- 1School of Zoology, Faculty of Life Sciences, Tel Aviv University, Tel Aviv, Israel
- 2Department of Marine Biology, the Leon H. Charney School of Marine Sciences, University of Haifa, Haifa, Israel
Climate change is leading to phase shifts in coral reefs worldwide. In many biogeographic regions, octocorals are now becoming the most abundant benthic components, due to their environmental resilience and ability to rapidly colonize reef surfaces. Regeneration abilities and asexual reproduction are highly important for this ability and probably contribute to the successful spread of certain octocorals, including invasive species. Regeneration, however, has been little investigated in octocorals. To achieve a deeper understanding of octocoral regeneration, we employed Xenia umbellata, a common octocoral in the Red Sea, as a novel experimental model for laboratory studies. Using single-polyp modules, we investigated its regeneration ability and polyp asexual reproduction (budding). Excised polyps successfully reattached to tissue-culture plates within 2-3 days and started budding within 10 days. Amputation of the oral disc led to full regeneration within 7-10 days, with budding continuing throughout this period. Moreover, amputated tentacles developed into polyps within 21 days, demonstrating an unusual capacity for whole-body regeneration. The regeneration abilities of this species imply high totipotency of all polyp parts and are likely important for its life cycle. Further research using this model is expected to enhance the ecological and molecular understanding of octocoral development and provide insights into phase shifts currently occurring in coral reefs. Our study also suggests that X. umbellata has potential as a model organism for integrative studies on regeneration, physiology, developmental biology, and more, encouraging its adoption as a novel colonial cnidarian model organism.
1 Introduction
Climate change and other anthropogenic stressors are leading to phase shifts across coral reefs worldwide, resulting in substantial biodiversity loss (Reverter et al., 2022). Past research has focused primarily on shifts to macroalgae-dominated communities, while animal-dominated ones have been significantly less studied (Norström et al., 2009). Recent research has revealed that octocoral-dominated communities are highly abundant in many biogeographic regions, leading to changes in biodiversity and ecosystem functioning (Ateweberhan et al., 2013; Reverter et al., 2022).
Octocorals constitute a significant component of reef frameworks and provide structural complexity, habitats for associated organisms, and the transfer of energy between plankton and benthos (Benayahu et al., 2019; Gili and Coma, 1998; van de Water et al., 2018). Many reef organisms are sensitive to increasing temperatures and other anthropogenic changes (Fabricius et al., 2011; Schubert et al., 2017), and the consequent phase shifts on coral reefs tend to favor the stress-tolerant, generalist, and weedy species (Darling et al., 2012). Several studies have shown that certain octocorals appear more resilient than scleractinians (reviewed in Schubert et al., 2017), showing resistance to ocean acidification (Inoue et al., 2013; Gabay et al., 2014; Enochs et al., 2016), rising temperatures (Goulet et al., 2017; Steinberg et al., 2022), eutrophication (Baum et al., 2016; Vollstedt et al., 2020), or extreme storms (Lasker et al., 2020). Phase shifts to octocoral dominance may therefore lead to alternative communities with greater resilience to climate change (Ruzicka et al., 2013; Lenz et al., 2015).
Regeneration is a ubiquitous biological process, (Birnbaum and Alvarado, 2008) ranging from cellular through tissue, organ, or body structure regeneration, up to whole-body regeneration (WBR) from small parts of the body (Bideau et al., 2021). In clonal, modular animals such as corals, regeneration is intimately connected to additional forms of agametic development, such as colony growth by clonal budding and asexual reproduction (Hiebert et al., 2021). For example, many corals regenerate from fragments as a form of asexual reproduction (reviewed in Fautin, 2002). Furthermore, modularity conduces to increased survival following partial colony mortality, due to the ability of the surviving ramets to regenerate the colony (Sánchez and Lasker, 2004). Indeed, colony growth, asexual propagation, and regeneration may be selected for together in disturbed habitats, as all three increase the fitness of the genet (Henry and Hart, 2005; Reusch et al., 2021). These factors play a decisive role in the success of benthic reef organisms over the course of invasions or shifts in community structure (Ruiz and Hewitt, 2002; Darling et al., 2012; Capel et al., 2017; Luz et al., 2021). Octocorals are known to colonize the reef substrate by means of rapid growth and diverse modes of asexual and sexual reproduction (Dahan & Benayahu, 1997; Perkol-Finkel & Benayahu, 2004), which may also favor their increased abundance, invasion, and phase shift on the reef (Kahng et al., 2008; Ateweberhan et al., 2013). However, the potential role of regeneration in these processes has not been investigated to date.
Regeneration is commonly examined through model organisms, which have been well studied and are relatively easy to maintain under laboratory conditions. Cnidarians were the first model organisms used for research on regeneration, beginning with Trembley’s pioneering (1744) research on hydra (reviewed in Galliot & Schmid, 2002; Vogg et al., 2019). Regeneration in corals has been investigated mostly at the colony level, such as wound-healing and fragmentation (Work and Aeby, 2010; Lizcano-Sandoval et al., 2018), with reports on WBR in several scleractinians (Kramarsky-Winter and Loya, 1996; Vizel et al., 2011; Luz et al., 2018; Luz et al., 2021). While studies exist on regeneration in octocorals (e.g. Henry et al., 2003; Fava et al., 2009), they have so far not involved the use of a model species. Development of a laboratory-grown octocoral would conduce to a mechanistic understanding of octocoral regeneration.
Among the octocorals, species in the Xeniidae family are particularly adept at reef colonization due to their asexual reproduction mode (Benayahu and Loya, 1981; Benayahu and Loya, 1985; Benayahu and Loya, 1987) and high fecundity (Benayahu and Loya, 1984; Ben-David-Zaslow et al., 1999). Xeniids are highly abundant on Indo-Pacific reefs (Benayahu et al., 2019). Some species exhibit an hypnotic pulsation motion (Lamarck, 1816), which has been shown to improve the photosynthesis of their algal symbionts (Kremien et al., 2013) and absorption of dissolved organic matter (Bednarz et al., 2012). Their life-cycle traits appear to have contributed to their invasive ability (Menezes et al., 2021; Ruiz-Allais and Lasso-Alcalá, 2021) and successful spread onto degraded reefs (Stemmer et al., 2013; Bruckner et al., 2015). Xeniids have also shown resilience to the effects of climate change (Gabay et al., 2014; Vollstedt et al., 2020; Steinberg et al., 2022), as well as possessing chemical defenses against predation (Hoang et al., 2015) and a high interspecific competitive ability (Benayahu and Loya, 1981; Sammarco et al., 1983). Consequently, it is likely that their abundance in reefs will increase following the predicted decline of the scleractinian component in the coming years (Hughes et al., 2018; Reverter et al., 2022).
Regeneration was observed in xeniids in early studies by Gohar, 1940a; Gohar, 1940b), who suggested potential WBR abilities in these corals. A recent study (Hu et al., 2020) on a lab-grown xeniid species utilized regeneration as a tool for single-cell RNAseq analyses and reported the regeneration of tentacles post-amputation, while also observing possible WBR from the amputated tentacles. It is thus apparent that xeniids are likely to possess significant regeneration capabilities, although no in-depth investigation of the regeneration process or of its relation to the known asexual profligacy of xeniids, has been conducted to date.
Here we propose the use of Xenia umbellata, a common octocoral in the Red Sea, as a new model organism for the study of octocoral regeneration. We developed a method for maintaining excised single polyps of this species as experimental modules and examined their regeneration ability. We first studied the morphogenetic cascade of events in the coral’s attachment to the substrate and subsequent polyp budding. We then followed the morphogenesis of regenerating excised polyps whose oral disc had been amputated. Amputated oral discs were also investigated for their attachment ability. In addition, we examined whether WBR was achievable from individual excised tentacles. Our findings revealed a unique ability of these polyp parts to regenerate into complete polyps, indicating high tissue plasticity and possible totipotency. The results also suggest that X. umbellata has potential as a model organism for integrative studies on regeneration and associated processes, encouraging its adoption as a novel colonial cnidarian model organism.
2 Materials and methods
2.1 Coral maintenance and formation of polyp modules
The study was conducted from December 2020 to July 2021. Colonies of X. umbellata were maintained in a flow-through water table at the Mevo’ot Yam Aquaculture Center (32.40440002909542 N, 34.86892245451319 S) at 23-25°C, pH 8.1 and salinity 38 ppt. Ethanol preserved samples were genetically verified as Xenia umbellata by Prof. C.S. McFadden as described before (Halász et al., 2019). The following procedures were used for regeneration experiments. Individual polyps were excised from the colony using scissors and 3 ml plastic pipettes, and introduced into 12-well tissue culture plates (Corning), for settlement in the aquaculture system (Supplementary Figure 1). Polyps were removed from X. umbellata colonies, around 1-2 cm at the base and 5 cm in diameter of the polyp crown. For each plate, polyps were selected from a different colony for each well to maximize variation in the system. Settlement was considered complete when these polyps had attached to the plates, forming a bulbous structure, hereafter referred to as the basal attachment disc. For all laboratory regeneration experiments the individual polyps were given one week to attach and acclimate in the water table, and the plates were then transported from Mevo’ot Yam to the laboratory at Tel Aviv University (TAU). Plates were kept either in incubators (MRC Bod Model 150) or in a temperature-controlled room at 24°C, 12L:12D light regime, and light level of 50-57 µmol m-2 s-1 or 42 µmol m-2 s-1, respectively, measured by LICOR LI-189 light meter. Half of the water volume of the polyp-containing plates was replaced every other day with 0.45 µm filtered seawater (FSW) collected from the aquaculture system. Regeneration experiments were performed after four days of acclimation in the incubators, to avoid excessive bud growth that might interfere with experimentation. The animals were also monitored by photography and video, using an Olympus SZX16 dissecting scope with an Olympus DP74 camera. Additional images of the colony (Supplementary Figure 1) were taken using a 48MP super quad camera on a Xiaomi Redmi personal cell phone.
2.2 Development of basal attachment disc in excised polyps
To observe the development of a basal attachment disc and subsequent polyp budding, excised polyps (n = 48) were photographed every 2-3 days in the water table for 17 days, and the development of primary and secondary buds was recorded.
2.3 Regeneration of oral disc
The oral disc was removed from the uppermost proximal part of each polyp’s body using Storz E3360 scissors (n=192). The amputated polyps, together with intact polyps (n=48) that served as a control, were maintained under the same conditions (see above) in a temperature-controlled room. Polyps were individually monitored for 10 days, and a subset of polyps (5-12 per regeneration stage) was photographed under a dissecting scope (see above) at designated stages of regeneration, namely days 0, 2, 3, 5, 7, 8, 9 and 10 post-amputation.
2.4 Ability of amputated oral discs to develop a basal attachment disc
A subset of amputated oral discs (n=71) from the above experiment was transferred to tissue culture plates and maintained in an incubator (see above) to determine their ability for attachment. The oral discs were monitored and photographed every 2-3 days for 9 days to monitor development of the basal attachment disc. Intact polyps served as a control (n=12).
2.5 Whole-body regeneration from tentacles and SEM protocol
In order to obtain excised individual tentacles, polyps grown in tissue culture plates were anesthetized with MgCl2 (Merck) solution in 0.45 FSW at a final concentration of 0.2 M, after which the tentacles(n=72) were excised using Vannas-Tubingen spring scissors (Bar Naor BN11-599-04W), and individually placed into wells. Intact polyps (n=12) served as a control. Regeneration of the tentacles into polyps and their attachment to the tissue-well substrate were monitored every 2-3 days for 21 days using photographs, and samples were taken for subsequent scanning electron microscopy (SEM). The samples were fixed in 4% glutaraldehyde in FSW and kept until processed at 4°C, then washed three times in PBS (Biological industries, 02-023-1A), dehydrated through a graded series of ethanol concentrations up to 100%, and critically point-dried with liquid CO2. Finally, the samples were coated with gold and examined under a JCM 6000 NeoScope Benchtop scanning electron microscope, LEOL Japan.
2.6 Image editing
Images of the oral disc regeneration presented varied backgrounds and colors. To reveal the gradual regeneration process, images were edited with Photoshop to remove the background and eliminate any distortion of the morphological features (original images supplied in Supplementary Figure 2). Tentacle regeneration SEM images were slightly edited to remove background texture.
3 Results
3.1 Formation of single polyp modules
Initially, we examined the potential of individual excised polyps to serve as laboratory modules. Such polyps were introduced into tissue-culture plates and photographed after 1 week in the Mevo’ot Yam water table (Supplementary Figure 1). All the excised polyps (n=84) settled on the plates and continued to pulsate at similar rates as the original colonies, around 30 pulsations per minute. These animals were consequently used as laboratory modules in the following experimental work.
3.2 Development of basal attachment disc from excised polyps
The excised polyps (n=48) had all attached to the bottom of the tissue culture plates by day three post-amputation. Their basal attachment disc had thickened, becoming bulbous (Figures 1A, B), and appeared to spread out on the bottom of the well. Polyp pulsation ceased immediately upon their removal from the colony but was observed to resume within several hours. On day 3, post-attachment to the wells, their pulsation was visually indistinguishable from that in intact colonies. By day 13, 21% of these polyps had started to bud daughter polyps from their basal attachment discs; and by day 17, 70% were budding, with 35% displaying two visible buds (Figure 1C). Notably, all polyps survived to this stage, upon which the experiment was terminated.
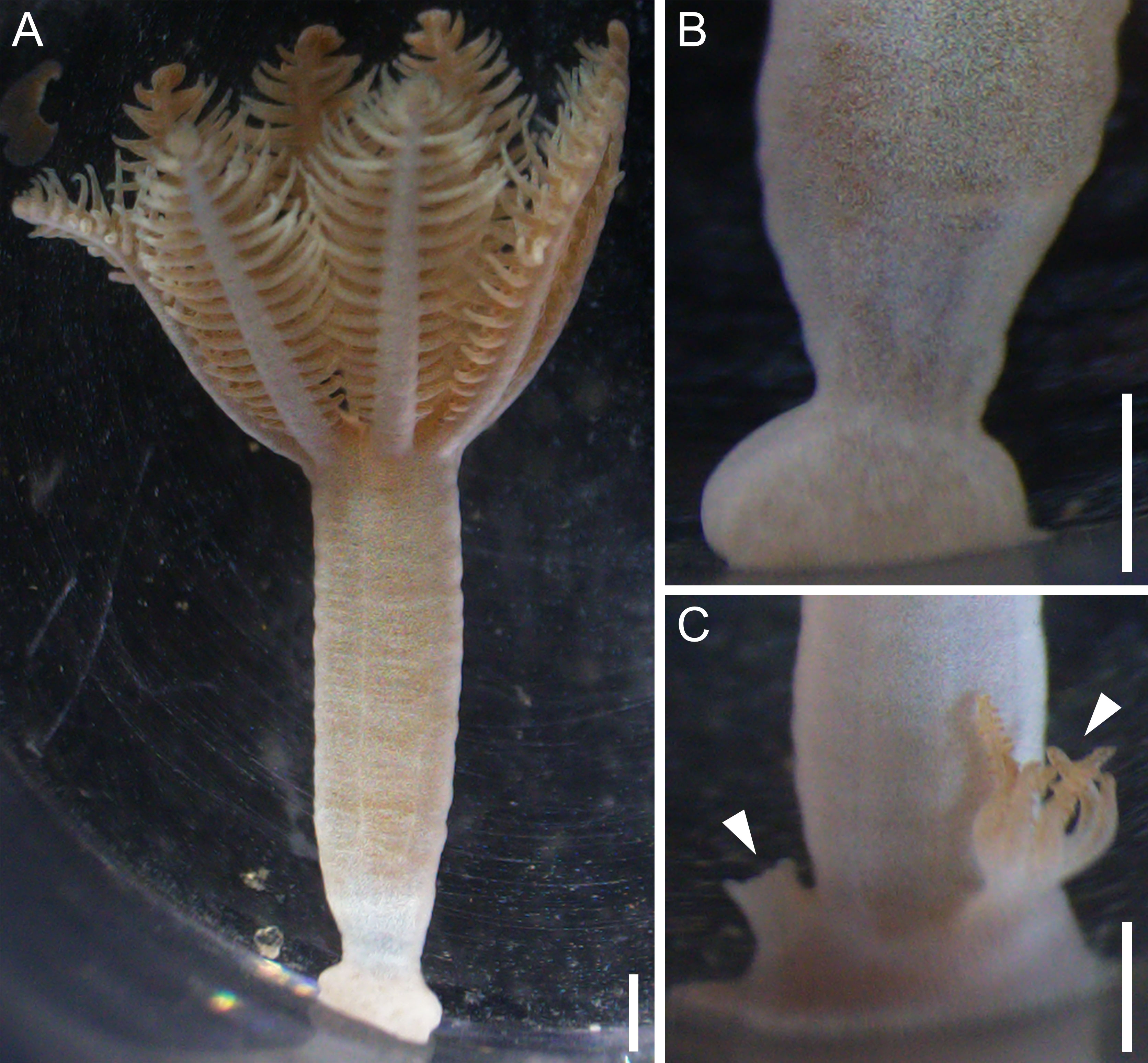
Figure 1 Polyp reattachment and budding in Xenia umbellata. (A) Polyp amputated from colony, reattached in tissue culture plate. (B) Close-up of basal attachment disc. (C) Daughter buds (arrowheads) forming from basal attachment disc. Scale bars 1 mm.
3.3 Oral disc regeneration
Following oral disc amputation (n=192), the polyp body regenerated a new disc in a characteristic series of morphogenetic events (Figure 2). These polyp bodies (Figure 2, day 0) seemed to contract initially, possibly preventing gastrovascular fluid from leaking from the wound region. Smooth tissue then formed over the amputated region, and the wound-region appeared closed by day 2 post-amputation. Tentacle buds subsequently developed and gradually elongated, and the external eight-fold symmetry became visible (Figure 2 days 3-5). On days 6-7 post-amputation, the tentacles elongated and pinnules gradually appeared along their margins. Movement of the tentacles started at this stage, although they did not pulsate regularly. By day 10 the polyps had further regrown their pinnules and 90% presented a fully synchronized, regular pulsation motion, as in the control polyps, at a rate of around 30 pulsations per minute, known to characterize intact polyps (Kremien et al., 2013; Vollstedt et al., 2020). At the end of the experiment (day 10), survival was 98% and all the polyps had regrown oral discs.
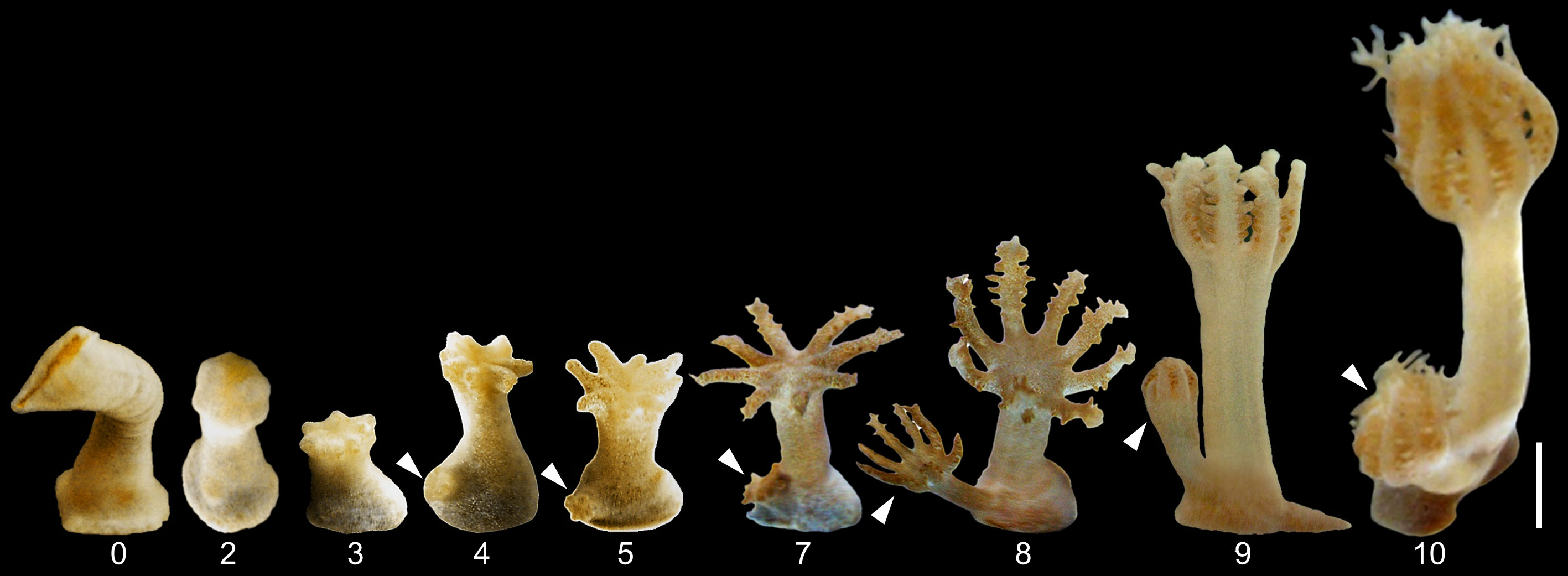
Figure 2 Timeline of oral disc regeneration of amputated polyps of Xenia umbellata from day 0 until regeneration into a fully functional polyp on day 10. Arrowheads indicate developing daughter buds showing similar morphogenesis. Scale bar 1 mm applies to all images.
Polyp budding took place throughout the entire regeneration process of this experiment, displaying similar stages as the regenerating oral discs (Figure 2, arrowheads). Namely, from the stage of tentacle bud formation to the gradual reestablishment of pulsation, all stages appeared extremely similar. The polyp body of the buds developed only after initial oral disc formation (Figure 2, day 8). Some buds had reached full pulsation at the end of the oral disc regeneration process on day 10 (Supplementary video 1).
3.4 Ability of amputated oral discs to develop a basal attachment disc
All the amputated oral discs (n = 71) were seen to survive on day 5 of the experiment, and by day 9, when the experiment was terminated, 86% still survived. Attachment to the wells was 54% by day 2 and 98% by day 9. The basal disc was seen to develop following initial attachment to the substrate (Figures 3A, B). At the end of the experiment, the basal part of the attached amputated polyps appeared flattened and thickened (Figure 3C), as in the previous experiment on development of the basal attachment disc. All the control animals survived until the end of the experiment and behaved normally.
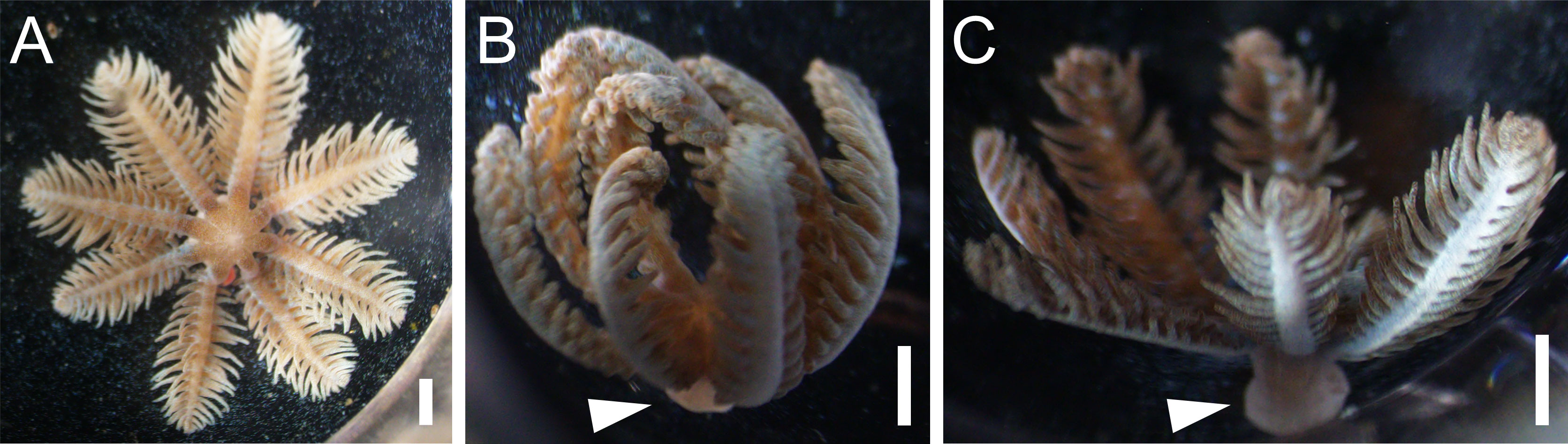
Figure 3 Oral discs at different stages of development of basal attachment disc. (A) Oral disc from amputated polyp, attached but basal disc not yet visible. (B) Oral disc with basal attachment disc forming. (C) Oral disc with fully formed basal attachment disc. Arrowheads indicate basal disc. Scale bars 1 mm.
3.5 Whole-body regeneration from tentacles
Excised tentacles (n=72) initially displayed a gradual retraction of the pinnules, which disappeared or were reabsorbed over several days (Figures 4A-D). During this period, attachment to the substrate was first noted, with 47% attached tentacles by day 5. By day 7 (Figure 4D), the external surface of the tentacles became smooth and amorphous, indicating that morphological dedifferentiation had taken place. These tentacles began developing polyp buds on day 10 (Figure 4E), which subsequently formed tentacles featuring pinnules along their margins (Figures 4F, G). Notably, regeneration was not morphologically uniform along the tentacles, with some growing multiple polyps and others revealing more slowly developing parts. However, by day 20 of the experiment all surviving tentacles (65.3%) had attached to the substrate and developed into pulsating polyps, except for one, which still was at the polyp bud stage. Final polyps were 1-2 mm long, about 10% the size of the original colony polyps (Figure 4G), but nonetheless appeared to be fully developed.
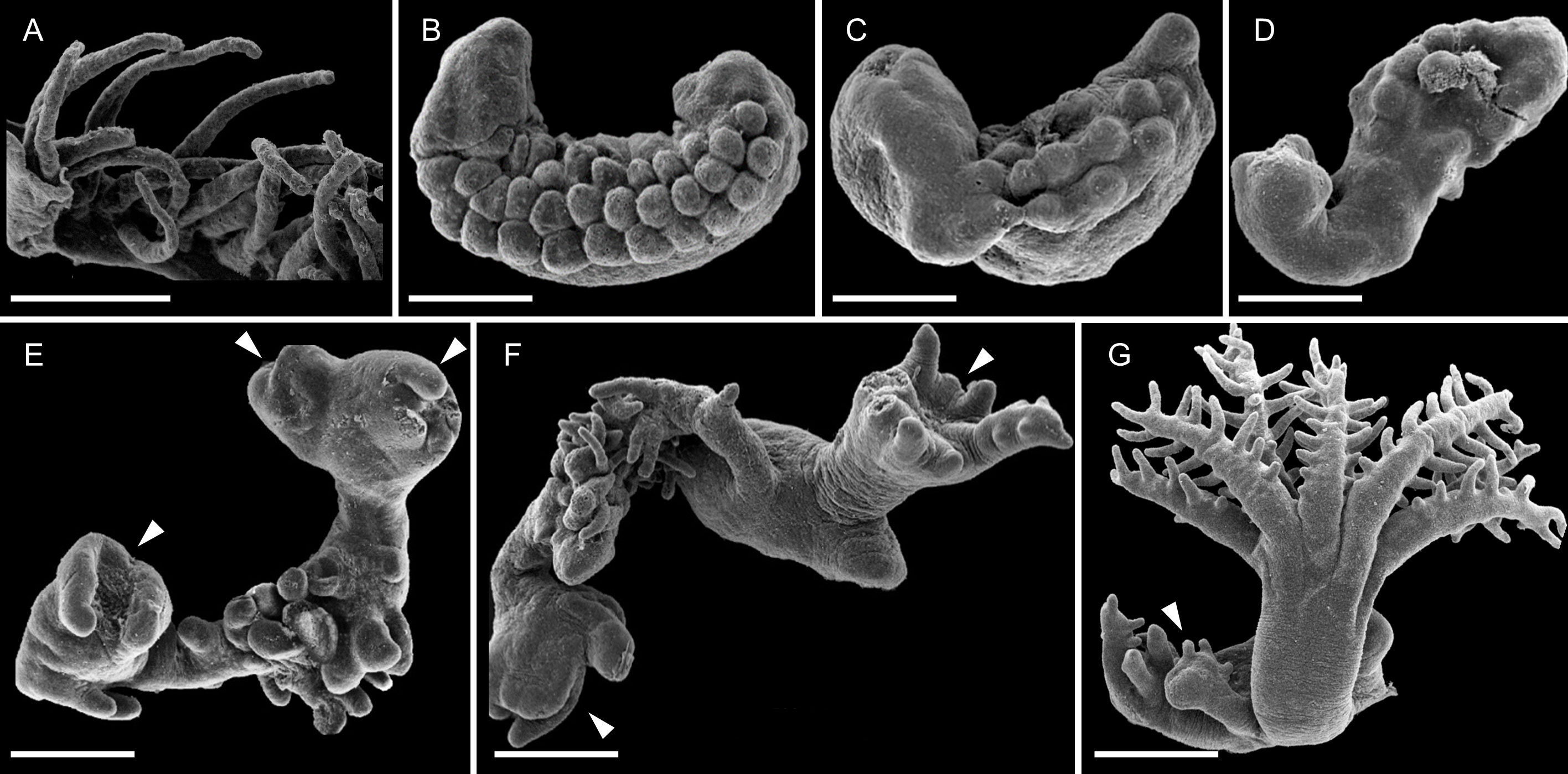
Figure 4 SEM images of whole-body-regeneration from excised tentacles of Xenia umbellata first into polyps and then into miniature colonies. (A) Day 0 post-amputation from polyp. Close-up of an amputated tentacle, showing elongated pinnules. (B-D) Days 3, 5, 7. Tentacles undergo morphological dedifferentiation to form smooth tissue. (E) Day 10. Polyp buds form on various parts of the tentacle. (F) Day 13, partially regenerated polyp with mouth visible and tentacles elongating. (G) Day 17, fully regenerated polyp with another bud forming. Scale bars 0.5 mm. Arrowheads indicate developing buds.
4 Discussion
This study reports the rapid regeneration abilities of the octocoral X. umbellata and presents the first investigation of whole-body regeneration in octocorals. All the tested body parts – the polyp body, oral disc, and tentacles – were capable of regenerating missing parts within a few days or weeks. We found that polyp budding resumed directly after development of the basal attachment disc and concurrent with the oral disc regeneration, suggesting high developmental plasticity. WBR took place also from the individual excised tentacles. Attachment ability was observed for detached polyps, oral discs, and tentacles. The remarkable regenerative capacity of X. umbellata recorded here suggests that this coral has potential as a useful laboratory model organism for furthering the understanding of octocoral regeneration.
In early studies on xeniids, Gohar (1940b) observed the formation of single-polyp colonies in Heteroxenia fuscescens, both by polyp amputation and transverse fission, in which a polyp became detached from the lower part of its body after constriction of the tissue. In both cases the polyps reattached themselves with a thickened basal attachment disc (syndete) that produced buds, and eventually developed into the colony base. This method of asexual reproduction is similar to that of polyp expulsion reported in scleractinians (Kramarsky-Winter et al., 1997), and suggests that the survival of detached single polyps and regeneration of the basal attachment disc is important in the life cycle of xeniids. In the current study, development of the polyp basal attachment disc occurred rapidly, completing attachment in 2-3 days (Figures 1, 3). Its reattachment and regeneration occurred either with or without most of the polyp body, indicating the high potency of the process. In both cases, the most aboral end of the polyp body became bulbous and flattened at the bottom, and subsequently budded new polyps, reestablishing the multi-polyp colonial state (Figure 1C).
Oral disc regeneration exhibited initial contraction of the polyp body, closure of the wound, and the ensuing formation of tentacle buds, similar to known regeneration processes in three model cnidarians – Hydra vulgaris, Exaiptasia pallida, and Nematostella vectensis (Bossert et al., 2013; Vogg et al., 2019; van der Burg et al., 2020). This suggests a conserved process of tissue remodeling during regeneration. The gradual reappearance of pulsation behavior by the regenerating oral disc may indicate a stepwise neurogenesis, as innervation of the regenerating tissue is necessary for regeneration in many metazoans (Brockes and Kumar, 2008; Boilly et al., 2017). Nerve-free hydra can still regenerate, although the disruption of neurogenesis appears to slow down regeneration in wild-type hydra (Miljkovic-Licina et al., 2007). Therefore, X. umbellata’s pulsation regeneration ability may provide a novel model for understanding the interaction between neurogenesis and regeneration, with a distinct behavioral output whose molecular mechanism is currently being studied.
The amputated tentacles of X. umbellata exhibited a distinctive example of WBR, also associated with subsequent polyp budding towards the formation of small colonies. Attachment to the substrate appears to be an important stage for WBR, as it began early during regeneration, concurrent with the morphological dedifferentiation stage, and occurred in almost all the surviving polyps. WBR from detached tentacles has been reported to date in only a few solitary cnidarians. For example, boloceroidid anemones regenerate from autotomized tentacles that are brooded in the animal’s gastrovascular cavity (Pearse, 2002). However, the anemone regenerates from the base of the tentacle that was originally attached to the oral disc, without dedifferentiating. Regeneration involving dedifferentiation from autotomized tentacles has been reported in the solitary scleractinian Euphilia glabrescens (Toh and Ng, 2016), the corallimorphian Ricordia yuma (Kaposi et al., 2022), and from scyphopolyps of Chrysaora sp. (Herouard, 1913) and Aurelia aurita (Lesh-Laurie and Corriel, 1973). For all but A. aurita, WBR presented a relatively similar series of events, with the detached tentacle first attaching to the substrate, then forming a mouth and polyp bud, and finally forming a new polyp. Notably, all these cnidarians took far longer (1-4 months) to regenerate in comparison to X. umbellata, and all regenerated into solitary organisms like the respective parental ones, as opposed to a colony. Interestingly, although H. vulgaris and N. vectensis possess remarkable WBR abilities (Johnston et al., 2021), even regeneration from dissociated cells in the hydra (Gierer et al., 1972), neither is capable of WBR from detached tentacles (Vogg et al., 2019; Röttinger, 2021). This suggests that in X. umbellata, adult polyp parts are potentially totipotent and can quickly remodel or regenerate the polyp even in tissues in which polyp budding does not occur in the intact colony state.
Regeneration is highly dependent on temperature, health state, and metabolic state, which reflect certain trade-offs between somatic growth, regeneration, and sexual reproduction (Henry and Hart, 2005). In the current study, excised polyps of X. umbellata were found to initiate budding immediately after formation of the new basal attachment disc and concurrent with the oral disc regeneration (Figures 1, 2). Such developmental duality indicates that the energy supply, even in a single-polyp form, was sufficient to support both processes, rather than being allocated to either regeneration or budding. Notably, budding was observed on day 5 of the oral disc regeneration experiment (Figure 2), 16 days post-amputation. This is well within the range observed for budding rate without amputation. The high dependence of this species on its symbiotic unicellular algae for nutrition (Fabricius & Alderslade, 2001; Gohar, 1940a) may be an important factor to consider, as regeneration is known to be metabolically costly (Bely and Nyberg, 2010; Lindsay, 2010), and frequently comes at the expense of other reproduction forms in corals (Kramarsky-Winter and Loya, 2000; Denis et al., 2013). As such, a high pluripotency, combined with a sufficient energy source, may expedite the concomitant regeneration and colony growth abilities of X. umbellata. Additionally, as many tentacles initially regenerated into multiple small polyps rather than large, individual polyps (Figure 4), it is suggested that there is some physiological advantage to directly regenerating into a colonial form (Hughes, 1983). Similarly, studies on the scleractinian Tubastrea showed that WBR frequently resulted in the simultaneous formation of two polyps (Luz et al., 2018; Luz et al., 2021), which was hypothesized to increase growth at later stages. As the colonial body plan possesses distinct ecological benefits in relation to survival, feeding, and growth (Hiebert et al., 2021), the formation of multiple polyps during early regeneration may increase fitness.
In summary, Xenia umbellata demonstrates rapid WBR, and constitutes a candidate for a novel model organism for studying octocoral regeneration and other associated physiological processes. In addition to its ease of laboratory manipulation and rapid regeneration, the unique characters of X. umbellata – coloniality, multiple forms of asexual reproduction, and its pulsation, indicate that it can be a successful model system to explore regeneration in an integrative manner. The ability of individual polyps or their tentacles to rapidly regenerate, reattach, and resume coloniality would appear to contribute to its successful colonization of the reef substrate. Combined with continued ecological research, the proposed model can help to elucidate the previously unexamined role of regeneration as a mechanism of octocoral colonization and invasion, providing a deeper understanding of octocoral phase shifts.
Data availability statement
The raw data supporting the conclusions of this article will be made available by the authors, without undue reservation.
Author contributions
EN designed, performed, and analyzed the experiments. YB and TL conceived the research and supervised the experimental work. EN wrote the manuscript with the help of YB and TL. All authors contributed to the article and approved the submitted version.
Acknowledgments
We thank Lindsay Weingart for her insight and enthusiastic assistance in experiments. We thank Yoavi Eyal, Nour Alhoda Akel, and Stav Vaknin for laboratory assistance, Vered Holdengreber for SEM imaging, Varda Wexler for image editing, Michal Weis for technical assistance and moral support, and Catherine S. McFadden (Harvey Mudd College, CA, USA) for genetic verification of the phylogenetic status of the study organism. We thank Raphael Yavetz for coral aquaculture maintenance and Mevo’ot Yam for sharing with us their seawater flow systems and support.
Conflict of interest
The authors declare that the research was conducted in the absence of any commercial or financial relationships that could be construed as a potential conflict of interest.
Publisher’s note
All claims expressed in this article are solely those of the authors and do not necessarily represent those of their affiliated organizations, or those of the publisher, the editors and the reviewers. Any product that may be evaluated in this article, or claim that may be made by its manufacturer, is not guaranteed or endorsed by the publisher.
Supplementary material
The Supplementary Material for this article can be found online at: https://www.frontiersin.org/articles/10.3389/fmars.2023.1021679/full#supplementary-material
References
Ateweberhan M., Feary D. A., Keshavmurthy S., Chen A., Schleyer M. H., Sheppard C. R. C. (2013). Climate change impacts on coral reefs: Synergies with local effects, possibilities for acclimation, and management implications. Mar. pollut. Bull. 74, 526–539. doi: 10.1016/J.MARPOLBUL.2013.06.011
Baum G., Januar I., Ferse S. C. A., Wild C., Kunzmann A. (2016). Abundance and physiology of dominant soft corals linked to water quality in Jakarta bay, Indonesia. PeerJ 2016, e2625. doi: 10.7717/PEERJ.2625/SUPP-2
Bednarz V. N., Naumann M. S., Niggl W., Wild C. (2012). Inorganic nutrient availability affects organic matter fluxes and metabolic activity in the soft coral genus Xenia. J. Exp. Biol. 215, 3672–3679. doi: 10.1242/jeb.072884
Bely A. E., Nyberg K. G. (2010). Evolution of animal regeneration: Re-emergence of a field. Trends Ecol. Evol. 25, 161–170. doi: 10.1016/J.TREE.2009.08.005
Benayahu Y., Bridge T. C. L., Colin P. L., Liberman R., McFadden C. S., Pizarro O., et al. (2019). Octocorals of the indo-pacific. Coral Reefs World 12, 709–728. doi: 10.1007/978-3-319-92735-0_38
Benayahu Y., Loya Y. (1981). Competition for space among coral-reef sessile organisms at eilat, red Sea. Bull. Mar. Sci 31 (3), 514–522.
Benayahu Y., Loya Y. (1984). Life history studies on the red sea soft coral Xenia macrospiculata gohar 1940. II. planulae shedding and post larval development. Biol. Bull. 166, 32–43. doi: 10.2307/1541429
Benayahu Y., Loya Y. (1985). Settlement and recruitment of a soft coral: why is Xenia macrospiculata a successful colonizer? Bull. Mar. Sci. 36, 177–188.
Benayahu Y., Loya Y. (1987). Long-term recruitment of soft-corals (Octocorallia: Alcyonacea) on artificial substrata at eilat (Red Sea). Mar. Ecol. Prog. Ser. 38, 161–167. doi: 10.3354/MEPS038161
Ben-David-Zaslow R., Henning G., Hofmann D. K., Benayahu Y. (1999). Reproduction in the red Sea soft coral heteroxenia fuscescens: Seasonality and long-term record, (1991 to 1997). Mar. Biol 133 (3), 553–559. doi: 10.1007/s002270050495
Bideau L., Kerner P., Hui J., Vervoort M., Gazave E. (2021). Animal regeneration in the era of transcriptomics. Cell. Mol. Life Sci. 78, 3941–3956. doi: 10.1007/S00018-021-03760-7/FIGURES/4
Birnbaum K. D., Alvarado A. S. (2008). Slicing across kingdoms: Regeneration in plants and animals. Cell 132, 697–710. doi: 10.1016/j.cell.2008.01.040
Boilly B., Faulkner S., Jobling P., Hondermarck H. (2017). Nerve dependence: From regeneration to cancer. Cancer Cell 31, 342–354. doi: 10.1016/j.ccell.2017.02.005
Bossert P. E., Dunn M. P., Thomsen G. H. (2013). A staging system for the regeneration of a polyp from the aboral physa of the anthozoan cnidarian nematostella vectensis. Dev. Dyn. 242, 1320–1331. doi: 10.1002/dvdy.24021
Brockes J. P., Kumar A. (2008). Comparative aspects of animal regeneration. Annu. Rev. Cell Dev. Biol. 24, 525–549. doi: 10.1146/ANNUREV.CELLBIO.24.110707.175336
Bruckner A. W., Dempsey A. C. (2015). “The Status, Threats, and Resilience of Reef-Building Corals of the Saudi Arabian Red Sea,” in The Red Sea Rasul N. M. A., Stewart I. C. F. Ed. (Springer, Berlin, Heidelberg: Springer Earth System Sciences), 471–486. doi: 10.1007/978-3-662-45201-1_27
Capel K. C. C., Toonen R. J., Rachid C. T. C. C., Creed J. C., Kitahara M. V., Forsman Z., et al. (2017). Clone wars: Asexual reproduction dominates in the invasive range of tubastraea spp. (Anthozoa: Scleractinia) in the south-Atlantic ocean. PeerJ 2017, e3873. doi: 10.7717/PEERJ.3873/SUPP-2
Dahan M., Benayahu Y. (1997). Clonal propagation by the azooxanthellate octocoral dendronephthya hemprichi. Coral Reefs 1997 161 16, 5–12. doi: 10.1007/S003380050053
Darling E. S., Alvarez-Filip L., Oliver T. A., Mcclanahan T. R., Côté I. M. (2012). Evaluating life-history strategies of reef corals from species traits. Ecol. Lett 15, 1378–1386. doi: 10.1111/J.1461-0248.2012.01861.X
Denis V., Guillaume M. M. M., Goutx M., de Palmas S., Debreuil J., Baker A. C., et al. (2013). Fast growth may impair regeneration capacity in the branching coral acropora muricata. PloS One 8, e72618. doi: 10.1371/JOURNAL.PONE.0072618
Enochs I. C., Manzello D. P., Wirshing H. H., Carlton R., Serafy J. (2016). Micro-CT analysis of the Caribbean octocoral eunicea flexuosa subjected to elevated pCO2. ICES J. Mar. Sci. 73, 910–919. doi: 10.1093/ICESJMS/FSV159
Fabricius K. E., Alderslade P. (2001). Soft corals and sea fans: A comprehensive guide to the tropical shallow water genera of the central-west pacific, the Indian ocean and the red Sea. PMB 3, Townsville MC, Queensland 4810, Australia: Australian Institute of Marine Science.
Fabricius K. E., Langdon C., Uthicke S., Humphrey C., Noonan S., De’ath G., et al. (2011). Losers and winners in coral reefs acclimatized to elevated carbon dioxide concentrations. Nat. Clim. Change 2011 13 1, 165–169. doi: 10.1038/nclimate1122
Fava F., Bavestrello G., Valisano L., Cerrano C. (2010). Survival, growth and regeneration in explants of four temperate gorgonian species in the Mediterranean Sea. Ital. J. Zool. 77, 44–52. doi: 10.1080/11250000902769680
Gabay Y., Fine M., Barkay Z., Benayahu Y. (2014). Octocoral tissue provides protection from declining oceanic pH. PloS One 9 (4), e91553. doi: 10.1371/journal.pone.0091553
Galliot B., Schmid V. (2002). Cnidarians as a model system for understanding evolution and regeneration. Int. J. Dev. Biol. 46, 39–48. doi: 10.1387/ijdb.11902686
Gierer A., Berking S., Bode H., David C. N., Flick K., Hansmann G., et al. (1972). Regeneration of hydra from reaggregated cells. Nat. New Biol. 239, 98–101. doi: 10.1038/newbio239098a0
Gili J.-M., Coma R. (1998). Benthic suspension feeders: Their paramount role in littoral marine food webs. Trends Ecol. Evol. 13, 316–321. doi: 10.1016/S0169-5347(98)01365-2
Gohar H. A. F. (1940a). Studies on the xeniidae of the red Sea: Their ecology, physiology, taxonomy and phylogeny. Publ. Mar. Biol. Stn. Ghardaqa 2, 24–118.
Gohar H. A. F. (1940b). The development of some xeniidae (Alcyonaria) (with some ecological aspects). Publ. Mar. Biol. Stn. Ghardaqa 3, 26–77.
Goulet T. L., Shirur K. P., Ramsby B. D., Iglesias-Prieto R. (2017). The effects of elevated seawater temperatures on Caribbean gorgonian corals and their algal symbionts, symbiodinium spp. PloS One 12, e0171032. doi: 10.1371/JOURNAL.PONE.0171032
Halász A., McFadden C. S., Toonen R., Benayahu Y. (2019). Re-description of type material of Xenia lamarck 1816 (Octocorallia: Xeniidae). Zootaxa 4652, 201–239. doi: 10.11646/zootaxa.4652.2.1
Henry L. A., Hart M. (2005). Regeneration from injury and resource allocation in sponges and corals - a review. Int. Rev. Hydrobiol. 90, 125–158. doi: 10.1002/iroh.200410759
Henry L. A., Kenchington E. L. R., Silvaggio A. (2003). Effects of mechanical experimental disturbance on aspects of colony responses, reproduction, and regeneration in the cold-water octocoral gersemia rubiformis. Can. J. Zool. 81, 1691–1701. doi: 10.1139/z03-161
Herouard E. (1913). Relations entre la depression et la formation de pseudoplanula tentaculaires chez le schyphistome. Comptes Rendus Hebd. Des. Sйances l’Acadйmie Des. Sci. 156, 1093–1095.
Hiebert L. S., Simpson C., Tiozzo S. (2021). Coloniality, clonality, and modularity in animals: The elephant in the room. J. Exp. Zool. Part B Mol. Dev. Evol. 336, 198–211. doi: 10.1002/JEZ.B.22944
Hoang B. X., Sawall Y., Al-Sofyani A., Wahl M. (2015). Chemical versus structural defense against fish predation in two dominant soft coral species (Xeniidae) in the red Sea. Aquat. Biol. 23, 129–137. doi: 10.3354/AB00614
Hughes R. N. (1983) Evolutionary ecology of colonial reef-organisms, with particular reference to corals. Available at: https://academic.oup.com/biolinnean/article/20/1/39/2666272.
Hughes T. P., Kerry J. T., Baird A. H., Connolly S. R., Dietzel A., Eakin C. M., et al. (2018). Global warming transforms coral reef assemblages. Nature 556 (7702), 492–496. doi: 10.1038/s41586-018-0041-2
Hu M., Zheng X., Fan C. M., Zheng Y. (2020). Lineage dynamics of the endosymbiotic cell type in the soft coral Xenia. Nature 582, 534–538. doi: 10.1038/s41586-020-2385-7
Inoue S., Kayanne H., Yamamoto S., Kurihara H. (2013). Spatial community shift from hard to soft corals in acidified water. Nat. Clim. Change 3, 683–687. doi: 10.1038/nclimate1855
Johnston H., Warner J. F., Amiel A. R., Nedoncelle K., Carvalho J. E., Röttinger E. (2021). Whole body regeneration deploys a rewired embryonic gene regulatory network logic. bioRxiv 658930. doi: 10.1101/658930
Kahng S., Benayahu Y., Wagner D., Rothe N. (2008). Sexual reproduction in the invasive octocoral carijoa riisei in Hawaii. Bull. Mar. Sci. 82, 1–17.
Kaposi K. L., Courtney R. L., Seymour J. E. (2022). Tentacle autotomy: An additional mode of asexual reproduction in ricordea yuma (Cnidaria, anthozoa, corallimorpharia). Mem. Queensl. Museum – Nat. 63, 84–91. doi: 10.17082/j.2204-1478.63.2022.2021-04
Kramarsky-Winter E., Fine M., Loya Y. (1997). Coral polyp expulsion. Nat. 1997 3876629 387, 137–137. doi: 10.1038/387137a0
Kramarsky-Winter E., Loya Y. (1996). Regeneration versus budding in fungiid corals: A trade-off. Mar. Ecol. Prog. Ser. 134, 179–185. doi: 10.3354/meps134179
Kramarsky-Winter E., Loya Y. (2000). Tissue regeneration in the coral fungia granulosa: The effect of extrinsic and intrinsic factors. Mar. Biol. 137, 867–873. doi: 10.1007/s002270000416
Kremien M., Shavit U., Mass T., Genin A. (2013). Benefit of pulsation in soft corals. Proc. Natl. Acad. Sci 110 (22), 8978–8983. doi: 10.1073/pnas.1301826110
Lamarck J. B. P. A. (1816). Histoire naturelle des animeaux sans vertèbres [Natural history of invertebrate animals], Verdiere, Paris Vol. 2. 409–410.
Lasker H. R., Martínez- Q., Bramanti L., Edmunds P. J. (2020). Resilience of octocoral forests to catastrophic storms. Sci. Rep. 2020 101 10, 1–8. doi: 10.1038/s41598-020-61238-1
Lenz E. A., Bramanti L., Lasker H. R., Edmunds P. J. (2015). Long-term variation of octocoral populations in st. John, US virgin islands. Coral Reefs 34, 1099–1109. doi: 10.1007/S00338-015-1315-X/FIGURES/5
Lesh-Laurie G., Corriel R. (1973). Scyphistoma regeneration from isolated tentacles in aurelia aurita. J. Mar. Biol. Assoc. United Kingdom 53, 885–894. doi: 10.1017/S0025315400022542
Lindsay S. M. (2010). Frequency of injury and the ecology of regeneration in marine benthic invertebrates. Integr. Comp. Biol. 50, 479–493. doi: 10.1093/ICB/ICQ099
Lizcano-Sandoval L. D., Londoño-Cruz E., Zapata F. A. (2018). Growth and survival of pocillopora damicornis (Scleractinia: Pocilloporidae) coral fragments and their potential for coral reef restoration in the tropical Eastern pacific. Mar. Biol. Res. 14, 887–897. doi: 10.1080/17451000.2018.1528011
Luz B. L. P., Capel K. C. C., Zilberberg C., Flores A. A. V., Migotto A. E., Kitahara M. V. (2018). A polyp from nothing: The extreme regeneration capacity of the Atlantic invasive sun corals tubastraea coccinea and t. tagusensis (Anthozoa, scleractinia). J. Exp. Mar. Bio. Ecol. 503, 60–65. doi: 10.1016/j.jembe.2018.02.002
Luz B. L. P., Miller D. J., Kitahara M. V. (2021). High regenerative capacity is a general feature within colonial dendrophylliid corals (Anthozoa, scleractinia). J. Exp. Zool. Part B Mol. Dev. Evol. 336, 281–292. doi: 10.1002/JEZ.B.23021
Menezes N. M., McFadden C. S., Miranda R. J., Nunes J. A. C. C., Lolis L., Barros F., et al. (2021). New non-native ornamental octocorals threatening a south-west Atlantic reef. J. Mar. Biol. Assoc. United Kingdom 101, 911–917. doi: 10.1017/S0025315421000849
Miljkovic-Licina M., Chera S., Ghila L., Galliot B. (2007). Head regeneration in wild-type hydra requires de novo neurogenesis. Development 134, 1191–1201. doi: 10.1242/dev.02804
Norström A., Nyström M., Lokrantz J., Folke C. (2009). Alternative states on coral reefs: beyond coral–macroalgal phase shifts. Mar. Ecol. Prog. Ser. 376, 295–306. doi: 10.3354/meps07815
Pearse V. B. (2002). Prodigies of propagation: The many modes of clonal replication in boloceroidid sea anemones (Cnidaria, anthozoa, actiniaria). Invertebr. Reprod. Dev. 41, 201–213. doi: 10.1080/07924259.2002.9652753
Perkol-Finkel S., Benayahu Y. (2004). Community structure of stony and soft corals on vertical unplanned artificial reefs in eilat (Red sea): Comparison to natural reefs. Coral Reefs 23, 195–205. doi: 10.1007/S00338-004-0384-Z/FIGURES/5
Reusch T. B. H., Baums I. B., Werner B. (2021). Evolution via somatic genetic variation in modular species. Trends Ecol. Evol. 36, 1083–1092. doi: 10.1016/J.TREE.2021.08.011
Reverter M., Helber S. B., Rohde S., de Goeij J. M., Schupp P. J. (2022). Coral reef benthic community changes in the anthropocene: Biogeographic heterogeneity, overlooked configurations, and methodology. Glob. Change Biol. 28, 1956–1971. doi: 10.1111/GCB.16034
Röttinger E. (2021). Nematostella vectensis, an emerging model for deciphering the molecular and cellular mechanisms underlying whole-body regeneration. Cells 10, 2692. doi: 10.3390/CELLS10102692
Ruiz-Allais J. P., Lasso-Alcalá O. M. (2021). The invasive octocoral unomia stolonifera (Alcyonacea, xeniidae) is dominating the benthos in the southeastern Caribbean Sea fundacion la tortuga. Mem. la Fund. La Salle Ciencias Nat 79 (187), 63–80. doi: 10.5281/zenodo.4784709
Ruiz G. M., Hewitt C. L. (2002). Toward understanding patterns of coastal marine invasions: A prospectus. Invasive Aquat. Species Eur. Distrib. Impacts Manage. 529–547. doi: 10.1007/978-94-015-9956-6_53
Ruzicka R. R., Colella M. A., Porter J. W., Morrison J. M., Kidney J. A., Brinkhuis V., et al. (2013). Temporal changes in benthic assemblages on Florida keys reefs 11 years after the 1997/1998 El niño. Mar. Ecol. Prog. Ser. 489, 125–141. doi: 10.3354/MEPS10427
Sammarco P. W., Coll J. C., La Barre S., Willis B. (1983). Competitive strategies of soft corals (Coelenterata: Octocorallia): Allelopathic effects on selected scleractinian corals. Coral Reefs 1983 13 1, 173–178. doi: 10.1007/BF00571194
Sánchez J. A., Lasker H. R. (2004). Do multi-branched colonial organisms exceed normal growth after partial mortality? Proc. R. Soc B Biol. Sci. 271, 117–120. doi: 10.1098/rsbl.2003.0108
Schubert N., Brown D., Rossi S. (2017). “Symbiotic versus nonsymbiotic octocorals: Physiological and ecological implications,” in Marine animal forests: The ecology of benthic biodiversity hotspots Cham, Switzerland: Springer International Publishing. doi: 10.1007/978-3-319-21012-4_54
Steinberg R. K., Ainsworth T. D., Moriarty T., Bednarek T., Dafforn K. A., Johnston E. L. (2022). Bleaching susceptibility and resistance of octocorals and anemones at the world’s southern-most coral reef. Front. Physiol. 13. doi: 10.3389/fphys.2022.804193
Stemmer K., Burghardt I., Mayer C., Reinicke G. B., Wägele H., Tollrian R., et al. (2013). Morphological and genetic analyses of xeniid soft coral diversity (Octocorallia; alcyonacea). Org. Divers. Evol. 13, 135–150. doi: 10.1007/s13127-012-0119-x
Toh T. C., Ng C. S. L. (2016). Tentacular autotomy and polyp regeneration in the scleractinian coral euphyllia glabrescens. Coral Reefs 35, 819–819. doi: 10.1007/s00338-016-1433-0
van der Burg C. A., Pavasovic A., Gilding E. K., Pelzer E. S., Surm J. M., Smith H. L., et al. (2020). The rapid regenerative response of a model Sea anemone species exaiptasia pallida is characterised by tissue plasticity and highly coordinated cell communication. Mar. Biotechnol. 22, 285–307. doi: 10.1007/s10126-020-09951-w
van de Water J. A. J. M., Allemand D., Ferrier-Pagès C. (2018). Host-microbe interactions in octocoral holobionts - recent advances and perspectives. Microbiome 6, 64. doi: 10.1186/s40168-018-0431-6
Vizel M., Loya Y., Downs C. A., Kramarsky-Winter E. (2011). A novel method for coral explant culture and micropropagation. Mar. Biotechnol. 13, 423–432. doi: 10.1007/s10126-010-9313-z
Vogg M. C., Galliot B., Tsiairis C. D. (2019). Model systems for regeneration: Hydra. Dev. 146. doi: 10.1242/dev.177212
Vollstedt S., Xiang N., Simancas-Giraldo S. M., Wild C. (2020). Organic eutrophication increases resistance of the pulsating soft coral Xenia umbellata to warming. PeerJ 2020, e9182. doi: 10.7717/peerj.9182
Keywords: cnidaria, soft coral, Xenia, whole-body regeneration, polyp amputation, morphogenesis, coral model
Citation: Nadir E, Lotan T and Benayahu Y (2023) Xenia umbellata (Octocorallia): A novel model organism for studying octocoral regeneration ability. Front. Mar. Sci. 10:1021679. doi: 10.3389/fmars.2023.1021679
Received: 17 August 2022; Accepted: 16 January 2023;
Published: 01 February 2023.
Edited by:
Daniel Wangpraseurt, University of California, San Diego, United StatesReviewed by:
Nina Yasuda, The University of Tokyo, JapanGiada Tortorelli, The University of Melbourne, Australia
Copyright © 2023 Nadir, Lotan and Benayahu. This is an open-access article distributed under the terms of the Creative Commons Attribution License (CC BY). The use, distribution or reproduction in other forums is permitted, provided the original author(s) and the copyright owner(s) are credited and that the original publication in this journal is cited, in accordance with accepted academic practice. No use, distribution or reproduction is permitted which does not comply with these terms.
*Correspondence: Elinor Nadir, ZWxpbm9ybmFkaXJAbWFpbC50YXUuYWMuaWw=