- 1Research Unit VEG-i-TEC, Department of Food Technology, Safety and Health, Faculty of Bioscience Engineering, Ghent University, Kortrijk, Belgium
- 2Flanders Research Institute for Agriculture, Fisheries and Food, Merelbeke, Belgium
- 3Department of Pathobiology, Pharmacology and Zoological Medicine, Faculty of Veterinary Medicine, Ghent University, Merelbeke, Belgium
- 4Department of Eco-Sustainable Marine Biotechnology, Stazione Zoologica Anthon Dorn, Naples, Italy
- 5School of Chemical and Pharmaceutical Sciences, Technological University Dublin, Dublin, Ireland
- 6School of Food Science and Environmental Health, Technological University Dublin, Dublin, Ireland
- 7Nofima, Tromsø, Norway
- 8Department of Biology and Biological Engineering, Food and Nutrition Science, Chalmers University of Technology, Göteborg, Sweden
A massive amount of crustaceans and bivalves are consumed each year, leading to millions of tons of processing side streams from the seafood industry. Considering the current trend of (bio)circular and zero-waste food production, crustacean and bivalve processing side streams (CBPS) seem a promising and emerging resource for producing high-value-added products. This paper highlights the general composition of CBPS with high commercial values, namely, protein, lipids, carotenoids, minerals and chitins. The extraction strategies of these fractions, including conventional chemical and environmentally friendly methods, are also discussed. This review presents and summarises CBPS as raw materials for developing fast time-to-market products complying with specific EU regulations, including animal feeds, bio-pesticide/stimulants, and cosmetic ingredients. This paper also provides insights into challenges of applying CBPS as raw materials to generate products for human consumption.
1 Introduction
Among the seafood captured for human consumption, the demand for shellfish steadily increased in the past decades (FAO, 2022). In the European Union (EU), shellfish, such as crab, shrimp, and mussels, are usually processed into muscle-based food products. Many processing side streams are generated during this type of production, accounting for up to 75% of the whole mass (Hamed et al., 2016). Considering the current goals for (bio)circular and zero-waste food production, there are strong reasons to valorise shellfish by-products into high-value-added products through biorefining. To support such developments, the EU has already issued regulations such as the Waste Framework Directive (EU, 2008; EU, 2018a), which lists measures to protect the environment and human health. These measures include reducing waste generation, improving waste management, and promoting the transition to a circular (bio)economy. More recently, the EU Bioeconomy Strategy (EU, 2018b) also emphasised the importance of circularity and sustainability, e.g., in food production (Stegmann et al., 2020).
Crustacean and bivalve processing side streams (CBPS) are important resources to upcycle because of their high content of valuable compounds with promising market potential (Özogul et al., 2019). Therefore, the exploration of the application potentials of CBPS has attracted great attention in the past 20 years, and there have been several review articles on the valorisation of CBPS (Harnedy and FitzGerald, 2012; Mao et al., 2017; Mathew et al., 2020; Mechri et al., 2020; Santos et al., 2020). However, these studies seldom assess whether the described potential applications can be commercialised or industrialised from a regulatory perspective. Therefore, the current review focuses on the general composition and extraction of key fractions from CBPS and on the discussion of the EU regulations that may have an impact on its valorisation. Also, some available fast time-to-market CBPS-derived products that require only 3 to 5 years from lab-scale discoveries to a place on the market are summarised.
2 General composition of marine crustacean and bivalve side streams and extraction methods for key fractions
2.1 Protein
CBPS are rich in proteins, although the exact content varies depending on the processing method and the origin. For instance, the protein content in some shrimp processing side streams (e.g., Penaeus semisulcatus head waste, Crangon crangon processing discards, and Penaeus vannamei by-products) reaches 40 - 65% of its dry weight (dw) (Synowiecki and Al-Khateeb, 2000; Mizani and Aminlari, 2007; Vazquez et al., 2017), while crab and mussel processing side streams (i.e., from Cancer irroratus and Paralithodes camtschaticus) contain approximately 20% (Mukhin and Novikov, 2001; Beaulieu et al., 2013) and 10% protein (dw) (Abdulkarim et al., 2013), respectively. The proteins in the actual crustacean shell may account for 16% of its fresh mass (Zhang et al., 2018). These proteins are tightly bound with chitin and are extensively calcified (Chen et al., 2008). In contrast, in bivalve shells with a low level of chitin, the proteins account only for 3 - 5% (dw) (Keith et al., 1993) and are embedded within their calcified layers (Marie et al., 2011). Therefore, a deproteinisation process is required to release proteins from the shells if targeting up the concentration of non-protein compounds such as chitin. This process can be conducted via chemical treatment or biological methods. Traditionally, strong alkali, such as NaOH, at different concentrations (from 0.125 to 5.0 M), at varying temperatures (up to 160°C) and treatment duration (from a few minutes up to a few days) is used to eliminate proteins from crustacean shells (Younes and Rinaudo, 2015). However, using such harsh treatments, the protein-rich fraction is rendered useless and abandoned (Shirai et al., 2001). Although denatured proteins could be of use in various feed applications (No et al., 1989) or hydrolysed into free amino acids, such investigation did not receive widespread attention. Alternatively, different added-value fractions could be better collected via a cascading principle in which different molecules are extracted step by step, starting with the most sensitive ones. Biotechnological methods, especially enzymatic hydrolysis and the so-called pH-shift process, have been studied to recover peptides and proteins, respectively, from CBPS. By adding commercial proteases under specific conditions, proteins from CBPS can be hydrolysed in an eco-friendly manner, and 64 - 96% of the proteins can be recovered in the form of a hydrolysate (Mao et al., 2017; Zou et al., 2021). Using the pH-shift process, i.e., acid and alkaline protein solubilisation followed by removal of non-soluble matter as shells and isoelectric precipitation of the purified proteins, lead to a recovery of 31 and 48% protein, respectively, from whole crushed blue mussels (Mytilus edulis) that were sorted away when packing mussels in nets (Vareltzis and Undeland, 2012). Using shrimp shells, a protein recovery of up to 25% was recently reported when applying alkaline pH shift processing with the incorporation of ultrasonication (Pezeshk et al., 2022). However, to the best of our knowledge, none of these two techniques is commercialised to valorise CBPS.
2.2 Lipids
CBPS can have a relatively high content of lipids rich in polyunsaturated fatty acids (PUFAs). The exact level depends on the species and the parts of the animal ending up in the side stream fraction. For example, the lipid content of snow crab (Chionoecetes opilio) side streams, consisting of cephalothorax shells, digestive systems, including hepatopancreas, and physiological liquid (hemolymph), was 14.8% (dw), and its fatty acid (FA) profile showed approximately 50% of monounsaturated fatty acids (MUFAs), followed by 20% of PUFAs (Beaulieu et al., 2009).
As summarised by Sánchez-Camargo et al. (2011), the lipid content of different shrimp processing side streams, which consists of heads and shells, varies from 0.76 - 7.14% (dw) depending on, e.g., the amount of tissue left. As reported from Pacific white shrimp side streams (exoskeleton and cephalothorax in roughly 1:1, w/w), PUFA content in the lipid fraction reached up to 37.5% of the total FA (Gulzar and Benjakul, 2018). In the lipid fraction of red-spotted shrimp by-products, even a higher PUFA content was reported; 61.36% of total FA, with the long-chained (LC) n-3 PUFAs eicosapentaenoic acid (EPA) and docosahexaenoic acid (DHA), contributing to 24% of total FA (Sánchez-Camargo et al., 2011). Therefore, these shrimp processing side streams can be regarded as a new source for LC n-3 PUFA extraction.
Compositional features of mussel processing side streams were recently reviewed by Naik and Hayes (2019). They categorised the potential side streams into seeds, mussels with broken shells, mussels with barnacles/fouled, and byssus threads. However, a fifth category can be the shell residue from steamed/peeled mussels, consisting of shells and adductor muscle. Uzcátegui et al. (2021) characterised lipids in the soluble and filtered fractions of industrial “mussel waste”, including the shells, and found 0.2 versus 0.4% (fw) lipids, respectively, with 25 and 15% PUFAs, respectively. Interestingly, unusual, but also very unstable furan FA’s with anti-inflammatory properties have been found in the lipid fraction of New Zeeland green-lipped mussels (Wakimoto et al., 2011), also calling for further exploration of mussel processing side streams.
Conventionally, the extraction method of lipids relies on employing organic solvents such as acetone, ethanol, ethyl acetate, isopropanol, hexane, and methyl ethyl ketone. Although some of these solvents are permitted in food industries (Takeungwongtrakul et al., 2015), the low extraction yield, high solvent consumption, and long processing time are the significant disadvantages of this method (Gulzar et al., 2020). To overcome these drawbacks, solvent extraction can be combined with physical techniques such as ultrasonic-assisted extraction (UAE) or replaced by supercritical fluid extraction (SFE). These newer approaches have been introduced to efficiently extract lipids from CBPS. For example, Gulzar and Benjakul (2019) applied n-hexane and isopropanol (1:1, v/v) plus UAE to extract lipids from pre-heated cephalothorax of Pacific white shrimp. UAE increased the extraction yield from 10.87% to 14.09% based on fw. Although a significant yield increase was obtained by UAE, oxidation and hydrolysis of the extracted lipids may occur during the process, e.g., as a result of temperature raises (Zhang et al., 2017b). Applying SFE for extraction of LC n-3 PUFA-rich lipids from CBPS can, in this respect, be more favourable, especially for pharmaceutical and nutraceutical industries, because of the heat-labile nature of the PUFAs. A recent review from Ahmadkelayeh and Hawboldt (2020) summarised that supercritical CO2 to recover lipids from CBPS gave a comparable yield to traditional solvent extraction methods without negative environmental and economic disadvantages.
2.3 Carotenoids
Carotenoids are another group of valuable compounds enriched in CBPS. The carotenoid content varies from 40 to 114 μg/g fw, depending on the species (Pattanaik et al., 2020). In crustacean shells, astaxanthin accounts for up to 75 - 95% of total carotenoids (Shahidi et al., 1998). Today, more than 95% of the astaxanthin used in the industry is supplied by synthetic routes (Panis and Carreon, 2016), but consumers have an increasing demand for natural food additives or ingredients. This fact together with the high cost of producing synthetic astaxanthin, have stimulated industries to find new astaxanthin sources with good potential for commercialisation.
Owing to their lipophilic nature, carotenoids are extracted by non-polar solvents such as acetone, chloroform, hexane, isopropanol, methylene chloride and diethyl ether (Saini and Keum, 2018). However, the recovery of carotenoids from CBPS by solvent extraction has been limited to analytical purposes during the past 30 years. The recovery yield may reach up to 70 µg/g dw using extraction with organic solvents but varies with methodology and specific source of raw material (Saini and Keum, 2018). Another conventional extraction method frequently studied is using edible vegetable oils as solvent. However, this method requires higher temperatures (60 - 90°C) which may influence the stability of astaxanthin (Rao et al., 2007). Moreover, the astaxanthin extracts are hard to concentrate when edible oils are used, leading to a relatively low recovery yield: 0.9 mg astaxanthin/g raw material versus 19.8 mg astaxanthin/g raw material acetone extraction (Dong et al., 2014). In order to reduce the environmental impacts and the consumption of organic solvents and energy, biotechnological methods such as enzymatic hydrolysis and microbial fermentation are studied to facilitate astaxanthin extraction from CBPS (Ahmadkelayeh and Hawboldt, 2020). In addition, astaxanthin can be recovered by SFE techniques, and by combining regular solvent extraction with UAE, the amounts of solvents used can be decreased. Details of the extraction procedures mentioned above and the recovery yield of astaxanthin from various CBPS have been thoroughly reviewed by Prameela et al. (2017).
2.4 Minerals
The mineral fraction of CBPS is well documented as a component of the characteristic shell nano-fibril structure. The nano-fibril consists of tightly wound strands of protein, organic polymer matrix - in this case, chitin - and mineral rods. The mineral component allows the thin shells to have enhanced rigidity, high relative tensile strength, and high resistance to shearing stress (El Knidri et al., 2018). The majority of the crustacean shell mineral is CaCO3 as calcite, amorphous CaCO3, and Ca3(PO4)2 as hydroxyapatite (Aklog et al., 2016).
Similarly, most of the minerals in bivalves are CaCO3 as calcite and aragonite crystal forms. The average mineral content of a fw basis is approximately 60% for brown crab, 50% for northern shrimp, and 50% for blue mussels (Beaulieu et al., 2009; Abdulkarim et al., 2013; Zhao et al., 2019). In the industry, CaCO3 from the organic matrix of CBPS is extracted by chemical demineralisation procedure by washing CBPS with HCl (0.275 - 2 M) at various temperatures (0 - 100°C) within 24 h (Percot et al., 2003). When washed with acid, calcium ions and CO2 are liberated (Younes and Rinaudo, 2015). The calcium, phosphate, and carbonate ions remain in the solution, whereas the insoluble chitin does not. Thus, using filtration, the minerals are separated from the chitin. The endpoint of the demineralisation reaction is deemed by the cessation of CO2 being released from the solution. Although chemical demineralisation is still the standard procedure to extract calcium salts from CBPS, it has some evitable disadvantages, such as high energy consumption and wastewater disposal problems. To solve these problems, new attempts using biological methods such as lactic acid fermentation have been studied to demineralise CBPS (Zou et al., 2021). However, this biological treatment is still conducted on a lab scale, not industrialised or commercialised.
2.5 Chitin and its derivatives
Chitin is a natural polysaccharide which is the second most abundant biopolymer after cellulose. This biopolymer is composed of β-1,4-N-acetyl glucosamine chains, which occur in the cell wall of some fungi, insect cuticles, and crustacean shells (Younes and Rinaudo, 2015). In industry, crustacean shells are the primary commercial sources of chitin due to their abundance. In general, the chitin content of crustacean shells is 17.8 - 75% (dw of exoskeletons) and differs by species (Hamed et al., 2016). However, the chitin fraction in the crustacean shells is tightly bonded to calcium salts and proteins, making it insoluble in conventional solvents (Kurita, 2006). Due to its insoluble nature, chitin cannot be recovered by traditional solvent extraction methods. Instead, eliminating proteins and calcium salts from the shells is required, making the processes complicated and laborious. Commercially, NaOH is used to eliminate the proteins (deproteinisation, as mentioned in section 2.1) and HCl to eliminate calcium salts (demineralisation, as mentioned in section 2.4), thereby allowing up-concentration of chitin. However, these processes are costly due to high energy consumption, and the discharged wastewater needs to be neutralised and detoxified before disposal.
Moreover, harsh chemical treatments may influence the final quality of chitin (Percot et al., 2003; Kjartansson et al., 2006). In order to tackle the mentioned problems, the development of more eco-friendly extraction methods has attracted great attention. For instance, applying biological methods such as enzymatic hydrolysis or microbial fermentation to extract chitin from CBPS was intensively studied and comparatively reviewed by Zou et al. (2021). Some new attempts, such as applying non-equilibrium plasmas, have also attracted great interest since they can efficiently modify various heat-liable polymers without affecting their properties (Delaux et al., 2016). Borić et al. (2018) reported using plasma generation by the dielectric barrier discharge to extract chitin from crustacean waste biomass. They concluded that this method could efficiently remove the proteins fast without influencing the chitin biopolymer. Also, this method did not require any solvents, and therefore no (solid and liquid) waste was formed. Later, they combined this method with lactic acid demineralisation, eliminating 90% of the proteins and completely removing the calcium salts from shrimp shells (Borić et al., 2020). Thus, advantages of this method are that it is scalable, efficient, sustainable and relatively cheap since the operation cost is mainly limited to electricity consumption. Also, no waste is generated during the process, which makes it even more appealing for the recovery of chitin and other valuable fractions such as proteins and CaCO3.
Chitosan is a deacetylated polymer composed of more than 50% of β-(1→4)-D-glucosamine units in the chain. Chitosan can be considered a soluble chitin version, explaining the particular interest in this molecule. Again, crustacean shells are considered the primary source for chitosan conversion from chitin. Commercially, demineralised and deproteinised crustaceans’ shells are treated with NaOH at high temperatures, allowing the hydrolysis of the acetyl groups. This treatment transforms the N-acetyl-D-glucosamine units into D-glucosamine units with free –NH2 groups. Like the chemical extraction of chitin from CBPS, the high energy and a large amount of concentrated alkali solution consumption do not conform to the “green chemistry” and circular economy trend. Therefore, biological alternatives such as using deacetylases to convert chitin to chitosan have been explored experimentally (Zhang et al., 2017a). Hybrid methods that can decrease the chitin crystallinity were also studied to increase the conversion rate and yield, including steam-explosion and SFE technology-aided enzymatic hydrolysis (Villa-Lerma et al., 2016). Although these methods are not commercialised yet, producing chitosan and interesting oligomers through this controllable enzymatic deacetylation process is an attractive and promising alternative.
3 Fast time-to-market products derived from CBPS and their EU regulations
As mentioned above, the potential applications of different fractions recovered from CBPS are well documented and discussed in several review articles. For instance, in a recent review study, Nirmal et al. (2020) concluded that the fractions extracted from shrimp waste (cephalothorax, shells, and processing/cooking water), namely, chitin/chitosan, carotenoids, protein hydrolysate, and PUFAs, may possess antioxidant, antimicrobial, antihypertensive, anti-inflammatory, and/or antiproliferative activities. Therefore, the authors believe these compounds could be used industrially as additives or functional food/feed ingredients, complying with the wish for a clean label. Another recent review from Santos et al. (2020) focused on chitin and chitosan extracted from seafood processing side streams, which could be used in the pharmaceutical, cosmetic, food, biomedical, chemical, and textile industries. However, it is essential to meet governmental regulations to bring these bioactive products to the market. In the EU, the by-product legislation [Regulation (EC) No. 1069/2009] (EU, 2009b) must be considered for an industrial application of CBPS unless they are handled in an entire food grade manner just as the main product, e.g., the peeled shrimp or mussel.
The by-product legislation legally classifies food by-products (in this review named as side streams) into different categories, as shown in Table 1. In general, many CBPS are classified as “Category 3 by-products” since some organic materials, such as the non-extractable meat tissues and hepatopancreas are still attached. These side streams could be contaminated and spoiled due to improper handling and storage, making them unsuitable for human consumption. Therefore, by-products with organic material handled in this manner could be used only under conditions determined by the competent authority, preventing public and animal health risks. In the following sections, the possibilities of using CBPS as raw material to produce fast time-to-market products and their relevant EU regulations are discussed, focusing on feed, agricultural/horticultural applications, and cosmetics. The possibility of valorising CBPS for human consumption, such as pharmaceutical applications, is not the focus of this review and will not be discussed further.

Table 1 Different categories of by-products with their risk levels, definitions, and legal applications according to EU regulation 1069/2009.
3.1 (Aquatic) feed and pet food
Aquaculture of carnivorous species depends on PUFAs from fishmeal and fish oil, but these often come from limited fisheries or fish slaughter by-products. Limited fisheries are fisheries where the resource withdrawal cannot be extended to meet the increased demand (Hamilton et al., 2020). Therefore, the continued growth of aquaculture will depend on developing more sustainable feeds with alternative ingredients or feeds of a low-trophic level. As mentioned in section 2, CBPS shows relatively high protein, astaxanthin, and lipid content with high levels of PUFAs (EPA and DHA). Previous studies have explored crustaceans and amphipods as alternatives in experimental diets for farmed fish (Moren et al., 2006; Opstad et al., 2006; Suontama et al., 2007), obtaining promising results. Especially for shrimp and salmonids, natural skin pigmentation maintenance is of great economic importance and is directly associated with acceptance or rejection by consumers (Prameela et al., 2017; Lim et al., 2018). Commercial products SeaPro® Shrimp Meal and SeaPro® Crab Meal from Bio-Oregon Protein, Inc. (OR, USA) are produced from the processing side streams of Northern shrimp (Pandalus jordani) and Dungeness crab (Metacarcinus magister). These products have already been used as ingredients in animal feed and pet food to balance nutrient profiles. It is worthy of mentioning that this company is vertically integrated within a seafood company, i.e. targeting food for human consumption (Pacific Seafood, OR, USA). This integration secures a stable supply chain of the raw materials and implements the FDA Food Safety Modernization Act (FSMA)/HACCP systems throughout the entire process to ensure the quality of the products. However, this type of integrated company does not yet exist in the EU, as far as we know.
At present, converting food by-products into animal feed and pet food is considered the most feasible and sustainable practice (Salemdeeb et al., 2017). In the EU, Regulation (EU) No. 2017/1017 (EU, 2017a) lists what raw materials can be used to produce animal feed and pet food. According to this regulation, CBPS are categorised as by-products from aquatic animals, crustacean meals, and mollusc meals. In general, any CBPS fulfil the requirements of “health rules as regards animal by-products and derived products not intended for human consumption” [Regulation (EC) No. 1069/2009 (EU, 2009b) and Regulation (EU) No. 142/2011 (EU, 2011)] and does not possess any risk of certain transmissible spongiform encephalopathies according to Regulation (EC) No. 999/2001 (EU, 2001). Thus, they can be used to produce aquatic feed and pet foods. It also deserves to be mentioned that Regulation (EU) No. 2017/1017 (EU, 2017a) only includes hydrolysed krill protein concentrate in the list; that said, other sources of protein hydrolysates such as crab and shrimp (and their by-products) cannot be used as raw material to produce animal feed and pet food yet. However, in section 13.2.8, the regulation mentions that glucosamine produced by the hydrolysis of crustaceans and other arthropod exoskeletons is legal to be used as feed material, making CBPS have broader application prospects.
If a nutraceutical (generated from food waste) is intended for animal nutrition, it must comply with Regulation (EC) No. 1831/2003 (EU, 2003) and (EU) No. 68/2013 (EU, 2013), and if it has pharmacological effects, Regulation (EU) 2019/6 (EU, 2019b) must be followed (Tedesco and Cagnardi, 2019).
3.2 Agricultural/horticultural products: Biofertilisers, biostimulants, and crop protection agents
In modern agriculture, the search for eco-friendly and sustainable alternatives to promote plant growth and protect plants from diseases and pests, enhancing crop productivity with lower environmental impact, is a priority. Chitin and its derivatives have shown promising safe alternatives to hazardous synthetic chemical pesticides/fungicides and fertilisers with negligible risk to human health and the environment (Shamshina et al., 2019).
Chitin is used in agriculture as a component for biofertilisers’ preparation and is considered an organic nitrogen fertiliser. Nitrogen fertilisers are typically used to promote leaf growth (Liu et al., 2014). The fertiliser effect of chitin or chitosan is due to the decomposition and convergence by chitinolytic microorganisms present in the soil into nitrogen forms useful for direct uptake by plants, like ammonia and nitrates (Manucharova et al., 2006; De Tender et al., 2019; Vandecasteele et al., 2021). Examples of commercial biofertilisers produced from CBPS are listed in Table 2. However, none of these commercial products are produced or available in the EU.
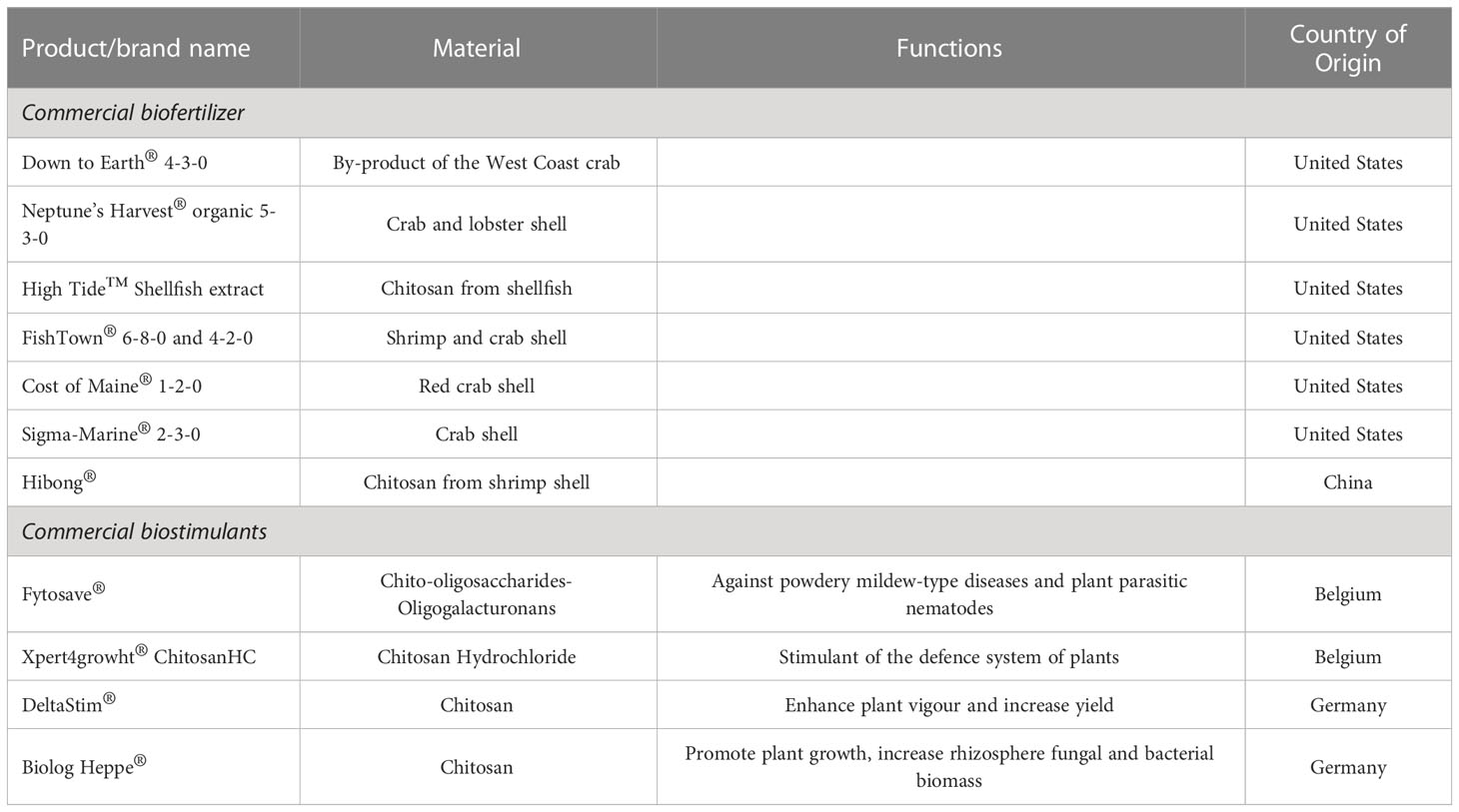
Table 2 Examples of commercial biofertilisers, biostimulants and biopesticides produced from crustaceans processing side streams.
In addition to fertiliser effects, chitin and its derivatives are also used to improve crop protection against plant pathogens and abiotic stress conditions. Crop protection is often an indirect activity mediated by beneficial symbiotic microorganisms, including plant growth-promoting rhizobacteria and fungi (De Tender, 2021; Maksimov et al., 2011). The presence of chitin enhances the proliferation of these microorganisms and facilitates plant-microorganism interactions. Plant growth-promoting rhizobacteria and fungi have been reported to improve plant growth by directly stimulating the plant or suppressing pathogens (Vandecasteele et al., 2021). In the presence of chitin, many of these microorganisms overproduce chitinolytic enzymes acting as natural fungicides/pesticides (Melent’ev et al., 2006). These findings suggest that biocontrol bacteria and fungi may be applied in combination with chitin or chitin-containing materials, like crustacean waste, or carrier materials supplemented with chitin-containing substances to protect plants. Chitin and its derivatives have been intensively studied as potential biopesticides in the past 20 years (Wang et al., 1999; Wang et al., 2002a; Wang et al., 2002b; Wang et al., 2005; Inderbitzin et al., 2018; Andreo-Jimenez et al., 2021). Recently, a Belgian company, Fytofend, successfully launched a product named Fytosave®, an elicitor of natural plant defence mechanisms. It is a soluble concentrate that contains 12.5 g/L COS-OGA (Chito-oligosaccharides-Oligogalacturonans). It provides good protection against powdery mildew on various crops and works against plant-parasitic nematodes (Singh et al., 2019). Another Belgian company, Xpert4growth, brings a new chitosan product to the market, which is authorised as a natural product. A German company, DeltaChem, produces the chitosan product DeltaStim® from shrimp shells, which can be used as a biostimulant to enhance plant vigour and increase yield. It can also be applied as a biopesticide against plant pathogens of the genus Phytophthora, Fusarium, and as an anthelmintic against plant-parasitic nematodes. Examples of commercial biostimulants and biopesticides produced from CBPS are listed in Table 2.
To reduce the consumers’ risk of exposure to pesticide residues in foods, the application of biopesticides in crop protection attracts great attention in modern agriculture. Biopesticides are usually inherently less toxic than conventional pesticides and generally affect only the target pest and closely related organisms (Czaja et al., 2015). In the EU, biopesticides are regulated under the plant protection Regulation (EC) No. 1107/2009 (EU, 2009c). This regulation is also carried out in conjunction with two other EU Regulations and Directives: 1) Regulation (EC) No. 396/2005 (EU, 2005), which focuses on the regulation of the maximum residue levels (MRLs) in food; 2) Directive 2009/128/EC (EU, 2009a) which promotes the application of Integrated Pest Management (IPM) and the use/development of alternatives to chemical pesticides. Although there is no regulatory category specified for biopesticides generated from CBPS, they should follow the general pesticide categories: “basic substances” or “low-risk substances” according to Regulation (EU) No. 2017/1432 (EU, 2017b).
Organic farming using natural preparations is highly promoted to reduce nitrogen-containing fertilisers used in agriculture and horticulture [Directive 91/676/EEC (EU, 1991)]. As an alternative to chemical ones, biofertilisers and biostimulants can improve plants’ general health, vitality, and growth and not pose many environmental and ecological risks (Pylak et al., 2019). According to Regulation (EU) No. 2019/1009 (EU, 2019c), a biostimulant is defined as a product stimulating plant nutrition processes independently of the product’s nutrient content with the sole aim of improving nutrient use efficiency, tolerance to abiotic stress, quality traits, and availability of confined nutrients in the soil or the plant rhizosphere. This new regulation lists specific types of bio-products which can be used in organic farming and specifies that a plant biostimulant “shall have the effects that are claimed on the label for the plants specified thereon”. Moreover, the European Biostimulants Industry Council (EBIC) has proposed some general guiding principles to follow, promoting and facilitating the launching of biostimulants in the EU market (Ricci et al., 2019).
Generally, from the regulation aspects, products valorised from CBPS, which are used as biopesticides, organic fertilisers, and biostimulants, do not face insurmountable legislation barriers. Moreover, evaluating low-risk bioactive ingredients takes 120 days, which is far shorter than 12 months for conventional active ingredients. Also, low-risk active ingredients are approved for 15 years instead of 10 years. However, the high registration cost can prohibit the commercialisation of new products, and products used as plant protection without authorisation are considered illegal. In order to tackle this problem, European legislation (Belgium as an example) provided a more straightforward procedure (FPS Public Health, Food Chain Safety and Environment, 2015), whereby basic substances such as marine sourced chitosan can be placed on a positive list.
3.3 Cosmetics
In cosmetics, chitosan and its derivatives have been used to manufacture skin, hair, and oral care products (Dutta et al., 2004; Morganti et al., 2011; Massironi et al., 2020). In recent years, chitin and chitosan applications in eco-friendly processes have been highlighted, along with chitosan’s advantages in the production of cosmetics (Casadidio et al., 2019). Specifically, marine-sourced chitosan is compatible with many cosmetic ingredients such as starch, fats, glucose, waxes, saccharose, polyols, oils, and acids, making it ideal for cosmetic formulations (Morin-Crini et al., 2019). An example of where crustacean-sourced chitosan is manufactured as a cosmetic ingredient in a commercial setting is Chitinor AS (https://chitinor.com), part of the Seagarden group Norway, which prepares various ingredients based on chitosan. This company uses varying molecular weight chitosan for applications in hair care, personal hygiene, and skin care. The use of chitin and chitosan derivatives in oral care and skincare products, including the antibacterial properties for oral healthcare and antioxidant effect in skincare, have been explored, and researchers have identified specific bacterial species that the chitosan mouthwash and oral care products can control (Aranaz et al., 2018). In addition, chitosan nanoparticle formulations have shown promise when loaded with fluoride as novel dental delivery systems to enhance oral hygiene (Nguyen et al., 2017).
The full potential of using CBPS in the cosmetic industry is yet to be explored. However, there are examples of routes to enhance the value added for the industry through innovation, such as cosmetic products developed by a Spanish company: Grup Barcelonesa, who applies glucosamine extracted from lobster, shrimp and crab shells. This company claims that the function of glucosamine in the cosmetic product is to provide moisture and elasticity to the skin resulting in an anti-ageing effect (Grup Barcelonesa, 2019). The cosmetic industry is a definite growth area for using CBPS as a multifunctional ingredient in the circular economy. A list of commercial cosmetic ingredients and products that contain chitosan is summarised in Table 3.
The EU Commission defines a cosmetic product as “any substance or mixture intended to be placed in contact with the external parts of the human body (epidermis, hair system, nails, lips, and external genital organs) or with the teeth and the mucous membranes of the oral cavity with a view exclusively or mainly to cleaning them, perfuming them, changing their appearance, protecting them, keeping them in good condition or correcting body odours.” In Europe, cosmetic products and their ingredients for the EU market are regulated under Cosmetic Product Regulation: Regulation (EC) No 1223/2009 (EU, 2009d). The European Commission also has a cosmetic ingredients database, CosIng, which maintains information on cosmetic substances and ingredients. More recently, in 2019, a glossary of common ingredient names for labelling cosmetic products placed on the market was put into action according to the EU Commission Decision 2019/701 (EU, 2019a).
The CosIng database currently lists eight products under a chitin search, including chitin and its derivatives (EU, 2022). It does not state the source of chitin but rather its function as an abrasive and bulking agent. Chitosan’s functions are described as film-forming and hair fixing on the CosIng database (EU, 2022). Fifty types of cosmetic ingredients are listed for chitosan and its derivatives which has grown from 44 products in 2018 (Aranaz et al., 2018). These ingredients have various applications for cosmetic products, including antimicrobial, antioxidant, hair and skin conditioning, surfactant, chelating, film-forming, skin protection and emulsifying, emollient, antistatic, binding, viscosity controlling. The increase in products would suggest that the development and use of chitosan derivatives is a growth area for the cosmetics industry. Regulation (EC) No. 1223/2009 (EU, 2009d) also indicates that cosmetic products shall not contain prohibited substances listed in Annex II. Prohibited substances include cadmium species that could be present in chitosan derived from crustaceans. However, there are no regulations on using chitosan-derived cosmetic products concerning allergic reactions.
A summary of EU regulations/legislations related to feed, agricultural/horticultural applications, and cosmetics is shown in Table 4.
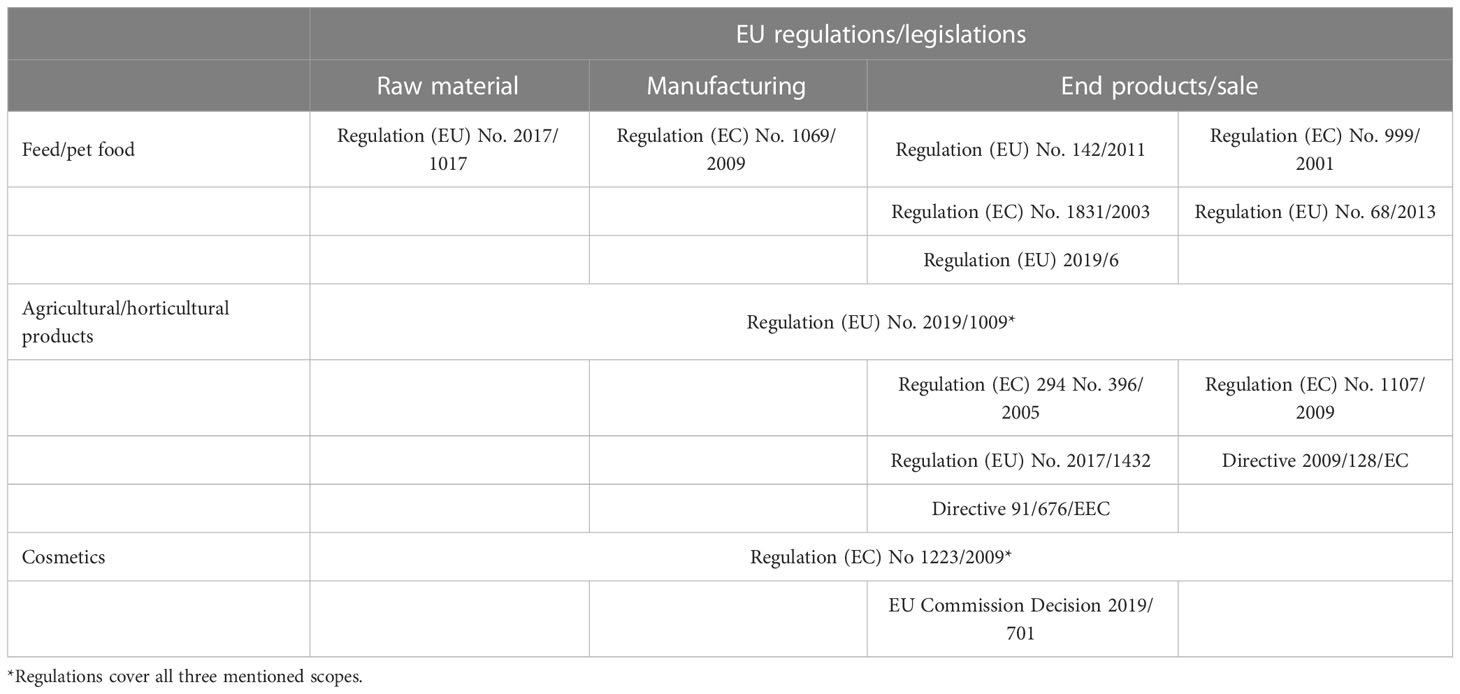
Table 4 Outline of the relevant EU regulations/legislations on three applications: feed/pet food, agricultural/horticultural products, and cosmetics; the regulatory scopes are divided into three subcategories: raw material, manufacturing, and end products/sale.
4 Challenges of valorising CBPS for human consumption
As discussed in the previous sections, the fast time-to-market products derived from CBPS are currently limited to animal feeds, bio-pesticide/stimulants, and cosmetic ingredients since CBPS usually are not handled in a food-grade manner but rather as by-products or even waste. Once CBPS are downgraded to by-products or waste, they can no longer be upgraded to food (ingredients). Therefore, to valorise CBPS for human consumption, food handling procedures must be in place throughout the entire value chain from catch to product, with Good Manufacturing Practice (GMP), Good Hygienic Practice (GHP) and Hazard Analysis and Critical Control Point (HACCP) principles applied. Additionally, the side streams must be considered safe to use as food with respect to microbiological and chemical criteria (including toxin levels and heavy metals) (de Boer and Bast, 2018). Due to the regulations mentioned in the previous sectors, the valorisation of CBPS to products intended for human consumption with quick market potential is currently challenging since processing and storage facilities are often non-fit for food handling and lack appropriate equipment. Extra labour cost is also a hurdle. In addition to technical and economic challenges, the market for food products derived from CBPS is not well developed; therefore, the knowledge about how to develop marketable products is insufficient. In addition, the tools for marketing and communication are underdeveloped (Olsen et al., 2014; Altintzoglou et al., 2021; Whitaker et al., 2021).
Another challenge of using CBPS for human consumption is consumer acceptance. This challenge can be mitigated by presenting products based on side streams as innovative food. Presenting products as innovative food could improve consumer acceptance by enhancing the ability of consumers to evaluate the products based on quality cues (Bhatt et al., 2018; Aschemann-Witzel and Peschel, 2019). However, consumers may also be concerned and resistant to environmental labels, such as sustainable and organic food, because of low trust in the labelling systems (Vittersø and Tangeland, 2015). Some studies showed that involvement with seafood and concerns about the marine environment positively affect the perception of the benefits of eating seafood (Jacobs et al., 2015).
Utilising side streams can lead to financial benefits for producers. However, this could lead to consumer scepticism towards the reasoning behind changes in production systems, even if a reduction of environmental impact is evident (Holloway et al., 2009). Additional studies in realistic environments, such as the consumers’ households, could support efforts towards reducing the latter scepticism. A decrease in scepticism could potentially increase consumer acceptance of products that include ingredients based on production methods aimed at reducing food waste. One example is providing consumers with skills and tools, such as explaining why by-products can help them plan their shopping routines and avoid food waste (Stefan et al., 2013). Buying labelled products could also help consumers make informed decisions and express their opinion about food production, such as ethics (Brom, 2000). Additional information about the benefits of using ingredients that stimulate the whole raw material in specific product categories, such as nutrition supplements, would increase consumer trust. The latter result is beneficial for consumers with high involvement in food waste reduction (Altintzoglou et al., 2021).
Although facing many challenges, there are still attempts trying to valorise CBPS into high-value-added products for human consumption. An EU project, BlueCC started in 2020, “takes underutilised CBPS to develop new eco-friendly marine ingredients and commercial products such as chitin/chitosan using a market acceptance approach” (BlueBio COFUND, 2020). In 2021, another project named “ReMeSS: Resource effective Multi-extraction of Sustainable high-value compounds from Shrimp production side-stream” started in Denmark. This project aims to “extract valuable compounds, namely, flavour products, calcium and collagen from shrimp processing side streams, considering the food value chain” (DTU, 2021). However, these projects can reach the Technology Readiness Level 7 at most, meaning there is still a relatively long procedure until real products are available on the market. On the other hand, these projects may build prototype demonstrations, providing valuable information to the industries, policymakers and stakeholders, which can accelerate commercialisation in the near future.
5 Conclusion
The composition of CBPS implies that these side streams could be valorised into value-added products instead of being landfilled or incinerated. However, because of the strict EU regulations, the valorisation potential of these side streams is not fully revealed, e.g., as long as they are not handled in a food grade manner, they still cannot be used to develop products for human consumption. Therefore, future actions on developing integrated facilities that properly handle CBPS as food-grade materials and subject them to biorefining for the production of multiple products should be promoted. In addition, further research on developing appropriate marketing strategies to influence consumer behaviours towards side stream related products should be conducted to promote the valorisation of CBPS.
Author contributions
KR, JR, YZ: Conceived the review. YZ: Wrote the paper. KR, JR, MH, JD, DP, PB, MG, CO’C, RS, KL, TA, RD, NS, IU: critically reviewed the paper. All authors contributed to the article and approved the submitted version.
Acknowledgments
This study was performed in the framework of the BlueShell project (Exploring Shellfish By-Products as source of Blue Bioactives) supported by ERA-NET MarineBiotech (ERA-MBT) and Fonds voor Wetenschappelijk Onderzoek – Vlaanderen (FWO).
Conflict of interest
The authors declare that the research was conducted in the absence of any commercial or financial relationships that could be construed as a potential conflict of interest.
Publisher’s note
All claims expressed in this article are solely those of the authors and do not necessarily represent those of their affiliated organizations, or those of the publisher, the editors and the reviewers. Any product that may be evaluated in this article, or claim that may be made by its manufacturer, is not guaranteed or endorsed by the publisher.
References
Abdulkarim A., Isa M. T., Abdulsalam S., Muhammad A. J., Ameh A. O. (2013). Extraction and characterisation of chitin and chitosan from mussel shell. Extraction 3, 108–114.
Ahmadkelayeh S., Hawboldt K. (2020). Extraction of lipids and astaxanthin from crustacean by-products: A review on supercritical CO2 extraction. Trends Food Sci. Technol. 103, 94–108. doi: 10.1016/j.tifs.2020.07.016
Aklog Y. F., Egusa M., Kaminaka H., Izawa H., Morimoto M., Saimoto H., et al. (2016). Protein/CaCO3/chitin nanofiber complex prepared from crab shells by simple mechanical treatment and its effect on plant growth. Int. J. Mol. Sci. 17, e1600. doi: 10.3390/ijms17101600
Altintzoglou T., Honkanen P., Whitaker R. D. (2021). Influence of the involvement in food waste reduction on attitudes towards sustainable products containing seafood by-products. J. Clean. Prod. 285, e125487. doi: 10.1016/j.jclepro.2020.125487
Andreo-Jimenez B., Schilder M. T., Nijhuis E. H., Te Beest D. E., Bloem J., Visser J. H. M., et al. (2021). Chitin- and keratin-rich soil amendments suppress rhizoctonia solani disease via changes to the soil microbial community. Appl. Environ. Microb. 87, 318–321. doi: 10.1128/AEM.00318-21
Aranaz I., Acosta N., Civera C., Elorza B., Mingo J., Castro C., et al. (2018). Cosmetics and cosmeceutical applications of chitin, chitosan and their derivatives. Polymers 10, e213. doi: 10.3390/polym10020213
Aschemann-Witzel J., Peschel A. O. (2019). How circular will you eat? the sustainability challenge in food and consumer reaction to either waste-to-value or yet underused novel ingredients in food. Food Qual. Prefer. 77, 15–20. doi: 10.1016/j.foodqual.2019.04.012
Beaulieu L., Thibodeau J., Bonnet C., Bryl P., Carbonneau M.É. (2013). Detection of antibacterial activity in an enzymatic hydrolysate fraction obtained from processing of Atlantic rock crab (Cancer irroratus) by-products. PharmaNutrition 1, 149–157. doi: 10.1016/j.phanu.2013.05.004
Beaulieu L., Thibodeau J., Bryl P., Carbonneau M. E. (2009). Characterization of enzymatic hydrolyzed snow crab (Chionoecetes opilio) by-product fractions: A source of high-valued biomolecules. Bioresour. Technol. 100, 3332–3342. doi: 10.1016/j.biortech.2009.01.073
Bhatt S., Lee J., Deutsch J., Ayaz H., Fulton B., Suri R. (2018). From food waste to value-added surplus products (VASP): Consumer acceptance of a novel food product category. J. Consum. Behav. 17, 57–63. doi: 10.1002/cb.1689
BlueBio COFUND (2020) Commercial exploitation of marine collagen and chitin from marine sources. Available at: https://bluebioeconomy.eu/commercial-exploitation-of-marine-collagen-and-chitin-from-marine-sources/ (Accessed February 14, 2023).
Borić M., Puliyalil H., Novak U., Likozar B. (2018). An intensified atmospheric plasma-based process for the isolation of the chitin biopolymer from waste crustacean biomass. Green Chem. 20, 1199–1204. doi: 10.1039/C7GC03735J
Borić M., Vicente F. A., Jurković D. L., Novak U., Likozar B. (2020). Chitin isolation from crustacean waste using a hybrid demineralization/DBD plasma process. Carbohydr. Polym. 246, e116648. doi: 10.1016/j.carbpol.2020.116648
Brom F. W. (2000). Food, consumer concerns, and trust: Food ethics for a globalizing market. J. Agric. Environ. Ethics. 12, 127–139. doi: 10.1023/A:1009586529518
Casadidio C., Peregrina D. V., Gigliobianco M. R., Deng S., Censi R., Di Martino P. (2019). Chitin and chitosans: characteristics, eco-friendly processes, and applications in cosmetic science. Mar. Drugs 17, e369. doi: 10.3390/md17060369
Chen P. Y., Lin A. Y., McKittrick J., Meyers M. A. (2008). Structure and mechanical properties of crab exoskeletons. Acta Biomater. 4, 587–596. doi: 10.1016/j.actbio.2007.12.010
Czaja K., Góralczyk K., Struciński P., Hernik A., Korcz W., Minorczyk M., et al. (2015). Biopesticides–towards increased consumer safety in the European union. Pest Manage. Sci. 71, 3–6. doi: 10.1002/ps.3829
de Boer A., Bast A. (2018). Demanding safe foods - safety testing under the novel food regulation, (2015/2283). Trends Food Sci. Technol. 72, 125–133. doi: 10.1016/j.tifs.2017.12.013
Delaux J., Nigen M., Fourré E., Tatibouët J.-M., Barakat A., Atencio L., et al. (2016). Fast and solvent free polymerization of carbohydrates induced by non-thermal atmospheric plasma. Green Chem. 18, 3013–3019. doi: 10.1039/C5GC02773J
De Tender C., Mesuere B., van der Jeugt F., Haegeman A., Ruttink T., Vandecasteele B., et al. (2019). Peat substrate amended with chitin modulates the n-cycle, siderophore and chitinase responses in the lettuce rhizobiome. Sci. Rep. 9, e9890. doi: 10.1038/s41598-019-46106-x
De Tender C., Vandecasteele B., Verstraeten B., Ommeslag S., De Meyer T., De Visscher J., et al. (2021). Chitin in strawberry cultivation: Foliar growth and defense response promotion, but reduced fruit yield and disease resistance by nutrient imbalances. Mol. Plant Microbe Interact. 34, 227–239. doi: 10.1094/MPMI-08-20-0223-R
Dong S., Huang Y., Zhang R., Wang S., Liu Y. (2014). Four different methods comparison for extraction of astaxanthin from green alga haematococcus pluvialis. Sci. World J. 2014, e694305. doi: 10.1155/2014/694305
DTU (2021) Resource effective multi-extraction of sustainable high-value compounds from shrimp production side-stream. Available at: https://orbit.dtu.dk/en/projects/resource-effective-multi-extraction-of-sustainable-high-value-com-3 (Accessed February 14, 2023).
Dutta P. K., Dutta J., Tripathi V. S. (2004). Chitin and chitosan: Chemistry, properties and applications. J. Sci. Ind. Res. 63, 20–31.
El Knidri H., Belaabed R., Addaou A., Laajeb A., Lahsini A. (2018). Extraction, chemical modification and characterization of chitin and chitosan. Int. J. Biol. Macromol. 120, 1181–1189. doi: 10.1016/j.ijbiomac.2018.08.139
EU (1991). Council directive 91/676/EEC of 12 December 1991 concerning the protection of waters against pollution caused by nitrates from agricultural sources (Luxembourg: Official Journal of the European Union), L375. Available at: https://eur-lex.europa.eu/legal-content/EN/TXT/?uri=CELEX%3A01991L0676-20081211.
EU (2001). Regulation (EC) no 999/2001 of the European parliament and of the council of 22 may 2001 laying down rules for the prevention, control and eradication of certain transmissible spongiform encephalopathies (Luxembourg: Official Journal of the European Union), L147. Available at: https://eur-lex.europa.eu/legal-content/EN/ALL/?uri=CELEX%3A32001R0999.
EU (2003). Regulation (EC) no 1831/2003 of the European parliament and of the council of 22 September 2003 on additives for use in animal nutrition (Text with EEA relevance) (Luxembourg: Official Journal of the European Union), L268. Available at: https://eur-lex.europa.eu/legal-content/EN/TXT/?uri=CELEX%3A32003R1831.
EU (2005). Regulation (EC) no 396/2005 of the European parliament and of the council of 23 February 2005 on maximum residue levels of pesticides in or on food and feed of plant and animal origin and amending council directive 91/414/EEC (Text with EEA relevance) (Luxembourg: Official Journal of the European Union), L70. Available at: https://eur-lex.europa.eu/legal-content/EN/TXT/?uri=CELEX%3A02005R0396-20220516.
EU (2008). Directive 2008/98/EC of the European parliament and of the council of 19 November 2008 on waste and repealing certain directives (Text with EEA relevance) (Luxembourg: Official Journal of the European Union), L312. Available at: https://eur-lex.europa.eu/legal-content/EN/TXT/?uri=CELEX%3A02008L0098-20180705.
EU (2009a). Directive 2009/128/EC of the European parliament and of the council of 21 October 2009 establishing a framework for community action to achieve the sustainable use of pesticides (Text with EEA relevance) (Luxembourg: Official Journal of the European Union), L309. Available at: https://eur-lex.europa.eu/legal-content/EN/TXT/?uri=CELEX%3A02009L0128-20190726.
EU (2009b). Regulation (EC) no 1069/2009 of the European parliament and of the council of 21 October 2009 laying down health rules as regards animal by-products and derived products not intended for human consumption and repealing regulation (EC) no 1774/2002 (Animal by-products regulation) (Luxembourg: Official Journal of the European Union), L300. Available at: https://eur-lex.europa.eu/legal-content/EN/TXT/?uri=celex:32009R1069.
EU (2009c). Regulation (EC) no 1107/2009 of the European parliament and of the council of 21 October 2009 concerning the placing of plant protection products on the market and repealing council directives 79/117/EEC and 91/414/EEC (Luxembourg: Official Journal of the European Union), L309. Available at: https://eur-lex.europa.eu/legal-content/EN/TXT/?uri=celex%3A32009R1107.
EU (2009d). Regulation (EC) no 1223/2009 of the European parliament and of the council of 30 November 2009 on cosmetic products (Text with EEA relevance) (Luxembourg: Official Journal of the European Union), L342. Available at: https://eur-lex.europa.eu/legal-content/EN/TXT/?uri=CELEX%3A02009R1223-20220301.
EU (2011). Commission regulation (EU) no 142/2011 of 25 February 2011 implementing regulation (EC) no 1069/2009 of the European parliament and of the council laying down health rules as regards animal by-products and derived products not intended for human consumption and implementing council directive 97/78/EC as regards certain samples and items exempt from veterinary checks at the border under that directive (Text with EEA relevance) (Luxembourg: Official Journal of the European Union), L54. Available at: https://eur-lex.europa.eu/eli/reg/2011/142/oj.
EU (2013). Commission regulation (EU) no 68/2013 of 16 January 2013 on the catalogue of feed materials (Text with EEA relevance) (Luxembourg: Official Journal of the European Union), L29. Available at: https://eur-lex.europa.eu/legal-content/EN/ALL/?uri=CELEX%3A32013R0068.
EU (2017a). Commission regulation (EU) 2017/1017 of 15 June 2017 amending regulation (EU) no 68/2013 on the catalogue of feed materials (Text with EEA relevance) (Luxembourg: Official Journal of the European Union), L159. Available at: https://eur-lex.europa.eu/eli/reg/2017/1017/oj.
EU (2017b). Commission regulation (EU) 2017/1432 of 7 august 2017 amending regulation (EC) no 1107/2009 of the European parliament and the council concerning the placing of plant protection products on the market as regards the criteria for the approval of low-risk active substances (Text with EEA relevance.) (Luxembourg: Official Journal of the European Union), L205. Available at: https://eur-lex.europa.eu/legal-content/EN/ALL/?uri=CELEX%3A32017R1432.
EU (2018a). Directive (EU) 2018/851 of the European parliament and of the council of 30 may 2018 amending directive 2008/98/EC on waste (Text with EEA relevance) (Luxembourg: Official Journal of the European Union), L150. Available at: https://eur-lex.europa.eu/legal-content/EN/TXT/?uri=celex%3A32018L0851.
EU (2018b). A sustainable bioeconomy for Europe: Strengthening the connection between economy, society and the environment: updated bioeconomy strategy (Publications Office). Available at: https://data.europa.eu/doi/10.2777/792130.
EU (2019a). Commission decision (EU) 2019/701 of 5 April 2019 establishing a glossary of common ingredient names for use in the labelling of cosmetic products (Text with EEA relevance) (Luxembourg: Official Journal of the European Union), L121. Available at: https://eur-lex.europa.eu/legal-content/en/TXT/?uri=CELEX:32019D0701.
EU (2019b). Regulation (EU) 2019/6 of the European parliament and of the council of 11 December 2018 on veterinary medicinal products and repealing directive 2001/82/EC (Text with EEA relevance) (Luxembourg: Official Journal of the European Union), L4. Available at: https://eur-lex.europa.eu/legal-content/EN/TXT/?uri=CELEX%3A02019R0006-20220128.
EU (2019c). Regulation (EU) 2019/1009 of the European parliament and of the council of 5 June 2019 laying down rules on the making available on the market of EU fertilising products and amending regulations (EC) no 1069/2009 and (EC) no 1107/2009 and repealing regulation (EC) no 2003/2003 (Text with EEA relevance) (Luxembourg: Official Journal of the European Union), L170. Available at: https://eur-lex.europa.eu/legal-content/EN/TXT/?uri=celex%3A32019R1009.
EU (2022) European Commission database for information on cosmetic substances and ingredients. Available at: https://ec.europa.eu/growth/tools-databases/cosing/index.cfm (Accessed August 10, 2022).
FAO (2022). The state of world fisheries and aquaculture 2022 - towards blue transformation (Rome: FAO).
FPS Public Health, Food Chain Safety and Environment (2015) Plant protection product. Available at: https://fytoweb.be/en/plant-protection-products/specific-products/basic-substances (Accessed February 14, 2023).
Grup Barcelonesa (2019) Crustacean shells for skin elasticity and moisturisation. Available at: https://www.grupbarcelonesa.com/en/blog/crustacean-shells-skin-elasticity-and-moisturisation/ (Accessed February 14, 2023).
Gulzar S., Benjakul S. (2018). Ultrasound waves increase the yield and carotenoid content of lipid extracted from cephalothorax of pacific white shrimp (Litopenaeus vannamei). Eur. J. Lipid Sci. Technol. 120, e1700495. doi: 10.1002/ejlt.201700495
Gulzar S., Benjakul S. J. L. (2019). Effect of pre-treatments on yield and properties of lipid extracted from cephalothorax of pacific white shrimp (Litopenaeus vannamei) by ultrasonic assisted process. LWT 100, 106–113. doi: 10.1016/j.lwt.2018.10.051
Gulzar S., Raju N., Nagarajarao R. C., Benjakul S. (2020). Oil and pigments from shrimp processing by-products: extraction, composition, bioactivities and its application - a review. Trends Food Sci. Technol. 100, 307–319. doi: 10.1016/j.tifs.2020.04.005
Hamed I., Özogul F., Regenstein J. M. (2016). Industrial applications of crustacean by-products (chitin, chitosan, and chitooligosaccharides): a review. Trends Food Sci. Technol. 48, 40–50. doi: 10.1016/j.tifs.2015.11.007
Hamilton H. A., Newton R., Auchterlonie N. A., Müller D. B. (2020). Systems approach to quantify the global omega-3 fatty acid cycle. Nat. Food. 1, 59–62. doi: 10.1038/s43016-019-0006-0
Harnedy P. A., FitzGerald R. (2012). Bioactive peptides from marine processing waste and shellfish: A review. J. Funct. Foods. 4, 6–24. doi: 10.1016/j.jff.2011.09.001
Holloway B. B., Wang S., Beatty S. E. (2009). Betrayal? relationship quality implications in service recovery. J. Serv. Mark. 23, 385–396. doi: 10.1108/08876040910985861
Inderbitzin P., Ward J., Barbella A., Solares N., Izyumin D., Burman P., et al. (2018). Soil microbiomes associated with verticillium wilt-suppressive broccoli and chitin amendments are enriched with potential biocontrol agents. Phytopathology 108, 31–43. doi: 10.1094/PHYTO-07-17-0242-R
Jacobs S., Sioen I., Pieniak Z., De Henauw S., Maulvault A. L., Reuver M., et al. (2015). Consumers’ health risk–benefit perception of seafood and attitude toward the marine environment: Insights from five European countries. Environ. Res. 143, 11–19. doi: 10.1016/j.envres.2015.02.029
Keith J., Stockwell S., Ball D., Remillard K., Kaplan D., Thannhauser T., et al. (1993). Comparative analysis of macromolecules in mollusc shells. Comp. Biochem. Physiol. B Biochem. Mol. Biol. 105, 487–496. doi: 10.1016/0305-0491(93)90078-J
Kjartansson G. T., Zivanovic S., Kristbergsson K., Weiss J. (2006). Sonication-assisted extraction of chitin from north Atlantic shrimps (Pandalus borealis). J. Agr. Food Chem. 54, 5894–5902. doi: 10.1021/jf060646w
Kurita K. (2006). Chitin and chitosan: functional biopolymers from marine crustaceans. Mar. Biotechnol. 8, 203–226. doi: 10.1007/s10126-005-0097-5
Lim K. C., Yusoff F. M., Shariff M., Kamarudin M. S. (2018). Astaxanthin as feed supplement in aquatic animals. Rev. Aquac. 10, 738–773. doi: 10.1111/raq.12200
Liu C. W., Sung Y., Chen B. C., Lai H. Y. (2014). Effects of nitrogen fertilizers on the growth and nitrate content of lettuce (Lactuca sativa l.). Int. J. Environ. Res. Public Health 11, 4427–4440. doi: 10.3390/ijerph110404427
Maksimov I., Abizgil’Dina R., Pusenkova L. (2011). Plant growth promoting rhizobacteria as alternative to chemical crop protectors from pathogens. Appl. Biochem. Microbiol. 47, 333–345. doi: 10.1134/S0003683811040090
Manucharova N. A., Yaroslavtsev A. M., Senchenko D. V (2006). Microbial transformation of chitin in soil under anaerobic conditions. Biol Bull Russ Acad Sci 33, 191–194. doi: 10.1134/S1062359006020154
Mao X., Guo N., Sun J., Xue C. (2017). Comprehensive utilization of shrimp waste based on biotechnological methods: a review. J. Clean. Prod. 143, 814–823. doi: 10.1016/j.jclepro.2016.12.042
Marie B., Le Roy N., Zanella-Cleon I., Becchi M., Marin F. (2011). Molecular evolution of mollusc shell proteins: Insights from proteomic analysis of the edible mussel mytilus. J. Mol. Evol. 72, 531–546. doi: 10.1007/s00239-011-9451-6
Massironi A., Morelli A., Puppi D., Chiellini F. (2020). Renewable polysaccharides micro/nanostructures for food and cosmetic applications. Molecules 25, e4886. doi: 10.3390/molecules25214886
Mathew G. M., Mathew D. C., Sukumaran R. K., Sindhu R., Huang C. C., Binod P., et al. (2020). Sustainable and eco-friendly strategies for shrimp shell valorization. Environ. pollut. 267, 115656. doi: 10.1016/j.envpol.2020.115656
Mechri S., Sellem I., Bouacem K., Jabeur F., Laribi-Habchi H., Mellouli L., et al. (2020). A biological clean processing approach for the valorization of speckled shrimp metapenaeus monoceros by-product as a source of bioactive compounds. Environ. Sci. pollut. Res. 27, 15842–15855. doi: 10.1007/s11356-020-08076-w
Melent’ev A., Helisto P., Kuz’mina L. Y., Galimzyanova N., Aktuganov G., Korpela T. (2006). Use of antagonistic bacilli for biocontrol of fungi degrading fresh wood. Appl. Biochem. Microbiol. 42, 62–66. doi: 10.1134/S0003683806010091
Mizani A. M., Aminlari B. M. (2007). “A new process for deproteinization of chitin from shrimp head waste,” in Proceedings of European Congress of Chemical Engineering (ECCE-6), Copenhagen, September 16-20. Technical University of Denmark, Kongens Lyngby, Denmark.
Moren M., Suontama J., Hemre G.-I., Karlsen Ø., Olsen R., Mundheim H., et al. (2006). Element concentrations in meals from krill and amphipods,–possible alternative protein sources in complete diets for farmed fish. Aquaculture 261, 174–181. doi: 10.1016/j.aquaculture.2006.06.022
Morganti P., Morganti G., Morganti A. (2011). Transforming nanostructured chitin from crustacean waste into beneficial health products: A must for our society. Nanotechnol. Sci. Appl. 4, 123. doi: 10.2147/NSA.S22459
Morin-Crini N., Lichtfouse E., Torri G., Crini G. (2019). Applications of chitosan in food, pharmaceuticals, medicine, cosmetics, agriculture, textiles, pulp and paper, biotechnology, and environmental chemistry. Environ. Chem. Lett. 17, 1667–1692. doi: 10.1007/s10311-019-00904-x
Mukhin V. A., Novikov V. (2001). Enzymatic hydrolysis of proteins from crustaceans of the barents Sea. Appl. Biochem. Microbiol. 37, 638–542. doi: 10.1023/A:1012371621575
Naik A. S., Hayes M. (2019). Bioprocessing of mussel by-products for value added ingredients. Trends Food Sci. Tech. 92, 111–121. doi: 10.1016/j.tifs.2019.08.013
Nguyen S., Escudero C., Sediqi N., Smistad G., Hiorth M. (2017). Fluoride loaded polymeric nanoparticles for dental delivery. Eur. J. Pharm Sci. 104, 326–334. doi: 10.1016/j.ejps.2017.04.004
Nirmal N. P., Santivarangkna C., Rajput M. S., Benjakul S. (2020). Trends in shrimp processing waste utilization: An industrial prospective. Trends Food Sci. Technol. 103, 20–35. doi: 10.1016/j.tifs.2020.07.001
No H. K., Meyers S. P., Lee K. S. (1989). Isolation and characterization of chitin from crawfish shell waste. J. Agr. Food Chem. 37, 575–579. doi: 10.1021/jf00087a001
Olsen R. L., Toppe J., Karunasagar I. (2014). Challenges and realistic opportunities in the use of by-products from processing of fish and shellfish. Trends Food Sci. Technol. 36, 144–151. doi: 10.1016/j.tifs.2014.01.007
Opstad I., Suontama J., Langmyhr E., Olsen R. (2006). Growth, survival, and development of Atlantic cod (Gadus morhua l.) weaned onto diets containing various sources of marine protein. ICES J. Mar. Sci. 63, 320–325. doi: 10.1016/j.icesjms.2005.11.014
Özogul F., Hamed I., Özogul Y., Regenstein J. M. (2019). “Crustacean by-products,” in Reference module in food science. Eds. Melton L., Shahidi F., Varelis P. (Oxford: Academic Press), 33–38.
Panis G., Carreon J. R. (2016). Commercial astaxanthin production derived by green alga haematococcus pluvialis: A microalgae process model and a techno-economic assessment all through production line. Algal Res. 18, 175–190. doi: 10.1016/j.algal.2016.06.007
Pattanaik S. S., Sawant P. B., Xavier K. M., Dube K., Srivastava P. P., Dhanabalan V., et al. (2020). Characterization of carotenoprotein from different shrimp shell waste for possible use as supplementary nutritive feed ingredient in animal diets. Aquaculture 515, e734594. doi: 10.1016/j.aquaculture.2019.734594
Percot A., Viton C., Domard A. (2003). Optimization of chitin extraction from shrimp shells. Biomacromolecules 4, 12–18. doi: 10.1021/bm025602k
Pezeshk S., Rezaei M., Hosseini H., Abdollahi M. (2022). Ultrasound-assisted alkaline pH-shift process effects on structural and interfacial properties of proteins isolated from shrimp by-products. Food Struct. 32, e100273. doi: 10.1016/j.foostr.2022.100273
Prameela K., Venkatesh K., Immandi S. B., Kasturi A. P. K., Rama Krishna C., Murali Mohan C. (2017). Next generation nutraceutical from shrimp waste: The convergence of applications with extraction methods. Food Chem. 237, 121–132. doi: 10.1016/j.foodchem.2017.05.097
Pylak M., Oszust K., Frąc M. (2019). Review report on the role of bioproducts, biopreparations, biostimulants and microbial inoculants in organic production of fruit. Rev. Environ. Sci. Biotechnol. 18, 597–616. doi: 10.1007/s11157-019-09500-5
Rao A. R., Sarada R., Ravishankar G. A. (2007). Stabilization of astaxanthin in edible oils and its use as an antioxidant. J. Sci. Food Agric. 87, 957–965. doi: 10.1002/jsfa.2766
Ricci M., Tilbury L., Daridon B., Sukalac K. (2019). General principles to justify plant biostimulant claims. Front. Plant Sci. 10, e494. doi: 10.3389/fpls.2019.00494
Saini R. K., Keum Y. S. (2018). Carotenoid extraction methods: a review of recent developments. Food Chem. 240, 90–103. doi: 10.1016/j.foodchem.2017.07.099
Salemdeeb R., Zu Ermgassen E. K., Kim M. H., Balmford A., Al-Tabbaa A. (2017). Environmental and health impacts of using food waste as animal feed: a comparative analysis of food waste management options. J. Clean. Prod. 140, 871–880. doi: 10.1016/j.jclepro.2016.05.049
Sánchez-Camargo A. P., Meireles M.Â.A., Lopes B. L. F., Cabral F. A. (2011). Proximate composition and extraction of carotenoids and lipids from Brazilian redspotted shrimp waste (Farfantepenaeus paulensis). J. Food Eng. 102, 87–93. doi: 10.1016/j.jfoodeng.2010.08.008
Santos V. P., Marques N. S., Maia P. C., Lima M. A. B. D., Franco L. D. O., Campos-Takaki G. M. D. (2020). Seafood waste as attractive source of chitin and chitosan production and their applications. Int. J. Mol. Sci. 21, e4290. doi: 10.3390/ijms21124290
Shahidi F., Metusalach, Brown J. A. (1998). Carotenoid pigments in seafoods and aquaculture. Crit. Rev. Food Sci. Nutr. 38, 1–67. doi: 10.1080/10408699891274165
Shamshina J. L., Oldham T., Rogers R. D. (2019). “Applications of chitin in agriculture,” in Sustainable agriculture reviews, vol. 36 . Eds. Crini G., Lichtfouse E. (Chem: Springer), 125–146.
Shirai K., Guerrero I., Huerta S., Saucedo G., Castillo A., Obdulia Gonzalez R., et al. (2001). Effect of initial glucose concentration and inoculation level of lactic acid bacteria in shrimp waste ensilation. Enzyme Microb. Technol. 28, 446–452. doi: 10.1016/S0141-0229(00)00338-0
Singh R. R., Chinnasri B., De Smet L., Haeck A., Demeestere K., Van Cutsem P., et al. (2019). Systemic defense activation by COS-OGA in rice against root-knot nematodes depends on stimulation of the phenylpropanoid pathway. Plant Physiol. Biochem. 142, 202–210. doi: 10.1016/j.plaphy.2019.07.003
Stefan V., van Herpen E., Tudoran A. A., Lähteenmäki L. (2013). Avoiding food waste by Romanian consumers: The importance of planning and shopping routines. Food Qual. Prefer. 28, 375–381. doi: 10.1016/j.foodqual.2012.11.001
Stegmann P., Londo M., Junginger M. (2020). The circular bioeconomy: Its elements and role in European bioeconomy clusters. Resour. Conserv. Recycl.: X. 6, e100029. doi: 10.1016/j.rcrx.2019.100029
Suontama J., Kiessling A., Melle W., Waagbø R., Olsen R. (2007). Protein from northern krill (Thysanoessa inermis), Antarctic krill (Euphausia superba) and the Arctic amphipod (Themisto libellula) can partially replace fish meal in diets to Atlantic salmon (Salmo salar) without affecting product quality. Aquac. Nutr. 13, 50–58. doi: 10.1111/j.1365-2095.2007.00453.x
Synowiecki J., Al-Khateeb N. A. A. Q. (2000). The recovery of protein hydrolysate during enzymatic isolation of chitin from shrimp crangon crangon processing discards. Food Chem. 68, 147–152. doi: 10.1016/S0308-8146(99)00165-X
Takeungwongtrakul S., Benjakul S., Santoso J., Trilaksani W., Nurilmala M. (2015). Extraction and stability of carotenoid-containing lipids from hepatopancreas of pacific white shrimp (Litopenaeus vannamei). J. Food Process. Preserv. 39, 10–18. doi: 10.1111/jfpp.12203
Tedesco D. E. A., Cagnardi P. (2019). “Regulatory guidelines for nutraceuticals in the European union,” in Nutraceuticals in veterinary medicine. Eds. Gupta R., Srivastava A., Lall R. (Cham: Springer), 793–805.
Uzcátegui L. U. M., Vergara K., Martínez Bordes G. (2021). Sustainable alternatives for by-products derived from industrial mussel processing: a critical review. Waste Manage. Res. 40, 123–138. doi: 10.1177/0734242X21996808
Vandecasteele B., Amery F., Ommeslag S., Vanhoutte K., Visser R., Robbens J., et al. (2021). Chemically versus thermally processed brown shrimp shells or Chinese mitten crab as a source of chitin, nutrients or salts and as microbial stimulant in soilless strawberry cultivation. Sci. Total Environ. 771, e145263. doi: 10.1016/j.scitotenv.2021.145263
Vareltzis P. K., Undeland I. (2012). Protein isolation from blue mussels (Mytilus edulis) using an acid and alkaline solubilisation technique–process characteristics and functionality of the isolates. J. Sci. Food Agric. 92, 3055–3064. doi: 10.1002/jsfa.5723
Vazquez J. A., Ramos P., Miron J., Valcarcel J., Sotelo C. G., Perez-Martin R. I. (2017). Production of chitin from penaeus vannamei by-products to pilot plant scale using a combination of enzymatic and chemical processes and subsequent optimization of the chemical production of chitosan by response surface methodology. Mar. Drugs 15, e180. doi: 10.3390/md15060180
Villa-Lerma G., Gonzalez-Marquez H., Gimeno M., Trombotto S., David L., Ifuku S., et al. (2016). Enzymatic hydrolysis of chitin pretreated by rapid depressurization from supercritical 1,1,1,2-tetrafluoroethane toward highly acetylated oligosaccharides. Bioresour. Technol. 209, 180–186. doi: 10.1016/j.biortech.2016.02.138
Vittersø G., Tangeland T. (2015). The role of consumers in transitions towards sustainable food consumption. the case of organic food in Norway. J. Clean. Prod. 92, 91–99. doi: 10.1016/j.jclepro.2014.12.055
Wakimoto T., Kondo H., Nii H., Kimura K., Egami Y., Oka Y., et al. (2011). Furan fatty acid as an anti-inflammatory component from the green-lipped mussel perna canaliculus. P. Natl. Acad. Sci. U.S.A. 108, 17533–17537. doi: 10.1073/pnas.1110577108
Wang S. L., Shih L., Wang C. H., Tseng K. C., Chang W. T., Twu Y. K., et al. (2002a). Production of antifungal compounds from chitin by bacillus subtilis. Enzyme Microb. Technol. 31, 321–328. doi: 10.1016/S0141-0229(02)00130-8
Wang S. L., Yen Y. H., Tsiao W. J., Chang W. T., Wang C. L. (2002b). Production of antimicrobial compounds by monascus purpureus CCRC31499 using shrimp and crab shell powder as a carbon source. Enzyme Microb. Technol. 31, 337–344. doi: 10.1016/S0141-0229(02)00135-7
Wang S. L., Yen Y. H., Tzeng G. C., Hsieh C. (2005). Production of antifungal materials by bioconversion of shellfish chitin wastes fermented by pseudomonas fluorescens K-188. Enzyme Microb. Technol. 36, 49–56. doi: 10.1016/j.enzmictec.2004.03.024
Wang S. L., Yieh T. C., Shih L. (1999). Production of antifungal compounds by pseudomonas aeruginosa K-187 using shrimp and crab shell powder as a carbon source. Enzyme Microb. Technol. 25, 142–148. doi: 10.1016/S0141-0229(99)00024-1
Whitaker R. D., Altintzoglou T., Lian K., Noriega Fernández E. N. (2021). Marine bioactive peptides in supplements and functional foods – a commercial perspective. Curr. Pharm. Des. 27, 1353–1364. doi: 10.2174/1381612824999201105164000
Younes I., Rinaudo M. (2015). Chitin and chitosan preparation from marine sources. structure, properties and applications. Mar. Drugs 13, 1133–1174. doi: 10.3390/md13031133
Zhang J., Feng M., Lu X., Shi C., Li X., Xin J., et al. (2018). Base-free preparation of low molecular weight chitin from crab shell. Carbohydr. Polym. 190, 148–155. doi: 10.1016/j.carbpol.2018.02.019
Zhang H., Yun S., Song L., Zhang Y., Zhao Y. (2017a). The preparation and characterization of chitin and chitosan under large-scale submerged fermentation level using shrimp by-products as substrate. Int. J. Biol. Macromol. 96, 334–339. doi: 10.1016/j.ijbiomac.2016.12.017
Zhang L., Zhou C. S., Wang B., Yagoub A. A., Ma H. L., Zhang X., et al. (2017b). Study of ultrasonic cavitation during extraction of the peanut oil at varying frequencies. Ultrason. Sonochem. 37, 106–113. doi: 10.1016/j.ultsonch.2016.12.034
Zhao D., Huang W.-C., Guo N., Zhang S., Xue C., Mao X. (2019). Two-step separation of chitin from shrimp shells using citric acid and deep eutectic solvents with the assistance of microwave. Polymers 11, e409. doi: 10.3390/polym11030409
Keywords: seafood industry, side streams, valorisation, regulation, by-products
Citation: Zou Y, Heyndrickx M, Debode J, Raes K, de Pascale D, Behan P, Giltrap M, O’Connor C, Solstad RG, Lian K, Altintzoglou T, Dragøy R, Scheers N, Undeland I and Robbens J (2023) Valorisation of crustacean and bivalve processing side streams for industrial fast time-to-market products: A review from the European Union regulation perspective. Front. Mar. Sci. 10:1068151. doi: 10.3389/fmars.2023.1068151
Received: 12 October 2022; Accepted: 20 February 2023;
Published: 01 March 2023.
Edited by:
Thomas Bartholomäus Brück, Technical University of Munich, GermanyReviewed by:
Maria Hayes, Teagasc Food Research Centre (Ireland), IrelandKonstantinos M Kasiotis, Benaki Phytopathological Institute, Greece
Copyright © 2023 Zou, Heyndrickx, Debode, Raes, de Pascale, Behan, Giltrap, O’Connor, Solstad, Lian, Altintzoglou, Dragøy, Scheers, Undeland and Robbens. This is an open-access article distributed under the terms of the Creative Commons Attribution License (CC BY). The use, distribution or reproduction in other forums is permitted, provided the original author(s) and the copyright owner(s) are credited and that the original publication in this journal is cited, in accordance with accepted academic practice. No use, distribution or reproduction is permitted which does not comply with these terms.
*Correspondence: Yang Zou, yang.zou@ugent.be