- 1Cascadia Research Collective, Olympia, WA, United States
- 2Naval Information Warfare Center Pacific, San Diego, CA, United States
- 3Pacific Islands Fisheries Science Center, National Marine Fisheries Service, National Oceanic and Atmospheric Administration, Honolulu, HI, United States
- 4National Marine Mammal Foundation, San Diego, CA, United States
- 5Marine Mammal Institute, Oregon State University, Newport, OR, United States
- 6Department of Fisheries, Wildlife, and Conservation Sciences, Oregon State University, Corvallis, OR, United States
In this assessment we incorporated published and unpublished information to delineate and score Biologically Important Areas (BIAs) for cetaceans in the Hawaiʻi region following standardized criteria. Twenty-six cetacean species have been documented in Hawaiʻi. Eleven odontocete species have distinct small populations resident to one or more island areas: rough-toothed dolphins, pantropical spotted dolphins, common bottlenose dolphins, spinner dolphins, short-finned pilot whales, false killer whales, pygmy killer whales, melon-headed whales, Blainville’s beaked whales, Cuvier’s beaked whales, and dwarf sperm whales. Eight species of mysticetes have been documented, although their occurrence and behavior are poorly understood, with the exception of breeding humpback whales and, more recently, common minke whales. Thirty-five BIAs were delineated or revised from the initial 2015 effort: 33 for small and resident odontocete populations and two for humpback whale reproductive areas. Hierarchical BIAs reflecting core areas of use or population-specific ranges were delineated for nine species. Reproductive watch list areas were designated for common minke whales in the main Hawaiian Islands (MHI) and humpback whales in the Northwestern Hawaiian Islands (NWHI); these areas did not meet the criteria for a BIA due to limited supporting information. All but three BIAs were in the MHI, reflecting the disparities in research effort between this region and the NWHI. Spatial extents of BIA boundaries ranged from 457 km2 to 138,001 km2 (median = 8,299 km2). Scores (range: 1-3) for Data Support and Boundary Certainty were moderate to high (mean = 2.40 and 2.43, respectively), while Intensity and Importance scores were slightly lower (mean = 1.94 and 1.89, respectively). Many of the Hawaiʻi species have been extensively studied over several decades; accordingly, this region ranks among the highest in terms of Data Support relative to other regions. BIAs presented here describe known ranges of small resident populations, intensities of use, and uncertainties in important areas for cetaceans in Hawaiʻi based on the best available data, and have also revealed knowledge gaps to guide future research efforts.
1 Introduction
Cetaceans in Hawaiian waters overlap with a number of anthropogenic activities that have the potential to result in both indirect and direct harmful population-level consequences. Threats include military operations (e.g., Martin et al., 2015; Henderson et al., 2019; Baird et al., 2021a; Durbach et al., 2021), commercial and recreational fishing (e.g., Baird and Webster, 2020; Baird et al., 2021b), tourism (e.g., Currie et al., 2021), shipping (e.g., Lammers et al., 2013), pollutants (e.g., Ylitalo et al., 2009; Bachman et al., 2014; Kratofil et al., 2020), protozoal disease from feral, non-native cats (Migaki et al., 1990; Landrau-Giovannetti et al., 2022), and marine debris (Currie et al., 2017). The range-resident behavior of many Hawaiian cetaceans (Baird, 2016) may further exacerbate risk from these threats; where site fidelity may have once been evolutionarily advantageous, this mechanism may become maladaptive in the Anthropocene (Merkle et al., 2022). To inform cetacean impact assessments and marine conservation and management efforts in this region, we identified and scored Biologically Important Areas (BIAs) for cetaceans in Hawaiʻi as part of a nationwide process coordinated by the National Marine Fisheries Service (NMFS; Harrison et al in review; Van Parijs et al., 2015).
BIAs represent areas and times (months or seasons) that are important to cetacean species, stocks, or populations for reproduction (R-BIAs), feeding (F-BIAs), or migration (M-BIAs; Harrison et al in review; Ferguson et al., 2015). BIAs may also be defined to encompass the range and core areas of small resident populations (S-BIAs). Fundamentally, BIAs are compilations of the best available science and have no inherent or direct regulatory authority. Several types of information relating to a species’ occurrence in a region are used to delineate BIAs, including (but not limited to) density or abundance, behavior, range size, and data availability. This effort builds upon the initial BIA effort (BIA I Van Parijs et al., 2015) by revising existing BIAs (Baird et al., 2015a), creating new BIAs based on new information, and scoring each BIA based on Intensity of use, Data Support, Importance, Spatiotemporal Variability, and Boundary Certainty (BIA II, hereafter; Harrison et al in review). Details on the BIA II delineation methodology applied in this study are available in Supplementary File A.
The archipelago spanning the main Hawaiian Islands (MHI) – from Hawaiʻi Island to Kauaʻi and Niʻihau (Kauaʻi/Niʻihau for brevity, hereafter) – to the shallow atolls of the Northwestern Hawaiian Islands (NWHI) is surrounded by an area of deep, unproductive waters (Doty and Oguri, 1956; Ziegler, 2002). In contrast to high-latitude regions, the central North Pacific is often characterized as a biological desert as it lacks an abundance of phytoplankton and zooplankton that typically underlie dynamic and productive ecological communities (Iverson, 1990; Polovina et al., 2008; Gove et al., 2016). Despite this, the Hawaiian Islands interrupt wind and ocean currents in a manner that produces biologically favorable conditions. This “island mass effect” involves several localized causative mechanisms, such as upwelling, precipitation patterns, and island-associated inputs (e.g., groundwater discharge), that collectively enhance productivity in the marine environment immediately surrounding the islands (Doty and Oguri, 1956; Gilmartin and Revelante, 1974; Gove et al., 2016). Seasonal variation in the Hawaiian environment is characterized by latitudinal shifts in trade winds and sea surface temperature (Flament, 1996). However, the magnitude of seasonal and dynamic or ephemeral environmental changes are not as drastic as those observed in high-latitude regions (e.g., the California Current System; Hickey, 1979; Checkley and Barth, 2009). Accordingly, evidence for spatiotemporal variation in habitat use by non-migratory cetaceans in this region is generally limited and only recently emerging (Ziegenhorn, 2022), but also less understood compared to those in some higher-latitude systems (e.g., Tynan et al., 2005; Becker et al., 2017).
As a result of the island mass effect and nearshore habitat provided by steep slopes of the volcanic islands, the Hawaiian Islands provide suitable habitat for many typically pelagic-ranging cetaceans (Baird, 2016). Not surprisingly, many of these deep-water species have formed island-associated populations resident to this region that are genetically distinct from offshore counterparts (Baird, 2016). The close proximity of deep-water habitat to accessible nearshore waters has enabled long-term studies on such species, primarily odontocetes, including a number of blackfish (e.g., false killer whale (Pseudorca crassidens), short-finned pilot whale (Globicephala macrorhynchus), pygmy killer whale (Feresa attenuata), melon-headed whale (Peponocephala electra)) and beaked whale species (Baird et al., 2013a; Baird, 2016). In addition, the shallow, warm waters created by island complexes and bays provide ideal nursery and breeding grounds for humpback whales and a protected refuge for small delphinids (Baird, 2016).
Sufficient information on population structure and occurrence is available to delineate BIAs for 12 of 26 cetacean species documented in Hawaiian waters (Baird, 2016; Baird et al., 2022). The majority (11) of these species are odontocetes found in nearshore regions of the MHI and/or NWHI and meet the criteria for small and resident population BIAs. Species with S-BIAs include the rough-toothed dolphin (Steno bredanensis), common bottlenose dolphin (Tursiops truncatus), pantropical spotted dolphin (Stenella attenuata), spinner dolphin (Stenella longirostris), pygmy killer whale, melon-headed whale, false killer whale, short-finned pilot whale, dwarf sperm whale (Kogia sima), Cuvier’s beaked whale (Ziphius cavirostris), and Blainville’s beaked whale (Mesoplodon densirostris). The remaining BIA is an R-BIA for humpback whales (Megaptera novaeangliae). Some of the BIAs were delineated for populations that are formally recognized as “stocks” by NMFS (Caretta et al., 2021), and others for populations that are not designated as stocks, but whose existence as a distinct population is supported by several lines of evidence that collectively warrant BIA delineation. For general purposes, throughout this assessment we use the term “population” in reference to the population unit (i.e., stock, population, or activity group, such as breeding population of humpback whales) that each BIA reflects, and only use “stock” when one is specifically recognized by NMFS. Our detailed definitions of stock, population, and other relevant terms in the context of this regional assessment are provided in Supplementary File A.
Studying many of these species in Hawaiʻi involves persistent challenges, primarily pertaining to the behavior of the species and limitations in data collection methods (Baird, 2016). For example, many species, such as beaked whales and dwarf sperm whales, are rare, cryptic, and long divers, and are therefore seldom visible to surface observers (Baird, 2016; Baird, 2019; Baird et al., 2021c). Additionally, many species often have an unpredictable occurrence compared to those with seasonal life history behaviors (e.g., breeding humpback whales) or move frequently and quickly among island areas (e.g., false killer whales; Baird et al., 2012). Further, poor working conditions outside of the islands’ protected leeward waters restricts direct observation using common methods (e.g., dedicated small-boat surveys; Baird et al., 2013a; Baird, 2016). The vast majority of information is available only for populations in the MHI. Although almost all of these species are known to occur in the NWHI (Bradford et al., 2021), its remoteness has precluded extensive research efforts that could provide a more comprehensive understanding of population structure, ranges, and abundance in the NWHI. Collectively, these factors drive variation among species in Hawaiʻi in the quality and quantity of data used to inform BIA boundaries and scores described herein.
For this BIA II effort, a synthesis of existing published and unpublished information was used to identify and characterize each population’s areas of importance following the new guidelines detailed by Harrison et al. (in review), in a manner consistent within the Hawaiʻi region and across regions in the nation. More specifically, the primary aims of this regional effort were: (1) to delineate S-BIA boundaries that encompass each population’s entire known spatial extent for those populations that meet the S-BIA criteria; (2) delineate new S-BIAs for populations that have gained recent recognition from ongoing research efforts; (3) use more contemporary and comprehensive sets of information to support BIA boundary delineations; (4) document all lines of available evidence to derive metrics of Intensity, Data Support, and associated uncertainties for all BIAs; and (5) identify watch list areas (i.e., areas not considered for BIAs at present, but added to a watch list for future consideration) and knowledge gaps to guide future research efforts.
In the following sections, we provide an overview of the methodology and data sources incorporated into the BIA delineation process for this region. For illustrative purposes, we present detailed examples of our approach for a sample of the Hawaiʻi BIAs with variable levels of information types and support. Watch list areas are detailed and the factors currently precluding their designation as BIAs are discussed. We then develop a summary of the BIAs described herein and discuss variation in all aspects of the new scoring process as it relates to current understanding of cetaceans in Hawaiʻi, as well as to other BIAs delineated across the nation. We conclude by drawing inferences from the resulting BIA products as they relate to conservation needs of cetaceans in Hawaiian waters, as well as remaining gaps to be filled by future research and associated recommendations that have been developed throughout this process. While only a sample of detailed examples of the Hawaiʻi BIAs is presented here, full descriptions of all BIAs (maps, supporting tables, scores, justifications) and detailed methodologies are provided as Supplementary Material and on the BIA website1.
2 Methods
BIAs for all seven regions around the U.S. were delineated and scored using consistent methodology, developed using principles of expert elicitation, and detailed in Harrison et al. (in review). The identification, delineation, and scoring of Hawaiʻi BIAs was overseen by a regional lead (RWB) with engagement from additional subject matter experts (all coauthors here). More specifically, within the Hawaiʻi region, the process involved an initial discussion with subject matter experts to identify species in Hawaiʻi for which BIAs could be delineated, data sources that were available and relevant, and analytical approaches for delineating BIA boundaries. Draft BIAs were then circulated to subject matter experts for review and contributions for each BIA account (i.e., writing, analysis, boundary delineation, scoring), before finalizing the BIA. BIA scoring metrics (Intensity, Data Support, Importance, Boundary Certainty, Spatiotemporal Variability) were assigned an integer value ranging from 1 (“low”) to 3 (“high”) except for the Spatiotemporal Variability indicator (static, dynamic, or ephemeral). Higher Intensity and Data Support scores correspond to a BIA characterized by factors indicating comparatively concentrated use (e.g., intensely used area, restricted range, small abundance) that is supported by more and/or higher quality information, respectively. These two scores are combined in the Importance matrix to determine the overall Importance score. High Boundary Certainty scores equate to high confidence in the location and timing of the BIA boundary. The Spatiotemporal Variability indicator characterizes the spatiotemporal variability of the BIA based on known links between the species’ distribution and underlying environment. BIAs with a resulting Importance score of 0 (i.e., Intensity = 1, Data Support = 1) were designated as watch list areas. Harrison et al. (in review) provide further details on the BIA methodology and discuss caveats and concerns relevant to all regions. All analyses involved in the development of Hawaiʻi BIAs were conducted in the program R v. 4.1.2 (R Core Team, 2021).
The available data types used to inform Hawaiʻi BIA boundaries and scores varied across species. Nevertheless, the information sources were generally consistent within taxonomic orders. All odontocete S-BIAs were predominantly supported by information curated by Cascadia Research Collective (CRC), involving dedicated odontocete studies in the Hawaiian Islands since 2000 (Baird, 2016; see Baird et al., 2013a for survey details). CRC data include sightings, photo-identification catalogs (information on re-sighting rates, number of identifications, etc.), and satellite tag data. Sightings data from large-scale ship-based line-transect surveys undertaken by NMFS from 2002-2020 were also available (see Barlow, 2006; Bradford et al., 2017; Yano et al., 2018; Yano et al., 2020). Additional data sources were used in delineating the humpback whale R-BIA, including satellite tag data from Oregon State University (OSU; Mate et al., 1998; Palacios et al., 2019; Palacios et al., 2020) and Naval Information Warfare Center Pacific (NIWC Pacific; Henderson et al., 2019; Henderson et al., 2021; Henderson et al., 2022), sightings from dedicated small-boat survey efforts conducted by NIWC Pacific and HDR, Inc., and sightings from aerial surveys conducted by Marine Mammal Research Consultants, Ltd. (MMRC; Mobley et al., 2001; Mobley, 2004) accessed from PacIOOS Voyager2. Lastly, acoustic detection data from both NMFS surveys and NIWC Pacific (Pacific Missile Range Facility, PMRF) served as the primary source for delineating the common minke whale (Balaenoptera acutorostrata; minke whale hereafter) watch list area, with all available, albeit limited, visual sightings included (NMFS, CRC, HDR, Inc.). For most of these species, there was also photographic/sighting information from community scientists in Hawaiʻi (e.g., ecotourism operators, fishermen). While these contributions rarely come with associated latitude and longitude to include in the boundary delineation process (typically only island or regional locality is provided), we incorporated relevant information on social structure and relative abundance into this assessment. We acknowledge that we have not included all potential sources of cetacean small-vessel and aerial survey effort from Hawaiian waters for BIA delineation purposes. While there have been large numbers of single-species (e.g., spinner dolphins, humpback whales) research efforts, these have typically been restricted to shallow leeward waters and off one or two islands, and are less relevant for determining BIA boundaries, although we used information from these studies in general support of BIA delineation and scoring. Similarly, sighting data from aerial surveys are available for many small cetaceans included here (e.g., Mobley et al., 2000), but population assignment cannot be determined from this source (i.e., identification photographs are required), and thus these data could not be attributed to population-specific odontocete BIAs.
Data sources, types, and quantification of effort for information used in the Hawaiʻi BIAs are summarized in Table 1. Background narratives introduce each species and corresponding population(s) and justify BIA delineation based on our current understanding of population structure and distribution from all relevant published studies, stock assessments (when applicable), technical reports, and/or unpublished information from the sources above. These narratives include details on types of available information, including, but not limited to, long-term photo-identification studies (e.g., re-sighting rates), relative abundance, movements observed from satellite tags or documented from sightings of unique individuals over time, and genetic analyses. Lastly, the narratives detail the development of the BIAs in this assessment compared to the earlier BIAs in Baird et al. (2015a).
The spatial extent of BIA boundaries varied by BIA type: the basis for S-BIA spatial boundaries were often represented by a polygon (e.g., minimum convex polygon (MCP)) encompassing all occurrence data (i.e., sightings, tag positions), as the intention of an S-BIA is to encompass the entire known range of the population (Harrison et alin review). In some cases, current stock boundaries were used as the basis for S-BIA boundaries (e.g., spinner dolphins) or incorporated into new S-BIA boundaries (e.g., Kauaʻi/Niʻihau/Oʻahu/Maui Nui common bottlenose dolphins). For populations with sufficient satellite tag data, location data were used to derive BIA boundaries through kernel density analysis (e.g., MHI short-finned pilot whales). In contrast, R-BIA boundary extents (MHI humpback whales) and the watch list areas (minke whales, NWHI humpback whales) were derived from the relative distribution of all available occurrence data with the intent to capture areas of noticeable importance (i.e., used by a substantial portion of the sample population) but not necessarily all points of occurrence. These were either represented through a polygon drawn around the relative distribution or an isobath that appropriately captured the distribution of importance. For all BIAs, the inside (i.e., shoreward) boundary of the BIA was defined by an isobath or proximity from land that was deemed reasonable for each species (e.g., 800-m isobath as inner boundary for deep-water dwelling Cuvier’s beaked whales; see Baird, 2019). In addition, for several BIA boundaries based on MCPs around locations from satellite tags, 3 km was added to the polygon to account for estimated satellite tag positional error that otherwise extended outside of the polygon (see Supplementary File B for details).
Hierarchical BIAs were delineated for several Hawaiʻi BIAs to either represent the core area of a population (i.e., area of intensified use for a reproductive BIA or for small resident populations) or to reflect the primary range of a community (i.e., localized group of interacting individuals within a population) or island-associated population within the broader range of a multi-island ranging population reflected by the parent BIA. As an example of a S-BIA core area, island-associated Cuvier’s beaked whales have a relatively large range around Hawaiʻi Island, but occurrence data indicate that they spend the majority of their time between the 2,000-m and 3,500-m isobaths off the leeward side of the island (Baird et al., 2013a; Baird, 2019). Here we considered the area between these isobaths to be their core range, represented by a child BIA (Supplementary File A). As for representing the primary range of a multi-island ranging population, although there are demographically distinct populations and recognized stocks of bottlenose dolphins associated with each of Kauaʻi/Niʻihau, Oʻahu, and Maui Nui (Maui Nui = Maui, Kahoʻolawe, Lānaʻi, Molokaʻi), movements of insular individuals are known to occur among these regions (e.g., Harnish, 2021). Therefore, we delineated one parent S-BIA to encompass the range of all three island-associated stocks, thus recognizing inter-island movements that create complexity in our understanding of their population structure, while child BIAs were delineated to represent the primary range for each island-associated stock (Supplementary File A). The spatial extents of child BIA boundaries were either determined using a polygon or isobath encompassing the majority of occurrence data, or kernel density analysis of satellite tag data to derive a 50% isopleth of the resulting utilization distribution, if sufficient data were available (see below). Potential child BIAs could not be added to the watch list, as they would inherently qualify as a BIA (i.e., within parent BIA) and watch list areas are not considered BIAs.
Satellite tag data included in the BIA delineation were processed in a common manner, detailed in Supplementary File B. Briefly, location data (Argos and/or Fastloc®-GPS) were first filtered to remove erroneous locations based on metrics such as unrealistic travel speeds and turning angles. Resultant locations were then fit to a continuous-time correlated random walk model using the package crawl (Johnson et al., 2008; Johnson and London, 2018). The crawl model predicted locations at regular time intervals while accounting for positional uncertainty that typifies tracking data. The crawl locations were re-routed around land as needed using the pathroutr package (London, 2021), either using a polygon representing the island with an added distance or a polygon representing an isobath to ensure re-routed locations were at a reasonable distance from shore or depth for the species.
Some BIAs involved kernel density estimation (KDE) of satellite tag data to generate a utilization distribution (UD) of the sample population (Worton, 1989). An isopleth of the UD served as the basis for the BIA boundary (e.g., 50% for core areas). A coarse timestep of crawl locations was used (e.g., 4-hour) in the KDE process to reduce autocorrelation. Crawl positions during periods of large transmission gaps (with a 1-day gap threshold) were removed from each individual’s track (where applicable) to avoid generating artificially “dense” areas resulting from interpolation over long periods without any underlying Argos/GPS data. One of each pair of tagged individuals moving in concert were removed when applicable to reduce pseudoreplication. All tag locations were pooled together. The contribution of each tag’s location was weighted to the overall kernel density based on deployment length, and the KDE was re-scaled to integrate to 1 (Hauser et al., 2014; Hill et al., 2019). As a result, locations from shorter deployments were given less weight than those from longer deployments, to mitigate bias associated with deployment locality and inherent variability in track duration among tags. Kernel densities were estimated using the bivariate plug-in bandwidth (or smoothing parameter) matrix (Duong and Hazelton, 2003; Duong and Hazelton, 2005; Duong, 2007) accessed through the ks package (Duong, 2021). The location weighting was completed using the weights argument within the ks package (Duong, 2021).
BIA scores were derived following the process in Harrison et al in review. Intensity scores for S-BIAs (which accounted for almost all of the BIAs in this region) were determined through the S-BIA Intensity scoring matrix that uses quantitative criteria on abundance and range size. Where recent and robust estimates of abundance specific to the BIA population were not available to inform Intensity scores, relative abundance from distinct photo-identified individuals and expert elicitation were used and associated uncertainties were documented. The area of each S-BIA was calculated using the sf package (Pebesma, 2018; Supplementary File B) to determine range size scores. Intensity scores for R-BIAs were assigned qualitatively based on a number of factors relating to the frequency of use, size, and density or relative abundance of the population that uses the BIA for activities associated with reproduction (see Harrison et al in review). For all BIAs, the remaining scores (Data Support, Importance, Boundary Certainty, Spatiotemporal Variability indicator) were derived based on the quantity and quality of information pertinent to each score as well as their biases and uncertainties, as detailed in Harrison et al. (in review).
3 Detailed BIA examples
Here we provide three detailed examples of BIAs in the Hawaiʻi region to illustrate our boundary delineation process across populations with varying types, quantities, and qualities of supporting information (in increasing order): (1) Hawaiʻi Island dwarf sperm whale S-BIA; (2) Kauaʻi/Niʻihau/Oʻahu rough-toothed dolphin S-BIA; and (3) MHI humpback whale R-BIA.
3.1 Dwarf sperm whales - Hawaiʻi island S-BIA
3.1.1 Background
A single stock of dwarf sperm whales is recognized by NMFS in Hawaiian waters (Caretta et al., 2021); however, the existence of a separate small, resident population associated with Hawaiʻi Island has long been acknowledged (Baird, 2005; Mahaffy et al., 2009; Oleson et al., 2013; Baird, 2016; Baird et al., 2021c). A recent study analyzing photo-identification data from 19 years of survey effort off the west coast of Hawaiʻi Island reported high site fidelity to slope waters and small geographical ranges within the study area (Baird et al., 2021c). Further, based on depth of sightings of individuals that were re-sighted versus those that were seen once, Baird et al. (2021c) suggested that the range of insular, resident dwarf sperm whales overlaps with that of dwarf sperm whales belonging to a broader pelagic population. While limited genetic samples (primarily from stranded animals) has precluded a genetic assessment of population structure, the lines of evidence derived from the best available data on this species support the existence of a small and resident population of dwarf sperm whales associated with Hawaiʻi Island.
3.1.2 BIA boundary delineation
Following Baird et al. (2015a), we delineated the parent BIA boundary for Hawaiʻi Island dwarf sperm whales based on CRC sighting data, using additional sighting locations obtained since the 2015 BIA assessment (Baird et al., 2021c). We excluded deep-water (> 2,000 m) areas where there were sightings of dwarf sperm whales, based on evidence that these offshore groups may be part of a pelagic population (Baird et al., 2021c). No satellite tag data were available for use in this process as this species has never been satellite tagged. In this assessment, we also estimated this population’s core range based on bathymetric depths with the greatest dwarf sperm whale sighting rates (500-1,000 m; Baird et al., 2013a; Baird et al., 2021c).
3.1.3 Sighting and photographic data
Sighting data were collected from non-systematic, dedicated small-boat surveys conducted by CRC off Hawaiʻi Island from April 2002 through November 2021 (Table 1, Figure 1; see Baird et al., 2013a for details on surveys). Six of the 89 total sightings were in waters greater than 2,000 m deep and suspected to be part of a broader pelagic population (Figure 1; Baird et al., 2021c); thus, they were excluded from the boundary delineation process. Community science photographic and sighting contributions also supplemented information on this population, with 26 sightings off Hawaiʻi Island spanning a period of 16 years (2004-2019), comprising approximately 20% of all identifications in CRC’s photo-identification catalog of Hawaiʻi Island dwarf sperm whales (CRC unpublished). Re-sightings of individuals photo-identified off this island span up to 15 years (Baird et al., 2021c). There were no dwarf sperm whale sightings from NMFS’s ship-based line-transect surveys around Hawaiʻi Island (Bradford et al., 2021).
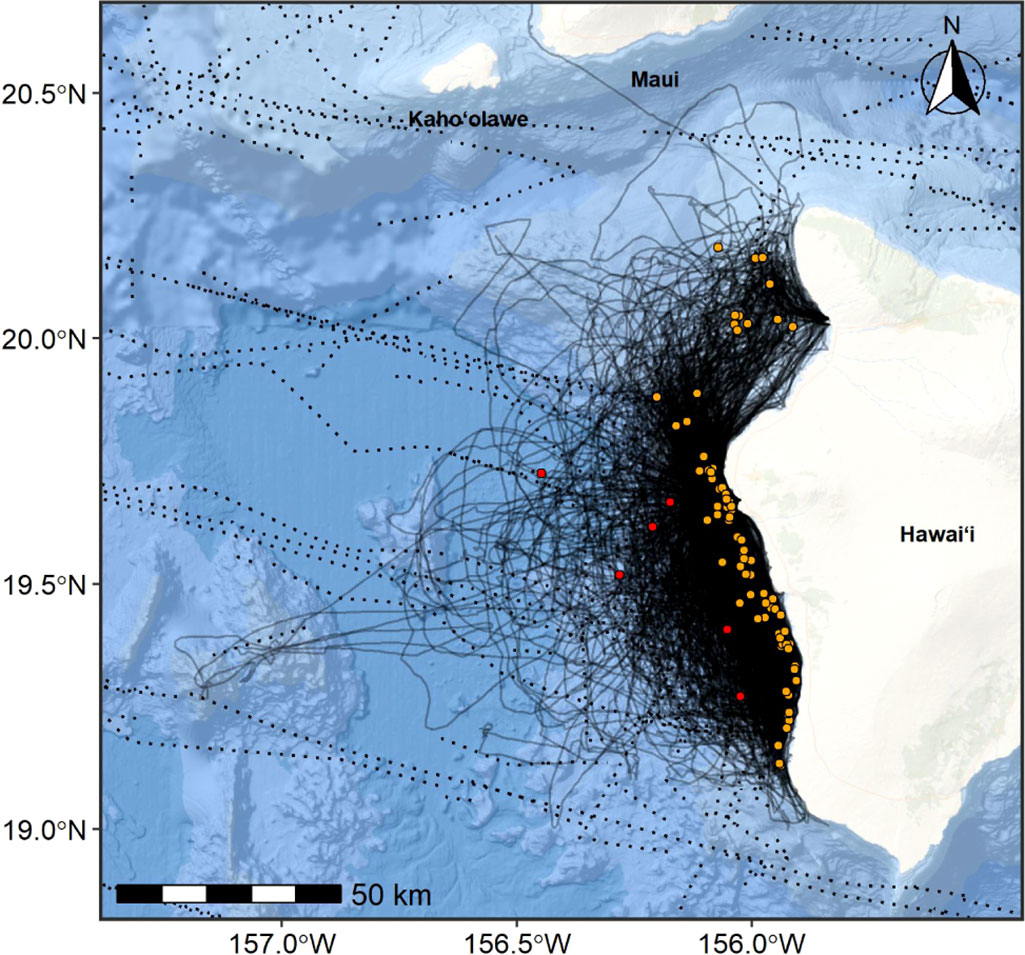
Figure 1 Dwarf sperm whale sighting locations (n=89) off Hawaiʻi Island overlaid on research vessel tracklines (CRC = solid lines, NMFS = dotted lines) from efforts conducted during 2002-2021 (97,438 km of effort CRC and 3,717 km of effort NMFS). Six sightings deeper than 2,000 m depth (included here, shown in red circles) were excluded from the BIA boundary determination as they were likely part of a broader pelagic population (final sample size = 83, orange circles; Baird et al., 2021c). Basemap image is the intellectual property of Esri and is used herein with permission. Copyright © 2022 Esri and its licensors. All rights reserved.
3.1.4 BIA boundary spatial extent
The basis of the parent BIA was an MCP encompassing all sighting locations shallower than the 2,000-m isobath (Figure 2). The inner boundary was defined as the 300-m isobath based on the shallowest sighting of dwarf sperm whales off this island (352 m). Based on sighting rates in relation to bathymetric depths (Baird et al., 2013a; Baird et al., 2021c), we designated the area between the 500-m and 1,000-m isobaths within the MCP as the child BIA (core range) of the population (Figure 2).
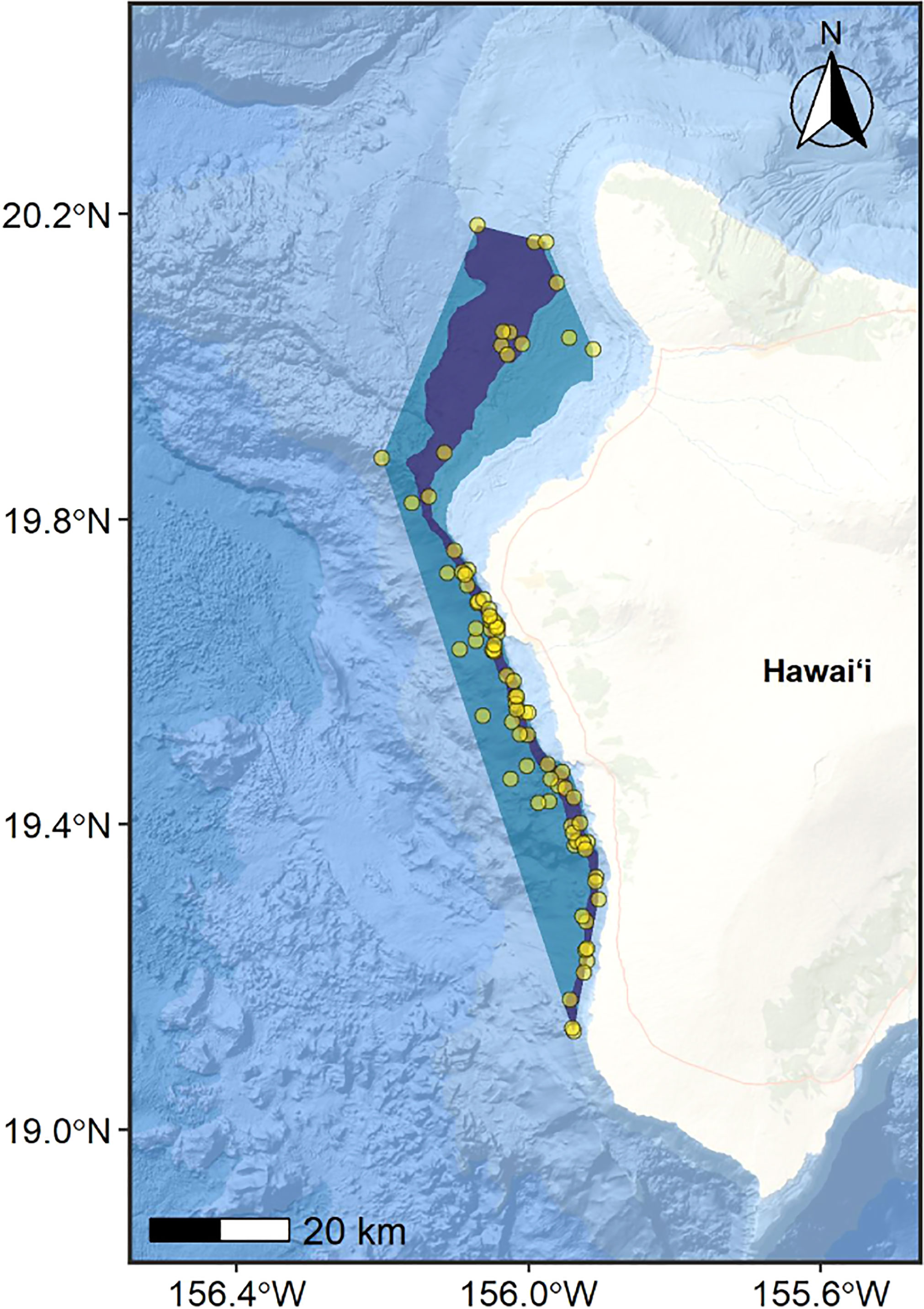
Figure 2 Parent BIA boundary (blue polygon) for the Hawaiʻi Island dwarf sperm whale population represented as a minimum convex polygon (MCP) encompassing all sighting locations in less than 2,000 m water depth (yellow circles), and child BIA boundary (core range; purple polygon) represented as the area between the 500-m and 1,000-m isobaths within the parent BIA. Points are partially transparent to highlight high density areas (i.e., where multiple points overlap). The inner (shoreward) boundary for both BIAs is defined as the 300-m isobath. Total area of the parent BIA = 1,341 km2 and child BIA = 457 km2. Basemap image is the intellectual property of Esri and is used herein with permission. Copyright © 2022 Esri and its licensors. All rights reserved.
3.1.5 BIA scoring
3.1.5.1 Intensity: Abundance and range size
This island-associated population is not formally recognized by NMFS, and no abundance estimate specific to this small, resident population is available. The most recent abundance estimate for the broader Hawaiʻi stock, derived from a shipboard line-transect survey within the U.S. Hawaiian Exclusive Economic Zone (EEZ) conducted in 2002, was 37,440 (CV=0.78) (Bradford et al., 2021). As of January 2021, CRC’s photo-identification catalog for the Hawaiʻi Island population of dwarf sperm whales (sighted in waters < 2,000 m deep) included a total of 84 individuals with slightly distinctive, distinctive, or very distinctive markings (from fair-, good-, or excellent-quality photographs; CRC unpublished). The high resighting rates of photo-identified individuals suggests that the population is small, and re-sightings span up to 15-years (2004 to 2019). Analysis of distances between re-sightings indicates their range is relatively small (Baird et al., 2021c). Photos from dedicated small boat survey effort span an 18-year period (2003-2020). Thus, it is likely that the catalog includes individuals that have died or been born into the population during this period, as well as individuals from a putative pelagic population (Baird et al., 2021c). Combined, these supporting lines of evidence suggest that the population is small; therefore, we assumed the population comprised 125 or fewer individuals (abundance score = 3) for the parent BIA scoring process. The area of the parent BIA is 1,341 km2, which falls within the range size intensity score bin (< 2,000 km2) of 3.
Combining the abundance score (3) and range size (3) results in an overall Intensity score of 3 for the parent BIA. Because the child BIA represents an area of intensified use relative to the entire range of this island-associated population, we assign an Intensity score of 3 for the child BIA. We assume the child BIA contains approximately 50% of the island-associated population, recognizing there are several sources of uncertainty associated with this estimate related to biases from survey effort and challenges in studying this species. A total of 55 sightings (66% of all sightings at < 2,000 m depth) were within the estimated core range (i.e., the child BIA).
3.1.5.2 Data support
Despite the fact that this population has not been formally recognized as a stock by NMFS for management purposes, its probable existence has long been acknowledged and is supported by long-term studies on photo-identified individuals off Hawaiʻi Island (Baird, 2005; Mahaffy et al., 2009; Oleson et al., 2013; Baird, 2016; Baird et al., 2021c). Although no abundance estimates specific to this population are available, long-term photo-identification analyses – with re-sightings of individuals up to 15 years – based on data collected from both dedicated and opportunistic efforts (over 20 years, and 16 years, respectively), provide evidence that this population is small and resident (Baird, 2005; Mahaffy et al., 2009; Baird, 2016; Baird et al., 2021c). No satellite tag data are available for this species (in this particular region or worldwide); therefore, their movements outside of the study area are unknown. It is also suspected that dwarf sperm whales encountered in deeper waters are part of a broader pelagic population and simply overlap with the range of insular, resident dwarf sperm whales. Thus, the resident, insular population’s range is much smaller than the entire geographical range in which all dwarf sperm whales (including pelagic) have been encountered, further supporting the biological importance of nearshore waters to this specific small and resident population (Baird et al., 2021c). Bias in both survey effort and the ability to detect this elusive species makes it challenging to estimate their true range. For example, their range may extend to waters off windward regions of the island where environmental conditions are not conducive to small boat surveys. Based on available information and associated biases, we have intermediate confidence (score = 2) in the Data Support for both the parent and child BIAs.
3.1.5.3 Importance
Combining the Intensity scores (parent = 3, child = 3) and Data Support scores (parent = 2, child = 2) results in Importance scores of 3 for both the parent and child Hawaiʻi Island dwarf sperm whale S-BIA (Harrison et al in review).
3.1.5.4 Boundary certainty
We have intermediate confidence (score = 2) in Boundary Certainty for both the parent and child BIA for Hawaiʻi Island dwarf sperm whales. The parent BIA boundary encompasses the entire population based on a long-term sighting dataset curated from extensive survey effort and community scientists (Figure 2). Resident dwarf sperm whales may use windward areas of the island where survey effort has been precluded; however, we have no evidence to assess this.
3.1.5.5 Spatiotemporal variability
The Spatiotemporal Variability indicator for both parent and child BIAs for Hawaiʻi Island dwarf sperm whales is static, as there is no information to suggest that these areas are used dynamically or ephemerally.
3.2 Rough-toothed dolphin - Kauaʻi/Niʻihau/Oʻahu S-BIA
3.2.1 Background
Although NMFS recognizes only a single stock of rough-toothed dolphins within the U.S. EEZ around the Hawaiian archipelago, there is evidence for considerable population structure within the archipelago, indicating the existence of several island-associated populations (Baird et al., 2008a; Oleson et al., 2013; Albertson et al., 2016; Baird, 2016). Genetic analysis of biopsy samples collected from rough-toothed dolphins revealed no significant differences in mtDNA or nuclear DNA for individuals off Kauaʻi/Niʻihau versus off Oʻahu, but did reveal differentiation from individuals sampled off Hawaiʻi Island (Albertson et al., 2016). Analyses of photo-identified individuals also indicate associations of individuals from Kauaʻi/Niʻihau and Oʻahu, although the degree of association appears to be limited; each island area has a well-defined social cluster of regularly associating individuals with only a single, mutual individual connecting them (Baird et al., 2021a). One satellite-tagged rough-toothed dolphin from Kauaʻi/Niʻihau moved to waters off west Oʻahu for a brief period, further supporting the existence of some association between the two island-associated populations (Baird, 2016; Baird et al., 2019a). Although satellite tags have never been deployed on rough-toothed dolphins off Oʻahu, photo-identification analyses indicate high site fidelity to the island, and movements to Kauaʻi/Niʻihau only rarely occur (CRC unpublished). Two rough-toothed dolphins have been documented moving from Kauaʻi to Hawaiʻi Island; however, those individuals were not associated with rough-toothed dolphins from the Hawaiʻi Island population (Baird et al., 2008) and were later documented back off Kauaʻi (Baird, 2016). In addition, a few inter-island movements among Hawaiʻi Island, Lānaʻi, and Molokaʻi have been documented through photo-identification data (CRC unpublished). The degree of association between traveling dolphins and residents from each island community is unclear due to limited information on this species off Maui Nui. Limited satellite tag-derived movement data preclude a better understanding of movements between Maui Nui and Hawaiʻi Island. Based on these independent lines of evidence, rough-toothed dolphins off Kauaʻi, Niʻihau, and Oʻahu meet the criteria of an S-BIA and are considered a single population (KNO hereafter) for the purposes of this BIA assessment, while recognizing each island-associated population may have different core ranges. Rough-toothed dolphins associated with Hawaiʻi Island and Maui Nui were assigned a separate S-BIA (Supplementary File A).
3.2.2 BIA boundary delineation
Baird et al. (2015a) did not delineate a BIA for this population; however, given the increased quantity and quality of information on rough-toothed dolphins in this region since the original assessment, a BIA for this population is warranted. Both sightings and satellite tag data were used to inform the parent BIA boundary for the KNO rough-toothed dolphin population. Because one satellite tagged dolphin moved to west Oʻahu and some individuals off Oʻahu have associated with those off Kauaʻi/Niʻihau, all Kauaʻi, Niʻihau, and Oʻahu sightings were included in the parent BIA. In addition, a child BIA was delineated to represent the core area of use for this population. It is worth noting that given the limited association between Oʻahu and Kauaʻi/Niʻihau dolphins, in addition to the Oʻahu community’s high site fidelity, there is likely a second core area for this population off Oʻahu that we do not have sufficient information to delineate a child BIA for at this time.
3.2.3 Sighting and photographic data
Sighting data were collected from non-systematic, dedicated small-boat surveys conducted by CRC off Kauaʻi and Niʻihau in 13 years spanning 2003-2021 and off Oʻahu in six years spanning 2002-2017 (Tables 1, 2; Figure 3; see Baird et al., 2013a; Baird et al., 2019a for details on surveys). Surveys off these islands resulted in a total of 295 rough-toothed dolphin sightings (Table 2; Figure 3). In addition, photographic contributions collected over 15 years by other researchers and community scientists (n=82 non-CRC sightings) have substantially supplemented what we know of these populations, particularly rough-toothed dolphins encountered off Oʻahu, given that CRC efforts there have been limited compared to those off Kauaʻi/Niʻihau. For example, 65% of the identifications included in CRC’s photo-identification catalog of the Oʻahu cluster of rough-toothed dolphins are attributed to non-CRC contributions. Additional sighting data were available from NMFS ship-based line-transect surveys (11 years between 2002-2020; Barlow, 2006; Bradford et al., 2017; Yano et al., 2018; Yano et al., 2020; Bradford et al., 2021). NMFS sightings with confirmed photographic assignment to the insular population or within the known range of the insular population were used in boundary determinations (n = 17; Figure 3); effort from these cruises in the area shown in Figure 3 total to 7,238 km (9 individual surveys between 2002-2020).
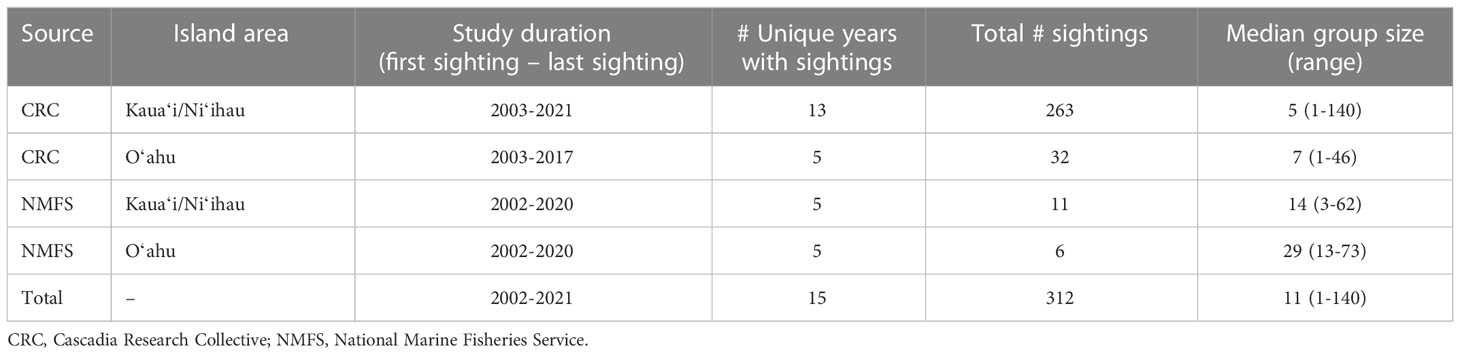
Table 2 Kauaʻi/Niʻihau/Oʻahu rough-toothed dolphin sighting data used in S-BIA boundary delineations.
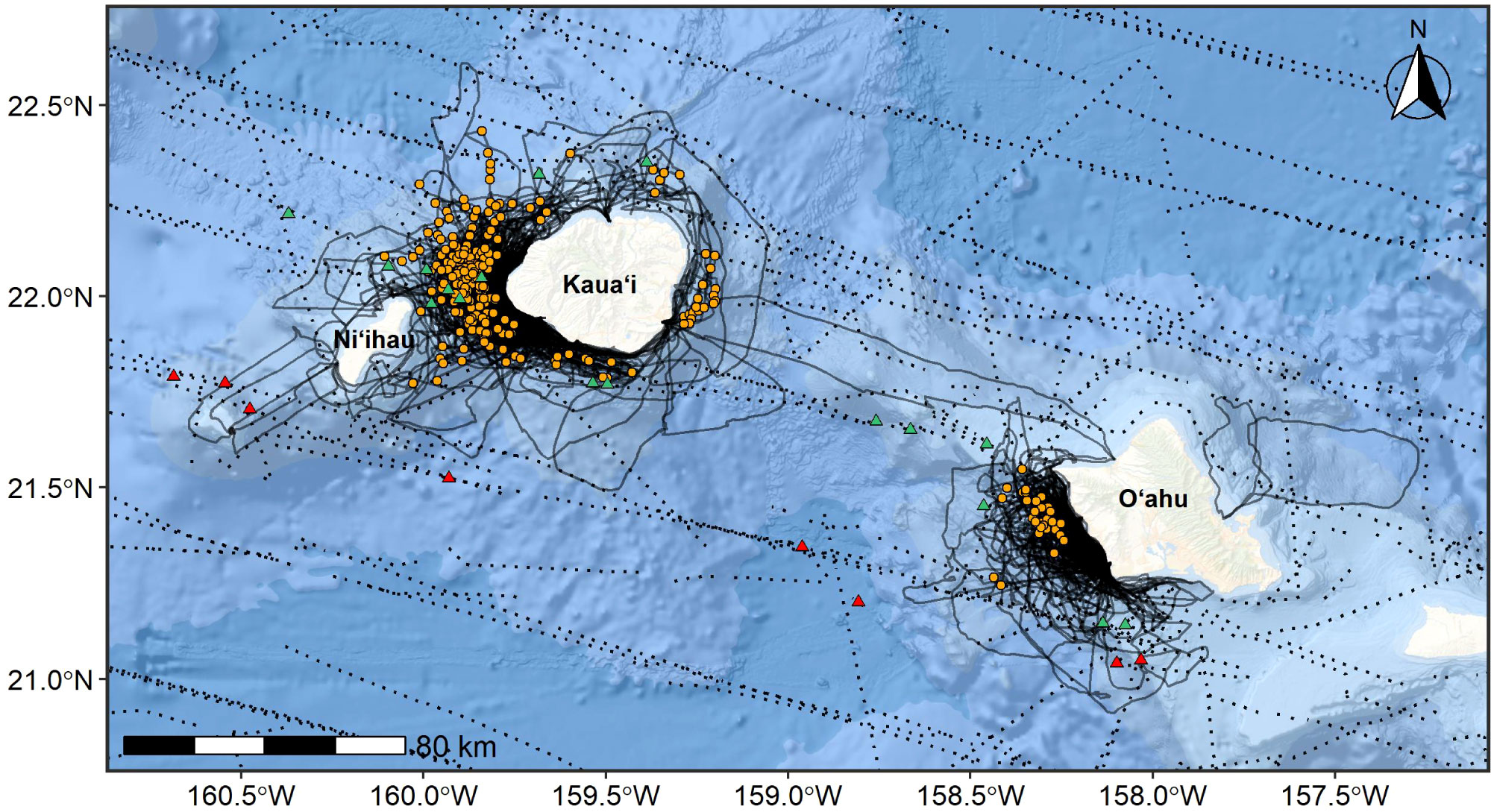
Figure 3 Rough-toothed dolphin sighting locations (orange circles = CRC, green triangles = NMFS) off Kauaʻi and Niʻihau (n=274) and Oʻahu (n=38) overlaid on CRC small boat survey research vessel tracklines (solid lines) from efforts conducted during 2002-2021 (33,850 km of effort; solid lines) and NMFS ship-based line-transect surveys (dotted lines) conducted during 2002-2020 (7,238 km of effort in the area mapped here; dotted lines). Red NMFS sighting locations (n=8) indicate sightings where population assignment is currently unknown and/or sightings are outside the known range of the insular population. Basemap image is the intellectual property of Esri and is used herein with permission. Copyright © 2022 Esri and its licensors. All rights reserved.
3.2.4 Satellite tag data
Satellite tags were deployed on a total of 19 rough-toothed dolphins during dedicated small-boat survey efforts off Kauaʻi and Niʻihau in eight years from 2011-2018 (Figure 4; Shaff and Baird, 2021). Median deployment duration was 12.5 days (range = 3.7-27.7 days), yielding a total of 3,642 Argos locations. Details on satellite tag data processing methods are provided as Supplementary Material and general methods are described in the Methods section. The model-estimated locations (crawl; Johnson and London, 2018) on land were re-routed around a polygon representing the 200-m isobath, based on the shallowest sighting of rough-toothed dolphins in survey effort at 265 m, using the pathroutr package (London, 2021).
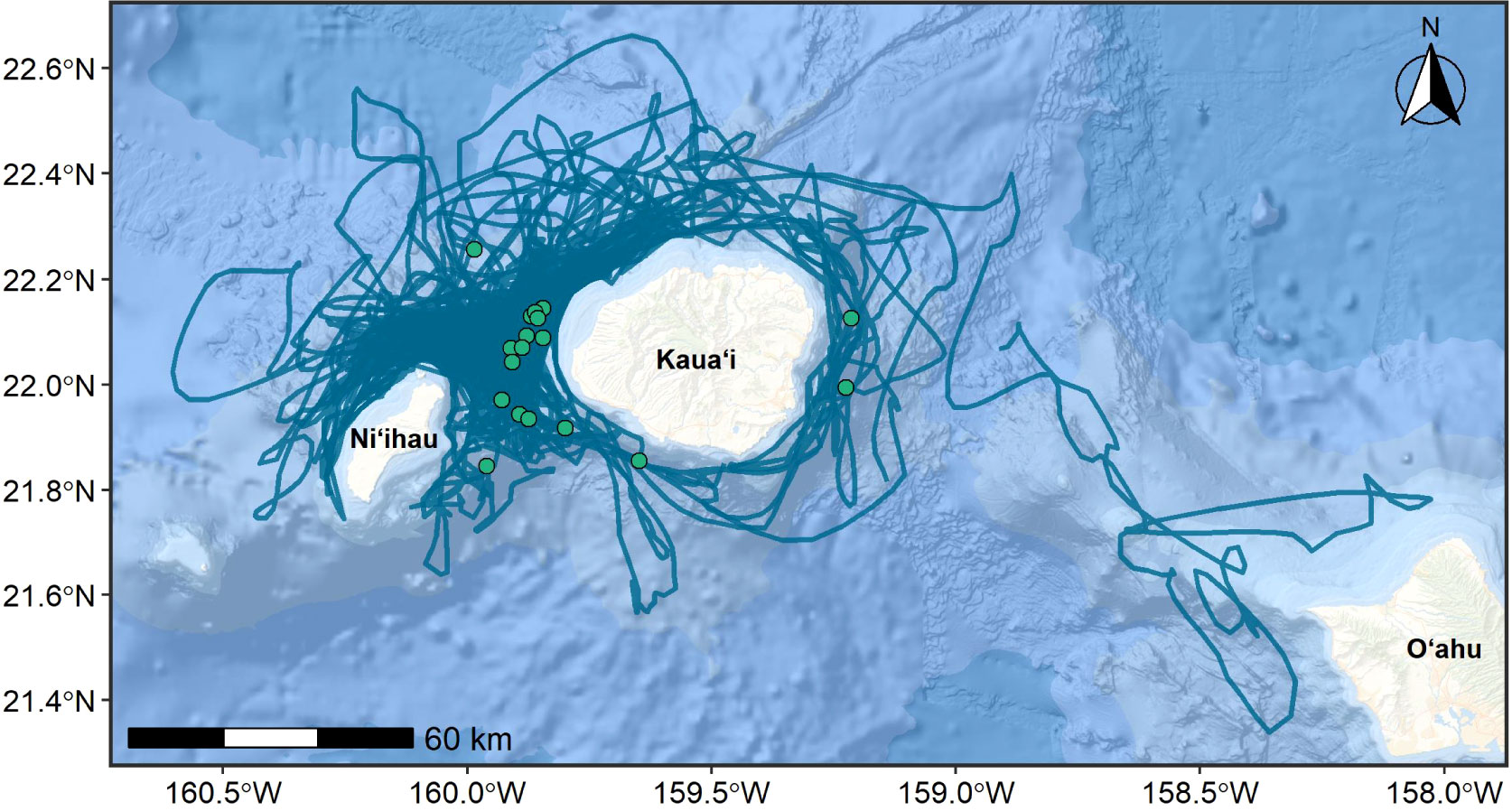
Figure 4 Tracklines of hourly crawl positions of satellite tagged rough-toothed dolphins (n=19), re-routed around the 200-m isobath where necessary to avoid tracks crossing land. Tag deployment locations are shown as green circles. Basemap image is the intellectual property of Esri and is used herein with permission. Copyright © 2022 Esri and its licensors. All rights reserved.
3.2.5 BIA boundary spatial extent
The basis for the parent BIA was an MCP around all sighting and satellite-tag derived crawl locations, with the inner boundary defined by the 200-m isobath (Figure 5). The BIA was established by adding 3-km to the outer boundary of the MCP to account for positional uncertainty in the locations estimated by crawl (Figure 5); this 3-km band captures most, but not all of the positional uncertainty generated by the model (mean crawl standard error in longitude and latitude approximately 2.5 km each; Supplementary File B). The basis for the child BIA was a 50% isopleth of a UD generated through kernel density analyses as described in the Methods and Supplementary File A, with the same 200-m isobath inner boundary as the parent BIA.
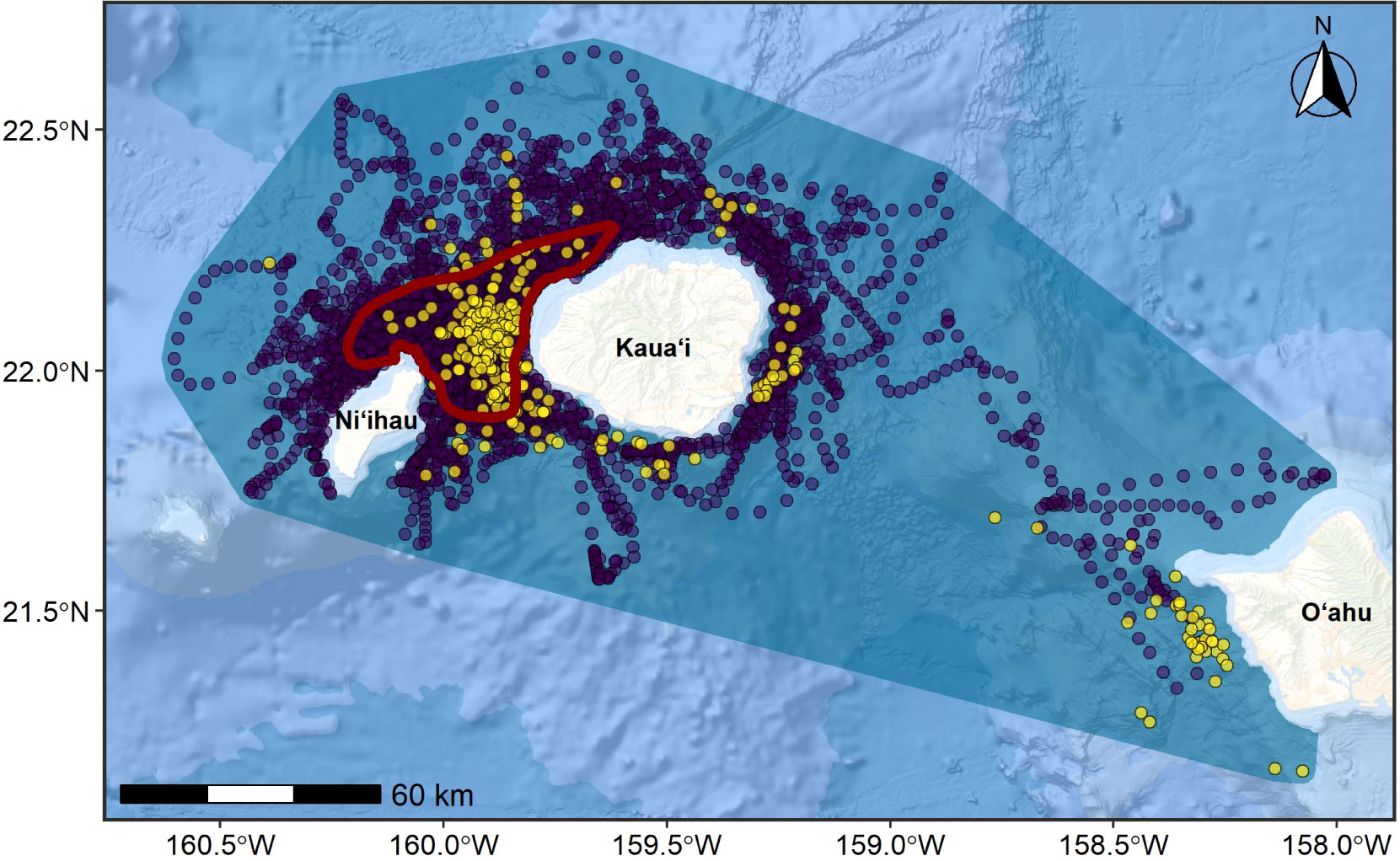
Figure 5 Parent BIA boundary (blue polygon) and child BIA boundary (core range; dark red polygon) for the KNO rough-toothed dolphin population, shown with all sighting locations (yellow circles) and hourly crawl-predicted tag locations (purple circles). Points are partially transparent to highlight high density areas (i.e., where multiple points overlap). The total area of the parent BIA = 25,083 km2 and child BIA = 1,098 km2. Basemap image is the intellectual property of Esri and is used herein with permission. Copyright © 2022 Esri and its licensors. All rights reserved.
3.2.6 BIA scoring
3.2.6.1 Intensity: Abundance and range size
NMFS does not formally recognize this population as a stock, and there is no population-specific abundance estimate for the KNO rough-toothed dolphin population. The latest abundance estimate for the entire Hawaiʻi stock of rough-toothed dolphins, derived from a shipboard line-transect survey within the U.S. Hawaiian EEZ conducted in 2017, was 76,375 dolphins (CV = 0.41; Bradford et al., 2021). The most recent estimate for rough-toothed dolphins associated with Kauaʻi/Niʻihau was reported by Baird et al. (2008a) at 1,665 dolphins (CV = 0.33), based on photo-identification data collected between 2003 and 2006. However, this estimate is dated and did not account for unmarked or Oʻahu animals, and hence underestimated the true KNO population size at that time. As of May 2021, the photo-identification catalog for this species includes 1,033 slightly distinctive, distinctive, or very distinctive individuals (from fair-, good-, or excellent-quality photographs) encountered off Kauaʻi, Niʻihau, and Oʻahu (CRC unpublished). The photo-identification catalog likely includes a number of individuals that have died or been born into the population, but for this assessment we assumed the population is within the 501-2,000 range (abundance score = 1) of the BIA criteria (Harrison et al in review). The resulting area of the parent BIA is 25,083 km2, thus the range size score is 1.
Combining the abundance score (1) and range size (1) results in an overall Intensity score of 1 for the parent BIA. We assigned an Intensity score of 2 for the child BIA because it represents an intensified area of use relative to the population’s entire range, but may not be used as frequently by a portion of the population (i.e., the Oʻahu community). Although we cannot provide a recent abundance estimate specific to this population (including Oʻahu animals), the distinct individual identification total, based on a long-term photo-ID catalog curated from both CRC survey effort and contributed sightings, falls in the (1) category and the overall parent BIA range size is relatively large considering the movements and sightings to west Oʻahu. Although the tag deployments were short, they were deployed over several years and during different seasons. A total of 190 sightings (61% of all sightings) were within the estimated core range. It is important to note that all tagged individuals used the core range. We assumed that approximately 50% of the population is contained within the core habitat (child BIA), although recognize that there is uncertainty associated with this value.
3.2.6.2 Data support
This population has been studied for 19 years (2002-2021). Additional photographic data supplied by other researchers and community science contributions span a 15-year period (2006-2020). CRC efforts have accrued 295 sightings, with an additional 17 sightings from NMFS ship-based line-transect surveys and 82 encounters from other researchers and community scientists since 2004, with re-sightings of individuals up to 17 years apart (2003 to 2020). Previous studies support genetic differentiation between this population and rough-toothed dolphins sampled off Hawaiʻi Island (Albertson et al., 2016). Movements from 19 satellite tag deployments (3,642 filtered Argos locations) transmitting for up to 28 days showed similar spatial use patterns (Kaulakahi Channel, windward sides of Kauaʻi/Niʻihau, circumnavigation of Kauaʻi), with the exception of the individual that moved to west Oʻahu over a period of 5 days. Tag positional uncertainty and irregularity was accounted for through the crawl model, and the parent BIA boundary encompasses nearly all of crawl standard error ellipses.
Despite the longevity and variety of information available on this population, no recent abundance estimates specific to this population are available, and the MCP boundary includes a large amount of space where no data points exist to support movements through the Kaʻieʻie Waho Channel between Kauaʻi and Oʻahu. In determining the spatial extent of the core range (child BIA) we attempted to account for bias associated with varying deployment durations and pseudoreplication (i.e., pairs of animals tagged together and acting in concert), using a widely used approach for estimating core range (KDEs). Therefore, we assigned a Data Support score of 2 for both the parent and child KNO rough-toothed dolphin BIAs.
3.2.6.3 Importance
Combining the Intensity scores (parent = 1, child = 2) and Data Support scores (parent = 2, child = 2) results in Importance scores of 1 for the parent BIA and 2 for the child BIA for KNO rough-toothed dolphins (Harrison et al in review).
3.2.6.4 Boundary certainty
We have intermediate confidence (score = 2) in Boundary Certainty for both the parent and child BIAs for KNO rough-toothed dolphins. The parent BIA boundary encompasses the entire population and we attempted to account for positional uncertainty in satellite tag data. The parent BIA boundary includes a large amount of space, but the extents are supported by the data using the MCP. Although there are some caveats associated with the kernel density analysis used to derive the child BIA, the estimated core range overlaps with concentrations of sightings and hourly satellite tag data. The core area was used by all tagged individuals, who were monitored by tags deployed during different years and seasons. As noted above, we attempted to account for some potential sources of bias in this analysis (e.g., tag deployment locality, spatial autocorrelation) by using coarser timesteps and a weighted KDE approach. However, tags used for this assessment did not transmit for longer than a month and nearly all were deployed in the same general region (within the core range) and during the same time of year (August and February), introducing a tagging bias.
3.2.6.5 Spatiotemporal variability
The Spatiotemporal Variability indicator for both parent and child BIAs for KNO rough-toothed dolphins is static, as there is no information to suggest that these areas are used dynamically or ephemerally.
3.3 Humpback whale – Main Hawaiian islands R-BIA
3.3.1 Background
The Hawaiian Archipelago is an important winter breeding ground for humpback whales in the North Pacific (Barlow et al., 2011). Although observations of births are rare (Patton and Lawless, 2021; Ransome et al., 2022), mating behaviors (e.g., singing, competitive groups) are common and newborn calves are regularly seen (Craig and Herman, 2000; Craig et al., 2002); Cartwright and Sullivan, 2009; Calambokidis et al. (2008) estimated that over 50% of the humpback whales in the North Pacific (approximately 21,000 individuals in total; see also Barlow et al., 2011) winter in Hawaiian waters. Although humpbacks can be found in Hawaiʻi as early as late fall and through spring, with stragglers into summer, numbers are high beginning in January, and peak in February and March (Mobley et al., 1999). In the MHI humpback whales are typically found in shallow waters, and particularly high densities of whales occur off Maui Nui (Mobley et al., 2001), although inter-island movements within the MHI are extensive (Cerchio et al., 1998; Calambokidis et al., 2008; Mate et al., 1998; ; Palacios et al., 2020; Henderson et al., 2021; Henderson et al., 2022). Habitat use varies between sexes; females with calves preferentially use shallower waters relative to groups without calves and adult males (Craig and Herman, 2000; Cartwright et al., 2012; Craig et al., 2014; Pack et al., 2018). Less is known on humpback whale presence in the NWHI largely due to their remoteness. However, visual sightings from shipboard surveys (Johnston et al., 2007; Yano et al., 2019), passive acoustic detections (Johnston et al., 2007; Lammers et al., 2011; Allen et al., 2021), and movements from a small proportion of humpback whales satellite tagged in the MHI (Henderson et al., 2019; Palacios et al., 2019; Palacios et al., 2020; Henderson et al., 2022) provide evidence on the importance of the NWHI for wintering humpback whales. The NWHI region may serve as a final breeding ground destination before whales begin their migration north to feeding grounds, or it may represent additional breeding habitat, although the degree of connectivity between the MHI and NWHI regions is poorly understood (Palacios et al., 2019; Palacios et al., 2020; Allen et al., 2021; Henderson et al., 2022). In this assessment, we revised the R-BIA for humpback whales in the MHI (detailed below) and defined a watch list area for humpback whale reproductive activities in the NWHI (Supplementary File A).
3.3.2 BIA boundary delineation
Baird et al. (2015a) delineated an R-BIA for humpback whales in the MHI based on areas with high densities of visual sightings. In this revised assessment, we used information from a large collection of satellite tag deployments to examine the proportion of time individual whales spent inside the established BIA boundaries (i.e., residence time) compared to outside the boundaries and used this to inform the adequacy of the 2015 boundaries. Sighting locations from several sources were also mapped to compare with the spatial distribution of satellite tagged whales. We restricted this revised assessment to the area around the Hawaiian Archipelago extending from the coastline to 50 km offshore, as described in Palacios et al. (2019); Palacios et al. (2020) and Henderson et al. (2022), which is hereafter considered the “breeding area perimeter”. This breeding area perimeter was informed by the relative distance from the islands at which satellite-tagged humpback whale movement behavior switched from area-restricted search (indicative of residence while in the breeding area) to directed travel (i.e., start of migration), as estimated by state-space models (Palacios et al., 2019; Palacios et al., 2020; Henderson et al., 2022). This particular R-BIA concerns important breeding areas within the MHI; therefore, we focused our assessment on the portion of the breeding area perimeter ranging from Middle Bank Seamount to Hawaiʻi Island (i.e., excluding the NWHI; Figure 6), which is also where most data are available. From here on, this area will be referred to as the MHI breeding area perimeter.
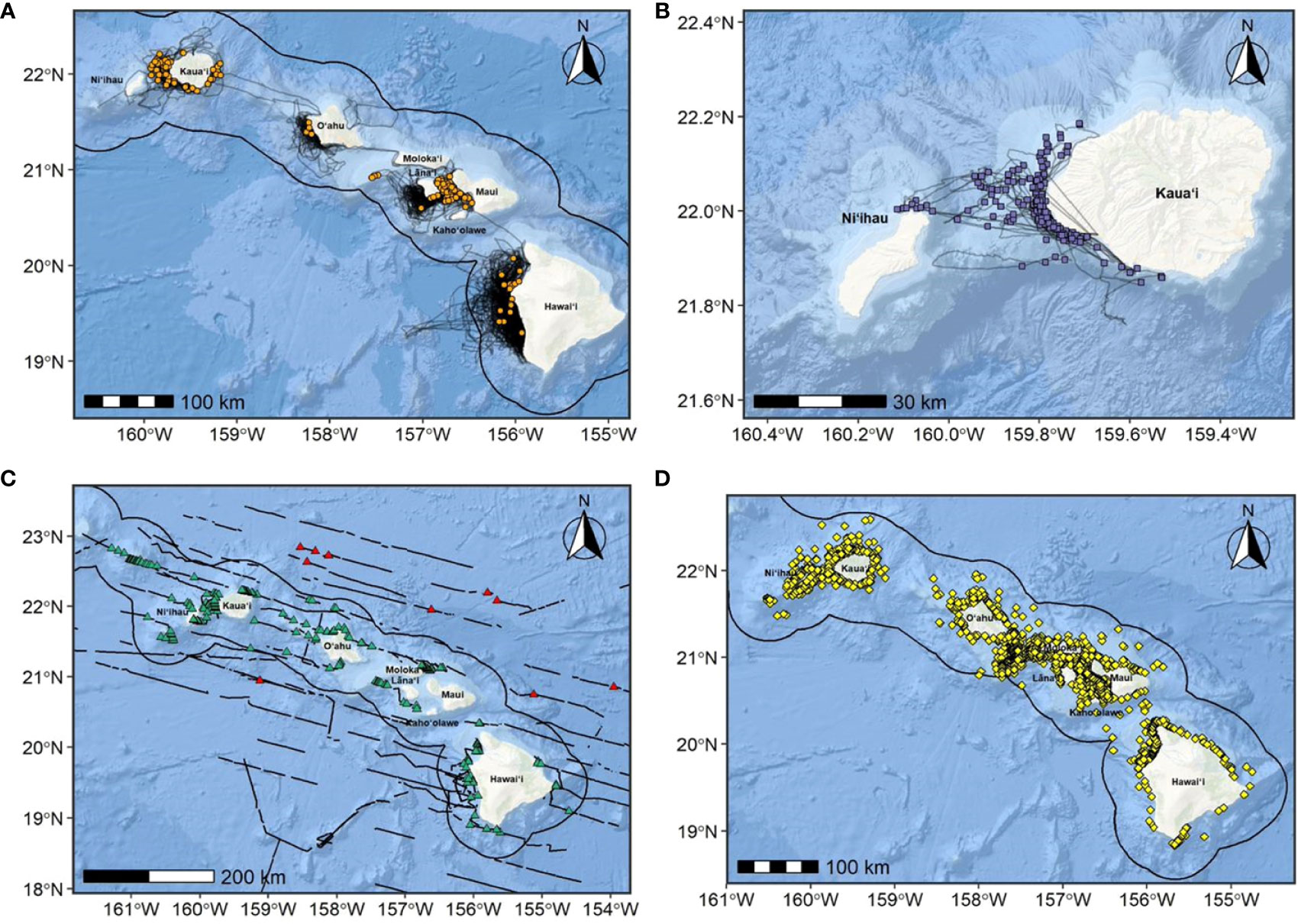
Figure 6 Humpback whale sighting locations overlaid on research vessel tracklines from (A) CRC during small boat surveys from 2000-2021 (orange circles; n=199); (B) NIWC Pacific and HDR, Inc. during small boat surveys from 2017-2019 (purple squares; n=202); (C) NMFS during ship-based line-transect surveys from 2002-2020 (green triangles; n=213; red triangles are sightings outside the MHI breeding area perimeter and excluded from BIA, n=11); and (D) MMRC aerial surveys from 1993-2003 (yellow diamonds, n=2,297; see Mobley et al., 2001; Mobley, 2004 for effort tracklines). The MHI breeding area perimeter considered in this revised assessment is shown as a solid black outline around the islands; only data collected within the perimeter boundary and around the main Hawaiian Islands were included in this revised assessment. Basemap image is the intellectual property of Esri and is used herein with permission. Copyright © 2022 Esri and its licensors. All rights reserved.
3.3.3 Sighting and photographic data
Sighting data during the December-May humpback whale breeding season used for this assessment were collected from four separate sources and in different manners: (1) opportunistically from non-systematic, small-boat surveys focusing on odontocetes conducted by CRC throughout the MHI from 2000 to 2021 (see Baird et al., 2013a for details on surveys); (2) ship-based line-transect surveys for cetaceans conducted by NMFS throughout the MHI and NWHI from 2002 to 2020, with humpback whale sightings within the MHI breeding area perimeter (during the December-May breeding season) in 2009, 2019, and 2020 (see Barlow, 2006; Bradford et al., 2017; Yano et al., 2018; Yano et al., 2020 for details on surveys); (3) dedicated small-boat survey efforts conducted by NIWC Pacific and HDR, Inc. off Kauaʻi/Niʻihau from 2017 to 2019; and (4) aerial surveys conducted throughout the MHI during February through April by MMRC from 1993 to 2003, collected by J. Mobley (Mobley et al., 2001; Mobley, 2004) and provided by PacIOOS Voyager (Table 3; Figure 6). There were 11 NMFS sightings outside of the MHI breeding area perimeter defined in this assessment; these sightings were excluded from the BIA revision process as they were outside the spatial scope of this BIA (MHI breeding area perimeter) and were likely whales that were migrating (Figure 6). Survey tracklines during the humpback whale breeding season and within the MHI breeding area perimeter considered in this BIA (December-May) from all four sources total to approximately 123,000 km of effort, with a total of 2,911 humpback whale sightings (1993-2021; Table 3).
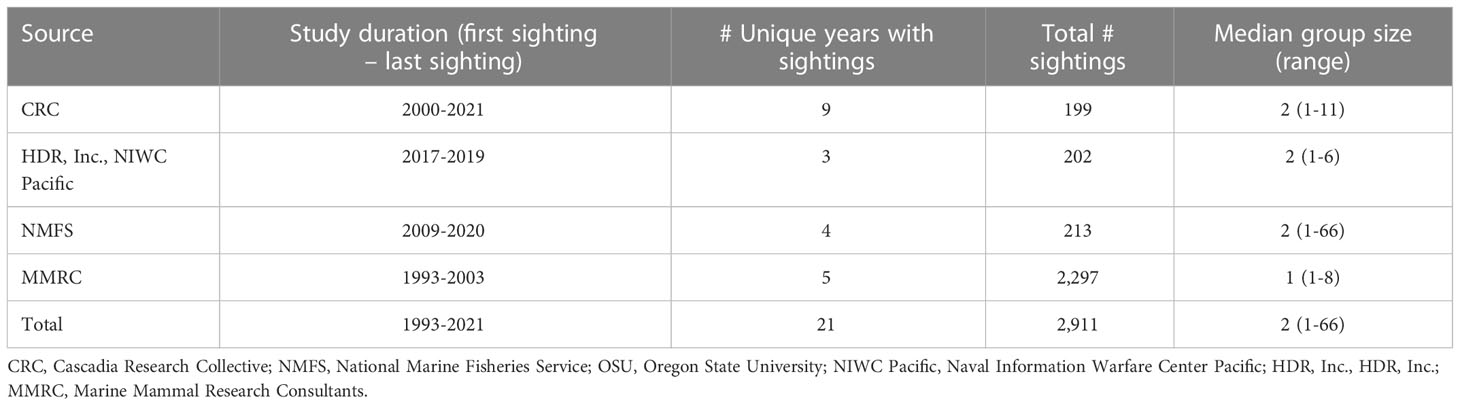
Table 3 Humpback whale sighting data during the breeding season (December-May) within the main Hawaiian Islands breeding area perimeter (see Figure 6).
3.3.4 Satellite tag data
Data from 84 satellite tags deployed on humpback whales off Maui (n=61) and Kauaʻi/Niʻihau (n=23) during dedicated efforts by OSU (1995-2019; Mate et al., 1998; Palacios et al., 2019; Palacios et al., 2020) and NIWC Pacific (2017-2019; Henderson et al., 2019; Henderson et al., 2022) in nine years were used for this assessment. Median tagging date and number of days of data within the MHI breeding area perimeter were February 7th and 13 days, respectively (ranges: December 13th to April 15th and 5-42 days, respectively). Detailed methods on satellite tag data processing methods are provided in Supplementary File B. Continuous-time correlated random walk models fit in crawl (Johnson and London, 2018) were used to predict locations at a fine temporal interval (10 minutes) for residence time calculations and locations were re-routed around a polygon representing the islands with an added 50-m distance using the pathroutr package (London, 2021) to prevent tracks from crossing over land.
Residence time within the 2015 BIA boundaries was calculated as the sum of 10-minute crawl locations contained within the BIA boundaries (rounded and expressed in units of days). Residence time outside of the 2015 BIA boundaries was calculated in the same manner, but was limited to those observations between the MHI breeding area perimeter and the 2015 BIA boundaries. Location data from satellite tags that had less than five days of data within the MHI breeding area perimeter were excluded from analyses to limit spatial bias associated with tag deployment locality; the resulting final sample size for this assessment was 71 satellite tags.
To visualize where tagged whales spent the most of their time (i.e., their occupancy pattern), we calculated a spatially explicit residence time for each tagged whale on a common hexagonal grid (cell diameter size = 10 km) encompassing the entire study area (i.e., the MHI breeding area perimeter) and summarized distributions across all tagged whales (Figure 7). Residence time was calculated by aggregating each whale’s 10-minute crawl locations into the hexagonal grid cells and summing the total number of locations contained within each cell (represented as time in cell, in days). To account for varying track durations and mitigate bias associated with short tracks near deployment sites, we weighted residence time in each cell by the whale’s track duration (within MHI breeding area perimeter) divided by the longest whale track duration in the dataset (within the MHI breeding area perimeter), following Möller et al. (2020). A full description of the weighted occupancy pattern modeling is available in the humpback whale section of Supplementary File A.
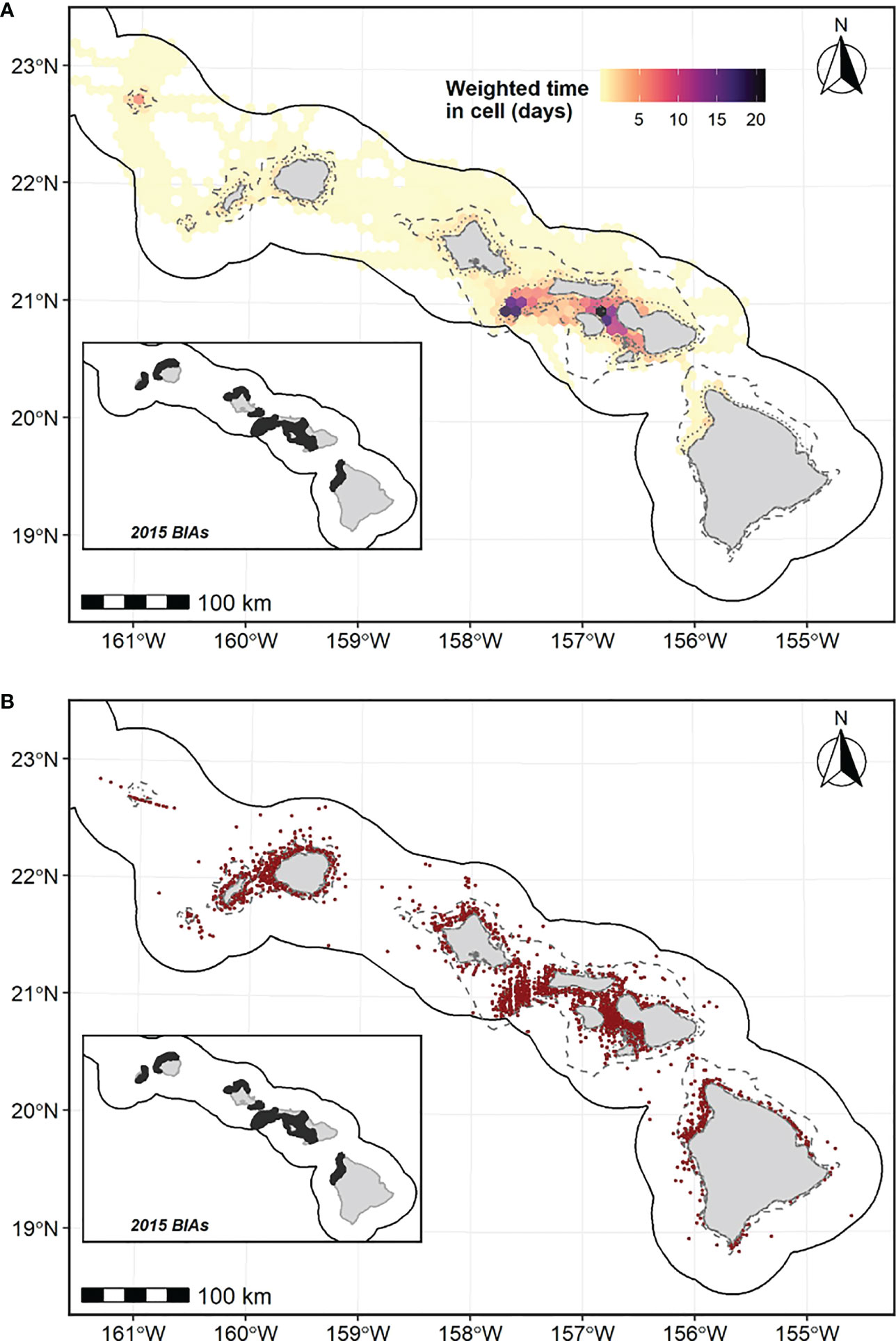
Figure 7 (A) Weighted occupancy pattern of satellite tagged humpback whales (n=71) throughout the main Hawaiian Islands; and (B) CRC, NIWC Pacific/HDR, Inc., NMFS, and MMRC visual sighting locations (n=2,911, dark red circles). The solid black line represents the breeding area perimeter and 1,000-m and 200-m isobaths are shown as the grey dashed lines and dotted lines, respectively, in each map. Established humpback whale R-BIA boundaries as described by Baird et al. (2015) are shown in the inset maps as black polygons.
Of the 69 tagged individuals that used any of the six BIAs from 2015, the mean time spent within BIA boundaries was 68% (SD=25%), with one individual spending 100% of its time (7.3 days) within a BIA (Maui Nui). The Maui Nui BIA was the most intensely used 2015 BIA (Figure 7); however, over 70% of all satellite tags used in this analysis were deployed in this region (Figure 7). Only one satellite tagged individual spent time in the 2015 Hawaiʻi Island BIA (Figure 7). Further details from the residence time analysis are provided in the humpback whale section of Supplementary File A.
3.3.5 BIA boundary spatial extent
Based on the spatial distribution of sightings and occupancy pattern of satellite-tagged whales (Figure 7), we revised the 2015 BIA by extending the boundary to the 1,000-m isobath around all MHI, including Middle Bank and Kaʻula (Figure 8). The revised BIA is hereafter referred as the ‘parent’ BIA (Figure 8). In contrast to the 2015 BIA boundary, the revised boundary encompasses a broader area used by humpback whales during the breeding season. The updated boundary also extends farther west to include areas of importance (e.g., Middle Bank) as indicated by both satellite tag and recent sighting data (Figure 7). In addition, we delineated a child BIA representing the ‘core range’ for this breeding population based on notably intensified use within the broader updated boundary (1,000-m isobath); we designated this area as the 200-m isobath because this isobath generally agreed with increased occupancy levels relative to the entire MHI breeding area perimeter and parent BIA, based on the distribution of all data sources (Figures 7, 8). For both parent and child BIA boundaries, the inner boundary was defined as a 50-m distance from shore (Figure 8). The area of the parent BIA is 23,042 km2 and the area of the child BIA is 6,679 km2. Satellite tag and sighting data used to inform revised BIA boundaries spanned the months of December through May (with locations occurring within the MHI breeding area perimeter). Thus, these boundaries likely encompass the most important reproductive areas for North Pacific humpback whales in the MHI from December through May.
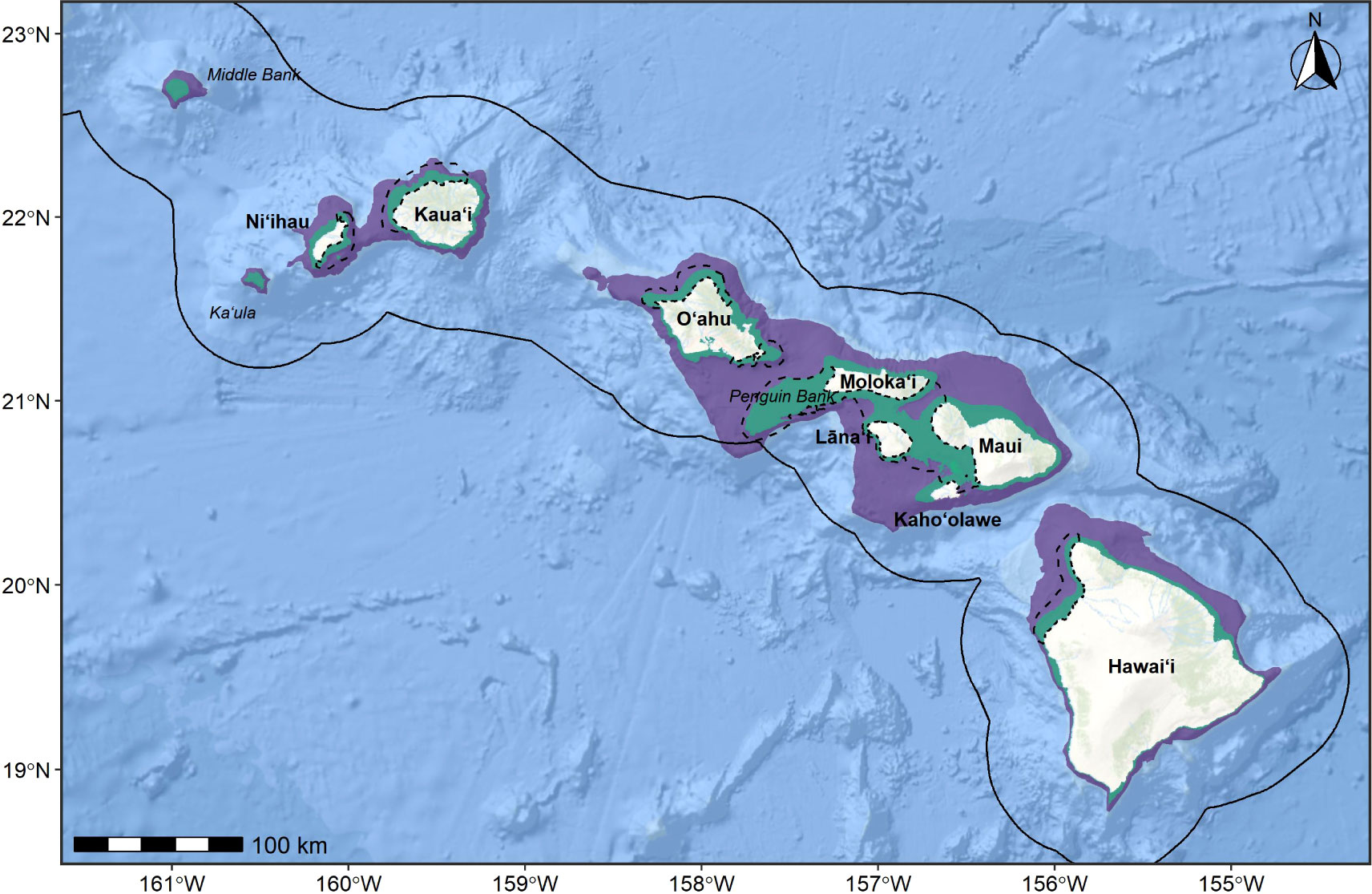
Figure 8 Revised parent BIA boundary (purple polygon) for humpback whales represented as the 1,000-m isobath around all main Hawaiian Islands as well as Middle Bank, and child BIA boundary (core range; green polygon) represented as the 200-m isobath within this area. BIAs span months December through May. The inner (shoreward) boundary for both BIAs is defined by a 50-m distance band from shore. Humpback whale breeding area perimeter is represented by the solid black line. Established R-BIA boundaries delineated in Baird et al. (2015) are shown in dashed black lines. Total area of the parent BIA = 23,042 km2 and child BIA is 6,679 km2. Basemap image is the intellectual property of Esri and is used herein with permission. Copyright © 2022 Esri and its licensors. All rights reserved.
3.3.6 BIA Scoring
3.3.6.1 Intensity
Humpback whales make extensive movements from high latitude feeding grounds to the tropical, shallow waters of the Hawaiian archipelago for breeding during winter months. Calambokidis et al. (2008) estimated that over 50% of North Pacific humpback whales use Hawaiian waters as breeding grounds, with an estimated abundance for the Hawaiʻi region at that time of 10,103 (no CV estimated) individuals. More recent model-based methods estimated an abundance of 11,278 humpback whales (CV=0.56) in the U.S. Hawaiian Islands EEZ during peak abundance (mid-February to -mid-March) in 2020; however, this may be an underestimate of all whales that overwinter in Hawaiian waters as it does not consider individuals outside of this peak period (Becker et al., 2022). Further, this estimate extends to both NWHI and MHI regions but was derived from survey data exclusively within the MHI region, so there remains uncertainty in the true EEZ-wide abundance during this period (Becker et al., 2022). Adult females with calves are known to preferentially use shallow waters of the Auʻau Channel (Craig and Herman, 2000; Cartwright et al., 2012; Craig et al., 2014; Pack et al., 2018), and this important nursery region is captured by the child BIA (Figure 8). High-density areas identified by satellite tag data (leeward Maui Nui, Penguin Bank; Figures 7, 8) agree with findings from previous photo-identification studies and aerial surveys (Mobley et al., 2001; Mobley, 2004), and additional areas (e.g., Kauaʻi/Niʻihau, Middle Bank) have been highlighted with the inclusion of more recent data (Henderson et al., 2019; Palacios et al., 2020; Yano et al., 2020; Henderson et al., 2022). Movements between island areas within the MHI occur frequently (Cerchio et al., 1998; Calambokidis et al., 2008; Palacios et al., 2020; Henderson et al., 2021; Henderson et al., 2022). Thus, some deeper water habitat/channels between islands are important for this species. No other reproductive BIA within U.S. waters is being delineated for North Pacific humpback whales, emphasizing the importance of this R-BIA in this basin (Harrison et al in review). Considering the above, we assigned Intensity scores of 2 and 3 for parent and child BIAs, respectively. We approximate that 75% of the population of breeding humpback whales in the MHI is contained within the child BIA (representing the core range). All tagged whales included in this assessment used the child BIA and the greatest weighted occupancy values occurred within the portion of the child BIA encompassing Penguin Bank and inner Maui Nui (Figures 7, 8). Additionally, 66% of the sightings (1,922 out of 2,911) were contained within the child BIA boundary, highlighting humpback whales’ known association with shallow waters for breeding. However, we acknowledge that there is uncertainty in this estimate.
3.3.6.2 Data support
The revised humpback whale BIAs presented here were informed by data on movements from 71 satellite tag deployments during nine unique years spanning 1995 to 2019. The maximum number of days transmitted within the MHI breeding area perimeter was 42 days. The area within which reproductive behavior is assumed (i.e., MHI breeding area perimeter) was informed by movement-model estimated behaviors on satellite tag tracks, identified as the switch from area restricted search to directed travel behavior (Palacios et al., 2019; Palacios et al., 2020; Henderson et al., 2022). The child BIA (core range) was supported by satellite tag data and all four sources of sighting data (CRC and NIWC Pacific/HDR, Inc. small-boat surveys, NMFS ship-based line-transect surveys, and MMRC aerial surveys). The revised boundaries are supported by satellite-tagged whale occupancy patterns and concentrations of sightings from both earlier (1990s to 2000s) and recent (2010s) survey efforts. Concentrations of sighting locations from all available efforts conducted throughout the MHI from 1993-2020 generally agree with revised BIA boundaries. Although there remains uncertainty in the most recent abundance estimate (Becker et al., 2022), it was derived from more recent data than the earlier estimate from Calambokidis et al. (2008), which can be considered a valid minimum estimate given recent evidence that the population has continued to increase in localized foraging regions (e.g., Alaska; Muto et al., 2019). Considering these lines of evidence and their strengths and weaknesses, we assigned Data Support scores of 2 for the parent BIA and 3 for the child BIA.
3.3.6.3 Importance
Combining the Intensity scores (parent = 2, child = 3) and Data Support scores (parent = 2, child = 3) results in Importance scores of 2 for the parent BIA and 3 for the child BIA for the MHI humpback whale R-BIA (Harrison et al in review).
3.3.6.4 Boundary certainty
We have intermediate confidence in Boundary Certainty for the parent BIA and high confidence in the Boundary Certainty for the child BIA. While the parent BIA boundary (1,000-m isobath) generally conforms to primary-use areas based on satellite tag data and sightings (Figures 7, 8), the majority of satellite tags used in this assessment were deployed off Maui Nui and the majority of sighting locations were collected over two decades ago during aerial surveys. In addition, only a small number of adult females with calves were satellite tagged (n=6) and thus the movements from available satellite tag data are biased towards adult males or adults without calves. We have high confidence in the child BIA boundary based on both supporting data used in this assessment and from previous studies.
3.3.6.5 Spatiotemporal variability
The Spatiotemporal Variability indicator for both parent and child BIAs for MHI humpback whales is static. There is no information to suggest either area is used dynamically or ephemerally; boundaries are based on static (bathymetric) features.
4 Watch list areas
Two watch list areas were delineated: one for humpback whale reproductive activities in the NWHI and one for minke whale reproductive activities in the MHI. Full descriptions of the watch list area boundary delineations and scoring are provided in Supplementary File A.
Baird et al. (2015) did not delineate an R-BIA for humpback whales in the NWHI due to limited supporting information at the time of the study. While evidence of humpback whale use of this region has increased since then, available supporting data is from (1) individual bottom-mounted acoustic receivers that do not provide much information on spatial distribution and relative abundance (Allen et al., 2021) and (2) movements from only a few satellite-tagged individuals, who spent little time within the NWHI (west of Middle Bank) before departing north (within 5 days; Henderson et al., 2019; Palacios et al., 2020; Henderson et al., 2022). There remains uncertainty in the intensity of use of the NWHI by all wintering humpback whales in the Hawaiian Archipelago, and notably, the proportion of humpbacks that divide their time between the MHI and the NWHI, versus those that may exclusively use the NWHI during the breeding season (Lammers et al., 2011). Therefore, we delineated a watch list area in the NWHI for humpback whale reproductive activities in this assessment. Future BIA efforts could consider transitioning this watch list area into a full BIA if additional studies address knowledge gaps in relative abundance and connectivity between the MHI and NWHI. Satellite tag deployments on humpback whales within the NWHI would greatly advance our ability to clarify their use of the NWHI and delineate a BIA in future efforts. The time period for this watch list area was assigned as December through May, which is supported by acoustic detection rates (Johnston et al., 2007; Lammers et al., 2011; Allen et al., 2021). We used suitable wintering habitat from spatial modeling (Johnston et al., 2007) to inform the watch list area boundary spanning Hōlanikū (Kure Atoll, westmost extent) to Nihoa (eastmost extent), and mapped available sighting locations and satellite tracking data as lines of support (Supplementary File A). Similar to the MHI humpback whale R-BIA, we considered a 50 km buffer around the NWHI to be the NWHI breeding area perimeter and area of interest in this assessment (Henderson et al., 2019; Palacios et al., 2019; Palacios et al., 2020; Henderson et al., 2022).
Minke whales are rarely observed in Hawaiian waters; their apparent offshore distribution, likely low density, and inconspicuous nature (e.g., cryptic surface behavior, typically travel alone or in small groups) makes visual survey efforts generally ineffective. However, their presence during winter and spring months has been documented from passive acoustic monitoring and limited visual sightings (Balcomb, 1987; Rankin and Barlow, 2005; Barlow, 2006; Rankin et al., 2007; Oswald et al., 2011; Norris et al., 2012; Bradford et al., 2017; Yano et al., 2018; Martin et al., 2020; Yano et al., 2020; Bradford et al., 2021). Minke whales have been acoustically detected in Hawaiʻi as early as October and as late as May, with the number of detections peaking from January through March (Thompson and Friedl, 1982; Oswald et al., 2011; Yano et al., 2018; Martin et al., 2020; Yano et al., 2020). Information from the small number of visual sightings has not noted the presence of calves or breeding behavior; however, it is believed that their seasonal presence in Hawaiian waters is linked to reproduction much like other migratory baleen whales (Baker and Herman, 1981; Oswald et al., 2011). Further, the unique “boing” call they produce during winter and spring in the North Pacific has been hypothesized to be produced by males engaged in courtship and reproduction, similar to other baleen whales (Croll et al., 2002; Rankin and Barlow, 2005; Herman et al., 2013). Although the majority of available data on minke whale presence in Hawaiʻi are from the MHI, they have also been acoustically detected and sighted in the Northwestern Hawaiian Islands (Shallenberger, 1981; Bradford et al., 2017; Yano et al., 2018; Bradford et al., 2021).
Baird et al. (2015a) did not delineate an R-BIA for minke whales in Hawaiʻi due to insufficient supporting information at that time. In this assessment, minke whale acoustic detection locations were used to inform the watch list area boundary for breeding grounds in the MHI during winter months. Based on acoustic detection rates (Thompson and Friedl, 1982; Oswald et al., 2011; Martin et al., 2020), we consider the period spanning October through April to be the minke whale breeding season for this watch list area. Available visual sightings of minke whales were also mapped in the assessment, although they did not play a role in determining the spatial extent of the watch list area because there were only a very small number of observations available.
Both watch list areas were assigned Importance scores of 0 (Intensity and Data Support =1). For minke whales, this was driven by (1) limited evidence to support the occurrence of reproductive behavior in Hawaiʻi, as existing evidence is predominantly passive acoustic; and (2) uncertainty in the extent of the watch list area boundary, which corresponds to the area covered during a single shipboard line-transect survey during which minke whales were frequently acoustically detected. The acoustic detections critical to defining the watch list area boundary do not resolve spatial patterns in minke whale density (e.g., number of whales per unit area), such that we were unable to identify areas of concentrated use within their broader range. For humpback whales in the NWHI, the scoring was driven by (1) limited information on the comparative importance of the NWHI for all humpback whales using the Hawaiian Archipelago for reproductive purposes; and (2) limited data to explicitly support spatial distribution of important areas in this region. For these reasons, we could not confidently define these two areas as BIAs.
Limited understanding of minke whale distribution and behavior in Hawaiʻi is largely due to the behavior of the species, while that for humpback whales in the NWHI is due to logistical challenges of studying cetaceans in this region. While several cryptic odontocetes have been studied extensively in Hawaiʻi (Baird, 2016; Baird, 2019), these species occur in more accessible, nearshore waters compared to the offshore waters where minke whales have been detected and documented. Minke whale presence has been studied more extensively on the PMRF off Kauaʻi (Martin et al., 2013; Martin et al., 2015; Martin et al., 2020); however, the area reported by these studies is only a fraction of the entire area encompassing their detections in Hawaiʻi (Martin et al., 2013; Martin et al., 2015; Martin et al., 2020). More information on their spatiotemporal patterns is needed to support a BIA for these two species and areas.
5 Regional summary
5.1 Overview
A total of 35 BIAs, including hierarchical BIAs (both parent and children), were delineated for cetaceans in Hawaiʻi (Figure 9; Table 4). Two of the 35 BIAs were R-BIAs for humpback whale breeding areas; the remaining 33 BIAs were all S-BIAs for odontocete populations. Only three BIAs were delineated in the NWHI (NWHI false killer whales and spinner dolphins), which is consistent with the limited research effort and thus understanding of cetaceans in that area. It is likely that if intensive studies, similar to those undertaken in the MHI, were conducted in the NWHI, more S-BIAs would be identified there. Nearly all BIAs delineated in the Hawaiʻi region were hierarchical (Table 4), highlighting the depth of available information and apparent heterogeneity of space use that these populations exhibit. Lastly, two watch list areas were designated for minke whale and humpback whale reproductive purposes in the MHI and NWHI, respectively.
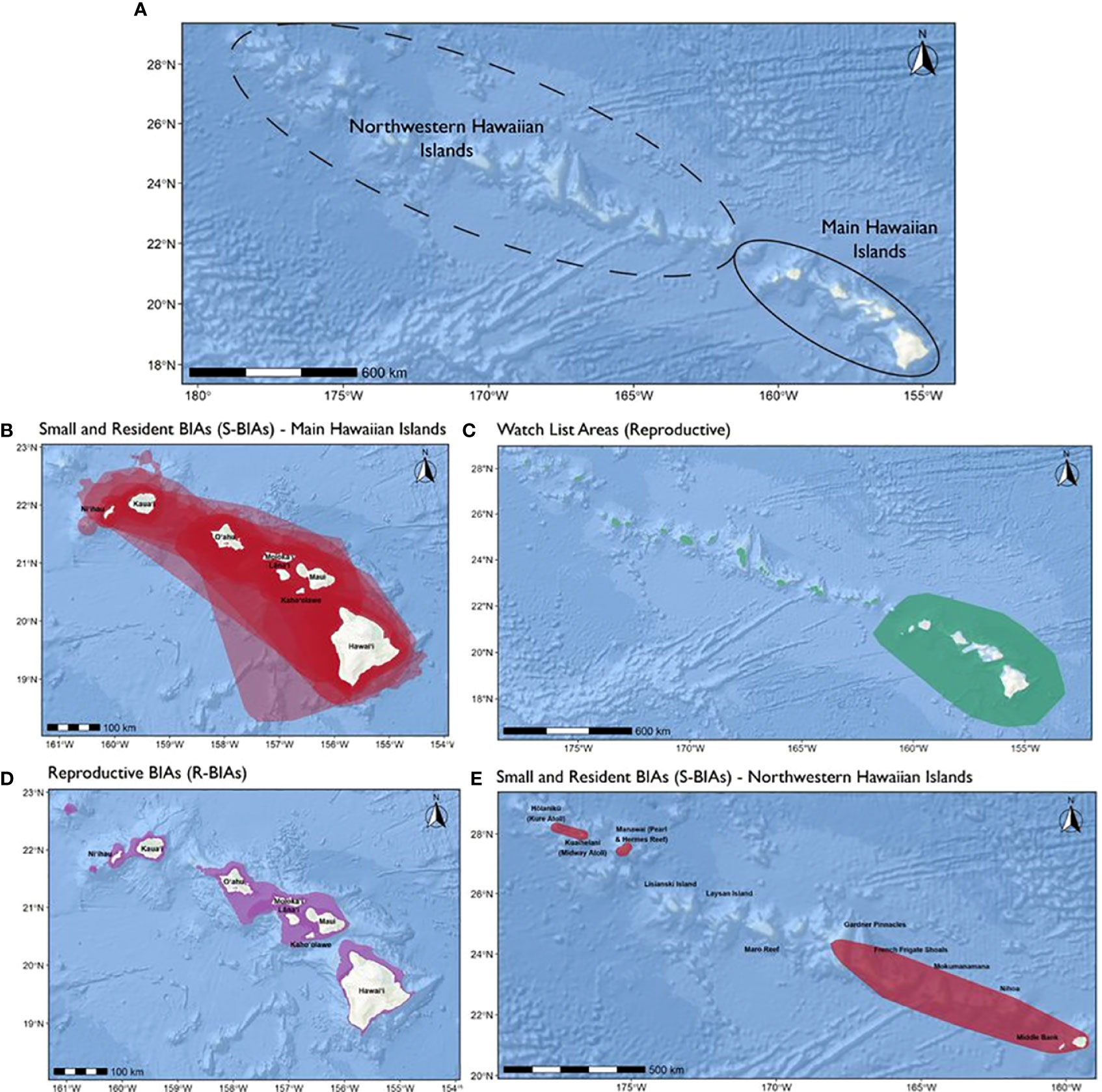
Figure 9 All Hawaiʻi BIAs by BIA type and region: (A) Locality of the Main Hawaiian Islands and Northwestern Hawaiian Islands within the Hawaiian Archipelago; (B) S-BIAs in the Main Hawaiian Islands (n=30, darker red areas indicate higher overlap among BIAs); (C) watch list areas (n=2; reproductive; (D) R-BIAs in the Main Hawaiian Islands (n=2)); (E) S-BIAs in the Northwestern Hawaiian Islands (n=3). Basemap image is the intellectual property of Esri and is used herein with permission. Copyright © 2022 Esri and its licensors. All rights reserved.
5.2 Scoring
Intensity scores were variable across BIAs (Figure 10). As the majority were S-BIAs, and Intensity scores were determined through the quantitative Intensity scoring matrix, these Intensity scores were strongly linked to the quantitative abundance and range size criteria (Table 4). Accordingly, the largest range sizes and highest abundance estimates had the lowest Intensity scores (Table 4). For several populations, robust abundance estimates were not available; Data Support scores assigned to these populations were lower to reflect such uncertainty.
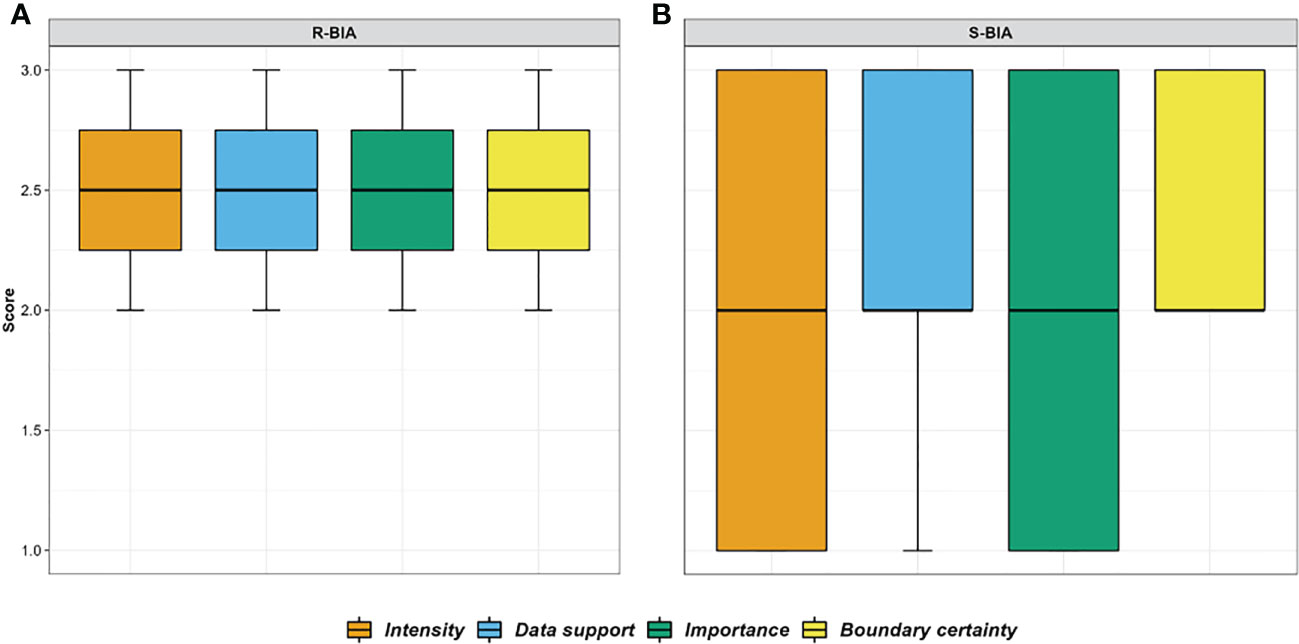
Figure 10 Hawaiʻi BIA score summary boxplots by BIA type (A: reproductive BIA; B: small and resident BIA). The thick black bar in the middle of each box plot represents the median value; the lower and upper hinges of the boxplots correspond to the first and third quartiles; the upper and lower whiskers extend from the quartiles to the largest (lowest) value no further than 1.5 times the interquartile range. Watch list area scores were not included in this summary.
Data Support scores were intermediate to high across all BIAs, with only two BIAs and the watch list areas having a Data Support score of 1 (Table 4; Figure 10). BIAs with low Data Support scores are characterized by comparatively few supporting lines of evidence: the NWHI spinner dolphin S-BIAs were supported by genetic differentiation (strong support) and some sighting records which were documented over two decades ago. The minke whale watch list area is almost entirely justified through acoustic detection data. Only a few sighting records have been documented, and although acoustic data suggest unique calls may be linked to mating behavior, visual observations of minke whales with calves have yet to be documented. Humpback whale occurrence in the NWHI is primarily supported by acoustic data as well, with few visual sightings and movements from tagged whales to inform spatial patterns. Many of the populations with BIAs in this assessment are among the most well-studied of their species throughout the world, due to extensive, consistent, long-term studies on their population structure and behavior utilizing a suite of methods (Baird, 2016). Geographic coverage and information from other published studies are extensive and involved a variety of research tools that collectively strengthen understanding of habitat use and population structure of cetaceans in Hawaiʻi (Table 1). Photographic methods have documented individual re-sighting histories over several decades for many species. Movements from satellite-tagged individuals have provided detailed information on spatial use. Genetic studies have revealed deeper context into population structure for many Hawaiian cetaceans and as such are a strength in defining distinct populations within species.
The only hierarchical BIA with an Importance score of 3 for both parent and child was the Hawaiʻi Island dwarf sperm whale S-BIA (Table 4). Given the small abundance and range size leading to the Importance score of 3, coupled with the apparent susceptibility of this species at large to a number of anthropogenic activities (e.g., high-intensity military sonars, interactions with fisheries; Simmonds and Lopez-Juardo, 1991; Hohn et al., 2006; Baird, 2016; Baird et al., 2021c), there are clear conservation concerns for this island-associated population. However, it should be noted that this high Importance score results directly from the information available on the population and the S-BIA scoring protocols. This population has a small range size and abundance based on long-term sighting histories from extensive survey effort; despite this, no genetic information or information on movements from satellite tag data were available to understand population structure or spatial use outside of the area surveyed, largely due to the cryptic and elusive nature of the species. Accordingly, the Data Support score for this BIA was a 2.
The BIA Importance score should not be interpreted directly as a measure of vulnerability or conservation priority (Harrison et al in review). The S-BIA quantitative scoring matrix can result in lower Importance scores for populations that may require immediate protective measures based on additional independent lines of evidence of status or stressors, or upon considering socioeconomic factors. For instance, the MHI insular false killer whale parent S-BIA had an Importance score of 1; although there was high Data Support (score = 3) for this population based on long-term photo-identification (Baird et al., 2008b; Baird et al., 2019b), satellite tracking (Baird et al., 2010; Baird et al., 2012; Baird et al., 2021b), and genetic studies (Chivers et al., 2007 ; Martien et al., 2014), their range size is large and the total estimated abundance is intermediate (approximately 167 individuals, Bradford et al., 2018) yielding an Intensity score of 1. Nevertheless, MHI insular false killer whales are exposed to a number of anthropogenic activities that pose a risk to their long-term viability, notably, interactions with nearshore fisheries (Oleson et al., 2010; Baird et al., 2015b; Baird et al., 2017; Baird et al., 2021b), and this stock was listed as endangered in 2012. This population may be arguably the most critical to protect in Hawaiian waters, yet such urgency is not reflected in the BIA scoring metrics alone. With these examples, we emphasize the need to consider the narratives that underlie these scores as well as external sources of information that detail population-specific conservation concerns.
Boundary Certainty scores were at least 2 for all BIAs (Table 4; Figure 10), as we had at least intermediate confidence in the placement and timing of the defined boundaries. A vast majority of the BIAs delineated in this region were S-BIAs and in many cases the occurrence of 100% of the population that has been sampled does not follow a natural feature (e.g., isobath or hydrographic feature) that could be used as the basis of the species’ BIA boundary. As such, boundaries were frequently defined by MCPs that more accurately meet the aim of S-BIAs, despite appearing decoupled from the physical environment. BIAs based on MCPs that contained expanses with no occurrence data (e.g., sightings, satellite tag locations) generally had intermediate Boundary Certainty scores (2) while others that had a relatively even distribution of occurrence data within the boundary were assigned a higher score (3). Although the Boundary Certainty score is intended to be considered independently of the Intensity and Data Support scores (Harrison et al in review), in many cases we made note of factors relating to our understanding of the distribution of the species (e.g., through satellite tracking) that may influence the score.
Spatiotemporal Variability indicator scores were static for all BIAs (Table 4). The magnitude of spatiotemporal variability in environmental phenomena in Hawaiʻi is considerably less than higher latitude regions. From available data, there is little information to suggest dynamic or ephemeral features drive spatiotemporal variation in the location of important areas for insular cetaceans in Hawaiian waters, although mesoscale processes (e.g., eddies, island lee zones) may influence their distribution (e.g., see Woodworth et al., 2011 for the potential influence on offshore populations). There is recent evidence for fine-scale shifts in foraging location for species that follow lunar patterns in migrating prey (e.g., short-finned pilot whales, see Owen et al., 2019), however, such shifts occur on a short time scale during the lunar cycle and do not result in complete abandonment of other suitable habitat; as such, these documented patterns do not warrant consideration in BIA delineation.
5.3 Comparison to national results
Compared to other regions, Hawaiʻi BIAs generally had higher Data Support and Intensity scores. This may reflect differences in the quantity and quality of supporting data in Hawaiʻi relative to other regions. Although some species within Hawaiian waters may be more data deficient than others, a meaningful proportion of the species are well understood and supported by several decades of extensive and dedicated research, spearheaded by CRC and supplemented by other researchers and an expansive network of community scientists. CRC efforts cover the entire MHI and although effort varies by island area, this has led to strong region-wide support for Hawaiʻi BIAs (Table 1). In addition, almost all of the Hawaiʻi BIAs were S-BIAs, which have a distinct quantitative scoring system (Harrison et al in review), and higher Intensity scores for parent BIAs are a direct product of abundance and range size. Higher child BIA Intensity scores reflect intensified area of use relative to the broader parent BIA. Combined, the Importance scores for Hawaiʻi BIAs were also higher. Boundary Certainty scores were generally high because for many Hawaiʻi BIAs they were defined as the extent of the known range of each population. While other regions often assigned “ephemeral” or “dynamic” Spatiotemporal Variability indicator scores for their BIAs, all Hawaiʻi BIAs were “static”, reflecting the comparatively limited spatiotemporal variation in Hawaiʻi compared to high latitude regions, and concurrent lack of evidence for strong spatiotemporal variation in distribution of the populations considered for BIAs in Hawaiʻi.
6 Conclusions
The BIAs detailed here characterize important areas for all species inhabiting Hawaiian waters for which sufficient information was available to conduct an assessment. As an indicator of progress, populations that Baird et al. (2015a) suggested should be considered for BIA designation have had BIAs delineated in this assessment, including rough-toothed dolphins off Kauaʻi/Niʻihau/Oʻahu, short-finned pilot whales from Lānaʻi to Niʻihau, and pygmy killer whales off Oʻahu. Existing S-BIAs were revised to align with definitions of S-BIAs where boundaries intend to encompass 100% of the population, rather than primary areas of use. The humpback whale R-BIA was revised based on a comprehensive assessment of spatial use within and outside of existing BIAs and was well-supported by several data sources, while also identifying emerging areas of importance towards the NWHI based on satellite tag data. For all BIAs, additional sources of data and/or new data of the same type (e.g., movements from new tag deployments, additional sightings since Baird et al. (2015a)) were incorporated into the delineation process and are documented in both the BIA website and Supplementary File A.
While we suggest these BIAs accurately identify areas of importance for the populations based on the best available data, there remain knowledge gaps for a number of populations in Hawaiian waters. Some factors driving uncertainty in the distribution of populations are related to challenges in observation: small-boat survey effort on windward sides of the islands has been limited due to typically poor working conditions. While ship-based line-transect surveys cover windward waters, these surveys do not typically cover nearshore habitat where many species here are likely to occur (e.g., small and resident populations). Similarly, the remoteness of the NWHI has limited the amount of knowledge on cetacean occurrence there relative to the MHI, and research efforts are unlikely to substantially increase there in the near future. A feasible alternative to surveying cetacean use of these areas is satellite tracking methods, which have been a great strength in many Hawaiʻi BIAs described herein. This includes humpback whales that, based on recent studies, move towards the northwestern region of the MHI before departing on their migration north (Palacios et al., 2019; Palacios et al., 2020; Henderson et al., 2022). Similarly, most NWHI false killer whales have previously been tagged off Kauaʻi/Niʻihau, revealing movements along the Northwestern Hawaiian Island chain (Baird et al., 2013b), and additional information on their movements would enhance our understanding of their BIAs. Johnston et al. (2007) also presented a relatively simple approach for providing an initial description of breeding humpback whale habitat use in the NWHI region, which could be applied to other cetaceans for similar purposes. A number of species with BIAs in this assessment have recent, albeit limited, documentation of larger-scale movements to other island areas (either through photo-ID or satellite tag data), including rough-toothed dolphins between Maui Nui and Hawaiʻi Island, Blainville’s beaked whales off Oʻahu and Hawaiʻi Island (Baird, 2019), and pantropical spotted dolphins between Oʻahu and Hawaiʻi Island (Kratofil et al., 2022). Accordingly, our understanding of the frequency of such inter-island movements and overall distribution of the species may benefit from future studies involving satellite tracking. For some cryptic and elusive species (e.g., dwarf sperm whales), this alternative is unlikely to be feasible, and continued efforts from small-boat surveys and non-invasive sampling methods (e.g., eDNA, drones) may be the most viable option moving forward (Baird et al., 2021c). Passive acoustic methods are also useful for documenting the spatiotemporal occurrence of cetaceans, especially in remote regions such as the NWHI (e.g., Allen et al., 2021). However, this method typically only provides information on occurrence at a single point in space which does not resolve spatial patterns. Further, attributing acoustic detections to specific island-associated populations is only possible for those who are known to have distinct “dialects” (Van Cise et al., 2018).
Little information is available on the occurrence and distribution of other baleen whale species in Hawaiʻi that could be used to support BIA designation, but several species were initially considered in this assessment, including Bryde’s whale (Balaenoptera edeni) F-BIA and/or R-BIA, sei whale (Balaenoptera borealis) R-BIA, and fin whale (Balaenoptera physalus) R-BIA. Visual observations of these species are few and sparse, largely due to the fact that these species occur in deep, offshore waters where limited survey effort has been undertaken (Baird, 2016). Large-scale ship-based line transect surveys in offshore waters have documented visual observations and acoustic detections of a number of these species (e.g., Yano et al., 2018); however, these surveys are often undertaken during summer months when some of these whales (e.g., sei, fin) are unlikely to use Hawaiian waters. While data are also sparse for species with watch list areas described here, there is ample evidence supporting the seasonal occurrence and comparatively more spatial data on these species than exists for Bryde’s, sei, and fin whales. Additional large-scale surveys undertaken during presumed breeding season for these species (e.g., Yano et al., 2020) may allow more information to be gained on their occurrence in Hawaiʻi.
Lastly, we encourage users of these BIAs to not consider the scores associated with BIAs on their own, but rather refer to the narratives that justify them for a more comprehensive interpretation (Supplementary File A). While the scores here intend to provide a consistent proxy of importance among different BIA species and types, current understanding – based on the amount of support, type of support, and uncertainties – is unique to each population and the accompanying narratives provide important context on the conservation needs of each species.
Data availability statement
The raw data supporting the conclusions of this article will be made available by the authors, without undue reservation.
Ethics statement
The animal studies that collected data used in this effort were reviewed and approved by: Cascadia Research Collective Institutional Animal Care and Use Committee (IACUC), NMFS Office of Protected Resources, Oregon State University IACUC.
Author contributions
MK developed the Hawaiʻi BIA methodology framework (in consultation with all coauthors), analyzed the data, and wrote the manuscript. AH contributed to the methodology, data analysis, and review of the manuscript. SDM contributed to the methodology, data analysis, and review of the manuscript. EH contributed data used in the analyses, contributed to the methodology, data analysis, and writing and review of the manuscript. AB contributed data used in the analyses, contributed to the methodology, and review of the manuscript. SWM contributed to the methodology and review of the manuscript. BL contributed to the methodology, data analysis, and review of the manuscript. DP contributed data used in the analyses, contributed to the methodology, data analysis, and review of the manuscript. EO contributed data used in the analyses, contributed to the methodology, and review of the manuscript. RB managed the project, obtained project funding, contributed data to the analyses, contributed to the methodology, and writing and review of the manuscript. All authors participated in expert elicitation at the national level (i.e., involvement in national BIA meetings) and at the regional level by providing feedback and review of all Hawaiʻi BIAs, including the background information used to justify BIA delineation, methodology implemented to determine the spatial boundary of each BIA, and scores corresponding to each BIA. All authors contributed to the article and approved the submitted version.
Funding
Funding for CRC field work during which many of the data were collected was received from NOAA Fisheries (Pacific Islands Fisheries Science Center (PIFSC), Southwest Fisheries Science Center (SWFSC), Northwest Fisheries Science Center (NWFSC), Bycatch Reduction Engineering Program, Ocean Acoustics Program), the U.S. Navy (LMR, ONR, Pacific Fleet), the Tides Foundation, and the Wild Whale Research Foundation. Dolphin Quest also supported CRC satellite tagging with a number of poorly known populations. NIWC Pacific humpback whale tagging and small boat surveys were funded by the NIWC Naval Innovative Science and Engineering program and COMPACFLT (award no. N0007021WR0EP8F). Funding for OSU’s humpback whale satellite tagging work was provided by the U.S. Navy’s Office of Naval Research (1995-2000), Pacific Life Foundation and Makana Aloha Foundation (2015), the U.S. Navy’s Commander, Pacific Fleet (CESU Cooperative Agreement No. N62473-19-2-0002), and private donors to the OSU Marine Mammal Institute Endowment. PIFSC ship surveys were funded by NMFS (PIFSC, SWFSC, National Take Reduction Program, Office of Science and Technology), the Bureau of Ocean Energy Management, and the U.S. Navy (Pacific Fleet, Chief of Naval Operations N45).
Acknowledgments
We thank Daniel Webster, Greg Schorr, Allan Ligon, and Colin Cornforth for assistance in deploying odontocete satellite tags included in this assessment and Marie Hill for curating the PIFSC satellite tag dataset. We also thank the many CRC volunteers (in the office and the field) for assistance in data collection and processing, as well as community scientists in Hawaiʻi who have contributed sighting and photographic data that supported many aspects of this BIA effort. Odontocete satellite tag deployments were performed under NMFS permit numbers 731-1774, 15330, and 20605 issued to Robin Baird/Cascadia Research Collective and 20311, 15240 issued to Pacific Islands Fisheries Science Center. NIWC Pacific humpback whale tagging and small boat surveys were conducted under permit number 16239 issued to HDR, Inc. OSU’s humpback whale satellite tagging work in Hawaiʻi was conducted under NMFS permit numbers 841, 369-1440, 14856, and 21585 issued to Bruce R. Mate. We thank Julie Rivers for NIWC Pacific humpback whale tagging funding and project support, the WARP team at NIWC Pacific, PMRF technicians, and Jon Winsley for acoustic support. We also thank Jessica Bredvik and Reagan Pablo at Naval Facilities Engineering Command Southwest for technical and administrative project support of OSU’s humpback whale tagging work (2018-2019). We are grateful to Kristen Alvstad for assistance with processing a portion of the satellite tag data incorporated in this study and Ladd Irvine for providing feedback and advice on kernel density analysis methods. We thank the survey coordinators, observers, acousticians, and the officers and crew aboard each of the SWFSC and PIFSC surveys (NMFS) that contributed data to this assessment. We are grateful to Ann Allen for her assistance with processing the minke whale acoustic detections. The SWFSC and PIFSC surveys were conducted under NMFS permit numbers 774-1437, 14097, 15240, and 20311. We appreciate Ei Fujioka and Sarah DeLand for their assistance with the BIA description tool website and for compiling BIA metadata. We thank Jolie Harrison, Megan Ferguson, Leslie New, and Hunter Stanke for feedback on early versions of the manuscript, and three additional reviewers for helpful comments on the manuscript.
Conflict of interest
The authors declare that the research was conducted in the absence of any commercial or financial relationships that could be construed as a potential conflict of interest.
Publisher’s note
All claims expressed in this article are solely those of the authors and do not necessarily represent those of their affiliated organizations, or those of the publisher, the editors and the reviewers. Any product that may be evaluated in this article, or claim that may be made by its manufacturer, is not guaranteed or endorsed by the publisher.
Supplementary material
The Supplementary Material for this article can be found online at: https://www.frontiersin.org/articles/10.3389/fmars.2023.1053581/full#supplementary-material
Footnotes
- ^ https://oceannoise.noaa.gov/biologically-important-areas
- ^ Data provided by PacIOOS (www.pacioos.org), which is part of the U.S. Integrated Ocean Observing System (IOOS), funded in part by National Oceanic and Atmospheric Administration (NOAA) Awards #NA11NOS0120039 and #NA16NOS0120024.
References
Albertson G. R., Baird R. W., Oremus M., Poole M. M., Martien K. K., Baker C. S. (2016). Staying close to home? genetic differentiation of rough-toothed dolphins near oceanic islands in the central Pacific ocean. Conserv. Genet. 18, 33–51. doi: 10.1007/s10592-016-0880-z
Allen A. N., Harvey M., Harrell L., Jansen A., Merkens K. P., Wall C. C., et al. (2021). A convolutional neural network for automated detection of humpback whale song in a diverse, long-term passive acoustic dataset. Front. Mar. Sci. 8. doi: 10.3389/fmars.2021.607321
Bachman M. J., Keller J. M., West K. L., Jensen B. A. (2014). Persistent organic pollutant concentrations in blubber of 16 species of cetaceans stranded in the Pacific islands from 1997 through 2011. Sci. Total. Environ. 488, 115–123. doi: 10.1016/j.scitotenv.2014.04.073
Baird R. W. (2005). Sightings of dwarf (Kogia sima) and pygmy (K. breviceps) sperm whales from the main Hawaiian islands. Pac. Sci. 59 (3), 461–466. doi: 10.1353/psc.2005/0031
Baird R. W. (2016). The lives of Hawaiʻi’s dolphins and whales: Natural history and conservation (Hawaiʻi: University of Hawaiʻi Press).
Baird R. W. (2019). “Behavior and ecology of not-so-social odontocetes: Cuvier’s and blainville’s beaked whales,” in Ethology and behavioral ecology of toothed whales and dolphins, the odontocetes. Ed. Würsig B. (New York, NY: Springer), 305–329.
Baird R. W., Anderson D. B., Kratofil M. A., Webster D. L. (2021b). Bringing the right fishermen to the table: indices of overlap between endangered false killer whales and nearshore fisheries in Hawaiʻi. Biol. Conserv. 255, 108975. doi: 10.1016/j.biocon.2021.108975
Baird R. W., Anderson D. B., Kratofil M. A., Webster D. L., Mahaffy S. D. (2019b). Cooperative conservation and long-term management of false killer whales in Hawaiʻi: geospatial analyses of fisheries and satellite tag data to understand fishery interactions (Olympia, Washington: Cascadia Research Collective).
Baird R. W., Cholewiak D., Webster D. L., Schorr G. S., Mahaffy S. D., Curtice C., et al. (2015a). Biologically important areas for cetaceans within U.S. waters – Hawaiʻi region. Aquat. Mamm. 41 (1), 54–64. doi: 10.1578/AM.41/1/2015.54
Baird R. W., Cornforth C. J., Jarvis S. M., DiMarzio N. A., Dolan K., Henderson E. E., et al. (2021a). Odontocete studies on the Pacific Missile Range Facility in February 2020: Satellite-tagging, photo-identification, and passive acoustic monitoring (Honolulu, HI: HDR Inc.).
Baird R. W., Gorgone A. M., McSweeney D. J., Webster D. L., Salden D. R., Deakos M. H., et al. (2008b). False killer whales (Pseudorca crassidens) around the main Hawaiian islands: Long-term site fidelity, inter-island movements, and association patterns. Mar. Mamm. Sci. 24, 591–612. doi: 10.1111/j.1748-7692.2008.00200.x
Baird R. W., Hanson M. B., Schorr G. S., Webster D. L., McSweeney D. J., Gorgone A. M., et al. (2012). Range and primary habitats of Hawaiian insular false killer whales: Informing determination of critical habitat. Endanger. Spec. Res. 18, 47–61. doi: 10.3354/esr00435
Baird R. W., James J., Mata C., Hughes M. (2022). Two gray whale sightings off Hawaiʻi island: The first records for the central tropical Pacific. Aquat. Mamm. 48 (5), 432–435. doi: 10.1578/AM.48.5.2022.432
Baird R. W., Mahaffy S. D., Gorgone A. M., Beach K. A., Cullins T., McSweeney D. J., et al. (2017). “Updated evidence of interactions between false killer whales and fisheries around the main Hawaiian islands,” in Assessment of mouthline and dorsal fin injuries (Honolulu, HI: Pacific Scientific Review Group). Available at: https://www.cascadiaresearch.org/files/publications/Bairdetal2017_FKW_PSRG.pdf.
Baird R. W., Mahaffy S. D., Gorgone A. M., Cullins T., McSweeney D. J., Oleson E. M., et al. (2015b). False killer whales and fisheries interactions in Hawaiian waters: Evidence for sex bias and variation among populations and social groups. Mar. Mamm. Sci. 31, 579–590. doi: 10.1111/mms.12177
Baird R. W., Mahaffy S. D., Lerma J. K. (2021c). Site fidelity, spatial use, and behavior of dwarf sperm whales in Hawaiian waters: Using small-boat surveys, photo-identification, and unmanned aerial systems to study a difficult-to-study species. Mar. Mamm. Sci. 38 (1), 326–348. doi: 10.1111/mms.12861
Baird R. W., Oleson E. M., Barlow J., Ligon A. D., Gorgone A. M., Mahaffy S. D. (2013b). Evidence of an island-associated population of false killer whales (Pseudorca crassidens) in the northwestern Hawaiian islands. Pac. Sci. 67 (4), 513–521. doi: 10.2984/67.4.2
Baird R. W., Schorr G. S., Webster D. L., McSweeney D. J., Hanson M. B., Andrews R. D. (2010). Movements and habitat use of satellite-tagged false killer whales around the main Hawaiian islands. Endanger. Spec. Res. 10, 107–121. doi: 10.3354/esr00258
Baird R. W., Webster D. L. (2020). Using dolphins to catch tuna: assessment of associations between pantropical spotted dolphins and yellowfin tuna hook and line fisheries in Hawaiʻi. Fish. Res. 230, 105652. doi: 10.1016/j.fishres.2020.105652
Baird R. W., Webster D. L., Aschettino J. M., Schorr G. S., McSweeney D. J. (2013a). Odontocete cetaceans around the main Hawaiian islands: Habitat use and relative abundance from small-boat sighting surveys. Aquat. Mamm. 39, 253–269. doi: 10.1578/AM.39.3.2013.253
Baird R. W., Webster D. L., Jarvis S. M., Henderson E. E., Watwood S. L., Mahaffy S. D., et al. (2019a). “Odontocete studies on the Pacific Missile Range Facility in August 2018: Satellite-tagging, photo-identification, and passive acoustic monitoring,” in Prepared for Commander, Pacific Fleet, under Contract No. N62470-15-D-8006 Task Order 6274218F0107 issued to (Honolulu, HI: HDR Inc.).
Baird R. W., Webster D. L., Mahaffy S. D., McSweeny D. J., Schorr G. S., Ligon A. D. (2008a). Site fidelity and association patterns in a deep-water dolphin: Rough-toothed dolphins (Steno bredanensis) in the Hawaiian archipelago. Mar. Mamm. Sci. 24 (3), 535–553. doi: 10.1111/j.1748-7692.2008.00201.x
Baker C. S., Herman L. M. (1981). Migration and local movement of humpback whales (Megaptera novaeangliae) through Hawaiian waters. Can. J. Zool. 59, 460–469. doi: 10.1139/z81-067
Balcomb K. M. (1987). The whales of Hawaii, including all species of marine mammals in Hawaiian and adjacent waters (California: Marine Mammal Fund).
Barlow J. (2006). Cetacean abundance in Hawaiian waters estimated from a summer/fall survey in 2002. Mar. Mamm. Sci. 22 (2), 446–464. doi: 10.1111/j.1748-7692.2006.00032.x
Barlow J., Calambokidis J., Falcone E. A., Baker C. S., Burdin A. M., Clapham P. J., et al. (2011). Humpback whale abundance in the north Pacific estimated by photographic capture-recapture with bias correction from simulation studies. Mar. Mamm. Sci. 27 (4), 793–818. doi: 10.1111/j.1748-7692.2010.00444.x
Becker E. A., Forney K. A., Oleson E. M., Bradford A. L., Hoopes R., Moore J. E., et al. (2022). “Abundance, distribution, and seasonality of cetaceans within the U.S. exclusive economic zone around the Hawaiian archipelago based on species distribution models,” in NOAA Technical memorandum, NOAA-TM-NMFS-PIFSC-131 (U.S. Department of Commerce). doi: 10.25923/9e50-d280
Becker E. A., Forney K. A., Thayre B. J., Debich A. J., Campbell G. S., Whitaker K., et al. (2017). Habitat-based density models for three cetacean species off southern California illustrate pronounced seasonal differences. Front. Mar. Sci. 4. doi: 10.3389/fmars.2017.00121
Bradford A. L., Baird R. W., Mahaffy S. D., Gorgone A. M., McSweeney D. J., Cullins T., et al. (2018). Abundance estimates for management of endangered false killer whales in the main Hawaiian islands. Endanger. Spec. Res. 36, 297–313. doi: 10.3354/esr00903
Bradford A. L., Forney K. A., Oleson E. M., Barlow J. (2017). Abundance estimates of cetaceans from a line-transect survey within the U.S. Hawaiian islands exclusive economic zone. Fish. Bull. 115, 129–142. doi: 10.7755/FB.115.2.1
Bradford A. L., Oleson E. M., Forney K. A., Moore J. E., Barlow J. (2021). “Line-transect abundance estimates of cetaceans in U.S. waters around the Hawaiian islands in 2002, 2010, and 2017,” in NOAA Technical memorandum, NMFS-PIFSC-115 (Honolulu, HI, Pacific Islands Fisheries Science Center, National Marine Fisheries Service: U.S. Department of Commerce). doi: 10.25923/daz4-kw84
Calambokidis J., Falcone E. A., Quinn T. J., Burdin A. M., Clapham P. J., Ford J. K. B., et al. (2008). “SPLASH: Structure of Populations, Levels of Abundance and Status of Humpback Whales in the North Pacific,” in Final report for Contract AB133F-03-RP-00078 (Western Administrative Center, Seattle, Washington, USA: U.S. Department of Commerce).
Caretta J. V., Oleson E. M., Forney K. A., Muto M. M., Weller D. W., Lang A. R., et al. (2021). “U.S. Pacific marine mammal stock assessments,” in 2020. NOAA technical memorandum, NOAA-TM-SWFSC-646 (La Jolla, CA, Southwest Fisheries Science Center, National Marine Fisheries Service: U.S. Department of Commerce).
Cartwright R., Gillespie B., LaBonte K., Mangold T., Venema A., Eden K., et al. (2012). Between a rock and a hard place: Habitat selection in female-calf humpback whale (Megaptera novaeangliae) pairs on the Hawaiian breeding grounds. PloS One 7 (5), e38004. doi: 10.1371/journal.pone.0038004
Cartwright R., Sullivan M. (2009). Behavioral ontogeny in humpback whale (Megaptera novaeangliae) calves during their residence in Hawaiian waters. Mar. Mamm. Sci. 25 (3), 659–680. doi: 10.1111/j.1748-7692.2009.00286.x
Cerchio S., Gabriele C. M., Norris T. F., Herman L. M. (1998). Movements of humpback whales between Kauai and Hawaii: Implications for population structure and abundance estimation in the Hawaiian islands. Mar. Ecol. Prog. Ser. 175, 13–22. doi: 10.3354/meps175013
Checkley D. M., Barth J. A. (2009). Patterns and processes in the California current system. Prog. Oceanogr. 83, 49–64. doi: 10.1016/j.pocean.2009.07.028
Chivers S. J., Baird R.W., McSweeney D. J., Webster D. L., Hedrick N. M., Salinas J. C., et al (2007). Genetic variation and evidence for population structure in eastern North Pacific false killer whales (Pseudorca crassidens). Can. J. Zool. 85, 783–794. doi: 10.1139/Z07-059
Craig A. S., Herman L. M. (2000). Habitat preferences of female humpback whales Megaptera novaeangliae in the Hawaiian islands are associated with reproductive status. Mar. Ecol. Prog. Ser. 193, 209–216. doi: 10.3354/meps193209
Craig A. S., Herman L. M., Pack A. A. (2002). Male mate choice and male-male competition coexist in the humpback whale (Megaptera novaeangliae). Can. J. Zool. 80, 745–755. doi: 10.1139/z02-050
Craig A. S., Herman L. M., Pack A. A., Waterman J. O. (2014). Habitat segregation by female humpback whales in Hawaiian waters: Avoidance of males? Behaviour 151, 613–631. doi: 10.1163/1568539X-00003151
Croll D. A., Clark C. W., Acevedo A., Tershy B. R., Flores R. S., Gedamke J., et al. (2002). Only male fin whales sing loud songs. Nature 417, 809–811. doi: 10.1038/417809a
Currie J. J., McCordic J. A., Olson G. L., Machernis A. F., Stack S. H. (2021). The impact of vessels on humpback whale behavior: the benefit of added whale watching guidelines. Front. Mar. Sci. 8. doi: 10.3389/fmars.2021.601433
Currie J. J., Stack S. H., McCordic J. A., Kaufman G. D. (2017). Quantifying the risk that marine debris poses to cetaceans in coastal waters of the 4-island region of Maui. Mar. pollut. Bull. 121, 69–77. doi: 10.1016/j.marpolbul.2017.05.031
Doty M. S., Oguri M. (1956). The island mass effect. ICES J. Mar. Sci. 22 (1), 33–37. doi: 10.1093/icesjms/22.1.33
Duong T. (2007). Ks: Kernel density estimation and kernel discriminant analysis for multivariate data in r. J. Stat. Software 21, 1–16. doi: 10.18637/jss.v021.i07
Duong T. (2021) Ks: Kernel smoothing. r package version 1.12.0. Available at: https://CRAN.R-project.org/package=ks.
Duong T., Hazelton M. L. (2003). Plug-in bandwidth matrices for bivariate kernel density estimation. J. Nonparametr. Stat. 15, 17–30. doi: 10.1080/10485250306039
Duong T., Hazelton M. L. (2005). Cross-validation bandwidth matrices for multivariate kernel density estimation. Scand. J. Statist. 32, 485–506. doi: 10.1111/j.1467-9469.2005.00445.x
Durbach I., Harris C., Martin C., Helble T. A., Henderson E. E., Ierley G., et al. (2021). Changes in the movement and calling behavior of minke whales (Balaenoptera acutorostrata) in response to navy training. Front. Mar. Sci. 8. doi: 10.3389/fmars.2021.660122
Ferguson M. C., Curtice C., Harrison J., Van Parijs S. M. (2015). Biologically important areas for cetaceans within U.S. waters – overview and rationale. Aquat. Mamm. 41 (1), 2–16. doi: 10.1578/AM.41.1.2015.2
Flament P. (1996). The ocean atlas of Hawaiʻi (University of Hawaiʻi). Available at: http://www.pacioos.hawaii.edu/eductation/ocean-atlas/.
Gilmartin M., Revelante N. (1974). The ‘island mass’ effect on the phytoplankton and primary production of the Hawaiian islands. J. Exp. Mar. Biol. Ecol. 16 (2), 181–204. doi: 10.1016/0022-0981(74)90019-7
Gove J. M., McManus M. A., Neuheimer A. B., Polovina J. J., Drazen J. C., Smith C. R., et al. (2016). Near-island biological hotpots in ocean basins. Nat. Commun. 7, 10581. doi: 10.1038/ncomms10581
Harnish A. E. (2021). Population structure, residency, and inter-island movements of common bottlenose dolphins (Tursiops truncatus) off Oʻahu and Maui nui (Olympia, WA: The Evergreen State College).
Harrison J., Ferguson M. C., New L., Cleary J., Curtice C., DeLand S., et al. Biologically important areas for cetaceans within U.S. and adjacent waters: Updates and the application of a new scoring system. Front. Mar. Sci. in review.
Hauser D. D. W., Laidre K. L., Suydam R. S., Richard P. R. (2014). Population-specific home ranges and migration timing of Pacific Arctic beluga whales (Delphinapterus leucas). Polar Biol. 37, 1171–1183. doi: 10.1007/s00300-014-1510-1
Henderson E. E., Aschettino J., Deakos M., Alongi G., Leota T. (2019). Quantifying the behavior of humpback whales (Megaptera novaeangliae) and potential responses to sonar. Aquat. Mamm. 45 (6), 612–631. doi: 10.1578/am.45.6.2019.612
Henderson E. E., Aschettino J., Engelhaupt D. (2021). Dive and movement behavior of humpback whale competitive group and a multiday association between a primary escort and female in Hawaiʻi. Mar. Mamm. Sci. 38 (2), 835–846. doi: 10.1111/mms.12891
Henderson E. E., Deakos M., Aschettino J., Engelhaupt D., Alongi G. (2022). Behavior and inter-island movements of satellite-tagged humpback whales in Hawaiʻi, USA. Mar. Ecol. Prog. Ser. 685, 197–213. doi: 10.3354/meps13976
Herman L. M., Pack A. A., Spitz S. S., Herman E. Y. K., Rose K., Hakala S., et al. (2013). Humpback whale song: Who sings? Behav. Ecol. Sociobiol. 67, 1653–1663. doi: 10.1007/s00265-013-1576-8
Hickey B. M. (1979). The California current system-hypotheses and facts. Prog. Oceanogr. 8 (4), 191–279. doi: 10.1016/0079-6611(79)90002-8
Hill M. C., Bendlin A. R., Van Cise A. M., Milette-Winfree A., Ligon A. D., Adam Ü.C., et al. (2019). Short-finned pilot whales (Globicephala macrorhynchus) of the Mariana archipelago: Individual affiliations, movements, and spatial use. Mar. Mamm. Sci. 35 (3), 797–824. doi: 10.1111/mms.12567
Hohn A. A., Rotstein D. S., Harms C. A., Southall B. L. (2006). “Report on marine mammal unusual mortality event UMESE0501Sp: Multispecies mass stranding of pilot whales (Globicephala macrorhynchus), minke whale (Balaenoptera acutorostrata), and dwarf sperm whales (Kogia sima) in North Carolina on 15-16 January 2005,” in NOAA Technical memorandum NMFS-SEFSC-537 (Miami, FL, Southeast Fisheries Science Center, National Marine Fisheries Service: U.S. Department of Commerce).
Iverson R. L. (1990). Control of marine fish production. Limnol. Oceanogr. 35 (7), 1593–1604. doi: 10.4319/lo.1990.35.7.1593
Johnson D. S., London J. M. (2018). Crawl: An r package for fitting continuous-time correlated random walk models to animal movement data (Zenodo). doi: 10.5281/zenodo.596464
Johnson D. S., London J. M., Lea M.-A., Durban J. W. (2008). Continuous-time correlated random walk model for animal telemetry data. Ecol 89, 1208–1215. doi: 10.1890/07-1032.1
Johnston D. W., Chapla M. E., Williams L. E., Mattila D. K. (2007). Identification of humpback whale Megaptera novaeangliae wintering habitat in the northwestern Hawaiian islands using spatial habitat modeling. End. Spec. Res. 3, 2490257. doi: 10.3354/esr00049
Kratofil M. A., Baird R. W., Webster D. L. (2022). Spatial use of pantropical spotted dolphins in relation to stock boundaries and environmental features in Hawaiian waters. In 24th Biennial Conference on the Biology of Marine Mammals (Palm Beach, Florida).
Kratofil M. A., Ylitalo G. M., Mahaffy S. D., West K. L., Baird R. W. (2020). Life history and social structure as drivers of persistent organic pollutant levels and stable isotopes in Hawaiian false killer whales (Pseudorca crassidens). Sci. Total Environ. 733, 138880. doi: 10.1016/j.scitotenv.2020.138880
Lammers M. O., Fisher-Pool P. I., Au W. W. L., Meyer C. G., Wong K. B., Brainard R. E. (2011). Humpback whale Megaptera novaeangliae song reveals wintering activity in the northwestern Hawaiian islands. Mar. Ecol. Prog. Ser. 423, 261–268. doi: 10.3354/meps08959
Lammers M., Pack A. A., Lyman E. G., Espiritu L. (2013). Trends in collisions between vessels and north Pacific humpback whales (Megaptera novaeangliae) in Hawaiian waters, (1975-2011). J. Cetacean Res. Manage. 13 (1), 73–80.
Landrau-Giovannetti N., Waltzek T. B., López-Orozco N., Su C., Rotstein D., Levine G., et al. (2022). Prevalence and genotype of Toxoplasma gondii in stranded Hawaiian cetaceans. Dis. Aquat. Org. 152, 27–36. doi: 10.3354/dao03699
London J. M. (2021). Pathroutr: an r package for (re-)routing paths around barriers (version v0.2.1) (Zenodo). doi: 10.5281/zenodo.4321827
Mahaffy S. D., Baird R. W., McSweeney D. J., Webster D. L., Schorr G. S. (2009)Individual photo-identification of dwarf sperm whales off the island of Hawaiʻi: Evidence of site fidelity and a small population size. 18th Biennial Conference on the Biology of Marine Mammals, Quebec.
Martien K. K., Chivers S. J., Baird R. W., Archer F. I., Gorgone A. M., Hancock-Hanser B. L., et al. (2014). Nuclear and mitochondrial patterns of population structure in north Pacific false killer whales (Pseudorca crassidens). J. Hered. 105, 611–626. doi: 10.1093/jhered/esu029
Martin C. R., Henderson E. E., Martin S. W., Helble T. A., Manzano-Roth R. A., Matsuyama B. M., et al. (2020). FY18 annual report on Pacific Missile Range Facility marine mammal monitoring. Technical Report 3202. (San Diego, CA: Naval Information Warfare Center, Pacific).
Martin S. W., Marques T. A., Thomas L., Morissey R. P., Jarvis S., DiMarzio N., et al. (2013). Estimating minke whale (Balaenoptera acutorostrata) boing sound density using passive acoustic sensors. Mar. Mamm. Sci. 29 (1), 142–158. doi: 10.1111/j.1748-7692.2011.00561.x
Martin S. W., Martin C. R., Matsuyama B. M., Henderson E. E. (2015). Minke whales (Balaenoptera acutorostrata) respond to navy training. J. Acoust. Soc Am. 137 (5), 2533–2541. doi: 10.1121/1.4919319
Mate B. R., Gisiner R., Mobley J. (1998). Local and migratory movements of Hawaiian humpback whales tracked by satellite telemetry. Can. J. Zool. 76, 863–868. doi: 10.1139/z98-008
Merkle J. A., Abrahms B., Armstrong J. B., Sawyer H., Costa D. P., Chalfoun A. D. (2022). Site fidelity as a maladaptive behavior in the anthropocene. Front. Ecol. Environ. 20 (3), 187–194. doi: 10.1002/fee.2456
Migaki G., Sawa T. R., Dubey J. P. (1990). Fatal disseminated toxoplasmosis in a spinner dolphin (Stenella longirostris). Vet. Pathol. 27, 463–464. doi: 10.1177/030098589902700615
Mobley J. R. (2004). Results of marine mammal surveys on U.S. navy underwater ranges in Hawaii and Bahamas. Final report submitted to (Honolulu, HI: Office of Naval Research, Marine Mammal Program), under award number #N000140210841.
Mobley J. R. Jr., Bauer G. B., Herman L. M. (1999). Changes over a ten-year interval in the distribution and relative abundance of humpback whales (Megaptera novaeangliae) wintering in Hawaiian waters. Aquat. Mamm. 25 (2), 63–72.
Mobley J. R., Spitz S. S., Forney K. A., Grotefendt R., Forestell P. H. (2000). Distribution and abundance of odontocete species in Hawaiian waters: Preliminary results of 1993-98 aerial surveys. NMFS-SWFSC Administrative Report LJ-00-14C.
Mobley J. R. Jr., Spitz S., Grotefendt R. (2001). Abundance of humpback whales in Hawaiian waters: Results of 1993-2000 aerial surveys (Honolulu, Hawaii: Hawaiian Islands Humpback Whale National Marine Sanctuary).
Möller L. M., Attard C. R. M., Bilgmann K., Andrews-Goff V., Jonsen I., Paton D., et al. (2020). Movements and behaviour of blue whales satellite tagged in an Australian upwelling system. Sci. Rep. 10, 21165. doi: 10.1038/s41598-020-78143-2
Muto M. M., Helker V. T., Delean G. J., Angliss R. P., Boveng P. L., Breiwick J. M., et al. (2019). Alaska marine mammal stock assessments, 2019. NOAA Technical Memorandum, NMFS-AFSC-404 (Seattle, WA, Alaska Fisheries Science Center, National Marine Fisheries Service: U.S. Department of Commerce).
Norris T., Martin S., Thomas L., Yack T., Oswald J. N., Nosal E.-M., et al. (2012). Acoustic ecology and behavior of minke whales in the Hawaiian and Marianas islands: Localization, abundance estimation, and characterization of minke whale “boings”. Adv. Exp. Med. Biol. 730, 149–153. doi: 10.1007/978-1-4419-7311-5_33
Oleson E. M., Baird R. W., Martien K. K., Taylor B. L. (2013). Island-associated stocks of odontocetes in the main Hawaiian islands: A synthesis of available information to facilitate evaluation of stock structure Document PSRG-2013-16 submitted to the (Del Mar, CA: Pacific Scientific Review Group). Available at https://cascadiaresearch.org/files/Oleson_etal_PSRG-2013-16.pdf.
Oleson E. M., Boggs C. H., Forney K. A., Hanson M. B., Kobayashi D. R., Taylor B. L., et al. (2010). “Status review of Hawaiian insular false killer whales (Pseudorca crassidens) under the endangered species act,” in NOAA Technical memorandum, NMFS-PIFSC-22 (Honolulu, HI, Pacific Islands Fisheries Science Center, National Marine Fisheries Service: U.S. Department of Commerce).
Oswald J. N., Au W. W. L., Duennebier F. (2011). Minke whale (Balaenoptera acutorostrata) boings detected at the station ALOHA cabled observatory. J. Acoust. Soc Am. 129, 3353–3360. doi: 10.1121/1.3575555
Owen K., Andrews R. D., Baird R. W., Schorr G. S., Webster D. L. (2019). Lunar cycles influence the diving behavior and habitat use of short-finned pilot whales around the main Hawaiian islands. Mar. Ecol. Prog. Ser. 629, 193–206. doi: 10.3354/meps13123
Pack A. A., Herman L. M., Craig A. S., Spitz S. S., Waterman J. O., Herman E. Y. K., et al. (2018). Comparing depth and seabed terrain preferences of individually identified female humpback whales (Megaptera novaeangliae), with and without calf, off Maui, Hawaii. Mar. Mamm. Sci. 34 (4), 1097–1110. doi: 10.1111/mms.12495
Palacios D. M., Mate B. R., Baker C. S., Hayslip C. E., Follett T. M., Steel D., et al. (2019). “Tracking North Pacific humpback whales to unravel their basin-wide movements,” in Final technical report prepared for Pacific Life Foundation (Oregon, USA: Marine Mammal Institute, Oregon State University, Newport). doi: 10.5399/osu/1117. Available at https://ir.library.oregonstate.edu/concern/technical_reports/z890s0924.
Palacios D. M., Mate B. R., Baker C. S., Lagerquist B. A., Irvine L. M., Follett T. M., et al. (2020). Humpback whale tagging in support of marine mammal monitoring across multiple navy training areas in the Pacific Ocean: Final report for the Hawaiian breeding area in Spring 2019, including historical data from previous tagging efforts. Prepared for Commander, US Pacific Fleet, and Commander, Naval Sea Systems Command (San Diego, California: Naval Facilities Engineering Command Southwest, San Diego, California, under Cooperative Ecosystem Studies Unit, Department of the Navy Cooperative). Agreement No. N62473-19-2-0002. 15 May 2020.
Patton D., Lawless S. (2021). Surface and underwater observation of a humpback whale (Megaptera novaeangliae) birth in progress off Lahaina, Maui, and subsequent encounter of the female with a healthy calf. Aquat. Mamm. 47 (6), 550–558. doi: 10.1578/AM.47.6.2021.550
Pebesma E. (2018). Simple features for r: Standardized support for spatial vector data. R J. 10 (1), 439–446. doi: 10.32614/RJ-2018-009
Polovina J. J., Howell E. A., Abecassis M. (2008). Ocean’s least productive waters are expanding. Geophys. Res. Lett. 35 (3), L03618. doi: 10.1029/2007GL031745
Rankin S., Barlow J. (2005). Source of the north Pacific “boing” sound attributed to minke whales. J. Acoust. Soc Am. 118, 3346–3351. doi: 10.1121/1.2046747
Rankin S., Norris T. F., Smultea M. A., Oedekoven C., Zoidis A. M., Sliva E., et al. (2007). A visual sighting and acoustic detections of minke whales, Balaenoptera acutorostrata (Cetacea: Balaenopteridae), in nearshore Hawaiian waters. Pac. Sci. 61 (3), 395–398. doi: 10.2984/1534-6188(2007)61[395:AVSAAD]2.0.CO;2
Ransome N., Bejder L., Jenner M., Penfold G., Brosig V. J., Kitson C., et al. (2022). Observations of parturition in humpback whales (Megaptera novaeangliae) and occurrence of escorting and competitive behavior around birthing females. Mar. Mamm. Sci. 38 (2), 408–432. doi: 10.1111/mms.12864
R Core Team (2021). R: A language and environment for statistical computing (Vienna, Austria: R Foundation for Statistical Computing). Available at: https://www.R-project.org/.
Shaff J. F., Baird R. W. (2021). Diel and lunar variation in diving behavior of rough-toothed dolphins (Steno bredanensis) off Kauaʻi, Hawaiʻi. Mar. Mamm. Sci. 37 (4), 1261–1276. doi: 10.1111/mms.12811
Shallenberger E. W. (1981). The status of Hawaiian cetaceans. marine mammal commission report no. MMC-77/23.
Simmonds M. P., Lopez-Juardo L. F. (1991). Whales and the military. Natur 351, 448. doi: 10.1038/351448a0
Thompson P. O., Friedl W. A. (1982). A long term study of low frequency sounds from several species of whales off Oahu, Hawaii. Cetology 45, 1–19.
Tynan C. T., Ainley D. G., Barth J. A., Cowles T. J., Pierce S. D., Spear L. B. (2005). Cetacean distributions relative to ocean processes in the northern California current system. Deep Sea. Res. 2, 52, 145–167. doi: 10.1016/j.dsr2.2004.09.024
Van Cise A. M., Mahaffy S. D., Baird R. W., Mooney T. A., Barlow J. (2018). Song of my people: Dialect differences among sympatric social groups of short-finned pilot whales in Hawaiʻi. behav. Ecol. Sociobiol. 72, 1–13. doi: 10.1007/s00265-018-2596-1
Van Parijs S. M., Curtice C., Ferguson M. (2015). Biologically important areas for cetaceans within U.S. waters. Aquat. Mammals 41 (1), 1–128. doi: 10.1578/AM.41.1.2015.1
Woodworth P. A., Schorr G. S., Baird R. W., Webster D. L., McSweeney D. J., Hanson M. B., et al. (2011). Eddies as offshore foraging grounds for melon-headed whales (Peponocephala electra). Mar. Mamm. Sci. 28 (3), 638–647. doi: 10.1111/j.1748-7692.2011.00509.x
Worton B. J. (1989). Kernel methods for estimating the utilization distribution in home-range studies. Ecol 70, 164–168. doi: 10.2307/1938423
Yano K. M., Oleson E. M., Hill M. C., Keating-McCullough J. L., Lammers M. O. (2019). “Spring survey reveals large numbers of humpback whales in the northwestern Hawaiian Islands,” in World Marine Mammal Conference, (Barcelona, Spain).
Yano K. M., Oleson E. M., Keating J. L., Ballance L. T., Hill M. C., Bradford A. L., et al. (2018). Cetacean and seabird data collected during the Hawaiian islands cetacean and ecosystem assessment survey (HICEAS). July-December 2017. NOAA Technical Memorandum, NOAA-TM-NMFS-PIFSC-72. (Honolulu, HI, Pacific Islands Fisheries Science Center, National Marine Fisheries Service: U.S. Department of Commerce). doi: 10.25923/7avn-gw82
Yano K. M., Oleson E. M., McCullough J. L. K., Hill M. C., Henry A. E. (2020). Cetacean and seabird data collected during the winter Hawaiian Islands Cetacean and Ecosystem Assessment Survey (Winter HICEAS). January-March 2020. NOAA Technical Memorandum, NOAA-TM-NMFS-PIFSC-111. (Honolulu, HI, Pacific Islands Fisheries Science Center, National Marine Fisheries Service: U.S. Department of Commerce). doi: 10.25923/ehfg-dp78
Ylitalo G. M., Baird R. W., Yanagida G. K., Webster D. L., Chivers S. J., Bolton J. L., et al. (2009). High levels of persistent organic pollutants measured in blubber of island-associated false killer whales (Pseudorca crassidens) around the main Hawaiian islands. Mar. pollut. Bull. 58, 1932–1937. doi: 10.1016/j.marpolbul.2009.08.029
Ziegenhorn M. (2022). Some like it cool: A study of odontocete ecology in the Hawaiian islands using passive acoustic monitoring (San Diego, CA: University of California San Diego).
Keywords: conservation, cetaceans, Hawaiʻi, whales, dolphins, management, habitat
Citation: Kratofil MA, Harnish AE, Mahaffy SD, Henderson EE, Bradford AL, Martin SW, Lagerquist BA, Palacios DM, Oleson EM and Baird RW (2023) Biologically Important Areas II for cetaceans within U.S. and adjacent waters – Hawaiʻi Region. Front. Mar. Sci. 10:1053581. doi: 10.3389/fmars.2023.1053581
Received: 25 September 2022; Accepted: 03 January 2023;
Published: 26 January 2023.
Edited by:
Sofie Van Parijs, Northeast Fisheries Science Center (NOAA), United StatesReviewed by:
Tiffini Brookens, Marine Mammal Commission, United StatesJulian A. Tyne, Department of Biodiversity, Conservation and Attractions (DBCA), Australia
Marc Lammers, Hawaiian Islands Humpback Whale National Marine Sanctuary, United States
Copyright © 2023 Kratofil, Harnish, Mahaffy, Henderson, Bradford, Martin, Lagerquist, Palacios, Oleson and Baird. This is an open-access article distributed under the terms of the Creative Commons Attribution License (CC BY). The use, distribution or reproduction in other forums is permitted, provided the original author(s) and the copyright owner(s) are credited and that the original publication in this journal is cited, in accordance with accepted academic practice. No use, distribution or reproduction is permitted which does not comply with these terms.
*Correspondence: Robin W. Baird, cndiYWlyZEBjYXNjYWRpYXJlc2VhcmNoLm9yZw==