pace with at least some climate effects for some species (e.g., Ostrea spp.: Gray et al., 2019; multiple oyster spp.: Waldbusser and Salisbury, 2014). In contrast, increasing temperatures, acidification, and climate-related extreme events pose immediate and arguably more dire threats to coral reefs. In comparison with oysters, most corals also have longer generation times and narrower tolerance envelopes, which may limit their adaptive capacities (Table 1; though see Dixon et al., 2015; Rinkevich, 2019). While reefs in warmer climates may harbor corals with greater capacity to adapt to rising temperatures, this response appears highly variable (Matz et al., 2020), and many coral species are already living at their upper thermal limits (Riegl et al., 2011). Globally, populations are also experiencing repeated, increasingly frequent climate-related mass mortality events including bleaching and disease, in combination with sexual recruitment failure (Hoegh-Guldberg et al., 2017; Hughes et al., 2018; Muller et al., 2018). This highlights that while aquaculture techniques show promise in conferring resilience to reef builders, there is a critical, immediate need to test the efficacy of their practical applications with more species and in more locations.
Various methods hold the potential to increase the resilience of reef building species and communities, but most are largely untested, especially in the wild Table 1B. We recommend monitoring to quantify differences between aquaculture-assisted and wild populations to track effects relative to selection occurring in the wild. In addition, the benefits of these techniques must continue to be carefully considered against their potential risks under changing environmental conditions. Culturing and outplanting individuals with phenotypes that display broader tolerances to future climate change conditions is one approach to increasing reef resilience (i.e., climate-adjusted provenancing, Prober et al., 2015). Transplanting and/or culturing individuals from warmer habitats and selectively increasing the abundance of extant species with broader tolerances has been tested with both corals and oysters (oysters: Burge et al., 2007; Fitzer et al., 2019; corals: Caruso et al., 2021; Humanes et al., 2021, although see Barott et al., 2020). Selective cross-breeding of individuals with heritable tolerance traits via an aquaculture program may also be used to confer resilience on a reef (oysters: Parker et al., 2012; Tan et al., 2020; corals: van Oppen et al., 2014; Howells et al., 2021) (Table 1B). However, selective breeding can decrease the overall genetic diversity of a reef, even when selection is from natural variation in the source population. In addition, selection for one “desirable” trait, such as increased thermal tolerance, can reduce the genetic variance in or phenotypic performance for other traits. This can have unintended consequences such as reducing the adaptive capacity and therefore resilience of reefs to other unpredictable stressors that (Proestou et al., 2016). We recommend that directed breeding be considered first for recovery of endangered/threatened coral and oyster species, and for reefs that are most at risk from severe climate effects. Any breeding techniques should also include careful consideration to maintain genetic diversity, likely requiring a thoughtful genetic management plan (e.g., Baums, 2008).
Stress conditioning of individuals or colonies - exposing them to predicted or current environmental conditions - is another method for increasing tolerances to some stressors, including diseases and climate effects (corals: Brown et al., 2015; van Oppen et al., 2015; De Merlis et al., 2022; oysters: Parker et al, 2011; Agnew et al., 2020; Pereira et al., 2020; Tan et al., 2020). Recent evidence suggests that conditioning of reef builders may also confer longer-term acclimatization to offspring via trans-generational plasticity (corals: Dixon et al., 2015; Putnam and Gates, 2015; Torda et al., 2017; Putnam et al., 2020; oysters: Gibbs, 2021; Parker et al., 2021). However, conditioning individuals to predicted future conditions is uncertain (Schoepf et al., 2019) and expensive, and it may primarily benefit the conditioned individual (Kleypas et al., 2021). This highlights a critical need for longer-term data on the efficacy of these techniques (Byrne et al., 2020). Thus, we recommend further investments into stress conditioning for reef building species that are longer lived, and for which there is evidence of trans-generational benefits of such aquaculture-induced stress conditioning relative wild populations.
In general, there are large gaps between the promising results of lab-based experiments into aquaculture methods that confer climate resilience and their application to support reef conservation goals. Because time is of the essence, we recommend investing in proof-of-concept efforts that test these findings and the efficacy of their methods on restored reefs. Particularly for corals, there is a need to validate improved thermal tolerance in most species, even under laboratory conditions (Humanes et al., 2021). Species that demonstrate increases in heat tolerance that are heritable (Dixon et al., 2015; Putnam and Gates, 2015) or that result from conditioning via temperature events on reefs (Ainsworth et al., 2016; Safaie et al., 2018) should be prioritized for this research. The mechanisms for supplementing wild populations with stress-conditioned individuals are still largely untested with either taxon. We recommend outplanting more tolerant species/individuals onto “source” reefs with high connectivity, so that larval spillover and recruitment will maximize the spatial scale of the effort over time (Hock et al., 2017; Walsworth et al., 2019). Again, it will also be critical to monitor whether the hatchery-selected genotypes or phenotypes increase measurably in wild populations at a reasonable spatial scale in the years following the intervention. In corals, symbionts offer an additional mechanism for increasing tolerance to coral bleaching, and thus mortality from extreme heat events (Mieog et al., 2009; Howells et al., 2011; Buerger et al., 2020) and need to be further explored not only in experimental settings, but also in situ on reefs. Finally, for both taxa, we recommend considering opportunities to use aquaculture methods to address the effects of multiple simultaneous stressors (e.g., disease, eutrophication, and sedimentation: Fitzer et al., 2019). For instance, the negative synergistic effects of climate change and diseases on both oysters (Burge et al., 2007; Burge et al., 2014) and corals (Muller et al., 2018) are clear, and aquaculture could potentially be used to address both.
8 Developing novel partnerships
The use of aquaculture for reef conservation can provide unique opportunities for important, but sometimes unexpected partnerships where shared goals can be reached. Collaborations with Indigenous communities, for example, can provide knowledge-sharing that leads to new or improved methods for managing and harvesting reefs. Partnerships with tourism interests and commercial shellfish growers, for example, can leverage untapped or underutilized infrastructure and resources, and increase the visibility of the need for reef conservation efforts to broader audiences. To ensure that project goals center on reef conservation and remain practical, however, the needs and capacity of the stakeholders must be carefully considered at the start of a partnership.
Indigenous communities have long used aquaculture techniques in their stewardship of reefs (Millin, 2020; The Nature Conservancy, 2021). Co-management of reefs with Indigenous communities, using both traditional and western science techniques (McLeod et al., 2018; No’kmaq et al., 2021; Jessen et al., 2022) can support the cultural values of reefs and the re-establishment of historical food sources for these communities. Some Indigenous groups have been more open to and proactive with aquaculture interventions due to the acute impact of lost coastal habitats and the services they provide to these communities, and some have led the way in revitalizing traditional aquaculture methods that help ameliorate climate change effects (e.g., Swinomish Indian Tribal Community clam gardens: Weinberger, 2021; Figure 6). Aquaculture of reef builders can also provide economic benefits to Indigenous communities by sustaining livelihoods (e.g., coral farming: Heeger et al., 1999) and maintaining traditional food ways (Thompson et al., 2020; Reeder-Myers et al., 2022). Unfortunately, there has been a long history of conservation groups excluding and disempowering Indigenous people with protectionist strategies, and tensions have been especially high where management plans prevent native people from exercising their hunting and gathering treaty rights (e.g., establishment of national parks: Colchester, 2004; Kumar Sunuwar, 2018; Sengupta et al., 2021). In addition to consulting Indigenous groups as part of the assessment stage of a project (see Section 2), we recommend implementing aquaculture with the full engagement of local Indigenous communities who are willing to share traditional aquaculture knowledge and techniques that have fostered sustainability. Any groups seeking to restore reefs can use this as an opportunity to restore, facilitate, or support the long-term stewardship of reefs by Indigenous communities, a practice which has been shown to increase positive conservation outcomes (Dawson et al., 2021; Gibbs et al., 2021; Figures 6A, B).
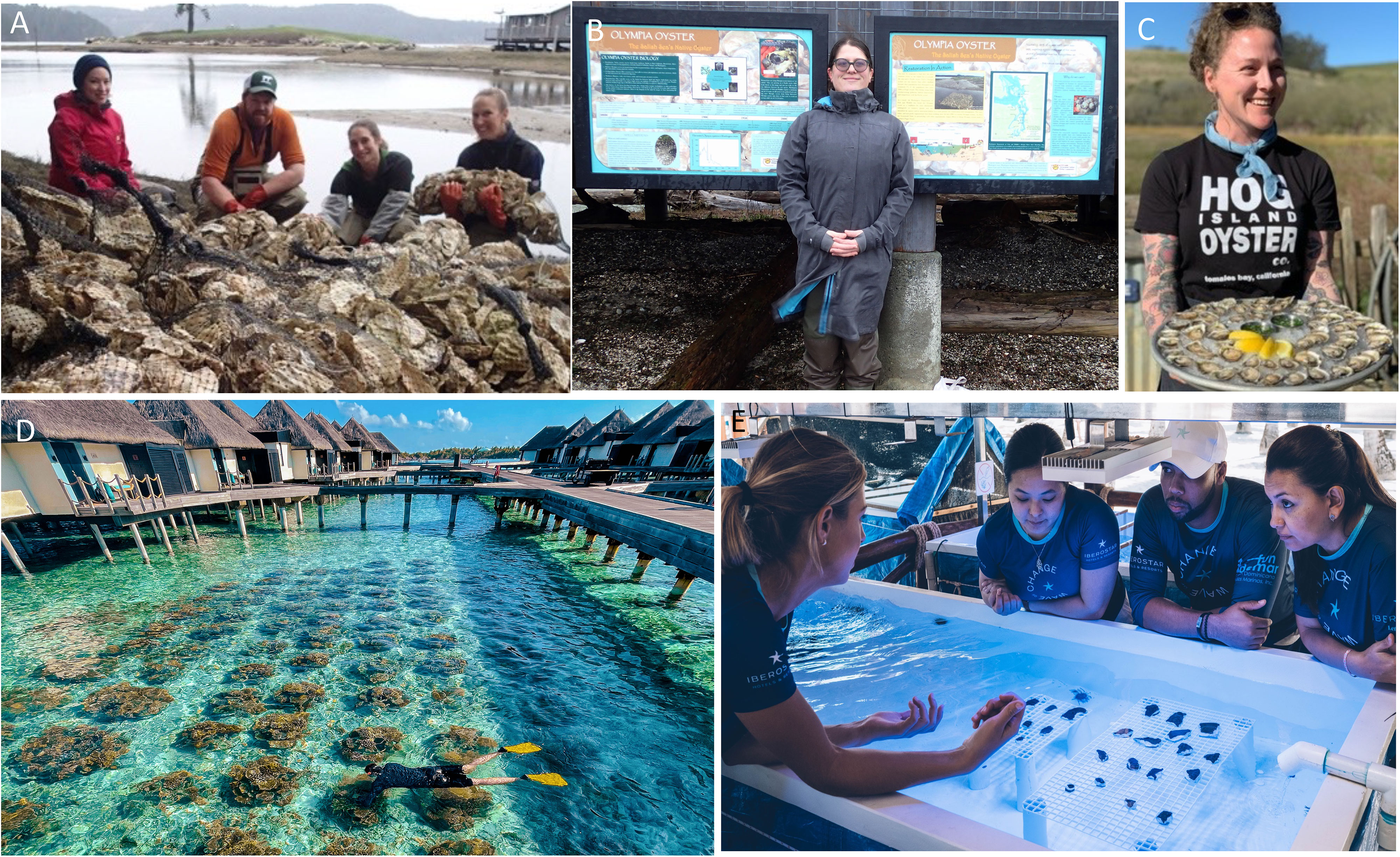
Figure 6 Aquaculture projects can restore the stewardship of reefs by Indigenous communities and facilitate novel partnerships. Top Row: Indigenous management and commercial partnerships restore Olympia oyster populations (A) Swinomish Tribal Community members deploy hatchery-raised oysters on Swinomish tidelands. Pictured: Sarah Grossman, James McArdle, Lindy Hunter, and Julie Barber. Photo credit: (J) Barber; (B) Swinomish Tribal community member in front of an educational kiosk about the oyster restoration projects the Tribe leads. Pictured: Jackie Dexter. Photo credit: (J) Barber; (C) Hog Island Oyster Company in California culture and market native oysters as a “farm-to-table” culinary experience connected to local restoration efforts. Pictured: Jamie Burgess. Photo Credit: (K) Wilkinson. Bottom Row: Coral restoration led by the tourism industry (D) A biologist from Reefsavers checks outplanted coral frames directly outside of a resort in the Maldives. Pictured : Reefsavers staff, with permission. Photo credit: Reefscapers; (E) Iberostar hotels worked closely with scientists to establish the lab and nursery they use to support coral restoration efforts in the Dominican Republic. Pictured: Macarena Blanco, Megan Morikawa, Alberto Silverio, Johanna Calle. Photo credit: Iberostar Hotels.
Increasingly, partnerships between commercial and conservation aquaculture interests are also emerging for reefs. For example, a large sector of coral restoration is now led by hotels or other tourism interests (Bayraktarov et al., 2020), some of whom have partnered with local resource managers to use aquaculture to rapidly restore reefs that directly support recreational activities (e.g., a reef near the hotel for tourists to dive or fish on: Hein et al., 2018; Figure 6D). Partnerships like these can leverage industry resources, incentivize the implementation of conservation aquaculture protocols, and produce innovative aquaculture technology for both sectors (e.g. The Kenneth K. Chew Center for Shellfish Research and Restoration industry partnerships, available at: restorationfund.org/programs/hatchery/; Blake and Bradbury, 2012). However, much of the coral gardening and site- or reef-specific coral culture to date has been done without the consultation of scientists or managers and without rigorous monitoring and reporting of the results (Boström-Einarsson et al., 2020; Ferse et al., 2021). We recommend the early engagement of scientists and managers in aquaculture-assisted reef restoration projects led by commercial industries (e.g., Punta Cana Ecological Foundation partnership with University of Miami, Lirman and Schopmeyer, 2016, Figure 6E) to promote conservation best practices, and minimize associated risks (Vaughan, 2021). The sharing of results in publicly accessible formats such as summaries and reports on websites (e.g., corals: Boström-Einarsson et al., 2020 database; oysters: Native Olympia Oyster Collaborative project profiles, available at: olympiaoysternet.ucdavis.edu), is critical to increasing communication about the methods and efficacy of these projects to partners and the public. We also recommend that academic partners synthesize these data in open-source scientific journals (e.g., Bayraktarov et al., 2020; Ferse et al., 2021; Ridlon et al., 2021a and reader-friendly outreach materials that can be accessed by partners outside of academic institutions, so that lessons learned can be shared widely. Likewise, we recommend having an established agreement in commercial-conservation partnerships that the aquaculture techniques used in every stage of production of reef builders are clearly driven by conservation goals for that species (e.g., The Nature Conservancy’s Principles of Corporate Engagement available at: nature.org) to address the risks associated with commercial production that are at odds with conservation goals, such as preserving the genetic diversity or adaptations to local conditions of reef organisms (Rhyne and Tlusty, 2012; Rhyne et al., 2012; Gjedrem and Robinson, 2014).
Finally, aquaculture of reef builders can capture public attention and promote broader awareness of conservation goals in a way that other restoration projects may not. Where commercial aquaculture of reef builders produces food for people, as with oysters, public education about the status and conservation of reefs can take the form of consumer marketing. For example, commercial oyster growers and restaurants can use a “slow food” or “farm to table” context for promoting the sale of a locally grown native oyster species and include information about its conservation and natural history in those efforts (e.g., Olympia oysters at Hog Island Oyster Company, available at: hogislandoysters.com/our-story/sustainability, Figure 6C). Recreational scuba divers and tourists that are captivated by coral reefs can effectively contribute to conservation aquaculture as citizen scientists employing relatively simple methods. The Seven Iconic Reefs program, for example, recently engaged divers through a network of “Blue Star Operators” (available at: Floridakeys.noaa.gov) to outplant over 1,300 Staghorn corals to “coral gardens”, and corals outplanted by recreational divers had the same survivorship as those outplanted by scientific experts (Hesley et al., 2017). Engaging the public in aquaculture-assisted coral restoration projects has been shown to reduce costs, create employment, and generate stewardship for local coral habitats (Dela Cruz et al., 2014; Lirman and Schopmeyer, 2016; Hein et al., 2018).
9 Actionable recommendations
Conservation aquaculture is a potentially powerful tool for restoring populations of reef builders, and it needs immediate development to contribute to the conservation of reefs globally in the coming century. Here, we have highlighted the urgent need to close the gap between the promise of aquaculture techniques and their practical implementation on reefs, especially given that research and development of restoration techniques can take many years. To that end, we provide seven recommendations for the timely development and implementation of reef aquaculture (Box 3 and Figure 1), illustrating that as with all restoration approaches, the processes involved are iterative. Each reef system should first be evaluated with respect to the risks, likely effectiveness, and practicality of implementing conservation aquaculture as compared with other tools (Section 2, Box 2). Intervening with aquaculture early, when the genetic resources of larger wild populations are available, and systems are relatively functioning and intact, may increase its success (Section 3, Figure 2). New methods for building complex reef systems (Section 4) and increasing the climate resilience of reef-builders (Section 5) need to be developed and tested, using proof-of concept pilot projects to determine which techniques work for which species and systems. Aquaculture-assisted projects can then be scaled to match conservation goals for a particular reef (Section 6), using both existing and novel methods (Section 7). Throughout this process, Indigenous communities and local partners should be engaged to set and reach conservation and human benefit goals (Section 8).
Reef building species create and maintain ecosystems and provide a wealth of services to human communities, including food provisioning, shoreline protection, increased water quality, and generation of economic and cultural benefits. Conservation aquaculture cannot replace proactive measures to preserve extant reef communities, or existing ecosystem integrity and functions. Instead, aquaculture can be implemented to conserve imperiled reefs while offering unique opportunities to directly maintain or increase the benefits that they provide to humans. Reefs are more vulnerable than ever to multiple stressors under changing environmental conditions, necessitating more active approaches to their conservation. Reef managers, scientists, and restoration practitioners need more available, effective aquaculture methods for timely and targeted implementation where they can best support conservation goals. The immediate development and use of reef conservation aquaculture approaches is thus critical to the future of resilient reef - and human - communities worldwide.
Ethics statement
Written informed consent was obtained from all individuals for the publication of any potentially identifiable images or data included in this article.
Author contributions
AR, KW, and EG led the conception and design of the study, to which all authors contributed through working group meetings. AR managed the literature review, and led the writing of all drafts of the manuscript. KW, EG, and BH wrote draft sections of the manuscript. All authors provided edits and suggested citations, contributed to manuscript revision, read, and approved the submitted version.
Acknowledgments
AR and her coordination of the working group of co-authors was generously supported by the Science for Nature and People Partnership and University of California, Davis. KW was supported by a grant from NOAA’s Office for Coastal Management to the Elkhorn Slough National Estuarine Research Reserve. EG was supported in part by the Alexander and Elizabeth Swantz Endowment. HF was supported by the University of California, Santa Barbara. We thank M. Colton, P. Harrison, and P. Mumby for their early contributions to the ideas presented here, and C. Kappel for facilitating working group meetings of co-authors. We are grateful to T. White at Johanna Villani Design for her help with the design of Figure 1. Figure photographs were generously provided by C. Zabin, P. Harrison, A. Goad and Reef Design Lab, E. Moore and Mars Incorporated, S. Ulrike Caroline Leib and the Grupo Puntacana Foundation, V. Galvin and Iberostar Hotels, and C. Doropoulos and the Commonwealth Scientific Industrial Research Organisation (CSIRO).
Conflict of interest
The authors declare that the research was conducted in the absence of any commercial or financial relationships that could be construed as a potential conflict of interest.
The reviewer AS declared a past co-authorship with one of the authors BH to the handling Editor.
Publisher’s note
All claims expressed in this article are solely those of the authors and do not necessarily represent those of their affiliated organizations, or those of the publisher, the editors and the reviewers. Any product that may be evaluated in this article, or claim that may be made by its manufacturer, is not guaranteed or endorsed by the publisher.
References
Abrina T. A. S., Bennett J. (2021). A benefit-cost comparison of varying scales and methods of coral reef restoration in the Philippines. Sci. Total Environ. 799, 149325. doi: 10.1016/j.scitotenv.2021.149325
Agnew M. V., Friedman C. S., Langdon C., Divilov K., Schoolfield B., Morga B., et al. (2020). Differential mortality and high viral load in naive pacific oyster families exposed to OSHV-1 suggests tolerance rather than resistance to infection. Pathog. 9, 1057. doi: 10.3390/pathogens9121057
Ainsworth T. D., Heron S. F., Ortiz J. C., Mumby P. J., Grech A., Ogawa D., et al. (2016). Climate change disables coral bleaching protection on the great barrier reef. Science 352, 338–342. doi: 10.1126/science.aac7125
Altieri A. H., Harrison S. B., Seemann J., Collin R., Diaz R. J., Knowlton N. (2017). Tropical dead zones and mass mortalities on coral reefs. Proc. Natl. Acad. Sci. U.S.A. 114, 3660–3665. doi: 10.1073/pnas.1621517114
Altizer S., Ostfeld R. S., Johnson P. T. J., Kutz S. (2013). And harvell, cClimate change and infectious diseases: From evidence to a predictive framework. D.Science 341, 514–519. doi: 10.1126/science.1239401
Anders P.J. (1998). Conservation aquaculture and endangered species. Fisheries 23 (11), 28–31. Available at: https://www.researchgate.net/profile/Paul-Anders-3/publication/285729796_Conservation_aquaculture_An_adaptive_approach_to_prevent_extinction_of_an_endangered_white_sturgeon_population/links/5818a13808aee7cdc685aacb/Conservation-aquaculture-An-adaptive-approach-to-prevent-extinction-of-an-endangered-white-sturgeon-population.pdf.
Anthony K. R. N., Helmstedt K. J., Bay L. K., Fidelman P., Hussey K. E., Lundgren P., et al. (2020). Interventions to help coral reefs under global change–a complex decision challenge. PloS One 15, e0236399. doi: 10.1371/journal.pone.0236399
Baria M. V. B., dela Cruz D. W., Villanueva R. D., Guest J. R. (2012). Spawning of three-year-old acropora millepora corals reared from larvae in northwestern philippines. Bull. Mar. Sci. 88, 61–62. doi: 10.5343/bms.2011.1075
Barott K. L., Huffmyer A. S., Davidson J. M., Lenz E. A., Matsuda S. B., Hancock J. R., et al. (2020). Bleaching resistant corals retain heat tolerance following acclimatization to environmentally distinct reefs. bioRxiv 2020, 9.25.314203. doi: 10.1101/2020.09.25.314203
Barton J. A., Willis B. L., Hutson K. S. (2017). Coral propagation: a review of techniques for ornamental trade and reef restoration. Rev. Aquac. 9, 238–256. doi: 10.1111/raq.12135
Baums I. B. (2008). A restoration genetics guide for coral reef conservation. Mol. Ecol. 17, 2796–2811. doi: 10.1111/j.1365-294x.2008.03787.x
Baums I. B., Baker A. C., Davies S. W., Ea A., Grottoli G., Kenkel C. D., et al. (2019). Considerations for maximizing the adaptive potential of restored coral populations in the western Atlantic. Ecol. Appl. 29, e01978. doi: 10.1002/eap.1978
Bayraktarov E., Banaszak A. T., Maya P. M., Kleypas J., Arias-Gonzalez J. E., Blanco M., et al. (2020). Coral reef restoration efforts in Latin American countries and territories. PloS One 15, e0228477. doi: 10.1371/journal.pone.0228477
Bayraktarov E., Stewart-Sinclair P. J., Brisbane S., Boström-Einarsson L., Saunders M. I., Lovelock C. E., et al. (2019). Motivations, success, and cost of coral reef restoration. Restor. Ecol. 27, 981–991. doi: 10.1111/rec.12977
Beck M. W., Brumbaugh R. D., Airoldi L., Carranza A., Coen L. D., Crawford C., et al. (2011). Oyster reefs at risk and recommendations for conservation, restoration, and management. Bioscience 61, 107–116. doi: 10.1525/bio.2011.61.2.5
Becker L. C., Mueller E. (2001). The culture, transplantation and storage of Montastraea faveolata, acropora cervicornis and Acropora palmata: What we have learned so far. Bull Mar Sci 69 (2), 881–896
Bellwood D. R., Hughes T. P., Folke C., Nyström M. (2004). Confronting the coral reef crisis. Nature 429, 827–33. doi: 10.1038/nature02691
Bellwood D. R., Pratchett M. S., Morrison T. H., Gurney G. G., Hughes T. P., Álvarez-Romero J. G., et al. (2019). Coral reef conservation in the anthropocene: Confronting spatial mismatches and prioritizing functions. Biol. Conserv. 236, 604–615. doi: 10.1016/j.biocon.2019.05.056
Ben-Horin T., Burge C. A., Bushek D., Groner M. L., Proestou D. A., Huey L. I., et al. (2018). Intensive oyster aquaculture can reduce disease impacts on sympatric wild oysters. Aquac. Environ. Interact. 10, 557–567. doi: 10.3354/aei00290
Bersoza Hernández A., Brumbaugh R. D., Frederick P., Grizzle R., Luckenbach M. W., Peterson C. H., et al. (2018). Restoring the eastern oyster: how much progress has been made in 53 years? Front. Ecol. Environ. 16, 463–471. doi: 10.1002/fee.1935
Blake B., Bradbury A. (2012). Washington Department of fish and wildlife plan for rebuilding Olympia oyster (Ostrea lurida) populations in puget sound with a historical and contemporary overview. Brinnon, WA: Washington department of fish and wildlife. 25.
Blomberg B. N., Pollack J. B., Montagna P. A., Yoskowitz D. W. (2018). Evaluating the U.S. estuary restoration act to inform restoration policy implementation: A case study focusing on oyster reef projects. Mar. Policy 91, 161–166. doi: 10.1016/j.marpol.2018.02.014
Boström-Einarsson L., Babcock R. C., Bayraktarov E., Ceccarelli D., Cook N., Ferse S. C. A., et al. (2020). Coral restoration – a systematic review of current methods, successes, failures and future directions. PloS One 15, e022663. doi: 10.1371/JOURNAL.PONE.0226631
Brown B. E., Dunne R. P., Edwards A. J., Sweet M. J., Phongsuwan N. (2015). Decadal environmental ‘memory’ in a reef coral? Mar. Biol. 162, 479–483. doi: 10.1007/s00227-014-2596-2
Bruce D. G., Cornwell J. C., Harris L., Ihde T. F., Lisa M., Knoche S., et al (2021). A synopsis of research on the ecosystem services provided by Large-scale oyster restoration in the Chesapeake bay. NOAA Tech. Memo. NMFS-OHC 8, 52.
Brumbaugh R. D., Sorabella L. A., Johnson C., Goldsborough W. J. (2000). Small scale aquaculture as a tool for oyster restoration in Chesapeake bay. Mar. Technol. Soc J. 34, 79–86. doi: 10.4031/mtsj.34.1.9
Bruno J. F., Selig E. R. (2007). Regional decline of coral cover in the indo-pacific: Timing, extent, and subregional comparisons. PloS One 2, 711. doi: 10.1371/journal.pone.0000711
Buerger P., Alvarez-Roa C., Coppin C. W., Pearce S. L., Chakravarti L. J., Oakeshott J. G., et al. (2020). Heat-evolved microalgal symbionts increase coral bleaching tolerance. Sci. Adv. 6, eaba2498. doi: 10.1126/sciadv.aba2498
Burge C. A., Judah L. R., Conquest L. L., Griffin F. J., Cheney D. P., Suhrbier A., et al. (2007). Summer seed mortality of the pacific oyster, Crassostrea gigas thunberg grown in tomales bay, California, USA: The influence of oyster stock, planting time, pathogens, and environmental stressors. J. Shellfish Res. 26 (1), 163–172. doi: 10.2983/0730-8000(2007)26[163:SSMOTP]2.0.CO;2
Burge C. A., Mark Eakin C., Friedman C. S., Froelich B., Hershberger P. K., Hofmann E. E., et al. (2014). Climate change influences on marine infectious diseases: Implications for management and society. Ann Rev Mar Sci 6, 249–77. doi: 10.1146/annurev-marine-010213-135029
Burke L., Reytar K., Spalding M., Perry A. (2011). Reefs at risk revisited (Washington DC USA: World resources institute).
Byrne M., Foo S. A., Ross P. M., Putnam H. M. (2020). Limitations of cross- and multigenerational plasticity for marine invertebrates faced with global climate change. Glob. Change Biol. 26, 80–102. doi: 10.1111/gcb.14882
Carne L., Kaufman L., Scavo K. (2006). “Measuring success for Caribbean acroporid restoration: key results from ten years of work in southern Belize,” in Proceedings 13th international coral reef symposium(Honolulu, HI).
Carranza A., Zu Ermgassen P. S. E. (2020). A global overview of restorative shellfish mariculture. Front. Mar. Sci. 7. doi: 10.3389/fmars.2020.00722
Caruso C., Hughes K., Drury C. (2021). Selecting heat-tolerant corals for proactive reef restoration. Front. Mar. Sci. 8. doi: 10.3389/fmars.2021.632027
Chakravarti L. J., Beltran V. H., van Oppen M. J. H. (2017). Rapid thermal adaptation in photosymbionts of reef building corals. Glob. Change Biol. 23, 4675–4688. doi: 10.1111/gcb.13702
Chamberland V. F., Petersen D., Guest J. R., Petersen U., Brittsan M., Vermeij M. J. A. (2017). New seeding approach reduces costs and time to outplant sexually propagated corals for reef restoration. Sci. Rep. 2017 71 7, 1–12. doi: 10.1038/s41598-017-17555-z
Chamberland V. F., Petersen D., Latijnhouwers K. R. W., Snowden S., Mueller B., Vermeij M. J. A. (2016). Four-year-old Caribbean Acropora colonies reared from field-collected gametes are sexually mature. Bull. Mar. Sci. 92, 263–264. doi: 10.5343/bms.2015.1074
Christianen M. J. A., Lengkeek W., Bergsma J. H., Coolen J. W. P., Didderen K., Dorenbosch M., et al. (2018). Return of the native facilitated by the invasive? population composition, substrate preferences and epibenthic species richness of a recently discovered shellfish reef with native European flat oysters (Ostrea edulis) in the north Sea. Mar. Biol. Res. 14, 590–597. doi: 10.1080/17451000.2018.1498520
Cinner J. E., Aswani S. (2007). Integrating customary management into marine conservation. Biol. Conserv. 140, 201–216. doi: 10.1016/J.BIOCON.2007.08.008
Cinner J. E., Marnane M. J., McClanahan T. R., Almany G. R. (2006). Periodic closures as adaptive coral reef management in the indo-pacific. Ecol. Soc 11.
Cinner J. E., McClanahan T. R., Daw T. M., Graham N. A. J., Maina J., Wilson S. K., et al. (2009). Linking social and ecological systems to sustain coral reef fisheries. Curr. Biol. 19, 206–212. doi: 10.1016/j.cub.2008.11.055
Combillet L., Fabregat-Malé S., Mena S., Marín-Moraga J. A., Gutierrez M., Alvarado J. J. (2022). Pocillopora spp. growth analysis on restoration structures in an Eastern tropical pacific upwelling area. PeerJ 10, e13248. doi: 10.7717/peerj.13248
Coon S. L., Bonar D. B., Weiner R. M. (1986). Chemical production of cultchless oyster spat using epinephrine and norepinephrine. Aquaculture 58, 255–262. doi: 10.1016/0044-8486(86)90090-6
Costanza R., de Groot R., Sutton P., van der Ploeg S., Anderson S. J., Kubiszewski I., et al. (2014). Changes in the global value of ecosystem services. Glob. Environ. Change 26, 152–158. doi: 10.1016/j.gloenvcha.2014.04.002
Darling E. S., Mcclanahan T. R., Côté I. M. (2010). Combined effects of two stressors on Kenyan coral reefs are additive or antagonistic, not synergistic. Conserv. Lett. 3, 122–130. doi: 10.1111/J.1755-263X.2009.00089.X
Dawson N. M., Coolsaet B., Sterling E. J., Loveridge R., Gross-Camp N. D., Wongbusarakum S., et al. (2021). The role of indigenous peoples and local communities in effective and equitable conservation. Ecol. Soc Publ. 26 (3), 19. doi: 10.5751/ES-12625-260319
De’ath G., Lough J. M., Fabricius K. E. (2009). Declining coral calcification on the great barrier reef. Science 323, 116–119. doi: 10.1126/science.1165283
DeFilippo L. B., McManus L. C., Schindler D. E., Pinsky M. L., Colton M. A., Fox H. E., et al. (2022). Assessing the potential for demographic restoration and assisted evolution to build climate resilience in coral reefs. Ecol. Appl. 32, e2650. doi: 10.1002/eap.2650
Dégremont L., Garcia C., Allen S. K. (2015). Genetic improvement for disease resistance in oysters: A review. J. Invertebr. Pathol. 131, 226–241. doi: 10.1016/j.jip.2015.05.010
Dela Cruz D. W., Villanueva R. D., Baria M. V. B. (2014). Community-based, low-tech method of restoring a lost thicket of Acropora corals. ICES J. Mar. Sci. 71)7, 1866–1875. doi: 10.1093/icesjms/fst228
dela Cruz D. W., Harrison P. L. (2017). Enhanced larval supply and recruitment can replenish reef corals on degraded reefs. Sci. Rep. 7 (1), pp.1–pp13. doi: 10.1038/s41598-017-14546-y
De Merlis A., Kirkland A., Kaufman M. L., Mayfield A. B., Formel N., Kolodziej G., et al. (2022). Pre-exposure to a variable temperature treatment improves the response of Acropora cervicornis to acute thermal stress. Coral Reefs 41, 435–445. doi: 10.1007/S00338-022-02232-Z/TABLES/6
Dixon G. B., Davies S. W., Aglyamova G. A., Meyer E., Bay L. K., Matz, et al. (2015). Genomic determinants of coral heat tolerance across latitudes. Science 348, 1460–1462. doi: 10.1126/science.1261224
Done T., Roelfsema C., Harvey A., Schuller L., Hill J., Schläppy M. L., et al. (2017). Reliability and utility of citizen science reef monitoring data collected by reef check australi–2015. Mar. pollut. Bull. 117, 148–155. doi: 10.1016/j.marpolbul.2017.01.054
Doropoulos C., Elzinga J., ter Hofstede R., van Koningsveld ,. M., Babcock R. C. (2019a). Optimizing industrial-scale coral reef restoration: comparing harvesting wild coral spawn slicks and transplanting gravid adult colonies. Restor. Ecol. 27, 758–767. doi: 10.1111/rec.12918
Doropoulos C., Vons F., Elzinga J., ter Hofstede R., Salee K., van Koningsveld M., et al. (2019b). Testing industrial-scale coral restoration techniques: Harvesting and culturing wild coral-spawn slicks. Front. Mar. Sci. 6, 658. doi: 10.3389/fmars.2019.00658
Duarte C. M., Conley D. J., Carstensen J., Sánchez-Camacho M. (2009). Return to neverland: Shifting baselines affect eutrophication restoration targets. Estuaries Coasts 32, 29–36. doi: 10.1007/s12237-008-9111-2
Elahi R., Edmunds P. J., Gates R. D., Kuffner I. B., Barnes B. B., Chollett I., et al. (2022). Scale dependence of coral reef oases and their environmental correlates. Ecol. Appl. 32, e2651. doi: 10.1002/eap.2651
Ferse S. C. A., Hein M. Y., Rölfer L. (2021). A survey of current trends and suggested future directions in coral transplantation for reef restoration. PloS One 16, e0249966. doi: 10.1371/journal.pone.0249966
Fitzer S. C., McGill R. A. R., Torres Gabarda S., Hughes B., Dove M., O’Connor W., et al. (2019). Selectively bred oysters can alter their biomineralization pathways, promoting resilience to environmental acidification. Glob. Change Biol. 25, 4105–4115. doi: 10.1111/gcb.14818
Fitzsimons J., Branigan S., Brumbaugh R. D., McDonald T., zu Ermgassen P. S. E. eds. (2019). Restoration guidelines for shellfish reefs. Arlington VA, USA: The Nature Conservancy.
Fitzsimons J. A., Branigan S., Gillies C. L., Brumbaugh R. D., Cheng J., DeAngelis B. M., et al. (2020). Restoring shellfish reefs: Global guidelines for practitioners and scientists. Conserv. Sci. Pract. 2, e198. doi: 10.1111/csp2.198
Food and Agriculture Organization (2010). Definition obtained from: FAO Term portal, Entry: 1345 - Collection: Aquaculture. Available at: https://www.fao.org/faoterm. Accessed April 4 2023.
Forsman Z., Page C., Toonen R., Vaughan D. (2015). Growing coral larger and faster: micro-colony-fusion as a strategy for accelerating coral cover. PeerJ 3, e1313. doi: 10.7717/peerj.1313
Froehlich H. E., Gentry R. R., Halpern B. S. (2017). Conservation aquaculture: Shifting the narrative and paradigm of aquaculture’s role in resource management. Biol. Conserv. 215, 162–168. doi: 10.1016/j.biocon.2017.09.012
Gardner T. A., Côté I. M., Gill J. A., Grant A., Watkinson A. R. (2005). Hurricanes and Caribbean coral reefs: impacts, recovery patterns, and role in long‐term decline. Ecology 86 (1), pp.174–pp.184. doi: 10.1890/04-0141
Gibbs M. T. (2021). Technology requirements, and social impacts of technology for at-scale coral reef restoration. Technol. Soc 66, 101622. doi: 10.1016/j.techsoc.2021.101622
Gibbs M. T., Gibbs B. L., Newlands M., Ivey J. (2021). Scaling up the global reef restoration activity: Avoiding ecological imperialism and ongoing colonialism. PloS One 16, e0250870. doi: 10.1371/journal.pone.0250870
Gilby B. L., Olds A. D., Peterson C. H., Connolly R. M., Voss C. M., Bishop M. J., et al. (2018). Maximizing the benefits of oyster reef restoration for finfish and their fisheries. Fish Fish 19, 931–947. doi: 10.1111/faf.12301
Gillies C. L., Castine S. A., Alleway H. K., Crawford C., Fitzsimons J. A., Hancock B., et al. (2020). Conservation status of the oyster reef ecosystem of southern and Eastern Australia. Glob. Ecol. Conserv. 22, e00988. doi: 10.1016/j.gecco.2020.e00988
Gjedrem T., Robinson N. (2014). Advances by selective breeding for aquatic species: A review. Agric. Sci. 5, 1152–1158. doi: 10.4236/as.2014.512125
Glynn P. W., Ault J. S. (2000). A biogeographic analysis and review of the far eastern pacific coral reef region. Coral Reefs 191 19, 1–23. doi: 10.1007/S003380050220
Goelz T., Vogt B., Hartley T. (2020). Alternative substrates used for oyster reef restoration: A review. J. Shellfish Res. 39 (1), pp.1–pp12. doi: 10.2983/035.039.0101
Goergen E. A., Schopmeyer S., Moulding A. L., Moura A., Kramer P., Viehman T. S. (2020). Coral reef restoration monitoring guide: Methods to evaluate restoration success from local to ecosystem scales. NOAA Technical Memorandum NOS NCCOS 279, Silver Spring, MD. 145 pp. doi: 10.25923/xndz-h538
Grabowski J. H., Brumbaugh R. D., Conrad R. F., Keeler A. G., Opaluch J. J., Peterson C. H., et al. (2012). Economic valuation of ecosystem services provided by oyster reefs. Bioscience 62, 900–909. doi: 10.1525/bio.2012.62.10.10
Graham N. A. J., Nash K. L. (2013). The importance of structural complexity in coral reef ecosystems. Coral Reefs 32, 315–326. doi: 10.1007/s00338-012-0984-y
Gray M. W., Chaparro O., Huebert K. B., O’Neill S. P., Couture T., Moreira A., et al. (2019). Life history traits conferring larval resistance against ocean acidification: The case of brooding oysters of the genus Ostrea. J. Shellfish Res. 38 (3), 751–761. doi: 10.2983/035.038.0326
Groner M. L., Burge C. A., Cox R., Rivlin N. D., Turner M., Van Alstyne ,. K. L., et al. (2018). Oysters and eelgrass: potential partners in a high pCO2 ocean. Ecology 99, 1802–1814. doi: 10.1002/ECY.2393
Gross K., Edmunds P. J., Holmes E. E. (2015). Stability of Caribbean coral communities quantified by long-term monitoring and autoregression models. Ecology 96, 1812–1822. doi: 10.1890/14-0941.1
Guest J. R., Edmunds P. J., Gates R. D., Kuffner I. B., Andersson A. J., Barnes B. B., et al. (2018). A framework for identifying and characterising coral reef “oases” against a backdrop of degradation. J. Appl. Ecol. 55, 2865–2875. doi: 10.1111/1365-2664.13179
Halpern B. S., Silliman B. R., Olden J. D., Bruno J. P., Bertness M. D. (2007). Incorporating positive interactions in aquatic restoration and conservation. Front. Ecol. Env. 5, 153–160. doi: 10.1890/1540-9295
Hancock B., Lewis K., Conklin E. (2017). “The restoration of coral reefs,” in Routledge handbook of ecological and environmental restoration. Eds. Allison S. K., Murphy S. D. (London/New York: Routledge).
Harrison P. L., Cameron K. A., Cabaitan P. C. (2021). Increased coral larval supply enhances recruitment for coral and fish habitat restoration. Front. Mar. Sci. 8. doi: 10.3389/fmars.2021.750210
Heeger T., Cashman M., Sotto F. (1999). “Coral farming as alternative livelihood, for sustainable natural resource management and coral reef rehabilitation,” in Proceedings of oceanology international, vol. Vol. 99. , 171–186.
Hein M. Y., Couture F., Scott C. M. (2018). “Ecotourism and coral reef restoration : Case studies from Thailand and the Maldives,” in Coral reefs : Tourism, conservation and management (Abingdon, UK: Routledge), 137–150.
Hesley D., Burdeno D., Drury C., Schopmeyer S., Lirman D. (2017). Citizen science benefits coral reef restoration activities. J. Nat. Conserv. 40, 94–99. doi: 10.1016/j.jnc.2017.09.001
Heyward A. J., Smith L. D., Rees M., Field S. N. (2002). Enhancement of coral recruitment by in situ mass culture of coral larvae. Mar. Ecol. Prog. Ser. 230, 113–118. doi: 10.3354/meps230113
Hock K., Wolff N. H., Ortiz J. C., Condie S. A., Anthony K. R. N., Blackwell P. G., et al. (2017). Connectivity and systemic resilience of the great barrier reef. PloS Biol. 15, e2003355. doi: 10.1371/journal.pbio.2003355
Hodgson G., Liebler J. (2002). The global coral reef crisis: trends and solutions. (Los Angeles: Reef Check Foundation). doi: 10.3/jquery-ui.js
Hoegh-Guldberg O., Poloczanska E. S., Skirving W., Dove S. (2017). Coral reef ecosystems under climate change and ocean acidification. Front. Mar. Sci. 4. doi: 10.3389/fmars.2017.00158/xml/nlm
Hog Island Oyster Company Sustainability: Native Olympia oyster restoration. hogislandoysters.com/our-story/sustainability (Accessed 11 September 2022).
Howells E. J., Abrego D., Liew Y. J., Burt J. A., Meyer E., Aranda M. (2021). Enhancing the heat tolerance of reef building corals to future warming. Sci. Adv. 7, eabg6070. doi: 10.1126/sciadv.abg6070
Howells E. J., Beltran V. H., Larsen N. W., Bay L. K., Willis B. L., van Oppen M. J. H. (2011). Coral thermal tolerance shaped by local adaptation of photosymbionts. Nat. Clim. Chang 2012 22 2, 116–120. doi: 10.1038/nclimate1330
Hughes T. P., Anderson K. D., Connolly S. R., Heron S. F., Kerry J. T., Lough J. M., et al. (2018). Spatial and temporal patterns of mass bleaching of corals in the anthropocene. Science 359, 80–83. doi: 10.1126/science.aan8048
Hughes T. P., Kerry J. T., Álvarez-Noriega M., Álvarez-Romero J. G., Anderson K. D., Baird A. H., et al. (2017). Global warming and recurrent mass bleaching of corals. Nat. 2017 5437645 543, 373–377. doi: 10.1038/nature21707
Hughes T. P., Tanner J. E. (2000). Recruitment failure, life histories, and long-term decline of Caribbean corals. Ecology 81, 2250–2263. doi: 10.1890/0012-9658
Humanes A., Beauchamp E. A., Bythell J. C., Carl M. K., Craggs J. R., Edwards A. J., et al. (2021). An experimental framework for selectively breeding corals for assisted evolution. Front. Mar. Sci. 8. doi: 10.3389/fmars.2021.669995
IUCN (2022) The IUCN red list of threatened species. version 2022-1. Available at: https://www.iucnredlist.org (Accessed 1 October 2022).
Jessen T. D., Ban N. C., Claxton N. X., Darimont C. T. (2022). Contributions of indigenous knowledge to ecological and evolutionary understanding. Front. Ecol. Environ. 20 (2), pp.93–pp101. doi: 10.1002/FEE.2435
Johnson M. E., Lustic C., Bartels E., Lirman D., Miller M. W., Nedimyer K., et al. (2011). Caribbean Acropora restoration guide: best practices for propagation and population enhancement (Arlington, VA: The Nature Conservancy).
Johnson M. D., Scott J. J., Leray M., Lucey N., Bravo L. M. R., Wied W. L., et al. (2021). Rapid ecosystem-scale consequences of acute deoxygenation on a Caribbean coral reef. Nat. Commun. 2021 121 12, 1–12. doi: 10.1038/s41467-021-24777-3
Kennedy V. S., Breitburg D. L., Christman M. C., Luckenbach M. W., Paynter K., Kramer J., et al. (2011). Lessons learned from efforts to restore oyster populations in Maryland and virgini To 2007. J. Shellfish Res. 30, 719–731. doi: 10.2983/035.030.0312
Kimbro D. L., White J. W., Grosholz E. D. (2019). The dynamics of open populations: integration of top–down, bottom–up and supply–side influences on intertidal oysters. Oikos 128, 584–595. doi: 10.1111/oik.05892
Kleypas J., Allemand D., Anthony K., Baker A. C., Beck M. W., Hale L. Z., et al. (2021). Designing a blueprint for coral reef survival. Biol. Conserv. 257, 109107. doi: 10.1016/j.biocon.2021.109107
Kumar Sunuwar D. (2018)Conservation’s impacts on indigenous peoples: A conversation with Victoria tauli-corpuz. Available at: https://www.culturalsurvival.org/publications/cultural-survival-quarterly/conservations-impacts-indigenous-peoples-conversation (Accessed 11 September 2022).
Ladd M. C., Burkepile D. E., Shantz A. A. (2019). Near-term impacts of coral restoration on target species, coral reef community structure, and ecological processes. Restor. Ecol. 27, 1166–1176. doi: 10.1111/rec.12939
Ladd M. C., Miller M. W., Hunt J. H., Sharp W. C., Burkepile D. E. (2018). Harnessing ecological processes to facilitate coral restoration. Front. Ecol. Environ. 16, 239–247. doi: 10.1002/fee.1792
Lafferty K. D., Harvell C. D., Conrad J. M., Friedman C. S., Kent M. L., Kuris A. M., et al. (2015). Infectious diseases affect marine fisheries and aquaculture economics. Ann. Rev. Mar. Sci. 7, 471–496. doi: 10.1146/annurev-marine-010814-015646
La Peyre M. K., Humphries A. T., Casas S. M., La Peyre J. F. (2014). Temporal variation in development of ecosystem services from oyster reef restoration. Ecol. Eng. 63, 34–44. doi: 10.1016/j.ecoleng.2013.12.001
La Peyre M. K., Marshall D. A., Buie S. C. L., Hijuelos A., Steyer G. D. (2022). Are we falling short on restoring oysters at a regional scale? Env. Man 70 (4), pp.581–pp.592. doi: 10.1007/s00267-022-01691-y
Lipcius R. N., Burke R. P., McCulloch D. N., Schreiber S. J., Schulte D. M., Seitz R. D., et al. (2015). Overcoming restoration paradigms: Value of the historical record and metapopulation dynamics in native oyster restoration. Front. Mar. Sci. 2. doi: 10.3389/fmars.2015.00065
Lirman D., Schopmeyer S. (2016). Ecological solutions to reef degradation: Optimizing coral reef restoration in the Caribbean and Western Atlantic. PeerJ 4, e2597. doi: 10.7717/peerj.2597
Lynch S. A., Flannery G., Hugh-Jones T., Hugh-Jones D., Culloty S. C. (2014). Thirty-year history of Irish (Rossmore) Ostrea edulis selectively bred for disease resistance to bonamia ostreae. Dis. Aquat. Organ 110, 113–121. doi: 10.3354/dao02734
Mann R., Powell E. N. (2007). Why oyster restoration goals in the chesapeake bay are not and probably cannot be achieved. J. Shellfish Res. 26, 905–917. doi: 10.2983/0730-8000
Maryland Oyster Restoration Interagency Workgroup (2020). Oyster reef monitoring report: Analysis of data from the ‘Ten tributaries’ sanctuary oyster restoration initiative in Maryland. 2021.
Matz M. V., Treml E. A., Haller B. C. (2020). Estimating the potential for coral adaptation to global warming across the indo-West pacific. Glob. Change Biol. 26, 3473–3481. doi: 10.1111/gcb.15060
McAfee D., Connell S. D. (2021). The global fall and rise of oyster reefs. Front. Ecol. Environ. 19 (2), 118–125. doi: 10.1002/fee.2291
McAfee D., O’Connor W. A., Bishop M. J. (2017). Fast-growing oysters show reduced capacity to provide a thermal refuge to intertidal biodiversity at high temperatures. J. Anim. Ecol. 86, 1352–1362. doi: 10.1111/1365-2656.12757
McClenachan G. M., Donnelly M. J., Shaffer M. N., Sacks P. E., Walters L. J. (2020). Does size matter? quantifying the cumulative impact of small-scale living shoreline and oyster reef restoration projects on shoreline erosion. Restor. Ecol. 28, 1365–1371. doi: 10.1111/rec.13235
McLeod I., Schmider J., Creighton C., Gillies C. (2018). Seven pearls of wisdom: Advice from traditional owners to improve engagement of local indigenous people in shellfish ecosystem restoration. Ecol. Manage. Restor. 19 (2), pp.98–pp101. doi: 10.1111/emr.12318
Melbourne-Thomas J., Johnson C. R., Fung T., Seymour R. M., Chérubin L. M., Ernesto Arias-González J., et al. (2011). Regional-scale scenario modeling for coral reefs: a decision support tool to inform management of a complex system. Ecol. Appl. 21, 1380–1398. doi: 10.1890/09-1564.1
Mieog J. C., Olsen J. L., Berkelmans R., Bleuler-Martinez S. A., Willis B. L., van Oppen M. J. H. (2009). The roles and interactions of symbiont, host and environment in defining coral fitness. PloS One 4, e6364. doi: 10.1371/journal.pone.0006364
Miller M. W., Latijnhouwers K. R. W., Bickel A., Mendoza-Quiroz S., Schick M., Burton K., et al. (2022). Settlement yields in large-scale in situ culture of Caribbean coral larvae for restoration. Restor. Ecol. 30, e13512. doi: 10.1111/rec.13512
Millin A. (2020). Indigenous aquaculture: A tool to support food security.Master thesis. (San Diego: Scripps Institution of Oceanography).
Montoya-Maya P. H., Smit K. P., Burt A. J., Frias-Torres S. (2016). Large-Scale coral reef restoration could assist natural recovery in Seychelles, Indian ocean. Nat. Conserv. 16 1-17 16, 1–17. doi: 10.3897/natureconservation.16.8604
Morrison T. H., Hughes T. P., Adger W. N., Brown K., Barnett J., Lemos, et al. (2019). Save reefs to rescue all ecosystems. Nat 573, 333–336doi: 10.1038/d41586-019-02737-8
Muller E. M., Bartels E., Baums I. B. (2018). Bleaching causes loss of disease resistance within the threatened coral species acropora cervicornis. Elife 7, e35066. doi: 10.7554/elife.35066
National Marine Fisheries Service (2015). Recovery plan for elkhorn (Acropora palmata) and staghorn (Acropora cervicornis) corals (Silver Spring, Maryland: Acropora Recovery Team for the National Marine Fisheries Service).
Native Olympia Oyster Collaborative (2018) Story map, project profiles, and reports. Available at: https://olympiaoysternet.ucdavis.edu (Accessed September 11, 2022]).
Nitsch C. K., Walters L. J., Sacks J. S., Sacks P. E., Chambers L. G. (2021). Biodegradable material for oyster reef restoration: First-year performance and biogeochemical considerations in a coastal lagoon. Sustain. 13, 7415. doi: 10.3390/su13137415
NOAA Coral Reef Watch Homepage and Near Real-Time Products Portal2019 Coral reef watch. Available at: https://coralreefwatch.noaa.gov/ (Accessed September 11, 2022).
NOAA Florida Keys National Marine Sanctuary2019Blue star program. In: Blue star operators. Available at: https://floridakeys.noaa.gov/onthewater/bluestar.html (Accessed September 11, 2022).
No’kmaq M., Marshall A., Beazley K. F., Hum J., Joudry S., Papadopoulos A., et al. (2021). Awakening the sleeping giant”: re-indigenization principles for transforming biodiversity conservation in Canada and beyond. FACETS 6, 839–869. doi: 10.1139/facets-2020-0083
Oliver E. C. J., Benthuysen J. A., Bindoff N. L., Hobday A. J., Holbrook N. J., Mundy C. N., et al. (2017). The unprecedented 2015/16 Tasman Sea marine heatwave. Nat. Commun. 2017 81 8, 1–12. doi: 10.1038/ncomms16101
Omori M. (2019). Coral restoration research and technical developments: what we have learned so far. Mar. Bio. Res. 15 (7), pp.377–pp.409. doi: 10.1080/17451000.2019.1662050
O’Neil K. L., Serafin R. M., Patterson J. T., Craggs J. R. K. (2021). Repeated ex situ spawning in two highly disease susceptible corals in the family Meandrinidae. Front. Mar. Sci. 8463. doi: 10.3389/fmars.2021.669976
Ozbay G., Reckenbeil B., Marenghi F., Erbland P. (2017). “Eastern Oyster (Crassostrea virginica) aquaculture and diversity of associated species,” in Oysters: Biology, consumption and ecological importance. marine biology (Hauppauge, NY: Nova Science Publishers, Incorporated), pp.1–pp54.
Parker M., Lipton D., Harrell R. M. (2020). Impact financing and aquaculture: Maryland oyster aquaculture profitability. J. World Aquac. Soc 51, 874–895. doi: 10.1111/jwas.12702
Parker L. M., Ross P. M., O’Connor W. A. (2011). Populations of the Sydney rock oyster, saccostrea glomerata, vary in response to ocean acidification. Mar. Biol. 158, 689–697. doi: 10.1007/s00227-010-1592-4
Parker L. M., Ross P. M., O’Connor W. A., Borysko L., Raftos D. A., Pörtner H. O. (2012). Adult exposure influences offspring response to ocean acidification in oysters. Glob. Change Biol. 18, 82–92. doi: 10.1111/j.1365-2486.2011.02520.x
Parker L. M., Scanes E., O’Connor W. A., Ross P. M. (2021). Transgenerational plasticity responses of oysters to ocean acidification differ with habitat. J. Exp. Biol. 224, jeb239269. doi: 10.1242/jeb.239269/238066
Pereira R. R. C., Scanes E., Gibbs M., Byrne M., Ross P. M. (2020). Can prior exposure to stress enhance resilience to ocean warming in two oyster species? PloS One 15, e0228527. doi: 10.1371/journal.pone.0228527
Pogoda B., Brown J., Hancock B., Preston J., Pouvreau S., Kamermans P., et al. (2019). The native oyster restoration alliance (NORA) and the Berlin oyster recommendation: bringing back a key ecosystem engineer by developing and supporting best practice in Europe. Aquat. Living Resour 32, 13. doi: 10.1051/alr/2019012
Poirier L. A., Quijón P. A. (2022). Natural attachment and size of cultured oysters limit mortality from a non‐indigenous predator. Aquac. Res. 53, 1121–1126. doi: 10.1111/are.15613
Pollock F. J., Katz S. M., van de Water J. A. J. M., Davies S. W., Hein M., Torda G., et al. (2017). Coral larvae for restoration and research: a large-scale method for rearing Acropora millepora larvae, inducing settlement, and establishing symbiosis. PeerJ 5, e3732. doi: 10.7717/peerj.3732
Precht W. E., Aronson R. B., Miller S. L., Keller B. D., Causey ,. B. (2005). The folly of coral restoration programs following natural disturbances in the Florida keys national marine sanctuary. Ecol. Restor. 23 (1), 24–28.
Prober S. M., Byrne M., McLean E. H., Steane D. A., Potts B. M., Vaillancourt R. E., et al. (2015). Climate-adjusted provenancing: a strategy for climate-resilient ecological restoration. Front. Ecol. Evol. 3. doi: 10.3389/fevo.2015.00065
Proestou D. A., Vinyard B. T., Corbett R. J., Piesz J., Allen S. K., Small J. M., et al. (2016). Performance of selectively-bred lines of eastern oyster, Crassostrea virginica, across eastern US estuaries. Aquaculture 464, 17–27. doi: 10.1016/j.aquaculture.2016.06.012
Puget Sound Restoration Fund The Kenneth k. chew center for shellfish research and restoration industry partnerships. Available at: https://restorationfund.org/programs/hatchery/ (Accessed 11 October 2022).
Putnam H. M., Gates R. D. (2015). Preconditioning in the reef building coral pocillopora damicornis and the potential for trans-generational acclimatization in coral larvae under future climate change conditions. J. Exp. Biol. 218, 2365–2372. doi: 10.1242/jeb.123018
Putnam H. M., Ritson-Williams R., Cruz J. A., Davidson J. M., Gates R. D. (2020). Environmentally-induced parental or developmental conditioning influences coral offspring ecological performance. Sci. Rep. 2020 101 10, 1–14. doi: 10.1038/s41598-020-70605-x
Randall C. J., Negri A. P., Quigley K. M., Foster T., Ricardo G. F., Webster N. S., et al. (2020). Sexual production of corals for reef restoration in the anthropocene. Mar. Ecol. Prog. Ser. 635, 203–232. doi: 10.3354/meps13206
Raymundo L. J., Maypa A. P. (2004). Getting bigger faster: mediation of size-specific mortality via fusion in juvenile coral transplants. Ecol. Appl. 14, 281–295. doi: 10.1890/02-5373
Razak T. B., Boström-Einarsson L., Alisa C. A. G., Vida R. T., Lamont T. A. C. (2022). Coral reef restoration in Indonesia: A review of policies and projects. Mar. Policy 137, 104940. doi: 10.1016/j.marpol.2021.104940
Reeder-Myers L., Braje T. J., Hofman C. A., Elliott Smith E. A., Garland C. J., Grone M., et al. (2022). Indigenous oyster fisheries persisted for millennia and should inform future management. Nat. Commun. 2022 131 13, 1–13. doi: 10.1038/s41467-022-29818-z
Reeves S. E., Renzi J. J., Fobert E. K., Silliman B. R., Hancock B., Gillies, et al. (2020). Facilitating better outcomes: How positive species interactions can improve oyster reef restoration. Front. Mar. Sci. 7. doi: 10.3389/fmars.2020.00656
Rhyne A., Tlusty M., Schofield PJ, Kaufman L, Morris JA Jr, Bruckner AW (2012). Revealing the appetite of the marine aquarium fish trade: the volume and biodiversity of fish imported into the United States. Plos One 7 (5), e35808. doi: 10.1371/journal.pone.0035808
Rhyne A. L., Tlusty M. F., Kaufman L. (2012). Long-term trends of coral imports into the united states indicate future opportunities for ecosystem and societal benefits. Conserv. Lett. 5, 478–485. doi: 10.1111/J.1755-263X.2012.00265.X
Ridlon A. D., Marks A., Zabin C. J., Zacherl D., Allen B., Crooks, et al. (2021a). Conservation of marine foundation species: Learning from native oyster restoration from California to British Columbia. Estuaries Coasts 44, 1723–1743. doi: 10.1007/s12237-021-00920-7
Ridlon A. D., Wasson K., Waters T., Adams J., Donatuto J., Fleener G., et al. (2021b). Conservation aquaculture as a tool for imperiled marine species: Evaluation of opportunities and risks for Olympia oysters. Ostrea lurida. PloS One 16, e0252810. doi: 10.1371/journal.pone.0252810
Riegl B. M., Purkis S. J., Al-Cibahy A. S., Abdel-Moati M. A., Hoegh-Guldberg O. (2011). Present limits to heat-adaptability in corals and population-level responses to climate extremes. PloS One 6 (9), e24802. doi: 10.1371/journal.pone.0024802
Rinkevich B. (2014). Rebuilding coral reefs: does active reef restoration lead to sustainable reefs? Curr. Opin. Environ. Sustain 7, 28–36. doi: 10.1016/j.cosust.2013.11.018
Rinkevich B. (2019). Coral chimerism as an evolutionary rescue mechanism to mitigate global climate change impacts. Glob. Change Biol. 25, 1198–1206. doi: 10.1111/gcb.14576
Safaie A., Silbiger N. J., McClanahan T. R., Pawlak G., Barshis D. J., Hench J. L., et al. (2018). High frequency temperature variability reduces the risk of coral bleaching. Nat. Commun. 2018 91 9, 1–12. doi: 10.1038/s41467-018-04074-2
Sax S. (2022)How the swinomish are reviving a native oyster and protecting eelgrass in puget sound. Available at: https://civileats.com/2022/01/04/aquaculture-and-ecosystem-protection-go-hand-in-hand-in-puget-sound/ (Accessed 11 September 2022).
Scanes E., Parker L. M., O’Connor W. A., Dove M. C., Ross P. M. (2020). Heatwaves alter survival of the Sydney rock oyster, Saccostrea glomerata. Mar. pollut. Bull. 158, 111389. doi: 10.1016/j.marpolbul.2020.111389
Schmidt-Roach S., Duarte C. M., Hauser C. A. E., Aranda M. (2020). Beyond reef restoration: Next-generation techniques for coral gardening, landscaping, and outreach. Front. Mar. Sci. 7. doi: 10.3389/fmars.2020.00672
Schoepf V., Carrion S. A., Pfeifer S. M., Naugle M., Dugal L., Bruyn J., et al. (2019). Stress-resistant corals may not acclimatize to ocean warming but maintain heat tolerance under cooler temperatures. Nat. Commun. 10, 4031. doi: 10.1038/s41467-019-12065-0
Schopmeyer S. A., Lirman D., Bartels E., Byrne J., Gilliam D. S., Hunt J., et al. (2012). In situ coral nurseries serve as genetic repositories for coral reef restoration after an extreme cold-water event. Restor. Ecol. 20, 696–703. doi: 10.1111/j.1526-100x.2011.00836.x
Schulte D. M., Burke R. P., Lipcius R. N. (2009). Unprecedented restoration of a native oyster metapopulation. Science 325, 1124–1128. doi: 10.1126/science.1176516
Sellares-Blasco R. I., Villalpando M. F., Guendulain-García S. D., Croquer A. (2021). Assisted coral reproduction in the Dominican republic: A successful story to replicate in the Caribbean. Front. Mar. Sci. 8. doi: 10.3389/fmars.2021.669505
Sengupta S., Einhorn C., Andreoni M. (2021)There’s a global plan to conserve nature. indigenous people could lead the way (Accessed 11 September 2022).
Serge A. (2013) Report on ecological and socio-economic conditions at ridge-to-reef project sites (Grenada). In Implementing a ‘Ridge to Reef’ approach to protecting biodiversity and ecosystem functions within and around protected areas in Grenada. Fisheries and Oceans Canada.
Shaver E. C., McLeod E., Hein M. Y., Palumbi S. R., Quigley K., Vardi T., et al. (2022). A roadmap to integrating resilience into the practice of coral reef restoration. Glob. Change Biol. 28, 4751–4764. doi: 10.1111/gcb.16212
Shaver E. C., Silliman B. R. (2017). Time to cash in on positive interactions for coral restoration. PeerJ 5, e3499. doi: 10.7717/peerj.3499
Smith A., Hancock B., Cook N., Vaughan D. (2021). “History of reef restoration,” in Active coral restoration: Techniques for a changing planet. Ed. Vaughan D. E. (Plantation FL USA: J. Ross Publishing).
Spalding M., Brumbaugh R. D., Landis E. (2016). Atlas of ocean wealth (Arlington VA USA: Nature Conservancy).
Stickney R. R. (2009). A global overview of aquaculture production. Food Rev. Intl 6, 299–315. doi: 10.1080/87559129009540874
Suzuki G., Yamashita H., Kai S., Hayashibara T., Suzuki K., et al. (2013). Early uptake of specific symbionts enhances the post-settlement survival of Acropora corals. Mar. Ecol. Prog. Ser. 494, 149–158. doi: 10.3354/meps10548
Tan K., Zhang H., Zheng H. (2020). Selective breeding of edible bivalves and its implication of global climate change. Rev. Aquac 12, 2559–2572. doi: 10.1111/raq.12458
The Nature Conservancy (2021). Global principles of restorative aquaculture (Arlington, VA). Available at: https://www.nature.org/.
The Nature Conservancy (2022) Principles of corporate engagement. Available at: https://www.nature.org/en-us/about-us/who-we-are/how-we-work/working-with-companies/corporate-principles/ (Accessed 11 September 2022).
Thompson V. D., Rick T., Garland C. J., Thomas D. H., Smith K. Y., Bergh S., et al. (2020). Ecosystem stability and native American oyster harvesting along the Atlantic coast of the united states. Sci. Adv. 6, aba9652. doi: 10.1126/sciadv.aba9652
Tillotson M. D., Barnett H. K., Bhuthimethee M., Koehler M. E., Quinn T. P. (2019). Artificial selection on reproductive timing in hatchery salmon drives a phenological shift and potential maladaptation to climate change. Evol. Appl. 12, 1344–1359. doi: 10.1111/eva.12730
Tlusty M. (2002). The benefits and risks of aquacultural production for the aquarium trade. Aquaculture 205, 203–219. doi: 10.1016/S0044-8486(01)00683-4
Torda G., Donelson J. M., Aranda M., Barshis D. J., Bay L., Berumen M. L., et al. (2017). Rapid adaptive responses to climate change in corals. Nat. Clim. Chang 2017 79 7, 627–636. doi: 10.1038/nclimate3374
Vanderklift M. A., Doropoulos C., Gorman D., Leal I., Minne A. J. P., Statton J., et al. (2020). Using propagules to restore coastal marine ecosystems. Front. Mar. Sci. 7. doi: 10.3389/fmars.2020.00724
van Oppen M. J. H., Gates R. D., Blackall L. L., Cantin N., Chakravarti L. J., Chan W. Y., et al. (2017). Shifting paradigms in restoration of the world’s coral reefs. Glob. Change Biol. 23, 3437–3448. doi: 10.1111/gcb.13647
van Oppen M. J., Oliver J. K., Putnam H. M., Gates R. D. (2015). Building coral reef resilience through assisted evolution. Proc. Natl. Acad. Sci. U. S. A. 112, 2307–2313. doi: 10.1073/pnas.1422301112
van Oppen M. J. H., Puill-Stephan E., Lundgren P., De’ath G., Bay L. K. (2014). First-generation fitness consequences of interpopulational hybridisation in a great barrier reef coral and its implications for assisted migration management. Coral Reefs 33, 607–611. doi: 10.1007/s00338-014-1145-2
van Woesik R., Scott W. J., Aronson R. B. (2014). Lost opportunities: Coral recruitment does not translate to reef recovery in the Florida keys. Mar. pollut. Bull. 88, 110–117. doi: 10.1016/j.marpolbul.2014.09.017
Vaughan D. E. (2021). Active coral restoration: Techniques for a changing planet (Plantation FL USA: J. Ross Publishing), 610, ISBN: ISBN: 978-1-60427-143-0.
Waldbusser G. G., Salisbury J. E. (2014). Ocean acidification in the coastal zone from an organism’s perspective: Multiple system parameters, frequency domains, and habitats. Annu. Rev. Mar. Sci. 6, 221–247. doi: 10.1146/annurev-marine-121211-172238
Walsworth T. E., Schindler D. E., Colton M. A., Webster M. S., Palumbi S. R., Mumby P. J., et al. (2019). Management for network diversity speeds evolutionary adaptation to climate change. Nat. Clim. Chang 2019 98 9, 632–636. doi: 10.1038/s41558-019-0518-5
Wasson K., Gossard D. J., Gardner L., Hain P. R., Zabin C. J., Fork S., et al. (2020). A scientific framework for conservation aquaculture: A case study of oyster restoration in central California. Biol. Conserv. 250, 108745. doi: 10.1016/j.biocon.2020.108745
Weinberger H. (2021) Swinomish tribe wants to resurrect U.S. clam gardening (Accessed 11 September 2022).
Williams D. E., Nedimyer K., Miller M. (2020). “Genotypic inventory of acropora palmata (elkhorn coral) populations in south Florida,” in Miami, NOAA National marine fisheries service southeast fisheries science center protected resources and biodiversity division report NOAA/SEFSC/PRBD-2020-1. Miami FL: NOAA National Marine Fisheries Service, Southeast Fisheries Science Center.
Williams S. L., Sur C., Janetski N., Hollarsmith J. A., Rapi S., Barron L., et al. (2019). Large-Scale coral reef rehabilitation after blast fishing in Indonesia. Restoration Ecol. 27 (2), 447–56. doi: 10.1111/rec.12866
Wolfe K., Anthony K., Babcock R. C., Bay L., Bourne D. G., Burrows D., et al. (2020). “Priority species to support the functional integrity of coral reefs,” in Oceanography and marine biology (Boca Raton FL USA: Taylor & Francis).
Young C. N., Schopmeyer S. A., Lirman D. (2012). A review of reef restoration and coral propagation using the threatened genus Acropora in the Caribbean and western Atlantic. Bull. Mar. Sci. 88, 1075–1098. doi: 10.5343/bms.2011.1143
Zabin C. J., Jurgens L. J., Bible J. M., Patten M. V., Chang A. L., Grosholz E. D., et al. (2022). Increasing the resilience of ecological restoration to extreme climatic events. Front. Ecol. Environ. 20, 310–318. doi: 10.1002/fee.2471
Zayasu Y., Suzuki G. (2019). Comparisons of population density and genetic diversity in artificial and wild populations of an arborescent coral, Acropora yongei: Implications for the efficacy of “artificial spawning hotspots. Restor. Ecol. 27, 440–446. doi: 10.1111/rec.12857
Keywords: conservation aquaculture, coral reef, oyster reef, reef resilience, reef recovery, restoration, coral culture, oyster aquaculture
Citation: Ridlon AD, Grosholz ED, Hancock B, Miller MW, Bickel A, Froehlich HE, Lirman D, Pollock FJ, Putnam HM, Tlusty MF, Waters TJ and Wasson K (2023) Culturing for conservation: the need for timely investments in reef aquaculture. Front. Mar. Sci. 10:1069494. doi: 10.3389/fmars.2023.1069494
Received: 13 October 2022; Accepted: 21 March 2023;
Published: 25 April 2023.
Edited by:
Brett W. Molony, Oceans and Atmosphere (CSIRO), AustraliaReviewed by:
Gary Jackson, Department of Primary Industries and Regional Development of Western Australia (DPIRD), AustraliaAshley Smyth, University of Florida, United States
Copyright © 2023 Ridlon, Grosholz, Hancock, Miller, Bickel, Froehlich, Lirman, Pollock, Putnam, Tlusty, Waters and Wasson. This is an open-access article distributed under the terms of the Creative Commons Attribution License (CC BY). The use, distribution or reproduction in other forums is permitted, provided the original author(s) and the copyright owner(s) are credited and that the original publication in this journal is cited, in accordance with accepted academic practice. No use, distribution or reproduction is permitted which does not comply with these terms.
*Correspondence: April D. Ridlon, ctenophores@gmail.com