- 1German Centre of Marine Biodiversity, Senckenberg am Meer, Wilhelmshaven, Germany
- 2Institut für Biologie und Umwelt, Carl von Ossietzky University Oldenburg, Oldenburg, Germany
- 3Zoological Museum, Moscow State University, Moscow, Russia
- 4Marine Invertebrates, Museums Victoria, Melbourne, VIC, Australia
- 5Biologiezentrum, OÖ Landes-Kultur GmbH, Linz, Austria
- 6Koltzov Institute of Developmental Biology, Russian Academy of Science (RAS), Moscow, Russia
- 7Department of Life Sciences, The Natural History Museum, London, United Kingdom
Introduction: The Clarion Clipperton Zone (CCZ) in the Northeast Pacific Ocean holds the largest deposits of polymetallic nodules at abyssal depths. These nodules are rock formations containing valuable metals and minerals targeted for mining. They further provide diverse habitat for a range of deep-sea species. Little is known so far on the taxonomy, natural history and biogeography of these deep-sea animals which is vital for accurate assessment of the risk of species extinctions from large-scale mining. One of the most abundant megafaunal groups in the CCZ is the Ophiuroidea (brittle stars), of which Ophiotholia is one of the more abundant genera found in the area. The genus Ophiotholia has a world-wide distribution and currently holds six species.
Methods: Material collected from seven scientific cruises to the CCZ was examined, morphologically, together with comparative material from all the known species. The small size and the damage caused during sampling often impeded their identification. The specimens were also genetically analyzed using a fragment of the mitochondrial COI gene. Scanning Electron Microscope images of the key microstructural characters were made using selected specimens from CCZ as well as from the comparative material.
Result and discussion: One morphotype was identified as the known species Ophiotholia supplicans Lyman, 1880, while the second is new to science and is described in this paper. The umbrella spines and the arrangement of their articulations on the lateral arm plate, were selected as the most relevant morphological characters in the taxonomy of the genus Ophiotholia and a revised identification key of all characters from all known Ophiotholia species is provided as a table in the supplement material. The identification and description of such a little-known genus improves the evaluation of the biodiversity not only in the CCZ but also for the deep sea.
1. Introduction
Deep-sea ecosystems are important reservoirs of biodiversity and provide key ecosystem services, such as carbon sequestration, chemosynthetic primary production and nutrient regeneration (Ramirez-Llodra et al., 2010; Armstrong et al., 2012; Thurber et al., 2014; Levin et al., 2016). Additionally, the deep sea contains a diverse range of habitats and harbors substantial untapped mineral deposits (Halbach and Fellerer, 1980; Halfar and Fujita, 2002; Wedding et al., 2015; Zeppilli et al., 2016). In the last decades, deep-sea related technologies have developed exponentially, boosting the sampling effort in the largest yet poorly studied biome on Earth (Amon et al., 2017; Ramirez-Llodra et al., 2010; Taylor et al., 2016; Vanreusel et al., 2016). Abyssal marine mineral resources have been known since the Challenger expedition (1872-4, Murray and Renard, 1891) and strategies for potential mining have been discussed since the 1960s (Bonatti and Nayudu, 1965; Mero, 1965; Halbach and Fellerer, 1980; Oebius et al., 2001; Wedding et al., 2015; Smith et al., 2021). Polymetallic nodules are rich in copper, cobalt, nickel, and manganese and therefore receive significant economic interest from governments and industry. The largest concentrations of polymetallic nodules are found in the Clarion Clipperton Zone (CCZ), located in the northeast Pacific. The CCZ also harbors a high marine biodiversity potentially resulting from habitat heterogeneity and energy availability (Buhl-Mortensen et al., 2010; Gillman and Wright, 2014; Kuhnz et al., 2014; Amon et al., 2016; Soetaert et al., 2016; Woolley et al., 2016; Zeppilli et al., 2016; Stratmann et al., 2021). Most recent biological studies dealing with the CCZ indicate the presence of new species, yet to be described, making proper assessments of biodiversity challenging (Amon et al., 2017; Simon-Lledó et al., 2019b; Bonifácio et al., 2020; Christodoulou et al., 2020; Rybakova et al., 2020; Durden et al., 2021; Lejzerowicz et al., 2021; Bribiesca-contreras et al., 2022).
Among taxa collected in the CCZ, the class Ophiuroidea (Echinodermata) are widespread and abundant (Simon-Lledó et al., 2019b; Christodoulou et al., 2020). Ophiuroids inhabit all kind of habitats, from the tropics to the poles and from shallow waters to deep-sea ecosystems (O’Hara, 2007; Stöhr et al., 2012; Bribiesca-Contreras et al., 2019; Laming et al., 2021). Because of their global distribution, ophiuroids serve as excellent model organisms for the understanding of large-scale diversity and evolution patterns (Hunter and Halanych, 2008; Cho and Shank, 2010; Galaska et al., 2017; Galaska et al., 2019; Jossart et al., 2019; O’Hara et al., 2019; Stöhr et al., 2020; Eichsteller et al., 2022). The world ophiuroid database (accessed 13.09.2022) lists around 2100 described species of which 100 exclusively dwell on the abyssal plains (Stöhr et al., 2012).
Christodoulou et al. (2020) identified 43 putative ophiuroid species in the CCZ using COI barcodes. The most abundant species were Ophiosphalma glabrum (Lütken & Mortensen, 1899) and Silax daleus (Lyman, 1879) followed by 3 unidentified species belonging to Ophiotholia spp. The genus Ophiotholia was erected by Lyman in 1880 to accommodate the type species of the genus, Ophiotholia supplicans, and was first placed in the family Ophiuridae. Although Lyman (1880) noticed the unique characters and body shape of this genus that set it apart from the other known genera of the family Ophiuridae, it was Perrier (1893) that created a new family, Ophiohelidae, to which Ophiotholia was assigned. Two more genera were then assigned to the family of Ophiohelidae: Ophiomyces Lyman, 1869 and Ophiohelus, Lyman, 1880 with the latter being the type of the family. In 1915, Clark transferred all three genera to the family of Ophicanthidae Ljungman, 1867, ignoring Ophiohelidae and Ophiomycetidae, a new family created to include the same genera by Verrill, 1899. Fell (1960) and Paterson (1985), although agreeing with Verrill (1899) that the three genera are quite distinct, assigned the genera to the family of Ophiacanthidae with Paterson (1985) reestablishing the subfamily Ophiohelinae (Perrier, 1891 amended) to include them. Martynov (2010) reviewed the segregating characters and suggested that the three genera, Ophiohelus, Ophiomyces, and Ophiotholia, be transferred from the Ophiacanthidae to new family but it was O’Hara et al. (2018), who re-established the family of Ophiohelidae within the order Ophioscolecida after restructuring the higher taxonomy of the class Ophiuroidea based on genetic and morphological data. Ophioscolecida are unique in having reduced radial shields concealed by disc scales or being absent (O’Hara et al., 2018). Ophiohelidae can be easily separated from the only other family in the order, Ophioscolecidae Lütken, 1869, by their unique body form, which form is perfectly adapted to living buried in the soft sediment with their arms protruding outside for feeding. The most unique character within this family is the so called “parasol-spine” on the posterior part of the arms. The function of these spines is unclear, but Lyman (1880) described them as supplementary spines or pedicellaria. Because of the species-specific structure of these spines, they are often used as a species delimitation character in the two genera in which they occur (Ophiohelus and Ophiotholia).
Within the genus Ophiotholia there are six extant species, Ophiotholia spathifer (Lyman, 1879); Ophiotholia supplicans Lyman, 1880; Ophiotholia mitrephora H.L. Clark, 1910; Ophiotholia montana Litvinova, 1981; Ophiotholia gibbosa Litvinova, 1992 and Ophiotholia odissea Litvinova, 1992. There is also one recently described extinct species Ophiotholia aurora Thuy and Meyer, 2013 that was found in the Hauptrogenstein Formation and coeval formations in Switzerland, dated to the middle Jurassic. Because all Ophiotholia species seem to be very rare and not well documented in the literature, the known distribution of the genus is limited between 60°N and 60°S (Lyman, 1882; Litvinova, 1992; Martynov, 2010). The depth in which the species have been collected previously varied between 216 m to 4100 m. Due to their fragile structure, they often get damaged during sampling (Litvinova, 1992), the parasol spines can be lost, and thus many specimens are only identified to higher taxonomic levels (Glover et al., 2016; Christodoulou et al., 2019; Christodoulou et al., 2020).
The present study aims to describe a new species within the genus Ophiotholia as well as reviewing the existing species of the genus using revised morphological characters. Besides morphology, mitochondrial COI sequences were used to explore the genetic diversity of Ophiotholia in the CCZ area.
2. Materials and methods
2.1. Specimen collection and processing
The present study is based on specimens included in the Christodoulou et al. (2019); Christodoulou et al. (2020) and the Glover et al. (2016) studies, as well as on additional acquired material (Figure 1 and Table S1). Ophiuroid specimens were collected during various cruises from the UKSRL, NORI-D and BGR licence areas, as well as the APEI3, using a Brenke-type epibenthic sledge (EBS) or Box Corer following standard deployment procedures (Brenke, 2005) and as described in detail by Christodoulou et al. (2020) for the EBS samples and by Glover et al. (2015) and Bonifácio et al., 2020 for the Box core samples. The voucher specimens of the Ophiotholia spp. collected from the different CCZ areas are kept in the German Centre of Marine Biodiversity Research (Wilhelmshaven), the Senckenberg Research Institute and Natural History Museum Frankfurt (SMF) and the Natural History Museum London (NHM), while the type material from the other extant species of Ophiotholia are deposited at either the Natural History Museum in London, UK, or at the Zoological Museum of Moscow University, Russia (ZMMU).
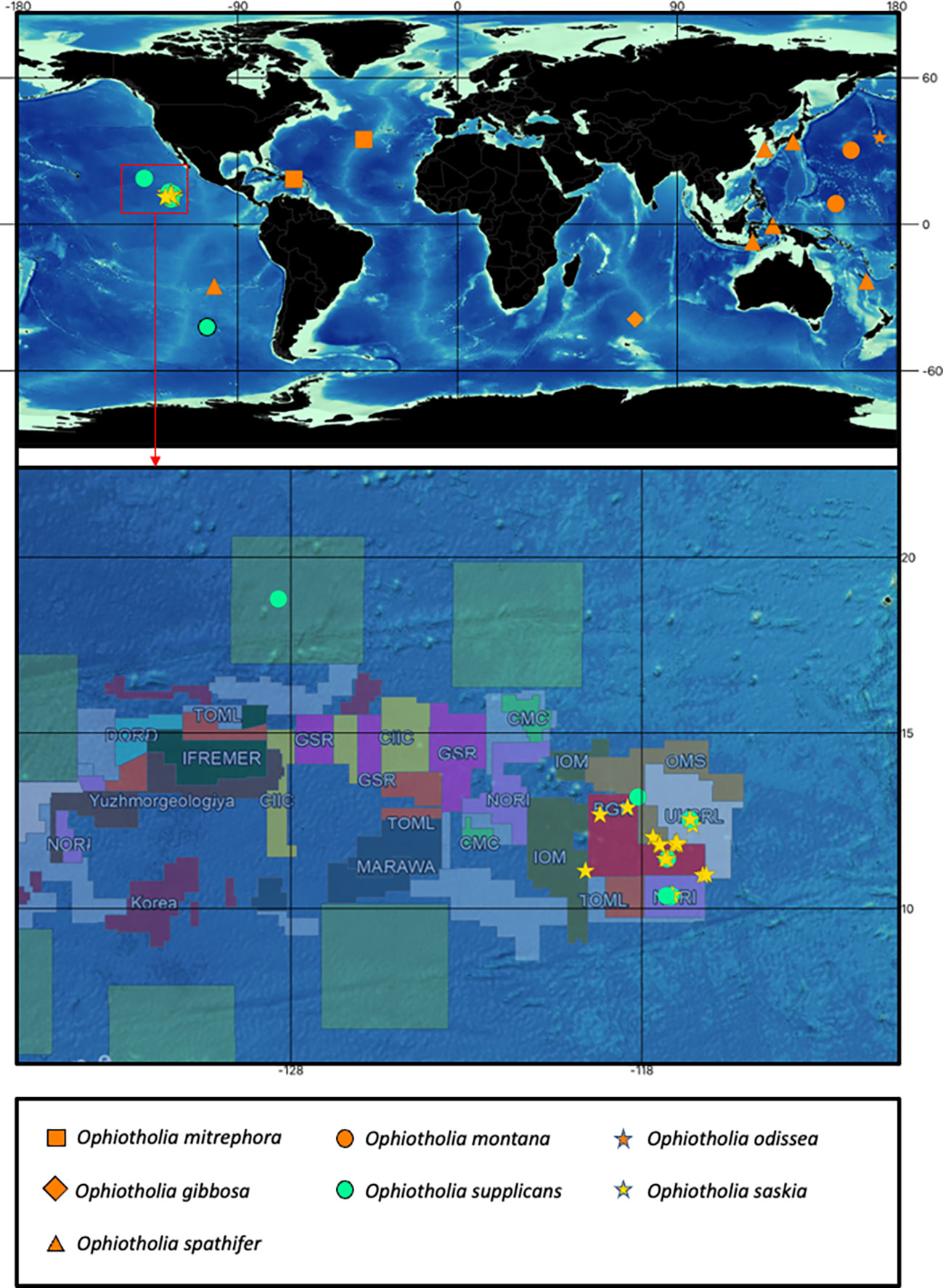
Figure 1 Map with the recorded distributions from all recent species of the genus Ophiotholia. The colour of the upper map indicates: orange, distributions recorded for species with just morphological data; yellow and turquoise, distributions recorded from species with morphological and molecular data. A unique combination of colour and symbol is assigned to each species. Detailed map of the Clarion Clipperton Zone showing the distribution of the cooccurring species Ophiotholia saskia sp. nov. and Ophiotholia supplicans. The distribution map of the Ophiotholia species was created using QGIS (version 3.16, http://www.qgis.org), considering the data of the International Seabed Authority (2020/2021).
2.2. Morphological analysis
Specimens were morphologically examined and photographed using a Leica M125 stereo microscope. Selected specimens were used for scanning electron microscope (SEM) analysis and essential micro-morphological characters were recorded either with a VEGA3 TESCAN, CamScan II, and JSM-6380 SEMs after the samples were gold-palladium-coated. Images of the whole body were taken after the selected specimens were cleaned with distilled water in order to remove any detritus. Ossicles were isolated by macerating arms or portions of arms in a mixture of (1:1) distilled water and household bleach (NaClO). In general, disintegration of the arm skeleton was completed within one hour following immersion. The disarticulated arm plates were rinsed in distilled water to remove any traces of NaClO. After rinsing, the plates were air-dried, and the selected ossicles, as well as the bodies, were mounted on aluminum stubs for SEM examination using a glue foil. The orientation of the plates on the stubs was chosen to best display the relevant characters on a single picture per view (ad/abradial, distal/proximal, dorsal/ventral). An image of each character per individual were taken if possible. The scanned habitus and ossicles mount on the stubs as well as the remaining ossicles of the disintegrated arms were deposited in the German Centre for Marine Biodiversity Research, Senckenberg, Wilhelmshaven, or the Zoological Museum of Moscow University. The images were edited and compiled with Adobe Photoshop Version 23.2.1. The most important characters were listed in Supplementary Table 2 and give an overview for each character for each species.
2.3. Molecular analysis
Cytochrome c oxidase I (COI) sequences analysed herein derive from Christodoulou et al. (2019), Christodoulou et al. (2020), Glover et al. (2016) and new COI sequences produced during this study. More specifically, the Ophiotholia dataset includes 42 sequences of O. saskia sp. nov. (Ophiotholia sp.1) and three sequences of O. supplicans (Ophiotholia sp.28) prepared by Christodoulou et al. (2019), Christodoulou et al. (2020). In addition, 18 new sequences of O. saskia sp. nov. and five sequences of O. supplicans were produced herein using methods described in Christodoulou et al. (2020); The arm tissue was extracted using E.Z.N.A DNA tissue Kit (Omega), the COI gene was amplified using the AccuStart PCR SuperMix polymerase (ThermoFisher Scientific) and the echinoderm specific LCOech1aF1 (Layton et al., 2016) forward primer paired with the universal jgHCO2198 reverse primer (Geller et al., 2013). Sequencing was executed at Macrogen (Amsterdam, Netherlands). Another 6 sequences were added from the NHM dataset (Glover et al., 2016), four specimens labeled as “NHM_076”, which can be assigned to O. supplicans, and two specimens referred as “NHM_303” which can be assigned to O. saskia sp. nov. Due to the age, the type of fixation or the insufficient amount of tissue, our efforts were unsuccessful in obtaining sequences from other species of the genus Ophiotholia. The COI sequences were assembled and edited using Geneious v.9.1.8 (Kearse et al., 2012), whereas all sequences were aligned using MAFFT v7.017 (Katoh et al., 2002) with the default settings. The sequences, trace files, collection data and photos for each specimen are listed in the dataset “Clarion Clipperton Zone - Specimens of the genus Ophiotholia (Echinodermata : Ophiuroidea)” (CCZOTHOL; doi: dx.doi.org/10.5883/DS-CCZOTHOL). The new sequences produced are also available in GenBank (Sayers et al., 2019), accession numbers: OP575327-OP575373, OQ152220, OQ152221 (Supplement Table 1).
2.4. Putative species delimitation and genetic distances
Different species delimitation methods were used to cluster Ophiotholia specimens into possible genetic species and classify the individuals based on the dataset of Christodoulou et al. (2020). (1) The method “General Mixed Yule Coalescent” (GMYC, Pons et al., 2006) was applied using the R package SPLITS (Fujisawa and Barraclough, 2013), with the single-threshold model. The required ultrametric tree based on bayesian analysis was produced using the software BEAST v.2.6.6 with parameters as set out in Christodoulou et al. (2020). Additionally, the only other available sequence of the genus Ophiotholia, (Ophiotholia spathifer, NIWA14067) was included. As an outgroup, three species of the close related genus Ophiomyces were used (AB1_EB4_11_22 “Ophiomyces sp.”, UF16941 “Ophiomyces frutectosus”, BP34 “Ophiomyces delata”. (2) Barcode index numbers (BINs) were assigned to the registered DNA dataset using the BOLD v.4 workbench (http://www.boldsystems.org; Ratnasingham and Hebert, 2013). The genetic distances between and within Ophiotholia species were calculated under the simple p-distance model in MEGA 11 (Tamura et al., 2021). The genetically delimited species were checked for morphological diagnostic characteristics to confirm the species definition by morphological evidence in parallel to genetic analysis. A minimum spanning haplotype network based on the COI sequences was produced in PopART (Version 1.7; available on: http://popart.otago.ac.nz/index.shtml).
3. Results
3.1. Taxonomy
Order Ophioscolecida O’Hara, Hugall, Thuy, Stöhr & Martynov, 2016
Family Ophiohelidae Perrier, 1893
Genus Ophiotholia Lyman, 1880
Diagnosis
Disc has a sac-like shape and is fully scaled. Radial shields are absent. Two to three large and flat teeth present. Jaws (oral plates) covered by numerous flat papillae. Lateral arm plate has a fragile ledge with a distalwards pointing ventral extension that separates all arm spine articulations from the distal edge and encloses the round to triangle shaped dorsal arm plate. Arm spine articulation with nearly vertical dorsal and ventral lobes, connected at their proximal ends, opening distal-wards into a horseshoe-shape, bordering a single muscle/nerve opening, dorsal and ventral lobe same shape (except dorsally bent in O. spathifer). Parasol-spine articulation with an elevated circular lobe and a single (possibly muscle or nerve) opening (Figure 2). Parasol-spines present on the distal part of the arm, with a thin shaft and one to two rings of teeth (one or two sprocket wheels), replacing arm spines partly or totally in some species.
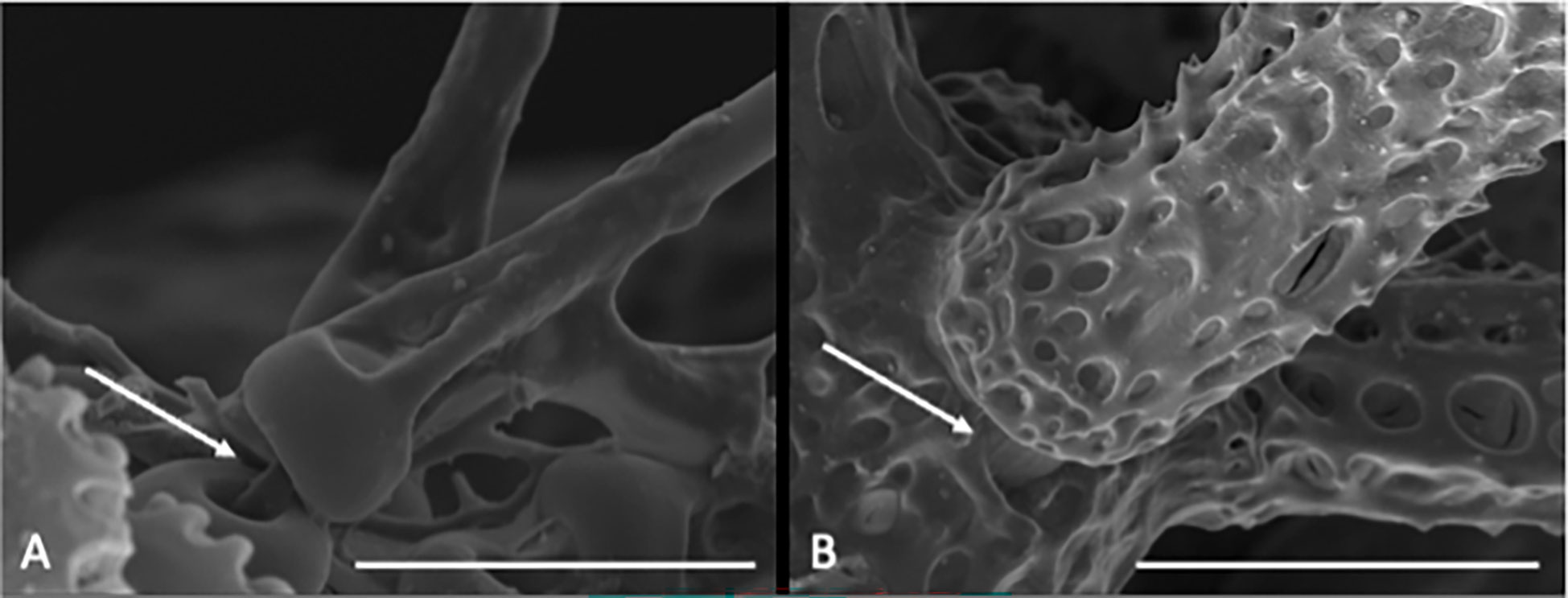
Figure 2 Scanning electron microscope images of close ups from the habitus of Ophiotholia saskia sp. nov. SO240_46_5. (A) Possible muscular connection between the parasol spine and its articulation. Scale = 50 µm (B) Muscular connection between arm spine and its articulation. Scale = 100µm.
Ophiotholia gibbosa Litvinova, 1992
(Figure 3)
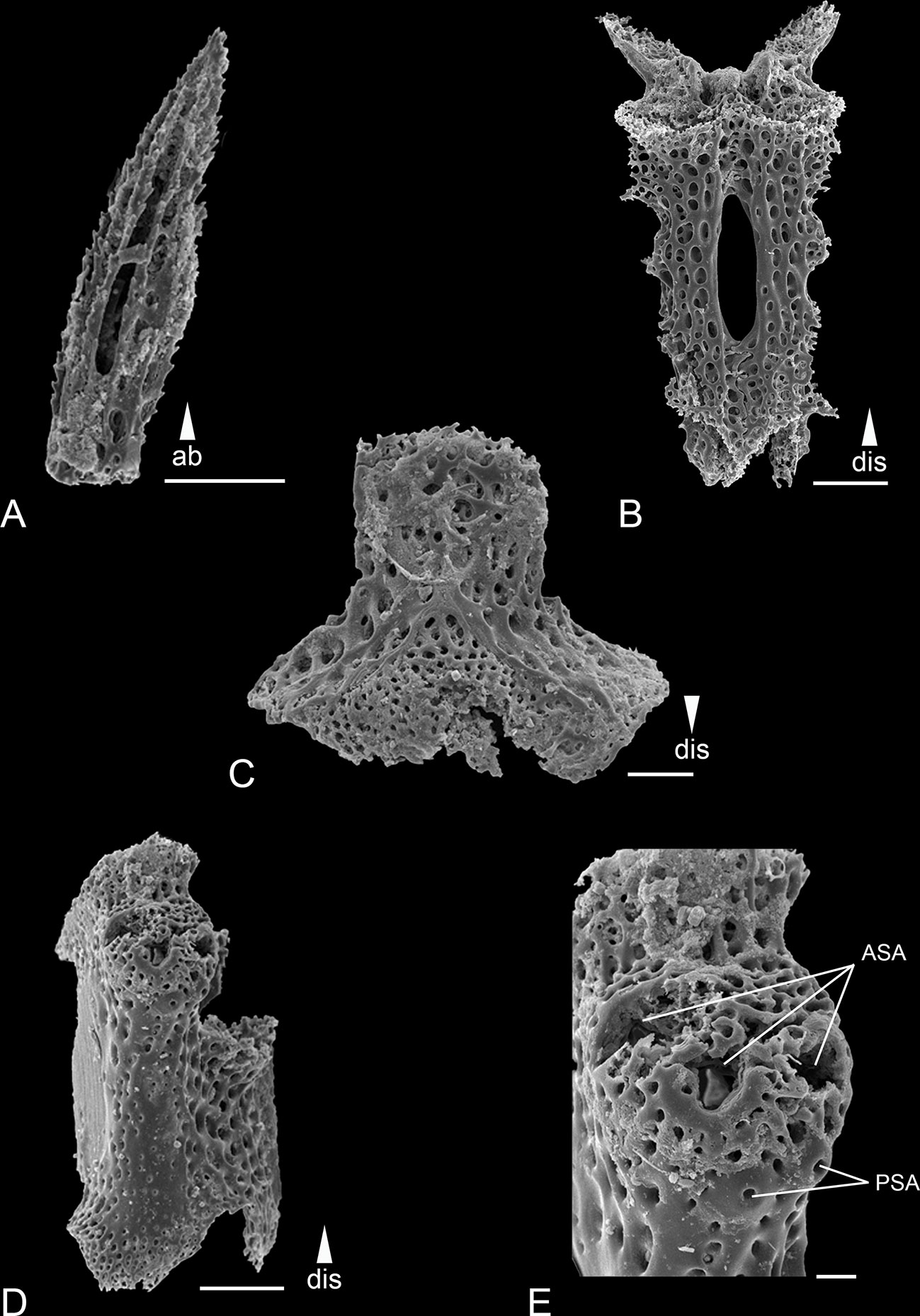
Figure 3 Scanning electron microscope images of the ossicles from Ophiotholia gibbosa. (A): Arm spine; (B): Ventral arm plate; (C): Arm vertebrae (ventral); (D): Lateral arm plate; (E): Lateral arm plate close up. Scales: 20 µm (E); 50 µm (B); 100 µm (A, C, D);. ab, abradial; ASA, arm spine articulation; dis, distal; PSA, parasol spine articulation.
Ophiotholia gibbosa. — Litvinova, 1992: Figs. 3 (A-E), 4 (Л,М)
Material: Holotype: ZMMU D-1009, Vityaz, stn 4893, Indian Ocean, 39°29.9’S 71°15.6’E, 420 m, 27/12/1960.
Distribution: This species was reported from the central Indian ocean in depths of 420 m (Litvinova, 1992).
Remarks: The species can be distinguished by the following characters. The disc is covered with large plates each provided with a long triangular spine (larger than in O. montana). There are 3-4 serrated arm spines that are shorter than an arm joint (Figure 3A) and are arranged slightly distal to the parasol spines. There can be up to six parasol spines distally, arranged in two rows (Figures 3D, E). The blunt parasol spine teeth are arranged in one ring. Two, pointed tentacle scales. The oral papillae were described as “very large”. The dental plate carries three teeth.
Ophiotholia mitrephora H.L. Clark, 1910
(Figure 4)
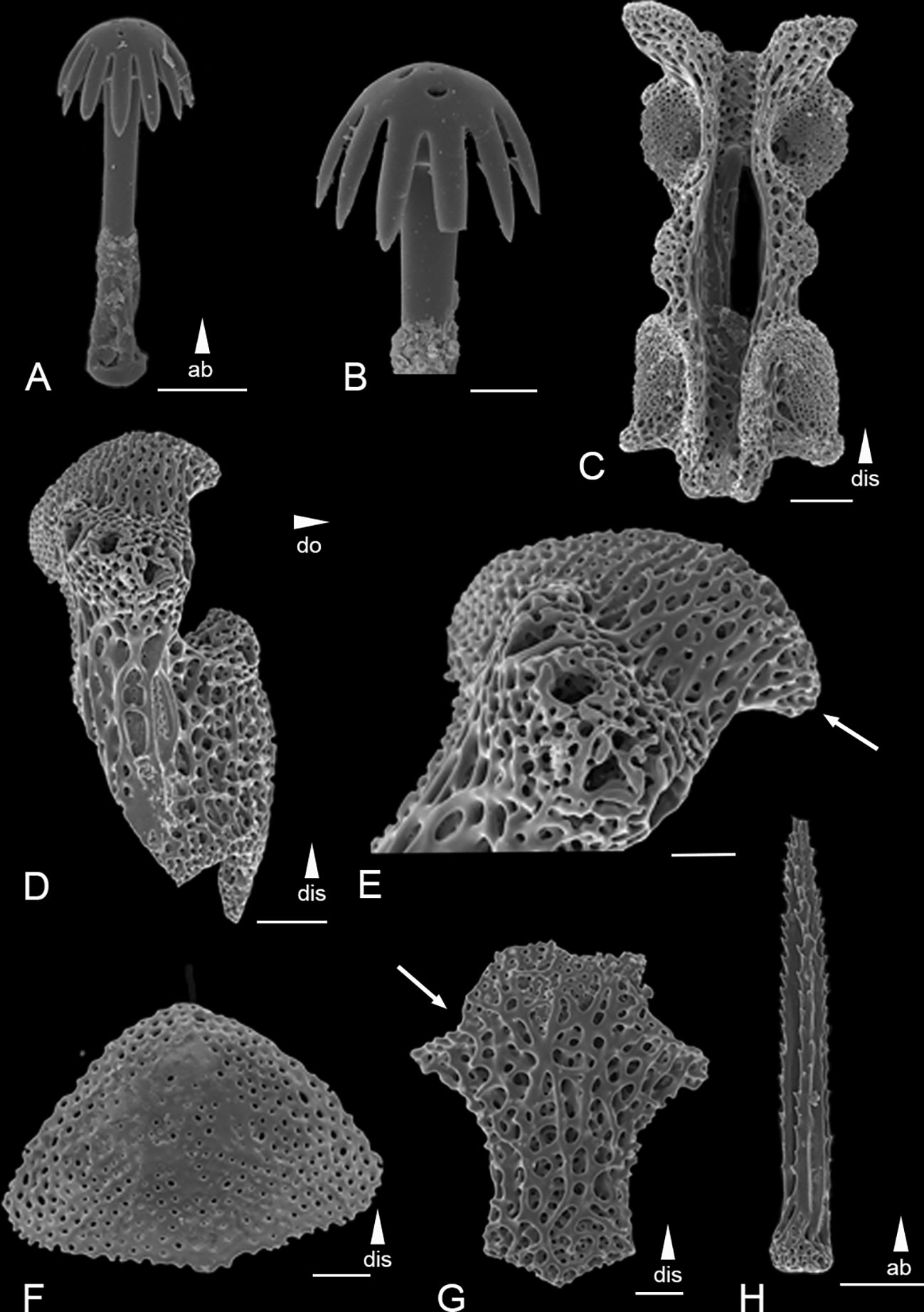
Figure 4 Scanning electron microscope images of the ossicles from Ophiotholia mitrephora. (A): Parasol-spine; (B): Head of parasol-spine; (C): Arm vertebrae (dorsal); (D): Lateral arm plate; (E): Close up lateral arm plate; (F): Dorsal arm plate; (G): Ventral arm plate; (H): Arm spine. Scales: 20 µm (B); 50 µm (A, E–G); 100 µm (C, D, H);. ab, abradial; dis, distal.
Ophiotholia mitrephora H. L. Clark, 1910: 665-666, Figs 1, 2. —Clark, 1915: 221. — Litvinova, 1992: 53, Fig. 4.
Material: ZMMU D-1010: Vityaz-II stn 161, Atlantic Ocean, 29°51.0’N 28°7’W, 3340–3440 m, 27/06/1982.
Distribution: This species has been reported from the east and west side of the North Atlantic in depths of 920-3440 m. (Type locality.—Albatross station 2750, east of the Danish West Indies; lat. 18°30’00”N.; long. 63° 31’ 00” W.; 496 fathoms; ~907 m).
Remarks: The species has 4-5 smooth arm spines at the arm base, that are as long as an arm joint (Figure 4H). The arm spines become confluent with a row of parasol spines distally (Figures 4D, E). The parasol spines start at the fourth to fifth arm joint and are around six times smaller than the arm spines. There are three to four parasol spines occurring on each lateral arm plate, each with one ring of broad but pointed teeth (Figures 4A, B). The dorsal arm plate is triangular shaped (Figure 4F). There are three tentacle scales, one on the adradial and two at the abradial side of basal tentacle pores. Each dental plate carries four teeth.
Ophiotholia montana Litvinova, 1981
(Figure 5)
Ophiotholia montana Litvinova, 1981: 127, Fig. 4 (3,5,6). — Litvinova, 1992: 53-54, Fig. 2 (Ж). — Martynov, 2010: Fig. 2 (19).
Material: Holotype: ZMMU D-1011, Dmitry Mendeleev Cruise 21 stn 1725, Dmitri Mendeleyev Seamount, 4°49’N 154°58’E, 1230-1370 m, 8/11/1978. Paratype: ZMMU D-1012, Vityaz stn 6369, 26°59’N 151°25’E, 3070m, 28/8/1970.
Distribution: This species was reported from the northwest in depths of 1230-3070 m (Litvinova, 1992).
Remarks: The disc has a conical shape, lower than in O. saskia sp. nov. and O. supplicans (Figure 5D), and is covered with long thin spines (shorter than in O. gibbosa). The jaw has one long, thin apical papilla and next to it on each side is a similar-shaped mouth papilla, followed by a gap and then by typically three long and thick, almost rectangular, mouth papillae arranged in a single row (Figure 5B). The dental plate carries two pointed teeth. There are five arm spines on the first four arm joints; followed by four arm spines in the subsequent arm joints, arm spines about 1.5 times the segment length (Figure 5C). These are in a row confluent with three parasol spines. The parasol spines are large and have two rings of about six teeth.
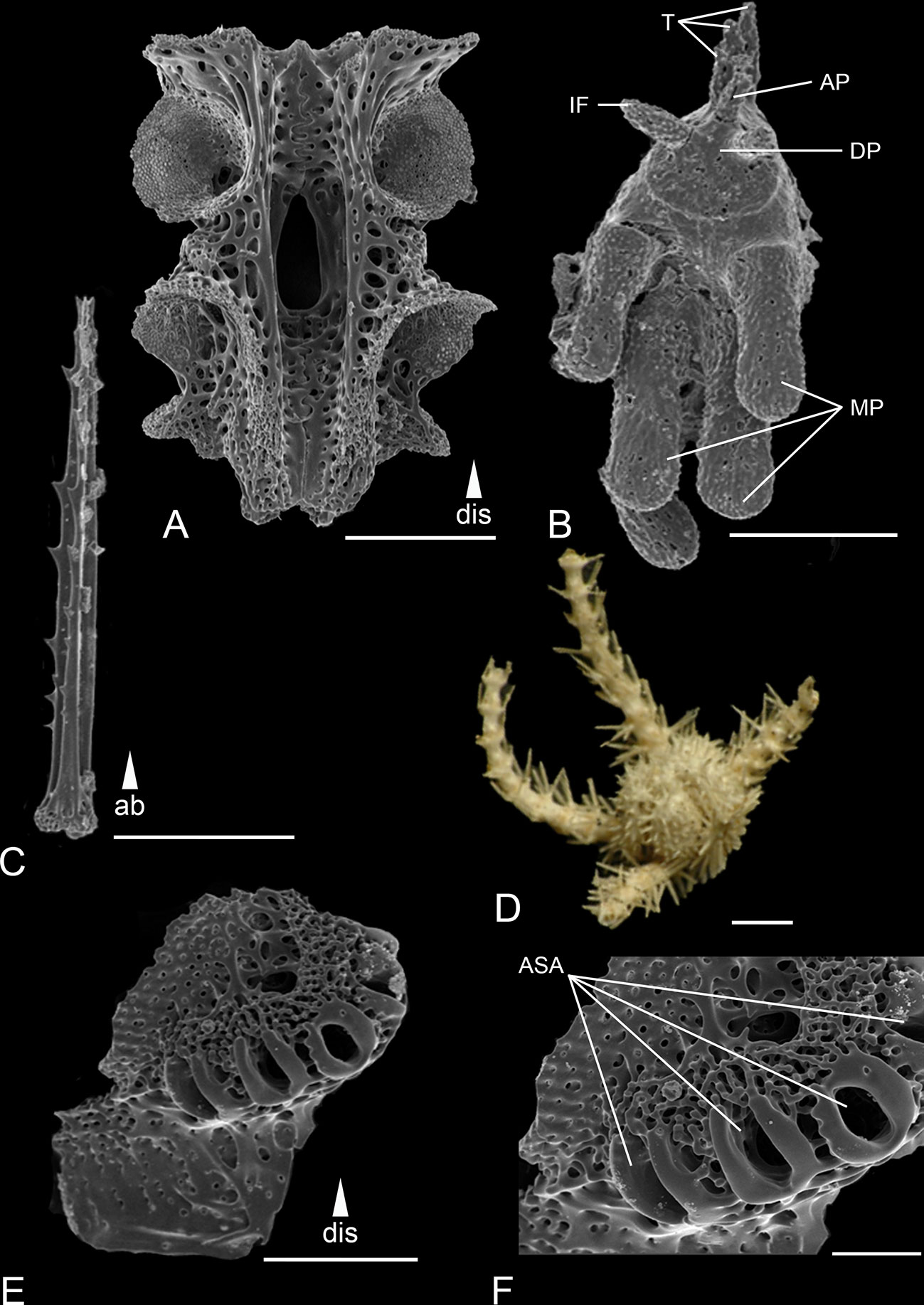
Figure 5 Scanning electron microscope images of the ossicles from Ophiotholia montana. (A): Arm vertebrae (ventral); (B): Jaw; (C): Arm spine; (D): Habitus; (E): Lateral arm plate; (F): Close up lateral arm plate. Scales: 30 µm (C); 80 µm (F); 200 µm (A, E); 400 µm (B); 1 mm (D). ab, abradial; AP, apical papillae; ASA, arm spine articulation; DP, dental plate; dis, distal; IF, infradental papillae; MP, mouth papillae; T, tooth.
Ophiotholia odissea Litvinova, 1992
(Figure 6)
Ophiotholia odissea Litvinova, 1992: Fig. 2 (З, И, Л, М); 4 (И, К)
Material: Holotype: ZMMU D-1013, Odissey Cruise 33 stn 36, Emperor Seamounts, 32°3’N 172°59’E, 700-750m, 21/8/1984.
Distribution: This species was reported from the northwest Pacific in depths of 700-750 m (Litvinova, 1992).
Remarks: The disc is cone shaped and it is covered with thin, fish-like scales that carry triangular, serrated spines (Figure 6E). It has four rounded arm spines (Figure 6D), 1.5 times the length of an arm joint. The ventral most spine is thicker than the others. The parasol spines start at the seventh to eight arm joint and form two alternating rows offset to each other. There are up to six per lateral arm plate. The arm spines are arranged slightly proximal to the parasol spines. Each parasol spine has two rings of short teeth (Figures 6A, B).
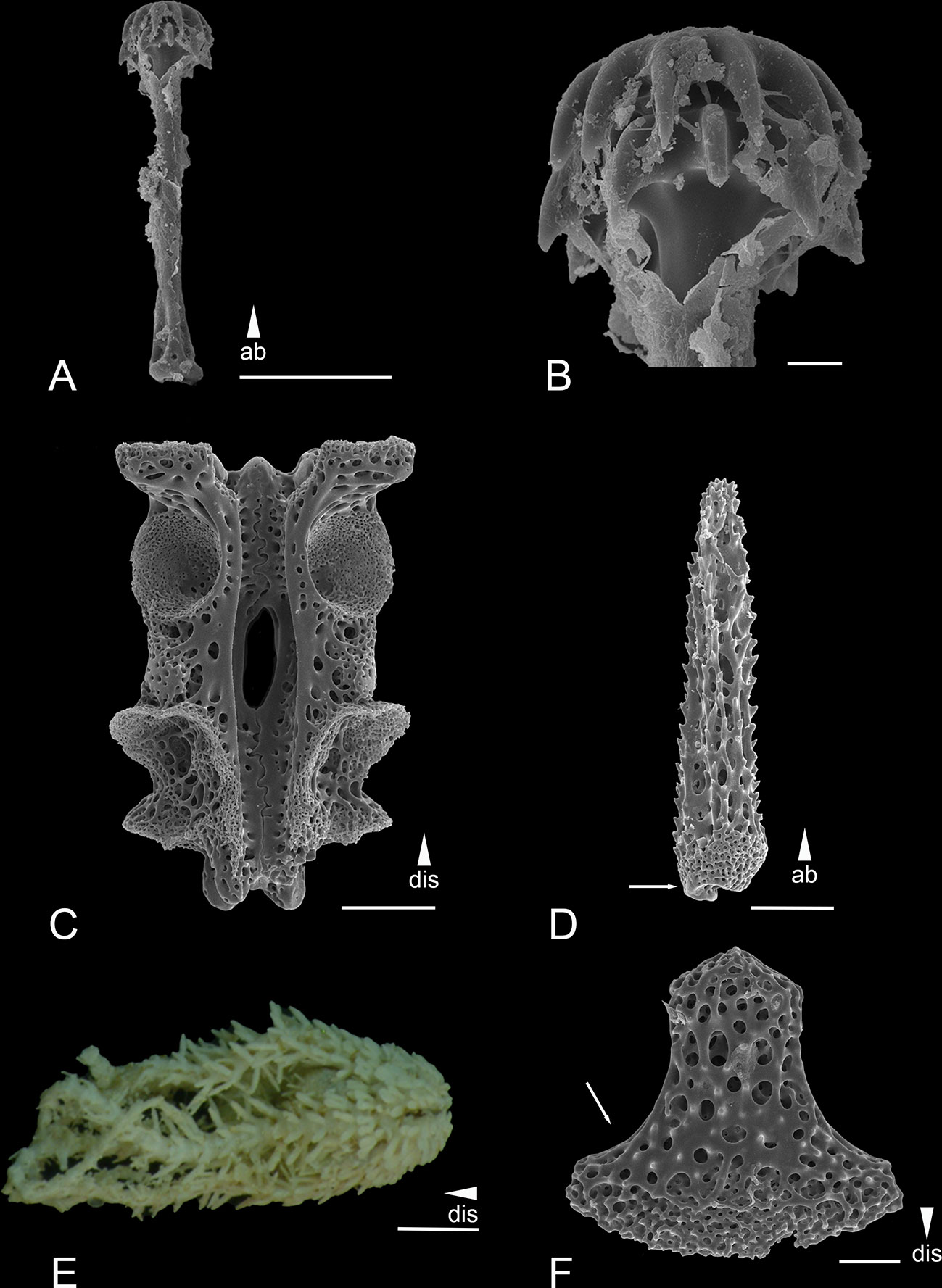
Figure 6 Scanning electron microscope images of the ossicles from Ophiotholia odissea (A) Parasol-spine; (B) Head of parasol-spine; (C) Arm vertebrae (ventral); (D) Arm spine; (E) Habitus; (F) Ventral arm plate. Scales: 10 µm (B); 50 µm (F); 100 µm (A, C, D); 1 mm (E) ab, abradial; dis, distal.
Ophiotholia saskia sp. nov.
Ophiotholia sp. Christodoulou et al., 2019: 1, Fig. 1.
Ophiotholia sp. 1 Christodoulou et al., 2020: 1850, Figs. 11, 12.
Material:
Holotype on SEM stub, Clarion Clipperton Fracture Zone, cruise: Mangan 2013, stn. 90, 11°49'43” N, 117°30'16” W 4340 m [SMF 6909 “MA13_90_18” in Christodoulou et al.]. Paratypes: Mangan 13, stn. 97: 1 spm, in 96% ethanol [SMF 6911 “MA13_97_1”]; Mangan 16 2016, stn 25: 2 spms, in 96% ethanol [SMF 6914 “KM16_25_2”; SMF 6913 “KM16_25_7”]; Mangan 14, stn 38: 1 spm, in 96% ethanol [SMF 6910 “MA14_38_13”]; SO239, stn 20: 1 spm, in 96% ethanol [SMF 6912 “SO239_20_11”]; C5A, stn. STM_050: 1 spm, in 80% ethanol [NHM UK 2022.111 “NHM_6653”]
Non type material: see Supplement Table 1.
Etymology: Ophiotholia saskia sp. nov. is dedicated to the isopod taxonomist Dr Saskia Brix who was the first author’s mentor at the start of her career. Dr Brix has contributed significantly to the research of deep-sea biodiversity and its conservation.
Description of the holotype: The description is based on the measurements of the holotype (SMF6909, Figure 7) with variation in the species represented by two additional specimens in Figure 8 (AB2_EB1_13_21) and Figure 9 (SO240_46_5). The disk diameter is 1.5 mm and its height 2.5 mm (when the arms are stretched out). The arms (without spines) are 0.35 mm in width and 4.7 mm in length. The mouth-angles are high and narrow, so that the mouth-slits between them are wide (Figures 7G, H): They have one pointed apical papilla with one pointed mouth papillae next to it on each side. The following 6-8 mouth papillae are broader and larger, almost rectangular in shape, and arranged in two rows on each jaw (Figures 7H, 9E). Three broad and serrated teeth are visible (Figure 9F). The oral plate is absent. The oval adoral shields are visible at the base of the jaws (Figure 9E). The dorsal arm plates are broad, almost as long as wide, and rounded triangle in shape (Figure 8I). They are well separated from each other by the contiguous lateral arm plates (Figure 9C). The lateral arm plates are longer than wide, and meet broadly below, and form an obvious spine-crest at their outer edge (Figures 7B, C, 8C, D, G). This spine-crest provides the articulation for the parasol-spines and is proximal to the arm spines (Figures 7C, 8D). The distal side of the lateral arm plate forms a fragile ledge with a distalward pointing ventral extension, which separates all arm spine articulations from the distal edge, and further encloses a dorsal arm plate. The ventral arm plates are elongated and are rounded to rectangular, wider than long. The disk has a high conical shape, set with rows of minute spines between the arms, each spine on one disc plate. The disc plates are irregular, round, and large (Figure 9A). No radial shields visible, which may account for the fact that the arms are raised vertically, encircling the high disk like a basket. Three to four pointed, slightly flattened, serrated arm-spines nearly as long as an arm segment, standing near the outer edge of lateral arm-plate and on a low spine-ridge, reduced to one spine in a row with the parasol-spines on distal arm segments (Figures 7C, D, 9A, C). The articulations of the parasol-spines are arranged in three to four visible rows (Figures 7C, 9C). From the third joint, there is a cluster up to 15, minute, parasol-spines on the inner side of spine-ridge, varying in length from 0.1 to 0.5 mm (Figures 7A, 9B–D). The number increases distally. They are shaped like long-handled parasols, with an elongate shaft, surmounted by a disk with two rings of alternating pointed teeth, with up to 15 in each ring, and with a slight bulb at the base, which is inserted into articulations on the spine-ridge (Figure 7A). Each tentacle pore on the first joints has one long, spine-like scale on its inner edge, about half the length of the arm spines (Figure 9A). The arm vertebrae are longer than wide, with small, fragile and oval muscle attachments but long appendages that serve to connect with other ossicles. The length to width ratio increases distally (Figure 7F).
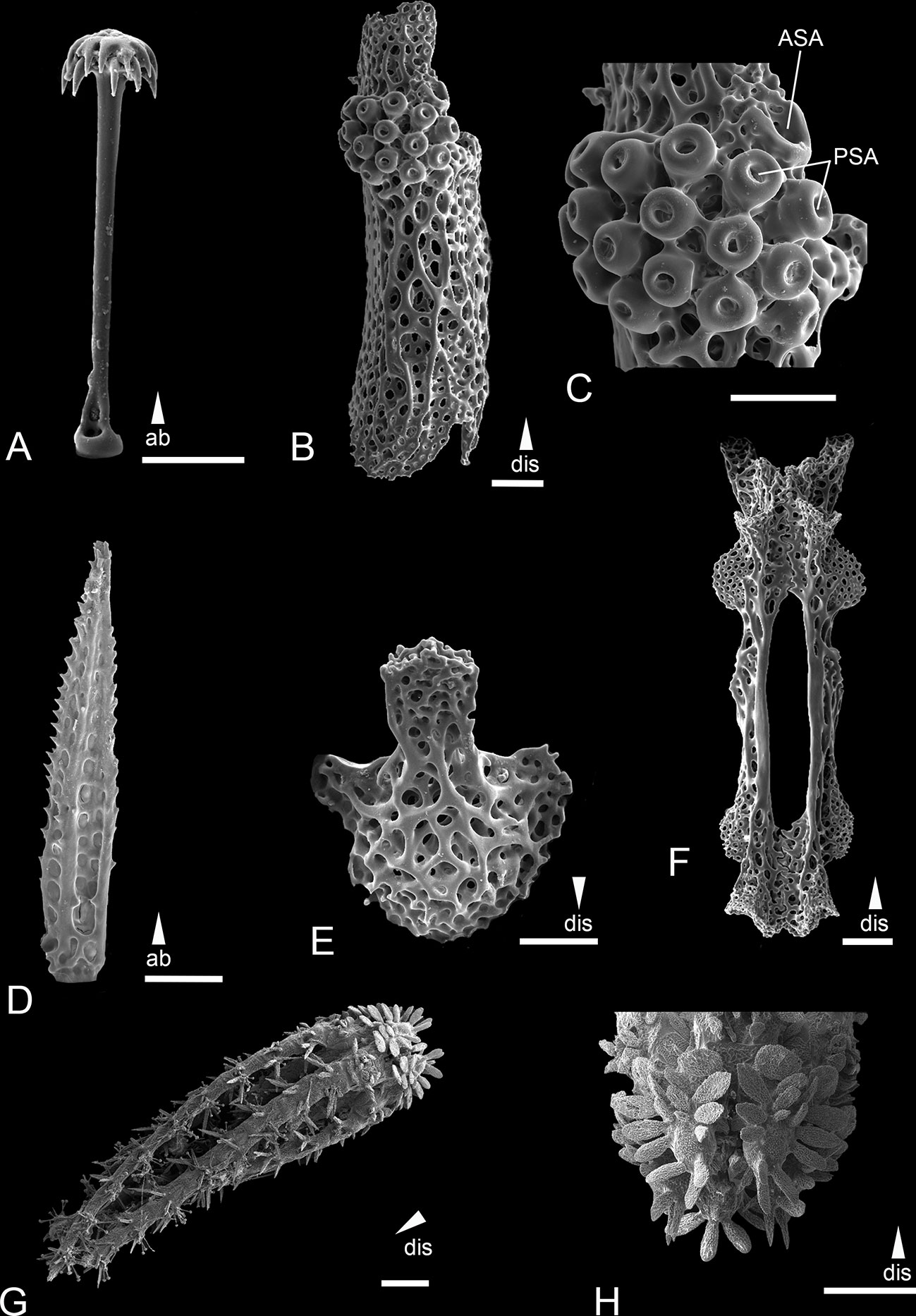
Figure 7 Scanning electron microscope images of the ossicles from Ophiotholia saskia sp. nov. (Holotype; SMF6909). (A): Parasol-spine; (B): Lateral arm plate; (C): Close up lateral arm plate; (D): Arm spine; (E): Ventral arm plate; (F): Arm Vertebrae; (G): Habitus; (H): Jaws. Scales: 50 µm (C); 100 µm (A, D, E); 200 µm (B, F); 500 µm (H); 1 mm (G). ab, abradial; ASA, arm spine articulation; dis, distal; PSA, parasol spine articulation.
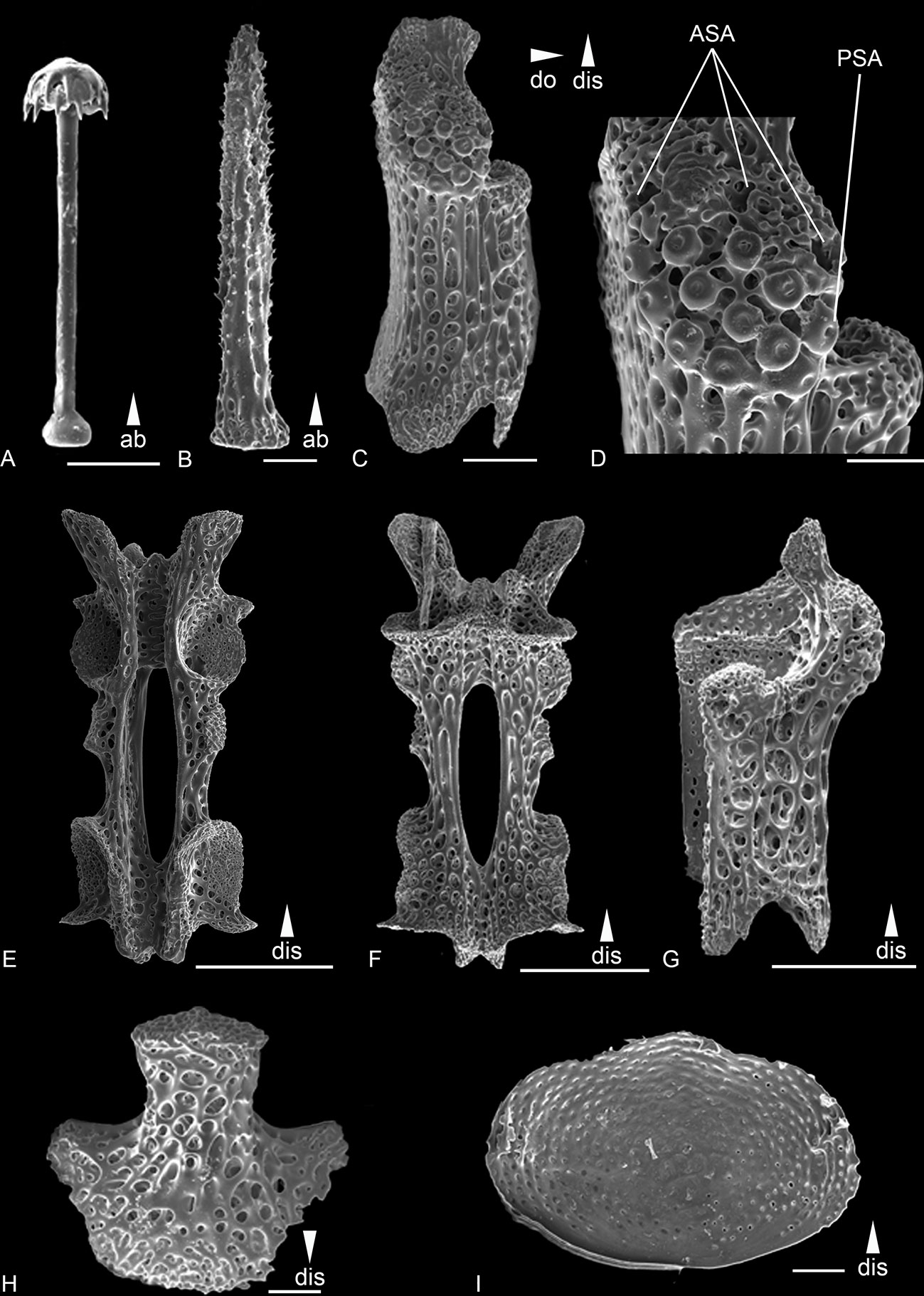
Figure 8 Scanning electron microscope images of the ossicles from Ophiotholia saskia sp. nov. (AB2_EB1_13_21). (A): Parasol-spine; (B): Arm spine; (C): Lateral arm plate lateral; (D): Close up lateral arm plate; (E): Arm Vertebrae ventral; (F): Arm Vertebrae dorsal; (G): Lateral arm plate dorsal; (H): Ventral arm plate. (I) dorsal arm plate ventral side. Scales: 50 µm (A, B, D, H, I); 200 µm (C, E–G);. ab, abradial; ASA, arm spine articulation; dis, distal; PSA, parasol spine articulation.
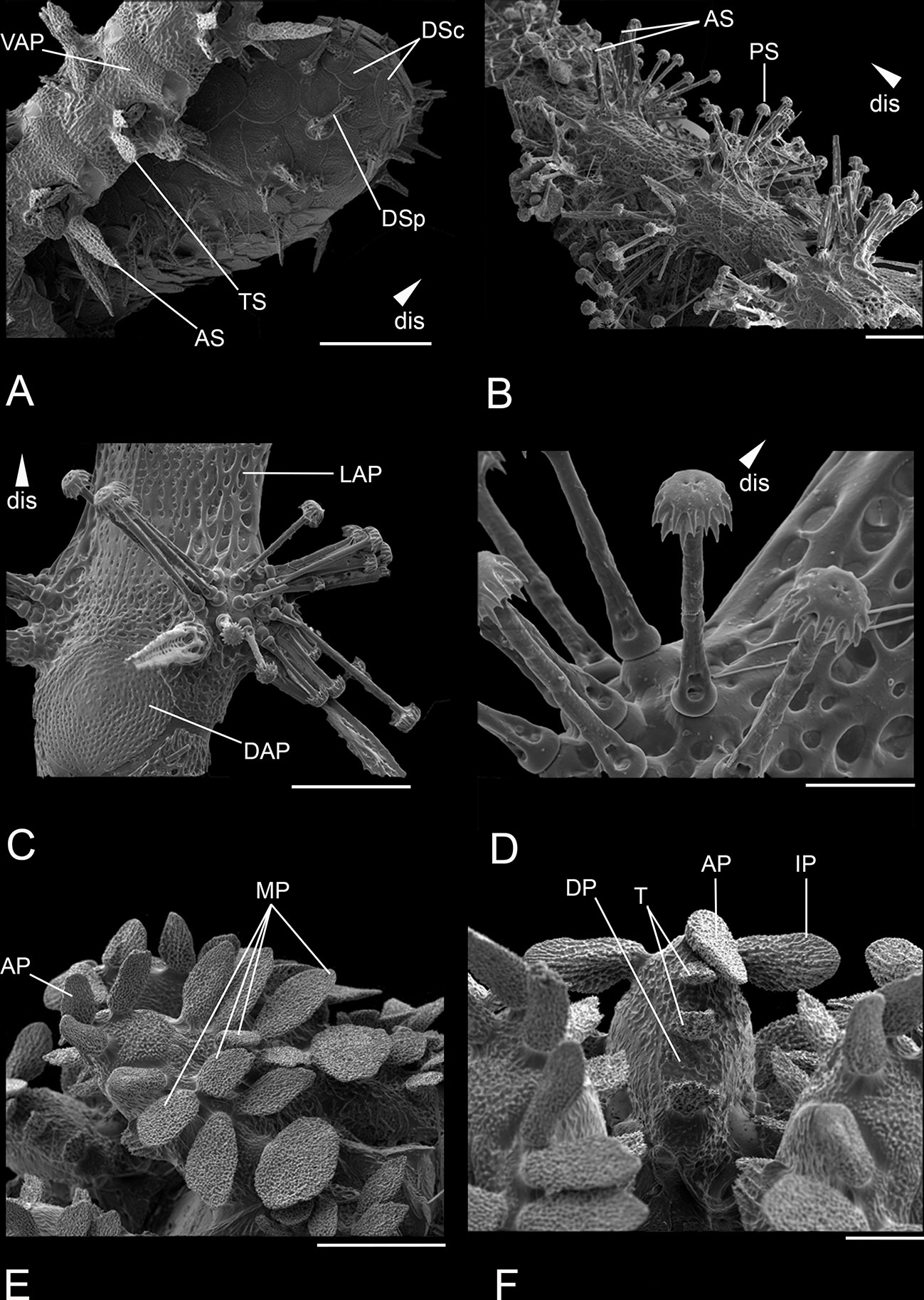
Figure 9 Scanning electron microscope images of close ups from the habitus of Ophiotholia saskia sp. nov. (SO240_46_5). (A): Disc with plates and proximal part of an arm; (B): Distal part of an arm; (C): Set up of an arm segment; (D): Set up of the articulations with inserted parasol-spines; (E): Set up of a jaw with papillae; (F): Teeth and papillae. Scales: 30 µm (C); 80 µm (F); 200 µm (A, E); 400 µm (B); 1 mm (D). ab, abradial; AP, apical papillae; AS, arm spine; ASA, arm spine articulation; DAP, dorsal arm plate; DP, dental plate; dis, distal; DSc, disc scale; DSp, disc spine; IP, infradental papillae; LAP, lateral arm plate; MP, mouth papillae; PS, parasol spine; PSA, parasol spine articulation; T, tooth; TS, tentacle scale; VAP, ventral arm plate.
Distribution. The species is only known so far from the Clarion Clipperton Zone in depths ranging from 4093 m to 4406 m (Christodoulou et al., 2020).
Remarks: The new species can be separated from the other species of the genus by the numerous parasol-spines which are found in clusters of up to 15, the highest number of parasol spines found in the genus. The tips of the parasol-spines have two rings of teeth, which also occur in O. odissea and O. montana, whereas O. spathifer, O. supplicans, O. mitrephora, and O. gibbosa just have one ring. The new species is most similar to O. montana based on the shape and arrangement of the apical- and infradental papillae and the two rings of teeth on the parasol spines, but it can be separated by the arrangement of the distal oral papillae. Ophiotholia saskia sp. nov. has two rows of broad and flat mouth papillae on each side while there is a single row of rectangular mouth papilla in O. montana. Further, the two species can be separated by the number of parasol spines, whereas O. saskia sp. nov. has up to 15 per lateral arm plate whereas O. montana has three (Litvinova, 1981).
Ophiotholia spathifer (Lyman, 1879).
Figure 10
Ophiomyces spathifer Lyman, 1879: 47, Plate 14 Figs 386–388a. — Lyman, 1882: 240, Plate 19, Figs 10–12. — Matsumoto 1917: 99. — Koehler, 1904: 101.
Ophiotholia multispina Koehler 1904: 99, Plate 33, Figs. 4, 5. — McKnight, 1967: 210, Figs. 4, 5. — Litvinova, 1992: 50, Pate 1, Figs A, B, b, D, H, M, O; Plate 2, Figs A–Γ, Η.
Ophiotholia spathifer — Fujita et al., 2010: 195, Fig. 6 — Thuy et al., 2012: 3, Figs. 2D, F.
Material: ZMMU D-1014 (1 spm): R/V Shtokman stn 2019, Pacific Ocean, 25°5.7’S 99°27.7’W, 750 m, 07/05/1987; ZMMU D-1015 (1 spm): Vityaz stn 3540, Pacific Ocean, 30°45.8’N 128°04.08’E, 500 m, 01/11/1955.
Distribution. Pacific Ocean. Previously known from off Japan, off New Caledonia, off Eastern Australia and the Indonesian archipelagos at depths of 216–1033 m (Lyman, 1879; Lyman, 1882; Koehler, 1904; McKnight, 1967; O’Hara unpublished material).
Remarks: The species was described by Lyman in, 1879 under the name Ophyomyces spathifer and was later transferred to the genus Ophiotholia by Litvinova, 1992 and synonymized with O. multispina. Fujita et al. (2010) have abolished the synominisation and assigned Ophiotholia spatifer as a species. Ophiotholia spathifer has six arm spines (Figure 10E), which are gradually replaced distally from the 15th arm joint by the parasol spines (Figures 10A, B). The parasol spines have one ring of thin pointed teeth (Figure 10C). The dorsal arm plate has a triangular shape (Figure 10F), whereas the ventral arm plate is rounded triangular, with convex distal edge and a thinner proximal lobe (Figure 10D). There are two tentacle scales, one on each side of the tentacle pore.
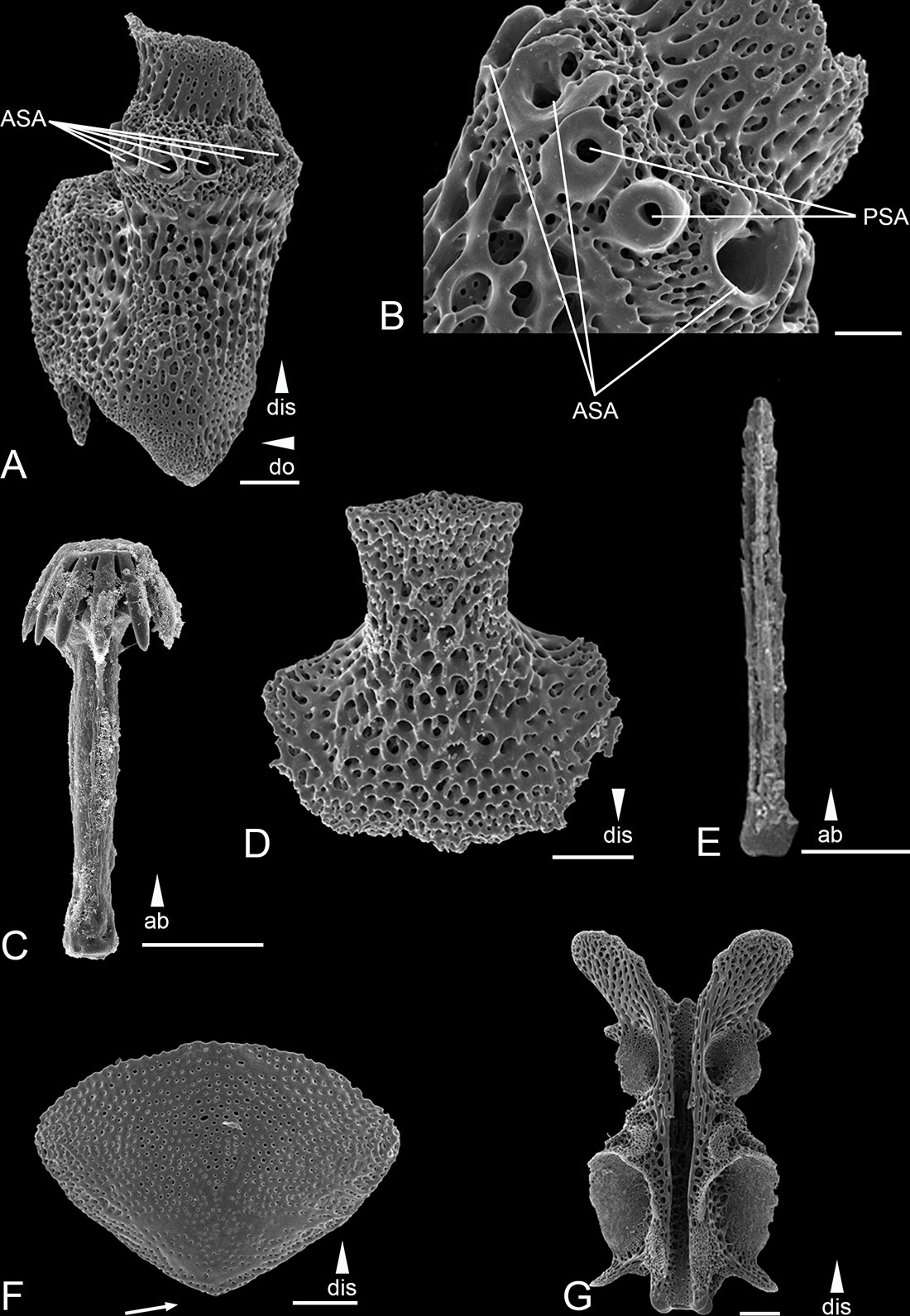
Figure 10 Scanning electron microscope images of the ossicles from Ophiotholia spathifer. (A): Lateral arm plate; (B): Close up lateral arm plate; (C): Parasol-spine; (D): Ventral arm plate; (E): Arm spine; (F): Dorsal arm plate; (G): Arm Vertebrae. Scales: 50 µm (D); 200 µm (B, C, F); 500 µm (A, E). ab, abradial; ASA, arm spine articulation; dis, distal; PSA, parasol spine articulation.
Ophiotholia supplicans Lyman, 1880
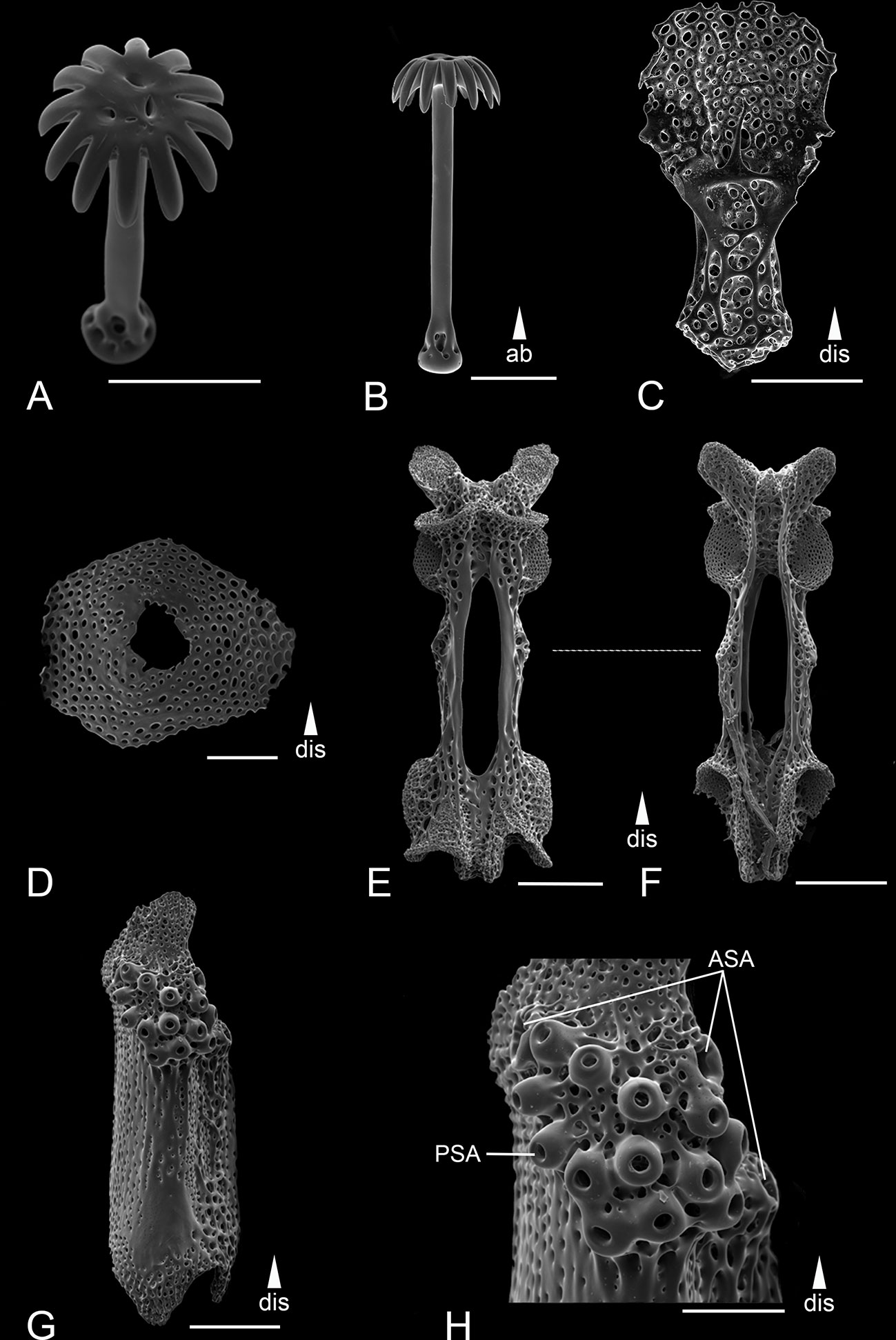
Figure 11 Scanning electron microscope images of the ossicles from Ophiotholia supplicans. (A): Head of parasol-spine; (B): Parasol-spine; (C): Ventral arm plate; (D): Dorsal arm plate; (E): Arm vertebrae (ventral); (F): Arm vertebrae (dorsal); (G): Lateral arm plate; (H): Close up lateral arm plate. Scales: 100 µm (A–D, H); 200 µm (E–G). ab, abradial; ASA, arm spine articulation; dis, distal; PSA, parasol spine articulation.
Ophiotholia supplicans Lyman, 1880: Fig. 5 (1-4). — H.L. Clark, 1915: 221. — Litvinova, 1992: 50.
Material:
Holotype. Lyman, 1880, Challenger stn. 296, 38° 6’ S., 88° 2’W, 1825 fathoms (~3300 m), NHM 1882.12.23.429.
Non type material: see Supplement Table 1.
Distribution: This species was reported from the southeast Pacific off Juan Fernandez Island in depths of 3285-4822 m and from the Clarion Clipperton Zone in depths ranging from 4093 m to 4406 m (Christodoulou et al., 2020).
Remarks: The species was used as the type species for Ophiotholia by Lyman in 1880 because of the uniqueness of its parasol-spines. Unfortunately, the holotype is so damaged that not all characters could be checked and consequently the type figures were also used to identify diagnostic characters. Ophiotholia supplicans can be distinguished from the other species by the following: The disc has a high cone shape and is sparsely covered by minute spines, which seem to stand on a small, fine disc scale. It has three arm spines, equal to the length of one arm segment. They are on the same flange of the lateral arm plate as the parasol spines (Figure 11H). The parasol-spine articulations visible are not in defined rows (different to all other species). The parasol-spines have a short shaft and possesses a single ring of widely separated, blunt teeth (Figures 11A, B). The ventral arm plate is elongated with a long and round structure on the distal side. The dorsal arm plate has an oval to triangle shape (Figure 11D). There is one thin and pointed apical papillae in a line with three to four lateral oral papillae of the same shape. The outermost three to four mouth papillae are large, flat, paddle-shaped, and arranged in two rows on each jaw. The adoral shield appear in an oval shape distal to the mouth papillae. The coexisting new species O. saskia sp. nov. differs in having two rings of parasol teeth and two rows of distal lateral oral papillae.
3.2. Species delimitation and genetic divergence
A total of 78 sequences were used for the genetic analyses of which 20 were novel sequences (BOLD dataset: DS-CCZOTHOL, 68 sequences; GenBank: 10 sequences) and ranging from 597 bp to 658 bp in length (87% have a length of 658 bp). These barcodes clustered into two clades in the baysian tree corresponding to the two species O. saskia sp. nov. and O. supplicans (Figure 12). The clustering is supported by a posterior probability value above 99%. Mean interspecific p-distance between the two species was 23.7% (σ=0.016) ranging from 21.7% to 24.9%, while mean intraspecific variability was 5% for O. supplicans and 1% for O. saskia sp. nov. So, a barcoding gap is recognizable. The BINs and GMYC both resulted in seven groups (Figure 12). Both methods also show the separation between the morphological assigned clusters O. saskia sp. nov. and O. supplicans. Within the cluster of O. saskia sp. nov. all specimens were considered to be one group. The cluster of O. supplicans is divided into six groups, one large group represented by seven specimens, three groups with two specimens and two singletons.
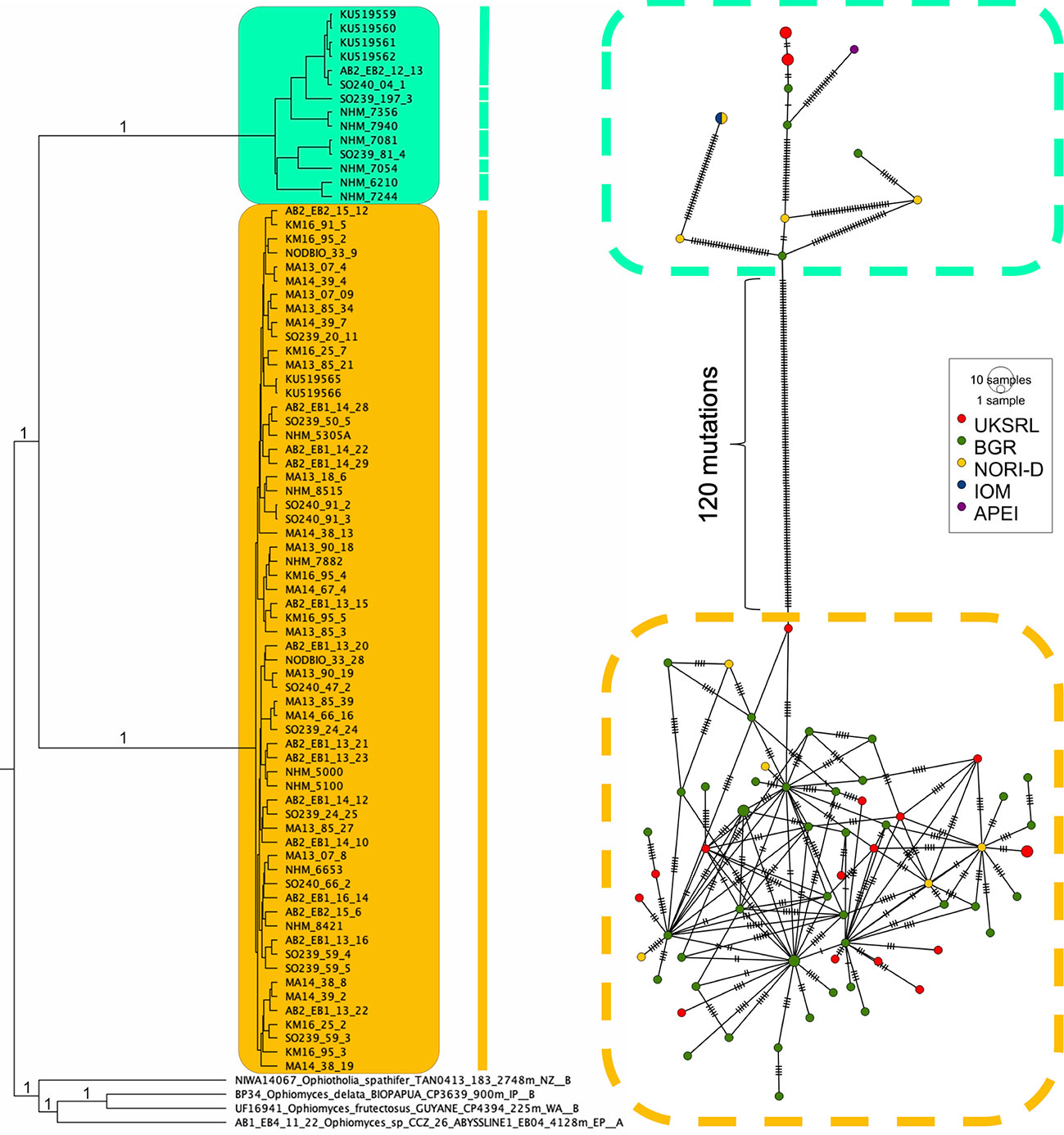
Figure 12 Ultrametric tree resulting from the Bayesian analysis of the CCZOTHOL dataset. The posterior support (≥ 99%) is indicated at the branch. The results of both species delimitation analyses (BINs, GMYC), are shown through the bar. The tree is combined with the haplotype network of Ophiotholia saskia sp. nov. and Ophiotholia supplicans; produced by PopART (Leigh and Bryant, 2015). The haplotype network is based on COI data of 75 samples. The size of the circle indicates the number of haplotypes. The colours are assigned to the different areas in the CCZ (red = UKSRL; green = BGR; yellow = NORI-D, blue = IOM, violet = APEI). The dashed boxes around each subnetwork indicate the morphological clusters in the Neighbour-Joining tree (Turquoise = Ophiotholia supplicans; Yellow= Ophiotholia saskia).
A total of 59 and 11 haplotypes were recovered out of the 62 and 14 individuals of O. saskia sp. nov. and O. supplicans respectively (Figure 12). The haplotype diversity (Hd) in the medium joining network was π = 0.0898503. The haplotypes of O. saskia sp. nov. were found in three different CCZ mining license areas (UKSRL, BGR and NORI-D), while haplotypes of O. supplicans were found in four different license areas (UKSRL, BGR, IOM and NORI-D) and one APEI (APEI3). The number of mutations separating the two species was 120. It should be noted that the haplotypes of O. supplicans are separated by a higher number of mutations (2-33) than the haplotypes of O. saskia sp.nov. (max. six mutations between haplotypes).
4. Discussion
In the current study, a new species of Ophiotholia from the CCZ is described using an consolidated taxonomic approach (morphological and molecular analyses). The remaining six species of the genus are revised based on morphological data and genetic data (for O. supplicans). Only freshly collected material belonging to O. saskia sp. nov. and O. supplicans was suitable for molecular work. Specimens of the five other known Ophiotholia species were too old or not properly preserved for sequencing. The holotype of O. supplicans was too damaged and fragile for tissue sampling or detailed morphological analyses. The presence of “one-ringed parasol spines” and the structure of the oral part of the species “Ophiotholia sp.28” (as reported by Christodoulou et al., 2020) is consistent with the drawings of Lyman (1880) in its original description. Consequently, we can confirm that “Ophiotholia sp. 28” is Ophiotholia supplicans. Additionally, Christodoulou et al. (2020) suggest the occurrence of a third Ophiotholia species (“Ophiotholia sp.41”) in the CCZ. However, because of the degraded condition and young age (postlarvae) of the specimens investigated, it was not possible to morphologically verify whether it is a known or new species, and its potential description as a new species will have to await the collection of more complete and adult specimens. The morphological study could show for the first time that there is a connection of each parasol-spines with the interior system of the ophiuroids (Figure 2). We suggest that it is a muscular connection, which allows the specimens to move the spines. The purpose of these spines is still unclear. In freshly collected specimens, of O. spathifer a curtain of mucus or a thin skin enclosing each parasol-spine was observed (personal observations Eichsteller and O’Hara). The parasol-spines could therefore have a respiratory or sensatory purpose, as they occur on the tips of the arms which are outside the sediment while the rest of the animal lives buried inside the sediment.
Species delimitation methods based on the COI gene have been widely used for ophiuroid species (e.g.Ward et al., 2008; Khodami et al., 2014; Boissin et al., 2017; O’Hara et al., 2019; Christodoulou et al., 2020; Eichsteller et al., 2022). The methods used (GMYC, BIN) corroborated the morphological distinction of O. saskia sp. nov. and O. supplicans in the present study. The separation of these two clades was well supported in the phylogenetic tree (posterior probability >99%). We also highlighted the presence of a barcode-gap (higher interspecific genetic distances compared to the intraspecific ones) as well as a high number of mutations between the two species in the haplotype network. The extended dataset “CCZOTHOL” did not support the third Ophiotholia species “Ophiotholia sp.41” found in Christodoulou et al. (2020) but instead included it in the cluster of O. supplicans. BOLD and GMYC have recovered six subdivisions within O. supplicans implying the presence of more species. However, it has been shown in previous studies (Boissin et al., 2017; Christodoulou et al., 2020) that GMYC and BIN can overestimate the number of species. The low intra-cluster divergence (2.2%) at the initial cluster step of refined single linkage (RESL) in BIN methodology (Ratnasingham and Hebert, 2013; Song et al., 2018) could be the reason why in some cases the BIN method overestimated species number. Furthermore, it can be that GMYC has not performed optimally as the ultrametric tree was estimated with a single locus. Finally, no morphological differences were found that could justify the presence of more species. While the mean intraspecific genetic variability within O. supplicans is 5% and, although arguable significantly higher than O. saskia which is 1%, it’s still within the limits of intraspecific variability in Ophiuroidea (Khodami et al., 2014; Boissin et al., 2017; Christodoulou et al., 2020), while species with higher intraspecific values are known from previous studies (eg. Layton et al., 2016; Boissin et al., 2017). The few individuals within these subdivisions come from different study areas. It has been shown in other studies that specimens of the same species can have a high variation in the haplotype analyses over a short distance (Pérez-Portela et al., 2013; Galaska et al., 2017; Weber et al., 2019). Additionally, the haplotype network of the specimens belonging to O. saskia sp. nov. shows a high haplotypes diversity, thus it can be expected that O. supplicans has a similar high haplotype diversity as well. To avoid unnecessarily splitting, we follow a conservative approach and classify the Ophiotholia specimens found in CCZ and, which are not O. saskia, as one species under O. supplicans until more data support otherwise. It is possible that our dataset of O. supplicans is too small to exhibit a stable number of species within this subdivision. Even if there is an accumulation of individuals aligning with species of Ophiotholia sp. 41 (Christodoulou et al., 2020), this subdivision is still closely related to O. supplicans. In many cases the animals were immature and damaged (sometimes only a single arm) which limited our ability to use morphology. A larger dataset is required to better resolve all the species boundaries within Ophiotholia.
Understanding the diversity of seafloor habitats and the associated fauna is critical to making informed decisions about deep-sea conservation (Brix et al., 2019; McQuaid et al., 2020; Jones et al., 2021; Washburn et al., 2021). In the CCZ, the possible extraction of the abundant and valuable nodules could not only result in loss of substratum but will also result in seabed upheaval and possible biodiversity loss, and perhaps an ongoing effect on the(Ratnasingham and Hebert, 2013) local food web (Levin et al., 2016; Niner et al., 2018; Brix et al., 2019; Simon-Lledó et al., 2019a; Stratmann et al., 2021). Although ophiuroids are reported to take the role of the “generalist” in recolonization after mining disturbance, this refers mostly to the large, mobile, deposit feeders recognized in image based analyses (Jones et al., 2017; Simon-Lledó et al., 2019a; Simon-Lledó et al., 2019b). Knowledge at species level is important to determining disturbance impacts. Species of the class Ophiuroidea represent all kinds of feeding types and show different lifestyles (e.g. Pearson and Gage, 1984; O’Hara et al., 2008; Mosher and Watling, 2009; Stöhr et al., 2012; Laming et al., 2021; Manjón-Cabeza et al., 2021). So, it is incorrect to generalize the behavior of the whole class. The smaller and fragile specimens of the genus Ophiotholia are living buried in the upper layer of the seafloor, with their arms erected into the water column. This behavior suggests a less mobile and possibly filter feeding lifestyle, as likely in other sessile fauna (Lyman, 1880; Litvinova, 1992). The impact on the sediment redeposition on the surrounding benthic fauna is not well understood, (Jones et al., 2017; Simon-Lledó et al., 2019a; Stratmann et al., 2021), so it is unclear what will happen to the benthic communities, including the Ophiotholia species. But it is most likely that not only the targeted area but also surrounding areas will be influenced by the sediment plume, for example benthic communities could be smothered or nutrients could be introduced to an otherwise nutrient poor area and hence alter the local community (Simon-Lledó et al., 2019a; Washburn et al., 2021). There is also a risk from mobilized toxic metals and machinery contaminates in- and outside the mining area (Peukert et al., 2018). In 1989 an area off Peru was disturbed in 4150 m during an intensive disturbance study DISCOL (disturbance and recolonization experiment, Thiel and Schriever, 1990). After revisiting the site more than 20 years later, studies on the biodiversity show little recovery in the disturbed area and only the presence of some mobile species, including large ophiuroids (Miljutin et al., 2011; Simon-Lledó et al., 2019a). Although not all circumstances of habitat condition and data collection are the same in both areas (CCZ and DISCOL), similar recovery can be expected. Thus, mobile, deposit feeding ophiuroids may recover, but ophiuroids with a different lifestyle, like species of Ophiotholia might not resettle.
To accurately assess the risk of species extinctions from large-scale mining, the knowledge of the taxonomy, genetic structure, biogeography and basic natural history of deep-sea species is crucial. In a recent review (Engel et al., 2021) the “Taxonomic impediment” is discussed and shows the need for additional taxonomic work in the time of biodiversity loss and study of lesser known habitats. Our study provides a better understanding of the specimens of the genus Ophiotholia as well as contributing to the list of species that occur in the CCZ and thus to the assessment of biodiversity in the area. A well-established taxonomic background supports the identification of common benthic communities with possible indicator species and can improve the models on e.g., food web analyses or ecosystem changes (Stratmann et al., 2021). Species descriptions and barcoding are also essential to informing future eDNA and imaging studies, as well as contributing to large scale analyses as shown in Woolley et al. (2021) and Uhlenkott et al. (2022).
Data availability statement
The datasets presented in this study can be found in online repositories. The names of the repository/repositories and accession number(s) can be found below: GenBank, OP575327-OP575373, OQ152220, OQ152221, and in the Barcode of Life Database: doi: dx.doi.org/10.5883/DS-CCZOTHOL.
Author contributions
The present study was conceptualized by AE, TO’H, MC and PMA. PMA provided the samples and acquired the funding for this project. GB-C also provided samples from the NHM London. AE, MC and GB-C planned and conducted all laboratory experiments. AE obtained the SEM images and configurated the plates of all species. AM and TK executed the data curation and SEM data obtaining of the specimens from the ZMMU. AE did the analyzing of the molecular data. TO’H and AM contributed with their taxonomic expertise in Ophiuroidea. AE wrote the initial draft of the manuscript; all authors contributed to the final version of the manuscript. All authors contributed to the article and approved the submitted version.
Funding
Research for this article was funded by Deutsche Forschungsgemeinschaft DFG, grant number MA2557/12-1 within the priority program “Taxon-Omics: New Approaches for Discovering and Naming Biodiversity” (SPP 1991). As well as funded by the EU JPIO-Oceans project MinigImpact-2 (German BMBF contract 03F0707E and 03F0812E). This is publication number 93 from the Senckenberg am Meer Molecular and Metabarcoding Laboratory. Also, funding was received from UK Seabed Resources and The Metals Company to the Natural History Museum, NORCE and University of Gothenburg to support the collection of samples and DNA sequencing made available to this study. GB-C has received support from TMC the metals company Inc. (The Metals Company) through its subsidiary Nauru Ocean Resources Inc. (NORI). NORI holds exploration rights to the NORI-D contract area in the CCZ regulated by the International Seabed Authority and sponsored by the government of Nauru. This is contribution TMC/NORI/D/005. The work of AM was performed under a research project of the MSU Zoological Museum (18-1-21 No. 121032300105-0). The work of TK was conducted under the Koltzov Institute of Developmental Biology RAS (IDB) basic research program in 2021 (No. 0088-2021-0019).
Acknowledgments
The authors would like to thank the crew and masters of all collecting expeditions (Abyssline 1 and 2, Mangan 2013, Mangan 2014, NODBIO, SO239 and SO240), in particular Carsten Rühlemann and Annemiek Vink for making the material of the BGR cruises available. A huge thank also goes to the team around Adrian G. Glover (NHM London) and Thomas G. Dahlgren (University Gothenburg) for providing supplementary specimens, data and scientific exchange. In particular, we would like to thank Helena Wiklund, who did some of the lab work. We would also like to thank Franziska Iwan (Senckenberg am Meer) for assistance in taking the SEM images. Jonathan Ablett (NHM London) for his assistance of the preparation of the Holotype from Ophiotholia supplicans. We also would like to thank Dr. Camille Moreau for his helpful input and suggestions on the manuscript. Finally, a special thanks to Wadim Jan for assisting the first author to translate Russian taxonomic papers.
Conflict of interest
The authors declare that the research was conducted in the absence of any commercial or financial relationships that could be construed as a potential conflict of interest.
Publisher’s note
All claims expressed in this article are solely those of the authors and do not necessarily represent those of their affiliated organizations, or those of the publisher, the editors and the reviewers. Any product that may be evaluated in this article, or claim that may be made by its manufacturer, is not guaranteed or endorsed by the publisher.
Supplementary material
The Supplementary Material for this article can be found online at: https://www.frontiersin.org/articles/10.3389/fmars.2023.1056282/full#supplementary-material
References
Amon D., Ziegler A., Dahlgren T., Glover A., Goineau A., et al. (2016). First insights into the abundance and diversity of abyssal megafauna in a polymetallic-nodule region in the eastern Clarion-Clipperton Zone. Sci. Rep. 6:30492. doi: 10.1038/srep30492
Amon D. J., Ziegler A. F., Kremenetskaia A., Mah C. L., Mooi R., O'Hara T., et al. (2017). Megafauna of the UKSRL exploration contract area and eastern clarion-clipperton zone in the pacific ocean: Echinodermata. Biodiversity Data J. 5).
Armstrong C. W., Foley N. S., Tinch R., Hove S. V. D. (2012). Services from the deep: Steps towards valuation of deep sea goods and services. Ecosyst. Serv. 2, 2–13. doi: 10.1016/j.ecoser.2012.07.001
Boissin E., Hoareau T. B., Paulay G., Bruggemann J. H. (2017). DNA Barcoding of reef brittle stars (Ophiuroidea, Echinodermata) from the southwestern Indian ocean evolutionary hot spot of biodiversity. Ecol. Evol. 7, 11197–11203. doi: 10.1002/ece3.3554
Bonatti E., Nayudu Y. R. (1965). The origin of manganese nodules on the ocean floor. Am. J. Sci. 263 (1), 17–39.
Bonifácio P., Martínez Arbizu P., Menot L. (2020). Alpha and beta diversity patterns of polychaete assemblages across the nodule province of the eastern clarion-clipperton fracture zone (equatorial pacific). Biogeosciences 17 (4), 865–886.
Brenke N. (2005). An epibenthic sledge for operations on marine soft bottom and bedrock. Mar. Technol. Soc. J. 2).
Bribiesca-Contreras G., Dahlgren T. G., Amon D. J., Cairns S., Drennan R., Durden J. M., et al. (2022). Benthic megafauna of the western clarion-clipperton zone, pacific ocean. ZooKeys 1113).
Bribiesca-Contreras G., Verbruggen H., Hugall A. F., O'Hara T. D. (2019). Global biogeographic structuring of tropical shallow-water brittle stars. J. Biogeography 46 (7), 1287–1299.
Brix S., Osborn K. J., Kaiser S., Truskey S. B., Schnurr S. M., Brenke N., et al. (2019). Adult life strategy affects distribution patterns in abyssal isopods – implications for conservation in pacific nodule areas. Biogeosciences 17 (23), 6163–6184. doi: 10.5194/bg-2019-358
Buhl-Mortensen L., Vanreusel A., Gooday A. J., Levin L. A., Priede I. G., Buhl-Mortensen P., et al. (2010). Biological structures as a source of habitat heterogeneity and biodiversity on the deep ocean margins. Mar. Ecol. 31 (1), 21–50.
Cho W., Shank T. M. (2010). Incongruent patterns of genetic connectivity among four ophiuroid species with differing coral host specificity on north Atlantic seamounts. Mar. Ecol. 31, 121–143.
Christodoulou M., O’Hara T. D., Hugall A., Khodami S., Rodrigues C. F., Hilario A., et al. (2020). Unexpected high abyssal ophiuroid diversity in polymetallic nodule fields of the northeast pacific ocean, and implications for conservation. Biogeosciences Discuss 17 (7), 1845–1876. doi: 10.5194/bg-2019-360
Christodoulou M., O’hara T. D., Hugall A. F., Arbizu P. M. (2019). Dark ophiuroid biodiversity in a prospective abyssal mine field. Curr. Biol. 29 (22), 3909–3912.
Clark H. L. (1915). Catalogue of recent ophiurans: based on the collection of the museum of comparative zoölogy.
Durden J. M., Putts M., Bingo S., Leitner A. B., Drazen J. C., Gooday A. J., et al. (2021). Megafaunal ecology of the western clarion clipperton zone. Front. Mar. Sci. 8, 671062.
Eichsteller A., Taylor J., Stöhr S., Brix S., Martìnez Arbizu P. (2022). DNA Barcoding of cold-water coral-associated ophiuroid fauna from the north Atlantic. Diversity 14, 1–16. doi: 10.3390/d14050358
Engel M. S., Ceríaco L. M. P., Daniel G. M., Dellapé P. M., Löbl I., Marinov M., et al. (2021). The taxonomic impediment: A shortage of taxonomists , not the lack of technical approaches. Zool. J. Linn. Soc 193, 381–387. doi: 10.1093/zoolinnean/zlab072
Fujisawa T., Barraclough T. G. (2013). Delimiting species using single-locus data and the generalized mixed Yule coalescent approach: a revised method and evaluation on simulated data sets. Systematic Biol. 62 (5), 707–724.
Fujita T., Ishida Y., Irimura S. (2010). “Ophiacanthidae (Echinodermata, ophiuroidea) collected from the sagami Sea, central Japan: A preliminary report,” in Echinoderms: Proceedings of the 12th international echinoderm conference. Ed. Durham-Harris (London: Taylor & Francis), 193–197.
Galaska M. P., Sands C. J., Santos S. R., Mahon A. R., Halanych K. M. (2017). Geographic structure in the southern ocean circumpolar brittle star ophionotus victoriae (ophiuridae) revealed from mtDNA and single- nucleotide polymorphism data. Ecology and evolution 475–485. doi: 10.1002/ece3.2617
Galaska M. P., Li Y., Kocot K. M., Mahon A. R., Halanych K. M. (2019). Conservation of mitochondrial genome arrangements in brittle stars (Echinodermata, ophiuroidea). Mol. Phylogenet. Evol. 130, 115–120.
Geller J., Meyer C., Parker M., Hawk H. (2013). Redesign of PCR primers for mitochondrial cytochrome c oxidase subunit I for marine invertebrates and application in all-taxa biotic surveys. Mol. Ecol. Resour. 13, 851–861. doi: 10.1111/1755-0998.12138
Gillman L. N., Wright S. D. (2014). Species richness and evolutionary speed: the influence of temperature, water and area. J. Biogeography 41 (1), 39–51.
Glover A. G., Wiklund H., Rabone M., Amon D. J., Smith C. R., O'Hara T., et al. (2016). Abyssal fauna of the UK-1 polymetallic nodule exploration claim, clarion-clipperton zone, central pacific ocean: Echinodermata. Biodiversity Data J. 4).
Halbach P., Fellerer R. (1980). The metallic minerals of the pacific seafloor. GeoJournal 4, 407–421.
Halfar J., Fujita R. M. (2002). Precautionary management of deep-sea mining. Mar. Policy 26 (2), 103–106.
Hunter R. L., Halanych K. M. (2008). Evaluating connectivity in the brooding brittle star astrotoma agassizii across the drake passage in the southern ocean. J. Heredity 99 (2), 137–148.
International Seabed Authority. (2020). Deep seabed minerals contractors. Available at: https://www.isa.org.jm/deep-seabed-minerals-contractors?qt-contractors_tabs_alt=0#qt-contractors_tabs_alt.
International Seabed Authority. (2021). Decision of the Council of the International Seabed Authority relating to the review of the environmental management plan for the Clarion-Clipperton Zone. Kingston, Jamaica.
Jones D. O. B., Kaiser S., Sweetman A. K., Smith C. R., Menot L., Vink A., et al. (2017). Biological responses to disturbance from simulated deep-sea polymetallic nodule mining. PLoS One 12, e0171750. doi: 10.1371/journal.pone.0171750
Jones D. O. B., Simon-Lledó E., Amon D. J., Bett B. J., Caulle C., Clément L., et al. (2021). Environment, ecology, and potential effectiveness of an area protected from deep-sea mining (Clarion clipperton zone, abyssal pacific). Prog. Oceanogr. 197, 102653. doi: 10.1016/j.pocean.2021.102653
Jossart Q., Sands C. J., Sewell M. A. (2019). Dwarf brooder versus giant broadcaster: combining genetic and reproductive data to unravel cryptic diversity in an Antarctic brittle star. Heredity 123 (5), 622–633.
Katoh K., Misawa K., Kuma K. I., Miyata T. (2002). MAFFT: A novel method for rapid multiple sequence alignment based on fast Fourier transform. Nucleic Acids Res. 30 (14), 3059–3066.
Kearse M., Moir R., Wilson A., Stones-Havas S., Cheung M., Sturrock S., et al. (2012). Geneious basic: an integrated and extendable desktop software platform for the organization and analysis of sequence data. Bioinformatics 28 (12), 1647–1649.
Khodami S., Martinez-Arbizu P., Stöhr S. (2014). Molecular species delimitation of icelandic brittle stars ( Echinodermata : Ophiuroidea ). Polish Polar Res. 35, 1–18. doi: 10.2478/popore
Kuhnz L. A., Ruhl H. A., Huffard C. L., Smith K. L. Jr. (2014). Rapid changes and long-term cycles in the benthic megafaunal community observed over 24 years in the abyssal northeast pacific. Prog. Oceanography 124, 1–11.
Laming S. R., Christodoulou M., Martinez Arbizu P., Hilário A. (2021). Comparative reproductive biology of deep-sea ophiuroids inhabiting polymetallic-nodule fields in the clarion-clipperton fracture zone. Frontiers in Marine Science. doi: 10.1101/2021.02.06.428832
Layton K. K. S., Corstorphine E. A., Hebert P. D. N. (2016). Exploring canadian echinoderm diversity through DNA barcodes. PLoS One 11, 1–16. doi: 10.1371/journal.pone.0166118
Leigh J. W., Bryant D. (2015). POPART: Full-feature software for haplotype network construction. Methods in ecology and evolution 6 (9), 1110–1116.
Lejzerowicz F., Gooday A. J., Barrenechea Angeles I., Cordier T., Morard R., Apothéloz-Perret-Gentil L., et al. (2021). Eukaryotic biodiversity and spatial patterns in the clarion-clipperton zone and other abyssal regions: Insights from sediment DNA and RNA metabarcoding. Front. Mar. Sci. 8, 671033.
Levin L. A., Mengerink K., Gjerde K. M., Rowden A. A., Van Dover C. L., Clark M. R., et al. (2016). Defining “serious harm” to the marine environment in the context of deep-seabed mining. Mar. Policy 74, 245–259. doi: 10.1016/j.marpol.2016.09.032
Litvinova N. M. (1981). “Ophiuroidea,” in Benthos of the submarine mountains Marcus -necker and adjacent pacific regions. Eds. Mironov A. N., Kuznetsov A. (Moscow: Academy of Sciences of the USSR P.P. Shirshov Institute of Oceanology), 113–130.
Litvinova N. M. (1992). Revision of the genus ophiotholia (Echinodermata, ophiuroidea). Zool. Zhjurnal 71, 47–57.
Ljungman A. V. (1867). Ophiuroidea viventia huc usque cognita enumerat. Öfversigt af Kongl. Vetenskaps-akademiens Forh. 23, 303–336.
Lütken C. (1869). “Additamenta ad historiam ophiuridarum,” in Beskrivende og kritiske bidrag til kundskab om slangestjernerne.
Lyman T. (1880). A new structural feature, hitherto unknown among Echinodermata, found in deep-Sea ophiurans. Anniv. Memories BostonSociety Nat. Hist. 50, 3–12.
Lyman T. (1869). Preliminary report on the ophiuridae and astrophytidae dredged in deep water between Cuba and the Florida reef. Bull. Museum Comp. Zool. 1, 309–354.
Lyman T. (1879). Ophiuridae and astrophytidae of the " challenger " expedition. Bull. Mus. Comp. Zool. 6, 17–84.
Lyman T. (1882). Report on the ophiuroidea dredged by HMS challenger during the years 1873-1876. Zoology 5, 1–386.
Manjón-Cabeza M. E., Ríos P., García-Guillén L. M., Macías-Ramírez A., Sánchez F., Rodríguez-Básalo A., et al. (2021). Asteroids and ophiuroids associated with sponge aggregations as a key to marine habitats. a comparative analysis between avilés canyons system and El cachucho, marine protected area. Front. Mar. Sci. 7. doi: 10.3389/fmars.2020.606749
Martynov A. (2010). Reassessment of the classification of the ophiuroidea (Echinodermata), based on morphological characters. i. general character evaluation and delineation of the families ophiomyxidae and ophiacanthidae. Zootaxa 2697 (1), 1–154.
Matsumoto H. (1917). A monograph of Japanese ophiuroidea, arranged according to a new classification. J. Coll. Science Imperial Univ. Tokyo 38, 1–408.
McKnight D. G. (1967). Additions to the echinoderm fauna of the chatham rise. New Z. J. Mar. Freshw. Res. 1 (3), 291–313.
McQuaid K. A., Attrill M. J., Clark M. R., Cobley A., Glover A. G., Smith C. R., et al. (2020). Using habitat classification to assess representativity of a protected area network in a Large, data-poor area targeted for deep-Sea mining. Front. Mar. Sci. 7. doi: 10.3389/fmars.2020.558860
Miljutin D. M., Miljutina M. A., Arbizu P. M., Galéron J. (2011). Deep-sea nematode assemblage has not recovered 26 years after experimental mining of polymetallic nodules (Clarion-clipperton fracture zone, tropical Eastern pacific). Deep Sea Res. Part I Oceanogr. Res. Pap. 58, 885–897. doi: 10.1016/j.dsr.2011.06.003
Mosher C. V., Watling L. (2009). Partners for life: A brittle star and its octocoral host. Mar. Ecol. Prog. Ser. 397, 81–88. doi: 10.3354/meps08113
Murray J., Renard A. F. (1891). Report on deep-sea deposits based on the specimens collected during the voyage of HMS challenger in the years 1872 to 1876. HM Stationery Office.
Niner H. J., Ardron J. A., Escobar E. G., Gianni M., Jaeckel A., Jones D. O. B., et al. (2018). Deep-sea mining with no net loss of biodiversity-an impossible aim. Front. Mar. Sci. 5. doi: 10.3389/fmars.2018.00053
O’Hara T. D., Hugall A. F., Stöhr S., Thuy B., Martynov A. (2018). Morphological diagnoses of higher taxa in ophiuroidea (Echinodermata) in support of a new classification. Eur. J. Taxonomy 2018 (416).
O’Hara T. D. (2007). Seamounts: Centres of endemism or species richness for ophiuroids? Global Ecol. Biogeography 16 (6), 720–732.
O’Hara T. D., Hugall A. F., Woolley S. N. C., Bribiesca-Contreras G., Bax N. J. (2019). Contrasting processes drive ophiuroid phylodiversity across shallow and deep seafloors. Nature 565, 636–639. doi: 10.1038/s41586-019-0886-z
O’Hara T. D., Rowden A. A., Williams A. (2008). Cold-water coral habitats on seamounts: Do they have a specialist fauna? Divers. Distrib. 14, 925–934. doi: 10.1111/j.1472-4642.2008.00495.x
Oebius H. U., Becker H. J., Rolinski S., Jankowski J. A. (2001). Parametrization and evaluation of marine environmental impacts produced by deep-sea manganese nodule mining. Deep Sea Res. Part II: Topical Stud. Oceanography 48 (17-18), 3453–3467.
Paterson G. L. (1985). The deep-sea ophiuroidea of the north Atlantic ocean. Bull. Br. Museum (Natural History) Zoology Ser. 49, 1–162.
Pearson M., Gage J. D. (1984). Diets of some deep-sea brittle stars in the rockall trough. Mar. Biol. 82, 247–258. doi: 10.1007/BF00392406
Pérez-Portela R., Almada V., Turon X. (2013). Cryptic speciation and genetic structure of widely distributed brittle stars (Ophiuroidea) in Europe. Zool. Scr. 42, 151–169. doi: 10.1111/j.1463-6409.2012.00573.x
Perrier E. (1891). Echinodermes de la mission scientifique du cap horn. Stellérides. Miss. Sci. Cap. Horn Zool 6, 1–168.
Peukert A., Schoening T., Alevizos E., Köser K., Kwasnitschka T., Greinert J. (2018). Understanding Mn-nodule distribution and evaluation of related deep-sea mining impacts using AUV-based hydroacoustic and optical data. Biogeosciences 15, 2525–2549. doi: 10.5194/bg-15-2525-2018
Pons J., Barraclough T. G., Gomez-Zurita J., Cardoso A., Duran D. P., Hazell S., et al. (2006). Sequence-based species delimitation for the DNA taxonomy of undescribed insects. Systematic Biol. 55 (4), 595–609.
Ramirez-Llodra E., Brandt A., Danovaro R., De Mol B., Escobar E., German C. R., et al. (2010). Deep, diverse and definitely different: Unique attributes of the world's largest ecosystem. Biogeosciences 7 (9), 2851–2899.
Ratnasingham S., Hebert P. D. N. (2013). A DNA-based registry for all animal species: The barcode index number (BIN) system. PLoS One 8 (7), e66213. doi: 10.1371/journal.pone.0066213
Rybakova E., Galkin S., Gebruk A., Sanamyan N., Martynov A. (2020). Vertical distribution of megafauna on the Bering Sea slope based on ROV survey. PeerJ 8, e8628.
Sayers E. W., Agarwala R., Bolton E. E., Brister J. R., Canese K., Clark K., et al. (2019). Database resources of the national center for biotechnology information. Nucleic Acids Res. 47 (Database issue), D23.
Simon-Lledó E., Bett B. J., Huvenne V. A. I., Köser K., Schoening T., Greinert J., et al. (2019a). Biological effects 26 years after simulated deep-sea mining. Sci. Rep. 9, 1–13. doi: 10.1038/s41598-019-44492-w
Simon-Lledó E., Bett B. J., Huvenne V. A. I., Schoening T., Benoist N. M. A., Jeffreys R. M., et al. (2019b). Megafaunal variation in the abyssal landscape of the clarion clipperton zone. Prog. Oceanogr. 170, 119–133. doi: 10.1016/j.pocean.2018.11.003
Smith C. R., Clark M. R., Goetze E., Glover A. G., Howell K. L. (2021). Biodiversity, connectivity and ecosystem function across the clarion-clipperton zone: A regional synthesis for an area targeted for nodule mining. Front. Mar. Sci. 8, 797516.
Song C., Lin X. L., Wang Q., Wang X. H. (2018). DNA Barcodes successfully delimit morphospecies in a superdiverse insect genus. Zool. Scr. 47, 311–324. doi: 10.1111/zsc.12284
Soetaert K., Mohn C., Rengstorf A., Grehan A., van Oevelen D. (2016). Ecosystem engineering creates a direct nutritional link between 600-m deep cold-water coral mounds and surface productivity. Sci. Rep. 6 (1), 35057.
Stöhr S., Martynov A. (2016). Paedomorphosis as an evolutionary driving force: insights from deep-sea brittle stars. PloS One 11 (11), e0164562.
Stöhr S., Weber A. A. T., Boissin E., Chenuil A. (2020). Resolving the ophioderma longicauda (Echinodermata: Ophiuroidea) cryptic species complex: five sisters, three of them new. Eur. J. Taxonomy 600).
Stöhr S., O’Hara T. D., Thuy B. (2012). Global diversity of brittle stars (Echinodermata : Ophiuroidea). Plos one 7. doi: 10.1371/journal.pone.0031940
Stratmann T., Soetaert K., Kersken D., van Oevelen D. (2021). Polymetallic nodules are essential for food-web integrity of a prospective deep-seabed mining area in pacific abyssal plains. Sci. Rep. 11, 1–11. doi: 10.1038/s41598-021-91703-4
Tamura K., Stecher G., Kumar S. (2021). MEGA11: Molecular evolutionary genetics analysis version 11. Mol. Biol. Evol. 38 (7), 3022–3027.
Taylor J., Krumpen T., Soltwedel T., Gutt J., Bergmann M. (2016). Regional-and local-scale variations in benthic megafaunal composition at the Arctic deep-sea observatory HAUSGARTEN. Deep Sea Res. Part I: Oceanographic Res. Papers 108, 58–72.
Thiel H., Schriever G. (1990). Deep-sea mining, environmental impact and the DISCOL project. Ambio 19, 245–250.
Thurber A. R., Sweetman A. K., Narayanaswamy B. E., Jones D. O., Ingels J., Hansman R. L. (2014). Ecosystem function and services provided by the deep sea. Biogeosciences 11 (14), 3941–3963.
Thuy B., Gale A. S., Kroh A., Kucera M., Numberger-Thuy L. D., Reich M., et al. (2012). Ancient origin of the modern deep-sea fauna.
Thuy B., Meyer C. A. (2013). The pitfalls of extrapolating modern depth ranges to fossil assemblages: New insights from middle Jurassic brittle stars (Echinodermata: Ophiuroidea) from Switzerland. Swiss J. Palaeontology 132 (1), 5–21.
Uhlenkott K., Simon-Lledó E., Martínez Arbizu P. (2022). Investigating the benthic megafauna in the eastern clarion clipperton fracture zone ( north − east pacific ) based on distribution models predicted with random forest. Sci. Rep. 12. Jg., Nr. 1, S. 8229. doi: 10.1038/s41598-022-12323-0
Vanreusel A., Hilario A., Ribeiro P. A., Menot L., Arbizu P. M. (2016). Threatened by mining, polymetallic nodules are required to preserve abyssal epifauna. Sci. Rep. 6 (1), 1–6.
Verrill A. E. (1899). North American ophiuroidea: I. revision of certain families and genera of West Indian ophiurans. II. a faunal catalogue of the known species of West Indian ophiurans (AE Verrill. Academy (printed by Tuttle, Morehouse and Taylor C).
Ward R. D., Holmes B. H., O’Hara T. D. (2008). DNA Barcoding discriminates echinoderm species. Mol. Ecol. 8, 1202–1211. doi: 10.1111/j.1755-0998.2008.02332.x
Washburn T. W., Menot L., Bonifácio P., Pape E., Błażewicz M., Bribiesca-Contreras G., et al. (2021). Patterns of macrofaunal biodiversity across the clarion-clipperton zone: An area targeted for seabed mining. Front. Mar. Sci. 8. doi: 10.3389/fmars.2021.626571
Weber A. A. T., Stöhr S., Chenuil A. (2019). Species delimitation in the presence of strong incomplete lineage sorting and hybridization: Lessons from ophioderma (Ophiuroidea: Echinodermata). Mol. Phylogenet. Evol. 131, 138–148. doi: 10.1016/j.ympev.2018.11.014
Wedding L. M., Reiter S. M., Smith C. R., Gjerde K. M., Kittinger J. N., Friedlander A. M., et al. (2015). Managing mining of the deep seabed. Science 349 (6244), 144–145.
Woolley S. N. C., Foster S. D., Bax N. J., Currie J. C., Dunn D. C., Hansen C., et al. (2021). Bioregions in marine environments: Combining biological and environmental data for management and scientific understanding. BioScience 70 (1), 48–59. doi: 10.1093/biosci/biz133
Woolley S. N., Tittensor D. P., Dunstan P. K., Guillera-Arroita G., Lahoz-Monfort J. J., Wintle B. A., et al. (2016). Deep-sea diversity patterns are shaped by energy availability. Nature 533 (7603), 393–396.
Keywords: Clarion Clipperton Zone, brittle stars, Ophiotholia saskia, COI, SEM, taxonomy
Citation: Eichsteller A, Martynov A, O’Hara TD, Christodoulou M, Korshunova T, Bribiesca-Contreras G and Martinez Arbizu P (2023) Ophiotholia (Echinodermata: Ophiuroidea): A little-known deep-sea genus present in polymetallic nodule fields with the description of a new species. Front. Mar. Sci. 10:1056282. doi: 10.3389/fmars.2023.1056282
Received: 28 September 2022; Accepted: 25 January 2023;
Published: 01 March 2023.
Edited by:
Eleonora Puccinelli, Université de Bretagne Occidentale, FranceReviewed by:
Mauricio Shimabukuro, Universidade Federal do Rio Grande, BrazilSergi Taboada, Autonomous University of Madrid, Spain
Copyright © 2023 Eichsteller, Martynov, O’Hara, Christodoulou, Korshunova, Bribiesca-Contreras and Martinez Arbizu. This is an open-access article distributed under the terms of the Creative Commons Attribution License (CC BY). The use, distribution or reproduction in other forums is permitted, provided the original author(s) and the copyright owner(s) are credited and that the original publication in this journal is cited, in accordance with accepted academic practice. No use, distribution or reproduction is permitted which does not comply with these terms.
*Correspondence: Angelina Eichsteller, YW5nZWxpbmEuZWljaHN0ZWxsZXJAc2VuY2tlbmJlcmcuZGU=