- 1Institute of Marine Sciences (LABOMAR), Federal University of Ceará (UFC), Fortaleza, Brazil
- 2Mar do Ceará, School Dive, Fortaleza, Brazil
- 3Institut de Ciència i Tecnologia Ambientals (ICTA), Universitat Autònoma de Barcelona (UAB), Cerdanyolla del Vallés, Barcelona, Spain
- 4Dipartimento di Scienze e Tecnologie Biologiche e Ambientali (DISTEBA), Via Monteroni s/n, Università del Salento, Lecce, Italy
- 5Reef Systems Group, Leibniz Centre for Tropical Marine Research (ZMT), Bremen, Germany
- 6Center for Marine and Environmental Studies (CMES), University of the Virgin Islands, Saint Thomas, US Virgin Islands
Tropical reefs can occur naturally under suboptimal environmental conditions, where few reef-building corals thrive. These unique reefs are especially important for understanding resistance to global warming, but they are understudied. We studied a coral bleaching event that occurred in turbid reefs (~ 19 m deep) in the equatorial southwestern Atlantic. Mass bleaching was observed in 91% of the Siderastrea stellata colonies in 2020, whereas only 7.7% of the colonies were bleached in 2019 and 10.9% in 2022. The year 2020 had the highest heat stress recorded in this century in this region according to the degree of heating weeks such as 17.6°C-week. In the first semester of 2020, the region also underwent three marine heatwaves (MHWs) above the average temperatures (1.3, 1.5, and 2.0°C). The lowest turbidity and wind speed matched long-lasting, repeated, and severe MHWs. These reef-building corals are dominant under moderate turbid waters and high sea temperature (26–29°C), however they are near the maximum tolerance limit. In this regard, these low-latitude reefs are warming twice as fast (0.2°C/decade) as other regions (e.g., Abrolhos and Coral Coast) (0.1 to 0.13°C/decade) in the South America reef system demonstrating that they cannot be considered climate-change refugia. These results suggest that even turbid marginal reefs and tolerant corals are highly susceptible to mass bleaching, especially when heatwaves and a decrease in turbidity occur simultaneously.
1 Introduction
Global climate change is a major and widespread cause of the decline in tropical coral reefs (Hughes et al., 2017; Hughes et al., 2018). Climate change with long-lasting and intense events such as marine heatwaves (MHWs) have caused a wide range of effects in these tropical areas, including mass coral bleaching (Hughes et al., 2018). Such marine heatwaves induce bleaching events that cause mortality and change the functioning of the reef ecosystems (e.g., Hughes et al., 2003; Hoegh-Guldberg et al., 2007). Stress-sensitive corals, in general, have low resistance and delayed recovery from heat stress events (Enríquez et al., 2017); thus, they are among the reef builders that have been harmed by MHWs and global warming during the last decades (Hughes et al., 2018). In this regard, fast-growing staghorn and tabular corals suffered a catastrophic die-off, transforming the three-dimensionality and ecological functioning of 29% of the 3,863 reefs (Hughes et al., 2018). Four life-history strategies (competitive, weedy, stress-tolerant and generalist taxa) of reef corals were identified. Reported shifts towards stress-tolerant, generalist and weedy species in reef communities are consistent with the expected responses of these life history strategies (Darling et al., 2012).
Turbid reefs have been suggested to provide climate-change refugia for some coral species adapted to suboptimal environmental conditions (e.g., moderate turbidity), but this hypothesis is under intense debate (Loiola et al., 2019; Teixeira et al., 2019; Evans et al., 2020; Sully and Van Woesik, 2020; Pereira et al., 2022). In this regard, marginal reefs are naturally occurring habitats in suboptimal environmental conditions allow only the persistence and dominance of a few resistant coral species (Soares et al., 2017; Bouwmeester et al., 2020; Soares et al., 2020). These marginal reef areas may resist bleaching events because of their lower light conditions (shading effect due to turbidity) (Sully and Van Woesik, 2020) and turbid-tolerant coral communities (Mies et al., 2020), even when temperatures rise above the bleaching threshold.
The South American Reef System stretches 4,000 km along the southwestern Atlantic (Carneiro et al., 2022). This large reef system is unique because it occurs in moderately turbid waters with high sedimentation rates (Segal and Castro, 2011) compared to clear-water coral reefs with less sedimentation, such as in several parts of the Indo-Pacific and Caribbean areas (Hughes et al., 2017; Hughes et al., 2018). This reef region has low coral richness (23 scleractinian species), but high endemism (~34% for reef-building corals) adapted to these suboptimal conditions for reef growth (Leão et al., 2016). The key to the potential responses of disturbance-tolerant species to bleaching is their tolerance to high-temperature conditions in medium light levels with respect to the transparent waters with high light levels found in the Caribbean and Indo-Pacific (Scheufen et al., 2017a; Scheufen et al., 2017b). In the Indo-Pacific (e.g., Australia’s Great Barrier Reef), mass coral bleaching events have been recorded in the last few decades (1997/1998, 2002, 2014/2017, and 2020), and are related to thermal stress (Hughes et al., 2017; Oliver et al., 2018; Pratchett et al., 2021). In this regard, massive corals in turbid reefs, such as the SW Atlantic, are considered more resistant to thermal stress than Caribbean and Indo-Pacific coral reefs (Mies et al., 2020; Soares et al., 2021). However, it is important to stress that they can be affected by repeated and intense marine heat waves (Duarte et al., 2020; Pereira et al., 2022), which is an understudied phenomenon in these habitats (Camp et al., 2018).
The bleaching events in the SW Atlantic reef region are related to high sea surface temperature anomalies, such as marine heatwaves during El Niño/Southern Oscillation (ENSO) conditions (Leão et al., 2008; Teixeira et al., 2019; Banha et al., 2020; Duarte et al., 2020; Pereira et al., 2022). In the last 26 years, bleaching events have been described for several reef species (Soares et al., 2021). The first bleaching event in the SW Atlantic reefs was recorded in 1994 (Migotto, 1995); thereafter, bleaching events were observed in 26 species of scleractinians, hydrocorals, octocorals, and zoantharians in Brazilian reefs (Amaral et al., 1998; Castro and Pires, 1999; Leão et al., 2008; Soares and Rabelo, 2014; Teixeira et al., 2019; Duarte et al., 2020; Soares et al., 2021) even in turbid waters. The variation in bleaching rates is a result of their photosynthetic performance, endosymbionts types, and ability to optimize light scattering (Enríquez and Pantoja-Reyes, 2005; Enríquez et al., 2017), which must be considered when analyzing bleaching effects (Schubert et al., 2017; Mies et al., 2020).
In 2016, 2019, and 2020, the SW Atlantic experienced intense heatwaves, with significant coral bleaching in high-latitude habitats (Banha et al., 2020) and mid-latitude habitats on the eastern Brazilian coast, such as the Abrolhos Reefs and Coral Coast (Teixeira et al., 2019; Duarte et al., 2020; Pereira et al., 2022). However, with few studies in low-latitude reefs. Together with the high temperatures and their small thermal variability, moderate turbidity characterizes the low-latitude sector of the South American reef system and makes it an iconic reef environment with suboptimal coral conditions (Soares et al., 2019). With the increase in wind speed, water turbidity is amplified in coastal regions (Knoppers et al., 1999) and can act as a protective barrier against solar irradiation, reducing light-heat photosynthetic stress (Baker et al., 2008; Teixeira et al., 2019). There is information on the mechanism underlying the effect of turbidity on coral bleaching; for instance, light shadowing is a key factor in bleaching resistance (Buddemeier et al., 2004; McClanahan et al., 2007; Van Woesik and McCaffrey, 2017). Understanding the bleaching events in other tropical coral reefs where water transparency is much lower (Hughes et al., 2003) is important such as in this current research.
We aimed to analyze the coral bleaching in a marginal low-latitude reef (SW Atlantic, Brazil) during the heat stress event of 2020 compared to 2019 and 2022 and assessed the environmental influence in terms of heat stress and turbidity. Our aim was to study the coral bleaching conditions before, during, and after the heat-light stress and investigate the ocean-environmental conditions (thermal stress, wind speed, and turbidity) during this event.
2 Material and methods
2.1 Study area
The low-latitude habitats in the South American Reef System harbor unique habitats, such as intertidal, shallow and mesophotic reefs, where the less diverse coral community is commonly dominated by the major reef-building Siderastrea spp. (Rabelo et al., 2013; Soares and Rabelo, 2014; Soares et al., 2019). Notably, the South American reef system is quite heterogeneous, and information about its low-latitude reefs, affected by coral bleaching, is still insufficient (Carneiro et al., 2022); hence, there is a need to describe and understand the equatorial reef habitats (Camp et al., 2018; Burt et al., 2020) that are crucial stepping stones connecting the eastern Brazilian reefs, the Amazon, and the Caribbean reefs (Carneiro et al., 2022).
The low latitude geographic position and absence of upwelling or other oceanographic processes in the area result in limited intra- and inter-annual temperature variations (Da Silva et al., 2017; Soares et al., 2019). The region has two different seasons: rainfall (January to June) and drought (July to December), with high sea temperatures between 26°C and 29°C (Cavalcanti et al., 2009). The winds are also bimodal between the seasons, with weaker westerly and southwestward winds from January to May (average monthly speed of 5.1 m/s in April), and stronger northwestward winds from August to December (average monthly speed of 8.8 m/s between August and September) (Da Silva et al., 2017) which makes oceanographic sampling difficult in the second half of the year.
2.2 Coral bleaching data collection
The surveys were conducted on a low-latitude reef on the Equatorial SW coast (Ceará State, Brazil) inside the Marine Protected Area Pedra da Risca do Meio (Soares et al., 2019) (Figure 1). This protected area is 33,20 km² and is the first and only state marine protected area in this equatorial region. Cabeço do Balanço is a flat sandstone reef that is dominated by algae, sponges and massive corals. The coral community is poor in species (~ 4) and dominated by Siderastrea stellata (Soares et al., 2019). Quantitative sampling was conducted in July 2019, July 2020, and May 2022. This reef site can only be accessed by SCUBA diving (18-19m depth). A record consisting of underwater photographs and videotransects recorded during citizen science activities was assembled (Bear, 2016; Stuart-Smith et al., 2017). Three dives were performed and recorded at the reef each year, resulting in six videos of approximately 20 min each year. The images were extracted from the video transects and focused on coral bleaching assessments. Corals were classified as “healthy” (no bleaching signals in the colony) and “ bleached” (when pigmentation was < 50% of the colony size).
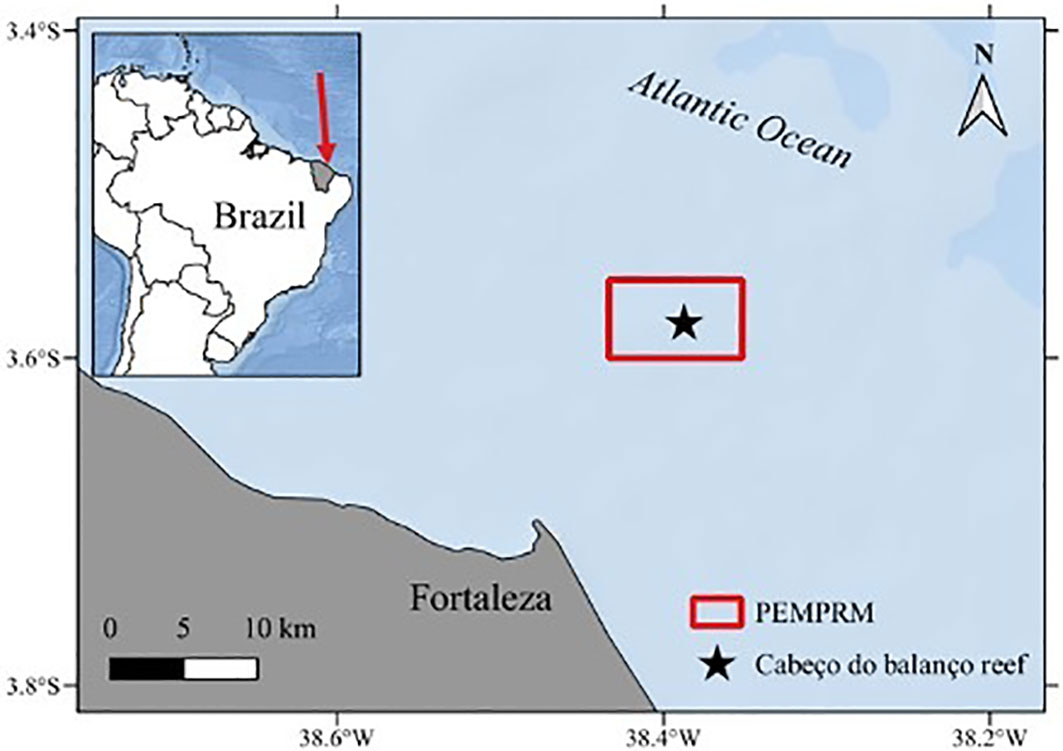
Figure 1 Study area (Equatorial Southwestern Atlantic coast, Ceará State, Brazil) with the localization of Cabeço do Balanço reef (18m deep) in Parque Estadual Marinho da Pedra da Risca do Meio marine protected area (PEMPRM) located at 12 nautical miles (~ 22km) from the Fortaleza coast.
The reef site was mapped using onboard vessel GPS, and coral species were identified using the scientific literature (Leão et al., 2016; Santos et al., 2016). The largest number of coral colonies were represented by the major reef-building Siderastrea stellata (~ 97% of the colonies). The number of colonies of other species was very low in the videos (Montastraea cavernosa-3 colonies, Mussismilia hispida-2 colonies, and Meandrina braziliensis-1 colony) in all years (2019, 2020, and 2022); therefore, the bleaching rate in these corals was not evaluated due to their lower abundance (Soares et al., 2017). We emphasize that with the COVID-19 pandemic and restrictions (e.g., lockdowns) to field surveys, it was impossible to collect mortality data exactly after mass bleaching in July 2020. However, we analyzed images from 2022 to determine whether S. stellata remains healthy in the coral community and with significant numbers of colonies as an indirect proxy for survival.
2.3 Remote sensing analysis: Climate and oceanographical characteristics
SST (Sea Surface temperature) and Kd490 were obtained through the Giovanni platform based on the MODIS Aqua sensor (4 km spatial resolution daily values) from 2003 to 2020. Kd490 has been used in coral reef studies to assess turbidity (Soares et al., 2019; Duarte et al., 2020; Sully and Van Woesik, 2020). The value of Kd490 (m-1) represents the rate at which light at 490 nm is attenuated with depth. A Kd490 of 0.1/m indicates that the light intensity will be reduced to one natural log within 10 m of water. Thus, for a Kd490 of 0.1, the attenuation length was 10 m. A higher Kd490 value indicates a smaller attenuation depth and lower clarity of ocean water.
Thermal stress was evaluated using indicators such as SST, sea surface temperature anomalies (SSTA), degree heating week (DHW), marine heatwaves (MHW), maximum monthly mean (MMM), and bleaching threshold (BT), particularly for the year 2020 (mass coral bleaching). To establish BT, we identified the warmest climatological monthly value for this area using MODIS Aqua data from 2003 to 2020, which is called the MMM SST. The BT was set as 1°C above the MMM for the study area.
DHW was extracted from the Global Coral Reef Watch (https://coralreefwatch.noaa.gov) at a resolution of 5 km and corresponded to the accumulation of heat over the previous three months. The DHW shows how much heat stress has accumulated in an area over the past 12 weeks based on the SSTAs larger than 1°C (Liu et al., 2014). The units for DHW are “degree Celsius-weeks” (or °C-weeks), combining the intensity and duration of the oceanic heat stress into one single number.
The MHW is an indicator produced from NOAA’s daily optimally interpolated sea surface temperature data and is available at http://www.marineheatwaves.org/tracker.html (Schlegel and Smit, 2018). It is emphasized that the remote sensing data are valid for assessing the thermal stress in this reef at 18 m depth because the water column is well mixed up to 30 m depth in this region (Soares et al., 2019; Barroso et al., 2023). Wind speeds (m.s-1) were obtained through the Brazilian Meteorological Database from an automatic meteorological station in Fortaleza (Ceará, NE Brazil). We conducted an analysis using the monthly average of turbidity and wind speed included in this study.
2.4 Data analysis
Statistical analysis of covariance was used to verify the relationship between the environmental factors (DHWs, SST, wind speed, and turbidity) evaluated in this study during the 2020 mass bleaching event. After using covariance, we used Pearson’s correlation (r) to indicate whether the relationship was strong or weak. We also used a determination coefficient to understand the variation between these factors. Monthly data were used for statistical analyses. Using the monthly DHW values for 2019 and 2020 we performed a t-student analysis to check for significant differences between the years. Finally, the coral bleaching rate among years (2019, 2020, and 2022) was examined using a chi-square test (X²) for equality of distributions comparing the distribution of the number of bleached versus pigmented S. stellata colonies. Statistical analyses were performed using GraphPad Prism software version 8.2.0.
3 Results
3.1 Mass bleaching of the major reef-building coral Siderastrea stellata
Through videos and photos, the total number of colonies of Siderastrea stellata (104 in 2019, 109 in 2020, and 112 in 2022) was counted, and the number of colonies bleached before (2019), during the bleaching event (2020), and two years after the event (2022) were analyzed. The number of colonies remained stable throughout the study period (2019, 2020, and 2022).
The bleaching event affected 91% of colonies, which corresponds to 99 fully bleached colonies in 2020 (Figure 2). Contrastingly, bleaching was observed in only 8 colonies out of 104 colonies in July 2019 (7.7% bleaching versus 92,3% pigmented colonies) and 12 colonies out of 110 colonies in May 2022 (10.9% bleaching versus 90,8% pigmented colonies). In other words, most of the S. stellata colonies are of pigmented color (92.3%) in 2019 (Figure 3) and in 2022 (89.1%). In this regard, a mass bleaching event occurred in July 2020 in the equatorial SW Atlantic in the dominant coral S. stellata (Figure 2). The number of bleached colonies in 2020 was statistically higher (X² chi-square test, p < 0.05) compared to 2019 and 2022 (which are equal to each other, p> 0.05) (Table 1).
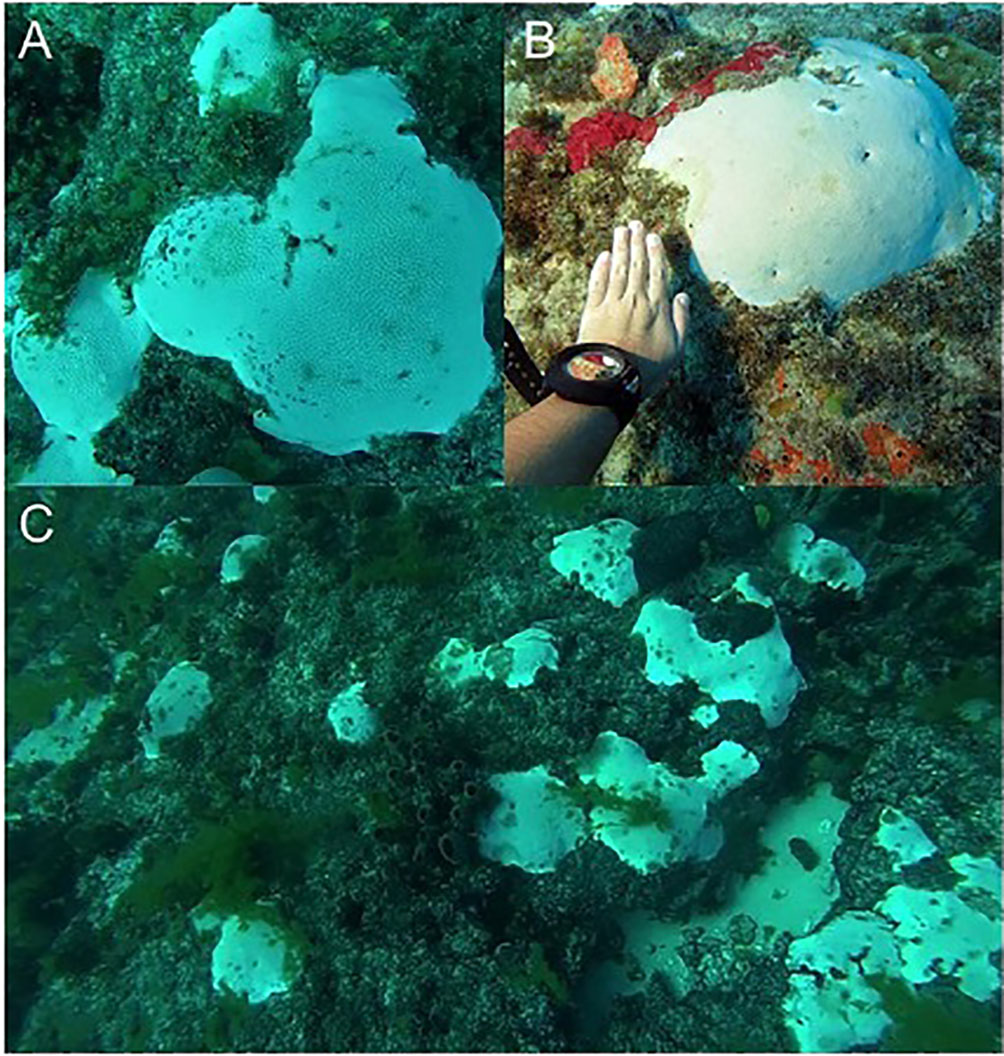
Figure 2 Siderastrea stellata coral colonies bleached in July 2020 (Equatorial Southwestern Atlantic, Ceará coast, Brazil). (A, B) – S. stellata encrusting colonies in the Cabeço do Balanço reef (18 m depth). (C) - Several fully bleached colonies of the major reef-building S. stellata.
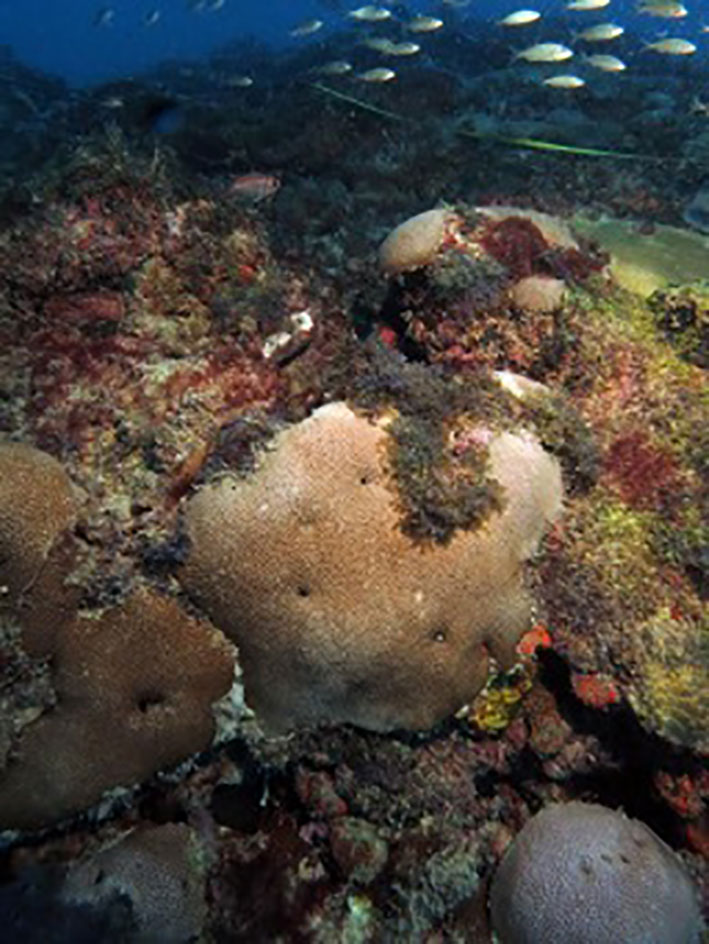
Figure 3 Siderastrea stellata coral colonies with normal color condition on low-latitude reefs in July 2019 (Equatorial Southwestern Atlantic, Ceará coast, Brazil).
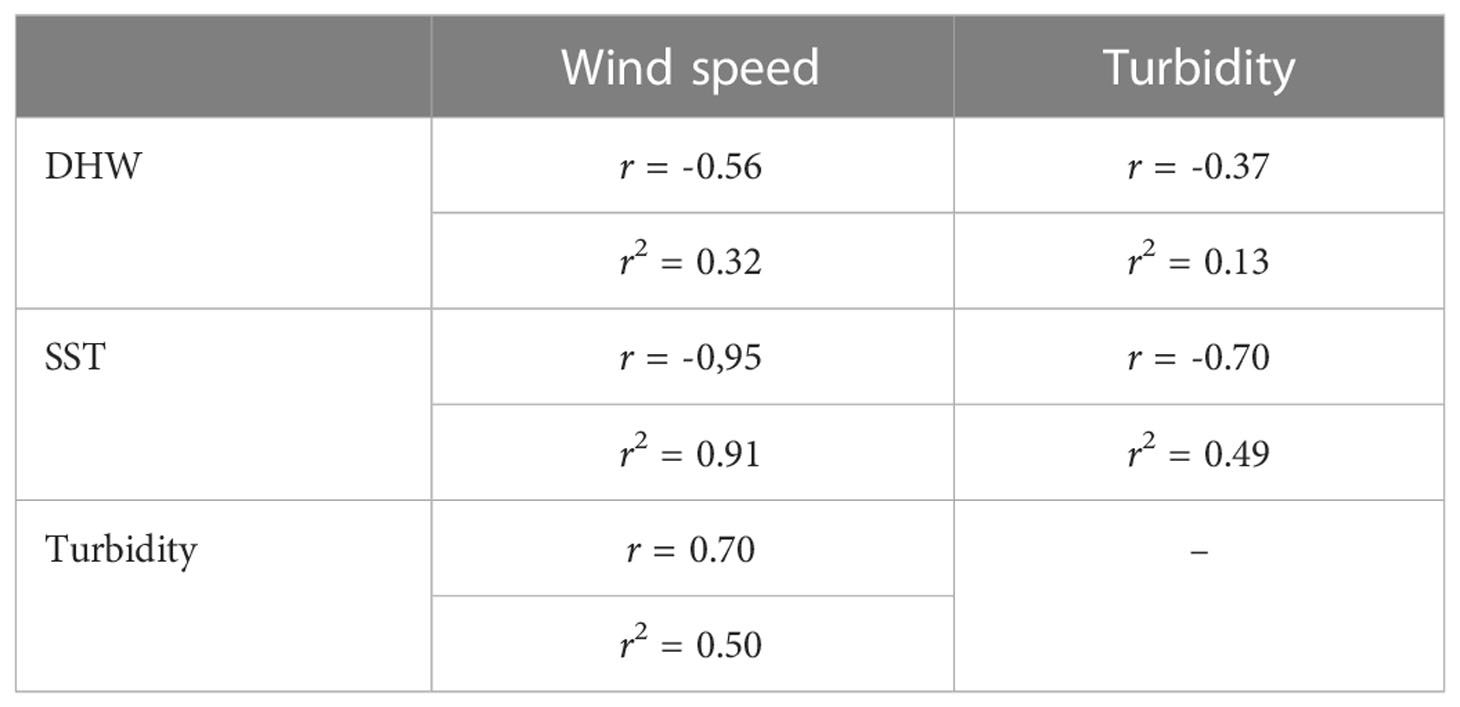
Table 1 Pearson correlation (r) and determination coefficient (r2) for environmental factors (SST, DHW, turbidity, and wind speed) for 2020 (Equatorial SW Atlantic, Brazil).
3.2 Heat stress
March of 2020 presented the highest (29.5°C) SST monthly averages (Figure 4) of the 18 years evaluated in this study (2003–2020). The average annual temperature in 2020 was approximately 0.6°C higher than the average annual temperature in 2003, the beginning of the Modis Aquaseries in this equatorial coral reef region. The MMM temperature was 28.8°C and occurred in March, indicating that the BT was 29.8°C (Figure 4). The average SST reached a maximum of 29.5°C in March 2020, close to the BT; however, bleaching was only observed in July 2020 during quantitative reef surveys. There were qualitative dives in April, May and June but no visual signs of mass bleaching. In February, March, April, and May 2020, SST remained above 29°C.
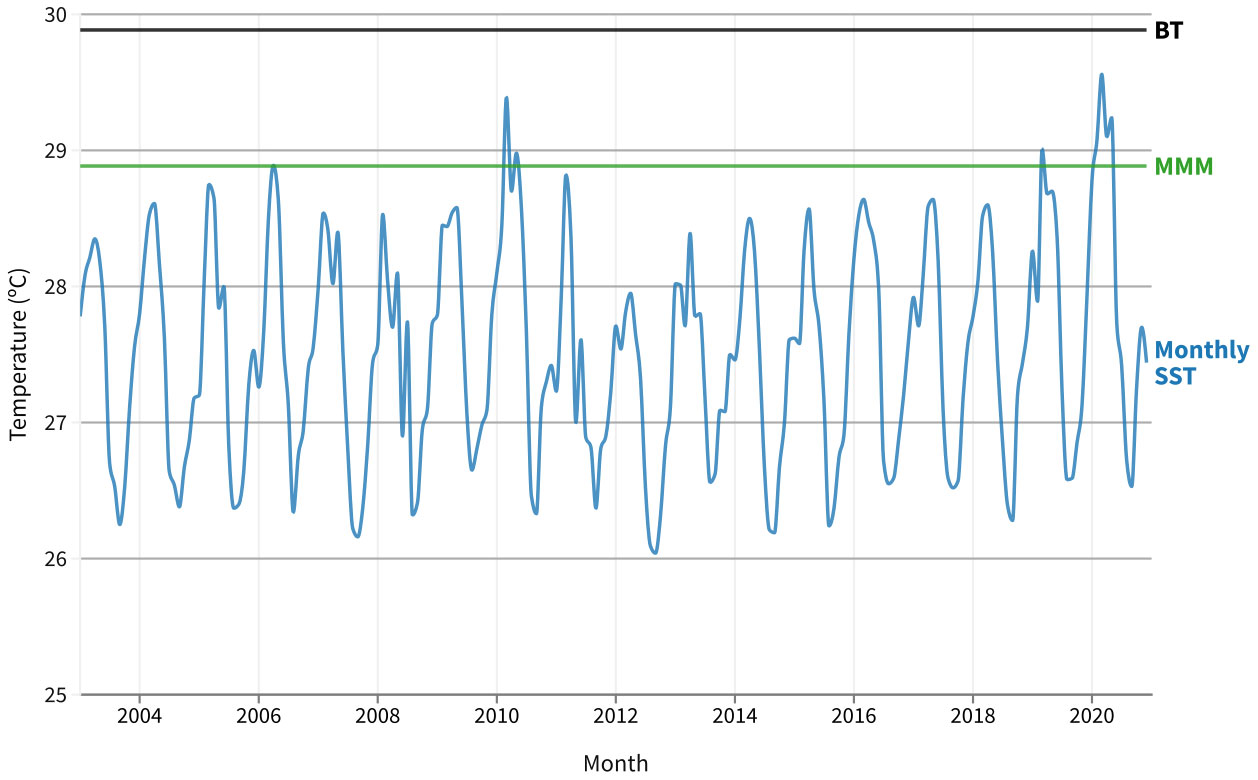
Figure 4 Heat stress indicators for the Equatorial Southwestern Atlantic coast (Ceará coast, Brazil) for the years 2003–2020. The solid blue line represents the monthly mean of sea surface temperature (MODIS Aqua Monthly SST). The green line represents the maximum monthly mean (MMM) climatological temperature. The solid black line represents the bleaching threshold (BT).
During the initial months of 2020, the SSTA reached a maximum of 0.7°C (Figure 5), and temperatures were above average. After the SSTA registered at the beginning of the year, the positive anomalies increased again in July (0.36°C; the month of the bleaching register) and again in September and October, but to a lesser degree when compared to the first six months of 2020 (Figure 5).
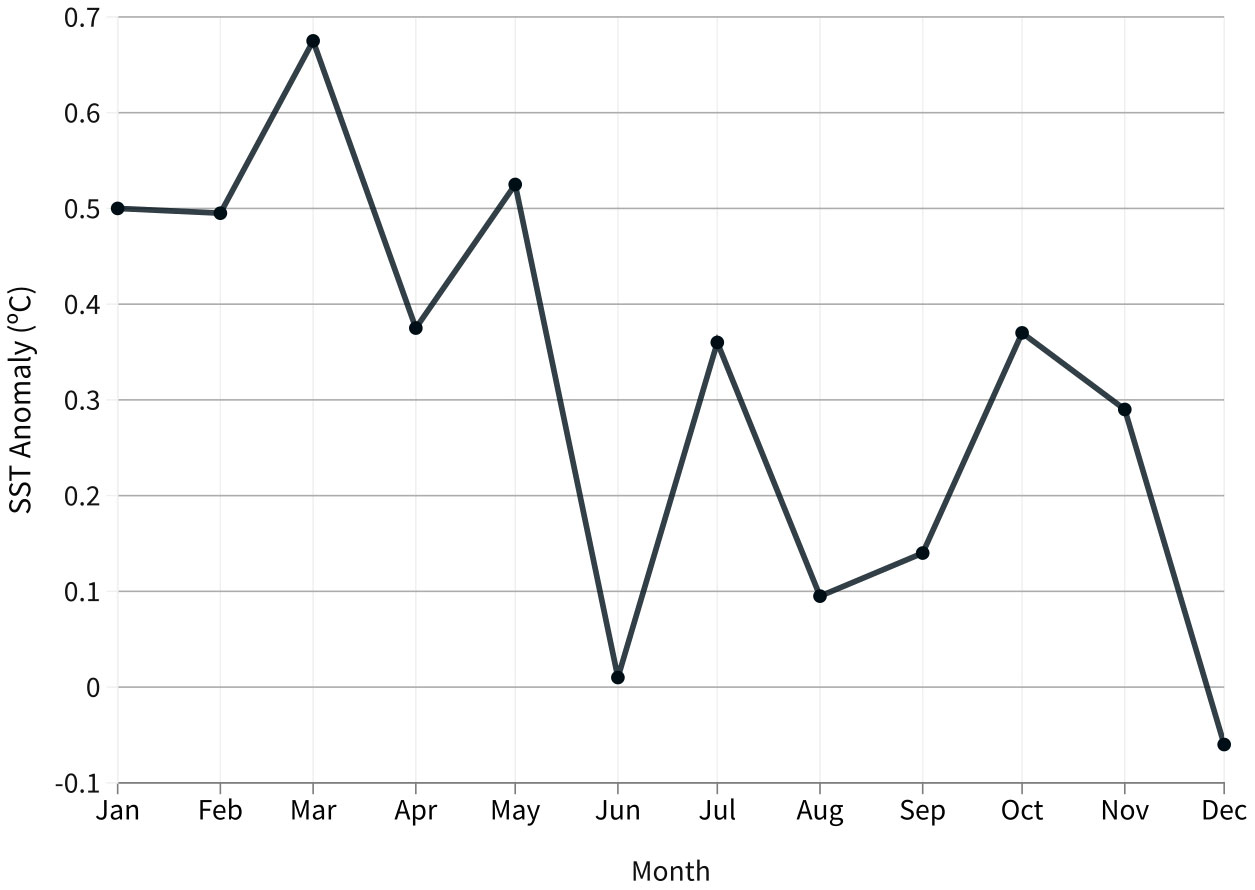
Figure 5 SSTA (°C) throughout 2020, showing that the largest anomalies occurred in the year’s initial months (Austral summer) (Equatorial Southwestern Atlantic, Ceará coast, Brazil).
An evaluation of the time series was carried out using the DHW and showed that the highest DHW record-breaking in this region occurred in May 2020 (17.6°C-week) (Figure 6). The reef region suffered from increasing thermal stress from January to May, but remained until August. The higher values of DHW were observed in April (14.8°C-week), May (17.6°C-week), and June (14.5°C-week) of 2020, before the mass bleaching record (Figure 6). In general, the DHW data showed that the accumulation of heat stress in the equatorial region increased during the first semester 2020, reached a maximum in May, and then decreased during the second semester (Figure 6). In contrast, DHW (0.1 – 5.3) was reduced in 2019 (Figure 6), which is compatible with the low bleaching rate in 2019 (7.7% bleached). Mean DHW was statistically higher in 2020 compared to 2019 (t student= 3.21, p=0.009).
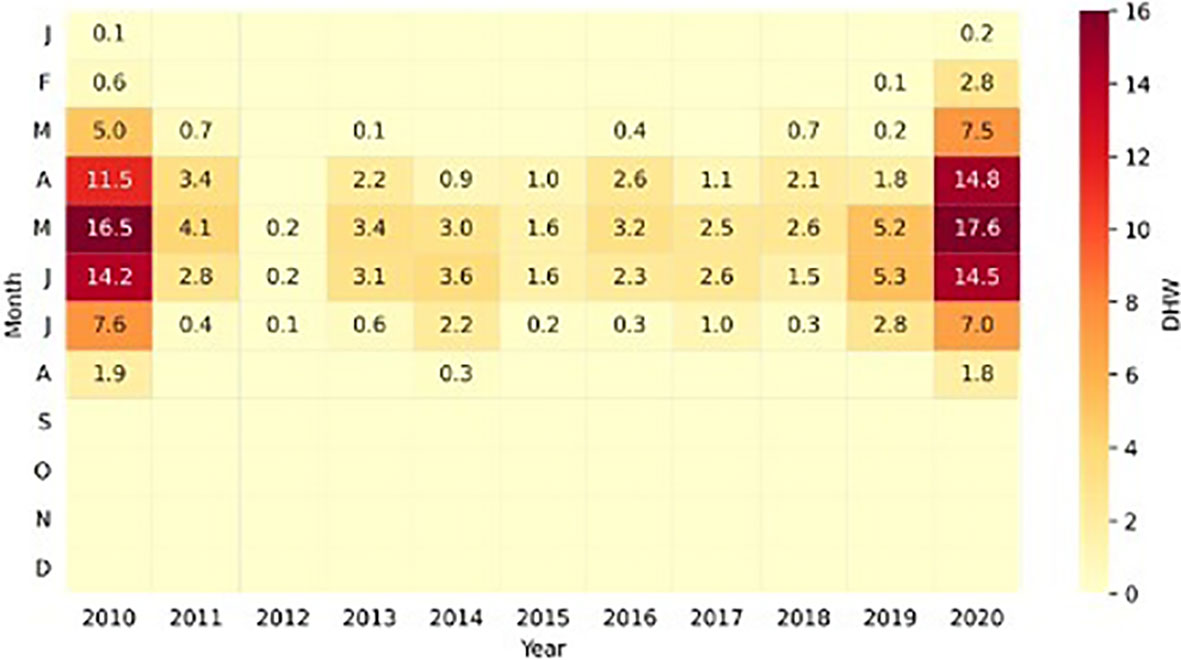
Figure 6 Heat map showing monthly Degree Heating Week (DHW) (°C/week) values (right side) between 2010 and 2020 in the Equatorial Southwestern Atlantic (Ceará coast, Brazil). Cells without values mean DHW is zero. Color changes with minimum values shown in light yellow and maximum values shown in dark red. Captions (left side): J (January), F (February), M (March), A (April), M (May), J (June), J (July), A (August), O (October), N (November), D (December).
We also used the MHW, a product for heatwave analysis that observes periods of thermal anomalies lasting at least five days in a given location. During the first six months of 2020, this reef region underwent three moments of MHW (1.54, 1.99, and 1.25°C above mean), totaling 157 days of elevated thermal stress (Figure 7 and Table 2). The longest single period lasted 76 days, from the end of February to the beginning of May (immediately before the coral bleaching observation). From May to June, the equatorial reefs experienced another 35-day extreme warming event (Figure 7). The second and third MHWs (Figure 7) probably explain the mass coral bleaching (Figure 2 and Table 2), together with the changes in turbidity and wind speed.
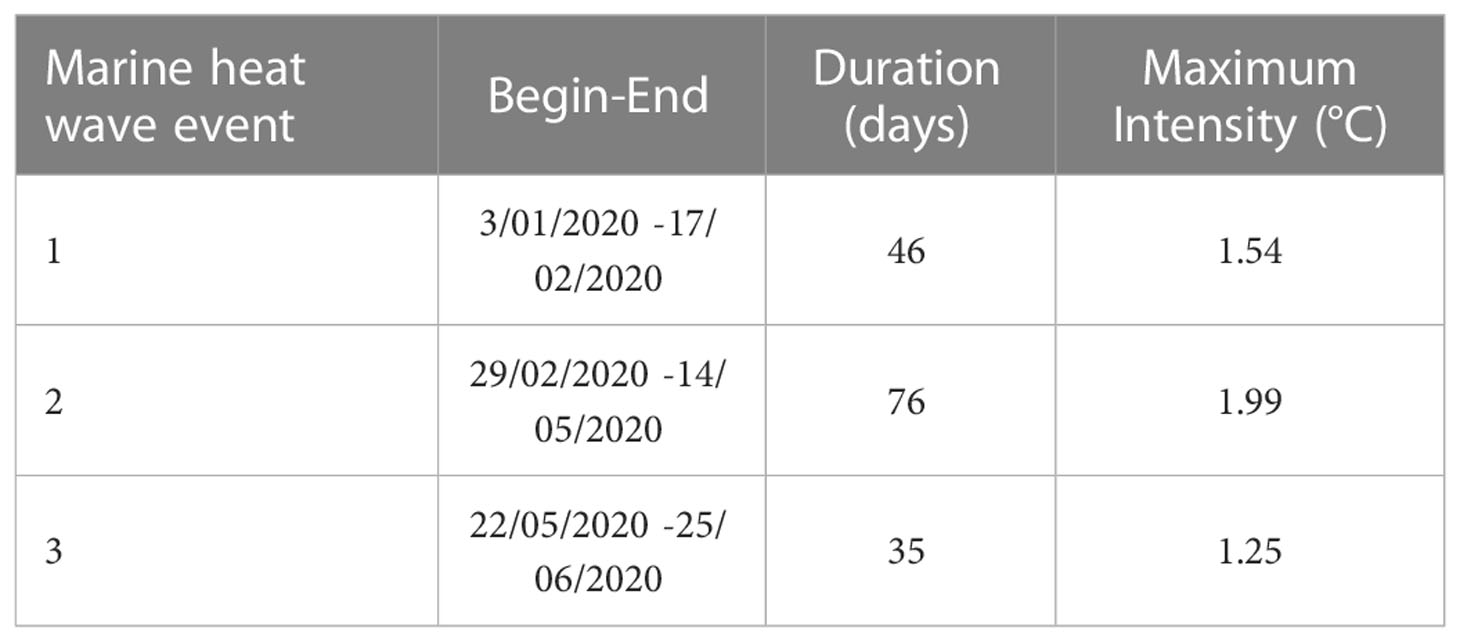
Table 2 Three marine heatwave events during the first half of 2020 are presented with their beginning and end dates, duration (days), and maximum intensity (°C) (Equatorial SW Atlantic, Brazil).
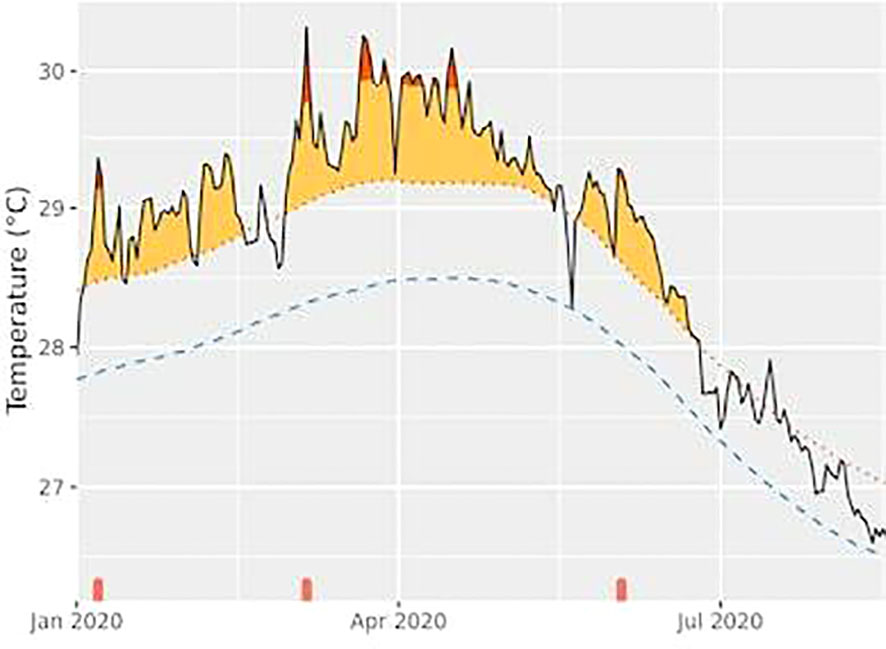
Figure 7 Three marine heatwave (MHW) events during the first half of 2020 are presented with their beginning and end dates, duration (days), and intensity (°C). The thermal stress MHW event is represented by orange areas. The lightest orange areas represent moderate MHW and the darkest, strong events.
3.3 Changes in turbidity and wind speed
In 2020, the mean wind speed increased from March (1 m/s) to August (3.4 m/s). Conversely, turbidity (Kd490) reached its minimum in March (0.041/m, before bleaching detection) and its maximum in November (0.056/m) (Figure 8). There was a slight turbidity increase in April possibly due to heavy rains on the coast (Figure 8). Therefore, the lowest average turbidity and wind speed, and the most intense heat stress (DHW and MHWs) were observed during the first six months, together with mass coral bleaching event (Figures 7, 8).
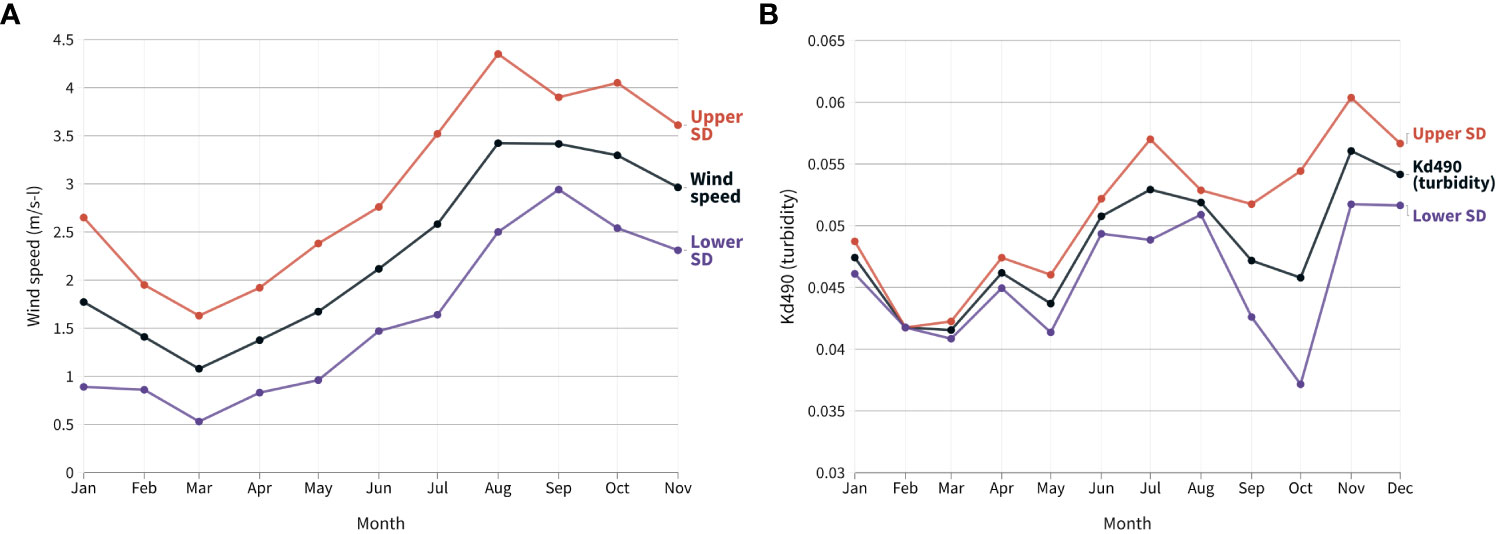
Figure 8 Monthly means and standard deviations (SD) of the wind speed (A) and light attenuation coefficient (Kd490) (B) on the Equatorial Southwestern Atlantic coast (Ceará coast, Brazil) during the mass bleaching event in 2020.
Using statistical analysis of covariance and Pearson’s correlation for the monthly data for 2020, it was possible to observe that the relationships between SST, turbidity, and wind speed were stronger. Among these, SST and turbidity (r = -0.70) and SST and wind speed were strong (Table 1) (r = -0,95) were inversely proportional, whereas turbidity and wind speed (r = 0.70) were directly proportional (Table 1). The Pearson correlation had a medium value for DHW and wind speed (-0.56) (Table 1). In other words, in 2020, when we had the highest thermal stress, we also had the lowest turbidity and wind speed.
4 Discussion
This study revealed that severe bleaching occurred in low-latitude turbid reefs in 2020. This year was marked by intense, repeated, and prolonged marine heatwaves, which, together with decreased shading due to weak trade winds and less turbid waters, may have resulted in the mass bleaching. In July 2020, more than 90% of S. stellata were bleached. This severe bleaching rate of 2020 was not detected in 2019 (~ 8% of fully bleached corals) and 2022 (~11% of bleached corals). All the thermal indicators utilized had high values during the first six months of 2020. The constant and positive SSTA must have favored the high DHW that reached the reef region during the first half of the year, triggering mass bleaching.
Similar mass-bleaching records for the same equatorial region were recorded in 2010. The first semester of 2010 have shown the highest levels of bleaching in surveys conducted in intertidal (Soares and Rabelo, 2014) and shallow reefs (Soares et al., 2019) in this region. In this regard, 2010 had the second-highest DHW recorded in the time series used in this study (May 2010; 14.9°C-week) (Figure 4). The first bleaching records occurred in 2010 for species such as S. stellata, Favia gravida, and Zoanthus sociatus. However, these species have recovered fully from intertidal reefs (Soares and Rabelo, 2014). In 2010, bleaching of S. stellata colonies in intertidal low-latitude reefs coincided with SSTA and higher SST (Soares and Rabelo, 2014). Bleaching was related to a thermal anomaly of 1.6°C in Rocas Atoll, located in the low-latitude South Atlantic region, in 2010 (Ferreira et al., 2013). Finally, there was also a record of S. stellata mass bleaching in 2010 in the same MPA as our research when temperatures ranged from 28.5 to 29.5°C (Soares et al., 2019).
The years 2010 and 2020 showed similar data for bleaching and thermal stress indicators in the equatorial SW Atlantic, indicating that 2020 bleaching was also triggered by thermal factors. We do not measure the mortality rate after 2020 due to lockdowns imposed by the pandemics of COVID-19 (virus Sars-Cov-2). However, we hypothesize a low mortality of S. stellata due to three main factors: 1) In 2010, despite the high bleaching rate (Soares et al., 2019), the species recovered, had low mortality, and remained dominant in the coral community in these equatorial reefs (Soares et al., 2017); 2) Footage images from 2022 showed that the species S. stellata remains dominant in the coral community in the study area with a stable number of colonies (see results); and 3) We clarify that for the last 40 years there are no records of mass mortality after intense bleaching for Siderastrea spp. in the Southwestern Atlantic (reviews in Leão et al., 2016; Mies et al., 2020; Soares et al., 2021) demonstrating the high survival potential and resilience of this weedy species until current days. The only records of mass coral mortality after severe bleaching in the South Atlantic include the species Millepora braziliensis, Millepora alcicornis, and Mussismilia hartii (Duarte et al., 2020; Soares et al., 2021; Pereira et al., 2022). The hydrocoral Millepora spp. is highly sensitive to temperature shifts (Duarte et al., 2020), probably because of its photosynthetic performance (Schubert et al., 2017).
Siderastrea spp., which are common at intertidal, shallow, and mesophotic depths, have adapted to thrive under suboptimal environmental conditions (Soares and Rabelo, 2014; Soares et al., 2019). This coral is also related to Symbiodinaceae clade C, which is recognized as one of the most widespread endosymbionts (Costa et al., 2008; Monteiro et al., 2013). In Brazil, Siderastrea spp. were associated with Symbiodinaceae strains B5, C3, C3 + B5, C46, C46 + B5, and C1 (Monteiro et al., 2013). In this regard, Symbiodinaceae clade B also seems to be relatively tolerant to higher light intensities. However, our study observed severe bleaching during thermal stress in equatorial and turbid reefs, as detected by thermal stress indicators.
Our results show a positive linear correlation between turbidity and wind speed and a negative linear correlation between turbidity and thermal indicators such as DHW as well as SST and wind speed. Massive corals in the equatorial SW Atlantic are adapted to lower light intensity with respect to corals in other areas with clearer waters (Soares et al., 2019), suggesting that adaptation may be constrained if harvested light increases (Schubert et al., 2017). Strong winds promote a mixture of the surface layers and increase the number of suspended particles in water, thus reducing the penetration of UV solar irradiation (Baker et al., 2008) and operating as anti-bleaching “shading” factors (Sully and Van Woesik, 2020) in turbid-tolerant species such as Siderastrea spp. (McClanahan et al., 2007). Therefore, our results corroborated the hypothesis that low turbidity, low wind speed, and intense thermal stress act together during the first half of the year and may have promoted mass bleaching of massive corals, even in turbid reefs.
DHW, MHWs, and SSTA are used to detect these temperature changes and are therefore good predictors of bleaching phenomena in this low-latitude region. Some reef regions (e.g., turbid-zone reefs) appear independent of the local thermal threshold (i.e., MMM+1°C) and suggest that other processes may drive the coral bleaching threshold (Eladawy et al., 2022). This may be the case for our study area. We hypothesize that the MMM+1°C threshold is not suitable for our low-latitude region where SST variability is small, but we need further studies on this topic.
Several studies on South Atlantic reefs have reported a link between DHW and coral bleaching (Soares et al., 2019; Teixeira et al., 2019; Duarte et al., 2020). Three shallow reefs (4–8 m) were analyzed during severe heat stress in 2019, and partial (15.4 to 28%) and total (13.3 to 32.2%) bleaching percentages for S. stellata were recorded (Duarte et al., 2020). According to the same study, the DHW reached higher values for the Abrolhos region and represented one of the most severe events on the Brazilian coast (Duarte et al., 2020). Severe bleaching (90.9%) was also detected in S. stellata colonies when DHW ranged from approximately 8°C-week in July 2010 (Soares et al., 2019). DHWs in the Abrolhos region reached 8.4, 6.7, and 9.7°C-week in 2014, 2015, and 2016, respectively (Teixeira et al., 2019). The bleaching event in 2016 was one of the most severe on record, affecting several species, including S. stellata, but with low coral mortality (Teixeira et al., 2019).
DHW is an important index calculated from the average of local temperatures and allows for comparison between different reef locations. The DHW observed in the present study (17.6° °C -week) was well above the established threshold for bleaching, even in weedy corals, such as S. stellata. A DHW equal to four generally results in bleaching of 30%–40%, and a DHW above eight generally results in bleaching of more than 70% of colonies (Liu et al., 2006; Kayanne, 2017). In addition to DHW, our results showed intense and repeated MHWs.
The MHW is one of the most recently derived indicators used to explain coral bleaching events. In addition to exhibiting non-patterned occurrences, MHWs vary widely in both frequency and magnitude across the oceans (Oliver et al., 2018). The bleaching of S. stellata was analyzed and correlated with ENSO and MHW in the Rocas Atoll (the same latitude as the region investigated in our study) in 2016, 2017, and 2019 (Gaspar et al., 2021). In this atoll, 2019 had the most intense and longest MHW events, with two events with an average duration of 31.5 days and a cumulative intensity of 31.51°C. The year 2019 also had the highest percentage of bleaching, which was likely intensified by MHW (Gaspar et al., 2021). Bleaching percentages were 11.71% in 2016, 1.52% in 2017, and 88% in 2019 (Gaspar et al., 2021). However, this atoll has clear waters, and S. stellata corals could occur in tide pools in this oceanic site, increasing the bleaching vulnerability of S. stellata to high heat-light stress. Analysis of MHW over large time scales can help monitor areas that are susceptible to bleaching (Donner et al., 2005; Oliver et al., 2021). The number of MHWs has doubled in the last century, and these phenomena have become the main drivers of coral bleaching worldwide, including turbid reefs (Oliver et al., 2018). MHW evaluations indicated that longer-lasting events were related to higher SSTA means, and these events also included weak winds and clear waters (Sen Gupta et al., 2020).
The results from other Brazilian reefs (Mies et al., 2020; Soares et al., 2021; Pereira et al., 2022) and our results show the severe bleaching of Siderastrea in different snapshots of time and low mortality of this coral. However, the increasing thermal stress predicted for the next decades undermines this short-term climate-change refugia and potential “winner” in the South Atlantic reefs. In this regard, there has been an increase in SST in all South Atlantic reef regions in the last 39 years (Soares et al., 2021). A clear trend of a 0.2°C increase per decade was detected in low-latitude equatorial reefs such as the low-latitude marine protected area analyzed here. Moreover, the results indicate 0.13°C increase in Abrolhos Bank and 0.1°C increase trend in the Coral Coast MPA (Soares et al., 2022). In other words, these low-latitude South Atlantic reefs are warming twice as fast as other tropical reefs in the same ocean basin (e.g., Abrolhos and Coral Coast), demonstrating that they are not climate change refugia under severe global change.
Our results and those of previous studies on warming projections in the study area (Soares et al., 2021; Soares et al., 2022) are congruent with recent modeling studies for the year 2100 (Principe et al., 2021). Modeling research with different greenhouse gas concentration scenarios and their effects on the future survival distribution of Siderastrea shows effects even on these turbid reefs (Principe et al., 2021). In this regard, modeling analysis indicates that Siderastrea can survive on these equatorial reefs in the optimistic (RCP–representative concentration pathway–2.6) and moderate (RCP 4.5) climate change scenarios but will lose the current studied area in the pessimistic scenario (RCP 8.5) (Principe et al., 2021). This is concerning, considering that Siderastrea dominates the majority of coral cover in low-latitude shallow and mesophotic habitats in the South American Reef System (Soares et al., 2017; Soares et al., 2019; Carneiro et al., 2022).
Overall, the SST has increased between 9.5% and 22% of the mean seasonal SST variability of each site in South Atlantic reef habitats, with the largest trends occurring at lower latitudes where the mean SST is higher and variability is smaller, such as the low-latitude habitats analyzed here (Soares et al., 2021; Soares et al., 2022). The average MHW frequency was close to three events per year from 1982 to 2020 in the South Atlantic reef regions. The frequency of MHWs events has increased in the last 39 years, and this increase is larger in low-latitude regions such as the equatorial region analyzed here. Finally, the duration of MHWs also increased in all South Atlantic reef location. The average SST anomalies caused by the MHWs range from 0.8 to 1°C, with maximum anomalies ranging from 2 to 2.2°C (Soares et al., 2021; Soares et al., 2022). The present study included periods of high thermal anomalies and weak trade winds before and during MHWs, suggesting that these conditions may have acted together to provoke mass coral bleaching even in turbid marginal reefs.
Turbid reefs are especially important for understanding global warming resistance (Camp et al., 2018; Burt et al., 2020; Sully and Van Woesik, 2020), but they are understudied. The lower vulnerability of South Atlantic corals to bleaching relative to the Caribbean and Indo-Pacific corals may be due to their deep bathymetric range, tolerance to nutrient discharge and turbidity, diversity of symbionts, and morphological characteristics such as massive growth form (Mies et al., 2020). Turbidity may alleviate intense light, especially in shallow areas where scleractinians (especially those that are turbid-tolerant) are better adapted to harvest light (Enríquez et al., 2017). However, the results of our study suggest that a match between lower turbidity and heat stress (DHW and MHW) could affect even the coral S. stellata, despite their resistance to heat and light stress (Leão et al., 2010; Soares et al., 2019).
In conclusion, the results of our research suggest that the main heat stress factors driving the 2020 bleaching event were MHWs and DHWs. It is apparent that the next stages in understanding the future climate change scenarios will be dependent on the photosynthetic performance of the weedy species. Understanding the survival of Siderastrea spp. on these turbid reefs would need research into chlorophyll concentration per surface (or biomass) unit, Symbiodinaceae density and diversity, and photosynthetic adaptability to seasonal changes. Climate projections for the equatorial southwestern Atlantic indicate hotter and drier weather. Therefore, it is necessary to monitor, investigate, and promote methods to reduce human pressure (local and global impacts) in these unique and rich low-latitude reef systems.
Data availability statement
The original contributions presented in the study are included in the article/supplementary material. Further inquiries can be directed to the corresponding authors.
Author contributions
CL: conceptualization, writing—original draft, writing—review and editing, methodology, and formal analysis. CT: writing—original draft, writing—review and editing, and formal analysis. MB: writing—original draft, writing—review and editing, and formal analysis. FJ: writing—original draft, writing—review and editing, and formal analysis. SP: writing—original draft, writing—review and editing, and formal analysis. AG: writing—original draft, writing—review and editing, and formal analysis. SR: conceptualization, writing—original draft, writing—review and editing, methodology, and formal analysis MS: conceptualization, writing—original draft, writing—review and editing, methodology, and formal analysis. All authors contributed to the article and approved the submitted version.
Funding
This study was sponsored by Brazilian Long Term Ecological Research (Brazilian LTER) at Costa Semiárida do Brasil (PELD-CSB/CNPq Proc. 442337/2020-5), Alexander Von Humboldt Foundation (CAPES/AVH, DFG), and Fulbright USA Commission. MS is CNPq (Grants 313518/2020-3) and FUNCAP research fellow (Chief Scientist Program). AG received a Msc. scholarship from CNPq and CL received a Msc. scholarship from CAPES. CT was supported by the Research Productivity Fellowship CNPQ grant 315289/2021-0 and the CAPES-Print project.
Acknowledgments
The authors thank the editor AC and the two reviewers for their constructive comments and recommendations that improved the manuscript. This study is a jointed contribution of the Brazilian LTER (PELD-CSB) and Institutional Program for Internationalization (CAPES – PrInt) from Federal University of Ceará and Università del Salento.
Conflict of interest
The authors declare that the research was conducted in the absence of any commercial or financial relationships that could be construed as a potential conflict of interest.
Publisher’s note
All claims expressed in this article are solely those of the authors and do not necessarily represent those of their affiliated organizations, or those of the publisher, the editors and the reviewers. Any product that may be evaluated in this article, or claim that may be made by its manufacturer, is not guaranteed or endorsed by the publisher.
References
Amaral F. D., Hudson M. M., Steiner A. Q. (1998). Note on the widespread bleaching observed at the Manuel luiz marine state park, maranhão, brazil. nota sobre o extenso branqueamento observado no parque estadual marinho do parcel do Manuel luiz, estado do maranhão. Arquivos Ciências do Mar. 39, 138–141. doi: 10.32360/acmar.v39i1-2.6383
Baker A. C., Glynn P. W., Riegl B. (2008). Climate change and coral reef bleaching: An ecological assessment of long-term impacts, recovery trends and future outlook. Estuar. Coast. Shelf Sci. 80(4), 435–471. doi: 10.1016/j.ecss.2008.09.003
Banha T. N. S., Capel K. C. C., Kitahara M. V., Francini-Filho R. B., Francini C. L. B., Sumida P. Y. G., et al. (2020). Low coral mortality during the most intense bleaching event ever recorded in subtropical southwestern Atlantic reefs. Coral Reefs 39, 3, 515–3, 521. doi: 10.1007/s00338-019-01856-y
Barroso H. S., Lima I. O., Bezerra A. D., Garcia T. M., Tavares T. C. L., Alves R. S., et al. (2023). Distribution of nutrients and chlorophyll across a marginal coral reef region: Insights on coastal gradients. Ocean Coast. Res. 71. doi: 10.1590/2675-2824071.22016hdsb
Bear M. (2016). Perspectives in marine citizen science. J. Microbiol. Biol. Educ. 17(1), 56–59. doi: 10.1128/jmbe.v17i1.1037
Bouwmeester J., Riera R., Range P., Ben-Hamadou R., Samimi-Namin K., Burt J. A. (2020). “Coral and reef fish communities in the thermally extreme Persian/Arabian gulf: Insights into potential climate change effects,” in Perspectives on the marine animal forests of the world. Eds. Rossi S., Bramanti L. (Cham: Springer).
Buddemeier R. W., Kleypas J. A., Aronson R. B. (2004). Coral reefs and global climate change. potential contributions of climate change to stresses on coral reef ecosystems (Arlington: Pew Center on Global Climate Change).
Burt J. A., Camp E. F., Enochs I. C., Johansen J. L., Morgan K. M., Riegl B., et al. (2020). Insights from extreme coral reefs in a changing world. Coral Reefs 39, 495–507. doi: 10.1007/s00338-020-01966-y
Camp E. F., Schoepf V., Mumby P. J., Hardtke L. A., Rodolfo-Metalpa R., David J., et al. (2018). The future of coral reefs subject to rapid climate change: Lessons from natural extreme environments. Front. Mar. Sci. 5. doi: 10.3389/fmars.2018.00004
Carneiro P. B. M., Ximenes Neto A. R., Jucá-Queiroz B., Teixeira C. E. P., Feitosa C. V., Barroso C. X., et al. (2022). Interconnected marine habitats forms a single continental-scale reef system in south America. Sci. Rep. 12, 17359. doi: 10.1038/s41598-022-21341-x
Castro C. B., Pires D. O. (1999). A bleaching event on a Brazilian coral reef. Braz. J. Oceanogr. 47(1), 87–90. doi: 10.1590/S1413-77391999000100008
Cavalcanti I. F. A., Ferreira N. J., Silva M. G. A. J., Dias M. A. F. S. (2009). Tempo e clima no brasil (São Paulo: Oficina de textos).
Costa F. C., Sassi R., Gorlach-Lira K. (2008). Zooxanthellae genotypes in the coral siderastrea stellata from coastal reefs in northeastern Brazil. J. Exp. Mar. Biol. Ecol. 367, 149–152. doi: 10.1016/j.jembe.2008.09.012
Da Silva G. K., Dos Santos A. C. S., Da Silva M. V. M. (2017). Estudo dos padrões de ventos offshore no litoral do ceará utilizando dados estimados pelo produto de satélites BSW. Rev. Bras. Meteorologia. 32, 579–690. doi: 10.1590/0102-7786324015
Darling E. S., Alvarez Filip L., Oliver T. A., McClanahan T. R., Côté I. M. (2012). Evaluating life history strategies of reef corals from species traits. Ecol. Letters 15 (12), 1378–1386. doi: 10.1111/j.1461-0248.2012.01861.x
Donner S. D., Skirving W. J., Little C. M., Oppenheimer M., Hoegh-Guldberg O. (2005). Global assessment of coral bleaching and required rates of adaptation under climate change. Global Change Biol. 11(12), 2251–2265. doi: 10.1111/j.1365-2486.2005.01073.x
Duarte G. A. S., Villela H. D. M., Deocleciano M., Silva D., Barno A., Cardoso P. M., et al. (2020). Heat waves are a major threat to turbid coral reefs in Brazil. Front. Mar. Sci. 7. doi: 10.3389/fmars.2020.00179
Eladawy A., Nakamura T., Shaltout M., Mohammed A., Nadaoka K., Fox M. D., et al. (2022). Appraisal of coral bleaching thresholds and thermal projections for the northern red Sea refugia. Front. Mar. Sci. 9. doi: 10.3389/fmars.2022.938454
Enríquez S., Méndez E. R., Hoegh-Guldberg O., Iglesias-Pietro R. (2017). Key functional role of the optical properties of coral skeletons in coral ecology and evolution. Proc. R. Soc Ser. B Biol. Sci. 284. doi: 10.1098/rspb.2016.1667
Enríquez S., Pantoja-Reyes N. I. (2005). Form-function analysis of the effect of canopy morphology on leaf self-shading in the seagrass Thalassia testudinum. Oecologia 145, 235–243. doi: 10.1007/s00442-005-0111-7
Evans R. D., Wilson S. K., Fisher R., Ryan N. M., Babcock R., Blakeway D., et al. (2020). Early recovery dynamics of turbid coral reefs after recurring bleaching events. J. Environ. Manage 268, 110666. doi: 10.1016/j.jenvman.2020.110666
Ferreira B. P., Costa M. B. S. F., Coxey M. S., Gaspar A. L. B., Veleda D., Araujo M. (2013). The effects of sea surface temperature anomalies on oceanic coral reef systems in the southwestern tropical Atlantic. Coral Reefs 32, 441–454. doi: 10.1007/s00338-012-0992-y
Gaspar T. L., Quimbayo J. P., Ozekoski R., Nunes L. T., Aued A. W., Mendes T. C., et al. (2021). Severe coral bleaching of Siderastrea stellata at the only atoll in the south Atlantic driven by sequential marine heatwaves. Biota Neotrop. 21 (2), e20201131. doi: 10.1590/1676-0611-BN-2020-1131
Hoegh-Guldberg O., Mumby P. J., Hooten A. J., Steneck R. S., Greenfield P., Gomez E., et al. (2007). Coral reefs under rapid climate change and ocean acidification. Science 318, 1737–1742. doi: 10.1126/science.1152509
Hughes T. P., Anderson K. D., Connolly S. R., Heron S. F., Kerry J. T., Lough J. M., et al. (2018). Spatial and temporal patterns of mass bleaching of corals in the anthropocene. Science 359 (6371), 80–83. doi: 10.1126/science.aan8048
Hughes T. P., Baird A. H., Bellwood D. R., Card M., Connolly S. R., Folke C., et al. (2003). Climate change, human impacts, and the resilience of coral reefs. Science 301 (5635), 929–933. doi: 10.1126/science.1085046
Hughes T. P., Kerry J. T., Álvarez-Noriega M., Álvarez-Romero J. G., Anderson K. D., Baird A. W., et al. (2017). Global warming and recurrent mass bleaching of corals. Nature 543 (7645), 373–377. doi: 10.1038/nature21707
Kayanne H. (2017). Validation of degree heating weeks as a coral bleaching index in the northwestern pacific. Coral Reefs 361, 63–70. doi: 10.1007/s00338-016-1524-y
Knoppers B., Ekau W., Figueiredo A. G. (1999). The coast and shelf of east and northeast Brazil and material transport. Geo-Mar. Lett. 19171–178. doi: 10.1007/s003670050106
Leão Z. M. A. N., De Kikuchi R. K. P., De Oliveira M. D. D. M. (2008). Coral bleaching in bahia reefs and its relation with sea surface temperature anomalies. Biota Neotrop. 8(3), 69–82. doi: 10.1590/S1676-06032008000300006
Leão Z. M. A. N., Kikuchi R. K. P., Oliveira M. D. M., Vasconcellos V. (2010). Status of eastern Brazilian coral reefs in time of climate changes. Pan-American J. Aquat. Sci. 5(2), 52–63.
Leão Z. M. A. N., Minervino-Neto A., Ferreira B. P., Feitosa C. V., Sampaio C. L. S., Sassi C. F. C., et al. (2016). “Monitoramento dos recifes e ecossistemas coralinos,” in Protocolos para o monitoramento de habitats bentônicos costeiros - rede de monitoramento de habitats bentônicos costeiros – ReBentos. Eds. Turra A., Denadai MárciaR. (São Paulo-SP: Instituto Oceanográfico da Universidade de São Paulo).
Liu G., Heron S. F., Eakin C. M., Muller-Karger F. E., Vega-Rodriguez M., Guild L. S., et al. (2014). Reef-scale thermal stress monitoring of coral ecosystems: New 5-km global products from NOAA coral reef watch. Remote Sens. 6, 11579–11606. doi: 10.3390/rs61111579
Liu G., Strong A. E., Skirving W., Arzayus L. F. (2006). “Overview of NOAA coral reef watch program’s near-real-time satellite global coral bleaching monitoring activities,” in Proceedings of the 10th International Coral Reef Symposium, Vol. 1793. 1783–1793.
Loiola M., Cruz I. C. S., Lisboa D. S., Mariano-Neto E., Leão Z. M. A. N., Oliveira M. D. M., et al. (2019). Structure of marginal coral reef assemblages under different turbidity regime. Mar. Environ. Res. 147, 138–148. doi: 10.1016/j.marenvres.2019.03.013
McClanahan T. R., Ateweberhan M., Muhando C. A., Maina J., Muhando C. (2007). Climate change and spatio-temporal variation in seawater temperature effects on coral bleaching and mortality. Ecol. Monogr. 77, 503–525. doi: 10.1890/06-1182.1
Mies M., Francini-Filho R. B., Zilberberg C., Garrido A. G., Longo G. O., Eduarda Laurentino E., et al. (2020). South Atlantic coral reefs are major global warming refugia and less susceptible to bleaching. Front. Mar. Sci. 7. doi: 10.3389/fmars.2020.00514
Migotto A. E. (1995). “Anthozoa bleaching on the southeastern coast of Brazil in the summer of 1994,” in Proceedings of the 6th International Conference on Coelenterate Biology, The Leeuwenhorst, Noordwijkerhout, The Netherlands. 329–335.
Monteiro J. G., Costa C. F., Gorlach-Lira K., Fitt W. K., Stefanni S. S., Sassi R., et al. (2013). Ecological and biogeographic implications of siderastrea symbiotic relationship with symbiodinium sp C46 in Sal island (Cape Verde, East Atlantic ocean). Mar. Biodivers 43, 261–272. doi: 10.1007/s12526-013-0153-8
Oliver E. C. J., Benthuysen J. A., Darmaraki S., Donat M. G., Hobday A. J., Holbrook N. J., et al. (2021). Marine heatwaves. Annu. Rev. Mar. Sci. 13, 313–342. doi: 10.1146/annurev-marine-032720-095144
Oliver E. C. J., Donat M. G., Burrows M. T., Moore P. J., Smale D. A., Alexander L. V., et al. (2018). Longer and more frequent marine heatwaves over the past century. Nat. Commun. 9(1), 1–12. doi: 10.1038/s41467-018-03732-9
Pereira P. H., Lima G., Pontes A., Silva L., Silva E., Sampaio C., et al. (2022). Unprecedented coral mortality on southwestern Atlantic (SWA) coral reefs following major thermal stress. Front. Mar. Sci. 9. doi: 10.3389/fmars.2022.725778
Pratchett M. S., Heron S. F., Mellin C., Cumming G. S. (2021). “Recurrent mass-bleaching and the potential for ecosystem collapse on australia’s great barrier reef,” in Ecosystem collapse and climate change. Eds. Canadell J. G., Jackson R. B. (Cham: Springer).
Principe S. C., Acosta A. L., Andrade J. E., Lotufo T. M. C. (2021). Predicted shifts in the distributions of Atlantic reef-building corals in the face of climate change. Front. Mar. Sci. 8. doi: 10.3389/fmars.2021.673086
Rabelo E. F., Soares M. O., Matthews-Cascon H. (2013). Competitive interactions among zoanthids (cnidaria: Zoanthidae) in an intertidal zone of northeastern Brazil. Braz. J. Oceanogr. 61 (1), 35–42. doi: 10.1590/S1679-87592013000100004
Santos M. E.A., Kitahara M. V., Lindner A., Reimer J. D. (2016). Overview of the order Zoantharia (Cnidaria: anthozoa) in Brazil. Mar. Biodiversity 46, 547–559. doi: 10.1007/s12526-015-0396-7
Scheufen T., Iglesias-Prieto R., Enríquez S. (2017a). Changes in the number of symbionts and symbiodinium cell pigmentation modulate differentially coral light absorption and photosynthetic performance. Front. Mar. Sci. 4. doi: 10.3389/fmars.2017.00309
Scheufen T., Kramer W. E., Iglesias-Prieto R., Enríquez S. (2017b). Seasonal variation modulates coral sensibility to heat-stress and explains annual changes in coral productivity. Sci. Rep. 7, 4937. doi: 10.1038/s41598-017-04927-8
Schlegel R. W., Smit A. J. (2018). HeatwaveR: A central algorithm for the detection of heatwaves and cold-spells. J. Open Source Software 3 (27), 821. doi: 10.21105/joss.00821
Schubert N., Brown D., Rossi S. (2017). “Symbiotic versus nonsymbiotic octocorals: physiological and ecological implications,” in Marine animal forests: the ecology of benthic biodiversity hotspots. Eds. Rossi S., Bramanti L., Gori A., Orejas C. (Switzerland: Springer).
Segal B., Castro C. B. (2011). Coral community structure and sedimentation at different distances from the coast of the abrolhos bank, Brazil. Braz. J. Oceanogr. 59, 119–129. doi: 10.1590/S1679-87592011000200001
Sen Gupta A., Thomsen M., Benthuysen J. A., Hobday A. J., Oliver E., Alexander L. V., et al. (2020). Drivers and impacts of the most extreme marine heatwaves events. Sci. Rep. 10 (1), 1–15. doi: 10.1038/s41598-020-75445-3
Soares M. O., Araújo J. T., Ferreira S. M. C., Santos B. A., Boavida J. R. H., Costantini F., et al. (2020). Why do mesophotic coral ecosystems have to be protected? Sci. Total Environ. 726, 138456. doi: 10.1016/j.scitotenv.2020.138456
Soares M. O., Rabelo E. F. (2014). Primeiro registro de branqueamento de corais no litoral do ceará (NE, brasil): Indicador das mudanças climáticas? Geociencias 33(1), 1–10.
Soares M. O., Rossi S., Gurgel A. R., Lucas C. C., Tavares T. C. L., Diniz B., et al. (2021). Impacts of a changing environment on marginal coral reefs in the tropical southwestern Atlantic. Ocean Coast. Manage 210, 105692. doi: 10.1016/j.ocecoaman.2021.105692
Soares M. O., Rossi S., Martins F. A. S., Carneiro P. B. M. (2017). The forgotten reefs: benthic assemblage coverage on a sandstone reef (Tropical south-western Atlantic). J. Mar. Biol. Assoc. 97 (8), 1585. doi: 10.1017/S0025315416000965
Soares M. O., Matos E., Diniz B., Paiva S. V., Gurgel A., Lucas C. C., et al. (2022). Climate change and local human impacts threaten Brazilian coral reefs. In Brazilian Coral Reefs. Eds, Turra A.. (Switzerland, Springer Nature), in press.
Soares M. O., Teixeira C. E. P., Ferreira S. M. C., Gurgel A. L. A. R., Paiva B. P., Menezes M. O. B., et al. (2019). Thermal stress and tropical reefs: mass coral bleaching in a stable temperature environment? Mar. Biodivers. 49 (6), 2921–2929. doi: 10.1007/s12526-019-00994-4
Stuart-Smith R. D., Edgar G. J., Barrett N. S., Bates A. E., Baker S. C., Bax N. J., et al. (2017). Assessing national biodiversity trends for rocky and coral reefs through the integration of citizen science and scientific monitoring programs. BioScience 67, 2. doi: 10.1093/biosci/biw180
Sully S., Van Woesik R. (2020). Turbid reefs moderate coral bleaching under climate-related temperature stress. Global Change Biol. 26(3), 1367–1373. doi: 10.1111/gcb.14948
Teixeira C. D., Leitão R. L. L., Ribeiro F. V., Moraes F. C., Neves L. M., Bastos A. C., et al. (2019). Sustained mass coral bleaching, (2016–2017) in Brazilian turbid-zone reefs: taxonomic, cross-shelf and habitat-related trends. Coral Reefs 38, 4, 801–4, 813. doi: 10.1007/s00338-019-01789-6
Keywords: coral bleaching, turbidity, coral reef, marine heatwave, marginal reef
Citation: Lucas CC, Teixeira CEP, Braga MDA, Júnior FC, Paiva SV, Gurgel AL, Rossi S and Soares MO (2023) Heatwaves and a decrease in turbidity drive coral bleaching in Atlantic marginal equatorial reefs. Front. Mar. Sci. 10:1061488. doi: 10.3389/fmars.2023.1061488
Received: 04 October 2022; Accepted: 02 February 2023;
Published: 21 February 2023.
Edited by:
Aldo Cróquer, The Nature Conservancy, Dominican RepublicReviewed by:
Cristiane Cassiolato Pires Hardoim, São Paulo State University, BrazilGonzalo Perez-Rosales, Woods Hole Oceanographic Institution, United States
Copyright © 2023 Lucas, Teixeira, Braga, Júnior, Paiva, Gurgel, Rossi and Soares. This is an open-access article distributed under the terms of the Creative Commons Attribution License (CC BY). The use, distribution or reproduction in other forums is permitted, provided the original author(s) and the copyright owner(s) are credited and that the original publication in this journal is cited, in accordance with accepted academic practice. No use, distribution or reproduction is permitted which does not comply with these terms.
*Correspondence: Caroline Costa Lucas, caroline.costalucas@gmail.com; Marcelo Oliveira Soares, marcelosoares@ufc.br