- 1Department of Marine and Environmental Sciences, Halmos College of Arts and Sciences and Guy Harvey Oceanographic Research Center, Nova Southeastern University, Dania Beach, FL, United States
- 2Center for Conservation & Research, San Antonio Zoo, San Antonio, TX, United States
Two of the main drivers of speciation among aquatic vertebrates are physical isolation (e.g., lakes and streams) and micro-niche availability (e.g., tropical reefs). In both regards, the mesopelagic domain of the open ocean, Earth’s second largest cumulative ecosystem (behind only the bathypelagic domain), would seem retardant. Ocean circulation makes isolation rare on both contemporary and geological time/space scales, and the lack of substrate precludes stable micro-niches. Paradoxically, some pelagic taxa demonstrate much higher-than-expected species richness on regional scales. A prime example is the dragonfish family Stomiidae, the most speciose family of mesopelagic fishes, owing largely to the subfamily Melanostomiinae (scaleless black dragonfishes), which contributes 222 of the 320 described species. Within genera, species are differentiated almost solely by form of the jugular-positioned, bioluminescent barbel, a structure putatively linked to feeding (via prey luring). The relationship between diversity (both systematic and morphological) and diet within the Melanostomiinae has not been previously examined, primarily due to sample size limitation. Herein, the diet and morphology of 16 species of melanostomiine dragonfishes from the Gulf of Mexico were examined to ascertain whether the diversification in this fish clade is based on prey specialization, as is the case with many other speciose fish families (e.g., Cichlidae). Gut content analysis revealed a rather small spectrum of prey taxa across a wide spectrum of predators, with most species exhibiting piscivory centered on the most regionally abundant lanternfishes (Myctophidae). Lesser numbers of species preyed upon bristlemouths (Gonostomatidae), oceanic basslets (Howellidae), bigscales (Melamphaidae), and other dragonfishes, while three species selected for cephalopod prey. No dragonfish species consumed macrocrustaceans (e.g., decapod shrimps), despite their numerical prevalence as potential prey. Regarding functional morphology, dissimilarity was driven mostly by barbel length, vertical oral gape, and horizontal maxillary oral gape. There were no robust morphological-dietary relationships amongst melanostomiines, with dietary diversity much lower than morphological diversity. These results suggest that other factors, perhaps conspecific recognition and/or sexual selection related to spawning, may be primary drivers of hyperspeciation in the micro-habitat-poor pelagic environment.
1 Introduction
With 320 described species, the dragonfish family Stomiidae is the most speciose in the mesopelagic (200 – 1000 m) zone of the World Ocean (Fricke et al., 2022). Stomiidae includes six subfamilies: Astronesthinae (snaggletooths), Chauliodontinae (viperfishes), Idiacanthinae (black dragonfishes), Malacosteinae (loosejaws), Stomiinae (scaly dragonfishes), and Melanostomiinae (scaleless dragonfishes). The Melanostomiinae, the focus of this study, is the most speciose subfamily, comprising 222 of the 320 stomiid species. Moreover, genus Eustomias within the subfamily Melanostomiinae accounts for approximately half of the species within the Stomiidae (Sutton and Hartel, 2004).
Despite the high species number, trophic studies generally pool dragonfish species by genus due to low sample sizes, excepting three dominant species (Chauliodus sloani, Photostomias guernei, and Stomias affinis), none of which are melanostomiines. In order to understand trophic ecological connections amongst taxa, the concept of feeding guilds, and groups within a guild, were established to demonstrate what taxa exploit the same prey resources. Of the three main feeding guilds for deep-pelagic species (i.e., micronektonivores, zooplanktivores, and generalists), dragonfishes are predatory fishes that prey upon micronekton, primarily lanternfishes (Family Myctophidae), which are the primary zooplanktivores in most oceanic food webs (Clarke, 1974; Hopkins and Gartner, 1992; Sutton and Hopkins, 1996a; Gartner et al., 1997). Lanternfishes are among the two dominant micronektonic fish taxa in the mesopelagic zone (Brodeur and Yamamura, 2005; De Forest and Drazen, 2009), the other being bristlemouths (Gonostomatidae) due to the preponderance of the genus Cyclothone. Lanternfishes and dragonfishes both undertake diel vertical migration (DVM), where they migrate to the epipelagic (0 – 200 m) zone at night to feed on the heightened influx of zooplankton and lanternfishes, respectively (reviewed in Drazen and Sutton, 2017). Dragonfishes and lanternfishes both are important mediators of organic carbon transfer between trophic levels within the water column and on continental margin benthic communities because stomiids and lanternfishes vertically migrate (Hidaka et al., 2001; Gartner et al., 2008). In a recent study, bioenergetic models suggested that lanternfishes and dragonfishes contributed greater than 53% and 12% of the active carbon flux for the entire Gulf of Mexico assemblage, respectively (Woodstock et al., 2022). By transporting carbon fixed in the surface waters to deeper depths, dragonfishes aid in deep-sea energy flow regulation and play an essential role in the interzonal energy transfer between the epipelagic, mesopelagic, and bathypelagic zones (Sutton and Hopkins, 1996a).
As vertical migrators, dragonfishes must balance energy consumption and expenditure. One way to maximize energy consumption is to capitalize on the larger prey items available. Certain morphological characters observed in dragonfishes, such as large mouths, fangs, and reduced ossification of anterior vertebrae, align with diet data showing that they do feed on larger prey (Borodulina, 1972; Sutton, 2005; Greven et al., 2009; Schnell et al., 2010; Kenaley, 2012; Schnell and Johnson, 2017). Of the available studies of dragonfish feeding, few can be considered quantitative with respect to prey type, prey size, and consumption rate (Clarke, 1982; Sutton and Hopkins, 1996a; Davison et al., 2013; Eduardo et al., 2020). While maximizing energy consumption is important, dragonfishes also need to minimize energy expenditure during predation to benefit from the energetically taxing process of DVM. Rather than actively pursue prey like many epipelagic predators, most dragonfishes adopt a sit and wait strategy, luring prey by use of a “chin” (mental or jugular) barbel bearing luminescent structures at the terminus. The length, shape and complexity of these barbels are highly conserved within species, but vary widely between melanostomiine species and genera (Figure 1). Morphological specializations for feeding in deep-sea fishes are well-documented in earlier trophic ecology studies (reviewed by Drazen and Sutton, 2017). Dragonfishes are known to be highly selective in their diet (Clarke, 1974; Sutton and Hopkins, 1996a), contrary to previous hypotheses that deep-sea fishes must have a wide range in diet, utilizing a generalist feeding strategy to survive in such a food-poor environment (Beebe and Crane, 1939; Haffner, 1952; Merrett and Roe, 1974). For example, Sutton and Hopkins (1996a) noted a correlation between the barbel structure and diet of dragonfishes, finding that species with reduced barbels preyed on zooplankton or larger invertebrates and species with more developed barbels preyed on fishes.
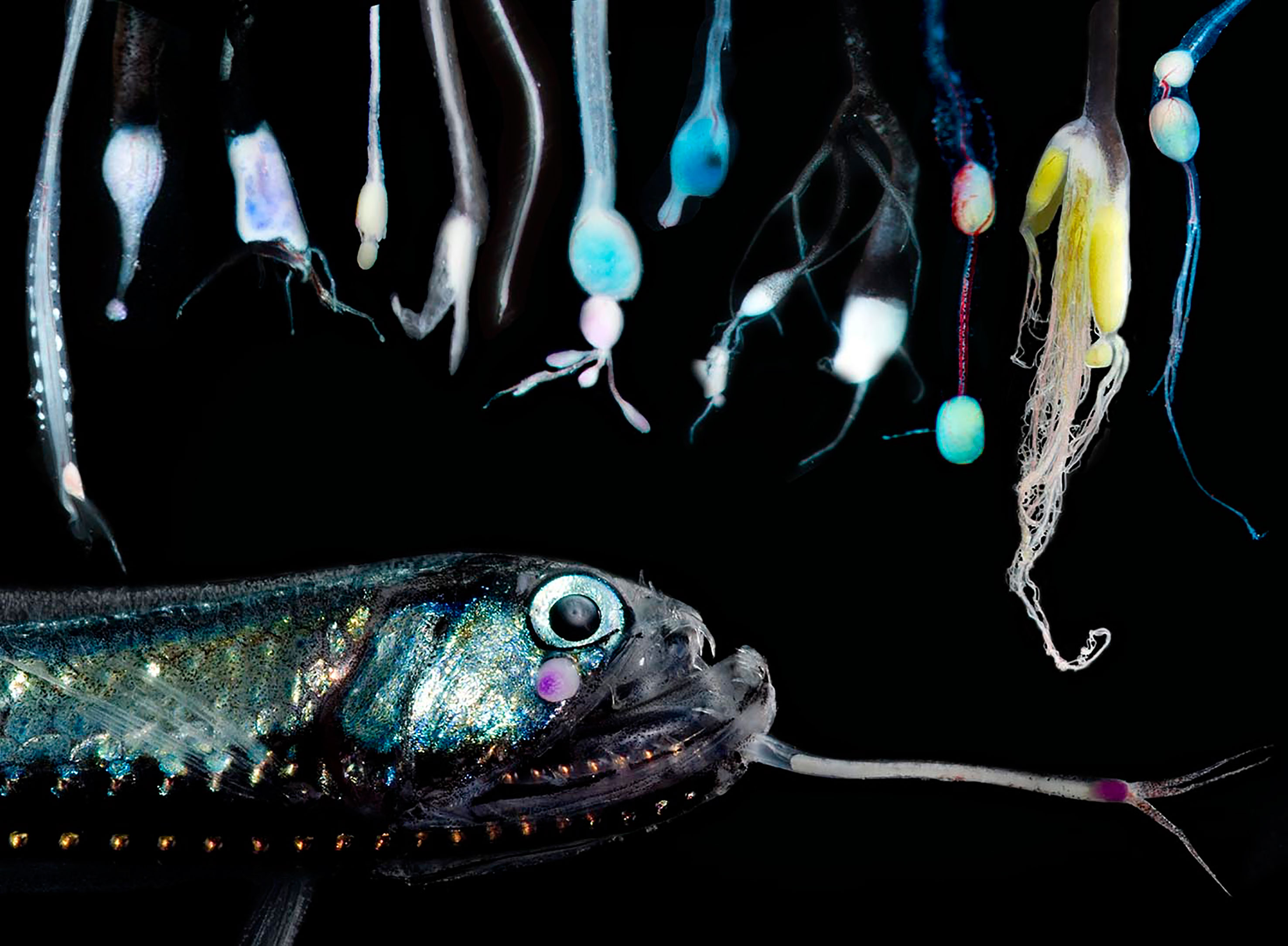
Figure 1 Examples of morphological variation in the barbels of dragonfishes. Clockwise from upper left corner to right: Melanostomias biseriatus, Photonectes leaucospilus, Photonectes margarita, Flagellostomias boureei, Melanostomias tentaculatus, Bathophilus pawneei, Echiostoma barbatum, Eustomias lipochirus, Eustomias fissibarbis, Eustomias hypopsilus, Eustomias schmidti, Eustomias bimargaritatus, and Stomias affinis (fish at bottom).
In this paper, we present the results of a detailed, species-specific analysis of the feeding morphology and diets of 16 species (from seven genera) of the dragonfish subfamily Melanostomiinae. A suite of morphological characteristics were analyzed to group species into morphotype clusters. Trophic analyses focused on prey composition and feeding selectivity. The trophic analysis conducted in this study is the most comprehensive to date of the Melanostomiinae. Morphotypes were compared to diet clusters to investigate the hypothesis that diversification in these taxa is driven by feeding specialization.
2 Methods
2.1 Sample collection and specimen processing
Seven research cruises were conducted during the Offshore Nekton Sampling and Analysis Program (ONSAP) in the Gulf of Mexico between 2010 and 2011 (Sutton et al., 2020). Two research vessels, the FRV Pisces and the M/V Meg Skansi, were utilized, each using specific gear types to collect deep-pelagic fishes and invertebrates during day and night. Four cruises on the NOAA FRV Pisces utilized a large, non-opening-closing high-speed rope trawl (HSRT) with a 165-m2 mouth area and graded mesh (3.2-m to 19-mm). HSRT trawls were designated “shallow” (0 to 700 m depth) and “deep” (0 to 1500 m). Three cruise series on the M/V Meg Skansi utilized a 10-m2 mouth area, 3-mm mesh Multiple Opening/Closing Net and Environmental Sensing System (MOCNESS; Wiebe et al., 1985). The MOCNESS comprised six nets that were opened and closed at targeted depths (Sutton et al., 2020). Each MOCNESS deployment produced up to five discrete-depth quantitative samples and one oblique tow from the surface to 1500 m. The HSRT and MOCNESS surveys were designed to catch larger- and smaller-sized pelagic organisms, respectively. Exact time, date, depth, and sampling locations of all samples can be found in Cook et al. (2020).
Collected specimens were formalin-fixed at sea and transported to the Oceanic Ecology Laboratory, Nova Southeastern University (NSU), where they were transferred to 70% ethanol:water, generally within a year of capture. The 16 most abundant dragonfish species were the subject of this study, listed in decreasing order of abundance: Eustomias schmidti, Echiostoma barbatum, Eustomias hypopsilus, Melanostomias melanops, Eustomias fissibarbis, Leptostomias gladiator, Melanostomias valdiviae, Eustomias brevibarbatus, Leptostomias bermudensis, Bathophilus pawneei, Photonectes margarita, Eustomias acinosus, Eustomias filifer, Flagellostomias boureei, Melanostomias tentaculatus, and Bathophilus longipinnis (Table 1).
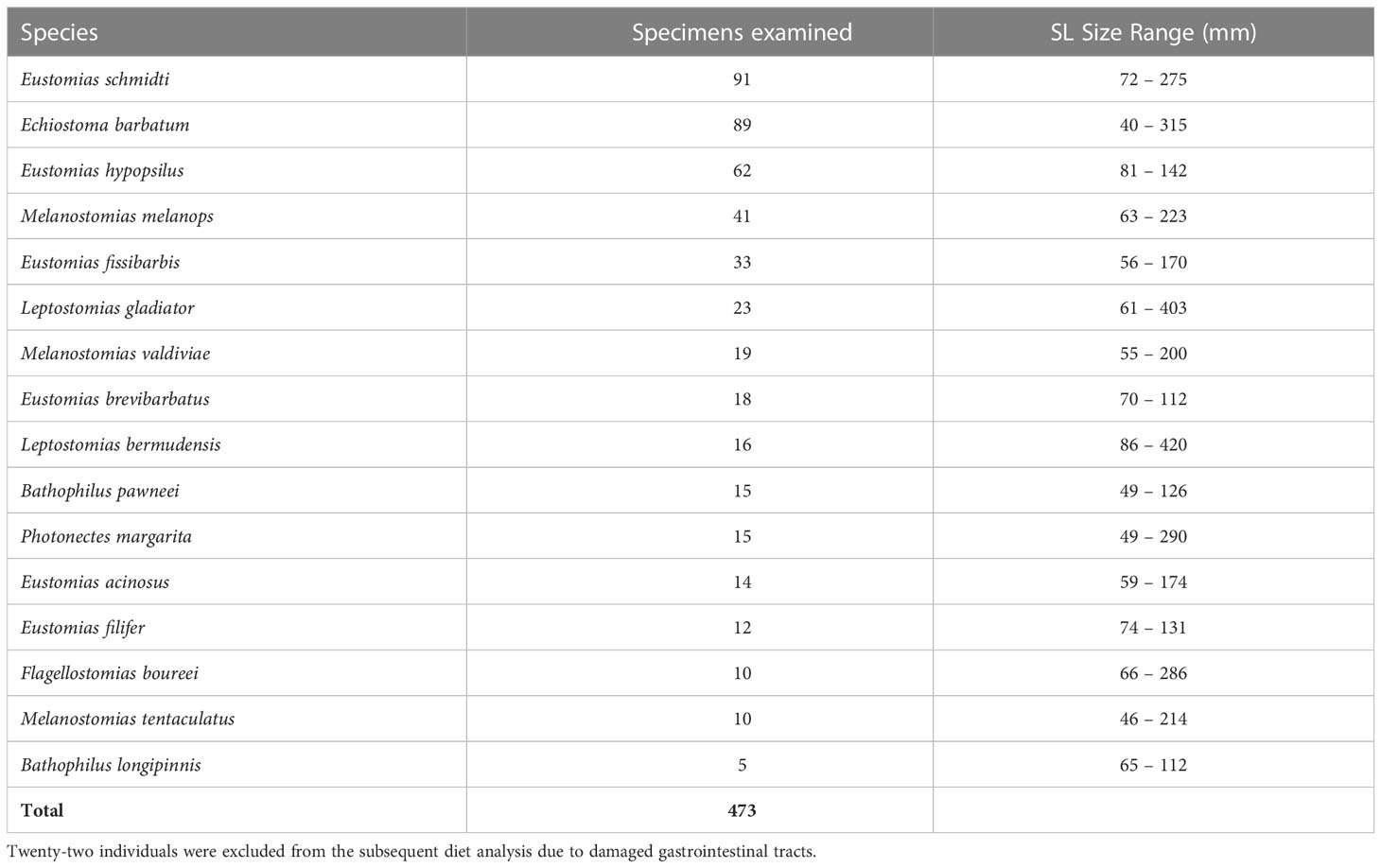
Table 1 Counts and standard length (SL) size range (mm) of melanostomiine dragonfish specimens used in this study for morphological analysis.
2.2 Diet analysis
Trophic analysis methods followed Sutton and Hopkins (1996a). Specimens were measured to the nearest 0.1 mm standard length (SL) and wet weights measured to the nearest 0.01 g after blotting dry. For diet analysis, the entire gastrointestinal (GI) tract was removed and the stomach and intestine separated. Only specimens with fully intact digestive tracts (i.e., no or minor trawl damage) were used in diet analysis, resulting in a slightly smaller sample size for diet than morphometric analyses. After stomach removal but prior to stomach dissection, stomach fullness was graded on a scale of 0 – 5, with zero being empty and five being completely full and distended. After dissection, prey items in the stomach and intestine were separated and identified to the lowest taxonomic level possible. Each prey item was measured to the nearest 0.1 mm SL and weighed to the nearest 0.1 g where applicable (Sutton and Hopkins, 1996a) and metrics of average prey size for each dragonfish species were calculated. Instantaneous ration was calculated as the weight of all prey from a single predator specimen divided by the weight of the predator specimen.
Feeding selectivity of dragonfishes was estimated using Ivlev Index (1961). This index has been successfully used to determine the prey selectivity of numerous fishes (e.g., Islam et al., 2006; Ribeiro and Nuñer, 2008), including mesopelagic predatory fishes (Feagans-Bartow and Sutton, 2014). Ivlev’s electivity index is defined as:
where r = percentage of a given prey taxon in the diet and p = percentage of that prey item in the environment. Values range from −1 to +1, with all values greater than zero indicating an overrepresentation of the prey relative to environmental abundance, zero indicating ambient representation, and all values less than zero indicating underrepresentation of the prey within the predator’s diet. The relative abundance of prey in the environment was estimated using quantitative catch data from 10-m2 MOCNESS sampling, a trawl best suited for catching prey-sized micronekton (Cook et al., 2020; Sutton et al., 2020).
For each specimen, morphometric analyses were based on standard length (SL), mouth gape size, dentition, head length, barbel length, lure complexity, and eye size. Most of the morphometric characteristics were measured using a Vernier caliper to the nearest 0.1 mm. Standard length was measured from the tip of the longest jaw (upper or lower) to the end of the hypural plate (Howe, 2002). Oral gape was characterized using three morphological measurements: the vertical oral gape, horizontal articular oral gape, and the horizontal maxillary oral gape. All measurements were taken with the mouth open maximally, just shy of head deformation. The vertical oral gape is the vertical distance between the anterior-most upper jaw and lower jaw, the horizontal articular oral gape is the distance between the two articular bones measured at the dorsoposterior margin, and the horizontal maxillary oral gape is the distance between the left and right maxilla-premaxilla complexes (Mihalitsis and Bellwood, 2017). Dentition measurements followed Gibbs et al. (1983), with only the longest premaxillary tooth and mandibular tooth measured. Head length was measured from the tip of the upper jaw to the posterior-most part of the fleshy operculum (Gibbs et al., 1983). Relative mouth size was represented by the ratio of each mouth gape size to head length. Eye size was measured as the diameter from the rostral to caudal ends of the orbit (de Busserolles et al., 2013). The barbels of dragonfishes have structures such as bulbs, filaments, and branching. Barbel length was measured from the barbel origin on the ventral head surface to the distal end of the distal bulb of the lure, excluding the filaments. Lure complexity was assessed by counting and reporting the number of main branches from the main stem of the barbel.
2.3 Statistical analysis
All statistical analyses were performed using the program PRIMER (v. 7.0.13; PRIMER-e (2017), Quest Research Limited). For analyses, a Bray-Curtis similarity index (Bray and Curtis, 1957) was computed after standardizing feeding data as a percentage of all prey items. Two multivariate techniques, (1) an unweighted pair-group method using arithmetic averages (UPGMA; Romesburg, 1990) cluster analysis and (2) non-parametric multi-dimensional scaling (MDS; Kruskal and Wish, 1978), were used to group melanostomiines into feeding guilds. Prey type was analyzed at two levels: 1) Infraclass or higher (Vertebrata, Crustacea, Cephalopoda, Annelida, Chaetognatha, Tunicata, Urochordata, Cnidaria, Ctenophora, and Sipuncula); and 2) Family for teleost consumers. For morphological analyses, a Bray-Curtis similarity index was computed using ratio values of morphological measurements. Similar to the trophic analyses, UPGMA clustering and non- parametric MDS analyses were also conducted to group melanostomiines into morphotypes. Groupings within each treatment (feeding and morphology) were defined by graphical (visual) concordance of the two analyses (UPGMA and MDS) (Sutton et al., 2008). In order to determine the morphological-dietary relationships of melanostomiines, graphical concordance of the treatment groupings was assessed using the same method.
3 Results
A total of 473 specimens were examined in the morphological analyses, representing 16 species from seven genera. Of those 473 specimens, 451 specimens were examined for diet (22 specimens were not included in trophic analysis due to damaged GI tracts).
3.1 Trophic ecology
3.1.1 Diet analysis
Of the 451 specimens examined, 29% were prey-positive (Table 2), with 151 prey items identified. Of the 151 prey items, 81% (n = 123) were identified to major prey taxon only (i.e., Teleostei or Cephalopoda – no other invertebrate taxa were consumed) due to digestion. Most of the dragonfishes displayed strict piscivorous behavior, with 13 of 16 species containing only teleost prey. The remaining three species, Echiostoma barbatum, Eustomias brevibarbatus, and Bathophilus longipinnis, had cephalopod prey items present in their diet, ranging from 8% – 50% of their total prey composition. Despite consumption of cephalopods, fishes comprised over 75% of the diet in both Echiostoma barbatum and Eustomias brevibarbatus. With respect to fishes consumed, 20.3% of fish prey items were identified to family, with a majority (72.4%) identified as lanternfishes (Myctophidae). Other fish families present in the diets were Howellidae (13.8%), Stomiidae (6.9%), Gonostomatidae (3.4%), and Melamphaidae (3.4%) (Table 3).
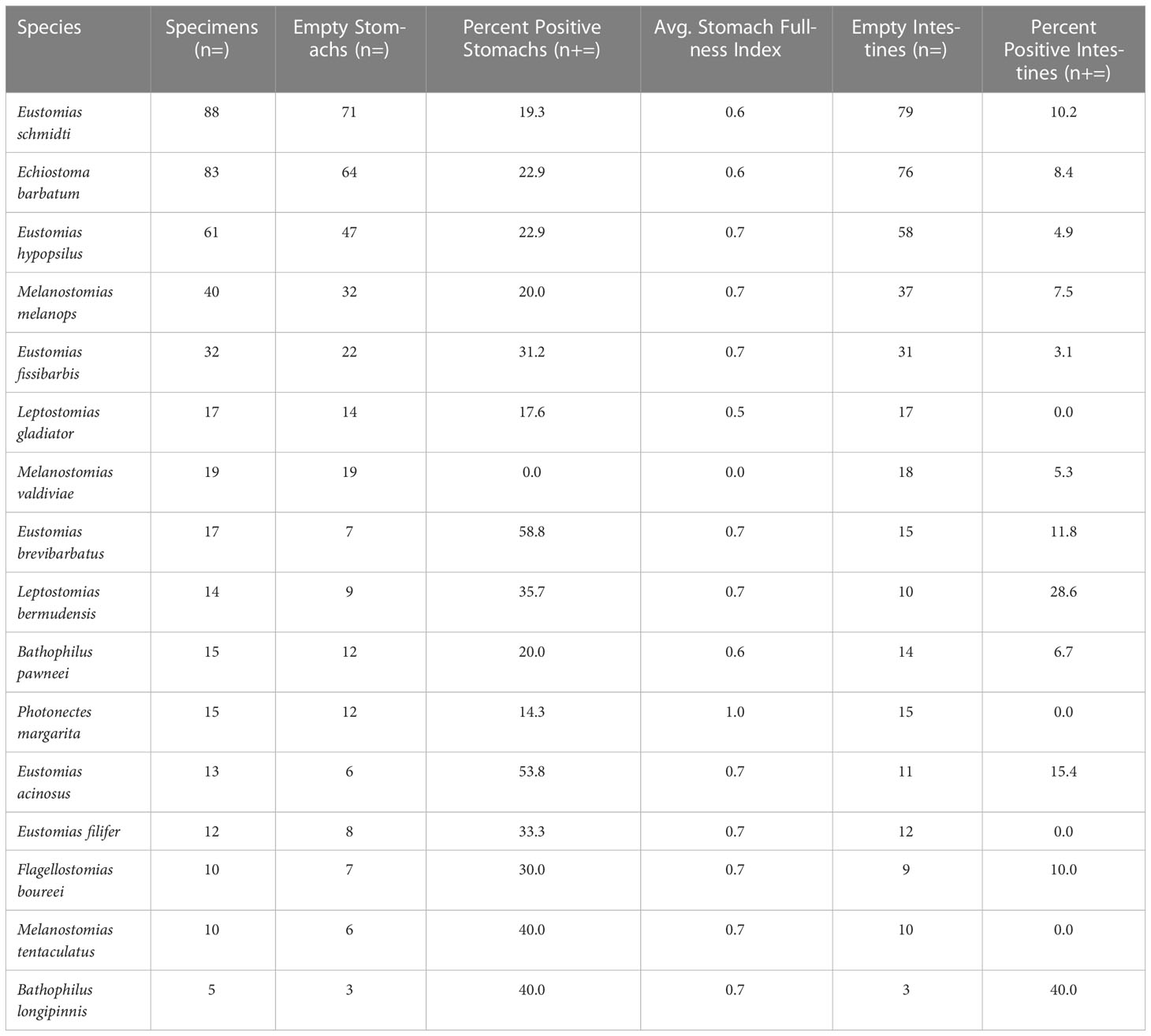
Table 2 Summary statistics of melanostomiine dragonfish feeding, including percentage of prey-positive stomachs and intestines and average stomach fullness index (values range from 0 [empty] to 5 [completely full]; see Section 2.2).
3.1.2 Feeding guilds
Collectively, the multivariate UPGMA and MDS analyses for diet composition of the 16 melanostomiine dragonfishes revealed two major feeding guilds by major prey taxon (Figure 2), with a tentative third guild (Feeding guild “a”) containing one species (B. longipinnis) with unique diet composition (high cephalopod consumption, Tables 3 and 4, Figures 2A, B), but very low sample size (n = 5, two prey-positive individuals). Our following treatment will then focus on the two major feeding guilds. Of these two, one was monospecific (Feeding Guild “b”), comprising Echiostoma barbatum, and was discriminated by UPGMA clustering (Figure 2A) but not MDS (Figure 2B). This guild/species was characterized by a high selectivity for cephalopods, a moderate selectivity for teleosts, and negative selectivity for all other potential invertebrate prey (Table 4). A teleost specialist feeding guild (Feeding Guild “c”) was the largest guild with 14 species in UPGMA and 15 species (including Echiostoma barbatum) in MDS (Figures 2A, B). This guild was highly selective for teleosts (Table 4). With this being the largest guild, a separate feeding selectivity analysis was conducted to establish feeding groups by prey fish family within this piscivorous guild. We note that despite the high relative abundance of mesopelagic macrocrustaceans (decapod shrimps, mysids, large euphausiids) in the Gulf of Mexico (Burdett et al., 2017; Frank et al., 2020), and their similar size to fish prey, this potential prey resource was never consumed by melanostomiine dragonfishes.
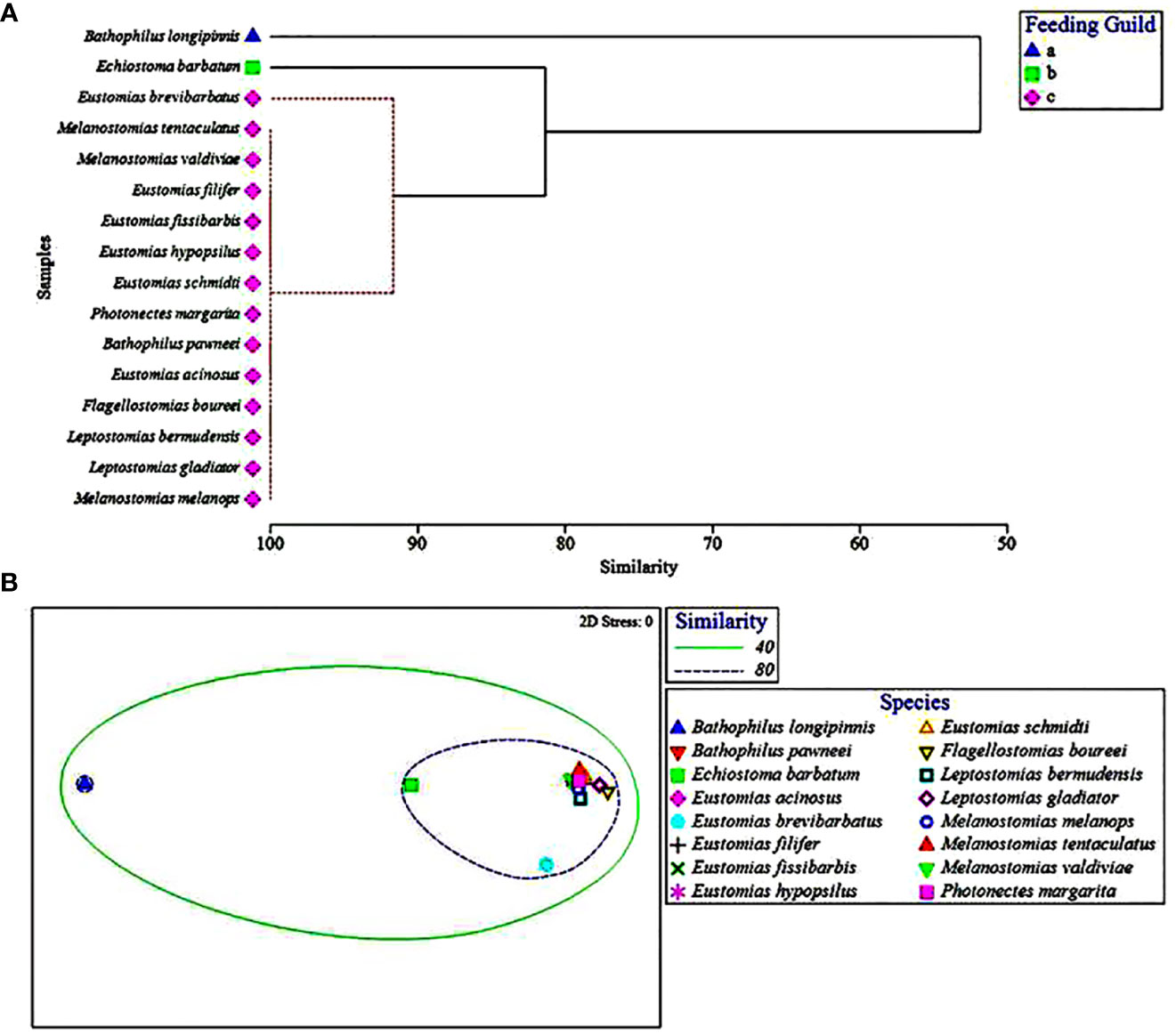
Figure 2 (A) Hierarchical classification and (B) non-metric multidimensional scaling depicting Bray-Curtis similarity of dragonfish diets by major prey taxon. Feeding guilds represented by symbols and ellipses, respectively. Dashed red lines in (A) represent that these species could not be distinguished from one another by major prey taxon consumed and therefore were placed into a feeding guild together.
Within the piscivorous feeding guild, the multivariate UPGMA and MDS analyses for diet composition of 13 species revealed four feeding groups by prey fish family (Figure 3). Two species from this guild were excluded from the analysis due to all prey being only identifiable to major prey taxon. Feeding Group “a” comprised two species, Eustomias acinosus and Eustomias filifer, and was classified as a howellid specialist group due to their high selectivity for Howella atlantica (Table 4). Feeding Group “b,” comprising Bathophilus pawneei was tentatively classified as a dragonfish specialist group (Table 4) due to the only prey item identifiable to family being another dragonfish, a rarity in this study, but low sample size precludes confidence in this assignment. Feeding Group “c” was classified as a lanternfish specialist group due to their high selectivity for that prey family (Table 4) and was the largest group (eight species in UPGMA clustering and 10 species in MDS). An opportunistic group (Feeding Group “d”) was only represented in UPGMA clustering and consisted of two species, Echiostoma barbatum and Melanostomias melanops (Figure 3A). These species had varying selectivity for different prey fish families. For example, E. barbatum was highly selective for melamphaids and strongly selective for lanternfishes. Melanostomias melanops was strongly selective for lanternfishes and only slightly selective against bristlemouths (as opposed to completely selected against as in all other dragonfishes; Table 4). Despite selectivity for certain fish families, the diet composition of both opportunistic predators had no family encompassing over 50% of their diet (Table 3). We note that in all cases of piscivory, the total absence as prey of the numerically dominant meso- and bathypelagic fish taxon in the Gulf of Mexico, Cyclothone spp., which alone comprise ~70% of the total fish assemblage (Sutton et al., 2020), will necessarily return a positive selectivity index for any fish prey other than Cyclothone.
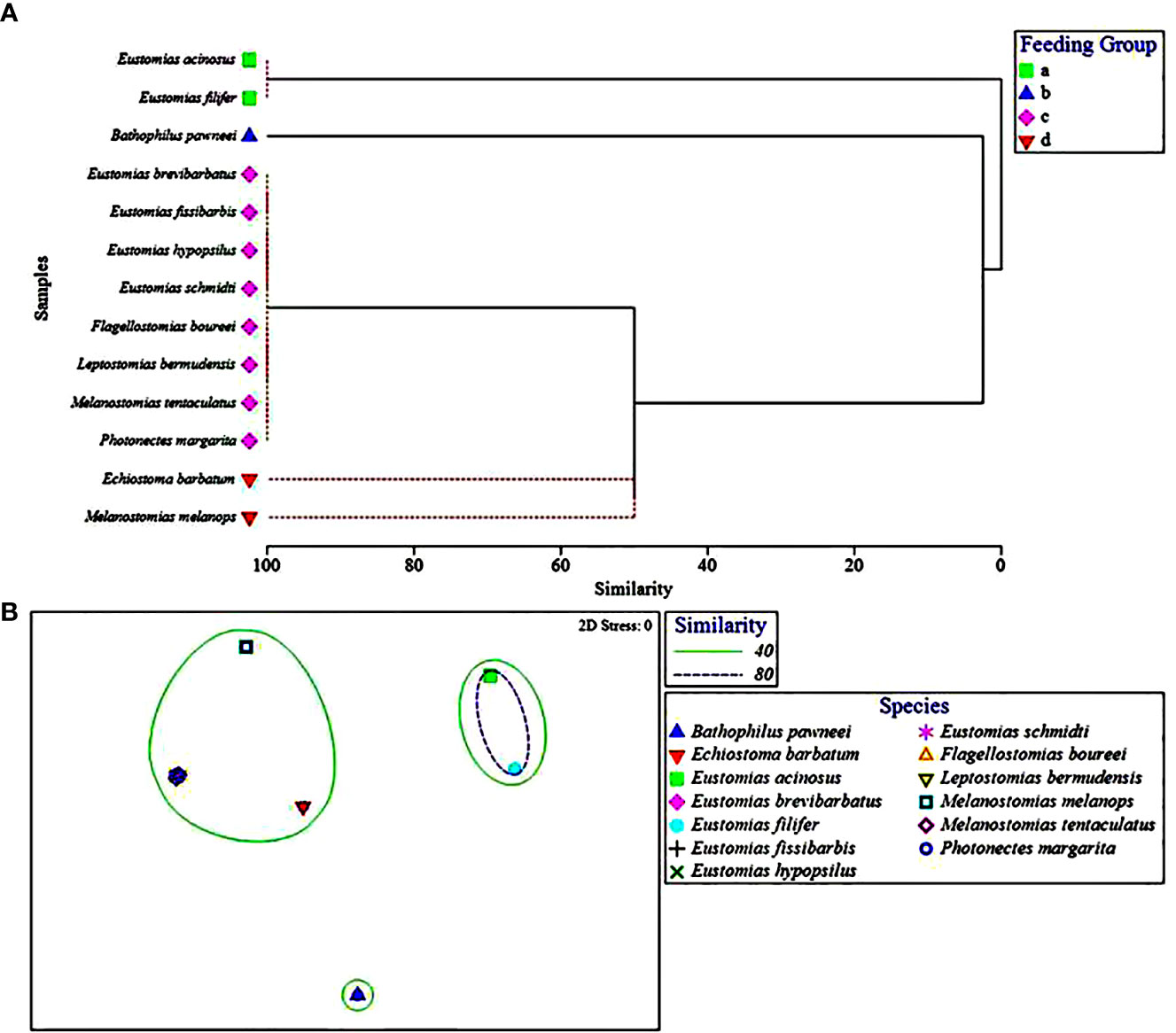
Figure 3 (A) Hierarchical classification and (B) non-metric multidimensional scaling depicting Bray-Curtis similarity of piscivorous dragonfish diets by prey fish family. Feeding group represented by symbols and ellipses, respectively. Dashed red lines in (A) represent that these species could not be distinguished from one another by prey fish family and therefore were placed into a feeding group together.
3.1.3 Dragonfish meal size
The measurable prey of dragonfishes averaged 7.2% of their own weight and 27.6% of their own length (Table 5), though these estimates should be considered minimal due to the effects of digestion. The largest relative prey weight consumed in one feeding bout was observed in Eustomias brevibarbatus (27.2% of body weight) and the largest relative prey size was observed in Melanostomias melanops (43.1% of body length) (Table 5).
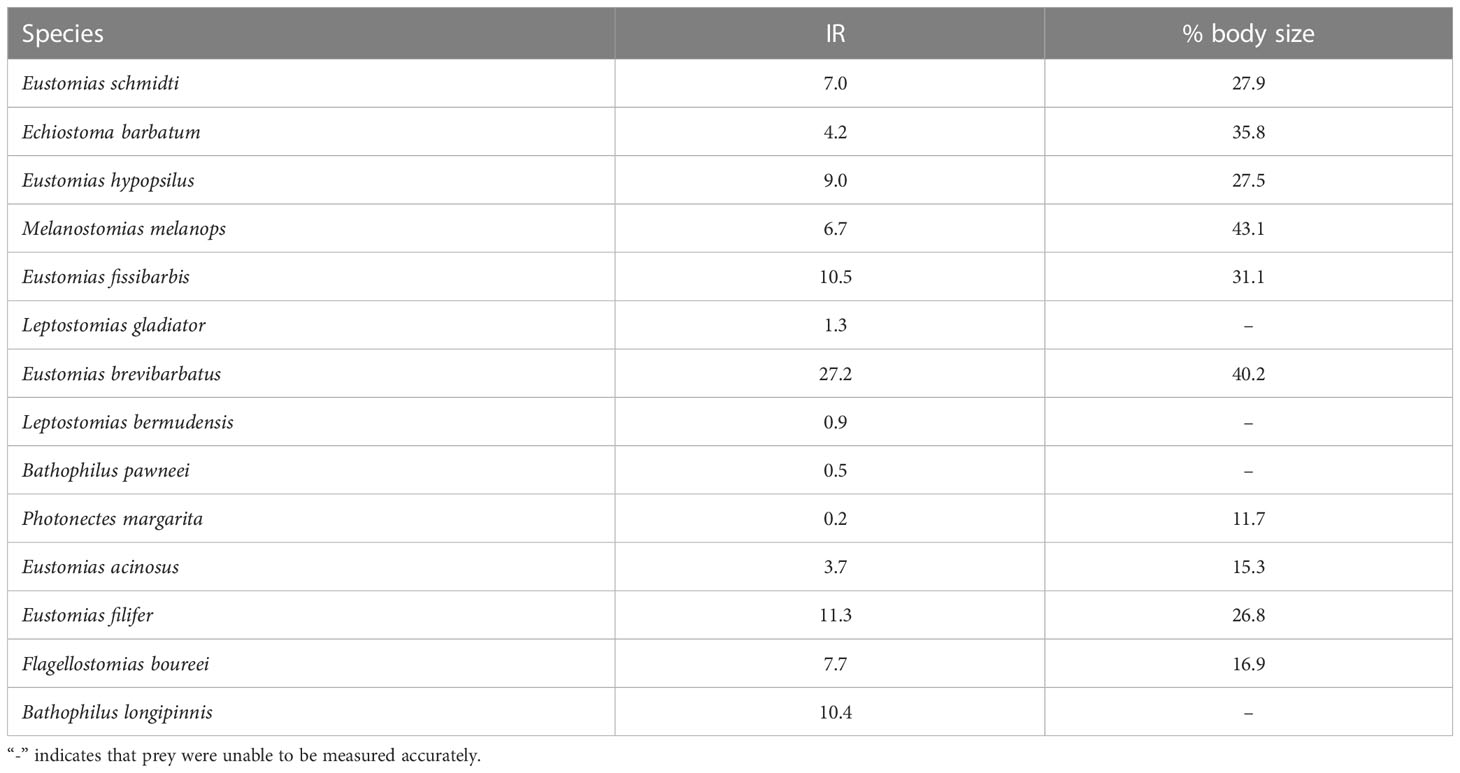
Table 5 Meal size of dragonfish species in terms of biomass (Instantaneous Ration [IR]; % of predator weight consumed per meal) and body size (% of predator length).
3.2 Morphology
3.2.1 Morphometrics
Seven morphological characteristics were measured on 473 specimens standardized as a percentage of head length. Dragonfish vertical oral gape ranged from 66.3% (E. brevibarbatus) to 125.3% (M. melanops), horizontal maxillary oral gape from 37.3% (Eustomias hypopsilus) to 79.0% (M. melanops), and horizontal articular oral gape from 30.7% (E. hypopsilus) to 67.1% (M. melanops). The longest premaxillary and mandibular teeth ranged from 9.7% (E. brevibarbatus) to 20.1% (Flagellostomias boureei) and 5.8% (Photonectes margarita) to 26.3% (F. boureei), respectively. Dragonfish eye size ranged from 18.5% (B. pawneei) to 26.2% (F. boureei). Dragonfishes barbel lengths ranged from 61.0% (P. margarita) to 997.1% (Leptostomias bermudensis). In terms of barbel branching, over 50% of the dragonfish species had barbels with zero primary branches on the main barbel stem. The number of primary branches on the main barbel stem of the other seven species ranged from 1 – 8 (Table 6).
3.2.2 Morphotypes
Multivariate analyses revealed five morphotypes at an 80% similarity level (a, b, c, d, and e) amongst the 16 species of dragonfishes (Figure 4), with dissimilarity driven mostly by barbel length, vertical oral gape, and horizontal maxillary gape. Morphotype “a,” comprising Leptostomias bermudensis, L. gladiator, and B. pawneei, was defined by a long barbel and relatively small vertical and horizontal gape. Morphotype “b” consisted of E. barbatum, M. valdiviae, P. margarita, E. schmidti, and E. fissibarbis and was defined by a short barbel and large vertical and horizontal gape. Morphotype “c” was the largest cluster and contained E. schmidti, E. barbatum, P. margarita, E. fissibarbis, and M. valdiviae. This morphotype was defined by a short barbel and medium vertical and horizontal gape. Morphotype “d” consisted of M. melanops, E. brevibarbatus, E. filifer, B. pawneei, and M. tentaculatus. This morphotype was defined by a long barbel and large vertical and horizontal gape. Morphotype “e” consisted of E. hypopsilus, E. acinosus, M. tentaculatus, B. pawneei, F. boureei, and L. gladiator. This morphotype was defined by a long barbel and medium vertical and horizontal gape. A comparison of morphotype clusters with dietary clusters revealed no relationships, with dietary diversity much lower than morphological diversity.
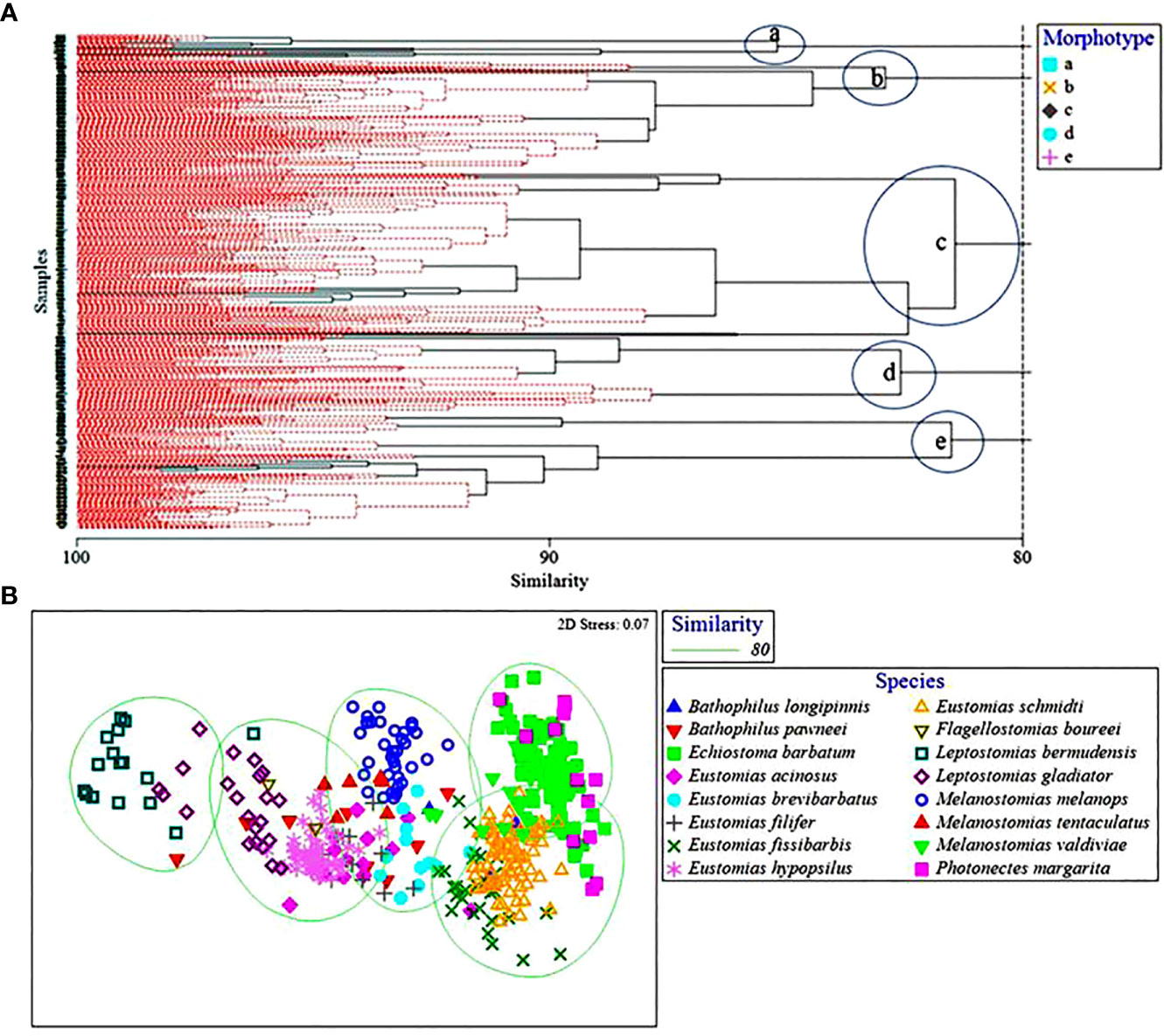
Figure 4 (A) Hierarchical classification and non-metric multidimensional scaling depicting Bray-Curtis similarity values of dragonfish morphotypes. Morphotypes represented by (A) symbols and clusters circled and (B) ellipses. Dashed red lines in (A) represent that these samples could not be distinguished from one another by morphology and therefore were placed into a morphotype together.
4 Discussion
4.1 Diet analysis and feeding guilds
This study represents the most extensive examination of the diet of the hyperdiverse mesopelagic fish subfamily Melanostomiinae. On a broader scale, dragonfishes have been classified as either micronektonivores or generalist feeders (Gartner et al., 1997; Drazen and Sutton, 2017). Congruent with previous studies by Borodulina (1972); Clarke (1982); Hopkins et al. (1996), and Sutton and Hopkins (1996a), the majority of dragonfish species in this study exhibited selective piscivorous feeding. The largest feeding guild, which included 14 or 15 of the 16 species examined, depending on multivariate analysis method, was defined by predation on teleost fishes. Two of the piscivorous species (Echiostoma barbatum and Eustomias brevibarbatus) also consumed cephalopods.
Previous data on dragonfish diets indicate predation primarily on lanternfishes, with a lesser portion of diets consisting of fishes from the families Gonostomatidae, Sternoptychidae, Bregmacerotidae, Argentinidae, the order Beryciformes (Clarke, 1982; Sutton and Hopkins, 1996a; Hopkins et al., 1996), and occasional cannibalism (e.g., Chauliodus sloani; Battaglia et al., 2018; Eduardo et al., 2020). Among the 13 predominantly piscivorous dragonfishes included in the prey fish family analysis, only four groups could be discerned statistically, with the largest being predation on the most common lanternfishes in the study area (Gartner et al., 1997; Sutton et al., 2020). Limited evidence of prey specialization was seen in two Eustomias species, E. acinosus and E. filifer, both of which consumed howellids (pelagic basses) out of proportion to their abundance in the environment. Howellids were also found in the diet of E. brevibarbatus in an earlier study (Sutton and Hopkins, 1996a). The only lack of concordance between the two multivariate analyses was with Echiostoma barbatum and Melanostomias melanops. Cluster analysis placed these two species into their own group, separate from the primarily lanternfish-eating group (Figure 3A). However, E. barbatum and M. melanops were placed into the lanternfish-eating group via MDS (Figure 3B). Echiostoma barbatum appeared to be the most opportunistic dragonfish, with a diet including several fish families (Myctophidae, Melamphaidae, and Stomiidae) as well as cephalopods. This finding agrees with Sutton and Hopkins (1996a), who reported a mixed diet for this species. Melanostomias melanops preyed upon the two numerically dominant fish families in the Gulf of Mexico, Gonostomatidae and Myctophidae, suggesting that M. melanops is a more opportunistic piscivore.
Overall, feeding data indicated that piscivorous dragonfishes are highly selective in their diet in terms of taking one prey fish family (Myctophidae) out of 169 potential prey fish families in the study area, many of which have species as or more abundant than individual myctophid species (e.g., Gonostomatidae, Sternoptychidae, Phosichthyidae) (Cook and Sutton, 2017a; Cook and Sutton, 2017b; Sutton et al., 2017a; Sutton et al., 2017b; Cook and Sutton, 2018; Cook and Sutton, 2019). This selectivity stands in contrast to earlier hypotheses that dragonfishes likely exhibit generalist feeding (Beebe and Crane, 1939; Haffner, 1952; Merrett and Roe, 1974). That said, there was very little evidence to suggest dragonfish specialization between species; taking the same prey across a range of predators was the rule rather than the exception.
4.2 Morphology
The feeding morphology of a species can be used to predict the relative prey size preference of a predator. The absolute mouth size and dentition of dragonfishes dictate how large of a prey item can be ingested (Sutton, 2005; Schnell et al., 2010; Schnell and Johnson, 2017). In the species examined, vertical oral gape was on average 86.0% of the head length, reflecting the capacity of this taxon to take large prey. With such a large mouth gape, dragonfishes have increased their chance at success in prey capture for each of the limited opportunities available to feed. Further, with the ability to ingest large prey, dragonfishes do not have to expend energy in vertically migrating every day (i.e., ‘asynchronous migration,’ where a portion of a species’ population migrates at night while the other portion stays at daytime depths; Sutton and Hopkins, 1996b) as larger prey items can sustain predators for longer than a day.
Dragonfishes typically possess fewer, longer jaw teeth than most midwater fishes. The fang-like teeth are associated with ingestion of large prey. In this study, the longest premaxillary and mandibular teeth were both on average 14.2% of the head length (Table 6). The opportunistic predators Echiostoma barbatum and Melanostomias melanops had larger teeth than most dragonfishes, supporting the notion of opportunistic behavior facilitated by larger teeth, which allow for a larger range in prey size. Two other genera that also possessed larger teeth than most dragonfishes were Flagellostomias and Leptostomias. These genera, unlike most dragonfishes, have dentary teeth that project at large angles (Fink, 1985). In terms of prey size, all prey items for these individuals were well digested, making prey size estimates imprecise. Bathophilus and Eustomias species are described as having smaller teeth closely set on the maxilla (Fink, 1985). Most Bathophilus and Eustomias species in this study possessed smaller teeth, except for B. longipinnis, which did demonstrate a wider range in diet; both teleosts and cephalopods were identified. Summarizing all species, Bathophilus and Eustomias species consumed relatively smaller prey sizes than the opportunistic predators.
Three morphological characters had the most influence in defining dragonfish morphotypes. The most influential character was barbel length, followed by vertical oral gape, and then horizontal maxillary gape. Dragonfishes are thought to use their bioluminescent lure, when present, to aid in their predatory lifestyle (Borodulina, 1972; Gibbs et al., 1983; Partridge and Douglas, 1995; Douglas et al., 1998). Barbel length was highly variable between species, ranging 61% to ~1000% of head length (HL), with an average of about 300% of head length for the entire species assemblage. Barbel length was observed at sizes smaller than the head length in Echiostoma barbatum and Photonectes margarita. Eustomias species had barbel lengths that ranged from just larger than the head length to nearly four times the head length. The largest barbel lengths were observed in Flagellostomias boureei (4.5 × head length), Leptostomias gladiator (5.8 × head length) and L. bermudensis (10 × head length). Barbels of these species may thus reach from midbody to the base of the caudal fin. Barbel diversity was also reflected in the number of primary branches from the barbel main stem. Species had a range in complexity, from no branches to up to eight primary branches, with these branches often bearing numerous secondary branches. Eustomias species possessed barbels that had no branches (one species), one branch (one species), two branches (one species) and three branches (three species). The largest amount of branching was observed in Flagellostomias boureei and Photonectes margarita, which possessed 4 – 5 and 6 – 8 main branches, respectively. These species were on the opposite ends of barbel length measurements; therefore, it is worth noting that there was no trend between barbel length and complexity. With respect to barbels and oral gapes, five morphotypes were distinguished: (1) long barbel with small vertical and horizontal maxillary gapes, (2) long barbel with medium-sized vertical horizontal maxillary gapes, (3) long barbel with large vertical and horizontal maxillary gapes, (4) short barbel with medium-sized vertical and horizontal maxillary gapes, and (5) short barbel with large vertical and large horizontal maxillary gapes. These character combinations did not appear to select for different prey taxa, as all dragonfish species consumed a common prey resource base, a relatively narrow suite of mesopelagic fishes, with no outstanding prey type exceptions (e.g., decapod shrimps, mysids, euphausiids, amphipods, chaetognaths, gelatinous zooplankton) or segregation of fish taxa (abundant fish families in the Gulf of Mexico other than lanternfishes, including other Stomiiformes [Sternoptychidae, Phosichthyidae, Gonostomatidae], Aulopiformes, Bathylagidae, Bregmacerotidae, or any epipelagic fishes). In short, most melanostomiine dragonfishes “do the same thing” trophically. This ecomorphological pattern stands in contrast to the plethora of studies that report that diet specialization is a putative driver of high species richness in other vertebrate taxa (Verwaijen et al., 2002; Santana et al., 2012; Collar et al., 2014; Olsen, 2017; Law et al., 2018).
While the primary function of dragonfish barbel-bulb combinations is thought to be predation, some studies have speculated that these structures could also be used for intraspecific communication. Davis et al. (2014) proposed that myctophids and dragonfishes with species-specific bioluminescent structures have rapidly speciated more than other taxa that use bioluminescence for predation or camouflage. Thus, barbel complexity (or lack thereof) may be as or more involved in mate recognition or selection than for luring specific prey taxa. The evolution of hypertrophied characters has been well documented in association with both mate attraction and in broadcasting cues, such as overall health and/or condition, to same sex rivals. Often, the exaggerated features come at considerable energetic and survivorship costs to the individuals bearing them; this is likely the case in dragonfishes, as extreme elongation (e.g., Leptostomias spp.) or otherwise ornate barbels (e.g., Eustomias spp.) surely impose hydrodynamic drag, hence restrictions, on their owners in what is considered an energy- (food)-limited environment. Yet, hypertrophied characters continue to persist through time and generations owing to the benefits that they confer in either reproductive success and/or successful competition for mates. For example, hypertrophied eyestalks have independently evolved in several fly families (Lande and Wilkinson, 1999), with the eye span of males exceeding their total body length (Wilkinson and Dodson, 1997). In species with strong sexual dimorphism, males with greater eye span are more attractive to females in spite of the fact that the exaggerated feature reduces flying performance in males (Wilkinson and Dodson, 1997; Lande and Wilkinson, 1999; Swallow et al., 2000). Multiple examples in fishes exist. For example, males of many species of the poeciliid genus Xiphophorus, the “swordtails,” often have elongations of the lower caudal fin, the “sword,” that likely increases visibility to predators and decreases swimming speeds – to the detriment of the males with the swords by way of increased risk of predation. However, the ornamentation results in greater female attraction in species with the swords and even (experimentally) in females of related species where males do not have the swords (Meyer et al., 1994; Schartl et al., 2021). Similarly, in other cyprinodontiform killifishes, larger fins decrease swimming performance but play large roles in mate attraction and male-male competition (Sowersby et al., 2022). In freshwater cichlids of the genus Amphilophus, a “nuchal forehead hump” is exaggerated during the breeding season. Larger male forehead humps are more attractive to females even though swimming abilities are decreased in males with larger humps (Rometsch et al., 2021). Given these numerous examples, and the finding here that the extreme morphological variation in the dragonfishes examined here does not scale with diet, we then hypothesize that the overall ‘bauplan’ of melanostomiine dragonfishes (mental barbel, large terminal mouth, large teeth) may allow for large-item piscivory in general, the feeding behavior (‘luring’ at night on vertically migrating fishes; sensu Sutton and Hopkins, 1996a) may select for a narrow range of prey (e.g., lanternfishes in most species), perhaps due to energetic constraints (i.e., cost-benefit ratio), and the exceptional barbel diversity may be tied to mate recognition, selection, and/or reproduction. The large unknown in this hypothesis at this time is vision – can dragonfishes see well enough in a scotopic environment to discern the subtleties of barbel form? All the examples listed previously occur in the photopic, colorized world. The visual acuity of melanostomiine dragonfishes is unknown, but a comprehensive review of deep-sea fish vision (de Busserolles et al., 2020) suggests that resolution is not likely high in dragonfishes (most species have an aphakic gap in the eye, which aids vision in dim light at the expense of image formation), but this is not to say that they cannot distinguish shapes, arrangements, and/or brightness of bulbs (or the lack thereof) when viewed up close to tell conspecifics part from other congeners. One can even imagine the possibility of more extensive behavior involving barbels for reproductive preparation (i.e., gamete synchronization) to increase the odds of successful fertilization when solitary predators in a dimly lit world finally meet a mate of the same species. Such conjecture is the stuff of future research, but herein we lay the foundation that the extraordinary diversity seen in both species number and barbel diversity of this group of deep-pelagic fishes may be driven by reproductive signaling using what has historically been considered a feeding appendage.
Data availability statement
The datasets presented in this study can be found in online repositories. The names of the repository/repositories and accession number(s) can be found below: Gulf of Mexico Research Initiative Information and Data Cooperative (GRIIDC) repository at: https://data.gulfresearchinitiative.org/.
Ethics statement
Ethical review and approval was not required for the animal study because Animals were collected under an institutionally approved permit from the Florida Atlantic University IACUC Committee.
Author contributions
RM and TS designed the study. RM and TS conducted the research and analyzed the data. All authors contributed to manuscript preparation. TS oversaw all aspects of this research, including collection and identification of specimens. All authors have read and approved of the submitted version of this manuscript.
Funding
This paper is a result of research funded in part by the National Oceanic and Atmospheric Administration’s RESTORE Science Program under award NA19NOS4510193 to Nova Southeastern University. Sample and data acquisition was also supported by grants from the Gulf of Mexico Research Initiative and the Gulf of Mexico Alliance.
Acknowledgments
The authors would like to thank the captains and crews aboard the FRV Pisces and M/V Meg Skansi and the scientific crews aboard for their services in sample collection and processing. We thank April Cook and Nina Pruzinsky for their assistance with database management. We also thank Drs. Heather Judkins and Michael Vecchione for their assistance in cephalopod prey identification.
Conflict of interest
The authors declare that the research was conducted in the absence of any commercial or financial relationships that could be construed as a potential conflict of interest.
Publisher’s note
All claims expressed in this article are solely those of the authors and do not necessarily represent those of their affiliated organizations, or those of the publisher, the editors and the reviewers. Any product that may be evaluated in this article, or claim that may be made by its manufacturer, is not guaranteed or endorsed by the publisher.
References
Battaglia P., Ammendolia G., Esposito V., Romeo T., Andaloro F. (2018). Few but relatively large prey: trophic ecology of Chauliodus sloani (Pisces: stomiidae) in deep waters of the central Mediterranean Sea. J. Ichthyology 58(1) pp, 8–16. doi: 10.1134/S0032945218010034
Beebe W., Crane J. (1939). Deep-sea fishes of the Bermuda oceanographic expeditions. family melanostomiatidae. Zoologica (NY) 24, 65–238. doi: 10.5962/p.203628
Borodulina O. D. (1972). The feeding of mesopelagic predatory fish in the open ocean. J. Ichthyology 12, 692–703.
Bray J. R., Curtis J. T. (1957). An ordination of the upland forest communities of southern Wisconsin. Ecol. Monogr. 27 (4), 325–349. doi: 10.2307/1942268
Brodeur R., Yamamura O. (2005). Micronekton of the north pacific. North Pacific Mar. Sci. Organ. (PICES) Sci. Rep. 30, 1–115.
Burdett E. A., Fine C. D., Sutton T. T., Cook A. B., Frank T. M. (2017). Geographic and depth distributions, ontogeny, and reproductive seasonality of decapod shrimps (Caridea: oplophoridae) from the northeastern gulf of Mexico. Bull. Mar. Sci. 93 (3), 743–767. doi: 10.5343/bms.2016.1083
Clarke T. A. (1974). Some aspects of ecology of stomiatoid fishes in pacific ocean near Hawaii. Fishery Bull. 72, 337–351.
Clarke T. A. (1982). Feeding habits of stomiatoid fishes from Hawaiian waters. Fishery Bull. US 80, 287–304.
Collar D. C., Reece J. S., Alfaro M. E., Wainwright P. C., Mehta R. S. (2014). Imperfect morphological convergence: variable changes in cranial structures underlie transitions to durophagy in moray eels. Am. Nat. 183 (6), E168–E184. doi: 10.1086/675810
Cook A. B., Bernard A. M., Boswell K. M., Bracken-Grissom H., D’Elia M., DeRada S., et al. (2020). A multidisciplinary approach to investigate deep-pelagic ecosystem dynamics in the gulf of Mexico following deepwater horizon. Front. Mar. Sci. 7, 548880. doi: 10.3389/fmars.2020.548880
Cook A., Sutton T. T. (2017a). Inventory of gulf oceanic fauna data including species, weight, and measurements. cruises DP01 may 1-8, 2015 and DP02 august 9-21, 2015 R/V on the point sur in the northern gulf of Mexico (Distributed by: gulf of Mexico research initiative information and data cooperative (GRIIDC)) (Corpus Christi, TX: Harte Research Institute, Texas A&M University). doi: 10.7266/N70P0X3T
Cook A., Sutton T. T. (2017b). Inventory of gulf of Mexico oceanic fauna data including species, weight, and measurements from R/V point sur (Cruises DP03 and DP04) may-august 2016. distributed by: gulf of Mexico research initiative information and data cooperative (GRIIDC) (Harte Research Institute, Texas A&M University: Corpus Christi). doi: 10.7266/N7XP7385
Cook A., Sutton T. T. (2018). Inventory of oceanic fauna data including species, weight, and measurements from R/V point sur (Cruise DP05) in the gulf of Mexico from 2017-05-01 to 2017-05-11. distributed by: gulf of Mexico research initiative information and data cooperative (GRIIDC) (Corpus Christi, TX: Harte Research Institute, Texas A&M University). doi: 10.7266/N7902234
Cook A., Sutton T. T. (2019). Inventory of oceanic fauna data including species, weight, and measurements from R/V point sur cruise PS19-04 (DP06) in the gulf of Mexico from 2018-07-19 to 2018-08-01. distributed by: gulf of Mexico research initiative information and data cooperative (GRIIDC) (Corpus Christi: Harte Research Institute, Texas A&M University). doi: 10.7266/n7-ac8e-0240
Davis M. P., Holcroft N. I., Wiley E. O., Sparks J. S., Smith W. L. (2014). Species-specific bioluminescence facilitates speciation in the deep sea. Mar. Biol. 161 (5), 1139–1148. doi: 10.1007/s00227-014-2406-x
Davison P. C., Checkley D. M. Jr., Koslow J. A., Barlow J. (2013). Carbon export mediated by mesopelagic fishes in the northeast pacific ocean. Prog. Oceanography 116, 14–30. doi: 10.1016/j.pocean.2013.05.013
de Busserolles F., Fitzpatrick J. L., Paxton J. R., Marshall N. J., Collin S. P. (2013). Eye-size variability in deep-sea lanternfishes (Myctophidae): an ecological and phylogenetic study. Public Library Sci. One 8 (3), 1–14. doi: 10.1371/journal.pone.0058519
de Busserolles F., Fogg L., Cortesi F., Marshall J. (2020). The exceptional diversity of visual adaptations in deep-sea teleost fishes. In Semin. Cell Dev. Biol. 106, 20–30). doi: 10.1016/j.semcdb.2020.05.027
De Forest L., Drazen J. (2009). The influence of a Hawaiian seamount on mesopelagic micronekton. Deep Sea Res. Part I: Oceanographic Res. Papers 56 (2), 232–250. doi: 10.1016/j.dsr.2008.09.007
Douglas R. H., Partridge J. C., Dulai K., Hunt D., Mullineaux C. W., Tauber A. Y., et al. (1998). Dragon fish see using chlorophyll. Nature 393 (6684), 423–424.
Drazen J. C., Sutton T. T. (2017). Dining in the deep: the feeding ecology of deep-sea fishes. Annu. Rev. Mar. Sci. 9, 337–366. doi: 10.1146/annurev-marine-010816-060543
Eduardo L. N., Lucena-Frédou F., Mincarone M. M., Soares A., Le Loc’h F., Frédou T., et al. (2020). Trophic ecology, habitat, and migratory behaviour of the viperfish Chauliodus sloani reveal a key mesopelagic player. Sci. Rep. 10 (1), 1–13. doi: 10.1038/s41598-020-77222-8
Feagans-Bartow J. N., Sutton T. T. (2014). Ecology of the oceanic rim: pelagic eels as key ecosystem components. Mar. Ecol. Prog. Series. 502, 257–266. doi: 10.3354/meps10707
Fink W. L. (1985). Phylogenetic interrelationships of the stomiid fishes (Teleostei: stomiiformes). Miscellaneous Publications Museum Zoology Univ. Michigan 171, 1–127.
Frank T. M., Fine C. D., Burdett E. A., Cook A. B., Sutton T. T. (2020). The vertical and horizontal distribution of deep-sea crustaceans in the order euphausiacea in the vicinity of the deepwater horizon oil spill. Front. Mar. Sci. 7, 99. doi: 10.3389/fmars.2020.00099
Fricke R., Eschmeyer W., Fong J. D. (2022) Catalogue of fishes (California Academy of Sciences) (Accessed 10 September 2022).
Gartner J. V. Jr., Crabtree R. E., Sulak K. J. (1997). “Feeding At depth,” in Deep-Sea fishes. Eds. Randall D. J., Farrell A. P. (Academic Press), 115–193.
Gartner J. V., Sulak K. J., Ross S. W., Necaise A. M. (2008). Persistent near-bottom aggregations of mesopelagic animals along the north Carolina and Virginia continental slopes. Mar. Biol. 153 (5), 825–841. doi: 10.1007/s00227-007-0855-1
Gibbs R. H. Jr., Clarke T. A., Gomon J. R. (1983). Taxonomy and distribution of the stomioid fish genus Eustomias (Melanostomiidae), I: subgenus nominostomias. Smithsonian Contributions to Zoology 380, 1–139. doi: 10.5479/si.00810282.380
Greven H., Walker Y., Zanger K. (2009). On the structure of teeth in the viperfish Chauliodus sloani Bloch & schneider 1801 (Stomiidae). Bull. Fish Biol. 11 (1/2), 87–98.
Haffner R. E. (1952). Zoogeography of the bathypelagic fish, Chauliodus. Systematic Zoology 1 (3), 113–133.
Hidaka K., Kawaguchi K., Murakami M., Takahashi M. (2001). Downward transport of organic carbon by diel migratory micronekton in the western equatorial pacific: its quantitative and qualitative importance. Deep Sea Res. Part I: Oceanographic Res. Papers 48 (8), 1923–1939.
Hopkins T. L., Gartner J. V. (1992). Resource-partitioning and predation impact of a low-latitude myctophid community. Mar. Biol. 114 (2), 185–197. doi: 10.1007/BF00349518
Hopkins T. L., Sutton T. T., Lancraft T. M. (1996). The trophic structure and predation impact of a low latitude midwater fish assemblage. Prog. Oceanography 38 (3), 205–239. doi: 10.1016/S0079-6611(97)00003-7
Howe J. C. (2002). Standard length: not quite so standard. Fisheries Res. 56 (1), 1–7. doi: 10.1016/S0165-7836(01)00312-5
Islam H. S., Hibino M., Tanaka M. (2006). Distribution and dietary relationships of the Japanese temperate bass Lateolabrax japonicus juveniles with two contrasting copepod assemblages in estuarine nursery grounds in the ariake Sea, Japan. J. Fish Biol. 68, 569–593. doi: 10.1111/j.0022-1112.2006.00943.x
Ivlev V. S. (1961). Experimental ecology on the feeding of fishes (New Haven: Yale University Press).
Kenaley C. P. (2012). Exploring feeding behaviour in deep-sea dragonfishes (Teleostei: stomiidae): jaw biomechanics and functional significance of a loosejaw. Biol. J. Linn. Soc. 106 (1), 224–240. doi: 10.1111/j.1095-8312.2012.01854.x
Lande R., Wilkinson G. S. (1999). Models of sex-ratio meiotic drive and sexual selection in stalk-eyed flies. Genet. Res. 74 (3), 245–253. doi: 10.1017/S0016672399004218
Law C. J., Duran E., Hung N., Richards E., Santillan I., Mehta R. S. (2018). Effects of diet on cranial morphology and biting ability in musteloid mammals. J. Evolutionary Biol. 31 (12), 1918–1931. doi: 10.1111/jeb.13385
Merrett N., Roe H. S. J. (1974). Patterns and selectivity in the feeding of certain mesopelagic fishes. Mar. Biol. 28 (2), 115–126. doi: 10.1007/BF00396302
Meyer A., Morrissey J. M., Schartl M. (1994). Recurrent origin of a sexually selected trait in Xiphophorus fishes inferred from a molecular phylogeny. Nature 368 (6471), 539–542.
Mihalitsis M., Bellwood D. R. (2017). A morphological and functional basis for maximum prey size in piscivorous fishes. Public Library Sci. One 12 (9), 1–19. doi: 10.1371/journal.pone.0184679
Olsen A. M. (2017). Feeding ecology is the primary driver of beak shape diversification in waterfowl. Funct. Ecol. 31 (10), 1985–1995. doi: 10.1111/1365-2435.12890
Partridge J. C., Douglas R. H. (1995). Far-red sensitivity of dragon fish. Nature 375 (6526), 21–22.
Ribeiro D. F. O., Nuñer A. P. O. (2008). Feed preferences of Salminus brasiliensis (Pisces, characidae) larvae in fish ponds. Aquaculture 274, 65–71. doi: 10.1016/j.aquaculture.2007.11.012
Romesburg H. C. (1990). Cluster analysis for researchers (Malabar, FL: Robert E Kreiger Publishing Company).
Rometsch S. J., Torres-Dowdall J., Machado-Schiaffino G., Karagic N., Meyer A. (2021). Dual function and associated costs of a highly exaggerated trait in a cichlid fish. Ecol. Evol. 11 (23), 17496–17508. doi: 10.1002/ece3.8383
Santana S. E., Grosse I. R., Dumont E. R. (2012). Dietary hardness, loading behavior, and the evolution of skull form in bats. Evolution: Int. J. Organic Evol. 66 (8), 2587–2598. doi: 10.1111/j.1558-5646.2012.01615.x
Schartl M., Kneitz S., Ormanns J., Schmidt C., Anderson J. L., Amores A., et al. (2021). The developmental and genetic architecture of the sexually selected male ornament of swordtails. Curr. Biol. 31 (5), 911–922. doi: 10.1016/j.cub.2020.11.028
Schnell N. K., Britz R., Johnson G. D. (2010). New insights into the complex structure and ontogeny of the occipito-vertebral gap in barbeled dragonfishes (Stomiidae, teleostei). J. Morphology 271 (8), 1 006–11022. doi: 10.1002/jmor.10858
Schnell N. K., Johnson G. D. (2017). Evolution of a functional head joint in deep-sea fishes (Stomiidae). PloS One 12 (2), e0170224. doi: 10.1371/journal.pone.0170224
Sowersby W., Eckerström-Liedholm S., Rowiński P. K., Balogh J., Eiler S., Upstone J. D., et al. (2022). The relative effects of pace of life-history and habitat characteristics on the evolution of sexual ornaments: a comparative assessment. Evolution 76 (1), 114–127. doi: 10.1111/evo.14358
Sutton T. T. (2005). Trophic ecology of the deep-sea fish Malacosteus niger (Pisces: stomiidae): an enigmatic feeding ecology to facilitate a unique visual system? Deep Sea Res. Part I: Oceanographic Res. Papers 52 (11), 2065–2076.
Sutton T. T., Cook A., Moore J. A., Frank T., Judkins H., Vecchione M., et al. (2017a). Inventory of gulf oceanic fauna data including species, weight, and measurements. Meg skansi cruises from jan. 25 – sept. 30, 2011 in the northern gulf of Mexico (Distributed by: gulf of Mexico research initiative information and data cooperative (GRIIDC)) (Corpus Christi, TX: Harte Research Institute, Texas A&M University). doi: 10.7266/N7VX0DK2
Sutton T. T., Cook A., Moore J. A., Frank T., Judkins H., Vecchione M., et al. (2017b). Inventory of gulf oceanic fauna data including species, weight, and measurements, Pisces cruises from dec. 1 2010 – sept. 29, 2011 in the northern gulf of Mexico (Distributed by: gulf of Mexico research initiative information and data cooperative (GRIIDC)) (Corpus Christi, TX: Harte Research Institute, Texas A&M University). doi: 10.7266/N7R49NTN
Sutton T. T., Frank T., Judkins H., Romero I. C. (2020). “As gulf oil extraction goes deeper, who is at risk? community structure, distribution, and connectivity of the deep-pelagic fauna,” in Scenarios and responses to future deep oil spills (Springer, Cham), 403–418.
Sutton T. T., Hartel K. E. (2004). New species of eustomias (Teleostei: stomiidae) from the western north Atlantic, with a review of the subgenus Neostomias. Copeia 2004 (1), 116–121.
Sutton T. T., Hopkins T. L. (1996a). Trophic ecology of the stomiid (Pisces: stomiidae) fish assemblage of the eastern gulf of Mexico: strategies, selectivity and impact of a top mesopelagic predator group. Mar. Biol. 127 (2), 179–192.
Sutton T. T., Hopkins T. L. (1996b). Species composition, abundance, and vertical distribution of the stomiid (Pisces: stomiiformes) fish assemblage of the gulf of Mexico. Bull. Mar. Sci. 59 (3), 530–542.
Sutton T. T., Porteiro F. M., Heino M., Byrkjedal I., Langhelle G., Anderson C. I. H., et al. (2008). Vertical structure, biomass and topographic association of deep-pelagic fishes in relation to a mid-ocean ridge system. Deep Sea Res. Part II: Topical Stud. Oceanography 55 (1-2), 161–184.
Swallow J. G., Wilkinson G. S., Marden J. H. (2000). Aerial performance of stalk-eyed flies that differ in eye span. J. Comp. Physiol. B 170 (7), 481–487. doi: 10.1007/s003600000124
Verwaijen D., Van Damme R., Herrel A. (2002). Relationships between head size, bite force, prey handling efficiency and diet in two sympatric lacertid lizards. Funct. Ecol. 16 (6), 842–850. doi: 10.1046/j.1365-2435.2002.00696.x
Wiebe P. H., Morton A. W., Bradley A. M., Backus R. H., Craddock J. E., Barber V., et al. (1985). New development in the MOCNESS, an apparatus for sampling zooplankton and micronekton. Mar. Biol. 87 (3), 313–323. doi: 10.1007/BF00397811
Wilkinson G. S., Dodson G. N. (1997). “Function and evolution of antlers and eye stalks in flies,” in Evolution of mating systems in insects and arachnids. Eds. Choe J., Crespi B. (Cambridge: Cambridge University Press), 310–328.
Keywords: Stomiidae, Melanostomiinae, trophic ecology, ecomorphology, morphological-dietary relationships, mesopelagic
Citation: McGonagle RP, Kerstetter DW, Fenolio D and Sutton TT (2023) Ecomorphology of a predatory deep-sea fish family: does trophic specialization drive hyperspeciation? Front. Mar. Sci. 10:1056094. doi: 10.3389/fmars.2023.1056094
Received: 28 September 2022; Accepted: 13 April 2023;
Published: 10 May 2023.
Edited by:
Alexandra Anh-Thu Weber, Swiss Federal Institute of Aquatic Science and Technology, SwitzerlandReviewed by:
Zuzana Musilova, Charles University, CzechiaNathan Vranken, Research Foundation Flanders (FWO), Belgium
Copyright © 2023 McGonagle, Kerstetter, Fenolio and Sutton. This is an open-access article distributed under the terms of the Creative Commons Attribution License (CC BY). The use, distribution or reproduction in other forums is permitted, provided the original author(s) and the copyright owner(s) are credited and that the original publication in this journal is cited, in accordance with accepted academic practice. No use, distribution or reproduction is permitted which does not comply with these terms.
*Correspondence: Tracey T. Sutton, dHN1dHRvbjFAbm92YS5lZHU=