- 1Departamento de Oceanografía Biológica, Centro de Investigación Científica y de Educación Superior de Ensenada, Baja California, Mexico
- 2Departamento de Oceanografía Física, Centro de Investigación Científica y de Educación Superior de Ensenada, Baja California, Mexico
Marine mammals are highly vulnerable to oil spills, although the effects at both individual and population levels are not fully understood. A first approximation to evaluate the possible consequences of oil spills on marine life is using ecological risk assessments, which are analytical tools used to assess the likelihood of adverse environmental effects due to exposure to stressors derived from human activities. We developed a semi-quantitative framework to evaluate the risk of oil spill exposure on marine mammals that combines the likelihood of exposure based on species-specific biological and ecological traits, and the feasibility of encounter, which considers not only the overlap between the distribution of the species and the total affected area by a spill but also considers the distribution of spilled oil within this area, thus reducing the uncertainty in the estimate. We applied our framework to assess the risk of exposure of eight cetaceans to scenarios of large heavy oil (API gravity<22) spills originating from three hypothetical deep-water wells in the western Gulf of Mexico. High habitat suitability areas obtained using the maximum entropy (MaxEnt) modeling approach were used as a proxy for the geographic regions where each species is likely to be distributed, and oil spill scenarios were generated using numerical models incorporating transport, dispersion, and oil degradation. The analysis allowed identifying those species for which there is a significant risk of exposure in each spill scenario. However, our results suggest that the risk does not appear to be high for any species under any scenario. The information generated by our risk assessment is key to developing management plans in those areas of the Gulf of Mexico where deep-water activities of the hydrocarbon industry are currently being developed or planned.
Introduction
The exploration, extraction, and transport of hydrocarbons threat biodiversity in different ways, from local effects (e.g., habitat degradation and noise pollution) to oil spills that can have severe long-term impacts that could affect wide areas of the marine environment (Kingston, 2002; Butt et al., 2013). Risk assessments are valuable tools for identifying those species or populations that could be of most concern during an oil spill, allowing decision-makers to prioritize management initiatives and recovery actions. Analytical approaches can be qualitative, semi-quantitative, or quantitative (Hobday et al., 2011; Holsman et al., 2017). Qualitative methods are fast and usually inexpensive evaluations based on qualitative data (e.g., expert opinion, workshops). Semi-quantitative approaches combine qualitative and quantitative data, and the values obtained are assigned qualitative scales (i.e., low, medium, or high) to describe the magnitude of the risk. Finally, quantitative approaches are based on models that explicitly describe error and probability profiles.
Marine mammals are a key group for assessing the health of aquatic ecosystems because changes in their populations reflect ecological variations at large spatial and temporal scales (Moore, 2008). This group is exposed to several anthropogenic disturbances that could negatively affect their populations, such as those related to the hydrocarbon industry (Davidson et al., 2012). They are highly vulnerable to oil, although the effects of spills, both at the individual and population levels, are not fully understood (Helm et al., 2015). Nevertheless, adverse impacts are undeniable. For example, the 2010 Deepwater Horizon oil spill, which occurred 70 km southeast of the Mississippi River delta in the northern Gulf of Mexico (GM), severely impacted coastal populations of bottlenose dolphin (Tursiops truncatus; Venn-Watson et al., 2015a) and presumably contributed to the death of hundreds of animals of other cetacean species (Williams et al., 2011; Litz et al., 2014). According to the Deepwater Horizon Natural Resource Damage Assessment Trustees (DHNRDAT, 2016), the impact on marine mammal populations included high rates of mortality, failed reproduction, and disease (e.g., kidney and lung damage). The bottlenose dolphin population of Barataria Bay, Louisiana, was perhaps the most affected, causing a 35% increase in deaths, a 46% increase in failed reproduction, and a 37% increase in illness compared to a healthy population. In addition, it is estimated that exposure to oil resulted in a 7% decline in the northern GM sperm whale (Physeter macrocephalus) population, and a 22% decline in the endemic northeastern GM population of Rice's whale (Balaenoptera ricei).
During a spill at sea, part of the oil rises to the surface, and the volatile components evaporate at a rate that depends both on the type of oil (light: API gravity > 38, medium: API gravity 22–38, or heavy: API gravity< 22; EIA, 2022) and the oceanographic and atmospheric conditions at the time of the incident (Lee et al., 2016). The greatest risk of oil exposure for marine mammals occurs near the surface, either through contact, inhalation, and/or ingestion (Harris et al., 2011; Jarvela Rosenberger et al., 2017). Methods have been designed to assess the likelihood of exposure of marine mammals to oil spills using their biological and ecological traits (e.g., Jarvela Rosenberger et al., 2017). However, the risk of exposure during a spill is also strongly influenced by the feasibility of the encounters between the animals and the oil, which is determined by the spatial and temporal distribution of the species and the evolution of the oil spilled (Fox et al., 2016).
The best way to evaluate the feasibility of encounter of any species is to use spatially explicit maps of its distribution. However, because marine mammals have a high displacement capacity, monitoring them can be expensive and logistically problematic; therefore, this information is not always available. An alternative is to characterize areas of high suitability as a proxy for the regions where the species of interest are likely to be found (Franklin, 2010). On the other hand, since each oil spill is unique, it is impossible to extrapolate the evolution and the impact from one spill to another, even when they occur in the same area and under the same initial blowout conditions (Abascal et al., 2010; Fingas, 2017). A practical way to determine the potential area affected by spills is to use numerical models to simulate the evolution under different environmental conditions (Pérez-Brunius et al., 2020; Romo-Curiel et al., 2022).
Here, we present a semi-quantitative framework for assessing the risk of exposure to oil spills of marine mammals that combines the evaluation of the likelihood of exposure and the feasibility of encounter. The first component is estimated using biological and ecological traits of a given species as an indicator of the likelihood of oil exposure for individuals. The second component is based on the overlap between the area potentially affected by the spill and the distribution of the species in the region of interest. The overall goal of this risk assessment is to generate a priori information regarding the species (or populations) that are likely to be impacted by oil spills in a given area that can be subsequently used for the early development of management plans. We used the western GM as a case study due to its diversity of marine mammals and the intense activity of the oil industry.
Materials and methods
Framework overview
The first step of our framework is to evaluate the likelihood of exposure of individuals (L), for which we used the exposure pathways proposed by Jarvela Rosenberger et al. (2017). This approach determines how likely it is for animals to encounter the oil, for which five exposure pathways are evaluated: 1) contact, 2) inhalation, 3) adhesion, 4) direct ingestion, and 5) indirect ingestion (Table 1; Supplementary Material S1). The scores assigned to each exposure pathway varied between 1 and 3, and the value of L is calculated as the arithmetic mean of the five pathway scores.
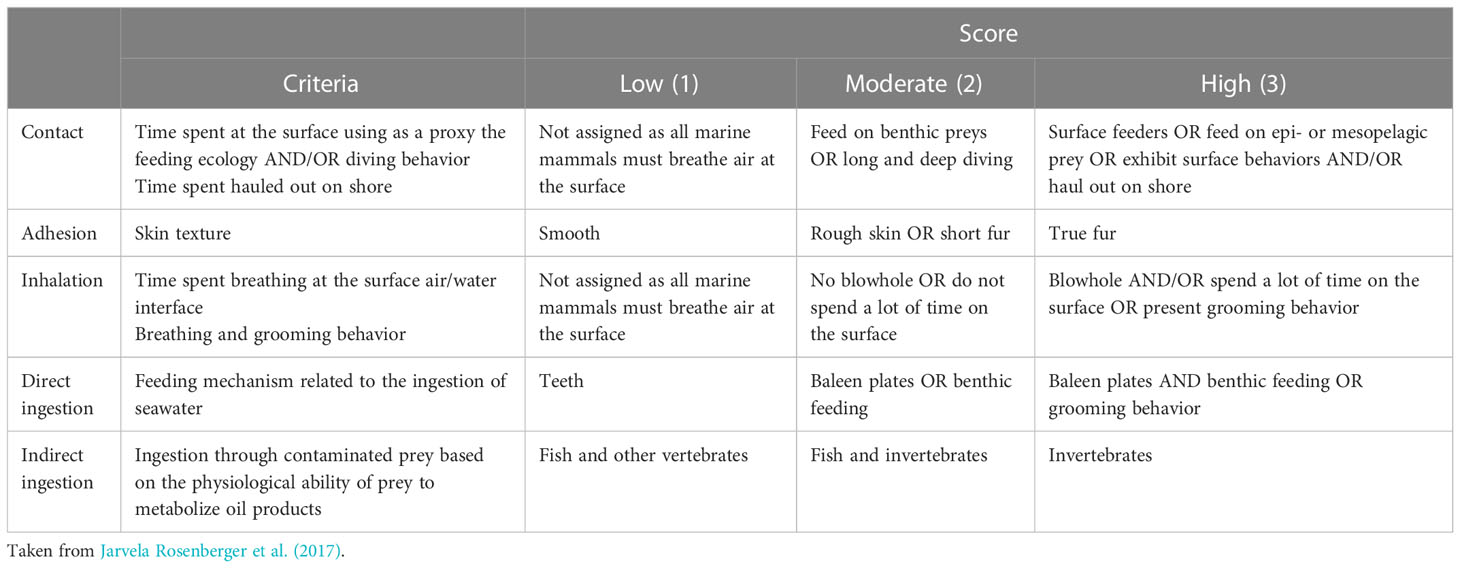
Table 1 Exposure pathways based on biological and ecological traits of marine mammals used to estimate the likelihood of exposure (L) to oil.
The second step is to calculate the feasibility of encounter between the species of interest and the spilled oil, for which we used an approach adapted from the method described by Fox et al. (2016). In a geographic grid, the spatial co-occurrence (S) is mapped between the cells where the species is distributed (D) and the cells with spilled oil (P); that is S = D ∩ P. The cells’ dimensions can be selected based on the ecosystem of interest and/or the spatial resolution of the species distribution maps.
The overlap (T) is obtained as
and it has values ranging from zero (no overlap, S = 0) to 1 (complete overlap, S = D). However, during a spill the oil is not evenly distributed but the amount of oil on the sea surface, O, varies between Omin and Omax, which are the minimum and maximum amount of oil observed, respectively. Therefore, each cell can be categorized using the 25th and 75th percentiles of O. Thus, Pl contains those cells with O values below the first quantile, Pm the cells with values in the interquartile range, and Ph the cells with values greater than the third quartile. Subsequently, the number of cells of Pl, Pm, and Ph that are contained in S are counted. That is:
but:
The feasibility of encounter (F) is quantified as:
This procedure gives more weight to those cells with a higher amount of oil. Note that if S = 0, then T = 0 and the feasibility of encounter is zero, but if T = 1 and SPh = S, then F = 3.00, which represents the highest feasibility of encounter.
Finally, the risk of exposure (R) is calculated by multiplying L by F, such that the values of R range from 0 (if S = 0) to 9 (if L = 3 and F = 3). The risk of exposure is classified in one of four categories: low (R< 2.25), moderate (2.25 ≤ R< 4.5), high (4.50 ≤ R< 6.75), and very high (R ≥ 6.75).
For example, suppose we want to assess the risk of exposure to Species 1 during a particular spill in a certain area. The hypothetical exposure pathway scores for Species 1 are: contact = 3, inhalation = 3, adhesion = 3, direct ingestion = 2, and indirect ingestion = 2; then L1 = 2.60, which suggests that Species 1 is highly susceptible to exposure. If the number of cells where Species 1 is distributed within the area of interest is equal to 190, and the number of cells where the distribution of Species 1 overlaps with the cells of the spill is 157, then D1 = 190, S1 = 157, and T1 = 0.83. Suppose also that the number of cells where the distribution of Species 1 overlapped with cells with a low amount of oil is 114, with a medium amount of oil is 25, and with a high amount of oil is 18. Then:
Since R=2.60×1.15=2.98 , then the risk of oil exposure during this particular spill is moderate for Species 1.
Study area and species assessed
The GM is a semi-enclosed marginal sea where the Exclusive Economic Zones (EEZs) of the United States, Mexico, and Cuba converge (Figure 1). Offshore oil production represents 17% of the total crude oil production of the United States (EIA, 2019), and 82% of the total production of Mexico (CNH, 2019), which places the GM as one of the most important regions for energy resources in North America as well as an area of high risk of oil incidents (Botello et al., 1997).
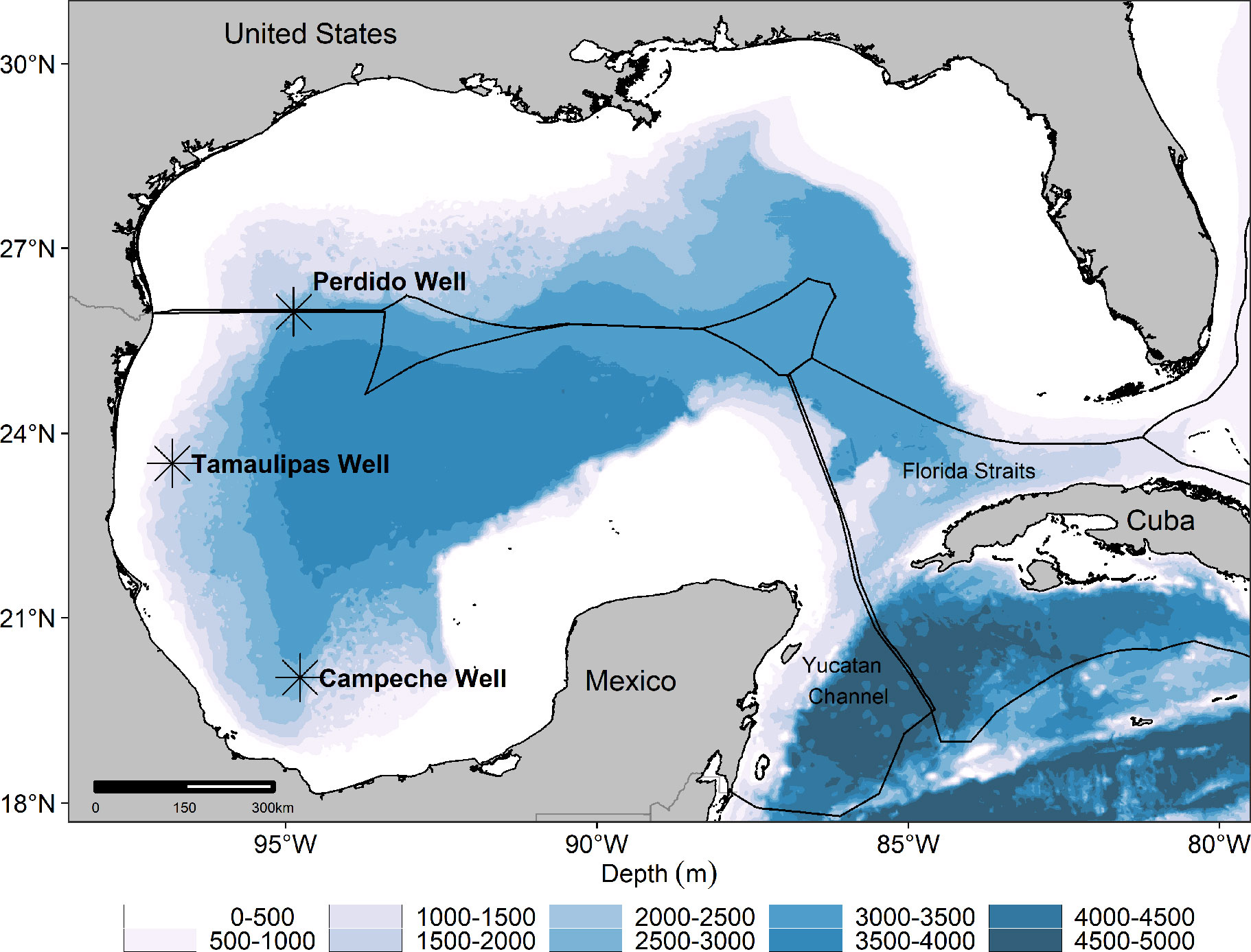
Figure 1 Gulf of Mexico and location of the three hypothetical deep-water wells used in our oil exposure risk assessment. Solid line indicates the delimitation of the Exclusive Economic Zones of Mexico, United States and Cuba.
The hydrocarbon extraction in the Mexican EEZ has been focused on the shallow (< 500 m depth) waters of the southwestern GM (CNH, 2019), but it is expanding into deep waters (Murawski et al., 2020). Recently, the Gulf of Mexico Research Consortium (CIGoM) generated oil spill scenarios for six hypothetical wells located in current or future drilling areas by PEMEX (the Mexican State-owned oil company) of the western GM using numerical models incorporating transport, dispersion, and oil degradation to identify the marine regions that could be affected by large-scale oil incidents (Pérez-Brunius et al., 2020; Supplemental Material S2). Given the high natural variability of both oceanic and atmospheric conditions, the fate of the oil during (and after) a spill will depend on the hydrodynamic conditions in which the incident occurs, which are unknown a priori. Thus, a spill scenario is defined as a statistical and probabilistic ensemble of a large number of simulations of individual spills under different oceanographic and atmospheric conditions in order to include as many outcomes as possible (Pérez-Brunius et al., 2020; Romo-Curiel et al., 2022).
The spill scenarios generated by CIGoM for these hypothetical wells were constructed using 20 years (1993-2012) of simulation of oceanographic and atmospheric conditions in the GM. All the individual spills started under the same initial conditions of the blowout on the seabed, and since each simulation began on the first day of each month, each scenario was built from 240 individual spills (Supplemental Material S2 for details). Initial conditions of the blowout were: oil flow of 40 kg/s, outlet diameter of 0.15 m, outlet temperature of 35°C, vertical release angle, and duration of 15 days. The simulations show the evolution of the oil up to two months after the start of the incident in the upper 20 m of the water column, since according to the modeling, most of the spilled oil accumulated in the surface layer of the sea. The scenarios are presented on maps that show the areas of possible affectation in the event of an accident, as well as the amount of oil expected within these areas; that is, each scenario generated for each well encloses the geographic area where oil is expected to be found from any individual spill occurring at any time of the year.
In our assessment, we used heavy oil spill scenarios from three of those wells (Figure 1; Table 2). The Perdido well is in the Perdido Foldbelt region in the northwestern GM, the Tamaulipas well is off the coast of the Mexican state of Tamaulipas, and the Campeche well is in the Bay of Campeche, in southwestern GM. As the scenarios indicate that the potential extent of the affected regions by large-scale spills can reach beyond the Mexican EZZ and into U.S. waters (see below), our study area covered the entire western GM, from the coastline to 90°W. Moreover, given that the metric used in the scenarios was the maximum average mass fraction Pmax (see Supplemental Material S2 for details), the cells within the affected area were categorized using the 25th and 75th percentiles; thus, Pl (low amounts of oil) includes cells with Pmax values< 1.54E-03, Pm (medium amounts of oil) cells with values in the interval [1.54E-03, 3.96E-03], and Ph (high amounts of oil) for cells with values > 3.96E-03.
The western GM marine mammal community includes at least 15 species of cetaceans (Ramírez-León et al., 2020) and the Antillean manatee (Trichechus manatus manatus; Morales-Vela, 2010) (Table 3). The Antillean manatee inhabits coastal areas along the Mexican states of Tamaulipas and Veracruz, the Bay of Campeche, and the Yucatán Peninsula (Morales-Vela, 2010). Unfortunately, we could not include this species in the assessment because its ecological information in the GM is too limited. We assessed eight odontocetes (i.e., toothed cetaceans), which are the most sighted species in the western and northern GM’s US and Mexican waters (Waring et al., 2016; Ramírez-León et al., 2020): the sperm whale, short-finned pilot whale (Globicephala macrorhynchus), rough toothed dolphin (Steno bredanensis), Risso’s dolphin (Grampus griseus), Clymene dolphin (Stenella clymene), pantropical spotted dolphin (Stenella attenuata), Atlantic spotted dolphin (Stenella frontalis), and bottlenose dolphin. Since there are no detailed distribution maps for any of these species in our study area, we used habitat suitability maps generated throughout the maximum entropy (MaxEnt; Phillips et al., 2006) modeling approach (Ramírez-León et al., 2021; Supplemental Material S3) for our oil exposure risk assessment.
The rationale of the modeling is to estimate the ecological niche of the species from occurrence records (presence-only data), which are discontinuous in nature, and contrast with environmental predictors to generate spatially continuous maps to predict the suitable habitat area (Peterson et al., 2011). MaxEnt assumes that the species are distributed uniformly (i.e., the maximum entropy distribution) on the modeling area, and the environmental values constrain this distribution at the presence of records locations (Phillips et al., 2006; Phillips et al., 2017). Thus, high suitability areas are defined as those sites where favorable environmental conditions are present for the long-term subsistence of the species of interest and, therefore, where it is expected to be distributed (Peterson and Soberón, 2012). Habitat suitability models of the eight odontocetes were built using sightings recorded during the last four decades, oceanographic data (sea surface temperature and chlorophyll-a concentration), and bathymetric variables (depth and slope of the seabed, and distance from the sightings to the 200 m isobath). Applying the precautionary principle, high suitability areas were defined as those cells of the geographic grid with suitability values > 0.56. For more details on the modeling process and the data used see Supplementary Material S3.
Results
The likelihood of oil exposure for all species was 2.00, except for the Clymene dolphin, which had an L value of 1.80 (Table 3). The eight species shared several biological and ecological traits, which is to be expected since they are all odontocetes. However, the scores that were assigned to the exposure pathways varied mainly due to differences in diving behavior and the type of prey (Table 4).
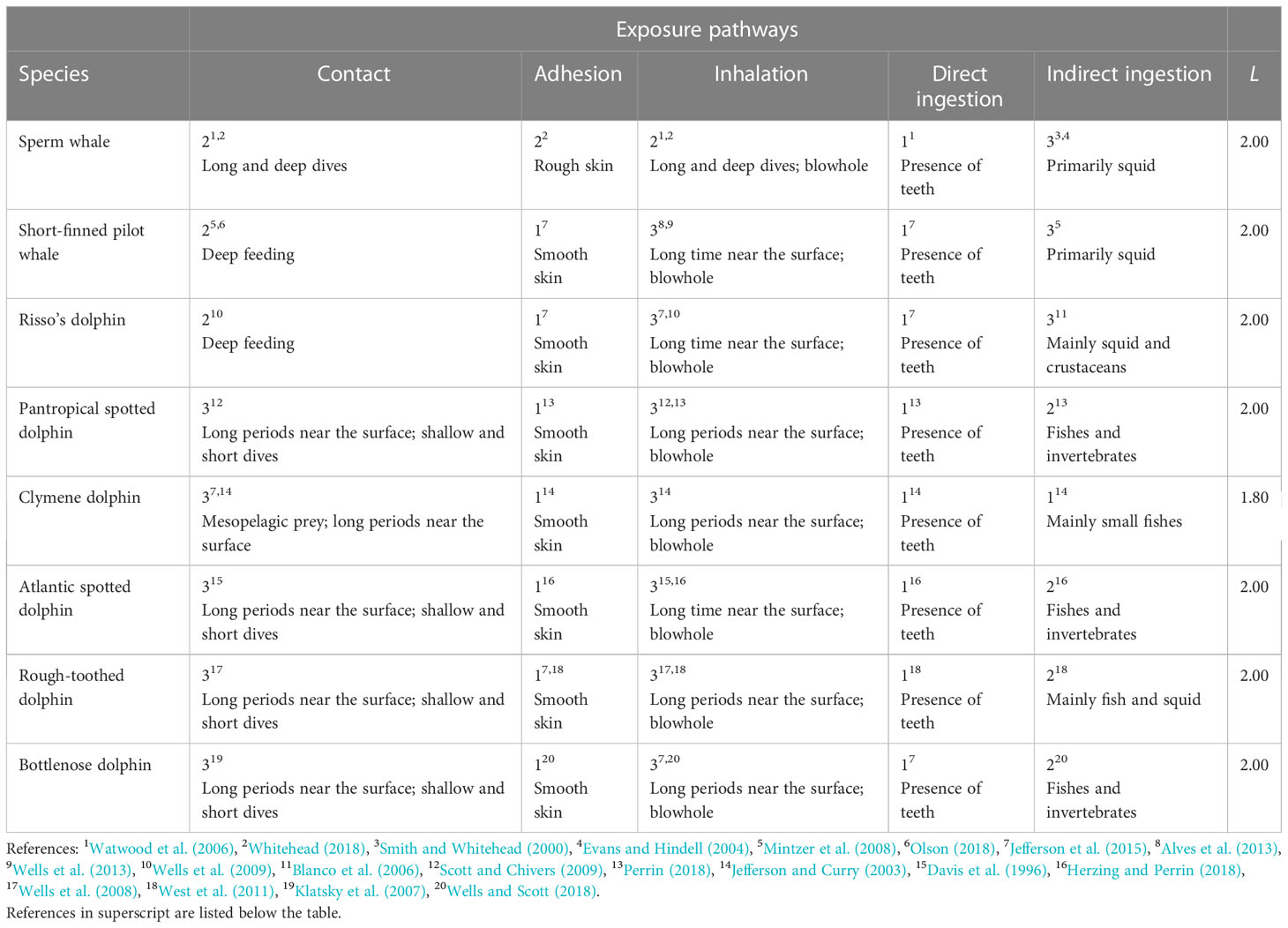
Table 4 Scores of the five exposure pathways and estimated likelihood of oil exposure (L) of eight odontocetes included in the study.
The contact exposure of the sperm whale, short-finned pilot whale, and Risso’s dolphin were scored as moderate because of their deep-diving behavior. In contrast, the remaining species perform short and shallow dives, increasing the likelihood of exposure through this pathway. Exposure by adhesion was low for most because odontocetes have smooth skin, except for the sperm whale, whose skin is slightly rough, and it was scored as moderate (Table 3). All species had a high score for inhalation exposure due to their tendency to remain on the surface for long periods, except for the sperm whale, which was classified as moderate because of its diving behavior. Exposure by direct ingestion was considered low for all species because the ingestion of contaminated water is expected to be minimal given their feeding mechanism. Exposure by indirect ingestion had the most variable scores between species because of the diversity of their preferred prey. The exposure was considered low for the Clymene dolphin since they feed mainly on fish, while it was scored as high for the sperm whale, short-finned pilot whale, and Risso’s dolphin due to their predation on squid; for the rest of the species, it was moderate because they feed both fish and invertebrates (i.e., cephalopods, decapods, or crustaceans).
High suitability areas for the eight species are shown in Figures 2, 3. The sperm whale, rough-toothed dolphin, Risso’s dolphin, and Clymene dolphin models (Figure 2) show high suitability along the northwestern continental slope, although for the first three, these areas also extend along the upper south-central slopes. The number of cells of high suitability in the study area (i.e., D) for these species was 230, 335, 242, and 219, respectively. The areas of high suitability for the pantropical dolphin were the most widespread (D = 495), covering the entire continental slope, and including the outer continental shelf (Figure 3A). Suitability areas for the short-finned pilot whale (D = 153) were located along the inner continental slope (Figure 3B). Finally, high suitability areas were located from the continental shelf to the upper slope for the Atlantic spotted dolphin (D = 240; Figure 3C), and for the bottlenose dolphin on shallow waters of the continental shelf, mainly north of 22°N (D = 234; Figure 3D).
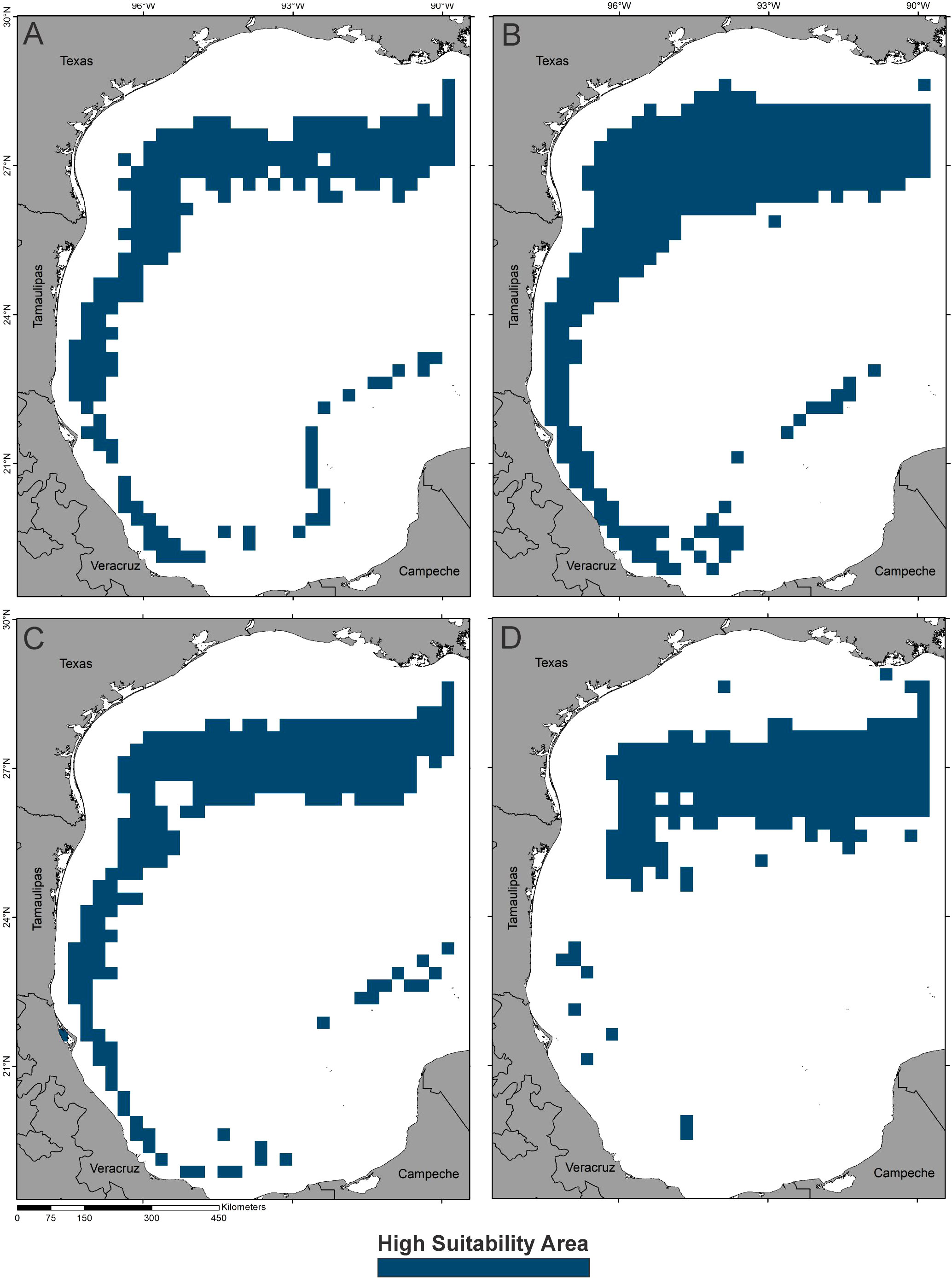
Figure 2 High suitability areas (in blue) in the western Gulf of Mexico for the sperm whale (A), rough-toothed dolphin (B), Risso’s dolphin (C), and Clymene dolphin (D). Based on Ramírez-León et al. (2021).
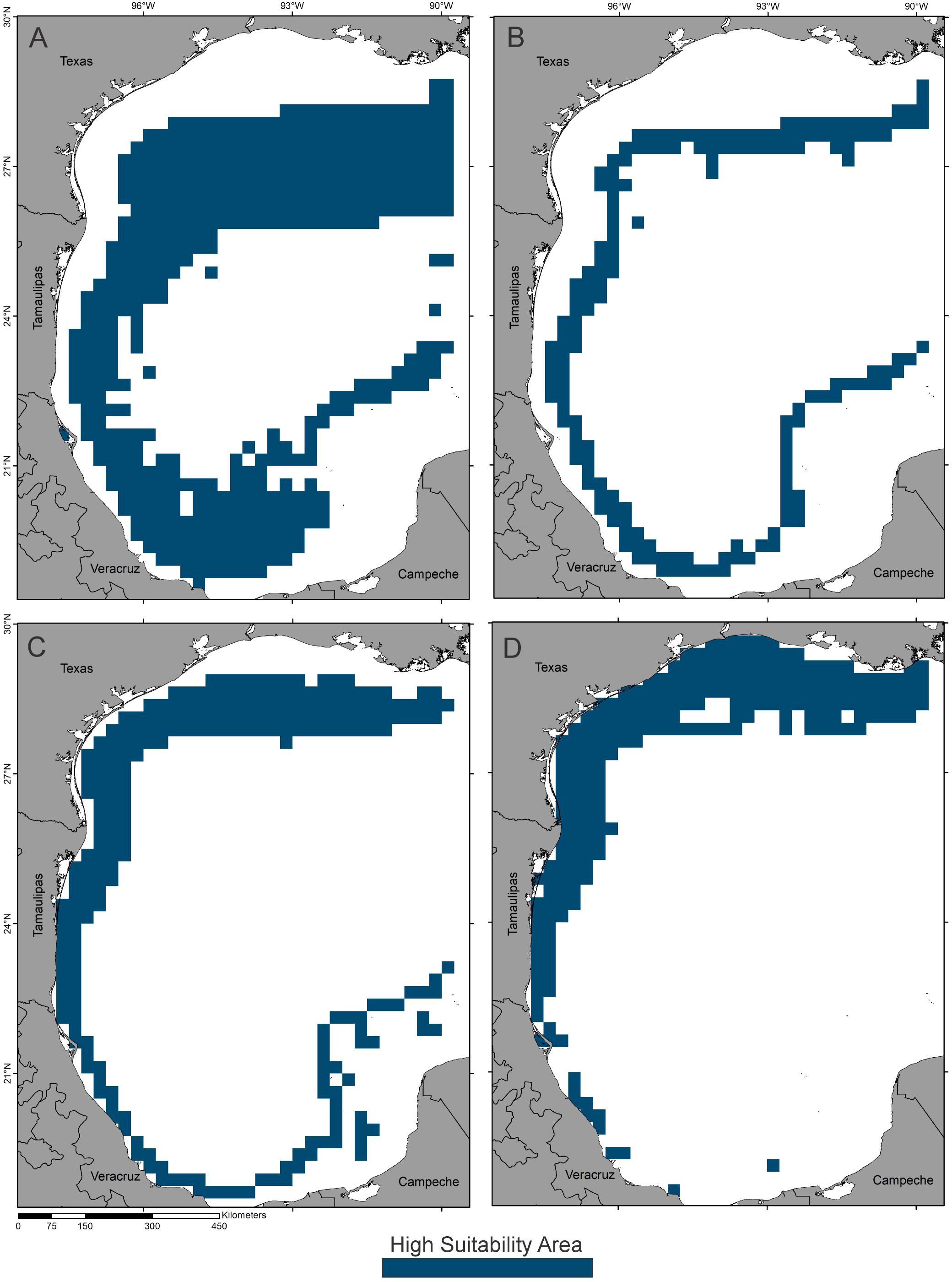
Figure 3 High suitability areas (in blue) in the western Gulf of Mexico for the pantropical spotted dolphin (A), short-finned pilot whale (B), Atlantic spotted dolphin (C), and bottlenose dolphin (D). Based on Ramírez-León et al. (2021).
The Perdido well spill scenario shows that the coast of Texas, U.S., could be affected and that the offshore region that could be impacted encompasses the entire northwestern GM, extending south to 23–24°N and west to ~91°W (Figure 4A). The number of cells with spilled oil (i.e., P) equals 451, where Pl = 129, Pm = 231, and Ph = 91. The values of F for each species under this scenario, as well as the parameters used in its calculation, are shown in Table 5. The greatest risk of exposure to oil occurs for the species whose habitat suitability areas are found mainly along the northwestern slope: the sperm whale, rough-toothed dolphin, Risso’s dolphin, and Clymene dolphin, although for all of them the risk was moderate (R range: 2.40–2.98; Figure 4B). The risk was low for the remaining species, ranging from 1.54 (short-finned pilot whale) to 2.04 (bottlenose dolphin).
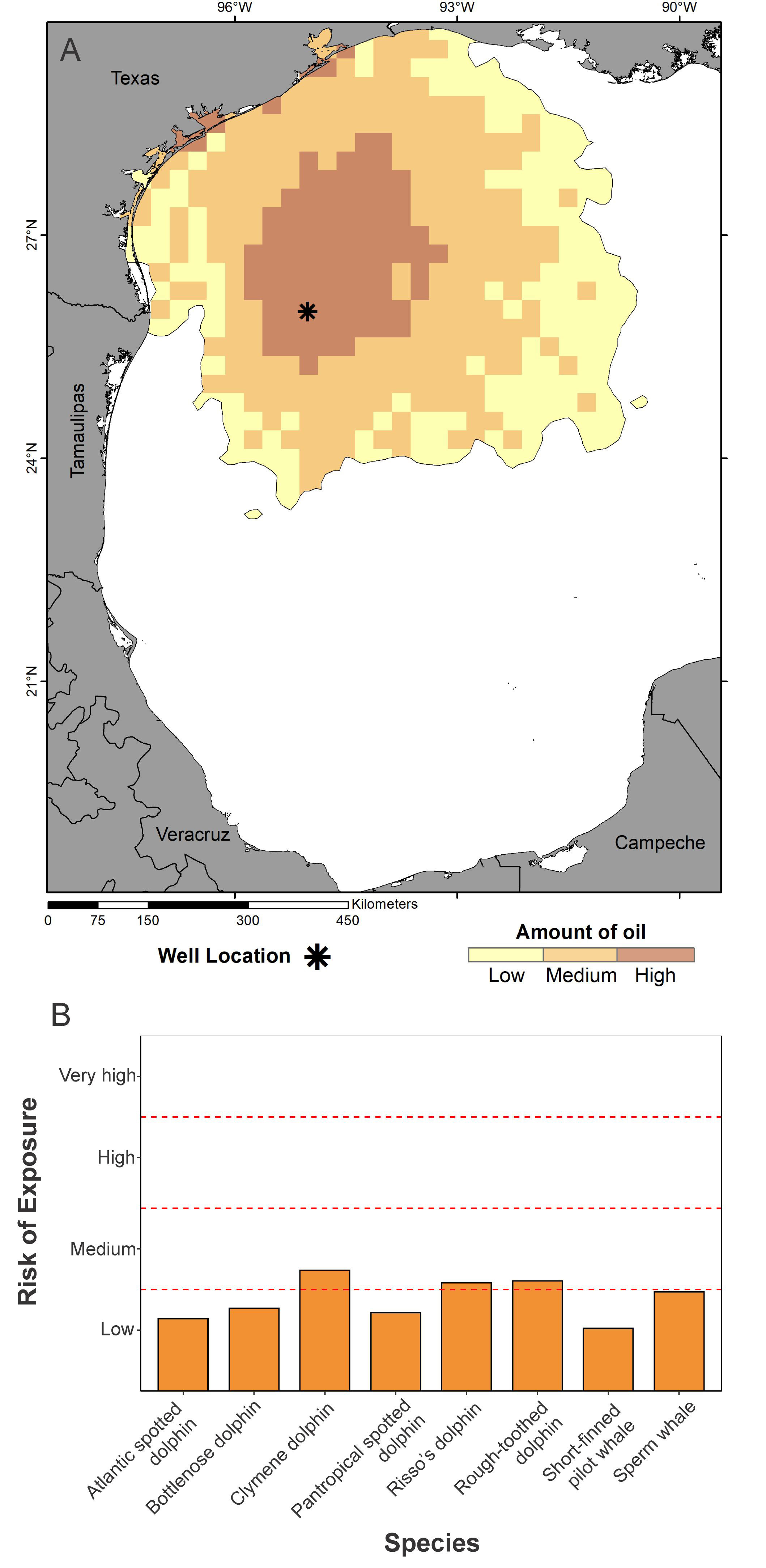
Figure 4 (A) Spill scenario for the Perdido well (black asterisk), northwestern Gulf of Mexico. The amount of oil is ranked as low, medium, and high using quantile breaks of the maximum average mass fraction (Pmax). Based on Pérez-Brunius et al. (2020). (B) Risk of oil exposure of eight species of cetaceans.
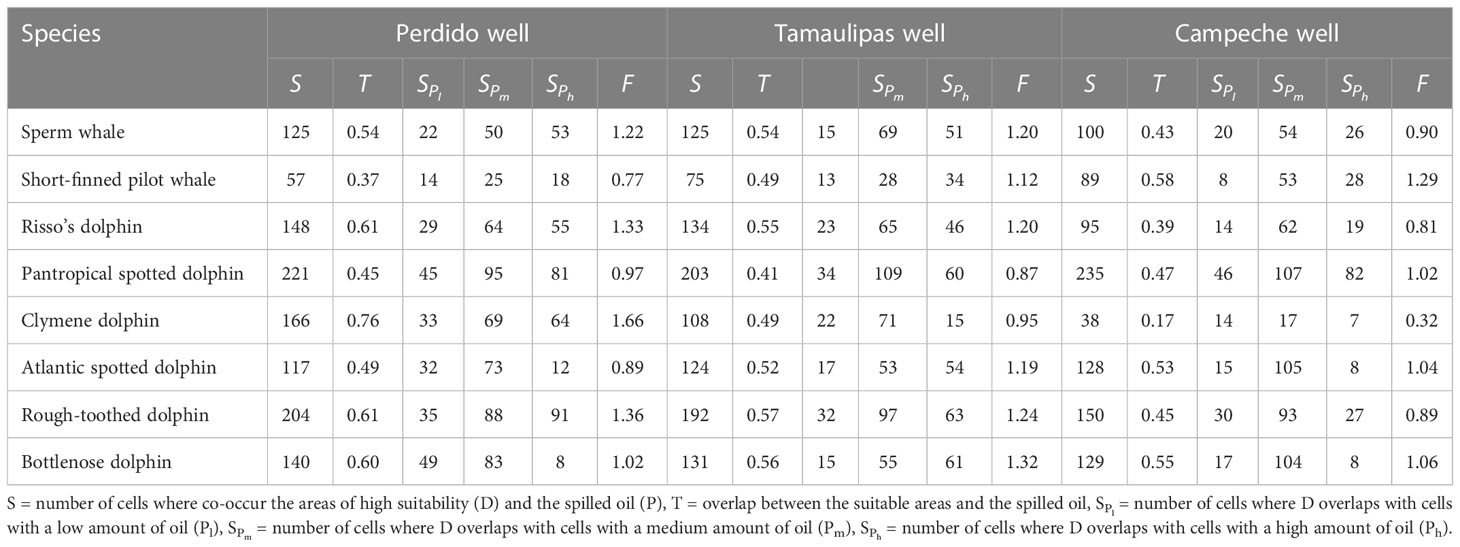
Table 5 Values of the parameters to estimate the feasibility of exposure to oil (F) of eight odontocetes from the Gulf of Mexico under three oil spill scenarios.
The Tamaulipas well spill scenario indicates that the coasts of Texas and Tamaulipas would be the most impacted; however, the northern coast off the state of Veracruz could also be impacted. The affected offshore region does not extend as far east as was the case for the Perdido scenario but did spread further south to 22°N (Figure 5A). For this scenario, P = 464, where Pl = 121, Pm = 220, and Ph = 123. F values varied between 0.87 (pantropical spotted dolphin) and 1.32 (bottlenose dolphin; Table 5). The risk of exposure to oil from spills in this well was moderate for the sperm whale, rough-toothed dolphin, Risso’s dolphin, Atlantic spotted dolphin, and bottlenose dolphin (R range: 2.38–2.63; Figure 5B). The risk was low for the remaining three species, ranging from 1.72 for the Clymene dolphin to 2.24 for the short-finned pilot whale.
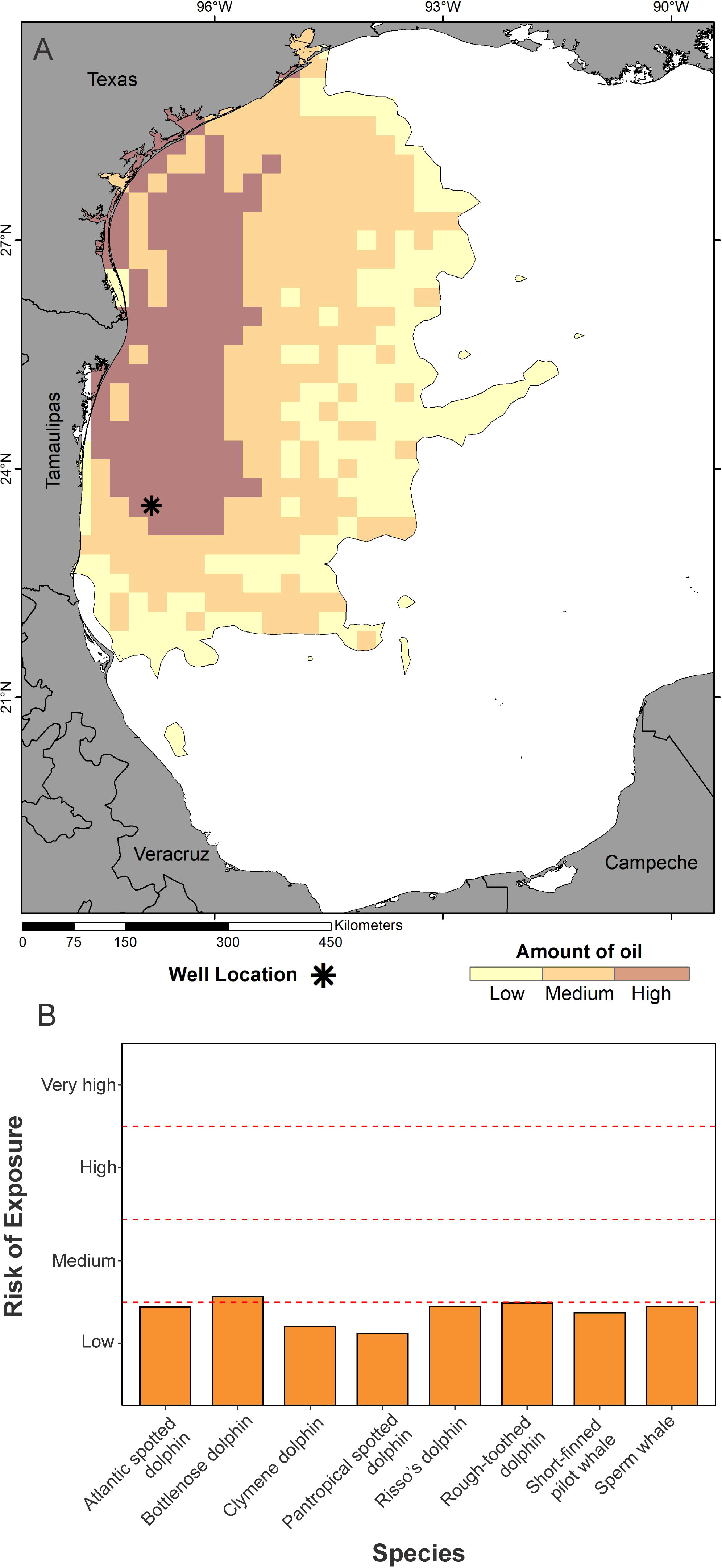
Figure 5 (A) Spill scenario for the Tamaulipas well (black asterisk), center-west of the Gulf of Mexico. The amount of oil is ranked as low, medium, and high using quantile breaks of the maximum average mass fraction (Pmax). Based on Pérez-Brunius et al. (2020). (B) Risk of oil exposure of eight species of cetaceans.
The Campeche well spill scenario shows that both the coasts of Veracruz and Tamaulipas could be affected, although the impact could also encompass the Texas coast. The offshore region that could be affected includes most of the Bay of Campeche and extends northward along the central-western slope and reaching the northwestern GM (Figure 6A). For this scenario, P = 480, where Pl = 99, Pm = 246, and Ph = 135. F values ranged from 0.32 (Clymene dolphin) to 1.29 (short-finned pilot whale; Table 5). Despite the large area that could be affected by spills from this well, the risk of exposure to oil was scored low for all species (ranging from 0.57 for the Clymene dolphin to 2.13 for the bottlenose dolphin), except for the short-finned pilot whale, for which it was moderate, R = 2.59 (Figure 6B).
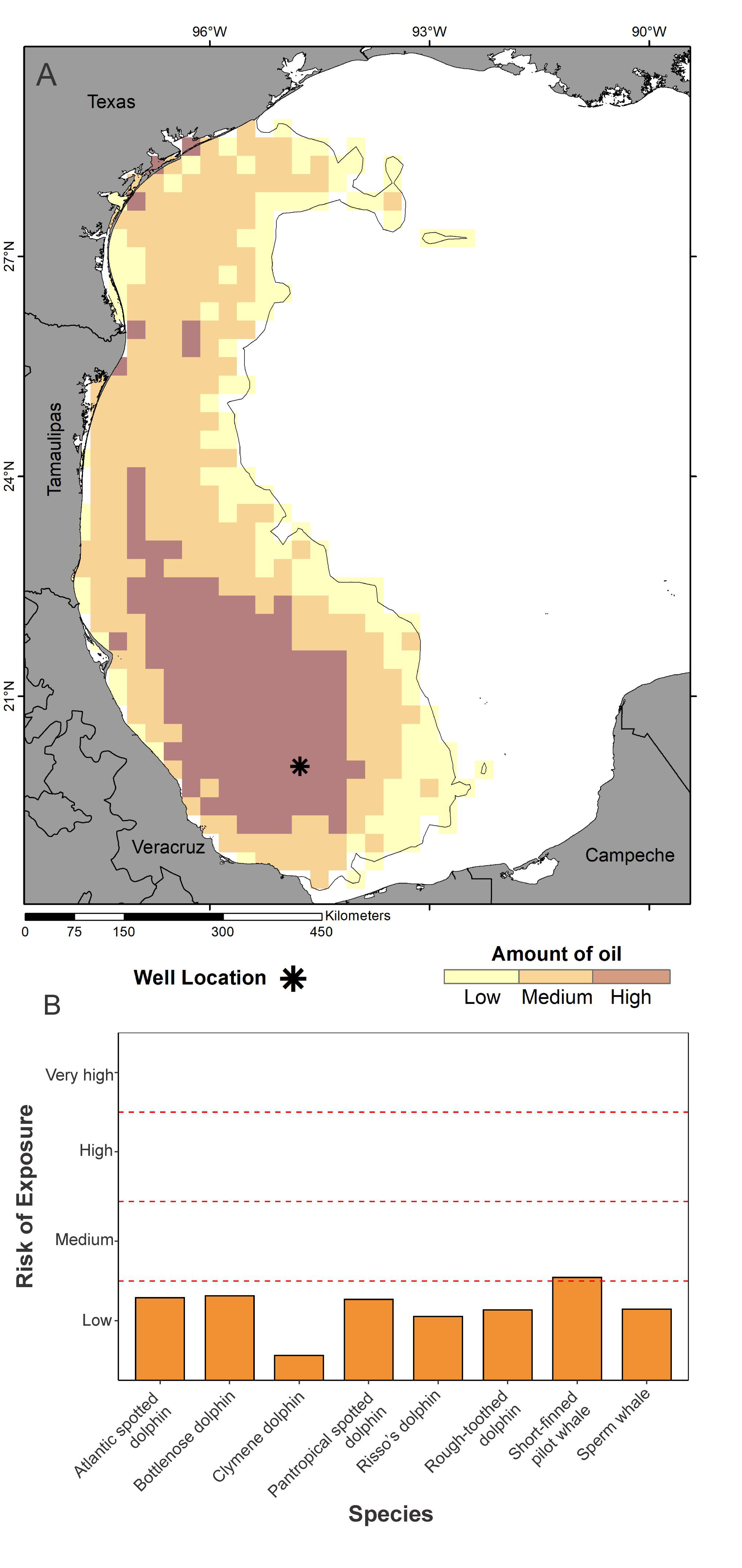
Figure 6 (A) Spill scenario for the Campeche well (black asterisk), southwestern Gulf of Mexico. The amount of oil is ranked as low, medium, and high using quantile breaks of the maximum average mass fraction (Pmax). Based on Pérez-Brunius et al. (2020). (B) Risk of oil exposure of eight species of cetaceans. places.
Discussion
The degree of exposure to oil spills of marine animals is strongly influenced by their morphology, feeding, diving behavior, and distribution, as well as the origin and evolution of the spill. Our framework developed to assess the risk of exposure of marine mammals to oil spills takes into account all of these factors using a semi-quantitative approach. The evaluation of the exposure pathways described by Jarvela Rosenberger et al. (2017) makes it possible to determine quickly and reliably how susceptible each species is to exposure to spilled oil. All the species evaluated in our study were odontocetes, so they share several biological, behavioral, and ecological traits, hence the likelihood of exposure was similar, although certain species may be more predisposed to exposure due to their diving behavior or type of prey. For example, deep-diving species that dive for long periods (e.g., the sperm whale; Whitehead, 2018) could reduce their likelihood of exposure via inhalation. In contrast, some small odontocetes (e.g., Clymene dolphins, rough-toothed dolphins, or bottlenose dolphins) perform shallow and short dives (Klatsky et al., 2007; Wells et al., 2008; Jefferson et al., 2015), spending a lot of time on the surface, which increases the likelihood of exposure. Likewise, diet influences exposure to oil. Those species that feed primarily on invertebrates (e.g., sperm whales and pilot whales) are more likely to be exposed than those that only feed on fish (e.g., the Clymene dolphin) because the detoxifying capacity of invertebrates is limited, and therefore, they bio-concentrate hydrocarbons, while vertebrates can metabolize the petroleum compounds more efficiently (Hylland, 2006).
The novelty of our method is in the estimation of the feasibility of the encounter, which not only considers the overlap between the distribution of the species and the total area affected but also considers the distribution and amount of spilled oil within this area. By incorporating this information, the uncertainty in estimating the feasibility of encounter is reduced, and, consequently, the exposure risk assessment yields more robust and reliable results. The main contribution and novelty of this work is the development of a new framework that can be used to assess the risk to any marine mammal species (i.e., cetaceans, pinnipeds, sea otters, and sirenians) in any habitat, but to apply it is essential to know a priori the distribution of the species (or at least the high suitability areas) and the most likely dispersion patterns of the spilled oil within the area of interest, which can be generated using numerical models.
We identified the odontocetes that might be most exposed under three large-scale heavy oil spill scenarios in the western GM, including the Bay of Campeche, where most of the Mexican offshore oil activity takes place. In all three scenarios, the overlap between the suitable areas for the odontocetes and the spilled oil was about 50% (range: 37-61%) since the slick was located along the western continental shelf and slope, which is where the evaluated species inhabit. The exception was the Clymene dolphin, whose values varied between 17 (Campeche well scenario) and 76% (Perdido well scenario). Although this species also inhabits neritic waters, its distribution seems to be limited exclusively to the northern slope (Ramírez-León et al., 2021). However, due that only a fraction of the predicted affected areas in the spill scenarios had a large amount of oil (range: 20-28%), the feasibility of encounter was not high for any species under any scenario (all F values were< 1.70), and our results indicate that the risk of exposure for the eight species ranged from low to moderate and, as expected, the species with the highest risk changed depending on well location.
In our case study, there were two possible sources of uncertainty in the estimation of the feasibility of encounter. First, we used spill scenario maps generated using the CIC-OIL three-dimensional model. Since the spill scenarios are statistical ensembles of hundreds of individual spills, what is evaluated is the mass of each individual spill that falls within the contour defined for the scenario (see Supplementary Material S2 for details). For the scenarios used in our study, it is true that more than 80% of the individual spills have more than 70% of the mass within the contour. This is a measure of error on the metrics used for the statistical scenarios, encompassing the variability in the possible evolution of a spill due to the large variability in the hydrodynamical conditions in the gulf (e.g., currents, wind, eddies; Pérez-Brunius et al., 2020). In other words, in the event of a spill in any of these wells, it is possible (although unlikely) that the oil slick (or a portion of it) would fall outside the area predicted by the scenario. Nevertheless, it is important to bear in mind that modeling oil spills is a complex endeavor which has several sources of uncertainties, discussed further in the Supplementary Material S2. Second, we used habitat suitability maps generated using the MaxEnt modeling approach as a proxy for the distribution of species. The performance of the models was good, which guarantees reliable results (see Supplementary Material S3). Nevertheless, it must be remembered that the maps do not represent either the abundance of a species or the probability of its presence (Peterson et al., 2011), but rather indicate the areas where favorable environmental conditions exist for its long-term subsistence (Peterson and Soberón, 2012). However, given the limited knowledge of the distribution of odontocetes in the study area, it is likely that due to omission errors (false absences) some sites used by the species have been classified as “unsuitable” (e.g., Beresford et al., 2011). Therefore, there is a possibility that the areas potentially occupied by these species could be slightly underestimated.
There have been two large-scale oil spills at GM: the Ixtoc-1 spill occurred in 1979 in the southwest, and the Deepwater Horizon spill in 2010 in the north (NOAA, 2022). The magnitude of the ecological impact of the Ixtoc-1 spill is unknown (Soto et al., 2014), but the damage caused by the Deepwater Horizon spill has been widely documented. For odontocetes, the data indicates that this spill caused severe adverse effects on the health of the populations, mainly impacting bottlenose dolphins in the north-central coastal areas and the northern stock of sperm whales (Ackleh et al., 2012; DHNRDAT, 2016). According to our findings, it is possible that the northwestern population of these two species could be affected by spills in the Perdido and Tamaulipas wells. It should be noted that the sperm whale is the only specie of the GM listed as at risk in the IUCN Red List of Threatened Species (Taylor et al., 2019). Moreover, because it is listed as endangered in the Endangered Species Act (ESA, 2019) of the U.S., the GM stock is considered strategic in the Marine Mammal Protection Act (Hayes et al., 2021). Northwestern populations of rough-toothed dolphin, Risso’s dolphin, Clymene dolphin, and Atlantic spotted dolphin could also be affected by spills in one of these wells. The only species that presented a significant risk of exposure to spills in the Campeche well was the short-finned pilot whale. However, these results should be examined considering that the estimation of the feasibility of encounter did not consider seasonal variability in the distribution of some species, such as the Atlantic spotted dolphin (Waring et al., 2016) and Risso’s dolphin (Mullin and Hoggard, 2000). Unfortunately, we were unable to incorporate this variability in our study due to a lack of enough sightings to model suitable areas by season. Nevertheless, it is expected that the risk of exposure varied throughout the year due to the seasonal movements of these species.
Oil spills threaten all marine species because exposure to hydrocarbons affects health, with immediate or long-term consequences on survival and/or reproduction (Venn-Watson et al., 2015a; Kellar et al., 2017; Smith et al., 2017). Even though the risk of exposure may not be high for a species or group of species in a specific area, as in our case study, the potential impact of large-scale oil spills on marine mammals should not be underestimated. Crude oil is a mixture of trace elements and organic compounds, some of them highly toxic, such as polycyclic aromatic hydrocarbons (PAHs; Neff, 1990). The routes of exposure of marine mammals to oil include contact both at the surface and within the water column, and ingestion of contaminated seawater and/or contaminated prey, but perhaps the greatest threat is the inhalation of volatile PAHs at the water-air interface (Ruberg et al., 2021). When these compounds enter the circulatory system, they can cause neurological disorders, such as disorientation and sleep-like behaviors (Wilkin et al., 2017), but also other physiological damage, such as brain and hematological injuries (Peterson, 2001), lung diseases (Venn-Watson et al., 2015b), liver damage (Geraci and St. Aubin, 1990), and impacts on the endocrine system, with the consequent effects on reproductive success (Lane et al., 2015). During an oil spill, a large number of animals can experience these deleterious effects, so the mortality rate may increase and the birth rate decrease. If the impact on these rates is significant, marine mammal populations may take decades to recover given their life-history features (e.g., longevity, late age of first reproduction, the prolonged interval between births; Wallace et al., 2017; Esler et al., 2018), as observed in populations of killer whales (Orcinus orca; Matkin et al., 2008) and sea otters (Enhydra lutris; Bodkin et al., 2002) in Alaskan waters after the Exxon Valdez oil spill in 1989.
The ability to assess and categorize risk is a critical part of oilfield incident response planning. Our risk assessment of marine mammal oil exposure generated key information for decision-makers to use in planning management actions in those areas of the western GM where activities of the hydrocarbon industry are currently being developed or planned. However, much remains to be done. The Deepwater Horizon accident highlighted the immediate and long-term impact of large-scale spills on marine mammals in the northern GM. The quantification of the damage and the subsequent planning of restoration measures was made possible due to the information on the status of the local populations before and after the spill. Unfortunately, ecological data for marine mammals in the western GM are virtually nonexistent, making robust vulnerability assessments currently impossible, and in the event of an oil incident, it would not be possible to reliably determine the level of damage. Therefore, it is necessary to focus research efforts to generate basic ecological information on the populations of this region of the GM, since only then can efficient contingency plans be developed. In the meantime, and as a precautionary measurement, is strongly recommended the application of preventive actions proposed by Frasier (2020) to reduce the sources of chronic stress of anthropogenic origin that could compromise the resilience of marine mammal populations, such as exposure to pollutants and noise, collisions with boats, and incidental by-catch in fishing gear.
Data availability statement
The raw data supporting the conclusions of this article will be made available by the authors, without undue reservation.
Author contributions
MRR-L: Visualization, formal analysis, data curation, writing, review, and editing. OS-N: Supervision, project administration, review, and editing. PP-B: Funding acquisition, methodology, visualization, writing, review, and editing. AER-C: Visualization, methodology, review, and editing. ZR-M: Formal analysis, data curation, review, and editing. AF-Y: Formal analysis, data curation, review, and editing. SH: Funding acquisition, project administration, review, and editing. MCG-A: Visualization, conceptualization, methodology, writing – original draft, writing, review, and editing. All authors contributed to the article and approved the submitted version.
Funding
This research was funded by the Mexican National Council for Science and Technology, Mexican Ministry of Energy, Hydrocarbon Fund: SENER-CONACYT/Hidrocarburos project. 201441.
Acknowledgments
This research contributes to the Gulf of Mexico Research Consortium (CIGoM) and was funded by the Mexican National Council for Science and Technology, Mexican Ministry of Energy, Hydrocarbon Fund: SENER-CONACYT/Hidrocarburos project 201441. We acknowledge PEMEX’s specific request to the Hydrocarbon Fund to address the environmental effects of oil spills in the Gulf of Mexico. We are grateful for the valuable contributions of J. Sheinbaum, K. Kotzakoulakis, J. Rodríguez-Outerelo, and F. Medrano. We are also thankful for the comments and suggestions provided by reviewers that greatly improved the quality of the manuscript.
Conflict of interest
The authors declare that the research was conducted in the absence of any commercial or financial relationships that could be construed as a potential conflict of interest.
Publisher’s note
All claims expressed in this article are solely those of the authors and do not necessarily represent those of their affiliated organizations, or those of the publisher, the editors and the reviewers. Any product that may be evaluated in this article, or claim that may be made by its manufacturer, is not guaranteed or endorsed by the publisher.
Supplementary material
The Supplementary Material for this article can be found online at: https://www.frontiersin.org/articles/10.3389/fmars.2023.1034647/full#supplementary-material
References
Abascal A. J., Castanedo S., Medina R., Liste M. (2010). Analysis of the reliability of a statistical oil spill response model. Mar. pollut. Bull. 60, 2099–2110. doi: 10.1016/j.marpolbul2010.07.008
Ackleh A. S., Ioup G. E., Ioup J. W., Ma B. L., Newcomb J. J., Pal N., et al. (2012). Assessing the deepwater horizon oil spill impact on marine mammal population trough acoustics: endangered sperm whales. J. Acoust. Soc Am. 131, 2306–2314. doi: 10.1121/1.3682042
Alves F., Dinis A., Ribeiro C., Nicolau C., Kaufmann M., Fortuna C. M., et al. (2013). Daytime dive characteristics from six short-finned pilot whales Globicephala macrorhynchus off Madeira island. Arquipelago 31, 1–8.
Beresford A. E., Buchanan G. M., Donald P. F., Butchart S. H. M., Fishpool L. D. C., Rondinini C. (2011). Poor overlap between the distribution of protected areas and globally threatened birds in Africa. Anim. Conserv. 14, 99–107. doi: 10.1111/j.1469-1795.2010.00398x
Blanco C., Raduán M. A., Raga J. A. (2006). Diet of risso’s dolphin (Grampus griseus) in the Western Mediterranean Sea. Sci. Mar. 70, 407–411. doi: 10.3989/scimar.2006.70n3407
Bodkin J. L., Ballachey B. E., Dean T. A., Fukuyama A. K., Jewett S. C., McDonald L., et al. (2002). Sea Otter population status and the process of recovery from the 1989 ‘Exxon valdez’ oil spill. Mar. Ecol. Prog. Ser. 241, 237–253. doi: 10.3354/meps241237
Botello A., Villanueva V. S., Diaz G. (1997). Petroleum pollution in the gulf of Mexico and Caribbean Sea. Rev. Environ. Contam. Toxicol. 153, 91–118. doi: 10.1007/978-1-4612-2302-3-3
Butt N., Beyer H. L., Bennett J. R., Biggs D., Maggini R., Mills M., et al. (2013). Biodiversity risks from fossil fuel extraction. Science 342, 425–426. doi: 10.1126/science.1237261
CNH (2019) Sistema de información de hidrocarburos. Available at: https://portal.cnih.cnh.gob.mx/ (Accessed January 17, 2022).
Davidson A. D., Boyer A. G., Hwahwan K., Pompa-Mansilla S., Hamilton M. J., Costa D. P., et al. (2012). Drivers and hotspots of extinction risk in marine mammals. Proc. Nat. Acad. Sci. U.S.A. 109, 3395–3400. doi: 10.1073/pnas.1121469109
Davis R. W., Worthy G. A. J., Würsig B., Lynn S. K., Townsend F. I. (1996). Diving behavior and at-sea movements of an Atlantic spotted dolphin in the gulf of Mexico. Mar. Mammal Sci. 12, 569–581. doi: 10.1111/j.1748-7692.1996.tb00069.x
DHNRDAT (2016) Deepwater horizon oil spill: Final programmatic damage assessment and restoration plan and final programmatic environmental impact statement. Available at: https://www.gulfspillrestoration/noaa.gov/restoration-planning/gulf-plan.
EIA (2019) Gulf of Mexico fact sheet. Available at: https://www.eia.gov/special/gulf_of_mexico/data.php (Accessed January 17, 2022).
EIA (2022) Petroleum & other liquids. Available at: https://www.eia.gov/dnav/pet/TblDefs/pet_pnp_crq_tbldef2.asp (Accessed October 23, 2022).
ESA (2019) Endangered species database. U.S. fish & wildlife service. Available at: https://www.fws.gov/endangered/species/ (Accessed January 17, 2022).
Esler D., Ballachey B. E., Matkin C., Cushing D., Kaler R., Bodkin J., et al. (2018). Timelines and mechanisms of wildlife population recovery following the Exxon Valdez oil spill. Deep-Sea Res. PT II 147, 36–42. doi: 10.1016/j.dsr2.2017.04.007
Evans K., Hindell M. A. (2004). The diet of sperm whales (Physeter macrocephalus) in southern Australian waters. ICES J. Mar. Sci. 61, 1313–1329. doi: 10.1016/j.icesjms.2004.07.026
Fox C. H., O’Hara P. D., Bertazzon S., Morgan K., Underwood F. E., Paquet P. C. (2016). A preliminary spatial assessment of risk: marine birds and chronic oil pollution in canada's pacific coast. Sci. Total Environ. 573, 799–809. doi: 10.1016/j.scitotenv.2016.08.145
Franklin J. (2010). Mapping species distributions: Spatial inference and prediction (Cambridge: Cambridge University Press).
Frasier K. E. (2020). “Evaluating impacts of deep oil spills on oceanic marine mammals,” in Scenarios and responses to future deep oil spills: Fighting the next war. Eds. Murawski S. A., Ainsworth C. H., Gilbert S., Hollander D. J., Paris C. B., Schlüter M., Wetzel D. L. (Cham, CH: Springer International Publishing), 419–444.
Geraci J. R., St. Aubin D. J. (1990). Summary and conclusions, in Sea mammals and oil: Confronting the risks. Eds. Geraci J. R., St. Aubin D. J. (San Diego, CA: Academic Press Inc), 253–256.
Harris K. A., Nichol L. M., Ross P. S. (2011). Hydrocarbon concentration and patterns in free-ranging sea otters (Enhydra lutris) from British Columbia, canada. environ. Toxicol. Chemi. 30, 2184–2193. doi: 10.1002/etc.627
Hayes S., Josephson E., Maze-Foley K., Rosel P. E., Turek J. (2021). “US Atlantic And gulf of Mexico marine mammal stock assessments 2020,” in NOAA Technical memorandum NMFS-NE-271(Woods Hole, MA: Silver, Spring). Available at: https://media.fisheries.noaa.gov/2021-07/Atlantic%202020%20SARs%20Final.pdf?null%09.
Helm R. C., Costa D. P., DeBruyn T. D., O’Shea T. J., Wells R. S., Williams T. M. (2015). “Overview of effects of oil spills on marine mammals,” in Handbook of oil spill science and technology. Ed. Fingas M. (Hoboken, NJ: John Wiley & Sons, Inc), 455–475. doi: 10.1002/9781118989982.ch18
Herzing D. L., Perrin W. F. (2018). “Atlantic Spotted dolphin: Stenella frontalis,” in Encyclopedia of marine mammals (Third edition). Eds. Würsig B., Thewissen J. G. M., Kovacs K. M. (San Diego, CA: Academic Press), 40–42. doi: 10.1016/B978-0-12-804327-1.00050-9
Hobday A. J., Smith A. D. M., Stobutzki I. C., Bulman C., Daley R., Dambacher J. M., et al. (2011). Ecological risk assessment for the effects of fishing. Fish. Res. 108, 372–384. doi: 10.1016/j.fishres.2011.01.013
Holsman K., Samhouri J., Cook G., Hazen E., Olsen E., Dillard M., et al. (2017). An ecosystem-based approach to marine risk assessment. Ecosyst. Health Sust. 3, e01256. doi: 10.1002/ehs2.1256
Hylland K. (2006). Polycyclic aromatic hydrocarbon (PAH) ecotoxicology in marine ecosystems. J. Toxicol. Environ. Health Part A. 69, 109–123. doi: 10.1080/15287390500259327
IUCN (2022) The IUCN red list of threatened species version 2022-1. Available at: https://www.iucnredlist.org (Accessed June 25, 2022).
Jarvela Rosenberger A. L., MacDuffee M., Rosenberger A. G. J., Ross P. S. (2017). Oil spills and marine mammals in British Columbia, Canada: development and application of a risk-based conceptual framework. arch. environ. Contam. Toxicol. 73, 131–153. doi: 10.1007/s00244-017-0408-7
Jefferson T. A., Webber M. A., Pitman R. L. (2015). “Marine mammals of the world,” in A comprehensive guide to their identification, 2nd ed (Cambridge, MA: Academic Press).
Kellar N. M., Speakman T. R., Smith C. R., Lane S. M., Balmer B. C., Trego M. L., et al. (2017). Low reproductive success rates of common bottlenose dolphins Tursiops truncatus in the northern gulf of Mexico following the Deepwater horizon disaster, (2010-2015). Endang. Species Res. 33, 143–158. doi: 10.3354/esr00775
Kingston P. F. (2002). Long-term environmental impact of oil spills. Spill Sci. Technol. Bull. 7, 53–61. doi: 10.1016/S1353-2561(02)00051-8
Klatsky L. J., Wells R. S., Sweeney J. C. (2007). Offshore bottlenose dolphins (Tursiops truncatus): movement and dive behavior near the Bermuda pedestal. J. Mammal. 88, 59–66. doi: 10.1644/05-MAMM-A-365R1.1
Lane S. M., Smith C. R., Mitchell J., Balmer B. C., Barry K. P., McDonald T., et al. (2015). Reproductive outcome and survival of common bottlenose dolphins sampled in barataria bay, lousiana, USA following the deepwater horizon oil spill. Proc. Biol. Sci. 282, 1–9.
Lee K., Boufadel M., Chen B., Foght J., Hodson P., Swanson S., et al. (2016). “High-priority research needs for oil spills in Canada: Summary of a royal society expert panel report on the behavior and environmental impacts of crude oil released into aqueous environments,” in Proceedings of the 39 AMOP technical seminar, environment and climate change(Ottawa, Canada: Environment Canada).
Litz J. A., Baran M. A., Bowen-Stevens S. R., Carmichael R. H., Colegrove K. M., Garrison L. P., et al. (2014). Review of historical unusual mortality events (UMEs) in the gulf of Mexico, (1990-2009): providing context for the multi-year northern gulf of Mexico cetacean UME declared in 2010. Dis. Aquat. Org. 112, 161–175. doi: 10.3354/dao02807
Matkin C. O., Saulitis E. L., Ellis G. M., Olesiuk P., Rice S. D. (2008). Ongoing population-level impacts on killer whales Orcinus orca following the Exxon Valdez oil spill in prince William sound, Alaska. Mar. Ecol. Prog. Ser. 356, 269–281. doi: 10.3354/meps07273
Mintzer V. J., Gannon D. P., Barros N. B., Read A. J. (2008). Stomach contents of mass-stranded short-finned pilot whales (Globicephala macrorhynchus) from north Carolina. Mar. Mamm. Sci. 24, 290–302. doi: 10.1111/j.1748-7692.2008.00189.x
Moore S. E. (2008). Marine mammals as ecosystem sentinels. J. Mamm. 89, 534–540. doi: 10.1644/07-MAMM-S-312R1.1
Morales-Vela B. (2010). “Conservación del manatí y su situación en méxico,” in Patrimonio natural de méxico, cien casos de Éxito. Eds. Carabias J., Saruhkán J., de la Maza J., Galindo C. (México, D.F: CONABIO), 70–71.
Mullin K. D., Hoggard W. (2000). “Visual surveys of cetaceans and sea turtles from aircraft and ships,” in Cetaceans, Sea turtles and seabirds in the northern gulf of Mexico: Distribution, abundance and habitat associations, vol. Volume II . Eds. Davis R. W., Evans W. E., Würsig B. (New Orleans, LA: NOAA Technical Report 346).
Murawski S. A., Ainsworth C. H., Gilbert S., Hollander D. J., Paris C. B., Schlüter M., et al. (2020). Deep oil spills: Facts, fate, and effects (Cham: Springer International Publishing). doi: 10.1007/978-3-030-11605-7
Neff J. M. (1990). “Composition and fate of petroleum and spill-treating agents in the marine environment,” in Sea Mammals and oil: Confronting the risks. Eds. Geraci J. R., Aubin D. J. (San Diego, CA: Academic Press), 1–33.
NOAA (2022) Incident news. Available at: https://incidentnews.noaa.gov/ (Accessed December 28, 2022).
Olson P. A. (2018). “Pilot whales: Globicephala melas and g. macrorhynchus,” in Encyclopedia of marine mammals, 3rd ed. Eds. Würsig B., Thewissen J. G. M., Kovacs K. M. (San Diego, CA: Academic Press), 701–705. doi: 10.1016/B978-0-12-804327-1.00194-1
Pérez-Brunius P., Beron-Vera F. J., Kotzakoulakis K., Lara-Hernández J. A., Miron P., Olascoaga M. J., et al. (2020). “Regiones posiblemente afectadas bajo escenarios de derrames de petróleo en las aguas profundas del golfo de méxico,” in Escenarios oceánicos y atmosféricos de un derrame de petróleo en aguas profundas del golfo de méxico. Eds. Pérez-Brunius P., Turrent-Thompson C., García-Carrillo P. (Ensenada, Baja California: CICESE), 15–130.
Perrin W. F. (2018). “Pantropical spotted dolphin: Stenella attenuata,” in Encyclopedia of marine mammals, 3rd ed. Eds. Würsig B., Thewissen J. G. M., Kovacs K. M. (San Diego, CA: Academic Press), 676–678. doi: 10.1016/B978-0-12-804327-1.00189-8
Peterson C. H. (2001). The Exxon Valdez oil spill in Alaska: acute, indirect and chronic effects on the ecosystem. Adv. Mar. Biol. 39, 1–103. doi: 10.1016/S0065-2881(01)39008-9
Peterson A. T., Soberón J. (2012). Species distribution modeling and ecological niche modeling: getting the concepts right. Nat. Conserv. 10, 102–107. doi: 10.4322/natcon.2012.019
Peterson C. H., Soberón J., Pearson R. G., Anderson R. P., Martínez-Meyer E., Nakamura M., et al. (2011). Ecological niches and geographic distributions (Princeton: Princeton University Press).
Phillips S. J., Anderson R. P., Schapire R. E. (2006). Maximum entropy modeling of species geographic distributions. ecol. Modell. 190, 231–259. doi: 10.1016/j.ecolmodel.2005.03.026
Phillips S. J., Anderson R. P., Dudík M., Schapire R. E., Blair M. E. (2017). Opening the black box: An open‐source release of Maxent. ECOGEG 40, 887–893. doi: 10.1111/ecog.03049
Ramírez-León M. R., García-Aguilar M. C., Aguayo-Lobo A., Fuentes-Allen I., Sosa-Nishizaki O. (2020). What do we know about cetaceans in the Mexican waters of the gulf of Mexico? a review. Aquat. Mamm. 46, 623–632. doi: 10.1578/AM.46.6.2020.623
Ramírez-León M. R., García-Aguilar M. C., Romo-Curiel A. E., Ramírez-Mendoza Z., Fajardo-Yamamoto A., Sosa-Nishizaki O. (2021). Habitat suitability of cetaceans in the gulf of Mexico using an ecological niche modeling approach. PeerJ 9, e10834. doi: 10.7717/peerj.10834
Romo-Curiel A. E., Ramírez-Mendoza Z., Fajardo-Yamamoto A., Ramírez-León M. R., García-Aguilar M. C., Herzka S. Z., et al. (2022). Assessing the exposure risk of large pelagic fish to oil spills scenarios in the deep waters of the gulf of Mexico. Mar. pollut. Bull. 176, 113434. doi: 10.1016/j.marpolbul.2022.113434
Ruberg E. J., Elliot J. E., Williams T. D. (2021). Review of petroleum toxicity and identifying common endpoints for future research on diluted bitumen toxicity in marine mammals. Ecotoxicology 30, 537–551. doi: 10.1007/s10646-021-02373-x
Scott M. D., Chivers S. J. (2009). Movements and diving behavior of pelagic spotted dolphins. Mar. Mamm. Sci. 25, 137–160. doi: 10.1111/j.1748-7692.2008.00241.x
Smith C. R., Rowles T. K., Hart L. B., Townsend F. I., Wells R. S., Zolman E. S., et al. (2017). Slow recovery of barataria bay dolphin health following the Deepwater horizon oil spill, (2013-2014), with evidence of persistent lung disease and impaired stress response. endanger. Species Res. 33, 127–142. doi: 10.3354/esr00778
Smith S. C., Whitehead H. (2000). The diet of galápagos sperm whales Physeter macrocephalus as indicated by fecal sample analysis. Mar. Mamm. Sci. 16, 315–325. doi: 10.1111/j.1748-7692.2000.tb00927.x
Soto L. A., Botello A. V., Licea-Durán S., Lizárraga-Partida M. L., Yáñez-Arancibia A. (2014). The environmental legacy of the ixtoc-I oil spill in campeche sound, southwestern gulf of Mexico. Front. Mar. Sci. 1. doi: 10.3389/fmars.2014.00057
Taylor B. L., Baird R., Barlow J., Dawson S. M., Ford J., Mead J. G., et al. (2019)Physeter macrocephalus (amended version of 2008 assessment). In: The IUCN red list of threatened species 2019 (Accessed 03 August 20).
Venn-Watson S., Colegrove K. M., Litz J., Kinsel M., Terio K., Saliki J., et al. (2015b). Adrenal gland and lung lesions in gulf of Mexico common bottlenose dolphins (Tursiops truncatus) found dead following the deepwater horizon oil spill. PloS One 10, e0126538. doi: 10.1371/journal.pone.0126538
Venn-Watson S., Garrison L., Litz J., Fougeres E., Mase B., Rappucci G., et al. (2015a). Demographic clusters identified within the northern gulf of Mexico common bottlenose dolphin (Tursiops truncatus) unusual mortality event: January 2010 - June 2013. PloS One 10, e0117248. doi: 10.1371/journal.pone.0117248
Wallace B. P., Brosnan T., McLamb D., Rowles T., Ruder E., Schroeder B., et al. (2017). Effects of the Deepwater horizon oil spill on protected marine species. endanger. Species Res. 33, 1–7. doi: 10.3354/esr00789
Waring G. T., Josephson E., Maze-Foley K., Rosel P. E. (2016). “US Atlantic And gulf of Mexico marine mammal stock assessment 2015,” in NOAA Technical memorandum NMFS-NE-238(Woods Hole, MA: NOAA technical memorandum). Available at: https://repository.library.noaa.gov/view/noaa/11985.
Watwood S. L., Miller P. J. O., Johnson M., Madsen P. T., Tyack P. L. (2006). Deep-diving foraging behavior of sperm whales (Physeter macrocephalus). J. Anim. Ecol. 75, 814–825. doi: 10.1111/j.1365-2656.2006.01101.x
Wells R. S., Early G. A., Gannon J. G., Lingenfelser R. G., Sweeney P. (2008). “Tagging and tracking of rough-toothed dolphins (Steno bredanensis) from the march 2005 mass stranding in the Florida keys,” in NOAA Technical memorandum NMFS-SEFSC-574(Miami, FL: NOAA technical memorandum). Available at: https://repository.library.noaa.gov/view/noaa/8656.
Wells R. S., Fougeres E. M., Cooper A. G., Stevens R. O., Brodsky M., Lingenfelser R., et al. (2013). Movements and dive patterns of short-finned pilot whales (Globicephala macrorhynchus) released from a mass stranding in the Florida keys. Aquat. Mamm. 39, 61–72. doi: 10.1578/AM.39.1.2013.61
Wells R. S., Manire C. A., Byrd L., Smith D. R., Gannon J. G., Fauquier D., et al. (2009). Movements and dive patterns of a rehabilitated risso’s dolphin, Grampus griseus, in the gulf of Mexico and Atlantic ocean. Mar. Mamm. Sci. 25, 420–429. doi: 10.1111/j.1748-7692.2008.00251.x
Wells R. S., Scott M. D. (2018). “Bottlenose dolphin, tursiops truncatus, common bottlenose dolphin,” in Encyclopedia of marine mammals, 3rd ed. Eds. Würsig B., Thewissen J. G. M., Kovacs K. M. (San Diego, CA: Academic Press), 118–125. doi: 10.1016/B978-0-12-804327-1.00072-8
West K. L., Mead J. M., White W. (2011). Steno bredanensis (Cetacea: Delphinidae). Mamm. Species 43, 177–189. doi: 10.1644/886.1
Whitehead H. (2018). “Sperm whale: Physeter macrocephalus,” in Encyclopedia of marine mammals, 3rd ed. Eds. Würsig B., Thewissen J. G. M., Kovacs K. M. (San Diego, CA: Academic Press), 919–925. doi: 10.1016/B978-0-12-804327-1.00242-9
Wilkin S. M., Rowles T. K., Stratton E., Adimey N., Field C. L., Wissman S., et al. (2017). Marine mammal response operations during the deepwater horizon oil spill. Endang. Species Res. 33, 107–118. doi: 10.3354/esr00811
Williams R., Gero S., Bejder L., Calambokidis J., Kraus S. D., Lusseau D., et al. (2011). Underestimating the damage: interpreting cetacean carcass recoveries in the context of the Deepwater Horizon/BP incident: low probability of cetacean carcass recovery. Conserv. Lett. 4, 228–233. doi: 10.1111/j.1755-263X.2011.00168.x
Keywords: likelihood of exposure, feasibility of exposure, hydrocarbon industry, ecological traits, geographical distribution, numerical modeling
Citation: Ramírez-León MR, Sosa-Nishizaki O, Pérez-Brunius P, Romo-Curiel AE, Ramírez-Mendoza Z, Fajardo-Yamamoto A, Herzka SZ and García-Aguilar MC (2023) Semi-quantitative risk assessment of marine mammal oil exposure: A case study in the western Gulf of Mexico. Front. Mar. Sci. 10:1034647. doi: 10.3389/fmars.2023.1034647
Received: 01 September 2022; Accepted: 09 January 2023;
Published: 27 January 2023.
Edited by:
Juan José Alava, Institute for the Oceans and Fisheries, University of British Columbia, CanadaReviewed by:
Igal Berenshtein, University of Haifa, IsraelCarmen Morales-Caselles, University of Cadiz, Spain
Copyright © 2023 Ramírez-León, Sosa-Nishizaki, Pérez-Brunius, Romo-Curiel, Ramírez-Mendoza, Fajardo-Yamamoto, Herzka and García-Aguilar. This is an open-access article distributed under the terms of the Creative Commons Attribution License (CC BY). The use, distribution or reproduction in other forums is permitted, provided the original author(s) and the copyright owner(s) are credited and that the original publication in this journal is cited, in accordance with accepted academic practice. No use, distribution or reproduction is permitted which does not comply with these terms.
*Correspondence: María C. García-Aguilar, gconcha99@gmail.com
†These authors have contributed equally to this work and share first authorship